- 1School of Medical and Life Sciences, Chengdu University of Traditional Chinese Medicine, Chengdu, Sichuan, China
- 2Translational Chinese Medicine Key Laboratory of Sichuan Province, Sichuan Academy of Chinese Medicine Sciences, Sichuan Institute for Translational Chinese Medicine, Chengdu, Sichuan, China
The dependence of tumor cells on glycolysis provides essential energy and raw materials for their survival and growth. Recent research findings have indicated that long chain non-coding RNAs (LncRNAs) have a key regulatory function in the tumor glycolytic pathway and offer new opportunities for cancer therapy. LncRNAs are analogous to a regulatory key during glycolysis. In this paper, we review the mechanisms of LncRNA in the tumor glycolytic pathway and their potential therapeutic strategies, including current alterations in cancer-related energy metabolism with lncRNA mediating the expression of key enzymes, lactate production and transport, and the mechanism of interaction with transcription factors, miRNAs, and other molecules. Studies targeting LncRNA-regulated tumor glycolytic pathways also offer the possibility of developing new therapeutic strategies. By regulating LncRNA expression, the metabolic pathways of tumor cells can be interfered with to inhibit tumor growth and metastasis, thus affecting the immune and drug resistance mechanisms of tumor cells. In addition, lncRNAs have the capacity to function as molecular markers and target therapies, thereby contributing novel strategies and approaches to the field of personalized cancer therapy and prognosis evaluation. In conclusion, LncRNA, as key molecules regulating the tumor glycolysis pathway, reveals a new mechanism of abnormal metabolism in cancer cells. Future research will more thoroughly investigate the specific mechanisms of LncRNA glycolysis regulation and develop corresponding therapeutic strategies, thereby fostering new optimism for the realization of precision medicine.
Introduction
Cancer is the most prevalent form of malignant tumor, resulting from genetic mutations that induce uncontrolled cell growth and division (1–3). Metabolic reprogramming is the distinctive biochemical signature shown by cancer cells, one of the “cancer hallmarks” (4, 5), which allows cancer cells to adapt to the microenvironment through plasticity and high flexibility in nutrient acquisition and utilization, particularly metabolic changes associated with key steps in cancer progression, such as proliferation, the initiation of metastasis, circulation, and colonization (6). This metabolic phenotype is typified by a preference for using glycolysis as a source of non-aerobic energy (7). This peculiar metabolic occurrence is frequently called the “Warburg effect” (8, 9). LncRNAs actively regulate the cancer epigenome and are significant participants in the regulation of the Warburg effect (10). The observation that a significant proportion of lncRNAs are specifically expressed in tumors and can regulate a diverse array of tumor biological processes has led to lncRNAs showing great promise in tumor therapy, especially lncRNA-mediated glucose metabolism signaling pathways and transcription factors, highlighting important recent insights into the mechanisms regulating cancer metabolism (11–13). Notwithstanding these recent advancements, there exists an insufficient comprehension of cancer cell metabolism, with a particular dearth of research in the domain of LncRNA-mediated regulation of cancer-associated metabolic reprogramming pertaining to glycolysis.
Long non-coding RNAs (lncRNAs) comprise a class of RNA molecules distinguished by their length in excess of 200 nucleotides and lack the ability to encode proteins. They may participate in a vast array of physiopathological processes associated with tumors. Their mechanisms of action include: i) lncRNAs control cellular processes by competitively adsorbing, as well as down-regulating miRNAs via base complementation. ii) lncRNAs attach to epigenetically associated proteins and modify their post-transcriptional translation. iii)lncRNAs can influence target gene expression through chromatin modifiers and transcription factors, among other regulatory proteins. iv) lncRNAs are capable of interacting with and modulating the activity of proteases. V) lncRNAs are capable of regulating cellular processes. VI) Triplets of lncRNAs can influence the transcription of certain genes when they bind to genomic DNA (14–17).
Recently, a genome-wide analysis of the human cancer transcriptome revealed that lncRNA expression is among the most prevalent transcriptional changes observed in cancer (18, 19). Consolidating data also suggest that chemoresistance is largely determined by the amount of ATP inside cells, and that lncRNAs can function as efficacious therapeutic targets for an array of malignancies (20). In this paper, we review the mechanisms of LncRNA in the tumor glycolytic pathway and their potential therapeutic strategies, including current alterations in cancer-related energy metabolism with lncRNA mediating the expression of key enzymes, lactate production and transport, and the mechanism of interaction with transcription factors, miRNAs, and other molecules. By regulating LncRNA expression, the metabolic pathways of tumor cells can be interfered with to inhibit tumor growth and metastasis, thus affecting the immune and drug resistance mechanisms of tumor cells. Furthermore, we discuss the clinical importance of these lncRNAs, including potential application scenarios as therapeutic biomarkers (21) (Figure 1).
Mechanisms governing the function of glycolysis-associated lncRNAs in cancer cells
Glucose metabolism, which includes processes such as oxidative phosphorylation, glucose uptake, and lactate production, serves as a crucial energy supply for cancer cells. Cancer cells alter glucose metabolism to encourage the development, survival, and medication resistance of tumor cells. LncRNAs exhibit aberrant expression across a broad spectrum of cancer cell types and are essential for a number of cancer’s hallmark characteristics (22, 23). LncRNAs play regulatory roles by means of interaction with proteins, mRNAs, ncRNAs, and DNA, serving as numerous functional molecules in numerous cellular processes, linked to cancer, such as signals, decoys, scaffolds, and guides (24). Here, we summarize how lncRNAs regulate glucose metabolism by modulating relevant signaling pathways, as well as mediating the activities of metabolism-associated transcription factors and enzymes, with an emphasis on understanding the mechanisms that govern the metabolism of malignancy.
Glycolysis-associated lncRNAs regulate cancer energy metabolism involved in signaling pathways
Alterations in metabolism-related proteins are correlated with modifications in metabolic pathways, which ultimately become carcinogenic signaling pathways or are triggered by oncogenes lncRNAs that can indirectly regulate metabolic pathways through post-translational modifications. Among the results noted, functional modifications in the adenosine monophosphate-activated protein kinase (AMPK) pathway, the metastasis-associated lung adenocarcinoma (LA) transcript 1 (MALAT1) pathway, and the Hippo/YAP pathway are particularly noticeable.
AMPK
As a significant energy sensor, AMPK is indispensable for regulating biological energy metabolism. AMPK activation can enhance glucose metabolism and antioxidant synthesis to control metabolic reprogramming and redox balance in response to metabolic stress. Recent research indicates that numerous signaling mechanisms regulate AMPK phosphorylation epigenetically, including LA-associated MACC1-AS1, mesenchymal stem cell-induced lncRNA HCP5, and UNC5B antisense lncRNA 1 (UNC5B-AS1). A widely known oncogene, MACC1, is the transcriptional regulator from which MACC1-AS1 is an antisense lncRNA transcript. Another study found that MACC1-AS1 overexpression promoted hexokinase 2 (HK2) and lactate dehydrogenase A (LDHA) activities, decreased reactive oxygen species (ROS) levels, and a nicotinamide adenine dinucleotide/nicotinamide adenine dinucleotide phosphate (NADP+/NAPDH) ratio, the upregulation of MACC1-AS1 was observed to enhance the expression of MACC1 in glioma cells through the activation of the AMPK pathway, hence exerting influence on glucose metabolism and redox balance, exerting a tumor-promoting effect (25). Furthermore, MSC-induced lncRNA HCP5 stimulates fatty acid oxidation through the miR-3619-5p/AMPK/PGC1α/CEBPB axis, promoting stemness and chemoresistance in gastric cancer (26). Suppressing UNC5B-AS1 can reduce the development and spread of colon cancer by upregulating the expression of miR-622 and blocking the AMPK and PI3K/AKT pathways (27). AMPK is a crucial regulator of glucose metabolism in tumor cells; it can reduce the energy supply of tumor cells by inhibiting the glycolytic pathway and transporter protein expression, while decreasing antioxidant capacity, thereby diminishing metabolic plasticity.
MALAT1
The lncRNA known as MALAT1 has been well recognized as a pivotal contributor to the progression of cancer. The lncRNA MALAT1 also engages in competitive interactions using natural microRNAs to adjust the downstream gene expression levels (28). Knockdown of MALAT1 inhibited MYBL2 expression and reduced the level of mammalian target of rapamycin (mTOR) pathway phosphorylation. The inhibition of MALAT1 and MYBL2 or the administration of rapamycin, a mTOR pathway inhibitor, resulted in a substantial suppression of prostate cancer (PCa) cell growth and a notable decrease in the Warburg effect. In addition, up-frameshift protein 1(UPF1) may regulate MALAT1, and the UPF1/MALAT1 pathways might potentially serve as a promising area of therapeutic exploration for the treatment of gastric cancer. The levels of UPF1 expression in healthy lung tissues were higher than in human LA tissues, suggesting that a reduction in nonsense-mediated decay (NMD) contributes to LA formation. UPF1 has additionally been documented as a tumor suppressor (29, 30). Another study found that, in combination with the translation process of lncRNA MALAT1 as well as its manifestation in cancer cells, the association between lncRNA-MALAT1 polymorphisms and carcinogenesis should be revealed. Nevertheless, it is imperative to validate this idea at subsequent stages by specific experiments. As an illustration, by studying the lncRNA-MALAT1’s inhibitory effect on metabolites and assessing the outcomes by measuring the spread or proliferation of cancer cells, the study of MALAT1 is important in inhibiting the proliferation of tumor cells.
Hippo/YAP
The Hippo/YAP pathway plays a pivotal role in various metabolic processes within the context of cancer, encompassing cell viability, proliferation, angiogenesis, and energy metabolism. Some lncRNAs, such as LINC00857, GHET1, and TNRC6C-AS1, are closely connected to the regulation of energy metabolism and yes-associated protein (YAP) phosphorylation in cancer. It has been shown that LncRNA GHET1 promotes hypoxia-induced triple-negative breast cancer (TNBC) proliferation, invasion, and glycolysis via the Hippo/YAP signaling pathway (31). Mechanistically, YAP was retained in the cytoplasm when lncRNA GHET1 was inhibited, leading to a rise in LATS1 and YAP phosphorylation levels. Conversely, hypoxia or overexpression of the lncRNA GHET1 promoted the growth of TNBC and the nuclear translocation of YAP. However, Lin et al. (32) demonstrated that ovarian cancer glycolysis and progression were controlled by LINC00857 by Spiking miR-486-5p, which up-regulated YAP1 and hence regulated the Hippo signaling pathway, which may potentially offer novel insights for therapeutic approaches targeting ovarian cancer. More information and mechanisms by which glycolysis-associated lncRNAs regulate cancer through signaling pathways are shown in Table 1.
In conclusion, Hippo-YAP signaling both regulates and is regulated by metabolism, thereby making a significant contribution to cellular metabolism. Consequently, the Hippo/YAP signaling network is utilized in a variety of environments and can influence the development of pancreatic β-cells involved in glucose metabolism, lipid metabolism, and autophagy. There are numerous putative LncRNA-mediated pathways associated with glycolysis, such as H19, NF-KB, and PTEN, the mechanisms of which remain unknown. We have only provided summaries of three representative signaling pathways. The correlation between intricate and adaptable signaling pathways and the energy metabolism of tumors must be substantiated through additional experiments.
Glycolysis-associated lncRNAs regulate cancer energy metabolism of transcription factors.
Considering that lncRNAs influence the regulation of targets by transcription factors, they also regulate the reprogramming of energy metabolism via glycolysis-associated transcription factors. LncRNAs exert regulatory control over the activity of these proteins via processes such as hydroxylation, phosphorylation, and ubiquitination, which enhances the manifestation of enzymes associated with metabolic processes. Additionally, lncRNAs facilitate the malignant evolution of neoplastic growths. Henceforth, we shall proceed to deliberate upon the recently documented mechanism of regulating representative factors (33).
