- 1Department of Medicine, University of Colorado Denver, Aurora, CO, United States
- 2Department of Research, Rocky Mountain Regional Veteran Affairs (VA) Medical Center, Aurora, CO, United States
Decades of evidence positioned IL-1β as a master regulatory cytokine in acute and chronic inflammatory diseases. Approved biologics aimed at inhibiting IL-1 signaling have shown efficacy but variable safety. More recently, targeting NLRP3 activation, an upstream mediator of IL-1β, has garnered the most attention. Aberrant NLRP3 activation has been demonstrated to participate in the progression of several pathological conditions from neurogenerative diseases to cardio-metabolic syndromes and cancer. Pharmacological and genetic strategies aimed to limit NLRP3 function have proven effective in many preclinical models of diseases. These evidences have lead to a significant effort in the generation and clinical testing of small orally active molecules that can target NLRP3. In this report, we discuss different properties of these molecules with translational potential and describe the technologies currently available to screen NLRP3 targeting molecules highlighting advantages and limitations of each method.
Introduction
NLRP3 belongs to the nucleotide-binding oligomerization domain (NOD)-like receptor (NLR) family of intracellular pattern-recognition receptors that sense infections or cellular perturbations. Stimulation of NLRP3, similarly to other NLRs, leads to the formation of intracellular macromolecular complexes termed inflammasomes. The initial event in the inflammasome formation process is the oligomerization of NLRP3 through homotypic interactions between NACHT domains with ATPase activity. The enzymatic activity of NLRP3’s NACHT domain has been shown to be vital for NLRP3 self-association and function (1). Next, is the recruitment of the inflammasome adaptor protein ASC [apoptosis-associated speck-like protein containing a caspase activation and recruitment domain (CARD)] through the PYD–PYD domain interactions. CARD-CARD domain interactions between ASC and caspase-1 mediate self-cleavage and activation of the inflammasome effector molecule caspase-1, which is responsible for the processing of the biologically inactive pro-IL-1β and pro-IL-18 into their biological active forms (2). Although NLRP3 function is critical for the host defense against infections, dysregulated NLRP3 activity leads to tissue damage. Currently, significant efforts are placed on investigations of the role of NLRP3 in disease progression, especially in sterile conditions like Alzheimer’s, Parkinson’s, cardio-metabolic syndromes and cancer where chronic inflammation has proven to mediate disease progression. This interest in NLRP3 is exemplified by the remarkable increase in scientific publications centered on this protein. In the past 5 years (2019–2023) 13’602 articles have been published on NLRP3 compared to the 4’347 articles published between 2014-2018 and the 1’212 listed from 2009 and 2013. This is likely driven by the attractive prospects of targeting NLRP3 for therapeutic development with small orally active molecules. In fact, numerous NLRP3 inhibitors with different chemotypes have been described and tested. This article reviews properties of these molecules and comments on current technologies to design and screen for potential candidates.
NLRP3 in health and disease
Activation of NLRP3 is triggered by a diverse set of endogenous and pathogen-associated stimuli. This large spectrum of activation signals posits NLRP3 as a prominent sensor of cellular and tissue homeostasis, thereby affording NLRP3 a crucial role in host defense and disease.
For example, NLRP3 activation is necessary for initiating immune responses required for resolving infections (3–8). On the other hand, NLRP3 activation promotes age-related chronic inflammatory diseases through production of IL-1β. Here, NLRP3 is activated through endogenous signals in a tissue specific manner in rheumatological, metabolic, neurodegenerative, cardiovascular diseases and cancer.
Evidence for the driving role of NLRP3 and NLRP3-mediated IL-1β production in the progression of cardiovascular disease was demonstrated in the CANTOS study, a randomized placebo-controlled and world-wide study in 10,067 patients with atherosclerosis. In this study, IL-1β neutralization with the anti-IL-1β antibody canakinumab reduced secondary cardiovascular events (9).
