- 1Department of Thoracic Surgery, The Affliated Hospital to Changchun University of Chinese Medicine, Changchun, China
- 2Department of Breast and Thyroid Surgery, The Affliated Hospital to Changchun University of Chinese Medicine, Changchun, China
- 3College of Acupuncture-Moxibustion and Tuina, Changchun University of Chinese Medicine, Changchun, China
- 4Laboratory Animal Center, College of Animal Science, Jilin University, Changchun, China
Breast cancer has a high incidence and a heightened propensity for metastasis. The absence of precise targets for effective intervention makes it imperative to devise enhanced treatment strategies. Exosomes, characterized by a lipid bilayer and ranging in size from 30 to 150 nm, can be actively released by various cells, including those in tumors. Exosomes derived from distinct subsets of immune cells have been shown to modulate the immune microenvironment within tumors and influence breast cancer progression. In addition, tumor-derived exosomes have been shown to contribute to breast cancer development and progression and may become a new target for breast cancer immunotherapy. Tumor immunotherapy has become an option for managing tumors, and exosomes have become therapeutic vectors that can be used for various pathological conditions. Edited exosomes can be used as nanoscale drug delivery systems for breast cancer therapy, contributing to the remodeling of immunosuppressive tumor microenvironments and influencing the efficacy of immunotherapy. This review discusses the regulatory role of exosomes from different cells in breast cancer and the latest applications of exosomes as nanoscale drug delivery systems and immunotherapeutic agents in breast cancer, showing the development prospects of exosomes in the clinical treatment of breast cancer.
1 Introduction
Despite advances in screening and treatment methods, breast cancer continues to be the predominant form of cancer for women (1). Breast cancer is the most commonly diagnosed cancer in women worldwide and the leading cause of cancer death, with an estimated 2.3 million new cancer cases and 685,000 cancer deaths in 2020. Breast cancer cases in women are expected to increase by 31% in 2040 compared to 2020 (2). Based on expression of estrogen receptor (ER), progesterone receptor (PR), and human epidermal growth factor receptor 2 (HER2), breast cancer can be categorized into the molecular subtypes of HER2-positive (ER-negative, PR-negative, and HER2-positive), luminal A (ER-positive and/or PR-positive and HER2-negative), luminal B (ER-positive and/or PR-positive and HER2-positve), and triple-negative (ER-negative, PR-negative, and HER2-negative) (3). In addition, the triple-negative breast cancer (TNBC) subtype tends to have a more aggressive clinical profile, constituting approximately 15% of all cases (4).
The current treatment for breast cancer varies according to the subtype and tumor stage. It is usually based on surgery and conventional therapy, followed by adjuvant or neoadjuvant therapy (chemotherapy, radiotherapy [RT], and immunotherapy) (5). Nevertheless, around 40% of TNBC tumors recur, with cancer metastasis being the primary factor contributing to mortality among patients with breast cancer (6). Due to clinicians’ inability to accurately predict patients’ risk of developing metastatic disease or how they will respond to treatment, up to 20% die from metastatic breast cancer (7). Given the limited overall survival rates of patients with breast cancer and the absence of universally defined molecular targets for treatment, there is a pressing need to explore and develop novel therapeutic approaches for patients with breast cancer (8).
Immunotarget therapies have become a fundamental approach in treating numerous cancers. “Hot tumors,” characterized by T cell infiltration, interferon (IFN), and elevated programmed cell death 1 ligand 1 (PD-L1) levels, show a more favorable response to immunotherapy than “cold tumors,” which lack T cells and other immune components within their tissue (9). Breast cancer is considered a “cold” tumor, and its immunotherapy and immune microenvironment still receive significant attention (10). There is an urgent need to expand the immune-targeted drugs for breast cancer and further understand the regulatory mechanism of its immune microenvironment.
The tumor microenvironment (TME) is formed by tumor stroma or mesenchyme, including immune cells, fibroblasts, extracellular matrix (ECM), blood vessels, and extracellular components (e.g., cytokines, chemokines, and exosomes) (11). The signal transduction pathway mediated by exosomes can regulate TME, which is an important way for exosomes to influence tumorigenesis and progression (12). Immunotherapy is a crucial approach in treating breast cancer, with unsatisfactory outcomes often attributed to an aberrant TME. Therefore, remodeling the TME to enhance the efficacy of breast cancer treatment and improve prognosis is greatly important (13). Enhancing the immunosuppressive microenvironment and manipulating immune cells and cytokines could contribute to optimizing antitumor therapy for breast cancer (14).
In recent years, the development and application of breast cancer nano-therapeutics have been constantly evolving to compensate for the inherent shortcomings of traditional therapies (15). Among them, exosomes have great potential to treat breast cancer because they have cell and tissue tropism, they can efficiently accumulate in tumors, and their contents or composition can be configured and modified (16). Exosomes are 30–150 nm nanoscale vesicles with phospholipid bilayer membranes secreted by eukaryotic cells (17). Endogenous exosomes can be used to enhance the delivery of drugs with low immunogenicity to tumors (18). In addition, exosomes can impact numerous vital cellular processes and the properties of breast cancer cells, including their proliferation, migration, invasion, and metastasis (19, 20). The RNA and protein contents of exosomes may be related to the reprogramming of receptor cells, such as immune cells, and have the potential for immune regulation (21). Exosomes are involved in antigen presentation and immune activation during cell communication, which may act as a medium for the interaction between cancer and immune cells. They may also be involved in avoiding immune surveillance during tumor development (22). Therefore, immune microenvironment-associated exosomes are promising therapeutic targets for early cancer diagnosis, immune regulation, and drug therapy. This review summarizes recent advancements in the research on exosomes for treating breast cancer, focusing on their regulatory impact within the tumor immune microenvironment, particularly those created by tumor and immune cells. It also discusses the potential of exosomes as therapeutic vectors to improve the efficacy of conventional cancer immunotherapy and novel therapies resulting from combining biomaterials and genetic engineering.
2 Exosomes influence tumor development
2.1 Exosomes from tumor cells promote breast cancer metastasis
Numerous studies have indicated that exosomes derived from cancer cells can guide immune cells to enhance tumor characteristics (Figure 1). These exosomes are crucial in promoting various aspects of tumor progression, such as stimulating tumorigenesis, infiltrating surrounding tissues, facilitating angiogenesis, inducing the formation of pre-metastatic niches, and promoting metastatic spread (23, 24).
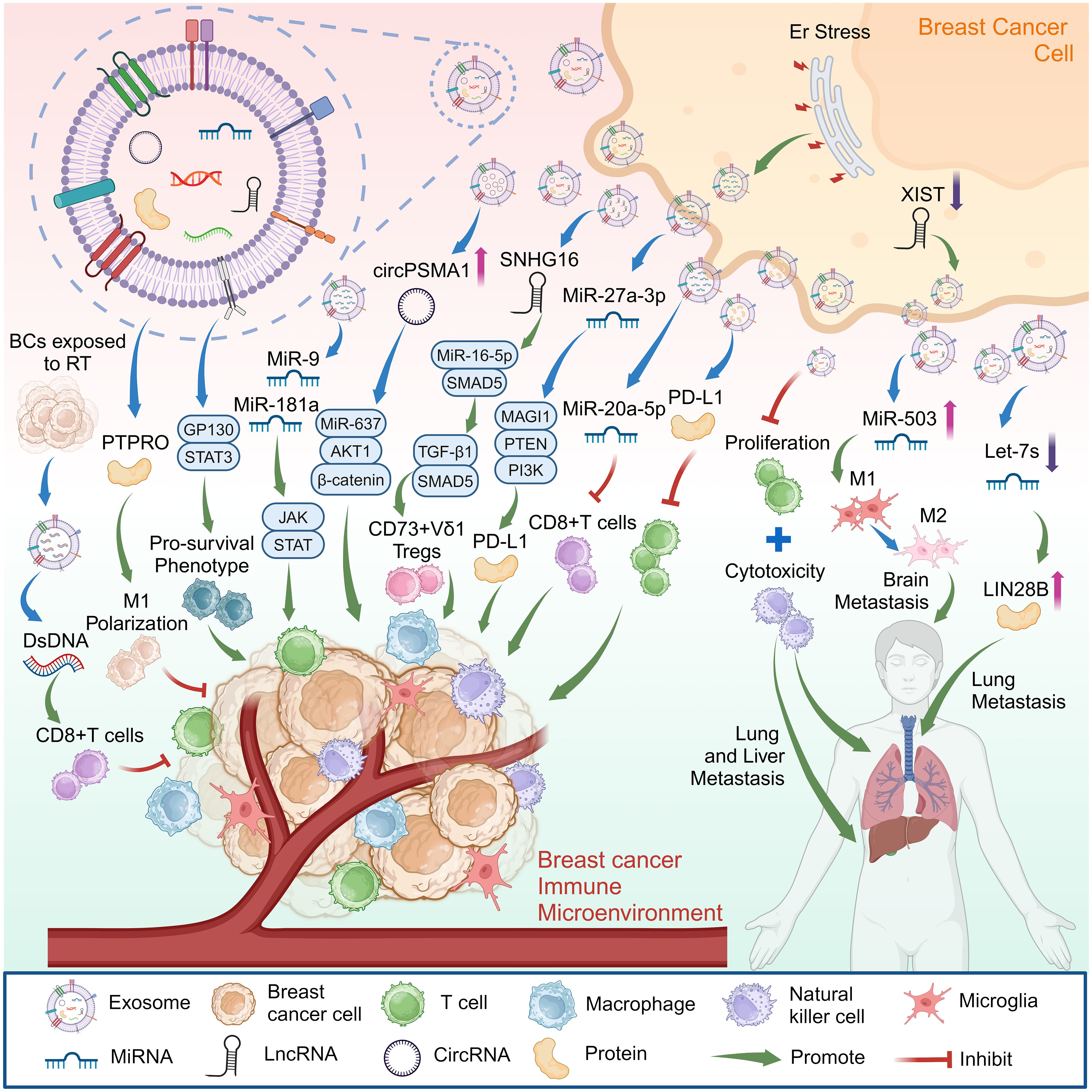
Figure 1 Exosomes from breast cancer cells can affect breast cancer progression by promoting cancer metastasis, regulating the immune microenvironment of breast cancer. Created with BioRender.com.
