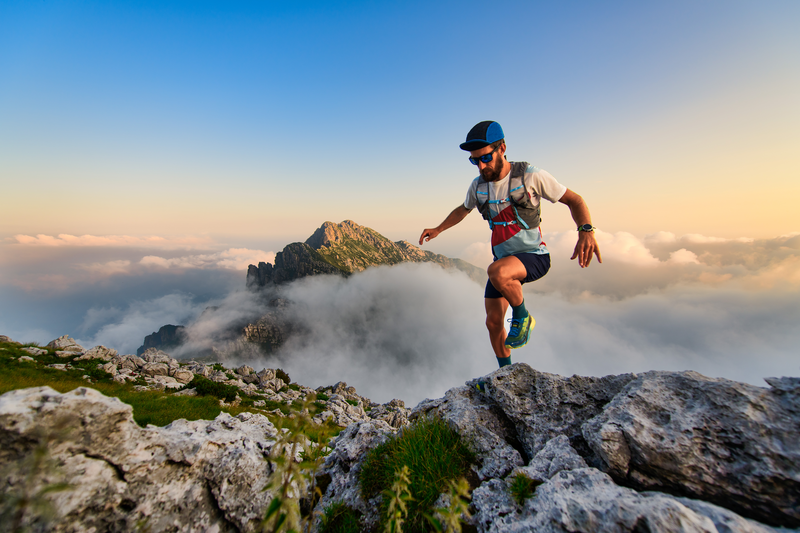
94% of researchers rate our articles as excellent or good
Learn more about the work of our research integrity team to safeguard the quality of each article we publish.
Find out more
REVIEW article
Front. Immunol. , 11 October 2024
Sec. Cancer Immunity and Immunotherapy
Volume 15 - 2024 | https://doi.org/10.3389/fimmu.2024.1427573
This article is part of the Research Topic Nanoparticles for Cancer Immunotherapy: from Basics to Clinics View all 12 articles
Ovarian cancer (OC) is one of the deadliest gynecological malignancies in the world and is the leading cause of cancer-related death in women. The complexity and difficult-to-treat nature of OC pose a huge challenge to the treatment of the disease, Therefore, it is critical to find green and sustainable drug treatment options. Natural drugs have wide sources, many targets, and high safety, and are currently recognized as ideal drugs for tumor treatment, has previously been found to have a good effect on controlling tumor progression and reducing the burden of metastasis. However, its clinical transformation is often hindered by structural stability, bioavailability, and bioactivity. Emerging technologies for the treatment of OC, such as photodynamic therapy, immunotherapy, targeted therapy, gene therapy, molecular therapy, and nanotherapy, are developing rapidly, particularly, nanotechnology can play a bridging role between different therapies, synergistically drive the complementary role of differentiated treatment schemes, and has a wide range of clinical application prospects. In this review, nanoscale natural drug delivery systems (NNDDS) for targeted drug delivery against OC were extensively explored. We reviewed the mechanism of action of natural drugs against OC, reviewed the morphological composition and delivery potential of drug nanocarriers based on the application of nanotechnology in the treatment of OC, and discussed the limitations of current NNDDS research. After elucidating these problems, it will provide a theoretical basis for future exploration of novel NNDDS for anti-OC therapy.
Worldwide, OC is the leading cause of death from malignant tumors in the female reproductive tract. According to data released by the International Agency for Research on Cancer (1), there will be 313,959 new cases of OC worldwide in 2020, and this number will reach 348,000 cases by 2025. The incidence of OC is increasing at an alarming rate in many parts of the world, and the disease burden remains high, particularly in low Human Development Index countries lacking quality cancer treatment and care, with OC incidence and mortality well above the threshold assessed in the Global Cancer Report. Not only that, the annual “economic toxicity” of OC is also devastating, costing more than $5.8 billion annually in the United States alone (2). OC always develops in an unpredictable direction, and a series of symptoms such as lower abdominal and pelvic pain, irregular vaginal bleeding, and changes in bowel and urination habits occur insidiously with the progression of the tumor. OC is not only difficult to diagnose in the early stage, highly aggressive and prone to multi-drug resistance (MDR), but the survival rate of patients will drop sharply during stages III and IV. Metastatic OC is largely considered incurable, and some patients have a low response rate to single-target traditional therapies, hoping that traditional treatments (surgery, chemotherapy) can cure gynecological tumors has become as difficult as starting a broken “machine” (3). Although in recent years, researchers have hoped to use immunotherapy and polyADP-ribose polymerase inhibitors to reduce OC recurrence and chemotherapy resistance through “synthetic lethal” and “PARP capture”, the therapeutic effect of these therapies is still limited and the toxic side effects on the gastrointestinal tract and blood are large. No significant difference has been observed in the overall survival of patients (4, 5). Therefore, safer and more effective drug regimens are needed to improve patient outcomes.
Natural medicines include secondary metabolites, such as Triptolide (TP), Doxorubicin (DOX) and other compounds that are isolated and extracted from natural plants, animals, microorganisms and other organisms, having high efficiency and better biocompatibility compared with synthetic drugs. Studies have shown that 32% of small-molecule drugs approved globally in the last 40 years were derived from natural products and their derivatives (6). Natural drugs have complex structures and a wide variety, including polysaccharides, flavonoids, alkaloids, quinones, terpenoids, sterols, and so on. Interestingly, these natural substance components can transmit their functions on the organism itself to the human body, playing a role in signaling and improving immune levels. Therefore, all kinds of natural products have been widely used in nutritional supplements and treatment of inflammation, cancer, cardiovascular and other diseases (7, 8). However, just as a coin has two sides, most natural drugs have defects in vivo targeting, poor stability and low bioavailability, while new drug development also faces challenges such as lead optimization and identification of bioactive compounds, seriously hitting pharmaceutical companies’ enthusiasm for natural drug development (9). A reasonable scheme is to modify the chemical structure of natural drugs, which is to add artificial design at the level of biological macromolecules, improving the stability of natural drugs in the human body and the ability of targeted therapy. However, when it comes to bioavailability, researchers such as Tang (10) have found that artificial design at the biological macromolecule level may not be a good solution. Another hot idea, which has emerged in recent years, is to design targeted drug delivery strategies, such as relevant targeted delivery systems for OC therapy including peptide/folate/aptamer-drug conjugates, antibody-drug conjugates (ADCs), polymer-drug conjugates, ligand-functionalized nanomedicines, and dual-targeted nanomedicines, etc (11).
Among many drug delivery strategies, the nanoscale drug delivery system is a drug synergist that has emerged in recent years. As a multifunctional system, it can regulate the distribution of packaged drugs in time, space and dose (12). In the past few decades, nanotechnology has been absorbing technical solutions from different subdisciplines and has been widely used, especially in the fields of biology, medicine, and materials. Nanoscale materials which can be used as a delivery agent for encapsulating drugs or attaching therapeutic drugs, are materials with at least one dimension ranging from 1-100 nm in three-dimensional space, which have unique advantages in structural characteristics, chemical properties, mechanical dynamics, electrical performance, and biological properties (13, 14). Compared with traditional drug delivery methods, nano-scale materials can maximize the delivery and release of drugs at specific targets in the human body, reduce the adverse reactions brought by drugs, extend the blood circulation time of compounds, and have better bioutilization efficiency (15–17). Surprisingly, in recent years, researchers have modified nanomaterials onto biomolecules with the aid of aptamer conjugating technology, which has greatly improved the selective targeting ability of materials, providing new opportunities for tumor-targeted therapy. At present, natural drug delivery systems have been used in cancer treatment fields including OC, such as Liposome-DOX and albumin-bound paclitaxel (PTX) (18, 19). In addition, smart drug delivery platforms (20) have been developed in the field of nanocarriers, while clinical trials have demonstrated that nanoscale drug delivery systems are effective in improving the efficiency of cancer treatment (21). More importantly, nanoscale drug delivery systems offer new solutions to oncology drug treatment bottlenecks, including side effects, efficacy, availability, and targeting, compared to problems with traditional chemotherapy regimens.
In this study, we comprehensively review and summarize the current recommendations for NNDDS as a promising targeted therapy and drug delivery system for OC and provide a detailed summary evaluation of the morphological composition and delivery potential of drug nanocarriers. Meanwhile, we describe the mechanisms of action of natural drugs against OC, clarify the key principles of the natural drug delivery system, summarize the latest application status of NNDDS, pointing out recognized challenges and prospects in this promising area of research. The purpose of this study is to provide a basis for the research and development of NNDDS anti-OC targeted therapy.
Currently, although the basic treatment options for OC are tumor reduction surgery and platinum-based chemotherapy, not all patients have the opportunity for surgery in clinical practice, and quite several patients are insensitive to chemotherapy or resistant to chemotherapy drugs in the later stage of treatment (22). In the early stages, even though the conventional treatment regimen is effective, the damage caused by the neurological and renal toxicity of chemotherapy drugs is difficult to estimate. At the same time, genetic mechanisms such as increased repair of DNA damage, disturbance of intracellular transduction pathways, and changes in cellular genes all act on the OC microenvironment and make the tumor resistant to drugs (23). Therefore, there is an urgent need to discover and develop new natural medicine preparations with alternative effects. In previous studies, we reviewed the independent predictive value of blood inflammatory complex markers for OC (24), and summarized and analyzed the specific mechanisms of natural polysaccharides against OC by intervening in inflammation and immune response, regulating tumor cell cycle, and affecting cell migration and invasion (25). However, in terms of the whole range of natural drugs, the mechanisms of anti-OC are more diverse, which is related to the complex and diverse structure of natural drugs (26). As expected, natural medicines remain an important source of compounds for the development of potential therapeutic agents for OC.
The biomolecular mechanism of anti-OC treatment with natural drugs can be divided into three parts, one is the direct treatment of OC cells, which means that natural drugs can inhibit the proliferation, invasion, and migration of tumor cells, block cell cycle and RNA expression, and induce apoptosis and autophagy; The second is the indirect anti-OC effect by improving the tumor microenvironment (TME), preventing neovascularization, regulating oxidative stress and DNA damage; The third is natural drugs as adjuvant chemotherapy drugs, can improve the sensitivity of chemotherapy drugs and reduce the side effects of chemotherapy drugs. The specific mechanism of natural products against ovarian cancer is shown in Figure 1.
Figure 1 The various cellular signaling pathways of natural drugs in anti-ovarian cancer. Natural drugs through Hedgehog, JAK-STAT, Wnt/β-catenin, NF-κB, PI3K/AKT/mTOR, and Ras/Raf/MEK/ERK signaling pathways regulate cell proliferation, apoptosis, migration, invasion, EMT, autophagy, metabolism and chemotherapy sensitivity, thereby inhibiting the occurrence and development of ovarian cancer.
An important characteristic of natural medicines compared to synthetic chemicals is that they contain a large variety of complex scaffolds, generally giving them higher molecular weight, more oxygen and carbon atoms, and fewer halogen and nitrogen atoms (27). Natural drugs against OC are mainly concentrated in flavonoids, polysaccharides, alkaloids, phenols, quinones, alcohols and other compounds, the rigid structure of which has a wide range of value in dealing with protein-protein interactions. This is often the key mechanism by which natural drugs exert their anti-tumor effects (28). Flavonoids are mostly low molecular weight compounds consisting of a tricyclic structure with various substituents, and their branching composition is often determined by the position of the intermediate epoxy group, the double bond between carbon atoms, or a partial hydroxyl group. These structures make it effective in anti-tumor proliferation, especially the hydroxylated structural pattern can increase the biological activity of flavonoids and promote the effect of inhibiting mast cell secretion (29, 30). In polysaccharides, the structure relationship of compounds is pretty clear, for example, most polysaccharides with anti-OC effects contain β-(1→3) -D-glucan main chain, and have similar alkaline glucan structure (31). It has been reported that β-(1→6)-glycoside and β-(1→3)-glycoside are the most active structures for anticancer activity, which may be related to the structural characteristics of the residual body (32). In alkaloids, the position of benzoyl, benzyl, and alkyl is related to their anti-tumor effect, and the structure that plays a key role or irreplaceable role in the compound is often called a “privileged scaffold”. Therefore, some active spatial structures are also regarded as the core content of the compound activity relationship (33–35). Phenols, quinones and alcohols are similar in structure, and there are few studies on their structural relationships. However, some studies have speculated that they all play similar roles in tumor prevention and regulation of carcinogen metabolism through hydroxymethyl-linked benzene rings (36, 37).
Berbamine, a bibenzyl isoquinoline alkaloid isolated from the plant Berberis amurensis Rupr. has previously been used for the treatment of leukemia in Asi (38). Zhang et al. (39) found that Berbamine could up-regulate the expression levels of caspase-3, caspase-9 and Bax by inhibiting the Wnt/β-catenin signaling pathway, inhibiting the proliferation activity of Human ovarian cancer SKOV-3 cells line(SKOV-3) and Human ovarian cancer ES-2 cells line(ES-2) cells. Baicalein is a natural medicine extracted from the root of Scutellaria baicalensis (SB). Yan et al. (40) demonstrated that Baicalein can inhibit the expression of matrix metalloproteinases (MMPs) in OC cells and significantly inhibit the invasion of OC cells. The biological mechanism may be related to the effect of Baicalein on p38 Mitogen‐activated protein kinase(MAPK)-dependent NF-κB signaling pathway. Scutellarein is another flavonoid substance derived from SB that has a different pharmacological effect due to the substitution of the hydroxyl group encoding position 7 in the molecular structure. Lang et al. (41) found that the proliferation rate of Human ovarian cancer A2780 cells line(A2780) and SKOV-3 cells treated with Scutellarein was significantly reduced, and the invasion and migration ability of OC cells were inhibited to the greatest extent when the concentration was 100 μM. In addition, many substances in SB, such as wogonin, Baicalin, Oroxylin A, etc. have been proven to be effective in the treatment of OC, suggesting that SB may be an important natural drug candidate for the treatment of OC.
The cell cycle, considered to be a key factor in tumor cell growth, is composed of G1, S, G2 and M cycles in mammals. Cyclin, cyclin-dependent kinase (CDK) and CDK inhibitors are the core substances that regulate the cell cycle. In OC, high expression of CDK 6 is often associated with a poor prognosis (42). Amentoflavone (AF) is a natural flavonoid derived from Selaginella tamariscina. Liu et al. (43) showed that AF can effectively inhibit the expression of S-phase kinase protein 2 (SKP2) in SKOV-3 cells and Human ovarian cancer OVCAR-3 cells line(OVCAR-3) cells through ROS/AMPK/mTOR signaling pathway, playing a role in blocking the cell cycle process. Astragalus polysaccharide (APS), a natural antitumor agent isolated from Astragalus membranaceus, has been shown to target the tumor suppressor F-box and WD-40 domain protein 7 through the regulation of miRNA, mediating miR-27a/FBXW 7 axis, which plays an anti-OC cell growth role in vitro (44). Deoxyschizandrin is the main active component of Schisandra berries, which has various biological activities such as hypoglycemic, antioxidant and anti-tumor. Lee et al. (45) found that Deoxyschizandrin can inhibit the expression of CD209 and CD163 in OC macrophages, promote the stagnation of the G0/G1 cell cycle of A2780 cells and play an anti-tumor role.
Apoptosis is an evolutionarily conserved form of programmed cell death in which cells actively die according to a specific genetic program to maintain the balance of tissues and organs. Apoptosis usually occurs in the process of organism development, tissue repair, immune response and tumor development (46). Zeylenone, a natural cyclohexanthin oxide derived from Uvaria grandiflora Roxb, was shown in a pilot study to reduce the expression of p-JAK and signal transduction and transcriptional activators of the Janus family of tyrosine kinases by increasing the mRNA levels of cytochrome C and apoptosis-inducing factors, promoting the apoptosis of SKOV-3 cells (47). Interestingly, the potential of Zeylenone to inhibit tumor cell proliferation and promote apoptosis has also been found in cervical cancer, of which the underlying mechanism is related to the inhibition of PI3K/AKT/mTOR and MAPK/ERK signaling pathways (48). Autophagy is a cell death pathway different from apoptosis. In the study of Che et al., the mechanism by which the natural metabolite grifolin induces autophagic death of OC cells has been extensively explored (49). The researchers treated A2780 cells and SKOV-3 cells with grifolin and found that it prevented tumor transformation at an early stage. Autophagy death of ovarian cancer cells was induced by up-regulating the expression of autophagy markers Beclin-1, LC3B, and Atg7, down-regulating the expression of P62, and inhibiting the expression of the Akt/mTOR/S6K pathway.
TME is a dynamic and complex acid-hypoxic system composed of malignant tumor cells, tumor-infiltrating immune cells, endothelial cells, extracellular matrix, vasculature, cytokines and chemokines. The mutual signaling between immune cells and OC can regulate the immune response and control disease progression (50). Natural drugs participate in multiple signaling pathways and molecular targets through metabolic crosstalk and indirectly act on tumor cells to exert anti-OC effects through metabolic regulation of TME cells. For example, resveratrol inhibits glycolysis levels and participates in regulating glycolysis and oxidation balance (51); Epigallocatechin gallate (EGCG) is one of the most bioactive catechins and has been found to have multi-target activity on endothelial cells. As revealed by the metabolic profile, EGCG interferes with the multi-pathway metabolism of tumor cells, and regulates oxidative stress and tumor angiogenesis, indirectly playing a role in anti-OC cell growth. Some studies integrating transcriptomics and metabolomics have also shown that EGCG has antioxidant stress effects on breast cancer and colon cancer (52, 53).
