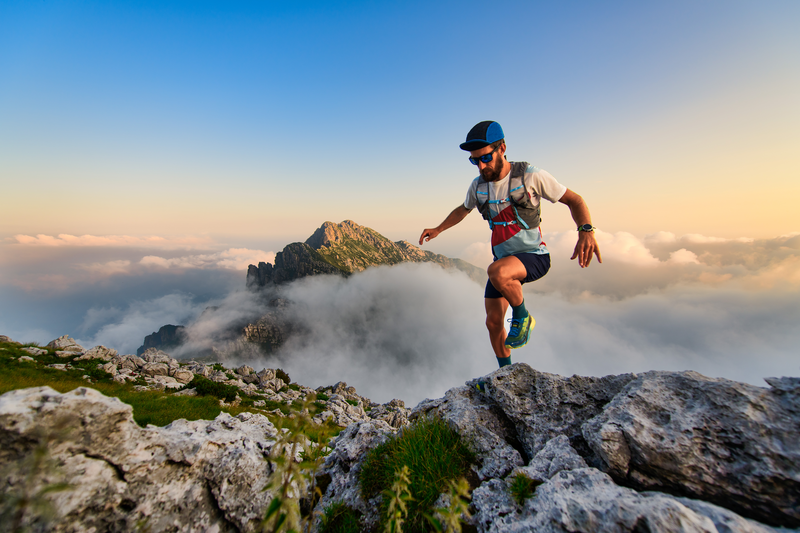
94% of researchers rate our articles as excellent or good
Learn more about the work of our research integrity team to safeguard the quality of each article we publish.
Find out more
REVIEW article
Front. Immunol. , 17 December 2024
Sec. Autoimmune and Autoinflammatory Disorders : Autoimmune Disorders
Volume 15 - 2024 | https://doi.org/10.3389/fimmu.2024.1455009
Bronchiolitis obliterans (BO) is a disease characterized by airway obstruction and fibrosis that can occur in all age groups. Bronchiolitis obliterans syndrome (BOS) is a clinical manifestation of BO in patients who have undergone lung transplantation or hematopoietic stem cell transplantation. Persistent inflammation and fibrosis of small airways make the disease irreversible, eventually leading to lung failure. The pathogenesis of BO is not entirely clear, but immune disorders are commonly involved, with various immune cells playing complex roles in different BO subtypes. Accordingly, the US Food and Drug Administration (FDA) has recently approved several new drugs that can alleviate chronic graft-versus-host disease (cGVHD) by regulating the function of immune cells, some of which have efficacy specifically with cGVHD-BOS. In this review, we will discuss the roles of different immune cells in BO/BOS, and introduce the latest drugs targeting various immune cells as the main target. This study emphasizes that immune dysfunction is an important driving factor in its pathophysiology. A better understanding of the role of the immune system in BO will enable the development of targeted immunotherapies to effectively delay or even reverse this condition.
Bronchiolitis obliterans (BO) is a chronic, irreversible pulmonary disease characterized by small airway obstruction and/or occlusion and peripheral and distal bronchiolar fibrosis (1). Typical signs and symptoms include dyspnea, wheezing, and hypoxemia. However, the patient may be asymptomatic, and BO can be diagnosed at an early stage by pulmonary function test (PFT) and computed tomography (CT) (2). The presence of obstructive airflow patterns in PFT and air trapping detected by CT during exhalation are characteristic indicators of BO (3). BO can occur in all ages, but its etiology differs in children and adults. Bronchiolitis obliterans syndrome (BOS) is a clinical manifestation of BO in patients who have undergone lung transplantation or hematopoietic stem cell transplantation (HSCT) (3). BO occurs in different diseases and is thus variously categorized under different medical terms. Chronic lung allograft dysfunction (CLAD)-BOS and chronic graft-versus-host disease (cGVHD)-BOS are the most common etiologies in adults (4), whereas post-infection-BO (PIBO) most commonly affects children (5).
PIBO is one of the rare complications of severe lower respiratory tract infections (6). Although PIBO can occur in patients of any age, it is more common in children, most often in association with adenovirus infection (7–10). However, other viruses (e.g., influenza, parainfluenza, respiratory syncytial virus, human metapneumovirus, human immunodeficiency virus-1, measles, cytomegalovirus, Sars-Cov-2) and bacteria (Mycoplasma pneumoniae, Legionella pneumophila, Bordetella pertussis) can also cause PIBO in the event of severe lower respiratory tract infection (11, 12). Based on histopathological features, BO can be categorized into two types: 1) proliferative BO, characterized by granulation tissue polyps obstructing the small airway, and 2) contractile BO, marked by peribronchiolar fibrosis resulting in varying degrees of lumenal constriction. As the disease progresses, the histological features of PIBO primarily exhibit a contractile pattern, accompanied by variable degrees of dilatation and airway obstruction (13). The pathological manifestations of patients with PIBO vary greatly, and the involvement of bronchioles is heterogeneous. Other signs of persistent airway disease in PIBO include bronchiolitis, mucus deposition, macrophage aggregation, and bronchiole distortion and expansion (13–15).
Lung transplantation is considered to be the best treatment option for patients with end-stage lung disease (16). CLAD is an umbrella term used to define a persistent (≥3 weeks) decline in pulmonary function (forced expiratory volume in 1 second (FEV 1), with or without a decline in forced vital capacity) of ≥10% from baseline (17). CLAD is the main cause of death one year after transplantation, and BOS is the most common CLAD phenotype. In the survival of lung transplant recipients, the prevalence rate of CLAD-BOS is 50% after 5 years and 76% after 10 years (16). From the perspective of histopathology, BOS is characterized by the accumulation of extracellular matrix under the mucosa, partial destruction of the original smooth muscle layer, or myocyte proliferation, and ultimately complete airway obstruction (3). Antibodies play an important role in the occurrence and development of CLAD-BOS. Graft-reactive antibodies can induce the activation of the complement system and the degradation of lung tissue, leading to the formation of CLAD-BOS (18).
BOS is a rare complication of allogeneic HSCT, characterized by fixed airflow obstruction after allogeneic HSCT. The incidence of BOS in allogeneic HSCT recipients is about 2-3%, but it can reach 6% in patients with cGVHD (19, 20). The early processes leading to BOS in cGVHD are different from those in CLAD, but the final histological changes are relatively similar (21). However, the etiology of BOS is still unknown. Allogeneic recognition of lung antigens may be the cause of this disease, and BOS involves allogeneic immunity, namely HSCT graft-versus-host (lung) disease. In fact, in cGVHD, lung epithelium may be a target of donor cytotoxic T cells, supporting the hypothesis that BOS is the manifestation of cGVHD in the lungs (22).
This paper summarizes research on patients with BO and animal models and reviews the roles of various immune cells, including macrophages, neutrophils, eosinophils, natural killer (NK) cells, B lymphocytes, and T lymphocytes, on BO onset and progression. The most common cause of PIBO is adenovirus infection. Castleman et al. (23) induced beagle dogs with adenovirus to establish a BO model that adequately reflects the pathophysiological process of BO formation caused by viral infection. However, owing to the considerable costs associated with large animal breeding and experimentation, the model is hindered from widespread adoption. Rodent models are therefore more commonly used, due to lower costs, simpler procedures, and easier reproducibility. However, because respiratory bronchioles are absent or rare in rodents, such studies cannot sufficiently reflect the complexity of clinical BO (24, 25). Hence, the relevance of virus-induced BO rodent models has yet to be validated. For non-transplantation models, diacetyl (26), nitric acid (27), chlorine (28), sulfur mustard (29), and papaverine (30) are currently used more frequently. Still, although these animal models can develop airway obstruction and even fibrosis, they cannot accurately reflect the pathophysiological process of BO. In turn, human research on BO relies on tissue specimens, but histological confirmation is often difficult due to insufficient diagnostic sensitivity of bronchoscopic biopsy specimens. Despite these caveats, animal models that effectively simulate human pathophysiological changes often provide detailed mechanistic insights, which are challenging to obtain in human research.
Monocytes and macrophages are multifunctional immune cells that exist or infiltrate tissues and crucially influence innate immunity, normal tissue development, homeostasis, and repair of damaged tissues (31). Macrophages are involved in various conditions and diseases, such as inflammation, tumors, and autoimmune disorders, and play essential roles in eliminating pathogens and regulating immune function (32, 33). Alveolar macrophages (AM) serve as the primary defenders for the airways and alveoli against pathogens, while pulmonary interstitial macrophages act as vital gatekeepers for the vascular system and pulmonary interstitium (34) (Figure 1).
Figure 1. Immune cells in bronchiolitis obliterans (BO). There are three main entities of BO: post infection BO; BO after stem cell transplantation and BO after lung transplantation. Macrophages, neutrophils, eosinophils, Th1 and Th17 cells may contribute to disease progression, while Th2, NK, and Tregs seem to have controversial roles. The balance between Tregs and Th17 cells is implicated in pulmonary fibrosis. MPO, Myeloperoxidase; ECM, Extracellular matrix; Treg cell, Regulatory T cell; NK cell, Natural killer cell; APC, Antigen-presenting cells; MT1H, Metallothionein-1H; NKG7, Natural killer cell granule protein 7.
Macrophages play a major role in the occurrence and development of BO. Duecker et al. (35) found that the percentage of macrophages in the sputum of patients with PIBO was significantly lower than that of healthy controls. Similarly, Palmer et al. (36) found that on day 1 post-induction, AM numbers in an animal model of BO were significantly higher than in the control group, while on days 3 and 7, AM abundance decreased slightly. O’Koren et al. (37) observed that AM levels rose during the initial phase and declined during the subsequent phase. In this model, infiltration of inflammatory cells, particularly monocytes and AM, preceded the development of intraluminal lesions, which manifested typically after 7 days of chlorine exposure. Meanwhile, suggesting the potential value of anti-TNF-α therapy in CLAD-BOS, it was further reported that classically activated macrophages adjacent to bronchial epithelial cells of lung transplant patients show increased secretion of TNF-α and IL-1β, in parallel with significantly enhanced epithelial-mesenchymal transition (EMT) driven by transforming growth factor beta-1 (TGF-β1) (38). This indicates that anti-TNF-α can inhibit macrophages driven EMT function and thus treat BOS.