In preclinical models of neurogenerative diseases, such as Alzheimer and Parkinson’s it has been shown that NLRP3 activation deteriorates cognitive and motor function in mice (10–12). Interestingly, recent investigations suggested a dispensable role for NLRP3 and caspase-1 in mouse models of Alzheimer’s (13). As also discussed by the authors, experimental differences in the model used including strain and age of the animals at analysis could have impacted the different outcomes. Another important example for the contribution of NLRP3 in the progression of sterile inflammation is in cancer. A pathological role for NLRP3/IL-1β has been demonstrated in several cancer models by promoting immunosuppression, tumor progression and reduced survival (14). The role of NLRP3 in other sterile conditions has been more extensively reviewed elsewhere (15–17). The consequences of deleterious NLRP3 activation are best demonstrated from patients with gain-of-function mutations in NLRP3. Cryopyrin-associated periodic syndromes (CAPS) are autoinflammatory diseases characterized by recurrent episodes of fever and systemic inflammation. These rare inherited autosomal dominant mutations in NLRP3 diseases are grouped in three different clinical phenotypes: neonatal-onset multisystem inflammatory disease/chronic infantile neurologic cutaneous articular syndrome (NOMID/CINCA) being the most severe form, Muckle–Wells syndrome (MWS) the intermediate phenotype and familial cold autoinflammatory syndrome (FCAS) the mildest form (18). IL-1 inhibitory agents have been shown to be effective in managing the clinical symptoms of these conditions. At present, NLRP3 inhibitors are under investigation in these rare diseases (Table 1).
Clinical development perspectives
The significant body of preclinical and clinical evidence of disease-promoting functions for NLRP3 and IL-1β has defined the rationale for testing NLRP3 inhibition in humans. NLRP3 inhibition presents several advantages compared to blocking IL-1 activity directly, most promising being the emergence of orally available NLRP3 inhibitors. Although numerous NLRP3 antagonist have been developed, no NLRP3 inhibitor has been approved in humans. As of today, several molecules are being tested in clinical trials. To the best of our knowledge, Table 1 summarizes current clinical trials using specific NLRP3 inhibitors. Although these diseases have very different etiology, the NLRP3/IL-1 dependent inflammatory onset can be considered as common ground. Contrary to acute conditions, the efficacy of NLRP3 inhibition for the treatment of chronic diseases, such as metabolic, cardiovascular, cancer, and neurodegenerative disorders, will require treatment for long dosing duration. While no comparative clinical data are available yet, the pharmacology of the NLRP3 inhibitor class suggest to have increased safety compared to anti-IL-1 biologics. This would be due, in part, to increased specificity and shorter half-life. Increased specificity afforded by directly blocking NLRP3 would not prevent activation of the other members of the NOD family, therefore, the risk of opportunist infection reported with anti-IL-1 biologics should be more limited with NLRP3 inhibitors. Shorter half-life could be advantageous in scenarios where immunosuppression can be detrimental. Immune modulation becomes particularly relevant in patients with cancer or undergoing bone marrow transplant that are severe immunocompromised. Anti-IL-1 biologics, albeit extremely efficient, have a mean terminal half-life up to 26 days (19). Whereas, NLRP3 inhibitors have a significantly shorter half-life depending on the molecule, therefore, treatment can be interrupted without lingering effect. In clinical trials reported to date for VTX2735, developed by Ventyx and dapansutrile developed by Olatec, treatment has generally been well tolerated with minimal side effects (NCT05812781; NCT03534297). Common side effects included mild gastrointestinal symptoms and mild to moderate headaches. Since NLRP3 inhibitors suppress parts of the immune response, there is a theoretical risk of increased susceptibility to infections. However, specific data on increased infection rates in patients treated with NLRP3 inhibitors are not yet available. The long-term safety profile of these molecules is still being evaluated. Ongoing trials (Table 1) are expected to provide more detailed safety information over extended periods. Overall, while the safety profile of NLRP3 inhibitors appear favorable in early-stage trials, ongoing monitoring and larger studies are necessary to fully understand their long-term safety and potential adverse effects.
Available technologies to screen NLRP3 inhibitors
Given the reported activity of NLRP3 in promoting the progression of several diseases, significant efforts have been devoted to developing effective NLRP3 inhibitors. This has led to a need for screening tests to evaluate potential candidates. Currently, the specificity and affinity of potential NLRP3 inhibitors can be tested in various ways. Here, we summarize and discuss the existing and recent advances in methodologies available for studying NLRP3 inhibitors.
