- 1Institute of Immunology and Microbiology, First Faculty of Medicine, Charles University, Prague, Czechia
- 2Department of Neonatology, Institute for the Care of Mother and Child, Prague, Czechia
- 3Pathology Department, University of Alabama at Birmingham, Birmingham, AL, United States
Introduction: The immune systems of both the mother and the newborn face significant challenges during birth. Proper immune regulation after birth is essential for the survival of neonates. Numerous studies have demonstrated that the neonatal immune system is relatively immature, particularly in its adaptive arm, placing the primary responsibility for immune surveillance on innate immunity.
Methods: Given the significant role of neutrophils in protecting the neonate after birth, we conducted a study investigating the properties of neutrophils in newborn cord blood using various methodological approaches.
Results: Our findings demonstrate the presence of immature low-density neutrophils in the cord blood, which are likely responsible for the observed elevated expression of genes coding for proteins essential to antimicrobial response, including myeloperoxidase, neutrophils elastase, and defensins.
Discussion: We propose that these cells function normally and support the protection of newborns early after birth. Furthermore, our results suggest that the mode of delivery might significantly influence the programming of neutrophil function. The presented findings emphasize the importance of distinct neutrophil subpopulations in neonatal immunity and their potential impact on early postnatal health.
1 Introduction
The neonatal immune system is characterized by its immaturity and generally lower functional capacity compared to the adult immune system. Neonatal adaptive immune responses are impaired since exposure to antigens in utero is limited (1). In the early days after birth, the newborn is primarily protected by antibodies of maternal origin, delivered either prenatally or postnatally through breastfeeding. The innate immune system, with neutrophils in the first line of defense, plays a crucial role in cellular immunity against potentially harmful pathogens in the early stages of infection. Despite this, due to the deficiency in immune functions, newborns are at a higher risk of neonatal sepsis, with preterm children being particularly vulnerable (2).
Interestingly, neonates delivered by caesarean section have more pronounced immaturity of the immune system, further increasing the risk of a severe course of infection. It is well-established that the mode of delivery plays a crucial role in the future health of the newborn, both in the early postnatal period and in the long term. For example, there is a higher incidence of childhood asthma (3) and inflammatory bowel disease (4, 5) in individuals born via caesarean section. Additionally, the delivery mode can significantly influence neutrophil functional response, namely reactive oxygen species (ROS) production (6) and migration (7) were increased in cord blood neutrophils of neonates delivered vaginally.
For a long time, neutrophils were considered to be a short-lived homogeneous cell population with functions limited to their suicidal nature at the site of inflammation. In recent years, the view of neutrophil biology has changed extensively. It was shown that neutrophils can live up to five days (8). Although this observation was challenged (9) it seems that the uncertainty about the length of neutrophil survival mainly stems from the limitation of techniques and methodological approaches used to evaluate neutrophil half-life in vivo (10). Neutrophils stably replenish their numbers in blood by exchange of mature neutrophils from the bone marrow and can influence the adaptive immune system either directly (11–13) or via the production of cytokines (14, 15). Neutrophils are now considered a heterogeneous population with an extensive range of functions (16–18).
Although neutrophil subpopulations may be distinguished based on multiple criteria including the presence of transcription factors (19), the cell separation method based on centrifugation in density gradient is most frequently employed. Neutrophils sedimenting in the erythrocyte fraction have been termed high-density neutrophils (HDN) and are typically considered “classical” pro-inflammatory neutrophils. Low-density neutrophils (LDN) in the peripheral blood mononuclear cells (PBMC) fraction were initially described in 1986 in patients with autoimmune disease (20). Since then, LDNs were found in increased numbers in various inflammatory conditions, including cancer, cardiovascular diseases, autoimmune diseases, and infections, suggesting their potential roles in inflammation (21–24). Hassani et al. (25) showed that LDN expand in patients with chronic inflammation and, following induction with LPS, they consist primarily of CD16dimCD62Lhigh neutrophils (25). LDN were shown to exert suppressive function during carcinogenesis (26) and pregnancy (27–29). Interestingly, LDN can exert pro-inflammatory function under specific circumstances, for example in patients with systemic lupus erythematosus (SLE) (16, 30) (for a detailed review of this topic see (31)). Villanueva and colleagues (32) showed an enhanced expression of proteins linked to neutrophil antimicrobial activity in systemic lupus erythematosus (SLE) [e.g., myeloperoxidase (MPO), neutrophil elastase (ELANE), alpha defensin 4 (DEFA4)].
However, the functional properties of LDNs remain contradictory. Different studies have reported conflicting findings, with some suggesting LDNs have T cell activating properties (30, 33) while others argue for suppressive effects (34–36). Similarly, LDNs have been linked to both pro-tumorigenic and anti-tumorigenic activities, pro-inflammatory and anti-inflammatory qualities, as well as variable phagocytic capacities and ROS production compared to normal-density neutrophils (37–39).
Previously, it was shown that neutrophils in cord blood display immature phenotype with impaired antimicrobial functions; however, the reported studies show low degree of consistency. Ssemaganda et al. (40) found that in cord blood (CB), LDN represented the most significant part of CD15+ neutrophils compared to peripheral blood (PB) of mothers and expressed low levels of CD16, suggesting their relative immaturity. A similar phenotype (low expression of CD16 with high expression of CD64) was observed in CB of children with neonatal sepsis (41). This group suggested that CB LDN are phenotypically distinct from adult LDN.
In this study, we investigate the phenotype and functional properties of neutrophils in umbilical cord blood, which can significantly impact the neonate’s early days after delivery and overall well-being. Our findings confirmed the presence of immature CD16low neutrophil subpopulations in umbilical cord blood. This population may be responsible for increased expression of genes associated with antimicrobial response, including defensins and myeloperoxidase. Interestingly, upon challenge with an inflammatory stimulus (Escherichia coli), both the cord blood and maternal peripheral blood neutrophils displayed normal levels of activation and oxidative burst.
2 Materials and methods
2.1 Sample collection
This study was approved by the Ethical Committee at the Institute for the Care of Mother and Child in Podolí, Prague, Czech Republic. All mothers and healthy volunteers signed a written informed consent before sample collection. Cord and peripheral blood were collected at the Institute for the Care of Mother and Child in Prague, the Czech Republic. Umbilical cord blood (20-30 ml) was drawn from the umbilical vein with a sterile needle into a 50 ml flask with heparin (10U/ml). During the standard pre-labour examination, maternal peripheral blood (2-5 ml) was drawn into K2EDTA or heparin-containing tubes. Blood samples were stored at RT in a dark box until pick-up. Peripheral blood (2-5 ml) of healthy women volunteers was drawn into K2EDTA or heparin-containing tubes. Details of the studied cohort of mothers and children are listed in Table 1.
2.2 Flow cytometry analysis
Whole blood was stained with anti-human CD14 (clone 61D3, Invitrogen, USA), CD15 (clone HI98, BioLegend, USA), CD16 (clone CB16, Invitrogen), CD62L (clone DREG-56, BioLegend, USA), and CD64 (clone 10.6, BioLegend, USA). After 15 min incubation in the dark, erythrocytes were lysed with 10x diluted Lysis Buffer (QIAGEN, Netherlands), and samples were washed twice with PBS. Samples were measured on BD FACS CantoTM II Flow Cytometer (BD Biosciences, USA) equipped with the BD FACS Diva Software v6.1.2 and analyzed with FlowJo v10.10.0. (FlowJo LLC, USA) software. Gating strategy is shown in Supplementary Figures 1, 2.
2.3 Cell isolation
For the isolation of the whole neutrophil population, the EasySepTM Direct Human Neutrophil Isolation Kit (STEMCELL Technologies, Canada) was used. Neutrophils were isolated according to the manufacturer’s instructions with slight modifications to increase cell yield and purity. In the first step, 50 μl of 0.5 M EDTA/1 ml blood was added, and the first incubation was extended to 6 min. Isolated cells were washed once with PBS and counted. Cell viability and purity were assessed by Trypan blue and flow cytometry, respectively. The purity of the isolated neutrophil population was higher than >96%. Mononuclear cell fraction was obtained as follows. The whole cord or peripheral blood was diluted 1:1 with PBS and layered carefully over Ficoll-PaqueTM PLUS (density 1.077 g/l) in a conical tube. Density-gradient centrifugation was performed at 500 x g for 30 min. After centrifugation, blood was separated into CBMC or PBMC-rich layer and granulocyte-rich erythrocyte pellet. Cells from both fractions were washed twice with PBS and counted. LDN were isolated from CBMC using EasySepTM Human CD15 Positive Selection Kit (STEMCELL Technologies, USA) according to the manufacturer’s instructions. HDN were isolated using EasySepTM Direct Human Neutrophil Isolation Kit (STEMCELL Technologies, USA) from the erythrocyte fraction as described above.
2.4 Quantitative PCR
Total RNA from isolated neutrophils was extracted using the RNeasy® Mini Kit (QIAGEN, Netherlands) according to the manufacturer’s instructions with the addition of the optional RNase-Free DNase I (QIAGEN) incubation step to prevent genomic DNA contamination. The purity and concentration of the RNA were determined using NanoDrop 1000 Spectrophotometer (Thermo Scientific, USA). Total RNA was reverse transcribed to cDNA using the High Capacity cDNA Reverse Transcription Kit (Applied Biosystems) according to the manufacturer’s instructions. The relative expression of selected genes was performed using the Luna® Universal qPCR Master Mix (New England BioLabs, UK) and TaqMan® probes (Applied Biosystems, USA) and measured on the LightCycler® 480 II Real-Time PCR System (Roche Molecular Systems, USA). All tested genes and their respective probe assay IDs are listed in Table 2.
