- Research Laboratory, Department of Obstetrics and Gynecology, University Medicine Greifswald, Greifswald, Germany
A well-balanced maternal immune system is crucial to maintain fetal tolerance in case of infections during pregnancy. Immune adaptations include an increased secretion of soluble mediators to protect the semi-allogeneic fetus from excessive pro-inflammatory response. B lymphocytes acquire a higher capacity to express CD83 and secrete soluble CD83 (sCD83) upon exposure to bacteria-derived components such as LPS. CD83 possesses immune modulatory functions and shows a promising therapeutic potential against inflammatory conditions. The administration of sCD83 to pregnant mice reduces LPS-induced abortion rates. The increased CD83 expression by endometrial B cells as compared to peripheral blood B cells suggests its modulatory role in the fetal tolerance, especially in the context of infection. We postulate that in pregnancy, CD83 expression and release is controlled by pregnancy-related hormones. The intra- and extracellular expression of CD83 in leukocytes from peripheral blood or decidua basalis and parietalis at term were analyzed by flow cytometry. After treatment with pregnancy-related hormones and LPS, ELISA and qPCR were performed to study sCD83 release and CD83 gene expression, respectively. Cleavage prediction analysis was used to find potential proteases targeting CD83. Expression of selected proteases was analyzed by ELISA. Higher levels of CD83 were found in CD11c+ dendritic cells, CD3+ T cells and CD19+ B cells from decidua basalis and decidua parietalis after LPS-stimulation in vitro. An increase of intracellular expression of CD83 was also detected in CD19+ B cells from both compartments. Stimulated B cells displayed significantly higher percentages of CD83+ cells than dendritic cells and T cells from decidua basalis and peripheral blood. Treatment of B lymphocytes with pregnancy-related molecules (E2, P4, TGF-β1 and hCG) enhanced the LPS-mediated increase of CD83 expression, while dexamethasone led to a reduction. Similarly, the release of sCD83 was increased under TGF-β1 treatment but decreased upon dexamethasone stimulation. Finally, we found that the hormonal regulation of CD83 expression is likely a result from a balance between gene transcription from CD83 and the modulation of the metalloproteinase MMP-7. Thus, data supports and complements our previous murine studies on hormonal regulation of CD83 expression, reinforcing its immunomodulatory relevance in anti-bacterial responses during pregnancy.
1 Introduction
The dynamic balance between pro- and anti-inflammatory mediators is essential for the establishment of pregnancy, its progression as well as for the onset of parturition (1–4). The immune system undergoes multiple adaptations that facilitate the accommodation of the fetus, foster tolerance against paternal antigens and adjust defense strategies to protect mother and fetus against pathogens (5–10). Still, the presence of pathogens can lead to excessive immune responses, breaking the delicate immune balance and resulting in pregnancy complications including preterm birth (2).
The immune system possesses several mechanisms to control such excessive immune responses, promoting tolerance and the maintenance of pregnancy (11–13). In previous studies, we observed that pregnant mice showed a reduced antigen presentation potential, a distinct immunoglobulin and cytokine secretory milieu and an improved capacity to secrete anti-inflammatory mediators, including soluble CD83 (sCD83) (14–17).
CD83 is a transmembrane glycoprotein that belongs to the immunoglobulin family (18). Originally described as a maturation marker for dendritic cells (DCs), CD83 expression has been detected in other immune cells including T and B cells, where immune modulatory functions have been observed (19–21). In DCs and B cells, CD83 stabilizes the major histocompatibility molecule type II (MHC-II) through negative regulation of the E3 ubiquitin-protein ligases MARCH1 and MARCH8 (22, 23). In CD4+ T cells, CD83 expression is associated with the promotion of a tolerogenic phenotype (24, 25).
Murine experiments permitted a vast characterization of CD83 on B cells. Uhde et al. showed that CD83 co-localizes with the B cell receptor (BCR) and the Toll-like receptor 4 (TLR4) (26). The expression is associated with a lower sensitivity of the BCR and an increase in marginal zone (MZ) B cell number (26, 27). Furthermore, CD83 overexpression improved the release of the anti-inflammatory cytokine IL-10 in lipopolysaccharide (LPS) stimulated lymphocytes (28).
CD83 has a soluble form, sCD83, which possesses strong anti-inflammatory functions as successfully evaluated in mouse models for autoimmunity, allograft transplantation, allergies and chronic inflammation (29–36). In this context, we were able to show that mice acquire a higher capacity to release sCD83 during pregnancy and that lower sCD83 serum levels are associated with disturbed murine pregnancies (15, 17). Additionally, it has recently been shown that sCD83 can protect pregnant mice from LPS-mediated fetal death (37). One major immunomodulatory mechanism of sCD83 concerns the blockade of LPS-TLR4/MD-2 interactions (38). These data point to a regulation of immune responses through sCD83 upon confrontation with bacteria, which could contribute to maintenance of pregnancy.
Although CD83 expression has been described in human endometrial B cells, its role during pregnancy is less known (39). A recent study described the levels of circulating sCD83 in human pregnancies (40). Analogously to our observations in mice, hormonal regulation of serum sCD83 levels is suggested for human pregnancies as well. The aim of this work was to evaluate the regulation and expression of CD83 in human pregnancies. We postulate that local and systemic pregnancy associated factors regulate CD83 expression and sCD83 release.
2 Materials and methods
2.1 Isolation of PBMCs (CD3+ and CD19+ cells)
Buffy coats from non-pregnant female donors at reproductive age (18-40 years) were obtained from the Institute of Transfusion Medicine of the University Medicine Greifswald with approval of the Ethics Committee of the University of Greifswald (Reg.Nr. BB 014/14). At the time of blood donation, all women felt healthy, were at least 4 weeks absent of febrile infectious disease, antibiotics or systemic corticosteroids intake, and had not been pregnant for the last 6 months. PBMCs were isolated using density centrifugation. Buffy coats were centrifuged at 1300 × g for 10 min without brake. The pellets were resuspended in PBS (Biochrom AG, Berlin, D) and layered on Lymphoprep™ (STEMCELL technologies Inc, Vancouver, Canada). After centrifugation at 800 × g for 30 min without brake and acceleration, mononuclear cells were collected, washed in PBS and centrifuged at 300 × g for 5 min.
CD3+ and CD19+ cells were isolated using the EasySep™ Human CD3 Positive Selection Kit II (STEMCELL technologies Inc, Vancouver, Canada) and the CD19 MicroBeads, human Kit (Miltenyi Biotec GmbH, Bergisch Gladbach, D) following manufacturer’s instructions.
2.2 Isolation of decidual mononuclear cells
Decidual mononuclear cells were isolated from decidua basalis and parietalis from term placentae (38-41 weeks) after caesarean section according to Xu et al., 2015. Experiments have been approved by the Ethic Committee of the University of Greifswald (BB 160/17a). Women included in this study did neither have diagnosed immunological nor acute or chronic inflammatory diseases. Their age ranged between 22-42. More detailed information can be found in Supplementary Table 1. Manually scraped tissue was enzymatically digested using collagenase D (1.67 mg/mL) (Merck, Darmstadt, D) and DNase I, Bovine Pankreas (0.5 mg/mL) (Merck, Darmstadt, D). Mechanical digestion was performed using the gentleMACS(TM) Dissociator system (program ‘m_heart_02’) (Miltenyi Biotec GmbH, Bergisch Gladbach, D). Afterwards the tubes were incubated for 60 min at 37°C and mixed regularly. To gather the cells, the suspension was filtered using a 0.4 µm cell strainer and centrifuged and 350 × g for 10 min. PBMC isolation was performed according to 5.1.
2.3 Cell culture
PBMCs from buffy coats and decidual tissue as well as CD3+ and CD19+ cells were cultured in RPMI 1640 (10% FBS, 1% PenStrep, 55 μM β-ME) media (Biochrome GmbH, Berlin, D). 1 × 106/mL PBMCs were cultured and stimulated on 24 well flat bottom suspension plates (Sarstedt AG&Co., Nümbrecht, D). For CD3+ and CD19+ cells, 4 × 106 cells/mL were seeded in 48 well flat bottom suspension plates (Sarstedt AG&Co., Nümbrecht, D). The PBMCs from buffy coat, CD3+ and CD19+ cells were stimulated with LPS (10 μg/mL)(Merck, Darmstadt, D) and additionally treated with human chorionic gonadotrophin (hCG) (100 UI/mL) (Merck, Darmstadt, D), TGF-β1 (20 ng/mL), (Bio-Techne, Minneapolis, USA), estradiol (20 ng/mL) (Merck, Darmstadt, D), progesterone (300 ng/mL) (Merck, Darmstadt, D) or 1 μM dexamethasone (400 ng/mL) (Merck, Darmstadt, D) for 48h. For the last 5 h PMA (50 ng/mL) (Merck, Darmstadt, D) and Ionomycin (500 ng/mL) (Merck, Darmstadt, D) were added. PBMCs from decidual tissue were stimulated with 10 μg/mL LPS for 43 h before 50 ng/mL PMA and 500 n/mL Ionomycin were added for additional 5 h.
2.4 Isolation of extracellular vesicles
For downstream analysis using flow cytometry, extracellular vesicles (EVs) were isolated from PBMC supernatant after 48 h stimulation using Total Exosome Isolation reagent (Invitrogen, Thermo Fisher Scientific, Waltham, Massachusetts, USA), following manual instructions. For CD83 ELISA, EVs were isolated from PBMC supernatant using EV Isolation Kit Pan (Miltenyi Biotec GmbH, Bergisch Gladbach, D), following manufacturer’s instructions.
