- 1National Reference Laboratory for Animal Schistosomiasis, Shanghai Veterinary Research Institute, Chinese Academy of Agricultural Sciences, Shanghai, China
- 2Key Laboratory of Animal Parasitology of Ministry of Agriculture and Rural Affairs, Shanghai Veterinary Research Institute, Chinese Academy of Agricultural Sciences, Shanghai, China
- 3State Key Laboratory for Animal Disease Control and Prevention, Lanzhou Veterinary Research Institute, Chinese Academy of Agricultural Science, Lanzhou, Gansu, China
- 4Key Laboratory of Veterinary Parasitology of Gansu Province, Lanzhou Veterinary Research Institute, Chinese Academy of Agricultural Science, Lanzhou, Gansu, China
Helminth infections are a major public health problem as they can cause long-term chronic infections in their hosts for which there is no effective vaccine. During the long-term interaction between helminths and their hosts, helminth-derived extracellular vesicles (EVs) can participate in host immunomodulatory processes by secreting bioactive molecules (BMAs). Growing data suggests that microRNAs (miRNAs) in helminth EVs have a significant impact on the host’s immune system. The let-7 family is highly conserved among helminth EVs and highly homologous in the host, and its function in host–parasite crosstalk may reflect active selection for compatibility with the host miRNA machinery. In-depth studies targeting this aspect may better elucidate the mechanism of parasite-host interactions. Hence, this review summarizes the current studies on the cross-species involvement of helminth EV-derived let-7 in host immune regulation and discusses the barriers to related research and potential applications of helminth EVs.
Introduction
Helminthiosis, caused by nematodes, cestodes, and trematodes, are a major public health concern and result in ∼60 million disability-adjusted life years (DALYs) globally (1). Recent estimates indicate that 2 billion people in low- and middle-income countries, mostly in endemic areas of Asia, the Americas, and Africa, have been infected by one or more parasitic worms (2). Unfortunately, there are currently no broad-spectrum anthelmintic vaccines available (3). Therefore, most helminth infections are controlled with the use of anthelmintic drugs, which do not prevent reinfection (4). However, prolonged and over use of these drugs can lead to the development of anthelmintic resistance (5–7). Hence, new control and prevention strategies against helminth infections are urgently needed.
As a result of complex life cycles and long-term symbiosis with the host, helminths release various mediators involved in host–parasite crosstalk, which promote immunomodulation, immune evasion, and pathogenesis (8, 9). Previous studies have primarily focused on helminth excretory/secretory products (ESPs) involved in regulating the host immune response (10, 11). However, as the study of host–parasite interactions has entered a deeper molecular realm, there has been a gradual emergence of helminth-derived microRNAs (miRNAs), mainly loaded in extracellular vesicles (EVs), involved in this intricate crosstalk (9, 12, 13).
EVs are small membrane-bounded secretory vesicles released by almost all cell types. EVs play essential roles in cell–cell communication via a wide variety of bio-active molecules (BMAs), including small RNAs, DNAs, messenger RNAs (mRNAs), proteins, lipids, and glycans (14, 15). These BMAs are encapsulated by EVs that are protected by a membrane to prevent degradation in the extracellular environment (16). Among these diverse BMAs conveyed by EVs, miRNAs are regarded as multifunctional regulators of various biological functions (17). MiRNAs play a pivotal role in regulating gene expression, affecting critical cellular functions such as growth, differentiation, and apoptosis (18). Their biogenesis begins with the transcription of primary miRNA (pri-miRNA) by RNA polymerase II. This pri-miRNA is processed in the nucleus by the Drosha-DGCR8 complex into precursor miRNA (pre-miRNA), which is then transported to the cytoplasm via Exportin-5. In the cytoplasm, Dicer further processes the pre-miRNA into mature miRNA. The mature miRNA is then integrated into the RNA-induced silencing complex (RISC), where it directs the repression of target mRNAs through sequence-specific binding, thereby modulating various biological processes and contributing to disease mechanisms (19). However, little is known about the mechanisms that drive and regulate the incorporation of miRNAs into helminth EVs and how specific miRNAs are preferentially selected as cargo (20). Nonetheless, both helminth EV-derived miRNAs and corresponding host targets are remarkably similar, suggesting that the packaging and release mechanisms of specific miRNAs during long-term host–parasite interactions and related immunomodulatory functions are evolutionarily conserved (13).
The most common miRNA clusters in helminth EVs are let-7, miR-2, miR-9, miR-10, miR-31, miR-71, miR-87, and miR-125 (21). As a conserved miRNA family that is highly homologous to the host, the function of helminth EV-derived let-7 in host–parasite interactions may reflect active selection for compatibility with the host miRNA machinery. This is because the let-7 miRNA from helminths and hosts may function similarly due to their similar sequences, and that the parasite miRNA, once taken up by the host cell, may rely on the host miRNA machinery to modulate gene expression (13). As one of the earliest identified miRNAs, let-7 is associated with reproductive development of Caenorhabditis elegans, while deficiency or overexpression of let-7 can lead to developmental abnormalities (22, 23). Although the importance in reproductive development of helminths remains unclear, let-7 can regulate host immune responses through EVs (24–27). Target gene prediction software is often used to analyze host target genes against the let-7 family-specific seed sequence (GAGGUAG) or the full-length sequence of helminth let-7 (26–29). Most of these target genes are associated with the TGF-β, MAPK, and Wnt signaling pathways, which play crucial roles not only in cell proliferation and differentiation but also in host immune and inflammatory reactions. This multiple involvement suggests different roles of helminth EV-derived let-7 in host–parasite crosstalk (13). Therefore, an in-depth study of helminth EV-derived let-7 will further enrich our understanding of this crosstalk and may further clarify the mechanisms underlying the crosstalk between helminth EVs and host cells.
