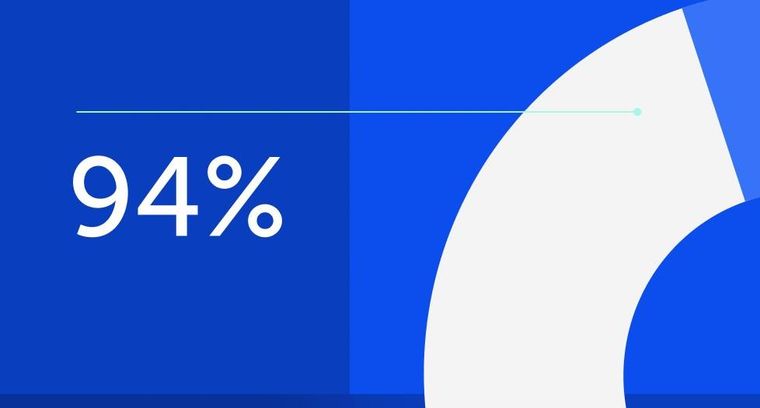
94% of researchers rate our articles as excellent or good
Learn more about the work of our research integrity team to safeguard the quality of each article we publish.
Find out more
ORIGINAL RESEARCH article
Front. Immunol., 02 August 2024
Sec. Microbial Immunology
Volume 15 - 2024 | https://doi.org/10.3389/fimmu.2024.1403789
Streptococcus suis causes diseases in pigs and has emerged as a zoonotic agent. When infected, the host develops an exacerbated inflammation that can lead to septic shock and meningitis. Although neutrophils greatly infiltrate the lesions, their dynamics during S. suis infection remain poorly described. Moreover, very few studies reported on the production and role of a key factor in the regulation of neutrophils: the colony-stimulating granulocyte factor (G-CSF). In this study, we characterized the G-CSF-neutrophil axis in the pathogenesis of S. suis induced disease. Using a mouse model of S. suis infection, we first evaluated the recruitment of neutrophils and their activation profile by flow cytometry. We found that infection provokes a massive neutrophil recruitment from the bone marrow to the blood and spleen. In both compartments, neutrophils displayed multiple activation markers. In parallel, we observed high systemic levels of G-CSF, with a peak of production coinciding with that of neutrophil recruitment. We then neutralized the effects of G-CSF and highlighted its role in the release of neutrophils from the bone marrow to the blood. However, it did not affect bacteremia nor the cytokine storm induced by S. suis. In conclusion, systemic G-CSF induces the release of neutrophils from the bone marrow to the blood, but its role in inflammation or bacterial clearance seems to be compensated by unknown factors. A better understanding of the role of neutrophils and inflammatory mediators could lead to better strategies for controlling the infection caused by S. suis.
The pig pathogen Streptococcus suis causes economic losses to the swine industry and represents a threat for humans due to its zoonotic potential (1). Several serotypes were described over the years, but the serotype 2 is considered the most prevalent and virulent worldwide (2). When S. suis invades the host, it causes septic disease manifested by endocarditis, meningitis, septic shock, and sudden death (1). These clinical manifestations arise from an exacerbated inflammation caused by the bacteria: while S. suis stimulates innate immune defenses, their incapacity to clear the bacteria leads to an outburst detrimental for the host (1). In this regard, studying the early events of the innate immune response against S. suis remains essential to understanding the pathogenesis of the disease caused by this bacterium.
Among the key cell types of the early immune response against pathogens, neutrophils quickly reach the site of infection under the influence of inflammatory signals (3, 4). When neutrophils reach the injured tissue, they strive to destroy pathogens with their microbicidal functions that include phagocytosis, neutrophil extracellular trap (NET) release, oxygen burst, and degranulation of antimicrobial cargo (3). The important role of neutrophils for pathogen clearance was demonstrated in a variety of infection models, including during S. suis infection (5–10). To perform their effector functions, neutrophils undergo phenotypical changes that turn them into an “activated” state (11, 12). This state can be visualized by changes in surface marker expression, including an enhanced expression of the integrin CD11b, the shedding of the L-selectin CD62L and the modulation of the CXC motif chemokine receptor 2 (CXCR2) (11–13). Although some studies report neutrophil recruitment in the blood, the brain and the lesions during S. suis infection in various animal models (10, 14–19), gaps exist concerning the precise dynamic of recruitment, the origin and the phenotypic changes of neutrophils during the early stages of S. suis-induced inflammation.
As mentioned, inflammatory signals dictate neutrophil intervention during infections. Of these, the granulocyte colony-stimulating factor (G-CSF), a glycoprotein involved in neutrophil differentiation, mobilization and activation, is amongst the most crucial (20, 21). In steady-state, G-CSF levels remain low, while infection leads to its production in various models (22–25). Several studies demonstrated that G-CSF participates in clearance of a variety of pathogens (26–28). Although such study was not conducted in a S. suis model of infection, a report evocated that administration of G-CSF extends the survival time of piglets challenged with S. suis (29). A second study showed that murine dendritic cells and macrophages produce G-CSF in response to S. suis via surface protein recognition (30). These results suggest that G-CSF could be of importance to fight S. suis in the early stages of the infection. However, information remains limited on the production and role of G-CSF during S. suis infection in vivo. In this study, we hypothesized that neutrophils are quickly mobilized and activated during S. suis infection, and that the G-CSF controls neutrophil mobilization, thereby participating in early immune response, bacterial clearance and the resolution of the infection.
The study was carried out accordingly with the recommendations of the guidelines and policies of the Canadian Council on Animal Care and the principles set forth in the Guide for the Care and Use of Laboratory Animals. The protocols and procedures were approved by the Animal Welfare Committee of the University of Montreal (protocols numbers Rech-1399 and Rech-1523), including euthanasia to minimize animal suffering, which was applied throughout this study when animals were seriously affected since mortality was not an endpoint measurement. Mice had free access to water and food pellets as well as enrichment such as diverse materials to use as bedding alongside access to a refuge and a rubber toy for gnawing.
The well-characterized and encapsulated strain P1/7 (serotype 2, sequence type 1) was used in this study. This strain was isolated from a pig with meningitis in the United Kingdom and its virulence was previously reported in experimental models of infection (31, 32). S. suis was grown overnight on Columbia agar plate supplemented with 5% sheep blood (Oxoid, Nepean, ON, Canada) at 37°C with 5% of CO2. Isolated colonies were used to inoculate 5 ml of Todd Hewitt broth (THB; Becton, Dickinson, Mississauga, ON, Canada) for 8 h. To prepare working solutions, 10 µl of a 10-3 dilution of the suspension were inoculated in 30 ml of THB for 16 h at 37°C with 5% of CO2. Bacteria were washed twice with pH 7.4 phosphate-buffered saline (PBS) and resuspended in THB at the appropriate dilution. To determine the bacterial concentration in CFU/ml, suspensions were diluted and plated on THB agar plates and colonies counted.
