- 1Department of Orthopaedic Surgery, The First Affiliated Hospital of Chongqing Medical University, Chongqing, China
- 2Orthopedic Laboratory of Chongqing Medical University, Chongqing, China
SAPHO syndrome is a complex inflammatory disorder affecting the skin and bones, characterized by osteomyelitis, acne, and pustulosis. Cytokines play a pivotal role in the pathogenesis of SAPHO syndrome, especially in inflammatory responses and immune regulation. This article reviews the cytokines involved in the pathogenesis of SAPHO syndrome, such as tumor necrosis factor α (TNF-α), interleukin 1β (IL-1β), IL-6, IL-10, and transforming growth factor-β (TGF-β), and discusses their potential as intervention points for treatment. These findings elucidate the intricate immune regulatory network of SAPHO syndrome and provide a theoretical foundation for the development of new targeted therapeutic strategies.
1 Introduction
SAPHO syndrome, a rare immune-mediated disorder, is characterized by its primary clinical manifestations: synovitis (inflammation of the synovial membrane, leading to joint pain and swelling), acne (severe acneiform eruptions, often resistant to conventional treatments), pustulosis (pustular skin lesions, commonly affecting the palms and soles), hyperostosis (excessive bone growth, particularly in the sternoclavicular region), and osteitis (inflammation of the bone, causing pain and structural changes), from which its acronym is derived. First described in 1987, the syndrome encapsulates a spectrum of diseases sharing similar clinical and radiological features (1). The clinical manifestations of SAPHO syndrome are diverse, ranging from dermatological symptoms like severe acne and pustulosis to complex osteoarticular symptoms including pain and swelling in the sternum, clavicle, and spine (2, 3).
SAPHO syndrome can affect individuals of any age, but it typically presents in young adults (4). There is no strong sex predilection, although some studies suggest a slight female predominance. The prevalence of SAPHO syndrome varies by region, with higher reporting rates in Europe and East Asia, potentially reflecting differences in diagnostic practices and awareness (5). The clinical course of SAPHO syndrome is often chronic and relapsing, significantly impacting patients’ quality of life. The dermatological and osteoarticular manifestations are frequently the most debilitating, necessitating a multidisciplinary approach to management (6).
Despite advancements in SAPHO syndrome research over the past decades, the precise etiology and pathogenesis of the disorder remain elusive. It is currently hypothesized that SAPHO syndrome could be linked to a variety of factors, including genetic predispositions, immune system abnormalities, and microbial infections. The role of cytokines in its pathogenesis is particularly emphasized, as these key signaling molecules regulate immune and inflammatory responses. Understanding their impact is critical for elucidating the mechanisms of the disease. Moreover, since existing treatments for SAPHO syndrome primarily address symptoms and lack targeted therapeutic approaches, developing novel treatments based on cytokine modulation is of paramount importance.
This review article aims to thoroughly examine the central role of cytokines in the pathogenesis of SAPHO syndrome. Pro-inflammatory cytokines like tumor necrosis factor α (TNF-α) and interleukin 1β (IL-1β) are crucial in promoting inflammatory responses, exacerbating tissue damage and pain by activating pathways such as nuclear factor κB (NF-κB). Conversely, anti-inflammatory cytokines such as IL-10 and transforming growth factor β (TGF-β) strive to restore immune balance by mitigating inflammatory responses. This discussion of cytokine involvement not only provides a theoretical basis for new targeted therapies but also explores directions for future treatment improvements to enhance clinical outcomes.
2 SAPHO syndrome
2.1 Pathophysiology of SAPHO syndrome
SAPHO syndrome encompasses a spectrum of inflammatory skin and skeletal disorders, primarily characterized by bone proliferation and osteitis. Commonly, these bone alterations manifest in the anterior chest wall, including the sternum and clavicle, and extend to the cervical vertebrae and sacroiliac joints. Typically, the lesions involve bone sclerosis and hypertrophy of the adjacent soft tissues (7). Pathologically, bone lesions in SAPHO syndrome may present as acute inflammatory responses, and as the condition progresses, bone marrow fibrosis and significant sclerosis of bone trabeculae may develop (8). These features distinguish SAPHO syndrome from other forms of osteitis, such as infectious osteitis (3, 9).
The dermatological manifestations of SAPHO syndrome vary, with the most prevalent being severe acne and pustular eczema, especially palmoplantar pustulosis. These skin lesions can manifest either before or concurrently with the skeletal symptoms (10). Skeletal symptoms typically include pain and swelling in the anterior chest wall and chronic pain, often associated with bone proliferation and inflammation near joints. This bone proliferation can impair the function of adjacent joints, adversely affecting the patient’s quality of life (3, 11, 12). Imaging examinations are vital for diagnosis, revealing characteristic changes in the affected bones, including bone proliferation and erosion (13).
2.2 Pathogenesis
SAPHO syndrome is an immune-mediated inflammatory disorder whose pathogenesis involves complex interactions between genetic and environmental factors. Although specific genetic markers have not been conclusively identified, genetic susceptibility plays a crucial role in SAPHO syndrome. Research has indicated that particular genetic variations, such as mutations in the PSTPIP2 and NOD2 genes, might be linked to the onset of SAPHO syndrome; however, these findings necessitate further confirmation (14). Environmental factors, especially infectious agents, are considered significant triggers for the syndrome. Notably, infection with Propionibacterium acnes is believed to potentially trigger inflammatory responses by activating the immune system (15–17).
The immune response in SAPHO syndrome is characterized by both autoimmune and inflammatory components. Studies show that the immune responses, particularly those mediated by neutrophils and T cells, are overly active in affected individuals. For instance, neutrophils in patients with SAPHO syndrome demonstrate excessive activation, releasing substantial quantities of pro-inflammatory cytokines like IL-8 and TNF-α (17). Furthermore, the signaling pathways involving IL-1 and TNF-α are central to the pathophysiology of SAPHO syndrome, supporting the use of biologic treatments such as TNF-α inhibitors and IL-1 receptor antagonists (6, 10, 18).
3 Biological basis of cytokines
3.1 Definition of cytokines
Cytokines are a class of small protein molecules secreted by cells, primarily involved in signal transmission between cells to regulate immune and inflammatory responses. They interact with specific cell surface receptors and modulate the differentiation, migration, and activation of immune cells. This class includes various types such as interleukins (ILs), tumor necrosis factors (TNFs), and interferons (IFNs), which are pivotal in both innate and adaptive immunity. Given the extensive bioactivity and overlapping functions of cytokines, a detailed biological understanding is essential for the development of therapeutic strategies against diverse diseases (7, 19, 20).
3.2 Cytokines and the immune system
Cytokines play a pivotal role in immune regulation by modulating the activities of various immune cells, such as T cells, B cells, and macrophages, thereby shaping the body’s response to pathogens. For example, IL-2, a crucial growth factor, is essential for the proliferation and survival of T cells, while TNF and IL-6 are key in initiating and sustaining inflammatory responses. Moreover, cytokines influence cell migration and localization within tissues, enhancing the efficiency and precision of immune responses (21). Recent studies have also shown that cytokines such as IL-10 and TGF-β possess anti-inflammatory properties that regulate immune responses, thus mitigating excessive immune activity and preventing damage to host tissues (22–24).
4 Role of cytokines in the SAPHO syndrome
4.1 TNF-α
In SAPHO syndrome, tumor necrosis factor α (TNF-α) serves as a key pro-inflammatory cytokine, significantly influencing inflammatory responses and bone remodeling. TNF-α regulates inflammation, immune responses, and cell survival by activating cellular signaling pathways, notably the nuclear factor κB (NF-κB) pathway (25). TNF-α operates via its receptors, TNFR1 and TNFR2, with TNFR1 activation being particularly critical. Upon binding, TNFR1 initiates the formation of a signaling complex by recruiting adaptor proteins such as TRADD, FADD, and RIP1. This complex activation leads to the phosphorylation and degradation of IκB, consequently releasing NF-κB to translocate into the nucleus and activate gen expression related to inflammation, cell proliferation, and survival (Figure 1). TNF-α also drives inflammatory and cellular responses by stimulating the MAPK pathway, which includes ERK, JNK, and p38 MAPK. Activation of these pathways increases the production of inflammatory cytokines, intensifying the inflammatory response, particularly in conditions like SAPHO syndrome (26–28). TNF-α impacts immune cells by promoting the recruitment of neutrophils and the activation of macrophages and T cells, thus enhancing the inflammatory milieu (Figure 2). TNF-α also affects bone cells, specifically osteoclasts and osteoblasts. TNF-α promotes osteoclast differentiation and activation, leading to increased bone resorption, while it inhibits osteoblast differentiation and function, impairing bone formation and repair. These dual actions on immune and bone cells underscore TNF-α’s pivotal role in the pathogenesis and progression of SAPHO syndrome, contributing to both inflammation and bone pathology (29–34).