2.5 Flow cytometry
After 48 h of cultivation, cells were washed in PBS and stained with 100 μl Fixable Viability dye eFlour 520 (1/1.000) (Thermo Fisher Scientific, Waltham, Massachusetts, USA) in PBS for live/dead distinction for 4°C for 30 min. Following a washing step, CD3 APC-Cy7 (BD, New Jersey, USA) CD4 PE-Cy7 (BD, New Jersey, USA), CD19 PerCP-Cy5.5 (BD, New Jersey, USA), CD14 FITC (BD, New Jersey, USA), CD16 FITC (BD, New Jersey, USA), CD56 FITC (Miltenyi Biotec GmbH, Bergisch Gladbach, D), CD83 APC (BD, New Jersey, USA), CD11c PE (BD, New Jersey, USA) antibodies were added for 30 min at 4° C. After incubation, the cells were washed with FACS buffer (1% BSA, 0,1% NaN3 in 1x PBS) and divided into two tubes to perform extra- and intracelluar staining. The cells for extracellar staining were directly stained with CD83 APC (BD, New Jersey, USA) antibody for 30 min at 4°C and measured afterwards. For intracellular staining, cells were previously fixated with 100 μL 1× eBioscience Fixation solution (Thermo Fisher Scientific, Waltham, Massachusetts, USA) for 20 min at room temperature kept in the dark. The cells were then permeabilized in 500 μl eBioscience Permabilization Buffer (Thermo Fisher Scientific, Waltham, Massachusetts, USA) for 5 min at 4°C and afterwards stained with CD83 APC (BD, New Jersey, USA) antibody for 30 min at 4°C.
Flow cytometric measurement was performed using the BD FACSCanto™ Clinical Flow Cytometry system. Data were analyzed in the FlowJo software (FlowJo V.10.1, FlowJo, LLC, Ashland, Oregon, USA). Samples were treated and measured in duplicates.
For flow cytometric analysis of EVs, Vybrant™ Dio Cell-Labeling Solution (Thermo Fisher Scientific, Waltham, Massachusetts, USA) was added to supernatants 15 min prior to collection of supernatants. Isolated EVs were stained with CD9-APC (Miltenyi Biotec GmbH, Bergisch Gladbach, D), CD63-PE-Cy7 (BD, New Jersey, USA) and CD83-PE (BD, New Jersey, USA) antibodies for 30 min at 4°C in the dark. PE Mouse IgG1 κ Isotype Control was used as CD83-negative control and Cytokeratin 7-APC (Miltenyi Biotec GmbH, Bergisch Gladbach, D) antibody was used as CD9 isotype control. Size reference of EVs was performed using Flow Cytometry Sub-Micron Size Reference Kit (Thermo Fisher Scientific, Waltham, Massachusetts, USA). Flow cytometric analysis was performed on the Attune NxT with Attune™ NxT Small Particle Side-Scatter Filter. Data were analyzed with FlowJo software (FlowJo V.10.10, FlowJo, LLC, Ashland, Oregon, USA).
2.6 ELISA
Cell culture supernatants were analyzed with a R&D DuoSet ELISA kit human sCD83 (DY2044-05) (R&D Systems, Minneapolis, USA) following manufacturer’s instructions. Samples were tested in duplicates.
2.7 Multiplex assay
CD3+ and CD19+ supernatants were characterized using an 8-plex Magnetic Bead Kit (MMP-2, MMP-7, MMP-9, MMP-13, TIMP-1, PD-L1, PD-L2, TGF-β; ThermoFisher Scientific, Schwerte, Germany) for detection in the Bio-Plex™ 200 System (Bio-Rad Laboratories, Hercules, USA). Analyte concentrations were determined in duplicates, the means are shown (pg/mL).
2.8 RNA extraction and quantitative real-time PCR
Cells were harvested in TRIzol® (ThermoFisher Scientific, Waltham, USA). Chloroform (> 99%; Chemsolute, Roskilde, Denmark) was added and phases were separated during 15 min centrifugation at 10,000 × g and 4°C. RNA was precipitated with Isopropanol (> 99%; Chemsolute, Roskilde, Denmark) for 1 h at –20°C, while RNA visibility was improved using Glyco Blue™ (ThermoFisher Scientific, Waltham, USA). After 20 min centrifugation at 10,000 × g and 4°C, RNA was washed with Ethanol (96%; Walter CMP, Kiel, Germany) centrifuged again for 6 min at 10,000 × g and 4°C and dried carefully. RNA was resupended in DEPC water. For reverse transcription in the PeqSTAR thermocycler (PEQLAB, Erlangen, Germany), the High-Capacity cDNA Reverse Transcription Kit with RNase Inhibitor (ThermoFisher Scientific, Waltham, USA) was used according to manufacturer’s instructions. Quantitative Real-Time PCR (qPCR) was performed in the 7300 Real Time PCR thermocycler (ThermoFisher Scientific, Waltham, USA) using the Power SYBR(R) Green PCR Master Mix (ThermoFisher Scientific, Waltham, USA) and primers flanking CD83 or ACTB, respectively. The cycling program was adjusted according to manufacturer’s protocol with 2 min at 50°C before pre-heating at 95°C for 10 min, followed by 41 PCR cycles with a denaturing temperature of 95°C for 15 s and annealing and elongation at 60°C for 1 min. The final PCR cycle was terminized with 95°C for 15 s. Relative quantification values for CD83 mRNA expression were calculated via comparative threshold cycle method (2-ΔΔCt) using ACTB (Beta-Actin) as an internal reference. The used oligonucleotides (ThermoFisher Scientific, Waltham, USA) are listed in Supplementary Table 2. The means of quadruplicates are shown for six biological replicates. Primer sequences are shown in Supplementary Table 1.
2.9 In silico cleavage analysis
A cleavage prediction analysis with Procleave (procleave.erc.monash.edu/Procleave_crf/webserver.html) was performed on the human CD83 molecule (GenBank: AAH30830.1) (41). Candidates targeting the extracellular domain of CD83 (residues 20-144) were identified and selected for further analysis when the predictive cleavage score was higher than 0.7 (see Supplementary Table 2).
2.10 Statistical analysis
Data represent the average of biological replicates obtained in independent experiments as described in the figure legends. Statistical analysis was performed using the GraphPad Prism 9 software. Data were tested for normal distribution before analysis. Statistical significance was determined using a one-way ANOVA with Dunnett’s post-hoc analysis and is defined as p < 0.05 (*), p < 0.01 (**), p < 0.001 (***), or p < 0.0001 (****).
3 Results
3.1 LPS increases CD83 expression in decidual leukocytes
To evaluate the involvement of CD83 in the tolerogenic responses of the human decidua, decidua basalis and decidua parietalis mononuclear cells were treated with LPS and the intracellular and extracellular expression of CD83 was evaluated by flow cytometry (Figure 1). After LPS treatment, a significant increase of CD83 expression could be detected in cells from decidua basalis and decidua parietalis intracellularly (iCD83) as well as extracellularly (mCD83). Particularly B lymphocytes experienced an upregulation in both extra and intracellular compartments, while T cells and DCs predominantly showed changes in mCD83 expression. These data indicate that leukocytes from both decidual compartments react to bacterial LPS via induction of CD83 expression.
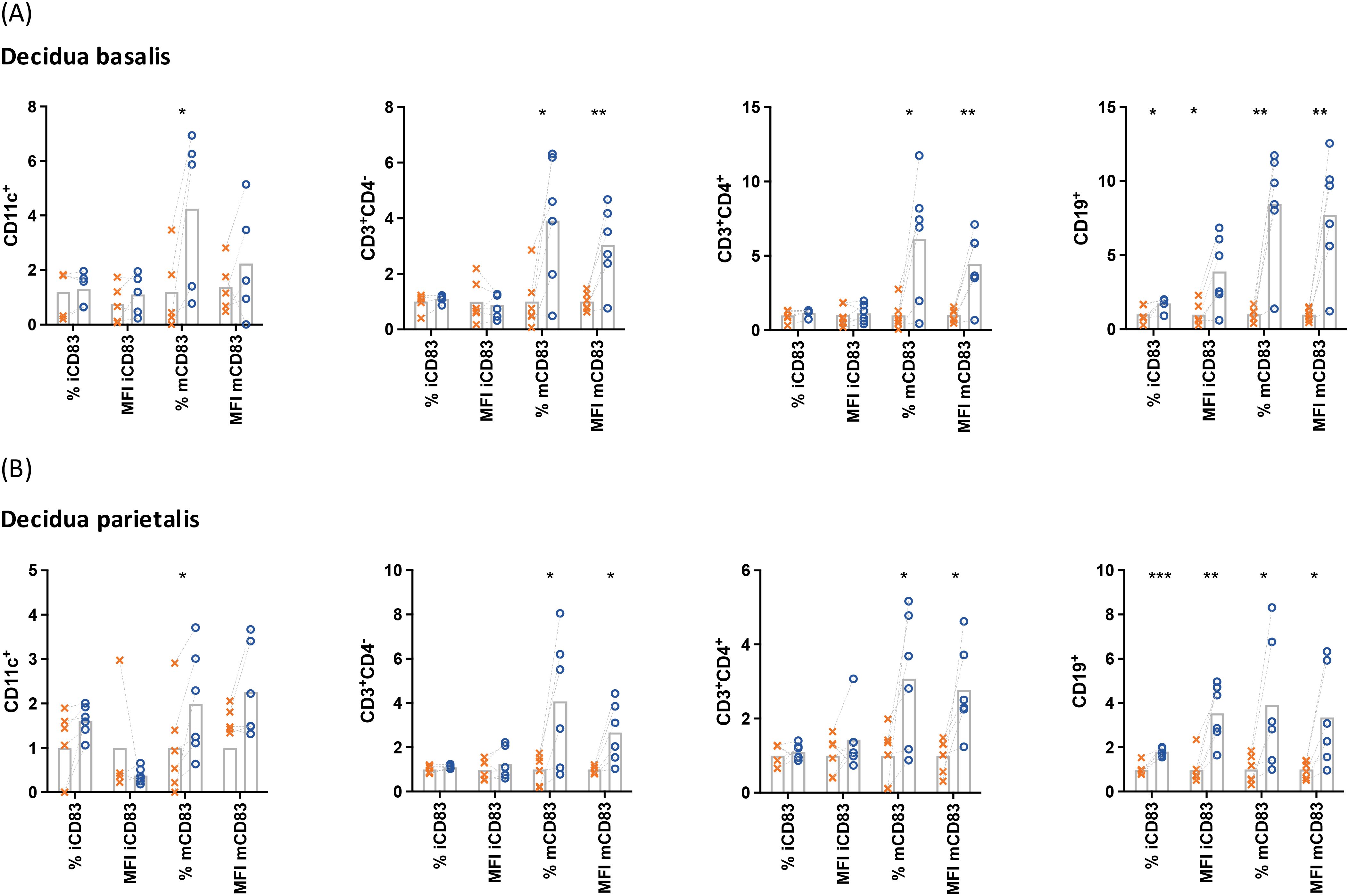
Figure 1. Mononuclear cells were isolated from the decidua basalis (A) or decidua parietalis (B) of term placentae, cultured in the presence (○) or absence (✕) of 10 µg/mL LPS, 50 ng/mL PMA, 500 ng/mL ionomycin and characterized by flow cytometry. Data were normalized to the respective mean of the control, analyzed by paired t-test, and presented as mean (n = 5). *p < 0.05; **p < 0.01; ***p < 0.001. MFI, mean fluorescence intensity; i, intracellular; m, membrane-bound (extracellular). Gating strategies are shown in Supplementary Figure 1.