Wild-type C57BL/6 mice (5 to 8 weeks of age) were obtained from Charles River Laboratories (Wilmington, MA, USA). Mice were housed under specific pathogen-free conditions in ventilated cages and had unlimited access to water and rodent chow. Mice were intraperitoneally infected with 1 ml of S. suis suspension containing 1 x 107 CFU. In this model, S. suis quickly reaches the bloodstream and spreads causing a systemic inflammatory response, as observed in pig natural infection (31). Control mice (mock-infected) were injected with the same volume of vehicle solution (THB). Clinical signs were observed every 6 h daily to monitor for severe clinical signs of infection. After different times post-infection, mice were euthanized to collect blood by intracardiac puncture, spleen, liver and/or bone marrow. Blood samples were collected from living mice at different time points (see below) via the caudal vein of the tail (to evaluate bacteremia) or the submandibular vein (to quantify pro-inflammatory mediators).
To neutralize the effect of the G-CSF, we applied an anti-mouse G-CSF receptor (G-CSFR) antibody (Ch5E2-VR81-mIgG1κ; CSL Innovation, Parkville, Australia) as previously described (33). Briefly, mice were intraperitoneally injected with 0.2 ml of the anti-mouse G-CSFR antibody (100 µg per dose) or the corresponding isotype control (mouse IgG1κ; either from Bioxcell, Lebanon, NH, USA or from Biolegend, San Diego, CA, USA). The dose of anti-mouse G-CSFR antibody was selected in pre-trials (data not shown). Mice that were infected for 72 h received four doses of antibodies at day -1, day 0 prior to infection and day +1 and day +2 after infection. Mice that were infected for less than 24 h received two doses of antibodies at day -1 and day 0 prior to infection. Mice were infected with S. suis as described above.
Five microliters of blood were collected at 12 h, 24 h, 48 h and 72 h post-infection through the caudal tail vein of mice. Samples were appropriately diluted in PBS, plated on THB agar and colonies counted to assess the blood bacterial load in infected mice.
The blood of infected mice was collected at different time points post-infection either by intracardiac puncture on euthanized mice or by the submandibular vein collection on living mice. Blood was collected in BD Microtainer™ serum separator tubes (BD Biosciences, Mississauga, ON, Canada) and centrifugated at 10,000 g for 2 min. The serum was collected at the surface of the gel and stored at -80°C until analysis. The spleen and the liver were homogenized using a POLYTRON PT 1200E system bundle (Kinematica, Lucerne, Switzerland) in an extraction buffer containing complete Mini, EDTA-free, protease inhibitor cocktail tablets (Roche Diagnostics GmbH, Mannheim, Germany), following the manufacturer’s instructions. The tibias of mice were flushed in 500 µl of extraction buffer. Homogenate supernatants were collected by centrifugation and stored at -80°C until analysis.
The concentrations of G-CSF, interleukin (IL)-6 and the C-X-C motif chemokine ligand 1 (CXCL1)/keratinocyte chemoattractant (KC), were determined by sandwich enzyme-linked immunosorbent assay (ELISA) using pair-matched antibodies from R&D Systems (Minneapolis, MN, USA). Cytokine measurements were performed according to the manufacturer’s recommendations, as previously described (34, 35). The concentrations of other cytokines and chemokines were measured using a Bio-Plex Pro assay (Bio-Rad, Mississauga, ON, Canada) according to the manufacturer’s instructions. The assay covered the G-CSF, the interferon-γ (IFN-γ), the IL-12p70, IL-6, C-C motif chemokine ligand 2 (CCL2)/MCP-1, CCL3/MIP-1α, CCL4/MIP-1β, CCL6/RANTES, CXCL9/MIG, and CXCL2/MIP-2. Acquisition was performed on the MAGPIX platform (Luminex, Toronto, ON, Canada), and data were analyzed using Bio-Plex Manager 6.1 software (Bio-Rad).
Blood was collected in tubes coated with lithium heparin (BD Biosciences). Organs freshly collected from euthanized mice were homogenized to obtain single-cell suspensions. In the samples, red cells were removed by suspending the cells in a commercial lysis buffer (Invitrogen, Burlington, ON, Canada). Suspensions were first stained with a Fixable viability Dye eFluor™ 506 (Invitrogen) to exclude the dead cells from the analysis. After washing with PBS containing 2% fetal bovine serum, surface Fc receptors - that non-specifically bind antibodies - were blocked using FcBlock (BD Biosciences) for 15 min before staining. The cells were then stained for the markers of interest (detailed below) for 30 min in PBS containing 2% fetal bovine serum before being fixed by suspending them for 15-30 min in cold paraformaldehyde-PBS (2% for extracellular staining and 4% for intracellular staining) and stored in PBS at 4°C until data acquisition. To count the cells, 10 µL of Countbright™ Absolute Counting Beads (Invitrogen) were added in 400 µL of cell suspension just before acquisition. Data were acquired on a BD Fortessa or a BD FACSAria Fusion using the BD FACSDiva software and analyzed using the Flow Jo software (BD Biosciences).
The different subsets of cells were identified as previously described (36) using the following antibodies: PE-conjugated anti-mouse CD45 (clone 30-F11), APC-conjugated anti-mouse CD11c (clone HL3), BV421-conjugated anti-mouse CD11b (clone M1/70), FITC-conjugated anti-mouse Ly6G (clone 1A8). In addition to neutrophil markers, cells were stained with the following antibodies: PE CF594-conjugated anti-mouse CXCR4 (clone 2B11/CXCR4), BV605-conjugated anti-mouse CD49d (clone R1-2), Alexa700-conjugated anti-mouse CD62L (clone MEL-14), BV711-conjugated anti-mouse CXCR2 (CD182) (clone V48-2310). For BV-conjugated antibodies, the BD Horizon™ Brilliant Stain Buffer was used. To stain intracellular G-CSF, cells were permeabilized by suspension in BD Perm/Wash™ Buffer for 15 min in the dark and stained with AlexaFluor700-conjugated anti-mouse G-CSF antibody (clone 67604; R&D Systems). Unless otherwise indicated, all antibodies and buffers were from BD Biosciences. The gating strategy is depicted in Supplementary Figure S1.
Experiments were repeated at least three times, and the number of animals varied from three to thirty-six depending on the condition or time point (see figure legends for details). For all data, the normality of distribution was verified using the Shapiro-Wilk test. Accordingly, parametric tests (unpaired t test) or nonparametric tests (Mann-Whitney rank sum test) were performed to evaluate statistical differences between groups. Data are presented as the mean +/- standard error of the mean (SEM). P < 0.05 was considered statistically significant.
