- 1Department of Nephrology, Blood Purification Center, Zhongshan People’s Hospital, Zhongshan, China
- 2Department of Nephrology, Guangdong Provincial People’s Hospital (Guangdong Academy of Medical Sciences), Southern Medical University, Guangzhou, China
Lung cancer is the leading cause of cancer-related death worldwide, especially non-small cell lung cancer. Early diagnosis and better treatment choices have already provided a more promising prognosis for cancer patients. In targeted therapy, antagonists target specific genes supporting cancer growth, proliferation and metastasis. With the incorporation of targeted therapies in routine cancer therapy, it is imperative that the array of toxicities associated with these agents must be well-recognized and managed, especially since these toxicities are distinct from those seen with conventional cytotoxic agents. Drug-related nephrotoxicity has attracted attention when initiating cancer therapy. Our review aims to summarize the adverse renal effects caused by targeted therapy during lung cancer treatment, mainly focusing on EGFR and ALK tyrosine kinase inhibitors. Also, we discuss the possible mechanism of the side effect and provide managements to help improve the renal function in clinical practice.
1 Introduction
Lung cancer remains the leading cause of cancer-related death worldwide, however, many patients diagnosed with advanced disease at their initial presentation with a high mortality rate. Based on microscopic evidence and histological features, lung cancer can be divided into three main subtypes: non-small cell lung cancer (NSCLC), small cell lung cancer (SCLC), and lung carcinoid tumors, of which non-small cell carcinoma accounts for up to 85% (1). Adenocarcinoma, squamous cell carcinoma and large cell carcinoma are the three most common forms of NSCLC (2). Lung cancer-related deaths accounted for 18.4% of total cancer deaths in 2018. More than 2000,000 new cases of lung cancer are expected to emerge, and up to 1761007 patients reach the death endpoint (3). The factors affecting the occurrence and progression of lung cancer mainly include smoking, air pollution, occupational exposure and genetic factors. Surgery, chemotherapy and radiation therapy are the traditional treatment choices for lung cancer. However, due to lack of specificity, chemotherapy and radiation therapy have also caused adverse effects such as bone marrow suppression, gastrointestinal reactions and local inflammation for cancer patients (4, 5).
Recent years, with the development of molecular biology technology, targeted therapies have provided us with more promising options for treating these diseases (6). Recent research has confirmed that folate-bovine serum albumin-coated ethoniosomes of pterostilbene allowed specific targeting of cancer tissues overexpressing folate receptors rather than healthy tissues. Since propolis is rich in a variety of different polyphenolic compounds, it can enhance antioxidant activity and regulate signaling pathways in cancer cells, and the propolis extract is loaded into albumin-folic acid to minimize the strong smell and taste of propolis and control its delivery, which in turn targets cancer cells (7, 8). For stage IVB cancer that has metastasized throughout the body, the cancer cells need to be tested for the presence of specific genetic mutations before any treatment. Correct screening of gene mutations in tumor patients is the premise of an individualized treatment plan. Compared with traditional treatment, targeted therapy has significantly improved the objective response rate (ORR) and progression free survival (PFS) of mutation-positive NSCLC, therefore the progress in molecular pathogenesis research of lung cancer is of great significance to targeted therapy with specific molecular changes (9, 10). Common gene mutations in lung cancer include the epidermal growth factor receptor (EGFR), vascular endothelial growth factor (VEGF), anaplastic lymphoma kinase (ALK), c-ros oncogene 1 (ROS1), Kirsten rat sarcoma viral oncogene homolog (KRAS), serine/threonine-protein kinase b-raf (BRAF), proto-oncogene tyrosine-protein kinase receptor Ret (RET), mesenchymal epithelial transition factor (MET). The EGFR mutation and ALK mutation are the most frequent oncogenic driver of NSCLC (11). We summarized these two molecular targeted therapies and common adverse events in Table 1.
The kidneys perform a vital role in the proper functioning of the body and in maintaining homeostasis. The kidneys excreted endogenous metabolic products as well as eliminated drugs and toxins. Nephrotoxicity is a common complication during many medical procedures, including those used for cancer treatment. Both chemotherapy and immunotherapy may cause deterioration of kidney function, which in turn leads to increased mortality in cancer patients. Antineoplastic drugs may damage the glomeruli, renal tubules or any other part of the nephron, leading to deterioration of renal function and the appearance of various clinical symptoms such as acute kidney injury, electrolyte disturbances, nephrotic syndrome and glomerulonephritis (12, 13). Previous review has usually focused on nephrotoxicity caused by a particular series of drugs or has not explored the specific mechanism and clinical management. In this review, in addition to the extensive clinical studies, we also included case reports and case series, and summarized different types of small molecule targeted drugs to provide effective clinical management by exploring the possible mechanisms of nephrotoxicity. In Table 2, we have summarized the details of approved EGFR and ALK-targeted therapies along with dosing in CKD patients. In this review, we aim to summarize the current literature on the nephrotoxicities of small molecule inhibitors, especially EGFR and ALK tyrosine kinase inhibitors, and provide possible managements of the renal adverse effect.
2 EGFR tyrosine kinase inhibitors
The EGFR belongs to the human epidermal growth factor receptor (HER) family. The physiological function of EGFR involves the development of epithelial tissue and maintenance of homeostasis. Dysregulation of these tyrosine kinases and their downstream signaling pathways is associated with cancer cell proliferation, angiogenesis, and metastasis (14). After ligand binding, EGFR tyrosine kinase activates the receptor by homologousing or heterodimering it and auto-phosphorylating the tyrosine-rich cytoplasmic region, which initiates two main downstream intermediate pathways (15). EGFR mutations account for nearly 10% to 60% in patients of NSCLC (16). EGFR mutations occur predominantly in exons 18-21 encoding the intracellular domain of tyrosine kinase. The in-frame deletion of exon 19 and the L858R missense mutation of exon 21 are the most common activating mutations in EGFR, accounting for more than 90% of the totals (17). Uncommon EGFR mutation, including G719X at exon 18, S768I at exon 20, and L861Q at exon 21 have reported in NSCLC patients (18). EGFR inhibitors, mainly including monoclonal antibodies and tyrosine kinase inhibitors, are one of the widely used targeted therapies in oncology (19). These drugs work by specifically targeting and inhibiting the EGFR, a type of protein found on the surface of some cancer cells that can promote cell growth, multiplication and angiogenesis. The development of these drugs has already significantly improved the prognosis of patients. EGFR tyrosine kinase inhibitors (TKIs), mainly including afatinib, erlotinib and gefitinib, are suitable for treating NSCLC harboring EGFR mutations. Erlotinib and gefitinib are widely used as the first-generation of anti-EGFR drugs, however, as the resistance to the treatment, the second-generation anti-EGFR drug, afatinib and the third-generation osimertinib, which targets the EGFR mutation T790M (20, 21). Erlotinib and gefitinib are reversibly inhibit EGFR, whereas afatinib is irreversible covalent binding to EGFR, HER2, and HER4 to inhibit all HER family signaling with a broader activity to overcome EGFR TKI-resistant mutations (22). Rash, diarrhea, hepatotoxicity, and less commonly, but important, interstitial lung disease are common adverse events (AEs) in clinical practice of first and second-generation of EGFR TKIs. About 6.1% of patients suffered treatment withdrawal due to the unpleasant adverse events, especially skin disorders, interstitial lung disease and hepatoxicity, but less frequent reported in osimertinib (23, 24).
In the kidney, EGFR was mainly expressed in renal tubules, especially in distal and collecting ducts, and to a lesser extent in glomerular capillary walls, proximal tubules and mesangial cells (25). EGF is essential in maintaining the integrity of renal tubules. EGFR activation results in the growth and regeneration of renal tubular epithelial cells after acute tubular necrosis (26). Renal side effects caused by EGFR tyrosine kinase inhibitors can differ among patients but commonly include electrolyte disturbances, such as hypomagnesemia, hypokalemia and hypophosphatemia. Also, proteinuria, hypertension, and acute kidney injury may occur, though uncommon, still reports showed indirect renal toxicity caused by diarrhea-induced dehydration may happen and it is pretty essential to recognize timely and take managements (27–29). Table 3 and Table 4 respectively summarizes the renal toxic events and possible mechanism of EGFR inhibitors in lung cancer. And the clinical managements have been listed in detail in Table 4.
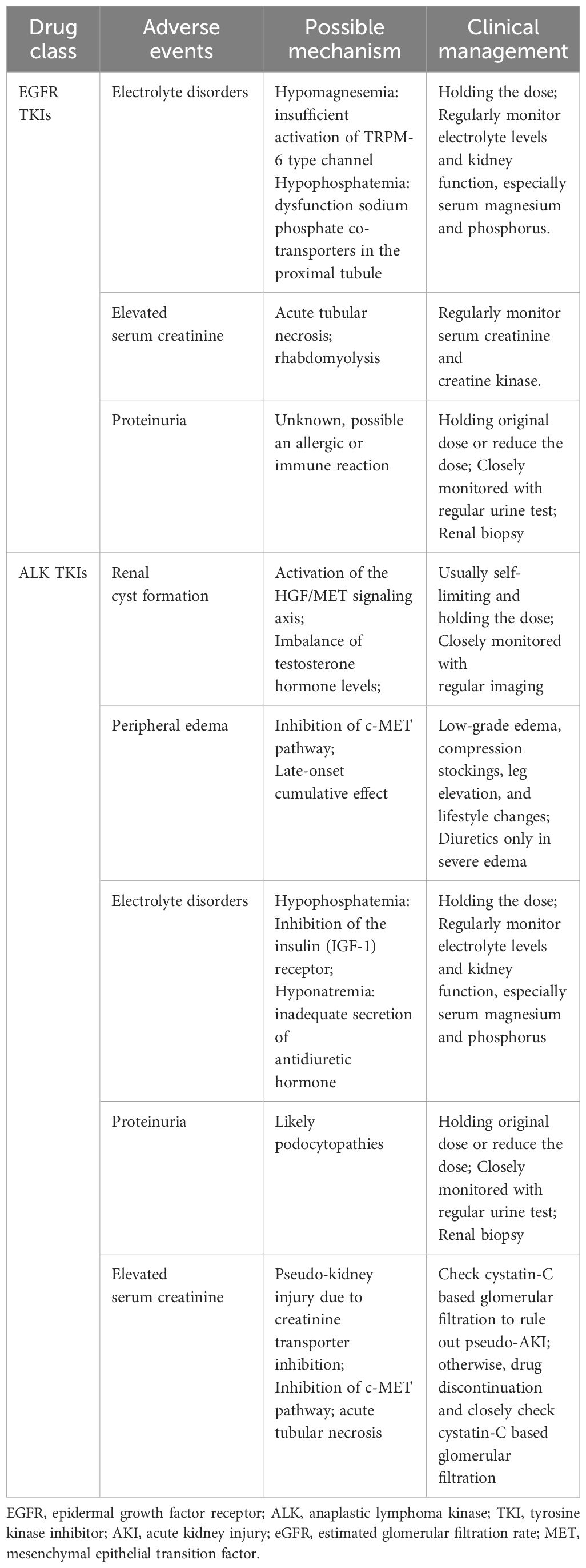
Table 4 Clinical management and possible mechanism to renal adverse events of EGFR and ALK targeted agents.
