- 1Pediatrics, “Grigore T. Popa” University of Medicine and Pharmacy, Iasi, Romania
- 2Clinical Medical Department, Faculty of Medicine and Pharmacy, “Dunarea de Jos” University, Galati, Romania
- 3Faculty of Medicine, “Grigore T. Popa” University of Medicine and Pharmacy, Iasi, Romania
- 4Pediatrics, “George Emil Palade” University of Medicine, Pharmacy, Science and Technology, Targu Mures, Romania
- 5Pediatrics, Nicolae Testemitanu State University of Medicine and Pharmacy, Chisinau, Moldova
- 6Pediatrics, Faculty of Medicine, “Ovidius” University, Constanta, Romania
Despite the early recognition of obesity as an epidemic with global implications, research on its pathogenesis and therapeutic approach is still on the rise. The literature of the 21st century records an excess weight found in up to 1/3 of children. Both the determining factors and its systemic effects are multiple and variable. Regarding its involvement in the potentiation of cardio-vascular, pulmonary, digestive, metabolic, neuro-psychic or even dermatological diseases, the information is already broadly outlined. The connection between the underlying disease and the associated comorbidities seems to be partially attributable to oxidative stress. In addition to these, and in the light of the recent COVID-19 pandemic, the role played by oxidative stress in the induction, maintenance and potentiation of chronic inflammation among overweight children and adolescents becomes a topic of interest again. Thus, this review’s purpose is to update general data on obesity, with an emphasis on the physiopathological mechanisms that underlie it and involve oxidative stress. At the same time, we briefly present the latest principles of pathology diagnosis and management. Among these, we will mainly emphasize the impact played by endogenous and exogenous antioxidants in the evolutionary course of pediatric obesity. In order to achieve our objectives, we will refer to the most recent studies published in the specialized literature.
1 Introduction
Being defined as an increase in the body mass index (BMI) above the 95th percentile/at +1-2 standard deviations compared to the reference average for age and sex, overweight or obesity is a chronic condition with multisystemic effects. Thus, it is frequently associated with hypertension, metabolic disorders (e.g., dyslipidemia, prediabetes or type 2 diabetes), neuro-psychological, gastroenterological (e.g., non-alcoholic fatty liver, gallstones, gastroesophageal reflux), renal, respiratory, orthopedic damage or dermatological (e.g., atopic dermatitis, acanthosis nigricans) (1–6). Also, a positive correlation was also identified with regard to sleep apnea or the increase in the flu severity score among children with obesity (7, 8).
Recent estimates according to the World Obesity Atlas 2023 place pediatric obesity as a chronic condition that affects 2.6 million children under the age of 5 and up to 175 million children between the ages of 5 and 19. The gender ratio in the second category is against girls (103 million cases) (9). Regarding the division by sex, it is certified that girls/women present an increased risk compared to boys partly due to hormonal variability (10). The highest incidence is noted in Western countries, while in low-income countries or in countries with a low development index, pediatric obesity is better represented among families with above-average income (11). An additive risk factor is identified among “medically complex” children, namely those children with chronic health conditions, significant functional limitations and increased risk of hospitalization (12). The prevalence of childhood obesity has grown rapidly in the last 40 years, doubling. According to current trends, it is predicted that in approximately 30 years, up to 25% of children will suffer from excessive weight gain (10, 13). In addition, the literature attests to the direct risk of developing obesity in adult life of the population with excess weight in childhood (14). In this situation, the pediatrician has a key role in reducing the global effects of obesity. Due to the nature of the population, he cares for, knowing and countering the pathological processes that disrupt the homeostasis of the internal environment in obesity and the associated comorbidities will lead, in the long term, to reducing the burden on adults and the medical system (15). To facilitate inclusion in risk groups and reduce childhood obesity, Sonoda R. et al. imagined a prediction model based on seven binary variables (16).
The defining imbalance of obesity is represented by a high caloric intake, doubled by a low consumption. Their main regulators are metabolic rate, appetite, eating habits and lifestyle dynamics. Genetic factors and individual characteristics (e.g., birth weight, breastfeeding period) may predispose to obesity, although a more important imprint is attributed to the environment. Therefore, the increase in the incidence of excess weight in recent years can be more correctly attributed to environmental changes, educational deficiencies or western-type diets. In this direction, current research attests to the involvement of numerous biomarkers (microRNA, adipocyte balance, oxidative stress, blood cell profile, nutrients and microbiota) determinants of the development of excess weight and systemic damage (2, 13, 17–19). Therefore, in addition to the disturbances stated above, excessive weight gain together with the accompanying chronic inflammation represents a continuous source of oxidative stress. The latter seems to be the crossroads in triggering the comorbidities that accompany obesity. Therefore, stimulating interest in research and countering it can be crucial initiatives, additive to new prevention strategies, to improve quality of life and ameliorate systemic decline (20, 21).
Therefore, knowing the global impact of obesity and its comorbidities, both in pediatric and adult age, we tried to broaden the horizons in terms of understanding the physiopathological mechanisms underlying the disturbances and the means by which they can be counteract. In this sense, we chose as a topic of interest the implications of oxidative stress in excessive weight gain. The antioxidant substances are multiple, they can be briefly distinguished into endogenous and exogenous. Although their importance is similar, in the management of the overweight patient, counteracting the main nutritional deficits is essential, both for the restoration of adequate levels, and from the perspective of the function of cofactors for the endogenous substances played by some of them. We caried out a narrative review of the scientific literature from the last decades, by accessing international databases (PubMed, Google Scholar, Web of Science, Scopus and Embase). To facilitate the search, we used terms such as “obesity”, “oxidative stress”, “antioxidant enzymes”, “exogenous antioxidants”, “antioxidant vitamins”, “antioxidant trace minerals” or “oxidative stress modulators”.
2 The impact of oxidative stress in the dynamics of obesity
Obesity has multiple causal determinations. Among these we note the environmental factors (stress, drugs, surgical interventions, chemical substances, diet, sleep schedule, physical activity), genetic predisposition, as well as the disruption of the homeostasis of the internal environment, objectified by oxidative stress reactions. Therefore, maintaining the redox balance in the adipose tissue is an important objective due to its implications in the decrease of the organic antioxidant capacity, the formation of free radicals and reactive species and in the pathogenesis of the metabolic syndrome associated with obesity (22–24).
Oxidative stress is defined as the disturbance of the balance between the production of oxidizing agents and the antioxidant defense, materialized by the formation of free radicals. Biochemical substrates that interfere with its production include the mitochondrial respiratory chain and enzymes (e.g., nicotinamide adenine dinucleotide phosphate oxidase, xanthine oxidase, lipoxygenases, cyclooxygenases, cytochrome P450 enzymes, or uncoupled nitric oxide synthases). This results in reactive species derived from oxygen and nitrogen (superoxide anion radicals, hydroxyl, alkoxyl, lipidic peroxyl, nitric oxide and peroxynitrite). It imprints a state of suffering on the body, sensitizing it. There is an alteration of the intra- and extra-cellular components. It is objectified by structural and functional imbalances of nucleic acids, proteins or lipids. Among its consequences, we note the over-expression of oncogenic genes, the generation of mutagenic compounds, the promotion of atherogenic activity or inflammation. Current research incriminates the existence of oxidative stress as a physiopathogenic mechanism in a variety of pathologies, depending on genetic susceptibility. Among these we highlight hypertension, atherosclerosis, obstructive pulmonary disease, diabetes, osteoporosis, cancer or even infertility (25–31).
The main method to combat the negative effects of oxidative stress is the detoxification of metabolic byproducts. This is done by enzymatic or non-enzymatic components. The first category includes superoxide dismutase (SOD), glutathione peroxidase (GPx), glutathione reductase, glutathione S-transferase, catalase (CAT), thioredoxin reductase, peroxiredoxins (Prx), ubiquinone oxidoreductase and heme oxygenase-1 (HO-1). Recently introduced in research, we find the paraoxonase family (PON) or aryl dialkyl phosphatases, enzymes strongly correlated with the accompanying pathologies of obesity (32). Another important determinant is nuclear factor erythroid 2 (Nrf2), defined as a regulator of cellular resistance to oxidants. Its action is manifested on a wide range of genes with implications in antioxidant modulation, immune or inflammatory responses, tissue remodeling, carcinogenesis and cognitive balance. Knowing and understanding its roles can lead to the explanation of the mechanisms underlying the correlation between oxidative stress and induced pathologies. At the same time, it lays the foundations for new therapeutic perspectives (33, 34). Melatonin is another magical compound, with functions in regulating the circadian rhythm, sleep, inhibiting cancer, but also detoxifying free radicals (35). Dietary antioxidant substances (non-enzymatic) such as vitamin A, C, E and other plant compounds (flavonoids, tannins, lignins) are added to all of these. Likewise, the trace elements (zinc, manganese, selenium) should not be lost sight of, substances assimilated as enzyme regulators (32).
Oxidative stress in obesity has been shown to be directly correlated with fat mass. Precipitating factors in this case include altered nutritional balance, hyperglycemia, hyperlipidemia and chronic inflammation. Also, the high-carbohydrate diet potentiates oxidative stress, an interaction objectified by increasing lipid peroxidation and protein carbonylation, doubled by the reduction of glutathione and antioxidant levels. Other classical risk factors incriminated are pollution, radiation, pesticides or other toxic substances, infections and surgical interventions. The consequences are diverse, among which we mention the influence of myocardial contractility, vascular remodeling, insulin resistance or adiponectin secretion. This explains the correlation between obesity, inflammation and various chronic pathologies that make up the metabolic syndrome (diabetes, heart disease) or not (carcinogenesis, asthma, COVID-19) (36–39). Summarizing the above, Figure 1 shows the enzymatic and non-enzymatic balance involved in the pathogenesis of oxidative stress in obesity. Imbalances at this level interfere, in addition to inflammation, with mitochondrial activity, adipogenesis, lipolysis and lipogenesis, appetite and iron metabolism.
2.1 Mitochondrial activity
The main roles of mitochondria are represented by production of energy (adenosine triphosphate – ATP) depending on cellular needs and fighting infections by promoting reactive oxygen species (40). In this sense, mitochondrial/peroxisomal oxidation of fatty acids, as well as excessive oxygen consumption can produce reactive oxygen species (41). Mainly, during the conversion to ATP, a small amount of high-energy electrons may deviate from the programmed path, thus generating superoxide radicals. They are enzymatically or spontaneously transformed into hydrogen peroxide and later into hydroxyl radicals, dangerous molecules (they can indicate cell apoptosis). The effective antioxidant mechanism in this sense is superoxide dismutase. The enzyme switches the process towards the formation of hydrogen peroxide. Later glutathione peroxidase will determine its transformation into water (42). Inflammation together with the overproduction of free radicals in obesity predispose to the appearance and maintenance of mitochondrial dysfunction. This is defined as decreased biogenesis, altered membrane potential, altered mitochondrial gene expression, and reduced ATP production (43). Another variable encountered in this case is the excess supply of nutrients. The consequence is the overwhelm of the Krebs cycle and the mitochondrial respiratory chain. This is how mitochondrial dysfunction is precipitated, which results in the formation of an increased amount of reactive oxygen species. The two mechanisms therefore intertwine, aggravating in parallel both mitochondrial dysfunction, as well as inflammation and increased insulin resistance specific to obesity (40, 42). The exposed changes, related to mitochondrial biogenesis, enzyme balance and the consequences of the two, were also reported by Zamora-Mendoza R. et al. (44). Summarizing the above, Figure 2 illustrates the way in which mitochondrial dysfunction induces an increase in oxidative status and disruption of the homeostasis of the internal environment.
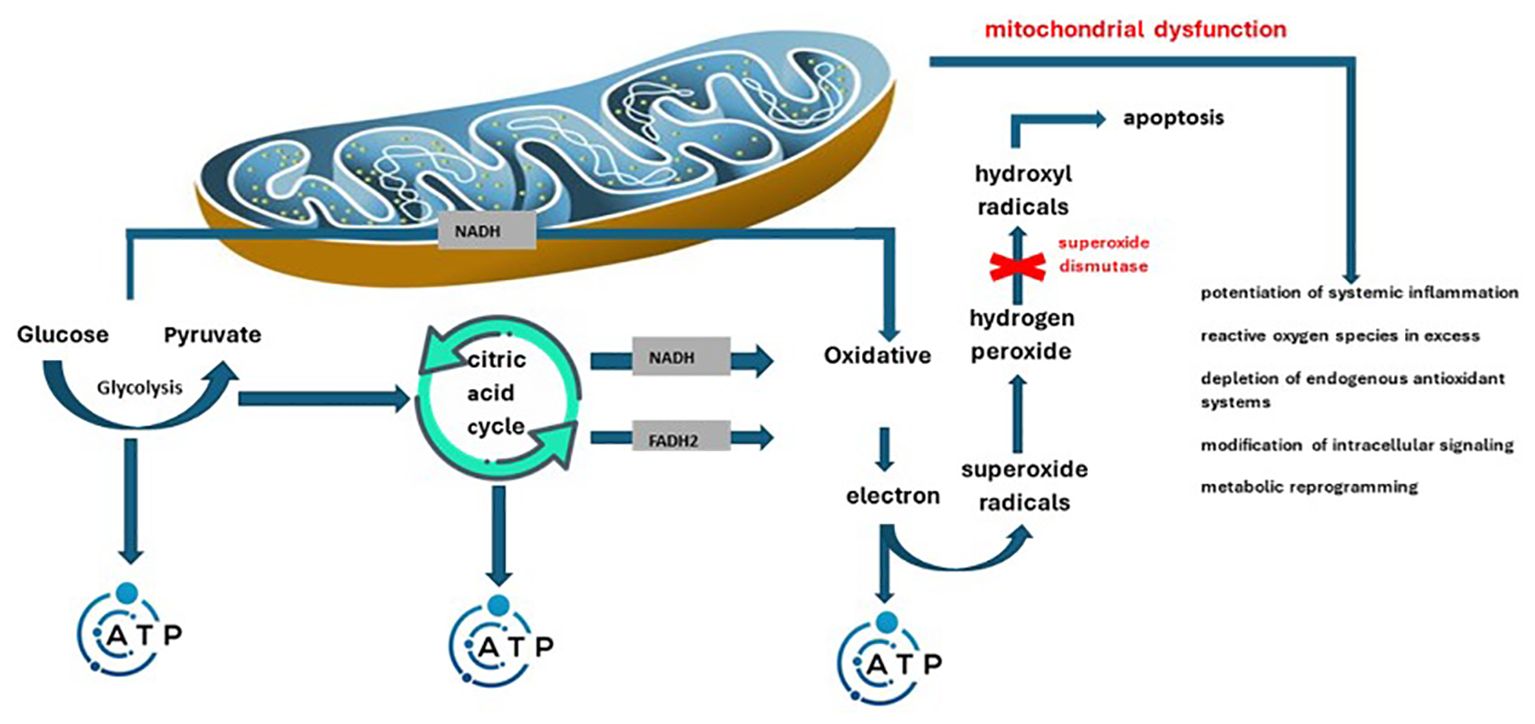
Figure 2 Pathological mechanisms through which mitochondrial dysfunction intervenes in the potentiation of the oxidative status and systemic damage.
