- Department of Microbiology Immunology, Georgetown University School of Medicine, Washington, DC, United States
IL-27, a member of the IL-6/IL-12 cytokine superfamily, is primarily secreted by antigen presenting cells, specifically by dendric cells, macrophages and B cells. IL-27 has antiviral activities and modulates both innate and adaptive immune responses against viruses. The role of IL-27 in the setting of viral infections is not well defined and both pro-inflammatory and anti-inflammatory functions have been described. Here, we discuss the latest advancements in the role of IL-27 in several viral infection models of human disease. We highlight important aspects of IL-27 expression regulation, the critical cell sources at different stages of the infection and their impact in cell mediated immunity. Lastly, we discuss the need to better define the antiviral and modulatory (pro-inflammatory vs anti-inflammatory) properties of IL-27 in the context of human chronic viral infections.
Introduction
The interleukin 12 (IL-12) family of cytokines formed by IL-12, IL-23, IL-27, IL-35 and IL-39, plays critical roles in the induction and regulation of innate and adaptive immune responses (1). IL-12, IL-23 and IL-27 are secreted by antigen presenting cells including B cells, monocytes/macrophages and dendritic cells (1–4). IL-12 and IL-23 are pro-inflammatory and are involved in the generation of the T helper subsets, Th1 and Th17 respectively. Additionally, IL-12 is an important factor for NK cell activation and IFNγ secretion (5). In contrast, IL-35 is produced by regulatory T (Treg) and B (Breg) cells and therefore exerts important immunoregulatory functions (6–8). The new member of this family, IL-39, has been shown to be involved in the pathogenesis of murine experimental lupus erythematosus, however, its role in human disease is still under evaluation (9).
IL-27 is a member of this family and has been shown to promote both pro-inflammatory and anti-inflammatory functions. IL-27 induces the development of Th1 cells in response to bacterial and parasitic infections (10–13). In addition, it facilitates the development of T follicular helper (TFH) cells via the induction of IL-21, regulating B cell function (14). The regulatory functions of IL-27 include inhibition of Th2 and Th17 cell differentiation, and the induction of IL-10-producing Type 1 regulatory cells (Tr1) cells controlling immunopathology in the setting of infection (15–18).
In the setting of viral infections, the role of IL-27 is not well understood. IL-27 has antiviral properties against human viruses including influenza, herpes simplex, human hepatitis B (HBV) and C (HCV), human immunodeficiency virus (HIV) and others viruses, which underscores the therapeutic potential of this cytokine (19–30). The antiviral effects of IL-27 has been recently reviewed in detail by Amsden et al. (30). In the present manuscript, we will discuss the role of IL-27 in T cell mediated immunity in the setting of viral infection.
IL-27/IL-27R signaling
The IL-12 family of cytokines are heterodimers that result from the combination of one of three alpha (α) chains (p19, p28 or p35), with one of two beta (β) chains (p40 or EBI3). The association of the α and β chains results in heterodimers that share a common element among the family members (Figure 1). IL-12 is composed by p35 and p40 subunits, and IL-23 results from the paring of p19 and p40 chains. IL-27 is formed by the association of p28 and EBI3. Lastly, EBI3 acts as the common element for IL-35 and IL-39 by pairing to p35 or p19 respectively (Figure 1) (10, 31–35).
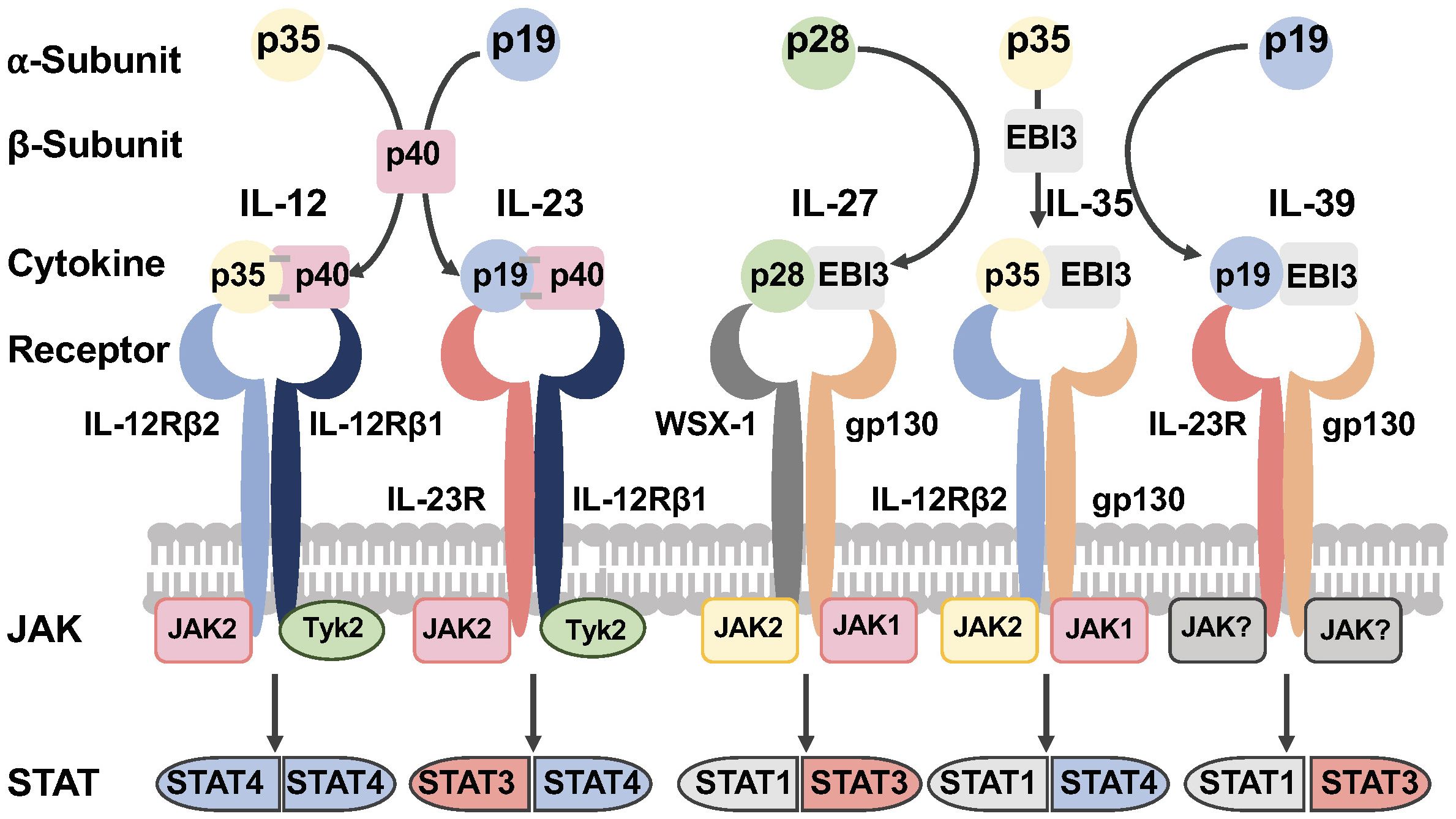
Figure 1 The IL-12 family of cytokines. The IL-12 family of cytokines are heterodimers that result from the combination of one of three alpha (α) chains (p19, p28 or p35), with one of two beta (β) chains (p40 or EBI3). The association of the α and β chains results in heterodimers that share a common element among the family members. The receptors of this family of cytokines are heterodimeric molecules shared by the family members. The cytokines of this family signal through the Janus Kinase (JAK)-Signal Transducer and Activator of Transcription (JAK/STAT) pathway (Figure was designed with BioRender.com).
A distinctive feature among the family members is that IL-12 and IL-23 are secreted as disulfide-linked heterodimers (10, 31, 36–40). In contrast, IL-27 and IL-35 are non-disulfide-linked, and it has been postulated this may impact the in vivo stability, resulting in lower secretion levels compared to disulfide-linked heterodimeric cytokines (10, 31, 40). The non-disulfide-linked features of IL-27 and IL-35 heterodimers present a challenge for their detection in tissues and measurements in the plasma.
The secretion of murine and human IL-27p28 shows interspecies differences. The folding and secretion of IL-27p28 depend on the formation of a disulfide-bond (Cys residue). In mice, the IL-27p28 subunit contains a single disulfide bond that stabilizes the protein and allows its independent secretion (10, 41–43). In contrast, the human IL-27p28 has only one cysteine residue and therefore lacks a disulfide-bond, instead requiring EBI3 binding for its secretion (10, 13, 43–46). The EBI3 subunit acts as chaperone-like protein and this property facilitates the secretion of non-disulfide-linked IL-27, IL-35, and IL-39 (45, 47).
In addition, computational studies of IL-27p28 Cys residue number and location across different species showed that in species with independent secretion of IL-27p28 such as mice, EBI3 is secretion incompetent and therefore retained inside the cells (43, 45, 48). This evolutionary feature of IL-27 has potential biological implications although it is not well understood and warrants further study.
IL-27 signals through a heterodimeric receptor composed of two subunits, the IL-27Rα (also known as WSX-1 or TCCR) and gp130 (IL6ST) (Figure 1) (10, 49, 50). The quaternary structure of IL-27 bound to its receptor resembles IL-6 and this structure reveals key interactions between IL-27Rα with IL-27p28 and the D2 domain of EBI3. In addition, a conserved tryptophan residue of IL-27p28 interacts with the D1 domain of gp130 (51, 52).
IL-27 binding activates the Janus Kinase (JAK)-Signal Transducer and Activator of Transcription (STAT1 and STAT3), and the mitogen activated protein kinase (MAPK) signaling pathway (Figure 1) (50, 53–59). IL-27 and IL-6 share a receptor subunit gp130 and both activate the transcription factor STAT3, however these cytokines exert different functions on target cells upon receptor binding. Studies in murine T cells using chromatin immunoprecipitation-sequencing in STAT deficient T cells showed that STAT3 plays an important role driving the overall transcriptome changes for both IL-27 and IL-6 (60). STAT1 was also found to be essential in providing the specific transcriptome normally induced by IL-27 signaling (60).
In human T cells, comparison of the global transcriptional changes induced by IL-27, IFNα and IL-6, showed that IL-27 clustered separately from IFNα and IL-6 signaling, but was closer to IL-6 than to IFNα. IL-27 induced a set of genes downstream of STAT1 that were also upregulated by IFNα and, to a lesser degree by IL-6 signaling (61).