In recent years, the ability of OC cells to repair DNA damage has been regarded as an important sign of its refractory, therefore the intervention of natural drugs in the DNA damage repair pathway has become a hot target for OC treatment (54). Existing in a variety of natural plant alkaloid components, Berberine is a natural antioxidant and anti-inflammatory drug, that has been proven to be effective against lung cancer (55), stomach cancer (56), colorectal cancer (57) and other tumors. Hou et al. confirmed that berberine can significantly down-regulate homologous recombination repair of OC cells and induce oxidative DNA damage, and the experiment also shows that berberine can increase the sensitivity of A2780 cells and Human ovarian cancer HO8910 cells line(HO8910) cells to Niraparib, which is a natural drug with a wide range of targets for the treatment of OC (58).
Cisplatin and PTX or carboplatin and PTX adjuvant chemotherapy is the conventional treatment for OC patients after receiving cell reduction therapy. Unfortunately, more than half of the patients will relapse after treatment and develop platinum drug resistance, which poses no small challenge to the chemical treatment of OC. After drug resistance, tumor clinicians often choose the standard regimen of PEGylated liposome DOX in combination with weekly PTX and topotecan, however, its therapeutic effectiveness is often limited (59). In recent years, studies have found that natural drugs have sensitizing effects on chemotherapy drugs to a large extent, and their mechanism is related to regulating immune cell response, regulating the expression level of immune molecules, and reversing MDR (60). LycopeneIt, a natural red carotenoid found in tomatoes, has excellent antioxidant properties. Holzapfel et al. (61) found that Lycopene could significantly reduce cancer-related factors and metastasis load in mice with OC. The lycopene plus PTX regimen was as effective as the PTX plus platinum regimen in reducing OC burden compared with placebo. The mechanism is related to reducing the production of integrin α5β1 heterodimer, stimulating the proliferation of immune cells, and enhancing the activity of macrophages and T cells (62).
Grape seed procyanidin (GSP) is a natural polyphenol with good antioxidant properties. Zhao et al. extensively explored the cytotoxic effects of GSP on PTX and DOX against drug-resistant A2780/T cells (63). Studies have shown that GSP can reverse MDR phenotype by inhibiting P-glycoprotein (P-gp) function, not only enhancing the therapeutic effect of PTX and DOX for OC but also interacting with P-gp through MAPK/ERK pathway to block nuclear translocation. The mechanism of action of natural drug ingredients against OC in recent years is summarized in Table 1.
The development of NNDDS enables targeted delivery of hydrophobic components of natural drugs driven by specific ligand functions, preferentially acting on OC cells and releasing the drug into tumor tissue. Nanoscale delivery systems enable better biocompatible distribution and pharmacokinetics of natural drugs compared to conventional delivery modes and amplify the therapeutic potential of natural drugs compared to non-functional delivery modes (156). To verify the effect of the nanoscale drug delivery system, Vandghanooni et al. (157) prepared nanoparticles of AS1411 anti-riboprotein-aptamer modified PEGylated poly (lactic-co-glycolic acid) and loaded them with a chemotherapeutic agent. Compared with traditional drug delivery methods, functionalized nanoparticles can strongly inhibit endogenous miR-21 expression through endocytosis. Therefore, targeted delivery of therapeutic drugs to Mir-21-inhibited OC cells using nanomedicine delivery systems can improve the mortality of tumor cells. Fang et al. (158) modified the amphiphilic block copolymer Pluronic F68 with conjugated linoleic acid, synthesized the F68-Linoleic acid (F68-LA) conjugate, and formed a drug delivery system by loading the natural drug Gambogic acid (GA), improving the therapeutic effect of OC. The study found that compared with the control group, the particle size and potential of GA-loaded F68-LA nano-spheres could remain unchanged for up to 6 days, and the cytotoxicity and pro-apoptotic effect of GA-loaded F68-LA nano-spheres was significantly enhanced on A2780 cells, which not only had high stability but also had the ability of targeted therapy on tumors. All these results suggest that nanoscale drug delivery systems are a promising prospect for therapeutic targeted intervention in OC.
Nanostructured carriers deliver drugs mainly through active delivery and passive delivery, in which drugs integrate into the inner cavity of the nanostructure with the help of hydrophobic effect, and realize the transfer of drugs from high concentration to low concentration at specific targets, and then release a specific amount of drugs. Meanwhile, nanomedicine materials designed in conjugates disengage from the carrier very quickly, making drug delivery easier (159). NNDDS changes the original pharmacokinetic characteristics of natural drugs to a certain extent and regulates the blood circulation of drugs by regulating the mechanism and rate of drug release reaction, improving the tumor treatment effect of nano combination (160). Yao et al. (161) have developed a novel targeted PTX-supported nanocore PTX-PEG-PLA-FA-NP for OC treatment, which connects Folic acid (FA) molecules to PEG-PLA to form block copolymers through covalent bonds between the hydroxyl group of FA molecules and the ends of polyethylene glycol and polylactic acid (PEG-PLA). SKOV-3 cells, HO8910 cells, and A2780 cells were used in the PTX-PEG-PLA-FA-NP to measure drug release and uptake in vitro, and pharmacokinetics and drug distribution in vivo were studied by high-performance liquid chromatography (HPLC). Ptx-peg-pla-fa-np has a stronger uptake of SKOV-3 cells in vitro than free PTX. Relevant studies on drug distribution in vivo confirmed that compared with the normal administration group, PTX loaded with nanocarriers contained 3 times more PTX drug concentration in tumor tissues, and had longer blood circulation time, playing a perfect drug release and anti-OC treatment at the same drug dose.
As mentioned in the previous introduction, NNDDS have shown a powerful function in the delivery of natural drugs to exert anti-tumor effects, not only improving the ability of targeted drug delivery to OC, but also controlling drug release, extending the blood circulation time of the drug, and maximizing the anti-tumor therapeutic effect. At present, the anti-tumor drug delivery of nano drug delivery system is mainly through active targeting and passive targeting. Active targeting uses the principle of ligand-receptor recognition to deliver natural or synthetic drugs to cells that promote drug capture and have specific receptor functions (162). Disorder and dilation are the normal conditions of new blood vessels, as the dense blood vessels of the tumor, the space of the blood vessel wall is relatively wide, and the phenomenon of lymphatic reflux is obstructed. This pathophysiological feature of tumors has selectivity and retention of macromolecular substances, in such cases, passive targeting came into being. Passive targeting refers to relying on the unique properties of nanoscale carriers to improve the permeability of drugs to tumor tissues so that drug concentration can accumulate. At the same time, the change of pathological microenvironment can reduce the kidney clearance rate of drugs, so that therapeutic drugs can enter and stay in tumor tissues (163). Physical encapsulation and front-end carrier connection are two major strategies for drug delivery systems. Physical encapsulated drug delivery systems include liposomes, polymer micelles, polymer-drug conjugates, nanosuspensions, etc., which are mostly associated with passive targeted drug delivery modes. The front-end carrier-linked drug delivery system involves ligands with high adaptation to tumor cell differentiation, of which the acceptors include FA receptors, EGFR receptors, HER2 receptors, etc., associated with active targeted drug delivery modes.
Lipid-based nanodrug delivery systems are mainly composed of liposomes and solid lipid nanoparticles (SLNs), currently widely used delivery carriers of lipid-based DDS. The combination with drugs is also the most common type of nanomedicine formulation currently approved (164). Liposome nanoparticles have long been regarded as carriers for the transport and management of various drugs into cells, and like other nanotechnology platforms, they can selectively improve the distribution and release efficiency of natural drugs in vivo. Liposome nanoparticles come from a wide range of sources and are the most used type in current tumor clinical trials, accounting for about 33% (165). The discovery of EXO, which are complex versions of liposomes, has led to advances in models of personalized medicine, especially in the fields of cancer immunotherapy and complementary and alternative medicine. It has received considerable attention, providing a broader perspective for the study of the biological activity, substantive capacity and targeted drug delivery of liposome nanoparticles in diseases (166). As lipids have the characteristics of hydrophilic head and hydrophobic tail, they can be used to deliver hydrophilic and hydrophobic drugs, and have a wide application prospect.
EV, composed of proteins such as four-transmembrane protein family, cellular endogenous proteins, skeleton proteins, non-coding RNA, mRNA and other substances, and bioactive lipids, is a nanoscale vesicular body with a diameter of about 20-200nm that is released by cells into the extracellular matrix. Now seen as a key mediator that plays an important role in intercellular communication, there is growing evidence that EVs are an important tool for targeted therapy of disease (167). In terms of drug delivery, EV has the advantages of strong biobarrier permeability, high biocompatibility, low toxicity and low immunogenicity, and is a natural lipid nanoparticle. EV plays a role in drug delivery mainly through receptor and ligand interaction, membrane fusion, cell phagocytosis and other forms, to transfer bioactive therapeutic drugs from the supplier to a specific target cell (168). According to the diameter and origin, EV can be divided into exosomes (EXO), microvesicles (MVS), apoptotic bodies, phagosomes, etc. EXO, a double-layer membrane-structure vesicle formed by the fusion of polyvesicles and plasma membrane, is formed from the plasma membrane in the way of budding, and apoptotic bodies are released after apoptosis.
EXO is relatively stable and widely sourced in circulation and can be selectively captured by tumor cells. It only plays the role of transportation like presenting “express parcels” without changing the function of the original drugs, as a promising nanoscale drug delivery carrier. In OC, EXO is often considered a biomarker of ovarian metastasis. According to the National Cancer Institute, 213 proteins are shared across 60 tumor cell lines from nine different cancers (93). In general, EXO derived from dendritic cells, macrophages, mesenchymal stem cells, B cells, and T cells can often be used as drug-carrying EXO, further promoting the application of natural drugs in anti-tumor therapy (169). The parental cell characteristics of EXO are reflected in surface markers, contents and cell activation pathways. It has significant advantages in the targeted recognition of tumor cells while avoiding systemic inflammation and immune system rejection (170).
Berries are rich in bioactive substances, especially anthocyanins with antioxidant, anti-inflammatory, and anti-tumor properties, which have been widely recognized for their importance to health. In the study of Munagala et al. (171), berry anthocyanins have anti-tumor cell proliferation effects on various cancer types such as lung, prostate, colon, breast, pancreas, and ovary. The antitumor function of anthocyanins in vitro has been fully explored, therefore anthocyanins have the potential to be developed as a therapeutic agent for OC. The daily dose required to supplement anthocyanins with berry intake is considerable, which is also impractical to scale up conversion in a population of patients with weakened OC (172). In addition, as there is ample evidence that oral anthocyanins have low bioavailability and stability, it is often futile to rely on free anthocyanins for the treatment of OC patients (173). In order to overcome these limitations, Farrukh et al. developed anthocyanins delivered via milk-derived EXO, including improving the passive diffusion absorption and availability of anthocyanins and using natural medicines to reduce platinum resistance in some OC patients (174). Studies have shown that even a suboptimal dose of anthocyanin EXO can significantly inhibit OC growth in nude mice, with better stability, higher cell uptake capacity and longer blood circulation time than free anthocyanin. In addition, this study also found that anthocyanin EXO preparation can reduce the corresponding chemotherapy dose threshold when OC cells develop cisplatin resistance, having the potential value of sensitizing drug-resistant OC cells.
TP is the main pharmacodynamic component of natural Chinese herbal medicine. Previous studies have found that TP can induce lethal autophagy and sensitizing chemotherapy drugs for cisplatin-resistant OC, and reduce the growth and metastasis of solid tumors. Therefore, to improve the poor solubility of TP, cytotoxic compounds, Liu et al. (175) developed a new bio-derived nanomaterial, Triptolide-loaded exosomes delivery system (TP-EXOS), a drug delivery system using EXO as a delivery carrier. This study evaluated the anti-OC effect of TP-EXOs in vivo and in vitro by nanoparticle tracking analysis, high-performance liquid chromatography, BrdU/DNA two-parameter flow cytometry, and other technologies. The results showed that TP-EXOS could block OC cells in the S phase, and the effect of inhibiting the proliferation of SKOV-3 cells was two times higher than that of carrier-free TP therapy. At the same time, TP-EXOs have a higher drug encapsulation rate, which can enrich natural drugs to the tumor site of tumor-bearing mice, and play an anti-OC role. Unfortunately, although the exosome delivery vector has endowed TP with the ability of targeted therapy, TP-EXOs also weaken the cytotoxicity and apoptosis-inducing effect of TP on SKOV-3 cells to a certain extent and does not completely reduce the liver toxicity of TP, which still needs to be further optimized around these problems to achieve the best therapeutic effect in the future.
In the study of Melzer et al., researchers isolated EXO containing PTX from mesenchymal stromal cells (MSC) treated with sublethal concentrations of PTX for 24 hours and applied it to lung, ovarian, and breast cancer cells (176). It was found that EXO containing PTX could play a specific role in drug delivery and accumulation in tumor tissues. Compared to the control group, the drug delivery system showed a strong tumor cell killing effect even with a 7.6-fold reduction in dose, demonstrating the usefulness of EXO derived from MSC to serve as a drug delivery vehicle to deliver chemotherapy compounds to multiple cancer cells.
MVS, a class of membranous vesicles ranging in diameter from 100 to 1000 nm, are released by the cell via budding during activation, injury, or apoptosis. Unlike EXO, MVS originates directly from the plasma membrane and is released into the extracellular space through a series of division processes (177, 178). As an important tool for intracellular communication in vivo, the unique bilayer phospholipid structure of MVS can effectively protect chemotherapy drugs in MVS from being metabolized before reaching the tumor site. In addition, MVS are excellent vectors for drug delivery, with good in vivo circulation stability, tumor targeting, physiological barrier permeability, and almost no immunogenicity and toxicity. Currently, in NNDDS for OC, there are few types of MVS as carriers, which may be related to the fact that MVS as drug carriers is still a new research field. At present, most of the existing studies on MVS as drug carriers focus on pancreatic cancer (179) and colon cancer (180), and the experimental results of different tumor cells have shown that MVS has good stability and immune tolerance. The existing experimental results have paved the way for MVS as an NNDDS for OC, which is worth looking forward to further research.
Chrysin, a natural flavonoid derived from Passiflora caerulea L., Oroxylum indicum (L.) Kurz and other plant sources, has previously been shown to have anti-tumor, anti-inflammatory and antioxidant effects. Chrysin and its derivatives have good anticancer activity against a variety of cancers. For example, the killing activity of its long-chain ester derivatives on liver cancer cell lines is 5.4 times that of Chrysin, and its porphyrin derivatives can be used for non-invasive photodynamic therapy of cervical cancer and gastric cancer (181). Chrysin is not an excellent drug in terms of its low bioavailability in the body, as low bioavailability is often indicative of low performance. In order to ameliorate this defect, a common approach is to produce flavonoid derivatives by biotransformation, such as microbial and enzymatic reactions, which does not substantially change their pharmacological action, but significantly improves their bioavailability due to structural changes. Although this recognized technique improves the performance of Chrysin, it is not the best solution to improve the bioavailability of Chrysin due to the limitations of complex technology and high cost (182). In order to solve the defects of poor absorption and rapid metabolism of Chrysin applications, Tarahomi et al. (183) designed and synthesized nanoliposomes Nano-niosomes containing chrysin (Chr-NiO) containing poplar. The liposomal drug delivery system was tested to improve the conversion performance in Chrysin and the toxic effect of Chr-NiO on SKOV-3 cells. The experimental results showed that, compared with conventional drugs, CR-NiO significantly inhibited the migration of SKOV-3 cells in vitro scratch test. The expression of the apoptosis gene in SKOV-3 cells was determined by real-time PCR. The researchers also found that CR-NiO could enhance the expression of pro-apoptotic genes Bax and caspase-3, and reduce the expression of the anti-apoptotic BCL-2 gene, which proved that CR-NiO is a cheap, stable and more effective drug delivery system against OC.
Curcumin, a fat-soluble polyphenol compound extracted from Amomum Tsao-Ko Crevostet, has a wide range of pharmacological activities such as antioxidant, anti-inflammatory and anti-tumor (80). As a natural medicine widely studied in clinical studies, the challenges restricting the clinical application of curcumin mainly come from its low stability and low solubility in solution, as well as poor absorption in the intestines and rapid metabolism after oral administration. Bondì et al. (184) embedded curcumin into SLNs to improve its bioavailability and antitumor activity. In this work, Nanostructured Lipid Carriers (NLCs) loaded with curcumin were prepared using precipitation techniques. By comparing the free curcumin with the NLCs loaded with curcumin, it was found that NLCs loaded with curcumin can induce the decrease of anti-apoptotic proteins such as Bcl-2, Mcl-1 and survivin, activate p38 MAPK to promote apoptosis and down-regulate the homeostasis of IL-6 in OC cells with superior efficacy. Surprisingly, curcumin was also found to inhibit the expression of β-catenin in A2780S cells and A2780CP cells, suggesting that β-catenin is a potential new target for curcumin anti-OC therapy.