Cytokine measurement
Immunoassays determine the concentration of molecules in cell supernatants or bodily fluid. These techniques can be used to quantify the levels of cytokines, antibodies, antigens, proteins, lipids and hormones in body fluid or samples. The most common and versatile immunoassay is the enzyme-linked immunosorbent assay or ELISA. ELISAs are highly specific for measuring mature IL-1β and IL-18 following NLRP3 inflammasome induction, for example using LPS and ATP or LPS and nigericin stimulations. For samples limited by low volume or that require multiple cytokine readouts, multiplex systems allow multiple cytokine readouts with less than 50µl per sample. As of today, many commercially and user friendly available platforms have been developed. IL-1β and IL-18, as well as other cytokines, may also be assessed in real-time or living cells, by using the so called “Lumit” system (20). This immunoassay approach relies on labeled antibodies and a detention reagent that generate luminescent which can be detected in living cells or supernatants without requiring media removal or lysate transfer.
Caspase-1 detection
Caspase-1 is the inflammasome effector enzyme responsible of the conversion of pro-IL-1β and pro-IL-18 into their respective biologically active forms. Therefore, a common measurement of inflammasome formation is detecting caspase-1 activity. Similar to IL-1β and IL-18, caspase-1 is originally synthesized as a precursor form which is activated by autocatalytic cleavage. The conversion of pro-caspase-1 into active caspase-1 can be determined by standard molecular technology like western blotting, measuring the levels of pro-caspase-1 (45KDa) and active caspase-1 (10 and 20KDa). Caspase-1 activation can be also measured by the addition to the testing system of a specific caspase-1 substrate, that once cleaved, releases measurable luminescence (21).
ASC specks
Following activation of NLRP3, recruitment of the inflammasome adaptor protein apoptosis-associated speck-like protein containing a CARD (ASC) is initiated. ASC self-associates, forming the so-called ASC specks or pyroptosome that can be used a readout for inflammasome formation (22–24). While the intracellular formation of ASC specks has been well described and documented, more recently it has been demonstrated that these structures can be also be detected extracellularly (25). This is particularly relevant in the context of clinical trials due to the increased feasibility of measuring ASC specks in plasma and cerebrospinal fluid rather than tissues.
Fluorescent resonance energy transfer
Another way of determining inflammasome formation is the determination of the association of the inflammasome sensor with the adaptor and/or the effector protein. A common technique used to achieve this goal is fluorescent resonance energy transfer (FRET) analysis. This technology permits the determination of the proximity between two molecules, for example NLRP3 with ASC and/or NLRP3 with caspase-1, within several nanometers, a distance sufficiently close for molecular interactions to occur. Typical fluorescence microscopy techniques rely upon the absorption by a fluorophore of light at one wavelength (excitation), followed by the subsequent emission of secondary fluorescence at a longer wavelength. Fluorescence resonance energy transfer is a process by which radiationless transfer of energy occurs from an excited state fluorophore to a second chromophore in close proximity. Because the range over which the energy transfer can take place is limited to approximately 10 nanometers (100 angstroms), and the efficiency of transfer is extremely sensitive to the separation distance between fluorophores, resonance energy transfer measurements can be a valuable tool for probing molecular interactions (26). Differently from measuring IL-1β and IL-18 processing, ASC specks formation and caspase-1 activity, FRET allows to determine directly the proximity of a specific inflammasome sensor with its adaptor protein increasing the specificity of the analysis.
NLRP3-engament assay NanoBRET
Albeit the majority of the techniques described are reliable tools to measure inflammasome activity, they are not necessarily specific for the NLRP3 inflammasome considering that ASC specks formation and caspase-1-mediated processing of IL-1β and IL-18 shared pathways among different NOD sensors.
