- 1Department of Head and Neck Surgery, Harbin Medical University Cancer Hospital, Harbin, China
- 2Department of Gastroenterology, Clinical Medical College and the First Affiliated Hospital of Chengdu Medical College, Chengdu, China
- 3Department of Pathology, Xi’an Daxing Hospital, Xi’an, China
- 4Laboratory of Medical Genetics, Harbin Medical University, Harbin, China
- 5Department of Pathology, Second Affiliated Hospital of Harbin Medical University, Harbin, China
- 6Department of General Surgery, Second Affiliated Hospital of Harbin Medical University, Harbin, China
- 7Department of Immunology, Harbin Medical University, Harbin, China
The interplay between immune components and the epithelium plays a crucial role in the development and progression of head and neck squamous cell carcinoma (HNSCC). Natural killer (NK) cells, one of the main tumor-killing immune cell populations, have received increasing attention in HNSCC immunotherapy. In this review, we explore the mechanism underlying the interplay between NK cells and HNSCC. A series of immune evasion strategies utilized by cancer cells restrict HNSCC infiltration of NK cells. Overcoming these limitations can fully exploit the antineoplastic potential of NK cells. We also investigated the tumor-killing efficacy of NK cell-based immunotherapies, immunotherapeutic strategies, and new results from clinical trials. Notably, cetuximab, the most essential component of NK cell-based immunotherapy, inhibits the epidermal growth factor receptor (EGFR) signaling pathway and activates the immune system in conjunction with NK cells, inducing innate effector functions and improving patient prognosis. In addition, we compiled information on other areas for the improvement of patient prognosis using anti-EGFR receptor-based monoclonal antibody drugs and the underlying mechanisms and prognoses of new immunotherapeutic strategies for the treatment of HNSCC.
1 Introduction
Head and neck squamous cell carcinoma (HNSCC) is the sixth most common malignancy worldwide, with 379,000 deaths annually (1, 2). HNSCC originates in the epithelium of the oral cavity, hypopharynx, oropharynx, and larynx (3) and tends to escape the immune system, with a poor prognosis (4). The use of immune checkpoint inhibitors has resulted in significant outcomes for some HNSCC patients. Although these drugs fail in most cases, there is still interest in targeting immune effector cells such as natural killer (NK) cells for immunotherapy. NK cells play an important role in tumor immune surveillance. Without prior sensitization, NK cells can quickly recognize and attack cancer cells (5). However, in some cases, intratumoral NK cells are dysfunctional and contribute to tumor growth. In contrast, when infiltrating activated NK cells increase in tumors, especially HNSCC, the clinical prognosis is significantly better (6). Therefore, NK cells play a crucial role in tumor management, prognosis, growth, and metastasis (7). In the last few years, NK cell immunotherapy has provided a different view of head and neck tumors. Cetuximab has received increasing attention because it can activate NK cells and produce durable responses. In this review, we introduce HNSCC-based NK cell immunotherapy from the perspective of modifying NK cell activation, proliferation, cell engineering, and inhibition of immune escape in HNSCC.
2 A brief introduction of NK cells
NK cells originating from CD34+ hematopoietic stem cells are the main inherent mediated antitumor and antiviral response lymphocyte subsets, and under a stimulus, they produce interferon γ (IFN-γ) and tumor necrosis factor α (TNF-α) (8, 9). NK cells express a variety of surface markers, such as CD56. According to the CD56 expression level, they could be divided into CD56dim and CD56bright subsets, which are mainly present in peripheral blood and tissues (10). CD16 is an NK cell surface marker. CD16 enables NK cells to exhibit antibody-dependent cell cytotoxicity (ADCC), as demonstrated by the minimal ADCC effect of CD56brightCD16− NK cells (11, 12). In contrast, CD56dim CD16+ NK cells can be mediated by infected cells and/or the continuous destruction of malignant cells, mainly through immune synapses that shed pre-assembled cellular lyses containing granzyme B and perforin, ultimately inducing target cell apoptosis (13).
Multiple receptors have been found to transmit signals that inhibit or activate NK cells. NKG2D is an agonist receptor on the NK cell surface, and its activation can be initiated by various NKG2D ligands (Table 1) (23). The immunotherapeutic approach of the NKG2D–NKG2DL axis is an appealing therapeutic target for cancer immunotherapy (24, 25). When cells are in a pathological state, surface HLA expression is usually downregulated to evade recognition by T cells. Target cells lacking HLA ligand expression require NK cells to inhibit the receptor and express ligands that activate the receptor, which may lead to NK cell activation without inhibitory signals (26). However, the role of NK cells in HNSCC remains unclear. This may be related to HNSCC cells producing various cytokines or modulating the signal transduction of different receptors, which is discussed in detail later in this review.
3 NK cell recognition and killing HNSCC
Major histocompatibility complex class I molecule (MHC I), also called human leukocyte antigen (HLA), is expressed on the surface of healthy cells and interacts with killer cell immunoglobulin-like receptor (KIR) on NK cell surfaces, inhibiting them through KIR–KIRL interactions (27). The KIR receptor binds to the ligand, and the intracellular ITIM sequence phosphorylates and enrolls SHP-1/SHP-2, which downregulates the phosphorylation of signaling molecules downstream of the activated receptor, thereby inhibiting NK cell function (28). Decreased MHC I expression on the surface of HNSCC cells leads to NK cell activation (29). NK cells are also auto-activated via the interplay between CD16 and the Fc structural domain of IgG antibodies and release cytokines and cytotoxic particles when interacting with tumor cells through the Fab structural domain of the antibodies (30). Furthermore, natural cytotoxicity receptor (NCR) is also a major activated receptor for NK cells, including NKp46, NKp44, and NKp30 (Figure 1) (31). NKp46 transmits activation signals via ITAM-associated receptors. Upon ligand binding, NKp46 binds to the junctional proteins CD3ze and FcϵRIγ, and the junctional ITAMs are phosphorylated and activated by SH2 recruitment. Tyrosine kinases activate downstream factors (such as PLC, PI3K, Vav1, Vav2, and Vav3) (32, 33). PI3K and Vav1 recruit small G proteins, and the PAK1 MEK–ERK signaling pathway induces a phosphorylation cascade that activates NK cells to kill HNSCC cells (28). Some HNSCC cells routinely overexpress heat shock protein 70 (Hsp70) and deliver it to the cell surface for recognition by pre-activated NK cells (34). These different effects of NK cells on tumor cells expressing Hsp70 or not expressing Hsp70 on the envelope were compared and analyzed. The results showed that tumor cells expressing Hsp70 were more sensitive to NK-mediated lysis (35). NK cells kill HNSCC cells expressing Hsp70 by releasing granzyme B. In 2014, Gehrmann et al. reported that Hsp70 may activate NK cells by inducing NKG2D expression (36).
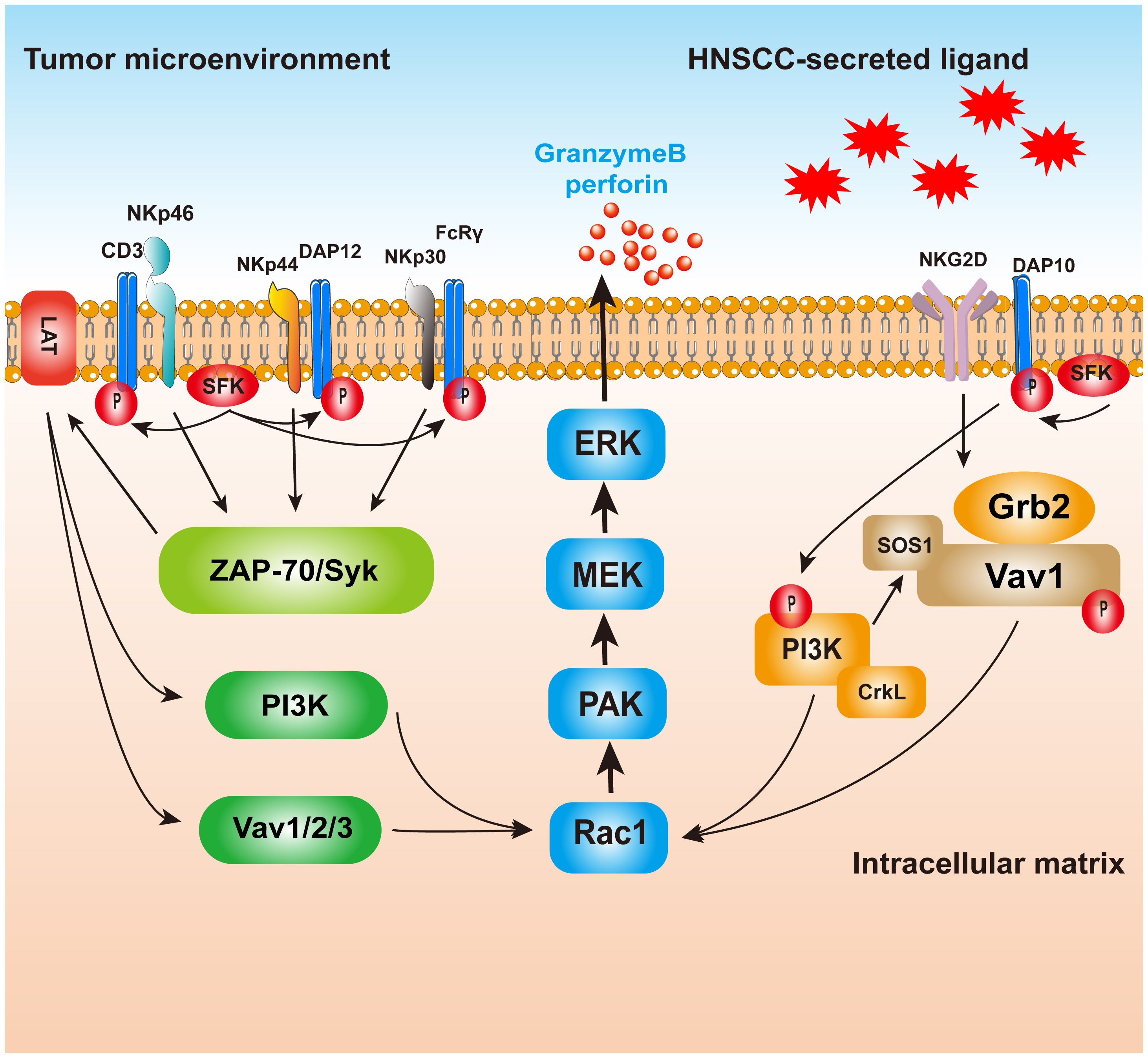
Figure 1. The killing mechanism of NK cells on HNSCC. Ligand binds to receptors on NK cells, and the NCR will raise and activate the downstream molecules with the help of the LAT; PAK1 MEK–Erk signaling pathway activation of MAPK signaling pathways induced enzyme release particles, HNSCC. NKp44, however, may be associated with inhibitory signaling, but the mechanism is still unclear. NKG2D activated, combined with PI3K and Grb2–Vav1–SOS1, raised the Ras family, and finally passed PAK1 MEK–Erk signaling pathway activation of MAPK signaling pathways in order to realize cell toxicity. NK, natural killer; HNSCC, head and neck squamous cell carcinoma; NCR, natural cytotoxicity receptor.
