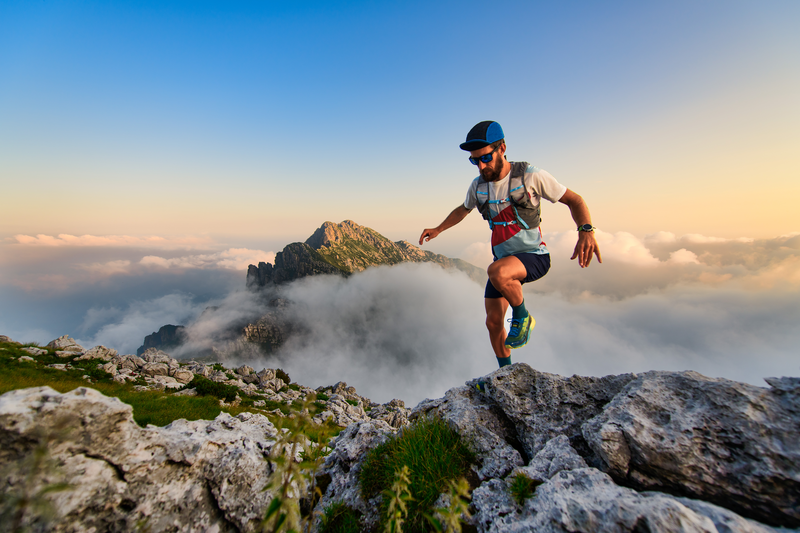
94% of researchers rate our articles as excellent or good
Learn more about the work of our research integrity team to safeguard the quality of each article we publish.
Find out more
EDITORIAL article
Front. Immunol. , 19 July 2024
Sec. Inflammation
Volume 15 - 2024 | https://doi.org/10.3389/fimmu.2024.1462563
This article is part of the Research Topic Immune dysfunction in acute pancreatitis: From Bench to Bedside Research View all 7 articles
Editorial on the Research Topic
Immune dysfunction in acute pancreatitis: from bench to bedside research
Acute pancreatitis is an common inflammatory gastrointestinal disease requiring acute admission to hospital (1). Most patients with acute pancreatitis have mild and self-limited symptoms, while up to 20% of patients develop severe disease requiring intensive care monitoring and support for organ failure (2).The incidence of AP is increasing at a rate of 2%-5% per year and varies between 3.4 and 73.4 cases per 100,000 worldwide (3). Despite the global burden of disease, there are currently no effective therapeutic agents to treat or prevent acute pancreatitis (4). Research into the pathogenesis of acute pancreatitis may help to identify novel cellular targets for potential drug development. There is increasing evidence that the severity and prognosis of acute pancreatitis are associated with uncontrolled or deregulated immune-system activation (4). This Research Topic highlights advances in the understanding of immune dysfunction in acute pancreatitis.
Normal gut mucosa is normally an effective barrier against the spread of toxins. Ischaemia and reperfusion of the intestinal mucosa during acute pancreatitis may damage the integrity of the intestinal barrier and increase intestinal permeability (5). The increased intestinal permeability could then lead to translocation of enteric bacteria and/or fungi into the pancreas, further contributing to the development of pancreatic necrosis and multiple organ failure. Reportedly, 68.8% of patients suffering from acute pancreatitis have bacterial DNA in their peripheral blood and over half (60.4%) display polymicrobial flora (6). Innate and adaptive immune cells stimulated by both pathogens and damage-associated molecular patterns (DAMPs) via pattern recognition receptors (PRRs) which including toll-like receptor-4 (TLR4) and dectin-1 (7), and the non-PRR DAMP receptors may be involved in amplifying proinflammatory cytokine responses and severe tissue injury (8). A mini-review by Otsuka et al. in this Research Topic summarises the role of fungus-induced activation of leucine-rich repeat kinase 2 (LRRK2) in the pathogenesis of severe acute pancreatitis. This study showed that the dectin-1-spleen tyrosine kinase (SYK)-leucine-rich repeat kinase 2 (LRRK2) axis, triggered by the detection of fungal cell wall elements, is capable of inducing the release of proinflammatory cytokines mediated by the nuclear translocation of NF-κB subunits.