More recently, the NLRP3 NanoBRET target engagement (TE) assay has been described as a method to measure direct NLRP3 engagement (27). Briefly, to quantify NLRP3 target occupancy, this assay uses an NLRP3-Nanuluc construct transfected into HEK293 cells, and a fluorescent tracer based on the structure of MCC950, an NLRP3 inhibitor that binds directly to the NACHT domain of NLRP3 (28–30). MCC950 is the most used preclinical NLRP3 inhibitor at the present time. Numerous studies have demonstrated the efficacy of MCC950 in a wide range of NLRP3-mediated inflammatory diseases. Mechanistically, MCC950 binds to NLRP3 with high affinity (KD=224nM) (29). In their screening assay, Teske et al. confirmed that MCC950 is a specific and potent NLRP3 inhibitor. The authors also tested six other NLRP3 inhibitors and concluded that of the seven NLRP3 inhibitor tested, one (Cy09) showed only partial engagement, and two (OXSI-2 and OLT1177) failed to show direct antagonism. These three compounds (Cy09, OXSI-2 and OLT1177) have extensive investigations demonstrating their inhibition on NLRP3 activation and NLRP3 inflammasome formation. Of these three, we are particularly familiar with OLT1177® (dapansutrile). Our group and other independent investigations have demonstrated that dapansutrile is a specific NLRP3 inhibitor. From the large body of evidence available, we emphasize that dapansutrile reduces NLRP3’s ATPase activity and directly binds NLRP3 with a KD of 1.2µM (31, 32). Compared to MCC950, dapansutrile has a lower affinity binding (0.224µM vs 1.2µM respectively) impacting the degree of inhibition, as also shown by our group in direct comparisons between the two molecules (31, 33). Since the NLRP3 NanoBRET TE assay is based on MCC950, we question the relevance of the assay for non-sulfonylurea chemotype based NLRP3 inhibitors with lower affinity binding. In this regard, we emphasize that such differences in chemotype and lower affinity are not a limitation but rather a safety advantage for clinical use in humans. Contrary to MCC950, non-sulfonylurea compounds like dapansutrile has shown a remarkable safe clinical profile and is conducting large phase 2/3 trials (Table 1) (31, 34, 35). As previously discussed in this article, with the expanding examples of the pathological role of NLRP3 in several chronic diseases, there is a clear need for safe NLRP3 inhibitors.
Teske et al. added to their TE assay the testing of the inhibitors on IL-1β and caspase-1 processing. Similar to what they observed in the TE assay, the authors reported no activity for OXSI-2 and OLT1177 on the processing of IL-1β and caspase-1 in J774A.1 cells, a macrophage cell line commonly used to test NLRP3 activity. We measured IL-1β levels using the same detection assay used by the authors under canonical NLRP3 stimulation (LPS 4 hours at 1µg/ml and nigericin 10µM for 60 minutes) as well as priming the cells overnight (LPS 500ng/ml) as performed by the authors. Each of the NLRP3 