- 1Department of Gastrointestinal Surgery, Union Hospital, Tongji Medical College, Huazhong University of Science and Technology, Wuhan, China
- 2Tongji Medical College, Huazhong University of Science and Technology, Wuhan, China
- 3Tianjin Key Laboratory of Technologies Enabling Development of Clinical Therapeutics and Diagnostics, School of Pharmacy, Tianjin Medical University, Tianjin, China
- 4Department of Oncology, Tongji Hospital, Tongji Medical College, Huazhong University of Science and Technology, Wuhan, China
Esophageal cancer (EC), a common type of malignant tumor, ranks as the sixth highest contributor to cancer-related mortality worldwide. Due to the condition that most patients with EC are diagnosed at advanced or metastatic status, the efficacy of conventional treatments including surgery, chemotherapy and radiotherapy is limited, resulting in a dismal 5-year overall survival rate. In recent years, the application of immune checkpoint inhibitors (ICIs) has presented a novel therapeutic avenue for EC patients. Both ICIs monotherapy and immunotherapy combined with chemotherapy or chemoradiotherapy (CRT) have demonstrated marked benefits for patients with advanced EC. Adjuvant or neoadjuvant therapy incorporating immunotherapy has also demonstrated promising prospects in the context of perioperative treatment. Nonetheless, due to the variable response observed among patients undergoing immunotherapy, it is of vital importance to identify predictive biomarkers for patient stratification, to facilitate identification of subgroups who may derive greater benefits from immunotherapy. In this review, we summarize validated or potential biomarkers for immunotherapy in EC in three dimensions: tumor-cell-associated biomarkers, tumor-immune microenvironment (TIME)-associated factors, and host-associated biomarkers, so as to provide a theoretical foundation to inform tailored therapy for individuals diagnosed with EC.
1 Introduction
Esophageal cancer (EC), including esophageal squamous cell carcinoma (ESCC) and esophageal adenocarcinoma (EAC), ranks as the eighth most common malignancy worldwide, with a mortality rate ranking sixth (1, 2). In China, EC, predominantly consisting of ESCC cases, poses a substantial disease burden, accounting for approximately half of the global annual incidence and mortality rates (3). Anatomically, ESCC mainly occurs in the upper or middle segment of the esophagus, whereas EAC tends to manifest in the distal region (4). Geographically, ESCC is more commonly found in Eastern Asia, Eastern Europe, and Southern and Eastern Africa, while EAC has a higher prevalence in North America, Central America, and Central Africa (3, 4).
Conventional treatment strategies for EC include surgery, radiotherapy, chemotherapy and targeted therapy (5), but the five-year survival rate still remained less than 20% until 2021, due to delayed diagnoses and high recurrence rates (3, 4). In recent years, the administration of immune checkpoint inhibitors (ICIs) in EC has attained significant advances, as ICIs can both suppress cancer cells by modulating anti-tumor immunity, as well as exerting a synergistic effects when combined with chemotherapy or/and radiotherapy (6) (Table 1). Immune checkpoint genes and cellular interactions contributing to tumor immunity are illustrated in Figure 1 (7, 8) (Figure 1). The phase III KEYNOTE-181 trial demonstrated that pembrolizumab, compared with chemotherapy, contributed to superior overall survival (OS), objective response rate (ORR), and lower incidence of high-grade treatment-related adverse events (9). Moreover, other phase III trials, such as CheckMate648 (10) and KEYNOTE-590 (11), have confirmed that the combination of immunotherapy and chemotherapy as first-line treatment significantly improves OS and progression-free survival (PFS) compared with chemotherapy alone. However, the benefits of immunotherapy vary among patients with patients, highlighting the significance of identifying reliable biomarkers that can predict their response to immunotherapy.
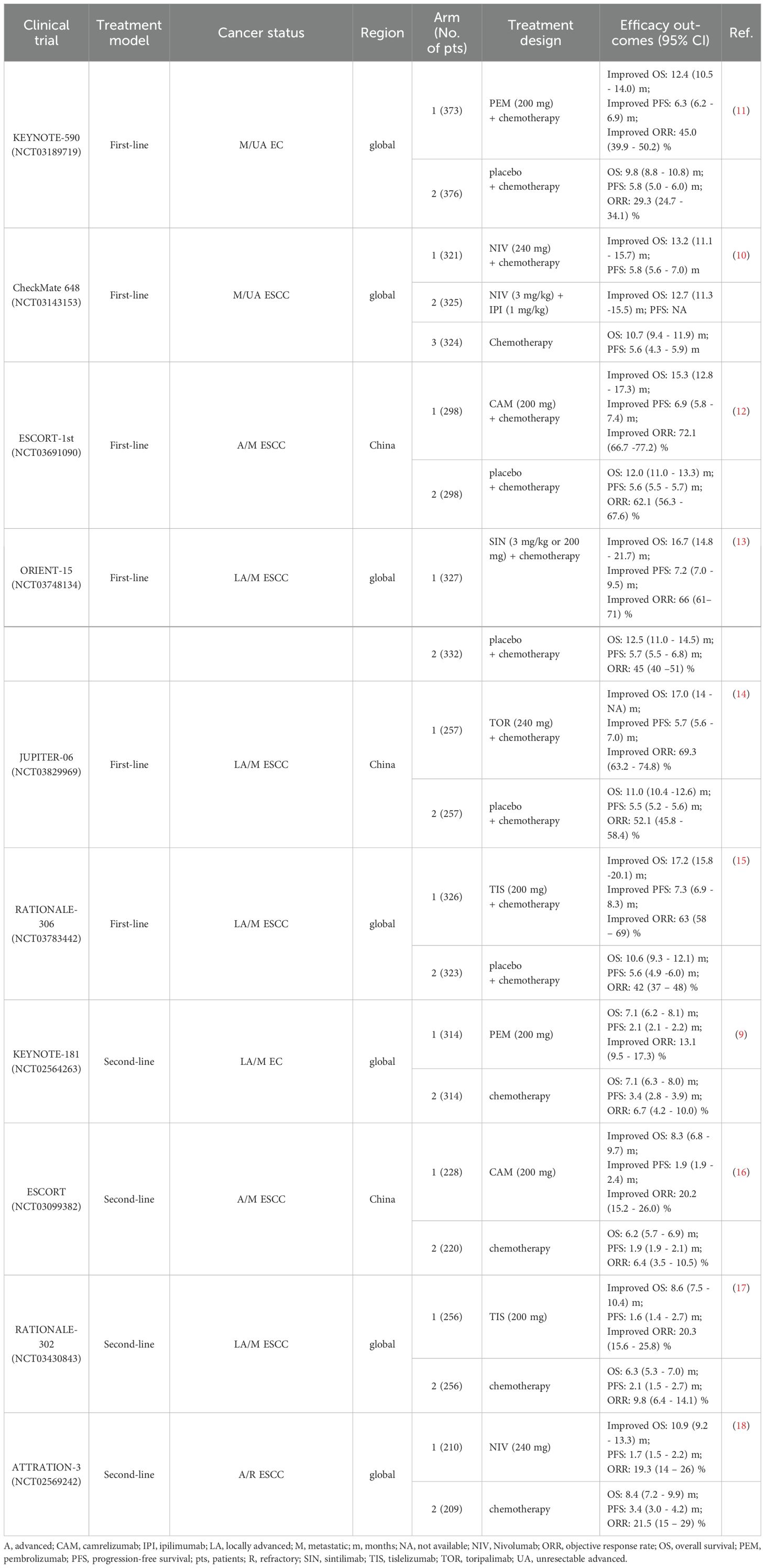
Table 1. The large-scale phase III clinical trials of immunotherapy for EC and efficacy outcomes in overall population regardless of PD-L1 expression.
Based on the latest advances in the field, this paper review categorizes prognostic biomarkers for patients with EC into three facets: tumor intrinsic factors, tumor-immune microenvironment (TIME)-associated factors, and host-related factors. Further research into these biomarkers, elucidating their mechanisms in mediating anti-tumor immunity and clarifying their clinical significance in EC immunotherapy, may aid in the identification of populations likely to benefit or in searching therapeutic targets that can enhance ICIs efficacy.
2 Tumor-cell-associated biomarkers
Tumor-cell-associated biomarkers, including tumor mutation burden (TMB) and high microsatellite instability (MSI-H), are established prognostic indicators for EC immunotherapy, while the evidence for programmed cell death ligand 1 (PD-L1) as a marker in this context has led to disputed conclusions (6). In this section, we summarize clinical findings relating both to these established biomarkers and to emerging markers, and explore the mechanisms potentially underlying related clinical phenomena.
2.1 PD-L1
The level of PD-L1 expression, generally assessed by combined positive score (CPS) or tumor proportion score (TPS), stands as a prognostic biomarker for predicting the efficacy of PD-1/PD-L1 inhibitors (19, 20). Many studies have reported correlations between elevated PD-L1 expression and adverse prognosis in patients with EC, including lymph node and distant metastasis, as well as poor OS (20). For example, Yagi et al. observed that high PD-L1 expression in patients with surgically resected EC indicated higher recurrence rate and shorter OS (21). The American Society of Clinical Oncology has issued a guideline pertaining to the use of immunotherapy for advanced gastroesophageal cancer, emphasizing the importance of PD-L1 testing and mismatch repair status assessment (22), which suggests conducting biomarker testing for individuals diagnosed with EC and gastroesophageal junction cancer, and formulating therapy models based on the results of CPS and TPS (22).
Although high PD-L1 expression tends to suggest the poor prognosis, some studies have shown that PD-1/PD-L1 inhibitors yield better therapeutic effects in patients with raised PD-L1 expression. In KEYNOTE-590 trial, pembrolizumab plus chemotherapy improved OS (p < 0.001) and PFS (p < 0.001) in ESCC participants with PD-L1 CPS ≥ 10 versus chemotherapy alone (11). CheckMate 648 study obtained similar results, showing that patients with elevated PD-L1 expression had superior OS and PFS after nivolumab treatment compared with the overall population (10). Nevertheless, clinical trials, such as ESCORT-1st (12), ORIENT-15 (13), and Jupiter-06 (14), have reported results indicating that PD-1 blockade agent efficacy is not correlated with PD-L1 level. In other words, immunotherapy plus chemotherapy was beneficial for patient OS regardless of PD-L1 status (Table 2). Therefore, whether PD-L1 can be considered a prognostic biomarker for immunotherapy in EC remains controversial.
Notably, some studies have reported that patients with heightened PD-L1 expression may exhibit favorable complete response rate (CRR), disease-free survival (DFS) and OS, but the statistical differences were not significant (p > 0.05) (23, 24). These contradicting findings regarding PD-L1 may be attributable to various factors including: 1) disease heterogeneity, such as variation in pathological types and stages; 2) differences in the ICIs administered; 3) variations in PD-L1 detection methods; 4) discrepancies in cut-off points; 5) inconsistency in the timing of PD-L1 detection (i.e., baseline versus post-treatment); 6) variations in sample sizes (25, 26). In the future, normalization and standardization of PD-L1 detection may contribute to clarifying its relationship with ICIs efficacy (27).