TLR4 is present in a wide range of cells and has been shown to have several functions in the evolution of acute pancreatitis, independently of lipopolysaccharide (LPS) (9, 10). The initial insult of pancreatitis results in the release of intracellular contents from damaged cells into the extracellular space as DAMPs (such as HMGB1 and heat shock proteins), which act as key ligands for TLR4 and contribute to sterile inflammation (11). On the other hand, increased intestinal permeability and the entry of bacteria and/or bacterial products into the circulation could lead to increased exposure of acinar cells to gut bacterial components and activation of the NLRP3 (NOD-like receptor family pyrin domain containing-3) inflammasome through signalling via toll-like receptor 4 (TLR4) (10). Activation of the NLRP3 inflammasome leads to membrane pore formation and release of mature interleukin (IL)-1β and IL-18 from the cytoplasm, and enhances the systemic immune response by promoting neutrophil maturation, infiltration and macrophage infiltration (12). A review by Mattke et al. in this Research Topic summarises the relationship between TLR4 and pancreatic injury and immune infiltration in acute pancreatitis. They suggest that TLR4 plays a role not only in inflammation within acinar cells, but also with endothelial cells, macrophage maturation and polarization, and neutrophil activation and lifespan. And the activation of TLR4 leads not only to pancreatic damage but also to complications in other organs in acute pancreatitis.
Hypercholesterolemia, mainly caused by a high cholesterol diet, has been identified as a risk factor for severe acute pancreatitis (SAP) (13). Cholesterol accumulation favours the activation of both innate and adaptive inflammatory pathways, including the upregulation of TLR4 (14). Hypercholesterolaemia is capable of causing damage to endothelial cells through the production of nitric oxide and, in this way, leading to dysfunction of endothelial progenitor cells. Moreover, this endothelial microinflammation can lead to a reduction in blood supply, thus exacerbating the severity of the disease (14, 15). Despite these potential molecular mechanisms, the genomic relationship between a high cholesterol diet and AP has not been investigated. The study by Qiu et al. in this Research Topic identified the Fabp5 gene as the common differentially expressed gene between high cholesterol diet and acute pancreatitis. The Authors propose that by regulating the expression of Fabp5, resulting in increased activation of TLR 4 signalling and the nuclear factor-κB pathway, this type of nutrition may influence the severity of acute pancreatitis.
It is thought that neutrophils are the first immune cells to be recruited to inflammatory tissues in the setting of acute inflammation (4). Their immune functions are phagocytic, ROS production, degranulation and neutrophil extracellular trap (NET) formation and release (16, 17)]. Although NETs have a protective role in mediating host defence by trapping and killing microorganisms, the contents of NETs (histones and cell-free DNA) can be a source of DAMPs during sterile inflammation, which can be recognised by pattern recognition receptors, leading to the release of proinflammatory cytokines and ROS, and increased vascular permeability (12, 18). It is well known that NETs could be involved in the pathogenesis of AP by inducing trypsin activation, tissue damage and accelerating systemic inflammatory responses (10, 12). However, little is known about the mechanisms that control NET formation in AP. The study by Xu et al. in this Research Topic suggests that high expression of P-selectin may induce neutrophil extracellular traps via the PSGL-1/Syk/Ca2+/PAD4 pathway to exacerbate acute pancreatitis.
Immunoglobulin G (IgG) is the most abundant antibody in plasma and is a key molecule of the humoral immune response, linking innate and adaptive immunity through its multiple roles (19). N-glycans attach to the conserved asparagine 297 in the fragment crystallizable (Fc) portion of this molecule (altering IgG conformation and interactions with various receptors) and act as a switch between pro- and anti-inflammatory IgG functionality (20). IgG N-glycosylation affects physiology and an altered profile of IgG N-glycans contributes to disease progression and has been implicated in autoimmune pancreatitis, type 2 diabetes and cardiovascular disease (21, 22). The study by Chen et al. in this Research Topic investigated the causality of genetically predicted IgG N-glycosylation traits on different forms of pancreatitis using Mendelian Randomisation (MR) analysis, which may be helpful in understanding the role of immune cells and the microenvironment in the pathogenesis of pancreatitis.
Evidence suggests that severe acute pancreatitis shares many clinical and biochemical features with sepsis syndrome and septic shock (23). In both severe acute pancreatitis and sepsis, the major cytokines involved in the development of complications are tumour necrosis factor-alpha, interleukin-1, interleukin-6 and interleukin-8 (23). Microbial translocation as a result of altered gut permeability may be a major cause of sepsis-related morbidity in patients presenting with AP (24).Therefore, it is hypothesised that acute pancreatitis and sepsis may share a common pathogenesis. The study by Liu et al. in this Research Topic investigated the causal relationship between acute pancreatitis and sepsis, as well as the causal association of 731 immune cell characteristics with acute pancreatitis and sepsis by Mendelian randomisation (MR) analysis. They indicated that memory CD8+ T cells may play a crucial role in the evolution from AP to sepsis.
Taken together, both innate and adaptive immune responses are implicated in the mechanisms underlying pancreatitis (25, 26). Despite the aforementioned advances in knowing the pathogenesis, there are currently no effective immunotherapeutic agents for the treatment of acute pancreatitis (4). Despite improvements in treatment and critical care, severe acute pancreatitis is still associated with high mortality (1), and there are currently no specific pharmacological therapies with proven efficacy (27). Therefore, further studies that elucidate the role of immune dysfunction during disease progression may open new avenues for immunomodulatory therapy in acute pancreatitis.