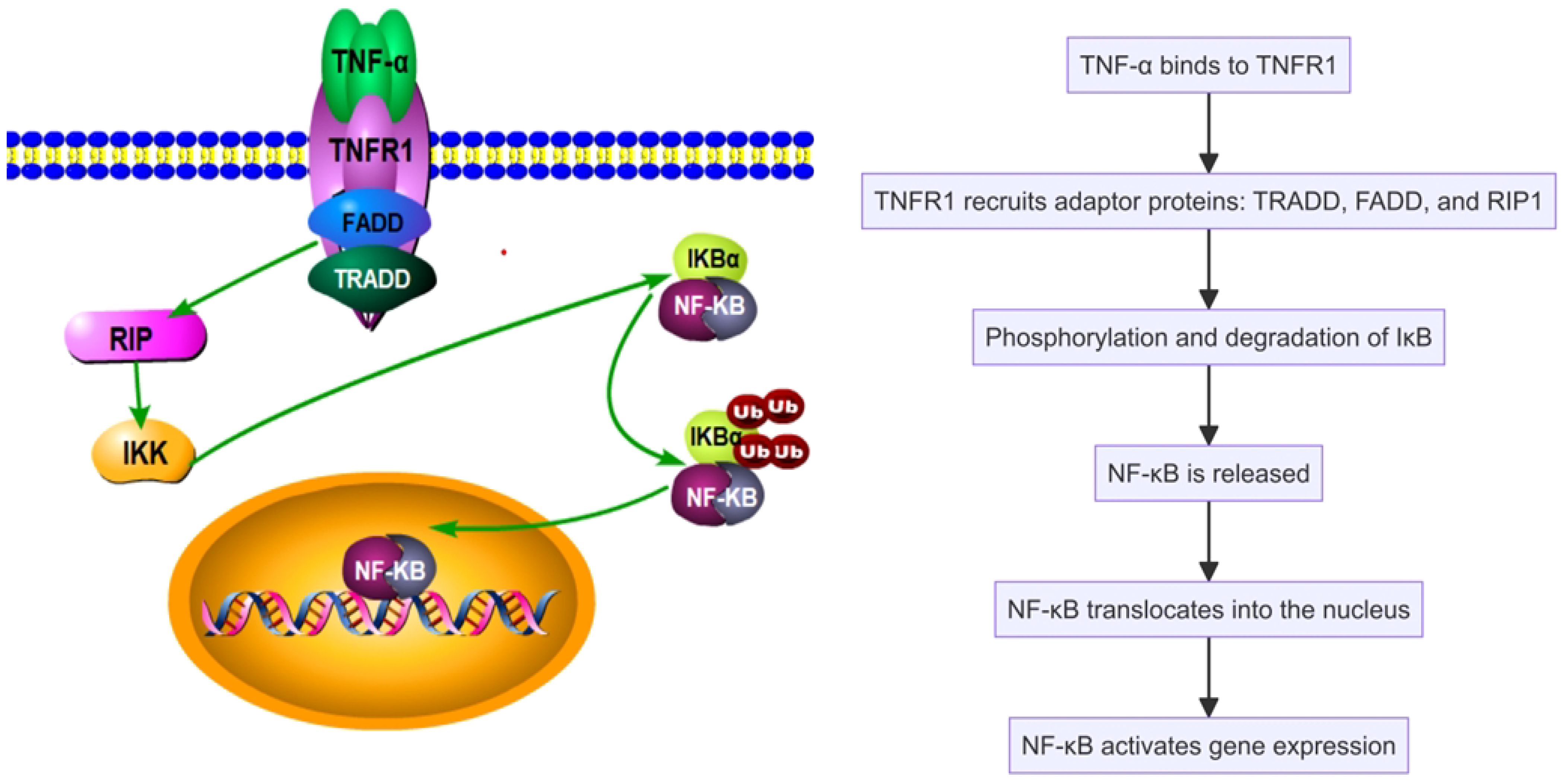
Figure 1 Tumor necrosis factor α (TNF-α) activates the nuclear factor κB (NF-κB) pathway in the SAPHO syndrome.
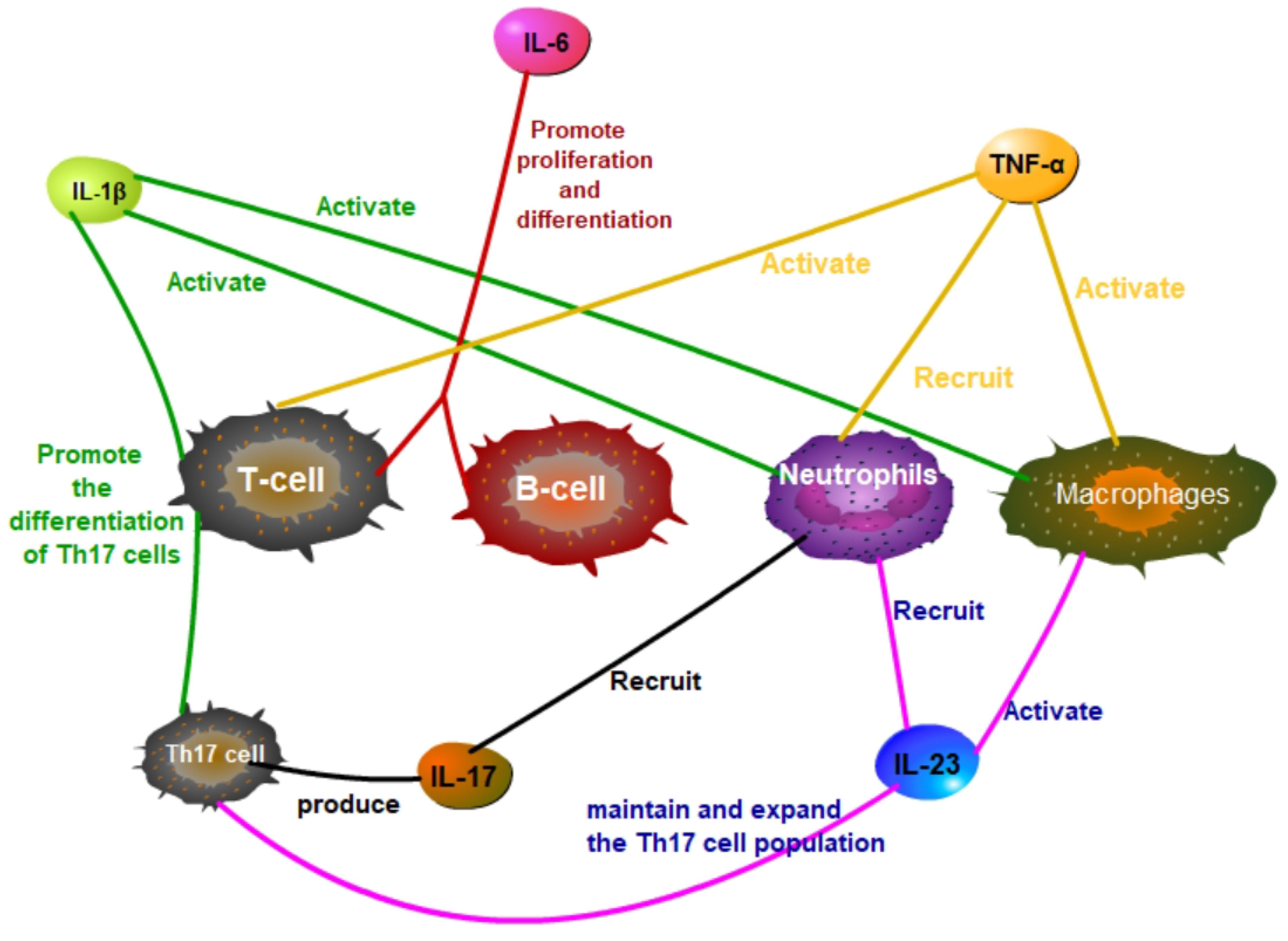
Figure 2 In SAPHO syndrome, IL-1β, IL-6, TNF-α, IL-17, and IL-23 have significant effects on T cells, B cells, macrophages, and neutrophils.
