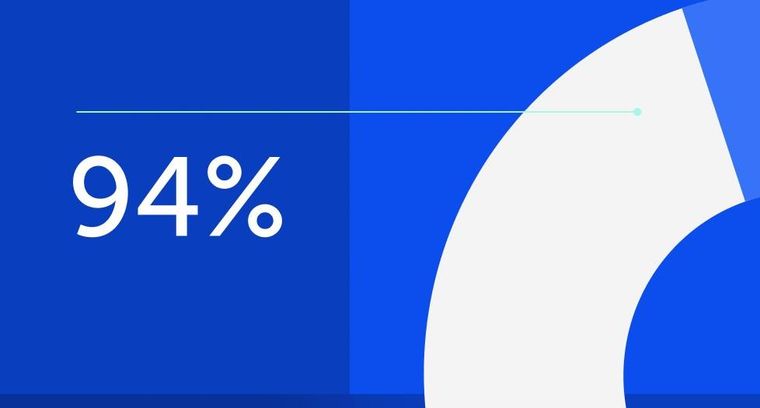
94% of researchers rate our articles as excellent or good
Learn more about the work of our research integrity team to safeguard the quality of each article we publish.
Find out more
REVIEW article
Front. Immunol., 19 July 2024
Sec. Cancer Immunity and Immunotherapy
Volume 15 - 2024 | https://doi.org/10.3389/fimmu.2024.1407449
This article is part of the Research TopicRegulators of the Immune-Tumor Microenvironment: A New Frontier for Cancer ImmunotherapyView all 18 articles
Innate immune cells in the colorectal cancer microenvironment mainly include macrophages, neutrophils, natural killer cells, dendritic cells and bone marrow-derived suppressor cells. They play a pivotal role in tumor initiation and progression through the secretion of diverse cytokines, chemokines, and other factors that govern these processes. Colorectal cancer is a common malignancy of the gastrointestinal tract, and understanding the role of innate immune cells in the microenvironment of CRC may help to improve therapeutic approaches to CRC and increase the good prognosis. In this review, we comprehensively explore the pivotal role of innate immune cells in the initiation and progression of colorectal cancer (CRC), alongside an extensive evaluation of the current landscape of innate immune cell-based immunotherapies, thereby offering valuable insights for future research strategies and clinical trials.
Colorectal cancer (CRC) is a global disease that poses a serious threat to human life and health, and is a common malignant tumor of the gastrointestinal tract. According to the latest cancer statistics, colorectal cancer has the third highest incidence rate globally and the second highest mortality rate after lung cancer (1). The tumor microenvironment refers to the complex surroundings in which tumor cells exist and grow. This includes the surrounding blood vessels, immune cells, fibroblasts, myeloid-derived inflammatory cells, a variety of signaling molecules, and the extracellular matrix (2). These cellular interactions facilitate immune evasion by tumor cells, thereby serving as a cytological mechanism underlying tumor progression and metastasis (3). There is increasing evidence that both innate and adaptive immune cells play a crucial role in the tumor microenvironment (TME) (Figure 1). These cellular components, encompassing macrophages, neutrophils, natural killer cells, dendritic cells, bone marrow-derived suppressor cells, T lymphocytes, and B lymphocytes, actively participate in tumorigenesis and the progression of tumors. In particular, innate immune cells secrete cytokines, chemokines, growth factors, and extracellular matrix (ECM) proteins through a complex communication network. These are regulated by interactions with colorectal cancer cells and affect the survival and progression of colorectal cancer. In addition to identifying the molecular events occurring in tumor cells, it is important to have a comprehensive understanding of the pathogenic mechanisms of CRC. This includes defects in the tumor microenvironment (TME), such as immune cell infiltration, immune defense, immune surveillance and immune homeostasis. It is crucial to not overlook these factors (3). This review focuses on innate immune cells in the tumor microenvironment of colorectal cancer. It also discusses the immune mechanisms that promote tumor progression. The aim is to provide ideas for finding new therapeutic approaches for CRC patients.
Macrophages are an important component of the tumor microenvironment and play an important role in maintaining the innate immune response, tissue homeostasis and inflammation. Macrophages are recruited around tumors to form tumor-associated macrophages (TAM), which can be induced into M1 and M2 types (4). M1 macrophages are also known as pro-inflammatory macrophages due to their secretion of large amounts of pro-inflammatory cytokines, including IL-1β and tumor necrosis factor-α (TNF-α). M1 macrophages are primarily induced by lipopolysaccharide (LPS) and interferon-γ (IFN-γ). In the presence of these substances, macrophages undergo a process of polarization, acquiring the M1 phenotype. M2 macrophages are known as anti-inflammatory macrophages due to their production of mainly anti-inflammatory factors, including IL-10, transforming growth factor-β (TGF-β), and arginase 1 (Arg1). M2 macrophages can be activated by interleukin (IL)-4 and IL-13.During carcinogenesis, macrophages initially exhibit an M1-like polarization with anti-tumor properties, leading to enhanced elimination of tumor cells. However, as the tumor progresses, macrophages undergo a shift towards an M2-like polarization state that promotes tumor progression. Studies have demonstrated that well-differentiated tumor-associated macrophages (TAMs) are associated with unfavorable prognosis and reduced overall survival (5).
Neutrophils are the most abundant white blood cells in the human circulation. During carcinogenesis, neutrophils are transformed into tumor-associated neutrophils (TAN) after migration to tumor tissue. TGF-β in the tumor microenvironment induces their differentiation into either N1 or N2 types (6). Neutrophils have cancer-inhibiting (7) and cancer-promoting effects. Tumor growth, proliferation, and aggressiveness are increased by neutrophil depletion.
Natural killer cells (NK cells) are immune cells derived from bone marrow lymphoid cells that play a crucial role in anti-tumor immunity and immunomodulation. The effectiveness of NK cells in attacking tumors depends on the receptors they express. These receptors are classified as either activating or inhibitory, and they regulate the activation of NK cells. Activated NK cells can kill tumor cells through two mechanisms: natural killer toxicity and the ADCC pathway.
Dendritic cells are a type of antigen-presenting cell in the immune system that link the adaptive and innate immune systems to initiate and maintain T-cell-mediated immunity against tumors. They have a close relationship with tumorigenesis and progression, and a high number of DCs infiltrating most solid tumors is associated with a good prognosis (8).
Myeloid derived suppressor cells have strong immunosuppressive activity. They are implicated in the regulation of immune responses under many pathological conditions and are strongly associated with poor clinical outcomes in cancer (9). Myeloid derived suppressor cells classified according to their origin as granulocyte/polymorphonuclear cell MDSCs (PMN-MDSCs) and monocyte MDSCs (M-MDSCs). Their main characteristic is the ability to suppress the immune response.
Macrophages can promote or suppress tumor immunity by polarizing into different types of tumors and play a key role in the tumor microenvironment. They produce various molecules that interact with other immune cells and tumor cells to further influence CRC progression (4). CRC cells secrete extracellular vesicles EV, and miRNAs carried by extracellular vesicles induce macrophage polarization, which plays an important role in regulating colorectal cancer cell migration and invasion (10). For instance, miR-195-5 regulates the NOTCH pathway, which affects interleukin 4 (IL-4)-associated M2-like TAM polarization in colorectal cancer (11). Other miRNAs also play an important role. The exosome circVCP derived from CRC cells regulates the miR-9-5p/NRP1 axis, promoting cell proliferation, migration, and invasion. Additionally, it induces macrophage M2 polarization and inhibits macrophage M1 polarization, thereby facilitating the progression of CRC (12). M2 macrophage-derived extracellular vesicle-mediated miR-186-5p targets DLC1 to promote colon cancer progression (13).
TAM induces epithelial-mesenchymal transition (EMT) through various pathways to enhance colorectal cancer migratory invasion. The EMT program is induced by TAM to enhance CRC migratory invasion by regulating the JAK2/STAT3/miR-506-3p/FoxQ1 axis. This leads to the production of chemokine 2 (CCL2), which promotes macrophage recruitment (14). Platelets promote tumor metastasis. Tumor cells interact with platelets to generate chimeric extracellular vesicles. These vesicles inhibit primary tumor growth through the activation of tumor-destroying macrophages, while promoting tumor metastasis through EMT and endothelial activation (15). The Metastasis-associated regenerative liver 3 protein phosphatase (PRL-3) promotes invasion and metastasis of colorectal cancer cells by activating the epithelial-mesenchymal transition through the MAPK pathway in TAMs. Additionally, PRL-3 promotes angiogenesis in CRC cells through activation of the NF-κB pathway (16). The SNAIL protein facilitates the transformation of epithelial tumors. Additionally, transformed mesenchymal stromal cells secrete CXCL2, which promotes the infiltration of M2 macrophages and the metastasis of tumor cells (17).
The secretion of chemokines can induce the polarization of M2-type macrophages. Most studies have focused on the Wnt5a/CaMKII/ERK/CCL2 axis. Additionally, HMGA2 directly binds to the STAT3 promoter, activating its transcription and subsequently inducing CCL2 secretion, which promotes macrophage recruitment (18). Wnt5a induces M2 polarization of TAMs by regulating interleukin 10 (IL-10) secretion through the CaMKII-ERK1/2-STAT3 pathway. This ultimately promotes tumor growth and metastasis in colorectal cancer (19). PIPkIγ promotes the activation of the PI3K-Akt-mTOR signaling pathway, leading to an increase in STAT3 phosphorylation levels. This, in turn, triggers CCL2 transcription, enhancing the recruitment of tumor-associated macrophages (20).The tissue inhibitory factor of matrix metalloproteinases 1 (TIMP1) promotes macrophage migration and participates in macrophage M2 polarization through the activation of ERK1/2/CLAM1 and CCL2 (21). The CCL2-CCR2 signaling pathway is involved in the polarization of M2-type macrophages. TCF4 enhances liver metastasis in colorectal cancer by regulating tumor-associated macrophages through CCL2/CCR2 signaling (22). Colorectal cancer growth is also promoted by the overexpression of transforming growth factor beta (TGF-β) in tumor-associated macrophages (23, 24). The secretion of chemokine 11 (CXCL11) increases and enhances the expression of transforming growth factor in tumor-associated macrophages, which in turn promotes the metastasis of colorectal cancer cells (25). The chemokines interleukins 4, 6, 8, and 10 promote the development of colorectal cancer by converting macrophages to tumor macrophage M2 type (11, 26–28). Other signaling pathways NK-κB (29, 30), I3K/AKT (31, 32), NOTCH (11) and tumor-associated macrophage M2 are involved in CRC development.
