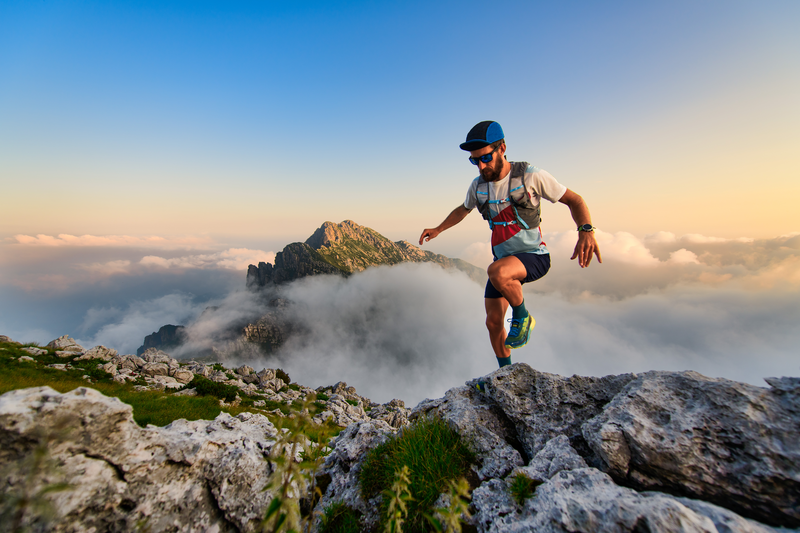
95% of researchers rate our articles as excellent or good
Learn more about the work of our research integrity team to safeguard the quality of each article we publish.
Find out more
REVIEW article
Front. Immunol. , 22 October 2024
Sec. Cancer Immunity and Immunotherapy
Volume 15 - 2024 | https://doi.org/10.3389/fimmu.2024.1483834
This article is part of the Research Topic Studying the immune microenvironment of liver cancer using artificial intelligence View all 9 articles
Hepatocellular carcinoma (HCC) is a prevalent form of liver cancer that poses significant challenges regarding morbidity and mortality rates. In the context of HCC, immune cells play a vital role, especially concerning the presentation of antigens. This review explores the intricate interactions among immune cells within HCC, focusing on their functions in antigen presentation and the modulation of T-cell responses. We begin by summarizing the strategies that HCC uses to escape immune recognition, emphasizing the delicate equilibrium between immune surveillance and evasion. Next, we investigate the specific functions of various types of immune cells, including dendritic cells, natural killer (NK) cells, and CD8+ T cells, in the process of antigen presentation. We also examine the impact of immune checkpoints, such as cytotoxic T-lymphocyte-associated protein 4 (CTLA-4) and the pathways involving programmed cell death protein 1 (PD-1) and programmed death ligand 1 (PD-L1), on antigen presentation, while taking into account the clinical significance of checkpoint inhibitors. The review further emphasizes the importance of immune-based therapies, including cancer vaccines and CAR-T cell therapy, in improving antigen presentation. In conclusion, we encapsulate the latest advancements in research, propose future avenues for exploration, and stress the importance of innovative technologies and customized treatment strategies. By thoroughly analyzing the interactions of immune cells throughout the antigen presentation process in HCC, this review provides an up-to-date perspective on the field, setting the stage for new therapeutic approaches.
Hepatocellular carcinoma (HCC), the predominant type of liver cancer, poses a considerable challenge to global health, illustrated by rising incidence figures and concerning mortality rates (1–3). The complex relationships between cancerous cells and the host’s immune system are crucial in influencing the development and progression of HCC. Understanding how immune cells participate in the antigen presentation process related to HCC is vital, as this insight is fundamental to improving our grasp of tumor immunology and formulating effective therapeutic strategies (4). This review seeks to explore the complex interactions between immune cells and HCC, emphasizing their functions in antigen presentation and the regulation of T cell responses (5). HCC is frequently associated with chronic liver conditions, such as viral hepatitis, alcoholic liver disease, and non-alcoholic fatty liver disease (5, 6). These risk factors lead to a microenvironment that fosters immune dysregulation, allowing tumor cells to escape immune detection and grow (7, 8).
The immune system’s ability to recognize and destroy abnormal cells is fundamental to cancer progression. In this context, the process of antigen presentation acts as a critical checkpoint (9, 10). Antigen-presenting cells (APCs), including dendritic cells (DCs) and macrophages, play a vital role in the capture of tumor-specific antigens and their subsequent delivery to CD8+ cytotoxic T cells. This process initiates a cascade of immune responses aimed at targeting cancerous cells. Nevertheless, the complex mechanisms linked to antigen presentation in HCC and their influence on the anti-tumor immune response remain subjects of ongoing research.
Understanding the immune dynamics related to HCC antigen presentation carries important therapeutic implications. While immune checkpoint inhibitors have achieved impressive results across various types of cancer, their effectiveness in HCC has been limited. This discovery suggests that gaining a deeper insight into the immune landscape within the microenvironment of HCC is essential for creating improved immunotherapy approaches (11, 12). Additionally, creating personalized treatment modalities, such as cancer vaccines and adoptive T cell therapies, relies on a clear understanding of the mechanisms of antigen presentation (13, 14).
As investigations reveal the complex interactions among immune cells and HCC, it is essential to present a thorough summary of the current understanding. This review aims to consolidate existing research to illuminate the varied functions of immune cells in relation to antigen presentation within HCC. By exploring the detailed communication among immune cells, tumor cells, and the tumor microenvironment, we seek to clarify the elements that contribute to immune evasion and identify potential pathways for enhancing anti-tumor immune responses. Through this analysis, the review enhances the overall comprehension of tumor immunology and establishes a foundation for developing innovative and focused immunotherapeutic approaches targeting HCC.
HCC is recognized as the main liver cancer that often arises in the context of ongoing liver inflammation, particularly associated with conditions such as viral hepatitis and liver damage from alcohol (15–17). The management of immune surveillance plays a crucial role in identifying and eliminating cancerous cells. Nevertheless, HCC has skillfully developed various strategies to avoid immune detection, promoting its unrestricted growth and advancement (18). An essential factor contributing to the immune evasion observed in HCC is the increased expression of inhibitory immune checkpoint proteins. Among these, programmed death ligand 1 (PD-L1) stands out prominently, alongside cytotoxic T-lymphocyte-associated protein 4 (CTLA-4) (19–21). These molecules interact with their corresponding receptors on T cells, hindering their activation and subsequent effector functions, thereby weakening the immune response aimed at cancerous cells.
Furthermore, the microenvironment surrounding tumors in hepatocellular carcinoma (HCC) frequently exhibits a suppressive immune profile, largely as a result of the buildup of regulatory T cells (Tregs) and myeloid-derived suppressor cells (MDSCs) (22–24). This aggregation of cells leads to the suppression of effector T cells and natural killer (NK) cells, ultimately undermining the immune response directed at malignant cells (25, 26). At the same time, HCC cells display a tendency to release a variety of immunosuppressive cytokines and chemokines, particularly transforming growth factor-beta (TGF-β) and interleukin-10 (IL-10), contributing to the overall reduction of anti-tumoral immune responses (Figure 1).
Figure 1. Hepatocellular carcinoma (HCC) cancer immunoediting is a dynamic and multifaceted process, comprising the stages of cancer initiation, elimination, equilibrium, and eventual escape. Immune surveillance initially destroys nascent cells, followed by immune equilibrium controlling growth. Over time, HCC evades detection, leading to progression. Understanding these stages reveals the complex immune-HCC relationship, emphasizing therapeutic potential.
Alterations in both genetic and epigenetic factors observed in HCC cells contribute to immune evasion, as demonstrated by changes in human leukocyte antigen (HLA) expression and modifications in mechanisms for antigen presentation (27–29). These irregularities enable HCC cells to avoid detection and destruction by cytotoxic T cells, crafting a sophisticated evasion strategy. Recently, clinical studies have highlighted the effectiveness of immunotherapeutic approaches aimed at these immune escape routes. In these initiatives, immune checkpoint blockers like anti-programmed cell death protein 1 (PD-1) and anti-PD-L1 antibodies have demonstrated effectiveness in certain groups of HCC patients by re-energizing the inhibited immune response directed towards the tumor. Furthermore, investigating combination therapies targeting various immune evasion pathways shows potential in enhancing the effectiveness of treatment option.
The complex interactions between various groups of immune cells and the tumor microenvironment (TME) in HCC represent a dynamic and multifaceted mechanism that significantly influences the progression of the disease (11, 30). A broad spectrum of research has uncovered important elements of this relationship, emphasizing the sophisticated ways in which distinct immune cell populations interact and communicate within the TME. Tumor-associated macrophages (TAMs) are key players in the immune milieu, predominantly exhibiting a pro-tumoral M2 phenotype in the context of HCC. This behavior fosters both tumor development and neovascularization by releasing factors such as vascular endothelial growth factor (VEGF) and IL-10 (31, 32). Tregs, another critical aspect of the immune response, build up in the TME and considerably influence antitumor immunity by effectively inhibiting cytotoxic T cells and establishing an immunosuppressive environment (33). Concurrently, MDSCs play a pivotal role in promoting immune evasion mechanisms, as they reduce T cell functionality and stimulate angiogenesis, further complicating the already intricate landscape of immune escape within the TME (34).
