- 1Chaum Life Center, CHA University, Seoul, Republic of Korea
- 2Department of Family Medicine, Yonsei University College of Medicine, Seoul, Republic of Korea
- 3Department of Biomedical Informatics, CHA University School of Medicine, CHA University, Seongnam, Republic of Korea
Resting heart rate (RHR), a simple physiological indicator, has been demonstrated to be associated with inflammation and even metabolic disorders. This study aimed to investigate whether RHR is associated with natural killer cell activity (NKA) in a large population of healthy adults using a novel assay to measure NKA. This cross-sectional study included 7,500 subjects in the final analysis. NKA was estimated by measuring the amount of interferon-gamma (IFN-γ) released by activated natural killer cells; low NKA was defined as IFN-γ level <500 pg/mL. Subjects were categorized into four groups according to RHR as follows: C1 (≤ 60 bpm), C2 (60–70 bpm), C3 (70–80 bpm), and C4 (≥ 80 bpm). Individuals with higher RHR exhibited poorer metabolic and inflammatory profiles, with the prevalence of low NKA being highest in the highest RHR category. Compared with C1 as reference, the fully adjusted odd ratios (ORs) [95% confidence intervals (CIs)] for low NKA were significantly higher in C3 (OR: 1.37, 95% CI: 1.08–1.75) and C4 (OR: 1.55, 95% CI: 1.20–2.00). In addition, RHR was shown to exert indirect effects on NKA upon consideration of the mediation effect of serum cortisol in path analysis. Our findings confirm a significant link between elevated RHR and low NKA, and suggest the usefulness of RHR, a simple indicator reflecting increased sympathetic nervous system activity and stress, in predicting reduced immune function.
1 Introduction
Resting heart rate (RHR) is a key physiological indicator that reflects the intrinsic pacemaker activity of the sinoatrial node simply measured by reliable methods like manual palpation or electrocardiography (1, 2). This simple yet critical vital sign is influenced by a myriad of factors, including autonomic nervous system activity (3), various hormonal regulations (4–6), age (7), fitness level (8), and overall cardiovascular health (9). Elevated RHR has been associated with a higher level of inflammation (10) and an increased risk of cardiovascular diseases, including hypertension (11), heart failure (12), atrial fibrillation (13), diabetes mellitus (14), metabolic syndrome (15), and even cognitive function (16), making it a valuable prognostic marker in clinical settings. Conversely, a lower RHR is often indicative of enhanced cardiovascular fitness and improved autonomic balance (17).
Natural Killer (NK) cells, key players in innate immunity, swiftly identify and eliminate virus-infected or abnormal cells without prior sensitization (18). Derived from bone marrow progenitors expressing CD56 and CD16, NK cells modulate immune responses through recognition of distressed cells (19). Their rapid response is crucial for early defense against infections and tumors, achieved via the release of cytotoxic granules and production of cytokines like interferon-gamma (IFN-γ) (20). NK cells influence adaptive immunity by interacting with dendritic cells and T cells, regulating antigen presentation and T cell balance which this intricate crosstalk enhances overall immune responsiveness and contributes to immune homeostasis (21). Reduced NK cell activity (NKA) has been associated with an increased susceptibility to infections in immunologically intact individuals and increased risk of various types of cancer development (22–24).
Previous studies have established that autonomic nervous system has a critical role in mediating interactions between the nervous and immune systems (25). Moreover, heart rate variability (HRV), reflecting the balance between the activities of the sympathetic and parasympathetic nervous systems, has been demonstrated to be associated with inflammation and infection (26). While majority of studies focus on the potential of HRV as a predictive marker of immunity (26, 27), there is no extensive body of research specifically linking between RHR, the simplest physiological indicator, and immune function. Highlighting the crucial role of NK cells in immune function, the aim of this study is to conduct an investigation whether resting heart rate is associated with NKA in a large population of healthy adults using a novel assay to measure NKA.
2 Materials and methods
2.1 Study population
This cross-sectional study analyzed data from Chaum Life Center, screening subjects who underwent the NKA assay between January 2016 and May 2022 (n = 8,154). The data were obtained from electronic medical records. Among the 8,154 eligible subjects, 15 subjects under 18 years of age were excluded. Further exclusions were made for 1) those with a history of malignant disease (n = 56); 2) those with a history of autoimmune disease such as rheumatoid arthritis or inflammatory bowel disease (n = 14); 3) recent use of steroids or immuno-suppressants (n = 5); 4) acute infectious disease (n = 6); 5) those with missing data on resting heart rate (n = 1); 6) those with a history of arrhythmia, resting heart rate > 100 or < 40 bpm (n = 161); and 7) those with a history of thyroid disease, thyroid stimulating hormone (TSH) > 4.94 or < 0.35 mIU/L (n = 396). Ultimately, a total of 7,500 subjects were included in the analysis (Figure 1). The study protocol was approved by the Institutional Review Board of CHA Bundang Medical Center (CHAMC 2020-10-006). The study was conducted in accordance with the principles of the Declaration of Helsinki.
