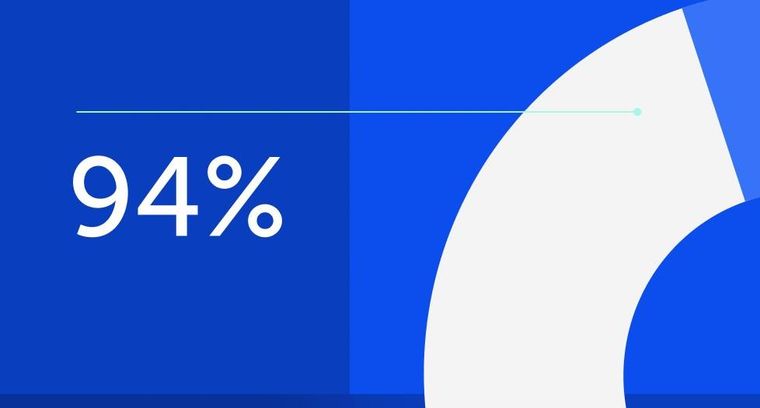
94% of researchers rate our articles as excellent or good
Learn more about the work of our research integrity team to safeguard the quality of each article we publish.
Find out more
REVIEW article
Front. Immunol., 08 August 2024
Sec. Viral Immunology
Volume 15 - 2024 | https://doi.org/10.3389/fimmu.2024.1430544
This article is part of the Research TopicCellular and host immune responses in the context of dual viral infectionView all 6 articles
Human Papillomavirus (HPV), an extensive family of DNA viruses, manifests as a persistent global health challenge. Persistent HPV infection is now firmly established as a significant aetiological factor for a spectrum of malignancies. In this review, we examine the latest insights into HPV biology and its intricate relationship with the host. We delve into the complex dynamics of co-infections involving HPV alongside other viruses, such as HIV, EBV, and HSV, as well as the burgeoning role of the microbiome in cancer development. We also explore recent advancements in understanding the specific contributions of HPV in the development of various cancers, encompassing cancers of the anogenital region, head and neck, as well as breast, lung, and prostate. Moreover, we focus on the current preventive strategies, including vaccination and screening methods, and therapeutic interventions that range from traditional approaches like surgery and chemotherapy to emerging modalities such as targeted therapies and immunotherapies. Additionally, we provide a forward-looking view on the future directions of HPV research, highlighting potential areas of exploration to further our understanding and management of HPV and its associated cancers. Collectively, this review is positioned to deepen readers’ understanding of HPV biology and its complex interplay with cancer biology. It presents innovative strategies for the prevention, management, and therapeutic intervention of HPV-associated malignancies.
Cancer is a significant global health concern and constitutes an enormous social burden. Theories aiming to describe the tumorigenesis have been long thought to be connected (1). Viral infections have been recognized as important risk factors for the development of various types of cancer (2–4). Oncogenic viruses can induce cellular transformation through several mechanisms, including the integration of viral DNA into the host genome, expression of viral oncoproteins, and modulation of host immune responses (5). Moreover, viral infections play a pivotal role in the anoikis resistance within tumour cells, thereby enabling their evasion of programmed cell death and promoting the spread and metastasis of malignant cells (6).
Approximately 12% of human cancers are caused by viral oncogenesis (7). Among these viruses, HPVs are an established etiological agent of human cancer. Most HPV infections are asymptomatic and resolve spontaneously within a couple of years. However, some infections can persist and lead to the development of various diseases. It is estimated that HPV causes approximately 610,000 cancers cases (8) and more than 250,000 deaths each year worldwide (9). It also causes approximately 40,000 cases of head and neck (particularly oropharyngeal) cancers each year (10). Certain high-risk HPV types, particularly HPV-16 and HPV-18, are responsible for the majority of cervical cancer cases. Besides, these high-risk types can also cause other anogenital cancers, including anal, vulvar, vaginal, and penile cancers. HPV has been increasingly recognized as a cause of head and neck cancers, specifically oropharyngeal cancer (11). Additionally, HPV DNA was detected in a significant proportion of prostate cancer (PCa) tissue samples, with a positive rate of 32.7% (12). HPV infection was implicated in the development of PCa metastases, potentially by modulating the expression of genes associated with anoikis resistance (13). This finding underscores the potential role of HPV in the aetiology of PCa and warrants further investigation into the mechanisms by which HPV may contribute to the development and progression of the disease. In this context, prevention and early detection are key to reducing the burden of HPV-associated cancers. The available preventive measures include HPV vaccination, regular screening, and practicing safe sex. Vaccination against HPV is the most effective preventive measure. The HPV vaccine, originally developed to shield against cervical dysplasias, has demonstrated efficacy in countering a spectrum of anogenital malignancies linked to high-risk HPV types 16 and 18.
In this review, we will provide an overview of the structure, organization of HPV genome and concentrate on metabolic deregulations induced by the HPV viral oncoproteins. We will also summarize the mechanisms by which it induces cellular transformation and cancer development, and the strategies that can be employed for prevention and treatment. This review provides a broader perspective on HPV and its significant role in global cancer epidemiology.
HPV is a small (50 to 60 nm in diameter and ~8 kb in length), non-enveloped, double-stranded DNA viruses (14). Currently, there are over 200 related variants of HPV that have been identified (15). These types are classified into five main genera, including alpha, beta, gamma, mu, and nu. Different types are associated with different disease and disease prevalence (16). The alpha genus infect both genital and oral mucosa, and can be associated with malignant disease, while the beta, gamma, mu, and nu genera target cutaneous that contribute to the formation of skin papillomas and skin warts (17). In addition, these types are often subdivided into low- and high-risk types based on their propensity to induce cancer. Among these, 14 types (HPV 16, 18, 31, 33, 35, 39, 45, 51, 52, 56, 58, 59, 66, and 68) are classified as high-risk due to their well-established association with different forms of cancer. These high-risk types, including HPV 16 and 18, are particularly notorious for their role in the pathogenesis of cancers such as cervical, oropharyngeal, anal, vulvar, vaginal, and penile cancers (18). In contrast, infection by low-risk HPV types, such as types 6, 11, 42, 43, and 44, is non carcinogenic and typically causes cutaneous genital lesions.
The genomic organization is highly conserved across the entire HPV family, contributing to the fundamental similarities in viral biology and pathogenesis among different HPV types. All HPV genomes are existed in infected cells as episomes. The icosahedral capsid is composed of 72 capsomers. HPV genome exists within the capsids, and are associated with cellular histones, forming chromatin-like structures.
Viral genomes harbors 8 open reading frames (ORFs) that is large enough to encode for a protein. The genome can be divided into three domains: an early gene coding region (E1, E2, E4, E5, E6 and E7), a late gene coding region (L1 and L2) and a long control region (LCR) (19).
Of these viral proteins, E1 and E2 are participated in initiating viral DNA replication. E2 is thoughted to be the most vital protein in HPV genome because of its important role in viral transcription, replication, and genome partitioning. E2 also tightly regulates the expression of E6 and E7 oncogenes. Loss of E2 function contributes to increased expression of E6 and E7, further promoting cell proliferation and survival (20). E4 is considered to assist in in virus synthesis and release by disrupting cellular keratin in the upper epithelium (21). Occasionally, E5 functions as an accessory oncogenes that can augment the transforming activity of E6 and E7, contributing to tumor progression (22). E6 and E7 are oncoproteins that can alter host cell functions and cause the onset of cancer. Conversely, the late genes encode the L1 major and L2 minor structural capsid proteins, respectively, which expressed during the later stages of infection. These proteins are structural in nature and are responsible for forming the viral capsid. The LCR, localized between L1 and E6, is a non-coding, regulatory DNA region that plays a crucial role in the replication and transcription of HPV. It contains important regulatory elements that control the expression of viral genes and is involved in the initiation of DNA replication (23). Both promoters and enhancers play a critical role in regulating RNA transcription. Two major promoters reside in the genomes of HPV-16: an early promoter (p97) located within the LCR and a late promoter (p670) within E7. Furthermore, the early polyadenylation sites (PAE) is situated at the termination of E5 ORF, and the late polyadenylation sites (PAL) is located at the termination of L1 ORF. The genomic organization is presented in Figure 1.
Figure 1 Genomic organization and functions of human papillomavirus type 16. HPV16 have approximately 8kb circular DNA genome containing eight open reading frames (ORFs) that are critical for different stages of the virus life cycle. The genome is divided into three main sections: the early region, containing the early genes(E1-E7); the late region, containing the late genes(L1and L2); and the long control region (LCR).
Integrating the HPV genome into the host cell’s DNA is a pivotal step in HPV-associated carcinogenesis. HPV infects epithelial basal cells, pivotal for regeneration, and expresses the oncoproteins E6 and E7, which subvert cell cycle controls (24). Integration of HPV DNA into the host genome can inactivate tumour suppressors such as p53 and Rb, initiating uncontrolled proliferation (25). This event may evade immune surveillance and induce genomic instability, promoting carcinogenesis (26). The integration is a critical step in the progression to cervical and anogenital cancers, linked to advanced lesion grades and poorer prognosis (27).
