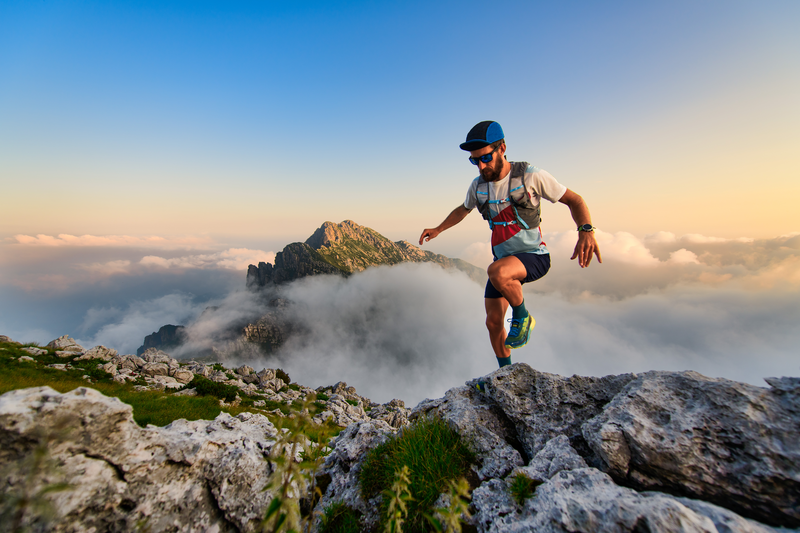
95% of researchers rate our articles as excellent or good
Learn more about the work of our research integrity team to safeguard the quality of each article we publish.
Find out more
ORIGINAL RESEARCH article
Front. Immunol. , 04 October 2024
Sec. Cancer Immunity and Immunotherapy
Volume 15 - 2024 | https://doi.org/10.3389/fimmu.2024.1448818
This article is part of the Research Topic Innate Immune Pathways as Targets for Developing Therapeutic Intervention against Human Cancers View all 6 articles
Background: Neutrophils have long been consistently adjudged to hold a dominant position in acute inflammation, which once led people to undervalue their role in chronic malignancy. It is now acknowledged that neutrophils also infiltrate into the tumor microenvironment in substantial quantities and form a highly abundant immune population within the tumor, known as tumor-associated neutrophils (TANs). There has been a surge of interest in researching the eminent heterogeneity and plasticity of TANs in recent years, and scholars increasingly cotton on to the multifaceted functions of TANs so that strenuous endeavors have been devoted to enunciating their potential as therapeutic targets. Yet it remains much left to translate TAN-targeted immunotherapies into clinical practice. Therefore, there is great significance to comprehensively appraise the research status, focal point, and evolution trend of TAN by using bibliometric analysis.
Methods: Publications related to TAN research from 2000 to 2024 are extracted from the Web of Science Core Collection. Bibliometric analysis and visualization were performed by tools encompassing Microsoft Excel, VOSviewer, CiteSpace, R-bibliometrix, and so on.
Results: The bibliometric analysis included a total of 788 publications authored by 5291 scholars affiliated with 1000 institutions across 58 countries/regions, with relevant articles published in 324 journals. Despite China’s maximum quantity of publications and top 10 institutions, the United States is the leading country with the most high-quality publications and is also the global cooperation center. FRONTIERS IN IMMUNOLOGY published the most papers, whereas CANCER RESEARCH is the highest co-cited journal. Israeli professor Fridlender, Zvi G. is the founder, pioneer, and cultivator with the highest citation counts and H-index in the TAN area. Our analysis prefigures the future trajectories: TAN heterogeneity, neutrophil extracellular trap, the crosstalk between TANs and immunocytes, and immunotherapy will likely be the focus of future research.
Conclusion: A comprehensive bibliometric and visual analysis is first performed to map the current landscape and intellectual structure of TAN, which proffers fresh perspectives for further research. The accurate identification of distinct TAN subpopulations and the precise targeting of key pro-tumor/anti-tumor subpopulations hold immense potential to develop into a TAN-targeted immunotherapy.
Comprehending the characteristics of immune cells in cancer is a progressive undertaking, with earlier studies mostly centered around adaptive immune cells. However, solely targeting the activating of T cells to boost the cytotoxic capacity of immune system has already demonstrated the miserable efficacy in addressing the underlying challenge of immunosuppression in the context of tumor (1, 2). Along with the deepening in perceptions of tumor microenvironment (TME), the attention of investigation has switched from adaptive immunity to innate immunity. As a fundamental component of the innate immune system, neutrophil is the predominant common population of polymorphonuclear leukocytes and accounts for 50-70% of circulating leukocytes in humans (3, 4). Neutrophil originates from granulocyte monocyte progenitor in bone marrow and is dispatched into circulation as mature cell distinguished by a segmented nucleus. As the front-line immunocyte defending against microbial infections, neutrophil holds a dominant position in the realm of inflammatory disorders, especially acute inflammation (5). Neutrophils possess two specific characteristics: an ephemeral lifespan and an inability to proliferate, which differentiates them from other immune cells. For instance, the half-life of circulating neutrophil is merely about 7 hours in humans. These peculiarities once led people to undervalue their role in tumors, which are chronic malignant diseases (6).
Nevertheless, as our perception of neutrophils has grown more profound, research has substantiated that their lifespan can extend up to 5.4 days through labeling with 2H2O in vivo. Neutrophils not only constitute the predominant population of circulating cells within the human bloodstream but also manifest a salient propensity for infiltration into the sophisticated milieu of the TME in substantial quantities, which could be actuated by external stimuli from TME, culminating in forming a highly abundant immune population within the TME, known as tumor-associated neutrophils (TANs) (7–10). TANs engage in TME with their phenotypic and functional plasticity. Specifically, the pro-tumor TANs are involved in tumor proliferation, angiogenesis, and immunosuppression within TME, thereby engaging in all stages of cancer progression. Whereas the anti-tumor TANs directly kill tumor cells with cytotoxicity or activate innate or adaptive immunities, thereby impeding cancer progression. Thus, there has been a surge of interest among scholars in researching the eminent heterogeneity and plasticity of TANs in recent years, and scholars increasingly cotton on to the multifaceted functions of TANs within the TME so that strenuous endeavors have been devoted to enunciating their potential as therapeutic targets (11–14). Correspondingly, the incipient boom of emerging TAN realm in recent years has achieved a series of scientific research outputs, which opens up a new perspective for perceiving the tumor immune microenvironment. Yet it remains much left to translate TAN-targeted immunotherapies into clinical practice. Therefore, there is great significance to comprehensively appraise the research status, focal point, and evolution trend of TAN.
Bibliometrics is an emerging interdisciplinary science that delivers a comprehensive and objective assessment of knowledge carriers by mathematics and statistics, in which the bibliographic analysis facilitates scholars to digest the progression of specific topics and evinces the evolution trend of this field. Nevertheless, bibliometric analysis on the TAN remains a void. Hence, this study aimed to map the current landscape and intellectual structure of TAN in the hope of proffering fresh clues and ideas for future research in the field of TAN.
Scientific output data was extracted from the Web of Science Core Collection (WoSCC) database (https://www.webofscience.com/wos/woscc/basic-search). Two reviewers independently searched original articles and reviews from inception to 2024 and downloaded information of these publications in plain text format in a single day (2024.06.01). The search formula was set as follows: TS = (“tumor associated neutrophil*” OR “tumor-associated neutrophil*” OR “tumour associated neutrophil*” OR “tumour-associated neutrophil*” OR “cancer associated neutrophil*” OR “cancer-associated neutrophil*” OR “tumor related neutrophil*” OR “tumor-related neutrophil*” OR “tumour related neutrophil*” OR “tumour-related neutrophil*” OR “cancer related neutrophil*” OR “cancer-related neutrophil*”) OR “tumor infiltrating neutrophil*” OR “tumor-infiltrating neutrophil*” OR “tumour infiltrating neutrophil*” OR “tumour-infiltrating neutrophil*” OR “cancer infiltrating neutrophil*” OR “cancer-infiltrating neutrophil*”). Among the various forms of relevant publications, the English articles and reviews were the ones analyzed. Finally, 788 publications were accessed and studied in total. The study flowchart for accessing and retrieving articles and reviews has been elaborated in Figure 1.