Macrophage dysfunction appears to be involved in the pathogenesis of BOS after lung transplantation, and potential therapeutic targets might be derived from the underlying mechanisms (39). It is controversial whether the main source of AM after transplantation comes from the donor or the recipient. Nayak et al. (40) demonstrated that >94% of AM in the bronchoalveolar lavage fluid (BALF) of human lung transplant recipients are sourced from the donor, being the predominant macrophage subset for at least 2 to 3 years after transplantation (41). The inflammatory cascade reaction caused by donor-derived AM leads to the injury of airway epithelium, which eventually leads to the obstructive airway disease of transplanted lung. However, Snyder et al. (42) found that about 2 years post-transplantation, the proportion of donor macrophages in lung recipients was only about 10.5%. Similarly, after HSCT, the majority of infiltrating AM appears to originate from the donor’s hematopoietic cells (43). Thus, there are conflicting data on the proportion of donor-derived AM in lung transplant recipients.
Based on the activation state and function of macrophages, these cells can be categorized into an M1 phenotype (classically activated macrophages) and an M2 phenotype (alternatively activated macrophages) (44). M1 macrophages are primarily involved in immune responses such as microbial killing and anti-tumor effects, while M2 macrophages are primarily involved in immune suppression and tissue repair. Both macrophage types play essential roles in the immune system, maintaining a dynamic equilibrium. Although the classification of macrophage phenotypes into a M1 and M2 category is oversimplified, the extreme heterogeneity of response to stimuli highlights the need for a better understanding of the function of macrophages in human pathology. The AM observed in the early phase of cGVHD-BOS are positive for CD68 and inducible nitric oxide synthase and negative for CD163 and CD206, suggesting an M1 phenotype. This suggests that donor-derived M1-AM may contribute to the pathogenesis of the early phase of cGVHD-BOS, while AM exhibiting M2 polarization potentially participate in fibrosis in the late phase (43).
During the formation of fibrotic scars, various cells secrete platelet-derived growth factor (PDGF) in response to injury, and many pro-inflammatory cytokines facilitate their mitogenic effects through the autocrine secretion of PDGF. Through immunohistochemical and in situ hybridization studies on tissue sections and BALF cells of patients with CLAD-BOS, it was found that AM are one of the cell sources of PDGF (45). TGF-β also plays a significant role in pulmonary fibrosis. During the acute complications of lung transplantation, AM are initially activated, leading to excessive secretion of interleukin-6 (IL-6), which mediates tissue repair (46). High airway TGF-β activity can enhance the expression of chemokine ligand 2 (CCL2) in AM, resulting in the development of BO mouse model driven by CCR2+ monocytes (47). It can thus be questioned whether the severity of BO rat model can be reduced by inhibiting the number and activation of AM. It was shown that macrophage depletion with GdCl3 significantly reduced the development of occlusive airway disease in experimental heterotopic tracheal allografts, resulting in a decrease in PDGF mRNA expression (48). In turn, inhibition of macrophage migration inhibitory factor (MIF) significantly prevented tracheal epithelial and luminal occlusions caused by fibrosis, reflecting blockade of MIF-related adverse immune reactions associated with allograft rejection (49).
Interestingly, Alexander et al. (50) reported that colony-stimulating factor 1 (CSF-1)-dependent donor derived macrophages mediate the occurrence of cGVHD in mouse models. Axatilimab is a hinge-stabilized IgG4κ antibody targeting the CSF-1 receptor, which is the first cGVHD therapeutic drug targeting disease-related macrophages (51). Among patients receiving approved doses of 0.3mg/kg niktimvo every two weeks (N=79), 75% of patients achieved an overall response rate (ORR) within the first six months of treatment. The pulmonary response rate was 50%, with 16 patients showing complete response (52). In 2024, the US Food and Drug Administration (FDA) has recently granted axatilimab orphan drug designation for patients with cGVHD and idiopathic pulmonary fibrosis.
During pulmonary infections, neutrophils are recruited to fend off further immune cell recruitment by engulfing necrotic cells. Concurrently, they facilitate tissue growth and neovascularization by releasing cytokines and synthesizing granule enzymes, playing an important role in tissue breakdown and repair (53). Neutrophils are persistently activated at sites of chronic inflammation, fueling the inflammatory process through the release of proteases, the formation of neutrophil extracellular traps (NETs), and the activation of other immune cells (54). As key players in chronic respiratory disorders, neutrophils are integral to the progression of conditions such as chronic obstructive pulmonary disease (COPD), asthma, and pulmonary fibrosis (55).
The characteristic airway fibrosis in BO is due to the inability of the airway epithelium to repair normally after injury (1). In this context, a large number of T cells and neutrophils functionally replace epithelial cells, followed by matrix degradation, collagen deposition, and fibroblast stimulation, ultimately leading to airway fibrosis (6). It was reported that patients with BO/BOS showed a significant increase in the percentage of neutrophils in BALF, sputum, and on histopathology (56–59).
Due to neutrophils’ capacity to induce ongoing airway remodeling and inflammation, their recruitment and activation may cause harm to the lung tissue of patients with CLAD-BOS (60, 61). Azithromycin can significantly reduce the number of airway neutrophils in patients with CLAD-BOS, which may be one of the mechanisms behind its therapeutic effects (62). The early increase of neutrophils in BALF has a predictive effect on the occurrence of BOS within 12 months after lung transplantation. By monitoring the number of neutrophils in BALF, CLAD-BOS can be determined earlier (63). Therefore, interrupting neutrophil-dependent pathways may mitigate tissue damage in BO/BOS. A phase I clinical trial of alvelestat (n = 7), an inhibitor of neutrophil elastase (NE), has been used in patients with cGVHD-BOS. In this trial, 6 patients had stable disease, while 1 patient had progression in the setting of pneumonia. Moreover, 2 patients had improvement in FEV1 of 9%, and 4 patients experienced improvement in symptoms (64).
Elevated blood counts of eosinophils, a type of innate immune cell, are closely linked to the severity of pulmonary fibrosis (65). Under the influence of inflammatory mediators, activated eosinophils induce fibroblasts to produce IL-6 and other pro-fibrotic cytokines (66). Through autocrine or paracrine mechanisms, these cytokines cause the fibroblasts to proliferate and differentiate into myofibroblasts. This results in excessive deposition of extracellular matrix (ECM) within the lung parenchyma, ultimately leading to pulmonary fibrosis. In patients with CLAD-BOS, eosinophil counts also rise, potentially serving as effector cells to exacerbate disease symptoms (67). Using a cut-off of ≥2% eosinophils, a BALF eosinophilia was recorded in patients with CLAD-BOS (25/79; 31.6%), compared to control lung transplant patients without BOS (41/277; 14.8%).
Montelukast is a potent, specific cysteinyl leukotriene receptor antagonist which can significantly decreases blood eosinophils (68). Suguru et al. (69) found that eosinophilia after allogeneic HSCT occurs before the onset of cGVHD, and early eosinophilia may predict the occurrence of cGVHD in children. A phase II clinical trial was shown that montelukast stabilized FEV1% predicted in all patients with cGVHD-BOS (n=23/23, 100%) at six months, improved compared to historical controls (60%) (70).
NK cells coordinate both innate and adaptive immune responses by stimulating the maturation of dendritic-like cells and B cells, the polarization of helper T cells, and the activation of cytokines in T cells (71). Notably, NK cells can exert pro-fibrotic (72) and anti-fibrotic (65, 73) effects and may play, through the production of inflammatory mediators, a crucial role in transplant rejection (74). In patients with CLAD-BOS, the number of NK cells in peripheral blood and BALF increases (75–77). Evidence indicates that activated NK cells can exacerbate the severity of CLAD-BOS, and were associated with long-term graft dysfunction and decreased CLAD-free survival (76). In this regard, NK cells were shown to exert harmful effects by interacting with donor-specific antibodies (78, 79), and to exacerbate airway obstruction in allogeneic transplants (80). In patients with CLAD-BOS, there was an increase in NK cells and cytotoxic molecules in NK cell derived exosomes. Injection of anti-NKG2D blocking antibody can alleviate the development of BO in allografted mice by inhibiting the activation of NK cells (81). Controversially, NK cells can also promote tolerance in solid organ allografts by killing donor antigen-presenting cells (APC), which correlates with improved survival (82). In turn, it was reported that NK cells can alleviate the severity of cGVHD-BOS by inhibiting the proliferation of transplanted T cells (83). Due to the important role of NK cells in cGVHD, some drugs are being developed with the intent of exploiting the above mechanisms (84, 85).
The role and function of B and T lymphocytes in BO pathophysiology are complex and controversial, and evidence suggests that their interaction may either promote or inhibit the progression of the disease. B cells function by producing antibodies, which mediate humoral immunity, and cytokines that contribute to immune modulation (86). Moreover, B cells can activate antigen-specific CD4+ and CD8+ T cells, and present antigens to already activated T cells. Traditionally, T cells are considered key cells involved in transplant rejection (87).
Lymphocyte infiltration, lymphoid follicles, and bronchus-associated lymphoid tissue hyperplasia are often observed in BOS (6). Several drugs with regulatory effects on lymphocyte function are being currently evaluated in clinical trials and have the potential to be applied in the treatment of various types of BOS. It is necessary to understand the mechanism of lymphocytes in BO disease for treatment.