The carcinogenic potential of Human Papillomavirus (HPV) lies in its ability to integrate its DNA into the host cell genome and produce viral oncoproteins that interfere with normal cellular processes. HPV oncoproteins E6 and E7 are crucial in the initiation and progression of carcinogenesis. The primary identified roles of E6 and E7 is their abilities to degradation of the tumor suppressors proteins p53 and the retinoblastoma protein (pRb), respectively, leading to uncontrolled cell growth.
E6 is small protein of approximately 150 amino acids and contains two zinc binding domains (28). The E6 of high-risk HPV strains possesses multiple activities that promote the development of cancer. E6-associated protein (E6AP), which functions as an E3 ubiquitin protein ligase, is essential for the degradation of specific target proteins and assists in the carcinogenic characteristics of HPV. E6 binds the tumor suppressor protein p53 leading to its degradation via E6AP-mediated ubiquitination (29). However, E6 needs to bind to E6AP firstly demonstrate that neither E6 nor E6AP interacts significantly with p53 in the absence of the other (30). Thus, degradation of p53 results in the inactivation of p21, which known as cyclin-dependent kinase inhibitor, further preventing G1-S phase entry of the cell cycle (31). This allows cells with DNA damage or mutations to proliferate unchecked, increasing the risk of cancer development.
E6 is also recognized to impact different cellular factors within the host and interfere with host signaling pathways. HPV E6 protein interacts with FADD and caspase-8 to dysregulate the extrinsic apoptosis pathway, which transmits extracellular apoptotic signals from the cell surface (32). One mechanism by which HPV E6 protein inhibits BAX and BAK is through the promotion of their degradation, similar to p53 (33, 34). The degradation of BAX and BAK prevents their activation and impairs their ability to induce apoptosis. Additionally, E6 directly regulates the immune system by down-regulating interferon regulatory factor 3(IRF-3), diminishing the immune reaction to HPV antigens (35). PDZ domain-containing proteins are cellular proteins engaged in various cellular processes, including cell signaling and protein-protein interactions. HPV E6 has been found to interact with PDZ domain-containing proteins through a specific motif present in its C-terminal region known as the PDZ-binding motif. These interactions disrupt the normal function of these proteins, which are involved in cell signaling pathways and cell adhesion complexes, contributing to the oncogenic properties of HPV (36). E6 can also inactivate the transcriptional coactivator complex p300/CBP, thereby regulating the progression and differentiation of the cell cycle, and proteins involved in apoptosis (37). The unbalanced expression of E6 can also be induced expression of human telomerase reverse transcriptase (hTERT), thereby achieving cell immortalization (38).
The E7 protein is approximately 100 amino acids in length and is characterized by the presence of conserved regions, including the CR1 and CR2 domains. CR-1 is required for transformation, whereas CR-2 contains the Rb binding domain. Similar to the E6 protein, the primary function of HPV E7 protein is to disrupt the cell cycle control mechanisms and promote cell proliferation. The E7 protein of high-risk HPV types primarily targets the pRb, another critical tumor suppressor protein. The interaction between E7 and pRb disrupts the Rb-E2F complex, leading to the release of E2F transcription factors. The released E2F factors then stimulate the transcription of genes involved in cell cycle progression, such as those encoding cyclins and cyclin-dependent kinases (CDKs). In addition to its interaction with pRb, HPV E7 protein also directly binds to and inhibits the activity of CDKs inhibitors such as p21 and p27, which normally function to inhibit cell cycle progression (39). By inhibiting CDKs, E7 further promotes cell cycle progression and and contributes to the development of HPV-associated cancers. One of the key mechanisms by which HPV E7 protein promotes carcinogenesis is through its interaction with the PKB/AKT pathway. HPV E7 protein enhances the activation of PKB/AKT by inhibiting PTEN, a known inhibitor of the PI3K (PI3 kinase) pathway. The activation of PKB/AKT promotes cell survival and proliferation, inhibits apoptosis, and contribute to the development and progression of HPV-associated cancers (40). Furthermore, E7 is also associated with a variety of other factors, such as Interleukin 6(IL-6) and Myeloid cell leukemia-1(Mcl-1), and causes the acceleration of cell cycle and cell division (40). Moreover, HPV E7 protein has mediated immune evading mechanism from IFN by binding to IRF-9 (41). The effect of HPV E6 and E7 in carcinogenesis is presented in Figure 2.
Figure 2 Molecular mechanisms of carcinogenesis by HPV E6 and E7 viral oncoproteins interacting with cellular protein. Left panel: the possible roles of HPV E6 in carcinogenesis. Right panel: the possible roles of HPV E7 in carcinogenesis. (E6AP, E6 associated protein; FADD, Fas-associated protein with death domain; BAK, Bcl-2 homologous antagonist/killer; BAX, Bcl-2-like protein 4; IRF, Interferon regulatory factors; NFX, X box-binding protein; hTERT, The TERT human protein; PDZ, Post-synaptic density proteins; CBP/300, CREB binding protein; pRb, Retinoblastoma protein; CDK, Cyclin-dependent kinase; IL-6, Interleukin 6; Mcl-1, Myeloid cell leukemia-1; PKB/AKT, Protein kinase B).
Additionally, the HPV E5 protein has also been reported to be involved in oncogenesis. One of its key functions is the activation of growth factor receptors, such as the epidermal growth factor receptor (EGFR). HPV E5 protein binds to EGFR and enhances its activation, resulting in continuous activation of downstream signaling pathways such as the mitogen-activated protein kinase (MAPK) and phosphatidylinositol 3-kinase (PI3K)/AKT pathways. This activation promotes cell proliferation, survival, and transformation, contributing to the development of tumor (42). E5 also disrupts the keratinocyte growth factor receptor signaling to inhibit autophagy and decrease the proliferation and differentiation of suprabasal keratinocytes (43). All these transformation events result in uncontrolled proliferation in tumor cells, which could be an initial stage in tumor development.
In the context of HPV infection, coinfections with other viruses and alterations in the bacterial microbiome can significantly influence the course of the disease (44, 45). Herpesviridae and Polyomaviridae, detected in HPV-positive tumors, suggest a role for co-infection in disease progression and oncogenesis (46). These viruses can compromise the immune system’s ability to clear HPV, leading to a higher likelihood of persistent infection and subsequent progression to cancer. Nahand et al. reported that the HPV/EBV coinfection could be a significant contributing factor in the progression of prostate cancer. Furthermore, it highlights a potential role for EBV in facilitating the integration of the HPV genome, thereby influencing carcinogenesis (3).
Concurrently, alterations in the bacterial microbiome can also impact HPV infection and disease progression. The microbiome plays a pivotal role in HPV-driven carcinogenesis and cancers (47). Recent studies have suggested a potential link between vaginal microbiome dysbiosis and an increased risk of HPV persistence and cervical cancer development (48). It is plausible that the depletion of the microbiome composition by Lactobacillus may lead to a pro-inflammatory environment, thus potentially increasing malignant cell proliferation and the expression of HPV E6 and E7 oncogenes (49).
The interaction of HPV with coinfections and the microbiome is a critical research frontier, potentially enhancing our understanding of disease progression and informing new preventive and therapeutic HPV strategies.
The prevalence and incidence of HPV-associated cancers are influenced by various factors including sexual behavior, immune status (such as HIV co-infection), smoking, and access to screening and vaccination services. The burden of HPV is not evenly distributed globally (50). Despite the availability of effective preventive measures, the burden of HPV remains high. This is due to several factors including low vaccine coverage in some regions, lack of regular cancer screening, and gaps in public awareness about HPV and its associated risks (51). Regions with the highest rates of HPV include Sub-Saharan Africa, Latin America, and the Caribbean (26). In addition, the most profound burden of HPV lies in its strong association with various forms of cancer (52). HPV-associated cancers often have long latency periods, which means that the impacts of these diseases are not only immediate but also long-term, affecting individuals’ quality of life and survival rates. The treatment of these cancers can be complex and costly, placing a significant economic burden on healthcare systems. Apart from cancers, HPV also causes non-malignant conditions such as genital warts and recurrent respiratory papillomatosis (53). While not life-threatening, these conditions can cause significant psychosocial distress, further contributing to the overall burden of HPV.
Head and neck squamous cell carcinomas (HNSCCs) are a diverse group of malignancies that arise from the mucosa lining the oral cavity, nasal cavity, larynx, hypopharynx, and oropharynx. These include oral squamous cell carcinomas (OSCC), oropharyngeal squamous cell carcinomas (OPSCC), laryngeal squamous cell carcinomas (LSCC) and nasal squamous cell carcinomas (NSCC). HPVs are responsible for a higher proportion of cases of OPSCC (33.6%) globally, while causing fewer cases of OSCC (22.2%) and LSCC (20.2%) (50). More than 90% of HPV-positive HNSCCs are mainly attributable to a single HPV type, HPV16 (54). In recent years, developed countries have seen a decrease in HPV prevalence due to the introduction of vaccination programs. However, HNSCC has now surpassed cervical cancer as the most common HPV related malignancy in these countries (10). Over the past few decades, there has been a significant increase in the incidence of OPSCCs, linked to HPV, particularly HPV type 16 in many developed countries (55). About 70% of OPSCCs are linked to HPV, this type of cancer is more common in men. Traditional risk factors for OPSCCs include tobacco use and heavy alcohol consumption, HPV-positive OPSCCs are often diagnosed in younger individuals with little to no history of tobacco or alcohol use. Instead, sexual behaviors, including early age at first sexual intercourse and a high number of sexual partners, particularly oral sex partners, have been linked to an increased risk of HPV-positive OPSCCs.