Additionally, the complex interactions of immune checkpoints in the HCC microenvironment trigger a series of sophisticated molecular exchanges that shape the trajectory of antitumor immune responses. Importantly, PD-1 found on T cells, when engaging with its corresponding ligand PD-L1 on tumor cells, induces a state of immune exhaustion, undermining the processes of effective tumor recognition and elimination (35, 36). In a similar vein, CTLA-4 inhibits the activation of antitumor T cell responses through its proficient suppression of costimulatory signals (37, 38). These diverse interactions collectively create a complex landscape dependent on a fragile balance between immune activation and inhibition, a balance that is significantly disrupted in the context of HCC.
Deepening our understanding of these complex interactions offers considerable potential for identifying new therapeutic avenues for HCC. Targeted strategies that modulate immune cell dynamics and interrupt the detailed network of immune evasion mechanisms may prove effective in halting tumor progression and enhancing antitumor immune responses. The rapid progress of breakthroughs in immunotherapy, including immune checkpoint blockers and adoptive T cell treatments, underscores the growing dynamism within this field. Furthermore, the integration of cutting-edge methodologies, including single-cell genomics and spatial transcriptomics, has the potential to reveal previously unexplored complexities within the immune environment of HCC (39). These revelations could lead to the creation of precision immunotherapeutic strategies specifically designed to address the unique immunological context of individual HCC patients, signaling a new era in the management of this challenging malignancy.
The intricate equilibrium between immune monitoring and evasion significantly impacts the advancement of HCC. A range of research efforts supports the complex interactions between tumor cells and the immune system of the host. While immune surveillance conducts a complex process to recognize and eliminate cancerous cells, HCC employs various strategies to evade detection and destruction by the immune system. The mechanisms of immune evasion in HCC are illustrated by the heightened expression of inhibitory immune checkpoint proteins, notably characterized by increased levels of PD-L1 (40, 41). This orchestrated interaction with PD-1 on T cells leads to their exhaustion and results in a weakened antineoplastic effector response (42). The TME within HCC acts as an architect, shaping an environment conducive to immunosuppression, which is significantly influenced by the recruitment of regulatory Tregs and MDSCs. The consolidation of these immunosuppressive entities ultimately suppresses the functionality of effector immune cells. This delicate equilibrium is further influenced by fluctuating levels of pro-inflammatory cytokines, notably IL-6 and tumor necrosis factor-alpha (TNF-α), which play dual roles by enhancing antitumoral immunity while also promoting inflammation within the tumor microenvironment (43–45).
Therapeutic strategies designed to counteract these complex mechanisms include immune checkpoint inhibitors and adoptive T cell therapies. The primary aim of these therapeutic approaches is to restore balance, thereby enhancing immune responses against HCC cells. Immune checkpoint inhibitors, known for their ability to disrupt immune inhibitory interactions, represent a powerful front in overcoming the immune-suppressive barriers established by HCC. In conjunction with this, adoptive T cell therapies provide a means to rejuvenate the functionality of effector T cells, thereby revitalizing the immune response. Together, these therapeutic modalities hold transformative potential, envisioning a therapeutic landscape that favors a recalibrated immune response against HCC and, consequently, a more effective therapeutic trajectory.
In the realm of HCC, the coordination of immune cells is vital to the intricate process of presenting antigens, which is essential for triggering adaptive immune responses aimed at combating cancer cells. Dendritic cells (DCs) are particularly important in this immune response, as they adeptly capture antigens from tumor cells, process them, and present tumor-derived peptides on their surface within the major histocompatibility complex (MHC) framework (46, 47). This essential interaction between antigens and MHC molecules is recognized by CD4+ and CD8+ T cells, which in turn triggers a specific immune response against HCC (48–50). However, HCC can evade this immune response through various mechanisms that interfere with antigen presentation, leading to immune resistance. Notably, tumor-infiltrating myeloid cells, including DCs, undergo changes that render them immunosuppressive, diminishing their ability to present antigens effectively. Moreover, HCC cells themselves can modulate MHC expression or suppress antigen processing and presentation, enabling them to escape detection by T cells. Therapeutic strategies, such as immune checkpoint inhibitors, are designed to counter these inhibitory signals and enhance antigen presentation, aiming to elicit robust antitumor immune responses, which holds promise in the treatment of HCC (Figure 2).
Figure 2. Immune cells play a pivotal role in HCC antigen presentation. (A) Antigen presentation by immune cells orchestrates anticancer responses. Dendritic cells (DCs) capture antigens and present to CD8+ T cells, with CD4+ T cells enhancing this process. HCC develops immune escape mechanisms, impairing antigen presentation. In-depth study may reveal new immunotherapy targets. (B) DCs capture and process antigens for presentation to CD8+ T cells. NK cells directly kill HCC cells. CD4+ T cells assist in antitumor immunity. HCC employs immune escape mechanisms; comprehensive understanding is crucial for treatment strategies.
As we delve deeper into this intricate environment, the expanding array of immune cell subsets within the HCC microenvironment significantly influences the dynamics of antigen presentation. Regulatory T cells (Tregs), known for their suppressive effects on immune responses, interact closely with antigen-presenting DCs, modulating the strength and effectiveness of immune activation (51) (Figure 2). MDSCs, characterized by their strong immunosuppressive properties, contribute to a complex interplay that dampens antigen presentation, creating a microenvironment that supports HCC progression. Understanding and targeting this complex interplay is crucial for developing new therapies that overcome immune suppression, enhance antigen presentation, and strengthen antitumor immune responses (52).
From a broader therapeutic perspective, the quest to enhance antigen presentation and reinvigorate immune responses is fraught with challenges. The inherent plasticity of the immune landscape, coupled with the complexity of the HCC microenvironment, complicates the development of effective interventions. While immune checkpoint inhibitors have shown promise, ongoing efforts must balance the enhancement of immune responses with the potential for immune-related adverse effects. The potential for combination therapies, including but not limited to immune checkpoint inhibitors, offers hope in dismantling the mechanisms of immune escape (53, 54). Identifying the most effective and lasting therapeutic approaches will require careful exploration through a multidisciplinary approach that integrates experimental findings with clinical observations.
DCs play a crucial role at the crossroads of the immune system, orchestrating key regulatory functions within both the innate and adaptive immune responses (55, 56). As specialized antigen-presenting cells, DCs are responsible for capturing, processing, and presenting antigens to T cells, thereby initiating and modulating a range of immune responses. In the complex environment of HCC, the essential role of DCs is evident in their ability to recognize tumor-derived antigens and initiate the priming of tumor-specific T cells (57, 58, 108). DCs utilize a variety of mechanisms, including phagocytosis, macropinocytosis, and receptor-mediated endocytosis, to capture tumor antigens. The antigens that have been captured are subsequently transformed into peptide fragments, which are displayed on the surface of DCs through major histocompatibility complex (MHC) molecules. This process of presenting antigens, aided by co-stimulatory signals from DCs, triggers the activation of naïve T cells, ultimately leading to the formation of tumor-specific cytotoxic CD8+ T cells and CD4+ T helper cells.
The functionality of DCs is closely tied to their maturation process, which is meticulously regulated. While immature DCs are highly efficient at capturing antigens, they have limited ability to stimulate T cells (59, 60). This changes when DCs are exposed to inflammatory signals, such as pathogen-associated molecular patterns (PAMPs) or danger-associated molecular patterns (DAMPs). These signals drive DCs into a mature state, characterized by increased surface expression of co-stimulatory molecules like CD80 and CD86, and the release of various cytokines. Maturation is critical for equipping DCs with the capacity to effectively prime T cells (61, 62). However, the tumor microenvironment in HCC can influence DC function, potentially leading to the development of tolerogenic DCs. These tolerogenic DCs exhibit reduced antigen presentation and diminished T cell activation, contributing to immune evasion by the tumor.
NK cells are a critical component of the innate immune defense, playing a vital role in the surveillance of HCC and other malignancies. These specialized lymphocytes have the unique ability to detect and eliminate target cells, including tumor cells, without requiring prior sensitization (63, 64). In HCC, NK cells are key players in antitumor immunity, primarily due to their capacity to recognize stress-induced ligands such as MHC class I-related chain A/B (MICA/B) and UL16-binding proteins (ULBPs) on the surface of cancer cells. The cytotoxic arsenal of activated NK cells, which includes perforin and granzymes, is instrumental in triggering apoptosis in tumor cells. Beyond direct cytotoxicity, NK cells also secrete immune-modulatory cytokines, notably interferon-gamma (IFN-γ), which amplify the immune response and contribute to a coordinated antitumor effect (65). Nonetheless, the function of NK cells in HCC is intricate and greatly influenced by the tumor microenvironment. The immunosuppressive nature of the HCC microenvironment can dampen NK cell activity through various mechanisms. A major factor is the upregulation of inhibitory ligands, such as PD-L1, on tumor cells, which interact with PD-1 receptors on NK cells, leading to functional exhaustion. Additionally, the presence of Tregs and MDSCs further suppresses NK cell function. To counteract these inhibitory influences and enhance NK cell activity in HCC, research is increasingly focused on innovative immunotherapies, including immune checkpoint inhibitors and adoptive NK cell therapies.
