- 1Department of Microbiology, School of Medicine, Hamadan University of Medical Sciences, Hamadan, Iran
- 2Department of Anatomical Sciences, School of Medicine, Hamadan University of Medical Sciences, Hamadan, Iran
- 3Department of Nutritional Sciences, School of Medicine, Hamadan University of Medical Sciences, Hamadan, Iran
- 4Nutrition Health Research Center, Hamadan University of Medical Sciences, Hamadan, Iran
Disruption in the wound-healing process is caused by the presence of bacteria and leads to major problems and delays in wound healing. The limitations of commonly used medicines for treating wound infections include drug toxicity, insufficient microbial coverage, poor penetration, and increased resistance. This study aimed to determine the effect of ciprofloxacin loaded in solid lipid nanoparticles (Cip-SLN) on Pseudomonas aeruginosa and ampiciliin-vancomycin loaded in solid lipid nanoparticles (Amp-Van-SLN) on Staphylococcus aureus in wounds. Antibiotics were encapsulated in SLNs using the double emulsion method and were characterized. The in-vitro effect of antibiotic-loaded nanoparticles on P. aeruginosa and S. aureus was assessed using well diffusion and MIC methods. Finally, the topical antibacterial activity of these nanoparticles against bacterial wound infection was measured in a mouse model. MIC results showed that in the first 24 hours, the free drug had a greater effect on inhibiting bacteria, and in 72 hours, the inhibitory effect of nanoparticles increased. There was no toxicity effect of 400 µg/mL of nanoparticles on cells. According to the findings, the groups treated with Cip-SLN and Amp-Van-SLN were more effective than the control group (untreated) in different concentrations. In the wound healing process, the group treated with solid lipid nanoparticles (SLNs) exhibited a greater epithelial thickness, indicating enhanced healing, compared to the group treated with the free drug. The use of SLN can increase the accumulation of antibiotics at the site of infection with a slow release of the drug due to its fatty nature, which leads to a significant inhibitory effect on bacteria and also improves wound healing.
1 Introduction
The skin serves as a vital barrier against microorganisms and pathogens, but when wounds occur, the risk of infection increases as normal flora and pathogens can enter the bloodstream (1). The medical field aims to expedite wound healing with fewer side effects to reduce infections, which remain a significant risk factor for disease and mortality despite advancements (2). Burn wounds, causing approximately 265,000 annual deaths worldwide, particularly affect low- and middle-income countries, often necessitating surgery and antimicrobial treatment for severe cases (3). Conversely, chronic ulcers are frequently linked to underlying conditions like diabetes or immunodeficiency, impairing the immune system’s ability to protect against infections which may lead to organ failure and death (4). Overall, approximately 10-20% of wounds become infected, posing considerable health risks, delaying healing, and increasing antibiotic usage, and treatment costs (5). The emergence of Methicillin-resistant S. aureus (MRSA) strains in response to methicillin during the 1960s raised concerns, forcing the adoption of vancomycin as the primary treatment. However, the rise of vancomycin-resistant S. aureus strains due to limited alternative treatment options became a global issue (6, 7). P. aeruginosa, found commonly in chronic wounds, poses challenges due to its resistance to various antibiotics, particularly in the presence of biofilms, hindering wound healing and leading to chronic conditions (8). Bacterial biofilms, containing pathogenic bacteria encased in an exopolysaccharide layer, exhibit increased resistance to immune responses and conventional antibiotics, complicating treatment (9). A biofilm structure contains pathogenic bacteria encapsulated in an exopolysaccharide layer, which communicate with each other through the secretion of signaling molecules that differentiate physiology, phenotype, and expression of altered genes compared to planktonic cells. Bacteria living in a biofilm are generally resistant to host immune responses and treatment (10). To enhance drug efficacy in wound management, Solid lipid nanoparticles (SLNs) have emerged as a promising drug delivery system to improve bioavailability and treatment efficiency (11). Nanoparticles, including SLNs, have shown potential in enhancing the effectiveness of antibiotics, particularly for wound healing applications when combined with antimicrobial (12). Nanocarriers carrying antibiotics have emerged as a promising option in drug delivery (13). Studies have shown that developing novel pharmaceuticals alone is ineffective due to the poor water solubility of certain drugs and the low viability of new drug molecules. Hence, focusing on developing drug delivery systems that can address these challenges is crucial. These carrier systems should be non-toxic, can encapsulate a sufficient amount of drug, and enable targeted and controlled drug release. Solid lipid nanoparticles (SLNs) have been investigated as a carrier system for various applications in recent studies. One of the key advantages of SLNs is that they are composed of natural, non-toxic, non-immunogenic, and biodegradable lipid molecules (14). The effectiveness of SLN loaded with antibiotics was evaluated in vitro and in vivo conditions to improve efficiency and minimize the use of conventional antibiotics in the treatment of bacterial wound infections. The study aimed to evaluate whether encapsulating antibiotics in solid lipid nanoparticles could improve their efficacy against wound infections by enhancing drug delivery, prolonging drug release, and reducing bacterial colonization in a mouse model.
2 Methods
2.1 Synthesis and evaluation of nanoparticles
The nanoparticle was synthesized by the double emulsion/melt dispersion technique. To find the optimal formulation, different percentages of lipid, surfactant, and antibiotic concentrations were investigated. Stearic acid was first heated to 70°C (5°C higher than the melting point), then pre-dissolved and warmed poloxamer 407/lecithin, and the antibiotics were added to the molten lipid, and magnetic stirring was used (150 rpm). After that, the mixture was homogenized with warm distilled water and the mixture was sonicated for 60 seconds at 45°C. In the second phase, heated tween-80 was added to the initial emulsion, and the mixture was homogenized by an ultrasonic device at 45°C for 2 minutes to obtain the second emulsion (15 seconds on, 5 seconds off). To stabilize the produced nanoparticles, the resultant mixture was gently added to cold distilled water (5°C) and disseminated in the solution for 5 minutes. For the preparation of free SLN, the same circumstances were observed (drug-free solid lipid nanoparticles). Finally, to separate the free drug, the nanoparticles were washed three times with distilled water using a high-speed centrifuge (25,000 rpm for 15 minutes). Almost 500 mg of nanoparticles was added to 1 mL glycerin and lyophilized at -80°C using a vacuum pump (lyophilizer). The nanoparticles were first dissolved in distilled water and sanitized with a 450 nm filter before being used in biological experiments (15).
2.2 Nanoparticle characterization and cytotoxicity assay
Mean particle size, PDI and zeta potential of nanoparticles were measured. The maximum wavelength (λ Max) of antibiotics was determined and diluted and the standard curve of the drug was plotted. The amount of loading and encapsulation of antibiotics in the synthesized nanoparticles was determined using a spectrophotometer (an indirect method). Briefly, 10 mg of nanoparticles were added to 10 mL of distilled water. Next, the resulting suspension was centrifuged at 1500 rpm for 15 minutes. The supernatant was analyzed using a spectrophotometer (2100 UV, USA) at the optimum wavelength for each antibiotic. The thermal behavior of optimal formulation and its components were evaluated by differential scanning calorimetry (DSC) (METTLER TOLEDO-DSC 1). For the spectroscopic examination of the samples, the synthesized lyophilized NP (optimal formulation) was mixed with some potassium bromide (KBr) simultaneously and converted into compact discs by a hydraulic compressor. The disks were then recorded in the path of infrared light in the middle IR range (400-400 cm-1) using an FTIR spectroscope (PerkinElmer, spectrum 400, America). Drug release from the synthesized nanoparticle was performed using the dialysis bag method (cut-off 12-14 kDa). Drug release was quantified by measuring the concentration of the released antibiotic in the surrounding solution at regular time intervals using a UV-visible spectrophotometer at the antibiotic’s maximum absorbance wavelength.
The stability of the optimal formulation in suspension was evaluated for the short-term (one week in terms of appearance and one month in terms of appearance and physicochemical properties) and long-term (6 to 12 months in terms of appearance and physicochemical properties). The emission scanning electron microscope (Fe-SEM) was used to examine the morphology of the nanoparticle. To evaluate the cytotoxicity of the synthesized nanoparticles and according to the instructions of the kit (Kiazist Kit/Iran), the human epidermoid carcinoma epithelial cell line (A431) was used. The dilutions studied were 25, 50, 100, 200 and 400, 800 µg of nanoparticles and free drugs. Cell viability was assessed using the MTT assay at 570 nm. The basis of comparisons was 100% survival of control samples.
2.3 Well diffusion and MIC
According to CLSI, recommended well diffusion and MIC methods for studied nanoparticle efficacy were used. For well diffusion assay, the bacterial suspension was diluted to yield 104 CFU/mL, which was then employed in the antibacterial studies. Using a sterile cotton swab, a lawn of bacterial culture prepared above was spread uniformly on the Muller–Hinton agar plates. Using a cylindrical glass tube were punched into the bacteria-coated Muller–Hinton agar plates. 100 µL of NP sample solution were added to each well and were incubated at 37°C for 18 h. The inhibition zone diameter which reflects the susceptibility of bacteria to the NPs, was measured (16).
