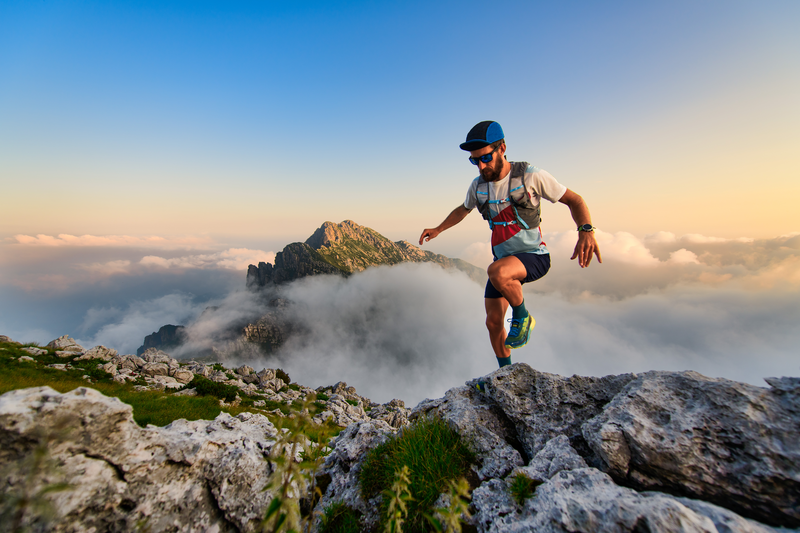
94% of researchers rate our articles as excellent or good
Learn more about the work of our research integrity team to safeguard the quality of each article we publish.
Find out more
SYSTEMATIC REVIEW article
Front. Immunol. , 25 June 2024
Sec. Molecular Innate Immunity
Volume 15 - 2024 | https://doi.org/10.3389/fimmu.2024.1399130
Background: Macrophages are innate immune cells that display remarkable phenotypic heterogeneity and functional plasticity. Due to their involvement in the pathogenesis of several human conditions, macrophages are considered to be an attractive therapeutic target. In line with this, platelet derivatives have been successfully applied in many medical fields and as active participants in innate immunity, cooperation between platelets and macrophages is essential. In this context, the aim of this review is to compile the current evidence regarding the effects of platelet derivatives on the phenotype and functions of macrophages to identify the advantages and shortcomings for feasible future clinical applications.
Methods: A total of 669 articles were identified during the systematic literature search performed in PubMed and Web of Science databases.
Results: A total of 27 articles met the inclusion criteria. Based on published findings, platelet derivatives may play an important role in inducing a dynamic M1/M2 balance and promoting a timely M1-M2 shift. However, the differences in procedures regarding platelet derivatives and macrophages polarization and the occasional lack of information, makes reproducibility and comparison of results extremely challenging. Furthermore, understanding the differences between human macrophages and those derived from animal models, and taking into account the peculiarities of tissue resident macrophages and their ontogeny seem essential for the design of new therapeutic strategies.
Conclusion: Research on the combination of macrophages and platelet derivatives provides relevant information on the function and mechanisms of the immune response.
Graphical Abstract Created with BioRender.com. TRM, tissue-resident macrophages.
Macrophages are innate immune cells present in all tissues. Beyond their central role in innate immunity, they are also crucial for organ development, inflammatory response, tissue remodelling and homeostasis (1, 2). They display a remarkably phenotypic heterogeneity and functional plasticity as they are epigenetically programmed in response to different microenvironmental cues (3–5).
Macrophages were first discovered by Elie Metchnikoff in the late 19th century (6). For decades, the concept of mononuclear phagocyte system (MPS), proposed by van Furth et al. (7, 8), has prevailed. This theory held the idea that tissue macrophages derived entirely from adult blood monocytes originating from bone marrow progenitors (9–11). However, accumulating evidence from fate-mapping mouse models and parabiosis studies have revised this paradigm regarding cellular ontogeny (12, 13). These data establish that most tissue-resident macrophages (TRM) arise from embryonic precursors and persist into adulthood due to its self-renewal ability and independently of adult hematopoietic stem cells (12, 14). Embryonic- and adult- derived macrophages coexist in certain tissues, and the contribution of each particular subset depends on the type of tissue (1, 11, 12) thus creating a complex scenario. Some tissues, such as dermis, gut and heart require a continuous blood monocyte replenishment during adulthood (10, 13, 15). Conversely, the microglia self-renewal is independent of adult haematopoiesis, deriving almost exclusively from embryonic progenitors (16, 17).
Macrophage polarization refers to a rigorously controlled process by which macrophages display different functional phenotypes in response to microenvironmental stimuli (18, 19). Overall, it is considered that macrophages can polarize into classically activated macrophages (M1) and alternatively activated macrophages (M2) (2, 20). This concept denotes an oversimplification of the M1/M2 paradigm as this is rather represented by a dynamically continuum of activation states (21, 22). M1 macrophages are polarized by bacterial endotoxins such as lipopolysaccharide (LPS) and Th1-related cytokines including interferon gamma (IFN-γ) and granulocyte-macrophage colony-stimulating factor (GM-CSF). As a result, they produce pro-inflammatory cytokines. Therefore, they exhibit a pro-inflammatory phenotype with antimicrobial and antitumoral activities (18, 20, 23). Moreover, M1 macrophages are involved in matrix degradation by direct and indirect production of matrix metalloproteinases (MMPs) and a variety of antifibrotic cytokines such as CXCL10 (18, 24, 25). Conversely, M2 macrophages are activated by Th2 cytokines including IL-4 and IL-13 and express high level of scavenger proteins such as mannose receptor (CD206) (18, 26, 27). M2 macrophages can be further subdivided in vitro into M2a, M2b, M2c and M2d, in accordance with their activation stimuli and gene expression profile (22, 28, 29). Functionally, M2 macrophages are associated with the resolution of inflammation and the promotion of angiogenesis and tissue repair (19, 30). Both phenotypes also differ in their metabolic profiles. The metabolism of M1 macrophages is rely on glycolysis, whereas M2 macrophages obtain their energy through fatty acid oxidation (19, 31). Macrophages are essential for maintaining tissue homeostasis, thus an imbalance between both phenotypes is present in many diseases. Due to their involvement in the pathogenesis of several human conditions, macrophages are considered as an attractive therapeutic target (32–34).
Regarding the use of platelets derivatives such as platelet-rich plasma (PRP), platelet lysate (PL) or platelet-rich fibrin (PRF), they have been successfully applied in many medical fields including dentistry, orthopedics, sports medicine, ophthalmology, dermatology and gynecology (35–40). The widespread clinical use of these platelet derivatives relies on their capacity to feature in different processes, beyond tissue hemostasis. They also exert their role in many biological processes, such as inflammation, immunity, angiogenesis and tissue regeneration (36, 41–43). Platelet derivatives provide growth factors, cytokines, chemokines and other biological mediators. They also contain clotting factors for developing a fibrin-based scaffold (36, 44). As active participants in innate immunity, cooperation between platelets and macrophages is essential (45). Platelets promote their recruitment and activation (46) and are involved in the macrophage NLRP3 inflammasome activation (47). Platelets express many immunomodulatory molecules such as adhesion receptors (P-selectin), or multiple pattern-recognition receptors including members of the Toll-like receptors (TLR) family (45, 48, 49). Moreover, they release a plethora of biological mediators including a large amount of chemokines (e.g., CXCL4) that may modulate the responses of macrophages (41, 42, 50). Nevertheless, the complexity of this partnership means that its mechanisms are not yet fully understood. Moreover, the lack of standardization for these platelets products leads to multiple preparation protocols with different concentration, composition or activation state resulting in biologically heterogeneous final products (51, 52) that may have an impact on the final response hindering the evaluation of clinical effectiveness.
As in regenerative medicine successful treatments usually emerge from the synergy of combining different treatments, the aim of this review is to gather the current evidence on the effects of platelet derivatives on the phenotype and functions of macrophages to identify the advantages and shortcomings for feasible future clinical applications.
For this narrative review, a systematic literature search was performed in PubMed and Web of Science database in August 2023, using the following search strategy: “(((((((platelet rich plasma) OR (PRP)) OR (platelet lysate)) OR (PL)) OR (platelet rich fibrin))) OR (PRF)) AND (macrophage polarization)”. Papers were excluded if: 1) the article was written in any language other than English or Spanish 2) duplicates 3) reviews, perspectives, editorials, commentaries, thesis, book chapters 4) no full-text available 5) out of scope 6) did not include macrophages 7) did not include PRP, PRF, PL or similar.
Studies that passed the initial title and abstract evaluation were retrieved for full-text review. For data extraction, an evidence table was created with Microsoft Excel. The following data were included: author and year of publication, study type, field of application, macrophages’ origin, platelet derivatives’ issues (type, origin, sample size, type of anticoagulant, conditions of centrifugation, presence or absence of leukocytes, type of activator), comparison groups, macrophage polarization and summary results.
The reporting quality and the risk of bias were assessed according to the criteria reported by Golbach et al. (53). The reporting quality was determined by the presence (“yes/partly”) or absence (“no”) of critical information. According to the answers (yes”, “partly”, or “no”), the risk of bias was divided into 3 categories: low, moderate and high, respectively.
The search strategy yielded a total of 669 articles from the two databases. Forty-three articles were removed as duplicates. After a properly screening, 27 studies (54–80) were finally included for the analysis in this review (Figure 1, Table 1).
Figure 1 Preferred Reporting Items for Systematic reviews and Meta-Analyses (PRISMA) Flow Diagram for Study Selection.