B cells can function as effector cells and regulatory cells in BOS, and it is important to balance these two roles in disease treatment. B lymphocytes promote CLAD-BOS by producing antibodies and presenting antigens through major histocompatibility complex (MHC) molecules (88). Due to antigen presentation, T cell co-activation, and the production of donor-specific antibodies, B cells are traditionally considered to be an important factor in chronic graft failure (89). B cells play an essential role in hyperacute rejection, which is caused by recognition of donor antigens by preexisting antibodies after organ transplantation (86). Regulatory B (Breg) cells secrete the anti-inflammatory factors IL-10 and TGF-β1, which inhibit the progression of CLAD-BOS (90). Smirnova et al. (91) found that B cells are the main source of local antibody production and a major contributor to CLAD-BOS. An animal study by Texier et al. (92) reported that allografts are infiltrated by a large number of B cells organized in germinal centers. These aggregates are strongly regulated in their IgG alloantibody response, and exhibit an inhibited and regulatory profile.
The accurate identification of biomarkers predictive of BOS can improve the prognosis for patients. Compared with patients without cGVHD, the percentage of CD19+CD21low B cells, as well as B cell-activating factor (BAFF) levels and the BAFF/CD19+ ratio, are notably elevated in patients with newly diagnosed BOS. Accordingly, an elevated frequency of CD19+CD21low B cells was proposed to represent a potential novel biomarker for predicting early risk of BOS in HSCT patients, potentially impacting prognosis (93). B cells play a pivotal role in antigen presentation in autoimmune diseases (94), and their function is particularly evident at low antigen thresholds. Cytokines can trigger an immune response to self-antigens, leading to the development of BO after administering anti-MHC antibodies. Nevertheless, B cells exhibit abnormal survival and maturation in BOS (95).
Srinivasan et al. (96) found that the development of BO in mouse models required the deposition of allogeneic antibodies from donor B cells and the formation of germinal centers. Ibrutinib, a small molecule drug that inhibits the B cell receptor pathway, has been approved by the FDA for the treatment of cGVHD (97). Although entospletinib combined with steroids as frontline treatment for cGVHD was terminated for lack of efficacy, fostamatinib (phase I trial) produced an ORR of 77% and allowed for a strong and durable steroid-sparing effect (98). Meanwhile, phase II clinical trials are currently ongoing to test the clinical efficacy of rituximab (the clinical response rate was 70%) (99) and ofatumumab (the ORR was 62.5%) (100), two monoclonal antibodies which are specific anti–B-cell therapy and beneficial for patients with steroidrefractory cGVHD.
The primary function of CD8+ T lymphocytes is to identify endogenous antigens, directly kill intracellular and extracellular pathogens, and eliminate infected, mutated, and cancerous cells (101). After lung transplantation, CD8+ T cells exhibit high cytotoxic activity and pro-inflammatory properties. A study described the presence of inflammatory infiltration of bronchioles in lung biopsies of 23 children with BO, and compared it with the infiltration of histologically normal airways (102). It was found that CD3+ T cells were the most common cell type in BO patients, represented mainly by the CD8+ T cell subtype. In addition, lung histopathology showed that CD4+ and CD8+ T cells were also significantly increased in animal models of BO (103).
BOS is associated with steroid resistance, down-regulation of CD28 expression in pro-inflammatory CD8+T cells in peripheral blood, and upregulation of selective costimulatory molecules (104). Increased abundance of cytotoxic/pro-inflammatory CD8+T cells, in association with exacerbated fibrosis, has been demonstrated in small distal airways in CLAD-BOS (105, 106). Khatri et al. (107) found that cytotoxic CD8+T cells not only accumulate in CLAD-BOS lungs, but also lead to targeted basal cell death in early CLAD-BOS airways. These findings suggest that infiltrating CD8+ T cells contribute importantly to the progression of BOS.
Patients with CLAD-BOS may exhibit relative resistance to immunosuppressive agents, including glucocorticoids (GC), which limits the effectiveness of immunosuppressive therapy to prevent pro-inflammatory cytokine secretion (108). Specifically, the stimulation and activation of CD8+ T cells during the disease process further accentuates this resistance, which may be associated with reduced GC receptor (GCR) expression in pro-inflammatory CD8+ T cells. Decrease expression of inhibiting p-glycoprotein-1 in GC-resistant T-cells would be further increased GCR in patients with CLAD-BOS. Therefore, upregulating GCR expression in CD8+ T cells may improve the prognosis of CLAD-BOS. T cells can induce epithelial cell apoptosis through the secretion of granzyme B, and inhibiting this enzyme can reduce the incidence of CLAD-BOS (18). Also, the upregulation of the gene encoding granzyme A in CD8+ T cells during homing to lymphoid tissues is linked to the activation of the non-canonical NF-κB pathway (95). However, gabexate mesylate, a synthetic serine protease inhibitor, and methylprednisolone, a synthetic GC, showed no impact on the production of granzyme B in CD8+ T cells in vitro, while tacrolimus and cyclosporine A, which inhibit IL-2 production, exhibited only modest effects (109). These findings indicate a clear need to identify effective T cell-targeted immunosuppressants for the prevention and treatment of CLAD-BOS (110).
In 1986, Mosmann et al. identified two different types of CD4+ Th cells (later defined as Th1 and Th2) that exhibited distinct cytokine profiles (111). One theory of immune modulation posits that Th1 and Th2 cells operate between states of stability (112), directing distinct immune response pathways through the production of cytokines. Th1 cells produce interferon (IFN)-, IL-2 and tumor necrosis factor (TNF)-, which cause phagocyte dependent inflammation. Th2 cells produce IL-4, IL-5, IL-6, IL-9, IL-10 and IL-13, which cause strong antibody response and inhibit the functions of phagocytes (113). Specifically, Th1 cells can stimulate macrophages through TNF and IFN, driving cellular immunity against viruses and other pathogens. It can also trigger delayed type hypersensitivity skin reactions. Th2 cells drive in turn humoral immunity, by inducing the production of antibodies by B cells to combat pathogens. While in healthy subjects the balance between Th1 and Th2 is in a state of dynamic equilibrium, alterations in either pathway can influence the other, and overactivation of either pathway can lead to the onset and progression of diseases. Indeed, research has shown that the Th1/Th2 balance plays a pivotal role in the progression of pulmonary diseases (114–116).
Lymphocytes infiltrating in BOS-affected lungs release a variety of inflammatory cytokines, especially Th1 cytokines (105, 117, 118). Th1 cytokines are significantly elevated in peripheral blood, BALF, and lung tissue of patients with BO/BOS (103, 109, 119–121). However, studies have shown that there was no significant difference in the levels of IFN-γ, IL-4, and IL-10 between the peripheral blood of children with PIBO and that of healthy controls, suggesting that Th1/Th2 imbalances may not be related to PIBO pathophysiology (122). In turn, whether Th2 cells mediate pro-fibrotic or anti-fibrotic effects in BO is controversial. Th2 cells can accelerate rejection by releasing pro-inflammatory and potent pro-fibrotic mediators such as IL-4, IL-6, and IL-13 (39, 123, 124). However, by releasing IL-10, Th2 cells can reduce the severity of cGVHD in animal models (125). These findings suggest that Th2 cells have different effects on BOS and fibrosis in different pathophysiological contexts.
A Phase II clinical trial (n =10) to assess aldesleukin, a recombinant analog of IL-2, was conducted for treatment of steroid-refractory cGVHD. The response rate was 80% when assessed by intent to treat in this trial (126). Besides, a potential Th2-targeted therapy is represented by romilkimab, a bispecific IL-4/IL-13 neutralizing antibody that is being evaluated in a phase II clinical trial as anti-fibrosis treatment in systemic sclerosis (127). Romilkimab (n = 47) resulted in a statistically significant decrease in modified Rodnan skin score (mRSS) from baseline to week 24 versus placebo (n = 48) in this trial. However, more research is needed to develop drugs targeting Th1-dependent inflammation, as TNF-α blockade failed to improve small airway obstruction in rheumatoid arthritis patients (128).
The process by which CD4+ T cells differentiate into Th cells is influenced by specific cytokines and costimulatory molecules acting on the T cell receptor (TCR) (129). Th17 cells, a subset of effector Th cells, secrete cytokines and exert effects that are distinct from those of Th1 and Th2 cells (130). Evidence shows that Th17 cells primarily contribute to the onset of various autoimmune and inflammatory diseases via secretion of IL-17, which acts on a variety of cells, including endothelial, mesenchymal, epithelial, and hematopoietic cells (131). Through IL-17-related mechanisms, Th17 cells participate in immune regulation and pathophysiological changes of lung diseases such as COPD, asthma, pulmonary fibrosis, pulmonary arterial hypertension, and lung cancer, among others (132).
During the development of CLAD-BOS, Th cells are induced to differentiate into immunomodulatory Th17 cells (133). After lung transplantation, IL-17 mRNA levels in the BALF of patients with CLAD-BOS were shown to be significantly increased compared to clinically stable lung transplant recipients. In mouse models, there is a correlation between IL-6 and IL-17 levels and tracheal obstruction, and blocking IL-6 can mitigate allograft fibrosis by decreasing the IL-17 transcripts (134). Th17 cells may indeed represent key regulators of airway fibrosis, as inhibiting the function of Th17 cells was shown to reduce the severity of airway fibrosis in BO (103, 135).
Th17 cells play also a deleterious role in cGVHD. Forcade et al. (136) showed that belumosudil, a rho-associated coiled-coil kinase 2 (ROCK2) inhibitor, decreases both severity of murine BO and clinical scores in sclerodermatous cGVHD through inhibition of STAT3 and activation of STAT5. Besides, belumosudil was associated with a best ORR of 32% for patients with early stages of cGVHD-BOS (137). In 2021, the FDA approved belumosudil for the treatment of cGVHD after failure of at least two prior lines of systemic therapy (97).
