- 1Department of Physiological Sciences, Faculty of Science, Stellenbosch University, Stellenbosch, South Africa
- 2MRCPCH Consultant Paediatric Neurodisability, Exeter, Devon, United Kingdom
- 3Department of Biochemistry and Systems Biology, Institute of Systems, Molecular and Integrative Biology, Faculty of Health and Life Sciences, University of Liverpool, Liverpool, United Kingdom
- 4The Novo Nordisk Foundation Centre for Biosustainability, Technical University of Denmark, Lyngby, Denmark
- 5Directorate of Respiratory Medicine, Manchester University Hospitals, Wythenshawe Hospital, Manchester, United Kingdom
- 6Long COVID department, Clinic St Georg, Bad Aibling, Germany
Myalgic Encephalomyelitis/Chronic Fatigue Syndrome (ME/CFS) is a chronic, debilitating disease characterised by a wide range of symptoms that severely impact all aspects of life. Despite its significant prevalence, ME/CFS remains one of the most understudied and misunderstood conditions in modern medicine. ME/CFS lacks standardised diagnostic criteria owing to variations in both inclusion and exclusion criteria across different diagnostic guidelines, and furthermore, there are currently no effective treatments available. Moving beyond the traditional fragmented perspectives that have limited our understanding and management of the disease, our analysis of current information on ME/CFS represents a significant paradigm shift by synthesising the disease’s multifactorial origins into a cohesive model. We discuss how ME/CFS emerges from an intricate web of genetic vulnerabilities and environmental triggers, notably viral infections, leading to a complex series of pathological responses including immune dysregulation, chronic inflammation, gut dysbiosis, and metabolic disturbances. This comprehensive model not only advances our understanding of ME/CFS’s pathophysiology but also opens new avenues for research and potential therapeutic strategies. By integrating these disparate elements, our work emphasises the necessity of a holistic approach to diagnosing, researching, and treating ME/CFS, urging the scientific community to reconsider the disease’s complexity and the multifaceted approach required for its study and management.
Introduction
Myalgic Encephalomyelitis/Chronic Fatigue Syndrome (ME/CFS) or Systemic Exertion Intolerance Disease (SEID) (1–3) (hereafter referred to as ME/CFS) is a debilitating chronic multisystem illness. ME/CFS is estimated to have a global prevalence ranging from 0.1-0.8% (4–7). It is thought to affect some 17 to 24 million people worldwide (8) and the United States (U.S.) Centres for Disease Control and Prevention (CDC) and the U.S. National Academy of Medicine estimate that there are approximately 836,000 to 2.5 million (2, 9) individuals diagnosed with ME/CFS in the U.S., with a quarter of these patients thought to be housebound or bedbound (10). Additionally, current data from the United Kingdom (U.K.) Biobank has indicated that there are more than 250,000 individuals suffering from ME/CFS in England and Wales (11), with a prevalence of 0.2% in three regions of England (6).
ME/CFS impacts all ages, races, and socioeconomic groups (4, 12). Most patients tend to be diagnosed around middle age (13), but diagnosis has been made in individuals as young as three years old and as old as 77 years (2, 12). Black and Hispanic populations appear to have a higher prevalence of ME/CFS with worse severity than other racial groups (9, 14). Women are affected 2-3 times more frequently than men (2, 4, 8, 11, 12).
Due to the disabling symptoms which include cognitive and physical impairment worsened by exertion (15–17), there is a significant economic burden created by ME/CFS (4) as many patients are unemployed and less than a fifth work full-time (11). It is thought that up to 75% of ME/CFS patients are unable to work (10). ME/CFS is thought to cost the U.S. between $18 and $24 billion annually (18), and £3.3 billion annually in the U.K (19).
In this review paper, we will focus on describing ME/CFS in terms of symptoms, severity and burden, diagnostic criteria, causes and triggers; followed by an overview of the complex pathophysiology and management of the condition. We will conclude by listing research priorities for the future. See Figure 1 for a content overview.
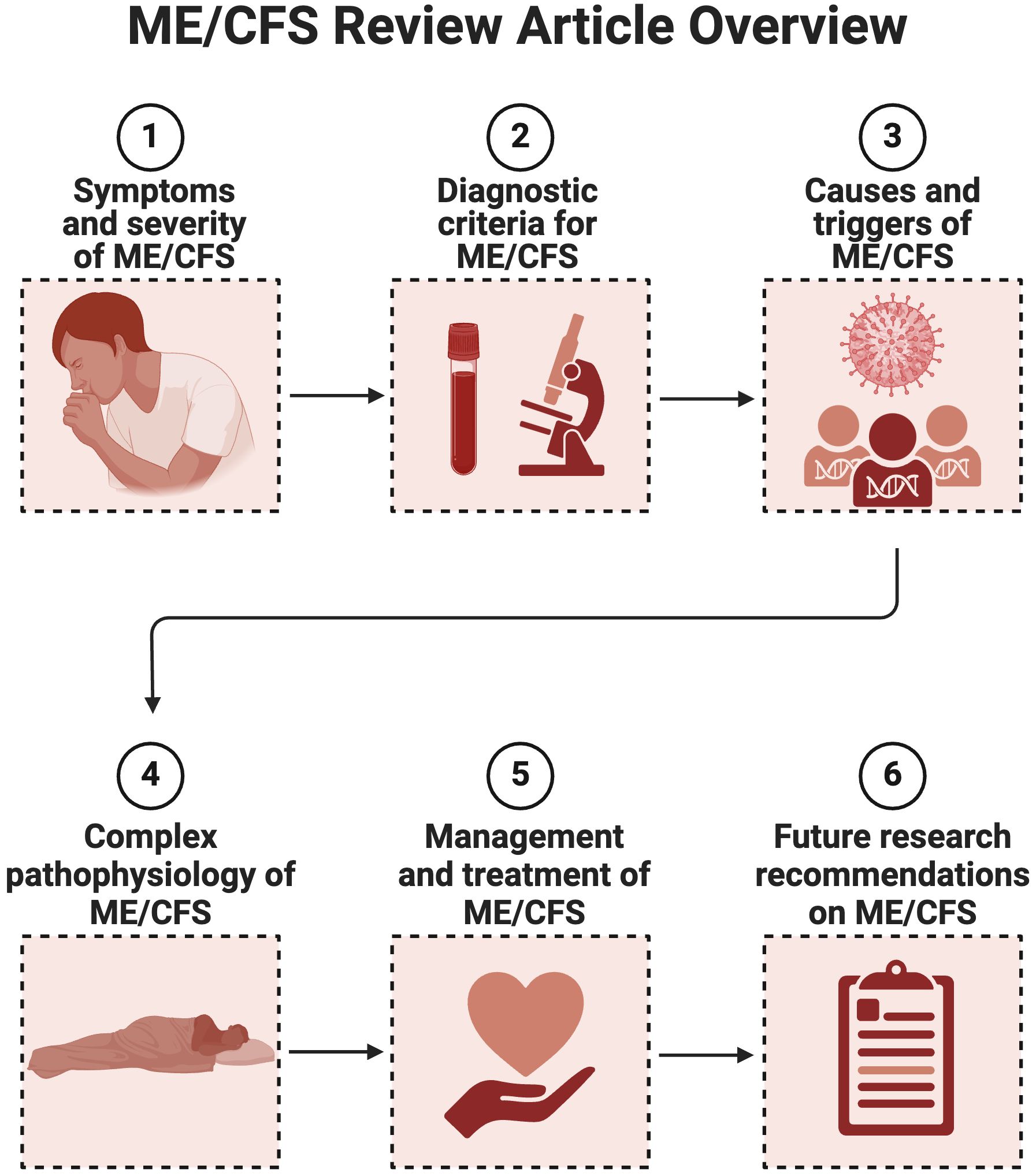
Figure 1 Overview review article of ME/CFS as a complex and multifactorial condition (Created with Biorender.com).
ME/CFS symptoms
ME/CFS is a heterogeneous disease (16, 20), meaning that patients display varying symptoms and several body systems are impacted (11). Although many of the symptoms of ME/CFS overlap with other diseases, one feature that sets ME/CFS apart is a worsening of symptoms in response to relatively minor physical, cognitive, orthostatic or even emotional exertion (13). This phenomenon is known as post-exertional malaise (PEM) or PESE (post exertional symptom exacerbation). Following exertion, patients experience reduced mental and physical stamina, accompanied by accelerated muscle and cognitive fatigue (15–17). PEM is characterised by a delayed onset, prolonged duration, and an intensity disproportionate to the precipitating exertion (15, 16). Fatigue is a prominent feature in most patients; in contrast to physiological tiredness, fatigue is not alleviated regardless of how much patients sleep or rest (2, 21). Common symptoms of ME/CFS are summarised in Figure 2.
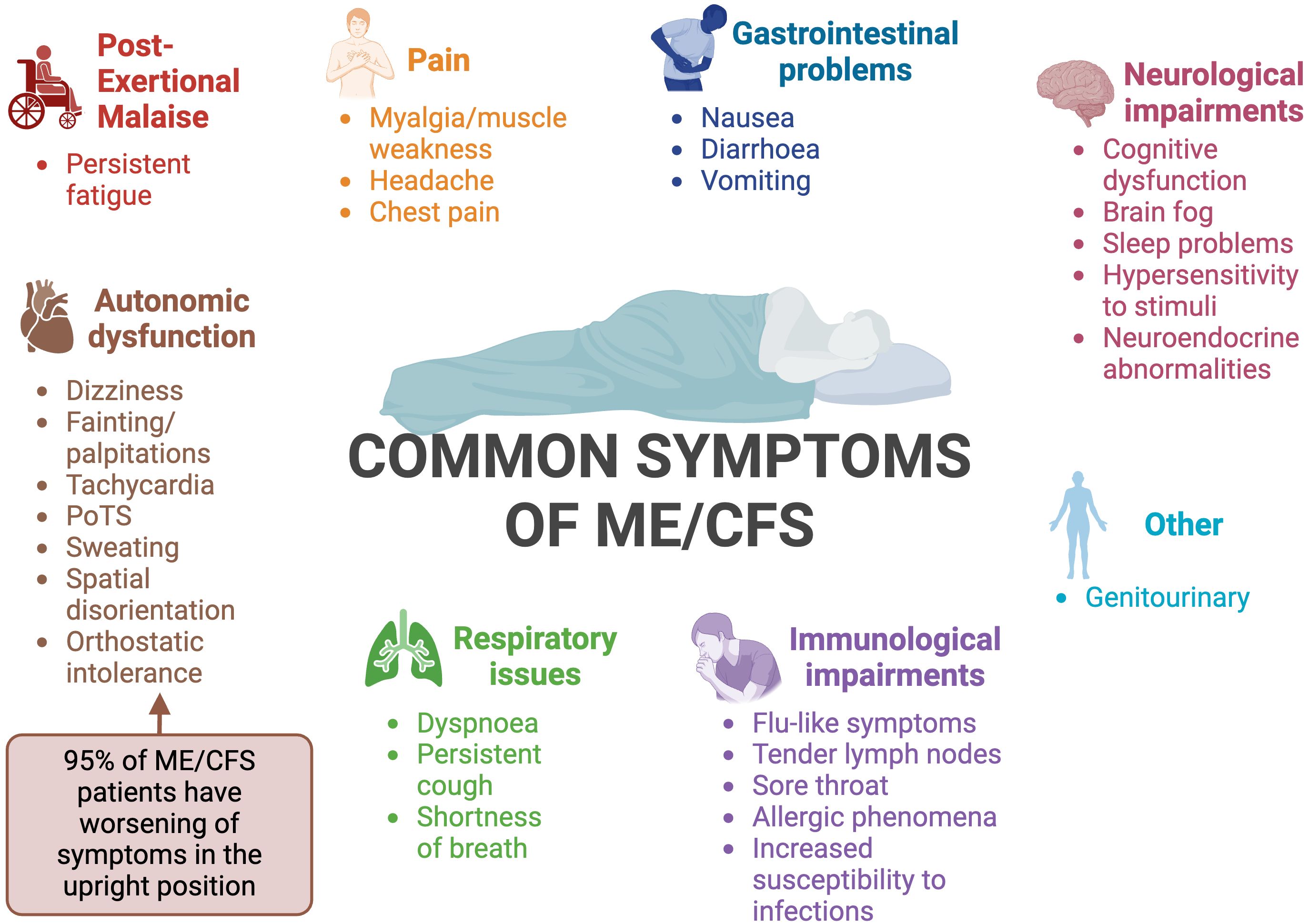
Figure 2 Summary of the common symptoms of ME/CFS (12, 15, 22–26). PoTS, postural orthostatic tachycardia syndrome. Created with Biorender.com.
ME/CFS severity classifications
The long-term outlook for ME/CFS patients varies significantly (11). An important prognostic factor is how the illness is managed in its initial stages (27). The chance of full recovery has been estimated to be only 5% (28) as most patients do not regain their full pre-ME/CFS function; a third of patients deteriorate to being house or bedbound (8). Hence, ME/CFS significantly reduces a patient’s quality of life (11, 24, 29–31), impacting activities of daily living, socialising, family life, emotional wellbeing, work, and education (11, 23). Numerous researchers have found that individuals suffering from ME/CFS have a lower quality of life than people with other chronic illnesses, including multiple sclerosis (MS) (32), lung disease (33), rheumatoid arthritis (RA) (33–35), and some forms of cancer (11, 33, 35). Similar to other disabling chronic illnesses, resultant depression and anxiety in ME/CFS are frequent (36) and there is a higher risk of suicide in ME/CFS (37).
Patients’ symptoms can usually be categorised into four levels of severity; mild, moderate, severe, and very severe (11, 29, 38). In mild severity ME/CFS, an individual is still able to attend work or education and perform light tasks (11). However, they are likely to experience difficulties with mobility, and their social life is impacted. In moderate ME/CFS, individuals experience impaired mobility, meaning they are likely unable to attend work or education, cannot undertake activities alone, have decreased sleep quality, and need frequent rest. People with severe ME/CFS are usually housebound (11) and have a functional capacity ranging from 5% to 15% of normal functioning (39). They also experience cognitive difficulties and are hypersensitive to external stimuli (11). Lastly, people with very severe ME/CFS are bedbound (11) and have a functional capacity of less than 5% (39). These individuals are usually completely dependent on others for care, require tube feeding, are unable to complete personal hygiene, and are highly sensitive to sensory stimuli (11). ME/CFS has been described as a fluctuating condition as symptoms change in nature and severity sporadically (40). 61% of patients have reported being bedbound on their worst days (41) and at least one-quarter of ME/CFS patients have recounted being housebound or bedbound at some point in their lives (2). Hence, patients must adapt to life with regular flare-ups and relapses (11, 42).
In one survey of 1418 ME/CFS patients (43), it was found that 98.5% (n=1397) of the ME/CFS participants struggled with performing daily tasks and more than half (n=775) were completely unable to complete their usual activities. Additionally, 93.9% (n=1331) of participants experienced moderate (n=976) or extreme (n=355) pain, 88.6% (n=1256) of patients had trouble with mobility, and 67.3% (n=954) experienced trouble with washing or dressing themselves. However, the severity of ME/CFS means that family members or caretakers are also severely impacted. Of 1418 respondent caretakers/family members, 96.1% (n=1362) felt worried in relation to their family member’s ME/CFS, 84.7% (n=1201) found it difficult to care for their family members suffering from ME/CFS, and 91.8% (n=1302) reported that their family activities were affected. Additionally, caring for a member with ME/CFS negatively impacts sleep, work, holidays, finances, and sex life (43).
Diagnostic criteria for ME/CFS
The original neurological classification of ME/CFS
In 1969, the World Health Organisation (WHO) classified ME/CFS as a neurological disease (44) based on the neurological features of the disease. Positron emission tomography (PET) brain imaging of ME/CFS patients also displays neuroinflammation (45–47). Additionally, abnormalities of the white (23, 48–50) and grey (23) matter have been noted. This neuroinflammation is likely to correlate with cognitive impairments such as lowered information processing speed, decreased reaction time, slower working memory, and reduced attention (51).
Available diagnostic criteria
Despite its high prevalence, there are still no universally accepted clinical criteria to characterise ME/CFS, making early and accurate diagnosis difficult (52). Different ME/CFS diagnostic criteria exist, as described in Figure 3. Additionally, many adult ME/CFS patients make use of the self-scoring Bell Chronic fatigue immune dysfunction syndrome (CFIDS) disability scale, a symptom scale created by Dr David Bell in 1995 (55). However, this classification is subjective, and scores may change due to the fluctuating nature of the disease; they may also vary depending on the physician doing the scoring. Another problem is that a patient may experience one score in one region, but a differing score in another region, making it difficult for the physician to make an overall decision. Despite the large number of criteria for the diagnosis of ME/CFS, it is still unclear which one is the most useful and validated. Current diagnostics rely on medical history, physical examination, and clinical observations, which often require many trips to the doctor and can be exhausting for a patient (11, 23, 56).
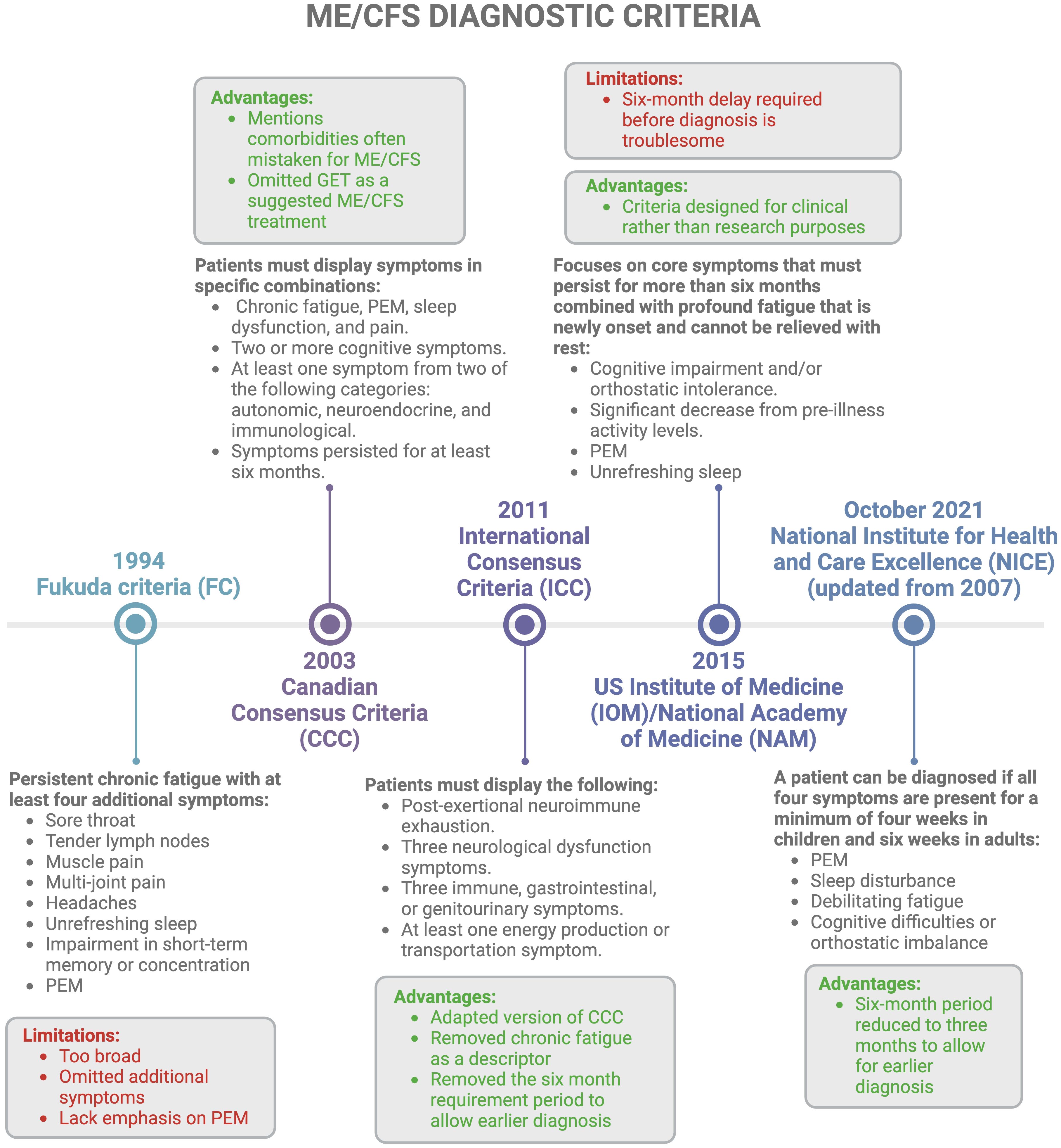
Figure 3 Different diagnostic criteria available for ME/CFS with a concise history of timelines (1, 2, 11, 15, 16, 22, 52–54). Created with Biorender.com. GET, graded exercise therapy; ME/CFS, Myalgic Encephalomyelitis/Chronic Fatigue Syndrome; PEM, post-exertional malaise.
Additionally, PEM which can vary from very minimal to extremely severe (e.g. affecting speaking, thinking, eating and drinking, and orthostatic tolerance) is considered a key diagnostic feature of ME/CFS; this is paradoxical as severe and very severe patients are incapable of even the slightest exertion (39). Moreover, diagnosis relies heavily on symptom-based exclusion of other disorders (2, 11, 15, 16, 22, 56, 57), since ME/CFS symptoms overlap with many other medical conditions (23). In essence, since ME/CFS can be a “diagnosis of last resort” (1, 26), it can take years for individuals to receive a diagnosis (22). This prolonged diagnostic process is compounded by differences in exclusion criteria across various definitions of ME/CFS, as highlighted by Jason et al., 2012 (54). Hence, re-evaluation of these diagnostic criteria is essential (57).
Probable predisposition and causes of ME/CFS
The diverse symptom presentation and patient heterogeneity indicate that ME/CFS is likely to have a multifactorial origin (23, 30, 53, 58), with multiple physiological processes implicated in the pathogenesis (52, 59). It is unlikely that one single protein or RNA transcript will be consistent across the disease spectrum (60). It has been proposed that stressful or traumatic incidents in genetically susceptible individuals may trigger the development of ME/CFS symptoms (58, 61). These stressors can include acute infections (61), emotional stress (62), and major life events (61). However, other stressors such as a quick walk, a glass of wine, or a temperature change have also been known to trigger relapses in ME/CFS (63). The next paragraphs will discuss genetic predisposition and susceptibility as an underlying risk factor for ME/CFS - the superimposition of external stressors and exposures (59) results in a state of “aberrant homeostasis”, where a temporary homeostatic state of functioning occurs, but functions at a less optimum level (64).
Genetic predisposition
Unfortunately, there is little consensus among researchers on the genetic, cellular, and molecular influences that alter the risk of developing ME/CFS (21). However, it is thought that ME/CFS may have a genetic predisposition (26, 29, 56, 61, 65–68) as members of the same family have frequently been diagnosed with ME/CFS (65, 67, 68), though they will also tend to share similar lifestyles and cultures. Similarly, genealogic analyses and twin studies have shown a genetic link between ME/CFS patients and their offspring, ensuing a heightened risk of developing ME/CFS in the offspring (26, 66, 69). As expected (70), these diagnoses do not seem to follow predictable Mendelian patterns, suggesting that there are multiple genes and alleles that increase the risk of developing ME/CFS (56). Various studies have found that some ME/CFS patients identified by the Fukuda criteria have a significant excess relatedness for close (first or second degree) and distant (third degree) relatives (66, 68). A large review (56) noted that three studies estimated the narrow-sense heritability (h2) in large cohorts, and two of these studies reported non-zero results, suggesting a heritability risk for ME/CFS. However, other studies have shown no evidence of heritability. One analysis of the U.S. health insurance resulted in a high narrow-sense heritability (h2 = 0.48) (71), while an analysis of U.K. Biobank self-reported ME/CFS patients approximated the h2 to be 0.08 with a low confidence (72). Furthermore, another large twin-based study produced inconclusive results (73).
Some polymorphisms in genes related to the immunomodulatory response have been identified (69, 74), such as an increase of TNF-857 TT and CT genotypes (p=0.002), and a significant decrease of IFN gamma low producers (A/A) (P=0.04) in ME/CFS patients in respect to controls (74). Polymorphisms impacting hormone action have also been noted (69, 75). For example, NR3C1-1F DNA methylation was found reduced in patients with ME/CFS and coincides with the hypothalamic pituitary-adrenal (HPA) axis hypofunction hypothesis (75). The metabolic kynurenic pathways is likely also implicated in ME/CFS, owing to common mutations in IDO2 (76) such as R248W and Y359STOP (77). However, these genetic associations are inconsistent as studies typically have quality-control issues (56). Additionally, the Gln27 mutation (a polymorphism in the B2dR genes) has been associated with adolescent ME/CFS (78). Such Gln27 mutations, along with Arg16 mutations in adults, can result in an unfavourable cardiovascular profile (79–81). On the other hand, genome-wide association studies (GWAS) studies performed on ME/CFS patient samples from the U.K. Biobank have found no DNA variant repeated in the multiple analyses (82). However, it is not entirely certain how many of these patients met ME/CFS criteria, suggesting that replication studies should be done in future to help us understand the genes, cellular processes, and tissues or cells that causally change the risk for developing ME/CFS. Additionally, pinpointing genetic risk factors for ME/CFS will help combat the disbelief some health professionals have towards ME/CFS (56).
There are also genetic predispositions that render certain individuals susceptible to developing autoimmune diseases (83) and even after Epstein-Barr virus (EBV) infection (30, 84), such as human leukocyte antigen (HLA) variants (30, 84, 85) that have experienced selective pressures whilst co-evolving with pathogens (86, 87). HLA proteins are crucial for the immune system as they distinguish foreign pathogens from self-cells (56). An increased frequency of HLA-DQA1 alleles and reduced expression of HLA-DRB1 was found associated with ME/CFS (88). Additionally, in patients diagnosed with the CCC, the HLA types HLA-C*07:04 or HLA-DQB1*03:03 were shown to be significantly linked to ME/CFS status when using the CCC (89). Since 10% of ME/CFS patients have these alleles, their risk is altered around 1.5-2.0-fold, meaning genetic differences in the immune system may change the risk of developing ME/CFS (89). Some haplotypes also appear to be less resistant to EBV infection, such as DR2-DQ6, DR3-DQ2, and DR4-DQ8, making them more likely to develop EBV-related disorders (90). Although, few researchers think that mitochondrial DNA variants can explain the prevalence of ME/CFS (91–93).
Viral triggers and reactivation
As in other chronic diseases, ME/CFS involves an asymptomatic predisposition stage, then a prodromal stage, followed by symptomatic disease (59). Infection (30, 61, 94–97) is often reported to be a common trigger for the development of ME/CFS, as many patients recognise that the onset of their symptoms coincided with an infectious episode (2, 58, 98). This is recounted by more than 80% of patients (98) and it has been estimated that two-thirds of ME/CFS cases arise following viral infection (85, 99). This is supported by the fact that several ME/CFS outbreaks have occurred in the same geographical region simultaneously (12). Clustering provides strong support for the involvement of infectious agents, as with some cases of MS that ‘appeared’ (100) in the Faroe Islands during World War II upon the arrival of foreign army corps, and was eventually shown to be due to EBV (101). Additionally, many ME/CFS patients experience symptoms similar to bacterial or viral infections which may correlate to specific regions. Infectious pathogens thought to promote the development of ME/CFS are summarised in Figure 4.
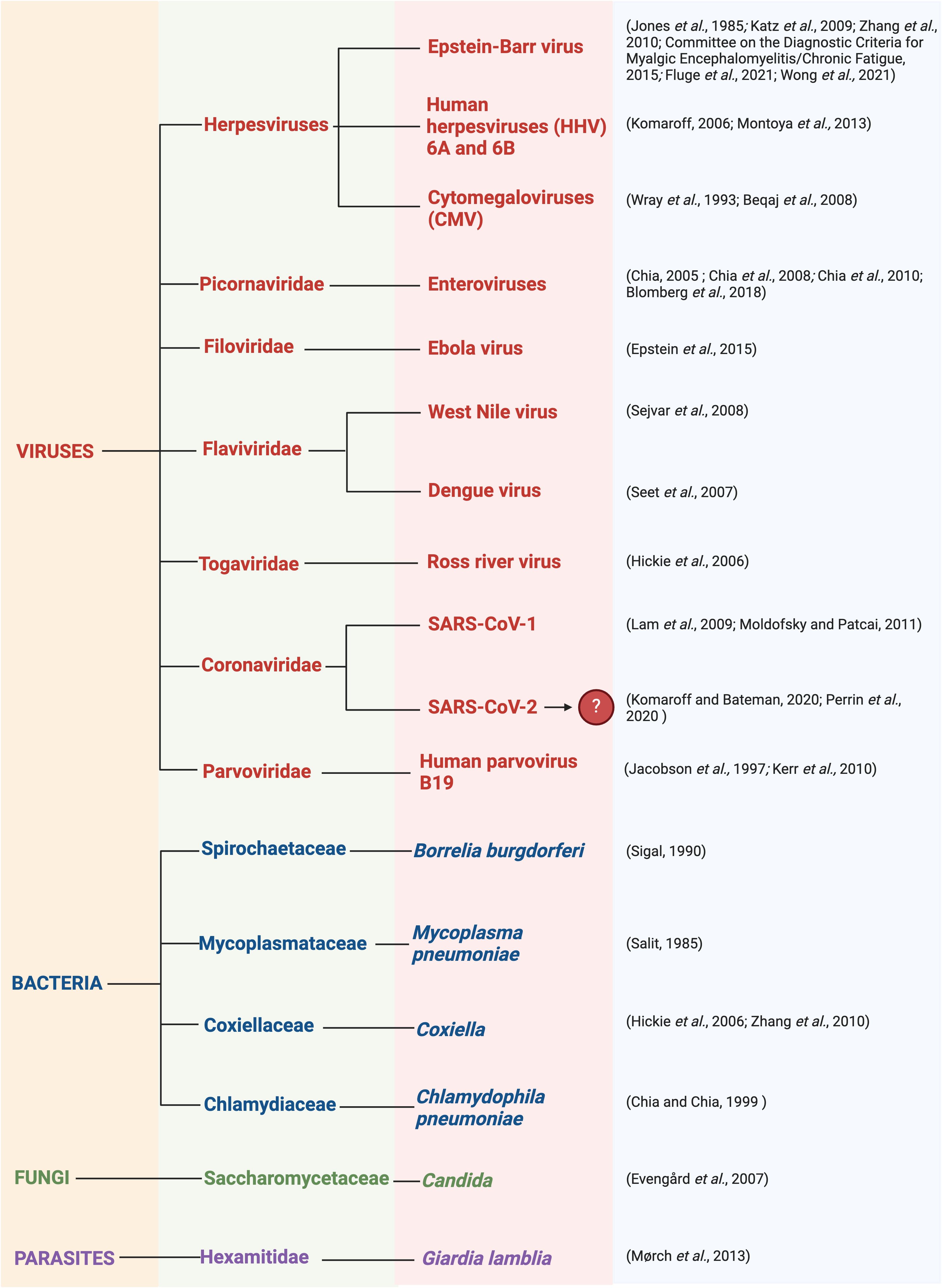
Figure 4 Infectious pathogens thought to promote the development of ME/CFS including viruses, bacteria, fungi, and parasites (2, 25, 29, 85, 102–126). Created with Biorender.com.
It is thought that many chronic illnesses, including ME/CFS, originate with reactivation of latent or dormant viruses that are present in the body, caused by a change in physiological conditions (127). During acute illness, direct consequences of viral reactivation or infection are thought to occur in localised areas such as the brain and neuronal cells, muscle cells, and cardiac myocytes [Extended Figure 8 in (127)]. Since these tissues are rich in mitochondria, it can result in neuronal and autonomic nervous system abnormalities, as well as immune modulation. However, with chronic illness, indirect consequences of viral reactivation or infection are proposed to occur. This occurs in peripheral circulation and can promote symptoms such as postural orthostatic tachycardia syndrome (PoTS), PEM, fatigue, endothelial cell dysfunction, platelet hyperactivation, mast cell activation, and connective tissue disorders. In this chronic phase, it is thought that auto recovery is difficult (127).
Viral pathogens that often persist in a dormant manner include herpesviruses such as the extensively studied EBV (52, 94, 95, 113). Reactivation of herpesvirus has interestingly been hypothesised as a key mechanism in the development of ME/CFS (128). In support of this theory, one paper investigated the possibility of EBV-encoded proteins existing in ME/CFS, such as BRRF1 and BLLF3, and the effects they may have (52). BRRF1 plays a role in the switch between latent and lytic EBV replication (129), while BLLF3 encodes a dUTPase and is expressed in the early phase of EBV lytic replication (52). Similarly, evidence for antibodies against HSV-1 (127), EBV (127, 130, 131), and HHV-6 (130) dUTPase proteins has been identified in ME/CFS. These dUTPase proteins, along with other viral dUTPases such as HSV-1 and HHV-6, are thought to promote cellular damage and promote autoimmune features, as well as promoting mitochondrial fusion and decreasing energy production (127). This antibody presence is thought to potentially elevate cytokine and chemokine levels, which is dependent on toll-like receptor (TLR)-2 signalling and causes NF-κB activation (132, 133). Hence, the EBV UTPase may act as a novel pathogen-associated molecular pattern (PAMP) ligand protein for TLR2 (132).
Although EBV proteins may play a role in the progression of ME/CFS (52), researchers have hypothesised that the virus has a third state termed the abortive/leaky/lytic replication, as has been suggested upon analysis of the EBV genome in T and NK cells of patients with chronic EBV infection (134–136). The presence of EBV dUTPases may cause neurological abnormalities in ME/CFS via changes in gene expression, resulting in modulated neurological circuits (137). Moreover, EBV dUTPases could also induce immune dysfunction (52).
However, earlier serological studies on EBV and ME/CFS using classical EBV antigens have resulted in contradicting results that illustrate no upregulation of EBV-encoded proteins (99, 138–147). More advanced studies using peptide microarray (148) and suspension multiplex immunoassay (149) have also not found a significantly higher EBV anti-immunoglobulin (Ig)-G response in ME/CFS patients in comparison to controls. Most studies have not reported a significant increase in the viral load in ME/CFS patients in comparison to controls. Due to this evidence, some researchers believe that herpesviruses as one of the causes of ME/CFS is a “fading” hypothesis (150). However, a trigger does not have to linger. Equally, heterogeneity in patient groups, the absence of uniformity in case definitions, and differences in reliability and precision could have resulted in there being no correlation between the viral load and serological data (61, 99, 103, 109).
That said, serological data are probably of limited use as an indicator of EBV reactivation (151). Although there is substantial evidence that ME/CFS has a viral trigger (94, 95), symptom severity and burden are often not related to the severity of the triggering viral infection and its symptomatology. Additionally, there are alternative theories differing to a post-viral causation, as noted later in the text.
