- 1Department of Infectious Diseases, Weifang People’s Hospital, Weifang, Shandong, China
- 2School of Basic Medicine, Fourth Military Medical University, Xi’an, China
Liver cancer is a major global health concern, ranking among the top causes of cancer-related deaths worldwide. Despite advances in medical research, the prognosis for liver cancer remains poor, largely due to the inherent limitations of current therapies. Traditional treatments like surgery, radiation, and chemotherapy often fail to provide long-term remission and are associated with significant side effects. Immunotherapy has emerged as a promising avenue for cancer treatment, leveraging the body’s immune system to target and destroy cancer cells. However, its application in liver cancer has been limited. One of the primary challenges is the liver’s unique immune microenvironment, which can inhibit the effectiveness of immunotherapeutic agents. This immune microenvironment creates a barrier, leading to drug resistance and reducing the overall efficacy of treatment. Recent studies have focused on understanding the immunological landscape of liver cancer to develop strategies that can overcome these obstacles. By identifying the specific factors within the liver that contribute to immune suppression and drug resistance, researchers aim to enhance the effectiveness of immunotherapy. Prospective strategies include combining immunotherapy with other treatments, using targeted therapies to modulate the immune microenvironment, and developing new agents that can bypass or counteract the inhibitory mechanisms in the liver. These advancements hold promise for improving outcomes in liver cancer treatment.
1 Introduction
Neoplasms remain the main killer worldwide (1–3). Among which, liver cancer, predominantly hepatocellular carcinoma (HCC), stands as one of the leading causes of cancer-related deaths worldwide (4–9). Despite advances in oncological therapies, the prognosis for liver cancer patients remains dire, especially in cases diagnosed at advanced stages (10). Traditional treatments, such as resection, transplantation, and systemic chemotherapy, offer limited efficacy and often come with significant side effects (11). This backdrop underscores the urgent need for innovative therapeutic approaches, among which immunotherapy has emerged as a promising candidate (12).
Immunotherapy, which harnesses the body’s immune system to fight cancer, has significantly transformed the treatment of various malignancies (13–16), marking a shift from traditional therapies by focusing on the interactions between cancer cells and the immune system (17). Applying immunotherapy in liver cancer, however, poses distinct challenges, primarily due to the liver’s unique immunological characteristics (18). The liver is not only a crucial metabolic organ but also plays a significant role in immunology (19). Its specialized microenvironment, inherently inclined towards tolerance for normal functioning, paradoxically provides a protective environment for tumor cells, complicating the effectiveness of immunotherapy in liver cancer (20).
The tolerogenic nature of the liver is characterized by a distinct array of immune cells and regulatory pathways (21). This environment is adept at maintaining immune homeostasis and preventing overactive responses to the myriad of antigens constantly presented to it, primarily from the gut via the portal circulation (22). In the context of HCC, this immunological landscape facilitates immune evasion, allowing cancer cells to thrive and proliferate under the radar of immune surveillance (23, 24).
Addressing these challenges requires a deep understanding of the liver’s immune milieu and the complex interplay between tumor biology and host immunity (25). This review aims to dissect the intricacies of the liver’s immune environment and explore how current and emerging immunotherapeutic strategies are being tailored to overcome these barriers (26). We delve into the latest research underscoring the potential of immunotherapy in liver cancer (27). This review not only highlights the progress in immunotherapy but also delves into the multifaceted nature of tumor drug resistance, exploring genetic alterations, immune evasion, and the influence of the tumor microenvironment.
2 Immunological landscape of liver cancer
The tumor microenvironment (TME) is a complex network comprising cancer cells, immune cells, fibroblasts, endothelial cells, and the extracellular matrix, actively influencing cancer progression and response to therapies like immunotherapy (28–31). The TME supports tumor growth through angiogenesis, immune evasion, and modifying drug responses, playing a critical role in immunotherapy tolerance by mechanisms such as cytokine secretion (e.g., TGF-β, IL-10) that suppress immune responses, and the expression of checkpoint molecules like PD-L1 that help tumors evade immune detection (32). Additionally, direct interactions between tumors and immune cells can deactivate effector immune cells, contributing to the TME’s immune-suppressive nature (33). Understanding and manipulating the TME is essential for developing effective cancer therapies, combining tumor-targeting strategies with approaches to alter the TME, aiming for improved therapeutic outcomes.
The liver’s immune system is uniquely adapted to its exposure to food antigens and gut-derived microbial products via the portal vein (34, 35). This exposure necessitates a predominantly tolerogenic environment to avoid an overactive immune response, which could lead to tissue damage and impaired liver function (36). The liver achieves this through a complex network of cells and signals that promote tolerance rather than immunity (37) (Figure 1).
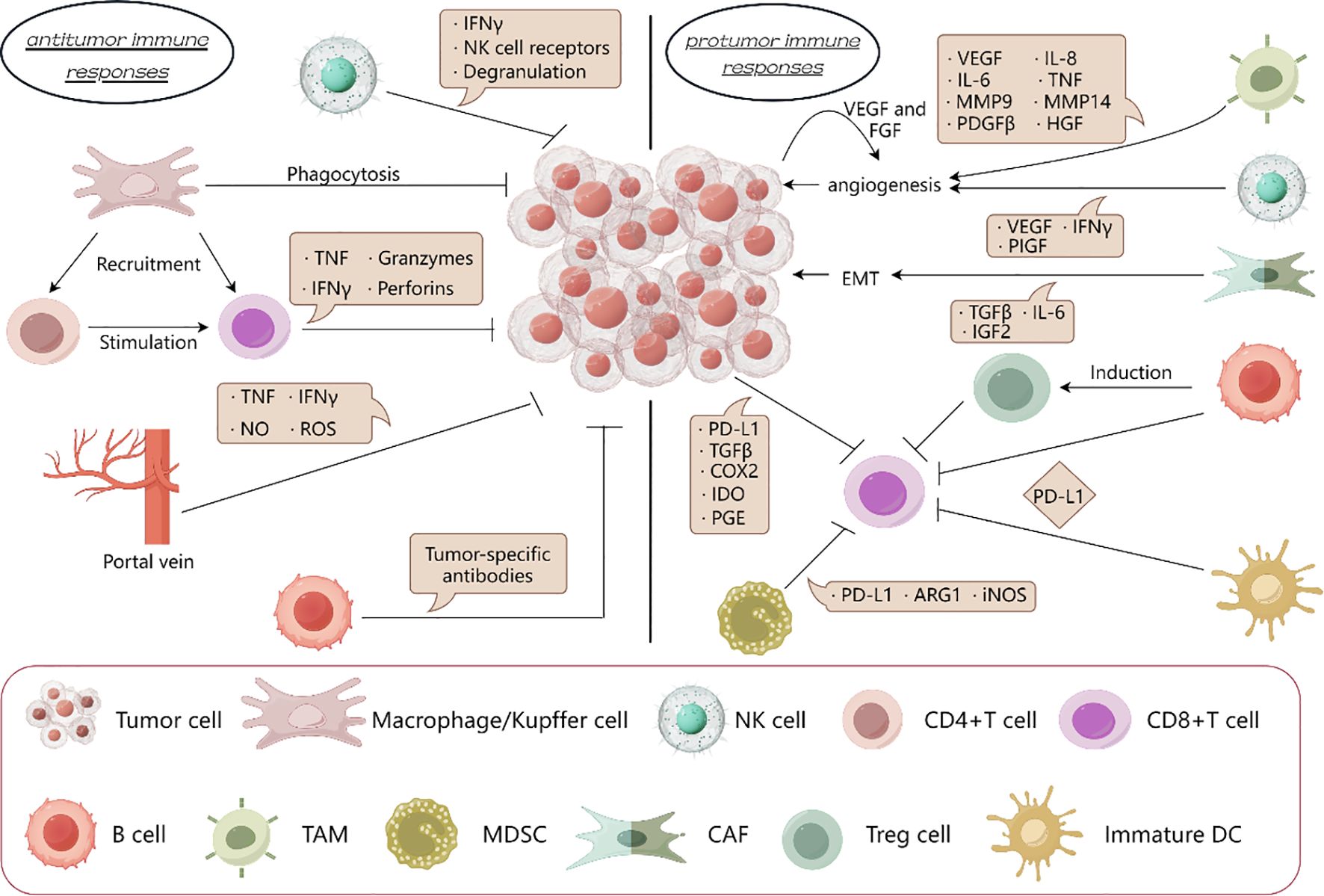
Figure 1. Protumor immune responses is dominant in liver cancer. In the immune microenvironment of liver cancer, the protumor immune response is superior to the anti-tumor immune response. By secreting cytokines, TAM and NK cells promote angiogenesis, CAF promotes epithelial mesenchymal transformation; Treg cells, immature DC cells, MDSCs and tumor cells suppresses the effect of CD8+T cells on tumors. And the killing effect on tumor by macrophages, NK cells and CD8+T cells is inhibited.
2.1 The antigenicity of HCC
The antigenic landscape of HCC is characterized by the presence of tumor-specific antigens (TSAs) and neoantigens, essential for the immune system’s recognition and attack on cancer cells (38). While TSAs, including alpha-fetoprotein (AFP), glypican-3 (GPC3), and melanoma-associated gene 1 (MAGE-1) in HCC, are predominantly cancer-centric, their presence, albeit in lower quantities, is not exclusive to cancer cells (39). The immune system, adept at identifying abnormalities, flags these antigens, especially when overexpressed or coupled with other tumor-associated signals (40). In contrast, neoantigens, borne out of tumor-specific genetic alterations such as point mutations and chromosomal rearrangements, are exclusive to cancer cells, rendering them precise targets for immune attacks (41).
Central to immune surveillance, TSAs and neoantigens underpin various immunotherapeutic strategies for HCC, including the deployment of cancer vaccines, the transfer of adoptive T cells, and the application of checkpoint blockade therapies (42, 43). However, targeting these antigens is fraught with challenges, given the liver’s natural inclination towards immune tolerance, the heterogeneous expression of antigens across tumors, and the cancer cells’ adeptness at evading immune detection (44, 45). Moreover, the liver’s altered immune landscape, often a consequence of underlying conditions like hepatitis or cirrhosis, can significantly impact the efficacy of antigen-targeted therapies (46). Thus, delving deep into the antigenic profile of HCC, through meticulous identification and functional analysis of TSAs and neoantigens, is imperative for refining immunotherapeutic approaches and enhancing treatment precision and effectiveness against liver cancer (47).
2.2 Specialized immune cell populations
The liver’s immune environment is intricately composed of various specialized cell types that play pivotal roles in maintaining immune homeostasis and regulating immune responses (48). In the context of liver cancer, particularly hepatocellular carcinoma (HCC), these cells contribute to a tolerogenic milieu that can impede effective immunotherapeutic interventions (49).
Cytotoxic T Cells (CTLs) are essential for the direct killing of cancer cells. In healthy immune responses, these cells recognize and destroy cells expressing specific antigens, including tumor cells. However, in HCC, the activity of CTLs is often suppressed due to the immunosuppressive signals within the liver (50). Factors such as the upregulation of PD-L1 on tumor cells and the secretion of immunosuppressive cytokines like IL-10 and TGF-beta inhibit CTL activation and proliferation (51). Moreover, the presence of regulatory elements such as Tregs and myeloid-derived suppressor cells (MDSCs) further dampens the CTL response, allowing tumor cells to evade immune detection (52).
