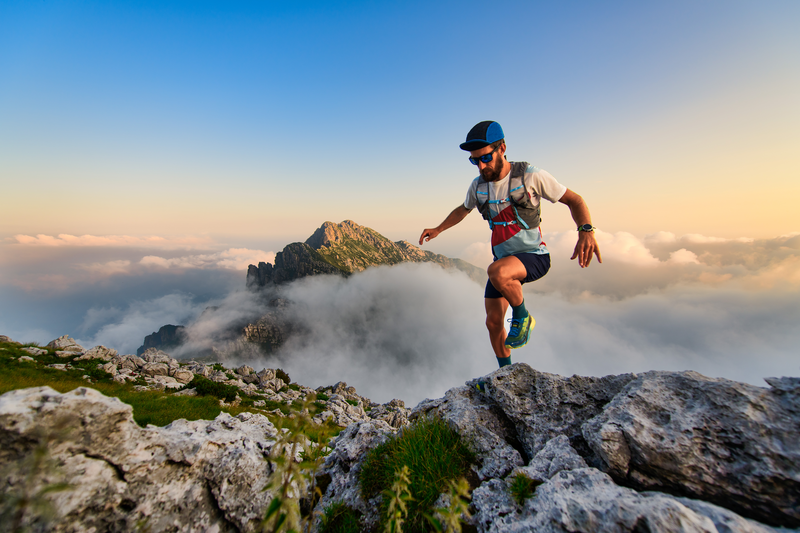
95% of researchers rate our articles as excellent or good
Learn more about the work of our research integrity team to safeguard the quality of each article we publish.
Find out more
ORIGINAL RESEARCH article
Front. Immunol. , 14 March 2024
Sec. Inflammation
Volume 15 - 2024 | https://doi.org/10.3389/fimmu.2024.1368448
This article is part of the Research Topic Community Series in Translational Insights into Mechanisms and Therapy of Organ Dysfunction in Sepsis and Trauma, volume III View all 20 articles
The pathogenic mechanisms of bacterial infections and resultant sepsis are partly attributed to dysregulated inflammatory responses sustained by some late-acting mediators including the procathepsin-L (pCTS-L). It was entirely unknown whether any compounds of the U.S. Drug Collection could suppress pCTS-L-induced inflammation, and pharmacologically be exploited into possible therapies. Here, we demonstrated that a macrophage cell-based screening of a U.S. Drug Collection of 1360 compounds resulted in the identification of progesterone (PRO) as an inhibitor of pCTS-L-mediated production of several chemokines [e.g., Epithelial Neutrophil-Activating Peptide (ENA-78), Monocyte Chemoattractant Protein-1 (MCP-1) or MCP-3] and cytokines [e.g., Interleukin-10 (IL-10) or Tumor Necrosis Factor (TNF)] in primary human peripheral blood mononuclear cells (PBMCs). In vivo, these PRO-entrapping 2,6-dimethal-β-cyclodextrin (DM-β-CD) nanoparticles (containing 1.35 mg/kg PRO and 14.65 mg/kg DM-β-CD) significantly increased animal survival in both male (from 30% to 70%, n = 20, P = 0.041) and female (from 50% to 80%, n = 30, P = 0.026) mice even when they were initially administered at 24 h post the onset of sepsis. This protective effect was associated with a reduction of sepsis-triggered accumulation of three surrogate biomarkers [e.g., Granulocyte Colony Stimulating Factor (G-CSF) by 40%; Macrophage Inflammatory Protein-2 (MIP-2) by 45%; and Soluble Tumor Necrosis Factor Receptor I (sTNFRI) by 80%]. Surface Plasmon Resonance (SPR) analysis revealed a strong interaction between PRO and pCTS-L (KD = 78.2 ± 33.7 nM), which was paralleled with a positive correlation between serum PRO concentration and serum pCTS-L level (ρ = 0.56, P = 0.0009) or disease severity (Sequential Organ Failure Assessment, SOFA; ρ = 0.64, P = 0.0001) score in septic patients. Our observations support a promising opportunity to explore DM-β-CD nanoparticles entrapping lipophilic drugs as possible therapies for clinical sepsis.
Bacterial infections and associated sepsis are probably the most prominent causes of death in hospitals, accounting for almost 20% of total deaths globally (1). Its pathogenic mechanisms partially attribute to dysregulated inflammatory responses to microbial infections that are initiated by early cytokines [e.g., interleukin-1 (IL-1) and tumor necrosis factor (TNF)] but sustained by late-acting mediators including high mobility group box 1 (HMGB1) (2, 3) and procathepsin-L (pCTS-L) (4, 5). For example, upon initial innate recognition of many “pathogen-associated molecular patterns molecules” (PAMPs, e.g., bacterial lipopolysaccharide, LPS) by corresponding pattern recognition receptors (PRRs) such as the toll-like receptor 4 (TLR4) (6), monocytes and macrophages sequentially produce “early” cytokines [e.g., TNF and interleukin-1β (IL-1β)] (7–9), toxic chemicals (e.g., lactate) (10–12), and late-acting mediators such as HMGB1 (2) and pCTS-L (4). In comparison with early cytokines, these late-acting mediators can be therapeutically targeted in delayed regimens (4, 13), thereby offering relatively wider therapeutic windows (5). It is therefore necessary to find small molecule drugs capable of suppressing pCTS-L-mediated dysregulated inflammation to develop potential therapeutic strategies for inflammatory diseases.
The US Drug Collection contains 1360 FDA-approved small molecule drugs that have reached clinical trials as evidenced by their assignment of the United States Adopted Names (USAN) and inclusion in the United States Pharmacopeia (USP) Dictionary, the authorized list of established names for drugs in the United States. These small molecule drugs can interact with specific protein targets in the body to confer a therapeutic effect, but possess distinct advantages such as oral administration convenience, target specificity, cell penetration, and cost-effectiveness. They serve as a basis for high throughput screening of established drugs for potential new activities (14). In this study, we developed a 96-well-based assay to screen for small molecule drugs that could suppress the pCTS-L-mediated dysregulated inflammation and confer protection against experimental sepsis. Here, we presented substantial evidence to suggest: i) a hormone, progesterone (PRO), as an inhibitor of pCTS-L-mediated dysregulated inflammation; and ii) a PRO-entrapping 2,6-dimethyl-β-cyclodextrin (DM-β-CD) nanoparticles as a potential therapy in a preclinical setting.
Murine macrophage RAW 264.7 cell line was purchased from the American Type Culture Collection (ATCC). Human blood samples were obtained from the New York Blood Center (Long Island City, NY, USA) to harvest primary human peripheral blood mononuclear cells (PBMCs) by density gradient centrifugation as previously described (4, 15, 16). Both macrophage cultures and PBMCs were routinely incubated in DMEM media containing 1% streptomycin/penicillin and 10% fecal bovine serum or 10% human serum. When cell densities reached 80-90% confluence, adherent macrophages or PBMCs were stimulated with bacterial endotoxins (lipopolysaccharides, LPS, E. coli 0111:B4, #L4130, Sigma-Aldrich) or recombinant human or murine pCTS-L protein in the absence or in the presence of each of the 1360 compounds in the U.S. Collection of Drug (10 mM in DMSO), as well as progesterone (Cat. # P0130, Sigma-Aldrich) solubilized in ethanol (5 mg/ml) or entrapped into 2,6-dimethyl-β-cyclodextrin (DM-β-CD, Cat. #H0513, Sigma-Aldrich) nanoparticles. The progesterone-carrying DM-β-CD nanoparticles (containing 84.4 mg progesterone per 1000 mg of the PRO/DM-β-CD complex) were also purchased from Sigma-Aldrich (Cat. # P7556). The extracellular levels of various cytokines and chemokines were respectively measured by using ELISA kits or Cytokine Antibody Arrays as previously described (4, 15, 16).