HPV-positive OPSCCs are often distinct from other head and neck cancers in terms of their risk factors, symptoms, progression, and response to treatment (56). HPV-positive OPSCCs often presents with a lump in the neck, which is a swollen lymph node containing metastatic cancer. Other symptoms can include persistent sore throat, difficulty swallowing, and changes in the voice. In comparison with HPV- negative HNSCCs, HPV-positive HNSCCs is easier to metastasize, and its mechanism has not yet been fully elucidated. Moreover, HPV-positive cancers tend to have a better prognosis and respond better to treatment than HPV-negative counterpart.
Since HPV-positive HNSCCs does not have an effective vaccine to prevent it like cervical cancer, early-stage detection and diagnosis of HPV-positive HNSCCs are very important. HPV detection by several methods (HPV16 E1 PCR, HPV RNA sequencing, HPV DNA sequencing, et al.) is widely used in determining HPV status (57–61). However, the methods and strategies of HPV detection have not been clearly stipulated, and approach for determining HPV status often differences among various studies (62). In addition, several studies have identified body fluids based biomarkers for HNSCCs. These biomarkers are proposed as possible novel diagnostic and prognostic strategy. Detection of serum HPV16 E6 and E7 antibodies are more clinically relevant in OPSCCs. A recent review and meta-analysis investigated that HPV16 E6 antibody is a highly sensitive and specific biomarker for the detection of HPV-related OPSCCs at diagnosis (63). In addition, a growing body of clinical evidence has suggested that pretreatment circulating neutrophil count (CNC), circulating monocyte count (CMC), and circulating lymphocyte count (CLC) can be used as early prognostic markers for HPV- related and HPV-unrelated OPC (64). In some studies, higher pretreatment concentrations of CD8 T cells predicted a response to induction chemotherapy in HPV16 positive OPC patients (65).
MicroRNA (miRNA) is a small non-coding RNA molecule that plays a vital role in regulating gene expression at the post-transcriptional level. Deregulation of miRNAs have been proposed as key players in numerous human malignancies, including HNSCCs. Extracellular miRNAs can be measured in many kinds of body fluids, including serum, plasma, and saliva, making it possible to act as minimally invasive biomarkers. The expression of miRNAs are difference between HPV-positive HNSCCs and HPV-negative HNSCCs. A number of studies have elucidated the association between HPV/p16 status and miRNA signatures. Furthermore, miRNAs level has been highly involved in HPV-positive HNSCC pathogenesis, immune response, invasion, chemoresistant or radioresistant phenotypes. Numerous studies have examined causal links between specific miRNAs and HPV-positive HNSCCs development or progression. Some of the most commonly up-regulated miRNAs in HPV-positive HNSCC tumors are miR-9, miR-127, miR-196, miR-222, miR-455 (66), while the down-regulated miRNAs are miR-122, miR-124, miR-146a (67). Table 1 summarizes body fluids based biomarkers in HPV-positive HNSCCs, including extracellular miRNA implicated as accurate biomarkers in HPV-positive HNSCCs. However, miRNA as a diagnostic biomarker has not been used as clinical practice to date. It’s important to note that the regulation between miRNAs and their target genes can be complex in different tumor microenvironments. Large-scale clinical practice and validation still required. A better understanding of the mechanisms could help clinicians choose appropriate HPV-positive HNSCCs treatments.
Cervical cancer continues to pose a significant public health challenge, particularly in settings with limited resources where screening and preventive measures may be less accessible. The incidence of other anogenital cancers, such as those of the anus, penis, vagina, and vulva, while comparatively rare, is exhibiting an upward trend.It is now clear that HPV plays a pivotal role in the etiology of cervical cancer and its precursor, cervical intraepithelial neoplasia (CIN) (68). High-risk HPV types, particularly HPV 16 and 18, are found in the majority of all high-grade CIN and invasive cervical cancers. The development of vaccines against HPV has been a milestone in the prevention of cervical cancer (69, 70). These vaccines are highly effective in preventing persistent infections with HPV and the development of CIN.
Studies have shown that over 90% of anal cancers are associated with HPV, making it the most significant risk factor for this type of cancer (71). HPV 16 has been found to be the most prevalent type in anal cancer. HPV is identified as a causative agent in approximately 25% of vulvar cancers (72), predominantly in younger women and particularly those with the high-grade squamous intraepithelial lesion, vulvar intraepithelial neoplasia (VIN). The majority of HPV-related vulvar cancers are associated with HPV type 16. Vaginal cancer is rarer than vulvar cancer, with HPV implicated in about 78% of cases (72). Similar to cervical and vulvar cancers, the primary high-risk HPV type associated with vaginal cancer is HPV 16.
HPV is a sexually transmitted infection that can infect the skin and mucous membranes, including those of the penis. It has been estimated that HPV is present in approximately 40-50% of penile cancers (73), with HPV 16 being the most commonly detected type. The virus can cause changes in the cells of the penis that may lead to penile intraepithelial neoplasia (PeIN), a precancerous condition, and eventually progress to invasive penile cancer. The majority of PeIN lesions are HPV positive, and they predominantly contain several HPV genotypes (HPV6, HPV11, or HPV16). A 2019 meta-analysis estimated that 98.6% of PeIN 1–2 lesions and 80.5% of PeIN 3 lesions were HPV positive (74). Risk factors for HPV-related penile cancer include a high number of sexual partners, history of genital warts, immunosuppression, smoking, poor personal hygiene, and uncircumcised status. Uncircumcised men are at a higher risk because the environment under the foreskin can favor HPV infection and persistence.
HPV’s role in anogenital, head and neck, and cervical cancer is established, yet its association with other malignancies, such as breast, lung, and prostate cancers, is controversial and under investigation. Breast cancer(BCa) is the second most prevalent cancer globally, posing a significant health challenge, particularly in developed countries. Mortality rates for breast cancer among women significantly exceed those attributable to lung and colorectal cancers (75). Evidence linking HPV to BCa was first reported in 1992, sparking ongoing research into the potential role of the virus in the etiology of this malignancy. International studies, spanning Italy, Iran, Qatar, USA, Poland, Congo, Sudan, Ethiopia, Egyptian, Lebanese, Peruvian, Brazil, Mexico, South Africa and Ahvaz, have consistently reported the presence of HPV in women diagnosed with BCa (4, 76–96). The prevalence of HPV infection in BCa exhibits significant variability across studies over the past five years, with reported frequencies ranging from 2.7% to 77.2%(Table 2). Additionally, inconsistencies in HPV infection rates have been noted among different molecular subtypes of BCa, suggesting potential differences in susceptibility or etiological mechanisms (109). Globally, HPV types 16, 18, and 33 are the most prevalent, accounting for approximately 70% of all HPV-associated BCa cases (108). Additionally, other HPV strains, such as HPV 31, 39, 45, 52, and 58, have been identified in BCa samples, contributing to the diversity of the viral presence in these malignancies. On the other hand, Oliveira et al.’s research (97) found no HPV DNA in BCa tissues, prompting further inquiry into HPV’s role in carcinogenesis.
Lung cancer(LCa) remains a leading cause of cancer-related mortality globally, with its aggressive nature and late diagnoses contributing to high fatality rates. LCa is primarily categorized into small-cell lung cancer(SCLC)and non-small-cell lung cancer (NSCLC), with SCLC constituting about 20% and NSCLC approximately 80% of cases. Globally, HPV has been identified in a significant proportion of LCa cases, with approximately 31% of these cases testing positive for the virus (110). Since Syrjanen’s 1979 proposal linking HPV to lung carcinogenesis (111), extensive research has explored potential mechanisms, reflecting ongoing global scientific inquiry. The HPV oncoprotein interacts with pRb, triggering E2F release and promoting cell cycle progression in infected lung cells. Evidence has consistently implicated HPV infection in a substantial proportion of LCa cases across various geographical regions, underscoring its potential role in the etiology and progression of the disease (100, 102, 103). The HPV types, including HPV16, HPV18, HPV33, HPV42, HPV44, HPV51, HPV56,and HPV58, have been identified in lung tissue samples (2, 99, 103, 104). However, in a study conducted in the Czech Republic, Jaworek et al. reported the absence of HPV in 80 primary NSCLCs (101). Similarly, Silva et al. observed the lack of HPV in 77 NSCLC patients (98).
Prostate cancer (PCa) is the most frequently diagnosed solid malignancy in men and is a leading cause of cancer-related mortality worldwide (112). The presence of HPV in prostate tissue was initially identified by McNicol et al. through the application of PCR method (113), marking a pivotal discovery in the study of HPV’s potential role in prostate cancer. High-risk HPV types 16 and 18 are implicated in the immortalisation and transformation of normal prostate cells into malignant counterparts (114, 115). A comprehensive review on the association between PCa and HPV encompassed 60 studies, highlighting diverse methodologies such as PCR, ELISA, hybridization, and IHC. Of these, 51 focused on HPV detection in tissue samples, while 13 examined blood samples. Notably, 11 studies, representing 18% of the total, demonstrated a positive correlation between HPV presence and the development of PCa (116). In contrast to other findings, a study by Khoury et al. (117), employing Next-Generation Sequencing (NGS) on data from The Cancer Genome Atlas (TCGA), reported the absence of HPV in 53 cases of PCa.