2.2 Data collection
Blood pressure (mmHg) was measured after at least 5 minutes of rest in a seated position. Systolic blood pressure (SBP) and diastolic blood pressure (DBP) were determined by averaging the last two of three measurements, with a minimum interval of 1 minute between each reading. Resting heart rate (RHR) was recorded from the right radial artery over a 60-second period. Blood samples were collected from the antecubital vein, in the morning after at least 8 hours of fasting. White blood cell (WBC) counts were quantified with XN-10 Hematology Analyzer (Sysmex, IL, USA). C-reactive protein (CRP) and fasting glucose were measured with the Hitachi 7600 Analyzer (Hitachi Co., Tokyo, Japan). Fasting insulin was measured by an electrochemiluminescence immunoassay using an Elecsys 2010 instrument (Roche, Mannheim, Germany). Insulin resistance was estimated using the homeostasis model assessment of insulin resistance (HOMA-IR) method by applying the following formula: HOMA-IR = fasting insulin (μIU/mL) × fasting glucose (mg/dL)/405 (28).
2.3 IFN-γ measurement for NKA
The IFN-γ released by activated NK cells was measured using a recently developed blood test (NK Vue® Kit, NKMAX, Sungnam, Korea). A 1 mL sample of whole blood was transferred directly into a specific blood collection tube containing a patented stimulatory cytokine for NKA analysis (Promoca®, NKMAX, Sungnam, Korea). The collection tube was gently and repeatedly mixed within 30 minutes of collection and then incubated for 20–24 hours in a 37.0°C chamber, following the manufacturer’s instructions. During incubation, the stimulatory cytokine induces IFN-γ secretion into the plasma. Although only NK cells secrete detectable amount of IFN-γ after Promoca® stimulation (29), interactions among activated immune cells might induce T cells and NKT cells to secrete IFN-γ. However, previous feasibility experiments have confirmed that the IFN-γ secretion predominantly occurs through NK cells rather than other innate or adaptive immune cells (29–31). After incubation, the supernatant was obtained and centrifuged at 3000 × g for 3 minutes. The supernatant was then loaded onto enzyme-linked immunosorbent assay (ELISA) plates, and the IFN-γ level was measured in pg/mL using the ELISA. Low NKA was defined as an IFN-γ level of less than 500 pg/mL (24, 32).
2.4 Statistical analysis
All data were presented as means ± standard deviations for continuous variables and as numbers (percentages) for categorical variables. Differences in clinical characteristics between participants with normal and low NKA were analyzed using the independent t-test for continuous variables and the chi-square test for categorical variables. Participants were categorized into four groups according to RHR as follows: C1 (≤ 60 bpm), C2 (60–70 bpm), C3 (70–80 bpm), and C4 (≥ 80 bpm). Comparisons of clinical characteristics among RHR categories were assessed using analysis of variance (ANOVA) for continuous variables and the chi-squared test for categorical variables. Post hoc analysis with the Bonferroni correction was conducted to determine the specific pairs of categories exhibiting significant differences after identifying significant differences among the categories. Multivariable logistic regression analyses were performed to calculate the odds ratios (ORs) and 95% confidence intervals (CIs) for low NKA, adjusting for age and sex in model 2, and for age, sex, DBP, WBC, CRP, and HOMA-IR in model 3. Additionally, path analysis was conducted to investigate the mediation effect of cortisol on NKA using the R statistical package (version 4.1.1; R Foundation for Statistical Computing, Vienna, Austria). The level of statistical significance for all analyses was set at p-value < 0.05. Statistical analyses were performed using SPSS statistical software (version 25.0, SPSS Inc., Chicago, IL, USA).
3 Results
3.1 Clinical characteristics of study populations
Table 1 presents the clinical characteristics of study population. Age, SBP, DBP, RHR, WBC counts, and CRP levels were significantly higher in subjects with low NKA than those with normal NKA. No significant differences in proportion of male sex, glucose, insulin, HOMA-IR, and proportion of participants with hypertension, diabetes, and dyslipidemia were observed between subjects with normal and low NKA.