The reporting quality showed large differences (Figure 2). The lack of information regarding sample size, PRP obtaining process and PRP composition had the greatest impact on the reporting quality as 70%, 66% and 63% of the studies, respectively, did provide partial or no information on these issues. Concerning the risk of bias, the risk associated with the selection bias was the highest detected risk, as only 36% of the articles did specify that animals were randomly assigned.
The great variability of PRP composition and preparation protocols leads to a myriad of biologically distinct products. This issue along with the lack of information in the literature, makes challenging the comparison of results (81, 82). In fact, details such as the sample size, the type of anticoagulant and activator, centrifugation conditions or the composition were often missing in this review. Fifty-nine percent of the articles used PRP in their assays compared to 19% and 15% that used PRF or PL, respectively (Figure 3A). Table 2 describes the conditions for obtaining the different platelet derivatives. In only 8 out 27 articles (55, 56, 64, 65, 72, 77–79) were these conditions fully defined. Of those, Cenni et al. (55), Escobar et al. (56), Nishio et al. (65) and Yu et al. (78) completely detailed the cellular composition which was also fully described in other articles (60, 66, 67, 74, 75, 80) where the obtaining protocol was not totally detailed. The origin was human or murine except in two of the articles that PRP from rabbit and mouse (60) or ovine (76) was used. Most used double centrifugation (n = 14) versus single (n = 6). Qian et al. (69) even used both centrifugation methods depending on the type of platelet derivative to be obtained. However, in this regard, conditions such as centrifugal force, speed and time were extremely varied, with as many different protocols as studies. Concerning the cellular composition, 33% of the articles did not provide information about the presence of leukocytes in their studies. Among those that did detail this issue (presence of leukocytes), the majority did not include leukocytes (n = 10), compared to the others (n=6) in which the leukocytes were included. Moreover, the study proposed by Uchiyama et al. (74) was the only one that had two types of platelet derivatives tested, with and without leukocytes, while Kargarpour et al. (61) evaluated several fractions from liquid PRF containing different composition.
Figure 3 Distribution of the selected publications according to the type of platelet derivative (A), field of application (B) and type of induced macrophage polarization (C*). *The graph reflects situations where two different states were reported in the same study. GraÇa et al. (57) reported a hybrid M1/M2 phenotype and a non-polarized state depending on the product studied. Tylek et al. (73) reported both non-polarized state and M1-like type according to the study conditions. Nishio et al. (65) showed polarization towards both phenotypes (M1 and M2) depending on the platelet derivative. NP, non-polarized state; H, hybrid phenotype.
Table 2 Description of the obtaining process of the platelet derivatives from the reviewed articles. Sample size and leukocyte inclusion are also detailed.
As reflected in the data shown in Table 1, most of the studies used murine macrophages (n = 15), compared to 41% that used human macrophages (n = 11). Only one article used macrophages of ovine origin (76). Regarding the type of macrophages in in vitro studies, tissue resident macrophages were used in only two articles (59, 61) compared to the rest of the studies that used monocyte derived macrophages. Macrophage isolation, culture, differentiation and polarization protocols greatly differed among studies (Table 3). The immunoregulatory effect of platelet derivatives was assessed in several fields of application such as tissue, bone and tendon regeneration, osteoarthritis, cardiovascular diseases, intervertebral disc degeneration, nerve and osteochondral regeneration and skin repair (Figure 3B). Although the reported results being diverse, the treatment with platelet derivatives stimulated macrophages to exhibit a reparative phenotype in most of the studies (n = 21) (Figure 3C).
Research in human macrophages were performed in 11 articles. In 8 of which a polarization towards the reparative phenotype (M2) was reported after treatment with the platelet derivatives (55, 56, 58, 63, 67, 71, 74, 77). Cenni et al. (55) specified that their PRP impaired osteoclast formation, which is typical of the M2 phenotype. On the contrary, a pro-inflammatory effect was described in only 2 articles (66, 73); however, certain peculiarities must be taken into account, as O´Donnell et al. (66) evaluated a PRP obtained from OA patients and the results reported by Tylek et al. (73) refer to the co-culture of macrophages with mesenchymal stem cells. Both, a hybrid M1/M2 phenotype and a non-polarized state was described by GraÇa et al. (57) according to the type of extracellular vesicle. All the research conducted in this section evaluated the effect of PRP in vitro. Only Yadav et al. (77) did it also in vivo.
Cenni et al. (55) evaluated the effect of bovine thrombin and calcium gluconate-activated PRP on human osteoclasts. Osteoclastogenesis is a multi-step process that requires the delicate coordination of osteoclast progenitors. In this sense, M-CSF (macrophage colony stimulating factor) promotes proliferation of the osteoclast precursor cells and RANKL (receptor activator of NFκB ligand) controls their differentiation (84, 85). Therefore, the authors analyzed the ability of PRP supernatant to influence on osteoclast precursors, both with and without RANKL and M-CSF. They concluded that PRP showed different effects depending on its concentration. Whereas 10% induced a similar number of tartrate-resistant acid phosphatase (TRACP)-positive multinucleated cells to the positive control, higher concentrations of PRP did not induce the generation of multinucleated cells positive for this enzyme considered as a marker of bone resorption. Nevertheless, even when the multinucleated cells were treated with 10%PRP, the bone resorptive activity was also significantly lower. According to the authors, these results could be explained by the fact that PRP at high concentration could recruit osteoclast precursors but inhibit their differentiation. They also suggested that the transforming growth factor-β (TGF-β), present in platelets, could be one of the factors responsible for this inhibition. Also regarding bone regeneration, He et al. (58) studied the effect of different medium suplementations (10% FBS, 10% human platelet lysate (hPL) and 5% hPL) on THP-1-derived macrophage polarization. They concluded that human platelet lysate was a better choice for M1 and M2 polarization as cells treated with 5% hPL exhibited a more consistent morphology with the expected phenotype. In fact, culturing with 10%FBS or with 5% hPL induced opposite patterns for M1 and M2 macrophages. The authors also analyzed the effect of jaw periosteal cells (JPCs) on macrophage polarization in direct coculture. They concluded that low concentration of hPL enhanced the ability of JPCs to inhibit M1 polarization compared to other supplementations. The authors also detected the recently discovered CD169+ macrophages. They concluded that the JPCs’ ability to regulate this macrophage population could be enhanced by a low concentration of hPL.
O´Donnell et al. (66) and Uchiyama et al. (74) conducted their research on osteoarthritis (OA), although with very different approaches. O´Donnell et al. (66) compared leukocyte-poor PRP from healthy young male donors with the PRP from older male patients with severe knee OA. They also grouped the samples according to the concentration of inflammatory mediators and growth factors into high and low. They reported that according to age and disease state the composition of PRP was different, as PRP from older OA patients showed increased levels of inflammatory cytokines and less growth factors and platelets. These differences were reflected in the response of macrophages to treatment with PRP from these two groups. OA-PRP upregulated mRNA for inflammatory proteins in human monocyte-derived macrophages, thus promoting the inflammatory macrophage phenotype. The authors therefore suggested that these two factors (age and disease condition) may influence the bioactivity of PRP and hence their clinical effect. On the other hand, Uchiyama et al. (74), compared two types of PRP purification kits that resulted in two PRP of different composition (leukocyte-poor PRP (LP-PRP) and leukocyte-rich PRP (APS LR-PRP)) on macrophage phenotypes. They found higher concentrations of both M1 and M2 macrophages related factors in APS. The addition of PRP supernatants decreased the expression of M1 macrophage markers, such as IL-1β, TNF-α, CD80 and CD86, when compared to the monocyte derived macrophages alone, while there were no detected differences in the purification kits. Regarding M2 markers, they showed higher expression of MRC1 when macrophages were cultured with both PRP supernatants with regard to negative control, while the gene expression of IL-10 and TGF-β was increased in cells treated with APS or LP-PRP, respectively. In contrast, cell surface markers of the M2 phenotype (CD163 and CD206) were not altered after PRP culture. They also reported that both types of PRP promoted the repolarization of monocyte-derived M1 macrophages to the M2 phenotype; however, according to the authors, they gave rise to different M2 subpopulations. While LP-PRP promoted polarization toward the M2c subset, mainly related to tissue repair, APS promoted towards M2a, related to anti-inflammatory activity. Nevertheless, this information should be interpreted with caution. M2 macrophages further divide into subsets based on their distinct gene expression profiles. However, many of these subpopulations share markers. Thus, M2a, M2c and M2d subsets are characterized by the secretion of IL-10 and TGF-β, making accurate classification difficult (28). The authors stated that an increase in the ratio M1/M2 macrophages leads to a progression in OA disease. Therefore, they concluded that PRP treatment could improve symptoms in these patients by reducing the imbalance of M1/M2 macrophages.