HIF-1α
In rapidly growing tumor tissues, hypoxia-inducible factor-1α (HIF-1α) performs an essential function in the metabolic reprogramming of tumor cells through the stimulation of gene transcription that encodes glycolytic enzymes and glucose transporters. For example, HIF-1α stimulates the transcription of lncRNA RAET1K via miR-100-5p to control the glycolysis induced by hypoxia in hepatocellular carcinoma cells (34). Furthermore, by creating a positive feedback loop involving AKT/HIF-1α signaling, the overexpression of lncRNA CASC9 under hypoxic settings enhances glycolysis and the epithelial-mesenchymal transition in pancreatic cancer (35, 36).
Interestingly, tumor-associated macrophages (Tam) deliver bone marrow-specific lncRNA through extracellular vesicles. HIF-1α-stabilizing long-stranded lncRNA HISLA increases resistance to apoptosis and aerobic glycolysis in breast cancer cells. Mechanistically, HISLA prevents PHD2 from interacting with HIF-1α and hinders HIF-1α’s hydroxylation and degradation. In turn, HISLA in macrophages is upregulated by lactate produced from glycolytic tumor cells, creating a feed-forward loop between tumor cells and tam. And restricting the release of HISLA from extracellular vesicles in breast cancer in vivo reduces glycolysis and chemoresistance (37). Meanwhile, Chen et al. demonstrated that the HIF-1α/glycolysis axis plays a role in FAM83A-AS1-mediated LA proliferation and metastasis, and the inhibition of tumor development and suppression of HIF-1α expression and glycolysis-related gene expression are seen in vivo with knockdown of FAM83A-AS1, making it a possible biomarker and treatment target for people with LA (38). However, given HIF-1α’s substantial influence on the expression of genes linked to the development of cancer and the inadequate effectiveness of chemotherapy, the future direction will be to create pharmaceuticals that target HIF-1 with more specificity by elucidating the molecular architecture of the structural domains that mediate HIF-1α’s critical activities.
c-MYC
Being a “master regulator” of cellular metabolism and cancer progression, c-MYC is among the most significant transcription factors in numerous types of cancer cells that are associated with reprogramming, proliferation, and resistance to chemotherapy. C-MYC exhibits both genetic and epigenetic changes in a wide variety of malignancies (39). There are reports indicating that lncRNAs influence the regulation of cancer energy metabolism via c-MYC post-translational modifications. Numerous lncRNAs exhibit substantial upregulation and serve as oncogenes within the colorectal or renal cancers, including the prostate cancer glycolysis-associated LncRNA SNHG7, the long intergenic noncoding RNA generated by energy stress FNCC1, mediated by the FoxO transcription factor, FILNC1, long intergenic noncoding RNA for IGF2BP2 stability (lncRNA LINRIS), and LINC00261. On a mechanical level, in prostate cancer PC-3 and DU-145 cells, SNHG7 stimulated glycolysis via the SRSF1/c-MYC axis; N6-methyladenosine (m6A) modification by methyltransferase-like 3 (METTL3) boosted SNHG7 stability (40).
Meanwhile, FILNC1 and LINRIS also regulate tumor cell proliferation. In renal tumors, knockdown of FILNC1 under circumstances of glucose deprivation only increases c-MYC protein levels and suggests that energy stress promotes the formation of tumors by regulating the FILNC1-auf1-c-MYC signaling axis through a minimum of two methods (41). In contrast, Wang et al. showed in vivo experiments that blocking LINRIS reduced the growth of tumors in both in situ and patient-derived xenograft (PDX) models. Mechanistically, LINRIS prevents IGF2BP2 from being stabilized by blocking its ubiquitination at the K139 site, a procedure that stops the autophagy-lysosomal pathway (alkaline phosphatase) from degrading IGF2BP2. Therefore, down-regulation of LINRIS mediated by MYC attenuates the downstream effects of IGF2BP2 and thus inhibits colorectal cancer (CRC) cell glycolysis (42). Furthermore, LINC00261 exerts its biological function by binding to miR-222-3p and triggering the HIPK2/ERK/c-MYC pathway. LINC00261 also inhibits the expression of c-MYC by sequestering IGF2BP1. It has been discovered that LINC00261 has unique epigenetic and post-transcriptional regulation mechanisms that act as tumor suppressors in pancreatic cancer, which could be beneficial for pancreatic cancer targeted treatment (43). In the moment, even though there are currently no c-MYC targeting medicines with any kind of clinical approval, more and more research is focused on addressing c-MYC targeting studies to develop viable approaches for treating tumors (44).
TGF-β1
Transforming growth factor-β1 (TGF-β1) exerts a wide range of activities during the process of developing an embryo, including differentiating cells and tissues, angiogenesis, wound healing, immunological response, and carcinogenesis. Several recent experiments have directly regulated tumor cell metabolism by mediating TGF-β1 through the glycolytic pathway. For example, TGF-β1 amplification stimulates the generation of ATP, lactate, and glucose absorption in primary hepatocellular carcinoma (HCC) cells. TGF-β1 serves as an upstream positive regulator of urothelial carcinoma-associated 1 (UCA1), which in turn is an upstream positive regulator of hexokinase-2 (HK2), and tumor-promoting lncRNA TGF-β1 tends to be up-regulated in tissues (45). Interestingly, quantitative reverse transcription polymerase chain reaction (qRT-PCR) results demonstrated that overexpression of exogenous lncRNA UCA1 upregulated HK2 and LDHA mRNA expression, whereas inhibition of lncRNA-UCA1 attenuated the glycolytic pathway, inhibiting prolactin (PRL) production and the proliferation of pituitary cancer cells (46). Meanwhile, the study has shown that LINC00973 has direct binding affinity towards LDHA, hence augmenting its enzymatic activity and facilitating glycolysis, ultimately leading to the proliferation of cancer cells (47, 48). Recent research indicates that LncDACH1 overexpression inhibits aberrant activation and collagen deposition induced by TGF-1, thereby preventing pulmonary fibrosis. Further, interestingly, the authors established that TGF-β1 facilitates the activation of cardiac fibroblasts, promotes the enhancement of glycolysis, and inhibits the expression of Linc00092. TGF-β1 is intimately associated with glycolysis and has the ability to modulate both lncRNA and related enzyme activity, making its regulation a very versatile and extensive study subject (49, 50). More information and mechanisms by which glycolysis-associated lncRNAs regulate cancer through transcription factors are shown in Table 2.
In summary, we found that the impact of glycolysis-associated transcription factors on tumors has received widespread attention. Transcription factors can regulate a variety of genes, even including some key enzymes, have important roles in metabolic shifts and the development of tumor cells, and can provide targets for the development of new tumor therapeutic strategies. Nevertheless, numerous challenges remain to be addressed when dealing with the intricate regulatory network. The interplay between various factors and the multifunctionality of transcription factors necessitate careful consideration. Additionally, the limitations imposed by detection technology pose a significant obstacle to the translation of these findings to clinical applications.
Glycolysis-associated lncRNAs regulate cancer energy metabolism of metabolic enzymes
During cancer development, lncRNAs can also regulate many glycolysis-related enzymes (51). Key regulators of glycolytic enzymes by lncRNAs include 6-phosphofructokinase1, glucose-6-phosphate dehydrogenase, pyruvate kinase 2 (PKM2), LDHA, HK, and glucose transporter protein 1 (GLUT1). Thus, aberrant regulation and expression of glycolytic pathway enzymes are essential for tumorigenesis. For example, the lncRNA TMEM105, which is related to glycolysis, exhibits upregulation of LDHA and facilitates the liver metastasis of breast cancer by acting as a miR-1208 sponge (52). The manifestation of G6PD is enhanced by ELFN1-AS1 via the promotion of TP53 degradation, leading to the activation of the pentose phosphate pathway (PPP). Zhang et al. identified that cellular migration, invasion, and proliferation of esophageal cancer tumor cells were inhibited when ELFN1-AS1 was suppressed. This was achieved by inhibiting miR-183-3p, which upregulated glutamine-fructose-6-phosphate transaminase 1 (GFPT1) (53).
PKM2 is an essential metabolic enzyme that converts phosphoenolpyruvate to pyruvate, playing a vital role in cellular metabolism and tumor proliferation. In recent studies, in order to increase aerobic glycolysis, SNHG6 and hnRNPA1 are lncRNAs that interact to promote the growth of colorectal cancer by regulating alternative splicing of PKM. Meanwhile, LncRNA HULC modulates the enzymatic activities of LDHA and PKM2 by increasing their phosphorylation levels and enhancing their interaction with the intracellular structural domain of the upstream kinase FGFR1 (54, 55). Meanwhile, glycolysis-associated enzymes represented by PKM2 are diverse and mechanistically complex, and they are closely linked to transcription factors and profoundly influence material metabolism, functioning in interaction with multiple lncRNAs.
Lactic acid, a major metabolite of glycolysis, has garnered considerable interest in recent years due to its role as a regulator that connects tumor progression and immunity. Zhao (56) et al. found that lncRNA HITT inhibited lactate production by suppressing the oligomerization of PKM2, thereby reducing tumor growth and macrophage polarization. Interestingly, hypoxic conditions have been found to drive the glycolytic activity of tumor-associated fibroblasts (CAF) through capillary dilatation ataxia mutated (ATM) oxidation, glucose transporter protein 1 phosphorylation, and PKM2 overexpression, and that lactic acid produced by CAF is ultimately used to drive breast cancer cell invasion through activation of the TGF1/p38 MAPK/MMP2/9 signaling axis and promotion of mitochondrial oxidative phosphorylation (57, 58). The Meanwhile, there exists a correlation between the spatial distribution of lactate concentration and the occurrence of lactation. Identifying several crucial lactation sites within the microenvironment of the tumor can aid in the recruitment of immune cells, induce modifications to the microenvironment, and collaborate with additional epigenetic modifications to stimulate tumorigenesis, progression, and direct modulation of gene expression associated with critical tumor pathways (59, 60). However, relevant studies are not focused enough and lack clinical validation, and further experimental data are urgently needed to prove their mechanisms. Additional research is warranted to explore the various functions and specific mechanisms underlying lactonization, as well as the regulatory enzymes involved in this process. Understanding the role of lactonization in exercise, lipolysis, neuroprotection, and angiogenesis is crucial, as it may offer important understandings for the creation of new medical diagnostics and treatment approaches for chronic diseases, including atherosclerosis and Alzheimer’s disease (61, 62). In a similar vein, it has been shown that lncRNAs such as UPF1, TUG1, and MALAT1 increase the activity of kinases connected to glycolysis, which has a facilitative effect on the glycolytic process (63). Meanwhile, from precancerous polyps to distant metastases, lncRNAs are frequently engaged in various phases of cancer and can be considered effective diagnostic biomarkers. More information and mechanisms by which glycolysis-associated lncRNAs regulate cancer through metabolic enzymes are shown in Table 3, Figure 2.
