- 1Cellular Biotechnology Unit, Instituto de Salud Carlos III, Madrid, Spain
- 2Universidad Nacional de Educación a (UNED), Madrid, Spain
- 3Instituto de Investigación de Enfermedades Raras (IIER) & Departamento de Desarrollo de Medicamentos de Terapias Avanzadas (DDMTA), Instituto de Salud Carlos III, Madrid, Spain
LIN28, a highly conserved RNA-binding protein that acts as a posttranscriptional modulator, plays a vital role in the regulation of T-cell development, reprogramming, and immune activity in infectious diseases and T-cell-based immunotherapies. LIN28 inhibit the expression of let-7 miRNAs, the most prevalent family of miRNAs in lymphocytes. Recently it has been suggested that let-7 enhances murine anti-tumor immune responses. Here, we investigated the impact of LIN28 upregulation on human T cell functions, focusing on its influence on CAR T cell therapy. LIN28 lentiviral transduction of human T cells led to a stable expression of LIN28 that significantly downregulated the let-7 miRNA family without affecting cell viability or expansion potential. LIN28 overexpression maintained human T cell phenotype markers and functionality but impaired the antitumoral cytotoxicity of NKG2D-CAR T cells both in vitro and in vivo. These findings highlight the intricate relationship between LIN28/let-7 axis and human T cell functionality, including in CAR T cell therapy.
1 Introduction
LIN28, an RNA-binding protein, is crucial in various cellular processes, such as cell self-renewal, differentiation, metabolism, tissue repair, and reprogramming (1). Initially identified in C. elegans, LIN28 is well-conserved among species underscoring its fundamental role (2). Its expression is dynamic, and tightly regulated during undifferentiated cell stages such as embryonic stem cells (3). For that reason, it has been used as a substitute for Yamanaka factors to induce pluripotent stem cells (4). On the other hand, LIN28 is increasingly being acknowledged for its role in the immune regulation of fetal lymphopoiesis.
LIN28 can regulate gene expression by directly interacting with mRNA sequences or blocking miRNA biogenesis, such as the lethal-7 (let-7) miRNA family (5). Let-7 miRNAs have been implicated in regulating the balance between effector T-cells and regulatory T-cell populations (6–8). In human fetal thymus, Lin28b stimulates invariant gd T cells (9). Moreover, studies demonstrate its capacity to reshape hematopoietic progenitors towards fetal-like lymphopoiesis, facilitating the development of different cell subsets, such as gd T lymphocytes and NK cells (10). In adults, murine T cells downregulate let-7 expression upon antigen stimulation, which promotes proliferation and differentiation of activated T cells into cytotoxic T lymphocytes (CTLs) (11). Let-7 suppression demonstrates enhanced cytotoxicity of murine CTLs in vitro, yet it fails to control tumor growth in vivo (11, 12). However, the exact mechanisms by which LIN28 and let-7 miRNAs act on human T cells are still unknown, including their immunomodulatory properties for potential therapeutic use.
T-cell-based immunotherapy has become a powerful tool to treat infections and cancer. Among these approaches, chimeric antigen receptor (CAR) T-cell therapy has expanded options for oncologic patients (13). Unlike traditional CAR T cells that target a single antigen, NKG2D-CAR T cells are designed to recognize multiple ligands expressed on the surface of a variety of tumor cells (NKG2DL). This broad targeting capability has shown promising efficacy in preclinical models of osteosarcoma (14). Considering this preclinical data, we are evaluating NKG2D-CAR T cell therapy in a phase I clinical trial with sarcoma patients (NCT06087341). Currently, more than 20 clinical trials using NKG2D-CAR as tumor therapy (clinicaltrials.gov).
In the present study, we investigated the impact of LIN28 upregulation on human NKG2D-CAR T-cell functionality, focusing on T-cell viability, expansion, and cytotoxicity. Our results demonstrated efficient overexpression of LIN28 into T cells using lentiviral vectors. Highlighting its regulatory role, LIN28 upregulation significantly downregulates the let-7 miRNA family in human T cells. Intriguingly, LIN28 upregulation impairs NKG2D-CAR T cell cytotoxicity both in vitro and in vivo, suggesting a critical role in modulating CAR T cell function and anti-tumor responses. These findings provide crucial insights into LIN28’s impact on human T cell functionality, particularly in the context of CAR T cell therapy.
2 Methods
2.1 Tumoral cell lines
The human lymphoma cell line K562 and human osteosarcoma (OS) cell lines 143B and Mnng_hOS were cultivated in Dulbecco’s Modified Eagle Medium (DMEM) supplemented with 10% fetal bovine serum (FBS), 100 IU/ml of penicillin/streptomycin, and 2 mM of glutamine. Cell lines were regularly tested for Mycoplasma detection (Lonza). Cells were authenticated by STR analysis (IIBM, Madrid).
2.2 Human T cells isolation
Human Leukocyte Reduction System cones were obtained from donors by the Biobank Hospital Universitario Puerta de Hierro Majadahonda (HUPHM)/Instituto de Investigación Sanitaria Puerta de Hierro-Segovia de Arana (IDIPHISA). They were processed under standard operating procedures with the appropriate approval of the Ethics and Scientific Committees. Human peripheral blood mononuclear cells (PBMCs) and Pan T cells were obtained as described previously (15).
2.3 Lentiviral vectors, human T cell transduction and ex vivo expansion
Lentiviral vectors were produced from the co-transfection HEK293T cells with plasmids encoding pLenti-SFFV-LIN28-PGK-GFP (Abm), PL-SIN-EF1α-EGFP (Addgene) and NKG2D-CAR construct (Supplementary Figure 2A) (14), the packaging plasmid (PsPAX2), and the envelope plasmid (VSV-G). The culture supernatants were collected 48 hours post-transfection, filtered through a 0.45 µm filter (Millipore), and subjected to ultracentrifugation at 23,000 rpm for 2 hours at 4°C.
Purified T cells were activated overnight using Dynabeads Human T-Activator CD3/CD28 (Gibco) at a 1:2 bead-to-T cell ratio in X-VIVO 15 media (Lonza) supplemented with 250 U/ml of IL-2 (Miltenyi Biotech). The following day activated T cells were transduced with lentiviral vectors at a multiple of infection (MOI) of 2. Lentiviral vectors were added at the same time in double-transductions.