Metastasis is the phenomenon where cancer cells disseminate to distant locations, and exosomes are recognized as essential mediators in forming the niches preceding metastasis (25). Experiments conducted with mouse models of breast cancer and samples from patients with cancer have indicated that cytokines including C-C motif chemokine ligand 2 (CCL2), interleukin-6 (IL-6), chemokine (C-X-C motif) ligand 1 (CXCL1), and granulocyte-macrophage colony-stimulating factor (GM-CSF) interact with cancer-derived exosomes in the TME by binding to the glycosaminoglycan side chains of their proteoglycans. This interaction influences the accumulation of organ-specific exosomes, contributing to the promotion of cancer metastasis (26). Besides, some studies have indicated increased circular RNA circPSMA1 levels in exosomes originating from TNBC cells. Exosomal circPSMA1 was found to enhance the processes of tumorigenesis, metastasis, and migration in TNBC by modulating the microRNA (miRNA)-637/AKT serine/threonine kinase 1 (AKT1)/β-catenin (CTNNB1; cyclin D1 [CCND1]) axis (27).
Additionally, research findings indicate that exosomes derived from breast cancer contribute to the development of an immunosuppressive microenvironment, facilitating breast cancer metastasis and colonization. Moreover, breast cancer exosomes can directly inhibit T cell proliferation and suppress the cytotoxicity of natural killer (NK) cells, potentially impeding the anti-cancer immune response in organs before metastasis (28). Furthermore, the pluripotent factor lin-28 homolog B (LIN28B) is highly expressed in breast tumors, and studies have shown that low-let-7s exosomes released by tumors are a prerequisite for LIN28B-induced immunosuppression. LIN28B promotes the lung metastasis of breast cancer by establishing an immunosuppressive pre-metastatic ecological niche, enabling neutrophil recruitment and pro-tumor (N2) transformation, and promoting cancer progression (29).
Additionally, approximately 30% of patients with metastatic breast cancer eventually develop brain metastasis, and tumor cells adapt to the brain microenvironment primarily by reprogramming cells within the brain metastasis niche, which is composed of astrocytes, microglia, and various immune cells (30). Microglia are the primary innate immune cells in the brain and are a vital component of the TME in brain metastasis. Like macrophages, activated microglia have both tumor-suppressing (M1) and tumor-promoting (M2) effects (31). Studies have shown that the long non-coding RNA (lncRNA) X inactive specific transcript (XIST) plays a crucial role in the brain metastasis of patients with breast cancer by affecting tumor cells and TME. Deleting XIST also increases the secretion of exosomal miRNA-503. It can potentially induce the M1-M2 polarization of microglia and suppress the proliferation of T cells, making it a promising target for effectively treating brain metastasis in breast cancer (32).
2.2 Exosomes from tumor cells regulate PD-1/PD-L1
PD-L1 is an immune surface protein that inhibits the antitumor activity of T cells by binding to the programmed cell death-1 (PD-1) receptor, effectively shielding tumors from immune surveillance (33). Research has shown that the PD-L1 carried in exosomes originating from human breast cancer cells plays a defensive role by actively impeding the ability of T cells to eliminate breast cancer cells, facilitating tumor growth (34).
TME stress that disrupts protein homeostasis produces endoplasmic reticulum (ER) stress. ER stress promotes exosome secretion and increased exosomal miR-27a-3p, which can promote immune escape by upregulating PD-L1 via the membrane-associated guanylate kinase, WW and PDZ domain-containing (MAGI1)/phosphatase and tensin homolog (PTEN)/phosphoinositide-3 kinase (PI3K) axis in breast cancer (35). Furthermore, combining PD-1 monoclonal antibodies with chemotherapy is currently widely used to treat patients with TNBC. However, clinical experience shows that most patients will develop resistance after prolonged treatment (36). Some studies have found that circulating miR-20a-5p released by TNBC cells through exosomes promotes cancer cell growth by inducing cluster of differentiation 8 (CD8+) T cell dysfunction, leading to immunosuppression. Modulating miR-20a-5p could emerge as a novel approach to overcome the resistance of TNBC to anti-PD-1 immunotherapy (37).
2.3 Exosomes from tumor cells regulate immune cells in the immune microenvironment
Gamma delta (γδ) T cells play an immunosuppressive role in various solid malignant tumors. They are the main component of breast cancer tumor-infiltrating lymphocytes, which are closely associated with poor pathological features and prognosis (38). Breast cancer cells have been shown to promote SMAD family member 5 (SMAD5) expression in γδT1 cells by transferring exosome lncRNA small nucleolar RNA host gene 16 (SNHG16), which acts as a competing endogenous RNA by sponging miR-16–5p, thereby enhancing the transforming growth factor (TGF)-β1/SMAD5 pathway and upregulating 5’-nucleotidase ecto (NT5E/CD73) levels. These results suggest that targeting CD73+ γδT1 cells or blocking tumor cell-derived exosomes may be potential strategies for TNBC therapy (10).
Additional research has demonstrated that miR-9 and miR-181a, originating from exosomes derived from breast cancer cells, activate the Janus kinase (JAK)/signal transducer and activator of transcription (STAT) signaling pathway. Through their respective targeting of suppressor of cytokine signaling 3 (SOCS3) and protein inhibitor of activated STAT 3 (PIAS3), these miRNAs help induce the proliferation of early myeloid suppressor cells within breast cancer and exert a potent inhibitory influence on T cell immunity in both mice and humans (39). Moreover, bone marrow-derived macrophages (BMDMs) can internalize exosomes originating from tumors. Studies have shown that exosomes derived from breast cancer cells induce a change in the macrophage phenotype through the IL-6 receptor beta (glycoprotein 130, GP130)/STAT3 signaling pathway, helping to establish a tumor-promoting TME (40).
There are crucial interactions between the cancer cells that were killed and molecules within the immune system, of which damage-associated molecular patterns (DAMPs) are frequently recognized as intracellular molecules able to play a pivotal role in immune stimulation, inducing a shift from a cold to a hot TME (41). Exosomal contents will change under diverse treatment conditions, stimulating the immune system through DAMPs. Therefore, studies have examined stimulating breast cancer cells through photothermal therapy to make them secrete exosomal DAMPs. They have found that exosomes released by dying cancer cells stimulate the immune system and inhibit tumor growth more effectively (9).
Moreover, RT leads to the accumulation of cytoplasmic double-stranded DNA (dsDNA) in cancer cells, which is one of the critical signals for the spontaneous activation of anti-immunogenic tumors by antitumor T cells and the initiation of T cells. The dsDNA activates type I IFN (IFN-I) through the cyclic GMP-AMP synthase (CGAS)/stimulator of interferon response cGAMP interactor 1 (STING1) pathway and induces multiple IFN-stimulating genes (42). Tumor-derived exosomes released by radiation-irradiated breast cancer cells were found to carry dsDNA that could induce dendritic cells (DCs) to produce IFN-I in a STING-dependent manner. Moreover, exosomal dsDNA was regulated by triple repair exonuclease 1 (TREX1) expression in parental cells, which induced tumor-specific CD8+ T cell responses and produced protective antitumor immunity in mice (43).
In addition, the protein tyrosine phosphatase receptor type O (PTPRO) is a tumor suppressor. Studies have shown that PTPRO in exosomes derived from tumor cells promoted the polarization of M1-like macrophages and modulated their associated functional phenotype. Furthermore, exosomal PTPRO from breast tumor cells inhibited the invasion and migration of breast cancer cells while concurrently deactivating STAT signaling in macrophages (44).
2.4 Exosomes from immune cells regulate the immune microenvironment
Immune cells can recognize neoantigens as well as endogenous and exogenous ligands and may be used for chronic inflammation and immune monitoring. Immune cells in normal breast tissue are mainly located in the epithelial components of the ductal lobules of the breast. Progression to breast cancer is characterized by increased infiltration of immune cells in the tumor parenchyma and stroma (45). Immune cell infiltration in breast cancer consists of a variety of cell subtypes, including T-cell cluster of differentiation 3 (CD3+), CD4+ and CD8+ cells, B cells, monocytes/macrophages, DCs, and NK cells (46).