2.2 TMB
TMB, characterized by the frequency of somatic mutations in the coding regions of tumor genomes, has emerged as a prognostic biomarker for ICIs therapy in various types of cancer, including EC (33–35). After transcription and translation, mutations in tumors generate neoantigens, which increase the immunogenicity of tumor cells and thus elicits an intenser anti-tumor immune activation induced by ICIs (34). Huang et al. found that ESCC patients with higher TMB (more than 60 missense mutations) showed improved clinical benefits in response to camrelizumab remedy (36). The KEYNOTE-158 study confirmed that patients with elevated TMB status (more than 10 mutations per megabase) in advanced solid tumors who received pembrolizumab had a significantly higher ORR than other patients (33), which led FDA to grant accelerated endorsement for the use of pembrolizumab for managing unresectable or metastatic solid tumors with TMB above 10 Mut/Mb in 2020 (37).
Tumors with enhanced TMB tend to generate a greater density of neoantigens, thereby inducing anti-tumor immune response characterized by recognition and cytotoxicity (38), which can be enhanced when ICIs block immune checkpoints to facilitate anti-tumor immunity. A multiomics analysis revealed a correlation between TMB and mismatch repair (MMR) status, as well as the infiltration of immune cells such as regulatory T cells (Tregs), monocytes, and T helper cells (Ths) in EC (39). Another bioinformatics analysis identified a positive correlation between nonsynonymous TMB level and the infiltration of resting NK cells (p = 0.028), Tregs (p = 0.064), and CD8+ T cells (p = 0.12) (40). Furthermore, elevated TMB also suggests a shorter distance between EC cells, especially PD-L1- tumor cells, to DCs and macrophages, which is associated with improved OS and PFS (41). This suggests that an increase in TMB leads to the distribution of antigen-presenting cells around the proximal tumor cells, thereby ameliorating the prognosis of patients undergoing immunotherapy.
2.3 MSI
MSI refers to the variations in the length of tandem repeats caused by defects in the DNA MMR system (42). Generally, tumors in which more than 30–40% of markers are mutated are termed as MSI-high (43). In a clinical study involving various types of solid malignancy, including gastroesophageal cancers, patients afflicted with MMR-deficient cancers and receiving pembrolizumab treatment exhibited favorable outcomes, with 53% achieving objective radiographic responses and 21% experiencing complete responses (44), indicating that ICIs are highly effective in the treatment of MSI-high patients regardless of the origin of the tumor tissue (44). Hence the FDA authorized the application of pembrolizumab in combating MSI-H solid tumors, marking the first time that the FDA has established a biomarker for treatment without restriction based on tumor type (45, 46).
Similar to the mechanism of TMB, MSI-H tumor cells generate more neoantigens, which in turn augments T cells’ proliferation and activation, making such tumors more susceptible to anti-tumor immune responses (44, 45). A study of adenocarcinoma of esophagogastric junction (AEG) demonstrated that MSI-H tumors were associated with elevated level of CD8+ T cells infiltration in both the intratumoral region and invasive margin, as well as higher PD-L1 expression level in AEG cells, compared with microsatellite stable (MSS) tumors (47). By contrast, although MSI-low tumors also showed increased level of CD8+ T cells in intratumoral area, no differences were observed in the invasive margin or in PD-L1 expression compared to MSS tumors (47). Similar results have also been observed in MSI-H colorectal cancer, which produces more neopeptides that are more readily recognized by the immune system than MSI-low tumor, and therefore eliciting an increased infiltration level of Th1 cells and CD8+ T cells (48).
2.4 Abnormal DNA methylation
DNA methylation is a common epigenetic modification, characterized in mammals by the methylation of the C5 position of cytosine to form 5-methylcytosine (49). Aberrant DNA methylation is a significant contributor to tumorigenesis and progression, and can serve as a prognostic biomarker for ESCC (50). For example, higher methylation levels in the promoters of miR129-2 and miR124-3 are associated with inferior response to neoadjuvant CRT of EC patients (51). However, studies discussing the relationship between DNA methylation and prognosis in the context of ICIs treatment are relatively limited. One study stratified 94 ESCC patients into two subgroups, S1 (n = 40) and S2 (n = 54), based on their DNA methylation and gene expression data (52). Genes differentially expressed between the two subgroups were identified as primarily involved in immune system regulation, immune cell activation, and cytokine function, according to KEGG/GO analysis. Taking their DNA methylation or gene expression data as the training set, the authors established a linear SVM model comprising 15 genes. Analysis of validation set data from 36 ESCC patients treated with PD-1/PD-L1 blockades plus chemotherapy was assembled. The final results demonstrated that the 15-gene expression signature exhibited a sensitivity of 68.8%, a specificity of 75%, and an efficacy of 72.2% in predicting responses to immunotherapy (52).
As mentioned previously, differences in DNA methylation and gene expression influenced the activation and immunological function of a wide range of immune cells in the S1 and S2 groups. The researchers investigated the condition of tumor microenvironment (TME) in each of the samples, observing that the patients in S2 group had higher levels of Tregs, resting memory CD4+ T cells, Ths, macrophages and activated mast cells, as well as higher levels of immune checkpoint molecules, including CTLA-4 and lymphocyte activation gene-3 (LAG-3) (52). These findings indicate that aberrant DNA methylation can result in T cell exhaustion and suppression of anti-tumor immunity. Given the significant role of DNA methylation in immune responses against cancers, it is anticipated to be a valuable biomarker for EC immunotherapy (53).
2.5 Amplification of chromosome 11q13
The amplification of genomic region in chromosome 11q13 is among the most common aberrations observed in various malignant tumors, including ESCC (54). Chromosome 11q13 amplification contributes to promoting lymphangiogenesis, lymphatic metastasis, and suppression of immune response within the TME, thus hindering anti-cancer therapies (54, 55). In a clinical study involving advanced ESCC patients undergoing toripalimab therapy, those without 11q13 amplification (n=26) demonstrated significantly higher ORR (p = 0.024) and longer PFS (p = 0.025) than those with 11q13 amplification (n=24) (56), suggesting that chromosome 11q13 amplification may serve as an unfavorable biomarker for ESCC treated with PD-1 inhibitors (56), and similar result were observed in unresectable hepatocellular carcinoma (HCC) (57).
Chromosome 11q13 amplification includes the amplification of miR-548k and Cyclin D1 (CCND1), among others (54, 55, 58). MiR-548k promotes lymphangiogenesis by stimulating VEGFC secretion and activating ADAMTS1/VEGFC/VEGFR3 signaling pathway (54). Additionally, MiR-548k facilitates nodal metastasis by regulating the LF10/EGFR axis (54). CCND1 amplification leads to a state of immune exhaustion in the TME, which manifests as decreased densities of CD8+ T cells, dendritic cells (DCs), and B cells and an elevated levels of Ths, Tregs, and myeloid-derived suppressor cells (MDSCs) (55). CCND1 exerts its function by activating CDK4/6 (57), and it is reported that the combined use of CDK4/6 inhibitors and anti-PD-1 antibodies significantly improves the survival outcomes in a mouse model of colon cancer (59). Moreover, chromosome 11q13 amplification is accompanied by an increased density of Foxp3+ Tregs, which may contribute to hyperprogressive disease, a condition characterized by primary resistance to immunotherapy (57).
2.6 Amplification of MCL-1
Myeloid cell leukemia 1 (MCL-1) was isolated from a human myeloid leukemia cell line and belongs to the Bcl-2 protein family (60). MCL-1 amplification and overexpression is associated with the proliferation, drug resistance and inferior prognosis in various tumors including ESCC (61, 62), and inhibition of CPEB4-mediated MCL-1 translation can reverse the resistance to cisplatin in ESCC (63). Both MCL-1 and Bcl-xL belong to the bcl-2 family (60). Combination therapy targeting MCL-1 and Bcl-xL effectively eliminates melanoma cells from patients who experience relapse after PD-1 or CTLA-4 remedy, and curtails the self-renewal capability of melanoma cells (64).
In EC-CRT-001 trial involving ESCC patients treated with toripalimab plus CRT, univariable analyses of 16 genes of interest were conducted and only MCL-1 level was significantly associated with shorter OS (p = 0.03) and PFS (p = 0.024) (23). In post-hoc analysis, it emerged that patients with MCL-1 amplification demonstrated elevated levels of PD-L1+ CD8+ T cells and PD-L1+ macrophages infiltration (23). In addition, a pan-cancer study found that the NANOG/HDAC1/MCL-1 axis mediates tumor resistance to PD-1 inhibitors, which could be reversed by silencing MCL-1 (65, 66). Collectively, these studies indicate a significant role for MCL-1 in mediating the immune-refractory state, thus suggesting the potential value of MCL-1 as both a biomarker and a therapeutic target in EC immunotherapy.
2.7 Long non-coding RNA (lncRNA)
long non-coding RNAs (lncRNAs), which is associated with tumor progression and immune evasion (67, 68), can be transcribed from both coding regions and regulatory regions of the tumor itself (69), or be delivered into cancer cells by TAMs via exosomes (70). Enhancers, as critical components of regulatory regions, are activated through demethylation and are subsequently transcribed into enhancer RNAs (eRNAs), a type of lncRNAs that are involved in the upregulation of corresponding target genes (71). Gao et al. constructed an EDRGS score model based on 12 target genes in ESCC and found that patients with higher EDRGS exhibited superior responses to anti-PD-1 regimen (p = 0.038) (71). Bulk RNA-seq and scRNA-seq analyses revealed that EDRGS-high group exhibited elevated infiltration of CD8+ T cells and NK cells, as well as upregulated levels of PD-1, LAG-3, TIM3, and TIGIT. In other words, EDRGS-high group denoted an immune-hot but immune-suppressive phenotype, accounting for improved response to ICIs (71). Additionally, it was found that eRNA AC005515.1 is co-expressed with several immune checkpoint genes, including CTLA4, Foxp3, and IDO1, and is positively correlated with the infiltration of CD8+ T cells and M1 macrophages (72). Interestingly, patients with higher expression of AC005515.1 had increased TIDE scores and worse survival outcomes (72). This bioinformatics-based inference appears to be inconsistent with Gao’s finding, highlighting the importance of further experiments and clinical studies to verify these results.
Another lncRNA, LINC02096, has been identified as a biomarker for ESCC immunotherapy by regulating immune evasion (73). Patients with elevated level of LINC02096 demonstrated inferior disease control rate (DCR) and ORR when undergoing anti-PD-1 monotherapy. In mouse model, knockdown of LINC02096 in TAMs upregulated the level of cytotoxic CD8+ T cells, which rescued tumor progression and enhanced the treatment efficacy of anti-PD-1 antibody (73). Further research confirmed that high levels of LINC02096 are accumulated in ESCC cells with the involvement of exosomes secreted by TAMs and TNF-α. In tumor cells, LINC02096 inhibits the ubiquitination of histone methyltransferase MLL1, which enhances the levels of H3K4me3 in the promoter regions of PD-L1 and IDO-1, thereby undermining anti-tumor immunity (73).
3 Tumor-immune-microenvironment-associated biomarkers
The whole tumor-immune microenvironment (TIME) can be viewed as a biomarker for immunotherapy. TIME-associated biomarkers encompass various components, such as tumor-infiltrating lymphocytes (TILs), tumor-associated macrophages (TAMs), and cytokines (74).
3.1 TILs
3.1.1 Conventional immune biomarkers
A meta-analysis has confirmed that TILs overall can serve as a prognostic biomarker for OS in patients with EC (75). For surgically resected EC, patients with TIL-positive status tend to achieve favorable OS and DFS (21). However, treatments in the analyzed studies were not solely confined to ICIs therapy. Additionally, there is evidence that different TILs subsets may have distinct prognostic values (75). Therefore, the predictive role of TILs in EC immunotherapy warrants further elucidation.