In vivo, certain proteins display tumorigenic activity and advance CRC progression by inducing M2 polarization of TAM. CRC cells activate STAT6 and the transcription factor KLF via the malignant fibrous histiocytoma amplicon sequence 1 (MFHAS1), which has tumorigenic activity, and induce M2 polarization of TAM, promoting CRC progression (12, 33, 34). Intercellular adhesion molecule-1 (ICAM-1) is a transmembrane glycoprotein that belongs to the immunoglobulin superfamily. It mediates cell-to-cell interactions and intra- and extracellular signaling during the immune response. ICAM-1 down-regulates cellular proliferation in the tumor microenvironment, inhibiting M2 macrophage polarization and metastatic tumor progression (35). Tumor-associated macrophages promote cancer cell migration and invasion by inducing serum amyloid A1 (SAA1) at the early colorectal cancer (CRC) invasion front (36). Immunoglobulin-like transcript 5 (ILT5) functions as a negative regulator of myeloid cell activation and directs M2-like polarization of tumor-associated macrophages (37).
Tumor-associated macrophages (TAMs) can inhibit the anti-tumor activity of T cells. Our research has shown that deletion of RNA N6-adenosylmethyltransferase Mettl14 in TAMs promotes CD8 T cell dysfunction and tumor growth (38). miR-21-5p and miR-200a work together to induce M2-like polarization of macrophages and increase PD-L1 expression by regulating the PTEN/AKT and SCOS1/STAT1 pathways. This leads to a decrease in CD8T cell activity and an increase in tumor growth (34). Additionally, Chemokine 8 (CXCL8) induces M2 macrophage polarization and inhibits CD8T cell infiltration, creating an immunosuppressive microenvironment in colorectal cancer (39). The p65/STAT3-CSN5-PD-L1 pathway is used by colorectal cancer cells to promote immune escape, with the assistance of macrophage-derived chemokine 5 (CCL5) (40).
Although macrophages in colorectal cancer (CRC) tend to convert to the M2 phenotype and act as tumor promoters, they can sometimes convert to the M1 phenotype and act as anti-tumor promoters. The protein Elastin microfibril interface 2 (EMILIN-2) triggers the activation of the Toll-like receptor 4/MyD88/NF-κB pathway and contributes to macrophage polarization towards the M1 phenotype (41). The axis between 1-acylglycerol-3-phosphate O-acyltransferase 4 (Agpat4) and lysophosphatidic acid (LPA) in CRC cells modulates p38/p65 signaling-dependent macrophage M1 polarization, promotes T-cell activation, and inhibits CRC progression (42). The extracellular matrix glycoprotein, spondin 2 (SPON2), derived from tumor cells, can inhibit tumor growth and invasion by indirectly inducing M1 poles. This is achieved through the up-regulation of cytokines expressed by IL2, CCL10, and colony-stimulating factor 2 (CSF2) in tumor cells (43). Mucin promotes the enrichment of M1-like macrophages both in vivo and in vitro. This process is dependent on the NOD-like receptor thermoprotein structural domain protein 3 (NLRP3). The absence of NLRP3 in macrophages attenuates the tumor suppressive effect of mucinophages (44). ILC1 promotes M1-like macrophage activation and inhibits colon cancer progression by secreting gamma interferon (IFN-γ) (45).
Chronic inflammation contributes to the progression of colorectal cancer. The development and progression of colorectal cancer (CRC) is closely linked to the interaction between the inflammatory factors interleukins (ILs) and neutrophils (46). The overexpression of IL-17 is linked to a poor prognosis in colorectal cancer, whereas the production of IL-22 is associated with a favorable clinical outcome (47, 48). IL-22 is produced by CD4 and CD8 multifunctional T cells, which also produce IL-17 and IFN-γ (48). The blockade of IL-17 has been demonstrated to result in a reduction in the recruitment of neutrophils to the intestinal mucosa, as well as a reduction in the expression and production of inflammatory cytokines and the expression of the transcription factor STAT3 in the intestinal mucosa (49). Furthermore, the combination of drugs targeting the IL-17 signaling pathway with T-cell checkpoint inhibitors has the potential to enhance cancer immunotherapy (50). CXCL8 is a potent chemokine that attracts neutrophils, myeloid-derived suppressor cells (MDSC) and monocytes (46). Apoptotic cancer cells release CXCL8, which attracts neutrophils into the tumor, interacts with macrophages and regulates the immune microenvironment of CRC.IL-8 regulates tumor stem cells (CSC) in colon and lung cancer cells via glucose transporter protein 3 (GLUT3) and glucosamine fructose-6-phosphate aminotransferase (GFAT) induced glucoramidic (O-GlcNAc) modification Sample Properties (51). The superoxide dismutase (SOD2)-CXCL8-neutrophil recruitment axis may be a potential factor in the progression of colorectal cancer (52). The presence of low non-red lineage protein (SPTAN1) in colorectal cancer (CRC) cells has been shown to result in the release of higher levels of IL-8 and the subsequent migration of neutrophils, leading to an increased infiltration of immune cells (53). The development of colon tumors is promoted by epithelial hypoxia-inducible factor 2 alpha, which recruits neutrophils through the CXCL1-CXCR2 signaling axis (54). SMAD4 is a crucial transcription factor in TGFβ signaling and functions as a tumor suppressor in colorectal cancer. SMAD4 deficiency promotes the progression of colorectal cancer by attracting tumor-associated neutrophils through the CXCL1/8-CXCR2 axis (55). Exosomal RNA derived from tumor stem-like cells stimulates neutrophils to promote colon cancer tumorigenesis by secreting CXCL1 and CXCL2. These chemokines recruit neutrophils to promote tumorigenesis in colorectal cancer cells via IL-1β (56). The inflammatory mediator prostaglandin E2 (PGE2) specifically promotes colorectal cancer formation through its E2-EP2 signaling pathway (57). Prostaglandin receptor 2 (EP2) has been reported to promote colon tumorigenesis by amplifying inflammation and shaping the tumor microenvironment in neutrophils and tumor-associated fibroblasts (58). In TME of CRC, tumor-associated neutrophils (TAN) secrete anterior gradient protein 2 (AGR2) to promote migration of CRC cells (59). Neutrophils promote the growth of liver metastases from colorectal cancer in mice through fibroblast growth factor 2 (FGF2)-dependent angiogenesis (60). Neutrophils create neutrophil extracellular traps (NETs) after necrosis. These NETs are important for directly promoting cancer growth (61). Clostridium nucleatum (Fn)-induced NETs promote tumor metastasis through the Toll-like receptor 4 (TLR4)-reactive oxygen species (ROS) signaling pathway and the NOD-like receptor (NOD1/2)-dependent signaling pathway (62). Defective aryl hydrocarbon receptor nuclear translocator (ARNT) induces neutrophil extracellular traps (NET) and promotes colorectal development (63). Neutrophil extracellular traps (NETs) promote T cell depletion in the tumor microenvironment (61). It has beenIsekai Cheat Magician reported that polymorphonuclear neutrophils (PMN) induce a homologous recombination-deficient colorectal cancer (CRC) phenotype (64). Defects in MIR4435-2HG, a long non-coding RNA that suppresses tumors, increase the number of polymorphonuclear myeloid-derived suppressor cells (PMN-MDSCs) that infiltrate tumors and enhance their immunosuppressive potential, promoting the growth of colorectal cancer (65). CCL2 enhances the number and function of PMN-MDSCs, promoting colorectal cancer (66).
Neutrophils not only secrete relevant molecules but also promote cancer development and interact with other immune cells to influence tumor development. They inhibit colon cancer tumor-infiltrating T cells through matrix metalloproteinase (MMP)-mediated activation of transforming factor β (TGFβ) (67). Some evidence suggests that interactions between macrophages and CRC cells lead to neutrophil recruitment and that SAA1 secreted by CRC cells activates neutrophils to promote invasion (68). Neutrophil extracellular traps (NETs) play a crucial role in promoting cancer growth by inhibiting T-cell responses through metabolic and functional depletion (61). Several studies have shown that tumor-associated neutrophils (TAN) promote the spread of cancer cells to distant organs. TAN promote tumor invasion and angiogenesis, as well as migration, through the production of matrix metalloproteinase-9 (MMP-9), vascular endothelial growth factor (VEGF), and hepatocyte growth factor (HGF) at both primary and metastatic sites (69).
In some cases, Neutrophils may also contribute to cancer suppression by inhibiting tumor growth through miR-155-dependent down-regulation of RAD51 (64).
Natural killer cells are rare in the tissues of colorectal cancer patients (70). Although at low levels, in colorectal cancer TME, activated NK cells can exert a killing effect on tumors, mainly through two mechanisms: direct killing action and the ADCC (antibody-dependent cytotoxic effect) pathway. Tissue protease binds to the cell membrane and exhibits ADCC potential. Colorectal cancer tumor cells express histone S on the surface, and the binding of Fsn0503h to the surface-associated histone S leads to the targeting of NK cells to tumor killing (71). The effect of NK cells on tumors is mainly determined by the receptors expressed on their surface. TIGIT is an inhibitory receptor found on all human NK cells and a wide range of T cells. The Fap2 protein of Fusarium nuclei directly interacts with TIGIT, resulting in the inhibition of NK cytotoxicity (72).
NK cells secrete chemokines and cytokines to exert immunomodulatory functions. Studies have shown that interleukin-15 (IL-15) promotes the growth and activation of cytotoxic CD8T and NK cells (73). Significantly increased interleukin-21 expression in NK cells in the colorectal cancer microenvironment has an increased survival time for CRC patients (74). Mesenchymal stem cells (mSCs) specifically target T/NK cells to chemokine ligand 9 (CXCL9), leading to local anti-tumor immunity (75). NLRP3 inflammatory vesicles inhibit metastatic growth of colorectal cancer in the liver by affecting hepatic NK cell maturation through the interleukin-18 pathway and surface expression of the death ligand FasL (76). Black raspberry enhances the expression of the transcriptional effector Smad4 in colonic epithelial cells and natural killer cells, thereby inhibiting colorectal cancer (77). Lactic acid induces apoptosis in NK cells by reducing intracellular pH, leading to mitochondrial dysfunction. Colorectal cancer liver metastases (CRLM) tumors produce lactic acid, which lowers the pH of the tumor microenvironment, inducing apoptosis (78). The combined treatment of MC38 mouse colon cancer with methotrexate nanocouplings and dendritic cells with down-regulated IL-10R expression has been shown to modulate the tumor microenvironment and enhance the systemic anti-tumor immune response (79).
