- 1Department of Geriatric Psychiatry, The Affiliated Mental Health Center of Jiangnan University, Wuxi Central Rehabilitation Hospital, Wuxi, Jiangsu, China
- 2School of Systems Biology, George Mason University, Fairfax, VA, United States
- 3Research Centre for Medical Genetics, Moscow, Russia
- 4Department of Psychiatry, The Affiliated Brain Hospital of Nanjing Medical University, Nanjing, China
- 5Institute of Neuropsychiatry, The Affiliated Brain Hospital of Nanjing Medical University, Nanjing, China
Introduction: The complex and unresolved pathogenesis of schizophrenia has posed significant challenges to its diagnosis and treatment. While recent research has established a clear association between immune function and schizophrenia, the causal relationship between the two remains elusive.
Methods: We employed a bidirectional two-sample Mendelian randomization approach to investigate the causal relationship between schizophrenia and 731 immune cell traits by utilizing public GWAS data. We further validated the causal relationship between schizophrenia and six types of white cell measures.
Results: We found the overall causal effects of schizophrenia on immune cell traits were significantly higher than the reverse ones (0.011 ± 0.049 vs 0.001 ± 0.016, p < 0.001), implying that disease may lead to an increase in immune cells by itself. We also identified four immune cell traits that may increase the risk of schizophrenia: CD11c+ monocyte %monocyte (odds ratio (OR): 1.06, 95% confidence interval (CI): 1.03~1.09, FDR = 0.027), CD11c+ CD62L- monocyte %monocyte (OR:1.06, 95% CI: 1.03~1.09, FDR = 0.027), CD25 on IgD+ CD38- naive B cell (OR:1.03, 95% CI:1.01~1.06, FDR = 0.042), and CD86 on monocyte (OR = 1.04, 95% CI:1.01~1.06, FDR = 0.042). However, we did not detect any significant causal effects of schizophrenia on immune cell traits. Using the white blood cell traits data, we identified that schizophrenia increases the lymphocyte counts (OR:1.03, 95%CI: 1.01-1.04, FDR = 0.007), total white blood cell counts (OR:1.02, 95%CI: 1.01-1.04, FDR = 0.021) and monocyte counts (OR:1.02, 95%CI: 1.00-1.03, FDR = 0.034). The lymphocyte counts were nominally associated with the risk of schizophrenia (OR:1.08,95%CI:1.01-1.16, P=0.019).
Discussion: Our study found that the causal relationship between schizophrenia and the immune system is complex, enhancing our understanding of the role of immune regulation in the development of this disorder. These findings offer new insights for exploring diagnostic and therapeutic options for schizophrenia.
Introduction
Schizophrenia is a severe mental disorder characterized by psychosis as a hallmark symptomatic manifestation (1). Approximately 1% of the global population will experience schizophrenia in their lifetime (2), and about 5% of these patients face increased physical health problems and elevated suicide rates (2, 3). The higher morbidity and disability rates associated with schizophrenia result in an average life expectancy that is 10-25 years shorter than that of the general population (4, 5), representing a profound loss for families and a substantial health burden on society (6). The complex etiology of schizophrenia complicates early detection and timely diagnosis, while the diversity of symptoms and conditions challenges the effective implementation of treatment strategies (7, 8). To achieve individualized and precise medical treatment for schizophrenia and facilitate the reintegration of patients into society, it is essential to explore the mechanisms of action and identify biomarkers related to its pathogenesis.
Inflammatory factors have a quantitative impact on brain development, typically aiding in processes such as neurogenesis, neuronal migration, and synapse formation (9–12). However, excessive inflammatory responses can disrupt these processes (13), and the release of large amounts of inflammatory factors in the brain increases the risk of psychiatric disorders, including epilepsy (14), Alzheimer’s disease (15), depression (16), and schizophrenia (17). Epidemiologic studies have long established an association between early-life infections and the development of autoimmune diseases and subsequent psychiatric disorders in adulthood (18). Numerous studies on chronic and first-episode schizophrenia have shown elevated levels of interleukin (IL)-1β, IL-6, IL-8, tumor necrosis factor (TNF), and C-reactive proteins in peripheral blood and cerebrospinal fluid (19–22), suggesting that neuroinflammation is a key pathophysiological mechanism of schizophrenia (21, 23). Genome-wide association studies (GWAS) have corroborated these findings, indicating a close relationship between genes regulating immune responses and schizophrenia (24, 25). Therefore, investigating the interactions between immune function and psychiatric disorders is crucial for understanding the pathogenesis of these conditions and may provide valuable insights for their treatment. Nevertheless, current findings on the association between immune inflammation and schizophrenia remain inconsistent, likely due to limitations in sample sizes, study design flaws, and uncontrolled confounding variables (26–28).
To mitigate confounding factors and reduce the effects of reverse causality commonly encountered in cross-sectional studies, we employed Mendelian randomization (MR) to analyze data from a GWAS of schizophrenia and two cell traits. This approach was widely used in recent studies on somatic (29–31) and mental disorders (32–37). We aim to uncover potential causal relationships between schizophrenia and immune function, thereby identifying potential biomarkers and facilitating individualized and precise treatment for schizophrenia.
Materials and methods
Overall study design
Using the GWAS public database, we conducted a bidirectional two-sample MR study. In the forward MR analysis, cell traits were used as exposures and schizophrenia as the outcome, and in the reverse MR analysis, schizophrenia was used as the exposure and cell traits as outcomes. The selection of instrumental variables adhered to the three core principles of MR research: 1) the genetic variant must be directly associated with the exposure; 2) the genetic variant should be independent of confounders related to the exposure or outcomes; and 3) the genetic variant must not influence the outcome except through its association with the exposure. Ethical review and informed consent were obtained for all GWAS study cohorts.
Data source of schizophrenia and two cell traits
The GWAS summary data on schizophrenia were acquired from the Psychiatric Genomics Consortium (PGC), including 53,386 cases and 77,258 controls (38). GWAS summary data for immune cell traits were collected from a cohort of 3,757 Sardinians by a team of Italian researchers, aiming to elucidate immune system function and dysfunction by assessing the impact of natural genetic variation on both quantitative and discrete immune-related traits (39). This study analyzed 731 immune cell traits, categorized into 4 types: absolute counts (AC, n=118), morphometric parameters (MP, n=32), median fluorescence intensity (MFI, n=389) analyzed by flow cytometry, and relative counts (RC, n=192) calculated using ratios between cell levels. The MFI, AC, and RC types included B cells, circulating dendritic cells (cDC), mature stage T cells, monocytes, myeloid cells, TBNK (T cells, B cells, natural killer cells), and Treg panels. The MP type encompassed only cDC and TBNK panels. This study cohort was adjusted for gender and age covariates, and its summary-level statistics are publicly available in the GWAS catalog (https://www.ebi.ac.uk/gwas/studies/) under accession numbers GCST0001391 to GCST0002121. GWAS summary data for six white blood cell traits were acquired from a large study which assessed the relevance of the omnigenic model to blood cell phenotypes in 563,085 participants (40).