When it comes to tissue regeneration or wound healing, several articles were also included. Both the study groups and the experimental design were also different in all studies. Escobar et al. (56) evaluated the biological effects of two platelet preparations on the phenotype of human monocyte-derived macrophages. They compared a leukocyte-depleted pure platelet-rich plasma without activation (P-PRP) and the supernatant released from that P-PRP after CaCl2 activation (S-PRP). Their findings showed that P-PRP and S-PRP generated different profiles of tissue-repair macrophages. Both preparations stimulated the expression of the M2 marker, CD206. In addition, only P-PRP enhanced the production of the potent anti-inflammatory cytokine IL-10. On the other hand, S-PRP also induced higher levels of expression of another M2 marker (CD163) compared with P-PRP; however, the expression of the pro-inflammatory marker CD86 was also enhanced after S-PRP treatment. Those results suggested different clinical regenerative potentials for both platelet preparations. Recent developments in platelet biology have led to new insights. In this regard, the platelet derived extracellular vesicles (EVs) and their role in intercellular communication are of interest (86). In this sense, the work of GraÇa et al. (57) evaluated the effect of two different EV populations (small EVs and medium EVs) derived from platelet lysates. Macrophage responses varied depending on the EV population they were treated with. sEVs induced polarization towards a hybrid M1/M2 phenotype, while mEVs induced a more non-polarized state. The authors stated that hybrid macrophages, which also exist in vivo, promote a more native-like extracellular matrix compared to a predominantly M2 macrophage phenotype. They also suggested that this difference in macrophage response to treatment could be useful as an effective strategy in modulating the process of tissue repair by sequentially using sEVs and mEVs in early and later healing stages, respectively. The immunoregulatory role of another platelet concentrate product in macrophage functional activities was also included (63). In this case, the authors tested different concentrations of conditioned medium (CCM) from concentrated growth factor (CGF). They reported reduced secretion of inflammatory cytokines (such as IL-1β) and enhanced chemokine production (such as RANTES) by CGF-stimulated macrophages, thus promoting M2 polarization. The Akt pathway was also determined to be involved in the immunoregulatory role of this concentrated growth factor. Papait el al (67). analyzed the effect of allogenic PRP mismatched for AB0 and Rh antigens on macrophages differentiation and function. Two types of preparations were used, standard (ST) and leucodepleted (LD). Macrophage cultures with GM-CSF, IL-4 and 10% FCS were referred as immature dendritic cells (iDC), while the addition of 5% ST-PRP or LD-PRP to that treatment were referred to as DC-ST or DC-LD, respectively. Combination of GM-CSF and IL-4 is generally used for the differentiation of human monocytes into dendritic cells (DC) (87, 88). The mononuclear phagocyte system (MPS) has been defined as a family of cells comprising monocytes, dendritic cells and macrophages. Their functional and phenotypical characteristics are often overlapping, making the distinction and classification of these cell types truly challenging. In fact, many of the proposed unique markers and functions are shared between cell types. This has added much confusion about their identity and function, opening the debate regarding which subsets represents distinct cell types and which are versions of the same cell type, thus leading to a possible misinterpretation of the results (89, 90). In fact, authors reported difficulty in clearly defining the type of DC induced by PRP. Their findings showed that PRP could inhibit macrophage differentiation to CD1a+ iDC; on the contrary, PRP induced type 2 macrophages, as they expressed high levels of CD163 and CD206. Moreover, release of IL-10 and PGE2 was also induced by PRP, even in the presence of LPS. Although both ST-PRP and LD-PRP induced DC with similar features, the authors stated that the inclusion of leukocytes did influence the secretion of those immunoregulatory factors, as more IL-10 and PGE-2 were reported with DC-LD, however, the white cell content was practically nil in both preparations. Taken together, data suggested that PRP promoted a regulatory anti-inflammatory phenotype thus supporting wound healing. The effects of platelet lysate (PL) on macrophages phenotype and functions were also examined in two other studies with different scopes (71, 73). Scopelliti et al. (71) used a commercial PL to conduct their assays in order to limit individual variation; however, as they stated, this also involved a drawback, as information about its composition was also missing. The authors exposed M1-polarized macrophages to 10% PL. They demonstrated that PL treatment reduced the expression of M1 markers (such as CD80 and CD86) and enhanced the expression of M2 markers (such as CD206, CD200R, PPAR and arginase). In addition, TNF-α and NFκB expression was significantly reduced. On the other hand, the release of TGF-β and VEGF, both largely involved in the wound healing process, was significantly increased along with the expression of STAT3, STAT6, SMAD2 and SMAD4. Therefore, the authors concluded that PL repolarized M1 macrophages towards a M2-like phenotype, thus favoring the regenerative process. Tylek et al. (73) evaluated another commercial platelet lysate as an alternative for in vitro culture of macrophages as well as for co-culture with hMSCs. The authors concluded that hPL, especially without adding heparin, was the best performing supplement both for the in vitro culture of human monocyte-derived macrophages and for the co-culture system, compared to human serum and fetal calf serum.
Finally, Yadav et al. (77) focused their research on nerve regeneration and conducted their assays both in vitro and in vivo. The authors studied, in vitro, the effect of different percentages of PRGF on M0 and M1 macrophage polarization. PRGF treatment inhibited the M1 phenotype. Consistent with Scopelliti et al. (71) they also showed a decrease in TNF-α, in addition to IL-1β and IL-6. However, in contrast to Scopelliti et al., who used a platelet lysate, the PRGF used in this experimental setting did increase IL-10 secretion. The therapeutic effects of PRGF were further investigated on nerve tissue regeneration by using a sciatic nerve transection model in rats. As was the case in the in vitro assays, the pro-inflammatory cytokines TNF-α, IL-1β and IL-6 were reduced in the PRGF group compared to the saline control group. In fact, treatment with the platelet preparation decreased M1-type macrophages, thus suggesting that its administration may modulate the inflammatory microenvironment to promote sciatic nerve regeneration via macrophage polarization among others.
Fifteen investigations were included in this section. In most of them (12 out of 15), the results were that treatment with platelet derivatives modulated the polarization of macrophages towards the M2 phenotype. Only one article (75) reported a stimulation towards the pro-inflammatory phenotype. Nishio et al. (65) described both situations, depending on the type of PRP used. No modulation of macrophage polarization was reported by Hudgens et al. (59). The studies covered different fields of application and responses were evaluated both in vitro and in vivo.
Five studies explored the in vitro response of murine macrophages to different platelet derivatives (54, 59, 61, 64, 75). Both Cao et al. (54) and Kargarpour et al. (61) focused on bone regeneration, and although the treatments and the approach were different, their outcomes supported the same conclusion that platelet derivatives possessed anti-inflammatory activity by promoting macrophage M2 polarization. Cao et al. (54) evaluated the immunomodulatory role of PRP in combination with methacrylated gelatin (GelMA) and methacrylated alginate (AlgMA) (GA) hydrogel alone or together with Laponite nanoparticles. The authors concluded that M1 and M2-type genes were decreased and increased, respectively, for both PRP-containing hydrogels. Different fractions of liquid PRF were studied by Kargarpour et al. (61): platelet poor plasma (PPP), the buffy coat (BC or C-PRF), the remaining red clot (RC) and albumin gels (Alb-gel) from heating PPP. The results of their research indicated that lysates of both BC and PPP inhibited the inflammatory response of macrophages exposed to different TLR agonists, as evidenced by the significant decrease of IL-6 and COX-2. This was further confirmed with a reduction in p65 phosphorylation and NFκB nuclear translocation. Both types of lysates repolarized the M1-like macrophages as deduced from the increased expression of M2 phenotype markers, ARG1 and YM1. Not only did these fractions exert a potent anti-inflammatory effect but they also inhibited osteoclastogenesis, finding that has also been reported by other authors (55) as already mentioned above.
The study performed by Hudgens et al. (59) compared the effect of PPP and PRP on macrophage polarization in tendon disorders. In contrast to most studies, they used resident macrophages, specifically rat resident peritoneal macrophages. They concluded that PRP had no obvious effect on modulating macrophage polarization. However, as they suggested, multiple PRP doses should have been evaluated as well as changes in protein expression beyond the gene response.
In the context of tissue regeneration, Nasirzade et al. (64) studied the effect of PRF lysates, produced by freeze-thawing the membranes, and the PRF conditioned medium, that is, its secretome, on macrophages exposed to saliva and LPS. Their findings were in line with those already discussed from Kargarpour et al. (61) (authors from the same working group). Briefly, PRF possessed anti-inflammatory activity and shifted the macrophage polarization from M1 toward M2 phenotype. Further, NF-κB p65 signalling activation was strongly reduced by PRF lysates. The authors reported that the transition between M1 and M2 was partially mediated via activation of TGF-β signalling. They also described that PRF could modulate the expression of lipoxygenases (ALOX5, ALOX12 and ALOX15) thus supporting the production of pro-resolving lipid mediators. Still in the field of wound healing, Ulivi et al. (75) showed that PL supports macrophages in a proinflammatory state, thus enhancing the key initial inflammatory response to the injury. However, it should be noted that the outcome described in this article is not a direct but a paracrine effect of the PL. That is, the collected conditioned media from MSCs exposed to PL were used to treat bone marrow-derived macrophages and to assay its effect on their phenotype.
In the field of bone healing, the M2 polarized macrophages are critical in the osteogenic microenvironment for effective bone regeneration (91). In this sense, Li et al. (62) developed hybrid hydrogels composed of injectable platelet-rich fibrin (i-PRF) and polycaprolactone/hydroxyapatite composite nanofibers by using enriched polydopamine (PDA) as linker (PnP-iPRF). To simulate the biodegradation process in vivo, i-PRF-containing hydrogels were treated with plasmin. RAW 264.7 macrophages were then incubated with those degradation products. The authors reported that the immunomodulatory activity of the PnP-iPRF could be attributed to the PDA component, as this hybrid hydrogel effectively induced M2 macrophage activation in a sustained manner. Cytokines secreted by these PnP-iPRF-treated macrophages also promoted the osteoblastic differentiation of BMSCs. PnP nanofibers were considered to be responsible for enhancing this osteogenic activity of i-PRF hydrogels. However, i-PRF hydrogel produced a moderate and unsustainable induction towards the M2 phenotype. The authors created a critical cranial defect in rats to further investigate the osteogenic effect of this hybrid hydrogel in vivo. The results were in line with those obtained in the in vitro assays. PnP-iPRF hydrogels could effectively induce M2 macrophage phenotype at 4 weeks post-surgery, as deduced from expression of CD206 and iNOS, markers of M2 and M1 respectively. Therefore, in this study, the added value was provided by the PnP rather than by the i-PRF.