2.1 Erlotinib
Erlotinib is the first-line treatment for patients with locally advanced or metastatic EGFR mutations with NSCLC, primarily as maintenance therapy for these patients (30). Erlotinib can be used in combination with VEGF antibodies, such as bevacizumab (31). The usual dose of erlotinib is 150 mg orally per day, and the drug binds to plasma proteins up to 95%. Its metabolites are excreted mainly in feces, while the proportion of renal elimination is less than 9% (32). FDA Adverse Event Reporting System (FAERS) review revealed that 63 patients with acute kidney injury and 8 patients developed hypomagnesemia (33). Recently, Crosnier et al. (27) indicated that 139/303(45.9%) patients present with acute kidney injury among the patients with lung cancer (271/303) by reviewing the VigiBase® the WHO Global Database, and diarrhea-related acute kidney injury was the leading cause.
In a small clinical trial of 17 patients with solid tumors treated with erlotinib and sorafenib, the incidence of hypophosphatemia was 76%. In comparison, another clinical trial that enrolled 25 patients diagnosed with glioma showed a 30% incidence of hypophosphatemia (34, 35). Erlotinib can affect magnesium hemostasis, but its effect on systemic magnesium concentrations does not appear to be as good as that observed in antibody-based EGFR inhibitors, and the erlotinib-induced hypomagnesemia may be corrected by magnesia supplementation (13). There are several proteins in the distal convoluted tubules that have been implicated in the transport of magnesium, including magnesium-permeable transient receptor potential cation channel, subfamily M, member 6 (TRPM6) and transient receptor potential cation channel, subfamily M, member 7 (TRPM7) (36). EGF is a magnesiotropic hormone that regulates magnesium levels by regulating the TRPM6 type channel. Mutation of EGF gene resulted in impaired basolateral sorting of pro-EGF, thereby the renal EGFR is inadequately stimulated, inducing insufficient activation of TRPM6 type channel and thus hypomagnesemia occurred, as described in Figure 1 (37).
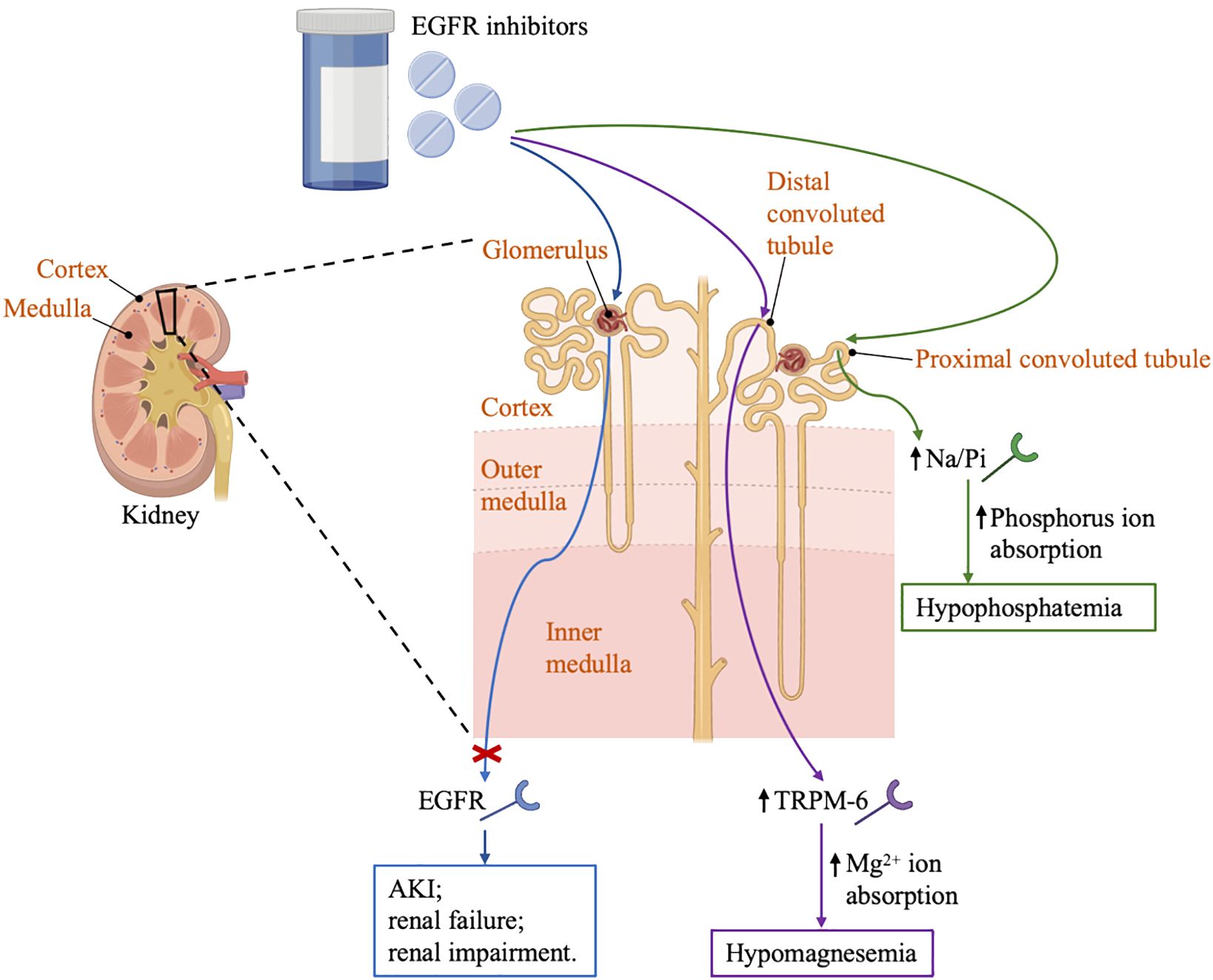
Figure 1 Renal effects of EGFR inhibitors. EGFR, the epidermal growth factor receptor; AKI, acute kidney injury; TRPM6, transient receptor potential cation channel; Na/Pi, sodium-phosphate co-transporter; GBM, glomerular basement membrane.
Therefore, it is recommended that all patients have their magnesium levels checked before starting treatment and then every 2 to 4 weeks, especially those with heart disease history. The severity of hypomagnesemia is closely related to treatment. Generally, EGFR TKIs induced hypomagnesemia may be corrected by extra magnesium supplementation. Hypomagnesemia can be divided into 5 grades according to the magnesium level (Grade 1 is 0.73-0.50 mmol/L, Grade 2 is 0.50-0.40 mmol/L, Grade 3 is 0.40-0.30 mmol/L, Grade 4 is <0.30 mmol/L, Grade 5 is death) (38). Grade 1 hypomagnesemia requires close monitoring of magnesium levels without medication. In grade 2 hypomagnesemia, magnesium supplementation, usually given intravenously with a dose of 4 grams of magnesium sulfate weekly, is highly recommended to avoid fatal arrhythmias, especially for those ineffective and poorly tolerated to oral magnesium supplementation (32, 39). Patients with grade 3/4 hypomagnesemia are advised to take 6-10 grams of magnesium sulfate twice a day twice a week, and monitor serum magnesium every other day until a stable state is reached. Another strategy may be to consider 2-month discontinuation of magnesium sulfate therapy in patients requiring frequent magnesium sulfate infusions (39).
In addition, hypophosphatemia has been reported in patients treated with EGFR inhibitors. Possible mechanism related to hypophosphatemia might involve sodium phosphate co-transporters in the proximal tubule (39). In general, hypophosphatemia should not be considered as a reason to discontinue aggressive cancer treatment unless it is recurrent and life-threatening, especially given that the use of these drugs may significantly improve life expectancy in many cancer patients. Intravenous rehydration should be considered in any symptomatic or severely exhausted patient (phosphate <1.0 mg/dL). Oral replacement therapy can be resumed after the patient has resolved symptoms or serum phosphate > 1.5 mg/dL (40). Therefore, it is advisable to check electrolyte levels before initiation of therapy, and then routinely monitor to prevent the development of severe complications caused by electrolyte disorders.
Erlotinib-related acute and chronic kidney dysfunction, though uncommon, still occur in clinical practice. Even for the same type of drug, patients may respond differently due to the different chemical structure of the drug. Maruyama et al (41) described that a 57-year-old patient with advanced lung adenocarcinoma developed nephrotic syndrome after taking gefitinib, which improved after discontinuation and switched to erlotinib without any kidney damage, and possible explanation is related to drug hypersensitivity reactions. Besides, another case reported that patient had only mild proteinuria on erlotinib, but developed nephrotic range proteinuria on gefitinib (42). While all EGFR TKIs have the potential to cause direct damage to glomerular podocytes, these drugs may also produce podocyte damage through indirect pathways. Different pathways of injury may explain the severity of podocyte injury in these EGFR TKIs (42). However, more researches are needed to explore the precise mechanism. Therefore, it is highly recommended to check the renal function before erlotinib therapy, and then check routinely for early recognition and treatment to prevent the development of severe complications.
2.2 Gefitinib
Gefitinib is another TKI that blocks the activity of the EGFR tyrosine kinase. Gefitinib is used across all lines of therapy for patients with locally advanced or metastatic NSCLC with activating mutations of EGFR (20, 28). The usual dose of gefitinib is 250 mg orally per day, and the drug binds to plasma proteins up to 90%. Its metabolites are excreted mainly in feces, while the proportion of renal elimination is less than 4% (32). The most common adverse effect caused by gefitinib included diarrhea, vomiting and skin rash. Also, renal dysfunction of gefitinib were observed in clinical studies, with literature reviewed that 6.6% patients with gefitinib therapy developed fluid retention (43, 44). According to the FAERS, 7 patients after gefitinib initiation developed acute kidney injury, with hypokalemia and hyponatremia as the second and third adverse renal effects (33).