WDH: Conceptualization, Data curation, Formal analysis, Funding acquisition, Investigation, Methodology, Project administration, Resources, Software, Supervision, Validation, Visualization, Writing – original draft, Writing – review & editing. MZ: Writing – original draft, Writing – review & editing. GW: Writing – review & editing. XJ: Writing – review & editing. WHH: Writing – review & editing. HG: Writing – review & editing.
The author(s) declare financial support was received for the research, authorship, and/or publication of this article. This work was supported by Zhejiang Medical and Health Science and Technology Plan Project (Number: 2022KY886).
The authors declare that the research was conducted in the absence of any commercial or financial relationships that could be construed as a potential conflict of interest.
All claims expressed in this article are solely those of the authors and do not necessarily represent those of their affiliated organizations, or those of the publisher, the editors and the reviewers. Any product that may be evaluated in this article, or claim that may be made by its manufacturer, is not guaranteed or endorsed by the publisher.
1. Boxhoorn L, Voermans RP, Bouwense SA, Bruno MJ, Verdonk RC, Boermeester MA, et al. Acute pancreatitis. Lancet. (2020) 396:726–34. doi: 10.1016/S0140-6736(20)31310-6
2. Trikudanathan G, Wolbrink DRJ, Van Santvoort HC, Mallery S, Freeman M, Besselink MG. Current concepts in severe acute and necrotizing pancreatitis: an evidence-based approach. Gastroenterology. (2019) 156:1994–2007.e1993. doi: 10.1053/j.gastro.2019.01.269
3. Tenner S, Vege SS, Sheth SG, Sauer B, Yang A, Conwell DL, et al. American college of gastroenterology guidelines: management of acute pancreatitis. Am J Gastroenterol. (2024) 119:419–37. doi: 10.14309/ajg.0000000000002645
4. Lee PJ, Papachristou GI. New insights into acute pancreatitis. Nat Rev Gastroenterol Hepatol. (2019) 16:479–96. doi: 10.1038/s41575-019-0158-2
5. Szatmary P, Grammatikopoulos T, Cai W, Huang W, Mukherjee R, Halloran C, et al. Acute pancreatitis: diagnosis and treatment. Drugs. (2022) 82:1251–76. doi: 10.1007/s40265-022-01766-4
6. Li Q, Wang C, Tang C, He Q, Li N, Li J. Bacteremia in patients with acute pancreatitis as revealed by 16S ribosomal RNA gene-based techniques*. Crit Care Med. (2013) 41:1938–50. doi: 10.1097/CCM.0b013e31828a3dba
7. Brown GD. Dectin-1: a signalling non-TLR pattern-recognition receptor. Nat Rev Immunol. (2005) 6:33–43. doi: 10.1038/nri1745
8. Huang Y, Jiang W, Zhou R. DAMP sensing and sterile inflammation: intracellular, intercellular and inter-organ pathways. Nat Rev Immunol. (2024). doi: 10.1038/s41577-024-01027-3
9. Sharif R, Dawra R, Wasiluk K, Phillips P, Dudeja V, Kurt-Jones E, et al. Impact of toll-like receptor 4 on the severity of acute pancreatitis and pancreatitis-associated lung injury in mice. Gut. (2009) 58:813–9. doi: 10.1136/gut.2008.170423
10. Watanabe T, Kudo M, Strober W. Immunopathogenesis of pancreatitis. Mucosal Immunol. (2017) 10:283–98. doi: 10.1038/mi.2016.101
11. Hoque R, Malik AF, Gorelick F, Mehal WZ. Sterile inflammatory response in acute pancreatitis. Pancreas. (2012) 41:353–7. doi: 10.1097/MPA.0b013e3182321500
12. Zhou Y, Huang X, Jin Y, Qiu M, Ambe PC, Basharat Z, et al. The role of mitochondrial damage-associated molecular patterns in acute pancreatitis. BioMed Pharmacother. (2024) 175:116690. doi: 10.1016/j.biopha.2024.116690
13. Hong W, Zimmer V, Basharat Z, Zippi M, Stock S, Geng W, et al. Association of total cholesterol with severe acute pancreatitis: A U-shaped relationship. Clin Nutr. (2020) 39:250–7. doi: 10.1016/j.clnu.2019.01.022
14. Tall AR, Yvan-Charvet L. Cholesterol, inflammation and innate immunity. Nat Rev Immunol. (2015) 15:104–16. doi: 10.1038/nri3793
15. Pirro M, Bagaglia F, Paoletti L, Razzi R, Mannarino MR. Review: Hypercholesterolemia-associated endothelial progenitor cell dysfunction. Ther Adv Cardiovasc Dis. (2008) 2:329–39. doi: 10.1177/1753944708094769