2.5 Myeloperoxidase activation assay
The phagocytic capacity of neutrophils quantified as relative myeloperoxidase (MPO) activity was tested by the FagoFlowExKit (EXBIO, CZ) according to the manufacturer’s instructions with the addition of anti-human anti-CD15 antibody (clone HI98, BD Biosciences) for precise determination of neutrophils. The method utilizes the oxidation of dihydrorhodamine (DHR) 123 to fluorescent rhodamine 123 by activated MPO. The fluorescence was measured using BD FACS CantoTM II Flow Cytometer (BD Biosciences, USA) equipped with the BD FACS Diva Software v6.1.2 and analyzed with FlowJo 7.6.5. (FlowJo LLC, USA) software. The gating strategy and representative histograms are shown in Supplementary Figure 3. The relative MPO activity was calculated based on mean fluorescent intensity (MFI) as follows:
(MFIS – MFI of sample stimulated with E. coli, MFIP – MFI of positive control, MFIN – MFI of negative control) to account for the background fluorescence.
2.6 Data analysis and statistical analysis
Flow cytometry results were standardized using Cytometer Setup and Tracking beads (BD Biosciences, USA) and analyzed by FlowJo software using appropriate compensation controls (single staining, compensation beads, isotype control) and fluorescence minus one (FMO) control to set proper gating strategy. Transcript expression of the target gene was quantified relative to endogenous control (housekeeping gene) actin beta (ACTB) mRNA levels using the 2-ΔΔCt method as described earlier (42). For flow cytometry analysis and qPCR analysis, parametric statistical tests (unpaired Students t-test) were utilized. Acquired data were statistically evaluated and graphically processed using GraphPad Prism 8 (GraphPad Software Inc.) software. Pearson correlation test was employed for the correlation of gene expression with neutrophil subpopulation. Statistically significant differences among the groups were considered when p-values were lower than 0.05.
3 Results
3.1 Umbilical cord blood has an increased number of CD16low neutrophils.
Based on previous reports indicating the presence of immature neutrophils in CB (40, 41), we performed phenotype analyses of selected neutrophil cell surface markers reflecting neutrophil maturational and activation status. Neutrophils in CB accounted for only about 40% of total cells compared with proportion of neutrophils in HV (68%) and PB (76%) (Figure 1A), whereas their frequency was only slightly elevated in the PB of mothers compared with HV (Figure 1A). The frequency of neutrophils identified as CD14-CD15+ granulocytes (43, 44) was lower in CB (Figure 1B), mainly due to the enrichment in CD14-CD15low granulocytes (Figure 1D), a granulocyte population not previously described (gating strategy in Supplementary Figures 1, 2). Monocytes, identified as CD14+CD15- cells (45), did not differ between the study groups (Figure 1C). Neutrophils were further gated based on the expression of CD16 (FcγRIIIB) and CD64 (FcγRI), markers considered to reflect neutrophil maturity, and CD62L (L-selectin). As previously reported (41), CD16lowCD64high neutrophils were increased in CB (Figure 1E), with a similar trend observed in the case of CD16lowCD62L+ (Figure 1F). Most of the neutrophils in the control group exhibited CD16+CD64low and CD16+CD62L+ phenotype (Figures 1E, F), suggesting a mature non-activated neutrophil population. Neutrophils in PB of mothers displayed increased expression of CD64 (Figure 1E), suggesting neutrophil activation during pregnancy. To address whether CD16low neutrophils have a low-density characteristics, identical staining and gating strategies were applied to isolated CBMC or PBMC. An increase in the frequency of CD16low neutrophils in CBMC was observed (Figures 2B, C), more prominent when cells were gated as CD16lowCD64high (Figure 2B). The other subpopulations did not differ between the experimental groups (Figures 2D, E), confirming the nature of CD16low neutrophils as LDN. To better identify the particular subpopulation based on the cell surface presence of CD16, alternative gating was performed (see Supplementary Figure 2) and three neutrophil subsets were identified according to CD16 (i.e. CD16 negative (CD16-); CD16 dim (CD16int) and CD16 bright (CD16+)). Proportion of particular neutrophil subsets based on CD16 distribution is presented in Supplementary Figure 4. This alternative gating provide us with remarkably elevated proportion of CD16-CD64high neutrophils within CD14-CD15+ cells in CB compared with HV. CD16intCD64high neutrophils within CD14-CD15+ cells were significantly increased in CB compared with HV. CD16+CD64high neutrophils were the most abundant in PB in comparison with HV and CB. Proportion of CD16+CD64high neutrophils was elevated in CB compared with HV. No difference was observed in proportion of CD16-CD64low among HV, PB and CB. On contrary, CD16+CD64low were significantly increased in HV compared to both PB and CB. However, CD16+CD64low was significantly elevated in CB compared to PB, Supplementary Figure 4A. No difference in proportion of CD16-CD62L+ and CD16intCD62L+ among HV, PB and CB has been detected. Subset of CD16+CD62L+ was significantly higher in HV compared to CB. CD16-CD62L- were significantly increased in CB compared with HV, Supplementary Figure 4B.
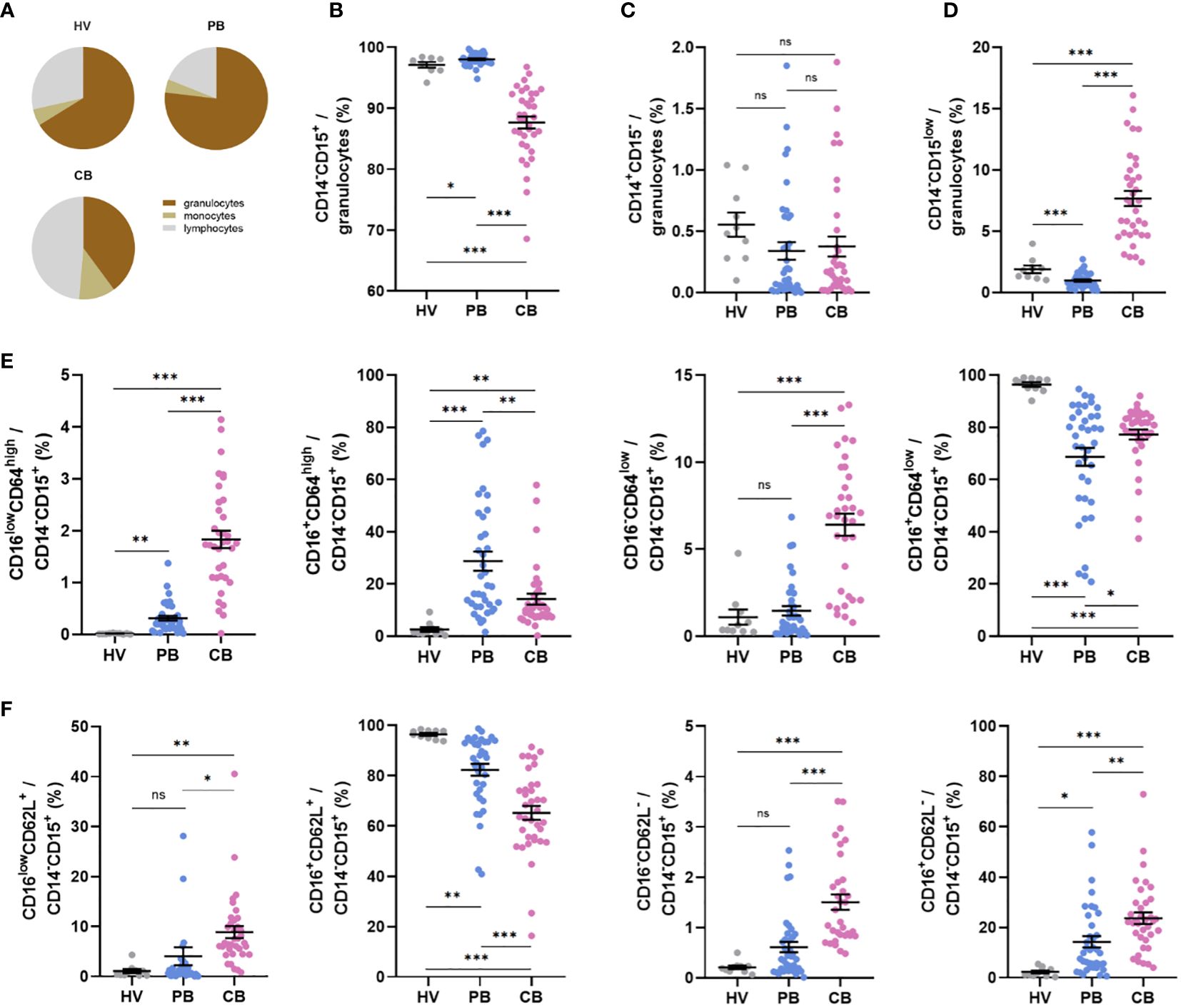
Figure 1 Expression of neutrophil cell surface markers in peripheral blood of healthy women volunteers (HV), maternal peripheral blood (PB), and umbilical cord blood (CB). (A) Relative proportion of granulocytes, monocytes, and lymphocytes. (B) The relative frequency of neutrophils, gated as CD14-CD15+. (C) The relative frequency of monocytes gated as CD14+CD15- cells. (D) Relative frequency of CD14-CD15low neutrophils. (E) Relative frequencies of CD16lowCD64high, CD16+CD64high, CD16-CD64low, and CD16+CD64low cells within CD14-CD15+ neutrophils. (F) Relative frequencies of CD16lowCD62L+, CD16+CD62L+, CD16lowCD62L- and CD16+CD62L- within CD14-CD15+ neutrophils. HV: n = 10, PB: n = 38, CB: n = 36. The data are shown as the mean ± SEM for the whole group. Asterisks indicate *p< 0.05, **p< 0.01, and ***p< 0.001, two-tailed unpaired Student’s t test. non-significant (ns) nsp > 0.05.