Pathological manifestations, including minimal change and mild IgA deposition, crescent formation, and tubular injury under microscopy have been reported in patients treated with EGFR inhibitors. A possible mechanism was proposed as an allergic or immune reaction as mild interstitial infiltration of lymphocytes was noted under the renal biopsy (44, 45). Kumasaka et al (44) reported a 76-year-old male presented with nephrotic syndrome after gefitinib therapy. Renal biopsy confirmed minimal change disease under electron microscopy, and her renal function discovered after discontinuation of gefitinib. Another case of acute renal failure due to thrombotic thrombocytopenic purpura/hemolytic uremic syndrome caused by gefitinib has been also reported. A 73-year-old male without potential precipitating factors for renal insufficiency has become uremic and acidosis after 16-day therapy of gefitinib. His renal function improved rapidly after gefitinib discontinuation (45). Kaneko et al (46) described the first case of gefitinib-related membranous nephropathy. Possible mechanisms include that gefitinib can act as a hapten attached to some native proteins, triggering an antibody response. Through long-term use, gefitinib may produce autoimmune reactions. In addition, gefitinib can locally bind to the increased phosphorylation of EGFR on podocytes, change its structure and induce antibodies, and thereby lead to subepithelial immune complexes (44, 46). During the first few weeks of gefitinib treatment, close monitoring of kidney function is required. When renal insufficiency is found, gefitinib should be discontinued immediately, and efforts should be made to find other secondary factors, and the next treatment should be determined until the cause is identified.
2.3 Afatinib
Afatinib, an orally administered irreversible EGFR TKI, is the first-line treatment for patients with EGFR mutation-positive advanced squamous NSCLC and patients harboring uncommon EGFR mutation, including G719X, S768I, and L861Q mutation (18, 47). Studies have shown that compared to first-generation EGFR TKIs, afatinib has a more favorable outcome, with significantly prolonged progression-free survival and time to treatment failure (47, 48). The recommended dose of afatinib is 40 mg orally per day. The drug binds mainly to plasma proteins and is excreted in feces, the proportion of which is excreted in the urine does not exceed 5% (32). Pharmacokinetic data indicate that in patients with mild or moderate renal impairment, the dosage of afatinib does not need to be adjusted, but 30 mg orally is prescribed to patients with severe renal impairment, even those patients under hemodialysis (49). In preliminary trials of afatinib, the incidence of hypokalemia was 34% (50). 26 patients under afatinib therapy developed acute kidney injury was noted in the FEARS report, which was followed by hypokalemia and hyponatremia (33). Koch et al (51) reported a case of hyponatremia in a 58-year-old female patient with EGFR-positive lung adenocarcinoma treated with afatinib. Serum electrolytes should be monitored closely in patients treated with EGFR TKIs and drugs should not be discontinued unless the symptoms are very severe.
2.4 Osimertinib
Osimertinib, a third-generation EGFR TKI, has been developed due to the acquired T790M mutation caused by resistance for advanced NSCLC EGFR mutant patients progressed after first-line EGFR TKI therapy (52). The T790M mutation is the most common mechanism of acquired resistance in NSCLC treatment, accounting for 50-60% of secondary resistance after first-line therapy (53). Usually, administration with osimertinib 20 mg once per day is chosen as the initiating dose, which is sufficient to inhibit EGFR T790M, while doses equivalent to 80 mg or more are expected to lead to profound inhibition of tumor growth (52). A clinical trial for patients with EGFR inhibitor-resistant NSCLC at doses of 20 to 240 mg once daily revealed that diarrhea is the most common toxicity, followed by rash, nausea, and decreased appetite (24). Research has found that adverse effect is more common in patients with impaired renal function, however, under close monitor, osimertinib can be safely administered to cancer patients undergoing regular hemodialysis (54). In another clinical trial with locally advanced or metastatic, MET-amplified, EGFR mutation-positive NSCLC, a case of acute renal failure was found in patients treated with osimertinib plus savolitinib (55). Recently, more cases related to renal toxicities were reported. Niitsu et al (56) reported a patient with NSCLC developed acute kidney injury associated with biopsy-proven mild IgA deposition, crescent formation, and tubular injury after initiation of osimertinib and his renal function recovered after reducing the dose of osimertinib (from 80 mg/day to 40 mg/day). Another case reported that a patient with advanced lung adenocarcinoma presented with myalgia, muscular weakness after 5-month treatment with osimertinib and bevacizumab. He was diagnosed with osimertinib-associated rhabdomyolysis and developed acute renal insufficiency, hyperuricemia, metabolic acidosis and electrolyte disorders. However, all symptoms recovered after discontinuation of osimertinib (57). Few cases of osimertinib-related kidney dysfunction were reported and more attention and follow-up are required.
3 ALK inhibitors
Besides EGFR tyrosine kinase, ALK has been another most frequently identified mutational driver of NSCLC. Due to chromosomal inversion, part of the ALK gene is fused with the echinoderm microtubule-associated protein-like 4 (EML4) gene in a small proportion of NSCLC patients, resulting in the activation and transformation of the EML4-ALK fusion protein compositionally, resulting in oncogene addiction. EML4-ALK fusion and other ALK rearrangements occur in 3-7% of patients with NSCLC. ALK inhibitors have already greatly improved prognosis in patients with advanced ALK-positive NSCLC (58, 59). To date, crizotinib and ceritinib are the ALK inhibitors most commonly used in clinical practice to treat advanced NSCLC (59). However, in clinical practice, most patients develop tolerance within 1 year of initiating crizotinib therapy. Compared to crizotinib, second-generation ALK TKIs, including alectinib, ceritinib, and brigatinib, have better sensitivity to ALK-positive NSCLC harboring a secondary mutation such as L1196M or G1269A (60).
With the widespread use of ALK inhibitors, the adverse events associated with these drugs have gradually attracted attention. A recently meta-analysis revealed that adverse events happened in most participants under ALK inhibitors therapy, among them serious adverse events occurred over 20%. Most of the adverse events of ALK TKIs are grade 1 to 2, which are generally tolerated by most patients. Diarrhea, vomiting, liver dysfunction, and vision disorder are common adverse events of ALK TKIs. Visual impairment and gastrointestinal symptoms, such as nausea and vomiting, were frequent adverse events of crizotinib. The most common renal adverse events include renal cysts, peripheral edema, elevated serum creatinine and proteinuria (61, 62). According to FAERS analysis, ALK inhibitors can cause acute or chronic renal insufficiency and may also cause electrolyte abnormalities such as hyponatremia and hypophosphatemia (33). Table 3 and Table 4 respectively summarizes the renal toxic events and possible mechanism of ALK inhibitors in lung cancer. Besides, the clinical managements have been listed in detail in Table 4.
3.1 Crizotinib
Crizotinib, the first-generation oral small molecule targeted therapy, inhibits multiple tyrosine kinase inhibitors, especially ALK, MET and ROS1. It has been shown to significantly improve outcomes compared with chemotherapy in both first-line and subsequent treatment in patients with ALK-positive advanced NSCLC. The recommended dose of crizotinib is 250 mg twice orally per day (63, 64).
According to the FDA, about 4% of patients develop complex renal cysts after initiation of crizotinib. Lin et al (65) revealed significant changes in renal cysts in 7 patients (22%) among 32 patients treated with crizotinib for ALK-positive advanced NSCLC, however, these may reverse after crizotinib discontinuation. An analysis of 255 patients treated with crizotinib for at least 6 months showed that 9% of patients acquired new cysts, and 2% of patients with pre-existing cysts developed new cysts and acquired enlargements in existing cysts (66). A retrospective study of 60 patients treated with crizotinib identified that female (p=0.008) and the presence of renal cysts on baseline scan (p=0.044) significantly related with renal cyst development or growth during initiation of crizotinib therapy (67). Also, Asians, especially Koreans tend to have higher odd ratios of developing de no renal cysts during crizotinib treatment (66).
Another adverse renal effect related to crizotinib is acute kidney injury. Camidge et al (68) have demonstrated that elevated serum creatinine with crizotinib occurs mainly in the first 12 weeks after initiation of crizotinib. There is little evidence of cumulative effects with long-term treatment, and renal function is largely restored after discontinuation within one week, suggesting that this may be primarily an effect on creatinine secretion rather than true nephrotoxicity, which is named pseudo acute kidney injury.
However, there were still case reports related to actual kidney injury. Gastaud et al (69) reported a case that a 49-year-old patient with previously normal renal function developed AKI after 3 weeks of crizotinib treatment, and renal function returned to normal on day 8 after discontinuation and renal biopsy showed diffuse acute tubular injury and tubular necrosis. Another case described by Izzedine et al (70) showed a patient diagnosed with NSCLC along with chronic kidney disease presented with progressive renal function worsening after 11-month crizotinib therapy, with kidney biopsy revealing features of acute tubular injury without interstitial cell infiltration and renal arteriolar myocyte vacuolization.
Electrolyte disorders have also occurred during clinical practice, including hypophosphatemia, hyponatremia, hypokalemia and hypocalcemia. Among them, hypophosphatemia has come to the first as a serious adverse event with up to 15% of all participants reporting during the treatment of crizotinib (71). Researches have revealed that hypophosphatemia is a sensitive prognostic factor related to the in-hospital day, severe complications or all-cause mortality. Still, the exact mechanism for these adverse events remains unclear, and there are no formal recommendations for guidance. Possible explanation for hypophosphatemia is associated with inhibiting the insulin-like growth factor-1 (IGF-1) located in the proximal tubules blocking the phosphate reabsorption, thus resulting in phosphaturia (40).
Renal cyst formation has been reported in patients treated with ALK inhibitors, especially those treated with the first-generation crizotinib. Yet, the potential causal relationship between crizotinib treatment and renal cyst formation has not been fully established. Hepatocyte growth factor receptor (HGF) is the only known ligand for MET and plays a vital role in embryonic development, tissue regeneration, and tumor progression. In the kidney, HGF is found in mesenchymal cells, while MET is expressed in non-mesenchymal cells. Activation of the HGF/MET signaling axis is associated with the development of renal cysts (66, 72). Another possible explanation is attributed to the imbalance of testosterone hormone levels, whose secretion decreased under crizotinib treatment but recovered after discontinuation of crizotinib (73). Routine ultrasound and renal function examination are needed when initiating ALK inhibitors.