In a clinical trial involving ESCC patients undergoing tislelizumab plus chemotherapy followed by esophagectomy, levels of CD8+ T cells, Ths, Tregs, and mature DCs were significantly increased in pathological complete response (pCR) group, while the density of B cells and neutrophils significantly decreased (76). Another clinical study of locally advanced ESCC also detected a statistically significant correlation between CD8+ T cells content, rather than other TIL subsets, and clinical response, with improved CRR (p = 0.004) and prolonged PFS (p = 0.005) (23). Another trial researching refractory EC demonstrated that patients with more abundant PD-1+ CD4+ T cells exhibited poorer radiological response (p = 0.035), and that the expression of PD-1 and T-cell immunoglobulin and mucin domain-3 (TIM-3) on CD4+ T cells suggested early progression (28). We consider that the infiltration of a certain quantity of CD8+ T cells is necessary for a physiological anti-tumor immune response; however, given the complex functions of diverse immune cells, how some immune cell types exert negative effects on EC immunotherapy requires further investigation.
3.1.2 Novel immune biomarkers
Novel immunotherapeutic targets have emerged in recent years, including LAG-3 and TIM-3, among others (5). However, due to the limited availability of clinical results related to these molecules, it is currently premature to reach conclusions regarding these factors. Therefore, we have included them as potential biomarkers and summarized relevant findings in the Discussion section of this review. TCF-1 expressed on CD8+ T cells may serve as a novel immune biomarker. A study investigating pembrolizumab combined with CRT in ESCC observed that patients in the pCR group exhibited a higher level of TCF-1+ cells than those in the non-pCR group (p = 0.01) (77). In melanoma, TCF-1+ CD8+ T cells are considered a positive biomarker, which will proliferate, self-renew, or differentiate into TCF-1- CD8+ T cells after treatment with ICIs (78). TCF-1 plays essential roles in maintaining the stem-like function of CD8+ T cells and in intratumoral immune responses (79). Even when the influx of new T cells is blocked, melanoma mice with elevated TCF-1+ TILs can still control tumor growth. Conversely, when TCF-1+ TILs are suppressed, tumor control ability is lost (79).
3.2 TAMs
TAMs are immune cells with crucial roles in the TIME, whose density, distribution, and subtypes are correlated with the prognosis of EC (80–82). In a clinical trial investigating toripalimab plus chemotherapy in patients with locally advanced ESCC, responders showed a lower density of M2-TAMs (31). Another study involving camrelizumab plus CRT demonstrated that higher density of PD-L1- macrophages in the baseline tumor compartment was associated with improved PFS (p = 0.032) and in the on-treatment compartment indicated superior OS (p = 0.018) and PFS (p = 0.028) (41, 83). Through spatial multi-immunofluorescence, it was found that PD-L1- macrophages are situated closer to tumor cells than PD-L1+ macrophages, which may account for better clinical outcomes, to some extent (41). This study also found that the spatial distribution of TAMs was correlated with TMB, while previous reports have confirmed positive correlation between TAMs and PD-L1 levels in EC (84), and shown that TAMs could also secrete a variety of chemokines to regulate the TIME (85, 86), suggesting that the prognostic significance of TAMs may need to be judged in conjunction with other biomarkers (84). Notably, this study did not differentiate between M1 and M2 TAMs, nor did it investigate any association between the expression of CD68 or CD163 on macrophages and immunotherapy efficacy, which are areas warranting further research.
3.3 Cytokines
3.3.1 Interferon-γ (IFN-γ)
The PERFECT study tested for a six-gene IFN-γ signature in EAC patients receiving neoadjuvant immunotherapy based on previous experience and found that responders had higher levels of the baseline IFN-γ signature (p = 0.043) (32). Researchers conducted gene expression profile analysis of melanoma patients treated with pembrolizumab, and identified an IFN-γ-related signature as a prognostic marker by comparing responders and non-responders, further confirming its association with PFS and ORR in head and neck squamous cell carcinoma (HNSCC) and gastric cancer (GC) (87). These findings may be attributable to the involvement of IFN-γ in antigen presentation, chemokines secretion, and cytotoxicity, all of which are essential for the efficacy of immunotherapy (87). The upregulation of PD-L1 in the TME is established as partly originating from the effects of IFN-γ released by CD8+ T cells, indicating that IFN-γ can affect other biomarkers to indirectly influence immunotherapy (88).
3.3.2 Chemokines
A study involving toripalimab plus chemotherapy in ESCC found that responders exhibited decreased CCL19 and elevated CXCL5 levels at baseline (31). CCL19 can activate the MEK1-ERK1/2 and PI3K-AKT pathways in M1-TAMs via CCR7, mediating their chemotaxis (89). However, although this finding regarding CXCL5 is consistent with the conclusion from a study of melanoma treated with nivolumab (90), the impact of CXCL5 on the TIME seems contradictory to its role as a positive biomarker, considering a study from skin cancers demonstrated that CXCL5 secreted by TAMs recruits MDSCs to the TME via the CXCR2-CXCL5 axis, exerting immunosuppressive effects (85). In addition, the infiltration of TAMs is reported to be modulated by the CCL2-CCR2 axis to affect PD-1 signaling pathway, leading to immune evasion (91), and CCL18 released by TAMs can promote tumor proliferation through activating the JAK2/STAT3 pathway (86), all of which contribute to the unsatisfactory prognosis of patients with ESCC.
4 Host-associated biomarkers
Host-associated biomarkers for cancer treatment include nutritional status (anemia, cachexia, etc.), peripheral blood immune substances, microbiomes, psychological disorders (including anxiety, depression, etc.), and endogenous hormones, among other factors. Promising results have been reported related to nutritional status, peripheral blood immune substances, antibiotic (ATB) use and its effects on microbiomes, and endogenous glucocorticoid in patients with EC.
4.1 Nutritional status
It is common for patients with upper gastrointestinal cancer to experience poor nutritional status, mainly due to reduced food intake and enhanced nutritional consumption by tumors (92). A recent retrospective study confirmed that the prognostic nutritional index (PNI) can serve as a biomarker for predicting the OS (p = 0.047) and PFS (p = 0.020) of EC patients undergoing anti-PD-1 inhibitors (93).
PNI, calculated from serum albumin content and total lymphocyte count, reflects the nutritional status and immunity of tumor patients (94). Albumin level reflects nutritional status, and a decrease in this factor indicates that the patient is in a state of malnutrition, which is a risk factor influencing prognosis (95). Lymphocytes exert anti-tumor immunity through cytokine-mediated cytotoxicity and insufficient lymphocytes results in lack of immune surveillance against tumors (96). Additionally, there are positive correlations between PNI and TILs status, CD8+ cells density and Foxp3+ cells density in EC patients, suggesting that lower PNI is associated with an unfavorable TIME, leading to poor response to immunotherapy (97).
4.2 Peripheral blood immune substances
Tumor-associated immunity can be categorized into immune responses within the TME and systemic immunity throughout the body, where the latter can be assessed by indicators such as neutrophil to lymphocyte ratio (NLR) and absolute neutrophil counts (ANC),among others (98). A real-world study of ESCC observed that an increase in NLR after ICIs treatment predicted decreased OS (p = 0.004) and inferior PFS (p = 0.019) (98). Similarly, the ORIENT-2 study showed that ESCC patients treated with sintilimab exhibited prolonged OS (p < 0.001) and PFS (p = 0.006) if the NLR was less than three at the sixth week (99). These findings may be attributable to the fact that neutrophils curtail the anti-tumor immunity mediated by NK cells and T cells, and secrete various cytokines, including IL-1 and IL-6, contributing to tumor proliferation (100–102).
Moreover, in neoadjuvant therapy combining toripalimab with chemotherapy for ESCC patients, although no relationship was observed between NLR and patients’ response, higher ANC and absolute natural killer cell counts were detected in responders (31). This phenomenon appears contradictory to the findings from patients with lung cancer, possibly due to the involvement of multiple chemotherapeutic agents in this study, which may have interfered with immunotherapy biomarker selection (103). Additionally, in ESCC patients treated with nivolumab, a rise in TIM-3+ CD4+ and TIM-3+ CD8+ T cells among peripheral blood mononuclear cells was observed in responders (104). Another study found that circulating CXCL10, interleukin 2 receptor α, and IL-6 were associated with pembrolizumab efficacy in treating EC, with favorable, unfavorable, and unfavorable relationships, respectively (28). These findings suggest that the prognostic significance of various immune substances in peripheral blood should not be neglected while TIME receives sufficient attention.
4.3 Antibiotic therapy and microbiomes
Microbiomes, including gut microbiota among others, can exert both protective and detrimental influences on cancer progression and therapeutic response (105). A meta-analysis incorporating retrospective studies of EC revealed that antibiotic (ATB) use from 60 days before to 30 days after ICIs treatment induced worse OS (P = 0.03) (106). This finding is consistent with the conclusion of a previous pan-cancer research (107), suggesting that ATB usage may lead to dysbiosis in patients’ microbiomes, affecting their immune function and response to ICIs (98, 106, 108, 109).
The relationships between gut microbiota and host immune function, tumor occurrence and progression, anti-tumor immunity, and patients’ prognosis are highly complex (105). Nevertheless, in general, the gut microbiota enhances antigen presentation mediated by DC cells, recruits and activates effector T cells to the TME, and reduces the density of Tregs and MDSCs (98), which provides a theoretical rationale for the beneficial role of normal gut microbiota in anti-tumor immunity. It is noteworthy that ATB use may not only affect patients’ prognosis by altering the composition and diversity of intestinal flora. Patients receiving ATB may have inherently compromised immune function and a state of infection or susceptibility to infection, which could also contribute to poor outcomes in immunotherapy. On the other hand, dysbiosis of the gut microbiota may not only be induced by ATB usage. A study investigating camrelizumab plus chemotherapy in ESCC revealed that patients experienced a decrease in gut microbiota diversity following treatment (110). Therefore, the relationship among ATB administration, alterations in both gut microbiota and intratumoral microbiomes, and response to immunotherapy warrants further investigation.
4.4 Endogenous glucocorticoid
Excessive glucocorticoid can suppress the proliferation and differentiation of naive T cells, inhibit the CD28-CD80/CD86 co-stimulatory pathway, and disrupt immune surveillance function by affecting tumor-infiltrating immune cells (111, 112). A retrospective study encompassing advanced or metastatic pan-cancer, including EC, found that patients with high baseline endogenous glucocorticoid levels tended to exhibit lower ORR (p < 0.001) and had poorer durable clinical benefits (DCB) (p = 0.001), as well as shorter OS (p < 0.001) and PFS (p < 0.001) (113). Further research indicated that elevated glucocorticoid level is associated with lower infiltration levels of lymphocytes, CD4+ T cells, and CD8+ T cells, as well as increased NLR (113).
In other malignancies, glucocorticoid-related signaling pathways are generally detrimental factors for immunotherapy (114, 115). In pancreatic ductal adenocarcinoma, researchers have confirmed that the glucocorticoid receptor (GR) can upregulate the expression of PD-L1 and downregulate the expression of MHC-1 in tumor cells, thereby impairing cytotoxic T cells’ infiltration and anti-tumor immune function (114). When GR is knocked down or inhibited, resistance to ICIs in mice will be reversed (114). In melanoma, HSD11B1 is an enzyme that can convert inert glucocorticoid into active glucocorticoid, and mice with high expression of HSD11B1 exhibit attenuated infiltration of CD4+ T cells and CD8+ T cells and are insensitive to PD-1 inhibitors (115). Blocking HSD11B1 leads to the decreased expression of CD206 and arginase-1 and the heightened expression of IL-12 in TAMs, as well as promoting secretion of IFN-γ by CD8+ T cells, thereby enhancing the efficacy of PD-1 blockades (115).