Therefore, the aim of this article is to summarize current studies on the cross-species involvement of helminth EV-derived let-7 in host immune regulation (Table 1), while addressing shortcomings of related research and potential applications of helminth EVs.
Helminth EV-derived let-7 in host-parasite crosstalk
Trematodes
Diseases caused by blood fluke (e.g. schistosomiasis), liver flukes (clonorchiosis, opisthorchiosis, fascioliosis etc.) and lung flukes (paragonimiosis) severely hamper health and productivity of humans and animals (24, 26, 30, 31). These diseases induce severe pathological impacts, including chronic inflammation, fibrosis, and extensive tissue damage in affected organs. Specifically, schistosomiasis and fascioliosis primarily target the liver, clonorchiosis and opisthorchiosis lead to biliary obstruction and elevate the risk of cholangiocarcinoma, while paragonimiosis causes pulmonary fibrosis and systemic symptoms due to parasite migration (32, 33).
Schistosomes are dioecious, presenting as separate male and female sexes (34). Following dialysis, ultrafiltration, and ultracentrifugation combined with a commercial kit, EVs derived from male and female S. japonicum were successfully enriched from the supernatant of in vitro cultures and contained a high abundance of sja-let-7 (24, 31, 35). Our group recently reported that SjEVs reduced expression of collagen type I alpha 2 chain (Col1α2), one of the host target genes of sja-let-7, thereby alleviating liver fibrosis (24). The same relationship between helminth let-7 and host Col1α2 was also reported in S. mansoni (21). Since sja-let-7 is not highly abundant in S. japonicum egg ESPs or EVs (36, 37), this regulatory effect might not be caused by eggs deposited in the host liver, but rather remotely mediated via worm-derived EVs, suggesting that worms can manipulate the host immune responses to alleviate pathological damage, thereby preventing expulsion and promoting a state of tolerance that benefits the parasite by prolonging the host’s life (12). Therefore, it is not surprising that helminth miRNAs can inhibit the pathological processes underlying the establishment of chronic infection (Figure 1). However, due to the large number of mRNAs targeted by miRNAs, the consequent biological functions may vary at different stages of infection (18). Fontenla et al. (30) reported that EVs from F. hepatica contained fhe-let-7 that could potentially interact with target genes related to different regulatory roles, although the specific mechanisms remain unclear. Notably, let-7 of C. sinensis promotes inflammation in the host (26). Yan et al. (26) isolated EVs from C. sinensis (CsEVs) by ultracentrifugation combined with iodixanol-based density gradient centrifugation. Further analysis revealed that csi-let-7a, a highly enriched miRNA delivered by CsEVs, plays a pivotal role in the activation of M1-like macrophages and subsequent proinflammatory responses to biliary injury by targeting the host genes suppressor of cytokine signaling 1 and c-type lectin domain containing 7A (26).