Selection of instrumental variables
We selected single nucleotide polymorphisms (SNPs) with large exposure-related effect sizes and high statistical significance as reliable instrumental variables (IVs) based on the principles of MR analysis. To avoid biased parameter estimates due to low statistical power and weak IVs during MR analysis, we applied different significance thresholds for associations under varying exposure conditions. For immune cell traits used as exposures, a significance level of 1 × 10⁻⁵ was employed for extracting IVs for each trait, in line with a precedent set in a prior study (41). For schizophrenia and blood cell traits used as exposures for IV extraction, a more stringent significance level of 5 × 10⁻⁸ was maintained. Independent SNPs were selected through linkage disequilibrium clumping (r² threshold < 0.001 within a 10,000 kb distance) based on the European 1000 Genomes reference panel. To satisfy the first assumption of MR and to estimate weak instrument bias and instrument strength, we calculated F-statistics (β²/SE²), recommending F-statistics > 10 for further MR analysis. Ultimately, we identified 3 to 640 independent IVs across various immune cell traits, along with 194 to 587 IVs in different blood cell traits and 147 IVs related to schizophrenia for subsequent analysis.
Statistical methods
We use the random-effects IVW method to assess the potential bidirectional causality between immune cell traits and schizophrenia, which guarantees robust causal estimates even in the presence of heterogeneity in genetic instruments (42). Other methods serve as complement methods to IVW like MR-Egger, which can enhance the reliability and validity of causal inferences in MR studies by addressing the potential confounding effects of pleiotropy (43). The weighted median also was used to provide ancillary support for the reliability of the causal results. Additionally, we conducted sensitivity analyses to evaluate pleiotropy and heterogeneity, aiming to avoid violating the second or third assumptions of MR. The MR-Egger intercept method was used to determine the presence of horizontal pleiotropy in the genetic instruments, and pleiotropy was considered to be absent when P > 0.05. Heterogeneity was tested using Cochran’s Q test, which indicated the existence of heterogeneity when P<0.05. A sensitivity analysis was conducted to further determine the effect of heterogeneity through the leave-one-out method and to assess the robustness of the final effects. The MR analysis in this study utilized the TwoSampleMR (44) package based on the R language. All P values and false discovery rate (FDR) are two-tailed in this study.
Results
Comparison of overall causal effect across different exposure conditions
We compared the causal effect sizes under different exposure conditions first. The overall causal effect sizes (beta) of the genetic component predisposing to schizophrenia on immune cell traits were significantly higher than that of the reverse MR (beta exposure SZ = 0.011 ± 0.049, beta exposure cell traits = 0.001 ± 0.016, w = 308435, p < 0.001; |beta| exposure SZ = 0.033 ± 0.038, |beta| exposure cell traits = 0.002 ± 0.016, w = 461306, p < 0.001). A comparison of effect values for different exposure conditions is depicted in Figure 1A. We then specifically analyzed the causal relationship between schizophrenia and the two types of blood cells.
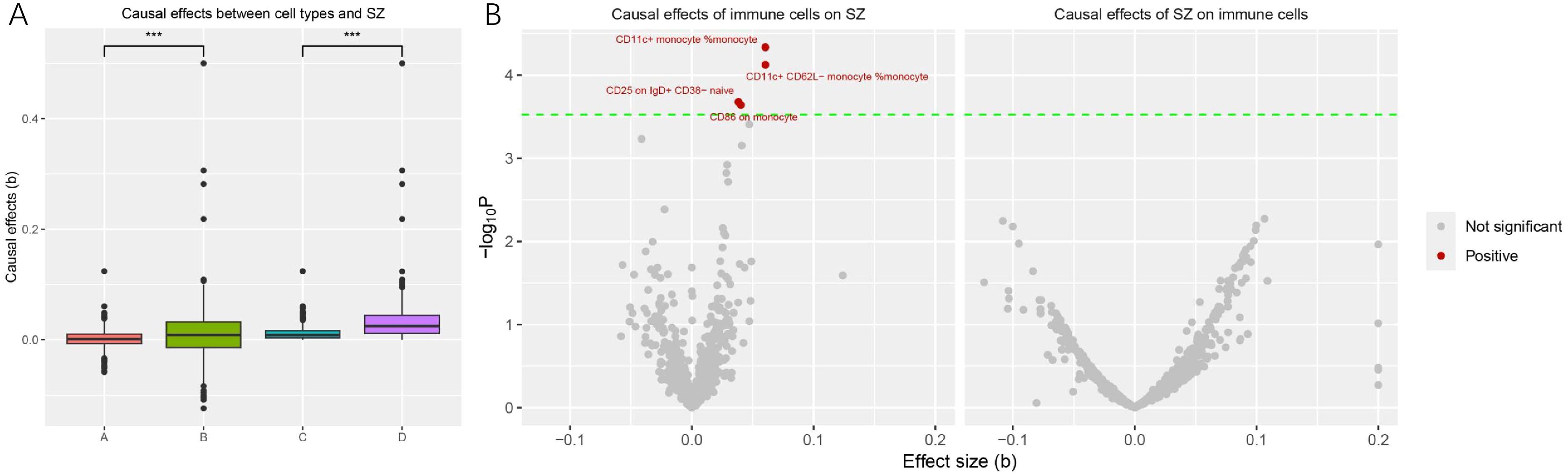
Figure 1. Comparison of causal effects size under different exposures and significant immune cell traits significantly associated with schizophrenia onset. (A) Each point in the box plots represents the causal effect of each exposure on the outcome. The horizontal coordinate A, B, C, and D represent the effect of cell traits on schizophrenia, the effect of schizophrenia on cell traits, the absolute effect of cell traits on schizophrenia, and the absolute effect of schizophrenia on cell traits, respectively. The vertical coordinate represents the magnitude of the causal effect size (beta value). (***, P < 0.001) (B) The volcano plot revealed four immune cell traits that were significantly and causally associated with the onset of schizophrenia. Significant cell traits are colored (red for positive relationships with schizophrenia, gray for not significant).