inhibitors were tested at 10µM. Contrary to the data reported by Teske et al, both OXSI-2 and dapansutrile decreased IL-1β secretion as detected by the Lumit Mouse IL-1β Immunoassay (Figures 1A, B). Western blotting analysis exhibited a reduction in the levels of active caspase-1 (p20) by dapansutrile when compared to cells treated with LPS and nigericin (Figure 1C). Consistent with the Teske et al, and our previous data, MCC950 showed the strongest inhibition (Figure 1).
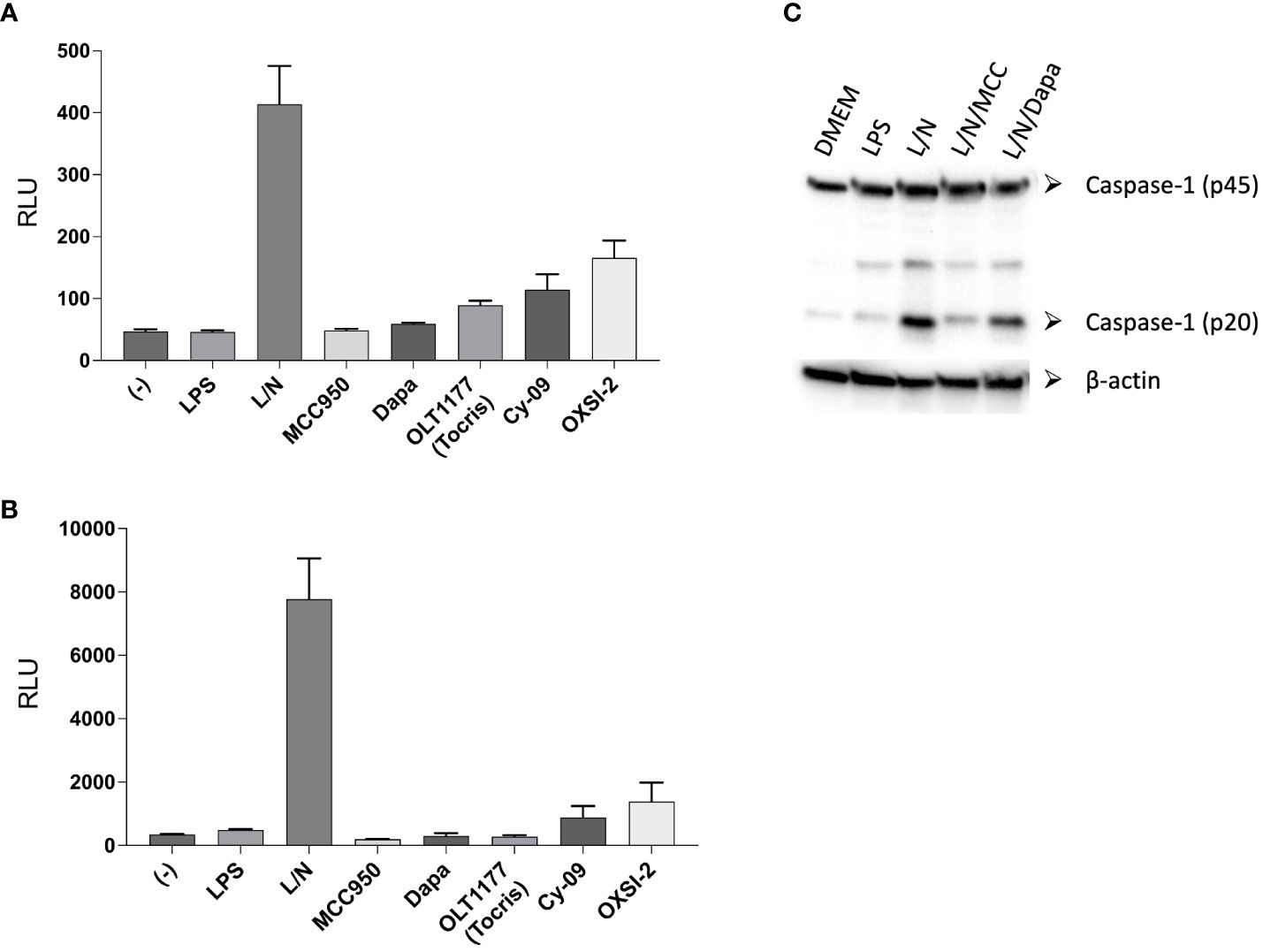
Figure 1. Lumit™ Mouse IL-1β Immunoassay under NLRP3 activating conditions. (A, B) J774A.1 cells were stimulated with LPS for 4 hours (A) or overnight (B) and nigericin for 60 minutes. NLRP3 inhibitors were added before nigericin. Lumit immunoassay was ran on whole cell culture as per manufacturer’s instructions. (C) Western blots for caspase-1 (p20 and p45) of cell lysates from J774A.1 cells stimulated with LPS and nigericin in the presence of MCC950 (MCC) and dapansutrile (Dapa).
As a result, although we recognize the potential of the TE method under certain conditions, the proposed applications of the TE assay have significant limitations. Regarding the effect of OLT1177 and OXSI-2 on NLRP3 activity, we report data that confirmed a significant reduction in NLRP3 activity using the same conditions used by Teske et al. Moreover, for dapansutrile the large available body of clinical and preclinical evidence strongly support that dapansutrile is a specific NLRP3 inhibitor.
Discussion
NLRP3 is emerging as an attractive therapeutic target to modulate the IL-1-mediated inflammation in several acute and chronic conditions. This is made possible by the development of specific NLRP3 inhibitors that present increased specificity compared to anti-IL-1 biologics and oral bioviability. To date, the most common chemotype for NLRP3 inhibitors is sulfonylurea compounds that have shown high potency and affinity binding but safety limitations. On the contrary, clinical and preclinical evidence suggest that non- sulfonylurea compounds present lower affinity but increased safety profile. Considering the reported role of NLRP3 in chronic conditions such as cancer, cardio-metabolic and neurodegenerative diseases, we believe this is particularly relevant considering the requirement of long-term treatment. With the increased interest in the development of effective and safe NLRP3 inhibitors, new specific and reliable screening tools are a clear need for the field. Albeit new technologies have been proposed (i.e. NLRP3 NanoBRET) they come with relevant caveats that could limit their use for non- sulfonylurea compounds. In this context, we recognize the need for additional technologies for functional and reliable screening assays for testing new anti-NLRP3 compounds entering this rapidly expanding field.
Data availability statement
The raw data supporting the conclusions of this article will be made available by the authors, without undue reservation.
