- 1Dermatology Center, Xinhua Hospital, Shanghai Jiaotong University School of Medicine, Shanghai, China
- 2Department of Dermatology, Xinhua Hospital, Shanghai Jiaotong University School of Medicine, Shanghai, China
- 3Institute of Dermatology, Shanghai Jiaotong University School of Medicine, Shanghai, China
Objectives: Numerous observational studies have reported associations between circulating cytokines and atopic dermatitis (AD); however, the causal relationships between them remain unclear. To explore the causal correlations and direction of causal effects between AD and levels of 91 circulating cytokines.
Methods: Two-sample Mendelian randomization (MR) analyses were conducted to examine the causal relationships between 91 circulating cytokines and AD using summary statistics from genome-wide association studies (GWAS). Reverse MR analyses were performed to investigate reverse causation. Pleiotropy and heterogeneity tests were conducted to assess the robustness of the findings. Additional transcriptome database and clinical peripheral blood mononuclear cells (PBMCs) samples were utilized to validate the results of MR analyses.
Results: Levels of interleukin (IL)-13, IL-18 Receptor 1, Tumor necrosis factor ligand superfamily member 14 (TNFSF14), TNF-related activation-induced cytokine (TRANCE), C-X-C motif chemokine (CXCL)11, IL-33, TNF-beta and CD5 were suggestively associated with the risk of AD (odds ratio, OR: 1.202, 95% CI: 1.018–1.422, p = 0.030; OR: 1.029, 95% CI: 1.029–1.157, p = 0.004; OR: 1.159, 95% CI: 1.018–1.320, p = 0.026; OR: 1.111, 95% CI: 1.016–1.214, p = 0.020; OR: 0.878, 95% CI: 0.783–0.984, p = 0.025; OR: 0.809, 95% CI: 0.661–0.991, p = 0.041; OR: 0.945, 95% CI: 0.896–0.997, p = 0.038; OR: 0.764, 95% CI: 0.652–0.895, p = 8.26e-04). In addition, levels of cytokines including Axin-1, CXCL5, CXCL10, Oncostatin-M (OSM), Sulfotransferase 1A1 (SULT1A1) and TNFSF14 were suggested to be consequences of AD (Beta: -0.080, p = 0.016; Beta: -0.062, p = 0.036; Beta: -0.066, p = 0.049; Beta: -0.073, p = 0.013; Beta: -0.089, p = 0.008; Beta: -0.079, p = 0.031). IL-13, IL-18R1, TNFSF14, and TRANCE were upregulated in both lesional skin biopsies and PBMCs from AD patients.
Conclusion: The study indicates that several cytokines, including IL-13, IL-18R1, TNFSF14, TRANCE, CXCL11, IL-33, TNF-beta, and CD5, are upstream of AD development, whereas a few circulating cytokines are potentially downstream in the development of AD.
1 Introduction
Atopic dermatitis (AD) is the most prevalent chronic inflammatory skin disease characterized by recurrent eczematous lesions and intense pruritus (1). It affects 15–20% of children and 5–10% of adults, making it the leading cause of the global burden of skin disease (2). The pathogenesis of AD is complex and involves various aspects such as genetic predisposition, epidermal barrier dysfunction, immune dysregulation, skin microbiome abnormalities and the neuroimmune system (3). Of note, the interaction between barrier dysfunction and allergic inflammation plays a key role in the pathogenesis of AD (4). However, the etiological factors driving AD still remain controversial (5), resulting in a paucity of effective and curative therapeutic targets. Therefore, gaining a thorough mechanistic insight into pathogenesis of AD is vital and urgent.
The current controversies pertaining to the pathogenesis of AD predominantly revolve around two prevailing hypotheses. One proposed “outside-to-inside” mechanism suggests that disrupted barrier serves as the initiating factor, releasing alarmins such as interleukin (IL)-33, thymic stromal lymphopoietin (TSLP) and IL-25, which recruit subsequent immune cells and elicit T helper cell type (Th)2-skewed inflammatory responses. TSLP promotes the chemotaxis of eosinophilia and enhances the expression level of IL-4, IL-5 and IL-13 (6). Overexpressing IL-33 in keratinocytes elevated circulating levels of IL-5 and IL-13 (7). Conversely, the “inside-to-outside” proponents argue that elevated inflammatory factors disturbing permeability barrier homeostasis are the etiologies of AD (8). For example, type 2 cytokines, IL-4 and IL-13 downregulate the expression of crucial proteins, such as filaggrin, involucrin and claudin-1 in keratinocytes (9, 10). Previous observational studies found elevated alarmins and inflammatory factors in AD patients (11). However, these studies may not capture the entire picture, and establishing definite causal correlations is challenging due to potential confounding factors or reverse causation. The question of whether the inflammatory cytokines or alarmins released by keratinocytes are the cause of AD remains debatable.
Mendelian randomization (MR) is an analytical approach used to infer the causal influence of an exposure on an outcome by employing genetic variations as instrumental variables (IVs) (12). By leveraging the random allocation of alleles during meiosis, MR can help alleviate reverse causation and is less susceptible to conventional confounding variables, thereby offering more robust evidence for causal inference (13). In the year 2023, Zhao et al. embarked upon a study employing MR to elucidate the causal effects of 58 circulating proteins, each with confirmed cis-protein quantitative trait loci (pQTLs) from their investigation, on 14 immune-mediated diseases (IMDs), including AD. Their work highlighted IL-18R1 as a driving protein in the pathogenesis of AD (14). Despite these contributions, the study confronted several constraints. Methodologically, the exclusive reliance on the GSMR method potentially introduced biases, particularly in the presence of pleiotropy. Moreover, the circumscribed focus on merely 58 proteins and the implementation of unidirectional MR analyses may have obscured other crucial disease mediators and reciprocal causal influences exerted by AD on cytokine profiles. The research emphasis was skewed towards ulcerative colitis, with no independent verification of findings within AD-centric datasets, thereby leaving the comprehensive understanding of the bidirectional causal network between circulating cytokines and AD incomplete.
To address these lacunae and attain a holistic understanding of the plasma protein involvement in AD, we conducted a bidirectional two-sample MR, utilizing genome-wide association study (GWAS) summary data of 91 inflammatory cytokines to investigate the potential bidirectional causal relationship between circulating inflammatory regulators and AD. To fortify the validity of our conclusions, we have corroborated our MR findings through replication in an additional GWAS dataset. Complementary to this, we have ventured into investigating the transcriptomic signatures of the identified cytokine drivers in AD, analyzing skin biopsy specimens and peripheral blood mononuclear cells (PBMCs) sourced from AD patients. This integrative strategy serves to refine our knowledge of the complex interplay between circulating cytokines and AD, offering a more nuanced and substantiated perspective on their causal interdependencies.
2 Materials and methods
2.1 Study design
The study design is presented schematically in Figure 1. Single nucleotide polymorphisms (SNPs) were treated as valid IVs. These IVs had to fulfil three core assumptions of MR analysis: relevance, independence, and exclusion restriction (15). It is assumed that the selected IVs are associated with the risk factor (relevance), but not with any confounders in the risk factor–outcome association (independence), and that they are not linked to the outcome via any pathways other than the risk factor of interest (exclusion restriction).