2.2 Adipogenesis
Adipose tissue normally represents up to 30% of body mass. Its roles in the body are variable, being briefly divided into structural (support) and functional. Depending on its distribution, it can be classified into subcutaneous adipose tissue and visceral adipose tissue. Its structural and functional characteristics facilitate its division into 3 categories: white, brown or beige adipose tissue. Functionally, the main role of white adipocytes is to store energy, while brown adipocytes intervene in thermogenesis. Thermogenesis occurs by stimulating uncoupling proteins, the result being the conversion to produce heat instead of ATP. Beige adipocytes share similar characteristics to brown ones, but being distributed in white adipose tissue deposits (45). Brown adipose tissue seems to have a metabolic activity directly correlated with the volume of muscle mass; an aspect partially explained by the presence of common precursors with muscle cells (myf5-positive precursors similar to myoblasts). Also, it is better represented in the pediatric population, unlike adults. The investigation recommended for its evaluation is positron emission tomography, although the results may be influenced by age, degree of sexual development, medication used, fat accumulation, disease state, plasma glucose concentration, radiotracer dose, season or temperature during examinations. Antioxidant supplementation has beneficial effects on the dynamics of brown adipose tissue (45–47). Current data from the literature attests to the discrepancy between white adipose tissue/brown adipose tissue in the pathogenesis of obesity. If the excessive distribution of the first one is found in the case of overweight people, the brown tissue protects against weight gain and its comorbidities (insulin resistance, dyslipidemia). However, the way of distribution of white adipose tissue should not be neglected. It differentiates pathological obesity from “metabolically healthy” obesity (Figure 3). The second case is characterized by the expansion of subcutaneous deposits, adipocyte hyperplasia and limited ectopic lipid deposition (48, 49).
The imbalance in adipose tissue, characteristic of obesity (hypertrophy, hyperplasia), is accompanied by a pro-inflammatory state, mitochondrial and endoplasmic reticulum dysfunction and, consequently, increased oxidative stress at this level. This in turn induces disturbances in the production of adipokines. The link between the two is strengthened by the direct correlation between oxidative damage markers and BMI, body fat percentage, low-density lipoprotein (LDL) oxidation, and triglyceride (TG) levels. The consequences are reflected in the increased risk of developing comorbidities in the medium and long term (50, 51). In agreement with the functional characteristics of adipose tissue, studies on adults attest to lower levels of oxidative stress in men, compared to women. At the same time, the correlation with environmental factors is certified (e.g., the use of oral contraceptives, hormonal therapies) (52). In turn, oxidative stress promotes inflammation by activating the components of innate immunity and modulating the activation of the nucleotide oligomerization domain protein-1 (NOD1). Following the activation of NOD1, there is an increase in NADPH oxidase (NOX) 1 and 4. From a therapeutic point of view, it seems that the manipulation in the descending direction of NOX1/4 decreases oxygen free radicals, in parallel with the increase in catalase-type antioxidant enzymes and SOD (53, 54).
2.3 Lipogenesis and lipolysis
Lipids fulfill important biological roles such as energy source, structural components or signaling mediators. The tissues involved in lipogenesis are the liver and adipose tissue (white/brown). This modulates, in addition to systemic energy homeostasis, the function of the immune and nervous systems. Thus, lipid metabolism disturbances can be accompanied by pathological consequences, partly due to membrane remodeling (55–57). The metabolic integrity of the body can be estimated by evaluating the ability of adipocytes to convert glucose into lipids (de novo lipogenesis), a process affected in the case of insulin resistance (58). Therefore, in obesity, the classic lipolytic pathway is switched to an alternative, inflammatory pathway. Reactive oxygen and nitrogen species can influence these pathways at different levels, with consequences dependent on concentration, reactivity and source (Figure 4). Mainly excessive and prolonged lipolysis (lipotoxicity) causes structural and functional changes in adipose tissue. The physiopathogenic cascade ends with the impairment of insulin sensitivity, maintaining the vicious circle of lipolysis. In conclusion, we emphasize the importance of antioxidants in restoring the homeostasis of the internal environment (59, 60).
2.4 Leptin – adiponectin balance
Adipose tissue also fulfills an endocrine role, secreting a series of bioactive molecules (adipokines) that influence the internal balance. Their production is influenced by insulin, catecholamines and adiposity. By this we mark another stage of the physio-pathological process influenced by oxidative stress, namely the leptin/adiponectin balance. The functions performed by the two molecules are contradictory (Figure 5). Leptin is involved in appetite regulation by modulating dopamine secretion by the limbic system. At the same time, it stimulates oxidative stress, inflammation, thrombogenic risk, arterial stiffness, angiogenesis, atherogenesis and lipolysis, inhibiting lipogenesis. This explains the increased cardiovascular risk characteristic of obesity. At the opposite pole, adiponectin is an anti-inflammatory molecule that increases sensitivity to insulin, reduces the development of atherosclerosis, cell apoptosis and the flow of free fatty acids, in parallel with the increase in their oxidation. Its action is mainly achieved through the interaction with ceramidase, the enzyme involved in the intracellular balance of ceramide. Oxidative stress suppresses its production, thus increasing the risk of associated comorbidities. Supplementation with antioxidants restores the circulatory balance and reduces morbidity. Other adipokines involved in the modulation of comorbidities are adipsin, resistin, visfatin, omentin and apelin (41, 61–64).
2.5 Iron metabolism
Iron metabolism has a double value in obesity, disturbances at this level can be both a consequence of overweight and a cause. The common point between the two entities seems to be represented by macrophages. On the one hand, it can be influenced by chronic inflammation, its deficit affecting the function of hemoproteins and non-heme proteins. In this case, iron deficiency is accompanied by a low value of ferritin, in contrast to that of hepcidin, and a negative correlation between transferrin saturation and adiposity. On the other hand, excess iron can induce the formation of oxygen free radicals (hydroxyl radical) through the Fenton reaction whose course and consequences are illustrated in Figure 6 (65, 66). Added to these are the increase in lipogenesis, the reduction of lipolysis and the promotion of mitochondrial dysfunction (67). This is how ferroptosis (regulated non-apoptotic cell death) occurs, characterized by lipid peroxidation when the endogenous antioxidant status of the cell is compromised. The remedies in this situation are represented by dietary restriction, the use of iron chelators, lipophilic antioxidants (vitamin E), ferostatin-1, liproxstatin-1 or polyphenols (68, 69).
2.6 Inflammation
Chronic, low-grade inflammation is the main mechanism incriminated in promoting obesity-induced damage. This is characterized by a continuous activation of the innate immune system (70). The cause is the accumulation of lipids in adipocytes, leading to adipocyte stress and local hypoxia following tissue hypertrophy and hyperplasia. Thus, necrosis and infiltration by macrophages occurs. There is an increase in the production of pro-inflammatory mediators (interleukin-6 and 1, tumor necrosis factor α, monocyte chemoattractant protein 1) and prothrombotic (plasminogen activator inhibitor-1). Additionally, deficiencies of the oxidizing and antioxidant system are noted. At the same time, the local inflammation spreads to the systemic level (42, 71, 72). Tumor necrosis factor α (TNF-α) has also been linked to endothelial dysfunction, increased atherogenic risk, development of insulin resistance and diabetes. This is achieved through four essential means, namely the activation of nuclear factor κB (NF-κB), increasing the release of free fatty acids in adipocytes, blocking the synthesis of adiponectin and modulating the phosphorylation of tyrosine residues, an essential substrate in intracellular signaling. Regarding IL-6, its levels are directly correlated with BMI, insulin resistance and carbohydrate intolerance. Besides these, it regulates the levels of adipokines (inhibits visfatin and adiponectin) (41).
In conclusion, we present the results obtained by Matusik P. et al. who reiterate, based on their research, the positive correlation between oxidative disturbances, sports, excess adipose tissue and its hormonal activity. However, he notes that in the case of patients practicing sports training (chronic physical activity), the antioxidant defense was more potent. In consensus, Huang CJ. et al. explains the connection between sport and the oxidant-antioxidant balance, emphasizing the dependence on the individual characteristics of the patient, the type of physical activity practiced, intensity and period. Also, a brief distinction is made of the mechanisms by which aerobic and anaerobic physical activity influence the internal balance. The final result is, in both situations, represented by the increase of stress markers (73, 74). Similar findings have been identified in the literature regarding non-alcoholic fatty liver disease, another component of the metabolic syndrome, frequently associated with obesity (75).
3 Antioxidant systems
Reactive oxygen species in excess can induce apoptosis, necrosis or autophagy. The main pathways targeted in this process are mTOR activity, adenosine monophosphate-activated protein kinase (AMPK), C-Jun-N-terminal kinase (JNK)/P53, and the balance between serine/threonine receptor-interacting protein kinase 3 (RIP3) and RIP1 (76). For these reasons, knowing and potentiating the main endogenous antioxidants is vital in the evolution of patients.
3.1 Enzymatic antioxidants
Superoxide dismutase and catalase are two key enzymes in returning the body to its equilibrium state. The two participate in the elimination of toxic radicals through the initial conversion to hydrogen peroxide (H2O2) by SOD, which will then be degraded by catalase into oxygen and water. Thus, to estimate the level of expression of the two in the peripheral sagin cells, Mohseni R. et al. they turned to real-time polymerase chain reaction (PCR). The results confirmed the low levels among obese children, inversely correlated with BMI, fasting blood glucose, insulin resistance, LDL-Cholesterol, TG and systolic blood pressure (77). SOD is divided into 3 isoforms in mammals. These are the cytosolic copper-zinc dimeric form (SOD1), mitochondrial manganese tetrameric SOD (SOD2), and extracellular Cu/Zn tetrameric SOD (SOD3). Among them Erdeve O. et al. demonstrates the involvement of SOD3 in the antioxidant response since childhood (78, 79). At the same time, Özgen İT. et al. underlines the role of genetic polymorphisms (respectively VV alleles) of SOD2 Ala16Val in dictating the risk of developing oxidative stress associated with increased HOMA-IR score (80). In turn, CAT is a peroxisomal antioxidant enzyme, crucial in adipogenesis. Studies on erythrocytes report the possible correlation of single nucleotide polymorphisms (promoter variant -844A/G) and post-translational modifications of CAT with the risk of developing obesity and its comorbidities. At the same time, the low levels of CAT observed in obesity are partially due to increased S-nitrosation of the enzyme (81–83). Paradoxically, it remains contradictory if CAT overexpression in adipose tissue offers any benefit in terms of improving the metabolic profile or the balance of adipogenesis (84).
The main disadvantage of antioxidant enzymes is the inability of exogenous variants to provide benefits to damaged tissues. The main cause is the low permeability of the membrane. Thus, in order to achieve results, recombinant formulas are used, made up of SOD and cell-penetrating proteins (SOD-CPP). This formula can even cross the blood-brain barrier, reducing the expression of inflammatory factors and inhibiting NF-κB signaling pathways (85). Another form used is the SOD mimetic manganese metalloporphyrin (86).
