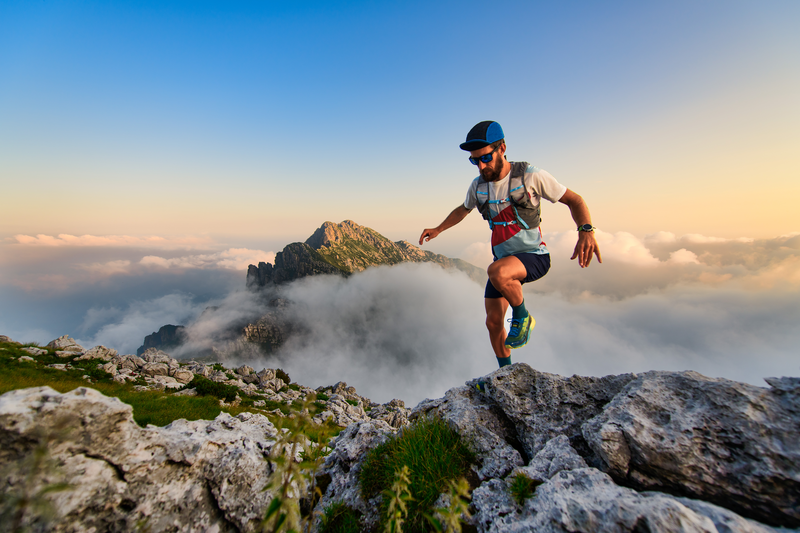
95% of researchers rate our articles as excellent or good
Learn more about the work of our research integrity team to safeguard the quality of each article we publish.
Find out more
HYPOTHESIS AND THEORY article
Front. Immunol. , 04 June 2024
Sec. Autoimmune and Autoinflammatory Disorders: Autoinflammatory Disorders
Volume 15 - 2024 | https://doi.org/10.3389/fimmu.2024.1393814
Systemic lupus erythematosus (SLE) is classified by instinctual classification criteria. A valid proclamation is that these formally accepted SLE classification criteria legitimate the syndrome as being difficult to explain and therefore enigmatic. SLE involves scientific problems linked to etiological factors and criteria. Our insufficient understanding of the clinical condition uniformly denoted SLE depends on the still open question of whether SLE is, according to classification criteria, a well-defined one disease entity or represents a variety of overlapping indistinct syndromes. Without rational hypotheses, these problems harm clear definition(s) of the syndrome. Why SLE is not anchored in logic, consequent, downstream interdependent and interactive inflammatory networks may rely on ignored predictive causality principles. Authoritative classification criteria do not reflect consequent causality criteria and do not unify characterization principles such as diagnostic criteria. We need now to reconcile legendary scientific achievements to concretize the delimitation of what SLE really is. Not all classified SLE syndromes are “genuine SLE”; many are theoretically “SLE-like non-SLE” syndromes. In this study, progressive theories imply imperative challenges to reconsider the fundamental impact of “the causality principle”. This may offer us logic classification and diagnostic criteria aimed at identifying concise SLE syndromes as research objects. Can a systems science approach solve this problem?
“Insanity is doing the same thing over and over and expecting different results”. Albert Einstein1
This study analyses what we understand and do not understand about systemic lupus erythematosus (SLE) and how we interpret and intellectualize current SLE classification criteria, definitions, and dogmas.
SLE is an enigmatic and consequently inexplicable syndrome, currently authorized by incomprehensible SLE classification criteria (1)—and by the vague and imprecise impact of the causality principle in theoretical and practical contexts. Such problems unveil SLE as a poly-phenotypical and poly-etiological syndrome that contrast—and argue against—the “one disease entity” paradigm [discussed in References (1, 2)]. In this study, research directions will be discussed with the perspective of transforming the enigmatic SLE into a more rational and explicable syndrome. The focus will be on, but not limited to, critical viewpoints on SLE classification criteria, the role and specificity of humoral autoimmunity, and the origin and pathophysiology of lupus nephritis as exemplified in a causality context.
Our insufficient understanding of the clinical condition denoted SLE depends on i) how we operate and interpret classification criteria that categorize SLE cohorts (1); ii) how and why we neglect relevant historical data on unique DNA structures, their immunogenicity, and relevant autoimmunity in rheumatological and clinical immunology contexts (2); and iii) how opposite and contradictory pathophysiological models, as is relevant for lupus nephritis, are left inconclusive and uninvestigated for decades. These points are the frames for the following theoretical discussion.
These problematic topics have provided us with inconsistent SLE cohorts and, consequently, insufficient research perspectives (1). For example, SLE as classified by established criteria may not constitute a “one disease entity” simply because the criteria do not reflect the causality principle that could lead to a more homogenous syndrome. Therefore, classified SLE cohorts may consist of patients that belong to the idiom “a one disease entity”—in other words described as “genuine SLE”. These are promoted by a central etiology. Other patients in an SLE cohort may belong to various groups of “poly-phenotypic SLE” or ultimately belong to a more diffuse group of patients classified by criteria—the “SLE-like non-SLE disorders”. The latter would be in terms of non-SLE diseases that imitate SLE.
In line with these terminologies, classification criteria authorize cohorts of classified SLE patients with or without anti-dsDNA antibodies, with and without lupus nephritis—in general with or without individual classification criteria (1). The clinical pictures of all these patients can be implemented in the same SLE cohort aimed to study etiology, pathogenesis, and, e.g., experimental therapies! Basically, the core question is whether all SLE classification criteria causally belong to—and identify—one dominant origin that promotes a “one disease entity” in harmony with “the causality principle”. Probably, they do not!
In order to transform SLE from being an enigmatic syndrome into a rational and explicable disorder, we have to re-introduce historical data on DNA structures and their unique auto-immunogenic potentials on one side and focus on links to the causality principle on the other. This means implementing causality to understand the etiology of consequent downstream inflammatory networks—i.e., interactive and interdependent symptoms (defined as criteria) that may explain the nature of SLE.
Miescher described nuclein in 1871 (3, 4), later named as DNA. In the aftermath of Miescher, scientific studies, experimental data, and biochemical analyses of DNA structures have reached an unconditioned status as central achievements in the history of science. The biochemical and biological impact of DNA belongs today to our most basic and quintessential knowledge related to the inheritance and reproduction of species. During decades after Miescher’s pioneering studies, a considerable amount of data have accumulated on structure–function relationship centered in dynamic DNA configurations—basically linked to gene expression and inheritance but also to their influence on clinical-related autoimmunity [as how immune responses are controlled, activated, and tolerated; reviewed in (2, 5–7)].
Considering the biological impact of DNA structures, it is unexpected and unpredicted to observe that a detailed description of DNA structure–functions as life’s most basic and vital molecular processes is largely ignored in modern autoimmunity, autoimmune pathogenesis, and rheumatology [see, e.g., References (8–10)]. Current mainstream thinking in DNA-associated clinical autoimmunity—and consequent praxis—is based on simplified models and theories that have resulted in dogmatic but invalid hypotheses, questionable and unclear scientific results, and biased interpretations.
If we consider the current literature, DNA in autoimmunity is handled as “ssDNA” and as “dsDNA”. The latter is clearly described in the central literature on autoimmunity and on SLE classification criteria impacted to classify and diagnose SLE2 (8–10). The dynamic transformation of intricate DNA structures, associated with their involvement in gene regulation, gene expression, DNA replication, and DNA repair (11), has not been considered important in contemporary studies on DNA immunogenicity (2). This provocative presentation is problematic and challenging to contemplate and articulate but necessary to perform.
In 1957, antibodies to DNA in SLE patients were reported in four independent studies (12–15). These reports authenticated the beginning of an intense scientific époque, which culminated in experiments that described molecular and cellular requirements explaining why these anti-DNA antibodies appear (5, 16–21) and why they were claimed unique in SLE as classification and diagnostic criteria (10, 22). The latter statement is mistrusted today because these requirements are universal in a physiological context and are relevant also for the normal immune system [discussed in References (23–25)]. That is, anti-B DNA3 antibodies are not unique to SLE. This statement harmonizes with the fact that anti-dsDNA antibodies were described in the context of infections already in 1938–1939 [discussed and reviewed in Reference (23)].
Many inconsistent scientific dogmas have hampered insight relevant to understanding DNA as an immunogen. Early experimental data promoted the concept that mammalian B DNA was non-immunogenic while ssDNA and synthetic DNA structures were potent immunogens (26–28). The dogma that settled B DNA as hapten-like and non-immunogenic progressed into a long-lasting principle that only recently became obsolete. While many versions of DNA (ssDNA, synthetic ssDNA/dsDNA, and even the left-handed Z DNA helix) were immunogenic in complex with the immunogenic carrier protein methylated bovine serum albumin [mBSA; see, e.g., References (29, 30)], mammalian B DNA remained indisputably immunologically inert (18, 26). During the 1980s, a confusing and disappointing conclusion emerged: mammalian B DNA was excluded from Sercarz’s hapten-carrier theorem (see below) by classifying B DNA as immunologically inert (26–28).
During the 1990s, this paradigm changed as a consequence of three new experimental concepts. The pioneering experiments in Tony Marion’s laboratory were a breakthrough in this context. He and his coworkers dismissed mBSA as a carrier protein for B DNA in the hapten-carrier paradigm and substituted it with a potent T-cell immunogenic peptide derived from the parasitic euglenoid (31) Trypanosoma cruzi—the Fus1 peptide. This artificial complex, B DNA-Fus, induced anti-mammalian B DNA antibodies, and the non-autoimmune experimental animals developed lupus-like nephritis4 (19, 32). This experiment teared down the dogma saying that mammalian B DNA was immunologically inert!
A similar in vivo model was developed in Rekvig/Moens laboratories. They introduced polyomavirus T antigen-expressing plasmids in mice and revealed that expressed T antigen associated with chromatin in T antigen-expressing dying cells, formed stable complexes, and induced antibodies to mammalian B DNA, histones, and transcription factors (20, 21, 33). Thus, the chromatin–T antigen complex stimulated B cells theoretically specific for any accessible ligand contained in the chromatin complex provided they presented (in this example) the complexed T antigen peptides to cognate helper T cells (20, 21, 33).
At the same time, Pisetsky et al. described the production of anti-bacterial DNA antibodies in normal mice in response to bacterial CpG-rich DNA-mBSA complex immunization and made an observation with key importance; the immunization protocol in pre-autoimmune mice accelerated production of potential pathogenic anti-mammalian B DNA (30, 34, 35). This implied that a ubiquitous source of immunogenic dsDNA, the bacteria, could induce autoimmunity to mammalian B DNA in pre-autoimmune animals. This brought infections as a central immunogenic source for anti-dsDNA autoimmunity into the SLE-related autoimmune forum. This paradigm shift is clinically important when we consider the predisposition of infections in SLE [principally discussed in References (22, 23)].