In conclusion, variations in study design and subject characteristics, such as sexual behaviour, racial genetic predispositions, sample sizes, and methodological approaches, likely contribute to the inconsistent findings on the relationship between HPV infection and the development of BCa, LCa and PCa. To elucidate the role of HPV in these specific cancers, there is a necessity for comprehensive research employing larger sample sizes and standardized diagnostic methodologies. Recent studies about the frequency of HPV detection globally in BCa, LCa and PCa is presented in Table 2 (105–107). Pathogenic mechanisms of HPV-associated cancers were summarized in Figure 3.
Prevention and early detection are key to reducing the burden of HPV-associated cancers. The available preventive measures include HPV vaccination, regular screening, and practicing safe sex.
Vaccination against HPV is the most effective preventive measure (118). Vaccination for both males and females are recommended to protect against HPV-related cancers and genital warts. Vaccination typically begins at age 11 or 12, with catch-up vaccinations for older individuals. Vaccination of individuals up to age 26, and sometimes even older, may be recommended depending on local guidelines. The vaccine also produces a higher immune response in preteens than in older adolescents.
There are several HPV vaccines available worldwide that protect against both high risk HPV types that can lead to cervical cancer and low risk types that cause genital warts. In the last decades, five vaccines are currently licensed. These vaccines are based on virus like particles (VLPs), which self-assemble from the major capsid HPV L1 protein. Gardasil is the original Gardasil vaccine. It protects against four types of HPV: the two types that cause most cervical cancers (16 and 18) and the two types that cause genital warts (6 and 11). Gardasil 9 is the most widely used HPV vaccine, and it protects against nine types of HPV (6, 11, 16, 18, 31, 33, 45, 52, and 58) (119). These include the two types that cause 70% of cervical cancers (16 and 18) and five other high-risk types (31, 33, 45, 52, 58), as well as two types that cause 90% of genital warts (6 and 11). Cervarix protects against HPV types 16 and 18 only (120). Unlike Gardasil, it does not protect against the HPV types that cause genital warts. It is not used as commonly as Gardasil, but is still used in some countries. Even though the new (9-valent) vaccine seems promising, next-generation vaccines as well as awareness programs associated with HPV vaccination and budget reinforcements for immunization are needed (43). Cecolin was licensed in China in 2020 and prequalified by the World Health Organization (WHO) in 2021 (121). More recently, a recombinant bivalent vaccine, targeting HPV-16 and HPV-18, developed by Shanghai Zerun Biotechnology, was also licensed in China in 2022 and prequalified by WHO in 2022 (122). The main features of currently licensed prophylactic HPV vaccines was listed in Table 3. In addition, emerging evidence suggests that HPV vaccines might also provide protection against oral HPV infections that could lead to oropharyngeal cancers (123). However, there is no clear evidence for licensed prophylactic HPV vaccines in the prevention of OPSCC as there are no identified precursor lesions that could be used as efficacy endpoint for OPSCCs (124). More research is needed to confirm this potential benefit.
The two primary methods used for HPV screening and early detection are the Papanicolaou (Pap) smear and HPV DNA testing (125). The Pap smear assesses cellular changes suggestive of pre-cancer, while HPV DNA testing identifies high-risk HPV strains. Early identification facilitates preventative measures, underscoring the necessity for regular screening, particularly among high-risk women.
Safe sex, including consistent condom use and limiting sexual partners, is pivotal in reducing HPV transmission risk (126). Proper use of condoms during any sexual activity, including vaginal, anal, or oral sex, can contribute to lowering the risk of HPV transmission (127). Monogamous relationships, particularly with uninfected partners, offer substantial protection against HPV acquisition.
HPV-associated cancers present a therapeutic challenge that necessitates a nuanced approach. Available interventions include surgery, radiation, and chemotherapy (128), each meticulously tailored to the cancer’s specific characteristics, including type, stage, and the patient’s health status. Surgery is a foundational treatment for HPV-associated cancers, particularly for those affecting the cervix, penis, and anus, ranging from excision of precancerous lesions to extensive tumor resections (129). Radiation therapy is integral to both primary and adjuvant treatment strategies, with a focus on targeting localized tumors or residual disease post-surgery, especially in oropharyngeal and anal cancers (130). The combination of radiation therapy with 5-FU, cisplatin, carboplatin, or mitomycin C, in single or combined regimens, significantly improves survival outcomes, independent of radiation scheduling (131). Intesity-Modulated Radiation Therapy (IMRT) has demonstrated significant advantages over conventional radiation techniques, primarily due to its ability to deliver precise radiation doses to tumours while sparing surrounding healthy tissues (132). HPV-positive HNCs have been observed to have better local control rates following radiation therapy, which is a significant factor in the overall prognosis of these patients. Studies have shown that patients with HPV-positive HNCs have improved overall survival and progression-free survival when treated with radiation therapy, compared to those with HPV-negative tumors (133). The immune response to HPV infection may play a role in the effectiveness of radiation therapy. The virus’s oncoproteins, such as E6 and E7, can disrupt the tumor’s ability to evade the immune system, potentially enhancing the therapeutic effect of radiation (134). Chemotherapy is critical for managing advanced or metastatic HPV-related cancers, often used in combination with radiation. Targeted therapies represent a paradigm shift in this therapeutic landscape, offering promising avenues for treatment (135), as illustrated by EGFR-targeted therapies in head and neck cancers.
Global HPV vaccine coverage is suboptimal, particularly in developing regions constrained by cost and logistics. The accuracy of HPV-related cancer screenings is inconsistent, with potential for false results, and their invasive nature can impede uptake. HPV testing struggles to distinguish between transient and precancerous infections. Despite HPV’s health risks, public awareness remains low, undermining prevention. The virus’s association with other STIs complicates diagnosis and treatment, and while early-stage cancers are manageable, advanced cases necessitate less invasive and more effective therapies. There is an urgent need for research into HPV’s carcinogenic mechanisms to foster innovative treatments and preventative strategies.
In conclusion, significant advances have been made in combating HPV-related cancers, yet challenges in prevention, diagnosis, and treatment remain. A multidisciplinary approach is essential for progress, including vaccine accessibility, screening improvements, public education, co-infection management, and research advancement.
MW: Conceptualization, Funding acquisition, Writing – original draft. HH: Writing – original draft. YT: Writing – original draft. XR: Writing – review & editing. XJ: Writing – review & editing. TM: Writing – review & editing, Funding acquisition, Validation. WL: Writing – review & editing, Funding acquisition, Supervision, Validation.
The author(s) declare financial support was received for the research, authorship, and/or publication of this article. This work was supported by China Postdoctoral Science Foundation (2022M721682), Nanjing Medical Science and Technology Development Foundation (ZKX20039) and Jiangsu Provincial Maternal and Child Health Research Institute (F202155).
The authors declare that the research was conducted in the absence of any commercial or financial relationships that could be construed as a potential conflict of interest.
All claims expressed in this article are solely those of the authors and do not necessarily represent those of their affiliated organizations, or those of the publisher, the editors and the reviewers. Any product that may be evaluated in this article, or claim that may be made by its manufacturer, is not guaranteed or endorsed by the publisher.