Treg cells (Tregs) are specialized CD4+ T cells typically defined by expression of FOXP3 and CD25 (IL-2 receptor alpha chain) (138). The inhibitory functions of Tregs are crucial to limit inflammation, maintain peripheral tolerance, and curtail the development of autoimmune and autoinflammatory diseases, allergies, acute and chronic infections, cancer, and metabolic inflammation (139). Ample evidence supports the involvement of Tregs in the regulation of immune cell interactions in lung diseases, including parasitic infections, pneumonia, COPD, asthma, fibrosis, and lung cancer (90, 140).
Tregs may enhance EMT and contribute to the progression of pulmonary fibrosis by secreting cytokines such as PDGF and TGF-β, or curb its progression by facilitating the repair of damaged epithelial cells, suppressing fibroblast accumulation, and inhibiting the production and activity of pro-inflammatory factors and cells (141).
However, the role of Tregs in lung fibrosis is controversial, as they were shown to alternatively promote and inhibit fibrosis by enhancing inflammation and contributing to tissue repair, respectively (142, 143). The balance between Tregs and Th17 cells is implicated in pulmonary fibrosis. Th17 mediate autoimmune responses and inflammation, whereas Tregs suppress inflammation and ensure immune homeostasis (144). It was reported that within 3 years after lung transplantation, the proportion of circulating Tregs was significantly higher in patients with BOS than in patients with non-BOS. The risk of BOS in patients with increased proportion of Tregs after transplantation was 2 times higher than that in patients without increased proportion of Tregs (145). Likewise, BO was more severe in animal models implanted with peripheral blood mononuclear cells depleted of CD4+ CD25high cells, which suggests that Tregs may have a protective effect on BOS. Accordingly, studies revealed that Tregs can reduce airway fibrosis in mice that developed BO after heterotopic bronchial transplantation (146, 147). However, other studies suggested that Tregs may exert instead pro-fibrotic roles. In mice with ischemic cardiomyopathy, Tregs ablation alleviated hypertrophy and myocardial fibrosis (148). Similarly, in renal fibrosis animal models, inhibiting Tregs differentiation was shown to reduce fibrosis (149). These findings suggest that Tregs have different effects on fibrosis in different pathophysiological contexts.
Extensive efforts are made to develop therapies targeting Tregs. Ruxolitinib, a JAK-STAT pathway inhibitor, can decrease collagen deposition and improve lung function in a mouse model of cGVHD by reducing the polarization of CD4+ T cells towards IFN-γ and IL-17A-producing cells and increasing their conversion to Tregs (150). A phase II multicenter trial of ruxolitinib to treat cGVHD-BOS showed that newly diagnosed BOS experienced more dynamic early changes in FEV1 while established BOS was stable, comparing PFTs at baseline. The best lung-specific ORR for the entire study is 34% (151). In 2021, the FDA approved ruxolitinib for the treatment of cGVHD after failure of one or two lines of systemic therapy (97).
Studies on patients and animal models indicate that immune dysregulation contributes to the onset and progression of airway obstruction and fibrosis in BO. Immune cells interact with each other and influence pathophysiological changes by secreting cytokines to regulate effector cells. In different BO subtypes, macrophages, neutrophils, eosinophils, Th1, and Th17 cells may contribute to disease progression, while Th2, NK, and Tregs seem to have controversial roles (Figure 1). cGVHD-BOS is treated with steroids – with ruxolitinib, belumosidil, and now potentially axatilimab for steroid refractory disease. In addition, systemic steroids, fluticasone-azithromycin-montelukast and inhaled long-acting bronchodilator are first-line treatment options for CLAD-BOS. However, none of these drugs are likely to reverse advanced fibrosis/severe obstruction. As research progresses on the complex interplay between immune cells shaping BO development, immune therapy and targeted immune modulators may emerge.
KD: Writing – original draft, Writing – review & editing. GL: Visualization, Writing – review & editing.
The author(s) declare financial support was received for the research, authorship, and/or publication of this article. This work was supported by both Research Foundation of Guangzhou Women and Children’s Medical Center for Clinical Doctor (No. 2020BS005) and Guangzhou Science and technology plan project (No. 202201011021).
The authors declare that the research was conducted in the absence of any commercial or financial relationships that could be construed as a potential conflict of interest.
All claims expressed in this article are solely those of the authors and do not necessarily represent those of their affiliated organizations, or those of the publisher, the editors and the reviewers. Any product that may be evaluated in this article, or claim that may be made by its manufacturer, is not guaranteed or endorsed by the publisher.
1. Kavaliunaite E, Aurora P. Diagnosing and managing bronchiolitis obliterans in children. Expert Rev Respir Med. (2019) 13:481–8. doi: 10.1080/17476348.2019.1586537
2. Shanthikumar S, Gower WA, Cooke KR, Bergeron A, Schultz KR, Barochia A, et al. Diagnosis of post-hematopoietic stem cell transplantation bronchiolitis obliterans syndrome in children: time for a rethink? Transplant Cell Ther. (2024) 30:760–9. doi: 10.1016/j.jtct.2024.05.012
3. Barker AF, Bergeron A, Rom WN, Hertz MI. Obliterative bronchiolitis. N Engl J Med. (2014) 370:1820–8. doi: 10.1056/NEJMra1204664
4. Kordjazy N, Amini S. A review of the therapeutic potential of the cysteinyl leukotriene antagonist Montelukast in the treatment of bronchiolitis obliterans syndrome following lung and hematopoietic-stem cell transplantation and its possible mechanisms. Ther Adv Respir Dis. (2024) 18:17534666241232284. doi: 10.1177/17534666241232284
5. Flanagan F, Casey A, Reyes-Mugica M, Kurland G. Post-infectious bronchiolitis obliterans in children. Paediatr Respir Rev. (2022) 42:69–78. doi: 10.1016/j.prrv.2022.01.007
6. Yu J. Postinfectious bronchiolitis obliterans in children: lessons from bronchiolitis obliterans after lung transplantation and hematopoietic stem cell transplantation. Korean J Pediatr. (2015) 58:459–65. doi: 10.3345/kjp.2015.58.12.459
7. Colom AJ, Teper AM, Vollmer WM, Diette GB. Risk factors for the development of bronchiolitis obliterans in children with bronchiolitis. Thorax. (2006) 61:503–6. doi: 10.1136/thx.2005.044909
8. Murtagh P, Giubergia V, Viale D, Bauer G, Pena HG. Lower respiratory infections by adenovirus in children. Clinical features and risk factors for bronchiolitis obliterans and mortality. Pediatr Pulmonol. (2009) 44:450–6. doi: 10.1002/ppul.20984
9. Castro-Rodriguez JA, Daszenies C, Garcia M, Meyer R, Gonzales R. Adenovirus pneumonia in infants and factors for developing bronchiolitis obliterans: a 5-year follow-up. Pediatr Pulmonol. (2006) 41:947–53. doi: 10.1002/ppul.20472
10. Lee E, Park S, Kim K, Yang HJ. Risk factors for the development of post-infectious bronchiolitis obliterans in children: A systematic review and meta-analysis. Pathogens. (2022) 11(11):1268. doi: 10.3390/pathogens11111268
11. Jerkic SP, Brinkmann F, Calder A, Casey A, Dishop M, Griese M, et al. Postinfectious bronchiolitis obliterans in children: diagnostic workup and therapeutic options: A workshop report. Can Respir J. (2020) 2020:5852827. doi: 10.1155/2020/5852827
12. Teper A, Colom AJ, Schubert R, Jerkic PS. Update in postinfectious bronchiolitis obliterans. Pediatr Pulmonol. (2023). doi: 10.1002/ppul.26570
13. Mauad T, Dolhnikoff M, São Paulo Bronchiolitis Obliterans Study Group. Histology of childhood bronchiolitis obliterans. Pediatr Pulmonol. (2002) 33:466–74. doi: 10.1002/ppul.10097
14. Ravaglia C, Poletti V. Bronchiolitis and bronchiolar disorders. Semin Respir Crit Care Med. (2020) 41:311–32. doi: 10.1055/s-0039-3402728
15. Swaminathan AC, Carney JM, Tailor TD, Palmer SM. Overview and challenges of bronchiolar disorders. Ann Am Thorac Soc. (2020) 17:253–63. doi: 10.1513/AnnalsATS.201907-569CME
16. Yusen RD, Edwards LB, Kucheryavaya AY, Benden C, Dipchand AI, Goldfarb SB, et al. The registry of the international society for heart and lung transplantation: thirty-second official adult lung and heart-lung transplantation report—2015; focus theme: early graft failure. J Heart Lung Transplantation. (2015) 34:1264–77. doi: 10.1016/j.healun.2015.08.014
17. Verleden GM, Raghu G, Meyer KC, Glanville AR, Corris P. A new classification system for chronic lung allograft dysfunction. J Heart Lung Transplantation. (2014) 33:127–33. doi: 10.1016/j.healun.2013.10.022
18. Hodge S, Hodge G, Ahern J, Liew CL, Hopkins P, Chambers DC, et al. Increased levels of T cell granzyme b in bronchiolitis obliterans syndrome are not suppressed adequately by current immunosuppressive regimens. Clin Exp Immunol. (2009) 158:230–6. doi: 10.1111/j.1365-2249.2009.04008.x
19. Dudek AZ, Mahaseth H Fau - DeFor TE, DeFor Te Fau - Weisdorf DJ, Weisdorf DJ. Bronchiolitis obliterans in chronic graft-versus-host disease: analysis of risk factors and treatment outcomes. Biol Blood Marrow Transplant. (2003) 9:657–66. doi: 10.1016/s1083-8791(03)00242-8
20. Marras TK, Chan Ck Fau - Lipton JH, Lipton Jh Fau - Messner HA, Messner Ha Fau - Szalai JP, Szalai Jp Fau - Laupacis A, Laupacis A. Long-term pulmonary function abnormalities and survival after allogeneic marrow transplantation. Bone Marrow Transplant. (2004) 33:509–17. doi: 10.1038/sj.bmt.1704377
21. Glanville AR, Benden C, Bergeron A, Cheng GS, Gottlieb J, Lease ED, et al. Bronchiolitis obliterans syndrome after lung or haematopoietic stem cell transplantation: current management and future directions. ERJ Open Res. (2022) 8(3):00185-2022. doi: 10.1183/23120541.00185-2022
22. Chien JW, Duncan S, Williams KM, Pavletic SZ. Bronchiolitis obliterans syndrome after allogeneic hematopoietic stem cell transplantation-an increasingly recognized manifestation of chronic graft-versus-host disease. Biol Blood Marrow Transplant. (2010) 16:S106–14. doi: 10.1016/j.bbmt.2009.11.002
23. Castleman WL. Bronchiolitis obliterans and pneumonia induced in young dogs by experimental adenovirus infection. Am J Pathol. (1985) 119:495–504.