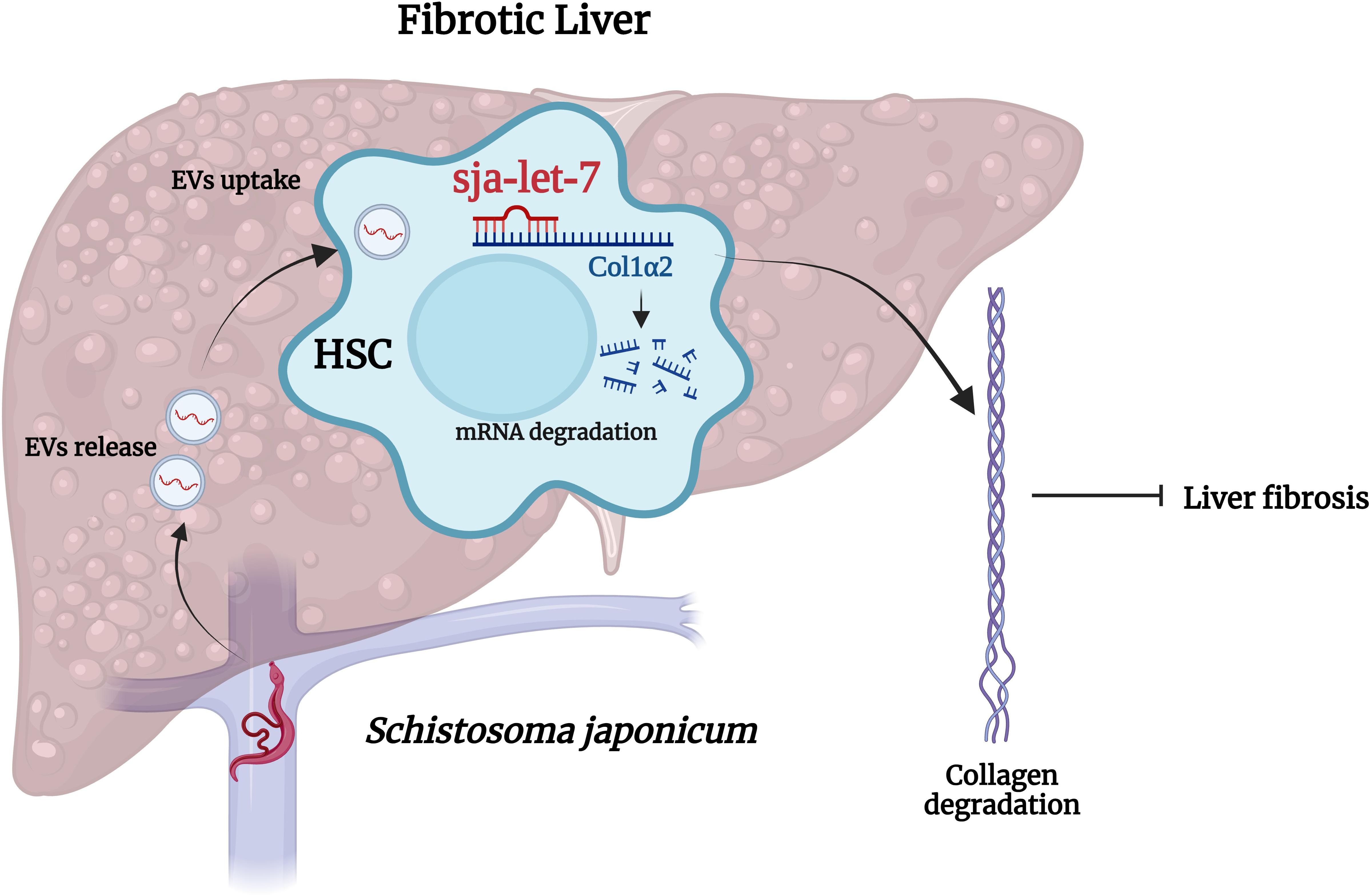
Figure 1. Cross-species sja-let-7 mediates host–parasite interactions in schistosome-induced liver fibrosis. Schistosoma japonicum worms residing in the host portal vein release SjEVs that contain sja-let-7, which alleviates schistosome-induced liver fibrosis through the regulation of Col1α2. This figure was created with Biorender.com.
Cestodes
Cestodes are zoonotic parasites that are usually widely spread in susceptible impoverished areas with poor hygiene practices, especially urban settings with livestock (38). In taenid cestodes such as Taenia solium, T. saginata, and Echinococcus spp., an intermediate host ingests contaminated vegetation or food containing cestode eggs, which hatch into larvae in the intestines. These larvae are then transported through the bloodstream to various tissues where they develop into cysts or metacestodes. When the definitive host consumes the infected meat, the infection is established. These metacestodes can cause chronic pathology, reflecting the parasite’s evolved mechanisms to evade the host’s immune response. In humans, T. solium can lead to cysticercosis, causing severe neurological damage, while Echinococcus spp. may result in hydatid disease with life-threatening cysts in the liver and lungs. In livestock, these infections cause economic losses due to reduced productivity, meat condemnation, and increased veterinary costs (39).
Cysticercus pisiformis, the larval stage of Taenia pisiformis, can infect rabbits and cause digestive disorders and growth retardation, resulting in great economic losses to the breeding industry. Co-incubation of RAW264.7 macrophages with EVs of T. pisiformis (TpEVs) collected by ultracentrifugation increased levels of tpi-let-7 in the cell, which reduced M1 phenotype expression and enhanced M2 phenotype polarization by inhibiting the target gene transcription factor CCAAT/enhancer-binding protein δ (27, 40). Likewise, co-incubation of rabbit peritoneal macrophages with TpEVs resulted in detectable levels of tpi-let-7 in the host cells, demonstrating the delivery of tpi-let-7 by TpEVs (25). Moreover, another potential target gene of tpi-let-7, nuclear factor kappa B subunit 2, was differentially expressed, as determined by proteomic analysis of the same cell samples, suggesting that the tpi-let-7/NFκB2 axis may also be involved in modulating the host immune response by TpEVs (25).
Echinococcosis is a zoonotic disease induced by adult or larval cestodes of the genus Echinococcus (41). Echinococcus granulosus is a medically significant cestode and a public health concern (42). EVs of E. granulosus collected by ultracentrifugation contained high levels of egr-let-7, which might play an immunomodulatory role by targeting insulin like growth factor 2 receptor, although the specific mechanisms remain unclear (43).
Nematodes
Nematodes are highly diverse, widely distributed, and well-adapted to almost all habitats. Based on complex and varied evolutionary histories, nematodes can be categorized into three main groups: free-living, saprophytic, and parasitic (44).