4.5 Circulating tumor DNA (ctDNA)
Circulating tumor DNA (ctDNA) refers to cell-free DNA present in body fluids such as blood, synovial fluid, and cerebrospinal fluid (116). As a non-invasive biomarker, ctDNA has been widely applied to treatment monitoring in recent years (116). While the ctDNA status before sintilimab treatment (i.e., the baseline ctDNA level) is not statistically significant with major pathological response (MPR) (p = 0.39), patients with undetectable ctDNA are more likely to achieve pCR (p = 0.008) (117). It is noteworthy that more attention is paid to the dynamic changes of ctDNA content compared to its baseline level (118). It was observed that patients with undetectable ctDNA or ctDNA clearance post-ICIs therapy tend to obtain prolonged recurrence-free survival (RFS) and OS (119). Similarly, the EC-CRT-001 trial found that patients with detectable ctDNA during or after treatment, rather than at baseline, exhibited shorter OS and PFS, as well as inferior clinical complete response (cCR) (120). ctDNA clearance represents neoantigen-specific T cell responses. In a clinical trial investigating neoadjuvant nivolumab alone or plus relatlimab treatment, two patients who achieved complete pathological response had ctDNA clearance following ICIs induction. Both of them exhibited expansions of neoantigen-specific T cell clones, which were not observed in patients with persistently detectable ctDNA (119). Currently, further studies are underway to explore the prognostic value of ctDNA in EC immunotherapy (121, 122).
5 Discussion
Despite variations in the most common pathological types across different geographical regions, EC poses significant threats and burdens to people worldwide (3). The emergence of ICIs has provided novel options for EC treatment, bringing promise for prolonged survival and improved quality of life for patients. ICIs are currently applied in first-line, second-line, adjuvant and neoadjuvant treatment patterns in EC (4, 123). However, not all EC patients can benefit equally from immunotherapy (5), highlighting the significance of identifying reliable biomarkers to discern subgroups who may respond better to immunotherapy. In this review we summarize tumor-cell-associated biomarkers, TIME-associated biomarkers, and host-associated biomarkers discovered in EC immunotherapy, as well as their possible underlying mechanisms (Table 3), with the goal of providing a theoretical basis for selection of suitable patients and administration of tailored treatments in the future.
In addition to summarizing well-established biomarkers such as PD-L1, TMB, MSI-H, and TILs, some relatively novel markers are also discussed in this review, including DNA methylation, amplification of chromosome 11q13 and specific genes, and cytokines’ levels. We found that different studies have yielded conflicting conclusions regarding the prognostic significance of certain biomarker. Regarding PD-L1, many clinical studies failed to establish a statistically significant association between its expression and the efficacy of ICIs (Table 1). Similarly, it is also reported that TMB is insufficient to predict the outcomes of immunotherapy (31). These discrepancies might be attributed to the differences in detection methods, cut-off points selection, sample sizes, and treatment patterns. Recently a team has developed copy number alteration (CNA)-corrected TMB to predict the efficacy of PD-1 inhibitors plus chemotherapy (124), suggesting that biomarker standardization and adjustment warrants further exploration. The timing of detecting biomarkers’ content and the attention to their dynamic changes are also crucial. When baseline levels of a biomarker are unrelated to efficacy, positive conclusions may be drawn from its post-treatment level, or from its dynamic alteration during therapy.
We have also summarized potential biomarkers for EC immunotherapy in this section based on the quality of evidence (Table 4). They fit into one of the following two features: 1) Although confirmed as associated with therapeutic efficacy in EC, evidence sources encompass all treatment methods, and are not confined to immunotherapy. Additionally, these markers affect prognosis through mechanisms involving the TIME or anti-tumor immunity; 2) Markers reported to be associated with the prognosis of immunotherapy in other types of cancers, primarily lung cancer and gastrointestinal cancers, while relevant research in EC is limited. We chose gastrointestinal tumors as a reference, since the esophagus and other gastrointestinal organs share similar histological structures and embryological origins. Lung cancer was also selected, both because of the anatomical proximity of the lungs and esophagus and considering the fact that studies of lung cancer are relatively abundant and advanced. There is a current trend in oncology research of validating results derived from lung cancer studies in other types of cancers.
Biomarkers summarized in the main sections of this review and potential biomarkers included in the Discussion section are illustrated in Figure 2. We found that various of these biomarkers can mutually interact to influence anti-tumor responses during immunotherapy. For instance, multiple biomarkers ultimately impact the responses of EC patients by mediating the quantity, activity, or phenotype of TILs. TAMs both influence the TIME by secreting chemokines to recruit MDSCs, and can also be modulated by chemokines, thereby assisting EC cells in evading anti-tumor immune responses (85, 86, 89, 91). These findings indicate that further research into the mechanisms underlying the activities of various biomarkers and to delineate their interactions will be of vital importance to provide comprehensive understanding of prognostic biomarkers in the context of EC immunotherapy.
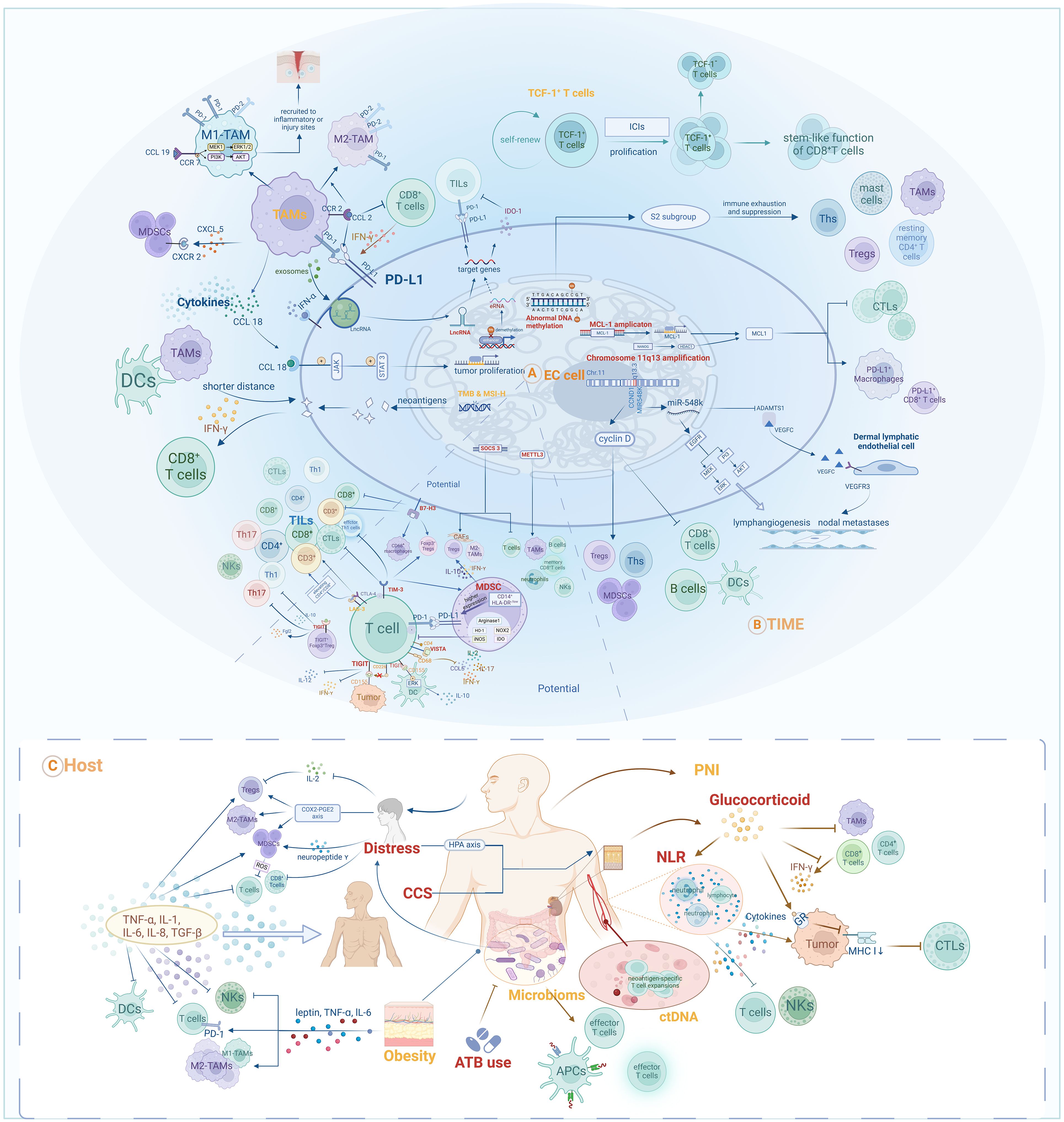
Figure 2. Prognostic and potential biomarkers for immunotherapy in EC and their interactive mechanisms: (A) Tumor-cell-associated biomarkers; (B) TIME-associated biomarkers; (C) Host-associated biomarkers.
Author contributions
XT: Writing – original draft, Writing – review & editing. MJ: Writing – original draft, Writing – review & editing. LW: Investigation, Methodology, Writing – review & editing. DZ: Investigation, Resources, Writing – review & editing. YY: Formal analysis, Supervision, Validation, Writing – review & editing. QS: Conceptualization, Formal analysis, Investigation, Resources, Supervision, Validation, Writing – review & editing.
Funding
The author(s) declare financial support was received for the research, authorship, and/or publication of this article. This work was supported by National Natural Science Foundation of China (No. 82203009 to QS), and No.82473175 to YY).
Conflict of interest
The authors declare that the research was conducted in the absence of any commercial or financial relationships that could be construed as a potential conflict of interest.
Publisher’s note
All claims expressed in this article are solely those of the authors and do not necessarily represent those of their affiliated organizations, or those of the publisher, the editors and the reviewers. Any product that may be evaluated in this article, or claim that may be made by its manufacturer, is not guaranteed or endorsed by the publisher.