4.2 IL-1β
Interleukin-1 beta (IL-1β), a key pro-inflammatory cytokine, plays a significant role in the pathogenesis of SAPHO syndrome. Produced primarily by activated macrophages and monocytes, IL-1β promotes the differentiation and activation of various immune cells, including macrophages, neutrophils, and T cells, enhancing the inflammatory response (Figure 2). IL-1β increases the production of other cytokines and chemokines, further amplifying the recruitment and activation of immune cells at inflammation sites (35–37). It activates multiple signaling pathways, including nuclear factor κB (NF-κB) and mitogen-activated protein kinase (MAPK), exacerbating SAPHO syndrome symptoms (38, 39). Upon binding to its specific receptor, IL-1R, IL-1β initiates MyD88-dependent signal transduction, activating downstream IRAK4 and IRAK1, which leads to the activation of TRAF6. TRAF6, a crucial signaling molecule, triggers TAK1, which subsequently activates the IKK complex and MAPKs. This activation sequence culminates in the phosphorylation and degradation of IκBα, releasing NF-κB to translocate into the nucleus and stimulate the expression of genes related to inflammation (Figure 3). Concurrently, activation of the MAPK pathway enhances the activity of the AP-1 transcription factor, intensifying the inflammatory response (40–45). Through these signaling pathways, IL-1β promotes the production of inflammatory mediators such as IL-6 and TNF-α and boosts the expression of chemokines and cell adhesion molecules that enhance the recruitment of inflammatory cells to inflammation sites. Additionally, in bone cells, IL-1β stimulates osteoclastogenesis, leading to increased bone resorption. IL-1β also inhibits osteoblast differentiation and function, impairing bone formation and repair. This dual action on immune and bone cells contributes to the chronic inflammation and bone pathology characteristic of SAPHO syndrome, exacerbating symptoms and disease progression (32, 42, 44, 46).
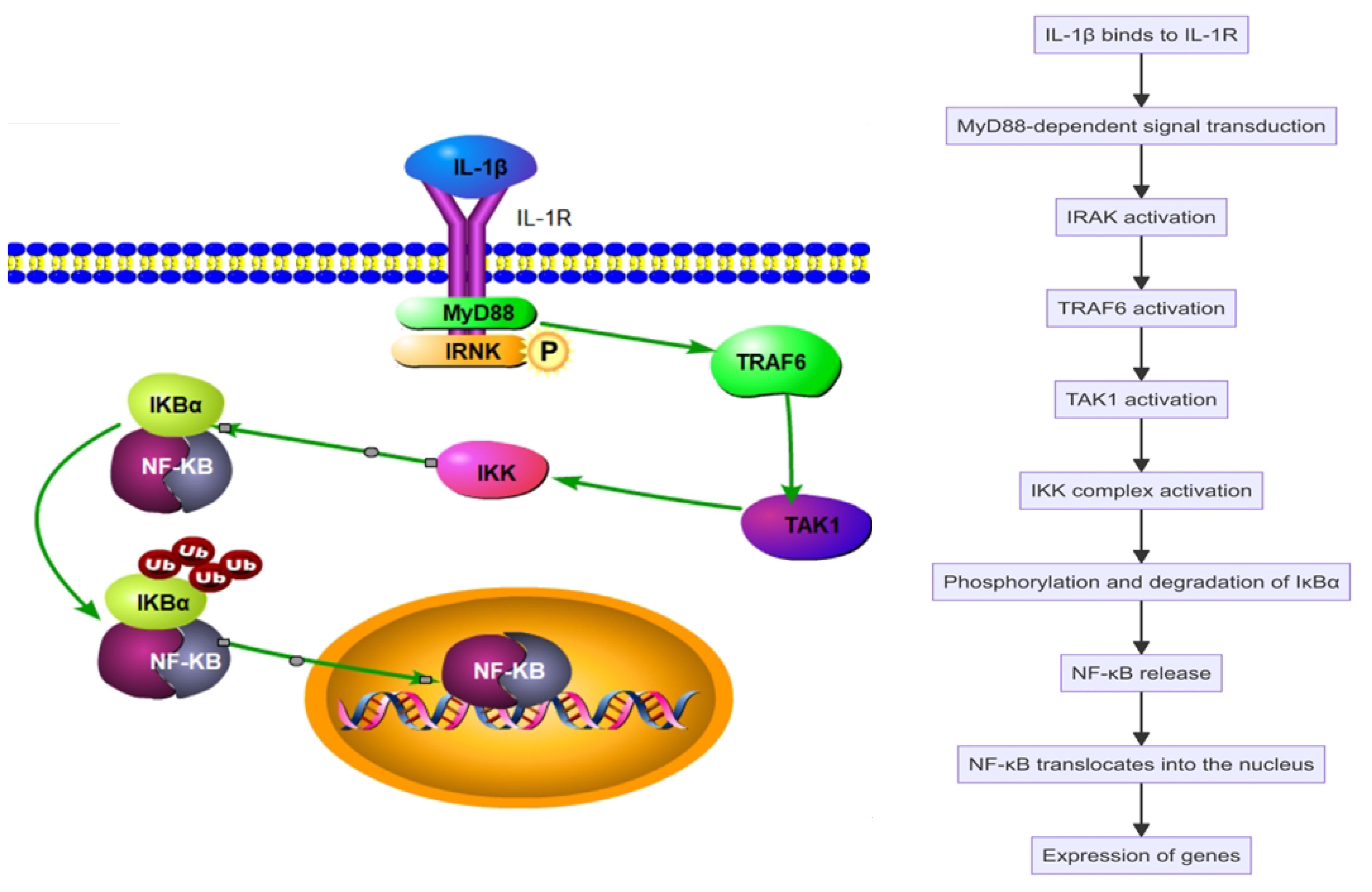
Figure 3 Interleukin-1 beta (IL-1β) activates the nuclear factor κB (NF-κB) pathway in the SAPHO syndrome.
4.3 IL-6
Interleukin-6 (IL-6) plays a pivotal role in SAPHO syndrome by promoting the proliferation and differentiation of B cells and T cells, thereby enhancing the immune response (Figure 2). IL-6 initiates intracellular signaling by binding to its specific receptor, IL-6 receptor (IL-6R), and pairing with the gp130 co-receptor. This interaction activates Janus kinase (JAK), leading to the phosphorylation of signal transducer and activator of transcription 3 (STAT3). The phosphorylated STAT3 then forms dimers and translocates to the nucleus to stimulate gene expression related to inflammation, cell survival, and proliferation (Figure 4). Additionally, IL-6 engages the MAPK and PI3K-Akt signaling pathways, further influencing cellular metabolism and functions (46–49). In SAPHO syndrome, excessive IL-6 expression is strongly linked to the development of osteitis and skin lesions. It promotes bone resorption by enhancing osteoclast differentiation and activity while concurrently inhibiting osteoblast functions, thereby disrupting bone remodeling balance. This imbalance contributes to the typical bone lesions and pain in patients with SAPHO syndrome. Furthermore, IL-6 drives the differentiation of naive T cells into Th17 cells, a subset of T cells that produce IL-17, a potent pro-inflammatory cytokine. This differentiation process exacerbates the inflammatory response, contributing to the chronic inflammation observed in SAPHO syndrome. IL-6 also enhances the activation and survival of T cells, further sustaining the inflammatory environment (32, 46–48, 50).
4.4 IL-23 and IL-17
In the pathogenesis of SAPHO syndrome, interleukin-23 (IL-23) and interleukin-17 (IL-17) form a critical cytokine axis significantly influencing inflammation and bone lesions. IL-23, secreted by dendritic cells and macrophages, primarily functions to maintain and expand the Th17 cell population, the principal producers of IL-17 (51, 52). IL-23 also enhances the recruitment and activation of neutrophils and macrophages, contributing to a robust inflammatory response (Figure 2). IL-23 binds to its receptor complex, consisting of IL-23R and the co-receptor IL-12Rβ1, thereby activating the JAK/STAT signaling pathway, particularly STAT3. This activation promotes the differentiation and survival of Th17 cells. Th17 cells produce IL-17, which, upon binding to IL-17R, triggers downstream signaling pathways including NF-κB and MAPK, notably the p38 and ERK pathways (Figure 5). This process enhances the production of inflammatory mediators such as TNF-α, IL-1β, and IL-6, intensifying inflammation and tissue damage. IL-17 also enhances the recruitment and activation of neutrophils and macrophages, contributing to a robust inflammatory response (53–55). In SAPHO syndrome, dysregulation of the IL-23/IL-17 axis is closely linked to the distinctive symptoms of skin and bone lesions. In bone cells, IL-17 directly stimulates osteoclastogenesis, leading to increased bone resorption. IL-17 inhibits the differentiation and function of osteoblasts, impairing bone formation and repair. The combined effect of increased osteoclast activity and reduced osteoblast function results in the characteristic bone lesions of SAPHO syndrome. By affecting both immune and bone cells, IL-17 plays a pivotal role in the pathogenesis of SAPHO syndrome, driving the inflammation and bone pathology that are hallmarks of the disease (56–59).
