- 1Department of Biology, Waseda University, Tokyo, Japan
- 2Department of R&D, BioPhenoMA Inc., Tokyo, Japan
- 3School of Pharmaceutical Sciences, Health Sciences University of Hokkaido, Hokkaido, Japan
- 4Graduate Institute of Medicine, Kaohsiung Medical University, Kaohsiung, Taiwan
Influenza virus, adenovirus, and respiratory syncytial virus cause major respiratory infections. These infections have similar initial symptoms making it difficult to differentiate them based on symptoms alone. PCR is currently used as the standard diagnostic test for these infections, however, it has its limitations such as non-specific and false-negative amplifications, high cost, and the inability to distinguish between a live or dead virus. Therefore, there is a need for alternative diagnostic methods that focus on protein. Here, we introduce TN-cyclon™, which is an enzyme-linked immunosorbent assay combined with thio-nicotinamide adenine dinucleotide cycling to amplify signals, rather than the protein itself. Using this method, we were able to detect extremely low levels of viruses such as influenza A, influenza B, adenovirus, and RS virus, with LODs of 2.96 × 10-18 moles/assay, 2.98 × 10-18 moles/assay, 2.36 × 10-18 moles/assay, and 3.55 × 10-18 moles/assay, respectively. Furthermore, we successfully detected viruses diluted with extract buffer, with a significant difference to the blank at concentrations of 3 pfu/mL for influenza A, 1000 pfu/mL for influenza B, 43.8 pfu/mL for adenovirus, and 125 pfu/mL for RS virus. This shows that our low-cost and easy-to-use technique has sufficient sensitivity in diagnosing respiratory infections.
1 Introduction
Influenza virus, adenovirus, and respiratory syncytial (RS) virus are the major viruses causing respiratory infections. The following is the common understanding regarding the symptoms of these infectious diseases. The typical window period for influenza between exposure to the virus and the onset of symptoms is 1 – 4 days (1). The initial symptoms are predominately non-specific, including fever, chills, and headaches. Adenovirus causes adenovirus infection, one of the respiratory tract infections (2). Onset is usually 2 – 14 days after exposure to the virus. Typical symptoms are those of a mild cold or similar to influenza (3). RS virus is a common cause of respiratory hospitalization in infants, and reinfection remains common in later life (4). The symptoms are nasal congestion, runny nose, cough, and low-grade fever (5). These suggest that it is difficult to distinguish these infections based on their symptoms alone, and thus detailed nucleic acid-level or protein-level analysis must be performed.
Although PCR is believed to be a gold standard for diagnosing these infections, it amplifies nucleic acid fractions regardless of amplification errors or whether the virus is alive or dead (6), and it has several challenges such as non-specific (i.e., false-positive) and false-negative amplifications, high cost, and difficulties in designing probe sequences (7, 8). Thus, alternative diagnostic methods such as an ultrasensitive antigen test, which is more sensitive than an immunochromatographic rapid test, are required for precise diagnoses (9). To develop this, we applied an ultrasensitive enzyme-linked immunosorbent assay (ELISA) combined with thio-nicotinamide adenine dinucleotide (thio-NAD) cycling for an increase in the detection sensitivity of antigens and the detection of the viruses. This detection method is called “TN-cyclon™”, and in this system, a sandwich ELISA is used in which the secondary antibody is labeled with alkaline phosphatase (ALP), and thio-NAD cycling consists of 3α-hydroxysteroid dehydrogenase (3α-HSD) and thio-NAD and NADH as the coenzymes (10).
In the present study, we describe the application of the TN-cyclon™ to the detection of recombinant proteins and inactive viruses for the above three kinds of viruses. We found that the results obtained by TN-cyclon™ showed sufficient detection sensitivity in diagnosing infection and compensated for the shortcomings of PCR.
2 Materials and methods
2.1 Reagents and chemicals
All the antibodies used in the present study were purchased from Bio Matrix Research, Inc. (Chiba, Japan). FLA-701 and FLA-2088 were used as the capture antibody and the detection antibody, respectively, for the detection of influenza virus type A. FLB-826 and F2B-3843 were used as the capture antibody and the detection antibody, respectively, for the detection of influenza virus type B. These 4 antibodies were produced against the nucleoproteins of influenza virus type A and B. AD1-1651 and AD1-2100 were used as the capture antibody and the detection antibody, respectively, for an adenovirus assay. These antibodies were produced against the hexon proteins of adenovirus. RS-398 and RS-186 were used as the capture antibody and the detection antibody, respectively, for an RS virus assay. These antibodies were produced against the fusion proteins of RS virus. Influenza A nucleoprotein (11675-V08B), Influenza B nucleoprotein (40438-V08B), and RS virus fusion protein (40037-V08B) were purchased from Sino Biological, Inc. (Beijing, China). Adenovirus hexon protein (#30R-AA006x) was purchased from Fitzgerald Industries International (Acton, MA, USA). The ALP labeling kit for the capture antibodies was purchased from Dojindo Laboratories (Kumamoto, Japan). NADH was purchased from Sigma-Aldrich (N1161-10VL; St. Louis, MO, USA), thio-NAD was from Oriental Yeast (44104001; Tokyo, Japan), and 3α-HSD (EC. 1.1.1.50) was from Asahi Kasei Pharma (T-58; Tokyo, Japan). The substrate, 17β-methoxy-5β-androstan-3α-ol 3-phosphate (A3P), was synthesized by one of the authors (T.Y.).