According to the CLSI recommendations, the minimum inhibitory concentration (MIC) was established using the microdilution technique. To summarize, all strains were cultivated on Brain Heart Infusion agar, and three or four colonies were floated in fresh sterile saline solution to achieve an initial concentration of 0.5 McFarland (1.5 108 CFU/mL). 100 µL of the 1:100 diluted cell suspensions were dispensed in each well. The MICs were established as the lowest dilution of nanoparticles capable of inhibiting observable bacterial growth after a 24-hour incubation period at 37°C (16).
2.4 Wound formation and treatment
In this study, male rats aged 6 to 8 weeks were utilized to create surgical wounds, with each group consisting of five rats. The rats with infectious wounds caused by P. aeruginosa were treated with various compounds, including; 1) Cip-SLN 2) free ciprofloxacin, 3) free SLN and 4) positive control (Wounded rats were untreated) and Rats with infectious wounds by Staphylococcus strains were treated with 1) Van-Amp-SLN, 2) Free Van-Amp, 3) Van-SLN. 4) Amp-SLN 6) free SLN and 7) positive control (Wounded rats were untreated) Ketamine (50 mg/g body weight) and xylazine (5 mg/g body weight) were injected intraperitoneally for anesthesia. The back of earea of each male rat’s neck was disinfected and the hair in this region was shaved in a one-centimeter circular area. Subsequently, a one-centimeter incision was made to create a wound at this site, and 1.5×106 of P. aeruginosa bacteria were inoculated into the wound. The survival of each rat was monitored daily. Upon detection of infection in the wounds, sterile adhesive dressings were applied half an hour after administering the drug treatment. Samples were collected using sterile swabs every two days to assess the bacterial load. The wound healing process and sample collection continued for two weeks following the identification of wound infection. Animal studies were performed following the ARRIVE guidelines. The ethics committee of Hamadan University of Medical Sciences gave its approval for this study, by the US National Institutes of Health guidelines.
The CFU counting method was used to assess the number of live P. aeruginosa and S. aureus bacteria in wound samples for up to 14 days (every other day). Following the observation of the infection, a sterile swab was used to collect the samples, which were then transferred to a flask containing 1 mL of sterile normal saline. Different dilutions were prepared from the sample suspension and then 100 μl of each dilution was cultured on a chocolate agar plate for 24 hours in an incubator at 37°C. The colonies’ units were then counted and the findings were reported as (CFU/mL) (17).
2.5 Histopathology
The macroscopic examinations were carried out by watching the wound’s appearance and the daily healing process. All wound samples were isolated and immersed in 10% formalin for 24 hours. Light microscopy was used to analyze skin morphology, and collagen synthesis after a 5-micron diameter incision was made with a microtome device and hematoxylin-eosin (18).
2.6 Ethical statement
The study was conducted under the ethics approval code IR.UMSHA.REC.1400.770.
2.7 Statistical analysis
The data is presented as a mean deviation with three repetitions. Data were analyzed using SPSS software version 20 and one-way ANOVA. A comparison of microbial load in different treatment groups was performed using the Tukey test. P <0.05 was considered significant.
3 Results
3.1 Properties of nanoparticles
The average size, zeta potential, and PDI of Cip-SLN were (300 ± 25 nm), (−18.5 ± 2.5 mV) and (0.295 ± 0.032), respectively. The amount of loading and encapsulation of ciprofloxacin in the synthesized nanoparticle was 18.1 ± 1.4% and 96.8 ± 3.2%, respectively. Particle size, PDI, zeta potential, drugs loading, and encapsulation efficiency of Amp-Van-SLN were 290 ± 31 nm, 0.350 ± 0.022, −17.9 ± 2.3 mV, 15.7 ± 1.5%, and 95.15 ± 2.6%, respectively. The results of the stability test showed that the size of the nanoparticles did not change much until the ninth month, but after 12 months, the size of the nanoparticles showed an increase of 11.5% (Table 1). The results of morphology analysis with Fe-SEM showed that the particles were spherical and had a smooth surface with homogeneous dispersion, as shown in Figure 1, the synthesized nanoparticle has a size of about 250 to 400 nm according to the scale bar.
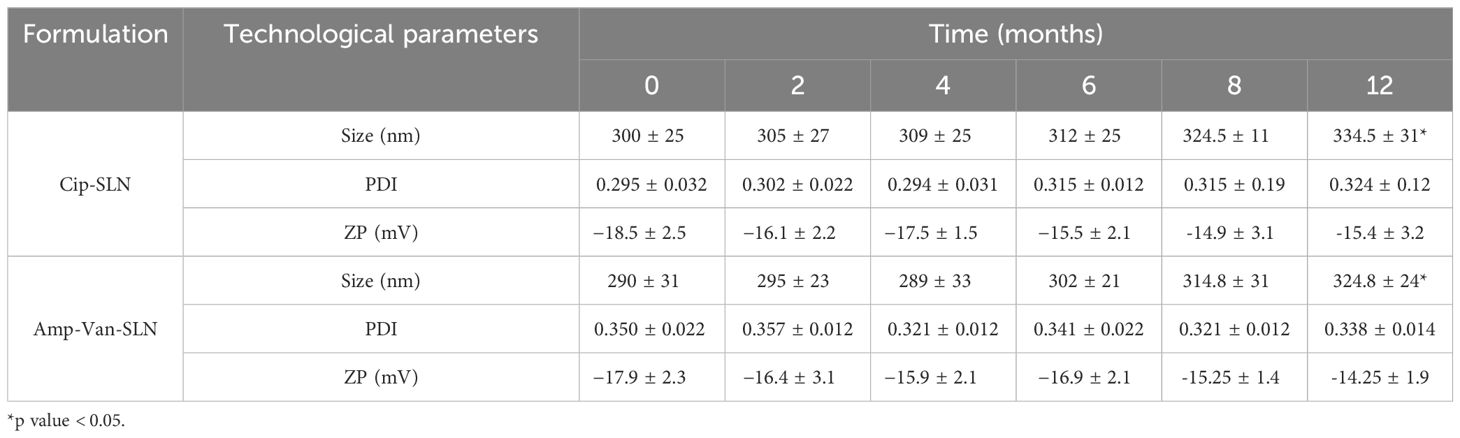
Table 1. Technological characteristics of NPs: average diameter, Polydispersity Index (PDI) and zeta potential (ZP) throughout stability study, (means ± SD, n = 3).
The results of FTIR and DSC showed that in the nanoparticle synthesis process, there was no chemical reaction that would create a new chemical compound, and drugs were placed in a molecular form in the lipid matrix and not in a crystalline and free form. In terms of the release time, the results showed that 82 hours are needed for 80% of drugs to be released from the nanoparticle (Figure 2).
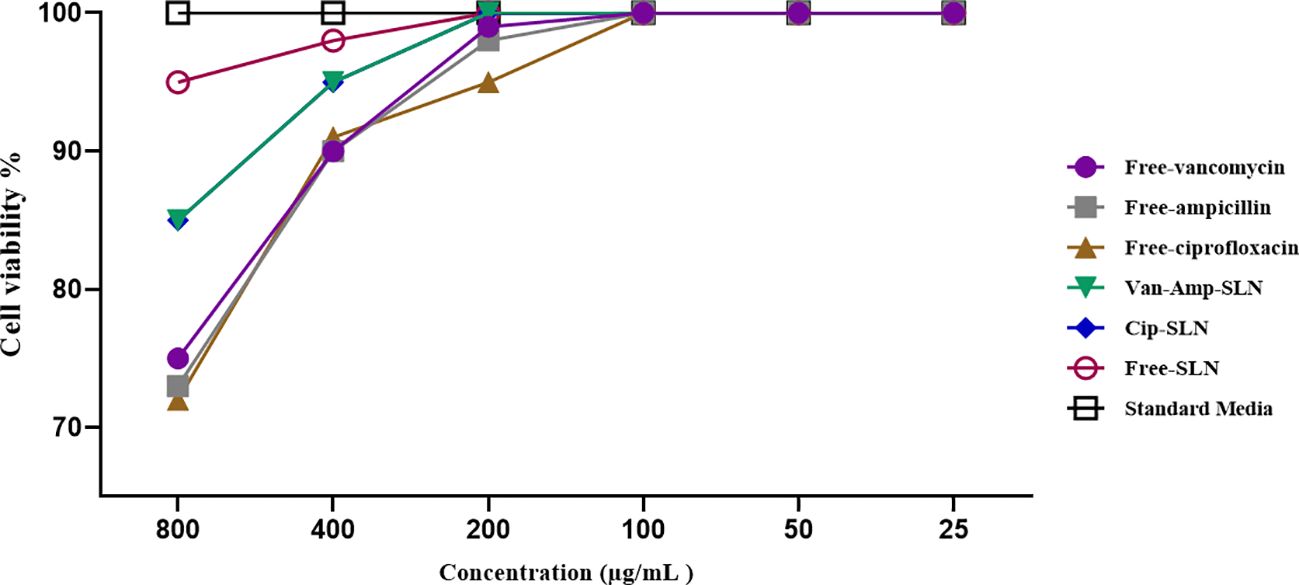
Figure 2. NPs and free drugs in vitro release profile from the SLN formulation in pH = 7.4 phosphate buffer (n = 3).
3.2 MIC and well diffusion results
According to agar well diffusion method data, free drugs had a greater effect on bacteria than nanoparticles. But, after 72 hours of incubation, the inhibition zone diameter of nanoparticles was increased gradually showing the slow release rate of nanoparticles (Tables 2, 3).