The breast cancer TME comprises not only tumor cells but also stromal cells, including different subpopulations of immune cells (47), exosomes from immune cells play an important role in the regulation of breast cancer progression (Figure 2). Cancer-associated fibroblasts (CAFs) are crucial contributors to the breast cancer TME. Their significant role lies in aiding breast cancer progression while inducing cancer cell migration and proliferation (48). Some studies have found that miR-92 expression significantly increases in breast cancer cells after treatment with CAF-derived exosomes. CAF-derived exosomes can significantly promote PD-L1 expression in breast cancer cells, inducing T cell apoptosis and impairing NK cell function (49). Additionally, PD-1 can be secreted as exosomes outside activated T cells and interact with the cell surface or PD-L1 remotely to weaken the inhibitory tumor immune microenvironment by attenuating the PD-L1-induced inhibition of tumor-specific cytotoxic T cell activity (50).
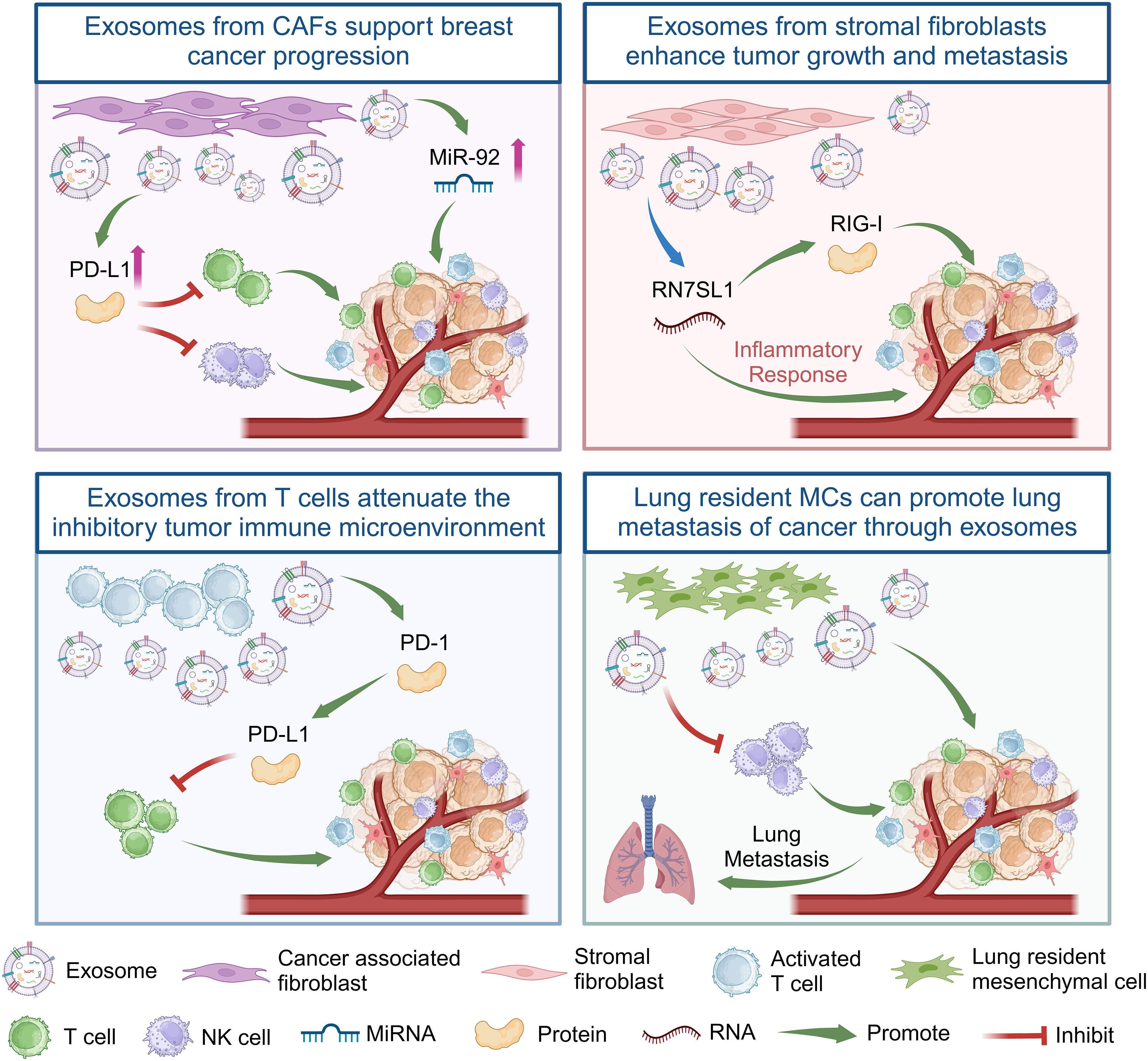
Figure 2 Exosomes from CAFs, stromal fibroblasts, T cells, and lung resident MCs regulate the immune response of T cells or NK cells in the immune microenvironment of breast cancer tumors by delivering non-coding RNA or proteins, thereby promoting the growth and metastasis of breast cancer. Created with BioRender.com.
In addition, the dynamic interplay between cancer and stromal cells within the TME is a notable aspect of regulated breast cancer (51). RNA component of signal recognition particle 7SL1 (RN7SL1), an endogenous lncRNA typically shielded by the RNA-binding signal recognition particles 9 (SRP9) and 14 (SRP14), is exposed within exosomes released by stromal fibroblasts. Following the transfer of these exosomes to immune cells, the unshielded RN7SL1 induces an inflammatory response. Upon breast cancer cell metastasis, the unshielded RN7SL1 activates the pattern recognition receptor retinoic acid-inducible gene I (RIGI), enhancing tumor growth, metastasis, and resistance to treatment (52). Furthermore, the environment of distant organs can potentially facilitate the metastasis of primary tumors (6). Research indicates that mesenchymal cells (MCs) in the lungs transport lipids to tumor and NK cells via exosome-like vesicles. This process results in enhanced tumor cell survival and proliferation, heightened NK cell dysfunction, the modulation of tumor cells and antitumor immunity, and ultimately promotes the lung metastasis of breast cancer (53). In summary, exosomes from different cells play an important role in the progression of breast cancer (Table 1).
3 Exosome-based breast cancer therapy
3.1 Targeted membrane-engineered exosome therapy for breast cancer
DCs are the most efficient antigen-presenting cells, which are pivotal in both triggering and orchestrating innate and adaptive immunity within the TME (54). Several vaccines designed to target DCs have been formulated and assessed in clinical trials, aiming to enhance the effectiveness of cancer immunotherapy (55). A targeted, specifically engineered exosome containing Hylton alcohol (a toll-like receptor 3 [TLR3] activator) and the immunogenic cell death-inducer human neutrophil elastase (ELANE) has been developed and optimized to form an in situ DC vaccine for breast cancer treatment, enhancing antitumor immunity against breast cancer (56).
The reduced efficiency of activating effector T cells results from the heightened immunosuppressive conditions in the TME (57). An approach that generates hybrids of macrophages and tumor cells has been developed to exploit the homing ability of circulating cancer cells to migrate back to the primary tumor site (58). This process involves the introduction of nuclei isolated from tumor cells into activated M1-like macrophages, resulting in the creation of chimeric exosomes termed aMT-exos. The aMT-exos can enter lymph nodes and activate T cells, targeting tumor sites, effectively inhibiting tumors, and prolonging the survival of primary breast cancer mouse models (59).
TNBC cells often overexpress the epidermal growth factor receptor (EGFR), with approximately 90% of patients with TNBC showing EGFR overexpression (60). An endogenous exosome carrying CD3 and a monoclonal antibody specific to cancer cell-associated EGFR has been designed and generated. The obtained exosomes could redirect and activate cytotoxic T cells to cancer cells to produce cancer-killing effects, not only inducing T cells to cross-link with EGFR-expressing breast cancer cells but also triggering effective antitumor immunity (61).
In addition, research has led to the creation of a synthetic polyvalent antibody retargeting exosome platform tailored to breast cancer expressing HER2. This platform redirects and activates cytotoxic T cells to target HER2-expressing breast cancer cells, achieved through the expression of anti-human CD3 and anti-human HER2 antibodies on the exosomes. The exosomes showed high efficiency and specific antitumor activity (62).
The aforementioned study showcases the potential preclinical viability of using natural exosomes for precise immunotherapy in treating breast cancer. Additionally, it highlights that genetically modified exosomes represent an innovative platform technology applicable to immune modulation (Figure 3).