ALK inhibitors have been associated with the occurrence of acute kidney injury and chronic kidney disease in clinical practice. Acute elevation of serum creatinine when initiating first-generation crizotinib may not be a reflection of true kidney injury, but rather a pseudo-kidney injury due to creatinine transporter inhibition, thereby interfering with creatinine secretion in the proximal tubule (58).
Still, cases of real acute kidney dysfunction are reported in the patients treated with ALK inhibitors. It is thought that MET is mainly expressed in the proximal convoluted tubule, proximal loop of Henle loop and distal convoluted tubule. Crizotinib competitively inhibits creatinine and water secretion by inhibiting the c-Met pathway, which may be the mechanism that predisposes to acute kidney injury (58, 70).
Recently, an analysis revealed that the calculated glomerular filtration rate in cancer patients undergoing TKIs was higher in almost all cases when using cystatin C (74). Therefore, it is important to recalculate with both serum creatinine and cystatin C to identify the actual situation when using crizotinib. Patients undergoing treatment with crizotinib should maintain proper hydration, regularly monitor kidney function, and promptly report any symptoms indicating potential kidney injury to healthcare professionals. Also, physicians should carefully assess the risk-benefit ratio before prescribing crizotinib to patients with known risk factors for kidney disease.
3.2 Ceritinib
Ceritinib, an oral small molecule, ATP-competitive tyrosine kinase inhibitor of ALK, is 20 times as potent as crizotinib in enzymatic assays. It is always used in patients harboring ALK mutation involving L1196M, G1296A, I1171T, and S1206Y mutations. The recommended dose of ceritinib is 750 mg orally per day (75, 76). The most common adverse events caused by ceritinib include gastrointestinal disorder (grade 1 or 2 diarrhea, nausea) or hepatic disorders among up to 5% of patients, which generally required essential approach and is reversible after drug modifications or discontinuation. Serum creatinine levels were elevated in 11% of patients, and hypomagnesemia occurred in 8% of patients undergo ceritinib therapy. Patients treated with ceritinib often present with hypophosphatemia and this is dose-dependent (75, 77). Hyponatremia is another common electrolyte disorder in patients treated with ceritinib therapy. More than 5% of patients developed hyponatremia during ceritinib therapy. A postulated explanation attributed hyponatremia in patients treated with ALK inhibitors to inadequate secretion of antidiuretic hormone in the collecting ducts and thus producing hyponatremia (78). Hypocalcemia and hypokalemia are also reported though in patients treated with ceritinib (59).
In conclusion, it is necessary to regularly monitor electrolyte levels and kidney function, recognize symptoms and signs early, and take relevant measures to intervene. Dose reductions have not usually been required (77).
3.3 Alectinib
Alectinib, a second-generation oral ALK TKIs, is highly selective and more potent against ALK. It has been used in patients with ALK mutations that cause resistance to crizotinib, such as L1196M and C1156Y (79). The recommended dose of alectinib is 600 mg twice orally per day. Alectinib has good brain barrier penetration, resulting in high concentrations in the cerebral blood fluid. The brain is the most common site of metastasis in patients with ALK-positive NSCLC treated with crizotinib, and many patients suffer recurrence of central nervous system involvement (80, 81). Common adverse effect caused by alectinib are constipation (35.6%), edema (33.6%) and myalgia (30.8%). The gastrointestinal and hepatobiliary disorders are the two most common vital adverse effects that calls for emergent attention and treatment (82).
Still, renal adverse events have been reported in patients treated with alectinib, commonly grade 1 or 2 nephrotoxicity. Grade 3 renal adverse events including elevated serum creatinine and neutropenia were reported in 26% of patients, and hypophosphatemia was reported in 2~4% of patients (81, 83). Ramachandran et al (84) reported that a 72-year-old patient with ALK-positive metastatic NSCLC developed acute kidney injury which necessitated emergency hemodialysis within 6-week treatment of alectinib. He recovered completely within 7-10 days on alectinib withdrawal. Recently, one case of biopsy-proven a mixed pattern of acute interstitial nephritis and acute tubular necrosis was reported with this agent for a 68-year-old patient with ALK-positive NSCLC stage IV. After initiation of corticotherapy, renal function in this patient returned to baseline. The patient was finally discharged from the hospital, the and treatment with alectinib was changed to lorlatinib, and renal function remained stable after 10 months of lorlatinib (85).
3.4 Brigatinib
Brigatinib, another highly selective ALK TKIs with a 12-fold stronger potent against ALK than crizotinib, has been widely applied in patients with regressive ALK-positive metastatic NSCLC or resistant to crizotinib. The recommended dose of brigatinib is 90 mg orally per day (86). Gastrointestinal disorder (nausea, diarrhea), fatigue and headache are the most common adverse effects and needed emergent attention and treatment (58).
Brigatinib-induced renal adverse events have been rarely reported in clinical practice. Wang et al (87) reported that a 39-year-old patient with advanced ALK-rearranged NSCLC developed tumor lysis syndrome after 22-day therapy of brigatinib, whose serum creatinine rose from 73 μmol/L to 320.2 μmol/L over 22 days of brigatinib treatment. Emergent hemodialysis is required; however, the patient and his family refused any other further interventions due to the worse prognosis, and finally he passed away within 24h.
3.5 Lorlatinib
Lorlatinib, a selective third-generation tyrosine kinase inhibitor, has been used to treat crizotinib-resistant ROS1-positive NSCLC and glioblastoma. Lorlatinib has shown high potency in crizotinib-resistant NSCLC with ROS1 G2032R mutation and the recommended dose of lorlatinib is 100 mg orally per day (88–90). Common adverse events of lorlatinib involve neurocognitive impairments, such as slowed speech and thinking and word-finding difficulty, which are dose-limited (91). Up to 50% of patients develop peripheral edema when treated with lorlatinib (10). Also, lorlatinib-related glomerular-toxicity may happen. Betton et al (92) reported that a 64-year-old female diagnosed with lung adenocarcinoma presented with diffuse edema and proteinuria after 1-month treatment with lorlatinib. She was diagnosed with lorlatinib-related nephrotic syndrome with a kidney-biopsy revealing diffuse podocytes foot process disappearance under electron microscopy and her symptoms relived after discontinuation of this drug. However, due to disease progression, the patient was restarted with lorlatinib and subsequently increased to 3.6 g/g proteinuria within 3 days of initiation (92). In addition, McGee et al (93) described a case of 63-year-old female with lung adenocarcinoma developed extinct hyperlipidemia, which may be caused by minimal change disease under renal biopsy. Another case reported by Lee et al (94) revealed a 68-year-old with ROS1 rearranged stage IV lung adenocarcinoma presented proteinuria after receiving lorlatinib, and her symptoms improved when reducing dose from 100 mg to 50 mg daily. However, the related mechanism remained unknown.
Peripheral edema seems to be the most common adverse event after ALK inhibitor therapy, occurring in nearly 50% of participants under the first-generation crizotinib and the third-generation lorlatinib, generally grade 1-2 adverse events (10). However, the exact mechanism remained unknown, but a postulated mechanism referred to the inhibition of the c-MET pathway. In the kidney, MET is expressed in the tubules, including proximal tubules, proximal loop of Henle and the distal tubules. Inhibition of this pathway may lead to electrolyte disorders (73). Peripheral edema generally appears late in the therapy of ALK inhibitors, which appears to be a late-onset cumulative effect. In patients with low-grade edema, compression stockings, leg elevation, and lifestyle changes should be advised first before starting dose adjustment (58). Also, it is important to monitor renal function regularly and take management with dose modification. Diuretics drugs may be a proper choice for cases with more resistant.
4 BRAF inhibitors
BRAF, one of the oncogenic drivers, accelerates the RAS-RAF-MEK-ERK pathway and induces cell growth and proliferation, which plays a critical role in cancer progression (95). Approximately 2-4% of patients with NSCLC have been shown to have mutations in the BRAF gene (96).
Vemurafenib, an oral selective BRAF inhibitor, has been used in treatment against NSCLC and melanoma harboring BRAF mutation (97, 98). Vemurafenib, which is highly protein-bound, is metabolized mainly in the liver and finally excreted in the feces (94%), rarely through urine (1%), so it may be possible to use it in patients with renal insufficiency at its usual doses. Similar to vemurafenib, dabrafenib is highly bound to protein and is widely distributed. However, dabrafenib is a higher drug for renal elimination than vemurafenib (71% fecal excretion and 23% urinary excretion). It can also be used for mild to moderate renal impairment, but the terminology for patients with severe renal impairment is lacking (99).
Proteinuria and decreased glomerular filtration rate are common renal adverse events reported by vemurafenib (100). Launay-Vacher et al (101) reported a case series about 8 patients that glomerular filtration rate decreased varied from 20 to 74% after vemurafenib therapy and concomitant use of other nephrotoxic drug, such as cisplatin and zoledronic acid, and a patient received renal biopsy revealing acute tubular necrosis. Electrolyte disorders are common renal adverse effects in clinical practice. In a phase 2 trial combined dabrafenib and trametinib in patients with BRAFV600E-mutant NSCLC, hypophosphatemia, hyponatremia and hypercalcemia has been reported in patients (102).
The mechanism of renal injury caused by BRAF inhibitors is not well understood, but may be related to their blocking of signal transduction of downstream cellular pathways and increased susceptibility of renal tubules to ischemic injury (103). When treating with dabrafenib and vemurafenib, serum creatinine and electrolytes must be routinely monitored and glomerular filtration rate calculated before the first dose. Still, the mechanism has remained unclear yet. Meanwhile, the associated cases are limited. Dabrafenib-related renal toxicity is limited, but more data and time are needed before concluding that these drugs are not nephrotoxic. Oncologists and nephrologists need to be aware of the nephrotoxicities of these agents.
5 VEGF inhibitors
After Folkman first proposed the angiogenesis theory of tumor growth and metastasis, researchers have continued to make intense efforts to target this pathway to stop tumor growth. One of the most effective promoters is involved in the VEGF pathway (104). The most widely used small-molecule drugs included bevacizumab, sorafenib, sunitinib, axitinib, and pazopanib (13). In the kidneys, VEGF is expressed in podocytes and maintains the normal function of the glomerular endothelium (105).