3.2 CD83 is preferentially increased in B lymphocytes after LPS treatment
In order to identify immune cell populations that preferentially express mCD83 upon LPS stimulation, cells from decidua basalis, decidua parietalis and peripheral blood from female non-pregnant donors were analyzed (Figure 2). Similar to our previous observations in multiple lymphoid organs in mice, decidua basalis B cells were the cells expressing mCD83+ (accounting for approximately 60% of B cells) most distinctly after LPS stimulation. Though less prominent, a similar pattern could be observed in decidua parietalis, while peripheral blood cells showed a clear predominance of CD83 expression in B cells among all studied leukocytes. These results suggest that B cells are the main source of sCD83 at the fetomaternal interface, especially in decidua basalis as compared to decidua parietalis.
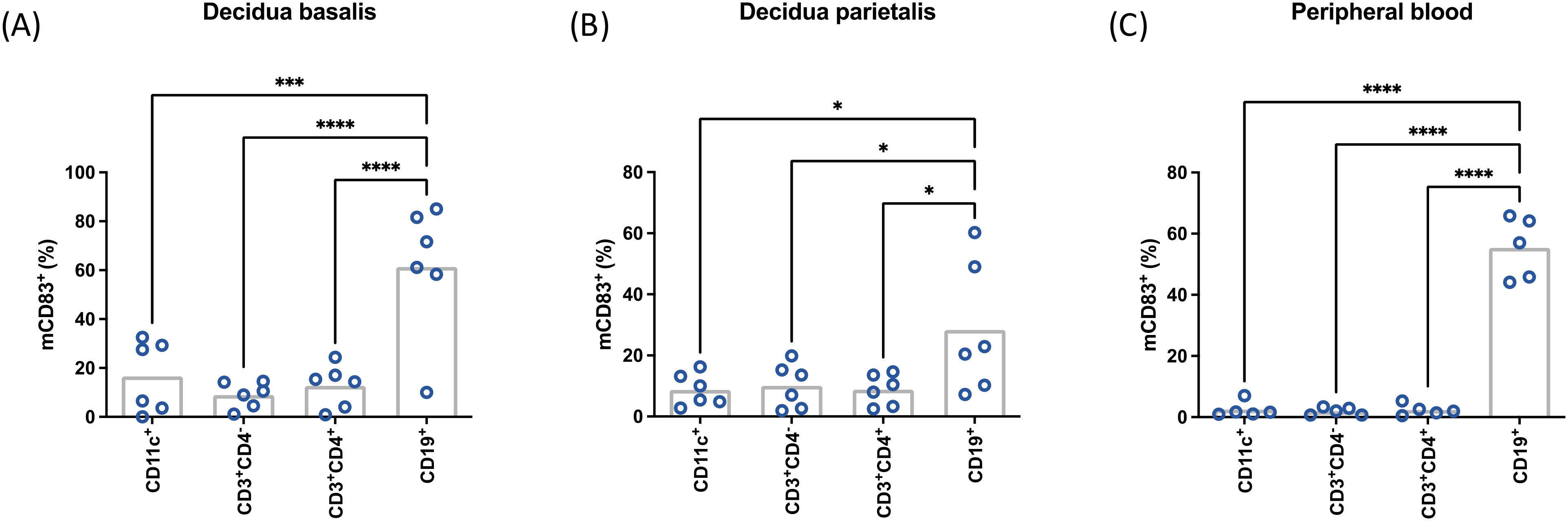
Figure 2. Mononuclear cells were isolated from term placental decidua basalis (A), decidua parietalis (B), or peripheral blood (C) stimulated with 10 µg/mL LPS, 50 ng/mL PMA, 500 ng/mL ionomycin, and characterized by flow cytometry. Data were analyzed by paired ANOVA with Tukey post-test (A) and presented as mean (a,b: n = 6; c: n = 5). *p < 0.05; ***p < 0.001; ***p < 0.0001. MFI, mean fluorescence intensity. Gating strategies are shown in Supplementary Figure 1.
3.3 Decidual leukocytes have a low capacity for sCD83 release
To evaluate the capacity of decidual leukocytes to release sCD83, the increment of mCD83 expression after LPS treatment was compared between cells from decidua basalis and peripheral blood from female non-pregnant donors (Figure 3). B cells from the decidua basalis showed a reduced capacity to increase mCD83 expression after LPS stimulation as compared to peripheral blood leukocytes. No significant differences were observed in T cells and DCs.
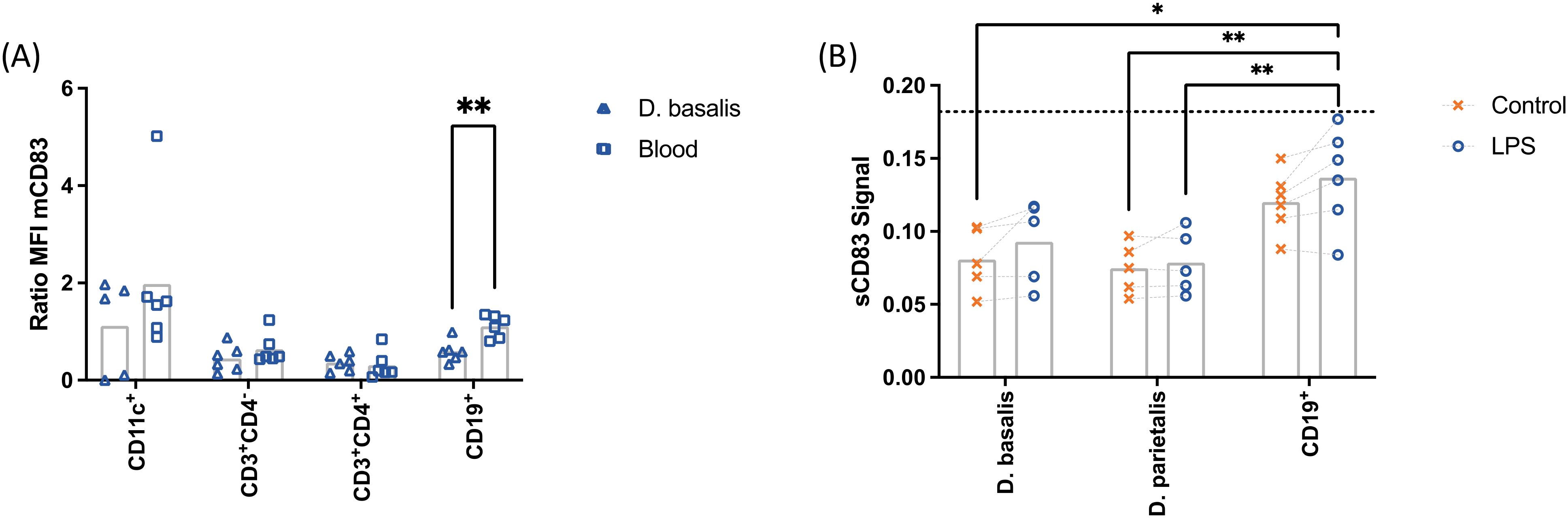
Figure 3. Mononuclear cells from decidua basalis (△) or peripheral blood (□) were isolated. Expression of mCD83 in CD11c+, CD3+CD4-, CD3+CD4+ and CD19+ cells after stimulation with 10 µg/mL LPS, 50 ng/mL PMA, 500 ng/mL ionomycin was compared using flow cytometry (ratio = stimulated:control; A). Mononuclear cells from decidua parietalis or decidua basalis and B cells from decidua basalis were isolated from term placentae and cultured in the presence (○) or absence (✕) of 10 µg/mL LPS, 50 ng/mL PMA, 500 ng/mL ionomycin. Cell culture supernatants were analyzed by ELISA (B). The dotted line represents the mean of the lowest standard. Data were analyzed by unpaired t-test (A) or ANOVA with Tukey (B) post-test and are presented as mean (a: n = 6; b: n = 4-6). *p < 0.05; **p < 0.01. Gating strategies are shown in Supplementary Figure 1.
Similar results were obtained for the analysis of sCD83 release. Conditioned media of the LPS-treated decidual leukocytes was analyzed by ELISA. The levels of sCD83 were detected below the lowest assay standard and therefore could not be quantified. Despite this, the obtained data was further analyzed to get insights into the relevance of decidual B lymphocytes on sCD83 release. The detected levels of sCD83 released by B cells isolated from decidua basalis were higher than in leukocytes from decidua basalis and decidua parietalis. Overall, these data suggest a local regulation of sCD83 release.
3.4 Pregnancy-related molecules modulate LPS-induced mCD83 expression and sCD83 release
The capacity of pregnancy-related molecules to modulate LPS-induced mCD83 expression and sCD83 release was analyzed by flow cytometry and ELISA (Figure 4). T cells showed a reduction of mCD83+ cells after treatment with estradiol and dexamethasone, as well as a reduction of sCD83 after treatment with solely dexamethasone. Progesterone, TGF-β1 and hCG lead to a determinable though statistically not significant reduction of mCD83 expression and sCD83 release.