In colorectal cancer, a study has shown that cancer-associated fibroblasts enhance the enrichment of tumor-associated macrophages and inhibit the function of NK cells, which are associated with other cells in the tumor microenvironment (80). The anti-tumor effects of activated NK cells and anti-heat shock protein 70 (Hsp70) CAR-T cells are comparable (81). Macrophage STING signaling promotes NK cell inhibition of colorectal cancer liver metastasis via 4-1BBL/4-1BB co-stimulation (82). Targeting epidermal growth factor receptor (EGFR) counteracts inhibition of natural killer cell function by colorectal tumor-associated fibroblasts (83). 肿Crosstalk between NK cells and CD8T cells in the tumor microenvironment benefits the prognosis of colorectal cancer patients (84).
Dendritic cells (DCs) play an important role in the colorectal cancer tumor microenvironment. It has been shown that a high density of DCs at the dermal site contributes to tumor antigen trapping and that local inflammation induces DC maturation and migration into draining lymph nodes (85), thereby participating in tumor progression. In DC, mixed-spectrum kinase structural domain-like protein (MLKL) attenuates colonic inflammation and colitis tumorigenesis by inhibiting the inflammatory response through activation of the MEK/ERK pathway (86). Vascular endothelial growth factor (VEGF) affects the tumor microenvironment by inhibiting dendritic cell (DC) differentiation and promoting the accumulation of myeloid-derived suppressor cells. Additionally, heterodimeric interleukin-15 (hetIL-15) induces the intratumoral accumulation of CD8+ T and natural killer (NK) cells, which helps to control tumor growth (73). Dendritic cells from various sources, along with associated cytokines, contribute to tumor progression. Dendritic cells, specifically tumor-associated dendritic cells (TADCs), that surround colorectal cancer express recombinant human chemokine ligand 5 (CCL5), which promotes the migration and invasion of colorectal cancer cells (87). Conventional type 1 dendritic cells (cDC1s) are a widely studied subpopulation of DCs that play a central role in anti-tumor immunity. Their presence in the tumor microenvironment has been associated with improved prognosis in cancer patients. cDC1s contain the dead cell sensing receptor (DNGR-1), which inhibits tumor-infiltrating type I conventional dendritic cells and limits Flt3L-mediated anti-tumor immunity (88). Plasma cell-like pre-dendritic cells (pDCs) are a type of dendritic cell that plays a crucial role in antiviral immunity. Studies have demonstrated that increased densities of tumor-infiltrating pDCs are linked to longer survival rates in patients with colon cancer (89). In the tumor microenvironment, pDCs induce apoptosis in innate lymphoid cells ILC3s through the CD95 pathway (90). The study found that Bone marrow-derived dendritic cell (BMDC) H3K79me2 up-regulates forkhead box transcription factor M1 (FOXM1) in colorectal cancer by inhibiting BMDC maturation phenotype and function through the Wnt5a signaling pathway leading to anti-tumor effects (91). SUMO-specific protease 3 (SENP3) senses oxidative stress to promote STING-dependent dendritic cell antitumor function (92).
Interactions between dendritic cells (DCs) and other cellular components within the tumor microenvironment play a pivotal role in driving tumor progression. Cancer-associated fibroblasts (CAFs) secrete WNT2, which exerts inhibitory effects on DC-mediated anti-tumor T-cell responses by suppressing the SOCS3/p-JAK2/p-STAT3 signaling pathway, thereby facilitating immune evasion by tumors (93). Extracellular vesicles (EVs) secreted by tumors that contain miR-424 have been found to inhibit the CD28-CD80/86 co-stimulatory pathway in tumor-infiltrating T cells and dendritic cells. This leads to resistance to immune checkpoint blockade. Additionally, dendritic cells that are triggered by exosomes rich in miR-155 have been found to inhibit the growth of colorectal cancer tumors (8). Most studies have found that the expression of programmed cell death protein ligand 1 (PD-L1) on T cells is associated with tumor progression. However, a recent study has shown that the expression of PD-L1 on dendritic cells (DCs) is associated with improved survival in stage III colon cancer (94).
Myeloid-derived suppressor cells (MDSCs) are a component of the tumor microenvironment (TME) and possess strong immunosuppressive capabilities. They modulate the immune response to cancer by expressing various protein molecules that interact with other molecules in the TME, thereby regulating colorectal carcinogenesis. MDSCs express a receptor for netrin-1, and netrin-1 enhances the immunosuppressive function of MDSCs through adenosine receptor 2B (A2BR), thereby promoting the development of colorectal cancer (9). Non-steroidal inflammatory drugs have a role in preventing colorectal cancer. Dectin-1 is a receptor for beta-glucan that plays a crucial role in host defense against fungi and in maintaining intestinal immune homeostasis. Blocking Dectin-1 can prevent colorectal cancer by inhibiting prostaglandin E2 production and enhancing the expression of interleukin-22 (IL-22)-binding proteins in myeloid-derived suppressor cells (95). Deletion of protease-activated receptor 2 (PAR2) in MDSCs promotes the progression of colorectal cancer by facilitating the production of reactive oxygen species mediated by transcription activator 3 (STAT3) (96). MDSC produces interleukin-10 (IL-10), which activates STAT3 to upregulate DNMT3b. This, in turn, silences the oncogene IRF8 in colonic epithelial cells, promoting colitis-associated colon tumorigenesis (97). The production of nitric oxide by MDSCs results in a decrease in interferon (IFN) responsiveness in immune cells (98). The STAT3 signaling pathway facilitates the accumulation of MDSCs, promoting colorectal cancer growth. G-CSF is implicated in this process (99). Inhibition of colorectal cancer growth can be achieved by inhibiting tumor neoangiogenesis and myeloid-derived suppressor cell aggregation through the use of reticulin 1 (ITLN1) (100). The junction protein CARD9 limits myeloid-derived suppressor cell expansion mediated by mycobacterial colonies, thereby inhibiting colon cancer (101). The tumor immunity suppression caused by GSK126, an inhibitor of histone methyltransferase EZH2, is due to its promotion of myeloid-derived suppressor cell production (102). S100A9 regulates the immunosuppression mediated by MDSCs through the RAGE and TLR4 signaling pathways in colorectal cancer (103). Fructose 1,6-bisphosphatase 1 (FBP1) inhibits colorectal tumorigenesis by suppressing the NF-κB pathway and mobilization of MDSCs (104).
Research indicates that myeloid-derived suppressor cells expressing chemokine receptor 2 (CXCR2) play a crucial role in promoting colitis-associated tumorigenesis (105). The promotion of colorectal cancer by Chemokine (CCL2) occurs through the enhancement of polymorphonuclear (PMN)-MDSC immunosuppressive features via the STAT3 pathway (66). The marker for gastrointestinal cancer stem cells, dicortin-like kinin (DCLK1), promotes the growth of colorectal cancer by inhibiting the function of tumor-specific cytotoxic T-lymphocytes through the recruitment of myeloid-derived suppressor cells (MDSCs) via the CXCL1-CXCR2 axis (106). Colorectal cancer progression is promoted by SMAD4 deficiency, which leads to the accumulation of MDSCs through the CCL15-CCR1 chemokine axis (107).
Additionally, other cells or molecules in the tumor microenvironment interact with myeloid-derived suppressor cells, which can influence colorectal cancer cell progression. Studies have shown that γδT17 cell infiltration positively correlates with colorectal cancer tumor stage and other clinicopathological features. γδT17 cells promote the accumulation and expansion of myeloid-derived suppressor cells in human colorectal cancer, which in turn promotes colorectal cancer growth (108). The activity of bone marrow-derived suppressor cells is promoted by mast cells, which contribute to the development of a microenvironment that is favorable to tumor growth (109). MDSCs promote tumor growth by suppressing CD8+ T cell cytotoxic activity (105). AlkB homologue 5 (ALKBH5) induces accumulation of MDSCs but reduces natural killer cells and cytotoxic CD8+ T cells, which in turn promotes colorectal carcinogenesis (110). Plasma cell-like dendritic cells (pDCs) can reduce colorectal cancer by modulating infiltration of myeloid-derived suppressor cells (111). The signaling of protein kinase 3 (RIPK3) in MDSCs promotes intestinal tumors by increasing tumor size. This is achieved through the expansion of interleukin-17 (IL-17)-producing T cells in MC38 tumors (112). Toll-like receptor agonists (TLR 7/8) reverse oxaliplatin resistance in colorectal cancer by directing myeloid-derived suppressor cells to tumor-killing M1 macrophages (113).
Toll-like receptor agonists (TLR 7/8) can reverse oxaliplatin resistance in colorectal cancer by directing myeloid-derived suppressor cells to tumor-killing M1 macrophages. Several drugs that act on MDSCs have been shown to clinically affect colorectal cancer development. Metformin, for example, activates the AMPK/mTOR pathway, leading to down-regulation of the mevalonate pathway, which reduces M2 macrophages and MDSCs and inhibits colorectal cancer development (114). Hydroxycumaric acid (MA) reduces the risk of colitis-associated colorectal cancer by inhibiting the recruitment of MDSCs through the suppression of IL-17 expression in γδT17 cells (115). Bojungikki-Tang (BJIKT) is a traditional Chinese herbal formulation used in Chinese medicine. When combined with anti-PD-L1 treatment, it significantly inhibits tumor growth, increases the proportion of cytotoxic T-lymphocytes and natural killer cells in tumor tissues, and suppresses the number of MDSCs in MC38-loaded mice (116).
MDSCs are associated with multiple metabolic pathways in relation to colorectal cancer. They produce nitric oxide which can lead to reduced interferon (IFN) responsiveness in immune cells (98). In human colorectal cancer, MDSCs promote tumor progression by inhibiting nitric oxide (NO) and reactive oxygen species (ROS) production through oxidative metabolism (117). Candida tropicalis fungus promotes colorectal carcinogenesis by inducing NLRP3 inflammasome activation through myeloid-derived inhibition of glycogen metabolism-dependent glycolysis and the JAK-STAT1 signaling pathway in cells (118) (Table 1).