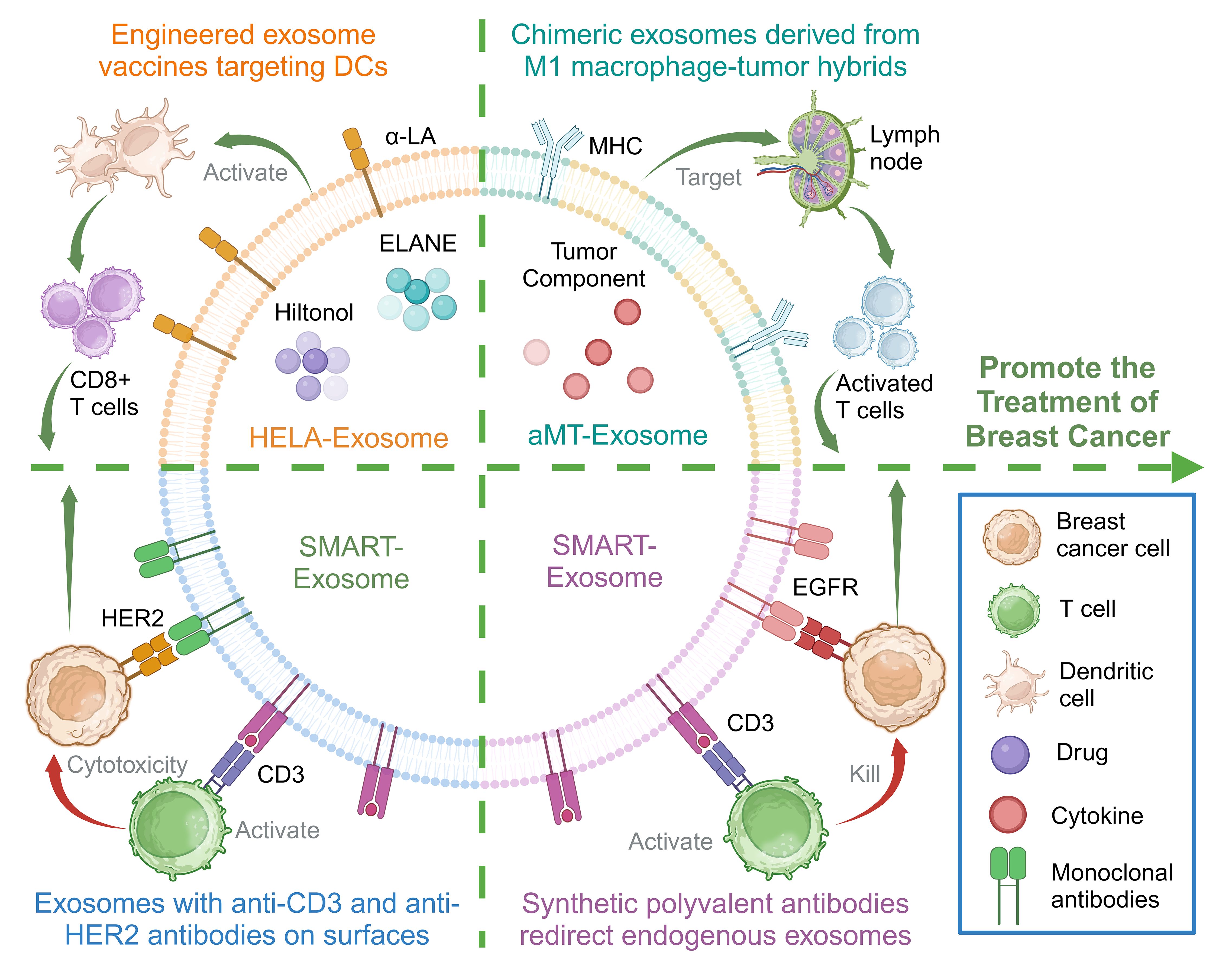
Figure 3 Membrane engineering on exosome surface enhances the targeting ability and immunogenicity to promote the treatment of breast cancer. Created with BioRender.com.
3.2 Exosomes targeting immune checkpoints to treat breast cancer
Cancer immunotherapy, which mainly uses immune checkpoint-targeting drugs, has gained wide attention (63) (Table 2). There are two well-established immune checkpoint pathways: the first involves the PD-1/PD-L1 pathway, inducing immune suppression; the second involves the tumor necrosis factor receptor superfamily member 4 (TNFRSF4/GP34/CD134/OX40)/tumor necrosis factor ligand superfamily member 4 (TNFSF4/OX40L) pathway, inducing immune activation (67). Stimulating the OX40/OX40L pathway can trigger adaptive immune cells, enabling them to exert an antitumor effect (68). Due to the vital role of the OX40/OX40L pathway in immunity, increasing research and drug development have focused on it (69). OX40L M1-exos, exosomes derived from M1-like macrophages overexpressing OX40L, have been developed to stimulate adaptive immunity by activating the OX40/OX40L pathway. These exosomes can also reprogram M2-like tumor-associated macrophages into M1-like macrophages. The OX40L M1-exos demonstrated significant therapeutic efficacy in a mouse breast cancer model, effectively suppressing both tumor growth and metastasis (64).
Another study created genetically modified exosomes to present antibodies and immunomodulatory proteins on their surfaces. These exosome membranes presented monoclonal antibodies targeting human T cell CD3, EGFR, and the immune checkpoint regulators PD-1 and OX40L. The resulting genetically engineered multifunctional immunomodulatory exosomes effectively inhibited EGFR-positive TNBC cells and elicited potent anti-cancer immune responses in vivo (65).
Tumor-draining lymph node (TDLN) metastases are the most common type of breast cancer. Since the TDLN is the primary immune organ that initiates the immune response against cancer, modulating its immunosuppressive microenvironment is critical to improving the prognosis of malignant tumors (70). One study developed a genetically engineered exosome carrying selectin L (SELL/CD62L; a protein that directs lymphocytes to home to lymph nodes) and OX40L in exosome donor cells. Treating mice with breast cancer using these exosomes activated effector T cells and inhibited regulatory T cells, promoting an antitumor immune response and inhibiting tumor development (66).
3.3 Genetically engineered drug-loaded exosomes to treat breast cancer
There is increasing research on exosomes modification to optimize breast cancer immunotherapy (Table 3). The immunosuppressive TME (ITM) is often considered the leading cause of many immunotherapy failures, in which the M2 tumor-associated macrophages play a critical tumor-promoting role in the ITM (78). Iron death is a form of cell death similar to pyrodeath that can induce a robust immune response and be used to enhance immunotherapy (79). Exosomes derived from M1 macrophages loaded with ferroptosis inducer RSL3 (RSL3-exo) spontaneously homed to tumors and M2-like macrophages, polarizing them into M1-like macrophages. The RSL3-exos could also disrupt redox homeostasis and induce iron death in breast cancer tumor cells, activating an immune response and having an antitumor effect (71).
Vaccines directed towards the TME can potentially enhance antitumor efficacy by transforming the immunosuppressive TME into an immune-enhancing environment. Most exosome-based cancer vaccines have been specifically designed to target the tumor’s parenchymal cells (80). CAFs are a significant cellular constituent within the TME. They help to facilitate tumor cell invasion and contribute to the persistent activation of signals driving tumor proliferation through ECM construction and remodeling. CAFs also help promote tumor angiogenesis and participate in the intricate interplay between cancer cells and other stromal cells (81). Fibroblast activation protein-alpha (FAP) is overexpressed in CAFs in >90% of human tumor tissues, and exosome-like nano-vesicles derived from tumor cells with FAP mutations (eNVs-FAP) have been developed. This vaccine inhibited tumor growth by eliciting a potent, specific cytotoxic T lymphocyte immune response against tumor cells and FAP-expressing CAFs, reshaping the immunosuppressive TME in a breast cancer model (72).
One study developed a docetaxel (DTX)-M1-exosomes drug delivery system, incorporating the chemotherapy drug DTX into exosomes derived from M1 macrophages (M1-EXO) with pro-inflammatory characteristics. Its findings indicated that DTX-M1-EXO could induce the polarization of M0 macrophages into M1 macrophages, sustaining a robust and prolonged M1 activation and further stimulating macrophage infiltration and activation in breast cancer (73).
Moreover, nanomaterial-based photothermal therapy has gained considerable attention as an emerging clinical approach for treating malignant tumors. Studies have indicated that gold nanorods have a direct cytotoxic effect on cancer cells in localized tumors through thermal ablation (82). A bionic hybrid system for specific drug delivery composed of tumor-derived exosomes and liposomes was designed to overcome the targeting defects of lipid carriers. A synergistic approach was devised using gold nanorod-polyethylene glycol and exosome-liposome paclitaxel (PTX) for the targeted treatment of recurrent tumors and distant metastases in patients with advanced breast cancer. Integrating thermal ablation, adaptive antitumor immunotherapy, and targeted PTX chemotherapy collectively enhanced the therapeutic efficacy against advanced breast cancer (74).
In addition, the mesenchymal-epithelial transition factor (c-Met) is a hepatocyte growth factor tyrosine kinase receptor important in promoting epithelial-mesenchymal transformation, angiogenesis, and cell proliferation (83). Based on its overexpression on the surface of TNBC cells, c-Met can potentially become an ideal target for TNBC antitumor drug delivery (84). A c-Met targeted exosome drug delivery system was developed using engineered exosome membrane-coated nanoparticles to package Doxorubicin (DOX)-loaded polylactic acid-glycolic acid nanoparticles into macrophage-derived exosomes. The engineered exosomes demonstrated significant tumor-targeting effects in vivo, inhibiting tumor growth and inducing tumor cell apoptosis, and are a promising drug delivery strategy for TNBC therapy (75).
Other studies have loaded sorafenib into NK cell-derived exosomes to enhance apoptosis in TNBC cells, which induced significant cytotoxicity in cancer cells (76). Furthermore, several miRNAs, including miR-155, let-7i, and miR-142, are involved in DC differentiation and maturation (85). Exosomes can serve as a vector for delivering any miRNA. miR-155, miR-142, and let-7i have been used to modify exosomes derived from breast cancer cells, enhancing their immunostimulatory potential and successfully prompting DCs maturation. These findings suggest that adapted exosomes hold substantial promise as a cell-free vaccine for cancer therapy (77). Engineered exosomes with therapeutic molecules may provide new insights into immune cell-based therapies or the development of novel vaccines for breast cancer (Figure 4).