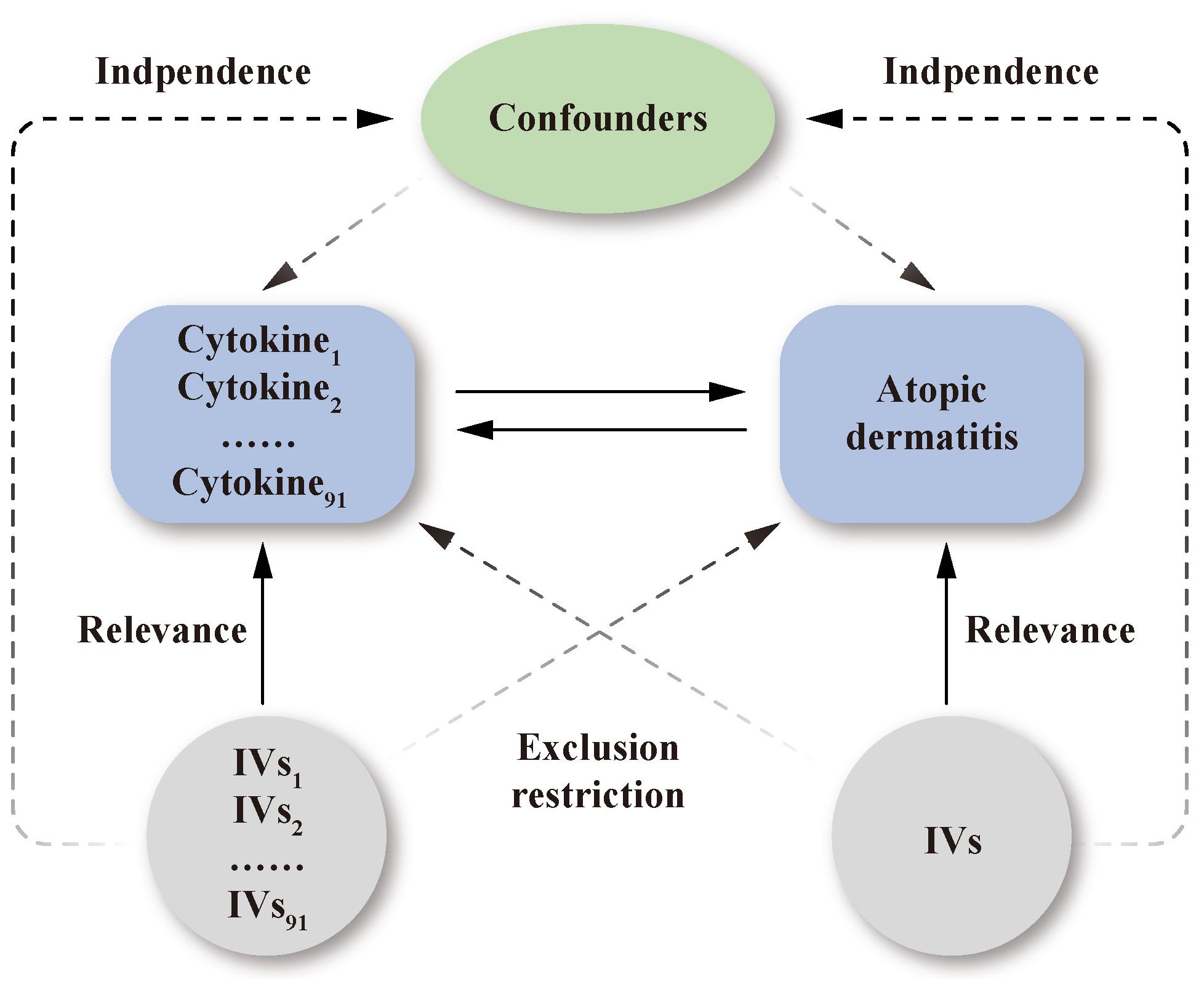
Figure 1 Schematic representation of the study design in this bidirectional Mendelian randomization analysis. Significant instrumental variables were selected for 91 inflammatory cytokines and atopic dermatitis. The bidirectional causal associations were then explored. Three core assumptions of MR analysis were presented in this causal directed acyclic graph: relevance, independence, and exclusion restrictions.
2.2 Data sources
This MR analysis utilized two datasets derived from publicly available summarized GWAS data. The detailed data for 91 circulating cytokine levels originated from a GWAS in 14,824 healthy subjects of European ancestry, which identified multiple common genetic variants influencing circulating cytokine levels (14). The summary statistics for AD were obtained from the Early Genetics and Lifecourse Epidemiology (EAGLE) Eczema consortium’s GWAS, encompassing 20 population-based cohorts and comprising 10,788 cases and 30,047 controls of European ancestry exclusively (16). An Additional AD GWAS data for validation in this study was obtained from the FinnGen consortium R8 release data, which comprised 11,964 cases and 306,909 controls of European ancestry. There was no overlap in the selection of populations between the exposure group and the outcome group.
2.3 Selection of IVs
A genome-wide statistical significance threshold of < 5.0 × 10-8 was established to extract the SNPs robustly associated with the studied exposures. Additionally, an r2 threshold of 0.001 and a clump window size of 10,000 kb were set to eliminate linkage disequilibrium (17). Based on these criteria, 74 cytokines were retained for analysis. In a secondary analysis, the genome-wide significance threshold was relaxed to 5.0 × 10-6 to include all 91 cytokines. The strength of IVs was evaluated using the F-statistic, which is calculated as follows: F = β2/SE2, where β represents the effect size of the allele and SE represents the standard error (18). An F-statistic greater than 10 suggests the absence of weak IV bias (19).
2.4 Statistical analysis
Three MR analysis methods were employed, including inverse variance weighted (IVW), MR-Egger and weighted median (WM). IVW was selected as the primary method for its ability to use inverse variances of each IV as weights in calculating a valid weighted average (20). MR-Egger, on the other hand, employs a form of weighted linear regression analysis. Estimates derived from this method are robust and independent of IV validity, although they might have lower statistical precision and be susceptible to outlying genetic variations (21). The WM method mitigates estimation precision variation by assigning inverse weights based on genetic variant variance, akin to the IVW method. It shows reliability despite violated causal effects (22). While significant causal correlations were evaluated using the IVW method, the MR egger and WM methods were utilized as supplementary methods for directional validation. Odds ratios (OR) with 95% confidence intervals (CI) were reported as effect estimates for cytokines on AD. Betas with 95% CI were reported as effect estimates for AD on cytokines.
Pleiotropy was assessed using MR-Egger regression. Additionally, the presence of pleiotropy and identification of outlying SNPs were determined through the MR-Pleiotropy RESidual Sum and Outlier (PRESSO) test (23). Heterogeneity was assessed using Cochran’s Q test, with a p-value < 0.05 indicating its presence.
When analyzing multiple outcomes, significance thresholds were adjusted using the Bonferroni method. Statistical significance was indicated by a p-value lower than the Bonferroni-corrected threshold. Correlations with p-values less than 0.05, but not reaching the Bonferroni-corrected significance level, were considered suggestively significant. All analyses were performed using R version 4.2.2, with the software packages “TwoSampleMR” and “MRPRESSO”.
2.5 Transcriptome analysis
Data of transcriptome profiles were extracted from Gene Expression Omnibus (GEO) accession no. GSE157194 consisting of 166 high-throughput RNA-sequencing expression profiles of lesional and non-lesional skin biopsies collected from 57 AD patients (24). Data cleaning and statistical analysis were applied with GEO2R software (25), and the comparison of gene expression between the lesional and non-lesional samples was applied with T-test.
2.6 Validation of the results in the PBMCs of AD patients
Blood samples (5 mL) were collected from hospitalized AD patients and healthy individuals undergoing physical examination at Xinhua Hospital, Shanghai Jiaotong University School of Medicine, anticoagulated with ethylenediamine tetraacetic acid, and sent to the laboratory within 2 h. The blood samples were centrifuged at 200 g/min 4°C for 5 min, and then the sediment was separated for preparing PBMC by standard Ficoll-Hypaque density centrifugation. In accordance with standard procedures, RNA was extracted from PBMCs and subjected to quality control and reverse transcription. The resulting cDNA was then amplified using specific primers for RT-qPCR. The internal reference gene GAPDH was used to calculate the relative expression levels of the target genes, based on the Ct values obtained from the RT-qPCR reaction. The relative mRNA expression was calculated based on the 2−ΔΔCt approach. Approval for the current study was granted by the Ethics Committee of Xinhua Hospital, Shanghai Jiaotong University School of Medicine. The primer sequences are listed in Table 1. The clinical sample information is presented in Supplementary Table S1.
3 Results
3.1 Causal effects of circulating cytokines on AD
Figure 2 presents the outcomes of the MR analysis on the causal relationship between circulating cytokines and AD at a significance threshold of < 5.0 × 10-8. The findings of the IVW method revealed that IL-10 receptor subunit beta (IL-10RB) (OR = 1.097, 95% CI = 1.009–1.192, p = 0.029), IL-18 receptor 1 (IL-18R1) (OR = 1.083, 95% CI = 1.018–1.153, p = 0.012) and TNF-related activation-induced cytokine (TRANCE) (OR = 1.119, 95% CI = 1.002–1.249, p = 0.045) were correlated with an elevated risk of AD. In addition, the IVW method showed T-cell surface glycoprotein CD5 (CD5) (OR = 0.766, 95% CI = 0.643–0.912, p = 0.003), C-X-C motif chemokine (CXCL) 11 (OR = 0.853, 95% CI = 0.731–0.995, p = 0.043), CXCL9 (OR = 0.727, 95% CI = 0.607–0.871, p = 5.35e-04) and Fms-related tyrosine kinase 3 ligand (FLT3LG) (OR = 0.873, 95% CI = 0.763–0.998, p = 0.047) were associated with the decreased risk of AD. Among these cytokines, CXCL9 met the Bonferroni-corrected threshold range (p = 0.05/51 = 9.80e-04), whereas the others did not. The scatter plots of MR analyses for above 7 cytokines are shown in Supplementary Figure S1. The specific SNP profiles, the pleiotropy and heterogeneity results of these 7 groups, are presented in Supplementary Tables S3 and S4. No evidence of heterogeneity and pleiotropy was observed (p > 0.05), and all F-statistics for the groups were >10, indicating the absence of weak IV bias. No outliers were found. Supplementary Table S5 presents the MR analyses involving 51 cytokines and AD.