T cells were transduced with pLenti-SFFV-LIN28-PGK-GFP to obtain Lin28T cells. T cells were maintained at a concentration of 0.5-1x106 in X-VIVO 15 media supplemented with 250 U/ml of IL-2, and the media was refreshed every 2-3 days. From day 5 post-transduction, beads were removed and different combinations of IL2, IL21 and IL15 (PeproTech) were used at 40 U/ml, 100 and 100 ng/ml respectively. Fold change expansion of Lin28T was calculated as the absolute number of T cells in a timepoint divided by the absolute number of T cells at the start of the expansion culture. For CAR studies, T cells were double-transduced with NKG2D-CAR and GFP construct in NKG2D-CAR T cells; and NKG2D-CAR and pLenti-SFFV-LIN28-PGK-GFP (Abm) in NKG2D-CAR Lin28T and maintained as well as T cells. After 5 days, CAR T cells were expanded with IL21 and IL15 at 100 ng/ml concentration. Functional experiments were conducted from day 10 after transduction.
2.4 RT-qPCR and Stem-loop RT-qPCR
Total RNAs were extracted from human T cells using RNeasy Mini kit (Qiagen). The stem-loop RT was done as previously described (16). Briefly, cDNA was generated with RETROscript Reverse Transcription Kit (Invitrogen) combined with 1ng of stem-loop RT primers and without them for conventional RT. qPCR was performed using SYBR Select Master Mix (Thermofisher) in QuantStudio (Thermofisher). The sequences of primers used in this study were listed in Supplementary Table 1. Expression level of GAPDH was used as an internal control and U6 for miRNA.
2.5 Western blot
Total protein was extracted from UTD, Lin28T, NKG2D-CAR T, and NKG2D-CAR Lin28T cells using CelLytic buffer (Merck Sigma) according to manufacturer’s instructions. The proteins were then separated using SDS-PAGE, transferred to PVDF membranes and blocked with 5% milk in TBS. LIN28a rabbit polyclonal (1:500 dilution; Proteintech) and β-actin mouse monoclonal (clone AC-15; 1:100,000 dilution; Merck Sigma) were used as primary antibodies. Secondary antibodies were polyclonal goat anti-rabbit and anti-mouse immunoglobulins conjugated to horseradish peroxidase (HRP; Agilent’s Dako). Chemiluminescent signal was detected using Immobilon Western Chemiluminescent HRP Substrate (Merck Millipore).
2.6 Flow cytometry analysis
T cells were analyzed on MACSQuant Analyzer 10 flow cytometer (Miltenyi). Anti–human fluorescence-conjugated antibodies were listed in Supplementary Table 2. Viability was determined using 7-amino-actinomycin D staining (Biolegend).
2.7 In vitro T cell cytotoxicity assay
The cytotoxicity of T cells against K562, 143B and Mnng_hOS were evaluated at the specified effector-to-target (E: T) ratio by performing a 4-hour CyQUANT LDH Cytotoxicity Assay Kit (ThermoFisher) in p96 plate. As well, cytotoxicity of NKG2D-CAR T cells was measured at different ratios (8:1, 4:1, 2:1 and 1:1) against 143B by the previously mentioned test and 8:1 ratio against Mnng_hOS. Cytotoxicity percentages were calculated by (treated LDH activity- spontaneous LDH activity)/(max LDH activity - spontaneous LDH activity) × 100.
2.8 In vivo studies
OS xenograft models were generated by injecting 1x106 143B cells subcutaneously in the right flank of NOD.Cg-Prkdcscid Il2rgtm1WjI/SzJ (NSG) mice (Jackson) of 8-12 weeks old. The experimental process and animal welfare were performed at the Instituto de Salud Carlos III in Madrid, Spain. All animal experiments were approved by Institutional Review Board of the ISCIII and Consejería de Medio Ambiente of Comunidad de Madrid (PROEX 133.7/21).
On day 9, the mice were inoculated intravenously with ex vivo expanded 3x106 T cells or PBS per mouse as a single dose of treatment. Tumor length (L), width (W), and height (H) were measured with a caliper periodically and tumor volume was calculated as (L × W × H)/2. Tumor growth was calculated by dividing the tumor volume by the initial tumor volume. The endpoint was established when the tumor volume was >1000 mm3.
2.9 Statistics
Data from the in vitro studies were analyzed using a two-way ANOVA comparison test followed by a post-hoc test. For in vivo studies, a one-way ANOVA with Tukey’s comparison tests was used. Statistical analyses were performed using GraphPad Prism Software V.8.
3 Results
3.1 LIN28 upregulation preserved the viability and expansion of human T cells in vitro
T lymphocytes from healthy human donors were activated and then transduced with a lentiviral vector expressing LIN28 and GFP as reporter gene. After 5 days of lentiviral transduction with LIN28 at MOI 2, we expanded T cells with three different cytokines combinations: IL2, IL2+IL21, and IL21+IL15 (Figure 1A). We tested different cytokine stimulations due to their recognized significance in influencing the activation, expansion, and quality of human T cells (17). The efficacy of transduction was first tested by flow cytometry with the IL2 condition at different time points to check whether transduction was stable over time. Our results showed a significant efficient transduction rate. LIN28 upregulation was significant and remained stable over time (Figure 1B). On the other hand, neither lentiviral transduction nor expansion with different cytokines combinations affected the viability of T cells (Figure 1C). As well, stimulation with different groups of cytokines did not alter LIN28 expression (Figure 1D). We counted LIN28 transduced (Lin28T) and untransduced (UTD) cells over time to determine if either cytokines or transduction changed the expansion kinetics of T lymphocytes. Results showed that Lin28T cell expansion in vitro followed the expansion of T cells (Figure 1E). Overall, similar kinetics of human T cell expansion were found between the different cytokine stimulations (Figure 1F).