Toxin and drug exposure
Some also hypothesise that toxin exposure could trigger ME/CFS (61), such as organophosphate compounds (152, 153) and heavy metals (154). In the early 1960s, researchers found that workers with chronic exposure to organophosphates, mainly in insecticides and after sheep dipping, experienced persistent central nervous system (CNS) changes (153). This included disabling fatigue exacerbated by exercise and associated with myalgia, excessive sleep, night sweats, irritable bowel syndrome (IBS) symptoms, and mental changes. It was hypothesised that the organophosphates induce various abnormalities such as an elevated prevalence of lymphoproliferative disorders associated with impaired natural killer (NK) cell and cytotoxic T cell function. Additionally, exposure to heavy metals, such as cadmium, may also contribute to the development of ME/CFS (154). Cadmium is a widespread environmental and occupational heavy metal pollutant and can potentially cause neurological symptoms. Cadmium induces neuronal death in cortical neurons through a combination of apoptosis and necrosis, involving reactive oxygen species (ROS) generation and lipid peroxidation (155). This action may explain decreased grey matter volume in ME/CFS (156), as well as certain effects on the CNS such as reduced attention levels and memory (157). Exposure to cadmium also potentially reduces cerebral blood flow (158) [particularly cortical blood flow (159)], as cadmium has a disruptive effect on angiogenesis (160). This reduced blood flow can result in neurological dysfunction and the abnormal neuroimaging observed in ME/CFS (158). Cadmium may accentuate inflammatory processes, which may in turn disrupt the HPA axis and trigger symptoms of ME/CFS (161, 162). However, the exact organ that cadmium toxicity targets is still unclear (154).
Additionally, cases of ME/CFS have also been recorded post-immunization (163) and many patients are fearful that vaccinations will worsen their already dysfunctional immune system and cause symptom exacerbation (164). More recently, ME/CFS development after the Sputnik V COVID-19 vaccination has been recorded (165). Some research about vaccination safety relates to adjuvant compounds used in some vaccinations to promote lasting immunization (166, 167). For example, aluminum hydroxide salts, often used as vaccine adjuvants, have been found to abnormally persist within immune cells at the site of previous immunization, resulting in macrophagic myofasciitis lesions (168). These inflammatory macrophage formations can result in associated microscopic muscle necrosis. While transient aberrant changes associated with aluminum hydroxide salts are acknowledged, the direct link between microscopic muscle necrosis and the mechanisms underlying ME/CFS requires further investigation. However, no indication of an increased risk of developing ME/CFS was found post-HPV vaccination (169). Additionally, ME/CFS patients were found to have similar humoral and cellular immune responses as healthy controls post-influenza vaccination (170) without worsening ME/CFS symptoms or causing adverse effects (164, 171).
Furthermore, various drug exposure has been found to trigger symptoms that are typically present in ME/CFS (172). For example, widely prescribed fluoroquinolone antibiotics are usually prescribed to treat various infections such as pneumonia and sinusitis (173–175). However, these fluoroquinolones have been found to increase tendinopathy involving oxidative stress and mitochondrial toxicity (176–180). Hence, the use of such drugs may have a multisystem effect and lead to the development of chronic illnesses such as ME/CFS.
The complex pathophysiology of ME/CFS
As a consequence of infection and other stressors, such as poly-trauma for example (181), a state of acute inflammation and aberrant immune activation may occur. A compensatory anti-inflammatory mechanism then typically follows (59), causing an imbalance in immune responses (58, 59) combined with hypothesised autoimmunity (30, 52, 85, 182). This may lead to subsequent physiological abnormalities including gut dysbiosis and systemic inflammation, eventually resulting in a pathological clotting system, chronic endothelialitis, vasoconstriction, and hypoxia, as found in similar conditions such as heat stroke (183). Additionally, dysfunctional energy metabolism (52, 184–186) along with oxidative stress (187, 188) are also hypothesised in the development of ME/CFS. It is hypothesised that these mechanisms occur in a spiralling, progressive way, toppling the host’s homeostatic equilibrium (59).
Gut dysbiosis
ME/CFS patients often have gastrointestinal (GI) symptoms (189) with gut inflammation (190), gut microbiome dysbiosis (57, 191) and changes in the gut microbiome (191, 192). A reduction in microbiome diversity has been identified (191), but it is likely that the microbiome composition will differ between ME/CFS patients as each patient has a unique infectious history (193). In ME/CFS, anti-inflammatory bacterial species such as Faecalibacterium (191, 194) and Bifidobacterium (191) are decreased, resulting in a decreased production of anti-inflammatory butyrate (191, 194). Butyrate is essential to maintain the mucosal barrier and immunomodulation (195), whilst having anti-inflammatory effects through downregulating pro-inflammatory cytokines (196). However, this decrease in Faecalibacterium is found in various disorders, and is not specific for ME/CFS (197). Similarly, some studies found an elevation in short chain fatty acids (SCFAs) butyrate, isovalerate, and valerate (198), contradicting these other articles. Conversely, proinflammatory Proteobacteria species have an elevated concentration in ME/CFS (191, 194). Proteobacteria are known as a “microbial signature of disease” (199) and one form, Enterobacteriaceae, is specifically increased in ME/CFS (191). This increased concentration of Enterobacteriaceae may result in increased transit time and IBS-like symptoms (200). It is also not yet known whether such dysbiosis leads to ME/CFS, or whether it is a consequence of the metabolic and immunologic changes that occur in the disease (201).
It is hypothesised that once an acute infection has dysregulated the host’s immune system, pathogens are capable of intracellular persistence where they escape immune surveillance (202). When metabolites and proteins expressed by these pathogens are created, they interfere with host transcription, translation, and DNA repair processes, leaving infected immune cells unable to express human metabolites. Since these pathogen proteins and metabolites are often similarly structured to ones created by the human host, such molecular mimicry makes it difficult for the host to recognise the foreign pathogen (193), resulting in the host’s immune system becoming increasingly sluggish and more susceptible to acquiring other infectious agents. This causes patients to become increasingly dysbiotic as their immune system weakens over time, and successive infections may explain the variability of symptoms experienced in ME/CFS (193).
Microbe-associated molecular patterns (MAMPs) are molecules found on bacterial surfaces (203) which interact with the immune cell receptors of the host to promote inflammation (204). Elevated levels of IgM and IgA antibodies to one potent MAMP- exotoxin lipopolysaccharide (LPS)- have been observed in ME/CFS (205), as well as higher blood levels of bacterial LPS, LPS-binding proteins, and soluble CD14 (191). LPS is a structural component in the outer membrane of many Gram-negative bacteria, and it has various immunostimulatory and procoagulant effects (206). LPS molecules have been identified as potent inflammagens (207–209) having cytotoxic and neurotoxic effects (210–214) and heightening the production of pro-inflammatory cytokines (215–218).
These inflammatory markers also indicate translocation of gut bacteria and toxins from the GI tract into the blood (191), which may result in systemic inflammation in ME/CFS (183). Specific gut inflammation (190) and gut hyper-permeability (219) have been identified, and microbe and virus communities may also persist in ME/CFS blood and brain tissue (193). Not only does the abundance of bacterial taxa correlate with the symptoms of pain and fatigue (191, 194); metabolomic results illustrate an expression of bacterial genes, rather than human (194). In essence, there appears to be a link between the microbiome, gut inflammation, and the symptoms of ME/CFS (201).
Additionally, the diverse intestinal virome that is established from birth (57) includes many prokaryotic viruses called bacteriophages (220). Bacteriophage richness was found elevated in one ME/CFS study, but it is limited owing to its small sample sise (191). Since bacteriophage predator-prey dynamics regulate the diversity and equilibrium of the bacterial microbiome (221), alterations in the virome can also cause intestinal microbial dysbiosis in different diseases (222–224) and alter microbiome homeostasis. Future studies need to include standardised techniques and analyses when doing further research into the virome as it is particularly understudied in ME/CFS (57).
External stressors like stressful or traumatic incidents, acute infection, and toxic stressors may result in widespread and chronic systemic and even neuroinflammation, driven by a variety of inflammatory molecules in circulation. Such molecules might have their direct origins from previous infections and the resulting gut dysbiosis, as summarised in Figure 5.
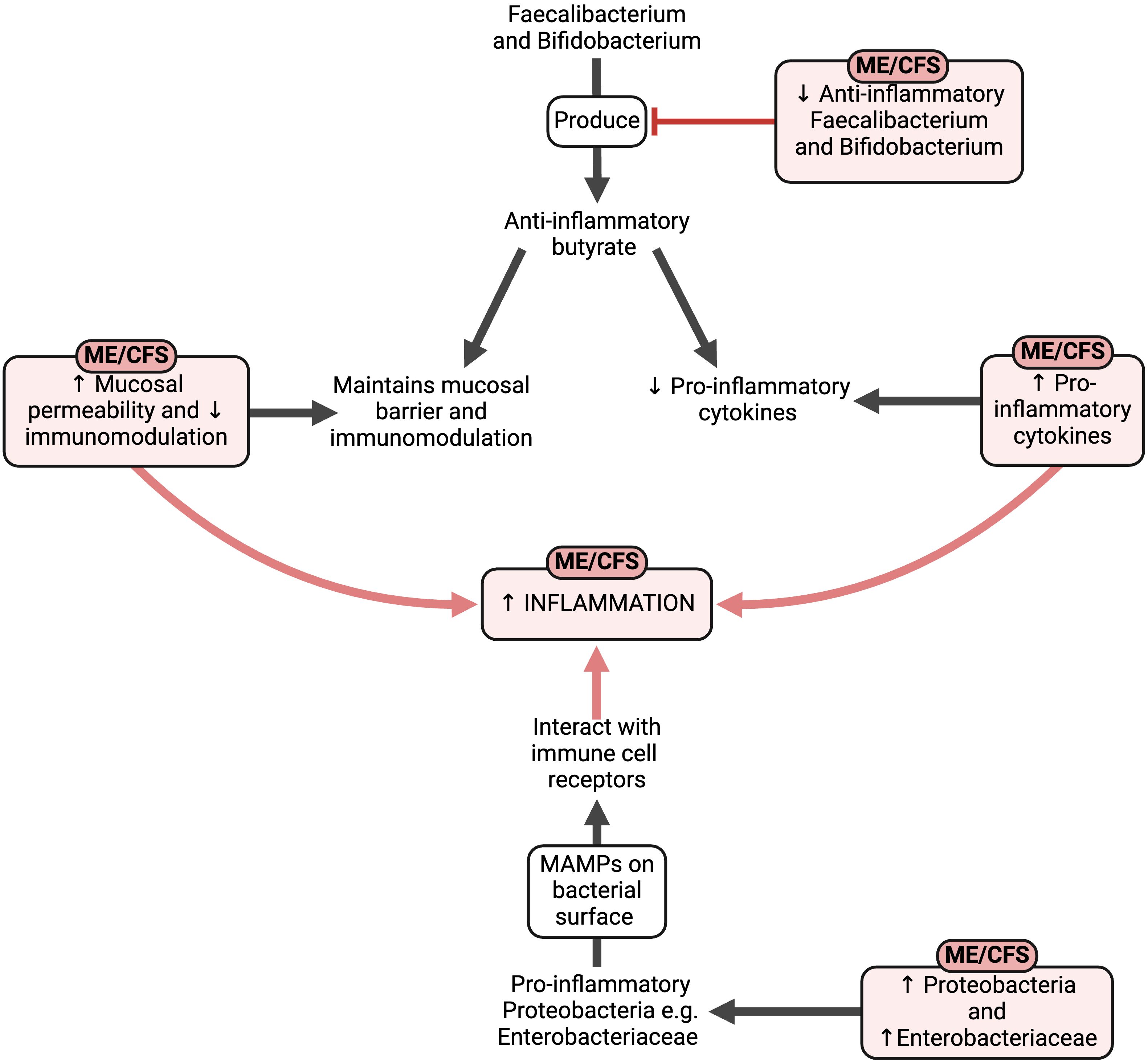
Figure 5 The effects of the altered gut composition in ME/CFS (57, 183, 190–196, 201, 203–209, 215–218). Created with Biorender.com. MAMP, microbe-associated molecular pattern; ME/CFS, Myalgic Encephalomyelitis/Chronic Fatigue Syndrome.
Systemic inflammation
Systemic inflammation (225) and neuroinflammation (45–47) are thought to play a major role in ME/CFS. Chronic inflammation is a crucial hallmark of persistent infection (193), as infections can alter proinflammatory and anti-inflammatory cytokine and chemokine expression, adjusting the inflammatory and immune responses (30). Although inflammation is reflected in selective biomarkers, traditional inflammatory markers such as C-reactive protein (CRP) and erythrocyte sedimentation rate have also shown an increased trend in ME/CFS, particularly in those with mild/moderate disease generally not raised in ME/CFS (226).
Circulating inflammatory molecules
Early ME/CFS has been associated with elevated proinflammatory cytokines (227–230) and a distinct cytokine inflammatory profile (225, 231). Heightened circulating inflammatory cytokines are crucial in driving the development of autoimmune diseases (232). These heightened cytokines are mainly related to Th1 and Th2-driven responses, but not all studies have found consistent results. However, as ME/CFS persists over several years, it is hypothesised that the inflammatory profile (231) and plasma immune signatures change with increasing disease duration (225). Perhaps this indicates that in the early stages of ME/CFS, the immune system actively attempts to target the infectious burden (193); however as the disease progresses, pathogens in the microbiome disable the immune response and “immune exhaustion” occurs (233, 234). In essence, acute pathogens can cause chronic symptoms in ME/CFS by existing in latent forms (193).
Cytokine activation has been noted in ME/CFS patients (225) and appears to increase along with disease severity (231). This suggests that patients with ME/CFS may struggle with an increasing infectious burden over time. One study noted 17 cytokines had a significant upward linear trend with the severity of ME/CFS: CCL11 (Eotaxin-1), CXCL1 (GROα), CXCL10 (IP-10), IFN (interferon)-y, interleukin (IL)-4, IL-5, IL-7, IL-12p70, IL-13, IL-17F, leptin, G-CSF, GM-CSF, LIF, NGF, SCF, and TGF-α (231). Out of these 17 cytokines, 13 are considered proinflammatory cytokines. Although these 17 cytokines are linearly correlated with ME/CFS increasing severity, they did not differ significantly between control and ME/CFS groups. This correlation of cytokine levels with severity may indicate that severity is a useful way to subgroup ME/CFS, as well as a dose-response defect in the metabolism or excretion of cytokines (231). A summary of the interactions between circulating inflammatory molecules can be found in Figure 6.
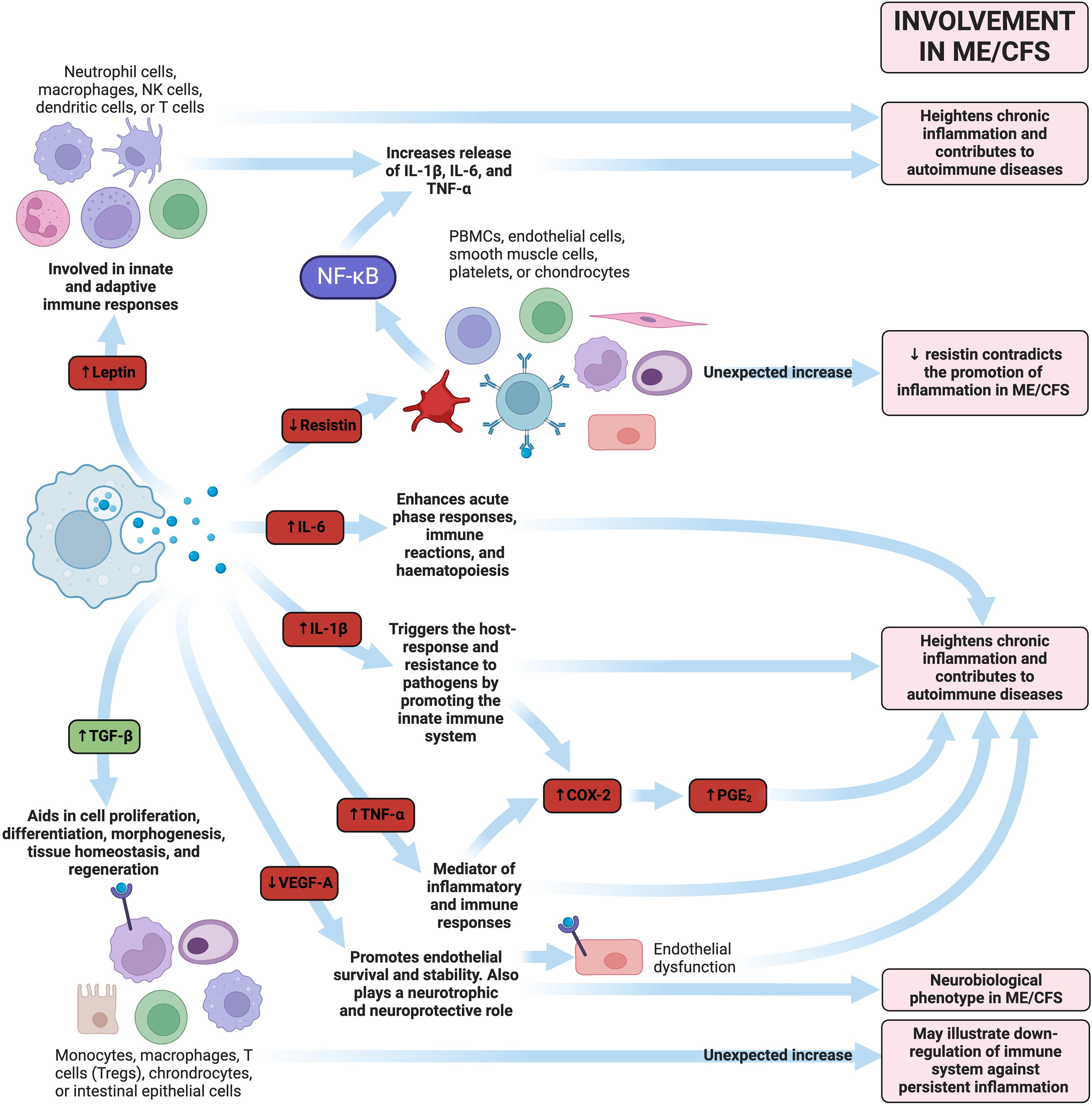
Figure 6 Examples of how dysregulated inflammatory molecules could play a pathological role in ME/CFS (113, 137, 225, 231, 235–247). Molecules highlighted in red represent pro-inflammatory molecules, whereas those written in green represent anti-inflammatory molecules. Created with Biorender.com. COX-2, cyclooxygenase-2; IL, interleukin; ME/CFS, Myalgic Encephalomyelitis/Chronic Fatigue Syndrome; NK, natural killer; PBMC, peripheral blood mononuclear cell; PGE2, prostaglandin-endoperoxide synthase 2; TGF-β, transforming growth factor-β; TNF-α, tumour necrosis factor-α; Treg, regulatory T cell; VEGF, vascular endothelial growth factor.
Redox imbalance and oxidative stress
Inflammation is bidirectionally linked to redox imbalances (248) as inflammation produces ROS and reactive nitrogen species (RNS), whilst redox imbalances cause cellular damage, evoking an inflammatory response (249, 250). Redox imbalances (251, 252), oxidative stress (182, 187, 253–255), nitrosative stress (182), and chronic ischaemia-reperfusion injury (256) have all been found to be central to ME/CFS. Markers of oxidative stress have been found in ME/CFS and, importantly, correlate with symptom severity (251) (Table 1).
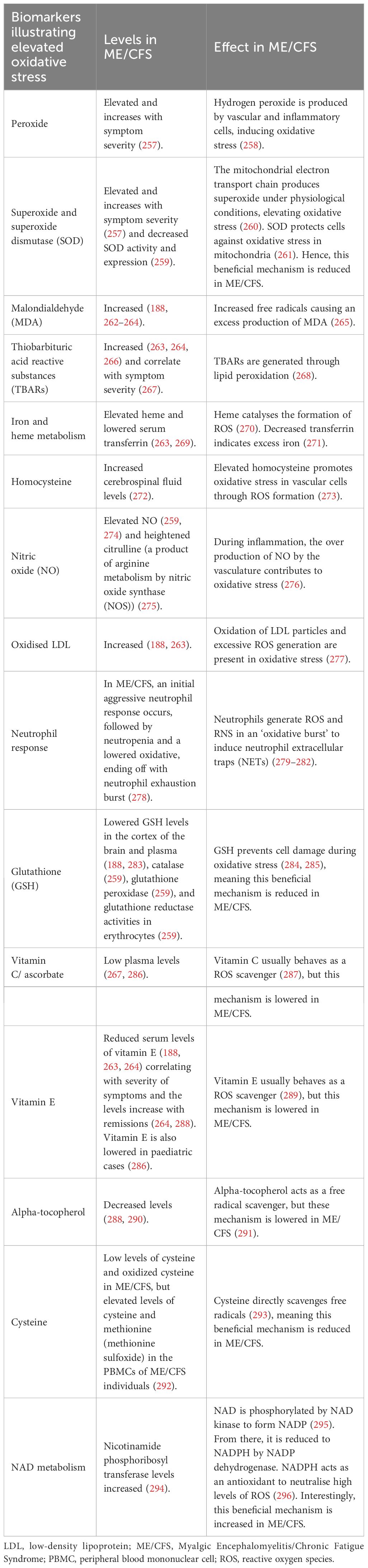
Table 1 Markers of oxidative stress present in ME/CFS (adapted from Paul et al., 2021) (188, 257–296).
Nitrosative stress is also present, as illustrated by markers of nitrosative stress such as increased NOS and NO (182, 297), peroxynitrite (182, 297), elevated NF-κβ production (182), and nitrate after exercise (182, 297). Even though NO is physiologically critical to vasodilation and neurotransmission (251), excess NO and RNS are damaging as they directly attack antioxidant enzymes such as catalase (298), promoting redox imbalance.
When muscle afferents are triggered by muscle fatigue, this triggers the production of heat shock proteins (HSPs) (299). HSPs protect muscle cells against any deleterious effects of ROS generated during exercise by activation of antioxidants (300, 301). In turn, the elevated antioxidant levels elevate the levels of plasma HSPs. However, prolonged activation of muscle afferents by oxidative stress due to low-grade exercise results in reduced HSP production (266). The formation of HSPs in ME/CFS individuals is reduced (302), and the responses of plasma HSP27 and HSP70 are delayed or lowered, while resting levels of plasma HSP70 are also decreased (303). Hence, this impaired HSP production (302) combined with oxidative and nitrosative stress, and low-grade inflammation could explain the exercise intolerance and muscle dysfunction seen in ME/CFS patients (303, 304).
Dysfunction of the vasculature, endothelium, and coagulation
Since persistent inflammation and immune cell activation is present in ME/CFS (305), vascular changes and endothelial damage (306, 307) will coexist due to the interplay between inflammation and vascular alterations. The altered autoregulation of blood flow cannot meet the metabolic demand of tissues in ME/CFS, leading to tissue hypoxia and subsequent ischaemia/reperfusion injury with its associated symptoms and signs (29).
Endothelial damage
Endothelial cells are important regulators of the immune system (308); endothelial dysfunction can promote oxidative stress and inflammation (309). The abnormal immune responses present in ME/CFS are thought to impact endothelial cell function (29) and patients show signs of endothelial dysfunction (306, 310). Endothelial dysfunction has been demonstrated in vivo (306, 311), in the large vessels of ME/CFS patients (307, 311) through flow-mediated dilation (FMD) and in the microcirculation through post-occlusive reactive hyperaemia.
FMD measures the dilation of blood vessels triggered by the release of NO from endothelial cells in response to shear stress (307, 311). It was also found that ME/CFS patients are unable to dilate their vessels adequately by endothelium-independent vasodilation when given sublingual nitroglycerin to promote relaxation of the vessels. Additionally, myocardial infarction associated transcript (MIAT) was found to be upregulated in PBMCs of ME/CFS patients, indicating endothelial dysfunction (312). Additionally, microclot presence (313) in ME/CFS may also cause damage to the endothelium. However, the endothelial damage observed in ME/CFS patients does not appear to correlate with the usual markers of endothelial dysfunction seen in cardiovascular disease such as increased levels of symmetric dimethylarginine (SDMA), asymmetric dimethylarginine (ADMA), and high-sensitivity C-reactive protein (hs-CRP), and reduced levels of arginine and homoarginine (311). This may suggest that a different mechanism is at play, which could relate to the abnormal immune response present in ME/CFS (311). Endothelial dysfunction can result in capillary leakage, accelerated inflammation, hypercoagulation, platelet aggregation, and decreased vascular tone (314).
MicroRNAs (miRNAs) are important to maintain endothelial function and altered miRNA profiles are often used to predict, diagnose, and monitor diseases (315). Studies have revealed interesting miRNA changes in ME/CFS. Silent information regulator 1 (Sirt1), an anti-inflammatory and anti-oxidative protein (316), increases the production of NO by activating endothelial NOS (eNOS) in endothelial cells in response to shear stress (317). The NO released by endothelial cells controls the vascular system to ensure sufficient blood and oxygen reaches tissues throughout the body. During inflammation and oxidative stress, eNOS uncoupling or reduction can occur (318), as well as decreased activity or expression of Sirt1 (316). In ME/CFS, a set of plasma miRNAs known to modulate the Sirt1/eNOS axis were analysed, showing elevation in miR-21, miR-34a, miR-92a, miR-126, and miR-200c (305). These five miRNAs have also been found increased in PBMCs from different cohorts. The functions of these miRNAs are illustrated in Figure 7; they relate to endothelial function signalling pathways, including oxygen regulation and oxidative stress. Hence, miRNAs may serve as a potential biomarker in ME/CFS, although they do not correspond with disease severity (305).
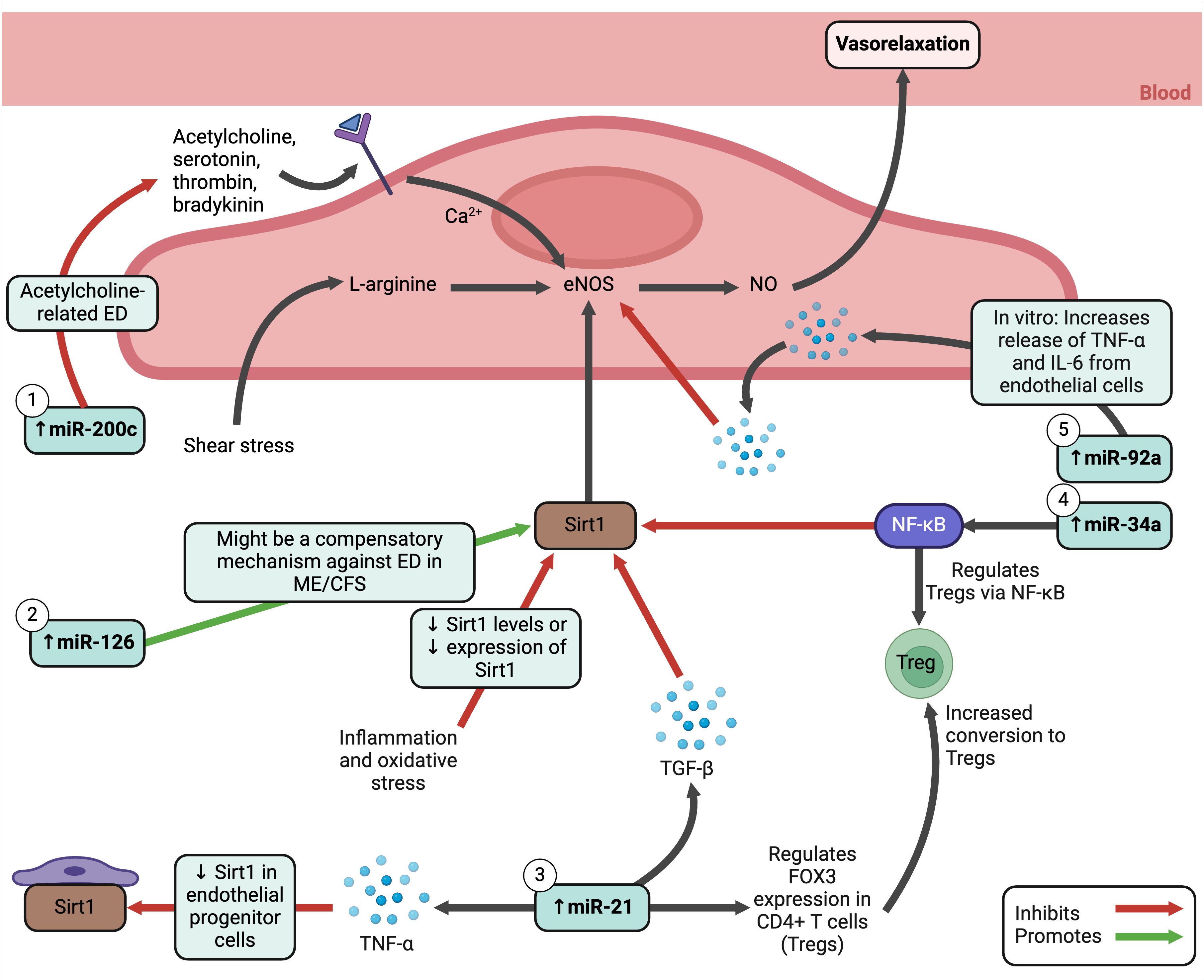
Figure 7 Elevated MicroRNAs in ME/CFS and how they influence endothelial cell functioning (305, 316, 319–328). Created with Biorender.com. ED, endothelial dysfunction; eNOS, endothelial nitric oxide synthase; Fox3, transcription factor forkhead box protein 3; IL, interleukin; ME/CFS, Myalgic Encephalomyelitis/Chronic Fatigue Syndrome; miRNA, micro ribonucleic acid; NF-κB, nuclear factor kappa B; NO, nitric oxide; Sirt1, silent information regulator 1; TGF-β, transforming growth factor beta; TNF-α, tumour necrosis factor alpha; Treg, regulatory T cell.
Autonomic dysfunction, vasoconstriction, and hypoxia
Many ME/CFS patients have a unique cardiovascular situation (58) and changes indicative of autonomic dysfunction (329–331). One cluster analysis of 131 ME/CFS patients diagnosed according to the Fukuda criteria revealed that 34% of patients experienced sympathetic symptoms with dysautonomia (and were associated with more severe disease severity), 5% from sympathetic symptoms alone, 21% parasympathetic, and 40% struggled with sympathovagal balance (331). However, studies assessing autonomic dysfunction in ME/CFS are equivocal (329). Since heart rate parameters often reflect changes in autonomic function, one meta-analysis analysed 64 studies to assess differences in heart rate parameters in ME/CFS (329). It was concluded that, in comparison with controls, patients with ME/CFS have a higher resting heart rate, lower maximal/peak heart rate, higher heart rate responses to head-up tilt testing and moving from sitting to standing, and a lower heart rate at submaximal exercise threshold. Moreover, the resting heart rate variability (HRV) parameters also differed in ME/CFS patients, with a higher low frequency power of HRV (LFP) and a lower high frequency power of HRV (HFP). This corresponds with other studies that suggest a decreased HRV is present in ME/CFS, indicative of a chronically high sympathetic tone (78, 330, 332–344). Hence, the results of the meta-analysis may indicate reduced vagal modulation in ME/CFS, along with increased sympathetic modulation of heart rate (329).
Autonomic changes in ME/CFS are likely to cause an overall effect of vasoconstriction and resultant hypoperfusion (58), which is central to the pathology of ME/CFS (183) (Figure 8). Additionally, vascular dysfunction, endothelial dysfunction, and microclot presence may also promote vasoconstriction and tissue hypoxia. When skeletal muscle is hypoperfused, metabolites accumulate and trigger muscle metaboreflex activation (MMA) (58) to increase the arterial pressure. If preload failure exists in ME/CFS, it is likely that MMA cannot achieve a sufficient rise in perfusion by increasing stroke volume; hence the only way to increase the blood pressure is via further vasoconstriction. This is worsened when ME/CFS patients are upright, as the baroreflex is activated (345), further promoting vasoconstriction in skeletal muscle. Additionally, when B2AdR is dysfunctional, it aggravates the situation by inhibiting vasodilation in skeletal muscle (58). Therefore, three factors cause excessive vasoconstriction: B2AdR dysfunction, excessive baroreflex activation due to hypovolemia, and excessive MMA activation due to the poor metabolic situation. With a chronically high sympathetic tone, there is then a need for sympatholysis in skeletal muscles to prevent vasoconstriction via the α1-adrenegeric receptors (58). Sympatholysis enhances the release of local endogenous, short-lived vasodilators such as adenosine, ATP, prostaglandins, prostacyclin, bradykinin, and protons to act as a compensatory mechanism (58). However, if these vasodilators enter the systemic circulation, they could contribute to ME/CFS symptoms such as fatigue, flu-like symptoms, pain, fever, and sleep disturbances, similar to the effects observed when vasodilators spill over in dysmenorrhea (346). An example of this effect can be observed with bradykinin (purple box within Figure 8) (347, 348), and preload failure experienced in ME/CFS may be correlated with the endogenous vasodilator substances produced in skeletal muscles.
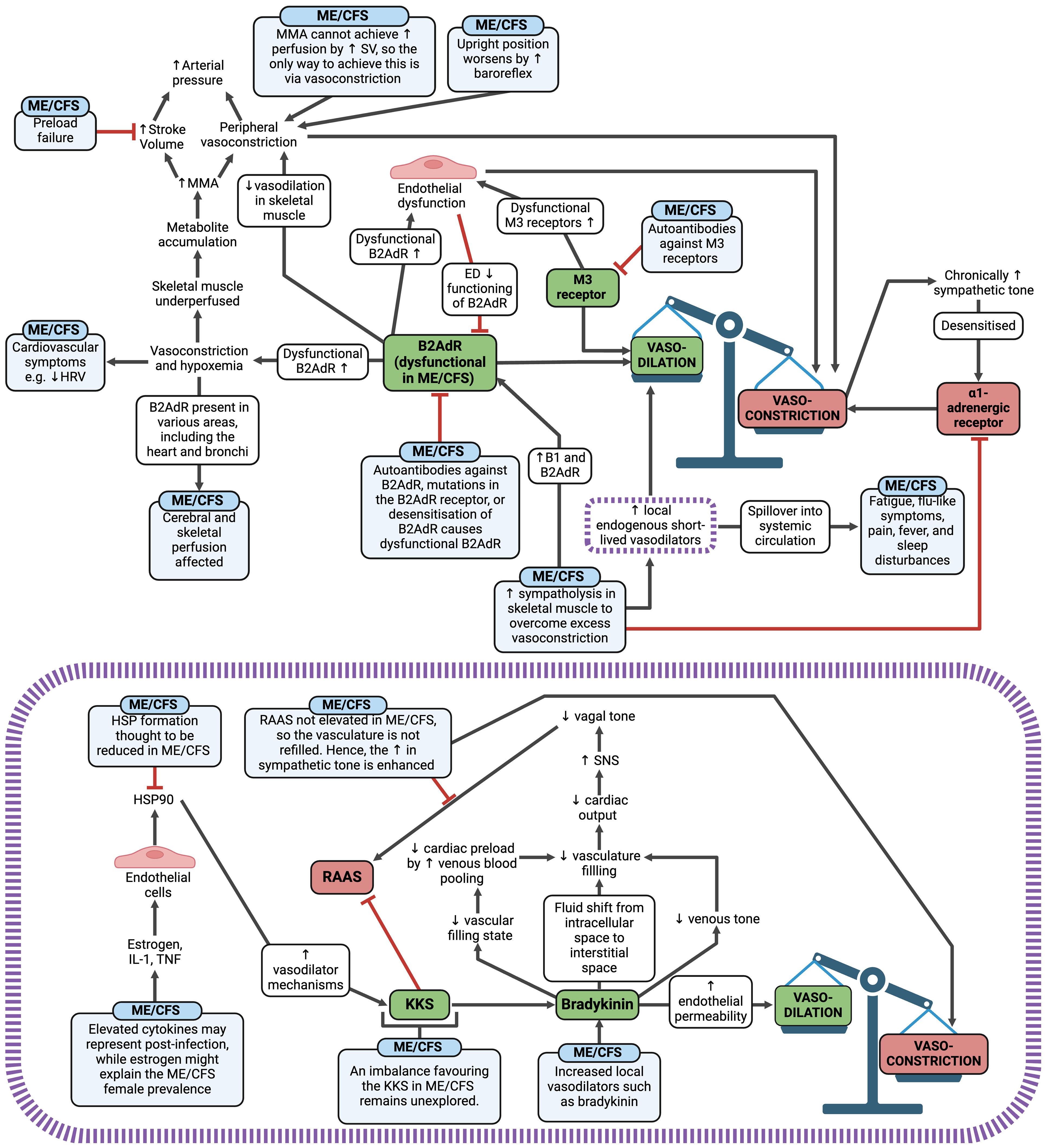
Figure 8 Vasoconstriction and hypoxia mechanisms in ME/CFS and an example of the potential effect of the local vasodilator bradykinin in ME/CFS (illustrated in the purple box) (58, 78, 183, 330, 332–348). Created with Biorender.com. B2AdR, beta-2 adrenergic receptor; ED, endothelial dysfunction; HRV, heart-rate variability; HSP, heat shock protein; IL, interleukin; KKS, kallikrein-kinin system; M3, muscarinic acetylcholine receptor M3; ME/CFS, Myalgic Encephalomyelitis/Chronic Fatigue Syndrome; MMA, muscle metaboreflex activation; RAAS, renin-angiotensin-aldosterone system; SNS, sympathetic nervous system; SV, stroke volume; TNF, tumour necrosis factor.