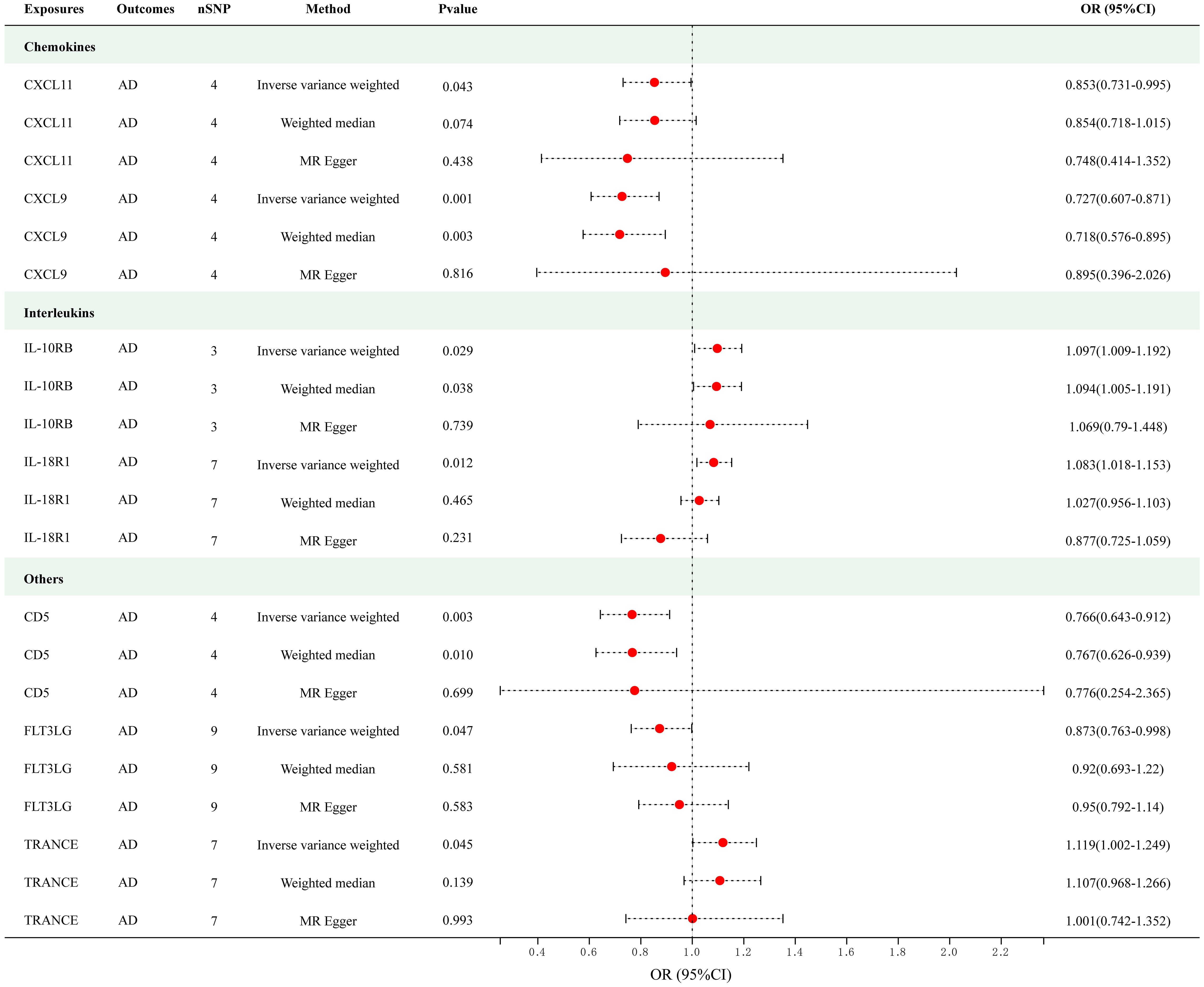
Figure 2 Causal correlations of 7 cytokines with atopic dermatitis (SNP associations reaching a significance level of p < 5×10-8). CXCL11, C-X-C motif chemokine 11; CXCL9, C-X-C motif chemokine 9; IL-10RB, interleukin-10 receptor subunit beta; IL-18R1, interleukin-18 receptor 1; CD5, T-cell surface glycoprotein CD5; FLT3LG, Fms-related tyrosine kinase 3 ligand; TRANCE, TNF-related activation-induced cytokine.
As presented in Figure 3, employing a significance threshold of <5.0 × 10-6, 8 sets of suggestively significant correlations (p < 0.05) were obtained. The findings of the IVW method revealed that elevated levels of IL-13 (OR = 1.202, 95% CI = 1.018–1.422, p = 0.030), IL-18R1 (OR = 1.029, 95% CI = 1.029–1.157, p = 0.004), tumor necrosis factor ligand superfamily member 14 (TNFSF14) (OR = 1.159, 95% CI = 1.018–1.320, p = 0.026) and TNF-related activation-induced cytokine (TRANCE) (OR = 1.111, 95% CI = 1.016–1.214, p = 0.020) were suggestively correlated with an increased risk of AD. In addition, the IVW method also revealed causal relationships between circulating levels of CXCL11 (OR = 0.878, 95% CI = 0.783–0.984, p = 0.025), IL-33 (OR = 0.809, 95% CI = 0.661–0.991, p = 0.041), TNF-beta (TNF-β) (OR = 0.945, 95% CI = 0.896–0.997, p = 0.038) and CD5 (OR = 0.764, 95% CI = 0.652–0.895, p = 8.26e-04) and a decreased risk of AD. After applying Bonferroni correction (p = 0.05/91 = 5.50e-04), none of the cytokines showed a statistically significant association with AD. The scatter plots of MR analyses for above 8 cytokines are shown in Supplementary Figure S2. Detailed information on the SNP profiles, pleiotropy, and heterogeneity are presented in the tables in the Supplementary Tables S6 and S7. No heterogeneity or pleiotropy was observed in the cytokines, and there was no evidence of weak IV bias. No outliers were found. The details of MR analyses involving 91 cytokines and AD are presented in Supplementary Table S8.
