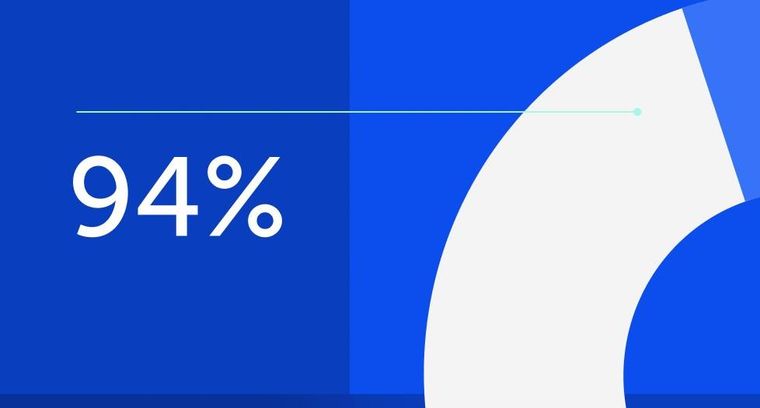
94% of researchers rate our articles as excellent or good
Learn more about the work of our research integrity team to safeguard the quality of each article we publish.
Find out more
ORIGINAL RESEARCH article
Front. Immunol., 04 October 2024
Sec. Viral Immunology
Volume 15 - 2024 | https://doi.org/10.3389/fimmu.2024.1434186
This article is part of the Research TopicChanges in T cell populations and cytokine production in SARS-CoV-2 infected individuals; their role in prognosisView all 20 articles
Background: IL-26 is a key mediator of pulmonary host defense given its abundant expression in human airways and its established antibacterial properties. Moreover, recent studies indicate that IL-26 can also inhibit viral replication. Along these lines, we have previously reported an increase in the plasma concentration of IL-26 among patients with acute COVID-19 that is linked to harmful hyperinflammation. Nevertheless, it is still unclear whether this systemic increase in IL-26 relates to disease severity, sex, comorbidities, viral load, or the innate immune response in acute COVID-19.
Methods: IL-26 was quantified using ELISA in plasma samples from a large cohort of well-characterized patients with acute COVID-19 (n=178) and healthy controls (n=30). The plasma concentrations of SARS-CoV-2 nucleocapsid and spike protein, as well as those of IFN-α2a, IFN-β, and IFN-γ, were determined using electrochemiluminescence immunoassay. The concentration of double-stranded DNA was determined using fluorometry.
Results: The plasma concentration of IL-26 was increased in patients with severe/critical COVID-19, particularly among males and patients with comorbid obstructive lung disease. Moreover, the concentration of IL-26 displayed positive correlations with length of hospital stay, as well as with systemic markers of viral load, antiviral immunity, and extracellular DNA.
Conclusions: Systemic IL-26 is involved in severe COVID-19, especially in males and patients with comorbid obstructive lung disease. These findings argue that systemic IL-26 has pathogenic and antiviral relevance, as well as biomarker potential.
Interleukin (IL)-26 is abundantly expressed in human airways (1), where it is thought to play a major role in host defense due to its potent anti-bacterial (2, 3) and neutrophil-mobilizing properties (4–6). Moreover, an expanding literature suggests that IL-26 participates in anti-viral immune responses. In fact, IL-26 was first identified in virally-infected T-lymphocytes (7), and IL-26 is often co-expressed with interferon (IFN)-γ in different lymphocyte subsets (8–10). Furthermore, IL-26 inhibits the replication of the Hepatitis C virus (HCV) in hepatocytes via direct binding to RNA replication intermediates (11). Given that both HCV and the severe acute respiratory syndrome coronavirus 2 (SARS-CoV-2) contain positive-sense single-stranded RNA genomes, it is plausible that IL-26 may play a comparable role in modulating the immune response against SARS-CoV-2. In line with this, we have previously demonstrated that stimulation of Toll-like receptors (TLRs) involved in the innate recognition of SARS-CoV-2 (i.e., TLR2, -3, -4, -7 and -8) triggers the release of IL-26 in different structural and immune cells isolated from human airways (3, 4, 12–17). In addition, we recently published evidence that systemic IL-26 is markedly increased in patients with acute coronavirus disease 2019 (COVID-19), and that this increase associates with hyperinflammation and tissue damage (18). Taken together, these findings highlight the potential of systemic IL-26 as a target for therapy in COVID-19. However, to evaluate this potential, we need to improve the understanding of how systemic IL-26 relates to disease severity and respiratory comorbidity.
In the present study, we utilized a large cohort of patients with acute COVID-19 and healthy controls to characterize how systemic IL-26 relates to disease severity, sex and comorbidities. We also addressed the corresponding associations with markers of viral load, the antiviral innate immune response, and a marker of NET formation and/or tissue damage. In doing so, we obtained evidence that systemic IL-26 associates with all the referred aspects of COVID-19, including respiratory comorbidity, thereby reinforcing the potential of systemic IL-26 as a target with clinical utility.