Microsoft Office Excel 2021 (Microsoft, Redmond, WA, USA) was the prime software used to construct a polynomial regression model for the number of publications. VOSviewer (Version 1.6.20) is a generally used bibliometric visualization software, which was utilized to conduct a co-authorship analysis of country/author/institution, co-citation analysis of journal, and keyword co-occurrence analysis. CiteSpace (Version 6.2.R6) is another favored visualization tool, which was chiefly applied to provide a visual representation of the co-citation network identifying key references and the references with the strongest citation bursts in this study. Additionally, a dual-map overlay of journals was also created by CiteSpace. R software (Version 4.3.3) is the language and environment, which is extensively utilized for statistical computing and graphics. In this study, the bibliometrix package 4.1.2 in R and two online platforms (https://bibliometric.com/ and https://flourish.studio/examples/) were used to perform the collaboration analysis among countries/regions, authors’ production over time, document citation analysis, keyword heatmaps, trend themes, and so on. The appropriate parameters are set to ensure that the clustering and grouping effects are clearly visible in the use of the aforementioned tools. Nodes with more significant connections will be classified as a cluster, and different clusters will be colored differently.
According to the search strategy (Figure 1), 788 eligible publications from 2000 to 2024 are retrieved. The current status of TAN research is described using R-bibliometrix in Figure 2. The included publications from 324 journals with an annual publication growth rate of 19.00%. There were 5291 authors, and a single author wrote 15 papers. Authors with international cooperation accounted for 25.63%. Each article had an average of 7-8 authors; 1485 author’s keywords were provided, and 38596 references were cited. The average life span of each paper from being noticed to being unknown was 4.38 years; each paper had been cited an average of 57-58 times. Additionally, these publications contained a total of 45,102 citations, with an average of 57.24 citations per paper. The H-index for all publications was 107.
Figure 2. Basic information of 788 relevant publications included. The period of included articles, the number of journal categories, the total number of articles, the annual growth rate, the total number of authors, the number of articles published by a single author, the proportion of international co-authors, the number of co-authors of an article, the keywords given by the author, the number of references cited, the average life span of each article, the average number of citations per article.
The change in the number of annual publications characterizes the pace and advancement of research on this topic and the level of attention paid to this area of research. Figure 3 illustrates the publication trend over the last 20 years, which reveals a circuitous and significant surge in the annual volume of publications in this field after 2015. With the fast increase in the number of annual publications, there were 713 articles on TAN published since 2015, accounting for 90.48% in the past two decades. The publication rate has remained high, with over 50 publications per year since 2019. What’s more, the polynomial curve shows a statistically significant and positive correlation between the number of annual/cumulative publications and the year of publication (R2 = 0.999 and R2 = 0.956, respectively). According to the fitting curve, the quantity of annual/cumulative publications will be 125 and 890, respectively, in 2024. These findings indicate that TAN is becoming a significant focus of TME research and theories about TAN are booming. Since TAN has gained increasing interest from more scholars in recent years, a spurt will occur shortly.
Figure 3. Annual and cumulative growth trend of publications. The red and orange dotted lines represent the trend-fitted curves using polynomial regression model. The correlation coefficients (R2) are displayed in the figure.
58 countries/regions have published papers on this topic, with China (N=255), the United States (N=213), and Germany (N=77) contributing the most (Figures 4A, B; Table 1). The interactive cooperation map plots the extensive cooperation relationships between countries/regions (Figures 4B, C). The top countries with the highest number of publications, such as China, the United States, Germany, and Italy, have notably close partnerships with other countries/regions, especially the closest relationship established between China and the United States among all countries. Despite producing more papers than the United States, China did not receive higher citations and total link strength (TLS) than the United States, partially because China recently has cut a striking figure as an emerging country in the realm according to Figure 4D. The United States is the international cooperation center with the highest TLS (N=151) in this field, but also had the highest citations (N=18496) as shown in Table 1.
Figure 4. (A) The trend of annual number of publications from top 10 countries/regions. (B) Geographic distribution of publications across different countries/regions and their international collaborations. The darker blue indicates more publications, and the thicker lines between countries/regions indicate stronger collaborations. (C) The international collaborations visualization of countries/regions. The thicker lines between countries/regions indicate stronger collaborations. (D) The overlay visualization of country/region co-authorship. The larger nodes represent more publications, and the thicker lines between countries/regions indicate stronger collaborations. The countries/regions in the blue cluster are considered pioneers, whereas the countries/regions in the yellow and green clusters began to publish papers in recent years. (E) The co-authorship network among institutions. The divergent colors represent divergent cooperative clusters, with institutions in the same color cluster exhibiting stronger co-authorships. (F) The overlay visualization of institution co-authorship. The institutions in the blue cluster are considered pioneers, whereas the institutions in the yellow and green clusters began to publish papers in recent years.
The units with the most publications related to TAN research are listed in Table 1. Fudan University held the top rank with the highest number of published papers. Nevertheless, the University of Pennsylvania is the institution with the highest total citations (N=5150). The 50 institutions with at least 6 papers were classified into 8 clusters according to the degree of collaboration (Figure 4E). These institutions exhibited close link strengths. The Harvard Medical School (N=128) and University of Pennsylvania (N=63) possess the highest TLS, indicative of active collaboration with other institutions in the field of TAN. However, the TLS of top publication institutions including Chinese Academy of Medical Sciences & Peking Union Medical College, Sun Yat-Sen University, and University of Duisburg-Essen were merely 46, 26, and 38 respectively (Table 1), which indicates their relatively weaker connections with other institutions. Wherein Chinese Academy of Medical Sciences & Peking Union Medical College and Sun Yat-Sen University are located in the yellow or chartreuse cluster (Figure 4F) and thereby they just initially set foot in the field in recent years, which at least partially explains their low TLS and citations in despite of the top publishments. Hence, it is advised that institutions with the highest publication volumes, such as those mentioned above, should aim to strengthen inter-agency cooperation beyond their current academic circles.
There were 5291 authors involved in the study of TAN. Scientific productivity through Lotka’s law shows that 89.1% of authors contributed solely single publication (Figure 5A). The 257 authors with co-authorship are depicted in Figure 5B. It is conspicuous from the figure that close collaboration and communication among distinct clusters are lacking, denoting again that the researchers in this field should strengthen their interagency and international cooperation efforts. When combined with the overlay visualization map of author co-authorship analysis (Figure 5C), it can be observed that the authors in the blue cluster are considered pioneers in the field of TAN, whereas the authors in the red and orange clusters began to publish papers in recent years.
Figure 5. (A) Productivity of authors through Lotka’s Law. The solid line represents the actual relationship between the proportion of authors and the quantity of publications they have published, while the dashed line represents the theoretical prediction according to Lotka’s Law. (B) The co-authorship network among authors. The larger nodes represent more publications, and the thicker lines between institutions indicate stronger collaborations. The divergent colors represent divergent cooperative clusters, with authors in the same color cluster exhibiting stronger co-authorships. (C) The overlay visualization of author co-authorship. The authors in the blue cluster are considered pioneers, whereas the authors in the yellow and green clusters began to publish papers in recent years. (D) The co-citation network among authors. The larger nodes denote more co-citations, and the thicker lines denote a stronger co-citation relationship. The authors in same color belong to the same cluster, indicating their work is frequently cited together. (E) The world’s publishing career of top 20 TAN researchers. The larger nodes indicate more annual publications, and the darker node shade denotes more annual citations.
Moreover, a co-citation analysis is performed to illustrate the functional and thematic influence of authors who have been cited more than 20 times in the field of TAN (Figure 5D), which generates a network of 401 interconnected authors. Apparently, Fridlender, Zvi G., Mantovani, Alberto, and Coffelt, Seth B. are the top three co-cited authors in this analysis, showing their dominance in this field. Furthermore, it is noteworthy that highly productive authors tend to appear together more than others.
Meanwhile, R-bibliometrix is employed to explore the authors’ local impact, and Table 2 highlights the top 10 authors in terms of the number of publications and total citations in which the local H-index attempts to evaluate the authors according to published papers in the TAN study. To further analyze the top 20 TAN researchers with the highest output, the number of papers published each year and the total amount of citations per year from 2000 to 2024 are depicted in the time graph (Figure 5E), through which the careers of the researchers can be seen directly. It is straightforward to deduce that the scientific research output of Fridlender, Zvi G. has been distributed discontinuously from 2009 to recent years, with a relatively high number of papers published and the number of citations, whereas the most other prolific authors have come to prominence in the past decade.
These publications were sourced from 324 journals. A total of 208 publications were covered in the top 10 prolific journals, representing 26.40% of all publications (Table 3). 5 of the top 10 prolific journals have an impact factor (IF) greater than 5, and 6 of the top 10 prolific journals were at the Q1 based on the 2023 Journal Citation Reports (JCR). Notably, 17 journals were identified as core journals using Bradford’s Law (Figure 6A), comprising 266 publications. The 69 journals with a minimum of 3 relevant publications created a citing network diagram and formed 5 clusters as shown in Figure 6B. Apparently, the journals in which TAN research results are published have active citation relationships. CANCER CELL had the highest citations (N=2950) and the second highest TLS (N=574) despite barely 5 publications, whereas FRONTIERS IN IMMUNOLOGY had the highest number both of publications (N=49) and TLS (N=828) (Figure 6B; Table 3), demonstrating their significant impacts in TAN field.