S. suis triggers an exaggerated inflammatory response in the host, including the production of multiple chemokines (31). Since those molecules participate in immune cell migration (37), we evaluated the changes in immune cell populations in the blood and the spleen at the early stages of S. suis infection. The markers and gating strategy allowed to distinguish leukocytes, lymphocytes, neutrophils and the pool of dendritic cells (DC), monocytes (Mo) and macrophages (Mph) (Supplementary Figure S1). During S. suis infection, the percentage of neutrophils significantly increased by 10-fold in the blood and by 3-fold in the spleen (Figures 1A, B). Other changes occurred in the blood: the percentage of lymphocytes decreased while the percentage of the pool DC-Mo-Mph increased (Figure 1A). However, no changes of these two cell populations were observed in the spleen (Figures 1B, C).
Figure 1 S. suis infection induces changes in immune cell populations. Mice were intraperitoneally infected with S. suis serotype 2 (1 x 107 CFU/ml) or mock-infected. After 6 h or 16 h of infection, blood (A) and spleen (B) were collected and cells analyzed by flow cytometry. DC-Mo-Mph stands for the pool of dendritic cells, monocytes and macrophages. Data are expressed as the percentage of each cell population among live cells in the blood (A) or in the spleen (B) expressed as mean +/- SEM (n = 3 to 10). *P < 0.05, indicates a significant difference between mock-infected and S. suis-infected mice. (C) Representative dot plots illustrating the gating strategy used to identify spleen immune cells in mock-infected (left) or S. suis-infected animals for 6 h (middle) and 16 h (right).
Since neutrophils are recruited in the blood and in the spleen during the infection, we characterized the kinetics of neutrophil mobilization in these organs as well as in the bone marrow, the headquarter of neutrophil production (38). Flow cytometry analyses revealed that neutrophil rates in the blood and spleen of mice increased as soon as 6 h post-infection (p.i.) and peaked at 12 h p.i. (Figure 2). A return to basal level was observed after 24 h of infection. In parallel, the bone marrow, in which neutrophils represent 20% of all leukocytes during homoeostasis, showed an important drop in percentages of neutrophils from 6 h to 24 h p.i. (Figure 2). From 48 h onwards, bone-marrow replenished its stock in neutrophils, returning to basal levels.
Figure 2 Neutrophils migrate from the bone marrow to the blood during S. suis infection. Mice were intraperitoneally infected with S. suis serotype 2 (1 x 107 CFU/ml) and the blood, spleen and bone marrow were collected at different time points to analyze cells by flow cytometry. C- represents mock-infected mice (all time points pooled). Data shows the percentage of neutrophils among live cells in the indicated organs expressed as mean +/- SEM (n = 3 to 28). *P < 0.05, indicates a significant difference between C- and S. suis-infected mice.
During infection, neutrophils undergo phenotypical changes to better respond to pathogen invasion (39). Most of the blood neutrophils expressed CD11b in steady state, but S. suis infection significantly increased this percentage from 85% to 96% (Figures 3A, G). In addition, neutrophils from infected mice presented higher levels of CD11b expression at their surface, as determined by the median fluorescence intensity (Figure 3B). On the contrary, infection reduced the percentage of neutrophils expressing CD62L and CXCR2 (Figures 3C, E, G). For CD62L, positive cells exhibited lower levels of molecule surface expression during the infection (Figure 3D), while expression levels remained unchanged for CXCR2+ cells (Figure 3F). An increased expression of CD11b was also observed in spleen neutrophils from infected mice. However, no statistical difference was observed in the percentage or expression levels of the other markers in spleen neutrophils during S. suis infection compared to controls. Nevertheless, the overall pattern of marker expression was similar between blood and spleen (Supplementary Figure S2). To compare the effect of a mild infection and a severe infection on neutrophil phenotype, we infected mice with a low dose (1 x 106 CFU) or a high dose (1 x 107 CFU, the dose used throughout this study) of S. suis. However, the phenotype of neutrophils was overall similar between the two doses (Supplementary Figure S3).
Figure 3 Neutrophils mobilized in the blood during S. suis infection change the expression of surface markers. Mice were infected intraperitoneally with 1 x 107 CFU/ml of S. suis serotype 2. At 16 h post-infection, blood was collected to analyze markers (CD11b, CD62L and CXCR2) at the surface of neutrophils by flow cytometry. Blood neutrophils were analyzed for the percentage of cells [mean +/- SEM (n = 6 to 30)] expressing the indicated marker (A, C, E) and the median fluorescence intensity (+/- SEM) for the indicated marker (B, D, F). *P < 0.05, indicates a significant difference between mock-infected and S. suis-infected mice. (G) Representative histograms of the surface markers expressed by blood neutrophils in mock-infected or S. suis-infected animals.
Among the factors produced during infections, the G-CSF represents the key cytokine involved in the control of neutrophils (21). Thus, we investigated if the G-CSF was produced in the serum and organs of S. suis-infected mice at different times post-infection. Important levels of G-CSF were produced in the spleen, the liver, the serum and the bone-marrow of infected mice with a peak of production at 12 h p.i. (Figure 4). Specifically, serum concentrations of G-CSF represented the highest of all evaluated cytokines at this time point (Supplementary Figure S4).
Figure 4 Infection by S. suis results in massive production of granulocyte colony-stimulating factor (G-CSF) in mice. The blood and organs of S. suis-infected mice (1 x 107 CFU/ml; intraperitoneally) were collected at different times and G-CSF analyzed in the spleen (A) and liver (B) by Luminex, or in the serum and bone marrow (C) by ELISA. C- refers to mock-infected mice (all time points pooled). The data represent the mean +/- SEM (n = 3 to 12). *P < 0.05, indicates a significant difference between C- and S. suis-infected mice.
The massive production of G-CSF during S. suis infection indicates that some cells, somehow, produce this factor. Since dendritic cells (DCs) and macrophages produce G-CSF in vitro when stimulated with S. suis (30), we hypothesized that immune cells could take part in G-CSF production in vivo. By quantifying intracellular G-CSF in immune cells, we found that G-CSF increases in blood leukocytes in response to S. suis infection (Figures 5A, C). Particularly, this rise occurs among both lymphocytes and the pool of DCs, monocytes, and macrophages. However, neutrophils, which express the highest levels of G-CSF at the basal state, decreased their intracellular levels during infection (Figure 5A). In the spleen, none of the hematopoietic cells showed an increase of intracellular G-CSF, but intracellular G-CSF decreased among the neutrophil population at 16 h p.i. (Figure 5B).