3.2 Comparison of clinical characteristics among RHR categories
Table 2 shows the comparison of clinical characteristics among RHR categories using post-hoc analyses. All variables, except SBP, showed statistically significant differences among the four categories (overall p-value < 0.05). Category 1 (RHR ≤ 60) had the lowest mean DBP, WBC counts, and HOMA-IR, and the highest mean age, IFN-γ levels, and proportion of male sex. Category 4 (RHR ≥ 80) had the highest mean DBP, WBC counts, CRP, insulin, and HOMA-IR, and the lowest mean age, IFN-γ levels, and proportion of male sex.
Figure 2 shows the proportion of subjects with low NKA according to four RHR categories. The prevalence of low NKA was 30.5%, 35.4%, 37.0%, and 40.7% in C1, C2, C3, and C4, respectively. The prevalence of low NKA was significantly higher in C4, compared to C1, C2, and C3.
3.3 Association between RHR categories and low NKA
Table 3 presents the results of multivariable logistic regression analysis of the relationship between RHR categories and low NKA. The unadjusted ORs (95% CIs) for low NKA of C2, C3, and C4 compared with C1 reference were 1.25 (1.05–1.50), 1.34 (1.12–1.60), and 1.56 (1.30–1.88), respectively. After adjusting for age, sex, DBP, WBC, CRP, and HOMA-IR, similar trends were observed. The fully adjusted ORs for low NKA of C3 and C4 were significantly higher compared with C1 (OR: 1.37, 95% CI: 1.08–1.75 for C3, and OR: 1.55, 95% CI: 1.20–2.00 for C4, respectively). In path analysis, RHR was shown to exert indirect effects on NKA upon consideration of the mediation effect of serum cortisol (Supplementary Figure S1).
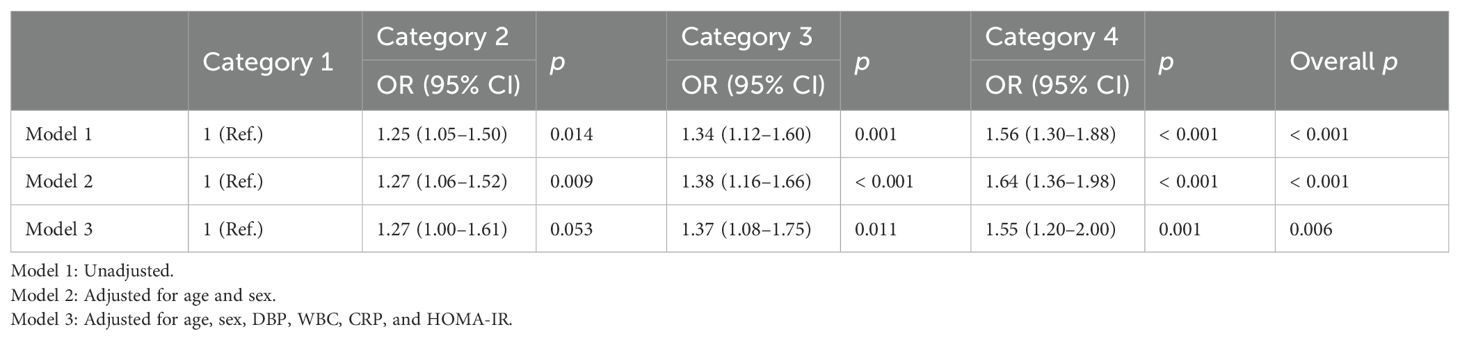
Table 3. Odds ratios and 95% confidence intervals of low NKA according to resting heart rate categories.
4 Discussion
This study demonstrated that a higher RHR is linked to low NKA. Individuals with elevated RHR exhibited poorer metabolic and inflammatory profiles, with the prevalence of low NKA being highest in those with higher RHR. Even after adjusting for various confounding factors, higher RHR remained significantly associated with greater odds of low NKA.