Figure 6. (A) 17 core journals based on Bradford’s Law. (B) The citing network among journals. The larger nodes denote more publications, and the thicker lines indicate stronger cross-citation relationships between two journals. (C) The co-cited network among journals. The larger nodes represent more co-citations. The journals in same color belong to the same cluster, and there is an analogous theme among journals in the same cluster. (D) The dual-map overlay of journals. The colored path from left to right depicts the citation pathways. The journals that cite articles are located on the left side, whereas the journals that are cited are on the right side. The labels close to the emitting region represent the respective disciplines, with each label centered around the cluster centroid of the corresponding journals. The longer the vertical axis of the ellipse, the more papers the journal published; and the longer the horizontal axis, the more authors it had.
All top 10 co-cited journals, each cited over 1000 times, with CANCER RESEARCH (N=2522 times) being the most co-cited (Table 3). Notably, all top 10 co-cited journals also had fairly high academic rankings, and almost all of them were in Q1 and had an IF of 10 or higher, with the highest being Nature, which had an IF of 50.5. Likewise, the journal co-citation network selecting journals with at least 100 citations illustrates the association between two journals (Figure 6C). The included 115 journals were classified into 3 clusters. There is an analogous theme between journals of the same color, especially for the red cluster. CANCER RESEARCH had the highest citations and TLS (citations = 2522, TLS = 357227), followed by JOURNAL OF IMMUNOLOGY (citations = 2075, TLS = 343577) and BLOOD (citations = 1965, TLS = 335179), which all had substantial contributions to TAN publications (Figure 6C).
A dual-map overlay of journals portrays the topic distribution of scientific journals and illustrates the primary citation connections between citing and cited journals, with the colored path from left to right depicting the citation pathways. As depicted in Figure 6D, the citing journals on the left side of the map are chiefly from Molecular/Biology/Immunology and Medicine/Medical/Clinical, called research frontiers; whereas the cited journals on the right side are chiefly from Molecular/Biology/Genetics, called the knowledge base.
The citation score of 788 documents was analyzed by the R-bibliometrix, and the top 20 local citation score (LCS) and global citation score (GCS) documents are listed in Table 4. Specifically, for a certain literature, LCS is the number of citations in the exported local database, which reflects the impact in this fields, whereas GCS is the total number of citations in the Web of Science database, which reveals the impact in all fields. There are 11 documents simultaneously listed in the top 20 of “Most Local Cited Documents” and “Most Global Cited Documents”. Of them, Fridlender, Zvi G. published the article (Polarization of tumor-associated neutrophil phenotype by TGF-beta: “N1” versus “N2” TAN) with the highest citation frequency both locally and globally (LCS: 396, GCS: 2290) in CANCER CELL in 2009, when the area just emerged. The above results illustrate that this document had a significant impact on the TAN field. The possible reason is that this incipient document concentrated on the basic biological characteristics of TAN within the TME and suggests that at least 2 different polarized populations of TANs are similar to what is seen in macrophages. In this paper, Fridlender, Zvi G. firstly introduced a classification scheme for TAN similar to that of tumor-associated macrophage: TAN can thus take an anti-tumorigenic (what we are calling an “N1-phenotype”) versus a pro-tumorigenic (“N2”) phenotype (15), and this paradigm could explain some of the apparent contradictions in the evaluation of the role of neutrophils in tumor biology, which proffers reference and theoretical basis for subsequent in-depth study in characteristics and biological functions of TAN within the TME. After that, scholars around the world conducted more and more research based on this classical research. What is more, Fridlender, Zvi G.’s next two publications in 2012 also ranked in the top 6 and top 15 most local cited documents, and won the high LCS scores of 133 and 70, respectively. In reality, combined with the world’s publishing career of the top TAN researchers mentioned above, we may safely conclude that Fridlender, Zvi G. is the founder, pioneer, and cultivator of the TAN area and his research outputs have been leading the trend and breakthrough in this area. Likewise, the citation relationship of 112 documents with at least 100 citations is also portrayed in the overlay visualization map (Figure 7A), while the impact of documents in the field is demonstrated in the density Figure 7B. Specifically, Figure 7C maps the relationship of the top 20 publications by local citation frequency.
Figure 7. (A) The overlay visualization map of document citation analysis. The larger nodes represent more citations, and the thicker lines indicate stronger cross-citation relationships between two documents. The color denotes the publication year. (B) The spectral density map of document citation. The redder color represents more citations. (C) Association between the top 20 citation bursts. The larger nodes represent more citations, and the arrows denote citing direction.
The reference co-citation network is depicted in Figure 8A, where a scaling factor k=15 was set and 676 cited literature references were extracted using g-index. The labels showed the first author with the thirty most cited references and the year. Additionally, considering the 10 most co-cited publications were also of interest to most TAN researchers, they are listed in Table 5.
Figure 8. (A) Co-citation analysis of the references. The larger nodes and labels are assigned to references with more co-citations, and the thicker lines represent stronger co-citation relationships. The thicker node periphery (purple circle) represents higher intermediate centricity. (B) Clustering network analysis of co-cited references. The clusters apply keywords of references as label source. The clusters are numbered in ascending order, and smaller numbers indicating more studies within the corresponding cluster. (C) The timeline view of co-cited references analysis. The nodes appear on the timelines in diverse positions and colors, based on the time of reference publication. The nodes on the left represent earlier references, whereas those on the right represent more recent ones. The nodes positions along the same line represent a cluster, identified by the label # on the right side; the length of the horizontal line and fore and aft ends are the duration for that category. (D) Top 30 references with the strongest citation bursts from 2000 to 2024. The green lines represent the period from 2000 to 2024, whereas the red lines demonstrate the duration of each citation burst during which the references were cited most.
The co-citation analysis forms the 17 clusters, and the modularity Q and the mean silhouette S were higher than 0.85, showing a significant clustering effect and a highly credible network (Figure 8B). The timeline view illustrates how research hotspots have evolved over time (Figure 8C). Overall, the rise in citations in this field and most of the highly cited literature emerged began in 2010, and many of the co-cited references continue to be widely cited, denoting the TAN area remains a prominent research topic that has been making a rapid advancement and a vast array of results. It can be observed that clusters #3 CD66b, #5 cervix carcinoma, #10 anti-tumor response, and #14 er stress are the initial topics of study in this field, but the hotspot has already shifted from them. Whereas the clusters #0 high-dose radiotherapy, #1 neutrophil extracellular trap (NET), #12 tumor microenvironment, and #15 pancreatic cancer are located at the line’s rightmost end, representing the current new hotspots in TAN research.
Herein, reference citation bursts are applied to spotlight the popularity and significance over time of specific references in the TAN field, and the top 30 references with the strongest citation bursts sorted by the beginning year of burst are depicted in Figure 8D. We can summarize that co-cited references with high citation bursts predominantly centered on literature with a high IF in which there are 11 reviews in total. The onset of the reference citation burst can be traced back to 2010, attributed to the paper authored by Fridlender, Zvi G., and the latest took place in 2022, with the longest burst spanning 5 years. The article entitled Eruslanov, Evgeniy B., 2014, J CLIN INVEST, V124, P5466 experienced the strongest burst (Strength=28.29). The nature and function of TANs within the TME are largely unclear, and Eruslanov, Evgeniy B. (2014) demonstrated that TANs are not immunosuppressive, but rather stimulate T cell responses in the earliest stages of lung cancer (16). Notably, wherein 3 references with the strongest citation bursts are from Fridlender, Zvi G., which again demonstrates he has made an eminent contribution to the TAN field and laid a solid foundation for subsequent trials. Meanwhile, the most recent burst is attributed to Shaul, Merav E. (2019), Giese, Morgan A. (2019), Jaillon, Sebastien (2020), and Teijeira, Alvaro (2020), which are still undergoing a citation explosion phase even to this day and have the current emergence of strong citation references. Wherein the reviews including Shaul, Merav E. (2019), Giese, Morgan A. (2019), and Jaillon, Sebastien (2020) were respectively published in the NATURE REVIEWS CLINICAL ONCOLOGY, BLOOD, and NATURE REVIEWS CANCER, which offer systematic summaries of the significant findings and concepts that have contributed to the field of TAN biology.