2. Hussen BM, Ahmadi G, Marzban H, Fard Azar ME, Sorayyayi S, Karampour R, et al. The role of hpv gene expression and selected cellular mirnas in lung cancer development. Microb Pathog. (2021) 150:104692. doi: 10.1016/j.micpath.2020.104692
3. Nahand JS, Khanaliha K, Mirzaei H, Moghoofei M, Baghi HB, Esghaei M, et al. Possible role of hpv/ebv coinfection in anoikis resistance and development in prostate cancer. BMC Cancer. (2021) 21:926. doi: 10.1186/s12885-021-08658-y
4. Khodabandehlou N, Mostafaei S, Etemadi A, Ghasemi A, Payandeh M, Hadifar S, et al. Human papilloma virus and breast cancer: the role of inflammation and viral expressed proteins. BMC Cancer. (2019) 19:61. doi: 10.1186/s12885-019-5286-0
5. Ameya G, Birri DJ. The molecular mechanisms of virus-induced human cancers. Microb Pathog. (2023) 183:106292. doi: 10.1016/j.micpath.2023.106292
6. Sobhi Amjad Z, Shojaeian A, Sadri Nahand J, Bayat M, Taghizadieh M, Rostamian M, et al. Oncoviruses: induction of cancer development and metastasis by increasing anoikis resistance. Heliyon. (2023) 9:e22598. doi: 10.1016/j.heliyon.2023.e22598
7. Mesri EA, Feitelson MA, Munger K. Human viral oncogenesis: A cancer hallmarks analysis. Cell Host Microbe. (2014) 15:266–82. doi: 10.1016/j.chom.2014.02.011
8. Cullen M, Boland JF, Schiffman M, Zhang X, Wentzensen N, Yang Q, et al. Deep sequencing of hpv16 genomes: A new high-throughput tool for exploring the carcinogenicity and natural history of hpv16 infection. Papillomavirus Res. (2015) 1:3–11. doi: 10.1016/j.pvr.2015.05.004
9. Lowy DR. Hpv vaccination to prevent cervical cancer and other hpv-associated disease: from basic science to effective interventions. J Clin Invest. (2016) 126:5–11. doi: 10.1172/JCI85446
10. Roman BR, Aragones A. Epidemiology and incidence of hpv-related cancers of the head and neck. J Surg Oncol. (2021) 124:920–2. doi: 10.1002/jso.26687
11. Lechner M, Liu J, Masterson L, Fenton TR. Hpv-associated oropharyngeal cancer: epidemiology, molecular biology and clinical management. Nat Rev Clin Oncol. (2022) 19:306–27. doi: 10.1038/s41571-022-00603-7
12. Sadri Nahand J, Esghaei M, Hamidreza Monavari S, Moghoofei M, Jalal Kiani S, Mostafaei S, et al. The assessment of a possible link between hpv-mediated inflammation, apoptosis, and angiogenesis in prostate cancer. Int Immunopharmacol. (2020) 88:106913. doi: 10.1016/j.intimp.2020.106913
13. Fatemipour M, Nahand JS, Fard Azar ME, Baghi HB, Taghizadieh M, Sorayyayi S, et al. Human papillomavirus and prostate cancer: the role of viral expressed proteins in the inhibition of anoikis and induction of metastasis. Microb Pathog. (2021) 152:104576. doi: 10.1016/j.micpath.2020.104576
14. Evande R, Rana A, Biswas-Fiss EE, Biswas SB. Protein-DNA interactions regulate human papillomavirus DNA replication, transcription, and oncogenesis. Int J Mol Sci. (2023) 24. doi: 10.3390/ijms24108493
15. Conway MJ, Meyers C. Replication and assembly of human papillomaviruses. J Dent Res. (2009) 88:307–17. doi: 10.1177/0022034509333446
16. Van Doorslaer K, Li Z, Xirasagar S, Maes P, Kaminsky D, Liou D, et al. The papillomavirus episteme: A major update to the papillomavirus sequence database. Nucleic Acids Res. (2017) 45:D499–506. doi: 10.1093/nar/gkw879
17. Cosper PF, Bradley S, Luo L, Kimple RJ. Biology of hpv mediated carcinogenesis and tumor progression. Semin Radiat Oncol. (2021) 31:265–73. doi: 10.1016/j.semradonc.2021.02.006
18. Garbuglia AR, Lapa D, Sias C, Capobianchi MR, Del Porto P. The use of both therapeutic and prophylactic vaccines in the therapy of papillomavirus disease. Front Immunol. (2020) 11:188. doi: 10.3389/fimmu.2020.00188
19. Tommasino M. The biology of beta human papillomaviruses. Virus Res. (2017) 231:128–38. doi: 10.1016/j.virusres.2016.11.013
20. Smith JA, White EA, Sowa ME, Powell ML, Ottinger M, Harper JW, et al. Genome-wide sirna screen identifies smcx, ep400, and brd4 as E2-dependent regulators of human papillomavirus oncogene expression. Proc Natl Acad Sci U.S.A. (2010) 107:3752–7. doi: 10.1073/pnas.0914818107
21. Doorbar J, Egawa N, Griffin H, Kranjec C, Murakami I. Human papillomavirus molecular biology and disease association. Rev Med Virol. (2015) 25 Suppl 1:2–23. doi: 10.1002/rmv.1822
22. Moody CA, Laimins LA. Human papillomavirus oncoproteins: pathways to transformation. Nat Rev Cancer. (2010) 10:550–60. doi: 10.1038/nrc2886
23. D'Abramo CM, Archambault J. Small molecule inhibitors of human papillomavirus protein - protein interactions. Open Virol J. (2011) 5:80–95. doi: 10.2174/1874357901105010080
24. Andersen AS, Koldjaer Solling AS, Ovesen T, Rusan M. The interplay between hpv and host immunity in head and neck squamous cell carcinoma. Int J Cancer. (2014) 134:2755–63. doi: 10.1002/ijc.28411
25. Munger K, Baldwin A, Edwards KM, Hayakawa H, Nguyen CL, Owens M, et al. Mechanisms of human papillomavirus-induced oncogenesis. J Virol. (2004) 78:11451–60. doi: 10.1128/JVI.78.21.11451-11460.2004
26. WHO. Human papillomavirus and cancer (2023). Available online at: https://www.who.int/news-room/fact-sheets/detail/human-papilloma-virus-and-cancer (Accessed October, 10, 2023).
27. Chao A, Huang HJ, Lai CH. Human papillomavirus research on the prevention, diagnosis, and prognosis of cervical cancer in Taiwan. Chang Gung Med J. (2012) 35:297–308. doi: 10.4103/2319-4170.106141
28. Mantovani F, Banks L. The human papillomavirus E6 protein and its contribution to Malignant progression. Oncogene. (2001) 20:7874–87. doi: 10.1038/sj.onc.1204869
29. Scheffner M, Huibregtse JM, Vierstra RD, Howley PM. The hpv-16 E6 and E6-ap complex functions as a ubiquitin-protein ligase in the ubiquitination of P53. Cell. (1993) 75:495–505. doi: 10.1016/0092-8674(93)90384-3
30. Huibregtse JM, Scheffner M, Howley PM. Cloning and expression of the cdna for E6-ap, a protein that mediates the interaction of the human papillomavirus E6 oncoprotein with P53. Mol Cell Biol. (1993) 13:775–84. doi: 10.1128/mcb.13.2.775-784.1993
31. Barr AR, Cooper S, Heldt FS, Butera F, Stoy H, Mansfeld J, et al. DNA damage during S-phase mediates the proliferation-quiescence decision in the subsequent G1 via P21 expression. Nat Commun. (2017) 8:14728. doi: 10.1038/ncomms14728
32. Filippova M, Johnson MM, Bautista M, Filippov V, Fodor N, Tungteakkhun SS, et al. The large and small isoforms of human papillomavirus type 16 E6 bind to and differentially affect procaspase 8 stability and activity. J Virol. (2007) 81:4116–29. doi: 10.1128/JVI.01924-06
33. Magal SS, Jackman A, Ish-Shalom S, Botzer LE, Gonen P, Schlegel R, et al. Downregulation of bax mrna expression and protein stability by the E6 protein of human papillomavirus 16. J Gen Virol. (2005) 86:611–21. doi: 10.1099/vir.0.80453-0
34. Thomas M, Banks L. Human papillomavirus (Hpv) E6 interactions with bak are conserved amongst E6 proteins from high and low risk hpv types. J Gen Virol. (1999) 80:1513–7. doi: 10.1099/0022-1317-80-6-1513
35. Shah M, Anwar MA, Park S, Jafri SS, Choi S. In silico mechanistic analysis of irf3 inactivation and high-risk hpv E6 species-dependent drug response. Sci Rep. (2015) 5:13446. doi: 10.1038/srep13446
36. Bezprozvanny I, Maximov A. Pdz domains: more than just a glue. Proc Natl Acad Sci U.S.A. (2001) 98:787–9. doi: 10.1073/pnas.98.3.787
37. Xie X, Piao L, Bullock BN, Smith A, Su T, Zhang M, et al. Targeting hpv16 E6-P300 interaction reactivates P53 and inhibits the tumorigenicity of hpv-positive head and neck squamous cell carcinoma. Oncogene. (2014) 33:1037–46. doi: 10.1038/onc.2013.25
38. Liu X, Dakic A, Zhang Y, Dai Y, Chen R, Schlegel R. Hpv E6 protein interacts physically and functionally with the cellular telomerase complex. Proc Natl Acad Sci U.S.A. (2009) 106:18780–5. doi: 10.1073/pnas.0906357106
39. Fischer M, Uxa S, Stanko C, Magin TM, Engeland K. Human papilloma virus E7 oncoprotein abrogates the P53-P21-dream pathway. Sci Rep. (2017) 7:2603. doi: 10.1038/s41598-017-02831-9
40. Santacroce L, Di Cosola M, Bottalico L, Topi S, Charitos IA, Ballini A, et al. Focus on hpv infection and the molecular mechanisms of oral carcinogenesis. Viruses. (2021) 13. doi: 10.3390/v13040559
41. Antonsson A, Payne E, Hengst K, McMillan NA. The human papillomavirus type 16 E7 protein binds human interferon regulatory factor-9 via a novel pest domain required for transformation. J Interferon Cytokine Res. (2006) 26:455–61. doi: 10.1089/jir.2006.26.455