Recombinant murine and human pCTS-L proteins containing an N-terminal 6×Histidine tag were respectively expressed in E. coli BL21 (DE3) pLysS cells and purified to homogeneity as previously described (4, 16). Briefly, upon sonication to disrupt the bacterial cell wall, the inclusion bodies containing pCTS-L proteins were harvested via differential centrifugation technique following sequential washings in 1% Triton X-100 dissolved in 1 × PBS buffer The purified inclusion bodies were subsequently dissolved in high concentration of urea solution (8.0 M), and then refolded by dialysis in Tris buffer (10 mM, pH 8.0). Afterward, recombinant pCTS-L proteins were purified by histidine-affinity chromatography technique and Triton X-114 extractions. The endotoxin content of recombinant pCTS-L proteins was estimated to be < 0.01 U per µg of pCTS-L protein.
We obtained the U.S. Collection of 1360 drugs (Supplementary Table 1, 10 mM in DMSO) from the MicroSource Discovery System Inc., and used this chemical library to search for potential pCTS-L inhibitors as previously described (16). Briefly, murine macrophage-like RAW 264.7 cells were challenged with murine pCTS-L protein in the absence or in the presence of each drug at several concentrations for 16 h, and levels of TNF in macrophage-conditioned medium was measured using specific TNF DuoSet ELISA kit (Cat# DY410, R&D Systems).
Murine Cytokine Antibody Array Kits (Cat.# AAM-CYT-3-8, RayBiotech Inc., Norcross, GA, USA) were employed to measure the relative levels of 62 cytokines/chemokines in macrophage cell culture-conditioned medium or murine serum as previously described (4, 15, 16). Similarly, human Cytokine Antibody C3 Array Kits (Cat.# AAH-CYT-3-8) were employed to measure the relative levels of 42 cytokines/chemokines in human PBMC-conditioned culture medium as previously described (4, 15, 16).
Adult male and female Balb/C mice (7-8 weeks old, 20-25 g body weight) were purchased from Charles River Laboratories (Wilmington, MA), housed in a temperature-controlled room on a 12-h light-dark cycle, and acclimated for at least 5-7 days before usage. Every attempt was made to limit the number of animals used in the present study as per the ARRIVE guidelines for reducing the number of animals in scientific research developed by the British National Centre for the Replacement, Refinement and Reduction of Animals in Research (NC3Rs). Additionally, all experiments were performed in accordance with the International Expert Consensus Initiative for Improvement of Animal Modeling in Sepsis - Minimum Quality Threshold in Pre-Clinical Sepsis Studies (MQTiPSS) (17), which includes practices such as randomization of animals in each experimental group, delayed therapeutic interventions with therapeutic agents (e.g., PRO-entrapping DM-β-CD nanoparticles) (18), establishment of specific criteria for euthanasia of moribund septic animals (e.g., labored breathing, minimized response to human touch, and immobility), as well as the administration of fluid resuscitation and antibiotics (19). This study was administratively approved by the IACUC of the Feinstein Institutes for Medical Research (FIMR, Protocol # 2017-003 Term II; Date of Approval, April 28th, 2020).
Adult male or female Balb/C mice aged 7-8 weeks and weighing 20-25 g underwent a surgical procedure referred to as “cecal ligation and puncture” (CLP) to induce experimental sepsis as previously outlined (4, 15, 16). Briefly, the cecum of Balb/C mice was surgically exposed, ligated approximately 5.0 mm from the cecal tip, and punctured once with a 22-gauge syringe needle. Prior to CLP surgery, all experimental animals received a buprenorphine injection (0.05 mg/kg, s.c.) to alleviate immediate surgical pain, because repetitive use of buprenorphine in the CLP model could paradoxically elevate sepsis surrogate markers and animal lethality (20, 21), leading to unnecessary use of more animals per experimental group. Additionally, a small dose of bupivacaine and lidocaine was locally injected around the incision site immediately after CLP surgery. Approximately 30 min post CLP surgery, all experimental animals were subcutaneously injected with imipenem/cilastatin (0.5 mg/mouse) (Primaxin, Merck & Co., Inc.), followed by resuscitation with sterile saline solution (20 ml/kg). Septic animals were only given a single dose of antibiotics before pharmacological administration of PRO-entrapping DM-β-CD nanoparticles at 24 h post CLP, worrying that subsequent antibiotics treatment may adversely affect the therapeutic efficacy of PRO-entrapping DM-β-CD nanoparticles. Before treatment, animals were randomly assigned to control vehicle and experimental groups, and PRO dissolved in sesame oil (Cat. #S3547, Sigma-Aldrich; 2.0 mg/ml) or water after complexation with DM-β-CD to form nanoparticles (containing 84.4 mg PRO per 1000 mg of PRO/DM-β-CD complex) was intraperitoneally injected to septic mice at various time points post CLP surgery. Animal survival was observed for two weeks to ensure no late death occurred. To elucidate the potential protective mechanisms of PRO-entrapping DM-β-CD nanoparticles, a separate group of Balb/C mice were subjected to CLP, and DM-β-CD vehicle (14.65 mg/kg) or PRO-entrapping DM-β-CD nanoparticles (containing 1.35 mg/kg PRO and 14.65 mg/kg DM-β-CD) were administered at 2 h and 20 h post CLP. At 24 h post CLP, animals were euthanized to collect blood and measure serum levels of various cytokines and chemokines using murine Cytokine Antibody Arrays or markers of tissue injury using specific colorimetric enzymatic assays.
Blood samples were harvested at 24 h post CLP following intraperitoneal administrations of DM-β-CD vehicle (14.65 mg/kg) or PRO-entrapping DM-β-CD nanoparticles (containing 1.35 mg/kg PRO and 14.65 mg/kg DM-β-CD) at 2 h and 20 h post CLP, and centrifuged at 3000 x g for 10 min to collect serum. Serum levels of liver injury markers such as aspartate aminotransferase (AST, Cat. No. 7561), and lactate dehydrogenase (LDH, Cat. No. 7572) were determined using specific colorimetric enzymatic assays (Pointe Scientific, Canton, MI) according to manufacturer’s instructions as previously described (15).
We employed the Nicoya Lifesciences’ gold-nanoparticle-based Open Surface Plasmon Resonance (OpenSPR) technology (Kitchener, ON, Canada) to characterize protein-drug interaction following the manufacturer’s instructions. Briefly, recombinant pCTS-L was immobilized on NTA sensor chip (Cat. # SEN-Au-100-10-NTA) as previously described (4, 22), and DMSO solution of PRO was applied as an analyte at different concentrations. The sensorgrams of the dynamic ligand-analyte interaction were recorded over time to estimate the equilibrium dissociation constant (KD) (Nicoya Lifesciences).
This study was administratively approved by the institutional review board (IRB) of the FIMR (IRB protocol #18-0184) and consented by all patients participants who were diagnosed with sepsis or septic shock based on the Sepsis-3 criteria (23). Small volume of blood samples (5.0 ml) was obtained from eleven septic patients recruited to the Long Island Jewish Medical Center or North Shore University Hospital between 2018-2019 at three time points: Time 0 (within 24 h of the initial diagnosis); Time 24 h (24 h post the initial diagnosis); and Time 72 h (72 h post the initial diagnosis). The demographics of these eleven septic patients have been reported previously (4), but briefly described in the Supplementary Table 2. These clinical samples were assayed for pCTS-L levels using human pCTS-L ELISA kit (Cat.# MBS7254442, MyBioSource.com) with reference to standard cure generated from using recombinant human pCTS-L. In parallel, the serum concentrations of progesterone were measured by using highly sensitive ELISA kit (Cat. # ADI-901-011, ENZO Life Sciences, Inc., Farmingdale, NY, USA).