Abnormal coagulation: the role of microclots and inflammagens in circulation that cause pathological clotting
It has been hypothesised that hypercoagulation plays a significant role in ME/CFS (349–351), but there are still discrepancies between studies (352). Hence, there is a need to investigate this matter in greater detail. In one study, ME/CFS blood samples displayed significant hypercoagulability in thromboelastography (TEG) analyses of both whole blood and platelet poor plasma (313). Platelet hyperactivation was also observed, as well as the presence of microclots containing fibrinogen and amyloid proteins. The area of these “fibrinaloid” microclots was found to be greater in ME/CFS samples in comparison to healthy controls (313).
As mentioned previously, elevated levels of LPS molecules in ME/CFS patients have been documented (191) with increased levels of IgM and IgA antibodies to LPS in serum (205). LPS can directly and acutely bind to plasma proteins such as fibrinogen to promote the formation of these “fibrinaloid” microclot deposits (353). Hence, not only does LPS induce chronic inflammation via cytokine production; it also has a hypercoagulatory effect by binding to plasma proteins. Since these “fibrinaloid” microclots are usually more resistant to fibrinolysis, they are hypothesised to linger in the circulation and have extended contact with the endothelium (313). Hence, the microclots may result in decreased circulation and blockage of the microcapillaries, resulting in ischemia and therefore many symptoms of ME/CFS, as seen in Figure 9.
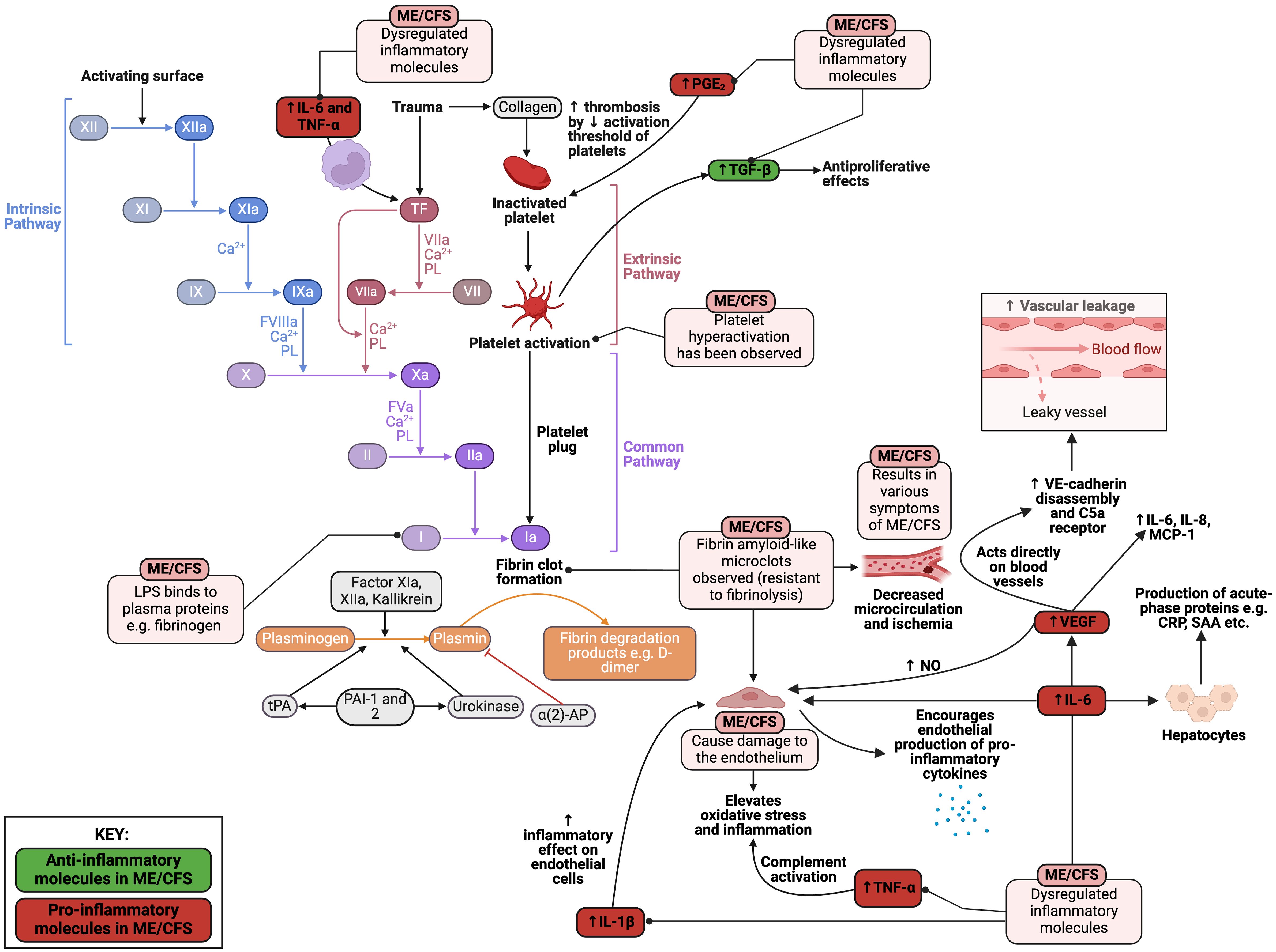
Figure 9 Pathological alterations in the coagulation cascade present in ME/CFS (191, 205, 313, 349–353). Created with Biorender.com. α (2)-AP, alpha-2-antiplasmin; CRP, C-reactive protein; IL, interleukin; LPS, lipopolysaccharide; MCP-1, monocyte chemoattractant protein-1; ME/CFS, Myalgic Encephalomyelitis/Chronic Fatigue Syndrome; NK, natural killer; NO, nitric oxide; PAI-1, plasminogen activator inhibitor 1; PBMC, peripheral blood mononuclear cell; PGE2, prostaglandin-endoperoxide synthase 2; SAA, serum amyloid A; TF, tissue factor; TGF-β, transforming growth factor beta; TNF-α, tumour necrosis factor alpha; tPA, tissue plasminogen activator; VEGF, vascular endothelial growth factor.
Neuroinflammation
PET imaging has displayed elevated cytokines in the spinal cord and brain (46, 354), as well as increased activation of astrocytes (46, 354) and microglia (46, 47, 354). Although cytokines are mainly produced by the immune system, there is also an unclear link between neuroinflammation and these peripheral proinflammatory cytokines (46) and some cytokines may also be produced in the CNS (201). These cytokines can promote cognitive dysfunction as they are able to disrupt the blood-brain barrier (BBB), allowing proinflammatory cytokines (52), cells (such as dendritic, B cells, and T cells) (52), and gut microbes or toxins (355) to translocate into the brain and promote inflammation (Figure 10). One in vitro study found that EBV dUTPase altered the expression of 34 genes with roles related to BBB integrity (137). Hence, EBV UTPase may alter the synaptic structure and function in ME/CFS, as well as dysregulate neuronal communication, influencing cognitive processes.
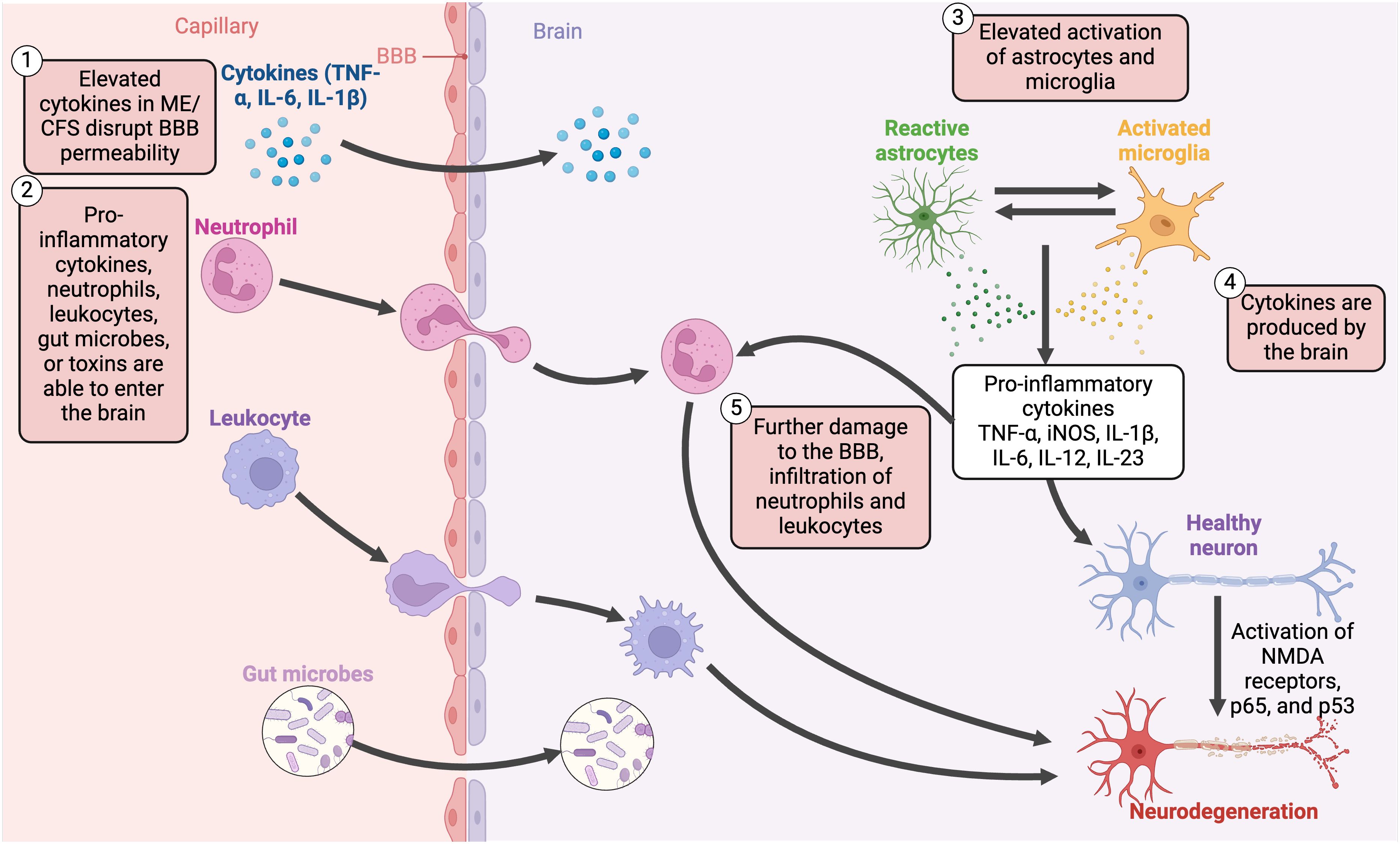
Figure 10 Disruption of the blood-brain barrier, the translocation of pro-inflammatory cytokines, and chronic activation of various non-neuronal cells contribute to neuroinflammatory mechanisms in ME/CFS (45, 47, 52, 201, 251, 354, 355). Created with Biorender.com. BBB, blood-brain barrier; IL, interleukin; iNOS, inducible nitric oxide synthase; ME/CFS, Myalgic Encephalomyelitis/Chronic Fatigue Syndrome; NMDA, N-methyl-D-aspartate; TNF-α, tumour necrosis factor alpha.
Such inflammatory changes potentially cause impaired autoregulation of blood flow (29), hypoperfusion in the brain stem (356–358), and brain tissue hypoxia (22, 23, 26, 29–101, 103, 109, 113, 127–234, 248–256, 266, 297–318, 329–359).
Tilt tests of ME/CFS patients have shown a reduced cerebral blood flow (360) and elevated intracranial pressure (361, 362). These changes may cause disordered sympathetic and parasympathetic activity (158, 360) as well as PEM and cognitive dysfunction (29). Likewise, such neuroinflammation can interact with neurotransmitters, elevate procoagulant activity and thrombosis, and cause endothelial damage, resulting in neurovascular coupling (NVC) dysfunction (363).
Impaired energy metabolism in ME/CFS
Even though an exact metabolic phenotype has not been established (185), a stressed metabolism is present in ME/CFS. When threats to homeostasis occur such as infection, hypoxia, and starvation (364, 365), they involve immune and inflammatory processes that influence energetics and metabolism (366, 367). In the case of ME/CFS, this energy strain may be a consequence of exertion-sensitive tissue hypoxia, leading to systemic patterns of metabolic adaptation and compensation (185). It is thought that over time, the disease shifts from an early hypermetabolic state to a hypometabolic state with decreased metabolites (251) and reduced energy production (59), correlating with patient clinical profiles (185).
Mitochondrial dysfunction is a prominent feature in ME/CFS (60, 368–375). It is known to exacerbate inflammation and redox imbalances (251) by triggering the NLR family pyrin domain containing 3 (NLRP3) inflammasome (251), increasing the release of inflammatory cytokines such as IL-1β and IL-18 (376). Additionally, damaged mitochondria release mtDNA into the cytosol, activating the innate immune system via damage-associated molecular patterns (DAMPs) (251, 377), modulating both innate and adaptive immune responses (377–379). Moreover, mitochondrial damage elevates ROS levels (380), which can in turn damage mtDNA and proteins that may be involved in the electron transport chain (ETC), resulting in lowered ATP production and decreased energy levels (381).
This mitochondrial dysfunction is a result of structural and functional changes. Structural mitochondrial abnormalities have been seen in muscle biopsies from ME/CFS patients (382), but this is not observed in other studies (383). More condensed mitochondrial cristae were observed in blood cells from ME/CFS patients, but the mitochondrial crista length, sise, shape, density, membrane potential, and enzymatic activities of the complexes inside the ETC remained intact (384). In CD4+ T cells, mitochondrial mass was also not altered in ME/CFS (254).
Metabolic abnormalities are evident in ME/CFS, such as reduced mitochondrial respiratory function in ME/CFS neutrophils (371, 375) and PBMCs (385). Although mitochondrial respiration was noted as unchanged in resting and stimulated CD4+ and CD8+ T cells, CD8+ T cells were found to have a reduction in proton leak, ATP synthesis, and mitochondrial membrane potential (254). Decreased ATP production has also been noted in lymphoblasts (386) and PBMCS (387). Additionally, decreased glycolysis has been observed in CD8+ T cells at rest and after activation (254), CD4+ T cells at rest (254), blood and urine samples (187), and PBMCs (388). Similarly, a decreased glycolytic reserve has been found in NK cells from ME/CFS patients (389) and metabolomic analyses have revealed compromised ATP production via the tricarboxylic acid (TCA) cycle (389). These dysfunctions may arise from impaired pyruvate dehydrogenase (PDH) function identified in muscle cells (390) and serum (184), as well as reduced plasma coenzyme Q10 (CoQ10) levels in ME/CFS blood and plasma (372, 391) that is inversely associated with fatigue severity (391). Abnormal oxidative phosphorylation may also occur in neutrophils (371, 375) and PBMCs (388).
If such aerobic metabolism is impaired, the body switches to anaerobic production which generates nominally 18 times less ATP per glucose molecule and produces more lactic acid (392). Two-day cardiopulmonary exercise tests (CPET) have practically demonstrated this reduced anaerobic threshold in ME/CFS (393, 394). Some clinical studies have found elevated lactate levels (395, 396) in ME/CFS patient blood, cerebrospinal fluid, and muscles, suggesting there is reduced oxidative phosphorylation and a switch to anaerobic glycolysis (392, 397–399). Moreover, elevated serum lactate dehydrogenase (LDH) has been observed (400), suggestive of tissue destruction, along with elevated lactic acid levels (47, 397, 401). However, the relationship between lactic acid levels and ME/CFS is still being investigated, as another study reported reduced lactic acid levels in urine, along with lower pyruvate and alanine (187). Additionally, it is worth considering that heightened lactic production, in conjunction with its clearance during rest and exercise, may function in a compensatory manner with lactic acid acting as an energy source. Skeletal muscle acidosis and dysregulated protons have also been found in patients during or after exercise (401–404).
On the other hand, there is also altered utilisation of amino acids and fatty acids as catabolic fuel (184, 186). These studies hypothesised that this impaired PDH activity in ME/CFS may result in increased amino acid utilisation as an energy source. Metabolomic studies have displayed this altered cellular energetics, but the affected metabolites differ between studies (186, 269, 405–407). Some affected metabolites link to amino acid metabolism and changes in key pathways such as amino acid metabolism has been observed (184, 187, 408, 409). Changes in blood glucose and lipids indicate a metabolic shift (405, 406, 410, 411) as there are reduced levels of acyl-carnitine and fatty acids (412), and amino acids from the urea cycle (187, 408). In essence, energy fuel storage, mobilisation, and utilisation may be altered in ME/CFS patients.
This energy impairment likely results in a hypometabolic state as the illness progresses (59, 401, 407, 413), called the “cell danger response” (414–416). This state may be secondary to a persisting stressor, such as a redox imbalance (251), persistent infection (201, 251), injury (201), insufficient nutrients (201), cold temperatures (201), or it could be due to a defect in the “switch” that turns off this protective state (251). However, the reduced blood flow and resulting ischaemia may also act as a stressor itself. This cell danger response is an evolutionary adaptation enabled to protect the cells and host from harm (414–416). At the level of the organism, this is called the “integrated stress response” (ISR) (417). In this process, non-essential energy-consuming mechanisms are reduced, allowing energy molecules to be used for mechanisms that are crucial for viability. Neuroinflammation or fever is presumed to also trigger ISR, as autoantibodies may target neural or immune systems and cause inflammation elsewhere (418). Since redox imbalance is a mechanism involved in ME/CFS, it may be an indication of systemic inflammation in response to persistent infection or injury (251).
Even though mitochondrial dysfunction appears evident in ME/CFS, the causes of such dysfunction are speculative (251) and there is inconsistent evidence correlating mitochondrial dysfunction and ME/CFS (254, 283, 386, 389, 419). In addition, mitochondria modulate intracellular calcium homeostasis and immune regulatory pathways (420, 421), which means these pathways may too be compromised in ME/CFS.
Hormonal alterations
Since stress has been described as a potential trigger for ME/CFS (422) and cause of symptom flare-ups (423, 424), it is likely that the HPA axis- the neurobiological stress system- may be implicated in ME/CFS (53, 425, 426), as well as abnormalities in growth hormone (GH) secretion and dysfunctional adrenergic metabolism (255). Immune and inflammatory responses in the blood are mediated by the HPA axis to prevent any autoimmune alterations (63). If the presence of an initial stressor is prolonged, the HPA axis will become chronically activated and trigger the overproduction of cortisol which, over time, will result in a reduction in cortisol levels (427). It is thought that hormonal changes such as this hypocortisolism may result in symptoms of fatigue experienced by patients (255, 428, 429). A potential negative feedback loop, often colloquially referred to as a “stress crash” or “adrenal burnout”, is likely to develop. However, it is important to note that “adrenal burnout” is not an official medical diagnosis, and the mechanisms involved may not directly involve the adrenals experiencing complete exhaustion (63). Chronic HPA activation elevates cortisol production, lowering immune responses and the production of proinflammatory cytokines. However, the HPA axis will then respond to these heightened cortisol levels by decreasing the production of cortisol over time. This will reduce the protection provided by the HPA axis, attenuating immune and inflammatory changes, and leaving ME/CFS patients more vulnerable to minor stressors (429). It is also possible that HPA sensitivity rather than HPA axis dysfunction exists in patients (63).
In one ME/CFS study, significantly lower levels of adrenocorticotropin (ACTH)/cortisol were found (430). GH peak/insulin-like growth factor-1 (IGF-1) were also significantly reduced in severe ME/CFS patients compared to controls and ME/CFS patients with mild disease. GH/IGF-1, and particularly IGF-1, play various roles in neurons such as neuroprotection, mitochondrial protection, antioxidant defence, and reduction in CNS inflammation (431). Hence, a reduction in these hormones would result in these beneficial mechanisms being reduced (430). Similarly, impaired GH release after exposure to dexamethasone was also found in patients exposed to organophosphates (153). GH is secreted from somatotrophs in the anterior pituitary gland and is influenced positively by growth hormone-releasing hormone (GHRH) and inhibited by somatostatin. Exercise and stress with B-adrenergic stimuli decrease GH secretion by elevating somatostatin tone. Hence, impaired release of GH in these toxin-exposed patients may suggest lowered responsivity of CNS type II glucocorticoid receptors (153).
Serotonin also plays a role in the CNS and controls many stress mechanisms such as the HPA axis through stimulation of corticotropin-releasing hormone (CRH) (432). It is proposed that the production and recycling of dopamine and serotonin is implicated in ME/CFS, which could also be triggered by the EBV dUTPase protein (137). Although an imbalance of these hormones is likely present in ME/CFS, it is unclear whether they are found in elevated or lowered concentrations. One article proposed that excessive serotonin levels could explain classic symptoms of ME/CFS (433), as it would promote the release of excess CRH, and therefore cause chronic reactivation of the HPA axis (434). Furthermore, this excess serotonin could eventually lead to dysregulation of its production (435). Excess serotonin can result in decreased control of various functions, including dysfunctional muscle contraction, migraines, sleep issues, dyspnea, hyperalgesia, and cognitive dysfunction (435). Heightened serotonin levels can also promote the release of dopamine and norepinephrine, resulting in changes to memory, GI problems, mood, and blood coagulation (436). However, lowered dopamine levels are associated with fatigue (437), a commonly present symptom of ME/CFS.
Exposure to toxins such as organophosphates, which are cholinesterase inhibitors, prolongs and amplifies the effects of acetylcholine (153). Acetylcholine is responsible for mood regulation, psychomotor activity, and sleep (438), by activation of central muscarinic receptors, rather than nicotinic receptors (153). Acetylcholine is also known to promote GH secretion (439). However, patients exposed to organophosphates experience a heightened GH response to pyridostigmine (153). It is possible that the somatotrophs developed increased sensitivity to GHRH as pyridostigmine causes intermediate stimulation of GHRH. Alternatively, the more supported hypothesis is that there could be hyper-responsivity of the cholinergic receptors at a hypothalamic level, causing a greater decrease in somatostatin tone, and elevated GH release from the anterior pituitary gland (153).
Various symptoms seen in ME/CFS, such as changes in body weight, appetite, fluid retention, and irregular menstruation, are also observed in hypothalamic dysfunction (440). Dysfunctional hypothalamic function can be seen in ME/CFS patients in the form of up-regulation of hypothalamic 5-hydroxytryptamine (5-HT) receptors (441) and abnormal arginine/vasopressin responses to deprivation tests and water loading (440). However, when the 5-HT-releasing agent D-fenfluramine is used in patients experiencing a neurobehavioral syndrome after exposure to organophosphates, elevated sensitivity of central 5-HT receptors is observed (442).
It is also possible that hypothalamic/pituitary autoimmunity may be present in ME/CFS- particularly in the more severe cases- as antipituitary and antihypothalamic antibodies have been identified (430). Additionally, if gut permeability is indeed increased in ME/CFS patients, microbes and antigens may be able to cross the epithelial barrier into surrounding tissue and blood, potentially crossing the BBB and altering the HPA axis (443). Hence, GI dysbiosis may also promote HPA axis activation (63).
Immune dysfunction
Since one of the most supported hypotheses for the origin of ME/CFS is bacterial or viral (85, 99, 444) infection (94); immune dysregulation has been linked to ME/CFS patients (201, 255) (Table 2) (Figure 11), it is possible that infectious organisms result in chronic symptoms by interfering with host gene expression, immunity, and metabolism (25). More evidence of an infectious aetiology can be seen in the alteration of the number and function of various immune cells, immune profiles, and autoimmune parameters (294, 486). It has been noted that these abnormal immune responses may be more pronounced within the first three years of the disease; as the disease becomes prolonged, these abnormalities appear to subside and T-cell exhaustion becomes evident (225, 254). This suggests that the overactive immune response eventually becomes exhausted or overcome by counter-regulatory mechanisms. Since a link between infections and autoimmune diseases has been well established, this correlation could explain the presence of autoimmune symptoms in ME/CFS (487). However, the measurement of the innate and adaptive immune responses in ME/CFS patients has resulted in conflicting abnormal results (235). Although immune dysfunction is clear in ME/CFS, further studies using defined cohorts, standardised assays, and new technologies are required to determine specific patterns (30). Furthermore, the use of antiviral drugs to treat ME/CFS has been unsuccessful (488), contradicting the hypothesis that ME/CFS could be caused by a clearance failure of the pathogenic microbe (99, 107). A further hypothesis is that ME/CFS could include misdirected immune responses to the initial infection, resulting in a chronic autoimmune disease with molecular mimicry (the “hit and run” hypothesis) (85).
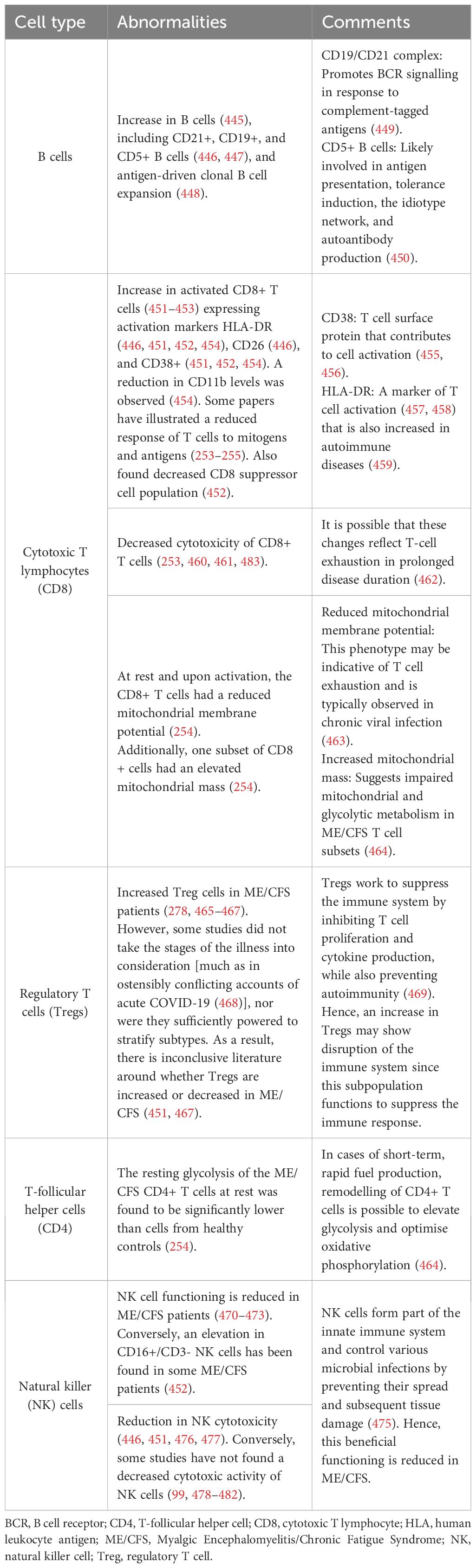
Table 2 Immune alterations present in ME/CFS and changes evident in B cells, T cells, and NK cells (99, 253–255, 278, 445–482).
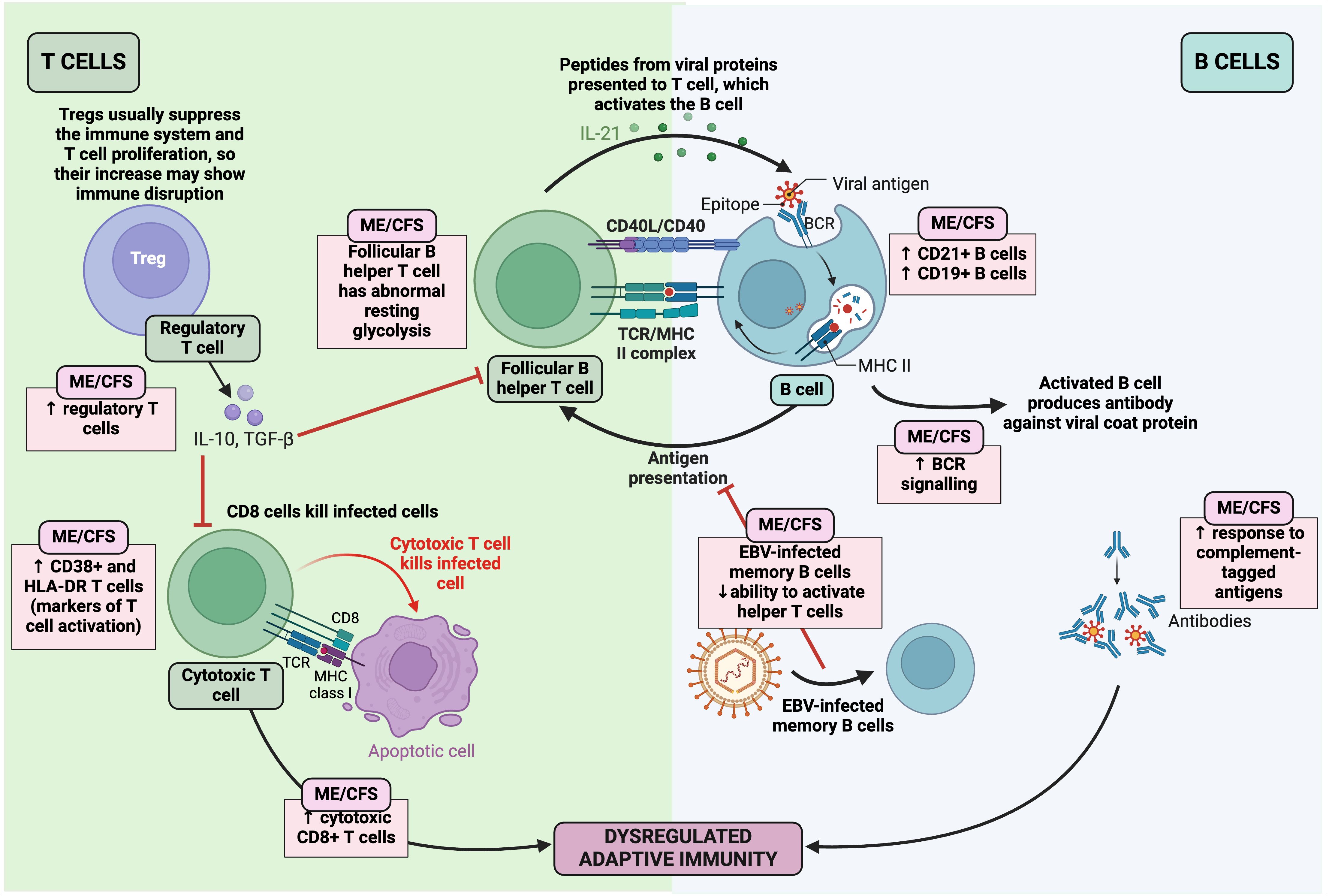
Figure 11 Changes in the adaptive immune system in ME/CFS (25, 52, 201, 278, 445–461, 465–467, 469, 483–485). Created with Biorender.com. BCR, B cell receptor; EBV, Epstein-Barr virus; HLA-DR, human leukocyte antigen- DR isotype; IL, interleukin; ME/CFS, Myalgic Encephalomyelitis/Chronic Fatigue Syndrome; MHC, major histocompatibility complex; TCR, T cell receptor; TGF-β, transforming growth factor-β; Treg, regulatory T cell.
Autoimmunity
Upregulation of autoimmune genes
As in other chronic disorders, some genes in ME/CFS patients have been found to be associated with autoimmunity, such as the HLA alleles (30). In ME/CFS, an increased prevalence of the class II major histocompatibility complex HLA-DQB*01 allele (88), along with two other variants of HLA-DQB1 in combination with two RAGE-374A variants (489) was found. Although some authors have tried to assess the mechanistic link between HLA-II allele expression and the development of ME/CFS, the lack of robust data makes it difficult to support this association (88, 490). Single nucleotide polymorphisms (SNPs) in receptors, enzymes, and transcription factors are also known to cause loss or gain of functions (30) that can increase the risk of autoimmune disease development (491–495). If such changes occur in T cell development, B cell activation and proliferation, or cytokine signalling, it may result in the development of autoimmune diseases. In ME/CFS, SNPs in TLR signalling pathways, the complement cascade, and cytokines have been identified (74, 496).
As mentioned, infectious mononucleosis caused by EBV is a risk factor for various autoimmune diseases (487, 497), and may play a potential role in ME/CFS (140, 498–500). After being infected by EBV, some ME/CFS patients have upregulation of the EBV-induced gene 2– an important gene in immune and CNS function (25). Hence, such gene induction may correlate with various neurological and immune-related symptoms of ME/CFS, as an estimated 38-55% of ME/CFS patients have symptoms that overlap with other autoimmune diseases (501). Similarly, one study illustrated an enhanced IgG reactivity against an EBV repeat sequence, EBNA-6, suggesting that homologous sequences of various human proteins with this repeat sequence might be useful targets for antigenic mimicry (85).
Autoantibody presence
It has been proposed that ME/CFS is a variant of an autoimmune mechanism (29). Typical autoimmune diseases have characteristic pathogenic IgGs correlated with inflammation, tissue injury, and complement activation. Such persistent autoantibodies are hypothesised to disturb vessel autoregulation, causing secondary metabolic and autonomic adaptations. Although these typical changes are not necessarily characteristic of ME/CFS, another autoimmune mechanism may exist in ME/CFS that affects the autonomic control of blood vessel tone and flow autoregulation. Hence, autoimmunity is considered important in the pathology of ME/CFS (30, 85, 182).
In some ME/CFS patients, autoantibodies have been identified, including those against antinuclear antibodies (502–505). These autoantibodies are hypothesised to target nuclear, membrane, and neurotransmitter receptor structures (30, 255). Double-stranded DNA antibodies have also been found in 12% of ME/CFS patients (506), although other studies have failed to find such antibodies in ME/CFS (0.7%) (507). Single-stranded DNA antibodies have also been identified (505), along with anti-ganglioside antibodies (508), autoantibodies against endothelial and neuronal cells (506), and phospholipid autoantibodies (506, 508, 509). Antibodies against cardiolipin were also found in 92-95% of ME/CFS patients (509, 510), although they were only 4% in another study (506). Additionally, antibodies have been identified against human nuclear dUTPase and nuclear envelope protein lamin B1 (511). Hence, this autoantibody presence especially targets the autonomic and central nervous systems (503), which may explain the dysautonomia and immune dysregulation present in ME/CFS (58).