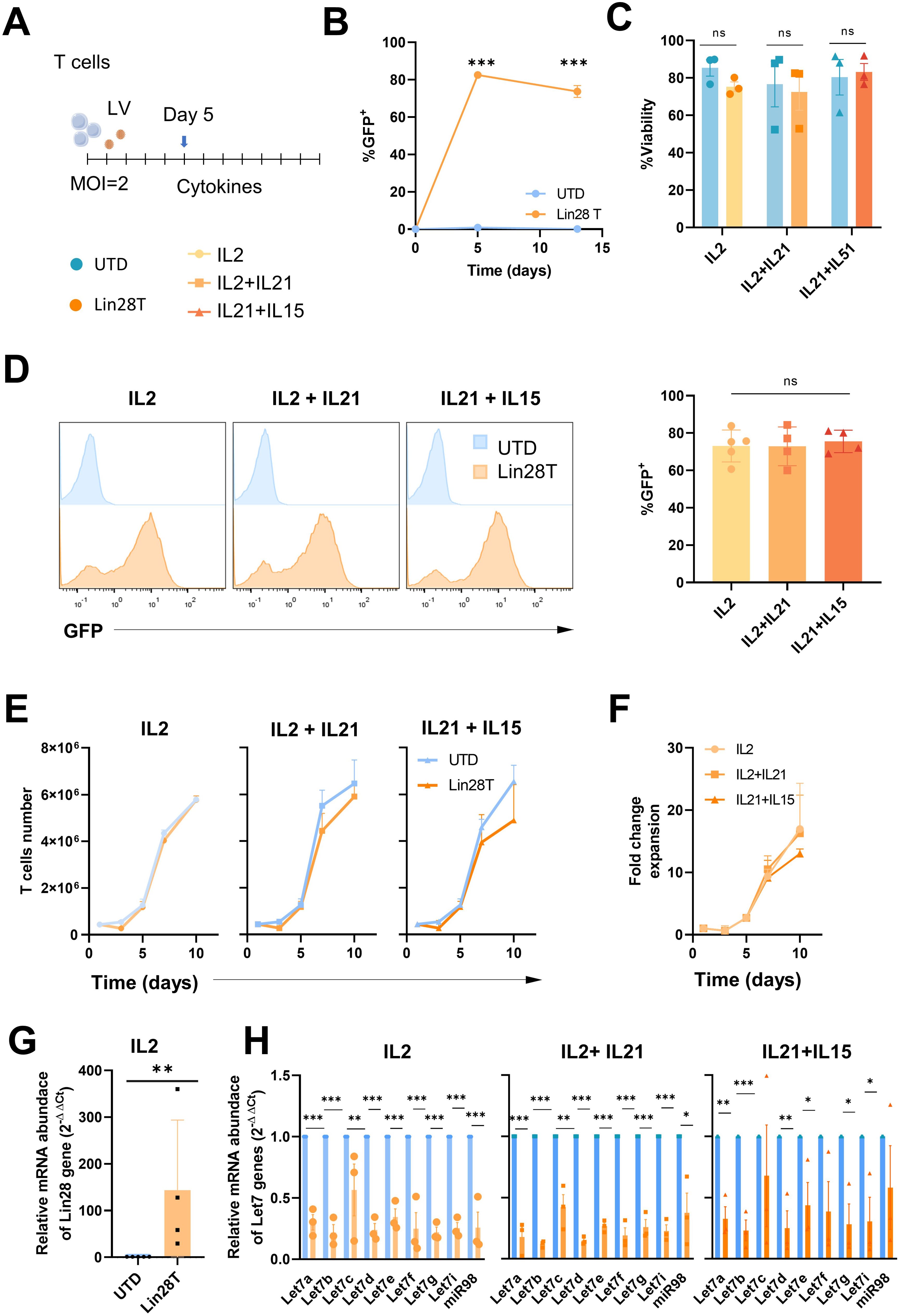
Figure 1. Upregulation of LIN28 down-regulates let-7 miRNAs in human T cells while maintaining their expansion and viability. (A) Schematic representation of the activation and transduction regimen for the ex vivo expansion of human T cells. (B) Lin28 transduction-reporter (GFP) expression was analyzed on days 5 and 12 post-transduction by flow cytometry. (C) The viability of Lin28T cells and untransduced cells after cytokines stimulation was analyzed by flow cytometry at day 12. (D) Histograms and percentage of GFP expression in transduced T cells after cytokine stimulation at day 12. (E) Kinetics of viable T cells overtime. (F) Fold change expansion of Lin28T overtime. (G) Relative mRNA abundance of the Lin28 gene was quantified by RT-qPCR in T cells expanded with IL2 and at day 12 post-transduction. (H) Relative miRNA abundance of the let-7 family was quantified by steep-loop RT-qPCR after different cytokines stimulations. The graphs represent the mean ± SD of three independent experiments (minimum n=3, healthy donors). The (***) indicates p < 0.001, (**) p < 0.01 and (*) p < 0.05, (ns) not significant compared to UTD by t-test analysis. LV: lentiviral vector; UTD: untraduced T cells (blue); Lin28T: LIN28 transduced T cells (orange).
3.2 Upregulation of LIN28 induced downregulation of let-7 miRNA family in human T cells ex vivo
In addition, LIN28 gene expression in transduced human T cells was analyzed by RT-qPCR. A significant increase in the relative abundance of LIN28 mRNA in transduced cells was obtained (Figure 1G and Supplementary Figure 2G), also in CAR T cells (Supplementary Figures 2F, G). To observe whether LIN28 has an impact on miRNAs, we tested the relative expression of the let-7 miRNA family. We used stem-loop RT-qPCR, a common molecular technique to identify and quantify individual small RNAs. Results showed that the let-7 miRNA family was significantly downregulated in Lin28T cells after cytokine stimulations (Figure 1H) and NKG2D-CAR Lin28T Supplementary Figure 2H).
3.3 Human T cell phenotype and cytotoxicity were maintained in vitro following LIN28 upregulation
After activation, lentiviral transduction with LIN28 construct, and expansion with different groups of cytokines, the phenotype of T cells was analyzed by flow cytometry. Results displayed comparable percentages of CD4+ and CD8+ T cells in Lin28T and UTD cell populations (Figure 2A). Considering that LIN28 is related to the regulation of T cell development early in life, we assessed whether LIN28 influences unconventional T cell subsets. These immune subpopulations, such as gd T cells and NKT-like cells, revealed no significant differences after LIN28 upregulation neither cytokine stimulations (Figures 2B, C). These results maintain that overexpression of LIN28 in human T cells does not affect their phenotype (Supplementary Figure 1A).
