- 1Africa Health Research Institute, Durban, South Africa
- 2Department of Infectious Diseases, Nelson R. Mandela School of Clinical Medicine, University of KwaZulu-Natal, Durban, South Africa
- 3Department of Infection and Immunity, University College London, London, United Kingdom
Introduction: Neutrophils play a complex and important role in the immunopathology of TB. Data suggest they are protective during early infection but become a main driver of immunopathology if infection progresses to active disease. Neutrophils are now recognized to exist in functionally diverse states, but little work has been done on how neutrophil states or subsets are skewed in TB disease.
Methods: To address this, we carried out comprehensive phenotyping by flow cytometry of neutrophils in the blood and airways of individuals with active pulmonary TB with and without HIV co-infection recruited in Durban, South Africa.
Results: Active TB was associated with a profound skewing of neutrophils in the blood toward phenotypes associated with activation and apoptosis, reduced phagocytosis, reverse transmigration, and immune regulation. This skewing was also apparently in airway neutrophils, particularly the regulatory subsets expressing PDL-1 and LOX-1. HIV co-infection did not impact neutrophil subsets in the blood but was associated with a phenotypic change in the airways and a reduction in key neutrophil functional proteins cathelicidin and arginase 1.
Discussion: Active TB is associated with profound skewing of blood and airway neutrophils and suggests multiple mechanisms by which neutrophils may exacerbate the immunopathology of TB. These data indicate potential avenues for reducing neutrophil-mediated lung pathology at the point of diagnosis.
Introduction
Tuberculosis (TB), caused by Mycobacterium tuberculosis (M.tb), remains a severe global health challenge. Infection primarily occurs via the lung mucosa through inhalation of aerosol droplets containing M.tb (1). In the alveolar space M.tb encounters immune cells such as alveolar macrophages, pneumocytes, and lung endothelial cells, leading to the recruitment of other immune subsets such as neutrophils, eosinophils, and dendritic cells. Of these, neutrophils are the first cell type to be recruited in abundance from the circulation and are regarded as a first line of defense against invading pathogens (2–4).
The role of neutrophils in TB immunity is complex and is not fully understood. On one hand they play a role in bacterial clearance by promoting the transition from innate to adaptive immunity through the release of cytokines and chemokines (5–10). Additionally, neutrophils can drive granuloma formation via the CXCR3 pathway, a key regulator of inflammatory responses (11). Depletion of neutrophils following vaccination with M. smegmatis in mice, for example, resulted in decreased T-cell responses and increased M.tb burden (12). Studies have also demonstrated an inverse correlation between blood neutrophil counts and the risk of active TB in humans (13, 14). On the other hand, in M.tb susceptible mice, neutrophils have been observed driving severe lung inflammation (15, 16), and they are thought to be central to TB immunopathology and lung cavitation in humans.
Neutrophils were historically considered as a single immune subset, but more recent studies indicate the existence of heterogenous populations of neutrophils whose functional capacity varies (13, 17–19). M.tb infection affects the phenotype and function of circulating neutrophils (20), and the skewing of neutrophil phenotypes in TB might play an important role in disease progression (15). In addition, TB has been linked with the release of banded and immature neutrophils into circulation, which have differential phenotypic and functional characteristics (21). Likewise, HIV infection has been reported to decrease neutrophil chemotaxis, phagocytosis, and bactericidal activity, which may result in failure of the host to control M.tb infection (22–25). However, detailed studies examining the heterogeneity of neutrophils induced during active TB disease and the impact of HIV co-infection are missing, and we still lack a clear picture of how they might contribute to immune control or pathogenesis. In addition, the limited studies examining this important cell type have focused primarily on the blood, and it is not clear whether the same neutrophils populations are present at the site of infection in the human lung. The aim of this study was thus to perform an extensive functional immunophenotyping of blood and sputum neutrophils in TB-infected individuals, as a proxy of the lung environment.
These data show that neutrophils are highly skewed by TB infection, and display evidence of immune activation, functional impairment and immunosuppressive phenotypes. Surprisingly, we detected no significant effect of HIV-coinfection on neutrophil subsets in blood. Neutrophils were found to be highly enriched in the sputum of TB infected subjects, and were also dominated by and activated and highly immunosuppressive phenotype. Unlike blood, however, we did observe significant difference in sputum neutrophils in the airways, including elevated expression of CD177 and LOX-1 and reduced expression of the antimicrobial protein cathelicidin and the immunosuppression molecule arginase 1.
Materials and methods
Cohort description
Patients were recruited at the clinic after presenting with symptoms of TB and were tested using GeneXpert and confirmed by liquid culture. All patients gave written informed consent for a blood draw and induced sputum collection. The study protocol, data collection tools and associated consent forms were approved by the University of KwaZulu-Natal biomedical research Ethics Committee (BE285/16). The study protocol for blood collection from healthy donors and patients with active TB was also approved (BE022/13).
Sputum and peripheral blood collection
Sputum samples were induced using PBS under supervision and samples were transported on ice to the lab and processing within one hour in a Biosafety level 3 facility. Blood samples were collected into EDTA tubes and processed within four hours of collection.
NDN and LDN isolation using a Percoll gradient
To isolate neutrophils from blood, 6 ml of whole blood was mixed with 4 ml 5% dextran in 0.9% NaCl and incubated at RT for 30 mins. The layer containing neutrophils was then harvested and washed twice with HBSS without Ca2+/Mg2+ at 250 xg for 5 mins at RT. Isolated neutrophils were then separated into NDNs and LDNs using a Percoll density gradient. Briefly, Percoll (PureGene, Zeiningen, Switzerland) was made at concentrations of 100%, 81% 70% and 55% respectively. The isolated neutrophil pellet was resuspended in 55% percoll which was overlayed onto a 70% Percoll layer which itself was overlayed onto an 81% Percoll layer. This was then centrifuged at 720xg without brake at RT for 30 mins. NDNs were siphoned off from the 70%/55% boundary, with LDNs being siphoned off from the 81%/70% boundary. Cells where then washed once with PBS at 300xg for 10 mins at RT, and stained using antibody panels and analyzed by flow cytometry.
Sputum processing
Induced sputum was mixed 1:2 (v/v) in 0.1% dithiothreitol (DTT, ThermoFisher Scientific) and incubated for 15 mins at 37 °C under agitation. Sputum/DTT mixture was mixed and passed through a 40 µm cell strainer. Cells were counted using an automated TC20 hemocytometer. Cells where then washed twice with PBS (300xg for 10 mins at RT), and stained using antibody panels and analyzed by flow cytometry.
Flow cytometry analysis
Between 1 – 5 million cells were used for each flow cytometry panel. Cells were pelleted (300xg for 10 mins at RT) and stained with antibody panels with FC blocking (Table 1) for 20 mins at RT in the dark. Stained cells were subsequently washed twice with PBS and subsequently resuspended in 200 µl 2% PFA in PBS. Samples were then analyzed using a BD FACS Aria Fusion III, with all data being analyzed using FlowJo v10.8.1. For dimensionality reduction (t-SNE) analysis, individual donor fcs files were used to generate expression matrices containing measured intensities for each antibody in each of the two antibody panels. A total of 500 000 cells were used to create a t-SNE map in all cases, with an equal number of cells from each donor. Cell populations were then sub-setted and relative frequencies tested non-parametric analysis.
Analysis of neutrophil protein levels in sputum and plasma
Neutrophil associated proteins were quantified by Enzyme-linked immunosorbent assays (ELISA) for both sputum and plasma. Sputum was processed as above, and then prepared for ELISA as per respective manufacturers instructions. Plasma was obtained from blood samples by centrifugation at 930xg for five mins and 1 ml plasma fractions were aliquoted and stored at -80°C. ELISAs for NGAL (HK330), MPO (HK324), Arginase-1 (HK386) and LL-37 (HK321) were purchased from Hycult Biotech (Hycult Biotech, Uden, Netherlands) while the TNFα quantikine kit was purchased from R&D systems (Bio-Techne, Minneapolis, United States). All ELISAs were run as per manufacturer’s instructions alongside standard protein curves.
Statistical analysis
All statistical analysis were carried out in Graphpad using one way ANOVA to compare groups with Dunnetts correction for multiple comparison.
Results
Pulmonary TB is associated with neutrophilia and increased frequency of low-density neutrophils
Full blood count data was obtained from participants with active TB disease (ATB), with and without HIV infection, attending TB clinics and sampled on the day of diagnosis. Control samples were obtained from non-TB infected volunteers without any signs or symptoms of TB disease. Neutrophil frequency and percentage were significantly higher in ATB regardless of HIV status compared to controls (Figures 1A, B). In addition, the neutrophil-lymphocyte ratio (NLR), a marker of systemic inflammation for several diseases (26, 27), was significantly elevated compared to controls in both ATB diseased groups (Figure 1C). Neutrophils are typically high-density cells that separated out with red blood cells during standard PBMC isolation procedures. However, a fraction of neutrophils separates out with the PBMC layer, due to a lower cell density. These low-density neutrophils are considered proinflammatory and are associated with several human diseases including sepsis. The frequency of low-density neutrophils (LDN) present was determined by centrifugation and was also found to be highly elevated in ATB regardless of HIV (Figure 1D). These data suggest a strong blood neutrophil response to ATB, consistent with other studies (28, 29).
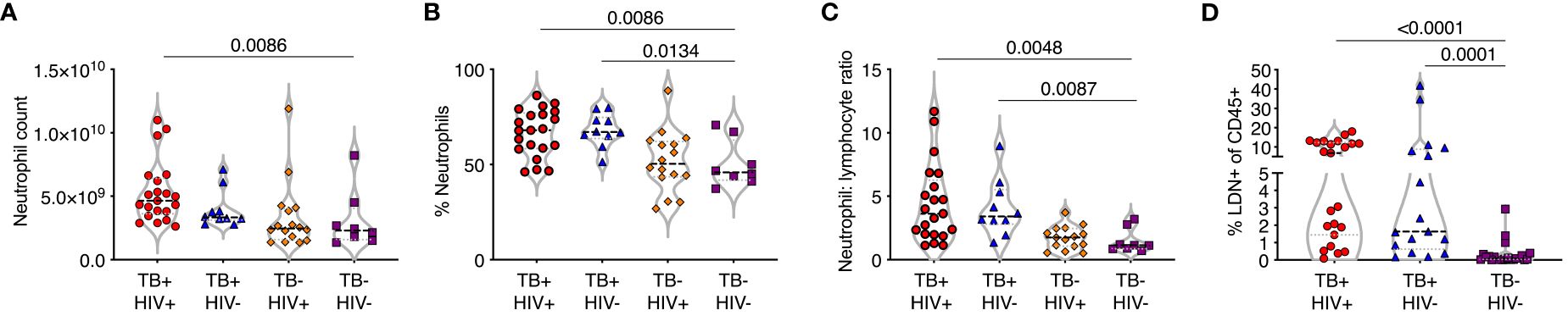
Figure 1 TB is associated with an expansion of normal and low-density neutrophils. (A–C) Whole blood counts from study participants with TB with and without HIV co-infection (TB+HIV+, TB+HIV-) with HIV alone (TB-HIV+) and controls with neither HIV or TB-HIV), showing (A) neutrophil count per ml whole blood, (B) percentage of nucleated cells (C) neutrophil:lymphocyte ratio. (D) Low density neutrophils (LDN) purified by Percol gradient and shown as a percentage of total CD45% cells. Only significant differences shown.