Heligmosomoides polygyrus is a gastrointestinal nematode that infects mice and belongs to the same nematode clade as C. elegans (45). During long-term interactions with the host, H. polygyrus may induce a strong Th2 response and simultaneously secrete immunomodulatory molecules, which can suppress the host immune response (46). EVs isolated from H. polygyrus (HpEVs) by ultracentrifugation were reported to suppress type 2 innate responses and reduce eosinophilia in mice (16). Subsequent microarray analysis revealed that hpo-let-7 from HpEVs could suppress expression of dual specificity phosphatase 1, which is a crucial regulator of inflammation and immunity, to manipulate host cells. In addition to H. polygyrus, Haemonchus contortus is another significant gastrointestinal nematode that affects livestock, particularly small ruminants such as sheep and goats (47). H. contortus is a major cause of parasitic gastroenteritis, leading to severe anemia, reduced productivity, and significant economic losses in the livestock industry (48). Although the specific role of let-7 in HcEVs remains underexplored, understanding its involvement in parasite-host interactions could open new avenues for developing targeted therapies and diagnostic tools for managing infections in livestock.
Interactions between helminth EV-derived let-7 and host targets have also been reported in other nematodes (49, 50). Brugia malayi is a pathogen of lymphatic filariasis, commonly known as elephantiasis, a neglected tropical disease that affects millions of people, and an important public health issue due to the high prevalence. Ricciardi et al. (50) isolated EVs from B. malayi with a commercial kit that were absorbed by human dendritic cells and monocytes, which downregulated the mammalian target of rapamycin pathway in host cells via the bma-let-7/eukaryotic translation initiation factor 4E axis. Ascaris suum is a prevalent parasitic nematode that infects pigs and was found to reduce nutrient utilization and weight gain, ultimately resulting in production loss (51). Through ultracentrifugation and size exclusion chromatography (SEC), EVs were obtained from A. suum adults, as well as L3 and L4 larvae, for miRNA profiling (49). The results of target gene prediction showed that asu-let-7 interacted with CD86, which facilitates T-cell activation, suggesting that this axis might be involved in host–parasite interactions (49).
In addition to these species, let-7 has been identified in the EVs of other helminths, although the specific target genes have not yet been identified (52, 53). Details can be found in Table 1.
Current challenges and future perspectives
Although current evidence confirms that the helminth EV-derived let-7 cluster is involved in various pathological and physiological processes, considerable challenges still exist to gain a deeper understanding of the roles of let-7 in host–parasite crosstalk.
First, helminth EVs are typically isolated from in vitro culture supernatants of a single formulation, such as Dulbecco’s modified Eagle’s medium or Roswell Park Memorial Institute 1640 medium supplemented with EV-free fetal bovine serum and antibiotics (24–26, 52–55). However, the viability of the worms is gradually decreased in these mediums, which may compromise the quality of helminth EVs. In addition, the evolutionary history of helminths is complex and the acquisition of essential substances from the host varies among parasitized stages. Whether there are differences between helminth EVs collected by in vitro culture and those involved in host–parasite interactions remain unknown. Recent studies have reported medium suitable for the reproductive development of some helminths in vitro (56, 57). Thus, the use of such a medium to obtain helminth EVs may be a direction for further research.
Second, in most studies, helminth EVs are collected by ultracentrifugation of ESPs as the primary separation and isolation method (25–27, 37, 39, 40, 53–55, 58). However, there has been a recent shift towards the use of other methods, such as SEC (59). While ultracentrifugation is commonly used to isolate EVs, it can compromise vesicle integrity due to high shear forces (60). SEC offers an alternative by separating molecules based on size through a column of porous beads, which enables high-purity isolation of EVs, preserving their biological activity and ensuring reproducibility. Additionally, SEC is scalable for larger volumes, making it ideal for studies requiring substantial quantities of purified EVs (61). Since the collection method influences the number, purity and type of enriched helminth EVs, each study should include detailed information based on a recent publication, titled “Special considerations for studies of extracellular vesicles from parasitic helminths: A community-led roadmap to increase rigor and reproducibility” (62).
Third, further studies are needed to determine whether helminth miRNAs are delivered to host cells as primary or precursor miRNAs, how miRNAs are processed and loaded in the EVs, and whether there are differences in the predicted target genes in vivo vs. in vitro. Investigating these mechanisms is crucial for advancing our understanding of host-parasite interactions. Future research should focus on exploring the molecular machinery involved in miRNA incorporation into EVs and identifying factors that determine the selectivity of miRNA cargo. These studies will provide valuable insights into how helminth EVs modulate host immune responses and contribute to parasite survival. To preliminarily confirm the interactions in vitro, our group conducted fluorescence in situ hybridization to label co-localized sja-let-7 and the related target gene Col1α2 in liver sections of mice with schistosome-induced liver fibrosis (24).
Fourth, to explore the biological functions of helminth EVs or miRNAs, recent studies have applied mimics either directly to a single cell type or directly to mice via tail vein injection. However, the use of mimics differs from natural helminth infection. Also, since miRNAs target a large number of mRNAs, potential side effects in the host should be considered. Interestingly, our group administered a mimic of sja-let-7, which resulted in a relatively high abundance miRNAs in SjEVs and an anti-fibrotic effect in mice with schistosome-induced liver fibrosis, as well as carbon tetrachloride-induced liver fibrosis, which alleviated pathological changes, demonstrating the potential application of helminth-derived miRNAs (63). Certainly, it is also necessary to assess safety in future studies.