4 Immune escape of NK cells
Various HNSCC-derived cytokines have been reported to inhibit NK cell function by inhibiting agonist receptor signaling or enhancing inhibitory receptor signaling (Figure 2) (37). HNSCC-derived IL-6 is one of the most crucial cytokines that mediate NK cell inactivation (38). IL-6 activates JAK/STAT3 signaling in NK cells to reduce NKG2D transcription, thereby inhibiting NK cells (39–41). TGF-β is also a well-known immunosuppressive factor, and in a 2004 trial in Oregon, the level of TGF-β was also higher in HNSCC tumors from 32 patients with sleep apnea than in five normal oropharyngeal tissues (42). TGF-β not only stimulates angiogenesis to promote HNSCC growth but immunizes HNSCC from NK cell immunosurveillance by inhibiting NKG2D (43). Increased binding of plasma-soluble major histocompatibility complex class I chain-associated peptide A (sMICA) to NKG2D inhibits its function in some patients with HNSCC. TGF-β1 potentiates negative regulatory sMICA effect on NKG2D expression in NK cells. In freshly purified patient and healthy NK cell control experiments, the human HNSCC cell line SCC-4 (ATCC: CRL-1624) showed synergistic inhibition of NKG2D expression under the action of multiple factors, especially TGF-β1 (44).
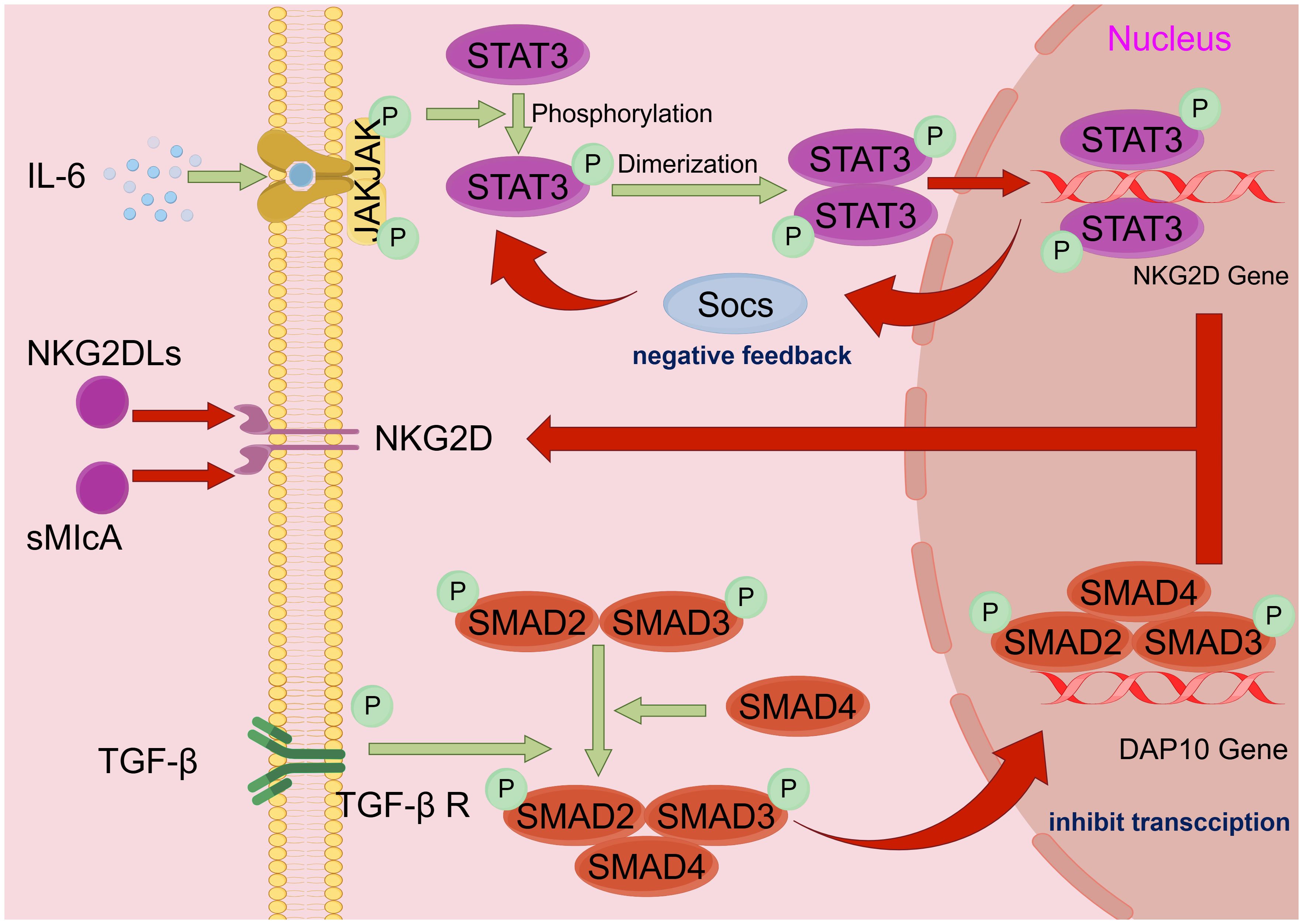
Figure 2. HNSCC immune escape mechanism. Schematic diagram of the mechanism by which HNSCC escapes NK cell immune surveillance: tumor cell escape mostly acts by affecting NKG2D, the surface receptor of NK cells. i) IL-6 activates the JAK/STAT3 pathway, causing STAT3 to phosphorylate and polymerize into a dimer into the nucleus, thereby inhibiting gene expression of the NKG2D receptor. ii) HNSCC secretes inhibitory ligands of NKG2D (e.g., NKG2DLs and sMIcA) to inactivate NKG2D. iii) TGF-β binds to TGF-βR on the NK cells surface to activate downstream SMAD2, SMAD3, and SMAD4 polymerization and then enters the nucleus to inhibit DAP 10 gene transcription, indirectly reducing the quantity of NKG2D on the cell surface. By Figdraw. HNSCC, head and neck squamous cell carcinoma; NK, natural killer.
Inhibition of NK cell surface agonist receptors enables immune escape of HNSCC cells. NKG2D is a C-type lectin-like activation receptor expressed on NK cells as well as TCRγδ+ and CD8+ TCRαβ+ T cells that stimulate naïve T-cell activation and provokes cytotoxicity without T-cell receptor (TCR) attachment (45). NKG2D-mediated activation signals can override the inhibitory signals of NK inhibitory receptors; thus, NKG2D is recognized as the main activating NK cell receptor, and NKG2D ligand expression (NKG2DLs) is strictly controlled. Based on plasma analysis of 44 HNSCC patients and 12 healthy volunteers, HNSCC may secrete NKG2DLs to inhibit the activation of NKG2D receptors on the NK cell surface, impair NK cell anti-tumor activity, and invade tumors, leading to immune escape (46, 47). Exosomes in the blood of HNSCC patients also inhibit ADCC in NK cells by suppressing the expression level of NKG2D. Co-culture of exosomes isolated from venous blood samples of patients with acute myeloid leukemia (AML) or HNSCC and healthy volunteers with NK cells revealed that they inhibited NKG2D expression in CD3−CD56+ NK cells and significantly decreased the NKG2D+ NK cell frequency (48). HNSCC patient-derived exosomes also significantly enhance CD8+ T-cell apoptosis and suppress NK cell proliferation and NKG2D expression (49).
The use of immune checkpoint inhibitors may be one of the reasons why HNSCC evades immune surveillance. TIM-3, an inhibitory receptor on the surface of NK cells, suppresses NK cell function, resulting in cancer metastasis or recurrence in patients (50). KIRs are also regarded as key receptors that affect human NK cell development and function (51). Drugs targeting NK cell inhibitory receptors (e.g., KIRs) (e.g., lirilumab) have been the focus of preclinical studies, either independently or in association with other immune checkpoint inhibitors (4). KIR3DL2, a member of the killer cell immunoglobulin-like receptor family, blocks NK cell activation and function upon contact with HLA-A3 or HLA-A11 and also contributes to immune escape from HNSCC (52).
In addition, HNSCC secretes other cytokines and immune components in the tumor microenvironment (TME) to suppress or activate T cells, B cells, and dendritic cells, directly or indirectly regulating NK cells (53–56).
5 NK cell-based immunotherapy in HNSCC
Numerous studies have indicated that the number and function of NK cells are associated with prognosis in patients with HNSCC. The role of NK cell checkpoints in neoplasm immunotherapy has attracted considerable interest. Currently, most NK cell-based immunotherapies use NK cell function as an entry point to strengthen the ADCC effect of NK cells by activating or inhibiting NK cell surface receptors (Table 2).