These results have clearly communicated to us the clinical impact of chromatin autoimmunity in diagnostic and pathogenic contexts. The data expand the impact of DNA from being a gene “bank” to, in a wider perspective, expanding our understanding of SLE and its contextual autoimmunity. From such data, chromatin autoimmunity as a principle has taught us basic regulatory principles of the immune system [reviewed and discussed in References (5, 7, 23, 36, 37)]. These progressive experimental studies inform us about the importance of new scientific research directions related to the autoimmune pathogenesis of lupus nephritis (25), and they taught us the lesson not to adhere to scientifically non-productive dogmas (see citation of Albert Einstein above).
The functional Sercarz’s hapten-carrier theorem for induction of anti-DNA antibodies (27, 28, 38) inherits a perpetual and self-explaining dogma: the anti-dsDNA antibodies cannot be specific for SLE. This statement is explained as follows: all people embody reactive DNA-specific B cells (39–41). They are not tolerant—rather, they are promiscuously responsive (42, 43). Helper T cells are tolerant of chromatin-associated autologous proteins (23). They can therefore not provide cognate T-cell help for DNA-specific B cells! However, in the context of, e.g. viral infections, DNA-binding viral proteins may associate with chromatin, thus creating a functional dsDNA (hapten-like) virus-derived (carrier–) protein complex. Since we all inherit DNA- and virus protein-responsive B and T cells, this hapten-carrier version explains why anti-dsDNA antibodies are not—and cannot—be specific for SLE (23).
Over decades, numerous studies have been published that attempted to describe the unique clinical impact of anti-B DNA antibodies in SLE. Surprisingly, most of these studies canceled or ignored experimental (19–21, 30, 35) and observational data that demonstrated the production of anti-dsDNA antibodies in the context of infections and malignancies [(23, 44) reviewed in Reference (23)] and sporadically in other conditions (45).
One central but disregarded science-based fact diminishes the impact of DNA in autoimmune pathogenicity and disease associations: the existence of disparate DNA structures, like ssDNA, bent dsDNA, elongated dsDNA, Z DNA, cruciform DNA, and bacterial and viral DNA, with theoretically unique and unrelated immunogenic potentials. This may raise critical questions around the clinical impact of “the anti-dsDNA antibody” and whether this specificity may remain “unique” for SLE [(8–10), discussed in References (2, 46)]. The definitive documentation of a clear immunogenic potential of any of the DNA structures alters this view [discussed in References (22, 35, 44, 47)]. The arguments in this study hint at why anti-dsDNA antibodies appear in SLE among many syndromes predisposed to conditions like infections and malignancies (23, 44, 48–53).
The latter observations basically confront the motivation for putting weight on anti-dsDNA antibodies in SLE classification criterion sets (8–10). It is obviously important to include non-SLE patients disposed for infections and malignancies as corresponding control groups.
Therefore, the term “anti-dsDNA antibody” [as in SLE classification criteria (8–10)] is a simplification that conceals the impact of antibodies on disparate, unique, and functional DNA structures.
Since the early 1870s, DNA structures and their biological operations have been investigated and are still a prevailing and central research focus [reviewed in Reference (2)]. These studies have identified a series of structurally unique DNA configurations that must be brought to this discussion forum. These unique DNA structures exert distinctive roles in gene biology and biochemistry but are still ignored in auto-immunological contexts.
Miescher et al. studied in the 1870s the nuclear substance nuclein and suggested that nuclein could have something to do with inheritance, and later, he theorized that a hereditary alphabet based on stereochemistry was hidden in the nuclein. This could explain how variation is generated [discussed in Lamm et al. (54)]. Mostly, Mischer’s concept of DNA as a carrier structure for inheritance has been ignored in this narrative (54). In 1909, the identification of the chemical nature and composition of DNA was described by Levene et al. (55). They determined that DNA was composed of the central nucleobases adenine, guanine, thymine, and cytosine.
Important scientific results on DNA structure emerged from central studies of Erwin Chargaff and Rosalind Franklin. Chargaff et al. (56, 57) defined the two Chargaff rules on the composition of double-stranded DNA, saying that the number of guanine equals the number of cytosine and that the number of adenine equals the number of thymine in all species [reviewed and discussed in Reference (2)]. In 1952, Franklin produced pioneering high-resolution photographs of crystallized DNA fibers and interpreted from these pictures that DNA was a double-stranded helical structure (58–60). This model harmonized with the Chargaff’s rules. Franklin and Chargaff were therefore the first to describe the basic structure of DNA as a double-stranded helix.
Essential in this context is that Franklin and Goslin were the first to describe two unique forms of DNA beyond its archetypical double-helix structure: the A and B DNA (58). The B DNA was later described as a dynamic fluctuating bi-structural DNA shape: elongated (61, 62) or bent dsDNA (63, 64). SsDNA (65, 66), Z DNA (26), cruciform DNA (67), and other structures were all described in the context of specific functions of DNA [see below, Figure 1, and References (2, 68, 69)]. Watson and Crick (70, 71) combined data from Chargaff’s studies and Franklin’s X-ray data and finally confirmed and settled the helical structure of DNA in which A pairs with T, and C pairs with G (corresponding to Chargaff’s rules).
Figure 1 Transcriptionally active DNA expresses distinct DNA structures—each structure is a unique antigen. (i) The B DNA helix is opened by single-stranded binding proteins (SSBPs; which stabilize ssDNA and polymerize, which are involved in replication and repair). (ii) Z DNA is a left-handed, high-energy, double helix. Z DNA forms during transcription as a result of torsional strains that depend on interaction with mobile polymerases. Z DNA is associated with linker DNA. (iii) Elongated (linker) DNA is a relaxed and stable, right-handed, low-energy form of B DNA. Cruciform DNA is another dsDNA structure (iv) and is different from B and Z DNA. Its formation requires that sequences (palindromes) in one strand are repeated on the other strand in opposite directions. The cruciform structures are, like Z DNA, higher-energy structures. Compacted B DNA as in core nucleosomes is defined as bent B DNA (v). Bent DNA is a compacted structure influenced by the histone octamer and histone H1. These structures (i–iv) are unique in terms of inducing highly specific antibodies with potential pathogenic impact if chromatin fragments are exposed in situ (see Figure 2). This figure demonstrates the unique immunogenic DNA structures [revised from Reference (2)].
Figure 2 Induction of anti-chromatin antibodies (A) and anti-DNA structure-specific antibodies (B)—demonstration of Sercarz’s hapten-carrier theorem involving expression of polyomavirus T antigen as immunogenic carrier protein. (A) Injection of non-autoimmune mice with plasmids expressing polyomavirus DNA-binding T antigen induces production of antibodies to DNA, T antigen, mammalian histones, and certain transcription factors like TATA-binding protein (TBP) and cAMP-responsive element-binding protein (CREB) by cognate interaction between chromatin specific B cells and polyomavirus T antigen peptide-specific helper T cells. (B) These antibodies bind chromatin-antigens exposed in GBM and promote nephritis. (C) Identical immunization regimes induce autoantibodies against elongated B DNA, bent B DNA, Z DNA, cruciform DNA, and ssDNA. (D) The anti-DNA structure-specific antibodies promote nephritis by binding exposed DNA antigens in GBM. Autoimmune B cells and operational immune T cells cooperate in this model. (E) All the induced anti-chromatin antibodies have pathogenic potentials if binding exposed chromatin, as is demonstrated in GBM as electron-dense structures (EDS in panels F, G). The induced autoantibodies (stained with 5-nm gold particles; F) bind chromatin fragments. Chromatin fragments are surrounded by GBM structures that bind anti-laminin antibodies added to the section in vitro (10-nm gold particles; G). (A–D) Modified from Reference (25).
Later, studies of DNA structure–function relationships in chromatin were performed by Olins and Olins (72), Kornberg et al. (73, 74), Richmond et al. and Luger et al. (75, 76), Laskey et al. (77), and others. They contributed to our understanding of the consequent interdependency of DNA structures and functions5 and the biology of chromatin-associated regulatory and chromatin compaction proteins—and a new view on concise DNA structure immunity (2).
DNA embodies all aspects of inheritance necessary to maintain the life and integrity of a species (69, 78–80).
Notably, the manifold of DNA structures (Figure 1) preserves a striking, still largely ignored relevance in an autoimmune context: each structure has a unique ability to induce highly specific anti-DNA antibodies within experimental and autoimmune frameworks (2).
Thus, from published studies over more than seven decades, it is clear that DNA structures and their functions tell a quite different story than the narrative about the sole existence of “the anti-DNA antibody” (2). This history contrasts general clinical viewpoints about anti-dsDNA antibodies operating as a single antibody specificity reacting with “the dsDNA” (8–10, 81–83). This somewhat naïve paradigm is not in agreement with the fact that each DNA structure has the potential to induce highly segregated and structure-specific anti-DNA antibodies [Figure 1 (2)].
“The anti-dsDNA antibody” holds an archaic position in SLE as a unique classification and diagnostic criterion. In light of what we know today, this position is principally wrong, as studies of disease association(s) of anti-dsDNA antibodies have been conducted without rational references to assay principles designed to detect individual DNA structure specificities (2).
Why do anti-dsDNA antibodies exist as a unique classification criterion in SLE (22)? During the decades following the 1957 discoveries of anti-DNA antibodies in SLE, two important aspects of the anti-B DNA antibody were comprehended: i) the promiscuous cross-reactive nature of anti-dsDNA antibodies (42, 84–89) and ii) the non-immunogenic nature of B DNA.
Cross-reactivity precipitated the dogma declaring that the existence of anti-B DNA antibodies depended on cross-stimulation by heterogeneous non-dsDNA structures, like phospholipids, different polypeptides, and non-mammalian bacterial DNA (23, 35, 42, 90–94).
If B cells were stimulated by a peptide, they were in immunological terms subjected to somatic hyper-mutations—affinity maturation—and the antibody-specificity converges toward the peptide immunogen. However, the clones were initially specific also for cross-stimulated dsDNA. Will these be further expanded by the proclaimed non-immunogenic, exposed chromatin-associated dsDNA, or will these DNA-specific clones passively expire? In other words, will cross-stimulated clonal anti-B DNA antibody responses be transient if occurring on a normal, non-autoimmune background, or can a peptide progressively expand and affinity-maturate DNA-specific clones? These concise questions remain unanswered.