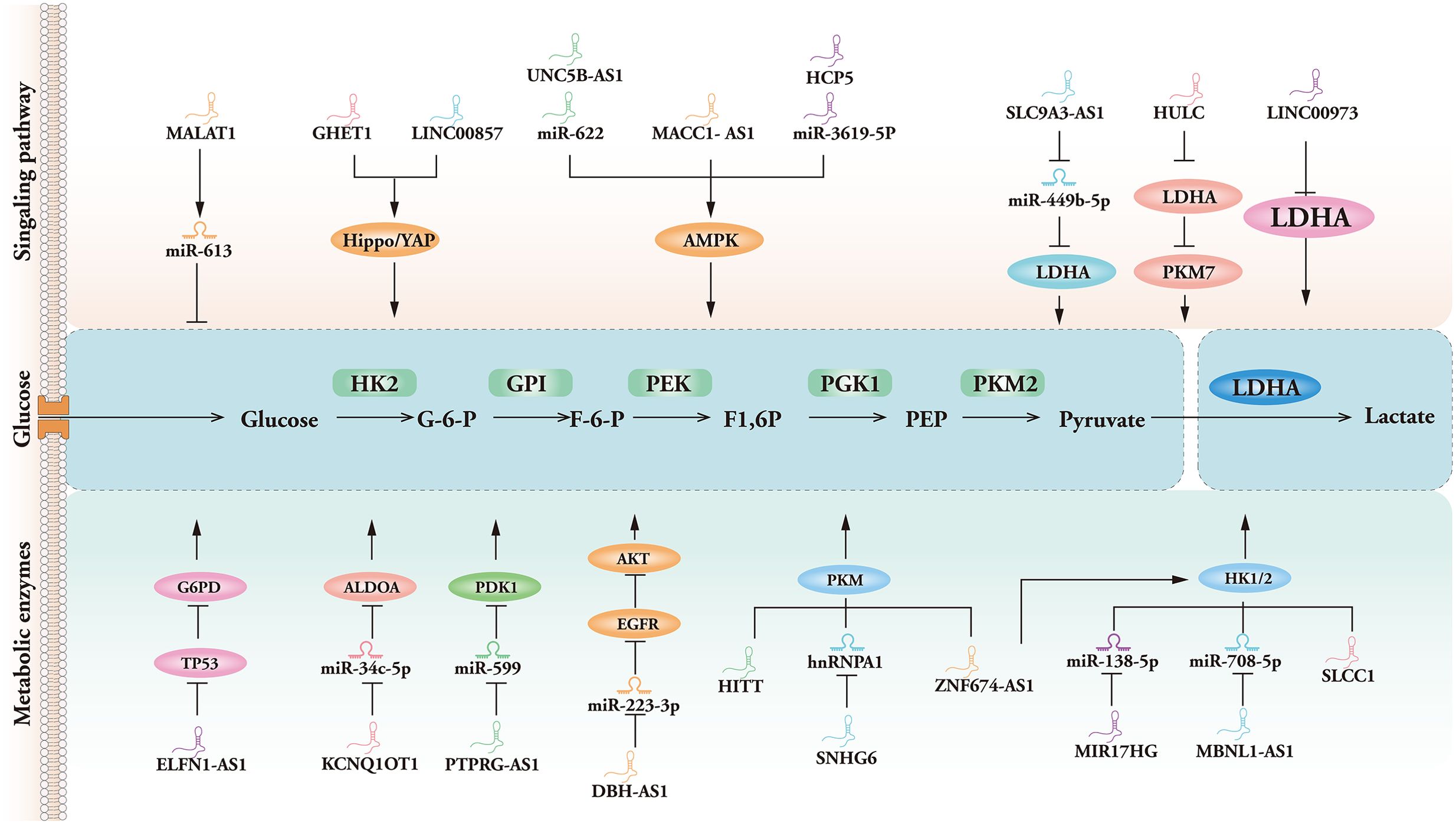
Figure 2. Mechanisms of glycolysis-associated LncRNA-mediated signaling pathways and enzymes of meditation in cancer.
In conclusion, experimental investigations are increasingly focused on the post-transcriptional regulation of lncRNAs and the competitive binding of miRNAs to affect the activity and expression of enzymes, with a particular emphasis on the role of lactate in metabolism. While initial studies may have identified a correlation between a particular LncRNA and a glycolysis-related enzyme, elucidating its precise significance within the context of the entire biological process remains a formidable task. In the past couple of years, a growing multitude of investigations have furnished empirical evidence supporting the involvement of lncRNA in the pathogenesis of cancer (64). In a word, the interaction between lncRNAs and metabolic enzymes is extensive and complex, necessitating further investigation and scholarly inquiry to fully comprehend the specific mechanisms and interconnections involved.
Multiple mediation of glycolysis-associated lncRNAs in tumor immune microenvironment
In the microenvironment of the tumor, the existence of immunological cells and immunological-related mediators plays an essential and pivotal role in facilitating tumor development (65, 66). Numerous research investigations have proven that glycolysis-associated lncRNAs are essential regulatory molecules that directly block the cancer immunity cycle, in addition to activating negative regulatory pathways to restrict tumor immunity. LncRNAs reshape the tumor microenvironment via the recruitment and activation of innate and adaptive lymphoid cells (67, 68). Subsequently, we concentrate on the correlation between glycolysis-associated lncRNAs, dendritic cells, T cells, B cells, and natural killer cells.
Dendritic cells
The glycolytic pathway plays a crucial role in the activation and function of DC cells. Recent studies have found that DC cells enhance glycolytic activity when stimulated by signals in the cancer immune microenvironment. The function of lncRNAs in regulation produced from macrophages in the modulation of glycolysis in tumor cells has been shown, and host lncRNA MIR4435-2HG in primary myeloid dendritic cells (mDCs) from elite controllers (ECs) responded to TLR3-stimulated increases in oxidative phosphorylation and glycolytic activity by mTOR signaling pathway participants through the enhancement of immunometabolism activity of primary myeloid dendritic cells in EC by using specific epigenetic alterations on constituents of the mTOR signaling pathway (69). In contrast, it has been found that DC cells migration is inhibited by lnc-DPF3 produced by the CCR7 chemokine receptor through the inhibition of glycolysis mediated by HIF-1α. Furthermore, the critical role of lnc-DPF3 in impeding the late phase of dendritic cell migration and preventing inflammatory pathogenesis while preserving immune homeostasis is its delayed and induced function (70). As the understanding of the importance of lncRNAs and glycolysis in DC cell function increases, researchers are exploring the possibility of applying this knowledge to cancer immunotherapy. By targeting specific lncRNAs or modulating glycolytic pathways, it is possible to enhance the anti-tumor capacity of DC cells, thereby improving the outcome of cancer patients.
T cells
Metabolic reprogramming is required for T cells to activate and perform their effector functions, and enhanced glycolysis can support T cells in high energy-demanding anti-tumor immune responses. Studies have shown that the cancer immunogenic lncRNA LIMIT, by targeting the LIMIT-GBP-HSF1 signaling axis, may restore MHC-I expression and function by cancer immunotherapy, which may also strengthen the T-cell-mediated immune response against cancers. As a result, lncRNA LIMIT may be considered an immunogenic target (71). Similarly, the researchers Zhao et al. made the observation that ovarian cancer cells exhibit elevated amounts of the microRNAs miR-101 and miR-26a. These microRNAs were shown to suppress the production of the methyltransferase EZH2. Consequently, EZH2 downregulation leads to glycolytic limitation in T cells and subsequent impairment of their functions (72). Meanwhile, numerous experiments have demonstrated that patients with grade II-III gliomas exhibit enhanced T lymphocyte infiltration, while Guo et al. used bioinformatics analysis and RT-qPCR to show that knockdown of lncRNA significantly reduces SHG-44 cell viability and proliferation and inhibits glycolysis, demonstrating the potential regulatory role of CRNDE in glucose metabolism in grade II-III gliomas. potential regulatory role of CRNDE in glucose metabolism in grade II-III gliomas (73–75). Furthermore, LncRNA NEAT1 plays a key role in the suppression of immune surveillance by T cells. NEAT1 is upregulated and positively modulates the expression of LDHA in prostate cancer. The anti-tumor effects are enhanced by the secretion of CD8+ T lymphocyte factors TNF-α, IFN-γ, and granzyme B, which is promoted by the knockdown of NEAT (76). The current study is still in its preliminary stages, but there is great potential for glycolysis-associated lncRNAs to be used in the cancer immune microenvironment. Future studies may further explore how these lncRNAs can be exploited clinically to enhance T cell-mediated anti-tumor immune responses, especially when used in combination with other immunotherapeutic approaches.
B cells
Although there have been fewer studies on the direct regulation of B-cell glycolysis by lncRNAs, a number of studies have begun to reveal potential mechanisms in this area. For example, Xie et al. demonstrated that PDL1+ B cells were induced by the exosome lncRNA HOTAIR in order to inhibit antitumor immunity in colorectal cancer. Mechanistically, PDL1 expression on B cells was increased by exosome HOTAIR, which prevented ubiquitination degradation of the PKM2 protein and caused PKM2 to stimulate STAT3 transcription (77). Notably, extracellular miR-122 from hepatocytes can be delivered to hepatic stellate cells (HSCs) to regulate their proliferation and gene expression, and ectopic miR-122 expression inhibits BCL2 expression in human HSC (LX-2) cells (78). Meanwhile, Zhao et al. demonstrated that the MYC-regulated lncRNA NEAT1 promotes B-cell proliferation and lymphangiogenesis through the miR-34b-5p-GLI1 pathway in diffuse large B-cell lymphoma (79). These lncRNAs may indirectly affect the glycolytic process of B cells by regulating key enzymes of glycolysis or metabolic signaling pathways, thereby altering the behavior of B cells in the cancer immune microenvironment.
Natural killer cells
The cytotoxic activity of NK cells can also be regulated by lncRNAs. NK cells are also one of the key immune components in the surveillance and eradication of neuroblastoma (NB). Within the neuroblastoma framework, data have demonstrated that the exosomal lncRNA EPB41L4A-AS1 translocates from CD56brightNK to CD56dimNK and inhibits glycolysis of target NKs. By means of exosomal transfer of the metabolism inhibitory lncRNA EPB41L4A-AS1, cross-talk between heterogeneous NK subpopulations was accomplished (80). Therefore, Exosomal lncRNA NEAT1 is up-regulated in multiple myeloma, and NEAT1 represses PBX1 by recruiting EZH2. Knockdown of PBX1 attenuates the effect of NEAT1-silenced exosomes on NK cells and multiple myeloma cells. Additionally, it enhances the expression of NKG2D, TNFα, and IFNγ in tumor tissues, hence promoting immune evasion by multiple myeloma cells (81). More information and mechanisms of glycolysis-associated lncRNAs regulating in tumor immune microenvironment are shown in Figure 3.
Notably, further research is warranted to determine whether lncRNAs regulate immune cell function synergistically, and future investigations may focus on identifying additional lncRNAs with potential combinatorial functions in immune cell behavior regulation.
Risk models of glycolysis-associated lncRNAs in tumor immune microenvironment
An increasing number of lncRNA risk models for immunization have been developed in recent years to improve disease progression and provide clinical decision-making for cancer patients. Representative models, including those related to glycolysis, are a signature consisting of seven lncRNAs developed by Feng’s team, BC-associated LRLPS, and a multistep model of lncRNA-lncRNA cooperation that synergistically regulates functional modules. For example, a prognostic signature comprising seven immune-associated lncRNAs, namely AL391832.3, LINC00892, LINC02207, LINC02416, and PSMB8, demonstrated significant predictive capabilities for the survival outcomes of individuals diagnosed with ovarian cancer. These findings hold potential for offering valuable indicators for the implementation of clinical stratified management and personalized treatment selection for this specific patient population (82). Meanwhile, Li et al. constructed a lactate-associated lncRNA prognostic signature (LRLPS) for breast cancer patients and created a unique LRLPS that targets BC and has the ability to forecast treatment response, immunological state, and prognosis (83). By combining multi-omics data, Shao et al. developed a multi-step model of lncRNA-lncRNA collaboration based on synergistic regulation of functional modules and systematically constructed and analyzed a cooperative network of lncRNA-lncRNAs (IC-LncRNAs) spanning a wide range of tumors, which helped to clarify the similarities and distinctions among tumor mechanisms. The IC-LncRNAs synergistic regulation of T cell activation may bring novel approaches to immunotherapy for tumors (84, 85).
To summarize, glycolysis-associated LncRNAs have a significant impact on the immunological microenvironment of tumors and can be used to develop prognostic models based on their specificity. Recent results indicate that glycolysis is significantly engaged in tumor cells’ immunological evasion since it enhances immunosuppression and resistance to tumor drugs. This finding highlights the potential use of targeting glycolysis as a unique strategy in immunotherapy. The findings offer valuable theoretical evidence for further elucidating the connection between immunity and metabolism (86, 87).
Implications of glycolysis-associated lncRNAs on tumor drug resistance
Chemotherapy is among the most critical therapeutic approaches in cancer treatment, and the glycolytic pathway of LncRNA transcription has been found to counteract tumor drug resistance (88, 89). For example, LncRNA GLTC promotes radioiodine resistance and the advancement of thyroid-like carcinoma via targeting increased succinylation and enzymatic activity of LDHA (90). The GLTC targets the enzymatic activity and succinylation of LDHA to facilitate the progression of thyroid cancer and promote resistance to radioiodine treatment.