42. Straight SW, Hinkle PM, Jewers RJ, McCance DJ. The E5 oncoprotein of human papillomavirus type 16 transforms fibroblasts and effects the downregulation of the epidermal growth factor receptor in keratinocytes. J Virol. (1993) 67:4521–32. doi: 10.1128/JVI.67.8.4521-4532.1993
43. Belleudi F, Leone L, Purpura V, Cannella F, Scrofani C, Torrisi MR. Hpv16 E5 affects the kgfr/fgfr2b-mediated epithelial growth through alteration of the receptor expression, signaling and endocytic traffic. Oncogene. (2011) 30:4963–76. doi: 10.1038/onc.2011.203
44. Kudela E, Liskova A, Samec M, Koklesova L, Holubekova V, Rokos T, et al. The interplay between the vaginal microbiome and innate immunity in the focus of predictive, preventive, and personalized medical approach to combat hpv-induced cervical cancer. EPMA J. (2021) 12:199–220. doi: 10.1007/s13167-021-00244-3
45. Wakabayashi R, Nakahama Y, Nguyen V, Espinoza JL. The host-microbe interplay in human papillomavirus-induced carcinogenesis. Microorganisms. (2019) 7. doi: 10.3390/microorganisms7070199
46. Guidry JT, Scott RS. The interaction between human papillomavirus and other viruses. Virus Res. (2017) 231:139–47. doi: 10.1016/j.virusres.2016.11.002
47. Lin D, Kouzy R, Abi Jaoude J, Noticewala SS, Delgado Medrano AY, Klopp AH, et al. Microbiome factors in hpv-driven carcinogenesis and cancers. PloS Pathog. (2020) 16:e1008524. doi: 10.1371/journal.ppat.1008524
48. Lebeau A, Bruyere D, Roncarati P, Peixoto P, Hervouet E, Cobraiville G, et al. Hpv infection alters vaginal microbiome through down-regulating host mucosal innate peptides used by lactobacilli as amino acid sources. Nat Commun. (2022) 13:1076. doi: 10.1038/s41467-022-28724-8
49. Kyrgiou M, Moscicki AB. Vaginal microbiome and cervical cancer. Semin Cancer Biol. (2022) 86:189–98. doi: 10.1016/j.semcancer.2022.03.005
50. de Martel C, Plummer M, Vignat J, Franceschi S. Worldwide burden of cancer attributable to hpv by site, country and hpv type. Int J Cancer. (2017) 141:664–70. doi: 10.1002/ijc.30716
51. Hirth J. Disparities in hpv vaccination rates and hpv prevalence in the United States: A review of the literature. Hum Vaccin Immunother. (2019) 15:146–55. doi: 10.1080/21645515.2018.1512453
52. Bloem P, Ogbuanu I. Vaccination to prevent human papillomavirus infections: from promise to practice. PloS Med. (2017) 14:e1002325. doi: 10.1371/journal.pmed.1002325
53. Ouda AM, Elsabagh AA, Elmakaty IM, Gupta I, Vranic S, Al-Thawadi H, et al. Hpv and recurrent respiratory papillomatosis: A brief review. Life (Basel). (2021) 11. doi: 10.3390/life11111279
54. Galati L, Chiocca S, Duca D, Tagliabue M, Simoens C, Gheit T, et al. Hpv and head and neck cancers: towards early diagnosis and prevention. Tumour Virus Res. (2022) 14:200245. doi: 10.1016/j.tvr.2022.200245
55. Liao CI, Francoeur AA, Kapp DS, Caesar MAP, Huh WK, Chan JK. Trends in human papillomavirus-associated cancers, demographic characteristics, and vaccinations in the us, 2001-2017. JAMA Netw Open. (2022) 5:e222530. doi: 10.1001/jamanetworkopen.2022.2530
56. Elrefaey S, Massaro MA, Chiocca S, Chiesa F, Ansarin M. Hpv in oropharyngeal cancer: the basics to know in clinical practice. Acta Otorhinolaryngol Ital. (2014) 34:299–309.
57. Shi W, Kato H, Perez-Ordonez B, Pintilie M, Huang S, Hui A, et al. Comparative prognostic value of hpv16 E6 mrna compared with in situ hybridization for human oropharyngeal squamous carcinoma. J Clin Oncol. (2009) 27:6213–21. doi: 10.1200/JCO.2009.23.1670
58. Bratman SV, Bruce JP, O'Sullivan B, Pugh TJ, Xu W, Yip KW, et al. Human papillomavirus genotype association with survival in head and neck squamous cell carcinoma. JAMA Oncol. (2016) 2:823–6. doi: 10.1001/jamaoncol.2015.6587
59. Connors KA, Abbott S, Jair K, Daniels J, Lintner M, Klein D, et al. Cross comparison of ampfire hpv genotyping assay and roche human papillomavirus (Hpv) linear array for hpv genotyping of anal swab samples. J Virol Methods. (2021) 292:114113. doi: 10.1016/j.jviromet.2021.114113
60. Tanaka H, Suzuki M, Takemoto N, Fukusumi T, Eguchi H, Takai E, et al. Performance of oral hpv DNA, oral hpv mrna and circulating tumor hpv DNA in the detection of hpv-related oropharyngeal cancer and cancer of unknown primary. Int J Cancer. (2022) 150:174–86. doi: 10.1002/ijc.33798
61. Chera BS, Kumar S, Beaty BT, Marron D, Jefferys S, Green R, et al. Rapid clearance profile of plasma circulating tumor hpv type 16 DNA during chemoradiotherapy correlates with disease control in hpv-associated oropharyngeal cancer. Clin Cancer Res. (2019) 25:4682–90. doi: 10.1158/1078-0432.CCR-19-0211
62. Westra WH. Detection of human papillomavirus (Hpv) in clinical samples: evolving methods and strategies for the accurate determination of hpv status of head and neck carcinomas. Oral Oncol. (2014) 50:771–9. doi: 10.1016/j.oraloncology.2014.05.004
63. Hibbert J, Halec G, Baaken D, Waterboer T, Brenner N. Sensitivity and specificity of human papillomavirus (Hpv) 16 early antigen serology for hpv-driven oropharyngeal cancer: A systematic literature review and meta-analysis. Cancers (Basel). (2021) 13. doi: 10.3390/cancers13123010
64. Huang SH, Waldron JN, Milosevic M, Shen X, Ringash J, Su J, et al. Prognostic value of pretreatment circulating neutrophils, monocytes, and lymphocytes in oropharyngeal cancer stratified by human papillomavirus status. Cancer. (2015) 121:545–55. doi: 10.1002/cncr.29100
65. Wansom D, Light E, Worden F, Prince M, Urba S, Chepeha DB, et al. Correlation of cellular immunity with human papillomavirus 16 status and outcome in patients with advanced oropharyngeal cancer. Arch Otolaryngol Head Neck Surg. (2010) 136:1267–73. doi: 10.1001/archoto.2010.211
66. Wan Y, Vagenas D, Salazar C, Kenny L, Perry C, Calvopina D, et al. Salivary mirna panel to detect hpv-positive and hpv-negative head and neck cancer patients. Oncotarget. (2017) 8:99990–100001. doi: 10.18632/oncotarget.21725
67. Salazar-Ruales C, Arguello JV, Lopez-Cortes A, Cabrera-Andrade A, Garcia-Cardenas JM, Guevara-Ramirez P, et al. Salivary micrornas for early detection of head and neck squamous cell carcinoma: A case-control study in the high altitude mestizo Ecuadorian population. BioMed Res Int. (2018) 2018:9792730. doi: 10.1155/2018/9792730
68. Tao L, Han L, Li X, Gao Q, Pan L, Wu L, et al. Prevalence and risk factors for cervical neoplasia: A cervical cancer screening program in beijing. BMC Public Health. (2014) 14:1185. doi: 10.1186/1471-2458-14-1185
69. Roden RBS, Stern PL. Opportunities and challenges for human papillomavirus vaccination in cancer. Nat Rev Cancer. (2018) 18:240–54. doi: 10.1038/nrc.2018.13
70. Simms KT, Hanley SJB, Smith MA, Keane A, Canfell K. Impact of hpv vaccine hesitancy on cervical cancer in Japan: A modelling study. Lancet Public Health. (2020) 5:e223–e34. doi: 10.1016/S2468-2667(20)30010-4
71. Krzowska-Firych J, Lucas G, Lucas C, Lucas N, Pietrzyk L. An overview of human papillomavirus (Hpv) as an etiological factor of the anal cancer. J Infect Public Health. (2019) 12:1–6. doi: 10.1016/j.jiph.2018.06.005
72. Bechini A, Moscadelli A, Velpini B, Bonito B, Orlando P, Putignano P, et al. Efficacy of hpv vaccination regarding vulvar and vaginal recurrences in previously treated women: the need for further evidence. Vaccines (Basel). (2023) 11. doi: 10.3390/vaccines11061084
73. Thomas A, Necchi A, Muneer A, Tobias-MaChado M, Tran ATH, Van Rompuy AS, et al. Penile cancer. Nat Rev Dis Primers. (2021) 7:11. doi: 10.1038/s41572-021-00246-5
74. Olesen TB, Sand FL, Rasmussen CL, Albieri V, Toft BG, Norrild B, et al. Prevalence of human papillomavirus DNA and P16(Ink4a) in penile cancer and penile intraepithelial neoplasia: A systematic review and meta-analysis. Lancet Oncol. (2019) 20:145–58. doi: 10.1016/S1470-2045(18)30682-X
75. Simoes PW, Medeiros LR, Simoes Pires PD, Edelweiss MI, Rosa DD, Silva FR, et al. Prevalence of human papillomavirus in breast cancer: A systematic review. Int J Gynecol Cancer. (2012) 22:343–7. doi: 10.1097/IGC.0b013e31823c712e