SLNs are mainly composed of solid lipid molecules such as fatty acids, triglycerides and phospholipids. When assembled with drugs, hydrophobic drugs can be dissolved in its hydrophobic core. With a particle size of 100-200 nm, SLNs can effectively cross the blood-brain barrier, and their unique biocompatible characteristics not only extend the circulation time of drugs in the body but also effectively avoid drug leakage (185). The unique feature is that SLNs can deliver physicochemically incompatible drugs, even those with poor pharmacokinetic profiles (186).
PTX is the most commonly used drug with SLNs used in the treatment of OC, due to early pharmacological studies on PTX and multiple drug delivery systems have been tried by researchers. Tarr et al. found that the solubility of PTX in general lipids is so low that conventional formulations are inadequate as a delivery system for PTX (187). Therefore, to solve the problem, Lee et al. developed a new PTX-loaded sterically stabilized SLN, which uses trimyristin as a lipid solid core and egg phosphatidylcholine and distearoylphosphatidyl-ethanolamine-N-poly-(ethylene glycol)2000 (PEG2000–PE) as a stabilizer changes the characterization of solid lipids in terms of morphology, charge, drug incorporation mode, and stability, making PTX-loaded sterically stabilized SLN a potential alternative to traditional drug delivery strategies (188). The researchers found that the load of PTX increased the average diameter of SLN from 128nm to 217nm, but did not affect the morphology and ideal potential of SLN. Meanwhile, the drug release curve in vitro indicated that PTX may bind to SLN through an association relationship and be absorbed into cells by granule administration, playing a slow release role. Tumor cell killing experiments showed that PTX-loaded sterically stabilized SLN showed dose-dependent cytotoxicity to SKOV-3 cells, with 15% higher lethal energy than PTX at the same dose, and the ICso value was about 20uM. In other tumor cells, SLN also acts as a coat to participate in a variety of natural drug conjugates, synergistically participating in targeted drug delivery and inducing tumor cell apoptosis. Kumar et al. evaluated the efficacy of chitosan-coated trans-resveratrol and ferulic acid-supported SLN in targeting colon cancer with FA conjugates. The results showed that natural drugs with SLN as the carrier had a significant anti-tumor effect, significantly inducing apoptosis of tumor cells compared with free drugs (189).
Chitosan, the product of alkaline deacetylation of chitin, is the main derivative component of chitin and a natural component of invertebrate exoskeletons. As it has many properties such as hemostasis, promoting wound healing, reducing blood cholesterol levels, and anti-tumor, Chitosan has become a widely used natural drug preparation in the biomedicine field in recent years (190). Since the molecular weight of chitosan is 20-200nm, which conforms to the typical nanostructure characteristics, it has good biodistribution and degradability of nanocarriers. In the treatment of OC, chitosan has been shown not only to inhibit the proliferation of OC cells and induce apoptosis in a dose-dependent manner but also to participate in gene and immunotherapy as a carrier of drug delivery (191). Therefore, compared with conventional carriers, chitosan drug delivery systems have unique advantages in nature, as both the shell and the internal-loaded drugs can play an anti-tumor therapeutic effect, proving to have a multiplier effect with half the effort (192).
DOX, a natural anthracycline derivative derived from the Streptomyces Peucetius, has extensive anti-tumor effects on lung cancer, breast cancer, OC, and other tumors (193). Due to the low bioavailability and short half-life of DOX, the key is that effective treatment often requires high drug doses, which often lead to an increase in side effects such as nephrotoxicity and cardiotoxicity. A combination of DOX and other chemotherapy drugs is seen as a promising approach. However, it can only reduce the toxic side effects of DOX, and cannot improve the tumor targeting and drug delivery ability of drug therapy. Using chitosan materials, HU et al. (194) designed and developed a novel acid-sensitive DOX drug delivery system, CMC-CBA-DOX nanoparticles, which passively released DOX in the acidic environment of tumor cells through the covalent bond between Carboxymethyl chitosan(CMC) and DOX. Yan et al. (195) prepared polyvinyl alcohol/chitosan nanofibers with complete biocompatibility for DOX delivery. The experimental results showed that the fiber material could inhibit the proliferation and adhesion of SKOV-3 cells and control the release of DOX, which was also found in a kind of magnetic ferric oxide nanoparticles coated with chitosan (196). Effectively reducing the toxic side effects of drugs is a key issue in the development of NNDDS. Although the toxic side effects of natural drugs are not so strong compared with synthetic drugs, the effect of a series of natural drugs represented by DOX on bone marrow suppression in the body still cannot be ignored. The chitosan nanoparticles developed by Youse et al. (197) have solved this problem well. By using succinic anhydride as a crosslinking agent for the combination of chitosan and DOX, the authors developed nanoparticles with narrow particle size distribution and a clear core-shell structure. Experimental results show that this novel chitosan nanostructure can distinguish between Her 2+ and Her 2- cells, and Her 2+ cells can selectively uptake it, which obviously weakens the cytotoxic effect of DOX on the body and enhances the potential application value of DOX. Meanwhile, the authors of this study also point out the potential application of PH-sensitive copulants in the development of drug delivery systems.
Camptotheca acuminata Decne. is a unique Chinese tree plant in the daucrate family, containing the bioactive ingredient, camptothecin (CPT), which has high medical value in pharmaceutical and biomedical fields. CPT possesses a unique 5-fused ring structure, can specifically block the synthesis of topoisomerase I, and has good anticancer activity against OC, gastric cancer, liver cancer and other tumors. However, the pharmacokinetics of CPT are poor and the toxicity of intravenous administration is high, so effectively breaking through the above limitations is the key to expanding clinical application. Li et al. (198) prepared a novel CPT local drug delivery system using chitosan and disodium hydrogen phosphate as carriers. A series of studies, such as degradation experiments, in vitro release experiments, and cytotoxicity experiments, have shown that the new drug delivery system is biocompatible and degradable, and the bioactivity of CPT loaded in it can be retained for more than 1 week. Of the drug release days included in the calculation, a total of 70% of CPT was released from the hydrogel within 18 days, and the drug delivery system maintained a good anti-tumor effect even at lower drug concentrations. In order to improve the low water solubility and severe side effects of CPT, Zhou et al. (199) established a safe and effective N-trimethyl chitosan (TMC) coated with the CPT drug delivery system, CPT-TMC. Human ovarian cancer SKOV-3 cells transfected by VEGF-D recombinant plasmid (SKOV-3/VEGF-D) cells were used to construct a lymphoid metastasis model of OC in nude mice to evaluate the anti-tumor and anti-metastatic activity of CPT-TMC and its side effects. The experimental results showed that CPT-TMC could significantly reduce tumor-related blood vessel and lymphangiogenesis, participate in the down-regulation of VEGF-D and MMP-9 expression, increase tumor apoptosis index, maximize the anti-tumor and anti-metastasis activity of CPT, and reduce systemic side effects caused by drugs.
In the past few decades, different types of polymer-based drug delivery systems have been developed, including polymer nanoparticles, polymer micelles, polymer drug couplings, dendritic polymers, etc., providing safer and more effective protocols for the clinical treatment of tumors. Polymer nanoparticles are the most widely used and effective polymer drug delivery system. By packaging and combining hydrophilic polymers of different proportions, polymer nanoparticles can achieve a constant rate of release of therapeutic drugs, of which the surface functional design can give drugs the ability of targeted therapy.
Kaempferol is a natural polyphenol compound, widely found in the daily consumption of vegetables, fruits and some Chinese herbs. Studies have shown that the natural dietary compound Kaempferol exerts anti-inflammatory effects by down-regulating the NF-κB pathway, inhibiting interleukin-4 and cyclo-oxygenase 2 expression (200). In terms of anti-OC treatment, Kaempferol has been reported to reduce the risk of OC risk events (201).
To better improve the bioavailability of Kaempferol, Luo et al. (202) developed five different types of polymer nanoparticles loaded with Kaempferol and evaluated its effect on the cell viability of OC. The results show that compared to other polymer drug delivery systems, Poly(DL-lactic acid-co-glycolic acid) (PLGA) nanoparticles incorporating kaempferol can reduce the vitality of OC cells without altering normal ones, indicating that it had cytoselective toxicity and could be a promising candidate for the prevention and treatment of OC.
Hypericin, a dianthranone compound, is a natural photosensitizer extracted from Hypericum perforatum L., which plays a role in inhibiting tumor cell growth in drug photodynamic therapy against cervical cancer, breast cancer, colon cancer, and liver cancer (203). In Labouebe’s (204) research, polymer nanoparticles based on PLA and PLGA were used as Hypericin drug delivery systems. It was found that free Hypericin is more inclined to passively diffuse into the cell membrane due to its hydrophobic properties, while the drug delivery system, affected by nanoparticle endocytosis, increases the concentration of the drug in the cell. Compared with free Hypericin, Hypericin-loaded PLA Nanoparticles present stronger in vitro photoactivity, which can significantly reduce the drug dose of Hypericin. Due to the unique hydrophobicity of Hypericin, it is quite difficult to avoid the poor encapsulation rate of the drug loading system, making it also difficult to prepare this drug loading system. Fortunately, the photoactivity of Hypericin is still maintained after nanoencapsulation. However, the full details of the mechanism of the Hypericin drug delivery system against OC were not disclosed in the original study, making its clinical application uncertain.
Genistein (GEN) is a kind of natural isoflavone existing in Glycine max (L.) Merr., which has the pharmacological effects of regulating steroid hormone receptors, regulating body metabolism, inhibiting inflammation, protecting nerves and anti-tumor (205). The anti-cancer effects of GEN against a variety of cancer types have been studied, but its low water solubility and bioavailability, rapid metabolism and excretion, and lack of specific targeting ability to tumor cells, limiting its clinical application. Arjun et al. (206) developed a GEN-containing FA receptor targeting and pegylated polynanoparticles (PLGA-PEG-FA NPs) that can deliver GEN targeting to OC cells. The study found that PLGA-PEG-FA NPs have the ability to continuously release GEN while increasing the uptake of the drug by SKOV-3 cells, with the potential for better specific delivery compared to non-targeted drugs. The development of this study provides a way for the research and development of GEN targeting nanoparticles, and provides a reference for the co-delivery of imaging agents based on diagnosis in the future.
Curcumin is one of the most widely studied natural drugs in the field of polymer nanoparticles. Sathish et al. (207) encapsulated curcumin in a hydrophilic polymer Poly(2-hydroxyethyl methacrylate) (PHEMA) using nanoprecipitation technology to enhance the anti-tumor targeting ability of curcumin.
The researchers increased the drug-carrying capacity of the drug carrier system to 26.4%. The anti-cancer activity experiment showed that the polymer drug carrier system had better regression activity of ovarian tumor cells than free curcumin, and could exert an anti-OC effect by significantly reducing G0/G1 phase cells, inhibiting NF-κB function, up-regulating the expression of Bax protein, and down-regulating the expression of Bcl-2 protein, anti-apoptotic survivin, VEGF and COX-2. Another interesting study showed that a polymer-loaded system of curcumin can double the uptake of cisplatin-resistant A2780CP cells and sixfold increase the uptake of metastatic MDA-MB-231 cells. Another interesting study showed that a polymer-loaded system of curcumin respectively increased drug uptake twice for cisplatin-resistant A2780CP cells and six times for metastatic MDA-MB-231 cells, of which the anti-tumor mechanism is related to the enhancement of tumor cell apoptosis induced by Nano-CUR6. The coupling of nanoparticles with anti-cancer antibodies also enables curcumin to achieve tumor-specific targeted delivery (208). In vivo experiments also showed that in the experimental group treated with curcumin nanoparticles, the animal ovary histology showed atypical hyperplasia, without tumor inflammatory cells, rough chromatin, and bleeding. Curcumin nanoparticles can inhibit the proliferation of tumor cells by down-regulating the JAK/STAT3 and PI3K/Akt signaling pathways, reducing the expression of JAK and reducing the concentration of TGF-β in ovarian tissue, which significantly affects the phosphorylation process of STAT3 (209). Unfortunately, among the original published studies of polymer drug delivery systems, clinical studies of curcumin against gynecological tumors have been ignored. Even though curcumin has been shown to have extremely high safe doses, its application from the laboratory to any clinical setting must be very cautious.
As a nano-delivery system, polymer micelles are self-assembled products of amphiphilic polymers in an aqueous environment, which are widely used to improve the water solubility of chemotherapy drugs in cancer therapy. Polymer micelles are one of the most biocompatible, non-immunogenic, stable and targeted drug delivery carriers with the ability to induce stealth of hydrophilic polymer brush on micelle surface and stable encapsulation capability provided by hydrophobic and rigid micelle core (210). In recent years, it has become a research hotspot that studies and develops ligand modifications, stimulatory responses and multifunctional polymer micelles for tumor drug-targeted therapy, improving micellar blood circulation time and stabilizing drug load (211).
Fisetin is a natural compound extracted from the leaves and stems of the sumac plant and found in a wide range of fruits and vegetables. At high doses, Fisetin has not shown adverse effects, making it an important drug for the study of aging and age-related diseases (212). Studies have shown that Fisetin can play an anti-OC role in various ways such as anti-inflammatory, anti-proliferation and apoptosis-inducing (213). However, the fact that Fisetin has very low water solubility (less than 1mg/g), and unmodified Fisetin has almost no efficacy to play a role, seriously hinders its application in the clinical treatment of OC. Xue prepared polymeric micelles encapsulating fisetin, which greatly improved the physical properties of Fisetin, and the characteristics of micellar carriers also enabled Fisetin to better gather into OC tissue (214). The experimental results showed that Fisetin could down-regulate the expression of Bcl-2 and up-regulate the expression of Bax, briking the balance between Bax and Bcl-2. Apoptosis of SKOV-3 cells was activated by the mitochondrial pathway, and the tumor size and weight were reduced, which effectively inhibited the growth of transplanted tumors in mice. Polymeric micelles encapsulating fisetin also showed a stronger inhibitory effect on tumor growth and prolonged survival compared with free fisetin.
Another interesting study found the ability of co-delivery of resveratrol and curcumin polymer micelles to reduce cardiotoxicity caused by DOX in vitro. In the study of Lisa et al. (215), polymer micelles made the water solubility of resveratrol and curcumin 29.6 times and 1617 times higher than their intrinsic solubility. The unique properties of micelles enhanced the retention time of drugs, not only acting as chemical sensitizers for DOX, but also delivering targeted drugs to reduce the occurrence of adverse reaction events. This study demonstrated the potential of co-delivery of two natural compounds in polymer micelles. Compared with conventional micelles, polymer micelles have the advantages of low toxicity, solubilization and structural stability, but the drugs encapsulated in the drug delivery process still have material selectivity. In the study of Sachin K. et al. (216), the researchers encapsulated ceramide with natural sweet tea side and prepared a nanomicelle with high bioavailability, demonstrating for the first time that natural nanomicelles can effectively deliver ceramide in vivo. It was found that compared with previous synthetic polymer micelles encapsulated ceramides, the new nanomicelles not only promoted drug delivery to OC cells, but also overcame tissue barriers, inhibited glycosylation, better participating in restoring tumor suppressor activity in OC cells carrying p53 mutations.
The three major advantages of metal and oxide nanoparticles in medicine are their strong stability, large surface area and good size effect. These advantages are not only reflected in modifying the surface of the nanoparticles to regulate the role they play in the body but also provide multiple surface binding sites for the drug, allowing the drug delivery system to better actively target therapy in the body (217). Gold, a precious and less toxic metal believed to have a calming and detoxifying effect, is also an ingredient in Angong Niuhuang pills in traditional Chinese medicine. In recent years, as gold has extremely high stability, more and more research on nanoparticles based on gold is being carried out.
Wang et al. (93) constructed a drug delivery system gold nanodots-PTX-polylysine (AuNDs-PTX-PLL) based on gold nanoparticles loaded with PTX, which has been proved by experiments to realize intelligent responsive drug delivery, effectively solving the problems of poor water solubility and drug resistance of PTX. In addition, the system reduces damage to normal tissue by intelligently delivering drugs in an acidic tumor environment. The multimodal imaging technology also shows that AuNDs-PTX-PLL nanosystem can also be used to assist tumor diagnosis, achieving accurate diagnosis and treatment of tumor. PTX has a wide range of anti-tumor efficacy against OC, breast cancer and other tumors, although the anti-tumor effect evaluated in the AuNDs-PTX-PLL nanosystem study was carried out on non-small cell lung cancer cell lines, this study also indicates the future research potential of gold nanodrug delivery systems.