Phenotyping of normal-density and low-density neutrophils using flow cytometry
Normal-density (NDN) and low-density neutrophils (LDN) were separated by centrifugation and stained with two antibody panels encompassing known phenotypic and functional neutrophil markers (Table 1). Except for CD177, these markers did not typically differentiate positive and negative populations but rather varied in expression levels. Therefore, differences in expression levels of these markers are displayed as Median Fluorescent Intensity (MFI) level. Markers displaying significant differences in MFI are shown in Figure 2, in which markers have been categorized according to function. It is important to note, however, that these categories are not always distinct, and several surface molecules are known to have overlapping functions.
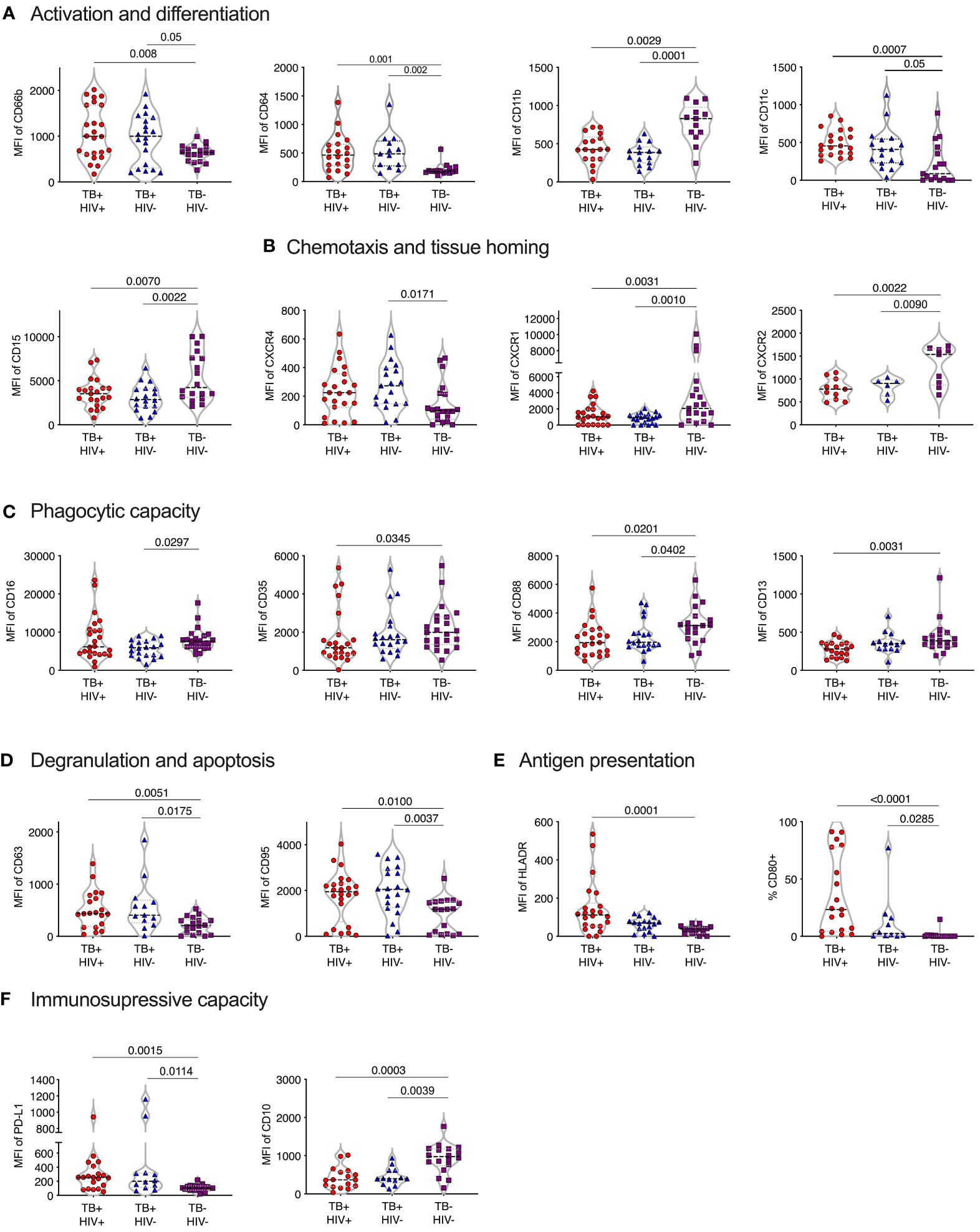
Figure 2 Phenotype of normal density blood neutrophils (NDN) highly skewed in active TB with and without HIV infection. Mean fluoresce intensity (MFI) for neutrophil surface markers grouped by functional category (A–F). Only markers with significant differences between groups shown, with p-values indicated by Kruskal-Wallis test.
Activation and differentiation
In general, NDN displayed an activated phenotype in ATB, compared to controls, with significantly elevated expression of surface CD66b and CD64 (30–33) (Figure 2A). In addition, CD11c was upregulated in ATB, which, although typically associated with dendritic cells, is expressed on activated neutrophils and, together with CD64, is an effective biomarker of sepsis in humans (34). However, the expression of CD11b (CR3), which is typically upregulated following neutrophil activation and during bacterial infection (35) was significantly lower in ATB. In addition, CD15 was significantly lower, potentially indicating the presence of more immature neutrophils.
Chemotaxis and homing markers
The expression of CXCR4, which is central for neutrophil retention in bone marrow via the SDF-1 gradient (36, 37), was significantly upregulated in ATB without HIV compared to controls (Figure 2B). In contrast, and again consistent with an activated phenotype (38), the expression of the two IL-8 receptors, CXCR1 and CXCR2, was downregulated in ATB. The relative upregulation of CXCR4 and reduction of CXCR2 is also consistent with the return of aging neutrophils back to the bone marrow (39).
Phagocytic molecules
The expression of CD35 (CR1), CD88 (C5aR), CD16 (FcRIII) and CD13 phagocytic receptors were all reduced in ATB, although this did not reach significance in all groups (Figure 2C). Down regulation of CD16 has been associated with reduced functional capacity and increased apoptosis (40), while CD35 and CD88 expression is reduced in chronic inflammation and correlates strongly with impaired phagocytic capacity (41–43). In addition, blocking of CD16 and CD35 has been shown to specifically reduce phagocytosis of Mtb by neutrophils (44). Together these data are consistent with a reduction of neutrophil phagocytic capacity in ATB.
Degranulation and apoptosis
The expression of the azurophillic granule protein CD63 on the surface of neutrophils, which is a marker of neutrophil activation and degranulation, was increased in ATB. CD95 expression was also significantly increased in ATB patients compared to controls, consistent with a pro-apoptotic phenotype (Figure 2D).
Expression of co-stimulatory and immunosuppressive receptors
Expression of the T-cell immunosuppressive molecule CD274 (PDL-1) was increased ATB, regardless of HIV status. However, CD10 expression was significantly reduced Figure 2F), which is indicative of immature neutrophils that activate T-cells (45, 46). Potentially in line with this, both HLA-DR and the co-stimulatory molecule CD80 were elevated in TB disease, consistent with an upregulation of antigen presentation activity (Figure 2E) (47, 48).
No significant differences in expression of the phenotypic markers measured were observed between ATB participants with and without HIV co-infection. Together these data indicate a profound change in neutrophil phenotype in TB disease, largely consistent with activation, degranulation and apoptosis, in addition to reduced phagocytic function, and increased cross talk with T-cells.
Comparison of NDN and LDN phenotypes in peripheral blood
Next, we examined the phenotype of LDN from the same subjects. Like NDNs, LDNs from ATB had an increased expression of HLA-DR, CXCR4, CD95, PDL-1 and CD80, and decreased expression of CD15, generally to a greater extent, but other phenotypic changes, such as for CXCR1 and 2 were not observed (Supplementary Figure S2). In addition, CD177, which is typically expressed by activated neutrophils (49) and was not differentially expressed on NDN in ATB, was highly upregulated on LDN in ATB. Likewise, LOX-1, which has been linked to immunosuppressive neutrophils and is upregulated during sepsis (50) was unchanged on NDN, but was highly upregulated on LDN in ATB. Together these data also suggest that the phenotype of activated and immunosuppressive neutrophils observed in NDN is largely reflected in LDN. However, the fact that key markers such as CD177 and LOX-1 were differentially expressed caused us to examine the general pattern of all neutrophil markers studied in NDN and LDN.
Comparison of NDNs and LDNs from controls
The expression level of opsonophagocytic receptors (CD88 and CD35), chemotaxis and tissue homing receptors (CXCR2, CD62L and CD177), antigen presentation markers (HLADR, CD11c) as well as the maturity and immunoregulatory receptor, CD10, were all significantly lower in LDNs compared to NDNs in healthy controls (Supplementary Figure S2). In contrast, CD66b, was higher in LDN, as was CD16 and CD15, in line with previous reports (20). Expression of CD63 was also higher in LDNs, supporting the hypothesis that LDNs represent or contain neutrophils that have degranulated. The other phenotypic markers measured were not significantly different between these two neutrophil subsets. Overall, these differences suggest that, under steady state conditions, LDNs are more degranulated and less functional than NDNs. However, the patterns of markers associated with other neutrophil states, such as activation, in both NDN and LDN were somewhat conflicting.
