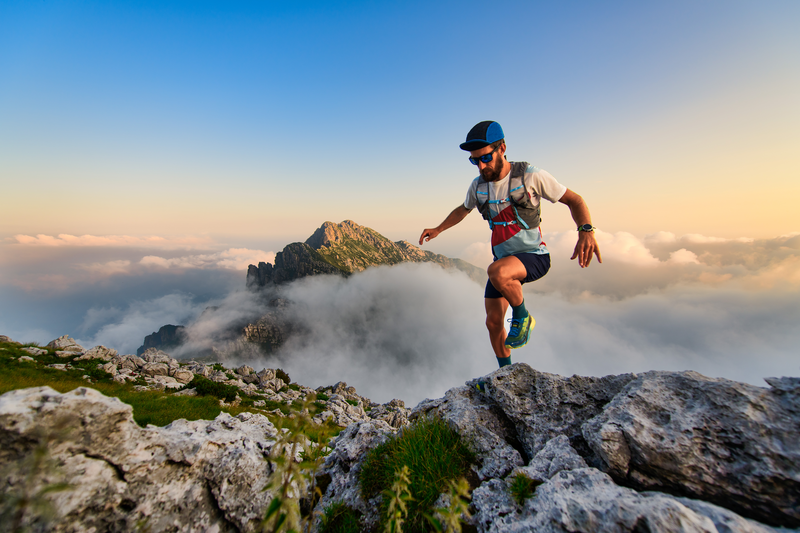
95% of researchers rate our articles as excellent or good
Learn more about the work of our research integrity team to safeguard the quality of each article we publish.
Find out more
ORIGINAL RESEARCH article
Front. Immunol. , 23 August 2024
Sec. Molecular Innate Immunity
Volume 15 - 2024 | https://doi.org/10.3389/fimmu.2024.1459213
Background: Lactiplantibacillus plantarum (L. plantarum) is known for its probiotic properties, including antioxidant and anti-inflammatory effects. Recent studies have highlighted the role of extracellular vesicles (EVs) from prokaryotic cells in anti-inflammatory effects.
Objective: This study aims to investigate the anti-inflammatory effects of extracellular vesicles derived from a newly isolated strain of L. plantarum (LP25 strain) and their role in macrophage polarization.
Methods: The LP25 strain and its extracellular vesicles were isolated and identified through genomic sequencing, transmission electron microscopy (TEM), and nanoparticle tracking analysis (NTA). RAW 264.7 cells were treated with lipopolysaccharide (LPS) and/or LP25-derived extracellular vesicles (LEV). Morphological changes in the cells were observed, and the expression levels of pro-inflammatory cytokines (TNF-α, IL-6)、iNOS and anti-inflammatory cytokines (IL-10) 、Arg-1 were measured using quantitative real-time PCR (qPCR). Flow cytometry was used to detect the expression of Arg-1 in the treated cells.
Results: Treatment with LP25 EVs led to significant morphological changes in RAW 264.7 cells exposed to LPS. LP25 EVs treatment resulted in increased expression of Arg-1 and anti-inflammatory cytokines IL-10, and decreased expression of iNOS and surface markers protein CD86. Flow cytometry confirmed the increased expression of the M2 macrophage marker Arg-1 in the LP25 EVs-treated group.
Conclusion: Extracellular vesicles from Lactiplantibacillus plantarum LP25 can suppress inflammatory responses and promote the polarization of macrophages toward the anti-inflammatory M2 phenotype. These findings provide new evidence supporting the anti-inflammatory activity of L. plantarum-derived EVs.
L. plantarum is a type of Gram-positive bacterium widely found in fermented foods, with a long history of safe consumption. Most strains of L. plantarum have been demonstrated to possess probiotic behaviors and functions, including antioxidant and anti-inflammatory activities (1, 2), as well as various physiological functions such as inhibiting intestinal pathogens, improving obesity, and regulating gut microbiota (1). Recent studies have shown that EVs from Gram-positive bacteria play a crucial role in health and disease (3), although Gram-positive bacteria were previously thought incapable of producing EVs due to their thick peptidoglycan cell wall.
Extracellular vesicles (EVs) are nanoscale vesicular structures secreted by both bacterial and eukaryotic cells, generated through the budding of cell membranes or organelle membranes (4). These vesicles possess a lipid bilayer membrane structure and encapsulate a variety of biomolecules including DNA, RNA, proteins, polysaccharides, lipids, various metabolites, and signaling molecules. The composition of EVs is complex, and their biological functions generally vary depending on the producing organism (5). Recently, EVs from L. plantarum APsulloc 331261, originally derived from green tea leaves, have been shown to trigger M2 macrophage polarization and induce an anti-inflammatory response in human skin (6). However, not all strains of L. plantarum exhibit anti-inflammatory effects and induce M2 macrophage polarization.
Macrophages are crucial host immune cells that play a central role in initiating and regulating inflammatory responses (7). Depending on local microenvironmental stimuli, macrophages can polarize into pro-inflammatory M1 and anti-inflammatory M2 phenotypes, which respectively trigger and suppress host inflammatory responses (8). M1 macrophages are primarily stimulated by interferon-γ (IFN-γ), lipopolysaccharides (LPS), and tumor necrosis factor-α (TNF-α). They secrete pro-inflammatory cytokines (TNF-α, IL-6, IL-1β), promote the production of monocyte chemoattractant protein-1 (MCP-1) and inducible nitric oxide synthase (iNOS), and express surface markers such as CD86. These molecules’ functions contribute to the development of inflammation, extracellular matrix degradation, cell apoptosis, and regulation of Th1-type immune responses (9, 10). Conversely, M2 macrophages are mainly stimulated by macrophage colony-stimulating factor (MCSF), IL-4, and IL-13. They secrete anti-inflammatory cytokines (IL-10), transforming growth factor-β (TGF-β), express arginase-1 (Arg-1), and promote the expression of the surface marker Arg-1. M2 macrophages inhibit T cell proliferation and activation, regulate Th2-type immune responses, and facilitate tissue remodeling (7). Notably, M1 and M2 macrophages can undergo reversible transformations under certain conditions (11). Therefore, effectively regulating monocyte/macrophage polarization is key to inhibiting inflammatory responses and promoting repair. Thus, L. plantarum strains that produce EVs promoting M2 macrophage polarization or reducing pro-inflammatory M1 expression are potential probiotics with anti-inflammatory effects.