2.2 Virus
Influenza type A (A/Puerto Rico/8/34(PR8) H1N1) virus was kindly gifted by Etsuhisa Takahashi at University of Tokushima. This strain was isolated in 1934 from a human patient in Puerto Rico and was deposited by the Centers for Disease Control and Prevention. Influenza type B (B/Washington/02/2019) (GISAID isolate ID EPI_ISL_353725) virus was isolated from a clinical specimen at National Institute of Infectious Diseases of Japan and kindly gifted. Human adenovirus 6 was purchased from ATCC (VR-6; Manassas, VA, USA). This product’s format is fluid and cell lysate from infected cultures. RS virus (RSV/S/NIID/2370/14) was kindly gifted by National Institute of Infectious Diseases of Japan (11). This virus was originally isolated from a nasal swab of 3-year old female, cultured on Hep-2 cell. Virus extract buffer was provided by BioPhenoMA Inc. (Tokyo, Japan). All viruses were inactivated by this virus extract buffer before use in the experiments.
2.3 Ultrasensitive ELISA with thio-NAD cycling (TN-cyclon™)
ALP labeled on the sandwich ELISA’s secondary antibody hydrolyzes 17β-methoxy-5β-androstan-3-ol 3-phosphate (Androsterone 3-phosphate) to 17β-methoxy-5β-androstan-3α-ol (i.e., Androsterone). This 17β-methoxy-5β-androstan-3α-ol is oxidized to 17β-methoxy-5β-androstan-3α-one (i.e., Androstane-3,17-dione) via 3α-hydroxysteroid dehydrogenase (3α-HSD) using thio-NAD as a coenzyme. In the reverse reaction, 17β-methoxy-5β-androstan-3α-one is reduced to 17β-methoxy-5β-androstan-3α-ol by 3α-HSD using NADH as a coenzyme. During these oxidation and reduction reactions, thio-NAD is reduced to thio-NADH during the oxidation reaction and NADH is changed to NAD during the reduction reaction. As the cycling of oxidation and reduction reactions repeats, thio-NADH accumulates in a triangular-number manner during the cycling reaction (10) (Figure 1). Accumulated thio-NADH can be measured directly by an increase in the absorbance at 400 nm (11,900 M−1 cm−1), e.g., 405 nm with a commercially available microplate reader, without any interference from other coenzymes such as thio-NAD, NAD and NADH, the absorbance maximums of which are all under 340 nm.
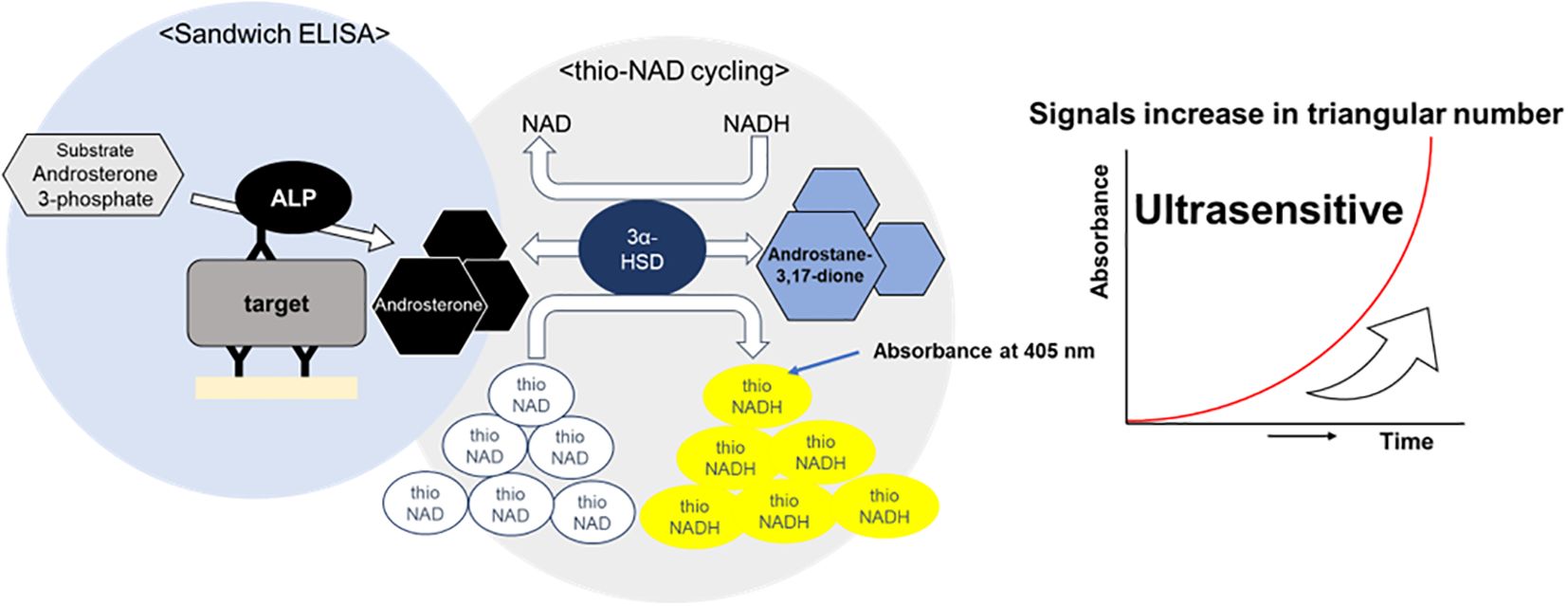
Figure 1. Schematic diagram of thio-NAD cycling ELISA. The signal generated by alkaline phosphatase is amplified through redox cycling via the enzyme 3α-hydroxysteroid dehydrogenase(3α-HSD) and its coenzymes, thio-NAD and NADH.