Distinct clusters of neutrophil subsets identified in NDN and LDN
Having observed complex and potentially conflicting neutrophil phenotypes, we next used an unsupervised t-SNE analysis of NDN and LDN to determine if these related to distinct neutrophil subsets. This approach identified 7 clusters for each antibody panel (Figures 3A, B, D; Supplementary Figures S3A, B, D). In Figure 3, cluster 1 forms a distinct subset distinguished by high expression of HLA-DR and CD66b and low expression of CD35 and CD88, consistent with a highly activated and functionally impaired subset. In line with the above observations, this subset was significantly expanded in both NDNs and LDNs in ATB (Figures 3C, E) (51). Cluster 4 was also highly expanded in ATB on NDN, and displayed elevated expression of CD11b, canonically associated with neutrophil activation and potentially explaining the unexpected overall reduction of CD11b. This cluster also displayed a reverse migratory phenotype of low CXCR1 and high CXCR4, potentially indicating neutrophils that have migrated out of the inflamed lung (52, 53). Interestingly, these cells retained expression of CD35 and CD88, which, like CD11b were downregulated overall. Again, consistent with the above data, cluster 6, which was significantly upregulated in LDN alone, was defined by unique expression of CD177. Cluster 2, 6 and 7 were the dominant subpopulations observed in NDN of uninfected controls, implying these represent steady state neutrophils subsets, of which cluster 2 was significantly down regulated in ATB. Similarly, cluster 2, 3 and 4 were by far the dominant subsets in healthy LDN, of which 2 and 3 were significantly reduced in ATB.
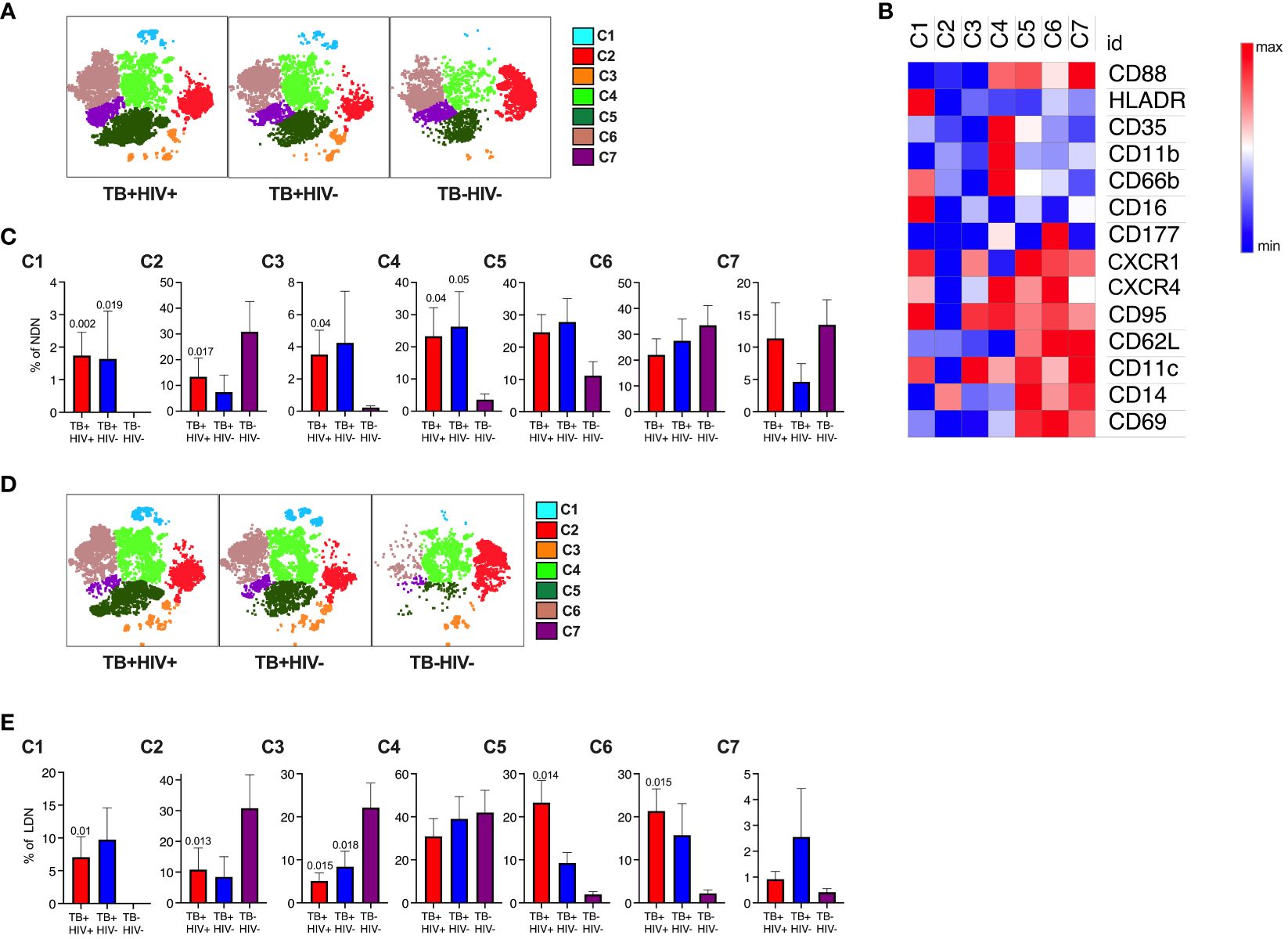
Figure 3 Subsets of NDN and LDN identified by tNSE analysis using FlowJo. Neutrophil subsets/phenotypes identified by clustering using unbiased dimensionality reduction tNSE analysis for NDN (A) and LDN (D). The heat map defining average expression value by MFI for marker is shown in panel (B). The mean frequency and SEM of each cluster shown for each group for NDN (C) and LDN (E), with p-value indicating significant differences to TB-HIV- controls by Kruskal-Wallis test.
In the second panel, the immunosuppressive phenotype is apparent (Figures S3C, E). Clusters 1 and 4 expressed high levels of PDL-1 and were both highly elevated in NDN and LDN from participants with ATB. In addition, cluster 1 expressed highly levels of CD10, consistent with a T-cell suppressive phenotype (46, 54), while the clusters lacking CD10 (3 and 7, consistent with a T-cell stimulatory phenotype) also lack PDL-1 expression. Furthermore, clusters 1 and 4 uniquely express CD80 as observed for tumor associated immunosuppressive neutrophils (55). Clusters 2 and 3 expressed elevated LOX-1, which has been linked with immunosuppressive activity, although this does not reach statistical significance. Interestingly, these populations express elevated CD137, which is linked to a protective response to Gram-positive but not Gram-negative bacteria (56). By far the dominant NDN cluster in controls is cluster 5, which is significantly reduced in ATB. In LDN this cluster is also dominant in controls, together with cluster 6, which uniquely expresses CD63, consistent with hypothesis that a proportion of LDN is made of degranulated neutrophils. Interestingly, however, this population is significantly reduced in LDN from ATB but expanded in NDNs.
For both panels, no significant differences were observed between HIV infected and uninfected participants. This unbiased sub clustering approach indicates diverse sub-populations or neutrophil states in blood, which are highly skewed by ATB. Overall, it confirms the association with highly activated and potentially dysfunctional neutrophils together with the expansion of separate populations of immunosuppressive neutrophils.
Neutrophil phenotypes in the lungs
Having observed profound differences in blood neutrophils in TB disease, we next determined if these changes were also reflected at the site of disease in the lung airways. To do this we studied the cellular content of sputum samples collected from participants attending the TB clinics at the time of diagnosis and before the initiation of TB treatment. Study participants had microbiologically confirmed TB by GeneXpert and liquid culture. In addition, samples were obtained from symptomatic TB negative participants who received a negative gene-expert result at the clinic and who were subsequently found to be culture negative. Sputum samples were collected into buffered media and transported to the lab within 1 hour of collection and incubated with DTT to break up mucins and liberate cells present. Subsequent flow cytometric analysis was then used to quantify cellular contents according to the gating strategy shown in Figure 4A. To confirm the cellular identity, CD3+ T-cells, CD14+ myeloid cells, CD206+ alveolar macrophages and CD66b+CD16+ neutrophils were sorted separately using a FACS ARIA contained with the BSL-3. Sorted populations were spun onto microscope slides and visualized, revealing the canonical morphologies associated with each cell type (Figure 4A).