In this study, we collected locally fermented dairy products, specifically milk tofu, from Inner Mongolia Autonomous Region, China. These samples were then transported to Jinzhou Medical University, Liaoning Province, through a cold chain. We screened and identified a strain of L. plantarum LP25 by analyzing the morphology of RAW 264.7 macrophages and the levels of the M2 macrophage-associated factor IL-10 induced by bacterial supernatants from 31 strains isolated from dairy products in Inner Mongolia, China. Subsequently, we isolated extracellular vesicles (EVs) from this strain and found that they can inhibit LPS-induced M1 polarization of RAW 264.7 macrophages and induce M2 polarization. Additionally, the effect of these EVs on macrophage M2 polarization was demonstrated through in vitro experiments.
Fermented dairy products were collected locally, specifically milk tofu, from Inner Mongolia Autonomous Region, China. These samples were then transported to Jinzhou Medical University in Liaoning Province via cold chain logistics. Using MRS agar medium (Qingdao Hope Bio-Technology Co., Ltd., China), which consists of 10 g/L proteose peptone, 8 g/L beef extract, 4 g/L yeast extract, 20 g/L glucose, 2 g/L dipotassium phosphate, 2 g/L diammonium hydrogen citrate, 5 g/L sodium acetate, 0.2 g/L magnesium sulfate, 0.04 g/L manganese sulfate, 14 g/L agar, and 1 g/L Tween 80, 31 strains of Lactobacillus plantarum were isolated. All strains were stored in 25% glycerol at -80°C. A bacterial suspension with a concentration of 109 CFU/mL was prepared and inoculated into 10 mL of MRS broth (Qingdao Hope Bio-Technology Co., Ltd., China), which has the same composition as the MRS agar medium but without the agar. The cultures were incubated at 37°C with shaking at 200 r/min for 72 hours. After incubation, the cultures were centrifuged at 4000 r/min at 4°C for 10 minutes. The supernatant was then filtered through a 0.22 µm sterile membrane filter, and the filtered supernatant was aliquoted and stored at -80°C.
BNCC299253, Lactiplantibacillus plantarum (Orla-Jensen) Bergey et al. (American Type Culture Collection), was cultured in same condition with the isolated strains.
RAW 264.7 murine macrophages were obtained from Meilun Biotechnology Co., Ltd. (Suzhou, China). The RAW 264.7 cells were cultured in high-glucose DMEM(Gibco)supplemented with 10% FBS (Zhejiang Tianhang Biotechnology Co., Ltd., China), 100 U/mL penicillin, and 100 μg/mL streptomycin (Caisson Labs, USA) at 37°C in a humidified incubator with 5% CO2. The cells were treated with supernatant (10μg/ml) of each of the 31 strains for 18 hours, cell morphology was observed using a Leica inverted microscope. Phase-contrast images were taken, and the long and short axes of each cell were measured using ImageJ software. The elongation factor was determined by the ratio of the two axes. Cell area was calculated by outlining the cell contours. To value the anti-inflammatroy effect of the LP25, the cells were treated with L. plantarum LP25 extracellular vesicles (LP25 EVs) (240 μg/ml) and LPS (1 μg/mL) (Escherichia coli serotype (O111:B4), Sigma-Aldrich) for 18 hours, with LPS-stimulated RAW 264.7 cells serving as the control.
Previous studies have shown that the production of EVs from L. plantarum NBRC 15891 varies with cultivation time, reaching the highest concentration at 72 hours (12). Accordingly, in this study, the supernatant of 72-hour cultures was used for LP25 EVs preparation. Following the polyethylene glycol (PEG) precipitation method (13), 109 CFU/mL of bacterial suspension was inoculated into 1 L of MRS broth. The culture was incubated at 37°C with shaking at 200 r/min for 72 hours. The culture was centrifuged at 500 g for 10 minutes at 4°C, and the supernatant was collected and centrifuged again at 2000 g for 30 minutes at 4°C. The supernatant was further centrifuged at 10,000 g for 30 minutes at 4°C and then filtered through a 0.22 μm vacuum filter. PEG-8000 (Sigma-Aldrich) was added to the supernatant to a final concentration of 5% and incubated overnight (at least 12 hours) at 4°C with shaking. The mixture was centrifuged at 10,000 g for 1 hour at 4°C, and the pellet was resuspended in sterile PBS and stored at -80°C. LP25 EVs was characterized using transmission electron microscopy (TEM) and nanoparticle tracking analysis (NTA) at Wuhan MaiSp Biotech Co., Ltd. The protein concentration of LP25 EVs was determined using a BCA Protein Assay Kit (Beyotime Biotechnology Co., Ltd., Shanghai, China.) according to the manufacturer’s instructions.