IL-27 expression in lymphoid and peripheral tissues
IL-27 is mainly produced by antigen presenting cells (APCs) including dendritic cells (DCs), plasma cells, macrophages, inflammatory monocytes, microglia, B cells and endothelial cells (62–66).
IL-27 mRNA expression showed high levels of EBI3 compared with IL-27p28 (10, 67). Similarly, in vitro experiments using LPS stimulation of monocyte-derived DCs showed that expression of IL-27p28 transcripts were transient compared to EBI3 which was more sustained (10). These observations suggest that the expression of heterodimeric IL-27p28 protein is regulated and highlights potential for other functions of EBI3.
The noncovalent nature of the IL-27 heterodimer and the independent secretion of the subunits had made it challenging to measure the bioactive heterodimeric form of IL-27 (10, 43, 45). In human tissues few studies have investigated the coexpression of both subunits to assess the main cell sources and expression dynamics in health and disease (Table 1). IL-27p28 protein expression was investigated in post-mortem human lymph nodes, and in lymph node biopsies from patients with granulomatous disease (sarcoidosis, tuberculosis and Crohn’s disease), which is associated with an epithelioid granuloma with accumulation of macrophages and CD4 Th1 cells (68, 69). IL-27p28 and EBI3 expression was observed CD68+ macrophages, blood and lymphatic endothelial cells and plasma cells (68, 69). EBI3 expression in lymph nodes was localized in follicles and inter-follicular areas, and in the T cell zone. In contrast IL-27p28 was expressed by plasma cells and fibroblasts, however coexpression of the subunits IL-27p28 and EBI3 was not observed in dendritic cells (69).
Interestingly, IL-27p28 expression partially overlapped with EBI3 in macrophages and endothelial cells (69). The partial coexpression of IL-27 subunits in tissues is consistent with a transient production of IL-27p28 mRNA transcripts observed in in vitro activated human DCs (10, 67). In addition, this observation may reflect the expression of other members of this family, IL-35 and IL-39, that share EBI3 (69).
IL-27 has also shown to modulate inflammatory responses in the brain (Table 1). IL-27 subunit expression was investigated in brain tissue obtained from individuals with Multiple Sclerosis (MS) and donors without clinical and neuropathological evidence of CNS disease (control samples) (72, 73). IL-27 subunits were mainly detected in astrocytes, microglia and macrophages; and their expression was increased in individuals with MS compared to the controls. Similar to the expression pattern reported in lymph nodes, in the brain, there was partial overlap of the IL-27 subunits coexpression (72, 73).
In tissues, IL-27 is produced by immune resident cell and cells recruited to the sites of inflammation (73–75). IL-27 signaling is involved in other pathological conditions such as atherosclerosis, non-small cell lung cancer (NSCLC) and other inflammatory diseases but the dynamics of IL-27 subunit coexpression in human tissues in the setting of health and viral infections remains scarce (76–80).
A common observation across tissue expression studies is the prominent expression of EBI3 and partial overlap with IL-27p28 subunits expression (Table 1). EBI3 is induced under inflammatory conditions and independently secreted, even in the absence of the subunits IL-27p28, IL-35p35 or IL-39p19. This has led to the hypothesis that EBI3 plays additional roles including a chaperone-like and regulatory functions (47, 67, 70, 71, 81, 82).
IL-27 signaling in the context of viral infections
Lymphocytic choriomeningitis virus
The murine model of infection with the Lymphocytic Choriomeningitis Virus clone 13 (LCMV Cl-13) establishes a chronic infection characterized by CD8 T cell immune exhaustion and high viral loads that mimics many features of human chronic viral infections including HIV, HBV, and HCV (83). LCMV-specific CD8 T cells are dysfunctional and express checkpoint receptors (84–88).
In this model, deficiency of IL-27 signaling (IL-27RA-/- mice) leads to higher viral titers and uncontrolled lifelong viremia compared to the wild type mice (89, 90). In the spleen of the infected mice, detectable IL27p28 mRNA transcripts were found in DCs subsets including plasmacytoid DCs (pDCs) and macrophages very early post infection (day 1 p.i) and returned to basal levels at day 8 p.i (89). In contrast, no changes were observed in Ebi3 mRNA transcripts were observed during infection, with only a slight reduction at a later time point (89). The rapid upregulation of IL-27 expression (IL-27p28) occurs with the early induction of Type I IFNs (91, 92). Indeed, IL-27RA deficient mice showed reduced Type I IFN production in both acute and chronic LCMV infection (89).
The T cell mediated responses in the absence of IL-27 signaling showed a significant early expansion of LCMVGp33-specific CD8 T cells (day 9 p.i) compared to the wild type mice, and returned to similar levels at day 30 p.i. Interestingly, despite lower expression of PD1 (a marker of exhaustion LCMV chronic infection), virus-specific CD8 T cells demonstrated similar ability to secrete cytokines to wild type T cells (89). Additionally, IL-27 signaling promoted survival of virus-specific CD4 T cells and viral control during early infection (89, 92, 93).
Moreover, IL-27 signaling is an important factor for a subset of exhausted memory CD8 T cells with stem-like properties (CXCR5+) that confers antiviral immunity during chronic LCMV infection (92, 94, 95). In these elegant studies, the authors showed that treatment with IL-27 significantly increased virus-specific CXCR5+CD8 T cells and reduced viral titers in the brain (92). IL-27 signaling was critical for CXCR5+CD8 T cell expansion, and this effect was in part mediated by STAT1-driven IRF1 expression preventing terminal differentiation and cell death.
In this model, B cell-derived IL-27 is a crucial factor for the survival and function of virus-specific CD4 T cells and TFH cells, enhancing humoral immunity and viral control at the later phase of the infection (89, 96). Altogether, these studies showed that in chronic LCMV infection, IL-27 is beneficial and plays an essential role in both T cell and humoral immunity.
Cytomegalovirus infection
The human cytomegalovirus (HCMV) infection establishes latency and persist at mucosal sites, including the salivary glands. In human and murine CMV infection, CD4 T cells are key players in the control of viral replication in the salivary glands (97, 98). Particularly, memory T cells control the virus in periods of viral reactivation preventing development of clinical disease (99). HCMV evades the immune system by modulating host responses including the induction IL-10 resulting in viral persistence (100–102). In addition, CMV infection has been associated with the expansion of virus-specific CD8 T cells with a senescent phenotype (103, 104).
The role of IL-27 was investigated in a murine model of CMV infection (MCMV). The studies showed that early in infection (2 days p.i), IL-27p28 is expressed in the spleen by myeloid cells (DCs, pDCs, macrophages and neutrophils) and B cells (98, 105). In contrast, in the salivary glands, its expression is detected during the viral persistence phase (day 14 p.i) (105). Induction of IL-27 was dependent on Type I IFN, as in vivo blockade of IFNAR reduced IL-27 expression by DCs, macrophages B cells and neutrophils (105).
IL-27 signaling regulated T cell mediated immunity by enhancing the expansion of virus-specific CD4 T cells including those that provide immunity during viral persistence. In contrast, these effects were not observed in CD8 T cells, which may be due to the lower IL-27Rα expression on CD8 T cells when compared with CD4 T cells (98). In the salivary glands, IL-27 signaling promoted virus-specific CD4 T cells that secrete IFNγ and IL-10 and resembled Tr1 cells, which contributed to viral persistence (98, 105–109).
In human CMV infection CD4 and CD8 virus-specific T cells play a critical role in controlling CMV, however whether IL-27 contributes to viral persistence is not well understood (110). Human CMV-specific CD4 T cells have a cytotoxic phenotype and express CX3CR1 suggesting a potential contribution to chronic endothelial inflammation and injury (111–115). Similar to the murine model, human CMVpp65 and CMVgb -specific CD4 T cells from peripheral blood and colon produce IL-10, although variable frequencies were present among individuals (105). The role of IL-27 in CMV infection requires further investigation to fully determine the modulatory effects in the context of CMV infection.
SIV infection
IL-27 has in vitro antiviral properties against HIV and other human viruses reviewed elsewhere (21, 22, 27, 30, 116, 117). Limited data of the coexpression of IL-27 subunits and kinetics of expression is available in the setting of SIV/HIV infection. In non-human primates infected with Simian Immunodeficiency Virus (SIV), interferon-stimulated genes were evaluated in the spleens of animals before and after infection at days 4, 7, 14, 21, and 56 p.i (118).
IL27p28 mRNA expression was induced in the acute phase similarly to IFNB transcripts, and both remained high compared to the uninfected animals during the chronic phase of infection (>56 days p.i). In addition, IL10 mRNA transcripts were also detected in the acute phase, however its expression was reduced to basal levels during the chronic phase (118).
The role of IL-27 in CD4 TFH cell differentiation was investigated in SIV infected rhesus macaques (RM) (119). This study evaluated mRNA of the IL-12 family subunits (p19, p40, p35, p28 and EBI3) in cell suspensions of mesenteric lymph nodes from SIV infected RM with high and low CD4 counts. mRNA transcripts of the subunits that form the cytokines IL-12, IL-23, and IL-35 were reduced in the infected animals with low CD4 counts. In contrast, no significant changes were observed for the expression of IL27p28 and EBI3 mRNA in the animals, irrespective of CD4 count or infection status (119).
In in vitro cultures of mesenteric lymph node cell suspensions, IL-27 induced downregulation of CXCR5 expression in CD4 TFH (CXCR5highPD1high) and increased the frequency of cells expressing CXCR5lowPD1high, where upregulation of Tbet expression and Th1-like function. This suggests that IL-27 may alter TFH differentiation during mucosal immune response against SIV infection (119).
HIV infection
There is no available data about IL-27 expression in tissues from people with HIV (PWH). Most reported studies have investigated plasma levels of IL-27 at distinct stages of the infection with no consistent conclusions about the potential in vivo regulation of IL-27 during HIV infection. A study reported no changes in circulating levels of IL-27 at several stages of infection, untreated, successfully suppressed viremia with ART (120). However, contradicting these results, another study showed IL-27 plasma levels were inversely correlated with viremia in naïve HIV monoinfected compared to HCV coinfected groups (121, 122). In PWH and CMV coinfection, IL-27 plasma levels were negatively correlated with viral load and positively associated with CD4 T cell counts suggesting a beneficial effect in CD4 T cell reconstitution in CMV infected PWH (123). Further, another study described a positive association between IL-27 plasma levels and provirus HIV-DNA in Peripheral Blood Mononuclear Cells (PBMCs) (124).
