- 1Department of Clinical and Toxicological Analyses, School of Pharmaceutical Sciences, University of São Paulo, São Paulo, Brazil
- 2Lagoa Federal Hospital, Rio de Janeiro, Rio de Janeiro, Brazil
- 3Galileo Biotech, Rio de Janeiro, Rio de Janeiro, Brazil
- 4Department of Morphology and Genetics, Federal University of São Paulo, São Paulo, São Paulo, Brazil
- 5Dante Pazzanese Institute of Cardiology of Sao Paulo, São Paulo, São Paulo, Brazil
Introduction: G-protein coupled receptors (GPCRs) expressed on neutrophils regulate their mobilization from the bone marrow into the blood, their half-live in the circulation, and their pro- and anti-inflammatory activities during inflammation. Chronic kidney disease (CKD) is associated with systemic inflammatory responses, and neutrophilia is a hallmark of CKD onset and progression. Nonetheless, the role of neutrophils in CKD is currently unclear.
Methods: Blood and renal tissue were collected from non-dialysis CKD (grade 3 - 5) patients to evaluate GPCR neutrophil expressions and functions in CKD development.
Results: CKD patients presented a higher blood neutrophil-to-lymphocyte ratio (NLR), which was inversely correlated with the glomerular filtration rate (eGFR). A higher frequency of neutrophils expressing the senescent GPCR receptor (CXCR4) and activation markers (CD18+CD11b+CD62L+) was detected in CKD patients. Moreover, CKD neutrophils expressed higher amounts of GPCR formyl peptide receptors (FPR) 1 and 2, known as neutrophil pro- and anti-inflammatory receptors, respectively. Cytoskeletal organization, migration, and production of reactive oxygen species (ROS) by CKD neutrophils were impaired in response to the FPR1 agonist (fMLP), despite the higher expression of FPR1. In addition, CKD neutrophils presented enhanced intracellular, but reduced membrane expression of the protein Annexin A1 (AnxA1), and an impaired ability to secrete it into the extracellular compartment. Secreted and phosphorylated AnxA1 is a recognized ligand of FPR2, pivotal in anti-inflammatory and efferocytosis effects. CKD renal tissue presented a low number of neutrophils, which were AnxA1+.
Conclusion: Together, these data highlight that CKD neutrophils overexpress GPCRs, which may contribute to an unbalanced aging process in the circulation, migration into inflamed tissues, and efferocytosis.
1 Introduction
Neutrophil homeostasis is an essential and multi-orchestrated process for host immune surveillance. In a steady state, approximately 1011 neutrophils are produced daily in the bone marrow and subsequently released into the circulation. Neutrophils have a short lifespan in the blood, around 7–10 hours in humans (1). Mature neutrophils in the bone marrow or circulation constitutively express membrane receptors that respond to chemical mediators to control their trafficking within body compartments and to prompt a response to aggression. Therefore, fine-tuned control and synchrony occur between the bone marrow, blood, and clearance tissues to maintain neutrophil homeostasis and support host defense (2, 3).
G-protein coupled receptors (GPCRs) consist of seven α-helical transmembrane regions, and their downstream signaling relies on the activation of heterotrimeric G proteins (4). GPCRs play a crucial role in a variety of physiological processes, and malfunctioning of their signaling is implicated in multiple diseases. Consequently, GPCR agonists and antagonists serve as important pharmaceutical tools to modulate inflammatory diseases (4, 5).
Production, recruitment, and activation of neutrophils rely on GPCR expression and activation (4). Fine-tuned GPCR activation orchestrates the release of mature bone marrow neutrophils into the circulation and their half-life. The binding of CXCR4 (G protein-coupled chemokine receptor) to stromal cell-derived factor 1 (SDF-1), also known as CXCL12 (chemokine-CX-C motif ligand 12), retains mature neutrophils in the bone marrow. During neutrophil delivery into the blood, the downregulation of CXCR4 and the upregulation of CXCR2 occur in response to CXCL2 (chemokine-CX-C motif ligand 2). Then, circulating aged neutrophils downregulate CXCR2 and upregulate CXCR4 to home back into the bone marrow in response to SDF-1 (6, 7). Moreover, GPCR formyl peptide receptors (FPRs) contribute to granulopoiesis (8–10) and are pivotal receptors in the pro- and anti-inflammatory actions of neutrophils during the host response. A vast range of ligands can activate FPR1, including peptides derived from invading pathogens or mitochondria from dead cells, leading to downstream intracellular pathways associated with cytoskeletal reorganization, adhesion, chemotaxis, degranulation, NET formation, and NADPH oxidase activation (11). Conversely, the activation of FPR2 by endogenous peptides and proteins, such as lipoxin A4 and Annexin A1 (AnxA1), triggers effects related to the resolution of inflammation, such as efferocytosis and tissue repair (12–15).
Systemic inflammation is a hallmark of chronic kidney disease (CKD), triggered by uremic toxins and mediators secreted by the renal epithelium and infiltrating leukocytes (16, 17). Cohort studies have linked blood biomarkers of acute inflammation, including peripheral neutrophil counts, with the progression of renal dysfunction and failure (17). Indeed, a high neutrophil-to-lymphocyte ratio (NLR) is a marker of CKD, associated with the disease’s severity and progression (18–20). Nonetheless, the role of neutrophils in renal lesion is unclear. Evidence supports that CKD peripheral neutrophils are activated cells, producing reactive oxygen species (ROS), releasing granular and nuclear contents, and secreting pro-inflammatory cytokines (16, 21, 22). These actions damage the vessels and worsen cardiovascular complications and diabetes, which are the main underlying diseases in CKD (21) (23). Pro-inflammatory neutrophils also induce renal damage and the development of fibrosis detected in the final stage of kidney disease (21, 22). Moreover, CKD circulating neutrophils have been described as primed and unresponsive cells, which are associated with impaired host defense, supporting the increased risk of infections found in CKD patients (24–27).
Here, we used blood and renal tissue from non-dialysis CKD (grades 3–5) patients to evaluate the profile of GPCR expression in neutrophils and GPCR-mediated functions. As expected, CKD patients presented a high neutrophil-to-lymphocyte ratio (NLR) correlated with the progression of the disease; nonetheless, neutrophils were not abundant in the CKD renal tissue. This may be reflected by the overexpression and reduced activation of GPCRs involved in neutrophil aging, chemotaxis, and tissue repair actions.
2 Materials and methods
2.1 Study design
Adults with CKD stages 3 to 5 were enrolled by informed consent from nephrology outpatient clinics at Lagoa Federal Hospital (Rio de Janeiro, Brazil) and at Dante Pazzanese Institute of Cardiology of Sao Paulo (São Paulo, Brazil). Inclusion criteria for the CKD 1–5 cohort were: (a) Age ≥ 18 years, (b) Diagnosis of CKD according to the criteria of the National Kidney Foundation (NFK) -NKF-KDOQI (2002) and NFK-KDIGO (2013) (28, 29), and clinical manifestations, (c) Not currently receiving chronic hemodialysis or peritoneal dialysis, (d) Not the recipient of a kidney transplant, (e) Not known to be positive for human immunodeficiency virus, hepatitis B virus or hepatitis C virus, (f) Not currently receiving immunosuppressive therapy.
Healthy volunteers referred as control (employees and professionals from the hospital itself) were invited and include in the control group of our study after accepting informed consent. Inclusion criteria for the no CKD cohort were as follows: (a) Age ≥ 18 years, (b) No active medical conditions, (c) Not taking any medication (over the counter or prescription) within 48 h of study (excluding contraceptives), (d) No current or previous history of kidney disease.
Clinical and medicines data were obtained from medical records maintained in a secure, password-protected, web-based clinical database by Hospital e-SUS, the SUS IT Department - DATASUS hospital management system.
2.2 Sample collection
To analyze leukogram and serum parameters, venous blood (3–5 mL) was sampled using metal-free Safety Vacutainer blood collecting tubes containing >1.5 mg K2EDTA obtained from Becton Dickinson (New Jersey, USA). For glucose measurement, BD Vacutainer Fluoride/EDTA tubes were used. For neutrophil isolation, blood was collected using Safety Vacutainer blood collecting tubes with spray-dried sodium heparin from Becton Dickinson. Plasma was separated by centrifugation, recovered, aliquoted for measuring biochemical parameters, and stored at −80°C until AnxA1 serum levels measurement.
2.3 Hemogram and serum parameter analysis
The hemogram analyses were performed using the Sysmex XN-10–51100 automated equipment (Sysmex, Illinois, USA). Urea, creatinine, glucose, albumin, uric acid, homocysteine, and cortisol levels were measured by the AU680 Chemistry Analyzer (Beckman Coulter Diagnostics, California, USA). Potassium, sodium, and bicarbonate were determined using the ABL800 FLEX blood gas analyzer (Radiometer America, California, USA). The levels of human AnxA1 in serum and neutrophil supernatant were quantified using a commercial kit from MyBiosource (California, USA), according to the supplier’s instructions. The estimated glomerular filtration rate (eGFR) was calculated using the CKD-EPI formula based on the Chronic Kidney Disease Epidemiology Collaboration according to NKF-KDOQI (2002) and NFK-KDIGO (2013) guidelines. The neutrophil-to-lymphocyte ratio (NLR) was calculated by the ratio between the number of circulating neutrophils and lymphocytes counted.