Figure 5 Blood hematopoietic cells produce G-CSF in response to S. suis infection, while neutrophils probably release their intracellular stock of G-CSF. Blood (A) and spleen (B) were collected from S. suis-infected (1 x 107 CFU/ml; intraperitoneal) or mock-infected mice at 6 h or 16 h post-infection. The cells were stained with surface antibodies to identify populations, permeabilized for intracellular staining of G-CSF and analyzed by flow cytometry. Graphs show the median fluorescence intensity (MFI) for G-CSF staining among each population. DC-Mo-Mph stands for the pool of dendritic cells, monocytes, and macrophages. Data represent the mean +/- SEM (n = 4 to 8). *P < 0.05, indicates a significant difference between mock-infected and S. suis-infected mice. (C) Representative plots of the G-CSF+ population among total leukocytes in the blood of mock-infected (left) or S. suis-infected (right) mice after 6 h.
Since G-CSF controls neutrophil recruitment (20), we neutralized its effect in a mouse model using an anti-G-CSFR antibody (αG-CSFR) prior to S. suis infection (Figure 6). At 12 h p.i., treatment with αG-CSFR prevented neutrophil recruitment in the blood, exhibiting similar levels to those of control mice (mock-infected) (Figures 6A, C). Simultaneously, the drop of the neutrophil percentage in the bone marrow caused by the infection did not occur during the G-CSF receptor blockade (Figure 6B). The stock of neutrophils was therefore maintained in the bone-marrow under this condition. The analysis of the different leukocyte populations revealed that mainly neutrophil population was affected by G-CSFR blockade (Supplementary Figure S5).
Figure 6 Granulocyte colony-stimulating factor (G-CSF) controls release of the neutrophils from the bone marrow to the blood during S. suis infection. To inhibit the effect of G-CSF in vivo, mice were treated with 100 µg of an antibody directed against the G-CSF receptor (αG-CSFR) or an isotype control. Mice received two doses of antibodies at day -1 and day 0 prior to intraperitoneal infection with S. suis serotype 2 (1 x 107 CFU/ml). Blood (A) and bone marrow (B) were collected at 12 h post-infection to quantify neutrophils by flow cytometry. Data represent the mean +/- SEM (n = 4 to 27). *P < 0.01, indicates a significant difference between the groups connected by a line. (C) Representative dot plots of the blood neutrophil population in mock-infected (left) or S. suis-infected for 12 h treated with isotype control (middle) or αG-CSFR (right).
Since G-CSF controls neutrophil egress from the bone marrow to the blood (Figure 6) and neutrophils promote host survival by controlling S. suis bacterial burden (10), we evaluated the role of the G-CSF in S. suis clearance from the blood. Results showed that the treatment with αG-CSFR did not affect the concentration of bacteria in the blood of S. suis-infected mice at any evaluated time (Figure 7).
Figure 7 The granulocyte colony-stimulating factor (G-CSF) does not participate in S. suis clearance during the infection. Mice were treated with 100 µg of the antibody anti-G-CSFR (αG-CSFR) or the isotype control as described in Materials and Methods. They were then infected with S. suis serotype 2 (1 x 107 CFU/ml; intraperitoneally). At different time points, blood was collected and the bacteremia evaluated. Each point corresponds to the colony forming unit (CFU) of bacteria per ml recovered from an individual mouse, with the black bars representing the mean +/- SEM (n = 6 to 21).
Neutrophils participate in the production of inflammatory mediators in systemic infection (10). Since G-CSFR blockade induced less neutrophil release from the bone marrow, there might also be an impact on the levels of pro-inflammatory mediators produced during inflammation. We quantified CXCL1 (KC) and IL-6 in the plasma of S. suis-infected mice at 12 h p.i., time at which most cytokine levels peak (40, 41). However, G-CSFR blockade did not significantly change levels induced by S. suis infection (Figure 8). We performed additional cytokine analyses by Luminex and showed that αG-CSFR treatment did not significantly modulate the plasmatic levels of most of the cytokines (despite an upward trend), but increased IFN-γ and MIP-1α production (Supplementary Figure S6).
Figure 8 Granulocyte colony-stimulating factor (G-CSF) does not modulate the production of pro-inflammatory cytokines during S. suis infection. To inhibit the effect of G-CSF in vivo, mice were treated with 100 µg of an antibody directed against the G-CSF receptor (αG-CSFR) or the isotype control. Mice received two doses of antibodies at day -1 and day 0 prior to intraperitoneal infection with S. suis serotype 2 (1 x 107 CFU/ml). At 12 h post-infection, blood was collected from the submandibular vein and the plasma analyzed by ELISA to quantify the keratinocyte chemoattractant/chemokine (C-X-C motif) ligand 1 (KC/CXCL1) (A) and the interleukin 6 (IL-6) (B). The data represent the mean +/- SEM (n = 12 to 36).
The bacterial pathogen S. suis causes major economic losses to the swine industry. Despite years of research in the field, uncertainties persist regarding how the immune system handles the bacterium. Herein, we proposed to study the recruitment of neutrophils during S. suis infection – from its establishment to its consequences, since these cells first respond to microbial invasions. We hypothesized that S. suis infection causes a rapid mobilization of neutrophils under the influence of G-CSF, thereby participating in inflammation and bacterial clearance.
Our results showed that neutrophil rates rise in both the blood and the spleen during the first hours of S. suis infection, in a time frame similar to those reported in other infection models (7, 27, 42, 43). Following a strict contrasting pattern, the percentage of neutrophils in the bone marrow decreases during the course of S. suis infection. Although other studies already described neutrophil increase in the blood in response to S. suis (16, 17), this work is the first to characterize neutrophil changes in the spleen and bone marrow. Results suggest that the neutrophils recruited in the blood and spleen come from the bone marrow, as was reported in the context of other infections, including Pseudomonas aeruginosa, Listeria monocytogenes and cecal ligation and puncture-induced sepsis (7, 27, 42). Thus, we showed that a strong immune response takes place in the first hours of S. suis infection. In parallel to this neutrophilia, S. suis causes a lymphopenia and an increase in the pool of DCs, monocytes and macrophages. The lymphopenia, frequently observed during infections, may be explained by the redistribution of the lymphocytes to the lymphoid tissue to engage the adaptative immune response (44–46), their distribution to other tissues or apoptosis (47). The increased proportion of DCs, monocytes and macrophages might be due to monocyte recruitment in the blood, which participate in bacterial clearance, as reported (48, 49). However, theses hypotheses remain to be confirmed by further investigations.