The effects of IL-27 in T cell immunity against HIV has been investigated in individuals with uncontrolled viral replication (125). In this report, Tregs were shown to secrete IL-27 inducing IL-10 production by monocytes, which resulted in blunted proliferation of HIV-specific CD4 T cells in vitro, although these effects were heterogenous among the individuals (125).
In the context of viral suppression by antiretroviral therapy (ART), T cells express higher levels of STAT1 which leads to enhanced STAT1 activation by IL-27 stimulation. This effect resulted in upregulation of T-bet expression by TIGIT+HIVGag-specific T cells and increased cytokine secretion and cytotoxic potential (expression of CD107a) (61). These results suggest that IL-27 can modulate the function of exhausted T cells during chronic HIV infection.
Moreover, in PWH and CMV coinfection, in vitro stimulation of PBMCs with CMVpp65 in the presence of IL-27 stimulation led to increased IFNγ secretion by CMVpp65-specific CD4 T cells in both CMV+PWH and CMV+PWOH (People Without HIV) (113, 123). IL-27 induced IL-10 secretion by IFNγ+CMV-specific CD4 T cells recapitulating Tr1 cells, but did not have an impact on the expansion of Tregs (CD25+FoxP3+) (105, 109, 123, 126).
Respiratory viruses
Respiratory infections are diverse in terms of the viral agent, severity of the disease and contribution to morbidity and mortality worldwide. Herein, we discuss those in which IL-27 has been reported to play a role.
Influenza
Seasonal influenza infection is a significant contributor of morbidity and mortality worldwide in young children and older adults (127). Influenza virus infection can drive massive pulmonary immune infiltration and immunopathology. In this setting, IL-10 plays an important role in regulating pro-inflammatory function of innate and adaptive cells to control immunopathology in the tissues (128).
Infection with a highly pathogenetic influenza strain in mice drove upregulation of the Il27p28 mRNA subunit and Il10 mRNA with similar kinetics in the lung that peaked at day 7 p.i. In contrast, Ebi3 transcripts were detected at baseline and no changes were observed through the course infection. Il27 mRNA subunits were highly expressed in the lungs compared to the spleens and the lymph nodes, and correlated with the lower viral load at day 7 p.i highlighting its potential antiviral effects (129).
IL-27 plays a critical role in limiting immunopathology by controlling neutrophil accumulation and reducing the inflammatory Th1 and Th17 cells by both IL-10-dependent and -independent mechanisms (130–133). IL-27 signaling promoted IL-10 production in IFNγ secreting virus-specific CD4 T cells and cytotoxic CD8 T cells (133).
In addition, the administration of IL-27 showed different effects, at the peak of the viral load (between 5–10 days p.i) it was beneficial and ameliorated the immunopathology by reducing the influx of pro-inflammatory cells into the lungs without impairing viral clearance (129). In contrast, when IL-27 was administered at early time point of the infection, 1–7 days p.i, disease worsened, albeit reducing immunopathology (129).
Murine parainfluenza Sendai virus
Respiratory paramyxoviruses are important causes of morbidity and mortality, particularly of infants and the elderly. Acute murine parainfluenza virus Sendai (SeV) infection is followed by a chronic type 2 immune pathology in the lung similar to that observed in humans (134). mRNA IL-27p28 transcripts are increased in the lungs during infection and IL-27 signaling promoted the generation of IL-10 secreting virus-specific CD8 T cells (135, 136). In addition, IL-27 promoted the development of IL-10 producing antiviral CD4 T cells resulting in the inhibition of the development of Th2 responses that mediates lung pathology during the chronic phase (137).
Human influenza infection
Limited data is available on dynamics of IL-27 in the setting of human influenza infection, IL-27 serum levels were reported to be increased in individuals infected with seasonal IAV (H3N2) and H1N1. The cellular sources IL-27 have been mainly attributed to APCs, however, lung epithelial cells in vitro produce IL-27 after infection with influenza A through a COX-2-derived prostaglandin E2 (PGE2) mechanism. Accordantly, serum levels of IL-27 in individuals infected with 2009 pandemic H1N1, and with seasonal H3N2 were positively correlated with PGE2 (30, 138).
SARS-CoV-2 infection
Severe acute respiratory syndrome coronavirus 2 (SARS-CoV-2) is a new strain of coronavirus that was first reported in December 2019. SARSCoV2 is the cause of the Coronavirus disease 2019 (COVID-19). The pathogenesis of severe disease is associated with an altered activation and regulation of the Type I IFN responses and an exacerbated inflammatory response, which contributes to the lung injury (139–142).
In individuals with COVID-19 disease, increased serum levels of IL-27 have been observed compared to healthy controls. In addition, lower serum levels were associated with severe disease in hospitalized patients in intensive care (143). Another study investigated levels of inflammatory cytokines and their potential association with lung damage and mortality at the time of hospital admission in 108 individuals. This study reported that low serum levels of IL-27 and higher levels of IL-1α and HGF were associated with poor clinical outcomes (144). Contrasting these observations, increased IL-27 subunit (p28 and EBI3) mRNA expression was observed in PBMCs and monocytes from individuals with severe disease (145). All of these findings suggest that the upregulation of IL-27 may contribute to antiviral immunity against SARS-CoV-2 by inducing interferon stimulated genes. However, whether IL-27 production is beneficial or detrimental to the progression to severe COVID-19 remains unclear.
Neurotropic mouse hepatitis virus (JHM strain)
Limiting immunopathology during central nervous system CNS viral infections is critical for the host survival. The murine hepatitis virus (JHMV) has a glia tropism and induces an acute demyelinating encephalomyelitis that leads to viral persistence and chronic demyelination (146). While local IL-10 production by infiltrating T cells limits tissue pathology, it also delayed viral control and elimination. IL-10+virus-specific CD8 T cells were highly activated, produce inflammatory cytokines, and have cytotoxic function suggesting that IL-10 may be a mechanism by which CD8 T cells regulate their own function at sites of tissue injury (147).
Mice deficient in the EBI3 subunit (shared by IL-27 and IL-35) and infected with JHMV showed immune mediated inflammation in the CNS. Accumulation of IFNγ secreting virus-specific CD4 and CD8 T cells lead to tissue damage and reduced survival. Interestingly, despite increased IFN-γ secretion by virus specific CD8 T cells the cytotoxic function was reduced (148). These studies imply that IL-27 and IL-35 may play a role in controlling immunopathology and disease severity by preventing recruitment of effector CD8 T cells.
In addition, IL-27 induces IL-10 production by virus specific CD4 but not CD8 T cells. IL-27R deficiency led to accumulation of IFN-γ secreting virus-specific CD4 and CD8 T cells and reduced IL-10 production by virus-specific CD4 T cells promoting viral control. In contrast, IL-10 production and cytolytic activity of virus-specific CD8 T cells was not reduced nor was there an effect on Tregs. The lack of IL-27 signaling did not enhance viral clearance in the CNS although the mice exhibited less severity of disease (149). These studies contrast with the protective role of IL-27 in limiting tissue pathology.
Discussion
IL-27 antiviral properties against several human viruses have highlighted its potential therapeutic use for the treatment of human viral infections (27, 30). However, how IL-27 mediates antiviral immunity leading to viral clearance and persistence is not well understood (Figure 2).
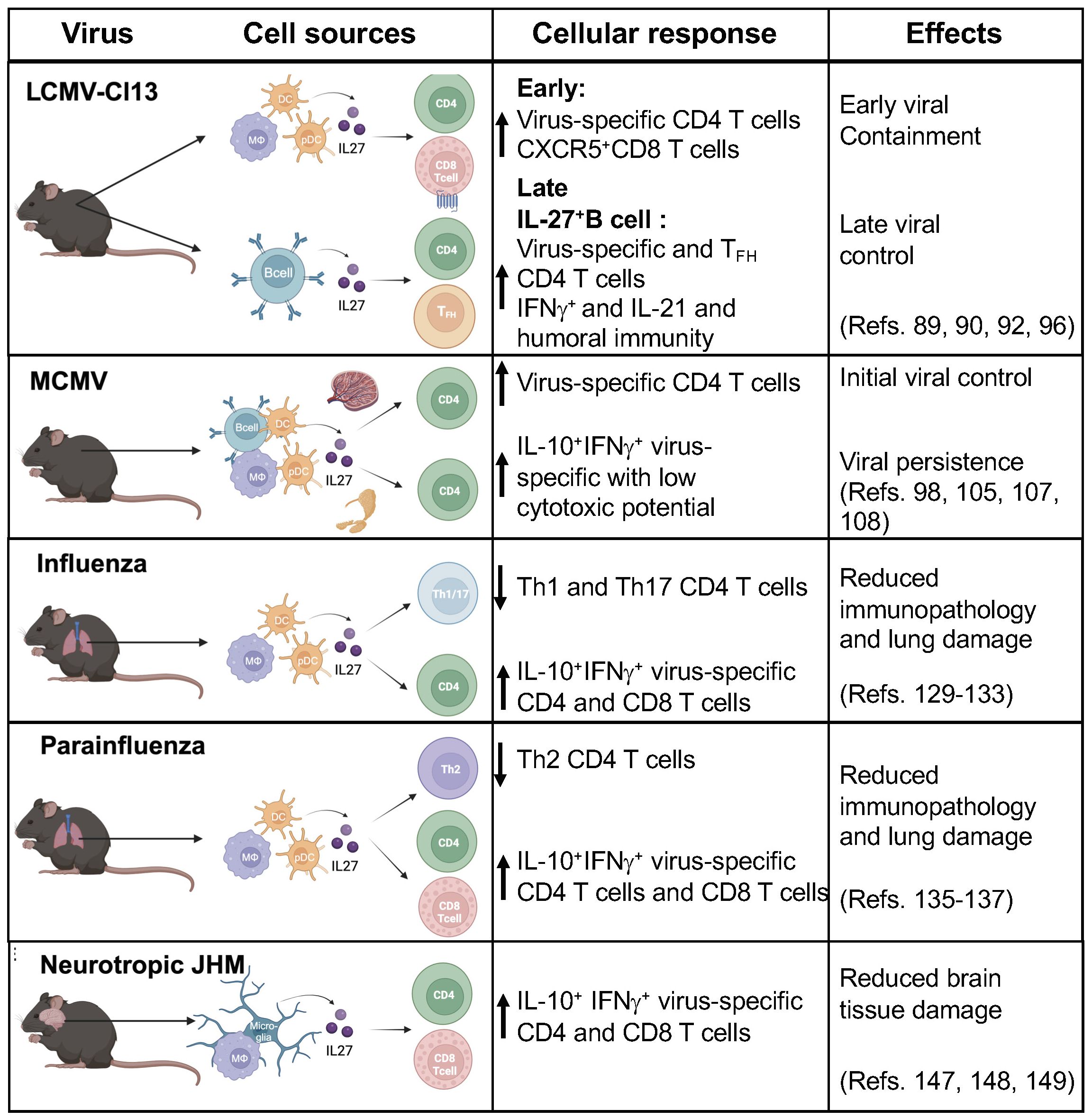
Figure 2 IL-27 cell sources and cellular responses during viral infection. IL-27 has antiviral activities that are prominent early after infection. IL-27 is mainly produced by antigen presenting cells (APCs) including dendritic cells (DCs), plasma cells, macrophages, monocytes, microglia, B cells and endothelial cells. IL-27 plays critical role by modulating adaptive cellular responses against viruses. The beneficial effects include reducing immunopathology and viral control, in contrast in some infections showed a predominant regulatory function leading to viral persistence. (Figure was created with BioRender.com).