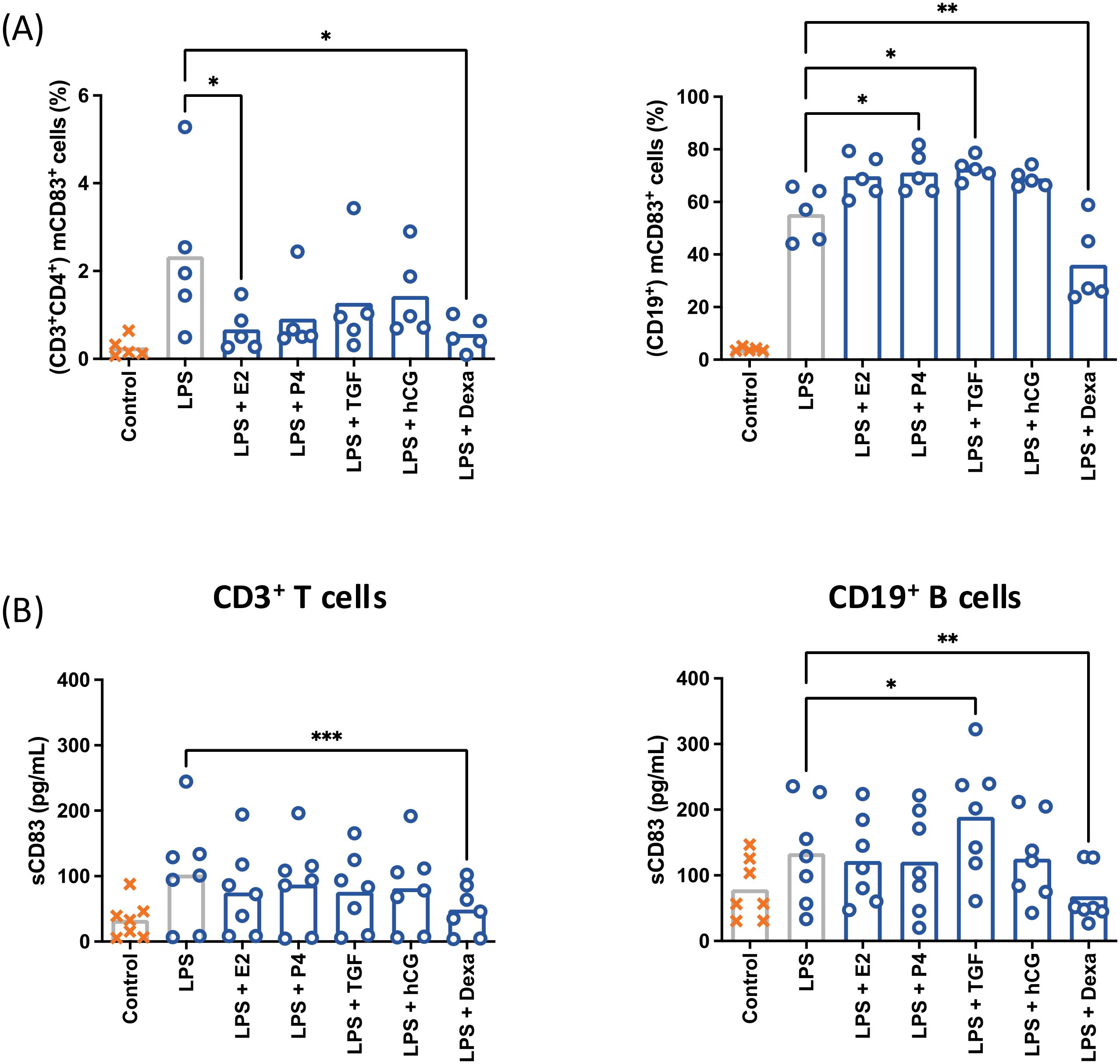
Figure 4. CD3+CD4+ T cells and CD19+ B cells from peripheral blood were characterized by flow cytometry after treatment with pregnancy-related factors (A). E2: estradiol (2 ng/mL), P4: progesterone (30 ng/mL), TGFβ: TGF-β1 (2 ng/mL), hCG (10 IU/mL) Dexa, dexamethasone (40 ng/mL). CD3+CD4+ and CD19+ cells were isolated by magnetic separation and stimulated with 10 µg/mL LPS, 50 ng/mL PMA, 500 ng/mL ionomycin (○) in the presence of pregnancy-related factors. The supernatant of treated cells was analyzed by ELISA (B). Data were analyzed by paired ANOVA with Dunnett post-test and are depicted as mean (a: n = 5; b: n = 7). *p < 0.05; **p < 0.01; ***p < 0.001. Gating strategies are shown in Supplementary Figure 2.
CD83-expressing B cells were increased after treatment with progesterone and TGF-β1 but reduced after dexamethasone treatment. There was also a significant increase in sCD83 release after treatment with TGF-β1 and a significant reduction of sCD83 release after dexamethasone treatment. Estradiol and hCG treatment induced a statistically non-significant increase of mCD83 expression, but had no effect on sCD83 release.
These data indicate that pregnancy-associated factors are able to modulate mCD83 expression and sCD83 release in leukocytes.
3.5 LPS induced increase of CD83 attributable to sCD83 and not EV-bound mCD83
The detection of sCD83 by ELISA in cell culture media of LPS-stimulated leukocytes could be attributed to the release of CD83 as a soluble protein or to the presence of the membrane-bound form in the surface of extracellular vesicles (EVs). To verify the latter, EVs were isolated from cell conditioned media and analyzed by flow cytometry (Figure 5) and ELISA (data not shown). Characterization of EVs was based on CD9- and CD63-expression on DiO-stained small particles, which were cross-checked by size reference beads (Figure 5A). We showed significantly higher number of isolated CD9+ exosomes from LPS stimulated PBMCs as compared to non-stimulated controls. This effect could be seen after 24 h as well as after 48 h stimulation with LPS, PMA and Ionomycin. Additionally, two populations of EVs were detected in unstimulated control samples, with one population encompassing exosomes up to 200 µm, and a second population of ectosomes in a size range of 200 – 500 µm. Upon LPS stimulation, samples showed less exosomes < 200 µm diameter, but a significant increase of EVs mostly within the size range of 200 – 1000 µm. EVs of LPS-stimulated samples additionally showed a significant increase of CD9-expressing DiO+ EVs and CD9 RFI (CD9 MFI of sample/CD9 MFI of isotype control) (Figure 5B). Interestingly, CD63-expressing DiO+ EVs were slightly increased in LPS-stimulated samples, yet CD63 MFI stagnated and tended to be decreased, implying a shift to CD9+CD63- ectosome- over CD9+CD63+ exosome-production.
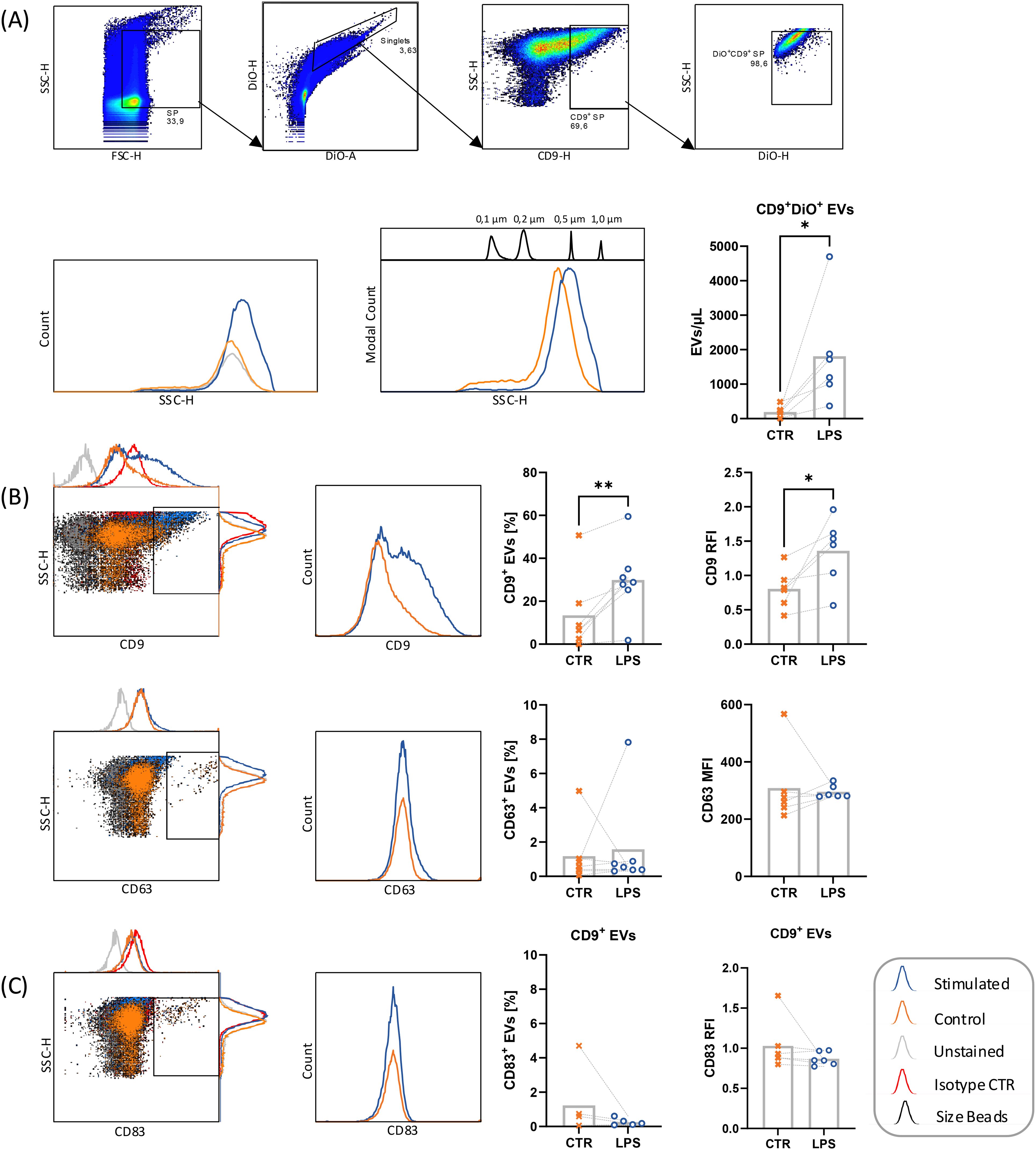
Figure 5. Flow cytometric analysis of EVs from PBMC supernatants before (CTR, ✕) and after stimulation with 10 µg/mL LPS, 50 ng/mL PMA, 500 ng/mL ionomycin (LPS, ○). DiO+ small particles (SP) of stimulated PBMC were analyzed by flow cytometry after EV isolation using Invitrogen Total Exosome Isolation reagent. (A) Small particles were gated for single events according to DiO-H to DiO-A ratio. Single events were first gated for CD9+ SP, followed by gating for DiO-stained SP, excluding high-SSC DiO-dye crystals. Henceforth, DiO+CD9+ SP were called extracellular vesicles (EVs). EVs were cross-checked with size reference beads. (B) DiO+ stained single events were investigated for their CD9 and CD63 expression. CD9 RFI calculated from CD9 MFI of sample in relation to isotype control. (C) DiO+CD9+ EVs were investigated for CD83 expression. RFI calculated from CD83 MFI of sample in relation to isotype control. Data were analyzed by paired t-test and are depicted as mean (n = 6). *p < 0.05; **p < 0.01. RFI, Relative Immunofluorescence.