76. De Carolis S, Storci G, Ceccarelli C, Savini C, Gallucci L, Sansone P, et al. Hpv DNA associates with breast cancer Malignancy and it is transferred to breast cancer stromal cells by extracellular vesicles. Front Oncol. (2019) 9:860. doi: 10.3389/fonc.2019.00860
77. Sher G, Salman NA, Kulinski M, Fadel RA, Gupta VK, Anand A, et al. Prevalence and type distribution of high-risk human papillomavirus (Hpv) in breast cancer: A Qatar based study. Cancers (Basel). (2020) 12. doi: 10.3390/cancers12061528
78. Balci FL, Uras C, Feldman SM. Is human papillomavirus associated with breast cancer or papilloma presenting with pathologic nipple discharge? Cancer Treat Res Commun. (2019) 19:100122. doi: 10.1016/j.ctarc.2019.100122
79. Biesaga B, Janecka-Widla A, Kolodziej-Rzepa M, Mucha-Malecka A, Slonina D, Ziobro M, et al. Low frequency of hpv positivity in breast tumors among patients from south-central Poland. Infect Agent Cancer. (2021) 16:67. doi: 10.1186/s13027-021-00405-z
80. Boumba ALM, Malanda Mboungou Moudiongui D, Ngatali SFC, Takale RP, Moukassa D, Peko JF. Oncogenic human papillomavirus in breast cancer: molecular prevalence in a group of congolese patients. Access Microbiol. (2021) 3:216. doi: 10.1099/acmi.0.000216
81. Elagali AM, Suliman AA, Altayeb M, Dannoun AI, Parine NR, Sakr HI, et al. Human papillomavirus, gene mutation and estrogen and progesterone receptors in breast cancer: A cross-sectional study. Pan Afr Med J. (2021) 38:43. doi: 10.11604/pamj.2021.38.43.22013
82. Alinezhadi M, Makvandi M, Kaydani GA, Jazayeri SN, Charostad J, Talaeizadeh AT, et al. Detection of high-risk human papillomavirus DNA in invasive ductal carcinoma specimens. Asian Pac J Cancer Prev. (2022) 23:3201–7. doi: 10.31557/APJCP.2022.23.9.3201
83. Golrokh Mofrad M, Sadigh ZA, Ainechi S, Faghihloo E. Detection of human papillomavirus genotypes, herpes simplex, varicella zoster and cytomegalovirus in breast cancer patients. Virol J. (2021) 18:25. doi: 10.1186/s12985-021-01498-z
84. Guo H, Idrovo JP, Cao J, Roychoudhury S, Navale P, Auguste LJ, et al. Human papillomavirus (Hpv) detection by chromogenic in situ hybridization (Cish) and P16 immunohistochemistry (Ihc) in breast intraductal papilloma and breast carcinoma. Clin Breast Cancer. (2021) 21:e638–e46. doi: 10.1016/j.clbc.2021.04.006
85. Gupta I, Jabeen A, Al-Sarraf R, Farghaly H, Vranic S, Sultan AA, et al. The co-presence of high-risk human papillomaviruses and epstein-barr virus is linked with tumor grade and stage in Qatari women with breast cancer. Hum Vaccin Immunother. (2021) 17:982–9. doi: 10.1080/21645515.2020.1802977
86. Metwally SA, Abo-Shadi MA, Abdel Fattah NF, Barakat AB, Rabee OA, Osman AM, et al. Presence of hpv, ebv and hmtv viruses among Egyptian breast cancer women: molecular detection and clinical relevance. Infect Drug Resist. (2021) 14:2327–39. doi: 10.2147/IDR.S313219
87. Nagi K, Gupta I, Jurdi N, Jabeen A, Yasmeen A, Batist G, et al. High-risk human papillomaviruses and epstein-barr virus in breast cancer in lebanese women and their association with tumor grade: A molecular and tissue microarray study. Cancer Cell Int. (2021) 21:308. doi: 10.1186/s12935-021-02009-4
88. Charostad J, Azaran A, Nakhaei M, Astani A, Kaydani GA, Motamedfar A, et al. Upregulation of interleukin-6 in hpv-positive breast cancer patients. Iran J Immunol. (2021) 18:315–30. doi: 10.22034/IJI.2021.89107.1930
89. El-Sheikh N, Mousa NO, Tawfeik AM, Saleh AM, Elshikh I, Deyab M, et al. Assessment of human papillomavirus infection and risk factors in Egyptian women with breast cancer. Breast Cancer (Auckl). (2021) 15:1178223421996279. doi: 10.1177/1178223421996279
90. Calderon G, Castaneda CA, Castillo M, Sanchez J, Bernabe L, Suarez N, et al. Human papillomavirus, cytomegalovirus infection and P16 staining in breast tumors from Peruvian women. Asian Pac J Cancer Prev. (2022) 23:1571–6. doi: 10.31557/APJCP.2022.23.5.1571
91. Gebregzabher E, Seifu D, Tigneh W, Bokretsion Y, Bekele A, Abebe M, et al. Detection of high- and low-risk hpv DNA in archived breast carcinoma tissues from Ethiopian women. Int J Breast Cancer. (2021) 2021:2140151. doi: 10.1155/2021/2140151
92. Gupta I, Al-Sarraf R, Farghaly H, Vranic S, Sultan AA, Al-Thawadi H, et al. Incidence of hpvs, ebv, and mmtv-like virus in breast cancer in Qatar. Intervirology. (2022) 65:188–94. doi: 10.1159/000525277
93. Maldonado-Rodriguez E, Hernandez-Barrales M, Reyes-Lopez A, Godina-Gonzalez S, Gallegos-Flores PI, Esparza-Ibarra EL, et al. Presence of human papillomavirus DNA in Malignant neoplasia and non-malignant breast disease. Curr Issues Mol Biol. (2022) 44:3648–65. doi: 10.3390/cimb44080250
94. Maroga N, Mokoena T, Ledibane N, Musekiwa A, Bida M, Kgomo M, et al. Profile of human papillomavirus genotypes in breast and oesophageal cancer patients in pretoria, South Africa. S Afr Med J. (2023) 113:49–54. doi: 10.7196/SAMJ.2023.v113i7.560
95. Tavakolian S, Faghihloo E. The prevalence of varicella zoster virus, herpes simplex virus type 2, and human papillomavirus in breast cancerous tissues and their adjacent ones in Iran. J Res Med Sci. (2023) 28:65. doi: 10.4103/jrms.jrms_475_22
96. Makvandi M, Rashno M, Faghihloo E, Bagheri S, Hesam S, Mirzaei H, et al. Low presence of papillomavirus and its lack of correlation with clinicopathological factors in breast cancer: A case control study. Iran J Microbiol. (2023) 15:585–93. doi: 10.18502/ijm.v15i4.13513
97. Oliveira ES, Ferreira MVP, Rahal P, Castelo Branco MB, Rabenhorst SHB. High frequency of epstein-barr virus and absence of papillomavirus in breast cancer patients from Brazilian northeast. Asian Pac J Cancer Prev. (2022) 23:2351–9. doi: 10.31557/APJCP.2022.23.7.2351
98. Silva EM, Mariano VS, Pastrez PRA, Pinto MC, Nunes EM, Sichero L, et al. Human papillomavirus is not associated to non-small cell lung cancer: data from a prospective cross-sectional study. Infect Agent Cancer. (2019) 14:18. doi: 10.1186/s13027-019-0235-8
99. He F, Xiong W, Yu F, Xiao R, Ye H, Li W, et al. Human papillomavirus infection maybe not associated with primary lung cancer in the fujian population of China. Thorac Cancer. (2020) 11:561–9. doi: 10.1111/1759-7714.13282
100. Rezaei M, Mostafaei S, Aghaei A, Hosseini N, Darabi H, Nouri M, et al. The association between hpv gene expression, inflammatory agents and cellular genes involved in emt in lung cancer tissue. BMC Cancer. (2020) 20:916. doi: 10.1186/s12885-020-07428-6
101. Jaworek H, Koudelakova V, Slavkovsky R, Drabek J, Hajduch M. The absence of high-risk human papillomavirus in czech non-small cell lung cancer cases. BioMed Pap Med Fac Univ Palacky Olomouc Czech Repub. (2020) 164:71–6. doi: 10.5507/bp.2018.079
102. Zou DJ, Zhao YB, Yang JH, Xu HT, Li QC, Wu GP. Expression and significance of hpv16 E6/E7 mrnas in the bronchial brush and tbna cells of patients with small cell lung cancer. Technol Cancer Res Treat. (2021) 20:15330338211019505. doi: 10.1177/15330338211019505
103. Wu Y, Yin Q, Zhou YL, He L, Zou ZQ, Dai XY, et al. Evaluation of micrornas as potential biomarkers in circulating hpv-DNA-positive non-small cell lung cancer patients. Cancer Biol Ther. (2021) 22:136–48. doi: 10.1080/15384047.2021.1872155
104. Sirera G, Videla S, Saludes V, Castella E, Sanz C, Ariza A, et al. Prevalence of hpv-DNA and E6 mrna in lung cancer of hiv-infected patients. Sci Rep. (2022) 12:13196. doi: 10.1038/s41598-022-17237-5
105. Khatami A, Nahand JS, Kiani SJ, Khoshmirsafa M, Moghoofei M, Khanaliha K, et al. Human papilloma virus (Hpv) and prostate cancer (Pca): the potential role of hpv gene expression and selected cellular mirnas in pca development. Microb Pathog. (2022) 166:105503. doi: 10.1016/j.micpath.2022.105503
106. Abumsimir B, Mrabti M, Laraqui A, Ameur A, Koraishi SI, Mzibri M, et al. Molecular characterization of human papillomavirus and mouse mammary tumor virus-like infections in prostate cancer tissue and relevance with tumor characteristics. Mol Clin Oncol. (2022) 16:97. doi: 10.3892/mco.2022.2530
107. Ahmed MY, Salman NA, Sandhu S, Cakir MO, Seddon AM, Kuehne C, et al. Detection of high-risk human papillomavirus in prostate cancer from a uk based population. Sci Rep. (2023) 13:7633. doi: 10.1038/s41598-023-34734-3
108. Haghshenas MR, Mousavi T, Moosazadeh M, Afshari M. Human papillomavirus and breast cancer in Iran: A meta- analysis. Iran J Basic Med Sci. (2016) 19:231–7.