5.1 Monoclonal antibody
5.1.1 Cetuximab
Cetuximab is an epidermal growth factor receptor (EGFR)-specific chimeric immunoglobulin G (IgG)1 isotype monoclonal antibody approved for the treatment of HNSCC (Figure 3). After co-culture with UM-22B HNSCC in charge of cetuximab, the NK cells activation was increased, and NK cells expressing different genotypes of FC-γRIIIa receptor (VV, VF, and FF) were differently activated (66). Furthermore, after co-culture of TU167 HNSCC with NK cells in charge of cetuximab, NK cells containing FcγRIIIa V allele had significantly enhanced ADCC ability compared to NK cells containing only the F allele. This V-allele advantage was also observed in the 012SCC cell line (67). In a 2008 randomized clinical trial (NCT00122460), 442 eligible patients with untreated HNSCC were randomly assigned to receive cetuximab treatment while receiving the same chemotherapy. Compared with chemotherapy alone, cetuximab improved progression-free survival (PFS) from 3.3 months to 5.6 months, while the response rate increased from 20% to 36% (p < 0.001) (68). In another single-arm, multicenter, phase II trial (jRCTs051200040), combination chemotherapy with paclitaxel and cetuximab was administered to 35 recurrent/metastatic (R/M)-HNSCC patients previously treated with platinum-based chemotherapy and PD-1 (programmed cell death protein 1) antibody. Outcome was evaluated in 33 of 35 patients, with objective response rate (ORR) of 69.6% (95% CI, 51.2% to 84.4%) and disease control rate (DCR) of 93.7% (95% CI, 79.7% to 99.2%) (69).
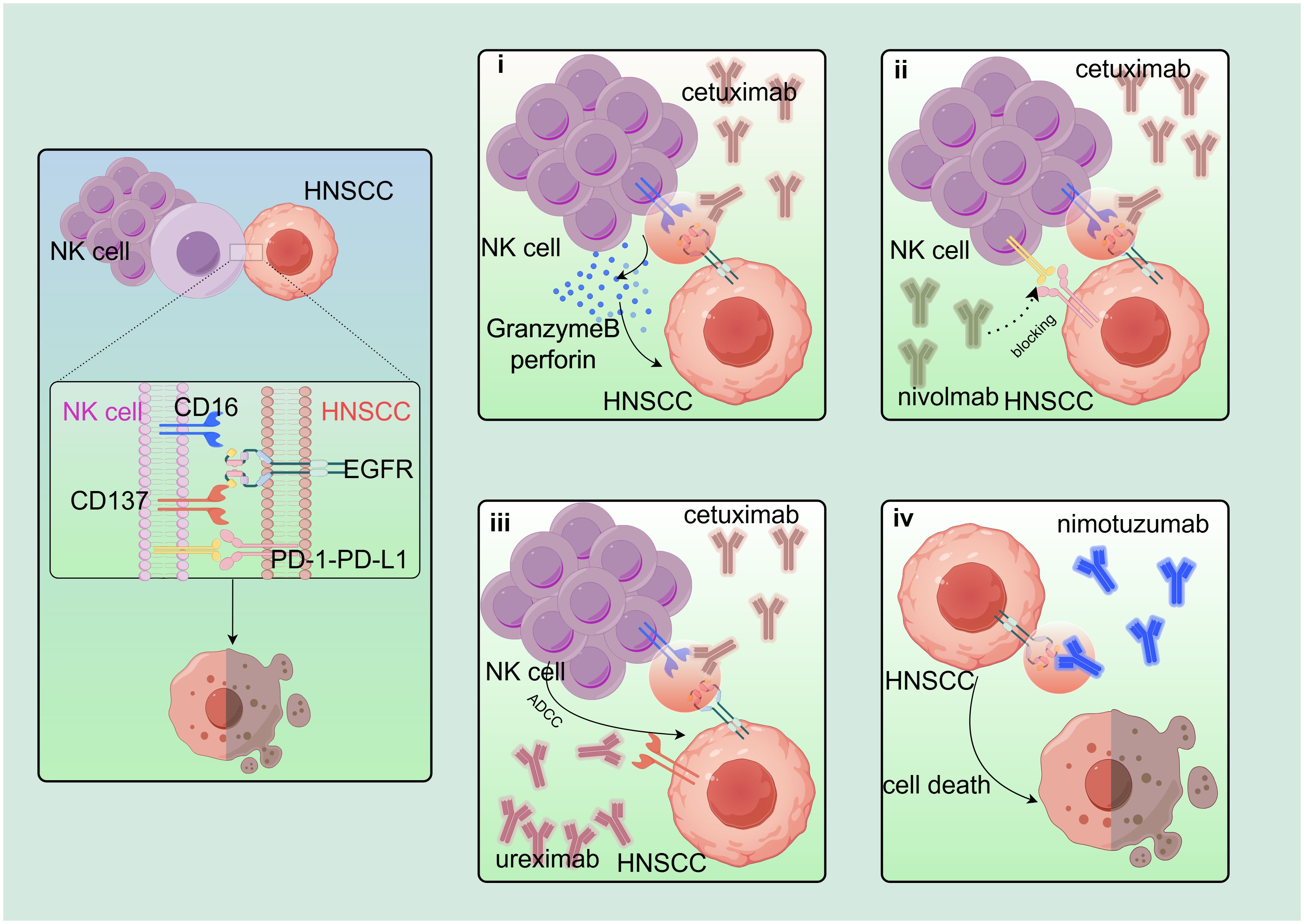
Figure 3. Based on the combination of cetuximab. EGFR-based combination therapy regimen: i) CD16 on NK cell surface is linked to EGFR on HNSCC surface via cetuximab to induce NK cells to secrete perforin and granzyme B to kill target cells. ii) Nivolumab blocks PD-1–PD-L1 axis and synergistically increases cetuximab treatment effect. iii) Cetuximab also stimulates NK cells to express CD137, and urelumab (agonist of CD137) acts synergistically with cetuximab. iv) Nimotuzumab binds to EGFR and blocks EGFR downstream signaling. By Figdraw. EGFR, epidermal growth factor receptor; HNSCC, head and neck squamous cell carcinoma.
5.1.2 Lirilumab
HLA-C missense mutations are frequently observed in patients who do not respond to cetuximab. Normal HLA-C binds to and inactivates KIRs, thereby activating NK cells. Lirilumab is targeted to activate NK cell KIR mAb, and in charge of lirilumab, NK cells were cultured in HNSCC JHU029 and 93 vu to improve the NK cell killing effect (70). Nivolumab and lirilumab in an open-label, single-arm, multicenter, phase II trial (NCT03341936). A pathological response to N + L was observed in 43% (12/28) of patients, a major response (tumor viability TV ≤ 10%) in 4/28 (14%), and a partial response (TV ≤ 50%) in 8/28 (29%). The median follow-up time was 22.8 months, and the 1-year disease-free survival (DFS) and overall survival (OS) were 55.2% (95% CI, 34.8 to 71.7) and 85.7% (95% CI, 66.3 to 94.4), respectively. Among patients with a pathological response, the 2-year DFS and OS rates were 64% and 80%, respectively. DFS was significantly improved in patients with a pathological response, and this regimen should be further validated (71).
5.1.3 Urelumab
Cetuximab also activates NK cells to upregulate the costimulatory receptor CD137. Cetuximab-coated JHU-029 HNSCC can increase CD137 expression on NK cell surface, and NK cells expressing FcγRIIIa VV/VF showed the most significant expression. Urelumab, a CD137 agonist, not only improved the survival rate of cetuximab-activated NK cells but also promoted dendritic cell (DC) maturation. Combination immunotherapy with cetuximab and CD137 agonists has shown beneficial effects (57).
5.1.4 Ipilimumab
Treg inhibited ADCC and/or NKG2D NK cells expression in a TGF-β1-dependent manner. In 22 patients treated with cetuximab, Tregs in the peripheral blood were increased by comparing peripheral venous blood from patients 7 days before and 1 month after treatment. JHU029 HNSCC cells were added to NK cells cultured with cetuximab in a 1:1 ratio with ipilimumab, which enhanced the ADCC of NK cells by targeting CTLA-4 to eliminate Tregs (58). Definitive radioimmunotherapy (RIT) with nivolumab and ipilimumab for locally advanced (LA) HNSCC was administered in a phase I trial involving 24 patients. The 3-year PFS was 74% (95% CI, 58% to 94%), and OS was 96% (95% CI, 88% to 100%). However, 21 (88%) patients reported treatment-related adverse events, and level 3 or higher was likely due to the small sample size. Based on the 3-year PFS and OS results, definitive RIT with nivolumab and ipilimumab had superior efficacy compared to standard chemoradiotherapy with high-dose cisplatin (72).
5.1.5 Nivolumab
JHU029 HNSCC cells were co-incubated with the treated (mAb) and freshly purified NK cells for 4 h. From the collected supernatant, we found that cetuximab-activated NK cells by PD-1 tended to be inactivated by the overexpression of PD-L1 (programmed death ligand 1) in the HNSCC TME. The effect of cetuximab was further inhibited by the downregulation of cell surface CD16. NK cells overexpressing PD-1 were co-cultured with three cell lines, SCC90 (PD-1−), JHU029 (PD-1low), and 93VU (PD-1high). It was found that nivolumab acted by blocking the PD-1-PD-L1 axis and significantly increased cetuximab-mediated killing (59). Nivolumab in combination with ipilimumab, with a complementary mode of action, demonstrates OS benefits and durable responses in a wide range of solid tumors. CheckMate 651 (NCT02741570) found that the median OS of nivolumab plus ipilimumab was 13.9 months (95% CI, 12.1 to 15.8). However, the OS for the EXTREME (≤6 cycles of cetuximab plus cisplatin/carboplatin plus fluorouracil, followed by maintenance cetuximab) was 13.5 months (95% CI, 12.6 to 15.2). CheckMate 651 does not meet the OS requirements, but compared with EXTREME, CheckMate 651 showed good security. Patients with R/M-HNSCC require new treatments (73).