Conclusions
This article summarized the roles of helminth EV-derived let-7 in host–parasite interactions. In addition to helminth let-7, in-depth studies are warranted to clarify the biological functions of other BMAs, such as miR-71 (21, 37) and miR-125 (43, 64), to further elucidate potential roles on host–parasite crosstalk and provide a theoretical basis for helminth prevention and control. Moreover, the potential therapeutic and diagnostic applications of let-7 are promising areas for future research. As understanding of the molecular mechanisms governing let-7 and its role in modulating host immune responses deepens, targeted therapies could be developed to inhibit or enhance specific pathways affected by let-7. Similarly, the presence of let-7 in extracellular vesicles could serve as a biomarker for parasitic infections, aiding in the early diagnosis and monitoring of disease progression.
Author contributions
HZ: Writing – original draft. GG: Writing – original draft. YJ: Writing – review & editing.
Funding
The author(s) declare that financial support was received for the research, authorship, and/or publication of this article. This work was supported by the Agricultural Science and Technology Innovation Program (ASTIP) (no. CAAS-ASTIP-2021-SHVRI), the Natural Science Foundation of Shanghai (no. 20ZR1469300) and partially funded by the Key Laboratory of Veterinary Etiological Biology, Ministry of Agricultural and Rural Affairs, China (no. XKQ2024002). The funders had no role in study design, data collection and analysis, decision to publish, or preparation of the manuscript.
Acknowledgments
We thank International Science Editing for revising the manuscript and their valuable comments.
Conflict of interest
The authors declare that the research was conducted in the absence of any commercial or financial relationships that could be construed as a potential conflict of interest.
Publisher’s note
All claims expressed in this article are solely those of the authors and do not necessarily represent those of their affiliated organizations, or those of the publisher, the editors and the reviewers. Any product that may be evaluated in this article, or claim that may be made by its manufacturer, is not guaranteed or endorsed by the publisher.
References
1. Anisuzzaman, Hossain MS, Hatta T, Labony SS, Kwofie KD, Kawada H, et al. Food- and vector-borne parasitic zoonoses: global burden and impacts. Adv Parasitol. (2023) 120:87–136. doi: 10.1016/bs.apar.2023.02.001
2. Weatherhead JE, Hotez PJ, Mejia R. The global state of helminth control and elimination in children. Pediatr Clinics North America. (2017) 64:867–77. doi: 10.1016/j.pcl.2017.03.005
3. Fissiha W, Kinde MZ. Anthelmintic resistance and its mechanism: A review. Infection Drug resistance. (2021) 14:5403–10. doi: 10.2147/idr.S332378
4. Shao B, Gui X, Lu Z, Lv R, Li H, Lu K, et al. Praziquantel promotes protection against schistosoma japonicum infection in mice. Acta tropica. (2023) 241:106874. doi: 10.1016/j.actatropica.2023.106874
5. Wang N, Peng HQ, Gao CZ, Cheng YH, Sun MT, Qu GL, et al. In vivo efficiency of praziquantel treatment of single-sex schistosoma japonicum aged three months old in mice. Int J Parasitol Drugs Drug Resist. (2022) 20:129–34. doi: 10.1016/j.ijpddr.2022.11.002
6. Liu Y, Luo X, Li J, Wang P, Teng B, Wang R, et al. Using feeding and motility patterns for ivermectin resistance detecting in haemonchus contortus larvae. Exp Parasitol. (2022) 238:108230. doi: 10.1016/j.exppara.2022.108230
7. Liu Y, Wang P, Wang R, Li J, Zhai B, Luo X, et al. An epidemiological investigation and drug-resistant strain isolation of nematodirus oiratianus in sheep in inner Mongolia, China. Animals. (2022) 13(1):30. doi: 10.3390/ani13010030
8. Mu Y, McManus DP, Hou N, Cai P. Schistosome infection and schistosome-derived products as modulators for the prevention and alleviation of immunological disorders. Front Immunol. (2021) 12:619776. doi: 10.3389/fimmu.2021.619776
9. Mu Y, McManus DP, Gordon CA, Cai P. Parasitic helminth-derived micrornas and extracellular vesicle cargos as biomarkers for helminthic infections. Front Cell infection Microbiol. (2021) 11:708952. doi: 10.3389/fcimb.2021.708952
10. Donnelly S, Dalton JP, Loukas A. Proteases in helminth- and allergen- induced inflammatory responses. Chem Immunol Allergy. (2006) 90:45–64. doi: 10.1159/000088880