2.4 Neutrophils isolation
Following plasma separation, the pellet containing leukocytes and erythrocytes was diluted with 0.9% NaCl (saline solution) at a 1:1 ratio and submitted to density gradient centrifugation using Histopaque (1077; Sigma Aldrich, Missouri, USA) as previously described. Mononuclear cells were then discarded, and the cell pellet containing erythrocytes and neutrophils was incubated with 3% Dextran (Sigma Aldrich) diluted in saline on ice for 30 minutes. Subsequently, the neutrophil-enriched supernatant was recovered and submitted to erythrocyte lysis. The cells were incubated with an ammonium chloride buffer at a 1:9 ratio for five minutes on ice, followed by centrifugation. This procedure was repeated until complete erythrocyte lysis was achieved. The neutrophils were then counted using a Neubauer chamber. For flow cytometry analysis, neutrophils were fixed in 2% paraformaldehyde (PFA), washed, resuspended in 1% bovine serum albumin (BSA; Gibco, Massachusetts, USA) in phosphate-buffered saline (PBS), and stored at 4°C until analysis. Fresh neutrophils were submitted to ex-vivo analysis, as described in the following sections. All buffers and solutions were prepared using salts acquired from Synth (São Paulo, Brazil).
2.5 Flow cytometry analysis
Neutrophils were characterized using specific antibodies for membrane and intracellular markers. For membrane markers, cells fixed with 2% PFA were washed with PBS containing 0.1M glycine, followed by washing with 1% BSA in PBS (BSA/PBS), and incubated with fluorophore-conjugated anti-human antibodies: CD18 (1:50; Southern Biotechnology Associates, Alabama, USA), CD11b (1:50; BD Pharmingen, New Jersey, USA), CD62L (1:100; Southern Biotechnology Associates), CXCR4 (1:200 PE/Cyanine 5; BioLegend, California, USA), CXCR2 (1:50; R&D Systems, Minnesota, USA), FPR1 (1:100; Bioss, Boston, MA, USA), human FPRL1/FPR2 (1:100; BD Biosciences, Minneapolis, MN, USA), and CD66b (1:75; BD Pharmingen) for 40 minutes in the dark at room temperature. The AnxA1 expression was assessed intracellularly by a permeabilization procedure using 0.1% Triton X-100 (Sigma-Aldrich) in PBS (15 minutes at room temperature) and at the membrane in non-permeabilized cells. For AnxA1 labeling, cells were washed once with PBS containing 0.1M glycine, twice with 1% BSA in PBS (BSA/PBS), and incubated with primary anti-human AnxA1 antibody (1:100; Invitrogen, Massachusetts, USA) for 1 hour at 37°C. Next, cells were washed twice with BSA/PBS and incubated with a secondary goat anti-rabbit antibody conjugated to Alexa Fluor 488 (1:500; Invitrogen) for 50 minutes in the dark at room temperature. Apoptosis was measured using Annexin V-FICT (1:100; Invitrogen) diluted in a binding buffer and incubated for 20 minutes in the dark at room temperature, followed by a single wash with PBS. The cells were analyzed using a BD Accuri™ C6 flow cytometer (BD Biosciences, New Jersey, USA), recording 10,000 or 50,000 events, and using BD CSampler™ Analysis software (version: 1.0.2641–21, BD Biosciences). Data were extracted using the gating strategy reported in Supplementary Figure 1 and reported as the frequency (%) or the expression by detecting median fluorescent intensity (MFI).
2.6 Boyden chamber assay
Neutrophils (2.5 × 106/ml) from control or CKD donors were resuspended in 30 μL Hanks balanced salt solution (HBSS) supplemented with 0.05% BSA and transferred to the upper compartment of a ChemoTx-101–8 microplate (Neuro Probe, Inc., Maryland, USA) (30). The bottom part of the microplate was filled with 300 μL of HBSS containing 50 or 100 nM N-formyl-methionyl-leucyl-phenylalanine (fMLP; Sigma Aldrich). The microplate was incubated under controlled conditions (37°C, 5% CO2) for 2 hours. Cell numbers in the bottom compartments were obtained via a hemocytometer and optical microscope. The assay was performed in triplicate.
2.7 Actin polymerization assay
Neutrophils from both control and CKD donors were used to determine actin polymerization through a flow cytometry assay. Cells were stimulated with 100 nM fMLP in RPMI supplemented with 10% heat-inactivated fetal bovine serum (FBS; Gibco, Massachusetts, USA) and 1% streptomycin/penicillin solution (Gibco) for 0.5, 1, and 3 minutes. The cells were then fixed with 100 μL 2% PFA buffered and incubated for 25 minutes at room temperature. After that, they were washed twice with BSA/PBS and permeabilized with 1 mL BD CytoFix/Wash buffer (BD Biosciences). Intracellular staining was done using FITC-phalloidin (1:100; Invitrogen) for 30 minutes at 37°C, followed by another wash with PBS. Finally, 30,000 events were acquired in a BD Accuri™ C6 flow cytometer. The data obtained were represented as MFI.
2.8 ROS measurement
Neutrophils from control and CKD donors were incubated in the absence and presence of 50 or 100 nM fMLP in complete Roswell Park Memorial Institute (RPMI) 1640 Medium (Gibco) for 30 minutes at 37°C. Subsequently, ROS production was measured by incubating neutrophils with 10 µM 2’,7’-dichlorofluorescin diacetate (DCFH-DA; Invitrogen). A total of 30,000 events were acquired using an Accuri C6 flow cytometer. The data obtained were represented as mean fluorescence intensity (MFI).
2.9 Apoptotic neutrophils
To induce neutrophil apoptosis, 5 × 105 cells from control and CKD donors were seeded in a 96-well plate (U-bottom; Corning, New York, USA) and incubated in serum-starved Dulbecco’s Modified Eagle Medium/Nutrient Mixture F-12 (DMEM/F12) medium (Gibco) for 18 hours. After incubation, apoptosis was measured by flow cytometry, and the free-cell supernatant recovered after centrifugation was stored at −80°C until AnxA1 levels measurement.
2.10 Biopsies
The study used biopsy samples from five CKD patients undergoing kidney cancer investigation submitted to partial or total nephrectomy. Among them, 4 patients were diagnosed with clear cell renal adenocarcinoma, and one with papillary-type renal cell carcinoma at different stages as described in the Supplementary Material. The analyzed regions were taken from tumor-free areas.
2.10.1 Tissue processing and staining
Kidney tissue was fixed with 10% buffered formalin at 4°C and histologically processed for paraffin embedding. Sections (5 μm) were stained with hematoxylin and eosin for morphological investigation. Periodic Acid-Schiff (PAS) staining was performed using a commercial kit following the manufacturer’s instructions (EasyPath; São Paulo, Brazil) to verify fibrosis.
2.10.2 Immunofluorescence
Briefly, sections were permeabilized with 1% Triton-X-100 (Sigma-Aldrich) in PBS at 4°C for 15 minutes. Sections were then blocked with 5% BSA (Sigma Aldrich) in PBS for 30 minutes at room temperature. Patients’ samples were incubated with anti-AnxA1 goat antibody (1:100) (AF3770; R&D SYSTEM) and anti-myeloperoxidase rabbit antibody (1:50; Biodesign International, Inc., Maine, USA) overnight at 4°C, followed by incubation with anti-goat Alexa Fluor 467 and anti-rabbit Alexa Fluor 488 secondary antibodies (1:200; Invitrogen). Negative controls were obtained by omitting the primary antibody. Immunofluorescence was analyzed using a Leica TCS SP8 CARS confocal microscope, and images were captured using the LAS X Life Science software.
2.11 Statistical analysis
Data were analyzed using GraphPad Prism version 9 (GraphPad Software®, California, United States). Data normality was performed using the Kolmogorov-Smirnov test. Next, we employed the parametric tests Welch’s t-test or one-factor analysis of variance (One-Way ANOVA) followed by Tukey post-hoc tests when appropriate. Pearson’s correlation tests were performed for the correlation analysis. Differences between assessed means and correlation coefficients were considered significant assuming p < 0.05. All results are shown as mean ± standard error of the mean.
3 Results
3.1 General data of the patients
The study included 36 patients and 11 control participants for comparison. As shown in Table 1, all 36 patients had varying stages of chronic kidney disease (CKD), with 47.20% in stage 3, another 47.20% in stage 4, and the remaining 5.50% in stage 5. The estimated Glomerular Filtration Rate (eGFR) of the CKD patients was approximately four times lower than normal, and high levels of urea and creatinine were observed. Additionally, homocysteine levels were found to be higher, and serum bicarbonate levels were lower in these patients. Annexin A1 (AnxA1) levels were elevated in the blood of CKD patients compared to control donors. Out of the CKD patients, 40% were also diagnosed with Type II Diabetes Mellitus, while 11% were smokers and 5.5% were alcoholics. All CKD patients were receiving conservative treatment, including medication and nutritional management. The main types of medication used were statins, fibrates, thiazide diuretics, and antihyperglycemics, as shown in Supplementary Table 1.
3.2 High neutrophil count and NLR are associated with CKD progression
Here, we confirmed that CKD patients in stages 3–5 presented leukocytosis (Figure 1A). Monocytes (Figure 1B) and lymphocytes (Figure 1C) showed slight increases. Circulating neutrophils were notably elevated (Figure 1D). Since a high neutrophil-to-lymphocyte ratio (NLR) is a hallmark of CKD progression, herein NLR was increased (Figures 1E, F), which correlated with a decreased eGFR (Figures 1G, H).