All COVID-19 patients admitted between February 2020 and January 2021 at the Department of Infectious Diseases, Sahlgrenska University Hospital (Gothenburg, Sweden) were eligible for inclusion. In parallel, a healthy control group was recruited for comparison Table 1. SARS-CoV-2 infection was confirmed via reverse transcriptase polymerase chain reaction (RT-PCR) from nasopharyngeal and throat aspirates, and all healthy controls had a negative test. Blood was collected at a single time-point and written informed consent was provided by all participants before sample collection. All procedures and handling of patient information were conducted in accordance with the World Medical Associations recommendations (the Declaration of Helsinki) and the ethical permit approved by the Swedish Ethical Review Authority (Diary No. 2020-01771). A subset of patients included in this study have been described previously (19–22).
The systemic protein concentration of IL-26 was assessed in plasma samples (1:2 dilution) via ELISA (15.6–4000 pg/ml detection range; Cusabio). The plasma concentrations of SARS-CoV-2 nucleocapsid and spike proteins (1:2 dilution), as well as the protein concentrations of IFN-α2a, IFN-β, and IFN-γ (all in undiluted samples), were determined using commercially available S-PLEX® kits (Meso Scale Discovery®) via electrochemiluminescence immunoassay (23). The detection ranges of these kits were as follows: 0.16 – 1100 pg/ml for SARS-CoV-2 nucleocapsid protein, 0.28 – 376 pg/ml for SARS-CoV-2 spike protein, 0.0053 – 33 pg/ml for IFN-α2a, 0.0065 – 33 pg/ml for IFN-β, and 0.0048 – 29 pg/ml for IFN-γ. The concentration of double-stranded DNA (dsDNA) in undiluted plasma samples was determined in a Qubit 3.0 Fluorometer using the Qubit 1X dsDNA BR Assay kit (20 – 20000 ng/ml detection range; both from ThermoFisher). Of note, the concentrations of viral antigens, interferons, and dsDNA could only be measured in 177, 149, and 176 samples, respectively, due to sample depletion.
Multiple comparisons were conducted via non-parametric Kruskal-Wallis test followed by Dunn’s post hoc test to compare each group against the others. Pairwise comparisons were performed by unpaired non-parametric Mann-Whitney test. Associations between two continuous variables were assessed by Spearman’s rank correlation test. Non-parametric methods were chosen given that all comparisons included at least one group that failed the D’Agostino & Pearson normality test. Pairwise comparisons of categorical data were conducted via chi-square (χ2) test. For the multivariable logistic regression analysis, all variables were transformed into categorical data as described in Table 2. For the transformation into categorical data of our results on viral antigens, IFNs, and dsDNA, we established whether each individual value was above or below the median of its corresponding COVID-19 severity group. The receiver operating characteristic (ROC) curve and its associated area under the curve were calculated to assess the prediction capacity of the generated logistic regression model.
One hundred and seventy-eight patients with COVID-19 and 30 age- and sex-matched healthy controls were enrolled in the study. The main characteristics of all study participants are summarized in Table 1 (see also Methods for more information). Disease severity was classified according to the COVID-19 Treatment Guidelines Panel of the National Institutes of Health (24). Mild disease included patients not requiring oxygen nor in-patient hospital care; moderate disease included hospitalized patients receiving oxygen therapy by mask or nasal cannula; and severe/critical disease included hospitalized patients in need of high flow nasal oxygen (HFNO) or mechanical ventilator. More than half of all patients with COVID-19 had at least one comorbidity regardless of disease severity, with hypertension, type 2 diabetes, and obstructive lung disease being the most frequent. In general, patients with severe/critical COVID-19 had prolonged length of hospital stay and a higher incidence of comorbidities.
The concentrations of IL-26 in plasma were higher in patients with severe/critical COVID-19 compared to those with mild and moderate disease, as well as to healthy controls (Figure 1A). Moreover, the number of plasma samples with a concentration of IL-26 above the lower limit of detection (LLOD) increased from 10% in samples from healthy controls and patients with mild COVID-19, to 30 and 55% in samples from patients with moderate and severe/critical COVID-19, respectively (Figure 1B). In addition, the concentration of IL-26 in plasma displayed positive correlations with disease severity (Figure 1C) and length of hospital stay (Figure 1D) in patients with COVID-19.
Figure 1. Relationship between the plasma concentration of IL-26 and severity of COVID-19. (A) Comparison of the plasma concentrations of IL-26 among healthy controls (n=30) and patients with mild (n=13), moderate (Mod.; n=96), or severe/critical (n=69) COVID-19 by Kruskal-Wallis test followed by Dunn’s post hoc test. Red horizontal lines represent the median. (B) Percentage of samples in which the plasma concentration of IL-26 fell below (black) or above (red) the lower limit of detection (LLOD) in each study group. Spearman correlation analyses of the plasma concentration of IL-26 with (C) the COVID-19 severity scale (1=mild, 2=moderate, and 3=severe/critical) and (D) the length of hospital stay of each study participant (n=178). *p < 0.05, **p < 0.005, ***p < 0.0005, ns, not significant.
Notably, the concentrations of IL-26 in plasma were higher in males than in females with COVID-19 (Figure 2A) and the number of plasma samples with a concentration of IL-26 above the LLOD were higher (χ2 test: p = 0.0187) in males than in females with COVID-19 (Figure 2B).