RAW264.7 cells were seeded in 24-well plates at a density of 2 × 105/well. The culture supernatant (10μg/ml) of each of the 31 strains was added for 18 hours, the supernatants were collected and the levels of IL-10 were determined by Mouse Interleukin 10 ELISA Kit (Beijing Chenglin Biotechnology Co., Ltd., China) following the manufacturers’ recommendations and quantified using ALLSHENG (Hangzhou, China) microplate reader at 450 nm immediately, and IL-10 concentrations were calculated based on a standard curve.
RNA was extracted using Trizol reagent (Vazyme). RNA concentration was measured with a UV spectrophotometer (HITACHI). Reverse transcription was performed using the ABScript Neo RT Master Mix kit (ABclonal). Quantitative polymerase chain reaction (qPCR) was carried out using Genious 2X SYBR Green Fast qPCR Mix (ABclonal) and analyzed with a qPCR instrument (Gentier 96, Tianlong). Primers used for qPCR are listed in Table 1. Gene expression was analyzed using the 2−ΔΔCt method.
RAW 264.7 macrophages were seeded in 6-well plates at a concentration of 2 × 10^6 cells/mL. After 24 hours, the medium was replaced with serum-free high-glucose DMEM, and the cells were treated with LPS (10 ng/mL) or LPS plus LP25 EVs for 18 hours. To obtain protein samples, cells were lysed in a lysis buffer consisting of NP40 (Solarbio, Beijing, China) and PMSF(100mM)(Phenylmethanesulfonyl fluoride) (Solarbio, Beijing, China)at a ratio of 100:1. Protein concentration was determined using a BCA Protein Assay Kit, and samples were denatured at 100°C for 10 minutes in 5× loading buffer. Proteins were separated by 15% SDS-PAGE and transferred onto PVDF membranes. The membranes were blocked with 5% non-fat milk at room temperature for 2 hours and incubated overnight at 4°C with primary antibodies against rabbit polyclonal antibody to Arg-1(1: 1000), IL-10(1: 1000), TNF-α(1: 500), CD86(1: 1000), and β-actin(1: 3000)(Affinity Biosciences, Cincinnati, USA). After washing with PBST, the membranes were incubated with anti-rabbit secondary antibodies (1: 8000)at room temperature for 2 hours and washed again with PBST. Protein bands were visualized using an enhanced chemiluminescence imaging system (CLiNX, Shanghai, China). Band intensities were quantified using ImageJ software.
Six-week-old male C57BL/6 mice (15 ± 2g) were obtained from Changsheng Biotechnology Co. (Liao Ning, China). The mice were allowed to live in controlled environmental conditions (ambient temperature, 23 ± 1°C; relative humidity, 50 ± 5%; 12-h light/dark cycle). The animals were freely allowed drinking water and a standard pelleted diet. All protocols for animal experiments were approved by the Animal Ethics Committee of Jinzhou Medical University (approval ID: 2022030601). Mice were maintained under standard laboratory conditions for one week prior to the experiments and then divided into two groups of 6–8 mice.
C57BL/6 mice received an intraperitoneal injection of 200 μl of LP25 EVs (5 mg/mL). After 8 hours, peritoneal cells were harvested by washing the peritoneal cavity with PBS. The collected cells were then centrifuged at 4°C, 1000 r/min for 3 minutes. Subsequently, the cells were treated with CD16/32 at 4°C for 30 minutes to block Fc receptors(2.4G2,BD Biosciences), followed by incubation with Apc-conjugated anti-mouse CD45.2(104,Biolegend), Apc-cy7-conjugated anti-mouse F4/80(BM8,Biolegend), and percp/cy5.5-conjugated anti-mouse CD11b antibodies(M1/70,Biolegend) or isotype-matched controls on ice for 15 minutes in the dark. Next, 300 μl of fixative (1X) was applied on ice for 30 minutes. This was followed by the addition of 700 μl of membrane breaking solution (1X), centrifugation at 4°C and 2000 r/min for 5 minutes. The cells were then incubated with FITC-conjugated anti-mouse Arg-1 antibody (IC5868F,R&D Systems) and PE-conjugated anti-mouse iNOS antibody (CXNFT, eBioscience) for 1 hour at room temperature. Finally, 1ml of membrane-breaking fluid was vortexed and centrifuged at 4°C, 2000 r/min for 5 minutes. Excess unbound antibodies were removed, and the labeled cells were analyzed using a FACSCanto™ II flow cytometer (BD Biosciences). Data on average fluorescence intensity were processed and quantified with FlowJo 10.8.1 software.
Results are presented as the mean ± SD from three independent experiments conducted in triplicate. Differences between conditions were evaluated using one-way ANOVA and two-tailed Student’s t-tests. A p-value of less than 0.05 was considered statistically significant. All calculations were performed using GraphPad Prism 10.0 software.
A total of 31 strains of lactic acid bacteria were isolated from fermented foods using selective MRS agar plates. Previous reports indicated that M2 macrophages exhibit an elongated shape compared to M1 macrophages (14). The morphology of RAW 264.7 macrophages treated with the supernatant from strain 25 was significantly more elongated compared to those treated with other strains (Figures 1A, B).To further evaluate the pro-inflammatory or anti-inflammatory effects of the strains, the concentration of IL-10 (an anti-inflammatory cytokine) in the supernatant was measured using ELISA (15). After 18 hours of treatment with the supernatant from strain 25,which is from Inner Mongolia Autonomous Region, China, the most significant changes in cell morphology and IL-10 concentration were observed (Figure 1C).