The radical paradigm shifts described above confirmed that B DNA is immunogenic, consistent with Sercarz’s hapten-carrier theorem. This, however, created some new intellectual problems: in view of the verifiable immunogenic potential of B DNA independent from an autoimmune background, are anti-dsDNA antibodies still specific for SLE? Do disparate anti-DNA structure-specific antibodies reflect the same clinical impact as the dogmatic anti-B DNA antibody? Are DNA or membrane ligands targeted by anti-dsDNA antibodies in lupus nephritis? Finally, why are theoretical, observational, and experimental scientific studies, basically aimed to understand SLE, ignoring i) classical and legendary data on DNA structures, ii) conflicting interpretations on lupus nephritis pathogenesis (see below), and iii) “the causality principle” in the context of the SLE classification criterion selection process. Problematically, these criteria promote today’s inconsistent SLE research strategies applied to potentially illogical SLE cohorts [discussed in Reference (1)].
In sum, it is now settled that DNA is not one “structurally dead” and inflexible macro-molecule. The dynamic and changing DNA structures are caused by histone mobility, enzymes, and regulatory proteins [reviewed by Zhang et al. (95)]. This means that over 7 decades of provokingly reflective and insightful high-impact research, DNA structures are well acquainted with relevant basic scientific disciplines linked to genetics, biochemistry, and regulation of gene transcription.
These scientific complications imply, so far, several consequences for SLE research: the insight into manifold DNA structures has expanded from an archetypical double helix structure into several concise and specialized function-related DNA structures (95). We must therefore bring to the discussion forum a concise proposition: the B DNA is immunogenic. Consequently, this relates also to unique DNA structures according to Sercarz’s theorem—they may all possess an immunogenic potential (2). This statement predicts the de facto existence of several concrete and unique anti-structural DNA antibody specificities (2). Notably, their individual immunopathogenic and clinical impacts have not been subjected to research in clinical hypothesis-driven contexts. We definitively need a more profound insight into assay principles that detect each of the clinically existing anti-structural DNA antibodies.
This leads to several new hypotheses:
1. Since B DNA is a weak immunogen (26), while, e.g., ssDNA or Z DNA is an effective immunogen (26, 39, 96), every structural DNA-specific antibody may have a unique impact as diagnostic or classification criterion, as is currently and historically relevant for the anti-mammalian B DNA antibody (8–10). The logic hypothesis imposed from this information could be that the easier the DNA structures promote immune responses, the less specific for a disorder will the emerging antibodies be. For example, immunity to ssDNA and Z DNA may inherit a lower diagnostic impact than, for example, antibodies against the bent mammalian dsDNA because of the relatively poor immunogenicity of bent dsDNA (2, 23).
2. Since anti-DNA antibodies are induced by DNA structures as those presented in chromatin, the produced antibodies must be able to bind the same cognate DNA structures when they are exposed as chromatin fragments in lupus nephritis. This must consequently mean that they are all potentially pathogenic, with one reservation: that the density of each target structure is sufficient in chromatin-associated DNA to bind adequate amounts of IgG anti-DNA structure-specific antibodies to mediate Fc gamma-dependent complement-activating inflammatory processes.
3. Importantly, the autoimmune responses as presented in Figures 1, 2 represent arguments against cross-reactivity of the disparate anti-dsDNA antibodies with membrane and matrix ligands to explain antibody-dependent lupus nephritis (see Figure 2). This whole repertoire of anti-chromatin antibodies may be involved in nephritis through cognate interaction with exposed chromatin ligands [Figure 2 (25, 97)] and not with cross-recognized membrane antigens. It is unlikely that all of a large group of anti-chromatin antibodies will, by chance, cross-react with a small number of membrane ligands (25, 53). Therefore, anti-chromatin antibodies are pathogenic if they bind exposed chromatin fragments in situ (see details discussed below).
4. In the absence of extracellular chromatin-associated DNA, an alternative inducer of anti-DNA antibodies may be mitochondrial DNA (Mit DNA) (98). Mit DNA has been reported to be involved in innate immunity (99) and inflammatory processes (98, 100, 101). Anti-Mit DNA antibodies are involved in disease processes (99). There are few studies that report the cross-reactivity of anti-nuclear antibodies with Mit DNA (101). Thus, whether anti-Mit DNA antibodies cross-react with exposed nuclear DNA is not firmly established. However, if Mit DNA is exposed in tissue, anti-Mit DNA antibodies may theoretically express pathogenic effects by binding Mit DNA in situ. This is, however, a speculation and is not based on experiments or descriptive analyses. In contrast, both viral DNA (102–105) and bacterial DNA (30, 34) have the potential to induce mammalian nuclear anti-dsDNA antibodies similar to such specificities in SLE (22, 23, 25).
To describe the origin of anti-dsDNA antibodies, the most probable explanation is founded on the release of (nuclear) chromatin as a result of inflammatory-mediated silencing of renal DNase 1 gene and loss of DNase 1 endonuclease activity. This may explain the efferent and afferent phases of immune response to nuclear DNA and may explain the basic processes in the chromatin model (see below—the “chromatin model” for induction of pathogenic anti-chromatin antibodies is discussed).
Anti-dsDNA antibodies are pathogenic in SLE. However, this statement is controversial, irrespective of whether alone or in combination with other anti-chromatin antibodies (Figure 2). What we need to comprehend from an enormous amount of studies (106) is to understand what makes the anti-DNA/anti-chromatin antibodies pathogenic—and in which contexts.
The archaic “anti-dsDNA antibody” terminology is founded on an outmoded comprehension in historical as well as contemporary contexts. Today, we may, in harmony with the authoritative attribution rules for SLE classification criteria (8–10), infer that any structure-specific anti-DNA antibody, detected in any anti-DNA antibody assay using any target dsDNA molecule, is valid as a criterion for SLE, as long as the target DNA is dsDNA (8–10).
We may formulate a simple and open-minded hypothesis: the more readily an antibody is induced, the less specific is the antibody for SLE. Any anti-dsDNA antibody may appear in different SLE/non-SLE conditions (2), while those DNA structures that are more effectively controlled by tolerance may induce antibodies with a closer connection to SLE ()?. In other words, antibodies against, e.g., ssDNA or Z DNA, may be less specific for SLE than anti-bent B DNA antibodies, as the former structures are more effective immunogens than the latter (2, 26, 107). This hypothesis remains open and still un-investigated.
The anti-dsDNA antibodies may inherit a nephritogenic potential by two incommensurable characteristics: their specificity for exposed chromatin-associated dsDNA, the chromatin model, or their cross-reactivity for inherent matrix/membrane ligands, the cross-reactive model.
In this section, the nephritogenic impact of anti-DNA/anti-chromatin antibodies (Figure 2) will be discussed. These antibodies may be specific for dsDNA without further definition [as incurred in the SLE classification criteria (8, 9, 82)], or for chromatin-associated dsDNA originated from apoptosis or neutrophil extracellular traps (NETs) (108–110), in addition to specificity for chromatin-associated proteins. Alternatively, they may cross-react with inherent membrane structures [see, e.g (23, 25, 42, 94, 111–115) and Table 2 in Reference (25)]. This dilemma is not comparatively investigated in terms of systems science (116) involving different congruent and corresponding scientific methods based on focused hypotheses.
Working hypothesis: Today, we are principally not able to unequivocally explain the nephritic process at a molecular level, although some detailed studies indicate relevance for the chromatin model (25, 53, 117–119).
Anti-chromatin antibodies may bind extracellular chromatin fragments exposed in glomeruli and form immune complexes—either in situ or trapped from circulation. These may be detected early and primarily be detected in the mesangial matrix (120, 121) and promote clinically mild or silent mesangial nephritis. This condition can be diagnosed by a combination of modest proteinuria and deposition of co-localized chromatin fragments and IgG antibodies in the mesangial matrix (120, 121). These IgG antibodies comprise specificities for dsDNA, histones, and transcription factors (20, 122–125). The model is consistent with a Type III immune complex-mediated inflammation [(126); see a model illustrated in Figure 2]. Mesangial nephritis promotes the progression of lupus nephritis into end-stage organ disease. This type of nephritis is characterized by heavy deposits of chromatin–IgG complexes in the Glomerulus basement membrane (GBM) [discussed in References (25, 53)].
The chromatin model is complex and evidently involves a wide spectrum of chromatin-specific antibodies. The model (illustrated in Figures 2A, C) exemplifies Sercarz’s hapten-carrier theorem: all B cells specific for chromatin-associated ligands (e.g., DNA, histones, non-histone regulatory proteins, and transcription factors) internalize in this model the immunogenic chromatin-associated polyomavirus T antigen (or auto-immunogenic histones) and present T antigen (or histone) peptides to non-tolerant helper T cells in context of HLA class II molecules (20, 21, 127). The net result is the production and binding of cognate chromatin-specific autoantibodies (see details in Figures 2B, D, E-G). Collectively, most of the various anti-chromatin antibodies have not been seriously considered as inducers of lupus nephritis, except for “the anti-dsDNA antibody” [as described in References (8–10)].
All anti-dsDNA antibodies (except anti-cruciform antibodies, which are not analyzed yet in clinical contexts) are induced in SLE or are experimentally inducible in vivo (2, 23). The chromatin structures (DNA and proteins) must therefore have been accessible to highly specific B-cell antigen receptors (2, 20). Then, it is likely that the antibodies access and recognize the same universe of DNA structures and chromatin-associated proteins when chromatin fragments are exposed in, e.g., glomeruli (Figures 2A–G).
It is in this context that it is unlikely that the whole spectrum of distinct anti-chromatin antibodies collectively cross-react with few inherent glomerulus matrix/membrane constituents.
In contrast to the cognate interaction of antibodies with dsDNA, a growing number of studies have demonstrated that anti-DNA antibodies may cross-react with glomerular matrix and membrane ligands, e.g., laminin, entactin, or collagen (25, 117). This pattern of antibody recognition gradually created a new paradigm: the anti-dsDNA antibodies promote nephritis by binding inherent GBM structures in vivo (86, 93, 112, 128–133), i.e., a model compatible with a Type II antibody-dependent inflammation (126).
The dual specificity of nephritogenic antibodies for dsDNA and non-dsDNA membrane ligands does not clarify which of the antigens are targeted in vivo.