Nano-silver, also known as colloidal silver or silver nanoparticles, is an excellent antimicrobial agent. In recent years, more and more studies have been conducted on its effects in organisms and cells, indicating that nano-silver has high research value in the medical field. In a study by Saratale et al. (218), a green silver nanoparticle (Li-AgNPs) prepared from Wheat straw lignin and AgNO3 was shown to be cytotoxic to SKOV-3 cells in vitro, suggesting that Li-AgNPs are a promising anti-OC agent.
Rhynchosia suaveolens (L.f.) DC. widely distributed in southern India, the leaves are rich in flavonoids and phenolic compounds with anti-cancer and antioxidant activities (219). Another interesting study reports a novel silver nanoparticle drug delivery system (RS-AgNPs) loaded with leaf extract of Rhynchosia suaveolens (L.f.) DC. In this drug loading system, loaded with extracts not only plays a major role in anticancer effects, but also reduces silver cations in silver nitrate solution, promoting the formation of bioderived silver nanoparticles during the interaction (220). Studies have confirmed that RS-AgNPs have anticancer activity similar to doxorubicin (DOX), and have targeted anti-proliferation and pro-apoptosis ability against SKOV-3 cells, playing an anticancer role through ROS production and apoptosis signaling pathway switching after DNA fragmentation.
Strictly speaking, this kind of method of reducing metal ions with plant extract to prepare nanoparticles does not belong to NNDDS, because these components only have a reduction reaction with metal ions, effectively remove free radicals, prevent the oxidation chain reaction, and then obtain highly active nanoparticles, which does not involve the category of drug loading. However, it should be noted that the types and components of natural drugs are different, so that the size, shape, and chemical properties of the synthesized nanoparticles are different. In these prepared nanoparticles, we can see the “shadow” of natural products and the properties they “inherit” from natural products, so a simple explanation is made in this part. The detailed anti-OC mechanism of NNDDS is shown in Table 2.
In this manuscript, we conduct a comprehensive summary and discussion of NNDDS targeted for drug delivery against OC. By analyzing its mechanism of action, application implications, and future potential, we reveal the importance and future prospects of this field. Our research not only expands the understanding of the field, but also provides useful guidance for future clinical treatment and drug development. Through in-depth analysis of the mechanism of action of NNDDS in anti-OC therapy, we found that this system can improve the stability and bioavailability of drugs by encapsulating drugs in nanoparticles, alter the pharmacokinetics and pharmacodynamics of drugs, and reduce the toxic side effects of drugs on healthy tissues. This is done primarily by (a) avoiding first-pass clearance increases circulating half-life and prolongs drug circulation time, (b) increasing the solubility of the natural drug, (c) overcoming chemotherapeutic drug resistance through intracellular delivery by endocytosis rather than diffusion, and (d) protecting the natural drug from interference by substances in the body such as various enzymes Figure 2. In addition, we also found that NNDDS can achieve selective drug release and improve therapeutic efficacy by targeting OC cell surface receptors and driving specific ligand functions (221).
Figure 2 The application advantages, structural simulation demonstration and comparison of advantages and disadvantages of various NNDDS. NNDDS can achieve the selective release of drugs and improve the therapeutic effect by (A) avoiding the first-pass clearance, (B) increasing the solubility of natural drugs, (C) overcoming chemotherapeutic drug resistance through endocytosis, and (D) protecting natural drugs from the intervention of substances in the body.
In order to maximize the anti-tumor potential of natural compounds, it is necessary to improve the efficiency of research and development actions, which is particularly important to focus on direct targets for targeted therapy of cancer. Direct targets of many of the natural compounds in this manuscript have been discovered, greatly promoting the treatment of OC and other malignant tumors. For example, the natural product Anibamine is a chemokine receptor CCR5 antagonist, and then new drug development based on this target has been carried out in ovarian and prostate cancer (64, 222). PCSK9, an enzyme involved in regulating lipid homeostasis by targeting low-density lipoprotein receptor degradation, has been found to play an important role in OC metabolism (223). In addition, Berberine, as a multi-target natural PCSK9 inhibitor, can greatly reduce the cost of drug synthesis (224). Curcumin is a novel p300/CREB binding protein specific acetyltransferase inhibitor and Pan HDAC inhibitor (225, 226). Recent studies have shown that HDAC inhibitors can induce OC sensitivity to cisplatin therapy and reverse the adverse effects of immunotherapy due to loss of homologous recombination defective functional phenotype (227, 228). Involved in numerous cellular processes including metabolism, oxidation, and inflammation, Nrf2 is a transcription factor that regulates different enzymes, receptors and miRNAs (229). Besides, It enhances cellular defense against undesirable stimuli by regulating the expression of related genes. In studies on OC, Nrf2 has been found to play an important regulatory role in the progression, proliferation and chemotherapy resistance of OC. In addition, Nrf2 has been found to regulate the expression of ERα and PGR in OC cells, as a consequence, Nrf2 is regarded as a promising target for future treatment of OC (230). Interestingly, our research has found that Nrf2 is the direct target of many natural compounds such as Carnosol, Resveratrol and Sulforaphane, which is undoubtedly beneficial for future research on the treatment of OC (231–233). Natural compounds, such as Voacamine, an emerging EGFR inhibitor, Withaferin A, a potent inhibitor of the transcription factor C/EBP-β, Quercetin, which suppresses JAK-STAT and telomerase activity, and Oroxylin A, a novel CDK9 and RON protein inhibitor, represent a diverse array of bioactive substances with potential therapeutic applications (234–238).
Many natural compound targets are well known, but the exciting thing is that there is not only one direct target for natural compounds. Dihydroartemisinin is a recognized STAT3 inhibitor and PTGS1 inhibitor (239, 240). Ginsenosides Rc is an activator of SIRT1, SIRT6, and FXR (241–243), and coincidentally, these targets are all linked to OC to some extent (244–246). Among the many natural compounds, these substances that have a direct intervention effect on OC are undoubtedly the first ones we consider to apply to NNDDS, which will save researchers a lot of time and effort in drug screening, enhancing the therapeutic potential of NNDDS against OC.
It can be predicted that NNDDS will become an important breakthrough in the field of cancer treatment. Future research can focus on the following aspects. First, the selection of the carrier of the nano drug delivery system. At present, the drug delivery system represented by PLGA nanoparticles, silica nanoparticles and liposome nanoparticles has been proven to help control the proportion of drug release. The encapsulation of carriers plays a key role in the delivery of natural medicines. The stability, solubility and bioavailability of natural drugs can be improved through rational selection and design of carriers, and the targeted delivery of drugs can be realized.
Second, the stability of the multi-drug co-delivery system. With the deepening of the application of natural drugs in OC, even though natural drugs have the advantage of low tumor resistance compared with synthetic drugs, the long-term application of natural drugs will inevitably make us face the problem of tumor drug resistance. Multi-drug co-delivery is an effective means to reduce the occurrence of drug resistance in tumors, but it requires higher requirements for the complex structure of the vector. It is not only necessary to use new synthetic methods to ensure the high uniformity and high-precision characterization of new materials, but also to consider the collection and production costs of drugs required in the co-delivery system (247, 248). A reasonable solution is to design an NNDDS with multi-target modification and multi-environment response, to realize the encapsulation of more than two response units, however, how to balance the delivery efficiency of the target is also worth considering.
Third, almost all current studies have been conducted in in vitro or in vivo animal models, and some experiments have shown that non-physiological concentrations of the drug are required to obtain some cellular effects, where the problem is not only the in vivo availability, but also the dose-response relationship for the treatment of OC. Limitations of natural product chemistry include low water solubility and limited availability of targeted organs, which may limit their therapeutic use in clinical settings (249–251). Therefore, the future clinical application of NNDDS still has a long way to go. The active ingredients of natural medicine are often low, and the choice of dosage has a direct impact on its therapeutic effect. Different drug doses and components may have differentiated anticancer and protective effects on the female reproductive system, so more effective pharmacodynamic evaluation methods are urgently needed to evaluate the effectiveness of NNDDS in clinical application.
Fourth, the green preparation and economic preparation of NNDDS. In the process of synthesis of some nanoparticles, a lot of chemicals need to be consumed, especially by chemical synthesis methods such as vapor deposition and liquid phase reduction. Therefore, the synthesis of targeted and synergical multi-drug nanocarrier materials using non-toxic and environmentally friendly green synthesis methods is also an important part of future development (252, 253). The nano particle synthesis with plant extract as the end agent and reducing agent can not only help to reduce the preparation cost, and improve the operability and scalability of NNDDS, but also make it safer and more environmentally friendly.
What is worth thinking about is that NNDDS needs a thorough biological evaluation and mechanism research before clinical application to ensure that it can play a normal physiological activity and role when entering cells. Not only drug delivery, but also nanomedicine is trying to find new ways to image, diagnose and identify tumors. It is believed that in the future, NNDDS can be used not only for drug delivery, but also for the implementation of new treatment strategies such as gene therapy and immunotherapy, which will provide new ideas and possibilities for personalized medical treatment and accurate treatment.
YL: Conceptualization, Writing – original draft. QS: Visualization, Writing – original draft. LF: Writing – original draft. CZ: Data curation, Writing – original draft. XJ: Data curation, Writing – original draft. FL: Data curation, Writing – original draft. BP: Funding acquisition, Supervision, Writing – review & editing.
The author(s) declare financial support was received for the research, authorship, and/or publication of this article. Funding was supported by the High Level Chinese Medical Hospital Promotion Project (No.HLCMHPP2023005), and the Scientific and Technological Innovation Project of China Academy of Chinese Medical Sciences (No.CI2021A01805; CI2021B009).
Thanks to BioRender (https://biorender.com/) for the drawing material.
The authors declare that the research was conducted in the absence of any commercial or financial relationships that could be construed as a potential conflict of interest.
All claims expressed in this article are solely those of the authors and do not necessarily represent those of their affiliated organizations, or those of the publisher, the editors and the reviewers. Any product that may be evaluated in this article, or claim that may be made by its manufacturer, is not guaranteed or endorsed by the publisher.
The Supplementary Material for this article can be found online at: https://www.frontiersin.org/articles/10.3389/fimmu.2024.1427573/full#supplementary-material
1. Sung H, Ferlay J, Siegel RL, Laversanne M, Soerjomataram I, Jemal A, et al. Global cancer statistics 2020: GLOBOCAN estimates of incidence and mortality worldwide for 36 cancers in 185 countries. CA Cancer J Clin. (2021) 71:209–49. doi: 10.3322/caac.21660
2. Carrera PM, Kantarjian HM, Blinder VS. The financial burden and distress of patients with cancer: Understanding and stepping-up action on the financial toxicity of cancer treatment. CA Cancer J Clin. (2018) 68:153–65. doi: 10.3322/caac.21443
3. Jiang Y, Wang C, Zhou S. Targeting tumor microenvironment in ovarian cancer: Premise and promise. Biochim Biophys Acta Rev Cancer. (2020) 1873:188361. doi: 10.1016/j.bbcan.2020.188361
4. Xie H, Wang W, Xia B, Jin W, Lou G. Therapeutic applications of PARP inhibitors in ovarian cancer. BioMed Pharmacother. (2020) 127:110204. doi: 10.1016/j.biopha.2020.110204
5. Francica P, Rottenberg S. Mechanisms of PARP inhibitor resistance in cancer and insights into the DNA damage response. Genome Med. (2018) 10:101. doi: 10.1186/s13073-018-0612-8
6. Newman DJ, Cragg GM. Natural products as sources of new drugs over the nearly four decades from 01/1981 to 09/2019. J Nat Prod. (2020) 83:770–803. doi: 10.1021/acs.jnatprod.9b01285
7. Gandhi GR, Antony PJ, Lana M, da Silva BFX, Oliveira RV, Jothi G, et al. Natural products modulating interleukins and other inflammatory mediators in tumor-bearing animals: A systematic review. Phytomedicine. (2022) 100:154038. doi: 10.1016/j.phymed.2022.154038
8. Jayakodi S, Senthilnathan R, Swaminathan A, Shanmugam VK, Shanmugam R, Krishnan A, et al. Bio-inspired nanoparticles mediated from plant extract biomolecules and their therapeutic application in cardiovascular diseases: A review. Int J Biol Macromol. (2023) 242:125025. doi: 10.1016/j.ijbiomac.2023.125025
9. Cragg GM, Pezzuto JM. Natural products as a vital source for the discovery of cancer chemotherapeutic and chemopreventive agents. Med Princ Pract. (2016) 25 Suppl 2:41–59. doi: 10.1159/000443404
10. Tang P, Shen T, Wang H, Zhang R, Zhang X, Li X, et al. Challenges and opportunities for improving the druggability of natural product: Why need drug delivery system? BioMed Pharmacother. (2023) 164:114955. doi: 10.1016/j.biopha.2023.114955
11. Wang Z, Meng F, Zhong Z. Emerging targeted drug delivery strategies toward ovarian cancer. Adv Drug Delivery Rev. (2021) 178:113969. doi: 10.1016/j.addr.2021.113969
12. Wicki A, Witzigmann D, Balasubramanian V, Huwyler J. Nanomedicine in cancer therapy: challenges, opportunities, and clinical applications. J Control Release. (2015) 200:138–57. doi: 10.1016/j.jconrel.2014.12.030
13. Patra JK, Das G, Fraceto LF, Campos EVR, Rodriguez-Torres MDP, Acosta-Torres LS, et al. Nano based drug delivery systems: recent developments and future prospects. J Nanobiotechnology. (2018) 16:71. doi: 10.1186/s12951-018-0392-8
14. Joseph RR, Venkatraman SS. Drug delivery to the eye: what benefits do nanocarriers offer? Nanomedicine (Lond). (2017) 12:683–702. doi: 10.2217/nnm-2016-0379
15. Zahin N, Anwar R, Tewari D, Kabir MT, Sajid A, Mathew B, et al. Nanoparticles and its biomedical applications in health and diseases: special focus on drug delivery. Environ Sci pollut Res Int. (2020) 27:19151–68. doi: 10.1007/s11356-019-05211-0
16. Duan L, Li X, Ji R, Hao Z, Kong M, Wen X, et al. Nanoparticle-based drug delivery systems: an inspiring therapeutic strategy for neurodegenerative diseases. Polymers (Basel). (2023) 15:2196. doi: 10.3390/polym15092196
17. Alqosaibi AI. Nanocarriers for anticancer drugs: Challenges and perspectives. Saudi J Biol Sci. (2022) 29:103298. doi: 10.1016/j.sjbs.2022.103298
18. Michlewska S, Garaiova Z, Subjakova V, Holota M, Kubczak M, Grodzicka M, et al. Lipid-coated ruthenium dendrimer conjugated with doxorubicin in anti-cancer drug delivery: Introducing protocols. Colloids Surf B Biointerfaces. (2023) 227:113371. doi: 10.1016/j.colsurfb.2023.113371
19. Chen H, Huang S, Wang H, Chen X, Zhang H, Xu Y, et al. Preparation and characterization of paclitaxel palmitate albumin nanoparticles with high loading efficacy: an in vitro and in vivo anti-tumor study in mouse models. Drug Deliv. (2021) 28:1067–79. doi: 10.1080/10717544.2021.1921078
20. Parayath NN, Stephan SB, Koehne AL, Nelson PS, Stephan MT. In vitro-transcribed antigen receptor mRNA nanocarriers for transient expression in circulating T cells in vivo. Nat Commun. (2020) 11:6080. doi: 10.1038/s41467-020-19486-2
21. Vergallo C, Hafeez MN, Iannotta D, Santos HA, D’Avanzo N, Dini L, et al. Conventional nanosized drug delivery systems for cancer applications. Adv Exp Med Biol. (2021) 1295:3–27. doi: 10.1007/978-3-030-58174-9_1
22. Dancey J. Targeted therapies and clinical trials in ovarian cancer. Ann Oncol. (2013) 24 Suppl 10:x59–63. doi: 10.1093/annonc/mdt473
23. Ottevanger PB. Ovarian cancer stem cells more questions than answers. Semin Cancer Biol. (2017) 44:67–71. doi: 10.1016/j.semcancer.2017.04.009
24. Zhang CL, Jiang XC, Li Y, Pan X, Gao MQ, Chen Y, et al. Independent predictive value of blood inflammatory composite markers in ovarian cancer: recent clinical evidence and perspective focusing on NLR and PLR. J Ovarian Res. (2023) 16:36. doi: 10.1186/s13048-023-01116-2
25. Li Y, Zhang C, Feng L, Shen Q, Liu F, Jiang X, et al. Application of natural polysaccharides and their novel dosage forms in gynecological cancers: therapeutic implications from the diversity potential of natural compounds. Front Pharmacol. (2023) 14:1195104. doi: 10.3389/fphar.2023.1195104
26. Jiao R, Liu Y, Gao H, Xiao J, So KF. The anti-oxidant and antitumor properties of plant polysaccharides. Am J Chin Med. (2016) 44:463–88. doi: 10.1142/S0192415X16500269
27. Atanasov AG, Zotchev SB, Dirsch VM, International Natural Product Sciences T, Supuran CT. Natural products in drug discovery: advances and opportunities. Nat Rev Drug Discovery. (2021) 20:200–16. doi: 10.1038/s41573-020-00114-z
28. Yang F, Yu XH, Qiao F, Cheng LH, Chen G, Long X, et al. Formulation and characterization of Brucea javanica oil microemulsion for improving safety. Drug Dev Ind Pharm. (2014) 40:266–77. doi: 10.3109/03639045.2012.756887
29. Xie C, Zhan T, Huang J, Lan J, Shen L, Wang H, et al. Functional characterization of nine critical genes encoding rate-limiting enzymes in the flavonoid biosynthesis of the medicinal herb Grona styracifolia. BMC Plant Biol. (2023) 23:299. doi: 10.1186/s12870-023-04290-z
30. Middleton E Jr., Kandaswami C, Theoharides TC. The effects of plant flavonoids on mammalian cells: implications for inflammation, heart disease, and cancer. Pharmacol Rev. (2000) 52:673–751.