Figure 2. Relationship between the plasma concentration of IL-26 and sex in patients with COVID-19. (A) Comparison of the plasma concentrations of IL-26 between female (n=53) and male (n=125) patients with COVID-19 by unpaired Mann-Whitney test. Red horizontal lines represent the median. (B) Percentage of samples in which the plasma concentration of IL-26 fell below (black) or above (red) the lower limit of detection (LLOD) in each study group. *p < 0.05.
The median time between onset of symptoms and sample collection was similar across groups (9 days; Table 1) and did not display a statistically significant correlation with the concentration of IL-26 when all samples were included (Figure 3A). Moreover, comparison of the IL-26 concentrations in samples collected before or after the median time from onset of symptoms revealed no statistically significant differences when all samples were included (Figures 3B, C). Furthermore, the number of samples with a concentration of IL-26 above the LLOD was similar (χ2 test: p = 0.5714; Supplementary Figure 1) in samples collected before or after the median time from onset of symptoms. However, the concentration of IL-26 displayed a negative correlation with the time between onset of symptoms and sample collection when all IL-26 measurements below the lower limit of detection were excluded (Figure 3D). In addition, exclusion of samples with an IL-26 concentration below the LLOD revealed that samples collected within 9 days from onset of symptoms had a higher IL-26 concentration than those collected afterwards in patients with severe/critical COVID-19 exclusively (Figures 3E, F). Of note, samples from patients with mild COVID-19 were excluded from this analysis given that only 1 of them had an IL-26 concentration above the lower limit of detection (Figure 1A).
Figure 3. Relationship between the plasma concentration of IL-26 and the time between onset of symptoms and sample collection in patients with COVID-19. (A, D) Spearman correlation analyses of the plasma concentrations of IL-26 with the time between onset of symptoms and sample collection in (A) all samples (n=178) and (D) only samples with an IL-26 concentration above the lower limit of detection (LLOD; n=67). Comparisons of the plasma concentrations of IL-26 between samples collected before or after the median time since onset of symptoms in patients with (B, E) moderate or (C, F) severe/critical COVID-19 including (B, C) all samples, or (E, F) only samples with an IL-26 concentration above the LLOD, by unpaired Mann-Whitney test. Red horizontal lines represent the median. **p < 0.005, ns, not significant.
We also investigated whether common comorbidities had an impact on the plasma concentration of IL-26 among all patients with COVID-19 and found that patients with a prior diagnosis of obstructive lung disease (asthma: n=22; COPD: n=4; asthma and COPD: n=1) displayed a higher plasma concentration of IL-26 (Figure 4A) and a clearly higher (χ2 test: p = 0.0370) number of samples with a concentration of IL-26 above the lower limit of detection (Figure 4B). In contrast, no matching differences were observed in patients with comorbid hypertension or diabetes type 2 (Supplementary Figure 2). A similar increase in IL-26 concentrations and number of samples above the LLOD (χ2 test: p = 0.0634) was observed for obstructive lung disease in patients with severe/critical COVID-19 (Figures 4C, D). Importantly, patients with COVID-19 who took corticosteroids as part of their treatment had similar plasma concentrations of IL-26 and a similar number of measurements above the LLOD (χ2 test: p = 0.3608) than those who did not receive corticosteroids (Supplementary Figure 3).
Figure 4. Relationship between the plasma concentration of IL-26 and the respiratory comorbidity obstructive lung disease in patients with COVID-19. (A, C) Comparisons of the plasma concentrations of IL-26 between patients with and without comorbid obstructive lung disease including (A) all patients with COVID-19 (n=178) or (C) only those with severe/critical COVID-19 (n=69) by (A) two-tailed or (C) one-tailed unpaired Mann-Whitney test. Red horizontal lines represent the median. (B, D) Percentage of samples in which the plasma concentration of IL-26 fell below (black) or above (red) the lower limit of detection (LLOD) in each study group including (B) all patients with COVID-19 (n=178) or (C) only those with severe/critical COVID-19. *p < 0.05, ns, not significant.
To determine whether a higher viral load was linked to an increase in IL-26, we first quantified the plasma concentrations of two SARS-CoV-2 antigens in patients with COVID-19. As expected, higher concentrations of SARS-CoV-2 nucleocapsid and spike protein corresponded with more severe disease (Figures 5A, B). Moreover, the plasma concentration of IL-26 displayed positive correlations with both markers of viral load in patients with COVID-19 (Figures 5C, D).
Figure 5. Relationship between plasma concentration of IL-26 and markers of viral load in patients with COVID-19. Comparison of the plasma concentrations of SARS-CoV-2 (A) nucleocapsid and (B) spike protein among patients with mild (n=13), moderate (n=95), or severe/critical (n=69) COVID-19 by Kruskal-Wallis test followed by Dunn’s post hoc test. Spearman correlation analyses of the plasma concentration of IL-26 with those of (C) SARS-CoV-2 nucleocapsid and (D) SARS-CoV-2 spike protein (n=177). Red horizontal lines represent the median. ***p < 0.0005, ****p < 0.0001.