To summarize, NK cells have an essential yet intricate function in the immune response to HCC. While they contribute significantly to tumor surveillance and destruction, the tumor microenvironment presents formidable challenges that undermine their effectiveness. Advances in immunotherapy that harness the full potential of NK cells may offer new therapeutic avenues and transform treatment strategies for HCC.
Cytotoxic T cells (CTLs), referred to as CD8+ T lymphocytes, play a crucial role in the immune system’s response to HCC and various other cancers (66). These cells are highly adept at recognizing and eliminating infected or transformed cells, making them crucial for maintaining immune surveillance. In the HCC microenvironment, their activation is initiated when their T cell receptors (TCRs) interact with tumor-associated antigens presented by major histocompatibility complex class I (MHC-I) molecules, typically facilitated by professional antigen-presenting cells such as dendritic cells (67). This interaction triggers the activation of CD8+ T cells, leading to their clonal expansion and differentiation into effector CTLs. These effector cells then migrate to the tumor site, where they deploy their cytotoxic arsenal, including perforin and granzymes, to induce apoptosis in cancer cells. Additionally, CD8+ T cells produce cytokines like IFN-γ, which not only enhance the antitumor immune response but also activate other immune cells, thereby contributing to the establishment of an immunogenic environment within the tumor microenvironment.
Despite their significant role, CD8+ T cells face numerous challenges within the HCC microenvironment. A major obstacle is the creation of an immunosuppressive environment, rich in soluble factors such as vascular endothelial growth factor (VEGF) and transforming growth factor-beta (TGF-β), which negatively impact CD8+ T cell activation and function (68). Moreover, HCC cells often downregulate MHC-I expression, rendering themselves less visible to CD8+ T cells and disrupting their ability to recognize and target malignant cells (69). To overcome these challenges, therapeutic strategies have been developed, focusing on immune checkpoints like the PD-1/PD-L1 axis (109). These approaches aim to block inhibitory pathways, thereby restoring the full functional capacity of CD8+ T cells and improving clinical outcomes for patients with HCC.
Besides the main immune cell subsets mentioned earlier, a varied range of additional immune cell populations contributes significantly to the intricate immune reaction against HCC (70, 71). Neutrophils, for instance, exhibit a dual role depending on their polarization, functioning both in promoting tumor growth and in exerting antitumor effects. Some subsets of neutrophils contribute to inflammation and cancer progression, while others support antitumor immunity by enhancing T cell infiltration and activation. Mast cells also significantly influence the tumor microenvironment by releasing various mediators that promote angiogenesis and recruit other immune cells. Although present in lower numbers within the HCC microenvironment, B cells contribute to antitumor immunity through antibody production and antigen presentation. Recent research has emphasized the role of γδ T cells and innate lymphoid cells (ILCs) in regulating the immune response in HCC, which may impact tumor development and alter treatment results.
The control of immune checkpoints while presenting antigens is vital for maintaining a balance between immune activation and tolerance in the context of HCC (37, 72). The intricate relationship involving immune checkpoints like PD-1 and its ligand, PD-L1, plays a significant role in influencing the immune response directed at cancer cells (73). Within the HCC environment, inflammatory signals promote the increased expression of PD-L1 on tumor cells, which aids in forming an immunosuppressive microenvironment. This process involves the interaction between CD8+ T cells and antigen-presenting cells (APCs), including dendritic cells. The interaction between PD-1 on T cells and PD-L1 on APCs results in T cell fatigue, which diminishes their ability to produce cytokines and perform cytotoxic functions. This mechanism, while preventing excessive immune activity, also allows tumor cells to evade immune detection.
Findings from clinical research underscore the promise of immune checkpoint blockade as a treatment strategy. Employing anti-PD-1/PD-L1 antibodies represents a potentially effective method to rejuvenate fatigued CD8+ T cells, thus amplifying the immune response toward tumors in patients with HCC (74). As our understanding of the delicate interplay between immune checkpoints, antigen presentation, and immune evasion in HCC expands, strategies aimed at deciphering and modifying this complex relationship offer the potential for substantial progress in boosting the effectiveness of antitumor immunotherapy. The active modulation of immune checkpoints during the process of antigen presentation highlights their essential function in influencing the immune response against HCC. Approaches focused on modifying immune checkpoint interactions could significantly enhance antitumor immunity and improve clinical outcomes for HCC patients.
The intricate involvement of the CTLA-4 and PD-1/PD-L1 pathways in HCC underscores their essential roles in regulating immune responses and significantly impacting tumor development (Figure 3A). CTLA-4, a key receptor expressed on activated T cells, competes with the CD28 receptor for binding to B7 ligands on antigen-presenting cells. By doing so, CTLA-4 functions as a negative regulator, effectively inhibiting T cell activation and acting as an immune checkpoint that prevents hyperactive immune responses, which could otherwise lead to autoimmune damage (75). In the context of HCC, CTLA-4 exerts its suppressive effects by curtailing the activity of tumor-infiltrating lymphocytes, thereby facilitating tumor immune evasion and contributing to the progression of the malignancy (Figure 3).
Figure 3. Combination therapies hold great potential for HCC. (A, B) Pancreatic cancer in the context of HCC often requires multifaceted treatment. The combination of immune checkpoint inhibitors with tyrosine kinase inhibitors or locoregional therapies is an attractive strategy, aiming to synergistically impede tumor growth, etc. This leverages complementary mechanisms of different treatments, laying the foundation for effective therapy. (C) Blocking CTLA-4 or PD-1 signaling is a promising approach in HCC immunotherapy. These checkpoints regulate T cell activity and are exploited by tumor cells to evade immune surveillance. Inhibitors targeting them release T cell responses. Clinical trials show encouraging results, potentially revolutionizing HCC treatment. Manipulating these pathways is a promising strategy to enhance immunity and improve outcomes.
Conversely, the PD-1 pathway serves a unique function in preserving peripheral tolerance and inhibiting autoimmunity. PD-1 is expressed on the surface of activated T cells, B cells, and myeloid cells, and interacts with its ligands, PD-L1 and PD-L2, which are expressed on a variety of cell types, including both neoplastic and immune cells (76, 77). This interaction is particularly relevant in the microenvironment of HCC, where the binding of PD-1 on T cells to PD-L1 on tumor or immune cells creates an immunosuppressive environment that promotes T cell exhaustion. The fatigue reduces T cells’ capability to efficiently target cancer cells, thereby undermining the immune system’s effectiveness in regulating tumor development.
Clinical studies have provided compelling evidence for the therapeutic potential of targeting these immune checkpoints in HCC. The development and application of immune checkpoint inhibitors, such as ipilimumab, which targets CTLA-4, and nivolumab, which targets the PD-1/PD-L1 axis, have shown promising results in enhancing antitumor immune responses. These inhibitors work by reinvigorating exhausted T cells, thereby restoring their ability to fight against tumor cells and leading to improved survival rates among HCC patients. The success of these therapies has opened new avenues for the treatment of HCC and has underscored the critical importance of continued research into the mechanisms of immune checkpoint regulation.
The clinical integration of immune checkpoint inhibitors has revolutionized the therapeutic landscape for HCC and various other malignancies (78). By strategically focusing on regulatory pathways like CTLA-4 and PD-1/PD-L1, these inhibitors activate the immune system’s natural ability to identify and destroy cancerous cells. In the context of HCC, these agents have shown significant promise. Notably, well-conducted clinical trials, including KEYNOTE-240 and CheckMate-040, have provided robust evidence of the efficacy and safety of immune checkpoint inhibitors like pembrolizumab and nivolumab in treating advanced HCC (79–81). These trials have demonstrated notable improvements in overall survival and sustained responses in a specific subset of patients. Combination therapies involving immune checkpoint inhibitors and other immunotherapies exhibit substantial potential in HCC. Evidence indicates that these combinations can synergistically enhance efficacy by activating the immune system through multiple pathways, thereby improving therapeutic outcomes (82, 83). As our understanding of HCC immunological mechanisms advances and new therapies continue to emerge, these combination strategies are likely to be further refined, offering improved survival benefits for HCC patients. The potential of combining immune checkpoint inhibitors with other therapeutic strategies, such as tyrosine kinase inhibitors and locoregional treatments, holds great promise for enhancing therapeutic outcomes (84). However, as this therapeutic approach continues to gain traction, several critical considerations must be addressed. Essential elements comprise the classification of patients according to significant clinical indicators, the discovery of predictive biomarkers, and the meticulous management of adverse immune-related effects. These aspects are vital for enhancing the clinical application of immune checkpoint inhibitors. The main challenges in implementing personalized immunotherapy for HCC include tumor heterogeneity, the complexity of immune escape mechanisms, and the lack of effective biomarkers for predicting therapeutic efficacy. Future studies should concentrate on a more thorough investigation of the immune evasion mechanisms in HCC, the identification of novel therapeutic targets and biomarkers, as well as the evaluation of the safety and effectiveness of new treatments via extensive clinical trials. The scientific community must continue to advance research efforts, focusing on strategies that effectively integrate immune checkpoint inhibitors with other treatments and identify prognostic markers. This ongoing research is essential to developing personalized treatment regimens tailored to the unique characteristics of individual patients.