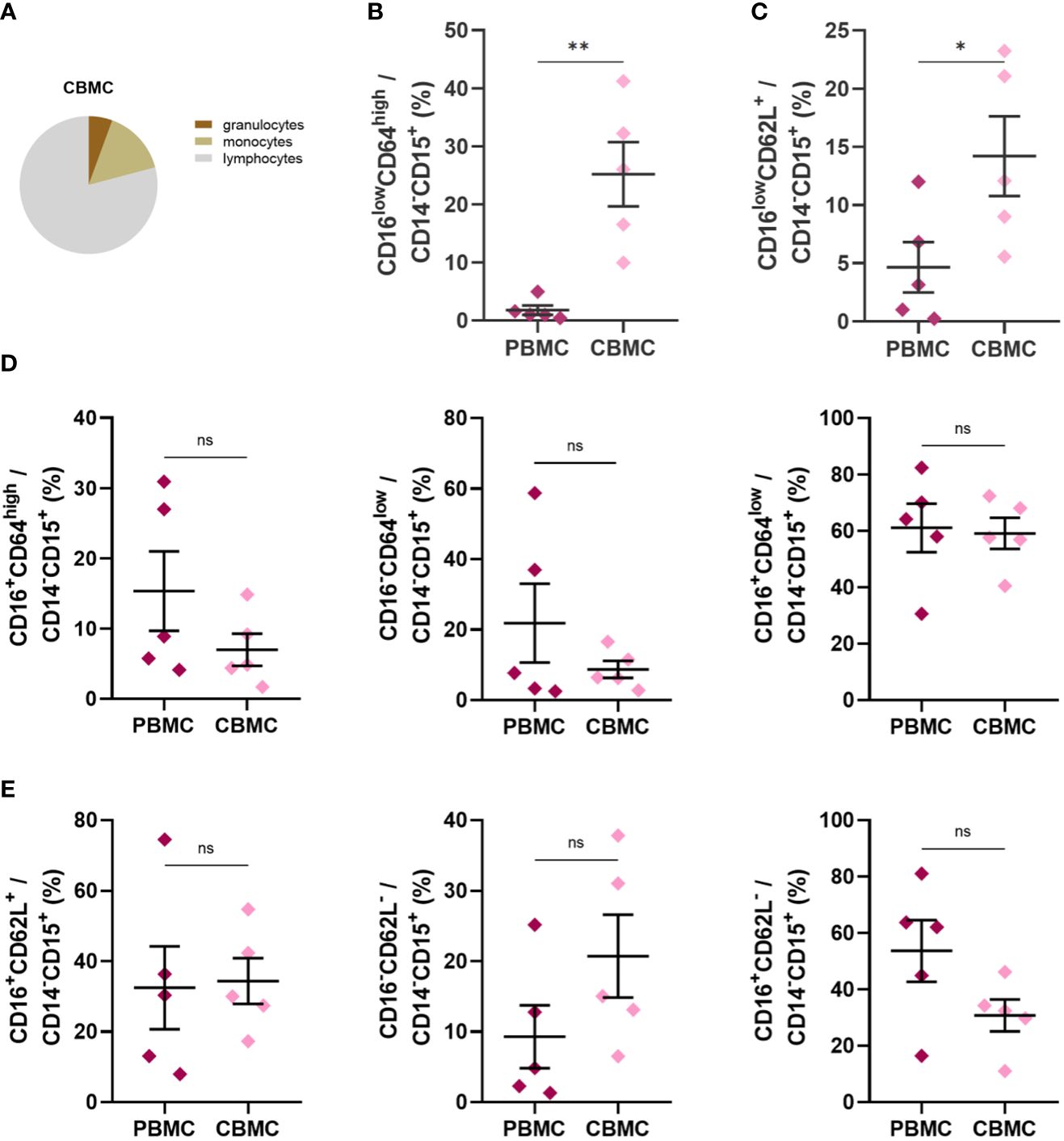
Figure 2 Expression of neutrophil cell surface markers in PB and CB mononuclear cell fraction after density gradient centrifugation. (A) Relative proportion of granulocytes, monocytes and lymphocytes. (B) CD16lowCD64highCD14-CD15+ (C) CD16lowCD64+CD14-CD15+ (D) Relative frequencies of CD16+CD64highCD14-CD15+, CD16-CD64lowCD14-CD15+ and CD16+CD64lowCD14-CD15+ (E) Relative frequencies of CD16+CD62L+CD14-CD15+, CD16-CD62L-CD14-CD15+ and CD16+CD62L-CD14-CD15+ (PBMC: n = 5, CBMC n = 5). The data are shown as the mean ± SEM for the whole group. Asterisks indicate *p< 0.05, **p< 0.01, two-tailed unpaired Student’s t test. non-significant (ns) nsp > 0.05.
3.2 Cord blood neutrophils display elevated expression of genes associated with antimicrobial function
Next, we determined the expression of genes linked to antimicrobial (Figure 3) or suppressive (Figure 4) functions in all three study groups. The association of human defensin alpha 3 in anti-infectious immunity has been highlighted (46). On the other hand, elevated presence of alpha defensin 3 (DEFA3) in vaginal fluid after premature preterm rupture of membranes pointed to neonatal inflammation (47). In our study, significantly increased gene expression of DEFA3 was observed in cord blood neutrophils compared to neutrophils of adults (both HV and PB). Importantly, PB neutrophils showed promoted gene expression of DEFA3 compared to HV suggesting increased readiness to fight against infection, Figure 3A. The marker linked with the function of neutrophils is myeloperoxidase which stabile levels during pregnancy can be considered as a biomarker of non-complicated course of pregnancy (48). Cord blood neutrophils shows superior gene expression of MPO compared to adult neutrophils (both HV and PB). Interestingly, PB neutrophils exerted elevated gene expression of MPO compared to HV confirming higher anti-microbial capacity, Figure 3B. There was no difference in the expression of MMP9 between the cord and maternal peripheral blood, possibly explained by the specific increase in the expression of metalloproteases during pregnancy and labour (49). Another peptide with antimicrobial capacity secreted by neutrophils is defensin alpha 4 (DEFA4) (50). We have observed significantly increased gene expression of DEFA4 in cord blood neutrophils compared to HV and PB. Neutrophil elastase (ELANE) is a marker of NET formation in health and disease (51). Here, we showed significantly elevated gene expression of ELANE in cord blood neutrophils compared to both HV and PB. Lactotransferrin (LTF) is playing a pivotal role in inflammatory homeostasis (52). Therefore, we have evaluated gene expression of LTF in cord blood neutrophils and confirmed superior expression of LTF in cord blood neutrophils compared with HV and PB highlighting prerequisite of cord blood neutrophils exert antimicrobial functions. PB neutrophils poses still elevated gene expression of LTF compared to HV neutrophils. In summary, cord blood neutrophils display highly increased expression of genes coding for proteins playing an important role in antimicrobial response (e.g., myeloperoxidase, defensins, and neutrophil elastase) compared to neutrophils from the mothers or healthy volunteers (Figures 3A, B, D–F).
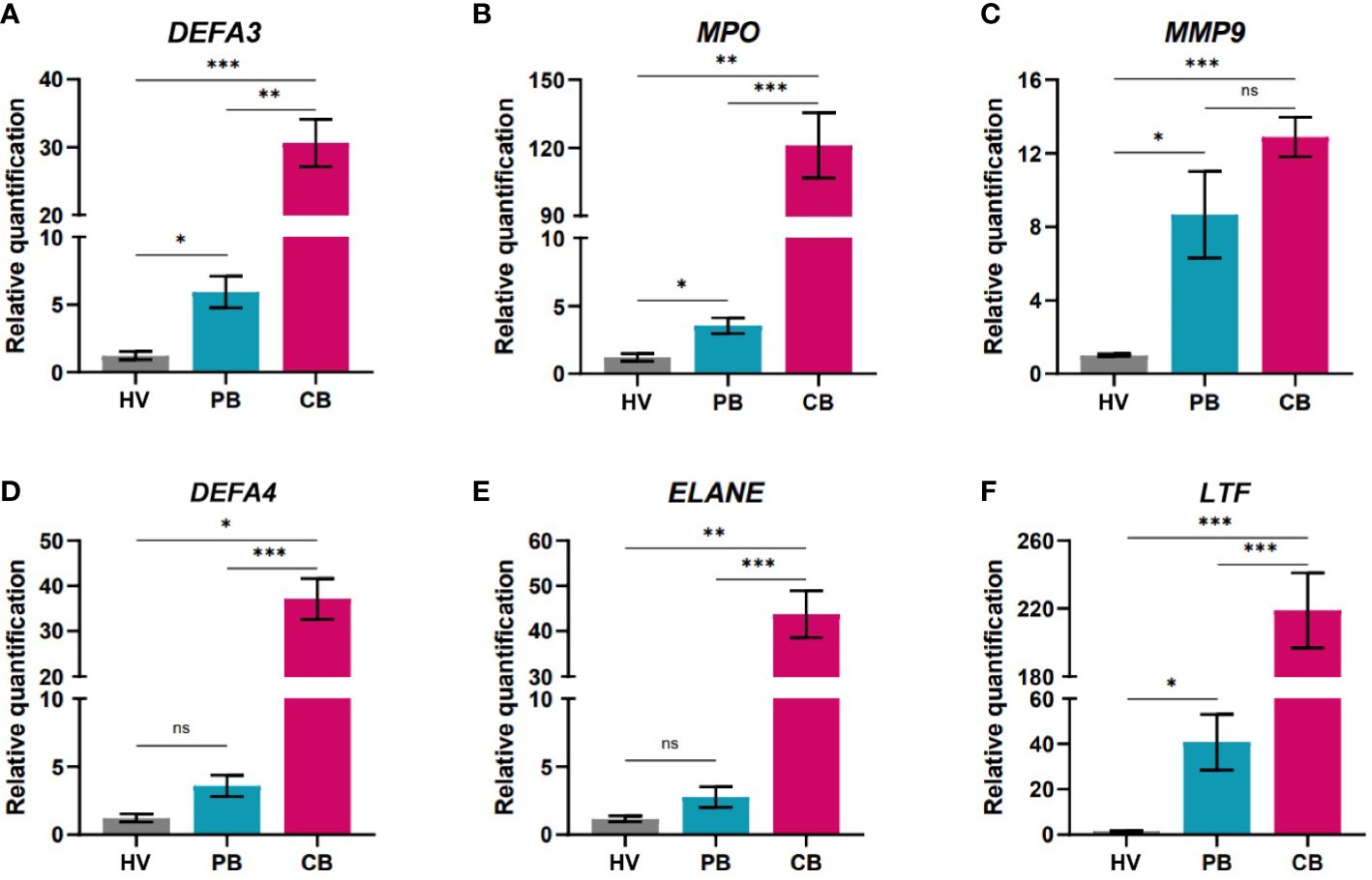
Figure 3 Quantification of gene expression in neutrophils isolated from peripheral blood of healthy women volunteers (HV), maternal peripheral blood (PB), and umbilical cord blood (CB). (A) alpha defensin 3 (B) myeloperoxidase (C) matrix metalloproteinase 9 (D) alpha defensin 4 (E) neutrophil elastase (F) lactotransferrin (HV: n = 6, PB: n = 16, CB: = 54). The data are shown as the mean ± SEM for the whole group. Asterisks indicate *p< 0.05, **p< 0.01, and ***p< 0.001, two-tailed unpaired Student’s t test. non-significant (ns) nsp > 0.05.