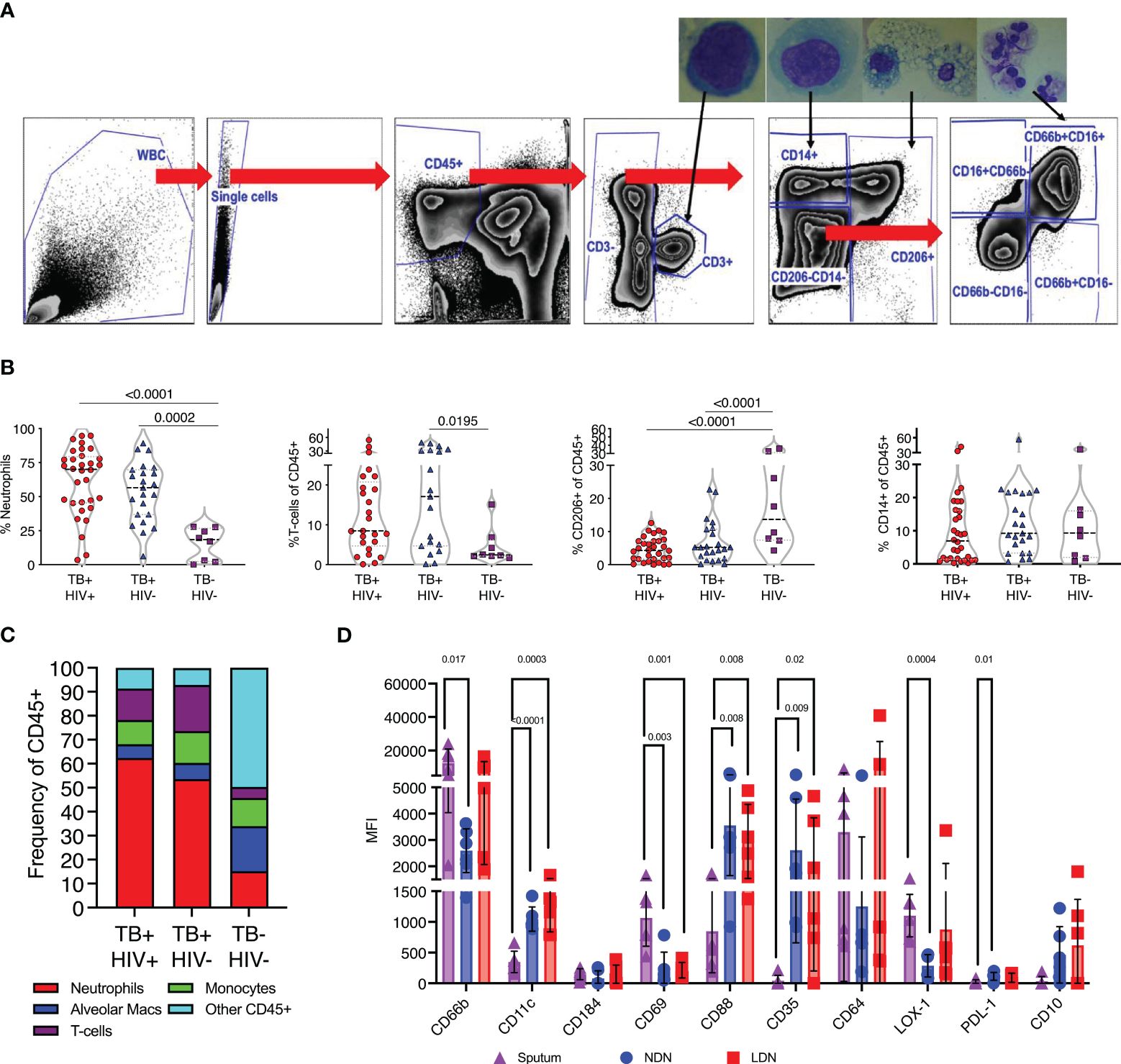
Figure 4 TB associated with high neutrophil abundance in sputum. (A) Flow gating strategy for identifying cell sub-sets within fresh sputum samples. Each of the main subsets was sorted using a FACS ARIA cell sorter and sorted cells spun on to microscope slides using a cytospin for visual confirmation of appropriate morphology, as shown. (B) The relative abundance of CD66b+ neutrophils, CD3+ T-cells, CD206+ alveolar macrophages and CD14+ monocytes. Significant differences indicated by p-values, by Kruskal-Wallis test. (C). A summary of the average cellular make of sputum samples from TB+HIV-, TB+HIV+ and TB and HIV uninfected controls. (D) Phenotypic characteristics of sputum neutrophils compared to NDN and LDN from matched blood samples from Non-TB Non-HIV infected controls, significant difference shown by p-value (Kruskal-Wallis).
Neutrophils accumulate in sputum during active TB
Quantification of sputum cellular contents based on these gates revealed a profound enrichment of neutrophils in ATB compared to controls, irrespective of HIV coinfection (Figure 4B). CD3+ve T-cells were also enriched compared to controls, consistent with a recruitment of these cells to TB diseased lung tissue, although this only reached significance for HIV co-infected participants (57). In contrast, the proportion made up by alveolar macrophages was significantly reduced in ATB, although maybe due to result from the enrichment of the neutrophils and T-cells rather than a loss of this important airway resident subset. From this data neutrophils were found to make up 50–60% of the cellular content of sputum of individuals with ATB, irrespective of HIV, but only 10% in respiratory symptomatic TB negative controls (Figure 4C).
First, we examined the phenotype of sputum neutrophils from TB negative controls compared to NDN and LDN from matched blood samples. It is important to note the non-TB controls in this case were symptomatic, and these neutrophil phenotypes may not represent a non-disease situation. None-the-less, this analysis clearly indicates differential neutrophil phenotypes in blood and sputum. Notably this includes increased expression of CD69, which is expressed on activated neutrophils (58), and higher expression of CD66b than NDN (Figure 4D). Sputum neutrophils expressed significantly lower levels of CD11c, and the phagocytic receptors CD35 and CD88, potentially suggested reduced functionality. They expressed higher levels of LOX-1 than blood NDN, consistent with an immunoregulatory role, but less PDL-1 and were negative for CD10. Markers not shown were not significantly different.
Sputum neutrophils have a distinct phenotype in TB infected individuals
Comparison of sputum neutrophils present in non-TB controls with those isolated from ATB revealed consistent and striking differences (Figure 5). In contrast to blood neutrophils during TB disease, sputum neutrophils expressed lower levels of CD66b compared to non-TB controls. Together with the reduced CD15 expression levels observed, this is consistent with a proapoptotic phenotype (59), although CD95 expression levels were not different. In addition, and similar to blood, sputum neutrophils from ATB expressed elevated levels of CXCR4, which is known to upregulate on aged neutrophils and is associated with migration back to the bone marrow (60). Again, as in blood, CXCR2 was significantly down regulated in ATB in sputum. Unlike blood, however, the frequency of CD177+ neutrophils was significantly elevated, as was the expression of CD31, which both promote neutrophil transmigration (61). Together these data suggest an accumulation of ageing and pro-apoptotic neutrophils in TB infected lungs with the potential to transmigrate out of the lung and back into the blood. Similar to blood, sputum neutrophils from TB infected participants expressed significantly lower levels of CD88, which impairs phagocytic capacity in general (42). However, CD35 expression was elevated compared to non-TB controls and CD16 was no longer reduced, as in the blood (Figure 5). As mentioned, together these molecules are crucial for phagocytosis of opsonized M.tb (44), suggesting that this capacity may be retained by sputum neutrophils during active disease.
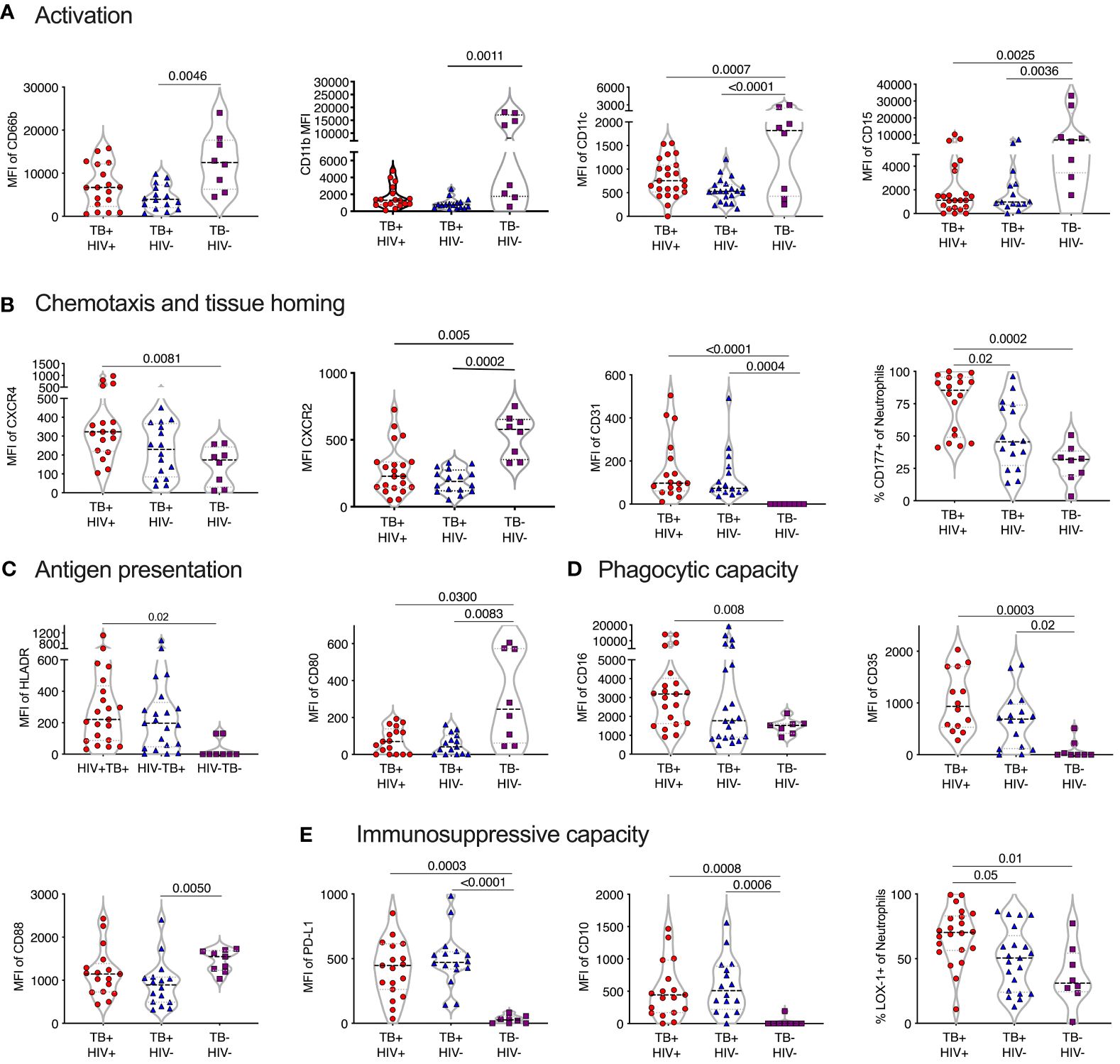
Figure 5 TB skewed phenotype of sputum. Mean fluoresce intensity (MFI) for neutrophil surface markers grouped by functional category (A–E), LDN and NDN were not distinguished in sputum. Only the markers with significant differences between groups are shown, with p-values indicated by Kruskal-Wallis test.
Strikingly, in TB-negative individuals, sputum neutrophils expressed lower levels of PDL-1 than in blood. However, in ATB this was highly elevated, consistent with an immunosuppressive phenotype. Sputum neutrophils from ATB also expressed significantly higher levels of CD10 and LOX-1 (Figure 5). Furthermore, the expression levels of PDL-1, CD10 and LOX-1 was higher than in match blood samples from TB diseased participants (Supplementary Figure 4). This immunosuppressive phenotype was further supported by the increased expression of the antigen presenting molecule HLA-DR, but decreased expression of the co-stimulatory receptor CD80, which was elevated in the blood. Therefore, sputum neutrophils in infected individuals express a consistent T-cell immunosuppressive phenotype. Overall, as in blood, neutrophil phenotype was similar in HIV infected and uninfected participants, although LOX-1+ and CD177+ neutrophils were significantly more frequent in individuals with HIV co-infection, suggesting a potential greater impact of HIV in the lung compartment.