Causal relationships between schizophrenia and 731 immune cell traits
We found that a total of 4 immune cell traits were causally associated with SZ. They are CD11c+ monocyte %monocyte (odds ratio (OR): 1.06, 95% confidence interval (CI): 1.03~1.09, FDR = 0.027), CD11c+ CD62L- monocyte %monocyte (OR:1.06, 95% CI: 1.03~1.09, FDR = 0.027), CD25 on IgD+ CD38- naive B cell (OR:1.03, 95% CI:1.01~1.06, FDR = 0.042), and CD86 on monocyte (OR = 1.04, 95%CI:1.01~1.06, FDR = 0.042), respectively (Table 1, Figure 2A). All of them were identified as risk factors for schizophrenia onset (Figure 1B). Sensitive analysis results can be found in Table 2 and Figure 2B. However, we did not find any causal effect of schizophrenia on immune cell traits (Figure 1B). Summary results of the causal relationship between schizophrenia and immune cell traits can be found in Supplementary Tables 1 and 2.
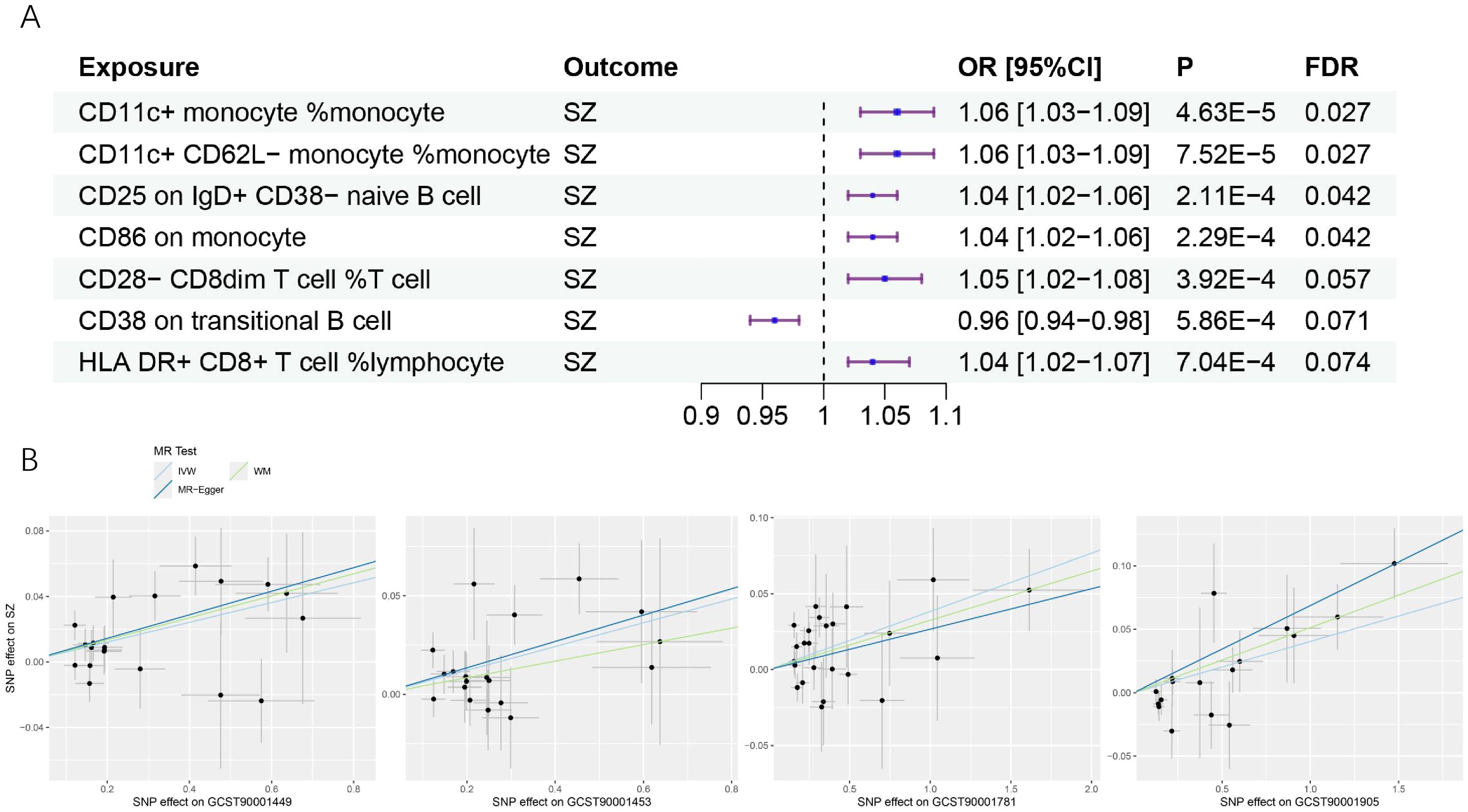
Figure 2. The causal effects of immune cell traits on schizophrenia. (A) Forest plots showed the causal associations of immune cell traits on schizophrenia. (B) Scatter plot showed the trends in the effect of CD11c+ monocyte %monocyte (trait ID: GCST90001449), CD11c+ CD62L- monocyte %monocyte (trait ID: GCST90001453), CD25 on IgD+ CD38- naive B cell (trait ID: GCST90001781), and CD86 on monocyte (trait ID: GCST90001905) on schizophrenia. SZ, schizophrenia; OR, Odds Ratio; CI, confidence interval; FDR, false discovery rate.
Causal relationships between schizophrenia and six white blood cell measures
In a manner similar to described above, we found that the genetic predisposition to higher lymphocyte count had a positive causal effect on schizophrenia, although this effect was of nominal significance (OR: 1.08, 95%CI:1.01-1.16, P=0.019). We also found that the genetic signature predisposing to schizophrenia increased the level of three immune cell indicators, namely, lymphocyte count (OR: 1.03, 95%CI: 1.01-1.04, FDR = 0.007), total white blood cell count (OR: 1.02, 95%CI: 1.01-1.04, FDR = 0.021) and monocyte count (OR: 1.02, 95%CI: 1.00-1.03, FDR = 0.034) (Figure 3, Table 3). However, we did not find any causal effects of the genetic components defining blood cell related traits on schizophrenia. The results describing effects of blood cell traits on schizophrenia are presented in Supplementary Tables 3, 4.