We also assessed whether the increase in plasma IL-26 associates with an increase in interferons—key markers of the antiviral innate immune response. Notably, the plasma concentrations of IFN-α2a, IFN-β, and IFN-γ were increased in patients with moderate or severe/critical COVID-19 compared to those with mild disease (Figures 6A–C). Moreover, the concentrations of these IFNs displayed positive correlations with that of IL-26 (Figures 6D–F).
Figure 6. Relationship between plasma concentration of IL-26 and production of interferons in patients with COVID-19. Comparison of the plasma concentrations of (A) IFN-α2a, (B) IFN-β, and (C) IFN-γ among patients with mild (n=12), moderate (n=76), or severe/critical (n=61) COVID-19 by Kruskal-Wallis test followed by Dunn’s post hoc test. Spearman correlation analyses of the plasma concentration of IL-26 with those of (D) IFN-α2a, (E) IFN-β, and (F) IFN-γ (n=149). Red horizontal lines represent the median. *p < 0.05, **p < 0.005, ***p< 0.0005, ns, not significant.
A higher concentration of dsDNA in plasma, which suggests increased NET production and/or tissue damage, was associated with more severe COVID-19 (Figure 7A) and a higher concentration of IL-26 (Figure 7B).
Figure 7. Relationship between the plasma concentration of IL-26 and double-stranded DNA (dsDNA) production in patients with COVID-19. (A) Comparison of the plasma concentrations of dsDNA among healthy controls (n=30) and patients with mild (n=13), moderate (n=94), or severe/critical (n=69) COVID-19 by Kruskal-Wallis test followed by Dunn’s post hoc test. (B) Spearman correlation analysis of the plasma concentration of IL-26 with that of dsDNA (n=176). Red horizontal lines represent the median. **p < 0.005, ****p < 0.0001, ns, not significant.
Finally, we performed a multivariable logistic regression analysis (Table 2) to determine whether each of the variables mentioned above associated with having a plasma concentration of IL-26 above the LLOD or not (Yes/No binary). We found that patients with severe/critical COVID-19 and males were 3.420 and 2.552 times more likely to have an IL-26 measurement (ELISA) above the LLOD than patients with mild/moderate COVID-19 and females, respectively. Notably, we found that patients with comorbid obstructive lung disease were 4.270 times more likely to have an IL-26 measurement above the LLOD than patients without this comorbidity. In addition, we found that patients with high plasma concentrations of IFN-γ and dsDNA (above their corresponding medians) were 2.107 and 2.532 times more likely to have an IL-26 measurement above the LLOD, respectively. On the other hand, time between onset of symptoms and sample collection, and the plasma concentrations of viral antigens, IFN-α2a or IFN-β were not associated with having IL-26 measurements above the LLOD. Importantly, all variables had a variance inflation factor (VIF) close to 1, which indicates low multicollinearity. Moreover, we calculated a ROC curve for this analysis (Supplementary Figure 4), and its associated area under the curve was 0.7881, which indicates that these variables had a good capacity to predict whether an IL-26 measurement would fall above the LLOD or not.
In essence, the results of the current study substantially expand the evidence that IL-26 participates in the immune response against SARS-CoV-2, as well as in the hyperinflammation that characterizes severe cases of COVID-19, in several different ways.
First, and most important, we show that the increase in the plasma concentrations of IL-26 is most pronounced in patients with severe/critical disease and that it displays a positive correlation with length of hospital stay. This is in contrast with a previous study by a different group which reported no differences in the serum concentration of IL-26 among patients with mild, moderate, and severe COVID-19 (25). Nevertheless, we think that the limited size (n = 27) of the study by Caterino M. et al., and the fact that it only included 6 study participants with severe disease, made it difficult to identify statistical differences among sub-groups of varying disease severity. For the very same reasons, we previously failed to detect a difference in the plasma concentration of IL-26 between patients with moderate and severe COVID-19—or a significant correlation with length of hospital stay—in a small cohort (n = 49) that we used in a recent pilot study (18).
Second, we found that the plasma concentration of IL-26 is higher in male patients with COVID-19 than in female patients. Notably, both the severity and mortality of COVID-19 are known to be higher in men than in women (26, 27), and this difference has been attributed to a number of genetic, hormonal, and physiological variations (28). Given that we also see an increase in systemic IL-26 in patients with severe/critical COVID-19, our results motivate further research into potential associations between increased IL-26 and patient mortality.
Third, we show that the plasma concentration of IL-26 is higher in COVID-19 patients with comorbid obstructive lung disease (i.e., asthma and/or COPD), which agrees with previous studies on asthma (29–31). Although we are not aware of corresponding studies on systemic IL-26 in COPD, our current findings are compatible with the fact that the concentration of IL-26 is enhanced in the airways of patients with COPD (32). Nevertheless, in our study we have focused on systemic alterations in IL-26 during acute COVID-19 and it is possible that the inflammatory profile in the airways of these patients might look different. In addition, even though we did not detect any differences in the plasma levels of IL-26 between patients with COVID-19 who received corticosteroids (a common treatment for COVID-19 and asthma) and those who did not, we cannot exclude the possibility that other drugs taken during sample collection might have had an impact on the systemic levels of IL-26 in certain patients.