Neutrophil associated protein levels in blood and sputum during ATB
Finally, we sought to confirm the presence of enriched neutrophils in the sputum of TB infected subjects by measuring neutrophil related proteins via ELISA (Figure 6). The same proteins were measured in matched plasma samples to compare the blood and lung compartments. Myeloperoxidase (MPO) is an important antimicrobial protein that is abundant in neutrophils and often used as a marker of this cell type, although it can also be found in myeloid and lymphoid cells (62). MPO was significantly increased in both plasma and sputum from TB participants irrespective of HIV, and this was especially pronounced in sputum and highest in HIV uninfected subjects (7.8x higher TB+HIV+, and 17x higher in TB+HIV- compared to TB-HIV-). Likewise, neutrophil gelatinase-associated lipocalin (NGAL), an antimicrobial peptide that limits iron availability and is highly expressed by neutrophils during inflammation, was elevated in both plasma and sputum in TB infected participants; but to a much greater extent in sputum (5.6x higher and 7x higher in TB+ HIV+ and TB+HIV-, respectively, compared to TB-HIV-). For cathelicidin (LL37), an antimicrobial protein which has been shown to be important in the killing of Mtb, levels were significantly elevated in plasma for TB+HIV+ participants only. In sputum, however, cathelicidin levels were only highly elevated in TB infected subjects without HIV (10x higher than TB-HIV-) and were significantly higher than TB infected subjects with HIV (5.9x higher than TB+HIV+). A similar trend was observed for arginase 1, an enzyme that enhances Mtb killing and limits pathogenic T-cell activation. As with cathelicidin, arginase 1 is significantly elevated in only the blood of TB+HIV+ participants, but in the sputum, it is only elevated in TB+HIV- participants compared to TB-HIV- (13.9x higher) and TB+HIV+ study subjects (7.2 x higher). No significant differences in TNF-a levels were seen in either blood or sputum of TB and non-TB controls. Overall, this data confirms the highly significant enrichment of neutrophil proteins in the sputum of TB infected subjects. Furthermore, our findings suggest reduced expression of important antimicrobial peptides in lungs of HIV co-infected individuals that are not reflected in the blood.
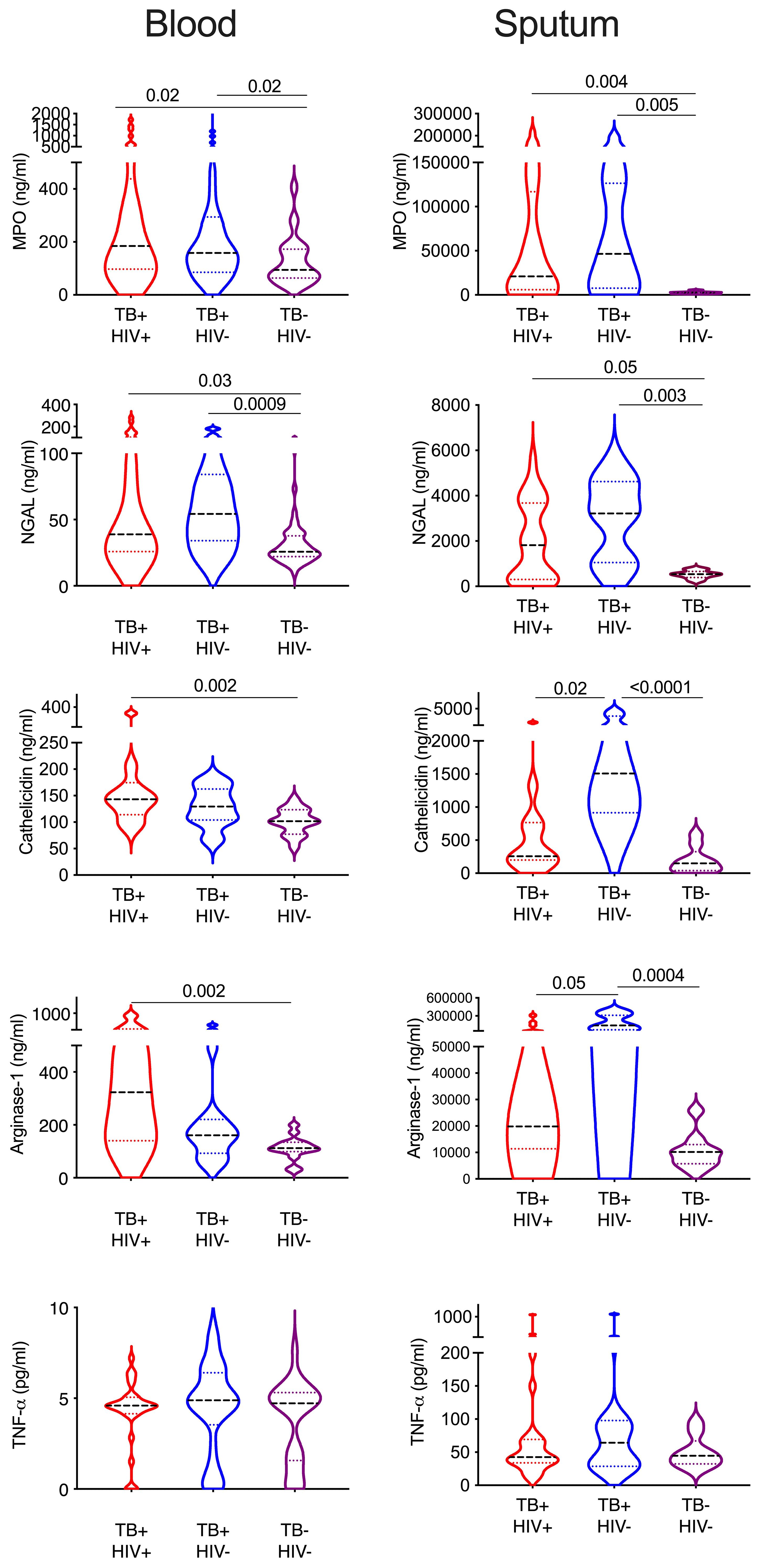
Figure 6 Sputum from HIV co-infected individual contains lower neutrophils proteins. Key neutrophil proteins were measured in matched blood plasma and decellularized sputum using commercial ELSIA assays. All significant differences are shown by p-value (Kruskal-Wallis test).
Discussion
Neutrophils play a complex role in the immunopathogenesis of TB that remains poorly understood, exacerbated by the growing appreciation that neutrophils exist in multiple distinct functional states. In this study we investigate the frequency and heterogeneity of neutrophils in blood and in the lung airways by sampling sputum. ATB was associated with an enrichment of neutrophils in both compartments, particularly in sputum, where they were by far the dominant cell type. In normal density neutrophils (NDN) from blood, ATB was associated with an activated and proapoptotic phenotype expressing changes consistent with reduced phagocytic capacity and increased immunoregulatory function. Low density neutrophils (LDN) were also significantly increased in frequency in ATB. This subset is generally considered to be proinflammatory and has reduced phagocytic capacity which was supported by comparison between NDN and LDN in controls. In addition, ATB was associated with further phenotypic changes in LDN consistent with those seen in NDN.
Using high dimensional clustering to visualize distinct neutrophil subsets confirmed these observations, showing significant upregulation of clusters with activated and functionally impaired phenotypes in ATB in both NDN and LDN. In addition, this analysis supports the enrichment of distinct immunosuppressive neutrophil subsets in ATB, some of which express high level of PDL-1 together with the T-cell co-stimulatory molecule CD80, and others which lack PDL-1 but express LOX-1. Expression of CD80 and HLA-DR on neutrophils is associated with the acquisition of antigen presenting functions on neutrophils (47), and, although not on the same panel, ATB was also associated with distinct HLA-DR high neutrophil subset. The expression of PDL-1, HLA-DR and CD80 is observed on tumor associated neutrophils and associated with suppression of T-cell activity. In addition, one subset of PDL-1 high neutrophils also expressed high levels of CD10, which has been shown to identify neutrophils that suppress T-cell activation (46). This suggests the active TB leads to the expansion of neutrophil subsets that directly inhibit Mtb specific T-cells via both the PDL-1 axis and the interaction between CD80 and CTLA4. Importantly, PDL-1 expression, whilst low on sputum neutrophils from TB-uninfected subjects was highly elevated in ATB, as was CD10, implying this T-cell suppressive phenotype is likely to be higher in the lung. M.tb infected mice lacking PD-1 had increased neutrophil recruitment and severe lung pathology which led to their early death compared to wild type mice (63), suggesting that PDL-1 upregulation is a negative feedback loop that limits excessive neutrophil recruitment. On the other hand, elevated PD-L1 expression may be counterproductive, as it could suppress the protective Mtb specific T-cell responses during infection (64, 65). In addition, PDL-1 expression by neutrophils has recently been shown to prolong neutrophil survival in patients with sepsis via activation of AKT dependent survival signaling (66). In conditional knockout mice, removal of PDL-1 improved survival by reducing neutrophil recruitment and subsequent tissue damage. Similarly, again in mice, PDL-1 expression was recently shown to promote neutrophil retention in lung tissue and enhance susceptibility to bacterial infection, which could be reversed by anti-PDL-1 treatment (67). PDL-1 expression on neutrophils was also found to prevent autophagy and thus exacerbate endotoxin induced lung injury by prolonging NETosis (68), a mechanism also thought to be important in TB pathology (69). Although LOX-1 has been associated with T-cell suppression by neutrophils, particularly in cancer (70), like PDL-1, LOX-1 is upregulated on neutrophils during sepsis, and deletion of LOX-1 reduced neutrophil driven lung pathology (50). Indeed, both LOX-1 and PDL-1 expressing neutrophils subsets were highly upregulated in blood and lung of patients with severe COVID (71). Thus, these neutrophil subsets may play dual roles in ATB, suppressing T-cell activity, whilst exacerbating lung immunopathology. For PDL-1 in particular, blockade of this molecule in experimental setting has reduced neutrophil associated lung injury associated with bacterial infection. The use of anti-PD1 blockade in cancer therapy has led to numerous cases of TB reactivation, indicating this pathway may be important in maintain latency (72). However, given the potential importance of PDL-1 in promoting neutrophil immunopathology in TB disease, blockade of the pathway might prove beneficial in conjunction with conventional anti-TB drugs.