Leukocyte rich PRP was select by Jiang et al. (60) for incorporation into the GelMA hydrogel on macrophages and to study its resulting influence on osteochondral regeneration. The addition of 20% PRP-GelMA significantly reduced the in vitro expression of several M1 markers (IL-1β, IL-6, iNOS, CCR7) in the LPS-treated macrophage culture when compared with pure GelMA or control. In this regard, M2 markers (Arg-1, IL-1ra, IL-10 and CD206) exhibited higher expression in PRP-GelMA than in the other groups. The authors indicated that the PRP-GelMA hydrogel not only inhibited the transition from M0 to M1 but also promoted M2 polarization. This hydrogel combination was further investigated in a rabbit model of osteochondral defect and showed early onset (12 weeks) and the persistence (18 weeks) of the M2c macrophage subset, and the reduction of M1 phenotype, thus suggesting that osteochondral regeneration was mediated by M2 polarization. Although not directly comparable, as the composition of PRP and hydrogel were different, the addition of the platelet derivative in this study did lead to an improvement in the performance of the hydrogel in contrast to that reported by Li et al. (62).
Macrophage infiltration and polarization have been increasingly associated with the degree of intervertebral disc degeneration (IDD) (92). Therefore, Qian et al. (70) addressed the underlying mechanisms involved in IDD pathology. They compared the effect of PRP with that of PRP-derived exosomes. The authors showed that both PRP and PRP-derived exosomes inhibited the polarization of M1-type macrophages through the inhibition of genes associated with this pro-inflammatory phenotype. This occurred via suppressing NF-κB and MAPK signalling by targeting TRAF6. The inhibitory ability was more pronounced in the case of PRP-derived exosomes. On the other hand, PRP-derived exosomes promoted M2 macrophage polarization via the STAT6 signaling pathway. The strongest inhibitory effect was exerted with respect to IL-1β, which led the authors to explore the effect of PRP-derived exosomes on NLRP3 inflammasome in macrophages. The NLRP3 inflammasome is a multiprotein complex critical in the innate immune system that assembles in response to pathogens and other stressors leading to the activation of caspase-1, the secretion of proinflammatory cytokines (Il-1β, IL-18) and the induction of pyroptosis (93–95). The authors reported the inhibition of NLRP3 inflammasome activation by PRP-derived exosomes. They also used rat models of IDD to address the issue in vivo. As it was reported in vitro, the expression of inflammasome-related proteins was also reduced in the PRP-derived exosomes group in vivo. The results of this study differ somewhat from those obtained by Graca et al. (57) where the extracellular vesicles did not clearly polarize towards the M2 phenotype. However, the comparison between these two studies is challenging, since the origin of the vesicles, PRP and macrophages is different, as well as the platelet derivative’s composition.
Several authors (72, 79, 80) have carried out studies in terms of wound healing and despite their different approaches, all results consistently showed that platelet derivatives stimulated polarization towards the M2 phenotype. Tang et al. (72) developed a thermosensitive injectable hydrogel known as the thermosensitive decellularized adipose tissue/platelet-rich plasma interpenetrating polymer network (t-DPI) hydrogel based on decellularized adipose tissue (DAT) and temperature-controlled platelet-rich plasma (t-PRP). All the treatments (t-PRP, DAT hydrogel and t-DPI hydrogel) promoted M2 macrophage polarization in vitro compared to the control. However, the t-DPI hydrogel group was the treatment that induced the highest polarization. Same results were obtained in vivo in a nude mouse model. The authors concluded that the biologically active ingredients present in the t-PRP and DAT contributed to the therapeutic effect of t-DPI hydrogel via M2 macrophage polarization, among others. On the other hand, Zhang et al. (79) concluded that i-PRF had greater anti-inflammatory response than whole blood (WB). In fact, i-PRF reduced the expression of IL-6, TNF-α, and INOS2 in LPS-treated murine-derived macrophage compared with WB. Moreover, p65 phosphorylation and TLR4 expression was also decreased after treatment with this platelet concentrate. The inhibition of NFκB signalling was consistent with that reported by other authors already included in this review (61, 64, 70, 71), that also showed the ability of the different platelet derivatives to stimulate the M2 phenotype. In this study, i-PRF also increased the M2-polarized macrophage phenotype-associated cytokines. Results also showed an anti-inflammatory response in vivo. i-PRF reduced the amount of local innate immune cells in a rat muscle defect model. Finally, Zhao et al. (80) evaluated PRP as an additive to develop an alginate-gelatin (AG) composite hydrogel bioink. Different concentrations of PRP were incorporated. Macrophages were seeded on the 3D bioprinted double-layered skin substitutes under inflammatory conditions (LPS). The addition of 5% PRP (AG-5P) led to greater decrease and increase of iNOS and Arg-1, respectively, than the control and AG groups. The same results were obtained in the in-situ 3D bioprinting repair of a rat dorsal full-thickness wound model. In addition, the AG-5P reduced the inflammatory response and macrophage infiltration after transplantation, as suggested by the lowest level of CD3, CD68 and MPO that were detected compared with control and AG group. Therefore, the authors concluded that the PRP incorporation regulated the immune response and tissue regeneration by promoting the macrophage polarization towards an M2 phenotype.
Four articles were included in this section. Despite the fact that the experimental design and the type and composition of PRP were different, 3 out of 4 studies concluded that platelet derivatives stimulated the anti-inflammatory phenotype. In the case of the study of Nishio et al. (65) polarization depended on the type of PRP, as LP-PRP induced the M2 phenotype, while LR-PRP induced the pro-inflammatory M1 phenotype.
In line with the studies of hybrid hydrogels, Qian et al. (69) reported the combination of PRP and lyophilized platelet-rich fibrin (Ly-PRF) with alginate-hyaluronic acid hydrogel as a novel approach for the treatment of myocardial infarction. They concluded that in this cardiovascular condition, Ly-PRF improved the immunological response by reducing the M1 macrophages and by stimulating their polarization towards the M2 phenotype.
Park et al. (68) evaluated the effect of PRP on an acute UVB-induced skin photodamage model in rats. They reported that the addition of PRP modulated the immune response depending on the stage of wound healing. That is, PRP enhanced the inflammatory or the repair response 7 days or 28 days after treatment, respectively, thus, finally reducing skin tissue inflammation. This regulation of macrophage polarization was performed via the activin receptor-follistatin system.
Two studies (65, 78) addressed tendon healing. As already mentioned, Nishio et al. (65) evaluated the influence of the leukocyte inclusion in the PRP on macrophage recruitment and polarization. They created full-thickness defects in the central third of patellar tendons in mice. As already described in the findings of Park et al. (68), macrophage modulation was dependent on the stage of tendon healing. They demonstrated that both types of PRP enhanced the tendon healing and promoted the recruitment of macrophages to injured tissue. However, leukocytes did influence the effect that PRP has on the balance between M1 and M2. In fact, LR-PRP preferentially stimulated the activity of the M1 phenotype, whereas LP-PRP did so with M2 macrophages. Nevertheless, the authors also noted that the effect of the PRP groups could be due to a foreign body reaction as the control group was not well-designed. On the other hand, Yu et al. (78) evaluated the effect of PRP releasate (released from platelets after PRP was activated) on the early stages of tendon healing. A rat model of Achilles tendon injury was used. In contrast to Nishio et al. (65), saline solution was used as a control. They reported lower levels of CD68+ (ED1+) macrophages, which have been suggested to stimulate tendon catabolism, in the samples treated with PRP as compared with the control group in the early healing stage (day 5 post injury), thus promoting tissue recovery.
Wessely-Szponder et al. (76) used ovine monocyte-derived macrophage cultures to assess the influence of autologous ovine PRP and rabbit antimicrobial peptide extracts. Macrophages were previously stimulated with LPS or dexamethasone. The proinflammatory cytokines superoxide and nitric oxide (NO) were increased in macrophage cultures after PRP treatment both alone and in combination with LPS, although the addition of the latter exacerbated this response. The use of dexamethasone reduced the proinflammatory response to PRP. Therefore, the authors concluded that PRP treatment induced a pro-inflammatory rather than repair phenotype rather than a repair one. Although this response might enhance antimicrobial activity, they suggested that the application of this platelet derivative should be restricted in cases of severe inflammation.
The therapeutic potential of promoting platelet-macrophage interactions is still a matter of debate. Macrophages are tissue-resident or infiltrated immune cells critical for innate immunity, normal tissue development, homeostasis, and tissue repair (5). The macrophage population in adults was originally thought to derive solely from circulating monocytes originating in the bone marrow. However, accumulating evidence has redefined this paradigm. Tissue-resident macrophages (TRMs) derive from yolk sac and fetal liver progenitors, and bone marrow-derived monocytes (12–14, 96). Therefore, ontogenetically distinct macrophages coexist in human adult tissues leading to a cellular mosaic that is dynamically modulated throughout life (9). Infiltrating monocyte-derived macrophages are functionally and phenotypically distinct from TRMs (34). In fact, when the former are recruited in a pathological process, they encounter a much more distinct milieu than TRMs (34). Under both normal and pathological conditions, the contribution of these distinct sources of macrophages varies in a tissue-specific manner (96). Macrophage function is a sum of their ontogeny, tissue-specific environmental signals, and the type of injuries to which they are exposed. All together contributes to shaping macrophage transcriptional regulation and functional specialization (5, 96, 97). Despite this macrophage heterogeneity, the cellular and molecular responses have been mainly studied on the monocyte-derived population, ignoring the peculiarities of tissue resident macrophages and their ontogeny. Therefore, it seems critical to identify reliable markers to distinguish the different subsets of TRMs and the infiltrating monocyte-derived macrophages to more precisely address the research of macrophages-mediated response and their multiple interactions (34). Most of the research available for this review studied the effects of the different platelet derivatives on the monocyte-derived macrophages, and those using resident macrophages (59, 61) also did not specify their ontogeny, thus, missing the final pieces of the puzzle.