Since the inflammation initiated by an infection might activate neutrophils, we evaluated surface molecule changes on the cells, as a reflection of their activation (12, 50). In S. suis-infected mice, blood neutrophils show an increased expression of CD11b, the shedding of the L-selectin CD62L and less cells were positive for CXCR2 expression, suggesting an activated phenotype for the neutrophils during the infection (13, 51). This first description of neutrophil phenotype during S. suis infection corroborates the observations made in other infection models, including in a mouse model of local Staphylococcus aureus infection and a primate model of simian immunodeficiency virus infection (52, 53). Similar results were observed in vitro with neutrophils stimulated with purified lipoproteins from Mycobacterium tuberculosis (51). Overall, our results indicate that S. suis infection do not alter the activated phenotype of blood neutrophils. Given the activated phenotype of neutrophils in the blood of S. suis-infected mice, exploring their in vivo functionality becomes imperative to gauge their effector potential in this model.
Neutrophil mobilization and activation depend on the inflammatory environment, including the effect of some pro-inflammatory factors. Among them, G-CSF seems to be critical for neutrophil control (21). Limited information exists on G-CSF production in the context of S. suis infection; however, we recently detected high levels of G-CSF in the plasma of S. suis-infected mice (30). We demonstrated that G-CSF is also detectable in the spleen and the liver of infected mice, the major filtration organs, as well as in the bone marrow, the source of neutrophil production. Moreover, we showed that the amounts of G-CSF produced in the plasma during S. suis infection exceed by far the levels of other cytokines, suggesting that G-CSF production constitutes an important feature of the immune response against S. suis. A previous work studied G-CSF production in vitro and showed that dendritic cells and macrophages produce G-CSF in response to S. suis stimulation (30). Our in vivo experiments showed that, in the blood, lymphocytes and the pool of DCs, monocytes and macrophages produce G-CSF throughout the infection, suggesting that they contribute to the high plasmatic levels observed in S. suis-infected mice. However, none of the spleen hematopoietic cells produced G-CSF in response to S. suis infection, foreshadowing the implication of other cell types in local production. Although neutrophils express the most G-CSF at a basal state, infection reduced their intracellular expression of G-CSF in both the blood and the spleen. We thus hypothesize that neutrophils stock G-CSF in their granules and release it during the infection, but this remains to be demonstrated. Non-hematopoietic cells can also produce G-CSF as demonstrated by Boettcher et al. (54), who identified in vivo that endothelial cells mainly produce G-CSF during a LPS challenge in mice. Thus, evaluating G-CSF production by endothelial cells or other non-hematopoietic cells could be a next step to study G-CSF production in the context of S. suis infection.
As previously mentioned, the impressive levels of G-CSF produced during S. suis infection might reflect its preponderant role during the infection. Therefore, we neutralized the G-CSFR with an antibody to investigate the role of G-CSF in neutrophil recruitment, bacterial clearance, and production of pro-inflammatory mediators – which characterize S. suis infection. Our results demonstrated for the first time that G-CSF causes the egress of neutrophils from the bone marrow to the blood during S. suis infection, supporting the observations made in other infection models (26–28, 55). Of note, most studies used knock-out mice models for G-CSF or its receptor, whose phenotypes present an altered basal production of neutrophils. Our model, using a neutralizing antibody, prevented this bias, with no alteration of neutrophil production in steady state.
Since neutrophils were recently shown to reduce bacteremia and produce cytokines during S. suis infection (10), we expected G-CSF-recruited neutrophils to exhibit the same characteristics. Surprisingly, G-CSF production does not impact bacteremia in our model, conflicting with other infection models in which G-CSF improved pathogen clearance (26–28, 56). However, our results corroborate those obtained in an infection model of Streptococcus pneumoniae, in which G-CSF does not affect bacterial load in the lungs at 48 h p.i (55, 57). Since bacteria colonize various organs of the infected host, it could be interesting to study if G-CSF-recruited neutrophils infiltered in the tissue better control bacterial load in the organs. Our data also showed that G-CSF does not significantly affect the production of pro-inflammatory mediators induced by S. suis, except for IFN-γ and MIP-1α, which were enhanced by G-CSF neutralization (Supplementary Figure S6). These two cytokines activate and recruit immune cells, particularly monocytes and macrophages. Reports in the literature suggest that G-CSF defects enhance cytokine production in response to various pathogens, with immune cells overproducing other cytokines to compensate for G-CSF neutralization, or that G-CSF could regulate cytokine production (26, 27, 56). Both the method to neutralize the G-CSF (antibody vs. knock-out) and the features of the studied pathogen might explain the differences observed. As such, more studies are required to untangle the mechanisms behind the pathogen-dependent effect of G-CSF on clearance and inflammatory mediator production. In our model, antibody-mediated neutralization of G-CSF did not significantly influence bacterial clearance and cytokine production during S. suis infection. A possible hypothesis would be that the “steady-state” neutrophils (the ones already in circulation before the G-CSF-driven recruitment) and the other immune cells can compensate for the altered G-CSF-driven recruitment of neutrophils.
We found that S. suis infection induces neutrophil recruitment and activation in the blood. Neutrophils egress from the bone marrow to the blood under the influence of G-CSF, a cytokine highly produced in response to the bacteria (Figure 9). This research provides insight into neutrophil recruitment and role during S. suis infection. Although G-CSF controls neutrophil recruitment, it is not crucial for bacterial clearance and cytokine production, suggesting that compensatory mechanisms exist within the immune system when G-CSF is neutralized. The progresses in fundamental knowledge constitute the groundwork for therapeutical avenues, with this study supporting the idea that the prophylactic administration of G-CSF shows limited benefits, as already evocated by Brockmeier et al. (29).
Figure 9 Suggested model for the production and role of the granulocyte colony-stimulating factor (G-CSF) during S. suis infection. S. suis infection induces a systemic production of G-CSF by blood leukocytes and possibly other cell types ①. G-CSF causes the release of bone marrow neutrophils into the blood, increasing the percentage of those cells in circulation ②. Blood neutrophils express activation markers ③. However, G-CSF-recruited neutrophils do not significantly participate in bacterial clearance ④ or inflammatory response ⑤.
The original contributions presented in the study are included in the article/Supplementary Material. Further inquiries can be directed to the corresponding author.
The animal study was approved by Animal Welfare Committee of the University of Montreal. The study was conducted in accordance with the local legislation and institutional requirements.
MB: Writing – review & editing, Writing – original draft, Visualization, Validation, Project administration, Investigation, Formal Analysis, Conceptualization. ML: Methodology, Writing – review & editing, Investigation, Formal Analysis. J-PA: Writing – review & editing, Investigation, Formal Analysis, Conceptualization. MG: Writing – review & editing, Supervision, Resources, Funding acquisition, Formal Analysis, Conceptualization. MS: Writing – review & editing, Visualization, Supervision, Resources, Project administration, Investigation, Funding acquisition, Formal Analysis, Conceptualization.