Considering the low CD63 expression upon LPS stimulation, mCD83 expression was also analyzed on DiO+CD9+ EVs (Figure 5C). Despite the increase in the numbers of DiO+CD9+ EVs after LPS stimulation, low mCD83 expression could be detected, which was even slightly decreased after LPS stimulation. Similarly, when magnetically purified CD63+, CD9+ and CD81+ EVs from 24 h LPS stimulated mononuclear cells were analyzed by ELISA, no CD83 could be detected.
These results indicate that the higher expression of CD83 in the culture media of LPS-stimulated mononuclear cells can be attributed to an increased release of sCD83 and not to an increase in the number of CD83+ EVs.
3.6 Pregnancy-related molecules regulate CD83-targeting protease MMP-7
As pregnancy-related factors modulated both mCD83 expression and sCD83 release, a possible induction of CD83 gene transcription was evaluated (Figure 6A). First, a promotion of CD83 mRNA expression in LPS-treated cells was observed after of TGF-β1 treatment.
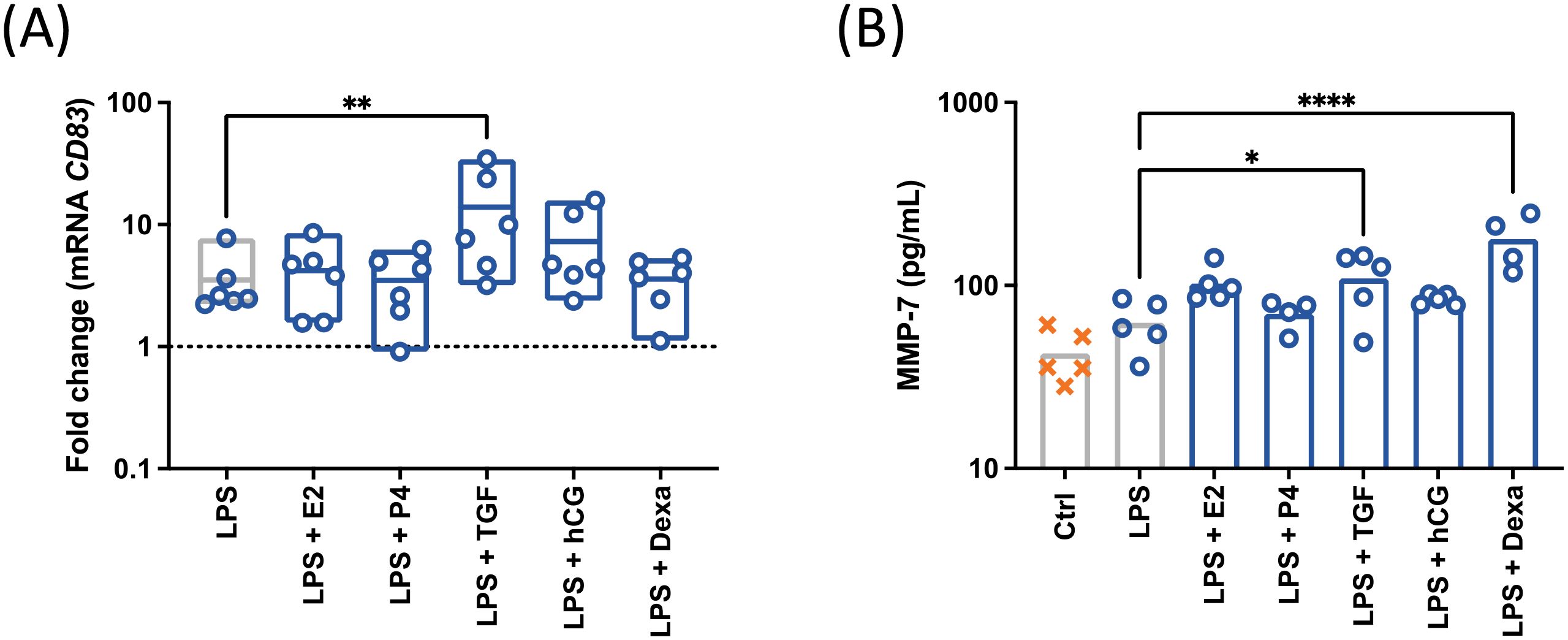
Figure 6. CD19+ cells were isolated by magnetic separation and stimulated with 10 µg/mL LPS, 50 ng/mL PMA, 500 ng/mL ionomycin (○) in the presence of pregnancy-related factors. E2, estradiol (2 ng/mL), P4: progesterone (30 ng/mL), TGFβ, TGF-β1 (2 ng/mL), hCG (10 IU/mL) Dexa, dexamethasone (40 ng/mL). The expression of CD83 was assessed by qPCR (A) and the secretion of MMP-7 was analyzed by ELISA (B). Data were analyzed by paired ANOVA with Dunnett post-test and are depicted as mean (n = 6). *p < 0.05; **p < 0.01; ****p < 0.0001.
Then, as the increase or reduction of sCD83 release could be caused by enhanced cleavage of the membrane-bound form or its degradation, respectively, modulations in protease activity were considered (Figure 6B). Based on cleavage prediction analysis of the extracellular structure of CD83 (Proclave), the highest predictive cleavage values (>0,700) were detected for MMP-2 (0,924), MMP-7 (0,721) and MMP-9 (0,703). As observed in our previous work, we identified TIMP-1 as a regulator of mCD83 expression. The MMPs targeted by TIMP1 (MMP-7 and MMP-9-) were therefore quantified in the conditioned media of treated cells. Both TGF-β1 and dexamethasone induced an increase of MMP-7 in supernatants of LPS-treated cells. Regarding MMP9 and TIMP1 mRNA levels, no significant modulation by pregnancy-related molecules could be observed (Supplementary Figure).
These data suggests that TGF-β1 and dexamethasone promote a MMP-7-mediated degradation of mCD83 which is overcome by an increment of gene transcription in the case of TGF-β1 (Supplementary Figure).
4 Discussion
The maternal immune responses against infections has to adapt in order to minimize the risk of compromising fetal tolerance during pregnancy (2, 42–44). The relevance of sCD83 in modulating excessive pro-inflammatory immune responses has been previously demonstrated (31, 32, 34–37, 45–48). Its role for pregnancy well-being however, has not been studied extensively. Murine studies suggest its relevance in response to inflammatory stimuli derived from bacterial components as LPS (15, 17). Human studies of CD83 during pregnancy are sparse but indicate a systemic control of sCD83 release (40).
In this work, we were able to characterize mCD83-expressing cells in decidua basalis and decidua parietalis of human pregnancies at term. Additionally, CD83-staining was performed after fixation and permeabilization of the cells (iCD83), providing data on the intracellular expression of the CD83 molecule. Although the extracellular expression of CD83 was not blocked before the intracellular staining, the iCD83 expression was significantly higher than the mCD83 staining. When the cells were stimulated, the increase of mCD83 expression was approximately 5 times higher than the iCD83 staining (control mCD83/iCD83 versus stimulated mCD83/iCD83 between 4.8 and 5.2). For this reason, we attribute the changes in CD83 mainly to a promotion of mCD83 expression rather than an increased de novo synthesis. However, further studies could address this in more detail.
In both decidua basalis and decidua parietalis, treatment with bacterial LPS led to an increased expression of mCD83 in dendritic cells, T cells and B cells. After this increment, mCD83+ cells were observed specially within the B cell subset. This observation was particularly distinctive in leukocytes isolated from the decidua basalis. Previous studies have described multiple differences between the composition of leukocytes isolated from decidua basalis and decidua parietalis (49). These differences might be related to the microenvironmental characteristics of each compartment. In particular, decidua basalis is exposed to higher levels of oxygen than decidua parietalis and stands in closer contact to other immunoregulatory cells such as decidual stroma cells and trophoblasts. Furthermore, the decidual leukocyte composition is influenced by blood derived immune cells.
In our study, B cells from decidua basalis contained a higher percentage of CD83+ cells than peripheral blood B cells after LPS Stimulation. However, the relative increase upon stimulation with LPS was lower in decidua basalis B cells. After evaluating the sCD83 secretory capacity, only low levels of sCD83 could be detected in the supernatants of cultured decidual mononuclear cells, hindering the quantification and further evaluation of the treatment with certainty. As similar number of B cells were stimulated in vitro, the data point to an individual decidual mCD83+ B cell compartment. In this context it is possible that decidual B cells experience a different regulatory environment than those in the periphery. There are several decidual immune cells with a specific decidual phenotype, which is partially determined by the role of hormones and other factors present at the fetomaternal interface (44, 50–54). As term deciduae were analyzed during this work, a regulated sCD83 release to facilitate inflammatory mechanisms during parturition cannot be discarded. However, considering the use of enzymatic digestion during decidual lymphocyte isolation and its possible influence on protease activity, this observation should be seen with caution.
Since peripheral B cells were obtained from blood from non-pregnant female donors, a modulation of CD83 expression by pregnancy-related factors was only evaluated in vitro. There was no effect of pregnancy-related hormones on baseline mCD83 expression (not shown). However, they clearly modulated the capacity of B-cells to express mCD83 and release sCD83 in the presence of pro-inflammatory triggers. These data support our previous observation from mouse models, where B cells acquired an enhanced capacity to express mCD83 and release sCD83 during pregnancy (15, 17).