Figure 1. Screening of anti-inflammatory Lactobacillus plantarum strains. The cells were treated with supernatant (10μg/ml) of each of the 31 strains for 18 hours, cell morphology was observed using a Leica inverted microscope. (A) Images of RAW 264.7 macrophages treated with the supernatant of screened partial bacterial strains (scale bar = 100 μm); (B) Quantification of the area for each group; (C) ELISA detection of IL-10 concentration after 18 hours of treatment with the partial bacterial supernatant. Error bars indicate mean ± SD for three separate experiments. *p < 0.05, **p < 0.01, ***p < 0.001, ****p < 0.0001 compared with the NC, which indicated as untreated cells.
When cultured on MRS agar at 37°C overnight, the colonies of strain LP25 were circular, milky white, smooth, convex, with neat edges, and opaque. Gram staining showed that the strain was Gram-positive (Figures 2A, B). The bacterial suspension was examined under a transmission electron microscope at Wuhan MaiSp Biotech Co., Ltd., revealing short, broad rod-shaped cells without pili or flagella (Figures 2C, D).
Figure 2. Morphological observation of Lactobacillus plantarum LP25. (A) Growth morphology of LP25 on MRS agar; (B) Gram staining of LP25; (C, D) Transmission electron microscopy images of LP25 at 500 nm and 200 nm magnification.
The growth curve of strain LP25 showed that the bacteria entered the stationary phase at 12 hours (Supplementary Figure S1A). The strain can grow in a microaerophilic environment with 5.0% CO2 and also thrives under both anaerobic and aerobic conditions. Furthermore, it can grow in a pH range of 2.0 to 10.0, with optimal growth at pH 7.0 (Supplementary Figure S1B). Additionally, the strain can grow at temperatures ranging from 10 to 45°C, with optimal growth at 37°C (Supplementary Figure S1C). Moreover, it can grow in salt concentrations up to 5%, with optimal growth at 0% salt (Supplementary Figure S1D). Finally, the 16S rRNA gene tree and core genome tree of the strain indicate that it is a strain of L. plantarum (Supplementary Figures S1E, F).
To assess if the anti-inflammatory effects in the bacterial supernatant are linked to extracellular vesicles (EVs), we extracted EVs from the LP25 strain. The size of the extracellular vesicles (EVs) was determined using both Transmission Electron Microscopy (TEM) and Nanoparticle Tracking Analysis (NTA). NTA offered a comprehensive size distribution analysis, reporting a median particle size (X50) of 145.8 nm and a peak size around 144.6 nm (Figure 3A). TEM provided detailed images showing the most frequent particle size, approximately 150 nm (Figure 3B).
Figure 3. Identification of LP25 extracellular vesicles and expression levels of TNF-α and IL-10 at different time points or concentrations. 109 CFU/mL of bacterial suspension was inoculated into 1 L of MRS broth. The culture was incubated at 37tiowith shaking at 200 r/min for 72 hours, LP25 EVs were isolated from the supernatants by PEG precipitation method. (A) Nanoparticle tracking analysis (NTA) of LP25 extracellular vesicles; (B) Transmission electron microscopy (TEM) image of LP25 extracellular vesicles; (C, D) At 18 hours post-treatment, qPCR analysis was conducted on LPS-induced RAW 264.7 macrophages treated with LEV at concentrations of 240 ng/ml, 60 ng/ml, and 24 ng/ml to assess the expression levels of the relevant genes TNF-α and IL-10; (E) qPCR detection of M1 macrophage phenotype-related genes TNF-α expression after 6 hours,12 hours,18 hours and 24 hours of LPS(1μg/ml) treatment or LPS(1μg/ml)/LEV (240 ng/ml) treatment. Error bars represent mean ± SD from three independent experiments. ####p<0.0001 indicates a significant difference from PBS group; *p<0.05, **p<0.01, ***p<0.001, ****p<0.0001 indicate significant differences from the LPS-treated group.
Quantitative analysis of TNF-α and IL-10 expression at different LP25 EVs concentrations was performed using qPCR, as shown in Figures 3C, D. The concentration titration of LP25 EVs revealed a notable decrease in TNF-α expression at 240 ng/ml (Figure 3C), along with an increase in IL-10 expression (Figure 3D). Subsequent the results indicated a significant inhibition of pro-inflammatory cytokine expression by LP25 EVs after 18 hours of LPS induction (Figure 3E). Following this, a LP25 EVs concentration of 240 ng/ml was used for an 18-hour culture period to perform subsequent experiments.
Additionally, we performed apoptosis assays using a one-step TUNEL apoptosis detection kit (Biyuntian, China) to compare with Lactobacillus plantarum 299253. The results showed that LP25 EVs did not induce apoptosis in RAW 264.7 macrophages and simultaneously reduced LPS-induced apoptosis (Supplementary Figure S2).
To investigate whether LP25 EVs could modulate M1 polarization in LPS-stimulated RAW264.7 cells (1 μg/mL), we used quantitative PCR (qPCR) to assess mRNA expression of different macrophage phenotypes and Western blotting to evaluate protein expression. LP25 EVs treatment (240 μg/mL) for 18 hours inhibited the expression of M1 phenotype markers TNF-α, IL-6, and iNOS in LPS-stimulated RAW 264.7 cells (Figures 4A–C). As shown in Figures 4D, E, LP25 EVs from the standard L. plantarum strain BNCC 299253 were used as a control. The expression of M1 phenotype markers TNF-α and surface markers protein CD86 was reduced in LPS-stimulated RAW 264.7 cells treated with LP25 EVs, but the 299253 strain did not exhibit the same trend.