The author(s) declare financial support was received for the research, authorship, and/or publication of this article. This work was mainly supported by the Natural Sciences and Engineering Research Council of Canada (NSERC) through grants to MS (no. 342150) and to MG (no. 04435). MB is the recipient of a Swine and Poultry Infectious Diseases Research Centre scholarship supported by the Fonds de recherche du Québec-Nature et technologies (grant no. RS-170946) and a Fonds de recherche du Québec-Nature et technologies (FRQ-NT) Doctoral Scholarship (no. 315194). MS is a holder of a Canada Research Chair -Tier 1.
We thank CSL Innovation (Parkville, Australia) for kindly provided the anti-mouse G-CSFR antibody (Ch5E2-VR81-mIgG1κ).
The authors declare that the research was conducted in the absence of any commercial or financial relationships that could be construed as a potential conflict of interest.
All claims expressed in this article are solely those of the authors and do not necessarily represent those of their affiliated organizations, or those of the publisher, the editors and the reviewers. Any product that may be evaluated in this article, or claim that may be made by its manufacturer, is not guaranteed or endorsed by the publisher.
The Supplementary Material for this article can be found online at: https://www.frontiersin.org/articles/10.3389/fimmu.2024.1403789/full#supplementary-material
αG-CSFR, antibody anti-G-CSF receptor; APC, allophycocyanin; BV, brillant violet; CD, cluster of differentiation; CFU, colony-forming unit; CCL, C-C motif chemokine ligand; CXCL, C-X-C motif chemokine ligand; CXCR, C-X-C motif chemokine receptor; DC, dendritic cells; ELISA, enzyme-linked immunosorbent assay; FITC, fluorescein isothiocyanate; G-CSF, granulocyte colony-stimulating; G-CSFR, granulocyte colony-stimulating receptor; IFN-γ, interferon-γ; IL, interleukin; i.p., intraperitoneal; KC, keratinocyte chemoattractant; LPS, lipopolysaccharide; MCP-1, monocyte chemoattractant protein-1; MIG, monokine induced by gamma interferon; MIP, macrophage inflammatory cytokine; Mo, monocyte; Mph, macrophages; NET, neutrophil extracellular trap; PBS, phosphate-buffered saline; PE, phycoerythrin; RANTES, regulated on activation; normal T cell expressed and secreted; SEM, standard error of the mean; THB, Todd Hewitt broth.
1. Gottschalk M, Segura M. Streptococcosis. In: Zimmerman JJ, Karriker LA, Ramirez A, Schwartz KJ, Stevenson GW, Zhang J, editors. Diseases of swine, 11th ed. Wiley-Blackwell, Hoboken, NJ, USA (2019). p. 934–54.
2. Goyette-Desjardins G, Auger JP, Xu J, Segura M, Gottschalk M. Streptococcus suis, an important pig pathogen and emerging zoonotic agent-an update on the worldwide distribution based on serotyping and sequence typing. Emerg Microbes Infect. (2014) 3:e45. doi: 10.1038/emi.2014.45
3. Amulic B, Cazalet C, Hayes GL, Metzler KD, Zychlinsky A. Neutrophil function: from mechanisms to disease. Annu Rev Immunol. (2012) 30:459–89. doi: 10.1146/annurev-immunol-020711-074942
4. Manz MG, Boettcher S. Emergency granulopoiesis. Nat Rev Immunol. (2014) 14:302–14. doi: 10.1038/nri3660
5. Witter AR, Okunnu BM, Berg RE. The essential role of neutrophils during infection with the intracellular bacterial pathogen Listeria monocytogenes. J Immunol. (2016) 197:1557–65. doi: 10.4049/jimmunol.1600599
6. Pino-Tamayo PA, Puerta-Arias JD, Lopera D, Uran-Jimenez ME, Gonzalez A. Depletion of neutrophils exacerbates the early inflammatory immune response in lungs of mice infected with Paracoccidioides brasiliensis. Mediators Inflamm. (2016) 2016:3183285. doi: 10.1155/2016/3183285
7. Navarini AA, Lang KS, Verschoor A, Recher M, Zinkernagel AS, Nizet V, et al. Innate immune-induced depletion of bone marrow neutrophils aggravates systemic bacterial infections. Proc Natl Acad Sci U S A. (2009) 106:7107–12. doi: 10.1073/pnas.0901162106
8. Fulurija A, Ashman RB, Papadimitriou JM. Neutrophil depletion increases susceptibility to systemic and vaginal candidiasis in mice, and reveals differences between brain and kidney in mechanisms of host resistance. Microbiol (Reading). (1996) 142:3487–96. doi: 10.1099/13500872-142-12-3487
9. Tate MD, Deng YM, Jones JE, Anderson GP, Brooks AG, Reading PC. Neutrophils ameliorate lung injury and the development of severe disease during influenza infection. J Immunol. (2009) 183:7441–50. doi: 10.4049/jimmunol.0902497
10. Auger JP, Rivest S, Benoit-Biancamano MO, Segura M, Gottschalk M. Inflammatory monocytes and neutrophils regulate Streptococcus suis-induced systemic inflammation and disease but are not critical for the development of central nervous system disease in a mouse model of infection. Infect Immun. (2020) 88:e00787–19. doi: 10.1128/IAI.00787-19
11. Silvestre-Roig C, Fridlender ZG, Glogauer M, Scapini P. Neutrophil diversity in health and disease. Trends Immunol. (2019) 40:565–83. doi: 10.1016/j.it.2019.04.012
12. Rosales C. Neutrophil: a cell with many roles in inflammation or several cell types? Front Physiol. (2018) 9:113. doi: 10.3389/fphys.2018.00113
13. Metzemaekers M, Gouwy M, Proost P. Neutrophil chemoattractant receptors in health and disease: double-edged swords. Cell Mol Immunol. (2020) 17:433–50. doi: 10.1038/s41423-020-0412-0
14. Sanford SE. Gross and histopathological findings in unusual lesions caused by Streptococcus suis in pigs. I. Cardiac lesions. Can J Vet Res. (1987) 51:481–5.
15. Sanford SE. Gross and histopathological findings in unusual lesions caused by Streptococcus suis in pigs. II. Central nervous system lesions. Can J Vet Res. (1987) 51:486–9.