Figures 9A, B depicts the word cloud of the top 60 Keywords Plus and Author Keywords, where the size of words shows their frequency of occurrence. The keyword “tumor-associated neutrophil” is in the center of Figure 9A, followed by “suppressor-cells,” “cancer,” and so on. Likewise, the results in Figure 9B show that “tumor-associated neutrophil” is the most prominent, and “neutrophil” and “tumor microenvironment” are close behind. Obviously, Keywords Plus is broader than Author Keywords. The heatmaps visualize the normalized frequency of Author Keywords from 2000-2024, wherein the annual frequency is on the left and the cumulative frequency is on the right (Figure 9C). Additionally, the top 30 most frequent All Keywords are presented in Table 6, which generally bespeaks the current focal research.
Figure 9. (A) The word cloud of top 60 Keywords Plus. The size of words positively correlates with their frequency of occurrence. (B) The word cloud of top 60 Author Keywords. The size of words positively correlates with their frequency of occurrence. (C) Heatmaps of the top 25 most frequent Author Keywords. Annual keyword frequency on the left and cumulative keyword frequency on the right. (D) Keyword-based research theme trend map. It presents each topic as a line whose span represents the duration, with the nodes denoting the most prevalent year for that particular topic. The larger nodes indicate the themes appear more frequently. (E) The alluvial diagram of thematic evolution. It unveils the changes and internal links of keywords in different periods. (F) The strategic diagram of sub-period. It displays the development trend and maturity level of keywords.
The R-bibliometrix visualizes the primary keywords over the past 20 years, analyzing comprehensively the research trends and hotspots. In Figure 9D, the research hotspots were relatively homogeneous before 2018, principally focusing on “survival,” “G-CSF,” and “CD66b”. Nevertheless, since 2018, the research hotspots manifested a sudden surge. Specifically, “pancreatic ductal adenocarcinoma,” “immunotherapy,” “neutrophil extracellular traps,” “neutrophil heterogeneity,” and “tumor microenvironment” continue to be the subject of intense research interest. What is more, the emergence of some new keywords in the top-left corner of Figure 9D, such as “immunotherapy,” “neutrophil extracellular traps,” “neutrophil heterogeneity,” etc., have gained prominence over the last 2 years, reflecting a discernible shift in the emphasis and direction of future research endeavors. In Figure 9E, the alluvial diagram manifests the evolution and internal links of keywords in divergent periods. Apparently, some new topics including “immune cells,” “neutrophil heterogeneity,” “immunosuppression,” “immunotherapy,” etc., have evolved into striking emergence in the past two years, which are basically consistent with the results of the above keyword-based research theme trend map. In Figure 9F, the strategic diagram of sub-period presents the development trend and maturity level of keywords. The clusters including “circulating neutrophil,” “oral cancer,” “oral squamous cell carcinoma,” etc., are located in the Motor Themes quadrant, denoting that the above keywords are the core themes with high maturity. Whereas the clusters including “invasion,” “myeloid-derived suppressor cell,” “IL-8,” “NF-kappa-B,” etc., are located in the Niche Themes quadrant, indicating the above keywords are well-developed but banal. Besides, “immunohistochemistry,” “survival analysis,” and “neutrophil infiltration” are located in the Emerging or Declining Themes quadrant, suggesting the above keywords are peripheral themes and have not developed well. On the flip side, the clusters including “tumor microenvironment,” “immunotherapy,” “NETs,” “pancreas,” “biomarker,” etc., are located in the Basic Themes quadrant, demonstrating that the above keywords are significant but the current research is not enough, therefore the above topics may become research hotspots or future development trends.
It is noteworthy that these findings generally align with the outcomes of co-cited reference analysis. Collectively, these findings identified research hotspots and illuminated trends in TAN, revealing a pronounced emphasis on molecular mechanisms in contemporary research.
More than 5 scientific tools were exploited to render a bibliometric map and visualization of 788 TAN-related publications extracted from WoSCC from 2000 to June 1, 2024. Our study systematically appraises the research status and promising future research interests in the field of TAN. To the best of our knowledge, this is the first bibliometric review, summary, and outlook of this field. We exerted quantitative, qualitative, and integrative assessments to identify trends and evolutions in research, which will be canvassed in detail in what follows, delineating a comprehensive overview of our findings.
The annual publication output wavily ascended over the years without a discernible upward trend until 2015, but there was a significant growth trend with an annual output exceeding 50 papers after 2019. Overall, the field of TAN has made great progress in the past 20 years, thanks to the progress of science and technology studies (especially single-cell multi-omic profiling) and the powerful support from the government. On another level, the yearly escalation in TAN publications is occasioned by the eminent contributions of countries/regions, institutions, authors, and journals.
In terms of geographical distribution, there are merely 58 countries/regions included in our study, and note that less than one-fifth of the countries/regions published more than 20 papers. Moreover, the distribution of institutions is in accordance with countries/regions based on geographical location, while the publication counts for most countries/regions and institutions are out of proportion to their citation counts in this field. Notably, China has emerged as the leading global producer of publications in the field, transcending all other countries/regions, and 6 out of the top 10 active institutions are also located in China. Intriguingly, the United States continues to dominate the field as a global cooperation center with overwhelming citation counts, which evinces the United States had conducted in-depth research in this field and the potentially greater leverage in their articles. As for cooperation between countries, most communication and collaboration were confined to Europe, North America, and a handful of Asian countries.
When it comes to authorship, Israeli professor Fridlender, Zvi G. from the Institute of Pulmonary Medicine, Hadassah-Hebrew University Medical Center is the founder, pioneer, and cultivator of the TAN area with the highest citation counts and local H-index and a significant presence of high LCS papers, therefore his research outputs have been leading the trend and breakthrough and offering reliable reference value for scholars in this field. Our analysis also discloses a disparity between productivity and impact, with some highly productive authors not having a high impact and vice versa. Specifically, just Fridlender, Zvi G. is concurrently in both the top 10 most prolific authors and top 10 most cited authors lists. Notably, Albelda, Steven M. had a high citation counts and local H-index but did not publish a great number of papers, reflecting that he sustains stringent quality control over his publications.
Regarding academic journals, FRONTIERS IN IMMUNOLOGY published the most papers about TAN, implying more meaningful findings are more likely to be published in this journal. In addition, CANCER CELL, FRONTIERS IN IMMUNOLOGY, and NATURE MEDICINE were cited more than 2000 times, with CANCER CELL possessing the most citations, which is indicative of the higher quality as well as better reference value of this journal in this area. Except for JOURNAL OF IMMUNOLOGY, all of the co-cited journals in Table 3 are located in Q1, demonstrating the significance of TAN in future research.
The performance of reference co-citation networks and keyword clustering outlines underlying research structure in the study of TAN. Through scrupulous examination of the above analyses, a wealth of beneficial insights can be gleaned, encompassing but not confined to TAN heterogeneity, NETs, the interactions between TANs and immunocytes, immunotherapy, and so on. These discoveries allow for the identification of emerging trends and research hotspots within the field of TAN. In subsequent sections, we will canvass their far-reaching meaning for this field of research and latent implications for future orientations.
Neutrophils consecutively differentiate from the bone marrow into divergent subpopulations, each possessing divergent functions. What is more, in the context of TME, TAN subpopulations display more multifaceted phenotypes and capacities, encompassing angiogenesis, extracellular matrix remodeling, metastasis, and immunomodulation (17–19). The research focuses on TANs have evolved from the macroscopic quantity and function to the microscopic genes and phenotypes. The categorization and detection of divergent phenotypes of TAN symbolize pivotal research hotspots in the field of TAN (20). It is worth noting that the conception of N1 and N2 subpopulations originally introduced by Fridlender, Zvi G. in 2009 to differentiate between anti-tumorigenic and pro-tumorigenic TANs is an oversimplification of their dual phenotypes (15). The latest investigations demonstrated that the simplistic dichotomy of tumor-infiltrating immune cells lacks the requisite specificity and precision to fully describe the heterogeneity of TANs and may not comprehensively depict the whole landscape (21, 22). To date, assorted subpopulations of TAN have been identified in tumors, evincing discrepancies in surface markers as well as functions (23–25). Accordingly, it is imperative to acknowledge the heterogeneity and plasticity of TANs during distinct phases and fathom their anomalous functions within the TME. Although TANs can be perceived as a consequence of neutrophil development and ensuing infiltration into the TME, the prevailing perception of subpopulation categorization remains fragmentary, and it remains hazy how these subpopulations arise from differentiation in the bone marrow, maturation in circulation, or reprogramming within the TME (26). Consequently, implementing a precise subpopulation analysis of TANs has emerged as a compelling focal point warranting further exploration. This ultimately hinges on novel technologies (e.g., genomics, single-cell, and spatial omics) for advancement, which are closely associated with the description of heterogenous transcriptional signatures and potential functional plasticity in TANs. Accurately delineating the provenance of TAN subpopulations and capturing their entire lifespan could furnish scholars with a holistic perception of TAN heterogeneity, which in turn optimizes the timing for interventions in TAN-targeted immunotherapy culminating in an improved patient prognosis. Moreover, a generally recognized separation and identification method for each TAN subpopulation, alongside further perspectives into their functions, is imperative (26). With well-defined notions, isolation procedures, and purification technologies for each TAN subpopulation, patients may be one step closer to the auspicious realm of TAN immunotherapy, akin to the triumph witnessed with CAR-T cell therapy (27–30).