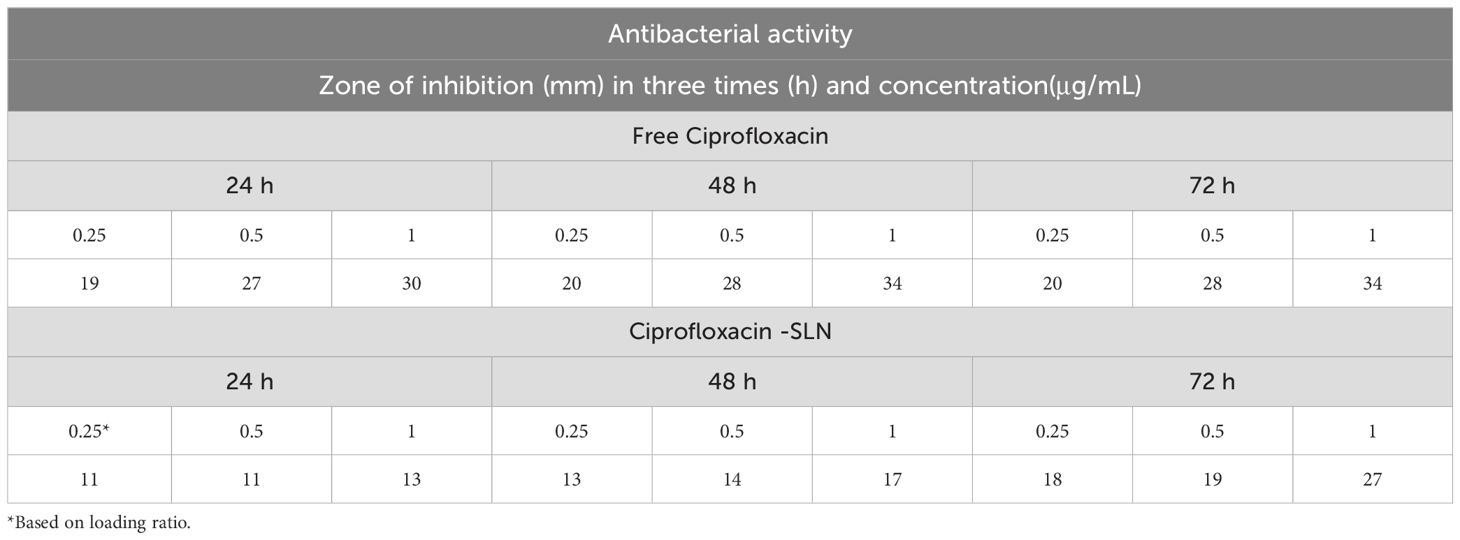
Table 2. Results of well diffusion test after Pseudomonas aeruginosa treatment with free ciprofloxacin and Cip-SLN.
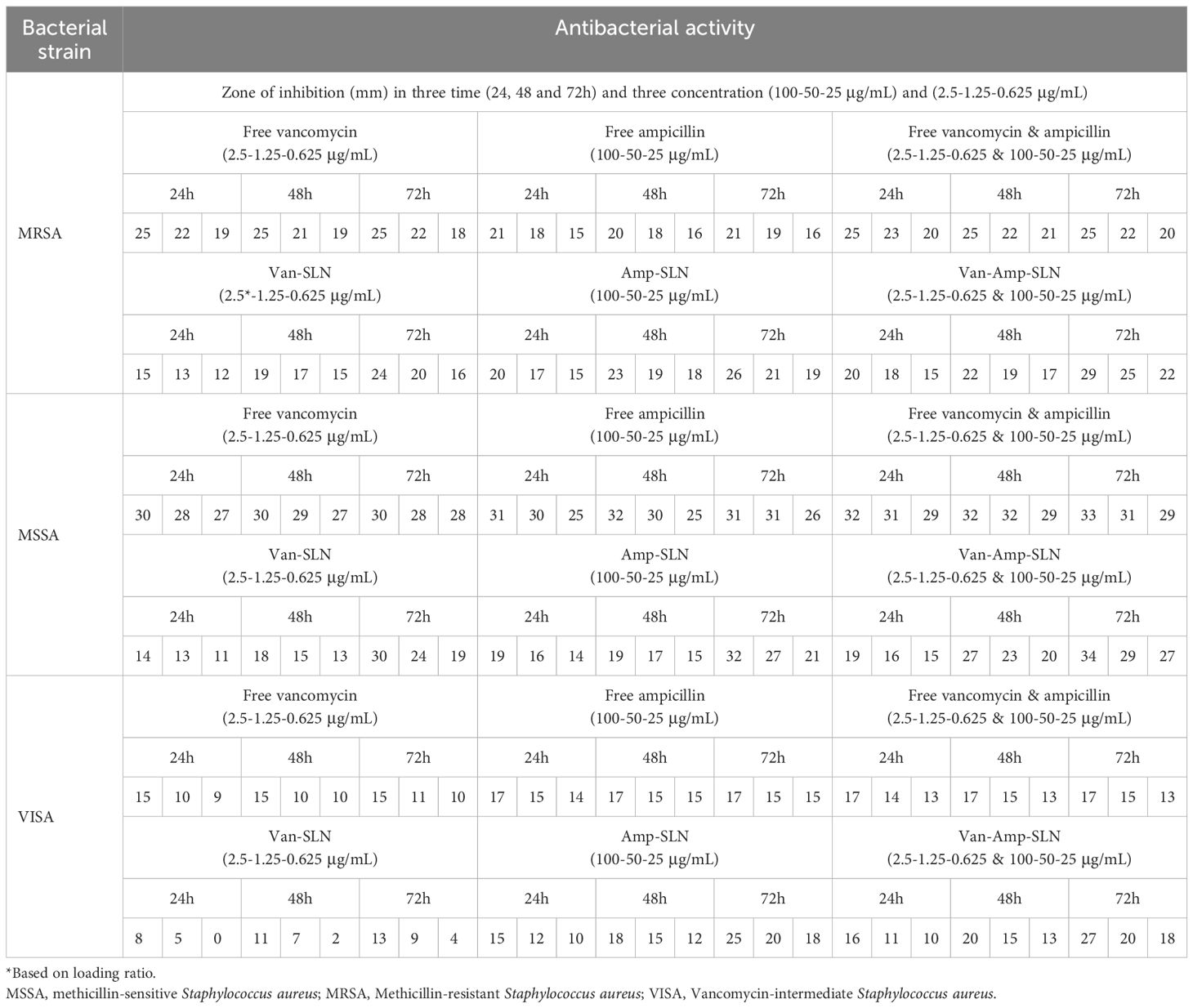
Table 3. Results of well diffusion and MIC test after Staphylococcus strains treatment with free drugs and NPs.
The results of MIC showed that in the first 24 hours, the free drug had a better effect on the bacteria by decreasing the mic, but it was equal to the passage time of the mic of the free drug and nanoparticle, and in 72 hours, the mic of the free drug increased (Figures 3, 4).
3.3 Cytotoxicity assay using MTT
Cytotoxicity investigation of different concentrations of free ciprofloxacin, free vancomycin, free ampicillin, Cip-SLN, Amp-Van-SLN, and free SLN on human epidermoid carcinoma epithelial cell line (A431) indicated that there was no toxic effect of the drugs and nanoparticles up to a concentration of 200 µg/mL (Figure 5).
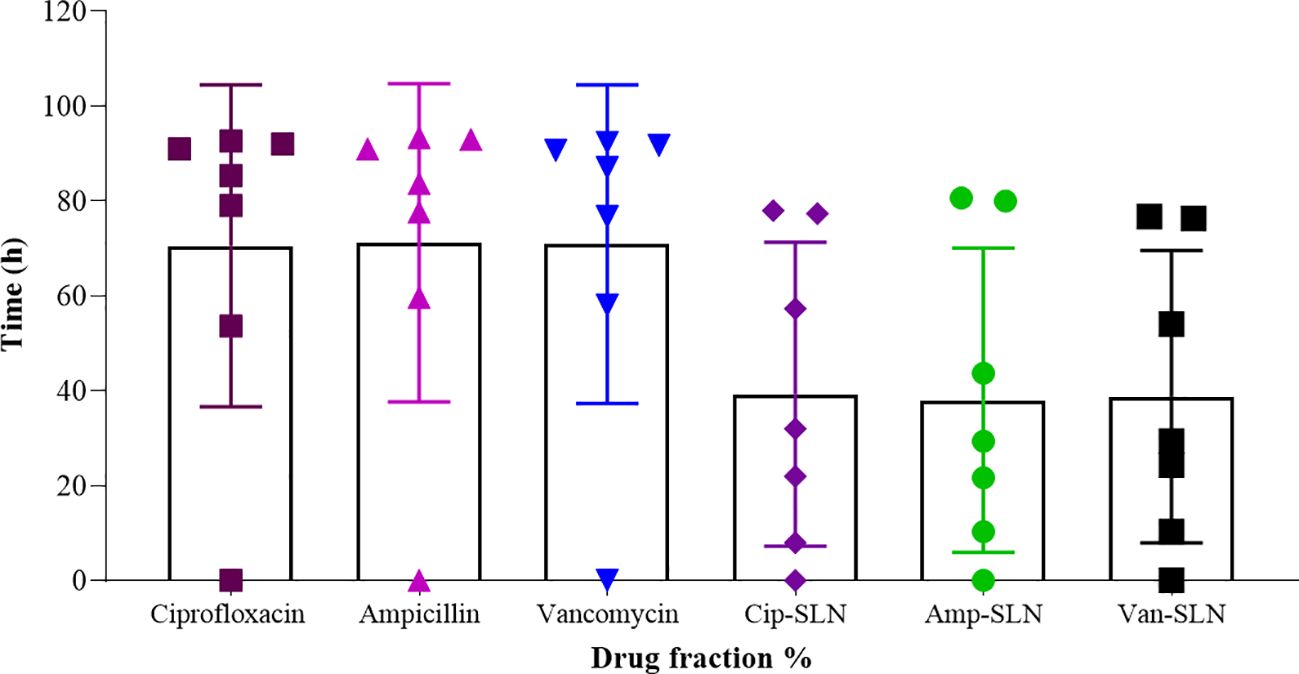
Figure 5. The effect of free drugs and NPs on fibroblast cell lines, the vertical axis shows the percentage of living cells and the horizontal axis shows the concentration of different formulations.