Fourth, we have previously shown that stimulation of TLRs involved in the innate recognition of SARS-CoV-2 triggers the release of IL-26 in several immune and structural cell types in vitro (3, 4, 16, 17), and we now report positive correlations between the plasma concentrations of IL-26 and two SARS-CoV-2 antigens in vivo. Nevertheless, our multivariable analysis does not support a direct association between having high systemic concentrations of SARS-CoV-2 antigens (above median) and having a plasma concentration of IL-26 above the LLOD. These seemingly contradictory findings suggest that although SARS-CoV-2 particles might be able to induce the production of IL-26 directly in the airways, the association between SARS-CoV-2 antigens and IL-26 production might be indirect at the systemic level. Importantly, SARS-CoV-2 particles can pass from the airways and into the bloodstream (viremia) where they trigger a potent and harmful hyperinflammatory response (33–37). In agreement with this, we found that patients with severe/critical COVID-19 had higher plasma levels of SARS-CoV-2 antigens. Thus, it seems likely that enhanced IL-26 production at the systemic level occurs because of the hyperinflammation associated with SARS-CoV-2 viremia and not because of direct SARS-CoV-2 recognition by pattern-recognition receptors (PRRs).
Fifth, it is known that virus-infected cells produce vast amounts of interferons, and we now report positive correlations between the plasma concentration of IL-26 and those of IFN-α2a, IFN-β, and IFN-γ. Notably, IFNs-α/β can modulate the expression of IFN-γ, while the genes of IFN-γ and IL-26 are located on the same chromosome, share an enhancer element that drives their expression, and are often co-expressed in different lymphocyte subsets (8–10, 38). In agreement with this, our multivariable analysis further supports the association between IL-26 and IFN-γ at the systemic level. Therefore, IL-26 seems likely to play complementary antiviral functions in COVID-19.
Sixth, we have now identified a positive correlation between the systemic concentration of IL-26 and that of extracellular dsDNA. Although extracellular dsDNA in plasma is a non-specific marker of NET formation that can also derive from tissue damage-related cell necrosis, there is a tight relationship between increased NET formation and tissue-damaging hyperinflammation. For instance, we and others have previously shown that patients with acute COVID-19 display increased neutrophil activation and NET formation, all of which correlates with harmful hyperinflammation in these patients (18, 39). Moreover, IL-26 is a neutrophil-mobilizing cytokine that can also bind and enhance the pro-inflammatory potential of extracellular DNA (4, 40, 41), and we have previously reported in a small pilot study that the plasma concentration of IL-26 correlates with several markers of hyperinflammation and tissue damage such as IL-8, TNFα, and lactate dehydrogenase (LDH) in patients with acute COVID-19 (18). Taken together, our findings support the notion that increased systemic IL-26 might enhance the harmful pro-inflammatory potential of extracellular dsDNA regardless of its origin during acute COVID-19.
In summary, our novel results link enhanced systemic IL-26 to severity of disease, male sex, and respiratory comorbidity in acute COVID-19. These results also associate enhanced systemic IL-26 with viral load and the magnitude of the classic antiviral immune response, plus extracellular dsDNA—an important sign of excessive neutrophil activation and tissue-damaging hyperinflammation in COVID-19. In view of the published evidence on the antimicrobial and neutrophil-mobilizing properties of IL-26, together with our recent finding that systemic IL-26 associates with hyperinflammation and tissue damage in COVID-19 (18), our novel results strongly forward IL-26 as an active player in acute COVID-19. Thus, IL-26 bears both antiviral and pathogenic relevance in COVID-19 and, given its accessibility in blood, it may constitute a potential target for clinical diagnosis, monitoring and treatment of this dangerous disease.
The original contributions presented in the study are included in the article/Supplementary Material. Further inquiries can be directed to the corresponding author.
The studies involving humans were approved by the Swedish Ethical Review Authority (Diary No. 2020-01771). The studies were conducted in accordance with the local legislation and institutional requirements. The participants provided their written informed consent to participate in this study.
EC: Data curation, Formal analysis, Investigation, Visualization, Writing – original draft, Writing – review & editing. JR: Data curation, Investigation, Writing – review & editing. SM: Investigation, Methodology, Resources, Writing – review & editing. JB: Investigation, Methodology, Resources, Writing – review & editing. MW: Investigation, Methodology, Resources, Writing – review & editing. MS: Investigation, Methodology, Resources, Writing – review & editing. GS: Investigation, Methodology, Resources, Writing – review & editing. JW: Investigation, Methodology, Resources, Writing – review & editing. MG: Conceptualization, Funding acquisition, Project administration, Resources, Supervision, Writing – review & editing. AL: Conceptualization, Funding acquisition, Project administration, Resources, Supervision, Writing – original draft, Writing – review & editing.