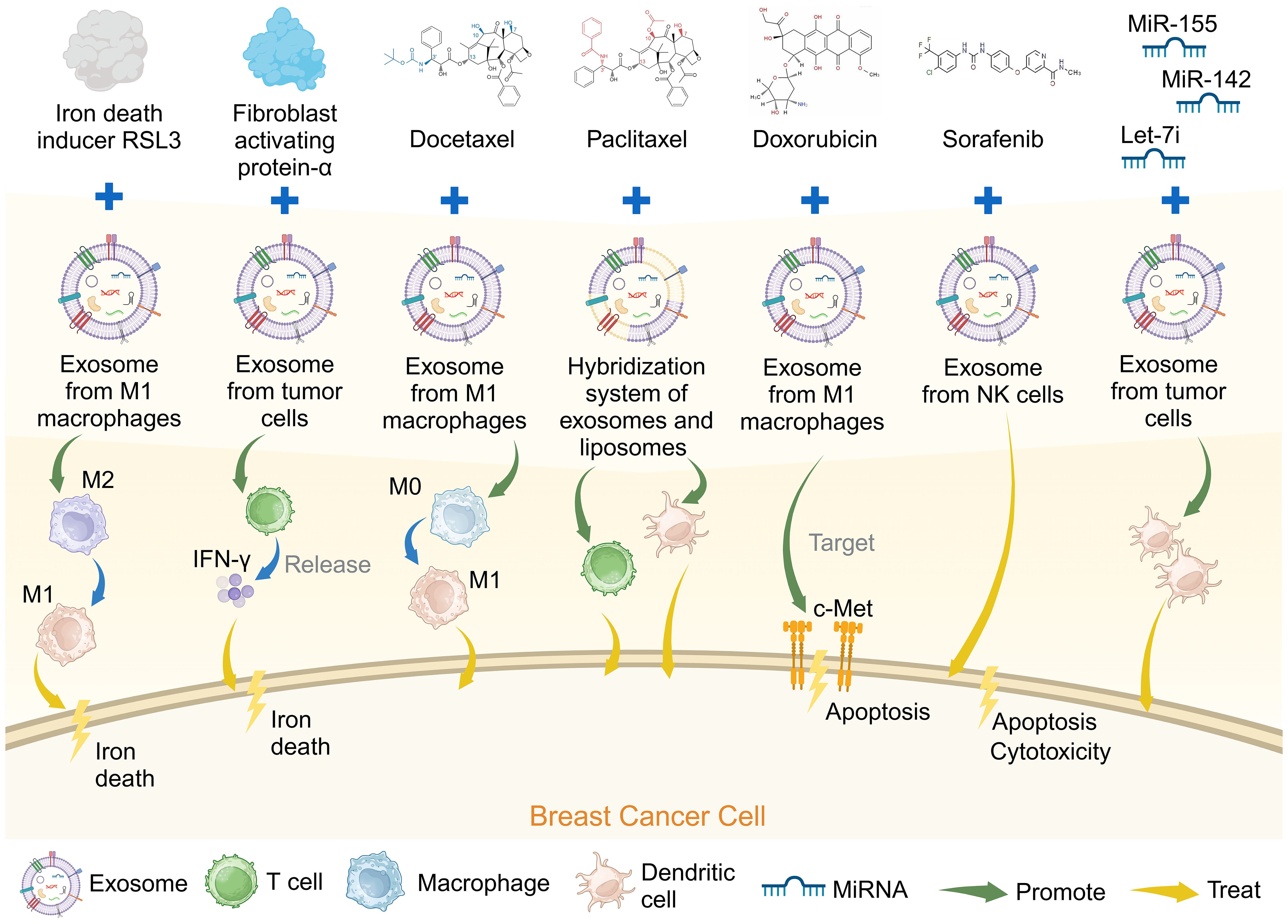
Figure 4 Genetically engineered exosomes loaded with breast cancer chemotherapy drugs can promote the treatment of breast cancer. Created with BioRender.com.
4 Discussion
The targeted therapy of immune checkpoints has shown promising results in clinical trials of various cancers. However, its efficacy in patients with breast cancer is generally unsatisfactory (86). Recent research has mainly explored potential ways to modulate the immunosuppressive TME while also inducing long-lasting antitumor activity in breast cancer. Therefore, developing appropriate tumor immune microenvironment delivery systems in preclinical studies is critical to improving the efficacy of combination therapy in patients with breast cancer. Exosomes have become key mediators of immunosuppression and immune evasion in the complex interactions between breast cancer tumors and normal cells (87). Tumor-derived exosomes may disrupt immune function and impair the efficacy of immunotherapy. In contrast, exosomes derived from immune cells may counteract these effects and promote immunotherapy, reflecting the crucial role of exosomes in regulating immune response. Therefore, they may serve as crucial therapeutic vectors targeting the tumor immune microenvironment.
The interactions between tumors and their microenvironment significantly contribute to tumor progression, metastasis, and resistance to treatment (88). Increasing evidence suggests that exosomes derived from tumor cells can systematically modulate or reprogram the TME by transferring molecules, including miRNAs, mRNAs, and proteins, from donor to recipient cells (89).
The bone, brain, liver, and lungs are the most frequent sites of metastasis in breast cancer (90). Metastasis is a significant characteristic of breast cancer, with approximately 20%–30% of patients diagnosed with early-stage breast cancer developing distant metastases. Unfortunately, complications arising from recurrent or metastatic disease contribute to the mortality of approximately 90% of patients (91).
Exosomes derived from tumors influence every phase of the invasion-metastasis cascade, and they actively contribute to the creation of pre-metastatic niches, engaging with and impeding the normal functions of immune cells, leading to the establishment of an immunosuppressive microenvironment (92). Studies have shown that interventions that interrupt exosome release or impede interactions between their contents and receptor molecules can partially reverse this remodeling of the immunosuppressive microenvironment.
In addition, immune cells such as CAFs, T cells, MCs, and NK cells can transfer exosomes to tumor cells to influence tumor fate. Since the antitumor immune response generated during immunotherapy is dynamic, the immune system must constantly rebuild its functional orientation to respond to different environmental developments. In addition, immune system elements can transmit exosomes to generate antitumor immunity or to promote tumor progression by shaping tumor immunogenicity (93). Enhancing the antitumor effect of exosomes and blocking their pro-tumor effect may be an important research target for breast cancer immunotherapy.
Immunotherapy is gaining widespread attention as a treatment option for breast cancer. Cancer immunotherapy commonly involves immune checkpoint inhibitors, cytokines, adoptive cell therapy, and cancer vaccines (94). Crucially, it is expected that exosomes originating from the TME could be highly efficient vaccines for breast cancer.
Numerous studies have shown the promising potential of exosomes as effective drug delivery carriers in anti-breast cancer treatment. These studies revealed that tumor-derived exosomes had favorable tumor-homing properties and low toxicity and immunogenicity in vivo (95). Modifying exosomes by genetic engineering could further enhance their targeting and therapeutic properties, expanding their clinical application.
Several studies have also designed hybrid exosomes that target immune cell activation to induce an immune response and promote the reversal of the immunosuppressive TME, which are more stable than cells and can be used after frozen storage (96). In addition, unlike molecular immunotherapies such as specific antibodies and immune checkpoint inhibitors, exosomes containing integrated immunomodulatory proteins may augment therapeutic efficacy by engaging in and regulating multiple immune checkpoint pathways. Approaches using membrane engineering to incorporate functional proteins into exosome membranes have shown enhanced stability and activity (65).
At present, most metastatic tumors are treated by systemic chemotherapy in the clinic. However, this poorly targeted treatment method can lead to drug resistance and side effects, reducing its efficacy. Exosome bioengineering methods can enhance targeted delivery to specific tissues or cells, and can be treated with smaller doses, facilitating their clinical application (97). Using exosomes as a drug delivery platform for combination therapy has shown better therapeutic effects in vivo, demonstrating that exosome-based targeted immune-chemotherapy is a promising candidate for treating breast cancer (74). Given the critical role of exosomes in breast cancer immunotherapy and TME regulation, it is necessary to further explore the relevant mechanisms and fully determine the safety and effectiveness of exosome-based immunotherapy, which is beneficial to developing targeted therapies for breast cancer.
5 Conclusions
Breast cancer is a challenging disease to cure. Therefore, new early diagnostic tools and more treatment options must be explored. The dynamic interactions between different cell types in the TME regulate various stages of breast cancer progression, and exosomes have been shown to mediate many regulatory processes. Exosomes from tumor and immune cells can promote an immunosuppressive microenvironment, tumor progression, and the immune response and inhibit tumor development. Compared to traditional nano-medical drugs, exosomes have unique advantages in drug delivery, especially genetically engineered exosomes or combining exosomes with chemotherapy drugs, which can significantly improve their targeting and therapeutic effect. Exosomes provide an opportunity for tumor immunotherapy, and factors such as the acquisition method of exosomes, biomarker detection, treatment method and drug selection, loading method of exosomes and appropriate dose for tumor treatment affect the clinical application of exosomes, which requires more extensive research and verification. In conclusion, exosomes play an essential role in breast cancer tumor immunity, modulating the immune microenvironment, underscoring the significance of investigating exosome-based immunotherapy for breast cancer.
Author contributions
YW: Writing – original draft, Writing – review & editing. QM: Writing – review & editing. TW: Writing – review & editing. JX: Writing – review & editing. QL: Writing – original draft, Writing – review & editing. DW: Writing – review & editing. GW: Writing – review & editing.