For measurements of the recombinant proteins and inactivated viruses, 100 µL of capture antibody solution diluted to 10 µg/mL in 50 mM Na2CO3 (pH 9.6) was first added to each well of microplates (469949; Thermo Scientific, Waltham, MA, USA), incubated for 1 h at room temperature, and washed 3 times with 300 µL of wash buffer consisting of 0.05% Tween20 in TBS. Next, 100 µL of 1% bovine serum albumin (BSA) in TBS was added for blocking, incubated for 1 h at room temperature, and washed 9 times with 300 µL of wash buffer. After blocking, 100 µL of recombinant protein solution, stepwise diluted in 0.1% BSA in TBS 2-fold from 1000 pg/mL to 31.25 pg/mL, was added and shaken for 1 h at room temperature. Measurements were performed in triplicates and repeated using 3 different microplates, that is, n = 9. For blanks, 100 µL of 0.1% BSA in TBS was added. It was also washed 9 times with wash buffer. The ALP-labelled detection antibody was then diluted to an antibody concentration of 100 ng/mL in TBS containing 0.1% BSA and 0.05% Tween 20, added in 100 µL portions, and shaken for 1 h at room temperature. It was also washed 9 times with wash buffer. Finally, 100 µL of 100 mM tris-HCl containing 10 U/mL 3α-HSD, 0.4 mM A3P, 1.0 mM NADH, and 2.0 mM thio-NAD were added, and measured in a microplate reader (Corona Electric SH-1000, Hitachinaka, Japan). The absorbance of 2 wavelengths was measured, 405 nm for the signal substance of thio-NADH and 660 nm for background correction, and the A405 – A660 value was used as the signal for statistical analysis. The same procedure was used to measure inactivated viruses. The viruses were diluted 2-fold from 100 pfu/mL to 3.125 pfu/mL for influenza virus A, 105 pfu/mL, 104 pfu/mL, 103 pfu/mL, 500 pfu/mL, 250 pfu/mL, 125 pfu/mL, for influenza virus B. For adenovirus, dilutions were made 2-fold from 700 pfu/mL to 21.9 pfu/mL; for RS virus, dilutions were made 2-fold from 1000 pfu/mL to 31.25 pfu/mL. A 100 µL of each solution was added to each well. The experimental absorbance data are expressed by subtracting the blank values from the target signals at the corresponding time and the concentration data points. The calibration curves using recombinant proteins were obtained within a range of concentrations that ensured linearity of absorbance.
2.4 Statistical analyses
The limit of detection (LOD) was estimated by employing the mean of the blanks, the standard deviation (SD) of the blanks, and a confidence factor of 3. In the same vein, the limit of quantitation (LOQ) was approximated with the same method used for LOD, but with a confidence factor of 10. The data are expressed as the mean ± SD. The significant difference was calculated using one-way ANOVA with a post-hoc Holm test in R (version 4.2.2) with P < 0.05.