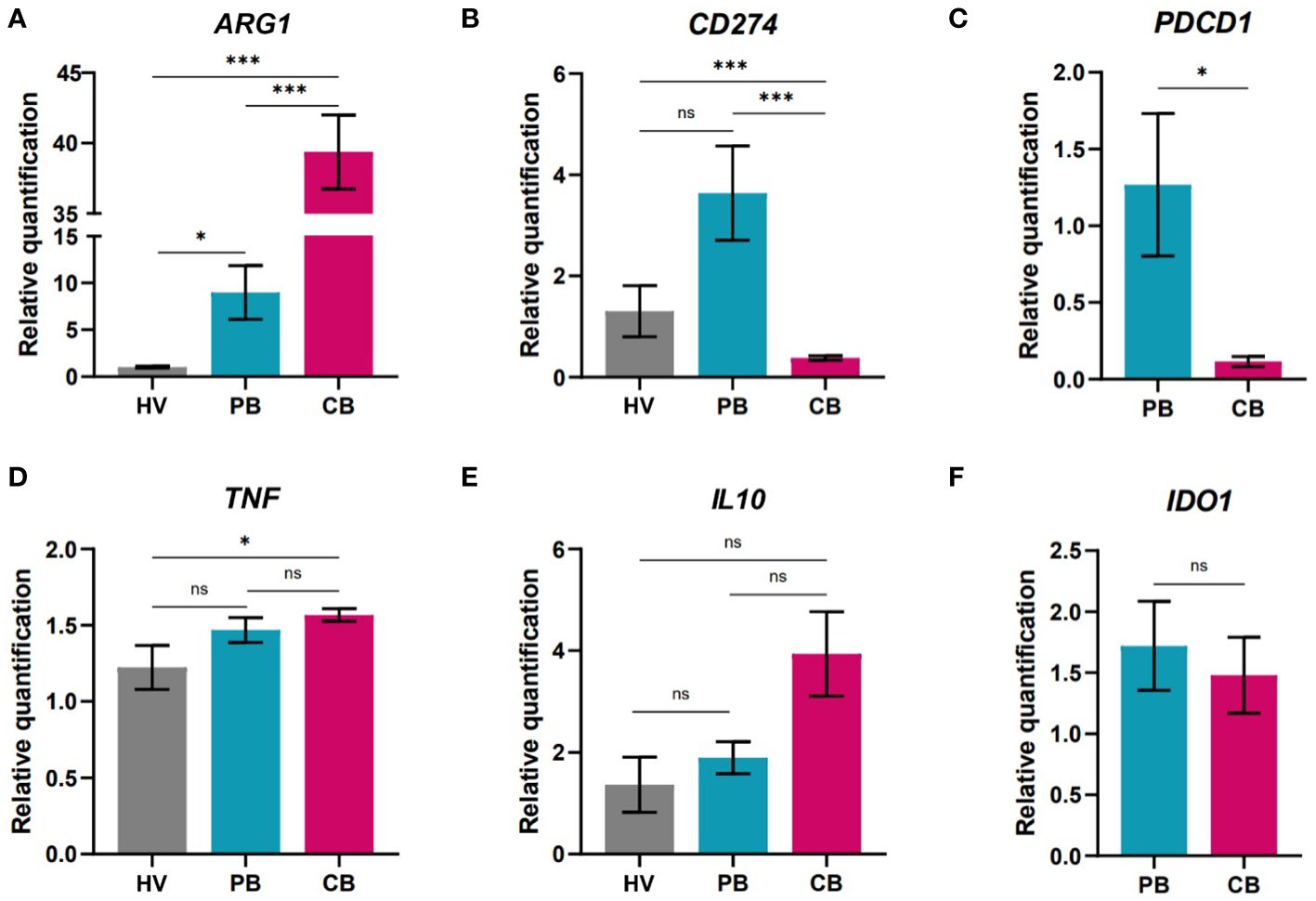
Figure 4 Gene expression analysis of neutrophils isolated from peripheral blood of healthy women volunteers (HV), maternal peripheral blood (PB), and umbilical cord blood (CB). (A) arginase (B) Programmed death-ligand 1 (PD-L1/CD274) (C) Programmed cell death 1 (PB: n = 4, CB: n = 4) (D) tumor necrosis factor alpha (E) interleukin 10 (F) indol amine 2,3 dioxygenase (PB: n = 10, CB = 11). (A, B, D, E HV: n = 6, PB = 9, CB = 17). The data are shown as the mean ± SEM for the whole group. Asterisks indicate *p< 0.05, ***p< 0.001, two-tailed unpaired Student’s t test. non-significant (ns) nsp > 0.05.
Successful pregnancies were associated with neutrophil subpopulations with prone expression of arginase (ARG1). Interestingly, in the case of genes associated with immune suppressive properties, there was an increase in the expression of ARG1 (Figure 4A) in CB neutrophils but limited expression of CD274 encoding PD-L1, that was highly elevated in neutrophils from maternal PB (Figure 4). PD-L1 is a marker identified on neutrophils and its expression is associated with undergoing inflammatory process (53, 54). Therefore, it is not surprising that only marginal PD-L1 expression has been detected in cord blood neutrophils of healthy neonates. Along with the increase in CD274, maternal neutrophils also displayed elevated expression of PDCD1 (Figure 4C), a gene translated to the receptor (Programmed cell death protein 1, PD-1) for PD-L1, potentially indicating a negative feedback loop exerted in maternal neutrophils. Only slight increase in the expression of tumor necrosis factor (TNF) in CB neutrophils was observed in comparison to HV neutrophils (Figure 4D), indicating elevated antimicrobial activity. We have focused on gene expression of immunoregulatory markers as well. Interleukin 10 (IL10) is well known cytokine with immunoregulatory/immunosuppressive function preventing undesirable immune responses (55). As another marker suggesting potential immunoregulatory capacity of neutrophils was selected indol amine 2,3 dioxygenase (IDO) which expression has been shown limited in more reactive cells (56). Surprisingly, no difference was observed in the expression of these genes of interest (Figures 4E, F). Next, the expression of genes of interest was compared in cord blood of children delivered either by vaginal delivery (VD) or caesarean section (CS) (Supplementary Figure 5). Interestingly, children delivered by CS displayed elevated expression of defensins and myeloperoxidase. In contrast, children delivered by VD displayed higher expression of genes coding for IL-10 and PD-L1 proteins, indicating elevated proinflammatory settings in children born by CS.
3.3 Elevated expression of genes associated with antimicrobial function correlates with the frequency of CD16low neutrophils in cord blood
Next, we set to elucidate whether the higher relative frequency of LDN in CB might be responsible for the elevated expression of genes associated with antimicrobial function. To this aim, we isolated LDN and HDN from CB and performed gene expression analysis of candidate genes of interest. The data did not reach statistical significance (Figures 5A, B), probably due to the low number of samples. Only non-significant tendency toward higher expression of genes associated with antimicrobial response was observed in LDN (Figure 5A). In order to overcome the difficulties associated with cell isolation, correlation analysis was performed. The frequency of CD16lowCD64high neutrophils in CB was correlated with the relative gene expression of examined genes in neutrophils isolated from whole CB (Figures 5C, D). Despite quite low value of correlation coefficient, the correlation reaches significance in three of the candidate genes (DEFA3, DEFA4 and MPO, Figure 5C) indicating that the presence of CD16low LDN is responsible for the observed increased expression of genes associated with antimicrobial response.