5.1.6 Monalizumab
The combination of monalizumab, a humanized anti-NKG2A antibody, with a PD-1-PD-L1 axis blocker enhances NK cell activity against a variety of tumor cells and rescues CD8+ T-cell function, a novel mechanism of checkpoint inhibition. It also promotes NK cell ADCC and cetuximab antitumor activity (74). The human HNSCC cell lines SNU-1041, SNU-1066, and SNU-1076 were cultured with NK cells. IFN-γ secreted via NK cells increased the expression of HLA-E on tumor cells. The HLA-E expression levels in SNU-1041 and SNU-1066 cells were higher than those in SNU-1076 cells. A combination of mAbs and cetuximab is more effective (60). In a phase II trial (NCT02643550), investigators evaluated the safety and efficacy of the combination of monalizumab and cetuximab in previously treated patients with recurrent or metastatic HNSCC. The midterm test results showed an ORR of 31%. The most common adverse events were fatigue (17%), fever (13%), and headache (10%). However, cetuximab monotherapy has limited activity in relapsed and/or metastatic HNSCC treatment, with an ORR of 13% (74).
5.1.7 Durvalumab
Durvalumab is a kind of immunoglobulin G1 kappa monoclonal antibody, as an antagonist of PD-L1 signal, often associated with other monoclonal antibodies for solid tumor treatment (75, 76). In a phase 2 study of cetuximab plus durvalumab (NCT00122460), NK cells were evaluated for the treatment of ADCC. Cetuximab increased NK cell cytotoxicity and had a synergistic effect when durvalumab was added to responders. The ORR was 39% (13/33), and the median response duration was 8.6 months (95% CI, 6.5 to 16.8). Median progression-free survival was 5.8 months (95% CI, 3.7 to 14.1), and overall survival was 9.6 months (95% CI, 4.8 to 16.3). Of note, patients who had received ICI (pembrolizumab or nivolumab) had better OS than those who had not (median OS 7.1 months; 95% CI, 2.8 to not reached (NR) vs. 13.9 months; 95% CI, 4.8 to NR) (77).
5.1.8 Avelumab
Thus, avelumab, combined with targeted therapy, may be a promising treatment option for cancer. After binding to tumor cells, avelumab activates NK cell ADCC to kill tumor cells by interacting with CD16 receptor/FcγRIII on NK cells by antibody Fc region (78). In a phase 3 trial, avelumab–cetuximab had an expected hazard ratio (HR) of 0.64 compared to cisplatin–RT. The HR for avelumab–cetuximab versus cetuximab was 0.62 (79). In another trial of avelumab plus chemoradiotherapy (NCT02952586), the median follow-up for progression-free survival with avelumab was 14.6 months [interquartile range (IQR) 8.5 to 19.6], and that of the placebo team was 14.8 months (11.6–18.8), which did not achieve the desired results (80).
5.1.9 Camrelizumab
In a single-center, prospective, randomized controlled trial, responders had significantly higher NK cell counts than non-responders when camrelizumab was co-administered with chemotherapy. CD56dim NK cell values can also be used to predict efficacy (81). In a single-center, single-group, phase 2 trial (chictr.org registry, ChiCTR1900025303), the PD-1 inhibitor camrelizumab was added to neoadjuvant chemotherapy. Thirty patients completed neoadjuvant therapy, with an ORR of 96.7% (29/30); 27 patients underwent immediate surgery, with an R0 removal rate of 92.6% (25/27) (82). In another phase I trial (NCT04393506), 20 patients with locally advanced resectable oral squamous cell carcinoma (OSCC) who received camrelizumab combined with apatinib had an 18-month locoregional recurrence rate of 10.5% (95% CI, 0% to 24.3%) and a survival rate of 95% (95% CI, 85.4%-100.0%), and major pathologic response (MPR) rate was 40% (8/20). Camrelizumab combined with apatinib showed promising MPR compared to pembrolizumab monotherapy (4.3%–20.5%), nivolumab monotherapy (5.9%), or nivolumab plus ipilimumab (20%) (83).
5.2 Interleukin
In addition to monoclonal antibodies, numerous cytokines have been found to augment NK cell function. Some of these interleukin family factors have been proven to upregulate NK cell activity and prolong NK cell survival in vivo.
Preclinical studies have demonstrated the anti-tumor immune potential of IL-2. In the early 1980s, IL-2-treated human peripheral blood mononuclear cells increased the number of killer cell populations (LAK), which were mainly composed of NK and T cells (84). IL-2, a confirmed activated NK cell ligand, is directly involved in NK cell antitumor activity. IL-2, the main component of the biological agent IRX-2, strengthens NK cell function by increasing the NKp30 and NKp46 expression levels (61, 85). Notably, high doses of IL-2 are life-threatening.
Over the past decade, IL-15 has gradually replaced IL-2. IL-15 can be used in NK cell therapy to increase lysis of antibody-coated target cells. ALT-803 is a novel compound consisting of genetically modified IL-15, and the IL-15 receptor alpha protein (IL15R) partially fused to IgG1 Fc. ALT-803 increased the ADCC effect in NK cells more strongly than IL-15 alone and, in combination with cetuximab, activated the JAK/STAT and MAPK signaling pathways in NK cells, resulting in stronger activation of cetuximab-coated HNSCC cells against NK cells. This treatment significantly reduced the size of tumors in mice (86). IL-21 also plays an important role in the regulation of T, B, and NK cells. Low concentrations of IL-21 and IL-15 synergistically promote NK cell growth, whereas high concentrations of IL-21 significantly inhibit NK cell growth. IL-21 induces humoral and cellular immune therapies and antitumor immune responses and causes no serious adverse reactions (87).
IL-12 has a similar structure and effect as IL-15, which can enhance NK cell ADCC in HNSCC cell lines and the dissolution activity of NK cells against cetuximab-coated cells simultaneously (62). Combined treatment with IL-2 and cetuximab significantly increases NK cell ADCC activity (88). Furthermore, IL-27 is an IL-12 family cytokine that enhances the ADCC of NK cells in vitro and in vivo and enhances NK cell viability in vitro (89).
5.3 PD-L1 t-haNK
Recently, increasing attention has been paid to the efficacy of engineered NK-92 cell lines in HNSCC therapy. Before clinical use, the NK-92 cell line derived from lymphoma patients must be subjected to deadly radiation. Adoptive transfer of the irradiated NK-92 cell line has proven to be safe and has shown preliminary clinical benefits in patients with cancer (90). NK-92 cells and their derivatives are designated as NK cells. aNK is modified to express IL-2 and CD16 with high affinity for PD-L1 (PD-L1 t-haNK). These cells not only preserve natural NK receptor expression but also contain high levels of granzymes and perforin granules (91, 92). PD-L1 t-ha NK cells induce cell lysis in vitro in a variety of human cancer cell lines (such as breast cancer, lung cancer, colon cancer, ovarian cancer, stomach cancer, and meningioma) (91). Furthermore, PD-1/PD-L1 pathways are involved in the immune escape mechanism of tumor cells and are key targets for cancer immunotherapy (93). PD-L1 t-haNKs also reduce the frequency of endogenous high PD-L1-expressing leukocytes in patients with HNSCC, an important complementary mechanism for killing tumor cells (94). Most importantly, the cytolytic ability of NK cells decreases under hypoxic conditions in most solid tumors, whereas haNK cells retain their cytolytic ability under hypoxic conditions (95). In addition to its direct HNSCC-killing effect, PD-L1 t-haNKs in vitro can be mediated by PD-L1 chimeric antigen receptor (CAR) to escape T cell-killing tumor cells (96). In vitro co-culture of peripheral blood leukocytes from advanced HNSCC patients with PD-L1 t-haNK for 24 h significantly reduced the number of macrophages and CD14+/CD15+ bone marrow cell subsets with high PD-L1 expression. The results showed that PD-L1 t-haNK holds therapeutic promise for patients with HNSCC with high PD-L1 expression (94).
5.4 Others
Toll-like receptors (TLRs) are key to the immune response to the medium, and TLR agonists are of great significance for enhancing the effect of HNSCC treatment (63). TLR8 is the main sensor of microbial invasion. The TLR8 agonist VTX-2337 (motolimod) can effectively activate TLR8, enhance NK cell function by indirectly activating NK cells through the stimulation of monocytes or myeloid DCs (mDCs), and increase tumor-directed ADCC in conjunction with cetuximab. The combination of VTX-2337 and cetuximab increased the clinical response rate in epidermal growth factor receptor-positive patients (97). In 2019, Wu et al. analyzed CAL27, CAL33, and UMSCC47 HNSCC after treatment with metformin and found that metformin prevented the development of HNSCC (98). In 2022, McKenzie Crist et al. found that the treatment of HNSCC patients with metformin-activated anti-tumor immunity enhanced ADCC, NK cell counts, STAT1 pathway stimulation, and subsequent NKG2D receptor expression. Metformin also increases NK cell cytotoxicity by inhibiting CXCL1 (65). Vitamin D deficiency is prevalent in HNSCC patients, and NK cell infiltration within the tumor and/or stroma significantly increases after oral vitamin D administration. In the study of Florian Bochen et al., NK cells were isolated from the peripheral blood samples of 11 vitamin D-deficient HNSCC patients, of whom nine vitamin D responders showed improvement in NK cell activity compared with pre-treatment (99). Vitamin D acts on NK cells to exert anti-tumor effects, but a 2013 in vitro study showed that vitamin D also inhibits HNSCC cell proliferation (100). Myeloid-derived suppressor cells (MDSCs) inhibit NK cell function in HNSCC, and orally bioavailable SX-682 inhibits MDSC trafficking, thereby significantly eliminating tumor MDSC accumulation and enhancing tumor invasion, activation, and NK cell efficacy (101). WEE1 kinase inhibitors and cell cycle checkpoints enhance the sensitivity of HNSCC cells to ADCC weakening of tumor cell resistance to granzyme B-induced cell death (102).