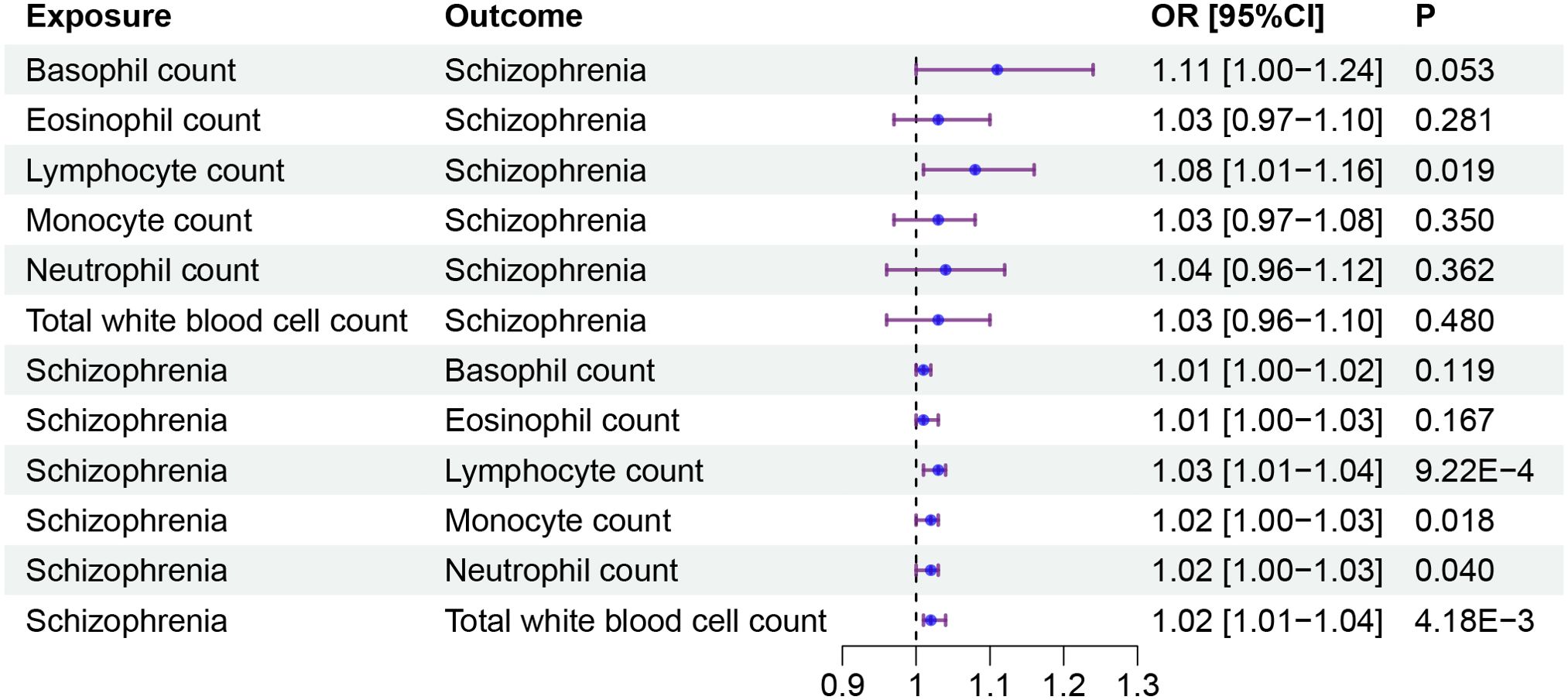
Figure 3. The causal effect between blood cell traits and schizophrenia. Forest plots showed the causal associations between blood cell traits and schizophrenia. OR, Odds Ratio; CI, confidence interval.
Discussion
By analyzing the causal relationships between schizophrenia and blood cell traits, we identified a complex association between this disease and the characteristics of the immune system. Although the traits describing four immune cell types were identified as potential risk factors for the development of schizophrenia, the macroscopic perspective suggests that schizophrenia has a greater impact on immune cells than the reverse, and blood cell-related results have confirmed this finding.
Specifically, the genetic component predisposing to schizophrenia has resulted in increased white blood cell (WBC) counts, total lymphocyte counts, and total monocyte counts. These observations are consistent with the results of a recent MR study of the genetic links between schizophrenia and immune cells (45). In patients with schizophrenia, clinical studies have identified alterations in immune cell indicators. In particular, both in first-episode and in chronic schizophrenia patients, their neutrophil and monocyte counts are elevated at baseline, then, after medication-induced symptom improvement, they return to normal (46). Recent cross-sectional study also reported that patients with schizophrenia had elevated eosinophil, lymphocyte, monocyte, and neutrophil counts when compared to the normal population, and that both the neutrophil/lymphocyte ratio (NLR) and the monocyte/lymphocyte ratio (MLR) were significantly and positively correlated with the severity of negative symptoms (47). Two previous studies analyzed by META, although not agreeing on the consequences of antipsychotic drug interventions on immune cells, also detected the presence of elevated monocyte counts or lymphocytes in patients with schizophrenia (48, 49).
The underlying reasons behind this may be related to the involvement of the autonomic nervous system in the connection between the brain and the immune system (50, 51) and the activation of the immune system by psychosocial stresses (52, 53). Lymphoid organs innervated by sympathetic and peptidergic nerve fibers are able to promote certain immune responses under the action of neurotransmitters such as norepinephrine and substance P (54). On the other hand, lymphocytes, monocytes, and granulocytes possess neurotransmitter receptors, and are subjected to norepinephrine regulation (55, 56). In addition, it is well-known that social stress activates the HPA axis, increasing the levels of circulating glucocorticoids and leading to suppression of immune function (57). Schizophrenia, especially in its acute exacerbations, is associated with elevated levels of norepinephrine and an increase in chronic psychosocial stress, both of which are known to increase susceptibility to infections, which may be reflected in altered levels of immune cells.
It is important to note that the relationships between schizophrenia and the immune system are expected to be bidirectional. This phenomenon has been elucidated in previous studies (45, 58, 59). The important role that neuroinflammation plays in the development of schizophrenia is self-evident. In particular, the link between autoimmune disorders and schizophrenia identified earlier on suggests that these two conditions share a common underlying pathway (60, 61) of an inflammatory immune response. In addition to its own effects on the brain cells, inflammation is thought to increase the permeability of the blood-brain barrier and contribute to the penetration of the immune system components into the brain parenchyma (62). When neuroinflammation entrenches, microglia releases the cytokines that bind to specific receptors on the neurons and affect the release of the neurotransmitters, the synaptic plasticity, and the cortisol concentrations, leading to the changes in mood, the cognition, and the behavior (63, 64). It is, however, important to remember that the immune system is an intricate functional network involving many different cell population, which may play distinct roles in the pathogenesis of schizophrenia. Understanding the interrelationship connecting schizophrenia and immune cell traits may further improve the treatment strategies for this important neuropsychiatric disorder.