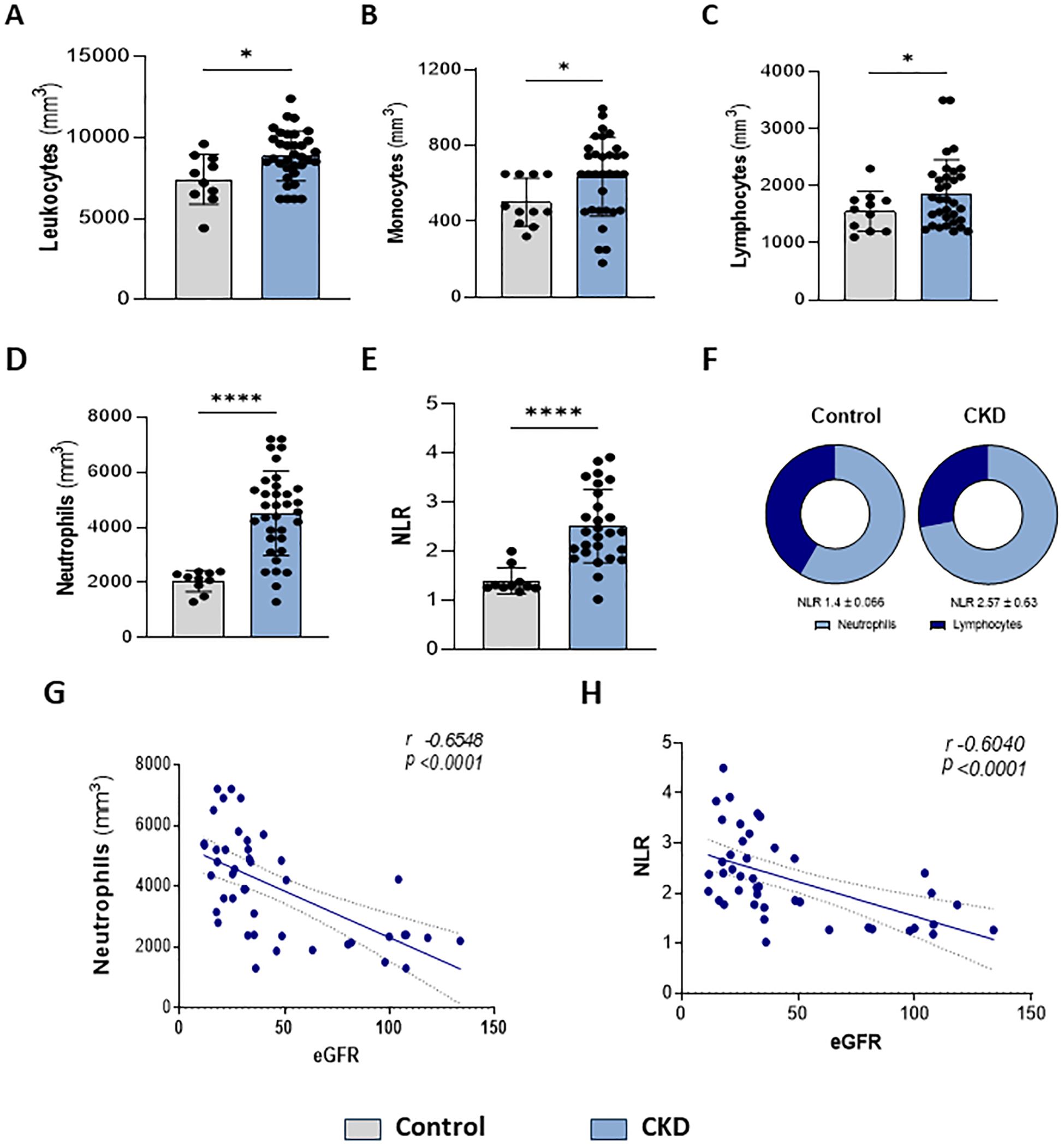
Figure 1. Higher neutrophil count is associated with renal dysfunction. Characterization of peripheral leukocytes. Count of total leukocytes (A), monocytes (B), lymphocytes (C), and neutrophils (D). Neutrophils-lymphocytes ratio (NLR) (E–F) (Control n= 11 and CKD n=36). Correlation of neutrophils (G) and NLR (H) with estimated Glomerular Filtration Rate (eGFR) *p<0.05; ****p<0.001 vs Control.
3.3 CKD neutrophils are activated and senescent
Neutrophilia occurs due to enhanced production of neutrophils from the bone marrow and is associated with systemic inflammation. Infiltrated neutrophils can display an enhanced half-life, which can result from cellular activation or impaired clearance processes. Quantification of membrane proteins involved in cell maturation, senescence, adhesion, and migration point out alterations in the trafficking of neutrophils in the body compartments. Here, we found that CKD neutrophils display a heterogeneous phenotype of proteins related to activation, senescence, and mobilization. These cells were characterized by a lower frequency but higher expression of CXCR2 (Figures 2A, B) and a higher frequency and expression of CXCR4, CD18, CD11b, and CD62L (Figures 2C–J).
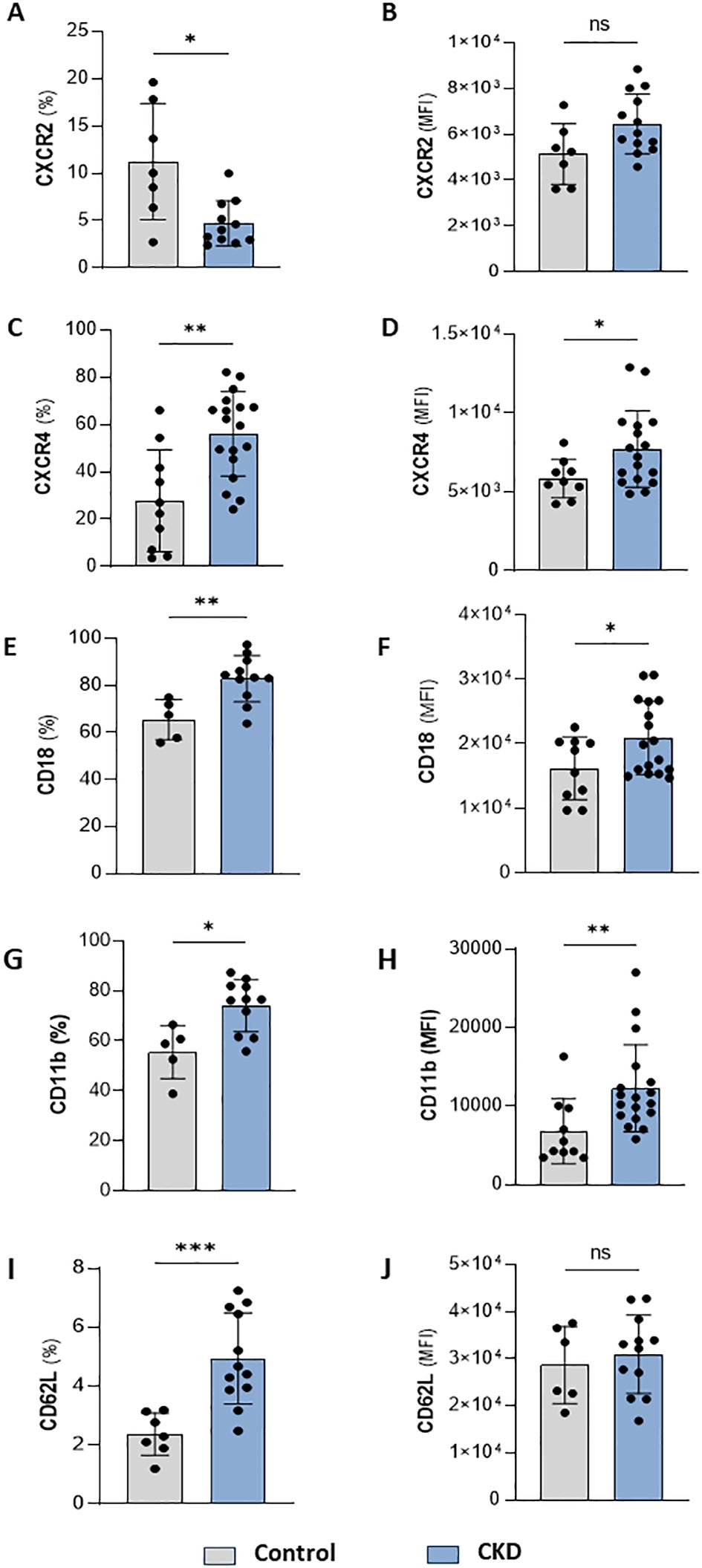
Figure 2. CKD neutrophils have heterogeneous phenotypes. Frequency of positive neutrophils and expression for CXCR2 (A, B), CXCR4 (C, D), CD18 (E, F), CD11b (G, H) and CD62L (I, J) from control and CKD donors. (Control n= 4–10 and CKD n=10–18) *p<0.05; **p<0.01; ***p<0.001 vs Control. ns, Not significant.