The analysis revealed significant differences in clinical characteristics among different RHR categories. Notably, individuals in the highest RHR category (≥80) had the poorest metabolic and inflammatory profiles, including higher DBP, WBC counts, CRP, insulin, and HOMA-IR, and lower IFN-γ levels suggesting that elevated RHR is a marker of both metabolic and cardiovascular risk. Previous studies have established a robust link between elevated RHR and metabolic syndrome (33, 34). Higher RHR was associated with increased metabolic syndrome prevalence and could predict its onset over time (33). Similarly, individuals with higher RHR were more likely to exhibit components of the metabolic syndrome, such as elevated blood pressure, dyslipidemia, and insulin resistance (34). The results of this study are consistent with these previous findings, as subjects with lower NKA showed significantly higher RHR, along with elevated WBC counts and CRP levels, indicating a state of chronic inflammation. This pro-inflammatory environment is known to contribute to insulin resistance and metabolic dysregulation, which are central to the development of metabolic syndrome. The association between RHR and inflammatory biomarkers is also well studied. For instance, Whelton et al. reported that higher RHR was linked to elevated levels of CRP, interleukin-6 (IL-6), and fibrinogen, markers that signify systemic inflammation (35). This inflammation can impair immune function, including NKA, potentially creating a feedback loop that exacerbates metabolic and cardiovascular risks.
Elevated RHR has been independently associated with increased cardiovascular and all-cause mortality, even after adjusting for inflammatory markers (36). The association of higher RHR with both low NKA and increased inflammatory markers in our study suggests that individuals with elevated RHR may be at a heightened risk for cardiovascular disease (CVD). Nanchen et al. found that higher RHR predicted incident heart failure and cardiovascular mortality in older adults, with inflammation and endothelial dysfunction playing significant roles (12). The link between NKA and CVD risk could be further explained by the role of chronic inflammation and immune dysfunction in atherosclerosis (37). Low NKA may reflect impaired immune surveillance, allowing for the progression of atherosclerotic plaques and increasing the likelihood of cardiovascular events.
The progressive increase in the prevalence of low NKA with higher RHR indicates a dose-response relationship, suggesting that elevated RHR correlates with a greater likelihood of impaired immune function. This relationship was further corroborated through multivariable logistic regression analysis, which confirmed that higher RHR categories are independently associated with increased odds of low NKA, even after adjusting for confounding variables. This robust association underscores the significance of elevated RHR as an independent predictor of reduced immune function. Path analysis identified a potential mechanism explaining the association between RHR and low NKA. Specifically, RHR was indirectly affecting NKA through elevated serum cortisol levels. This finding suggests that increased RHR may reflect heightened sympathetic nervous system activity, which is associated with stress and subsequently increased cortisol levels. While cortisol is essential for managing acute stress, chronically elevated levels due to prolonged stress can suppress immune function by activating the hypothalamic-pituitary-adrenal (HPA) axis (38).
Autonomic nervous system (ANS), one of the main homeostatic regulatory systems in the body, plays a crucial role in RHR. The two interacting branches of ANS, the sympathetic nervous system (SNS) and parasympathetic nervous system (PNS), must be in balance to maintain the physiological homeostasis (39). ANS balance is maintained through complex mechanisms involving central and peripheral regulation, reciprocal inhibition, and baroreceptor reflex (40). However, aging, acute and chronic stress, organic and idiopathic causes lead to ANS imbalance, characterized by increased SNS activity and reduced PNS activity at rest (41). Increased SNS activity releases norepinephrine and epinephrine, which bind to β1-adrenergic receptors on the pacemaker cells of SA node, activates intracellular signaling pathways that increase the production of cyclic adenosine monophosphate (cAMP), accelerates the depolarization of the pacemaker cells, and consequently increases RHR (42, 43). Stress can lead to ANS imbalance and increased heart rate, both of which are associated with suppressed NK cell activity and elevated pro-inflammatory cytokines (44). This supports the idea that stress-induced increases in RHR may compromise immune function. Suh et al. reported that elevated serum cortisol levels are associated with lower NKA, reinforcing the notion that chronic stress negatively impacts immune function through hormonal pathways (45). Also, Seiler et al. highlighted that everyday stressors can lead to chronic activation of the HPA axis, resulting in sustained high cortisol levels, which in turn can suppress immune function and reduce NKA (46). Moreover, Li et al. found that NK cell apoptosis is increased in conditions of oxidative stress, which is prevalent in chronic stress situations (47). This oxidative stress further contributes to the reduction in NKA observed in individuals with higher RHR. The balance of apoptosis and cell proliferation during heart development is crucial for normal cardiac function, and disruptions in these processes, potentially influenced by NKA, may impact RHR by altering the structural and functional integrity of the heart (48).