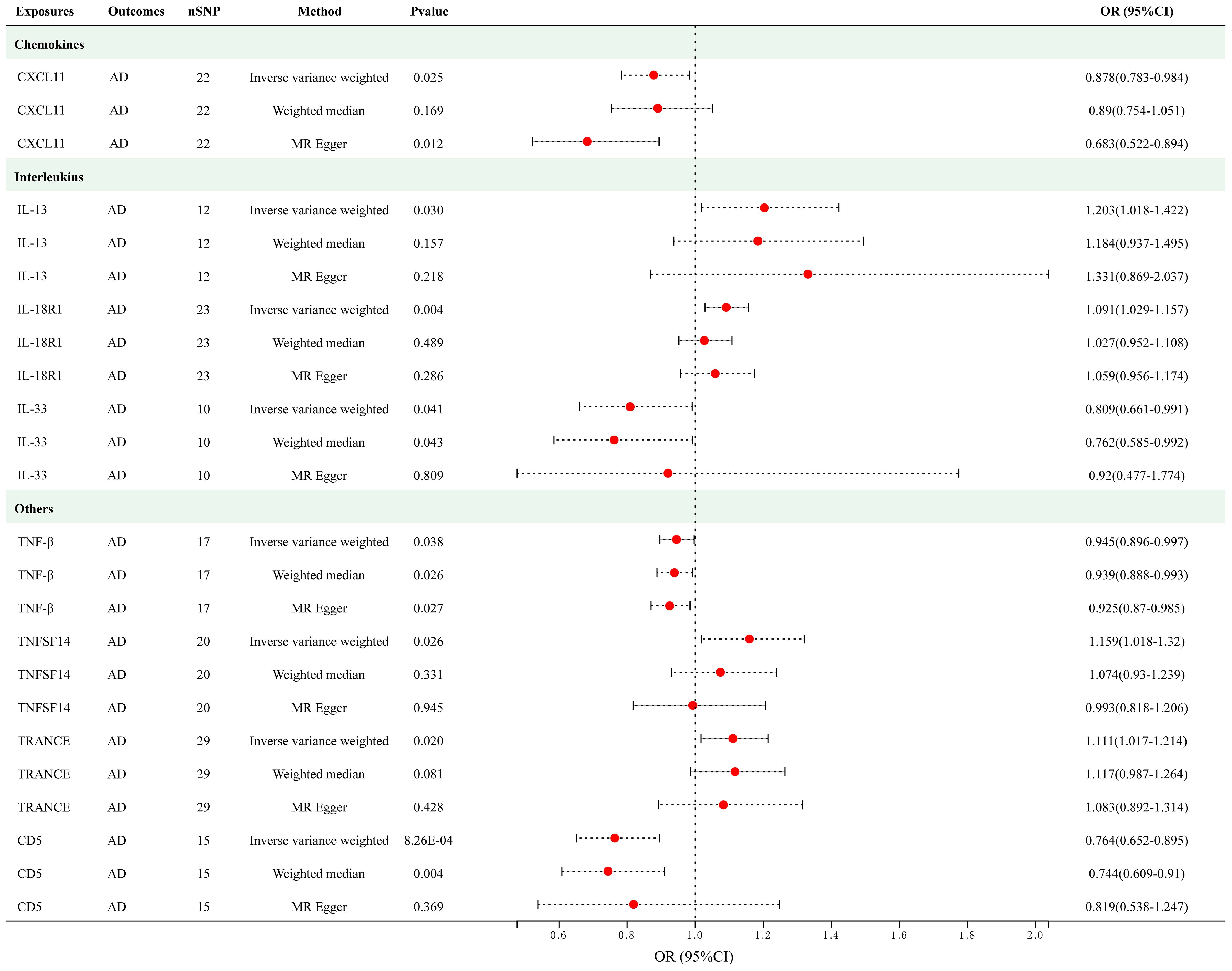
Figure 3 Causal correlations of 8 cytokines with atopic dermatitis (SNP associations reaching a significance level of p < 5×10-6). CXCL11, C-X-C motif chemokine 11; IL-13, interleukin-13; IL-18 R1, interleukin-18 receptor 1; IL-33, interleukin-33; TNF-β, TNF-beta; TNFSF14, Tumor necrosis factor ligand superfamily member 14; TRANCE, TNF-related activation-induced cytokine; CD5, T-cell surface glycoprotein CD5.
3.2 Causal effects of AD on circulating cytokines
Twelve significant SNPs were extracted as the IVs for AD. Figure 4 presents the results of the MR analysis investigating the causality between AD and inflammatory cytokines. The findings of the IVW method revealed that AD was suggestively associated with an decreased level of Axin-1 (Beta = -0.080, 95% CI = -0.144–0.015, p = 0.016), CXCL5 (Beta = -0.062, 95% CI = -0.119–0.004, p = 0.036), CXCL10 (Beta = -0.062, 95% CI = -0.132–1.572e-04, p = 0.016), Oncostatin-M (OSM) (Beta = -0.073, 95% CI = -0.13–0.015, p = 0.013), Sulfotransferase 1A1 (SULT1A1) (Beta = -0.089, 95% CI = -0.154–0.023, p = 0.008) and TNFSF14 (Beta = -0.079, 95% CI = -0.151–0.007, p = 0.031). The scatter plots of MR analyses for above results are shown in Supplementary Figure S3. Supplementary Tables S9 and S10 include information about the SNPs, heterogeneity, and pleiotropy for these groups. No heterogeneity or pleiotropy was observed, and the F-statistics indicated the absence of weak IV bias. No outliers were found. Detailed results of reverse MR analyses are presented in Supplementary Table S11.
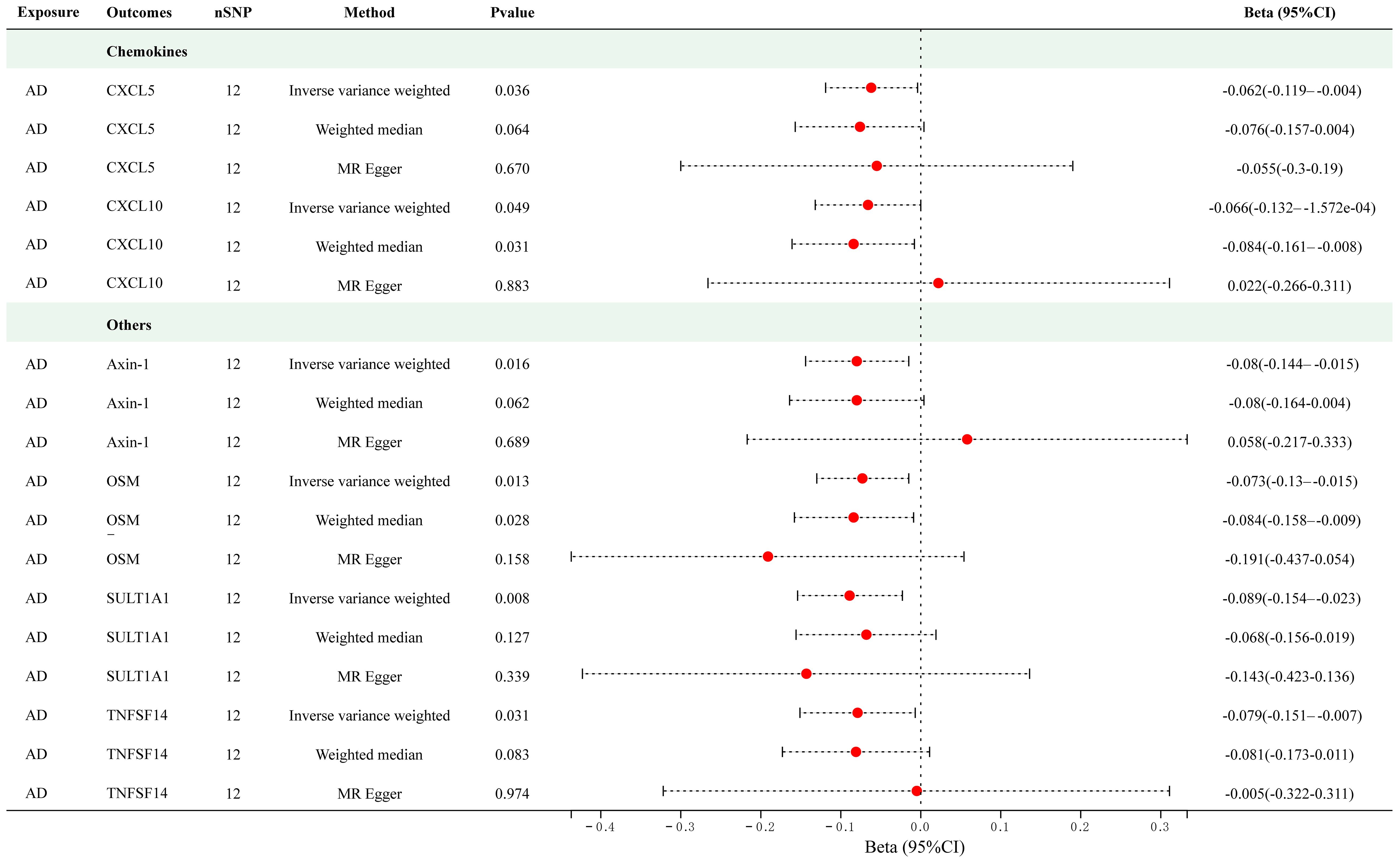
Figure 4 Causal correlations of atopic dermatitis with 6 cytokines (SNP associations reaching a significance level of p < 5×10-8). CXCL5, C-X-C motif chemokine 5; CXCL10, C-X-C motif chemokine 10; OSM, Oncostatin-M; SULT1A1, Sulfotransferase 1A1; TNFSF14, Tumor necrosis factor ligand superfamily member 14.