3.4 Antimicrobial test results
The effect of different formulations on reducing the number of S.aureus colonies in infectious wounds of different groups of rats is shown in Table 4. According to the results, the most efficacy was related to Amp-Van-SLN which significantly reduced 66% of the S.aureus colony count at the site of infection (P <0.05). Comparing the treated groups, it was found that nanoparticles containing two antibiotics have a better effect than free antibiotics and have significantly reduced the number of bacteria at the site of infection. The effect of Cip-SLN on reducing the number of P.aeruginosa colonies in infected wounds of rats in different groups is shown in Figure 6. According to the results, Cip-SLN was more effective than the control group (infection without treatment) in different concentrations, which caused a 64% reduction in the number of bacteria at the site of infection, which was remarkably significant (p<0.05). Also, Cip-SLN is more effective than free drugs and leads to a bacterial burden reduction at the site of infection.
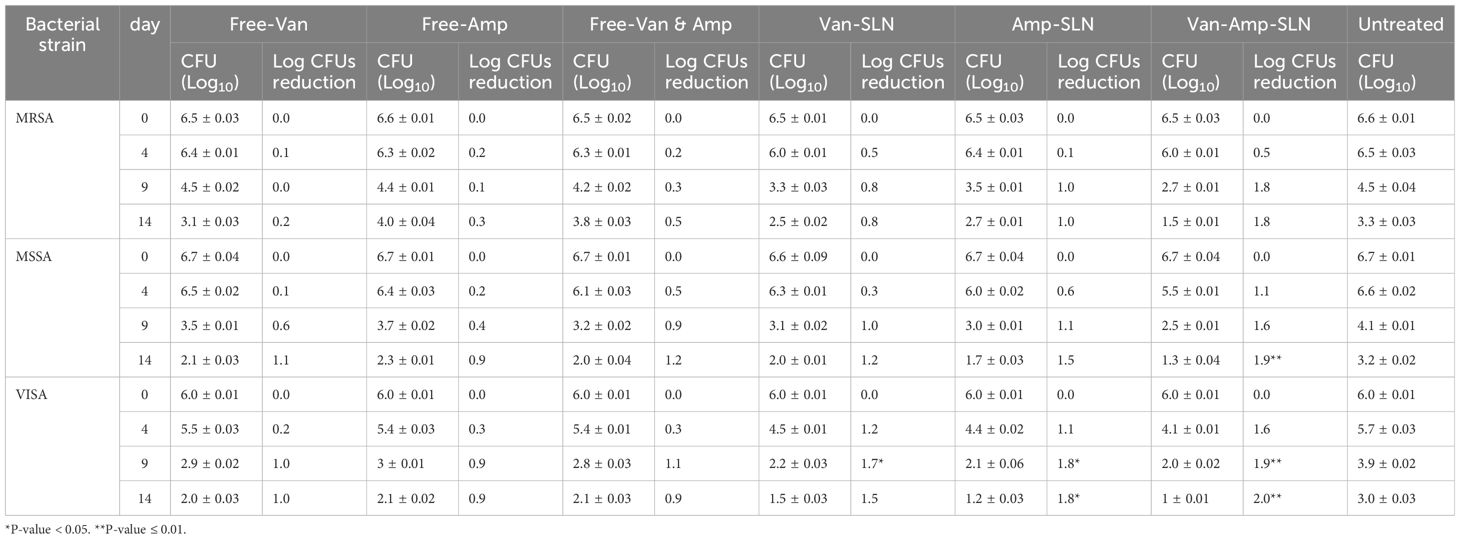
Table 4. Bacterial counts (CFU Log10) of rat skin at 4, 9, and 14 days before and after treatment with free drugs and NPs.
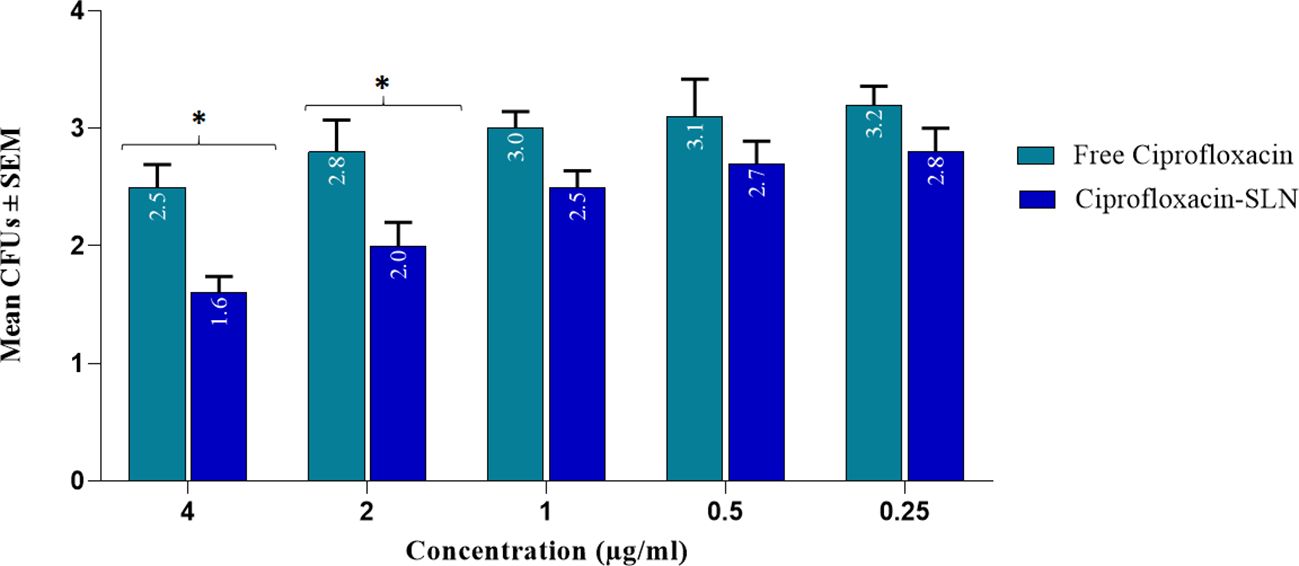
Figure 6. Bacterial counts (CFU Log10) of rat skin at 0, 4, 9, and 14 days after treatment with free ciprofloxacin and Cip-SLN, the vertical axis shows the average bacterial colony count (CFUs) and the horizontal axis shows the concentration value of free ciprofloxacin and ciprofloxacin-SLN. *p-value < 0.05.
3.5 Wound healing
The wound healing process in the Amp-Van-SLN groups compared with the control group (untreated infection) during two weeks is shown in Figure 7. Due to the effect of Amp-Van-SLN on the ability to reduce the number of bacteria at the site of infection, wound healing occurred earlier. In general, after 2 weeks of wound formation, the wounds healed in all treatment and control groups. The wound healing process of groups receiving Cip-SLN on different days compared to the control group (infection without treatment) is shown in Figure 8. Because Cip-SLN can reduce the number of bacteria at the site of infection, wound healing occurs earlier. In general, after two weeks of wound formation, the healing process occurred in all treated and control groups.