6 Future perspectives
Car-NK follows the success of Car T-cell therapy (103). Recent clinical trials investigated the use of Car in different cancer treatments to determine its efficacy (104). Car-NK not only has the disadvantages of Car-T but also has the advantages of easy access, short production time, and inhibition of the immune escape of cancer cells (105). The role of allogeneic NK cell infusion in hematological tumors has also been validated (27). However, the expansion of NK cells in vitro faces many challenges and has limited infiltration into solid tumors (29). The short half-life of NK cells and the decrease in their cytotoxic activity with age are also reasons for the limitations of CAR-NK cells (106).
The solid tumor-associated antigen EphA2, a receptor tyrosine kinase, is overexpressed in many solid tumors and weakly expressed in normal human tissues (107). In an in vitro experiment, the UM-SCC9 HNSCC cell line with EphA2-CAR ML NK cells after training improved significantly compared with the control group EphA2-CAR ML NK cells of HNSCC lethality (108). Trophoblastic cell surface antigen 2 (TROP2) is a transmembrane glycoprotein. Trop2 overexpression is associated with disease progression and poor prognosis in various cancers. The experimenter designed a TROP2 specificity of CAR (T-CAR), NK-92 cells and PCI-13 HNSCC tumor cell lines after the training, activate the E7-NK-92 cells-double expression of TCR/T-CAR. T-CAR enhanced E7-TCR-mediated activation and cytotoxicity in TCR-engineered NK92-derived cell lines (109). In another trial, anti-HER1 (EGFR) CAR-NK-92 cells showed enhanced killing, apoptosis, and degranulation of HNSCC cells (110).
CD44v6, a subtype of CD44, is a potential target for HNSCC therapy (111). Anti-CD44v6 CAR-NK cells were prepared from peripheral blood-derived NK cells from healthy donors using a γ retroviral vector. Compared with the original generation of undecorated NK cells, CAR-NK cells for a variety of HNSCC cell lines (UT-SCC-14, UT-SCC-42B, SCC-25) increased by two to three times (112).
7 Conclusions
NK cells are special immune cells that respond swiftly to tumor cells. Tumors have a much harder time escaping immune surveillance by NK cells than T cells. However, the treatment of HNSCC with NK cells still faces many challenges. Interactions between immune cells in the TME affect the number of NK cells and ADCC. To enhance NK cell effects, NK cell expansion and infusion in vitro and antibody–drug coupling (ADC) have shown great promise and will be further investigated in future trials. Synergistic therapy between monoclonal antibodies also needs further trials to verify its effectiveness and safety. In addition, the combination of mAbs with other therapies may involve adjusting the dose of mAbs to reduce the probability of adverse events.
The CAR structures of CAR NK cells have undergone many iterations and have made good progress in the treatment of hematological tumors. Compared to CAR-T cells, CAR-NK cells are highly non-specific and do not cause graft-versus-host disease (GVHD). However, it lags behind the treatment of solid tumors, especially HNSCC. However, the number of donor cells was too small for treatment with CAR-NK cells. The safety and effectiveness of amplification also need to be further investigated in future studies.
Recently, NK cell immunotherapy has become a popular research topic. NK cell-based immunotherapy has important implications for surgical resection, preservation of maxillofacial function, tumor metastasis, and recurrence in patients with HNSCC.
Author contributions
SD: Data curation, Writing – original draft, Writing – review & editing. MZ: Data curation, Writing – original draft, Writing – review & editing. JZ: Data curation, Methodology, Writing – original draft, Writing – review & editing. TL: Writing – original draft, Writing – review & editing. MY: Writing – original draft, Writing – review & editing. KX: Writing – original draft, Writing – review & editing. PL: Writing – original draft, Writing – review & editing. SY: Conceptualization, Supervision, Writing – original draft, Writing – review & editing. JM: Conceptualization, Writing – original draft, Writing – review & editing. HH: Conceptualization, Writing – original draft, Writing – review & editing.
Funding
The author(s) declare financial support was received for the research, authorship, and/or publication of this article. China Primary Care Health Foundation (2023-clinical special). China Primary Care Health Foundation (2022–2026).
Acknowledgments
We thank all those who contributed to this manuscript.
Conflict of interest
The authors declare that the research was conducted in the absence of any commercial or financial relationships that could be construed as a potential conflict of interest.
Publisher’s note
All claims expressed in this article are solely those of the authors and do not necessarily represent those of their affiliated organizations, or those of the publisher, the editors and the reviewers. Any product that may be evaluated in this article, or claim that may be made by its manufacturer, is not guaranteed or endorsed by the publisher.
Abbreviations
NK, natural killer; HNSCC, head and neck squamous cell carcinoma; EGFR, epidermal growth factor receptor; IFN-γ, interferon-gamma; TNF, tumor necrosis factor; MHC I, major histocompatibility complex I; CAR, chimeric antigen receptor; IL, interleukin; ADCC, antibody-dependent cell cytotoxicity; HLA, human leukocyte antigen; NCR, natural cytotoxicity receptor; MDSC, myeloid-derived suppressor cell; Hsp 70, heat shock protein 70; sMICA, soluble major histocompatibility complex class I chain-associated peptide A; TCR, T-cell receptor; KIR, killer cell immunoglobulin-like receptor; TLR, Toll-like receptor; ADC, antibody–drug coupling; MPR, major pathologic response.
References
1. Johnson DE, Burtness B, Leemans CR, Lui VWY, Bauman JE, Grandis JR, et al. Head and neck squamous cell carcinoma. Nat Rev Dis Primers. (2020) 6(1):92. doi: 10.1038/s41572-020-00233-2
2. Rebecca LS, Angela NG, Ahmedin J. Cancer statistics, 2024. CA Cancer J Clin. (2024) 74(2):203. doi: 10.3322/caac.21830
3. Sun Z, Sun X, Chen Z, Du J, Wu Y. Head and neck squamous cell carcinoma: risk factors, molecular alterations, immunology and peptide vaccines. Int J Pept Res Ther. (2021) 28. doi: 10.1007/s10989-021-10334-5
4. Mandal R, Senbabaoglu Y, Desrichard A, Havel JJ, Dalin MG, Riaz N, et al. The head and neck cancer immune landscape and its immunotherapeutic implications. JCI Insight. (2016) 1:e89829. doi: 10.1172/jci.insight.89829
5. Imai K, Matsuyama S, Miyake S, Suga K, Nakachi . Natural cytotoxic activity of peripheral-blood lymphocytes and cancer incidence: an 11-year follow-up study of a general population. Lancet (London England). (2000) 356:1795–9. doi: 10.1016/S0140-6736(00)03231-1
6. Moreno-Nieves U, Tay JK, Saumyaa S, Horowitz NB, Shin JH, Mohammad IA, et al. Landscape of innate lymphoid cells in human head and neck cancer reveals divergent NK cell states in the tumor microenvironment. Proc Natl Acad Sci U S A. (2021) 118. doi: 10.1073/pnas.2101169118
7. Chen X, Jiang L, Liu X. Natural killer cells: the next wave in cancer immunotherapy. Front Immunol. (2022) 13:954804. doi: 10.3389/fimmu.2022.954804
8. Abel A, Yang C, Thakar MS, Malarkannan S. Natural killer cells: development, maturation, and clinical utilization. Front Immunol. (2018) 9:1869. doi: 10.3389/fimmu.2018.01869
9. Spits H, Artis D, Colonna M, Diefenbach A, Di Santo JP, Eberl G, et al. Innate lymphoid cells–a proposal for uniform nomenclature. Nat Rev Immunol. (2013) 13(2):145–9. doi: 10.1038/nri3365
10. Melsen JE, Lugthart G, Lankester AC, Schilham MW. Human circulating and tissue-resident CD56(bright) natural killer cell populations. Front Immunol. (2016) 7:262. doi: 10.3389/fimmu.2016.00262
11. Michel T, Poli A, Cuapio A, Briquemont B, Iserentant G, Ollert M, et al. Human CD56bright NK cells: an update. J Immunol. (2016) 196(7):2923–31. doi: 10.4049/jimmunol.1502570
12. Beziat V, Duffy D, Quoc S. N, Le Garff-Tavernier M, Decocq J, Combadiere B, et al. CD56brightCD16+ NK cells: a functional intermediate stage of NK cell differentiation. J Immunol. (2011) 186(12):6753–61. doi: 10.4049/jimmunol.1100330
13. Prager I, Liesche C, van Ooijen H, Urlaub D, Verron Q, Sandstrom N, et al. NK cells switch from granzyme B to death receptor-mediated cytotoxicity during serial killing. J Exp Med. (2019) 216(9):2113–27. doi: 10.1084/jem.20181454