In other autoimmune diseases, natural antibodies are found against adrenergic, muscarinergic, and other G protein coupled receptors (GPCR) (30, 512). In some ME/CFS patients, such antibodies against neurotransmitter receptors have been identified, such as against the muscarinic M1AChR (504), M3AChR (58, 359, 503), and the adrenergic B2AdR (58, 359, 503). The presence of these autoantibodies potentially results in dysfunction in these receptors (58, 513). However, no difference between ME/CFS patients and controls was found with respect to autoantibodies against serotonin, angiotensin, endothelin, mu-opioid, and dopamine (503, 504). Although, autoantibodies against serotonin have been associated with ME/CFS (506, 508).
Various general autoantibodies have also been identified in ME/CFS, such as those against cellular components including anchorage molecules (514), HSP-60 (515), microtubule associated protein 2 (516), cardiolipin in 92-95% of ME/CFS patients in two studies (509, 510) but only 4% in another study (506), and neo-antigens (517). Moreover, 30% of ME/CFS patients in one study were identified to have antibodies against endothelial cells (506). If autoimmunity is present in ME/CFS, it may increase intestinal permeability (518) and explain the various GI manifestations (190).
Soluble autoimmunity markers
B lymphocyte activating factor (BAFF) has been identified in many autoimmune diseases (519) as it regulates survival and maturation of B cells to control the IL-10 production of regulatory B cells (520, 521). Some ME/CFS patients have displayed elevated BAFF, but the gene expression of the BAFF receptor (TNFRSF13C) has been shown to be reduced in ME/CFS patients, suggesting that the elevated serum BAFF is a compensatory mechanism. However, the link between BAFF and autoantibodies in ME/CFS is yet to be investigated (30). Members of the TGF-β family- Activin A and B- are known to control inflammation and muscle mass (522). Heightened levels of activin A and B have been found in ME/CFS with an elevated ratio of activin A or B to the binding protein follostatin (523). Although no causal role has been established, activin A is a pleiotropic cytokine known to influence immune regulation and is altered in various autoimmune and inflammatory diseases (524). IL-21 is also a pleiotropic cytokine (525) important for differentiation of follicular helper T cells that are essential for the germinal centre antibody response (526). When activin A (527) and IL-21’s (528) processes are dysregulated, it is hypothesised to promote autoimmune and inflammatory diseases, although a causal role is still not shown (527).
Management of ME/CFS
As well as there being insufficient diagnostic testing available for ME/CFS patients, there are also no effective therapies (12, 26, 60, 488, 529–531) and few established non-pharmacological treatments for ME/CFS (11, 29). The lack of awareness (60), paucity of diagnostic tools (60), heterogeneity between patients (26, 60, 529), disbelief from health care workers (60), unpredictable relapses, and multiplicity of symptoms have made it difficult to formulate a treatment for ME/CFS. Hence current advice is aimed at symptom management and lifestyle changes (11). The current available treatments/lifestyle modifications are summarised in Figure 12.
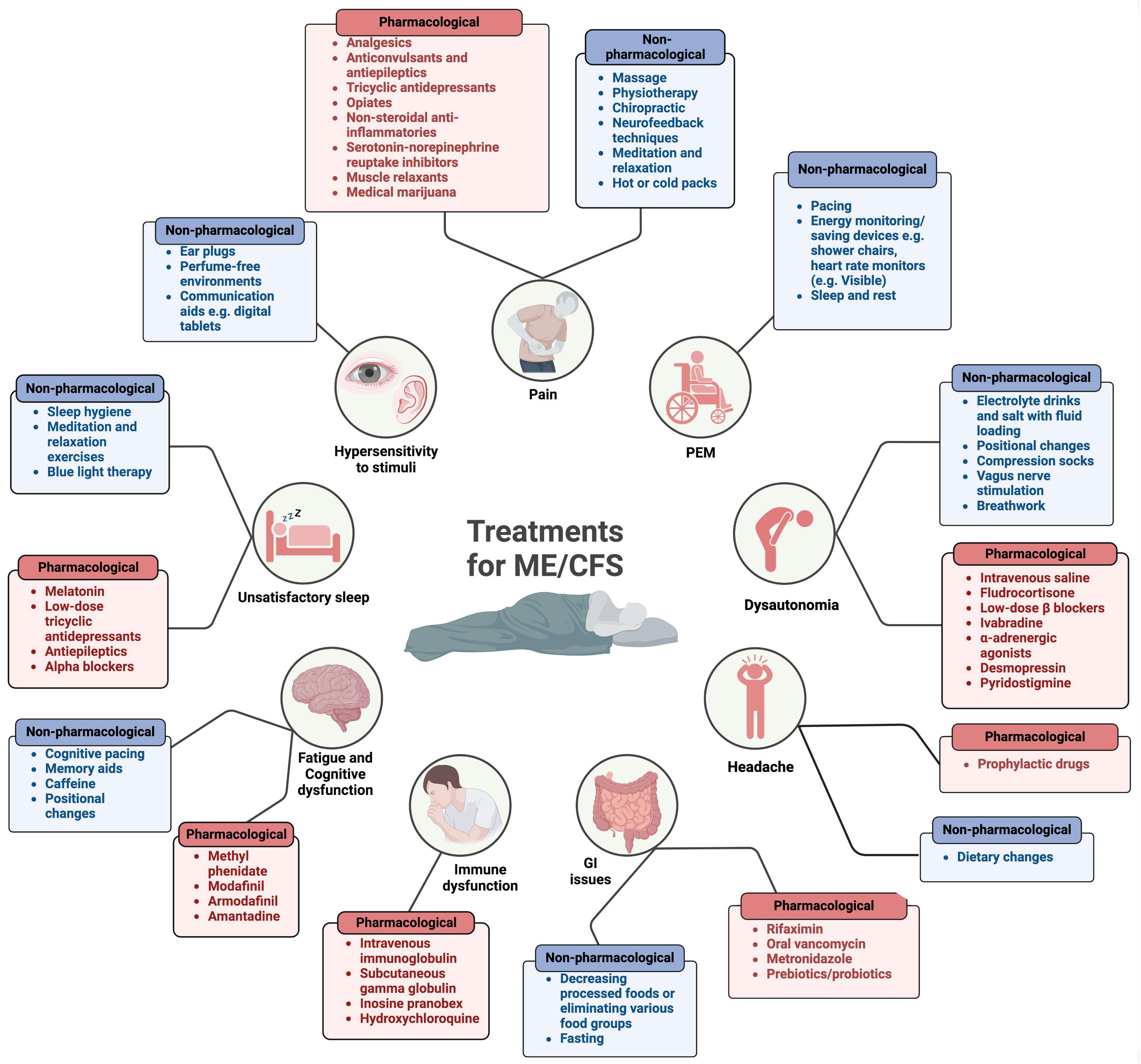
Figure 12 Pharmacological and non-pharmacological treatments currently available for ME/CFS (11, 23, 60, 532). Created with Biorender.com. GI, gastrointestinal; PEM, post-exertional malaise.
Disbelief of doctors
Since not enough is known about the risk factors of ME/CFS, it makes primary prevention unlikely (53). However, secondary prevention is possible to reduce diagnostic delay, the incidence of severe and prolonged disease, and costs of care. One way to minimise diagnostic delays is to rectify the disbelief of doctors and the stigmatization around ME/CFS (53, 533, 534). In 1969, the WHO classified ME/CFS as a neurological disease (44) based on the neurological features of the disease. Following this, epidemic cases of ME/CFS were described as “mass hysteria” in 1970 by two psychiatrists, McEvedy and Beard (535). Although these psychiatrists were faulted for inadequately investigating the patients they described (536) and their conclusions were discredited (537–539), this sparked stigmatization of ME/CFS.
Another mistake that doctors sometimes make is to second-guess their initial diagnosis (39). This scenario may arise when doctors experience uncertainty or apprehension about their assessment, potentially leading to ME/CFS patients feeling neglected. This uncertainty or hesitation may prompt doctors to seek confirmation from other professionals, which may create a traumatic experience for the patient and prolong their diagnosis. Although, since ME/CFS is currently diagnosed based on exclusion criteria and subjective symptom assessment, it is sometimes necessary for patients to be referred to other professionals to exclude other possible diagnoses. For example, referral to psychiatrists may be helpful since ME/CFS seems to coexist with anxiety disorders, symptoms of ME/CFS overlap with Major Depressive Disorder (MDD), and patients with severe ME/CFS may also be at risk of developing secondary MDD (36, 532). However, it can also be detrimental referring ME/CFS patients to psychiatrists (39), not because it excludes other diagnoses, but rather if patients are labelled as hypochondriacs and their condition is attributed solely to psychosomatic origins (23). Hence, validation of the patient’s experiences is important. Additionally, friends and family must offer support to patients, such as helping them acquire handicap placards, attain work and school accommodations, make nutritional adjustments, and apply for disability and housing benefits (23).
To manage and live with ME/CFS, it is also important that patients schedule regular trips to their physician to manage their symptoms, as well as discuss complementary approaches the patient may have adopted- such as new supplements (23). This communication is important to minimise any adverse side effects or drug interactions that may occur (540). Additionally, the reassurance of doctors is important, and they should openly address questions related to a patient’s prognosis. This will help patients maximise their functioning and enhance their quality of life (23). It is worth pointing out that more severe patients are likely to tolerate visits to their doctor poorly, and home visits should be undertaken for them.
Pacing/energy management
Several guidelines have been established to guide the management of ME/CFS. However, some of the recommendations have possibly resulted in more harm than good. One controversial form of self-help is physical activity. When ME/CFS was still believed by many to be a psychological disease (23), doctors often prescribed inappropriate “treatments” such as cognitive behavior therapy (CBT) (39, 530), graded exercise therapy (GET) (39, 530), or the Lightning Process (11) to ME/CFS patients. In 2007 for example, the National Institute for Health and Care Excellence (NICE) released a guideline for clinicians and patients where GET was recommended as a treatment (541). GET first establishes a patient’s baseline of physical activity, and this is then stepped up in fixed increments (11). However, these “treatment” strategies have now been strongly criticised for the harm they have caused (542–544), and in October 2021, GET was removed from the revised NICE guidelines (11). Although, it is important to note that while CBT may not be appropriate as a cure for ME/CFS itself, it could be applied in ME/CFS patients to address symptoms or secondary disorders such as MDD (545).
Furthermore, one misleading and now entirely discredited study (546) was the 2011 PACE (Pacing, Graded Activity, and Cognitive Behavior therapy; a Randomised Evaluation) trial (547). In this paper, the therapies were described as safe with 22% of participants recovering and 60-61% of patients experiencing symptom improvement (547, 548). However, there were specific flaws identified in this paper. Without any clear rationale, the study outcome measures were purposefully modified midtrial to alter the findings in favour of their hypothesis (549, 550). Additionally, patients could worsen during the study and still be classified as “recovered” and the study was unblinded with subjective outcomes. Subsequent review of the raw data revealed that the improvement and recovery rates were not significantly different from the control participants. Moreover, 54% to 74% of patients revealed that they experienced harm after GET (543).
Instead, energy management (pacing) is now emphasised for symptom control (11). Pacing is an individualised approach that monitors energy expenditure to reduce the occurrence, duration, and severity of PEM (23). Energy expenditure encompasses physical, social, cognitive, and emotional activity (11). Avoidance of PEM can help decrease fatigue, cognitive difficulties, sleep disruption, and other symptoms of ME/CFS (551). Some patients use energy monitoring and saving devices such as shower chairs and heart rate monitors (552). Although many commercially available trackers such as Fitbit and Garmin encourage exercise and exertion, the Visible application is a recently designed activity monitor for those with chronic illness (553). According to the amended NICE guidelines, an individualised approach is more appropriate for ME/CFS patients (11). If an ME/CFS patient wishes to incorporate physical activity, it should be closely monitored by a team of specialists as over-exertion has the potential to worsen the symptoms of ME/CFS. Hence, it is the patient’s responsibility to gauge their activity levels while a physiotherapist or occupational therapist oversees the activity. Additionally, patients should be wary of potential relapses or flare-ups to prevent worsening of symptoms.
Nutraceuticals and pharmacological approaches
Many ME/CFS patients take nutritional supplements (530). Vitamin D supplementation can help prevent a vitamin D deficiency (11, 39). If bedbound for extended periods of time, bisphosphonates can also help reduce the risk of osteoporosis (39). If toxin exposure is thought to have triggered the development of ME/CFS, nutritional supplementation of zinc and magnesium may be beneficial in the prophylaxis and therapy of cadmium exposure (154). More research is required around nutraceuticals to optimise their dosages.
Despite there being no U.S. Food and Drug Administration (FDA)-approved treatments for ME/CFS (40), in many cases the symptoms and comorbidities experienced by patients can be treated (24). However, it is important that any medication should be introduced at low dosages as many ME/CFS patients are extremely drug sensitive (23), making them vulnerable to adverse side effects. Since ME/CFS was psychologised at first, many patients were initially treated with antidepressants (60). This misdiagnosis resulted in adverse side effects and dependence on the drug. However, antidepressants are still sometimes prescribed to help if patients are experiencing depression (39).
Treating comorbidities
ME/CFS displays an overlap with several autoimmune or immune-mediated diseases that also have chronic fatigue as a main symptom, such as Hashimoto’s thyroiditis (530), fibromyalgia (FM) (532, 554–557), Mast Cell Activation Disorder (532), sleep apnoea (532), IBS (532), secondary depression or anxiety (532), Ehlers-Danlos syndrome (532), and PoTS (532, 558–563). One ME/CFS speciality clinic found 84% of 960 patients presented with at least one other comorbid condition, often resulting in worsened health (3). Hence, more research on ME/CFS is required to separate it from other diseases that are also associated with chronic fatigue. Treating some of these comorbidities will not cure ME/CFS but might alleviate some of their symptoms and improve some quality of life (23).
Potential therapies
Although some researchers have attempted to establish a treatment for ME/CFS and no reproducible results have been found (60), some treatments seem promising in ME/CFS (Figure 13). Additionally, not many trials meet the clinical design standards to be considered credible. Although no causation has been established for ME/CFS, as the knowledge around the disease expands, so will the potential treatment avenues. Hence, pathways and mechanisms need to be investigated for possible pharmacological intervention. Considering the heterogeneity among ME/CFS patients, however, it is unlikely that one treatment will suit all ME/CFS patients.
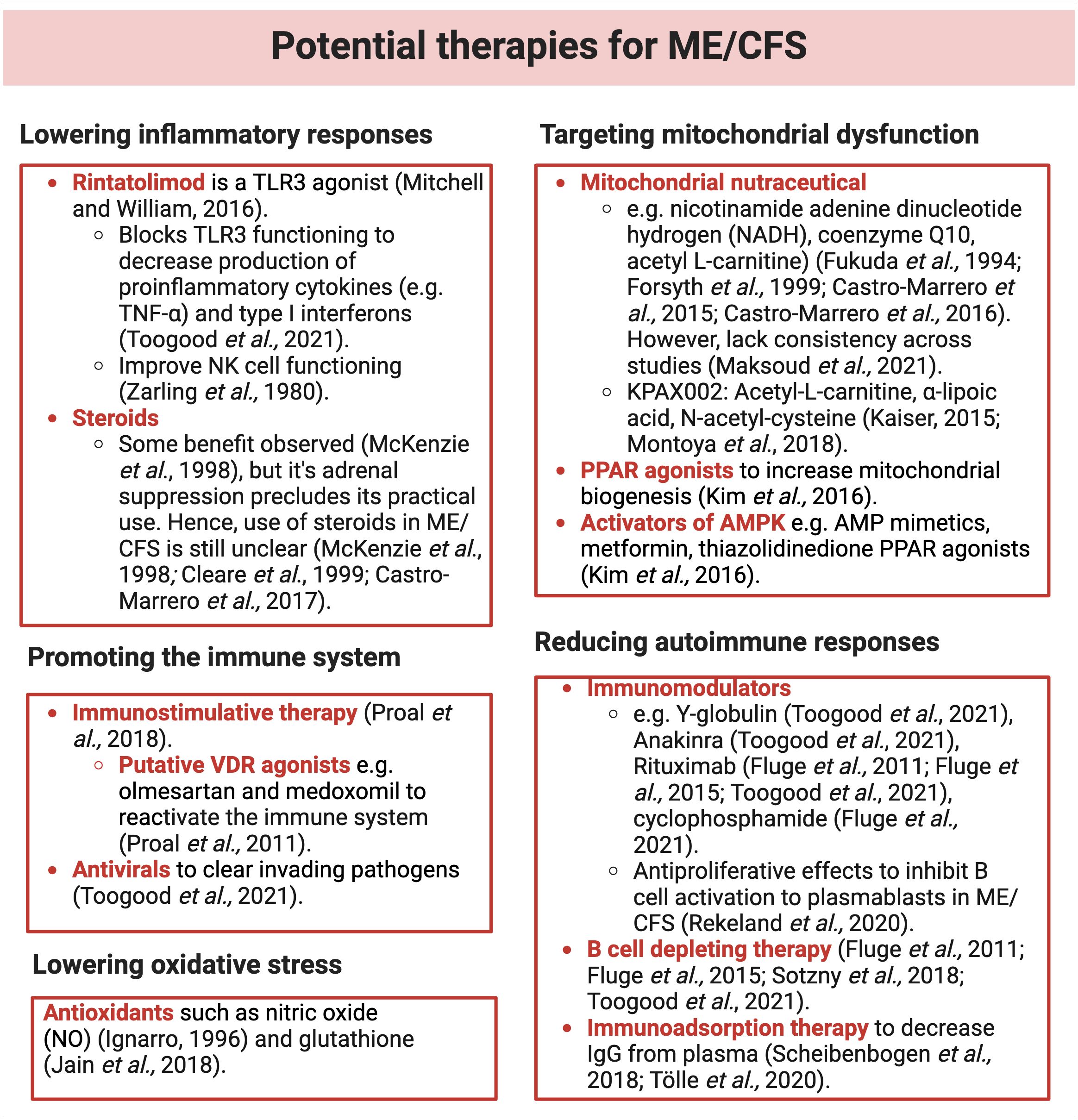
Figure 13 Potential therapies that could be beneficial in the treatment of ME/CFS (22, 29, 29, 30, 84, 193, 530, 564–580). Created with Biorender.com. IgG, immunoglobulin G; ME/CFS, Myalgic Encephalomyelitis/Chronic Fatigue Syndrome; NK, natural killer; NO, nitric oxide; PPAR, peroxisome proliferator-activated receptor; TLR3, toll-like receptor 3; VDR, vitamin D receptor.
Future research recommendations
Most of the research and clinical studies conducted on ME/CFS have inconsistent outcomes and use different study parameters, making the results difficult to compare or combine (11). Most studies are limited owing to their insufficient sample sises, short follow-up times, high dropout rates, inappropriate definitions of recovery, and inclusion of patients with varying conditions (581, 582). Additionally, the small cohort in many studies along with limited comparison groups with other fatiguing illnesses cause inconsistent findings (201). To improve their reproducibility, studies need to use uniform clinical and research criteria, standardise sample collection, and use thorough statistical analyses (305).
Although the research surrounding ME/CFS has increased in the last decade (29), there is still a significant lack of funding for research surrounding ME/CFS (60). In the U.S., the National Institutes of Health (NIH) receives an overall budget of 42 billion dollars. However, in 2019, they only budgeted 15 million dollars towards ME/CFS research, whereas 111 million dollars and 94 million dollars was donated to MS and RA research, respectively (both of which already have drugs on the market). More research is required to define and diagnose ME/CFS as a serious chronic illness (25), as it is uncertain how many patients diagnosed with ME/CFS have an accurate diagnosis (57). Not only does the diagnosis of ME/CFS need to be more accurate, but it also needs to be timely to ensure an earlier diagnosis before a prolonged disease progression occurs (11). Standardisation in diagnosis and disease severity is required to create robust and reproducible results. In combination, more effort needs to be made to include correctly matched controls. For example, since age, lifestyle, medication, geography, and diet are all able to impact microbiome function and composition (583–585), detailed descriptions of food consumption at least 48 hours before the sample collection should be included. Moreover, no defined clinical criteria for ME/CFS makes it even more complicated to compare various study results (52). Additionally, a large GWAS in ME/CFS is desperately needed to assess the biomolecular mechanisms of ME/CFS (56). One study aiming to achieve this is the DecodeME study that launched September 2022 (586). This study aims to pinpoint genetic causes of ME/CFS by testing more than 25,000 DNA samples (586), as a large cohort will help achieve statistical power with reproducible and rigorous results (52). Similarly, identifying microbiomes in ME/CFS blood and brain tissue should be prioritised in ME/CFS research (193). Since some of the main components of ME/CFS include redox imbalance, chronic inflammation, and defective energy metabolism, it is also important to investigate how these components interact bidirectionally (201).
One thing to consider is that because ME/CFS is a severely disabling disease, many patients are house or bedbound and are unable to visit the clinics multiple times for follow-up analyses (39), even though these very severe cases are estimated to be around 25% of the ME/CFS cases (11, 39, 587). It is important to make research more available for this percentage of ME/CFS cases, since they are the most severely affected by the disease and these severe cases are rarely studied (10). It is likely that as the condition worsens, so the probability of identifiable biomarkers for the disease increases, making it crucial to study severely ill patients. ME/CFS needs to be more patient-centered, involving patients in clinical decisions regarding their treatment (11, 53). There is also concern that the COVID-19 pandemic may increase the number of people suffering from ME/CFS (588). Similarly, with the growing emergence of Long COVID cases, many Long COVID symptoms are coinciding with those present in ME/CFS (29). More research is required to determine whether these symptoms are caused by subtle organ damage from the viral infection, or whether these Long COVID patients have a postinfectious immune disturbance whose pathomechanisms is like that in ME/CFS.
It is also important to break the negative stigma that is associated with ME/CFS. ME/CFS is a remarkably misunderstood disease, and many patients have experienced judgement, prejudice, and disbelief from healthcare professionals (11, 39) as some refuse to view ME/CFS as an indisputable clinical entity (39) and question its legitimacy (25). This disbelief and misjudgment (40, 58) delays any possible early diagnosis for the patients and has also resulted in psychologisation of the disease (11, 40, 53). Health and social care professionals need to acknowledge that their patients are living with ME/CFS, and how they have symptoms that severely affect them (11). Hence, they should take time to build empathetic relationships with their patients.
Finally, it is imperative to provide increased support to the ME/CFS community, a necessity that is steadily growing alongside the establishment of research groups and charitable organisations dedicated to aiding ME/CFS patients. One multidisciplinary team consisting of ME/CFS researchers and health professionals, the European Network on ME/CFS (EUROMENE), aims to evaluate healthcare for ME/CFS in Europe, improve research and services in the field, and grasp socioeconomic and clinical dimensions of the disease to issue recommendations accordingly (589). The Open Medicine Foundation is another collaborative effort dedicated to advancing medical research on ME/CFS, fostering engagement within the patient community, and promoting education about the condition (590). The Charité Fatigue Centre in Berlin not only conducts clinical research, but also offers support to both patients and doctors in diagnosing and treating ME/CFS, making it an invaluable interdisciplinary network (591). Additionally, registered charities such as the Austrian “WE&ME” Foundation are dedicated to financing ME/CFS research endeavors and raising awareness about the condition (592). Similarly, “Action for M.E.” in England and Wales aim to spread awareness and education around ME/CFS and raise funds to support these patients and their families. They have also recently worked together with the James Lind Alliance non-profit organisation, ME/CFS clinicians, and ME/CFS sufferers and caretakers to identify ME/CFS research priorities (593). The top three research priorities were found to be (1) understanding the biological mechanism behind PEM and how this can be treated or managed (2), which existing drugs used for other conditions would be useful in treating ME/CFS, and (3) finding accurate and reliable diagnostic testing for ME/CFS. Hence, future research should include recommendations such as these with personal input from ME/CFS sufferers.
Author contributions
EP: Conceptualization, Funding acquisition, Supervision, Writing – review & editing, Resources, Validation. HA: Project administration, Validation, Visualization, Writing – original draft. MK: Writing – review & editing. BJ: Writing – review & editing. BM: Writing – review & editing. DK: Writing – review & editing.
Funding
The author(s) declare financial support was received for the research, authorship, and/or publication of this article. HA thanks the Harry Crossley Foundation for research funding. DK thanks the Novo Nordisk Foundation for funding (grant NNF20CC0035580) and Balvi (grant 18). The funders had no role in study design, data collection and analysis, decision to publish, or preparation of the manuscript. EP thanks the NRF of South Africa (grant number 142142) and SA MRC (self-initiated research (SIR) grant). The content and findings reported and illustrated are the sole deduction, view and responsibility of the researchers and do not reflect the official position and sentiments of the funders.
Conflict of interest
The authors declare that the research was conducted in the absence of any commercial or financial relationships that could be construed as a potential conflict of interest.
Publisher’s note
All claims expressed in this article are solely those of the authors and do not necessarily represent those of their affiliated organizations, or those of the publisher, the editors and the reviewers. Any product that may be evaluated in this article, or claim that may be made by its manufacturer, is not guaranteed or endorsed by the publisher.
References
1. Clayton EW. Beyond myalgic encephalomyelitis/chronic fatigue syndrome: an IOM report on redefining an illness. Jama. (2015) 313:1101–2. doi: 10.1001/jama.2015.1346
2. S. Committee on the Diagnostic Criteria for Myalgic Encephalomyelitis/Chronic Fatigue, P. Board on the Health of Select and M. Institute of, The National Academies Collection. Reports funded by national institutes of health, beyond myalgic encephalomyelitis/Chronic fatigue syndrome: redefining an illness, national academies press (US). Washington (DC: National Academy of Sciences (2015).
3. Bateman L, Darakjy SS, Klimas NG, Peterson DL, Levine SM, Allen A, et al. Chronic fatigue syndrome and co-morbid and consequent conditions: evidence from a multi-site clinical epidemiology study. Fatigue: Biomedicine Health Behav. (2015) 3:1–15. doi: 10.1080/21641846.2014.978109
4. Valdez AR, Hancock EE, Adebayo S, Kiernicki DJ, Proskauer D, Attewell JR, et al. Demographics, and costs of ME/CFS using large scale medical claims data and machine learning. Front Pediatr. (2018) 6:412. doi: 10.3389/fped.2018.00412
5. Jason LA, Katz BZ, Sunnquist M, Torres C, Cotler J, Bhatia S. The prevalence of pediatric myalgic encephalomyelitis/chronic fatigue syndrome in a community−Based sample. Child Youth Care Forum. (2020) 49:563–79. doi: 10.1007/s10566-019-09543-3
6. Nacul LC, Lacerda EM, Pheby D, Campion P, Molokhia M, Fayyaz S, et al. Prevalence of myalgic encephalomyelitis/chronic fatigue syndrome (ME/CFS) in three regions of England: a repeated cross-sectional study in primary care. BMC Med. (2011) 9:91. doi: 10.1186/1741-7015-9-91
7. Bakken IJ, Tveito K, Gunnes N, Ghaderi S, Stoltenberg C, Trogstad L, et al. Two age peaks in the incidence of chronic fatigue syndrome/myalgic encephalomyelitis: a population-based registry study from Norway 2008-2012. BMC Med. (2014) 12:167. doi: 10.1186/PREACCEPT-1843684980131041
8. Lim E-J, Son C-G. Review of case definitions for myalgic encephalomyelitis/chronic fatigue syndrome (ME/CFS). J Trans Med. (2020) 18:289. doi: 10.1186/s12967-020-02455-0
9. Jason LA, Richman JA, Rademaker AW, Jordan KM, Plioplys AV, Taylor RR, et al. A community-based study of chronic fatigue syndrome. Arch Intern Med. (1999) 159:2129–37. doi: 10.1001/archinte.159.18.2129
10. Pendergrast T, Brown A, Sunnquist M, Jantke R, Newton JL, Strand EB, et al. Housebound versus nonhousebound patients with myalgic encephalomyelitis and chronic fatigue syndrome. Chronic Illn. (2016) 12:292–307. doi: 10.1177/1742395316644770
11. NICE. Myalgic encephalomyelitis (or encephalopathy)/chronic fatigue syndrome: diagnosis and management (NICE guideline [NG206]). (2021).
12. Rowe PC, Underhill RA, Friedman KJ, Gurwitt A, Medow MS, Schwartz MS, et al. Myalgic encephalomyelitis/chronic fatigue syndrome diagnosis and management in young people: A primer. Front Pediatr. (2017) 5:121. doi: 10.3389/fped.2017.00121
13. Centers for Disease Control and Prevention. Myalgic encephalomyelitis/chronic fatigue syndrome. U.S. Department of Health & Human Services. (2023).
14. Bhui KS, Dinos S, Ashby D, Nazroo J, Wessely S, White PD. Chronic fatigue syndrome in an ethnically diverse population: the influence of psychosocial adversity and physical inactivity. BMC Med. (2011) 9:26. doi: 10.1186/1741-7015-9-26
15. Carruthers BM, Jain AK, De Meirleir KL, Peterson DL, Klimas NG, Lerner AM, et al. Myalgic encephalomyelitis/chronic fatigue syndrome. J Chronic Fatigue Syndrome. (2003) 11:7–115. doi: 10.1300/J092v11n01_02
16. Carruthers BM, Sande MIvde, Meirleir KLDe, Klimas NG, Broderick G, Mitchell T, et al. Myalgic encephalomyelitis: international consensus criteria. J Intern Med. (2011) 270:327–38. doi: 10.1111/j.1365-2796.2011.02428.x
17. Keech A, Sandler CX, Vollmer-Conna U, Cvejic E, Lloyd AR, Barry BK. Capturing the post-exertional exacerbation of fatigue following physical and cognitive challenge in patients with chronic fatigue syndrome. J Psychosomatic Res. (2015) 79:537–49. doi: 10.1016/j.jpsychores.2015.08.008
18. Jason LA, Benton MC, Valentine L, Johnson A, Torres-Harding S. The economic impact of ME/CFS: individual and societal costs. Dyn Med. (2008) 7:6. doi: 10.1186/1476-5918-7-6
19. The Optimum Health Clinic (OHC). Counting the cost: chronic fatigue syndrome/myalgic encephalomyelitis. (2017).
20. Hickie I, Lloyd A, Hadzi-Pavlovic D, Parker G, Bird K, Wakefield D. Can the chronic fatigue syndrome be defined by distinct clinical features? Psychol Med. (1995) 25:925–35. doi: 10.1017/S0033291700037417
21. Edwards JC, McGrath S, Baldwin A, Livingstone M, Kewley A. The biological challenge of myalgic encephalomyelitis/chronic fatigue syndrome: a solvable problem. Fatigue. (2016) 4:63–9. doi: 10.1080/21641846.2016.1160598
22. Fukuda K, Straus SE, Hickie I, Sharpe MC, Dobbins JG, Komaroff A. The chronic fatigue syndrome: a comprehensive approach to its definition and study International Chronic Fatigue Syndrome Study Group. Ann Intern Med. (1994) 121:953–9. doi: 10.7326/0003-4819-121-12-199412150-00009
23. Bateman L, Bested AC, Bonilla HF, Chheda BV, Chu L, Curtin JM, et al. Myalgic encephalomyelitis/chronic fatigue syndrome: essentials of diagnosis and management. Mayo Clin Proc. (2021) 96:2861–78. doi: 10.1016/j.mayocp.2021.07.004
24. Chang CJ, Hung LY, Kogelnik AM, Kaufman D, Aiyar RS, Chu AM, et al. A comprehensive examination of severely ill ME/CFS patients. Healthcare (Basel). 9. (2021). doi: 10.3390/healthcare9101290
25. Wong TL, Weitzer DJ, Long COVID. and myalgic encephalomyelitis/chronic fatigue syndrome (ME/CFS)-A systemic review and comparison of clinical presentation and symptomatology. Medicina (Kaunas). (2021) 57. doi: 10.3390/medicina57050418
26. Bested AC, Marshall LM. Review of Myalgic Encephalomyelitis/Chronic Fatigue Syndrome: an evidence-based approach to diagnosis and management by clinicians. Rev Environ Health. (2015) 30:223–49. doi: 10.1515/reveh-2015-0026
27. Ramsay MA. Myalgic encephalomyelitis: A baffling syndrome with a tragic aftermath. ME Assoc. (1981).
28. Cairns R, Hotopf M. A systematic review describing the prognosis of chronic fatigue syndrome. Occup Med (Lond). (2005) 55:20–31. doi: 10.1093/occmed/kqi013
29. Fluge Ø., Tronstad KJ, Mella O. Pathomechanisms and possible interventions in myalgic encephalomyelitis/chronic fatigue syndrome (ME/CFS). J Clin Invest 131. (2021). doi: 10.1172/JCI150377
30. Sotzny F, Blanco J, Capelli E, Castro-Marrero J, Steiner S, Murovska M, et al. Myalgic Encephalomyelitis/Chronic Fatigue Syndrome - Evidence for an autoimmune disease. Autoimmun Rev. (2018) 17:601–9. doi: 10.1016/j.autrev.2018.01.009
31. Brittain E, Muirhead N, Finlay AY, Vyas J. Myalgic encephalomyelitis/chronic fatigue syndrome (ME/CFS): major impact on lives of both patients and family members. Medicina (Kaunas) 57. (2021). doi: 10.3390/medicina57010043
32. Kingdon CC, Bowman EW, Curran H, Nacul L, Lacerda EM. Functional status and well-being in people with myalgic encephalomyelitis/chronic fatigue syndrome compared with people with multiple sclerosis and healthy controls. Pharmacoecon Open. (2018) 2:381–92. doi: 10.1007/s41669-018-0071-6
33. Nacul LC, Lacerda EM, Campion P, Pheby D, M.d.L. Drachler JC, Poland F, et al. Molokhia, The functional status and well being of people with myalgic encephalomyelitis/chronic fatigue syndrome and their carers. BMC Public Health. (2011) 11:402. doi: 10.1186/1471-2458-11-402
34. Núñez M, Nunez E, Del Val JL, J. fernandez-huerta C, Alegre M, Roig D, et al. Health-related quality of life in chronic fatigue syndrome versus rheumatoid arthritis as control group. J Chronic Fatigue Syndrome. (2008) 14:31–43. doi: 10.1300/J092v14n02_04
35. Falk Hvidberg M, Brinth LS, Olesen AV, Petersen KD, Ehlers L. The health-related quality of life for patients with myalgic encephalomyelitis/chronic fatigue syndrome (ME/CFS). PloS One. (2015) 10:e0132421. doi: 10.1371/journal.pone.0132421
36. Stein E. Assessment and treatment of patients with ME/CFS: Clinical guidelines for psychiatrists. Hentet. (2005) 11:13.
37. Roberts E, Wessely S, Chalder T, Chang C-K, Hotopf M. Mortality of people with chronic fatigue syndrome: a retrospective cohort study in England and Wales from the South London and Maudsley NHS Foundation Trust Biomedical Research Centre (SLaM BRC) Clinical Record Interactive Search (CRIS) Register. Lancet. (2016) 387:1638–43. doi: 10.1016/S0140-6736(15)01223-4
38. Cox DL. Management of CFS: development and evaluation of a service. Br J Ther Rehabil. (1998) 5:205–9. doi: 10.12968/bjtr.1998.5.4.14088
40. Williams LR, Isaacson-Barash C. Three cases of severe ME/CFS in adults. Healthcare (Basel) 9. (2021). doi: 10.3390/healthcare9020215
41. US Food and Drug Administration. The voice of the patient. In: A series of reports from the U.S. Food and Drug Administration’s (FDA’s) patient-focused drug development initiative. Center for Drug Evaluation and Research (CDER) and Center for Biologics Evaluation and Research (CBER) U.S. Food and Drug Administration (FDA) (2013).