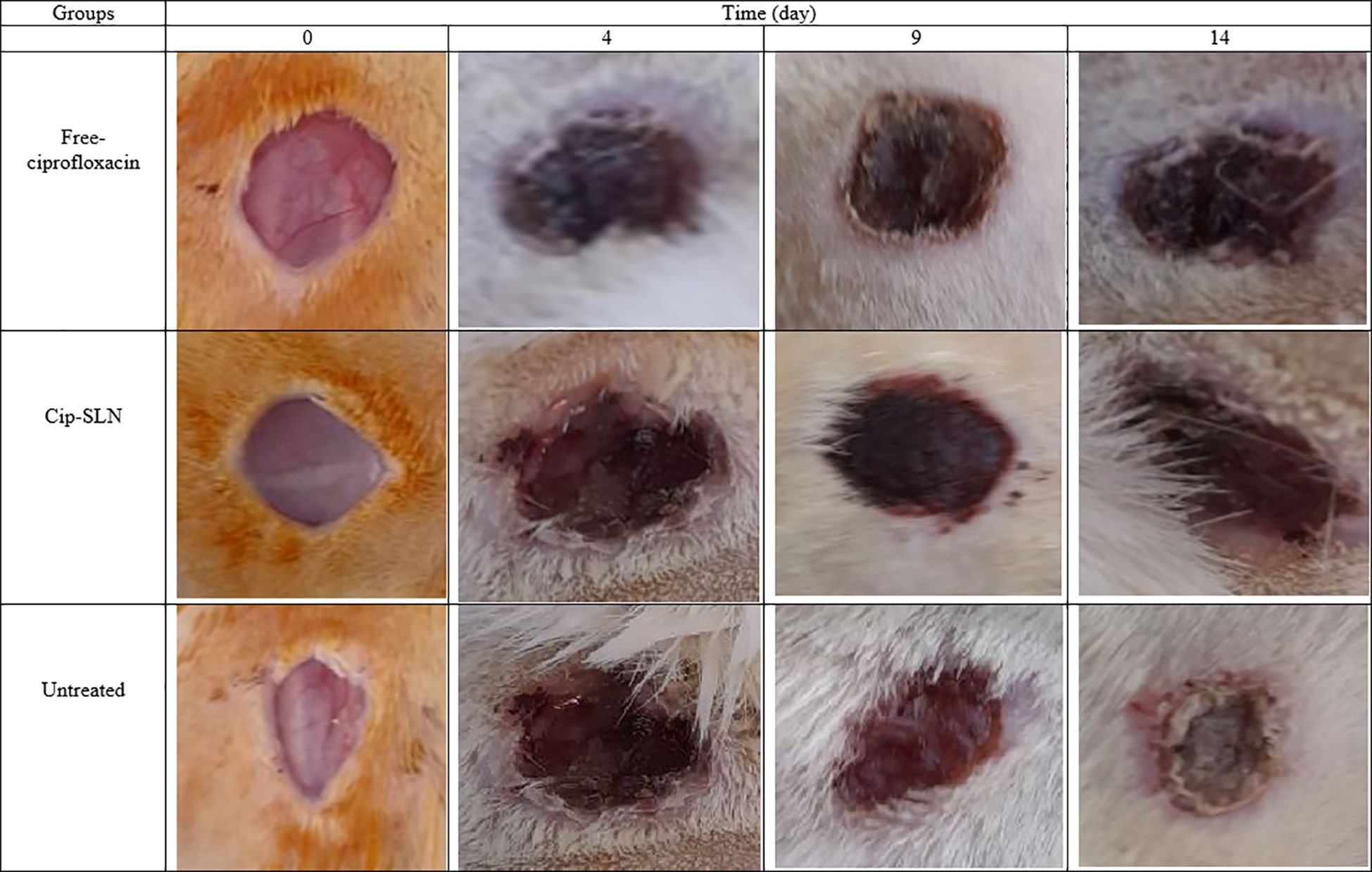
Figure 7. Representative images of change in skin and wound healing after treatment by free-Van-Amp and Van-Amp-SLN on days 0, 4, 9 and 14.
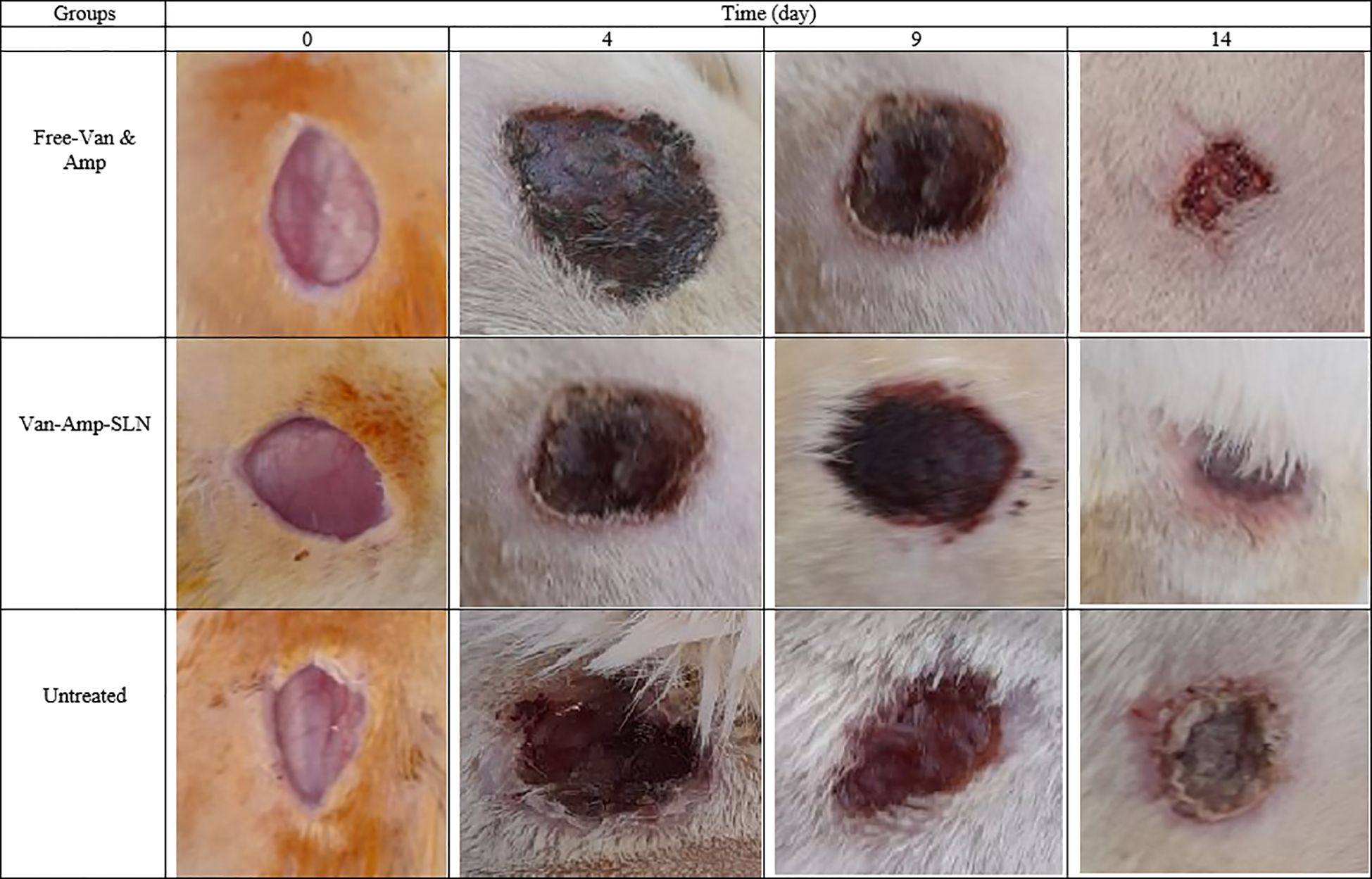
Figure 8. Representative images of change in skin and wound healing after treatment by free ciprofloxacin and Cip-SLN on days 0, 4, 9 and 14.
3.6 Histological analysis
The results showed that the healing process and regeneration of skin layers in all groups of rats were faster than in the control group. A comparison of the rate of epithelial cell formation in the treated groups is shown in Figure 9. It has been confirmed that using Van-Amp-SLN has about 50% greater epithelial diameter, confirming faster healing than other groups. Figure 10 indicated that the use of Cip-SLN at the same time has 150% more epithelial diameter, which confirms faster recovery and healing than the group receiving the free drug.
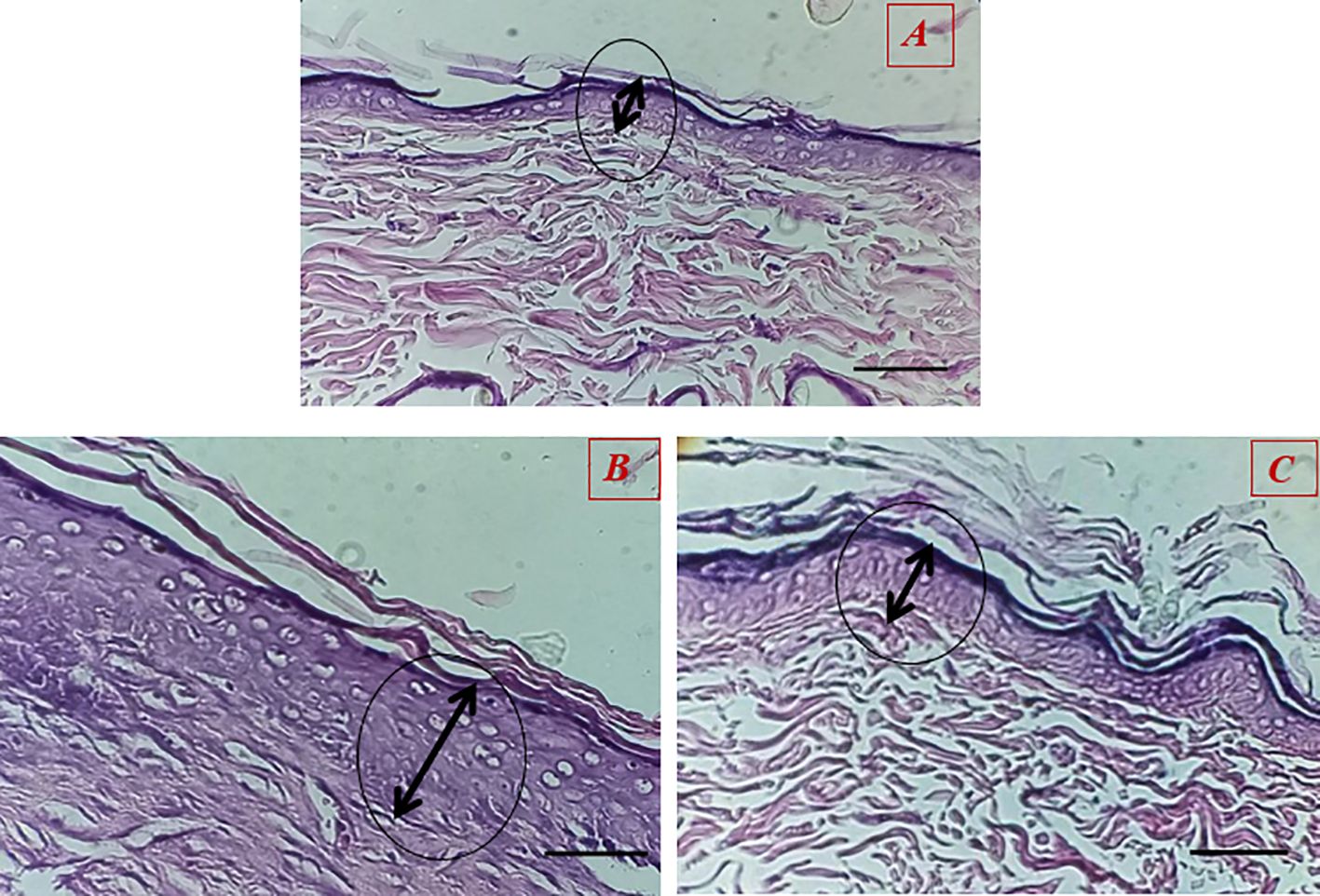
Figure 9. Histopathology of skin at day 14 stained with H&E, Epithelial layer thickness in treated groups (A) untreated groups, (B) Treated with Van-Amp-SLN, (C) Treated with free-Van-Amp.