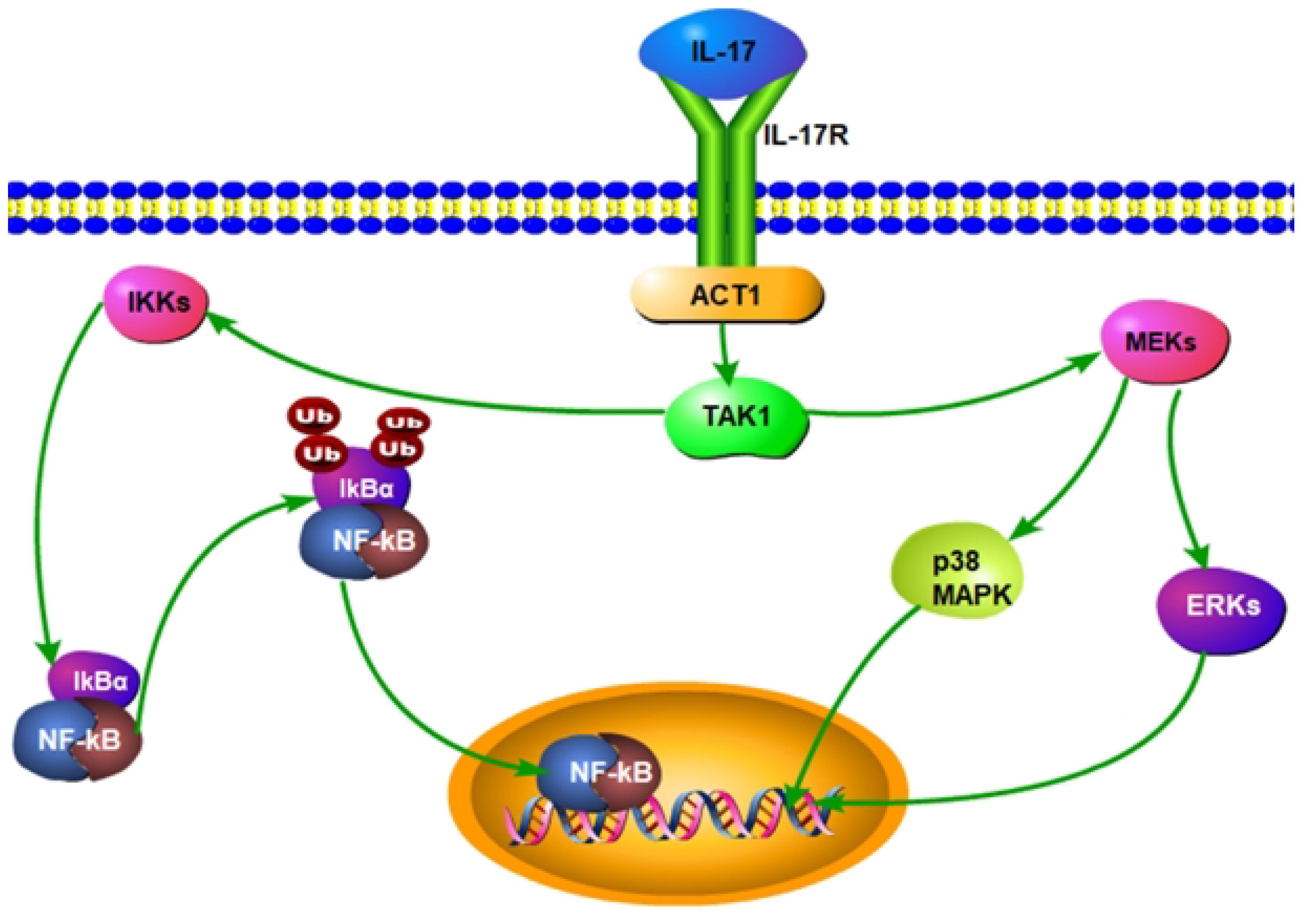
Figure 5 Interleukin-17 (IL-17) activates the nuclear factor κB (NF-κB) and Mitogen-Activated Protein Kinase(MAPK) pathways in SAPHO syndrome.
4.5 IL-8
In SAPHO syndrome, IL-8 primarily recruits and activates neutrophils at inflammation sites. This chemokine not only attracts neutrophils but also enhances their adherence to endothelial cells, facilitating transendothelial migration. Once activated, neutrophils release enzymes and reactive oxygen species that contribute to inflammatory damage in SAPHO syndrome, evident in bone resorption and skin lesions (60). Beyond its chemotactic role, IL-8 also stimulates the production of other inflammatory cytokines. Specifically, IL-8 has been shown to upregulate the production of TNF-α and IL-1β, creating a feedback loop that amplifies the inflammatory response, which is crucial for sustaining chronic inflammation characteristic of SAPHO syndrome (17). IL-8 acts through multiple signaling pathways, primarily by binding to its receptors, CXCR1 and CXCR2, on neutrophils. This binding activates downstream signaling pathways including MAPK, NF-κB, and PI3K/Akt, leading to transcriptional activation of genes involved in inflammation, survival, and migration of immune cells, further contributing to the disease’s pathogenesis (61–63).
4.6 IL-18
Interleukin-18 (IL-18) plays a critical role in the inflammatory and pathogenic mechanisms of SAPHO syndrome, affecting both innate and adaptive immune responses. IL-18 enhances the activity of macrophages and dendritic cells, leading to increased production of pro-inflammatory cytokines such as TNF-α, IL-1β, and IL-6. This amplifies the inflammatory response, promoting chronic inflammation characteristic of SAPHO syndrome (14). IL-18 is key in activating Th1 and NK cell responses, enhancing the production of IFN-γ by these cells, thereby exacerbating the inflammatory response in SAPHO syndrome. Elevated levels of IL-18, observed in conditions with similar inflammatory profiles, suggest its involvement in sustained inflammatory processes in SAPHO syndrome (64, 65). IL-18 signals primarily through the IL-18 receptor (IL-18R), engaging the NF-κB signaling pathway, a central pathway in inflammatory responses. Activation of NF-κB leads to the transcription of various pro-inflammatory genes, amplifying the inflammatory response and contributing to tissue damage and bone remodeling observed in SAPHO syndrome (66, 67). Additionally, IL-18 activates crucial signaling molecules like MAPKs and the PI3K/AKT pathway, which are essential for propagating the inflammatory response. Besides its immune-stimulatory roles, IL-18 indirectly influences bone resorption in bone cells by promoting the production of RANKL, a key factor in osteoclast differentiation and activation. This results in increased osteoclast activity and bone resorption, contributing to the bone lesions typical of SAPHO syndrome. By affecting both immune and bone cells, IL-18 plays a critical role in the pathogenesis of SAPHO syndrome, exacerbating the inflammatory and bone remodeling processes (65, 67, 68).
4.7 IL-10
IL-10 is a potent anti-inflammatory cytokine known to inhibit the activation of various immune cells and the release of cytokines. In SAPHO syndrome, IL-10’s regulatory function potentially alleviates chronic inflammation and autoimmune responses by reducing the production of pro-inflammatory cytokines such as tumor necrosis factor α (TNF-α) and interleukin-1β (IL-1β) (18). Moreover, IL-10 promotes the differentiation of B cells and antibody production, enhancing immune regulation against infections and inflammation (69, 70). IL-10 activates STAT3 via the JAK-STAT signaling pathway, increasing the expression of anti-inflammatory genes such as SOCS3, which inhibits the activation of immune cells like macrophages and T cells, as well as the production of pro-inflammatory factors (71–73). Additionally, IL-10 enhances its anti-inflammatory effects through the PI3K/Akt pathway by activating mTOR, which inhibits NF-κB activation and the expression of downstream inflammatory genes (74).
4.8 TGF-β
Transforming Growth Factor-beta (TGF-β), another key anti-inflammatory cytokine, helps maintain tissue homeostasis and immune tolerance. It reduces inflammation by inhibiting the activation of macrophages and T cells and supports immune balance by enhancing the differentiation and function of regulatory T cells, crucial for controlling autoimmune disease progression (75–78). TGF-β initially binds to the type II TGF-β receptor on the cell surface. This interaction facilitates the recruitment and binding of the type I TGF-β receptor, forming a receptor complex. Upon TGF-β binding, TβRII undergoes autophosphorylation and subsequently phosphorylates TβRI. This phosphorylation promotes the activation of Smad2 and Smad3, which then associate with Smad4 and translocate to the nucleus to regulate genes related to anti-inflammation and immune tolerance (79) (Figure 6). TGF-β also activates other signaling pathways such as MAPK and PI3K, further regulating cell survival, proliferation, and differentiation, thereby playing a multifaceted role in immune regulation (80, 81). In SAPHO syndrome, IL-10 and TGF-β collaboratively modulate immune cell activity and inflammatory response intensity, helping to control disease activity and progression. The functions of these cytokines extend beyond merely inhibiting inflammatory responses to include promoting tissue repair and regeneration, essential for the long-term management and treatment of patients with SAPHO syndrome (6, 18).