All data were initially evaluated for normality by the Shapiro-Wilk test before conducting appropriate statistical tests. The Student’s t test was used to compare two independent experimental groups. For comparison among multiple groups with non-normal (skewed) distribution (as assessed by the Shapiro-Wilk test), the statistical difference was evaluated with the non-parametric Kruskal-Wallis ANOVA test followed by the Dunn’s test. The Kaplan-Meier method was employed to compare the differences in mortality rates between two different groups along with the nonparametric log-rank post hoc test. Finally, the Spearman rank correlation coefficient test was used to evaluate associations between two quantitative variables that exhibited non-normal distribution. Statistical significance was defined as a P value less than 0.05.
To explore novel pCTS-L inhibitors, we adapted a macrophage cell-based bioassay that we recently developed (16) to screen a U.S. Collection of 1360 drugs supplied as 10.0 mM Dimethyl Sulfoxide (DMSO) solutions in seventeen 96-well microplates for possible activities to inhibit pCTS-L-stimulated TNF secretion (Figure 1A). We optimized the experimental conditions by respectively titrating the concentration of pCTS-L protein (to 1.0 μg/ml) and the confluence of macrophage cultures (to 80-90%). A complete screening of 1360 drugs of the U.S. Collection resulted in the identification of progesterone (PRO) and three analogs (i.e., dydrogesterone, exemestane, and medroxyprogesterone acetate; Figure 1B) as inhibitors of pCTS-L-stimulated TNF production. When dissolved in DMSO, PRO dose-dependently attenuated pCTS-L-mediated TNF secretion with an estimated IC around 20.0 µM (Figure 1B) without affecting mitochondrial metabolic activity (MTT assay) or cell viability (Trypan blue uptake, Supplementary Figure 1).
Figure 1 High-throughput screening of a U.S. Collection of 1360 Drugs for potential inhibitors of pCTS-L-mediated TNF production. (A) The scheme for the 96-well plate-based high-throughput screening of potential pCTS-L inhibitors was adapted from our recent publication (16). Murine macrophage-like RAW 264.7 cells were cultured until 80-90% confluence and challenged with recombinant murine pCTS-L in the absence or in the presence of each of the 1360 compounds at different concentrations for 16 h. The levels of TNF in the macrophage-conditioned medium were measured by using ELISA. (B) Effects of progesterone and three analogs on pCTS-L-induced TNF secretion by murine macrophage cultures. Note that all four lead compounds dose-dependently inhibited pCTS-L-induced TNF secretion by murine macrophage cultures. *, P < 0.05 versus “- pCTS-L”; #, P < 0.05 versus “+ pCTS-L”, non-parametric Kruskal-Wallis ANOVA test.
To confirm PRO’s pCTS-L-inhibitory activities, we first dissolved it in ethanol before testing its effects on pCTS-L-stimulated production of 42 cytokines and chemokines in primary human PBMCs. In agreement with our earlier report (4), pCTS-L markedly stimulated the secretion of a few chemokines (e.g., ENA-78, GRO, and MCP-1) and cytokines (e.g., TNF, IL-6 and IL-10) (Figure 2). However, the pCTS-L-mediated TNF production was markedly inhibited by the co-administration of PRO at a relatively high dose (40 μM, Figure 2), an almost most effective dose that suppressed pCTS-L-induced TNF secretion in dose-response studies using murine macrophages (Figure 1B) as well as primary PBMCs from several different donors. Likewise, the pCTS-L-triggered secretion of several other cytokines (e.g., IL-10) and chemokines (e.g., ENA-78 and MCP-1) was similarly suppressed by PRO (Figure 2). Consistent with our earlier reports (16, 24), endotoxins effectively stimulated the secretion of several chemokines (MCP-1, ENA-78 and GRO) and cytokines (e.g., TNF, IL-6 and IL-10) in primary human PBMCs (Figure 2). However, PRO was unable to affect LPS-induced production of any cytokines/chemokines (Figure 2) even at the concentration that markedly inhibited pCTS-L-mediated cytokine/chemokine production, suggesting that PRO selectively inhibited pCTS-L-mediated inflammation.
Figure 2 Progesterone selectively suppressed the pCTS-L-mediated productions of several cytokines/chemokines in primary human peripheral blood mononuclear cells (PBMCs). Human PBMCs were challenged with human pCTS-L (2.0 μg/ml) or LPS (0.2 μg/ml) in the absence or in the presence of PRO (40 μM in ethanol) for 16 h. The levels of various cytokines and chemokines in human PBMC-conditioned medium were measured by using Cytokine Antibody Array kits (in arbitrary units, AU). *, P < 0.05 versus “- pCTS-L”; #, P < 0.05 versus “+ pCTS-L”, non-parametric Kruskal-Wallis ANOVA test.
The β-cyclodextrin (β-CD) is defined as cyclic oligosaccharides of seven glucopyranoses in its β-chair conformation (Figure 3A), thereby displaying the shape of truncated cone with an outer hydrophilic surface that render it water-soluble and an inner hydrophobic core (25) that can entrap hydrophobic molecules (e.g., PRO). To increase the water-solubility of β-CD, the hydroxyl groups of the inner hydrophobic cavity (position 2) and the outer hydrophilic surface (position 6) of β-CD can be substituted with hydrophobic methyl moieties to produce the 2,6-dimethyl-β-cyclodextrin (DM-β-CD, Figure 3A), which still displayed the shape of cone with slightly less symmetry and smaller inner cavity (Figure 3A). As a lipophilic sterol, PRO could be inserted into the hydrophobic inner cavity of two DM-β-CD molecules at 1:2 molar ratio (Figure 3A). These PRO-carrying DM-β-CD nanoparticles dose-dependently suppressed pCTS-L-mediated production of a few cytokines (e.g., TNF, IL-6 and IL-10) and chemokines (GRO, IL-8 and MCP-3) in human PBMCs (Figure 3B), confirming that PRO-carrying DM-β-CD nanoparticles maintained pCTS-L-inhibiting properties of PRO under pharmacological conditions.
Figure 3 PRO-carrying DM-β-CD nanoparticles significantly inhibited pCTS-L-stimulated production of several cytokines and chemokines in human PBMCs. (A) Structures of DM-β-CD and PRO as well as the inclusion complex of these two molecules. Given its lipophilic nature, PRO can easily be intercalated and embedded into the hydrophobic inner cavity of the truncated cone structure of two DM-β-CD molecules. (B) PRO significantly inhibited pCTS-L-stimulated production of several cytokines and chemokines. Human PBMCs were challenged with recombinant human pCTS-L in the absence or in the presence of DM-β-CD/PRO complexes at different concentrations (µM) for 16 h, and extracellular concentrations of cytokines and chemokines were measured by using Cytokine Antibody Arrays. *, P < 0.05 versus “- pCTS-L”; #, P < 0.05 versus “+ pCTS-L”, non-parametric Kruskal-Wallis ANOVA test.