Regulatory T Cells (Tregs) play a critical role in maintaining immune tolerance by suppressing autoimmunity and excessive immune responses that could damage host tissues. In liver cancer, Tregs are recruited and expanded within the tumor microenvironment, where they inhibit the function of CTLs and NK cells through the secretion of suppressive cytokines like TGF-beta and IL-10 (53). This suppression helps the tumor evade immune surveillance. The enrichment of Tregs in the liver is also facilitated by the liver’s exposure to antigens from the gut, which promotes a generally tolerogenic environment (54).
As the liver’s resident macrophages, Kupffer cells are involved in clearing pathogens and cellular debris. However, in HCC, their role shifts towards promoting tumor growth and survival. They achieve this by secreting pro-tumorigenic cytokines and growth factors that enhance tumor cell proliferation, angiogenesis, and metastasis (55). Kupffer cells also contribute to the immunosuppressive environment by producing IL-10 and TGF-beta, which inhibit the functions of dendritic cells and CTLs. Additionally, they engage in crosstalk with hepatic stellate cells and cancer-associated fibroblasts to remodel the extracellular matrix, further facilitating tumor progression (56).
Dendritic Cells (DCs) are crucial for antigen presentation and the activation of T cells. However, in the liver tumor microenvironment, the function of DCs is often compromised. They are either numerically decreased or functionally impaired, which hampers their ability to present tumor antigens effectively and initiate a robust anti-tumor immune response (57). The impaired functionality of DCs in HCC is partly due to the suppressive cytokines produced by other immune cells and the tumor cells themselves.
Natural Killer (NK) and NKT Cells are important for their roles in immune surveillance and the early response to tumor formation. These cells can recognize and kill transformed cells without the need for prior sensitization to specific antigens. In liver cancer, however, their cytotoxic activity is often inhibited by the immunosuppressive cytokines in the microenvironment and by direct interactions with tumor cells that express inhibitory molecules (58).
Each of these cell populations plays a significant role in the immunological landscape of liver cancer, contributing to the complexity and challenge of developing effective immunotherapeutic strategies. Understanding and manipulating the functions and interactions of these cells is key to enhancing the immune response against liver cancer (Figure 2).
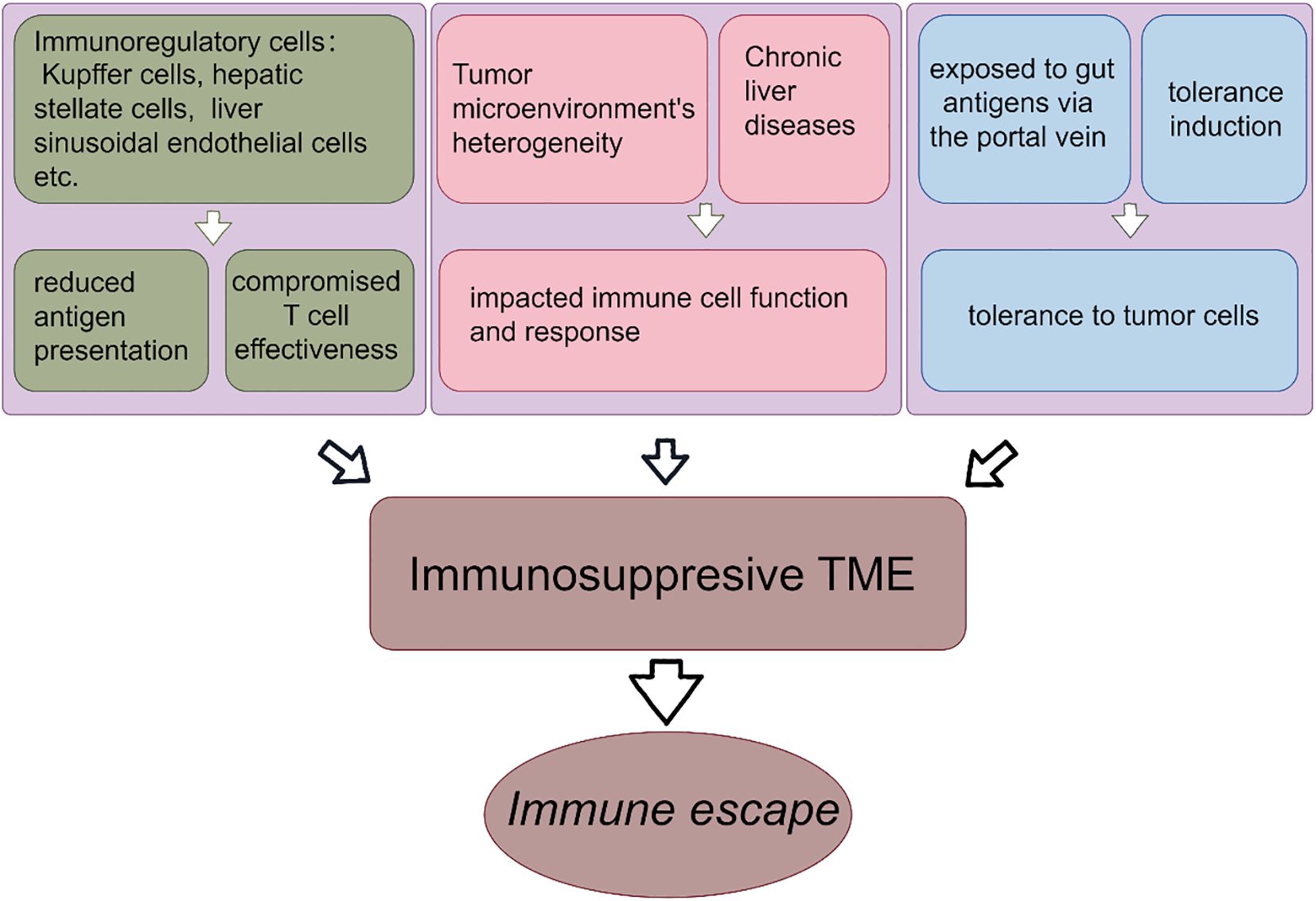
Figure 2. Formation of liver immunosuppressive tumor microenvironment. The formation of immunosuppressive microenvironment of liver cancer promotes the immune escape. The reduced antigen presentation by immunoregulatory cells leads to the impaired T cell effects. The heterogeneity of TME and chronic liver diseases lead to the suppression of immune function and immune response. Liver is exposed to intestinal antigens and obtains immune tolerance.
2.3 Key cytokines in immune suppression
Among the cytokines that play critical roles in the liver’s immune landscape, TGF-beta and IL-10 stand out due to their potent immunosuppressive effects. TGF-beta is a multifunctional cytokine that primarily facilitates an immunosuppressive environment conducive to tumor growth and metastasis in the context of liver cancer. It acts by inhibiting the proliferation and activation of T cells and by promoting the conversion of effector T cells into regulatory T cells, thus enhancing immune tolerance (59).
IL-10, another immunosuppressive cytokine, further contributes to the complexity of the immune landscape in liver cancer by inhibiting the synthesis of pro-inflammatory cytokines, thus reducing the effectiveness of the immune response against tumor cells. It also promotes the differentiation of Tregs and hampers the antigen-presenting capabilities of dendritic cells, reducing the overall immune surveillance in HCC (60).
2.4 Impact of liver microenvironment on immune surveillance
The liver’s unique immunological landscape, pivotal for metabolism and detoxification, significantly shapes liver cancer immunotherapy (61). Tasked with tolerance induction, the liver, constantly exposed to gut antigens via the portal vein, distinguishes between harmful and benign substances (62). This mechanism, however, may inadvertently extend tolerance to tumor cells, complicating immunotherapy (63).
Immunoregulatory cells such as the liver sinusoidal endothelial cells, the Kupffer cells, and the hepatic stellate cells, integral to the liver’s immune tolerance, can suppress the immune response against HCC cells (64). This suppression leads to reduced antigen presentation and compromises T cell effectiveness in targeting cancer cells (65). Additionally, the liver harbors unique immune cells like NK, NKT, and γδ T cells, each with specific roles in immune surveillance, offering avenues for immunotherapy (66).
The roles of specific immune cells such as macrophages, neutrophils, and regulatory T cells (Tregs) are critical in mediating immunotherapy resistance, particularly to immune checkpoint inhibitors (ICIs). Within the liver TME, macrophages contribute significantly to ICI resistance. Their interaction with tumor cells often results in the secretion of various chemokines and cytokines that not only protect the tumor from immune attack but also enhance the recruitment of other immunosuppressive cell types. This activity establishes a feedback loop that sustains and amplifies immune suppression, diminishing the therapeutic efficacy of ICIs (67). The role of neutrophils extends beyond traditional pathogen defense to influencing the balance of the immune response in the TME. They support a suppressive environment by interacting with other immune cells and modulating their activity towards tolerance rather than immunity. Their presence in the TME correlates with poorer outcomes in immunotherapy, suggesting their potential as therapeutic targets to enhance ICI response (68). Tregs directly impact the effectiveness of ICIs by maintaining a high threshold for T cell activation. They utilize various mechanisms to suppress effector T cell function, crucially dampening the immune response against the tumor. Manipulating Treg activity or selectively reducing their numbers within the TME could potentially restore immune activity and improve responses to immunotherapies (69).
The liver tumor microenvironment’s heterogeneity, influenced by individual patient factors, affects immunotherapy’s effectiveness (70). Chronic liver diseases, often precursors to liver cancer, alter the immune landscape, impacting immune cell function and response (71).
The challenge in liver cancer immunotherapy lies in effectively activating an anti-tumor immune response without disrupting the liver’s essential tolerance mechanisms (72). Understanding the intricate balance of liver immunity is crucial for designing effective immunotherapeutic strategies (73). This involves identifying targets within the liver’s immune milieu that can be modulated to enhance the immune response against HCC cells while preserving the liver’s vital functions (74).
In conclusion, the liver’s immunological microenvironment, with its unique cellular composition and tolerance-promoting mechanisms, presents both a challenge and an opportunity for the development of effective immunotherapies for liver cancer (75). Strategies that can navigate and modulate this complex environment hold the key to successful immunotherapeutic interventions in HCC (76).
3 Current immunotherapeutic approaches for liver cancer
3.1 Genetic and molecular landscape of liver cancer
Liver cancer, particularly HCC, is characterized by distinct genetic mutations that influence both tumor behavior and interaction with the immune system. Key mutations often involve genes like TP53, known for its role in cell cycle regulation and apoptosis, and CTNNB1, which affects the Wnt/beta-catenin signaling pathway (77). These genetic abnormalities are not only pivotal for cancer progression but also modulate the tumor microenvironment to favor immune evasion and resistance to therapy.
TP53, the most commonly mutated gene in human cancers, plays a crucial role in DNA repair, cell cycle regulation, and apoptosis. Mutations in TP53 are associated with poor prognosis in liver cancer and can lead to an accumulation of genomic instability, making tumors more aggressive and resistant to conventional therapies (78).
