- 1Huzhou Key Laboratory of Medical and Environmental Applications Technologies, School of Life Sciences, Huzhou University, Huzhou, China
- 2Key Laboratory of Vector Biology and Pathogen Control of Zhejiang Province, Huzhou University, Huzhou, China
Inflammation is a normal immune response in organisms, but it often triggers chronic diseases such as colitis and arthritis. Currently, the most widely used anti-inflammatory drugs are non-steroidal anti-inflammatory drugs, albeit they are accompanied by various adverse effects such as hypertension and renal dysfunction. Bioactive peptides (BAPs) provide therapeutic benefits for inflammation and mitigate side effects. Herein, this review focuses on the therapeutic effects of various BAPs on inflammation in different body parts. Emphasis is placed on the immunomodulatory mechanisms of BAPs in treating inflammation, such as regulating the release of inflammatory mediators, modulating MAPK and NF-κB signaling pathways, and reducing oxidative stress reactions for immunomodulation. This review aims to provide a reference for the function, application, and anti-inflammation mechanisms of BAPs.
1 Introduction
Inflammation is a normal immune response of the body’s innate and adaptive immune systems to infections (1), which can protect the body from damage caused by external toxins and stimuli (2). It is a way to self-heal, repair damaged tissues, and combat pathogens (3). However, the attack of inflammatory factors will result in cellular necrosis and the reduction of metabolic and immune functions, eventually leading to tissue damage and organ dysfunction. The duration of inflammation is different, which could be divided into acute and chronic inflammation (4). Many chronic diseases are associated with inflammation, including arthritis, inflammatory bowel disease (5), cardiovascular diseases (6), osteoporosis (7), cancer (8), and obesity (9). Therefore, combating inflammatory damage is one of the major health challenges of the 21st century. Non-steroidal anti-inflammatory drugs (NSAIDs), such as aspirin and ibuprofen, are a class of chemically synthesized anti-inflammatory drugs that do not contain steroid structures (10). They are the most widely used anti-inflammatory drugs. However, numerous studies have shown that NSAIDs have various side effects on the host, including hypertension, nephrotic syndrome, cardiovascular toxicity, acute renal failure, and gastrointestinal complications (1). Additionally, antibiotics can be used to treat inflammation, but they can induce to the emergence of antibiotic-resistant superbugs. Therefore, there is an urgent need to explore new strategies for anti-inflammation. Since the first antimicrobial peptide Cecropins was discovered in 1981, the antibacterial and anti-inflammatory activity of peptides has attracted more and more attention from academia (11). Research has described that the peptide GPETAFLR possessed anti-inflammatory activity, effectively inhibiting neuroinflammation and maintaining stability in the central nervous system (12).
BAPs refer to short-chain amino acid sequences with active biological functions within organisms, typically consisting of 2 to 20 amino acid residues interconnected by peptide or amide bonds (13). The arrangement and combination of these amino acid residues are different and can form linear or cyclic structures (13). The sources of BAPs are diverse, mainly including animals, plants, microorganisms, marine organisms, soy products, milk, and fermented products (14). When BAPs remain inactive within parent proteins, they can become active upon enzymatic release through peptide cleavage (15). Apart from being generated through the hydrolysis of parent proteins, BAPs can also be produced via microbial fermentation. In order to obtain BAPs with specific activity, specific proteases with a wide range of functions are usually used for hydrolysis (16).
Peptides offer several advantages over traditional drugs in disease treatment (17). For example, their low molecular weight allows them to penetrate membranes effectively (18, 19), making them more potent (20). Furthermore, bioactive peptides (BAPs) have the potential for targeted therapy with minimal or negligible toxicity, even at low concentrations (21). Inflammation occurs after the activation of inflammatory pathways by triggering factors, leading to the release of inflammatory agents (22). Concurrently, the anti-inflammatory characteristics of BAPs may be influenced by molecular weight, amino acid composition (hydrophobic amino acids, positively charged amino acids, specific amino acids), and amino acid position (3).
This review provides a detailed overview of the research status of BAPs in the treatment of skin inflammation, intestinal inflammation, pulmonary inflammatory disease, arthritis, and ocular inflammation. Subsequently, it delves into the immunomodulatory mechanisms employed by BAPs in the treatment of inflammation, such as regulating the release of inflammatory mediators, modulating mitogen-activated protein kinase (MAPK) and nuclear factor κB (NF-κB) signaling pathways, and reducing oxidative stress response for immunomodulation. The aim is to seek new strategies for inflammation treatment and provide references for the development and application of anti-inflammatory peptides.
2 The functions of BAPs
BAPs exhibit a wide array of functions including antimicrobial, antioxidative, anti-inflammatory, memory-enhancing, antithrombotic and antihypertensive activities, regulation of gastrointestinal absorption, appetite suppression, opioid modulation, immune modulation, and cell regulation. According to different functions, BAPs are mainly divided into anti-inflammatory peptides, antimicrobial peptides (AMPs), antioxidant active peptides, anticancer active peptides, antihypertensive peptides, and neuropeptides (Table 1). Anti-inflammatory peptides can modulate immune responses and alleviate inflammation. They can suppress the production of pro-inflammatory cytokines and the activation of inflammatory pathways, or directly interact with immune cells. BAPs with antibacterial activity are called AMPs.The activity of AMPs may be attributed to their ability to effectively disrupt bacterial cell walls or membranes with a strong negative charge, exerting their action with cations and their hydrophobic effect (15). They may also attack microbial membranes or cytoplasmic components, altering their cellular functions and leading to cell death (23). AMPs can inhibit the synthesis of cell walls, nucleic acids, and proteins by engaging various enzymes within target cells (23). AMPs possess minimal to provoke resistance (24), thereby conferring a natural advantage over antibiotics for combating microbial infections. Han et al. (25) discovered that AMPs containing tryptophan can downregulate the expression of DNA replication initiation genes in cells, consequently demonstrating efficacy in combating multidrug-resistant Pseudomonas aeruginosa.
The antioxidant effect of BAPs can slow down or prevent cellular damage (26). With the disturbance of the prevailing environment, oxidative stress reactions occur, resulting in the release of free radicals, which may contribute to health issues, including cancer, cardiovascular, and other diseases (27). These peptides primarily consist of 5-16 hydrophobic amino acids (27). They typically include tyrosine, whose phenolic side chain serves as an important scavenger of free radicals (28). Hydrophobic amino acids can increase the penetration rate of peptides to cell membranes, and enhance the ability of peptides to reach mitochondria, which is one of the main sites of free radical production (29, 30). An important feature of the antioxidant activity of BAPs is their hydrophobicity. It helps protect the polyunsaturated fatty acids and other lipophilic targets from oxidation (29, 30). Teng et al. (31) reported that jellyfish peptides (JPHT-2) were effective antioxidants which could scavenge free radicals. The peptides enhanced the levels of superoxide dismutase (SOD) and inhibited oxidative damage by H2O2. Gao et al. (32) reported a new anti-inflammatory peptide from sturgeon muscle, and found that it can effectively inhibit the release of NO, IL-6 and IL-1β, increase the SOD activity in the LPS-induced RAW264.7 cells, and down-regulate MAPK pathway. Zhou et al. (33) described that milk casein-derived peptide OEPVL could regulate the release of nitric oxide (NO) and the production of cytokines IL-4, IL-10, IFN-γ, and TNF-α in vivo, thereby achieving the purpose of inhibiting LPS-induced inflammation.
3 Anti-inflammation of BAPs
As infection affects or damages different organs within the body, an inflammatory response occurs to combat infection, address injury, and facilitate self-repair. However, inflammatory factors can attack cells, leading to cell death, reduced cellular metabolism, and compromised immune function (3). BAPs can treat skin, intestine, lung, joint and eye inflammation, etc (Figure 1). BAPs can regulate the inflammatory pathways, the levels of cytokines or gut microbiota, and alleviate oxidative stress (Table 2).
3.1 Skin inflammation
The skin serves as a physical barrier between internal and external environments (107). Various factors can induce inflammatory responses in the skin, primarily due to immune dysregulation caused by internal diseases, infections, and allergic reactions. Skin inflammation is a primary manifestation of chronic autoimmune inflammatory diseases such as psoriasis, atopic dermatitis (AD), and lupus erythematosus (108). Approximately, 60 million people suffer from psoriasis, a chronic, systemic, immune-mediated inflammatory skin disease (109). As previously described, the synthetic peptide LKEKK (150-500 μg) combined with Aldara cream containing 5% imiquimod was applied to the ears of the imiquimod-induced psoriasis mouse model (80). After 6 days of treatment, the thickness of mouse ears was significantly reduced, indicating that the development of inflammation was effectively inhibited. Traditional medications for AD often yield unsatisfactory results. Lee et al. (83) reported a short peptide TPS240 and investigated its therapeutic effect in a DNCB-induced AD mouse model. The control group was treated with the same concentration of dexamethasone. Finally, it was found that the symptoms of AD in the TPS240 group were alleviated, and the skin damage was significantly restored by using 5 mg/kg TPS240. The body weight of mice treated with 5 mg/kg dexamethasone decreased and the organs contracted abnormally. TPS240 exerts its anti-AD effect by inhibiting the activation of NF-κB and STAT3, which is similar to dexamethasone and has no side effects. These results indicated that TPS240 would be a safe and effective drug for AD. Systemic lupus erythematosus (SLE) is an autoimmune disease that can promote chronic inflammation (110). It has been reported that the artificial peptide pConsensus, which blocks the PD-1/PD-1 ligand 1 pathway in untreated mice, promotes tolerance and inhibits SLE (111). Schall et al. (112) reported the peptide P140 could clear harmful T and B cells, and normalize the immune response in lupus-susceptible mice.
Additionally, due to the skin’s susceptibility to various injuries, wounds disrupt its environmental barrier, leading to a cascade of inflammatory responses. Controlling inflammation is crucial for maintaining skin health. Li et al. (113) demonstrated that the peptide OA-RD17 extracted from Odorrana-andersonii skin tissue could activate MAPK to promote macrophage proliferation and migration, block inflammation and propel wound healing by inhibiting NF-κB. OA-RD17 could accelerate the regeneration of full-thickness skin wounds in mice, showing that the repair rate of skin wounds was nearly 100%. At the same time, it had a certain repair effect on deep second-degree burns and isolated skin wounds of diabetic patients. OA-RD17 could up-regulate the expression of miR-632 and promote the regeneration of full-thickness skin wounds in rats, and the repair rate reached 92.4%. Therefore, BAPs with their antimicrobial and immune-modulating functions offer efficacious therapeutic approaches for wound healing and skin inflammation.