Beyond the aforementioned ontogeny, comparison of results was also very challenging for several reasons. Firstly, the great variability of protocols for obtaining platelet derivatives leads to products with different compositions; that information was also missing in many studies, thus further confusing the interpretation of the results. Protocols for obtaining M1 and M2 phenotypes, including inducer molecules and treatment times, also varied widely among studies which could influence the secretome released by polarized macrophages thus affecting the outcome of the immune challenge. The origin of species might be another intrinsic factor that can modulate the biological response of macrophages. Two different species have been mainly studied in this review: human and murine. Mice are the experimental system of choice for most immunological studies. In fact, they have contributed extensively to the understanding of the human immune system, with the genomes of both species being highly conserved. However, there are also significant differences in their immune systems in terms of development, activation and response. Therefore, while these mouse models will continue to be used to provide new knowledge on the subject, there is also an increasing need for a cross-species approach to determine the potential limitations in translating data to humans (98). On the other hand, among rodent models, both rats and mice of different strains are used. It has already been reported that macrophages from different strains differ in the type of immune response (99, 100). C57BL/6 and Balb/c mice are two of the most commonly used strains, as also reflected in the current review, that differ in their immune responses. The former is more reactive, giving prototypical Th1-biased immune responses whereas Balb/c mice give Th2-biased immune responses (101, 102). This further complicates the interpretation of the data. The type of cells used to generate macrophages could also add a further level of difficulty.
Despite the challenges of comparing data, the studies covered in this review mostly showed that platelet derivatives promoted macrophage polarization towards the pro-repair M2 phenotype. There was no apparent difference in immune response among the different types of platelet derivatives (PRP, PL and PRF). Nor did the composition of various preparations showed clear differences in terms of leukocyte inclusion. In other words, in the light of the studies included in this review, the inclusion of white cells did not induce a M1 phenotype to a greater extent. However, assumptions on this issue should be assessed with caution, as many of the studies did not specify whether they included leukocytes or not. Furthermore, in studies that did provide details, the conditions under which the platelet-derived products were obtained varied greatly, further complicating the comparison between them. Ideally, every study should have included the same platelet derivative with and without leukocytes for the results to be reliable. However, this only occurred in a total of 3 articles (65, 67, 74), of which only Nishio et al. (65) found different outcomes between the two products, as leukocyte-rich PRP mainly enhanced the effects of M1 macrophages compared to leukocyte-poor product that stimulated M2 phenotype. Furthermore, the white cell content reported by Papait et al. (67) was practically nil in both preparations. On the other hand, the M1/M2 dichotomy is an over-simplification, and therefore might not serve as a potency testing to truly assess the effect of leukocytes on platelet derivatives in vivo, in which a “continuum” of activation states exists.
Several studies (54, 60, 62, 69, 72, 80) have also focused on developing hybrid hydrogels that combine platelet derivatives with biomaterials from different origins, with the aim of complementing and optimising their immunoregulatory functions. In all cases, the results of the combination were similar, that is, stimulation towards the M2 phenotype. All the authors considered the incorporation of the platelet derivatives as an added value in the composite hydrogel development to improve the immunoresponse, except for Li et al. who attributed the immunomodulatory activity to the PDA component. Nevertheless, only two of the articles (62, 72) included the platelet derivative as a control group in their study, so that the relative contribution of the blood derivative to the composite could be deduced.
As already mentioned, the widespread use of M1/M2 nomenclature is an over-simplified description of macrophage heterogeneity. This model was introduced to describe the two different macrophage responses, reflecting the T-helper cell nomenclature (103). However, macrophage polarization is a complex dynamic process with this M1-M2 binary model representing only the extremes of the spectrum (104). Nowadays the identification of other subsets of unconventional macrophages such as tumor associated macrophages (TAMs), macrophages expressing T cell receptors (TCR) and CD169 has added complexity to the issue (105, 106). In fact, a small group of macrophage biologists met at the International Congress of Immunology in Milan in August 2013 and proposed a common framework for macrophage activation nomenclature (107) in an attempt to resolve areas of confusion and to establish an initial set of experimental guidelines. Recommendations encompassed the following: a reproducible in vitro experimental standard, minimal reporting standards, definition of the activator, avoidance of certain terms and inclusion of markers of activation. However, even though most of the articles included in this review provided fairly complete information on in vitro assays (Table 3), some studies lacked details on cell density, culture medium, etc. The focus on whether a certain treatment stimulates the M1 or M2 phenotype does not clearly reflect the biological complexity, given that M1-like macrophages may participate in the tissue repair process, depending on the circumstances in which they occur and the length of time that they remain. Thus, it may be more informative to focus on the ratio or balance between the two phenotypes or on the ability of a given treatment to shift polarization towards the M2 phenotype.
Macrophage polarization is a tightly controlled process that is regulated by multiple signalling cascades and transcription factors to achieve an optimum and dynamic balance between macrophage subpopulations (24, 108). In this review, several mechanisms have been proposed to be involved in the immunoregulatory role of platelet derivatives in controlling macrophage polarization. Both human and murine macrophages share signalling pathways. For the former, polarization towards M2 phenotype was reported via PI3K/AKT signalling pathway activation (63), or by reducing the expression of NF-κB, or by increasing STAT3 and STAT6 and SMAD2 and SMAD4 expression (71), thus involving TLRs/NF-κB, JAK-STAT, and TGF−β signalling pathways, respectively. Regarding murine macrophages, the main transcription factor involved in the inflammatory response, namely NF-κB, was also reported its signalling reduction in several studies (61, 64, 70, 79), thus promoting the polarization of M2-like macrophages. Besides the TLR/NF-κB signalling pathway, Qian et al. (70) also described the involvement of the JNK and JAK-STAT signalling pathways in M1 and M2 phenotypes, respectively. In fact, they showed that PRP-derived exosomes inhibited M1 macrophage polarization by inactivating NF-κB and MAPK pathways and targeting TRAF6 and promoted the polarization toward M2-type macrophage via the STAT6 signalling pathway. Nasirzade et al. (64) also showed that the transition from M1 to M2 was partially due to an activation of TGF-β. This growth factor-related signalling pathway was also involved in the regulatory role of PRP through affecting activin activity (68).
In summary, macrophages are extremely plastic immune cells involved in tissue homeostasis and pathological conditions. As a consequence, they represent relevant therapeutic targets. To this end, several approaches have been proposed, including pharmacological interventions, transplantation of specific subsets of macrophages, epigenetic modifications, genetic engineering or depletion of NLRP3 inflammasome (109, 110). In this sense, platelet derivatives that have been successfully applied in many medical fields for decades, might be another option to consider. However, the heterogeneity of these biological therapies, due to differences in preparation protocols, cell content or platelet activation status, and inconsistences in nomenclature, has contributed to different clinical outcomes (111). In addition, most of the published studies do not provide all the information necessary for protocol reproducibility. In the same vein, the standardization and reproducibility of macrophage isolation, activation and polarization should be a requirement for the development of new therapies. Furthermore, understanding the differences between human macrophages and those derived from animal models seems essential for the effective design of new therapeutic strategies (105). Similarly, monocyte-derived macrophages do not fully represent what occurs in humans; thus, understanding the complex relationships between tissue-resident macrophages and infiltrating monocyte-derived macrophages and their relative contribution to homeostasis and disease might provide critical insights to platelet derivatives’ immunomodulatory response. Reprograming macrophage phenotypes is considered to be a promising strategy for designing novel therapies rather than those focusing on eliminating these immune cells (33). In this regard, and on the basis of the results derived from this review, platelet derivatives could play an important role in inducing a dynamic M1/M2 balance and promoting a timely M1-M2 shift. Therefore, despite the issues that remain to be resolved, combination of macrophages and platelet derivatives provides relevant information on the function and mechanisms of the immune response.
EA: Conceptualization, Supervision, Writing – review & editing. MT: Data curation, Formal analysis, Investigation, Methodology, Software, Writing – original draft, Writing – review & editing. MA: Conceptualization, Supervision, Writing – review & editing.
The author(s) declare that no financial support was received for the research, authorship, and/or publication of this article.
The authors declare the following competing financial interests: EA is the Scientific Director of and MT and MA are scientists at BTI Biotechnology Institute, a dental implant company that investigates in the fields of oral implantology and PRGF-Endoret technology.
The remaining authors declare that the research was conducted in the absence of any commercial or financial relationships that could be constructed as a potential conflict of interest.
All claims expressed in this article are solely those of the authors and do not necessarily represent those of their affiliated organizations, or those of the publisher, the editors and the reviewers. Any product that may be evaluated in this article, or claim that may be made by its manufacturer, is not guaranteed or endorsed by the publisher.