Funding
The author(s) declare financial support was received for the research, authorship, and/or publication of this article. This work was supported by the Jilin Province University Key Laboratory Scientific Research Program under Grant (2019) No. 004, the Jilin Education Department Program under Grant JJKH20230259KJ, the Jilin Province Development and Reform Commission Program under Grant 2023C028-6, the Graduate Innovation Fund of Jilin University under Grant 2023CX139, and the Jilin Scientific and Technological Development Program under Grant 20210101010JC, 20220505033ZP and 20230203077SF.
Acknowledgments
Appreciate BioRender.com and other participants for their contributions.
Conflict of interest
The authors declare that the research was conducted in the absence of any commercial or financial relationships that could be construed as a potential conflict of interest.
Publisher’s note
All claims expressed in this article are solely those of the authors and do not necessarily represent those of their affiliated organizations, or those of the publisher, the editors and the reviewers. Any product that may be evaluated in this article, or claim that may be made by its manufacturer, is not guaranteed or endorsed by the publisher.
References
1. Britt KL, Cuzick J, Phillips KA. Key steps for effective breast cancer prevention. Nat Rev Cancer. (2020) 20:417–36. doi: 10.1038/s41568–020-0266-x
2. Sedeta ET, Jobre B, Avezbakiyev B. Breast cancer: global patterns of incidence, mortality, and trends. J Clin Oncol. (2023) 41:10528. doi: 10.1200/JCO.2023.41.16_suppl.10528
3. Bae MS. Impact of molecular subtype definitions on ai classification of breast cancer at mri. Radiology. (2023) 307:e223041. doi: 10.1148/radiol.223041
4. Howell A, Cuzick J, Baum M, Buzdar A, Dowsett M, Forbes JF, et al. Results of the atac (Arimidex, tamoxifen, alone or in combination) trial after completion of 5 years' Adjuvant treatment for breast cancer. Lancet. (2005) 365:60–2. doi: 10.1016/S0140–6736(04)17666–6
5. Fraguas-Sanchez AI, Martin-Sabroso C, Fernandez-Carballido A, Torres-Suarez AI. Current status of nanomedicine in the chemotherapy of breast cancer. Cancer Chemother Pharmacol. (2019) 84:689–706. doi: 10.1007/s00280–019-03910–6
6. Chaffer CL, Weinberg RA. A perspective on cancer cell metastasis. Science. (2011) 331:1559–64. doi: 10.1126/science.1203543
7. Siegel R, Ma J, Zou Z, Jemal A. Cancer statistics, 2014. CA Cancer J Clin. (2014) 64:9–29. doi: 10.3322/caac.21208
8. Hsu JY, Chang CJ, Cheng JS. Survival, treatment regimens and medical costs of women newly diagnosed with metastatic triple-negative breast cancer. Sci Rep. (2022) 12:729. doi: 10.1038/s41598–021-04316–2
9. Najaflou M, Bani F, Khosroushahi AY. Immunotherapeutic effect of photothermal-mediated exosomes secreted from breast cancer cells. Nanomedicine (Lond). (2023) 18:1535–52. doi: 10.2217/nnm-2023–0014
10. Ni C, Fang QQ, Chen WZ, Jiang JX, Jiang Z, Ye J, et al. Breast cancer-derived exosomes transmit lncrna snhg16 to induce cd73+Gammadelta1 treg cells. Signal Transduct Target Ther. (2020) 5:41. doi: 10.1038/s41392–020-0129–7
11. Yuan YY, Li HM, Pu W, Chen LL, Guo D, Jiang HF, et al. Cancer metabolism and tumor microenvironment: fostering each other? Sci China Life Sci. (2022) 65:236–79. doi: 10.1007/s11427–021-1999–2
12. Xie JD, Zheng ZH, Tuo L, Deng XP, Tang HL, Peng C, et al. Recent advances in exosome-based immunotherapy applied to cancer. Front Immunol. (2023) 14:1296857. doi: 10.3389/fimmu.2023.1296857
13. Fan Y, He S. The characteristics of tumor microenvironment in triple negative breast cancer. Cancer Manag Res. (2022) 14:1–17. doi: 10.2147/CMAR.S316700
14. Liu Y, Hu Y, Xue J, Li J, Yi J, Bu J, et al. Advances in immunotherapy for triple-negative breast cancer. Mol Cancer. (2023) 22:145. doi: 10.1186/s12943–023-01850–7
15. Torres Quintas S, Canha-Borges A, Oliveira MJ, Sarmento B, Castro F. Special issue: nanotherapeutics in women's health emerging nanotechnologies for triple-negative breast cancer treatment. Small. (2023):e2300666. doi: 10.1002/smll.202300666
16. Wu D, Si M, Xue HY, Wong HL. Nanomedicine applications in the treatment of breast cancer: current state of the art. Int J Nanomedicine. (2017) 12:5879–92. doi: 10.2147/IJN.S123437
17. Parlatan U, Ozen MO, Kecoglu I, Koyuncu B, Torun H, Khalafkhany D, et al. Label-free identification of exosomes using raman spectroscopy and machine learning. Small. (2023) 19:e2205519. doi: 10.1002/smll.202205519
18. Gomari H, Forouzandeh Moghadam M, Soleimani M. Targeted cancer therapy using engineered exosome as a natural drug delivery vehicle. Onco Targets Ther. (2018) 11:5753–62. doi: 10.2147/OTT.S173110
19. Tang W, Xia M, Liao Y, Fang Y, Wen G, Zhong J. Exosomes in triple negative breast cancer: from bench to bedside. Cancer Lett. (2022) 527:1–9. doi: 10.1016/j.canlet.2021.12.009
20. Tutanov O, Orlova E, Proskura K, Grigor'eva A, Yunusova N, Tsentalovich Y, et al. Proteomic analysis of blood exosomes from healthy females and breast cancer patients reveals an association between different exosomal bioactivity on non-tumorigenic epithelial cell and breast cancer cell migration in vitro. Biomolecules. (2020) 10:495. doi: 10.3390/biom10040495
21. Guo W, Gao Y, Li N, Shao F, Wang C, Wang P, et al. Exosomes: new players in cancer (Review). Oncol Rep. (2017) 38:665–75. doi: 10.3892/or.2017.5714
22. Mao X, Xu J, Wang W, Liang C, Hua J, Liu J, et al. Crosstalk between cancer-associated fibroblasts and immune cells in the tumor microenvironment: new findings and future perspectives. Mol Cancer. (2021) 20:131. doi: 10.1186/s12943–021-01428–1
23. Sceneay J, Smyth MJ, Moller A. The pre-metastatic niche: finding common ground. Cancer Metastasis Rev. (2013) 32:449–64. doi: 10.1007/s10555–013-9420–1
24. Tutanov O, Proskura K, Kamyshinsky R, Shtam T, Tsentalovich Y, Tamkovich S. Proteomic profiling of plasma and total blood exosomes in breast cancer: A potential role in tumor progression, diagnosis, and prognosis. Front Oncol. (2020) 10:580891. doi: 10.3389/fonc.2020.580891
25. Lobb RJ, Lima LG, Moller A. Exosomes: key mediators of metastasis and pre-metastatic niche formation. Semin Cell Dev Biol. (2017) 67:3–10. doi: 10.1016/j.semcdb.2017.01.004
26. Lima LG, Ham S, Shin H, Chai EPZ, Lek ESH, Lobb RJ, et al. Tumor microenvironmental cytokines bound to cancer exosomes determine uptake by cytokine receptor-expressing cells and biodistribution. Nat Commun. (2021) 12:3543. doi: 10.1038/s41467–021-23946–8
27. Yang SJ, Wang DD, Zhong SL, Chen WQ, Wang FL, Zhang J, et al. Tumor-derived exosomal circpsma1 facilitates the tumorigenesis, metastasis, and migration in triple-negative breast cancer (Tnbc) through mir-637/akt1/beta-catenin (Cyclin D1) axis. Cell Death Dis. (2021) 12:420. doi: 10.1038/s41419–021-03680–1
28. Wen SW, Sceneay J, Lima LG, Wong CS, Becker M, Krumeich S, et al. The biodistribution and immune suppressive effects of breast cancer-derived exosomes. Cancer Res. (2016) 76:6816–27. doi: 10.1158/0008–5472.CAN-16–0868
29. Qi M, Xia Y, Wu Y, Zhang Z, Wang X, Lu L, et al. Lin28b-high breast cancer cells promote immune suppression in the lung pre-metastatic niche via exosomes and support cancer progression. Nat Commun. (2022) 13:897. doi: 10.1038/s41467–022-28438-x
30. Lowery FJ, Yu D. Brain metastasis: unique challenges and open opportunities. Biochim Biophys Acta Rev Cancer. (2017) 1867:49–57. doi: 10.1016/j.bbcan.2016.12.001
31. Hu X, Leak RK, Shi Y, Suenaga J, Gao Y, Zheng P, et al. Microglial and macrophage polarization-new prospects for brain repair. Nat Rev Neurol. (2015) 11:56–64. doi: 10.1038/nrneurol.2014.207