For example, monocytes, which are derived from hematopoietic stem cells embed in the bone marrow, are integral to the innate immune response, protecting the host from pathogen invasion and playing a crucial role in adaptive immunity (49). Elevated circulating monocyte levels have been observed in patients with schizophrenia, supported by the prevalence of mononucleosis in first-episode psychosis (65). A recent MR study further corroborates the pathogenic risk that monocytes pose to schizophrenia (45). Circulating monocytes are also suggested as indirect markers of microglia activation in the brain, with a noted correlation between increased monocyte levels and microglia activation (66, 67). It is proposed that microglia activation under pathological conditions may be part of the systemic activation of the mononuclear phagocyte system, leading to the activation of circulating monocytes (66, 68). The increased risk of schizophrenia associated with monocytes may be explained by the abnormal growth, differentiation, and dysfunction of neuronal circuits in the brain caused by early-life inflammatory activation (69), and/or the hyperactivation of microglia due to adverse environmental influences during adolescence (70).
B cells serve multiple functions in the adaptive immune system, including the production of immunoglobulins and the presentation of antigens to CD4+ T cells via the MHC-II complex (71). They also produce proinflammatory cytokines such as IL-6 and influence the recruitment of chemokines by monocytes and neutrophils (72, 73). IL-6 is particularly significant in B cell-related immune activation, with elevated levels often associated with schizophrenia (74). Autoreactive B cells also can produce autoantibodies, a process potentially triggered by environmental factors such as bacterial or viral infections, posing a risk factor for schizophrenia (60). Additionally, a distinct B cell subtype, B-1A cells, plays a crucial role in axonal myelin formation by stimulating oligodendrocyte production, which may also be implicated in the pathogenesis of schizophrenia (75).
Previous studies have cursorily explored the potential influence of immune cells on the pathophysiology of schizophrenia. The present study refines the understanding of the specific types of immune cells involved and establishes a causal relationship between immune cells and schizophrenia. These findings will inform future research on the interaction between immune function and the development of schizophrenia. However, this study has several limitations. First, the schizophrenia data cohort was derived from a European population, and caution is needed when applying the results to other populations. Second, the lack of demographic information prevented analysis of schizophrenia stratified by age and gender, limiting our understanding of how causality may vary across different age groups and sex. Third, the data describing the immune cell traits were derived from the general population of Sardinia and lacked specific information related to the status of general health in these individuals. Epidemiologic evidence suggests that due to known genetic drift, autoimmune diseases in this population are more common than in other Europeans populations (76). This should be considered when interpreting the conclusions of our study. In addition, we should not remain satisfied with current picture of the complex causal relationship between schizophrenia and immune cell traits, but endeavor on more in-depth studies such as exploring key genes and pathways linking the two, for example with the help of scPagwas methods (77) and scRNAseq data (78). Future gene- and pathway-level dissection should aid us in understanding the molecular mechanisms behind the uncovered causal relationships between the blood cell traits and the psychiatric diseases.
In conclusion, the causal relationship between schizophrenia and the immune system is complex, with the disease having a greater effect on immune cells, while the elevated risk of schizophrenia brought about by immune cells traits may be secondary. This finding enhances our understanding of the role of immune regulation in the development of this disorder and brings new insights for those exploring diagnostic and therapeutic options for schizophrenia.
Data availability statement
The original contributions presented in the study are included in the article/Supplementary Material. Further inquiries can be directed to the corresponding authors.
Author contributions
JD: Writing – review & editing, Writing – original draft, Visualization, Project administration, Methodology, Formal Analysis, Conceptualization. AB: Writing – review & editing, Validation, Supervision, Methodology. GZ: Writing – review & editing, Validation, Supervision, Project administration, Methodology. FZ: Writing – review & editing, Validation, Supervision, Project administration, Data curation.
Funding
The author(s) declare that no financial support was received for the research, authorship, and/or publication of this article.
Acknowledgments
The authors thank all investigators and participants from the groups for sharing these data.
Conflict of interest
The authors declare that the research was conducted in the absence of any commercial or financial relationships that could be construed as a potential conflict of interest.
Publisher’s note
All claims expressed in this article are solely those of the authors and do not necessarily represent those of their affiliated organizations, or those of the publisher, the editors and the reviewers. Any product that may be evaluated in this article, or claim that may be made by its manufacturer, is not guaranteed or endorsed by the publisher.