Antitumor immunotherapy signifies a groundbreaking method for treating cancer, improving the presentation of antigens and bolstering the immune system’s reaction to malignant cells. This method leverages the complex interactions among immune cells, tumor antigens, and the tumor microenvironment to thwart immune evasion and reinstate effective immune surveillance. In the context of HCC and various other malignancies, treatment approaches seek to enhance the pathways for antigen presentation, thereby promoting the activation of CTLs along with other essential immune cells.
A fundamental component of antitumor immunotherapy is the deployment of immune checkpoint inhibitors (ICIs). These inhibitors target suppressive molecules on immune cells—for instance, PD-1 on T cells and its ligand PD-L1 on tumor cells (85, 86). By inhibiting these interactions, ICIs remove inhibitory controls on the immune system, enhancing T cells’ ability to recognize and eliminate cancer cells more effectively. Clinical research, including the KEYNOTE-240 and CheckMate-040 trials, has substantiated the effectiveness of ICIs like pembrolizumab and nivolumab in boosting antigen presentation and fostering antitumor immunity in advanced HCC cases. These treatments not only reactivate exhausted CD8+ T cells but also increase tumor-infiltrating lymphocytes (TILs) and amplify tumor antigen-specific immune responses (87–89).
Another strategy to augment antigen presentation involves cancer vaccines, which are designed to elicit a targeted immune response against tumor antigens (90). Comprising either tumor-associated antigens (TAAs) or neoantigens from mutated cancer-specific proteins, these vaccines are pivotal in initiating an immune reaction. DCs are crucial in the process of capturing, processing, and presenting antigens to T cells. Clinical trials investigating various cancer vaccine modalities, including peptide-based, protein-based, and nucleic acid-based vaccines in HCC, have demonstrated encouraging results in enhancing antigen-specific T cell responses and improving clinical outcomes. Additionally, adoptive T cell therapy (ACT) involves the ex vivo expansion and modification of patient-derived T cells to boost their antigen recognition and cytotoxic activity (91–93). Chimeric antigen receptor (CAR) T cell therapy, a subset of ACT, involves engineering T cells to express CARs that target specific tumor antigens. While CAR T cell therapy has achieved significant success in treating hematological malignancies, its application in HCC is under investigation. ACT enhances antigen presentation by directly infusing highly specific and potent T cells into the patient, counteracting tumor-induced immunosuppression and enhancing the potential for tumor eradication.
The development of immunotherapy is propelled by cancer vaccines that harness the immune system’s ability to recognize and destroy tumor cells (94, 95). Specifically tailored to induce targeted immune responses against cancer-specific antigens, these vaccines activate CTLs and foster the development of immunological memory, thereby enhancing antigen presentation and triggering robust immune responses in HCC and other malignancies, which may improve clinical outcomes.
Various types of cancer vaccines exist, including peptide-based, protein-based, nucleic acid-based, and whole-cell vaccines (96, 97). Peptide-based vaccines administer short peptides derived from tumor antigens to prime T cells for cancer cell recognition and targeting. Protein-based vaccines use entire proteins or their fragments from tumor cells to provoke immune reactions. Vaccines that utilize nucleic acids deliver either DNA or RNA encoding tumor antigens, which aids in the synthesis of these proteins and stimulates the immune response. Whole-cell vaccines employ either intact tumor cells or their lysates to prompt immune recognition of a wide array of tumor antigens. At the core of these vaccination approaches are DCs, which take up, process, and present antigens to T cells, thereby initiating the immune response. In clinical settings, the use of cancer vaccines in HCC has been evaluated, such as in a phase III trial of the peptide-based vaccine adjuvant MelCancerVac, which targets melanoma-associated antigens in HCC patients. Although primary endpoints were not met, the trial underscored the potential to induce tumor-specific immune responses. Additionally, early-phase trials of the TERT-encoding DNA vaccine GRANITE-001 have yielded promising results by stimulating T cell responses against telomerase reverse transcriptase (TERT), an antigen prevalent in various cancers, including HCC.
The future of cancer vaccines in HCC appears promising but is not without its challenges. The heterogeneity of tumor antigens and immune responses among patients calls for the identification of both unique and common antigens to optimize vaccine design. Additionally, the tumor microenvironment’s immunosuppressive characteristics and possible mechanisms of immune tolerance might reduce the effectiveness of vaccines. To overcome these barriers, combination strategies, including the integration of cancer vaccines with immune checkpoint inhibitors, are under investigation to enhance therapeutic outcomes. The potential of cancer vaccines to improve antigen presentation and target specific immune responses against cancer cells underscores their role in advancing personalized immunotherapy approaches in HCC. Ongoing investigations and clinical studies are crucial for the advancement and enhancement of cancer vaccine approaches, offering the potential to greatly influence the treatment of HCC.
CAR-T cell therapy, a pivotal advancement in immunotherapy, has profoundly influenced antigen presentation and the immune response to cancers, including HCC. This therapy involves T lymphocytes engineered to express chimeric antigen receptors (CARs) that merge the antigen-binding domain of an antibody with T cell signaling domains (98). These engineered receptors allow CAR-T cells to identify specific tumor-associated antigens without the need for major histocompatibility complex (MHC) presentation, circumventing conventional antigen presentation pathways. In practice, T cells are harvested from patients, genetically modified to bear CARs that target tumor-specific antigens, and then reinfused (99). These CAR-T cells then recognize and bind to tumor cells displaying these antigens, triggering direct cytotoxic attacks and cytokine release, which not only promotes tumor cell destruction but also enhances antigen presentation through direct T-cell engagement.
Research in the clinical field highlights the considerable potential of CAR-T cell therapy for the treatment of HCC. A noteworthy investigation centered on CAR-T cells targeting glypican-3 (GPC3), a unique antigen commonly found in advanced HCC cases, revealed impressive antitumor effectiveness, with several patients showing marked tumor reduction and increased survival duration (100). Additional investigations into CAR-T cells targeting other antigens like alpha-fetoprotein (AFP) and mesothelin in preclinical and early clinical phases further attest to the adaptability of this approach in HCC. However, challenges such as the immunosuppressive nature of the tumor microenvironment, tumor antigen variability, and potential antigen escape mechanisms pose hurdles to the lasting effectiveness of CAR-T cell therapy. Additionally, immune checkpoint inhibitors and CAR-T cell therapies are associated with certain adverse effects, including skin toxicity, gastrointestinal reactions, and cytokine release syndrome. To address these issues, strategies that combine CAR-T therapy with immune checkpoint inhibitors are being explored to counteract immunosuppression and enhance therapeutic results. Adverse reactions can be effectively mitigated through close monitoring, appropriate medication, and supportive care, thereby ensuring patient safety. Ongoing research into identifying optimal antigens and developing effective and safe CAR constructs is critical to refining and expanding the utility of CAR-T cell therapy in HCC treatment.
Recent advancements have significantly enhanced our understanding of immune cells in both healthy and diseased states, including HCC. Single-cell RNA sequencing (scRNA-seq) has proven to be a powerful tool for delineating gene expression at the individual cell level (101). In HCC, scRNA-seq has exposed the diversity within immune cell populations in the tumor microenvironment, offering insights into their functional states and interactions (90). Further, developments in high-dimensional flow cytometry and mass cytometry have enabled the simultaneous measurement of multiple markers on individual cells, improving our grasp of immune cell phenotypes and functionalities. Spatial transcriptomics have also been employed to map immune cells within tumors, illuminating their spatial distribution and intercellular communication. These technological breakthroughs collectively provide a detailed picture of the dynamic immune responses and the mechanisms of immune escape in HCC, setting the stage for more targeted therapeutic approaches.
The transition to personalized medicine is increasingly evident in the realm of HCC immunotherapy, underscored by the unique immune profiles and tumor characteristics exhibited by each patient (102). Detailed profiling of tumor antigens and immune cell infiltrates enhances the formulation of customized treatment plans (103). The identification of neoantigens through genomic sequencing has facilitated the creation of personalized vaccines and adoptive T cell therapies (104). Additionally, the discovery of biomarkers that predict responses to immune checkpoint inhibitors aids in selecting appropriate treatments, reducing ineffective approaches and improving patient outcomes. The combination of various omics data, such as genomic, transcriptomic, and proteomic analyses, assists in identifying the key molecular factors and pathways that contribute to immune dysfunction in HCC (105, 106). Looking ahead, the amalgamation of these insights with cutting-edge immunotherapies promises to refine clinical results by aligning interventions with the specific immunological profiles of HCC patients.
Despite substantial progress, numerous pivotal challenges and directions remain in the domain of HCC immunotherapy. Initially, deciphering the intricate relationships between immune cells and the tumor microenvironment remains a challenging endeavor. It is crucial to comprehend the diverse functionalities of immune cells and their communication networks to devise potent combination therapies. Secondly, addressing the immunosuppression prevalent within the HCC microenvironment demands innovative approaches. The synergy of immune checkpoint inhibitors, immune modulators, and targeted therapies presents a promising route to counteract immune escape mechanisms (107). Thirdly, the enhancement of adoptive T cell therapies, such as CAR-T cells, hinges on refining engineering techniques to boost tumor specificity and longevity. Additionally, the development of universally applicable CAR-T cells or allogeneic methods could mitigate logistical and manufacturing hurdles (7). Lastly, the quest for biomarkers that can stratify patients and predict treatment responses is critical. Effective predictive markers are essential to tailor therapy choices, minimizing adverse effects while maximizing therapeutic efficacy.