3.2 Intestinal inflammation
The intestine plays a crucial role in human health, serving as a site for digestion and nutrient absorption, and the largest organ of the immune system (114). The intestinal barrier is essential for separating the external environment from the host’s internal environment. As the intestine is exposed to pathogens or other toxic substances, inflammatory responses occur under the influence of harmful stimuli (115). Enteritis is a prevalent inflammatory bowel disease. So far, the main methods used clinically for enteritis treatment include drug therapy, dietary interventions and surgical treatment. However, the treatment outcomes are often unsatisfactory. Therefore, it is very important to find a better and safer treatment method. BAPs have immunomodulatory and anti-inflammatory effects, making it possible to effectively treat intestinal inflammation and provide a new treatment for enteritis. Zhi et al. (116) reported that walnut-derived peptide leucine-proline-phenylalanine (LPF) could promote the repair of the intestinal epithelial barrier, reduce levels of pro-inflammatory cytokines, and exert protective and restorative effects on DSS-induced colitis in mice. It was found that the number of apoptotic cells in the treatment group was significantly less than that in the DSS group. The percentages of reduction in the three groups of DSS + 50 mg/kg LPF, DSS + 100 mg/kg LPF, and DSS + 200 mg/kg LPF on the 10th day were 50.00%, 41.18%, and 57.35%, respectively. In addition, 16S rDNA sequencing results showed that 100 mg/kg LPF had a regulatory effect on the intestinal flora of colitis mice. Additionally, Rahabi et al. (117) reported that fish collagen peptide Naticol®Gut could also be used to treat colitis. It directly acts on macrophages, polarizing them into an anti-inflammatory, immunotolerant, and antioxidative phenotype through an MR-dependent mechanism. For enteritis, antibiotics are often used for treatment, but their long-term use can lead to increased antibiotic resistance, posing a significant challenge. Sun et al. (118) reported that AMP R7I with anti-proteolytic properties could reduce inflammatory factors and maintain intestinal barrier function. The histological examination of the intestine showed that the tissue structure in the 20 mg/kg R7I group was basically normalized with only a small amount of isolated epithelial cells, and R7I could restore the normal morphology of the intestine. In addition, this peptide plays a crucial role in the treatment of murine bacterial enteritis and is helpful in finding effective strategies for the treatment of enteritis.
3.3 Lung inflammation
Pneumonia is a prevalent respiratory illness that involves inflammation in the lungs (119). Its occurrence is associated with respiratory viruses, common gram-negative or gram-positive bacteria, and mycobacterium (120, 121). Pneumonia has a complex etiology, and traditional treatment methods mainly involve the use of antibiotics, which can effectively reduce the incidence and mortality of pneumonia. However, issues such as antibiotic resistance, low bioavailability, and strong side effects exist (92, 122). Therefore, there is a necessity to discover novel treatment approaches. BAPs as a novel therapeutic drug may have potential in the treatment of pneumonia. Zhao et al. (92) reported that 7-amino acid peptide (7P), as a synthetic analog peptide, could effectively reduce bronchial contraction, inhibit acute inflammatory cytokines (TNFα, IL-1β and IL-6) and Th2 cytokine responses (IL-5, IL-4 and IL-13), and has certain effects on relieving airway hyperresponsiveness, airway inflammation and Th2 response. The results inferred that 7P could reduce allergic lung inflammation. It made a new option for addressing allergic pulmonary inflammation. Additionally, peptide modification can also be employed to improve the therapeutic effects. Moreira et al. (123) pegylated the synthetic peptide LyeTx I-b derived from natural LyeTx I, and reported that pegylated LyeTx I-b exhibited significant therapeutic effects against multidrug-resistant Acinetobacter baumannii-induced pneumonia. LyeTx I-bPEG increased the anti-biofilm activity. At 16 μM and 32 μM, LyeTx I-bPEG reduced the carbapenem-resistant Acinetobacter baumannii biofilm by 33 ± 4% and 26 ± 8%, respectively, compared with untreated cells. Furthermore, Jin et al. (124) designed two derived peptides GHbK4R and GHb3K based on the maternal peptide GHb. Vancomycin reduced lung bacteria in mice to 7.8 × 107 CFU/g, whereas GHb3K and GHbK4R decreased lung bacteria to 5.3 × 105 and 5.4 × 105 CFU/g. These results demonstrated that these peptides had significant therapeutic effects in a mouse model of acute pneumonia caused by Staphylococcus aureus infection. PS1-2 peptide is active against fluconazole-resistant Candida albicans, can inhibit the activity of TLR-2 and the expression of TNF-α, and has anti-fungal and anti-inflammatory functions for intratracheal infection induced by Candida albicans (91). However, there is limited research on the use of BAPs for the treatment of human pneumonia. It still needs a good strategy to treat pneumonia.
3.4 Joint inflammation
Arthritis is a common inflammatory disease which affects the joints and surrounding tissues. It can be acute or chronic, leading to joint pain, swelling and difficulty movement in severe cases. Arthritis has a high prevalence and encompasses various types, including osteoarthritis, rheumatoid arthritis, and psoriatic arthritis (125). Osteoarthritis is a progressive disease and a major cause of chronic disability (126). Peptides offer a new therapeutic approach for osteoarthritis. Wu et al. (127) validated that the anti-inflammatory capacity of skipjack tuna elastin peptides in a zebrafish model could inhibit the JAK2/STAT3 signaling pathway, suppress inflammation and protect cartilage. Rheumatoid arthritis is an autoimmune disease that can lead to joint and bone damage (128, 129). For rheumatoid arthritis, Kim et al. (96) reported that a synthetic peptide AESIS-1 could inhibit STAT3-mediated signaling by upregulating SOCS3 expression, resulting in the decrease of Th17 cells. Psoriatic arthritis is a chronic systemic inflammatory disease affecting the skin, joints, and tendons (130). Wixler et al. (131) discovered small splenic peptides (SSPs) in the spleen, which could target dendritic cells and transforming them into tolerant cells, thus differentiating naive CD4 cells into regulatory T cells expressing Foxp3. SSPs had anti-inflammatory effects in vivo, and restore peripheral tolerance, effectively inhibiting the development of psoriatic arthritis. In addition, ankylosing spondylitis and gouty arthritis could be treated by using BAPs. Ankylosing spondylitis is an immune-mediated chronic inflammatory rheumatic disease that most commonly affects the spine (132). Liu et al. (99) reported that BAPs IQW could treat mice with ankylosing spondylitis, delay disease progression, alleviate inflammation in the intervertebral joints, and reduce the concentration of pro-inflammatory factors. Gouty arthritis is caused by inflammation triggered by the deposition of urate crystals in the joints and surrounding tissues (108). Commonly used medications include colchicine, corticosteroids, NSAIDs, and adrenocorticotropic hormone, but these drugs have certain side effects such as nausea and gastrointestinal toxicity. Therefore, there is an urgent need to develop new drugs to treat gouty arthritis (133). Yan et al. (134) described that BAPs mastoparan M (Mast-M) extracted from wasp venom could inhibit the MAPK/NF-κB signaling pathway and reduce oxidative stress, thereby blocking the activation of the NLRP3 inflammasome and effectively treating gouty arthritis. Hence, BAPs have good therapeutic effect on joint inflammation.
3.5 Eyes inflammation
Eye inflammation is a common ocular condition that can occur from the surface of the eye to intraocular tissues (135). As threatened by inflammation, the eye tissues can sustain damage over the short or long term (136). The causes of eye inflammation are varied, including pathogen infections such as bacterial, fungal, and viral infections, as well as non-infectious factors like external environmental stimuli and allergic reactions (137). The treatment of eye inflammation mainly involves the use of anti-inflammatory drugs and antibiotics for medication or surgical methods. However, these approaches have certain drawbacks such as drug side effects and long recovery times. In recent years, more BAPs with therapeutic potential have emerged. Lu et al. (102) designed a peptide called WP-17, which targeted the toll-like receptor 4 (TLR4) to inhibit the activation of the NF-κB pathway. The highest dose of WP‐17 (10 μg/eye) strikingly decreased the protein levels of TNF‐α and IL‐6 in the aqueous humor of rats by 77.26% and 85.67%, respectively. WP-17 has shown promising therapeutic effects in rat uveitis. Similarly, Ho et al. (138) reported that a 29-mer peptide derived from pigment epithelium-derived factor could inhibit the expression of matrix metalloproteinase-9 and pro-inflammatory cytokines on murine dry eye. In addition, Zeng et al. (103) described that tilapia skin peptides (TSP) impeded the generation and development of dry eye disease via inhibition of apoptosis (19.4%), inflammation, and oxidative stress.
3.6 Other inflammation
The oral cavity is an important part of the human body and serves as the starting point of the digestive system. The oral cavity harbors a rich microbial population, constituting the second abundant microbial community in the human body after the gut, with over 700 identified oral microbial species (139, 140). Disruption of the oral microbiota can lead to an increase in local TH17 cells, which are associated with oral immunity and inflammation (141). Dysbiosis of the oral microbiota can lead to periodontitis, a common oral disease caused by pathogens invading the periodontal tissues such as the gums (142, 143). BAPs can inhibit bacterial growth and reduce inflammation. Akiyama et al. (104) reported the role of peptide P140 in a mouse model of periodontitis, and found that treatment with P140 effectively alleviated inflammation in gingival tissues, reduced lymphocyte infiltration, and lowered the expression of pro-inflammatory mediators. In addition, liver injury can also be treated by bioactive peptides. Zhu et al. (144) described a peptide HEPFYGNEGALR isolated and identified from Apostichopus japonicus. This peptide can activate the Nrf2/HO-1 pathway, block the nuclear translocation of NF-κB, alleviate oxidative stress and inflammation, and alleviate acute alcoholic liver injury caused by excessive alcohol intake. Besides, BAPs have a certain ability in the treatment of myocarditis. Cortistatin is a small molecule bioactive peptide (145). Delgado-Maroto et al. (146) reported the therapeutic effect of cortistatin in experimental autoimmune myocarditis, and found that it could inhibit the inflammatory response driven by cardiomyogenic T cells.
3.7 Clinical application of BAPs
Peptides and peptidomimetics are emerging as an important class of clinic therapeutics (147). However, their application is hindered by their poor stability, short half-life, and low retention rate (148). It was reported that cyclic peptide structures had high topological flexibility, and their shape changes without transforming the amino acid composition sequence could not alter their properties (149). Therefore, molecular grafting is a good choice. It has been demonstrated that bradykinin antagonists were conjugated onto cyclic peptide scaffolds for the inflammation treatment (150). And sustained-release peptide analogues can be used for clinical treatment (151). BAPs are widely used to regulate inflammatory pathways and inflammatory factors to treat inflammation in clinics. Brimapitide (XG-102), a peptide bound to the N-terminal sequence of c-Jun, inhibits JNK by competing with endogenous c-Jun. In this way, it suppresses inflammation caused by JNK. This drug is currently under Phase III (149). Thymosin alpha-1 is an immunostimulatory peptide. It can regulate the immune system, enhance T cell function, inhibit the release of pro-inflammatory cytokines, and promote the production of anti-inflammatory cytokines (152). It is clinically used to treat hepatitis B (153). Since one century ago, more than 80 peptide drugs have reached the market for a wide range of diseases, including diabetes, cancer, osteoporosis, multiple sclerosis, HIV infection and chronic pain (154). However, there are still few peptides as clinical drugs for the treatment of inflammation.
4 Anti-inflammatory mechanism of BAPs
4.1 Regulation of the release of inflammatory mediators
Chemical substances released by cells or produced by body fluids during the inflammatory process, which participate in or cause the inflammatory reaction, are referred to as inflammatory mediators. They mainly include prostaglandins, NO, cytokines like interleukins (IL) (e.g., IL-1β, 2, 6, and 8), chemokines, etc. (155). As activated through toll-like receptors (TLR), these innate immune cells induce the release of IL-6 and TNF-α, along with transforming growth factor-β, which facilitates cell proliferation (156). The NF-κB and MAPK are also key pro-inflammatory intermediaries that are produced after TLR activation (157). Cytokines are low molecular weight glycoproteins produced and secreted by different cells, which can regulate the proliferation and differentiation of immune cells (158). They can be divided into two major categories: pro-inflammatory and anti-inflammatory factors. Pro-inflammatory factors such as IL-1β and TNF-α further induce the inflammatory response, while anti-inflammatory factors such as IL-10 can promote the resolution of the inflammatory response (159). Many studies show that BAPs can regulate the release of inflammatory mediators. Tornatore et al. (157) isolated four peptides from eggs white and these peptides exhibited anti-inflammatory activities in colitis mice by inhibiting the production of TNF-α and IL-6 as well as reducing the mRNA-expressions TNF-α, IL-6, IL17, IL-1β, IFN-γ, and MCP-1. Xing et al. (160) reported that bovine bone gelatin peptides could alleviate the additional secretion of inflammatory factors IL-6, NO, and TNF-α induced by lipopolysaccharide (LPS) in RAW264.7 cells to mitigate DSS-induced colitis. Cresti et al. (161) conducted efficacy studies on the synthetic peptide SET-M33 targeting gram-negative bacteria by using an LPS-induced pneumonia model. They found that the peptide effectively reduced the production of pro-inflammatory cytokines KC, MIP-1α, IP-10, MCP-1, and TNF-α.