Another striking observation from the in-depth phenotyping of blood and airway neutrophils comes from the expression of molecules associated with migration. In both blood and sputum, ATB is associated with significant upregulation of CXCR4 and downregulation of CXCR1 and CXCR2. The latter are receptors for IL-8 and promote release of neutrophils from bone and recruitment to site of inflammation. CXCR2 in particular has been shown to be essential from recruitment of neutrophils to the lung (10). Down regulation of both receptors has been associated with neutrophil activation in numerous disease settings including COPD (73). Likewise, CXCR4 is upregulated in chronic inflammatory lung disease and is associated with recruitment of apoptotic neutrophils from the lung back to the bone marrow (74). Consistent, with this, high dimensional analysis of blood neutrophils revealed a distinct population of CXCR4hi CXCR1lo neutrophils that also expressed CD11b, CD66b and CD95, consistent with highly activated, proapoptotic neutrophils that may be recruited back to the bone marrow. In the lung airways, the same down regulation of CXCR1 and upregulation of CXCR4 was observed. In addition, this is accompanied by upregulation of CD31 (PECAM-1), which is important for neutrophil migration into the airways via homophilic binding of CD31 (75). Interestingly, CD177 was also upregulated on sputum neutrophils in ATB, which is known promote transmigration also via binding to CD31 (61). This data suggests that CD31 may play an important role in the recruitment of neutrophils to the lung alveolar space during active TB. Like alveolar macrophages, neutrophils can reverse migrate from airways back into tissue during infection (76). Reverse migration is thought to be important event in several settings, including sepsis, where it may help to resolve inflammation or promote tissue damage (77). Given that sputum neutrophils are known to be heavily infected with mTB during ATB (78), it is possible that neutrophils play a role on disseminating Mtb within lung tissue and systemically. Indeed, as CXCR4hi CXCR1lo neutrophils are recruited back to the bone marrow, it is also possible that this is a mechanism by which Mtb is translocated to bone marrow, where it can be found even following TB cure (79).
The functionality of neutrophils in blood and sputum was not directly assessed in this study. However, blood neutrophils expressed significantly lower levels of the phagocytic markers CD16, CD35, CD88 and CD13. As mentioned, blockade of CD16 and CD35 has been shown to reduce phagocytosis of Mtb (44). In that study blockade of CD88 had no effect, however, in critically ill patients with suspected pneumonia, only surface CD88 expression was found to correlated with reduced phagocytic capacity of blood neutrophils (42). Similarly, cross linking of CD13 has recently been shown to induce phagocytosis on neutrophils (80). The FCyR CD64, in contrast, is highly upregulated in ATB, consistent with its potential useful role as a clinically useful marker of bacterial infection (81), including Mtb (82). However, despite facilitating phagocytosis, elevated CD64 in tuberculosis is associated with decreased phagocytic potential (83). This is potentially explained by our data showing a down regulation of most phagocytic receptors during ATB, consistent with an overall reduction in phagocytic capacity. Interestingly, down regulation of CD88 in the above study, compared to controls, was only observed in blood and not bronchioalveolar lavage fluid (42). Here, we did observe a significant down regulation of CD88 in sputum neutrophils from TB+HIV- participants compared to controls, but it was modest. In addition, CD16 and CD35 expression increased. This data suggests that airway neutrophils are likely to retain phagocytic activity, which is consistent with the observation that they are the most abundantly infected cell type in this compartment. However, whether they are able to kill phagocytosed Mtb is not clear and the data is conflicting (84).
Finally, we did not observe many phenotypic differences between ATB with and without HIV co-infection in blood. However, in sputum the expression of CD177 and LOX-1 was significantly elevated and two key neutrophil associated proteins, cathelicidin and arginase-1 were both significantly reduced in ATB co-infected with HIV. The frequency of neutrophils in these same sputum samples was not significantly different and, indeed tended to be higher in co-infected subjects. This suggests that HIV co-infection may lead to further functional impairment of neutrophils in the lung compartment, which might contribute to immunopathology in these individuals.
In conclusion, detailed phenotyping of blood and sputum neutrophils revealed profound skewing of this important immune subset in ATB in both HIV infected and uninfected individuals. The enrichment of highly activated and immunoregulatory subsets is broadly consistent between the blood and airways. Importantly, these phenotypic changes suggest diverse ways by which the skewing of neutrophils in the lung may exacerbate the immunopathology of TB. These data also suggest potential avenues for reducing neutrophil mediated lung pathology at the point of diagnosis.
Data availability statement
The raw data supporting the conclusions of this article will be made available by the authors, without undue reservation.
Ethics statement
The studies involving humans were approved by University of KwaZulu Natal Biomedical Research Ethics Committee (BREC). The studies were conducted in accordance with the local legislation and institutional requirements. The participants provided their written informed consent to participate in this study.
Author contributions
SN: Formal analysis, Investigation, Methodology, Writing – original draft. MC: Data curation, Formal analysis, Investigation, Visualization, Writing – original draft. LN: Conceptualization, Writing – review & editing. FK: Project administration, Writing – review & editing. MM: Project administration, Writing – review & editing. ZM: Project administration, Writing – review & editing. LM: Project administration, Writing – review & editing. YM: Project administration, Writing – review & editing. SM: Formal analysis, Writing – review & editing. MH: Project administration, Writing – review & editing. AL: Conceptualization, Methodology, Supervision, Writing – original draft, Writing – review & editing.
Funding
The author(s) declare financial support was received for the research, authorship, and/or publication of this article. This work was jointly sponsored by the South African National Science Foundation and the National Institute for Allergy and Infectious Diseases, National Institutes of Health, and administered by CRDF Global (OISE-16-62060-1). AL was supported by the Wellcome Trust (210662/Z/18/Z).
Conflict of interest
The authors declare that the research was conducted in the absence of any commercial or financial relationships that could be construed as a potential conflict of interest.
Publisher’s note
All claims expressed in this article are solely those of the authors and do not necessarily represent those of their affiliated organizations, or those of the publisher, the editors and the reviewers. Any product that may be evaluated in this article, or claim that may be made by its manufacturer, is not guaranteed or endorsed by the publisher.
Supplementary material
The Supplementary Material for this article can be found online at: https://www.frontiersin.org/articles/10.3389/fimmu.2024.1422836/full#supplementary-material
References
1. Russell DG, Barry CE, Flynn JL. Tuberculosis: what we don’t know can, and does, hurt us. Science. (2010) 328:852–6. doi: 10.1126/science
2. Monin L, Khader SA. Chemokines in tuberculosis: the good, the bad and the ugly. Semin Immunol. (2014) 26:552–8. doi: 10.1016/j.smim.2014
3. Bohrer AC, Castro E, Hu Z, Queiroz ATL, Tocheny CE, Assmann M, et al. Eosinophils are part of the granulocyte response in tuberculosis and promote host resistance in mice. J Exp Med. (2021) 218:e20210469. doi: 10.1084/jem.20210469
4. Pai M, Behr MA, Dowdy D, Dheda K, Divangahi M, Boehme CC, et al. Tuberculosis. Nat Rev Dis Primers. (2016) 2:16076. doi: 10.1038/nrdp.2016.76
5. Tecchio C, Cassatella MA. Neutrophil-derived chemokines on the road to immunity. Semin Immunol. (2016) 28:119–28. doi: 10.1016/j.smim.2016.04.003
6. Petrofsky M, Bermudez LE. Neutrophils from mycobacterium avium-infected mice produce TNF-alpha, IL-12, and IL-1 beta and have a putative role in early host response. Clin Immunol. (1999) 91:354–8. doi: 10.1006
7. Tecchio C, Micheletti A, Cassatella MA. Neutrophil-derived cytokines: facts beyond expression. Front Immunol. (2014) 5:508. doi: 10.3389/fimmu.2014.00508
8. Scapini P, Lapinet-Vera JA, Gasperini S, Calzetti F, Bazzoni F, Cassatella MA. The neutrophil as a cellular source of chemokines. Immunol Rev. (2000) 177:195–203. doi: 10.1034/j.1600-065x.2000.17706.x
9. Tecchio C, Cassatella MA. Neutrophil-derived chemokines on the road to immunity. Semin Immunol. (2016) 28:119–28. doi: 10.1016/j.smim.2016.04.003
10. Metzemaekers M, Gouwy M, Proost P. Neutrophil chemoattractant receptors in health and disease: double-edged swords. Cell Mol Immunol. (2020) 17:433–50. doi: 10.1038/s41423-020-0412-0
11. Seiler P, Aichele P, Bandermann S, Hauser AE, Lu B, Gerard NP, et al. Early granuloma formation after aerosol mycobacterium tuberculosis infection is regulated by neutrophils via CXCR3-signaling chemokines. Eur J Immunol. (2003) 33:2676–86. doi: 10.1002/eji.200323956
12. Trentini MM, de Oliveira FM, Kipnis A, Junqueira-Kipnis AP. The role of neutrophils in the induction of specific Th1 and Th17 during vaccination against tuberculosis. Front Microbiol. (2016) 7:898. doi: 10.3389/fmicb.2016.00898
13. Martineau AR, Newton SM, Wilkinson KA, Kampmann B, Hall BM, Nawroly N, et al. Neutrophil-mediated innate immune resistance to mycobacteria. J Clin Invest. (2007) 117:1988–94. doi: 10.1172/JCI31097
14. Miyahara R, Piyaworawong S, Naranbhai V, Prachamat P, Kriengwatanapong P, Tsuchiya N, et al. Predicting the risk of pulmonary tuberculosis based on the neutrophil-to-lymphocyte ratio at TB screening in HIV-infected individuals. BMC Infect Dis. (2019) 19:667. doi: 10.1186/s12879-019-4292-9
15. Eruslanov EB, Lyadova IV, Kondratieva TK, Majorov KB, Scheglov IV, Orlova MO, et al. Neutrophil responses to mycobacterium tuberculosis infection in genetically susceptible and resistant mice. Infect Immun. (2005) 73:1744–53. doi: 10.1128/IAI.73.3.1744-1753.2005
16. Ong CWM, Elkington PT, Brilha S, Ugarte-Gil C, Tome-Esteban MT, Tezera LB, et al. Neutrophil-derived MMP-8 drives AMPK-dependent matrix destruction in human pulmonary tuberculosis. PloS Pathog. (2015) 11:e1004917. doi: 10.1371/journal.ppat.1004917
17. Beyrau M, Bodkin JV, Nourshargh S. Neutrophil heterogeneity in health and disease: a revitalized avenue in inflammation and immunity. Open Biol. (2012) 2:120134. doi: 10.1098/rsob.120134
18. Sagiv JY, Michaeli J, Assi S, Mishalian I, Kisos H, Levy L, et al. Phenotypic diversity and plasticity in circulating neutrophil subpopulations in cancer. Cell Rep. (2015) 10:562–73. doi: 10.1016/j.celrep.2014.12.039
19. Scapini P, Marini O, Tecchio C, Cassatella MA. Human neutrophils in the saga of cellular heterogeneity: insights and open questions. Immunol Rev. (2016) 273:48–60. doi: 10.1111/imr.12448
20. La Manna MP, Orlando V, Paraboschi EM, Tamburini B, Di Carlo P, Cascio A, et al. Mycobacterium tuberculosis drives expansion of low-density neutrophils equipped with regulatory activities. Front Immunol. (2019) 10:2761. doi: 10.3389/fimmu.2019.02761
21. Panteleev AV, Nikitina IY, Burmistrova IA, Kosmiadi GA, Radaeva TV, Amansahedov RB, et al. Severe tuberculosis in humans correlates best with neutrophil abundance and lymphocyte deficiency and does not correlate with antigen-specific CD4 t-cell response. Front Immunol. (2017) 8:96. doi: 10.3389/fimmu.2017.0096
22. Ellis M, Gupta S, Galant S, Hakim S, VandeVen C, Toy C, et al. Impaired neutrophil function in patients with AIDS or AIDS-related complex: a comprehensive evaluation. J Infect Dis. (1988) 158:1268–76. doi: 10.1093/infdis/158.6.1268
23. Roilides E, Mertins S, Eddy J, Walsh TJ, Pizzo PA, Rubin M. Impairment of neutrophil chemotactic and bactericidal function in children infected with human immunodeficiency virus type 1 and partial reversal after in vitro exposure to granulocyte-macrophage colony-stimulating factor. J Pediatr. (1990) 117:531–40. doi: 10.1016/s0022-3476(05)80684-5
24. Elbim C, Prevot MH, Bouscarat F, Franzini E, Chollet-Martin S, Hakim J, et al. Polymorphonuclear neutrophils from human immunodeficiency virus-infected patients show enhanced activation, diminished fMLP-induced l-selectin shedding, and an impaired oxidative burst after cytokine priming. Blood. (1994) 84:2759–66.