This problem further escalated into an intellectual conflict that still is not investigated in comparative studies, is not resolved, and is currently largely ignored as a problem! Today, there is no visible research program on the horizon that may solve the problem of whether, and how, cross-reacting anti-dsDNA antibodies are involved in lupus nephritis (see a concise hypothesis-based discussion in the next section).
How do the chromatin and the cross-reacting models comply with the clinical course of lupus nephritis? Implementation of the causality principle must in this context be considered.
The chromatin model is principally characterized by a progressive profile that implements two immunopathological aspects: Phase 1 and Phase 2 (Figures 3A, B). Phase 1 is caused by an early and modest production of anti-dsDNA/anti-chromatin antibodies (117, 120, 121, 134). The pure presence of the anti-dsDNA antibody causes accumulation of immune complexes in the mesangial matrix—observed by immune electron microscopy as electron-dense structures [EDSs; consisting of chromatin–IgG complexes (121)] and clinically silent or mild mesangial nephritis with low-graded proteinuria (Figure 3A).
Figure 3 Theoretical disease profiles differ due to the molecular specificities of the autoantibodies. (A) Anti-dsDNA antibodies form immune complexes with assumed circulating small chromatin fragments that accumulate in glomerulus mesangium (121). This promotes mild, early mono-phasic, and transient lupus nephritis (Phase 1). Under certain conditions, mesangial inflammation promotes silencing of the renal DNase 1 endonuclease (121). In reflection of loss of DNase 1 enzyme activity, chromatin released from dead cells accumulates as undigested chromatin fragments in complex with anti-chromatin antibodies in mesangial matrix and in GBM [see Figure 2 (121)]. This promotes end-stage nephritis (B; Phase 2)—a second-phased progression of lupus nephritis. This model contrasts with the cross-reacting model (described in panel C). Here, a cross-reacting anti-DNA antibody binds inherent membrane antigens (like laminin, collagen, and entactin) shared between the mesangial matrix and GBM. Therefore, the nephritis profile is mono-phasic, as the mesangium and GBM are simultaneously affected by antibodies. This mono-phasic nephritis is complementary to nephritis in Goodpasture syndrome (D). Goodpasture-type nephritis is caused by anti-collagen IV antibodies that bind collagen structures shared by the mesangial matrix and the GBM. The antibodies therefore promote a mono-phasic profile of the nephritis in cross-reacting lupus nephritis models and Goodpasture syndrome.
These mesangial immune complexes may incite a local inflammation that is associated with an abrupt silencing of the renal DNase 1 gene, the dominant renal endonuclease (109, 135). This is consistent with increased glomerular accumulation of undigested extracellular chromatin fragments in complex with IgG anti-dsDNA antibodies (108, 121, 123, 135–137) in both the mesangial matrix and GBM (Phase 2, Figure 3B). This pattern reflects the progression of lupus nephritis (and severe proteinuria) into end-stage organ disease (121, 137). The chromatin model linked to progressive loss of renal DNase 1 explains Phase 2 of the bi-phasic progressive lupus nephritis (see details in Figures 3B, 4).
Figure 4 Severe proteinuria correlates positively with chromatin fragment deposits [observed as electron-dense structures (EDS) in GBM] and inversely with renal Dnase 1 mRNA levels. Renal tissue was collected from female (NZBxNZW)F1 (BW) and female age-matched BALB/c mice (Jackson Laboratory, Bar Harbor, ME, USA) sacrificed approximately every second week (n = 3) from the age of 4 weeks until development of end-stage disease in the BW mice, clinically defined when severe proteinuria developed (≥20 g/L). Tissue was snap-frozen for protein extraction, preserved according to Tokuyasu for immune electron microscopy (138), or preserved in RNAlater (Ambion Inc., Austin, TX, USA) for mRNA analyses. Serum and urine samples were collected at 2–3-week intervals and stored at −80°C. No correlation between degree of proteinuria and levels of anti-dsDNA antibody titers was observed (A). To analyze if loci for electron-dense structure (EDS) deposits had impact on proteinuria, data on proteinuria and presence of EDS in the mesangial matrix (weighted 1 in B) or in the GBM (weighted 2 to make a visual distinction from mesangial deposits) were combined for each mouse and sorted by ascending values of proteinuria. Severe proteinuria (≥20 g/L) was, except for one mouse with intermediate proteinuria (≤3 g/L), exclusively observed in mice with EDS in GBM (B), while intermediate or mild proteinuria was observed in mice with mesangial matrix deposits only (B). In panel C, the degree of proteinuria and renal Dnase 1 mRNA levels were paired and sorted by ascending proteinuria levels. Severe proteinuria (≥20 g/L) correlated with a substantial loss of Dnase 1 mRNA (and enzyme activity; (C). Thus, in mice selected for proteinuria ≥20 g/L, renal Dnase 1 mRNA was nearby lost in all mice but one (C), and deposits of chromatin–IgG complexes (observed as EDS) in GBM were observed only in these mice. This instructive figure is copied from Reference (121). For statistical analyses, see Table 1 in Reference (121).
In Figure 4A, it is demonstrated that the degree of proteinuria in mesangial as well as in end-stage lupus nephritis does not correlate with fluctuations in anti-dsDNA antibody titers. In contrast, the progression of proteinuria correlated significantly with the progressive accumulation of EDS [representing chromatin–IgG complexes (25, 120, 121)] in the mesangial matrix and subsequently in GBM (Figure 4B). A striking inverse correlation was observed between the degree of proteinuria and loss of renal DNase 1 gene expression (Figure 4C). Similar murine characteristics of progressive lupus nephritis are observed in human SLE (25, 108, 136, 139).
The cross-reacting lupus nephritis model is characterized by a quite different dynamic profile (Figure 3C). This is basically determined by the equal distribution of the target membrane ligands for the antibodies in the mesangial matrix and the GBM [reviewed in References (25, 53)]. The target molecules, such as laminin, collagen, or entactin, are shared by membranes in glomeruli and alveoli on one side (140) and by glomerular matrix and membranes on the other (141–144). This opens for the intriguing concept that the cross-reacting lupus nephritis model (if true) may principally represent a mirror image of the autoimmune Goodpasture syndrome (indicated and compared in Figure 3C, the cross-reacting model, and Figure 3D, Goodpasture nephritis). The two latter pathogenic situations are principally similar, as the target antigens in both situations are shared between the glomerular mesangial matrix and basement membranes (145–147).
In a corresponding argumentation, the glomerular immunofluorescence pattern of IgG deposits in Goodpasture-type nephritis is continuous and linear, as would be expected also for the pattern promoted by the cross-reacting model. However, in the chromatin model, the deposits in the mesangial matrix and GBM are granular and discontinuous (121). These differences account for two alternative aspects of lupus nephritis: the mono-phasic nephritis caused by cross-reacting anti-dsDNA antibodies and the bi-phasic nephritis caused by immune complex deposition as predicted—and observed—for the chromatin model (Figures 2, 3). Data so far on lupus nephritis are compatible with the chromatin model (25).
A third distinction between the chromatin and the cross-reacting models relies on the term “cross-reaction” itself. If we dissect the chromatin model, it is complex, as a manifold of anti-chromatin antibodies is produced in SLE [Figure 2 (23, 148)]. Many of these antibodies bind in, and are eluted from, nephritic kidneys ( (123–125, 149, 150). This fact has one implication: the anti-chromatin antibodies access chromatin-associated nucleic acids and proteins in chromatin structures exposed in glomeruli. Ergo, the B-cell antigen receptor, as well as anti-chromatin antibodies, bind the same accessible antigens in chromatin fragments (151–153).
The manifold of potentially nephritogenic anti-chromatin antibodies (Figure 2) is per se a clear argument against the cross-reacting model. It is unlikely that the high number of potentially nephritogenic anti-chromatin antibodies together, by chance, cross-react with the relatively few glomerular matrix and membrane proteins. This does not, however, exclude that single, individual anti-chromatin antibodies may contribute to nephritis by cross-binding membrane/matrix constituents, although the majority of the antibodies distinctly cause a Phase 1 nephritis and a subsequent Phase 2 nephritis according to the chromatin model (see Figures 3A, B). This causation cannot be explained by the cross-reacting model.
The conflicting arguments favoring Type II or Type III nephritis derive from studies over decades, preliminary conclusions, and a lack of international consensus [see, e.g., contrasting viewpoints in References (25, 97, 119, 131–133, 154–156)]. In light of this, it is strange to observe that systematic comparative studies have not been prioritized and are still awaited in the context of the systems science algorithm (157). We still need definitive experimental and descriptive evidence for either of the two models, although arguments favoring the chromatin model are relatively strong.
Clinical-related research on dsDNA/chromatin autoimmunity is undisputedly one of the most prominent disciplines in the SLE research field. These research efforts are basically resulting in confusing and inconclusive data when our focus is concerned with diagnostic, as well as pathogenic implications of autoimmunity. We still, after all these years, misinterpret the diagnostic impact of anti-dsDNA antibodies (they are not unique to SLE), and we still do not agree on what these antibodies bind in glomeruli (2), whether dsDNA (as in chromatin) or intrinsic membrane ligands.
We have to rethink, and critically develop, new strategies to gain relevant insight into the delimitation of SLE classification criteria. Are they all causally related to SLE (1)? Are we able to describe pathogenic mechanisms at a molecular level that are operational in SLE (1)? The basis for our contemporary investigations relies on classification criteria that are based i) on criteria that are established by the same procedures today as in 1971 (8–10, 81–83), except that immunological parameters were not implemented in 1971 (8–10, 81–83); ii) the majority of criteria are reiterated over the last 50 years (158); iii) the causality principle has not been implemented in the classification criteria (158); and iv) there are modest explanations that link these dogmatic criteria to SLE as “a one disease entity”. These problems incarnate Albert Schweitzer’s “insanity dogma” cited in the Introduction of this study.
Indeed, we have to develop new hypotheses and research strategies to develop a causality principle-based armamentarium of parameters that is (relatively)? specific for SLE as a “one disease entity”. Basically, we need strategies to separate SLE categories, as like a “one disease entity”, or polyphenotypic and poly-etiological SLE, and to identify disorders that mimic SLE: the “SLE-like non-SLE disorders”—the latter in terms of non-SLE diseases that imitate SLE (1, 158). Today’s SLE classification criteria do not have logical and understandable rules to distinguish these alternative SLE definitions from each other (158).