Mutations in CTNNB1, which encodes beta-catenin, are prevalent in liver cancer. These mutations lead to the activation of the Wnt/beta-catenin signaling pathway, promoting cell proliferation and survival. Importantly, beta-catenin activation is linked to immune evasion mechanisms, such as the suppression of cytokine production and inhibition of T cell infiltration into the tumor microenvironment (79).
The genetic makeup of HCC significantly influences the effectiveness of immunotherapeutic approaches. Tumors with extensive mutational burdens may present a higher number of neoantigens, potentially enhancing their visibility to the immune system. However, the same mutations often enhance the expression of immune checkpoints like PD-L1, contributing to an immunosuppressive tumor milieu (80).
3.2 Checkpoint inhibitors
The use of drugs targeting immune checkpoints like PD-L1, PD-1, and CTLA-4, has been a significant development in the treatment of liver cancer, particularly hepatocellular carcinoma (HCC) (81). These immune checkpoint inhibitors (ICIs) are designated to inhibit cancer cells’ mechanisms to evade the immune system (82).
Nivolumab was among the first PD-1 inhibitors being utilized in HCC (83). Clinical trials have shown it effective in patients with advanced HCC, particularly in those who had previous treatment with the standard treatment sorafenib (84). The response rates in these trials varied but showed promising results, with some patients experiencing significant tumor reduction and prolonged survival (85).
Pembrolizumab has also been tested in HCC patients, especially those who did not respond to first-line therapies like sorafenib (86). Clinical trials reported moderate response rates, with a certain subset of patients achieving durable responses (87). Unfortunately, the overall effectiveness and best patient selection criteria for pembrolizumab in HCC are still areas of active research (88).
Atezolizumab has been studied in combination with bevacizumab, an anti-angiogenic agent. This combination has shown enhanced effectiveness compared to atezolizumab alone or other standard therapies in HCC (89). This combination has shown a promising response rate and satisfying survival rate in patients, leading to changes in first-line treatment recommendations for some HCC patients (90).
Ipilimumab, a CTLA4 monoclonal antibody, often used in combination with nivolumab, has shown effectiveness in HCC, particularly in patients who failed to respond to previous treatments. The combination of nivolumab and ipilimumab appears to have significant synergistic effect, leading to higher response rates compared to either drug alone (91).
While the response rates for ICIs in HCC vary, a significant number of patients have shown partial or complete responses. These drugs have also been associated with improved overall survival rates in certain patient groups (92). Importantly, ICIs tend to have a lasting effect for those who do respond, leading to longer periods of disease control (93).
Various clinical trials are focused on optimizing the utilization of ICIs in liver cancer, including determining the best combinations of drugs, the ideal sequencing of therapies, and identifying biomarkers to predict the most likely group of patients to benefit from these treatments (94).
3.3 Adoptive cell therapy
CAR-T cell therapy, a form of immunotherapy that genetically engineers the patients’ T cells to express a Chimeric Antigen Receptor (CAR) to be more capable of recognizing and killing cancer cells, is becoming a potential candidate as a treatment option for liver cancer, including hepatocellular carcinoma (HCC) (95). The current application of CAR-T cell therapy in liver cancer is primarily in the research and clinical trial phases (96). Various studies are focused on identifying suitable targets specific to liver carcinoma cells and engineering CAR-T cells to recognize these targets (97). These targets might include, for instance, GPC3 (glypican-3), which is often overexpressed in HCC (98). While still in the early stages, initial results from clinical trials suggest potential for CAR-T cell therapy in treating liver cancer. The field of CAR-T cell therapy for liver cancer is rapidly evolving, and future findings from ongoing basic and clinical research and trials are expected to provide more insights to the effectiveness and practical application of this therapy in liver cancer treatment.
3.4 Vaccine-based therapies
Therapeutic vaccines for liver cancer are an emerging area of research, focusing on stimulating the immune system itself to generate potent inhibition of cancer. These vaccines differ from traditional vaccines; instead of preventing disease, they are designed to treat existing cancer (42, 99).
Oncolytic virus vaccines attracted great attention of researchers and clinicians. The development of these vaccines involves genetically modifying viruses that selectively infect the cancer cells and kill them (100). Once the virus infects the tumor cells, it triggers an immune response not only against the virus but also against the tumor cells. This dual action helps in directly destroying the cancer cells and also in priming the immune system to recognize the cancer cells and induce cell death in the tumor (101).
Peptide-based vaccines serve as another strategy to treat cancer. These vaccines use specific peptides (short chains of amino acids) that are found on the outer membrane of cancer cells. After injecting these peptides, the immune system is trained to recognize and kill cells displaying these peptides, which, in most cases, are typically tumor cells in HCC (102).
The primary function of therapeutic vaccines in liver cancer is to boost the immune system’s capacity to identify and destroy cancer cells. They work by either introducing specific antigens associated with liver cancer into the body or by modifying existing immune cells to be more effective against cancer cells (42, 103). The general idea is to trigger specifically targeted immune response that leads to the destruction of cancer cells while sparing normal tissue. These therapeutic vaccines stand for a promising area of research in the treatment of liver cancer, offering potential benefits such as targeted therapy with fewer side effects compared to traditional treatments. However, most of these vaccines are still in clinical trials, and more research is needed for us to better understand their efficacy and safety in treating liver cancer.
3.5 Clinical trials
Several significant clinical trials have been conducted focusing on novel immunotherapeutic approaches for liver cancer, particularly hepatocellular carcinoma (HCC). Here’s an overview of some key trials and their preliminary results:
CheckMate 040 and 459 evaluated the efficacy of nivolumab, a PD-1 inhibitor, in advanced HCC patients. CheckMate 040 reported encouraging results in terms of overall response rate (ORR) and survival benefits in HCC patients, including those previously treated with sorafenib. CheckMate 459 compared first-line use of nivolumab with sorafenib. Although it failed to meet the pre-set primary endpoint of improved overall survival, nivolumab demonstrated a favorable safety profile (104, 105).
KEYNOTE-224, -240, and -394 focused on pembrolizumab in HCC. KEYNOTE-224 trial demonstrated encouraging results for pembrolizumab in sorafenib-treated patients. KEYNOTE-240 and KEYNOTE-394 trials aimed to confirm these findings in a larger cohort. The results demonstrated a better overall survival (OS) and progression-free survival (PFS), although the statistical significance varied (106–108).
The IMbrave150 trial, a pivotal phase III study, demonstrated significant advancements in treating advanced hepatocellular carcinoma (HCC) by combining atezolizumab, an anti-PD-L1 antibody, with bevacizumab, an anti-VEGF antibody, showcasing superior overall survival and progression-free survival compared to the standard treatment with sorafenib. This combination leverages dual mechanisms to modulate the tumor microenvironment, enhancing immune cell infiltration and activity while simultaneously inhibiting angiogenesis crucial for tumor growth. Despite its effectiveness, challenges such as therapy resistance—mediated by alternative immune pathways or adaptive resistance mechanisms within the tumor—persist, highlighting the need for predictive biomarkers to identify likely responders and optimize treatment regimens. Future directions include exploring synergies with other therapies and tailoring approaches based on comprehensive molecular profiling to overcome immunotolerance and improve outcomes in liver cancer treatment (109, 110).
Various ongoing trials have been exploring CAR-T cell therapy targeting specific antigens in liver cancer, such as GPC3 (111, 112). These trials are still in early phases, and results are awaited to understand the efficacy and, importantly, safety of CAR-T cells in HCC.
Trials are ongoing for vaccines targeting tumor antigens in liver cancer, such as AFP. Early-phase trials have shown some promise, but more intensive exploration is needed to establish their foundation in HCC treatment (103).
In addition, recent clinical trial updates from prominent oncology conferences, such as ASCO and ESMO, showed that the field of immunotherapy in liver cancer is rapidly advancing. The EMERALD-1 trial, combining durvalumab with bevacizumab and TACE, has shown encouraging results, significantly elongating PFS compared to TACE monotherapy (113). This underscores the potential of combining ICIs with locoregional therapies to enhance therapeutic outcomes. Furthermore, the LEAP-002 study highlights the effectiveness of combining lenvatinib with pembrolizumab, marking a step forward in dual-therapy regimens (114). Additionally, the innovative approach of the REVERT LIVER CANCER Phase 2 trial, exploring, a STAT3 inhibitor, as monotherapy and in combination, opens new avenues in targeting the liver’s immunosuppressive environment (115, 116). These developments reflect a growing exploration of the liver’s immune tolerance mechanisms and the potential of tailored combination therapies to overcome these barriers, offering new hope for patients battling liver cancer.
The most recent 2024 ASCO annual meeting reported updates on important clinical trials, such as KEYNOTE-224 and EMERALD-1, showing encouraging survival data (117, 118). CAR-T cell therapies showed promising efficacy, especially in heavily treated advanced HCC cases (119). Additionally, the oncolytic virus VG161 reported substantial disease control rates in refractory HCC (120). These studies emphasize the ongoing shift towards precision medicine, leveraging advanced genomic profiling and novel therapeutic combinations to improve outcomes for HCC patients. There are currently more than 70 ongoing clinical trials regarding immunotherapy in liver cancer (Table 1).
4 Strategies to overcome the tolerogenic microenvironment
4.1 Combination therapies
The rationale for combining other therapies with immunotherapy in liver cancer treatment stems from several key factors. Firstly, immunotherapies alone might not be fully effective due to the liver’s immune-tolerant nature and the complex tumor microenvironment in conditions like hepatocellular carcinoma (HCC) (121). Combining these therapies can enhance overall efficacy and overcome the resistance that often develops against single-treatment modalities. Moreover, liver cancer involves various biological pathways, and a combination approach allows for a more comprehensive targeting of the disease. Such combinations can also produce synergistic effects; for instance, certain chemotherapy induces immunogenic cell death, potentially enhancing the immune system’s recognition and attack on tumor cells (122). Additionally, this strategy might allow for lower dosages of each treatment, potentially reducing side effects while maintaining or improving efficacy. Finally, certain therapies can modify the tumor’s immune microenvironment, which becomes more susceptible to an immune attack, thus supporting the effectiveness of immunotherapy. This multi-modal approach is central to current research in liver cancer, aiming to significantly improve patient outcomes.
Combining Immunotherapy with Chemotherapy leverages the direct tumor-killing effect of chemotherapy and the immune-modulating properties of immunotherapy. Chemotherapy can release cancer antigens, making tumor cells easier to recognize by the immune system, while immunotherapy can strengthen the immune response against these exposed antigens.