31. Rioux LE, Turgeon SL, Beaulieu M. Structural characterization of laminaran and galactofucan extracted from the brown seaweed Saccharina longicruris. Phytochemistry. (2010) 71:1586–95. doi: 10.1016/j.phytochem.2010.05.021
32. Tzianabos AO. Polysaccharide immunomodulators as therapeutic agents: structural aspects and biologic function. Clin Microbiol Rev. (2000) 13:523–33. doi: 10.1128/CMR.13.4.523
33. Garg P, Awasthi S, Horne D, Salgia R, Singhal SS. The innate effects of plant secondary metabolites in preclusion of gynecologic cancers: Inflammatory response and therapeutic action. Biochim Biophys Acta Rev Cancer. (2023) 1878:188929. doi: 10.1016/j.bbcan.2023.188929
34. Mize BK, Salvi A, Ren Y, Burdette JE, Fuchs JR. Discovery and development of botanical natural products and their analogues as therapeutics for ovarian cancer. Nat Prod Rep. (2023) 40:1250–70. doi: 10.1039/D2NP00091A
35. Vergara D, De Domenico S, Tinelli A, Stanca E, Del Mercato LL, Giudetti AM, et al. Anticancer effects of novel resveratrol analogues on human ovarian cancer cells. Mol Biosyst. (2017) 13:1131–41. doi: 10.1039/C7MB00128B
36. Feng J, Zhang XL, Li YY, Cui YY, Chen YH. Pinus massoniana bark extract: structure-activity relationship and biomedical potentials. Am J Chin Med. (2016) 44:1559–77. doi: 10.1142/S0192415X16500877
37. Nan Y, Su H, Zhou B, Liu S. The function of natural compounds in important anticancer mechanisms. Front Oncol. (2022) 12:1049888. doi: 10.3389/fonc.2022.1049888
38. Zhao Y, Tan Y, Wu G, Liu L, Wang Y, Luo Y, et al. Berbamine overcomes imatinib-induced neutropenia and permits cytogenetic responses in Chinese patients with chronic-phase chronic myeloid leukemia. Int J Hematol. (2011) 94:156–62. doi: 10.1007/s12185-011-0887-7
39. Zhang H, Jiao Y, Shi C, Song X, Chang Y, Ren Y, et al. Berbamine suppresses cell proliferation and promotes apoptosis in ovarian cancer partially via the inhibition of Wnt/beta-catenin signaling. Acta Biochim Biophys Sin (Shanghai). (2018) 50:532–9. doi: 10.1093/abbs/gmy036
40. Yan H, Xin S, Wang H, Ma J, Zhang H, Wei H. Baicalein inhibits MMP-2 expression in human ovarian cancer cells by suppressing the p38 MAPK-dependent NF-kappaB signaling pathway. Anticancer Drugs. (2015) 26:649–56. doi: 10.1097/CAD.0000000000000230
41. Lang X, Chen Z, Yang X, Yan Q, Xu M, Liu W, et al. Scutellarein induces apoptosis and inhibits proliferation, migration, and invasion in ovarian cancer via inhibition of EZH2/FOXO1 signaling. J Biochem Mol Toxicol. (2021) 35:e22870. doi: 10.1002/jbt.22870
42. Milde-Langosch K, Riethdorf S. Role of cell-cycle regulatory proteins in gynecological cancer. J Cell Physiol. (2003) 196:224–44. doi: 10.1002/jcp.10286
43. Liu H, Yue Q, He S. Amentoflavone suppresses tumor growth in ovarian cancer by modulating Skp2. Life Sci. (2017) 189:96–105. doi: 10.1016/j.lfs.2017.09.026
44. Guo Y, Zhang Z, Wang Z, Liu G, Liu Y, Wang H. Astragalus polysaccharides inhibit ovarian cancer cell growth via microRNA-27a/FBXW7 signaling pathway. Biosci Rep. (2020) 40:BSR20193396. doi: 10.1042/BSR20193396
45. Lee K, Ahn JH, Lee KT, Jang DS, Choi JH. Deoxyschizandrin, isolated from schisandra berries, induces cell cycle arrest in ovarian cancer cells and inhibits the protumoural activation of tumour-associated macrophages. Nutrients. (2018) 10:91. doi: 10.3390/nu10010091
46. Cheng X, Ferrell JE Jr. Apoptosis propagates through the cytoplasm as trigger waves. Science. (2018) 361:607–12. doi: 10.1126/science.aah4065
47. Xu X, Shi J, Gao H, Li Q. Zeylenone inhibits proliferation and promotes apoptosis in ovarian carcinoma cells via Janus kinase 2/signal transducers and activators of transcription 3 pathways. J Obstet Gynaecol Res. (2018) 44:1451–7. doi: 10.1111/jog.13690
48. Zhang L, Huo X, Liao Y, Yang F, Gao L, Cao L. Zeylenone, a naturally occurring cyclohexene oxide, inhibits proliferation and induces apoptosis in cervical carcinoma cells via PI3K/AKT/mTOR and MAPK/ERK pathways. Sci Rep. (2017) 7:1669. doi: 10.1038/s41598-017-01804-2
49. Che X, Yan H, Sun H, Dongol S, Wang Y, Lv Q, et al. Grifolin induces autophagic cell death by inhibiting the Akt/mTOR/S6K pathway in human ovarian cancer cells. Oncol Rep. (2016) 36:1041–7. doi: 10.3892/or.2016.4840
50. Luo X, Xu J, Yu J, Yi P. Shaping immune responses in the tumor microenvironment of ovarian cancer. Front Immunol. (2021) 12:692360. doi: 10.3389/fimmu.2021.692360
51. Brockmueller A, Sameri S, Liskova A, Zhai K, Varghese E, Samuel SM, et al. Resveratrol’s anti-cancer effects through the modulation of tumor glucose metabolism. Cancers (Basel). (2021) 13:188. doi: 10.3390/cancers13020188
52. Zhang Z, Zhang S, Yang J, Yi P, Xu P, Yi M, et al. Integrated transcriptomic and metabolomic analyses to characterize the anti-cancer effects of (-)-epigallocatechin-3-gallate in human colon cancer cells. Toxicol Appl Pharmacol. (2020) 401:115100. doi: 10.1016/j.taap.2020.115100
53. Gan RY, Li HB, Sui ZQ, Corke H. Absorption, metabolism, anti-cancer effect and molecular targets of epigallocatechin gallate (EGCG): An updated review. Crit Rev Food Sci Nutr. (2018) 58:924–41. doi: 10.1080/10408398.2016.1231168
54. Huang TT, Lampert EJ, Coots C, Lee JM. Targeting the PI3K pathway and DNA damage response as a therapeutic strategy in ovarian cancer. Cancer Treat Rev. (2020) 86:102021. doi: 10.1016/j.ctrv.2020.102021
55. Achi IT, Sarbadhikary P, George BP, Abrahamse H. Multi-target potential of berberine as an antineoplastic and antimetastatic agent: A special focus on lung cancer treatment. Cells. (2022) 11:3433. doi: 10.3390/cells11213433
56. Liu Q, Tang J, Chen S, Hu S, Shen C, Xiang J, et al. Berberine for gastric cancer prevention and treatment: Multi-step actions on the Correa’s cascade underlie its therapeutic effects. Pharmacol Res. (2022) 184:106440. doi: 10.1016/j.phrs.2022.106440
57. Sun Q, Yang H, Liu M, Ren S, Zhao H, Ming T, et al. Berberine suppresses colorectal cancer by regulation of Hedgehog signaling pathway activity and gut microbiota. Phytomedicine. (2022) 103:154227. doi: 10.1016/j.phymed.2022.154227
58. Hou D, Xu G, Zhang C, Li B, Qin J, Hao X, et al. Berberine induces oxidative DNA damage and impairs homologous recombination repair in ovarian cancer cells to confer increased sensitivity to PARP inhibition. Cell Death Dis. (2017) 8:e3070. doi: 10.1038/cddis.2017.471
59. Disis ML, Taylor MH, Kelly K, Beck JT, Gordon M, Moore KM, et al. Efficacy and safety of avelumab for patients with recurrent or refractory ovarian cancer: phase 1b results from the JAVELIN solid tumor trial. JAMA Oncol. (2019) 5:393–401. doi: 10.1001/jamaoncol.2018.6258
60. Wu J, Zhou T, Wang Y, Jiang Y, Wang Y. Mechanisms and advances in anti-ovarian cancer with natural plants component. Molecules. (2021) 26:5949. doi: 10.3390/molecules26195949
61. Holzapfel NP, Shokoohmand A, Wagner F, Landgraf M, Champ S, Holzapfel BM, et al. Lycopene reduces ovarian tumor growth and intraperitoneal metastatic load. Am J Cancer Res. (2017) 7:1322–36.
62. Milani A, Basirnejad M, Shahbazi S, Bolhassani A. Carotenoids: biochemistry, pharmacology and treatment. Br J Pharmacol. (2017) 174:1290–324. doi: 10.1111/bph.13625
63. Zhao BX, Sun YB, Wang SQ, Duan L, Huo QL, Ren F, et al. Grape seed procyanidin reversal of p-glycoprotein associated multi-drug resistance via down-regulation of NF-kappaB and MAPK/ERK mediated YB-1 activity in A2780/T cells. PloS One. (2013) 8:e71071. doi: 10.1371/journal.pone.0071071
64. Zhang Y, Arnatt CK, Zhang F, Wang J, Haney KM, Fang X. The potential role of anibamine, a natural product CCR5 antagonist, and its analogues as leads toward development of anti-ovarian cancer agents. Bioorg Med Chem Lett. (2012) 22:5093–7. doi: 10.1016/j.bmcl.2012.05.127
65. Ren L, Cao QX, Zhai FR, Yang SQ, Zhang HX. Asiatic acid exerts anticancer potential in human ovarian cancer cells via suppression of PI3K/Akt/mTOR signalling. Pharm Biol. (2016) 54:2377–82. doi: 10.3109/13880209.2016.1156709
66. Chen J, Li Z, Chen AY, Ye X, Luo H, Rankin GO, et al. Inhibitory effect of baicalin and baicalein on ovarian cancer cells. Int J Mol Sci. (2013) 14:6012–25. doi: 10.3390/ijms14036012
67. He Z, Li B, Rankin GO, Rojanasakul Y, Chen YC. Selecting bioactive phenolic compounds as potential agents to inhibit proliferation and VEGF expression in human ovarian cancer cells. Oncol Lett. (2015) 9:1444–50. doi: 10.3892/ol.2014.2818
68. Li Y, Wang D, Liu J, Li Y, Chen D, Zhou L, et al. Baicalin attenuates YAP activity to suppress ovarian cancer stemness. Onco Targets Ther. (2020) 13:7151–63. doi: 10.2147/OTT.S254607
69. Liu L, Fan J, Ai G, Liu J, Luo N, Li C, et al. Berberine in combination with cisplatin induces necroptosis and apoptosis in ovarian cancer cells. Biol Res. (2019) 52:37. doi: 10.1186/s40659-019-0243-6
70. Li J, Zhang S, Wu L, Pei M, Jiang Y. Berberine inhibited metastasis through miR-145/MMP16 axis in vitro. J Ovarian Res. (2021) 14:4. doi: 10.1186/s13048-020-00752-2
71. Chuang TC, Wu K, Lin YY, Kuo HP, Kao MC, Wang V, et al. Dual down-regulation of EGFR and ErbB2 by berberine contributes to suppression of migration and invasion of human ovarian cancer cells. Environ Toxicol. (2021) 36:737–47. doi: 10.1002/tox.23076
72. Yu Z, Wan Y, Liu Y, Yang J, Li L, Zhang W. Curcumin induced apoptosis via PI3K/Akt-signalling pathways in SKOV3 cells. Pharm Biol. (2016) 54:2026–32. doi: 10.3109/13880209.2016.1139601
73. Watson JL, Greenshields A, Hill R, Hilchie A, Lee PW, Giacomantonio CA, et al. Curcumin-induced apoptosis in ovarian carcinoma cells is p53-independent and involves p38 mitogen-activated protein kinase activation and downregulation of Bcl-2 and survivin expression and Akt signaling. Mol Carcinog. (2010) 49:13–24. doi: 10.1002/mc.20571
74. Saydmohammed M, Joseph D, Syed V. Curcumin suppresses constitutive activation of STAT-3 by up-regulating protein inhibitor of activated STAT-3 (PIAS-3) in ovarian and endometrial cancer cells. J Cell Biochem. (2010) 110:447–56. doi: 10.1002/jcb.22558
75. Seo JA, Kim B, Dhanasekaran DN, Tsang BK, Song YS. Curcumin induces apoptosis by inhibiting sarco/endoplasmic reticulum Ca2+ ATPase activity in ovarian cancer cells. Cancer Lett. (2016) 371:30–7. doi: 10.1016/j.canlet.2015.11.021
76. Zhao SF, Zhang X, Zhang XJ, Shi XQ, Yu ZJ, Kan QC. Induction of microRNA-9 mediates cytotoxicity of curcumin against SKOV3 ovarian cancer cells. Asian Pac J Cancer Prev. (2014) 15:3363–8. doi: 10.7314/APJCP.2014.15.8.3363
77. Sun S, Fang H. Curcumin inhibits ovarian cancer progression by regulating circ-PLEKHM3/miR-320a/SMG1 axis. J Ovarian Res. (2021) 14:158. doi: 10.1186/s13048-021-00916-8
78. Barinda AJ, Arozal W, Sandhiutami NMD, Louisa M, Arfian N, Sandora N, et al. Curcumin Prevents Epithelial-to Mesenchymal Transition-Mediated Ovarian Cancer Progression through NRF2/ETBR/ET-1 Axis and Preserves Mitochondria Biogenesis in Kidney after Cisplatin Administration. Adv Pharm Bull. (2022) 12:128–41. doi: 10.34172/apb.2022.014
79. Liu J, Liu X, Ma W, Kou W, Li C, Zhao J. Anticancer activity of cucurbitacin-A in ovarian cancer cell line SKOV3 involves cell cycle arrest, apoptosis and inhibition of mTOR/PI3K/Akt signaling pathway. J BUON. (2018) 23:124–8.