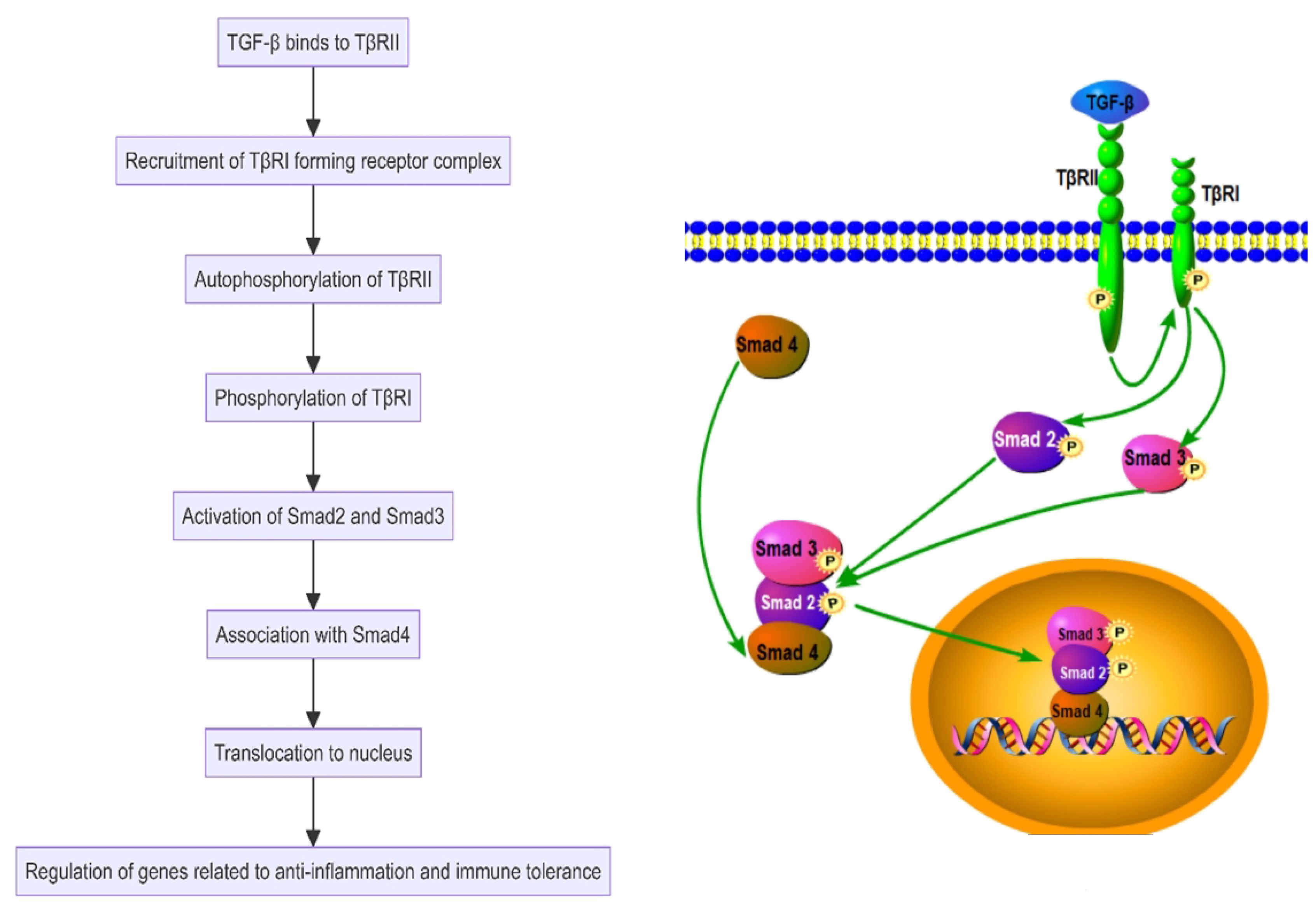
Figure 6 Transforming Growth Factor-beta (TGF-β) activates the Smad-dependent pathway in the SAPHO syndrome.
4.9 Cytokines and skin lesions connection
Numerous studies have investigated the connection between cytokines and skin lesions in SAPHO syndrome. Research has consistently demonstrated that cytokines, including TNF-α, IL-1, IL-6, IL-17, and IL-23, are abnormally expressed in the skin lesions of patients, often characterized by severe pustulosis and acne (18).
One particular study on patients with SAPHO syndrome showed that treatment with Secukinumab, an IL-17 inhibitor, significantly alleviated skin lesions and joint pain, indicating a crucial role of IL-17 in the associated skin manifestations. Furthermore, other research has revealed that anti-TNF-α treatments can provoke skin lesions in some patients, highlighting the influential role of TNF-α in these dermatological manifestations (56, 82).
Additionally, there is evidence suggesting that skin lesions in SAPHO syndrome may be linked to anomalies in the IL-23 pathway (83). For instance, paradoxical skin reactions have been observed in some patients following IL-17 inhibitor therapy, pointing to complex interactions between the IL-23 and IL-17 signaling pathways in affecting the syndrome’s dermatological features (84, 85).
In conclusion, skin lesions in SAPHO syndrome are intimately associated with the aberrant expression of various inflammatory cytokines, which intensify skin pathology by impacting inflammatory responses and immune regulatory mechanisms. Therefore, elucidating the roles and specific mechanisms of these cytokines in SAPHO syndrome is essential for developing precise therapeutic strategies.
5 The potential of cytokine targeted therapy for SAPHO syndrome
The diversity of clinical manifestations in SAPHO syndrome complicates its treatment. Current therapeutic strategies primarily focus on symptom relief, utilizing non-steroidal anti-inflammatory drugs (NSAIDs), corticosteroids, disease-modifying anti-rheumatic drugs (DMARDs), and bisphosphonates. Recent studies have underscored the effectiveness of bisphosphonates, such as zoledronic acid, in alleviating pain and reducing inflammatory lesions in patients with SAPHO syndrome. Cytokine-targeted therapy, aimed at specific inflammatory mediators integral to its pathogenesis, represents the cutting edge of treatments for SAPHO syndrome. IL-1 and TNF-α are considered critical to the inflammatory processes of the syndrome, with clinical trials of inhibitors like anakinra and infliximab demonstrating benefits in reducing symptoms and enhancing patient quality of life (10, 61, 86). Treatment of SAPHO syndrome continues to face significant challenges, particularly in target development. Recent studies have concentrated on identifying new biomarkers and therapies that influence the disease’s progression. For instance, the IL-23/Th 17 pathway has been recognized for its potential therapeutic value in SAPHO syndrome. Biologics targeting IL-23 and Th 17, such as Ustekinumab and Secukinumab, have proven somewhat effective, especially in ameliorating skin symptoms. Although the impact of these agents on osteoarticular symptoms remains uncertain, the research provides a scientific foundation for developing new therapeutic targets (56, 61, 85).
Furthermore, increasing evidence suggests a role for the microbiome in the pathogenesis of SAPHO syndrome. Propionibacterium acnes, for example, is thought to potentially exacerbate the condition by triggering host immune responses, offering a theoretical basis for the use of antibiotics, despite ongoing debates about their efficacy. Future research should continue to explore the interaction between the microbiome and the disease, aiming to develop targeted treatments based on this mechanism (15).
6 Conclusion
Cytokines are central to the pathogenesis of SAPHO syndrome, encompassing synovitis, acne, pustulosis, hyperostosis, and osteitis. Studies have indicated that pro-inflammatory cytokines, including tumor necrosis factor α (TNF-α), interleukin 1β (IL-1β), IL-6, and IL-8, are highly expressed in this disorder and contribute to heightened inflammatory responses. These cytokines drive the progression of SAPHO syndrome by influencing inflammation, inducing acute phase responses, and facilitating chemotaxis. Conversely, anti-inflammatory cytokines such as IL-10 and transforming growth factor-β (TGF-β) play protective roles by modulating immune responses and curbing excessive inflammation. Specifically, IL-10 mitigates symptoms by inhibiting the activities of TNF-α and IL-1, while TGF-β helps maintain tissue homeostasis and immune tolerance, thus decelerating the disease’s progression. These insights not only offer new therapeutic targets for SAPHO syndrome but also enhance understanding of its complex pathophysiological mechanisms.
Despite recent advances in cytokine research related to SAPHO syndrome, numerous unresolved issues persist. The precise pathogenesis, particularly the specific roles and interactions of various cytokines, remains elusive and necessitates further investigation. Moreover, while various biologics have demonstrated therapeutic promise, their clinical efficacy varies among individuals, highlighting the need for additional data to validate their long-term safety and effectiveness.