Figure 4. Expression of M1 macrophage markers in LPS-stimulated RAW 264.7 cells treated with LP25 EVs. (A–C) qPCR detection of M1 macrophage phenotype-related genes TNF-α、IL-6 and iNOS expression after 18 hours of LPS (1μg/ml) treatment or LPS (1μg/ml)/LP25 EVs (240 ng/ml) treatment; (D, E) Representative Western blot and average quantitative data from three independent experiments of TNF-α, CD86, and β-actin expression after 18 hours of LPS(1μg/ml) treatment or LPS/LP25 EVs treatment. Error bars represent mean ± SD from three independent experiments. ####p<0.0001 indicates a significant difference from NC,NC indicate LPS-untreated cells; *p < 0.05,**p < 0.01, ***p < 0.001, ****p < 0.0001 indicate significant differences from the LPS alone.
Currently, ultracentrifugation is regarded as the “gold standard” for exosome isolation (13). Therefore, we compared LP25 EVs obtained by PEG precipitation with those obtained by ultracentrifugation. LP25 EVs (240 μg/mL) were used to treat LPS-stimulated RAW 264.7 macrophages, and the expression of the M2 polarization marker Arg-1 protein was assessed. No significant differences between the LP25 EVs from two methods that induced RAW 264.7 macrophage polarization were observed (Supplementary Figures S3A, B).
To investigate whether LP25 EVs could regulate the polarization of LPS-stimulated RAW264.7 cells, we assessed the mRNA and protein expression of M2 phenotype macrophages using qPCR and Western blotting. As shown in Figure 5, LP25 EVs treatment for 18 hours increased the levels of M2 phenotype markers IL-10 and Arg-1 in LPS-stimulated RAW264.7 cells (Figures 5A, B). The protein expression of IL-10 and Arg-1 was also increased, consistent with the qPCR results (Figures 5C, D).
Figure 5. LP25 EVs modulates the expression of M2 macrophage markers. (A, B) qPCR detection of IL-10 and Arg-1 expression after 18 hours of LPS(1μg/ml)treatment or LPS(1μg/ml)/LP25 EVs(240ng/ml)treatment; (C, D) Representative Western blot and average quantitative data from three independent experiments of IL-10, Arg-1, and β-actin expression. Error bars represent mean ± SD from three independent experiments. #p<0.05, ####p<0.0001 indicates a significant difference from NC, NC indicate LPS-untreated cells; *p < 0.05,**p < 0.01, ***p < 0.001, ****p < 0.0001 indicate significant differences from the LPS alone.
Arg-1 serves as a marker for the M2 phenotype of macrophage (9). Flow cytometry results showed that the Arg-1 expression level was significantly upregulated in mice intraperitoneally injected with 1 mg/ml LP25 EVs compared to untreated group at 8 hours (Figures 6A–C).Simultaneous intraperitoneal injection of LP25 EVs with LPS (2.5 mg/kg) resulted in a downward trend of iNOS compared to the LPS group at 8 hours (Figures 6D, E). These data collectively indicate that LP25 EVs can modulate the polarization of RAW 264.7 cells stimulated by LPS, promoting the formation of the M2 phenotype and reducing the expression of the M1 marker iNOS.
Figure 6. Expression of iNOS and Arg-1 in mouse peritoneal macrophages after intraperitoneal injection of LPS with or without LP25 EVs treatment. C57BL/6 mice received an intraperitoneal injection of 200 μl of LP25 EVs (5 mg/mL). After 8 hours, peritoneal cells were harvested by washing the peritoneal cavity with PBS. The collected cells polarization level were detected by Flow Cytometry. (A) Density plot of F4/8-CD11b macrophages; (B, C) Representative flow cytometry histograms and average relative mean fluorescence intensity (MFI) of Arg-1 at 8 hours from two independent experiments; (D) Expression of iNOS in peritoneal lavage cells (d’-d’’’ represent NC, LPS, LPS+LP25 EVs groups respectively); (E) Percentage of iNOS at 8 hours from two independent experiments. Error bars represent mean ± SD from two independent experiments. ###p<0.001 indicates a significant difference from NC,NC indicate LPS-untreated cells, *p < 0.05, **p < 0.01 indicate significant differences from the LPS alone.
In this study, we screened various strains of L. plantarum for anti-inflammatory properties, focusing on changes in RAW 264.7 macrophage morphology and IL-10 expression after LPS stimulation. The LP25 strain was identified as having significant anti-inflammatory activity. Further in vitro analysis demonstrated that treatment with LPS and LP25-derived extracellular vesicles (LEVs) led to decreased expression of M1 macrophage markers (TNF-α, IL-6, iNOS, and CD86) and increased expression of M2 macrophage markers (Arg-1 and IL-10). These findings were corroborated by in vivo experiments, which showed higher Arg-1 expression in mice treated with LEVs compared to control groups. This study provides compelling evidence that LEVs can modulate macrophage polarization, promoting anti-inflammatory M2 phenotypes and reducing inflammation.