109. Ma H, Wang Y, Sullivan-Halley J, Weiss L, Marchbanks PA, Spirtas R, et al. Use of four biomarkers to evaluate the risk of breast cancer subtypes in the women's contraceptive and reproductive experiences study. Cancer Res. (2010) 70:575–87. doi: 10.1158/0008-5472.CAN-09-3460
110. Karnosky J, Dietmaier W, Knuettel H, Freigang V, Koch M, Koll F, et al. Hpv and lung cancer: A systematic review and meta-analysis. Cancer Rep (Hoboken). (2021) 4:e1350. doi: 10.1002/cnr2.1350
111. Syrjanen KJ. Condylomatous changes in neoplastic bronchial epithelium. Rep Case Respiration. (1979) 38:299–304. doi: 10.1159/000194095
112. Sung H, Ferlay J, Siegel RL, Laversanne M, Soerjomataram I, Jemal A, et al. Global cancer statistics 2020: globocan estimates of incidence and mortality worldwide for 36 cancers in 185 countries. CA Cancer J Clin. (2021) 71:209–49. doi: 10.3322/caac.21660
113. McNicol PJ, Dodd JG. High prevalence of human papillomavirus in prostate tissues. J Urol. (1991) 145:850–3. doi: 10.1016/s0022-5347(17)38476-8
114. Rhim JS, Webber MM, Bello D, Lee MS, Arnstein P, Chen LS, et al. Stepwise immortalization and transformation of adult human prostate epithelial cells by a combination of hpv-18 and V-ki-ras. Proc Natl Acad Sci U.S.A. (1994) 91:11874–8. doi: 10.1073/pnas.91.25.11874
115. Schutze DM, Snijders PJ, Bosch L, Kramer D, Meijer CJ, Steenbergen RD. Differential in vitro immortalization capacity of eleven (Probable) [Corrected] high-risk human papillomavirus types. J Virol. (2014) 88:1714–24. doi: 10.1128/JVI.02859-13
116. Opeyemi Bello R, Willis-Powell L, James O, Sharma A, Marsh E, Ellis L, et al. Does human papillomavirus play a causative role in prostate cancer? A systematic review using bradford hill's criteria. Cancers (Basel). (2023) 15. doi: 10.3390/cancers15153897
117. Khoury JD, Tannir NM, Williams MD, Chen Y, Yao H, Zhang J, et al. Landscape of DNA virus associations across human Malignant cancers: analysis of 3,775 cases using rna-seq. J Virol. (2013) 87:8916–26. doi: 10.1128/JVI.00340-13
118. Kechagias KS, Kalliala I, Bowden SJ, Athanasiou A, Paraskevaidi M, Paraskevaidis E, et al. Role of human papillomavirus (Hpv) vaccination on hpv infection and recurrence of hpv related disease after local surgical treatment: systematic review and meta-analysis. BMJ. (2022) 378:e070135. doi: 10.1136/bmj-2022-070135
119. Joura EA, Giuliano AR, Iversen OE, Bouchard C, Mao C, Mehlsen J, et al. A 9-valent hpv vaccine against infection and intraepithelial neoplasia in women. N Engl J Med. (2015) 372:711–23. doi: 10.1056/NEJMoa1405044
120. Monie A, Hung CF, Roden R, Wu TC. Cervarix: A vaccine for the prevention of hpv 16, 18-associated cervical cancer. Biologics. (2008) 2:97–105.
121. Markowitz LE, Schiller JT. Human papillomavirus vaccines. J Infect Dis. (2021) 224:S367–S78. doi: 10.1093/infdis/jiaa621
122. Illah O, Olaitan A. Updates on hpv vaccination. Diagn (Basel). (2023) 13. doi: 10.3390/diagnostics13020243
123. Lehtinen M, Apter D, Eriksson T, Harjula K, Hokkanen M, Lehtinen T, et al. Effectiveness of the as04-adjuvanted hpv-16/18 vaccine in reducing oropharyngeal hpv infections in young females-results from a community-randomized trial. Int J Cancer. (2020) 147:170–4. doi: 10.1002/ijc.32791
124. Diana G, Corica C. Human papilloma virus vaccine and prevention of head and neck cancer, what is the current evidence? Oral Oncol. (2021) 115:105168. doi: 10.1016/j.oraloncology.2020.105168
125. Chrysostomou AC, Kostrikis LG. Methodologies of primary hpv testing currently applied for cervical cancer screening. Life (Basel). (2020) 10. doi: 10.3390/life10110290
126. Redd DS, Jensen JL, Hughes SJ, Pogue K, Sloan-Aagard CD, Miner DS, et al. Effects of religious practice and teachings about sexual behavior on intent to vaccinate against human papillomavirus. Vaccines (Basel). (2022) 10. doi: 10.3390/vaccines10030397
127. FitzGerald S, Cornally N, Hegarty J. Men's perspectives on cancer prevention behaviors associated with hpv. Psychooncology. (2018) 27:484–91. doi: 10.1002/pon.4515
128. Gong X, Chi H, Xia Z, Yang G, Tian G. Advances in hpv-associated tumor management: therapeutic strategies and emerging insights. J Med Virol. (2023) 95:e28950. doi: 10.1002/jmv.28950
129. Mydlarz WK, Chan JY, Richmon JD. The role of surgery for hpv-associated head and neck cancer. Oral Oncol. (2015) 51:305–13. doi: 10.1016/j.oraloncology.2014.10.005
130. Salzillo TC, Taku N, Wahid KA, McDonald BA, Wang J, van Dijk LV, et al. Advances in imaging for hpv-related oropharyngeal cancer: applications to radiation oncology. Semin Radiat Oncol. (2021) 31:371–88. doi: 10.1016/j.semradonc.2021.05.001
131. Shin DM, Khuri FR. Advances in the management of recurrent or metastatic squamous cell carcinoma of the head and neck. Head Neck. (2013) 35:443–53. doi: 10.1002/hed.21910
132. Duprez F, Madani I, Bonte K, Boterberg T, Vakaet L, Derie C, et al. Intensity-modulated radiotherapy for recurrent and second primary head and neck cancer in previously irradiated territory. Radiother Oncol. (2009) 93:563–9. doi: 10.1016/j.radonc.2009.10.012
133. Chen L, Riaz N, Lee N, McBride S. Current considerations for radiotherapy in hpv-associated head and neck cancer. J Surg Oncol. (2021) 124:945–51. doi: 10.1002/jso.26689
134. Eberhardt CS, Kissick HT, Patel MR, Cardenas MA, Prokhnevska N, Obeng RC, et al. Functional hpv-specific pd-1(+) stem-like cd8 T cells in head and neck cancer. Nature. (2021) 597:279–84. doi: 10.1038/s41586-021-03862-z
135. Mukerjee N, Maitra S, Gorai S, Ghosh A, Alexiou A, Thorat ND. Revolutionizing human papillomavirus (Hpv)-related cancer therapies: unveiling the promise of proteolysis targeting chimeras (Protacs) and proteolysis targeting antibodies (Protabs) in cancer nano-vaccines. J Med Virol. (2023) 95:e29135. doi: 10.1002/jmv.29135
Keywords: HPV, HPV-associated cancers, HPV vaccines, microRNA, cancer prevention
Citation: Wu M, Huang H, Tang Y, Ren X, Jiang X, Tian M and Li W (2024) Unveiling the multifaceted realm of human papillomavirus: a comprehensive exploration of biology, interactions, and advances in cancer management. Front. Immunol. 15:1430544. doi: 10.3389/fimmu.2024.1430544
Received: 10 May 2024; Accepted: 24 July 2024;
Published: 08 August 2024.
Edited by:
Srivastava Vartika, Cleveland Clinic, United StatesReviewed by:
Marcela Lizano, National Cancer Institute, MexicoCopyright © 2024 Wu, Huang, Tang, Ren, Jiang, Tian and Li. This is an open-access article distributed under the terms of the Creative Commons Attribution License (CC BY). The use, distribution or reproduction in other forums is permitted, provided the original author(s) and the copyright owner(s) are credited and that the original publication in this journal is cited, in accordance with accepted academic practice. No use, distribution or reproduction is permitted which does not comply with these terms.
*Correspondence: Wei Li, d2VpbGkxMjZAMTI2LmNvbQ==; Man Tian, dG1zd2VldEAxNjMuY29t
†These authors have contributed equally to this work
Disclaimer: All claims expressed in this article are solely those of the authors and do not necessarily represent those of their affiliated organizations, or those of the publisher, the editors and the reviewers. Any product that may be evaluated in this article or claim that may be made by its manufacturer is not guaranteed or endorsed by the publisher.
Research integrity at Frontiers
Learn more about the work of our research integrity team to safeguard the quality of each article we publish.