To assess the PRO’s therapeutic efficacy, we first dissolved PRO in Sesame oil containing various unsaturated fatty acids that could emulsify and dissolve lipophilic PRO in the form of micelles (26–30). When dissolved in sesame oil, PRO did not show any protection against sepsis within a dose range of 1.0 mg/kg to 8.0 mg/kg (Figure 4A, Left Panel). When given intraperitoneally at a relatively high dose (16 mg/kg) at 2 h and 24 h post CLP, PRO promoted a marked protection against sepsis, significantly increasing animal survival rates from 30% to 70% (Figure 4A). In an effort to enhance its pharmacological efficacy, PRO was entrapped into DM-β-CD nanoparticles to enhance its water-solubility and bioavailability, and further tested in an animal model of experimental sepsis induced by cecal ligation and puncture (CLP). The PRO-carrying DM-β-CD nanoparticles was given at 24 h after CLP, which was a time point when circulating pCTS-L plateaued and some animals started to succumb to death (4). As a vehicle control, DM-β-CD (14.65 mg/kg) itself did not affect animal survival rate when it was given at 24 h and 48 h post CLP surgery (Figure 4A). In contrast, the PRO-carrying DM-β-CD nanoparticles significantly rescued both male and female mice from infections even when the 1st dose was intraperitoneally administered at 24 h post CLP (Figure 4A). This effective protective dose of PRO entrapped in the DM-β-CD nanoparticles (even when initially given at 24 h post CLP) was almost 10-fold lower than the dosage of progesterone dissolved in sesame oil (when first given at 2 h post CLP.) The calculated molar concentration of PRO (MW = 314.47 Daltons; 1.4 mg/kg) intraperitoneally injected into septic animals was estimated to be > 4.5 μM and comparable to the effective concentrations (10 µM) of PRO in inhibiting pCTS-L-induced dysregulated inflammation in vitro (Figure 3B).
Figure 4 PRO-carrying DM-β-CD nanoparticles rescued mice from sepsis partially by attenuating sepsis-triggered inflammation. (A) PRO-containing sesame oil or PRO-entrapping DM-β-CD nanoparticles protected or rescued mice from experimental sepsis. Male (M) and/or female (F) Balb/C mice were subjected to CLP, and PRO-containing sesame oil or PRO/DM-β-CD nanoparticles were intraperitoneally administered at indicated doses and time points. *, P < 0.05 versus saline or vehicle (sesame oil or DM-β-CD) controls. (B, C) PRO-carrying DM-β-CD nanoparticles attenuated CLP-triggered systemic inflammation and tissue injury. Balb/C mice were subjected to CLP, and PRO/DM-β-CD nanoparticles (containing 1.35 mg/kg PRO and 14.65 mg/kg DM-β-CD) or DM-β-CD vehicle (14.65 mg/kg) were administered at 2 h and 20 h post CLP, and then euthanized at 24 h post CLP to collect blood and measure serum levels of various cytokines and chemokines (in arbitrary units, AU) as well as markers of tissue injury (AST and LDH) using specific colorimetric enzymatic assays. *, P < 0.05 versus “ - CLP”; #, P < 0.05 versus “+ CLP”, non-parametric Kruskal-Wallis ANOVA test.
To elucidate the underlying protective mechanisms of PRO, we assessed its impact on CLP sepsis-triggered inflammation and tissue injury. In agreement with our previous report (4), experimental sepsis markedly elevated blood levels of G-CSF, MIP-2 and sTNFR1 (Figure 4B). In contrast to DM-β-CD vehicle, which did not affect sepsis-triggered systemic accumulation of any cytokines or chemokines, PRO-carrying DM-β-CD nanoparticles significantly reduced sepsis-triggered elevation of MIP-2 and sTNFRI (Figure 4B). Furthermore, in contrast to DM-β-CD vehicle, which did not affect sepsis-triggered release of liver enzymes (such as AST) or other general tissue injury markers such as LDH, PRO-carrying DM-β-CD nanoparticles significantly reduced the levels of AST and LDH in septic animals (Figure 4C), suggesting that PRO-entrapping DM-β-CD nanoparticles protect mice against infections partly by suppressing sepsis-mediated dysregulated inflammation and tissue injury.
To gain further insight into PRO’s protective mechanisms, we examined the possible interaction and relationship between systemic accumulation PRO and pCTS-L in clinical sepsis. SPR analysis revealed a strong interaction between PRO and pCTS-L, as evidenced by the relatively low equilibrium dissociation constant (KD, Figure 5A) for PRO-pCTS-L interaction, suggesting that PRO might bind pCTS-L to inhibit its proinflammatory properties under pharmacological conditions. In agreement with previous findings of a marked (1 - 5 folds) elevation of blood PRO levels in septic animals (31) or patients with severe sepsis (32) or septic shock (33), we found a significant increase of serum PRO levels in septic mice (Figure 5B), as well as a parallel positive correlation with Sequential Organ Failure Assessment (SOFA, Supplementary Table 1) score and serum pCTS-L concentrations in patients with clinical sepsis (Figure 5C), confirming a possible progesterone upregulation as a potential protective mechanism against infections.
Figure 5 PRO interacted and positively correlated with pCTS-L in clinical sepsis. (A) SPR analysis of PRO/pCTS-L interaction. Recombinant pCTS-L with an N-terminal 6 × His tag was immobilized onto nitrilotriacetic acid (NTA)-conjugated chip, and an analyte of PRO solution (in DMSO) was injected at several increasing concentrations (6.25, 12.5, 25.0, 50.0 and 100.0 µM) to estimate the KD of PRO-pCTS-L interaction. PRO was injected for a contact time of 250 seconds at increasing concentrations, and dissociation was monitored for 300 seconds. The 1:1 model fit to the raw data is shown as solid black lines. The KD was shown as the mean ± SEM of three independent experiments. (B) Elevation of serum PRO levels in septic mice at 24 h post CLP surgery. Male Balb/C mice were subjected to CLP surgery, and animals were sacrificed at 24 h post CLP to harvest blood and to compare serum PRO levels between normal healthy (“N”) and septic (“S”) mice. *, P < 0.05 versus normal healthy mice (N), non-parametric Kruskal-Wallis ANOVA test. (C) Correlation between serum PRO concentrations and sequential organ failure assessment (SOFA) score as well as serum pCTS-L levels in septic patients.
Currently, there is no effective therapies for clinical sepsis other than some adjunctive care such as administration of antibiotics and resuscitation of fluid (34–36). Therefore, it might still be important to explore small-molecule inhibitors to attenuate late-acting pathogenic mediators that may have relatively wider therapeutic windows (5). Here, we showed that a lipophilic hormone, PRO, selectively impaired pCTS-L-triggered production of several chemokines (e.g., ENA-78, MCP-1 or MCP-3) and cytokines (e.g., TNF, IL-6 or IL-10) without affecting LPS-induced TNF production. Our findings were in agreement with previous reports that PRO failed to inhibit LPS-stimulated TNF production in human PBMCs (37, 38). Nevertheless, it has been shown that PRO could inhibit LPS-stimulated production and secretion of TNF, IL-6, IL-12 or nitric oxide in other types of immune cells such as macrophages (39–43) and microglial cells (44, 45) potentially via PRO receptor-independent mechanisms (42). The discrepancies between divergent effects of progesterone on LPS-induced responses in different cell types remain a subject of future investigation. Nevertheless, as a steroid hormone produced primarily by the ovaries in women and, to a lesser extent, by the adrenal glands in both men and women, PRO occupies a crucial role in regulating menstrual cycles of the female reproductive system as well as innate immune responses against microbial infections.
Consequently, PRO-carrying DM-β-CD nanoparticles rescued both male and female mice from microbial infections even when they were initially given at 24 hours post the disease onset. This finding mirrored previous observations that systemic administration of PRO attenuated endotoxin-induced hypotension (46), CLP-induced systemic inflammation (47), as well as dysregulated inflammatory responses elicited by viral infections with SARS-CoV-2 (48, 49) and hepatitis C virus (50). When given locally, however, subcutaneous implanting PRO adversely thinned the cervicovaginal epithelium and adversely enhanced vaginal transmission of Simian immunodeficiency virus (SIV) (51), genital herpes infection (52) or HIV infection (53). It will thus be important to determine whether systemic administration of PRO at pharmacological doses will confer universal protection against all bacterial and viral infections in future preclinical and clinical investigations.