Glutathione peroxidase is an antioxidant enzyme found at the cytoplasmic and mitochondrial level in mammals. The entire superfamily brings together eight isoforms, of which types 1-4 and 6 are selenoproteins, the rest based on cysteine. In humans, the most common isoform involved in oxidative balance is GPx1 (87). As a means of action, it maintains the balance between the necessary/damaging level of cellular oxidants by reducing hydrogen peroxide and soluble lipid hydroperoxides. The source of reducing equivalents used is glutathione. Enzyme balance is important because low levels of hydrogen peroxide are required for physiological processes such as growth factor-mediated signaling, formation of protein-disulfide bonds, and regulation of normal mitochondrial function. At the same time, a high level of GPx1 can induce dysregulation of islet insulin production and secretion, culminating in diabetes-like phenotypes. The repercussions at the pancreatic level consist of depletion of murine regenerating islet-derived protein 2 (REG2). Thus, maintaining optimal levels of GPx1 is crucial in modulating systemic inflammation and preventing associated metabolic, neurological, cardio-vascular and oncological risks (88–91). In this process, an important role is attributed to glutathione reductase. The enzyme is the main supplier of reduced glutathione, a necessary substrate for the proper development of the redox process (92). Independent of other variables that can influence the redox balance, it seems that seasonal factors (e.g., vitamin D) can modulate the reactions based on glutathione (93). In this sense, vitamin D supplementation showed benefits in increasing glutathione, GPx1 and SOD, reducing oxidative stress and suppressing ferroptosis (by activating the Nrf2 signaling pathway). In parallel, the neuroprotective effect exerted following the hypoxic-ischemic stimulus is noted (94).
Peroxiredoxins represent an enzyme superfamily, dependent on cysteine. Prx reduce over 90% of cellular peroxides. These have six subfamilies in their composition (95). They demonstrated their role in regulating adipogenesis and the metabolic/inflammatory implications of obesity. Among these we note diabetes, atherosclerosis, liver, cardiac or even reproductive damage (spermatogenesis) (96–100). Similar implications are noted in the case of paraoxodaxa-1, the thioredoxin/thioredoxin reductase system (Trx/TrxR), adipokines or heme oxygenase-1 (101–105).
3.2 Non-enzymatic antioxidants
Among the non-enzymatic molecules involved in the clearance of reactive oxygen species and the reduction of their harmful effects, we find vitamins (A, E and C) and other plant compounds (flavonoids, tannins, lignins, carotene, alpha-lipoic acid), chemicals such as coenzyme Q/ubiquinone (MitoQ), N-acetylcysteine (NAC) or trace elements (zinc, manganese, selenium). Compounds with special properties such as melatonin and taurine are added to these (32, 43, 76).
3.2.1 Vitamins
Fat-soluble vitamins (A and E) are dietary constituents whose intestinal passage (absorption/elimination) occurs passively, without energy consumption. Vitamin A can be found as two forms, preformed (all-trans-retinol and its esters) and provitamin A (β-carotene). Of these, β-carotene, similar to other dietary carotenoid products, acts as an antioxidant substrate (106). Similarly, the distribution of vitamin E brings together 8 isoforms (four tocopherols and four tocotrienols - α -, β-, γ-). Vitamin E is ubiquitous in the body’s sites, although it lacks the possibility of endogenous synthesis. The functions performed by it spread over the allergic, inflammatory, atherogenic, metabolic (glycemic, lipidic), oncological balance, being also an important pillar in cardioprotection and neuroprotection. It is believed that the antioxidant activity of vitamin E is dependent on the number of methyl groups on the chroman ring. The antioxidant power includes α, β, γ and δ isoforms in descending order, while tocopherol is stronger than tocotrienol (107).
Based on previous findings, Guerendiain M. et al. certifies the involvement of the correlation between optimal levels of fat-soluble vitamins and reduced adiposity, greater weight loss and improved cardio-metabolic profile (108). In addition, Gama Oliveira MN. et al. include in the discussion another predisposing variable, namely the Q223R leptin receptor polymorphism (109). Another concern brought into discussion by Gajewska J. et al. is the risk of hypovitaminosis E or A in obese children, dependent on the BMI curve for weight loss through lifestyle interventions (110).
Vitamin C (ascorbic acid) fulfills in the body both a role in the antioxidant and immune balance (innate or adaptive) and as an enzyme cofactor. Through its functions, it promotes the integrity of the skin barrier, increases chemotaxis, phagocytosis, the generation of reactive oxygen species and microbial killing, reduces necrosis and post-infectious tissue damage and promotes the differentiation and proliferation of T and B lymphocytes. Thus, in inflammations and infections, subject to important reactive oxygen species, vitamin C has a double valence. While its deficiency induces the disruption of immune defense mechanisms, the infections themselves promote the deficiency due to inflammation and nutritional requirements, thus maintaining the vicious circle (111, 112). Current literature attests to the benefit of ascorbic acid in oncology, cardiovascular (hypertension, stroke, atherosclerosis) and nutritional (obesity) conditions. However, we emphasize the existence of gender influence, marked by a negative correlation between vitamin C intake and abdominal obesity in women. The mechanisms probably involved in this are represented by the modulation of adipocyte lipolysis, adrenal glucocorticoid levels, glucose metabolism (improves blood sugar and decreases glycosylation) and leptin secretion and reduces the inflammatory response (113–115). It is known that the optimal intake for a person of approximately 60 kg is 110 mg/day. Carr AC. et al. note that, to avoid vitamin C deficiencies, it is necessary to supplement with 10 mg/day for every 10 kg of excess weight (116).
Comparing the three vitamins, Xie D. et al. notes an increased antioxidant power of vitamin A compared to vitamin C. At the same time, vitamin E is more potent than vitamin A, the combination of the two not registering additional antioxidant benefits (117).
Vitamin D (25-hydroxyvitamin D) is another fat-soluble vitamin with a strong role in homeostasis of the internal environment. The main functions are based on the inflammatory, oxidative and mitochondrial balance. Its balance is influenced by a multitude of physical and environmental factors. In obesity in particular, hypovitaminosis D seems to be due to insufficient food intake, reduced exposure to sunlight due to the inclination towards a sedentary lifestyle, decreased intestinal absorption, hydroxylation in adipose tissue or accumulation in fat. To these is added the genetic predisposition marked by the variation of the vitamin D receptor gene (118–120). Studies on murine models attest to the beneficial effects of vitamin D supplementation on oxidative stress and inflammatory markers in the overweight group (121). Similar findings were reported by Ionica M. et al., in the study on the adult, overweight and obese population. They also record the inverse correlation between the serum level of the vitamin and the amplitude of adipose oxidative stress (122). Continuing the reasoning, Usman M. et al. objectifies, by studying obese pediatric patients aged between 10-18 years, the positive link between adipose markers and oxidative DNA damage. In conclusion, the three entities (obesity, hypovitaminosis D and DNA damage) represent predictive markers of genomic instabilities (123). In conclusion, Filgueiras MS. et al. underlines the importance of screening for vitamin D status in light of the association with non-conventional cardiometabolic markers (C-reactive protein, IL-6, cathepsin S, vascular cell adhesion molecule-1, malondialdehyde, myeloperoxidase, 3-nitrotyrosine and SOD) in the pediatric population (124). It is estimated that increasing vitamin D by approximately 9 ng/ml would counterbalance this negative effect of visceral adiposity (125).
3.2.2 Microelements
Microelements are important chemical structures in the balance of the internal environment. By their nature, they fulfill multiple functions, entering into the composition of enzymes, vitamins, hormones and pigments. By far the most important role is that of an enzymatic cofactor, modulating organic biochemical processes through their level. Currently, the medical literature attests to the presence of deficiency in trace elements in children and adolescents with obesity. Among these, the biggest shortage seems to be recorded in what concerns copper (126). Although the relationship between obesity and nutritional deficiencies remains open to research, Błoniarz J. et al. links the metabolism of carbohydrates, fats and implicitly the appearance of secondary obesity to the balance of chromium, zinc, copper, manganese, iron or nickel (127). In addition, Luque-Díaz MJ. et al. underlines the existence of hyperzincuria, hypocupruria, hypozincemia and hypercupremia in groups of obese patients, unlike controls (128). Not being the object of the current study, we choose to expose in what follows its implications from the perspective of oxidative stress.
We therefore consider zinc, manganese, selenium and magnesium as the main elements involved in antioxidant regulation. The function of zinc includes a wide range of biological processes such as cell proliferation, immune function and defense against free radicals (through the synthesis of metallothioneins - they reduce hydroxyl radicals and sequester reactive oxygen species), cellular response to oxidative stress, DNA repair, cycle regulation cellular, membrane stabilization, inhibition of the enzyme nicotinamide adenine dinucleotide phosphate oxidase (NADPH-oxidase) and apoptosis. The zinc deficiency, doubled by the high-fat diet, is blamed for the occurrence of cardiac hypertrophy related to obesity. Regarding the role of enzyme cofactor, it influences various structures such as SOD or zinc-α2-glycoprotein (ZAG). The latter is an adipokine with an anti-inflammatory role and in the mobilization of lipids found in reduced quantities in obese patients (129–133). The level of zinc must therefore be kept within normal limits, its marginal deficit may increase the risk of obesity (134). At the same time, Mendes Garrido Abregú F. et al. discuss the importance of adequate zinc levels during breastfeeding. They emphasize that adequate post-weaning zinc diet has a reduced role in preventing cardio-metabolic changes induced by perinatal restriction (135).
Selenium supplementation had, similar to zinc, a beneficial effect in regulating body weight, metabolic and oxidative profile (136, 137). In addition to its antioxidant (main cofactor of GPx) and anti-inflammatory implications, selenium intervenes beneficially in non-alcoholic fatty liver disease induced by obesity. It promotes the synthesis of selenoprotein P1 (SEPP1) which further regulates the Kelch-like ECH-associated protein 1 (KEAP1)/Nrf2 pathway (138, 139). The advantages of exposure to selenium were also documented by Abo El-Magd NF. et al. (140). Last but not least, manganese influences the body’s antioxidant balance. It is a redox-active component with a key role in cellular adaptation to oxidative stress. The main functions are cofactor for SOD and substrate for the formation of manganese non-proteinaceous antioxidants. In these processes, the “adversary” is the iron (141, 142).
3.2.3 Other compounds with an antioxidant role
Flavonoids are plant compounds found in fruits and vegetables, known for their strong antioxidant properties. They are estimated to have a diversity that includes six subgroups made up of up to 5000 substances with a common characteristic (skeletal structures with 15 carbon atoms, two phenyl rings and a heterocyclic ring). The most abundant flavonols are quercetin, catechins and kaempferol. They can be used in the prevention or management of obesity and associated diabetes. It exerts its functions on peripheral tissues and pancreatic beta cells, improving insulin secretion and sensitivity. It also regulates inflammation by acting on NF-κB through mitogen-activated protein kinase (MAPK) pathways. Quercetin can inhibit macrophage inflammatory response by activating AMPK phosphorylation and sirtuin 1 (SIRT1) expression (143–145). Besides these, flavonoids modulate thermogenesis, lipogenesis (decrease), lipolysis (increase), energy consumption, food and nutritional intake, B-oxidation of fatty acids and carbohydrate balance. In the oxidizing balance, flavonoids can clean reactive species of oxygen or nitrogen by direct or indirect mechanism (146–148). A special role is attributed to the ability to stabilize the intestinal microbial ecosystem. Thus, we previously demonstrated that intestinal dysbiosis is a process that can be the basis of a wide range of diseases starting from obesity, the associated comorbidities and culminating in gastrointestinal, cardiovascular, renal, atopy or autoimmunity pathologies. The link between them is attributed to the intestines-vital organs axes, intensively studied at the present time (149–156). Finally, Gentile D. et al. underlines the importance of a diet rich in flavonoids in modulating the systemic inflammatory and oxidative balance, with the aim of avoiding obesity and its associated comorbidities (157).
Alpha-lipoic acid (ALA) is another vegetable component with antioxidant effects. Although it is still under study, research on adults attests that supplementation with 600 mg intravenously for 2 weeks improves insulin sensitivity and reduces mitochondrial functional manifestations. Also, the levels of free fatty acids, dyslipidemia and oxidative/inflammatory markers were reduced, in parallel with adiponectin, which registered an increase. To these is added the positive impact in the recycling of vitamins C and E and the chelation of toxic metals (158–161). Research on murine models is more advanced, indicating similar results. Looking specifically at inflammation and oxidative stress, ALA caused reductions in prostaglandin E2, leukotrienes B4 and C4, T cell proliferation and IL-2 production, and levels of lipid peroxidation products, doubled by increased SOD2 and reduced intracellular glutathione (161–164).
Coenzyme Q10 (ubiquinol/ubiquinone) is a key component in the mitochondrial electron transport chain. One of the causes of ubiquinone deficiency is attributed to the statin-induced lipid-lowering effect by inhibiting the conversion of 3-hydroxy-3-methyl-glutaryl-coenzyme A reductase (HMG-CoA) to mevalonate. The main antioxidant mechanisms attributed to coenzyme Q10 are its action as a cofactor and activator of mitochondrial uncoupling proteins, the ability to accept and donate electrons, the inhibition of lipid and protein peroxidation, the prevention of LDL oxidation and the improvement of the availability of other antioxidants (vitamin C, E, beta-carotene). Thus, a diet with foods rich in coenzyme Q10 (100-150 mg/day) or its supplementation has proven effective in regulating carbohydrate metabolism, improving inflammation and oxidative stress characteristic of obesity/metabolic syndrome (165–168).
The benefits of N-acetyl cysteine (NAC) are multiple and beyond doubt. NAC acts against the complications induced by obesity both by reducing the abnormal pro-inflammatory response and limiting oxidative damage, as well as by inhibiting lipid accumulation by targeting adipogenic transcription factors. Studies on murine models have also demonstrated an effective effect on hyperglycemia, dyslipidemia and oxidative stress (increase the expression of endogenous antioxidant enzymes) occurring consecutively to a diet rich in sucrose (169–172).