Ethics statement
Ethical approval was not required for the studies on animals in accordance with the local legislation and institutional requirements because only commercially available established cell lines were used.
Author contributions
IT: Investigation, Formal analysis, Data curation, Writing – review & editing, Writing – original draft, Methodology, Conceptualization. MB: Writing – review & editing, Methodology, Investigation, Formal analysis. CD: Writing – review & editing, Writing – original draft, Visualization, Supervision, Conceptualization. CM: Methodology, Writing – review & editing, Writing – original draft, Visualization, Supervision, Conceptualization.
Funding
The author(s) declare financial support was received for the research, authorship, and/or publication of this article. These studies were supported by Wings of Hope for Pancreatic Cancer Research to CM; American Cancer Society (ACSIRG#22-154-59) to CM; Cancer League of Colorado (AW#240395) to CM and VA-BX00614701A2 to CM.
Conflict of interest
IT serves as consultant for Olatec and receives compensation. CD serves as Chairman of Olatec’s Scientific Advisory Board, is co-Chief Scientific Officer, receives compensation, and has equity in Olatec. CM serves as Director for Olatec’s Innovative Science Program and has equity in Olatec.
The remaining author declares that the research was conducted in the absence of any commercial or financial relationships that could be construed as a potential conflict of interest.
The author(s) declared that they were an editorial board member of Frontiers, at the time of submission. This had no impact on the peer review process and the final decision.
Publisher’s note
All claims expressed in this article are solely those of the authors and do not necessarily represent those of their affiliated organizations, or those of the publisher, the editors and the reviewers. Any product that may be evaluated in this article, or claim that may be made by its manufacturer, is not guaranteed or endorsed by the publisher.
References
1. Duncan JA, Bergstralh DT, Wang Y, Willingham SB, Ye Z, Zimmerman AG, et al. Cryopyrin/NALP3 binds ATP/dATP, is an ATPase, and requires ATP binding to mediate inflammatory signaling. Proc Natl Acad Sci U.S.A. (2007) 104:8041–6. doi: 10.1073/pnas.0611496104
2. Swanson KV, Deng M, Ting JP. The NLRP3 inflammasome: molecular activation and regulation to therapeutics. Nat Rev Immunol. (2019) 19:477–89. doi: 10.1038/s41577-019-0165-0
3. Kumar H, Kumagi Y, Tsuchida T, Koenig PA, Satoh T, Guo Z, et al. Involvement of the NLRP3 inflammasome in innate and humoral adaptive immune responses to fungal beta-glucan. J Immunol. (2009) 183:8061–7. doi: 10.4049/jimmunol.0902477
4. Lamkanfi M, Malireddi RK, Kanneganti TD. Fungal zymosan and mannan activate the cryopyrin inflammasome. J Biol Chem. (2009) 284:20574–81. doi: 10.1074/jbc.M109.023689
5. Allen IC, Scull MA, Moore CB, Holl EK, McElvania-TelKippe E, Taxman DJ, et al. The NLRP3 inflammasome mediates in vivo innate immunity to influenza A virus through recognition of viral RNA. Immunity. (2009) 30:556–65. doi: 10.1016/j.immuni.2009.02.005
6. Kanneganti TD, Body-Malapel M, Amer A, Park JH, Whitfield J, Franchi L, et al. Critical role for Cryopyrin/Nalp3 in activation of caspase-1 in response to viral infection and double-stranded RNA. J Biol Chem. (2006) 281:36560–8. doi: 10.1074/jbc.M607594200
7. Ichinohe T, Lee HK, Ogura Y, Flavell R, Iwasaki A. Inflammasome recognition of influenza virus is essential for adaptive immune responses. J Exp Med. (2009) 206:79–87. doi: 10.1084/jem.20081667