According to recent studies, epigenetic regulation of different signaling molecules can reduce the sensitivity of cancer cells to cisplatin. LncRNA XIST, LncRNA-DANCR, and LncRNA-SARCC associated with LA promote chemoresistance through the glycolytic pathway. For example, it was demonstrated that suppressing the lncRNA XIST significantly decreased the expression of glycolysis-critical enzymes while increasing the expression of miR-101-3p, which reduced the rate of extracellular acidification, glucose uptake, and lactic acid products and increased lung cancer’s resistance to cisplatin. In contrast, the upregulation of the PKM2/PKM1 ratio via the LncRNA XIST/miR-137 axis was found to increase glycolysis and chemotherapy tolerance in colorectal cancer (91, 92). In the meantime, Shi et al. illustrated how LncRNA-DANCR disrupted the miR-125b-5p/HK2 axis, thereby inducing anaerobic glycolysis and desensitizing colorectal cancer cells to cisplatin (93). Interestingly, Wen et al. illustrated how LncRNA-SARCC could increase osteosarcoma sensitivity to cisplatin by targeting HK2 via mir-143 to inhibit glycolysis (94). Furthermore, there is an increasing body of data showing that elevated glycolysis rates are positively correlated with chemotherapy resistance, and the glycolysis of tumors has been identified as a prospective target for the creation of anticancer medications.
Several studies have shown evidence for the substantial participation of lncRNAs in the control of glucose metabolism via influencing the HIF signaling pathway, which can promote drug sensitivity. Chen et al. established that suppressing HISLA via interference-mediated targeting of Tam-specific lncRNA could potentially stabilize HIF-1α and offer a more practical method for inhibiting glycolysis and apoptosis resistance in tumor cells. This finding underscores the appeal of lncRNA as a target in the field of tumor therapy (37). Meanwhile, LncRNA HIF1a - AS1 facilitates the interaction between y-box binding protein 1 (YB1) and serine/threonine kinase (AKT) by regulating the AKT/YB1/HIF1a pathway, which promotes the phosphorylation of YB1 (pYB1) and enhances glycolysis, thereby contributing to gemcitabine resistance in pancreatic cancer (95, 96).
The resistance mechanism of cancer cells is not only related to chemotherapy but also inextricably linked to the targeted therapy of traditional Chinese medicine. Recent studies have shown that tretinoin lactone (TP) is implicated in the development of hepatocellular carcinoma. Nevertheless, the precise biochemical network that is controlled by TP remains unknown. LncRNAs MBNL1-AS1 and SLC9A3 play roles in various pathological progressions. In contrast to MBNL1-AS1, which is down-regulated in HCC tissues and increases the sensitivity of hepatocellular carcinoma to tretinoin via regulation of glycolysis mediated by miR-708-5p, the lncRNA SLC9A3-AS1 exhibits up-regulation in both hepatocellular carcinoma tissue samples and cells. Mechanistically, the inhibition of SLC9A3-AS1 resulted in a considerable reduction in the glycolysis rate mediated by the miR-449b-5p/LDHA axis, causing Huh7 cells’ susceptibility to TP to decline (97, 98).
Consequently, the function of glycolysis-associated lncRNA in the tumor immune microenvironment and the mechanisms of drug resistance in cancer cells are combined. The efficacy of targeting glycolysis has been shown by the regulation of tumor development and the augmentation of anti-cancer treatment. Finally, other non-coding RNAs associated with glycolysis, including lncRNAs and circRNAs, should additionally be investigated, as this would enable the enrichment of molecular networks that elucidate the etiology of malignancies (99). More information and mechanisms by which glycolysis-associated lncRNAs regulate cancer through other pathways are shown in Table 4.
Other regulatory functions and clinical significance of glycolysis-associated lncRNAs
Application of glycolysis-associated lncRNAs as biomarkers
Biomarkers contribute significantly to various fields such as personalized medicine, drug development, clinical care, and molecular breeding, which can expedite the process of medication development, serve as a preliminary marker of enhanced clinical responsiveness, and improve the level of patient safety. LncRNAs are an important direction of research at the molecular level (100, 101). Glycolysis-associated lncRNAs are more stable compared to proteins in cancer research and demonstrate a high degree of cell-tissue specificity and targeted therapeutic potential, constantly revealing new molecular regulatory networks. In contrast, in the treatment of cancer, protein molecules are susceptible to denaturation or inactivation by environmental conditions; some exogenous proteins or protein drugs may trigger an immune response, which can lead to adverse reactions or reduced efficacy; and protein molecules are structurally complex with limited targeting (102, 103).
Numerous lncRNAs are prospective biomarkers in the investigation of tumor cell suppression and glycolysis. LncRNA AFAP1-AS1 was also considerably expressed in patients with colon cancer. Patients exhibiting elevated levels of AFAP1-AS1 demonstrated a worse duration of survival and exhibited a strong association with tumor volume, local lymph node metastasis, disease stage, and overall survival. Accordingly, it is probable that LncRNA AFAP1-AS1 could function as a therapeutic target and diagnostic marker for colon cancer (104). In their study, Yang et al. observed a simultaneous upregulation of LncRNA H19 in both oral cancer cell lines and cancer associated fibroblasts (CAFs). The lncRNA H19/miR-675-5p/PFKFB3 axis promoted glycolysis in oral CAFs; lncRNA H19 inhibition in oral CAFs impeded glucose metabolism, cell proliferation, and migration. Furthermore, scientific investigations have revealed that lncRNA H19 influences tumor cell proliferation, metastasis, and apoptosis inhibition and that up-regulation of lncRNA H19 stimulates cancer cell resistance to chemotherapy and radiotherapy; thus, H19 not only provides new biomarkers for molecular diagnostics, but also serves as a new target for anti-tumor therapy (105–107). Furthermore, the lncRNA EGOT is frequently used to predict the prognosis of multiple or gastrointestinal tumors, and it potentially participates in numerous biological processes, such as autophagy and glycolysis (11, 108). Thus, despite the increasing convenience it provides, biomarker research remains far from complete and serves as a compass for future investigations into cancer treatments.
Construction of prognostic models by glycolysis-associated lncRNAs
Since the diagnostic power of a single circulating lncRNA is not superior to that of a model consisting of multiple lncRNAs, an increasing number of prognostic models are undergoing growth with the advancement of bioinformatics, both for predicting disease progression as well as for early intervention and prevention, while providing new ideas and helping to make initial therapeutic decisions for cancer treatment (109, 110). Predictive models based on lncRNAs and clinical features are effective in predicting renal cell carcinoma with clear cells (111). Ma et al. constructed an alternative marker for clear cell renal cell carcinoma consisting of seven lncRNAs, which was able to precisely Predict the prognosis of individuals diagnosed with clear cell renal cell carcinoma, and there was a substantial correlation between distant metastasis, tumor size, stage, and grade. The experimental prognostic characteristics provide a foundation for tracking the effectiveness of mTOR inhibitors or learning more about how they work in renal cell carcinoma (112). In their study, Ma et al. created a unique bladder cancer prognosis model by examining nine lncRNAs associated with glycolysis. These lncRNAs are involved in various signaling pathways, including lymphocyte receptor signaling, the nuclear factor-k-gene binding pathway, the Notch signaling pathway, the P53 pathway, chemokine signaling, and oxidative phosphorylation, which provides a newer and more comprehensive approach for cancer research (112, 113).
Therapeutic targeting of malignancy by glycolysis-associated lncRNAs
Inhibiting proto-oncogene expression decreases tumor cell growth and metastasis while increasing treatment susceptibility and apoptosis. In an in vitro investigation, scholars noted that lncRNA HOTAIRM1 silencing inhibited glucose consumption and lactate production in non-small cell lung cancer. Western blot analysis revealed that lncRNA HOTAIRM1 inactivation prevented the HK2 protein from being expressed, and the miR-498 inhibitor impeded this suppression. In another in vitro investigation, lncRNA KCNQ1OT1 was found to stimulate cell proliferation and inhibit apoptosis while promoting osteosarcoma cell development in vivo. lncRNA KCNQ1OT1 sponged miR-34c-5p in the capacity of a competitive endogenous RNA (ceRNA), hence inhibiting the development of osteosarcoma cells in vivo, and prevented the in vivo proliferation of osteosarcoma cells by directly targeting its 3’Untranslated. Region to inhibit aldolase A expression (99, 114). In vivo studies, knockdown of the lncRNAs MALAT-1, HULC, DANCR, and AP000695.2 revealed inhibition of tumor cell growth. Similarly, upregulation of oncogenes is an efficacious strategy to combat tumor development.
Moreover, the LncRNA ELFN1-AS1 has been observed to promote the proliferation of colorectal cancer cells and impede apoptosis by enhancing G6PD activity (53). In a separate investigation, Fusarium promotes carcinogenesis by enhancing glucose metabolism in colorectal cancer cells. One way Fusarium promotes lncRNA ENO1-IT1 transcription is by increasing the binding efficiency of transcription factor SP1 to the promoter region of this gene. Raise ENO1-IT levels alter histone modification patterns on ENO1 and other target genes by interacting with KAT7 histone acetyltransferase, thereby altering the biological function of colorectal cancer; therefore, targeting the pathway may be advantageous in the treatment of patients with CRC (11). Therefore, despite the increasing accessibility of biomarker research, it is still far from complete and serves as a compass for future cancer treatment research.
Conclusions and discussions
Glycolysis has become more well-known in cancer research in recent years. LncRNAs play a significant role in the modulation of several crucial biological processes and tumor development through the glycolytic pathway, including regulating the manifestation of key enzymes of glycolysis, modulating lactate production and transport, engaging in glycolysis-associated immune escape from tumors, or forming complexes with transcription factors that regulate gene expression, which in turn affects the expression of related genes. Here we focus on the complex and multi-functional network of energy metabolism regulators controlled by post-translational modifications mediated by LncRNA. This network serves as a central node for numerous cell signaling pathways and is among the quickest ways in which cells react to both internal and external stimuli. Nevertheless, the present comprehension of its particular mode of action remains rather restricted, necessitating more investigation to clarify the complicated processes and regulatory pathways linked to its exact activity.
In conclusion, lncRNAs in tumors are similar to a regulatory key during glycolysis, and lncRNAs can turn on or off switches for key genes, signaling pathways, or cellular functions in cancer cells. They can be involved as regulators not only in the normal regulation of cells but also in immunity and drug resistance. The existence of such amazing regulatory keys has shed light on the mechanisms of cancer development, providing new ideas and potential therapeutic targets for early diagnosis, treatment, and prognostic evaluation of cancer. However, these molecular mechanisms and their interactions require further studies to elucidate.
Perspectives
Regarding human genes, only 2% can code for proteins, of which 98% are non-coding sequences that form a complex regulatory network. A significant proportion of these DNA sequences, which lack protein-coding potential, undergo the process of transcription to become RNA molecules. This group includes a substantial number of microRNAs (miRNAs) as well as lncRNAs, numbering in the thousands for each category. As discussed herein, many studies have shown that lncRNAs act by regulating specific miRNAs downstream of them. However, individual lncRNAs are not limited to regulating only one miRNA, so it is critical to conduct a more extensive investigation into the myriad targets or signaling pathways that follow lncRNAs. As an illustration, LncRNA EGOT-mediated glycolysis also involves autophagy-associated regulation. Whether lncRNAs regulate these pathways necessitates additional research and discourse. On one side, the regulatory mechanisms behind these non-glycolytic reactions must be clarified; on the other side, clarifying the relationship between glycolysis and various non-glycolytic activities is of paramount importance so as to examine in greater detail how lncRNA regulation of tumor cell glycolysis affects the immune system’s monitoring mechanism for tumor cells. Further investigation of the intricate lncRNA-miRNA-mRNA competing endogenous RNA networks is warranted since they constitute a sophisticated and tightly controlled mechanism for governing gene expression and cellular processes and may help to address the etiology of diseases such as glioblastoma, osteoarthritis, neuroblastoma, heart disease, lung cancer, pancreatic cancer, and inflammation.