The roles of melatonin are vast and still incompletely elucidated. Besides the well-known implications of melatonin in dictating the circadian rhythm, the balance of the intestinal microbiota, sleep disorders and the opioidergic system, it has recently come to the attention of research as a regulatory factor (modulating the activity of melatonin 1 and 2 membrane receptors) of the lipid and tension profile, of glucose metabolism, oxidative stress, inflammation and adipose tissue. Thus, melatonin supplementation between 1-20 mg/day demonstrates a beneficial role in pediatric obesity by reducing associated mitochondrial damage, regulating glycemic homeostasis and increasing the volume/activity of brown adipose tissue. Also, no serious adverse effects were recorded (173–176). At the same time, the use of melatonin as an antioxidant has demonstrated its effectiveness in reducing muscle damage after physical activity in overweight people (177).
Although research on taurine (2-aminoethanesulfonic acid) is limited, its implications in modulating oxidative/metabolic stress, inflammation, insulin sensitivity and vascular remodeling cannot be denied. Thus, its balance can influence the appearance of the affections as well as the predisposition towards the development of associated comorbidities. For estimation purposes, the current scientific literature considers taurine excretion/24 hours inversely correlated with BMI, blood pressure profile and cholesterolemia (178–181). Also, taurine supplementation (3 g/day) doubled by sustained physical exercises, with/without nutritional counseling, demonstrated benefits in reducing inflammation, oxidative stress, preventing endothelial dysfunction induced by a high-fat diet and improving the plasticity of subcutaneous adipose tissue (182–184).
The oral and intestinal microbiota also represent a broad field of research. As I mentioned, its disturbances can be found as risk factors in various pathologies. In particular, at the local level, intestinal dysbiosis can be involved in the pathogenesis of irritable bowel, pancreatitis and celiac disease. It thus achieves a pathogenic interrelation of autoimmunities (see systemic lupus erythematosus). Therefore, the current literature states that the dietary modulation of the intestinal microbiota as well as the transplantation of fecal matter can represent prophylactic and curative strategies in childhood obesity (185–189). Its evaluation currently represents a pinnacle of research. It is known that it is in permanent change since the perinatal period, being influenced by both maternal and individual factors. The first three years are the most important for its optimal definition, the disruptive factors being cesarean birth, artificial feeding, antibiotic therapy (190, 191). Consequently, due to the multiple causal associations with obesity, it is necessary to emphasize the possible beneficial implications of prebiotics/probiotics or symbiotics in its therapeutic modulation. The ways in which they interact with the homeostasis of the internal environment playing the role of antioxidants are vast, from the clearance of reactive substances to the stimulation of signaling with the increase of the cytoprotective capacity of the host (192, 193). They have proven benefits in the co-adjuvant therapy of obesity, insulin resistance, diabetes and non-alcoholic fatty liver disease (194). The observation was demonstrated in studies on adult populations, when supplemented with Saccharomyces boulardii and SOD for two months. In addition, the increase in the level of vitamin D was noted (195).
Table 1 illustrates the main means of action of enzymatic and non-enzymatic antioxidants in mitigating the oxidative stress present in obesity.
4 The role of diet in obesity management
Continuing the appropriate screening of the population at risk, both the targeted therapeutic approach (pharmacological or surgical) and the adjunctive management of pediatric obesity (sleep hygiene, balanced diet, avoiding sedentary lifestyle, sustained physical exercise) is a broad topic of ongoing research. According to the current consensus, multidisciplinary management aims to prevent and counteract the underlying pathology and associated comorbidities, efforts aimed at maintaining the psychosocial integrity of the child (196–198).
In practical terms, international guidelines currently support the approach of a minimum of 60 minutes/day of moderate to vigorous physical exercises. The most effective method to eliminate excess weight and reduce waist circumference has proven to be the combination of aerobics and resistance training. An important factor in this approach is the school. Studies have shown that encouraging physical activity in schools has led to improvements in BMI, waist circumference in women, skinfold thickness and body fat (197, 199). Undoubtedly, in order to be effective, physical activity must be a part of changing the entire lifestyle. Thus, it was proven that the lack of breakfast, the frequent consumption of snacks, the increase in the amount of fat and carbohydrates in the diet and a narrow variability of healthy food options (e.g., fruits, vegetables and dairy) were precipitating factors of childhood obesity. Added to these are the consumption of sugar-sweetened beverages or fast food (199–201).
The pharmacological intervention possibilities are more restrictive compared to those in the adult population. The main consideration resides in the lack of substance certification studies. Therefore, the pharmacological substances currently approved for obesity are Orlestin, glucagon-like peptide-1 analogues, Liraglutide, Setmelanotide and Metreleptin. The means of action, dosage and indications are varied, thereby ensuring the possibility of an individualized therapy. We also note Semaglutide and Exenatide as therapeutic options in research regarding the utility in obesity (199).
Bariatric surgery represents a therapeutic alternative dedicated to severe cases of obesity, refractory to the previously described hygienic-dietary and pharmacological measures and which associate comorbidities. Its indications are defined depending on the value of the body mass index. We thus recognize the usefulness of bariatric surgery when BMI >40 kg/m2 or BMI >35 kg/m2 with significant comorbidities. The possible surgical techniques are quite varied, and BMI reduction seems to be effective in a follow-up interval of 1-5 years post-procedural. Additionally, this has proven to be beneficial in improving associated comorbidities (e.g., diabetes, hypertension, dyslipidemia, and proteinuria). The studies that will unequivocally certify the role of bariatric surgery and the superiority of each technique are currently underway (199, 201). One such example is a multicenter study undertaken by Järvholm K. et al. (202).
Finally, in accordance with what was stated previously, Stabouli S. et al. support the need for future research on the early detection of risk factors and the elucidation of the mechanisms underlying pediatric obesity (203). Of these, we detailed throughout the manuscript the pathophysiology implications of antioxidant substances in the evolutionary pattern of obesity. Therefore, in order to add practical utility to the work, Table 2 refers to the main foods with a high content of antioxidants, beneficial in the diet of children and obese adolescents.
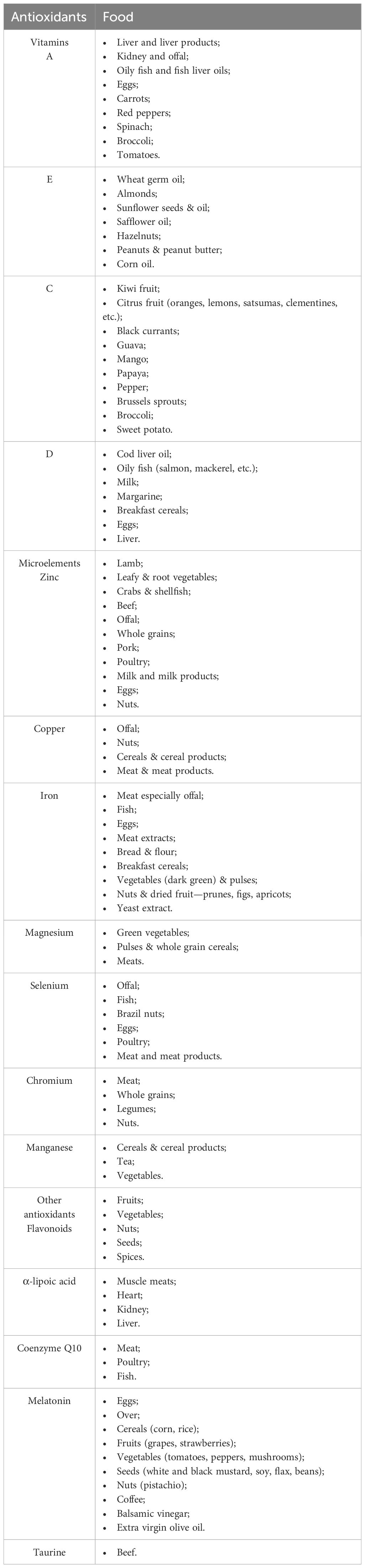
Table 2 Food sources rich in antioxidants (adapted from Webster-Gandy J. et al., Kozłowska A. et al., Alam MA. et al., Meng X. et al., Wu G. et al. and Shay KP. et al.) (165, 204–208).
5 Conclusions
Obesity is found both in childhood/adolescence and in adulthood. Many pathological processes compete with its induction and maintenance. Therefore, the unbalanced lifestyle is only the tip of the iceberg. The current work achieved its goal of bringing the concept of pediatric obesity and accompanying comorbidities up to date. Most of the obese children, without therapeutic interventions, will evolve further into obese adults that can also associate metabolic syndrome. In order to improve/stop the systemic decline, measures aimed at lifestyle modification, pharmacotherapy or surgical therapy are considered. Knowing the impact played by released free radicals as a consequence of oxidative stress, we discussed the main endogenous or exogenous antioxidant substances that interfere with the pathological process, briefly detailing the roles of each. Although the implications of antioxidants in childhood obesity are certified by specialized medical literature, we identified in our search a reporting bias of the main food sources rich in substances with an antioxidant role. Consequently, we chose to do a brief review of them. In conclusion, the current study places the obesity-oxidative stress-antioxidants relationship in a different light. We consider it opportune to widen the horizons in this direction by designing and implementing screening programs and individualized supplementation of nutritional deficiencies in antioxidant substances with the aim of reducing the incidence and burden of pediatric obesity.
Author contributions
AL: Conceptualization, Investigation, Writing – original draft. SF: Investigation, Methodology, Writing – original draft. EJ: Investigation, Methodology, Writing – original draft. IS: Investigation, Software, Writing – original draft. II: Investigation, Software, Writing – original draft. AK: Validation, Visualization, Writing – review & editing. DS: Software, Validation, Writing – review & editing. MS: Validation, Visualization, Writing – review & editing. OC: Investigation, Software, Writing – original draft. NR: Supervision, Validation, Writing – review & editing. CMM: Writing – review & editing, Visualization. VL: Methodology, Supervision, Writing – review & editing. AN: Project administration, Validation, Writing – review & editing.
Funding
The author(s) declare that no financial support was received for the research, authorship, and/or publication of this article.
Conflict of interest
The authors declare that the research was conducted in the absence of any commercial or financial relationships that could be construed as a potential conflict of interest.
Publisher’s note
All claims expressed in this article are solely those of the authors and do not necessarily represent those of their affiliated organizations, or those of the publisher, the editors and the reviewers. Any product that may be evaluated in this article, or claim that may be made by its manufacturer, is not guaranteed or endorsed by the publisher.
References
1. Valerio G, Maffeis C, Saggese G, Ambruzzi MA, Balsamo A, Bellone S, et al. Diagnosis, treatment and prevention of pediatric obesity: consensus position statement of the Italian Society for Pediatric Endocrinology and Diabetology and the Italian Society of Pediatrics. Ital J Pediatr. (2018) 44:88. doi: 10.1186/s13052-018-0525-6
3. Iturriaga C, Bustos MF, Le Roy C, Rodríguez R, Cifuentes L, Silva-Valenzuela S, et al. Association between obesity and atopic dermatitis in children: A case-control study in a high obesity prevalence population. Pediatr Dermatol. (2023) 40:64–8. doi: 10.1111/pde.15110
4. Mill JG. Obesity and risk of hypertension: A growing problem in children and adolescents. Arq Bras Cardiol. (2023) 120:e2022094. doi: 10.36660/abc.20220940
5. Nur Zati Iwani AK, Jalaludin MY, Roslan FA, Mansor F, Md Zain F, Hong JYH, et al. Cardiometabolic risk factors among children who are affected by overweight, obesity and severe obesity. Front Public Health. (2023) 11:1097675. doi: 10.3389/fpubh.2023.1097675
6. van Dam MJCM, Pottel H, Vreugdenhil ACE. Relation between obesity-related comorbidities and kidney function estimation in children. Pediatr Nephrol. (2023) 38:1867–76. doi: 10.1007/s00467-022-05810-z
7. Ersu R, Chen ML, Ehsan Z, Ishman SL, Redline S, Narang I. Persistent obstructive sleep apnoea in children: treatment options and management considerations. Lancet Respir Med. (2023) 11:283–96. doi: 10.1016/S2213-2600(22)00262-4
8. Vitoratou DI, Milas GP, Korovessi P, Kostaridou S, Koletsi P. Obesity as a risk factor for severe influenza infection in children and adolescents: a systematic review and meta-analysis. Eur J Pediatr. (2023) 182:363–74. doi: 10.1007/s00431-022-04689-0
9. World Obesity Federation. World Obesity Atlas 2023 . Available online at: https://data.worldobesity.org/publications/?cat=19 (Accessed 25 December 2023).