32. Xing F, Liu Y, Wu SY, Wu K, Sharma S, Mo YY, et al. Loss of xist in breast cancer activates msn-C-met and reprograms microglia via exosomal mirna to promote brain metastasis. Cancer Res. (2018) 78:4316–30. doi: 10.1158/0008–5472.CAN-18–1102
33. Zou W, Wolchok JD, Chen L. Pd-L1 (B7-H1) and pd-1 pathway blockade for cancer therapy: mechanisms, response biomarkers, and combinations. Sci Transl Med. (2016) 8:328rv4. doi: 10.1126/scitranslmed.aad7118
34. Yang Y, Li CW, Chan LC, Wei Y, Hsu JM, Xia W, et al. Exosomal pd-L1 harbors active defense function to suppress T cell killing of breast cancer cells and promote tumor growth. Cell Res. (2018) 28:862–4. doi: 10.1038/s41422–018-0060–4
35. Yao X, Tu Y, Xu Y, Guo Y, Yao F, Zhang X. Endoplasmic reticulum stress-induced exosomal mir-27a-3p promotes immune escape in breast cancer via regulating pd-L1 expression in macrophages. J Cell Mol Med. (2020) 24:9560–73. doi: 10.1111/jcmm.15367
36. Haratake N, Toyokawa G, Tagawa T, Kozuma Y, Matsubara T, Takamori S, et al. Positive conversion of pd-L1 expression after treatments with chemotherapy and nivolumab. Anticancer Res. (2017) 37:5713–7. doi: 10.21873/anticanres.12009
37. Li W, Han G, Li F, Bu P, Hao Y, Huang L, et al. Cancer cell-derived exosomal mir-20a-5p inhibits cd8(+) T-cell function and confers anti-programmed cell death 1 therapy resistance in triple-negative breast cancer. Cancer Sci. (2023) 115:347–56.. doi: 10.1111/cas.16036
38. Peng G, Wang HY, Peng W, Kiniwa Y, Seo KH, Wang RF. Tumor-infiltrating gammadelta T cells suppress T and dendritic cell function via mechanisms controlled by a unique toll-like receptor signaling pathway. Immunity. (2007) 27:334–48. doi: 10.1016/j.immuni.2007.05.020
39. Jiang M, Zhang W, Zhang R, Liu P, Ye Y, Yu W, et al. Cancer exosome-derived mir-9 and mir-181a promote the development of early-stage mdscs via interfering with socs3 and pias3 respectively in breast cancer. Oncogene. (2020) 39:4681–94. doi: 10.1038/s41388–020-1322–4
40. Ham S, Lima LG, Chai EPZ, Muller A, Lobb RJ, Krumeich S, et al. Breast cancer-derived exosomes alter macrophage polarization via gp130/stat3 signaling. Front Immunol. (2018) 9:871. doi: 10.3389/fimmu.2018.00871
41. Krysko DV, Garg AD, Kaczmarek A, Krysko O, Agostinis P, Vandenabeele P. Immunogenic cell death and damps in cancer therapy. Nat Rev Cancer. (2012) 12:860–75. doi: 10.1038/nrc3380
42. Woo SR, Fuertes MB, Corrales L, Spranger S, Furdyna MJ, Leung MY, et al. Sting-dependent cytosolic DNA sensing mediates innate immune recognition of immunogenic tumors. Immunity. (2014) 41:830–42. doi: 10.1016/j.immuni.2014.10.017
43. Diamond JM, Vanpouille-Box C, Spada S, Rudqvist NP, Chapman JR, Ueberheide BM, et al. Exosomes shuttle trex1-sensitive ifn-stimulatory dsdna from irradiated cancer cells to dcs. Cancer Immunol Res. (2018) 6:910–20. doi: 10.1158/2326–6066.CIR-17–0581
44. Dong H, Xie C, Jiang Y, Li K, Lin Y, Pang X, et al. Tumor-derived exosomal protein tyrosine phosphatase receptor type O polarizes macrophage to suppress breast tumor cell invasion and migration. Front Cell Dev Biol. (2021) 9:703537. doi: 10.3389/fcell.2021.703537
45. Goff SL, Danforth DN. The role of immune cells in breast tissue and immunotherapy for the treatment of breast cancer. Clin Breast Cancer. (2021) 21:e63–73. doi: 10.1016/j.clbc.2020.06.011
46. Azizi E, Carr AJ, Plitas G, Cornish AE, Konopacki C, Prabhakaran S, et al. Single-cell map of diverse immune phenotypes in the breast tumor microenvironment. Cell. (2018) 174:1293–308 e36. doi: 10.1016/j.cell.2018.05.060
47. Noy R, Pollard JW. Tumor-associated macrophages: from mechanisms to therapy. Immunity. (2014) 41:49–61. doi: 10.1016/j.immuni.2014.06.010
48. Houthuijzen JM, Jonkers J. Cancer-associated fibroblasts as key regulators of the breast cancer tumor microenvironment. Cancer Metastasis Rev. (2018) 37:577–97. doi: 10.1007/s10555–018-9768–3
49. Dou D, Ren X, Han M, Xu X, Ge X, Gu Y, et al. Cancer-associated fibroblasts-derived exosomes suppress immune cell function in breast cancer via the mir-92/pd-L1 pathway. Front Immunol. (2020) 11:2026. doi: 10.3389/fimmu.2020.02026
50. Qiu Y, Yang Y, Yang R, Liu C, Hsu JM, Jiang Z, et al. Activated T cell-derived exosomal pd-1 attenuates pd-L1-induced immune dysfunction in triple-negative breast cancer. Oncogene. (2021) 40:4992–5001. doi: 10.1038/s41388–021-01896–1
51. Kalluri R. The biology and function of fibroblasts in cancer. Nat Rev Cancer. (2016) 16:582–98. doi: 10.1038/nrc.2016.73
52. Nabet BY, Qiu Y, Shabason JE, Wu TJ, Yoon T, Kim BC, et al. Exosome rna unshielding couples stromal activation to pattern recognition receptor signaling in cancer. Cell. (2017) 170:352–66 e13. doi: 10.1016/j.cell.2017.06.031
53. Gong Z, Li Q, Shi J, Liu ET, Shultz LD, Ren G. Lipid-laden lung mesenchymal cells foster breast cancer metastasis via metabolic reprogramming of tumor cells and natural killer cells. Cell Metab. (2022) 34:1960–76 e9. doi: 10.1016/j.cmet.2022.11.003
54. Wculek SK, Cueto FJ, Mujal AM, Melero I, Krummel MF, Sancho D. Dendritic cells in cancer immunology and immunotherapy. Nat Rev Immunol. (2020) 20:7–24. doi: 10.1038/s41577–019-0210-z
55. Andtbacka RH, Kaufman HL, Collichio F, Amatruda T, Senzer N, Chesney J, et al. Talimogene laherparepvec improves durable response rate in patients with advanced melanoma. J Clin Oncol. (2015) 33:2780–8. doi: 10.1200/JCO.2014.58.3377
56. Huang L, Rong Y, Tang X, Yi K, Qi P, Hou J, et al. Engineered exosomes as an in situ dc-primed vaccine to boost antitumor immunity in breast cancer. Mol Cancer. (2022) 21:45. doi: 10.1186/s12943–022-01515-x
57. Gajewski TF, Schreiber H, Fu YX. Innate and adaptive immune cells in the tumor microenvironment. Nat Immunol. (2013) 14:1014–22. doi: 10.1038/ni.2703
58. Sancho-Albero M, Rubio-Ruiz B, Perez-Lopez AM, Sebastian V, Martin-Duque P, Arruebo M, et al. Cancer-derived exosomes loaded with ultrathin palladium nanosheets for targeted bioorthogonal catalysis. Nat Catal. (2019) 2:864–72. doi: 10.1038/s41929–019-0333–4
59. Wang S, Li F, Ye T, Wang J, Lyu C, Qing S, et al. Macrophage-tumor chimeric exosomes accumulate in lymph node and tumor to activate the immune response and the tumor microenvironment. Sci Transl Med. (2021) 13:eabb6981. doi: 10.1126/scitranslmed.abb6981
60. Changavi AA, Shashikala A, Ramji AS. Epidermal growth factor receptor expression in triple negative and nontriple negative breast carcinomas. J Lab Physicians. (2015) 7:79–83. doi: 10.4103/0974–2727.163129
61. Cheng Q, Shi X, Han M, Smbatyan G, Lenz HJ, Zhang Y. Reprogramming exosomes as nanoscale controllers of cellular immunity. J Am Chem Soc. (2018) 140:16413–7. doi: 10.1021/jacs.8b10047
62. Shi X, Cheng Q, Hou T, Han M, Smbatyan G, Lang JE, et al. Genetically engineered cell-derived nanoparticles for targeted breast cancer immunotherapy. Mol Ther. (2020) 28:536–47. doi: 10.1016/j.ymthe.2019.11.020
63. Galon J, Bruni D. Approaches to treat immune hot, altered and cold tumours with combination immunotherapies. Nat Rev Drug Discovery. (2019) 18:197–218. doi: 10.1038/s41573–018-0007-y
64. Yu Y, Li T, Ou M, Luo R, Chen H, Ren H, et al. Ox40l-expressing M1-like macrophage exosomes for cancer immunotherapy. J Control Release. (2024) 365:469–79. doi: 10.1016/j.jconrel.2023.11.051
65. Cheng Q, Dai Z, Smbatyan G, Epstein AL, Lenz HJ, Zhang Y. Eliciting anti-cancer immunity by genetically engineered multifunctional exosomes. Mol Ther. (2022) 30:3066–77. doi: 10.1016/j.ymthe.2022.06.013
66. Ji P, Yang Z, Li H, Wei M, Yang G, Xing H, et al. Smart exosomes with lymph node homing and immune-amplifying capacities for enhanced immunotherapy of metastatic breast cancer. Mol Ther Nucleic Acids. (2021) 26:987–96. doi: 10.1016/j.omtn.2021.10.009