4.2 Regulation of inflammatory signaling pathways
Inducers like LPS can stimulate and activate key proteins or genes involved in cellular signaling pathways such as NF-κB pathway (162) and MAPK pathway (163). The anti-inflammatory peptides inhibit cell inflammatory responses mainly through the MAPK and NF-κB pathways. NF-κB pathway is the most important way to regulate the transcription of pro-inflammatory cytokines such as IL-6, IL-1β and TNF-α, and also plays a vital role in the expressions of inducible nitric oxide synthase (iNOS) and COX-2 (164). NF-κB is a family of transcription factor proteins, including five subunits: p65 (RelA), p50, p52, Rel, and RelB. After dimer p65/p50 is released into the cytosol, it can be translocated into the nucleus and initiates target gene transcription for pro-inflammatory factors, causing inflammation (164, 165). MAPK can regulate many cellular activities, including proliferation, differentiation, death and immune response. The stimulus and MAP3K phosphorylation can mediate the phosphorylation of the downstream MAP2K and MAPK, which contain three subfamilies: p38, extracellular signal-regulated kinases (ERK1 and ERK2), and c-Jun N-terminal kinase (JNK). In unstimulated cells, JNK mainly exists in the cytoplasm and partly distributes in the nucleus. After being stimulated, JNK accumulates in the nucleus and causes the corresponding gene (IL-1 and TNF-α) expression, resulting in an inflammatory response (166). BAPs inhibit the expression of inflammatory genes by blocking NF-κB and MAPK signaling pathways (Figure 2). The JAK-STAT pathway is also important for inflammatory response, which can regulate hematopoietic cell development and inflammatory cytokines (167). Phosphorylation of JAK and STATs can form the dimer translocated to the nucleus (168). In addition, the peptide transporter PepT1 can transport small BAPs to the bloodstream. Therefore, the role of PepT1 is vital to the bioactivity of BAPs (167). Chei et al. (169) described that acid-hydrolyzed silk peptide (SP) inhibited LPS-induced inflammation by modulating the TLR4 signaling pathway, while clam peptide MMV2 reduced the mRNA levels of inflammation-related genes induced by LPS in adult zebrafish (170). Formyl peptide receptors (FPRs), members of the GPCR family with seven transmembrane domains (171), play important roles in antimicrobial host defence mechanisms. FPRs recognize formylated peptides, non-formylated peptides, synthetic small molecules, and formyl analogs from bacteria and mitochondria to regulate inflammatory responses that lead to chemotaxis, degranulation, and oxidative bursts (172). Jin et al. (173) reported that VLATSGPG (VLA), a DPP-IV inhibitory peptide isolated from the skin of Salmo Salar, could inhibit the activation of PERK through the AKT signaling pathway, and increase the expression of IκBα mRNA through the PERK/IκBα pathway, leading to blocking the activation of NF-κB p65 and further cell inflammation. Tsuruki et al. (174) isolated some immunostimulating peptides from soy protein, which had specific binding sites on mouse or human macrophages and could stimulate their phagocytic activity.
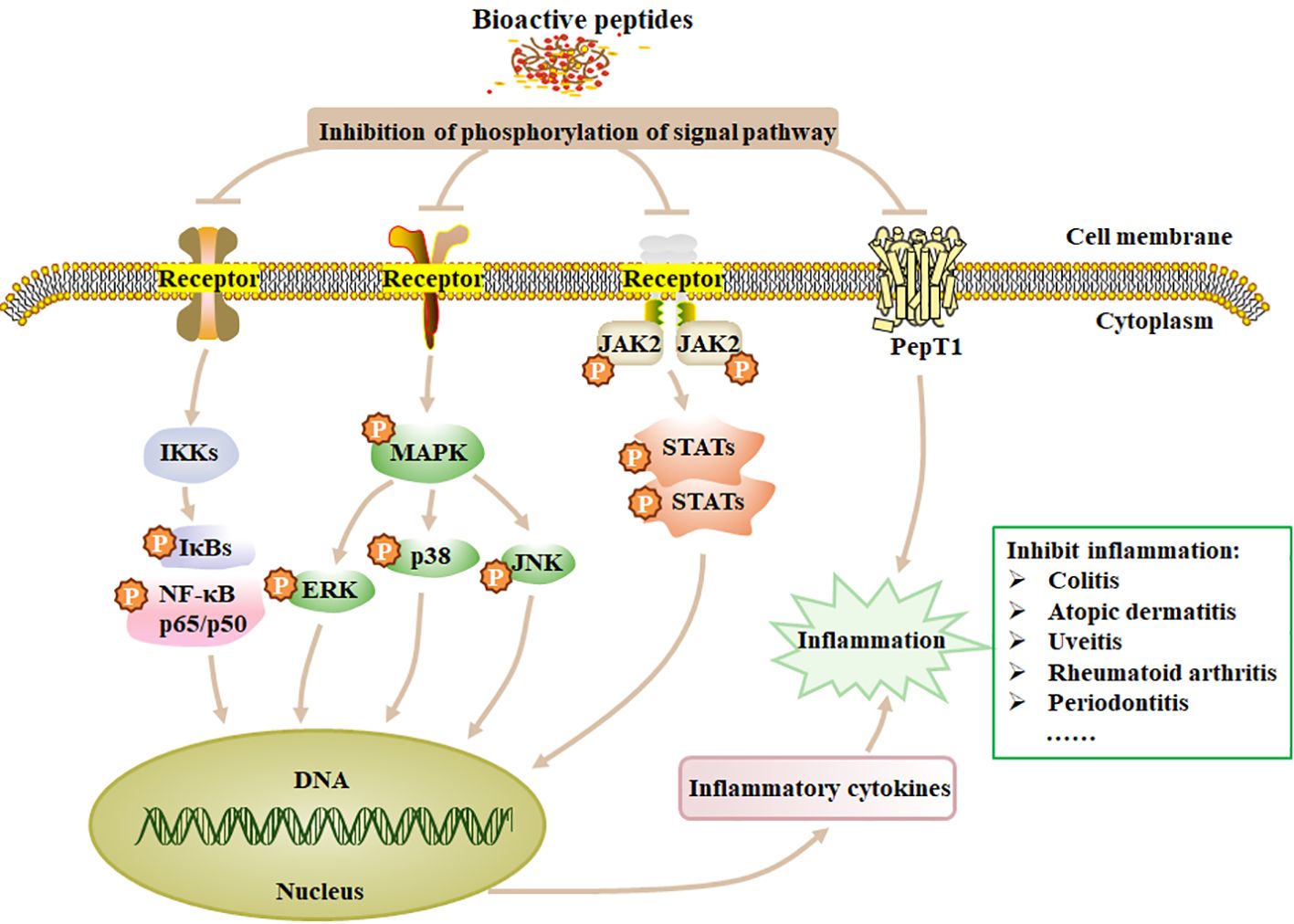
Figure 2. The mechanism of anti-inflammation of BAPs. Treatment of inflammation by modulating the four signaling pathways, such as NF-κB, MAPK, JAK and STATs. p, phosphorylation; Ikks, inhibitor of kappa B kinase. Adapted from previous reports (167).
4.3 Regulation of reduced oxidative stress response
Oxidative stress is a significant pathological factor that contributes to various inflammatory diseases. Inflammatory responses trigger the excessive generation of reactive oxygen species (ROS) within cells, disrupting the body’s free radical metabolism and leading to oxidative stress. Moreover, during oxidative metabolism, excessive ROS can attack cells or tissues, causing structural and functional damage and exacerbating inflammatory reactions (175, 176). BAPs can reduce the generation of ROS. Lee et al. (177) isolated the peptide PPY1 from Pyropia yezoensis, and stated that PPY1 significantly decreased the ROS levels in LPS-induced macrophages. Oxidative stress and inflammation are closely related, which can elucidate why NF-κB is the initial mammalian transcription factor to be influenced by oxidation (178). NF-κB plays a crucial role in mediating inflammatory responses and is regulated by various mediators, including H2O2 and ROS (178). ROS can modulate NF-κB through both the Canonical and Noncanonical pathways (Figure 3). Malondialdehyde (MDA) and glutathione (GSH) are important markers of oxidative stress. MDA is the final product of ROS-induced lipid peroxidation, while GSH is an intracellular antioxidant that protects cells from oxidative stress damage. Peng et al. (54) identified an active peptide, GGAW, which exhibits excellent antioxidant functionality. This peptide effectively reduces the production of ROS, MDA and lactate dehydrogenase (LDH), and increases the activity of SOD and glutathione peroxidase (GSH-PX). Consequently, it enhances cell viability and protects IEC-6 cells from H2O2-induced oxidative damage. The Kelch-like ECH-associated protein 1-(Keap1) Nrf2-antioxidant response element is the main antioxidant signaling pathway that prevents oxidative stress and helps maintain the optimum redox steady state in vivo (179). Hence, the Nrf2 antioxidant signaling pathway can be stimulated to suppress oxidative stress within the body (167). Fernando et al. (180) reported that AMVDAIAR, a peptide isolated from pepsin hydrolysate of krill enhanced antioxidant enzymes SOD, CAT and GPx, thereby suppressing the oxidative stress in H2O2-induced hepatocytes and increasing the expression of Nrf2.