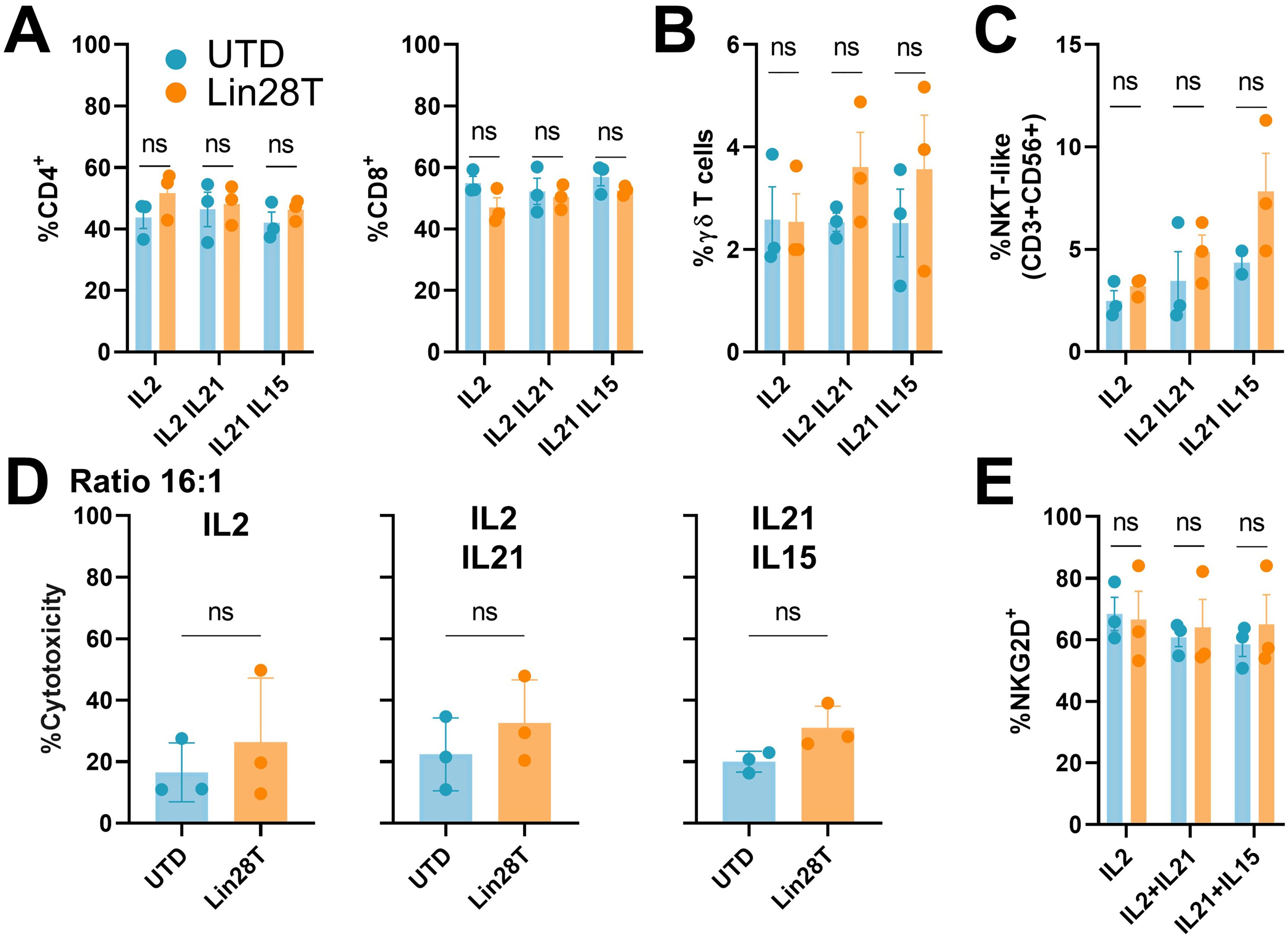
Figure 2. LIN28 upregulated T cells preserve T cells phenotype and cytotoxicity in vitro. Lin28T cells phenotype and cytotoxicity were analyzed 12 days after transduction and expansion. (A) Percentage of CD4+ and CD8+ T cells, (B) gd T cells (C) NKT-like cells (CD3+ CD56+). (D) Percentage of cytotoxicity measured by LDH assay on K562 cells exposure to T cells in 16:1 effector: target ratio for 4h. (E) Percentage of NKG2D+ cells. All data are shown as the mean ± SD. (ns) not significant. All results are representative of at least 3 independent experiments from different healthy donors. UTD: untraduced T cells (blue); Lin28T: transduced T cells (orange).
To perform functional analysis, we cocultured Lin28T cells with different tumoral cell lines. First, we wanted to isolate the cytotoxicity of NKT-like cells against a cell line that lacks MHC I molecules, such as K562. Results revealed no significant differences in vitro between Lin28T cells and UTD (Figure 2D). Moreover, the cytotoxicity of human T cells expanded with different groups of cytokines showed no difference among the tested conditions. It is described that NKT cells degranulated in response to NKG2D engagement independently of their invariant TCR stimulation (18). We described no discernible variance of NKG2D between the two T subpopulations (Figure 2E, Supplementary Figure 1B). Similarly, we cocultured Lin28T against 143B cells in different effector ratios to test the cytotoxicity of CD8 T cells, resulting in similar results between conditions. Although T cells cytotoxicity was slightly increased in ratio-dependent manner, no significant differences in cytotoxicity between UTD and Lin28T were observed (Figure 3A). The absence of significant differences in cytotoxicity between Lin28T and UTD, regardless of the type of cytokine stimulation used, suggests that the LIN28 overexpression does not notably affect T cell cytotoxicity.