24. Jungraithmayr W, Jang JH, Schrepfer S, Inci I, Weder W. Small animal models of experimental obliterative bronchiolitis. Am J Respir Cell Mol Biol. (2013) 48:675–84. doi: 10.1165/rcmb.2012-0379TR
25. Baldassi D, Gabold B, Merkel O. Air-liquid interface cultures of the healthy and diseased human respiratory tract: promises, challenges and future directions. Adv Nanobiomed Res. (2021) 1(6):2000111. doi: 10.1002/anbr.202000111
26. Beckett EM, Cyrs WD, Abelmann A, Monnot AD, Gaffney SH, Finley BL. Derivation of an occupational exposure limit for diacetyl using dose-response data from a chronic animal inhalation exposure study. J Appl Toxicol. (2019) 39:688–701. doi: 10.1002/jat.3757
27. Yang T, Xu C, Ding N, Luo S, Wu B, Jin S, et al. p14ARF ameliorates inflammation and airway remodeling in nitric acid aerosol inhalation-induced bronchiolitis obliterans. Exp Lung Res. (2023) 49:27–38. doi: 10.1080/01902148.2022.2163322
28. Hoyle GW, Svendsen ER. Persistent effects of chlorine inhalation on respiratory health. Ann N Y Acad Sci. (2016) 1378:33–40. doi: 10.1111/nyas.13139
29. McGraw MD, Dysart MM, Hendry-Hofer TB, Houin PR, Rioux JS, Garlick RB, et al. Bronchiolitis obliterans and pulmonary fibrosis after sulfur mustard inhalation in rats. Am J Respir Cell Mol Biol. (2018) 58:696–705. doi: 10.1165/rcmb.2017-0168OC
30. Svetlecic J, Molteni A, Herndon B. Bronchiolitis obliterans induced by intratracheal papaverine: a novel animal model. Lung. (2004) 182:119–34. doi: 10.1007/s00408-003-1049-3
31. Oishi Y, Sohrabi Y, Xiao P. Editorial: Metabolic regulation of macrophage functions in inflammation. Front Immunol. (2024) 15:1369896. doi: 10.3389/fimmu.2024.1369896
32. Hou F, Xiao K, Tang L, Xie L. Diversity of macrophages in lung homeostasis and diseases. Front Immunol. (2021) 12:753940. doi: 10.3389/fimmu.2021.753940
33. Watanabe S, Alexander M, Misharin AV, Budinger GRS. The role of macrophages in the resolution of inflammation. J Clin Invest. (2019) 129:2619–28. doi: 10.1172/JCI124615
34. Aegerter H, Lambrecht BN, Jakubzick CV. Biology of lung macrophages in health and disease. Immunity. (2022) 55:1564–80. doi: 10.1016/j.immuni.2022.08.010
35. Duecker RP, De Mir Messa I, Jerkic SP, Kochems A, Gottwald G, Moreno-Galdo A, et al. Epigenetic regulation of inflammation by microRNAs in post-infectious bronchiolitis obliterans. Clin Transl Immunol. (2022) 11:e1376. doi: 10.1002/cti2.1376
36. Palmer SM, Flake GP, Kelly FL, Zhang HL, Nugent JL, Kirby PJ, et al. Severe airway epithelial injury, aberrant repair and bronchiolitis obliterans develops after diacetyl instillation in rats. PLoS One. (2011) 6:e17644. doi: 10.1371/journal.pone.0017644
37. O'Koren EG, Hogan BL, Gunn MD. Loss of basal cells precedes bronchiolitis obliterans-like pathological changes in a murine model of chlorine gas inhalation. Am J Respir Cell Mol Biol. (2013) 49:788–97. doi: 10.1165/rcmb.2012-0369OC
38. Borthwick LA, Corris PA, Mahida R, Walker A, Gardner A, Suwara M, et al. TNFalpha from classically activated macrophages accentuates epithelial to mesenchymal transition in obliterative bronchiolitis. Am J Transplant. (2013) 13:621–33. doi: 10.1111/ajt.12065
39. Bos S, Milross L, Filby AJ, Vos R, Fisher AJ. Immune processes in the pathogenesis of chronic lung allograft dysfunction: identifying the missing pieces of the puzzle. Eur Respir Rev. (2022) 31(165):220060. doi: 10.1183/16000617.0060-2022
40. Nayak DK, Zhou F, Xu M, Huang J, Tsuji M, Hachem R, et al. Long-term persistence of donor alveolar macrophages in human lung transplant recipients that influences donor-specific immune responses. Am J Transplant. (2016) 16:2300–11. doi: 10.1111/ajt.13819
41. Kopecky BJ, Frye C, Terada Y, Balsara KR, Kreisel D, Lavine KJ. Role of donor macrophages after heart and lung transplantation. Am J Transplant. (2020) 20:1225–35. doi: 10.1111/ajt.15751
42. Snyder ME, Weisberg SP, Connors T, Benvenuto L, Shah L, Robbins HJ, et al. Persisting donor alveolar macrophages have increased expression of scavenger receptor CD206 compared to graft-infiltrating recipient-derived macrophages following lung transplantation. J Heart Lung Transplant. (2019) 38(4):220060. doi: 10.1016/j.healun.2019.01.378
43. Kuroi T, Fujii N, Ichimura K, Seike K, Yamamoto A, Kambara Y, et al. Characterization of localized macrophages in bronchiolitis obliterans after allogeneic hematopoietic cell transplantation. Int J Hematol. (2021) 114:701–8. doi: 10.1007/s12185-021-03214-7
44. Yang H, Cheng H, Dai R, Shang L, Zhang X, Wen H. Macrophage polarization in tissue fibrosis. PeerJ. (2023) 11:e16092. doi: 10.7717/peerj.16092
45. Hertz MI, Henke CA, Nakhleh RE, Harmon KR, Marinelli WA, Fox JM, et al. Obliterative bronchiolitis after lung transplantation: a fibroproliferative disorder associated with platelet-derived growth factor. Proc Natl Acad Sci U S A. (1992) 89:10385–9. doi: 10.1073/pnas.89.21.10385
46. Magnan. A, Mege. JL, Escallier. JC, Brisse. J, Capo. C, Reynaud. M, et al. Balance between alveolar macrophage IL-6 and TGF-beta in lung-transplant recipients. Marseille and Montreíal Lung Transplantation Group. Am J Respir Crit Care Med. (1996) 153:1431–6. doi: 10.1164/ajrccm.153.4.8616577
47. Liu Z, Liao F, Zhu J, Zhou D, Heo GS, Leuhmann HP, et al. Reprogramming alveolar macrophage responses to TGF-beta reveals CCR2+ monocyte activity that promotes bronchiolitis obliterans syndrome. J Clin Invest. (2022) 132(19):e159229. doi: 10.1172/JCI159229
48. Oyaizu T, Okada Y, Shoji W, Matsumura Y, Shimada K, Sado T, et al. Reduction of recipient macrophages by gadolinium chloride prevents development of obliterative airway disease in a rat model of heterotopic tracheal transplantation. Transplantation. (2003) 76:1214–20. doi: 10.1097/01.TP.0000088672.48259.F1
49. Sumida. Y, Yamashita. H, Inoue. M, Ohsawa. K, Sato. H, Nanashima. A, et al. Anti-macrophage migration inhibitory factor antibody suppresses chronic rejection of heterotopically transplanted trachea in rats. Acta Med. (2006) 51:51–6. doi: 10.1016/j.tibs.2018.10.011
50. Alexander KA, Flynn R, Lineburg KE, Kuns RD, Teal BE, Olver SD, et al. CSF-1-dependant donor-derived macrophages mediate chronic graft-versus-host disease. J Clin Invest. (2014) 124:4266–80. doi: 10.1172/JCI75935
51. Crescioli S, Kaplon H, Chenoweth A, Wang L, Visweswaraiah J, Reichert JM. Antibodies to watch in 2024. MAbs. (2024) 16:2297450. doi: 10.1080/19420862.2023.2297450
52. Wolff D, Cutler C, Lee SJ, Pusic I, Bittencourt H, White J, et al. Axatilimab in recurrent or refractory chronic graft-versus-host disease. N Engl J Med. (2024) 391:1002–14. doi: 10.1056/NEJMoa2401537
53. Peiseler M, Kubes P. More friend than foe: the emerging role of neutrophils in tissue repair. J Clin Invest. (2019) 129:2629–39. doi: 10.1172/JCI124616
54. Herrero-Cervera A, Soehnlein O, Kenne E. Neutrophils in chronic inflammatory diseases. Cell Mol Immunol. (2022) 19:177–91. doi: 10.1038/s41423-021-00832-3
55. Ham J, Kim J, Ko YG, Kim HY. The dynamic contribution of neutrophils in the chronic respiratory diseases. Allergy Asthma Immunol Res. (2022) 14:361–78. doi: 10.4168/aair.2022.14.4.361