The cell sources of IL-27 in human lymph nodes in health and disease, showed that IL-27p28 and EBI3 subunits are coexpressed by macrophages, monocytes, B cells and endothelial cells, however IL-27p28 was not expressed in DCs (68, 69). One consistent observation in lymph nodes and other tissues is the high expression of EBI3 compared to IL-27p28 (Table 1). This has led to the hypothesis that EBI3 may play additional roles including chaperone-like function and/or regulatory functions (47, 67, 70, 71, 81, 82). In humans, in vitro studies showed that IL-27 can be produced by CD4 TFH cells, in the setting of chronic HBV infection, and by Tregs in untreated HIV infection and uninfected controls (125, 150).
More importantly, murine in vivo studies using chronic LCMV infection have revealed important insights to its temporal expression, cell sources and impact in mediating innate and adaptive immune responses. During chronic LCMV infection, DCs and myeloid cells are the predominant sources of IL-27, mediating early viral control. Where lack of IL-27 signaling during this stage of infection leads to higher viral titers compared with wild type animals. IL-27 promotes expansion of virus-specific CXCR5+CD8 T cell and virus-specific CD4 T cell function. In contrast, IL-27 secreted by B cells was relevant at later phase of the infection and facilitated maintenance and function of virus-specific CD4 T cells as well as CD4 TFH function, which promoted humoral immunity and viral clearance (89, 92, 96).
The regulatory functions of IL-27 in the setting of viral infection involves both IL-10 dependent and independent mechanisms. IL-27 induces IL-10+ secretion by IFNγ+virus-specific T cells (Tr1-like cells), controlling exacerbated inflammation and conferring host protection from immunopathology in the lung during influenza infection (129, 133). In contrast, in the setting of MCMV, expression of IL-27 and induction of IL-10 by Tr1-like cells contributed to viral persistence in the latent phase of the infection (98, 105).
In the context of human infections, the role of IL-27 is not well defined and studies of the kinetics of IL-27 expression in vitro and in vivo studies are limited. The detection of IL-27 in serum and plasma showed contradictory results, likely because the measurements of circulating levels of IL-27 has been challenging due to the non-disulfide-linked nature of IL-27 (IL-27p28 and EBI3) (31). Some ELISAs use polyclonal capture antibodies and reported cross-reactivity with recombinant EIB3, given there are potentially other cytokines that express this subunit this data is confounding (41, 46, 151, 152). More reliable tools to detect the heterodimer may allow us better understand its role in human viral infections.
IL-27 may have a potential clinical applications in the setting of viral infections, while its antiviral effects seem broad and synergistic with Type I IFNs, its modulatory effects require further investigation in the setting of viral infections. Current clinical applications of targeting IL-27 are more advanced in the setting of cancer and focus on blocking immunosuppressive activities (49, 153). A human monoclonal (IgG1) antibody against the IL-27p28 subunit CHS388 (former SRF388), that blocks IL-27/IL-27RA interaction is under consideration for cancer treatment, where a Phase I study has already been shown to be safe in humans (1, 154–158). However other studies, focus on the anti-tumor activities of IL-27 (159, 160).
IL-27 signaling modulates several immune cells and there is a need to better define its temporal expression and its cell sources during viral infections. It will be important to fill this gap in knowledge to dissect IL-27’s antiviral effects and modulatory (regulatory vs stimulatory) functions. Determining the outcome of this balance will be beneficial in the setting of human chronic infections including HIV, HBC, HCV and others.
Author contributions
MC: Writing – review & editing, Writing – original draft. FA-M: Writing – original draft. CJ: Visualization, Writing – review & editing.
Funding
The author(s) declare financial support was received for the research, authorship, and/or publication of this article. Research reported in this publication was supported by the National Institute of Allergy and Infectious Diseases of the National Institutes of Health under award number NIH R01AI145549-02.
Acknowledgments
We thank the District of Columbia Center for AIDS Research, an NIH funded program (P30AI117970) which is supported by the following NIH Co-Funding and Participating Institutes and Centers: NIAID, NCI, NICHD, NHLBI, NIDA, NIMH, NIA, NIDDK, NIMHD, NIDCR, NINR, FIC and OAR.
Conflict of interest
The authors declare that the research was conducted in the absence of any commercial or financial relationships that could be construed as a potential conflict of interest.
Publisher’s note
All claims expressed in this article are solely those of the authors and do not necessarily represent those of their affiliated organizations, or those of the publisher, the editors and the reviewers. Any product that may be evaluated in this article, or claim that may be made by its manufacturer, is not guaranteed or endorsed by the publisher.
References
1. Tait Wojno ED, Hunter CA, Stumhofer JS. The immunobiology of the interleukin-12 family: room for discovery. Immunity. (2019) 50:851–70. doi: 10.1016/j.immuni.2019.03.011
2. Ma X, Trinchieri G. Regulation of interleukin-12 production in antigen-presenting cells. Adv Immunol. (2001) 79:55–92.
3. O'Shea JJ, Paul WE. Regulation of T(H)1 differentiation–controlling the controllers. Nat Immunol. (2002) 3:506–8. doi: 10.1038/ni0602-506
4. Hunter CA. New IL-12-family members: IL-23 and IL-27, cytokines with divergent functions. Nat Rev. (2005) 5:521–31. doi: 10.1038/nri1648
5. Vignali DA, Kuchroo VK. IL-12 family cytokines: immunological playmakers. Nat Immunol. (2012) 13:722–8. doi: 10.1038/ni.2366
6. Collison LW, Delgoffe GM, Guy CS, Vignali KM, Chaturvedi V, Fairweather D, et al. The composition and signaling of the IL-35 receptor are unconventional. Nat Immunol. (2012) 13:290–9. doi: 10.1038/ni.2227
7. Shen P, Roch T, Lampropoulou V, O'Connor RA, Stervbo U, Hilgenberg E, et al. IL-35-producing B cells are critical regulators of immunity during autoimmune and infectious diseases. Nature. (2014) 507:366–70. doi: 10.1038/nature12979
8. Wang RX, Yu CR, Dambuza IM, Mahdi RM, Dolinska MB, Sergeev YV, et al. Interleukin-35 induces regulatory B cells that suppress autoimmune disease. Nat Med. (2014) 20:633–41. doi: 10.1038/nm.3554
9. Wang X, Liu X, Zhang Y, Wang Z, Zhu G, Han G, et al. Interleukin (IL)-39 [IL-23p19/Epstein-Barr virus-induced 3 (Ebi3)] induces differentiation/expansion of neutrophils in lupus-prone mice. Clin Exp Immunol. (2016) 186:144–56. doi: 10.1111/cei.12840
10. Pflanz S, Timans JC, Cheung J, Rosales R, Kanzler H, Gilbert J, et al. IL-27, a heterodimeric cytokine composed of EBI3 and p28 protein, induces proliferation of naive CD4+ T cells. Immunity. (2002) 16:779–90. doi: 10.1016/S1074-7613(02)00324-2
11. Villarino AV, Stumhofer JS, Saris CJ, Kastelein RA, Sauvage FJ, Hunter CA. IL-27 limits IL-2 production during Th1 differentiation. J Immunol. (2006) 176:237–47. doi: 10.4049/jimmunol.176.1.237
12. Diveu C, McGeachy MJ, Boniface K, Stumhofer JS, Sathe M, Joyce-Shaikh B, et al. IL-27 blocks RORc expression to inhibit lineage commitment of Th17 cells. J Immunol. (2009) 182:5748–56. doi: 10.4049/jimmunol.0801162