Common renal adverse effects related to VEGF inhibitors in lung cancer therapy include proteinuria, acute renal failure and hypertension (106). Zhu et al (107) have shown that the incidence of all-grade hypertension was 21% and a significantly higher incidence in patients with renal cell carcinoma in a meta-analysis of total 4609 patients treated with sunitinib. In the treatment of lung cancer, bevacizumab is more likely to induce nephrotoxicity than other VEGF inhibitors (108, 109).
Bevacizumab, a recombinant human monoclonal antibody that binds to all known vascular endothelial growth factor A (VEGF-A) subtypes and exerts antiangiogenic effects by blocking the binding of VEGF-A to VEGF receptors (primarily VEGFR-1 and VEGFR-2) on the surface of endothelial cells, as VEGF-A binds to VEGFR-1 and VEGFR-2 to promote endothelial cell proliferation, activate survival pathways, and form new blood vessels. Therefore, bevacizumab plays an important role in the treatment of advanced cancer, including NSCLC (109, 110). The most common renal impairment of bevacizumab is proteinuria in clinical practice (111). In a pooled analysis of bevacizumab-treated patients including patients diagnosed NSCLC, the incidence of proteinuria of any grade was 8.2% and 4.6% in the bevacizumab and control groups, respectively, while the incidence of grade 3/4 proteinuria was 1.4% and 0.2%, respectively (108). Another population-based retrospective cohort study revealed that patients treated with bevacizumab had a significantly 1.35-fold higher risk of CKD than those who did not receive bevacizumab (109).
The main mechanisms related to proteinuria may include the interference with podocytes endothelial VEGF axis signaling, increased intraglomerular pressure caused by secondary hypertension and subacute glomerular thrombotic microangiopathy. The most common histopathological manifestations of the kidney caused by VEGF inhibitors is thrombotic microangiopathy, followed by glomerular lesions and interstitial nephritis (112, 113).
Inhibition of the VEGF pathway may lead to hypertension. A possible mechanism is that VEGF is a mediator of endothelium-dependent vasodilation, leading to upregulation of nitric oxide synthase. Meanwhile, capillary rarefaction and increased prostacyclin production play a role in exacerbating hypertension (112, 114). Monitoring of proteinuria during treatment is also important, especially during the treatment with bevacizumab. Patients with proteinuria ≥2.0 g/24 h need to suspend the medication. And if there is a proteinuria > 3.0 g/24 h or if nephrotic syndrome occurs, permanent discontinuation of the medication is required. When this happens, we may consider switching to other drugs. For example, in patients with nephrotic syndrome associated with gefitinib therapy, we may consider erlotinib as a potential treatment option. Acute kidney injury and other renal dysfunction may occur during the treatment, so creatinine and urea levels should be determined. Increased monitoring frequency is required when creatinine levels increase >1 to 1.5-fold baseline. If the creatinine concentration increases 1.5-fold baseline, administration of methylprednisolone is required. A renal biopsy should also be considered. The administration of drugs should be delayed when creatinine levels increase >3-fold baseline (111).
6 Discussion
In targeted therapy for lung cancer, especially EGFR and ALK inhibitors, may damage the glomeruli, renal tubules or any other part of the nephron, leading to deterioration of renal function and the appearance of various clinical symptoms such as AKI, electrolyte disturbances, nephrotic syndrome and glomerulonephritis (12). EGFR was expressed in the kidney, so EGFR inhibitors may cause AKI, renal failure or renal impairment. Mutation of EGF gene also resulted in the activation of TRPM-6 type channel and thus hypomagnesemia occurred. In addition, EGFR inhibitors may cause hypophosphatemia and hypocalcemia by affecting the Na/Pi (sodium-phosphate) co-transporter channels (26). The ALK inhibitors may cause peripheral edema by inhibiting the c-MET pathway and associate with an acute increase in serum creatinine by inhibiting of a creatinine transporter, thus interfering with the secretion of creatinine in the proximal tubule (73).
The kidney is vulnerable to injury from the targeted therapy used to treat lung cancer, so we recommend routine physical examinations, imaging, serum or urine monitoring in these patients. It is important to improve awareness of the factors that that enhance nephrotoxic risk. These factors include specific patient characteristics, nephrotoxicity of the substance itself and renal handling of the causative substance. Electrolyte disorders including hypophosphatemia, hyponatremia, hypokalemia and hypocalcemia can be life-threatening.
Therefore, it is advisable to check electrolyte levels prior to the initiation of therapy, and then routinely monitor the electrolyte levels to prevent the development of severe complications caused by electrolyte disorders. Drugs should not be discontinued unless the symptoms are very severe.
7 Conclusion
In recent years, significant progress has been made in the early diagnosis and treatment of cancer, which has a significant impact on prolonging the survival of patients. However, nephrotoxicity may happen after initiation of the therapy and this may force reduction or discontinuation of the medication. Nephrotoxicities induced by targeted therapy cause manifestations of various forms, ranging from nephrotic syndrome to acute kidney injury. Currently, there is limited data on many kidney-related toxicities, especially new drugs released in recent years. Yet, the relevant mechanism has remained unclear. It is necessary to further study the potential mechanism of the effect of these drugs on the kidney. It is important to monitor the renal function when initiating the targeted therapy and pay close attention and regular follow-up. In the future, further studies should be carried out and animal experiments are needed to explore the potential mechanism of these drugs on the kidney and reduce renal adverse events.
Author contributions
QL: Conceptualization, Writing – original draft, Writing – review & editing, Methodology, Resources, Validation. JL: Conceptualization, Methodology, Resources, Validation, Writing – original draft, Writing – review & editing. GH: Conceptualization, Writing – review & editing. AX: Conceptualization, Writing – review & editing. SL: Conceptualization, Writing – review & editing. BT: Conceptualization, Writing – review & editing.
Funding
The author(s) declare financial support was received for the research, authorship, and/or publication of this article. This work was supported by the Natural Science Foundation of Guangdong Province (No. 2022A1515012374 and 2023A1515010024), the Zhongshan Science and Technology Bureau Project (No. 2023B1025 and 2023B1026).
Conflict of interest
The authors declare that the research was conducted in the absence of any commercial or financial relationships that could be construed as a potential conflict of interest.
Publisher’s note
All claims expressed in this article are solely those of the authors and do not necessarily represent those of their affiliated organizations, or those of the publisher, the editors and the reviewers. Any product that may be evaluated in this article, or claim that may be made by its manufacturer, is not guaranteed or endorsed by the publisher.
Abbreviations
ALK, anaplastic lymphoma kinase; BRAF, murine sarcoma viral oncogene homolog B1; EGF, epidermal growth factor; EGFR, epidermal growth factor receptor; EML4, echinoderm microtubule-associated protein-like 4; FAERS, FDA Adverse Event Reporting System; HGF, Hepatocyte growth factor; IGF-1, insulin-like growth factor-1; KRAS, Kirsten rat sarcoma viral oncogene homolog; MET, mesenchymal epithelial transition factor; NSCLC, non-small cell lung cancer; ORR, objective response rate; PFS, progression free survival; RET, rearranged during transfection; ROS1, c-ros oncogene 1; TKI, tyrosine kinase inhibitors; TRPM6, transient receptor potential cation channel, subfamily M, member 6; TRPM7, transient receptor potential cation channel, subfamily M, member 7; VEGF, vascular endothelial growth factor.