Our study has several limitations. First, this study is a cross-sectional design, which cannot reveal causality. Further longitudinal prospective studies are needed to confirm the predictive value of RHR for low NKA. Second, despite a relatively large sample size, our results have limited generalizability as our data were collected from a single hospital in South Korea. Third, lifestyle factors such as physical activity, medical conditions, and medications that could affect RHR and NKA were not fully evaluated. Fourth, RHR was measured by physician, not by echocardiogram. Lastly, the evaluation of NKA was done only using an IFN-γ assay. Our study did not incorporate techniques for directly quantifying NK cells or cytokines other than IFN-γ. Nevertheless, this new assay we employed has the advantage of simplicity, which can be more appropriate for large-scale studies. Despite these weaknesses, our study has the strength of being the first, to our knowledge, to demonstrate a significant association between high RHR and low NKA in a relatively large sample size. We also demonstrated a possible explanation for our results by showing the indirect effect of RHR on NKA upon consideration of the median effect of serum cortisol by path analysis.
In conclusion, this study found a significant link between higher RHR and reduced NKA, even after accounting for various confounding factors. Individuals with higher RHR had poorer metabolic and inflammatory profiles, with the lowest NKA levels seen in those with the highest RHR. Elevated RHR, which reflects increased sympathetic nervous system activity and stress, results in higher cortisol levels that suppress immune function. This finding underscores the importance of RHR as an independent predictor of reduced immune function and highlights its association with metabolic and cardiovascular risks. Thus, interventions aimed at lowering stress, RHR, and cortisol levels may help improve immune function and reduce these risks.
Data availability statement
The raw data supporting the conclusions of this article will be made available by the authors, without undue reservation.
Ethics statement
The studies involving humans were approved by Institutional Review Board of CHA Bundang Medical Center. The studies were conducted in accordance with the local legislation and institutional requirements. The participants provided their written informed consent to participate in this study.
Author contributions
HO: Writing – review & editing, Writing – original draft, Methodology, Investigation, Data curation, Conceptualization. A-RC: Writing – review & editing, Writing – original draft, Methodology, Investigation, Formal analysis, Conceptualization. J-HJ: Writing – review & editing, Writing – original draft, Methodology, Investigation, Data curation, Conceptualization. ES: Writing – review & editing, Writing – original draft, Methodology, Investigation, Data curation, Conceptualization. JM: Writing – review & editing, Writing – original draft, Methodology, Investigation, Data curation, Conceptualization. BC: Writing – review & editing, Writing – original draft, Supervision, Methodology, Investigation, Funding acquisition, Conceptualization. Y-KL: Writing – review & editing, Writing – original draft, Supervision, Methodology, Investigation, Funding acquisition, Conceptualization.
Funding
The author(s) declare financial support was received for the research, authorship, and/or publication of this article. This research was supported by the Institute of Information & Communications Technology Planning & Evaluation (IITP) grant funded by the Korea government(MSIT) (No. RS-2024-00357879, AI-based Biosignal Fusion and Generation Technology for Intelligent Personalized Chronic Disease Management) and NKMAX Co., Ltd. (grant number 2021-09-047). The funder was not involved in the study design, collection, analysis, interpretation of data, the writing of this article or the decision to submit it for publication.
Acknowledgments
We thank all researchers and subjects who participated in this study.
Conflict of interest
The authors declare that the research was conducted in the absence of any commercial or financial relationships that could be construed as a potential conflict of interest.
Publisher’s note
All claims expressed in this article are solely those of the authors and do not necessarily represent those of their affiliated organizations, or those of the publisher, the editors and the reviewers. Any product that may be evaluated in this article, or claim that may be made by its manufacturer, is not guaranteed or endorsed by the publisher.
Supplementary material
The Supplementary Material for this article can be found online at: https://www.frontiersin.org/articles/10.3389/fimmu.2024.1465953/full#supplementary-material
Supplementary Figure 1 | Path analysis to identify the direct and indirect effects of RHR on NKA upon consideration of the mediation effect of cortisol.