8. Thomas PG, Dash P, Aldridge JR, Ellebedy AH, Reynolds C, Funk AJ, et al. The intracellular sensor NLRP3 mediates key innate and healing responses to influenza A virus via the regulation of caspase-1. Immunity. (2009) 30:566–75. doi: 10.1016/j.immuni.2009.02.006
9. Ridker PM, Everett BM, Thuren T, MacFayden JG, Chang WH, Ballantyne C, et al. Antiinflammatory therapy with canakinumab for atherosclerotic disease. N Engl J Med. (2017) 377:1119–31. doi: 10.1056/NEJMoa1707914
10. Lonnemann N, Hosseini S, Marchetti C, Skouras DB, Stefanoni D, D'Alessandro A, et al. The NLRP3 inflammasome inhibitor OLT1177 rescues cognitive impairment in a mouse model of Alzheimer's disease. Proc Natl Acad Sci USA. (2020) 117:32145–54. doi: 10.1073/pnas.2009680117
11. Heneka MT, Kummer MP, Stutz A, Delekate A, Schwartz S, Viera-Saecker A, et al. NLRP3 is activated in Alzheimer's disease and contributes to pathology in APP/PS1 mice. Nature. (2013) 493:674–8. doi: 10.1038/nature11729
12. Amo-Aparicio J, Daly J, Hojen JF, Dinarello CA. Pharmacologic inhibition of NLRP3 reduces the levels of alpha-synuclein and protects dopaminergic neurons in a model of Parkinson's disease. J Neuroinflamm. (2023) 20:147. doi: 10.1186/s12974-023-02830-w
13. Srinivasan S, Kancheva D, De Ren S, Saito T, Jans M, Boone F, et al. Inflammasome signaling is dispensable for β-amyloid-induced neuropathology in preclinical models of Alzheimer's disease. Front Immunol. (2024) 15:1323409. doi: 10.3389/fimmu.2024.1323409
14. Tengesdal IW, Dinarello CA, Marchetti C. NLRP3 and cancer: Pathogenesis and therapeutic opportunities. Pharmacol Ther. (2023) 251:108545. doi: 10.1016/j.pharmthera.2023.108545
15. Heneka MT, Kummer MP, Latz E. Innate immune activation in neurodegenerative disease. Nat Rev Immunol. (2014) 14:463–77. doi: 10.1038/nri3705
16. Abbate A, Toldo S, Marchetti C, Kron J, Van Tassell BW, Dinarello CA. Interleukin-1 and the inflammasome as therapeutic targets in cardiovascular disease. Circ Res. (2020) 126:1260–80. doi: 10.1161/CIRCRESAHA.120.315937
17. Dinarello CA. The IL-1 family of cytokines and receptors in rheumatic diseases. Nat Rev Rheumatol. (2019) 15:612–32. doi: 10.1038/s41584-019-0277-8
18. Moltrasio C, Romagnuolo M, Marzano AV. NLRP3 inflammasome and NLRP3-related autoinflammatory diseases: From cryopyrin function to targeted therapies. Front Immunol. (2022) 13:1007705. doi: 10.3389/fimmu.2022.1007705
20. Brian Hwang B, Alves J, Lazar D, Nath N, Engel L, O'Brien M, et al. Lumit: A homogeneous bioluminescent immunoassay for detecting diverse analytes and intracellular protein targets. Methods Mol Biol. (2023) 2612:195–224. doi: 10.1007/978-1-0716-2903-1_15
21. O'Brien M, Moehring D, Munoz-Planillo R, Nunez G, Callaway J, Ting J, et al. A bioluminescent caspase-1 activity assay rapidly monitors inflammasome activation in cells. J Immunol Methods. (2017) 447:1–13. doi: 10.1016/j.jim.2017.03.004
22. Lu A, Magupalli VG, Ruan J, Yin Q, Atianand MK, Vos MR, et al. Unified polymerization mechanism for the assembly of ASC-dependent inflammasomes. Cell. (2014) 156:1193–206. doi: 10.1016/j.cell.2014.02.008
23. Fernandes-Alnemri T, Wu J, Yu JW, Datta P, Miller B, Jankowski W, et al. The pyroptosome: a supramolecular assembly of ASC dimers mediating inflammatory cell death via caspase-1 activation. Cell Death Differ. (2007) 14:1590–604. doi: 10.1038/sj.cdd.4402194
24. Stutz A, Horvath GL, Monks BG, Latz E. ASC speck formation as a readout for inflammasome activation. Methods Mol Biol. (2013) 1040:91–101. doi: 10.1007/978-1-62703-523-1_8
25. Franklin BS, Latz E, Schmidt FI. The intra- and extracellular functions of ASC specks. Immunol Rev. (2018) 281:74–87. doi: 10.1111/imr.12611