Because the lipophilic PRO is not soluble in water, we employed either sesame oil to emulsify it into micelles or DM-β-CD to complex with it to produce water-soluble PRO-carrying DM-β-CD-based nanoparticles. In the water solution, DM-β-CD displays the shape of truncated cone with a hydrophilic outer surface that renders it water-soluble and a hydrophobic inner cavity that entraps small hydrophobic molecules such as PRO (54, 55). This combined internal hydrophobic cavity along with external hydrophilicity enables DM-β-CD to form “host-guest” complexes with hydrophobic PRO via van der Waals forces and hydrophobic interactions (56). Although both a partial and a complete inclusion may occur, free-energy calculations favored the partial inclusion event for DM-β-CD and PRO (56), in which the complexation was initiated by inclusion of PRO into the first DM-β-CD to produce the 1:1 complex, but continued with the engagement of the second DM-β-CD to generate the more stable 1:2 complex (55, 57). Therefore, the hydrophobic part of PRO can be hidden within the inner hydrophobic cores of two DM-β-CD molecules, thereby potentially improving the solubility, stability, as well as bioavailability of the PRO in vivo (58). However, the entrapment of this hydrophobic PRO by DM-β-CD is reversible, enabling rapid dissociation of PRO from the complexes upon their dilution in biological fluids (58, 59). PRO was released from DM-β-CD within hours (59), and the dissociated PRO could potentially bind pCTS-L to interrupt some of its proinflammatory properties. The free DM-β-CDs could be gradually removed from the circulation within 36 h, destining to organs with increased blood flow velocities such as the spleen, liver, and kidney (60), where it can be metabolized (60) or excreted via glomerular filtration (61).
Regardless of whether PRO was solubilized in organic solvents (e.g., DMSO or ethanol) or entrapped in DM-β-CD-based nanoparticles, it similarly suppressed pCTS-L-induced secretion of several chemokines (e.g., ENA-78, MCP-1, GRO, or MCP-3) and cytokines (e.g., TNF or IL-10) in human PBMCs. The intricate mechanisms of PRO-mediated suppression of pCTS-L-stimulated inflammation will be an interesting subject for future investigations. In light of the essential involvement of TLR4 and RAGE in pCTS-L-induced inflammation (4), it will be interesting to determine whether PRO interacts with pCTS-L to interfere with its interaction with TLR4 or RAGE receptors. This is plausible, because pCTS-L-neutralizing antibodies inhibited pCTS-L-stimulated inflammation by disrupting pCTS-L interaction with PRRs such as TLR4 and RAGE (4).
To our best knowledge, the DM-β-CD-based nanoparticle technology has not yet been used to explore the therapeutic potential of PRO in any animal models of microbial infections. Consistent with its inhibitory activity in inhibiting late-acting mediator pCTS-L-induced cytokine/chemokine production in human PBMCs, we found that delayed administration of PRO-carrying DM-β-CD nanoparticles effectively rescued both male and female mice from microbial infections even when they were initially given at 24 h post onset of infections. Currently, the mechanism for PRO-mediated protection remains elusive, but appeared to attribute to its attenuation of sepsis- or pCTS-L-induced dysregulated inflammation and tissue injury. Indeed, PRO-carrying DM-β-CD nanoparticles significantly attenuated sepsis-triggered accumulation of G-CSF, sTNFRI and MIP-2/GRO-β, three pCTS-L-inducible surrogate markers of experimental sepsis (20, 62, 63). Our findings were in agreement with an earlier report (47), and support the possibility that PRO protects mice against sepsis partially by suppressing sepsis-triggered dysregulated inflammation. In light of the pathogenic involvement of pCTS-L in other inflammatory diseases such as pancreatitis (64), atherosclerosis (65), renal disease (66), vascular intimal hyperplasia (67), arthritis (68) and colitis (69), it will be interesting to explore the therapeutic potential of PRO-carrying DM-β-CD nanoparticles in other inflammatory diseases.
There are a few limitations in the current study: (i) We did not assess systemic inflammatory cytokine profiles at later stages of sepsis, because many septic animals in the control group might have succumbed to sepsis between 24 - 48 h post CLP (as depicted in Figure 4A), rendering blood sampling at later time points after the death of some septic animals in the control vehicle group practically infeasible. Even if post-mortem blood collection was still feasible, post-mortem tissue decomposition could lead to the release of cellular contents (including cytokines) into the surrounding environment, resulting in artificially elevated cytokine levels in post-mortem samples. Thus, sampling at later time points particularly after the death of septic animals in the control group might introduce confounding variables that hinder the interpretability of the systemic inflammatory profile, rendering them ineligible for the inclusion of comprehensive assessment of systemic inflammation at later points (e.g., 48 h post CLP). (ii) We do not know if PRO-entrapping DM-β-CD nanoparticles are orally active and protective against sepsis and other bacterial or viral infections. (iii) We do not know why progesterone selectively inhibits pCTS-L-mediated inflammation, although the robust interaction between progesterone and pCTS-L may contribute to the observed selectivity, allowing progesterone to inhibit pCTS-L-mediated inflammation without impacting LPS-mediated cytokine/chemokine production. (iv) It remains elusive why PRO-entrapping DM-β-CD nanoparticles exhibited a bell-shaped dose-response curve in inhibiting pCTS-L-mediated inflammatory response, although this type of bell-shaped dose-response curve has also been observed in the context of PRO-mediated protection against cerebral ischemic injury (70, 71), wherein a higher dose (32 mg/kg) exhibited reduced efficacy compared to lower doses (e.g., 8 mg/kg or 16 mg/kg). Bell-shaped concentration-response curves typically indicate more complex biological effects, such as receptor saturation or dual mechanisms of action with multiple binding sites or targets of DM-β-CD complexes. For instance, at extremely higher concentrations (5 - 50 mM), β-CD and derivatives (e.g., DM-β-CD) can extract lipophilic molecules such as cholesterol from cytoplasmic membranes (72, 73), thereby disrupting lipid rafts to interfere with innate immune responses to microbial infections (74, 75). Similarly, at extremely high concentrations (in the ranges of mM), another β-CD derivative, 2-Hydroxypropyl-β-cyclodextrin, also exhibited some pro-inflammatory properties as evidenced by the increased expression and release of cytokines (such as TNF and CCL2/MCP-1) by murine macrophages (75). At present, it is not yet known whether DM-β-CD possesses similar weak proinflammatory properties in complexation with PRO that could be attributed to the bell-shaped dose-response cure of PRO/DM-β-CD complexes. Despite these limitations of this study, our findings of PRO as a selective inhibitor of pCTS-L-mediated dysregulated inflammation have supported a promising opportunity of developing novel DM-β-CD-based nanoparticles to treat microbial infections. Therefore, it will be critical to further develop PRO-entrapping DM-β-CD-based nanoparticles and further translate our pre-clinical research into clinical treatment of bacterial infections.
The original contributions presented in the study are included in the article/Supplementary Materials, further inquiries can be directed to the corresponding author/s.
This study was administratively approved by the institutional review board (IRB) of the FIMR (IRB protocol #18-0184). The studies were conducted in accordance with the local legislation and institutional requirements. The participants provided their written informed consent to participate in this study. Our animal study was approved by the Institutional Animal Care and Use Committee (IACUC) of the FIMR (Protocol # 2017-003 Term II, approved on April 28th, 2020). The study was conducted in accordance with the local legislation and institutional requirements.
XQ: Writing – review & editing, Methodology, Investigation, Formal analysis, Data curation. WC: Writing – review & editing, Methodology, Investigation, Data curation. CZ: Writing – review & editing, Methodology, Investigation, Data curation. JL: Writing – review & editing, Resources. TQ: Writing – review & editing, Investigation, Data curation. LL: Writing – review & editing, Investigation, Formal analysis. PW: Writing – review & editing, Conceptualization. KT: Writing – review & editing, Resources. HW: Writing – review & editing, Writing – original draft, Supervision, Project administration, Funding acquisition, Formal analysis, Conceptualization.