Another important strategy is to combine immunotherapy and targeted therapy. Targeted therapies work by acting on specific molecular targets related to cancer. When combined with immunotherapy, these therapies can disrupt cancer cell mechanisms that suppress the antitumor immunity, enhancing the function of immunotherapeutic agents. Overcoming resistance to immune checkpoint inhibitors (ICIs) is significantly enhanced by incorporating anti-angiogenic drugs, which target the vascular endothelial growth factor (VEGF) pathway (123). These drugs aid in normalizing the tumor’s abnormal vasculature, which not only improves blood flow and oxygenation within the tumor, thereby reducing hypoxia, but also facilitates the infiltration of effector T cells into the tumor microenvironment (124). This process enhances the immune system’s capacity to target and destroy tumor cells. Additionally, anti-angiogenic therapies help reduce the recruitment of immunosuppressive cells such as regulatory T cells (Tregs) and myeloid-derived suppressor cells (MDSCs) to the tumor site and alter immune-related signaling, including the modulation of PD-L1 expression on tumor and immune cells (125). The combination of the immune checkpoint inhibitor atezolizumab (PD-L1 inhibitor) with bevacizumab (VEGF inhibitor) has become the recommended first-line systemic treatment for advanced HCC (109). A case study reported the successful treatment of brain metastasis in intrahepatic cholangiocarcinoma with a combination of the PD-1 inhibitor camrelizumab and a multi-kinase inhibitor lenvatinib. The patient showed a complete response (CR) and a PFS of 17.5 months without serious side effects, suggesting the potential of this combination therapy (126).
Dual Immune Checkpoint Inhibition is another widely-used approach. Using two different immune checkpoint inhibitors can have a synergistic effect. This combination can enhance T-cell activation and more effectively attack cancer cells than single-agent therapy (127). In the HIMALAYA study, Tremelimumab and durvalumab show potential in treating unresectable, advanced liver cancer, offering a new choice for inflammation-driven cancer (128).
Techniques like radiofrequency ablation (RFA) or transarterial chemoembolization (TACE) can also be combined with immunotherapy (129, 130). These local treatments can increase antigen presentation and inflammation, potentially making immunotherapy more effective (131).
In addition, therapeutic cancer vaccines can be combined with immunotherapies to enhance the immune response specifically against liver cancer cells (132).
These combination therapies aim to capitalize on the strengths of each treatment modality, aiming for a more robust and targeted attack on liver cancer cells (12). Clinical trials are still being carried out to find out the most effective combinations and protocols (42).
Preclinical studies also gave sight to novel strategies to enhance the effect of immunotherapy. For instance, a recent study reported that antitumor immunity can be enhanced by targeting cGas/STING pathway (133). Targeting fibrinogen-like protein 1 can also enhance immunotherapy in hepatocellular carcinoma (134).
4.2 Personalized medicine by immune classification
Personalized approaches, including the development of biomarkers for the prediction of immunotherapy outcome, are increasingly important in liver cancer treatment, allowing for more targeted and effective treatments (135).
The immune microenvironment of liver cancer can be classified based on molecular features and immunogenicity into distinct types, reflecting the heterogeneity and complexity of tumor immune interactions (22). Based on the differentiated infiltration of the cytotoxic immune cells, primary liver cancers are categorized into inflamed tumors, which are immunologically active, and non-inflamed tumors, which are immunologically inactive (136). Recent studies further identified four immune subclasses of liver cancer according to their immunosuppression mechanisms and genomic alterations, namely, 1) Tumor-associated macrophage (TAM): This subclass shows increased levels of extracellular matrix genes, and is associated with poor survival (137). 2) CTNNB1: characterized by CTNNB1 mutations (138). 3) Cytolytic activity (CYT): Represents inflamed tumors with high cytolytic activity (139). 4) Regulatory T cell (Treg): Also represents inflamed tumors but with increased presence of Treg cells (140). The TAM and CTNNB1 subclasses are seen as non-inflamed, while the CYT and Treg subclasses represent inflamed tumors (141). Further classification based on immunogenomic features has led to the identification of three HCC subtypes based on immune characteristics: immunity high (referred as Immunity_H), medium (Immunity_M), and low (Immunity_L). This classification is effectively predictive of patient prognosis, with the Immunity_H subtype indicating a better survival rate due to higher immune and stromal scores (85, 89).
The classifications of the liver cancer immune microenvironment based on molecular features and immunogenicity enabled personalized therapeutic strategies (142). Understanding the specific immune subclass of a liver tumor allows for selecting patients more likely to respond to immunotherapies, as well as developing targeted therapies (143). For instance, patients with inflamed tumors might have a higher responding rate to Immunotherapies due to the presence of active immune cells in the tumor (144). Tumors in the TAM subclass might benefit from therapies targeting TAMs or the extracellular matrix to reverse immunosuppression and enhance immune activity against the tumor (145). Moreover, the identification of Immunity subclasses can serve as predictive biomarkers for patient prognosis. Patients with the Immunity_H subtype, characterized by higher stromal and immune scores, have a better survival rate, indicating that these patients might respond better to immunotherapies (137, 146). This information is crucial for clinical decision-making and modifying treatment approaches based on individual tumor features (99, 147). By identifying the specific mechanisms of immune resistance in different liver cancer subclasses, therapies can be tailored to counteract these mechanisms (148). For instance, if a tumor employs specific checkpoint pathways to evade immune surveillance, checkpoint inhibitors targeting those pathways can be used (149).
Strategies based on the immune classification enables a more precise and personalized approach to liver cancer treatment (150). By understanding the molecular and immunological landscape of individual tumors, treatments can be tailored to target specific pathways and immune cells involved in tumor progression, leading to more effective and less toxic treatment options (151).
5 Conclusion and future directions
Recent advances in liver cancer immunotherapy, particularly in HCC, have highlighted several key findings, including the efficacy of novel ICIs, the potential of combination therapies, and the importance of personalized approaches based on biomarkers (152). These developments suggest a future where liver cancer treatments are more tailored and effective (153). The focus is shifting toward understanding the liver’s unique immune environment and developing therapies to overcome its inherent challenges (Figure 3). The future outlook for liver cancer immunotherapy is promising, with ongoing research aimed at improving response rates and patient outcomes through more targeted, personalized treatment. Future research in immunotherapy for liver cancer should focus on combination therapies that merge different immunotherapeutic strategies or pair them with traditional treatments to overcome the immunosuppressive tumor microenvironment. Personalized immunotherapies based on genomic profiling, alongside the development of predictive biomarkers, could tailor treatments to individual patient profiles for improved efficacy. Targeting regulatory T cells, exploring new immunotherapeutic targets, and enhancing T cell responsiveness within the suppressive liver environment are promising directions. Studies should also address inherent or acquired resistance mechanisms to optimize therapeutic outcomes. Innovative clinical trial designs that incorporate dynamic endpoints and real-time biomarker analysis can expedite the advancement of effective treatments. An integrative approach combining genomic, proteomic, and clinical data might offer a comprehensive understanding of disease mechanisms and therapy interactions, paving the way for breakthroughs in liver cancer immunotherapy.
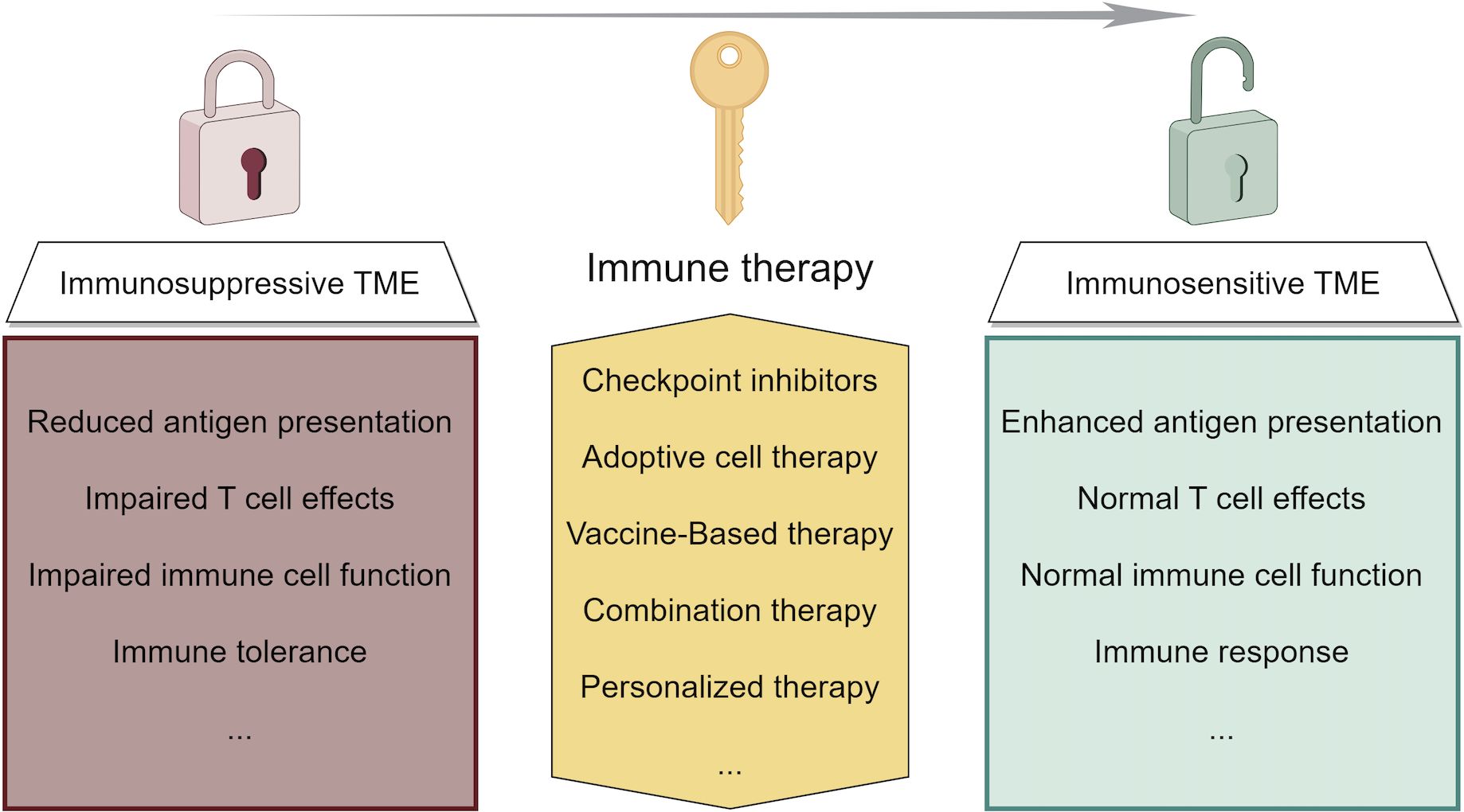
Figure 3. Strategies of immunotherapy in liver cancer and their function of modifying the tumor microenvironment.
Author contributions
YL: Investigation, Writing – original draft. HY: Data curation, Investigation, Writing – original draft. TL: Methodology, Supervision, Writing – review & editing. NZ: Conceptualization, Data curation, Formal analysis, Funding acquisition, Investigation, Methodology, Project administration, Resources, Software, Supervision, Validation, Visualization, Writing – review & editing.
Funding
The author(s) declare that no financial support was received for the research, authorship, and/or publication of this article.
Conflict of interest
The authors declare that the research was conducted in the absence of any commercial or financial relationships that could be construed as a potential conflict of interest.
Publisher’s note
All claims expressed in this article are solely those of the authors and do not necessarily represent those of their affiliated organizations, or those of the publisher, the editors and the reviewers. Any product that may be evaluated in this article, or claim that may be made by its manufacturer, is not guaranteed or endorsed by the publisher.