3.4 CKD neutrophils are hyporesponsive to FPR1 agonist
The effective response of neutrophils during host defense relies on GPCR chemosensors, which recognize a repertoire of plasma and inflammatory mediators. Here, we tested whether CKD neutrophils could respond to a specific FPR1 agonist. fMLP (N-Formyl-Met-Leu-Phe) is a formyl peptide derived from invading pathogens or mitochondria from dead host cells, binding to FPR1 to signal pro-inflammatory pathways in neutrophils. CKD neutrophils expressed higher levels of FPR1 than the control group (Figure 3A). However, in CKD neutrophils, fMLP did not induce phalloidin expression, chemotaxis, and ROS production (Figures 3B–D). Altogether, the data obtained here show that CKD neutrophils are hyporesponsive to FPR1-mediated actions.
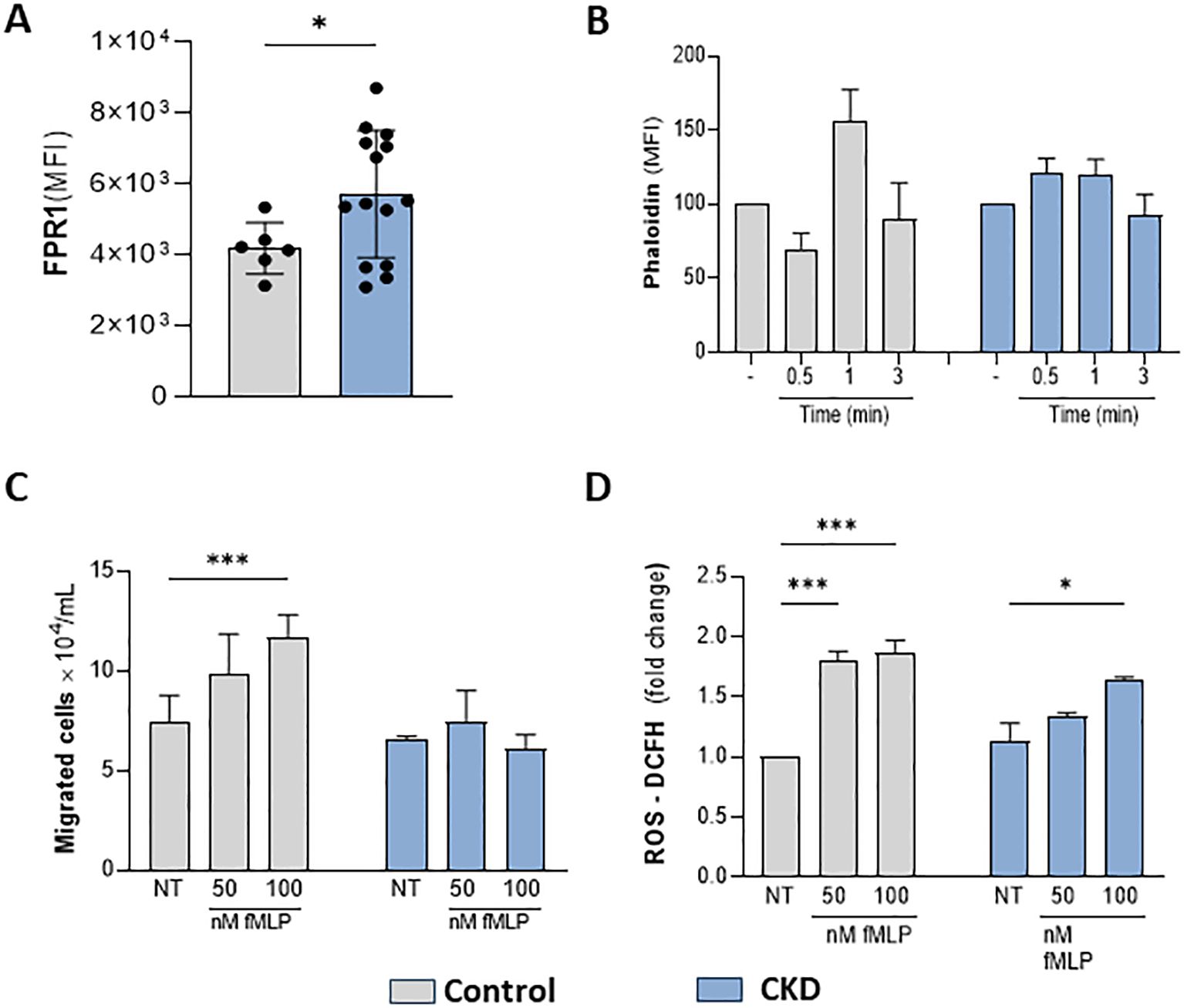
Figure 3. CKD neutrophils did not respond to the FPR1 agonist fMLP. FPR1 expression in neutrophils (A). Chemotaxis (B); Phalloidin expression (C) and ROS production (D) were decreased in CKD neutrophils stimulated with fMLP. (Control n= 3–5 and CKD n= 3–18). *p<0.05 vs Control or NT-control. ***p<0.001 vs NT- Control; *p<0.05 vs 50 nM fMLP-CKD vs 50 nM fMLP Control.
3.5 Intracellular AnxA1 is overexpressed in CKD neutrophils but is not secreted
Meanwhile, FPR1 agonism by formyl peptides triggers pro-inflammatory effects in neutrophils. Conversely, the binding of endogenous peptides and proteins, such as Lipoxin A4 and AnxA1, to FPR2, expressed on neutrophil membranes, evokes anti-inflammatory and efferocytosis effects (13, 31, 32). Interestingly, CKD neutrophils also expressed higher levels of FPR2 (Figure 4A). Endogenous AnxA1 is secreted and phosphorylated to induce autocrine anti-inflammatory effects elicited by FPR2 activation (12). Here, we detected that CKD neutrophils contain higher levels of intracellular AnxA1 expression but lower levels of membrane-bound AnxA1 compared to the control group (Figures 4B, C). Although CKD neutrophils overexpress AnxA1, neutrophils incubated for 18 hours secrete similar levels of AnxA1 as the control group (Figure 4D), despite the high rate of apoptotic neutrophils (Figure 4E), which is a requirement for AnxA1 secretion during efferocytosis (33, 34).
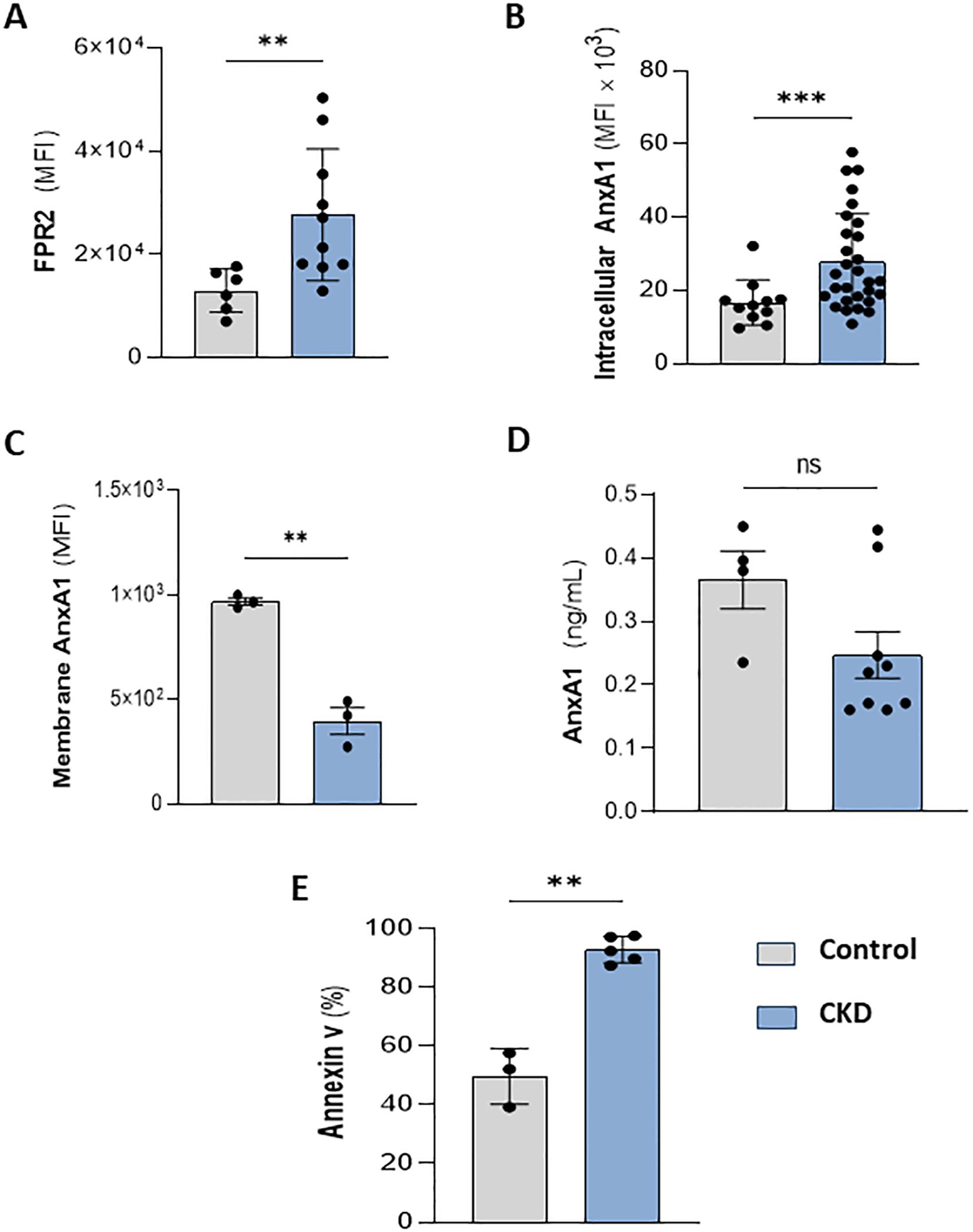
Figure 4. CKD neutrophils overexpress intracellular AnxA1. Increased FPR2 expression in CKD neutrophils (A). AnxA1 expression in neutrophils at intracellular (B) and membrane compartments (C). AnxA1 secretion from neutrophils (D) and apoptotic neutrophils (E) cultured for 18 hours under serum starvation conditions. (Control n= 3–11 and CKD n= 3–36) **p<0.01; ***p<0.001 CKD vs Control. ns, Not significant.