80. Vergara D, Simeone P, Bettini S, Tinelli A, Valli L, Storelli C, et al. Antitumor activity of the dietary diterpene carnosol against a panel of human cancer cell lines. Food Funct. (2014) 5:1261–9. doi: 10.1039/c4fo00023d
81. Liu Y, Gao S, Zhu J, Zheng Y, Zhang H, Sun H. Dihydroartemisinin induces apoptosis and inhibits proliferation, migration, and invasion in epithelial ovarian cancer via inhibition of the hedgehog signaling pathway. Cancer Med. (2018) 7:5704–15. doi: 10.1002/cam4.1827
82. Huh SW, Bae SM, Kim YW, Lee JM, Namkoong SE, Lee IP, et al. Anticancer effects of (-)-epigallocatechin-3-gallate on ovarian carcinoma cell lines. Gynecol Oncol. (2004) 94:760–8. doi: 10.1016/j.ygyno.2004.05.031
83. Yan C, Yang J, Shen L, Chen X. Inhibitory effect of Epigallocatechin gallate on ovarian cancer cell proliferation associated with aquaporin 5 expression. Arch Gynecol Obstet. (2012) 285:459–67. doi: 10.1007/s00404-011-1942-6
84. Zhang Z, Zhang Q, Yu Y, Su S. Epigallocatechin gallate inhibits ovarian cancer cell growth and induces cell apoptosis via activation of FOXO3A. In Vitro Cell Dev Biol Anim. (2023) 59:739–46. doi: 10.1007/s11626-023-00830-x
85. Qin J, Fu M, Wang J, Huang F, Liu H, Huangfu M, et al. PTEN/AKT/mTOR signaling mediates anticancer effects of epigallocatechin−3−gallate in ovarian cancer. Oncol Rep. (2020) 43:1885–96. doi: 10.3892/or.2020.7571
86. Lu J, Xu Y, Wei X, Zhao Z, Xue J, Liu P. Emodin inhibits the epithelial to mesenchymal transition of epithelial ovarian cancer cells via ILK/GSK-3beta/slug signaling pathway. BioMed Res Int. (2016) 2016:6253280. doi: 10.1155/2016/6253280
87. Park S, Bazer FW, Lim W, Song G. The O-methylated isoflavone, formononetin, inhibits human ovarian cancer cell proliferation by sub G0/G1 cell phase arrest through PI3K/AKT and ERK1/2 inactivation. J Cell Biochem. (2018) 119:7377–87. doi: 10.1002/jcb.27041
88. Gao L, Ouyang Y, Li R, Zhang X, Gao X, Lin S, et al. Icaritin inhibits migration and invasion of human ovarian cancer cells via the Akt/mTOR signaling pathway. Front Oncol. (2022) 12:843489. doi: 10.3389/fonc.2022.843489
89. Liu T, Zhao L, Zhang Y, Chen W, Liu D, Hou H, et al. Ginsenoside 20(S)-Rg3 targets HIF-1alpha to block hypoxia-induced epithelial-mesenchymal transition in ovarian cancer cells. PloS One. (2014) 9:e103887. doi: 10.1371/journal.pone.0103887
90. Liu T, Zhao L, Hou H, Ding L, Chen W, Li X. Ginsenoside 20(S)-Rg3 suppresses ovarian cancer migration via hypoxia-inducible factor 1 alpha and nuclear factor-kappa B signals. Tumour Biol. (2017) 39:1010428317692225. doi: 10.1177/1010428317692225
91. Liu D, Liu T, Teng Y, Chen W, Zhao L, Li X. Ginsenoside Rb1 inhibits hypoxia-induced epithelial-mesenchymal transition in ovarian cancer cells by regulating microRNA-25. Exp Ther Med. (2017) 14:2895–902. doi: 10.3892/etm.2017.4889
92. Zhao L, Sun W, Zheng A, Zhang Y, Fang C, Zhang P. Ginsenoside Rg3 suppresses ovarian cancer cell proliferation and invasion by inhibiting the expression of lncRNA H19. Acta Biochim Pol. (2021) 68:575–82. doi: 10.18388/abp.2020_5343
93. Zhang R, Li L, Li H, Bai H, Suo Y, Cui J, et al. Ginsenoside 20(S)-Rg3 reduces KIF20A expression and promotes CDC25A proteasomal degradation in epithelial ovarian cancer. J Ginseng Res. (2024) 48:40–51. doi: 10.1016/j.jgr.2023.06.008
94. Chen HY, Huang TC, Shieh TM, Wu CH, Lin LC, Hsia SM. Isoliquiritigenin induces autophagy and inhibits ovarian cancer cell growth. Int J Mol Sci. (2017) 18:2025. doi: 10.3390/ijms18102025
95. Shen JJ, Zhu XF, Xu J, Wang ZF, Gu WJ, Chen Y. Oroxylin A exerts anticancer effects on human ovarian cancer cells via the PPARgamma−dependent reversal of the progesterone receptor membrane component 1/2 expression profile. Oncol Rep. (2020) 43:1309–18. doi: 10.3892/or.2020.7509
96. Gao J, Zhu H, Wan H, Zou X, Ma X, Gao G. Harmine suppresses the proliferation and migration of human ovarian cancer cells through inhibiting ERK/CREB pathway. Oncol Rep. (2017) 38:2927–34. doi: 10.3892/or.2017.5952
97. Jeong M, Kim HM, Kim HJ, Choi JH, Jang DS. Kudsuphilactone B, a nortriterpenoid isolated from Schisandra chinensis fruit, induces caspase-dependent apoptosis in human ovarian cancer A2780 cells. Arch Pharm Res. (2017) 40:500–8. doi: 10.1007/s12272-017-0902-5
98. Yin J, Yu C, Yang Z, He JL, Chen WJ, Liu HZ, et al. Tetramethylpyrazine inhibits migration of SKOV3 human ovarian carcinoma cells and decreases the expression of interleukin-8 via the ERK1/2, p38 and AP-1 signaling pathways. Oncol Rep. (2011) 26:671–9. doi: 10.3892/or.2011.1334
99. Ren MX, Deng XH, Ai F, Yuan GY, Song HY. Effect of quercetin on the proliferation of the human ovarian cancer cell line SKOV-3 in vitro. Exp Ther Med. (2015) 10:579–83. doi: 10.3892/etm.2015.2536
100. Zhou J, Gong J, Ding C, Chen G. Quercetin induces the apoptosis of human ovarian carcinoma cells by upregulating the expression of microRNA-145. Mol Med Rep. (2015) 12:3127–31. doi: 10.3892/mmr.2015.3679
101. Teekaraman D, Elayapillai SP, Viswanathan MP, Jagadeesan A. Quercetin inhibits human metastatic ovarian cancer cell growth and modulates components of the intrinsic apoptotic pathway in PA-1 cell line. Chem Biol Interact. (2019) 300:91–100. doi: 10.1016/j.cbi.2019.01.008
102. Yi L, Zongyuan Y, Cheng G, Lingyun Z, Guilian Y, Wei G. Quercetin enhances apoptotic effect of tumor necrosis factor-related apoptosis-inducing ligand (TRAIL) in ovarian cancer cells through reactive oxygen species (ROS) mediated CCAAT enhancer-binding protein homologous protein (CHOP)-death receptor 5 pathway. Cancer Sci. (2014) 105:520–7. doi: 10.1111/cas.12395
103. Vergara D, Simeone P, Toraldo D, Del Boccio P, Vergaro V, Leporatti S, et al. Resveratrol downregulates Akt/GSK and ERK signalling pathways in OVCAR-3 ovarian cancer cells. Mol Biosyst. (2012) 8:1078–87. doi: 10.1039/c2mb05486h
104. Mikula-Pietrasik J, Sosinska P, Ksiazek K. Resveratrol inhibits ovarian cancer cell adhesion to peritoneal mesothelium in vitro by modulating the production of alpha5beta1 integrins and hyaluronic acid. Gynecol Oncol. (2014) 134:624–30. doi: 10.1016/j.ygyno.2014.06.022
105. Park SY, Jeong KJ, Lee J, Yoon DS, Choi WS, Kim YK, et al. Hypoxia enhances LPA-induced HIF-1alpha and VEGF expression: their inhibition by resveratrol. Cancer Lett. (2007) 258:63–9. doi: 10.1016/j.canlet.2007.08.011
106. Kim SC, Choi B, Kwon Y. Thiol-reducing agents prevent sulforaphane-induced growth inhibition in ovarian cancer cells. Food Nutr Res. (2017) 61:1368321. doi: 10.1080/16546628.2017.1368321
107. Park S, Lim W, Jeong W, Bazer FW, Lee D, Song G. Sideroxylin (Callistemon lanceolatus) suppressed cell proliferation and increased apoptosis in ovarian cancer cells accompanied by mitochondrial dysfunction, the generation of reactive oxygen species, and an increase of lipid peroxidation. J Cell Physiol. (2018) 233:8597–604. doi: 10.1002/jcp.26540
108. Lee D, Ko H, Kim YJ, Kim SN, Choi KC, Yamabe N, et al. Inhibition of A2780 human ovarian carcinoma cell proliferation by a rubus component, sanguiin H-6. J Agric Food Chem. (2016) 64:801–5. doi: 10.1021/acs.jafc.5b05461
109. Ruibin J, Bo J, Danying W, Chihong Z, Jianguo F, Linhui G. Therapy effects of wogonin on ovarian cancer cells. BioMed Res Int. (2017) 2017:9381513. doi: 10.1155/2017/9381513
110. Zhao Q, Chang W, Chen R, Liu Y. Anti-proliferative effect of wogonin on ovary cancer cells involves activation of apoptosis and cell cycle arrest. Med Sci Monit. (2019) 25:8465–71. doi: 10.12659/MSM.917823
111. Yang L, Zheng XL, Sun H, Zhong YJ, Wang Q, He HN, et al. Catalase suppression-mediated H(2)O(2) accumulation in cancer cells by wogonin effectively blocks tumor necrosis factor-induced NF-kappaB activation and sensitizes apoptosis. Cancer Sci. (2011) 102:870–6. doi: 10.1111/j.1349-7006.2011.01874.x
112. Chen SS, Corteling R, Stevanato L, Sinden J. Polyphenols inhibit indoleamine 3,5-dioxygenase-1 enzymatic activity–a role of immunomodulation in chemoprevention. Discovery Med. (2012) 14:327–33.
113. Kim KK, Singh AP, Singh RK, Demartino A, Brard L, Vorsa N, et al. Anti-angiogenic activity of cranberry proanthocyanidins and cytotoxic properties in ovarian cancer cells. Int J Oncol. (2012) 40:227–35. doi: 10.3892/ijo.2011.1198
114. Liu J, Bai J, Jiang G, Li X, Wang J, Wu D, et al. Anti-Tumor Effect of Pinus massoniana Bark Proanthocyanidins on Ovarian Cancer through Induction of Cell Apoptosis and Inhibition of Cell Migration. PloS One. (2015) 10:e0142157. doi: 10.1371/journal.pone.0142157
115. Opipari AW Jr., Tan L, Boitano AE, Sorenson DR, Aurora A, Liu JR. Resveratrol-induced autophagocytosis in ovarian cancer cells. Cancer Res. (2004) 64:696–703. doi: 10.1158/0008-5472.CAN-03-2404
116. Zhong LX, Zhang Y, Wu ML, Liu YN, Zhang P, Chen XY, et al. Resveratrol and STAT inhibitor enhance autophagy in ovarian cancer cells. Cell Death Discovery. (2016) 2:15071. doi: 10.1038/cddiscovery.2015.71
117. Zhong LX, Nie JH, Liu J, Lin LZ. Correlation of ARHI upregulation with growth suppression and STAT3 inactivation in resveratrol-treated ovarian cancer cells. Cancer biomark. (2018) 21:787–95. doi: 10.3233/CBM-170483
118. Ferraresi A, Esposito A, Girone C, Vallino L, Salwa A, Ghezzi I, et al. Resveratrol contrasts LPA-induced ovarian cancer cell migration and platinum resistance by rescuing hedgehog-mediated autophagy. Cells. (2021) 10:3213. doi: 10.3390/cells10113213
119. Lang F, Qin Z, Li F, Zhang H, Fang Z, Hao E. Apoptotic cell death induced by resveratrol is partially mediated by the autophagy pathway in human ovarian cancer cells. PloS One. (2015) 10:e0129196. doi: 10.1371/journal.pone.0129196
120. Fong MY, Jin S, Rane M, Singh RK, Gupta R, Kakar SS. Withaferin A synergizes the therapeutic effect of doxorubicin through ROS-mediated autophagy in ovarian cancer. PloS One. (2012) 7:e42265. doi: 10.1371/journal.pone.0042265
121. Liu LD, Pang YX, Zhao XR, Li R, Jin CJ, Xue J, et al. Curcumin induces apoptotic cell death and protective autophagy by inhibiting AKT/mTOR/p70S6K pathway in human ovarian cancer cells. Arch Gynecol Obstet. (2019) 299:1627–39. doi: 10.1007/s00404-019-05058-3
122. Zheng X, Chen W, Hou H, Li J, Li H, Sun X, et al. Ginsenoside 20(S)-Rg3 induced autophagy to inhibit migration and invasion of ovarian cancer. BioMed Pharmacother. (2017) 85:620–6. doi: 10.1016/j.biopha.2016.11.072
123. Li J, Liu T, Zhao L, Chen W, Hou H, Ye Z, et al. Ginsenoside 20(S)−Rg3 inhibits the Warburg effect through STAT3 pathways in ovarian cancer cells. Int J Oncol. (2015) 46:775–81. doi: 10.3892/ijo.2014.2767
124. Zheng X, Zhou Y, Chen W, Chen L, Lu J, He F, et al. Ginsenoside 20(S)-rg3 prevents PKM2-targeting miR-324-5p from H19 sponging to antagonize the warburg effect in ovarian cancer cells. Cell Physiol Biochem. (2018) 51:1340–53. doi: 10.1159/000495552
125. Lu J, Wang L, Chen W, Wang Y, Zhen S, Chen H, et al. miR-603 targeted hexokinase-2 to inhibit the Malignancy of ovarian cancer cells. Arch Biochem Biophys. (2019) 661:1–9. doi: 10.1016/j.abb.2018.10.014
126. Gwak H, Kim S, Dhanasekaran DN, Song YS. Resveratrol triggers ER stress-mediated apoptosis by disrupting N-linked glycosylation of proteins in ovarian cancer cells. Cancer Lett. (2016) 371:347–53. doi: 10.1016/j.canlet.2015.11.032
127. Tino AB, Chitcholtan K, Sykes PH, Garrill A. Resveratrol and acetyl-resveratrol modulate activity of VEGF and IL-8 in ovarian cancer cell aggregates via attenuation of the NF-kappaB protein. J Ovarian Res. (2016) 9:84. doi: 10.1186/s13048-016-0293-0
128. Chen J, Huang ST, Chen JG, He JH, Lin WM, Huang ZH, et al. Resveratrol reduces lactate production and modifies the ovarian cancer immune microenvironment. Neoplasma. (2022) 69:1129–37. doi: 10.4149/neo_2022_220414N410
129. Li J, Zou Y, Pei M, Zhang Y, Jiang Y. Berberine inhibits the Warburg effect through TET3/miR-145/HK2 pathways in ovarian cancer cells. J Cancer. (2021) 12:207–16. doi: 10.7150/jca.48896
130. Yallapu MM, Maher DM, Sundram V, Bell MC, Jaggi M, Chauhan SC. Curcumin induces chemo/radio-sensitization in ovarian cancer cells and curcumin nanoparticles inhibit ovarian cancer cell growth. J Ovarian Res. (2010) 3:11. doi: 10.1186/1757-2215-3-11
131. Zhang J, Liu J, Xu X, Li L. Curcumin suppresses cisplatin resistance development partly via modulating extracellular vesicle-mediated transfer of MEG3 and miR-214 in ovarian cancer. Cancer Chemother Pharmacol. (2017) 79:479–87. doi: 10.1007/s00280-017-3238-4
132. Muhanmode Y, Wen MK, Maitinuri A, Shen G. Curcumin and resveratrol inhibit chemoresistance in cisplatin-resistant epithelial ovarian cancer cells via targeting P13K pathway. Hum Exp Toxicol. (2022) 41:9603271221095929. doi: 10.1177/09603271221095929
133. Huang SL, Chang TC, Sun NK. Curcumin reduces paclitaxel resistance in ovarian carcinoma cells by upregulating SNIP1 and inhibiting NFkappaB activity. Biochem Pharmacol. (2023) 212:115581. doi: 10.1016/j.bcp.2023.115581
134. Liu Y, Shen Z, Zhu T, Lu W, Fu Y. Curcumin enhances the anti-cancer efficacy of paclitaxel in ovarian cancer by regulating the miR-9-5p/BRCA1 axis. Front Pharmacol. (2022) 13:1014933. doi: 10.3389/fphar.2022.1014933
135. Engelke LH, Hamacher A, Proksch P, Kassack MU. Ellagic acid and resveratrol prevent the development of cisplatin resistance in the epithelial ovarian cancer cell line A2780. J Cancer. (2016) 7:353–63. doi: 10.7150/jca.13754
136. Hasan AA, Kalinina E, Nuzhina J, Volodina Y, Shtil A, Tatarskiy V. Potentiation of cisplatin cytotoxicity in resistant ovarian cancer SKOV3/cisplatin cells by quercetin pre-treatment. Int J Mol Sci. (2023) 24:10960. doi: 10.3390/ijms241310960
137. Li S, Yi Z, Li M, Zhu Z. Baicalein improves the chemoresistance of ovarian cancer through regulation of CirSLC7A6. J Ovarian Res. (2023) 16:212. doi: 10.1186/s13048-023-01285-0
138. Marverti G, Ligabue A, Lombardi P, Ferrari S, Monti MG, Frassineti C, et al. Modulation of the expression of folate cycle enzymes and polyamine metabolism by berberine in cisplatin-sensitive and -resistant human ovarian cancer cells. Int J Oncol. (2013) 43:1269–80. doi: 10.3892/ijo.2013.2045