References
1. Sung H, Ferlay J, Siegel RL, Laversanne M, Soerjomataram I, Jemal A, et al. Global cancer statistics 2020: GLOBOCAN estimates of incidence and mortality worldwide for 36 cancers in 185 countries. CA Cancer J Clin. (2021) 71:209–49. doi: 10.3322/caac.21660
2. Morgan E, Soerjomataram I, Rumgay H, Coleman HG, Thrift AP, Vignat J, et al. The global landscape of esophageal squamous cell carcinoma and esophageal adenocarcinoma incidence and mortality in 2020 and projections to 2040: new estimates from GLOBOCAN 2020. Gastroenterology. (2022) 163:649–58 e2. doi: 10.1053/j.gastro.2022.05.054
3. Li J, Xu J, Zheng Y, Gao Y, He S, Li H, et al. Esophageal cancer: Epidemiology, risk factors and screening. Chin J Cancer Res. (2021) 33:535–47. doi: 10.21147/j.issn.1000-9604.2021.05.01
4. Petrillo A, Smyth EC. Immunotherapy for squamous esophageal cancer: A review. J Pers Med. (2022) 12:862. doi: 10.3390/jpm12060862
5. Zhou X, Ren T, Zan H, Hua C, Guo X. Novel immune checkpoints in esophageal cancer: from biomarkers to therapeutic targets. Front Immunol. (2022) 13:864202. doi: 10.3389/fimmu.2022.864202
6. Li Q, Liu T, Ding Z. Neoadjuvant immunotherapy for resectable esophageal cancer: A review. Front Immunol. (2022) 13:1051841. doi: 10.3389/fimmu.2022.1051841
7. Marin-Acevedo JA, Kimbrough EO, Lou Y. Next generation of immune checkpoint inhibitors and beyond. J Hematol Oncol. (2021) 14:45. doi: 10.1186/s13045-021-01056-8
8. Morad G, Helmink BA, Sharma P, Wargo JA. Hallmarks of response, resistance, and toxicity to immune checkpoint blockade. Cell. (2022) 185:576. doi: 10.1016/j.cell.2022.01.008
9. Kojima T, Shah MA, Muro K, Francois E, Adenis A, Hsu CH, et al. Randomized phase III KEYNOTE-181 study of pembrolizumab versus chemotherapy in advanced esophageal cancer. J Clin Oncol. (2020) 38:4138–48. doi: 10.1200/JCO.20.01888
10. Doki Y, Ajani JA, Kato K, Xu J, Wyrwicz L, Motoyama S, et al. Nivolumab combination therapy in advanced esophageal squamous-cell carcinoma. N Engl J Med. (2022) 386:449–62. doi: 10.1056/NEJMoa2111380
11. Sun JM, Shen L, Shah MA, Enzinger P, Adenis A, Doi T, et al. Pembrolizumab plus chemotherapy versus chemotherapy alone for first-line treatment of advanced oesophageal cancer (KEYNOTE-590): a randomised, placebo-controlled, phase 3 study. Lancet. (2021) 398:759–71. doi: 10.1016/S0140-6736(21)01234-4
12. Luo H, Lu J, Bai Y, Mao T, Wang J, Fan Q, et al. Effect of camrelizumab vs placebo added to chemotherapy on survival and progression-free survival in patients with advanced or metastatic esophageal squamous cell carcinoma: the ESCORT-1st randomized clinical trial. JAMA. (2021) 326:916–25. doi: 10.1001/jama.2021.12836
13. Lu Z, Wang J, Shu Y, Liu L, Kong L, Yang L, et al. Sintilimab versus placebo in combination with chemotherapy as first line treatment for locally advanced or metastatic oesophageal squamous cell carcinoma (ORIENT-15): multicentre, randomised, double blind, phase 3 trial. BMJ. (2022) 377:e068714. doi: 10.1136/bmj-2021-068714
14. Wang ZX, Cui C, Yao J, Zhang Y, Li M, Feng J, et al. Toripalimab plus chemotherapy in treatment-naive, advanced esophageal squamous cell carcinoma (JUPITER-06): A multi-center phase 3 trial. Cancer Cell. (2022) 40:277–88 e3. doi: 10.1016/j.ccell.2022.02.007
15. Xu J, Kato K, Raymond E, Hubner RA, Shu Y, Pan Y, et al. Tislelizumab plus chemotherapy versus placebo plus chemotherapy as first-line treatment for advanced or metastatic oesophageal squamous cell carcinoma (RATIONALE-306): a global, randomised, placebo-controlled, phase 3 study. Lancet Oncol. (2023) 24:483–95. doi: 10.1016/S1470-2045(23)00108-0
16. Huang J, Xu J, Chen Y, Zhuang W, Zhang Y, Chen Z, et al. Camrelizumab versus investigator’s choice of chemotherapy as second-line therapy for advanced or metastatic oesophageal squamous cell carcinoma (ESCORT): a multicentre, randomised, open-label, phase 3 study. Lancet Oncol. (2020) 21:832–42. doi: 10.1016/S1470-2045(20)30110-8
17. Shen L, Kato K, Kim SB, Ajani JA, Zhao K, He Z, et al. Tislelizumab versus chemotherapy as second-line treatment for advanced or metastatic esophageal squamous cell carcinoma (RATIONALE-302): A randomized phase III study. J Clin Oncol. (2022) 40:3065–76. doi: 10.1200/JCO.21.01926
18. Kato K, Cho BC, Takahashi M, Okada M, Lin CY, Chin K, et al. Nivolumab versus chemotherapy in patients with advanced oesophageal squamous cell carcinoma refractory or intolerant to previous chemotherapy (ATTRACTION-3): a multicentre, randomised, open-label, phase 3 trial. Lancet Oncol. (2019) 20:1506–17. doi: 10.1016/S1470-2045(19)30626-6
19. Liu D, Wang S, Bindeman W. Clinical applications of PD-L1 bioassays for cancer immunotherapy. J Hematol Oncol. (2017) 10:110. doi: 10.1186/s13045-017-0479-y
20. Liu Z, Sun L, Cai L, Guo M, Xu G, Liu S, et al. Clinicopathological and prognostic values of PD-L1 expression in oesophageal squamous cell carcinoma: a meta-analysis of 31 studies with 5368 patients. Postgrad Med J. (2022) 98:948–57. doi: 10.1136/postgradmedj-2021-140029
21. Yagi T, Baba Y, Ishimoto T, Iwatsuki M, Miyamoto Y, Yoshida N, et al. PD-L1 expression, tumor-infiltrating lymphocytes, and clinical outcome in patients with surgically resected esophageal cancer. Ann Surg. (2019) 269:471–8. doi: 10.1097/SLA.0000000000002616
22. Shah MA, Kennedy EB, Alarcon-Rozas AE, Alcindor T, Bartley AN, Malowany AB, et al. Immunotherapy and targeted therapy for advanced gastroesophageal cancer: ASCO guideline. J Clin Oncol. (2023) 41:1470–91. doi: 10.1200/JCO.22.02331
23. Zhu Y, Wen J, Li Q, Chen B, Zhao L, Liu S, et al. Toripalimab combined with definitive chemoradiotherapy in locally advanced oesophageal squamous cell carcinoma (EC-CRT-001): a single-arm, phase 2 trial. Lancet Oncol. (2023) 24:371–82. doi: 10.1016/S1470-2045(23)00060-8
24. Park S, Sun JM, Choi YL, Oh D, Kim HK, Lee T, et al. Adjuvant durvalumab for esophageal squamous cell carcinoma after neoadjuvant chemoradiotherapy: a placebo-controlled, randomized, double-blind, phase II study. ESMO Open. (2022) 7:100385. doi: 10.1016/j.esmoop.2022.100385
25. Hansen AR, Siu LL. PD-L1 testing in cancer: challenges in companion diagnostic development. JAMA Oncol. (2016) 2:15–6. doi: 10.1001/jamaoncol.2015.4685
26. Doroshow DB, Bhalla S, Beasley MB, Sholl LM, Kerr KM, Gnjatic S, et al. PD-L1 as a biomarker of response to immune-checkpoint inhibitors. Nat Rev Clin Oncol. (2021) 18:345–62. doi: 10.1038/s41571-021-00473-5
27. Cooper DL, Manago J, Patel V, Schaar D, Krimmel T, McGrath MK, et al. Incorporation of posttransplant cyclophosphamide as part of standard immunoprophylaxis for all allogeneic transplants: a retrospective, single institution study. Bone Marrow Transplant. (2021) 56:1099–105. doi: 10.1038/s41409-020-01144-2
28. de Klerk LK, Patel AK, Derks S, Pectasides E, Augustin J, Uduman M, et al. Phase II study of pembrolizumab in refractory esophageal cancer with correlates of response and survival. J Immunother Cancer. (2021) 9:e002472. doi: 10.1136/jitc-2021-002472
29. Zhu M, Chen C, Foster NR, Hartley C, Mounajjed T, Salomao MA, et al. Pembrolizumab in combination with neoadjuvant chemoradiotherapy for patients with resectable adenocarcinoma of the gastroesophageal junction. Clin Cancer Res. (2022) 28:3021–31. doi: 10.1158/1078-0432.CCR-22-0413
30. Liu J, Yang Y, Liu Z, Fu X, Cai X, Li H, et al. Multicenter, single-arm, phase II trial of camrelizumab and chemotherapy as neoadjuvant treatment for locally advanced esophageal squamous cell carcinoma. J Immunother Cancer. (2022) 10:e004291corr1. doi: 10.1136/jitc-2021-004291
31. He W, Leng X, Mao T, Luo X, Zhou L, Yan J, et al. Toripalimab plus paclitaxel and carboplatin as neoadjuvant therapy in locally advanced resectable esophageal squamous cell carcinoma. Oncologist. (2022) 27:e18–28. doi: 10.1093/oncolo/oyab011
32. van den Ende T, de Clercq NC, van Berge Henegouwen MI, Gisbertz SS, Geijsen ED, Verhoeven RHA, et al. Neoadjuvant chemoradiotherapy combined with atezolizumab for resectable esophageal adenocarcinoma: A single-arm phase II feasibility trial (PERFECT). Clin Cancer Res. (2021) 27:3351–9. doi: 10.1158/1078-0432.Ccr-20-4443
33. Marabelle A, Fakih M, Lopez J, Shah M, Shapira-Frommer R, Nakagawa K, et al. Association of tumour mutational burden with outcomes in patients with advanced solid tumours treated with pembrolizumab: prospective biomarker analysis of the multicohort, open-label, phase 2 KEYNOTE-158 study. Lancet Oncol. (2020) 21:1353–65. doi: 10.1016/S1470-2045(20)30445-9
34. Chan TA, Yarchoan M, Jaffee E, Swanton C, Quezada SA, Stenzinger A, et al. Development of tumor mutation burden as an immunotherapy biomarker: utility for the oncology clinic. Ann Oncol. (2019) 30:44–56. doi: 10.1093/annonc/mdy495
35. Cao J, Yang X, Chen S, Wang J, Fan X, Fu S, et al. The predictive efficacy of tumor mutation burden in immunotherapy across multiple cancer types: A meta-analysis and bioinformatics analysis. Transl Oncol. (2022) 20:101375. doi: 10.1016/j.tranon.2022.101375
36. Huang J, Xu B, Mo H, Zhang W, Chen X, Wu D, et al. Safety, activity, and biomarkers of SHR-1210, an anti-PD-1 antibody, for patients with advanced esophageal carcinoma. Clin Cancer Res. (2018) 24:1296–304. doi: 10.1158/1078-0432.CCR-17-2439
37. Subbiah V, Solit DB, Chan TA, Kurzrock R. The FDA approval of pembrolizumab for adult and pediatric patients with tumor mutational burden (TMB) >/=10: a decision centered on empowering patients and their physicians. Ann Oncol. (2020) 31:1115–8. doi: 10.1016/j.annonc.2020.07.002
38. Sholl LM, Hirsch FR, Hwang D, Botling J, Lopez-Rios F, Bubendorf L, et al. The promises and challenges of tumor mutation burden as an immunotherapy biomarker: A perspective from the international association for the study of lung cancer pathology committee. J Thorac Oncol. (2020) 15:1409–24. doi: 10.1016/j.jtho.2020.05.019