42. Vink M, Vink-Niese F. Work rehabilitation and medical retirement for myalgic encephalomyelitis/chronic fatigue syndrome patients. A review and appraisal of diagnostic strategies. Diagnostics (Basel). (2019) 9. doi: 10.3390/diagnostics9040124
43. Vyas J, Muirhead N, Singh R, Ephgrave R, Finlay AY. Impact of myalgic encephalomyelitis/chronic fatigue syndrome (ME/CFS) on the quality of life of people with ME/CFS and their partners and family members: an online cross-sectional survey. BMJ Open. (2022) 12:e058128. doi: 10.1136/bmjopen-2021-058128
44. World Health Organization. Manual of the international statistical classification of diseases, injuries, and causes of death based on the recommendations of the eighth revision conference. Geneva: World Health Organization (1969). p. 173.
45. Nakatomi Y, Kuratsune H, Watanabe Y. [Neuroinflammation in the brain of patients with myalgic encephalomyelitis/chronic fatigue syndrome]. Brain Nerve. (2018) 70:19–25. doi: 10.11477/mf.1416200945
46. Nakatomi Y, Mizuno K, Ishii A, Wada Y, Tanaka M, Tazawa S, et al. Neuroinflammation in patients with chronic fatigue syndrome/myalgic encephalomyelitis: an ¹¹C-(R)-PK11195 PET study. J Nucl Med. (2014) 55:945–50. doi: 10.2967/jnumed.113.131045
47. Mueller C, Lin JC, Sheriff S, Maudsley AA, Younger JW. Evidence of widespread metabolite abnormalities in Myalgic encephalomyelitis/chronic fatigue syndrome: assessment with whole-brain magnetic resonance spectroscopy. Brain Imaging Behav. (2020) 14:562–72. doi: 10.1007/s11682-018-0029-4
48. Zeineh MM, Kang J, Atlas SW, Raman MM, Reiss AL, Norris JL, et al. Right arcuate fasciculus abnormality in chronic fatigue syndrome. Radiology. (2015) 274:517–26. doi: 10.1148/radiol.14141079
49. Buchwald D, Cheney PR, Peterson DL, Henry B, Wormsley SB, Geiger A, et al. A chronic illness characterised by fatigue, neurologic and immunologic disorders, and active human herpesvirus type 6 infection. Ann Intern Med. (1992) 116:103–13. doi: 10.7326/0003-4819-116-2-103
50. Barnden LR, Shan ZY, Staines DR, Marshall-Gradisnik S, Finegan K, Ireland T, et al. Hyperintense sensorimotor T1 spin echo MRI is associated with brainstem abnormality in chronic fatigue syndrome. NeuroImage Clin. (2018) 20:102–9. doi: 10.1016/j.nicl.2018.07.011
51. Cockshell SJ, Mathias JL. Cognitive functioning in chronic fatigue syndrome: a meta-analysis. psychol Med. (2010) 40:1253–67. doi: 10.1017/S0033291709992054
52. Ariza ME. Myalgic encephalomyelitis/chronic fatigue syndrome: the human herpesviruses are back! Biomolecules. (2021) 11. doi: 10.3390/biom11020185
53. Pheby DFH, Friedman KJ, Murovska M, Zalewski P. Turning a corner in ME/CFS research. Medicina (Kaunas) 57. (2021). doi: 10.3390/medicina57101012
54. Jason LA, Brown A, Clyne E, Bartgis L, Evans M, Brown M. Contrasting case definitions for chronic fatigue syndrome, Myalgic Encephalomyelitis/chronic fatigue syndrome and myalgic encephalomyelitis. Eval Health Prof. (2012) 35:280–304. doi: 10.1177/0163278711424281
55. Bell DS. The doctor’s guide to chronic fatigue syndrome: understanding, treating, and living with Cfids. (Boston, Massachusetts: Da Capo Press) (1994).
56. Dibble JJ, McGrath SJ, Ponting CP. Genetic risk factors of ME/CFS: a critical review. Hum Mol Genet 29. (2020) 29, R117–r124. doi: 10.1093/hmg/ddaa169
57. Newberry F, Hsieh SY, Wileman T, Carding SR. Does the microbiome and virome contribute to myalgic encephalomyelitis/chronic fatigue syndrome? Clin Sci (Lond). (2018) 132:523–42. doi: 10.1042/CS20171330
58. Wirth K, Scheibenbogen C. A Unifying Hypothesis of the Pathophysiology of Myalgic Encephalomyelitis/Chronic Fatigue Syndrome (ME/CFS): Recognitions from the finding of autoantibodies against ß2-adrenergic receptors. Autoimmun Rev. (2020) 19:102527. doi: 10.1016/j.autrev.2020.102527
59. Nacul L, O’Boyle S, Palla L, Nacul FE, Mudie K, Kingdon CC, et al. How myalgic encephalomyelitis/chronic fatigue syndrome (ME/CFS) progresses: the natural history of ME/CFS. Front Neurol. (2020) 11:826doi: 10.3389/fneur.2020.00826
60. Toogood PL, Clauw DJ, Phadke S, Hoffman D. Myalgic encephalomyelitis/chronic fatigue syndrome (ME/CFS): Where will the drugs come from? Pharmacol Res. (2021) 165:105465. doi: 10.1016/j.phrs.2021.105465
61. Chu L, Valencia IJ, Garvert DW, Montoya JG. Onset patterns and course of myalgic encephalomyelitis/chronic fatigue syndrome. Front Pediatr. (2019) 7:12. doi: 10.3389/fped.2019.00012
62. Salit IE. Precipitating factors for the chronic fatigue syndrome. J Psychiatr Res. (1997) 31:59–65. doi: 10.1016/S0022-3956(96)00050-7
63. Tate W, Walker M, Sweetman E, Helliwell A, Peppercorn K, Edgar C, et al. Molecular mechanisms of neuroinflammation in ME/CFS and long COVID to sustain disease and promote relapses. Front Neurol. (2022) 13:877772. doi: 10.3389/fneur.2022.877772
64. Klimas NG, Broderick G, Fletcher MA. Biomarkers for chronic fatigue. Brain Behav Immun. (2012) 26:1202–10. doi: 10.1016/j.bbi.2012.06.006
65. Walsh CM, Zainal NZ, Middleton SJ, Paykel ES. A family history study of chronic fatigue syndrome. Psychiatr Genet. (2001) 11:123–8. doi: 10.1097/00041444-200109000-00003
66. Albright F, Light K, Light A, Bateman L, Cannon-Albright LA. Evidence for a heritable predisposition to Chronic Fatigue Syndrome. BMC Neurol. (2011) 11:62. doi: 10.1186/1471-2377-11-62
67. Buchwald D, Herrell R, Ashton S, Belcourt M, Schmaling K, Sullivan P, et al. A twin study of chronic fatigue. Psychosom Med. (2001) 63:936–43. doi: 10.1097/00006842-200111000-00012
68. Underhill R, O’Gorman R. Prevalence of chronic fatigue syndrome and chronic fatigue within families of CFS patients. J Chronic Fatigue Syndrome. (2006) 13:3–13. doi: 10.1300/J092v13n01_02
69. Perez M, Jaundoo R, Hilton K, Del Alamo A, Gemayel K, Klimas NG, et al. Genetic predisposition for immune system, hormone, and metabolic dysfunction in myalgic encephalomyelitis/chronic fatigue syndrome: A pilot study. Front Pediatr. (2019) 7:206.doi: 10.3389/fped.2019.00206
70. Maher B. Personal genomes: The case of the missing heritability. Nature. (2008) 456:18–21. doi: 10.1038/456018a
71. Lakhani CM, Tierney BT, Manrai AK, Yang J, Visscher PM, Patel CJ. Repurposing large health insurance claims data to estimate genetic and environmental contributions in 560 phenotypes. Nat Genet. (2019) 51:327–34. doi: 10.1038/s41588-018-0313-7
72. Canela-Xandri O, Rawlik K, Tenesa A. An atlas of genetic associations in UK Biobank. Nat Genet. (2018) 50:1593–9. doi: 10.1038/s41588-018-0248-z
73. Sullivan PF, Evengård B, Jacks A, Pedersen NL. Twin analyses of chronic fatigue in a Swedish national sample. Psychol Med. (2005) 35:1327–36. doi: 10.1017/S0033291705005222
74. Carlo-Stella N, Badulli C, De Silvestri A, Bazzichi L, Martinetti M, Lorusso L, et al. A first study of cytokine genomic polymorphisms in CFS: Positive association of TNF-857 and IFNgamma 874 rare alleles. Clin Exp Rheumatol. (2006) 24:179–82.
75. Vangeel E, Van Den Eede F, Hompes T, Izzi B, Del Favero J, Moorkens G, et al. Chronic fatigue syndrome and DNA hypomethylation of the glucocorticoid receptor gene promoter 1F region: associations with HPA axis hypofunction and childhood trauma. Psychosomatic Med 77. (2015). doi: 10.1097/PSY.0000000000000224
76. Kashi AA, Davis RW, Phair RD. The IDO metabolic trap hypothesis for the etiology of ME/CFS. Diagnostics (Basel) 9. (2019). doi: 10.3390/diagnostics9030082
77. Metz R, Duhadaway JB, Kamasani U, Laury-Kleintop L, Muller AJ, Prendergast GC. Novel tryptophan catabolic enzyme IDO2 is the preferred biochemical target of the antitumor indoleamine 2,3-dioxygenase inhibitory compound D-1-methyl-tryptophan. Cancer Res. (2007) 67:7082–7. doi: 10.1158/0008-5472.CAN-07-1872
78. Sommerfeldt L, Portilla H, Jacobsen L, Gjerstad J, Wyller VB. Polymorphisms of adrenergic cardiovascular control genes are associated with adolescent chronic fatigue syndrome. Acta Paediatrica. (2011) 100:293–8. doi: 10.1111/j.1651-2227.2010.02072.x
79. Eisenach JH, Wittwer ED. β-Adrenoceptor gene variation and intermediate physiological traits: prediction of distant phenotype. Exp Physiol. (2010) 95:757–64. doi: 10.1113/expphysiol.2009.048330
80. Wittwer ED, Liu Z, Warner ND, Schroeder DR, Nadeau AM, Allen AR, et al. Beta-1 and beta-2 adrenergic receptor polymorphism and association with cardiovascular response to orthostatic screening. Autonomic Neurosci. (2011) 164:89–95. doi: 10.1016/j.autneu.2011.07.004
81. Snyder EM, Beck KC, Dietz NM, Eisenach JH, Joyner MJ, Turner ST, et al. Arg16Gly polymorphism of the beta2-adrenergic receptor is associated with differences in cardiovascular function at rest and during exercise in humans. J Physiol. (2006) 571:121–30. doi: 10.1113/jphysiol.2005.098558
82. Tanigawa Y, Li J, Justesen JM, Horn H, Aguirre M, DeBoever C, et al. Components of genetic associations across 2,138 phenotypes in the UK Biobank highlight adipocyte biology. Nat Commun. (2019) 10:4064. doi: 10.1038/s41467-019-11953-9
83. Li YR, Li J, Zhao SD, Bradfield JP, Mentch FD, Maggadottir SM, et al. Meta-analysis of shared genetic architecture across ten pediatric autoimmune diseases. Nat Med. (2015) 21:1018–27. doi: 10.1038/nm.3933
84. Fluge Ø, Risa K, Lunde S, Alme K, Rekeland IG, Sapkota D, et al. B-lymphocyte depletion in myalgic encephalopathy/chronic fatigue syndrome. An open-label phase II study with rituximab maintenance treatment. PloS One. (2015) 10:e0129898. doi: 10.1371/journal.pone.0129898
85. Blomberg J, Gottfries CG, Elfaitouri A, Rizwan M, Rosén A. Infection elicited autoimmunity and myalgic encephalomyelitis/chronic fatigue syndrome: an explanatory model. Front Immunol. (2018) 9:229. doi: 10.3389/fimmu.2018.00229
86. Meyer D, C.A. VR, Bitarello BD, C.B. DY, Nunes K. A genomic perspective on HLA evolution. Immunogenetics. (2018) 70:5–27. doi: 10.1007/s00251-017-1017-3
87. Yasukochi Y, Satta Y. A human-specific allelic group of the MHC DRB1 gene in primates. J Physiol Anthropol. (2014) 33:14. doi: 10.1186/1880-6805-33-14
88. Smith J, Fritz EL, Kerr JR, Cleare AJ, Wessely S, Mattey DL. Association of chronic fatigue syndrome with human leucocyte antigen class II alleles. J Clin Pathol. (2005) 58:860–3. doi: 10.1136/jcp.2004.022681
89. Lande A, Fluge Ø, Strand EB, Flåm ST, Sosa DD, Mella O, et al. Human Leukocyte Antigen alleles associated with Myalgic Encephalomyelitis/Chronic Fatigue Syndrome (ME/CFS). Sci Rep. (2020) 10:5267. doi: 10.1038/s41598-020-62157-x
90. Ruiz-Pablos M, Paiva B, Montero-Mateo R, Garcia N, Zabaleta A. Epstein-barr virus and the origin of myalgic encephalomyelitis or chronic fatigue syndrome. Front Immunol. (2021) 12:656797. doi: 10.3389/fimmu.2021.656797
91. Schoeman EM, van der Westhuisen FH, Erasmus E, van Dyk E, Knowles CV, Al-Ali S, et al. Clinically proven mtDNA mutations are not common in those with chronic fatigue syndrome. BMC Med Genet. (2017) 18:29. doi: 10.1186/s12881-017-0387-6
92. Billing-Ross P, Germain A, Ye K, Keinan A, Gu Z, Hanson MR. Mitochondrial DNA variants correlate with symptoms in myalgic encephalomyelitis/chronic fatigue syndrome. J Transl Med. (2016) 14:19. doi: 10.1186/s12967-016-0771-6
93. Venter M, Tomas C, Pienaar IS, Strassheim V, Erasmus E, Ng WF, et al. MtDNA population variation in Myalgic encephalomyelitis/Chronic fatigue syndrome in two populations: a study of mildly deleterious variants. Sci Rep. (2019) 9:2914. doi: 10.1038/s41598-019-39060-1
94. Ortega-Hernandez OD, Shoenfeld Y. Infection, vaccination, and autoantibodies in chronic fatigue syndrome, cause or coincidence? Ann N Y Acad Sci. (2009) 1173:600–9. doi: 10.1111/j.1749-6632.2009.04799.x
95. Arnett SV, Alleva LM, Korossy-Horwood R, Clark IA. Chronic fatigue syndrome–a neuroimmunological model. Med Hypotheses. (2011) 77:77–83. doi: 10.1016/j.mehy.2011.03.030
96. Lacerda EM, Geraghty K, Kingdon CC, Palla L, Nacul L. A logistic regression analysis of risk factors in ME/CFS pathogenesis. BMC Neurol. (2019) 19:275. doi: 10.1186/s12883-019-1468-2
97. Prins JB, van der Meer JW, Bleijenberg G. Chronic fatigue syndrome. Lancet. (2006) 367:346–55. doi: 10.1016/S0140-6736(06)68073-2
98. Naess H, Sundal E, Myhr KM, Nyland HI. Postinfectious and chronic fatigue syndromes: clinical experience from a tertiary-referral centre in Norway. In Vivo. (2010) 24:185–8.
99. Rasa S, Nora-Krukle Z, Henning N, Eliassen E, Shikova E, Harrer T, et al. Chronic viral infections in myalgic encephalomyelitis/chronic fatigue syndrome (ME/CFS). J Transl Med. (2018) 16:268. doi: 10.1186/s12967-018-1644-y
100. Sospedra M, Martin R. Immunology of multiple sclerosis. Annu Rev Immunol. (2005) 23:683–747. doi: 10.1146/annurev.immunol.23.021704.115707
101. Wekerle H. Epstein-Barr virus sparks brain autoimmunity in multiple sclerosis. Nature. (2022) 603:230–2. doi: 10.1038/d41586-022-00382-2
102. Montoya JG, Kogelnik AM, Bhangoo M, Lunn MR, Flamand L, Merrihew LE, et al. Randomized clinical trial to evaluate the efficacy and safety of valganciclovir in a subset of patients with chronic fatigue syndrome. J Med Virol (2013) 85:2101–9.
103. Katz BZ, Shiraishi Y, Mears CJ, Binns HJ, Taylor R. Chronic fatigue syndrome after infectious mononucleosis in adolescents. Pediatrics. (2009) 124:189–93. doi: 10.1542/peds.2008-1879
104. Chia JK, Chia AY. Chronic fatigue syndrome is associated with chronic enterovirus infection of the stomach. J Clin Pathol (2008) 61:43–8.
105. Kerr JR, Gough J, Richards SC, Main J, Enlander D, McCreary M, et al. Antibody to parvovirus B19 nonstructural protein is associated with chronic arthralgia in patients with chronic fatigue syndrome/myalgic encephalomyelitis. J Gen Virol (2010) 91:893–7.
106. Jacobson SK, Daly JS, Thorne GM, McIntosh K. Chronic parvovirus B19 infection resulting in chronic fatigue syndrome: case history andf review. Clin Infect Dis (1997) 24:1048–51.
107. Chia J, Chia A, Voeller M, Lee T, Chang R. Acute enterovirus infection followed by myalgic encephalomyelitis/chronic fatigue syndrome (ME/CFS) and viral persistence. J Clin Pathol. (2010) 63:165–8. doi: 10.1136/jcp.2009.070466
109. Hickie I, Davenport T, Wakefield D, Vollmer-Conna U, Cameron B, Vernon SD, et al. Post-infective and chronic fatigue syndromes precipitated by viral and non-viral pathogens: prospective cohort study. Bmj. (2006) 333:575. doi: 10.1136/bmj.38933.585764.AE
110. Perrin R, Riste L, Hann M, Walther A, Mukherjee A, Heald A. Into the looking glass: Post-viral syndrome post COVID-19. Med Hypotheses (2020) 144:110055.
111. Komaroff AL, Bateman L. Will COVID-19 Lead to Myalgic Encephalomyelitis/Chronic Fatigue Syndrome? Front Med (Lausanne) (2020) 7:606824.
112. Chia JK, Chia LY. Chronic Chlamydia pneumoniae infection: a treatable cause of chronic fatigue syndrome. Clin Infect Dis (1999) 29:452–3.
113. Zhang L, Gough J, Christmas D, Mattey DL, Richards SC, Main J, et al. Microbial infections in eight genomic subtypes of chronic fatigue syndrome/myalgic encephalomyelitis. J Clin Pathol. (2010) 63:156–64. doi: 10.1136/jcp.2009.072561
114. Komaroff AL. Is human herpesvirus-6 a trigger for chronic fatigue syndrome? J Clin Virol (2006) 37 Suppl 1:S39–46.
115. Wray B, Gaughf C, Chandler F Jr., Berry S, Latham J, Wood L, et al. Detection of Epstein-Barr virus and cytomegalovirus in patients with chronic fatigue. Ann Allergy (1993) 71:223–6.
116. Jones JF, Ray CG, Minnich LL, Hicks MJ, Kibler R, Lucas DO. Evidence for active Epstein-Barr virus infection in patients with persistent, unexplained illnesses: elevated anti-early antigen antibodies. Ann Intern Med (1985) 102:1–7.
117. Sejvar JJ, Curns AT, Welburg L, Jones JF, Lundgren LM, Capuron L, et al. Neurocognitive and functional outcomes in persons recovering from West Nile virus illness. J Neuropsychol (2008) 2:477–99.
118. Evengård B, Gräns H, Wahlund E, Nord CE. Increased number of Candida albicans in the faecal microflora of chronic fatigue syndrome patients during the acute phase of illness. Scand J Gastroenterol (2007) 42:1514–5.
119. Moldofsky H, Patcai J. Chronic widespread musculoskeletal pain, fatigue, depression and disordered sleep in chronic post-SARS syndrome; a case-controlled study. BMC Neurol (2011) 11:37.
120. Epstein L, Wong KK, Kallen AJ, Uyeki TM. Post-Ebola Signs and Symptoms in U. S. Survivors. N Engl J Med (2015) 373:2484–6.
121. Seet RC, Quek AM, Lim EC. Post-infectious fatigue syndrome in dengue infection. J Clin Virol (2007) 38:1–6.
123. Mørch K, Hanevik K, Rivenes AC, Bødtker JE, Næss H, Stubhaug B, et al. Chronic fatigue syndrome 5 years after giardiasis: differential diagnoses, characteristics and natural course. BMC Gastroenterol (2013) 13:28.
124. Beqaj SH, Lerner AM, Fitzgerald JT. Immunoassay with cytomegalovirus early antigens from gene products p52 and CM2 (UL44 and UL57) detects active infection in patients with chronic fatigue syndrome. J Clin Pathol (2008) 61:623–6.
125. Lam MH, Wing YK, Yu MW, Leung CM, Ma RC, Kong AP, et al. Mental morbidities and chronic fatigue in severe acute respiratory syndrome survivors: long-term follow-up. Arch Intern Med (2009) 169:2142–7.
126. Sigal LH. Summary of the first 100 patients seen at a lyme disease referral center. Am J Med (1990) 88:577–81.
127. Liu Z, Hollmann C, Kalanidhi S, Grothey A, Keating S, Mena-Palomo I, et al. Increased circulating fibronectin, depletion of natural IgM and heightened EBV, HSV-1 reactivation in ME/CFS and long COVID. medRxiv. (2023). doi: 10.1101/2023.06.23.23291827
128. Banko A, Miljanovic D, Cirkovic A. Systematic review with meta-analysis of active herpesvirus infections in patients with COVID-19: Old players on the new field. Int J Infect Dis. (2023) 130:108–25. doi: 10.1016/j.ijid.2023.01.036
129. Hagemeier SR, Barlow EA, Kleman AA, Kenney SC. The Epstein-Barr virus BRRF1 protein, Na, induces lytic infection in a TRAF2- and p53-dependent manner. J Virol. (2011) 85:4318–29. doi: 10.1128/JVI.01856-10
130. Halpin P, Williams MV, Klimas NG, Fletcher MA, Barnes Z, Ariza ME. Myalgic encephalomyelitis/chronic fatigue syndrome and gulf war illness patients exhibit increased humoral responses to the herpesviruses-encoded dUTPase: Implications in disease pathophysiology. J Med Virol. (2017) 89:1636–45. doi: 10.1002/jmv.24810
131. Lerner AM, Ariza ME, Williams M, Jason L, Beqaj S, Fitzgerald JT, et al. Antibody to Epstein-Barr virus deoxyuridine triphosphate nucleotidohydrolase and deoxyribonucleotide polymerase in a chronic fatigue syndrome subset. PloS One. (2012) 7:e47891. doi: 10.1371/journal.pone.0047891
132. Ariza ME, Rivailler P, Glaser R, Chen M, Williams MV. Epstein-Barr virus encoded dUTPase containing exosomes modulate innate and adaptive immune responses in human dendritic cells and peripheral blood mononuclear cells. PloS One. (2013) 8:e69827. doi: 10.1371/journal.pone.0069827
133. Ariza ME, Glaser R, Williams MV. Human herpesviruses-encoded dUTPases: a family of proteins that modulate dendritic cell function and innate immunity. Front Microbiol. (2014) 5:504. doi: 10.3389/fmicb.2014.00504
134. Murata T, Okuno Y, Sato Y, Watanabe T, Kimura H. Oncogenesis of CAEBV revealed: Intragenic deletions in the viral genome and leaky expression of lytic genes. Rev Med Virol. (2020) 30:e2095. doi: 10.1002/rmv.2095
135. Okuno Y, Murata T, Sato Y, Muramatsu H, Ito Y, Watanabe T, et al. Defective Epstein-Barr virus in chronic active infection and haematological Malignancy. Nat Microbiol. (2019) 4:404–13. doi: 10.1038/s41564-018-0334-0
136. Laichalk LL, Thorley-Lawson DA. Terminal differentiation into plasma cells initiates the replicative cycle of Epstein-Barr virus in vivo. J Virol. (2005) 79:1296–307. doi: 10.1128/JVI.79.2.1296-1307.2005
137. Williams Ph DM, Cox B, Lafuse Ph DW, Ariza ME. Epstein-barr virus dUTPase induces neuroinflammatory mediators: implications for myalgic encephalomyelitis/chronic fatigue syndrome. Clin Ther. (2019) 41:848–63. doi: 10.1016/j.clinthera.2019.04.009
138. Buchwald D, Ashley RL, Pearlman T, Kith P, Komaroff AL. Viral serologies in patients with chronic fatigue and chronic fatigue syndrome. J Med Virol. (1996) 50:25–30. doi: 10.1002/(ISSN)1096-9071
139. Buchwald D, Sullivan JL, Komaroff AL. Frequency of ‘chronic active Epstein-Barr virus infection’ in a general medical practice. Jama. (1987) 257:2303–7. doi: 10.1001/jama.257.17.2303
140. DuBois RE, Seeley JK, Brus I, Sakamoto K, Ballow M, Harada S, et al. Chronic mononucleosis syndrome. South Med J. (1984) 77:1376–82. doi: 10.1097/00007611-198411000-00007
141. Tobi M, Morag A, Ravid Z, Chowers I, Feldman-Weiss V, Michaeli Y, et al. Prolonged atypical illness associated with serological evidence of persistent Epstein-Barr virus infection. Lancet. (1982) 1:61–4. doi: 10.1016/S0140-6736(82)90210-0
142. Holmes GP, Kaplan JE, Stewart JA, Hunt B, Pinsky PF, Schonberger LB. A cluster of patients with a chronic mononucleosis-like syndrome. Is Epstein-Barr Virus cause? JAMA. (1987) 257:2297–302. doi: 10.1001/jama.257.17.2297
143. Hellinger WC, Smith TF, Van Scoy RE, Spitzer PG, Forgacs P, Edson RS. Chronic fatigue syndrome and the diagnostic utility of antibody to Epstein-Barr virus early antigen. Jama. (1988) 260:971–3. doi: 10.1001/jama.260.7.971
144. Straus SE, Dale JK, Tobi M, Lawley T, Preble O, Blaese RM, et al. Acyclovir treatment of the chronic fatigue syndrome Lack of efficacy in a placebo-controlled trial. . N Engl J Med. (1988) 319:1692–8. doi: 10.1056/NEJM198812293192602
145. Gold D, Bowden R, Sixbey J, Riggs R, Katon WJ, Ashley R, et al. Chronic fatigue. A prospective clinical and virologic study. Jama. (1990) 264:48–53. doi: 10.1001/jama.264.1.48
146. Levine PH, Jacobson S, Pocinki AG, Cheney P, Peterson D, Connelly RR, et al. Clinical, epidemiologic, and virologic studies in four clusters of the chronic fatigue syndrome. Arch Intern Med. (1992) 152:1611–6. doi: 10.1001/archinte.152.8.1611
147. Cameron B, Flamand L, Juwana H, Middeldorp J, Naing Z, Rawlinson W, et al. Serological and virological investigation of the role of the herpesviruses EBV, CMV and HHV-6 in post-infective fatigue syndrome. J Med Virol. (2010) 82:1684–8. doi: 10.1002/jmv.21873
148. Loebel M, Eckey M, Sotzny F, Hahn E, Bauer S, Grabowski P, et al. Serological profiling of the EBV immune response in Chronic Fatigue Syndrome using a peptide microarray. PloS One. (2017) 12:e0179124. doi: 10.1371/journal.pone.0179124
149. Blomberg J, Rizwan M, Böhlin-Wiener A, Elfaitouri A, Julin P, Zachrisson O, et al. Antibodies to human herpesviruses in myalgic encephalomyelitis/chronic fatigue syndrome patients. Front Immunol. (2019) 10:1946. doi: 10.3389/fimmu.2019.01946
150. Soto NE, Straus SE. Chronic fatigue syndrome and herpesviruses: the fading evidence. Herpes. (2000) 7:46–50.
151. Gärtner BC, Fischinger JM, Roemer K, Mak M, Fleurent B, Mueller-Lantzsch N. Evaluation of a recombinant line blot for diagnosis of Epstein-Barr Virus compared with ELISA, using immunofluorescence as reference method. J Virol Methods. (2001) 93:89–96. doi: 10.1016/S0166-0934(00)00301-3
152. Tahmaz N, Soutar A, Cherrie JW. Chronic fatigue and organophosphate pesticides in sheep farming: a retrospective study amongst people reporting to a UK pharmacovigilance scheme. Ann Occup Hyg. (2003) 47:261–7. doi: 10.1093/annhyg/meg042
153. Behan PO. Chronic fatigue syndrome as a delayed reaction to chronic low-dose organophosphate exposure. J Nutr Environ Med. (1996) 6:341–50. doi: 10.3109/13590849609007262
154. Pacini S, Fiore MG, Magherini S, Morucci G, Branca JJ, Gulisano M, et al. Could cadmium be responsible for some of the neurological signs and symptoms of Myalgic Encephalomyelitis/Chronic Fatigue Syndrome. Med Hypotheses. (2012) 79:403–7. doi: 10.1016/j.mehy.2012.06.007
155. López E, Arce C, Oset-Gasque MJ, Cañadas S, González MP. Cadmium induces reactive oxygen species generation and lipid peroxidation in cortical neurons in culture. Free Radic Biol Med. (2006) 40:940–51. doi: 10.1016/j.freeradbiomed.2005.10.062
156. de Lange FP, Kalkman JS, Bleijenberg G, Hagoort P, van der Meer JWM, Toni I. Gray matter volume reduction in the chronic fatigue syndrome. NeuroImage. (2005) 26:777–81. doi: 10.1016/j.neuroimage.2005.02.037
157. Hart RP, Rose CS, Hamer RM. Neuropsychological effects of occupational exposure to cadmium. J Clin Exp Neuropsychol. (1989) 11:933–43. doi: 10.1080/01688638908400946
158. Biswal B, Kunwar P, Natelson BH. Cerebral blood flow is reduced in chronic fatigue syndrome as assessed by arterial spin labeling. J Neurol Sci. (2011) 301:9–11. doi: 10.1016/j.jns.2010.11.018
159. Yoshiuchi K, Farkas J, Natelson BH. Patients with chronic fatigue syndrome have reduced absolute cortical blood flow. Clin Physiol Funct Imaging. (2006) 26:83–6. doi: 10.1111/j.1475-097X.2006.00649.x
160. Pacini S, Punzi T, Morucci G, Gulisano M, Ruggiero M. A paradox of cadmium: a carcinogen that impairs the capability of human breast cancer cells to induce angiogenesis. J Environ Pathol Toxicol Oncol. (2009) 28:85–8. doi: 10.1615/JEnvironPatholToxicolOncol.v28.i1
161. Stejskal VD, Danersund A, Lindvall A, Hudecek R, Nordman V, Yaqob A, et al. Metal-specific lymphocytes: biomarkers of sensitivity in man. Neuro Endocrinol Lett. (1999) 20:289–98.
162. Valentine-Thon E, Müller K, Guzzi G, Kreisel S, Ohnsorge P, Sandkamp M. LTT-MELISA is clinically relevant for detecting and monitoring metal sensitivity. Neuro Endocrinol Lett. (2006) 27 Suppl 1:17–24.
163. Gherardi RK, Crépeaux G, Authier F-J. Myalgia and chronic fatigue syndrome following immunization: macrophagic myofasciitis and animal studies support linkage to aluminum adjuvant persistency and diffusion in the immune system. Autoimmun Rev. (2019) 18:691–705. doi: 10.1016/j.autrev.2019.05.006
164. Sleigh KM, Marra FH, Stiver HG. Influenza vaccination: is it appropriate in chronic fatigue syndrome? Am J Respir Med. (2002) 1:3–9. doi: 10.1007/BF03257157
165. Manysheva K, Sherman M, Zhukova N, Kopishinskaia S. Myalgic encephalomyelitis/chronic fatigue syndrome: first described complication after gam-COVID-vac vaccine. Psychiatr Danub. (2022) 34:189–90.
166. Gherardi RK. [Lessons from macrophagic myofasciitis: towards definition of a vaccine adjuvant-related syndrome]. Rev Neurol (Paris). (2003) 159:162–4.
167. Shoenfeld Y, Agmon-Levin N. ‘ASIA’ - autoimmune/inflammatory syndrome induced by adjuvants. J Autoimmun. (2011) 36:4–8. doi: 10.1016/j.jaut.2010.07.003
168. Gherardi RK, Coquet M, Cherin P, Belec L, Moretto P, Dreyfus PA, et al. Macrophagic myofasciitis lesions assess long-term persistence of vaccine-derived aluminum hydroxide in muscle. Brain. (2001) 124:1821–31. doi: 10.1093/brain/124.9.1821
169. Feiring B, Laake I, Bakken IJ, Greve-Isdahl M, Wyller VB, Håberg SE, et al. HPV vaccination and risk of chronic fatigue syndrome/myalgic encephalomyelitis: A nationwide register-based study from Norway. Vaccine. (2017) 35:4203–12. doi: 10.1016/j.vaccine.2017.06.031
170. Prinsen H, de Vries IJM, Torensma R, Pots JM, Mulder SF, van Herpen CML, et al. Humoral and cellular immune responses after influenza vaccination in patients with chronic fatigue syndrome. BMC Immunol. (2012) 13:71. doi: 10.1186/1471-2172-13-71
171. Sleigh KM, Danforth DG, Hall RT, Fleming JA, Stiver HG. Double-blind, randomised study of the effects of influenza vaccination on the specific antibody response and clinical course of patients with chronic fatigue syndrome. Can J Infect Dis. (2000) 11:267–73. doi: 10.1155/2000/602862
172. Golomb BA, Koslik HJ, Redd AJ. Fluoroquinolone-induced serious, persistent, multisymptom adverse effects. BMJ Case Rep 2015. (2015). doi: 10.1136/bcr-2015-209821
173. Balfour JA, Wiseman LR. Moxifloxacin. Drugs. (1999) 57:363–73. doi: 10.2165/00003495-199957030-00007
174. Lipsky BA, Dorr MB, Magner DJ, Talbot GH. Safety profile of sparfloxacin, a new fluoroquinolone antibiotic. Clin Ther. (1999) 21:148–59. doi: 10.1016/S0149-2918(00)88275-2
175. Wimer SM, Schoonover L, Garrison MW. Levofloxacin: a therapeutic review. Clin Ther. (1998) 20:1049–70. doi: 10.1016/S0149-2918(98)80104-5
176. Kaleagasioglu F, Olcay E. Fluoroquinolone-induced tendinopathy: etiology and preventive measures. Tohoku J Exp Med. (2012) 226:251–8. doi: 10.1620/tjem.226.251
178. Sendzik J, Shakibaei M, Schäfer-Korting M, Lode H, Stahlmann R. Synergistic effects of dexamethasone and quinolones on human-derived tendon cells. Int J Antimicrob Agents. (2010) 35:366–74. doi: 10.1016/j.ijantimicag.2009.10.009
179. Kim GK. The risk of fluoroquinolone-induced tendinopathy and tendon rupture: what does the clinician need to know? J Clin Aesthet Dermatol. (2010) 3:49–54.