56. Rosewich M, Zissler UM, Kheiri T, Voss S, Eickmeier O, Schulze J, et al. Airway inflammation in children and adolescents with bronchiolitis obliterans. Cytokine. (2015) 73:156–62. doi: 10.1016/j.cyto.2014.10.026
57. Jerkic SP, Michel F, Donath H, Herrmann E, Schubert R, Rosewich M, et al. Calprotectin as a new sensitive marker of neutrophilic inflammation in patients with bronchiolitis obliterans. Mediators Inflamm. (2020) 2020:4641585. doi: 10.1155/2020/4641585
58. Riise GC, Williams A, Kjellstrom C, Schersten H, Andersson BA, Kelly FJ. Bronchiolitis obliterans syndrome in lung transplant recipients is associated with increased neutrophil activity and decreased antioxidant status in the lung. Eur Respir J. (1998) 12:82–8. doi: 10.1183/09031936.98.12010082
59. Gassas A, Krueger J, Zaidman I, Schechter T, Craig-Barnes H, Ali M, et al. Infections and neutrophils in the pathogenesis of bronchiolitis obliterans syndrome in children after allogeneic stem cell transplantation. Pediatr Transplant. (2016) 20:303–6. doi: 10.1111/petr.12657
60. Elssner A, Vogelmeier C. The role of neutrophils in the pathogenesis of obliterative bronchiolitis after lung transplantation. Transpl Infect Dis. (2001) 3:168–76. doi: 10.1034/j.1399-3062.2001.003003168.x
61. Sato M, Keshavjee S. Bronchiolitis obliterans syndrome: alloimmune-dependent and -independent injury with aberrant tissue remodeling. Semin Thorac Cardiovasc Surg. (2008) 20:173–82. doi: 10.1053/j.semtcvs.2008.05.002
62. Verleden GM, Vanaudenaerde BM, Dupont LJ, Van Raemdonck DE. Azithromycin reduces airway neutrophilia and interleukin-8 in patients with bronchiolitis obliterans syndrome. Am J Respir Crit Care Med. (2006) 174:566–70. doi: 10.1164/rccm.200601-071OC
63. Devouassoux. G, Drouet. C, Pin. I, Brambilla. C, Brambilla. E, Colle. P-E, et al. Alveolar neutrophilia is a predictor for the bronchiolitis obliterans syndrome, and increases with degree of severity. Transpl Immunol. (2002) 10:303–10. doi: 10.1016/s0966-3274(02)00074-6
64. Im A, Holtzman NG, Curtis LM, Parsons-Wandell L, Nashed J, Peer C, et al. Phase 1 study of alvelestat, an oral neutrophil elastase inhibitor, in patients with bronchiolitis obliterans syndrome after hematopoietic cell transplantation. Blood. (2020) 136:18–9. doi: 10.1182/blood-2020-140481
65. Xu Y, Lan P, Wang T. The role of immune cells in the pathogenesis of idiopathic pulmonary fibrosis. Medicina (Kaunas). (2023) 59(11):e159229. doi: 10.3390/medicina59111984
66. Aoki A, Hirahara K, Kiuchi M, Nakayama T. Eosinophils: Cells known for over 140 years with broad and new functions. Allergol Int. (2021) 70:3–8. doi: 10.1016/j.alit.2020.09.002
67. Verleden SE, Ruttens D, Vandermeulen E, Vaneylen A, Dupont LJ, Van Raemdonck DE, et al. Bronchiolitis obliterans syndrome and restrictive allograft syndrome: do risk factors differ? Transplantation. (2013) 95:1167–72. doi: 10.1097/TP.0b013e318286e076
68. Pizzichini E, Leff JA, Reiss TF, Hendeles L, Boulet LP, Wei LX, et al. Montelukast reduces airway eosinophilic inflammation in asthma: a randomized, controlled trial. Eur Respir J. (1999) 14:12–8. doi: 10.1034/j.1399-3003.1999.14a04.x
69. Uemura S, Kishimoto K, Fujikawa T, Hyodo S, Kozaki A, Saito A, et al. Early elevation of absolute eosinophil count after allogeneic hematopoietic stem cell transplantation is associated with chronic graft-versus-host disease in children. Blood. (2023) 142:6995. doi: 10.1182/blood-2023-182599
70. Williams KM, Pavletic SZ, Lee SJ, Martin PJ, Farthing DE, Hakim FT, et al. Prospective Phase II Trial of Montelukast to Treat Bronchiolitis Obliterans Syndrome after Hematopoietic Cell Transplantation and Investigation into Bronchiolitis Obliterans Syndrome Pathogenesis. Transplant Cell Ther. (2022) 28:264.e1–e9. doi: 10.1016/j.jtct.2022.01.021
71. Mace EM. Human natural killer cells: Form, function, and development. J Allergy Clin Immunol. (2023) 151:371–85. doi: 10.1016/j.jaci.2022.09.022
72. Huang E, Peng N, Xiao F, Hu D, Wang X, Lu L. The roles of immune cells in the pathogenesis of fibrosis. Int J Mol Sci. (2020) 21(15):5203. doi: 10.3390/ijms21155203
73. Yang M, Vanderwert E, Kimchi ET, Staveley-O'Carroll KF, Li G. The important roles of natural killer cells in liver fibrosis. Biomedicines. (2023) 11(5):1391. doi: 10.3390/biomedicines11051391
74. Hamada S, Dubois V, Koenig A, Thaunat O. Allograft recognition by recipient's natural killer cells: Molecular mechanisms and role in transplant rejection. HLA. (2021) 98:191–9. doi: 10.1111/tan.14332
75. Hagen N, Hitz A, Bellmàs-Sanz R, Chichelnitskiy E, Beushausen K, Keil J, et al. Longitudinal dynamics of the NK cell repertoire after lung transplantation and their potential role for control in CMV reactivation. J Heart Lung Transplantation. (2024) 43:S318–S9. doi: 10.1016/j.healun.2024.02.473
76. Calabrese DR, Chong T, Singer JP, Rajalingam R, Hays SR, Kukreja J, et al. CD16(+) natural killer cells in bronchoalveolar lavage are associated with antibody-mediated rejection and chronic lung allograft dysfunction. Am J Transplant. (2023) 23:37–44. doi: 10.1016/j.ajt.2022.10.006
77. Zamir MR, Shahi A, Salehi S, Amirzargar A. Natural killer cells and killer cell immunoglobulin-like receptors in solid organ transplantation: Protectors or opponents? Transplant Rev (Orlando). (2022) 36:100723. doi: 10.1016/j.trre.2022.100723
78. Zafarani A, Taghavi-Farahabadi M, Razizadeh MH, Amirzargar MR, Mansouri M, Mahmoudi M. The role of NK cells and their exosomes in graft versus host disease and graft versus leukemia. Stem Cell Rev Rep. (2023) 19:26–45. doi: 10.1007/s12015-022-10449-2
79. Calabrese DR, Chong T, Singer JP, Hays SR, Golden JA, Leard LE, et al. Natural killer cells in bronchoalveolar lavage are associated with antibody-mediated rejection, CLAD, and death in lung transplant recipients. J Heart Lung Transplantation. (2020) 39:S105–S6. doi: 10.1016/j.healun.2020.01.965
80. Kawakami T, Ito K, Matsuda Y, Noda M, Sakurada A, Hoshikawa Y, et al. Cytotoxicity of natural killer cells activated through NKG2D contributes to the development of bronchiolitis obliterans in a murine heterotopic tracheal transplant model. Am J Transplant. (2017) 17:2338–49. doi: 10.1111/ajt.14257
81. Itabashi Y, Ravichandran R, Bansal S, Bharat A, Hachem R, Bremner R, et al. Decline in club cell secretory proteins, exosomes induction and immune responses to lung self-antigens, kalpha1 tubulin and collagen V, leading to chronic rejection after human lung transplantation. Transplantation. (2021) 105:1337–46. doi: 10.1097/TP.0000000000003428
82. Oberholtzer N, Atkinson C, Nadig SN. Adoptive transfer of regulatory immune cells in organ transplantation. Front Immunol. (2021) 12:631365. doi: 10.3389/fimmu.2021.631365
83. Sheng L, Mu Q, Wu X, Yang S, Zhu H, Wang J, et al. Cytotoxicity of donor natural killer cells to allo-reactive T cells are related with acute graft-vs.-host-disease following allogeneic stem cell transplantation. Front Immunol. (2020) 11:1534. doi: 10.3389/fimmu.2020.01534
84. Pradier A, Papaserafeim M, Li N, Rietveld A, Kaestel C, Gruaz L, et al. Small-molecule immunosuppressive drugs and therapeutic immunoglobulins differentially inhibit NK cell effector functions in vitro. Front Immunol. (2019) 10:556. doi: 10.3389/fimmu.2019.00556