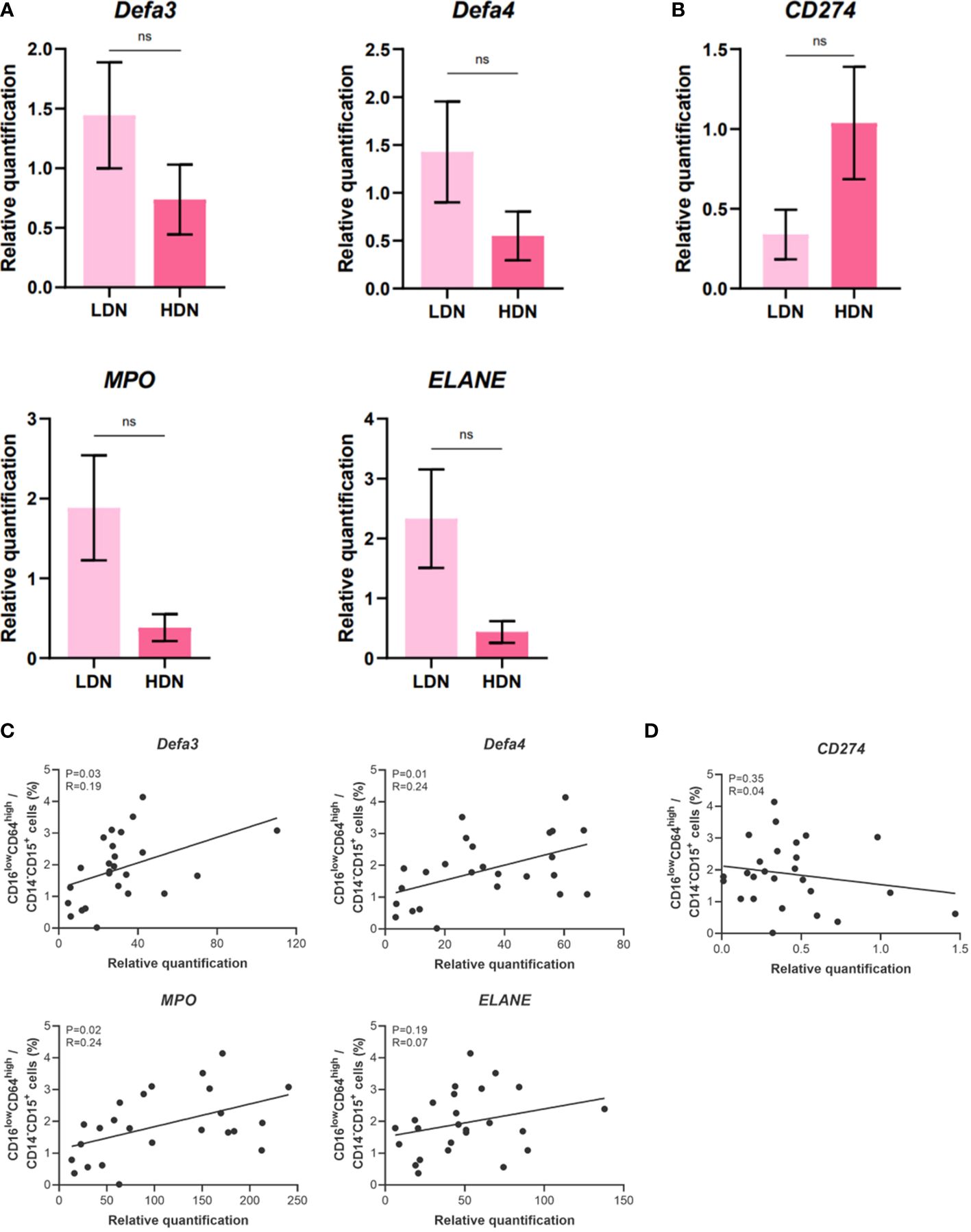
Figure 5 Gene expression analysis of low-density neutrophils (LDN) and high-density neutrophils (HDN) isolated from umbilical cord blood (CB). (A) alpha defensin 3, alpha defensin 4, myeloperoxidase, neutrophil elastase. (B) Programmed death-ligand 1 (PD-L1/CD274). (C) Correlation analysis between the relative gene expression of genes coding for antimicrobial substances and percentage of the neutrophil population (CD14-CD15+CD16lowCD64high). (D) Correlation analysis between the relative gene expression of CD274 and percentage of the neutrophil population (CD14-CD15+CD16lowCD64high). The data are shown as the mean ± SEM for the whole group. Asterisks indicate nsp > 0.05, two-tailed unpaired Student’s t test. Pearson correlation analysis was performed for (C, D). Pearson correlation coefficient and p-value were obtained and p-value less than 0.05 (p< 0.05) was considered statistically significant. non-significant (ns) nsp > 0.05.
3.4 Neutrophils from umbilical cord blood do not exert impaired activation of myeloperoxidase
To elucidate whether the presence of CD16low neutrophils affects the functional properties of neutrophils in CB, we studied their activation after stimulation with inflammatory stimulus Escherichia coli. To this end, we utilised FagoFlowEx Kit (EXBIO) employing dihydrorhodamine 123 oxidation to fluorescent rhodamine 123 by activated MPO (representative histograms shown at Figure 6A). Staining for CD15 employed for higher stringency of gating of neutrophil population did not exert any effect on their activation status (Figure 6B). As depicted in Figure 6C, the activation of neutrophils by inflammatory stimulus did not differ among the study groups.
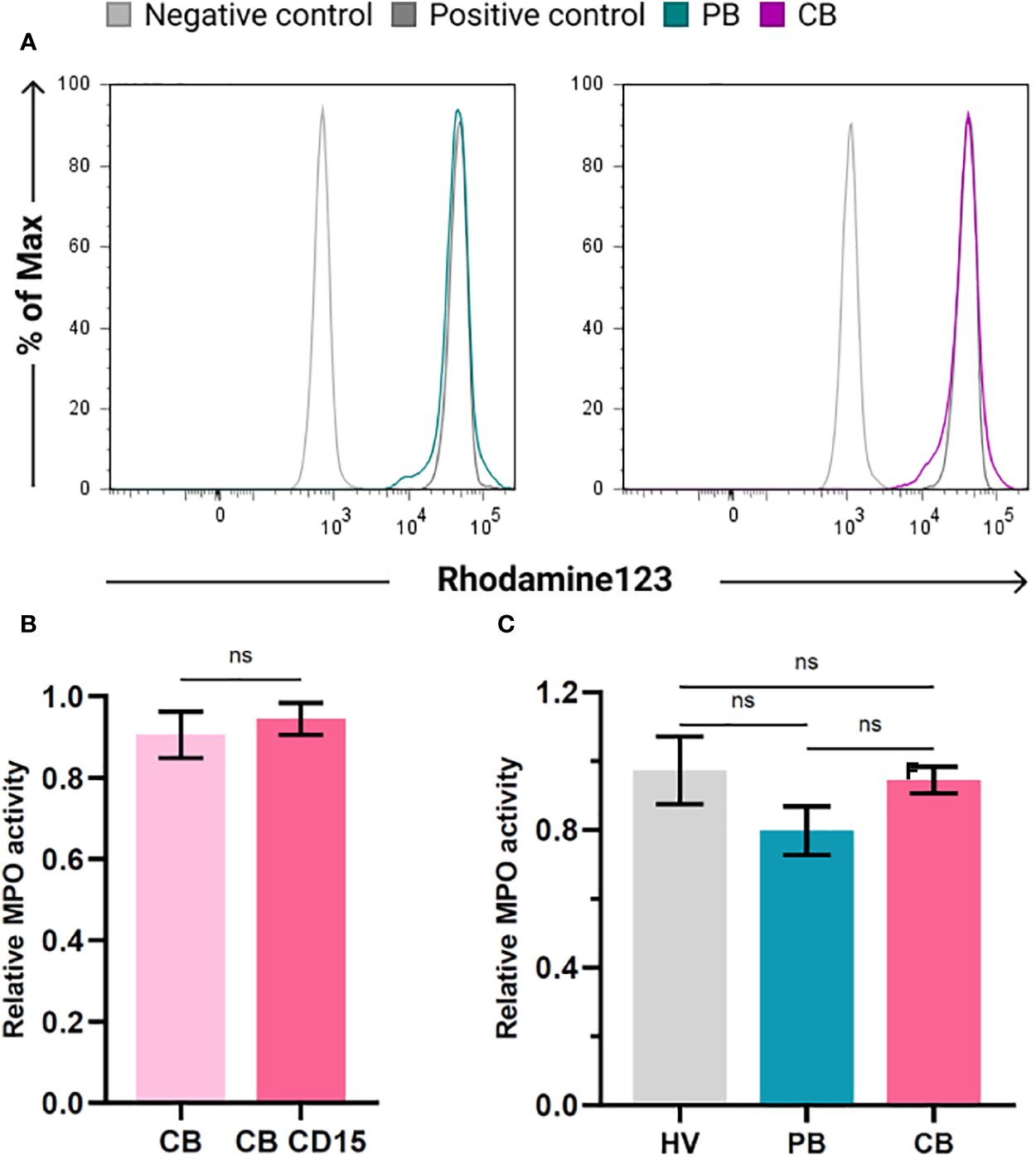
Figure 6 Activation of myeloperoxidase (MPO) in neutrophils of healthy volunteers (HV), peripheral blood (PB) and cord blood (CB). (A) Representative histograms documenting MPO activity in PB and CB using FagoFlowExKit. Oxidation of Dihydrorhodamine123 to fluorescent Rhodamine123 in cells stimulated with E. coli (green (PB) and violet (CB), nonstimulated neutrophils (grey line) and neutrophils stimulated with PMA (dark grey) - positive control. (B) Comparison of MPO activity in whole population of granulocytes and CD15+ granulocytes (C) comparison of relative MPO activity of neutrophils of HV, PB and CB. The data are shown as the mean ± SEM for the whole group. ns p > 0.05, two-tailed unpaired Student’s t test.
4 Discussion
In this study, we analysed the relative proportion of granulocytes to other cell types in cord and peripheral blood. We found that the proportion of granulocytes to other cell types in CB, accounting for about 40% of all cells, was significantly lower compared to that of peripheral blood from mothers and healthy volunteers, where granulocytes account for about 70-80% of all cells. The lower frequency of granulocytes was accompanied by an increase in the absolute number of lymphocytes in CB, possibly accounting for the decreased proportion of granulocytes. Previous publications focusing on the relative representation of immune cell populations in CB described a similar increase in lymphocytes with immature phenotypical properties (57, 58).