References
1. Tang Z, Dong H, Li T, Wang N, Wei X, Wu H, et al. The synergistic reducing drug resistance effect of cisplatin and ursolic acid on osteosarcoma through a multistep mechanism involving ferritinophagy. Oxid Med Cell Longev 2021. (2021), 5192271. doi: 10.1155/2021/5192271
2. Hu W, Yang Y, Fan C, Ma Z, Deng C, Li T, et al. Clinical and pathological significance of N-Myc downstream-regulated gene 2 (NDRG2) in diverse human cancers. Apoptosis. (2016) 21:675–82. doi: 10.1007/s10495-016-1244-3
3. Hu C, Cao Y, Li P, Tang X, Yang M, Gu S, et al. Oleanolic acid induces autophagy and apoptosis via the AMPK-mTOR signaling pathway in colon cancer. J Oncol. (2021) 2021:8281718. doi: 10.1155/2021/8281718
4. Siegel RL, Giaquinto AN, Jemal A. Cancer statistics, 2024. CA Cancer J Clin. (2024) 74:12–49. doi: 10.3322/caac.21820
5. Zongyi Y, Xiaowu L. Immunotherapy for hepatocellular carcinoma. Cancer Lett. (2020) 470:8–17. doi: 10.1016/j.canlet.2019.12.002
6. Wang J, Peng Y, Jing S, Han L, Li T, Luo J. A deep-learning approach for segmentation of liver tumors in magnetic resonance imaging using UNet+. BMC Cancer. (2023) 23:1060. doi: 10.1186/s12885-023-11432-x
7. Fu XT, Qie JB, Chen JF, Gao Z, Li XG, Feng SR, et al. Inhibition of SIRT1 relieves hepatocarcinogenesis via alleviating autophagy and inflammation. Int J Biol Macromol. (2024) 278(Pt 1):134120. doi: 10.1016/j.ijbiomac.2024.134120
8. Qu X, Zhang L, Li S, Li T, Zhao X, Wang N, et al. m(6)A-related angiogenic genes to construct prognostic signature, reveal immune and oxidative stress landscape, and screen drugs in hepatocellular carcinoma. Oxid Med Cell Longev. (2022) 2022:8301888. doi: 10.1155/2022/8301888
9. Luo J, Huang Z, Wang M, Li T, Huang J. Prognostic role of multiparameter MRI and radiomics in progression of advanced unresectable hepatocellular carcinoma following combined transcatheter arterial chemoembolization and lenvatinib therapy. BMC Gastroenterol. (2022) 22:108. doi: 10.1186/s12876-022-02129-9
10. Rashid T, Bennett JE, Muller DC, Cross AJ, Pearson-Stuttard J, Asaria P, et al. Mortality from leading cancers in districts of England from 2002 to 2019: a population-based, spatiotemporal study. Lancet Oncol. (2024) 25:86–98. doi: 10.1016/S1470-2045(23)00530-2
11. Maki H, Hasegawa K. Advances in the surgical treatment of liver cancer. Biosci Trends. (2022) 16:178–88. doi: 10.5582/bst.2022.01245
12. Llovet JM, Kelley RK, Villanueva A, Singal AG, Pikarsky E, Roayaie S, et al. Hepatocellular carcinoma. Nat Rev Dis Primers. (2021) 7:6. doi: 10.1038/s41572-020-00240-3
13. Li T, Qiao T. Unraveling tumor microenvironment of small-cell lung cancer: Implications for immunotherapy. Semin Cancer Biol. (2022) 86:117–25. doi: 10.1016/j.semcancer.2022.09.005
14. Guo W, Qiao T, Dong B, Li T, Liu Q, Xu X. The effect of hypoxia-induced exosomes on anti-tumor immunity and its implication for immunotherapy. Front Immunol. (2022) 13:915985. doi: 10.3389/fimmu.2022.915985
15. Zeng L, Liang L, Fang X, Xiang S, Dai C, Zheng T, et al. Glycolysis induces Th2 cell infiltration and significantly affects prognosis and immunotherapy response to lung adenocarcinoma. Funct Integr Genomics. (2023) 23:221. doi: 10.1007/s10142-023-01155-4
16. Guo W, Qiao T, Li T. The role of stem cells in small-cell lung cancer: evidence from chemoresistance to immunotherapy. Semin Cancer Biol. (2022) 87:160–9. doi: 10.1016/j.semcancer.2022.11.006
17. Oliveira G, Wu CJ. Dynamics and specificities of T cells in cancer immunotherapy. Nat Rev Cancer. (2023) 23:295–316. doi: 10.1038/s41568-023-00560-y
18. Yi M, Li T, Niu M, Mei Q, Zhao B, Chu Q, et al. Exploiting innate immunity for cancer immunotherapy. Mol Cancer. (2023) 22:187. doi: 10.1186/s12943-023-01885-w
19. Heymann F, Tacke F. Immunology in the liver–from homeostasis to disease. Nat Rev Gastroenterol Hepatol. (2016) 13:88–110. doi: 10.1038/nrgastro.2015.200
20. Gottwick C, Carambia A, Herkel J. Harnessing the liver to induce antigen-specific immune tolerance. Semin Immunopathol. (2022) 44:475–84. doi: 10.1007/s00281-022-00942-8
21. Zou Y, Ye F, Kong Y, Hu X, Deng X, Xie J, et al. The single-cell landscape of intratumoral heterogeneity and the immunosuppressive microenvironment in liver and brain metastases of breast cancer. Adv Sci (Weinh). (2023) 10:e2203699. doi: 10.1002/advs.202203699
22. Rizvi S, Gores GJ. Pathogenesis, diagnosis, and management of cholangiocarcinoma. Gastroenterology. (2013) 145:1215–29. doi: 10.1053/j.gastro.2013.10.013
23. Prieto J, Melero I, Sangro B. Immunological landscape and immunotherapy of hepatocellular carcinoma. Nat Rev Gastroenterol Hepatol. (2015) 12:681–700. doi: 10.1038/nrgastro.2015.173
24. Kim E, Viatour P. Hepatocellular carcinoma: old friends and new tricks. Exp Mol Med. (2020) 52:1898–907. doi: 10.1038/s12276-020-00527-1
25. Yahoo N, Dudek M, Knolle P, Heikenwälder M. Role of immune responses in the development of NAFLD-associated liver cancer and prospects for therapeutic modulation. J Hepatol. (2023) 79:538–51. doi: 10.1016/j.jhep.2023.02.033
26. Foerster F, Gairing SJ, Ilyas SI, Galle PR. Emerging immunotherapy for HCC: A guide for hepatologists. Hepatology. (2022) 75:1604–26. doi: 10.1002/hep.32447
27. Geh D, Leslie J, Rumney R, Reeves HL, Bird TG, Mann DA. Neutrophils as potential therapeutic targets in hepatocellular carcinoma. Nat Rev Gastroenterol Hepatol. (2022) 19:257–73. doi: 10.1038/s41575-021-00568-5
28. Giovannetti E, Li T. Extracellular vesicles in cancer: Shaping the intricate network of metabolic reprogramming and immune interactions in the tumor microenvironment. Cytokine Growth Factor Rev. (2023) 73:1–2. doi: 10.1016/j.cytogfr.2023.09.001
29. Zhang X, Yu S, Li X, Wen X, Liu S, Zu R, et al. Research progress on the interaction between oxidative stress and platelets: Another avenue for cancer? Pharmacol Res. (2023) 191:106777. doi: 10.1016/j.phrs.2023.106777
30. Bao G, Li T, Guan X, Yao Y, Liang J, Xiang Y, et al. Development of a prognostic alternative splicing signature associated with tumor microenvironment immune profiles in lung adenocarcinoma. Front Oncol. (2022) 12:880478. doi: 10.3389/fonc.2022.880478
31. Wu G, Ma Z, Cheng Y, Hu W, Deng C, Jiang S, et al. Targeting Gas6/TAM in cancer cells and tumor microenvironment. Mol Cancer. (2018) 17:20. doi: 10.1186/s12943-018-0769-1
32. Mellman I, Chen DS, Powles T, Turley SJ. The cancer-immunity cycle: Indication, genotype, and immunotype. Immunity. (2023) 56:2188–205. doi: 10.1016/j.immuni.2023.09.011
33. Bader JE, Voss K, Rathmell JC. Targeting metabolism to improve the tumor microenvironment for cancer immunotherapy. Mol Cell. (2020) 78:1019–33. doi: 10.1016/j.molcel.2020.05.034
34. Zhang W, He H, Zang M, Wu Q, Zhao H, Lu LL, et al. Genetic features of aflatoxin-associated hepatocellular carcinoma. Gastroenterology. (2017) 153:249–262.e2. doi: 10.1053/j.gastro.2017.03.024
35. Sharma A, Seow JJW, Dutertre CA, Pai R, Blériot C, Mishra A, et al. Onco-fetal reprogramming of endothelial cells drives immunosuppressive macrophages in hepatocellular carcinoma. Cell. (2020) 183:377–394.e21. doi: 10.1016/j.cell.2020.08.040
36. Pfister D, Núñez NG, Pinyol R, Govaere O, Pinter M, Szydlowska M, et al. NASH limits anti-tumour surveillance in immunotherapy-treated HCC. Nature. (2021) 592:450–6. doi: 10.1038/s41586-021-03362-0
37. Wu YQ, Zhang CS, Xiong J, Cai DQ, Wang CZ, Wang Y, et al. Low glucose metabolite 3-phosphoglycerate switches PHGDH from serine synthesis to p53 activation to control cell fate. Cell Res. (2023) 33:835–50. doi: 10.1038/s41422-023-00874-4
38. He J, Xiong X, Yang H, Li D, Liu X, Li S, et al. Defined tumor antigen-specific T cells potentiate personalized TCR-T cell therapy and prediction of immunotherapy response. Cell Res. (2022) 32:530–42. doi: 10.1038/s41422-022-00627-9
39. Pang BY, Leng Y, Wang X, Wang YQ, Jiang LH. A meta-analysis and of clinical values of 11 blood biomarkers, such as AFP, DCP, and GP73 for diagnosis of hepatocellular carcinoma. Ann Med. (2023) 55:42–61. doi: 10.1080/07853890.2022.2153163
40. Grosjean I, Roméo B, Domdom MA, Belaid A, D'Andréa G, Guillot N, et al. Autophagopathies: from autophagy gene polymorphisms to precision medicine for human diseases. Autophagy. (2022) 18:2519–36. doi: 10.1080/15548627.2022.2039994
41. Gao Q, Zhu H, Dong L, Shi W, Chen R, Song Z, et al. Integrated proteogenomic characterization of HBV-related hepatocellular carcinoma. Cell. (2019) 179:561–577.e22. doi: 10.1016/j.cell.2019.08.052
42. Llovet JM, Zucman-Rossi J, Pikarsky E, Sangro B, Schwartz M, Sherman M, et al. Hepatocellular carcinoma. Nat Rev Dis Primers. (2016) 2:16018. doi: 10.1038/nrdp.2016.18
43. Dong S, Guo X, Han F, He Z, Wang Y. Emerging role of natural products in cancer immunotherapy. Acta Pharm Sin B. (2022) 12:1163–85. doi: 10.1016/j.apsb.2021.08.020