14. Murad S, Michen S, Becker A, Fussel M, Schackert G, Tonn T, et al. NKG2C+ NK cells for immunotherapy of glioblastoma multiforme. Int J Mol Sci. (2022) 23(10):5857. doi: 10.3390/ijms23105857
15. Xiang RF, Li S, Ogbomo H, Stack D, Mody CH. beta1 Integrins Are Required To Mediate NK Cell Killing of Cryptococcus neoformans. J Immunol (Baltimore Md.: 1950). (2018) 201:2369–76. doi: 10.4049/jimmunol.1701805
16. Li SS, Ogbomo H, Mansour MK, Xiang RF, Szabo L, Munro F. Identification of the fungal ligand triggering cytotoxic PRR-mediated NK cell killing of Cryptococcus and Candida. Nat Commun. (2018) 9:751. doi: 10.1038/s41467-018-03014-4
17. Gaggero S, Bruschi M, Petretto A, Parodi M, Del Zotto G, Lavarello C, et al. Nidogen-1 is a novel extracellular ligand for the NKp44 activating receptor. Oncoimmunology. (2018) 7:e1470730. doi: 10.1080/2162402X.2018.1470730
18. Jarahian M, Fiedler M, Cohnen A, Djandji D, Hämmerling GJ, Gati C, et al. Modulation of NKp30- and NKp46-mediated natural killer cell responses by poxviral hemagglutinin. PLoS Pathog. (2011) 7:e1002195. doi: 10.1371/journal.ppat.1002195
19. Hecht ML, Rosental B, Horlacher T, Hershkovitz O, De Paz JL, Noti C, et al. Natural cytotoxicity receptors NKp30, NKp44 and NKp46 bind to different heparan sulfate/heparin sequences. J Proteome Res. (2009) 8:712–20. doi: 10.1021/pr800747c
20. Mandelboim O, Lieberman N, Lev M, Paul L, Arnon TI, Bushkin Y, et al. Recognition of haemagglutinins on virus-infected cells by NKp46 activates lysis by human NK cells. Nature. (2001) 409:1055–60. doi: 10.1038/35059110
21. Battin C, Kaufmann G, Leitner J, Tobias J, Wiedermann U, Rölle A, et al. NKG2A-checkpoint inhibition and its blockade critically depends on peptides presented by its ligand HLA-E. Immunology. (2022) 166:507–21. doi: 10.1111/imm.13515
22. Pende D, Falco M, Vitale M, Cantoni C, Vitale C, Munari E, et al. Killer ig-like receptors (KIRs): their role in NK cell modulation and developments leading to their clinical exploitation. Front Immunol. (2019) 10:1179. doi: 10.3389/fimmu.2019.01179
23. Zingoni A, Molfetta R, Fionda C, Soriani A, Paolini R, Cippitelli M, et al. NKG2D and its ligands: "One for all, all for one. Front Immunol. (2018) 9. doi: 10.3389/fimmu.2018.00476
24. Lewis L L. NKG2D receptor and its ligands in host defense. Cancer Immunol Res. (2015) 3(6):575–82. doi: 10.1158/2326-6066.CIR-15-0098
25. Mercedes Beatriz F, Carolina Inés D, Norberto Walter Z. Leveraging NKG2D ligands in immuno-oncology. Front Immunol. (2021) 12. doi: 10.3389/fimmu.2021.713158
26. Wei W, et al. NK cell-mediated antibody-dependent cellular cytotoxicity in cancer immunotherapy. Front Immunol. (2015) 6. doi: 10.3389/fimmu.2015.00368
27. Rupert H, Peter L, Maya C A. Exploitation of natural killer cells for the treatment of acute leukemia. Blood. (2016) 127(26):3341–9. doi: 10.1182/blood-2015-12-629055
28. Chen YY, Lu D, Churov A, Fu R. Research progress on NK cell receptors and their signaling pathways. Mediators Inflammation. (2020) 2020:6437057. doi: 10.1155/2020/6437057
29. Liu S, Galat V, Galat Y, Lee YKA, Wainwright D, Wu J, et al. NK cell-based cancer immunotherapy: from basic biology to clinical development. J Hematol Oncol. (2021) 14(1). doi: 10.1186/s13045-020-01014-w
30. Baysal H, De Pauw I, Zaryouh H, Peeters M, Vermorken JB, Lardon F, et al. The right partner in crime: unlocking the potential of the anti-EGFR antibody cetuximab via combination with natural killer cell chartering immunotherapeutic strategies. Front Immunol. (2021) 12. doi: 10.3389/fimmu.2021.737311
31. Bottino C, Biassoni R, Millo R, Moretta L, Moretta A. The human natural cytotoxicity receptors (NCR) that induce HLA class I-independent NK cell triggering. Hum Immunol. (2000) 61(1). doi: 10.1016/S0198-8859(99)00162-7
32. Alexander David B, Claudia Jane M, Marco C. The natural cytotoxicity receptors in health and disease. Front Immunol. (2019) 10. doi: 10.3389/fimmu.2019.00909
33. Brumbaugh KM, Binstadt BA, Billadeau DD, Schoon RA, Dick CJ, Ten RM, et al. Functional role for Syk tyrosine kinase in natural killer cell-mediated natural cytotoxicity. J Exp Med. (1998) 186(12):1965–74. doi: 10.1084/jem.186.12.1965
34. Stangl S, Tontcheva N, Sievert W, Shevtsov M, Niu M, Schmid TE, et al. Heat shock protein 70 and tumor-infiltrating NK cells as prognostic indicators for patients with squamous cell carcinoma of the head and neck after radiochemotherapy: A multicentre retrospective study of the German Cancer Consortium Radiation Oncology Group (DKTK-ROG). Int J Cancer. (2018) 142:1911–25. doi: 10.1002/ijc.31213
35. Multhoff G, Mizzen L, Winchester CC, Milner CM, Wenk S, Eissner G, et al. Heat shock protein 70 (Hsp70) stimulates proliferation and cytolytic activity of natural killer cells. Exp Hematol. (1999) 27:1627–36. doi: 10.1016/S0301-472X(99)00104-6
36. Gehrmann M, Specht HM, Bayer C, Brandstetter M, Chizzali B, Duma M, et al. Hsp70–a biomarker for tumor detection and monitoring of outcome of radiation therapy in patients with squamous cell carcinoma of the head and neck. Radiat Oncol (London England). (2014) 9:131. doi: 10.1186/1748-717X-9-131
37. Robert L F. Immunology and immunotherapy of head and neck cancer. J Clin Oncol. (2015) 33(29):3293–304. doi: 10.1200/JCO.2015.61.1509
38. Cheng FD, Wang HW, Cuenca A, Huang M, Ghansah T, Brayer J, et al. A critical role for Stat3 signaling in immune tolerance. Immunity. (2003) 19(3):425–36. doi: 10.1016/S1074-7613(03)00232-2
39. Witalisz-Siepracka A, Klein K, Zdarsky B, Stoiber D. The multifaceted role of STAT3 in NK-cell tumor surveillance. Front Immunol. (2022) 13. doi: 10.3389/fimmu.2022.947568
40. Hua Y, Marcin K, Drew P. Crosstalk between cancer and immune cells: role of STAT3 in the tumour microenvironment. Nat Rev Immunol. (2006) 7(1):41–51. doi: 10.1038/nri1995
41. Sampath S, Won HJ, Massarelli E, Li M, Frankel P, Vora N, et al. Combined modality radiation therapy promotes tolerogenic myeloid cell populations and STAT3-related gene expression in head and neck cancer patients. Oncotarget. (2018) 9(13):11279–90. doi: 10.18632/oncotarget.v9i13
42. Lu SL, Reh D, Li AG, Woods J, Corless CL, Kulesz-Martin M, et al. Overexpression of transforming growth factor beta1 in head and neck epithelia results in inflammation, angiogenesis, and epithelial hyperproliferation. Cancer Res. (2004) 64(13):4405–10. doi: 10.1158/0008-5472.CAN-04-1032
43. Mariya L, Alexander S. Impairment of NKG2D-mediated tumor immunity by TGF-β. Front Immunol. (2019) 10. doi: 10.3389/fimmu.2019.02689
44. Kloss S, Chambron N, Gardlowski T, Weil S, Koch J, Esser R, et al. Cetuximab reconstitutes pro-inflammatory cytokine secretions and tumor-infiltrating capabilities of sMICA-inhibited NK cells in HNSCC tumor spheroids. Front Immunol. (2015) 6. doi: 10.3389/fimmu.2015.00543
45. Ashiru O, Boutet P, Fernandez-Messina L, Aguera-Gonzalez S, Skepper JN, Vales-Gomez M, et al. Natural killer cell cytotoxicity is suppressed by exposure to the human NKG2D ligand MICA*008 that is shed by tumor cells in exosomes. Cancer Res. (2010) 70(2):481–9. doi: 10.1158/0008-5472.CAN-09-1688
46. Payal D, Jennifer D W. NKG2D and its ligands in cancer. Curr Opin Immunol. (2018) 51:55–61. doi: 10.1016/j.coi.2018.02.004
47. Weil S, Memmer S, Lechner A, Huppert V, Giannattasio A, Becker T, et al. Natural killer group 2D ligand depletion reconstitutes natural killer cell immunosurveillance of head and neck squamous cell carcinoma. Front Immunol. (2017) 8. doi: 10.3389/fimmu.2017.00387
48. Hong CS, Funk S, Muller L, Boyiadzis M, Whiteside TL. Isolation of biologically active and morphologically intact exosomes from plasma of patients with cancer. J Extracell Vesicles. (2016) 5:29289. doi: 10.3402/jev.v5.29289
49. Ludwig S, Floros T, Theodoraki MN, Hong CS, Jackson EK, Lang S, et al. Suppression of lymphocyte functions by plasma exosomes correlates with disease activity in patients with head and neck cancer. Clin Cancer Res. (2017) 23(16):4843–54. doi: 10.1158/1078-0432.CCR-16-2819
50. Mei Z, Huang J, Qiao B, Lam AK. Immune checkpoint pathways in immunotherapy for head and neck squamous cell carcinoma. Int J Oral Sci. (2020) 12(1):16. doi: 10.1038/s41368-020-0084-8
51. Barani S, Khademi B, Ashouri E, Ghaderi A. KIR2DS1, 2DS5, 3DS1 and KIR2DL5 are associated with the risk of head and neck squamous cell carcinoma in Iranians. Hum Immunol. (2018) 79(4):218–23. doi: 10.1016/j.humimm.2018.01.012