180. Lowes DA, Wallace C, Murphy MP, Webster NR, Galley HF. The mitochondria targeted antioxidant MitoQ protects against fluoroquinolone-induced oxidative stress and mitochondrial membrane damage in human Achilles tendon cells. Free Radic Res. (2009) 43:323–8. doi: 10.1080/10715760902736275
181. Keel M, Trentz O. Pathophysiology of polytrauma. Injury. (2005) 36:691–709. doi: 10.1016/j.injury.2004.12.037
182. Morris G, Berk M, Galecki P, Maes M. The emerging role of autoimmunity in myalgic encephalomyelitis/chronic fatigue syndrome (ME/cfs). Mol Neurobiol. (2014) 49:741–56. doi: 10.1007/s12035-013-8553-0
183. Stanculescu D, Sepúlveda N, Lim CL, Bergquist J. Lessons from heat stroke for understanding myalgic encephalomyelitis/chronic fatigue syndrome. Front Neurol. (2021) 12:789784. doi: 10.3389/fneur.2021.789784
184. Fluge Ø, Mella O, Bruland O, Risa K, Dyrstad SE, Alme K, et al. Metabolic profiling indicates impaired pyruvate dehydrogenase function in myalgic encephalopathy/chronic fatigue syndrome. JCI Insight. (2016) 1:e89376. doi: 10.1172/jci.insight.89376
185. Hoel F, Hoel A, Pettersen IK, Rekeland IG, Risa K, Alme K, et al. A map of metabolic phenotypes in patients with myalgic encephalomyelitis/chronic fatigue syndrome. JCI Insight 6. (2021). doi: 10.1172/jci.insight.149217
186. Germain A, Ruppert D, Levine SM, Hanson MR. Metabolic profiling of a myalgic encephalomyelitis/chronic fatigue syndrome discovery cohort reveals disturbances in fatty acid and lipid metabolism. Mol Biosyst. (2017) 13:371–9. doi: 10.1039/C6MB00600K
187. Armstrong CW, McGregor NR, Lewis DP, Butt HL, Gooley PR. Metabolic profiling reveals anomalous energy metabolism and oxidative stress pathways in chronic fatigue syndrome patients. Metabolomics. (2015) 11:1626–39. doi: 10.1007/s11306-015-0816-5
188. Kennedy G, Spence VA, McLaren M, Hill A, Underwood C, Belch JJ. Oxidative stress levels are raised in chronic fatigue syndrome and are associated with clinical symptoms. Free Radic Biol Med. (2005) 39:584–9. doi: 10.1016/j.freeradbiomed.2005.04.020
189. Steinsvik EK, Hausken T, Fluge Ø, Mella O, Gilja OH. Gastric dysmotility and gastrointestinal symptoms in myalgic encephalomyelitis/chronic fatigue syndrome. Scandinavian J Gastroenterol. (2023) 58:718–25. doi: 10.1080/00365521.2023.2173533
190. Lakhan SE, Kirchgessner A. Gut inflammation in chronic fatigue syndrome. Nutr Metab (Lond). (2010) 7:79. doi: 10.1186/1743-7075-7-79
191. Giloteaux L, Goodrich JK, Walters WA, Levine SM, Ley RE, Hanson MR. Reduced diversity and altered composition of the gut microbiome in individuals with myalgic encephalomyelitis/chronic fatigue syndrome. Microbiome. (2016) 4:30. doi: 10.1186/s40168-016-0171-4
192. Lupo GFD, Rocchetti G, Lucini L, Lorusso L, Manara E, Bertelli M, et al. Potential role of microbiome in Chronic Fatigue Syndrome/Myalgic Encephalomyelits (CFS/ME). Sci Rep. (2021) 11:7043. doi: 10.1038/s41598-021-86425-6
193. Proal A, Marshall T. Myalgic encephalomyelitis/chronic fatigue syndrome in the era of the human microbiome: persistent pathogens drive chronic symptoms by interfering with host metabolism, gene expression, and immunity. Front Pediatr. (2018) 6:373. doi: 10.3389/fped.2018.00373
194. Nagy-Szakal D, Williams BL, Mishra N, Che X, Lee B, Bateman L, et al. Fecal metagenomic profiles in subgroups of patients with myalgic encephalomyelitis/chronic fatigue syndrome. Microbiome. (2017) 5:44. doi: 10.1186/s40168-017-0261-y
195. Machiels K, Joossens M, Sabino J, De Preter V, Arijs I, Eeckhaut V, et al. A decrease of the butyrate-producing species Roseburia hominis and Faecalibacterium prausnitzii defines dysbiosis in patients with ulcerative colitis. Gut. (2014) 63:1275–83. doi: 10.1136/gutjnl-2013-304833
196. Segain JP, Raingeard de la Blétière D, Bourreille A, Leray V, Gervois N, Rosales C, et al. Butyrate inhibits inflammatory responses through NFkappaB inhibition: implications for Crohn’s disease. Gut. (2000) 47:397–403. doi: 10.1136/gut.47.3.397
197. Ríos-Covián D, Ruas-Madiedo P, Margolles A, Gueimonde M, de Los Reyes-Gavilán CG, Salazar N. Intestinal short chain fatty acids and their link with diet and human health. Front Microbiol. (2016) 7:185. doi: 10.3389/fmicb.2016.00185
198. Armstrong CW, McGregor NR, Lewis DP, Butt HL, Gooley PR. The association of fecal microbiota and fecal, blood serum and urine metabolites in myalgic encephalomyelitis/chronic fatigue syndrome. Metabolomics. (2017) 13:1–13. doi: 10.1007/s11306-016-1145-z
199. Rizzatti G, Lopetuso LR, Gibiino G, Binda C, Gasbarrini A. Proteobacteria: A common factor in human diseases. BioMed Res Int. (2017) 2017:9351507. doi: 10.1155/2017/9351507
200. Carroll IM, Ringel-Kulka T, Siddle JP, Ringel Y. Alterations in composition and diversity of the intestinal microbiota in patients with diarrhea-predominant irritable bowel syndrome. Neurogastroenterol Motil. (2012) 24:521–30.e248. doi: 10.1111/j.1365-2982.2012.01891.x
201. Komaroff AL, Lipkin WI. Insights from myalgic encephalomyelitis/chronic fatigue syndrome may help unravel the pathogenesis of postacute COVID-19 syndrome. Trends Mol Med. (2021) 27:895–906. doi: 10.1016/j.molmed.2021.06.002
202. Ercoli G, Fernandes VE, Chung WY, Wanford JJ, Thomson S, Bayliss CD, et al. Intracellular replication of Streptococcus pneumoniae inside splenic macrophages serves as a reservoir for septicaemia. Nat Microbiol. (2018) 3:600–10. doi: 10.1038/s41564-018-0147-1
203. Baldelli V, Scaldaferri F, Putignani L, Del Chierico F. The role of enterobacteriaceae in gut microbiota dysbiosis in inflammatory bowel diseases. Microorganisms 9. (2021). doi: 10.3390/microorganisms9040697
204. Scales BS, Dickson RP, Huffnagle GB. A tale of two sites: how inflammation can reshape the microbiomes of the gut and lungs. J Leukoc Biol. (2016) 100:943–50. doi: 10.1189/jlb.3MR0316-106R
205. Maes M, Mihaylova I, Leunis JC. Increased serum IgA and IgM against LPS of enterobacteria in chronic fatigue syndrome (CFS): indication for the involvement of gram-negative enterobacteria in the etiology of CFS and for the presence of an increased gut-intestinal permeability. J Affect Disord. (2007) 99:237–40. doi: 10.1016/j.jad.2006.08.021
206. Page MJ, Kell DB, Pretorius E. The role of lipopolysaccharide-induced cell signalling in chronic inflammation. Chronic Stress (Thousand Oaks). (2022) 6:24705470221076390. doi: 10.1177/24705470221076390
207. Tan Y, Kagan JC. A cross-disciplinary perspective on the innate immune responses to bacterial lipopolysaccharide. Mol Cell. (2014) 54:212–23. doi: 10.1016/j.molcel.2014.03.012
208. Płóciennikowska A, Hromada-Judycka A, Borzęcka K, Kwiatkowska K. Co-operation of TLR4 and raft proteins in LPS-induced pro-inflammatory signaling. Cell Mol Life Sci. (2015) 72:557–81. doi: 10.1007/s00018-014-1762-5
209. Rutherford NJ, Sacino AN, Brooks M, Ceballos-Diaz C, Ladd TB, Howard JK, et al. Studies of lipopolysaccharide effects on the induction of α-synuclein pathology by exogenous fibrils in transgenic mice. Mol Neurodegener. (2015) 10:32. doi: 10.1186/s13024-015-0029-4
210. Kell DB, Pretorius E. On the translocation of bacteria and their lipopolysaccharides between blood and peripheral locations in chronic, inflammatory diseases: the central roles of LPS and LPS-induced cell death. Integr Biol (Camb). (2015) 7:1339–77. doi: 10.1039/c5ib00158g
211. Liu M, Bing G. Lipopolysaccharide animal models for Parkinson’s disease. Parkinsons Dis. (2011) 2011:327089. doi: 10.4061/2011/327089
212. Zielen S, Trischler J, Schubert R. Lipopolysaccharide challenge: immunological effects and safety in humans. Expert Rev Clin Immunol. (2015) 11:409–18. doi: 10.1586/1744666X.2015.1012158
213. Frey D, Jung S, Brackmann F, Richter-Kraus M, Trollmann R. Hypoxia potentiates LPS-mediated cytotoxicity of BV2 microglial cells in vitro by synergistic effects on glial cytokine and nitric oxide system. Neuropediatrics. (2015) 46:321–8. doi: 10.1055/s-00000041
214. Hoshino K, Takeuchi O, Kawai T, Sanjo H, Ogawa T, Takeda Y, et al. Cutting edge: Toll-like receptor 4 (TLR4)-deficient mice are hyporesponsive to lipopolysaccharide: evidence for TLR4 as the Lps gene product. J Immunol. (1999) 162:3749–52. doi: 10.4049/jimmunol.162.7.3749
215. Fujimoto Y, Shimoyama A, Suda Y, Fukase K. Synthesis and immunomodulatory activities of Helicobacter pylori lipophilic terminus of lipopolysaccharide including lipid A. Carbohydr Res. (2012) 356:37–43. doi: 10.1016/j.carres.2012.03.013
216. Medzhitov R. Origin and physiological roles of inflammation. Nature. (2008) 454:428–35. doi: 10.1038/nature07201
217. Rossol M, Heine H, Meusch U, Quandt D, Klein C, Sweet MJ, et al. LPS-induced cytokine production in human monocytes and macrophages. Crit Rev Immunol. (2011) 31:379–446. doi: 10.1615/CritRevImmunol.v31.i5
218. Savage CD, Lopez-Castejon G, Denes A, Brough D. NLRP3-inflammasome activating DAMPs stimulate an inflammatory response in glia in the absence of priming which contributes to brain inflammation after injury. Front Immunol. (2012) 3:288. doi: 10.3389/fimmu.2012.00288
219. Maes M, Coucke F, Leunis JC. Normalization of the increased translocation of endotoxin from gram negative enterobacteria (leaky gut) is accompanied by a remission of chronic fatigue syndrome. Neuro Endocrinol Lett. (2007) 28:739–44.
220. Paez-Espino D, Eloe-Fadrosh EA, Pavlopoulos GA, Thomas AD, Huntemann M, Mikhailova N, et al. Uncovering earth’s virome. Nature. (2016) 536:425–30. doi: 10.1038/nature19094
221. De Sordi L, Lourenço M, Debarbieux L. “I will survive”: A tale of bacteriophage-bacteria coevolution in the gut. Gut Microbes. (2019) 10:92–9. doi: 10.1080/19490976.2018.1474322
222. Wagner J, Maksimovic J, Farries G, Sim WH, Bishop RF, Cameron DJ, et al. Bacteriophages in gut samples from pediatric Crohn’s disease patients: metagenomic analysis using 454 pyrosequencing. Inflammation Bowel Dis. (2013) 19:1598–608. doi: 10.1097/MIB.0b013e318292477c
223. Pérez-Brocal V, García-López R, Vázquez-Castellanos JF, Nos P, Beltrán B, Latorre A, et al. Study of the viral and microbial communities associated with Crohn’s disease: a metagenomic approach. Clin Transl Gastroenterol. (2013) 4:e36. doi: 10.1038/ctg.2013.9
224. Wang W, Jovel J, Halloran B, Wine E, Patterson J, Ford G, et al. Metagenomic analysis of microbiome in colon tissue from subjects with inflammatory bowel diseases reveals interplay of viruses and bacteria. Inflammation Bowel Dis. (2015) 21:1419–27. doi: 10.1097/MIB.0000000000000344
225. Hornig M, Montoya JG, Klimas NG, Levine S, Felsenstein D, Bateman L, et al. Distinct plasma immune signatures in ME/CFS are present early in the course of illness. Sci Adv 1. (2015). doi: 10.1126/sciadv.1400121
226. Nacul L, de Barros B, Kingdon CC, Cliff JM, Clark TG, Mudie K, et al. Evidence of clinical pathology abnormalities in people with myalgic encephalomyelitis/chronic fatigue syndrome (ME/CFS) from an analytic cross-sectional study. Diagnostics (Basel) 9. (2019). doi: 10.3390/diagnostics9020041
227. Lorusso L, Mikhaylova SV, Capelli E, Ferrari D, Ngonga GK, Ricevuti G. Immunological aspects of chronic fatigue syndrome. Autoimmun Rev. (2009) 8:287–91. doi: 10.1016/j.autrev.2008.08.003
228. Hornig M, Gottschalk G, Peterson DL, Knox KK, Schultz AF, Eddy ML, et al. Cytokine network analysis of cerebrospinal fluid in myalgic encephalomyelitis/chronic fatigue syndrome. Mol Psychiatry. (2016) 21:261–9. doi: 10.1038/mp.2015.29
229. Broderick G, Fuite J, Kreitz A, Vernon SD, Klimas N, Fletcher MA. A formal analysis of cytokine networks in chronic fatigue syndrome. Brain Behav Immun. (2010) 24:1209–17. doi: 10.1016/j.bbi.2010.04.012
230. Kuratsune H. [Overview of chronic fatigue syndrome focusing on prevalence and diagnostic criteria]. Nihon Rinsho. (2007) 65:983–90.
231. Montoya JG, Holmes TH, Anderson JN, Maecker HT, Rosenberg-Hasson Y, Valencia IJ, et al. Cytokine signature associated with disease severity in chronic fatigue syndrome patients. Proc Natl Acad Sci U.S.A. (2017) 114:E7150–e7158. doi: 10.1073/pnas.1710519114
232. Moudgil KD, Choubey D. Cytokines in autoimmunity: role in induction, regulation, and treatment. J Interferon Cytokine Res. (2011) 31:695–703. doi: 10.1089/jir.2011.0065
233. McKinney EF, Smith KG. T cell exhaustion and immune-mediated disease-the potential for therapeutic exhaustion. Curr Opin Immunol. (2016) 43:74–80. doi: 10.1016/j.coi.2016.09.005
234. Sen DR, Kaminski J, Barnitz RA, Kurachi M, Gerdemann U, Yates KB, et al. The epigenetic landscape of T cell exhaustion. Science. (2016) 354:1165–9. doi: 10.1126/science.aae0491
235. Blundell S, Ray KK, Buckland M, White PD. Chronic fatigue syndrome and circulating cytokines: A systematic review. Brain Behav Immun. (2015) 50:186–95. doi: 10.1016/j.bbi.2015.07.004
238. Almehed K, d'Elia HF, Bokarewa M, Carlsten H. Role of resistin as a marker of inflammation in systemic lupus erythematosus. Arthritis Res Ther (2008) 10:R15.
239. Huang X, Yang Z. Resistin's, obesity and insulin resistance: the continuing disconnect between rodents and humans. J Endocrinol Invest (2016) 39:607–15.
240. Idriss HT, Naismith JH. TNF alpha and the TNF receptor superfamily: structure-function relationship(s). Microsc Res Tech (2000) 50:184–95.
241. Landi A, Broadhurst D, Vernon SD, Tyrrell DL, Houghton M. Reductions in circulating levels of IL-16, IL-7 and VEGF-a in myalgic encephalomyelitis/chronic fatigue syndrome. Cytokine (2016) 78:27–36.
243. Miyamoto H, Saura R, Harada T, Doita M, Mizuno K. The role of cyclooxygenase-2 and inflammatory cytokines in pain induction of herniated lumbar intervertebral disc. Kobe J Med Sci (2000) 46:13–28.
244. Procaccini C, Jirillo E, Matarese G. Leptin as an immunomodulator. Mol Aspects Med (2012) 33:35–45.
245. Procaccini C, La Rocca C, Carbone F, De Rosa V, Galgani M, Matarese G. Leptin as immune mediator: Interaction between neuroendocrine and immune system. Dev Comp Immunol (2017) 66:120–9.
246. Simons M, Gordon E, Claesson-Welsh L. Mechanisms and regulation of endothelial VEGF receptor signalling. Nat Rev Mol Cell Biol (2016) 17:611–25.
247. Tanaka T, Narazaki M, Kishimoto T. IL-6 in inflammation, immunity, and disease. Cold Spring Harb Perspect Biol (2014) 6:a016295.
248. Muri J, Kopf M. Redox regulation of immunometabolism. Nat Rev Immunol. (2021) 21:363–81. doi: 10.1038/s41577-020-00478-8
249. Mittal M, Siddiqui MR, Tran K, Reddy SP, Malik AB. Reactive oxygen species in inflammation and tissue injury. Antioxid Redox Signal. (2014) 20:1126–67. doi: 10.1089/ars.2012.5149
250. Biswas SK. Does the interdependence between oxidative stress and inflammation explain the antioxidant paradox? Oxid Med Cell Longev. (2016) 2016:5698931. doi: 10.1155/2016/5698931
251. Paul BD, Lemle MD, Komaroff AL, Snyder SH. Redox imbalance links COVID-19 and myalgic encephalomyelitis/chronic fatigue syndrome. Proc Natl Acad Sci U.S.A. (2021) 118. doi: 10.1073/pnas.2024358118
252. Nijs J, Nees A, Paul L, De Kooning M, Ickmans K, Meeus M, et al. Altered immune response to exercise in patients with chronic fatigue syndrome/myalgic encephalomyelitis: a systematic literature review. Exerc Immunol Rev. (2014) 20:94–116.
253. Brenu EW, van Driel ML, Staines DR, Ashton KJ, Ramos SB, Keane J, et al. Immunological abnormalities as potential biomarkers in Chronic Fatigue Syndrome/Myalgic Encephalomyelitis. J Transl Med. (2011) 9:81. doi: 10.1186/1479-5876-9-81
254. Mandarano AH, Maya J, Giloteaux L, Peterson DL, Maynard M, Gottschalk CG, et al. Myalgic encephalomyelitis/chronic fatigue syndrome patients exhibit altered T cell metabolism and cytokine associations. J Clin Invest. (2020) 130:1491–505. doi: 10.1172/JCI132185
255. Cortes Rivera M, Mastronardi C, Silva-Aldana CT, Arcos-Burgos M, Lidbury BA. Myalgic encephalomyelitis/chronic fatigue syndrome: A comprehensive review. Diagnostics (Basel). (2019) 9. doi: 10.3390/diagnostics9030091
256. Kell DB, Pretorius E. The potential role of ischaemia-reperfusion injury in chronic, relapsing diseases such as rheumatoid arthritis, Long COVID, and ME/CFS: evidence, mechanisms, and therapeutic implications. Biochem J. (2022) 479:1653–708. doi: 10.1042/BCJ20220154
257. Maes M, Kubera M, Uytterhoeven M, Vrydags N, Bosmans E. Increased plasma peroxides as a marker of oxidative stress in myalgic encephalomyelitis/chronic fatigue syndrome (ME/CFS). Med. Sci. Monit. (2011) 17:Sc11–5
258. Coyle CH, Kader KN. Mechanisms of H2O2-induced oxidative stress in endothelial cells exposed to physiologic shear stress. Asaio J (2007) 53:17–22.
259. Kurup RK, Kurup PA. Hypothalamic digoxin, cerebral chemical dominance and myalgic encephalomyelitis. Int J Neurosci (2003) 113:683–701.
260. Indo HP, Yen HC, Nakanishi I, Matsumoto K, Tamura M, Nagano Y, et al. A mitochondrial superoxide theory for oxidative stress diseases and aging. J Clin Biochem Nutr (2015) 56:1–7.
261. Younus H. Therapeutic potentials of superoxide dismutase. Int J Health Sci (Qassim) (2018) 12:88–93.
262. Brkic S, Tomic S, Maric D, Novakov Mikic A, Turkulov V. Lipid peroxidation is elevated in female patients with chronic fatigue syndrome. Med Sci Monit (2010) 16:Cr628–32.
263. Keenoy BM, Moorkens G, Vertommen J, De Leeuw I. Antioxidant status and lipoprotein peroxidation in chronic fatigue syndrome. Life Sci (2001) 68:2037–49.
264. Vecchiet J, Cipollone F, Falasca K, Mezzetti A, Pizzigallo E, Bucciarelli T, et al. Relationship between musculoskeletal symptoms and blood markers of oxidative stress in patients with chronic fatigue syndrome. Neurosci Lett (2003) 335:151–4.
265. Gaweł S, Wardas M, Niedworok E, Wardas P. [Malondialdehyde (MDA) as a lipid peroxidation marker]. Wiad Lek (2004) 57:453–5.
266. Jammes Y, Retornaz F. Understanding neuromuscular disorders in chronic fatigue syndrome. F1000Res. (2019) 8. doi: 10.12688/f1000research
267. Fenouillet E, Vigouroux A, Steinberg JG, Chagvardieff A, Retornaz F, Guieu R, et al. Association of biomarkers with health-related quality of life and history of stressors in myalgic encephalomyelitis/chronic fatigue syndrome patients. J Transl Med (2016) 14:251.
268. Catalán V, Frühbeck G, Gómez-Ambrosi J. Chapter 8 - inflammatory and oxidative stress markers in skeletal muscle of obese subjects. In: del Moral AM, Aguilera García CM, editors. Obesity. Academic Press (2018).
269. Germain A, Ruppert D, Levine SM, Hanson MR. Prospective biomarkers from plasma metabolomics of myalgic encephalomyelitis/chronic fatigue syndrome implicate redox imbalance in disease symptomatology. Metabolites. (2018) 8. doi: 10.3390/metabo8040090
270. Chiabrando D, Vinchi F, Fiorito V, Mercurio S, Tolosano E. Heme in pathophysiology: a matter of scavenging, metabolism and trafficking across cell membranes. Front Pharmacol (2014) 5:61.
271. Ogun AS, Adeyinka A. Biochemistry, transferrin. In: StatPearls. Treasure Island (FL: StatPearls Publishing (2023).
272. Regland B, Andersson M, Abrahamsson L, Bagby J, Dyrehag LE, Gottfries CG. Increased concentrations of homocysteine in the cerebrospinal fluid in patients with fibromyalgia and chronic fatigue syndrome. Scand J Rheumatol (1997) 26:301–7.
273. Weiss N. Mechanisms of increased vascular oxidant stress in hyperhomocys-teinemia and its impact on endothelial function. Curr Drug Metab (2005) 6:27–36.
274. Morris G, Berk M, Klein H, Walder K, Galecki P, Maes M. Nitrosative stress, hypernitrosylation, and autoimmune responses to nitrosylated proteins: New pathways in neuroprogressive disorders including depression and chronic fatigue syndrome. Mol Neurobiol (2017) 54:4271–91.
275. Pall ML. Levels of nitric oxide synthase product citrulline are elevated in sera of chronic fatigue syndrome patients. J Chronic Fatigue Syndrome (2002) 10:37–41.
276. Lubos E, Handy DE, Loscalzo J. Role of oxidative stress and nitric oxide in atherothrombosis. Front Biosci (2008) 13:5323–44.
277. Poznyak AV, Nikiforov NG, Markin AM, Kashirskikh DA, Myasoedova VA, Gerasimova EV, et al. Overview of OxLDL and its impact on cardiovascular health: Focus on atherosclerosis. Front Pharmacol (2021) 11.
278. Brenu EW, Huth TK, Hardcastle SL, Fuller K, Kaur M, Johnston S, et al. Role of adaptive and innate immune cells in chronic fatigue syndrome/myalgic encephalomyelitis. Int Immunol (2014) 26:233–42.
279. Laforge M, Elbim C, Frère C, Hémadi M, Massaad C, Nuss P, et al. Tissue damage from neutrophil-induced oxidative stress in COVID-19. Nat Rev Immunol (2020) 20:515–6.
280. Middleton EA, He XY, Denorme F, Campbell RA, Ng D, Salvatore SP, et al. Neutrophil extracellular traps contribute to immunothrombosis in COVID-19 acute respiratory distress syndrome. Blood (2020) 136:1169–79.
281. Zuo Y, Yalavarthi S, Shi H, Gockman K, Zuo M, Madison JA, et al. Neutrophil extracellular traps in COVID-19. JCI Insight (2020) 5.
282. Veenith T, Martin H, Le Breuilly M, Whitehouse T, Gao-Smith F, Duggal N, et al. High generation of reactive oxygen species from neutrophils in patients with severe COVID-19. Sci Rep (2022) 12:10484.
283. Shungu DC, Weiduschat N, Murrough JW, Mao X, Pillemer S, Dyke JP, et al. Increased ventricular lactate in chronic fatigue syndrome. III. Relationships to cortical glutathione and clinical symptoms implicate oxidative stress in disorder pathophysiology. NMR BioMed. (2012) 25:1073–87. doi: 10.1002/nbm.2772
284. Song J, Kang SM, Lee WT, Park KA, Lee KM, Lee JE. Glutathione protects brain endothelial cells from hydrogen peroxide-induced oxidative stress by increasing nrf2 expression. Exp Neurobiol (2014) 23:93–103.
285. Kwon DH, Cha HJ, Lee H, Hong SH, Park C, Park SH, et al. Protective effect of glutathione against oxidative stress-induced cytotoxicity in RAW 264.7 macrophages through activating the nuclear factor erythroid 2-related factor-2/Heme oxygenase-1 pathway. . Antioxidants (Basel) (2019) 8.
286. Kennedy G, Khan F, Hill A, Underwood C, Belch JJ. Biochemical and vascular aspects of pediatric chronic fatigue syndrome. Arch Pediatr Adolesc Med (2010) 164:817–23.
287. Kawashima A, Sekizawa A, Koide K, Hasegawa J, Satoh K, Arakaki T, et al. Vitamin c induces the reduction of oxidative stress and paradoxically stimulates the apoptotic gene expression in extravillous trophoblasts derived from first-trimester tissue. Reprod Sci (2015) 22:783–90.
288. Miwa K, Fujita M. Fluctuation of serum vitamin e (alpha-tocopherol) concentrations during exacerbation and remission phases in patients with chronic fatigue syndrome. Heart Vessels (2010) 25:319–23.
289. Surai PF. Natural antioxidants in avian nutrition and reproduction. Nottingham University Press Nottingham (2002).
290. Miwa K, Fujita M. Increased oxidative stress suggested by low serum vitamin e concentrations in patients with chronic fatigue syndrome. Int J Cardiol (2009) 136:238–9.
291. Tucker JM, Townsend DM. Alpha-tocopherol: roles in prevention and therapy of human disease. BioMed Pharmacother (2005) 59:380–7.
292. Aoki T, Miyakoshi H, Usuda Y, Herberman RB. Low NK syndrome and its relationship to chronic fatigue syndrome. Clin Immunol Immunopathol (1993) 69:253–65.
293. Fra A, Yoboue ED, Sitia R. Cysteines as redox molecular switches and targets of disease. Front Mol Neurosci (2017) 10:167.
294. Sweetman E, Ryan M, Edgar C, MacKay A, Vallings R, Tate W. Changes in the transcriptome of circulating immune cells of a New Zealand cohort with myalgic encephalomyelitis/chronic fatigue syndrome. Int J Immunopathol Pharmacol. (2019) 33:2058738418820402. doi: 10.1177/2058738418820402
295. Feng D, Xu D, Murakoshi N, Tajiri K, Qin R, Yonebayashi S, et al. Nicotinamide phosphoribosyltransferase (Nampt)/Nicotinamide adenine dinucleotide (NAD) axis suppresses atrial fibrillation by modulating the calcium handling pathway. Int J Mol Sci (2020) 21.
296. Tedeschi PM, Bansal N, Kerrigan JE, Abali EE, Scotto KW, Bertino JR. NAD+ kinase as a therapeutic target in cancer. Clin Cancer Res (2016) 22:5189–95.
297. Suárez A, Guillamó E, Roig T, Blázquez A, Alegre J, Bermúdez J, et al. Nitric oxide metabolite production during exercise in chronic fatigue syndrome: a case-control study. J Womens Health (Larchmt). (2010) 19:1073–7. doi: 10.1089/jwh.2008.1255
298. Pérez-Torres I, Manzano-Pech L, Rubio-Ruíz ME, Soto ME, Guarner-Lans V. Nitrosative stress and its association with cardiometabolic disorders. Molecules. (2020) 25:2555. doi: 10.3390/molecules25112555
299. Jammes Y, Steinberg JG, Olivier M, Brerro-Saby C, Condo J, Ravailhe S, et al. The mechanisms of the widespread production of phosphorylated HSP25 after fatiguing muscle stimulation. J Exp Biol. (2013) 216:3620–6. doi: 10.1242/jeb.088898
300. Whitham M, Fortes MB. Heat shock protein 72: release and biological significance during exercise. Front Biosci. (2008) 13:1328–39. doi: 10.2741/2765
301. Noble E. Heat shock proteins and their induction with exercise. Exercise and stress response: the role of stress proteins. Barcelona: Boca Raton. (2002), 43–78. doi: 10.1201/CRCEXEPHYSIO
302. Jammes Y, Steinberg JG, Delliaux S, Brégeon F. Chronic fatigue syndrome combines increased exercise-induced oxidative stress and reduced cytokine and Hsp responses. J Intern Med. (2009) 266:196–206. doi: 10.1111/j.1365-2796.2009.02079.x
303. Jammes Y, Steinberg JG, Delliaux S. Chronic fatigue syndrome: acute infection and history of physical activity affect resting levels and response to exercise of plasma oxidant/antioxidant status and heat shock proteins. J Intern Med. (2012) 272:74–84. doi: 10.1111/j.1365-2796.2011.02488.x
304. Gerwyn M, Maes M. Mechanisms explaining muscle fatigue and muscle pain in patients with myalgic encephalomyelitis/chronic fatigue syndrome (ME/CFS): a review of recent findings. Curr Rheumatol Rep. (2017) 19:1. doi: 10.1007/s11926-017-0628-x
305. Blauensteiner J, Bertinat R, León LE, Riederer M, Sepúlveda N, Westermeier F. Altered endothelial dysfunction-related miRs in plasma from ME/CFS patients. Sci Rep. (2021) 11:10604. doi: 10.1038/s41598-021-89834-9
306. Scherbakov N, Szklarski M, Hartwig J, Sotzny F, Lorenz S, Meyer A, et al. Peripheral endothelial dysfunction in myalgic encephalomyelitis/chronic fatigue syndrome. ESC Heart Fail. (2020) 7:1064–71. doi: 10.1002/ehf2.12633
307. Newton DJ, Kennedy G, Chan KK, Lang CC, Belch JJ, Khan F. Large and small artery endothelial dysfunction in chronic fatigue syndrome. Int J Cardiol. (2012) 154:335–6. doi: 10.1016/j.ijcard.2011.10.030
308. Pober JS, Sessa WC. Evolving functions of endothelial cells in inflammation. Nat Rev Immunol. (2007) 7:803–15. doi: 10.1038/nri2171
309. Bai B, Yang Y, Wang Q, Li M, Tian C, Liu Y, et al. NLRP3 inflammasome in endothelial dysfunction. Cell Death Dis. (2020) 11:776. doi: 10.1038/s41419-020-02985-x
310. Newton J, Okonkwo O, Sutcliffe K, Seth A, Shin J, Jones D. Symptoms of autonomic dysfunction in chronic fatigue syndrome. QJM: Int J Med. (2007) 100:519–26. doi: 10.1093/qjmed/hcm057
311. Sørland K, Sandvik MK, Rekeland IG, Ribu L, Småstuen MC, Mella O, et al. Reduced endothelial function in myalgic encephalomyelitis/chronic fatigue syndrome-results from open-label cyclophosphamide intervention study. Front Med (Lausanne). (2021) 8:642710. doi: 10.3389/fmed.2021.642710
312. Yang C-A, Bauer S, Ho Y-C, Sotzny F, Chang J-G, Scheibenbogen C. The expression signature of very long non-coding RNA in myalgic encephalomyelitis/chronic fatigue syndrome. J Trans Med. (2018) 16:231. doi: 10.1186/s12967-018-1600-x
313. Nunes JM, Kruger A, Proal A, Kell DB, Pretorius E. The occurrence of hyperactivated platelets and fibrinaloid microclots in myalgic encephalomyelitis/chronic fatigue syndrome (ME/CFS). Pharm (Basel) 15. (2022). doi: 10.21203/rs.3.rs-1727226/v2
314. Martin L, Koczera P, Zechendorf E, Schuerholz T. The endothelial glycocalyx: new diagnostic and therapeutic approaches in sepsis. BioMed Res Int. (2016) 2016:3758278. doi: 10.1155/2016/3758278
315. Santulli G. MicroRNAs and endothelial (Dys) function. J Cell Physiol. (2016) 231:1638–44. doi: 10.1002/jcp.v231.8
316. Kauppinen A, Suuronen T, Ojala J, Kaarniranta K, Salminen A. Antagonistic crosstalk between NF-κB and SIRT1 in the regulation of inflammation and metabolic disorders. Cell Signal. (2013) 25:1939–48. doi: 10.1016/j.cellsig.2013.06.007
317. Mattagajasingh I, Kim CS, Naqvi A, Yamamori T, Hoffman TA, Jung SB, et al. SIRT1 promotes endothelium-dependent vascular relaxation by activating endothelial nitric oxide synthase. Proc Natl Acad Sci U.S.A. (2007) 104:14855–60. doi: 10.1073/pnas.0704329104
318. Farah C, Michel LYM, Balligand JL. Nitric oxide signalling in cardiovascular health and disease. Nat Rev Cardiol. (2018) 15:292–316. doi: 10.1038/nrcardio.2017.224
319. Fan W, Fang R, Wu X, Liu J, Feng M, Dai G, et al. Shear-sensitive microRNA-34a modulates flow-dependent regulation of endothelial inflammation. J Cell Sci (2015) 128:70–80.
320. Gou L, Zhao L, Song W, Wang L, Liu J, Zhang H, et al. Inhibition of miR-92a suppresses oxidative stress and improves endothelial function by upregulating heme oxygenase-1 in db/db mice. Antioxid Redox Signal (2018) 28:358–70.
321. Guo Y, Li P, Gao L, Zhang J, Yang Z, Bledsoe G, et al. Kallistatin reduces vascular senescence and aging by regulating microRNA-34a-SIRT1 pathway. Aging Cell (2017) 16:837–46.
322. Murugaiyan G, da Cunha AP, Ajay AK, Joller N, Garo LP, Kumaradevan S, et al. MicroRNA-21 promotes Th17 differentiation and mediates experimental autoimmune encephalomyelitis. J Clin Invest (2015) 125:1069–80.
323. Rouas R, Fayyad-Kazan H, El Zein N, Lewalle P, Rothé F, Simion A, et al. Human natural treg microRNA signature: role of microRNA-31 and microRNA-21 in FOXP3 expression. Eur J Immunol (2009) 39:1608–18.