A systems science approach6 may provide solutions to solve the basic problems discussed in this study. Basically, systems science brings together various science disciplines and scientists relevant to an actual, often fundamental, problem (116, 157). This means that all aspects are approached to identify and investigate the complexity of a problem. The causality principle must be a central scientific element. This means that scientists representing diverse, complementary disciplines must come together, agree to—and define—the problem in order to implement systems science principles to describe the complexity of the problem—and logically to solve it.
The original contributions presented in the study are included in the article/supplementary material. Further inquiries can be directed to the corresponding author.
OR: Conceptualization, Data curation, Formal analysis, Investigation, Methodology, Project administration, Resources, Software, Validation, Visualization, Writing – original draft, Writing – review & editing.
The author(s) declare financial support was received for the research, authorship, and/or publication of this article. Open access payment was supported by the Open access fund, UiT-The Arctic University of Norway.
The system thinking presented in this study is influenced by years of curiosity and by important discussions with professors David Isenberg, University College London, Pier Luigi Meroni, University of Milano, David S. Pisetsky University of Durham and Marko Radic, University of Tennessee. They have formed my intuitive understanding of SLE and SLE-associated autoimmunity: origin and pathogenicity. Their influence on my considerations has toned down some of my more radical theories. I sincerely thank Associate Professor Gunnar Rekvig, UiT-The Arctic University of Norway, for the critical and patient reading and constructive logic and semantic comments on the manuscript. I am deeply thankful to Rod Wolstenholme, Section for Dissemination Services, Faculty of Health Sciences, UiT, for the expert help in preparing the figures.
The author declares that the research was conducted in the absence of any commercial or financial relationships that could be construed as a potential conflict of interest.
All claims expressed in this article are solely those of the authors and do not necessarily represent those of their affiliated organizations, or those of the publisher, the editors and the reviewers. Any product that may be evaluated in this article, or claim that may be made by its manufacturer, is not guaranteed or endorsed by the publisher.
1. Rekvig OP. SLE classification criteria: Science-based icons or algorithmic distractions - an intellectually demanding dilemma. Front Immunol. (2022) 13:1011591. doi: 10.3389/fimmu.2022.1011591
2. Rekvig OP. The anti-DNA antibodies: their specificities for unique DNA structures and their unresolved clinical impact-A system criticism and a hypothesis. Front Immunol. (2021) 12:808008. doi: 10.3389/fimmu.2021.808008
3. Miescher F. Ueber die chemische Zusammensetzung der Eiterzellen. Medizinisch-Chemische Untersuchungen. (1871) 4:441–60. doi: 10.1017/S000708742000062X
4. Dahm R. Friedrich miescher and the discovery of DNA. Dev Biol. (2005) 278:274–88. doi: 10.1016/j.ydbio.2004.11.028
5. Radic MZ, Weigert M. Genetic and structural evidence for antigen selection of anti- DNA antibodies. Annu Rev Immunol. (1994) 12:487–520. doi: 10.1146/annurev.iy.12.040194.002415
6. Chen C, Radic MZ, Erikson J, Camper SA, Litwin S, Hardy RR, et al. Deletion and editing of B cells that express antibodies to DNA. J Immunol. (1994) 152:1970–82. doi: 10.4049/jimmunol.152.4.1970
7. Radic MZ, Zouali M. Receptor editing, immune diversification, and self-tolerance. Immunity. (1996) 5:505–11. doi: 10.1016/s1074–7613(00)80266–6
8. Tan EM, Cohen AS, Fries JF, Masi AT, McShane DJ, Rothfield NF, et al. The 1982 revised criteria for the classification of systemic lupus erythematosus. Arthritis Rheum. (1982) 25:1271–7. doi: 10.1002/art.1780251101
9. Petri M, Orbai AM, Alarcon GS, Gordon C, Merrill JT, Fortin PR, et al. Derivation and validation of the Systemic Lupus International Collaborating Clinics classification criteria for systemic lupus erythematosus. Arthritis Rheum. (2012) 64:2677–86. doi: 10.1002/art.34473
10. Aringer M, Costenbader K, Daikh D, Brinks R, Mosca M, Ramsey-Goldman R, et al. 2019 European league against rheumatism/American college of rheumatology classification criteria for systemic lupus erythematosus. Arthritis Rheumatol. (2019) 71:1400–12. doi: 10.1136/annrheumdis-2018–214819
11. Wang G, Vasquez KM. Dynamic alternative DNA structures in biology and disease. Nat Rev Genet. (2023) 24:211–34. doi: 10.1038/s41576–022-00539–9
12. Robbins WC, Holman HR, Deicher H, Kunkel HG. Complement fixation with cell nuclei and DNA in lupus erythematosus. Proc Soc Exp Biol Med. (1957) 96:575–9. doi: 10.3181/00379727–96-23545
13. Ceppellini R, Polli E, Celada F. A DNA-reacting factor in serum of a patient with lupus erythematosus diffusus. Proc Soc Exp Biol Med. (1957) 96:572–4. doi: 10.3181/00379727–96-23544
14. Miescher P, Strassle R. New serological methods for the detection of the L.E. factor. Vox Sang. (1957) 2:283–7. doi: 10.1159/000478330
15. Seligmann M. [Demonstration in the blood of patients with disseminated lupus erythematosus a substance determining a precipitation reaction with desoxyribonucleic acid.]. C R Hebd Seances Acad Sci. (1957) 245:243–5
16. Stollar BD. The origin and pathogenic role of anti-DNA autoantibodies. Curr Opin Immunol. (1989) 2:607–12. doi: 10.1016/0952-7915(90)90019-D
17. Zouali M, Stollar BD, Schwartz RS. Origin and diversification of anti-DNA antibodies. Immunol Rev. (1988) 105:137–59. doi: 10.1111/j.1600-065X.1988.tb00770.x
18. Madaio MP, Hodder S, Schwartz RS, Stollar BD. Responsiveness of autoimmune and normal mice to nucleic acid antigens. J Immunol. (1984) 132:872–6. doi: 10.4049/jimmunol.132.2.872
19. Desai DD, Krishnan MR, Swindle JT, Marion TN. Antigen-specific induction of antibodies against native mammalian DNA in nonautoimmune mice. J Immunol. (1993) 151:1614–26. doi: 10.4049/jimmunol.151.3.1614
20. Rekvig OP, Moens U, Sundsfjord A, Bredholt G, Osei A, Haaheim H, et al. Experimental expression in mice and spontaneous expression in human SLE of polyomavirus T-antigen. A molecular basis for induction of antibodies to DNA and eukaryotic transcription factors. J Clin Invest. (1997) 99:2045–54. doi: 10.1172/JCI119373
21. Moens U, Seternes OM, Hey AW, Silsand Y, Traavik T, Johansen B, et al. In vivo expression of a single viral DNA-binding protein generates systemic lupus erythematosus-related autoimmunity to double-stranded DNA and histones. Proc Natl Acad Sci U.S.A. (1995) 92:12393–7. doi: 10.1073/pnas.92.26.12393
22. Pisetsky DS. Anti-DNA antibodies - quintessential biomarkers of SLE. Nat Rev Rheumatol. (2016) 12:102–10. doi: 10.1038/nrrheum.2015.151
23. Rekvig OP. The anti-DNA antibody: origin and impact, dogmas and controversies. Nat Rev Rheumatol. (2015) 11:530–40. doi: 10.1038/nrrheum.2015.69
24. Rekvig OP, Moens U, Fredriksen K, Traavik T. Human polyomavirus BK and immunogenicity of mammalian DNA: a conceptual framework. Methods. (1997) 11:44–54. doi: 10.1006/meth.1996.0386
25. Rekvig OP. The dsDNA, Anti-dsDNA Antibody, and Lupus Nephritis: What We Agree on, What Must Be Done, and What the Best Strategy Forward Could Be. Front Immunol. (2019) 10:1104. doi: 10.3389/fimmu.2019.01104
26. Stollar BD. Why the difference between B-DNA and Z-DNA? Lupus. (1997) 6:327–8. doi: 10.1177/096120339700600327
27. Eardley DD, Sercarz EE. Modulation of help and suppression in a hapten-carrier system. J Immunol. (1976) 116:600–5. doi: 10.4049/jimmunol.116.3.600
28. Ando DG, Sercarz EE, Hahn BH. Mechanisms of T and B cell collaboration in the in vitro production of anti-DNA antibodies in the NZB/NZW F1 murine SLE model. J Immunol. (1987) 138:3185–90. doi: 10.4049/jimmunol.138.10.3185
29. Lafer EM, Moller A, Nordheim A, Stollar BD, Rich A. Antibodies specific for left-handed Z-DNA. Proc Natl Acad Sci U.S.A. (1981) 78:3546–50. doi: 10.1073/pnas.78.6.3546
30. Gilkeson GS, Ruiz P, Howell D, Lefkowith JB, Pisetsky DS. Induction of immune-mediated glomerulonephritis in normal mice immunized with bacterial DNA. Clin Immunol Immunopathol. (1993) 68:283–92. doi: 10.1006/clin.1993.1129
31. Linton EW, Hittner D, Lewandowski C, Auld T, Triemer RE. A molecular study of euglenoid phylogeny using small subunit rDNA. J Eukaryot Microbiol. (1999) 46:217–23. doi: 10.1111/j.1550-7408.1999.tb04606.x
32. Krishnan MR, Marion TN. Comparison of the frequencies of arginines in heavy chain CDR3 of antibodies expressed in the primary B-cell repertoires of autoimmune-prone and normal mice. Scand J Immunol. (1998) 48:223–32. doi: 10.1046/j.1365-3083.1998.00426.x