10. Alfaris N, Alqahtani AM, Alamuddin N, Rigas G. Global impact of obesity. Gastroenterol Clin North Am. (2023) 52:277–93. doi: 10.1016/j.gtc.2023.03.002
11. Dinsa GD, Goryakin Y, Fumagalli E, Suhrcke M. Obesity and socioeconomic status in developing countries: a systematic review. Obes Rev. (2012) 13:1067–79. doi: 10.1111/j.1467-789X.2012.01017.x
12. Peinado Fabregat MI, Saynina O, Sanders LM. Obesity and overweight among children with medical complexity. Pediatrics. (2023) 151:e2022058687. doi: 10.1542/peds.2022-058687
13. Verduci E, Bronsky J, Embleton N, Gerasimidis K, Indrio F, Köglmeier J, et al. ESPGHAN committee on nutrition. Role of dietary factors, food habits, and lifestyle in childhood obesity development: A position paper from the european society for paediatric gastroenterology, hepatology and nutrition committee on nutrition. J Pediatr Gastroenterol Nutr. (2021) 72:769–83. doi: 10.1097/MPG.0000000000003075
14. Simmonds M, Llewellyn A, Owen CG, Woolacott N. Predicting adult obesity from childhood obesity: a systematic review and meta-analysis. Obes Rev. (2016) 17:95–107. doi: 10.1111/obr.12334
15. Rodríguez Delgado J. The key role of primary care pediatrics in the management of childhood obesity. Pediatr (Engl Ed). (2021) 95:137–8. doi: 10.1016/j.anpede.2021.02.008
16. Sonoda R, Tokiya M, Touri K, Tanomura Y, Yada K, Funakoshi Y, et al. A point system to predict the future risk of obesity in 10-year-old children. Environ Health Prev Med. (2023) 28:25. doi: 10.1265/ehpm.22-00270
17. Endalifer ML, Diress G. Epidemiology, predisposing factors, biomarkers, and prevention mechanism of obesity: A systematic review. J Obes. (2020) 2020:6134362. doi: 10.1155/2020/6134362
18. Sun J, Jin X, Yang L, Ma X, Xi B, Song S, et al. Association between gut microbiota and obesity combined with high carotid intima-media thickness among Chinese children. Asia Pac J Clin Nutr. (2023) 32:93–105. doi: 10.6133/apjcn.202303_32(1).0014
19. Jia P, Shi Y, Jiang Q, Dai S, Yu B, Yang S, et al. Environmental determinants of childhood obesity: a meta-analysis. Lancet Glob Health. (2023) 11:S7. doi: 10.1016/S2214-109X(23)00092-X
20. Li H, Ren J, Li Y, Wu Q, Wei J. Oxidative stress: The nexus of obesity and cognitive dysfunction in diabetes. Front Endocrinol (Lausanne). (2023) 14:1134025. doi: 10.3389/fendo.2023.1134025
21. Deal BJ, Huffman MD, Binns H, Stone NJ. Perspective: childhood obesity requires new strategies for prevention. Adv Nutr. (2020) 11:1071–8. doi: 10.1093/advances/nmaa040
22. Kappes C, Stein R, Körner A, Merkenschlager A, Kiess W. Stress, stress reduction and obesity in childhood and adolescence. Horm Res Paediatr. (2023) 96:88–96. doi: 10.1159/000519284
23. Furukawa S, Fujita T, Shimabukuro M, Iwaki M, Yamada Y, Nakajima Y, et al. Increased oxidative stress in obesity and its impact on metabolic syndrome. J Clin Invest. (2004) 114:1752–61. doi: 10.1172/JCI21625
24. Korac B, Kalezic A, Pekovic-Vaughan V, Korac A, Jankovic A. Redox changes in obesity, metabolic syndrome, and diabetes. Redox Biol. (2021) 42:101887. doi: 10.1016/j.redox.2021.101887
25. Forman HJ, Zhang H. Targeting oxidative stress in disease: promise and limitations of antioxidant therapy. Nat Rev Drug Discov. (2021) 20:689–709. doi: 10.1038/s41573-021-00233-1
26. Pisoschi AM, Pop A. The role of antioxidants in the chemistry of oxidative stress: A review. Eur J Med Chem. (2015) 97:55–74. doi: 10.1016/j.ejmech.2015.04.040
27. Kimball JS, Johnson JP, Carlson DA. Oxidative stress and osteoporosis. J Bone Joint Surg Am. (2021) 103:1451–61. doi: 10.2106/JBJS.20.00989
28. Kattoor AJ, Pothineni NVK, Palagiri D, Mehta JL. Oxidative stress in atherosclerosis. Curr Atheroscler Rep. (2017) 19:42. doi: 10.1007/s11883-017-0678-6
29. Sinha N, Dabla PK. Oxidative stress and antioxidants in hypertension-a current review. Curr Hypertens Rev. (2015) 11:132–42. doi: 10.2174/1573402111666150529130922
30. Bisht S, Faiq M, Tolahunase M, Dada R. Oxidative stress and male infertility. Nat Rev Urol. (2017) 14:470–85. doi: 10.1038/nrurol.2017.69
31. Griendling KK. ATVB in focus: redox mechanisms in blood vessels. Arterioscler Thromb Vasc Biol. (2005) 25:272–3. doi: 10.1161/01.ATV.0000153515.72375.3b
32. Pérez-Torres I, Castrejón-Téllez V, Soto ME, Rubio-Ruiz ME, Manzano-Pech L, Guarner-Lans V. Oxidative stress, plant natural antioxidants, and obesity. Int J Mol Sci. (2021) 22:1786. doi: 10.3390/ijms22041786
33. Ma Q. Role of nrf2 in oxidative stress and toxicity. Annu Rev Pharmacol Toxicol. (2013) 53:401–26. doi: 10.1146/annurev-pharmtox-011112-140320
34. Hybertson BM, Gao B, Bose SK, McCord JM. Oxidative stress in health and disease: the therapeutic potential of Nrf2 activation. Mol Aspects Med. (2011) 32:234–46. doi: 10.1016/j.mam.2011.10.006
35. Reiter RJ, Tan DX, Galano A. Melatonin: exceeding expectations. Physiol (Bethesda). (2014) 29:325–33. doi: 10.1152/physiol.00011.2014
36. Zhou Y, Li H, Xia N. The interplay between adipose tissue and vasculature: role of oxidative stress in obesity. Front Cardiovasc Med. (2021) 8:650214. doi: 10.3389/fcvm.2021.650214
37. Rani V, Deep G, Singh RK, Palle K, Yadav UC. Oxidative stress and metabolic disorders: Pathogenesis and therapeutic strategies. Life Sci. (2016) 148:183–93. doi: 10.1016/j.lfs.2016.02.002
38. Grasemann H, Holguin F. Oxidative stress and obesity-related asthma. Paediatr Respir Rev. (2021) 37:18–21. doi: 10.1016/j.prrv.2020.05.004
39. Moreno-Fernandez J, Ochoa J, Ojeda ML, Nogales F, Carreras O, Díaz-Castro J. Inflammation and oxidative stress, the links between obesity and COVID-19: a narrative review. J Physiol Biochem. (2022) 78:581–91. doi: 10.1007/s13105-022-00887-4
40. Andrieux P, Chevillard C, Cunha-Neto E, Nunes JPS. Mitochondria as a cellular hub in infection and inflammation. Int J Mol Sci. (2021) 22:11338. doi: 10.3390/ijms222111338
41. Fernández-Sánchez A, Madrigal-Santillán E, Bautista M, Esquivel-Soto J, Morales-González A, Esquivel-Chirino C, et al. Inflammation, oxidative stress, and obesity. Int J Mol Sci. (2011) 12:3117–32. doi: 10.3390/ijms12053117
42. Prasun P. Mitochondrial dysfunction in metabolic syndrome. Biochim Biophys Acta Mol Basis Dis. (2020) 1866:165838. doi: 10.1016/j.bbadis.2020.165838
43. Bhatti JS, Bhatti GK, Reddy PH. Mitochondrial dysfunction and oxidative stress in metabolic disorders - A step towards mitochondria based therapeutic strategies. Biochim Biophys Acta Mol Basis Dis. (2017) 1863:1066–77. doi: 10.1016/j.bbadis.2016.11.010
44. Zamora-Mendoza R, Rosas-Vargas H, Ramos-Cervantes MT, Garcia-Zuniga P, Perez-Lorenzana H, Mendoza-Lorenzo P, et al. Dysregulation of mitochondrial function and biogenesis modulators in adipose tissue of obese children. Int J Obes (Lond). (2018) 42:618–24. doi: 10.1038/ijo.2017.274
45. Frigolet ME, Gutiérrez-Aguilar R. The colors of adipose tissue. Gac Med Mex. (2020) 156:142–9. doi: 10.24875/GMM.M20000356
46. Ponrartana S, Hu HH, Gilsanz V. On the relevance of brown adipose tissue in children. Ann N Y Acad Sci. (2013) 1302:24–9. doi: 10.1111/nyas.12195
47. Cui X, Xiao W, You L, Zhang F, Cao X, Feng J, et al. Age-induced oxidative stress impairs adipogenesis and thermogenesis in brown fat. FEBS J. (2019) 286:2753–68. doi: 10.1111/febs.14838
48. Gilsanz V, Hu HH, Kajimura S. Relevance of brown adipose tissue in infancy and adolescence. Pediatr Res. (2013) 73:3–9. doi: 10.1038/pr.2012.141
49. Vishvanath L, Gupta RK. Contribution of adipogenesis to healthy adipose tissue expansion in obesity. J Clin Invest. (2019) 129:4022–31. doi: 10.1172/JCI129191
50. Marseglia L, Manti S, D'Angelo G, Nicotera A, Parisi E, Di Rosa G, et al. Oxidative stress in obesity: a critical component in human diseases. Int J Mol Sci. (2014) 16:378–400. doi: 10.3390/ijms16010378
51. Codoñer-Franch P, Valls-Bellés V, Arilla-Codoñer A, Alonso-Iglesias E. Oxidant mechanisms in childhood obesity: the link between inflammation and oxidative stress. Transl Res. (2011) 158:369–84. doi: 10.1016/j.trsl.2011.08.004
52. León J, Sáenz JM, Artacho-Cordón F, Fernández MF, Martin-Olmedo P, Salamanca-Fernández E, et al. Contribution of sociodemographic, occupational, lifestyle and dietary characteristics to the oxidative stress microenvironment in adipose tissue. Environ Res. (2019) 175:52–62. doi: 10.1016/j.envres.2019.04.035
53. Sharma A, Singh S, Ahmad S, Gulzar F, Schertzer JD, Tamrakar AK. NOD1 activation induces oxidative stress via NOX1/4 in adipocytes. Free Radic Biol Med. (2021) 162:118–28. doi: 10.1016/j.freeradbiomed.2020.11.036
54. Shin SK, Cho HW, Song SE, Im SS, Bae JH, Song DK. Oxidative stress resulting from the removal of endogenous catalase induces obesity by promoting hyperplasia and hypertrophy of white adipocytes. Redox Biol. (2020) 37:101749. doi: 10.1016/j.redox.2020.101749
55. Jeon YG, Kim YY, Lee G, Kim JB. Physiological and pathological roles of lipogenesis. Nat Metab. (2023) 5:735–59. doi: 10.1038/s42255-023-00786-y
56. Wallace M, Metallo CM. Tracing insights into de novo lipogenesis in liver and adipose tissues. Semin Cell Dev Biol. (2020) 108:65–71. doi: 10.1016/j.semcdb.2020.02.012
57. GianFrancesco MA, Paquot N, Piette J, Legrand-Poels S. Lipid bilayer stress in obesity-linked inflammatory and metabolic disorders. Biochem Pharmacol. (2018) 153:168–83. doi: 10.1016/j.bcp.2018.02.022
58. Hsiao WY, Guertin DA. De novo lipogenesis as a source of second messengers in adipocytes. Curr Diabetes Rep. (2019) 19:138. doi: 10.1007/s11892-019-1264-9
59. Abou-Rjeileh U, Contreras GA. Redox regulation of lipid mobilization in adipose tissues. Antioxidants (Basel). (2021) 10:1090. doi: 10.3390/antiox10071090
60. Yazıcı D, Sezer H. Insulin resistance, obesity and lipotoxicity. Adv Exp Med Biol. (2017) 960:277–304. doi: 10.1007/978-3-319-48382-5_12
61. Katsiki N, Mikhailidis DP, Banach M. Leptin, cardiovascular diseases and type 2 diabetes mellitus. Acta Pharmacol Sin. (2018) 39:1176–88. doi: 10.1038/aps.2018.40
62. Matsuda M, Shimomura I. Roles of adiponectin and oxidative stress in obesity-associated metabolic and cardiovascular diseases. Rev Endocr Metab Disord. (2014) 15:1–10. doi: 10.1007/s11154-013-9271-7
63. Fang H, Judd RL. Adiponectin regulation and function. Compr Physiol. (2018) 8:1031–63. doi: 10.1002/cphy.c170046
64. Than A, Zhang X, Leow MK, Poh CL, Chong SK, Chen P. Apelin attenuates oxidative stress in human adipocytes. J Biol Chem. (2014) 289:3763–74. doi: 10.1074/jbc.M113.526210
65. González-Domínguez Á, Visiedo-García FM, Domínguez-Riscart J, González-Domínguez R, Mateos RM, Lechuga-Sancho AM. Iron metabolism in obesity and metabolic syndrome. Int J Mol Sci. (2020) 21:5529. doi: 10.3390/ijms21155529
66. Ikeda Y, Funamoto M, Tsuchiya K. The role of iron in obesity and diabetes. J Med Invest. (2022) 69:1–7. doi: 10.2152/jmi.69.1
67. Song CC, Pantopoulos K, Chen GH, Zhong CC, Zhao T, Zhang DG, et al. Iron increases lipid deposition via oxidative stress-mediated mitochondrial dysfunction and the HIF1α-PPARγ pathway. Cell Mol Life Sci. (2022) 79:394. doi: 10.1007/s00018-022-04423-x
68. Kajarabille N, Latunde-Dada GO. Programmed cell-death by ferroptosis: antioxidants as mitigators. Int J Mol Sci. (2019) 20:4968. doi: 10.3390/ijms20194968
69. Horinouchi Y, Ikeda Y, Tamaki T. [Body iron accumulation in obesity, diabetes and its complications, and the possibility of therapeutic application by iron regulation]. Nihon Yakurigaku Zasshi. (2019) 154:316–21. doi: 10.1254/fpj.154.316
70. Monteiro R, Azevedo I. Chronic inflammation in obesity and the metabolic syndrome. Mediators Inflamm. (2010) 2010:289645. doi: 10.1155/2010/289645
71. Engin A. The pathogenesis of obesity-associated adipose tissue inflammation. Adv Exp Med Biol. (2017) 960:221–45. doi: 10.1007/978-3-319-48382-5_9
72. Bondia-Pons I, Ryan L, Martinez JA. Oxidative stress and inflammation interactions in human obesity. J Physiol Biochem. (2012) 68:701–11. doi: 10.1007/s13105-012-0154-2
73. Matusik P, Prokopowicz Z, Norek B, Olszanecka-Glinianowicz M, Chudek J, Malecka-Tendera E. Oxidative/Antioxidative status in obese and sport trained children: a comparative study. BioMed Res Int. (2015) 2015:315747. doi: 10.1155/2015/315747
74. Huang CJ, McAllister MJ, Slusher AL, Webb HE, Mock JT, Acevedo EO. Obesity-related oxidative stress: the impact of physical activity and diet manipulation. Sports Med Open. (2015) 1:32. doi: 10.1186/s40798-015-0031-y
75. Farzanegi P, Dana A, Ebrahimpoor Z, Asadi M, Azarbayjani MA. Mechanisms of beneficial effects of exercise training on non-alcoholic fatty liver disease (NAFLD): Roles of oxidative stress and inflammation. Eur J Sport Sci. (2019) 19:994–1003. doi: 10.1080/17461391.2019.1571114
76. He L, He T, Farrar S, Ji L, Liu T, Ma X. Antioxidants maintain cellular redox homeostasis by elimination of reactive oxygen species. Cell Physiol Biochem. (2017) 44:532–53. doi: 10.1159/000485089
77. Mohseni R, Arab Sadeghabadi Z, Goodarzi MT, Teimouri M, Nourbakhsh M, Razzaghy Azar M. Evaluation of Mn-superoxide dismutase and catalase gene expression in childhood obesity: its association with insulin resistance. J Pediatr Endocrinol Metab. (2018) 31:727–32. doi: 10.1515/jpem-2017-0322
78. Erdeve O, Siklar Z, Kocaturk PA, Dallar Y, Kavas GO. Antioxidant superoxide dismutase activity in obese children. Biol Trace Elem Res. (2004) 98:219–28. doi: 10.1385/BTER:98:3:219
79. Mondola P, Damiano S, Sasso A, Santillo M. The cu, zn superoxide dismutase: not only a dismutase enzyme. Front Physiol. (2016) 7:594. doi: 10.3389/fphys.2016.00594
80. Özgen İT, Torun E, Ergen A, Cesur Y, Karagedik H, Zeybek Ü, et al. Myeloperoxidase 463 G>A and superoxide dismutase Ala16Val gene polymorphisms in obese children. Turk J Pediatr. (2014) 56:511–7.