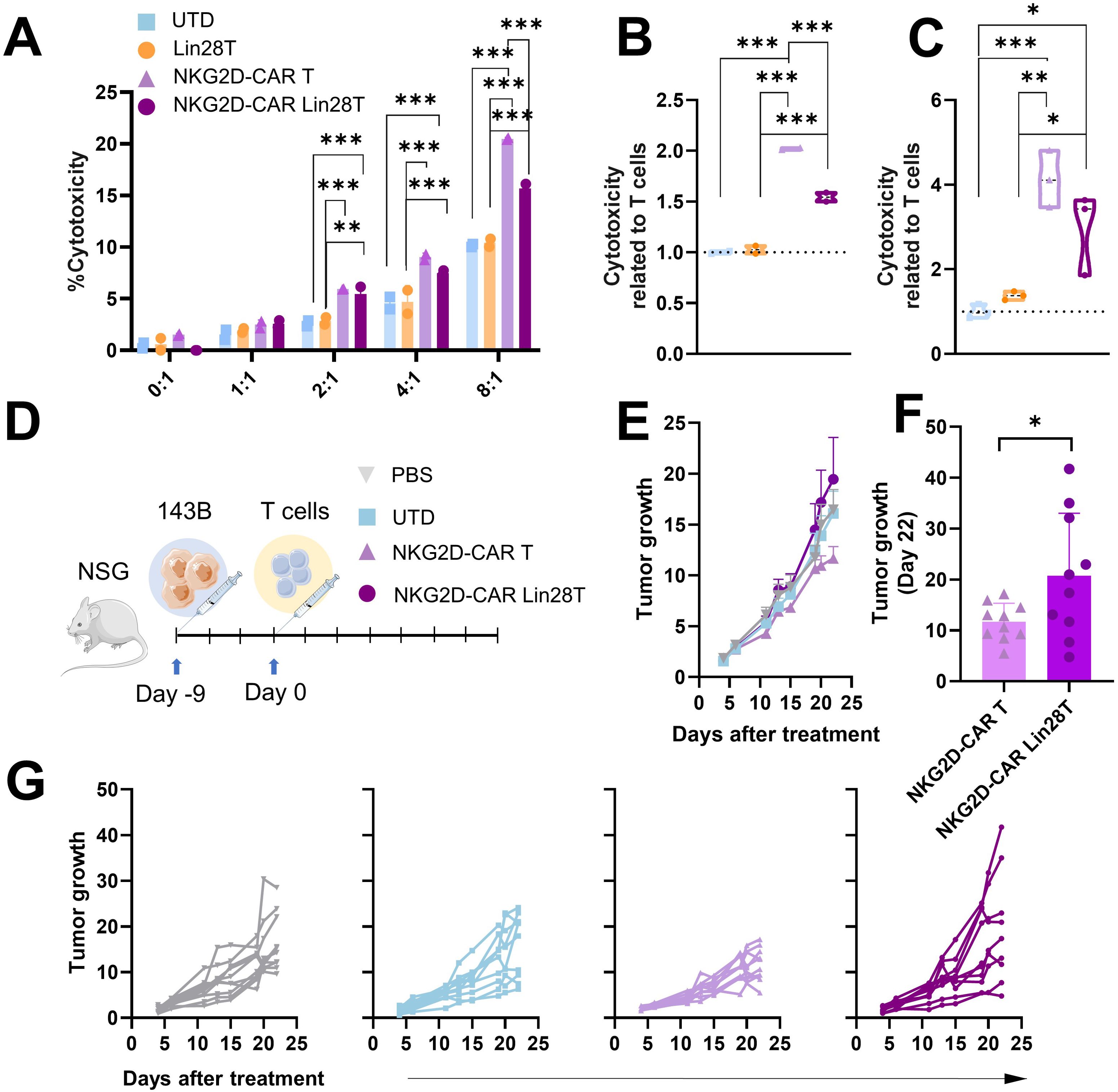
Figure 3. NKG2D-CAR Lin28 T cells impair NKG2D-CAR T cells cytotoxicity in vitro and in vivo. (A) Cytotoxicity assay in vitro, cells were coculture at different effector-target ratios (1:1-8:1). Cytotoxicity was measured 4h later using LDH cytotoxicity assay. (B) Cytotoxicity was related to T cells at 4h at 8:1 ratio in 143B and in (C) Mnng_hOS. The data shown represents mean ± SD. One-way ANOVA followed by Tukey’s test (n=2-3). *p<0.05, **p<0.01, ***p<0.001. (D) Schematic illustration of in vivo experimental setting. (E) Tumor growth of mice treated represented as mean + SEM (n=10). (F) Comparison of tumor growth of mice treated with NKG2D-CAR T and NKG2D-CAR Lin28T represented as mean ± SD (n=10) at day 22 post-treatment. *p<0.05 difference was found following a t-test statistical analysis. (G) Follow-up of tumor growth in mice treated with PBS (grey), UTD (T cells), NKG2D-CAR T (purple) and NKG2D-CAR Lin28T (dark purple) represented as individual values. Data were analyzed by One-way ANOVA followed by Tukey’s test (n=10).
3.4 Upregulation of LIN28 expression impaired NKG2D-CAR T cytotoxicity activity
The impact of LIN28 upregulation on NKG2D-CAR T cells cytotoxicity both in vitro and in vivo was investigated. We did a double-transduction of T cells with NKG2D-CAR and LIN28, demonstrating a high effectivity of transduction of both transgenes (Supplementary Figures 2B–E). In vitro cytotoxicity was measured by LDH assay, with cocultures against osteosarcoma 143B and Mnng_hOS cell lines. Previously, we confirmed the expression of NKG2D ligands in the 143B and Mnng_hOS cell lines. Different E:T ratios (1:1, 2:1, 4:1 and 8:1) were tested at 4h of coculture. We observed a dose-dependent effect (Figure 3A). Relevantly, we observed a significant decrease of cytotoxicity in NKG2D-CAR Lin28T cells compared with NKG2D-CAR T cells (Figures 3B, C).
Moving to in vivo settings, an OS xenograft model was established, and treatments were i.v. administrated (PBS, UTD, NKG2D-CAR T, and NKG2D-CAR Lin28T) (Figure 3D). Subsequent monitoring of tumor growth revealed that mice treated with NKG2D-CAR Lin28T cells showed slight promotion of tumor growth compared to NKG2D-CAR T (Figures 3E–G). These findings collectively suggest that the upregulation of LIN28 into NKG2D-CAR T cells might compromise their cytotoxic potential both in vitro and in vivo, highlighting the critical role of LIN28 in modulating CAR T cell function and anti-tumor responses.
4 Discussion
LIN28 serves as a critical controller orchestrating the transition from pluripotency to differentiated cell lineages (19). According to these roles, LIN28 demonstrates proficiency in reprogramming somatic cells into pluripotent states (20). Within murine T lymphocytes, LIN28 significantly shapes early development and functionality (21). In the present study, we investigated the impact of LIN28 transduction upregulation on human T cells function, including CAR T immunotherapies.
It was published that transgenic upregulation of LIN28 in murine models leads to an aggressive peripheral T cell lymphoma (22). In humans, high LIN28B expression is associated with juvenile myelomonocytic leukemias and specific subtypes of pediatric leukemias (23, 24). However, our lentiviral transduction of LIN28 into T cells effectively induced stable expression of LIN28 without compromising cell viability or the expansion potential of human T cells in vitro. It is important to note that neonatal and adult T cells are derived from different progenitor cells (25). These differences in the stage of development of LIN28 overexpression might have a differential impact on their proliferative effect.
According to the literature, our findings reveal the downstream consequences of LIN28 overexpression, particularly on let-7 miRNA family expression (26). As shown with murine T cells, LIN28 upregulation in human peripheral blood-derived T cells report a regulatory role in controlling miRNA-mediated pathways, potentially implicating LIN28 in broader regulatory networks governing T cell function and differentiation. Several lines of evidence suggest that the level of let-7 expression appears to inversely correlate with the activation status of the T cells (27). Once T cells are exposed to antigens, T cells rapidly down-regulate let-7 expression. This enhances the proliferation and differentiation of activated T cells into effector lymphocyte subsets (11). On the other hand, the same group has recently reported that let-7 downregulation promotes the generation of a terminal effector population that becomes vulnerable to exhaustion and cell death in immunosuppressive environments and fails to reject tumors (12). In addition, let-7 downregulation in fetal CD4+ T cells mediate homeostasis and self-tolerance through increased signaling through the TGF-β pathway and differentiation into Tregs (28). Considering these controversies, we wanted to investigate the role of LIN28 in T-cell-based cancer treatment.