References
1. Olshansky B, Ricci F, Fedorowski A. Importance of resting heart rate. Trends Cardiovasc Med. (2023) 33:502–15. doi: 10.1016/j.tcm.2022.05.006
2. Vogel CU, Wolpert C, Wehling M. How to measure heart rate? Eur J Clin Pharmacol. (2004) 60:461–6. doi: 10.1007/s00228-004-0795-3
3. Agorastos A, Heinig A, Stiedl O, Hager T, Sommer A, Müller JC, et al. Vagal effects of endocrine HPA axis challenges on resting autonomic activity assessed by heart rate variability measures in healthy humans. Psychoneuroendocrinology. (2019) 102:196–203. doi: 10.1016/j.psyneuen.2018.12.017
4. Blake EF, Eagan LE, Ranadive SM. Heart rate variability between hormone phases of the menstrual and oral contraceptive pill cycles of young women. Clin Auton Res. (2023) 33:533–7. doi: 10.1007/s10286-023-00951-z
5. Yildirir A, Kabakci G, Yarali H, Aybar F, Akgul E, Bukulmez O, et al. Effects of hormone replacement therapy on heart rate variability in postmenopausal women. Ann Noninvasive Electrocardiol. (2001) 6:280–4. doi: 10.1111/j.1542-474x.2001.tb00119.x
6. Kim KH, Lee J, Ahn CH, Yu HW, Choi JY, Lee HY, et al. Association between thyroid function and heart rate monitored by wearable devices in patients with hypothyroidism. Endocrinol Metab (Seoul). (2021) 36:1121–30. doi: 10.3803/EnM.2021.1216
7. Kumral D, Schaare HL, Beyer F, Reinelt J, Uhlig M, Liem F, et al. The age-dependent relationship between resting heart rate variability and functional brain connectivity. Neuroimage. (2019) 185:521–33. doi: 10.1016/j.neuroimage.2018.10.027
8. Cornelissen VA, Verheyden B, Aubert AE, Fagard RH. Effects of aerobic training intensity on resting, exercise and post-exercise blood pressure, heart rate and heart-rate variability. J Hum Hypertens. (2010) 24:175–82. doi: 10.1038/jhh.2009.51
9. Brito Díaz B, Alemán Sánchez JJ, Cabrera de León A. Resting heart rate and cardiovascular disease. Med Clin (Barc). (2014) 143:34–8. doi: 10.1016/j.medcli.2013.05.034
10. Al-Rashed F, Sindhu S, Al Madhoun A, Ahmad Z, AlMekhled D, Azim R, et al. Elevated resting heart rate as a predictor of inflammation and cardiovascular risk in healthy obese individuals. Sci Rep. (2021) 11:13883. doi: 10.1038/s41598-021-93449-5
11. Colangelo LA, Yano Y, Jacobs DR Jr., Lloyd-Jones DM. Association of resting heart rate with blood pressure and incident hypertension over 30 years in black and white adults: the CARDIA study. Hypertension. (2020) 76:692–8. doi: 10.1161/hypertensionaha.120.15233
12. Nanchen D, Stott DJ, Gussekloo J, Mooijaart SP, Westendorp RG, Jukema JW, et al. Resting heart rate and incident heart failure and cardiovascular mortality in older adults: role of inflammation and endothelial dysfunction: the PROSPER study. Eur J Heart Fail. (2013) 15:581–8. doi: 10.1093/eurjhf/hfs195
13. Siland JE, Geelhoed B, Roselli C, Wang B, Lin HJ, Weiss S, et al. Resting heart rate and incident atrial fibrillation: A stratified Mendelian randomization in the AFGen consortium. PloS One. (2022) 17:e0268768. doi: 10.1371/journal.pone.0268768
14. Aune D, ÓH B, Vatten LJ. Resting heart rate and the risk of type 2 diabetes: A systematic review and dose–response meta-analysis of cohort studies. Nutr Metab Cardiovasc Dis. (2015) 25:526–34. doi: 10.1016/j.numecd.2015.02.008
15. Liu X, Luo X, Liu Y, Sun X, Han C, Zhang L, et al. Resting heart rate and risk of metabolic syndrome in adults: a dose-response meta-analysis of observational studies. Acta Diabetol. (2017) 54:223–35. doi: 10.1007/s00592-016-0942-1
16. Mao M, Liu R, Dong Y, Wang C, Ren Y, Tian N, et al. Resting heart rate, cognitive function, and inflammation in older adults: a population-based study. Aging Clin Exp Res. (2023) 35:2821–9. doi: 10.1007/s40520-023-02576-8
17. Franklin BA, Chaddha A. Resting heart rate and blood pressure as indices of cardiovascular and mortality risk: IS LOWER INVARIABLY BETTER? J Cardiopulm Rehabil Prev. (2018) 38:353–7. doi: 10.1097/hcr.0000000000000376