Current immunotherapies have improved the survival of some colorectal cancer patients. Anti-tumor immunity requires organized and spatially subtle interactions between the components of the tumor immune microenvironment (Figure 2).
Immunotherapy for macrophages is currently under investigation using immune checkpoint inhibitors, monoclonal antibodies, cellular relay therapies, and combination therapies. The aim is to directly reduce the number of tumor-associated macrophages (TAMs), prevent their recruitment, enhance phagocytosis and killing, or target surface molecules of TAMs. Numerous studies have shown that TAMs are potential targets for immunotherapy of colorectal cancer (CRC) (119, 120). The study found that certain clinical drugs, such as metformin, Yunnan Garcinia Cambogia, and Mongolian Astragalus-Yulin, can decrease the recruitment of TAMs (114, 121, 122). The PD-1/PD-L1 immune checkpoint inhibitor enhances the phagocytosis of tumor-associated macrophages (TAM) and reduces tumor load (123). Several combination therapies are currently in progress. Liposomes (TAT-BLZmlips) with magnetic navigation and magnetic heat therapy (MHT) can repolarize M2, inhibiting tumor growth and recurrence (124). The combination of cryophotothermal therapy (PTT) and anti-PD-L1 immunotherapy has been reported to improve efficacy against colorectal cancer (125). Combined furazinotinib chemotherapy and anti-PD-1 therapy promotes an anti-tumor immune response (123).
Neutrophils are a cellular target of immunotherapy. Current research on tumor-associated neutrophils (TANs) aims to reduce their numbers and inhibit their accumulation in tumors. Studies have shown that increased accumulation of TANs in tumors hinders the response to anti-PD-1 drug therapy (126). The combination of oxaliplatin chemotherapy and immunotherapy has been found to enhance lipid A immunotherapy efficacy by recruiting neutrophils (127). Further research is needed to develop other immunotherapies targeting neutrophils.
In recent years, there has been an increase in research on NK cell immunotherapy. However, there has been limited research on its effectiveness in treating colorectal cancer. One way to enhance the activity of NK cells and improve their ability to kill tumors is through cytokine supplementation. For instance, the survival time of colorectal cancer patients can be prolonged by supplementing with IL-12 (74). Additionally, NK cells have been utilized in genetic engineering. The CAR-NK cells, which are genetically engineered immune cells equipped with chimeric antigen receptors (CARs) for precise targeting of tumor-specific antigens, have exhibited promising outcomes in preclinical investigations focusing on colorectal cancer (128).
Dendritic cells play a crucial role in anti-tumor immunity. Relevant immunotherapies include in vivo activation of DCs, chemotherapy combination therapy, in vivo expansion, and DC vaccines. Clotrimazole can activate DCs through the lactate-lysosome axis, promote antigen cross-presentation, and enhance the effect of anti-PD-1 immunotherapy (129). The use of immune adjuvant nanocomplexes and dendritic vaccines, in combination with immune checkpoint blockade, has been shown to be effective in cancer therapy (130). Electrothermal therapy and dendritic cell immunotherapy have been used to improve the immune-tumor microenvironment (131). Although initial results in glioblastoma and metastatic melanoma are promising, DC vaccines have not yet been fully explored in colorectal cancer (132).
Modifying the suppressive effects of myeloid-derived suppressor cells (MDSCs) is currently considered a promising cancer therapy in colorectal cancer. A study has shown that histamine-targeted depletion of MDSCs enhances the efficacy of PD-L1 blockade in colorectal cancer (133). Additionally, supramolecular tadalafil nanovaccines have been used in cancer therapy to attenuate MDSCs and enhance immunogenicity (134). Chemotherapeutic agents, such as paclitaxel, reduce tumor progression by inhibiting reactive oxygen species and nitric oxide produced by MDSC cells (135). Rhythmic therapy targeting poly ADP-ribose polymerase-1 (PARP-1) modulates MDSC inhibition and enhances anti-PD-1 immunotherapy in colon cancer (136). Antagonizing the signaling of the prostaglandin E2 axis effectively reduces recruitment and differentiation of MDSCs, thereby controlling tumor growth (95).
Immunotherapy is a therapeutic approach that aims to harness the immune system to fight cancer. As illustrated above, immune checkpoint inhibitors (ICIs) regulate the interactions between T cells, antigen-presenting cells (APCs) and tumor cells, thereby facilitating the release of suppressed immune responses. Immunotherapy is currently effective in patients with metastatic colorectal cancer (mCRC) who have defective mismatch repair (dMMR) or high microsatellite instability (MSI-H) (referred to as dMMR/MSI-H mCRC). Many clinical trials have also been conducted in patients with defective mismatch repair (pMMR) or microsatellite stabilization (MSS) or low microsatellite instability (MSI-L) (referred to as pMMR/MSI/MSI-L mCRC) in patients with mCRC (Table 2).
In the tumor microenvironment, innate immune cells play a crucial role in the development of colorectal cancer. Depending on the microenvironment and stimuli, they can either promote or suppress the growth of colorectal cancer. Innate immune cells can promote inflammation, angiogenesis, drug resistance, and cancer growth by secreting certain substances or undergoing changes in phenotype. Tumor development is closely linked to the reconfiguration of cellular metabolic processes. A hallmark of cancerous cells’ metabolism is their capacity to secure vital nutrients from environments that are typically nutrient-deficient. They utilize these nutrients not only to sustain their survival but also to produce additional cellular material. Changes in both the internal and external metabolic substances can occur alongside the metabolic reprogramming associated with cancer, significantly influencing gene expression, cell differentiation, and the surrounding environment of the tumor. Although the current treatment for colorectal cancer (CRC) patients is primarily based on surgery, radiotherapy, and chemotherapy, immunotherapy is gradually being introduced as a treatment option. Therefore, it is essential to have a comprehensive understanding of how innate immune cells and related molecular mechanisms impact the development of CRC. Current immunotherapy has significantly improved the survival rate of colorectal cancer patients. However, it also has several limitations, including immune adverse reactions and enhanced drug resistance. Therefore, in the future, we should explore the mechanism of immune cells on colorectal cancerIsekai Cheat Magician development, search for more accurate tumor markers, and investigate new methods of combination therapy. This will enable us to formulate more effective and individualized therapeutic strategies.
WD: Writing – review & editing. WL: Writing – original draft. TK: Writing – original draft. LL: Writing – original draft.
The author(s) declare financial support was received for the research, authorship, and/or publication of this article. This work was supported by grants from National Natural Science Foundation of China (No. 82172855). The funding bodies played no role in the design of the study and collection, analysis, and interpretation of data and in writing the manuscript.
The authors declare that the research was conducted in the absence of any commercial or financial relationships that could be construed as a potential conflict of interest.
All claims expressed in this article are solely those of the authors and do not necessarily represent those of their affiliated organizations, or those of the publisher, the editors and the reviewers. Any product that may be evaluated in this article, or claim that may be made by its manufacturer, is not guaranteed or endorsed by the publisher.
1. Bray F, Ferlay J, Soerjomataram I, Siegel RL, Torre LA, Jemal A. Global cancer statistics 2018: GLOBOCAN estimates of incidence and mortality worldwide for 36 cancers in 185 countries[J]. CA Cancer J Clin. (2018) 68:394–424. doi: 10.3322/caac.21492
2. Anderson NM, Simon MC. The tumor microenvironment. Curr Biol. (2020) 30:R921–r925. doi: 10.1016/j.cub.2020.06.081
3. Guo L, Wang C, Qiu X, Pu X, Chang P. Colorectal cancer immune infiltrates: significance in patient prognosis and immunotherapeutic efficacy[J]. Front Immunol. (2020) 11:1052. doi: 10.3389/fimmu.2020.01052
4. Mantovani A, Sozzani S, Locati M, Allavena P, Sica A. Macrophage polarization: tumor-associated macrophages as a paradigm for polarized M2 mononuclear phagocytes[J]. Trends Immunol. (2002) 23:549–55. doi: 10.1016/S1471-4906(02)02302-5
5. Bingle L, Brown NJ, Lewis CE. The role of tumor-associated macrophages in tumor progression: implications for new anticancer therapies[J]. J Pathol. (2002) 196:254–65. doi: 10.1002/path.1027
6. Fridlender ZG, Sun J, Kim S, Kapoor V, Cheng G, Ling L, et al. Polarization of tumor-associated neutrophil phenotype by TGF-beta: "N1" versus "N2" TAN[J]. Cancer Cell. (2009) 16:183–94. doi: 10.1016/j.ccr.2009.06.017
7. Oberg HH, Wesch D, Kalyan S, Kabelitz D. Regulatory interactions between neutrophils, tumor cells and T cells[J]. Front Immunol. (2019) 10:1690. doi: 10.3389/fimmu.2019.01690
8. Asadirad A, Baghaei K, Hashemi SM, Dehnavi S, Ghanbarian H, Mortaz E, et al. Dendritic cell immunotherapy with miR-155 enriched tumor-derived exosome suppressed cancer growth and induced antitumor immune responses in murine model of colorectal cancer induced by CT26 cell line[J]. Int Immunopharmacol. (2022) 104:108493. doi: 10.1016/j.intimp.2021.108493