Current cancer immunotherapies focus on strengthening the cytotoxicity function of CTLs by preventing an exhausted state of effector cells. One way to optimize manufacturing of T cells in immunotherapy is by using different types of cytokines, such as IL2, IL7, IL15, IL18 and IL21 (17). As our results, IL2, IL15 and IL21 lead the ex vivo expansion. Although we observed no differences between T-cell stimulation with different cytokines, other research shows differences in the differentiation status of T-cells when stimulated with different types of cytokines (29). IL2 induced a more differentiated state, whereas IL15 stimulates ex vivo expansion and IL21 younger phenotype (29, 30). Upon no activation differences were observed, we expanded CAR T cells with IL15 and IL21 because recent studies show that combined expression of IL-15 and IL-21 result in a less differentiated profile and longer survival in repeated exposures to tumor cells (31).
Other way to potent cytotoxicity function of T cells is via blockade of immunosuppressive ligand-receptor interactions in the tumor microenvironment (32). Some studies demonstrated that LIN28 has a potential role in tumor progression and mediated immune checkpoint upregulation, such as PD-L1 expression (33). Moreover, in this study treatment with a LIN28 inhibitor, C1632, significantly augmented the tumor-killing activity of the GPC3-CAR T cells against hepatocellular carcinoma cell (HCC) lines in vitro and in vivo through inhibition of PD-L1 and IDO in the tumor. However, our findings revealed that ectopic LIN28 expression in human T cells leads to sustained PD1 expression. Combining these results, one could speculate that the observed effect in these HCC models could also be attributed to the inhibition of LIN28 in CAR T cells and the subsequent upregulation of let-7, potentially enhancing their antitumoral activity.
We have analyzed the consequences of upregulated LIN28, and subsequent downregulation of let-7 expression, within NKG2D-CAR T cells. Notably, we observed that the upregulation of LIN28 in CAR T cells attenuates their cytotoxic capabilities. Contrary to what we first hypothesized; our findings coincide with the growing interest in using miRNA let-7 stimulators as a therapeutic tool (34). Thus, we speculate now that upregulation of let-7 would potentially enhance the anti-tumor activity of CAR T cells. Importantly, this strategy differs from other methodologies described that potentiate let-7 into tumor cells, which can result in unwanted effects on adjacent non-target cells, such as tumor-associated macrophages. Indeed, overexpression of let-7c has been associated with a shift towards an M2 phenotype associated with poor immunotherapy responses (35). Selectively potentiating let-7 within CAR T cells might mitigate the side effects in broader systemic applications and potent its cytotoxicity. Overall, this strategy may diminish early exhaustion and improve persistent memory of CAR T.
In conclusion, our work provides a comprehensive insight into the complex interplay between LIN28 and T cell functionality. Our initial hypothesis was that Lin28 overexpression could enhance the functionality of CAR-T cells; however, our findings highlighted its potential impairment in CAR T cell functionality. Future research should continue to understand the role of upregulation let-7 within CAR T cells, potentially opening avenues for improving the efficacy of CAR T cell therapies.
Data availability statement
The original contributions presented in the study are included in the article/Supplementary Material. Further inquiries can be directed to the corresponding author.
Ethics statement
The studies involving humans were approved by Biobank Hospital Universitario Puerta de Hierro Majadahonda (HUPHM)/Instituto de Investigación Sanitaria Puerta de Hierro-Segovia de Arana (IDIPHISA). The studies were conducted in accordance with the local legislation and institutional requirements. The participants provided their written informed consent to participate in this study. The animal study was approved by Institutional Review Board of the ISCIII and Consejería de Medio Ambiente of Comunidad de Madrid (PROEX 133.7/21). The study was conducted in accordance with the local legislation and institutional requirements.
Author contributions
PG-R: Conceptualization, Data curation, Formal analysis, Investigation, Methodology, Project administration, Software, Validation, Visualization, Writing – original draft, Writing – review & editing. LH: Conceptualization, Data curation, Formal analysis, Methodology, Supervision, Validation, Visualization, Writing – original draft, Writing – review & editing. MR-M: Formal analysis, Methodology, Project administration, Resources, Writing – original draft, Writing – review & editing. BS-C: Data curation, Formal analysis, Methodology, Writing – original draft, Writing – review & editing. JG-C: Conceptualization, Formal analysis, Funding acquisition, Investigation, Project administration, Resources, Supervision, Visualization, Writing – original draft, Writing – review & editing.
Funding
The author(s) declare that financial support was received for the research, authorship, and/or publication of this article. This research was funded by Instituto de Salud Carlos III (ISCIII): PI20CIII-00040, PI23CIII/00024 and RD21/0017/0005, Red Española de Terapias Avanzadas TERAV-ISCIII (NextGenerationEU; Plan de Recuperación Transformación y Resiliencia), Nueva generación de inmunoterapias STAB y CAR-T multidianas (P2022/BMD-7225; NEXT GENERATION CART MAD), the Asociación Pablo Ugarte, the Fundación Oncohematología Infantil and AFANION for grants support. Grant PID2022-137510OB-I00 and CPP2022-009535 funded by MCIN/AEI/10.13039/501100011033 and, as appropriate, by “ERDF A way of making Europe”, by the “European Union” or by the “European Union NextGenerationEU/PRTR”. PG-R is beneficiary of PhD ISCIII-PFIS program (FI22CIII/00004) and enrolling the Doctoral Program in Biomedical Sciences and Public Health as a trainee researcher at the UNED International Doctoral School.
Acknowledgments
NK2G2D CAR encoding plasmid was kindly provided by Dr. Antonio Pérez Martínez and Dr. Lucía Fernández from Hospital Universitario La Paz, Madrid. The authors wish to thank the donors and the Biobank Hospital Universitario Puerta de Hierro Majadahonda (HUPHM)/Instituto de Investigación Sanitaria Puerta de Hierro-Segovia de Arana (IDIPHISA) (PT17/0015/0020 in the Spanish National Biobanks Network) for the human specimens used in this study. Images for the graphical scheme of experiments were obtained and modified from SMART—Servier Medical Art under a Creative Common Attribution 3.0 Unported License.