52. Chi H, Xie X, Yan Y, Peng G, Strohmer DF, Lai G, et al. Natural killer cell-related prognosis signature characterizes immune landscape and predicts prognosis of HNSCC. Front Immunol. (2022) 13. doi: 10.3389/fimmu.2022.1018685
53. Camacho M, Leon X, Fernandez-Figueras MT, Quer M, Vila L. Prostaglandin E(2) pathway in head and neck squamous cell carcinoma. Head Neck. (2008) 30(9):1175–81. doi: 10.1002/hed.20850
54. Yoshihiro K, Masahisa J, Motohiro T. Clinical significance of macrophage heterogeneity in human Malignant tumors. Cancer Sci. (2013) 105(1):1–8. doi: 10.1111/cas.12314
55. Suzanne O-R, Pratima S. Myeloid-derived suppressor cells: linking inflammation and cancer. J Immunol. (2009) 182(8):4499–506. doi: 10.4049/jimmunol.0802740
56. Seiwert TY, Cohen EEW. Targeting angiogenesis in head and neck cancer. Semin Oncol. (2008) 35(3):274–85. doi: 10.1053/j.seminoncol.2008.03.005
57. Srivastava RM, Trivedi S, Concha-Benavente F, Gibson SP, Reeder C, Ferrone S, et al. CD137 stimulation enhances cetuximab-induced natural killer: dendritic cell priming of antitumor T-cell immunity in patients with head and neck cancer. Clin Cancer Res. (2017) 23:707–16. doi: 10.1158/1078-0432.CCR-16-0879
58. Jie HB, Schuler PJ, Lee SC, Srivastava RM, Argiris A, Ferrone S, et al. CTLA-4+ Regulatory T cells increased in cetuximab-treated head and neck cancer patients suppress NK cell cytotoxicity and correlate with poor prognosis. Cancer Res. (2015) 75:2200–10. doi: 10.1158/0008-5472.CAN-14-2788
59. Concha-Benavente F, Kansy B, Moskovitz J, Moy J, Chandran U, Ferris RL, et al. PD-L1 mediates dysfunction in activated PD-1 NK cells in head and neck cancer patients. Cancer Immunol Res. (2018) 6:1548–60. doi: 10.1158/2326-6066.CIR-18-0062
60. Lee J, Keam B, Park HR, Park JE, Kim S, Kim M, et al. Monalizumab efficacy correlates with HLA-E surface expression and NK cell activity in head and neck squamous carcinoma cell lines. J Cancer Res Clin Oncol. (2023) 149:5705–15. doi: 10.1007/s00432-022-04532-x
61. Wolf GT, Moyer JS, Kaplan MJ, Newman JG, Egan JE, Berinstein NL, et al. IRX-2 natural cytokine biologic for immunotherapy in patients with head and neck cancers. Onco Targets Ther. (2018) 11:3731–46. doi: 10.2147/OTT
62. Luedke E, Jaime-Ramirez AC, Bhave N, Roda J, Choudhary MM, Kumar B, et al. Cetuximab therapy in head and neck cancer: immune modulation with interleukin-12 and other natural killer cell-activating cytokines. Surgery. (2012) 152:431–40. doi: 10.1016/j.surg.2012.05.035
63. Chow LQM, Morishima C, Eaton KD, Baik CS, Goulart BH, Anderson LN, et al. Phase ib trial of the toll-like receptor 8 agonist, motolimod (VTX-2337), combined with cetuximab in patients with recurrent or metastatic SCCHN. Clin Cancer Res. (2017) 23:2442–50. doi: 10.1158/1078-0432.CCR-16-1934
64. Ferris RL, Saba NF, Gitlitz BJ, Haddad R, Sukari A, Neupane P, et al. Effect of adding motolimod to standard combination chemotherapy and cetuximab treatment of patients with squamous cell carcinoma of the head and neck: the active8 randomized clinical trial. JAMA Oncol. (2018) 4:1583–8. doi: 10.1001/jamaoncol.2018.1888
65. Crist M, Yaniv B, Palackdharry S, Lehn MA, Medvedovic M, Stone T, et al. Metformin increases natural killer cell functions in head and neck squamous cell carcinoma through CXCL1 inhibition. J immunotherapy Cancer. (2022) 10(11):e005632. doi: 10.1136/jitc-2022-005632
66. Lopez-Albaitero A, Lee SC, Morgan S, Grandis JR, Gooding WE, Ferrone S, et al. Role of polymorphic Fc gamma receptor IIIa and EGFR expression level in cetuximab mediated, NK cell dependent in vitro cytotoxicity of head and neck squamous cell carcinoma cells. Cancer Immunol Immunother. (2009) 58(11):1853–64. doi: 10.1007/s00262-009-0697-4
67. Taylor RJ, Chan SL, Wood A, Voskens CJ, Wolf JS, Lin W, et al. FcgammaRIIIa polymorphisms and cetuximab induced cytotoxicity in squamous cell carcinoma of the head and neck. Cancer Immunol Immunother. (2009) 58(7):997–1006. doi: 10.1007/s00262-008-0613-3
68. Vermorken JB, Mesia R, Rivera F, Remenar E, Kawecki A, Rottey S, et al. Platinum-based chemotherapy plus cetuximab in head and neck cancer. N Engl J Med. (2008) 359(11):1116–27. doi: 10.1056/NEJMc082096
69. Koyama T, Kiyota N, Boku S, Imamura Y, Shibata N, Satake H, et al. A phase II trial of paclitaxel plus biweekly cetuximab for patients with recurrent or metastatic head and neck cancer previously treated with both platinum-based chemotherapy and anti-PD-1 antibody. ESMO Open. (2024) 9(6):103476. doi: 10.1016/j.esmoop.2024.103476
70. Faden DL, Concha-Benavente F, Chakka AB, McMichael EL, Chandran U, Ferris RL, et al. Immunogenomic correlates of response to cetuximab monotherapy in head and neck squamous cell carcinoma. Head Neck. (2019) 41(8):2591–601. doi: 10.1002/hed.25726
71. Hanna JG, O'Neill A, Shin K-Y, Wong K, Jo VY, Quinn CT, et al. Neoadjuvant and adjuvant nivolumab and lirilumab in patients with recurrent, resectable squamous cell carcinoma of the head and neck. Clin Cancer Res. (2022) 28(3):468–78. doi: 10.1158/1078-0432.CCR-21-2635
72. Johnson JM, Vathiotis IA, Harshyne LA, Ali A, Bar Ad V, Axelrod R, et al. Nivolumab and ipilimumab in combination with radiotherapy in patients with high-risk locally advanced squamous cell carcinoma of the head and neck. J immunotherapy Cancer. (2023) 11(8):e007141. doi: 10.1136/jitc-2023-007141
73. Haddad R, Harrington K, Tahara MM, Ferris RL, Gillison M, Fayette J, et al. Nivolumab plus ipilimumab versus EXTREME regimen as first-line treatment for recurrent/metastatic squamous cell carcinoma of the head and neck: the final results of checkMate 651. J Clin Oncol. (2023) 41:2166–80. doi: 10.1200/JCO.22.00332
74. André P, Denis C, Soulas C, Bourbon-Caillet C, Lopez J, Arnoux T, et al. Anti-NKG2A mAb is a checkpoint inhibitor that promotes anti-tumor immunity by unleashing both T and NK cells. Cell. (2018) 175:1731–43.e13. doi: 10.1016/j.cell.2018.10.014
75. Patel SP, Alonso-Gordoa T, Banerjee S, Wang D, Naidoo J, Standifer NE, et al. Phase 1/2 study of monalizumab plus durvalumab in patients with advanced solid tumors. J immunotherapy Cancer. (2024) 12(2):e007340. doi: 10.1136/jitc-2023-007340
76. Simon F, Yahiya Y S. Durvalumab: A review in advanced biliary tract cancer. Target Oncol. (2023) 18(6):965–72. doi: 10.1007/s11523-023-01007-y
77. Gulati S, Crist M, Riaz MK, Takiar V, Lehn M, Monroe I, et al. Durvalumab plus cetuximab in patients with recurrent or metastatic head and neck squamous cell carcinoma: an open-label, nonrandomized, phase II clinical trial. Clin Cancer Res. (2023) 29(10):1906–15. doi: 10.1158/1078-0432.CCR-22-3886
78. Jean B, Bourhis J, Stein Al, Paul de Boer J, Van Den Eynde M, Gold KA, Stintzing S, et al. Avelumab and cetuximab as a therapeutic combination: An overview of scientific rationale and current clinical trials in cancer. Cancer Treat Rev. (2021) 97:102172. doi: 10.1016/j.ctrv.2021.102172
79. Tao YG, Aupérin A, Sun XS, Sire C, Martin L, Coutte A, et al. Avelumab-cetuximab-radiotherapy versus standards of care in locally advanced squamous-cell carcinoma of the head and neck: The safety phase of a randomised phase III trial GORTEC 2017-01 (REACH). Eur J Cancer. (2020) 141:21–29. doi: 10.1016/j.ejca.2020.09.008
80. Lee NY, Ferris RL, Psyrri A, Haddad RI, Tahara M, Bourhis J, et al. Avelumab plus standard-of-care chemoradiotherapy versus chemoradiotherapy alone in patients with locally advanced squamous cell carcinoma of the head and neck: a randomised, double-blind, placebo-controlled, multicentre, phase 3 trial. Lancet Oncol. (2021) 22(4):450–62. doi: 10.1016/S1470-2045(20)30737-3
81. Wang S, Xu GH, Li MB, Zheng JY, Wang YT, Feng XY, et al. M1 macrophage predicted efficacy of neoadjuvant camrelizumab combined with chemotherapy vs chemotherapy alone for locally advanced ESCC: A pilot study. Front Oncol. (2023) 13. doi: 10.3389/fonc.2023.1139990
82. Zhang ZJ, Wu B, Peng G, Xiao GX, Huang J, Ding Q, et al. Neoadjuvant chemoimmunotherapy for the treatment of locally advanced head and neck squamous cell carcinoma: A single-arm phase 2 clinical trial. Clin Cancer Res. (2022) 28(15):3268–76. doi: 10.1158/1078-0432.CCR-22-0666
83. Ju WT, Xia RH, Zhu DW, Dou SJ, Zhu GP, Dong MJ, et al. A pilot study of neoadjuvant combination of anti-PD-1 camrelizumab and VEGFR2 inhibitor apatinib for locally advanced resectable oral squamous cell carcinoma. Nat Commun. (2022) 13(1):5378. doi: 10.1038/s41467-022-33080-8
84. Girmm EA, Mazumder A, Zhang HZ, Rosenberg SA. Lymphokine-activated killer cell phenomenon. Lysis of natural killer-resistant fresh solid tumor cells by interleukin 2-activated autologous human peripheral blood lymphocytes. J Exp Med. (1982) 155(6):1823–41. doi: 10.1084/jem.155.6.1823