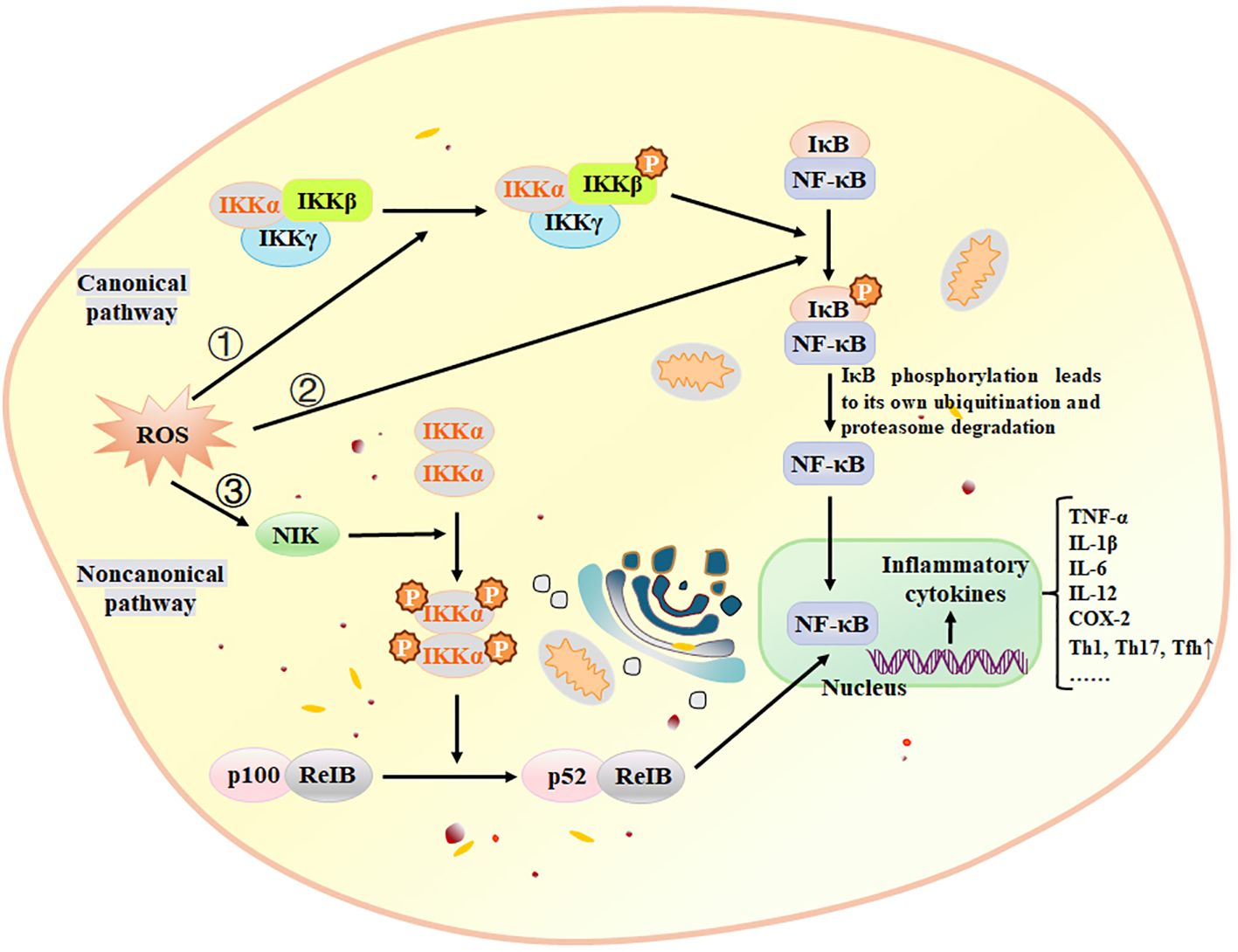
Figure 3. ROS activate NF-κB through three pathways. ① Canonical pathway: ROS activates the IKK complex, phosphorylating IκBα. Phosphorylation leads to ubiquitination and proteasomal degradation of IκBα, resulting in nuclear translocation of the NF-κB complex and gene expression through high-affinity binding to κB components, ② ROS directly phosphorylate IκBα, subsequently following the same pathway as the canonical pathway, ③ Noncanonical pathway: NIK is activated by ROS through inhibition of phosphatases and oxidation of cysteine residues. The NF-κB activation pathway relies on IKKα and activates the p52/RelB complex by triggering proteolytic cleavage of the p52/p100 precursor. IKK, IκB kinase; NIK, NF-κB-inducing kinase. Adapted from previous reports (181).
5 Conclusions and prospects
BAPs are widely employed in the treatment of inflammation. This review summarizes the therapeutic effects of BAPs on various inflammatory diseases such as pulmonary, gastrointestinal, dermatological, arthritic, oral and ocular inflammations. It also outlines the anti-inflammatory mechanisms of action of BAPs, which include modulation of inflammatory mediators’ release, regulation of inflammatory signaling pathways (NF-κB, MAPK, and JAK-STAT), and reduction of oxidative stress reactions to influence the development of inflammation.
BAPs have promising prospects for the preparation of anti-inflammatory drugs. However, BAPs are commonly implicated with several challenges, encompassing a short half-life, susceptibility to proteases, instability, potential toxicities, and other processing-related issues. Attempts can be made to modify or transform the BAPs, such as by attaching metal ions, targeting groups or nanomaterials to maximize their effectiveness. However, before using BAPs to treat various inflammatory diseases, more experiments are needed to obtain additional data on dosages, pharmacodynamics and pharmacokinetics. Studies should also investigate the differential effects of BAPs on different populations to better understand their efficacy. Furthermore, the anti-inflammatory mechanisms of various types of BAPs require investigation to ensure their safety in clinical applications. Additionally, many peptides face challenges in maintaining stability and functional activity in vivo due to inherent limitations of amino acids. BAPs can be encapsulated within nanoparticles to improve their stability. Future efforts should concentrate on finding more methods to overcome these challenges to maximize the efficacy of BAPs. In conclusion, BAPs hold great promise as potential inflammatory therapy. Further research and clinical data are necessary to support their widespread and safe application.
Author contributions
HL: Writing – original draft. LZ: Writing – original draft. JY: Conceptualization, Writing – review & editing. SS: Conceptualization, Writing – review & editing.
Funding
The author(s) declare that no financial support was received for the research, authorship, and/or publication of this article.
Conflict of interest
The authors declare that the research was conducted in the absence of any commercial or financial relationships that could be construed as a potential conflict of interest.
Publisher’s note
All claims expressed in this article are solely those of the authors and do not necessarily represent those of their affiliated organizations, or those of the publisher, the editors and the reviewers. Any product that may be evaluated in this article, or claim that may be made by its manufacturer, is not guaranteed or endorsed by the publisher.
References
1. Dutta P, Sahu RK, Dey T, Lahkar MD, Manna P, Kalita J. Beneficial role of insect-derived bioactive components against inflammation and its associated complications (colitis and arthritis) and cancer. Chemico-Biological Interactions. (2019) 313:108824. doi: 10.1016/j.cbi.2019.108824
2. Velnar T, Bailey T, Smrkoli V. The wound healing process: An overview of the cellular and molecular mechanisms. J Int Med Res. (2009) 37:1528–42. doi: 10.1177/147323000903700531
3. Liu WL, Chen XW, Li H, Zhang J, JL An, Liu XQ. Anti-inflammatory function of plant-derived bioactive peptides: A review. Foods. (2022) 11:2361. doi: 10.3390/foods11152361
4. Yi ZJ, Gong JP, Zhang W. Transcriptional co-regulator RIP140: An important mediator of the inflammatory response and its associated diseases. Mol Med Rep. (2017) 16:994–1000. doi: 10.3892/mmr.2017.6683
5. Fernández-Tomé S, Hernández-Ledesma B, Chaparro M, Indiano-Romacho P, Bernardo D, Gisbert JP. Role of food proteins and bioactive peptides in inflammatory bowel disease. Trends Food Sci Technol. (2019) 88:194–206. doi: 10.1016/j.tifs.2019.03.017
6. Lammi C, Aiello G, Boschin G, Arnoldi A. Multifunctional peptides for the prevention of cardiovascular disease: A new concept in the area of bioactive food-derived peptides. J Funct Foods. (2019) 55:135–45. doi: 10.1016/j.jff.2019.02.016
7. Yuan XY, Bao XL, Feng GX, Zhang ML, Ma S. Effects of peptide-calcium complexes from sunflower seeds and peanuts on enhancing bone mineral density. Int J Food Sci Technol. (2020) 55:2942–53. doi: 10.1111/ijfs.14555
8. Daghero H, Massó JRF, Astrada S, Vallespí MG, Bollati-Fogolín M. The anticancer peptide CIGB-552 exerts anti-inflammatory and anti-angiogenic effects through COMMD1. Molecules. (2021) 26:152. doi: 10.3390/molecules26010152
9. Wang GL, Yang XY, Wang J, Zhong DY, Zhang RG, Zhang YN, et al. Walnut green husk polysaccharides prevent obesity, chronic inflammatory responses, nonalcoholic fatty liver disease and colonic tissue damage in high-fat diet fed rats. Int J Biol Macromolecules. (2021) 182:879–98. doi: 10.1016/j.ijbiomac.2021.04.047
10. Brennan R, Wazaify M, Shawabkeh H, Boardley I, McVeigh J, Van Hout MC. A scoping review of non-medical and extra-medical use of non-steroidal anti-inflammatory drugs (NSAIDs). Drug Safety. (2021) 44:917–28. doi: 10.1007/s40264-021-01085-9
11. Steiner H, Hultmark D, Engström Å, Bennich H, Boman HG. Sequence and specificity of two antibacterial proteins involved in insect immunity. Nature. (1981) 292:246–8. doi: 10.1038/292246a0
12. Lemus-Conejo A, Millan-Linares MD, Toscano R, Millan F, Pedroche J, Muriana FJG, et al. GPETAFLR, a peptide from Lupinus angustifolius L. prevents inflammation in microglial cells and confers neuroprotection in brain. Nutr Neurosci. (2022) 25:472–84. doi: 10.1080/1028415x.2020.1763058
13. Karami Z, Akbari-adergani B. Bioactive food derived peptides: a review on correlation between structure of bioactive peptides and their functional properties. J Food Sci Technology-Mysore. (2019) 56:535–47. doi: 10.1007/s13197-018-3549-4
14. Purohit K, Reddy N, Sunna A. Exploring the potential of bioactive peptides: From natural sources to therapeutics. Int J Mol Sci. (2024) 25:1391. doi: 10.3390/ijms25031391
15. Chelliah R, Wei S, Daliri EBM, Elahi F, Yeon SJ, Tyagi A, et al. The role of bioactive peptides in diabetes and obesity. Foods. (2021) 10:2220. doi: 10.3390/foods10092220
16. Jakubczyk A, Karas M, Rybczynska-Tkaczyk K, Zielinska E, Zielinski D. Current trends of bioactive peptides-new sources and therapeutic effect. Foods. (2020) 9:846. doi: 10.3390/foods9070846
17. Diao H, Lu YH, Ling Y, Shen YJ, Yu JM, Ma K. Peptide-based self-assembly: Design, bioactive properties, and its applications. Curr Pharm Design. (2023) 29:640–51. doi: 10.2174/1381612829666230213152259
18. Anjum K, Abbas SQ, Akhter N, Shagufta BI, Shah SAA, ul Hassan SS. Emerging biopharmaceuticals from bioactive peptides derived from marine organisms. Chem Biol Drug Design. (2017) 90:12–30. doi: 10.1111/cbdd.12925
19. Zhang L, Yao L, Zhao F, Yu ALC, Zhou YR, Wen QM, et al. Protein and peptide-based nanotechnology for enhancing stability, bioactivity, and delivery of anthocyanins. Advanced Healthcare Materials. (2023) 12:2300473. doi: 10.1002/adhm.202300473
20. Zhang L, Hao ML, Yao L, Xing C, Wen QM, Zhang ZN, et al. Sericin "hairpin structure"-based multifunctional anthocyanin nanoencapsulation for remodeling ROS-dependent cutaneous wound healing. Chem Eng J. (2023) 475:145863. doi: 10.1016/j.cej.2023.145863
21. Bouglé D, Bouhallab S. Dietary bioactive peptides: Human studies. Crit Rev Food Sci Nutr. (2017) 57:335–43. doi: 10.1080/10408398.2013.873766
22. Nathan C, Ding AH. Nonresolving inflammation. Cell. (2010) 140:871–82. doi: 10.1016/j.cell.2010.02.029
23. Feijó CJA, Gonçalves EA, Melo N, Bittencourt LF. Fundamentals on the molecular mechanism of action of antimicrobial peptides. Materialia. (2019) 8:100494–. doi: 10.1016/j.mtla.2019.100494
24. Li WY, Separovic F, O'Brien-Simpson NM, Wade JD. Chemically modified and conjugated antimicrobial peptides against superbugs. Chem Soc Rev. (2021) 50:4932–73. doi: 10.1039/d0cs01026j
25. Han X, Kou ZR, Jiang FQ, Sun XM, Shang DJ. Interactions of designed Trp-containing antimicrobial peptides with DNA of multidrug-resistant. Pseudomonas aeruginosa DNA Cell Biol. (2021) 40:414–24. doi: 10.1089/dna.2019.4874
26. Garmidolova A, Desseva I, Mihaylova D, Lante A. Bioactive peptides from Lupinus spp. seed proteins-state-of-the-art and perspectives. Appl Sciences-Basel. (2022) 12:3766. doi: 10.3390/app12083766
27. Ucak I, Afreen M, Montesano D, Carrillo C, Tomasevic I, Simal-Gandara J, et al. Functional and bioactive properties of peptides derived from marine side streams. Mar Drugs. (2021) 19:71. doi: 10.3390/md19020071
28. Kati E, Nina G, Katrin S, Henning S. The ACE inhibitory dipeptide Met-Tyr diminishes free radical formation in human endothelial cells via induction of heme oxygenase-1 and ferritin. J Nutr. (2006) 136:2148–52. doi: 10.1093/jn/136.8.2148