In spite of the hormone-based increase of mCD83/sCD83 on stimulated leukocytes, it is remarkable that the release of sCD83 from decidual mononuclear cells was considerably low. As the release of sCD83 was LPS-triggered, we postulate that sCD83-mediated regulation predominantly modulates systemically, but less effectively in confrontation to local infections. This might result advantageous during infections during pregnancy, as immune responses distant from the fetomaternal interface will be accompanied by a boost of sCD83 release, contributing to pregnancy maintenance. Our data, on the other hand, do not support a local sCD83-mediated regulation of immune responses towards infections at the fetomaternal interface. However, a sCD83-dependent regulation at the fetomaternal interface might occur though the influence of systemic sCD83 or by infiltrating leukocytes. Alternatively, this might represent an advantage to prioritize inflammation to foster immune responses against local infections.
It remains unknow which factors account for the differential sCD83 secretory capacity between peripheral and decidual B cells. Differences in B cell phenotype between both compartments have been observed previously and include a higher CD83 expression on resting endometrial B cells (39). However, in this and in our study, B cell subpopulations were not analyzed in detail. In peripheral blood and decidua, B cells represent a heterogeneous cell type which can be subdivided in two major groups: B1 (B1-a, B1-b) and B2 subsets (Marginal zone and follicular zone B cells, plasma cells, etc) (55, 56). Murine and human studies have shown that pregnancy induces changes in the development and function of several B cell subtypes (5, 56–63). Among these adaptations, an increased development of marginal zone B cells over follicular zone B cells could be observed. Although the expression and relevance of CD83 on B2 development and function has been well investigated, less is known about its relevance of B1 B cell biology (21, 27, 64). Therefore, as the distribution of each subset varies between peripheral blood and decidua, it is possible that these differences reflect the CD83-behavior we observed on total CD19+ B cells (65, 66).
Our results indicate that sCD83 might protect pregnancy from systemic but not local infections. While local leukocytes have a reduced sCD83 secretion capacity, blood derived leukocytes remain a main source of sCD83, although not through the secretion of CD83+ EVs. To evaluate how pregnancy alters mCD83 and sCD83 release on peripheral leukocytes, we performed a series of in vitro experiments in the presence of hormones and further pregnancy-related factors.
Hormones exert regulatory roles shaping the dynamics of the immune system during the different stages of pregnancy. In general terms, hormones including E2, P4 and hCG promote tolerogenic responses that facilitate fetal tolerance and strengthen immune balance (67–70). In our experiments, an increment of CD83 expression could be observed after treatment with E2, P4 and hCG, but no considerable increment of sCD83 release was detected. This observation suggests that if mCD83 represents a source of sCD83, the membrane expression and the release of a soluble form might be independently modulated by hormones during pregnancy. Indeed, as we discussed in a previous work, the modulation of proteinases of the stromal tissue might exert an important role in the sCD83 release (17). This is a strong limitation in our work, as we evaluated the role of hormones in an in vitro set-up with peripheral blood mononuclear cells. A closer look into the modulation of proteolytic enzymes that target mCD83 has been discussed below. Nevertheless, it is still possible that the increase in CD83 expression on B cells enhances further tolerogenic adaptations, such as IL-10 release and the promotion marginal zone cell development (26–28).
TGF-β1 is an important modulator of immune cell function at the fetomaternal interface and its circulation increases during pregnancy (71, 72). TGF-β1 induces immunosuppressive regulatory T cells (Tregs), regulates the function of decidual NK cells and promotes M2-like macrophages (73). In our study, TGF-β1 showed a clear induction of CD83 production in mRNA level, membrane expression and release.
To evaluate the role of hormonal factors linked to late pregnancy and parturition, we analyzed the effects of glucocorticoids (dexamethasone) in vitro. The treatment led to a reduced mCD83 expression and sCD83 release from T cells and B cells. This could prevent sCD83 from interfering with the pro-inflammatory cascade occurring in the context of labor (74–77). Additionally, based on these data, sCD83 levels could be expected to be reduced in pregnant women experiencing high levels of stress, therefore high levels of cortisol. A possible role of stress/cortisol-mediated sCD83 reduction in preterm birth should be addressed in further studies (78).
As reported previously in mouse models, the bioavailability of mCD83 and subsequently sCD83 are likely to be subjects of hormone-dependent regulation of the proteolytic machinery (17). In an earlier work, we had found that negative regulation through the inhibitor of metalloproteinases TIMP-1 induces mCD83 expression and release, pointing to a proteinase-related appearance. The protease families ADAM and MMPs are modulated by TIMP-1 and strongly regulated during pregnancy (79). However, we had not found evidence of ADAM involvement in CD83 in our previous work and looked into MMPs closer in this study.
TIMP-1 is known to modulate MMP-1, MMP-3, MMP-7 and MMP-9 (80). After performing an in silico degradation of the external membrane segments of CD83, we found MMP-7 and MMP-9 to be feasible candidates for CD83 cleavage. Both enzymes displayed a cleavage “predictive score” higher than 0.7 (41). MMP-7 showed an inverse correlation to mCD83 expression and sCD83 release after treatment with dexamethasone, suggesting an MMP-7 mediated degradation of mCD83. However, after TGF-β1 treatment, increased levels of mCD83 and sCD83 were observed in the presence of high levels of MMP-7. This phenomenon could be explained through the compensatory upregulation of CD83 mRNA transcription. A summary of the relative effects of hormone treatment on mCD83, sCD83, CD83 mRNA and MMP-7 levels is listed in the supplements (Supplementary Figure 3).
The results of this study encourage a deeper characterization of mCD83/sCD83 during pregnancy. While the most relevant data of this study relies on in vitro observations, further studies could engage a larger number of patients to better reflect in vivo data. This will be particularly interesting during the different stages of pregnancy, which is accompanied by systemic changes in the hormonal levels. Furthermore, this study did not include samples from deciduae after spontaneous labor, which could depict further regulations of CD83 during the pro-inflammatory process of parturition. The effect of hormones and further immune modulatory factors could be studied in non-pregnant women in more detail. Although we only studied samples from healthy women in reproductive age, data concerning hormonal intake is missing. Larger studies could evaluate the role of menopause, contraceptives or hormonal therapy on CD83 expression and also provide further interesting data, including stress levels, demographics, etc.
In this work, we showed that mCD83 expression and sCD83 release is induced by LPS treatment in the fetomaternal interface. However, the contribution of systemic sCD83 might be stronger and subjected to a modulation by pregnancy-related molecules. This regulation is associated with changes in the production of CD83 and the modulation of proteolytic activity. In summary, the regulation of mCD83 and sCD83 release fits the model of tolerogenic mechanisms that prevails during mid pregnancy but declines towards the end of pregnancy.
Data availability statement
The data presented in the study are deposited in the Figshare repository, DOI number 10.6084/m9.figshare.26947969.
Ethics statement
The studies involving humans were approved by Ethic Committee of the University of Greifswald (Reg.-Nr. BB 014/14 and BB 160/17a). The studies were conducted in accordance with the local legislation and institutional requirements. The human samples used in this study were acquired from primarily isolated as part of your previous study for which ethical approval was obtained. Written informed consent for participation was not required from the participants or the participants’ legal guardians/next of kin in accordance with the national legislation and institutional requirements.
Author contributions
PK: Data curation, Formal analysis, Investigation, Validation, Visualization, Writing – original draft, Writing – review & editing. HW: Writing – review & editing, Data curation, Formal analysis, Investigation, Validation, Visualization, Writing – original draft. LZ: Data curation, Formal analysis, Investigation, Validation, Visualization, Writing – original draft, Writing – review & editing, Methodology. MZ: Writing – review & editing, Funding acquisition, Resources, Supervision. DM: Data curation, Formal analysis, Investigation, Validation, Visualization, Writing – original draft, Writing – review & editing, Conceptualization, Funding acquisition, Methodology, Project administration, Resources, Supervision.
Funding
The author(s) declare financial support was received for the research, authorship, and/or publication of this article. This study was supported by the Deutsche Forschungsgemeinschaft (DFG), grant awarded to DM (MU 4404/3-1) and intramural funding from the University Medicine Greifswald.
Acknowledgments
We thank the Landesgraduiertenförderung M-V and the Studienstiftung des Deutschen Volkes for supporting HW.
Conflict of interest
The authors declare that the research was conducted in the absence of any commercial or financial relationships that could be construed as a potential conflict of interest.
Publisher’s note
All claims expressed in this article are solely those of the authors and do not necessarily represent those of their affiliated organizations, or those of the publisher, the editors and the reviewers. Any product that may be evaluated in this article, or claim that may be made by its manufacturer, is not guaranteed or endorsed by the publisher.