81. González-Domínguez Á, Visiedo F, Domínguez-Riscart J, Durán-Ruiz MC, Saez-Benito A, Lechuga-Sancho AM, et al. Catalase post-translational modifications as key targets in the control of erythrocyte redox homeostasis in children with obesity and insulin resistance. Free Radic Biol Med. (2022) 191:40–7. doi: 10.1016/j.freeradbiomed.2022.08.017
82. Rupérez AI, Olza J, Gil-Campos M, Leis R, Mesa MD, Tojo R, et al. Are catalase -844A/G polymorphism and activity associated with childhood obesity? Antioxid Redox Signal. (2013) 19:1970–5. doi: 10.1089/ars.2013.5386
83. Nitta Y, Muraoka-Hirayama S, Sakurai K. Catalase is required for peroxisome maintenance during adipogenesis. Biochim Biophys Acta Mol Cell Biol Lipids. (2020) 1865:158726. doi: 10.1016/j.bbalip.2020.158726
84. Croft AJ, Kelly C, Chen D, Haw TJ, Sverdlov AL, Ngo DTM. Overexpression of Mitochondrial Catalase within Adipose Tissue Does Not Confer Systemic Metabolic Protection against Diet-Induced Obesity. Antioxidants (Basel). (2023) 12:1137. doi: 10.3390/antiox12051137
85. Wang XL, Jiang RW. Therapeutic potential of superoxide dismutase fused with cell- penetrating peptides in oxidative stress-related diseases. Mini Rev Med Chem. (2022) 22:2287–98. doi: 10.2174/1389557522666220228150127
86. Coudriet GM, Delmastro-Greenwood MM, Previte DM, Marré ML, O'Connor EC, Novak EA, et al. Treatment with a catalytic superoxide dismutase (SOD) mimetic improves liver steatosis, insulin sensitivity, and inflammation in obesity-induced type 2 diabetes. Antioxidants (Basel). (2017) 6:85. doi: 10.3390/antiox6040085
87. Brigelius-Flohé R, Flohé L. Regulatory phenomena in the glutathione peroxidase superfamily. Antioxid Redox Signal. (2020) 33:498–516. doi: 10.1089/ars.2019.7905
88. Handy DE, Loscalzo J. The role of glutathione peroxidase-1 in health and disease. Free Radic Biol Med. (2022) 188:146–61. doi: 10.1016/j.freeradbiomed.2022.06.004
89. Huang JQ, Zhou JC, Wu YY, Ren FZ, Lei XG. Role of glutathione peroxidase 1 in glucose and lipid metabolism-related diseases. Free Radic Biol Med. (2018) 127:108–15. doi: 10.1016/j.freeradbiomed.2018.05.077
90. Yan X, Zhao Z, Weaver J, Sun T, Yun JW, Roneker CA, et al. Role and mechanism of REG2 depletion in insulin secretion augmented by glutathione peroxidase-1 overproduction. Redox Biol. (2022) 56:102457. doi: 10.1016/j.redox.2022.102457
91. Wang XD, Vatamaniuk MZ, Wang SK, Roneker CA, Simmons RA, Lei XG. Molecular mechanisms for hyperinsulinaemia induced by overproduction of selenium-dependent glutathione peroxidase-1 in mice. Diabetologia. (2008) 51:1515–24. doi: 10.1007/s00125-008-1055-3
92. Couto N, Wood J, Barber J. The role of glutathione reductase and related enzymes on cellular redox homoeostasis network. Free Radic Biol Med. (2016) 95:27–42. doi: 10.1016/j.freeradbiomed.2016.02.028
93. Montero D, Vicente-Salar N, Herranz M, Micol V, Walther G, Pérez-Martín A, et al. Glutathione-dependent enzyme activities of peripheral blood mononuclear cells decrease during the winter season compared with the summer in normal-weight and severely obese adolescents. J Physiol Biochem. (2019) 75:321–7. doi: 10.1007/s13105-019-00693-5
94. Cai Y, Li X, Tan X, Wang P, Zhao X, Zhang H, et al. Vitamin D suppresses ferroptosis and protects against neonatal hypoxic-ischemic encephalopathy by activating the Nrf2/HO-1 pathway. Transl Pediatr. (2022) 11:1633–44. doi: 10.21037/tp-22-397
95. Perkins A, Nelson KJ, Parsonage D, Poole LB, Karplus PA. Peroxiredoxins: guardians against oxidative stress and modulators of peroxide signaling. Trends Biochem Sci. (2015) 40:435–45. doi: 10.1016/j.tibs.2015.05.001
96. Gateva AT, Velikova TV, Kamenov ZA. Peroxiredoxin 4 levels in patients with PCOS and/or obesity. J Gynecol Obstet Hum Reprod. (2019) 48:739–43. doi: 10.1016/j.jogoh.2019.04.002
97. Shin HJ, Lee SW, Kim MH, Park YH, Lee HJ, Lee DS. Mitochondrial peroxiredoxin 5 overexpression suppresses insulin-induced adipogenesis by downregulating the phosphorylation of p38. Biosci Biotechnol Biochem. (2023) 87:696–706. doi: 10.1093/bbb/zbad040
98. Kim MH, Kim JY, Kim JH, Lee HS, Huh JW, Lee DS. Peroxiredoxin 2 deficiency reduces white adipogenesis due to the excessive ROS generation. Cell Biol Int. (2020) 44:2086–93. doi: 10.1002/cbin.11417
99. Arriga R, Pacifici F, Capuani B, Coppola A, Orlandi A, Scioli MG, et al. Peroxiredoxin 6 is a key antioxidant enzyme in modulating the link between glycemic and lipogenic metabolism. Oxid Med Cell Longev. (2019) 2019:9685607. doi: 10.1155/2019/9685607
100. Xu GL, Ye XL, Vashisth MK, Zhao WZ. Correlation between PRDX2 and spermatogenesis under oxidative stress. Biochem Biophys Res Commun. (2023) 656:139–45. doi: 10.1016/j.bbrc.2023.03.050
101. Tinkov AA, Bjørklund G, Skalny AV, Holmgren A, Skalnaya MG, Chirumbolo S, et al. The role of the thioredoxin/thioredoxin reductase system in the metabolic syndrome: towards a possible prognostic marker? Cell Mol Life Sci. (2018) 75:1567–86. doi: 10.1007/s00018-018-2745-8
102. Das SK, Sharma NK, Hasstedt SJ, Mondal AK, Ma L, Langberg KA, et al. An integrative genomics approach identifies activation of thioredoxin/thioredoxin reductase-1-mediated oxidative stress defense pathway and inhibition of angiogenesis in obese nondiabetic human subjects. J Clin Endocrinol Metab. (2011) 96:E1308–13. doi: 10.1210/jc.2011-0101
103. Kota SK, Meher LK, Kota SK, Jammula S, Krishna SV, Modi KD. Implications of serum paraoxonase activity in obesity, diabetes mellitus, and dyslipidemia. Indian J Endocrinol Metab. (2013) 17:402–12. doi: 10.4103/2230-8210.111618
104. Fülöp P, Harangi M, Seres I, Paragh G. Paraoxonase-1 and adipokines: Potential links between obesity and atherosclerosis. Chem Biol Interact. (2016) 259:388–93. doi: 10.1016/j.cbi.2016.04.003
105. Tu TH, Joe Y, Choi HS, Chung HT, Yu R. Induction of heme oxygenase-1 with hemin reduces obesity-induced adipose tissue inflammation via adipose macrophage phenotype switching. Mediators Inflamm. (2014) 2014:290708. doi: 10.1155/2014/290708
106. Dawson MI. The importance of vitamin A in nutrition. Curr Pharm Des. (2000) 6:311–25. doi: 10.2174/1381612003401190
107. Miyazawa T, Burdeos GC, Itaya M, Nakagawa K, Miyazawa T. Vitamin E: regulatory redox interactions. IUBMB Life. (2019) 71:430–41. doi: 10.1002/iub.2008
108. Guerendiain M, Mayneris-Perxachs J, Montes R, López-Belmonte G, Martín-Matillas M, Castellote AI, et al. Relation between plasma antioxidant vitamin levels, adiposity and cardio-metabolic profile in adolescents: Effects of a multidisciplinary obesity programme. Clin Nutr. (2017) 36:209–17. doi: 10.1016/j.clnu.2015.11.001
109. Gama Oliveira MN, Rosa G, João Mansure J, Wagner Ramos V, Godoy PH. Anthropometric profile, serum leptin, antioxidant vitamins, LEPR Q223R polymorphism and cardiovascular risk factors in adolescents. Int J Vitam Nutr Res. (2018) 88:27–38. doi: 10.1024/0300-9831/a000274
110. Gajewska J, Ambroszkiewicz J, Szamotulska K, Klemarczyk W, Weker H, Chełchowska M. Associations between antioxidant vitamin status, dietary intake, and retinol-binding protein 4 levels in prepubertal obese children after 3-month weight loss therapy. J Clin Res Pediatr Endocrinol. (2021) 13:187–97. doi: 10.4274/jcrpe.galenos.2020.2020.0207
111. Carr AC, Maggini S. Vitamin C and immune function. Nutrients. (2017) 9:1211. doi: 10.3390/nu9111211
112. Lykkesfeldt J, Tveden-Nyborg P. The pharmacokinetics of vitamin C. Nutrients. (2019) 11(10):2412. doi: 10.3390/nu11102412
113. Garcia-Diaz DF, Lopez-Legarrea P, Quintero P, Martinez JA. Vitamin C in the treatment and/or prevention of obesity. J Nutr Sci Vitaminol (Tokyo). (2014) 60:367–79. doi: 10.3177/jnsv.60.367
114. Ellulu MS. Obesity, cardiovascular disease, and role of vitamin C on inflammation: a review of facts and underlying mechanisms. Inflammopharmacology. (2017) 25:313–28. doi: 10.1007/s10787-017-0314-7
115. Choi MK, Song HJ, Paek YJ, Lee HJ. Gender differences in the relationship between vitamin C and abdominal obesity. Int J Vitam Nutr Res. (2013) 83:377–84. doi: 10.1024/0300-9831/a000179
116. Carr AC, Block G, Lykkesfeldt J. Estimation of vitamin C intake requirements based on body weight: implications for obesity. Nutrients. (2022) 14:1460. doi: 10.3390/nu14071460
117. Xie D, Hu J, Yang Z, Wu T, Xu W, Meng Q, et al. Vitamin supplementation protects against nanomaterial-induced oxidative stress and inflammation damages: A meta-analysis of in vitro and in vivo studies. Nutrients. (2022) 14:2214. doi: 10.3390/nu14112214
118. Migliaccio S, Di Nisio A, Mele C, Scappaticcio L, Savastano S, Colao A, et al. Obesity and hypovitaminosis D: causality or casualty? Int J Obes Suppl. (2019) 9:20–31. doi: 10.1038/s41367-019-0010-8
119. Wimalawansa SJ. Vitamin D deficiency: effects on oxidative stress, epigenetics, gene regulation, and aging. Biol (Basel). (2019) 8:30. doi: 10.3390/biology8020030
120. Ruiz-Ojeda FJ, Anguita-Ruiz A, Leis R, Aguilera CM. Genetic factors and molecular mechanisms of vitamin D and obesity relationship. Ann Nutr Metab. (2018) 73:89–99. doi: 10.1159/000490669
121. Farhangi MA, Mesgari-Abbasi M, Hajiluian G, Nameni G, Shahabi P. Adipose tissue inflammation and oxidative stress: the ameliorative effects of vitamin D. Inflammation. (2017) 40:1688–97. doi: 10.1007/s10753-017-0610-9
122. Ionica M, Aburel OM, Vaduva A, Petrus A, Raţiu S, Olariu S, et al. Vitamin D alleviates oxidative stress in adipose tissue and mesenteric vessels from obese patients with subclinical inflammation. Can J Physiol Pharmacol. (2020) 98:85–92. doi: 10.1139/cjpp-2019-0340
123. Usman M, Woloshynowych M, Britto JC, Bilkevic I, Glassar B, Chapman S, et al. Obesity, oxidative DNA damage and vitamin D as predictors of genomic instability in children and adolescents. Int J Obes (Lond). (2021) 45:2095–107. doi: 10.1038/s41366-021-00879-2
124. Filgueiras MS, Rocha NP, Novaes JF, Bressan J. Vitamin D status, oxidative stress, and inflammation in children and adolescents: A systematic review. Crit Rev Food Sci Nutr. (2020) 60:660–9. doi: 10.1080/10408398.2018.1546671
125. Samouda H, De Beaufort C, Gilson G, Schritz A, Vaillant M, Ghaddhab C, et al. Relationship of oxidative stress to visceral adiposity in youth and role played by vitamin D. Pediatr Diabetes. (2020) 21:758–65. doi: 10.1111/pedi.13055
126. Calcaterra V, Verduci E, Milanta C, Agostinelli M, Todisco CF, Bona F, et al. Micronutrient deficiency in children and adolescents with obesity-A narrative review. Children (Basel). (2023) 10:695. doi: 10.3390/children10040695
127. Błoniarz J, Zareba S. Selected microelements (Cr, Zn, Cu, Mn, Fe, Ni) in slimming preparations. Rocz Panstw Zakl Hig. (2007) 58:165–70.