33. Rekvig OP, Fredriksen K, Brannsether B, Moens U, Sundsfjord A, Traavik T. Antibodies to eukaryotic, including autologous, native DNA are produced during BK virus infection, but not after immunization with non-infectious BK DNA. Scand J Immunol. (1992) 36:487–95. doi: 10.1111/j.1365-3083.1992.tb02964.x
34. Gilkeson GS, Grudier JP, Karounos DG, Pisetsky DS. Induction of anti-double stranded DNA antibodies in normal mice by immunization with bacterial DNA. J Immunol. (1989) 142:1482–6. doi: 10.4049/jimmunol.142.5.1482
35. Gilkeson GS, Pippen AM, Pisetsky DS. Induction of cross-reactive anti-dsDNA antibodies in preautoimmune NZB/NZW mice by immunization with bacterial DNA. J Clin Invest. (1995) 95:1398–402. doi: 10.1172/JCI117793
36. Khan SN, Witsch EJ, Goodman NG, Panigrahi AK, Chen C, Jiang Y, et al. Editing and escape from editing in anti-DNA B cells. Proc Natl Acad Sci U.S.A. (2008) 105:3861–6. doi: 10.1073/pnas.0800025105
37. Radic M, Marion TN. Neutrophil extracellular chromatin traps connect innate immune response to autoimmunity. Semin Immunopathol. (2013) 35:465–80. doi: 10.1007/s00281–013-0376–6
38. Singh RR, Kumar V, Ebling FM, Southwood S, Sette A, Sercarz EE, et al. T cell determinants from autoantibodies to DNA can upregulate autoimmunity in murine systemic lupus erythematosus. J Exp Med. (1995) 181:2017–27. doi: 10.1084/jem.181.6.2017
39. Stollar BD. The experimental induction of antibodies to nucleic acids. Methods Enzymol. (1980) 70:70–85. doi: 10.1016/s0076–6879(80)70042–3
40. Stollar BD. The antigenic potential and specificity of nucleic acids, nucleoproteins, and their modified derivatives. Arthritis Rheum. (1981) 24:1010–8. doi: 10.1002/art.1780240806
41. Andreassen K, Bendiksen S, Kjeldsen E, Van Ghelue M, Moens U, Arnesen E, et al. T cell autoimmunity to histones and nucleosomes is a latent property of the normal immune system. Arthritis Rheum. (2002) 46:1270–81. doi: 10.1002/art.10254
42. Lafer EM, Rauch J, Andrzejewski C Jr., Mudd D, Furie B, Furie B, et al. Polyspecific monoclonal lupus autoantibodies reactive with both polynucleotides and phospholipids. J Exp Med. (1981) 153:897–909. doi: 10.1084/jem.153.4.897
43. Andrzejewski C Jr., Rauch J, Lafer E, Stollar BD, Schwartz RS. Antigen-binding diversity and idiotypic cross-reactions among hybridoma autoantibodies to DNA. J Immunol. (1981) 126:226–31. doi: 10.4049/jimmunol.126.1.226
44. Pisetsky DS, Vrabie IA. Antibodies to DNA: infection or genetics? Lupus. (2009) 18:1176–80. doi: 10.1177/0961203309106492
45. Granito A, Muratori L, Tovoli F, Muratori P. Diagnostic role of anti-dsDNA antibodies: do not forget autoimmune hepatitis. Nat Rev Rheumatol. (2021) 17:244. doi: 10.1038/s41584–021-00573–7
46. Rekvig OP. Anti-dsDNA antibodies as a classification criterion and a diagnostic marker for systemic lupus erythematosus: critical remarks. Clin Exp Immunol. (2015) 179:5–10. doi: 10.1111/cei.12296
47. Gilkeson GS, Pritchard AJ, Pisetsky DS. Cellular requirements for anti-DNA production induced in mice by immunization with bacterial DNA. Eur J Immunol. (1990) 20:1789–94. doi: 10.1002/eji.1830200825
48. Pego-Reigosa JM, Nicholson L, Pooley N, Langham S, Embleton N, Marjenberg Z, et al. The risk of infections in adult patients with systemic lupus erythematosus: systematic review and meta-analysis. Rheumatol (Oxford). (2021) 60:60–72. doi: 10.1093/rheumatology/keaa478
49. bu-Shakra M, Buskila D, Ehrenfeld M, Conrad K, Shoenfeld Y. Cancer and autoimmunity: autoimmune and rheumatic features in patients with Malignancies. Ann Rheum Dis. (2001) 60:433–41. doi: 10.1136/ard.60.5.433
50. Martin DN, Mikhail IS, Landgren O. Autoimmunity and hematologic Malignancies: associations and mechanisms. Leuk Lymphoma. (2009) 50:541–50. doi: 10.1080/10428190902780677
51. Ngalamika O, Zhang Y, Yin H, Zhao M, Gershwin ME, Lu Q. Epigenetics, autoimmunity and hematologic Malignancies: a comprehensive review. J Autoimmun. (2012) 39:451–65. doi: 10.1016/j.jaut.2012.09.002
52. Younus J, Ahmed AR. Autoimmunity and Malignancy. J Am Acad Dermatol. (1990) 23:720–3. doi: 10.1016/0190-9622(90)70280-U
53. Rekvig OP. Autoimmunity and SLE: factual and semantic evidence-based critical analyses of definitions, etiology, and pathogenesis. Front Immunol. (2020) 11:569234. doi: 10.3389/fimmu.2020.569234
54. Lamm E, Harman O, Veigl SJ. Before watson and crick in 1953 came friedrich miescher in 1869. Genetics. (2020) 215:291–6. doi: 10.1534/genetics.120.303195
55. Levene PA. On the chemistry of the chromatin substance of the nerve cell. J Med Res. (1903) 10:204–11
56. Chargaff E, Zamenhof S, Green C. Composition of human desoxypentose nucleic acid. Nature. (1950) 165:756–7. doi: 10.1038/165756b0
57. Elson D, Chargaff E. On the desoxyribonucleic acid content of sea urchin gametes. Experientia. (1952) 8:143–5. doi: 10.1007/BF02170221
58. Franklin RE, Gosling RG. Evidence for 2-chain helix in crystalline structure of sodium deoxyribonucleate. Nature. (1953) 172:156–7. doi: 10.1038/172156a0
59. Maddox B. Rosalind Franklin: The dark Lady of DNA. HarperCollins (1957). Ref Type: Serial (Book,Monograph)
60. Elkin LO. Rosalind Franklin and the double helix. Phys Today. (2003) 56:42. doi: 10.1063/1.1570771
61. Bosaeus N, Reymer A, Beke-Somfai T, Brown T, Takahashi M, Wittung-Stafshede P, et al. A stretched conformation of DNA with a biological role? Q Rev Biophys. (2017) 50:e11. doi: 10.1017/S0033583517000099
62. Veloso A, Kirkconnell KS, Magnuson B, Biewen B, Paulsen MT, Wilson TE, et al. Rate of elongation by RNA polymerase II is associated with specific gene features and epigenetic modifications. Genome Res. (2014) 24:896–905. doi: 10.1101/gr.171405.113
63. Nomidis SK, Caraglio M, Laleman M, Phillips K, Skoruppa E, Carlon E. Twist-bend coupling, twist waves, and the shape of DNA loops. Phys Rev E. (2019) 100:22402. doi: 10.1103/PhysRevE.100.022402
64. Caraglio M, Skoruppa E, Carlon E. Overtwisting induces polygonal shapes in bent DNA. J Chem Phys. (2019) 150:135101. doi: 10.1063/1.5084950
65. Ashton NW, Bolderson E, Cubeddu L, O’Byrne KJ, Richard DJ. Human single-stranded DNA binding proteins are essential for maintaining genomic stability. BMC Mol Biol. (2013) 14:9. doi: 10.1186/1471–2199-14–9
66. Richard DJ, Bolderson E, Khanna KK. Multiple human single-stranded DNA binding proteins function in genome maintenance: structural, biochemical and functional analysis. Crit Rev Biochem Mol Biol. (2009) 44:98–116. doi: 10.1080/10409230902849180
67. Brazda V, Laister RC, Jagelska EB, Arrowsmith C. Cruciform structures are a common DNA feature important for regulating biological processes. BMC Mol Biol. (2011) 12:33. doi: 10.1186/1471–2199-12–33
68. Ghosh A, Bansal M. A glossary of DNA structures from A to Z. Acta Crystallogr D Biol Crystallogr. (2003) 59:620–6. doi: 10.1107/s0907444903003251
69. Dickerson RE. DNA structure from A to Z. Methods Enzymol. (1992) 211:67–111. doi: 10.1016/0076–6879(92)11007–6
70. Watson JD, Crick FH. The structure of DNA. Cold Spring Harb Symp Quant Biol. (1953) 18:123–31. doi: 10.1101/sqb.1953.018.01.020
71. Watson JD, Crick FH. Genetical implications of the structure of deoxyribonucleic acid. Nature. (1953) 171:964–7. doi: 10.1038/171964b0
72. Olins AL, Olins DE. Spheroid chromatin units (v bodies). Science. (1974) 183:330–2. doi: 10.1126/science.183.4122.330
73. Kornberg RD, Lorch Y. Chromatin-modifying and -remodeling complexes. Curr Opin Genet Dev. (1999) 9:148–51. doi: 10.1016/S0959–437X(99)80022–7
74. Kornberg RD, Lorch Y. Twenty-five years of the nucleosome, fundamental particle of the eukaryote chromosome. Cell. (1999) 98:285–94. doi: 10.1016/s0092–8674(00)81958–3
75. Richmond TJ, Finch JT, Rushton B, Rhodes D, Klug A. Structure of the nucleosome core particle at 7 A resolution. Nature. (1984) 311:532–7. doi: 10.1038/311532a0
76. Luger K, Mader AW, Richmond RK, Sargent DF, Richmond TJ. Crystal structure of the nucleosome core particle at 2.8 A resolution [see comments]. Nature. (1997) 389:251–60. doi: 10.1038/38444
77. Laskey RA, Mills AD, Philpott A, Leno GH, Dilworth SM, Dingwall C. The role of nucleoplasmin in chromatin assembly and disassembly. Philos Trans R Soc Lond B Biol Sci. (1993) 339:263–9. doi: 10.1098/rstb.1993.0024
78. Minchin S, Lodge J. Understanding biochemistry: structure and function of nucleic acids. Essays Biochem. (2019) 63:433–56. doi: 10.1042/EBC20180038
79. van Steensel B. Chromatin: constructing the big picture. EMBO J. (2011) 30:1885–95. doi: 10.1038/emboj.2011.135
80. Kaushik M, Kaushik S, Roy K, Singh A, Mahendru S, Kumar M, et al. A bouquet of DNA structures: Emerging diversity. Biochem Biophys Rep. (2016) 5:388–95. doi: 10.1016/j.bbrep.2016.01.013
81. Cohen AS, Reynolds WE, Franklin EC, Kulka JP, Ropes MW, Shulman LE, et al. Preliminary classification criteria for Systemic lupus erythematosus. Bull Rheum Dis. (1971) 21:643–8