39. Li L, Bai L, Lin H, Dong L, Zhang R, Cheng X, et al. Multiomics analysis of tumor mutational burden across cancer types. Comput Struct Biotechnol J. (2021) 19:5637–46. doi: 10.1016/j.csbj.2021.10.013
40. Li J, Chen H, Guo H, Qiu M, Yang F. Characterization of gene expression profiles of esophageal cancer patients with different nonsynonymous tumor mutation burden. Thorac Cancer. (2020) 11:2270–8. doi: 10.1111/1759-7714.13537
41. Ma X, Guo Z, Wei X, Zhao G, Han D, Zhang T, et al. Spatial distribution and predictive significance of dendritic cells and macrophages in esophageal cancer treated with combined chemoradiotherapy and PD-1 blockade. Front Immunol. (2021) 12:786429. doi: 10.3389/fimmu.2021.786429
42. Styk J, Pos Z, Pos O, Radvanszky J, Turnova EH, Buglyo G, et al. Microsatellite instability assessment is instrumental for Predictive, Preventive and Personalised Medicine: status quo and outlook. EPMA J. (2023) 14:143–65. doi: 10.1007/s13167-023-00312-w
43. Lefler DS, Snook AE, Bashir B. Immune checkpoint inhibitors in luminal gastrointestinal Malignancies: going beyond MSI-H/dMMR, TMB and PD-L1. Immunotherapy. (2022) 14:885–902. doi: 10.2217/imt-2022-0012
44. Le DT, Durham JN, Smith KN, Wang H, Bartlett BR, Aulakh LK, et al. Mismatch repair deficiency predicts response of solid tumors to PD-1 blockade. Science. (2017) 357:409–13. doi: 10.1126/science.aan6733
45. Lemery S, Keegan P, Pazdur R. First FDA approval agnostic of cancer site - when a biomarker defines the indication. N Engl J Med. (2017) 377:1409–12. doi: 10.1056/NEJMp1709968
46. Lin EI, Tseng LH, Gocke CD, Reil S, Le DT, Azad NS, et al. Mutational profiling of colorectal cancers with microsatellite instability. Oncotarget. (2015) 6:42334–44. doi: 10.18632/oncotarget.5997
47. Imamura Y, Toihata T, Haraguchi I, Ogata Y, Takamatsu M, Kuchiba A, et al. Immunogenic characteristics of microsatellite instability-low esophagogastric junction adenocarcinoma based on clinicopathological, molecular, immunological and survival analyses. Int J Cancer. (2021) 148:1260–75. doi: 10.1002/ijc.33322
48. Jin Z, Yoon HH. The promise of PD-1 inhibitors in gastro-esophageal cancers: microsatellite instability vs. PD-L1. J Gastrointest Oncol. (2016) 7:771–88. doi: 10.21037/jgo.2016.08.06
49. Moore LD, Le T, Fan G. DNA methylation and its basic function. Neuropsychopharmacology. (2013) 38:23–38. doi: 10.1038/npp.2012.112
50. Xi Y, Lin Y, Guo W, Wang X, Zhao H, Miao C, et al. Multi-omic characterization of genome-wide abnormal DNA methylation reveals diagnostic and prognostic markers for esophageal squamous-cell carcinoma. Signal Transduct Target Ther. (2022) 7:53. doi: 10.1038/s41392-022-00873-8
51. Macedo-Silva C, Constancio V, Miranda-Goncalves V, Leite-Silva P, Salta S, Lobo J, et al. DNA methylation biomarkers accurately detect esophageal cancer prior and post neoadjuvant chemoradiation. Cancer Med. (2023) 12:8777–88. doi: 10.1002/cam4.5623
52. Zheng Y, Gao Q, Su X, Xiao C, Yu B, Huang S, et al. Genome-wide DNA methylation and gene expression profiling characterizes molecular subtypes of esophagus squamous cell carcinoma for predicting patient survival and immunotherapy efficacy. Cancers (Basel). (2022) 14:4970. doi: 10.3390/cancers14204970
53. Cao J, Yan Q. Cancer epigenetics, tumor immunity, and immunotherapy. Trends Cancer. (2020) 6:580–92. doi: 10.1016/j.trecan.2020.02.003
54. Zhang W, Hong R, Li L, Wang Y, Du P, Ou Y, et al. The chromosome 11q13.3 amplification associated lymph node metastasis is driven by miR-548k through modulating tumor microenvironment. Mol Cancer. (2018) 17:125. doi: 10.1186/s12943-018-0871-4
55. Chen Y, Huang Y, Gao X, Li Y, Lin J, Chen L, et al. CCND1 amplification contributes to immunosuppression and is associated with a poor prognosis to immune checkpoint inhibitors in solid tumors. Front Immunol. (2020) 11:1620. doi: 10.3389/fimmu.2020.01620
56. Wang F, Ren C, Zhao Q, Xu N, Shen L, Dai G, et al. Association of frequent amplification of chromosome 11q13 in esophageal squamous cell cancer with clinical benefit to immune check point blockade. J Clin Oncol. (2019) 37:4036–. doi: 10.1200/JCO.2019.37.15_suppl.4036
57. Yan K, Zhang D, Chen Y, Lu W, Huang M, Cai J, et al. Chromosome 11q13 amplification correlates with poor response and prognosis to PD-1 blockade in unresectable hepatocellular carcinoma. Front Immunol. (2023) 14:1116057. doi: 10.3389/fimmu.2023.1116057
58. Ying J, Shan L, Li J, Zhong L, Xue L, Zhao H, et al. Genome-wide screening for genetic alterations in esophageal cancer by aCGH identifies 11q13 amplification oncogenes associated with nodal metastasis. PloS One. (2012) 7:e39797. doi: 10.1371/journal.pone.0039797
59. Zhang J, Bu X, Wang H, Zhu Y, Geng Y, Nihira NT, et al. Cyclin D-CDK4 kinase destabilizes PD-L1 via cullin 3-SPOP to control cancer immune surveillance. Nature. (2018) 553:91–5. doi: 10.1038/nature25015
60. Yamaguchi R, Lartigue L, Perkins G. Targeting Mcl-1 and other Bcl-2 family member proteins in cancer therapy. Pharmacol Ther. (2019) 195:13–20. doi: 10.1016/j.pharmthera.2018.10.009
61. Wang H, Guo M, Wei H, Chen Y. Targeting MCL-1 in cancer: current status and perspectives. J Hematol Oncol. (2021) 14:67. doi: 10.1186/s13045-021-01079-1
62. Lin J, Fu D, Dai Y, Lin J, Xu T. Mcl-1 inhibitor suppresses tumor growth of esophageal squamous cell carcinoma in a mouse model. Oncotarget. (2017) 8:114457–62. doi: 10.18632/oncotarget.18772
63. Liu Z, Gu S, Wu K, Li L, Dong C, Wang W, et al. CircRNA-DOPEY2 enhances the chemosensitivity of esophageal cancer cells by inhibiting CPEB4-mediated Mcl-1 translation. J Exp Clin Cancer Res. (2021) 40:361. doi: 10.1186/s13046-021-02149-5
64. Mukherjee N, Skees J, Todd KJ, West DA, Lambert KA, Robinson WA, et al. MCL1 inhibitors S63845/MIK665 plus Navitoclax synergistically kill difficult-to-treat melanoma cells. Cell Death Dis. (2020) 11:443. doi: 10.1038/s41419-020-2646-2
65. Oh SJ, Lee HJ, Song KH, Kim S, Cho E, Lee J, et al. Targeting the NANOG/HDAC1 axis reverses resistance to PD-1 blockade by reinvigorating the antitumor immunity cycle. J Clin Invest. (2022) 132:e147908. doi: 10.1172/JCI147908
66. Song KH, Choi CH, Lee HJ, Oh SJ, Woo SR, Hong SO, et al. HDAC1 upregulation by NANOG promotes multidrug resistance and a stem-like phenotype in immune edited tumor cells. Cancer Res. (2017) 77:5039–53. doi: 10.1158/0008-5472.Can-17-0072
67. Adhikary S, Roy S, Chacon J, Gadad SS, Das C. Implications of enhancer transcription and eRNAs in cancer. Cancer Res. (2021) 81:4174–82. doi: 10.1158/0008-5472.Can-20-4010
68. Jiang W, Pan S, Chen X, Wang Z-w, Zhu X. The role of lncRNAs and circRNAs in the PD-1/PD-L1 pathway in cancer immunotherapy. Mol Cancer. (2021) 20:116. doi: 10.1186/s12943-021-01406-7
69. Lam MTY, Cho H, Lesch HP, Gosselin D, Heinz S, Tanaka-Oishi Y, et al. Rev-Erbs repress macrophage gene expression by inhibiting enhancer-directed transcription. Nature. (2013) 498:511–5. doi: 10.1038/nature12209
70. Liu J, Liu Z-X, Li J-J, Zeng Z-L, Wang J-H, Luo X-J, et al. The macrophage-associated lncRNA MALR facilitates ILF3 liquid–liquid phase separation to promote HIF1α Signaling in esophageal cancer. Cancer Res. (2023) 83:1476–89. doi: 10.1158/0008-5472.Can-22-1922
71. Gao W, Liu S, Wu Y, Wei W, Yang Q, Li W, et al. Enhancer demethylation-regulated gene score identified molecular subtypes, inspiring immunotherapy or CDK4/6 inhibitor therapy in oesophageal squamous cell carcinoma. eBioMedicine. (2024) 105:105177. doi: 10.1016/j.ebiom.2024.105177
72. Jia L, Chen J, Zhao J, Yang J. Identification of enhancer RNA AC005515.1 as a novel biomarker for prognosis in esophageal cancer and predictors of immunotherapy response. Trans Cancer Res. (2023) 12:3266–83. doi: 10.21037/tcr-23-777
73. Liu J, Zhou WY, Luo XJ, Chen YX, Wong CW, Liu ZX, et al. Long noncoding RNA Regulating ImMune Escape regulates mixed lineage leukaemia protein-1-H3K4me3-mediated immune escape in oesophageal squamous cell carcinoma. Clin Trans Med. (2023) 13:e1410. doi: 10.1002/ctm2.1410
74. Baba Y, Nomoto D, Okadome K, Ishimoto T, Iwatsuki M, Miyamoto Y, et al. Tumor immune microenvironment and immune checkpoint inhibitors in esophageal squamous cell carcinoma. Cancer Sci. (2020) 111:3132–41. doi: 10.1111/cas.14541
75. Zheng X, Song X, Shao Y, Xu B, Hu W, Zhou Q, et al. Prognostic role of tumor-infiltrating lymphocytes in esophagus cancer: a meta-analysis. Cell Physiol Biochem. (2018) 45:720–32. doi: 10.1159/000487164
76. Yao G, Fan H, Wang R, Zhang Y, Du C, Chen B, et al. 15P Prediction for pCR after neoadjuvant immunotherapy combined with chemotherapy using single-cell RNA sequencing in patients with locally advanced esophageal squamous cell carcinoma (escc): A single-arm phase II clinical trial. Immuno-Oncol Technol. (2022) 16:100120. doi: 10.1016/j.iotech.2022.100120
77. Li C, Zhao S, Zheng Y, Han Y, Chen X, Cheng Z, et al. Preoperative pembrolizumab combined with chemoradiotherapy for oesophageal squamous cell carcinoma (PALACE-1). Eur J Cancer. (2021) 144:232–41. doi: 10.1016/j.ejca.2020.11.039
78. Held W, Siddiqui I, Schaeuble K, Speiser DE. Intratumoral CD8(+) T cells with stem cell-like properties: Implications for cancer immunotherapy. Sci Transl Med. (2019) 11:eaay6863. doi: 10.1126/scitranslmed.aay6863
79. Siddiqui I, Schaeuble K, Chennupati V, Fuertes Marraco SA, Calderon-Copete S, Pais Ferreira D, et al. Intratumoral tcf1(+)PD-1(+)CD8(+) T cells with stem-like properties promote tumor control in response to vaccination and checkpoint blockade immunotherapy. Immunity. (2019) 50:195–211 e10. doi: 10.1016/j.immuni.2018.12.021
80. Li J, Xie Y, Wang X, Li F, Li S, Li M, et al. Prognostic impact of tumor-associated macrophage infiltration in esophageal cancer: a meta-analysis. Future Oncol. (2019) 15:2303–17. doi: 10.2217/fon-2018-0669
81. Wang XL, Liu K, Liu JH, Jiang XL, Qi LW, Xie YF, et al. High infiltration of CD68-tumor associated macrophages, predict poor prognosis in Kazakh esophageal cancer patients. Int J Clin Exp Pathol. (2017) 10:10282–92.