26. Sekar RB, Periasamy A. Fluorescence resonance energy transfer (FRET) microscopy imaging of live cell protein localizations. J Cell Biol. (2003) 160:629–33. doi: 10.1083/jcb.200210140
27. Teske KA, Corona C, Wilkinson J, Mamott D, Good DA, Zambrano D, et al. Interrogating direct NLRP3 engagement and functional inflammasome inhibition using cellular assays. Cell Chem Biol. (2024) 31:349–360 e346. doi: 10.1016/j.chembiol.2023.09.016
28. Tapia-Abellan A, Angosto-Bazarra D, Martinez-Banaclocha H, de Torre-Minguela C, Ceron-Carrasco JP, Perez-Sanchez H, et al. MCC950 closes the active conformation of NLRP3 to an inactive state. Nat Chem Biol. (2019) 15:560–4. doi: 10.1038/s41589-019-0278-6
29. Coll RC, Hill JR, Day CJ, Zamoshnikova A, Boucher D, Massey NL, et al. MCC950 directly targets the NLRP3 ATP-hydrolysis motif for inflammasome inhibition. Nat Chem Biol. (2019) 15:556–9. doi: 10.1038/s41589-019-0277-7
30. Coll RC, Robertson AA, Chae JJ, Higgins SC, Munoz-Planillo R, Inserra MC, et al. A small-molecule inhibitor of the NLRP3 inflammasome for the treatment of inflammatory diseases. Nat Med. (2015) 21:248–55. doi: 10.1038/nm.3806
31. Marchetti C, Swartzwelter B, Gamboni F, Neff CP, Richter K, Azam T, et al. OLT1177, a beta-sulfonyl nitrile compound, safe in humans, inhibits the NLRP3 inflammasome and reverses the metabolic cost of inflammation. Proc Natl Acad Sci U.S.A. (2018) 115:E1530–9. doi: 10.1073/pnas.1716095115
32. Tengesdal IW, Menon DR, Osborne DG, Neff CP, Powers NE, Gamboni F, et al. Targeting tumor-derived NLRP3 reduces melanoma progression by limiting MDSCs expansion. Proc Natl Acad Sci USA. (2021) 118:e2000915118. doi: 10.1073/pnas.2000915118
33. Amo-Aparicio J, Dominguez A, Atif SM, Dinarello A, Azam T, Alula KM, et al. Pancreatic ductal adenocarcinoma cells regulate NLRP3 activation to generate a tolerogenic microenvironment. Cancer Res Commun. (2023) 3:1899–911. doi: 10.1158/2767-9764.CRC-23-0065
34. Wohlford GF, Van Tassell BW, Billingsley HE, Kadariya D, Canada JM, Carbone S, et al. A phase IB, randomized, double-blinded, dose escalation, single center, repeat-dose safety and pharmacodynamics study of the oral NLRP3 inhibitor dapansutrile in subjects with NYHA II-III systolic heart failure. J Cardiovasc Pharmacol. (2020) 77:49–60. doi: 10.1097/FJC.0000000000000931
35. Kluck V, Jansen T, Janssen M, Comarinuceanu A, Efde M, Tengesdal IW, et al. Dapansutrile, an oral selective NLRP3 inflammasome inhibitor, for treatment of gout flares: an open-label, dose-adaptive, proof-of-concept, phase 2a trial. Lancet Rheumatol. (2020) 2:e270–80. doi: 10.1016/S2665-9913(20)30065-5
Keywords: NLRP3, small molecule inhibitor, inflammasome, IL-1, screening method
Citation: Tengesdal IW, Banks M, Dinarello CA and Marchetti C (2024) Screening NLRP3 drug candidates in clinical development: lessons from existing and emerging technologies. Front. Immunol. 15:1422249. doi: 10.3389/fimmu.2024.1422249
Received: 23 April 2024; Accepted: 11 July 2024;
Published: 30 July 2024.
Edited by:
Jonathan A. Harton, Albany Medical College, United StatesReviewed by:
Ivana Kawikova, University of Hartford, United StatesCopyright © 2024 Tengesdal, Banks, Dinarello and Marchetti. This is an open-access article distributed under the terms of the Creative Commons Attribution License (CC BY). The use, distribution or reproduction in other forums is permitted, provided the original author(s) and the copyright owner(s) are credited and that the original publication in this journal is cited, in accordance with accepted academic practice. No use, distribution or reproduction is permitted which does not comply with these terms.
*Correspondence: Carlo Marchetti, carlo.marchetti@cuanschutz.edu