29. Freitas AC, Andrade JC, Silva FM, Rocha-Santos TAP, Duarte AC, Gomes AM. Antioxidative peptides: Trends and perspectives for future research. Curr Medicinal Chem. (2013) 20:4575–94. doi: 10.2174/09298673113209990147
30. López-García G, Dublan-García O, Arizmendi-Cotero D, Oliván LMG. Antioxidant and antimicrobial peptides derived from food proteins. Molecules. (2022) 27:1343. doi: 10.3390/molecules27041343
31. Teng LC, Wang XQ, Yu HH, Li RF, Geng H, Xing RE, et al. Jellyfish peptide as an alternative source of antioxidant. Antioxidants. (2023) 12:742. doi: 10.3390/antiox12030742
32. Gao R, Shu W, Shen Y, Sun Q, Bai F, Wang J, et al. Sturgeon protein-derived peptides exert anti-inflammatory effects in LPS-stimulated RAW264.7 macrophages via the MAPK pathway. J Funct Foods. (2020) 72:104044. doi: 10.1016/j.jff.2020.104044
33. Zhou JH, Ma LL, Xu HH, Gao Y, Jin YK, Zhao L, et al. Immunomodulating effects of casein-derived peptides QEPVL and QEPV on lymphocytes in vitro and in vivo. Food Funct. (2014) 5:2061–9. doi: 10.1039/c3fo60657k
34. Milán-Linares MD, Millán F, Pedroche J, Yust MD. GPETAFLR: A new anti-inflammatory peptide from Lupinus angustifolius L. protein hydrolysate. J Funct Foods. (2015) 18:358–67. doi: 10.1016/j.jff.2015.07.016
35. Chen JL, Bai WB, Cai DB, Yu ZL, Xu BJ. Characterization and identification of novel anti-inflammatory peptides from Baijiao sea bass (Lateolabrax maculatus). Lwt-Food Sci Technol. (2021) 147:111521. doi: 10.1016/j.lwt.2021.111521
36. Moronta J, Smaldini PL, Docena GH, Añón MC. Peptides of amaranth were targeted as containing sequences with potential anti-inflammatory properties. J Funct Foods. (2016) 21:463–73. doi: 10.1016/j.jff.2015.12.022
37. Mesquita JX, de Brito TV, Fontenelle TPC, Damasceno ROS, de Souza M, de Souza Lopes JL, et al. Lectin from red algae amansia multifida lamouroux: Extraction, characterization and anti-inflammatory activity. Int J Biol Macromol. (2021) 170:532–9. doi: 10.1016/j.ijbiomac.2020.12.203
38. Chen X, Sun L, Li D, Lai X, Wen S, Chen R, et al. Green tea peptides ameliorate diabetic nephropathy by inhibiting the TGF-β/Smad signaling pathway in mice. Food Funct. (2022) 13:3258–70. doi: 10.1039/d1fo03615g
39. Shi Z, Dun B, Wei Z, Liu C, Tian J, Ren G, et al. Peptides released from extruded adzuki bean protein through simulated gastrointestinal digestion exhibit anti-inflammatory activity. J Agric Food Chem. (2021) 69:7028–36. doi: 10.1021/acs.jafc.1c01712
40. Chen YP, Liang CH, Wu HT, Pang HY, Chen C, Wang GH, et al. Antioxidant and anti-inflammatory capacities of collagen peptides from milkfish (Chanos chanos) scales. J Food Sci Technology-Mysore. (2018) 55:2310–7. doi: 10.1007/s13197-018-3148-4
41. García-Mora P, Frías J, Peñas E, Zieliński H, Giménez-Bastida JA, Wiczkowski W, et al. Simultaneous release of peptides and phenolics with antioxidant, ACE-inhibitory and anti-inflammatory activities from pinto bean (Phaseolus vulgaris L. var. pinto) proteins by subtilisins. J Funct Foods. (2015) 18:319–32. doi: 10.1016/j.jff.2015.07.010
42. Peng ZL, Gao JL, Su WM, Cao WH, Zhu GP, Qin XM, et al. Purification and identification of peptides from oyster (Crassostrea hongkongensis) protein enzymatic hydrolysates and their anti-skin photoaging effects on UVB-irradiated HaCaT cells. Mar Drugs. (2022) 20:749. doi: 10.3390/md20120749
43. Hansen IKO, Lövdahl T, Simonovic D, Hansen KO, Andersen AJC, Devold H, et al. Antimicrobial activity of small synthetic peptides based on the marine peptide turgencin a: Prediction of antimicrobial peptide sequences in a natural peptide and strategy for optimization of potency. Int J Mol Sci. (2020) 21:5460. doi: 10.3390/ijms21155460
44. Oh R, Lee MJ, Kim YO, Nam BH, Kong HJ, Kim JW, et al. Myticusin-beta, antimicrobial peptide from the marine bivalve, Mytilus coruscus. Fish Shellfish Immunol. (2020) 99:342–52. doi: 10.1016/j.fsi.2020.02.020
45. Ye ZM, Zhou XW, Xi XP, Zai Y, Zhou M, Chen XL, et al. In vitro & in vivo studies on identifying and designing temporin-1CEh from the skin secretion of Rana chensinensis as the optimised antibacterial prototype drug. Pharmaceutics. (2022) 14:604. doi: 10.3390/pharmaceutics14030604
46. Pellegrini A, Thomas U, Bramaz N, Hunziker P, von Fellenberg R. Isolation and identification of three bactericidal domains in the bovine α-lactalbumin molecule. Biochim Biophys Acta (BBA) - Gen Subjects. (1999) 1426:439–48. doi: 10.1016/S0304-4165(98)00165-2
47. Beaulieu L, Bondu S, Doiron K, Rioux L-E, Turgeon SL. Characterization of antibacterial activity from protein hydrolysates of the macroalga Saccharina longicruris and identification of peptides implied in bioactivity. J Funct Foods. (2015) 17:685–97. doi: 10.1016/j.jff.2015.06.026
48. Lesiuk M, Paduszynska M, Greber KE. Synthetic antimicrobial immunomodulatory peptides: Ongoing studies and clinical trials. Antibiotics-Basel. (2022) 11:1062. doi: 10.3390/antibiotics11081062
49. Liu Y, Shi DN, Wang J, Chen XL, Zhou M, Xi XP, et al. A novel amphibian antimicrobial peptide, phylloseptin-PV1, exhibits effective anti-staphylococcal activity without inducing either hepatic or renal toxicity in mice. Front Microbiol. (2020) 11:565158. doi: 10.3389/fmicb.2020.565158
50. Aguilar-Toalá JE, Deering AJ, Liceaga AM. New insights into the antimicrobial properties of hydrolysates and peptide fractions derived from chia seed (Salvia hispanica L.). Probiotics Antimicrobial Proteins. (2020) 12:1571–81. doi: 10.1007/s12602-020-09653-8
51. Outman A, Deracinois B, Flahaut C, Diab MA, Dhaouefi J, Gressier B, et al. Comparison of the bioactive properties of human and bovine hemoglobin hydrolysates obtained by enzymatic hydrolysis: Antimicrobial and antioxidant potential of the active peptide α137-141. Int J Mol Sci. (2023) 24:13055. doi: 10.3390/ijms241713055
52. Ma B, Guo Y, Fu X, Jin Y. Identification and antimicrobial mechanisms of a novel peptide derived from egg white ovotransferrin hydrolysates. LWT. (2020) 131:109720. doi: 10.1016/j.lwt.2020.109720
53. Czelej M, Czernecki T, Garbacz K, Wawrzykowski J, Jamiol M, Michalak K, et al. Egg yolk as a new source of peptides with antioxidant and antimicrobial properties. Foods. (2023) 12:3394. doi: 10.3390/foods12183394
54. Peng B, Cai BN, Pan JY. Octopus-derived antioxidant peptide protects against hydrogen peroxide-induced oxidative stress in IEC-6 cells. Food Sci Nutr. (2022) 10:4049–58. doi: 10.1002/fsn3.3000
55. Hernández-Ledesma B, Quirós A, Amigo L, Recio I. Identification of bioactive peptides after digestion of human milk and infant formula with pepsin and pancreatin. Int Dairy J. (2007) 17:42–9. doi: 10.1016/j.idairyj.2005.12.012
56. Solieri L, Valentini M, Cattivelli A, Sola L, Helal A, Martini S, et al. Fermentation of whey protein concentrate by Streptococcus thermophilus strains releases peptides with biological activities. Process Biochem. (2022) 121:590–600. doi: 10.1016/j.procbio.2022.08.003
57. Yang XR, Zhao YQ, Qiu YT, Chi CF, Wang B. Preparation and characterization of gelatin and antioxidant peptides from gelatin hydrolysate of skipjack tuna (Katsuwonus pelamis) bone stimulated by in vitro gastrointestinal digestion. Mar Drugs. (2019) 17:78. doi: 10.3390/md17020078
58. Chi C-F, Wang B, Hu F-Y, Wang Y-M, Zhang B, Deng S-G, et al. Purification and identification of three novel antioxidant peptides from protein hydrolysate of bluefin leatherjacket (Navodon septentrionalis) skin. Food Res Int. (2015) 73:124–9. doi: 10.1016/j.foodres.2014.08.038
59. Zhang F, Qu J, Thakur K, Zhang J-G, Mocan A, Wei Z-J. Purification and identification of an antioxidative peptide from peony (Paeonia suffruticosa Andr.) seed dreg. Food Chem. (2019) 285:266–74. doi: 10.1016/j.foodchem.2019.01.168
60. Jun S-Y, Park P-J, Jung W-K, Kim S-K. Purification and characterization of an antioxidative peptide from enzymatic hydrolysate of yellowfin sole (Limanda aspera) frame protein. Eur Food Res Technol. (2004) 219:20–6. doi: 10.1007/s00217-004-0882-9
61. Silva F, Hernández-Ledesma B, Amigo L, FM N, Miralles B. Identification of peptides released from flaxseed (Linum usitatissimum) protein by Alcalase® hydrolysis: Antioxidant activity. LWT - Food Sci Technol. (2017) 76:140–6. doi: 10.1016/j.lwt.2016.10.049
62. Agrawal H, Joshi R, Gupta M. Purification, identification and characterization of two novel antioxidant peptides from finger millet (Eleusine coracana) protein hydrolysate. Food Res Int. (2019) 120:697–707. doi: 10.1016/j.foodres.2018.11.028
63. Sheih IC, Wu T-K, Fang TJ. Antioxidant properties of a new antioxidative peptide from algae protein waste hydrolysate in different oxidation systems. Bioresource Technol. (2009) 100:3419–25. doi: 10.1016/j.biortech.2009.02.014
64. Mune MAM, Miyabe Y, Shimizu T, Matsui W, Kumagai Y, Kishimura H. Characterisation of bioactive peptides from red alga Gracilariopsis chorda. Mar Drugs. (2023) 21:49. doi: 10.3390/md21010049
65. Rival SG, Fornaroli S, Boeriu CG, Wichers HJ. Caseins and casein hydrolysates. 1. Lipoxygenase inhibitory properties. J Agric Food Chem. (2001) 49:287–94. doi: 10.1021/jf000392t
66. Ibrahim SRM, Min CC, Teuscher F, Ebel R, Kakoschke C, Lin W, et al. Callyaerins A–F and H, new cytotoxic cyclic peptides from the Indonesian marine sponge Callyspongia aerizusa. Bioorganic Medicinal Chem. (2010) 18:4947–56. doi: 10.1016/j.bmc.2010.06.012
67. García-Gasca T, García-Cruz M, Hernandez-Rivera E, López-Matínez J, Castañeda-Cuevas AL, Yllescas-Gasca L, et al. Effects of tepary bean (Phaseolus acutifolius) protease inhibitor and semipure lectin fractions on cancer cells. Nutr Cancer-an Int J. (2012) 64:1269–78. doi: 10.1080/01635581.2012.722246
68. Zampella A, Sepe V, Luciano P, Bellotta F, Monti MC, D'Auria MV, et al. an anti-HIV cyclodepsipeptide from the sponge Homophymia sp. J Organic Chem. (2008) 73:5319–27. doi: 10.1021/jo800583b