Conflict of interest
The authors declare that the research was conducted in the absence of any commercial or financial relationships that could be construed as a potential conflict of interest.
Publisher’s note
All claims expressed in this article are solely those of the authors and do not necessarily represent those of their affiliated organizations, or those of the publisher, the editors and the reviewers. Any product that may be evaluated in this article, or claim that may be made by its manufacturer, is not guaranteed or endorsed by the publisher.
Supplementary material
The Supplementary Material for this article can be found online at: https://www.frontiersin.org/articles/10.3389/fimmu.2024.1462796/full#supplementary-material
Supplementary Figure 1 | LIN28 upregulation conserves T cell phenotype. (A) Mean fluorescence intensity (MFI) of PD1 expression on T cells. (B) MFI of NKG2D in CD4+ and CD8+ T cells. All data are shown as the mean ± SD. All results are representative of at least 2 independent experiments from different healthy donors. UTD: untraduced T cells (blue); Lin28T: transduced T cells (orange).
Supplementary Figure 2 | Efficacy of double-transduction to generate NKG2D-CAR T cells and upregulate Lin28T. (A) Schematic representation for the CAR construct used. (B) Histogram of NKG2D expression on T cells from PBMCs. (C) Percentage of NKG2D expressing T cells. (D) Histogram of GFP expression on T cells from PBMCs. (E) Percentage of GFP expressing T cells. The data shown are representative of three independent experiments, mean ± SD. One-way ANOVA followed by Tukey’s test. **p<0.01, ***p<0.001. (F) Relative mRNA abundance of the Lin28 gene was quantified by RT-qPCR in CAR T cells at day 10 post-transduction, analyzed with t-test, **p<0.01 (G) LIN28 and actin expression analyzed by Western blot analysis at 10 days post-transduction. (H) Relative miRNA abundance of the let-7 family was quantified by steep-loop RT-qPCR in CAR T cells, analyzed with t-test, **p<0.01. UTD: untraduced T cells (blue); NKG2D-CAR T (light purple); NKG2D-CAR Lin28T (dark purple).
Supplementary Table 1 | Stem-loop RT primers and qPCR primers.
Supplementary Table 2 | Flow cytometer antibodies.
References
1. Wu K, Ahmad T, Eri R. LIN28A: A multifunctional versatile molecule with future therapeutic potential. World J Biol Chem. (2022) 13:35–46. doi: 10.4331/wjbc.v13.i2.35
2. Ilbay O, Nelson C, Ambros VC. elegans LIN-28 controls temporal cell fate progression by regulating LIN-46 expression via the 5’ UTR of lin-46 mRNA. Cell Rep. (2021) 36:109670. doi: 10.1016/j.celrep.2021.109670
3. Krsnik D, Marić T, Bulić-Jakuš F, Sinčić N, Bojanac AK. LIN28 family in testis: control of cell renewal, maturation, fertility and aging. Int J Mol Sci. (2022) 23:7245. doi: 10.3390/ijms23137245
4. Yang SG, Wang XW, Qian C, Zhou FQ. Reprogramming neurons for regeneration: The fountain of youth. Prog Neurobiol. (2022) 214:102284. doi: 10.1016/j.pneurobio.2022.102284
5. Heo I, Joo C, Kim YK, Ha M, Yoon MJ, Cho J, et al. TUT4 in concert with Lin28 suppresses microRNA biogenesis through pre-microRNA uridylation. Cell. (2009) 138:696–708. doi: 10.1016/j.cell.2009.08.002
6. Swaminathan S, Suzuki K, Seddiki N, Kaplan W, Cowley MJ, Hood CL, et al. Differential regulation of the Let-7 family of microRNAs in CD4+ T cells alters IL-10 expression. J Immunol. (2012) 188:6238–46. doi: 10.4049/jimmunol.1101196
7. Pobezinsky LA, Etzensperger R, Jeurling S, Alag A, Kadakia T, McCaughtry TM, et al. Let-7 microRNAs target the lineage-specific transcription factor PLZF to regulate terminal NKT cell differentiation and effector function. Nat Immunol. (2015) 16:517–24. doi: 10.1038/ni.3146
8. Okoye IS, Coomes SM, Pelly VS, Czieso S, Papayannopoulos V, Tolmachova T, et al. MicroRNA-containing T-regulatory-cell-derived exosomes suppress pathogenic T helper 1 cells. Immunity. (2014) 41:89–103. doi: 10.1016/j.immuni.2014.05.019
9. Tieppo P, Papadopoulou M, Gatti D, McGovern N, Chan JKY, Gosselin F, et al. The human fetal thymus generates invariant effector γδ T cells. J Exp Med. (2020) 217:jem.20190580. doi: 10.1084/jem.20190580
10. Yuan J, Nguyen CK, Liu X, Kanellopoulou C, Muljo SA. Lin28b reprograms adult bone marrow hematopoietic progenitors to mediate fetal-like lymphopoiesis. Science. (2012) 335:1195–200. doi: 10.1126/science.1216557
11. Wells AC, Daniels KA, Angelou CC, Fagerberg E, Burnside AS, Markstein M, et al. Modulation of let-7 miRNAs controls the differentiation of effector CD8 T cells. Elife. (2017) 6:e26398. doi: 10.7554/eLife.26398
12. Wells AC, Hioki KA, Angelou CC, Lynch AC, Liang X, Ryan DJ, et al. Let-7 enhances murine anti-tumor CD8 T cell responses by promoting memory and antagonizing terminal differentiation. Nat Commun. (2023) 14:5585. doi: 10.1038/s41467-023-40959-7
13. June CH, O’Connor RS, Kawalekar OU, Ghassemi S, Milone MC. CAR T cell immunotherapy for human cancer. Science. (2018) 359:1361–5. doi: 10.1126/science.aar6711
14. Fernández L, Metais JY, Escudero A, Vela M, Valentín J, Vallcorba I, et al. Memory T cells expressing an NKG2D-CAR efficiently target osteosarcoma cells. Clin Cancer Res. (2017) 23:5824–35. doi: 10.1158/1078-0432.CCR-17-0075
15. Hidalgo L, Somovilla-Crespo B, Garcia-Rodriguez P, Morales-Molina A, Rodriguez-Milla MA, Garcia-Castro J. Switchable CAR T cell strategy against osteosarcoma. Cancer Immunol Immunother. (2023) 72:2623–33. doi: 10.1007/s00262-023-03437-z