3 Results
3.1 Measurements of recombinant proteins
Linear calibration curves were obtained using viral recombinant proteins. The absorbance at 55 min of thio-NAD cycling was used for influenza A and B, that of 60 min was used for adenovirus, and that of 50 min was used for RS virus. The cycling time was chosen to provide optimal sensitivity. The linear calibration curves are as follows: y = 8.43 × 10-4x (R2 = 0.999) for influenza A, y = 7.63 × 10-4x (R2 = 0.997) for influenza B, y = 5.55 × 10-4x (R2 = 0,999) for adenovirus, and y = 6.30 × 10-4x (R2 = 0.999) for RS virus (Figure 2). The LODs were 1.68 pg/mL (2.96 × 10-18 moles/assay) for influenza A, 1.85 pg/mL (2.98 × 10-18) for influenza B, 2.55 pg/mL (2.36 × 10-18) for adenovirus, and 2.25 pg/mL (3.55 × 10-18) for RS virus. One assay volume used was 100 μL. The LOQs were 5.59 pg/mL (9.86 × 10-18 moles/assay) for influenza A, 6.18 pg/mL (9.95 × 10-18 moles/assay) for influenza B, 8.49 pg/mL (7.86 × 10-18 moles/assay) for adenovirus, and 7.48 pg/mL (1.18 × 10-17 moles/assay) for RS virus (see Table 1 for the comparison of LOD and LOQ).
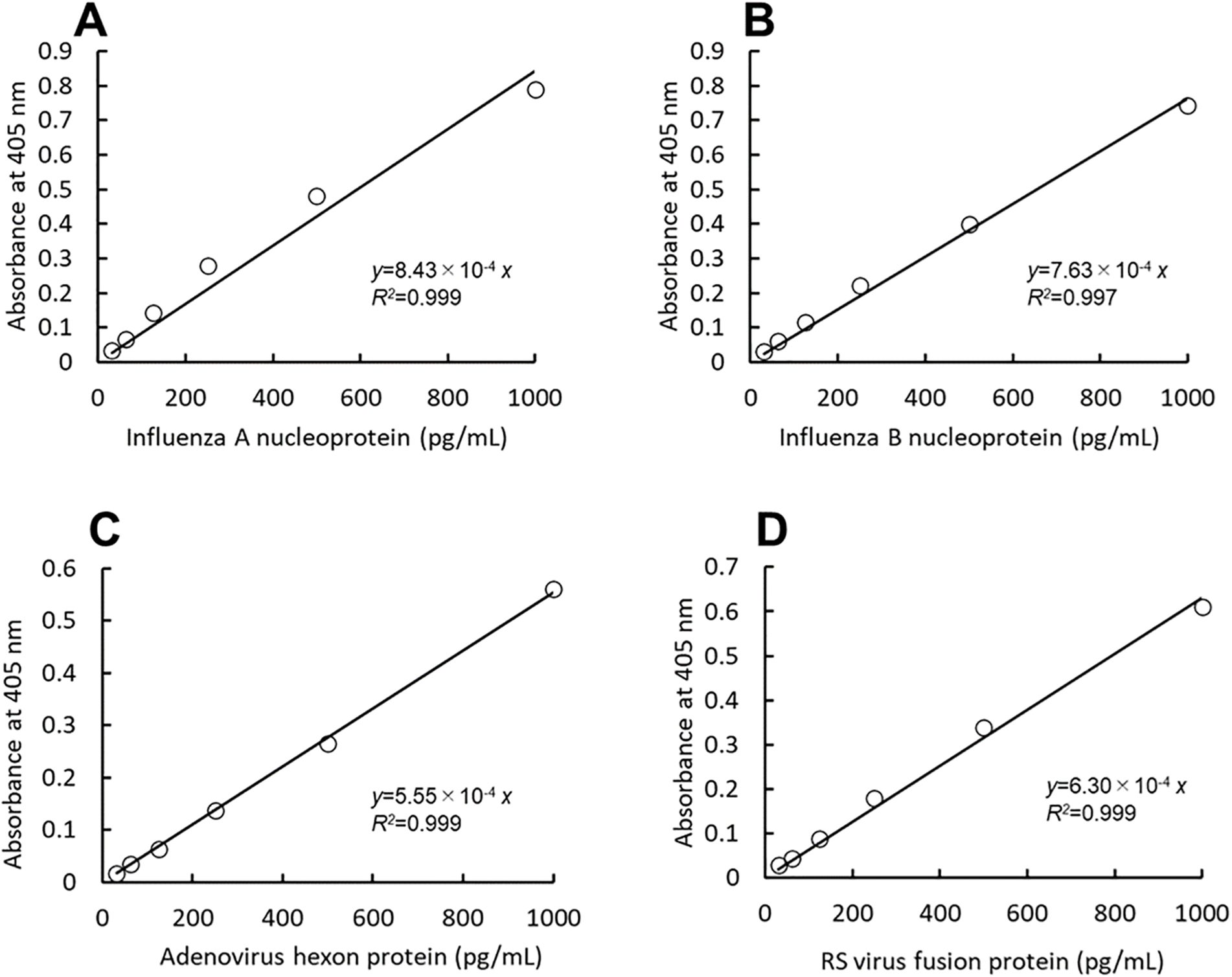
Figure 2. A thio-NAD cycling ELISA was used to measure the calibration curves for the nucleoproteins of influenza A (A) and B (B), the hexon protein of adenovirus (C), and the fusion protein of RS virus (D). The absorbance was measured at the following time points of thio-AND cycling: 55 min for influenza A, 55 min for influenza B, 60 min for adenovirus, and 50 min for RS virus (n = 3 each). Each of the time points was determined before the saturation of the reaction absorbance. The antigen was applied within a range of 31.25 – 1000 pg/mL.
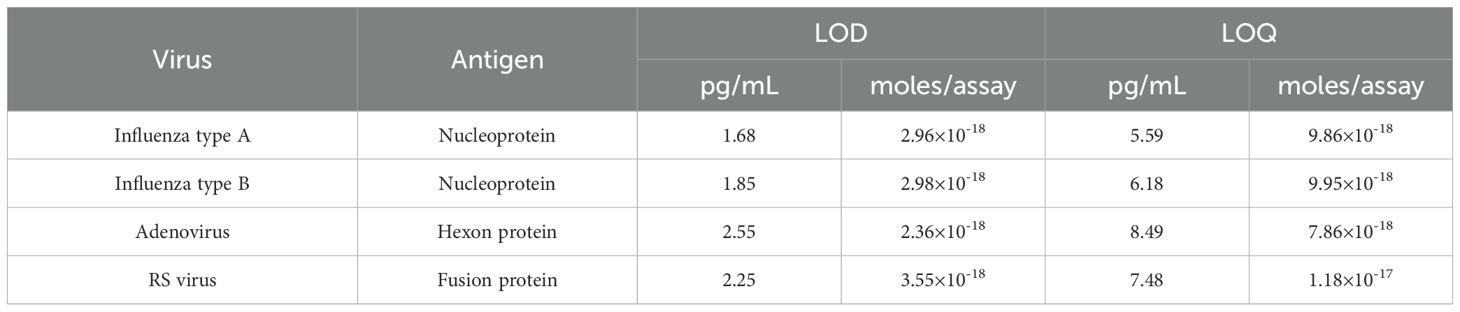
Table 1. LOD and LOQ of influenza type A nucleoprotein, type B nucleoprotein, adenovirus hexon protein, and RS virus fusion protein.
3.2 Measurements of viruses
The viruses were measured using a technique equivalent to that used to measure recombinant proteins. Three investigators conducted measurements for each virus. For influenza A, all three investigators showed a significant difference in signals between 3.13 pfu/mL and the blank (Figure 3). For influenza B, a significant difference in signals between 1000 pfu/mL and the blank was observed by the two investigators, and that between 500 pfu/mL and the blank was observed by the one investigator (Figure 4). For adenovirus, all three investigators observed a significant difference in signals between 43.8 pfu/mL and the blank (Figure 5). For the RS virus, there was a significant difference in signals between 125 pfu/mL and the blank by all three investigators (Figure 6).