Macrophages play a critical role in innate immune surveillance, and their proper activation in response to environmental cues is crucial for tissue homeostasis (6). Macrophages exhibit pleiotropy and functional diversity, capable of altering their activation state (M1 or M2) in response to growth factors and external stimuli, such as cytokines, chemokines, microorganisms, and microbial products (16). Probiotics have been shown to modulate inflammation in a strain-specific manner (17). As a representative probiotic species, L. plantarum is commonly used in studies of anti-inflammatory activity (18). Different strains of L. plantarum have demonstrated significant anti-inflammatory and immunomodulatory effects. Research indicates that L. plantarum A106 enhances antioxidant and anti-inflammatory activities by improving mitochondrial function, significantly reducing TNF-α and IL-6 levels in LPS-induced RAW 264.7 macrophages, and regulating the expression of related genes (19). In a rat model of peripheral neuropathy, L. plantarum mitigates neuropathic pain by promoting macrophage M2 polarization, increasing short-chain fatty acid (SCFA) levels, and enhancing the release of anti-inflammatory cytokines (20). Another strain, L. plantarum MWFLp-182, improves D-galactose-induced cognitive impairment by modulating the gut-brain axis, increasing the expression of anti-inflammatory cytokines, and decreasing the expression of pro-inflammatory cytokines (21). Additionally, L. plantarum T10 enhances intestinal barrier function both in vitro and in vivo through its exopolysaccharide products, significantly alleviating DSS-induced colitis (22). Moreover, postbiotic fractions of L. plantarum 299v show remarkable immunomodulatory effects, effectively activating the anti-inflammatory cytokine IL-10 and modulating the IL-18-related response, thereby controlling inflammation and protecting the intestinal epithelial barrier (23). These studies suggest that different strains of Lactiplantibacillus plantarum have potential clinical applications in anti-inflammatory and immunomodulatory therapies. Recently, EVs derived from Gram-positive bacteria have gained increasing attention due to their impact on human health (24).This study reports that EVs derived from L. plantarum LP25 can reduce the expression of inflammatory cytokines and proteins in LPS-stimulated RAW 264.7 macrophages and induce the differentiation of these cells toward the M2 phenotype.
The anti-inflammatory activity of EVs is primarily observed in non-pathogenic species like probiotics. Choi et al. (25) found that EVs from Lactobacillus paracasei reduced the expression of LPS-induced cytokines (IL-1α, IL-1β, IL-2, and TNF-α). Rezende et al. (26) demonstrated that EVs from the Gram-positive probiotic Propionibacterium freudenreichii regulated NF-κB transcription factor activity and IL-8 release, alleviating inflammation both in vitro and in vivo. Additionally, lipoteichoic acid from Lactobacillus reuteri DSMZ 8533 inhibited induced inflammation in macrophage-like cells (27). Other studies have found that peptidoglycans (PGN) from L. plantarum strains CAU1054, CAU1055, CAU1064, and CAU1301 significantly inhibited LPS-induced inflammation in RAW 264.7 mouse macrophages by regulating iNOS, COX-2, IL-6, and TNF-α expression (28). These findings highlight the significant interactions between probiotics and the host immune system. The findings of this study have significant implications for the development of probiotic therapies. The ability of LEVs from LP25 to modulate macrophage polarization and reduce inflammation positions them as a promising candidate for anti-inflammatory probiotic treatments. Compared to conventional anti-inflammatory drugs, probiotics and their derivatives like LEVs offer a natural, potentially safer alternative with fewer side effects. Moreover, the targeted modulation of immune responses by LEVs could provide therapeutic benefits for a range of inflammatory conditions, including inflammatory bowel disease, arthritis, and skin inflammation.
The mechanism by which LEVs induce macrophage polarization remains unclear. Comprehensive analysis of macrophage-related cell markers, chemokines, and cytokine gene expression revealed that LEV-treated THP1 macrophages primarily differentiated into M2 macrophages, particularly the M2b subtype, which is known for its strong immunoregulatory and anti-inflammatory properties, thus modulating the breadth and depth of immune responses (29). Extracellular vesicles (EVs) from L. plantarum species, have shown potential in modulating immune responses through various mechanisms, including the regulation of endoplasmic reticulum (ER) stress pathways. Research indicates that L. paracasei-derived EVs can attenuate inflammation by augmenting the ER stress pathway, leading to a reduction in pro-inflammatory cytokines and an increase in anti-inflammatory cytokines like IL-10 (25). TRIM29, an E3 ubiquitin ligase, plays a critical role in regulating immune responses by modulating the activation of macrophages and the production of pro-inflammatory cytokines (30). Furthermore, PERK, a key protein in the ER stress response, is crucial for the metabolic reprogramming of M2 macrophages, promoting their immunosuppressive functions (31). TRIM29 has been shown to interact with PERK, promoting its stability and enhancing ER stress responses, which are essential for the polarization and function of M2 macrophages (32).The interplay between TRIM29 and PERK-mediated signaling pathways suggests that L. plantarum-derived EVs could potentially regulate TRIM29 expression, thereby influencing PERK activity and promoting M2 macrophage polarization. This mechanism would contribute to the anti-inflammatory effects observed with these EVs, making them a promising therapeutic approach for inflammatory diseases.
In conclusion, our study demonstrates that extracellular vesicles (EVs) from the LP25 strain of Lactiplantibacillus plantarum effectively modulate macrophage polarization, promoting an anti-inflammatory M2 phenotype and reducing inflammation. These findings highlight the significant potential of these EVs as anti-inflammatory agents in probiotic therapies. By enhancing our understanding of the underlying mechanisms, particularly involving TRIM29 and PERK-mediated ER stress signaling pathways, future research can pave the way for novel, natural treatments for various inflammatory diseases, including inflammatory bowel disease, arthritis, and skin inflammation.
The datasets presented in this study can be found in online repositories. The names of the repository/repositories and accession number(s) can be found in the article/Supplementary Material.
The animal study was approved by ethical committee of Jinzhou Medical University. The study was conducted in accordance with the local legislation and institutional requirements.
SG: Methodology, Software, Writing – original draft, Writing – review & editing, Data curation, Validation, Visualization. LL: Writing – review & editing, Conceptualization, Investigation, Supervision. RW: Writing – review & editing, Visualization. MX: Writing – review & editing, Conceptualization, Visualization. HD: Writing – review & editing, Validation, Visualization. ZW: Writing – review & editing, Validation, Visualization. RZ: Writing – review & editing, Investigation, Resources, Supervision. YZ: Writing – review & editing, Conceptualization, Formal analysis, Investigation, Supervision, Visualization, Writing – original draft.