3.3 Validation of the results in FinnGen GWAS data
To further verify our results, we utilized an additional AD GWAS dataset from the FinnGen consortium R8 release data, which comprised 11,964 cases and 306,909 controls of European ancestry. As presented in Supplementary Figure S4, employing the IVW methodology, we observed a reduced risk of AD associated with CD5 (OR = 0.860, 95% CI = 0.778–0.952, p = 0.004). Furthermore, the IVW analysis revealed an elevated AD risk correlated with IL-18R1 (OR = 1.103, 95% CI = 1.048–1.161, p = 0.046). While the IVW method did not yield uniformly conclusive results, alternative methodologies, specifically the MR Egger (OR = 1.160, 95% CI = 1.021–1.317, p = 0.034) and the WM method (OR = 1.183, 95% CI = 1.051–1.332, p = 0.005), suggested potential correlations implicating TNFSF14 with a heightened risk of AD. Also, the MR Egger analysis reinforced an augmented AD risk in connection with TRANCE (OR = 1.139, CI =1.011–1.283, p = 0.044). The scatter plots of MR analyses for above results are shown in Supplementary Figure S5. Detailed results of the MR analyses for validation are presented in Supplementary Table 12. No heterogeneity or pleiotropy was observed, and no outliers were found. Collectively, these outcomes fortify the probable causative roles of CD5, IL-18R1, TNFSF14, and TRANCE in AD etiology, significantly bolstering the rigor and reproducibility of our initial discoveries to a considerable extent.
3.4 Expression patterns of protein drivers in AD patients
To gain more mechanistic insights into AD, we undertook a more in-depth investigation into four inflammatory factors that have emerged as elevating risks for AD, namely IL-13, IL-18R1, TNFSF14, and TRACNE. Considering that circulating inflammatory factors primarily originate from tissues and cellular components within the circulation, we sought to investigate the expression profiles of these four factors in both skin tissue and PBMCs to further validate our research outcomes. To this end, we compared the expression levels of these factors in lesional and non-lesional skin biopsies from 57 AD patients utilizing transcriptome databases. We observed that the gene expression of IL18R1 (Figure 5A), TNFSF14 (Figure 5B), TRANCE (Figure 5C) and IL13 (Figure 5D) was significantly increased in AD lesional samples compared with non-lesional samples, which is consistent with the results of our MR analysis. Augmenting these bioinformatic analyses, we executed qPCR assays on PBMCs derived from AD patients and healthy volunteers to contrast the expression patterns of the four inflammatory markers. Our results showed that all of them were elevated in PBMCs from AD patients compared to healthy individuals (Figures 5E–H). These combined approaches serve to robustly corroborate and fortify the conclusions drawn from the MR study.
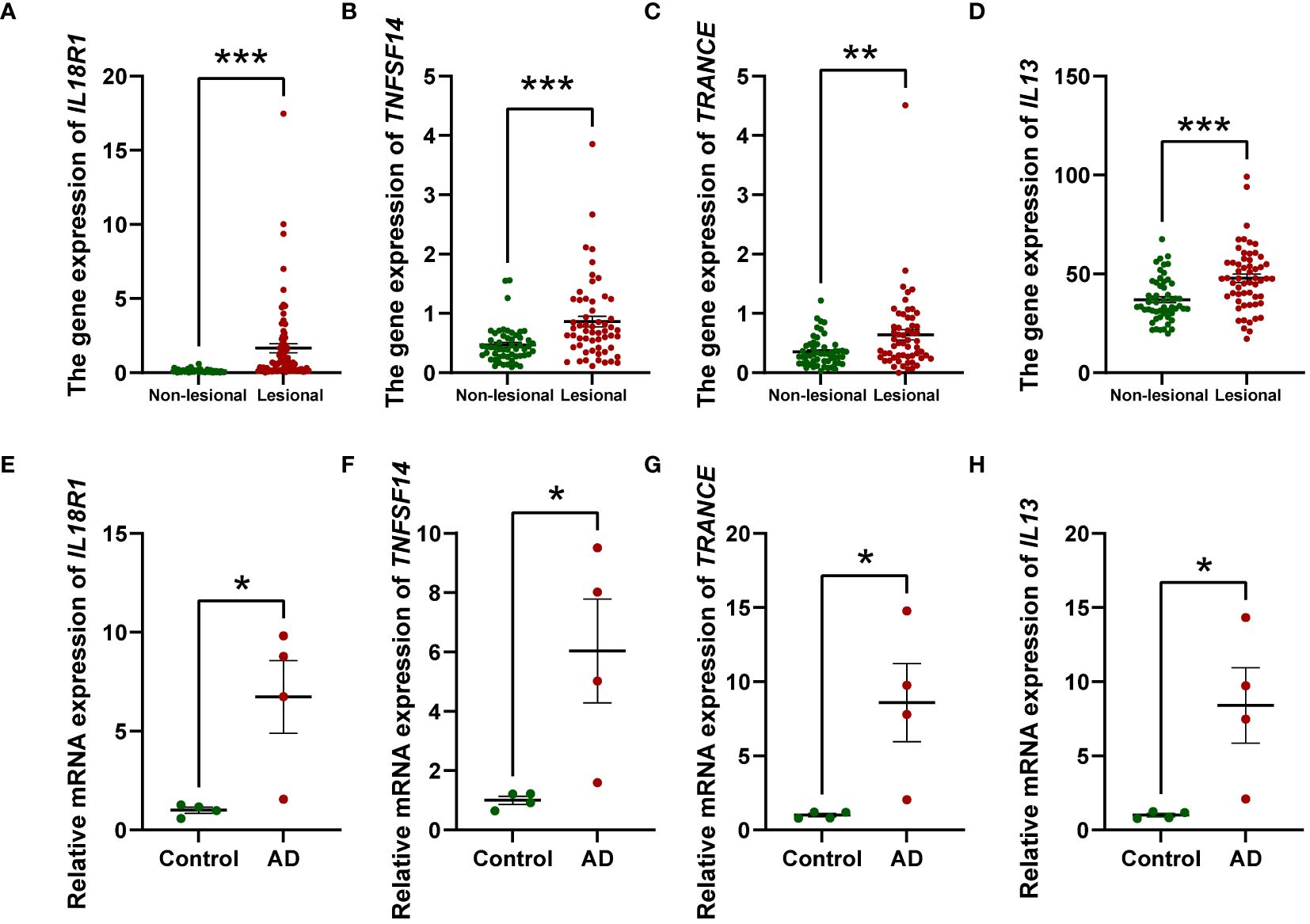
Figure 5 Relative mRNA levels of IL18R1, TNFSF14, TRANCE, and IL13 in AD lesional samples compared to non-lesional samples (A–D) and PBMCs from AD patients compared to healthy individuals (n = 4) (E–H) investigated by RT-qPCR. The relative mRNA expression was calculated based on the 2−ΔΔCt approach. Data are presented as mean ± SEM of three independent experiments. The significance level was set at p ≤ 0.05 (*), p ≤ 0.01 (**), and p ≤ 0.001 (***).
4 Discussion
AD is a systemic inflammatory disease characterized by variational levels of inflammatory cytokines in the serum of affected individuals (26). The roles of circulating inflammatory cytokines in AD, however, are subject to ongoing debate. It remains unclear whether they serve as primary drivers of AD or if they are instead downstream players during progression of the disease. The “outside-to-inside” mechanism suggests that the dysfunction of the barrier is the primary etiology of AD and subsequently induces immunological alterations (27). On the contrary, the “inside-to-outside” proposal insists inflammatory factors serve as initial pathological causes to disturb permeability barrier homeostasis. To advance our understanding, there is an imperative need for meticulous studies, such as MR studies to furnish empirical evidence supporting either hypothesis.
Until now, several studies have explored the casual effects of circulating cytokines and skin diseases utilizing MR. Li et al. investigated the causal relationship between inflammatory cytokines and three immunoinflammatory dermatoses, and revealed inhibitory roles of IL-4 and IL-1RA in the risk of developing AD (28). The findings of this study diverge from ours, potentially attributable to variations in GWAS selections and dissimilarities in study designs. This unidirectional MR study confined its scope to an examination of only 41 inflammatory mediators and did not validate the results in clinical samples, thereby imparting a considerable degree of limitation to the conclusions drawn. Another work featured an extensive pQTL analysis employing the Olink Target platform, alongside MR investigations utilizing 58 plasma proteins with cis-pQTLs, to clarify the causal roles of plasma proteins in AD (14). And their recognition of IL-18R1 as a driver of AD resonates with our findings. Despite the breadth of this investigation, its analytical limitations and inadequate focus on AD might hindered the dissection of the intricate causal dynamics between inflammatory cytokines and AD. To unravel the complex etiological mechanisms of AD, we capitalized on the GWAS data encompassing 91 plasma cytokines from the original study, conducting a bidirectional two-sample MR analysis to scrutinize causal links between these cytokines and AD. To surmount prior methodological limitations, we adopted a trio of MR approaches – IVW, MR-Egger, and WM – to mitigate biases effectively. To cover all 91 cytokines, we set a genome-wide significance threshold of <10-6 when discussing the results. When considering circulating cytokines as the exposures, our analysis showed that IL-13, IL-18R1, TNFSF14 and TRANCE were potential upstream causal contributors to the development of AD, while CXCL11, IL-33, TNF-β and CD5 appeared to be associated with the decreased risk of AD. Additionally, when using AD as the exposure in our MR analysis, we found that AD was associated with reduced circulating levels of Axin-1, CXCL5, CXCL10, OSM, SULT1A1 and TNFSF14 through causative mechanisms. To validate these findings, we capitalized on additional GWAS data from the FinnGen biobank, reinforcing the significant causal relationships between AD and CD5, IL-18R1, TNFSF14, and TRANCE. Our investigation further disclosed elevated expression of four driver cytokines in lesional versus non-lesional skin biopsies from AD patients. Complementary RT-qPCR assays in PBMCs from AD patients demonstrated elevated expression of these cytokines relative to healthy controls, consolidating the validity of our research conclusions through an integrated strategy.