82. Svensson MC, Svensson M, Nodin B, Borg D, Hedner C, Hjalmarsson C, et al. High infiltration of CD68+/CD163- macrophages is an adverse prognostic factor after neoadjuvant chemotherapy in esophageal and gastric adenocarcinoma. J Innate Immun. (2022) 14:615–28. doi: 10.1159/000524434
83. Zhang W, Yan C, Zhang T, Chen X, Dong J, Zhao J, et al. Addition of camrelizumab to docetaxel, cisplatin, and radiation therapy in patients with locally advanced esophageal squamous cell carcinoma: a phase 1b study. Oncoimmunology. (2021) 10:1971418. doi: 10.1080/2162402X.2021.1971418
84. Yagi T, Baba Y, Okadome K, Kiyozumi Y, Hiyoshi Y, Ishimoto T, et al. Tumour-associated macrophages are associated with poor prognosis and programmed death ligand 1 expression in oesophageal cancer. Eur J Cancer. (2019) 111:38–49. doi: 10.1016/j.ejca.2019.01.018
85. Fujimura T, Aiba S. Significance of immunosuppressive cells as a target for immunotherapies in melanoma and non-melanoma skin cancers. Biomolecules. (2020) 10:1087. doi: 10.3390/biom10081087
86. Sui X, Chen C, Zhou X, Wen X, Shi C, Chen G, et al. Integrative analysis of bulk and single-cell gene expression profiles to identify tumor-associated macrophage-derived CCL18 as a therapeutic target of esophageal squamous cell carcinoma. J Exp Clin Cancer Res. (2023) 42:51. doi: 10.1186/s13046-023-02612-5
87. Ayers M, Lunceford J, Nebozhyn M, Murphy E, Loboda A, Kaufman DR, et al. IFN-gamma-related mRNA profile predicts clinical response to PD-1 blockade. J Clin Invest. (2017) 127:2930–40. doi: 10.1172/JCI91190
88. Spranger S, Spaapen RM, Zha Y, Williams J, Meng Y, Ha TT, et al. Up-regulation of PD-L1, IDO, and T(regs) in the melanoma tumor microenvironment is driven by CD8(+) T cells. Sci Transl Med. (2013) 5:200ra116. doi: 10.1126/scitranslmed.3006504
89. Xuan W, Qu Q, Zheng B, Xiong S, Fan GH. The chemotaxis of M1 and M2 macrophages is regulated by different chemokines. J Leukoc Biol. (2015) 97:61–9. doi: 10.1189/jlb.1A0314-170R
90. Fujimura T, Sato Y, Tanita K, Lyu C, Kambayashi Y, Amagai R, et al. Association of baseline serum levels of CXCL5 with the efficacy of nivolumab in advanced melanoma. Front Med (Lausanne). (2019) 6:86. doi: 10.3389/fmed.2019.00086
91. Yang H, Zhang Q, Xu M, Wang L, Chen X, Feng Y, et al. CCL2-CCR2 axis recruits tumor associated macrophages to induce immune evasion through PD-1 signaling in esophageal carcinogenesis. Mol Cancer. (2020) 19:41. doi: 10.1186/s12943-020-01165-x
92. Mariette C, De Botton ML, Piessen G. Surgery in esophageal and gastric cancer patients: what is the role for nutrition support in your daily practice? Ann Surg Oncol. (2012) 19:2128–34. doi: 10.1245/s10434-012-2225-6
93. Wu M, Zhu Y, Chen X, Wang X, Lin X, Yan X, et al. Prognostic nutritional index predicts the prognosis of patients with advanced esophageal cancer treated with immune checkpoint inhibitors: a retrospective cohort study. J Gastrointest Oncol. (2023) 14:54–63. doi: 10.21037/jgo-23-48
94. Xue Y, Zhou X, Xue L, Zhou R, Luo J. The role of pretreatment prognostic nutritional index in esophageal cancer: A meta-analysis. J Cell Physiol. (2019) 234:19655–62. doi: 10.1002/jcp.28565
95. Fearon K, Strasser F, Anker SD, Bosaeus I, Bruera E, Fainsinger RL, et al. Definition and classification of cancer cachexia: an international consensus. Lancet Oncol. (2011) 12:489–95. doi: 10.1016/S1470-2045(10)70218-7
96. Ray-Coquard I, Cropet C, Van Glabbeke M, Sebban C, Le Cesne A, Judson I, et al. Lymphopenia as a prognostic factor for overall survival in advanced carcinomas, sarcomas, and lymphomas. Cancer Res. (2009) 69:5383–91. doi: 10.1158/0008-5472.CAN-08-3845
97. Okadome K, Baba Y, Yagi T, Kiyozumi Y, Ishimoto T, Iwatsuki M, et al. Prognostic nutritional index, tumor-infiltrating lymphocytes, and prognosis in patients with esophageal cancer. Ann Surg. (2020) 271:693–700. doi: 10.1097/SLA.0000000000002985
98. Kim JH, Ahn B, Hong SM, Jung HY, Kim DH, Choi KD, et al. Real-world efficacy data and predictive clinical parameters for treatment outcomes in advanced esophageal squamous cell carcinoma treated with immune checkpoint inhibitors. Cancer Res Treat. (2022) 54:505–16. doi: 10.4143/crt.2020.1198
99. Xu J, Li Y, Fan Q, Shu Y, Yang L, Cui T, et al. Clinical and biomarker analyses of sintilimab versus chemotherapy as second-line therapy for advanced or metastatic esophageal squamous cell carcinoma: a randomized, open-label phase 2 study (ORIENT-2). Nat Commun. (2022) 13:857. doi: 10.1038/s41467-022-28408-3
100. Gregory AD, Houghton AM. Tumor-associated neutrophils: new targets for cancer therapy. Cancer Res. (2011) 71:2411–6. doi: 10.1158/0008-5472.CAN-10-2583
101. Shau HY, Kim A. Suppression of lymphokine-activated killer induction by neutrophils. J Immunol. (1988) 141:4395–402. doi: 10.4049/jimmunol.141.12.4395
102. Balkwill F, Mantovani A. Inflammation and cancer: back to Virchow? Lancet. (2001) 357:539–45. doi: 10.1016/S0140-6736(00)04046-0
103. Ancel J, Dormoy V, Raby BN, Dalstein V, Durlach A, Dewolf M, et al. Soluble biomarkers to predict clinical outcomes in non-small cell lung cancer treated by immune checkpoints inhibitors. Front Immunol. (2023) 14:1171649. doi: 10.3389/fimmu.2023.1171649
104. Kato R, Yamasaki M, Urakawa S, Nishida K, Makino T, Morimoto-Okazawa A, et al. Increased Tim-3(+) T cells in PBMCs during nivolumab therapy correlate with responses and prognosis of advanced esophageal squamous cell carcinoma patients. Cancer Immunol Immunother. (2018) 67:1673–83. doi: 10.1007/s00262-018-2225-x
105. Hanahan D. Hallmarks of cancer: new dimensions. Cancer Discovery. (2022) 12:31–46. doi: 10.1158/2159-8290.CD-21-1059
106. Zhou J, Huang G, Wong WC, Hu DH, Zhu JW, Li R, et al. The impact of antibiotic use on clinical features and survival outcomes of cancer patients treated with immune checkpoint inhibitors. Front Immunol. (2022) 13:968729. doi: 10.3389/fimmu.2022.968729
107. Khan U, Ho K, Hwang EK, Pena C, Brouwer J, Hoffman K, et al. Impact of use of antibiotics on response to immune checkpoint inhibitors and tumor microenvironment. Am J Clin Oncol. (2021) 44:247–53. doi: 10.1097/COC.0000000000000813
108. Greally M, Chou JF, Chatila WK, Margolis M, Capanu M, Hechtman JF, et al. Clinical and molecular predictors of response to immune checkpoint inhibitors in patients with advanced esophagogastric cancer. Clin Cancer Res. (2019) 25:6160–9. doi: 10.1158/1078-0432.CCR-18-3603
109. Guo JC, Lin CC, Lin CY, Hsieh MS, Kuo HY, Lien MY, et al. Neutrophil-to-lymphocyte ratio and use of antibiotics associated with prognosis in esophageal squamous cell carcinoma patients receiving immune checkpoint inhibitors. Anticancer Res. (2019) 39:5675–82. doi: 10.21873/anticanres.13765
110. Xu L, Qi Y, Jiang Y, Ji Y, Zhao Q, Wu J, et al. Crosstalk between the gut microbiome and clinical response in locally advanced thoracic esophageal squamous cell carcinoma during neoadjuvant camrelizumab and chemotherapy. Ann Trans Med. (2022) 10:325–. doi: 10.21037/atm-22-1165
111. Giles AJ, Hutchinson MND, Sonnemann HM, Jung J, Fecci PE, Ratnam NM, et al. Dexamethasone-induced immunosuppression: mechanisms and implications for immunotherapy. J Immunother Cancer. (2018) 6:51. doi: 10.1186/s40425-018-0371-5
112. Yang H, Xia L, Chen J, Zhang S, Martin V, Li Q, et al. Stress-glucocorticoid-TSC22D3 axis compromises therapy-induced antitumor immunity. Nat Med. (2019) 25:1428–41. doi: 10.1038/s41591-019-0566-4
113. Cui Y, Han X, Liu H, Xie Q, Guan Y, Yin B, et al. Impact of endogenous glucocorticoid on response to immune checkpoint blockade in patients with advanced cancer. Front Immunol. (2023) 14:1081790. doi: 10.3389/fimmu.2023.1081790
114. Deng Y, Xia X, Zhao Y, Zhao Z, Martinez C, Yin W, et al. Glucocorticoid receptor regulates PD-L1 and MHC-I in pancreatic cancer cells to promote immune evasion and immunotherapy resistance. Nat Commun. (2021) 12:7041. doi: 10.1038/s41467-021-27349-7
115. Martins Nascentes Melo L, Herrera-Rios D, Hinze D, Loffek S, Oezel I, Turiello R, et al. Glucocorticoid activation by HSD11B1 limits T cell-driven interferon signaling and response to PD-1 blockade in melanoma. J Immunother Cancer. (2023) 11:e004150. doi: 10.1136/jitc-2021-004150
116. Wan JCM, Massie C, Garcia-Corbacho J, Mouliere F, Brenton JD, Caldas C, et al. Liquid biopsies come of age: towards implementation of circulating tumour DNA. Nat Rev Cancer. (2017) 17:223–38. doi: 10.1038/nrc.2017.7
117. Chen X, Xu X, Wang D, Liu J, Sun J, Lu M, et al. Neoadjuvant sintilimab and chemotherapy in patients with potentially resectable esophageal squamous cell carcinoma (KEEP-G 03): an open-label, single-arm, phase 2 trial. J ImmunoTher Cancer. (2023) 11:e005830. doi: 10.1136/jitc-2022-005830
118. He Q, Shi X, Yan J, Wu M, Gu C, Yu X. Circulating tumor DNA serial monitoring of relapse and responses to tislelizumab immunotherapy as second−line monotherapy for metastatic esophageal squamous cell carcinoma: A prospective study. Mol Clin Oncol. (2024) 20:29. doi: 10.3892/mco.2024.2727