Future research should aim for a more comprehensive understanding of cytokine roles in SAPHO syndrome, especially their interplay and impact on disease progression. Additionally, there is a need to develop more personalized treatment strategies, potentially by customizing treatment plans according to individual cytokine expression profiles, to enhance therapeutic efficacy and safety.
Author contributions
YY: Writing – original draft, Data curation. QC: Resources, Writing – review & editing. WZ: Conceptualization, Writing – original draft, Writing – review & editing.
Funding
The author(s) declare financial support was received for the research, authorship, and/or publication of this article. This study was supported by the Chongqing Nature Science Foundation Project (CSTB2022NSCQ-MSX0070), the Hospital Training Funding (PYJJ2 0 1 9 -0 8), and the program for Youth Innovation in Future of Chongqing Medical Universi ty (W 0 0 1 2). Funding bodies were not involved in the design, data collection, analysis, interpretation, or writing of the manuscript.
Conflict of interest
The authors declare that the research was conducted in the absence of any commercial or financial relationships that could be construed as a potential conflict of interest.
Publisher’s note
All claims expressed in this article are solely those of the authors and do not necessarily represent those of their affiliated organizations, or those of the publisher, the editors and the reviewers. Any product that may be evaluated in this article, or claim that may be made by its manufacturer, is not guaranteed or endorsed by the publisher.
References
1. Gao S, Deng X, Zhang L, Song L. The comparison analysis of clinical and radiological features in SAPHO syndrome. Clin Rheumatol. (2021) 40:349–57. doi: 10.1007/s10067-020-05187-0
2. Ruan DD, Wang RL, Hu YN, Lin X, Luo JW, Yu QH, et al. Clinical and imaging features of six Han patients with SAPHO syndrome. Acta Radiol. (2024) 65:211–24. doi: 10.1177/02841851221142783
3. Cao Y, Li C, Yang Q, Wu N, Xu P, Li Y, et al. Three patterns of osteoarticular involvement in SAPHO syndrome: a cluster analysis based on whole body bone scintigraphy of 157 patients. Rheumatol (Oxford). (2019) 58:1047–55. doi: 10.1093/rheumatology/key415
4. Cao Y, Li C, Xu W, Wu X, Sun X, Zhang W, et al. Spinal and sacroiliac involvement in SAPHO syndrome: A single center study of a cohort of 354 patients. Semin Arthritis Rheumatism. (2019) 48:990–6. doi: 10.1016/j.semarthrit.2018.09.004
5. Van Doornum S, Barraclough D, McColl G, Wicks I. SAPHO: Rare or just not recognized? Semin Arthritis Rheumatism. (2000) 30:70–7. doi: 10.1053/sarh.2000.8371
6. Firinu D, Garcia-Larsen V, Manconi PE, Del Giacco SR. SAPHO syndrome: current developments and approaches to clinical treatment. Curr Rheumatol Rep. (2016) 18(6):35. doi: 10.1007/s11926-016-0583-y
7. Hussain A, Gondal M, Abdallah N, Yousuf H, Iqbal M. Synovitis, acne, pustulosis, hyperostosis, osteitis (SAPHO); an interesting clinical syndrome. Cureus J Med Sci. (2020) 12(9):e10184. doi: 10.7759/cureus.10184
8. Shao S, Wang W, Qian Y. SAPHO syndrome involving the mandible treated with <SUP>99</SUP>Tc-MDP. J Craniofacial Surg. (2020) 31:510–2. doi: 10.1097/scs.0000000000006161
9. Reith JD, Bauer TW, Schils JP. Osseous manifestations of SAPHO (synovitis, acne, pustulosis, hyperostosis, osteitis) syndrome. Am J Surg Pathology. (1996) 20:1368–77. doi: 10.1097/00000478-199611000-00008
10. Adamo S, Nilsson J, Krebs A, Steiner U, Cozzio A, French LE, et al. Successful treatment of SAPHO syndrome with apremilast. Br J Dermatol. (2018) 179:959–62. doi: 10.1111/bjd.16071
11. Cotten A, Flipo RM, Mentre A, Delaporte E, Duquesnoy B, Chastanet P. SAPHO SYNDROME. Radiographics. (1995) 15:1147–54. doi: 10.1148/radiographics.15.5.7501856
12. Leone A, Cassar-Pullicino VN, Casale R, Magarelli N, Semprini A, Colosimo C. The SAPHO syndrome revisited with an emphasis on spinal manifestations. Skeletal Radiology. (2015) 44:9–24. doi: 10.1007/s00256-014-2025-0
13. Vekic DA, Woods J, Lin P, Cains GD. SAPHO syndrome associated with hidradenitis suppurativa and pyoderma gangrenosum successfully treated with adalimumab and methotrexate: a case report and review of the literature. Int J Dermatol. (2018) 57:10–8. doi: 10.1111/ijd.13740
14. Hurtado-Nedelec M, Chollet-Martin S, Chapeton D, Hugot J-P, Hayem G, Gerard B. Genetic susceptibility factors in a cohort of 38 patients with SAPHO syndrome: A study of PSTPIP2, NOD2, and LPIN2 genes. J Rheumatol. (2010) 37:401–9. doi: 10.3899/jrheum.090456
15. Assmann G, Simon P. The SAPHO syndrome–are microbes involved? Best Pract Res Clin Rheumatol. (2011) 25:423–34. doi: 10.1016/j.berh.2011.01.017
16. Luzzati M, Simonini G, Filippeschi C, Giani T, Trapani S. SAPHO syndrome: the supposed trigger by isotretinoin, the efficacy of adalimumab and the specter of depressive disorder: a case report. Ital J Pediatr. (2020) 46:169. doi: 10.1186/s13052-020-00933-1
17. Hurtado-Nedelec M, Chollet-Martin S, Nicaise-Roland P, Grootenboer-Mignot S, Ruimy R, Meyer O, et al. Characterization of the immune response in the synovitis, acne, pustulosis, hyperostosis, osteitis (SAPHO) syndrome. Rheumatology. (2008) 47:1160–7. doi: 10.1093/rheumatology/ken185
18. Zhang S, Li C, Zhang S, Li L, Zhang W, Dong Z, et al. Serum levels of proinflammatory, anti-inflammatory cytokines, and RANKL/OPG in synovitis, acne, pustulosis, hyperostosis, and osteitis (SAPHO) syndrome. Mod Rheumatol. (2019) 29:523–30. doi: 10.1080/14397595.2018.1469580
19. Shachar I, Karin N. The dual roles of inflammatory cytokines and chemokines in the regulation of autoimmune diseases and their clinical implications. J Leukocyte Biol. (2013) 93:51–61. doi: 10.1189/jlb.0612293
20. Zeng J, Xie K, Wu H, Zhang BY, Huang CH. Identification and functional study of cytokines and chemokines involved in tumorigenesis. Combinatorial Chem High Throughput Screening. (2012) 15:276–85. doi: 10.2174/138620712799218608
21. Charles KA, Kulbe H, Soper R, Escorcio-Correia M, Lawrence T, Schultheis A, et al. The tumor-promoting actions of TNF-α involve TNFR1 and IL-17 in ovarian cancer in mice and humans. J Clin Invest. (2009) 119:3011–23. doi: 10.1172/jci39065
22. Hetta HF, Mwafey IM, Batiha GE-S, Alomar SY, Mohamed NA, Ibrahim MA, et al. CD19+ CD24hi CD38hi regulatory B cells and memory B cells in periodontitis: association with pro-inflammatory and anti-inflammatory cytokines. Vaccines. (2020) 8(2):340. doi: 10.3390/vaccines8020340
23. Chen Y, Li C, Lu Y, Zhuang H, Gu W, Liu B, et al. IL-10-producing CD1dhiCD5+ Regulatory B cells may play a critical role in modulating immune homeostasis in silicosis patients. Front Immunol. (2017) 8:110. doi: 10.3389/fimmu.2017.00110
24. Becker-Merok A, Eilertsen GO, Nossent JC. Levels of transforming growth factor-β Are low in systemic lupus erythematosus patients with active disease. J Rheumatol. (2010) 37:2039–45. doi: 10.3899/jrheum.100180
25. Schmidlin PR, Ramenzoni LL. Inflammatory stimulation of osteoblasts and keratinocytes from a SAPHO patient for implant risk evaluation. Swiss Dent J. (2023) 133:297–303. doi: 10.61872/sdj-2023-05-947
26. Choy EHS, Panayi GS. Mechanisms of disease: Cytokine pathways and joint inflammation in rheumatoid arthritis. New Engl J Med. (2001) 344:907–16. doi: 10.1056/nejm200103223441207
27. Webster JD, Vucic D. The balance of TNF mediated pathways regulates inflammatory cell death signaling in healthy and diseased tissues. Front Cell Dev Biol. (2020) 8:365. doi: 10.3389/fcell.2020.00365
28. Ben Abdelghani K, Dran DG, Gottenberg J-E, Morel J, Sibila J, Combe B. Tumor necrosis factor-α Blockers in SAPHO syndrome. J Rheumatol. (2010) 37:1699–704. doi: 10.3899/jrheum.091086
29. Kitaura H, Marahleh A, Ohori F, Noguchi T, Nara Y, Pramusita A, et al. Role of the interaction of tumor necrosis factor-α and tumor necrosis factor receptors 1 and 2 in bone-related cells. Int J Mol Sci. (2022) 23(3):1481. doi: 10.3390/ijms23031481
30. Lam J, Takeshita S, Barker JE, Kanagawa O, Ross FP, Teitelbaum SL. TNF-α induces osteoclastogenesis by direct stimulation of macrophages exposed to permissive levels of RANK ligand. J Clin Invest. (2000) 106:1481–8. doi: 10.1172/jci11176
31. Nanes MS. Tumor necrosis factor-α:: molecular and cellular mechanisms in skeletal pathology. Gene. (2003) 321:1–15. doi: 10.1016/s0378-1119(03)00841-2
32. Souza PPC, Lerner UH. The role of cytokines in inflammatory bone loss. Immunol Investigations. (2013) 42:555–622. doi: 10.3109/08820139.2013.822766
33. Jang DI, Lee AH, Shin HY, Song HR, Park JH, Kang TB, et al. The role of tumor necrosis factor alpha (TNF-α) in autoimmune disease and current TNF-α Inhibitors in therapeutics. Int J Mol Sci. (2021) 22(5):2719. doi: 10.3390/ijms22052719
34. Zhao X, Zhang G, Wu L, Tang Y, Guo C. Inhibition of ER stress-activated JNK pathway attenuates TNF-α-induced inflammatory response in bone marrow mesenchymal stem cells. Biochem Biophys Res Commun. (2021) 541:8–14. doi: 10.1016/j.bbrc.2020.12.101
35. Xu D, Liu X, Lu C, Luo J, Wang C, Gao C, et al. Reduction of peripheral natural killer cells in patients with SAPHO syndrome. Clin Exp Rheumatol. (2019) 37:12–8.