9. Xia X, Mao Z, Wang W, Ma J, Tian J, Wang S, et al. Netrin-1 promotes the immunosuppressive activity of MDSCs in colorectal cancer[J]. Cancer Immunol Res. (2023) 11:600–13. doi: 10.1158/2326-6066.CIR-22-0658
10. Lan J, Sun L, Xu F, Liu L, Hu F, Song D, et al. M2 macrophage-derived exosomes promote cell migration and invasion in colon cancer[J]. Cancer Res. (2019) 79:146–58. doi: 10.1158/0008-5472.CAN-18-0014
11. Lin X, Wang S, Sun M, Zhang C, Wei C, Yang C, et al. miR-195-5p/NOTCH2-mediated EMT modulates IL-4 secretion in colorectal cancer to affect M2-like TAM polarization[J]. J Hematol Oncol. (2019) 12:20. doi: 10.1186/s13045-019-0708-7
12. Tang Y, Hu S, Li T, Qiu X. Tumor cells-derived exosomal circVCP promoted the progression of colorectal cancer by regulating macrophage M1/M2 polarization[J]. Gene. (2023) 870:147413. doi: 10.1016/j.gene.2023.147413
13. Guo J, Wang X, Guo Q, Zhu S, Li P, Zhang S, et al. M2 macrophage derived extracellular vesicle-mediated transfer of miR-186-5p promotes colon cancer progression by targeting DLC1[J]. Int J Biol Sci. (2022) 18:1663–76. doi: 10.7150/ijbs.69405
14. Wei C, Yang C, Wang S, Shi D, Zhang C, Lin X, et al. Crosstalk between cancer cells and tumor associated macrophages is required for mesenchymal circulating tumor cell-mediated colorectal cancer metastasis[J]. Mol Cancer. (2019) 18:64. doi: 10.1186/s12943-019-0976-4
15. Plantureux L, Mège D, Crescence L, Carminita E, Robert S, Cointe S, et al. The interaction of platelets with colorectal cancer cells inhibits tumor growth but promotes metastasis[J]. Cancer Res. (2020) 80:291–303. doi: 10.1158/0008-5472.CAN-19-1181
16. Zhang T, Liu L, Lai W, Zeng Y, Xu H, Lan Q, et al. Interaction with tumor−associated macrophages promotes PRL−3−induced invasion of colorectal cancer cells via MAPK pathway−induced EMT and NF−κB signaling−induced angiogenesis[J]. Oncol Rep. (2019) 41:2790–802. doi: 10.3892/or
17. Bao Z, Zeng W, Zhang D, Wang L, Deng X, Lai J, et al. SNAIL induces EMT and lung metastasis of tumors secreting CXCL2 to promote the invasion of M2-type immunosuppressed macrophages in colorectal cancer[J]. Int J Biol Sci. (2022) 18:2867–81. doi: 10.7150/ijbs.66854
18. Wang X, Wang J, Zhao J, Wang H, Chen J, Wu J. HMGA2 facilitates colorectal cancer progression via STAT3-mediated tumor-associated macrophage recruitment[J]. Theranostics. (2022) 12:963–75. doi: 10.7150/thno.65411
19. Liu Q, Yang C, Wang S, Shi D, Wei C, Song J. Wnt5a-induced M2 polarization of tumor-associated macrophages via IL-10 promotes colorectal cancer progression[J]. Cell Commun Signal. (2020) 18:51. doi: 10.1186/s12964-020-00557-2
20. Xue J, Ge X, Zhao W, Xue L, Dai C, Lin F, et al. PIPKIγ Regulates CCL2 expression in colorectal cancer by activating AKT-STAT3 signaling[J]. J Immunol Res. (2019) 2019:3690561. doi: 10.1155/2019/3690561
21. Li J, Yang J, Xing R, Wang Y. A novel inflammation-related signature for predicting prognosis and characterizing the tumor microenvironment in colorectal cancer[J]. Aging (Albany NY). (2023) 15:2554–81. doi: 10.18632/aging.v15i7
22. Tu W, Gong J, Zhou Z, Tian D, Wang Z. TCF4 enhances hepatic metastasis of colorectal cancer by regulating tumor-associated macrophage via CCL2/CCR2 signaling[J]. Cell Death Dis. (2021) 12:882. doi: 10.1038/s41419-021-04166-w
23. Liu C, Zhang W, Wang J, Si T, Xing W. Tumor-associated macrophage-derived transforming growth factor-β promotes colorectal cancer progression through HIF1-TRIB3 signaling[J]. Cancer Sci. (2021) 112:4198–207. doi: 10.1111/cas.15101
24. Jiang X, Zhang H, Zhang H, Wang F, Wang X, Ding T, et al. Microcystin-LR-induced interaction between M2 tumor-associated macrophage and colorectal cancer cell promotes colorectal cancer cell migration through regulating the expression of TGF-β1 and CST3[J]. Int J Mol Sci. (2023) 24(13). doi: 10.3390/ijms241310527
25. Liu M, Fu X, Jiang L, Ma J, Zheng X, Wang S, et al. Colon cancer cells secreted CXCL11 via RBP-Jκ to facilitated tumor-associated macrophage-induced cancer metastasis[J]. J Cell Mol Med. (2021) 25:10575–90. doi: 10.1111/jcmm.16989
26. Wunderlich CM, Ackermann PJ, Ostermann AL, Adams-Quack P, Vogt MC, Tran ML, et al. Obesity exacerbates colitis-associated cancer via IL-6-regulated macrophage polarisation and CCL-20/CCR-6-mediated lymphocyte recruitment[J]. Nat Commun. (2018) 9:1646. doi: 10.1038/s41467-018-03773-0
27. Xu H, Lai W, Zhang Y, Liu L, Luo X, Zeng Y, et al. Tumor-associated macrophage-derived IL-6 and IL-8 enhance invasive activity of LoVo cells induced by PRL-3 in a KCNN4 channel-dependent manner[J]. BMC Cancer. (2014) 14:330. doi: 10.1186/1471-2407-14-330
28. Zhang Y, Sime W, Juhas M, Sjölander A. Crosstalk between colon cancer cells and macrophages via inflammatory mediators and CD47 promotes tumor cell migration[J]. Eur J Cancer. (2013) 49:3320–34. doi: 10.1016/j.ejca.2013.06.005
29. Yang M, Shao JH, Miao YJ, Cui W, Qi YF, Han JH, et al. Tumor cell-activated CARD9 signaling contributes to metastasis-associated macrophage polarization[J]. Cell Death Differ. (2014) 21:1290–302. doi: 10.1038/cdd.2014.45
30. Jiang X, Cao G, Gao G, Wang W, Zhao J, Gao C. Triptolide decreases tumor-associated macrophages infiltration and M2 polarization to remodel colon cancer immune microenvironment via inhibiting tumor-derived CXCL12[J]. J Cell Physiol. (2021) 236:193–204. doi: 10.1002/jcp.29833
31. Deng Z, Wu N, Suo Q, Wang J, Yue Y, Geng L, et al. Fucoidan, as an immunostimulator promotes M1 macrophage differentiation and enhances the chemotherapeutic sensitivity of capecitabine in colon cancer. Int J Biol Macromol. (2022) 222:562–72. doi: 10.1016/j.ijbiomac.2022.09.201
32. Yue L, Xu X, Dai S, Xu F, Zhao W, Gu J, et al. Orosomucoid 1 promotes colorectal cancer progression and liver metastasis by affecting PI3K/AKT pathway and inducing macrophage M2 polarization[J]. Sci Rep. (2023) 13:14092. doi: 10.1038/s41598-023-40404-1
33. Chen W, Xu Y, Zhong J, Wang H, Weng M, Cheng Q, et al. MFHAS1 promotes colorectal cancer progress by regulating polarization of tumor-associated macrophages via STAT6 signaling pathway[J]. Oncotarget. (2016) 7:78726–35. doi: 10.18632/oncotarget.v7i48
34. Yin Y, Liu B, Cao Y, Yao S, Liu Y, Jin G, et al. Colorectal cancer-derived small extracellular vesicles promote tumor immune evasion by upregulating PD-L1 expression in tumor-associated macrophages[J]. Adv Sci (Weinh). (2022) 9:2102620. doi: 10.1002/advs.202102620
35. Yang M, Liu J, Piao C, Piao C, Shao J, Du J. ICAM-1 suppresses tumor metastasis by inhibiting macrophage M2 polarization through blockade of efferocytosis[J]. Cell Death Dis. (2015) 6:e1780. doi: 10.1038/cddis.2015.144
36. Sudo G, Aoki H, Yamamoto E, Takasawa A, Niinuma T, Yoshido A, et al. Activated macrophages promote invasion by early colorectal cancer via an interleukin 1β-serum amyloid A1 axis[J]. Cancer Sci. (2021) 112:4151–65. doi: 10.1111/cas.15080
37. Shi W, Zhang F, Chen X, Wang S, Zhang H, Yang Z, et al. Tumor-derived immunoglobulin like transcript 5 induces suppressive immunocyte infiltration in colorectal cancer[J]. Cancer Sci. (2022) 113:1939–54. doi: 10.1111/cas.15360
38. Dong L, Chen C, Zhang Y, Guo P, Wang Z, Li J, et al. The loss of RNA N(6)-adenosine methyltransferase Mettl14 in tumor-associated macrophages promotes CD8(+) T cell dysfunction and tumor growth[J]. Cancer Cell. (2021) 39:945–957.e10. doi: 10.1016/j.ccell.2021.04.016
39. Shao Y, Lan Y, Chai X, Gao S, Zheng J, Huang R, et al. CXCL8 induces M2 macrophage polarization and inhibits CD8(+) T cell infiltration to generate an immunosuppressive microenvironment in colorectal cancer[J]. FASEB J. (2023) 37:e23173. doi: 10.1096/fj.202201982RRR
40. Liu C, Yao Z, Wang J, Zhang W, Yang Y, Zhang Y, et al. Macrophage-derived CCL5 facilitates immune escape of colorectal cancer cells via the p65/STAT3-CSN5-PD-L1 pathway[J]. Cell Death Differ. (2020) 27:1765–81. doi: 10.1038/s41418-019-0460-0
41. Andreuzzi E, Fejza A, Polano M, Poletto E, Camicia L, Carobolante G, et al. Colorectal cancer development is affected by the ECM molecule EMILIN-2 hinging on macrophage polarization via the TLR-4/MyD88 pathway[J]. J Exp Clin Cancer Res. (2022) 41:60. doi: 10.1186/s13046-022-02271-y
42. Zhang D, Shi R, Xiang W, Kang X, Tang B, Li C, et al. The Agpat4/LPA axis in colorectal cancer cells regulates antitumor responses via p38/p65 signaling in macrophages[J]. Signal Transduct Target Ther. (2020) 5:24. doi: 10.1038/s41392-020-0117-y
43. Huang C, Ou R, Chen X, Zhang Y, Li J, Liang Y, et al. Tumor cell-derived SPON2 promotes M2-polarized tumor-associated macrophage infiltration and cancer progression by activating PYK2 in CRC[J]. J Exp Clin Cancer Res. (2021) 40:304. doi: 10.1186/s13046-021-02108-0
44. Fan L, Xu C, Ge Q, Lin Y, Wong CC, Qi Y, et al. Muciniphila suppresses colorectal tumorigenesis by inducing TLR2/NLRP3-mediated M1-like TAMs. Cancer Immunol Res. (2021) 9:1111–24. doi: 10.1158/2326-6066.CIR-20-1019
45. Zhang Y, Ma S, Li T, Tian Y, Zhou H, Wang H, et al. ILC1-derived IFN-γ regulates macrophage activation in colon cancer[J]. Biol Direct. (2023) 18:56. doi: 10.1186/s13062-023-00401-w
46. Li E, Yang X, Du Y, Wang G, Chan DW, Wu D, et al. CXCL8 associated dendritic cell activation marker expression and recruitment as indicators of favorable outcomes in colorectal cancer[J]. Front Immunol. (2021) 12:667177. doi: 10.3389/fimmu.2021.667177
47. Dmitrieva-Posocco O, Dzutsev A, Posocco DF, Hou V, Yuan W, Thovarai V, et al. Cell-type-specific responses to interleukin-1 control microbial invasion and tumor-elicited inflammation in colorectal cancer[J]. Immunity. (2019) 50:166–180.e7. doi: 10.1016/j.immuni.2018.11.015