11. Hewitson JP, Grainger JR, Maizels RM. Helminth immunoregulation: the role of parasite secreted proteins in modulating host immunity. Mol Biochem Parasitol. (2009) 167:1–11. doi: 10.1016/j.molbiopara.2009.04.008
12. He X, Pan W. Host-parasite interactions mediated by cross-species micrornas. Trends Parasitol. (2022) 38(6):478–88. doi: 10.1016/j.pt.2022.02.011
13. Chowdhury S, Sais D, Donnelly S, Tran N. The knowns and unknowns of helminth–host mirna cross-kingdom communication. Trends Parasitol. (2024) 40:176–91. doi: 10.1016/j.pt.2023.12.003
14. Deolindo P, Evans-Osses I, Ramirez MI. Microvesicles and exosomes as vehicles between protozoan and host cell communication. Biochem Soc Trans. (2013) 41:252–7. doi: 10.1042/BST20120217
15. van Niel G, D’Angelo G, Raposo G. Shedding light on the cell biology of extracellular vesicles. Nat Rev Mol Cell Biol. (2018) 19:213–28. doi: 10.1038/nrm.2017.125
16. Buck AH, Coakley G, Simbari F, McSorley HJ, Quintana JF, Le Bihan T, et al. Exosomes secreted by nematode parasites transfer small rnas to mammalian cells and modulate innate immunity. Nat Commun. (2014) 5:5488. doi: 10.1038/ncomms6488
17. Zhong H, Jin Y. Multifunctional roles of micrornas in schistosomiasis. Front Microbiol. (2022) 13:925386. doi: 10.3389/fmicb.2022.925386
18. Bartel DP. Micrornas: genomics, biogenesis, mechanism, and function. Cell. (2004) 116:281–97. doi: 10.1016/s0092-8674(04)00045-5
19. Bartel DP. Micrornas: target recognition and regulatory functions. Cell. (2009) 136:215–33. doi: 10.1016/j.cell.2009.01.002
20. Ding J, He G, Wu J, Yang J, Guo X, Yang X, et al. Mirna-seq of echinococcus multilocularis extracellular vesicles and immunomodulatory effects of mir-4989. Front Microbiol. (2019) 10:2707. doi: 10.3389/fmicb.2019.02707
21. Ovchinnikov VY, Kashina EV, Mordvinov VA, Fromm B. Ev-transported micrornas of schistosoma mansoni and fasciola hepatica: potential targets in definitive hosts. Infection Genet evolution: J Mol Epidemiol evolutionary Genet Infect Dis. (2020) 85:104528. doi: 10.1016/j.meegid.2020.104528
22. Ding XC, Slack FJ, Grosshans H. The let-7 microrna interfaces extensively with the translation machinery to regulate cell differentiation. Cell Cycle. (2008) 7:3083–90. doi: 10.4161/cc.7.19.6778
23. Reinhart BJ, Slack FJ, Basson M, Pasquinelli AE, Bettinger JC, Rougvie AE, et al. The 21-nucleotide let-7 rna regulates developmental timing in caenorhabditis elegans. Nature. (2000) 403:901–6. doi: 10.1038/35002607
24. Zhong H, Dong B, Zhu D, Fu Z, Liu J, Jin Y. Sja-let-7 suppresses the development of liver fibrosis via schistosoma japonicum extracellular vesicles. PloS Pathog. (2024) 20(4):e1012153. doi: 10.1371/journal.ppat.1012153
25. Wang L, Liu T, Pu G, Chen G, Li H, Zhang S, et al. Global profiling of mirna and protein expression patterns in rabbit peritoneal macrophages treated with exosomes derived from taenia pisiformis cysticercus. Genomics. (2023) 115:110690. doi: 10.1016/j.ygeno.2023.110690
26. Yan C, Zhou Q-Y, Wu J, Xu N, Du Y, Li J, et al. Csi-let-7a-5p delivered by extracellular vesicles from a liver fluke activates M1-like macrophages and exacerbates biliary injuries. Proc Natl Acad Sci. (2021) 118(46):e2102206118. doi: 10.1073/pnas.2102206118
27. Wang L, Liu T, Chen G, Li Y, Zhang S, Mao L, et al. Exosomal microrna let-7-5p from taenia pisiformis cysticercus prompted macrophage to M2 polarization through inhibiting the expression of C/ebp delta. Microorganisms. (2021) 9(7):1403. doi: 10.3390/microorganisms9071403
28. Krüger J, Rehmsmeier M. Rnahybrid: microrna target prediction easy, fast and flexible. Nucleic Acids Res. (2006) 34:W451–4. doi: 10.1093/nar/gkl243
29. Peterson SM, Thompson JA, Ufkin ML, Sathyanarayana P, Liaw L, Congdon CB. Common features of microrna target prediction tools. Front Genet. (2014) 5:23. doi: 10.3389/fgene.2014.00023
30. Fontenla S, Langleib M, de la Torre-Escudero E, Domínguez MF, Robinson MW, Tort J. Role of fasciola hepatica small rnas in the interaction with the mammalian host. Front Cell infection Microbiol. (2022) 11:812141. doi: 10.3389/fcimb.2021.812141
31. Zhu L, Liu J, Dao J, Lu K, Li H, Gu H, et al. Molecular characterization of S. Japonicum exosome-like vesicles reveals their regulatory roles in parasite-host interactions. Sci Rep. (2016) 6:25885. doi: 10.1038/srep25885