The author(s) declare that financial support was received for the research, authorship, and/or publication of this article. Our work was partly supported by the National Institutes of Health (NIH) grants R01AT005076 and R35GM145331.
The authors declare that the research was conducted in the absence of any commercial or financial relationships that could be construed as a potential conflict of interest.
All claims expressed in this article are solely those of the authors and do not necessarily represent those of their affiliated organizations, or those of the publisher, the editors and the reviewers. Any product that may be evaluated in this article, or claim that may be made by its manufacturer, is not guaranteed or endorsed by the publisher.
The Supplementary Material for this article can be found online at: https://www.frontiersin.org/articles/10.3389/fimmu.2024.1368448/full#supplementary-material
1. Rudd KE, Johnson SC, Agesa KM, Shackelford KA, Tsoi D, Kievlan DR, et al. Global, regional, and national sepsis incidence and mortality, 1990-2017: analysis for the global burden of disease study. Lancet. (2020) 395:200–11. doi: 10.1016/S0140-6736(19)32989-7
2. Wang H, Bloom O, Zhang M, Vishnubhakat JM, Ombrellino M, Che J, et al. Hmg-1 as a late mediator of endotoxin lethality in mice. Science. (1999) 285:248–51. doi: 10.1126/science.285.5425.248
3. Tang D, Kang R, Zeh HJ, Lotze MT. The multifunctional protein hmgb1: 50 years of discovery. Nat Rev Immunol. (2023) 23:824–41. doi: 10.1038/s41577-023-00894-6
4. Zhu CS, Qiang X, Chen W, Li J, Lan X, Yang H, et al. Identification of procathepsin L (Pcts-L)-neutralizing monoclonal antibodies to treat potentially lethal sepsis. Sci Adv. (2023) 9:eadf4313. doi: 10.1126/sciadv.adf4313
5. Li J, Zhu CS, He L, Qiang X, Chen W, Wang H. A two-decade journey in identifying high mobility group box 1 (Hmgb1) and procathepsin L (Pcts-L) as potential therapeutic targets for sepsis. Expert Opin Ther Targets. (2023) 27:575–91. doi: 10.1080/14728222.2023.2239495
6. Poltorak A, He X, Smirnova I, Liu MY, Huffel CV, Du X, et al. Defective lps signaling in C3h/hej and C57bl/10sccr mice: mutations in tlr4 gene. Science. (1998) 282:2085–8. doi: 10.1126/science.282.5396.2085
7. Tracey KJ, Fong Y, Hesse DG, Manogue KR, Lee AT, Kuo GC, et al. Anti-cachectin/tnf monoclonal antibodies prevent septic shock during lethal bacteraemia. Nature. (1987) 330:662–4. doi: 10.1038/330662a0
8. Dinarello CA. Biologic basis for interleukin-1 in disease. Blood. (1996) 87:2095–147. doi: 10.1182/blood.V87.6.2095.bloodjournal8762095
9. Song Y, Shi Y, Ao LH, Harken AH, Meng XZ. Tlr4 mediates lps-induced ho-1 expression in mouse liver: role of tnf-alpha and il-1beta. World J Gastroenterol. (2003) 9:1799–803. doi: 10.3748/wjg.v9.i8.1799
10. Fan M, Yang K, Wang X, Chen L, Gill PS, Ha T, et al. Lactate promotes endothelial-to-mesenchymal transition via snail1 lactylation after myocardial infarction. Sci Adv. (2023) 9:eadc9465. doi: 10.1126/sciadv.adc9465
11. Yang K, Fan M, Wang X, Xu J, Wang Y, Tu F, et al. Lactate promotes macrophage hmgb1 lactylation, acetylation, and exosomal release in polymicrobial sepsis. Cell Death Differ. (2022) 29:133–46. doi: 10.1038/s41418-021-00841-9
12. Yang L, Xie M, Yang M, Yu Y, Zhu S, Hou W, et al. Pkm2 regulates the warburg effect and promotes hmgb1 release in sepsis. Nat Commun. (2014) 5:4436. doi: 10.1038/ncomms5436.:4436
13. Yang H, Ochani M, Li J, Qiang X, Tanovic M, Harris HE, et al. Reversing established sepsis with antagonists of endogenous high-mobility group box 1. Proc Natl Acad Sci U.S.A. (2004) 101:296–301. doi: 10.1073/pnas.2434651100
14. Zhang MM, Qiao Y, Ang EL, Zhao H. Using natural products for drug discovery: the impact of the genomics era. Expert Opin Drug Discovery. (2017) 12:475–87. doi: 10.1080/17460441.2017.1303478
15. Chen W, Qiang X, Wang Y, Zhu S, Li J, Babaev A, et al. Identification of tetranectin-targeting monoclonal antibodies to treat potentially lethal sepsis. Sci Transl Med. (2020) 12:12/539. doi: 10.1126/scitranslmed.aaz3833
16. Chen W, Zhu CS, Qiang X, Chen S, Li J, Wang P, et al. Development of procathepsin L (Pcts-L)-inhibiting lanosterol-carrying liposome nanoparticles to treat lethal sepsis. Int J Mol Sci. (2023) 24. doi: 10.3390/ijms24108649
17. Osuchowski MF, Ayala A, Bahrami S, Bauer M, Boros M, Cavaillon JM, et al. Minimum quality threshold in pre-clinical sepsis studies (Mqtipss): an international expert consensus initiative for improvement of animal modeling in sepsis. Shock. (2018) 50:377–80. doi: 10.1097/shk.0000000000001212
18. Zingarelli B, Coopersmith CM, Drechsler S, Efron P, Marshall JC, Moldawer L, et al. Part I: minimum quality threshold in preclinical sepsis studies (Mqtipss) for study design and humane modeling endpoints. Shock. (2019) 51:10–22. doi: 10.1097/shk.0000000000001243
19. Hellman J, Bahrami S, Boros M, Chaudry IH, Fritsch G, Gozdzik W, et al. Part iii: minimum quality threshold in preclinical sepsis studies (Mqtipss) for fluid resuscitation and antimicrobial therapy endpoints. Shock. (2019) 51:33–43. doi: 10.1097/shk.0000000000001209
20. Chen W, Brenner M, Aziz M, Chavan SS, Deutschman CS, Diamond B, et al. Buprenorphine markedly elevates a panel of surrogate markers in a murine model of sepsis. Shock. (2019) 52:550–3. doi: 10.1097/SHK.0000000000001361
21. Cotroneo TM, Hugunin KM, Shuster KA, Hwang HJ, Kakaraparthi BN, Nemzek-Hamlin JA. Effects of buprenorphine on a cecal ligation and puncture model in C57bl/6 mice. J Am Assoc Lab Anim Sci. (2012) 51:357–65.
22. Zhu SC, Li J, Wang. H. Use of open surface plasma resonance (Openspr) to characterize teh binding affinity of protein-protein interactions. Bio-Protocol. (2023) 13. doi: 10.21769/BioProtoc.4795
23. Shankar-Hari M, Phillips GS, Levy ML, Seymour CW, Liu VX, Deutschman CS, et al. Developing a new definition and assessing new clinical criteria for septic shock: for the third international consensus definitions for sepsis and septic shock (Sepsis-3). JAMA. (2016) 315:775–87. doi: 10.1001/jama.2016.0289
24. Zhang M, Caragine T, Wang H, Cohen PS, Botchkina G, Soda K, et al. Spermine inhibits proinflammatory cytokine synthesis in human mononuclear cells: A counterregulatory mechanism that restrains the immune response. J Exp Med. (1997) 185:1759–68. doi: 10.1084/jem.185.10.1759
25. Duchêne D, Bochot A. Thirty years with cyclodextrins. Int J Pharm. (2016) 514:58–72. doi: 10.1016/j.ijpharm.2016.07.030
26. Auger CJ, Jessen HM, Auger AP. Microarray profiling of gene expression patterns in adult male rat brain following acute progesterone treatment. Brain Res. (2006) 1067:58–66. doi: 10.1016/j.brainres.2005.10.033
27. Russo SJ, Sun WL, Minerly AC, Weierstall K, Nazarian A, Festa ED, et al. Progesterone attenuates cocaine-induced conditioned place preference in female rats. Brain Res. (2008) 1189:229–35. doi: 10.1016/j.brainres.2007.10.057
28. Sun WL, Luine VN, Zhou L, Wu HB, Weierstall KM, Jenab S, et al. Acute progesterone treatment impairs spatial working memory in intact male and female rats. Ethn Dis. (2010) 20:S1–83-7.