18. Hazeldine J, Lord JM. The impact of ageing on natural killer cell function and potential consequences for health in older adults. Ageing Res Rev. (2013) 12:1069–78. doi: 10.1016/j.arr.2013.04.003
19. Smyth MJ, Hayakawa Y, Takeda K, Yagita H. New aspects of natural-killer-cell surveillance and therapy of cancer. Nat Rev Cancer. (2002) 2:850–61. doi: 10.1038/nrc928
20. Morvan MG, Lanier LL. NK cells and cancer: you can teach innate cells new tricks. Nat Rev Cancer. (2016) 16:7–19. doi: 10.1038/nrc.2015.5
21. Vivier E, Tomasello E, Baratin M, Walzer T, Ugolini S. Functions of natural killer cells. Nat Immunol. (2008) 9:503–10. doi: 10.1038/ni1582
22. Ogata K, An E, Shioi Y, Nakamura K, Luo S, Yokose N, et al. Association between natural killer cell activity and infection in immunologically normal elderly people. Clin Exp Immunol. (2001) 124:392–7. doi: 10.1046/j.1365-2249.2001.01571.x
23. Imai K, Matsuyama S, Miyake S, Suga K, Nakachi K. Natural cytotoxic activity of peripheral-blood lymphocytes and cancer incidence: an 11-year follow-up study of a general population. Lancet. (2000) 356:1795–9. doi: 10.1016/s0140-6736(00)03231-1
24. Lee J, Park KH, Ryu JH, Bae HJ, Choi A, Lee H, et al. Natural killer cell activity for IFN-gamma production as a supportive diagnostic marker for gastric cancer. Oncotarget. (2017) 8:70431–40. doi: 10.18632/oncotarget.19712
25. Kenney MJ, Ganta CK. Autonomic nervous system and immune system interactions. Compr Physiol. (2014) 4:1177–200. doi: 10.1002/cphy.c130051
26. Adam J, Rupprecht S, Künstler ECS, Hoyer D. Heart rate variability as a marker and predictor of inflammation, nosocomial infection, and sepsis - A systematic review. Auton Neurosci. (2023) 249:103116. doi: 10.1016/j.autneu.2023.103116
27. Luo H, Wei J, Yasin Y, Wu SJ, Barszczyk A, Feng ZP, et al. Stress determined through heart rate variability predicts immune function. Neuroimmunomodulation. (2019) 26:167–73. doi: 10.1159/000500863
28. Matthews DR, Hosker JP, Rudenski AS, Naylor BA, Treacher DF, Turner RC. Homeostasis model assessment: insulin resistance and beta-cell function from fasting plasma glucose and insulin concentrations in man. Diabetologia. (1985) 28:412–9. doi: 10.1007/bf00280883
29. Lee SB, Cha J, Kim IK, Yoon JC, Lee HJ, Park SW, et al. A high-throughput assay of NK cell activity in whole blood and its clinical application. Biochem Biophys Res Commun. (2014) 445:584–90. doi: 10.1016/j.bbrc.2014.02.040
30. Nederby L, Jakobsen A, Hokland M, Hansen TF. Quantification of NK cell activity using whole blood: Methodological aspects of a new test. J Immunol Methods. (2018) 458:21–5. doi: 10.1016/j.jim.2018.04.002
31. Koo KC, Shim DH, Yang CM, Lee SB, Kim SM, Shin TY, et al. Reduction of the CD16(-)CD56bright NK cell subset precedes NK cell dysfunction in prostate cancer. PloS One. (2013) 8:e78049. doi: 10.1371/journal.pone.0078049
32. Lee YK, Haam JH, Cho SH, Kim YS. Cross-sectional and time-dependent analyses on inflammatory markers following natural killer cell activity. Diagnostics (Basel). (2022) 12(2):448. doi: 10.3390/diagnostics12020448
33. Jiang X, Liu X, Wu S, Zhang GQ, Peng M, Wu Y, et al. Metabolic syndrome is associated with and predicted by resting heart rate: a cross-sectional and longitudinal study. Heart. (2015) 101:44–9. doi: 10.1136/heartjnl-2014-305685
34. Rogowski O, Steinvil A, Berliner S, Cohen M, Saar N, Ben-Bassat OK, et al. Elevated resting heart rate is associated with the metabolic syndrome. Cardiovasc Diabetol. (2009) 8:55. doi: 10.1186/1475-2840-8-55
35. Whelton SP, Narla V, Blaha MJ, Nasir K, Blumenthal RS, Jenny NS, et al. Association between resting heart rate and inflammatory biomarkers (high-sensitivity C-reactive protein, interleukin-6, and fibrinogen) (from the Multi-Ethnic Study of Atherosclerosis). Am J Cardiol. (2014) 113:644–9. doi: 10.1016/j.amjcard.2013.11.009