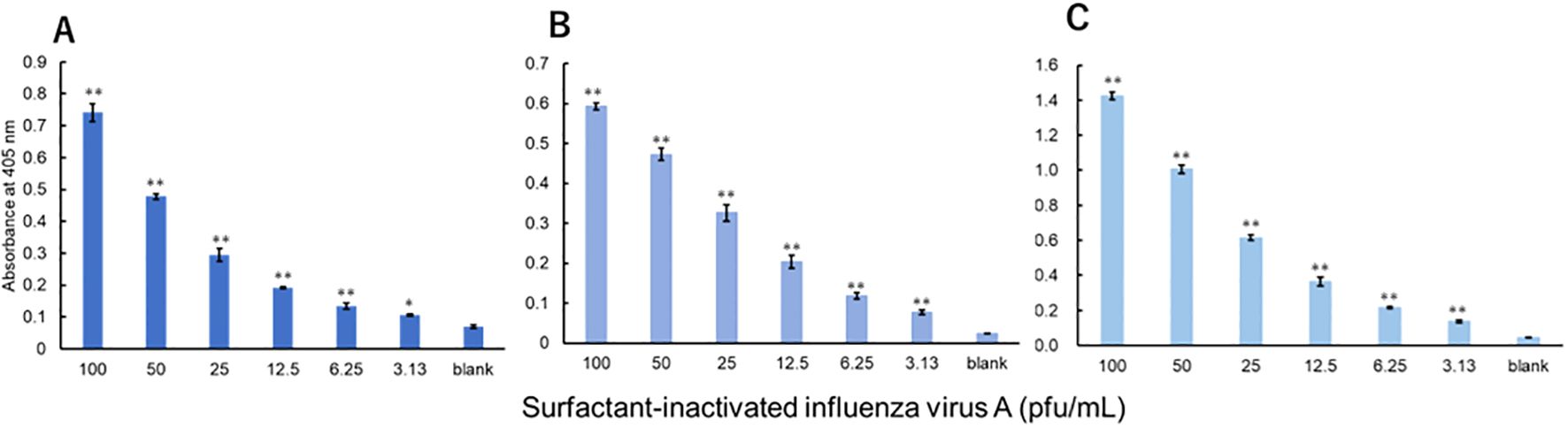
Figure 3. Detection of nucleoproteins in surfactant-inactivated influenza virus type A. Three datasets were measured by three investigators and are presented as (A–C). Triplicate measurements were performed at 60 min of thio-NAD cycling by each investigator. All datasets show that the signals were significantly higher than the blank values at concentrations over 3.13 pfu/mL (*P < 0.05, **P < 0.01).
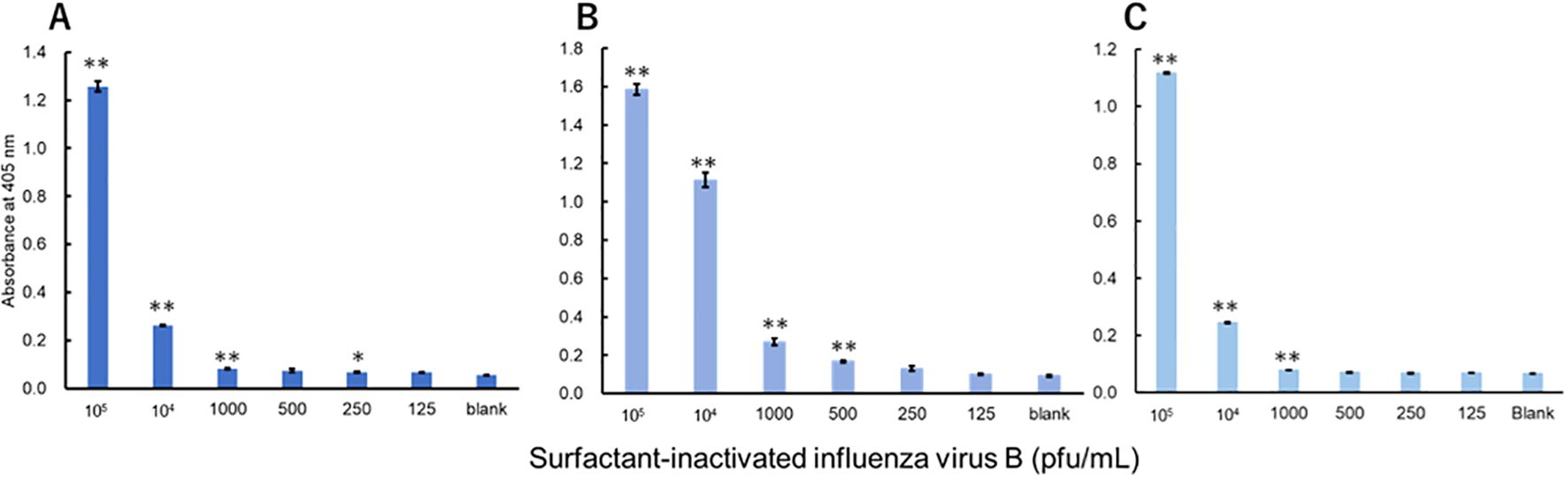
Figure 4. Detection of nucleoproteins in surfactant-inactivated influenza virus type B. Three datasets were measured by three investigators and are presented as (A–C). Triplicate measurements were performed at 60 min of thio-NAD cycling by each investigator. All datasets show that the signals were significantly higher than the blank values at concentrations over 1000 pfu/mL (*P < 0.05, **P < 0.01).