References
1. Essa ML, El-Kemary MA, Ebrahem Saied EM, Leporatti S, Nemany Hanafy NA. Nano targeted Therapies Made of Lipids and Polymers have Promising Strategy for the Treatment of Lung Cancer. Mater (Basel). (2020) 13:5397. doi: 10.3390/ma13235397
2. Lu T, Yang X, Huang Y, Zhao M, Li M, Ma K, et al. Trends in the incidence, treatment, and survival of patients with lung cancer in the last four decades. Cancer Manag Res. (2019) 11:943–53. doi: 10.2147/CMAR
3. Bray F, Ferlay J, Soerjomataram I, Siegel RL, Torre LA, Jemal A. Global cancer statistics 2018: GLOBOCAN estimates of incidence and mortality worldwide for 36 cancers in 185 countries. CA Cancer J Clin. (2018) 68:394–424. doi: 10.3322/caac.21492
4. Ettinger DS, Wood DE, Aisner DL, Akerley W, Bauman J, Chirieac LR, et al. Non-small cell lung cancer, version 5.2017, NCCN clinical practice guidelines in oncology. J Natl Compr Canc Netw. (2017) 15:504–35. doi: 10.6004/jnccn.2017.0050
5. Molina JR, Yang P, Cassivi SD, Schild SE, Adjei AA. Non-small cell lung cancer: epidemiology, risk factors, treatment, and survivorship. Mayo Clin Proc. (2008) 83:584–94. doi: 10.1016/S0025-6196(11)60735-0
6. Wang M, Herbst RS, Boshoff C. Toward personalized treatment approaches for non-small-cell lung cancer. Nat Med. (2021) 27:1345–56. doi: 10.1038/s41591-021-01450-2
7. Ghazy MGM, Hanafy NAN. Targeted therapies for breast and lung cancers by using Propolis loaded albumin protein nanoparticles. Int J Biol Macromol. (2024) 260:129338. doi: 10.1016/j.ijbiomac.2024.129338
8. Hanafy NAN, Abdelbadea RH, Abdelaziz AE, Mazyed EA. Formulation and optimization of folate-bovine serum albumin-coated ethoniosomes of pterostilbene as a targeted drug delivery system for lung cancer: In vitro and in vivo demonstrations. Cancer Nanotechnol. (2023) 14:1–31. doi: 10.1186/s12645-023-00197-4
9. Duma N, Santana-Davila R, Molina JR. Non-small cell lung cancer: epidemiology, screening, diagnosis, and treatment. Mayo Clin Proc. (2019) 94:1623–40. doi: 10.1016/j.mayocp.2019.01.013
10. Shaw AT, Bauer TM, de Marinis F, Felip E, Goto Y, Liu G, et al. First-line lorlatinib or crizotinib in advanced ALK-positive lung cancer. N Engl J Med. (2020) 383:2018–29. doi: 10.1056/NEJMoa2027187
11. Rotow J, Bivona TG. Understanding and targeting resistance mechanisms in NSCLC. Nat Rev Cancer. (2017) 17:637–58. doi: 10.1038/nrc.2017.84
12. Perazella MA. Renal vulnerability to drug toxicity. Clin J Am Soc Nephrol. (2009) 4:1275–83. doi: 10.2215/CJN.02050309
13. Jhaveri KD, Wanchoo R, Sakhiya V, Ross DW, Fishbane S. Adverse renal effects of novel molecular oncologic targeted therapies: A narrative review. Kidney Int Rep. (2017) 2:108–23. doi: 10.1016/j.ekir.2016.09.055
14. Modjtahedi H, Cho BC, Michel MC, Solca F. A comprehensive review of the preclinical efficacy profile of the ErbB family blocker afatinib in cancer. Naunyn Schmiedebergs Arch Pharmacol. (2014) 387:505–21. doi: 10.1007/s00210-014-0967-3
15. Hynes NE, Lane HA. ERBB receptors and cancer: the complexity of targeted inhibitors. Nat Rev Cancer. (2005) 5:341–54. doi: 10.1038/nrc1609
16. Barlesi F, Mazieres J, Merlio JP, Debieuvre D, Mosser J, Lena H, et al. Routine molecular profiling of patients with advanced non-small-cell lung cancer: results of a 1-year nationwide programme of the French Cooperative Thoracic Intergroup (IFCT). Lancet. (2016) 387:1415–26. doi: 10.1016/S0140-6736(16)00004-0
17. Kobayashi S, Canepa HM, Bailey AS, Nakayama S, Yamaguchi N, Goldstein MA, et al. Compound EGFR mutations and response to EGFR tyrosine kinase inhibitors. J Thorac Oncol. (2013) 8:45–51. doi: 10.1097/JTO.0b013e3182781e35
18. Yang JC, Sequist LV, Geater SL, Tsai CM, Mok TS, Schuler M, et al. Clinical activity of afatinib in patients with advanced non-small-cell lung cancer harbouring uncommon EGFR mutations: a combined post-hoc analysis of LUX-Lung 2, LUX-Lung 3, and LUX-Lung 6. Lancet Oncol. (2015) 16:830–8. doi: 10.1016/S1470-2045(15)00026-1
19. Scaltriti M, Baselga J. The epidermal growth factor receptor pathway: a model for targeted therapy. Clin Cancer Res. (2006) 12:5268–72. doi: 10.1158/1078-0432.CCR-05-1554
20. Karachaliou N, Fernandez-Bruno M, Bracht JWP, Rosell R. EGFR first- and second-generation TKIs-there is still place for them in EGFR-mutant NSCLC patients. Transl Cancer Res. (2019) 8:S23–47. doi: 10.21037/tcr.2018.10.06
21. Tan CS, Kumarakulasinghe NB, Huang YQ, Ang YLE, Choo JR, Goh BC, et al. Third generation EGFR TKIs: current data and future directions. Mol Cancer. (2018) 17:29. doi: 10.1186/s12943-018-0778-0
22. Akula S, Kamasani S, Sivan SK, Manga V, Vudem DR, Kancha RK. Computational analysis of epidermal growth factor receptor mutations predicts differential drug sensitivity profiles toward kinase inhibitors. J Thorac Oncol. (2018) 13:721–6. doi: 10.1016/j.jtho.2018.01.003
23. Takeda M, Okamoto I, Nakagawa K. Pooled safety analysis of EGFR-TKI treatment for EGFR mutation-positive non-small cell lung cancer. Lung Cancer. (2015) 88:74–9. doi: 10.1016/j.lungcan.2015.01.026
24. Janne PA, Yang JC, Kim DW, Planchard D, Ohe Y, Ramalingam SS, et al. AZD9291 in EGFR inhibitor-resistant non-small-cell lung cancer. N Engl J Med. (2015) 372:1689–99. doi: 10.1056/NEJMoa1411817
25. Izzedine H, Rixe O, Billemont B, Baumelou A, Deray G. Angiogenesis inhibitor therapies: focus on kidney toxicity and hypertension. Am J Kidney Dis. (2007) 50:203–18. doi: 10.1053/j.ajkd.2007.04.025
26. Rayego-Mateos S, Rodrigues-Diez R, Morgado-Pascual JL, Valentijn F, Valdivielso JM, Goldschmeding R, et al. Role of epidermal growth factor receptor (EGFR) and its ligands in kidney inflammation and damage. Mediators Inflamm. (2018) 2018:8739473. doi: 10.1155/2018/8739473
27. Crosnier A, Abbara C, Cellier M, Lagarce L, Babin M, Bourneau-Martin D, et al. Renal safety profile of EGFR targeted therapies: A study from vigiBase((R)) the WHO global database of individual case safety reports. Cancers (Basel). (2021) 13:5907. doi: 10.3390/cancers13235907
28. Abourehab MAS, Alqahtani AM, Youssif BGM, Gouda AM. Globally approved EGFR inhibitors: insights into their syntheses, target kinases, biological activities, receptor interactions, and metabolism. Molecules. (2021) 26:6677. doi: 10.3390/molecules26216677
29. Fagerberg L, Hallstrom BM, Oksvold P, Kampf C, Djureinovic D, Odeberg J, et al. Analysis of the human tissue-specific expression by genome-wide integration of transcriptomics and antibody-based proteomics. Mol Cell Proteomics. (2014) 13:397–406. doi: 10.1074/mcp.M113.035600
30. Zhou C, Wu YL, Chen G, Feng J, Liu XQ, Wang C, et al. Erlotinib versus chemotherapy as first-line treatment for patients with advanced EGFR mutation-positive non-small-cell lung cancer (OPTIMAL, CTONG-0802): a multicentre, open-label, randomised, phase 3 study. Lancet Oncol. (2011) 12:735–42. doi: 10.1016/S1470-2045(11)70184-X
31. Zhou Q, Xu CR, Cheng Y, Liu YP, Chen GY, Cui JW, et al. Bevacizumab plus erlotinib in Chinese patients with untreated, EGFR-mutated, advanced NSCLC (ARTEMIS-CTONG1509): A multicenter phase 3 study. Cancer Cell. (2021) 39:1279–91 e3. doi: 10.1016/j.ccell.2021.07.005
32. Cosmai L, Gallieni M, Porta C. Renal toxicity of anticancer agents targeting HER2 and EGFR. J Nephrol. (2015) 28:647–57. doi: 10.1007/s40620-015-0226-9
33. Jhaveri KD, Sakhiya V, Wanchoo R, Ross D, Fishbane S. Renal effects of novel anticancer targeted therapies: a review of the Food and Drug Administration Adverse Event Reporting System. Kidney Int. (2016) 90:706–7. doi: 10.1016/j.kint.2016.06.027
34. Duran I, Hotte SJ, Hirte H, Chen EX, MacLean M, Turner S, et al. Phase I targeted combination trial of sorafenib and erlotinib in patients with advanced solid tumors. Clin Cancer Res. (2007) 13:4849–57. doi: 10.1158/1078-0432.CCR-07-0382
35. Broniscer A, Baker SJ, Stewart CF, Merchant TE, Laningham FH, Schaiquevich P, et al. Phase I and pharmacokinetic studies of erlotinib administered concurrently with radiotherapy for children, adolescents, and young adults with high-grade glioma. Clin Cancer Res. (2009) 15:701–7. doi: 10.1158/1078-0432.CCR-08-1923
36. de Baaij JHF. Magnesium reabsorption in the kidney. Am J Physiol Renal Physiol. (2023) 324:F227–F44. doi: 10.1152/ajprenal.00298.2022
37. Workeneh BT, Uppal NN, Jhaveri KD, Rondon-Berrios H. Hypomagnesemia in the cancer patient. Kidney360. (2021) 2:154–66. doi: 10.34067/KID.0005622020
38. U.S. Department Of Health And Human Services, National Institutes of Health, National Cancer Institute. Common Terminology Criteria for Adverse Events (CTCAE) Version 4.0[EB/OL]. (2009-05-28)[2014-01-10] . Available online at: http://evs.nci.nih.gov/ftp1/CTCAE/CTCAE_4.03_2010-06-14_QuickReference_5x7.pdf.