NETs released by neutrophils consist of decondensed chromatin DNA filaments coated with granule proteins responsible for trapping and killing extracellular pathogens with microbicidal bioactivity via binding to their DNA structures (31, 32), consequently playing a protective role in the antimicrobial defense. Recently, there is growing evidence, however, that NETs also can be induced by tumors through the secretion of varied tumor- and infection-derived molecules, encompassing the overexpression of granulocyte colony-stimulating factor (G-CSF) generally observed in cancer and nicotinamide phosphoribosyl transferase (NAMPT), thereby playing pro-tumorigenic roles in the scenario of cancer-associated inflammation in the majority but not all conditions (33, 34). Wherein NETs induced by G-CSF manifest diversified biomedical behaviors such as capture of circulating tumor cells, epithelial-to-mesenchymal transition, and increment of vascular permeability, which promote cancer-associated thrombosis, tumor intravasation, and metastasis, as well as laminin remodeling and immunosuppressive behaviors, which conduce to tumor proliferation and metastasis (35). On the other hand, NAMPT can stimulate NET formation derived from aged TANs expressing high chemokine receptors 4 and low CD62L, thereby facilitating tumor metastasis (36).
The underlying mechanism of NETs in tumor growth, metastasis formation, and cancer-associated angiogenesis are currently being intensively investigated in full swing. The DNA ingredients of NETs (NET-DNA) in the liver are chemotactic for tumor cells through interplay with high affinity with the coiled-coil domain containing protein 25 (CCDC25) that is a transmembrane protein expressed on tumor cells so that DNA-CCDC25 interplay triggers an intracellular signaling cascade facilitating tumor cell directional migration and metastasis formation (37). Additionally, inflammation facilitates the transition between dormancy and awakening of tumor cells. Specifically, dormant tumor cells adhere to the extracellular matrix protein laminin in murine models with breast and prostate cancers. Repetitive instillation of two NET inducers, including lipopolysaccharide or nicotine (38), bolsters neutrophil inflammation as well as NET formation in the lung. Two NET-related proteases, including neutrophil elastase and matrix metalloproteinase-9, successively cleave laminin exposing an epitope that actuates dormant tumor cell proliferation via integrin activation (38). Furthermore, NETs wrap and coat tumor cells and shield them from the cytotoxicity mediated by CD8+ T cells and natural killer (NK) cells (39). Notably, NET-DNA binds transmembrane and coiled-coil domains 6 on CD8+ T cells to impede antineoplastic immunity, thereby boosting progression of hepatocellular carcinoma (40). Moreover, as a potential therapeutic target for angiogenesis, angiopoietin induces NETs and thereby elevates tube length and loop quantity in human vascular endothelial cells (HUVECs) (41). The NET-DNA receptor CCDC25 is expressed in HUVECs, proffering a platform for NETs to accelerate HUVEC proliferation, migration, and tubulation (42). NAMPT/SIRT pathway also contributes a lot to NET-associated tumor angiogenesis (43), in which SIRT3 is capable of regulating endothelial cells, thereby stimulating the ROS generation and modulating the hypoxia-inducible factor, culminating in the preferment of angiogenesis (44).
While it is unambiguous that NETs contribute to tumorigenesis, several researchers have begun highlighting also the anti-tumorigenic role of NETs. There is some experimental evidence that NETs suppress the proliferation of colon cancer cells and exert cytotoxic effects on melanoma cells (45, 46). Additionally, NETs forecast elevated survival in patients with head and neck squamous cell carcinoma (47). Whether variations in the quality and quantity of NETs play pro- or anti-tumorigenic roles in divergent types and divergent phases of tumors requires further exploration.
TAN is an integral part of the TME, and its high diversity, heterogeneity and plasticity allow TANs to perform their dual function in tumor immunity via forming an intricate crosstalk with immunocytes. Wherein the crosstalk between TAN and T cell was initially disclosed within the TME. For instance, a distinctive subpopulation of N1 TAN, induced by granulocyte-macrophage colony stimulating factor (GM-CSF) and interferon-γ (IFN-γ) and characterized by CD86 and HLA-DR expression, was identified as possessing antigen-presentation facilities that augment the antineoplastic adaptive immunity of both CD8+ T cells and CD4+ T cells through MHC-TCR binding (48). Another specific subpopulation of TANs discerned in sarcoma and expressing augmented CD11b and CD54, along with diminished CD62L, has been shown an exceptional cytokine secretion profile, encompassing C-X-C Motif Chemokine Ligand (CXCL)10, interleukin (IL)-23a, and arginase-1, which in conjunction with IL-12 secreted by macrophages synergistically robustly boost the CD4−CD8− unconventional αβ T cell polarization and their IFN-γ secretion, thereby triggering a type I immune response against tumor (49). By contrast, there is also literature indicating that TANs can repress the antineoplastic function of T cells and facilitate tumor metastasis (50). Moreover, another subpopulation of programmed cell death 1 ligand+ (PD-L1+) TANs, differentiated by lactate, can curb T cell cytotoxicity against tumors (51, 52). Given the dual regulatory effect on T cells by TANs, it is imperative to exactly differentiate the specific subpopulations for the exploitation of immunotherapies that complement T-cell anti-tumor immunity.
TANs can also communicate with tumor-infiltrating B cells in varied cancers, resulting in consequential transitions in the behavior of plasma cells (53). TANs secrete the cytokine B-cell-activating factor (BAFF) and a proliferation-inducing ligand (APRIL), which conduce to B cell recruitment but also the IgM generation, along with its switching to IgG or IgA (54). Noticeably, except for molecules encompassing cytokine BAFF, APRIL, and IL-21, TNF-α secreted by TANs also plays a role in inducing B cell chemotaxis, which bolsters the movement and migration of B cells along with CXCL12 or CXCL13 (31, 55). Furthermore, TANs are implicated in the neoplastic B cell differentiation, which also can be mediated by the aforementioned pertinent molecules, like APRIL. Given the manifold effects of B cells in anti-tumor immunity, encompassing direct antineoplastic function via antibody-dependent cellular cytotoxicity and indirect function via activating other immune cells (e.g., T cells and NK cells) (56, 57), it is imperative to investigate whether and how TANs participate in these interactions. Simultaneously, it introduces an intriguing problem pertaining to whether TANs are related to the immunosuppressive function of B cells, encompassing tumor angiogenesis, lymphotoxin generation, and T cell suppression (58–60).
The convoluted interplays between TANs and other innate cells mostly are presumed to exert immunosuppressive effects, while the latent mechanism remains substantially unexplored and warrants further investigations. Fascinatingly, TANs repress tumor metastasis without NK cell, while the crosstalk between TANs and NK cells may facilitate breast cancer metastasis (61). Moreover, the dynamic interaction between TANs and tumor-associated macrophages within the TME collaboratively advances the progression of intrahepatic cholangiocarcinoma via activating signal transducer and activator of transcription 3 (62). Another subpopulation of CCL3+CCL4+ TAN induced by GM-CSF and IL-6 recruits macrophages by secreting chemokines and thereby promoting tumor metastasis, albeit the ambiguous phenotypic characteristics of the above macrophages (51).