Extensive research has explored the correlations between inflammatory factors and AD, which supported the results of our study. IL-13, predominantly secreted by Th2 cells and group 2 innate lymphoid cells (ILC2s) in the dermis, has emerged as a cardinal type 2 cytokine driving inflammatory processes in AD (29). Its pathogenic role encompasses a broad spectrum of effects on cutaneous biology, notably the recruitment of inflammatory cells, modulation of the skin microbiome, and compromise of the epidermal barrier (29). IL-18, initially characterized as an “interferon-γ-inducing factor”, is synthesized by a diverse array of cell types, inclusive of keratinocytes, dermal macrophages, and dendritic cells (30). The level of IL-18 in AD lesions was significantly higher in comparison to healthy controls and was correlated with AD severity (31). The binding of IL-18 to its receptor IL-18R1 on mast cells and basophils amplifies the concentrations of IL-4 and IL-13 within AD lesions, culminating in inflammatory cascades (32). TNFSF14, chiefly synthesized by CD4+ and CD8+ T cells, has garnered attention in the context of AD (33). Compared to healthy counterparts, AD patients exhibit markedly elevated serum levels of TNFSF14, positioning it as a potential biomarker for assessing disease severity (34). The role of TNFSF14 in AD is multifaceted, with implications for keratinocyte stimulation. Conditional ablation of TNFSF14 receptors in keratinocytes has been shown to confer protection against allergen-induced skin inflammation in murine models of AD. Furthermore, exposure of human keratinocytes to recombinant TNFSF14 triggers transcriptional alterations in genes pertinent to AD pathogenesis (35). Our study suggested causal associations between these cytokines and AD, which further substantiates their roles as upstream factors in the pathogenesis of AD. Limited studies have investigated the role of TRANCE in the pathogenesis of AD. TRANCE, a member of the TNF superfamily, is mainly expressed by stromal cells, activated T cells and B cells (36, 37). Our MR analysis found that elevated levels of circulating TRANCE were associated with an increased risk of AD, providing new mechanistic insights into the pathogenesis of AD. The TRANCE-involved pathways have been reported to enhance the secretion of inflammatory cytokines in dendritic cells (38), and the characteristic expansion of inflammatory dendritic cells in AD lesions has been substantiated through several single-cell RNA sequencing analyses (39, 40). Therefore, we hypothesize that TRANCE may instigate AD by priming dendritic cells to adopt a pro-inflammatory phenotype.
Acknowledging the significance of targeting pivotal upstream cytokines in the management of AD, recent advancements have indeed underscored their therapeutic potential. Notably, the approval of dupilumab, an antagonist of IL-4 and IL-13, for treating patients with moderate-to-severe AD exemplifies this promising approach (41). Our research findings bear considerable therapeutic implications, as they unveil novel targets that may permit targeted intervention like biologicals on the very origin of AD pathology. Rigorous validation and well-designed clinical trials will be essential in ascertaining the therapeutic efficacy and safety of targeting these newly identified cytokines in patient populations.
In addition, our MR analysis revealed negative causal associations between several circulating cytokines and the risk of AD, including TNF-β, CD5, CXCL11, and IL-33. Previous research has explored the roles of these cytokines. For instance, it has been reported that TNF-β downregulates the expression of IL-5 and IL-13 in PBMCs, and the blood levels of TNF-β were significantly lower in AD patients compared to normal levels (42), which aligns with our results. In addition, TNF-β is required for efficient regulatory T cells (Tregs) migration and suppressive function (43, 44), which might be helpful in treating AD as Tregs are essential in controlling type 2 inflammation (45). Although the down-regulatory roles of some factors in inflammatory responses were well-established, their associations with AD have received little attention. CD5, a transmembrane receptor (46), has been identified as a negative regulator of the T-cell receptor signaling pathway in both thymocytes and T lymphocytes (47–49). CD5 deficiency leads to an enhanced phosphorylation of Signal Transducer and Activator of Transcription 3 (STAT3) in T lymphocytes and primes them for an increased production of Interleukin-17A (50), a cytokine that has been substantiated as pivotal in the pathogenesis of AD, particularly in its more severe manifestations (51). Furthermore, intravenous immune globulin (IVIG) therapy increased the serum CD5 levels in patients with AD, along with the improvement of disease severity (52). The chemokine CXCL11 binds to CXCR3 receptors on CD4+ T cells (53) and inhibits inflammatory autoimmune responses (54). Further investigation is required to explore associations between these cytokines and AD. Notably, we found that higher levels of IL-33 genetic prediction were associated with a lower risk of atopic dermatitis, which seemingly contradicts prior research findings. As an alarmin, IL-33 recruits various cells to the dermis, including ILC2, which produce type 2 cytokines like IL-5 and IL-13 (55, 56). However, the primary site where IL-33 functions as an alarmin is predominantly limited to the skin, rather than the bloodstream. In addition, it is noteworthy that IL-33 antibody failed to demonstrate any benefits in a Phase II double-blind randomized controlled study involving patients with moderate-to-severe AD (57). Therefore, the role of circulating IL-33 in AD patients may be less prominent than initially hypothesized, and its precise function within the disease context remains an intriguing question awaiting further exploration.
In clinical practice, the restoration of immune cell populations and functionality through cytokine therapy presents a promising avenue for the treatment of inflammatory diseases. A case in point is the utilization of low-dose IL-2 therapy, given its pivotal role in expanding the Treg population (58). This approach has been successfully employed in the management of various inflammatory diseases, notably systemic lupus erythematosus and rheumatoid arthritis (59). Our research findings provide the potential for employing low-dose injection of these key cytokines as a therapeutic strategy for AD, and further foundational and clinical investigations are expected to substantiate the efficacy and ascertain the safety of such an intervention.
When AD was defined as the exposure in the MR analysis, it suggestively resulted in decreased circulating levels of Axin-1, CXCL5, CXCL10, OSM, SULT1A1 and TNFSF14 through causative pathways. The roles of several aforementioned cytokines in AD are sparingly investigated. For example, current research investigating Axin-1, a scaffold protein, and SULT1A1, an essential isoform of phenol SULT enzymes, is mainly focused on their roles in oncogenesis in different tissue (60–63). Further clinical and basic studies are imperative to validate our findings. In contrast, some of these inflammatory factors are tightly linked with AD, according to previous studies. OSM, a pro-inflammatory cytokine released by macrophages, monocytes, dendritic cells, and T lymphocytes (64), is up-regulated in the AD lesions (65). OSM can sensitize sensory neurons during episodes of inflammatory pruritus (66). CXCL5, a neutrophil-attracting chemokine, and CXCL10, a member of Th1 chemokine, are significantly up-regulated in skin biopsies of AD patients (67, 68). Of note, our results revealed that AD can decrease the serum levels of TNFSF14 in a causal pathway, while in turn, TNFSF14 is an etiology of AD according to our study when it is considered as an exposure. It is possible that AD reduces the levels of these cytokines through a negative feedback mechanism. Additional research is necessary to explore their underlying mechanistic roles in AD.