1. Mass E, Nimmerjahn F, Kierdorf K, Schlitzer A. Tissue-specific macrophages: how they develop and choreograph tissue biology. Nat Rev Immunol. (2023) 23:563–79. doi: 10.1038/s41577-023-00848-y
2. Wang C, Ma C, Gong L, Guo Y, Fu K, Zhang Y, et al. Macrophage polarization and its role in liver disease. Front Immunol. (2021) 12:803037. doi: 10.3389/fimmu.2021.803037
3. Chang CF, Goods BA, Askenase MH, Beatty HE, Osherov A, DeLong JH, et al. Divergent functions of tissue-resident and blood-derived macrophages in the hemorrhagic brain. Stroke. (2021) 52:1798–808. doi: 10.1161/STROKEAHA.120.032196
4. Jenkins SJ, Allen JE. The expanding world of tissue-resident macrophages. Eur J Immunol. (2021) 51:1882–96. doi: 10.1002/eji.202048881
5. Watanabe S, Alexander M, Misharin AV, Budinger GRS. The role of macrophages in the resolution of inflammation. J Clin Invest. (2019) 129:2619–28. doi: 10.1172/JCI124615
6. Merien F. A journey with elie metchnikoff: from innate cell mechanisms in infectious diseases to quantum biology. Front Public Health. (2016) 4:125. doi: 10.3389/fpubh.2016.00125
7. van Furth R, Cohn ZA. The origin and kinetics of mononuclear phagocytes. J Exp Med. (1968) 128:415–35. doi: 10.1084/jem.128.3.415
8. van Furth R, Cohn ZA, Hirsch JG, Humphrey JH, Spector WG, Langevoort HL. The mononuclear phagocyte system: a new classification of macrophages, monocytes, and their precursor cells. Bull World Health Organ. (1972) 46:845–52.
9. Bleriot C, Chakarov S, Ginhoux F. Determinants of resident tissue macrophage identity and function. Immunity. (2020) 52:957–70. doi: 10.1016/j.immuni.2020.05.014
10. Franklin RA, Li MO. Ontogeny of tumor-associated macrophages and its implication in cancer regulation. Trends Cancer. (2016) 2:20–34. doi: 10.1016/j.trecan.2015.11.004
11. Varol C, Mildner A, Jung S. Macrophages: development and tissue specialization. Annu Rev Immunol. (2015) 33:643–75. doi: 10.1146/annurev-immunol-032414-112220
12. Epelman S, Lavine KJ, Randolph GJ. Origin and functions of tissue macrophages. Immunity. (2014) 41:21–35. doi: 10.1016/j.immuni.2014.06.013
13. Hoeffel G, Ginhoux F. Ontogeny of tissue-resident macrophages. Front Immunol. (2015) 6:486. doi: 10.3389/fimmu.2015.00486
14. Ginhoux F, Guilliams M. Tissue-resident macrophage ontogeny and homeostasis. Immunity. (2016) 44:439–49. doi: 10.1016/j.immuni.2016.02.024
15. Italiani P, Boraschi D. Development and functional differentiation of tissue-resident versus monocyte-derived macrophages in inflammatory reactions. Results Probl Cell Differ. (2017) 62:23–43. doi: 10.1007/978-3-319-54090-0_2
16. Nicolás-Ávila JA, Hidalgo A, Ballesteros I. Specialized functions of resident macrophages in brain and heart. J Leukoc Biol. (2018) 104:743–56. doi: 10.1002/JLB.6MR0118-041R
17. Li Q, Barres BA. Microglia and macrophages in brain homeostasis and disease. Nat Rev Immunol. (2018) 18:225–42. doi: 10.1038/nri.2017.125
18. Chen X, Tang J, Shuai W, Meng J, Feng J, Han Z. Macrophage polarization and its role in the pathogenesis of acute lung injury/acute respiratory distress syndrome. Inflammation Res. (2020) 69:883–95. doi: 10.1007/s00011-020-01378-2
19. Perez S, Rius-Perez S. Macrophage polarization and reprogramming in acute inflammation: A redox perspective. Antioxidants (Basel). (2022) 11. doi: 10.3390/antiox11071394
20. Peng H, Xian D, Liu J, Pan S, Tang R, Zhong J. Regulating the polarization of macrophages: A promising approach to vascular dermatosis. J Immunol Res. (2020) 2020:8148272. doi: 10.1155/2020/8148272
21. Atri C, Guerfali FZ, Laouini D. Role of human macrophage polarization in inflammation during infectious diseases. Int J Mol Sci. (2018) 19. doi: 10.3390/ijms19061801
22. Mantovani A, Sica A, Sozzani S, Allavena P, Vecchi A, Locati M. The chemokine system in diverse forms of macrophage activation and polarization. Trends Immunol. (2004) 25:677–86. doi: 10.1016/j.it.2004.09.015
23. Cotechini T, Atallah A, Grossman A. Tissue-resident and recruited macrophages in primary tumor and metastatic microenvironments: potential targets in cancer therapy. Cells. (2021) 10. doi: 10.3390/cells10040960
24. Deng L, Jian Z, Xu T, Li F, Deng H, Zhou Y, et al. Macrophage polarization: an important candidate regulator for lung diseases. Molecules. (2023) 28. doi: 10.3390/molecules28052379
25. Zerrouk N, Alcraft R, Hall BA, Auge F, Niarakis A. Large-scale computational modelling of the M1 and M2 synovial macrophages in rheumatoid arthritis. NPJ Syst Biol Appl. (2024) 10:10. doi: 10.1038/s41540-024-00337-5
26. Viola A, Munari F, Sanchez-Rodriguez R, Scolaro T, Castegna A. The metabolic signature of macrophage responses. Front Immunol. (2019) 10:1462. doi: 10.3389/fimmu.2019.01462
27. Takiguchi H, Yang CX, Yang CWT, Sahin B, Whalen BA, Milne S, et al. Macrophages with reduced expressions of classical M1 and M2 surface markers in human bronchoalveolar lavage fluid exhibit pro-inflammatory gene signatures. Sci Rep. (2021) 11:8282. doi: 10.1038/s41598-021-87720-y
28. Roszer T. Understanding the mysterious M2 macrophage through activation markers and effector mechanisms. Mediators Inflammation. (2015) 2015:816460. doi: 10.1155/2015/816460
29. Wang LX, Zhang SX, Wu HJ, Rong XL, Guo J. M2b macrophage polarization and its roles in diseases. J Leukoc Biol. (2019) 106:345–58. doi: 10.1002/JLB.3RU1018-378RR
30. Lyu L, Cai Y, Zhang G, Jing Z, Liang J, Zhang R, et al. Exosomes derived from M2 macrophages induce angiogenesis to promote wound healing. Front Mol Biosci. (2022) 9:1008802. doi: 10.3389/fmolb.2022.1008802
31. Zhang C, Yang M, Ericsson AC. Function of macrophages in disease: current understanding on molecular mechanisms. Front Immunol. (2021) 12:620510. doi: 10.3389/fimmu.2021.620510
32. Ponzoni M, Pastorino F, Di Paolo D, Perri P, Brignole C. Targeting macrophages as a potential therapeutic intervention: impact on inflammatory diseases and cancer. Int J Mol Sci. (2018) 19. doi: 10.3390/ijms19071953
33. Ardura JA, Rackov G, Izquierdo E, Alonso V, Gortazar AR, Escribese MM. Targeting macrophages: friends or foes in disease? Front Pharmacol. (2019) 10:1255. doi: 10.3389/fphar.2019.01255
34. Park MD, Silvin A, Ginhoux F, Merad M. Macrophages in health and disease. Cell. (2022) 185:4259–79. doi: 10.1016/j.cell.2022.10.007
35. Anitua E, Troya M, Zalduendo M, Orive G. Personalized plasma-based medicine to treat age-related diseases. Mater Sci Eng C Mater Biol Appl. (2017) 74:459–64. doi: 10.1016/j.msec.2016.12.040
36. Orive G, Anitua E. Platelet-rich therapies as an emerging platform for regenerative medicine. Expert Opin Biol Ther. (2021) 21:1603–8. doi: 10.1080/14712598.2021.1936495
37. De Pascale MR, Sommese L, Casamassimi A, Napoli C. Platelet derivatives in regenerative medicine: an update. Transfus Med Rev. (2015) 29:52–61. doi: 10.1016/j.tmrv.2014.11.001
38. Meftahpour V, Malekghasemi S, Baghbanzadeh A, Aghebati-Maleki A, Pourakbari R, Fotouhi A, et al. Platelet lysate: a promising candidate in regenerative medicine. Regener Med. (2021) 16:71–85. doi: 10.2217/rme-2020-0065
39. Deeb MA. Role of platelet-rich fibrin (PRF) and platelet-rich plasma (PRP) in oro-facial tissue regeneration: A narrative review. J Advanced Oral Res. (2020) 11:5–11. doi: 10.1177/2320206819895836
40. Kawase T. Platelet-rich plasma and its derivatives as promising bioactive materials for regenerative medicine: basic principles and concepts underlying recent advances. Odontology. (2015) 103:126–35. doi: 10.1007/s10266-015-0209-2
41. van der Meijden PEJ, Heemskerk JWM. Platelet biology and functions: new concepts and clinical perspectives. Nat Rev Cardiol. (2019) 16:166–79. doi: 10.1038/s41569-018-0110-0
42. Nurden AT. The biology of the platelet with special reference to inflammation, wound healing and immunity. Front Biosci (Landmark Ed). (2018) 23:726–51. doi: 10.2741/4613
43. Anitua E, Prado R, Azkargorta M, Rodriguez-Suarez E, Iloro I, Casado-Vela J, et al. High-throughput proteomic characterization of plasma rich in growth factors (PRGF-Endoret)-derived fibrin clot interactome. J Tissue Eng Regener Med. (2015) 9:E1–12. doi: 10.1002/term.v9.11
44. Scopelliti F, Cattani C, Dimartino V, Mirisola C, Cavani A. Platelet derivatives and the immunomodulation of wound healing. Int J Mol Sci. (2022) 23. doi: 10.3390/ijms23158370
45. Mantovani A, Garlanda C. Platelet-macrophage partnership in innate immunity and inflammation. Nat Immunol. (2013) 14:768–70. doi: 10.1038/ni.2666
46. Derler M, Teubenbacher T, Carapuig A, Nieswandt B, Fessler J, Kolb D, et al. Platelets induce endoplasmic reticulum stress in macrophages in vitro. J Thromb Haemost. (2024) 22:1475–88. doi: 10.1016/j.jtha.2024.01.009
48. Li JL, Zarbock A, Hidalgo A. Platelets as autonomous drones for hemostatic and immune surveillance. J Exp Med. (2017) 214:2193–204. doi: 10.1084/jem.20170879