48. Tosti N, Cremonesi E, Governa V, Basso C, Kancherla V, Coto-Llerena M, et al. Infiltration by IL22-producing T cells promotes neutrophil recruitment and predicts favorable clinical outcome in human colorectal cancer[J]. Cancer Immunol Res. (2020) 8:1452–62. doi: 10.1158/2326-6066.CIR-19-0934
49. Delgado-Ramirez Y, Baltazar-Perez I, Martinez Y, Callejas BE, Medina-Andrade I, Olguín JE, et al. STAT1 Is Required for Decreasing Accumulation of Granulocytic Cells via IL-17 during Initial Steps of Colitis-Associated Cancer[J]. . Int J Mol Sci. (2021) 22(14). doi: 10.3390/ijms22147695
50. Llosa NJ, Luber B, Tam AJ, Smith KN, Siegel N, Awan AH, et al. Intratumoral adaptive immunosuppression and type 17 immunity in mismatch repair proficient colorectal tumors[J]. Clin Cancer Res. (2019) 25:5250–9. doi: 10.1158/1078-0432.CCR-19-0114
51. Shimizu M, Tanaka N. IL-8-induced O-GlcNAc modification via GLUT3 and GFAT regulates cancer stem cell-like properties in colon and lung cancer cells[J]. Oncogene. (2019) 38:1520–33. doi: 10.1038/s41388-018-0533-4
52. Su H, Cai T, Zhang S, Yan X, Zhou L, He Z, et al. Identification of hub genes associated with neutrophils infiltration in colorectal cancer[J]. J Cell Mol Med. (2021) 25:3371–80. doi: 10.1111/jcmm.16414
53. Ackermann A, Lafferton B, Plotz G, Zeuzem S, Brieger A. Expression and secretion of the pro−inflammatory cytokine IL−8 is increased in colorectal cancer cells following the knockdown of non−erythroid spectrin αII[J]. Int J Oncol. (2020) 56:1551–64. doi: 10.3892/ijo
54. Triner D, Xue X, Schwartz AJ, Jung I, Colacino JA, Shah YM. Epithelial hypoxia-inducible factor 2α Facilitates the progression of colon tumors through recruiting neutrophils[J]. Mol Cell Biol. (2017) 37(5). doi: 10.1128/MCB.00481-16
55. Ogawa R, Yamamoto T, Hirai H, Hanada K, Kiyasu Y, Nishikawa G, et al. Loss of SMAD4 promotes colorectal cancer progression by recruiting tumor-associated neutrophils via the CXCL1/8-CXCR2 axis[J]. Clin Cancer Res. (2019) 25:2887–99. doi: 10.1158/1078-0432.CCR-18-3684
56. Hwang WL, Lan HY, Cheng WC, Huang SC, Yang MH. Tumor stem-like cell-derived exosomal RNAs prime neutrophils for facilitating tumorigenesis of colon cancer[J]. J Hematol Oncol. (2019) 12:10. doi: 10.1186/s13045-019-0699-4
57. Aoki T, Narumiya S. Prostaglandin E(2)-EP2 signaling as a node of chronic inflammation in the colon tumor microenvironment[J]. Inflammation Regener. (2017) 37:4. doi: 10.1186/s41232-017-0036-7
58. Ma X, Aoki T, Tsuruyama T, Narumiya S. Definition of prostaglandin E2-EP2 signals in the colon tumor microenvironment that amplify inflammation and tumor growth[J]. Cancer Res. (2015) 75:2822–32. doi: 10.1158/0008-5472.CAN-15-0125
59. Tian S, Chu Y, Hu J, Ding X, Liu Z, Fu D, et al. Tumor-associated neutrophils secrete AGR2 to promote colorectal cancer metastasis via its receptor CD98hc-xCT[J]. Gut. (2022) 71:2489–501. doi: 10.1136/gutjnl-2021-325137
60. Gordon-Weeks AN, Lim SY, Yuzhalin AE, Jones K, Markelc B, Kim KJ, et al. Neutrophils promote hepatic metastasis growth through fibroblast growth factor 2-dependent angiogenesis in mice[J]. Hepatology. (2017) 65:1920–35. doi: 10.1002/hep.29088
61. Kaltenmeier C, Yazdani HO, Morder K, Geller DA, Simmons RL, Tohme S, et al. Neutrophil extracellular traps promote T cell exhaustion in the tumor microenvironment[J]. Front Immunol. (2021) 12:785222. doi: 10.3389/fimmu.2021.785222
62. Kong X, Zhang Y, Xiang L, You Y, Duan Y, Zhao Y, et al. Fusobacterium nucleatum-triggered neutrophil extracellular traps facilitate colorectal carcinoma progression[J]. J Exp Clin Cancer Res. (2023) 42:236. doi: 10.1186/s13046-023-02817-8
63. Bi Y, Yang Q, Li Z, Wang Y, Wang Y, Jia A, et al. Aryl hydrocarbon receptor nuclear translocator limits the recruitment and function of regulatory neutrophils against colorectal cancer by regulating the gut microbiota[J]. J Exp Clin Cancer Res. (2023) 42:53. doi: 10.1186/s13046-023-02627-y
64. Bui TM, Butin-Israeli V, Wiesolek HL, Zhou M, Rehring JF, Wiesmüller L, et al. Neutrophils alter DNA repair landscape to impact survival and shape distinct therapeutic phenotypes of colorectal cancer[J]. Gastroenterology. (2021) 161:225–238.e15. doi: 10.1053/j.gastro.2021.03.027
65. Yu H, Chen C, Han F, Tang J, Deng M, Niu Y, et al. Long noncoding RNA MIR4435-2HG suppresses colorectal cancer initiation and progression by reprogramming neutrophils[J]. Cancer Immunol Res. (2022) 10:1095–110. doi: 10.1158/2326-6066.CIR-21-1011
66. Chun E, Lavoie S, Michaud M, Gallini CA, Kim J, Soucy G, et al. CCL2 promotes colorectal carcinogenesis by enhancing polymorphonuclear myeloid-derived suppressor cell population and function[J]. Cell Rep. (2015) 12:244–57. doi: 10.1016/j.celrep.2015.06.024
67. Germann M, Zangger N, Sauvain MO, Sempoux C, Bowler AD, Wirapati P, et al. Neutrophils suppress tumor-infiltrating T cells in colon cancer via matrix metalloproteinase-mediated activation of TGFβ[J]. EMBO Mol Med. (2020) 12:e10681. doi: 10.15252/emmm.201910681
68. Yoshido A, Sudo G, Takasawa A, Aoki H, Kitajima H, Yamamoto E, et al. Serum amyloid A1 recruits neutrophils to the invasive front of T1 colorectal cancers[J]. J Gastroenterol Hepatol. (2023) 38:301–10. doi: 10.1111/jgh.16055
69. Mizuno R, Kawada K, Itatani Y, Ogawa R, Kiyasu Y, Sakai Y. The role of tumor-associated neutrophils in colorectal cancer[J]. Int J Mol Sci. (2019) 20(3). doi: 10.3390/ijms20030529
70. Halama N, Braun M, Kahlert C, Spille A, Quack C, Rahbari N, et al. Natural killer cells are scarce in colorectal carcinoma tissue despite high levels of chemokines and cytokines[J]. Clin Cancer Res. (2011) 17:678–89. doi: 10.1158/1078-0432.CCR-10-2173
71. Kwok HF, Buick RJ, Kuehn D, Gormley JA, Doherty D, Jaquin TJ, et al. Antibody targeting of Cathepsin S induces antibody-dependent cellular cytotoxicity[J]. Mol Cancer. (2011) 10:147. doi: 10.1186/1476-4598-10-147
72. Gur C, Ibrahim Y, Isaacson B, Yamin R, Abed J, Gamliel M, et al. Binding of the Fap2 protein of Fusobacterium nucleatum to human inhibitory receptor TIGIT protects tumors from immune cell attack[J]. Immunity. (2015) 42:344–55. doi: 10.1016/j.immuni.2015.01.010
73. Bergamaschi C, Pandit H, Nagy BA, Stellas D, Jensen SM, Bear J, et al. Heterodimeric IL-15 delays tumor growth and promotes intratumoral CTL and dendritic cell accumulation by a cytokine network involving XCL1, IFN-γ, CXCL9 and CXCL10[J]. J Immunother Cancer. (2020) 8(1). doi: 10.1136/jitc-2020-000599
74. Cui G, Yuan A, Zhu L, Florholmen J, Goll R. Increased expression of interleukin-21 along colorectal adenoma-carcinoma sequence and its predicating significance in patients with sporadic colorectal cancer[J]. Clin Immunol. (2017) 183:266–72. doi: 10.1016/j.clim.2017.09.003
75. Yin P, Gui L, Wang C, Yan J, Liu M, Ji L, et al. Targeted delivery of CXCL9 and OX40L by mesenchymal stem cells elicits potent antitumor immunity[J]. Mol Ther. (2020) 28:2553–63. doi: 10.1016/j.ymthe.2020.08.005
76. Dupaul-Chicoine J, Arabzadeh A, Dagenais M, Douglas T, Champagne C, Morizot A, et al. The nlrp3 inflammasome suppresses colorectal cancer metastatic growth in the liver by promoting natural killer cell tumoricidal activity[J]. Immunity. (2015) 43:751–63. doi: 10.1016/j.immuni.2015.08.013
77. Huang YW, Lin CW, Pan P, Shan T, Echeveste CE, Mo YY, et al. Black raspberries suppress colorectal cancer by enhancing smad4 expression in colonic epithelium and natural killer cells[J]. Front Immunol. (2020) 11:570683. doi: 10.3389/fimmu.2020.570683
78. Harmon C, Robinson MW, Hand F, Almuaili D, Mentor K, Houlihan DD, et al. Lactate-mediated acidification of tumor microenvironment induces apoptosis of liver-resident NK cells in colorectal liver metastasis[J]. Cancer Immunol Res. (2019) 7:335–46. doi: 10.1158/2326-6066.CIR-18-0481
79. Szczygieł A, Węgierek-Ciura K, Wróblewska A, Mierzejewska J, Rossowska J, Szermer-Olearnik B, et al. Combined therapy with methotrexate nanoconjugate and dendritic cells with downregulated IL-10R expression modulates the tumor microenvironment and enhances the systemic anti-tumor immune response in MC38 murine colon carcinoma[J]. Front Immunol. (2023) 14:1155377. doi: 10.3389/fimmu.2023.1155377
80. Zhang R, Qi F, Zhao F, Li G, Shao S, Zhang X, et al. Cancer-associated fibroblasts enhance tumor-associated macrophages enrichment and suppress NK cells function in colorectal cancer[J]. Cell Death Dis. (2019) 10:273. doi: 10.1038/s41419-019-1435-2
81. Bashiri Dezfouli A, Yazdi M, Benmebarek MR, Schwab M, Michaelides S, Miccichè A, et al. CAR T cells targeting membrane-bound hsp70 on tumor cells mimic hsp70-primed NK cells[J]. Front Immunol. (2022) 13:883694. doi: 10.3389/fimmu.2022.883694
82. Sun Y, Hu H, Liu Z, Xu J, Gao Y, Zhan X, et al. Macrophage STING signaling promotes NK cell to suppress colorectal cancer liver metastasis via 4-1BBL/4-1BB co-stimulation[J]. J Immunother Cancer. (2023) 11(3). doi: 10.1136/jitc-2022-006481
83. Costa D, Venè R, Benelli R, Romairone E, Scabini S, Catellani S, et al. Targeting the epidermal growth factor receptor can counteract the inhibition of natural killer cell function exerted by colorectal tumor-associated fibroblasts[J]. Front Immunol. (2018) 9:1150. doi: 10.3389/fimmu.2018.01150
84. Sconocchia G, Eppenberger S, Spagnoli GC, Tornillo L, Droeser R, Caratelli S, et al. NK cells and T cells cooperate during the clinical course of colorectal cancer[J]. Oncoimmunology. (2014) 3:e952197. doi: 10.4161/21624011.2014.952197
85. Bonnotte B, Gough M, Phan V, Ahmed A, Chong H, Martin F, et al. Intradermal injection, as opposed to subcutaneous injection, enhances immunogenicity and suppresses tumorigenicity of tumor cells[J]. Cancer Res. (2003) 63:2145–9.