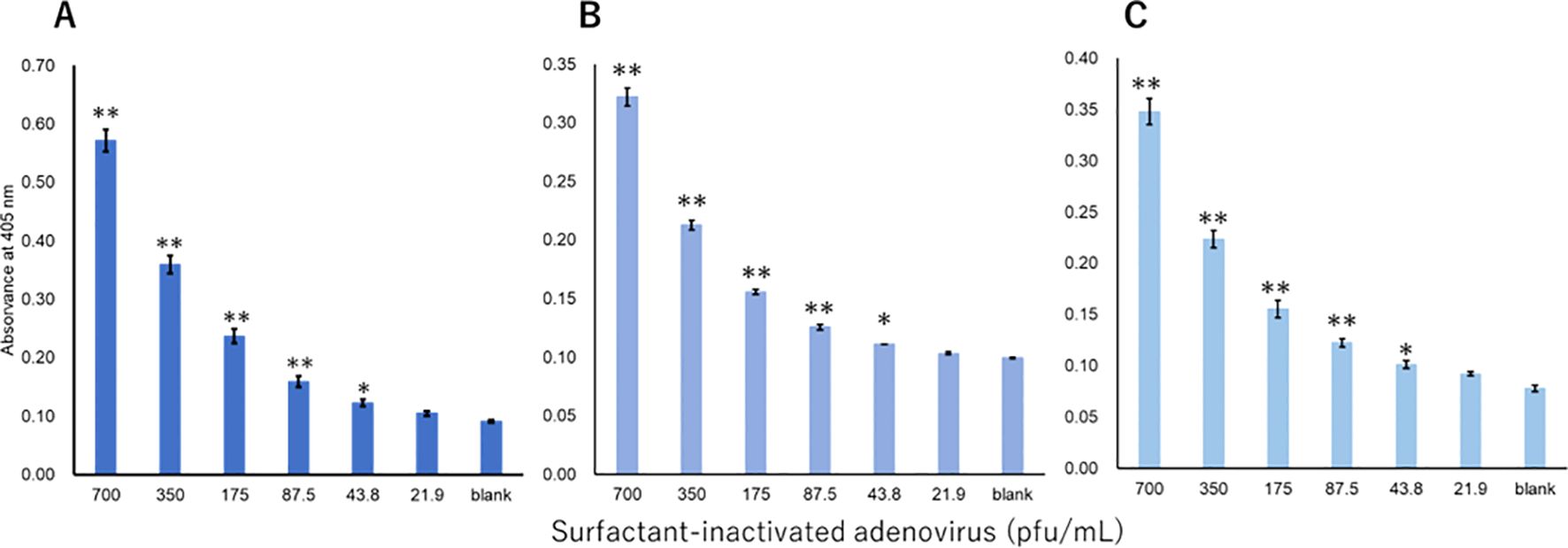
Figure 5. Detection of hexon proteins in surfactant-inactivated adenovirus. Three datasets were measured by three investigators and are presented as (A–C). Triplicate measurements were performed at 60 min of thio-NAD cycling by each investigator. All datasets show that the signals were significantly higher than the blank values at the concentrations over 43.8 pfu/mL (*P < 0.05, **P < 0.01).
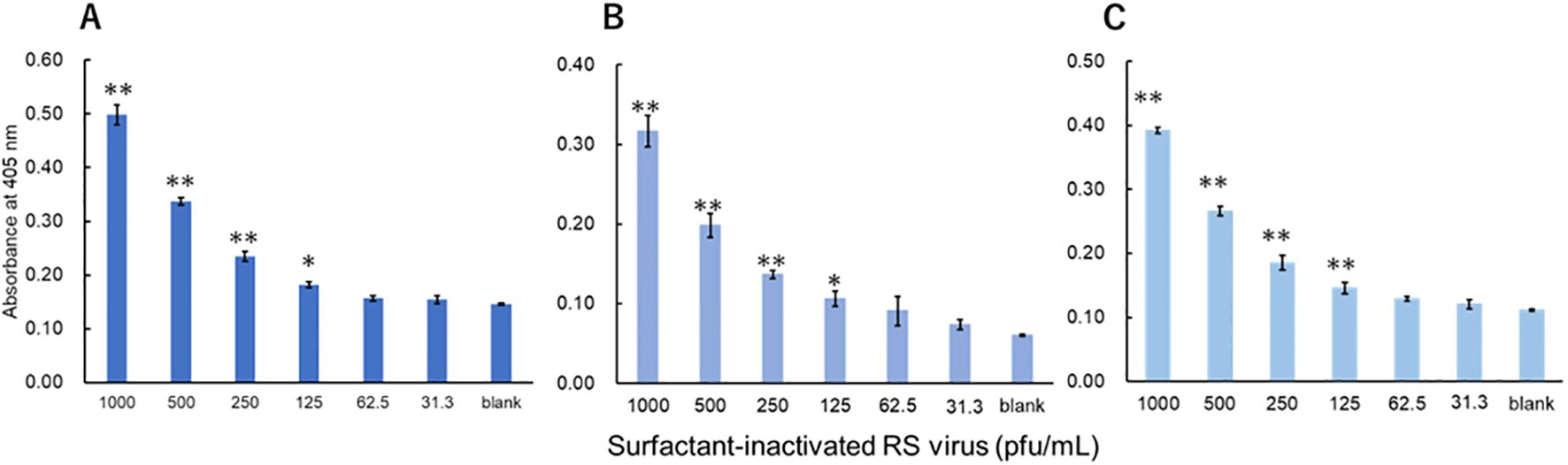
Figure 6. Detection of fusion proteins in surfactant-inactivated RS virus. Three datasets were measured by three investigators and are presented as (A–C). Triplicate measurements were performed at 60 min of thio-NAD cycling by each investigator. All datasets show that the signals were significantly higher than the blank values at concentrations over 125 pfu/mL (*P < 0.05, **P < 0.01).
4 Discussion
4.1 Measurements of recombinant proteins
For the measurements of recombinant proteins, we compare our results with those of previous high-sensitivity measurements. About the measurement of recombinant influenza type A nucleoproteins, the digital ELISA assay combining ELISA and a surface plasmon resonance system had the LOD of 4 ± 1 fM [(4 ± 1) × 10-19 moles/100 μL] (12). An amplified luminescent proximity homogeneous assay linked immunosorbent assay (AlphaLISA) to detect influenza B nucleoproteins had an LOD of 240 pg/mL (13). It can be seen that our detection sensitivity is two orders of magnitude higher. Regarding the antibodies used in this study, the manufacturers stated that antibodies to influenza A, B, adenovirus and RS viruses do not cross-react with each other (14–17).