13. Hunter CA, Kastelein R. Interleukin-27: balancing protective and pathological immunity. Immunity. (2012) 37:960–9. doi: 10.1016/j.immuni.2012.11.003
14. Batten M, Ramamoorthi N, Kljavin NM, Ma CS, Cox JH, Dengler HS, et al. IL-27 supports germinal center function by enhancing IL-21 production and the function of T follicular helper cells. J Exp Med. (2010) 207:2895–906. doi: 10.1084/jem.20100064
15. Murugaiyan G, Mittal A, Lopez-Diego R, Maier LM, Anderson DE, Weiner HL. IL-27 is a key regulator of IL-10 and IL-17 production by human CD4+ T cells. J Immunol. (2009) 183:2435–43. doi: 10.4049/jimmunol.0900568
16. Jankovic D, Kugler DG, Sher A. IL-10 production by CD4+ effector T cells: a mechanism for self-regulation. Mucosal Immunol. (2010) 3:239–46. doi: 10.1038/mi.2010.8
17. Awasthi A, Carrier Y, Peron JP, Bettelli E, Kamanaka M, Flavell RA, et al. A dominant function for interleukin 27 in generating interleukin 10-producing anti-inflammatory T cells. Nat Immunol. (2007) 8:1380–9. doi: 10.1038/ni1541
18. Batten M, Kljavin NM, Li J, Walter MJ, Sauvage FJ, Ghilardi N. Cutting edge: IL-27 is a potent inducer of IL-10 but not FoxP3 in murine T cells. J Immunol. (2008) 180:2752–6. doi: 10.4049/jimmunol.180.5.2752
19. Pirhonen J, Siren J, Julkunen I, Matikainen S. IFN-alpha regulates Toll-like receptor-mediated IL-27 gene expression in human macrophages. J Leukoc Biol. (2007) 82:1185–92. doi: 10.1189/jlb.0307157
20. Chen Q, Swaminathan S, Yang D, Dai L, Sui H, Yang J, et al. Interleukin-27 is a potent inhibitor of cis HIV-1 replication in monocyte-derived dendritic cells via a type I interferon-independent pathway. PloS One. (2013) 8:e59194. doi: 10.1371/journal.pone.0059194
21. Dai L, Lidie KB, Chen Q, Adelsberger JW, Zheng X, Huang D, et al. IL-27 inhibits HIV-1 infection in human macrophages by down-regulating host factor SPTBN1 during monocyte to macrophage differentiation. J Exp Med. (2013) 210:517–34. doi: 10.1084/jem.20120572
22. Poudyal D, Yang J, Chen Q, Goswami S, Adelsberger JW, Das S, et al. IL-27 posttranslationally regulates Y-box binding protein-1 to inhibit HIV-1 replication in human CD4+ T cells. AIDS (London England). (2019) 33:1819–30. doi: 10.1097/QAD.0000000000002288
23. Cao Y, Zhang R, Zhang W, Zhu C, Yu Y, Song Y, et al. IL-27, a cytokine, and IFN-lambda1, a type III IFN, are coordinated to regulate virus replication through type I IFN. J Immunol. (2014) 192:691–703. doi: 10.4049/jimmunol.1300252
24. Frank AC, Zhang X, Katsounas A, Bharucha JP, Kottilil S, Imamichi T. Interleukin-27, an anti-HIV-1 cytokine, inhibits replication of hepatitis C virus. J Interferon Cytokine Res. (2010) 30:427–31. doi: 10.1089/jir.2009.0093
25. Heikkila O, Nygardas M, Paavilainen H, Ryodi E, Hukkanen V. Interleukin-27 inhibits herpes simplex virus type 1 infection by activating STAT1 and 3, interleukin-6, and chemokines IP-10 and MIG. J Interferon Cytokine Res. (2016) 36:617–29. doi: 10.1089/jir.2016.0015
26. Swaminathan S, Hu X, Zheng X, Kriga Y, Shetty J, Zhao Y, et al. Interleukin-27 treated human macrophages induce the expression of novel microRNAs which may mediate anti-viral properties. Biochem Biophys Res Commun. (2013) 434:228–34. doi: 10.1016/j.bbrc.2013.03.046
27. Swaminathan S, Dai L, Lane HC, Imamichi T. Evaluating the potential of IL-27 as a novel therapeutic agent in HIV-1 infection. Cytokine Growth Factor Rev. (2013) 24:571–7. doi: 10.1016/j.cytogfr.2013.07.001
28. Tan G, Xiao Q, Song H, Ma F, Xu F, Peng D, et al. Type I IFN augments IL-27-dependent TRIM25 expression to inhibit HBV replication. Cell Mol Immunol. (2018) 15:272–81. doi: 10.1038/cmi.2016.67
29. Kwock JT, Handfield C, Suwanpradid J, Hoang P, McFadden MJ, Labagnara KF, et al. IL-27 signaling activates skin cells to induce innate antiviral proteins and protects against Zika virus infection. Sci Adv. (2020) 6:eaay3245. doi: 10.1126/sciadv.aay3245
30. Amsden H, Kourko O, Roth M, Gee K. Antiviral activities of interleukin-27: A partner for interferons? Front Immunol. (2022) 13:902853. doi: 10.3389/fimmu.2022.902853
31. Jones LL, Vignali DA. Molecular interactions within the IL-6/IL-12 cytokine/receptor superfamily. Immunol Res. (2011) 51:5–14. doi: 10.1007/s12026-011-8209-y
32. Collison LW, Vignali DA. Interleukin-35: odd one out or part of the family? Immunol Rev. (2008) 226:248–62. doi: 10.1111/j.1600-065X.2008.00704.x
33. Collison LW, Workman CJ, Kuo TT, Boyd K, Wang Y, Vignali KM, et al. The inhibitory cytokine IL-35 contributes to regulatory T-cell function. Nature. (2007) 450:566–9. doi: 10.1038/nature06306
34. Niedbala W, Wei XQ, Cai B, Hueber AJ, Leung BP, McInnes IB, et al. IL-35 is a novel cytokine with therapeutic effects against collagen-induced arthritis through the expansion of regulatory T cells and suppression of Th17 cells. Eur J Immunol. (2007) 37:3021–9. doi: 10.1002/eji.200737810
35. Oppmann B, Lesley R, Blom B, Timans JC, Xu Y, Hunte B, et al. Novel p19 protein engages IL-12p40 to form a cytokine, IL-23, with biological activities similar as well as distinct from IL-12. Immunity. (2000) 13:715–25. doi: 10.1016/S1074-7613(00)00070-4
36. Floss DM, Moll JM, Scheller J. IL-12 and IL-23-close relatives with structural homologies but distinct immunological functions. Cells. (2020) 9. doi: 10.3390/cells9102184
37. Lupardus PJ, Garcia KC. The structure of interleukin-23 reveals the molecular basis of p40 subunit sharing with interleukin-12. J Mol Biol. (2008) 382:931–41. doi: 10.1016/j.jmb.2008.07.051
38. Beyer BM, Ingram R, Ramanathan L, Reichert P, Le HV, Madison V, et al. Crystal structures of the pro-inflammatory cytokine interleukin-23 and its complex with a high-affinity neutralizing antibody. J Mol Biol. (2008) 382:942–55. doi: 10.1016/j.jmb.2008.08.001
39. Yoon C, Johnston SC, Tang J, Stahl M, Tobin JF, Somers WS. Charged residues dominate a unique interlocking topography in the heterodimeric cytokine interleukin-12. EMBO J. (2000) 19:3530–41. doi: 10.1093/emboj/19.14.3530
40. Egwuagu CE, Yu CR, Sun L, Wang R. Interleukin 35: Critical regulator of immunity and lymphocyte-mediated diseases. Cytokine Growth Factor Rev. (2015) 26:587–93. doi: 10.1016/j.cytogfr.2015.07.013
41. Crabe S, Guay-Giroux A, Tormo AJ, Duluc D, Lissilaa R, Guilhot F, et al. The IL-27 p28 subunit binds cytokine-like factor 1 to form a cytokine regulating NK and T cell activities requiring IL-6R for signaling. J Immunol. (2009) 183:7692–702. doi: 10.4049/jimmunol.0901464
42. Garbers C, Spudy B, Aparicio-Siegmund S, Waetzig GH, Sommer J, Holscher C, et al. An interleukin-6 receptor-dependent molecular switch mediates signal transduction of the IL-27 cytokine subunit p28 (IL-30) via a gp130 protein receptor homodimer. J Biol Chem. (2013) 288:4346–54. doi: 10.1074/jbc.M112.432955
43. Muller SI, Aschenbrenner I, Zacharias M, Feige MJ. An interspecies analysis reveals molecular construction principles of interleukin 27. J Mol Biol. (2019) 431:2383–93. doi: 10.1016/j.jmb.2019.04.032
44. Liu J, Guan X, Ma X. Regulation of IL-27 p28 gene expression in macrophages through MyD88- and interferon-gamma-mediated pathways. J Exp Med. (2007) 204:141–52. doi: 10.1084/jem.20061440
45. Muller SI, Friedl A, Aschenbrenner I, Bieren Esser-von J, Zacharias M, Devergne O, et al. A folding switch regulates interleukin 27 biogenesis and secretion of its alpha-subunit as a cytokine. Proc Natl Acad Sci United States America. (2019) 116:1585–90.