Supplementary material
The Supplementary Material for this article can be found online at: https://www.frontiersin.org/articles/10.3389/fimmu.2024.1452879/full#supplementary-material
References
1. Robertson SA, Moldenhauer LM. Immunological determinants of implantation success. Int J Dev Biol. (2014) 58:205–17. doi: 10.1387/ijdb.140096sr
2. Green ES, Arck PC. Pathogenesis of preterm birth: bidirectional inflammation in mother and fetus. Semin Immunopathol. (2020) 42:413–29. doi: 10.1007/s00281-020-00807-y
3. Gomez-Lopez N, StLouis D, Lehr MA, Sanchez-Rodriguez EN, Arenas-Hernandez M. Immune cells in term and preterm labor. Cell Mol Immunol. (2014) 11:571–81. doi: 10.1038/cmi.2014.46
4. Mor G, Cardenas I. The immune system in pregnancy: a unique complexity. Am J Reprod Immunol. (2010) 63:425–33. doi: 10.1111/j.1600-0897.2010.00836.x
5. Muzzio DO, Soldati R, Ehrhardt J, Utpatel K, Evert M, Zenclussen AC, et al. B cell development undergoes profound modifications and adaptations during pregnancy in mice. Biol Reprod. (2014) 91:115. doi: 10.1095/biolreprod.114.122366
6. Solano ME. Decidual immune cells: Guardians of human pregnancies. Best Pract Res Clin Obstet Gynaecol. (2019) 60:3–16. doi: 10.1016/j.bpobgyn.2019.05.009
7. Zenclussen AC. Adaptive immune responses during pregnancy. Am J Reprod Immunol. (2013) 69:291–303. doi: 10.1111/aji.12097
8. Robertson SA, Care AS, Moldenhauer LM. Regulatory T cells in embryo implantation and the immune response to pregnancy. J Clin Invest. (2018) 128:4224–35. doi: 10.1172/JCI122182
9. Erlebacher A. Immunology of the maternal-fetal interface. Annu Rev Immunol. (2013) 31:387–411. doi: 10.1146/annurev-immunol-032712-100003
10. Blois SM, Klapp BF, Barrientos G. Decidualization and angiogenesis in early pregnancy: unravelling the functions of DC and NK cells. J Reprod Immunol. (2011) 88:86–92. doi: 10.1016/j.jri.2010.11.002
11. Tsuda S, Nakashima A, Shima T, Saito S. New paradigm in the role of regulatory T cells during pregnancy. Front Immunol. (2019) 10:573. doi: 10.3389/fimmu.2019.00573
12. Wang L-L, Li Z-H, Wang H, Kwak-Kim J, Liao A-H. Cutting edge: the regulatory mechanisms of macrophage polarization and function during pregnancy. J Reprod Immunol. (2022) 151:103627. doi: 10.1016/j.jri.2022.103627
13. Huhn O, Zhao X, Esposito L, Moffett A, Colucci F, Sharkey AM. How do uterine natural killer and innate lymphoid cells contribute to successful pregnancy? Front Immunol. (2021) 12:607669. doi: 10.3389/fimmu.2021.607669
14. Einenkel R, Ehrhardt J, Hartmann K, Krüger D, Muzzio DO, Zygmunt M. Hormonally controlled ILC antigen presentation potential is reduced during pregnancy. Reproduction. (2020) 160:155–69. doi: 10.1530/REP-19-0554
15. Packhäuser KR, Roman-Sosa G, Ehrhardt J, Krüger D, Zygmunt M, Muzzio DO. A kinetic study of CD83 reveals an upregulation and higher production of sCD83 in lymphocytes from pregnant mice. Front Immunol. (2017) 8:486. doi: 10.3389/fimmu.2017.00486
16. Fröhlich C, Ehrhardt J, Krüger D, Trojnarska D, Zygmunt M, Muzzio DO. Pregnancy status alters IL-21-mediated effects on murine B lymphocytes. Reproduction. (2020) 159:351–9. doi: 10.1530/REP-19-0407
17. Einenkel R, Packhäuser KR, Ehrhardt J, Tüngler A, Zygmunt M, Muzzio DO. CD83 is locally regulated and differentially expressed in disturbed murine pregnancy. Reproduction. (2019) 158:323–33. doi: 10.1530/REP-19-0171
18. Zhou LJ, Schwarting R, Smith HM, Tedder TF. A novel cell-surface molecule expressed by human interdigitating reticulum cells, Langerhans cells, and activated lymphocytes is a new member of the Ig superfamily. J Immunol. (1992) 149:735–42. doi: 10.4049/jimmunol.149.2.735
19. Grosche L, Knippertz I, König C, Royzman D, Wild AB, Zinser E, et al. The CD83 molecule - an important immune checkpoint. Front Immunol. (2020) 11:721. doi: 10.3389/fimmu.2020.00721
20. Zhou LJ, Tedder TF. Human blood dendritic cells selectively express CD83, a member of the immunoglobulin superfamily. J Immunol. (1995) 154:3821–35. doi: 10.4049/jimmunol.154.8.3821
21. Breloer M, Fleischer B. CD83 regulates lymphocyte maturation, activation and homeostasis. Trends Immunol. (2008) 29:186–94. doi: 10.1016/j.it.2008.01.009
22. Bannard O, McGowan SJ, Ersching J, Ishido S, Victora GD, Shin J-S, et al. Ubiquitin-mediated fluctuations in MHC class II facilitate efficient germinal center B cell responses. J Exp Med. (2016) 213:993–1009. doi: 10.1084/jem.20151682
23. Liu H, Jain R, Guan J, Vuong V, Ishido S, La Gruta NL, et al. Ubiquitin ligase MARCH 8 cooperates with CD83 to control surface MHC II expression in thymic epithelium and CD4 T cell selection. J Exp Med. (2016) 213:1695–703. doi: 10.1084/jem.20160312
24. Kreiser S, Eckhardt J, Kuhnt C, Stein M, Krzyzak L, Seitz C, et al. Murine CD83-positive T cells mediate suppressor functions in vitro and in vivo. Immunobiology. (2015) 220:270–9. doi: 10.1016/j.imbio.2014.08.005
25. Reinwald S, Wiethe C, Westendorf AM, Breloer M, Probst-Kepper M, Fleischer B, et al. CD83 expression in CD4+ T cells modulates inflammation and autoimmunity. J Immunol. (2008) 180:5890–7. doi: 10.4049/jimmunol.180.9.5890
26. Uhde M, Kuehl S, Richardt U, Fleischer B, Osterloh A. Differential regulation of marginal zone and follicular B cell responses by CD83. Int Immunol. (2013) 25:507–20. doi: 10.1093/intimm/dxt021
27. Lüthje K, Kretschmer B, Fleischer B, Breloer M. CD83 regulates splenic B cell maturation and peripheral B cell homeostasis. Int Immunol. (2008) 20:949–60. doi: 10.1093/intimm/dxn054
28. Kretschmer B, Lüthje K, Guse AH, Ehrlich S, Koch-Nolte F, Haag F, et al. CD83 modulates B cell function in vitro: increased IL-10 and reduced Ig secretion by CD83Tg B cells. PloS One. (2007) 2:e755. doi: 10.1371/journal.pone.0000755
29. Eckhardt J, Kreiser S, Döbbeler M, Nicolette C, DeBenedette MA, Tcherepanova IY, et al. Soluble CD83 ameliorates experimental colitis in mice. Mucosal Immunol. (2014) 7:1006–18. doi: 10.1038/mi.2013.119
30. Ge W, Arp J, Lian D, Liu W, Baroja ML, Jiang J, et al. Immunosuppression involving soluble CD83 induces tolerogenic dendritic cells that prevent cardiac allograft rejection. Transplantation. (2010) 90:1145–56. doi: 10.1097/TP.0b013e3181f95718
31. Peckert-Maier K, Schönberg A, Wild AB, Royzman D, Braun G, Stich L, et al. Pre-incubation of corneal donor tissue with sCD83 improves graft survival via the induction of alternatively activated macrophages and tolerogenic dendritic cells. Am J Transplant. (2022) 22:438–54. doi: 10.1111/ajt.16824
32. Royzman D, Andreev D, Stich L, Rauh M, Bäuerle T, Ellmann S, et al. Soluble CD83 triggers resolution of arthritis and sustained inflammation control in IDO dependent manner. Front Immunol. (2019) 10:633. doi: 10.3389/fimmu.2019.00633
33. Starke C, Steinkasserer A, Voll RE, Zinser E. Soluble human CD83 ameliorates lupus in NZB/W F1 mice. Immunobiology. (2013) 218:1411–5. doi: 10.1016/j.imbio.2013.06.002
34. Wu Y-J, Song Y-N, Geng X-R, Ma F, Mo L-H, Zhang X-W, et al. Soluble CD83 alleviates experimental allergic rhinitis through modulating antigen-specific Th2 cell property. Int J Biol Sci. (2020) 16:216–27. doi: 10.7150/ijbs.38722
35. Xiong L, Wang D, Lin S, Wang Y, Luo M, Gao L. Soluble CD83 inhibits acute rejection by up regulating TGF-β and IDO secretion in rat liver transplantation. Transpl Immunol. (2021) 64:101351. doi: 10.1016/j.trim.2020.101351
36. Zinser E, Naumann R, Wild AB, Michalski J, Deinzer A, Stich L, et al. Endogenous expression of the human CD83 attenuates EAE symptoms in humanized transgenic mice and increases the activity of regulatory T cells. Front Immunol. (2019) 10:1442. doi: 10.3389/fimmu.2019.01442
37. Huo S, Wu F, Zhang J, Wang X, Li W, Cui D, et al. Porcine soluble CD83 alleviates LPS-induced abortion in mice by promoting Th2 cytokine production, Treg cell generation and trophoblast invasion. Theriogenology. (2020) 157:149–61. doi: 10.1016/j.theriogenology.2020.07.026
38. Horvatinovich JM, Grogan EW, Norris M, Steinkasserer A, Lemos H, Mellor AL, et al. Soluble CD83 inhibits T cell activation by binding to the TLR4/MD-2 complex on CD14+ Monocytes. J Immunol. (2017) 198:2286–301. doi: 10.4049/jimmunol.1600802
39. Shen M, Child T, Mittal M, Sarodey G, Salim R, Granne I, et al. B cell subset analysis and gene expression characterization in mid-luteal endometrium. Front Cell Dev Biol. (2021) 9:709280. doi: 10.3389/fcell.2021.709280
40. Vinketova K, Koleva V, Puncheva E, Nashar S, Oreshkova T. A sustained decrease in serum CD83 in pregnant women. J Reprod Immunol. (2022) 154:103762. doi: 10.1016/j.jri.2022.103762
41. Li F, Leier A, Liu Q, Wang Y, Xiang D, Akutsu T, et al. Procleave: predicting protease-specific substrate cleavage sites by combining sequence and structural information. Genomics Proteomics Bioinf. (2020) 18:52–64. doi: 10.1016/j.gpb.2019.08.002
42. Sykes L, MacIntyre DA, Yap XJ, Teoh TG, Bennett PR. The Th1:th2 dichotomy of pregnancy and preterm labour. Mediators Inflammation. (2012) 2012:967629. doi: 10.1155/2012/967629