128. Luque-Díaz MJ, Dean-Guelbenzu M, Culebras-Poza JM. Alteraciones del metabolismo del hierro, cobre y zinc en la obesidad [Changes in the metabolism of iron, copper and zinc in obesity]. Rev Esp Fisiol. (1982) 38:155–8.
129. Sharif R, Thomas P, Zalewski P, Fenech M. The role of zinc in genomic stability. Mutat Res. (2012) 733:111–21. doi: 10.1016/j.mrfmmm.2011.08.009
130. Severo JS, Morais JBS, Beserra JB, Dos Santos LR, de Sousa Melo SR, de Sousa GS, et al. Role of zinc in zinc-α2-glycoprotein metabolism in obesity: a review of literature. Biol Trace Elem Res. (2020) 193:81–8. doi: 10.1007/s12011-019-01702-w
131. Zhang H, Cai L. Zinc homeostasis plays an important role in the prevention of obesity-induced cardiac inflammation, remodeling and dysfunction. J Trace Elem Med Biol. (2020) 62:126615. doi: 10.1016/j.jtemb.2020.126615
132. Marreiro DD, Cruz KJ, Morais JB, Beserra JB, Severo JS, de Oliveira AR. Zinc and oxidative stress: current mechanisms. Antioxidants (Basel). (2017) 6:24. doi: 10.3390/antiox6020024
133. Morais JB, Severo JS, Santos LR, de Sousa Melo SR, de Oliveira Santos R, de Oliveira AR, et al. Role of magnesium in oxidative stress in individuals with obesity. Biol Trace Elem Res. (2017) 176:20–6. doi: 10.1007/s12011-016-0793-1
134. Li Y, Li M, Liu X, Wu M, Yang L, Yang L, et al. [Effects of marginal zinc deficiency on obesity]. Wei Sheng Yan Jiu. (2017) 46:373–7.
135. Mendes Garrido Abregú F, Caniffi C, Arranz CT, Tomat AL. Impact of zinc deficiency during prenatal and/or postnatal life on cardiovascular and metabolic diseases: experimental and clinical evidence. Adv Nutr. (2022) 13:833–45. doi: 10.1093/advances/nmac012
136. Mahmoud AH, AbdElMonem HA, Abbas MM. The role of selenium and zinc oxide nanoparticles on mitigating side effects of obesity in rats. Braz J Biol. (2022) 84:e264004. doi: 10.1590/1519-6984.264004
137. Zavros A, Giannaki CD, Aphamis G, Roupa Z, Andreou E. The effects of zinc and selenium supplementation on body composition and thyroid function in individuals with overweight or obesity: A systematic review. J Diet Suppl. (2023) 20:643–71. doi: 10.1080/19390211.2022.2072044
138. Wang Y, Liu B, Wu P, Chu Y, Gui S, Zheng Y, et al. Dietary selenium alleviated mouse liver oxidative stress and NAFLD induced by obesity by regulating the KEAP1/NRF2 pathway. Antioxidants (Basel). (2022) 11:349. doi: 10.3390/antiox11020349
139. Tinkov AA, Ajsuvakova OP, Filippini T, Zhou JC, Lei XG, Gatiatulina ER, et al. Selenium and selenoproteins in adipose tissue physiology and obesity. Biomolecules. (2020) 10:658. doi: 10.3390/biom10040658
140. Abo El-Magd NF, Barbosa PO, Nick J, Covalero V, Grignetti G, Bermano G. Selenium, as selenite, prevents adipogenesis by modulating selenoproteins gene expression and oxidative stress-related genes. Nutrition. (2022) 93:111424. doi: 10.1016/j.nut.2021.111424
141. Aguirre JD, Culotta VC. Battles with iron: manganese in oxidative stress protection. J Biol Chem. (2012) 287:13541–8. doi: 10.1074/jbc.R111.312181
142. Li L, Yang X. The essential element manganese, oxidative stress, and metabolic diseases: links and interactions. Oxid Med Cell Longev. (2018) 2018:7580707. doi: 10.1155/2018/7580707
143. Dinda B, Dinda M, Roy A, Dinda S. Dietary plant flavonoids in prevention of obesity and diabetes. Adv Protein Chem Struct Biol. (2020) 120:159–235. doi: 10.1016/bs.apcsb.2019.08.006
144. García-Barrado MJ, Iglesias-Osma MC, Pérez-García E, Carrero S, Blanco EJ, Carretero-Hernández M, et al. Role of flavonoids in the interactions among obesity, inflammation, and autophagy. Pharm (Basel). (2020) 13:342. doi: 10.3390/ph13110342
145. Kawser Hossain M, Abdal Dayem A, Han J, Yin Y, Kim K, Kumar Saha S, et al. Molecular mechanisms of the anti-obesity and anti-diabetic properties of flavonoids. Int J Mol Sci. (2016) 17:569. doi: 10.3390/ijms17040569
146. Oliveira AKS, de Oliveira E Silva AM, Pereira RO, Santos AS, Barbosa Junior EV, Bezerra MT, et al. Anti-obesity properties and mechanism of action of flavonoids: A review. Crit Rev Food Sci Nutr. (2022) 62:7827–48. doi: 10.1080/10408398.2021.1919051
147. Mahboob A, Samuel SM, Mohamed A, Wani MY, Ghorbel S, Miled N, et al. Role of flavonoids in controlling obesity: molecular targets and mechanisms. Front Nutr. (2023) 10:1177897. doi: 10.3389/fnut.2023.1177897
148. Song D, Cheng L, Zhang X, Wu Z, Zheng X. The modulatory effect and the mechanism of flavonoids on obesity. J Food Biochem. (2019) 43:e12954. doi: 10.1111/jfbc.12954
149. Naomi R, Teoh SH, Halim S, Embong H, Hasain Z, Bahari H, et al. Unraveling obesity: transgenerational inheritance, treatment side effects, flavonoids, mechanisms, microbiota, redox balance, and bioavailability-A narrative review. Antioxidants (Basel). (2023) 12:1549. doi: 10.3390/antiox12081549
150. Lupu VV, Adam Raileanu A, Mihai CM, Morariu ID, Lupu A, Starcea IM, et al. The implication of the gut microbiome in heart failure. Cells. (2023) 12:1158. doi: 10.3390/cells12081158
151. Bozomitu L, Miron I, Adam Raileanu A, Lupu A, Paduraru G, Marcu FM, et al. The gut microbiome and its implication in the mucosal digestive disorders. Biomedicines. (2022) 10:3117. doi: 10.3390/biomedicines10123117
152. Pantazi AC, Mihai CM, Balasa AL, Chisnoiu T, Lupu A, Frecus CE, et al. Relationship between gut microbiota and allergies in children: A literature review. Nutrients. (2023) 15:2529. doi: 10.3390/nu15112529
153. Lupu VV, Trandafir LM, Raileanu AA, Mihai CM, Morariu ID, Starcea IM, et al. Advances in understanding the human gut microbiota and its implication in pediatric celiac disease-A narrative review. Nutrients. (2023) 15:2499. doi: 10.3390/nu15112499
154. Lupu A, Jechel E, Mihai CM, Mitrofan EC, Fotea S, Starcea IM, et al. The footprint of microbiome in pediatric asthma-A complex puzzle for a balanced development. Nutrients. (2023) 15:3278. doi: 10.3390/nu15143278
155. Mocanu A, Bogos RA, Lazaruc TI, Trandafir LM, Lupu VV, Ioniuc I, et al. Exploring a complex interplay: kidney–gut axis in pediatric chronic kidney disease. Nutrients. (2023) 15:3609. doi: 10.3390/nu15163609
156. Pantazi AC, Kassim MAK, Nori W, Tuta LA, Mihai CM, Chisnoiu T, et al. Clinical perspectives of gut microbiota in patients with chronic kidney disease and end-stage kidney disease: where do we stand? Biomedicines. (2023) 11:2480. doi: 10.3390/biomedicines11092480
157. Gentile D, Fornai M, Pellegrini C, Colucci R, Blandizzi C, Antonioli L. Dietary flavonoids as a potential intervention to improve redox balance in obesity and related co-morbidities: a review. Nutr Res Rev. (2018) 31:239–47. doi: 10.1017/S0954422418000082
158. Zhang Y, Han P, Wu N, He B, Lu Y, Li S, et al. Amelioration of lipid abnormalities by α-lipoic acid through antioxidative and anti-inflammatory effects. Obes (Silver Spring). (2011) 19:1647–53. doi: 10.1038/oby.2011.121
159. El Amrousy D, El-Afify D. Effects of alpha lipoic acid as a supplement in obese children and adolescents. Cytokine. (2020) 130:155084. doi: 10.1016/j.cyto.2020.155084
160. Moura FA, de Andrade KQ, dos Santos JC, Goulart MO. Lipoic Acid: its antioxidant and anti-inflammatory role and clinical applications. Curr Top Med Chem. (2015) 15:458–83. doi: 10.2174/1568026615666150114161358
161. Tibullo D, Li Volti G, Giallongo C, Grasso S, Tomassoni D, Anfuso CD, et al. Biochemical and clinical relevance of alpha lipoic acid: antioxidant and anti-inflammatory activity, molecular pathways and therapeutic potential. Inflamm Res. (2017) 66:947–59. doi: 10.1007/s00011-017-1079-6
162. Sztolsztener K, Hodun K, Chabowski A. α-lipoic acid ameliorates inflammation state and oxidative stress by reducing the content of bioactive lipid derivatives in the left ventricle of rats fed a high-fat diet. Biochim Biophys Acta Mol Basis Dis. (2022) 1868:166440. doi: 10.1016/j.bbadis.2022.166440
163. Dajnowicz-Brzezik P, Żebrowska E, Maciejczyk M, Zalewska A, Chabowski A. The effect of α-lipoic acid on oxidative stress in adipose tissue of rats with obesity-induced insulin resistance. Cell Physiol Biochem. (2022) 56:239–53. doi: 10.33594/000000528
164. Cui J, Huang D, Zheng Y. Ameliorative effects of α-lipoic acid on high-fat diet-induced oxidative stress and glucose uptake impairment of T cells. Free Radic Res. (2016) 50:1106–15. doi: 10.1080/10715762.2016.1210140
165. Alam MA, Rahman MM. Mitochondrial dysfunction in obesity: potential benefit and mechanism of Co-enzyme Q10 supplementation in metabolic syndrome. J Diabetes Metab Disord. (2014) 13:60. doi: 10.1186/2251-6581-13-60
166. Gutierrez-Mariscal FM, Arenas-de Larriva AP, Limia-Perez L, Romero-Cabrera JL, Yubero-Serrano EM, López-Miranda J. Coenzyme Q10 supplementation for the reduction of oxidative stress: clinical implications in the treatment of chronic diseases. Int J Mol Sci. (2020) 21:7870. doi: 10.3390/ijms21217870
167. Raygan F, Rezavandi Z, Dadkhah Tehrani S, Farrokhian A, Asemi Z. The effects of coenzyme Q10 administration on glucose homeostasis parameters, lipid profiles, biomarkers of inflammation and oxidative stress in patients with metabolic syndrome. Eur J Nutr. (2016) 55:2357–64. doi: 10.1007/s00394-015-1042-7
168. Dabbaghi Varnousfaderani S, Musazadeh V, Ghalichi F, Kavyani Z, Razmjouei S, Faghfouri AH, et al. Alleviating effects of coenzyme Q10 supplements on biomarkers of inflammation and oxidative stress: results from an umbrella meta-analysis. Front Pharmacol. (2023) 14:1191290. doi: 10.3389/fphar.2023.1191290
169. Dludla PV, Mazibuko-Mbeje SE, Nyambuya TM, Mxinwa V, Tiano L, Marcheggiani F, et al. The beneficial effects of N-acetyl cysteine (NAC) against obesity associated complications: A systematic review of pre-clinical studies. Pharmacol Res. (2019) 146:104332. doi: 10.1016/j.phrs.2019.104332
170. Diniz YS, Rocha KK, Souza GA, Galhardi CM, Ebaid GM, Rodrigues HG, et al. Effects of N-acetylcysteine on sucrose-rich diet-induced hyperglycaemia, dyslipidemia and oxidative stress in rats. Eur J Pharmacol. (2006) 543:151–7. doi: 10.1016/j.ejphar.2006.05.039