86. Zhao Q, Yu X, Li M, Liu Y, Han Y, Zhang X, et al. MLKL attenuates colon inflammation and colitis-tumorigenesis via suppression of inflammatory responses[J]. Cancer Lett. (2019) 459:100–11. doi: 10.1016/j.canlet.2019.05.034
87. Kan JY, Wu DC, Yu FJ, Wu CY, Ho YW, Chiu YJ, et al. Chemokine (C-C motif) ligand 5 is involved in tumor-associated dendritic cell-mediated colon cancer progression through non-coding RNA MALAT-1[J]. J Cell Physiol. (2015) 230:1883–94. doi: 10.1002/jcp.24918
88. Cueto FJ, Del Fresno C, Brandi P, Combes AJ, Hernández-García E, Sánchez-Paulete AR, et al. DNGR-1 limits Flt3L-mediated antitumor immunity by restraining tumor-infiltrating type I conventional dendritic cells[J]. J Immunother Cancer. (2021) 9(5). doi: 10.1136/jitc-2020-002054
89. Kießler M, Plesca I, Sommer U, Wehner R, Wilczkowski F, Müller L, et al. Tumor-infiltrating plasmacytoid dendritic cells are associated with survival in human colon cancer[J]. J Immunother Cancer. (2021) 9. doi: 10.1136/jitc-2020-001813
90. Ghirelli C, Reyal F, Jeanmougin M, Zollinger R, Sirven P, Michea P, et al. Breast cancer cell-derived GM-CSF licenses regulatory th2 induction by plasmacytoid predendritic cells in aggressive disease subtypes[J]. Cancer Res. (2015) 75:2775–87. doi: 10.1158/0008-5472.CAN-14-2386
91. Zhou Z, Chen H, Xie R, Wang H, Li S, Xu Q, et al. Epigenetically modulated FOXM1 suppresses dendritic cell maturation in pancreatic cancer and colon cancer[J]. Mol Oncol. (2019) 13:873–93. doi: 10.1002/1878-0261.12443
92. Hu Z, Teng XL, Zhang T, Yu X, Ding R, Yi J, et al. SENP3 senses oxidative stress to facilitate STING-dependent dendritic cell antitumor function[J]. Mol Cell. (2021) 81:940–952.e5. doi: 10.1016/j.molcel.2020.12.024
93. Huang TX, Tan XY, Huang HS, Li YT, Liu BL, Liu KS, et al. Targeting cancer-associated fibroblast-secreted WNT2 restores dendritic cell-mediated antitumor immunity[J]. Gut. (2022) 71:333–44. doi: 10.1136/gutjnl-2020-322924
94. Miller TJ, Anyaegbu CC, Lee-Pullen TF, Spalding LJ, Platell CF, McCoy MJ. PD-L1+ dendritic cells in the tumor microenvironment correlate with good prognosis and CD8+ T cell infiltration in colon cancer[J]. Cancer Sci. (2021) 112:1173–83. doi: 10.1111/cas.14781
95. Tang C, Sun H, Kadoki M, Han W, Ye X, Makusheva Y, et al. Blocking Dectin-1 prevents colorectal tumorigenesis by suppressing prostaglandin E2 production in myeloid-derived suppressor cells and enhancing IL-22 binding protein expression[J]. Nat Commun. (2023) 14:1493. doi: 10.1038/s41467-023-37229-x
96. Ke Z, Wang C, Wu T, Wang W, Yang Y, Dai Y, et al. PAR2 deficiency enhances myeloid cell-mediated immunosuppression and promotes colitis-associated tumorigenesis[J]. Cancer Lett. (2020) 469:437–46. doi: 10.1016/j.canlet.2019.11.015
97. Ibrahim ML, Klement JD, Lu C, Redd PS, Xiao W, Yang D, et al. Myeloid-derived suppressor cells produce IL-10 to elicit DNMT3b-dependent IRF8 silencing to promote colitis-associated colon tumorigenesis[J]. Cell Rep. (2018) 25:3036–3046.e6. doi: 10.1016/j.celrep.2018.11.050
98. Mundy-Bosse BL, Lesinski GB, Jaime-Ramirez AC, Benninger K, Khan M, Kuppusamy P, et al. Myeloid-derived suppressor cell inhibition of the IFN response in tumor-bearing mice[J]. Cancer Res. (2011) 71:5101–10. doi: 10.1158/0008-5472.CAN-10-2670
99. Li W, Zhang X, Chen Y, Xie Y, Liu J, Feng Q, et al. G-CSF is a key modulator of MDSC and could be a potential therapeutic target in colitis-associated colorectal cancers[J]. Protein Cell. (2016) 7:130–40. doi: 10.1007/s13238-015-0237-2
100. Chen L, Jin XH, Luo J, Duan JL, Cai MY, Chen JW, et al. ITLN1 inhibits tumor neovascularization and myeloid derived suppressor cells accumulation in colorectal carcinoma[J]. Oncogene. (2021) 40:5925–37. doi: 10.1038/s41388-021-01965-5
101. Wang T, Fan C, Yao A, Xu X, Zheng G, You Y, et al. The adaptor protein CARD9 protects against colon cancer by restricting mycobiota-mediated expansion of myeloid-derived suppressor cells[J]. Immunity. (2018) 49:504–514.e4. doi: 10.1016/j.immuni.2018.08.018
102. Huang S, Wang Z, Zhou J, Huang J, Zhou L, Luo J, et al. EZH2 inhibitor GSK126 suppresses antitumor immunity by driving production of myeloid-derived suppressor cells[J]. Cancer Res. (2019) 79:2009–20. doi: 10.1158/0008-5472.CAN-18-2395
103. Huang M, Wu R, Chen L, Peng Q, Li S, Zhang Y, et al. S100A9 regulates MDSCs-mediated immune suppression via the RAGE and TLR4 signaling pathways in colorectal carcinoma[J]. Front Immunol. (2019) 10:2243. doi: 10.3389/fimmu.2019.02243
104. Zhu W, Chu H, Zhang Y, Luo T, Yu H, Zhu H. Fructose-1,6-bisphosphatase 1 dephosphorylates IκBα and suppresses colorectal tumorigenesis[J]. Cell Res. (2023) 33:245–57. doi: 10.1038/s41422-022-00773-0
105. Katoh H, Wang D, Daikoku T, Sun H, Dey SK, Dubois RN. CXCR2-expressing myeloid-derived suppressor cells are essential to promote colitis-associated tumorigenesis[J]. Cancer Cell. (2013) 24:631–44. doi: 10.1016/j.ccr.2013.10.009
106. Yan R, Li J, Xiao Z, Fan X, Liu H, Xu Y, et al. DCLK1 suppresses tumor-specific cytotoxic T lymphocyte function through recruitment of MDSCs via the CXCL1-CXCR2 axis[J]. Cell Mol Gastroenterol Hepatol. (2023) 15:463–85. doi: 10.1016/j.jcmgh.2022.10.013
107. Inamoto S, Itatani Y, Yamamoto T, Minamiguchi S, Hirai H, Iwamoto M, et al. Loss of SMAD4 promotes colorectal cancer progression by accumulation of myeloid-derived suppressor cells through the CCL15-CCR1 chemokine axis[J]. Clin Cancer Res. (2016) 22:492–501. doi: 10.1158/1078-0432.CCR-15-0726
108. Wu P, Wu D, Ni C, Ye J, Chen W, Hu G, et al. γδT17 cells promote the accumulation and expansion of myeloid-derived suppressor cells in human colorectal cancer[J]. Immunity. (2014) 40:785–800. doi: 10.1016/j.immuni.2014.03.013
109. Danelli L, Frossi B, Gri G, Mion F, Guarnotta C, Bongiovanni L, et al. Mast cells boost myeloid-derived suppressor cell activity and contribute to the development of tumor-favoring microenvironment[J]. Cancer Immunol Res. (2015) 3:85–95. doi: 10.1158/2326-6066.CIR-14-0102
110. Zhai J, Chen H, Wong CC, Peng Y, Gou H, Zhang J, et al. ALKBH5 drives immune suppression via targeting AXIN2 to promote colorectal cancer and is a target for boosting immunotherapy[J]. Gastroenterology. (2023) 165:445–62. doi: 10.1053/j.gastro.2023.04.032
111. Hong EH, Cho J, Ahn JH, Kwon BE, Kweon MN, Seo SU, et al. Plasmacytoid dendritic cells regulate colitis-associated tumorigenesis by controlling myeloid-derived suppressor cell infiltration[J]. Cancer Lett. (2020) 493:102–12. doi: 10.1016/j.canlet.2020.08.007
112. Jayakumar A, Bothwell ALM. RIPK3-induced inflammation by I-MDSCs promotes intestinal tumors[J]. Cancer Res. (2019) 79:1587–99. doi: 10.1158/0008-5472.CAN-18-2153
113. Liu Z, Xie Y, Xiong Y, Liu S, Qiu C, Zhu Z, et al. TLR 7/8 agonist reverses oxaliplatin resistance in colorectal cancer via directing the myeloid-derived suppressor cells to tumoricidal M1-macrophages[J]. Cancer Lett. (2020) 469:173–85. doi: 10.1016/j.canlet.2019.10.020
114. Kang J, Lee D, Lee KJ, Yoon JE, Kwon JH, Seo Y, et al. Tumor-suppressive effect of metformin via the regulation of M2 macrophages and myeloid-derived suppressor cells in the tumor microenvironment of colorectal cancer[J]. Cancers (Basel). (2022) 14(12). doi: 10.3390/cancers14122881