16. Yang C, Chen B, Zhao J, Lin L, Han L, Pan S, et al. TREM-1 signaling promotes host defense during the early stage of infection with highly pathogenic Streptococcus suis. Infect Immun. (2015) 83:3293–301. doi: 10.1128/IAI.00440-15
17. Xu L, Lu X, Xiao P, Liu R, Xia KL, Wu MZ, et al. Interleukin-17A contributes to bacterial clearance in a mouse model of streptococcal toxic shock-like syndrome. Pathogens. (2021) 10:766. doi: 10.3390/pathogens10060766
18. Ni C, Gao S, Zheng Y, Liu P, Zhai Y, Huang W, et al. Annexin A1 attenuates neutrophil migration and IL-6 expression through Fpr2 in a mouse model of Streptococcus suis-induced meningitis. Infect Immun. (2021) 89:e00680–20. doi: 10.1128/IAI.00680-20
19. Sun Q, Li N, Jia L, Guo W, Jiang H, Liu B, et al. Ribosomal protein SA-positive neutrophil elicits stronger phagocytosis and neutrophil extracellular trap formation and subdues pro-inflammatory cytokine secretion against Streptococcus suis serotype 2 infection. Front Immunol. (2020) 11:585399. doi: 10.3389/fimmu.2020.585399
20. Bendall LJ, Bradstock KF. G-CSF. from granulopoietic stimulant to bone marrow stem cell mobilizing agent. Cytokine Growth Factor Rev. (2014) 25:355–67. doi: 10.1016/j.cytogfr.2014.07.011
21. Chousterman BG, Arnaud M. Is there a role for hematopoietic growth factors during sepsis? Front Immunol. (2018) 9:1015. doi: 10.3389/fimmu.2018.01015
22. Panopoulos AD, Watowich SS. Granulocyte colony-stimulating factor: molecular mechanisms of action during steady state and 'emergency' hematopoiesis. Cytokine. (2008) 42:277–88. doi: 10.1016/j.cyto.2008.03.002
23. Cheers C, Haigh AM, Kelso A, Metcalf D, Stanley ER, Young AM. Production of colony-stimulating factors (CSFs) during infection: separate determinations of macrophage-, granulocyte-, granulocyte-macrophage-, and multi-CSFs. Infect Immun. (1988) 56:247–51. doi: 10.1128/iai.56.1.247-251.1988
24. Balamayooran G, Batra S, Theivanthiran B, Cai S, Pacher P, Jeyaseelan S. Intrapulmonary G-CSF rescues neutrophil recruitment to the lung and neutrophil release to blood in Gram-negative bacterial infection in MCP-1-/- mice. J Immunol. (2012) 189:5849–59. doi: 10.4049/jimmunol.1200585
25. Kragsbjerg P, Holmberg H, Vikerfors T. Dynamics of blood cytokine concentrations in patients with bacteremic infections. Scand J Infect Dis. (1996) 28:391–8. doi: 10.3109/00365549609037926
26. Hermesh T, Moran TM, Jain D, Lopez CB. Granulocyte colony-stimulating factor protects mice during respiratory virus infections. PloS One. (2012) 7:e37334. doi: 10.1371/journal.pone.0037334
27. Gregory AD, Hogue LA, Ferkol TW, Link DC. Regulation of systemic and local neutrophil responses by G-CSF during pulmonary Pseudomonas aeruginosa infection. Blood. (2007) 109:3235–43. doi: 10.1182/blood-2005-01-015081
28. Lieschke GJ, Grail D, Hodgson G, Metcalf D, Stanley E, Cheers C, et al. Mice lacking granulocyte colony-stimulating factor have chronic neutropenia, granulocyte and macrophage progenitor cell deficiency, and impaired neutrophil mobilization. Blood. (1994) 84:1737–46. doi: 10.1182/blood.V84.6.1737.1737
29. Brockmeier SL, Loving CL, Eberle KC, Hau SJ, Mou KT, Kehrli ME Jr. Administration of granulocyte-colony stimulating factor (G-CSF) to pigs results in a longer mean survival time after exposure to Streptococcus suis. Vet Microbiol. (2019) 231:116–9. doi: 10.1016/j.vetmic.2019.03.010
30. Bleuze M, Auger JP, Lavagna A, Gisch N, Gottschalk M, Segura M. In vitro characterization of granulocyte-colony stimulating factor (G-CSF) production by dendritic cells and macrophages during Streptococcus suis infection. Immunobiology. (2020) 225:151979. doi: 10.1016/j.imbio.2020.151979
31. Auger JP, Fittipaldi N, Benoit-Biancamano MO, Segura M, Gottschalk M. Virulence studies of different sequence types and geographical origins of Streptococcus suis serotype 2 in a mouse model of infection. Pathogens. (2016) 5:48. doi: 10.3390/pathogens5030048
32. Lavagna A, Auger JP, Dumesnil A, Roy D, Girardin SE, Gisch N, et al. Interleukin-1 signaling induced by Streptococcus suis serotype 2 is strain-dependent and contributes to bacterial clearance and inflammation during systemic disease in a mouse model of infection. Vet Res. (2019) 50:52. doi: 10.1186/s13567-019-0670-y
33. Campbell IK, Leong D, Edwards KM, Rayzman V, Ng M, Goldberg GL, et al. Therapeutic targeting of the G-CSF receptor reduces neutrophil trafficking and joint inflammation in antibody-mediated inflammatory arthritis. J Immunol. (2016) 197:4392–402. doi: 10.4049/jimmunol.1600121
34. Lecours MP, Segura M, Fittipaldi N, Rivest S, Gottschalk M. Immune receptors involved in Streptococcus suis recognition by dendritic cells. PloS One. (2012) 7:e44746. doi: 10.1371/journal.pone.0044746
35. Auger JP, Payen S, Roy D, Dumesnil A, Segura M, Gottschalk M. Interactions of Streptococcus suis serotype 9 with host cells and role of the capsular polysaccharide: comparison with serotypes 2 and 14. PloS One. (2019) 14:e0223864. doi: 10.1371/journal.pone.0223864
36. OuYang X, Guo J, Lv Q, Jiang H, Zheng Y, Liu P, et al. TRIM32 drives pathogenesis in streptococcal toxic shock-like syndrome and Streptococcus suis meningitis by regulating innate immune responses. Infect Immun. (2020) 88:e00957–19. doi: 10.1128/IAI.00957-19
37. Griffith JW, Sokol CL, Luster AD. Chemokines and chemokine receptors: positioning cells for host defense and immunity. Annu Rev Immunol. (2014) 32:659–702. doi: 10.1146/annurev-immunol-032713-120145
38. Lawrence SM, Corriden R, Nizet V. The ontogeny of a neutrophil: mechanisms of granulopoiesis and homeostasis. Microbiol Mol Biol Rev. (2018) 82:e00057–17. doi: 10.1128/MMBR.00057-17
39. Garley M, Jablonska E. Heterogeneity among neutrophils. Arch Immunol Ther Exp (Warsz). (2018) 66:21–30. doi: 10.1007/s00005-017-0476-4