29. O'Connor CA, Cernak I, Johnson F, Vink R. Effects of progesterone on neurologic and morphologic outcome following diffuse traumatic brain injury in rats. Exp Neurol. (2007) 205:145–53. doi: 10.1016/j.expneurol.2007.01.034
30. Uphouse L, Guptarak J, Hiegel C. Progesterone reduces the inhibitory effect of a serotonin 1b receptor agonist on lordosis behavior. Pharmacol Biochem Behav. (2010) 97:317–24. doi: 10.1016/j.pbb.2010.08.017
31. Dembek K, Timko K, Moore C, Johnson L, Frazer M, Barr B, et al. Longitudinal assessment of adrenocortical steroid and steroid precursor response to illness in hospitalized foals. Domest Anim Endocrinol. (2023) 82:106764. doi: 10.1016/j.domaniend.2022.106764
32. Angstwurm MW, Gaertner R, Schopohl J. Outcome in elderly patients with severe infection is influenced by sex hormones but not gender. Crit Care Med. (2005) 33:2786–93. doi: 10.1097/01.ccm.0000190242.24410.17
33. Feng JY, Liu KT, Abraham E, Chen CY, Tsai PY, Chen YC, et al. Serum estradiol levels predict survival and acute kidney injury in patients with septic shock–a prospective study. PloS One. (2014) 9:e97967. doi: 10.1371/journal.pone.0097967
34. Buchman TG, Simpson SQ, Sciarretta KL, Finne KP, Sowers N, Collier M, et al. Sepsis among medicare beneficiaries: 1. The burdens of sepsis, 2012-2018. Crit Care Med. (2020) 48:276–88. doi: 10.1097/CCM.0000000000004224
35. Tindal EW, Armstead BE, Monaghan SF, Heffernan DS, Ayala A. Emerging therapeutic targets for sepsis. Expert Opin Ther Targets. (2021) 25:175–89. doi: 10.1080/14728222.2021.1897107
36. Reinhart K, Daniels R, Kissoon N, MaChado FR, Schachter RD, Finfer S. Recognizing sepsis as a global health priority - a who resolution. N Engl J Med. (2017) 377:414–7. doi: 10.1056/NEJMp1707170
37. Bouman A, Schipper M, Heineman MJ, Faas M. 17beta-estradiol and progesterone do not influence the production of cytokines from lipopolysaccharide-stimulated monocytes in humans. Fertil Steril. (2004) 82 Suppl 3:1212–9. doi: 10.1016/j.fertnstert.2004.05.072
38. Amory J, Lawler R, Shields L. Hydroxyprogesterone caproate and progesterone increase tumor necrosis factor-alpha production in lipopolysaccharide stimulated whole blood from non-pregnant women. J Perinat Med. (2005) 33:506–9. doi: 10.1515/jpm.2005.089
39. Miller L, Hunt JS. Regulation of tnf-alpha production in activated mouse macrophages by progesterone. J Immunol. (1998) 160:5098–104. doi: 10.4049/jimmunol.160.10.5098
40. Jones LA, Anthony JP, Henriquez FL, Lyons RE, Nickdel MB, Carter KC, et al. Toll-like receptor-4-mediated macrophage activation is differentially regulated by progesterone via the glucocorticoid and progesterone receptors. Immunology. (2008) 125:59–69. doi: 10.1111/j.1365-2567.2008.02820.x
41. Su L, Sun Y, Ma F, Lü P, Huang H, Zhou J. Progesterone inhibits toll-like receptor 4-mediated innate immune response in macrophages by suppressing nf-kappab activation and enhancing socs1 expression. Immunol Lett. (2009) 125:151–5. doi: 10.1016/j.imlet.2009.07.003
42. Wolfson ML, Schander JA, Bariani MV, Correa F, Franchi AM. Progesterone modulates the lps-induced nitric oxide production by a progesterone-receptor independent mechanism. Eur J Pharmacol. (2015) 769:110–6. doi: 10.1016/j.ejphar.2015.11.005
43. Miller L, Alley EW, Murphy WJ, Russell SW, Hunt JS. Progesterone inhibits inducible nitric oxide synthase gene expression and nitric oxide production in murine macrophages. J Leukoc Biol. (1996) 59:442–50. doi: 10.1002/jlb.59.3.442
44. Müller E, Kerschbaum HH. Progesterone and its metabolites 5-dihydroprogesterone and 5-3-tetrahydroprogesterone decrease lps-induced no release in the murine microglial cell line, bv-2. Neuro Endocrinol Lett. (2006) 27:675–8.
45. Lei B, Mace B, Dawson HN, Warner DS, Laskowitz DT, James ML. Anti-inflammatory effects of progesterone in lipopolysaccharide-stimulated bv-2 microglia. PloS One. (2014) 9:e103969. doi: 10.1371/journal.pone.0103969
46. Zöllner J, Howe LG, Edey LF, O'Dea KP, Takata M, Leiper J, et al. Lps-induced hypotension in pregnancy: the effect of progesterone supplementation. Shock. (2020) 53:199–207. doi: 10.1097/shk.0000000000001343
47. Aksoy AN, Toker A, Celık M, Aksoy M, Halıcı Z, Aksoy H. The effect of progesterone on systemic inflammation and oxidative stress in the rat model of sepsis. Indian J Pharmacol. (2014) 46:622–6. doi: 10.4103/0253-7613.144922
48. Yuan L, Zhu H, Wu K, Zhou M, Ma J, Chen R, et al. Female sex hormone, progesterone, ameliorates the severity of sars-cov-2-caused pneumonia in the Syrian hamster model. Signal Transduct Target Ther. (2022) 7:47. doi: 10.1038/s41392-021-00860-5
49. Ghandehari S, Matusov Y, Pepkowitz S, Stein D, Kaderi T, Narayanan D, et al. Progesterone in addition to standard of care vs standard of care alone in the treatment of men hospitalized with moderate to severe covid-19: A randomized, controlled pilot trial. Chest. (2021) 160:74–84. doi: 10.1016/j.chest.2021.02.024
50. Tayel SS, Helmy AA, Ahmed R, Esmat G, Hamdi N, Abdelaziz AI. Progesterone suppresses interferon signaling by repressing tlr-7 and mxa expression in peripheral blood mononuclear cells of patients infected with hepatitis C virus. Arch Virol. (2013) 158:1755–64. doi: 10.1007/s00705-013-1673-z
51. Cohen J. Siv transmission. Monkey study prompts high-level public health response. Science. (1996) 272:805. doi: 10.1126/science.272.5263.805
52. Kaushic C, Ashkar AA, Reid LA, Rosenthal KL. Progesterone increases susceptibility and decreases immune responses to genital herpes infection. J Virol. (2003) 77:4558–65. doi: 10.1128/jvi.77.8.4558-4565.2003
53. Mingjia L, Short R. How oestrogen or progesterone might change a woman's susceptibility to hiv-1 infection. Aust N Z J Obstet Gynaecol. (2002) 42:472–5. doi: 10.1111/j.0004-8666.2002.00472.x
54. Kurkov SV, Loftsson T. Cyclodextrins. Int J Pharm. (2013) 453:167–80. doi: 10.1016/j.ijpharm.2012.06.055
55. Liu FY, Kildsig DO, Mitra AK. Beta-cyclodextrin/steroid complexation: effect of steroid structure on association equilibria. Pharm Res. (1990) 7:869–73. doi: 10.1023/a:1015973218303