171. Faghfouri AH, Zarezadeh M, Tavakoli-Rouzbehani OM, Radkhah N, Faghfuri E, Kord-Varkaneh H, et al. The effects of N-acetylcysteine on inflammatory and oxidative stress biomarkers: A systematic review and meta-analysis of controlled clinical trials. Eur J Pharmacol. (2020) 884:173368. doi: 10.1016/j.ejphar.2020.173368
172. Lee YS, Kim AY, Choi JW, Kim M, Yasue S, Son HJ, et al. Dysregulation of adipose glutathione peroxidase 3 in obesity contributes to local and systemic oxidative stress. Mol Endocrinol. (2008) 22:2176–89. doi: 10.1210/me.2008-0023
173. Guan Q, Wang Z, Cao J, Dong Y, Chen Y. Mechanisms of melatonin in obesity: A review. Int J Mol Sci. (2021) 23:218. doi: 10.3390/ijms23010218
174. Prado NJ, Ferder L, Manucha W, Diez ER. Anti-inflammatory effects of melatonin in obesity and hypertension. Curr Hypertens Rep. (2018) 20:45. doi: 10.1007/s11906-018-0842-6
175. Genario R, Cipolla-Neto J, Bueno AA, Santos HO. Melatonin supplementation in the management of obesity and obesity-associated disorders: A review of physiological mechanisms and clinical applications. Pharmacol Res. (2021) 163:105254. doi: 10.1016/j.phrs.2020.105254
176. Szewczyk-Golec K, Rajewski P, Gackowski M, Mila-Kierzenkowska C, Wesołowski R, Sutkowy P, et al. Melatonin supplementation lowers oxidative stress and regulates adipokines in obese patients on a calorie-restricted diet. Oxid Med Cell Longev. (2017) 2017:8494107. doi: 10.1155/2017/8494107
177. Ben Dhia I, Maaloul R, Marzougui H, Ghroubi S, Kallel C, Driss T, et al. Melatonin reduces muscle damage, inflammation and oxidative stress induced by exhaustive exercise in people with overweight/obesity. Physiol Int. (2022) 109(4):524–6. doi: 10.1556/2060.2022.00126
178. Ito T, Schaffer SW, Azuma J. The potential usefulness of taurine on diabetes mellitus and its complications. Amino Acids. (2012) 42:1529–39. doi: 10.1007/s00726-011-0883-5
179. Abebe W, Mozaffari MS. Role of taurine in the vasculature: an overview of experimental and human studies. Am J Cardiovasc Dis. (2011) 1:293–311.
180. Gentile CL, Nivala AM, Gonzales JC, Pfaffenbach KT, Wang D, Wei Y, et al. Experimental evidence for therapeutic potential of taurine in the treatment of nonalcoholic fatty liver disease. Am J Physiol Regul Integr Comp Physiol. (2011) 301:R1710–22. doi: 10.1152/ajpregu.00677.2010
181. Murakami S. Role of taurine in the pathogenesis of obesity. Mol Nutr Food Res. (2015) 59:1353–63. doi: 10.1002/mnfr.201500067
182. Oharomari LK, Garcia NF, Freitas EC, Jordão Júnior AA, Ovídio PP, Maia AR, et al. Exercise training and taurine supplementation reduce oxidative stress and prevent endothelium dysfunction in rats fed a highly palatable diet. Life Sci. (2015) 139:91–6. doi: 10.1016/j.lfs.2015.08.015
183. De Carvalho FG, Brandao CFC, Muñoz VR, Batitucci G, Tavares MEA, Teixeira GR, et al. Taurine supplementation in conjunction with exercise modulated cytokines and improved subcutaneous white adipose tissue plasticity in obese women. Amino Acids. (2021) 53:1391–403. doi: 10.1007/s00726-021-03041-4
184. Rosa FT, Freitas EC, Deminice R, Jordão AA, Marchini JS. Oxidative stress and inflammation in obesity after taurine supplementation: a double-blind, placebo-controlled study. Eur J Nutr. (2014) 53:823–30. doi: 10.1007/s00394-013-0586-7
185. Lupu VV, Ghiciuc CM, Stefanescu G, Mihai CM, Popp A, Sasaran MO, et al. Emerging role of the gut microbiome in post-infectious irritable bowel syndrome: A literature review. World J Gastroenterol. (2023) 29:3241–56. doi: 10.3748/wjg.v29.i21.3241
186. Lupu VV, Bratu RM, Trandafir LM, Bozomitu L, Paduraru G, Gimiga N, et al. Exploring the microbial landscape: gut dysbiosis and therapeutic strategies in pancreatitis—A narrative review. Biomedicines. (2024) 12:645. doi: 10.3390/biomedicines12030645
187. Lupu VV, Butnariu LI, Fotea S, Morariu ID, Badescu MC, Starcea IM, et al. The disease with a thousand faces and the human microbiome-A physiopathogenic intercorrelation in pediatric practice. Nutrients. (2023) 15:3359. doi: 10.3390/nu15153359
188. Lupu VV, Jechel E, Mihai CM, Mitrofan EC, Lupu A, Starcea IM, et al. Connection between celiac disease and systemic lupus erythematosus in children-A development model of autoimmune diseases starting from what we inherit to what we eat. Nutrients. (2023) 15:2535. doi: 10.3390/nu15112535
189. Wang W, Yan Y, Yu F, Zhang W, Su S. Role of oral and gut microbiota in childhood obesity. Folia Microbiol (Praha). (2023) 68:197–206. doi: 10.1007/s12223-023-01033-3
190. Pantazi AC, Balasa AL, Mihai CM, Chisnoiu T, Lupu VV, Kassim MAK, et al. Development of gut microbiota in the first 1000 days after birth and potential interventions. Nutrients. (2023) 15:3647. doi: 10.3390/nu15163647
191. Lupu VV, Miron IC, Raileanu AA, Starcea IM, Lupu A, Tarca E, et al. Difficulties in Adaptation of the Mother and Newborn via Cesarean Section versus Natural Birth—A Narrative Review. Life. (2023) 13:300. doi: 10.3390/life13020300
192. Zolotukhin PV, Prazdnova EV, Chistyakov VA. Methods to assess the antioxidative properties of probiotics. Probiotics Antimicrob Proteins. (2018) 10:589–99. doi: 10.1007/s12602-017-9375-6
193. Tang C, Kong L, Shan M, Lu Z, Lu Y. Protective and ameliorating effects of probiotics against diet-induced obesity: A review. Food Res Int. (2021) 147:110490. doi: 10.1016/j.foodres.2021.110490
194. Sáez-Lara MJ, Robles-Sanchez C, Ruiz-Ojeda FJ, Plaza-Diaz J, Gil A. Effects of probiotics and synbiotics on obesity, insulin resistance syndrome, type 2 diabetes and non-alcoholic fatty liver disease: A review of human clinical trials. Int J Mol Sci. (2016) 17:928. doi: 10.3390/ijms17060928
195. Rondanelli M, Miraglia N, Putignano P, Castagliuolo I, Brun P, Dall'Acqua S, et al. Effects of 60-day saccharomyces boulardii and superoxide dismutase supplementation on body composition, hunger sensation, pro/antioxidant ratio, inflammation and hormonal lipo-metabolic biomarkers in obese adults: A double-blind, placebo-controlled trial. Nutrients. (2021) 13:2512. doi: 10.3390/nu13082512
196. Styne DM, Arslanian SA, Connor EL, Farooqi IS, Murad MH, Silverstein JH, et al. Pediatric obesity-assessment, treatment, and prevention: an endocrine society clinical practice guideline. J Clin Endocrinol Metab. (2017) 102:709–57. doi: 10.1210/jc.2016-2573
197. Jebeile H, Kelly AS, O'Malley G, Baur LA. Obesity in children and adolescents: epidemiology, causes, assessment, and management. Lancet Diabetes Endocrinol. (2022) 10:351–65. doi: 10.1016/S2213-8587(22)00047-X
198. Calcaterra V, Rossi V, Mari A, Casini F, Bergamaschi F, Zuccotti GV, et al. Medical treatment of weight loss in children and adolescents with obesity. Pharmacol Res. (2022) 185:106471. doi: 10.1016/j.phrs.2022.106471
199. Apperley LJ, Blackburn J, Erlandson-Parry K, Gait L, Laing P, Senniappan S. Childhood obesity: A review of current and future management options. Clin Endocrinol (Oxf). (2022) 96:288–301. doi: 10.1111/cen.14625
200. Alman KL, Lister NB, Garnett SP, Gow ML, Aldwell K, Jebeile H. Dietetic management of obesity and severe obesity in children and adolescents: A scoping review of guidelines. Obes Rev. (2021) 22:e13132. doi: 10.1111/obr.13132
201. Wong G, Srivastava G. Obesity management in children and adolescents. Gastroenterol Clin North Am. (2023) 52:443–55. doi: 10.1016/j.gtc.2023.03.011
202. Järvholm K, Janson A, Peltonen M, Neovius M, Gronowitz E, Engström M, et al. Metabolic and bariatric surgery versus intensive non-surgical treatment for adolescents with severe obesity (AMOS2): a multicentre, randomised, controlled trial in Sweden. Lancet Child Adolesc Health. (2023) 7:249–60. doi: 10.1016/S2352-4642(22)00373-X
203. Stabouli S, Erdine S, Suurorg L, Jankauskienė A, Lurbe E. Obesity and eating disorders in children and adolescents: the bidirectional link. Nutrients. (2021) 13:4321. doi: 10.3390/nu13124321
204. Webster-Gandy J, Madeen A, Holdsworth M. Oxford handbook of nutrition and dietetics. Oxford Univ Press. (2006) 1:55–152.
205. Kozłowska A, Szostak-Wegierek D. Flavonoids–food sources and health benefits. Rocz Panstw Zakl Hig. (2014) 65:79–85.
206. Meng X, Li Y, Li S, Zhou Y, Gan RY, Xu DP, et al. Dietary sources and bioactivities of melatonin. Nutrients. (2017) 9:367. doi: 10.3390/nu9040367
207. Wu G. Important roles of dietary taurine, creatine, carnosine, anserine and 4-hydroxyproline in human nutrition and health. Amino Acids. (2020) 52:329–60. doi: 10.1007/s00726-020-02823-6
Keywords: oxidative stress, endogenous antioxidant systems, exogenous antioxidants, obesity, diet, immunity, child
Citation: Lupu A, Fotea S, Jechel E, Starcea IM, Ioniuc I, Knieling A, Salaru DL, Sasaran MO, Cirstea O, Revenco N, Mihai CM, Lupu VV and Nedelcu AH (2024) Is oxidative stress - antioxidants imbalance the physiopathogenic core in pediatric obesity? Front. Immunol. 15:1394869. doi: 10.3389/fimmu.2024.1394869
Received: 02 March 2024; Accepted: 23 July 2024;
Published: 08 August 2024.
Edited by:
Ujjawal Sharma, Central University of Punjab, IndiaReviewed by:
Aruna Pal, West Bengal University of Animal and Fishery Sciences, IndiaDeeksha Pal, Maharishi Markandeshwar University, Mullana, India
Copyright © 2024 Lupu, Fotea, Jechel, Starcea, Ioniuc, Knieling, Salaru, Sasaran, Cirstea, Revenco, Mihai, Lupu and Nedelcu. This is an open-access article distributed under the terms of the Creative Commons Attribution License (CC BY). The use, distribution or reproduction in other forums is permitted, provided the original author(s) and the copyright owner(s) are credited and that the original publication in this journal is cited, in accordance with accepted academic practice. No use, distribution or reproduction is permitted which does not comply with these terms.
*Correspondence: Silvia Fotea, silvia_ghimpu@yahoo.com; Elena Jechel, elena.jechel@yahoo.com; Vasile Valeriu Lupu, valeriulupu@yahoo.com
†These authors have contributed equally to this work