139. Liu S, Fang Y, Shen H, Xu W, Li H. Berberine sensitizes ovarian cancer cells to cisplatin through miR-21/PDCD4 axis. Acta Biochim Biophys Sin (Shanghai). (2013) 45:756–62. doi: 10.1093/abbs/gmt075
140. Zhao Y, Cui L, Pan Y, Shao D, Zheng X, Zhang F, et al. Berberine inhibits the chemotherapy-induced repopulation by suppressing the arachidonic acid metabolic pathway and phosphorylation of FAK in ovarian cancer. Cell Prolif. (2017) 50:e12393. doi: 10.1111/cpr.12393
141. Zhao Y, Yang X, Zhao J, Gao M, Zhang M, Shi T, et al. Berberine inhibits chemotherapy-exacerbated ovarian cancer stem cell-like characteristics and metastasis through GLI1. Eur J Pharmacol. (2021) 895:173887. doi: 10.1016/j.ejphar.2021.173887
142. Go WJ, Ryu JH, Qiang F, Han HK. Evaluation of the flavonoid oroxylin A as an inhibitor of P-glycoprotein-mediated cellular efflux. J Nat Prod. (2009) 72:1616–9. doi: 10.1021/np9003036
143. Chen H, Landen CN, Li Y, Alvarez RD, Tollefsbol TO. Enhancement of Cisplatin-Mediated Apoptosis in Ovarian Cancer Cells through Potentiating G2/M Arrest and p21 Upregulation by Combinatorial Epigallocatechin Gallate and Sulforaphane. J Oncol. (2013) 2013:872957. doi: 10.1155/2013/872957
144. Wang X, Jiang P, Wang P, Yang CS, Wang X, Feng Q. EGCG enhances cisplatin sensitivity by regulating expression of the copper and cisplatin influx transporter CTR1 in ovary cancer. PloS One. (2015) 10:e0125402. doi: 10.1371/journal.pone.0125402
145. Chen H, Landen CN, Li Y, Alvarez RD, Tollefsbol TO. Epigallocatechin gallate and sulforaphane combination treatment induce apoptosis in paclitaxel-resistant ovarian cancer cells through hTERT and Bcl-2 down-regulation. Exp Cell Res. (2013) 319:697–706. doi: 10.1016/j.yexcr.2012.12.026
146. Chen S, Cooper M, Jones M, Madhuri TK, Wade J, Bachelor A, et al. Combined activity of oridonin and wogonin in advanced-stage ovarian cancer cells: sensitivity of ovarian cancer cells to phyto-active chemicals. Cell Biol Toxicol. (2011) 27:133–47. doi: 10.1007/s10565-010-9176-0
147. Xing F, Sun C, Luo N, He Y, Chen M, Ding S, et al. Wogonin increases cisplatin sensitivity in ovarian cancer cells through inhibition of the phosphatidylinositol 3-kinase (PI3K)/Akt pathway. Med Sci Monit. (2019) 25:6007–14. doi: 10.12659/MSM.913829
148. Vinay DS, Ryan EP, Pawelec G, Talib WH, Stagg J, Elkord E, et al. Immune evasion in cancer: Mechanistic basis and therapeutic strategies. Semin Cancer Biol. (2015) 35 Suppl:S185–S98. doi: 10.1016/j.semcancer.2015.03.004
149. Zhang Y, Chen S, Wei C, Rankin GO, Ye X, Chen YC. Dietary compound proanthocyanidins from Chinese bayberry (Myrica rubra Sieb. et Zucc.) leaves attenuate chemotherapy-resistant ovarian cancer stem cell traits via targeting the Wnt/beta-catenin signaling pathway and inducing G1 cell cycle arrest. Food Funct. (2018) 9:525–33. doi: 10.1039/C7FO01453H
150. Zhang Y, Chen S, Wei C, Rankin GO, Rojanasakul Y, Ren N, et al. Dietary Compound Proanthocyanidins from Chinese bayberry (Myrica rubra Sieb. et Zucc.) leaves inhibit angiogenesis and regulate cell cycle of cisplatin-resistant ovarian cancer cells via targeting Akt pathway. J Funct Foods. (2018) 40:573–81. doi: 10.1016/j.jff.2017.11.045
151. Pellegrini E, Multari G, Gallo FR, Vecchiotti D, Zazzeroni F, Condello M, et al. A natural product, voacamine, sensitizes paclitaxel-resistant human ovarian cancer cells. Toxicol Appl Pharmacol. (2022) 434:115816. doi: 10.1016/j.taap.2021.115816
152. Wang G, Li L, Li Y, Zhang LH. Toosendanin reduces cisplatin resistance in ovarian cancer through modulating the miR-195/ERK/beta-catenin pathway. Phytomedicine. (2023) 109:154571. doi: 10.1016/j.phymed.2022.154571
153. Zhang T, Xu C, Zheng P, Zhang X, Qiu C, Wu F, et al. Glaucocalyxin B attenuates ovarian cancer cell growth and cisplatin resistance in vitro via activating oxidative stress. Oxid Med Cell Longev. (2022) 2022:6324292. doi: 10.1155/2022/6324292
154. Yun UJ, Lee JH, Koo KH, Ye SK, Kim SY, Lee CH, et al. Lipid raft modulation by Rp1 reverses multidrug resistance via inactivating MDR-1 and Src inhibition. Biochem Pharmacol. (2013) 85:1441–53. doi: 10.1016/j.bcp.2013.02.025
155. Deng S, Wong CKC, Lai HC, Wong AST. Ginsenoside-Rb1 targets chemotherapy-resistant ovarian cancer stem cells via simultaneous inhibition of Wnt/beta-catenin signaling and epithelial-to-mesenchymal transition. Oncotarget. (2017) 8:25897–914. doi: 10.18632/oncotarget.v8i16
156. Barani M, Bilal M, Sabir F, Rahdar A, Kyzas GZ. Nanotechnology in ovarian cancer: Diagnosis and treatment. Life Sci. (2021) 266:118914. doi: 10.1016/j.lfs.2020.118914
157. Vandghanooni S, Eskandani M, Barar J, Omidi Y. AS1411 aptamer-decorated cisplatin-loaded poly(lactic-co-glycolic acid) nanoparticles for targeted therapy of miR-21-inhibited ovarian cancer cells. Nanomedicine (Lond). (2018) 13:2729–58. doi: 10.2217/nnm-2018-0205
158. Fang X, Xu Y, Wang S, Wan J, He C, Chen M. Pluronic F68-linoleic acid nano-spheres mediated delivery of gambogic acid for cancer therapy. AAPS PharmSciTech. (2017) 18:147–55. doi: 10.1208/s12249-015-0473-z
159. Tang F, Li L, Chen D. Mesoporous silica nanoparticles: synthesis, biocompatibility and drug delivery. Adv Mater. (2012) 24:1504–34. doi: 10.1002/adma.201104763
160. Chrastina A, Massey KA, Schnitzer JE. Overcoming in vivo barriers to targeted nanodelivery. Wiley Interdiscip Rev Nanomed Nanobiotechnol. (2011) 3:421–37. doi: 10.1002/wnan.143
161. Yao S, Li L, Su XT, Wang K, Lu ZJ, Yuan CZ, et al. Development and evaluation of novel tumor-targeting paclitaxel-loaded nano-carriers for ovarian cancer treatment: in vitro and in vivo. J Exp Clin Cancer Res. (2018) 37:29. doi: 10.1186/s13046-018-0700-z
162. Li Y, Gao Y, Zhang X, Guo H, Gao H. Nanoparticles in precision medicine for ovarian cancer: From chemotherapy to immunotherapy. Int J Pharm. (2020) 591:119986. doi: 10.1016/j.ijpharm.2020.119986
163. Frank D, Indermun S, Govender M, Kumar P, Choonara YE, du Toit LC, et al. Antineoplastic nano-lipobubbles for passively targeted ovarian cancer therapy. Colloids Surf B Biointerfaces. (2019) 177:160–8. doi: 10.1016/j.colsurfb.2019.01.049
164. Fan Y, Marioli M, Zhang K. Analytical characterization of liposomes and other lipid nanoparticles for drug delivery. J Pharm BioMed Anal. (2021) 192:113642. doi: 10.1016/j.jpba.2020.113642
165. Tenchov R, Bird R, Curtze AE, Zhou Q. Lipid Nanoparticles horizontal line From Liposomes to mRNA Vaccine Delivery, a Landscape of Research Diversity and Advancement. ACS Nano. (2021) 15:16982–7015. doi: 10.1021/acsnano.1c04996
166. Tenchov R, Sasso JM, Wang X, Liaw WS, Chen CA, Zhou QA. Exosomes horizontal line Nature’s Lipid Nanoparticles, a Rising Star in Drug Delivery and Diagnostics. ACS Nano. (2022) 16:17802–46. doi: 10.1021/acsnano.2c08774
167. Srivastava A, Babu A, Filant J, Moxley KM, Ruskin R, Dhanasekaran D, et al. Exploitation of exosomes as nanocarriers for gene-, chemo-, and immune-therapy of cancer. J BioMed Nanotechnol. (2016) 12:1159–73. doi: 10.1166/jbn.2016.2205
168. Croft PK, Sharma S, Godbole N, Rice GE, Salomon C. Ovarian-cancer-associated extracellular vesicles: microenvironmental regulation and potential clinical applications. Cells. (2021) 10:2272. doi: 10.3390/cells10092272
169. Wiklander OP, Nordin JZ, O’Loughlin A, Gustafsson Y, Corso G, Mager I, et al. Extracellular vesicle in vivo biodistribution is determined by cell source, route of administration and targeting. J Extracell Vesicles. (2015) 4:26316. doi: 10.3402/jev.v4.26316
170. Wen C, Seeger RC, Fabbri M, Wang L, Wayne AS, Jong AY. Biological roles and potential applications of immune cell-derived extracellular vesicles. J Extracell Vesicles. (2017) 6:1400370. doi: 10.1080/20013078.2017.1400370
171. Munagala R, Aqil F, Jeyabalan J, Agrawal AK, Mudd AM, Kyakulaga AH, et al. Exosomal formulation of anthocyanidins against multiple cancer types. Cancer Lett. (2017) 393:94–102. doi: 10.1016/j.canlet.2017.02.004
172. Slavin JL, Lloyd B. Health benefits of fruits and vegetables. Adv Nutr. (2012) 3:506–16. doi: 10.3945/an.112.002154
173. Stoner GD. Foodstuffs for preventing cancer: the preclinical and clinical development of berries. Cancer Prev Res (Phila). (2009) 2:187–94. doi: 10.1158/1940-6207.CAPR-08-0226
174. Aqil F, Jeyabalan J, Agrawal AK, Kyakulaga AH, Munagala R, Parker L, et al. Exosomal delivery of berry anthocyanidins for the management of ovarian cancer. Food Funct. (2017) 8:4100–7. doi: 10.1039/C7FO00882A
175. Liu H, Shen M, Zhao D, Ru D, Duan Y, Ding C, et al. The effect of triptolide-loaded exosomes on the proliferation and apoptosis of human ovarian cancer SKOV3 cells. BioMed Res Int. (2019) 2019:2595801. doi: 10.1155/2019/2595801
176. Melzer C, Rehn V, Yang Y, Bahre H, von der Ohe J, Hass R. Taxol-loaded MSC-derived exosomes provide a therapeutic vehicle to target metastatic breast cancer and other carcinoma cells. Cancers (Basel). (2019) 11:798. doi: 10.3390/cancers11060798
177. van Niel G, D’Angelo G, Raposo G. Shedding light on the cell biology of extracellular vesicles. Nat Rev Mol Cell Biol. (2018) 19:213–28. doi: 10.1038/nrm.2017.125
178. Minciacchi VR, Freeman MR, Di Vizio D. Extracellular vesicles in cancer: exosomes, microvesicles and the emerging role of large oncosomes. Semin Cell Dev Biol. (2015) 40:41–51. doi: 10.1016/j.semcdb.2015.02.010
179. Ghasemzadeh T, Hasannia M, Abnous K, Taghdisi SM, Nekooei S, Nekooei N, et al. Preparation of targeted theranostic red blood cell membranes-based nanobubbles for treatment of colon adenocarcinoma. Expert Opin Drug Deliv. (2023) 20:131–43. doi: 10.1080/17425247.2022.2152792
180. Pascucci L, Cocce V, Bonomi A, Ami D, Ceccarelli P, Ciusani E, et al. Paclitaxel is incorporated by mesenchymal stromal cells and released in exosomes that inhibit in vitro tumor growth: a new approach for drug delivery. J Control Release. (2014) 192:262–70. doi: 10.1016/j.jconrel.2014.07.042
181. Stompor-Goracy M, Bajek-Bil A, Machaczka M. Chrysin: perspectives on contemporary status and future possibilities as pro-health agent. Nutrients. (2021) 13:2038. doi: 10.3390/nu13062038
182. Naz S, Imran M, Rauf A, Orhan IE, Shariati MA, Iahtisham Ul H, et al. Chrysin: Pharmacological and therapeutic properties. Life Sci. (2019) 235:116797. doi: 10.1016/j.lfs.2019.116797
183. Tarahomi M, Firouzi Amandi A, Eslami M, Yazdani Y, Salek Farrokhi A, Ghorbani F, et al. Niosomes nanoparticles as a novel approach in drug delivery enhances anticancer properties of chrysin in human ovarian carcinoma cells (SKOV3): an in vitro study. Med Oncol. (2023) 40:87. doi: 10.1007/s12032-023-01952-8
184. Bondi ML, Emma MR, Botto C, Augello G, Azzolina A, Di Gaudio F, et al. Biocompatible lipid nanoparticles as carriers to improve curcumin efficacy in ovarian cancer treatment. J Agric Food Chem. (2017) 65:1342–52. doi: 10.1021/acs.jafc.6b04409
185. Zhang X, Lu T, Ma Y, Li R, Pang Y, Mao H, et al. Novel nanocomplexes targeting STAT3 demonstrate promising anti-ovarian cancer effects in vivo. Onco Targets Ther. (2020) 13:5069–82. doi: 10.2147/OTT.S247398
186. Sivadasan D, Ramakrishnan K, Mahendran J, Ranganathan H, Karuppaiah A, Rahman H. Solid lipid nanoparticles: applications and prospects in cancer treatment. Int J Mol Sci. (2023) 24:6199. doi: 10.3390/ijms24076199
187. Tarr BD, Sambandan TG, Yalkowsky SH. A new parenteral emulsion for the administration of taxol. Pharm Res. (1987) 4:162–5. doi: 10.1023/A:1016483406511
188. Lee MK, Lim SJ, Kim CK. Preparation, characterization and in vitro cytotoxicity of paclitaxel-loaded sterically stabilized solid lipid nanoparticles. Biomaterials. (2007) 28:2137–46. doi: 10.1016/j.biomaterials.2007.01.014
189. Senthil Kumar C, Thangam R, Mary SA, Kannan PR, Arun G, Madhan B. Targeted delivery and apoptosis induction of trans-resveratrol-ferulic acid loaded chitosan coated folic acid conjugate solid lipid nanoparticles in colon cancer cells. Carbohydr Polym. (2020) 231:115682. doi: 10.1016/j.carbpol.2019.115682
190. Shariatinia Z. Carboxymethyl chitosan: Properties and biomedical applications. Int J Biol Macromol. (2018) 120:1406–19. doi: 10.1016/j.ijbiomac.2018.09.131
191. Chamani M, Maleki Dana P, Chaichian S, Moazzami B, Asemi Z. Chitosan is a potential inhibitor of ovarian cancer: Molecular aspects. IUBMB Life. (2020) 72:687–97. doi: 10.1002/iub.2206
192. Han HD, Mangala LS, Lee JW, Shahzad MM, Kim HS, Shen D, et al. Targeted gene silencing using RGD-labeled chitosan nanoparticles. Clin Cancer Res. (2010) 16:3910–22. doi: 10.1158/1078-0432.CCR-10-0005
193. Peter S, Alven S, Maseko RB, Aderibigbe BA. Doxorubicin-based hybrid compounds as potential anticancer agents: A review. Molecules. (2022) 27:4478. doi: 10.3390/molecules27144478
194. Hu R, Zheng H, Cao J, Davoudi Z, Wang Q. Synthesis and in vitro characterization of carboxymethyl chitosan-CBA-doxorubicin conjugate nanoparticles as pH-sensitive drug delivery systems. J BioMed Nanotechnol. (2017) 13:1097–105. doi: 10.1166/jbn.2017.2407
195. Yan E, Fan Y, Sun Z, Gao J, Hao X, Pei S, et al. Biocompatible core-shell electrospun nanofibers as potential application for chemotherapy against ovary cancer. Mater Sci Eng C Mater Biol Appl. (2014) 41:217–23. doi: 10.1016/j.msec.2014.04.053
196. Javid A, Ahmadian S, Saboury AA, Kalantar SM, Rezaei-Zarchi S. Chitosan-coated superparamagnetic iron oxide nanoparticles for doxorubicin delivery: synthesis and anticancer effect against human ovarian cancer cells. Chem Biol Drug Des. (2013) 82:296–306. doi: 10.1111/cbdd.12145
197. Yousefpour P, Atyabi F, Vasheghani-Farahani E, Movahedi AA, Dinarvand R. Targeted delivery of doxorubicin-utilizing chitosan nanoparticles surface-functionalized with anti-Her2 trastuzumab. Int J Nanomedicine. (2011) 6:1977–90. doi: 10.2147/IJN.S21523
198. Li X, Kong X, Zhang J, Wang Y, Wang Y, Shi S, et al. A novel composite hydrogel based on chitosan and inorganic phosphate for local drug delivery of camptothecin nanocolloids. J Pharm Sci. (2011) 100:232–41. doi: 10.1002/jps.22256