43. Orefice R. Immunology and the immunological response in pregnancy. Best Pract Res Clin Obstet Gynaecol. (2021) 76:3–12. doi: 10.1016/j.bpobgyn.2020.07.013
44. Abu-Raya B, Michalski C, Sadarangani M, Lavoie PM. Maternal immunological adaptation during normal pregnancy. Front Immunol. (2020) 11:575197. doi: 10.3389/fimmu.2020.575197
45. Song W, Li H, Jia B, Wang Z, Liu Q, Yang G, et al. Soluble CD83 suppresses experimental food allergy via regulating aberrant T helper 2 responses. Immunol Res. (2020) 68:141–51. doi: 10.1007/s12026-020-09133-9
46. Royzman D, Andreev D, Stich L, Peckert-Maier K, Wild AB, Zinser E, et al. The soluble CD83 protein prevents bone destruction by inhibiting the formation of osteoclasts and inducing resolution of inflammation in arthritis. Front Immunol. (2022) 13:936995. doi: 10.3389/fimmu.2022.936995
47. Royzman D, Peckert-Maier K, Stich L, König C, Wild AB, Tauchi M, et al. Soluble CD83 improves and accelerates wound healing by the induction of pro-resolving macrophages. Front Immunol. (2022) 13:1012647. doi: 10.3389/fimmu.2022.1012647
48. Feng M, Wang X, Zhou S, Li M, Liu T, Wei X, et al. CD83+ B cells alleviate uveitis through inhibiting DCs by sCD83. Immunology. (2023) 170:134–53. doi: 10.1111/imm.13654
49. Solders M, Gorchs L, Gidlöf S, Tiblad E, Lundell A-C, Kaipe H. Maternal adaptive immune cells in decidua parietalis display a more activated and coinhibitory phenotype compared to decidua basalis. Stem Cells Int. (2017) 2017:8010961. doi: 10.1155/2017/8010961
50. Wang X-Q, Li D-J. The mechanisms by which trophoblast-derived molecules induce maternal-fetal immune tolerance. Cell Mol Immunol. (2020) 17:1204–7. doi: 10.1038/s41423-020-0460-5
51. Cerdeira AS, Rajakumar A, Royle CM, Lo A, Husain Z, Thadhani RI, et al. Conversion of peripheral blood NK cells to a decidual NK-like phenotype by a cocktail of defined factors. J Immunol. (2013) 190:3939–48. doi: 10.4049/jimmunol.1202582
52. Zhang Y-H, Aldo P, You Y, Ding J, Kaislasuo J, Petersen JF, et al. Trophoblast-secreted soluble-PD-L1 modulates macrophage polarization and function. J Leukoc Biol. (2020) 108:983–98. doi: 10.1002/JLB.1A0420-012RR
53. Jin X, Mao L, Zhao W, Liu L, Li Y, Li D, et al. Decidualization-derived cAMP promotes decidual NK cells to be angiogenic phenotype. Am J Reprod Immunol. (2022) 88:e13540. doi: 10.1111/aji.13540
54. Lee C-L, Vijayan M, Wang X, Lam KK, Koistinen H, Seppala M, et al. Glycodelin-A stimulates the conversion of human peripheral blood CD16-CD56bright NK cell to a decidual NK cell-like phenotype. Hum Reprod. (2019) 34:689–701. doi: 10.1093/humrep/dey378
55. Leng Y, Romero R, Xu Y, Galaz J, Slutsky R, Arenas-Hernandez M, et al. Are B cells altered in the decidua of women with preterm or term labor? Am J Reprod Immunol. (2019) 81:e13102. doi: 10.1111/aji.13102
56. Ziegler KB, Muzzio DO, Matzner F, Bommer I, Ventimiglia MS, Malinowsky K, et al. Human pregnancy is accompanied by modifications in B cell development and immunoglobulin profile. J Reprod Immunol. (2018) 129:40–7. doi: 10.1016/j.jri.2018.07.003
57. Muzzio DO, Ziegler KB, Ehrhardt J, Zygmunt M, Jensen F. Marginal zone B cells emerge as a critical component of pregnancy well-being. Reproduction. (2016) 151:29–37. doi: 10.1530/REP-15-0274
58. Medina KL, Smithson G, Kincade PW. Suppression of B lymphopoiesis during normal pregnancy. J Exp Med. (1993) 178:1507–15. doi: 10.1084/jem.178.5.1507
59. Valeff N, Muzzio DO, Matzner F, Dibo M, Golchert J, Homuth G, et al. B cells acquire a unique and differential transcriptomic profile during pregnancy. Genomics. (2021) 113:2614–22. doi: 10.1016/j.ygeno.2021.06.016
60. Valeff NJ, Ventimiglia MS, Dibo M, Markert UR, Jensen F. Splenic B1 B cells acquire a proliferative and anti-inflamatory profile during pregnancy in mice. Front Immunol. (2022) 13:873493. doi: 10.3389/fimmu.2022.873493
61. Rizzuto G, Brooks JF, Tuomivaara ST, McIntyre TI, Ma S, Rideaux D, et al. Establishment of fetomaternal tolerance through glycan-mediated B cell suppression. Nature. (2022) 603:497–502. doi: 10.1038/s41586-022-04471-0
62. Bhat NM, Mithal A, Bieber MM, Herzenberg LA, Teng NN. Human CD5+ B lymphocytes (B-1 cells) decrease in peripheral blood during pregnancy. J Reprod Immunol. (1995) 28:53–60. doi: 10.1016/0165-0378(94)00907-o
63. Benner M, Feyaerts D, García CC, Inci N, López SC, Fasse E, et al. Clusters of tolerogenic B cells feature in the dynamic immunological landscape of the pregnant uterus. Cell Rep. (2020) 32:108204. doi: 10.1016/j.celrep.2020.108204
64. Kretschmer B, Weber J, Hutloff A, Fleischer B, Breloer M, Osterloh A. Anti-CD83 promotes IgG1 isotype switch in marginal zone B cells in response to TI-2 antigen. Immunobiology. (2015) 220:964–75. doi: 10.1016/j.imbio.2015.02.008
65. Wang L, Jiang P, Zhao S, Liu H, Liu L, Mor G, et al. The dynamic profile and potential function of B-cell subsets during pregnancy. Cell Mol Immunol. (2021) 18:1082–4. doi: 10.1038/s41423-020-00535-1
66. Slawek A, Lorek D, Kedzierska AE, Chelmonska-Soyta A. Regulatory B cells with IL-35 and IL-10 expression in a normal and abortion-prone murine pregnancy model. Am J Reprod Immunol. (2020) 83:e13217. doi: 10.1111/aji.13217
67. Muzzio D, Zygmunt M, Jensen F. The role of pregnancy-associated hormones in the development and function of regulatory B cells. Front Endocrinol (Lausanne). (2014) 5:39. doi: 10.3389/fendo.2014.00039
68. Schumacher A, Costa S-D, Zenclussen AC. Endocrine factors modulating immune responses in pregnancy. Front Immunol. (2014) 5:196. doi: 10.3389/fimmu.2014.00196
69. Schumacher A. Human chorionic gonadotropin as a pivotal endocrine immune regulator initiating and preserving fetal tolerance. Int J Mol Sci. (2017) 18(10):2166. doi: 10.3390/ijms18102166
70. Piccinni M-P, Raghupathy R, Saito S, Szekeres-Bartho J. Cytokines, hormones and cellular regulatory mechanisms favoring successful reproduction. Front Immunol. (2021) 12:717808. doi: 10.3389/fimmu.2021.717808
71. Sotoodeh Jahromi A, Sanie MS, Yusefi A, Zabetian H, Zareian P, Hakimelahi H, et al. Association of tumor growth factor-β and interferon-γ Serum levels with insulin resistance in normal pregnancy. Glob J Health Sci. (2015) 8:25–32. doi: 10.5539/gjhs.v8n6p25
72. Horvat Mercnik M, Schliefsteiner C, Sanchez-Duffhues G, Wadsack C. TGFβ signalling: a nexus between inflammation, placental health and preeclampsia throughout pregnancy. Hum Reprod Update. (2024) 30:442–71. doi: 10.1093/humupd/dmae007
73. Yang D, Dai F, Yuan M, Zheng Y, Liu S, Deng Z, et al. Role of transforming growth factor-β1 in regulating fetal-maternal immune tolerance in normal and pathological pregnancy. Front Immunol. (2021) 12:689181. doi: 10.3389/fimmu.2021.689181
74. Romero R, Espinoza J, Gonçalves LF, Kusanovic JP, Friel LA, Nien JK. Inflammation in preterm and term labour and delivery. Semin Fetal Neonatal Med. (2006) 11:317–26. doi: 10.1016/j.siny.2006.05.001
75. Gimeno-Molina B, Muller I, Kropf P, Sykes L. The role of neutrophils in pregnancy, term and preterm labour. Life (Basel). (2022) 12(10):1512. doi: 10.3390/life12101512
76. Kelly RW. Inflammatory mediators and parturition. Rev Reprod. (1996) 1:89–96. doi: 10.1530/ror.0.0010089
77. Norman JE, Bollapragada S, Yuan M, Nelson SM. Inflammatory pathways in the mechanism of parturition. BMC Pregnancy Childbirth. (2007) 7 Suppl 1:S7. doi: 10.1186/1471-2393-7-S1-S7
78. Giurgescu C. Are maternal cortisol levels related to preterm birth? J Obstet Gynecol Neonatal Nurs. (2009) 38:377–90. doi: 10.1111/j.1552-6909.2009.01034.x
79. Jing M, Chen X, Qiu H, He W, Zhou Y, Li D, et al. Insights into the immunomodulatory regulation of matrix metalloproteinase at the maternal-fetal interface during early pregnancy and pregnancy-related diseases. Front Immunol. (2022) 13:1067661. doi: 10.3389/fimmu.2022.1067661
Keywords: pregnancy, tolerance, CD83, lipopolysaccharide, hormones
Citation: Krupa P, Wein H, Zemmrich LS, Zygmunt M and Muzzio DO (2024) Pregnancy-related factors induce immune tolerance through regulation of sCD83 release. Front. Immunol. 15:1452879. doi: 10.3389/fimmu.2024.1452879
Received: 21 June 2024; Accepted: 21 August 2024;
Published: 12 September 2024.
Edited by:
Vanesa Hauk, Universidad de Buenos Aires, ArgentinaReviewed by:
Federico Jensen, University of Buenos Aires, ArgentinaSoledad Gori, Universidad de Buenos Aires, Argentina
Copyright © 2024 Krupa, Wein, Zemmrich, Zygmunt and Muzzio. This is an open-access article distributed under the terms of the Creative Commons Attribution License (CC BY). The use, distribution or reproduction in other forums is permitted, provided the original author(s) and the copyright owner(s) are credited and that the original publication in this journal is cited, in accordance with accepted academic practice. No use, distribution or reproduction is permitted which does not comply with these terms.
*Correspondence: Damián Oscar Muzzio, ZGFtaWFuLm11enppb0BtZWQudW5pLWdyZWlmc3dhbGQuZGU=