36. Sun Y, Li C, Zhu M, Zhang S, Cao Y, Yang Q, et al. Enhanced migration and adhesion of peripheral blood neutrophils from SAPHO patients revealed by RNA-Seq. Orphanet J Rare Dis. (2019) 14(1):192. doi: 10.1186/s13023-019-1169-3
37. Nemetz A, Toth M, Garcia-Gonzalez MA, Zagoni T, Feher J, Pena AS, et al. Allelic variation at the interleukin 1beta gene is associated with decreased bone mass in patients with inflammatory bowel diseases. Gut. (2001) 49:644–9. doi: 10.1136/gut.49.5.644
38. Przepiera-Będzak H, Fischer K, Brzosko M. Serum IL-6 and IL-23 levels and their correlation with angiogenic cytokines and disease activity in ankylosing spondylitis, psoriatic arthritis, and SAPHO syndrome. Mediators Inflammation. (2015) 2015:785705. doi: 10.1155/2015/785705
39. Cai Y, Xue F, Quan C, Qu M, Liu N, Zhang Y, et al. A critical role of the IL-1β-IL-1R signaling pathway in skin inflammation and psoriasis pathogenesis. J Invest Dermatol. (2019) 139:146–56. doi: 10.1016/j.jid.2018.07.025
40. Dinarello CA. Immunological and inflammatory functions of the interleukin-1 family. Annu Rev Immunol. (2009) 27:519–50. doi: 10.1146/annurev.immunol.021908.132612
41. Wagner EF, Eferl R. Fos/AP-1 proteins in bone and the immune system. Immunol Rev. (2005) 208:126–40. doi: 10.1111/j.0105-2896.2005.00332.x
42. Yang J, Wang J, Liang X, Zhao H, Lu J, Ma Q, et al. IL−1β increases the expression of inflammatory factors in synovial fluid−derived fibroblast−like synoviocytes via activation of the NF−κB−mediated ERK−STAT1 signaling pathway. Mol Med Rep. (2019) 20:4993–5001. doi: 10.3892/mmr.2019.10759
43. Rasheed Z, Rasheed N, Abdulmonem WA, Khan MI. MicroRNA-125b-5p regulates IL-1β induced inflammatory genes via targeting TRAF6-mediated MAPKs and NF-κB signaling in human osteoarthritic chondrocytes. Sci Rep. (2019) 9:6882. doi: 10.1038/s41598-019-42601-3
44. Lin S, Zhao XL, Wang Z. TANK-binding kinase 1 mediates osteoclast differentiation by regulating NF-κB, MAPK and Akt signaling pathways. Immunol Cell Biol. (2021) 99:223–33. doi: 10.1111/imcb.12401
45. Shouval DS, Biswas A, Kang YH, Griffith AE, Konnikova L, Mascanfroni ID, et al. Interleukin 1β Mediates intestinal inflammation in mice and patients with interleukin 10 receptor deficiency. Gastroenterology. (2016) 151:1100–4. doi: 10.1053/j.gastro.2016.08.055
46. Wendling D, Prati C, Aubin F. Anakinra treatment of SAPHO syndrome: short-term results of an open study. Ann Rheumatic Diseases. (2012) 71:1098–100. doi: 10.1136/annrheumdis-2011-200743
47. Schett G. Physiological effects of modulating the interleukin-6 axis. Rheumatol (Oxford). (2018) 57:ii43–50. doi: 10.1093/rheumatology/kex513
48. Tanaka T, Narazaki M, Kishimoto T. IL-6 in inflammation, immunity, and disease. Cold Spring Harb Perspect Biol. (2014) 6:a016295. doi: 10.1101/cshperspect.a016295
49. Huang B, Lang X, Li X. The role of IL-6/JAK2/STAT3 signaling pathway in cancers. Front Oncol. (2022) 12:1023177. doi: 10.3389/fonc.2022.1023177
50. Kishimoto T. Interleukin-6: from basic science to medicine–40 years in immunology. Annu Rev Immunol. (2005) 23:1–21. doi: 10.1146/annurev.immunol.23.021704.115806
51. Navarro-Compán V, Puig L, Vidal S, Ramírez J, Llamas-Velasco M, Fernández-Carballido C, et al. The paradigm of IL-23-independent production of IL-17F and IL-17A and their role in chronic inflammatory diseases. Front Immunol. (2023) 14:1191782. doi: 10.3389/fimmu.2023.1191782
52. Liu T, Li S, Ying S, Tang S, Ding Y, Li Y, et al. The IL-23/IL-17 pathway in inflammatory skin diseases: from bench to bedside. Front Immunol. (2020) 11:594735. doi: 10.3389/fimmu.2020.594735
53. Sherlock JP, Joyce-Shaikh B, Turner SP, Chao CC, Sathe M, Grein J, et al. IL-23 induces spondyloarthropathy by acting on ROR-γt+ CD3+CD4-CD8- entheseal resident T cells. Nat Med. (2012) 18:1069–76. doi: 10.1038/nm.2817
54. Hueber W, Patel DD, Dryja T, Wright AM, Koroleva I, Bruin G, et al. Effects of AIN457, a fully human antibody to interleukin-17A, on psoriasis, rheumatoid arthritis, and uveitis. Sci Transl Med. (2010) 2:52ra72. doi: 10.1126/scitranslmed.3001107
55. Schinocca C, Rizzo C, Fasano S, Grasso G, La Barbera L, Ciccia F, et al. Role of the IL-23/IL-17 pathway in rheumatic diseases: an overview. Front Immunol. (2021) 12:637829. doi: 10.3389/fimmu.2021.637829
56. Wendling D, Aubin F, Verhoeven F, Prati C. IL-23/Th17 targeted therapies in SAPHO syndrome. A Case series. Joint Bone Spine. (2017) 84:733–5. doi: 10.1016/j.jbspin.2017.05.016
57. Shukla P, Mansoori MN, Singh D. Efficacy of anti-IL-23 monotherapy versus combination therapy with anti-IL-17 in estrogen deficiency induced bone loss conditions. Bone. (2018) 110:84–95. doi: 10.1016/j.bone.2018.01.027
58. Cauli A, Piga M, Floris A, Mathieu A. Current perspective on the role of the interleukin-23/interleukin-17 axis in inflammation and disease (chronic arthritis and psoriasis). Immunotargets Ther. (2015) 4:185–90. doi: 10.2147/itt.S62870
59. Wang Z, Wei Y, Lei L, Zhong J, Shen Y, Tan J, et al. RANKL expression of primary osteoblasts is enhanced by an IL-17-mediated JAK2/STAT3 pathway through autophagy suppression. Connect Tissue Res. (2021) 62:411–26. doi: 10.1080/03008207.2020.1759562
60. Pignatti P, Moscato G, Casarini S, Delmastro M, Poppa M, Brunetti G, et al. Downmodulation of CXCL8/IL-8 receptors on neutrophils after recruitment in the airways. J Allergy Clin Immunol. (2005) 115:88–94. doi: 10.1016/j.jaci.2004.08.048
61. Daoussis D, Konstantopoulou G, Kraniotis P, Sakkas L, Liossis SN. Biologics in SAPHO syndrome: A systematic review. Semin Arthritis Rheumatol. (2019) 48:618–25. doi: 10.1016/j.semarthrit.2018.04.003
62. Cesta MC, Zippoli M, Marsiglia C, Gavioli EM, Mantelli F, Allegretti M, et al. The role of interleukin-8 in lung inflammation and injury: implications for the management of COVID-19 and hyperinflammatory acute respiratory distress syndrome. Front Pharmacol. (2021) 12:808797. doi: 10.3389/fphar.2021.808797