56. Cai W, Sun T, Liu P, Chipot C, Shao X. Inclusion mechanism of steroid drugs into beta-cyclodextrins. Insights from free energy calculations. J Phys Chem B. (2009) 113:7836–43. doi: 10.1021/jp901825w
57. Zoppetti G, Puppini N, Ospitali F, Fini A. Solid state characterization of progesterone in a freeze dried 1:2 progesterone/hpbcd mixture. J Pharm Sci. (2007) 96:1729–36. doi: 10.1002/jps.20671
58. Scavone C, Bonagura AC, Fiorentino S, Cimmaruta D, Cenami R, Torella M, et al. Efficacy and safety profile of diclofenac/cyclodextrin and progesterone/cyclodextrin formulations: A review of the literature data. Drugs R D. (2016) 16:129–40. doi: 10.1007/s40268-016-0123-2
59. Memişoğlu E, Bochot A, Sen M, Duchêne D, Hincal AA. Non-surfactant nanospheres of progesterone inclusion complexes with amphiphilic beta-cyclodextrins. Int J Pharm. (2003) 251:143–53. doi: 10.1016/s0378-5173(02)00593-8
60. Mu K, Jiang K, Wang Y, Zhao Z, Cang S, Bi K, et al. The biological fate of pharmaceutical excipient Β-cyclodextrin: pharmacokinetics, tissue distribution, excretion, and metabolism of Β-cyclodextrin in rats. Molecules. (2022) 27. doi: 10.3390/molecules27031138
61. Loftsson T, Moya-Ortega MD, Alvarez-Lorenzo C, Concheiro A. Pharmacokinetics of cyclodextrins and drugs after oral and parenteral administration of drug/cyclodextrin complexes. J Pharm Pharmacol. (2016) 68:544–55. doi: 10.1111/jphp.12427
62. Osuchowski MF, Welch K, Siddiqui J, Remick DG. Circulating cytokine/inhibitor profiles reshape the understanding of the sirs/cars continuum in sepsis and predict mortality. J Immunol. (2006) 177:1967–74. doi: 10.4049/jimmunol.177.3.1967
63. Bozza FA, Salluh JI, Japiassu AM, Soares M, Assis EF, Gomes RN, et al. Cytokine profiles as markers of disease severity in sepsis: A multiplex analysis. Crit Care. (2007) 11:R49. doi: 10.1186/cc5783
64. Wartmann T, Mayerle J, Kahne T, Sahin-Toth M, Ruthenburger M, Matthias R, et al. Cathepsin L inactivates human trypsinogen, whereas cathepsin L-deletion reduces the severity of pancreatitis in mice. Gastroenterology. (2010) 138:726–37. doi: 10.1053/j.gastro.2009.10.048
65. Kitamoto S, Sukhova GK, Sun J, Yang M, Libby P, Love V, et al. Cathepsin L deficiency reduces diet-induced atherosclerosis in low-density lipoprotein receptor-knockout mice. Circulation. (2007) 115:2065–75. doi: 10.1161/CIRCULATIONAHA.107.688523
66. Cao Y, Liu X, Li Y, Lu Y, Zhong H, Jiang W, et al. Cathepsin L activity correlates with proteinuria in chronic kidney disease in humans. Int Urol Nephrol. (2017) 49:1409–17. doi: 10.1007/s11255-017-1626-7
67. Cai J, Zhong H, Wu J, Chen RF, Yang H, Al-Abed Y, et al. Cathepsin L promotes vascular intimal hyperplasia after arterial injury. Mol Med. (2017) 23:92–100. doi: 10.2119/molmed.2016.00222
68. Schurigt U, Eilenstein R, Gajda M, Leipner C, Sevenich L, Reinheckel T, et al. Decreased arthritis severity in cathepsin L-deficient mice is attributed to an impaired T helper cell compartment. Inflammation Res. (2012) 61:1021–9. doi: 10.1007/s00011-012-0495-x
69. Bauer C, Duewell P, Mayer C, Lehr HA, Fitzgerald KA, Dauer M, et al. Colitis induced in mice with dextran sulfate sodium (Dss) is mediated by the nlrp3 inflammasome. Gut. (2010) 59:1192–9. doi: 10.1136/gut.2009.197822
70. Wali B, Ishrat T, Won S, Stein DG, Sayeed I. Progesterone in experimental permanent stroke: A dose-response and therapeutic time-window study. Brain. (2014) 137:486–502. doi: 10.1093/brain/awt319
71. Yousuf S, Atif F, Sayeed I, Tang H, Stein DG. Progesterone in transient ischemic stroke: A dose-response study. Psychopharmacol (Berl). (2014) 231:3313–23. doi: 10.1007/s00213-014-3556-8
72. Stelzl D, Nielsen TT, Hansen T, di Cagno M. Β-cd-dextran polymer for efficient sequestration of cholesterol from phospholipid bilayers: mechanistic and safe-toxicity investigations. Int J Pharm. (2015) 496:896–902. doi: 10.1016/j.ijpharm.2015.10.041
73. Kiss T, Fenyvesi F, Bácskay I, Váradi J, Fenyvesi E, Iványi R, et al. Evaluation of the cytotoxicity of beta-cyclodextrin derivatives: evidence for the role of cholesterol extraction. Eur J Pharm Sci. (2010) 40:376–80. doi: 10.1016/j.ejps.2010.04.014
74. Horlock AD, Ormsby TJR, Clift MJD, Santos JEP, Bromfield JJ, Sheldon IM. Cholesterol supports bovine granulosa cell inflammatory responses to lipopolysaccharide. Reproduction. (2022) 164:109–23. doi: 10.1530/rep-22-0032
Keywords: innate immune cells, procathepsin-L, progesterone, 2,6-dimethyl-β-cyclodextrin, sepsis
Citation: Qiang X, Chen W, Zhu CS, Li J, Qi T, Lou L, Wang P, Tracey KJ and Wang H (2024) Therapeutic potential of procathepsin L-inhibiting and progesterone-entrapping dimethyl-β-cyclodextrin nanoparticles in treating experimental sepsis. Front. Immunol. 15:1368448. doi: 10.3389/fimmu.2024.1368448
Received: 10 January 2024; Accepted: 04 March 2024;
Published: 14 March 2024.
Edited by:
Christoph Thiemermann, Queen Mary University of London, United KingdomReviewed by:
Jianmin Chen, Queen Mary University of London, United KingdomCopyright © 2024 Qiang, Chen, Zhu, Li, Qi, Lou, Wang, Tracey and Wang. This is an open-access article distributed under the terms of the Creative Commons Attribution License (CC BY). The use, distribution or reproduction in other forums is permitted, provided the original author(s) and the copyright owner(s) are credited and that the original publication in this journal is cited, in accordance with accepted academic practice. No use, distribution or reproduction is permitted which does not comply with these terms.
*Correspondence: Haichao Wang, aHdhbmdAbm9ydGh3ZWxsLmVkdQ==
†These authors have contributed equally to this work
Disclaimer: All claims expressed in this article are solely those of the authors and do not necessarily represent those of their affiliated organizations, or those of the publisher, the editors and the reviewers. Any product that may be evaluated in this article or claim that may be made by its manufacturer is not guaranteed or endorsed by the publisher.
Research integrity at Frontiers
Learn more about the work of our research integrity team to safeguard the quality of each article we publish.