Our findings seem to align with the “inside-to-outside” hypothesis as several inflammatory cytokines have been implicated as etiological factors in AD, whereas none of the alarmins show a causal association with AD. However, our study has several limitations. First, cytokines analyzed in this study were quantified in plasma rather than in skin tissue. Proteomic analyses revealed that increases in inflammatory proteins in AD-affected skin compared to healthy controls were more pronounced than those found in the blood, suggesting that the skin exhibits the most evident inflammatory profile in AD (69). Therefore, circulating inflammatory factors may not accurately reflect the inflammatory status of the skin tissue, and a sole focus on inflammatory factors in the blood may neglect other crucial factors and fail to provide a comprehensive understanding of AD. Currently, there is relatively limited GWAS data on inflammatory factors in skin tissue, making it challenging to infer causal relationships between cutaneous inflammatory factors and AD using MR. Second, our findings may primarily apply to European populations due to the origin of the dataset, failing to capture the whole picture. Recent research indicated that AD exhibits distinct pathological mechanisms among various ethnicities and age groups (70). Th2 and Th17 immune pathways has been reportedly activated in Asian patients, while patients of European descent with atopic dermatitis exhibit predominantly Th2 pathway activation (71). In the future, more heterogeneous GWAS data, or those specifically stratified by age or disease severity in AD patients may be utilized as better sources for such MR analysis to identify more precise causal relationships between inflammatory factors and AD. Finally, since cytokine levels as dynamic biomarkers are subject to fluctuations, our analysis failed to capture the temporal variability of cytokines. Therefore, although the causative roles of circulating cytokines in AD support the “inside-to-outside” proposal, the “outside-to-inside” theory cannot be dismissed. Further biological experiments and clinical trials are expected to verify these hypotheses and provide more specific mechanistic insights.
5 Conclusion
Figure 6 depicts the findings of our study, which implicates elevated serum concentrations of IL-13, IL-18R1, TNFSF14 and TRANCE as being suggestive of an elevated risk for AD. This associative link is corroborated by observations of elevated gene expression levels in both lesional skin tissues and PBMCs derived from AD patients, whereas TNF-β, CD5, CXCL11 and IL-33 levels were negatively associated with the risk of AD. In contrast, AD was associated with reduced circulating levels of Axin-1, CXCL5, CXCL10, OSM, SULT1A1 and TNFSF14. Our research findings carry considerable therapeutic potential, as they unveil novel targets that may permit targeted intervention like biologicals on the very origin of AD pathology. Also, these discoveries herald the prospect of employing injections of immunoregulatory cytokines as a promising and efficacious therapeutic strategy. By identifying these critical junctures in the disease’s progression, we lay the groundwork for treatments that modulate the body’s intrinsic cytokine network, potentially leading to more potent and tailored interventions for AD patients.
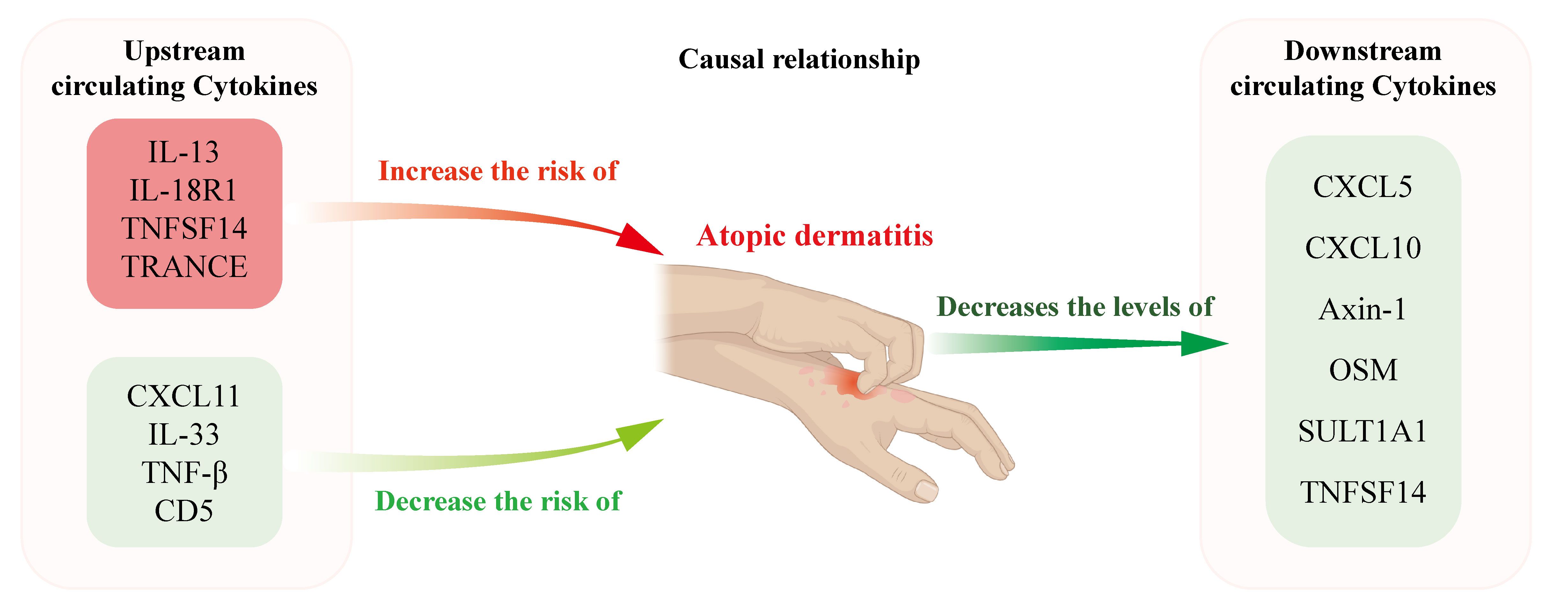
Figure 6 Schematic representation illustrating the postulated causal relationships and direction of causal effects between AD and the levels of circulating cytokines. Elevated circulating levels of IL-13, IL-18R1, TNFSF14, and TRACNE were suggestively associated with an increased risk of AD, whereas TNF-β, CD5, CXCL11, and IL-33 levels were negatively associated with the risk of AD. In contrast, AD was associated with reduced circulating levels of Axin-1, CXCL5, CXCL10, OSM, SULT1A1 and TNFSF14.
Data availability statement
Publicly available datasets were analyzed in this study. This data can be found here: The data that support this study are openly available. GWAS data of AD for exploration could be downloaded from ieu open gwas project (https://gwas.mrcieu.ac.uk/datasets/ieu-a-996). GWAS data of AD for validation could be downloaded from the FinnGen consortium R8 release data (https://console.cloud.google.com/storage/browser/finngen-public-data-r8/summary_stats). Summary statistics of 91 circulating cytokines could be downloaded from GWAS Catalog (https://www.ebi.ac.uk/gwas/publications/37563310).
Ethics statement
The studies involving humans were approved by Xinhua Hospital, Shanghai Jiaotong University School of Medicine. The studies were conducted in accordance with the local legislation and institutional requirements. The participants provided their written informed consent to participate in this study.
Author contributions
ZX: Conceptualization, Data curation, Supervision, Writing – original draft. XC: Methodology, Supervision, Writing – original draft. WZ: Data curation, Methodology, Writing – original draft. YS: Data curation, Formal Analysis, Writing – original draft. ZS: Formal Analysis, Writing – original draft. HZ: Writing – original draft, Writing – review & editing. ZY: Supervision, Writing – review & editing.
Funding
The author(s) declare financial support was received for the research, authorship, and/or publication of this article. This work was supported by funds from the National Nature Science Foundation of China (81903197, 82173413), and the most important clinical discipline in Shanghai (2017ZZ2016–02).
Acknowledgments
We sincerely thanks for the researcher who provided the public GWAS data used in this study.
Conflict of interest
The authors declare that the research was conducted in the absence of any commercial or financial relationships that could be construed as a potential conflict of interest.
Publisher’s note
All claims expressed in this article are solely those of the authors and do not necessarily represent those of their affiliated organizations, or those of the publisher, the editors and the reviewers. Any product that may be evaluated in this article, or claim that may be made by its manufacturer, is not guaranteed or endorsed by the publisher.
Supplementary material
The Supplementary Material for this article can be found online at: https://www.frontiersin.org/articles/10.3389/fimmu.2024.1367958/full#supplementary-material
Abbreviations
AD, Atopic dermatitis; IL, interleukin; TSLP thymic stromal lymphopoietin; Th, T helper cell type; MR, Mendelian randomization; IVs, instrumental variables; GWAS, genome-wide association study; SNPs, single nucleotide polymorphisms; IVW, inverse variance weighted; WM, weighted median; OR, Odds ratios; CI, confidence intervals; PRESSO, Pleiotropy RESidual Sum and Outlier; TRANCE, TNF-related activation-induced cytokine; CXCL, C-X-C motif chemokine; FLT3LG, Fms-related tyrosine kinase 3 ligand; TNFSF14, tumor necrosis factor ligand superfamily member 14; TNF-β, TNF-beta; OSM, Oncostatin-M; SULT1A1, Sulfotransferase 1A1.