49. Linke B, Schreiber Y, Picard-Willems B, Slattery P, Nusing RM, Harder S, et al. Activated platelets induce an anti-inflammatory response of monocytes/macrophages through cross-regulation of PGE(2) and cytokines. Mediators Inflammation. (2017) 2017:1463216. doi: 10.1155/2017/1463216
50. Anitua E, Troya M, Zalduendo M, Tejero R, Orive G. Progress in the use of autologous regenerative platelet-based therapies in implant dentistry. Curr Pharm Biotechnol. (2016) 17:402–13. doi: 10.2174/1389201017666160127110300
51. Anitua E, Padilla S, Prado R, Alkhraisat MH. Platelet-rich plasma: are the obtaining methods, classification and clinical outcome always connected? Regener Med. (2022) 17:887–90. doi: 10.2217/rme-2022-0118
52. Magalon J, Brandin T, Francois P, Degioanni C, De Maria L, Grimaud F, et al. Technical and biological review of authorized medical devices for platelets-rich plasma preparation in the field of regenerative medicine. Platelets. (2021) 32:200–8. doi: 10.1080/09537104.2020.1832653
53. Golbach LA, Portelli LA, Savelkoul HF, Terwel SR, Kuster N, de Vries RB, et al. Calcium homeostasis and low-frequency magnetic and electric field exposure: A systematic review and meta-analysis of in vitro studies. Environ Int. (2016) 92–93:695–706. doi: 10.1016/j.envint.2016.01.014
54. Cao B, Lin J, Tan J, Li J, Ran Z, Deng L, et al. 3D-printed vascularized biofunctional scaffold for bone regeneration. Int J Bioprint. (2023) 9:702. doi: 10.18063/ijb.v9i3
55. Cenni E, Avnet S, Fotia C, Salerno M, Baldini N. Platelet-rich plasma impairs osteoclast generation from human precursors of peripheral blood. J Orthop Res. (2010) 28:792–7. doi: 10.1002/jor.21073
56. Escobar G, Escobar A, Ascui G, Tempio FI, Ortiz MC, Pérez CA, et al. Pure platelet-rich plasma and supernatant of calcium-activated P-PRP induce different phenotypes of human macrophages. Regenerative Med. (2018) 13:427–41. doi: 10.2217/rme-2017-0122
57. Graca AL, Gomez-Florit M, Osorio H, Rodrigues MT, Domingues RMA, Reis RL, et al. Controlling the fate of regenerative cells with engineered platelet-derived extracellular vesicles. Nanoscale. (2022) 14:6543–56. doi: 10.1039/D1NR08108J
58. He F, Umrath F, Reinert S, Alexander D. Jaw periosteum-derived mesenchymal stem cells regulate THP-1-derived macrophage polarization. Int J Mol Sci. (2021) 22. doi: 10.3390/ijms22094310
59. Hudgens JL, Sugg KB, Grekin JA, Gumucio JP, Bedi A, Mendias CL. Platelet-rich plasma activates proinflammatory signaling pathways and induces oxidative stress in tendon fibroblasts. Am J Sports Med. (2016) 44:1931–40. doi: 10.1177/0363546516637176
60. Jiang G, Li S, Yu K, He B, Hong J, Xu T, et al. A 3D-printed PRP-GelMA hydrogel promotes osteochondral regeneration through M2 macrophage polarization in a rabbit model. Acta Biomater. (2021) 128:150–62. doi: 10.1016/j.actbio.2021.04.010
61. Kargarpour Z, Nasirzade J, Panahipour L, Miron RJ, Gruber R. Liquid PRF reduces the inflammatory response and osteoclastogenesis in murine macrophages. Front Immunol. (2021) 12:636427. doi: 10.3389/fimmu.2021.636427
62. Li N, Liu L, Wei C, Ren S, Liu X, Wang X, et al. Immunomodulatory blood-derived hybrid hydrogels as multichannel microenvironment modulators for augmented bone regeneration. ACS Appl Mater Interfaces. (2022) 14:53523–34. doi: 10.1021/acsami.2c16774
63. Luo H, Liu W, Zhou Y, Jiang X, Liu Y, Yang Q, et al. Concentrated growth factor regulates the macrophage-mediated immune response. Regener Biomater. (2021) 8:rbab049. doi: 10.1093/rb/rbab049
64. Nasirzade J, Kargarpour Z, Hasannia S, Strauss FJ, Gruber R. Platelet-rich fibrin elicits an anti-inflammatory response in macrophages in vitro. J Periodontol. (2020) 91:244–52. doi: 10.1002/JPER.19-0216
65. Nishio H, Saita Y, Kobayashi Y, Takaku T, Fukusato S, Uchino S, et al. Platelet-rich plasma promotes recruitment of macrophages in the process of tendon healing. Regener Ther. (2020) 14:262–70. doi: 10.1016/j.reth.2020.03.009
66. O’Donnell C, Migliore E, Grandi FC, Koltsov J, Lingampalli N, Cisar C, et al. Platelet-rich plasma (PRP) from older males with knee osteoarthritis depresses chondrocyte metabolism and upregulates inflammation. J Orthop Res. (2019) 37:1760–70. doi: 10.1002/jor.24322
67. Papait A, Cancedda R, Mastrogiacomo M, Poggi A. Allogeneic platelet-rich plasma affects monocyte differentiation to dendritic cells causing an anti-inflammatory microenvironment, putatively fostering wound healing. J Tissue Eng Regener Med. (2018) 12:30–43. doi: 10.1002/term.2361
68. Park G, Qian W, Zhang MJ, Chen YH, Ma LW, Zeng N, et al. Platelet-rich plasma regulating the repair of ultraviolet B-induced acute tissue inflammation: adjusting macrophage polarization through the activin receptor-follistatin system. Bioengineered. (2021) 12:3125–36. doi: 10.1080/21655979.2021.1944026
69. Qian B, Yang Q, Wang M, Huang S, Jiang C, Shi H, et al. Encapsulation of lyophilized platelet-rich fibrin in alginate-hyaluronic acid hydrogel as a novel vascularized substitution for myocardial infarction. Bioact Mater. (2022) 7:401–11. doi: 10.1016/j.bioactmat.2021.05.042
70. Qian J, Wang X, Su G, Shu X, Huang Z, Jiang H, et al. Platelet-rich plasma-derived exosomes attenuate intervertebral disc degeneration by promoting NLRP3 autophagic degradation in macrophages. Int Immunopharmacol. (2022) 110:108962. doi: 10.1016/j.intimp.2022.108962
71. Scopelliti F, Caterina C, Valentina D, Gianfranco C, Concetta M, Andrea C. Platelet lysate converts M (IFNgamma+LPS) macrophages in CD206(+) TGF-beta(+) arginase(+) M2-like macrophages that affect fibroblast activity and T lymphocyte migration. J Tissue Eng Regener Med. (2021) 15:788–97. doi: 10.1002/term.3229
72. Tang J, Li H, Peng H, Zhang Z, Liu C, Cheng Y, et al. Pre-clinical evaluation of thermosensitive decellularized adipose tissue/platelet-rich plasma interpenetrating polymer network hydrogel for wound healing. Mater Today Bio. (2022) 17:100498. doi: 10.1016/j.mtbio.2022.100498
73. Tylek T, Schilling T, Schlegelmilch K, Ries M, Rudert M, Jakob F, et al. Platelet lysate outperforms FCS and human serum for co-culture of primary human macrophages and hMSCs. Sci Rep. (2019) 9:3533. doi: 10.1038/s41598-019-40190-9
74. Uchiyama R, Toyoda E, Maehara M, Wasai S, Omura H, Watanabe M, et al. Effect of platelet-rich plasma on M1/M2 macrophage polarization. Int J Mol Sci. (2021) 22. doi: 10.3390/ijms22052336
75. Ulivi V, Tasso R, Cancedda R, Descalzi F. Mesenchymal stem cell paracrine activity is modulated by platelet lysate: induction of an inflammatory response and secretion of factors maintaining macrophages in a proinflammatory phenotype. Stem Cells Dev. (2014) 23:1858–69. doi: 10.1089/scd.2013.0567
76. Wessely-Szponder J, Szponder T, Bobowiec R, Michalska J. Changes in the activity of ovine blood-derived macrophages stimulated with antimicrobial peptide extract (AMP) or platelet-rich plasma (PRP). J Vet Res. (2019) 63:235–42. doi: 10.2478/jvetres-2019-0021
77. Yadav A, Ramasamy TS, Lin SC, Chen SH, Lu J, Liu YH, et al. Autologous platelet-rich growth factor reduces M1 macrophages and modulates inflammatory microenvironments to promote sciatic nerve regeneration. Biomedicines. (2022) 10. doi: 10.3390/biomedicines10081991
78. Yu TY, Pang JS, Lin LP, Cheng JW, Liu SJ, Tsai WC. Platelet-rich plasma releasate promotes early healing in tendon after acute injury. Orthop J Sports Med. (2021) 9:2325967121990377. doi: 10.1177/2325967121990377
79. Zhang J, Yin C, Zhao Q, Zhao Z, Wang J, Miron RJ, et al. Anti-inflammation effects of injectable platelet-rich fibrin via macrophages and dendritic cells. J BioMed Mater Res A. (2020) 108:61–8. doi: 10.1002/jbm.a.36792
80. Zhao M, Wang J, Zhang J, Huang J, Luo L, Yang Y, et al. Functionalizing multi-component bioink with platelet-rich plasma for customized in-situ bilayer bioprinting for wound healing. Mater Today Bio. (2022) 16:100334. doi: 10.1016/j.mtbio.2022.100334
81. DeLong JM, Russell RP, Mazzocca AD. Platelet-rich plasma: the PAW classification system. Arthroscopy. (2012) 28:998–1009. doi: 10.1016/j.arthro.2012.04.148
82. Chahla J, Cinque ME, Piuzzi NS, Mannava S, Geeslin AG, Murray IR, et al. A call for standardization in platelet-rich plasma preparation protocols and composition reporting: A systematic review of the clinical orthopaedic literature. J Bone Joint Surg Am. (2017) 99:1769–79. doi: 10.2106/JBJS.16.01374
83. Kon E, Di Matteo B, Delgado D, Cole BJ, Dorotei A, Dragoo JL, et al. Platelet-rich plasma for the treatment of knee osteoarthritis: an expert opinion and proposal for a novel classification and coding system. Expert Opin Biol Ther. (2020) 20:1447–60. doi: 10.1080/14712598.2020.1798925
84. Wei W, Wan Y. Chapter 10 - Regulation of Bone Resorption by PPARγ. Karsenty G, editor. San Diego: Academic Press (2013). doi: 10.1016/B978-0-12-415784-2.00010-5
85. Choi Y, Faccio R, Teitelbaum SL, Takayanagi H. Chapter 4 - Osteoclast Biology: Regulation of Formation and Function. Lorenzo J, Horowitz MC, Choi Y, Takayanagi H, Schett G, editors. San Diego: Academic Press (2016).