44. Shiraki K, Yamanaka T, Inoue H, Kawakita T, Enokimura N, Okano H, et al. Expression of TNF-related apoptosis-inducing ligand in human hepatocellular carcinoma. Int J Oncol. (2005) 26:1273–81. doi: 10.3892/ijo
45. Cheng AL, Hsu C, Chan SL, Choo SP, Kudo M. Challenges of combination therapy with immune checkpoint inhibitors for hepatocellular carcinoma. J Hepatol. (2020) 72:307–19. doi: 10.1016/j.jhep.2019.09.025
46. Pinter M, Jain RK, Duda DG. The current landscape of immune checkpoint blockade in hepatocellular carcinoma: A review. JAMA Oncol. (2021) 7:113–23. doi: 10.1001/jamaoncol.2020.3381
47. Sun Y, Wu P, Zhang Z, Wang Z, Zhou K, Song M, et al. Integrated multi-omics profiling to dissect the spatiotemporal evolution of metastatic hepatocellular carcinoma. Cancer Cell. (2024) 42:135–156.e17. doi: 10.1016/j.ccell.2023.11.010
48. Ringelhan M, Pfister D, O'Connor T, Pikarsky E, Heikenwalder M. The immunology of hepatocellular carcinoma. Nat Immunol. (2018) 19:222–32. doi: 10.1038/s41590-018-0044-z
49. Oura K, Morishita A, Tani J, Masaki T. Tumor immune microenvironment and immunosuppressive therapy in hepatocellular carcinoma: A review. Int J Mol Sci. (2021) 22. doi: 10.3390/ijms22115801
50. Zheng C, Zheng L, Yoo JK, Guo H, Zhang Y, Guo X, et al. Landscape of infiltrating T cells in liver cancer revealed by single-cell sequencing. Cell. (2017) 169:1342–1356.e16. doi: 10.1016/j.cell.2017.05.035
51. Lu C, Rong D, Zhang B, Zheng W, Wang X, Chen Z, et al. Current perspectives on the immunosuppressive tumor microenvironment in hepatocellular carcinoma: challenges and opportunities. Mol Cancer. (2019) 18:130. doi: 10.1186/s12943-019-1047-6
52. Santagata S, Rea G, Castaldo D, Napolitano M, Capiluongo A, D'Alterio C, et al. Hepatocellular carcinoma (HCC) tumor microenvironment is more suppressive than colorectal cancer liver metastasis (CRLM) tumor microenvironment. Hepatol Int. (2024) 18:568–81. doi: 10.1007/s12072-023-10537-6
53. Lee JC, Mehdizadeh S, Smith J, Young A, Mufazalov IA, Mowery CT, et al. Regulatory T cell control of systemic immunity and immunotherapy response in liver metastasis. Sci Immunol. (2020) 5. doi: 10.1126/sciimmunol.aba0759
54. Riaz F, Wei P, Pan F. Fine-tuning of regulatory T cells is indispensable for the metabolic steatosis-related hepatocellular carcinoma: A review. Front Cell Dev Biol. (2022) 10:949603. doi: 10.3389/fcell.2022.949603
55. Liu W, Zhou X, Yao Q, Chen C, Zhang Q, Ding K, et al. In situ expansion and reprogramming of Kupffer cells elicit potent tumoricidal immunity against liver metastasis. J Clin Invest. (2023) 133. doi: 10.1172/JCI157937
56. Tacke F. Targeting hepatic macrophages to treat liver diseases. J Hepatol. (2017) 66:1300–12. doi: 10.1016/j.jhep.2017.02.026
57. Sun Y, Wu L, Zhong Y, Zhou K, Hou Y, Wang Z, et al. Single-cell landscape of the ecosystem in early-relapse hepatocellular carcinoma. Cell. (2021) 184:404–421.e16. doi: 10.1016/j.cell.2020.11.041
58. Zhang S, Saha B, Kodys K, Szabo G. IFN-γ production by human natural killer cells in response to HCV-infected hepatoma cells is dependent on accessory cells. J Hepatol. (2013) 59:442–9. doi: 10.1016/j.jhep.2013.04.022
59. Bissell DM. Chronic liver injury, TGF-beta, and cancer. Exp Mol Med. (2001) 33:179–90. doi: 10.1038/emm.2001.31
60. Shiri AM, Zhang T, Bedke T, Zazara DE, Zhao L, Lücke J, et al. IL-10 dampens antitumor immunity and promotes liver metastasis via PD-L1 induction. J Hepatol. (2024) 80:634–44. doi: 10.1016/j.jhep.2023.12.015
61. Cogliati B, Yashaswini CN, Wang S, Sia D, Friedman SL. Friend or foe? The elusive role of hepatic stellate cells in liver cancer. Nat Rev Gastroenterol Hepatol. (2023) 20:647–61. doi: 10.1038/s41575-023-00821-z
62. Vandierendonck A, Degroote H, Vanderborght B, Verhelst X, Geerts A, Devisscher L, et al. NOX1 inhibition attenuates the development of a pro-tumorigenic environment in experimental hepatocellular carcinoma. J Exp Clin Cancer Res. (2021) 40:40. doi: 10.1186/s13046-021-01837-6
63. Umemura A, Park EJ, Taniguchi K, Lee JH, Shalapour S, Valasek MA, et al. Liver damage, inflammation, and enhanced tumorigenesis after persistent mTORC1 inhibition. Cell Metab. (2014) 20:133–44. doi: 10.1016/j.cmet.2014.05.001
64. Ji G, Ma L, Yao H, Ma S, Si X, Wang Y, et al. Precise delivery of obeticholic acid via nanoapproach for triggering natural killer T cell-mediated liver cancer immunotherapy. Acta Pharm Sin B. (2020) 10:2171–82. doi: 10.1016/j.apsb.2020.09.004
65. Zhou C, Weng J, Liu C, Liu S, Hu Z, Xie X, et al. Disruption of SLFN11 deficiency-induced CCL2 signaling and macrophage M2 polarization potentiates anti-PD-1 therapy efficacy in hepatocellular carcinoma. Gastroenterology. (2023) 164:1261–78. doi: 10.1053/j.gastro.2023.02.005
66. Sun H, Sun C, Xiao W, Sun R. Tissue-resident lymphocytes: from adaptive to innate immunity. Cell Mol Immunol. (2019) 16:205–15. doi: 10.1038/s41423-018-0192-y
67. Casari M, Siegl D, Deppermann C, Schuppan D. Macrophages and platelets in liver fibrosis and hepatocellular carcinoma. Front Immunol. (2023) 14:1277808. doi: 10.3389/fimmu.2023.1277808
68. Scheiner B, Pinato DJ. Tumor-infiltrating neutrophils: gatekeepers in liver cancer immune control. Gastroenterology. (2023) 164:1338–9. doi: 10.1053/j.gastro.2023.01.025
69. Ji D, Song C, Li Y, Xia J, Wu Y, Jia J, et al. Combination of radiotherapy and suppression of Tregs enhances abscopal antitumor effect and inhibits metastasis in rectal cancer. J Immunother Cancer. (2020) 8. doi: 10.1136/jitc-2020-000826
70. Lee TK, Guan XY, Ma S. Cancer stem cells in hepatocellular carcinoma - from origin to clinical implications. Nat Rev Gastroenterol Hepatol. (2022) 19:26–44. doi: 10.1038/s41575-021-00508-3
71. Seitz HK, Bataller R, Cortez-Pinto H, Gao B, Gual A, Lackner C, et al. Alcoholic liver disease. Nat Rev Dis Primers. (2018) 4:16. doi: 10.1038/s41572-018-0014-7
72. Cai Z, Su X, Qiu L, Li Z, Li X, Dong X, et al. Personalized neoantigen vaccine prevents postoperative recurrence in hepatocellular carcinoma patients with vascular invasion. Mol Cancer. (2021) 20:164. doi: 10.1186/s12943-021-01467-8
73. Zhu Y, Yang J, Xu D, Gao XM, Zhang Z, Hsu JL, et al. Disruption of tumour-associated macrophage trafficking by the osteopontin-induced colony-stimulating factor-1 signalling sensitises hepatocellular carcinoma to anti-PD-L1 blockade. Gut. (2019) 68:1653–66. doi: 10.1136/gutjnl-2019-318419
74. Finkin S, Yuan D, Stein I, Taniguchi K, Weber A, Unger K, et al. Ectopic lymphoid structures function as microniches for tumor progenitor cells in hepatocellular carcinoma. Nat Immunol. (2015) 16:1235–44. doi: 10.1038/ni.3290
75. Craig AJ, von Felden J, Garcia-Lezana T, Sarcognato S, Villanueva A. Tumour evolution in hepatocellular carcinoma. Nat Rev Gastroenterol Hepatol. (2020) 17:139–52. doi: 10.1038/s41575-019-0229-4
76. Yang XM, Wang XQ, Hu LP, Feng MX, Zhou YQ, Li DX, et al. Nucleolar HEAT repeat containing 1 up-regulated by the mechanistic target of rapamycin complex 1 signaling promotes hepatocellular carcinoma growth by dominating ribosome biogenesis and proteome homeostasis. Gastroenterology. (2023) 165:629–46. doi: 10.1053/j.gastro.2023.05.029
77. Comprehensive and integrative genomic characterization of hepatocellular carcinoma. Cell. (2017) 169:1327–1341.e23. doi: 10.1016/j.cell.2017.05.046
78. Hussain SP, Schwank J, Staib F, Wang XW, Harris CC. TP53 mutations and hepatocellular carcinoma: insights into the etiology and pathogenesis of liver cancer. Oncogene. (2007) 26:2166–76. doi: 10.1038/sj.onc.1210279
79. Calderaro J, Ziol M, Paradis V, Zucman-Rossi J. Molecular and histological correlations in liver cancer. J Hepatol. (2019) 71:616–30. doi: 10.1016/j.jhep.2019.06.001
80. Losic B, Craig AJ, Villacorta-Martin C, Martins-Filho SN, Akers N, Chen X, et al. Intratumoral heterogeneity and clonal evolution in liver cancer. Nat Commun. (2020) 11:291. doi: 10.1038/s41467-019-14050-z
81. Greten TF, Villanueva A, Korangy F, Ruf B, Yarchoan M, Ma L, et al. Biomarkers for immunotherapy of hepatocellular carcinoma. Nat Rev Clin Oncol. (2023) 20:780–98. doi: 10.1038/s41571-023-00816-4
82. Dong LQ, Peng LH, Ma LJ, Liu DB, Zhang S, Luo SZ, et al. Heterogeneous immunogenomic features and distinct escape mechanisms in multifocal hepatocellular carcinoma. J Hepatol. (2020) 72:896–908. doi: 10.1016/j.jhep.2019.12.014
83. Kang YK, Chen LT, Ryu MH, Oh DY, Oh SC, Chung HC, et al. Nivolumab plus chemotherapy versus placebo plus chemotherapy in patients with HER2-negative, untreated, unresectable advanced or recurrent gastric or gastro-oesophageal junction cancer (ATTRACTION-4): a randomised, multicentre, double-blind, placebo-controlled, phase 3 trial. Lancet Oncol. (2022) 23:234–47. doi: 10.1016/S1470-2045(21)00692-6