In summary, a detailed investigation of immune cell dynamics and antigen presentation within HCC has revealed complex interaction layers that critically affect tumor progression, immune reactions, and therapeutic results. The immune evasion tactics utilized by HCC illustrate the tumor’s capacity to circumvent immune surveillance and underscore the importance of immune checkpoints like CTLA-4 and PD-1/PD-L1 in regulating the intricate equilibrium between immune activation and suppression. Various immune cells, including DCs, NK cells, and CD8+ T cells, engage in a sophisticated interaction within the tumor microenvironment. This interaction influences antigen presentation, immune infiltration, and ultimately, tumor control. The introduction of novel approaches such as cancer vaccines and CAR-T cell therapies opens promising pathways to enhance antigen presentation and empower the immune system to more effectively recognize and combat cancer cells.
Clinical investigations, such as the KEYNOTE-240 and CheckMate-040 trials, have corroborated the significant clinical advantages of immune checkpoint inhibitors in reinvigorating immune responses and enhancing outcomes for advanced HCC. Nevertheless, hurdles in patient stratification, biomarker identification, and the management of immune-related side effects persist. The integration of innovative technologies, such as single-cell RNA sequencing and high-dimensional flow cytometry, has afforded profound insights into the functionality of immune cells. This technological prowess has unraveled the complexities of immune cell populations and their interplay within HCC, thereby facilitating the crafting of personalized treatment modalities that leverage unique patient characteristics to maximize therapeutic efficacy. Furthermore, advancements in CAR-T cell therapy underscore its potential in augmenting antigen presentation, thus spearheading a new frontier in immunotherapy for HCC and potentially other malignancies.
Looking forward, the unraveling of the complex interactions between immune cells and tumors within the HCC microenvironment is imperative. This understanding is critical for the informed development of combination therapies aimed at counteracting immunosuppression. The quest for biomarkers that accurately predict treatment responses continues to be a focal point, promising to refine therapy selection to enhance patient outcomes and minimize side effects. Ongoing research is essential to perfect adoptive T cell therapies and address challenges such as immunosuppression, tumor heterogeneity, and scalability of manufacturing processes.
In summary, the comprehensive analysis of immune cell function and antigen presentation within HCC has laid a robust groundwork for innovative cancer immunotherapy strategies. The synergy of cutting-edge technologies, tailored treatment approaches, and a nuanced comprehension of the immune landscape is poised to transform the management of HCC, thereby reshaping the therapeutic paradigm for patients in dire need.
JN: Conceptualization, Investigation, Visualization, Writing – original draft, Writing – review & editing. YW: Conceptualization, Investigation, Project administration, Resources, Software, Writing – original draft, Writing – review & editing. ZT: Conceptualization, Investigation, Project administration, Resources, Software, Visualization, Writing – original draft, Writing – review & editing.
The author(s) declare that no financial support was received for the research, authorship, and/or publication of this article.
Here, we would like to express our gratitude to Jingyi Zhang for her support during the process of writing the article.
The authors declare that the research was conducted in the absence of any commercial or financial relationships that could be construed as a potential conflict of interest.
All claims expressed in this article are solely those of the authors and do not necessarily represent those of their affiliated organizations, or those of the publisher, the editors and the reviewers. Any product that may be evaluated in this article, or claim that may be made by its manufacturer, is not guaranteed or endorsed by the publisher.
1. Vogel A, Meyer T, Sapisochin G, Salem R, Saborowski A. Hepatocellular carcinoma. Lancet. (2022) 400(10360):1345–62. doi: 10.1016/S0140-6736(22)01200-4
2. Chidambaranathan-Reghupaty S, Fisher PB, Sarkar D. Hepatocellular carcinoma (HCC): Epidemiology, etiology and molecular classification. Adv Cancer Res. (2021) 149:1–61. doi: 10.1016/bs.acr.2020.10.001
3. Ganesan P, Kulik LM. Hepatocellular carcinoma: new developments. Clin Liver Dis. (2023) 27:85–102. doi: 10.1016/j.cld.2022.08.004
4. Hartke J, Johnson M, Ghabril M. The diagnosis and treatment of hepatocellular carcinoma. Semin Diagn Pathol. (2017) 34:153–9. doi: 10.1053/j.semdp.2016.12.011
5. Galle PR, Dufour JF, Peck-Radosavljevic M, Trojan J, Vogel A. Systemic therapy of advanced hepatocellular carcinoma. Future Oncol. (2021) 17(10):1237–51. doi: 10.2217/fon-2020-0758
6. Rajendran L, Ivanics T, Claasen MP, Muaddi H, Sapisochin G. The management of post-transplantation recurrence of hepatocellular carcinoma. Clin Mol Hepatol. (2022) 28(1):1–16. doi: 10.3350/cmh.2021.0217
7. Alizadeh D, Wong RA, Yang X, Wang D, Pecoraro JR, Kuo CF, et al. IL15 enhances CAR-T cell antitumor activity by reducing mTORC1 activity and preserving their stem cell memory phenotype. Cancer Immunol Res. (2019) 7(5):759–72. doi: 10.1158/2326-6066.CIR-18-0466
8. Chen X, Wang Z, Han S, Wang Z, Zhang Y, Li X, et al. Targeting SYK of monocyte-derived macrophages regulates liver fibrosis via crosstalking with Erk/Hif1α and remodeling liver inflammatory environment. Cell Death Dis. (2021) 12(12):1123. doi: 10.1038/s41419-021-04403-2
9. Zhang H, Zhang N, Wu W, Wang Z, Dai Z, Liang X, et al. Pericyte mediates the infiltration, migration, and polarization of macrophages by CD163/MCAM axis in glioblastoma. iScience. (2022) 25(9):104918. doi: 10.1016/j.isci.2022.104918
10. Chew GL, Campbell AE, De Neef E, Sutliff NA, Shadle SC, Tapscott SJ, et al. DUX4 suppresses MHC class I to promote cancer immune evasion and resistance to checkpoint blockade. Dev Cell. (2019) 50(5):658–671.e7. doi: 10.1016/j.devcel.2019.06.011
11. Zhang Z, Zeng X, Wu Y, Liu Y, Zhang X, Song Z. Cuproptosis-related risk score predicts prognosis and characterizes the tumor microenvironment in hepatocellular carcinoma. Front Immunol. (2022) 13:925618. doi: 10.3389/fimmu.2022.925618
12. Wei CY, Zhu MX, Zhang PF, Huang XY, Wan JK, Yao XZ, et al. PKCα/ZFP64/CSF1 axis resets the tumor microenvironment and fuels anti-PD1 resistance in hepatocellular carcinoma. J Hepatol. (2022) 77(1):163–76. doi: 10.1016/j.jhep.2022.02.019
13. Zuo B, Zhang Y, Zhao K, Wu L, Qi H, Yang R, et al. Universal immunotherapeutic strategy for hepatocellular carcinoma with exosome vaccines that engage adaptive and innate immune responses. J Hematol Oncol. (2022) 15(1):46. doi: 10.1186/s13045-022-01266-8
14. Lu Z, Zuo B, Jing R, Gao X, Rao Q, Liu Z, et al. Dendritic cell-derived exosomes elicit tumor regression in autochthonous hepatocellular carcinoma mouse models. J Hepatol. (2017) 67(4):739–48. doi: 10.1016/j.jhep.2017.05.019
15. Wen N, Cai Y, Li F, Ye H, Tang W, Song P, et al. The clinical management of hepatocellular carcinoma worldwide: A concise review and comparison of current guidelines: 2022 update. Biosci Trends. (2022) 16(1):20–30. doi: 10.5582/bst.2022.01061
16. Heller M, Parikh ND, Fidelman N, Owen D. Frontiers of therapy for hepatocellular carcinoma. Abdom Radiol (NY). (2021) 46(8):3648–59. doi: 10.1007/s00261-021-03065-0