46. Shimozato O, Sato A, Kawamura K, Chiyo M, Ma G, Li Q, et al. The secreted form of p28 subunit of interleukin (IL)-27 inhibits biological functions of IL-27 and suppresses anti-allogeneic immune responses. Immunology. (2009) 128:e816–825. doi: 10.1111/j.1365-2567.2009.03088.x
47. Watanabe A, Mizoguchi I, Hasegawa H, Katahira Y, Inoue S, Sakamoto E, et al. A chaperone-like role for EBI3 in collaboration with calnexin under inflammatory conditions. Front Immunol. (2021) 12:757669. doi: 10.3389/fimmu.2021.757669
48. Hildenbrand K, Aschenbrenner I, Franke FC, Devergne O, Feige MJ. Biogenesis and engineering of interleukin 12 family cytokines. Trends Biochem Sci. (2022) 47:936–49. doi: 10.1016/j.tibs.2022.05.005
49. Figueiredo ML, Neto Figueiredo M, Salameh JW, Decker RE, Letteri R, Chan-Seng D, et al. Ligand-mediated targeting of cytokine interleukin-27 enhances its bioactivity In Vivo. Mol Ther Methods Clin Dev. (2020) 17:739–51. doi: 10.1016/j.omtm.2020.03.022
50. Pflanz S, Hibbert L, Mattson J, Rosales R, Vaisberg E, Bazan JF, et al. WSX-1 and glycoprotein 130 constitute a signal-transducing receptor for IL-27. J Immunol. (2004) 172:2225–31. doi: 10.4049/jimmunol.172.4.2225
51. Caveney NA, Glassman CR, Jude KM, Tsutsumi N, Garcia KC. Structure of the IL-27 quaternary receptor signaling complex. Elife. (2022) 11. doi: 10.7554/eLife.78463
52. Jin Y, Fyfe PK, Gardner S, Wilmes S, Bubeck D, Moraga I. Structural insights into the assembly and activation of the IL-27 signaling complex. EMBO Rep. (2022) 23:e55450. doi: 10.15252/embr.202255450
53. Lucas S, Ghilardi N, Li J, de Sauvage FJ. IL-27 regulates IL-12 responsiveness of naive CD4+ T cells through Stat1-dependent and -independent mechanisms. Proc Natl Acad Sci United States America. (2003) 100:15047–52. doi: 10.1073/pnas.2536517100
54. Takeda A, Hamano S, Yamanaka A, Hanada T, Ishibashi T, Mak TW, et al. Cutting edge: role of IL-27/WSX-1 signaling for induction of T-bet through activation of STAT1 during initial Th1 commitment. J Immunol. (2003) 170:4886–90. doi: 10.4049/jimmunol.170.10.4886
55. Villarino A, Hibbert L, Lieberman L, Wilson E, Mak T, Yoshida H, et al. The IL-27R (WSX-1) is required to suppress T cell hyperactivity during infection. Immunity. (2003) 19:645–55. doi: 10.1016/S1074-7613(03)00300-5
56. Kastelein RA, Hunter CA, Cua DJ. Discovery and biology of IL-23 and IL-27: related but functionally distinct regulators of inflammation. Annu Rev Immunol. (2007) 25:221–42. doi: 10.1146/annurev.immunol.22.012703.104758
57. Hibbert L, Pflanz S, De Waal Malefyt R, Kastelein RA. IL-27 and IFN-alpha signal via Stat1 and Stat3 and induce T-Bet and IL-12Rbeta2 in naive T cells. J Interferon Cytokine Res. (2003) 23:513–22. doi: 10.1089/10799900360708632
58. Owaki T, Asakawa M, Morishima N, Mizoguchi I, Fukai F, Takeda K, et al. STAT3 is indispensable to IL-27-mediated cell proliferation but not to IL-27-induced Th1 differentiation and suppression of proinflammatory cytokine production. J Immunol. (2008) 180:2903–11. doi: 10.4049/jimmunol.180.5.2903
59. Kamiya S, Owaki T, Morishima N, Fukai F, Mizuguchi J, Yoshimoto T. An indispensable role for STAT1 in IL-27-induced T-bet expression but not proliferation of naive CD4+ T cells. J Immunol. (2004) 173:3871–7. doi: 10.4049/jimmunol.173.6.3871
60. Hirahara K, Onodera A, Villarino AV, Bonelli M, Sciume G, Laurence A, et al. Asymmetric action of STAT transcription factors drives transcriptional outputs and cytokine specificity. Immunity. (2015) 42:877–89. doi: 10.1016/j.immuni.2015.04.014
61. Cheng J, Myers TG, Levinger C, Kumar P, Kumar J, Goshu BA. IL-27 induces IFN/STAT1-dependent genes and enhances function of TIGIT(+) HIVGag-specific T cells. iScience. (2022) 25:103588. doi: 10.1016/j.isci.2021.103588
62. Yoshida H, Hunter CA. The immunobiology of interleukin-27. Annu Rev Immunol. (2015) 33:417–43. doi: 10.1146/annurev-immunol-032414-112134
63. Hall AO, Silver JS, Hunter CA. The immunobiology of IL-27. Adv Immunol. (2012) 115:1–44. doi: 10.1016/B978-0-12-394299-9.00001-1
64. Kalliolias GD, Ivashkiv LB. IL-27 activates human monocytes via STAT1 and suppresses IL-10 production but the inflammatory functions of IL-27 are abrogated by TLRs and p38. J Immunol. (2008) 180:6325–33. doi: 10.4049/jimmunol.180.9.6325
65. Devergne O, Hummel M, Koeppen H, Beau Le MM, Nathanson EC, Kieff E, et al. A novel interleukin-12 p40-related protein induced by latent Epstein-Barr virus infection in B lymphocytes. J Virol. (1996) 70:1143–53. doi: 10.1128/jvi.70.2.1143-1153.1996
66. Yoshida H, Hamano S, Senaldi G, Covey T, Faggioni R, Mu S, et al. WSX-1 is required for the initiation of Th1 responses and resistance to L. major infection. Immunity. (2001) 15:569–78. doi: 10.1016/S1074-7613(01)00206-0
67. Coulomb-L'Hermine A, Larousserie F, Pflanz S, Bardel E, Kastelein RA, Devergne O. Expression of interleukin-27 by human trophoblast cells. Placenta. (2007) 28:1133–40. doi: 10.1016/j.placenta.2007.06.004
68. Morandi F, Di Carlo E, Ferrone S, Petretto A, Pistoia V, Airoldi I. IL-27 in human secondary lymphoid organs attracts myeloid dendritic cells and impairs HLA class I-restricted antigen presentation. J Immunol. (2014) 192:2634–42. doi: 10.4049/jimmunol.1302656
69. Larousserie F, Pflanz S, Coulomb-L'Hermine A, Brousse N, Kastelein R, Devergne O. Expression of IL-27 in human Th1-associated granulomatous diseases. J Pathol. (2004) 202:164–71. doi: 10.1002/path.1508
70. Devergne O, Coulomb-L'Hermine A, Capel F, Moussa M, Capron F. Expression of Epstein-Barr virus-induced gene 3, an interleukin-12 p40-related molecule, throughout human pregnancy: involvement of syncytiotrophoblasts and extravillous trophoblasts. Am J Pathol. (2001) 159:1763–76. doi: 10.1016/S0002-9440(10)63023-4
71. Cassatella MA, Gardiman E, Arruda-Silva F, Bianchetto-Aguilera F, Gasperini S, Bugatti M, et al. Human neutrophils activated by TLR8 agonists, with or without IFNgamma, synthesize and release EBI3, but not IL-12, IL-27, IL-35, or IL-39. J Leukoc Biol. (2020) 108:1515–26. doi: 10.1002/JLB.3MA0520-054R
72. Senecal V, Deblois G, Beauseigle D, Schneider R, Brandenburg J, Newcombe J, et al. Production of IL-27 in multiple sclerosis lesions by astrocytes and myeloid cells: Modulation of local immune responses. Glia. (2016) 64:553–69.
73. Lalive PH, Kreutzfeldt M, Devergne O, Metz I, Bruck W, Merkler D, et al. Increased interleukin-27 cytokine expression in the central nervous system of multiple sclerosis patients. J Neuroinflamm. (2017) 14:144. doi: 10.1186/s12974-017-0919-1
74. Tanida S, Yoshitomi H, Ishikawa M, Kasahara T, Murata K, Shibuya H, et al. IL-27-producing CD14(+) cells infiltrate inflamed joints of rheumatoid arthritis and regulate inflammation and chemotactic migration. Cytokine. (2011) 55:237–44. doi: 10.1016/j.cyto.2011.04.020
75. Nortey AN, Garces KN, Hackam AS. Exploring the role of interleukin-27 as a regulator of neuronal survival in central nervous system diseases. Neural Regener Res. (2022) 17:2149–52. doi: 10.4103/1673-5374.336134
76. Hirase T, Hara H, Miyazaki Y, Ide N, Nishimoto-Hazuku A, Fujimoto H, et al. Interleukin 27 inhibits atherosclerosis via immunoregulation of macrophages in mice. Am J Physiol Heart Circulatory Physiol. (2013) 305:H420–429. doi: 10.1152/ajpheart.00198.2013
77. Airoldi I, Tupone MG, Esposito S, Russo MV, Barbarito G, Cipollone G, et al. Interleukin-27 re-educates intratumoral myeloid cells and down-regulates stemness genes in non-small cell lung cancer. Oncotarget. (2015) 6:3694–708. doi: 10.18632/oncotarget.v6i6
78. Kourko O, Seaver K, Odoardi N, Basta S, Gee K. IL-27, IL-30, and IL-35: A cytokine triumvirate in cancer. Front Oncol. (2019) 9:969. doi: 10.3389/fonc.2019.00969
79. Wang Q, Li D, Cao G, Shi Q, Zhu J, Zhang M, et al. IL-27 signalling promotes adipocyte thermogenesis and energy expenditure. Nature. (2021) 600:314–8. doi: 10.1038/s41586-021-04127-5
80. Wang T, Chen Y, Nie H, Huang Y, Zhao Y, Yang J. IL-27 inhibits non-small-cell lung cancer cell metastasis by miR-935 in vitro. OncoTargets Ther. (2019) 12:1447–54. doi: 10.2147/OTT
81. Gonin J, Larousserie F, Bastard C, Picquenot JM, Couturier J, Radford-Weiss I, et al. Epstein-Barr virus-induced gene 3 (EBI3): a novel diagnosis marker in Burkitt lymphoma and diffuse large B-cell lymphoma. PloS One. (2011) 6:e24617. doi: 10.1371/journal.pone.0024617