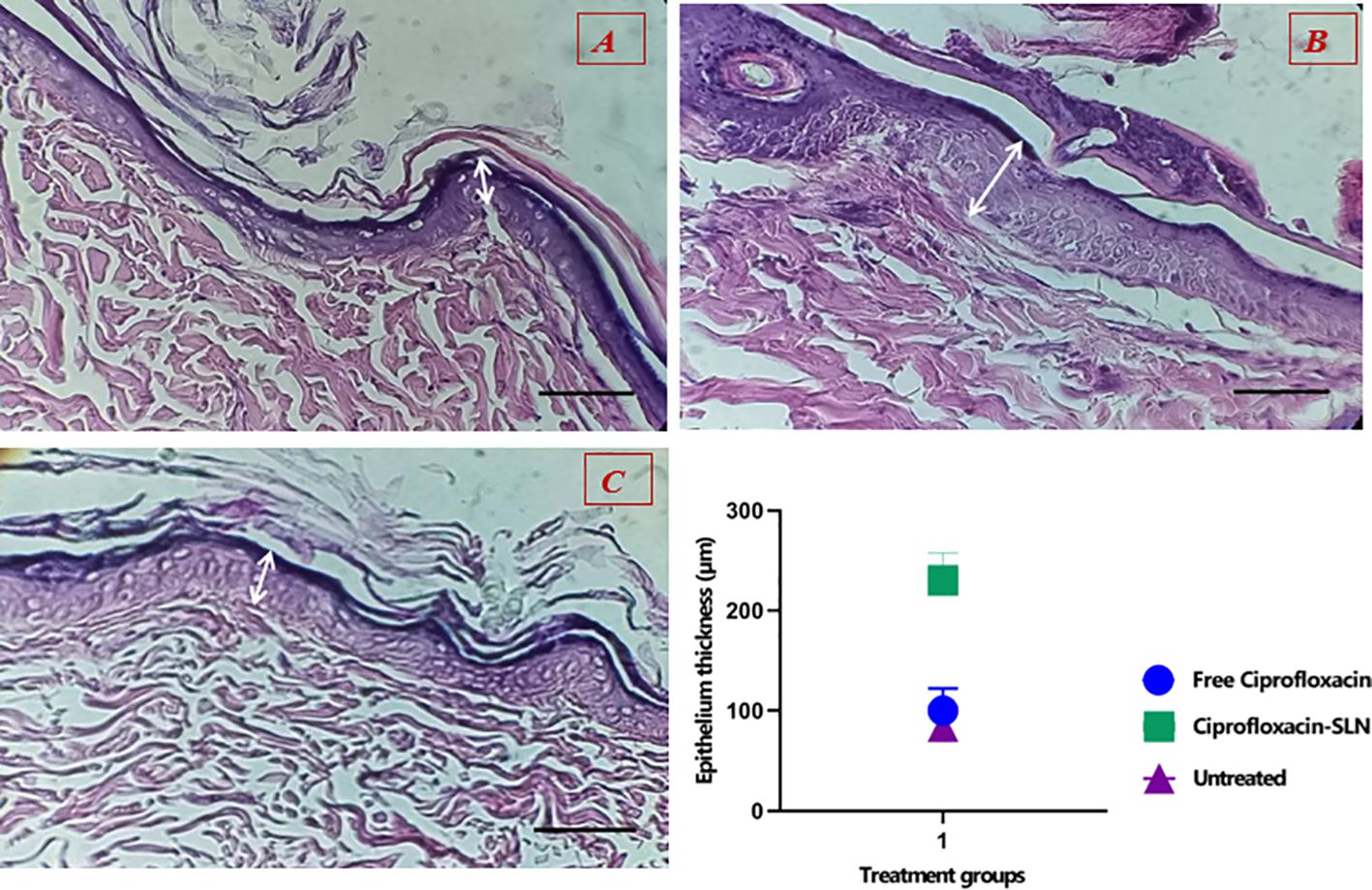
Figure 10. Histopathology of skin at day 14 stained with H&E, Epithelial layer thickness in treated groups (A) untreated groups, (B) Treated with Cip-SLN, (C) Treated with free ciprofloxacin.
4 Discussion
Antimicrobial resistance is a global health concern, and antibiotics have become ineffective against resistant infectious pathogens. MDR bacterial infections are estimated to kill 10 million people a year by 2050. Despite the availability of diverse antibiotic treatment options, their efficacy is constrained by the prevalence of drug-resistant bacteria. Moreover, this resistance contributes to economic repercussions, disease spread, and treatment failures, necessitating the development of innovative therapeutic approaches (19).
In this research, the double emulsion method was used to prepare nanoparticles which is a simple, inexpensive, and repeatable technique. This method needs excellent solvents and few amounts of surfactant. This method is a suitable structural model for encapsulating hydrophilic and lipophilic drugs such as ciprofloxacin, vancomycin, ampicillin, streptomycin, doxycycline, gentamicin and rifampicin (20–24). As the PDI value became closer to zero, the particles became more homogeneous. High zeta potential increases the stability of nanoparticles during treatment. Zeta potential is an important physical and chemical parameter affecting nanostructure stability (25). In the present study, the electric charge on the surface of the zeta potential particles was -18.5 ± 2.5 mV. This finding shows the high encapsulation efficiency without using organic solvents with less toxicity.
Stearic acid is a medium-chain fatty acid (molecular weight 284.48 g/mol) with a negative charge surface at neutral pH. Compared with the properties of any solid lipid, it is clear that stearic acid is the best choice for ciprofloxacin due to its good solubility. This lipid has a high drug-loading potential (26). In the optimal formulation, 600 mg of stearic acid was used, and the particle size was between 250 and 350 nm, but in the formulation that used 1200 mg, the particle size reached more than 400 nm.
Poloxamer 407 is a non-ionic surfactant that produces nanoparticles with a smaller size and less toxicity than Poloxamer 188, which prevents lipid matrix degradation in vivo (27). However, in this study, the use of Poloxamer 407 alone increased the size, PDI, and drug leakage. For this reason, a co-surfactant was used to solve this problem. Lipoid S-100 (derived from soy lecithin) was used in this study as a co-surfactant, which resulted in a smaller size, increased stability, and drug loading in the lipid matrix. Lipoid S-100 is a biodegradable and non-toxic phospholipid capable of emulsifying lipids and drugs to form water-in-oil or oil-in-water emulsions, which have been reported to be useful in various nanocarriers (27, 28). The present study confirmed that without the use of Lipoid S-100, the size of nanoparticles was higher than 700 nm, but with its use, the size was reduced to 250-350 nm.
According to the DSC results, it was determined that the drug is molecularly placed inside the lipid matrix. The disappearance of the peak related to ciprofloxacin in the DSC thermogram in the synthesized nanoparticle indicates the interaction of the drug with the lipid because the lipid tends to dissolve at 65°C. In the case of lyophilized nanoparticles, the peak shift of the drug to a lower temperature confirms its placement inside the solid lipid nanoparticles. One of the most important goals of the present study was the slow release of the drug from the nano-drug. The results showed that 82 hours are needed for 80% of the drug to be released from the synthesized nanoparticle. The nature of the lipid matrix, the concentration of surfactant and cosurfactant, and the parameters involved in the production of Cip-SLN are important and effective on the release of the drug from the nanoparticles, and the smaller the size of the nanoparticles, the duration of the release of the drug decreases due to the more contact of nanoparticles surface.