25. Flø RW, Naess A, Nilsen A, Harthug S, Solberg CO. A longitudinal study of phagocyte function in HIV-infected patients. AIDS. (1994) 8:771–7. doi: 10.1097/00002030-199406000-00008
26. Guthrie GJK, Charles KA, Roxburgh CSD, Horgan PG, McMillan DC, Clarke SJ. The systemic inflammation-based neutrophil-lymphocyte ratio: experience in patients with cancer. Crit Rev Oncol Hematol. (2013) 88:218–30. doi: 10.1016/j.critrevonc.2013.03.010
27. Naess A, Nilssen SS, Mo R, Eide GE, Sjursen H. Role of neutrophil to lymphocyte and monocyte to lymphocyte ratios in the diagnosis of bacterial infection in patients with fever. Infection. (2017) 45:299–307. doi: 10.1007/s15010-016-0972-1
28. Ndlovu LN, Peetluk L, Moodley S, Nhamoyebonde S, Ngoepe AT, Mazibuko M, et al. Increased neutrophil count and decreased neutrophil CD15 expression correlate with TB disease severity and treatment response irrespective of HIV co-infection. Front Immunol. (2020) 11:1872. doi: 10.3389/fimmu.2020.01872
29. Alemán M, García A, Saab MA, de la Barrera SS, Finiasz M, Abbate E, et al. Mycobacterium tuberculosis-induced activation accelerates apoptosis in peripheral blood neutrophils from patients with active tuberculosis. Am J Respir Cell Mol Biol. (2002) 27:583–92. doi: 10.1165/rcmb.2002-0038OC
30. Opasawatchai A, Amornsupawat P, Jiravejchakul N, Chan-In W, Spoerk NJ, Manopwisedjaroen K, et al. Neutrophil activation and early features of NET formation are associated with dengue virus infection in human. Front Immunol. (2018) 9:3007. doi: 10.3389/fimmu.2018.03007
31. Sack U. CD64 expression by neutrophil granulocytes. Cytometry B Clin Cytom. (2017) 92:189–91. doi: 10.1002/cyto.b.21216
32. Davis RE, Sharma S, Conceição J, Carneiro P, Novais F, Scott P, et al. Phenotypic and functional characteristics of HLA-DR+ neutrophils in brazilians with cutaneous leishmaniasis. J Leukoc Biol. (2017) 101:739–49. doi: 10.1189/jlb.4A0915-442RR
33. Silvestre-Roig C, Fridlender ZG, Glogauer M, Scapini P. Neutrophil diversity in health and disease. Trends Immunol. (2019) 40:565–83. doi: 10.1016/j.it.2019.04.012
34. Lewis SM, Treacher DF, Edgeworth J, Mahalingam G, Brown CS, Mare TA, et al. Expression of CD11c and EMR2 on neutrophils: potential diagnostic biomarkers for sepsis and systemic inflammation. Clin Exp Immunol. (2015) 182:184–94. doi: 10.1111/cei.12679
35. Nuutila J, Hohenthal U, Laitinen I, Kotilainen P, Rajamäki A, Nikoskelainen J, et al. Quantitative analysis of complement receptors, CR1 (CD35) and CR3 (CD11b), on neutrophils improves distinction between bacterial and viral infections in febrile patients: comparison with standard clinical laboratory data. J Immunol Methods. (2006) 315:191–201. doi: 10.1016/j.jim.2006.07.021
36. De Filippo K, Rankin SM. CXCR4, the master regulator of neutrophil trafficking in homeostasis and disease. Eur J Clin Invest. (2018) 48 Suppl 2:e12949. doi: 10.1111/eci.12949
37. Summers C, Rankin SM, Condliffe AM, Singh N, Peters AM, Chilvers ER. Neutrophil kinetics in health and disease. Trends Immunol. (2010) 31:318–24. doi: 10.1016/j.it.2010.05.006
38. Sabroe I, Jones EC, Whyte MKB, Dower SK. Regulation of human neutrophil chemokine receptor expression and function by activation of toll-like receptors 2 and 4. Immunology. (2005) 115:90–8. doi: 10.1111/j.1365-2567.2005.02133
39. Martin C, Burdon PCE, Bridger G, Gutierrez-Ramos JC, Williams TJ, Rankin SM. Chemokines acting via CXCR2 and CXCR4 control the release of neutrophils from the bone marrow and their return following senescence. Immunity. (2003) 19:583–93. doi: 10.1016/s1074-7613(03)00263-2
40. Muthas D, Reznichenko A, Balendran CA, Böttcher G, Clausen IG, Kärrman Mårdh C, et al. Neutrophils in ulcerative colitis: a review of selected biomarkers and their potential therapeutic implications. Scand J Gastroenterol. (2017) 52:125–35. doi: 10.1080/00365521.2016.1235224
41. Furebring M, Håkansson LD, Venge P, Nilsson B, Sjölin J. Expression of the C5a receptor (CD88) on granulocytes and monocytes in patients with severe sepsis. Crit Care. (2002) 6:363–70. doi: 10.1186/cc1524
42. Conway Morris A, Kefala K, Wilkinson TS, Dhaliwal K, Farrell L, Walsh T, et al. C5a mediates peripheral blood neutrophil dysfunction in critically ill patients. Am J Respir Crit Care Med. (2009) 180:19–28. doi: 10.1164/rccm.200812-1928OC
43. Sadallah S, Hess C, Miot S, Spertini O, Lutz H, Schifferli JA. Elastase and metalloproteinase activities regulate soluble complement receptor 1 release. Eur J Immunol. (1999) 29:3754–61. doi: 10.1002/(SICI)1521-4141(199911)29:11<3754::AID-IMMU3754>3.0.CO;2-5
44. Bangani N, Nakiwala J, Martineau AR, Wilkinson RJ, Wilkinson KA, Lowe DM. Brief report: HIV-1 infection impairs CD16 and CD35 mediated opsonophagocytosis of mycobacterium tuberculosis by human neutrophils. J Acquir Immune Defic Syndr. (2016) 73:263–7. doi: 10.1097/QAI.0000000000001103
45. McNab FW, Berry MPR, Graham CM, Bloch SAA, Oni T, Wilkinson KA, et al. Programmed death ligand 1 is over-expressed by neutrophils in the blood of patients with active tuberculosis. Eur J Immunol. (2011) 41:1941–7. doi: 10.1002/eji.201141421
46. Marini O, Costa S, Bevilacqua D, Calzetti F, Tamassia N, Spina C, et al. Mature CD10+ and immature CD10- neutrophils present in g-CSF-treated donors display opposite effects on t cells. Blood. (2017) 129:1343–56. doi: 10.1182/blood-2016-04-713206
47. Vono M, Lin A, Norrby-Teglund A, Koup RA, Liang F, Loré K. Neutrophils acquire the capacity for antigen presentation to memory CD4+ t cells in vitro and ex vivo. Blood. (2017) 129:1991–2001. doi: 10.1182/blood-2016-10-744441
48. van de Vyver A, Delport E, Visser A. Decreased CD10 expression in the bone marrow neutrophils of HIV positive patients. Mediterr J Hematol Infect Dis. (2010) 2:e2010032. doi: 10.4084/MJHID.2010.032
49. Lévy Y, Wiedemann A, Hejblum BP, Durand M, Lefebvre C, Surénaud M, et al. CD177, a specific marker of neutrophil activation, is associated with coronavirus disease 2019 severity and death. iScience. (2021) 24:102711. doi: 10.1016/j.isci.2021.102711
50. Wu Z, Sawamura T, Kurdowska AK, Ji H-L, Idell S, Fu J. LOX-1 deletion improves neutrophil responses, enhances bacterial clearance, and reduces lung injury in a murine polymicrobial sepsis model. Infect Immun. (2011) 79:2865–70. doi: 10.1128/IAI.01317-10
51. Epstein SP, Gadaria-Rathod N, Wei Y, Maguire MG, Asbell PA. HLA-DR expression as a biomarker of inflammation for multicenter clinical trials of ocular surface disease. Exp Eye Res. (2013) 111:95–104. doi: 10.1016/j.exer.2013.03.018
52. Margraf A, Ley K, Zarbock A. Neutrophil recruitment: From model systems to tissue-specific patterns. Trends Immunol. (2019) 40:613–34. doi: 10.1016/j.it.2019.04.010
53. Buckley CD, Ross EA, McGettrick HM, Osborne CE, Haworth O, Schmutz C, et al. Identification of a phenotypically and functionally distinct population of long-lived neutrophils in a model of reverse endothelial migration. J Leukoc Biol. (2006) 79:303–11. doi: 10.1189/jlb.0905496
54. Minns D, Smith KJ, Hardisty G, Rossi AG, Gwyer Findlay E. The outcome of neutrophil-t cell contact differs depending on activation status of both cell types. Front Immunol. (2021) 12:633486. doi: 10.3389/fimmu.2021.633486
55. Margaroli C, Cardenas MA, Jansen CS, Moon Reyes A, Hosseinzadeh F, Hong G, et al. The immunosuppressive phenotype of tumor-infiltrating neutrophils is associated with obesity in kidney cancer patients. Oncoimmunology. (2020) 9:1747731. doi: 10.1080/2162402X.2020.1747731
56. Nguyen Q-T, Nguyen T-HT, Ju S-A, Lee Y-S, Han SH, Lee S-C, et al. CD137 expressed on neutrophils plays dual roles in antibacterial responses against gram-positive and gram-negative bacterial infections. Infect Immun. (2013) 81:2168–77. doi: 10.1128/IAI.00115-13