324. Xu F, Zhou F. Inhibition of microRNA-92a ameliorates lipopolysaccharide-induced endothelial barrier dysfunction by targeting ITGA5 through the PI3K/Akt signaling pathway in human pulmonary microvascular endothelial cells. Int Immunopharmacol (2020) 78:106060.
325. Yang HH, Chen Y, Gao CY, Cui ZT, Yao JM. Protective effects of MicroRNA-126 on human cardiac microvascular endothelial cells against Hypoxia/Reoxygenation-induced injury and inflammatory response by activating PI3K/Akt/eNOS signaling pathway. Cell Physiol Biochem (2017) 42:506–18.
326. Zhang H, Liu J, Qu D, Wang L, Luo JY, Lau CW, et al. Inhibition of miR-200c restores endothelial function in diabetic mice through suppression of COX-2. Diabetes (2016) 65:1196–207.
327. Guo Y, Li P, Bledsoe G, Yang ZR, Chao L, Chao J. Kallistatin inhibits TGF-β-induced endothelial-mesenchymal transition by differential regulation of microRNA-21 and eNOS expression. Exp Cell Res (2015) 337:103–10.
328. Hart M, Walch-Rückheim B, Friedmann KS, Rheinheimer S, Tänzer T, Glombitza B, et al. miR-34a: a new player in the regulation of t cell function by modulation of NF-κB signaling. Cell Death Dis (2019) 10:46.
329. Nelson MJ, Bahl JS, Buckley JD, Thomson RL, Davison K. Evidence of altered cardiac autonomic regulation in myalgic encephalomyelitis/chronic fatigue syndrome: A systematic review and meta-analysis. Med (Baltimore). (2019) 98:e17600. doi: 10.1097/MD.0000000000017600
330. Meeus M, Goubert D, De Backer F, Struyf F, Hermans L, Coppieters I, et al. Heart rate variability in patients with fibromyalgia and patients with chronic fatigue syndrome: A systematic review. Semin Arthritis Rheumatism. (2013) 43:279–87. doi: 10.1016/j.semarthrit.2013.03.004
331. Słomko J, Estévez-López F, Kujawski S, Zawadka-Kunikowska M, Tafil-Klawe M, Klawe JJ, et al. Autonomic phenotypes in chronic fatigue syndrome (CFS) are associated with illness severity: A cluster analysis. J Clin Med. (2020) 9. doi: 10.3390/jcm9082531
332. Martı́nez-Martı́nez L-A, Mora T, Vargas A, Fuentes-Iniestra M, Martı́nez-Lavı́n M. Sympathetic nervous system dysfunction in fibromyalgia, chronic fatigue syndrome, irritable bowel syndrome, and interstitial cystitis: a review of case-control studies. JCR: J Clin Rheumatol (2014) 20:146–50. doi: 10.1097/RHU.0000000000000089
333. Orjatsalo M, Alakuijala A, Partinen M. Autonomic nervous system functioning related to nocturnal sleep in patients with chronic fatigue syndrome compared to tired controls. J Clin Sleep Med (2018) 14:163–71. doi: 10.5664/jcsm.6924
334. Sisto SA, Tapp W, Drastal S, Bergen M, DeMasi I, Cordero D, et al. Vagal tone is reduced during paced breathing in patients with the chronic fatigue syndrome. Clin Autonomic Res. (1995) 5:139–43. doi: 10.1007/BF01826195
335. Jones DEJ, Hollingsworth KG, Taylor R, Blamire AM, Newton JL. Abnormalities in pH handling by peripheral muscle and potential regulation by the autonomic nervous system in chronic fatigue syndrome. J Internal Med. (2010) 267:394–401. doi: 10.1111/j.1365-2796.2009.02160.x
336. Stewart JM. Autonomic nervous system dysfunction in adolescents with postural orthostatic tachycardia syndrome and chronic fatigue syndrome is characterised by attenuated vagal baroreflex and potentiated sympathetic vasomotion. Pediatr Res. (2000) 48:218–26. doi: 10.1203/00006450-200008000-00016
337. Beaumont A, Burton AR, Lemon J, Bennett BK, Lloyd A, Vollmer-Conna U. Reduced cardiac vagal modulation impacts on cognitive performance in chronic fatigue syndrome. PloS One. (2012) 7:e49518. doi: 10.1371/journal.pone.0049518
338. Wyller VB, Saul JP, Walløe L, Thaulow E. Sympathetic cardiovascular control during orthostatic stress and isometric exercise in adolescent chronic fatigue syndrome. Eur J Appl Physiol. (2008) 102:623–32. doi: 10.1007/s00421-007-0634-1
339. De Becker P, Dendale P, De Meirleir K, Campine I, Vandenborne K, Hagers Y. Autonomic testing in patients with chronic fatigue syndrome. Am J Med. (1998) 105:22S–6S. doi: 10.1016/S0002-9343(98)00168-5
340. Rimes KA, Lievesley K, Chalder T. Stress vulnerability in adolescents with chronic fatigue syndrome: experimental study investigating heart rate variability and skin conductance responses. J Child Psychol Psychiatry. (2017) 58:851–8. doi: 10.1111/jcpp.12711
341. Van Cauwenbergh D, Nijs J, Kos D, Van Weijnen L, Struyf F, Meeus M. Malfunctioning of the autonomic nervous system in patients with chronic fatigue syndrome: a systematic literature review. Eur J Clin Invest. (2014) 44:516–26. doi: 10.1111/eci.12256
342. Frith J, Zalewski P, Klawe JJ, Pairman J, Bitner A, Tafil-Klawe M, et al. Impaired blood pressure variability in chronic fatigue syndrome—a potential biomarker. QJM: Int J Med. (2012) 105:831–8. doi: 10.1093/qjmed/hcs085
343. Burton AR, Rahman K, Kadota Y, Lloyd A, Vollmer-Conna U. Reduced heart rate variability predicts poor sleep quality in a case–control study of chronic fatigue syndrome. Exp Brain Res. (2010) 204:71–8. doi: 10.1007/s00221-010-2296-1
344. Yataco A, Talo H, Rowe P, Kass DA, Berger RD, Calkins H. Comparison of heart rate variability in patients with chronic fatigue syndrome and controls. Clin Autonomic Res. (1997) 7:293–7. doi: 10.1007/BF02267720
345. Kaur J, Krishnan AC, Senador D, Alvarez A, Hanna HW, O’Leary DS. Altered arterial baroreflex-muscle metaboreflex interaction in heart failure. Am J Physiol Heart Circ Physiol (2018). doi: 10.1152/ajpheart.00338.2018
346. Ylikorkala O, Dawood MY. New concepts in dysmenorrhea. Am J Obstetrics Gynecology. (1978) 130:833–47. doi: 10.1016/0002-9378(78)90019-4
347. Mullins RJ, Malias MA, Hudgens RW. Isoproterenol inhibits the increase in microvascular membrane permeability produced by bradykinin. J Trauma. (1989) 29:1053–63. doi: 10.1097/00005373-198908000-00002
348. Szabó G, Magyar Z. Effect of histamine and bradykinin on lymph and tissue fluid composition in the rabbit hindlimb: evidence for two compartments in tissue fluid. Acta Physiol Acad Sci Hung. (1982) 59:15–27.
349. Berg D, Berg LH, Couvaras J, Harrison H. Chronic fatigue syndrome and/or fibromyalgia as a variation of antiphospholipid antibody syndrome: an explanatory model and approach to laboratory diagnosis. Blood Coagul Fibrinolysis. (1999) 10:435–8. doi: 10.1097/00001721-199910000-00006
350. Hannan KL, Berg DE, Baumzweiger W, Harrison HH, Berg LH, Ramirez R, et al. Activation of the coagulation system in Gulf War Illness: a potential pathophysiologic link with chronic fatigue syndrome. A Lab approach to diagnosis. Blood Coagul Fibrinolysis (2000) 11:673–8. doi: 10.1097/00001721-200010000-00013
351. Brewer JH, Berg D. Hypercoaguable state associated with active human herpesvirus-6 (HHV-6) viremia in patients with chronic fatigue syndrome. J Chronic Fatigue Syndrome. (2001) 8:111–6. doi: 10.1300/J092v08n03_10
352. Kennedy G, Norris G, Spence V, McLaren M, Belch JJ. Is chronic fatigue syndrome associated with platelet activation? Blood Coagul Fibrinolysis (2006) 17:89–92. doi: 10.1097/01.mbc.0000214705.80997.73
353. Pretorius E, Mbotwe S, Bester J, Robinson CJ, Kell DB. Acute induction of anomalous and amyloidogenic blood clotting by molecular amplification of highlysubstoichiometric levels of bacterial lipopolysaccharide. J R Soc Interface (2016) 13. doi: 10.1101/053538
354. Natelson BH, Weaver SA, Tseng CL, Ottenweller JE. Spinal fluid abnormalities in patients with chronic fatigue syndrome. Clin Diagn Lab Immunol (2005) 12:52–5. doi: 10.1128/CDLI.12.1.52-55.2005
355. Sfera A, Osorio C, Zapata Martı́n Del Campo CM, Pereida S, Maurer S, Maldonado JC, et al. Endothelial senescence and chronic fatigue syndrome, a COVID-19 based hypothesis. Front Cell Neurosci (2021) 15:673217. doi: 10.3389/fncel.2021.673217
356. Costa DC, Tannock C, Brostoff J. Brainstem perfusion is impaired in chronic fatigue syndrome. Qjm. (1995) 88:767–73.
357. Tirelli U, Chierichetti F, Tavio M, Simonelli C, Bianchin G, Zanco P, et al. Brain positron emission tomography (PET) in chronic fatigue syndrome: preliminary data. Am J Med (1998) 105. doi: 10.1016/S0002-9343(98)00179-X
358. Kuratsune H, Yamaguti K, Lindh G, Evengård B, Hagberg G, Matsumura K, et al. Brain regions involved in fatigue sensation: reduced acetylcarnitine uptake into the brain. Neuroimage. (2002) 17:1256–65. doi: 10.1006/nimg.2002.1260
359. Wirth KJ, Scheibenbogen C. Pathophysiology of skeletal muscle disturbances in Myalgic Encephalomyelitis/Chronic Fatigue Syndrome (ME/CFS). J Transl Med (2021) 19:162. doi: 10.1186/s12967-021-02833-2
360. van Campen C, Verheugt FWA, Rowe PC, Visser FC. Cerebral blood flow is reduced in ME/CFS during head-up tilt testing even in the absence of hypotension or tachycardia: A quantitative, controlled study using Doppler echography. Clin Neurophysiol Pract (2020) 5:50–8. doi: 10.1016/j.cnp.2020.01.003
361. Bragée B, Michos A, Drum B, Fahlgren M, Szulkin R, Bertilson BC. Signs of intracranial hypertension, hypermobility, and craniocervical obstructions in patients with myalgic encephalomyelitis/chronic fatigue syndrome. Front Neurol (2020) 11:828. doi: 10.3389/fneur.2020.00828
362. Hulens M, Rasschaert R, Vansant G, Stalmans I, Bruyninckx F, Dankaerts W. The link between idiopathic intracranial hypertension, fibromyalgia, and chronic fatigue syndrome: exploration of a shared pathophysiology. J Pain Res (2018) 11:3129–40. doi: 10.2147/JPR
363. Tohidpour A, Morgun AV, Boitsova EB, Malinovskaya NA, Martynova GP, Khilazheva ED, et al. Neuroinflammation and infection: molecular mechanisms associated with dysfunction of neurovascular unit. Front Cell Infect Microbiol (2017) 7:276. doi: 10.1146/annurev.nutr.26.061505.111258
364. Hotamisligil GS. Inflammation, metaflammation and immunometabolic disorders. Nature. (2017) 542:177–85. doi: 10.1038/nature21363
366. van Hall G. The physiological regulation of skeletal muscle fatty acid supply and oxidation during moderate-intensity exercise. Sports Med (2015) 45 Suppl 1:S23–32. doi: 10.1007/s40279-015-0394-8
367. Wagenmakers AJ. Muscle amino acid metabolism at rest and during exercise: role in human physiology and metabolism. Exerc Sport Sci Rev (1998) 26:287–314. doi: 10.1249/00003677-199800260-00013
368. Myhill S, Booth NE, McLaren-Howard J. Chronic fatigue syndrome and mitochondrial dysfunction. Int J Clin Exp Med (2009) 2:1–16.
369. Holden S, Maksoud R, Eaton-Fitch N, Cabanas H, Staines D, Marshall-Gradisnik S. A systematic review of mitochondrial abnormalities in myalgic encephalomyelitis/chronic fatigue syndrome/systemic exertion intolerance disease. J Transl Med (2020) 18:290. doi: 10.1186/s12967-020-02452-3
370. Booth NE, Myhill S, McLaren-Howard J. Mitochondrial dysfunction and the pathophysiology of Myalgic Encephalomyelitis/Chronic Fatigue Syndrome (ME/CFS). Int J Clin Exp Med (2012) 5:208–20.
371. Castro-Marrero J, Cordero MD, Sáez-Francas N, Jimenez-Gutierrez C, Aguilar- Montilla FJ, Aliste L, et al. Could mitochondrial dysfunction be a differentiating marker between chronic fatigue syndrome and fibromyalgia? Antioxid Redox Signal (2013) 19:1855–60. doi: 10.1089/ars.2013.5346
372. Myhill S, Booth NE, McLaren-Howard J. Targeting mitochondrial dysfunction in the treatment of Myalgic Encephalomyelitis/Chronic Fatigue Syndrome (ME/CFS)-a clinical audit. Int J Clin Exp Med (2013) 6:1.
373. Morris G, Maes M. Mitochondrial dysfunctions in myalgic encephalomyelitis/ chronic fatigue syndrome explained by activated immuno-inflammatory, oxidative and nitrosative stress pathways. Metab Brain Dis (2014) 29:19–36. doi: 10.1007/s11011-013-9435-x
374. Missailidis D, Annesley SJ, Fisher PR. Pathological mechanisms underlying myalgic encephalomyelitis/chronic fatigue syndrome. Diagnostics (Basel). (2019) 9. doi: 10.20944/preprints201907.0196.v1
375. Maes M, Twisk FNM, Ringel K. Inflammatory and cell-mediated immune biomarkers in myalgic encephalomyelitis/chronic fatigue syndrome and depression: inflammatory markers are higher in myalgic encephalomyelitis/chronic fatigue syndrome than in depression. Psychother Psychosomatics. (2012) 81:286–95. doi: 10.1159/000336803
376. Sadatomi D, Nakashioya K, Mamiya S, Honda S, Kameyama Y, Yamamura Y, et al. Mitochondrial function is required for extracellular ATP-induced NLRP3= inflammasome activation. J Biochem (2017) 161:503–12. doi: 10.1093/jb/mvw098
377. Mills EL, Kelly B, O’Neill LAJ. Mitochondria are the powerhouses of immunity. Nat Immunol (2017) 18:488–98. doi: 10.1038/ni.3704
378. Breda CNS, Davanzo GG, Basso PJ, Saraiva Câmara NO, Moraes-Vieira PMM. Mitochondria as central hub of the immune system. Redox Biol (2019) 26:101255. doi: 10.1016/j.redox.2019.101255
379. Weinberg SE, Sena LA, Chandel NS. Mitochondria in the regulation of innate and adaptive immunity. Immunity. (2015) 42:406–17. doi: 10.1016/j.immuni.2015.02.002
380. Murphy MP. How mitochondria produce reactive oxygen species. Biochem J (2009) 417:1–13. doi: 10.1042/BJ20081386
381. Abate G, Vezzoli M, Sandri M, Rungratanawanich W, Memo M, Uberti D. Mitochondria and cellular redox state on the route from ageing to Alzheimer’s disease. Mech Ageing Dev (2020) 192:111385. doi: 10.1016/j.mad.2020.111385
382. Behan WM, More IA, Behan PO. Mitochondrial abnormalities in the postviral fatigue syndrome. Acta Neuropathol. (1991) 83:61–5. doi: 10.1007/BF00294431
383. Plioplys AV, Plioplys S. Electron-microscopic investigation of muscle mitochondria in chronic fatigue syndrome. Neuropsychobiology. (1995) 32:175–81. doi: 10.1159/000119233
384. Lawson N, Hsieh CH, March D, Wang X. Elevated energy production in chronic fatigue syndrome patients. J Nat Sci. (2016) 2.
385. Tomas C, Brown A, Strassheim V, Elson JL, Newton J, Manning P. Cellular bioenergetics is impaired in patients with chronic fatigue syndrome. PloS One. (2017) 12:e0186802. doi: 10.1371/journal.pone.0186802
386. Missailidis D, Annesley SJ, Allan CY, Sanislav O, Lidbury BA, Lewis DP, et al. An isolated complex V inefficiency and dysregulated mitochondrial function in immortalised lymphocytes from ME/CFS patients. Int J Mol Sci 21. (2020). doi: 10.20944/preprints201909.0043.v2
387. Sweetman E, Kleffmann T, Edgar C, de Lange M, Vallings R, Tate W. A SWATH-MS analysis of Myalgic Encephalomyelitis/Chronic Fatigue Syndrome peripheral blood mononuclear cell proteomes reveals mitochondrial dysfunction. J Transl Med. (2020) 18:365. doi: 10.1186/s12967-020-02533-3
388. Tomas C, Elson JL, Strassheim V, Newton JL, Walker M. The effect of myalgic encephalomyelitis/chronic fatigue syndrome (ME/CFS) severity on cellular bioenergetic function. PloS One. (2020) 15:e0231136. doi: 10.1371/journal.pone.0231136
389. Nguyen T, Staines D, Johnston S, Marshall-Gradisnik S. Reduced glycolytic reserve in isolated natural killer cells from Myalgic encephalomyelitis/chronic fatigue syndrome patients: A preliminary investigation. Asian Pac J Allergy Immunol. (2019) 37:102–8. doi: 10.12932/AP-011117-0188. PMID: 29981562
390. Rutherford G, Manning P, Newton JL. Understanding muscle dysfunction in chronic fatigue syndrome. J Aging Res. (2016) 2016:2497348. doi: 10.1155/2016/2497348
391. Maes M, Twisk FN. Why myalgic encephalomyelitis/chronic fatigue syndrome (ME/CFS) may kill you: disorders in the inflammatory and oxidative and nitrosative stress (IO&NS) pathways may explain cardiovascular disorders in ME/CFS. Neuro Endocrinol Lett. (2009) 30:677–93.
392. Bartee L, Anderson C. Mt Hood Community College Biology 101: An overview of cellular respiration, Open Oregon Educational Resources. (2016).
393. van Campen CLM, Rowe PC, Visser FC. Two-day cardiopulmonary exercise testing in females with a severe grade of myalgic encephalomyelitis/chronic fatigue syndrome: comparison with patients with mild and moderate disease. Healthcare (Basel) 8. (2020). doi: 10.3390/healthcare8030192
394. Stevens S, Snell C, Stevens J, Keller B, VanNess JM. Cardiopulmonary exercise test methodology for assessing exertion intolerance in myalgic encephalomyelitis/chronic fatigue syndrome. Front Pediatr. (2018) 6:242. doi: 10.3389/fped.2018.00242
395. Murrough JW, Mao X, Collins KA, Kelly C, Andrade G, Nestadt P, et al. Increased ventricular lactate in chronic fatigue syndrome measured by 1H MRS imaging at 3.0 T. II: comparison with major depressive disorder. NMR BioMed. (2010) 23:643–50. doi: 10.1002/nbm.1512
396. Mathew SJ, Mao X, Keegan KA, Levine SM, Smith EL, Heier LA, et al. Ventricular cerebrospinal fluid lactate is increased in chronic fatigue syndrome compared with generalised anxiety disorder: an in vivo 3. 0 T (1)H MRS Imaging study. NMR BioMed. (2009) 22:251–8. doi: 10.1002/nbm.1315
397. Lien K, Johansen B, Veierød MB, Haslestad AS, Bøhn SK, Melsom MN, et al. Abnormal blood lactate accumulation during repeated exercise testing in myalgic encephalomyelitis/chronic fatigue syndrome. Physiol Rep. (2019) 7:e14138. doi: 10.14814/phy2.14138
398. Snell CR, Stevens SR, Davenport TE, Van Ness JM. Discriminative validity of metabolic and workload measurements for identifying people with chronic fatigue syndrome. Phys Ther. (2013) 93:1484–92. doi: 10.2522/ptj.20110368
399. Vermeulen RC, Vermeulen van Eck IW. Decreased oxygen extraction during cardiopulmonary exercise test in patients with chronic fatigue syndrome. J Transl Med. (2014) 12:20. doi: 10.1186/1479-5876-12-20
400. Fegan KG, Behan PO, Bell EJ. Myalgic encephalomyelitis–report of an epidemic. J R Coll Gen Pract. (1983) 33:335–7.
401. Tomas C, Newton J. Metabolic abnormalities in chronic fatigue syndrome/myalgic encephalomyelitis: a mini-review. Biochem Soc Trans. (2018) 46:547–53. doi: 10.1042/BST20170503
402. Jones DE, Hollingsworth KG, Jakovljevic DG, Fattakhova G, Pairman J, Blamire AM, et al. Loss of capacity to recover from acidosis on repeat exercise in chronic fatigue syndrome: a case-control study. Eur J Clin Invest. (2012) 42:186–94. doi: 10.1111/j.1365-2362.2011.02567.x
403. Chaudhuri A, Behan PO. In vivo magnetic resonance spectroscopy in chronic fatigue syndrome. Prostaglandins Leukot Essent Fatty Acids. (2004) 71:181–3. doi: 10.1016/j.plefa.2004.03.009
404. Lengert N, Drossel B. In silico analysis of exercise intolerance in myalgic encephalomyelitis/chronic fatigue syndrome. Biophys Chem. (2015) 202:21–31. doi: 10.1016/j.bpc.2015.03.009
405. Germain A, Barupal DK, Levine SM, Hanson MR. Comprehensive circulatory metabolomics in ME/CFS reveals disrupted metabolism of acyl lipids and steroids. Metabolites. (2020) 10. doi: 10.3390/metabo10010034
406. Nagy-Szakal D, Barupal DK, Lee B, Che X, Williams BL, Kahn EJR, et al. Insights into myalgic encephalomyelitis/chronic fatigue syndrome phenotypes through comprehensive metabolomics. Sci Rep. (2018) 8:10056. doi: 10.1038/s41598-018-28477-9
407. Naviaux RK, Naviaux JC, Li K, Bright AT, Alaynick WA, Wang L, et al. Metabolic features of chronic fatigue syndrome. Proc Natl Acad Sci U.S.A. (2016) 113:E5472–80. doi: 10.1073/pnas.1607571113
408. Yamano E, Sugimoto M, Hirayama A, Kume S, Yamato M, Jin G, et al. Index markers of chronic fatigue syndrome with dysfunction of TCA and urea cycles. Sci Rep. (2016) 6:34990. doi: 10.1038/srep34990
409. Niblett SH, King KE, Dunstan RH, Clifton-Bligh P, Hoskin LA, Roberts TK, et al. Hematologic and urinary excretion anomalies in patients with chronic fatigue syndrome. Exp Biol Med (Maywood). (2007) 232:1041–9. doi: 10.3181/0702-RM-44
410. Maloney EM, Boneva RS, Lin JM, Reeves WC. Chronic fatigue syndrome is associated with metabolic syndrome: results from a case-control study in Georgia. Metabolism. (2010) 59:1351–7. doi: 10.1016/j.metabol.2009.12.019
411. Kitami T, Fukuda S, Kato T, Yamaguti K, Nakatomi Y, Yamano E, et al. Deep phenotyping of myalgic encephalomyelitis/chronic fatigue syndrome in Japanese population. Sci Rep. (2020) 10:19933. doi: 10.1038/s41598-020-77105-y
412. Reuter SE, Evans AM. Long-chain acylcarnitine deficiency in patients with chronic fatigue syndrome. Potential involvement of altered carnitine palmitoyltransferase-I activity. J Internal Med. (2011) 270:76–84. doi: 10.1111/j.1365-2796.2010.02341.x
413. Huth TK, Eaton-Fitch N, Staines D, Marshall-Gradisnik S. A systematic review of metabolomic dysregulation in Chronic Fatigue Syndrome/Myalgic Encephalomyelitis/Systemic Exertion Intolerance Disease (CFS/ME/SEID). J Transl Med. (2020) 18:198. doi: 10.1186/s12967-020-02356-2
414. Naviaux RK. Incomplete healing as a cause of aging: the role of mitochondria and the cell danger response. Biol (Basel). (2019) 8. doi: 10.3390/biology8020027
415. Naviaux RK. Perspective: Cell danger response Biology-The new science that connects environmental health with mitochondria and the rising tide of chronic illness. Mitochondrion. (2020) 51:40–5. doi: 10.1016/j.mito.2019.12.005
416. Naviaux RK. Metabolic features of the cell danger response. Mitochondrion. (2014) 16:7–17. doi: 10.1016/j.mito.2013.08.006
417. Costa-Mattioli M, Walter P. The integrated stress response: From mechanism to disease. Science. (2020) 368. doi: 10.1126/science.aat5314
418. Poon DC, Ho YS, Chiu K, Wong HL, Chang RC. Sickness: From the focus on cytokines, prostaglandins, and complement factors to the perspectives of neurons. Neurosci Biobehav Rev. (2015) 57:30–45. doi: 10.1016/j.neubiorev.2015.07.015
419. Missailidis D, Sanislav O, Allan CY, Annesley SJ, Fisher PR. Cell-based blood biomarkers for myalgic encephalomyelitis/chronic fatigue syndrome. Int J Mol Sci. (2020) 21. doi: 10.20944/preprints202002.0029.v1
420. Pivovarova NB, Andrews SB. Calcium-dependent mitochondrial function and dysfunction in neurons. FEBS J. (2010) 277:3622–36. doi: 10.1111/j.1742-4658.2010.07754.x
421. Angajala A, Lim S, Phillips JB, Kim JH, Yates C, You Z, et al. Diverse roles of mitochondria in immune responses: novel insights into immuno-metabolism. Front Immunol. (2018) 9:1605. doi: 10.3389/fimmu.2018.01605
422. Johnston SC, Staines DR, Marshall-Gradisnik SM. Epidemiological characteristics of chronic fatigue syndrome/myalgic encephalomyelitis in Australian patients. Clin Epidemiol. (2016) 8:97–107. doi: 10.2147/CLEP
423. Lattie EG, Antoni MH, Fletcher MA, Penedo F, Czaja S, Lopez C, et al. Stress management skills, neuroimmune processes and fatigue levels in persons with chronic fatigue syndrome. Brain Behav Immun. (2012) 26:849–58. doi: 10.1016/j.bbi.2012.02.008
424. Balinas C, Eaton-Fitch N, Maksoud R, Staines D, Marshall-Gradisnik S. Impact of life stressors on myalgic encephalomyelitis/chronic fatigue syndrome symptoms: an Australian longitudinal study. Int J Environ Res Public Health. (2021) 18. doi: 10.3390/ijerph182010614
425. Tomas C, Newton J, Watson S. A review of hypothalamic-pituitary-adrenal axis function in chronic fatigue syndrome. ISRN Neurosci. (2013) 2013:784520. doi: 10.1155/2013/784520
426. Tak LM, Cleare AJ, Ormel J, Manoharan A, Kok IC, Wessely S, et al. Meta-analysis and meta-regression of hypothalamic-pituitary-adrenal axis activity in functional somatic disorders. Biol Psychol. (2011) 87:183–94. doi: 10.1016/j.biopsycho.2011.02.002
427. Hannibal KE, Bishop MD. Chronic stress, cortisol dysfunction, and pain: a psychoneuroendocrine rationale for stress management in pain rehabilitation. Phys Ther. (2014) 94:1816–25. doi: 10.2522/ptj.20130597
428. Torres-Harding S, Sorenson M, Jason L, Maher K, Fletcher MA, Reynolds N, et al. The associations between basal salivary cortisol and illness symptomatology in chronic fatigue syndrome. J Appl Biobehav Res. (2008) 13:157–80. doi: 10.1111/j.1751-9861.2008.00033.x
429. Morris G, Anderson G, Maes M. Hypothalamic-pituitary-adrenal hypofunction in myalgic encephalomyelitis (ME)/chronic fatigue syndrome (CFS) as a consequence of activated immune-inflammatory and oxidative and nitrosative pathways. Mol Neurobiol. (2017) 54:6806–19. doi: 10.1007/s12035-016-0170-2
430. De Bellis A, Bellastella G, Pernice V, Cirillo P, Longo M, Maio A, et al. Hypothalamic-pituitary autoimmunity and related impairment of hormone secretions in chronic fatigue syndrome. J Clin Endocrinol Metab. (2021) 106:e5147–55. doi: 10.1210/clinem/dgab429
431. Berwaerts J, Moorkens G, Abs R. Secretion of growth hormone in patients with chronic fatigue syndrome. Growth Horm IGF Res 8 Suppl B. (1998), 127–9. doi: 10.1016/S1096-6374(98)80036-1
432. Pan J, Ma N, Zhong J, Yu B, Wan J, Zhang W. Age-associated changes in microglia and astrocytes ameliorate blood-brain barrier dysfunction. Mol Ther Nucleic Acids. (2021) 26:970–86. doi: 10.1016/j.omtn.2021.08.030
433. Akalin A, Kormaksson M, Li S, Garrett-Bakelman FE, Figueroa ME, Melnick A, et al. methylKit: a comprehensive R package for the analysis of genome-wide DNA methylation profiles. Genome Biol. (2012) 13:R87. doi: 10.1186/gb-2012-13-10-r87
434. Dinan TG. Serotonin and the regulation of hypothalamic-pituitary-adrenal axis function. Life Sci. (1996) 58:1683–94. doi: 10.1016/0024-3205(96)00066-5
435. Pereira G, Gillies H, Chanda S, Corbett M, Vernon SD, Milani T, et al. Acute corticotropin-releasing factor receptor type 2 agonism results in sustained symptom improvement in myalgic encephalomyelitis/chronic fatigue syndrome. Front Syst Neurosci. (2021) 15:698240. doi: 10.3389/fnsys.2021.698240
436. Johnson C. Health rising. (2018). Available at: https://www.healthrising.org [Accessed 17 January 2024].
437. Dobryakova E, Genova HM, DeLuca J, Wylie GR. The dopamine imbalance hypothesis of fatigue in multiple sclerosis and other neurological disorders. Front Neurol. (2015) 6:52. doi: 10.3389/fneur.2015.00052
438. Janowsky DS, Davis JM. Psychological effects of cholinomimetic agents. In: Davis KL, Berger PA, editors. Brain acetylcholine and neuropsychiatric disease. Springer US, Boston, MA (1979). p. 3–14.
439. Casanueva FF, Betti R, Cella SG, Müller EE, Mantegazza P. Effect of agonists and antagonists of cholinergic neurotransmission on growth hormone release in the dog. Acta Endocrinol (Copenh). (1983) 103:15–20. doi: 10.1530/acta.0.1030015
440. Bakheit AM, Behan PO, Watson WS, Morton JJ. Abnormal arginine-vasopressin secretion and water metabolism in patients with postviral fatigue syndrome. Acta Neurol Scand. (1993) 87:234–8. doi: 10.1111/j.1600-0404.1993.tb04108.x
441. Bakheit AM, Behan PO, Dinan TG, Gray CE, O’Keane V. Possible upregulation of hypothalamic 5-hydroxytryptamine receptors in patients with postviral fatigue syndrome. Bmj. (1992) 304:1010–2. doi: 10.1136/bmj.304.6833.1010
442. Cleare AJ, Bearn J, Allain T, McGregor A, Wessely S, Murray RM, et al. Contrasting neuroendocrine responses in depression and chronic fatigue syndrome. J Affect Disord. (1995) 34:283–9. doi: 10.1016/0165-0327(95)00026-J
443. Misiak B, Łoniewski I, Marlicz W, Frydecka D, Szulc A, Rudzki L, et al. The HPA axis dysregulation in severe mental illness: Can we shift the blame to gut microbiota? Prog Neuropsychopharmacol Biol Psychiatry. (2020) 102:109951. doi: 10.1016/j.pnpbp.2020.109951
444. R.a.P. Moss-Morris K. Chronic fatigue syndrome. 1st ed. Milton Park, Abingdon-on-Thames, Oxfordshire, England, UK: Routledge (2000).
445. Bradley AS, Ford B, Bansal AS. Altered functional b cell subset populations in patients with chronic fatigue syndrome compared to healthy controls. Clin Exp Immunol (2013) 172:73–80.
446. Klimas NG, Salvato FR, Morgan R, Fletcher MA. Immunologic abnormalities in chronic fatigue syndrome. J Clin Microbiol (1990) 28:1403–10.
447. Tirelli U, Marotta G, Improta S, Pinto A. Immunological abnormalities in patients with chronic fatigue syndrome. Scandinavian J Immunol (1994) 40:601–8.
448. Milivojevic M, Che X, Bateman L, Cheng A, Garcia BA, Hornig M, et al. Plasma proteomic profiling suggests an association between antigen driven clonal b cell expansion and ME/CFS. PloS One (2020) 15:e0236148.
449. Cherukuri A, Cheng PC, Sohn HW, Pierce SK. The CD19/CD21 complex functions to prolong b cell antigen receptor signaling from lipid rafts. Immunity (2001) 14:169–79.
450. Pers JO, Jamin C, Predine-Hug F, Lydyard P, Youinou P. The role of CD5-expressing b cells in health and disease (review). Int J Mol Med (1999) 3:239–45.
451. Rivas JL, Palencia T, Fernández G, García M. Association of t and NK cell phenotype with the diagnosis of myalgic Encephalomyelitis/Chronic fatigue syndrome (ME/CFS). Front Immunol (2018) 9:1028.
452. Landay AL, Jessop C, Lennette ET, Levy JA. Chronic fatigue syndrome: clinical condition associated with immune activation. Lancet (1991) 338:707–12.
453. Cliff JM, King EC, Lee JS, Sepúlveda N, Wolf AS, Kingdon C, et al. Cellular immune function in myalgic Encephalomyelitis/Chronic fatigue syndrome (ME/CFS). Front Immunol (2019) 10:796.
454. Barker E, Fujimura SF, Fadem MB, Landay AL, Levy JA. Immunologic abnormalities associated with chronic fatigue syndrome. Clin Infect Dis (1994) 18 Suppl 1:S136–41.
455. Deterre P, Berthelier V, Bauvois B, Dalloul A, Schuber F, Lund F. CD38 in t- and b-cell functions. Chem Immunol (2000) 75:146–68.
456. Morra M, Zubiaur M, Terhorst C, Sancho J, Malavasi F. CD38 is functionally dependent on the TCR/CD3 complex in human t cells. FASEB J (1998) 12:581–92.
457. Baecher-Allan C, Wolf E, Hafler DA. MHC class II expression identifies functionally distinct human regulatory t cells. J Immunol (2006) 176:4622–31.
458. Viallard JF, Blanco P, André M, Etienne G, Liferman F, Neau D, et al. CD8+HLA-DR+ t lymphocytes are increased in common variable immunodeficiency patients with impaired memory b-cell differentiation. Clin Immunol (2006) 119:51–8.
459. Viallard JF, Bloch-Michel C, Neau-Cransac M, Taupin JL, Garrigue S, Miossec V, et al. HLA-DR expression on lymphocyte subsets as a marker of disease activity in patients with systemic lupus erythematosus. Clin Exp Immunol (2001) 125:485–91.