84. Abdu S, Juaid N, Amin A, Moulay M, Miled N. Therapeutic effects of crocin alone or in combination with sorafenib against hepatocellular carcinoma: in vivo & In vitro insights. Antioxidants (Basel). (2022) 11. doi: 10.3390/antiox11091645
85. Llovet JM, Castet F, Heikenwalder M, Maini MK, Mazzaferro V, Pinato DJ, et al. Immunotherapies for hepatocellular carcinoma. Nat Rev Clin Oncol. (2022) 19:151–72. doi: 10.1038/s41571-021-00573-2
86. Janjigian YY, Kawazoe A, Bai Y, Xu J, Lonardi S, Metges JP, et al. Pembrolizumab plus trastuzumab and chemotherapy for HER2-positive gastric or gastro-oesophageal junction adenocarcinoma: interim analyses from the phase 3 KEYNOTE-811 randomised placebo-controlled trial. Lancet. (2023) 402:2197–208. doi: 10.1016/S0140-6736(23)02033-0
87. Llovet JM, De Baere T, Kulik L, Haber PK, Greten TF, Meyer T, et al. Locoregional therapies in the era of molecular and immune treatments for hepatocellular carcinoma. Nat Rev Gastroenterol Hepatol. (2021) 18:293–313. doi: 10.1038/s41575-020-00395-0
88. Sangro B, Sarobe P, Hervás-Stubbs S, Melero I. Advances in immunotherapy for hepatocellular carcinoma. Nat Rev Gastroenterol Hepatol. (2021) 18:525–43. doi: 10.1038/s41575-021-00438-0
89. Zhu AX, Abbas AR, de Galarreta MR, Guan Y, Lu S, Koeppen H, et al. Molecular correlates of clinical response and resistance to atezolizumab in combination with bevacizumab in advanced hepatocellular carcinoma. Nat Med. (2022) 28:1599–611. doi: 10.1038/s41591-022-01868-2
90. Hong TS, Wo JY, Yeap BY, Ben-Josef E, McDonnell EI, Blaszkowsky LS, et al. Multi-institutional phase II study of high-dose hypofractionated proton beam therapy in patients with localized, unresectable hepatocellular carcinoma and intrahepatic cholangiocarcinoma. J Clin Oncol. (2016) 34:460–8. doi: 10.1200/JCO.2015.64.2710
91. Gordan JD, Kennedy EB, Abou-Alfa GK, Beg MS, Brower ST, Gade TP, et al. Systemic therapy for advanced hepatocellular carcinoma: ASCO guideline. J Clin Oncol. (2020) 38:4317–45. doi: 10.1200/JCO.20.02672
92. El Zarif T, Nassar AH, Adib E, Fitzgerald BG, Huang J, Mouhieddine TH, et al. Safety and activity of immune checkpoint inhibitors in people living with HIV and cancer: A real-world report from the cancer therapy using checkpoint inhibitors in people living with HIV-international (CATCH-IT) consortium. J Clin Oncol. (2023) 41:3712–23. doi: 10.1200/JCO.22.02459
93. Meyers BM, Knox JJ, Liu DM, McLeod D, Ramjeesingh R, Tam VC, et al. The evolution of immune checkpoint inhibitor combinations in advanced hepatocellular carcinoma - A systematic review. Cancer Treat Rev. (2023) 118:102584. doi: 10.1016/j.ctrv.2023.102584
94. Dal Bo M, De Mattia E, Baboci L, Mezzalira S, Cecchin E, Assaraf YG, et al. New insights into the pharmacological, immunological, and CAR-T-cell approaches in the treatment of hepatocellular carcinoma. Drug Resist Update. (2020) 51:100702. doi: 10.1016/j.drup.2020.100702
95. Fu Q, Zheng Y, Fang W, Zhao Q, Zhao P, Liu L, et al. RUNX-3-expressing CAR T cells targeting glypican-3 in patients with heavily pretreated advanced hepatocellular carcinoma: a phase I trial. EClinicalMedicine. (2023) 63:102175. doi: 10.1016/j.eclinm.2023.102175
96. Rimassa L, Pressiani T, Merle P. Systemic treatment options in hepatocellular carcinoma. Liver Cancer. (2019) 8:427–46. doi: 10.1159/000499765
97. Sun L, Gao F, Gao Z, Ao L, Li N, Ma S, et al. Shed antigen-induced blocking effect on CAR-T cells targeting Glypican-3 in Hepatocellular Carcinoma. J Immunother Cancer. (2021) 9. doi: 10.1136/jitc-2020-001875
98. Yu M, Luo H, Fan M, Wu X, Shi B, Di S, et al. Development of GPC3-specific chimeric antigen receptor-engineered natural killer cells for the treatment of hepatocellular carcinoma. Mol Ther. (2018) 26:366–78. doi: 10.1016/j.ymthe.2017.12.012
99. Global prevalence, cascade of care, and prophylaxis coverage of hepatitis B in 2022: a modelling study. Lancet Gastroenterol Hepatol. (2023) 8:879–907. doi: 10.1016/S2468-1253(23)00197-8
100. Park R, Eshrat F, Al-Jumayli M, Saeed A, Saeed A. Immuno-oncotherapeutic approaches in advanced hepatocellular carcinoma. Vaccines (Basel). (2020) 8. doi: 10.3390/vaccines8030447
101. Ilyas FZ, Beane JD, Pawlik TM. The state of immunotherapy in hepatobiliary cancers. Cells. (2021) 10. doi: 10.3390/cells10082096
102. Bauer J, Köhler N, Maringer Y, Bucher P, Bilich T, Zwick M, et al. The oncogenic fusion protein DNAJB1-PRKACA can be specifically targeted by peptide-based immunotherapy in fibrolamellar hepatocellular carcinoma. Nat Commun. (2022) 13:6401. doi: 10.1038/s41467-022-33746-3
103. Zuo B, Zhang Y, Zhao K, Wu L, Qi H, Yang R, et al. Universal immunotherapeutic strategy for hepatocellular carcinoma with exosome vaccines that engage adaptive and innate immune responses. J Hematol Oncol. (2022) 15:46. doi: 10.1186/s13045-022-01266-8
104. Yau T, Park JW, Finn RS, Cheng AL, Mathurin P, Edeline J, et al. Nivolumab versus sorafenib in advanced hepatocellular carcinoma (CheckMate 459): a randomised, multicentre, open-label, phase 3 trial. Lancet Oncol. (2022) 23:77–90. doi: 10.1016/S1470-2045(21)00604-5
105. El-Khoueiry AB, Sangro B, Yau T, Crocenzi TS, Kudo M, Hsu C, et al. Nivolumab in patients with advanced hepatocellular carcinoma (CheckMate 040): an open-label, non-comparative, phase 1/2 dose escalation and expansion trial. Lancet. (2017) 389:2492–502. doi: 10.1016/S0140-6736(17)31046-2
106. Zhu AX, Finn RS, Edeline J, Cattan S, Ogasawara S, Palmer D, et al. Pembrolizumab in patients with advanced hepatocellular carcinoma previously treated with sorafenib (KEYNOTE-224): a non-randomised, open-label phase 2 trial. Lancet Oncol. (2018) 19:940–52. doi: 10.1016/S1470-2045(18)30351-6
107. Finn RS, Ryoo BY, Merle P, Kudo M, Bouattour M, Lim HY, et al. Pembrolizumab as second-line therapy in patients with advanced hepatocellular carcinoma in KEYNOTE-240: A randomized, double-blind, phase III trial. J Clin Oncol. (2020) 38:193–202. doi: 10.1200/JCO.19.01307
108. Merle P, Kudo M, Edeline J, Bouattour M, Cheng AL, Chan SL, et al. Pembrolizumab as second-line therapy for advanced hepatocellular carcinoma: longer term follow-up from the phase 3 KEYNOTE-240 trial. Liver Cancer. (2023) 12:309–20. doi: 10.1159/000529636
109. Finn RS, Qin S, Ikeda M, Galle PR, Ducreux M, Kim TY, et al. Atezolizumab plus bevacizumab in unresectable hepatocellular carcinoma. N Engl J Med. (2020) 382:1894–905. doi: 10.1056/NEJMoa1915745
110. Cheng AL, Qin S, Ikeda M, Galle PR, Ducreux M, Kim TY, et al. Updated efficacy and safety data from IMbrave150: Atezolizumab plus bevacizumab vs. sorafenib for unresectable hepatocellular carcinoma. J Hepatol. (2022) 76:862–73. doi: 10.1016/j.jhep.2021.11.030
111. Waldman AD, Fritz JM, Lenardo MJ. A guide to cancer immunotherapy: from T cell basic science to clinical practice. Nat Rev Immunol. (2020) 20:651–68. doi: 10.1038/s41577-020-0306-5
112. Shah NN, Fry TJ. Mechanisms of resistance to CAR T cell therapy. Nat Rev Clin Oncol. (2019) 16:372–85. doi: 10.1038/s41571-019-0184-6
113. Fishbane S, Schiller B, Locatelli F, Covic AC, Provenzano R, Wiecek A, et al. Peginesatide in patients with anemia undergoing hemodialysis. N Engl J Med. (2013) 368:307–19. doi: 10.1056/NEJMoa1203165
114. Llovet JM, Kudo M, Merle P, Meyer T, Qin S, Ikeda M, et al. Lenvatinib plus pembrolizumab versus lenvatinib plus placebo for advanced hepatocellular carcinoma (LEAP-002): a randomised, double-blind, phase 3 trial. Lancet Oncol. (2023) 24:1399–410. doi: 10.1016/S1470-2045(23)00469-2
115. Lan L, Evan T, Li H, Hussain A, Ruiz EJ, Zaw Thin M, et al. GREM1 is required to maintain cellular heterogeneity in pancreatic cancer. Nature. (2022) 607:163–8. doi: 10.1038/s41586-022-04888-7
116. Bharadwaj U, Kasembeli MM, Robinson P, Tweardy DJ. Targeting janus kinases and signal transducer and activator of transcription 3 to treat inflammation, fibrosis, and cancer: rationale, progress, and caution. Pharmacol Rev. (2020) 72:486–526. doi: 10.1124/pr.119.018440
117. Finn RS, Kudo M, Borbath I, Edeline J, Cattan S, Vlierberghe HV, et al. Pembrolizumab (pembro) in patients (pts) with sorafenib-treated (cohort 1) and treatment (tx)-naive (cohort 2) advanced hepatocellular carcinoma (aHCC) after additional follow-up in the phase 2 KEYNOTE-224 study. J Clin Oncol. (2024) 42:4100–0. doi: 10.1200/JCO.2024.42.16_suppl.4100
118. Chan SL, Sangro B, Kudo M, Erinjeri JP, Qin S, Ren Z, et al. Safety analysis by treatment periods from EMERALD-1: A phase 3, randomized, placebo-controlled study of transarterial chemoembolization with durvalumab with/without bevacizumab in participants with embolization-eligible unresectable hepatocellular carcinoma. J Clin Oncol. (2024) 42:4122–2. doi: 10.1200/JCO.2024.42.16_suppl.4122