The key implication of TANs in the immunotherapy realm has been preliminarily expounded via several retrospective cohort studies. Patients afflicted with hepatocellular carcinoma were observed a high expression of programmed cell death protein 1 (PD-1) in both intratumoral and peritumoral TANs, exceeding that detected in circulating neutrophil populations (63). These particular TANs repressed T cell proliferation and activation, thereby accentuating their necessity in the case of PD-1-based immunotherapies. Nevertheless, fascinatingly, the intratumoral TAN counts in melanoma patients treated with the anti-PD-1 antibody, nivolumab, did not significantly deviate from those of non-users (64). Additionally, the administration of nivolumab was correlated with escalated infiltration density of TANs in patients afflicted with pancreatic ductal adenocarcinoma, which pertains to worse overall survival (65). The latest scientific endeavors have proffered a more nuanced insight, positing that the hitherto conventional beliefs may have been somewhat unilateral and that certain particular TAN subpopulations exert a conspicuous imprint on immunotherapy efficacy. For instance, the efficacious immunotherapy provoked a fleeting upsurge in the TAN abundance, which outlined a characteristic cohort of therapy-induced TANs expressing dominantly an interferon gene signature and is a peculiarity regarded integral for the immunotherapeutic regimens (66). Furthermore, albeit the deficiency of target antigen Trp1 in the bulk of melanoma cells, T-cell therapy efficaciously annihilated these immune-escaped melanoma cells with the aid of a subpopulation of TANs possessing the capacity to secrete nitric oxide in a murine model (64). The exact ramifications of TANs in immunotherapies are still contentious. Nevertheless, we can presume that the immunotherapeutic efficacy may be remarkably impacted by particular TAN subpopulations. The precise mechanisms underlying the interplay between immunotherapy and TANs within the TME are yet to be allsidedly studied. Collectively, TAN is an attractive and fresh immunotherapeutic target (12, 67–70), and a precise delineation as well as accurate targeting of TAN subpopulations doubtlessly hold promise as vital parameters of optimized current immunotherapeutic efficacy. Perhaps the eventual remaining destination in the realm is to translate TAN-targeted immunotherapies into clinical practice.
This study inevitably is not without its limitations. First, the search was limited to publications in the core dataset of the Web of Science database, which means certain pertinent papers in other data sources (PubMed, Embase, Scopus, etc.) possibly have been excluded. Secondly, the focus was only on English-language literature so that high-quality articles published in other languages may have been omitted, introducing selection bias. Thirdly, the recently published high-quality literature with a low citation rate may not fully encapsulate its academic merit and not appear in our analysis due to the temporal restriction of our analysis. Lastly, the bibliometric techniques utilized here are limited to metadata rather than full-text analysis so that we may have missed critical insights only present in the full articles, such as author perspectives and outlook on the field. Although the above constraints would not change the results of this study, future work should expand the research base to include non-English studies and the latest outstanding publications.
A comprehensive bibliometric and visual analysis is first performed to map the current landscape and intellectual structure of TAN. Based on the systematical analysis, it is irrefutable that TANs play a significant role in the TME. The number of publications about TANs has been significantly surging in the past 20 years, denoting that research on TAN is experiencing a vibrant and rapidly evolving stage. Despite China’s maximum quantity of publications and top 10 institutions, the United States is the leading country with the highest impact and is also the global cooperation center. Israeli professor Fridlender, Zvi G. is the founder, pioneer, and cultivator in the TAN area. Our analysis prefigures the future trajectories: TAN heterogeneity, NETs, the crosstalk between TANs and immunocytes, and immunotherapy will likely be the focal points of future research in the field of TAN. Most of all, wherein the TAN heterogeneity in the context of tumor-related pathology exerts a pivotal influence on NETs, the crosstalk between TANs and immunocytes, and immunotherapy, therefore divergent TAN subpopulations could have completely divergent NETs, intercellular crosstalk, and immunotherapeutic implication. Hence, future endeavors must precisely delineate the origin of TAN subpopulations and comprehend their complete lifespan. The accurate identification of distinct TAN subpopulations and the precise targeting of key pro-tumor/anti-tumor subpopulations hold immense potential to develop into a TAN-targeted immunotherapy.
The original contributions presented in the study are included in the article/supplementary material. Further inquiries can be directed to the corresponding author.
CX: Conceptualization, Data curation, Formal analysis, Investigation, Methodology, Project administration, Resources, Software, Validation, Visualization, Writing – original draft. XF: Data curation, Investigation, Methodology, Project administration, Resources, Software, Validation, Visualization, Writing – original draft. WA: Data curation, Formal analysis, Investigation, Methodology, Project administration, Software, Visualization, Writing – original draft. ZZ: Formal analysis, Investigation, Methodology, Project administration, Visualization, Writing – original draft. GD: Data curation, Investigation, Project administration, Resources, Writing – original draft. YG: Conceptualization, Funding acquisition, Project administration, Supervision, Writing – review & editing.
The author(s) declare financial support was received for the research, authorship, and/or publication of this article. This work was supported by National Natural Science Foundation of China (No. 82150006); Chinese Society of Clinical Oncology Research Foundation (Nos. Y-HR2017-117, Y-HH202102-0060); Beijing Medical and Health Foundation (No. YWJKJJHKYJJ-F3046D); Qujiang District Quzhou City Life Oasis Public Service Center (No. BJHA-CRP-040); Wu Jieping Medical Foundation (No. 320.6750.2023-05-10) to YG.
The authors declare that the research was conducted in the absence of any commercial or financial relationships that could be construed as a potential conflict of interest.
All claims expressed in this article are solely those of the authors and do not necessarily represent those of their affiliated organizations, or those of the publisher, the editors and the reviewers. Any product that may be evaluated in this article, or claim that may be made by its manufacturer, is not guaranteed or endorsed by the publisher.
APRIL, A proliferation-inducing ligand; BAFF, B-cell-activating factor; CCDC25, coiled-coil domain containing protein 25; CXCL, C-X-C Motif Chemokine Ligand; GCS, global citation score; G-CSF, granulocyte colony-stimulating factor; GM-CSF, granulocyte-macrophage colony stimulating factor; HUVECs, human vascular endothelial cells; IF, impact factor; IFN-γ, interferon-γ; IL, interleukin; JCR, Journal Citation Reports; LCS, local citation score; NAMPT, nicotinamide phosphoribosyl transferase; NET, neutrophil extracellular trap; NET-DNA, the DNA ingredients of NETs; NK cells, Natural killer cells; PD-1, programmed cell death protein 1; PD-L1, programmed cell death 1 ligand; TAN, tumor-associated neutrophil; TLS, total link strength; TME, tumor microenvironment; WoSCC, Web of Science Core Collection.
1. Goliwas KF, Deshane JS, Elmets CA, Athar M. Moving immune therapy forward targeting TME. Physiol Rev. (2021) 101:417–25. doi: 10.1152/physrev.00008.2020