The author(s) declare financial support was received for the research, authorship, and/or publication of this article. This work was supported by the National Natural Science Foundation of China (Approval Number: No.32171151); Department of Science and Technology of Liaoning Province (Approval Number: No.2019-MS-143); College Students’ Innovation and Training Program of Jinzhou Medical University (Approval Number: No. S202310160017).
We express our sincere gratitude to the Flow Cytometry Core Facility of the Department of Immunology for their assistance with flow cytometry analysis, to the Animal Experiment Center of our institution for their support with animal experiments, and to Wuhan MaiSp Biotech Co., Ltd. for their help with exosome analysis.
The authors declare that the research was conducted in the absence of any commercial or financial relationships that could be construed as a potential conflict of interest.
All claims expressed in this article are solely those of the authors and do not necessarily represent those of their affiliated organizations, or those of the publisher, the editors and the reviewers. Any product that may be evaluated in this article, or claim that may be made by its manufacturer, is not guaranteed or endorsed by the publisher.
The Supplementary Material for this article can be found online at: https://www.frontiersin.org/articles/10.3389/fimmu.2024.1459213/full#supplementary-material
1. Fidanza M, Panigrahi P, Kollmann TR. Lactiplantibacillus plantarum-nomad and ideal probiotic. Front Microbiol. (2021) 12:712236. doi: 10.3389/fmicb.2021.712236
2. Han KJ, Lee JE, Lee NK, Paik HD. Antioxidant and anti-inflammatory effect of probiotic lactobacillus plantarum KU15149 derived from Korean homemade diced-radish kimchi. J Microbiol Biotechnol. (2020) 30:591–8. doi: 10.4014/jmb.2002.02052
3. Hou Y, Liu Y, Liang S, Ru D, Mo S, Yan D, et al. The novel target: exosoms derived from M2 macrophage. Int Rev Immunol. (2021) 40:183–96. doi: 10.1080/08830185.2020.1800687
4. Pegtel DM, Gould SJ. Exosomes. Annu Rev Biochem. (2019) 88:487–514. doi: 10.1146/annurev-biochem-013118-111902
5. Bose S, Aggarwal S, Singh DV, Acharya N. Extracellular vesicles: An emerging platform in gram-positive bacteria. Microb Cell. (2020) 7:312–22. doi: 10.15698/mic
6. Kim W, Lee EJ, Bae IH, Myoung K, Kim ST, Park PJ, et al. Lactobacillus plantarum-derived extracellular vesicles induce anti-inflammatory M2 macrophage polarization. vitro. J Extracell Vesicles. (2020) 9:1793514. doi: 10.1080/20013078.2020.1793514
7. Zhang YH, He M, Wang Y, Liao AH. Modulators of the balance between M1 and M2 macrophages during pregnancy. Front Immunol. (2017) 8:120. doi: 10.3389/fimmu.2017.00120
8. Isidro RA, Appleyard CB. Colonic macrophage polarization in homeostasis, inflammation, and cancer. Am J Physiol Gastrointest Liver Physiol. (2016) 311:G59–73. doi: 10.1152/ajpgi.00123.2016
9. Fukui S, Iwamoto N, Takatani A, Igawa T, Shimizu T, Umeda M, et al. M1 and M2 monocytes in rheumatoid arthritis: A contribution of imbalance of M1/M2 monocytes to osteoclastogenesis. Front Immunol. (2018) 8:1958. doi: 10.3389/fimmu.2017.01958
10. Kong X, Gao J. Macrophage polarization: a key event in the secondary phase of acute spinal cord injury. J Cell Mol Med. (2017) 21:941–54. doi: 10.1111/jcmm.13034
11. Murray PJ. Macrophage polarization. Annu Rev Physiol. (2017) 79:541–66. doi: 10.1146/annurev-physiol-022516-034339
12. Yamasaki-Yashiki S, Kawashima F, Saika A, Hosomi R, Kunisawa J, Katakura Y. RNA-based anti-inflammatory effects of membrane vesicles derived from lactiplantibacillus plantarum. Foods. (2024) 13:967. doi: 10.3390/foods13060967
13. Rider MA, Hurwitz SN, Meckes DG Jr. ExtraPEG: A polyethylene glycol-based method for enrichment of extracellular vesicles. Sci Rep. (2016) 6:23978. doi: 10.1038/srep23978
14. McWhorter FY, Wang T, Nguyen P, Chung T, Liu WF. Modulation of macrophage phenotype by cell shape. Proc Natl Acad Sci USA. (2013) 110:17253–8. doi: 10.1073/pnas.1308887110
15. Lobo-Silva D, Carriche GM, Castro AG, Roque S, Saraiva M. Interferon-β regulates the production of IL-10 by toll-like receptor-activated microglia. Glia. (2017) 65:1439–51. doi: 10.1002/glia.23172
16. Anderson CF, Mosser DM. A novel phenotype for an activated macrophage: the type 2 activated macrophage. J Leukoc Biol. (2002) 72:101–6. doi: 10.1189/jlb.72.1.101
17. Foligne B, Nutten S, Grangette C, Dennin V, Goudercourt D, Poiret S, et al. Correlation between in vitro and in vivo immunomodulatory properties of lactic acid bacteria. World J Gastroenterol. (2007) 13:236–43. doi: 10.3748/wjg.v13.i2.236
18. van Baarlen P, Troost FJ, van Hemert S, van der Meer C, de Vos WM, de Groot PJ, et al. Differential NF-kappaB pathways induction by Lactobacillus plantarum in the duodenum of healthy humans correlating with immune tolerance. Proc Natl Acad Sci USA. (2009) 106:2371–6. doi: 10.1073/pnas.0809919106