32. Yongvanit P, Pinlaor S, Loilome W. Risk biomarkers for assessment and chemoprevention of liver fluke-associated cholangiocarcinoma. J hepato-biliary-pancreatic Sci. (2014) 21:309–15. doi: 10.1002/jhbp.63
33. Mas-Coma S, Bargues MD, Valero MA. Human fascioliasis infection sources, their diversity, incidence factors, analytical methods and prevention measures. Parasitology. (2018) 145:1665–99. doi: 10.1017/s0031182018000914
34. van Hellemond JJ, van Balkom BW, Tielens AG. Schistosome biology and proteomics: progress and challenges. Exp Parasitol. (2007) 117:267–74. doi: 10.1016/j.exppara.2007.05.004
35. Du P, Giri BR, Liu J, Xia T, Grevelding CG, Cheng G. Proteomic and deep sequencing analysis of extracellular vesicles isolated from adult male and female schistosoma japonicum. PloS Negl Trop Dis. (2020) 14:e0008618. doi: 10.1371/journal.pntd.0008618
36. Zhu S, Wang S, Lin Y, Jiang P, Cui X, Wang X, et al. Release of extracellular vesicles containing small rnas from the eggs of schistosoma japonicum. Parasit Vectors. (2016) 9:574. doi: 10.1186/s13071-016-1845-2
37. Wang L, Liao Y, Yang R, Yu Z, Zhang L, Zhu Z, et al. Sja-mir-71a in schistosome egg-derived extracellular vesicles suppresses liver fibrosis caused by schistosomiasis via targeting semaphorin 4d. J Extracell Vesicles. (2020) 9:1785738. doi: 10.1080/20013078.2020.1785738
38. Craig P, Ito A. Intestinal cestodes. Curr Opin Infect Dis. (2007) 20:524–32. doi: 10.1097/QCO.0b013e3282ef579e
39. Ancarola ME, Marcilla A, Herz M, Macchiaroli N, Perez M, Asurmendi S, et al. Cestode parasites release extracellular vesicles with micrornas and immunodiagnostic protein cargo. Int J Parasitol. (2017) 47:675–86. doi: 10.1016/j.ijpara.2017.05.003
40. Wang L-Q, Liu T-L, Liang P-H, Zhang S-H, Li T-S, Li Y-P, et al. Characterization of exosome-like vesicles derived from taenia pisiformis cysticercus and their immunoregulatory role on macrophages. Parasites Vectors. (2020) 13(1):318. doi: 10.1186/s13071-020-04186-z
41. Wen H, Vuitton L, Tuxun T, Li J, Vuitton DA, Zhang W, et al. Echinococcosis: advances in the 21st century. Clin Microbiol Rev. (2019) 32(2):e00075-18. doi: 10.1128/cmr.00075-18
42. McManus DP, Gray DJ, Zhang W, Yang Y. Diagnosis, treatment, and management of echinococcosis. BMJ (Clinical Res ed). (2012) 344:e3866. doi: 10.1136/bmj.e3866
43. Zhang X, Gong W, Cao S, Yin J, Zhang J, Cao J, et al. Comprehensive analysis of non-coding rna profiles of exosome-like vesicles from the protoscoleces and hydatid cyst fluid of echinococcus granulosus. Front Cell infection Microbiol. (2020) 10:316. doi: 10.3389/fcimb.2020.00316
44. Antonopoulos A, Gilleard JS, Charlier J. Next-generation sequencing technologies for helminth diagnostics and surveillance in ruminants: shifting diagnostic barriers. Trends Parasitol. (2024) 40(6):511–26. doi: 10.1016/j.pt.2024.04.013
45. Blaxter ML, De Ley P, Garey JR, Liu LX, Scheldeman P, Vierstraete A, et al. A molecular evolutionary framework for the phylum nematoda. Nature. (1998) 392:71–5. doi: 10.1038/32160
46. Finney CA, Taylor MD, Wilson MS, Maizels RM. Expansion and activation of cd4(+)Cd25(+) regulatory T cells in heligmosomoides polygyrus infection. Eur J Immunol. (2007) 37:1874–86. doi: 10.1002/eji.200636751
47. Liu Y, Wang X, Luo X, Wang R, Zhai B, Wang P, et al. Transcriptomics and proteomics of haemonchus contortus in response to ivermectin treatment. Animals. (2023) 13(5):919. doi: 10.3390/ani13050919
48. Tuersong W, Zhou C, Wu S, Qin P, Wang C, Di W, et al. Comparative analysis on transcriptomics of ivermectin resistant and susceptible strains of haemonchus contortus. Parasites Vectors. (2022) 15(1):159. doi: 10.1186/s13071-022-05274-y
49. Hansen EP, Fromm B, Andersen SD, Marcilla A, Andersen KL, Borup A, et al. Exploration of extracellular vesicles from ascaris suum provides evidence of parasite-host cross talk. J Extracell Vesicles. (2019) 8:1578116. doi: 10.1080/20013078.2019.1578116
50. Ricciardi A, Bennuru S, Tariq S, Kaur S, Wu W, Elkahloun AG, et al. Extracellular vesicles released from the filarial parasite brugia malayi downregulate the host mtor pathway. PloS Negl Trop Dis. (2021) 15:e0008884. doi: 10.1371/journal.pntd.0008884