119. Kelly RJ, Landon BV, Zaidi AH, Singh D, Canzoniero JV, Balan A, et al. Neoadjuvant nivolumab or nivolumab plus LAG-3 inhibitor relatlimab in resectable esophageal/gastroesophageal junction cancer: a phase Ib trial and ctDNA analyses. Nat Med. (2024) 30(4):1023–34. doi: 10.1038/s41591-024-02877-z
120. Chen B, Liu S, Zhu Y, Wang R, Cheng X, Chen B, et al. Predictive role of ctDNA in esophageal squamous cell carcinoma receiving definitive chemoradiotherapy combined with toripalimab. Nat Commun. (2024) 15:1919. doi: 10.1038/s41467-024-46307-7
121. Wang H, Zhang X, Zhao X, Song C, Deng W, Shen W. Minimal residual disease guided radical chemoradiotherapy combined with immunotherapy after neoadjuvant immunochemotherapy followed by adjuvant immunotherapy for esophageal squamous cell cancer (ECMRD-001): a study protocol for a prospective cohort study. Front Immunol. (2024) 14:1330928. doi: 10.3389/fimmu.2023.1330928
122. Chen Y, Zhang J, Han G, Tang J, Guo F, Li W, et al. Efficacy and safety of XELOX combined with anlotinib and penpulimab vs XELOX as an adjuvant therapy for ctDNA-positive gastric and gastroesophageal junction adenocarcinoma: a protocol for a randomized, controlled, multicenter phase II clinical trial (EXPLORING study). Front Immunol. (2023) 14:1232858. doi: 10.3389/fimmu.2023.1232858
123. Schizas D, Charalampakis N, Kole C, Mylonas KS, Katsaros I, Zhao M, et al. Immunotherapy for esophageal cancer: a 2019 update. Immunotherapy. (2020) 12:203–18. doi: 10.2217/imt-2019-0153
124. Chen YX, Wang ZX, Jin Y, Zhao Q, Liu ZX, Zuo ZX, et al. An immunogenic and oncogenic feature-based classification for chemotherapy plus PD-1 blockade in advanced esophageal squamous cell carcinoma. Cancer Cell. (2023) 41:919–32 e5. doi: 10.1016/j.ccell.2023.03.016
125. Dai L, Tao Y, Shi Z, Liang W, Hu W, Xing Z, et al. SOCS3 acts as an onco-immunological biomarker with value in assessing the tumor microenvironment, pathological staging, histological subtypes, therapeutic effect, and prognoses of several types of cancer. Front Oncol. (2022) 12:881801. doi: 10.3389/fonc.2022.881801
126. Ge F, Li Z, Hu J, Pu Y, Zhao F, Kong L. METTL3/m(6)A/IFIT2 regulates proliferation, invasion and immunity in esophageal squamous cell carcinoma. Front Pharmacol. (2022) 13:1002565. doi: 10.3389/fphar.2022.1002565
127. Xia TL, Yan SM, Yuan L, Zeng MS. Upregulation of METTL3 expression predicts poor prognosis in patients with esophageal squamous cell carcinoma. Cancer Manag Res. (2020) 12:5729–37. doi: 10.2147/CMAR.S245019
128. Zhou Y, Guo S, Li Y, Chen F, Wu Y, Xiao Y, et al. METTL3 is associated with the Malignancy of esophageal squamous cell carcinoma and serves as a potential immunotherapy biomarker. Front Oncol. (2022) 12:824190. doi: 10.3389/fonc.2022.824190
129. Wang L, Cao NN, Wang S, Man HW, Li PF, Shan BE. Roles of coinhibitory molecules B7-H3 and B7-H4 in esophageal squamous cell carcinoma. Tumour Biol. (2016) 37:2961–71. doi: 10.1007/s13277-015-4132-5
130. Chen L, Chen J, Xu B, Wang Q, Zhou W, Zhang G, et al. B7-H3 expression associates with tumor invasion and patient’s poor survival in human esophageal cancer. Am J Transl Res. (2015) 7:2646–60.
131. Gebauer F, Krämer M, Bruns C, Schlößer HA, Thelen M, Lohneis P, et al. Lymphocyte activation gene-3 (LAG3) mRNA and protein expression on tumour infiltrating lymphocytes (TILs) in oesophageal adenocarcinoma. J Cancer Res Clin Oncol. (2020) 146:2319–27. doi: 10.1007/s00432-020-03295-7
132. Tawbi HA, SChadendorf D, Lipson EJ, Ascierto PA, Matamala L, Castillo Gutierrez E, et al. Relatlimab and nivolumab versus nivolumab in untreated advanced melanoma. N Engl J Med. (2022) 386:24–34. doi: 10.1056/NEJMoa2109970
133. Wang W, Chen D, Zhao Y, Zhao T, Wen J, Mao Y, et al. Characterization of LAG-3, CTLA-4, and CD8(+) TIL density and their joint influence on the prognosis of patients with esophageal squamous cell carcinoma. Ann Transl Med. (2019) 7:776. doi: 10.21037/atm.2019.11.38
134. Zhang Y, Liu YD, Luo YL, Liu BL, Huang QT, Wang F, et al. Prognostic value of lymphocyte activation gene-3 (LAG-3) expression in esophageal squamous cell carcinoma. J Cancer. (2018) 9:4287–93. doi: 10.7150/jca.26949
135. Das M, Zhu C, Kuchroo VK. Tim-3 and its role in regulating anti-tumor immunity. Immunol Rev. (2017) 276:97–111. doi: 10.1111/imr.12520
136. Wang P, Chen Y, Long Q, Li Q, Tian J, Liu T, et al. Increased coexpression of PD-L1 and TIM3/TIGIT is associated with poor overall survival of patients with esophageal squamous cell carcinoma. J Immunother Cancer. (2021) 9:e002836. doi: 10.1136/jitc-2021-002836
137. Joller N, Lozano E, Burkett PR, Patel B, Xiao S, Zhu C, et al. Treg cells expressing the coinhibitory molecule TIGIT selectively inhibit proinflammatory Th1 and Th17 cell responses. Immunity. (2014) 40:569–81. doi: 10.1016/j.immuni.2014.02.012
138. Xiao K, Xiao K, Li K, Xue P, Zhu S. Prognostic role of TIGIT expression in patients with solid tumors: A meta-analysis. J Immunol Res. (2021) 2021:5440572. doi: 10.1155/2021/5440572
139. Yu X, Harden K, Gonzalez LC, Francesco M, Chiang E, Irving B, et al. The surface protein TIGIT suppresses T cell activation by promoting the generation of mature immunoregulatory dendritic cells. Nat Immunol. (2009) 10:48–57. doi: 10.1038/ni.1674
140. Loeser H, Kraemer M, Gebauer F, Bruns C, Schroder W, Zander T, et al. The expression of the immune checkpoint regulator VISTA correlates with improved overall survival in pT1/2 tumor stages in esophageal adenocarcinoma. Oncoimmunology. (2019) 8:e1581546. doi: 10.1080/2162402X.2019.1581546
141. Wang Y, Zhang H, Liu C, Wang Z, Wu W, Zhang N, et al. Immune checkpoint modulators in cancer immunotherapy: recent advances and emerging concepts. J Hematol Oncol. (2022) 15:111. doi: 10.1186/s13045-022-01325-0
142. Huang H, Zhang G, Li G, Ma H, Zhang X. Circulating CD14(+)HLA-DR(-/low) myeloid-derived suppressor cell is an indicator of poor prognosis in patients with ESCC. Tumour Biol. (2015) 36:7987–96. doi: 10.1007/s13277-015-3426-y
143. Zhao Y, Du J, Shen X. Targeting myeloid-derived suppressor cells in tumor immunotherapy: Current, future and beyond. Front Immunol. (2023) 14:1157537. doi: 10.3389/fimmu.2023.1157537
144. Guo H, Lin XY, Feng S, Wang C, Yuan LQ, Sheng XG, et al. Prognostic value of obesity in patients with cancer treated with immune checkpoint inhibitors: An updated meta−analysis and systematic review. Mol Clin Oncol. (2024) 20:5. doi: 10.3892/mco.2023.2703
145. Wang Z, Aguilar EG, Luna JI, Dunai C, Khuat LT, Le CT, et al. Paradoxical effects of obesity on T cell function during tumor progression and PD-1 checkpoint blockade. Nat Med. (2019) 25:141–51. doi: 10.1038/s41591-018-0221-5
146. Editorial O. Erratum to cancer cachexia syndrome and clinical outcome in patients with metastatic non-small cell lung cancer treated with PD-1/PD-L1 inhibitors: results from a prospective, observational study. Transl Lung Cancer Res. (2023) 12:2146–7. doi: 10.21037/tlcr-2023-3
147. Rounis K, Makrakis D, Gioulbasanis I, Ekman S, De Petris L, Mavroudis D, et al. Cancer cachexia and antitumor immunity: common mediators and potential targets for new therapies. Life (Basel). (2022) 12:880. doi: 10.3390/life12060880
148. Yu S, Li W, Tang L, Fan X, Yao S, Zhang X, et al. Depression in breast cancer patients: Immunopathogenesis and immunotherapy. Cancer Lett. (2022) 536:215648. doi: 10.1016/j.canlet.2022.215648
149. Bi Z, Li W, Zhao J, Pang L, Jing Y, Zhang X, et al. Negative correlations of psychological distress with quality of life and immunotherapy efficacy in patients with advanced NSCLC. Am J Cancer Res. (2022) 12:805–15.
150. Aldea M, Craciun L, Tomuleasa C, Crivii C. The role of depression and neuroimmune axis in the prognosis of cancer patients. J Buon. (2014) 19:5–14.
Keywords: esophageal cancer, immunotherapy, biomarkers, ICIs, TIME
Citation: Tong X, Jin M, Wang L, Zhang D, Yin Y and Shen Q (2024) Prognostic biomarkers for immunotherapy in esophageal cancer. Front. Immunol. 15:1420399. doi: 10.3389/fimmu.2024.1420399
Received: 20 April 2024; Accepted: 05 September 2024;
Published: 30 September 2024.
Edited by:
Prakash Radhakrishnan, University of Nebraska Medical Center, United StatesReviewed by:
Chao Song, Harbin Medical University, ChinaPei-Yu Huang, Sun Yat-sen University Cancer Center (SYSUCC), China
Copyright © 2024 Tong, Jin, Wang, Zhang, Yin and Shen. This is an open-access article distributed under the terms of the Creative Commons Attribution License (CC BY). The use, distribution or reproduction in other forums is permitted, provided the original author(s) and the copyright owner(s) are credited and that the original publication in this journal is cited, in accordance with accepted academic practice. No use, distribution or reproduction is permitted which does not comply with these terms.
*Correspondence: Qian Shen, c2hlbnFpYW5AdGpoLnRqbXUuZWR1LmNu; Yuping Yin, eWlueXVwaW5nMjAxN0BodXN0LmVkdS5jbg==
†These authors have contributed equally to this work and share first authorship