57. Ogongo P, Tezera LB, Ardain A, Nhamoyebonde S, Ramsuran D, Singh A, et al. Tissue-resident-like CD4+ t cells secreting IL-17 control mycobacterium tuberculosis in the human lung. J Clin Invest. (2021) 131:e142014. doi: 10.1172/JCI142014
58. Gavioli R, Risso A, Smilovich D, Baldissarro I, Capra MC, Bargellesi A, et al. CD69 molecule in human neutrophils: its expression and role in signal-transducing mechanisms. Cell Immunol. (1992) 142:186–96. doi: 10.1016/0008-8749(92)90279-x
59. Hart SP, Ross JA, Ross K, Haslett C, Dransfield I. Molecular characterization of the surface of apoptotic neutrophils: implications for functional downregulation and recognition by phagocytes. Cell Death Differ. (2000) 7:493–503. doi: 10.1038/sj.cdd.4400680
60. Zhang D, Chen G, Manwani D, Mortha A, Xu C, Faith JJ, et al. Neutrophil ageing is regulated by the microbiome. Nature. (2015) 525:528–32. doi: 10.1038/nature15367
61. Sachs UJH, Andrei-Selmer CL, Maniar A, Weiss T, Paddock C, Orlova VV, et al. The neutrophil-specific antigen CD177 is a counter-receptor for platelet endothelial cell adhesion molecule-1 (CD31). J Biol Chem. (2007) 282:23603–12. doi: 10.1074/jbc.M701120200
62. Khan AA, Alsahli MA, Rahmani AH. Myeloperoxidase as an active disease biomarker: Recent biochemical and pathological perspectives. Med Sci (Basel). (2018) 6:33. doi: 10.3390/medsci6020033
63. Lázár-Molnár E, Chen B, Sweeney KA, Wang EJ, Liu W, Lin J, et al. Programmed death-1 (PD-1)-deficient mice are extraordinarily sensitive to tuberculosis. Proc Natl Acad Sci USA. (2010) 107:13402–7. doi: 10.1073/pnas.1007394107
64. Jurado JO, Alvarez IB, Pasquinelli V, Martínez GJ, Quiroga MF, Abbate E, et al. Programmed death (PD)-1:PD-ligand 1/PD-ligand 2 pathway inhibits t cell effector functions during human tuberculosis. J Immunol. (2008) 181:116–25. doi: 10.4049/jimmunol.181.1.116
65. Alvarez IB, Pasquinelli V, Jurado JO, Abbate E, Musella RM, de la Barrera SS, et al. Role played by the programmed death-1-programmed death ligand pathway during innate immunity against mycobacterium tuberculosis. J Infect Dis. (2010) 202:524–32. doi: 10.1086/654932
66. Wang J-F, Wang Y-P, Xie J, Zhao Z-Z, Gupta S, Guo Y, et al. Upregulated PD-L1 delays human neutrophil apoptosis and promotes lung injury in an experimental mouse model of sepsis. Blood. (2021) 138:806–10. doi: 10.1182/blood.2020009417
67. Thanabalasuriar A, Chiang AJ, Morehouse C, Camara M, Hawkins S, Keller AE, et al. PD-L1+ neutrophils contribute to injury-induced infection susceptibility. Sci Adv. (2021) 7:eabd9436. doi: 10.1126/sciadv.abd9436
68. Zhu C-L, Xie J, Zhao Z-Z, Li P, Liu Q, Guo Y, et al. PD-L1 maintains neutrophil extracellular traps release by inhibiting neutrophil autophagy in endotoxin-induced lung injury. Front Immunol. (2022) 13:949217. doi: 10.3389/fimmu.2022.949217
69. Moreira-Teixeira L, Stimpson PJ, Stavropoulos E, Hadebe S, Chakravarty P, Ioannou M, et al. Type i IFN exacerbates disease in tuberculosis-susceptible mice by inducing neutrophil-mediated lung inflammation and NETosis. Nat Commun. (2020) 11:5566. doi: 10.1038/s41467-020-19412-6
70. Condamine T, Dominguez GA, Youn J-I, Kossenkov AV, Mony S, Alicea-Torres K, et al. Lectin-type oxidized LDL receptor-1 distinguishes population of human polymorphonuclear myeloid-derived suppressor cells in cancer patients. Sci Immunol. (2016) 1:aaf8943. doi: 10.1126/sciimmunol.aaf8943
71. Combadière B, Adam L, Guillou N, Quentric P, Rosenbaum P, Dorgham K, et al. LOX-1-Expressing immature neutrophils identify critically-ill COVID-19 patients at risk of thrombotic complications. Front Immunol. (2021) 12:752612. doi: 10.3389/fimmu.2021.752612
72. Ahmed M, Tezera LB, Elkington PT, Leslie AJ. The paradox of immune checkpoint inhibition re-activating tuberculosis. Eur Respir J. (2022) 60:2102512. doi: 10.1183/13993003.02512-2021
73. Kaur M, Singh D. Neutrophil chemotaxis caused by chronic obstructive pulmonary disease alveolar macrophages: the role of CXCL8 and the receptors CXCR1/CXCR2. J Pharmacol Exp Ther. (2013) 347:173–80. doi: 10.1124/jpet.112.201855
74. Yamada M, Kubo H, Kobayashi S, Ishizawa K, He M, Suzuki T, et al. The increase in surface CXCR4 expression on lung extravascular neutrophils and its effects on neutrophils during endotoxin-induced lung injury. Cell Mol Immunol. (2011) 8:305–14. doi: 10.1038/cmi.2011.8
75. Privratsky JR, Newman DK, Newman PJ. PECAM-1: conflicts of interest in inflammation. Life Sci. (2010) 87:69–82. doi: 10.1016/j.lfs.2010.06.001
76. Robinson E, Herbert JA, Palor M, Ren L, Larken I, Patel A, et al. Trans-epithelial migration is essential for neutrophil activation during RSV infection. J Leukoc Biol. (2023) 113:354–64. doi: 10.1093/jleuko/qiad011
77. Ji J, Fan J. Neutrophil in reverse migration: Role in sepsis. Front Immunol. (2021) 12:656039. doi: 10.3389/fimmu.2021.656039
78. Eum S-Y, Kong J-H, Hong M-S, Lee Y-J, Kim J-H, Hwang S-H, et al. Neutrophils are the predominant infected phagocytic cells in the airways of patients with active pulmonary TB. Chest. (2010) 137:122–8. doi: 10.1378/chest.09-0903
79. Das B, Kashino SS, Pulu I, Kalita D, Swami V, Yeger H, et al. CD271(+) bone marrow mesenchymal stem cells may provide a niche for dormant mycobacterium tuberculosis. Sci Transl Med. (2013) 5:170ra13. doi: 10.1126/scitranslmed.3004912
80. Pérez-Figueroa E, Álvarez-Carrasco P, Ortega E. Crosslinking of membrane CD13 in human neutrophils mediates phagocytosis and production of reactive oxygen species, neutrophil extracellular traps and proinflammatory cytokines. Front Immunol. (2022) 13:994496. doi: 10.3389/fimmu.2022.994496
81. Wang X, Li Z-Y, Zeng L, Zhang A-Q, Pan W, Gu W, et al. Neutrophil CD64 expression as a diagnostic marker for sepsis in adult patients: a meta-analysis. Crit Care. (2015) 19:245. doi: 10.1186/s13054-015-0972-z
82. Corrêa R da S, Rodrigues LS, Pereira LHL, Nogueira OC, Leung J, Sousa M da S, et al. Neutrophil CD64 expression levels in IGRA-positive individuals distinguish latent tuberculosis from active disease. Mem Inst Oswaldo Cruz. (2019) 114:e180579. doi: 10.1590/0074-02760180579
83. Nancy Hilda J, Das S. Neutrophil CD64, TLR2 and TLR4 expression increases but phagocytic potential decreases during tuberculosis. Tuberculosis (Edinb). (2018) 111:135–42. doi: 10.1016/j.tube.2018.06.010
Keywords: neutrophil, tuberculosis, HIV, blood, sputum, PDL-1
Citation: Nhamoyebonde S, Chambers M, Ndlovu L, Karim F, Mazibuko M, Mhlane Z, Madziwa L, Moosa Y, Moodley S, Hoque M and Leslie A (2024) Detailed phenotyping reveals diverse and highly skewed neutrophil subsets in both the blood and airways during active tuberculosis infection. Front. Immunol. 15:1422836. doi: 10.3389/fimmu.2024.1422836
Received: 24 April 2024; Accepted: 21 May 2024;
Published: 14 June 2024.
Edited by:
Nathalie Winter, Institut National de recherche pour l’agriculture, l’alimentation et l’environnement (INRAE), FranceReviewed by:
Danielle Oliveira Nascimento, Federal Rural University of Rio de Janeiro, BrazilDebora Decote-Ricardo, Federal Rural University of Rio de Janeiro, Brazil
Copyright © 2024 Nhamoyebonde, Chambers, Ndlovu, Karim, Mazibuko, Mhlane, Madziwa, Moosa, Moodley, Hoque and Leslie. This is an open-access article distributed under the terms of the Creative Commons Attribution License (CC BY). The use, distribution or reproduction in other forums is permitted, provided the original author(s) and the copyright owner(s) are credited and that the original publication in this journal is cited, in accordance with accepted academic practice. No use, distribution or reproduction is permitted which does not comply with these terms.
*Correspondence: Alasdair Leslie, al.leslie@ahri.org