3.6 Renal infiltrated neutrophils are AnxA1+
Selected biopsies from five patients with CKD (Cr 1.3 ± 0.12 mg/dL; eGFR 52.4 ± 8.08) were analyzed. As observed by H&E staining, the first patient’s sample showed normal tissue architecture characterized by intact glomeruli of the renal corpuscle (G); distal convoluted tubules (DTC); and proximal convoluted tubules (PCT) (Figure 5A). In Figures 5B–E, the samples presented CKD features such as interstitial nephritis characterized by the presence of inflammatory infiltrate in the renal interstitium (asterisks and insert - Figure 5D). A thickened basement membrane was detected in renal corpuscles and convoluted tubules (arrowheads - Figure 5F), as observed by PAS staining. Additionally, it highlights the basement membrane in the parietal layer of the renal corpuscle Bowman’s capsule and in the proximal and distal convoluted tubules (arrowheads - Figures 5F-J). Some regions showed tissue fibrosis (f) and tubules in the process of degeneration (t) (Figures 5G, J). By immunofluorescence, neutrophils were identified by myeloperoxidase (MPO) labeling. As observed in Figures 5K–O, neutrophils were found in all samples, and the number of infiltrated neutrophils varied among CKD biopsies. Further analysis showed that neutrophils were also labeled for AnxA1.
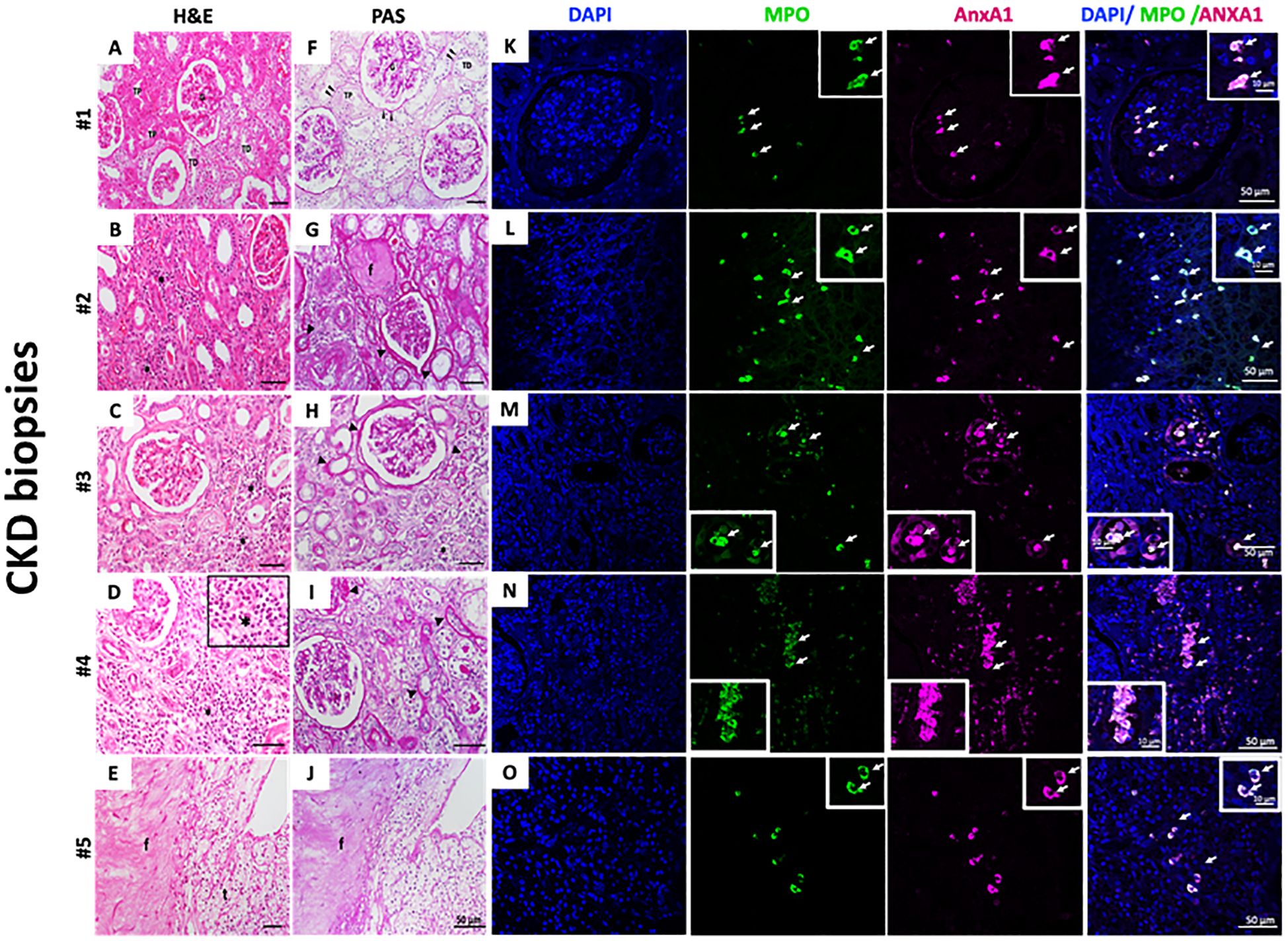
Figure 5. Infiltrate neutrophils in CKD kidney biopsies are AnxA+. H&E (A–E) and PAS staining (F–J). Myeloperoxidase (MPO) and AnxA1 labeling (K–O). Five CKD biopsies were selected and stained for morphological, fibrosis visualization, and immunofluorescence. Figure 5D – Insert: * immune cells infiltrated; MPO and AnxA1 labeling. The image is representative of at least three fields analyzed on each biopsy.
4 Discussion
Chronic Kidney Disease (CKD) is a global health burden, affecting 13.4% of the world ‘s population (35, 36). CKD progression leads to severe morbidity and high mortality, which can be attributed to impaired renal function during end-stage kidney disease (ESKD) or to cardiovascular diseases and secondary infections (36, 37). Neutrophils contribution to CKD and related diseases development is an inconclusive subject, despite high NLR be a clinical indicator of disease progression and a robust predictor of all-cause mortality in severe patients (18–20, 38, 39). We herein show high frequency of activated cells in the pool of circulating neutrophils of 3–5 grade CKD patients, and a variable number of neutrophils in the damaged renal tissue. Our unprecedented data, showing CKD conditions disturb neutrophil GPCR expression and the response against infectious stimulus, point out that the imbalance in GPCRs contributes to an aged pool of neutrophils in the blood and an inefficient host defense.
The GPCR CXCR4 is considered the master regulator receptor of neutrophils trafficking in steady–state and host defense, as its expression regulates the bone marrow fate of pre-delivery mature and old homing neutrophils (7). CXCR4 up-regulation was initially observed in aged neutrophils (cultured for 20 hours) before the apoptosis marker Annexin V (AnxV) was expressed. Meanwhile, CXCR4+ neutrophils migrate in response to SDF-1, homing to the bone marrow, whereas CXCR4+AnxV+ cells are apoptotic and unresponsive to SDF-1 (40, 41). Here, CKD neutrophils demonstrated high expression of CXCR4+ and showed an increased frequency of CKD neutrophils positive for AnxV, indicating that CKD promotes the aging and death of blood neutrophils.
CXCR4 is widely expressed in several tissues and it plays a key role in modulating the survival, proliferation, and migration of epithelial and endothelial cells (42). In CKD, the overexpression of CXCR4 by epithelial cells has been associated with the development of renal fibrosis, making it a potential target for drug development in CKD (43–46). Hence, these data support our novel findings, highlighting that higher CXCR4 expression by different cells may be involved in CKD.
Activation of the GPCRs FPR1 and FPR2 in neutrophils during infections lead to FPR1-fMLP binding inducing pro-inflammatory actions, while the FPR2 agonist activation, primarily by endogenous mediators, favor efferocytosis and tissue repair (12, 13, 47). Although FPR1 and FPR2 share a high structural and amino acids composition similarity, there is a distinct preference to formylpeptides ligands. A wide range of endogenous and exogenous sources, which act as full or partial agonists, can trigger FPR1 activation. Conversely, FPR2 display a promiscuous nature, binding to a wide range of agonists, including serum amyloid A protein or living bacteria that induces neutrophil migration and the secretion of the pro-inflammatory cytokine CXCL2 (48, 49). This dual function of FPRs in modulating pro-inflammatory or pro-resolutive actions has been elucidated by robust studies that highlight the concept of biased agonism in FPRs. These studies demonstrate that the agonist’s nature and its interaction with the receptor can lead to specific conformational changes, triggering distinct downstream signaling pathways (50–52). Here, we observed that CKD neutrophils express a unique profile of FPRs expressions, characterized by higher expression of both FPR1 and FPR2 receptors. Despite this higher expression, CKD neutrophils were hyporesponsive to FPR1 activation by fMLP.