82. Aringer M, Brinks R, Dorner T, Daikh D, Mosca M, Ramsey-Goldman R, et al. European League Against Rheumatism (EULAR)/American College of Rheumatology (ACR) SLE classification criteria item performance. Ann Rheum Dis. (2021) 80:775–81. doi: 10.1136/annrheumdis-2020–219373
83. Aringer M, Johnson SR. New lupus criteria: a critical view. Curr Opin Rheumatol. (2021) 33:205–10. doi: 10.1097/BOR.0000000000000771
84. Shoenfeld Y, Rauch J, Massicotte H, Datta SK, Andre Schwartz J, Stollar BD, et al. Polyspecificity of monoclonal lupus autoantibodies produced by human-human hybridomas. N Engl J Med. (1983) 308:414–20. doi: 10.1056/NEJM198302243080802
85. Eilat D. Cross-reactions of anti-DNA antibodies and the central dogma of lupus nephritis. Immunol Today. (1985) 6:123–7. doi: 10.1016/0167–5699(85)90077–5
86. Mageed RA, Zack DJ. Cross-reactivity and pathogenicity of anti-DNA autoantibodies in systemic lupus erythematosus. Lupus. (2002) 11:783–6. doi: 10.1191/0961203302lu317oa
87. Diamond B, Scharff MD. Somatic mutation of the T15 heavy chain gives rise to an antibody with autoantibody specificity. Proc Natl Acad Sci U.S.A. (1984) 81:5841–4. doi: 10.1073/pnas.81.18.5841
88. DeGiorgio LA, Konstantinov KN, Lee SC, Hardin JA, Volpe BT, Diamond B. A subset of lupus anti-DNA antibodies cross-reacts with the NR2 glutamate receptor in systemic lupus erythematosus. Nat Med. (2001) 7:1189–93. doi: 10.1038/nm1101–1189
89. Ray SK, Putterman C, Diamond B. Pathogenic autoantibodies are routinely generated during the response to foreign antigen: a paradigm for autoimmune disease. Proc Natl Acad Sci U.S.A. (1996) 93:2019–24. doi: 10.1073/pnas.93.5.2019
90. Putterman C, Limpanasithikul W, Edelman M, Diamond B. The double edged sword of the immune response: mutational analysis of a murine anti-pneumococcal, anti-DNA antibody. J Clin Invest. (1996) 97:2251–9. doi: 10.1172/JCI118666
91. Putterman C, Diamond B. Immunization with a peptide surrogate for double-stranded DNA (dsDNA) induces autoantibody production and renal immunoglobulin deposition. J Exp Med. (1998) 188:29–38. doi: 10.1084/jem.188.1.29
92. Deocharan B, Zhou Z, Antar K, Siconolfi-Baez L, Angeletti RH, Hardin J, et al. Alpha-actinin immunization elicits anti-chromatin autoimmunity in nonautoimmune mice. J Immunol. (2007) 179:1313–21. doi: 10.4049/jimmunol.179.2.1313
93. Zhao Z, Weinstein E, Tuzova M, Davidson A, Mundel P, Marambio P, et al. Cross-reactivity of human lupus anti-DNA antibodies with alpha-actinin and nephritogenic potential. Arthritis Rheum. (2005) 52:522–30. doi: 10.1002/art.20862
94. Beger E, Deocharan B, Edelman M, Erblich B, Gu Y, Putterman C. A peptide DNA surrogate accelerates autoimmune manifestations and nephritis in lupus-prone mice. J Immunol. (2002) 168:3617–26. doi: 10.4049/jimmunol.168.7.3617
95. Zhang Y, Pan V, Li X, Yang X, Li H, Wang P, et al. Dynamic DNA structures. Small. (2019) 15:e1900228. doi: 10.1002/smll.201900228
96. Edgington SM, Stollar BD. Immunogenicity of Z-DNA depends on the size of polynucleotide presented in complexes with methylated BSA. Mol Immunol. (1992) 29:609–17. doi: 10.1016/0161–5890(92)90197–6
97. Wang X, Xia Y. Anti-double stranded DNA antibodies: origin, pathogenicity, and targeted therapies. Front Immunol. (2019) 10:1667. doi: 10.3389/fimmu.2019.01667
98. Riley JS, Tait SW. Mitochondrial DNA in inflammation and immunity. EMBO Rep. (2020) 21:e49799. doi: 10.15252/embr.201949799
99. Hu MM, Shu HB. Mitochondrial DNA-triggered innate immune response: mechanisms and diseases. Cell Mol Immunol. (2023) 20:1403–12. doi: 10.1038/s41423-023-01086-x
100. West AP, Shadel GS. Mitochondrial DNA in innate immune responses and inflammatory pathology. Nat Rev Immunol. (2017) 17:363–75. doi: 10.1038/nri.2017.21
101. Reimer G, Rubin RL, Kotzin BL, Tan EM. Anti-native DNA antibodies from autoimmune sera also bind to DNA in mitochondria. J Immunol. (1984) 133:2532–6. doi: 10.4049/jimmunol.133.5.2532
102. Flaegstad T, Fredriksen K, Dahl B, Traavik T, Rekvig OP. Inoculation with BK virus may break immunological tolerance to histone and DNA antigens. Proc Natl Acad Sci U.S.A. (1988) 85:8171–5. doi: 10.1073/pnas.85.21.8171
103. Fredriksen K, Brannsether B, Traavik T, Rekvig OP. Antibodies to viral and mammalian native DNA in response to BK virus inoculation and subsequent immunization with calf thymus DNA. Scand J Immunol. (1991) 34:109–19. doi: 10.1111/j.1365-3083.1991.tb01526.x
104. Cerutti ML, Zarebski LM, de Prat GG, Goldbaum FA. A viral DNA-binding domain elicits anti-DNA antibodies of different specificities. Mol Immunol. (2005) 42:327–33. doi: 10.1016/j.molimm.2004.09.003
105. Sundar K, Jacques S, Gottlieb P, Villars R, Benito ME, Taylor DK, et al. Expression of the Epstein-Barr virus nuclear antigen-1 (EBNA-1) in the mouse can elicit the production of anti-dsDNA and anti-Sm antibodies. J Autoimmun. (2004) 23:127–40. doi: 10.1016/j.jaut.2004.06.001
106. Koo M. Systemic lupus erythematosus research: A bibliometric analysis over a 50-year period. Int J Environ Res Public Health. (2021) 18:7095. doi: 10.3390/ijerph18137095
107. Pancer LB, Bell DA, Singhal SK. Induction of anti-ssDNA antibodies in normal and preautoimmune mice in vivo. J Immunol. (1980) 124:939–46. doi: 10.4049/jimmunol.124.2.939
108. Kalaaji M, Fenton KA, Mortensen ES, Olsen R, Sturfelt G, Alm P, et al. Glomerular apoptotic nucleosomes are central target structures for nephritogenic antibodies in human SLE nephritis. Kidney Int. (2007) 71:664–72. doi: 10.1038/sj.ki.5002133
109. Thiyagarajan D, Fismen S, Seredkina N, Jacobsen S, Elung-Jensen T, Kamper AL, et al. Silencing of renal DNaseI in murine lupus nephritis imposes exposure of large chromatin fragments and activation of toll like receptors and the clec4e. PloS One. (2012) 7:e34080. doi: 10.1371/journal.pone.0034080
110. Gupta S, Kaplan MJ. The role of neutrophils and NETosis in autoimmune and renal diseases. Nat Rev Nephrol. (2016) 12:402–13. doi: 10.1038/nrneph.2016.71
111. Mostoslavsky G, Fischel R, Yachimovich N, Yarkoni Y, Rosenmann E, Monestier M, et al. Lupus anti-DNA autoantibodies cross-react with a glomerular structural protein: a case for tissue injury by molecular mimicry. Eur J Immunol. (2001) 31:1221–7. doi: 10.1002/(ISSN)1521-4141
112. Deocharan B, Qing X, Lichauco J, Putterman C. Alpha-actinin is a cross-reactive renal target for pathogenic anti-DNA antibodies. J Immunol. (2002) 168:3072–8. doi: 10.4049/jimmunol.168.6.3072
113. Zhang W, Reichlin M. A possible link between infection with burkholderia bacteria and systemic lupus erythematosus based on epitope mimicry. Clin Dev Immunol. (2008) 2008:683489. doi: 10.1155/2008/683489
114. Qureshi F, Yang Y, Jaques SM, Johnson MP, Naparstek Y, Ulmansky R, et al. Anti-DNA antibodies cross-reacting with laminin inhibit trophoblast attachment and migration: implications for recurrent pregnancy loss in SLE patients. Am J Reprod Immunol. (2000) 44:136–42. doi: 10.1111/j.8755-8920.2000.440302.x
115. Yadav P, Carr MT, Yu R, Mumbey-Wafula A, Spatz LA. Mapping an epitope in EBNA-1 that is recognized by monoclonal antibodies to EBNA-1 that cross-react with dsDNA. Immun Inflammation Dis. (2016) 4:362–75. doi: 10.1002/iid3.119
116. Mobus GE, Kalton MC. Principles of Systems Science. New York: Springer (2015). doi: 10.1007/978-1-4939-1920-8
117. van Bavel CC, van der Vlag J, Berden JH. Glomerular binding of anti-dsDNA autoantibodies: the dispute resolved? Kidney Int. (2007) 71:600–1. doi: 10.1038/sj.ki.5002126
118. van Bavel CC, Fenton KA, Rekvig OP, van der Vlag J, Berden JH. Glomerular targets of nephritogenic autoantibodies in systemic lupus erythematosus. Arthritis Rheum. (2008) 58:1892–9. doi: 10.1002/art.23626
119. Seredkina N, van der Vlag J, Berden J, Mortensen E, Rekvig OP. Lupus nephritis: enigmas, conflicting models and an emerging concept. Mol Med. (2013) 19:161–9. doi: 10.2119/molmed.2013.00010
120. Fenton KA, Tommeras B, Marion TN, Rekvig OP. Pure anti-dsDNA mAbs need chromatin structures to promote glomerular mesangial deposits in BALB/c mice. Autoimmunity. (2010) 43:179–88. doi: 10.3109/08916930903305633
121. Fenton K, Fismen S, Hedberg A, Seredkina N, Fenton C, Mortensen ES, et al. Anti-dsDNA antibodies promote initiation, and acquired loss of renal Dnase1 promotes progression of lupus nephritis in autoimmune (NZBxNZW)F1 mice. PloS One. (2009) 4:e8474. doi: 10.1371/journal.pone.0008474
122. Kalaaji M, Sturfelt G, Mjelle JE, Nossent H, Rekvig OP. Critical comparative analyses of anti-alpha-actinin and glomerulus-bound antibodies in human and murine lupus nephritis. Arthritis Rheum. (2006) 54:914–26. doi: 10.1002/art.21622
123. Xie C, Liang Z, Chang S, Mohan C. Use of a novel elution regimen reveals the dominance of polyreactive antinuclear autoantibodies in lupus kidneys. Arthritis Rheum. (2003) 48:2343–52. doi: 10.1002/art.11092