69. Pan X, Zhao YQ, Hu FY, Chi CF, Wang B. Anticancer activity of a hexapeptide from skate (Raja porosa) cartilage protein hydrolysate in HeLa cells. Mar Drugs. (2016) 14:153. doi: 10.3390/md14080153
70. Oh JY, Je JG, Lee HG, Kim EA, Kang SI, Lee JS, et al. Anti-hypertensive activity of novel peptides identified from olive flounder (Paralichthys olivaceus) surimi. Foods. (2020) 9:647. doi: 10.3390/foods9050647
71. Chi-Kang TB, Wei-Wen K, Hsuan DC, Jine-Yuan HD, Chia-Hua K, Jayasimharayalu D, et al. The soybean bioactive peptide VHVV alleviates hypertension-induced renal damage in hypertensive rats via the SIRT1-PGC1α/Nrf2 pathway. J Funct Foods. (2020) 75:104255. doi: 10.1016/j.jff.2020.104255
72. Jahandideh F, Chakrabarti S, Davidge ST, Wu JP. Egg white hydrolysate shows insulin mimetic and sensitizing effects in 3T3-F442A pre-adipocytes. PloS One. (2017) 12:e0185653. doi: 10.1371/journal.pone.0185653
73. Moayedi A, Mora L, Aristoy MC, Safari M, Hashemi M, Toldrá F. Peptidomic analysis of antioxidant and ACE-inhibitory peptides obtained from tomato waste proteins fermented using Bacillus subtilis. Food Chem. (2018) 250:180–7. doi: 10.1016/j.foodchem.2018.01.033
74. Wongngam W, Mitani T, Katayama S, Nakamura S, Yongsawatdigul J. Production and characterization of chicken blood hydrolysate with antihypertensive properties. Poultry Science. (2020) 99:5163–74. doi: 10.1016/j.psj.2020.07.006
75. Mora L, Escudero E, Arihara K, Toldrá F. Antihypertensive effect of peptides naturally generated during Iberian dry-cured ham processing. Food Res Int. (2015) 78:71–8. doi: 10.1016/j.foodres.2015.11.005
76. Koch TL, Torres JP, Baskin RP, Salcedo PF, Chase K, Olivera BM, et al. A toxin-based approach to neuropeptide and peptide hormone discovery. Front Mol Neurosci. (2023) 16:1176662. doi: 10.3389/fnmol.2023.1176662
77. Li CY, Zhang L, Li J, Qi CL, Li DY, Liu X, et al. Effect of endogenous arginine-vasopressin arising from the paraventricular nucleus on learning and memory functions in vascular dementia model rats. BioMed Res Int. (2017) 2017:3214918. doi: 10.1155/2017/3214918
78. Blázquez E, Alvarez E, Navarro M, Roncero I, Rodríguez-Fonseca F, JA C, et al. Glucagon-like peptide-1 (7-36) amide as a novel neuropeptide. Mol neurobiol. (1998) 18:157–73. doi: 10.1007/bf02914270
79. Ong KL, Lam KSL, Cheung BMY. Urotensin II: Its function in health and its role in disease. Cardiovasc Drugs Ther. (2005) 19:65–75. doi: 10.1007/s10557-005-6899-x
80. Navolotskaya EV, Sadovnikov VB, Zinchenko DV, Murashev AN. Effect of the synthetic peptide LKEKK on psoriasis. Russian J Bioorganic Chem. (2023) 49:1346–52. doi: 10.1134/s1068162023060158
81. Ju N, Shimamura M, Hayashi H, Ikeda Y, Yoshida S, Nakamura A, et al. Preventative effects of the partial RANKL peptide MHP1-AcN in a mouse model of imiquimod-induced psoriasis. Sci Rep. (2019) 9:15434. doi: 10.1038/s41598-019-51681-0
82. Kim MS, Song J, Park S, Kim TS, Park HJ, Cho D. The wound healing peptide, AES16-2M, ameliorates atopic dermatitis in vivo. Molecules. (2021) 26:1168. doi: 10.3390/molecules26041168
83. Lee D, Hwang-Bo J, Veerappan K, Moon H, Park J, Chung H. Anti-atopic dermatitis effect of TPS240, a novel therapeutic peptide, via suppression of NF-κB and STAT3 activation. Int J Mol Sci. (2023) 24:15814. doi: 10.3390/ijms242115814
84. Nguyen HL, Peng G, Trujillo-Paez JV, Yue HA, Ikutama R, Takahashi M, et al. The antimicrobial peptide AMP-IBP5 suppresses dermatitis-like lesions in a mouse model of atopic dermatitis through the low-density lipoprotein receptor-related protein-1 receptor. Int J Mol Sci. (2023) 24:5200. doi: 10.3390/ijms24065200
85. Huang B, Jiang JT, Luo BW, Zhu W, Liu YQ, Wang ZS, et al. Non-erythropoietic erythropoietin-derived peptide protects mice from systemic lupus erythematosus. J Cell Mol Med. (2018) 22:3330–9. doi: 10.1111/jcmm.13608
86. Xu CL, Guo Y, Qiao L, Ma L, Cheng YY. Recombinant expressed vasoactive intestinal peptide analogue ameliorates TNBS-induced colitis in rats. World J Gastroenterol. (2018) 24:706–15. doi: 10.3748/wjg.v24.i6.706
87. Su YZ, Sun TT, Gao JH, Zhang CX, Liu XS, Bi CP, et al. Anti-proteolytic peptide R7I protects the intestinal barrier and alleviates fatty acid malabsorption in Salmonella typhimurium-infected mice. Int J Mol Sci. (2023) 24:16409. doi: 10.3390/ijms242216409
88. Hong ZS, Xie J, Wang XF, Dai JJ, Mao JY, Bai YY, et al. Moringa oleifera Lam. peptide remodels intestinal mucosal barrier by inhibiting JAK-STAT activation and modulating gut microbiota in colitis. Front Immunol. (2022) 13:924178. doi: 10.3389/fimmu.2022.924178
89. Xiang XW, Zhou XL, Wang R, Shu CH, Zhou YF, Ying XG, et al. Protective effect of tuna bioactive peptide on dextran sulfate sodium-induced colitis in mice. Mar Drugs. (2021) 19:127. doi: 10.3390/md19030127
90. Wei XB, Zhang LL, Zhang RJ, Koci M, Si DY, Ahmad B, et al. A novel cecropin-LL37 hybrid peptide protects mice against EHEC infection-mediated changes in gut microbiota, intestinal inflammation, and impairment of mucosal barrier functions. Front Immunol. (2020) 11:1361. doi: 10.3389/fimmu.2020.01361
91. Lee JK, Park S, Kim YM, Guk T, Choi JK, Kim JY, et al. Antifungal and anti-inflammatory activities of PS1-2 peptide against fluconazole-resistant Candida albicans. Antibiotics-Basel. (2022) 11:1779. doi: 10.3390/antibiotics11121779
92. Zhao WZ, Mi YH, Zhao YY, Deng C, Yu RH, Mei QB, et al. 7-Amino acid peptide (7P) decreased airway inflammation and hyperresponsiveness in a murine model of asthma. Eur J Pharmacol. (2021) 912:174576. doi: 10.1016/j.ejphar.2021.174576
93. Wuerth KC, Falsafi R, Hancock REW. Synthetic host defense peptide IDR-1002 reduces inflammation in Pseudomonas aeruginosa lung infection. PloS One. (2017) 12:e0187565. doi: 10.1371/journal.pone.0187565
94. Wu GS, Wang JJ, Luo PF, Li A, Tian S, Jiang HL, et al. Hydrostatin-SN1, a sea snake-derived bioactive peptide, reduces inflammation in a mouse model of acute lung injury. Front Pharmacol. (2017) 8:246. doi: 10.3389/fphar.2017.00246
95. Wang K, Li YY, Dai YF, Han LH, Zhu YJ, Xue CH, et al. Peptides from antarctic krill (Euphausia superba) improve osteoarthritis via inhibiting HIF-2α-mediated death receptor apoptosis and metabolism regulation in osteoarthritic mice. J Agric Food Chem. (2019) 67:3125–33. doi: 10.1021/acs.jafc.8b05841
96. Kim KE, Jeon S, Song J, Kim TS, Jung MK, Kim MS, et al. The novel synthetic peptide AESIS-1 exerts a preventive effect on collagen-induced arthritis mouse model via STAT3 suppression. Int J Mol Sci. (2020) 21:378. doi: 10.3390/ijms21020378
97. Chuang KC, Lai YW, Ko CH, Yen CC, Chen HL, Lan YW, et al. Therapeutic effects of kefir peptides on adjuvant-induced arthritis in rats through anti-inflammation and downregulation of matrix metalloproteinases. Life Sci. (2023) 317:121411. doi: 10.1016/j.lfs.2023.121411
98. Meng M, Wang LF, Yao Y, Lin DM, Wang CY, Yao JL, et al. Ganoderma lucidum polysaccharide peptide (GLPP) attenuates rheumatic arthritis in rats through inactivating NF-κB and MAPK signaling pathways. Phytomedicine. (2023) 119:155010. doi: 10.1016/j.phymed.2023.155010
99. Liu G, Ma Y, Yang Q, Deng S. Modulation of inflammatory response and gut microbiota in ankylosing spondylitis mouse model by bioactive peptide IQW. J Appl Microbiol. (2020) 128:1669–77. doi: 10.1111/jam.14588
100. Ding W, Miao ZY, Feng XK, Luo AS, Tan WF, Li P, et al. Alamandine, a new member of the renin-angiotensin system (RAS), attenuates collagen-induced arthritis in mice via inhibiting cytokine secretion in synovial fibroblasts. Peptides. (2022) 154:170816. doi: 10.1016/j.peptides.2022.170816
101. Ahmed CM, Patel AP, Ildefonso CJ, Johnson H, Lewin AS. Corneal application of R9-SOCS1-KIR peptide alleviates endotoxin-induced uveitis. Trans Vision Sci Technol. (2021) 10:25. doi: 10.1167/tvst.10.3.25
102. Lu Y, Wang RA, Jin HY, Xie JM, Gu Q, Yang XL. A novel peptide derived from the mannose binding lectin inhibits LPS-activated TLR4/NF-κB signaling and suppresses ocular inflammation. Cell Biol Int. (2023) 47:1614–26. doi: 10.1002/cbin.12058
103. Zeng J, Hu C, Lin C, Zhang S, Deng K, Du J, et al. Tilapia skin peptides Inhibit apoptosis, inflammation, and oxidative stress to improve dry eye disease in vitro and in vivo. J Food Biochem. (2023) 2023:6761792. doi: 10.1155/2023/6761792
104. Akiyama K, Aung KT, Talamini L, Huck O, Kuboki T, Muller S. Therapeutic effects of peptide P140 in a mouse periodontitis model. Cell Mol Life Sci. (2022) 79:518. doi: 10.1007/s00018-022-04537-2
105. Wang HY, Lin L, Fu W, Yu HY, Yu N, Tan LS, et al. Preventive effects of the novel antimicrobial peptide Nal-P-113 in a rat periodontitis model by limiting the growth of Porphyromonas gingivalis and modulating IL-1β and TNF-α production. BMC Complementary Altern Med. (2017) 17:426. doi: 10.1186/s12906-017-1931-9
106. Wu W, Li GQ, Dong S, Chu CH, Ma SS, Zhang ZW, et al. Bomidin attenuates inflammation of periodontal ligament stem cells and periodontitis in mice via inhibiting ferroptosis. Int Immunopharmacol. (2024) 127:111423. doi: 10.1016/j.intimp.2023.111423
107. Song YL, Wu CY, Zhang XH, Bian WX, Liu NX, Yin SG, et al. A short peptide potentially promotes the healing of skin wound. Bioscience Rep. (2019) 39:Bsr20181734. doi: 10.1042/bsr20181734
108. Zou FM, Li XF, Yang R, Zhang RW, Zhao X. Effects and underlying mechanisms of food polyphenols in treating gouty arthritis: A review on nutritional intake and joint health. J Food Biochem. (2022) 46:e14072. doi: 10.1111/jfbc.14072
109. Hawkins P, Earl K, Tektonidis TG, Fallaize R. The role of diet in the management of psoriasis: a scoping review. Nutr Res Rev. (2023), 1–35. doi: 10.1017/s0954422423000185. (prepublish).