GPCR activation often leads to the removal of receptors from the cell surface by internalization, and internalized receptors are either recycled to the cell surface or degraded in lysosomes, frequently resulting in downregulation (53). Moreover, GPCRs undergo desensitization and cross-desensitization, which can affect agonist responses. These phenomena have been well established for FPR1-induced chemotaxis. For instance, fMLP-induced chemotaxis occurs over a range of concentrations, and FPR1-fMLP binding desensitizes the C5a receptor, inhibiting neutrophil calcium mobilization and chemotaxis induced by C5a or IL-8 (54–56). The data presented here indicate that CKD may impair GPCR turnover in the cell membrane, resulting in increased expression of CXCR4 and FPRs. Alternatively, FPR1 may undergo cross-desensitization by CKD compounds in the blood, rendering neutrophils unresponsive to specific agonists. Indeed, CKD neutrophils respond differently based on the agonist profile. For instance, studies demonstrated that CKD neutrophils challenged with LPS showed a higher secretion of TNF-α and IL-1β and upregulation, but downregulation of mRNA expression of SOD2 and IL-1a (57, 58). On the other hand, CKD neutrophils were responsive to liposoluble molecules such as n-3 fatty acids and coenzyme Q-10. These molecules reduced the levels of pro-inflammatory leukotrienes and myeloperoxidase and increased the levels of LTB5 (59, 60). Based on these data and findings, it is clear that CKD neutrophils’ responsiveness is dependent on ligands nature. It requires further investigation to better understand how CKD and related disorders disrupt the fine-tuning balance in neutrophil biology.
The mobilization of subsets of intracellular granules to the plasma membrane occurs at the onset of neutrophil responsiveness. Previous data have shown that FPR1 is intracellularly co-localized with CD11b/CD18 and is mobilized to the external surface of the cell to mediate adhesion to the endothelium and subsequent migration into inflamed tissue (61). We also demonstrated that CD11b/CD18 expression is upregulated in CKD neutrophils, indicating an exacerbated ability of neutrophil degranulation in CKD. Although we aimed to address the impaired function of neutrophils in CKD patients, one of the limitations of our study was the small sample size, which did not allow us to categorize how GPCR expression and function are affected by CKD stage progression. Additionally, all subjects in our study were non-dialysis patients undergoing conservative therapies to attenuate base diseases, such as diabetes and higher blood pressure.
Among leukocytes, neutrophils have the highest content of AnxA1, which is secreted, phosphorylated, and displays autocrine, juxtacrine, and paracrine anti-inflammatory properties by binding to FPR2 (12). Glucocorticoids and cytokines stimulate AnxA1 synthesis and release to halt and resolve the acute inflammatory response. In resting conditions, AnxA1 is mainly stored in neutrophil gelatinase granules and rapidly released after neutrophil stimulation using ABC transport or microvesicles (12, 62). Indeed, AnxA1 microvesicles are the major subset released during an inflammatory response (63, 64). Our data show high intracellular content of AnxA1 in CKD neutrophils and low levels of expression at the membrane or secretion into the supernatant, pointing out the inability of CKD neutrophils to release the protein. This inability suggests an inefficient function of neutrophils in the resolution of inflammation, as apoptotic neutrophils express membrane AnxA1 as an “eat me” signal to phagocytic macrophages (65, 66).
The contribution of neutrophils as pro or anti-inflammatory players in renal tissue damage remains unclear. Recently, it was proposed that migrated neutrophils switch to a SiglecF+ phenotype due to actions of inflammatory mediators released by damaged epithelial cells. This suggests the presence of a neutrophil subset as pivotal to fibrosis development (67). The analysis of kidney tissue from fibrotic CKD patients revealed a variation in the number of neutrophils among biopsy samples, with some positive for AnxA1. Given previous data indicating the inability of CKD neutrophils to secrete the protein, the contribution of AnxA1 to tissue damage remains to be elucidated. Moreover, AnxA1 is secreted by renal epithelial cells in CKD patients and experimental models of kidney injury; however, the data obtained thus far are controversial (68–71).
5 Conclusion
Associated, the data presented herein corroborates the line of evidence suggesting that CKD renders neutrophils unresponsive and indicates that the unbalanced expression of GPCRs at the membrane contributes to the impaired responses in the host defense.
Data availability statement
The raw data supporting the conclusions of this article will be made available by the authors, without undue reservation.
Ethics statement
The studies involving humans were approved by Committee Ethical from the Faculty of Pharmaceutical Sciences – University of São Paulo (CAAE: 29434120.7.0000.0067); Dante Pazzanese Institute of Cardiology of Sao Paulo (CAAE: 29434120.7.3002.5462). The studies were conducted in accordance with the local legislation and institutional requirements. The participants provided their written informed consent to participate in this study.
Author contributions
PS: Conceptualization, Data curation, Investigation, Writing – original draft, Writing – review & editing. SS: Conceptualization, Data curation, Investigation, Writing – original draft, Writing – review & editing. FR: Data curation, Resources (human samples), Writing – review & editing. LFX: Investigation, Writing – review & editing. DG: Writing – review & editing. RC-S: Investigation, Writing – review & editing. PF: Resources (human samples), Writing – review & editing. CG: Writing – review & editing. SF: Supervision, Funding acquisition, Writing – original draft, Writing – review & editing.
Funding
The author(s) declare financial support was received for the research, authorship, and/or publication of this article. This work was supported by FAPESP (Fundação de Amparo á Pesquisa do Estado de São Paulo), grant number 2014/07328–4; SF is a fellow researcher of CNPq (Conselho Nacional de Pesquisa); FR was post-doctoral fellows of CAPES (Coordenação de Aperfeiçoamento de Pessoal de Nível Superior); PS is a PhD fellow of the FAPESP, grant number 2020/14368–3. LFX; PhD fellow of the CNPq.
Acknowledgments
We would also like to acknowledge the nurses and staff from Lagoa Federal Hospital and Dante Pazzanese Institute of Cardiology of Sao Paulo for their support. Lastly, we are extremely grateful to the patients and their families who consented to donate their biospecimens.
Conflict of interest
The authors declare that the research was conducted in the absence of any commercial or financial relationships that could be construed as a potential conflict of interest.
Publisher’s note
All claims expressed in this article are solely those of the authors and do not necessarily represent those of their affiliated organizations, or those of the publisher, the editors and the reviewers. Any product that may be evaluated in this article, or claim that may be made by its manufacturer, is not guaranteed or endorsed by the publisher.