Supplementary material
The Supplementary Material for this article can be found online at: https://www.frontiersin.org/articles/10.3389/fimmu.2024.1452214/full#supplementary-material
References
1. Tandon R, Gaebel W, Barch DM, Bustillo J, Gur RE, Heckers S, et al. Definition and description of schizophrenia in the DSM-5. Schizophr Res. (2013) 150:3–10. doi: 10.1016/j.schres.2013.05.028
3. Hor K, Taylor M. Review: Suicide and schizophrenia: a systematic review of rates and risk factors. J Psychopharmacol. (2010) 24:81–90. doi: 10.1177/1359786810385490
4. Laursen TM, Nordentoft M, Mortensen PB. Excess early mortality in schizophrenia. Annu Rev Clin Psychol. (2014) 10:425–48. doi: 10.1146/annurev-clinpsy-032813-153657
5. Wang H, Naghavi M, Allen C, Barber RM, Bhutta ZA, Carter A, et al. Global, regional, and national life expectancy, all-cause mortality, and cause-specific mortality for 249 causes of death, 1980–2015: a systematic analysis for the Global Burden of Disease Study 2015. Lancet. (2016) 388:1459–544. doi: 10.1016/S0140-6736(16)31012-1
6. Solmi M, Seitidis G, Mavridis D, Correll CU, Dragioti E, Guimond S, et al. Incidence, prevalence, and global burden of schizophrenia - data, with critical appraisal, from the Global Burden of Disease (GBD) 2019. Mol Psychiatry. (2023) 28:5319–27. doi: 10.1038/s41380-023-02138-4
7. Nucifora FC, Woznica E, Lee BJ, Cascella N, Sawa A. Treatment resistant schizophrenia: Clinical, biological, and therapeutic perspectives. Neurobiol Dis. (2019) 131:104257. doi: 10.1016/j.nbd.2018.08.016
8. Gaebel W, Zielasek J. Schizophrenia in 2020: Trends in diagnosis and therapy. Psychiatry Clin Neurosci. (2015) 69:661–73. doi: 10.1111/pcn.12322
9. Cordeiro CN, Tsimis M, Burd I. Infections and brain development. Obstetrical Gynecological Survey. (2015) 70:644–55. doi: 10.1097/OGX.0000000000000236
10. Lenz KM, Nelson LH. Microglia and beyond: innate immune cells as regulators of brain development and behavioral function. Front Immunol. (2018) 9:698. doi: 10.3389/fimmu.2018.00698
11. Butler CA, Popescu AS, Kitchener EJA, Allendorf DH, Puigdellívol M, Brown GC. Microglial phagocytosis of neurons in neurodegeneration, and its regulation. J Neurochemistry. (2021) 158:621–39. doi: 10.1111/jnc.15327
12. Harry GJ. Microglia during development and aging. Pharmacol Ther. (2013) 139:313–26. doi: 10.1016/j.pharmthera.2013.04.013
13. Hohlfeld R, Kerschensteiner M, Meinl E. Dual role of inflammation in CNS disease. Neurology. (2007) 68:S58–63. doi: 10.1212/01.wnl.0000275234.43506.9b
14. Flammer J, Neziraj T, Rüegg S, Pröbstel A-K. Immune mechanisms in epileptogenesis: update on diagnosis and treatment of autoimmune epilepsy syndromes. Drugs. (2023) 83:135–58. doi: 10.1007/s40265-022-01826-9
15. Heneka MT, Carson MJ, Khoury JE, Landreth GE, Brosseron F, Feinstein DL, et al. Neuroinflammation in alzheimer’s disease. Lancet Neurol. (2015) 14:388–405. doi: 10.1016/S1474-4422(15)70016-5
16. Drevets WC, Wittenberg GM, Bullmore ET, Manji HK. Immune targets for therapeutic development in depression: towards precision medicine. Nat Rev Drug Discovery. (2022) 21:224–44. doi: 10.1038/s41573-021-00368-1
17. Birnbaum R, Weinberger DR. A genetics perspective on the role of the (Neuro)Immune system in schizophrenia. Schizophr Res. (2020) 217:105–13. doi: 10.1016/j.schres.2019.02.005
18. Martyn CN. Infection in childhood and neurological diseases in adult life. Br Med Bull. (1997) 53:24–39. doi: 10.1093/oxfordjournals.bmb.a011603
19. Hayes LN, Severance EG, Leek JT, Gressitt KL, Rohleder C, Coughlin JM, et al. Inflammatory molecular signature associated with infectious agents in psychosis. Schizophr Bull. (2014) 40:963–72. doi: 10.1093/schbul/sbu052
20. Na K-S, Jung H-Y, Kim Y-K. The role of pro-inflammatory cytokines in the neuroinflammation and neurogenesis of schizophrenia. Prog Neuropsychopharmacol Biol Psychiatry. (2014) 48:277–86. doi: 10.1016/j.pnpbp.2012.10.022
21. Vallée A. Neuroinflammation in schizophrenia: the key role of the WNT/β-catenin pathway. IJMS. (2022) 23:2810. doi: 10.3390/ijms23052810
22. Ermakov EA, Mednova IA, Boiko AS, Buneva VN, Ivanova SA. Chemokine dysregulation and neuroinflammation in schizophrenia: A systematic review. IJMS. (2023) 24:2215. doi: 10.3390/ijms24032215
23. Conen S, Gregory CJ, Hinz R, Smallman R, Corsi-Zuelli F, Deakin B, et al. Neuroinflammation as measured by positron emission tomography in patients with recent onset and established schizophrenia: implications for immune pathogenesis. Mol Psychiatry. (2021) 26:5398–406. doi: 10.1038/s41380-020-0829-y
24. Corvin A, Morris DW. Genome-wide association studies: findings at the major histocompatibility complex locus in psychosis. Biol Psychiatry. (2014) 75:276–83. doi: 10.1016/j.biopsych.2013.09.018
25. Zhang Y, Tao S, Coid J, Wei W, Wang Q, Yue W, et al. The role of total white blood cell count in antipsychotic treatment for patients with schizophrenia. Curr Neuropharmacol. (2024) 22:159–67. doi: 10.2174/1570159X21666230104090046
26. Fang X, Yu L, Wang D, Chen Y, Wang Y, Wu Z, et al. Association between SIRT1, cytokines, and metabolic syndrome in schizophrenia patients with olanzapine or clozapine monotherapy. Front Psychiatry. (2020) 11:602121. doi: 10.3389/fpsyt.2020.602121
27. Williams JA, Burgess S, Suckling J, Lalousis PA, Batool F, Griffiths SL, et al. Inflammation and brain structure in schizophrenia and other neuropsychiatric disorders: A mendelian randomization study. JAMA Psychiatry. (2022) 79:498. doi: 10.1001/jamapsychiatry.2022.0407
28. Hartwig FP, Borges MC, Horta BL, Bowden J, Davey Smith G. Inflammatory biomarkers and risk of schizophrenia: A 2-sample mendelian randomization study. JAMA Psychiatry. (2017) 74:1226. doi: 10.1001/jamapsychiatry.2017.3191