119. Zhang Q, Fu Q, Cao W, Wang H, Xu X, Huang J, et al. Phase I study of C-CAR031, a GPC3-specific TGFβRIIDN armored autologous CAR-T, in patients with advanced hepatocellular carcinoma (HCC). J Clin Oncol. (2024) 42:4019–9. doi: 10.1200/JCO.2024.42.16_suppl.4019
120. Shen Y, Liang X, Jin X, Tan Q, Zhao R, Wei G, et al. Clinical outcomes from a phase I clinical trial of a novel oncolytic virus VG161 in patients with hepatocellular carcinoma (HCC) refractory after 2 prior lines of therapy including checkpoint inhibitors (CPI). J Clin Oncol. (2024) 42:4105–5. doi: 10.1200/JCO.2024.42.16_suppl.4105
121. Duan H, Liu Y, Gao Z, Huang W. Recent advances in drug delivery systems for targeting cancer stem cells. Acta Pharm Sin B. (2021) 11:55–70. doi: 10.1016/j.apsb.2020.09.016
122. Khemlina G, Ikeda S, Kurzrock R. The biology of Hepatocellular carcinoma: implications for genomic and immune therapies. Mol Cancer. (2017) 16:149. doi: 10.1186/s12943-017-0712-x
123. Lee WS, Yang H, Chon HJ, Kim C. Combination of anti-angiogenic therapy and immune checkpoint blockade normalizes vascular-immune crosstalk to potentiate cancer immunity. Exp Mol Med. (2020) 52:1475–85. doi: 10.1038/s12276-020-00500-y
124. Song Y, Fu Y, Xie Q, Zhu B, Wang J, Zhang B. Anti-angiogenic agents in combination with immune checkpoint inhibitors: A promising strategy for cancer treatment. Front Immunol. (2020) 11:1956. doi: 10.3389/fimmu.2020.01956
125. McDonald PC, Chafe SC, Dedhar S. Overcoming hypoxia-mediated tumor progression: combinatorial approaches targeting pH regulation, angiogenesis and immune dysfunction. Front Cell Dev Biol. (2016) 4:27. doi: 10.3389/fcell.2016.00027
126. Xie P, Guo L, Zhang B, Xu Y, Song Q, Shi H, et al. Case report: immunotherapy successfully treated brain metastasis in intrahepatic cholangiocarcinoma and literature review. Front Oncol. (2022) 12. doi: 10.3389/fonc.2022.911202
127. Rimassa L, Finn RS, Sangro B. Combination immunotherapy for hepatocellular carcinoma. J Hepatol. (2023) 79:506–15. doi: 10.1016/j.jhep.2023.03.003
128. de Castria TB, Khalil DN, Harding JJ, O'Reilly EM, Abou-Alfa GK. Tremelimumab and durvalumab in the treatment of unresectable, advanced hepatocellular carcinoma. Future Oncol. (2022) 18:3769–82. doi: 10.2217/fon-2022-0652
129. Takayama T, Hasegawa K, Izumi N, Kudo M, Shimada M, Yamanaka N, et al. Surgery versus radiofrequency ablation for small hepatocellular carcinoma: A randomized controlled trial (SURF trial). Liver Cancer. (2022) 11:209–18. doi: 10.1159/000521665
130. Tan J, Fan W, Liu T, Zhu B, Liu Y, Wang S, et al. TREM2(+) macrophages suppress CD8(+) T-cell infiltration after transarterial chemoembolisation in hepatocellular carcinoma. J Hepatol. (2023) 79:126–40. doi: 10.1016/j.jhep.2023.02.032
131. Chen S, Huang C, Liao G, Sun H, Xie Y, Liao C, et al. Distinct single-cell immune ecosystems distinguish true and de novo HBV-related hepatocellular carcinoma recurrences. Gut. (2023) 72:1196–210. doi: 10.1136/gutjnl-2022-328428
132. Llovet JM, Pinyol R, Yarchoan M, Singal AG, Marron TU, Schwartz M, et al. Adjuvant and neoadjuvant immunotherapies in hepatocellular carcinoma. Nat Rev Clin Oncol. (2024) 21:294–311. doi: 10.1038/s41571-024-00868-0
133. Lv H, Zong Q, Chen C, Lv G, Xiang W, Xing F, et al. TET2-mediated tumor cGAS triggers endothelial STING activation to regulate vasculature remodeling and anti-tumor immunity in liver cancer. Nat Commun. (2024) 15:6. doi: 10.1038/s41467-023-43743-9
134. Lin M, He J, Zhang X, Sun X, Dong W, Zhang R, et al. Targeting fibrinogen-like protein 1 enhances immunotherapy in hepatocellular carcinoma. J Clin Invest. (2023) 133. doi: 10.1172/JCI164528
135. Yang X, Yang C, Zhang S, Geng H, Zhu AX, Bernards R, et al. Precision treatment in advanced hepatocellular carcinoma. Cancer Cell. (2024) 42:180–97. doi: 10.1016/j.ccell.2024.01.007
136. Donne R, Lujambio A. The liver cancer immune microenvironment: Therapeutic implications for hepatocellular carcinoma. Hepatology. (2023) 77:1773–96. doi: 10.1002/hep.32740
137. Ruf B, Bruhns M, Babaei S, Kedei N, Ma L, Revsine M, et al. Tumor-associated macrophages trigger MAIT cell dysfunction at the HCC invasive margin. Cell. (2023) 186:3686–3705.e32. doi: 10.1016/j.cell.2023.07.026
138. Liang B, Zhou Y, Qian M, Xu M, Wang J, Zhang Y, et al. TBX3 functions as a tumor suppressor downstream of activated CTNNB1 mutants during hepatocarcinogenesis. J Hepatol. (2021) 75:120–31. doi: 10.1016/j.jhep.2021.01.044
139. Tang TWH, Chen HC, Chen CY, Yen CYT, Lin CJ, Prajnamitra RP, et al. Loss of gut microbiota alters immune system composition and cripples postinfarction cardiac repair. Circulation. (2019) 139:647–59. doi: 10.1161/CIRCULATIONAHA.118.035235
140. Wang H, Zhang H, Wang Y, Brown ZJ, Xia Y, Huang Z, et al. Regulatory T-cell and neutrophil extracellular trap interaction contributes to carcinogenesis in non-alcoholic steatohepatitis. J Hepatol. (2021) 75:1271–83. doi: 10.1016/j.jhep.2021.07.032
141. Tao J, Krutsenko Y, Moghe A, Singh S, Poddar M, Bell A, et al. Nuclear factor erythroid 2-related factor 2 and β-Catenin Coactivation in Hepatocellular Cancer: Biological and Therapeutic Implications. Hepatology. (2021) 74:741–59. doi: 10.1002/hep.31730
142. Jia W, Xie G, Jia W. Bile acid-microbiota crosstalk in gastrointestinal inflammation and carcinogenesis. Nat Rev Gastroenterol Hepatol. (2018) 15:111–28. doi: 10.1038/nrgastro.2017.119
143. Yang C, Zhang H, Zhang L, Zhu AX, Bernards R, Qin W, et al. Evolving therapeutic landscape of advanced hepatocellular carcinoma. Nat Rev Gastroenterol Hepatol. (2023) 20:203–22. doi: 10.1038/s41575-022-00704-9
144. Sia D, Jiao Y, Martinez-Quetglas I, Kuchuk O, Villacorta-Martin C, Castro de Moura M, et al. Identification of an immune-specific class of hepatocellular carcinoma, based on molecular features. Gastroenterology. (2017) 153:812–26. doi: 10.1053/j.gastro.2017.06.007
145. Cassetta L, Pollard JW. Targeting macrophages: therapeutic approaches in cancer. Nat Rev Drug Discov. (2018) 17:887–904. doi: 10.1038/nrd.2018.169
146. Feng Z, Li H, Liu Q, Duan J, Zhou W, Yu X, et al. CT radiomics to predict macrotrabecular-massive subtype and immune status in hepatocellular carcinoma. Radiology. (2023) 307:e221291. doi: 10.1148/radiol.221291
147. Calderaro J, Couchy G, Imbeaud S, Amaddeo G, Letouzé E, Blanc JF, et al. Histological subtypes of hepatocellular carcinoma are related to gene mutations and molecular tumour classification. J Hepatol. (2017) 67:727–38. doi: 10.1016/j.jhep.2017.05.014
148. Li H, Li CW, Li X, Ding Q, Guo L, Liu S, et al. MET inhibitors promote liver tumor evasion of the immune response by stabilizing PDL1. Gastroenterology. (2019) 156:1849–1861.e13. doi: 10.1053/j.gastro.2019.01.252
149. Rebouissou S, Nault JC. Advances in molecular classification and precision oncology in hepatocellular carcinoma. J Hepatol. (2020) 72:215–29. doi: 10.1016/j.jhep.2019.08.017
150. Choi WM, Yip TC, Kim WR, Yee LJ, Brooks-Rooney C, Curteis T, et al. Chronic hepatitis B baseline viral load and on-treatment liver cancer risk: A multinational cohort study of HBeAg-positive patients. Hepatology. (2024) 80(2):428–39. doi: 10.1097/HEP.0000000000000884
151. Ho DW, Tsui YM, Chan LK, Sze KM, Zhang X, Cheu JW, et al. Single-cell RNA sequencing shows the immunosuppressive landscape and tumor heterogeneity of HBV-associated hepatocellular carcinoma. Nat Commun. (2021) 12:3684. doi: 10.1038/s41467-021-24010-1
152. Kim SC, Kim DW, Cho EJ, Lee JY, Kim J, Kwon C, et al. A circulating cell-free DNA methylation signature for the detection of hepatocellular carcinoma. Mol Cancer. (2023) 22:164. doi: 10.1186/s12943-023-01872-1
Keywords: immunotherapy, liver cancer, cancer microenvironment, combinational therapy, therapeutic advances
Citation: Liu Y, Yang H, Li T and Zhang N (2024) Immunotherapy in liver cancer: overcoming the tolerogenic liver microenvironment. Front. Immunol. 15:1460282. doi: 10.3389/fimmu.2024.1460282
Received: 05 July 2024; Accepted: 21 August 2024;
Published: 03 September 2024.
Edited by:
Yue Wang, Second Military Medical University, ChinaReviewed by:
Gautam Sethi, National University of Singapore, SingaporeYongsheng Xiao, Fudan University, China
Copyright © 2024 Liu, Yang, Li and Zhang. This is an open-access article distributed under the terms of the Creative Commons Attribution License (CC BY). The use, distribution or reproduction in other forums is permitted, provided the original author(s) and the copyright owner(s) are credited and that the original publication in this journal is cited, in accordance with accepted academic practice. No use, distribution or reproduction is permitted which does not comply with these terms.
*Correspondence: Tian Li, fmmult@foxmail.com; Na Zhang, na.zhang.weifang@outlook.com