The author(s) declare financial support was received for the research, authorship, and/or publication of this article. This study was supported by project grants from the Swedish state, under an agreement between the Swedish government and the county councils (ALF agreement JR: #ALFGBG-942707; MG: #ALFGBG-965885); the Knut and Alice Wallenberg Foundation via SciLifeLab (MG: #2020.0182 & #2020.0241); the Swedish Research Council (MG: #2021-05045 & #2021-06545; A.L. #2021-01527); the Swedish Heart-Lung Foundation (A.L.: #20210286); Region Stockholm (AL: #20180088); the Swedish Society for Respiratory Medicine (AL); the King Gustaf V and Queen Victoria Foundation (MG); and federal funding from Karolinska Institutet (AL). The authors declare that this study received an unrestricted research grant from CHIESI AB, Sweden (AL). The funder was not involved in the study design, collection, analysis, interpretation of data, the writing of this article, or the decision to submit it for publication.
Authors SM, JB, MW, MS, GS, and JW are employed by Meso Scale Diagnostics, LLC., Rockville, MD, USA.
The remaining authors declare that the research was conducted in the absence of any commercial or financial relationships that could be construed as a potential conflict of interest.
All claims expressed in this article are solely those of the authors and do not necessarily represent those of their affiliated organizations, or those of the publisher, the editors and the reviewers. Any product that may be evaluated in this article, or claim that may be made by its manufacturer, is not guaranteed or endorsed by the publisher.
The Supplementary Material for this article can be found online at: https://www.frontiersin.org/articles/10.3389/fimmu.2024.1434186/full#supplementary-material
COPD, Chronic Obstructive Pulmonary Disease; COVID-19, Coronavirus disease 2019; dsDNA, Double-stranded DNA; ELISA, Enzyme-linked immunosorbent assay; HCV, Hepatitis C virus; HFNO, High flow nasal oxygen; IFN, Interferon; LLOD, Lower limit of detection; NET, Neutrophil extracellular trap; RT-PCR, Reverse transcriptase polymerase chain reaction; SARS-CoV-2, Severe acute respiratory syndrome coronavirus 2; TLR, Toll-like receptor.
1. Cardenas EI, Che KF, Konradsen JR, Bao A, Linden A. Il-26 in asthma and copd. Expert Rev Respir Med. (2022) 16:293–301. doi: 10.1080/17476348.2022.2045197
2. Meller S, Di Domizio J, Voo KS, Friedrich HC, Chamilos G, Ganguly D, et al. T(H)17 cells promote microbial killing and innate immune sensing of DNA via interleukin 26. Nat Immunol. (2015) 16:970–9. doi: 10.1038/ni.3211
3. Che KF, Paulsson M, Piersiala K, Sax J, Mboob I, Rahman M, et al. Complex involvement of interleukin-26 in bacterial lung infection. Front Immunol. (2021) 12:761317. doi: 10.3389/fimmu.2021.761317
4. Che KF, Tengvall S, Levanen B, Silverpil E, Smith ME, Awad M, et al. Interleukin-26 in antibacterial host defense of human lungs. Effects on neutrophil mobilization. Am J Respir Crit Care Med. (2014) 190:1022–31. doi: 10.1164/rccm.201404-0689OC
5. Bao A, Che KF, Bozinovski S, Ji J, Gregory JA, Kumlien Georen S, et al. Recombinant human il-26 facilitates the innate immune response to endotoxin in the bronchoalveolar space of mice in vivo. PloS One. (2017) 12:e0188909. doi: 10.1371/journal.pone.0188909
6. Paulsson M, Cardenas EI, Che KF, Brundin B, Smith M, Qvarfordt I, et al. Tlr4-mediated release of heparin-binding protein in human airways: A co-stimulatory role for il-26. Front Immunol. (2023) 14:1178135. doi: 10.3389/fimmu.2023.1178135
7. Knappe A, Hor S, Wittmann S, Fickenscher H. Induction of a novel cellular homolog of interleukin-10, ak155, by transformation of T lymphocytes with herpesvirus saimiri. J Virol. (2000) 74:3881–7. doi: 10.1128/jvi.74.8.3881-3887.2000