Our study confirms prior observation by Weinhage et al. (41) that CB contains a high number of LDN, which they identified as CD15+CD16lowCD64high neutrophils with an immature phenotype. The origin of these immature cells is unclear since it was previously reported that CD16lowCD62L+ LDN can be induced upon inflammatory stimulus (43) and during long-term chronic inflammation (25). The presence of inflammation during term labour (59, 60) may drive the expansion of the observed immature neutrophil populations in neonatal immune system.
Cord blood neutrophils have been previously reported to display impaired ability to release NETs (41) with CB CD15+CD16lowCD64high LDN exerting lower phagocytic capacity. In our results, the activation of CB neutrophils by E. coli was similar to neutrophils in PB and HV, indicating that CB neutrophils have lower phagocytic capacity but are still fully capable of activation and bacteria clearance. Previous studies have reported inconsistency in the impairment of neutrophil function in cord blood, with most studies pointing to greater impairment in neutrophils in preterm newborns (7). It has been suggested that neutrophils of term children do not display impaired intracellular functions, including generation of ROS, as opposed to children born preterm or with sepsis (61). However, they are unable to properly generate NETs (62). These findings suggest that neutrophils in term CB have an impaired ability to eliminate bacteria by NETosis but can efficiently phagocytize and kill infectious agents intracellularly.
It is important to mention that cord blood neutrophils can be influenced by the mother’s treatment before and during birth, e.g., by the application of anaesthetics (7, 63). While detailed studies on this theme are lacking, it would be interesting to elucidate whether the treatment of the mother has an impact on the composition and function of neutrophil populations. The myeloperoxidase activation assay used in this study is advantageous compared to previous studies (63, 64) as it does not require neutrophil isolation prior to the analysis, decreasing the risk of inadvertent neutrophil activation and loss of their ability to respond to stimuli.
We present a novel observation that CB neutrophils express higher levels of genes associated with antimicrobial function. This finding is consistent with previous studies reporting increased expression of MPO, ELANE, DEFA4, and MMP8 genes in LDN isolated from SLE patients (32). The presented results suggest that LDN might be responsible for the observed increase in gene expression. We have to consider that MPO and other antimicrobial peptides tested in our study could be released during degranulation of LDN as described by Ssemaganda et al. (40). Possibly, elevated gene expression of antimicrobial peptides and MPO is a compensatory mechanism trying to renew the levels of these proteins. Mathias et al. (65) conducted a genome-wide expression profile of CB neutrophils from term neonates and found that unstimulated CB neutrophils produce higher levels of inflammatory cytokines such as IFN-γ, IL-1β, and TNF-α. Further, they showed that LPS treatment has a reduced capacity to upregulate genes linked to neutrophil activation, phagocytosis, and chemotaxis compared to adult peripheral blood neutrophils (65). This might suggest that CB neutrophils exert lower response to infectious agents to prevent harmful overt activation in the early stages after birth. This effect may be dependent on neutrophil composition since the population frequencies are different at birth (66). Neutrophil numbers normalize to adult levels by about four weeks of age (67).
Jimenez et al. (35) discovered that the accumulation of C-reactive protein (CRP) triggers the release of MDSC from mouse bone marrow, leading to an increase in ROS production and suppression of T cell proliferation. Similar effect was also seen in isolated human neutrophils. While CRP is commonly used as a biomarker for acute inflammation, it is not as sensitive as neutrophil CD64 expression when diagnosing neonatal sepsis (68). Injarabian et al. (69) addressed the effect of metabolic pathways and microenvironment on neutrophil differentiation and phenotype, with the metabolic shift during inflammation potentially leading to the formation of LDN. Our study found that CD15+CD16lowCD64high and CD15+CD16lowCD62L+ neutrophils were enriched in the low-density compartment of CBMC. The high expression of CD64 on immature CD16low neutrophil subpopulation in CB and their further recruitment during infection could contribute to the high sepsis rates observed in neonates.
Gene expression analysis revealed that CB neutrophils displayed increased expression of genes related to antimicrobial functions compared to maternal PB and PB of healthy volunteers. Maternal PB neutrophils exhibited slightly higher expression of several genes, potentially facilitating neutrophils’ role in dissolving fetal membranes via the production of MMPs during labour (70). Both CB and maternal PB neutrophils displayed elevated levels of the Arg1 gene, which is, along with IL-10 and PD-L1, associated with suppressive function of PMN-MDSC (71, 72). PD-L1 expression was found to be associated with immune suppressive mechanisms in pregnancy, and its upregulation was observed in maternal PB neutrophils, suggesting regulatory mechanisms that differ from those in CB. The mode of delivery was found to affect neutrophil gene expression, with children delivered by CS showing increased expression of genes related to antimicrobial response, while children delivered by VD displayed increased expression of suppressive molecules.
5 Conclusions
Our study highlights a significant aspect of the complex neonatal immune system, particularly the role of umbilical cord blood neutrophils. It confirms the presence of CD16low neutrophil subpopulation in cord blood, exhibiting an immature phenotype. CD16low neutrophil subpopulation plays a crucial role in the heightened expression of genes associated with antimicrobial responses, including myeloperoxidase, defensins, and neutrophil elastase. Interestingly, cord blood neutrophils demonstrate proper activation and oxidative burst when challenged with an inflammatory stimulus compared to the peripheral blood neutrophils of adults.
Importantly, our findings suggest that the mode of delivery can influence neonatal immune properties. Although we recognize the limitations of our study, we hope that the results presented here will provide valuable insights into the immune landscape of newborns, emphasizing the importance of distinct neutrophil subpopulations in neonatal immunity and their potential impact on early postnatal health. Further research into these immune mechanisms may help develop effective neonatal care and infection prevention strategies.
Data availability statement
The original contributions presented in the study are included in the article/Supplementary Materials, further inquiries can be directed to the corresponding author/s.
Ethics statement
The studies involving humans were approved by Ethical Committee of Institute for the Care of Mother and Child. The studies were conducted in accordance with the local legislation and institutional requirements. Written informed consent for participation in this study was provided by the participants’ legal guardians/next of kin.
Author contributions
EM: Methodology, Investigation, Formal analysis, Writing – review & editing, Writing – original draft, Conceptualization. VČ: Writing – review & editing, Methodology, Investigation. ON: Methodology, Investigation, Writing – review & editing. PP: Writing – review & editing, Methodology, Investigation. KB: Project administration, Writing – review & editing, Investigation. ZH: Supervision, Funding acquisition, Writing – review & editing, Writing – original draft. JH: Resources, Conceptualization, Writing – review & editing, Writing – original draft, Supervision, Funding acquisition.
Funding
The author(s) declare financial support was received for the research, authorship, and/or publication of this article. This research was funded by Czech Health Research Council NV15-26877A, NV23-05-00462 and Charles University research program Cooperatio IMMU207032. ZH was supported by R01HL129878, R01DK108353, and R01DK135413.
Acknowledgments
We would like to thank the women who generously provided their peripheral and cord blood samples, as well as the healthy women volunteers who dedicated their time and cooperation.
Conflict of interest
The authors declare that the research was conducted in the absence of any commercial or financial relationships that could be construed as a potential conflict of interest.
Publisher’s note
All claims expressed in this article are solely those of the authors and do not necessarily represent those of their affiliated organizations, or those of the publisher, the editors and the reviewers. Any product that may be evaluated in this article, or claim that may be made by its manufacturer, is not guaranteed or endorsed by the publisher.