82. Nishino R, Takano A, Oshita H, Ishikawa N, Akiyama H, Ito H, et al. Identification of Epstein-Barr virus-induced gene 3 as a novel serum and tissue biomarker and a therapeutic target for lung cancer. Clin Cancer Res. (2011) 17:6272–86. doi: 10.1158/1078-0432.CCR-11-0060
83. Brooks DG, McGavern DB, Oldstone MB. Reprogramming of antiviral T cells prevents inactivation and restores T cell activity during persistent viral infection. J Clin Invest. (2006) 116:1675–85. doi: 10.1172/JCI26856
84. Wherry EJ, Ha SJ, Kaech SM, Haining WN, Sarkar S, Kalia V, et al. Molecular signature of CD8+ T cell exhaustion during chronic viral infection. Immunity. (2007) 27:670–84. doi: 10.1016/j.immuni.2007.09.006
85. Shin H, Wherry EJ. CD8 T cell dysfunction during chronic viral infection. Curr Opin Immunol. (2007) 19:408–15. doi: 10.1016/j.coi.2007.06.004
86. Blackburn SD, Wherry EJ. IL-10, T cell exhaustion and viral persistence. Trends Microbiol. (2007) 15:143–6. doi: 10.1016/j.tim.2007.02.006
87. Matloubian M, Concepcion RJ, Ahmed R. CD4+ T cells are required to sustain CD8+ cytotoxic T-cell responses during chronic viral infection. J Virol. (1994) 68:8056–63. doi: 10.1128/jvi.68.12.8056-8063.1994
88. Elsaesser H, Sauer K, Brooks DG. IL-21 is required to control chronic viral infection. Sci (New York N.Y. (2009) 324:1569–72. doi: 10.1126/science.1174182
89. Harker JA, Wong KA, Dallari S, Bao P, Dolgoter A, Jo Y, et al. Interleukin-27R signaling mediates early viral containment and impacts innate and adaptive immunity after chronic lymphocytic choriomeningitis virus infection. J Virol. (2018) 92. doi: 10.1128/JVI.02196-17
90. Harker JA, Dolgoter A, Zuniga EI. Cell-intrinsic IL-27 and gp130 cytokine receptor signaling regulates virus-specific CD4(+) T cell responses and viral control during chronic infection. Immunity. (2013) 39:548–59. doi: 10.1016/j.immuni.2013.08.010
91. Teijaro JR, Ng C, Lee AM, Sullivan BM, Sheehan KC, Welch M, et al. Persistent LCMV infection is controlled by blockade of type I interferon signaling. Sci (New York N.Y. (2013) 340:207–11. doi: 10.1126/science.1235214
92. Huang Z, Zak J, Pratumchai I, Shaabani N, Vartabedian VF, Nguyen N, et al. IL-27 promotes the expansion of self-renewing CD8(+) T cells in persistent viral infection. J Exp Med. (2019) 216:1791–808. doi: 10.1084/jem.20190173
93. Harker JA, Greene TT, Barnett BE, Bao P, Dolgoter A, Zuniga EI. IL-6 and IL-27 play both distinct and redundant roles in regulating CD4 T-cell responses during chronic viral infection. Front Immunol. (2023) 14:1221562. doi: 10.3389/fimmu.2023.1221562
94. Im SJ, Hashimoto M, Gerner MY, Lee J, Kissick HT, Burger MC, et al. Defining CD8+ T cells that provide the proliferative burst after PD-1 therapy. Nature. (2016) 537:417–21. doi: 10.1038/nature19330
95. Utzschneider DT, Charmoy M, Chennupati V, Pousse L, Ferreira DP, Calderon-Copete S, et al. T cell factor 1-expressing memory-like CD8(+) T cells sustain the immune response to chronic viral infections. Immunity. (2016) 45:415–27. doi: 10.1016/j.immuni.2016.07.021
96. Pratumchai I, Zak J, Huang Z, Min B, Oldstone MBA, Teijaro JR. B cell-derived IL-27 promotes control of persistent LCMV infection. Proc Natl Acad Sci United States America. (2022) 119. doi: 10.1073/pnas.2116741119
97. Walton SM, Mandaric S, Torti N, Zimmermann A, Hengel H, Oxenius A. Absence of cross-presenting cells in the salivary gland and viral immune evasion confine cytomegalovirus immune control to effector CD4 T cells. PloS Pathog. (2011) 7:e1002214. doi: 10.1371/journal.ppat.1002214
98. Wehrens EJ, Wong KA, Gupta A, Khan A, Benedict CA, Zuniga EI. IL-27 regulates the number, function and cytotoxic program of antiviral CD4 T cells and promotes cytomegalovirus persistence. PloS One. (2018) 13:e0201249. doi: 10.1371/journal.pone.0201249
99. Griffiths P, Reeves M. Pathogenesis of human cytomegalovirus in the immunocompromised host. Nat Rev Microbiol. (2021) 19:759–73. doi: 10.1038/s41579-021-00582-z
100. Poole E, Neves TC, Oliveira MT, Sinclair J, da Silva MCC. Human cytomegalovirus interleukin 10 homologs: facing the immune system. Front Cell infection Microbiol. (2020) 10:245. doi: 10.3389/fcimb.2020.00245
101. Kotenko SV, Saccani S, Izotova LS, Mirochnitchenko OV, Pestka S. Human cytomegalovirus harbors its own unique IL-10 homolog (cmvIL-10). Proc Natl Acad Sci United States America. (2000) 97:1695–700. doi: 10.1073/pnas.97.4.1695
102. Walton SM, Wyrsch P, Munks MW, Zimmermann A, Hengel H, Hill AB, et al. The dynamics of mouse cytomegalovirus-specific CD4 T cell responses during acute and latent infection. J Immunol. (2008) 181:1128–34. doi: 10.4049/jimmunol.181.2.1128
103. Soto-Heredero G, Gomez de Las Heras MM, Escrig-Larena JI, Mittelbrunn M. Extremely differentiated T cell subsets contribute to tissue deterioration during aging. Annu Rev Immunol. (2023) 41:181–205. doi: 10.1146/annurev-immunol-101721-064501
104. Leng SX, Margolick JB. Aging, sex, inflammation, frailty, and CMV and HIV infections. Cell Immunol. (2020) 348:104024. doi: 10.1016/j.cellimm.2019.104024
105. Clement M, Marsden M, Stacey MA, Abdul-Karim J, Gimeno Brias S, Costa Bento D, et al. Cytomegalovirus-specific IL-10-producing CD4+ T cells are governed by type-I IFN-induced IL-27 and promote virus persistence. PloS Pathog. (2016) 12:e1006050. doi: 10.1371/journal.ppat.1006050
106. Pot C, Apetoh L, Awasthi A, Kuchroo VK. Molecular pathways in the induction of interleukin-27-driven regulatory type 1 cells. J Interferon Cytokine Res. (2010) 30:381–8. doi: 10.1089/jir.2010.0047
107. Jost NH, Abel S, Hutzler M, Sparwasser T, Zimmermann A, Roers A, et al. Regulatory T cells and T-cell-derived IL-10 interfere with effective anti-cytomegalovirus immune response. Immunol Cell Biol. (2014) 92:860–71. doi: 10.1038/icb.2014.62
108. Humphreys IR, de Trez C, Kinkade A, Benedict CA, Croft M, Ware CF, et al. Cytomegalovirus exploits IL-10-mediated immune regulation in the salivary glands. J Exp Med. (2007) 204:1217–25. doi: 10.1084/jem.20062424
109. Chihara N, Madi A, Karwacz K, Awasthi A, Kuchroo VK. Differentiation and characterization of tr1 cells. Curr Protoc Immunol / edited by John E. Coligan … [et al.]. (2016) 113:3 27 21–23 27 10. doi: 10.1002/0471142735.im0327s113
110. Sylwester AW, Mitchell BL, Edgar JB, Taormina C, Pelte C, Ruchti F, et al. Broadly targeted human cytomegalovirus-specific CD4+ and CD8+ T cells dominate the memory compartments of exposed subjects. J Exp Med. (2005) 202:673–85. doi: 10.1084/jem.20050882
111. Casazza JP, Betts MR, Price DA, Precopio ML, Ruff LE, Brenchley JM, et al. Acquisition of direct antiviral effector functions by CMV-specific CD4+ T lymphocytes with cellular maturation. J Exp Med. (2006) 203:2865–77. doi: 10.1084/jem.20052246
112. Pachnio A, Ciaurriz M, Begum J, Lal N, Zuo J, Beggs A, et al. Cytomegalovirus infection leads to development of high frequencies of cytotoxic virus-specific CD4+ T cells targeted to vascular endothelium. PloS Pathog. (2016) 12:e1005832. doi: 10.1371/journal.ppat.1005832
113. Bolovan-Fritts CA, Trout RN, Spector SA. Human cytomegalovirus-specific CD4+-T-cell cytokine response induces fractalkine in endothelial cells. J Virol. (2004) 78:13173–81. doi: 10.1128/JVI.78.23.13173-13181.2004
114. Bolovan-Fritts CA, Trout RN, Spector SA. High T-cell response to human cytomegalovirus induces chemokine-mediated endothelial cell damage. Blood. (2007) 110:1857–63. doi: 10.1182/blood-2007-03-078881
115. Bolovan-Fritts CA, Spector SA. Endothelial damage from cytomegalovirus-specific host immune response can be prevented by targeted disruption of fractalkine-CX3CR1 interaction. Blood. (2008) 111:175–82. doi: 10.1182/blood-2007-08-107730
116. Imamichi T, Yang J, Huang DW, Brann TW, Fullmer BA, Adelsberger JW, et al. IL-27, a novel anti-HIV cytokine, activates multiple interferon-inducible genes in macrophages. AIDS (London England). (2008) 22:39–45. doi: 10.1097/QAD.0b013e3282f3356c
117. Fakruddin JM, Lempicki RA, Gorelick RJ, Yang J, Adelsberger JW, Garcia-Pineres AJ, et al. Noninfectious papilloma virus-like particles inhibit HIV-1 replication: implications for immune control of HIV-1 infection by IL-27. Blood. (2007) 109:1841–9.