8. Collins PL, Henderson MA, Aune TM. Lineage-specific adjacent ifng and il26 genes share a common distal enhancer element. Genes Immun. (2012) 13:481–8. doi: 10.1038/gene.2012.22
9. Eyerich S, Eyerich K, Pennino D, Carbone T, Nasorri F, Pallotta S, et al. Th22 cells represent a distinct human T cell subset involved in epidermal immunity and remodeling. J Clin Invest. (2009) 119:3573–85. doi: 10.1172/JCI40202
10. Geremia A, Arancibia-Carcamo CV, Fleming MP, Rust N, Singh B, Mortensen NJ, et al. Il-23-responsive innate lymphoid cells are increased in inflammatory bowel disease. J Exp Med. (2011) 208:1127–33. doi: 10.1084/jem.20101712
11. Beaumont E, Larochette V, Preisser L, Miot C, Pignon P, Blanchard S, et al. Il-26 inhibits hepatitis C virus replication in hepatocytes. J Hepatol. (2022) 76:822–31. doi: 10.1016/j.jhep.2021.12.011
12. Zheng M, Karki R, Williams EP, Yang D, Fitzpatrick E, Vogel P, et al. Tlr2 senses the sars-cov-2 envelope protein to produce inflammatory cytokines. Nat Immunol. (2021) 22:829–38. doi: 10.1038/s41590-021-00937-x
13. Zhao Y, Kuang M, Li J, Zhu L, Jia Z, Guo X, et al. Publisher correction: sars-cov-2 spike protein interacts with and activates tlr4. Cell Res. (2021) 31:825. doi: 10.1038/s41422-021-00501-0
14. Bortolotti D, Gentili V, Rizzo S, Schiuma G, Beltrami S, Strazzabosco G, et al. Tlr3 and tlr7 rna sensor activation during sars-cov-2 infection. Microorganisms. (2021) 9:1–14. doi: 10.3390/microorganisms9091820
15. Salvi V, Nguyen HO, Sozio F, Schioppa T, Gaudenzi C, Laffranchi M, et al. Sars-cov-2-associated ssrnas activate inflammation and immunity via tlr7/8. JCI Insight. (2021) 6:1–15. doi: 10.1172/jci.insight.150542
16. Che KF, Kaarteenaho R, Lappi-Blanco E, Levanen B, Sun J, Wheelock A, et al. Interleukin-26 production in human primary bronchial epithelial cells in response to viral stimulation: modulation by th17 cytokines. Mol Med. (2017) 23:247–57. doi: 10.2119/molmed.2016.00064
17. Che KF, Sun J, Linden A. Pharmacological modulation of endotoxin-induced release of il-26 in human primary lung fibroblasts. Front Pharmacol. (2019) 10:956. doi: 10.3389/fphar.2019.00956
18. Cardenas EI, Ekstedt S, Piersiala K, Petro M, Karlsson A, Kagedal A, et al. Increased il-26 associates with markers of hyperinflammation and tissue damage in patients with acute covid-19. Front Immunol. (2022) 13:1016991. doi: 10.3389/fimmu.2022.1016991
19. Marklund E, Leach S, Axelsson H, Nystrom K, Norder H, Bemark M, et al. Serum-igg responses to sars-cov-2 after mild and severe covid-19 infection and analysis of igg non-responders. PloS One. (2020) 15:e0241104. doi: 10.1371/journal.pone.0241104
20. Robertson J, Gostner JM, Nilsson S, Andersson LM, Fuchs D, Gisslen M. Serum neopterin levels in relation to mild and severe covid-19. BMC Infect Dis. (2020) 20:942. doi: 10.1186/s12879-020-05671-7
21. Kanberg N, Simren J, Eden A, Andersson LM, Nilsson S, Ashton NJ, et al. Neurochemical signs of astrocytic and neuronal injury in acute covid-19 normalizes during long-term follow-up. EBioMedicine. (2021) 70:103512. doi: 10.1016/j.ebiom.2021.103512
22. Lundgren A, Leach S, Axelsson H, Isakson P, Nystrom K, Scharf L, et al. Plasmablasts in previously immunologically naive covid-19 patients express markers indicating mucosal homing and secrete antibodies cross-reacting with sars-cov-2 variants and other beta-coronaviruses. Clin Exp Immunol. (2023) 213:173–89. doi: 10.1093/cei/uxad044
23. Lippi G, Henry BM, Montagnana M, Plebani M. Diagnostic accuracy of the ultrasensitive S-plex sars-cov-2 N electrochemiluminescence immunoassay. Clin Chem Lab Med. (2022) 60:e121–e4. doi: 10.1515/cclm-2022-0155
24. (NIH) NIoH. Coronavirus Disease 2019 (Covid-19) Treatment Guidelines (2023). Available online at: https://www.covid19treatmentguidelines.nih.gov/ (Accessed March 17, 2021).