39. Izzedine H, Bahleda R, Khayat D, Massard C, Magne N, Spano JP, et al. Electrolyte disorders related to EGFR-targeting drugs. Crit Rev Oncol Hematol. (2010) 73:213–9. doi: 10.1016/j.critrevonc.2009.03.012
40. Adhikari S, Mamlouk O, Rondon-Berrios H, Workeneh BT. Hypophosphatemia in cancer patients. Clin Kidney J. (2021) 14:2304–15. doi: 10.1093/ckj/sfab078
41. Maruyama K, Chinda J, Kuroshima T, Kabara M, Nakagawa N, Fujino T, et al. Minimal change nephrotic syndrome associated with gefitinib and a successful switch to erlotinib. Intern Med. (2015) 54:823–6. doi: 10.2169/internalmedicine.54.3661
42. Latcha S, Jaimes EA, Gutgarts V, Seshan S. Case of proteinuria, worsening hypertension, and glomerular endotheliosis with erlotinib and gefitinib. Kidney Int Rep. (2018) 3:1477–81. doi: 10.1016/j.ekir.2018.07.005
43. Kim ES, Hirsh V, Mok T, Socinski MA, Gervais R, Wu YL, et al. Gefitinib versus docetaxel in previously treated non-small-cell lung cancer (INTEREST): a randomised phase III trial. Lancet. (2008) 372:1809–18. doi: 10.1016/S0140-6736(08)61758-4
44. Kumasaka R, Nakamura N, Shirato K, Osawa H, Takanashi S, Hasegawa Y, et al. Side effects of therapy: case 1. Nephrotic syndrome associated with gefitinib therapy. J Clin Oncol. (2004) 22:2504–5. doi: 10.1200/JCO.2004.09.064
45. Wan HL, Yao NS. Acute renal failure associated with gefitinib therapy. Lung. (2006) 184:249–50. doi: 10.1007/s00408-005-2581-0
46. Kaneko T, Shimizu A, Aoki M, Tsuruoka S. A case of gefitinib-associated membranous nephropathy in treatment for pulmonary adenocarcinoma. CEN Case Rep. (2015) 4:31–7. doi: 10.1007/s13730-014-0135-0
47. Lu S, Shih JY, Jang TW, Liam CK, Yu Y. Afatinib as first-line treatment in asian patients with EGFR mutation-positive NSCLC: A narrative review of real-world evidence. Adv Ther. (2021) 38:2038–53. doi: 10.1007/s12325-021-01696-9
48. Keating GM. Afatinib: A review in advanced non-small cell lung cancer. Target Oncol. (2016) 11:825–35. doi: 10.1007/s11523-016-0465-2
49. Imai H, Kaira K, Naruse I, Hayashi H, Iihara H, Kita Y, et al. Successful afatinib treatment of advanced non-small-cell lung cancer patients undergoing hemodialysis. Cancer Chemother Pharmacol. (2017) 79:209–13. doi: 10.1007/s00280-016-3201-9
50. Miller VA, Hirsh V, Cadranel J, Chen YM, Park K, Kim SW, et al. Afatinib versus placebo for patients with advanced, metastatic non-small-cell lung cancer after failure of erlotinib, gefitinib, or both, and one or two lines of chemotherapy (LUX-Lung 1): a phase 2b/3 randomised trial. Lancet Oncol. (2012) 13:528–38. doi: 10.1016/S1470-2045(12)70087-6
51. Koch M, Utpatel K, Schulz C. Hyponatremia in a 58-year-old female patient with EGFR-positive lung adenocarcinoma. Internist (Berl). (2018) 59:384–7. doi: 10.1007/s00108-017-0321-1
52. Cross DA, Ashton SE, Ghiorghiu S, Eberlein C, Nebhan CA, Spitzler PJ, et al. AZD9291, an irreversible EGFR TKI, overcomes T790M-mediated resistance to EGFR inhibitors in lung cancer. Cancer Discovery. (2014) 4:1046–61. doi: 10.1158/2159-8290.CD-14-0337
53. Yu HA, Arcila ME, Rekhtman N, Sima CS, Zakowski MF, Pao W, et al. Analysis of tumor specimens at the time of acquired resistance to EGFR-TKI therapy in 155 patients with EGFR-mutant lung cancers. Clin Cancer Res. (2013) 19:2240–7. doi: 10.1158/1078-0432.CCR-12-2246
54. Fujiwara Y, Makihara R, Hase T, Hashimoto N, Naito T, Tsubata Y, et al. Pharmacokinetic and dose-finding study of osimertinib in patients with impaired renal function and low body weight. Cancer Sci. (2023) 114:2087–97. doi: 10.1111/cas.15736
55. Sequist LV, Han JY, Ahn MJ, Cho BC, Yu H, Kim SW, et al. Osimertinib plus savolitinib in patients with EGFR mutation-positive, MET-amplified, non-small-cell lung cancer after progression on EGFR tyrosine kinase inhibitors: interim results from a multicentre, open-label, phase 1b study. Lancet Oncol. (2020) 21:373–86. doi: 10.1016/S1470-2045(19)30785-5
56. Niitsu T, Hayashi T, Uchida J, Yanase T, Tanaka S, Kuroyama M, et al. Drug-induced kidney injury caused by osimertinib: report of a rare case. Nephron. (2022) 146:58–63. doi: 10.1159/000518774
57. Li Y, Liu Y, Zhao Z, Zhang Y. Rhabdomyolysis in a patient with advanced lung cancer treated with osimertinib: a case report. Transl Lung Cancer Res. (2023) 12:629–36. doi: 10.21037/tlcr
58. Bonilla M, Jhaveri KD, Izzedine H. Anaplastic lymphoma kinase inhibitors and their effect on the kidney. Clin Kidney J. (2022) 15:1475–82. doi: 10.1093/ckj/sfac062
59. Golding B, Luu A, Jones R, Viloria-Petit AM. The function and therapeutic targeting of anaplastic lymphoma kinase (ALK) in non-small cell lung cancer (NSCLC). Mol Cancer. (2018) 17:52. doi: 10.1186/s12943-018-0810-4
60. Gainor JF, Dardaei L, Yoda S, Friboulet L, Leshchiner I, Katayama R, et al. Molecular mechanisms of resistance to first- and second-generation ALK inhibitors in ALK-rearranged lung cancer. Cancer Discovery. (2016) 6:1118–33. doi: 10.1158/2159-8290.CD-16-0596
61. Hou H, Sun D, Liu K, Jiang M, Liu D, Zhu J, et al. The safety and serious adverse events of approved ALK inhibitors in Malignancies: a meta-analysis. Cancer Manag Res. (2019) 11:4109–18. doi: 10.2147/CMAR
62. Gainor JF, Tan DS, De Pas T, Solomon BJ, Ahmad A, Lazzari C, et al. Progression-free and overall survival in ALK-positive NSCLC patients treated with sequential crizotinib and ceritinib. Clin Cancer Res. (2015) 21:2745–52. doi: 10.1158/1078-0432.CCR-14-3009
63. Solomon BJ, Mok T, Kim DW, Wu YL, Nakagawa K, Mekhail T, et al. First-line crizotinib versus chemotherapy in ALK-positive lung cancer. N Engl J Med. (2014) 371:2167–77. doi: 10.1056/NEJMoa1408440
64. Solomon BJ, Kim DW, Wu YL, Nakagawa K, Mekhail T, Felip E, et al. Final overall survival analysis from a study comparing first-line crizotinib versus chemotherapy in ALK-mutation-positive non-small-cell lung cancer. J Clin Oncol. (2018) 36:2251–8. doi: 10.1200/JCO.2017.77.4794
65. Lin YT, Wang YF, Yang JC, Yu CJ, Wu SG, Shih JY, et al. Development of renal cysts after crizotinib treatment in advanced ALK-positive non-small-cell lung cancer. J Thorac Oncol. (2014) 9:1720–5. doi: 10.1097/JTO.0000000000000326
66. Schnell P, Bartlett CH, Solomon BJ, Tassell V, Shaw AT, de Pas T, et al. Complex renal cysts associated with crizotinib treatment. Cancer Med. (2015) 4:887–96. doi: 10.1002/cam4.437
67. Halpenny DF, McEvoy S, Li A, Hayan S, Capanu M, Zheng J, et al. Renal cyst formation in patients treated with crizotinib for non-small cell lung cancer-Incidence, radiological features and clinical characteristics. Lung Cancer. (2017) 106:33–6. doi: 10.1016/j.lungcan.2017.01.010
68. Camidge DR, Kim EE, Usari T, Polli A, Lewis I, Wilner KD. Renal effects of crizotinib in patients with ALK-positive advanced NSCLC. J Thorac Oncol. (2019) 14:1077–85. doi: 10.1016/j.jtho.2019.02.015
69. Gastaud L, Ambrosetti D, Otto J, Marquette CH, Coutts M, Hofman P, et al. Acute kidney injury following crizotinib administration for non-small-cell lung carcinoma. Lung Cancer. (2013) 82:362–4. doi: 10.1016/j.lungcan.2013.08.007
70. Izzedine H, Brocheriou I, Amoura Z, Mathian A. Acute tubular injury and renal arterial myocyte vacuolization following crizotinib administration. Kidney Int Rep. (2021) 6:526–8. doi: 10.1016/j.ekir.2020.11.029
71. Shaw AT, Ou SH, Bang YJ, Camidge DR, Solomon BJ, Salgia R, et al. Crizotinib in ROS1-rearranged non-small-cell lung cancer. N Engl J Med. (2014) 371:1963–71. doi: 10.1056/NEJMoa1406766
72. Horie S, Higashihara E, Nutahara K, Mikami Y, Okubo A, Kano M, et al. Mediation of renal cyst formation by hepatocyte growth factor. Lancet. (1994) 344:789–91. doi: 10.1016/S0140-6736(94)92344-2
73. Izzedine H, El-Fekih RK, Perazella MA. The renal effects of ALK inhibitors. Invest New Drugs. (2016) 34:643–9. doi: 10.1007/s10637-016-0379-y
74. Chen MF, Harada G, Liu D, DeMatteo R, Falcon C, Wilhelm C, et al. Brief report: tyrosine kinase inhibitors for lung cancers that inhibit MATE-1 can lead to “False” Decreases in renal function. J Thorac Oncol. (2023) 19:153–9. doi: 10.1016/j.jtho.2023.09.1444
75. Shaw AT, Kim TM, Crino L, Gridelli C, Kiura K, Liu G, et al. Ceritinib versus chemotherapy in patients with ALK-rearranged non-small-cell lung cancer previously given chemotherapy and crizotinib (ASCEND-5): a randomised, controlled, open-label, phase 3 trial. Lancet Oncol. (2017) 18:874–86. doi: 10.1016/S1470-2045(17)30339-X
76. Friboulet L, Li N, Katayama R, Lee CC, Gainor JF, Crystal AS, et al. The ALK inhibitor ceritinib overcomes crizotinib resistance in non-small cell lung cancer. Cancer Discovery. (2014) 4:662–73. doi: 10.1158/2159-8290.CD-13-0846
77. Califano R, Greystoke A, Lal R, Thompson J, Popat S. Management of ceritinib therapy and adverse events in patients with ALK-rearranged non-small cell lung cancer. Lung Cancer. (2017) 111:51–8. doi: 10.1016/j.lungcan.2017.06.004
78. Hill J, Shields J, Passero V. Tyrosine kinase inhibitor-associated syndrome of inappropriate secretion of anti-diuretic hormone. J Oncol Pharm Pract. (2016) 22:729–32. doi: 10.1177/1078155215592023