29. Jin Y, Yu X, Li J, Su M, Li X. Causal effects and immune cell mediators between prescription analgesic use and risk of infectious diseases: a Mendelian randomization study. Front Immunol. (2023) 14:1319127. doi: 10.3389/fimmu.2023.1319127
30. Chen Y, Tang S. Gut microbiota and immune mediation: a Mendelian randomization study on granulomatosis with polyangiitis. Front Immunol. (2023) 14:1296016. doi: 10.3389/fimmu.2023.1296016
31. Qin C, Yu Q, Deng Z, Zhang Y, Chen M, Wang X, et al. Causal relationship between the immune cells and ankylosing spondylitis: univariable, bidirectional, and multivariable Mendelian randomization. Front Immunol. (2024) 15:1345416. doi: 10.3389/fimmu.2024.1345416
32. Baranova A, Chandhoke V, Cao H, Zhang F. Shared genetics and bidirectional causal relationships between type 2 diabetes and attention-deficit/hyperactivity disorder. Gen Psychiatr. (2023) 36:e100996. doi: 10.1136/gpsych-2022-100996
33. Cao H, Baranova A, Zhao Q, Zhang F. Bidirectional associations between mental disorders, antidepressants and cardiovascular disease. BMJ Ment Health. (2024) 27:e300975. doi: 10.1136/bmjment-2023-300975
34. Baranova A, Zhao Q, Cao H, Chandhoke V, Zhang F. Causal influences of neuropsychiatric disorders on Alzheimer’s disease. Transl Psychiatry. (2024) 14:114. doi: 10.1038/s41398-024-02822-1
35. Baranova A, Zhao Y, Cao H, Zhang F. Causal associations between major depressive disorder and COVID-19. Gen Psychiatr. (2023) 36:e101006. doi: 10.1136/gpsych-2022-101006
36. Tang C, Lei X, Ding Y, Yang S, Ma Y, He D. Causal relationship between immune cells and neurodegenerative diseases: a two-sample Mendelian randomisation study. Front Immunol. (2024) 15:1339649. doi: 10.3389/fimmu.2024.1339649
37. Ma Z, Zhao M, Zhao H, Qu N. Causal role of immune cells in generalized anxiety disorder: Mendelian randomization study. Front Immunol. (2023) 14:1338083. doi: 10.3389/fimmu.2023.1338083
38. Trubetskoy V, Pardiñas AF, Qi T, Panagiotaropoulou G, Awasthi S, Bigdeli TB, et al. Mapping genomic loci implicates genes and synaptic biology in schizophrenia. Nature. (2022) 604:502–8. doi: 10.1038/s41586-022-04434-5
39. Orrù V, Steri M, Sidore C, Marongiu M, Serra V, Olla S, et al. Complex genetic signatures in immune cells underlie autoimmunity and inform therapy. Nat Genet. (2020) 52:1036–45. doi: 10.1038/s41588-020-0684-4
40. Vuckovic D, Bao EL, Akbari P, Lareau CA, Mousas A, Jiang T, et al. The polygenic and monogenic basis of blood traits and diseases. Cell. (2020) 182:1214–1231.e11. doi: 10.1016/j.cell.2020.08.008
41. Wang C, Zhu D, Zhang D, Zuo X, Yao L, Liu T, et al. Causal role of immune cells in schizophrenia: Mendelian randomization (MR) study. BMC Psychiatry. (2023) 23:590. doi: 10.1186/s12888-023-05081-4
42. Bowden J, Holmes MV. Meta-analysis and Mendelian randomization: A review. Res Synth Methods. (2019) 10:486–96. doi: 10.1002/jrsm.1346
43. Burgess S, Thompson SG. Interpreting findings from Mendelian randomization using the MR-Egger method. Eur J Epidemiol. (2017) 32:377–89. doi: 10.1007/s10654-017-0255-x
44. Hemani G, Zheng J, Elsworth B, Wade KH, Haberland V, Baird D, et al. The MR-Base platform supports systematic causal inference across the human phenome. Elife. (2018) 7:e34408. doi: 10.7554/eLife.34408
45. Leung PBM, Liu Z, Zhong Y, Tubbs JD, Di Forti M, Murray RM, et al. Bidirectional two-sample Mendelian randomization study of differential white blood cell counts and schizophrenia. Brain Behavior Immun. (2024) 118:22–30. doi: 10.1016/j.bbi.2024.02.015
46. Steiner J, Frodl T, Schiltz K, Dobrowolny H, Jacobs R, Fernandes BS, et al. Innate immune cells and C-reactive protein in acute first-episode psychosis and schizophrenia: relationship to psychopathology and treatment. Schizophr Bull. (2019) 46:363–73. doi: 10.1093/schbul/sbz068
47. Šagud M, Madžarac Z, Nedic Erjavec G, Šimunović Filipčić I, Mikulić FL, Rogić D, et al. The associations of neutrophil-lymphocyte, platelet-lymphocyte, monocyte-lymphocyte ratios and immune-inflammation index with negative symptoms in patients with schizophrenia. Biomolecules. (2023) 13:297. doi: 10.3390/biom13020297
48. Miller BJ, Gassama B, Sebastian D, Buckley P, Mellor A. Meta-analysis of lymphocytes in schizophrenia: clinical status and antipsychotic effects. Biol Psychiatry. (2013) 73:993–9. doi: 10.1016/j.biopsych.2012.09.007
49. Mazza MG, Capellazzi M, Lucchi S, Tagliabue I, Rossetti A, Clerici M. Monocyte count in schizophrenia and related disorders: a systematic review and meta-analysis. Acta Neuropsychiatr. (2020) 32:229–36. doi: 10.1017/neu.2020.12
50. Ader R, Cohen N, Felten D. Psychoneuroimmunology: interactions between the nervous system and the immune system. Lancet. (1995) 345:99–103. doi: 10.1016/s0140-6736(95)90066-7
51. Felten SY, Felten DL. Innervation of lymphoid tissue. In: Psychoneuroimmunology. (Cham, Switzerland: Academic Press Elsevier (1991). p. 27–69. doi: 10.1016/B978-0-12-043780-1.50006-3
52. Sheridan JF, Dobbs C, Brown D, Zwilling B. Psychoneuroimmunology: stress effects on pathogenesis and immunity during infection. Clin Microbiol Rev. (1994) 7:200–12. doi: 10.1128/CMR.7.2.200
53. Cohen S, Williamson GM. Stress and infectious disease in humans. Psychol Bull. (1991) 109:5–24. doi: 10.1037/0033-2909.109.1.5
54. Strell C, Sievers A, Bastian P, Lang K, Niggemann B, Zänker KS, et al. Divergent effects of norepinephrine, dopamine and substance P on the activation, differentiation and effector functions of human cytotoxic T lymphocytes. BMC Immunol. (2009) 10:62. doi: 10.1186/1471-2172-10-62
55. Pavlov VA, Tracey KJ. The cholinergic anti-inflammatory pathway. Brain Behav Immun. (2005) 19:493–9. doi: 10.1016/j.bbi.2005.03.015
56. Scanzano A, Cosentino M. Adrenergic regulation of innate immunity: a review. Front Pharmacol. (2015) 6:171. doi: 10.3389/fphar.2015.00171