25. Caterino M, Gelzo M, Sol S, Fedele R, Annunziata A, Calabrese C, et al. Dysregulation of lipid metabolism and pathological inflammation in patients with covid-19. Sci Rep. (2021) 11:2941. doi: 10.1038/s41598-021-82426-7
26. Alwani M, Yassin A, Al-Zoubi RM, Aboumarzouk OM, Nettleship J, Kelly D, et al. Sex-based differences in severity and mortality in covid-19. Rev Med Virol. (2021) 31:e2223. doi: 10.1002/rmv.2223
27. Prinelli F, Trevisan C, Noale M, Franchini M, Giacomelli A, Cori L, et al. Sex- and gender-related differences linked to sars-cov-2 infection among the participants in the web-based epicovid19 survey: the hormonal hypothesis. Maturitas. (2022) 158:61–9. doi: 10.1016/j.maturitas.2021.11.015
28. Paschou SA, Psaltopoulou T, Halvatsiotis P, Raptis A, Vlachopoulos CV, Dimopoulos MA. Gender differences in covid-19. Maturitas. (2022) 161:72–3. doi: 10.1016/j.maturitas.2022.03.004
29. Salhi M, Tizaoui K, Louhaichi S, Lahmar O, Hamzaoui K, Hamzaoui A. Il-26 gene variants and protein expression in Tunisian asthmatic patients. Cytokine. (2020) 134:155206. doi: 10.1016/j.cyto.2020.155206
30. Louhaichi S, Mlika M, Hamdi B, Hamzaoui K, Hamzaoui A. Sputum il-26 is overexpressed in severe asthma and induces proinflammatory cytokine production and th17 cell generation: A case-control study of women. J Asthma Allergy. (2020) 13:95–107. doi: 10.2147/JAA.S229522
31. Kovach M, Käck U, Che KF, Brundin B, Konradsen J, Lindén A. Systemic il-26 correlates with improved asthma control in children sensitized to dog allergen. Respir Res. (2024) 25(1):163. doi: 10.1186/s12931-024-02773-7
32. Che KF, Tufvesson E, Tengvall S, Lappi-Blanco E, Kaarteenaho R, Levanen B, et al. The neutrophil-mobilizing cytokine interleukin-26 in the airways of long-term tobacco smokers. Clin Sci (Lond). (2018) 132:959–83. doi: 10.1042/CS20180057
33. Jacobs JL, Bain W, Naqvi A, Staines B, Castanha PMS, Yang H, et al. Severe acute respiratory syndrome coronavirus 2 viremia is associated with coronavirus disease 2019 severity and predicts clinical outcomes. Clin Infect Dis. (2022) 74:1525–33. doi: 10.1093/cid/ciab686
34. Rovito R, Bono V, Augello M, Tincati C, Mainoldi F, Beaudoin-Bussieres G, et al. Association between sars-cov-2 rnaemia and dysregulated immune response in acutely ill hospitalized covid-19 patients. Sci Rep. (2022) 12:19658. doi: 10.1038/s41598-022-23923-1
35. Chen X, Zhao B, Qu Y, Chen Y, Xiong J, Feng Y, et al. Detectable serum severe acute respiratory syndrome coronavirus 2 viral load (Rnaemia) is closely correlated with drastically elevated interleukin 6 level in critically ill patients with coronavirus disease 2019. Clin Infect Dis. (2020) 71:1937–42. doi: 10.1093/cid/ciaa449
36. Augello M, Bono V, Rovito R, Tincati C, Bianchi S, Taramasso L, et al. Association between sars-cov-2 rnaemia, skewed T cell responses, inflammation, and severity in hospitalized covid-19 people living with hiv. iScience. (2024) 27:108673. doi: 10.1016/j.isci.2023.108673
37. Li Y, Schneider AM, Mehta A, Sade-Feldman M, Kays KR, Gentili M, et al. Sars-cov-2 viremia is associated with distinct proteomic pathways and predicts covid-19 outcomes. J Clin Invest. (2021) 131:1–12. doi: 10.1172/JCI148635
38. Nguyen KB, Cousens LP, Doughty LA, Pien GC, Durbin JE, Biron CA. Interferon alpha/beta-mediated inhibition and promotion of interferon gamma: stat1 resolves a paradox. Nat Immunol. (2000) 1:70–6. doi: 10.1038/76940
39. Ekstedt S, Piersiala K, Petro M, Karlsson A, Kagedal A, Kumlien Georen S, et al. A prolonged innate systemic immune response in covid-19. Sci Rep. (2022) 12:9915. doi: 10.1038/s41598-022-13986-5
40. Poli C, Augusto JF, Dauve J, Adam C, Preisser L, Larochette V, et al. Il-26 confers proinflammatory properties to extracellular DNA. J Immunol. (2017) 198:3650–61. doi: 10.4049/jimmunol.1600594
Keywords: IL-26, COVID-19, SARS-CoV-2, asthma, COPD
Citation: Cardenas EI, Robertson J, Misaghian S, Brown J, Wang M, Stengelin M, Sigal G, Wohlstadter J, Gisslén M and Lindén A (2024) Systemic increase in IL-26 is associated with severe COVID-19 and comorbid obstructive lung disease. Front. Immunol. 15:1434186. doi: 10.3389/fimmu.2024.1434186
Received: 17 May 2024; Accepted: 17 September 2024;
Published: 04 October 2024.
Edited by:
Athanasia Mouzaki, University of Patras, GreeceReviewed by:
Matteo Augello, University of Milan, ItalyCopyright © 2024 Cardenas, Robertson, Misaghian, Brown, Wang, Stengelin, Sigal, Wohlstadter, Gisslén and Lindén. This is an open-access article distributed under the terms of the Creative Commons Attribution License (CC BY). The use, distribution or reproduction in other forums is permitted, provided the original author(s) and the copyright owner(s) are credited and that the original publication in this journal is cited, in accordance with accepted academic practice. No use, distribution or reproduction is permitted which does not comply with these terms.
*Correspondence: Eduardo I. Cardenas, ZWR1YXJkby5jYXJkZW5hc0BraS5zZQ==
†ORCID: Eduardo I. Cardenas, orcid.org/0000-0001-5973-5675
Disclaimer: All claims expressed in this article are solely those of the authors and do not necessarily represent those of their affiliated organizations, or those of the publisher, the editors and the reviewers. Any product that may be evaluated in this article or claim that may be made by its manufacturer is not guaranteed or endorsed by the publisher.
Research integrity at Frontiers
Learn more about the work of our research integrity team to safeguard the quality of each article we publish.