79. Kinoshita K, Asoh K, Furuichi N, Ito T, Kawada H, Hara S, et al. Design and synthesis of a highly selective, orally active and potent anaplastic lymphoma kinase inhibitor (CH5424802). Bioorg Med Chem. (2012) 20:1271–80. doi: 10.1016/j.bmc.2011.12.021
80. Kodama T, Tsukaguchi T, Satoh Y, Yoshida M, Watanabe Y, Kondoh O, et al. Alectinib shows potent antitumor activity against RET-rearranged non-small cell lung cancer. Mol Cancer Ther. (2014) 13:2910–8. doi: 10.1158/1535-7163.MCT-14-0274
81. Gadgeel SM, Gandhi L, Riely GJ, Chiappori AA, West HL, Azada MC, et al. Safety and activity of alectinib against systemic disease and brain metastases in patients with crizotinib-resistant ALK-rearranged non-small-cell lung cancer (AF-002JG): results from the dose-finding portion of a phase 1/2 study. Lancet Oncol. (2014) 15:1119–28. doi: 10.1016/S1470-2045(14)70362-6
82. Paik J, Dhillon S. Alectinib: A review in advanced, ALK-positive NSCLC. Drugs. (2018) 78:1247–57. doi: 10.1007/s40265-018-0952-0
83. Seto T, Kiura K, Nishio M, Nakagawa K, Maemondo M, Inoue A, et al. CH5424802 (RO5424802) for patients with ALK-rearranged advanced non-small-cell lung cancer (AF-001JP study): a single-arm, open-label, phase 1-2 study. Lancet Oncol. (2013) 14:590–8. doi: 10.1016/S1470-2045(13)70142-6
84. Ramachandran P, Morcus R, Tahir M, Onukogu I, Spinowitz B, Wang JC. Alectinib (Alecensa)-induced reversible grade IV nephrotoxicity: a case report and review of the literature. J Med Case Rep. (2018) 12:303. doi: 10.1186/s13256-018-1849-y
85. Prado-Mel E, Ciudad-Gutierrez P, Sanchez-Martin A, Martin LA. Alectinib - induced acute renal failure. J Oncol Pharm Pract. (2023). doi: 10.1177/10781552231167808
86. Zhang S, Anjum R, Squillace R, Nadworny S, Zhou T, Keats J, et al. The potent ALK inhibitor brigatinib (AP26113) overcomes mechanisms of resistance to first- and second-generation ALK inhibitors in preclinical models. Clin Cancer Res. (2016) 22:5527–38. doi: 10.1158/1078-0432.CCR-16-0569
87. Wang Y, Wang T, Xue J, Jia Z, Liu X, Li B, et al. Fatal tumour lysis syndrome induced by brigatinib in a lung adenocarcinoma patient treated with sequential ALK inhibitors: A case report. Front Pharmacol. (2021) 12:809467. doi: 10.3389/fphar.2021.809467
88. Awad MM, Katayama R, McTigue M, Liu W, Deng Y-L, Brooun A, et al. Acquired resistance to crizotinib from a mutation in CD74–ROS1. New Engl J Med. (2013) 368:2395–401. doi: 10.1056/NEJMoa1215530
89. Gainor JF, Tseng D, Yoda S, Dagogo-Jack I, Friboulet L, Lin JJ, et al. Patterns of metastatic spread and mechanisms of resistance to crizotinib in ROS1-positive non-small-cell lung cancer. JCO Precis Oncol. (2017) 2017:1–13. doi: 10.1200/PO.17.00063
90. Zou HY, Li Q, Engstrom LD, West M, Appleman V, Wong KA, et al. PF-06463922 is a potent and selective next-generation ROS1/ALK inhibitor capable of blocking crizotinib-resistant ROS1 mutations. Proc Natl Acad Sci U S A. (2015) 112:3493–8. doi: 10.1073/pnas.1420785112
91. Shaw AT, Felip E, Bauer TM, Besse B, Navarro A, Postel-Vinay S, et al. Lorlatinib in non-small-cell lung cancer with ALK or ROS1 rearrangement: an international, multicentre, open-label, single-arm first-in-man phase 1 trial. Lancet Oncol. (2017) 18:1590–9. doi: 10.1016/S1470-2045(17)30680-0
92. Betton M, Gounant V, Sannier A, Hanouna G, Goujon J-M, Brosseau S, et al. Minimal change disease induced by lorlatinib. J Thorac Oncol. (2018) 13:e154–e6. doi: 10.1016/j.jtho.2018.03.033
93. McGee K, Stone NJ, Wadhwani S, Kanwar YS, Villaflor V, Akhter N. A possible mechanism of hyperlipidemia in a patient with metastatic non-small cell lung cancer on lorlatinib therapy. J Oncol Pharm Pract. (2021) 27:2010–3. doi: 10.1177/10781552211004698
94. Lee CS, Wanchoo R, Seetharamu N. Lorlatinib induced proteinuria: A case report. J Oncol Pharm Pract. (2021) 27:1037–9. doi: 10.1177/1078155220961549
95. Upadhya A, Yadav KS, Misra A. Targeted drug therapy in non-small cell lung cancer: Clinical significance and possible solutions-Part I. Expert Opin Drug Deliv. (2021) 18:73–102. doi: 10.1080/17425247.2021.1825377
96. Paik PK, Arcila ME, Fara M, Sima CS, Miller VA, Kris MG, et al. Clinical characteristics of patients with lung adenocarcinomas harboring BRAF mutations. J Clin Oncol. (2011) 29:2046–51. doi: 10.1200/JCO.2010.33.1280
97. Mazieres J, Cropet C, Montane L, Barlesi F, Souquet PJ, Quantin X, et al. Vemurafenib in non-small-cell lung cancer patients with BRAF(V600) and BRAF(nonV600) mutations. Ann Oncol. (2020) 31:289–94. doi: 10.1016/j.annonc.2019.10.022
98. Hyman DM, Puzanov I, Subbiah V, Faris JE, Chau I, Blay JY, et al. Vemurafenib in multiple nonmelanoma cancers with BRAF V600 mutations. N Engl J Med. (2015) 373:726–36. doi: 10.1056/NEJMoa1502309
99. Wanchoo R, Jhaveri KD, Deray G, Launay-Vacher V. Renal effects of BRAF inhibitors: a systematic review by the Cancer and the Kidney International Network. Clin Kidney J. (2016) 9:245–51. doi: 10.1093/ckj/sfv149
100. Uthurriague C, Thellier S, Ribes D, Rostaing L, Paul C, Meyer N. Vemurafenib significantly decreases glomerular filtration rate. J Eur Acad Dermatol Venereol. (2014) 28:978–9. doi: 10.1111/jdv.12322
101. Launay-Vacher V, Zimner-Rapuch S, Poulalhon N, Fraisse T, Garrigue V, Gosselin M, et al. Acute renal failure associated with the new BRAF inhibitor vemurafenib: a case series of 8 patients. Cancer. (2014) 120:2158–63. doi: 10.1002/cncr.28709
102. Planchard D, Besse B, Groen HJM, Souquet PJ, Quoix E, Baik CS, et al. Dabrafenib plus trametinib in patients with previously treated BRAF(V600E)-mutant metastatic non-small cell lung cancer: an open-label, multicentre phase 2 trial. Lancet Oncol. (2016) 17:984–93. doi: 10.1016/S1470-2045(16)30146-2
103. Hurabielle C, Pillebout E, Stehle T, Pages C, Roux J, Schneider P, et al. Mechanisms underpinning increased plasma creatinine levels in patients receiving vemurafenib for advanced melanoma. PloS One. (2016) 11:e0149873. doi: 10.1371/journal.pone.0149873
104. Folkman J. Tumor angiogenesis: therapeutic implications. N Engl J Med. (1971) 285:1182–6. doi: 10.1056/NEJM197111182852108
105. Breen EC. VEGF in biological control. J Cell Biochem. (2007) 102:1358–67. doi: 10.1002/jcb.21579
106. Gurevich F, Perazella MA. Renal effects of anti-angiogenesis therapy: update for the internist. Am J Med. (2009) 122:322–8. doi: 10.1016/j.amjmed.2008.11.025
107. Zhu X, Stergiopoulos K, Wu S. Risk of hypertension and renal dysfunction with an angiogenesis inhibitor sunitinib: systematic review and meta-analysis. Acta Oncol. (2009) 48:9–17. doi: 10.1080/02841860802314720
108. Lafayette RA, McCall B, Li N, Chu L, Werner P, Das A, et al. Incidence and relevance of proteinuria in bevacizumab-treated patients: pooled analysis from randomized controlled trials. Am J Nephrol. (2014) 40:75–83. doi: 10.1159/000365156
109. Lye LF, Chou RH, Wu TK, Chuang WL, Tsai SC, Lin HJ, et al. Administration of bevacizumab and the risk of chronic kidney disease development in Taiwan residents: A population-based retrospective cohort study. Int J Mol Sci. (2023) 25:340. doi: 10.3390/ijms25010340
110. Garcia J, Hurwitz HI, Sandler AB, Miles D, Coleman RL, Deurloo R, et al. Bevacizumab (Avastin(R)) in cancer treatment: A review of 15 years of clinical experience and future outlook. Cancer Treat Rev. (2020) 86:102017. doi: 10.1016/j.ctrv.2020.102017
111. Jagiela J, Bartnicki P, Rysz J. Nephrotoxicity as a complication of chemotherapy and immunotherapy in the treatment of colorectal cancer, melanoma and non-small cell lung cancer. Int J Mol Sci. (2021) 22:4618. doi: 10.3390/ijms22094618
112. Yogi A, O’Connor SE, Callera GE, Tostes RC, Touyz RM. Receptor and nonreceptor tyrosine kinases in vascular biology of hypertension. Curr Opin Nephrol Hypertens. (2010) 19:169–76. doi: 10.1097/MNH.0b013e3283361c24
113. Cosmai L, Gallieni M, Liguigli W, Porta C. Renal toxicity of anticancer agents targeting vascular endothelial growth factor (VEGF) and its receptors (VEGFRs). J Nephrol. (2017) 30:171–80. doi: 10.1007/s40620-016-0311-8
Keywords: lung cancer, targeted therapy, EGFR TKIs, ALK TKIs, nephrotoxicity
Citation: Li Q, Lin J, Hao G, Xie A, Liu S and Tang B (2024) Nephrotoxicity of targeted therapy used to treat lung cancer. Front. Immunol. 15:1369118. doi: 10.3389/fimmu.2024.1369118
Received: 11 January 2024; Accepted: 21 June 2024;
Published: 04 July 2024.
Edited by:
Mohammed Abu El-Magd, Kafrelsheikh University, EgyptReviewed by:
Shikha Kumari, Virginia Tech, United StatesShuo-Yan Gau, Chung Shan Medical University, Taiwan
Amr Adel Elkelish, Suez Canal University, Egypt
Nemany A. N. Hanafy, Kafrelsheikh University, Egypt
Copyright © 2024 Li, Lin, Hao, Xie, Liu and Tang. This is an open-access article distributed under the terms of the Creative Commons Attribution License (CC BY). The use, distribution or reproduction in other forums is permitted, provided the original author(s) and the copyright owner(s) are credited and that the original publication in this journal is cited, in accordance with accepted academic practice. No use, distribution or reproduction is permitted which does not comply with these terms.
*Correspondence: Shuangxin Liu, MTM1NDM0NTY0NDZAMTYzLmNvbQ==; Bin Tang, YmluYmluMDY4QHFxLmNvbQ==
†These authors have contributed equally to this work