85. Jalali S, Stankovic S, Westall GP, Reading PC, Sullivan LC, Brooks AG. Examining the impact of immunosuppressive drugs on antibody-dependent cellular cytotoxicity (ADCC) of human peripheral blood natural killer (NK) cells and gamma delta (gammadelta) T cells. Transpl Immunol. (2024) 82:101962. doi: 10.1016/j.trim.2023.101962
86. Heeger PS, Haro MC, Jordan S. Translating B cell immunology to the treatment of antibody-mediated allograft rejection. Nat Rev Nephrol. (2024) 20:218–32. doi: 10.1038/s41581-023-00791-0
87. Jiang H, Fu D, Bidgoli A, Paczesny S. T cell subsets in graft versus host disease and graft versus tumor. Front Immunol. (2021) 12:761448. doi: 10.3389/fimmu.2021.761448
88. Jaramillo A, Fernandez FG, Kuo EY, Trulock EP, Patterson GA, Mohanakumar T. Immune mechanisms in the pathogenesis of bronchiolitis obliterans syndrome after lung transplantation. Pediatr Transplant. (2005) 9:84–93. doi: 10.1111/j.1399-3046.2004.00270.x
89. Zhao Y, Gillen JR, Meher AK, Burns JA, Kron IL, Lau CL. Rapamycin prevents bronchiolitis obliterans through increasing infiltration of regulatory B cells in a murine tracheal transplantation model. J Thorac Cardiovasc Surg. (2016) 151:487–96.e3. doi: 10.1016/j.jtcvs.2015.08.116
90. Lao P, Chen J, Tang L, Zhang J, Chen Y, Fang Y, et al. Regulatory T cells in lung disease and transplantation. Biosci Rep. (2023) 11(5):1391. doi: 10.1042/BSR20231331
91. Smirnova NF, Riemondy K, Bueno M, Collins S, Suresh P, Wang X, et al. Single-cell transcriptome mapping identifies a local, innate B cell population driving chronic rejection after lung transplantation. JCI Insight. (2022) 7(18):e156648. doi: 10.1172/jci.insight.156648
92. Le Texier L, Thebault P, Lavault A, Usal C, Merieau E, Quillard T, et al. Long-term allograft tolerance is characterized by the accumulation of B cells exhibiting an inhibited profile. Am J Transplantation. (2011) 11:429–38. doi: 10.1111/j.1600-6143.2010.03336.x
93. Kuzmina Z, Krenn K, Petkov V, Körmöczi U, Weigl R, Rottal A, et al. CD19+CD21low B cells and patients at risk for NIH-defined chronic graft-versus-host disease with bronchiolitis obliterans syndrome. Blood. (2013) 121:1886–95. doi: 10.1182/blood-2012-06-435008
94. Fukami N, Ramachandran S, Takenaka M, Weber J, Subramanian V, Mohanakumar T. An obligatory role for lung infiltrating B cells in the immunopathogenesis of obliterative airway disease induced by antibodies to MHC class I molecules. Am J Transplantation. (2012) 12:867–76. doi: 10.1111/j.1600-6143.2011.03917.x
95. Gutor SS, Richmond BW, Du RH, Wu P, Lee JW, Ware LB, et al. Characterization of immunopathology and small airway remodeling in constrictive bronchiolitis. Am J Respir Crit Care Med. (2022) 206:260–70. doi: 10.1164/rccm.202109-2133OC
96. Srinivasan M, Flynn R, Price A, Ranger A, Browning JL, Taylor PA, et al. Donor B-cell alloantibody deposition and germinal center formation are required for the development of murine chronic GVHD and bronchiolitis obliterans. Blood. (2012) 119:1570–80. doi: 10.1182/blood-2011-07-364414
97. Zeiser R, Lee SJ. Three US Food and Drug Administration-approved therapies for chronic GVHD. Blood. (2022) 139:1642–5. doi: 10.1182/blood.2021014448
98. Lin C, DiCioccio RA, Haykal T, McManigle WC, Li Z, Anand SM, et al. A phase I trial of SYK inhibition with fostamatinib in the prevention and treatment of chronic graft-versus-host disease. Transplant Cell Ther. (2023) 29:179.e1–e10. doi: 10.1016/j.jtct.2022.12.015
99. Cutler C, Miklos D, Kim HT, Treister N, Woo SB, Bienfang D, et al. Rituximab for steroid-refractory chronic graft-versus-host disease. Blood. (2006) 108:756–62. doi: 10.1182/blood-2006-01-0233
100. Lazaryan A, Lee S, Arora M, Kim J, Betts BC, Khimani F, et al. A phase 2 multicenter trial of ofatumumab and prednisone as initial therapy for chronic graft-versus-host disease. Blood Adv. (2022) 6:259–69. doi: 10.1182/bloodadvances.2021005552
101. Hodge G, Hodge S, Reynolds P, Holmes M. Intracellular cytokines in blood T cells in lung transplant patients–a more relevant indicator of immunosuppression than drug levels. Clin Exp Immunol. (2005) 139:159–64. doi: 10.1111/j.1365-2249.2005.02671.x
102. Mauad T, van SChadewijk A, Schrumpf J, Hack CE, Fernezlian S, Garippo AL, et al. Lymphocytic inflammation in childhood bronchiolitis obliterans. Pediatr Pulmonol. (2004) 38:233–9. doi: 10.1002/ppul.20064
103. Xu KY, Tong S, Wu CY, Ding XC, Chen JL, Ming Y, et al. Nlrp3 inflammasome inhibitor MCC950 ameliorates obliterative bronchiolitis by inhibiting th1/th17 response and promoting treg response after orthotopic tracheal transplantation in mice. Transplantation. (2020) 104:e151–e63. doi: 10.1097/TP.0000000000003208
104. Hodge G, Hodge S, Ahern J, Holmes-Liew CL, Reynolds PN, Holmes M. Up-regulation of alternate co-stimulatory molecules on proinflammatory CD28null T cells in bronchiolitis obliterans syndrome. Clin Exp Immunol. (2013) 173:150–60. doi: 10.1111/cei.12081
105. Hodge G, Hodge S, Liu H, Nguyen P, Holmes-Liew CL, Holmes M. Bronchiolitis obliterans syndrome is associated with increased senescent lymphocytes in the small airways. J Heart Lung Transplant. (2021) 40:108–19. doi: 10.1016/j.healun.2019.12.005
106. Hodge G, Hodge S, Yeo A, Nguyen P, Hopkins E, Holmes-Liew CL, et al. BOS is associated with increased cytotoxic proinflammatory CD8 T, NKT-like, and NK cells in the small airways. Transplantation. (2017) 101:2469–76. doi: 10.1097/TP.0000000000001592
107. Khatri A, Todd JL, Kelly FL, Nagler A, Ji Z, Jain V, et al. JAK-STAT activation contributes to cytotoxic T cell-mediated basal cell death in human chronic lung allograft dysfunction. JCI Insight. (2023) 8(6):e167082. doi: 10.1172/jci.insight.167082
108. Hodge G, Hodge S, Nguyen PT, Yeo A, Sarkar P, Badiei A, et al. Bronchiolitis obliterans syndrome is associated with increased p-glycoprotein expression and loss of glucocorticoid receptor from steroid-resistant proinflammatory CD8(+) T cells. Clin Exp Immunol. (2018) 192:242–50. doi: 10.1111/cei.13103
109. Hodge G, Hodge S, Holmes-Liew C-L, Reynolds PN, Holmes M. Bronchiolitis obliterans syndrome is associated with increased peripheral blood natural killer and natural killer T-like granzymes, perforin, and T-helper-type 1 pro-inflammatory cytokines. J Heart Lung Transplantation. (2012) 31:888–95. doi: 10.1016/j.healun.2012.04.007
110. Arjuna A, Olson MT, Walia R, Bremner RM, Smith MA, Mohanakumar T. An update on current treatment strategies for managing bronchiolitis obliterans syndrome after lung transplantation. Expert Rev Respir Med. (2021) 15:339–50. doi: 10.1080/17476348.2021.1835475
111. Mosmann TR, Cherwinski H, Bond MW, Giedlin MA, Coffman RL. Two types of murine helper T cell clone. I. Definition according to profiles of lymphokine activities and secreted proteins. J Immunol. (1986) 136:2348–57. doi: 10.4049/jimmunol.136.7.2348
112. Kidd P. Th1/Th2 balance: the hypothesis, its limitations, and implications for health and disease. Altern Med Rev. (2003) 8:223–46.