67. Yi Y, Yu M, Li W, Zhu D, Mei L, Ou M. Vaccine-like nanomedicine for cancer immunotherapy. J Control Release. (2023) 355:760–78. doi: 10.1016/j.jconrel.2023.02.015
68. Webb GJ, Hirschfield GM, Lane PJ. Ox40, ox40l and autoimmunity: A comprehensive review. Clin Rev Allergy Immunol. (2016) 50:312–32. doi: 10.1007/s12016–015-8498–3
69. Alves Costa Silva C, Facchinetti F, Routy B, Derosa L. New pathways in immune stimulation: targeting ox40. ESMO Open. (2020) 5:e000573. doi: 10.1136/esmoopen-2019–000573
70. Munn DH, Mellor AL. The tumor-draining lymph node as an immune-privileged site. Immunol Rev. (2006) 213:146–58. doi: 10.1111/j.1600–065X.2006.00444.x
71. Wang D, Qiu G, Zhu X, Wang Q, Zhu C, Fang C, et al. Macrophage-inherited exosome excise tumor immunosuppression to expedite immune-activated ferroptosis. J Immunother Cancer. (2023) 11. doi: 10.1136/jitc-2022–006516
72. Hu S, Ma J, Su C, Chen Y, Shu Y, Qi Z, et al. Engineered exosome-like nanovesicles suppress tumor growth by reprogramming tumor microenvironment and promoting tumor ferroptosis. Acta Biomater. (2021) 135:567–81. doi: 10.1016/j.actbio.2021.09.003
73. Zhao Y, Zheng Y, Zhu Y, Li H, Zhu H, Liu T. Docetaxel-loaded M1 macrophage-derived exosomes for a safe and efficient chemoimmunotherapy of breast cancer. J Nanobiotechnology. (2022) 20:359. doi: 10.1186/s12951–022-01526–2
74. Huang H, Shao L, Chen Y, Tang L, Liu T, Li J, et al. Synergistic strategy with hyperthermia therapy based immunotherapy and engineered exosomes-liposomes targeted chemotherapy prevents tumor recurrence and metastasis in advanced breast cancer. Bioeng Transl Med. (2022) 7:e10284. doi: 10.1002/btm2.10284
75. Li S, Wu Y, Ding F, Yang J, Li J, Gao X, et al. Engineering macrophage-derived exosomes for targeted chemotherapy of triple-negative breast cancer. Nanoscale. (2020) 12:10854–62. doi: 10.1039/d0nr00523a
76. Hashemi ZS, Ghavami M, Kiaie SH, Mohammadi F, Barough MS, Khalili S, et al. Novel delivery of sorafenib by natural killer cell-derived exosomes-enhanced apoptosis in triple-negative breast cancer. Nanomedicine (Lond). (2023) 18:437–53. doi: 10.2217/nnm-2022–0237
77. Taghikhani A, Hassan ZM, Ebrahimi M, Moazzeni SM. Microrna modified tumor-derived exosomes as novel tools for maturation of dendritic cells. J Cell Physiol. (2019) 234:9417–27. doi: 10.1002/jcp.27626
78. Daurkin I, Eruslanov E, Stoffs T, Perrin GQ, Algood C, Gilbert SM, et al. Tumor-associated macrophages mediate immunosuppression in the renal cancer microenvironment by activating the 15-lipoxygenase-2 pathway. Cancer Res. (2011) 71:6400–9. doi: 10.1158/0008–5472.CAN-11–1261
79. Zhao L, Zhou X, Xie F, Zhang L, Yan H, Huang J, et al. Ferroptosis in cancer and cancer immunotherapy. Cancer Commun (Lond). (2022) 42:88–116. doi: 10.1002/cac2.12250
80. Rao Q, Zuo B, Lu Z, Gao X, You A, Wu C, et al. Tumor-derived exosomes elicit tumor suppression in murine hepatocellular carcinoma models and humans in vitro. Hepatology. (2016) 64:456–72. doi: 10.1002/hep.28549
81. Gaggioli C, Hooper S, Hidalgo-Carcedo C, Grosse R, Marshall JF, Harrington K, et al. Fibroblast-led collective invasion of carcinoma cells with differing roles for rhogtpases in leading and following cells. Nat Cell Biol. (2007) 9:1392–400. doi: 10.1038/ncb1658
82. Xu W, Qian J, Hou G, Wang Y, Wang J, Sun T, et al. A dual-targeted hyaluronic acid-gold nanorod platform with triple-stimuli responsiveness for photodynamic/photothermal therapy of breast cancer. Acta Biomater. (2019) 83:400–13. doi: 10.1016/j.actbio.2018.11.026
83. Raghav KP, Wang W, Liu S, Chavez-MacGregor M, Meng X, Hortobagyi GN, et al. Cmet and phospho-cmet protein levels in breast cancers and survival outcomes. Clin Cancer Res. (2012) 18:2269–77. doi: 10.1158/1078–0432.CCR-11–2830
84. Zagouri F, Bago-Horvath Z, Rossler F, Brandstetter A, Bartsch R, Papadimitriou CA, et al. High met expression is an adverse prognostic factor in patients with triple-negative breast cancer. Br J Cancer. (2013) 108:1100–5. doi: 10.1038/bjc.2013.31
85. Zhou H, Wu L. The development and function of dendritic cell populations and their regulation by mirnas. Protein Cell. (2017) 8:501–13. doi: 10.1007/s13238–017-0398–2
86. Deepak KGK, Vempati R, Nagaraju GP, Dasari VR, S N, Rao DN, et al. Tumor microenvironment: challenges and opportunities in targeting metastasis of triple negative breast cancer. Pharmacol Res. (2020) 153:104683. doi: 10.1016/j.phrs.2020.104683
87. Yue M, Hu S, Sun H, Tuo B, Jia B, Chen C, et al. Extracellular vesicles remodel tumor environment for cancer immunotherapy. Mol Cancer. (2023) 22:203. doi: 10.1186/s12943–023-01898–5
88. Quail DF, Joyce JA. Microenvironmental regulation of tumor progression and metastasis. Nat Med. (2013) 19:1423–37. doi: 10.1038/nm.3394
89. Milane L, Singh A, Mattheolabakis G, Suresh M, Amiji MM. Exosome mediated communication within the tumor microenvironment. J Control Release. (2015) 219:278–94. doi: 10.1016/j.jconrel.2015.06.029
90. Berman AT, Thukral AD, Hwang WT, Solin LJ, Vapiwala N. Incidence and patterns of distant metastases for patients with early-stage breast cancer after breast conservation treatment. Clin Breast Cancer. (2013) 13:88–94. doi: 10.1016/j.clbc.2012.11.001
91. Chen W, Hoffmann AD, Liu H, Liu X. Organotropism: new insights into molecular mechanisms of breast cancer metastasis. NPJ Precis Oncol. (2018) 2:4. doi: 10.1038/s41698–018-0047–0
92. Guo Y, Ji X, Liu J, Fan D, Zhou Q, Chen C, et al. Effects of exosomes on pre-metastatic niche formation in tumors. Mol Cancer. (2019) 18:39. doi: 10.1186/s12943–019-0995–1
93. Dunn GP, Old LJ, Schreiber RD. The three es of cancer immunoediting. Annu Rev Immunol. (2004) 22:329–60. doi: 10.1146/annurev.immunol.22.012703.104803
94. Li Z, Qiu Y, Lu W, Jiang Y, Wang J. Immunotherapeutic interventions of triple negative breast cancer. J Transl Med. (2018) 16:147. doi: 10.1186/s12967–018-1514–7
95. Taghikhani A, Farzaneh F, Sharifzad F, Mardpour S, Ebrahimi M, Hassan ZM. Engineered tumor-derived extracellular vesicles: potentials in cancer immunotherapy. Front Immunol. (2020) 11:221. doi: 10.3389/fimmu.2020.00221
96. Zhang Y, Bi J, Huang J, Tang Y, Du S, Li P. Exosome: A review of its classification, isolation techniques, storage, diagnostic and targeted therapy applications. Int J Nanomedicine. (2020) 15:6917–34. doi: 10.2147/IJN.S264498
Keywords: breast cancer, exosomes, immunity, tumor microenvironment, tumor immunotherapy
Citation: Wang Y, Ma Q, Wang T, Xing J, Li Q, Wang D and Wang G (2024) The involvement and application potential of exosomes in breast cancer immunotherapy. Front. Immunol. 15:1384946. doi: 10.3389/fimmu.2024.1384946
Received: 11 February 2024; Accepted: 29 April 2024;
Published: 21 May 2024.
Edited by:
Zhirui Zeng, Guizhou Medical University, ChinaReviewed by:
Peng Liu, Sun Yat-sen University, ChinaSankar Bhattacharyya, Sidho Kanho Birsha University, India
Svetlana Tamkovich, Novosibirsk State University, Russia
Copyright © 2024 Wang, Ma, Wang, Xing, Li, Wang and Wang. This is an open-access article distributed under the terms of the Creative Commons Attribution License (CC BY). The use, distribution or reproduction in other forums is permitted, provided the original author(s) and the copyright owner(s) are credited and that the original publication in this journal is cited, in accordance with accepted academic practice. No use, distribution or reproduction is permitted which does not comply with these terms.
*Correspondence: Gang Wang, d2FuZ2dhbmdAY2N1Y20uZWR1LmNu