Supplementary material
The Supplementary Material for this article can be found online at: https://www.frontiersin.org/articles/10.3389/fimmu.2024.1368624/full#supplementary-material
References
1. PrabhuDas M, Adkins B, Gans H, King C, Levy O, Ramilo O, et al. Challenges in infant immunity: implications for responses to infection and vaccines. Nat Immunol. (2011) 12:189–94. doi: 10.1038/ni0311-189
2. Lukacs SL, Schrag SJ. Clinical sepsis in neonates and young infants, United States, 1988-2006. J Pediatr. (2012) 160:960–5. doi: 10.1016/j.jpeds.2011.12.023
3. Darabi B, Rahmati S, HafeziAhmadi MR, Badfar G, Azami M. The association between caesarean section and childhood asthma: an updated systematic review and meta-analysis. Allergy Asthma Clin Immunol Off J Can Soc Allergy Clin Immunol. (2019) 15:62. doi: 10.1186/s13223-019-0367-9
4. Bager P, Simonsen J, Nielsen NM, Frisch M. Cesarean section and offspring’s risk of inflammatory bowel disease: a national cohort study. Inflammation Bowel Dis. (2012) 18:857–62. doi: 10.1002/ibd.21805
5. Andersen V, Möller S, Jensen PB, Møller FT, Green A. Caesarean delivery and risk of chronic inflammatory diseases (Inflammatory bowel disease, rheumatoid arthritis, coeliac disease, and diabetes mellitus): A population based registry study of 2,699,479 births in Denmark during 1973-2016. Clin Epidemiol. (2020) 12:287–93. doi: 10.2147/CLEP
6. Sundqvist M, Osla V, Jacobsson B, Rudin A, Sävman K, Karlsson A. Cord blood neutrophils display a galectin-3 responsive phenotype accentuated by vaginal delivery. BMC Pediatr. (2013) 13:128. doi: 10.1186/1471-2431-13-128
7. Birle A, Nebe CT, Hill S, Hartmann K, Poeschl J, Koch L. Neutrophil chemotaxis in cord blood of term and preterm neonates is reduced in preterm neonates and influenced by the mode of delivery and anaesthesia. PloS One. (2015) 10:e0120341. doi: 10.1371/journal.pone.0120341
8. Pillay J, den Braber I, Vrisekoop N, Kwast LM, de Boer RJ, Borghans JAM, et al. In vivo labeling with 2H2O reveals a human neutrophil lifespan of 5.4 days. Blood. (2010) 116:625–7. doi: 10.1182/blood-2010-01-259028
9. Tofts PS, Chevassut T, Cutajar M, Dowell NG, Peters AM. Doubts concerning the recently reported human neutrophil lifespan of 5.4 days. Blood. (2011) 117:6050–2; author reply 6053-6054. doi: 10.1182/blood-2010-10-310532
10. Koenderman L, Tesselaar K, Vrisekoop N. Human neutrophil kinetics: a call to revisit old evidence. Trends Immunol. (2022) 43:868–76. doi: 10.1016/j.it.2022.09.008
11. van Gisbergen KPJM, Sanchez-Hernandez M, Geijtenbeek TBH, van Kooyk Y. Neutrophils mediate immune modulation of dendritic cells through glycosylation-dependent interactions between Mac-1 and DC-SIGN. J Exp Med. (2005) 201:1281–92. doi: 10.1084/jem.20041276
12. Hufford MM, Richardson G, Zhou H, Manicassamy B, García-Sastre A, Enelow RI, et al. Influenza-infected neutrophils within the infected lungs act as antigen presenting cells for anti-viral CD8(+) T cells. PloS One. (2012) 7:e46581. doi: 10.1371/journal.pone.0046581
13. Meinderts SM, Baker G, van Wijk S, Beuger BM, Geissler J, Jansen MH, et al. Neutrophils acquire antigen-presenting cell features after phagocytosis of IgG-opsonized erythrocytes. Blood Adv. (2019) 3:1761–73. doi: 10.1182/bloodadvances.2018028753
14. Bennouna S, Bliss SK, Curiel TJ, Denkers EY. Cross-talk in the innate immune system: neutrophils instruct recruitment and activation of dendritic cells during microbial infection. J Immunol Baltim Md. (2003) 171:6052–8. doi: 10.4049/jimmunol.171.11.6052
15. Tecchio C, Micheletti A, Cassatella MA. Neutrophil-derived cytokines: facts beyond expression. Front Immunol. (2014) 5:508. doi: 10.3389/fimmu.2014.00508
16. Silvestre-Roig C, Fridlender ZG, Glogauer M, Scapini P. Neutrophil diversity in health and disease. Trends Immunol. (2019) 40:565–83. doi: 10.1016/j.it.2019.04.012
17. Montaldo E, Lusito E, Bianchessi V, Caronni N, Scala S, Basso-Ricci L, et al. Cellular and transcriptional dynamics of human neutrophils at steady state and upon stress. Nat Immunol. (2022) 23:1470–83. doi: 10.1038/s41590-022-01311-1
18. McLaren AS, Fetit R, Wood CS, Falconer J, Steele CW. Single cell sequencing of neutrophils demonstrates phenotypic heterogeneity and functional plasticity in health, disease, and cancer. Chin Clin Oncol. (2023) 12:18. doi: 10.21037/cco
19. Xie X, Shi Q, Wu P, Zhang X, Kambara H, Su J, et al. Single-cell transcriptome profiling reveals neutrophil heterogeneity in homeostasis and infection. Nat Immunol. (2020) 21:1119–33. doi: 10.1038/s41590-020-0736-z
20. Hacbarth E, Kajdacsy-Balla A. Low density neutrophils in patients with systemic lupus erythematosus, rheumatoid arthritis, and acute rheumatic fever. Arthritis Rheumatol. (1986) 29:1334–42. doi: 10.1002/art.1780291105
21. Hsu BE, Tabariès S, Johnson RM, Andrzejewski S, Senecal J, Lehuédé C, et al. Immature low-density neutrophils exhibit metabolic flexibility that facilitates breast cancer liver metastasis. Cell Rep. (2019) 27:3902–3915.e6. doi: 10.1016/j.celrep.2019.05.091
22. Yiu JYT, Hally KE, Larsen PD, Holley AS. Increased levels of low density neutrophils (LDNs) in myocardial infarction. Acta Cardiol. (2023) 78:47–54. doi: 10.1080/00015385.2021.2015145
23. Tay SH, Celhar T, Fairhurst AM. Low-density neutrophils in systemic lupus erythematosus. Arthritis Rheumatol Hoboken NJ. (2020) 72:1587–95. doi: 10.1002/art.41395
24. Morrissey SM, Geller AE, Hu X, Tieri D, Ding C, Klaes CK, et al. A specific low-density neutrophil population correlates with hypercoagulation and disease severity in hospitalized COVID-19 patients. JCI Insight. (2021) 6:e148435. doi: 10.1172/jci.insight.148435
25. Hassani M, Hellebrekers P, Chen N, van Aalst C, Bongers S, Hietbrink F, et al. On the origin of low-density neutrophils. J Leukoc Biol. (2020) 107:809–18. doi: 10.1002/JLB.5HR0120-459R
26. Sagiv JY, Michaeli J, Assi S, Mishalian I, Kisos H, Levy L, et al. Phenotypic diversity and plasticity in circulating neutrophil subpopulations in cancer. Cell Rep. (2015) 10:562–73. doi: 10.1016/j.celrep.2014.12.039
27. Köstlin N, Kugel H, Spring B, Leiber A, Marmé A, Henes M, et al. Granulocytic myeloid derived suppressor cells expand in human pregnancy and modulate T-cell responses. Eur J Immunol. (2014) 44:2582–91. doi: 10.1002/eji.201344200
28. Nair RR, Sinha P, Khanna A, Singh K. Reduced myeloid-derived suppressor cells in the blood and endometrium is associated with early miscarriage. Am J Reprod Immunol N Y N. (2015) 73:479–86. doi: 10.1111/aji.2015.73.issue-6
29. Ren J, Zeng W, Tian F, Zhang S, Wu F, Qin X, et al. Myeloid-derived suppressor cells depletion may cause pregnancy loss via upregulating the cytotoxicity of decidual natural killer cells. Am J Reprod Immunol N Y N. (2019) 81:e13099. doi: 10.1111/aji.13099
30. Rahman S, Sagar D, Hanna RN, Lightfoot YL, Mistry P, Smith CK, et al. Low-density granulocytes activate T cells and demonstrate a non-suppressive role in systemic lupus erythematosus. Ann Rheum Dis. (2019) 78:957–66. doi: 10.1136/annrheumdis-2018-214620
31. Wang X, Qiu L, Li Z, Wang XY, Yi H. Understanding the multifaceted role of neutrophils in cancer and autoimmune diseases. Front Immunol. (2018) 9:2456. doi: 10.3389/fimmu.2018.02456
32. Villanueva E, Yalavarthi S, Berthier CC, Hodgin JB, Khandpur R, Lin AM, et al. Netting neutrophils induce endothelial damage, infiltrate tissues, and expose immunostimulatory molecules in systemic lupus erythematosus. J Immunol Baltim Md. (2011) 187:538–52. doi: 10.4049/jimmunol.1100450
33. Matthews NC, Burton CS, Alfred A. Low-density neutrophils in chronic graft versus host disease (cGVHD) are primarily immature CD10- and enhance T cell activation. Clin Exp Immunol. (2021) 205:257–73. doi: 10.1111/cei.13612
34. Vlkova M, Chovancova Z, Nechvatalova J, Connelly AN, Davis MD, Slanina P, et al. Neutrophil and granulocytic myeloid-derived suppressor cell-mediated T cell suppression significantly contributes to immune dysregulation in common variable immunodeficiency disorders. J Immunol Baltim Md. (2019) 202:93–104. doi: 10.4049/jimmunol.1800102
35. Jimenez RV, Kuznetsova V, Connelly AN, Hel Z, Szalai AJ. C-reactive protein promotes the expansion of myeloid derived cells with suppressor functions. Front Immunol. (2019) 10:2183. doi: 10.3389/fimmu.2019.02183
36. Condamine T, Dominguez GA, Youn JI, Kossenkov AV, Mony S, Alicea-Torres K, et al. Lectin-type oxidized LDL receptor-1 distinguishes population of human polymorphonuclear myeloid-derived suppressor cells in cancer patients. Sci Immunol. (2016) 1:aaf8943. doi: 10.1126/sciimmunol.aaf8943
37. Veglia F, Sanseviero E, Gabrilovich DI. Myeloid-derived suppressor cells in the era of increasing myeloid cell diversity. Nat Rev Immunol. (2021) 21:485–98. doi: 10.1038/s41577-020-00490-y
38. Blanco-Camarillo C, Alemán OR, Rosales C. Low-density neutrophils in healthy individuals display a mature primed phenotype. Front Immunol. (2021) 12:672520. doi: 10.3389/fimmu.2021.672520