86. Anitua E, Troya M, Falcon-Pérez JM, López-Sarrio S, González E, Alkhraisat MH. Advances in platelet rich plasma-derived extracellular vesicles for regenerative medicine: A systematic-narrative review. Int J Mol Sci. (2023) 24. doi: 10.3390/ijms241713043
87. Hiasa M, Abe M, Nakano A, Oda A, Amou H, Kido S, et al. GM-CSF and IL-4 induce dendritic cell differentiation and disrupt osteoclastogenesis through M-CSF receptor shedding by up-regulation of TNF-alpha converting enzyme (TACE). Blood. (2009) 114:4517–26. doi: 10.1182/blood-2009-04-215020
88. Lellahi SM, Azeem W, Hua Y, Gabriel B, Paulsen Rye K, Reikvam H, et al. GM-CSF, Flt3-L and IL-4 affect viability and function of conventional dendritic cell types 1 and 2. Front Immunol. (2022) 13:1058963. doi: 10.3389/fimmu.2022.1058963
89. Guilliams M, Ginhoux F, Jakubzick C, Naik SH, Onai N, Schraml BU, et al. Dendritic cells, monocytes and macrophages: a unified nomenclature based on ontogeny. Nat Rev Immunol. (2014) 14:571–8. doi: 10.1038/nri3712
90. Gottschalk C, Kurts C. The debate about dendritic cells and macrophages in the kidney. Front Immunol. (2015) 6:435. doi: 10.3389/fimmu.2015.00435
91. Zhang D, Dang Y, Deng R, Ma Y, Wang J, Ao J, et al. Research progress of macrophages in bone regeneration. J Tissue Eng Regenerative Med. (2023) 2023:1–13. doi: 10.1155/2023/1512966
92. Li XC, Luo SJ, Fan W, Zhou TL, Tan DQ, Tan RX, et al. Macrophage polarization regulates intervertebral disc degeneration by modulating cell proliferation, inflammation mediator secretion, and extracellular matrix metabolism. Front Immunol. (2022) 13:922173. doi: 10.3389/fimmu.2022.922173
93. Kelley N, Jeltema D, Duan Y, He Y. The NLRP3 inflammasome: an overview of mechanisms of activation and regulation. Int J Mol Sci. (2019) 20. doi: 10.3390/ijms20133328
94. Swanson KV, Deng M, Ting JPY. The NLRP3 inflammasome: molecular activation and regulation to therapeutics. Nat Rev Immunol. (2019) 19:477–89. doi: 10.1038/s41577-019-0165-0
95. Blevins HM, Xu Y, Biby S, Zhang S. The NLRP3 inflammasome pathway: A review of mechanisms and inhibitors for the treatment of inflammatory diseases. Front Aging Neurosci. (2022) 14:879021. doi: 10.3389/fnagi.2022.879021
96. Dey A, Allen J, Hankey-Giblin PA. Ontogeny and polarization of macrophages in inflammation: blood monocytes versus tissue macrophages. Front Immunol. (2014) 5:683. doi: 10.3389/fimmu.2014.00683
97. Lavin Y, Mortha A, Rahman A, Merad M. Regulation of macrophage development and function in peripheral tissues. Nat Rev Immunol. (2015) 15:731–44. doi: 10.1038/nri3920
98. Mestas J, Hughes CC. Of mice and not men: differences between mouse and human immunology. J Immunol. (2004) 172:2731–8. doi: 10.4049/jimmunol.172.5.2731
99. Orecchioni M, Ghosheh Y, Pramod AB, Ley K. Macrophage Polarization: Different Gene Signatures in M1(LPS+) vs. Classically and M2(LPS-) vs. Alternatively Activated Macrophages. Front Immunol. (2019) 10:1084. doi: 10.3389/fimmu.2019.01084
100. De Cunto G, Cavarra E, Bartalesi B, Lungarella G, Lucattelli M. Alveolar macrophage phenotype and compartmentalization drive different pulmonary changes in mouse strains exposed to cigarette smoke. COPD. (2020) 17:429–43. doi: 10.1080/15412555.2020.1783648
101. Ghosh P, Sinha S, Katkar GD, Vo D, Taheri S, Dang D, et al. Machine learning identifies signatures of macrophage reactivity and tolerance that predict disease outcomes. EBioMedicine. (2023) 94:104719. doi: 10.1016/j.ebiom.2023.104719
103. Mills CD, Kincaid K, Alt JM, Heilman MJ, Hill AM. Pillars article: M-1/M-2 macrophages and the th1/Th2 paradigm. J Immunol 2000. (2017) 164:6166–73. doi: 10.4049/jimmunol.164.12.6166
104. Strizova Z, Benesova I, Bartolini R, Novysedlak R, Cecrdlova E, Foley LK, et al. M1/M2 macrophages and their overlaps - myth or reality? Clin Sci (Lond). (2023) 137:1067–93. doi: 10.1042/CS20220531
105. Chavez-Galan L, Olleros ML, Vesin D, Garcia I. Much More than M1 and M2 Macrophages, There are also CD169(+) and TCR(+) Macrophages. Front Immunol. (2015) 6:263. doi: 10.3389/fimmu.2015.00263
106. Zhou J, Tang Z, Gao S, Li C, Feng Y, Zhou X. Tumor-associated macrophages: recent insights and therapies. Front Oncol. (2020) 10:188. doi: 10.3389/fonc.2020.00188
107. Murray PJ, Allen JE, Biswas SK, Fisher EA, Gilroy DW, Goerdt S, et al. Macrophage activation and polarization: nomenclature and experimental guidelines. Immunity. (2014) 41:14–20. doi: 10.1016/j.immuni.2014.06.008
108. Vergadi E, Ieronymaki E, Lyroni K, Vaporidi K, Tsatsanis C. Akt signaling pathway in macrophage activation and M1/M2 polarization. J Immunol. (2017) 198:1006–14. doi: 10.4049/jimmunol.1601515
109. Na YR, Kim SW, Seok SH. A new era of macrophage-based cell therapy. Exp Mol Med. (2023) 55:1945–54. doi: 10.1038/s12276-023-01068-z
110. Sansonetti M, Al Soodi B, Thum T, Jung M. Macrophage-based therapeutic approaches for cardiovascular diseases. Basic Res Cardiol. (2024) 119:1–33. doi: 10.1007/s00395-023-01027-9
Keywords: macrophages, polarization, platelet rich plasma, platelet lysate, platelet rich fibrin
Citation: Anitua E, Troya M and Alkhraisat MH (2024) Immunoregulatory role of platelet derivatives in the macrophage-mediated immune response. Front. Immunol. 15:1399130. doi: 10.3389/fimmu.2024.1399130
Received: 11 March 2024; Accepted: 07 June 2024;
Published: 25 June 2024.
Edited by:
Carlos Rosales, National Autonomous University of Mexico, MexicoReviewed by:
Ricardo Goncalves, Federal University of Minas Gerais, BrazilCopyright © 2024 Anitua, Troya and Alkhraisat. This is an open-access article distributed under the terms of the Creative Commons Attribution License (CC BY). The use, distribution or reproduction in other forums is permitted, provided the original author(s) and the copyright owner(s) are credited and that the original publication in this journal is cited, in accordance with accepted academic practice. No use, distribution or reproduction is permitted which does not comply with these terms.
*Correspondence: Eduardo Anitua, ZWR1YXJkb0BmdW5kYWNpb25lZHVhcmRvYW5pdHVhLm9yZw==
Disclaimer: All claims expressed in this article are solely those of the authors and do not necessarily represent those of their affiliated organizations, or those of the publisher, the editors and the reviewers. Any product that may be evaluated in this article or claim that may be made by its manufacturer is not guaranteed or endorsed by the publisher.
Research integrity at Frontiers
Learn more about the work of our research integrity team to safeguard the quality of each article we publish.