Early detection of cancer can increase survival or cure rates. Nevertheless, despite lncRNAs’ promising future as biomarkers in medical research, they still face challenges of uncertainty in functional interpretation, variability and dynamics in expression levels, standardization and technical differences, lack of validation and validation sets for clinical applications, and bioinformatics analysis and interpretation, and solving these problems requires additional research and methodological improvements. The use of multiple lncRNAs in model construction can greatly enhance diagnostic and predictive capabilities, but further extensive research is required in order to identify circulating biomarkers that possess the capability to consistently detect malignancies in their early stages.
Author contributions
XZ: Conceptualization, Formal analysis, Writing – original draft. YZ: Investigation, Supervision, Writing – original draft. QL: Methodology, Validation, Writing – original draft. AZ: Writing – review & editing. LS: Writing – review & editing.
Funding
The author(s) declare financial support was received for the research, authorship, and/or publication of this article. The work is supported by National Natural Science Foundation of China (82305011).
Conflict of interest
The authors declare that the research was conducted in the absence of any commercial or financial relationships that could be construed as a potential conflict of interest.
Publisher’s note
All claims expressed in this article are solely those of the authors and do not necessarily represent those of their affiliated organizations, or those of the publisher, the editors and the reviewers. Any product that may be evaluated in this article, or claim that may be made by its manufacturer, is not guaranteed or endorsed by the publisher.
Glossary
AMPK: adenosine monophosphate-activated protein kinase
ATP: adenosine-triphosphate
CAF: cancer associated fibroblasts
CircRNAs: CircularRNA
c-MYC: cellular-myelocytomatosis viral oncogene
CRC: Colorectal cancer
DNA: Deoxyribonucleic acid
DC: Dendritic cells
ENO1: enolase 1
FILNC1: FoxO-induced long non-coding RNA 1
FoxO: Forkhead Box O
G6PD: Glucose-6-Phosphate Dehydrogenase
GLUT1: glucose transporter isoform 1
HCC: hepatocellular carcinoma
HIF-1α: hypoxia-inducible factor-1α
HISLA: HIF-1α-stabilizing lncRNA
HK2: hexokinase 2
HOTAIR: HOX antisense intergenic RNA
HOTAIRM1: HOX antisense intergenic RNA myeloid 1
HULC: highly up-regulated in liver cancer
IGF2BP2: insulin-like growth factor 2 mRNA-binding protein 2
LDHA: lactate dehydrogenase A
lincRNA: long intergenic noncoding RNA
LINRIS: long intergenic noncoding RNA for IGF2BP2 stability
Lnc RNA: Long non-coding RNA
lncRNA HITT: HIF-1α inhibitor at translation level
m6A: N6-methyladenosine
MACC1: metastasis-associated in colon cancer-1
MALAT1: metastasis-associated lung adenocarcinoma transcript 1
miRNA: microRNA
miRNA: MicroRNA: mRNA-binding, protein 2 stability (LINRIS) metastasis-associated in colon
mTOR: mammalian target of rapamycin
NADH: nicotinamide adenine dinucleotide
NADPH: nicotinamide adenine dinucleotide phosphate
NK: Natural killer cells
NSCLC: non-small cell lung cancer
OXPHOS: oxidative phosphorylation
PCa: prostate cancer
PFK1: phosphofructokinase-1;PFKFB3,6-phosphofructo-2-kinase/fructose-2,6-bisphosphatase3
PGK1: phosphoglycerate kinase 1
PHD2: proline hydroxylase domain 2
PI3K: phosphoinositide-3-kinase
PKM2: pyruvate kinase muscle isozyme M2
PKM2: Pyruvate kinase muscle isozyme M2
Tam: tumor-associated macrophages
TNBC: triple-negative breast cancer
TP53: Tumor Protein P53
UPF1: Up-frameshift protein 1
YAP: yes-associated protein
References
1. Faubert B, Solmonson A, DeBerardinis RJ. Metabolic reprogramming and cancer progression. Science. (2020) 368(6487):eaaw5473. doi: 10.1126/science.aaw5473
2. Shigeta K, Hasegawa M, Hishiki T, Naito Y, Baba Y, Mikami S, et al. Idh2 stabilizes hif-1α-induced metabolic reprogramming and promotes chemoresistance in urothelial cancer. EMBO J. (2023) 42:e110620. doi: 10.15252/embj.2022110620
3. Jin HR, Wang J, Wang ZJ, Xi MJ, Xia BH, Deng K, et al. Lipid metabolic reprogramming in tumor microenvironment: from mechanisms to therapeutics. J Hematol Oncol. (2023) 16:103. doi: 10.1186/s13045-023-01498-2
4. Chakravarti D, LaBella KA, DePinho RA. Telomeres: history, health, and hallmarks of aging. Cell. (2021) 184:306–22. doi: 10.1016/j.cell.2020.12.028
5. Hanahan D, Weinberg RA. Hallmarks of cancer: the next generation. Cell. (2011) 144:646–74. doi: 10.1016/j.cell.2011.02.013
6. Yang L, Lin C, Jin C, Yang JC, Tanasa B, Li W, et al. Lncrna-dependent mechanisms of androgen-receptor-regulated gene activation programs. Nature. (2013) 500:598–602. doi: 10.1038/nature12451
7. Ganapathy-Kanniappan S, Geschwind JF. Tumor glycolysis as a target for cancer therapy: progress and prospects. Mol Cancer. (2013) 12:152. doi: 10.1186/1476-4598-12-152
8. Zhou Y, Lin F, Wan T, Chen A, Wang H, Jiang B, et al. Zeb1 enhances warburg effect to facilitate tumorigenesis and metastasis of hcc by transcriptionally activating pfkm. Theranostics. (2021) 11:5926–38. doi: 10.7150/thno.56490
9. Jing Z, Liu Q, He X, Jia Z, Xu Z, Yang B, et al. Ncapd3 enhances warburg effect through C-myc and E2f1 and promotes the occurrence and progression of colorectal cancer. J Exp Clin Cancer Res. (2022) 41:198. doi: 10.1186/s13046-022-02412-3
10. Yang F, Zhang H, Mei Y, Wu M. Reciprocal regulation of hif-1α and lincrna-P21 modulates the warburg effect. Mol Cell. (2014) 53:88–100. doi: 10.1016/j.molcel.2013.11.004
11. Hong J, Guo F, Lu SY, Shen C, Ma D, Zhang X, et al. F. Nucleatum targets lncrna eno1-it1 to promote glycolysis and oncogenesis in colorectal cancer. Gut. (2021) 70:2123–37. doi: 10.1136/gutjnl-2020-322780
12. Li Z, Zhu T, Yao F, Shen X, Xu M, Fu L, et al. Linc00665 promotes glycolysis in lung adenocarcinoma cells via the let-7c-5p/hmmr axis. J Bioenerg Biomembr. (2024) 56:181–91. doi: 10.1007/s10863-024-10004-3
13. Su M, Liang Z, Shan S, Gao Y, He L, Liu X, et al. Long non-coding rna neat1 promotes aerobic glycolysis and progression of cervical cancer through wnt/B-catenin/pdk1 axis. Cancer Med. (2024) 13:e7221. doi: 10.1002/cam4.7221
14. Wu T, Ji Z, Lin H, Wei B, Xie G, Ji G, et al. Noncoding rna pvt1 in osteosarcoma: the roles of lncrna pvt1 and circpvt1. Cell Death Discovery. (2022) 8:456. doi: 10.1038/s41420-022-01192-1
15. Lin YH. Crosstalk of lncrna and cellular metabolism and their regulatory mechanism in cancer. Int J Mol Sci. (2020) 21:2497. doi: 10.3390/ijms21082947
16. Zhang C, Liu N. Noncoding rnas in the glycolysis of ovarian cancer. Front Pharmacol. (2022) 13:855488. doi: 10.3389/fphar.2022.855488
17. Ren Z, Yu Y, Chen C, Yang D, Ding T, Zhu L, et al. The triangle relationship between long noncoding rna, rig-I-like receptor signaling pathway, and glycolysis. Front Microbiol. (2021) 12:807737. doi: 10.3389/fmicb.2021.807737
18. Du Z, Fei T, Verhaak RG, Su Z, Zhang Y, Brown M, et al. Integrative genomic analyses reveal clinically relevant long noncoding rnas in human cancer. Nat Struct Mol Biol. (2013) 20:908–13. doi: 10.1038/nsmb.2591
19. Iyer MK, Niknafs YS, Malik R, Singhal U, Sahu A, Hosono Y, et al. The landscape of long noncoding rnas in the human transcriptome. Nat Genet. (2015) 47:199–208. doi: 10.1038/ng.3192
20. Wang SY, Zeng FR, Liang SM, Wang QP, Wen Y, Wang QY, et al. Lncrna linc00173 modulates glucose metabolism and multidrug chemoresistance insclc: potentialmolecular panel for targeted therapy. Mol Ther. (2022) 30:2868. doi: 10.1016/j.ymthe.2022.06.014
21. Yang B, Zhang L, Cao Y, Chen S, Cao J, Wu D, et al. Overexpression of lncrna igfbp4-1 reprograms energy metabolism to promote lung cancer progression. Mol Cancer. (2017) 16:154. doi: 10.1186/s12943-017-0722-8
22. Xu Z, Chen Y, Ma L, Chen Y, Liu J, Guo Y, et al. Role of exosomal non-coding rnas from tumor cells and tumor-associated macrophages in the tumor microenvironment. Mol Ther. (2022) 30:3133–54. doi: 10.1016/j.ymthe.2022.01.046
23. Ye L, Jiang Y, Zhang M. Crosstalk between glucose metabolism, lactate production and immune response modulation. Cytokine Growth Factor Rev. (2022) 68:81–92. doi: 10.1016/j.cytogfr.2022.11.001
24. Wang L, Cho KB, Li Y, Tao G, Xie Z, Guo B. Long noncoding rna (Lncrna)-mediated competing endogenous rna networks provide novel potential biomarkers and therapeutic targets for colorectal cancer. Int J Mol Sci. (2019) 20:5758. doi: 10.3390/ijms20225758
25. Zheng D, Che D, Lin F, Wang X, Lu L, Chen J, et al. Lncrna macc1-as1/macc1 enhances the progression of glioma via regulating metabolic plasticity. Cell Cycle. (2020) 19:2286–97. doi: 10.1080/15384101.2020.1795595
26. Wu H, Liu B, Chen Z, Li G, Zhang Z. Msc-induced lncrna hcp5 drove fatty acid oxidation through mir-3619-5p/ampk/pgc1α/cebpb axis to promote stemness and chemo-resistance of gastric cancer. Cell Death Dis. (2020) 11:233. doi: 10.1038/s41419-020-2426-z
27. Zhang Y, Li Z, Lan Z. Silencing unc5b antisense lncrna 1 represses growth and metastasis of human colon cancer cells via raising mir-622. Artif Cells Nanomed Biotechnol. (2020) 48:60–7. doi: 10.1080/21691401.2019.1699809
28. Hao L, Wu W, Xu Y, Chen Y, Meng C, Yun J, et al. Lncrna-malat1: A key participant in the occurrence and development of cancer. Molecules. (2023) 28:2126. doi: 10.3390/molecules28052126
29. Mu X, Shen Z, Lin Y, Xiao J, Xia K, Xu C, et al. Lncrna-malat1 regulates cancer glucose metabolism in prostate cancer via mybl2/mtor axis. Oxid Med Cell Longev. (2022) 2022:8693259. doi: 10.1155/2022/8693259
30. Hwang HJ, Park Y, Kim YK. Upf1: from mrna surveillance to protein quality control. Biomedicines. (2021) 9:995. doi: 10.3390/biomedicines9080995
31. Wang Y, Liu S. Lncrna ghet1 promotes hypoxia-induced glycolysis, proliferation, and invasion in triple-negative breast cancer through the hippo/yap signaling pathway. Front Cell Dev Biol. (2021) 9:643515. doi: 10.3389/fcell.2021.643515
32. Lin X, Feng D, Li P, Lv Y. Lncrna linc00857 regulates the progression and glycolysis in ovarian cancer by modulating the hippo signaling pathway. Cancer Med. (2020) 9:8122–32. doi: 10.1002/cam4.3322
33. Tan YT, Lin JF, Li T, Li JJ, Xu RH, Ju HQ. Lncrna-mediated posttranslational modifications and reprogramming of energy metabolism in cancer. Cancer Commun (Lond). (2021) 41:109–20. doi: 10.1002/cac2.12108
34. Zhou Y, Huang Y, Hu K, Zhang Z, Yang J, Wang Z. Hif1a activates the transcription of lncrna raet1k to modulate hypoxia-induced glycolysis in hepatocellular carcinoma cells via mir-100-5p. Cell Death Dis. (2020) 11:176. doi: 10.1038/s41419-020-2366-7
35. Zhang Z, Fang E, Rong Y, Han H, Gong Q, Xiao Y, et al. Hypoxia-induced lncrna casc9 enhances glycolysis and the epithelial-mesenchymal transition of pancreatic cancer by a positive feedback loop with akt/hif-1α Signaling. Am J Cancer Res. (2021) 11:123–37.