113. Romagnani S. T-cell subsets (Th1 versus Th2). Ann Allergy Asthma Immunol. (2000) 85:9–18. doi: 10.1016/S1081-1206(10)62426-X
114. Spagnolo P, Tonelli R, Samarelli AV, Castelli G, Cocconcelli E, Petrarulo S, et al. The role of immune response in the pathogenesis of idiopathic pulmonary fibrosis: far beyond the Th1/Th2 imbalance. Expert Opin Ther Targets. (2022) 26:617–31. doi: 10.1080/14728222.2022.2114897
115. Sun J, Liu T, Yan Y, Huo K, Zhang W, Liu H, et al. The role of Th1/Th2 cytokines played in regulation of specific CD4 (+) Th1 cell conversion and activation during inflammatory reaction of chronic obstructive pulmonary disease. Scand J Immunol. (2018) 88:e12674. doi: 10.1111/sji.12674
116. Zhang T, Wang G, Li Q, Yan P, Sun J, Jin Y. Relationship between serum Th1/Th2 imbalance and depression in elderly patients with COPD and its clinical implications. Technol Health Care. (2023) 31:2047–58. doi: 10.3233/THC-230665
117. Wu Z, Chen X, Wu S, Liu Z, Li H, Mai K, et al. Transcriptome analysis reveals the impact of NETs activation on airway epithelial cell EMT and inflammation in bronchiolitis obliterans. Sci Rep. (2023) 13:19226. doi: 10.1038/s41598-023-45617-y
118. Mazenq J, Dubus J-C, Chanez P, Gras D. Post viral bronchiolitis obliterans in children: A rare and potentially devastating disease. Paediatric Respir Rev. (2024). doi: 10.1016/j.prrv.2024.04.003
119. Hodge G, Hodge S, Chambers D, Reynolds PN, Holmes M. Bronchiolitis obliterans syndrome is associated with absence of suppression of peripheral blood Th1 proinflammatory cytokines. Transplantation. (2009) 88:211–8. doi: 10.1097/TP.0b013e3181ac170f
120. Kastelijn EA, Rijkers GT, Van Moorsel CH, Zanen P, Kwakkel-van Erp JM, Van De Graaf EA, et al. Systemic and exhaled cytokine and chemokine profiles are associated with the development of bronchiolitis obliterans syndrome. J Heart Lung Transplant. (2010) 29:997–1008. doi: 10.1016/j.healun.2010.04.013
121. Xu W, Yang H, Liu H, Tang X, Xu H, Li H, et al. Bronchoalveolar lavage T cell cytokine profiles and their association with lung function in children with Mycoplasma pneumoniae -associated bronchiolitis obliterans. Pediatr Pulmonology. (2020) 55:2033–40. doi: 10.1002/ppul.24882
122. Costa ML, Stein RT, Bauer ME, MaChado DC, Jones MH, Bertotto C, et al. Levels of Th1 and Th2 cytokines in children with post-infectious bronchiolitis obliterans. Ann Trop Paediatr. (2005) 25:261–6. doi: 10.1179/146532805X72403
123. Foley JE, Jung U, Miera A, Borenstein T, Mariotti J, Eckhaus M, et al. Ex vivo rapamycin generates donor Th2 cells that potently inhibit graft-versus-host disease and graft-versus-tumor effects via an IL-4-dependent mechanism. J Immunol. (2005) 175:5732–43. doi: 10.4049/jimmunol.175.9.5732
124. Fu J, Heinrichs J, Yu XZ. Helper T-cell differentiation in graft-versus-host disease after allogeneic hematopoietic stem cell transplantation. Arch Immunol Ther Exp (Warsz). (2014) 62:277–301. doi: 10.1007/s00005-014-0284-z
125. Henden AS, Hill GR. Cytokines in graft-versus-host disease. J Immunol. (2015) 194:4604–12. doi: 10.4049/jimmunol.1500117
126. Salhotra A, Talley M, Wu X, Tsai W, Mokhtari S, Qin H, et al. Clinical and immunologic responses to extracorporeal photopheresis and low-dose IL-2 in patients with steroid refractory chronic graft-versus host disease. Bone Marrow Transplant. (2022) 57:1045–7. doi: 10.1038/s41409-022-01671-0
127. Allanore Y, Wung P, Soubrane C, Esperet C, Marrache F, Bejuit R, et al. A randomised, double-blind, placebo-controlled, 24-week, phase II, proof-of-concept study of romilkimab (SAR156597) in early diffuse cutaneous systemic sclerosis. Ann Rheum Dis. (2020) 79:1600–7. doi: 10.1136/annrheumdis-2020-218447
128. Yamazaki. R, Kurasawa. K, Nagasawa. J, Tanaka. A, Arai. S, Owada. T, et al. Tumor necrosis factor blockade fails to improve small airway obstruction in rheumatoid arthritis. Dokkyo Med J. (2022) 1:285–91. doi: 10.51040/dkmj.2022-013
129. Korn T, Bettelli E, Oukka M, Kuchroo VK. IL-17 and th17 cells. Annu Rev Immunol. (2009) 27:485–517. doi: 10.1146/annurev.immunol.021908.132710
130. Schnell A, Littman DR, Kuchroo VK. T(H)17 cell heterogeneity and its role in tissue inflammation. Nat Immunol. (2023) 24:19–29. doi: 10.1038/s41590-022-01387-9
131. Huangfu L, Li R, Huang Y, Wang S. The IL-17 family in diseases: from bench to bedside. Signal Transduct Target Ther. (2023) 8:402. doi: 10.1038/s41392-023-01620-3
132. Ritzmann F, Lunding LP, Bals R, Wegmann M, Beisswenger C. IL-17 cytokines and chronic lung diseases. Cells. (2022) 11(14):e167082. doi: 10.3390/cells11142132
133. Vanaudenaerde BM, De Vleeschauwer SI, Vos R, Meyts I, Bullens DM, Reynders V, et al. The role of the IL23/IL17 axis in bronchiolitis obliterans syndrome after lung transplantation. Am J Transplant. (2008) 8:1911–20. doi: 10.1111/j.1600-6143.2008.02321.x
134. Nakagiri T, Inoue M, Morii E, Minami M, Sawabata N, Utsumi T, et al. Local IL-17 production and a decrease in peripheral blood regulatory T cells in an animal model of bronchiolitis obliterans. Transplantation. (2010) 89:1312–9. doi: 10.1097/TP.0b013e3181d8ea16
135. Fan L, Benson HL, Vittal R, Mickler EA, Presson R, Fisher AJ, et al. Neutralizing IL-17 prevents obliterative bronchiolitis in murine orthotopic lung transplantation. Am J Transplant. (2011) 11:911–22. doi: 10.1111/j.1600-6143.2011.03482.x
136. Flynn R, Paz K, Du J, Reichenbach DK, Taylor PA, Panoskaltsis-Mortari A, et al. Targeted Rho-associated kinase 2 inhibition suppresses murine and human chronic GVHD through a Stat3-dependent mechanism. Blood. (2016) 127:2144–54. doi: 10.1182/blood-2015-10-678706
137. DeFilipp Z, Kim HT, Yang Z, Noonan J, Blazar BR, Lee SJ, et al. Clinical response to belumosudil in bronchiolitis obliterans syndrome: a combined analysis from 2 prospective trials. Blood Adv. (2022) 6:6263–70. doi: 10.1182/bloodadvances.2022008095
138. Sakaguchi S, Miyara M, Costantino CM, Hafler DA. FOXP3+ regulatory T cells in the human immune system. Nat Rev Immunol. (2010) 10:490–500. doi: 10.1038/nri2785
139. Josefowicz SZ, Lu LF, Rudensky AY. Regulatory T cells: mechanisms of differentiation and function. Annu Rev Immunol. (2012) 30:531–64. doi: 10.1146/annurev.immunol.25.022106.141623
140. Singh R, Alape D, de Lima A, Ascanio J, Majid A, Gangadharan SP. Regulatory T cells in respiratory health and diseases. Pulm Med. (2019) 2019:1907807. doi: 10.1155/2019/1907807
141. Wang F, Xia H, Yao S. Regulatory T cells are a double-edged sword in pulmonary fibrosis. Int Immunopharmacol. (2020) 84:106443. doi: 10.1016/j.intimp.2020.106443
142. Deng L, Huang T, Zhang L. T cells in idiopathic pulmonary fibrosis: crucial but controversial. Cell Death Discovery. (2023) 9:62. doi: 10.1038/s41420-023-01344-x
143. Wu KJ, Qian QF, Zhou JR, Sun DL, Duan YF, Zhu X, et al. Regulatory T cells (Tregs) in liver fibrosis. Cell Death Discovery. (2023) 9:53. doi: 10.1038/s41420-023-01347-8
144. Galati D, De Martino M, Trotta A, Rea G, Bruzzese D, Cicchitto G, et al. Peripheral depletion of NK cells and imbalance of the Treg/Th17 axis in idiopathic pulmonary fibrosis patients. Cytokine. (2014) 66:119–26. doi: 10.1016/j.cyto.2013.12.003
145. Durand M, Lacoste P, Danger R, Jacquemont L, Brosseau C, Durand E, et al. High circulating CD4(+)CD25(hi)FOXP3(+) T-cell sub-population early after lung transplantation is associated with development of bronchiolitis obliterans syndrome. J Heart Lung Transplant. (2018) 37:770–81. doi: 10.1016/j.healun.2018.01.1306
146. Sommer W, Knofel AK, Madrahimov N, Avsar M, Jonigk D, Salman J, et al. Allogeneic CD4+CD25high T cells regulate obliterative bronchiolitis of heterotopic bronchus allografts in both porcinized and humanized mouse models. Transplantation. (2015) 99:482–91. doi: 10.1097/TP.0000000000000632
147. Siemeni T, Knofel AK, Madrahimov N, Sommer W, Avsar M, Salman J, et al. In vivo development of transplant arteriosclerosis in humanized mice reflects alloantigen recognition and peripheral treg phenotype of lung transplant recipients. Am J Transplant. (2016) 16:3150–62. doi: 10.1111/ajt.13905
148. Bansal SS, Ismahil MA, Goel M, Zhou G, Rokosh G, Hamid T, et al. Dysfunctional and proinflammatory regulatory T-lymphocytes are essential for adverse cardiac remodeling in ischemic cardiomyopathy. Circulation. (2019) 139:206–21. doi: 10.1161/CIRCULATIONAHA.118.036065
149. Estes JD, Wietgrefe S, Schacker T, Southern P, Beilman G, Reilly C, et al. Simian immunodeficiency virus-induced lymphatic tissue fibrosis is mediated by transforming growth factor beta 1-positive regulatory T cells and begins in early infection. J Infect Dis. (2007) 195:551–61. doi: 10.1086/510852
150. Spoerl S, Mathew NR, Bscheider M, Schmitt-Graeff A, Chen S, Mueller T, et al. Activity of therapeutic JAK 1/2 blockade in graft-versus-host disease. Blood. (2014) 123:3832–42. doi: 10.1182/blood-2013-12-543736
Keywords: bronchiolitis obliterans, bronchiolitis obliterans syndrome, immune dysregulation, immune cells, fibrosis
Citation: Deng K and Lu G (2024) Immune dysregulation as a driver of bronchiolitis obliterans. Front. Immunol. 15:1455009. doi: 10.3389/fimmu.2024.1455009
Received: 26 June 2024; Accepted: 29 November 2024;
Published: 17 December 2024.
Edited by:
Lazaros Ignatios Sakkas, University of Thessaly, GreeceReviewed by:
Chih-Ru Lin, Kaohsiung Medical University, TaiwanCopyright © 2024 Deng and Lu. This is an open-access article distributed under the terms of the Creative Commons Attribution License (CC BY). The use, distribution or reproduction in other forums is permitted, provided the original author(s) and the copyright owner(s) are credited and that the original publication in this journal is cited, in accordance with accepted academic practice. No use, distribution or reproduction is permitted which does not comply with these terms.
*Correspondence: Gen Lu, bHVnZW41NjYzMzMwQHNpbmEuY29t
Disclaimer: All claims expressed in this article are solely those of the authors and do not necessarily represent those of their affiliated organizations, or those of the publisher, the editors and the reviewers. Any product that may be evaluated in this article or claim that may be made by its manufacturer is not guaranteed or endorsed by the publisher.
Research integrity at Frontiers
Learn more about the work of our research integrity team to safeguard the quality of each article we publish.