110. Nichilatti LP, Fernandes JMC, Marques CPC. Physiopathology of pain in systemic erythematosus lupus. Lupus. (2020) 29:721–6. doi: 10.1177/0961203320919872
111. Amarilyo G, Hahn B, Cava AL. Preclinical studies with synthetic peptides in systemic lupus erythematosus. FBL. (2012) 17:1940–7. doi: 10.2741/4030
112. Schall N, Talamini L, Wilhelm M, Jouvin-Marche E, Muller S. P140 peptide leads to clearance of autoreactive lymphocytes and normalizes immune response in lupus-prone mice. Front Immunol. (2022) 13:904669. doi: 10.3389/fimmu.2022.904669
113. Li C, Fu Z, Jin T, Liu YX, Liu NX, Yin SG, et al. A frog peptide provides new strategies for the intervention against skin wound healing. Cell Mol Biol Letters. (2023) 28:61. doi: 10.1186/s11658-023-00468-3
114. Mowat AM, Agace WW. Regional specialization within the intestinal immune system. Nat Rev Immunol. (2014) 14:667–85. doi: 10.1038/nri3738
115. Potrykus M, Czaja-Stolc S, Stankiewicz M, Kaska L, Malgorzewicz S. Intestinal microbiota as a contributor to chronic inflammation and its potential modifications. Nutrients. (2021) 13:3839. doi: 10.3390/nu13113839
116. Zhi TX, Hong D, Zhang ZJ, Li ST, Xia JX, Wang C, et al. Anti-inflammatory and gut microbiota regulatory effects of walnut protein derived peptide LPF in vivo. Food Res Int. (2022) 152:110875. doi: 10.1016/j.foodres.2021.110875
117. Rahabi M, Salon M, Bruno-Bonnet C, Prat M, Jacquemin G, Benmoussa K, et al. Bioactive fish collagen peptides weaken intestinal inflammation by orienting colonic macrophages phenotype through mannose receptor activation. Eur J Nutr. (2022) 61:2051–66. doi: 10.1007/s00394-021-02787-7
118. Sun TT, Liu XS, Su YZ, Wang ZH, Cheng BJ, Dong N, et al. The efficacy of anti-proteolytic peptide R7I in intestinal inflammation, function, microbiota, and metabolites by multi-omics analysis in murine bacterial enteritis. Bioengineering Trans Med. (2023) 8:e10446. doi: 10.1002/btm2.10446
119. Chou CC, Shen CF, Chen SJ, Chen HM, Wang YC, Chang WS, et al. Recommendations and guidelines for the treatment of pneumonia in Taiwan. J Microbiol Immunol Infection. (2019) 52:172–99. doi: 10.1016/j.jmii.2018.11.004
120. Long ME, Mallampalli RK, Horowitz JC. Pathogenesis of pneumonia and acute lung injury. Clin Science. (2022) 136:747–69. doi: 10.1042/cs20210879
121. Mei XZ, Zhang YC, Wang S, Wang H, Chen R, Ma K, et al. Necroptosis in pneumonia: Therapeutic strategies and future perspectives. Viruses-Basel. (2024) 16:94. doi: 10.3390/v16010094
122. Adamo R, Margarit I. Fighting antibiotic-resistant Klebsiella pneumoniae with "sweet" immune targets. Mbio. (2018) 9:e00874–18. doi: 10.1128/mBio.00874-18
123. Brito JCM, Lima WG, Resende JM, de Assis DCS, Boff D, Cardoso VN, et al. Pegylated LyeTx I-b peptide is effective against carbapenem-resistant Acinetobacter baumannii in an in vivo model of pneumonia and shows reduced toxicity. Int J Pharmaceutics. (2021) 609:121156. doi: 10.1016/j.ijpharm.2021.121156
124. Jin X, Hu XY, Jiang SJ, Zhao T, Zha YM, Wei SS, et al. Temporin-ghb-derived peptides exhibit potent antibacterial and antibiofilm activities against Staphylococcus aureus in vitro and protect mice from acute infectious pneumonia. ACS Infect Diseases. (2023) 9:840–55. doi: 10.1021/acsinfecdis.2c00544
125. Tang CH. Research of pathogenesis and novel therapeutics in arthritis. Int J Mol Sci. (2019) 20:1646. doi: 10.3390/ijms20071646
126. Mei XL, Villamagna IJ, Nguyen T, Beier F, Appleton CT, Gillies ER. Polymer particles for the intra-articular delivery of drugs to treat osteoarthritis. Biomed Materials. (2021) 16:042006. doi: 10.1088/1748-605X/abee62
127. Wu QL, Liu B, Yu RX, Sun XL, Wang ZY, Zhou J, et al. Studies on blocking the JAK2/STAT3 signaling pathway with elastin peptides from skipjack tuna (Katsuwonus pelamis) bulbus cordis to alleviate osteoarthritis. Food Bioscience. (2023) 56:103253. doi: 10.1016/j.fbio.2023.103253
128. Tobón GJ, Youinou P, Saraux A. The environment, geo-epidemiology, and autoimmune disease: Rheumatoid arthritis. J Autoimmunity. (2010) 35:10–4. doi: 10.1016/j.jaut.2009.12.009
129. Ciofoaia EI, Pillarisetty A, Constantinescu F. Health disparities in rheumatoid arthritis. Ther Adv Musculoskeletal Dis. (2022) 14:1759720x221137127. doi: 10.1177/1759720x221137127
130. Karmacharya P, Chakradhar R, Ogdie A. The epidemiology of psoriatic arthritis: A literature review. Best Pract Res Clin Rheumatol. (2021) 35:101692. doi: 10.1016/j.berh.2021.101692
131. Wixler V, Zaytsev IZ, Dantas RL, Schied T, Boergeling Y, Lührmann V, et al. Small spleen peptides prevent development of psoriatic arthritis via restoration of peripheral tolerance. Mol Ther. (2022) 30:745–62. doi: 10.1016/j.ymthe.2021.08.030
132. Mohanakrishnan R, Beier S, Deodhar A. Tofacitinib for the treatment of active ankylosing spondylitis in adults. Expert Rev Clin Immunol. (2022) 18:273–80. doi: 10.1080/1744666x.2022.2038134
133. Katturajan R, Sabina EP. Joint inflammation: Insights of osteoarthritis, gouty and rheumatoid arthritis and its prevalence, mechanism, medications and remedies. Indian J Pharm Sci. (2021) 83:886–98. doi: 10.36468/pharmaceutical-sciences.840
134. Yan YB, Yu LQ, Chen BY, Cao CA, Zhao HR, Wang Q, et al. Mastoparan M suppressed NLRP3 inflammasome activation by inhibiting MAPK/NF-κB and oxidative stress in gouty arthritis. J Inflammation Res. (2023) 16:6179–93. doi: 10.2147/jir.S434587
135. Li J, Du L, He JN, Chu KO, Guo CL, Wong MOM, et al. Anti-inflammatory effects of GTE in eye diseases. Front Nutr. (2021) 8:753955. doi: 10.3389/fnut.2021.753955
136. Epps SJ, Boldison J, Stimpson ML, Khera TK, Lait PJP, Copland DA, et al. Re-programming immunosurveillance in persistent non-infectious ocular inflammation. Prog Retinal Eye Res. (2018) 65:93–106. doi: 10.1016/j.preteyeres.2018.03.001
137. Zhou LB, Ho BM, Chan HYE, Tong Y, Du L, He JN, et al. Emerging roles of cGAS-STING signaling in mediating ocular inflammation. J Innate Immunity. (2023) 15:739–50. doi: 10.1159/000533897
138. Ho TC, Fan NW, Yeh SI, Chen SL, Tsao YP. The therapeutic effects of a PEDF-derived short peptide on murine experimental dry eye involves suppression of MMP-9 and inflammation. Trans Vision Sci Technol. (2022) 11:12. doi: 10.1167/tvst.11.10.12
139. Pathak JL, Yan YY, Zhang QB, Wang LP, Ge LH. The role of oral microbiome in respiratory health and diseases. Respir Med. (2021) 185:106475. doi: 10.1016/j.rmed.2021.106475
140. Wang JY, Gao B. Mechanisms and potential clinical implications of oral microbiome in oral squamous cell carcinoma. Curr Oncol. (2024) 31:168–82. doi: 10.3390/curroncol31010011
141. Dutzan N, Kajikawa T, Abusleme L, Greenwell-Wild T, Zuazo CE, Ikeuchi T, et al. A dysbiotic microbiome triggers TH17 cells to mediate oral mucosal immunopathology in mice and humans. Sci Trans Med. (2018) 10:eaat0797. doi: 10.1126/scitranslmed.aat0797
142. Sui LH, Wang JL, Xiao ZX, Yang YQ, Yang ZC, Ai KL. ROS-scavenging nanomaterials to treat periodontitis. Front Chem. (2020) 8:595530. doi: 10.3389/fchem.2020.595530
143. Sirisereephap K, Maekawa T, Tamura H, Hiyoshi T, Domon H, Isono T, et al. Osteoimmunology in periodontitis: Local proteins and compounds to alleviate periodontitis. Int J Mol Sci. (2022) 23:5540. doi: 10.3390/ijms23105540
144. Zhu QL, Zhuo HL, Yang LM, Ouyang HH, Chen J, Liu B, et al. A peptide HEPFYGNEGALR from Apostichopus japonicus alleviates acute alcoholic liver injury by enhancing antioxidant response in male C57BL/6J mice. Molecules. (2022) 27:5839. doi: 10.3390/molecules27185839
145. Liang J, Bai Y, Chen WJ, Fu Y, Liu Y, Yin XH. Cortistatin, a novel cardiovascular protective peptide. Cardiovasc Diagnosis Ther. (2019) 9:394–9. doi: 10.21037/cdt.2018.12.08
146. Delgado-Maroto V, Falo CP, Forte-Lago I, Adan N, Morell M, Maganto-Garcia E, et al. The neuropeptide cortistatin attenuates experimental autoimmune myocarditis via inhibition of cardiomyogenic T cell-driven inflammatory responses. Br J Pharmacol. (2017) 174:267–80. doi: 10.1111/bph.13682
147. Tang J, Chen HF, He YD, Sheng WJ, Bai QQ, Wang H. Peptide-guided functionalization and macrocyclization of bioactive peptidosulfonamides by Pd(II)-catalyzed late-stage C-H activation. Nat Commun. (2018) 9:3383. doi: 10.1038/s41467-018-05440-w
148. Shang YN, Zhu QR, Ding JM, Zhao L, Zhang F, JY Lu, et al. Bioactive peptide relieves glucocorticoid-induced osteoporosis by giant macrocyclic encapsulation. J Controlled Release. (2024) 369:75–87. doi: 10.1016/j.jconrel.2024.02.048