36. Masoud GN, Li W. Hif-1α Pathway: role, regulation and intervention for cancer therapy. Acta Pharm Sin B. (2015) 5:378–89. doi: 10.1016/j.apsb.2015.05.007
37. Chen F, Chen J, Yang L, Liu J, Zhang X, Zhang Y, et al. Extracellular vesicle-packaged hif-1α-stabilizing lncrna from tumour-associated macrophages regulates aerobic glycolysis of breast cancer cells. Nat Cell Biol. (2019) 21:498–510. doi: 10.1038/s41556-019-0299-0
38. Chen Z, Hu Z, Sui Q, Huang Y, Zhao M, Li M, et al. Lncrna fam83a-as1 facilitates tumor proliferation and the migration via the hif-1α/glycolysis axis in lung adenocarcinoma. Int J Biol Sci. (2022) 18:522–35. doi: 10.7150/ijbs.67556
39. Fatma H, Maurya SK, Siddique HR. Epigenetic modifications of C-myc: role in cancer cell reprogramming, progression and chemoresistance. Semin Cancer Biol. (2022) 83:166–76. doi: 10.1016/j.semcancer.2020.11.008
40. Liu J, Yuan JF, Wang YZ. Mettl3-stabilized lncrna snhg7 accelerates glycolysis in prostate cancer via srsf1/C-myc axis. Exp Cell Res. (2022) 416:113149. doi: 10.1016/j.yexcr.2022.113149
41. Xiao ZD, Han L, Lee H, Zhuang L, Zhang Y, Baddour J, et al. Energy stress-induced lncrna filnc1 represses C-myc-mediated energy metabolism and inhibits renal tumor development. Nat Commun. (2017) 8:783. doi: 10.1038/s41467-017-00902-z
42. Wang Y, Lu JH, Wu QN, Jin Y, Wang DS, Chen YX, et al. Lncrna linris stabilizes igf2bp2 and promotes the aerobic glycolysis in colorectal cancer. Mol Cancer. (2019) 18:174. doi: 10.1186/s12943-019-1105-0
43. Zhai S, Xu Z, Xie J, Zhang J, Wang X, Peng C, et al. Epigenetic silencing of lncrna linc00261 promotes C-myc-mediated aerobic glycolysis by regulating mir-222-3p/hipk2/erk axis and sequestering igf2bp1. Oncogene. (2021) 40:277–91. doi: 10.1038/s41388-020-01525-3
44. Gao FY, Li XT, Xu K, Wang RT, Guan XX. C-myc mediates the crosstalk between breast cancer cells and tumor microenvironment. Cell Commun Signal. (2023) 21:28. doi: 10.1186/s12964-023-01043-1
45. Hu ML, Wang XY, Chen WM. Tgf-B1 upregulates the expression of lncrna uca1 and its downstream hxk2 to promote the growth of hepatocellular carcinoma. Eur Rev Med Pharmacol Sci. (2018) 22:4846–54. doi: 10.26355/eurrev_201808_15620
46. Liu G, Wang L, Li Y. Inhibition of lncrna-uca1 suppresses pituitary cancer cell growth and prolactin (Prl) secretion via attenuating glycolysis pathway. In Vitro Cell Dev Biol Anim. (2020) 56:642–9. doi: 10.1007/s11626-020-00494-x
47. Wang H, Lin K, Zhu L, Zhang S, Li L, Liao Y, et al. Oncogenic lncrna linc00973 promotes warburg effect by enhancing ldha enzyme activity. Sci Bull (Beijing). (2021) 66:1330–41. doi: 10.1016/j.scib.2021.01.001
48. de Streel G, Lucas S. Targeting immunosuppression by tgf-B1 for cancer immunotherapy. Biochem Pharmacol. (2021) 192:114697. doi: 10.1016/j.bcp.2021.114697
49. Sun J, Jin T, Niu Z, Guo J, Guo Y, Yang R, et al. Lncrna dach1 protects against pulmonary fibrosis by binding to srsf1 to suppress ctnnb1 accumulation. Acta Pharm Sin B. (2022) 12:3602–17. doi: 10.1016/j.apsb.2022.04.006
50. Chen ZT, Zhang HF, Wang M, Wang SH, Wen ZZ, Gao QY, et al. Long non-coding rna linc00092 inhibits cardiac fibroblast activation by altering glycolysis in an erk-dependent manner. Cell Signal. (2020) 74:109708. doi: 10.1016/j.cellsig.2020.109708
51. Hu B, Zhao X, Wang E, Zhou J, Li J, Chen J, et al. Efficient heterologous expression of cytochrome P450 enzymes in microorganisms for the biosynthesis of natural products. Crit Rev Biotechnol. (2023) 43:227–41. doi: 10.1080/07388551.2022.2029344
52. Han J, Chen X, Wang J, Liu B. Glycolysis-related lncrna tmem105 upregulates ldha to facilitate breast cancer liver metastasis via sponging mir-1208. Cell Death Dis. (2023) 14:80. doi: 10.1038/s41419-023-05628-z
53. Wu F, Zhang W, Wei H, Ma H, Leng G, Zhang Y. Lncrna elfn1-as1 promotes proliferation, migration and invasion and suppresses apoptosis in colorectal cancer cells by enhancing G6pd activity. Acta Biochim Biophys Sin (Shanghai). (2023) 55:649–60. doi: 10.3724/abbs.2023010
54. Lan Z, Yao X, Sun K, Li A, Liu S, Wang X. The interaction between lncrna snhg6 and hnrnpa1 contributes to the growth of colorectal cancer by enhancing aerobic glycolysis through the regulation of alternative splicing of pkm. Front Oncol. (2020) 10:363. doi: 10.3389/fonc.2020.00363
55. Wang C, Li Y, Yan S, Wang H, Shao X, Xiao M, et al. Interactome analysis reveals that lncrna hulc promotes aerobic glycolysis through ldha and pkm2. Nat Commun. (2020) 11:3162. doi: 10.1038/s41467-020-16966-3
56. Zhao K, Wang X, Zhao D, Lin Q, Zhang Y, Hu Y. Lncrna hitt inhibits lactate production by repressing pkm2 oligomerization to reduce tumor growth and macrophage polarization. Res (Wash D C). (2022) 2022:9854904. doi: 10.34133/2022/9854904
57. Frisardi V, Canovi S, Vaccaro S, Frazzi R. The significance of microenvironmental and circulating lactate in breast cancer. Int J Mol Sci. (2023) 24:15369. doi: 10.3390/ijms242015369
58. Sun K, Tang S, Hou Y, Xi L, Chen Y, Yin J, et al. Oxidized atm-mediated glycolysis enhancement in breast cancer-associated fibroblasts contributes to tumor invasion through lactate as metabolic coupling. EBioMedicine. (2019) 41:370–83. doi: 10.1016/j.ebiom.2019.02.025
59. Wang T, Ye Z, Li Z, Jing DS, Fan GX, Liu MQ, et al. Lactate-induced protein lactylation: A bridge between epigenetics and metabolic reprogramming in cancer. Cell Prolif. (2023) 56:e13478. doi: 10.1111/cpr.13478
60. Li X, Yang Y, Zhang B, Lin X, Fu X, An Y, et al. Lactate metabolism in human health and disease. Signal Transduct Target Ther. (2022) 7:305. doi: 10.1038/s41392-022-01151-3
61. Certo M, Tsai CH, Pucino V, Ho PC, Mauro C. Lactate modulation of immune responses in inflammatory versus tumour microenvironments. Nat Rev Immunol. (2021) 21:151–61. doi: 10.1038/s41577-020-0406-2
62. Hui S, Ghergurovich JM, Morscher RJ, Jang C, Teng X, Lu W, et al. Glucose feeds the tca cycle via circulating lactate. Nature. (2017) 551:115–8. doi: 10.1038/nature24057
63. Zhang Y, Mao Q, Xia Q, Cheng J, Huang Z, Li Y, et al. Noncoding rnas link metabolic reprogramming to immune microenvironment in cancers. J Hematol Oncol. (2021) 14:169. doi: 10.1186/s13045-021-01179-y
64. Larionova I, Patysheva M, Iamshchikov P, Kazakova E, Kazakova A, Rakina M, et al. Pfkfb3 overexpression in monocytes of patients with colon but not rectal cancer programs pro-tumor macrophages and is indicative for higher risk of tumor relapse. Front Immunol. (2022) 13:1080501. doi: 10.3389/fimmu.2022.1080501
65. Geri JB, Oakley JV, Reyes-Robles T, Wang T, McCarver SJ, White CH, et al. Microenvironment mapping via dexter energy transfer on immune cells. Science. (2020) 367:1091–7. doi: 10.1126/science.aay4106
66. Petroni G, Buqué A, Coussens LM, Galluzzi L. Targeting oncogene and non-oncogene addiction to inflame the tumour microenvironment. Nat Rev Drug Discovery. (2022) 21:440–62. doi: 10.1038/s41573-022-00415-5
67. Zhan DT, Xian HC. Exploring the regulatory role of lncrna in cancer immunity. Front Oncol. (2023) 13:1191913. doi: 10.3389/fonc.2023.1191913
68. He R, He Y, Du R, Liu C, Chen Z, Zeng A, et al. Revisiting of tams in tumor immune microenvironment: insight from nf-Kb signaling pathway. BioMed Pharmacother. (2023) 165:115090. doi: 10.1016/j.biopha.2023.115090
69. Hartana CA, Rassadkina Y, Gao C, Martin-Gayo E, Walker BD, Lichterfeld M, et al. Long noncoding rna mir4435-2hg enhances metabolic function of myeloid dendritic cells from hiv-1 elite controllers. J Clin Invest. (2021) 131:e146136. doi: 10.1172/jci146136
70. Liu J, Zhang X, Chen K, Cheng Y, Liu S, Xia M, et al. Ccr7 chemokine receptor-inducible lnc-dpf3 restrains dendritic cell migration by inhibiting hif-1α-mediated glycolysis. Immunity. (2019) 50:600–15.e15. doi: 10.1016/j.immuni.2019.01.021
71. Li G, Kryczek I, Nam J, Li X, Li S, Li J, et al. Limit is an immunogenic lncrna in cancer immunity and immunotherapy. Nat Cell Biol. (2021) 23:526–37. doi: 10.1038/s41556-021-00672-3
72. Zhao E, Maj T, Kryczek I, Li W, Wu K, Zhao L, et al. Cancer mediates effector T cell dysfunction by targeting micrornas and ezh2 via glycolysis restriction. Nat Immunol. (2016) 17:95–103. doi: 10.1038/ni.3313
73. Yang T, Zhang R, Cui Z, Zheng B, Zhu X, Yang X, et al. Glycolysis−Related lncrna may be associated with prognosis and immune activity in grade ii−Iii glioma. Oncol Lett. (2024) 27:238. doi: 10.3892/ol.2024.14371
74. D’Angelo F, Ceccarelli M, Tala, Garofano L, Zhang J, Frattini V, et al. The molecular landscape of glioma in patients with neurofibromatosis 1. Nat Med. (2019) 25:176–87. doi: 10.1038/s41591-018-0263-8
75. Guo X, Pan Y, Xiong M, Sanapala S, Anastasaki C, Cobb O, et al. Midkine activation of cd8(+) T cells establishes a neuron-immune-cancer axis responsible for low-grade glioma growth. Nat Commun. (2020) 11:2177. doi: 10.1038/s41467-020-15770-3
76. Xia KG, Wang CM, Shen DY, Song XY, Mu XY, Zhou JW, et al. Lncrna neat1-associated aerobic glycolysis blunts tumor immunosurveillance by T cells in prostate cancer. Neoplasma. (2022) 69:594–602. doi: 10.4149/neo_2022_211021N1497