118. Williams DW, Engle EL, Shirk EN, Queen SE, Gama L, Mankowski JL, et al. Splenic damage during SIV infection: role of T-cell depletion and macrophage polarization and infection. Am J Pathol. (2016) 186:2068–87. doi: 10.1016/j.ajpath.2016.03.019
119. Moukambi F, Rabezanahary H, Fortier Y, Rodrigues V, Clain J, Benmadid-Laktout G, et al. Mucosal T follicular helper cells in SIV-infected rhesus macaques: contributing role of IL-27. Mucosal Immunol. (2019) 12:1038–54. doi: 10.1038/s41385-019-0174-0
120. Swaminathan S, Hu Z, Rupert AW, Higgins JM, Dewar RL, Stevens R, et al. Plasma interleukin-27 (IL-27) levels are not modulated in patients with chronic HIV-1 infection. PloS One. (2014) 9:e98989. doi: 10.1371/journal.pone.0098989
121. He L, Zhao J, Wang MH, Siu KK, Gan YX, Chen L, et al. Interleukin-27 is differentially associated with HIV viral load and CD4+ T cell counts in therapy-naive HIV-mono-infected and HIV/HCV-co-infected Chinese. PloS One. (2014) 9:e96792. doi: 10.1371/journal.pone.0096792
122. Zheng YH, Xiao SL, He B, He Y, Zhou HY, Chen Z, et al. The role of IL-27 and its receptor in the pathogenesis of HIV/AIDS and anti-viral immune response. Curr HIV Res. (2017) 15:279–84. doi: 10.2174/1570162X15666170517130339
123. Garg A, Trout R, Spector SA. Human immunodeficiency virus type-1 myeloid derived suppressor cells inhibit cytomegalovirus inflammation through interleukin-27 and B7-H4. Sci Rep. (2017) 7:44485. doi: 10.1038/srep44485
124. Ruiz-Riol M, Berdnik D, Llano A, Mothe B, Galvez C, Perez-Alvarez S, et al. Identification of interleukin-27 (IL-27)/IL-27 receptor subunit alpha as a critical immune axis for In Vivo HIV Control. J Virol. (2017) 91. doi: 10.1128/JVI.00441-17
125. Kwon DS, Angin M, Hongo T, Law KM, Johnson J, Porichis F, et al. CD4+ CD25+ regulatory T cells impair HIV-1-specific CD4 T cell responses by upregulating interleukin-10 production in monocytes. J Virol. (2012) 86:6586–94. doi: 10.1128/JVI.06251-11
126. Clement M, Ladell K, Miners KL, Marsden M, Chapman L, Cardus Figueras A, et al. Inhibitory IL-10-producing CD4(+) T cells are T-bet-dependent and facilitate cytomegalovirus persistence via coexpression of arginase-1. Elife. (2023) 12. doi: 10.7554/eLife.79165
127. Uyeki TM, Hui DS, Zambon M, Wentworth DE, Monto AS. Influenza. Lancet. (2022) 400:693–706. doi: 10.1016/S0140-6736(22)00982-5
128. Moore KW, de Waal Malefyt R, Coffman RL, O'Garra A. Interleukin-10 and the interleukin-10 receptor. Annu Rev Immunol. (2001) 19:683–765. doi: 10.1146/annurev.immunol.19.1.683
129. Liu FD, Kenngott EE, Schroter MF, Kuhl A, Jennrich S, Watzlawick R, et al. Timed action of IL-27 protects from immunopathology while preserving defense in influenza. PloS Pathog. (2014) 10:e1004110. doi: 10.1371/journal.ppat.1004110
130. Sun J, Madan R, Karp CL, Braciale TJ. Effector T cells control lung inflammation during acute influenza virus infection by producing IL-10. Nat Med. (2009) 15:277–84. doi: 10.1038/nm.1929
131. Abbott CA, Freimayer EL, Tyllis TS, Norton TS, Alsharifi M, Heng AHS, et al. Determination of Tr1 cell populations correlating with distinct activation states in acute IAV infection. Mucosal Immunol. (2023). doi: 10.1016/j.mucimm.2023.06.003
132. Mayer KD, Mohrs K, Reiley W, Wittmer S, Kohlmeier JE, Pearl JE, et al. Cutting edge: T-bet and IL-27R are critical for in vivo IFN-gamma production by CD8 T cells during infection. J Immunol. (2008) 180:693–7. doi: 10.4049/jimmunol.180.2.693
133. Sun J, Dodd H, Moser EK, Sharma R, Braciale TJ. CD4+ T cell help and innate-derived IL-27 induce Blimp-1-dependent IL-10 production by antiviral CTLs. Nat Immunol. (2011) 12:327–34. doi: 10.1038/ni.1996
134. Garcia GL, Valenzuela A, Manzoni T, Vaughan AE, Lopez CB. Distinct chronic post-viral lung diseases upon infection with influenza or parainfluenza viruses differentially impact superinfection outcome. Am J Pathol. (2020) 190:543–53. doi: 10.1016/j.ajpath.2019.11.003
135. Kim WK, Jain D, Sanchez MD, Koziol-White CJ, Matthews K, Ge MQ, et al. Deficiency of melanoma differentiation-associated protein 5 results in exacerbated chronic postviral lung inflammation. Am J Respir Crit Care Med. (2014) 189:437–48. doi: 10.1164/rccm.201307-1338OC
136. Perona-Wright G, Kohlmeier JE, Bassity E, Freitas TC, Mohrs K, Cookenham T, et al. Persistent loss of IL-27 responsiveness in CD8+ memory T cells abrogates IL-10 expression in a recall response. Proc Natl Acad Sci United States America. (2012) 109:18535–40. doi: 10.1073/pnas.1119133109
137. Muallem G, Wagage S, Sun Y, DeLong JH, Valenzuela A, Christian DA, et al. IL-27 limits type 2 immunopathology following parainfluenza virus infection. PloS Pathog. (2017) 13:e1006173. doi: 10.1371/journal.ppat.1006173
138. Liu L, Cao Z, Chen J, Li R, Cao Y, Zhu C, et al. Influenza A virus induces interleukin-27 through cyclooxygenase-2 and protein kinase A signaling. J Biol Chem. (2012) 287:11899–910. doi: 10.1074/jbc.M111.308064
139. Hadjadj J, Yatim N, Barnabei L, Corneau A, Boussier J, Smith N, et al. Impaired type I interferon activity and inflammatory responses in severe COVID-19 patients. Sci (New York N.Y. (2020) 369:718–24. doi: 10.1126/science.abc6027
140. Galani IE, Rovina N, Lampropoulou V, Triantafyllia V, Manioudaki M, Pavlos E, et al. Untuned antiviral immunity in COVID-19 revealed by temporal type I/III interferon patterns and flu comparison. Nat Immunol. (2021) 22:32–40. doi: 10.1038/s41590-020-00840-x
141. Domizio JD, Gulen MF, Saidoune F, Thacker VV, Yatim A, Sharma K, et al. The cGAS-STING pathway drives type I IFN immunopathology in COVID-19. Nature. (2022) 603:145–51. doi: 10.1038/s41586-022-04421-w
142. Lamers MM, Haagmans BL. SARS-coV-2 pathogenesis. Nat Rev Microbiol. (2022) 20:270–84. doi: 10.1038/s41579-022-00713-0
143. Zamani B, Najafizadeh M, Motedayyen H, Arefnezhad R. Predicting roles of IL-27 and IL-32 in determining the severity and outcome of COVID-19. Int J Immunopathol Pharmacol. (2022) 36:3946320221145827. doi: 10.1177/03946320221145827
144. Tamayo-Velasco A, Martinez-Paz P, Penarrubia-Ponce MJ, Fuente I, Perez-Gonzalez S, Fernandez I, et al. HGF, IL-1alpha, and IL-27 are robust biomarkers in early severity stratification of COVID-19 patients. J Clin Med. (2021) 10. doi: 10.21203/rs.3.rs-374650/v1
145. Valdes-Lopez JF, Urcuqui-Inchima S. Antiviral response and immunopathogenesis of interleukin 27 in COVID-19. Arch Virol. (2023) 168:178. doi: 10.1007/s00705-023-05792-9
146. Matthews AE, Weiss SR, Paterson Y. Murine hepatitis virus–a model for virus-induced CNS demyelination. J Neurovirol. (2002) 8:76–85. doi: 10.1080/13550280290049534
147. Trandem K, Zhao J, Fleming E, Perlman S. Highly activated cytotoxic CD8 T cells express protective IL-10 at the peak of coronavirus-induced encephalitis. J Immunol. (2011) 186:3642–52. doi: 10.4049/jimmunol.1003292
148. Tirotta E, Duncker P, Oak J, Klaus S, Tsukamoto MR, Gov L, et al. Epstein-Barr virus-induced gene 3 negatively regulates neuroinflammation and T cell activation following coronavirus-induced encephalomyelitis. J Neuroimmunol. (2013) 254:110–6. doi: 10.1016/j.jneuroim.2012.10.005
149. de Aquino MT, Kapil P, Hinton DR, Phares TW, Puntambekar SS, Savarin C, et al. IL-27 limits central nervous system viral clearance by promoting IL-10 and enhances demyelination. J Immunol. (2014) 193:285–94. doi: 10.4049/jimmunol.1400058
150. Khanam A, Ayithan N, Tang L, Poonia B, Kottilil S. IL-21-deficient T follicular helper cells support B cell responses through IL-27 in patients with chronic hepatitis B. Front Immunol. (2020) 11:599648.
151. Tormo AJ, Meliani Y, Beaupre LA, Sharma M, Fritz JH, Elson G, et al. The composite cytokine p28/cytokine-like factor 1 sustains B cell proliferation and promotes plasma cell differentiation. J Immunol. (2013) 191:1657–65. doi: 10.4049/jimmunol.1201595
152. Stumhofer JS, Tait ED, Quinn WJ 3rd, Hosken N, Spudy B, Goenka R, et al. A role for IL-27p28 as an antagonist of gp130-mediated signaling. Nat Immunol. (2010) 11:1119–26. doi: 10.1038/ni.1957
153. Figueiredo ML. Editorial: IL-27 expression following TLR activation in bone: sounding the alarm for repair. J Leukoc Biol. (2017) 101:1276–9. doi: 10.1189/jlb.3CE1016-442RR
154. Skladanowska K, Bloch Y, Strand J, White KF, Hua J, Aldridge D, et al. Structural basis of activation and antagonism of receptor signaling mediated by interleukin-27. Cell Rep. (2022) 41:111490. doi: 10.1016/j.celrep.2022.111490
155. Patnaik A, Morgensztern D, Mantia C, Tannir NM, Harshman LC, Hill J, et al. Results of a phase 1 study of SRF388, a first-in-human, first-in-class, high-affinity anti-IL-27 antibody in advanced solid tumors. J Clin Oncol. (2021) 39. doi: 10.1200/JCO.2021.39.15_suppl.2551
156. Andrews C, McLean MH, Durum SK. Interleukin-27 as a novel therapy for inflammatory bowel disease: A critical review of the literature. Inflamm Bowel Dis. (2016) 22:2255–64. doi: 10.1097/MIB.0000000000000818
157. DeLong JH, Hall AO, Konradt C, Coppock GM, Park J, Harms Pritchard G, et al. Cytokine- and TCR-mediated regulation of T cell expression of ly6C and sca-1. J Immunol. (2018) 200:1761–70. doi: 10.4049/jimmunol.1701154
158. Chihara N, Madi A, Kondo T, Zhang H, Acharya N, Singer M, et al. Induction and transcriptional regulation of the co-inhibitory gene module in T cells. Nature. (2018) 558:454–9. doi: 10.1038/s41586-018-0206-z
159. Hisada M, Kamiya S, Fujita K, Belladonna ML, Aoki T, Koyanagi Y, et al. Potent antitumor activity of interleukin-27. Cancer Res. (2004) 64:1152–6. doi: 10.1158/0008-5472.CAN-03-2084
Keywords: IL-27 (interleukin 27), CD8 T cells, IL-27 viral infection, IL-27 viral immunity, IL-27/IL-27R
Citation: Andres-Martin F, James C and Catalfamo M (2024) IL-27 expression regulation and its effects on adaptive immunity against viruses. Front. Immunol. 15:1395921. doi: 10.3389/fimmu.2024.1395921
Received: 04 March 2024; Accepted: 03 June 2024;
Published: 20 June 2024.
Edited by:
Vikas Duhan, The University of Queensland, AustraliaReviewed by:
Melissa Govender, University of Oxford, United KingdomYongping Chen, First Affiliated Hospital of Wenzhou Medical University, China
Copyright © 2024 Andres-Martin, James and Catalfamo. This is an open-access article distributed under the terms of the Creative Commons Attribution License (CC BY). The use, distribution or reproduction in other forums is permitted, provided the original author(s) and the copyright owner(s) are credited and that the original publication in this journal is cited, in accordance with accepted academic practice. No use, distribution or reproduction is permitted which does not comply with these terms.
*Correspondence: Marta Catalfamo, mc2151@georgetown.edu