2. Xiao C, Xiong W, Xu Y, Zou J, Zeng Y, Liu J, et al. Immunometabolism: a new dimension in immunotherapy resistance. Front Med. (2023) 17:585–616. doi: 10.1007/s11684-023-1012-z
3. Hidalgo A, Chilvers ER, Summers C, Koenderman L. The neutrophil life cycle. Trends Immunol. (2019) 40:584–97. doi: 10.1016/j.it.2019.04.013
4. Awasthi D, Sarode A. Neutrophils at the crossroads: unraveling the multifaceted role in the tumor microenvironment. Int J Mol Sci. (2024) 25:2929. doi: 10.3390/ijms25052929
5. Kolaczkowska E, Kubes P. Neutrophil recruitment and function in health and inflammation. Nat Rev Immunol. (2013) 13:159–75. doi: 10.1038/nri3399
6. Summers C, Rankin SM, Condliffe AM, Singh N, Peters AM, Chilvers ER. Neutrophil kinetics in health and disease. Trends Immunol. (2010) 31:318–24. doi: 10.1016/j.it.2010.05.006
7. Granot Z. Neutrophils as a therapeutic target in cancer. Front Immunol. (2019) 10:1710. doi: 10.3389/fimmu.2019.01710
8. Pillay J, den Braber I, Vrisekoop N, Kwast LM, de Boer RJ, Borghans JA, et al. In vivo labeling with 2H2O reveals a human neutrophil lifespan of 5.4 days. Blood. (2010) 116:625–7. doi: 10.1182/blood-2010-01-259028
9. Wang X, Juncker-Jensen A, Huang G, Nagy ML, Lu X, Cheng L, et al. Spatial relationship of tertiary lymphoid structures and tumor-associated neutrophils in bladder cancer and prognostic potential for anti-PD-L1 immunotherapy. Cancer Commun (Lond). (2024) 44:499–503. doi: 10.1002/cac2.12491
10. Liu L, Xu L, Wu D, Zhu Y, Li X, Xu C, et al. Impact of tumour stroma-immune interactions on survival prognosis and response to neoadjuvant chemotherapy in bladder cancer. EBioMedicine. (2024) 104:105152. doi: 10.1016/j.ebiom.2024.105152
11. Bejarano L, Jordāo MJC, Joyce JA. Therapeutic targeting of the tumor microenvironment. Cancer Discov. (2021) 11:933–59. doi: 10.1158/2159-8290.Cd-20-1808
12. Yang S, Jia J, Wang F, Wang Y, Fang Y, Yang Y, et al. Targeting neutrophils: Mechanism and advances in cancer therapy. Clin Transl Med. (2024) 14:e1599. doi: 10.1002/ctm2.1599
13. Wu J, Ma T, Zhu M, Mu J, Huang T, Xu D, et al. A pluripotential neutrophil-mimic nanovehicle modulates immune microenvironment with targeted drug delivery for augmented antitumor chemotherapy. ACS Nano. (2024) 18:5864–77. doi: 10.1021/acsnano.3c12694
14. Bodac A, Mayet A, Rana S, Pascual J, Bowler AD, Roh V, et al. Bcl-xL targeting eliminates ageing tumor-promoting neutrophils and inhibits lung tumor growth. EMBO Mol Med. (2024) 16:158–84. doi: 10.1038/s44321-023-00013-x
15. Fridlender ZG, Sun J, Kim S, Kapoor V, Cheng G, Ling L, et al. Polarization of tumor-associated neutrophil phenotype by TGF-beta: “N1” versus “N2” TAN. Cancer Cell. (2009) 16:183–94. doi: 10.1016/j.ccr.2009.06.017
16. Eruslanov EB, Bhojnagarwala PS, Quatromoni JG, Stephen TL, Ranganathan A, Deshpande C, et al. Tumor-associated neutrophils stimulate T cell responses in early-stage human lung cancer. J Clin Invest. (2014) 124:5466–80. doi: 10.1172/jci77053
17. Ng MSF, Kwok I, Tan L, Shi C, Cerezo-Wallis D, Tan Y, et al. Deterministic reprogramming of neutrophils within tumors. Science. (2024) 383:eadf6493. doi: 10.1126/science.adf6493
18. Wang J, Cao X. The tumor niche can reprogram long-lived protumorigenic neutrophils. Trends Immunol. (2024) 45:155–7. doi: 10.1016/j.it.2024.02.001
19. Cassatella MA, Scapini P, Tamassia N. How murine neutrophils are hijacked within the microenvironment of pancreatic cancer. J Leukoc Biol. (2024) 115:585–8. doi: 10.1093/jleuko/qiae042
20. Hedrick CC, Malanchi I. Neutrophils in cancer: heterogeneous and multifaceted. Nat Rev Immunol. (2022) 22:173–87. doi: 10.1038/s41577-021-00571-6
21. Horvath L, Puschmann C, Scheiber A, Martowicz A, Sturm G, Trajanoski Z, et al. Beyond binary: bridging neutrophil diversity to new therapeutic approaches in NSCLC. Trends Cancer. (2024) 10:457–74. doi: 10.1016/j.trecan.2024.01.010
22. Hu T, Cheng B, Matsunaga A, Zhang T, Lu X, Fang H, et al. Single-cell analysis defines highly specific leukemia-induced neutrophils and links MMP8 expression to recruitment of tumor associated neutrophils during FGFR1 driven leukemogenesis. Exp Hematol Oncol. (2024) 13:49. doi: 10.1186/s40164-024-00514-6
23. Wang L, Liu Y, Dai Y, Tang X, Yin T, Wang C, et al. Single-cell RNA-seq analysis reveals BHLHE40-driven pro-tumour neutrophils with hyperactivated glycolysis in pancreatic tumour microenvironment. Gut. (2023) 72:958–71. doi: 10.1136/gutjnl-2021-326070
24. Bui TM, Yalom LK, Ning E, et al. Tissue-specific reprogramming leads to angiogenic neutrophil specialization and tumor vascularization in colorectal cancer. J Clin Invest. (2024) 134:e174545. doi: 10.1172/jci174545
25. Wu Y, Ma J, Yang X, Nan F, Zhang T, Ji S, et al. Neutrophil profiling illuminates anti-tumor antigen-presenting potency. Cell. (2024) 187:1422–39.e24. doi: 10.1016/j.cell.2024.02.005
26. Silvestre-Roig C, Fridlender ZG, Glogauer M, Scapini P. Neutrophil diversity in health and disease. Trends Immunol. (2019) 40:565–83. doi: 10.1016/j.it.2019.04.012
27. Que H, Fu Q, Lan T, Tian X, Wei X. Tumor-associated neutrophils and neutrophil-targeted cancer therapies. Biochim Biophys Acta Rev Cancer. (2022) 1877:188762. doi: 10.1016/j.bbcan.2022.188762
28. Depil S, Duchateau P, Grupp SA, Mufti G, Poirot L. [amp]]lsquo;Off-the-shelf’ allogeneic CAR T cells: development and challenges. Nat Rev Drug Discov. (2020) 19:185–99. doi: 10.1038/s41573-019-0051-2
29. Tay HM, Dalan R, Li KHH, Boehm BO, Hou HW. A novel microdevice for rapid neutrophil purification and phenotyping in type 2 diabetes mellitus. Small. (2018) 14:10.1002/smll.201702832. doi: 10.1002/smll.201702832
30. Blanter M, Cambier S, De Bondt M, Vanbrabant L, Pörtner N, Abouelasrar Salama S, et al. Method matters: effect of purification technology on neutrophil phenotype and function. Front Immunol. (2022) 13:820058. doi: 10.3389/fimmu.2022.820058
31. Hidalgo A, Libby P, Soehnlein O, Aramburu IV, Papayannopoulos V, Silvestre-Roig C. Neutrophil extracellular traps: from physiology to pathology. Cardiovasc Res. (2022) 118:2737–53. doi: 10.1093/cvr/cvab329
32. Herre M, Cedervall J, Mackman N, Olsson AK. Neutrophil extracellular traps in the pathology of cancer and other inflammatory diseases. Physiol Rev. (2023) 103:277–312. doi: 10.1152/physrev.00062.2021
33. Liu S, Wu W, Du Y, Yin H, Chen Q, Yu W, et al. The evolution and heterogeneity of neutrophils in cancers: origins, subsets, functions, orchestrations and clinical applications. Mol Cancer. (2023) 22:148. doi: 10.1186/s12943-023-01843-6
34. Cristinziano L, Modestino L, Antonelli A, Marone G, Simon HU, Varricchi G, et al. Neutrophil extracellular traps in cancer. Semin Cancer Biol. (2022) 79:91–104. doi: 10.1016/j.semcancer.2021.07.011
35. Chen Y, Hu H, Tan S, Dong Q, Fan X, Wang Y, et al. The role of neutrophil extracellular traps in cancer progression, metastasis and therapy. Exp Hematol Oncol. (2022) 11:99. doi: 10.1186/s40164-022-00345-3
36. Yang C, Wang Z, Li L, Zhang Z, Jin X, Wu P, et al. Aged neutrophils form mitochondria-dependent vital NETs to promote breast cancer lung metastasis. J Immunother Cancer. (2021) 9:e002875. doi: 10.1136/jitc-2021-002875
37. Yang L, Liu Q, Zhang X, Liu X, Zhou B, Chen J, et al. DNA of neutrophil extracellular traps promotes cancer metastasis via CCDC25. Nature. (2020) 583:133–8. doi: 10.1038/s41586-020-2394-6
38. Albrengues J, Shields MA, Ng D, Park CG, Ambrico A, Poindexter ME, et al. Neutrophil extracellular traps produced during inflammation awaken dormant cancer cells in mice. Science. (2018) 361:eaao4227. doi: 10.1126/science.aao4227
39. Teijeira Á, Garasa S, Gato M, Alfaro C, Migueliz I, Cirella A, et al. CXCR1 and CXCR2 chemokine receptor agonists produced by tumors induce neutrophil extracellular traps that interfere with immune cytotoxicity. Immunity. (2020) 52:856–71.e8. doi: 10.1016/j.immuni.2020.03.001
40. Song M, Zhang C, Cheng S, Ouyang D, Ping Y, Yang J, et al. DNA of neutrophil extracellular traps binds TMCO6 to impair CD8+ T-cell immunity in hepatocellular carcinoma. Cancer Res. (2024) 84:1613–29. doi: 10.1158/0008-5472.Can-23-2986