39. McLeish KR, Shrestha R, Vashishta A, Rane MJ, Barati MT, Brier ME, et al. Differential functional responses of neutrophil subsets in severe COVID-19 patients. Front Immunol. (2022) 13:879686. doi: 10.3389/fimmu.2022.879686
40. Ssemaganda A, Kindinger L, Bergin P, Nielsen L, Mpendo J, Ssetaala A, et al. Characterization of neutrophil subsets in healthy human pregnancies. PloS One. (2014) 9:e85696. doi: 10.1371/journal.pone.0085696
41. Weinhage T, Kölsche T, Rieger-Fackeldey E, Schmitz R, Antoni AC, Ahlmann M, et al. Cord blood low-density granulocytes correspond to an immature granulocytic subset with low expression of S100A12. J Immunol Baltim Md. (2020) 205:56–66. doi: 10.4049/jimmunol.1901308
42. Livak KJ, Schmittgen TD. Analysis of relative gene expression data using real-time quantitative PCR and the 2(-Delta Delta C(T)) Method. Methods San Diego Calif. (2001) 25:402–8. doi: 10.1006/meth.2001.1262
43. Pillay J, Tak T, Kamp VM, Koenderman L. Immune suppression by neutrophils and granulocytic myeloid-derived suppressor cells: similarities and differences. Cell Mol Life Sci CMLS. (2013) 70:3813–27. doi: 10.1007/s00018-013-1286-4
44. Gustafson MP, Lin Y, Maas ML, Van Keulen VP, Johnston PB, Peikert T, et al. A method for identification and analysis of non-overlapping myeloid immunophenotypes in humans. PloS One. (2015) 10:e0121546. doi: 10.1371/journal.pone.0121546
45. Merah-Mourah F, Cohen SO, Charron D, Mooney N, Haziot A. Identification of novel human monocyte subsets and evidence for phenotypic groups defined by interindividual variations of expression of adhesion molecules. Sci Rep. (2020) 10:4397. doi: 10.1038/s41598-020-61022-1
46. Son GH, Choi SY, Ju YJ, Lee KY, Lee JJ, Song JE, et al. Whole blood RNA sequencing reveals a differential transcriptomic profile associated with cervical insufficiency: a pilot study. Reprod Biol Endocrinol RBE. (2021) 19:32. doi: 10.1186/s12958-021-00715-2
47. Lucovnik M, Kornhauser-Cerar L, Premru-Srsen T, Gmeiner-Stopar T, Derganc M. Neutrophil defensins but not interleukin-6 in vaginal fluid after preterm premature rupture of membranes predict fetal/neonatal inflammation and infant neurological impairment. Acta Obstet Gynecol Scand. (2011) 90:908–16. doi: 10.1111/j.1600-0412.2011.01177.x
48. Kalva-Borato DC, Ribas JT, Parabocz GC, Borba LM, Maciel MAS, Santos FAD, et al. Biomarkers in non-complicated pregnancy: insights about serum myeloperoxidase and ultrasensitive C-reactive protein. Exp Clin Endocrinol Diabetes Off J Ger Soc Endocrinol Ger Diabetes Assoc. (2019) 127:585–9. doi: 10.1055/a-0777-2090
49. Li W, Mata KM, Mazzuca MQ, Khalil RA. Altered matrix metalloproteinase-2 and -9 expression/activity links placental ischemia and anti-angiogenic sFlt-1 to uteroplacental and vascular remodeling and collagen deposition in hypertensive pregnancy. Biochem Pharmacol. (2014) 89:370–85. doi: 10.1016/j.bcp.2014.03.017
50. Tirone C, Boccacci S, Inzitari R, Tana M, Aurilia C, Fanali C, et al. Correlation of levels of α–defensins determined by HPLC-ESI-MS in bronchoalveolar lavage fluid with the diagnosis of pneumonia in premature neonates. Pediatr Res. (2010) 68:140–4. doi: 10.1203/PDR.0b013e3181e5c242
51. Islam MM, Takeyama N. Role of neutrophil extracellular traps in health and disease pathophysiology: recent insights and advances. Int J Mol Sci. (2023) 24:15805. doi: 10.3390/ijms242115805
52. Rosa L, Cutone A, Lepanto MS, Paesano R, Valenti P. Lactoferrin: A natural glycoprotein involved in iron and inflammatory homeostasis. Int J Mol Sci. (2017) 18:1985. doi: 10.3390/ijms18091985
53. Wang JF, Wang YP, Xie J, Zhao ZZ, Gupta S, Guo Y, et al. Upregulated PD-L1 delays human neutrophil apoptosis and promotes lung injury in an experimental mouse model of sepsis. Blood. (2021) 138:806–10. doi: 10.1182/blood.2020009417
54. Fallon EA, Biron-Girard BM, Chung CS, Lomas-Neira J, Heffernan DS, Monaghan SF, et al. A novel role for coinhibitory receptors/checkpoint proteins in the immunopathology of sepsis. J Leukoc Biol. (2018) 103:1151–64. doi: 10.1002/JLB.2MIR0917-377R
55. Súkeníková L, Černý V, Novotná O, Petrásková P, Boráková K, Kolářová L, et al. Different capacity of in vitro generated myeloid dendritic cells of newborns of healthy and allergic mothers to respond to probiotic strain E. coli O83:K24:H31. Immunol Lett. (2017) 189:82–9. doi: 10.1016/j.imlet.2017.05.013
56. Hrdý J, Novotná O, Kocourková I, Prokešová L. Gene expression of subunits of the IL-12 family cytokines in moDCs derived in vitro from the cord blood of children of healthy and allergic mothers. Folia Biol (Praha). (2014) 60:74–82.
57. D’Arena G, Musto P, Cascavilla N, Di Giorgio G, Fusilli S, Zendoli F, et al. Flow cytometric characterization of human umbilical cord blood lymphocytes: immunophenotypic features. Haematologica. (1998) 83:197–203.
58. Chirumbolo S, Ortolani R, Veneri D, Raffaelli R, Peroni D, Pigozzi R, et al. Lymphocyte phenotypic subsets in umbilical cord blood compared to peripheral blood from related mothers. Cytometry B Clin Cytom. (2011) 80:248–53. doi: 10.1002/cyto.b.20588
59. Romero R, Espinoza J, Gonçalves LF, Kusanovic JP, Friel LA, Nien JK. Inflammation in preterm and term labour and delivery. Semin Fetal Neonatal Med. (2006) 11:317–26. doi: 10.1016/j.siny.2006.05.001
60. Gomez-Lopez N, StLouis D, Lehr MA, Sanchez-Rodriguez EN, Arenas-Hernandez M. Immune cells in term and preterm labor. Cell Mol Immunol. (2014) 11:571–81. doi: 10.1038/cmi.2014.46
61. Shigeoka AO, Santos JI, Hill HR. Functional analysis of neutrophil granulocytes from healthy, infected, and stressed neonates. J Pediatr. (1979) 95:454–60. doi: 10.1016/S0022-3476(79)80535-1
62. Yost CC, Cody MJ, Harris ES, Thornton NL, McInturff AM, Martinez ML, et al. Impaired neutrophil extracellular trap (NET) formation: a novel innate immune deficiency of human neonates. Blood. (2009) 113:6419–27. doi: 10.1182/blood-2008-07-171629
63. Billert H, Czerniak K, Bednarek E, Kulińska K. Effects of local anesthetics on the respiratory burst of cord blood neutrophils in vitro. Pediatr Res. (2016) 80:258–66. doi: 10.1038/pr.2016.68
64. Makoni M, Eckert J, Anne Pereira H, Nizet V, Lawrence SM. Alterations in neonatal neutrophil function attributable to increased immature forms. Early Hum Dev. (2016) 103:1–7. doi: 10.1016/j.earlhumdev.2016.05.016
65. Mathias B, Mira JC, Rehfuss JP, Rincon JC, Ungaro R, Nacionales DC, et al. LPS stimulation of cord blood reveals a newborn-specific neutrophil transcriptomic response and cytokine production. Shock Augusta Ga. (2017) 47:606–14. doi: 10.1097/SHK.0000000000000800
66. Prabhu SB, Rathore DK, Nair D, Chaudhary A, Raza S, Kanodia P, et al. Comparison of human neonatal and adult blood leukocyte subset composition phenotypes. PloS One. (2016) 11:e0162242. doi: 10.1371/journal.pone.0162242
67. Lawrence SM, Corriden R, Nizet V. Age-appropriate functions and dysfunctions of the neonatal neutrophil. Front Pediatr. (2017) 5:23. doi: 10.3389/fped.2017.00023
68. Hashem HE, Abdel Halim RM, El Masry SA, Mokhtar AM, Abdelaal NM. The utility of neutrophil CD64 and presepsin as diagnostic, prognostic, and monitoring biomarkers in neonatal sepsis. Int J Microbiol. (2020) 2020:8814892. doi: 10.1155/2020/8814892
69. Injarabian L, Devin A, Ransac S, Marteyn BS. Neutrophil Metabolic Shift during their Lifecycle: Impact on their Survival and Activation. Int J Mol Sci. (2019) 21:287. doi: 10.3390/ijms21010287
70. Chen J, Khalil RA. Matrix metalloproteinases in normal pregnancy and preeclampsia. Prog Mol Biol Transl Sci. (2017) 148:87–165. doi: 10.1016/bs.pmbts.2017.04.001
71. Bowers NL, Helton ES, Huijbregts RPH, Goepfert PA, Heath SL, Hel Z. Immune suppression by neutrophils in HIV-1 infection: role of PD-L1/PD-1 pathway. PloS Pathog. (2014) 10:e1003993. doi: 10.1371/journal.ppat.1003993
Keywords: neutrophils, cord blood, myeloperoxidase, defensins, oxidative burst
Citation: Miková E, Černý V, Novotná O, Petrásková P, Boráková K, Hel Z and Hrdý J (2024) Immature neutrophils in cord blood exert increased expression of genes associated with antimicrobial function. Front. Immunol. 15:1368624. doi: 10.3389/fimmu.2024.1368624
Received: 10 January 2024; Accepted: 15 March 2024;
Published: 26 March 2024.
Edited by:
Aleksander M. Grabiec, Jagiellonian University, PolandReviewed by:
Sumanta Kumar Naik, Washington University in St. Louis, United StatesIsabella Forasteiro Tavares, Oswaldo Cruz Foundation (FIOCRUZ), Brazil
Copyright © 2024 Miková, Černý, Novotná, Petrásková, Boráková, Hel and Hrdý. This is an open-access article distributed under the terms of the Creative Commons Attribution License (CC BY). The use, distribution or reproduction in other forums is permitted, provided the original author(s) and the copyright owner(s) are credited and that the original publication in this journal is cited, in accordance with accepted academic practice. No use, distribution or reproduction is permitted which does not comply with these terms.
*Correspondence: Jiří Hrdý, amlyaS5ocmR5QGxmMS5jdW5pLmN6