85. Wrangle JM, Patterson A, Johnson CB, Neitzke DJ, Mehrotra S, Denlinger CE, et al. IL-2 and beyond in cancer immunotherapy. J Interferon Cytokine Res. (2018) 38:45–68. doi: 10.1089/jir.2017.0101
86. Pinette A, McMichael E, Courtney NB, Duggan M, Benner BN, Choueiry F, et al. An IL-15-based superagonist ALT-803 enhances the NK cell response to cetuximab-treated squamous cell carcinoma of the head and neck. Cancer immunology immunotherapy: CII. (2019) 68:1379–89. doi: 10.1007/s00262-019-02372-2
87. Nakano H, Kishida T, Asada H, Shin-Ya M, Shinomiya T, Imanishi J, et al. Interleukin-21 triggers both cellular and humoral immune responses leading to therapeutic antitumor effects against head and neck squamous cell carcinoma. J Gene Med. (2006) 8:90–9. doi: 10.1002/jgm.817
88. McMichael EL, Benner B, Atwal LS, Courtney NB, Mo X, Davis ME, et al. A phase I/II trial of cetuximab in combination with interleukin-12 administered to patients with unresectable primary or recurrent head and neck squamous cell carcinoma. Clin Cancer Res. (2019) 25:4955–65. doi: 10.1158/1078-0432.CCR-18-2108
89. Matsui M, Kishida T, Nakano H, Yoshimoto K, Shin-Ya M, Shimada T, et al. Interleukin-27 activates natural killer cells and suppresses NK-resistant head and neck squamous cell carcinoma through inducing antibody-dependent cellular cytotoxicity. Cancer Res. (2009) 69:2523–30. doi: 10.1158/0008-5472.CAN-08-2793
90. Jochems C, Hodge JW, Fantini M, Fujii R, Morillon YM, Greiner JW, et al. An NK cell line (haNK) expressing high levels of granzyme and engineered to express the high affinity CD16 allele. Oncotarget. (2016) 7:86359–73. doi: 10.18632/oncotarget.13411
91. Fabian K, Padget MR, Donahue RN, Solocinski K, Robbins Y, Allen CT, et al. PD-L1 targeting high-affinity NK (t-haNK) cells induce direct antitumor effects and target suppressive MDSC populations. J immunotherapy Cancer. (2020) 8(1):e000450. doi: 10.1136/jitc-2019-000450
92. Jochems C, Hodge JW, Fantini M, Tsang KY, Vandeveer AJ, Gulley JL, et al. ADCC employing an NK cell line (haNK) expressing the high affinity CD16 allele with avelumab, an anti-PD-L1 antibody. Int J Cancer. (2017) 141:583–93. doi: 10.1002/ijc.30767
93. Hamanishi J, Mandai M, Matsumura N, Abiko K, Baba T, Konishi I, et al. PD-1/PD-L1 blockade in cancer treatment: perspectives and issues. Int J Clin Oncol. (2016) 21:462–73. doi: 10.1007/s10147-016-0959-z
94. Robbins Y, Greene S, Friedman J, Clavijo PE, Van Waes C, Fabian KP, et al. Tumor control via targeting PD-L1 with chimeric antigen receptor modified NK cells. eLife. (2020) 9:e54854. doi: 10.7554/eLife.54854
95. Solocinski K, Padget MR, Fabian KP, Wolfson B, Cecchi F, Hembrough T, et al. Overcoming hypoxia-induced functional suppression of NK cells. J immunotherapy Cancer. (2020) 8(1):e000246. doi: 10.1136/jitc-2019-000246
96. Lee M, Robbins Y, Sievers C, Friedman J, Abdul Sater H, Clavijo PE, et al. Chimeric antigen receptor engineered NK cellular immunotherapy overcomes the selection of T-cell escape variant cancer cells. J immunotherapy Cancer. (2021) 9(3):e002128. doi: 10.1136/jitc-2020-002128
97. Stephenson RM, Lim CM, Matthews M, Dietsch G, Hershberg R, Ferris RL, et al. TLR8 stimulation enhances cetuximab-mediated natural killer cell lysis of head and neck cancer cells and dendritic cell cross-priming of EGFR-specific CD8+ T cells. Cancer immunology immunotherapy: CII. (2013) 62:1347–57. doi: 10.1007/s00262-013-1437-3
98. Wu X, Yeerna H, Goto Y, Ando T, Wu VH, Zhang X, et al. Metformin inhibits progression of head and neck squamous cell carcinoma by acting directly on carcinoma-initiating cells. Cancer Res. (2019) 79:4360–70. doi: 10.1158/0008-5472.CAN-18-3525
99. Bochen F, Balensiefer B, Körner S, Bittenbring JT, Neumann F, Koch A, et al. Vitamin D deficiency in head and neck cancer patients - prevalence, prognostic value and impact on immune function. Oncoimmunology. (2018) 7:e1476817. doi: 10.1080/2162402X.2018.1476817
100. Chiang KC, Yeh CN, Hsu JT, Chen LW, Kuo SF, Sun CC, et al. MART-10, a novel vitamin D analog, inhibits head and neck squamous carcinoma cells growth through cell cycle arrest at G0/G1 with upregulation of p21 and p27 and downregulation of telomerase. J Steroid Biochem Mol Biol. (2013) 138:427–34. doi: 10.1016/j.jsbmb.2013.09.002
101. Greene S, Robbins Y, Mydlarz WK, Huynh AP, Schmitt NC, Friedman J, et al. Inhibition of MDSC trafficking with SX-682, a CXCR1/2 inhibitor, enhances NK-cell immunotherapy in head and neck cancer models. Clin Cancer Res. (2020) 26:1420–31. doi: 10.1158/1078-0432.CCR-19-2625
102. Friedman J, Morisada M, Sun L, Moore EC, Padget M, Hodge JW, et al. Inhibition of WEE1 kinase and cell cycle checkpoint activation sensitizes head and neck cancers to natural killer cell therapies. J immunotherapy Cancer. (2018) 6:59. doi: 10.1186/s40425-018-0374-2
103. Myers J, Miller J. Exploring the NK cell platform for cancer immunotherapy. Nat Rev Clin Oncol. (2021) 18:85–100. doi: 10.1038/s41571-020-0426-7
104. Heipertz EL, Zynda ER, Stav-Noraas TE, Hungler AD, Boucher SE, Kaur N, et al. Current perspectives on "Off-the-shelf" Allogeneic NK and CAR-NK cell therapies. Front Immunol. (2021) 12:732135. doi: 10.3389/fimmu.2021.732135
105. Rezvani K, Rouce R, Liu E, Shpall E. Engineering natural killer cells for cancer immunotherapy. Mol Ther. (2017) 25(8):1769–81. doi: 10.1016/j.ymthe.2017.06.012
106. Zhang Y, Wallace DL, de Lara MC, Ghattas H, Asquith B, Worth A, et al. In vivo kinetics of human natural killer cells: the effects of ageing and acute and chronic viral infection. Immunology. (2007) 121(2):258–65. doi: 10.1111/j.1365-2567.2007.02573.x
107. Wu ZX, Doondeea JB, Gholami AM, Janning MC, Lemeer S, Kramer K, et al. Quantitative chemical proteomics reveals new potential drug targets in head and neck cancer. Mol Cell Proteomics. (2011) 10(12):M111.011635. doi: 10.1074/mcp.M111.011635
108. Jacobs MT, Wong P, Zhou AY, Becker-Hapak M, Marin ND, Marsala L, et al. Memory-like differentiation, tumor-targeting mAbs, and chimeric antigen receptors enhance natural killer cell responses to head and neck cancer. Clin Cancer Res. (2023) 29(20):4196–208. doi: 10.1158/1078-0432.CCR-23-0156
109. Poorebrahim M, Quiros-Fernandez I, Marmé F, Burdach SE, Cid-Arregui A. A costimulatory chimeric antigen receptor targeting TROP2 enhances the cytotoxicity of NK cells expressing a T cell receptor reactive to human papillomavirus type 16 E7. Cancer Lett. (2023) 566:216242. doi: 10.1016/j.canlet.2023.216242
110. Nowak J, Bentele M, Kutle I, Zimmermann K, Lühmann JL, Steinemann D, et al. CAR-NK cells targeting HER1 (EGFR) show efficient anti-tumor activity against head and neck squamous cell carcinoma (HNSCC). Cancers. (2023) 15(12):3169. doi: 10.3390/cancers15123169
111. Haist C, Schulte E, Bartels N, Bister A, Poschinski Z, Ibach TC, et al. CD44v6-targeted CAR T-cells specifically eliminate CD44 isoform 6 expressing head/neck squamous cell carcinoma cells. Oral Oncol. (2021) 116:105259. doi: 10.1016/j.oraloncology.2021.105259
Keywords: NK cell, interleukin, HNSCC, immunotherapy, cetuximab
Citation: Dong S, Zhao M, Zhu J, Li T, Yan M, Xing K, Liu P, Yu S, Ma J and He H (2024) Natural killer cells: a future star for immunotherapy of head and neck squamous cell carcinoma. Front. Immunol. 15:1442673. doi: 10.3389/fimmu.2024.1442673
Received: 02 June 2024; Accepted: 15 July 2024;
Published: 21 August 2024.
Edited by:
Haitao Wang, National Cancer Institute (NIH), United StatesReviewed by:
Mark Elia Issa, Massachusetts General Hospital and Harvard Medical School, United StatesRan Wei, Changzhou University, China
Yang Wang, Changchun University of Chinese Medicine, China
Copyright © 2024 Dong, Zhao, Zhu, Li, Yan, Xing, Liu, Yu, Ma and He. This is an open-access article distributed under the terms of the Creative Commons Attribution License (CC BY). The use, distribution or reproduction in other forums is permitted, provided the original author(s) and the copyright owner(s) are credited and that the original publication in this journal is cited, in accordance with accepted academic practice. No use, distribution or reproduction is permitted which does not comply with these terms.
*Correspondence: Shan Yu, eXVzaGFuQGhyYm11LmVkdS5jbg==; Jian Ma, am1hQGhyYm11LmVkdS5jbg==; Hongjiang He, aGVob25namlhbmdAZW1zLmhyYm11LmVkdS5jbg==
†These authors have contributed equally to this work and share first authorship