124. Van Bruggen MC, Kramers C, Hylkema MN, Smeenk RJ, Berden JH. Significance of anti-nuclear and anti-extracellular matrix autoantibodies for albuminuria in murine lupus nephritis; a longitudinal study on plasma and glomerular eluates in MRL/l mice. Clin Exp Immunol. (1996) 105:132–9. doi: 10.1046/j.1365-2249.1996.d01-731.x
125. Winfield JB, Faiferman I, Koffler D. Avidity of anti-DNA antibodies in serum and IgG glomerular eluates from patients with systemic lupus erythematosus. Association of high avidity antinative DNA antibody with glomerulonephritis. J Clin Invest. (1977) 59:90–6. doi: 10.1172/JCI108626
127. Rekvig OP, Bendiksen S, Moens U. Immunity and autoimmunity induced by polyomaviruses: clinical, experimental and theoretical aspects. Adv Exp Med Biol. (2006) 577:117–47. doi: 10.1007/0–387-32957–9_9
128. Termaat RM, Assmann KJ, van Son JP, Dijkman HB, Koene RA, Berden JH. Antigen-specificity of antibodies bound to glomeruli of mice with systemic lupus erythematosus-like syndromes. Lab Invest. (1993) 68:164–73
129. Amital H, Heilweil M, Ulmansky R, Szafer F, Bar-Tana R, Morel L, et al. Treatment with a laminin-derived peptide suppresses lupus nephritis. J Immunol. (2005) 175:5516–23. doi: 10.4049/jimmunol.175.8.5516
130. Krishnan MR, Wang C, Marion TN. Anti-DNA autoantibodies initiate experimental lupus nephritis by binding directly to the glomerular basement membrane in mice. Kidney Int. (2012) 82:184–92. doi: 10.1038/ki.2011.484
131. Marion TN, Postlethwaite AE. Chance, genetics, and the heterogeneity of disease and pathogenesis in systemic lupus erythematosus. Semin Immunopathol. (2014) 36:495–517. doi: 10.1007/s00281-014-0440-x
132. Madaio MP. The relevance of antigen binding to the pathogenicity of lupus autoantibodies. Kidney Int. (2012) 82:125–7. doi: 10.1038/ki.2012.159
133. Goilav B, Putterman C. The role of anti-DNA antibodies in the development of lupus nephritis: A complementary, or alternative, viewpoint? Semin Nephrol. (2015) 35:439–43. doi: 10.1016/j.semnephrol.2015.08.005
134. Van Bruggen MC, Walgreen B, Rijke TP, Tamboer W, Kramers K, Smeenk RJ, et al. Antigen specificity of anti-nuclear antibodies complexed to nucleosomes determines glomerular basement membrane binding in vivo. Eur J Immunol. (1997) 27:1564–9. doi: 10.1002/eji.1830270636
135. Seredkina S, Rekvig OP. Acquired loss of renal nuclease activity is restricted to DNaseI and is an organ-selective feature in murine lupus nephritis. Am J Pathol. (2011) 179:1120–8. doi: 10.1016/j.ajpath.2011.05.011
136. Pedersen HL, Horvei KD, Thiyagarajan D, Norby GE, Seredkina N, Moroni G, et al. Lupus nephritis: low urinary DNase I levels reflect loss of renal DNase I and may be utilized as a biomarker of disease progression. J Pathol Clin Res. (2018) 4:193–203. doi: 10.1002/cjp2.99
137. Seredkina N, Zykova SN, Rekvig OP. Progression of murine lupus nephritis is linked to acquired renal Dnase1 deficiency and not to up-regulated apoptosis. Am J Pathol. (2009) 175:97–106. doi: 10.2353/ajpath.2009.080943
138. Tokuyasu KT. Application of cryoultramicrotomy to immunocytochemistry. J Microsc. (1986) 143:139–49. doi: 10.1111/j.1365-2818.1986.tb02772.x
139. Pedersen HL, Horvei KD, Thiyagarajan D, Seredkina N, Rekvig OP. Murine and human lupus nephritis: pathogenic mechanisms and theoretical strategies for therapy. Semin Nephrol. (2015) 35:427–38. doi: 10.1016/j.semnephrol.2015.08.004
140. Jandl K, Mutgan AC, Eller K, Schaefer L, Kwapiszewska G. The basement membrane in the cross-roads between the lung and kidney. Matrix Biol. (2022) 105:31–52. doi: 10.1016/j.matbio.2021.11.003
141. Randles MJ, Humphries MJ, Lennon R. Proteomic definitions of basement membrane composition in health and disease. Matrix Biol. (2017) 57–58:12–28. doi: 10.1016/j.matbio.2016.08.006
142. Randles MJ, Woolf AS, Huang JL, Byron A, Humphries JD, Price KL, et al. Genetic background is a key determinant of glomerular extracellular matrix composition and organization. J Am Soc Nephrol. (2015) 26:3021–34. doi: 10.1681/ASN.2014040419
143. Miner JH. Renal basement membrane components. Kidney Int. (1999) 56:2016–24. doi: 10.1046/j.1523-1755.1999.00785.x
144. Kruegel J, Miosge N. Basement membrane components are key players in specialized extracellular matrices. Cell Mol Life Sci. (2010) 67:2879–95. doi: 10.1007/s00018-010-0367-x
145. Pedchenko V, Kitching AR, Hudson BG. Goodpasture’s autoimmune disease - A collagen IV disorder. Matrix Biol. (2018) 71–72:240–9. doi: 10.1016/j.matbio.2018.05.004
146. Borza DB, Neilson EG, Hudson BG. Pathogenesis of Goodpasture syndrome: a molecular perspective. Semin Nephrol. (2003) 23:522–31. doi: 10.1053/s0270–9295(03)00131–1
147. Saxena R, Bygren P, Butkowski R, Wieslander J. Specificity of kidney-bound antibodies in Goodpasture’s syndrome. Clin Exp Immunol. (1989) 78:31–6
148. Fu SM, Dai C, Zhao Z, Gaskin F. Anti-dsDNA Antibodies are one of the many autoantibodies in systemic lupus erythematosus. F1000Res. (2015) 4:939. doi: 10.12688/f1000research.6875.1
149. Amoura Z, Chabre H, Koutouzov S, Lotton C, Cabrespines A, Bach JF, et al. Nucleosome-restricted antibodies are detected before anti-dsDNA and/or antihistone antibodies in serum of MRL-Mp lpr/lpr and +/+ mice, and are present in kidney eluates of lupus mice with proteinuria. Arthritis Rheum. (1994) 37:1684–8. doi: 10.1002/art.1780371118
150. Elouaai F, Lule J, Benoist H, Appolinaire-Pilipenko S, Atanassov C, Muller S, et al. Autoimmunity to histones, ubiquitin, and ubiquitinated histone H2A in NZB x NZW and MRL-lpr/lpr mice. Anti-histone antibodies are concentrated in glomerular eluates of lupus mice. Nephrol Dial Transplant. (1994) 9:362–6
151. Maeshima K, Iida S, Tamura S. Physical nature of chromatin in the nucleus. Cold Spring Harb Perspect Biol. (2021) 13. doi: 10.1101/cshperspect.a040675
152. Hansen JC. Conformational dynamics of the chromatin fiber in solution: determinants, mechanisms, and functions. Annu Rev Biophys Biomol Struct. (2002) 31:361–92. doi: 10.1146/annurev.biophys.31.101101.140858
153. Hansen JC, Ausio J. Chromatin dynamics and the modulation of genetic activity. Trends Biochem Sci. (1992) 17:187–91. doi: 10.1016/0968-0004(92)90264-A
154. Mohan C, Putterman C. Genetics and pathogenesis of systemic lupus erythematosus and lupus nephritis. Nat Rev Nephrol. (2015) 11:329–41. doi: 10.1038/nrneph.2015.33
155. Bourse CN, Orquevaux P, Giusti D, Gatouillat G, Tabary T, Tonye LM, et al. Absence of anti-glomerular basement membrane antibodies in 200 patients with systemic lupus erythematosus with or without lupus nephritis: results of the GOODLUPUS study. Front Immunol. (2020) 11:597863. doi: 10.3389/fimmu.2020.597863
156. Rekvig OP, Thiyagarajan D, Pedersen HL, Horvei KD, Seredkina N. Future perspectives on pathogenesis of lupus nephritis: facts, problems, and potential causal therapy modalities. Am J Pathol. (2016) 186:2772–82. doi: 10.1016/j.ajpath.2016.06.026
157. Warfield JN. A proposal for systems science. Syst Res Behav Sci. (2010) 20:507–20. doi: 10.1002/sres.528
158. Rekvig OP. SLE classification criteria: Is “The causality principle” integrated and operative - and do the molecular and genetical network, on which criteria depend on, support the definition of SLE as “a one disease entity” - A theoretical discussion. Autoimmun Rev. (2023) 22:103470. doi: 10.1016/j.autrev.2023.103470
Keywords: systemic lupus erythematosus, SLE, SLE classification criteria, unique DNA structures, the causality principle, lupus nephritis, system science
Citation: Rekvig OP (2024) SLE: a cognitive step forward—a synthesis of rethinking theories, causality, and ignored DNA structures. Front. Immunol. 15:1393814. doi: 10.3389/fimmu.2024.1393814
Received: 29 February 2024; Accepted: 09 May 2024;
Published: 04 June 2024.
Edited by:
Pier Luigi Meroni, Italian Auxological Institute (IRCCS), ItalyReviewed by:
Charis Pericleous, Imperial College London, United KingdomCopyright © 2024 Rekvig. This is an open-access article distributed under the terms of the Creative Commons Attribution License (CC BY). The use, distribution or reproduction in other forums is permitted, provided the original author(s) and the copyright owner(s) are credited and that the original publication in this journal is cited, in accordance with accepted academic practice. No use, distribution or reproduction is permitted which does not comply with these terms.
*Correspondence: Ole Petter Rekvig, b3ByMDAwQHVpdC5ubw==
Disclaimer: All claims expressed in this article are solely those of the authors and do not necessarily represent those of their affiliated organizations, or those of the publisher, the editors and the reviewers. Any product that may be evaluated in this article or claim that may be made by its manufacturer is not guaranteed or endorsed by the publisher.
Research integrity at Frontiers
Learn more about the work of our research integrity team to safeguard the quality of each article we publish.