17. von Felden J, Garcia-Lezana T, Schulze K, Losic B, Villanueva A. Liquid biopsy in the clinical management of hepatocellular carcinoma. Gut. (2020) 69(11):2025–34. doi: 10.1136/gutjnl-2019-320282
18. Chen QF, Li W, Wu PH, Shen LJ, Huang ZL. Significance of tumor-infiltrating immunocytes for predicting prognosis of hepatitis B virus-related hepatocellular carcinoma. World J Gastroenterol. (2019) 25(35):5266–82. doi: 10.3748/wjg.v25.i35.5266
19. Lu LG, Zhou ZL, Wang XY, Liu BY, Lu JY, Liu S, et al. PD-L1 blockade liberates intrinsic antitumourigenic properties of glycolytic macrophages in hepatocellular carcinoma. Gut. (2022) 71(12):2551–60. doi: 10.1136/gutjnl-2021-326350
20. Li Q, Zhang L, You W, Xu J, Dai J, Hua D, et al. PRDM1/BLIMP1 induces cancer immune evasion by modulating the USP22-SPI1-PD-L1 axis in hepatocellular carcinoma cells. Nat Commun. (2022) 13(1):7677. doi: 10.1038/s41467-022-35469-x
21. Sové RJ, Verma BK, Wang H, Ho WJ, Yarchoan M, Popel AS. Virtual clinical trials of anti-PD-1 and anti-CTLA-4 immunotherapy in advanced hepatocellular carcinoma using a quantitative systems pharmacology model. J Immunother Cancer. (2022) 10(11). doi: 10.1136/jitc-2022-005414
22. Zheng C, Zheng L, Yoo JK, Guo H, Zhang Y, Guo X, et al. Landscape of infiltrating T cells in liver cancer revealed by single-cell sequencing. Cell. (2017) 169(7):1342–56.e16. doi: 10.1016/j.cell.2017.05.035
23. Zakeri N, Hall A, Swadling L, Pallett LJ, Schmidt NM, Diniz MO, et al. Characterisation and induction of tissue-resident gamma delta T-cells to target hepatocellular carcinoma. Nat Commun. (2022) 13(1):1372. doi: 10.1038/s41467-022-29012-1
24. Ma J, Zheng B, Goswami S, Meng L, Zhang D, Cao C, et al. PD1(Hi) CD8(+) T cells correlate with exhausted signature and poor clinical outcome in hepatocellular carcinoma. J Immunother Cancer. (2019) 7(1):331. doi: 10.1186/s40425-019-0814-7
25. Wang S, Wu Q, Chen T, Su R, Pan C, Qian J, et al. Blocking CD47 promotes antitumour immunity through CD103(+) dendritic cell-NK cell axis in murine hepatocellular carcinoma model. J Hepatol. (2022) 77(2):467–78. doi: 10.1016/j.jhep.2022.03.011
26. Yu W, He J, Wang F, He Q, Shi Y, Tao X, et al. NR4A1 mediates NK-cell dysfunction in hepatocellular carcinoma via the IFN-γ/p-STAT1/IRF1 pathway. Immunology. (2023) 169(1):69–82. doi: 10.1111/imm.13611
27. Catamo E, Zupin L, Crovella S, Celsi F, Segat L. Non-classical MHC-I human leukocyte antigen (HLA-G) in hepatotropic viral infections and in hepatocellular carcinoma. Hum Immunol. (2014) 75(12):1225–31. doi: 10.1016/j.humimm.2014.09.019
28. Matsui M, Machida S, Itani-Yohda T, Akatsuka T. Downregulation of the proteasome subunits, transporter, and antigen presentation in hepatocellular carcinoma, and their restoration by interferon-gamma. J Gastroenterol Hepatol. (2002) 17(8):897–907. doi: 10.1046/j.1440-1746.2002.02837.x
29. Tang PM, Bui-Xuan NH, Wong CK, Fong WP, Fung KP. Pheophorbide a-mediated photodynamic therapy triggers HLA class I-restricted antigen presentation in human hepatocellular carcinoma. Transl Oncol. (2010) 3(2):114–22. doi: 10.1593/tlo.09262
30. Donne R, Lujambio A. The liver cancer immune microenvironment: Therapeutic implications for hepatocellular carcinoma. Hepatology. (2023) 77(5):1773–96. doi: 10.1002/hep.32740
31. Lu Z, Liu R, Wang Y, Jiao M, Li Z, Wang Z, et al. Ten-eleven translocation-2 inactivation restrains IL-10-producing regulatory B cells to enable antitumor immunity in hepatocellular carcinoma. Hepatology. (2023) 77(3):745–59. doi: 10.1002/hep.32442
32. Barooah P, Saikia S, Kalita MJ, Bharadwaj R, Sarmah P, Bhattacharyya M, et al. IL-10 polymorphisms and haplotypes predict susceptibility to hepatocellular carcinoma occurrence in patients with hepatitis C virus infection from northeast India. Viral Immunol. (2020) 33(6):457–67. doi: 10.1089/vim.2019.0170
33. Sakaguchi S, Yamaguchi T, Nomura T, Ono M. Regulatory T cells and immune tolerance. Cell. (2008) 133(5):775–87. doi: 10.1016/j.cell.2008.05.009
34. Gabrilovich DI, Nagaraj S. Myeloid-derived suppressor cells as regulators of the immune system. Nat Rev Immunol. (2009) 9:162–74. doi: 10.1038/nri2506
35. Hu B, Yu M, Ma X, Sun J, Liu C, Wang C, et al. IFNα Potentiates anti-PD-1 efficacy by remodeling glucose metabolism in the hepatocellular carcinoma microenvironment. Cancer Discov. (2022) 12(7):1718–41. doi: 10.1158/2159-8290.CD-21-1022
36. Tan Z, Chiu MS, Yang X, Yue M, Cheung TT, Zhou D, et al. Isoformic PD-1-mediated immunosuppression underlies resistance to PD-1 blockade in hepatocellular carcinoma patients. Gut. (2023) 72(8):1568–80. doi: 10.1136/gutjnl-2022-327133
37. Shang BB, Chen J, Wang ZG, Liu H. Significant correlation between HSPA4 and prognosis and immune regulation in hepatocellular carcinoma. PeerJ. (2021) 9:e12315. doi: 10.7717/peerj.12315
38. Kudo M. Combination cancer immunotherapy with molecular targeted agents/anti-CTLA-4 antibody for hepatocellular carcinoma. Liver Cancer. (2019) 8:1–11. doi: 10.1159/000496277
39. Bidkhori G, Benfeitas R, Klevstig M, Zhang C, Nielsen J, Uhlen M, et al. Metabolic network-based stratification of hepatocellular carcinoma reveals three distinct tumor subtypes. Proc Natl Acad Sci USA. (2018) 115(50):E11874–e83. doi: 10.1073/pnas.1807305115
40. Cheng AL, Qin S, Ikeda M, Galle PR, Ducreux M, Kim TY, et al. Updated efficacy and safety data from IMbrave150: Atezolizumab plus bevacizumab vs. sorafenib for unresectable hepatocellular carcinoma. J Hepatol. (2022) 76(4):862–73. doi: 10.1016/j.jhep.2021.11.030
41. Du SS, Chen GW, Yang P, Chen YX, Hu Y, Zhao QQ, et al. Radiation Therapy Promotes Hepatocellular Carcinoma Immune Cloaking via PD-L1 Upregulation Induced by cGAS-STING Activation. Int J Radiat Oncol Biol Phys. (2022) 112(5):1243–55. doi: 10.1016/j.ijrobp.2021.12.162
42. Teng MW, Ngiow SF, Ribas A, Smyth MJ. Classifying cancers based on T-cell infiltration and PD-L1. Cancer Res. (2015) 75(11):2139–45. doi: 10.1158/0008-5472.CAN-15-0255
43. Yin Z, Ma T, Lin Y, Lu X, Zhang C, Chen S, et al. IL-6/STAT3 pathway intermediates M1/M2 macrophage polarization during the development of hepatocellular carcinoma. J Cell Biochem. (2018) 119(11):9419–32. doi: 10.1002/jcb.27259
44. Zhang W, Liu Y, Yan Z, Yang H, Sun W, Yao Y, et al. IL-6 promotes PD-L1 expression in monocytes and macrophages by decreasing protein tyrosine phosphatase receptor type O expression in human hepatocellular carcinoma. J Immunother Cancer. (2020) 8(1). doi: 10.1136/jitc-2019-000285
45. Dai Z, Wang X, Peng R, Zhang B, Han Q, Lin J, et al. Induction of IL-6Rα by ATF3 enhances IL-6 mediated sorafenib and regorafenib resistance in hepatocellular carcinoma. Cancer Lett. (2022) 524:161–71. doi: 10.1016/j.canlet.2021.10.024
46. Fujiwara K, Higashi T, Nouso K, Nakatsukasa H, Kobayashi Y, Uemura M, et al. Decreased expression of B7 costimulatory molecules and major histocompatibility complex class-I in human hepatocellular carcinoma. J Gastroenterol Hepatol. (2004) 19:1121–7. doi: 10.1111/j.1440-1746.2004.03467.x
47. Haber PK, Castet F, Torres-Martin M, Andreu-Oller C, Puigvehí M, Miho M, et al. Molecular markers of response to anti-PD1 therapy in advanced hepatocellular carcinoma. Gastroenterology. (2023) 164(1):72–88.e18. doi: 10.1053/j.gastro.2022.09.005
48. Magen A, Hamon P, Fiaschi N, Soong BY, Park MD, Mattiuz R, et al. Intratumoral dendritic cell-CD4(+) T helper cell niches enable CD8(+) T cell differentiation following PD-1 blockade in hepatocellular carcinoma. Nat Med. (2023) 29(6):1389–99. doi: 10.1038/s41591-023-02345-0
49. Ma C, Kesarwala AH, Eggert T, Medina-Echeverz J, Kleiner DE, Jin P, et al. NAFLD causes selective CD4(+) T lymphocyte loss and promotes hepatocarcinogenesis. Nature. (2016) 531(7593):253–7. doi: 10.1038/nature16969