77. Xie Z, Xia J, Jiao M, Zhao P, Wang Z, Lin S, et al. Exosomal lncrna hotair induces pdl1(+) B cells to impede anti-tumor immunity in colorectal cancer. Biochem Biophys Res Commun. (2023) 644:112–21. doi: 10.1016/j.bbrc.2023.01.005
78. Teng KY, Barajas JM, Hu P, Jacob ST, Ghoshal K. Role of B cell lymphoma 2 in the regulation of liver fibrosis in mir-122 knockout mice. Biol (Basel). (2020) 9:157. doi: 10.3390/biology9070157
79. Qian CS, Li LJ, Huang HW, Yang HF, Wu DP. Myc-regulated lncrna neat1 promotes B cell proliferation and lymphomagenesis via the mir-34b-5p-gli1 pathway in diffuse large B-cell lymphoma. Cancer Cell Int. (2020) 20:87. doi: 10.1186/s12935-020-1158-6
80. Chai W, Wang X, Lu Z, Zhang S, Wang W, Wang H, et al. Elevated exosome-transferrable lncrna epb41l4a-as1 in cd56(Bright) nk cells is responsible for the impaired nk function in neuroblastoma patients by suppressing cell glycolysis. Clin Immunol. (2023) 250:109322. doi: 10.1016/j.clim.2023.109322
81. Wang QM, Lian GY, Sheng SM, Xu J, Ye LL, Min C, et al. Exosomal lncrna neat1 inhibits nk-cell activity to promote multiple myeloma cell immune escape via an ezh2/pbx1 axis. Mol Cancer Res. (2024) 22:125–36. doi: 10.1158/1541-7786.Mcr-23-0282
82. Feng J, Yu Y, Yin W, Qian S. Development and verification of a 7-lncrna prognostic model based on tumor immunity for patients with ovarian cancer. J Ovarian Res. (2023) 16:31. doi: 10.1186/s13048-023-01099-0
83. Li J, Zhang Y, Li C, Wu H, Feng C, Wang W, et al. A lactate-related lncrna model for predicting prognosis, immune landscape and therapeutic response in breast cancer. Front Genet. (2022) 13:956246. doi: 10.3389/fgene.2022.956246
84. Shao T, Xie Y, Shi J, Yang C, Zou H, Li Y, et al. Surveying lncrna-lncrna cooperations reveals dominant effect on tumor immunity cross cancers. Commun Biol. (2022) 5:1324. doi: 10.1038/s42003-022-04249-0
85. Xiao J, Wang X, Liu Y, Liu X, Yi J, Hu J. Lactate metabolism-associated lncrna pairs: A prognostic signature to reveal the immunological landscape and mediate therapeutic response in patients with colon adenocarcinoma. Front Immunol. (2022) 13:881359. doi: 10.3389/fimmu.2022.881359
86. Wu L, Liu J, Li W. Prognostic significance of a 4-lncrna glycolysis-related signature in oral squamous cell carcinoma. J Dent Sci. (2022) 17:991–1000. doi: 10.1016/j.jds.2021.11.002
87. Tao H, Zhong X, Zeng A, Song L. Unveiling the veil of lactate in tumor-associated macrophages: A successful strategy for immunometabolic therapy. Front Immunol. (2023) 14:1208870. doi: 10.3389/fimmu.2023.1208870
88. Hou J, Zhang G, Wang X, Wang Y, Wang K. Functions and mechanisms of lncrna malat1 in cancer chemotherapy resistance. biomark Res. (2023) 11:23. doi: 10.1186/s40364-023-00467-8
89. Davern M, Lysaght J. Cooperation between chemotherapy and immunotherapy in gastroesophageal cancers. Cancer Lett. (2020) 495:89–99. doi: 10.1016/j.canlet.2020.09.014
90. Shi L, Duan R, Sun Z, Jia Q, Wu W, Wang F, et al. Lncrna gltc targets ldha for succinylation and enzymatic activity to promote progression and radioiodine resistance in papillary thyroid cancer. Cell Death Differ. (2023) 30:1517–32. doi: 10.1038/s41418-023-01157-6
91. Hua G, Zeng ZL, Shi YT, Chen W, He LF, Zhao GF. Lncrna xist contributes to cisplatin resistance of lung cancer cells by promoting cellular glycolysis through sponging mir-101-3p. Pharmacology. (2021) 106:498–508. doi: 10.1159/000512621
92. Zheng H, Zhang M, Ke X, Deng X, Li D, Wang Q, et al. Lncrna xist/mir-137 axis strengthens chemo-resistance and glycolysis of colorectal cancer cells by hindering transformation from pkm2 to pkm1. Cancer biomark. (2021) 30:395–406. doi: 10.3233/cbm-201740
93. Shi H, Li K, Feng J, Liu G, Feng Y, Zhang X. Lncrna-dancr interferes with mir-125b-5p/hk2 axis to desensitize colon cancer cells to cisplatin vis activating anaerobic glycolysis. Front Oncol. (2020) 10:1034. doi: 10.3389/fonc.2020.01034
94. Wen JF, Jiang YQ, Li C, Dai XK, Wu T, Yin WZ. Lncrna-sarcc sensitizes osteosarcoma to cisplatin through the mir-143-mediated glycolysis inhibition by targeting hexokinase 2. Cancer biomark. (2020) 28:231–46. doi: 10.3233/cbm-191181
95. Xu F, Huang M, Chen Q, Niu Y, Hu Y, Hu P, et al. Lncrna hif1a-as1 promotes gemcitabine resistance of pancreatic cancer by enhancing glycolysis through modulating the akt/yb1/hif1α Pathway. Cancer Res. (2021) 81:5678–91. doi: 10.1158/0008-5472.Can-21-0281
96. Wang Q, Zeng A, Zhu M, Song L. Dual inhibition of egfr−Vegf: an effective approach to the treatment of advanced non−Small cell lung cancer with egfr mutation (Review). Int J Oncol. (2023) 62:26. doi: 10.3892/ijo.2023.5474
97. Zhang H, Zhao L, Ren P, Sun X. Lncrna mbnl1-as1 knockdown increases the sensitivity of hepatocellular carcinoma to tripterine by regulating mir-708-5p-mediated glycolysis. Biotechnol Genet Eng Rev. (2023) 23:1–18. doi: 10.1080/02648725.2023.2193776
98. Zhao L, Zhang H, Ren P, Sun X. Lncrna slc9a3-as1 knockdown increases the sensitivity of liver cancer cell to triptolide by regulating mir-449b-5p-mediated glycolysis. Biotechnol Genet Eng Rev. (2023) 22:1–17. doi: 10.1080/02648725.2023.2193775
99. Shen Y, Xu J, Pan X, Zhang Y, Weng Y, Zhou D, et al. Lncrna kcnq1ot1 sponges mir-34c-5p to promote osteosarcoma growth via aldoa enhanced aerobic glycolysis. Cell Death Dis. (2020) 11:278. doi: 10.1038/s41419-020-2485-1
100. Pierrakos C, Vincent JL. Sepsis biomarkers: A review. Crit Care. (2010) 14:R15. doi: 10.1186/cc8872
101. Vernooij LM, van Klei WA, Moons KG, Takada T, van Waes J, Damen JA. The comparative and added prognostic value of biomarkers to the revised cardiac risk index for preoperative prediction of major adverse cardiac events and all-cause mortality in patients who undergo noncardiac surgery. Cochrane Database Syst Rev. (2021) 12:Cd013139. doi: 10.1002/14651858.CD013139.pub2
102. Badowski C, He B, Garmire LX. Blood-derived lncrnas as biomarkers for cancer diagnosis: the good, the bad and the beauty. NPJ Precis Oncol. (2022) 6:40. doi: 10.1038/s41698-022-00283-7
103. Wang Y, Lin Y, Wu S, Sun J, Meng Y, Jin E, et al. Bioka: A curated and integrated biomarker knowledgebase for animals. Nucleic Acids Res. (2023) 52(D1):1121–30. doi: 10.1093/nar/gkad873
104. Bo H, Fan L, Li J, Liu Z, Zhang S, Shi L, et al. High expression of lncrna afap1-as1 promotes the progression of colon cancer and predicts poor prognosis. J Cancer. (2018) 9:4677–83. doi: 10.7150/jca.26461
105. Yang J, Shi X, Yang M, Luo J, Gao Q, Wang X, et al. Glycolysis reprogramming in cancer-associated fibroblasts promotes the growth of oral cancer through the lncrna H19/mir-675-5p/pfkfb3 signaling pathway. Int J Oral Sci. (2021) 13:12. doi: 10.1038/s41368-021-00115-7
106. Zhang X, Luo M, Zhang J, Guo B, Singh S, Lin X, et al. The role of lncrna H19 in tumorigenesis and drug resistance of human cancers. Front Genet. (2022) 13:1005522. doi: 10.3389/fgene.2022.1005522
107. Hashemi M, Moosavi MS, Abed HM, Dehghani M, Aalipour M, Heydari EA, et al. Long non-coding rna (Lncrna) H19 in human cancer: from proliferation and metastasis to therapy. Pharmacol Res. (2022) 184:106418. doi: 10.1016/j.phrs.2022.106418
108. Liao T, Lu Y, Li W, Wang K, Zhang Y, Luo Z, et al. Construction and validation of a glycolysis-related lncrna signature for prognosis prediction in stomach adenocarcinoma. Front Genet. (2022) 13:794621. doi: 10.3389/fgene.2022.794621
109. How J, Hobbs GS. A practical guide for using myelofibrosis prognostic models in the clinic. J Natl Compr Canc Netw. (2020) 18:1271–8. doi: 10.6004/jnccn.2020.7557
110. Reeve K, On BI, Havla J, Burns J, Gosteli-Peter MA, Alabsawi A, et al. Prognostic models for predicting clinical disease progression, worsening and activity in people with multiple sclerosis. Cochrane Database Syst Rev. (2023) 9:Cd013606. doi: 10.1002/14651858.CD013606.pub2
111. Li T, Tong H, Zhu J, Qin Z, Yin S, Sun Y, et al. Identification of a three-glycolysis-related lncrna signature correlated with prognosis and metastasis in clear cell renal cell carcinoma. Front Med (Lausanne). (2021) 8:777507. doi: 10.3389/fmed.2021.777507
112. Ma W, Zhong M, Liu X. Identification of a glycolysis-related lncrna prognostic signature for clear cell renal cell carcinoma. Biosci Rep. (2021) 41:BSR20211451. doi: 10.1042/bsr20211451
113. Zheng Z, Lai C, Li W, Zhang C, Ma K, Yao Y. Identification of a novel glycolysis-related lncrna signature for predicting overall survival in patients with bladder cancer. Front Genet. (2021) 12:720421. doi: 10.3389/fgene.2021.720421
114. Chen D, Li Y, Wang Y, Xu J. Lncrna hotairm1 knockdown inhibits cell glycolysis metabolism and tumor progression by mir-498/abce1 axis in non-small cell lung cancer. Genes Genomics. (2021) 43:183–94. doi: 10.1007/s13258-021-01052-9
115. Wang S, Wang T, Liu D, Kong H. Lncrna malat1 aggravates the progression of non-small cell lung cancer by stimulating the expression of commd8 via targeting mir-613. Cancer Manag Res. (2020) 12:10735–47. doi: 10.2147/cmar.S263538
116. Zhang ZL, Fang EH, Rong YP, Han H, Gong Q, Xiao YY, et al. Hypoxia-induced lncrna casc9 enhances glycolysis and the epithelial-mesenchymal transition of pancreatic cancer by a positive feedback loop with akt/hif-1α Signaling [Retraction. Am J Cancer Res. (2021) 11:3377.