16. Wang Y, Zhou J, Chen Y, Wang C, Wu E, Fu L, et al. Quantification of distinct let-7 microRNA family members by a modified stem-loop RT-qPCR. Mol Med Rep. (2018) 17:3690–6. doi: 10.3892/mmr.2017.8297
17. Silveira CRF, Corveloni AC, Caruso SR, Macêdo NA, Brussolo NM, Haddad F, et al. Cytokines as an important player in the context of CAR-T cell therapy for cancer: Their role in tumor immunomodulation, manufacture, and clinical implications. Front Immunol. (2022) 13:947648. doi: 10.3389/fimmu.2022.947648
18. Kuylenstierna C, Björkström NK, Andersson SK, Sahlström P, Bosnjak L, Paquin-Proulx D, et al. NKG2D performs two functions in invariant NKT cells: direct TCR-independent activation of NK-like cytolysis and co-stimulation of activation by CD1d. Eur J Immunol. (2011) 41:1913–23. doi: 10.1002/eji.200940278
19. Tsialikas J, Romer-Seibert J. LIN28: roles and regulation in development and beyond. Development. (2015) 142:2397–404. doi: 10.1242/dev.117580
20. Zhang J, Ratanasirintrawoot S, Chandrasekaran S, Wu Z, Ficarro SB, Yu C, et al. LIN28 regulates stem cell metabolism and conversion to primed pluripotency. Cell Stem Cell. (2016) 19:66–80. doi: 10.1016/j.stem.2016.05.009
21. Dong M, Mallet Gauthier È, Fournier M, Melichar HJ. Developing the right tools for the job: Lin28 regulation of early life T-cell development and function. FEBS J. (2022) 289:4416–29. doi: 10.1111/febs.16045
22. Beachy SH, Onozawa M, Chung YJ, Slape C, Bilke S, Francis P, et al. Enforced expression of Lin28b leads to impaired T-cell development, release of inflammatory cytokines, and peripheral T-cell lymphoma. Blood. (2012) 120:1048–59. doi: 10.1182/blood-2012-01-401760
23. Helsmoortel HH, Bresolin S, Lammens T, Cavé H, Noellke P, Caye A, et al. LIN28B overexpression defines a novel fetal-like subgroup of juvenile myelomonocytic leukemia. Blood. (2016) 127:1163–72. doi: 10.1182/blood-2015-09-667808
24. Helsmoortel HH, De Moerloose B, Pieters T, Ghazavi F, Bresolin S, Cavé H, et al. LIN28B is over-expressed in specific subtypes of pediatric leukemia and regulates lncRNA H19. Haematologica. (2016) 101:e240–4. doi: 10.3324/haematol.2016.143818
25. Wang J, Wissink EM, Watson NB, Smith NL, Grimson A, Rudd BD. Fetal and adult progenitors give rise to unique populations of CD8+ T cells. Blood. (2016) 128:3073–82. doi: 10.1182/blood-2016-06-725366
26. Lin Z, Radaeva M, Cherkasov A, Dong X. Lin28 regulates cancer cell stemness for tumour progression. Cancers (Basel). (2022) 14. doi: 10.3390/cancers14194640
27. Wells AC, Pobezinskaya EL, Pobezinsky LA. Non-coding RNAs in CD8 T cell biology. Mol Immunol. (2020) 120:67–73. doi: 10.1016/j.molimm.2020.01.023
28. Bronevetsky Y, Burt TD, McCune JM. Lin28b regulates fetal regulatory T cell differentiation through modulation of TGF-β Signaling. J Immunol. (2016) 197:4344–50. doi: 10.4049/jimmunol.1601070
29. Xu XJ, Song DG, Poussin M, Ye Q, Sharma P, Rodríguez-García A, et al. Multiparameter comparative analysis reveals differential impacts of various cytokines on CART cell phenotype and function ex vivo and in vivo. Oncotarget. (2016) 7:82354–68. doi: 10.18632/oncotarget.v7i50
30. Battram AM, Bachiller M, Lopez V, Fernández de Larrea C, Urbano-Ispizua A, Martín-Antonio B. IL-15 enhances the persistence and function of BCMA-targeting CAR-T cells compared to IL-2 or IL-15/IL-7 by limiting CAR-T cell dysfunction and differentiation. Cancers (Basel). (2021) 13:3534. doi: 10.3390/cancers13143534
31. Batra SA, Rathi P, Guo L, Courtney AN, Fleurence J, Balzeau J, et al. Glypican-3-specific CAR T cells coexpressing IL15 and IL21 have superior expansion and antitumor activity against hepatocellular carcinoma. Cancer Immunol Res. (2020) 8:309–20. doi: 10.1158/2326-6066.CIR-19-0293
32. Shiravand Y, Khodadadi F, Kashani SMA, Hosseini-Fard SR, Hosseini S, Sadeghirad H, et al. Immune checkpoint inhibitors in cancer therapy. Curr Oncol. (2022) 29:3044–60. doi: 10.3390/curroncol29050247
33. Patra T, Cunningham DM, Meyer K, Toth K, Ray RB, Heczey A, et al. Targeting Lin28 axis enhances glypican-3-CAR T cell efficacy against hepatic tumor initiating cell population. Mol Ther. (2023) 31:715–28. doi: 10.1016/j.ymthe.2023.01.002
34. Gilles ME, Slack FJ. Let-7 microRNA as a potential therapeutic target with implications for immunotherapy. Expert Opin Ther Targets. (2018) 22:929–39. doi: 10.1080/14728222.2018.1535594
Keywords: LIN28, let-7, CAR T, immunotherapy, osteosarcoma
Citation: Garcia-Rodriguez P, Hidalgo L, Rodriguez-Milla MA, Somovilla-Crespo B and Garcia-Castro J (2024) LIN28 upregulation in primary human T cells impaired CAR T antitumoral activity. Front. Immunol. 15:1462796. doi: 10.3389/fimmu.2024.1462796
Received: 10 July 2024; Accepted: 30 September 2024;
Published: 16 October 2024.
Edited by:
John Kyung Lee, Fred Hutchinson Cancer Research Center, United StatesCopyright © 2024 Garcia-Rodriguez, Hidalgo, Rodriguez-Milla, Somovilla-Crespo and Garcia-Castro. This is an open-access article distributed under the terms of the Creative Commons Attribution License (CC BY). The use, distribution or reproduction in other forums is permitted, provided the original author(s) and the copyright owner(s) are credited and that the original publication in this journal is cited, in accordance with accepted academic practice. No use, distribution or reproduction is permitted which does not comply with these terms.
*Correspondence: Javier Garcia-Castro, amdjYXN0cm9AaXNjaWlpLmVz