19. Qin M, Xing Y, Sun M, Ma L, Li X, Ma F, et al. An Exploration of the Antioxidative and Anti-Inflammatory Role of Lactiplantibacillus plantarum 106 via Improving Mitochondrial Function. Foods. (2024) 13:1981. doi: 10.3390/foods13131981
20. Huang CT, Wang LK, Lue JH, Chen SH, Tsai YJ. Lactobacillus Plantarum intake mitigates neuropathic pain behavior via enhancing macrophage M2 polarization in a rat model of peripheral neuropathy. BioMed Pharmacother. (2024) 175:116769. doi: 10.1016/j.biopha.2024.116769
21. Nie H, Wang X, Luo Y, Kong F, Mu G, Wu X. Mechanism Explanation on Improved Cognitive Ability of D-Gal Inducing Aged Mice Model by Lactiplantibacillus plantarum MWFLp-182 via the Microbiota-Gut-Brain Axis. J Agric Food Chem. (2024) 72:9795–806. doi: 10.1021/acs.jafc.3c09675
22. Tao T, Zhang L, Yu T, Ma J, Lu S, Ren J, et al. Exopolysaccharide production by Lactobacillus plantarum T10 is responsible for the probiotic activity in enhancing intestinal barrier function in vitro and in vivo. Food Funct. (2024) 15:3583–99. doi: 10.1039/D4FO00526K
23. Magryś A, Pawlik M. Postbiotic Fractions of Probiotics Lactobacillus plantarum 299v and Lactobacillus rhamnosus GG Show Immune-Modulating Effects. Cells. (2023) 12:2538. doi: 10.3390/cells12212538
24. Liu Y, Defourny KAY, Smid EJ, Abee T. Gram-positive bacterial extracellular vesicles and their impact on health and disease. Front Microbiol. (2018) 9:1502. doi: 10.3389/fmicb.2018.01502
25. Choi JH, Moon CM, Shin TS, Kim EK, McDowell A, Jo MK, et al. Lactobacillus paracasei-derived extracellular vesicles attenuate the intestinal inflammatory response by augmenting the endoplasmic reticulum stress pathway. Exp Mol Med. (2020) 52:423–37. doi: 10.1038/s12276-019-0359-3
26. de Rezende Rodovalho V, da Luz BSR, Nicolas A, do Carmo FLR, Jardin J, Briard-Bion V, et al. Environmental conditions modulate the protein content and immunomodulatory activity of extracellular vesicles produced by the probiotic. Propionibacterium freudenreichii. Appl Environ Microbiol. (2021) 87:e02263–20. doi: 10.1128/AEM.02263-20
27. Lu Q, Guo Y, Yang G, Cui L, Wu Z, Zeng X, et al. Structure and anti-inflammation potential of lipoteichoic acids isolated from lactobacillus strains. Foods. (2022) 11:1610. doi: 10.3390/foods11111610
28. Choi SH, Lee SH, Kim MG, Lee HJ, Kim GB. Lactobacillus plantarum CAU1055 ameliorates inflammation in lipopolysaccharide-induced RAW264.7 cells and a dextran sulfate sodium-induced colitis animal model. J Dairy Sci. (2019) 102:6718–25. doi: 10.3168/jds.2018-16197
29. Wang LX, Zhang SX, Wu HJ, Rong XL, Guo J. M2b macrophage polarization and its roles in diseases. J Leukoc Biol. (2019) 106:345–58. doi: 10.1002/JLB.3RU1018-378RR
30. Xing J, Weng L, Yuan B, Wang Z, Jia L, Jin R, et al. Identification of a role for TRIM29 in the control of innate immunity in the respiratory tract. Nat Immunol. (2016) 17:1373–80. doi: 10.1038/ni.3580
31. Raines LN, Zhao H, Wang Y, Chen HY, Gallart-Ayala H, Hsueh PC, et al. PERK is a critical metabolic hub for immunosuppressive function in macrophages. Nat Immunol. (2022) 23:431–45. doi: 10.1038/s41590-022-01145-x
Keywords: Lactiplantibacillus plantarum, extracellular vesicles, lipopolysaccharide, macrophages, anti-inflammatory
Citation: Gong S, Zeng R, Liu L, Wang R, Xue M, Dong H, Wu Z and Zhang Y (2024) Extracellular vesicles from a novel Lactiplantibacillus plantarum strain suppress inflammation and promote M2 macrophage polarization. Front. Immunol. 15:1459213. doi: 10.3389/fimmu.2024.1459213
Received: 03 July 2024; Accepted: 02 August 2024;
Published: 23 August 2024.
Edited by:
Junji Xing, Houston Methodist Research Institute, United StatesReviewed by:
Yaling Dou, Texas A&M Health Science Center, United StatesCopyright © 2024 Gong, Zeng, Liu, Wang, Xue, Dong, Wu and Zhang. This is an open-access article distributed under the terms of the Creative Commons Attribution License (CC BY). The use, distribution or reproduction in other forums is permitted, provided the original author(s) and the copyright owner(s) are credited and that the original publication in this journal is cited, in accordance with accepted academic practice. No use, distribution or reproduction is permitted which does not comply with these terms.
*Correspondence: Yibo Zhang, emhhbmd5YkBqem11LmVkdS5jbg==
Disclaimer: All claims expressed in this article are solely those of the authors and do not necessarily represent those of their affiliated organizations, or those of the publisher, the editors and the reviewers. Any product that may be evaluated in this article or claim that may be made by its manufacturer is not guaranteed or endorsed by the publisher.
Research integrity at Frontiers
Learn more about the work of our research integrity team to safeguard the quality of each article we publish.