117. Liu J, Zhu Y, Wang H, Han C, Wang Y, Tang R. Linc00629, a hoxb4-downregulated long noncoding rna, inhibits glycolysis and ovarian cancer progression by destabilizing C-myc. Cancer Sci. (2024) 115:804–19. doi: 10.1111/cas.16049
118. Zeng Z, Wang J, Xu F, Hu P, Hu Y, Zhuo W, et al. The M6a modification-mediated positive feedback between glycolytic lncrna slc2a1-dt and C-myc promotes tumorigenesis of hepatocellular carcinoma. Int J Biol Sci. (2024) 20:1744–62. doi: 10.7150/ijbs.86658
119. Zhang C, Wang H, Liu Q, Dai S, Tian G, Wei X, et al. Lncrna ccat1 facilitates the progression of gastric cancer via ptbp1-mediated glycolysis enhancement. J Exp Clin Cancer Res. (2023) 42:246. doi: 10.1186/s13046-023-02827-6
120. Bian Z, Zhang J, Li M, Feng Y, Wang X, Zhang J, et al. Lncrna-fezf1-as1 promotes tumor proliferation and metastasis in colorectal cancer by regulating pkm2 signaling. Clin Cancer Res. (2018) 24:4808–19. doi: 10.1158/1078-0432.Ccr-17-2967
121. Li D, Xie Y, Sun J, Zhang L, Jiang W. Lncrna znf674-as1 hinders proliferation and invasion of hepatic carcinoma cells through the glycolysis pathway. J Oncol. (2022) 2022:8063382. doi: 10.1155/2022/8063382
122. Cui K, Wu H, Fan J, Zhang L, Li H, Guo H, et al. The mixture of ferulic acid and P-coumaric acid suppresses colorectal cancer through lncrna 495810/pkm2 mediated aerobic glycolysis. Int J Mol Sci. (2022) 23:12106. doi: 10.3390/ijms232012106
123. Guan YF, Huang QL, Ai YL, Chen QT, Zhao WX, Wang XM, et al. Nur77-activated lncrna wfdc21p attenuates hepatocarcinogenesis via modulating glycolysis. Oncogene. (2020) 39:2408–23. doi: 10.1038/s41388-020-1158-y
124. Bian Z, Yang F, Xu P, Gao G, Yang C, Cao Y, et al. Linc01852 inhibits the tumorigenesis and chemoresistance in colorectal cancer by suppressing srsf5-mediated alternative splicing of pkm. Mol Cancer. (2024) 23:23. doi: 10.1186/s12943-024-01939-7
125. Yao Y, Chen X, Wang X, Li H, Zhu Y, Li X, et al. Glycolysis related lncrna snhg3/mir-139-5p/pkm2 axis promotes castration-resistant prostate cancer (Crpc) development and enzalutamide resistance. Int J Biol Macromol. (2024) 260:129635. doi: 10.1016/j.ijbiomac.2024.129635
126. Fei M, Li X, Liang S, Zhou S, Wu H, Sun L, et al. Lncrna pwrn1 inhibits the progression of hepatocellular carcinoma by activating pkm2 activity. Cancer Lett. (2024) 584:216620. doi: 10.1016/j.canlet.2024.216620
127. Jin Y, Jiang A, Sun L, Lu Y. Long noncoding rna tmpo-as1 accelerates glycolysis by regulating the mir-1270/pkm2 axis in colorectal cancer. BMC Cancer. (2024) 24:238. doi: 10.1186/s12885-024-11964-w
128. Yan T, Shen C, Jiang P, Yu C, Guo F, Tian X, et al. Risk snp-induced lncrna-slcc1 drives colorectal cancer through activating glycolysis signaling. Signal Transduct Target Ther. (2021) 6:70. doi: 10.1038/s41392-020-00446-7
129. Duan BX, Geng XR, Wu YQ. Lncrna rncr2 facilitates cell proliferation and epithelial-mesenchymal transition in melanoma through hk2-mediated warburg effect via targeting mir-495-3p. Neoplasma. (2021) 68:692–701. doi: 10.4149/neo_2021_201120N1255
130. Zhao S, Guan B, Mi Y, Shi D, Wei P, Gu Y, et al. Lncrna mir17hg promotes colorectal cancer liver metastasis by mediating a glycolysis-associated positive feedback circuit. Oncogene. (2021) 40:4709–24. doi: 10.1038/s41388-021-01859-6
131. Huang SL, Huang ZC, Zhang CJ, Xie J, Lei SS, Wu YQ, et al. Lncrna snhg5 promotes the glycolysis and proliferation of breast cancer cell through regulating bach1 via targeting mir-299. Breast Cancer. (2022) 29:65–76. doi: 10.1007/s12282-021-01281-6
132. Guo T, Liu D, Peng S, Wang M, Li Y. A positive feedback loop of lncrna mir31hg-mir-361-3p -yy1 accelerates colorectal cancer progression through modulating proliferation, angiogenesis, and glycolysis. Front Oncol. (2021) 11:684984. doi: 10.3389/fonc.2021.684984
133. Chi JR, Yu ZH, Liu BW, Zhang D, Ge J, Yu Y, et al. Snhg5 promotes breast cancer proliferation by sponging the mir-154-5p/pcna axis. Mol Ther Nucleic Acids. (2019) 17:138–49. doi: 10.1016/j.omtn.2019.05.013
134. Xu S, Cheng Z, Du B, Diao Y, Li Y, Li X. Lncrna ap000695.2 promotes glycolysis of lung adenocarcinoma via the mir-335-3p/tead1 axis. Acta Biochim Biophys Sin (Shanghai). (2023) 55:1592–605. doi: 10.3724/abbs.2023227
135. Fang K, Xu ZJ, Jiang SX, Tang DS, Yan CS, Deng YY, et al. Lncrna fgd5−As1 promotes breast cancer progression by regulating the hsa−Mir−195−5p/nuak2 axis. Mol Med Rep. (2021) 23:460. doi: 10.3892/mmr.2021.12099
136. Hu Y, Sun H, Hu J, Zhang X. Lncrna dlx6-as1 promotes the progression of neuroblastoma by activating stat2 via targeting mir-506-3p. Cancer Manag Res. (2020) 12:7451–63. doi: 10.2147/cmar.S252521
137. Lu L, Huang J, Mo J, Da X, Li Q, Fan M, et al. Exosomal lncrna tug1 from cancer-associated fibroblasts promotes liver cancer cell migration, invasion, and glycolysis by regulating the mir-524-5p/six1 axis. Cell Mol Biol Lett. (2022) 27:17. doi: 10.1186/s11658-022-00309-9
138. Zhang Z, Yang W, Li N, Chen X, Ma F, Yang J, et al. Lncrna mcf2l-as1 aggravates proliferation, invasion and glycolysis of colorectal cancer cells via the crosstalk with mir-874-3p/foxm1 signaling axis. Carcinogenesis. (2021) 42:263–71. doi: 10.1093/carcin/bgaa093
139. Cheng Z, Luo C, Guo Z. Lncrna-xist/microrna-126 sponge mediates cell proliferation and glucose metabolism through the irs1/pi3k/akt pathway in glioma. J Cell Biochem. (2020) 121:2170–83. doi: 10.1002/jcb.29440
140. Wan T, Zheng J, Yao R, Yang S, Zheng W, Zhou P. Lncrna ddx11-as1 accelerates hepatocellular carcinoma progression via the mir-195-5p/macc1 pathway. Ann Hepatol. (2021) 20:100258. doi: 10.1016/j.aohep.2020.09.003
141. Xu S, Jia G, Zhang H, Wang L, Cong Y, Lv M, et al. Lncrna hoxb-as3 promotes growth, invasion and migration of epithelial ovarian cancer by altering glycolysis. Life Sci. (2021) 264:118636. doi: 10.1016/j.lfs.2020.118636
142. Shi L, Li B, Zhang Y, Chen Y, Tan J, Chen Y, et al. Exosomal lncrna mir100hg derived from cancer stem cells enhance glycolysis and promote metastasis of lung adenocarcinoma through mircrorna-15a-5p/31-5p. Cell Commun Signal. (2023) 21:248. doi: 10.1186/s12964-023-01281-3
143. Wu K, Wang Z, Huang Y, Yao L, Kang N, Ge W, et al. Lncrna ptprg-as1 facilitates glycolysis and stemness properties of esophageal squamous cell carcinoma cells through mir-599/pdk1 axis. J Gastroenterol Hepatol. (2022) 37:507–17. doi: 10.1111/jgh.15719
144. Xing TR, Chen P, Wu JM, Gao LL, Yang W, Cheng Y, et al. Upf1 participates in the progression of endometrial cancer by inhibiting the expression of lncrna pvt1. Onco Targets Ther. (2020) 13:2103–14. doi: 10.2147/ott.S233149
145. Hu Y, Tang J, Xu F, Chen J, Zeng Z, Han S, et al. A reciprocal feedback between N6-methyladenosine reader ythdf3 and lncrna dicer1-as1 promotes glycolysis of pancreatic cancer through inhibiting maturation of mir-5586-5p. J Exp Clin Cancer Res. (2022) 41:69. doi: 10.1186/s13046-022-02285-6
146. Liao M, Liao W, Xu N, Li B, Liu F, Zhang S, et al. Lncrna epb41l4a-as1 regulates glycolysis and glutaminolysis by mediating nucleolar translocation of hdac2. EBioMedicine. (2019) 41:200–13. doi: 10.1016/j.ebiom.2019.01.035
147. Su X, Xue C, Xie C, Si X, Xu J, Huang W, et al. Lncrna-let regulates glycolysis and glutamine decomposition of esophageal squamous cell carcinoma through mir-93-5p/mir-106b-5p/socs4. Front Oncol. (2022) 12:897751. doi: 10.3389/fonc.2022.897751
148. Chen XX, Zhang N, Fu XF, Jiang Y, Wang MY. Lncrna dbh-as1 facilitates the tumorigenesis of melanoma by targeting mir-233-3p via igf-1r/akt signaling. Eur Rev Med Pharmacol Sci. (2020) 24:7698–708. doi: 10.26355/eurrev_202007_22272
149. Zhao X, Zhu X, Xiao C, Hu Z. Lncrna sh3bp5-as1 promotes hepatocellular carcinoma progression by sponging mir-6838-5p and activation of ptpn4. Aging (Albany NY). (2024) 16:8511–23. doi: 10.18632/aging.205811
150. Chan YT, Wu J, Lu Y, Li Q, Feng Z, Xu L, et al. Loss of lncrna linc01056 leads to sorafenib resistance in hcc. Mol Cancer. (2024) 23:74. doi: 10.1186/s12943-024-01988-y
Keywords: cancer, glycolysis, immune, lncRNA, metabolism
Citation: Zhang X, Zhang Y, Liu Q, Zeng A and Song L (2024) Glycolysis-associated lncRNAs in cancer energy metabolism and immune microenvironment: a magic key. Front. Immunol. 15:1456636. doi: 10.3389/fimmu.2024.1456636
Received: 28 June 2024; Accepted: 27 August 2024;
Published: 13 September 2024.
Edited by:
Leonard Maggi, Washington University in St. Louis, United StatesReviewed by:
Gopinath Prakasam, University of Texas Southwestern Medical Center, United StatesDehai Yu, First Affiliated Hospital of Jilin University, China
Copyright © 2024 Zhang, Zhang, Liu, Zeng and Song. This is an open-access article distributed under the terms of the Creative Commons Attribution License (CC BY). The use, distribution or reproduction in other forums is permitted, provided the original author(s) and the copyright owner(s) are credited and that the original publication in this journal is cited, in accordance with accepted academic practice. No use, distribution or reproduction is permitted which does not comply with these terms.
*Correspondence: Linjiang Song, c29uZ2xpbmppYW5nQGNkdXRjbS5lZHUuY24=; Anqi Zeng, emVuZzYwMDJhcUAxNjMuY29t