149. Anand U, Bandyopadhyay A, Jha NK, de la Lastra JMP, Dey A. Translational aspect in peptide drug discovery and development: An emerging therapeutic candidate. Biofactors. (2023) 49:251–69. doi: 10.1002/biof.1913
150. Wong CTT, Rowlands DK, Wong CH, Lo TWC, Nguyen GKT, Li HY, et al. Orally active peptidic bradykinin B1 receptor antagonists engineered from a cyclotide scaffold for inflammatory pain treatment. Angewandte Chemie-International Edition. (2012) 51:5620–4. doi: 10.1002/anie.201200984
151. Xie ZX, Shen Q, Xie C, Lu WY, Peng CM, Wei XL, et al. Retro-inverso bradykinin opens the door of blood-brain tumor barrier for nanocarriers in glioma treatment. Cancer Letters. (2015) 369:144–51. doi: 10.1016/j.canlet.2015.08.010
152. Tao NA, Xu X, Ying YY, Hu SY, Sun QR, Lv GY, et al. Thymosin α1 and its role in viral infectious diseases: The mechanism and clinical application. Molecules. (2023) 28:3539. doi: 10.3390/molecules28083539
153. Wu XN, Jia JD, You H. Thymosin alpha-1 treatment in chronic hepatitis B. Expert Opin Biol Ther. (2015) 15:S129–S32. doi: 10.1517/14712598.2015.1007948
154. Muttenthaler M, King GE, Adams DJ, Alewood PE. Trends in peptide drug discovery. Nat Rev Drug Discov. (2021) 20:309–25. doi: 10.1038/s41573-020-00135-8
155. Patil KR, Mahajan UB, Unger BS, Goyal SN, Belemkar S, Surana SJ, et al. Animal models of inflammation for screening of anti-inflammatory drugs: Implications for the discovery and development of phytopharmaceuticals. Int J Mol Sci. (2019) 20:4367. doi: 10.3390/ijms20184367
156. Grivennikov SI, Greten FR, Karin M. Immunity, inflammation, and cancer. Cell. (2010) 140:883–99. doi: 10.1016/j.cell.2010.01.025
157. Tornatore L, Thotakura AK, Bennett J, Moretti M, Franzoso G. The nuclear factor kappa B signaling pathway: integrating metabolism with inflammation. Trends Cell Biol. (2012) 22:557–66. doi: 10.1016/j.tcb.2012.08.001
158. Fu-jia Y, Xu C, Mu-chen H, Qian Y, Xi-xi C, Xuan C, et al. Molecular characteristics and structure–activity relationships of food-derived bioactive peptides. J Integr Agricult. (2021) 20:2313–32. doi: 10.1016/S2095-3119(20)63463-3
159. María L-P, A. ZA, M. LJ, Mercedes C, Daniel F. A review on bioactive peptides derived from meat and by-products: Extraction methods, biological activities, applications and limitations. Meat Science. (2023) 204:109278. doi: 10.1016/j.meatsci.2023.109278
160. Xing LJ, Fu LJ, Cao SM, Yin YT, Wei LL, Zhang WG. The anti-inflammatory effect of bovine bone-gelatin-derived peptides in LPS-induced RAW264.7 macrophages cells and dextran sulfate sodium-induced C57BL/6 mice. Nutrients. (2022) 14:1479. doi: 10.3390/nu14071479
161. Cresti L, Cappello G, Vailati S, Melloni E, Brunetti J, Falciani C, et al. In vivo efficacy and toxicity of an antimicrobial peptide in a model of endotoxin-induced pulmonary inflammation. Int J Mol Sci. (2023) 24:7967. doi: 10.3390/ijms24097967
162. Diao JJ, Chi ZP, Guo ZW, Zhang LP. Mung bean protein hydrolysate modulates the immune response through NF-κB pathway in lipopolysaccharide-stimulated RAW 264.7 macrophages. J Food Science. (2019) 84:2652–7. doi: 10.1111/1750-3841.14691
163. Yao LJ, Yang P, Luo WQ, Li SM, Wu Y, Cai N, et al. Macrophage-stimulating activity of European eel (Anguilla Anguilla) peptides in RAW264.7 cells mediated via NF-κB and MAPK signaling pathways. Food Funct. (2020) 11:10968–78. doi: 10.1039/d0fo02497j
164. T PP, F SG. NF-kappaB: a key role in inflammatory diseases. J Clin Invest. (2001) 107:7–11. doi: 10.1172/JCI11830
165. Chakrabarti S, Jahandideh F, Wu JP. Food-derived bioactive peptides on inflammation and oxidative stress. BioMed Res Int. (2014) 2014:608979. doi: 10.1155/2014/608979
166. Zhu WY, Ren LY, Zhang L, Qiao QQ, Farooq MZ, Xu QB. The potential of food protein-derived bioactive peptides against chronic intestinal inflammation. Mediators Inflammation. (2020) 2020:6817156. doi: 10.1155/2020/6817156
167. Qiao QQ, Chen L, Li X, Lu XY, Xu QB. Roles of dietary bioactive peptides in redox balance and metabolic disorders. Oxid Med Cell Longevity. (2021) 2021:5582245. doi: 10.1155/2021/5582245
168. Li S, Bu T, Zheng J, Liu L, He G, Wu J. Preparation, bioavailability, and mechanism of emerging activities of Ile-Pro-Pro and Val-Pro-Pro. Compr Rev Food Sci Food Saf. (2019) 18:1097–110. doi: 10.1111/1541-4337.12457
169. Chei S, Oh HJ, Lee K, Jin H, Lee JY, Lee BY. Dietary silk peptide inhibits LPS-induced inflammatory responses by modulating toll-like receptor 4 (TLR4) signaling. Biomolecules. (2020) 10:771. doi: 10.3390/biom10050771
170. Ila J, Shaik MH, Abdul NR. A Meretrix meretrix visceral mass derived peptide inhibits lipopolysaccharide-stimulated responses in RAW264.7 cells and adult zebrafish model. Int Immunopharmacol. (2020) 90:107140. doi: 10.1016/j.intimp.2020.107140
171. Zhu JH, LF Li, Ding J, Huang JY, Shao AW, Tang B. The role of formyl peptide receptors in neurological diseases via regulating inflammation. Front Cell Neurosci. (2021) 15:753832. doi: 10.3389/fncel.2021.753832
172. Ye RD, Boulay F, Wang JM, Dahlgren C, Gerard C, Parmentier M, et al. International union of basic and clinical pharmacology. LXXIII. nomenclature for the formyl peptide receptor (FPR) family. Pharmacol Rev. (2009) 61:119–61. doi: 10.1124/pr.109.001578
173. Jin RT, Aweya JJ, Lin R, Weng WY, Shang JQ, Wang DF, et al. The bioactive peptide VLATSGPG regulates the abnormal lipid accumulation and inflammation induced by free fatty acids in HepG2 cells via the PERK signaling pathway. J Funct Foods. (2023) 104:105515. doi: 10.1016/j.jff.2023.105515
174. Tsuruki T, Kishi K, Takahashi M, Tanaka M, Matsukawa T, Yoshikawa MJFL. Soymetide, an immunostimulating peptide derived from soybean β-conglycinin, is an fMLP agonist. FEBS Letters. (2003) 540:206–10. doi: 10.1016/S0014-5793(03)00265-5
175. Li Y, Ma QS, Liu GQ, Wang CF. Effects of donkey milk on oxidative stress and inflammatory response. J Food Biochem. (2022) 46:e13935. doi: 10.1111/jfbc.13935
176. Yu JM, Xu J, Jiang RL, Yuan QL, Ding YY, Ren J, et al. Versatile chondroitin sulfate-based nanoplatform for chemo-photodynamic therapy against triple-negative breast cancer. Int J Biol Macromolecules. (2024) 265:130709. doi: 10.1016/j.ijbiomac.2024.130709
177. Lee HA, Kim IH, Nam TJ. Bioactive peptide from Pyropia yezoensis and its anti-inflammatory activities. Int J Mol Med. (2015) 36:1701–6. doi: 10.3892/ijmm.2015.2386
178. Schreck R, Rieber P, Baeuerle PA. Reactive oxygen intermediates as apparently widely used messengers in the activation of the NF-kappa B transcription factor and HIV-1. EMBO J. (1991) 10:2247–58. doi: 10.1002/j.1460-2075.1991.tb07761.x
179. Rungratanawanich W, Memo M, Uberti D. Redox homeostasis and natural dietary compounds: Focusing on antioxidants of rice (Oryza sativa L.). Nutrients. (2018) 10:1605. doi: 10.3390/nu10111605
180. Fernando IPS, Park SY, Han EJ, Kim HS, Kang DS, Je JY, et al. Isolation of an antioxidant peptide from krill protein hydrolysates as a novel agent with potential hepatoprotective effects. J Funct Foods. (2020) 67:103889. doi: 10.1016/j.jff.2020.103889
Keywords: bioactive peptides, inflammation, immunomodulation, inflammatory mediators, pathways
Citation: Liu H, Zhang L, Yu J and Shao S (2024) Advances in the application and mechanism of bioactive peptides in the treatment of inflammation. Front. Immunol. 15:1413179. doi: 10.3389/fimmu.2024.1413179
Received: 06 April 2024; Accepted: 06 August 2024;
Published: 23 August 2024.
Edited by:
Danila Cianciosi, Università Politecnica delle Marche, ItalyReviewed by:
Gabriela Calin Mihalache, Apollonia University, RomaniaFei Xu, McMaster University, Canada
Jingjing Qian, South China University of Technology, China
Copyright © 2024 Liu, Zhang, Yu and Shao. This is an open-access article distributed under the terms of the Creative Commons Attribution License (CC BY). The use, distribution or reproduction in other forums is permitted, provided the original author(s) and the copyright owner(s) are credited and that the original publication in this journal is cited, in accordance with accepted academic practice. No use, distribution or reproduction is permitted which does not comply with these terms.
*Correspondence: Jingmou Yu, eWptMTAxNkBob3RtYWlsLmNvbQ==; Shengwen Shao, c2hhc2hlOThAMTYzLmNvbQ==
†These authors have contributed equally to this work