460. Hardcastle SL, Brenu EW, Johnston S, Nguyen T, Huth T, Wong N, et al. Characterisation of cell functions and receptors in chronic fatigue Syndrome/Myalgic encephalomyelitis (CFS/ME). BMC Immunol (2015) 16:35.
461. Theorell J, Bileviciute-Ljungar I, Tesi B, Schlums H, Johnsgaard MS, Asadi-Azarbaijani B, et al. Unperturbed cytotoxic lymphocyte phenotype and function in myalgic Encephalomyelitis/Chronic fatigue syndrome patients. Front Immunol (2017) 8.
462. Gao Z, Feng Y, Xu J, Liang J. T-cell exhaustion in immune-mediated inflammatory diseases: New implications for immunotherapy. Front Immunol 13 (2022).
463. Callender LA, Carroll EC, Bober EA, Akbar AN, Solito E, Henson SM. Mitochondrial mass governs the extent of human t cell senescence. Aging Cell (2020) 19:e13067.
464. Hornig M. Can the light of immunometabolism cut through "brain fog"? J Clin Invest (2020) 130:1102–5.
465. Brenu EW, van Driel ML, Staines DR, Ashton KJ, Ramos SB, Keane J, et al. Immunological abnormalities as potential biomarkers in chronic fatigue Syndrome/Myalgic encephalomyelitis. J Transl Med (2011) 9:81.
466. Curriu M, Carrillo J, Massanella M, Rigau J, Alegre J, Puig J, et al. And t-cell phenotype and function in patients suffering from chronic fatigue syndrome. J Transl Med (2013) 11:68.
467. Ramos S, Brenu E, Broadley S, Kwiatek R, Ng J, Nguyen T, et al. Natural killer t and γδ t cells in multiple sclerosis and chronic fatigue syndrome/myalgic encephalomyelitis: a comparison. Asian Pac J Allergy Immunol (2016) 34:300–5.
468. Grobler C, Maphumulo SC, Grobbelaar LM, Bredenkamp JC, Laubscher GJ, Lourens PJ, et al. Covid-19: The rollercoaster of Fibrin(Ogen), d-dimer, von willebrand factor, p-selectin and their interactions with endothelial cells, platelets and erythrocytes. Int J Mol Sci (2020) 21.
469. Kondlková K, Vokurková D, Krejsek J, Borská L, Fiala Z, Ctirad A. Regulatory t cells (TREG) and their roles in immune system with respect to immunopathological disorders. Acta Med (Hradec Kralove) (2010) 53:73–7.
470. Brenu EW, Hardcastle SL, Atkinson GM, van Driel ML, Kreijkamp-Kaspers S, Ashton KJ, et al. Natural killer cells in patients with severe chronic fatigue syndrome. Auto Immun Highlights (2013) 4:69–80.
471. Caligiuri M, Murray C, Buchwald D, Levine H, Cheney P, Peterson D, et al. Phenotypic and functional deficiency of natural killer cells in patients with chronic fatigue syndrome. J Immunol (1987) 139:3306–13.
472. Fletcher MA, Zeng XR, Maher K, Levis S, Hurwitz B, Antoni M, et al. Biomarkers in chronic fatigue syndrome: evaluation of natural killer cell function and dipeptidyl peptidase IV/CD26. PloS One (2010) 5:e10817.
473. Natelson BH, Haghighi MH, Ponzio NM. Evidence for the presence of immune dysfunction in chronic fatigue syndrome. Clin Diagn Lab Immunol (2002) 9:747–52.
474. Rasa S, Nora-Krukle Z, Henning N, Eliassen E, Shikova E, Harrer T, et al. Chronic viral infections in myalgic encephalomyelitis/chronic fatigue syndrome (ME/CFS). J Transl Med (2018) 16:268.
475. Vivier E, Tomasello E, Baratin M, Walzer T, Ugolini S. Functions of natural killer cells. Nat Immunol (2008) 9:503–10.
476. Brenu EW, van Driel ML, Staines DR, Ashton KJ, Hardcastle SL, Keane J, et al. Longitudinal investigation of natural killer cells and cytokines in chronic fatigue syndrome/myalgic encephalomyelitis. J Transl Med (2012) 10:88.
477. Eaton-Fitch N, du Preez S, Cabanas H, Staines D, Marshall-Gradisnik S. A systematic review of natural killer cells profile and cytotoxic function in myalgic encephalomyelitis/chronic fatigue syndrome. Syst Rev (2019) 8:279.
478. Borish L, Schmaling K, DiClementi JD, Streib J, Negri J, Jones JF. Chronic fatigue syndrome: identification of distinct subgroups on the basis of allergy and psychologic variables. J Allergy Clin Immunol (1998) 102:222–30.
479. Corbitt M, Eaton-Fitch N, Staines D, Cabanas H, Marshall-Gradisnik S. A systematic review of cytokines in chronic fatigue syndrome/myalgic encephalomyelitis/systemic exertion intolerance disease (CFS/ME/SEID). BMC Neurol (2019) 19:207.
480. Giloteaux L, O'Neal A, Castro-Marrero J, Levine SM, Hanson MR. Cytokine profiling of extracellular vesicles isolated from plasma in myalgic encephalomyelitis/chronic fatigue syndrome: a pilot study. J Transl Med (2020) 18:387.
481. Gupta S, Aggarwal S, See D, Starr A. Cytokine production by adherent and non-adherent mononuclear cells in chronic fatigue syndrome. J Psychiatr Res (1997) 31:149–56.
482. Yang T, Yang Y, Wang D, Li C, Qu Y, Guo J, et al. The clinical value of cytokines in chronic fatigue syndrome. J Trans Med (2019) 17:213.
483. Brenu EW, Broadley S, Nguyen T, Johnston S, Ramos S, Staines D, et al. A preliminary comparative assessment of the role of CD8+ t cells in chronic fatigue Syndrome/Myalgic encephalomyelitis and multiple sclerosis. J Immunol Res (2016) 2016:9064529.
484. Cortes Rivera M, Mastronardi C, Silva-Aldana CT, Arcos-Burgos M, Lidbury BA. Myalgic Encephalomyelitis/Chronic fatigue syndrome: A comprehensive review. Diagnostics (Basel) 9 (2019).
485. Mandarano AH, Maya J, Giloteaux L, Peterson DL, Maynard M, Gottschalk CG, et al. Myalgic encephalomyelitis/chronic fatigue syndrome patients exhibit altered t cell metabolism and cytokine associations. J Clin Invest (2020) 130:1491–505.
486. Curriu M, Carrillo J, Massanella M, Rigau J, Alegre J, Puig J, et al. and T-cell phenotype and function in patients suffering from Chronic Fatigue Syndrome. J Transl Med. (2013) 11:68. doi: 10.1186/1479-5876-11-68
487. Ascherio A, Munger KL. EBV and autoimmunity. Epstein Barr Virus Volume 1: One Herpes Virus: Many Dis. (2015) 390:365–85. doi: 10.1007/978-3-319-22822-8_15
488. Richman S, Morris MC, Broderick G, Craddock TJA, Klimas NG, Fletcher MA. Pharmaceutical interventions in chronic fatigue syndrome: A literature-based commentary. Clin Ther. (2019) 41:798–805. doi: 10.1016/j.clinthera.2019.02.011
489. Carlo-Stella N, Bozzini S, De Silvestri A, Sbarsi I, Pizzochero C, Lorusso L, et al. Molecular study of receptor for advanced glycation endproduct gene promoter and identification of specific HLA haplotypes possibly involved in chronic fatigue syndrome. Int J Immunopathol Pharmacol. (2009) 22:745–54. doi: 10.1177/039463200902200320
490. Underhill JA, Mahalingam M, Peakman M, Wessely S. Lack of association between HLA genotype and chronic fatigue syndrome. Eur J Immunogenet. (2001) 28:425–8. doi: 10.1046/j.1365-2370.2001.00235.x
491. Kochi Y. Genetics of autoimmune diseases: perspectives from genome-wide association studies. Int Immunol. (2016) 28:155–61. doi: 10.1093/intimm/dxw002
492. Frederiksen B, Liu E, Romanos J, Steck A, Yin X, Kroehl M, et al. Investigation of the vitamin D receptor gene (VDR) and its interaction with protein tyrosine phosphatase, non-receptor type 2 gene (PTPN2) on risk of islet autoimmunity and type 1 diabetes: the Diabetes Autoimmunity Study in the Young (DAISY). J Steroid Biochem Mol Biol. (2013) 133:51–7. doi: 10.1016/j.jsbmb.2012.08.012
493. Sigurdsson S, Nordmark G, Garnier S, Grundberg E, Kwan T, Nilsson O, et al. A risk haplotype of STAT4 for systemic lupus erythematosus is over-expressed, correlates with anti-dsDNA and shows additive effects with two risk alleles of IRF5. Hum Mol Genet. (2008) 17:2868–76. doi: 10.1093/hmg/ddn184
494. Hirschfield GM, Liu X, Xu C, Lu Y, Xie G, Lu Y, et al. Primary biliary cirrhosis associated with HLA, IL12A, and IL12RB2 variants. New Engl J Med. (2009) 360:2544–55. doi: 10.1056/NEJMoa0810440
495. Ting W-H, Chien M-N, Lo F-S, Wang C-H, Huang C-Y, Lin C-L, et al. Association of cytotoxic T-lymphocyte-associated protein 4 (CTLA4) gene polymorphisms with autoimmune thyroid disease in children and adults: case-control study. PloS One. (2016) 11:e0154394. doi: 10.1371/journal.pone.0154394
496. Rajeevan MS, Dimulescu I, Murray J, Falkenberg VR, Unger ER. Pathway-focused genetic evaluation of immune and inflammation related genes with chronic fatigue syndrome. Hum Immunol. (2015) 76:553–60. doi: 10.1016/j.humimm.2015.06.014
497. Niller HH, Wolf H, Ay E, Minarovits J. Epigenetic dysregulation of epstein-barr virus latency and development of autoimmune disease. Epigenetic Contributions Autoimmune Dis. (2011) 711, 82–102. doi: 10.1007/978-1-4419-8216-2_7
498. Manian FA. Simultaneous measurement of antibodies to Epstein-Barr virus, human herpesvirus 6, herpes simplex virus types 1 and 2, and 14 enteroviruses in chronic fatigue syndrome: is there evidence of activation of a nonspecific polyclonal immune response? Clin Infect Dis. (1994) 19:448–53. doi: 10.1093/clinids/19.3.448
499. Loebel M, Eckey M, Sotzny F, Hahn E, Bauer S, Grabowski P, et al. Serological profiling of the EBV immune response in Chronic Fatigue Syndrome using a peptide microarray. PloS One. (2017) 12:e0179124. doi: 10.1371/journal.pone.0179124
500. Loebel M, Strohschein K, Giannini C, Koelsch U, Bauer S, Doebis C, et al. Deficient EBV-specific B-and T-cell response in patients with chronic fatigue syndrome. PloS One. (2014) 9:e85387. doi: 10.1371/journal.pone.0085387
501. Kerr JR. Epstein-barr virus induced gene-2 upregulation identifies a particular subtype of chronic fatigue syndrome/myalgic encephalomyelitis. Front Pediatr. (2019) 7:59. doi: 10.3389/fped.2019.00059
502. Nishikai M, Tomomatsu S, Hankins RW, Takagi S, Miyachi K, Kosaka S, et al. Autoantibodies to a 68/48 kDa protein in chronic fatigue syndrome and primary fibromyalgia: a possible marker for hypersomnia and cognitive disorders. Rheumatology. (2001) 40:806–10. doi: 10.1093/rheumatology/40.7.806
503. Loebel M, Grabowski P, Heidecke H, Bauer S, Hanitsch LG, Wittke K, et al. Antibodies to β adrenergic and muscarinic cholinergic receptors in patients with Chronic Fatigue Syndrome. Brain Behav Immun. (2016) 52:32–9. doi: 10.1016/j.bbi.2015.09.013
504. Tanaka S, Kuratsune H, Hidaka Y, Hakariya Y, Tatsumi K-I, Takano T, et al. Autoantibodies against muscarinic cholinergic receptor in chronic fatigue syndrome. Int J Mol Med. (2003) 12:225–30. doi: 10.3892/ijmm
505. Mikecz AV, Konstantinov K, Buchwald DS, Gerace L, Tan EM. High frequency of autoantibodies to insoluble cellular antigens in patients with chronic fatigue syndrome. Arthritis Rheumatism: Off J Am Coll Rheumatol. (1997) 40:295–305. doi: 10.1002/art.1780400215
506. Ortega-Hernandez OD, Cuccia M, Bozzini S, Bassi N, Moscavitch S, Diaz-Gallo LM, et al. Autoantibodies, polymorphisms in the serotonin pathway, and human leukocyte antigen class II alleles in chronic fatigue syndrome: are they associated with age at onset and specific symptoms? Ann New York Acad Sci. (2009) 1173:589–99. doi: 10.1111/j.1749-6632.2009.04802.x
507. De Beéck KO, Vermeersch P, Verschueren P, Westhovens R, Mariën G, Blockmans D, et al. Antinuclear antibody detection by automated multiplex immunoassay in untreated patients at the time of diagnosis. Autoimmun Rev. (2012) 12:137–43. doi: 10.1016/j.autrev.2012.02.013
508. Klein R, Berg P. High incidence of antibodies to 5-hydroxytryptamine, gangliosides and phospholipids in patients with chronic fatigue and fibromyalgia syndrome and their relatives: evidence for a clinical entity of both disorders. Eur J Med Res. (1995) 1:21–6.
509. Hokama Y, Empey-Campora C, Hara C, Higa N, Siu N, Lau R, et al. Acute phase phospholipids related to the cardiolipin of mitochondria in the sera of patients with chronic fatigue syndrome (CFS), chronic Ciguatera fish poisoning (CCFP), and other diseases attributed to chemicals, Gulf War, and marine toxins. J Clin Lab Anal. (2008) 22:99–105. doi: 10.1002/jcla.20217
510. Hokama Y, Campora CE, Hara C, Kuribayashi T, Le Huynh D, Yabusaki K. Anticardiolipin antibodies in the sera of patients with diagnosed chronic fatigue syndrome. J Clin Lab Anal. (2009) 23:210–2. doi: 10.1002/jcla.20325
511. Konstantinov K, von Mikecz A, Buchwald D, Jones J, Gerace L, Tan EM. Autoantibodies to nuclear envelope antigens in chronic fatigue syndrome. J Clin Invest. (1996) 98:1888–96. doi: 10.1172/JCI118990
512. Cabral-Marques O, Marques A, Giil LM, De Vito R, Rademacher J, Günther J, et al. GPCR-specific autoantibody signatures are associated with physiological and pathological immune homeostasis. Nat Commun. (2018) 9:5224. doi: 10.1038/s41467-018-07598-9
513. Hartwig J, Sotzny F, Bauer S, Heidecke H, Riemekasten G, Dragun D, et al. IgG stimulated β2 adrenergic receptor activation is attenuated in patients with ME/CFS. Brain Behavior Immun - Health. (2020) 3:100047. doi: 10.1016/j.bbih.2020.100047
514. Maes M, Mihaylova I, Kubera M, Leunis J-C, Twisk FNM, Geffard M. IgM-mediated autoimmune responses directed against anchorage epitopes are greater in Myalgic Encephalomyelitis/Chronic Fatigue Syndrome (ME/CFS) than in major depression. Metab Brain Dis. (2012) 27:415–23. doi: 10.1007/s11011-012-9316-8
515. Elfaitouri A, Herrmann B, Bölin-Wiener A, Wang Y, Gottfries C-G, Zachrisson O, et al. Epitopes of microbial and human heat shock protein 60 and their recognition in myalgic encephalomyelitis. PloS One. (2013) 8:e81155. doi: 10.1371/journal.pone.0081155
516. Vernon SD, Reeves WC. Evaluation of autoantibodies to common and neuronal cell antigens in Chronic Fatigue Syndrome. J Autoimmune Dis. (2005) 2:5. doi: 10.1186/1740-2557-2-5
517. Maes M, Mihaylova I, Leunis JC. Chronic fatigue syndrome is accompanied by an IgM-related immune response directed against neopitopes formed by oxidative or nitrosative damage to lipids and proteins. Neuro Endocrinol Lett. (2006) 27:615–21.
518. Fasano A. All disease begins in the (leaky) gut: role of zonulin-mediated gut permeability in the pathogenesis of some chronic inflammatory diseases. F1000Research. (2020) 9. doi: 10.12688/f1000research
519. Moisini I, Davidson A. BAFF: a local and systemic target in autoimmune diseases. Clin Exp Immunol. (2009) 158:155–63. doi: 10.1111/j.1365-2249.2009.04007.x
520. Mackay F, Schneider P. Cracking the BAFF code. Nat Rev Immunol. (2009) 9:491–502. doi: 10.1038/nri2572
521. Saulep-Easton D, Vincent FB, Quah PS, Wei A, Ting SB, Croce CM, et al. The BAFF receptor TACI controls IL-10 production by regulatory B cells and CLL B cells. Leukemia. (2016) 30:163–72. doi: 10.1038/leu.2015.174
522. Hedger MP, de Kretser DM. The activins and their binding protein, follistatin—diagnostic and therapeutic targets in inflammatory disease and fibrosis. Cytokine Growth factor Rev. (2013) 24:285–95. doi: 10.1016/j.cytogfr.2013.03.003
523. Lidbury BA, Kita B, Lewis DP, Hayward S, Ludlow H, Hedger MP, et al. Activin B is a novel biomarker for chronic fatigue syndrome/myalgic encephalomyelitis (CFS/ME) diagnosis: a cross sectional study. J Trans Med. (2017) 15:1–10. doi: 10.1186/s12967-017-1161-4
524. Cox BS, Alharshawi K, Mena-Palomo I, Lafuse WP, Ariza ME. EBV/HHV-6A dUTPases contribute to myalgic encephalomyelitis/chronic fatigue syndrome pathophysiology by enhancing TFH cell differentiation and extrafollicular activities. JCI Insight. (2022) 7. doi: 10.1172/jci.insight.158193
525. Spolski R, Leonard WJ. Interleukin-21: a double-edged sword with therapeutic potential. Nat Rev Drug Discovery. (2014) 13:379–95. doi: 10.1038/nrd4296
526. Crotty S. T follicular helper cell biology: A decade of discovery and diseases. Immunity. (2019) 50:1132–48. doi: 10.1016/j.immuni.2019.04.011
527. Chen W, Ten Dijke P. Immunoregulation by members of the TGFβ superfamily. Nat Rev Immunol. (2016) 16:723–40. doi: 10.1038/nri.2016.112
528. Chang PP, Barral P, Fitch J, Pratama A, Ma CS, Kallies A, et al. Identification of Bcl-6-dependent follicular helper NKT cells that provide cognate help for B cell responses. Nat Immunol. (2011) 13:35–43. doi: 10.1038/ni.2166
529. Smith MB, Haney E, McDonagh M, Pappas M, Daeges M, Wasson N, et al. Treatment of myalgic encephalomyelitis/chronic fatigue syndrome: a systematic review for a National Institutes of Health Pathways to Prevention Workshop. Ann Internal Med. (2015) 162:841–50. doi: 10.7326/M15-0114
530. Castro-Marrero J, Sáez-Francàs N, Santillo D, Alegre J. Treatment and management of chronic fatigue syndrome/myalgic encephalomyelitis: all roads lead to Rome. Br J Pharmacol. (2017) 174:345–69. doi: 10.1111/bph.13702
531. Kim DY, Lee JS, Park SY, Kim SJ, Son CG. Systematic review of randomised controlled trials for chronic fatigue syndrome/myalgic encephalomyelitis (CFS/ME). J Transl Med. (2020) 18:7. doi: 10.1186/s12967-019-02196-9
532. U.S. ME/CFS clinician coalition, diagnosing and treating myalgic encephalomyelitis/chronic fatigue syndrome (ME/CFS). (U.S. ME/CFS CLinician Coalition) (2020).
533. Cullinan J, Pheby DFH, Araja D, Berkis U, Brenna E, de Korwin JD, et al. Perceptions of european ME/CFS experts concerning knowledge and understanding of ME/CFS among primary care physicians in europe: A report from the european ME/CFS research network (EUROMENE). Medicina (Kaunas). (2021) 57. doi: 10.3390/medicina57030208
534. Hng KN, Geraghty K, Pheby DFH. An audit of UK hospital doctors’ Knowledge and experience of myalgic encephalomyelitis. Medicina (Kaunas). (2021) 57. doi: 10.3390/medicina57090885
535. McEvedy CP, Beard AW. Concept of benign myalgic encephalomyelitis. Br Med J. (1970) 1:11–5. doi: 10.1136/bmj.1.5687.11
536. Hooper M. Myalgic encephalomyelitis: a review with emphasis on key findings in biomedical research. J Clin Pathol. (2007) 60:466–71. doi: 10.1136/jcp.2006.042408
537. Evengård B, Schacterle RS, Komaroff AL. Chronic fatigue syndrome: new insights and old ignorance. J Intern Med. (1999) 246:455–69. doi: 10.1046/j.1365-2796.1999.00513.x
538. David AS, Wessely S, Pelosi AJ. Postviral fatigue syndrome: time for a new approach. Br Med J (Clin Res Ed). (1988) 296:696–9. doi: 10.1136/bmj.296.6623.696
539. Stricklin A, Sewell M, Austad C. Objective measurement of personality variables in epidemic neuromyasthenia patients. S Afr Med J. (1990) 77:31–4.
540. American geriatrics society, american geriatrics society 2019 updated AGS beers criteria® for potentially inappropriate medication use in older adults. J Am Geriatr Soc. (2019) 67:674–94. doi: 10.1111/jgs.15767
541. National Institute for Health and Care Excellence - NICE, Chronic fatigue syndrome/myalgic encephalomyelitis (or encephalopathy): diagnosis and management (2007).
542. Jason LA, Brown M, Brown A, Evans M, Flores S, Grant-Holler E, et al. Energy conservation/envelope theory interventions to help patients with myalgic encephalomyelitis/chronic fatigue syndrome. Fatigue. (2013) 1:27–42. doi: 10.1080/21641846.2012.733602
543. Geraghty K, Hann M, Kurtev S. Myalgic encephalomyelitis/chronic fatigue syndrome patients’ reports of symptom changes following cognitive behavioural therapy, graded exercise therapy and pacing treatments: Analysis of a primary survey compared with secondary surveys. J Health Psychol. (2019) 24:1318–33. doi: 10.1177/1359105317726152
544. Kindlon T. Reporting of harms associated with graded exercise therapy and cognitive behavioural therapy in myalgic encephalomyelitis/chronic fatigue syndrome. Bull IACFS/ME. (2011) 19:59–111.
545. Psychotherapy for major depressive disorder and generalised anxiety disorder: A health technology assessment. Ont Health Technol Assess Ser. (2017) 17:1–167.
546. ME Association. TRIAL BY ERROR: the troubling case of the PACE chronic fatigue syndrome study| investigation by david tuller (Accessed 21 October 2015).
547. White PD, Goldsmith KA, Johnson AL, Potts L, Walwyn R, DeCesare JC, et al. Comparison of adaptive pacing therapy, cognitive behavior therapy, graded exercise therapy, and specialist medical care for chronic fatigue syndrome (PACE): a randomised trial. Lancet. (2011) 377:823–36. doi: 10.1016/S0140-6736(11)60096-2
548. White PD, Goldsmith K, Johnson AL, Chalder T, Sharpe M. Recovery from chronic fatigue syndrome after treatments given in the PACE trial. Psychol Med. (2013) 43:2227–35. doi: 10.1017/S0033291713000020
549. Wilshire CE, Kindlon T. Response: Sharpe, Goldsmith and Chalder fail to restore confidence in the PACE trial findings. BMC Psychol. (2019) 7:19. doi: 10.1186/s40359-019-0296-x
550. Wilshire CE, Kindlon T, Courtney R, Matthees A, Tuller D, Geraghty K, et al. Rethinking the treatment of chronic fatigue syndrome-a reanalysis and evaluation of findings from a recent major trial of graded exercise and CBT. BMC Psychol. (2018) 6:6. doi: 10.1186/s40359-018-0218-3
552. Campbell B. Pacing tutorial. In: ME/CFS and fibromyalgia self-help program. (USA: ME/CFS and Fibromyalgia Self-Help) (2020).
553. Visible, visible. (2024). Available at: https://www.makevisible.com
554. Aaron LA, Herrell R, Ashton S, Belcourt M, Schmaling K, Goldberg J, et al. Comorbid clinical conditions in chronic fatigue: a co-twin control study. J Gen Intern Med. (2001) 16:24–31. doi: 10.1046/j.1525-1497.2001.03419.x
555. Natelson BH. Myalgic encephalomyelitis/chronic fatigue syndrome and fibromyalgia: definitions, similarities, and differences. Clin Ther. (2019) 41:612–8. doi: 10.1016/j.clinthera.2018.12.016
556. Goldenberg DL, Simms RW, Geiger A, Komaroff AL. High frequency of fibromyalgia in patients with chronic fatigue seen in a primary care practice. Arthritis Rheum. (1990) 33:381–7. doi: 10.1002/art.1780330311
557. McKay PG, Walker H, Martin CR, Fleming M. Exploratory study into the relationship between the symptoms of chronic fatigue syndrome (CFS)/myalgic encephalomyelitis (ME) and fibromyalgia (FM) using a quasiexperimental design. BMJ Open. (2021) 11:e041947. doi: 10.1136/bmjopen-2020-041947
558. Reynolds GK, Lewis DP, Richardson AM, Lidbury BA. Comorbidity of postural orthostatic tachycardia syndrome and chronic fatigue syndrome in an Australian cohort. J Intern Med. (2014) 275:409–17. doi: 10.1111/joim.12161
559. Hoad A, Spickett G, Elliott J, Newton J. Postural orthostatic tachycardia syndrome is an under-recognised condition in chronic fatigue syndrome. Qjm. (2008) 101:961–5. doi: 10.1093/qjmed/hcn123
560. Jones JF, Nicholson A, Nisenbaum R, Papanicolaou DA, Solomon L, Boneva R, et al. Orthostatic instability in a population-based study of chronic fatigue syndrome. Am J Med. (2005) 118:1415. doi: 10.1016/j.amjmed.2005.06.013
561. Dahan S, Tomljenovic L, Shoenfeld Y. Postural Orthostatic Tachycardia Syndrome (POTS)–A novel member of the autoimmune family. Lupus. (2016) 25:339–42. doi: 10.1177/0961203316629558
562. Shanks L, Jason LA, Evans M, Brown A. Cognitive impairments associated with CFS and POTS. Front Physiol. (2013) 4:113. doi: 10.3389/fphys.2013.00113
563. Roerink ME, Lenders JW, Schmits IC, Pistorius AM, Smit JW, Knoop H, et al. Postural orthostatic tachycardia is not a useful diagnostic marker for chronic fatigue syndrome. J Intern Med. (2017) 281:179–88. doi: 10.1111/joim.12564
564. Mitchell WM. Efficacy of rintatolimod in the treatment of chronic fatigue syndrome/myalgic encephalomyelitis (CFS/ME). Expert Rev Clin Pharmacol (2016) 9:755–70.
565. Zarling JM, Schlais J, Eskra L, Greene JJ, Ts'o PO, Carter WA. Augmentation of human natural killer cell activity by polyinosinic acid-polycytidylic acid and its nontoxic mismatched analogues. J Immunol (1980) 124:1852–7.
566. Kim J, Yang G, Kim Y, Kim J, Ha J. AMPK activators: mechanisms of action and physiological activities. Exp Mol Med (2016) 48:e224–4.
567. Toogood PL, Clauw DJ, Phadke S, Hoffman D. Myalgic encephalomyelitis/chronic fatigue syndrome (ME/CFS): Where will the drugs come from? Pharmacol Res (2021) 165:105465.
568. Scheibenbogen C, Loebel M, Freitag H, Krueger A, Bauer S, Antelmann M, et al. Immunoadsorption to remove ß2 adrenergic receptor antibodies in Chronic Fatigue Syndrome CFS/ME. PloS One (2018) 13:e0193672.
569. Proal AD, Albert PJ, Blaney GP, Lindseth IA, Benediktsson C, et al. Immunostimulation in the era of the metagenome. Cell Mol Immunol (2011) 8:213–25.
570. Tölle M, Freitag H, Antelmann M, Hartwig J, Schuchardt M, van der Giet ,M, et al. Myalgic Encephalomyelitis/Chronic Fatigue Syndrome: Efficacy of Repeat Immunoadsorption. J Clin Med (2020) 9.
571. Rekeland IG, Fosså A, Lande A, Ktoridou-Valen I, Sørland K, Holsen M, et al. Intravenous Cyclophosphamide in Myalgic Encephalomyelitis/Chronic Fatigue Syndrome. An Open-Label Phase II Study. Front Med (Lausanne) (2020) 7:162.
572. Jain SK, Parsanathan R, Achari AE, Kanikarla-Marie P, Bocchini JA Jr.. Glutathione Stimulates Vitamin D Regulatory and Glucose-Metabolism Genes, Lowers Oxidative Stress and Inflammation, and Increases 25-Hydroxy-Vitamin D Levels in Blood: A Novel Approach to Treat 25-Hydroxyvitamin D Deficiency. Antioxid Redox Signal (2018) 29:1792–807.
574. Kaiser JD. A prospective, proof-of-concept investigation of KPAX002 in chronic fatigue syndrome. Int J Clin Exp Med (2015) 8:11064–74.
575. Montoya JG, Anderson JN, Adolphs DL, Bateman L, Klimas NG, Levine SM, et al. KPAX 002 as a treatment for Myalgic Encephalomyelitis / Chronic Fatigue Syndrome ( ME / CFS ) : a prospective , randomized trial. (2018).
576. Castro-Marrero J, Sáez-Francàs N, Segundo MJ, Calvo N, Faro M, Aliste L, et al. Effect of coenzyme Q10 plus nicotinamide adenine dinucleotide supplementation on maximum heart rate after exercise testing in chronic fatigue syndrome - A randomized, controlled, double-blind trial. Clin Nutr (2016) 35:826–34.
577. Castro-Marrero J, Cordero MD, Segundo MJ, Sáez-Francàs N, Calvo N, Román-Malo L, et al. Does oral coenzyme Q10 plus NADH supplementation improve fatigue and biochemical parameters in chronic fatigue syndrome? Antioxid Redox Signal (2015) 22:679–85.
578. Forsyth LM, Preuss HG, MacDowell AL, Chiazze L Jr., Birkmayer GD, Bellanti JA. Therapeutic effects of oral NADH on the symptoms of patients with chronic fatigue syndrome. Ann Allergy Asthma Immunol (1999) 82:185–91.
579. Maksoud R, Balinas C, Holden S, Cabanas H, Staines D, Marshall-Gradisnik S. A systematic review of nutraceutical interventions for mitochondrial dysfunctions in myalgic encephalomyelitis/chronic fatigue syndrome. J Transl Med (2021) 19:81.
580. Fluge Ø, Bruland O, Risa K, Storstein A, Kristoffersen EK, Sapkota D, et al. Benefit from b-lymphocyte depletion using the anti-CD20 antibody rituximab in chronic fatigue syndrome. a double-blind and placebo-controlled study. PloS One (2011) 6(10):e26358. doi: 10.1371/journal.pone.0026358
581. Adamowicz JL, Caikauskaite I, Friedberg F. Defining recovery in chronic fatigue syndrome: a critical review. Qual Life Res. (2014) 23:2407–16. doi: 10.1007/s11136-014-0705-9
582. Devendorf AR, Jackson CT, Sunnquist M. Defining and measuring recovery from myalgic encephalomyelitis and chronic fatigue syndrome: the physician perspective. Disabil Rehabil. (2019) 41:158–65. doi: 10.1080/09638288.2017.1383518
583. Koenig JE, Spor A, Scalfone N, Fricker AD, Stombaugh J, Knight R, et al. Succession of microbial consortia in the developing infant gut microbiome. Proc Natl Acad Sci U.S.A. (2011) 108 Suppl 1:4578–85. doi: 10.1073/pnas.1000081107
584. Tamburini S, Shen N, Wu HC, Clemente JC. The microbiome in early life: implications for health outcomes. Nat Med. (2016) 22:713–22. doi: 10.1038/nm.4142
585. Palmer C, Bik EM, DiGiulio DB, Relman DA, Brown PO. Development of the human infant intestinal microbiota. PloS Biol. (2007) 5:e177. doi: 10.1371/journal.pbio.0050177
586. DecodeME. (2023). Available at: https://www.decodeme.org.uk
587. Shan ZY, Barnden LR, Kwiatek RA, Bhuta S, Hermens DF, Lagopoulos J. Neuroimaging characteristics of myalgic encephalomyelitis/chronic fatigue syndrome (ME/CFS): a systematic review. J Transl Med. (2020) 18:335. doi: 10.1186/s12967-020-02506-6
588. Friedman KJ, Murovska M, Pheby DFH, Zalewski P. Our evolving understanding of ME/CFS. Medicina (Kaunas) 57. (2021). doi: 10.3390/medicina57030200
589. Nacul L, Authier FJ, Scheibenbogen C, Lorusso L, Helland IB, Martin JA, et al. European network on myalgic encephalomyelitis/chronic fatigue syndrome (EUROMENE): expert consensus on the diagnosis, service provision, and care of people with ME/CFS in europe. Medicina (Kaunas) 57. (2021). doi: 10.3390/medicina57050510
593. Tyson S, Stanley K, Gronlund TA, Leary S, Emmans Dean M, Dransfield C, et al. Research priorities for myalgic encephalomyelitis/chronic fatigue syndrome (ME/CFS): the results of a James Lind alliance priority setting exercise. Fatigue: Biomedicine Health Behav. (2022) 10:200–11. doi: 10.1080/21641846.2022.2124775
Keywords: myalgic encephalomyelitis/chronic fatigue syndrome, pathology, treatment, pathophysiology, diagnostic criteria
Citation: Arron HE, Marsh BD, Kell DB, Khan MA, Jaeger BR and Pretorius E (2024) Myalgic Encephalomyelitis/Chronic Fatigue Syndrome: the biology of a neglected disease. Front. Immunol. 15:1386607. doi: 10.3389/fimmu.2024.1386607
Received: 15 February 2024; Accepted: 11 April 2024;
Published: 03 June 2024.
Edited by:
Aleksandra Piechota-Polanczyk, Jagiellonian University, PolandReviewed by:
Pawel Zalewski, Nicolaus Copernicus University in Toruń, PolandShuhei Sakakibara, Jikei University of Health Care Sciences, Japan
Lorenzo Lorusso, ASST Lecco, Italy
Copyright © 2024 Arron, Marsh, Kell, Khan, Jaeger and Pretorius. This is an open-access article distributed under the terms of the Creative Commons Attribution License (CC BY). The use, distribution or reproduction in other forums is permitted, provided the original author(s) and the copyright owner(s) are credited and that the original publication in this journal is cited, in accordance with accepted academic practice. No use, distribution or reproduction is permitted which does not comply with these terms.
*Correspondence: Etheresia Pretorius, cmVzaWFwQHN1bi5hYy56YQ==; Douglas B. Kell, ZGJrQGxpdi5hYy51aw==
‡ORCID: Hayley E. Arron, orcid.org/0000-0002-6427-1278
Douglas B. Kell, orcid.org/0000-0001-5838-7963
B. Asad khan, orcid.org/0000-0003-1838-9002
Etheresia Pretorius, orcid.org/0000-0002-9108-2384