40. Dominguez-Punaro MC, Segura M, Plante MM, Lacouture S, Rivest S, Gottschalk M. Streptococcus suis serotype 2, an important swine and human pathogen, induces strong systemic and cerebral inflammatory responses in a mouse model of infection. J Immunol. (2007) 179:1842–54. doi: 10.4049/jimmunol.179.3.1842
41. Dominguez-Punaro Mde L, Segura M, Radzioch D, Rivest S, Gottschalk M. Comparison of the susceptibilities of C57BL/6 and A/J mouse strains to Streptococcus suis serotype 2 infection. Infect Immun. (2008) 76:3901–10. doi: 10.1128/IAI.00350-08
42. Delano MJ, Kelly-Scumpia KM, Thayer TC, Winfield RD, Scumpia PO, Cuenca AG, et al. Neutrophil mobilization from the bone marrow during polymicrobial sepsis is dependent on CXCL12 signaling. J Immunol. (2011) 187:911–8. doi: 10.4049/jimmunol.1100588
43. Sun Z, Huang W, Zheng Y, Liu P, Yang W, Guo Z, et al. Fpr2/CXCL1/2 controls rapid neutrophil infiltration to inhibit Streptococcus agalactiae infection. Front Immunol. (2021) 12:786602. doi: 10.3389/fimmu.2021.786602
44. Agnello L, Giglio RV, Bivona G, Scazzone C, Gambino CM, Iacona A, et al. The value of a complete blood count (CBC) for sepsis diagnosis and prognosis. Diagnostics (Basel). (2021) 11(10):1881. doi: 10.3390/diagnostics11101881
45. Hohlstein P, Gussen H, Bartneck M, Warzecha KT, Roderburg C, Buendgens L, et al. Prognostic relevance of altered lymphocyte subpopulations in critical illness and sepsis. J Clin Med. (2019) 8(3):353. doi: 10.3390/jcm8030353
46. Guo Z, Zhang Z, Prajapati M, Li Y. Lymphopenia caused by virus infections and the mechanisms beyond. Viruses. (2021) 13(9):1876. doi: 10.3390/v13091876
47. Luperto M, Zafrani L. T cell dysregulation in inflammatory diseases in ICU. Intensive Care Med Exp. (2022) 10:43. doi: 10.1186/s40635-022-00471-6
48. Xiong H, Pamer EG. Monocytes and infection: modulator, messenger and effector. Immunobiology. (2015) 220:210–4. doi: 10.1016/j.imbio.2014.08.007
49. Okura M, Auger JP, Shibahara T, Goyette-Desjardins G, Van Calsteren MR, Maruyama F, et al. Capsular polysaccharide switching in Streptococcus suis modulates host cell interactions and virulence. Sci Rep. (2021) 11:6513. doi: 10.1038/s41598-021-85882-3
50. Vogt KL, Summers C, Chilvers ER, Condliffe AM. Priming and de-priming of neutrophil responses in vitro and in vivo. Eur J Clin Invest. (2018) 48 Suppl 2:e12967. doi: 10.1111/eci.12967
51. Neufert C, Pai RK, Noss EH, Berger M, Boom WH, Harding CV. Mycobacterium tuberculosis 19-kDa lipoprotein promotes neutrophil activation. J Immunol. (2001) 167:1542–9. doi: 10.4049/jimmunol.167.3.1542
52. Hampton HR, Bailey J, Tomura M, Brink R, Chtanova T. Microbe-dependent lymphatic migration of neutrophils modulates lymphocyte proliferation in lymph nodes. Nat Commun. (2015) 6:7139. doi: 10.1038/ncomms8139
53. Lemaitre J, Cosma A, Desjardins D, Lambotte O, Le Grand R. Mass cytometry reveals the immaturity of circulating neutrophils during SIV infection. J Innate Immun. (2020) 12:170–81. doi: 10.1159/000499841
54. Boettcher S, Gerosa RC, Radpour R, Bauer J, Ampenberger F, Heikenwalder M, et al. Endothelial cells translate pathogen signals into G-CSF-driven emergency granulopoiesis. Blood. (2014) 124:1393–403. doi: 10.1182/blood-2014-04-570762
55. Wang H, Aloe C, Wilson N, Bozinovski S. G-CSFR antagonism reduces neutrophilic inflammation during pneumococcal and influenza respiratory infections without compromising clearance. Sci Rep. (2019) 9:17732. doi: 10.1038/s41598-019-54053-w
56. Basu S, Hodgson G, Zhang HH, Katz M, Quilici C, Dunn AR. "Emergency" granulopoiesis in G-CSF-deficient mice in response to Candida albicans infection. Blood. (2000) 95:3725–33.
57. Knapp S, Hareng L, Rijneveld AW, Bresser P, van der Zee JS, Florquin S, et al. Activation of neutrophils and inhibition of the proinflammatory cytokine response by endogenous granulocyte colony-stimulating factor in murine pneumococcal pneumonia. J Infect Dis. (2004) 189:1506–15. doi: 10.1086/382962
Keywords: Streptococcus suis, neutrophils, granulocyte colony-stimulating factor (G-CSF), cytokines, systemic inflammation, infection, immune cell recruitment, bacteremia
Citation: Bleuzé M, Lehoux M, Auger J-P, Gottschalk M and Segura M (2024) The granulocyte colony-stimulating factor produced during Streptococcus suis infection controls neutrophil recruitment in the blood without affecting bacterial clearance. Front. Immunol. 15:1403789. doi: 10.3389/fimmu.2024.1403789
Received: 19 March 2024; Accepted: 01 July 2024;
Published: 02 August 2024.
Edited by:
René Köffel, Zurich University of Applied Sciences, SwitzerlandReviewed by:
Shallu Tomer, University of California, Los Angeles, United StatesCopyright © 2024 Bleuzé, Lehoux, Auger, Gottschalk and Segura. This is an open-access article distributed under the terms of the Creative Commons Attribution License (CC BY). The use, distribution or reproduction in other forums is permitted, provided the original author(s) and the copyright owner(s) are credited and that the original publication in this journal is cited, in accordance with accepted academic practice. No use, distribution or reproduction is permitted which does not comply with these terms.
*Correspondence: Mariela Segura, bWFyaWVsYS5zZWd1cmFAdW1vbnRyZWFsLmNh
Disclaimer: All claims expressed in this article are solely those of the authors and do not necessarily represent those of their affiliated organizations, or those of the publisher, the editors and the reviewers. Any product that may be evaluated in this article or claim that may be made by its manufacturer is not guaranteed or endorsed by the publisher.
Research integrity at Frontiers
Learn more about the work of our research integrity team to safeguard the quality of each article we publish.