50. Dai Z, Wang Z, Lei K, Liao J, Peng Z, Lin M, et al. Irreversible electroporation induces CD8(+) T cell immune response against post-ablation hepatocellular carcinoma growth. Cancer Lett. (2021) 503:1–10. doi: 10.1016/j.canlet.2021.01.001
51. Guermonprez P, et al. Antigen presentation and T cell stimulation by dendritic cells. Annu Rev Immunol. (2002) 20:621–67. doi: 10.1146/annurev.immunol.20.100301.064828
52. Xing R, et al. Strategies to improve the antitumor effect of immunotherapy for hepatocellular carcinoma. Front Immunol. (2021) 12:783236. doi: 10.3389/fimmu.2021.783236
53. Cheng AL, et al. Challenges of combination therapy with immune checkpoint inhibitors for hepatocellular carcinoma. J Hepatol. (2020) 72:307–19. doi: 10.1016/j.jhep.2019.09.025
54. El Dika I, Khalil DN, Abou-Alfa GK. Immune checkpoint inhibitors for hepatocellular carcinoma. Cancer. (2019) 125:3312–9. doi: 10.1002/cncr.v125.19
55. Théry C, Amigorena S. The cell biology of antigen presentation in dendritic cells. Curr Opin Immunol. (2001) 13:45–51. doi: 10.1016/S0952-7915(00)00180-1
56. Unanue ER, Cerottini JC. Antigen presentation. FASEB J. (1989) 3:2496–502. doi: 10.1096/fasebj.3.13.2572499
57. Knight SC, Burke F, Bedford PA. Dendritic cells, antigen distribution and the initiation of primary immune responses to self and non-self antigens. Semin Cancer Biol. (2002) 12:301–8. doi: 10.1016/S1044-579X(02)00016-0
58. Argüello RJ, Reverendo M, Gatti E, Pierre P. Regulation of protein synthesis and autophagy in activated dendritic cells: implications for antigen processing and presentation. Immunol Rev. (2016) 272(1):28–38. doi: 10.1111/imr.12427
59. Edelson RL. Cutaneous T cell lymphoma: the helping hand of dendritic cells. Ann N Y Acad Sci. (2001) 941:1–11. doi: 10.1111/j.1749-6632.2001.tb03705.x
60. Mbongue JC, Nieves HA, Torrez TW, Langridge WH. The role of dendritic cell maturation in the induction of insulin-dependent diabetes mellitus. Front Immunol. (2017) 8:327. doi: 10.3389/fimmu.2017.00327
61. Scales HE, Meehan GR, Hayes AJ, Benson RA, Watson E, Walters A, et al. A novel cellular pathway of antigen presentation and CD4 T cell activation in vivo. Front Immunol. (2018) 9:2684. doi: 10.3389/fimmu.2018.02684
62. Knight SC. Dendritic cell-T-cell circuitry in health and changes in inflammatory bowel disease and its treatment. Dig Dis. (2016) 34:51–7. doi: 10.1159/000442926
63. Erokhina SA, Streltsova MA, Kanevskiy LM, Grechikhina MV, Sapozhnikov AM, Kovalenko EI. HLA-DR-expressing NK cells: Effective killers suspected for antigen presentation. J Leukoc Biol. (2021) 109(2):327–37. doi: 10.1002/JLB.3RU0420-668RR
64. Nakamura T, Sato T, Endo R, Sasaki S, Takahashi N, Sato Y, et al. STING agonist loaded lipid nanoparticles overcome anti-PD-1 resistance in melanoma lung metastasis via NK cell activation. J Immunother Cancer. (2021) 9(7). doi: 10.1136/jitc-2021-002852
65. Costa-García M, Ataya M, Moraru M, Vilches C, López-Botet M, Muntasell A. Human cytomegalovirus antigen presentation by HLA-DR+ NKG2C+ Adaptive NK cells specifically activates polyfunctional effector memory CD4+ T lymphocytes. Front Immunol. (2019) 10:687. doi: 10.3389/fimmu.2019.00687
66. Pishesha N, Harmand TJ, Ploegh HL. A guide to antigen processing and presentation. Nat Rev Immunol. (2022) 22:751–64. doi: 10.1038/s41577-022-00707-2
67. Moustaki A, Crawford JC, Alli S, Fan Y, Boi S, Zamora AE, et al. Antigen cross-presentation in young tumor-bearing hosts promotes CD8(+) T cell terminal differentiation. Sci Immunol. (2022) 7(68):eabf6136. doi: 10.1126/sciimmunol.abf6136
68. MacNabb BW, Tumuluru S, Chen X, Godfrey J, Kasal DN, Yu J, et al. Dendritic cells can prime anti-tumor CD8(+) T cell responses through major histocompatibility complex cross-dressing. Immunity. (2022) 55(6):982–97.e8. doi: 10.1016/j.immuni.2022.04.016
69. Kilian M, Sheinin R, Tan CL, Friedrich M, Krämer C, Kaminitz A, et al. MHC class II-restricted antigen presentation is required to prevent dysfunction of cytotoxic T cells by blood-borne myeloids in brain tumors. Cancer Cell. (2023) 41(2):235–51.e9. doi: 10.1016/j.ccell.2022.12.007
70. Zhu X, Mulcahy LA, Mohammed RA, Lee AH, Franks HA, Kilpatrick L, et al. IL-17 expression by breast-cancer-associated macrophages: IL-17 promotes invasiveness of breast cancer cell lines. Breast Cancer Res. (2008) 10(6):R95. doi: 10.1186/bcr2195
71. Franco OE, Jiang M, Strand DW, Peacock J, Fernandez S, Jackson RS 2nd, et al. Altered TGF-β signaling in a subpopulation of human stromal cells promotes prostatic carcinogenesis. Cancer Res. (2011) 71(4):1272–81. doi: 10.1158/0008-5472.CAN-10-3142
72. Kong P, Yang H, Tong Q, Dong X, Yi MA, Yan D. Expression of tumor-associated macrophages and PD-L1 in patients with hepatocellular carcinoma and construction of a prognostic model. J Cancer Res Clin Oncol. (2023) 149:10685–700. doi: 10.1007/s00432-023-04949-y
73. Morad G, Helmink BA, Sharma P, Wargo JA. Hallmarks of response, resistance, and toxicity to immune checkpoint blockade. Cell. (2021) 184(21):5309–37. doi: 10.1016/j.cell.2021.09.020
74. Yao H, Shen N, Ji G, Huang J, Sun J, Wang G, et al. Cisplatin nanoparticles promote intratumoral CD8(+) T cell priming via antigen presentation and T cell receptor crosstalk. Nano Lett. (2022) 22(8):3328–39. doi: 10.1021/acs.nanolett.2c00478
75. de Vries NL, van de Haar J, Veninga V, Chalabi M, Ijsselsteijn ME, van der Ploeg M, et al. γδ T cells are effectors of immunotherapy in cancers with HLA class I defects. Nature. (2023) 613(7945):743–50. doi: 10.1038/s41586-022-05593-1
76. Hack SP, Zhu AX, Wang Y. Augmenting anticancer immunity through combined targeting of angiogenic and PD-1/PD-L1 pathways: challenges and opportunities. Front Immunol. (2020) 11:598877. doi: 10.3389/fimmu.2020.598877
77. Minnar CM, Chariou PL, Horn LA, Hicks KC, Palena C, Schlom J, et al. Tumor-targeted interleukin-12 synergizes with entinostat to overcome PD-1/PD-L1 blockade-resistant tumors harboring MHC-I and APM deficiencies. J Immunother Cancer. (2022) 10(6). doi: 10.1136/jitc-2022-004561
78. Xu S, Zheng Y, Ye M, Shen T, Zhang D, Li Z, et al. Comprehensive pan-cancer analysis reveals EPHB2 is a novel predictive biomarker for prognosis and immunotherapy response. BMC Cancer. (2024) 24(1):1064. doi: 10.1186/s12885-024-12843-0
79. Mei K, Qin S, Chen Z, Liu Y, Wang L, Zou J. Camrelizumab in combination with apatinib in second-line or above therapy for advanced primary liver cancer: cohort A report in a multicenter phase Ib/II trial. J Immunother Cancer. (2021) 9(3). doi: 10.1136/jitc-2020-002191
80. Finn RS, Ryoo BY, Merle P, Kudo M, Bouattour M, Lim HY, et al. Pembrolizumab as second-line therapy in patients with advanced hepatocellular carcinoma in KEYNOTE-240: A randomized, double-blind, phase III trial. J Clin Oncol. (2020) 38(3):193–202. doi: 10.1200/JCO.19.01307
81. Guo M, Qi F, Rao Q, Sun J, Du X, Qi Z, et al. Serum LAG-3 predicts outcome and treatment response in hepatocellular carcinoma patients with transarterial chemoembolization. Front Immunol. (2021) 12:754961. doi: 10.3389/fimmu.2021.754961
82. Baysal H, Siozopoulou V, Zaryouh H, Hermans C, Lau HW, Lambrechts H, et al. The prognostic impact of the immune signature in head and neck squamous cell carcinoma. Front Immunol. (2022) 13:1001161. doi: 10.3389/fimmu.2022.1001161
83. Miao Y, Yuan Q, Wang C, Feng X, Ren J, Wang C. Comprehensive characterization of RNA-binding proteins in colon adenocarcinoma identifies a novel prognostic signature for predicting clinical outcomes and immunotherapy responses based on machine learning. Comb Chem High Throughput Screen. (2023) 26(1):163–82. doi: 10.2174/1386207325666220404125228