36. Jensen MT, Marott JL, Allin KH, Nordestgaard BG, Jensen GB. Resting heart rate is associated with cardiovascular and all-cause mortality after adjusting for inflammatory markers: the Copenhagen City Heart Study. Eur J Prev Cardiol. (2012) 19:102–8. doi: 10.1177/1741826710394274
37. Lee YK, Suh E, Oh H, Haam JH, Kim YS. Decreased natural killer cell activity as a potential predictor of hypertensive incidence. Front Immunol. (2024) 15:1376421. doi: 10.3389/fimmu.2024.1376421
38. Cain DW, Cidlowski JA. Immune regulation by glucocorticoids. Nat Rev Immunol. (2017) 17:233–47. doi: 10.1038/nri.2017.1
39. Wehrwein EA, Orer HS, Barman SM. Overview of the anatomy, physiology, and pharmacology of the autonomic nervous system. Compr Physiol. (2016) 6:1239–78. doi: 10.1002/cphy.c150037
40. McCorry LK. Physiology of the autonomic nervous system. Am J Pharm Educ. (2007) 71:78. doi: 10.5688/aj710478
41. Bairey Merz CN, Elboudwarej O, Mehta P. The autonomic nervous system and cardiovascular health and disease: a complex balancing act. JACC Heart Fail. (2015) 3:383–5. doi: 10.1016/j.jchf.2015.01.008
42. Gordan R, Gwathmey JK, Xie LH. Autonomic and endocrine control of cardiovascular function. World J Cardiol. (2015) 7:204–14. doi: 10.4330/wjc.v7.i4.204
43. Behar J, Ganesan A, Zhang J, Yaniv Y. The Autonomic Nervous System Regulates the Heart Rate through cAMP-PKA Dependent and Independent Coupled-Clock Pacemaker Cell Mechanisms. Front Physiol. (2016) 7:419. doi: 10.3389/fphys.2016.00419
44. Owen N, Steptoe A. Natural killer cell and proinflammatory cytokine responses to mental stress: associations with heart rate and heart rate variability. Biol Psychol. (2003) 63:101–15. doi: 10.1016/s0301-0511(03)00023-1
45. Suh E, Cho AR, Haam JH, Gil M, Lee YK, Kim YS. Relationship between serum cortisol, dehydroepiandrosterone sulfate (DHEAS) levels, and natural killer cell activity: A cross-sectional study. J Clin Med. (2023) 12(12):4027. doi: 10.3390/jcm12124027
46. Seiler AF, Fagundes CP, Christian LM. The impact of everyday stressors on the immune system and health. In: Choukèr A, editor. Stress Challenges and Immunity in Space. Cham: Springer (2020). p. 231–45.
47. Li W, Lidebjer C, Yuan XM, Szymanowski A, Backteman K, Ernerudh J, et al. NK cell apoptosis in coronary artery disease: relation to oxidative stress. Atherosclerosis. (2008) 199:65–72. doi: 10.1016/j.atherosclerosis.2007.10.031
Keywords: RESTING HEART RATE, cortisol, natural killer cell, natural killer cell activity, immunity
Citation: Oh H, Cho A-R, Jeon J-H, Suh E, Moon J, Cho BH and Lee Y-K (2024) Association between resting heart rate and low natural killer cell activity: a cross-sectional study. Front. Immunol. 15:1465953. doi: 10.3389/fimmu.2024.1465953
Received: 17 July 2024; Accepted: 02 September 2024;
Published: 27 September 2024.
Edited by:
Lan Wu, Vanderbilt University Medical Center, United StatesReviewed by:
Vasilios E Papaioannou, Democritus University of Thrace, GreeceVera Usuelli, University of Milan, Italy
Copyright © 2024 Oh, Cho, Jeon, Suh, Moon, Cho and Lee. This is an open-access article distributed under the terms of the Creative Commons Attribution License (CC BY). The use, distribution or reproduction in other forums is permitted, provided the original author(s) and the copyright owner(s) are credited and that the original publication in this journal is cited, in accordance with accepted academic practice. No use, distribution or reproduction is permitted which does not comply with these terms.
*Correspondence: Baek Hwan Cho, YmFla2h3YW4uY2hvQGNoYS5hYy5rcg==; Yun-Kyong Lee, eWtsZWVmbUBjaGEuYWMua3I=
†These authors have contributed equally to this work and share first authorship