51. Nansen P, Roepstorff A. Parasitic helminths of the pig: factors influencing transmission and infection levels. Int J Parasitol. (1999) 29:877–91. doi: 10.1016/s0020-7519(99)00048-x
52. Bernal D, Trelis M, Montaner S, Cantalapiedra F, Galiano A, Hackenberg M, et al. Surface analysis of dicrocoelium dendriticum. The molecular characterization of exosomes reveals the presence of mirnas. J Proteomics. (2014) 105:232–41. doi: 10.1016/j.jprot.2014.02.012
53. Wu J, Liao Y, Li D, Zhu Z, Zhang L, Wu Z, et al. Extracellular vesicles derived from trichinella spiralis larvae promote the polarization of macrophages to M2b type and inhibit the activation of fibroblasts. Front Immunol. (2022) 13:974332. doi: 10.3389/fimmu.2022.974332
54. Kondo Y, Ito D, Taniguchi R, Tademoto S, Horie T, Otsuki H. Extracellular vesicles derived from spirometra erinaceieuropaei plerocercoids inhibit activation of murine macrophage raw264.7 cells. Parasitol Int. (2023) 95:102742. doi: 10.1016/j.parint.2023.102742
55. Nowacki FC, Swain MT, Klychnikov OI, Niazi U, Ivens A, Quintana JF, et al. Protein and small non-coding rna-enriched extracellular vesicles are released by the pathogenic blood fluke schistosoma mansoni. J Extracell Vesicles. (2015) 4:28665. doi: 10.3402/jev.v4.28665
56. Wang J, Chen R, Collins JJ 3rd. Systematically improved in vitro culture conditions reveal new insights into the reproductive biology of the human parasite schistosoma mansoni. PloS Biol. (2019) 17:e3000254. doi: 10.1371/journal.pbio.3000254
57. You Y, Chen X, Huo L, Chen L, Chen G, Gu M, et al. An improved medium for in vitro studies of female reproduction and oviposition in schistosoma japonicum. Parasites Vectors. (2024) 17(1):116. doi: 10.1186/s13071-024-06191-y
58. Wang Y, Gong W, Zhou H, Hu Y, Wang L, Shen Y, et al. A novel mirna from egg-derived exosomes of schistosoma japonicum promotes liver fibrosis in murine schistosomiasis. Front Immunol. (2022) 13:860807. doi: 10.3389/fimmu.2022.860807
59. Li C, Li X. Exosome-derived circ_0094343 promotes chemosensitivity of colorectal cancer cells by regulating glycolysis via the mir-766-5p/trim67 axis. Contrast Media Mol Imaging. (2022) 2022:2878557. doi: 10.1155/2022/2878557
60. Baranyai T, Herczeg K, Onódi Z, Voszka I, Módos K, Marton N, et al. Isolation of exosomes from blood plasma: qualitative and quantitative comparison of ultracentrifugation and size exclusion chromatography methods. PloS One. (2015) 10:e0145686. doi: 10.1371/journal.pone.0145686
61. Konoshenko MY, Lekchnov EA, Vlassov AV, Laktionov PP. Isolation of extracellular vesicles: general methodologies and latest trends. BioMed Res Int. (2018) 2018:8545347. doi: 10.1155/2018/8545347
62. White R, Sotillo J, Ancarola ME, Borup A, Boysen AT, Brindley PJ, et al. Special considerations for studies of extracellular vesicles from parasitic helminths: A community-led roadmap to increase rigour and reproducibility. J Extracellular Vesicles. (2023) 12(1):e12298. doi: 10.1002/jev2.12298
63. Zhong H, Dong B, Zhu D, Li H, Lu K, Fu Z, et al. Sja-let-7 attenuates carbon tetrachloride-induced liver fibrosis in a mouse model via col1α2. Biology. (2023) 12(12):1465. doi: 10.3390/biology12121465
Keywords: helminth, extracellular vesicles, microRNA, Let-7 family, host-parasite interaction
Citation: Zhong H, Guan G and Jin Y (2024) Roles of helminth extracellular vesicle-derived let-7 in host–parasite crosstalk. Front. Immunol. 15:1449495. doi: 10.3389/fimmu.2024.1449495
Received: 15 June 2024; Accepted: 06 September 2024;
Published: 28 October 2024.
Edited by:
Anisuzzaman Anisuzzaman, Bangladesh Agricultural University, BangladeshReviewed by:
Nahla Galal Metwally, Bernhard Nocht Institute for Tropical Medicine (BNITM), GermanyAnita Rani Dey, Bangladesh Agricultural University, Bangladesh
Copyright © 2024 Zhong, Guan and Jin. This is an open-access article distributed under the terms of the Creative Commons Attribution License (CC BY). The use, distribution or reproduction in other forums is permitted, provided the original author(s) and the copyright owner(s) are credited and that the original publication in this journal is cited, in accordance with accepted academic practice. No use, distribution or reproduction is permitted which does not comply with these terms.
*Correspondence: Yamei Jin, yameijin@shvri.ac.cn