Supplementary material
The Supplementary Material for this article can be found online at: https://www.frontiersin.org/articles/10.3389/fimmu.2024.1387566/full#supplementary-material
References
1. Palomino-Segura M, Sicilia J, Ballesteros I, Hidalgo A. Strategies of neutrophil diversification. Nat Immunol. (2023) 24:575–84. doi: 10.1038/s41590-023-01452-x
2. Mei J, Liu Y, Dai N, Hoffmann C, Hudock KM, Zhang P, et al. Cxcr2 and Cxcl5 regulate the IL-17/G-CSF axis and neutrophil homeostasis in mice. J Clin Invest. (2012) 122:974–86. doi: 10.1172/JCI60588
3. Bugl S, Wirths S, Radsak MP, Schild H, Stein P, André MC, et al. Steady-state neutrophil homeostasis is dependent on TLR4/TRIF signaling. Blood. (2013) 121:723–33. doi: 10.1182/blood-2012-05-429589
4. Dahlgren C, Lind S, Mårtensson J, Björkman L, Wu Y, Sundqvist M, et al. G protein coupled pattern recognition receptors expressed in neutrophils: Recognition, activation/modulation, signaling and receptor regulated functions. Immunol Rev. (2023) 314:69–92. doi: 10.1111/imr.13151
5. Jiang H, Galtes D, Wang J, Rockman HA. G protein-coupled receptor signaling: transducers and effectors. Am J Physiol Cell Physiol. (2022) 323:C731–C48. doi: 10.1152/ajpcell.00210.2022
6. Strydom N, Rankin SM. Regulation of circulating neutrophil numbers under homeostasis and in disease. J Innate Immun. (2013) 5:304–14. doi: 10.1159/000350282
7. De Filippo K, Rankin SM. CXCR4, the master regulator of neutrophil trafficking in homeostasis and disease. Eur J Clin Invest. (2018) 48(Suppl 2):e12949. doi: 10.1111/eci.12949
8. Machado ID, Spatti M, Hastreiter A, Santin JR, Fock RA, Gil CD, et al. Annexin A1 is a physiological modulator of neutrophil maturation and recirculation acting on the CXCR4/CXCL12 pathway. J Cell Physiol. (2016) 231:2418–27. doi: 10.1002/jcp.25346
9. Barbosa CMV, Fock RA, Hastreiter AA, Reutelingsperger C, Perretti M, Paredes-Gamero EJ, et al. Extracellular annexin-A1 promotes myeloid/granulocytic differentiation of hematopoietic stem/progenitor cells via the Ca2+/MAPK signalling transduction pathway. Cell Death Discovery. (2019) 5:135–46. doi: 10.1038/s41420-019-0215-1
10. Kim HS, Park MY, Lee SK, Park JS, Lee HY, Bae YS. Activation of formyl peptide receptor 2 by WKYMVm enhances emergency granulopoiesis through phospholipase C activity. BMB Rep. (2018) 51:418–23. doi: 10.5483/BMBREP.2018.51.8.080
11. Weiß E, Kretschmer D. Formyl-peptide receptors in infection, inflammation, and cancer. Trends Immunol. (2018) 39(10):815–29. doi: 10.1016/j.it.2018.08.005
12. Perretti M, D’Acquisto F. Annexin A1 and glucocorticoids as effectors of the resolution of inflammation. Nat Rev Immunol. (2009) 9:62–70. doi: 10.1038/nri2470
13. Dalli J, Consalvo AP, Ray V, Di Filippo C, D’Amico M, Mehta N, et al. Proresolving and tissue-protective actions of annexin A1–based cleavage-resistant peptides are mediated by formyl peptide receptor 2/lipoxin A4 receptor. J Immunol. (2013) 190:6478–87. doi: 10.4049/jimmunol.1203000
14. Singhal A, Kumar S. Neutrophil and remnant clearance in immunity and inflammation. Immunology. (2022) 165:22–43. doi: 10.1111/imm.13423
15. Loh W, Vermeren S. Anti-inflammatory neutrophil functions in the resolution of inflammation and tissue repair. Cells. (2022) 11:4076–91. doi: 10.3390/cells11244076
16. Tinti F, Lai S, Noce A, Rotondi S, Marrone G, Mazzaferro S, et al. Chronic kidney disease as a systemic inflammatory syndrome: Update on mechanisms involved and potential treatment. Life. (2021) 11:419–35. doi: 10.3390/life11050419
17. Zoccali C, Mallamaci F. Innate immunity system in patients with cardiovascular and kidney disease. Circ Res. (2023) 132:915–32. doi: 10.1161/CIRCRESAHA.122.321749
18. Zhao WM, Tao SM, Liu GL. Neutrophil-to-lymphocyte ratio in relation to the risk of all-cause mortality and cardiovascular events in patients with chronic kidney disease: a systematic review and meta-analysis. Ren Fail. (2020) 42:1059–66. doi: 10.1080/0886022X.2020.1832521
19. Lano G, Sallee M, Pelletier M, Bataille S, Fraisse M, Mckay N, et al. Neutrophil:lymphocyte ratio correlates with the uremic toxin indoxyl sulfate and predicts the risk of death in patients on hemodialysis. Nephrol Dialysis Transplant. (2022) 37:2528–37. doi: 10.1093/ndt/gfab350
20. Lee TW, Bae W, Choi J, Bae E, Jang HN, Chang SH, et al. The neutrophil-to-lymphocyte ratio may indicate when to start hemodialysis. Ren Fail. (2022) 44:1401–8. doi: 10.1080/0886022X.2022.2110894
21. Bronze-Da-Rocha E, Santos-Silva A. Neutrophil elastase inhibitors and chronic kidney disease. Int J Biol Sci. (2018) 14:1341–60. doi: 10.7150/ijbs.26111
22. Kim IS, Kim DH, Lee HW, Kim SG, Kim YK, Kim JK. Role of increased neutrophil extracellular trap formation on acute kidney injury in COVID-19 patients. Front Immunol. (2023) 14:1122510. doi: 10.3389/fimmu.2023.1122510
23. Ulasi II, Awobusuyi O, Nayak S, Ramachandran R, Musso CG, Depine SA, et al. Chronic kidney disease burden in low-resource settings: regional perspectives. Semin Nephrol. (2022) 42:151336. doi: 10.1016/j.semnephrol.2023.151336
24. Heinzelmann M, Mercer-Jones MA, Passmore JC. Neutrophils and renal failure. Am J Kidney Dis. (1999) 34:384–99. doi: 10.1016/S0272-6386(99)70375-6
25. Sela S, Shurtz-Swirski R, Cohen-Mazor M, Mazor R, Chezar J, Shapiro G, et al. Primed peripheral polymorphonuclear leukocyte: A culprit underlying chronic low-grade inflammation and systemic oxidative stress in chronic kidney disease. J Am Soc Nephrol. (2005) 16:2431–8. doi: 10.1681/ASN.2004110929
26. Yilmaz H, Cakmak M, Inan O, Darcin T, Akcay A. Can neutrophil-lymphocyte ratio be independent risk factor for predicting acute kidney injury in patients with severe sepsis? Ren Fail. (2015) 37:225–9. doi: 10.3109/0886022X.2014.982477
27. Xie T, Xin Q, Chen R, Zhang X, Zhang F, Ren H, et al. Clinical value of prognostic nutritional index and neutrophil-to-lymphocyte ratio in prediction of the development of sepsis-induced kidney injury. Dis Markers. (2022) 2022:1449758. doi: 10.1155/2022/1449758
28. Milik A, Hrynkiewicz E. KDIGO 2012 clinical practice guideline for the evaluation and management of chronic kidney disease. Kidney international supplements. J Int Soc Nephrol. (2013) 3:1–150. doi: 10.1038/kisup.2012.73
29. Levey AS, Coresh J, Bolton K, Culleton B, Harvey KS, Ikizler TA, et al. K/DOQI clinical practice guidelines for chronic kidney disease: Evaluation, classification, and stratification. Am J Kidney Dis. (2002) 39:S1–266.
30. Sandri S, Hebeda CB, Loiola RA, Calgaroto S, Uchiyama MK, Araki K, et al. Direct effects of poly(ϵ-caprolactone) lipid-core nanocapsules on human immune cells. Nanomedicine. (2019) 14:1429–42. doi: 10.2217/nnm-2018-0484
31. Galvão I, Melo EM, de Oliveira VLS, Vago JP, Queiroz-Junior C, de Gaetano M, et al. Therapeutic potential of the FPR2/ALX agonist AT-01-KG in the resolution of articular inflammation. Pharmacol Res. (2021) 165:7–15. doi: 10.1016/j.phrs.2021.105445
32. Wu M, Ge Y, Wang E, Liao Q, Ren Z, Yu Y, et al. Enhancement of efferocytosis through biased FPR2 signaling attenuates intestinal inflammation. EMBO Mol Med. (2023) 15. doi: 10.15252/emmm.202317815
33. Sugimoto MA, Vago JP, Teixeira MM, Sousa LP. Annexin A1 and the resolution of inflammation: modulation of neutrophil recruitment, apoptosis, and clearance. J Immunol Res. (2016). doi: 10.1155/2016/8239258
34. Nadkarni S, Smith J, Sferruzzi-Perri AN, Ledwozyw A, Kishore M, Haas R, et al. Neutrophils induce proangiogenic T cells with a regulatory phenotype in pregnancy. Proc Natl Acad Sci U.S.A. (2016) 113:E8415–24. doi: 10.1073/pnas.1611944114
35. Lv JC, Zhang LX. Prevalence and disease burden of chronic kidney disease. Adv Exp Med Biol. (2019) 1165:3–15. doi: 10.1007/978-981-13-8871-2_1
36. Kovesdy CP. Epidemiology of chronic kidney disease: an update 2022. Kidney Int Suppl. (2022) 12:7–11. doi: 10.1016/j.kisu.2021.11.003
37. Roth GA, Mensah GA, Johnson CO, Addolorato G, Ammirati E, Baddour LM, et al. Global burden of cardiovascular diseases and risk factors, 1990-2019: update from the GBD 2019 study. J Am Coll Cardiol. (2020) 76:2982–3021. doi: 10.1016/j.jacc.2020.11.010
38. Ouellet G, Malhotra R, Lars Penne E, Usvyat L, Levin NW, Kotanko P. Neutrophil-lymphocyte ratio as a novel predictor of survival in chronic hemodialysis patients. Clin Nephrol. (2016) 85:191–8. doi: 10.5414/CN108745
39. Rong WX, Yuan L, Shi R, Li H, Guang WD, Gui W. Predictors of coronary artery calcification and its association with cardiovascular events in patients with chronic kidney disease. Ren Fail. (2021) 43:1172–9. doi: 10.1080/0886022X.2021.1953529