41. Poto R, Cristinziano L, Modestino L, de Paulis A, Marone G, Loffredo S, et al. Neutrophil extracellular traps, angiogenesis and cancer. Biomedicines. (2022) 10:431. doi: 10.3390/biomedicines10020431
42. Yang S, Sun B, Li J, Li N, Zhang A, Zhang X, et al. Neutrophil extracellular traps promote angiogenesis in gastric cancer. Cell Commun Signal. (2023) 21:176. doi: 10.1186/s12964-023-01196-z
43. Pylaeva E, Harati MD, Spyra I, Bordbari S, Strachan S, Thakur BK, et al. NAMPT signaling is critical for the proangiogenic activity of tumor-associated neutrophils. Int J Cancer. (2019) 144:136–49. doi: 10.1002/ijc.31808
44. He X, Zeng H, Chen JX. Emerging role of SIRT3 in endothelial metabolism, angiogenesis, and cardiovascular disease. J Cell Physiol. (2019) 234:2252–65. doi: 10.1002/jcp.27200
45. Arelaki S, Arampatzioglou A, Kambas K, Papagoras C, Miltiades P, Angelidou I, et al. Gradient infiltration of neutrophil extracellular traps in colon cancer and evidence for their involvement in tumour growth. PloS One. (2016) 11:e0154484. doi: 10.1371/journal.pone.0154484
46. Schedel F, Mayer-Hain S, Pappelbaum KI, Metze D, Stock M, Goerge T, et al. Evidence and impact of neutrophil extracellular traps in Malignant melanoma. Pigment Cell Melanoma Res. (2020) 33:63–73. doi: 10.1111/pcmr.12818
47. Millrud CR, Kågedal Å, Kumlien Georén S, Winqvist O, Uddman R, Razavi R, et al. NET-producing CD16(high) CD62L(dim) neutrophils migrate to tumor sites and predict improved survival in patients with HNSCC. Int J Cancer. (2017) 140:2557–67. doi: 10.1002/ijc.30671
48. Singhal S, Bhojnagarwala PS, O’Brien S, Moon EK, Garfall AL, Rao AS, et al. Origin and role of a subset of tumor-associated neutrophils with antigen-presenting cell features in early-stage human lung cancer. Cancer Cell. (2016) 30:120–35. doi: 10.1016/j.ccell.2016.06.001
49. Ponzetta A, Carriero R, Carnevale S, Barbagallo M, Molgora M, Perucchini C, et al. Neutrophils driving unconventional T cells mediate resistance against murine sarcomas and selected human tumors. Cell. (2019) 178:346–60.e24. doi: 10.1016/j.cell.2019.05.047
50. Coffelt SB, Kersten K, Doornebal CW, Weiden J, Vrijland K, Hau CS, et al. IL-17-producing γδ T cells and neutrophils conspire to promote breast cancer metastasis. Nature. (2015) 522:345–8. doi: 10.1038/nature14282
51. Xue R, Zhang Q, Cao Q, Kong R, Xiang X, Liu H, et al. Liver tumour immune microenvironment subtypes and neutrophil heterogeneity. Nature. (2022) 612:141–7. doi: 10.1038/s41586-022-05400-x
52. Deng H, Kan A, Lyu N, He M, Huang X, Qiao S, et al. Tumor-derived lactate inhibit the efficacy of lenvatinib through regulating PD-L1 expression on neutrophil in hepatocellular carcinoma. J Immunother Cancer. (2021) 9:e002305. doi: 10.1136/jitc-2020-002305
53. Thompson E, Taube JM, Elwood H, Sharma R, Meeker A, Warzecha HN, et al. The immune microenvironment of breast ductal carcinoma in situ. Mod Pathol. (2016) 29:249–58. doi: 10.1038/modpathol.2015.158
54. Puga I, Cols M, Barra CM, He B, Cassis L, Gentile M, et al. B cell-helper neutrophils stimulate the diversification and production of immunoglobulin in the marginal zone of the spleen. Nat Immunol. (2011) 13:170–80. doi: 10.1038/ni.2194
55. Shaul ME, Zlotnik A, Tidhar E, Schwartz A, Arpinati L, Kaisar-Iluz N, et al. Tumor-associated neutrophils drive B-cell recruitment and their differentiation to plasma cells. Cancer Immunol Res. (2021) 9:811–24. doi: 10.1158/2326-6066.Cir-20-0839
56. Hansen MH, Nielsen HV, Ditzel HJ. Translocation of an intracellular antigen to the surface of medullary breast cancer cells early in apoptosis allows for an antigen-driven antibody response elicited by tumor-infiltrating B cells. J Immunol. (2002) 169:2701–11. doi: 10.4049/jimmunol.169.5.2701
57. Downs-Canner SM, Meier J, Vincent BG, Serody JS. B cell function in the tumor microenvironment. Annu Rev Immunol. (2022) 40:169–93. doi: 10.1146/annurev-immunol-101220-015603
58. Inoue S, Leitner WW, Golding B, Scott D. Inhibitory effects of B cells on antitumor immunity. Cancer Res. (2006) 66:7741–7. doi: 10.1158/0008-5472.Can-05-3766
59. Yang C, Lee H, Pal S, Jove V, Deng J, Zhang W, et al. B cells promote tumor progression via STAT3 regulated-angiogenesis. PloS One. (2013) 8:e64159. doi: 10.1371/journal.pone.0064159
60. Ammirante M, Luo JL, Grivennikov S, Nedospasov S, Karin M. B-cell-derived lymphotoxin promotes castration-resistant prostate cancer. Nature. (2010) 464:302–5. doi: 10.1038/nature08782
61. Li P, Lu M, Shi J, Hua L, Gong Z, Li Q, et al. Dual roles of neutrophils in metastatic colonization are governed by the host NK cell status. Nat Commun. (2020) 11:4387. doi: 10.1038/s41467-020-18125-0
62. Zhou Z, Wang P, Sun R, Li J, Hu Z, Xin H, et al. Tumor-associated neutrophils and macrophages interaction contributes to intrahepatic cholangiocarcinoma progression by activating STAT3. J Immunother Cancer. (2021) 9:e001946. doi: 10.1136/jitc-2020-001946
63. He G, Zhang H, Zhou J, et al. Peritumoural neutrophils negatively regulate adaptive immunity via the PD-L1/PD-1 signalling pathway in hepatocellular carcinoma. J Exp Clin Cancer Res. (2015) 34:141. doi: 10.1186/s13046-015-0256-0
64. Krieg C, Nowicka M, Guglietta S, et al. High-dimensional single-cell analysis predicts response to anti-PD-1 immunotherapy. Nat Med. (2018) 24:144–53. doi: 10.1038/nm.4466
65. Li K, Tandurella JA, Gai J, et al. Multi-omic analyses of changes in the tumor microenvironment of pancreatic adenocarcinoma following neoadjuvant treatment with anti-PD-1 therapy. Cancer Cell. (2022) 40:1374–91.e7. doi: 10.1016/j.ccell.2022.10.001
66. Gungabeesoon J, Gort-Freitas NA, Kiss M, et al. A neutrophil response linked to tumor control in immunotherapy. Cell. (2023) 186:1448–64.e20. doi: 10.1016/j.cell.2023.02.032
67. Singhal S, Rao AS, Stadanlick J, et al. Human tumor-associated macrophages and neutrophils regulate antitumor antibody efficacy through lethal and sublethal trogocytosis. Cancer Res. (2024) 84:1029–47. doi: 10.1158/0008-5472.Can-23-2135
68. Montemagno C, Jacquel A, Pandiani C, et al. Unveiling CXCR2 as a promising therapeutic target in renal cell carcinoma: exploring the immunotherapeutic paradigm shift through its inhibition by RCT001. J Exp Clin Cancer Res. (2024) 43:86. doi: 10.1186/s13046-024-02984-2
69. Kumbhojkar N, Prakash S, Fukuta T, et al. Neutrophils bearing adhesive polymer micropatches as a drug-free cancer immunotherapy. Nat BioMed Eng. (2024) 8:579–92. doi: 10.1038/s41551-024-01180-z
Keywords: tumor-associated neutrophil, cancer, immunology, bibliometrics, visualization, VOSviewer, CiteSpace, R-bibliometrix
Citation: Xiao C, Feng X, Aini W, Zhao Z, Ding G and Gao Y (2024) Knowledge landscape of tumor-associated neutrophil: a bibliometric and visual analysis from 2000-2024. Front. Immunol. 15:1448818. doi: 10.3389/fimmu.2024.1448818
Received: 13 June 2024; Accepted: 20 September 2024;
Published: 04 October 2024.
Edited by:
Geeta Rai, Banaras Hindu University, IndiaReviewed by:
Ke Tang, Huazhong University of Science and Technology, ChinaCopyright © 2024 Xiao, Feng, Aini, Zhao, Ding and Gao. This is an open-access article distributed under the terms of the Creative Commons Attribution License (CC BY). The use, distribution or reproduction in other forums is permitted, provided the original author(s) and the copyright owner(s) are credited and that the original publication in this journal is cited, in accordance with accepted academic practice. No use, distribution or reproduction is permitted which does not comply with these terms.
*Correspondence: Yawen Gao, eWdhbzZAY3N1LmVkdS5jbg==
Disclaimer: All claims expressed in this article are solely those of the authors and do not necessarily represent those of their affiliated organizations, or those of the publisher, the editors and the reviewers. Any product that may be evaluated in this article or claim that may be made by its manufacturer is not guaranteed or endorsed by the publisher.
Research integrity at Frontiers
Learn more about the work of our research integrity team to safeguard the quality of each article we publish.