57. Juruena MF, Eror F, Cleare AJ, Young AH. The role of early life stress in HPA axis and anxiety. Adv Exp Med Biol. (2020) 1191:141–53. doi: 10.1007/978-981-32-9705-0_9
58. Perry BI, Upthegrove R, Kappelmann N, Jones PB, Burgess S, Khandaker GM. Associations of immunological proteins/traits with schizophrenia, major depression and bipolar disorder: A bi-directional two-sample mendelian randomization study. Brain Behav Immun. (2021) 97:176–85. doi: 10.1016/j.bbi.2021.07.009
59. Severance EG, Yolken RH. Deciphering microbiome and neuroactive immune gene interactions in schizophrenia. Neurobiol Dis. (2020) 135:104331. doi: 10.1016/j.nbd.2018.11.016
60. Khandaker GM, Cousins L, Deakin J, Lennox BR, Yolken R, Jones PB. Inflammation and immunity in schizophrenia: implications for pathophysiology and treatment. Lancet Psychiatry. (2015) 2:258–70. doi: 10.1016/S2215-0366(14)00122-9
61. Benros ME, Nielsen PR, Nordentoft M, Eaton WW, Dalton SO, Mortensen PB. Autoimmune diseases and severe infections as risk factors for schizophrenia: a 30-year population-based register study. Am J Psychiatry. (2011) 168:1303–10. doi: 10.1176/appi.ajp.2011.11030516
62. Engelhardt B, Sorokin L. The blood–brain and the blood–cerebrospinal fluid barriers: function and dysfunction. Semin Immunopathol. (2009) 31:497–511. doi: 10.1007/s00281-009-0177-0
63. Dantzer R, O’Connor JC, Freund GG, Johnson RW, Kelley KW. From inflammation to sickness and depression: when the immune system subjugates the brain. Nat Rev Neurosci. (2008) 9:46–56. doi: 10.1038/nrn2297
64. Miller AH, Maletic V, Raison CL. Inflammation and its discontents: the role of cytokines in the pathophysiology of major depression. Biol Psychiatry. (2009) 65:732–41. doi: 10.1016/j.biopsych.2008.11.029
65. Drexhage RC, Weigelt K, Van Beveren N, Cohen D, Versnel MA, Nolen WA, et al. Immune and neuroimmune alterations in mood disorders and schizophrenia. In: Int Rev Neurobiol. (2011) 101:169–01. doi: 10.1016/B978-0-12-387718-5.00007-9
66. Beumer W, Gibney SM, Drexhage RC, Pont-Lezica L, Doorduin J, Klein HC, et al. The immune theory of psychiatric diseases: a key role for activated microglia and circulating monocytes. J Leukocyte Biol. (2012) 92:959–75. doi: 10.1189/jlb.0212100
67. Müller N, Wagner JK, Krause D, Weidinger E, Wildenauer A, Obermeier M, et al. Impaired monocyte activation in schizophrenia. Psychiatry Res. (2012) 198:341–6. doi: 10.1016/j.psychres.2011.12.049
68. Prinz M, Priller J. Microglia and brain macrophages in the molecular age: from origin to neuropsychiatric disease. Nat Rev Neurosci. (2014) 15:300–12. doi: 10.1038/nrn3722
69. Bergink V, Gibney SM, Drexhage HA. Autoimmunity, inflammation, and psychosis: A search for peripheral markers. Biol Psychiatry. (2014) 75:324–31. doi: 10.1016/j.biopsych.2013.09.037
70. De Picker LJ, Morrens M, Chance SA, Boche D. Microglia and brain plasticity in acute psychosis and schizophrenia illness course: A meta-review. Front Psychiatry. (2017) 8:238. doi: 10.3389/fpsyt.2017.00238
71. Crawford A, Macleod M, Schumacher T, Corlett L, Gray D. Primary T cell expansion and differentiation in vivo requires antigen presentation by B cells. J Immunol. (2006) 176:3498–506. doi: 10.4049/jimmunol.176.6.3498
72. Barr TA, Shen P, Brown S, Lampropoulou V, Roch T, Lawrie S, et al. B cell depletion therapy ameliorates autoimmune disease through ablation of IL-6-producing B cells. J Exp Med. (2012) 209:1001–10. doi: 10.1084/jem.20111675
73. Zouggari Y, Ait-Oufella H, Bonnin P, Simon T, Sage AP, Guérin C, et al. B lymphocytes trigger monocyte mobilization and impair heart function after acute myocardial infarction. Nat Med. (2013) 19:1273–80. doi: 10.1038/nm.3284
74. Smith SEP, Li J, Garbett K, Mirnics K, Patterson PH. Maternal immune activation alters fetal brain development through interleukin-6. J Neurosci. (2007) 27:10695–702. doi: 10.1523/JNEUROSCI.2178-07.2007
75. Tanabe S, Yamashita T. B-1a lymphocytes promote oligodendrogenesis during brain development. Nat Neurosci. (2018) 21:506–16. doi: 10.1038/s41593-018-0106-4
76. Urru SA, Antonelli A, Sechi GM, MS Working Group. Prevalence of multiple sclerosis in Sardinia: A systematic cross-sectional multi-source survey. Mult Scler. (2020) 26:372–80. doi: 10.1177/1352458519828600
77. Ma Y, Deng C, Zhou Y, Zhang Y, Qiu F, Jiang D, et al. Polygenic regression uncovers trait-relevant cellular contexts through pathway activation transformation of single-cell RNA sequencing data. Cell Genom. (2023) 3:100383. doi: 10.1016/j.xgen.2023.100383
Keywords: schizophrenia, immune cell, immunology, psychiatry, Mendelian randomization analysis
Citation: Du J, Baranova A, Zhang G and Zhang F (2024) The causal relationship between immune cell traits and schizophrenia: a Mendelian randomization analysis. Front. Immunol. 15:1452214. doi: 10.3389/fimmu.2024.1452214
Received: 20 June 2024; Accepted: 09 September 2024;
Published: 27 September 2024.
Edited by:
Chuan Shi, Peking University Sixth Hospital, ChinaReviewed by:
Yunlong Ma, University of Pennsylvania, United StatesMassimo Tusconi, University of Cagliari, Italy
Copyright © 2024 Du, Baranova, Zhang and Zhang. This is an open-access article distributed under the terms of the Creative Commons Attribution License (CC BY). The use, distribution or reproduction in other forums is permitted, provided the original author(s) and the copyright owner(s) are credited and that the original publication in this journal is cited, in accordance with accepted academic practice. No use, distribution or reproduction is permitted which does not comply with these terms.
*Correspondence: Guofu Zhang, emhhbmdndW9mdXd4QDE2My5jb20=; Fuquan Zhang, emhhbmdmcUBuam11LmVkdS5jbg==