16. Kolaczkowska E, Kubes P. Neutrophil recruitment and function in health and inflammation. Nat Rev Immunol. (2013) 13:159–75. doi: 10.1038/nri3399
17. Zhang Z, Niu R, Zhao L, Wang Y, Liu G. Mechanisms of neutrophil extracellular trap formation and regulation in cancers. Int J Mol Sci. (2023) 24:10265. doi: 10.3390/ijms241210265
18. van Zyl M, Cramer E, Sanders J-SF, Leuvenink HGD, Lisman T, Van Rooy M-J, et al. The role of neutrophil extracellular trap formation in kidney transplantation: Implications from donors to the recipient. Am J Transplant. (2024). doi: 10.1016/j.ajt.2024.04.018
19. Štambuk J, Vučković F, Habazin S, Hanić M, Novokmet M, Nikolaus S, et al. Distinct longitudinal changes in immunoglobulin G N-glycosylation associate with therapy response in chronic inflammatory diseases. Int J Mol Sci. (2022) 23:8473. doi: 10.3390/ijms23158473
20. Liu D, Chu X, Wang H, Dong J, Ge S-Q, Zhao Z-Y, et al. The changes of immunoglobulin G N-glycosylation in blood lipids and dyslipidaemia. J Trans Med. (2018) 16(1):235. doi: 10.1186/s12967-018-1616-2
21. Chen MX, Su H-H, Shiao C-Y, Chang Y-T, Chang M-C, Kao C-C, et al. Affinity purification coupled to stable isotope dilution LC-MS/MS analysis to discover IgG4 glycosylation profiles for autoimmune pancreatitis. Int J Mol Sci. (2021) 22:11527. doi: 10.3390/ijms222111527
22. Birukov A, Plavša B, Eichelmann F, Kuxhaus O, Hoshi RA, Rudman N, et al. Immunoglobulin G N-glycosylation signatures in incident type 2 diabetes and cardiovascular disease. Diabetes Care. (2022) 45:2729–36. doi: 10.2337/dc22-0833
23. Wilson PG, Manji M, Neoptolemos JP. Acute pancreatitis as a model of sepsis. J Antimicrobial Chemotherapy. (1998) 41:51–63. doi: 10.1093/jac/41.suppl_1.51
24. Solomkin JS, Umanskiy K. Intraabdominal sepsis: newer interventional and antimicrobial therapies for infected necrotizing pancreatitis. Curr Opin Crit Care. (2003) 9:424–7. doi: 10.1097/00075198-200310000-00013
25. Fonteh P, Smith M, Brand M. Adaptive immune cell dysregulation and role in acute pancreatitis disease progression and treatment. Archivum Immunologiae Therapiae Experimentalis. (2017) 66:199–209. doi: 10.1007/s00005-017-0495-1
26. Peng C, Li Z, Yu X. The role of pancreatic infiltrating innate immune cells in acute pancreatitis. Int J Med Sci. (2021) 18:534–45. doi: 10.7150/ijms.51618
Keywords: acute pancreatitis, damage associated molecular pattern, toll-like receptor, hypercholesterolemia, innate immune, adaptive immune
Citation: Hong W, Zippi M, Wang G, Jin X, He W and Goyal H (2024) Editorial: Immune dysfunction in acute pancreatitis: from bench to bedside research. Front. Immunol. 15:1462563. doi: 10.3389/fimmu.2024.1462563
Received: 10 July 2024; Accepted: 12 July 2024;
Published: 19 July 2024.
Edited and Reviewed by:
Pietro Ghezzi, University of Urbino Carlo Bo, ItalyCopyright © 2024 Hong, Zippi, Wang, Jin, He and Goyal. This is an open-access article distributed under the terms of the Creative Commons Attribution License (CC BY). The use, distribution or reproduction in other forums is permitted, provided the original author(s) and the copyright owner(s) are credited and that the original publication in this journal is cited, in accordance with accepted academic practice. No use, distribution or reproduction is permitted which does not comply with these terms.
*Correspondence: Wandong Hong, eGhuay1od2RAMTYzLmNvbQ==
Disclaimer: All claims expressed in this article are solely those of the authors and do not necessarily represent those of their affiliated organizations, or those of the publisher, the editors and the reviewers. Any product that may be evaluated in this article or claim that may be made by its manufacturer is not guaranteed or endorsed by the publisher.
Research integrity at Frontiers
Learn more about the work of our research integrity team to safeguard the quality of each article we publish.