The findings of MIC and Well diffusion showed that there is no significant difference in the effect of free drug and nanoparticle on the studied bacterial strains, considering that in these methods, bacteria are directly exposed to the drug, so in the first 24 to 48 hours free form of antibiotics had a better effect than nanoparticles, but after 72 hours, the growth inhibition zone and the minimum inhibitory amount of nanoparticles and free form of antibiotics were not significantly different. It was similar to the results of the studies by Öztürk et al., which examined the properties of clarithromycin incorporated into SLN (29).
Another aim of this study was to investigate the effect of prepared nanoparticles on P. aeruginosa causing wound infection in laboratory animals. A comparison of the number of bacterial colonies in different treatment groups revealed that on the first day of the bacteria inoculation into the wounds, a large number of bacteria were seen at the site of infection. Sampling continued on days 4, 9, and 14. An important point and an interesting finding were that the rats that did not receive any drug, whether a free drug or nano-drug, recovered after 14 days and the number of bacteria in the wound site was significantly reduced. In the comparison between the drug-receiving groups and the untreated group, it was found that the bacterial colonies had a significant decrease (P<0.05). The results of comparing the treatment of infected wounds using nanoparticles and free drugs, it was found that nano-drugs have a better effect than free drugs and have reduced the number of bacteria at the site of infection. Cip-SLN had the best effect, which caused a 64% reduction of P. aeruginosa at the site of infection compared to the untreated group (P<0.05). The results of comparing the treatment of infectious wounds using Van-Amp-SLNs and free antibiotics showed that Van-Amp-SLNs have a better effect than free antibiotics and have reduced the number of bacteria at the site of infection. The best finding was related to Van-Amp-SLN, which reduced S. aureus by 66% at the site of infection compared to the untreated group (P <0.05).
These findings were consistent with the results of the study by Hajiahmadi et al. This group used liposomes loaded with vancomycin and lysostaphin to reduce the number of bacteria at the site of wounds infected with MRSA (17). Their findings showed that the use of liposomes loaded with vancomycin and lysostaphin significantly reduces the number of bacteria at the site of infection. One of the reasons for this is the slow and continuous release of the drug loaded in the nanoparticle matrix. Slow release results in the accumulation of more antibiotics at the infection site and may be more efficient than free medication. Wounds indicate a major health problem. Epithelialization, contraction, and deposition of connective tissue are processes that are effective in wound healing. The healing process largely depends on the regulated biosynthesis and deposition of new collagens and their subsequent maturation. In the process of tissue repair, inflammatory cells encourage the migration and proliferation of endothelial cells, leading to the neovascularization of connective tissue cells that synthesize extracellular matrices including collagen and keratinocytes, leading to re-epithelialization of the wounded tissue. Inflammation, collagen maturation, and scar formation are some of the multiple stages of wound healing that occur simultaneously but independently of each other (3, 17, 30).
Monitoring of the wounds infected by P. aeruginosa and different strains of S. aureus showed that after the injection of bacteria in the wound site, it takes 3 to 4 days for infected wounds to develop. The relative healing of the wound in all groups after two weeks of wound formation indicates wound healing in healthy rats. However, an important point was the healing time of wounds in different treated groups. The results showed that the lower number of bacteria in the wound could help the faster healing process. As seen in Figures 7 and 8, the wounds of rats treated with nanoparticles were cured 5 days earlier. Jun Tian and colleagues used silver nanoparticles to cure burns in rats and their findings showed that the use of silver nanoparticles caused burn healing 10 days earlier compared to free drugs and routine drugs used for burns (31).
Nikpasand et al. used titanium dioxide nanoparticles/gelatin to heal infected skin wounds and determined by daily measurement of the diameter of the created wounds that the use of these nanoparticles leads to faster healing of the wounds (32). This finding is consistent with the results of the present study. According to the results of pathology studies, it was found that the process of making epithelium happens faster in treated rats compared to untreated ones. According to Figures 9 and 10, the diameter of the epithelium formed in the group of rats receiving nanoparticles is significantly increased compared to rats receiving free drugs.
Xiaoxiao Wen et al. used silver sulfadiazine nanoparticles to treat burn infection wounds caused by E.coli and P. aeruginosa, their results showed that the use of nanoparticles has a greater effect on inhibiting bacteria and also causes faster healing of the wound, which is confirmed by our study (33). Other studies have also used nanoparticles to treat wound infections (34–37). However, the use of SLNs showed promising results in wound healing due to their high persistence on the wound surface. In the future, it is suggested to conduct studies using antibiotics or natural compounds such as curcumin using solid lipid nanoparticles in the treatment of infected wounds.
5 Conclusion
The results of this study revealed that by reducing the number of bacteria in infected wounds, the wound healing process happens faster. The use of SLNs due to their fatty nature and sufficient drug loading can increase the number of antibiotics at the site of infection with slow drug release, which leads to greater effects on reducing the number of bacteria and faster wound healing.
Data availability statement
The datasets presented in this study can be found in online repositories. The names of the repository/repositories and accession number(s) can be found in the article/supplementary material.
Ethics statement
The study was conducted under the ethics approval code IR.UMSHA.REC.1400.770. The study was conducted in accordance with the local legislation and institutional requirements.
Author contributions
MT: Methodology, Writing – original draft, Data curation, Investigation. MA: Investigation, Methodology, Writing – original draft. FK: Data curation, Investigation, Methodology, Software, Writing – original draft. SA: Formal analysis, Validation, Writing – original draft. MA: Methodology, Writing – original draft. SH: Conceptualization, Funding acquisition, Methodology, Validation, Writing – original draft, Writing – review & editing.
Funding
The author(s) declare that financial support was received for the research, authorship, and/or publication of this article. Hamadan University of Medical Sciences, Hamadan, Iran, funded this study (Grant No. 140011199612).
Acknowledgments
The authors are thankful to the Hamadan University of Medical Sciences for the financial support.
Conflict of interest
The authors declare that the research was conducted in the absence of any commercial or financial relationships that could be construed as a potential conflict of interest.
The author(s) declared that they were an editorial board member of Frontiers, at the time of submission. This had no impact on the peer review process and the final decision.
Publisher’s note
All claims expressed in this article are solely those of the authors and do not necessarily represent those of their affiliated organizations, or those of the publisher, the editors and the reviewers. Any product that may be evaluated in this article, or claim that may be made by its manufacturer, is not guaranteed or endorsed by the publisher.
References
1. Maheswary T, Nurul AA, Fauzi MB. The insights of microbes’ roles in wound healing: a comprehensive review. Pharmaceutics. (2021) 13:981. doi: 10.3390/pharmaceutics13070981
2. Gao J, Wang H, Liu X, Song X, Zhong X. Surgical site wound infection, and other postoperative problems after coronary artery bypass grafting in subjects with chronic obstructive pulmonary disease: A meta-analysis. Int Wound J. (2022) 20:302–12. doi: 10.1111/iwj.13877
3. Jahromi MAM, Zangabad PS, Basri SMM, Zangabad KS, Ghamarypour A, Aref AR, et al. Nanomedicine and advanced technologies for burns: Preventing infection and facilitating wound healing. Advanced Drug Delivery Rev. (2018) 123:33–64. doi: 10.1016/j.addr.2017.08.001
4. Schuijt TJ, Lankelma JM, Scicluna BP, e Melo F, Roelofs JJ, de Boer JD, et al. The gut microbiota plays a protective role in the host defence against pneumococcal pneumonia. Gut. (2016) 65:575–83. doi: 10.1136/gutjnl-2015-309728
5. Dieckmann R, Boone I, Brockmann SO, Hammerl JA, Kolb-Mäurer A, Goebeler M, et al. The risk of bacterial infection after tattooing: a systematic review of the literature. Deutsches Ärzteblatt Int. (2016) 113:665.