40. Wolach B, van der Laan LJW, Maianski NA, Tool ATJ, van Bruggen R, Roos D, et al. Growth factors G-CSF and GM-CSF differentially preserve chemotaxis of neutrophils aging. vitro. Exp Hematol. (2007) 35:541–50. doi: 10.1016/j.exphem.2006.12.008
41. Eash KJ, Means JM, White DW, Link DC. CXCR4 is a key regulator of neutrophil release from the bone marrow under basal and stress granulopoiesis conditions. Blood. (2009) 113:4711–9. doi: 10.1182/blood-2008-09-177287
42. Bianchi ME, Mezzapelle R. The chemokine receptor CXCR4 in cell proliferation and tissue regeneration. Front Immunol. (2020) 11:2109. doi: 10.3389/fimmu.2020.02109
43. Yuan A, Lee Y, Choi U, Moeckel G, Karihaloo A. Chemokine receptor Cxcr4 contributes to kidney fibrosis via multiple effectors. Am J Physiol Renal Physiol. (2015) 308:F459–72. doi: 10.1152/ajprenal.00146.2014
44. Jackson EK, Zhang Y, Gillespie DD, Zhu X, Cheng D, Jackson TC. SDF-1α (stromal cell-derived factor 1α) induces cardiac fibroblasts, renal microvascular smooth muscle cells, and glomerular mesangial cells to proliferate, cause hypertrophy, and produce collagen. J Am Heart Assoc. (2017) 6. doi: 10.1161/JAHA.117.007253
45. Liu Y, Feng Q, Miao J, Wu Q, Zhou S, Shen W, et al. C-X-C motif chemokine receptor 4 aggravates renal fibrosis through activating JAK/STAT/GSK3β/β-catenin pathway. J Cell Mol Med. (2020) 24:3837–55. doi: 10.1111/jcmm.14973
46. Mo H, Ren Q, Song D, Xu B, Zhou D, Hong X, et al. CXCR4 induces podocyte injury and proteinuria by activating β-catenin signaling. Theranostics. (2022) 12:767–81. doi: 10.7150/thno.65948
47. Gröper J, König GM, Kostenis E, Gerke V, Raabe CA, Rescher U. Exploring biased agonism at FPR1 as a means to encode danger sensing. Cells. (2020) 9. doi: 10.3390/cells9041054
48. Lentini G, De Gaetano GV, Famà A, Galbo R, Coppolino F, Mancuso G, et al. Neutrophils discriminate live from dead bacteria by integrating signals initiated by Fprs and TLRs. EMBO J. (2022) 41. doi: 10.15252/embj.2021109386
49. Abouelasrar Salama S, Gouwy M, Van Damme J, Struyf S. Acute-serum amyloid A and A-SAA-derived peptides as formyl peptide receptor (FPR) 2 ligands. Front Endocrinol (Lausanne). (2023) 14:1119227. doi: 10.3389/fendo.2023.1119227
50. Cooray SN, Gobbetti T, Montero-Melendez T, McArthur S, Thompson D, Clark AJL, et al. Ligand-specific conformational change of the G-protein-coupled receptor ALX/FPR2 determines proresolving functional responses. Proc Natl Acad Sci U.S.A. (2013) 110:18232–7. doi: 10.1073/pnas.1308253110
51. Zhuang Y, Wang L, Guo J, Sun D, Wang Y, Liu W, et al. Molecular recognition of formylpeptides and diverse agonists by the formylpeptide receptors FPR1 and FPR2. Nat Commun. (2022) 13. doi: 10.1038/s41467-022-28586-0
52. Raabe CA, Gröper J, Rescher U. Biased perspectives on formyl peptide receptors. Biochim Biophys Acta Mol Cell Res. (2019) 1866:305–16. doi: 10.1016/j.bbamcr.2018.11.015
53. Gainetdinov RR, Premont RT, Bohn LM, Lefkowitz RJ, Caron MG. Desensitization of G protein-coupled receptors and neuronal functions. Annu Rev Neurosci. (2004) 27:107–44. doi: 10.1146/annurev.neuro.27.070203.144206
54. Tomhave ED, Richardson RM, Didsbury JR MLSRAH. Cross-desensitization of receptors for peptide chemoattractants. Characterization of a new form of leukocyte regulation. J Immunol. (1994) 153:3267–75. doi: 10.4049/jimmunol.153.7.3267
55. Blackwood RA, Hartiala KT, Kwoh EE, Transue AT, Brower RC. Unidirectional heterologous receptor desensitization between both the fMLP and C5a receptor and the IL-8 receptor. J Leukoc Biol. (1996) 60:88–93. doi: 10.1002/jlb.60.1.88
56. Sabroe I, Williams TJ, Hébert CA, Collins PD. Chemoattractant cross-desensitization of the human neutrophil IL-8 receptor involves receptor internalization and differential receptor subtype regulation. J Immunol. (1997) 158:1361–9. doi: 10.4049/jimmunol.158.3.1361
57. Olsson J, Jacobson TAS, Paulsson JM, Dadfar E, Moshfegh A, Jacobson SH, et al. Expression of neutrophil SOD2 is reduced after lipopolysaccharide stimulation: A potential cause of neutrophil dysfunction in chronic kidney disease. Nephrol Dialysis Transplant. (2011) 26:2195–201. doi: 10.1093/ndt/gfq673
58. Buemi M, Arena A, Stassi G, Iannello D, Gazzara D, Calapai M, et al. Both IL-1β and TNF-α regulate NGAL expression in polymorphonuclear granulocytes of chronic hemodialysis patients. Mediators Inflammation. (2010) 2010. doi: 10.1155/2010/613937
59. Maaløe T, Schmidt EB, Svensson M, Aardestrup IV, Christensen JH. The effect of n-3 polyunsaturated fatty acids on leukotriene B4 and leukotriene B5 production from stimulated neutrophil granulocytes in patients with chronic kidney disease. Prostaglandins Leukot Essent Fatty Acids. (2011) 85:37–41. doi: 10.1016/j.plefa.2011.04.004
60. Barden AE, Shinde S, Burke V, Puddey IB, Beilin LJ, Irish AB, et al. The effect of n-3 fatty acids and coenzyme Q10 supplementation on neutrophil leukotrienes, mediators of inflammation resolution and myeloperoxidase in chronic kidney disease. Prostaglandins Other Lipid Mediat. (2018) 136:1–8. doi: 10.1016/j.prostaglandins.2018.03.002
61. English D, Graves V. Simultaneous mobilization of Mac-1 (CD11b/CD18) and formyl peptide chemoattractant receptors in human neutrophils. Blood. (1992) 80:776–87.
62. Perretti M, Dalli J. Resolution pharmacology: focus on pro-resolving annexin A1 and lipid mediators for therapeutic innovation in inflammation. Annu Rev Pharmacol Toxicol. (2023) 63:449–69. doi: 10.1146/annurev-pharmtox-051821-042743
63. Headland SE, Jones HR, Norling LV, Kim A, Souza PR, Corsiero E, et al. Neutrophil-derived microvesicles enter cartilage and protect the joint in inflammatory arthritis. Sci Transl Med. (2015) 7. doi: 10.1126/scitranslmed.aac5608
64. Rhys HI, Dell’Accio F, Pitzalis C, Moore A, Norling LV, Perretti M. Neutrophil microvesicles from healthy control and rheumatoid arthritis patients prevent the inflammatory activation of macrophages. EBioMedicine. (2018) 29:60–9. doi: 10.1016/j.ebiom.2018.02.003
65. Scannell M, Flanagan MB, deStefani A, Wynne KJ, Cagney G, Godson C, et al. Annexin-1 and peptide derivatives are released by apoptotic cells and stimulate phagocytosis of apoptotic neutrophils by macrophages. J Immunol. (2007) 178:4595–605. doi: 10.4049/jimmunol.178.7.4595
66. Galvão I, Vago JP, Barroso LC, Tavares LP, Queiroz-Junior CM, Costa VV, et al. Annexin A1 promotes timely resolution of inflammation in murine gout. Eur J Immunol. (2017) 47:585–96. doi: 10.1002/eji.201646551
67. Ryu S, Shin JW, Kwon S, Lee J, Kim YC, Bae YS, et al. Siglec-F–expressing neutrophils are essential for creating a profibrotic microenvironment in renal fibrosis. J Clin Invest. (2022) 132. doi: 10.1172/JCI156876
68. Neymeyer H, Labes R, Reverte V, Saez F, Stroh T, Dathe C, et al. Activation of annexin A1 signalling in renal fibroblasts exerts antifibrotic effects. Acta Physiologica. (2015) 215:144–58. doi: 10.1111/apha.12586
69. Zhao SQ, Shen ZC, Gao BF, Han P. microRNA-206 overexpression inhibits epithelial-mesenchymal transition and glomerulosclerosis in rats with chronic kidney disease by inhibiting JAK/STAT signaling pathway. J Cell Biochem. (2019) 120:14604–17. doi: 10.1002/jcb.28722
70. Wu L, Liu C, Chang D-Y, Zhan R, Zhao M, Man Lam S, et al. The attenuation of diabetic nephropathy by annexin A1 via regulation of lipid metabolism through the AMPK/PPARα/CPT1b pathway. Diabetes. (2021) 70:2192–203. doi: 10.2337/db21-0050
Keywords: neutrophil-to-lymphocyte ratio, CXCR4, FPR, Annexin A1, fMLP
Citation: Scharf P, Sandri S, Rizzetto F, Xavier LF, Grosso D, Correia-Silva RD, Farsky PS, Gil CD and Farsky SHP (2024) GPCRs overexpression and impaired fMLP-induced functions in neutrophils from chronic kidney disease patients. Front. Immunol. 15:1387566. doi: 10.3389/fimmu.2024.1387566
Received: 18 February 2024; Accepted: 02 August 2024;
Published: 26 August 2024.
Edited by:
Pietro Ghezzi, University of Urbino Carlo Bo, ItalyReviewed by:
Gareth S. D. Purvis, University of Oxford, United KingdomUrsula Rescher, University of Münster, Germany
Copyright © 2024 Scharf, Sandri, Rizzetto, Xavier, Grosso, Correia-Silva, Farsky, Gil and Farsky. This is an open-access article distributed under the terms of the Creative Commons Attribution License (CC BY). The use, distribution or reproduction in other forums is permitted, provided the original author(s) and the copyright owner(s) are credited and that the original publication in this journal is cited, in accordance with accepted academic practice. No use, distribution or reproduction is permitted which does not comply with these terms.
*Correspondence: Sandra Helena Poliselli Farsky, c2ZhcnNreUB1c3AuYnI=
†These authors share first authorship