4.2 Measurements of viruses
About the previous reports for the detection of influenza A virus, combined immunomagnetic beads and biotin-nanoparticle-based detection assay targeting the nucleoproteins had the LODs of 3 × 103 pfu/mL (H1N1) and 4 × 104 pfu/mL (H3N2) (18). Using a fluorescence immunochromatographic assay with a quantum dot nanobead has shown that the LOD for influenza A viruses was 50 pfu/mL (19). Using a digital ELISA method has shown that the LOD for influenza A virus [A/Puerto Rico/8/1934(H1N1)] was 3.0×104 pfu/mL (20). Comparing the results of these influenza A measurements, our results are comparable in sensitivity. However, our TN-cyclon™ which is modified from sandwich ELISA is overwhelmingly easy-to-use and inexpensive, so it is a very suitable measurement technology. In a study combining sandwich ELISA assay and nano-based immunosensing, adenovirus was detected with a LOD of 100 pfu/mL (21). This result is similar to ours. To our knowledge, no papers were found that discussed the detection limits for the RS virus.
4.3 Application of TN-cyclon™ to clarification of infection mechanism
The TN-cyclon™ method that we developed has recently begun to demonstrate its power in elucidating the infection mechanism of coronavirus disease 2019 (COVID-19). We have applied the TN-cyclon method to severe acute respiratory syndrome coronavirus 2 (SARS-CoV-2) S1 protein and achieved a LOD of 0.205 pg/mL (22). We have also applied the method to SARS-CoV-2 nucleocapsid protein and achieved a LOD of 14 pg/mL (23). Based on the results of ultrasensitive protein detection (24), women are less susceptible than men to COVID-19, which might be due to the female steroid hormone 17β-estradiol. We hypothesized that 17β-estradiol removes the soluble portion of angiotensin-converting enzyme 2 (sACE2) to which SARS-CoV-2 binds in host cells and that sACE2 then binds to the virus, thereby reducing the infectivity. Using the TN-cyclon™, we succeeded in demonstrating that the soluble portion of ACE2, which was removed from 17β-estradiol-treated VeroE6/TMPRSS2 cells, bound to the spike proteins of SARS-CoV-2, thereby reducing COVID-19 infectivity.
5 Conclusion
Using the TN-cyclon™ method, all recombinant proteins for infectious viruses could be measured at the pg/mL level. This is a sufficiently sensitive measurement compared to other methods. Our TN-cyclon™ method gives better or at least equal detection sensitivity to other viral detection methods.
Data availability statement
The original contributions presented in the study are included in the article/supplementary material. Further inquiries can be directed to the corresponding author.
Author contributions
YKo: Investigation, Methodology, Writing – original draft. YKy: Investigation, Methodology, Writing – review & editing. RO: Investigation, Writing – review & editing. KO: Investigation, Writing – review & editing. TY: Funding acquisition, Methodology, Writing – review & editing. EI: Conceptualization, Funding acquisition, Methodology, Supervision, Writing – original draft.
Funding
The author(s) declare that financial support was received for the research, authorship, and/or publication of this article. This research received financial support from BioPhenoMA Inc. The funder was not involved in the study design, collection, analysis, interpretation of data, the writing of this article of the decision to submit it for publication.
Acknowledgments
We appreciate Etsuhisa Takahashi at Tokushima University for giving us influenza type A virus cultures.
Conflict of interest
The authors YKo, TY and EI declare a conflict of interest with BioPhenoMA Inc. YKo and EI have received honoraria from BioPhenoMA Inc. TY and EI have received research funding from BioPhenoMA Inc.
The remaining authors declare that the research was conducted in the absence of any commercial or financial relationships that could be construed as a potential conflict of interest.
The author(s) declared that they were an editorial board member of Frontiers, at the time of submission. This had no impact on the peer review process and the final decision.
Publisher’s note
All claims expressed in this article are solely those of the authors and do not necessarily represent those of their affiliated organizations, or those of the publisher, the editors and the reviewers. Any product that may be evaluated in this article, or claim that may be made by its manufacturer, is not guaranteed or endorsed by the publisher.
References
1. Centers for Disease Control and Prevention. Key Facts About Influenza (Flu). Available online at: https://www.cdc.gov/flu/about/keyfacts.htm (Accessed April 2, 2024).
2. Centers for Disease Control and Prevention. Clinical Overview. Available online at: https://www.cdc.gov/adenovirus/hcp/clinical-overview.html (Accessed April 2, 2024).
3. Centers for Disease Control and Prevention. Symptoms. Available online at: https://www.cdc.gov/adenovirus/symptoms.html (Accessed April 2, 2024).
4. Centers for Disease Control and Prevention. RSV Transmission. Available online at: https://www.cdc.gov/rsv/about/transmission.html (Accessed April 2, 2024).
5. Centers for Disease Control and Prevention. Symptoms and Care. Available online at: https://www.cdc.gov/rsv/about/symptoms.html (Accessed April 2, 2024).