199. Zhou L, Du L, Chen X, Li X, Li Z, Wen Y, et al. The antitumor and antimetastatic effects of N-trimethyl chitosan-encapsulated camptothecin on ovarian cancer with minimal side effects. Oncol Rep. (2010) 24:941–8. doi: 10.3892/or.2010.941
200. Cortes JR, Perez GM, Rivas MD, Zamorano J. Kaempferol inhibits IL-4-induced STAT6 activation by specifically targeting JAK3. J Immunol. (2007) 179:3881–7. doi: 10.4049/jimmunol.179.6.3881
201. Duthie G, Crozier A. Plant-derived phenolic antioxidants. Curr Opin Clin Nutr Metab Care. (2000) 3:447–51. doi: 10.1097/00075197-200011000-00006
202. Luo H, Jiang B, Li B, Li Z, Jiang BH, Chen YC. Kaempferol nanoparticles achieve strong and selective inhibition of ovarian cancer cell viability. Int J Nanomedicine. (2012) 7:3951–9. doi: 10.2147/IJN.S33670
203. Dong X, Zeng Y, Zhang Z, Fu J, You L, He Y, et al. Hypericin-mediated photodynamic therapy for the treatment of cancer: a review. J Pharm Pharmacol. (2021) 73:425–36. doi: 10.1093/jpp/rgaa018
204. Zeisser-Labouebe M, Lange N, Gurny R, Delie F. Hypericin-loaded nanoparticles for the photodynamic treatment of ovarian cancer. Int J Pharm. (2006) 326:174–81. doi: 10.1016/j.ijpharm.2006.07.012
205. Mukund V, Mukund D, Sharma V, Mannarapu M, Alam A. Genistein: Its role in metabolic diseases and cancer. Crit Rev Oncol Hematol. (2017) 119:13–22. doi: 10.1016/j.critrevonc.2017.09.004
206. Patra A, Satpathy S, Naik PK, Kazi M, Hussain MD. Folate receptor-targeted PLGA-PEG nanoparticles for enhancing the activity of genistein in ovarian cancer. Artif Cells Nanomed Biotechnol. (2022) 50:228–39. doi: 10.1080/21691401.2022.2118758
207. Kumar SS, Surianarayanan M, Vijayaraghavan R, Mandal AB, MacFarlane DR. Curcumin loaded poly(2-hydroxyethyl methacrylate) nanoparticles from gelled ionic liquid–in vitro cytotoxicity and anti-cancer activity in SKOV-3 cells. Eur J Pharm Sci. (2014) 51:34–44. doi: 10.1016/j.ejps.2013.08.036
208. Yallapu MM, Gupta BK, Jaggi M, Chauhan SC. Fabrication of curcumin encapsulated PLGA nanoparticles for improved therapeutic effects in metastatic cancer cells. J Colloid Interface Sci. (2010) 351:19–29. doi: 10.1016/j.jcis.2010.05.022
209. Sandhiutami NMD, Arozal W, Louisa M, Rahmat D, Wuyung PE. Curcumin nanoparticle enhances the anticancer effect of cisplatin by inhibiting PI3K/AKT and JAK/STAT3 pathway in rat ovarian carcinoma induced by DMBA. Front Pharmacol. (2020) 11:603235. doi: 10.3389/fphar.2020.603235
210. Mahmud A, Xiong XB, Aliabadi HM, Lavasanifar A. Polymeric micelles for drug targeting. J Drug Targeting. (2007) 15:553–84. doi: 10.1080/10611860701538586
211. Talelli M, Rijcken CJ, van Nostrum CF, Storm G, Hennink WE. Micelles based on HPMA copolymers. Adv Drug Delivery Rev. (2010) 62:231–9. doi: 10.1016/j.addr.2009.11.029
212. Maher P. How fisetin reduces the impact of age and disease on CNS function. Front Biosci (Schol Ed). (2015) 7:58–82. doi: 10.2741/s425
213. Rais J, Khan H, Arshad M. The role of phytochemicals in cancer prevention: A review with emphasis on baicalein, fisetin, and biochanin A. Curr Top Med Chem. (2023) 23:1123–35. doi: 10.2174/1568026623666230516161827
214. Xiao X, Zou J, Fang Y, Meng Y, Xiao C, Fu J, et al. Fisetin and polymeric micelles encapsulating fisetin exhibit potent cytotoxic effects towards ovarian cancer cells. BMC Complement Altern Med. (2018) 18:91. doi: 10.1186/s12906-018-2127-7
215. Carlson LJ, Cote B, Alani AW, Rao DA. Polymeric micellar co-delivery of resveratrol and curcumin to mitigate in vitro doxorubicin-induced cardiotoxicity. J Pharm Sci. (2014) 103:2315–22. doi: 10.1002/jps.24042
216. Khiste SK, Liu Z, Roy KR, Uddin MB, Hosain SB, Gu X, et al. Ceramide-rubusoside nanomicelles, a potential therapeutic approach to target cancers carrying p53 missense mutations. Mol Cancer Ther. (2020) 19:564–74. doi: 10.1158/1535-7163.MCT-19-0366
217. Jiao M, Zhang P, Meng J, Li Y, Liu C, Luo X, et al. Recent advancements in biocompatible inorganic nanoparticles towards biomedical applications. Biomater Sci. (2018) 6:726–45. doi: 10.1039/C7BM01020F
218. Saratale RG, Saratale GD, Ghodake G, Cho SK, Kadam A, Kumar G, et al. Wheat straw extracted lignin in silver nanoparticles synthesis: Expanding its prophecy towards antineoplastic potency and hydrogen peroxide sensing ability. Int J Biol Macromol. (2019) 128:391–400. doi: 10.1016/j.ijbiomac.2019.01.120
219. Rammohan A, Gunasekar D, Reddy NV, Vijaya T, Devillee A, Bodo B. Structure elucidation and antioxidant activity of the phenolic compounds from Rhynchosia suaveolens. Nat Prod Commun. (2015) 10:609–11. doi: 10.1177/1934578X1501000418
220. Bethu MS, Netala VR, Domdi L, Tartte V, Janapala VR. Potential anticancer activity of biogenic silver nanoparticles using leaf extract of Rhynchosia suaveolens: an insight into the mechanism. Artif Cells Nanomed Biotechnol. (2018) 46:104–14. doi: 10.1080/21691401.2017.1414824
221. Schluep T, Gunawan P, Ma L, Jensen GS, Duringer J, Hinton S, et al. Polymeric tubulysin-peptide nanoparticles with potent antitumor activity. Clin Cancer Res. (2009) 15:181–9. doi: 10.1158/1078-0432.CCR-08-1848
222. Zhang X, Haney KM, Richardson AC, Wilson E, Gewirtz DA, Ware JL, et al. Anibamine, a natural product CCR5 antagonist, as a novel lead for the development of anti-prostate cancer agents. Bioorg Med Chem Lett. (2010) 20:4627–30. doi: 10.1016/j.bmcl.2010.06.003
223. Jacome Sanz D, Raivola J, Karvonen H, Arjama M, Barker H, Murumagi A, et al. Evaluating targeted therapies in ovarian cancer metabolism: novel role for PCSK9 and second generation mTOR inhibitors. Cancers (Basel). (2021) 13:3727. doi: 10.3390/cancers13153727
224. Coppinger C, Pomales B, Movahed MR, Marefat M, Hashemzadeh M. Berberine: A multi-target natural PCSK9 inhibitor with the potential to treat diabetes, Alzheimer’s, cancer and cardiovascular disease. Curr Rev Clin Exp Pharmacol. (2024) 19:312–26. doi: 10.2174/0127724328250471231222094648
225. Balasubramanyam K, Varier RA, Altaf M, Swaminathan V, Siddappa NB, Ranga U, et al. Curcumin, a novel p300/CREB-binding protein-specific inhibitor of acetyltransferase, represses the acetylation of histone/nonhistone proteins and histone acetyltransferase-dependent chromatin transcription. J Biol Chem. (2004) 279:51163–71. doi: 10.1074/jbc.M409024200
226. Soflaei SS, Momtazi-Borojeni AA, Majeed M, Derosa G, Maffioli P, Sahebkar A. Curcumin: A natural pan-HDAC inhibitor in cancer. Curr Pharm Des. (2018) 24:123–9. doi: 10.2174/1381612823666171114165051
227. Rodrigues Moita AJ, Bandolik JJ, Hansen FK, Kurz T, Hamacher A, Kassack MU. Priming with HDAC inhibitors sensitizes ovarian cancer cells to treatment with cisplatin and HSP90 inhibitors. Int J Mol Sci. (2020) 21:8300. doi: 10.3390/ijms21218300
228. Yang D, Huang FX, Wei W, Li QQ, Wu JW, Huang Y, et al. Loss of HRD functional phenotype impedes immunotherapy and can be reversed by HDAC inhibitor in ovarian cancer. Int J Biol Sci. (2023) 19:1846–60. doi: 10.7150/ijbs.79654
229. Tossetta G, Marzioni D. Natural and synthetic compounds in Ovarian Cancer: A focus on NRF2/KEAP1 pathway. Pharmacol Res. (2022) 183:106365. doi: 10.1016/j.phrs.2022.106365
230. Tossetta G, Fantone S, Montanari E, Marzioni D, Goteri G. Role of NRF2 in ovarian cancer. Antioxidants (Basel). (2022) 11:663. doi: 10.3390/antiox11040663
231. Li X, Zhang Q, Hou N, Li J, Liu M, Peng S, et al. Carnosol as a nrf2 activator improves endothelial barrier function through antioxidative mechanisms. Int J Mol Sci. (2019) 20:880. doi: 10.3390/ijms20040880
232. Kim EN, Lim JH, Kim MY, Ban TH, Jang IA, Yoon HE, et al. Resveratrol, an Nrf2 activator, ameliorates aging-related progressive renal injury. Aging (Albany NY). (2018) 10:83–99. doi: 10.18632/aging.v10i1
233. Moon SJ, Jhun J, Ryu J, Kwon JY, Kim SY, Jung K, et al. The anti-arthritis effect of sulforaphane, an activator of Nrf2, is associated with inhibition of both B cell differentiation and the production of inflammatory cytokines. PloS One. (2021) 16:e0245986. doi: 10.1371/journal.pone.0245986
234. Chen Y, Yang J, Zuo Y, Zhang C, Pu Y, Ren Q, et al. Voacamine is a novel inhibitor of EGFR exerting oncogenic activity against colorectal cancer through the mitochondrial pathway. Pharmacol Res. (2022) 184:106415. doi: 10.1016/j.phrs.2022.106415
235. Falkenberg KD, Jakobs A, Matern JC, Dorner W, Uttarkar S, Trentmann A, et al. a natural compound with anti-tumor activity, is a potent inhibitor of transcription factor C/EBPbeta. Biochim Biophys Acta Mol Cell Res. (2017) 1864:1349–58. doi: 10.1016/j.bbamcr.2017.05.003
236. Zalpoor H, Nabi-Afjadi M, Forghaniesfidvajani R, Tavakol C, Farahighasreaboonasr F, Pakizeh F, et al. Quercetin as a JAK-STAT inhibitor: a potential role in solid tumors and neurodegenerative diseases. Cell Mol Biol Lett. (2022) 27:60. doi: 10.1186/s11658-022-00355-3
237. Yao JY, Xu S, Sun YN, Xu Y, Guo QL, Wei LB. Novel CDK9 inhibitor oroxylin A promotes wild-type P53 stability and prevents hepatocellular carcinoma progression by disrupting both MDM2 and SIRT1 signaling. Acta Pharmacol Sin. (2022) 43:1033–45. doi: 10.1038/s41401-021-00708-2
238. Chen Y, Zheng J, Mo L, Chen F, Li R, Wang Y, et al. Oroxylin A suppresses breast cancer-induced osteoclastogenesis and osteolysis as a natural RON inhibitor. Phytomedicine. (2024) 129:155688. doi: 10.1016/j.phymed.2024.155688
239. Jia L, Song Q, Zhou C, Li X, Pi L, Ma X, et al. Dihydroartemisinin as a putative STAT3 inhibitor, suppresses the growth of head and neck squamous cell carcinoma by targeting Jak2/STAT3 signaling. PloS One. (2016) 11:e0147157. doi: 10.1371/journal.pone.0147157
240. Ni L, Zhu X, Zhao Q, Shen Y, Tao L, Zhang J, et al. Dihydroartemisinin, a potential PTGS1 inhibitor, potentiated cisplatin-induced cell death in non-small cell lung cancer through activating ROS-mediated multiple signaling pathways. Neoplasia. (2024) 51:100991. doi: 10.1016/j.neo.2024.100991
241. Huang Q, Su H, Qi B, Wang Y, Yan K, Wang X, et al. A SIRT1 activator, ginsenoside rc, promotes energy metabolism in cardiomyocytes and neurons. J Am Chem Soc. (2021) 143:1416–27. doi: 10.1021/jacs.0c10836
242. Yang Z, Yu Y, Sun N, Zhou L, Zhang D, Chen H, et al. Ginsenosides Rc, as a novel SIRT6 activator, protects mice against high fat diet induced NAFLD. J Ginseng Res. (2023) 47:376–84. doi: 10.1016/j.jgr.2020.07.005
243. Zhong Y, Chen Y, Pan Z, Tang K, Zhong G, Guo J, et al. Ginsenoside Rc, as an FXR activator, alleviates acetaminophen-induced hepatotoxicity via relieving inflammation and oxidative stress. Front Pharmacol. (2022) 13:1027731. doi: 10.3389/fphar.2022.1027731
244. Li D, Bi FF, Chen NN, Cao JM, Sun WP, Zhou YM, et al. A novel crosstalk between BRCA1 and sirtuin 1 in ovarian cancer. Sci Rep. (2014) 4:6666. doi: 10.1038/srep06666
245. Sun X, Wang S, Li Q. Comprehensive analysis of expression and prognostic value of sirtuins in ovarian cancer. Front Genet. (2019) 10:879. doi: 10.3389/fgene.2019.00879
246. Rezen T, Rozman D, Kovacs T, Kovacs P, Sipos A, Bai P, et al. The role of bile acids in carcinogenesis. Cell Mol Life Sci. (2022) 79:243. doi: 10.1007/s00018-022-04278-2
247. Cheng Z, Li Y, Zhao D, Zhao W, Wu M, Zhang W, et al. Nanocarriers for intracellular co-delivery of proteins and small-molecule drugs for cancer therapy. Front Bioeng Biotechnol. (2022) 10:994655. doi: 10.3389/fbioe.2022.994655
248. Guo X, Zhao Z, Chen D, Qiao M, Wan F, Cun D, et al. Co-delivery of resveratrol and docetaxel via polymeric micelles to improve the treatment of drug-resistant tumors. Asian J Pharm Sci. (2019) 14:78–85. doi: 10.1016/j.ajps.2018.03.002
249. Bose S, Banerjee S, Mondal A, Chakraborty U, Pumarol J, Croley CR, et al. Targeting the JAK/STAT signaling pathway using phytocompounds for cancer prevention and therapy. Cells. (2020) 9:1451. doi: 10.3390/cells9061451
250. Li Y, Wang X, Ma X, Liu C, Wu J, Sun C. Natural polysaccharides and their derivates: A promising natural adjuvant for tumor immunotherapy. Front Pharmacol. (2021) 12:621813. doi: 10.3389/fphar.2021.621813
251. Yang M, Li J, Gu P, Fan X. The application of nanoparticles in cancer immunotherapy: Targeting tumor microenvironment. Bioact Mater. (2021) 6:1973–87. doi: 10.1016/j.bioactmat.2020.12.010
252. Wu S, Yang X, Lu Y, Fan Z, Li Y, Jiang Y, et al. A green approach to dual-drug nanoformulations with targeting and synergistic effects for cancer therapy. Drug Deliv. (2017) 24:51–60. doi: 10.1080/10717544.2016.1228716
Keywords: ovarian cancer, nanoparticles, nanoscale natural drug delivery system, targeted drug delivery, chemical compound
Citation: Li Y, Shen Q, Feng L, Zhang C, Jiang X, Liu F and Pang B (2024) A nanoscale natural drug delivery system for targeted drug delivery against ovarian cancer: action mechanism, application enlightenment and future potential. Front. Immunol. 15:1427573. doi: 10.3389/fimmu.2024.1427573
Received: 04 May 2024; Accepted: 22 July 2024;
Published: 11 October 2024.
Edited by:
Paola Allavena, University of Milan, ItalyCopyright © 2024 Li, Shen, Feng, Zhang, Jiang, Liu and Pang. This is an open-access article distributed under the terms of the Creative Commons Attribution License (CC BY). The use, distribution or reproduction in other forums is permitted, provided the original author(s) and the copyright owner(s) are credited and that the original publication in this journal is cited, in accordance with accepted academic practice. No use, distribution or reproduction is permitted which does not comply with these terms.
*Correspondence: Bo Pang, ZHJwYW5nYm9AZ21haWwuY29t
†These authors have contributed equally to this work
Disclaimer: All claims expressed in this article are solely those of the authors and do not necessarily represent those of their affiliated organizations, or those of the publisher, the editors and the reviewers. Any product that may be evaluated in this article or claim that may be made by its manufacturer is not guaranteed or endorsed by the publisher.
Research integrity at Frontiers
Learn more about the work of our research integrity team to safeguard the quality of each article we publish.