84. Yau T, Park JW, Finn RS, Cheng AL, Mathurin P, Edeline J, et al. Nivolumab versus sorafenib in advanced hepatocellular carcinoma (CheckMate 459): a randomised, multicentre, open-label, phase 3 trial. Lancet Oncol. (2022) 23(1):77–90. doi: 10.1016/S1470-2045(21)00604-5
85. Sperandio RC, Pestana RC, Miyamura BV, Kaseb AO. Hepatocellular carcinoma immunotherapy. Annu Rev Med. (2022) 73:267–78. doi: 10.1146/annurev-med-042220-021121
86. Sung PS, Park DJ, Roh PR, Mun KD, Cho SW, Lee GW, et al. Intrahepatic inflammatory IgA(+)PD-L1(high) monocytes in hepatocellular carcinoma development and immunotherapy. J Immunother Cancer. (2022) 10(5). doi: 10.1136/jitc-2021-003618
87. Zheng X, Jin W, Wang S, Ding H. Progression on the roles and mechanisms of tumor-infiltrating T lymphocytes in patients with hepatocellular carcinoma. Front Immunol. (2021) 12:729705. doi: 10.3389/fimmu.2021.729705
88. Lu C, Rong D, Zhang B, Zheng W, Wang X, Chen Z, et al. Current perspectives on the immunosuppressive tumor microenvironment in hepatocellular carcinoma: challenges and opportunities. Mol Cancer. (2019) 18(1):130. doi: 10.1186/s12943-019-1047-6
89. Kim HD, Song GW, Park S, Jung MK, Kim MH, Kang HJ, et al. Association between expression level of PD1 by tumor-infiltrating CD8(+) T cells and features of hepatocellular carcinoma. Gastroenterology. (2018) 155(6):1936–50.e17. doi: 10.1053/j.gastro.2018.08.030
90. Zhang Q, He Y, Luo N, Patel SJ, Han Y, Gao R, et al. Landscape and dynamics of single immune cells in hepatocellular carcinoma. Cell. (2019) 179(4):829–45.e20. doi: 10.1016/j.cell.2019.10.003
91. Krishna S, Lowery FJ, Copeland AR, Bahadiroglu E, Mukherjee R, Jia L, et al. Stem-like CD8 T cells mediate response of adoptive cell immunotherapy against human cancer. Science. (2020) 370(6522):1328–34. doi: 10.1126/science.abb9847
92. Castella M, Caballero-Baños M, Ortiz-Maldonado V, González-Navarro EA, Suñé G, Antoñana-Vidósola A, et al. Point-of-care CAR T-cell production (ARI-0001) using a closed semi-automatic bioreactor: experience from an academic phase I clinical trial. Front Immunol. (2020) 11:482. doi: 10.3389/fimmu.2020.00482
93. Nguyen A, Ho L, Hogg R, Chen L, Walsh SR, Wan Y. HDACi promotes inflammatory remodeling of the tumor microenvironment to enhance epitope spreading and antitumor immunity. J Clin Invest. (2022) 132(19). doi: 10.1172/JCI159283
94. Cai Z, Su X, Qiu L, Li Z, Li X, Dong X, et al. Personalized neoantigen vaccine prevents postoperative recurrence in hepatocellular carcinoma patients with vascular invasion. Mol Cancer. (2021) 20(1):164. doi: 10.1186/s12943-021-01467-8
95. Löffler MW, Gori S, Izzo F, Mayer-Mokler A, Ascierto PA, Königsrainer A, et al. Phase I/II multicenter trial of a novel therapeutic cancer vaccine, hepaVac-101, for hepatocellular carcinoma. Clin Cancer Res. (2022) 28(12):2555–66. doi: 10.1158/1078-0432.CCR-21-4424
96. Chen H, Li Z, Qiu L, Dong X, Chen G, Shi Y, et al. Personalized neoantigen vaccine combined with PD-1 blockade increases CD8(+) tissue-resident memory T-cell infiltration in preclinical hepatocellular carcinoma models. J Immunother Cancer. (2022) 10(9). doi: 10.1136/jitc-2021-004389
97. Guo ZS, Lu B, Guo Z, Giehl E, Feist M, Dai E, et al. Vaccinia virus-mediated cancer immunotherapy: cancer vaccines and oncolytics. J Immunother Cancer. (2019) 7(1):6. doi: 10.1186/s40425-018-0495-7
98. Shi D, Shi Y, Kaseb AO, Qi X, Zhang Y, Chi J, et al. Chimeric antigen receptor-glypican-3 T-cell therapy for advanced hepatocellular carcinoma: results of phase I trials. Clin Cancer Res. (2020) 26(15):3979–89. doi: 10.1158/1078-0432.CCR-19-3259
99. Makkouk A, Yang XC, Barca T, Lucas A, Turkoz M, Wong JTS, et al. Off-the-shelf Vδ1 gamma delta T cells engineered with glypican-3 (GPC-3)-specific chimeric antigen receptor (CAR) and soluble IL-15 display robust antitumor efficacy against hepatocellular carcinoma. J Immunother Cancer. (2021) 9(12). doi: 10.1136/jitc-2021-003441
100. Sun Y, Dong Y, Sun R, Liu Y, Wang Y, Luo H, et al. Chimeric anti-GPC3 sFv-CD3ϵ receptor-modified T cells with IL7 co-expression for the treatment of solid tumors. Mol Ther Oncolytics. (2022) 25:160–73. doi: 10.1016/j.omto.2022.04.003
101. Sun Y, Wu L, Zhong Y, Zhou K, Hou Y, Wang Z, et al. Single-cell landscape of the ecosystem in early-relapse hepatocellular carcinoma. Cell. (2021) 184(2):404–21.e16. doi: 10.1016/j.cell.2020.11.041
102. Siwowska K, Guzik P, Domnanich KA, Monné Rodríguez JM, Bernhardt P, Ponsard B, et al. Therapeutic potential of (47)Sc in comparison to (177)Lu and (90)Y: preclinical investigations. Pharmaceutics. (2019) 11(8). doi: 10.3390/pharmaceutics11080424
103. Piñero F, Dirchwolf M, Pessôa MG. Biomarkers in hepatocellular carcinoma: diagnosis, prognosis and treatment response assessment. Cells. (2020) 9. doi: 10.3390/cells9061370
104. Tan DJH, Ng CH, Lin SY, Pan XH, Tay P, Lim WH, et al. Clinical characteristics, surveillance, treatment allocation, and outcomes of non-alcoholic fatty liver disease-related hepatocellular carcinoma: a systematic review and meta-analysis. Lancet Oncol. (2022) 23(4):521–30. doi: 10.1016/S1470-2045(22)00078-X
105. Liu P, Zhou Q, Li J. Integrated multi-omics data analysis reveals associations between glycosylation and stemness in hepatocellular carcinoma. Front Oncol. (2022) 12:913432. doi: 10.3389/fonc.2022.913432
106. Cui D, Li W, Jiang D, Wu J, Xie J, Wu Y. Advances in multi-omics applications in HBV-associated hepatocellular carcinoma. Front Med (Lausanne). (2021) 8:754709. doi: 10.3389/fmed.2021.754709
107. Verduin M, Zindler JD, Martinussen HM, Jansen RL, Croes S, Hendriks LE, et al. Use of systemic therapy concurrent with cranial radiotherapy for cerebral metastases of solid tumors. Oncologist. (2017) 22(2):222–35. doi: 10.1634/theoncologist.2016-0117
108. Zhao G, Wang Y, Fan Z, Xiong J, Ertas YN, Ashammakhi N, et al. Nanomaterials in crossroad of autophagy control in human cancers: Amplification of cell death mechanisms. Cancer Lett. (2024) 591:216860. doi: 10.1016/j.canlet.2024.216860
Keywords: hepatocellular carcinoma, dendritic cells, natural killer cells, CTLA-4, PD-1/PD-L1
Citation: Ning J, Wang Y and Tao Z (2024) The complex role of immune cells in antigen presentation and regulation of T-cell responses in hepatocellular carcinoma: progress, challenges, and future directions. Front. Immunol. 15:1483834. doi: 10.3389/fimmu.2024.1483834
Received: 20 August 2024; Accepted: 30 September 2024;
Published: 22 October 2024.
Edited by:
Bing Yang, Tianjin Medical University, ChinaReviewed by:
Jun Hu, Tianjin Medical University Cancer Institute and Hospital, ChinaCopyright © 2024 Ning, Wang and Tao. This is an open-access article distributed under the terms of the Creative Commons Attribution License (CC BY). The use, distribution or reproduction in other forums is permitted, provided the original author(s) and the copyright owner(s) are credited and that the original publication in this journal is cited, in accordance with accepted academic practice. No use, distribution or reproduction is permitted which does not comply with these terms.
*Correspondence: Yutao Wang, cHVtY2hfeXR3YW5nQDEyNi5jb20=; Zijia Tao, emp0YW9AY211LmVkdS5jbg==
Disclaimer: All claims expressed in this article are solely those of the authors and do not necessarily represent those of their affiliated organizations, or those of the publisher, the editors and the reviewers. Any product that may be evaluated in this article or claim that may be made by its manufacturer is not guaranteed or endorsed by the publisher.
Research integrity at Frontiers
Learn more about the work of our research integrity team to safeguard the quality of each article we publish.