6. Motley MP, Bennett-Guerrero E, Fries BC, Spitzer ED. Review of viral testing (polymerase chain reaction) and antibody/serology testing for severe acute respiratory syndrome-coronavirus-2 for the intensivist. Crit Care Explorations. (2020) 2:6. doi: 10.1097/CCE.0000000000000154
7. Kyosei Y, Yamura S, Namba M, Yoshimura T, Watabe S, Ito E. Antigen tests for COVID-19. Biophys Physicobiol. (2021) 10:18. doi: 10.2142/biophysico.bppb-v18.004
8. Li X, Wang H, Qi X, Ji Y, Li F, Chen X, et al. PCR independent strategy-based biosensors for RNA detection. Biosensors. (2024) 14:200. doi: 10.3390/bios14040200
9. Chen PK, Chang JH, Ke LY, Kao JK, Chen CH, Yang RC, et al. Advanced detection method for dengue NS1 protein using ultrasensitive ELISA with thio-NAD cycling. Viruses. (2023) 15:1894. doi: 10.3390/v15091894
10. Watabe S, Kodama H, Kaneda M, Morikawa M, Nakaishi K, Yoshimura T, et al. Ultrasensitive enzyme-linked immunosorbent assay (ELISA) of proteins by combination with the thio-NAD cycling method. Biophysics. (2014) 5:10. doi: 10.2142/biophysics.10.49
11. Suwa R, Kume Y, Kawase M, Chishiki M, Ono T, Norito S, et al. Practical validation of United States centers for disease control and prevention assays for the detection of human respiratory syncytial virus in pediatric inpatients in Japan. Pathogens. (2022) 11:754. doi: 10.3390/pathogens11070754
12. Leirs K, Tewari Kumar P, Decrop D, Pérez-Ruiz E, Leblebici P, Van Kelst B, et al. Bioassay development for ultrasensitive Detection of influenza A nucleoprotein using digital ELISA. Anal Chem. (2016) 88:17. doi: 10.1021/acs.analchem.6b00502
13. Zong H, Zhang S, Shang X, Jiang H, Zhao Z, Chen S, et al. Development of an AlphaLISA assay for sensitive and accurate detection of influenza B virus. Front Med. (2023) 10:1155551. doi: 10.3389/fmed.2023.1155551
14. Bio Matrix Research, Inc. Anti-Influenza A Virus NP Monoclonal Antibodies. Available online at: https://www.biomatrix.co.jp/images/page/01-Anti-Influenza_A_virus_NP_Monoclonal_Antibodies.pdf (Accessed September 25, 2024).
15. Bio Matrix Research, Inc. Anti-Influenza B Virus NP Monoclonal Antibodies. Available online at: https://www.biomatrix.co.jp/images/page/02-Anti-Influenza_B_virus_Monoclonal_Antibodies.pdf (Accessed September 25, 2024).
16. Bio Matrix Research, Inc. Anti-Adenovirus Hexon Monoclonal Aantibodies. Available online at: https://www.biomatrix.co.jp/images/page/04-Anti-Adenovirus_Monoclonal_Monoclonal_Antibodies.pdf (Accessed September 25, 2024).
17. Bio Matrix Research, Inc. Anti-RSV F Protein Monoclonal Antibodes. Available online at: https://www.biomatrix.co.jp/images/page/05-Anti-RSV_F-protein_Monoclonal_Antibodies.pdf (Accessed September 25, 2024).
18. Farre C, Viezzi S, Wright A, Robin P, Lejal N, Manzano M, et al. Specific and sensitive detection of Influenza A virus using a biotin-coated nanoparticle enhanced immunomagnetic assay. Anal Bioanal Chem. (2022) 414:265–76. doi: 10.1007/s00216-020-03081-x
19. Wang C, Yang X, Zheng S, Cheng X, Xiao R, Li Q, et al. Development of an ultrasensitive fluorescent immunochromatographic assay based on multilayer quantum dot nanobead for simultaneous detection of SARS-CoV-2 antigen and influenza A virus. Sensors actuators: B Chemical. (2021) 345:130372. doi: 10.1016/j.snb.2021.130372
20. Minagawa Y, Ueno H, Tabata KV, Noji H. Mobile imaging platform for digital influenza virus counting. Lab Chio. (2019) 19:2678–87. doi: 10.1039/C9LC00370C
21. Aloraij Y, Alsheikh A, Alyousef RA, Alhamlan F, Suaifan GARY, Muthana S, et al. Development of a rapid immuno-based screening assay for the detection of adenovirus in eye infections. ACS Omega. (2022) 7:21. doi: 10.1021/acsomega.1c07022
22. Kyosei Y, Namba M, Makioka D, Kokubun A, Watabe S, Yoshimura T, et al. Ultrasensitive detection of SARS-CoV-2 spike proteins using the thio-NAD cycling reaction: A preliminary study before clinical trials. Microorganisms. (2021) 9:2214. doi: 10.3390/microorganisms9112214
23. Kyosei Y, Namba M, Yamura S, Watabe S, Yoshimura T, Sasaki T, et al. Improved detection sensitivity of an antigen test for SARS-CoV-2 nucleocapsid proteins with thio-NAD cycling. Biol Pharm Bull. (2021) 44:1332–6. doi: 10.1248/bpb.b21-00387
Keywords: adenovirus, influenza virus, protein detection assay, respiratory syncytial virus, thio-NAD cycling, ultrasensitive ELISA
Citation: Kobayashi Y, Kyosei Y, Ogawa R, Okita K, Yoshimura T and Ito E (2024) Ultrasensitive protein-level detection for respiratory infectious viruses. Front. Immunol. 15:1445771. doi: 10.3389/fimmu.2024.1445771
Received: 11 June 2024; Accepted: 14 November 2024;
Published: 02 December 2024.
Edited by:
Mohan Tulapurkar, University of Maryland, United StatesReviewed by:
Nazly Shafagati, Quest Diagnostics, United StatesZhenhua Zheng, Chinese Academy of Sciences (CAS), China
Copyright © 2024 Kobayashi, Kyosei, Ogawa, Okita, Yoshimura and Ito. This is an open-access article distributed under the terms of the Creative Commons Attribution License (CC BY). The use, distribution or reproduction in other forums is permitted, provided the original author(s) and the copyright owner(s) are credited and that the original publication in this journal is cited, in accordance with accepted academic practice. No use, distribution or reproduction is permitted which does not comply with these terms.
*Correspondence: Etsuro Ito, ZWl0b0B3YXNlZGEuanA=