6. Hosseini SM, Farmany A, Arabestani MR. Effect of doxycycline-loaded solid lipid nanoparticles on serum level of trace elements, biochemical and hematological parameters in acute and chronic brucellosis. Biol Trace Element Res. (2020) 194:463–71. doi: 10.1007/s12011-019-01798-0
7. Hosseini SM, Farmany A, Alikhani MY, Taheri M, Asl SS, Alamian S, et al. Co-delivery of doxycycline and hydroxychloroquine using CdTe-labeled solid lipid nanoparticles for treatment of acute and chronic brucellosis. Front Chem. (2022) 10:890252. doi: 10.3389/fchem.2022.890252
8. Vanderwoude J, Fleming D, Azimi S, Trivedi U, Rumbaugh KP, Diggle SP. The evolution of virulence in Pseudomonas aeruginosa during chronic wound infection. Proc R Soc B. (2020) 287:20202272. doi: 10.1098/rspb.2020.2272
9. Gajula B, Munnamgi S, Basu S. How bacterial biofilms affect chronic wound healing: A narrative review. IJS Global Health. (2020) 3:e16. doi: 10.1097/GH9.0000000000000016
10. González JF, Hahn MM, Gunn JS. Chronic biofilm-based infections: skewing of the immune response. Pathog Dis. (2018) 76:fty023. doi: 10.1093/femspd/fty023
11. Pandya NT, Jani P, Vanza J, Tandel H. Solid lipid nanoparticles as an efficient drug delivery system of olmesartan medoxomil for the treatment of hypertension. Colloids Surfaces B: Biointerfaces. (2018) 165:37–44. doi: 10.1016/j.colsurfb.2018.02.011
12. Negut I, Grumezescu V, Grumezescu AM. Treatment strategies for infected wounds. Molecules. (2018) 23:2392. doi: 10.3390/molecules23092392
13. Singh A, Gautam PK, Verma A, Singh V, Shivapriya PM, Shivalkar S, et al. Green synthesis of metallic nanoparticles as effective alternatives to treat antibiotics resistant bacterial infections: A review. Biotechnol Rep. (2020) 25:e00427. doi: 10.1016/j.btre.2020.e00427
14. Salvi VR, Pawar P. Nanostructured lipid carriers (NLC) system: A novel drug targeting carrier. J Drug Delivery Sci Technol. (2019) 51:255–67. doi: 10.1016/j.jddst.2019.02.017
15. Peres LB, Peres LB, de Araújo PHH, Sayer C. Solid lipid nanoparticles for encapsulation of hydrophilic drugs by an organic solvent free double emulsion technique. Colloids Surfaces B: Biointerf. (2016) 140:317–23. doi: 10.1016/j.colsurfb.2015.12.033
16. Shariati A, Asadian E, Fallah F, Azimi T, Hashemi A, Yasbolaghi Sharahi J, et al. Evaluation of Nano-curcumin effects on expression levels of virulence genes and biofilm production of multidrug-resistant Pseudomonas aeruginosa isolated from burn wound infection in Tehran, Iran. Infection Drug Resistance. (2019) 12:2223–35. doi: 10.2147/IDR.S213200
17. Hajiahmadi F, Alikhani MY, Shariatifar H, Arabestani MR, Ahmadvand D. The bactericidal effect of lysostaphin coupled with liposomal vancomycin as a dual combating system applied directly on methicillin-resistant Staphylococcus aureus infected skin wounds in mice. Int J Nanomed. (2019) 14:5943. doi: 10.2147/IJN.S214521
18. Hosseini SM, Farmany A, Abbasalipourkabir R, Soleimani Asl S, Nourian A, Arabestani MR. Doxycycline-encapsulated solid lipid nanoparticles for the enhanced antibacterial potential to treat the chronic brucellosis and preventing its relapse: in vivo study. Ann Clin Microbiol Antimicrobials. (2019) 18:1–10. doi: 10.1186/s12941-019-0333-x
19. Thappeta KR, Vikhe YS, Yong AM, Chan-Park MB, Kline KA. Combined efficacy of an antimicrobial cationic peptide polymer with conventional antibiotics to combat multidrug-resistant pathogens. ACS Infect Dis. (2020) 6:1228–37. doi: 10.1021/acsinfecdis.0c00016
20. Pignatello R, Leonardi A, Fuochi V, Petronio G, Greco AS, Furneri PM. A method for efficient loading of ciprofloxacin hydrochloride in cationic solid lipid nanoparticles: Formulation and microbiological evaluation. Nanomaterials. (2018) 8:304. doi: 10.3390/nano8050304
21. Ibrahim UH, Devnarain N, Omolo CA, Mocktar C, Govender T. Biomimetic pH/lipase dual responsive vitamin-based solid lipid nanoparticles for on-demand delivery of vancomycin. Int J Pharmaceut. (2021) 607:120960. doi: 10.1016/j.ijpharm.2021.120960
22. Alihosseini F, Azarmi S, Ghaffari S, Haghighat S, Sorkhabadi SMR. Synergic antibacterial effect of curcumin with ampicillin; free drug solutions in comparison with SLN dispersions. Advanced Pharm Bull. (2016) 6:461. doi: 10.15171/apb.2016.060
23. Karimitabar Z, Chegini Z, Shokoohizadeh L, Moez NM, Arabestani MR, Hosseini SM. Use of the quantum dot-labeled solid lipid nanoparticles for delivery of streptomycin and hydroxychloroquine: A new therapeutic approach for treatment of intracellular Brucella abortus infection. Biomed Pharmacother. (2023) 158:114116. doi: 10.1016/j.biopha.2022.114116
24. Hosseini SM, Abbasalipourkabir R, Jalilian FA, Asl SS, Farmany A, Roshanaei G, et al. Doxycycline-encapsulated solid lipid nanoparticles as promising tool against Brucella melitensis enclosed in macrophage: a pharmacodynamics study on J774A. 1 Cell Line Antimicrobial Resistance Infection Control. (2019) 8:1–12. doi: 10.1186/s13756-019-0504-8
25. Hassanzadeh S, Nematollahzadeh A, Mirzayi B, Kaboli SF. Protein-based nanoparticles synthesized at a high shear rate and optimized for drug delivery applications. J Mol Liquids. (2021) 335:116133. doi: 10.1016/j.molliq.2021.116133
26. Ghaderkhani J, Yousefimashouf R, Arabestani M, Roshanaei G, Asl SS, Abbasalipourkabir R. Improved antibacterial function of Rifampicin-loaded solid lipid nanoparticles on Brucella abortus. Artif Cells Nanomed Biotechnol. (2019) 47:1181–93. doi: 10.1080/21691401.2019.1593858
27. Hippalgaonkar K, Majumdar S, Kansara V. Injectable lipid emulsions—advancements, opportunities and challenges. AAPS Pharmscitech. (2010) 11:1526–40. doi: 10.1208/s12249-010-9526-5
28. Singh RP, Gangadharappa H, Mruthunjaya K. Phospholipids: Unique carriers for drug delivery systems. J Drug Delivery Sci Technol. (2017) 39:166–79. doi: 10.1016/j.jddst.2017.03.027
29. Öztürk AA, Aygül A, Şenel B. Influence of glyceryl behenate, tripalmitin and stearic acid on the properties of clarithromycin incorporated solid lipid nanoparticles (SLNs): Formulation, characterization, antibacterial activity and cytotoxicity. J Drug Delivery Sci Technol. (2019) 54:101240. doi: 10.1016/j.jddst.2019.101240
30. Shende P, Gupta H. Formulation and comparative characterization of nanoparticles of curcumin using natural, synthetic and semi-synthetic polymers for wound healing. Life Sci. (2020) 253:117588. doi: 10.1016/j.lfs.2020.117588
31. Tian J, Wong KK, Ho CM, Lok CN, Yu WY, Che CM, et al. Topical delivery of silver nanoparticles promotes wound healing. ChemMedChem: Chem Enabling Drug Discovery. (2007) 2:129–36. doi: 10.1002/cmdc.200600171
32. Nikpasand A, Parvizi MR. Evaluation of the effect of titatnium dioxide nanoparticles/gelatin composite on infected skin wound healing; an animal model study. Bull Emergency Trauma. (2019) 7:366. doi: 10.29252/beat-070405
33. Wen X, Zheng Y, Wu J, Yue L, Wang C, Luan J, et al. In vitro and in vivo investigation of bacterial cellulose dressing containing uniform silver sulfadiazine nanoparticles for burn wound healing. Prog Natural Sci: Mater Int. (2015) 25:197–203. doi: 10.1016/j.pnsc.2015.05.004
34. Chang RYK, Das T, Manos J, Kutter E, Morales S, Chan H-K. Bacteriophage PEV20 and ciprofloxacin combination treatment enhances removal of Pseudomonas aeruginosa biofilm isolated from cystic fibrosis and wound patients. AAPS J. (2019) 21:1–8. doi: 10.1208/s12248-019-0315-0
35. Leu J-G, Chen S-A, Chen H-M, Wu W-M, Hung C-F, Yao Y-D, et al. The effects of gold nanoparticles in wound healing with antioxidant epigallocatechin gallate and α-lipoic acid. Nanomed: Nanotechnol Biol Med. (2012) 8:767–75. doi: 10.1016/j.nano.2011.08.013
36. Masson-Meyers DS, Andrade TA, Caetano GF, Guimaraes FR, Leite MN, Leite SN, et al. Experimental models and methods for cutaneous wound healing assessment. Int J Exp Pathol. (2020) 101:21–37. doi: 10.1111/iep.v101.1-2
Keywords: wound healing, ciprofloxacin, vancomycin, solid lipid nanoparticles, Pseudomonas aeruginosa, Staphylococcus aureus
Citation: Taheri M, Arabestani MR, Kalhori F, Soleimani Asl S, Asgari M and Hosseini SM (2024) Antibiotics-encapsulated nanoparticles as an antimicrobial agent in the treatment of wound infection. Front. Immunol. 15:1435151. doi: 10.3389/fimmu.2024.1435151
Received: 19 May 2024; Accepted: 08 October 2024;
Published: 29 October 2024.
Edited by:
José M. Lanao, University of Salamanca, SpainReviewed by:
Vivian Angelica Salazar Montoya, Catalan Institute of Nanoscience and Nanotechnology (CIN2), SpainPayal Gupta, Graphic Era University, India
Copyright © 2024 Taheri, Arabestani, Kalhori, Soleimani Asl, Asgari and Hosseini. This is an open-access article distributed under the terms of the Creative Commons Attribution License (CC BY). The use, distribution or reproduction in other forums is permitted, provided the original author(s) and the copyright owner(s) are credited and that the original publication in this journal is cited, in accordance with accepted academic practice. No use, distribution or reproduction is permitted which does not comply with these terms.
*Correspondence: Seyed Mostafa Hosseini, c21ob3NlaW55ODhAeWFob28uY29t