115. Yun X, Zhang Q, Fang Y, Lv C, Chen Q, Chu Y, et al. Madecassic acid alleviates colitis-associated colorectal cancer by blocking the recruitment of myeloid-derived suppressor cells via the inhibition of IL-17 expression in γδT17 cells[J]. Biochem Pharmacol. (2022) 202:115138. doi: 10.1016/j.bcp.2022.115138
116. Chun J, Park SM, Yi JM, Ha IJ, Kang HN, Jeong MK. Bojungikki-tang improves response to PD-L1 immunotherapy by regulating the tumor microenvironment in MC38 tumor-bearing mice[J]. Front Pharmacol. (2022) 13:901563. doi: 10.3389/fphar.2022.901563
117. Ouyang LY, Wu XJ, Ye SB, Zhang RX, Li ZL, Liao W, et al. Tumor-induced myeloid-derived suppressor cells promote tumor progression through oxidative metabolism in human colorectal cancer[J]. J Transl Med. (2015) 13:47. doi: 10.1186/s12967-015-0410-7
118. Zhang Z, Chen Y, Yin Y, Chen Y, Chen Q, Bing Z, et al. Candida tropicalis induces NLRP3 inflammasome activation via glycogen metabolism-dependent glycolysis and JAK-STAT1 signaling pathway in myeloid-derived suppressor cells to promote colorectal carcinogenesis[J]. Int Immunopharmacol. (2022) 113:109430. doi: 10.1016/j.intimp.2022.109430
119. Inagaki K, Kunisho S, Takigawa H, Yuge R, Oka S, Tanaka S, et al. Role of tumor-associated macrophages at the invasive front in human colorectal cancer progression[J]. Cancer Sci. (2021) 112:2692–704. doi: 10.1111/cas.14940
120. Mao Y, Chen L, Wang F, Zhu D, Ge X, Hua D, et al. Cancer cell-expressed B7-H3 regulates the differentiation of tumor-associated macrophages in human colorectal carcinoma[J]. Oncol Lett. (2017) 14:6177–83. doi: 10.3892/ol
121. Sui H, Tan H, Fu J, Song Q, Jia R, Han L, et al. The active fraction of Garcinia yunnanensis suppresses the progression of colorectal carcinoma by interfering with tumorassociated macrophage-associated M2 macrophage polarization in vivo and in vitro[J]. FASEB J. (2020) 34:7387–403. doi: 10.1096/fj.201903011R
122. Gu J, Sun R, Tang D, Liu F, Chang X, Wang Q. Astragalus mongholicus Bunge-Curcuma aromatica Salisb. suppresses growth and metastasis of colorectal cancer cells by inhibiting M2 macrophage polarization via a Sp1/ZFAS1/miR-153-3p/CCR5 regulatory axis[J]. Cell Biol Toxicol. (2022) 38:679–97. doi: 10.1007/s10565-021-09679-w
123. Wang Y, Wei B, Gao J, Cai X, Xu L, Zhong H, et al. Combination of fruquintinib and anti-PD-1 for the treatment of colorectal cancer[J]. J Immunol. (2020) 205:2905–15. doi: 10.4049/jimmunol.2000463
124. Fang Y, He Y, Wu C, Zhang M, Gu Z, Zhang J, et al. Magnetism-mediated targeting hyperthermia-immunotherapy in "cold" tumor with CSF1R inhibitor[J]. Theranostics. (2021) 11:6860–72. doi: 10.7150/thno.57511
125. Wang S, Song Y, Cao K, Zhang L, Fang X, Chen F, et al. Photothermal therapy mediated by gold nanocages composed of anti-PDL1 and galunisertib for improved synergistic immunotherapy in colorectal cancer[J]. Acta Biomater. (2021) 134:621–32. doi: 10.1016/j.actbio.2021.07.051
126. Nebot-Bral L, Hollebecque A, Yurchenko AA, de Forceville L, Danjou M, Jouniaux JM, et al. Overcoming resistance to αPD-1 of MMR-deficient tumors with high tumor-induced neutrophils levels by combination of αCTLA-4 and αPD-1 blockers[J]. J Immunother Cancer. (2022) 10(7). doi: 10.1136/jitc-2022-005059
127. Seignez C, Martin A, Rollet CE, Racoeur C, Scagliarini A, Jeannin JF, et al. Senescence of tumor cells induced by oxaliplatin increases the efficiency of a lipid A immunotherapy via the recruitment of neutrophils[J]. Oncotarget. (2014) 5:11442–51. doi: 10.18632/oncotarget.v5i22
128. Rezvani K, Rouce R, Liu E, Shpall E. Engineering natural killer cells for cancer immunotherapy[J]. Mol Ther. (2017) 25:1769–81. doi: 10.1016/j.ymthe.2017.06.012
129. Wang Z, Xu F, Hu J, Zhang H, Cui L, Lu W, et al. Modulation of lactate-lysosome axis in dendritic cells by clotrimazole potentiates antitumor immunity[J]. J Immunother Cancer. (2021) 9(5). doi: 10.1136/jitc-2020-002155
130. Vo MC, Ahn SY, Chu TH, Uthaman S, Pillarisetti S, Uong TNT, et al. A combination of immunoadjuvant nanocomplexes and dendritic cell vaccines in the presence of immune checkpoint blockade for effective cancer immunotherapy[J]. Cell Mol Immunol. (2021) 18:1599–601. doi: 10.1038/s41423-021-00666-z
131. Tsang YW, Huang CC, Yang KL, Chi MS, Chiang HC, Wang YS, et al. Improving immunological tumor microenvironment using electro-hyperthermia followed by dendritic cell immunotherapy[J]. BMC Cancer. (2015) 15:708. doi: 10.1186/s12885-015-1690-2
132. Van Willigen WW, Bloemendal M, Gerritsen WR, Schreibelt G, de Vries IJM, Bol KF. Dendritic cell cancer therapy: vaccinating the right patient at the right time[J]. Front Immunol. (2018) 9:2265. doi: 10.3389/fimmu.2018.02265
133. Tang Y, Zhou C, Li Q, Cheng X, Huang T, Li F, et al. Targeting depletion of myeloid-derived suppressor cells potentiates PD-L1 blockade efficacy in gastric and colon cancers[J]. Oncoimmunology. (2022) 11:2131084. doi: 10.1080/2162402X.2022.2131084
134. Zhang T, Xiong H, Ma X, Gao Y, Xue P, Kang Y, et al. Supramolecular tadalafil nanovaccine for cancer immunotherapy by alleviating myeloid-derived suppressor cells and heightening immunogenicity[J]. Small Methods. (2021) 5:e2100115. doi: 10.1002/smtd.202100115
135. Lee YH, Lee YR, Park CS, Baccatin III. a precursor for the semisynthesis of paclitaxel, inhibits the accumulation and suppressive activity of myeloid-derived suppressor cells in tumor-bearing mice[J]. Int Immunopharmacol. (2014) 21:487–93. doi: 10.1016/j.intimp.2014.06.012
Keywords: innate immune cells, colorectal cancer, tumor microenvironment, immunotherapy, immune cell
Citation: Liu W, Kuang T, Liu L and Deng W (2024) The role of innate immune cells in the colorectal cancer tumor microenvironment and advances in anti-tumor therapy research. Front. Immunol. 15:1407449. doi: 10.3389/fimmu.2024.1407449
Received: 26 March 2024; Accepted: 25 June 2024;
Published: 19 July 2024.
Edited by:
Noha Mousaad Elemam, University of Sharjah, United Arab EmiratesReviewed by:
Anne Jarry, Institut National de la Santé et de la Recherche Médicale (INSERM), FranceCopyright © 2024 Liu, Kuang, Liu and Deng. This is an open-access article distributed under the terms of the Creative Commons Attribution License (CC BY). The use, distribution or reproduction in other forums is permitted, provided the original author(s) and the copyright owner(s) are credited and that the original publication in this journal is cited, in accordance with accepted academic practice. No use, distribution or reproduction is permitted which does not comply with these terms.
*Correspondence: Wenhong Deng, d2VuaG9uZ2RlbmdAd2h1LmVkdS5jbg==
Disclaimer: All claims expressed in this article are solely those of the authors and do not necessarily represent those of their affiliated organizations, or those of the publisher, the editors and the reviewers. Any product that may be evaluated in this article or claim that may be made by its manufacturer is not guaranteed or endorsed by the publisher.
Research integrity at Frontiers
Learn more about the work of our research integrity team to safeguard the quality of each article we publish.