63. Van den Blink B, Branger J, Weijer S, Gupta A, Van Deventer SJH, Peppelenbosch MP, et al. P38 mitogen activated protein kinase is involved in the downregulation of granulocyte CXC chemokine receptors 1 and 2 during human endotoxemia. J Clin Immunol. (2004) 24:37–41. doi: 10.1023/b:Joci.0000018061.58504.75
64. Wu Z, Giudice V, Chen J, Sun W, Lin Z, Keyvanfar K, et al. Interleukin-18 plays a dispensable role in murine and likely also human bone marrow failure. Exp Hematol. (2019) 69:54–64.e2. doi: 10.1016/j.exphem.2018.10.003
65. Li Z, Yu X, Werner J, Bazhin AV, D'Haese JG. The role of interleukin-18 in pancreatitis and pancreatic cancer. Cytokine Growth Factor Rev. (2019) 50:1–12. doi: 10.1016/j.cytogfr.2019.11.001
66. Ahmed MG. CLINICAL SIGNIFICANCE OF INTERLEUKIN-18 AND INTERLEUKIN-6 ON DISEASE COURSE OF ADULT-ONSET STILL'S DISEASE. Ann Rheumatic Diseases. (2018) 77:1162–. doi: 10.1136/annrheumdis-2018-eular.6951
67. Shimizu M, Takei S, Mori M, Yachie A. Pathogenic roles and diagnostic utility of interleukin-18 in autoinflammatory diseases. Front Immunol. (2022) 13:951535. doi: 10.3389/fimmu.2022.951535
68. Assmann G, Bittenbring JT, Wagner AD, Schreiber M, Christofyllakis K, Hueffmeier U, et al. IMPACT OF INTERLEUKIN 17 BLOCKING AGENT ON CLINICAL OUTCOME IN SAPHO PATIENTS. Ann Rheumatic Diseases. (2019) 78:875–6. doi: 10.1136/annrheumdis-2019-eular.4145
69. Nagata K, Nishiyama C. IL-10 in mast cell-mediated immune responses: anti-inflammatory and proinflammatory roles. Int J Mol Sci. (2021) 22(9):4972. doi: 10.3390/ijms22094972
70. Goswami B, Rajappa M, Mallika V, Shukla DK, Kumar S. TNF-α/IL-10 ratio and C-reactive protein as markers of the inflammatory response in CAD-prone North Indian patients with acute myocardial infarction. Clinica Chimica Acta. (2009) 408:14–8. doi: 10.1016/j.cca.2009.06.029
71. Islam H, Jackson GS, Yoon JSJ, Cabral-Santos C, Lira FS, Mui AL, et al. Sex differences in IL-10's anti-inflammatory function: greater STAT3 activation and stronger inhibition of TNF-α production in male blood leukocytes ex vivo. Am J Physiol Cell Physiol. (2022) 322:C1095–c104. doi: 10.1152/ajpcell.00091.2022
72. Bharath LP, Nikolajczyk BS. The intersection of metformin and inflammation. Am J Physiol Cell Physiol. (2021) 320:C873–c9. doi: 10.1152/ajpcell.00604.2020
73. Duncan SA, Sahu R, Dixit S, Singh SR, Dennis VA. Suppressors of cytokine signaling (SOCS)1 and SOCS3 proteins are mediators of interleukin-10 modulation of inflammatory responses induced by chlamydia muridarum and its major outer membrane protein (MOMP) in mouse J774 macrophages. Mediators Inflamm. (2020) 2020:7461742. doi: 10.1155/2020/7461742
74. Giomarelli P, Scolletta S, Borrelli E, Biagioli B. Myocardial and lung injury after cardiopulmonary bypass: Role of interleukin (IL)-10. Ann Thorac Surgery. (2003) 76:117–23. doi: 10.1016/s0003-4975(03)00194-2
75. Winston BW, Chan ED, Johnson GL, Riches DW. Activation of p38mapk, MKK3, and MKK4 by TNF-alpha in mouse bone marrow-derived macrophages. J Immunol. (1997) 159:4491–7. doi: 10.4049/jimmunol.159.9.4491
76. Li MO, Flavell RA. Contextual regulation of inflammation: a duet by transforming growth factor-beta and interleukin-10. Immunity. (2008) 28:468–76. doi: 10.1016/j.immuni.2008.03.003
77. Batlle E, Massagué J. Transforming growth factor-β Signaling in immunity and cancer. Immunity. (2019) 50:924–40. doi: 10.1016/j.immuni.2019.03.024
78. Prado DS, Cattley RT, Shipman CW, Happe C, Lee M, Boggess WC, et al. Synergistic and additive interactions between receptor signaling networks drive the regulatory T cell versus T helper 17 cell fate choice. J Biol Chem. (2021) 297:101330. doi: 10.1016/j.jbc.2021.101330
79. Matsumura S, Shibakusa T, Fujikawa T, Yamada H, Matsumura K, Inoue K, et al. Intracisternal administration of transforming growth factor-β evokes fever through the induction of cyclooxygenase-2 in brain endothelial cells. Am J Physiol-Regul Integr Comp Physiol. (2008) 294:R266–R75. doi: 10.1152/ajpregu.00181.2007
80. Hanada T, Yoshimura A. Regulation of cytokine signaling and inflammation. Cytokine Growth Factor Rev. (2002) 13:413–21. doi: 10.1016/s1359-6101(02)00026-6
81. Luo K. Signaling cross talk between TGF-β/smad and other signaling pathways. Cold Spring Harb Perspect Biol. (2017) 9(1):a022137. doi: 10.1101/cshperspect.a022137
82. Li C, Wu X, Cao Y, Zeng Y, Zhang W, Zhang S, et al. Paradoxical skin lesions induced by anti-TNF-α agents in SAPHO syndrome. Clin Rheumatol. (2019) 38:53–61. doi: 10.1007/s10067-018-4083-5
83. Licata G, Gambardella A, Calabrese G, Alfano R, Argenziano G. SAPHO syndrome successful treated with tildrakizumab. Dermatologic Ther. (2021) 34(1):e14758. doi: 10.1111/dth.14758
84. Li Y, Meng F, Jiang H, Peng Q, Fu J, Yin D, et al. Paradoxical skin lesions induced by IL-17 inhibitors in SAPHO syndrome. J Dermatolog Treat. (2024) 35:2295239. doi: 10.1080/09546634.2023.2295239
85. Firinu D, Murgia G, Lorrai MM, Barca MP, Peralta MM, Manconi PE, et al. Biological treatments for SAPHO syndrome: an update. Inflammation Allergy Drug Targets. (2014) 13:199–205. doi: 10.2174/1871528113666140520100402
Keywords: SAPHO syndrome, cytokines, TNF-α, IL-1β, inflammatory response, immune regulation
Citation: Yang Y, Chen Q and Zhong W (2024) The role of cytokines in the pathogenesis of SAPHO syndrome. Front. Immunol. 15:1427784. doi: 10.3389/fimmu.2024.1427784
Received: 04 May 2024; Accepted: 09 July 2024;
Published: 02 September 2024.
Edited by:
Riadh Ben Mansour, Université de Sfax, TunisiaReviewed by:
Masanori A. Murayama, Kansai Medical University, JapanYoshiro Horai, Sasebo City General Hospital, Japan
Copyright © 2024 Yang, Chen and Zhong. This is an open-access article distributed under the terms of the Creative Commons Attribution License (CC BY). The use, distribution or reproduction in other forums is permitted, provided the original author(s) and the copyright owner(s) are credited and that the original publication in this journal is cited, in accordance with accepted academic practice. No use, distribution or reproduction is permitted which does not comply with these terms.
*Correspondence: Weiyang Zhong, NDkyNDY3MTEyQHFxLmNvbQ==