References
1. Mennini M, Di Nardo G, Fiocchi AG. Atopic dermatitis: time for tailored therapy. Lancet. (2022) 400:252–3. doi: 10.1016/S0140-6736(22)01331-9
2. Langan SM, Irvine AD, Weidinger S. Atopic dermatitis. Lancet. (2020) 396:345–60. doi: 10.1016/S0140-6736(20)31286-1
3. Li H, Zhang Z, Zhang H, Guo Y, Yao Z. Update on the pathogenesis and therapy of atopic dermatitis. Clin Rev Allergy Immunol. (2021) 61:324–38. doi: 10.1007/s12016-021-08880-3
4. Hatano Y, Elias PM. "Outside-to-inside," "inside-to-outside," and "Intrinsic" Endogenous pathogenic mechanisms in atopic dermatitis: keratinocytes as the key functional cells involved in both permeability barrier dysfunction and immunological alterations. Front Immunol. (2023) 14:1239251. doi: 10.3389/fimmu.2023.1239251
5. Leung DYM, Berdyshev E, Goleva E. Cutaneous barrier dysfunction in allergic diseases. J Allergy Clin Immunol. (2020) 145:1485–97. doi: 10.1016/j.jaci.2020.02.021
6. Sano Y, Masuda K, Tamagawa-Mineoka R, Matsunaka H, Murakami Y, Yamashita R, et al. Thymic stromal lymphopoietin expression is increased in the horny layer of patients with atopic dermatitis. Clin Exp Immunol. (2013) 171:330–7. doi: 10.1111/cei.12021
7. Imai Y, Yasuda K, Sakaguchi Y, Haneda T, Mizutani H, Yoshimoto T, et al. Skin-specific expression of il-33 activates group 2 innate lymphoid cells and elicits atopic dermatitis-like inflammation in mice. Proc Natl Acad Sci U.S.A. (2013) 110:13921–6. doi: 10.1073/pnas.1307321110
8. Elias PM, Steinhoff M. "Outside-to-inside" (and now back to "Outside") pathogenic mechanisms in atopic dermatitis. J Invest Dermatol. (2008) 128:1067–70. doi: 10.1038/jid.2008.88
9. Gruber R, Börnchen C, Rose K, Daubmann A, Volksdorf T, Wladykowski E, et al. Diverse regulation of claudin-1 and claudin-4 in atopic dermatitis. Am J Pathol. (2015) 185:2777–89. doi: 10.1016/j.ajpath.2015.06.021
10. Howell MD, Kim BE, Gao P, Grant AV, Boguniewicz M, Debenedetto A, et al. Cytokine modulation of atopic dermatitis filaggrin skin expression. J Allergy Clin Immunol. (2007) 120:150–5. doi: 10.1016/j.jaci.2007.04.031
12. Wootton RE, Richmond RC, Stuijfzand BG, Lawn RB, Sallis HM, Taylor GMJ, et al. Evidence for causal effects of lifetime smoking on risk for depression and schizophrenia: A mendelian randomisation study. Psychol Med. (2020) 50:2435–43. doi: 10.1017/S0033291719002678
13. Miao L, Deng GX, Yin RX, Nie RJ, Yang S, Wang Y, et al. No causal effects of plasma homocysteine levels on the risk of coronary heart disease or acute myocardial infarction: A mendelian randomization study. Eur J Prev Cardiol. (2021) 28:227–34. doi: 10.1177/2047487319894679
14. Zhao JH, Stacey D, Eriksson N, Macdonald-Dunlop E, Hedman ÅK, Kalnapenkis A, et al. Genetics of circulating inflammatory proteins identifies drivers of immune-mediated disease risk and therapeutic targets. Nat Immunol. (2023) 24:1540–51. doi: 10.1038/s41590-023-01588-w
15. Hartwig FP, Davies NM, Hemani G, Davey Smith G. Two-sample mendelian randomization: avoiding the downsides of a powerful, widely applicable but potentially fallible technique. Int J Epidemiol. (2016) 45:1717–26. doi: 10.1093/ije/dyx028
16. Paternoster L, Standl M, Waage J, Baurecht H, Hotze M, Strachan DP, et al. Multi-ancestry genome-wide association study of 21,000 cases and 95,000 controls identifies new risk loci for atopic dermatitis. Nat Genet. (2015) 47:1449–56. doi: 10.1038/ng.3424
17. Sanna S, van Zuydam NR, Mahajan A, Kurilshikov A, Vich Vila A, Võsa U, et al. Causal relationships among the gut microbiome, short-chain fatty acids and metabolic diseases. Nat Genet. (2019) 51:600–5. doi: 10.1038/s41588-019-0350-x
18. Bowden J, Del Greco MF, Minelli C, Davey Smith G, Sheehan NA, Thompson JR. Assessing the suitability of summary data for two-sample mendelian randomization analyses using mr-egger regression: the role of the I2 statistic. Int J Epidemiol. (2016) 45:1961–74. doi: 10.1093/ije/dyw220
19. Burgess S, Thompson SG. Avoiding bias from weak instruments in mendelian randomization studies. Int J Epidemiol. (2011) 40:755–64. doi: 10.1093/ije/dyr036
20. Burgess S, Butterworth A, Thompson SG. Mendelian randomization analysis with multiple genetic variants using summarized data. Genet Epidemiol. (2013) 37:658–65. doi: 10.1002/gepi.21758
21. Bowden J, Davey Smith G, Burgess S. Mendelian randomization with invalid instruments: effect estimation and bias detection through egger regression. Int J Epidemiol. (2015) 44:512–25. doi: 10.1093/ije/dyv080
22. Bowden J, Davey Smith G, Haycock PC, Burgess S. Consistent estimation in mendelian randomization with some invalid instruments using a weighted median estimator. Genet Epidemiol. (2016) 40:304–14. doi: 10.1002/gepi.21965
23. Verbanck M, Chen CY, Neale B, Do R. Detection of widespread horizontal pleiotropy in causal relationships inferred from mendelian randomization between complex traits and diseases. Nat Genet. (2018) 50:693–8. doi: 10.1038/s41588-018-0099-7
24. Möbus L, Rodriguez E, Harder I, Stölzl D, Boraczynski N, Gerdes S, et al. Atopic dermatitis displays stable and dynamic skin transcriptome signatures. J Allergy Clin Immunol. (2021) 147:213–23. doi: 10.1016/j.jaci.2020.06.012
25. Barrett T, Wilhite SE, Ledoux P, Evangelista C, Kim IF, Tomashevsky M, et al. Ncbi geo: archive for functional genomics data sets–update. Nucleic Acids Res. (2013) 41:D991–5. doi: 10.1093/nar/gks1193
26. Thijs JL, Strickland I, Bruijnzeel-Koomen C, Nierkens S, Giovannone B, Knol EF, et al. Serum biomarker profiles suggest that atopic dermatitis is a systemic disease. J Allergy Clin Immunol. (2018) 141:1523–6. doi: 10.1016/j.jaci.2017.12.991
27. Elias PM, Hatano Y, Williams ML. Basis for the barrier abnormality in atopic dermatitis: outside-inside-outside pathogenic mechanisms. J Allergy Clin Immunol. (2008) 121:1337–43. doi: 10.1016/j.jaci.2008.01.022
28. Li J, Lu Y, Zhao X. Exploring the causal relationship between inflammatory cytokines and immunoinflammatory dermatoses: A mendelian randomization study. Front Med (Lausanne). (2024) 11:1263714. doi: 10.3389/fmed.2024.1263714
29. Bieber T. Interleukin-13: targeting an underestimated cytokine in atopic dermatitis. Allergy. (2020) 75:54–62. doi: 10.1111/all.13954
30. Tsutsui H, Mizutani H, Nakanishi K. Contribution of interleukin 18 to the development of infection-associated atopic dermatitis. Curr problems Dermatol. (2011) 41:93–103. doi: 10.1159/000323302
31. Andersson AM, Sølberg J, Koch A, Skov L, Jakasa I, Kezic S, et al. Assessment of biomarkers in pediatric atopic dermatitis by tape strips and skin biopsies. Allergy. (2022) 77:1499–509. doi: 10.1111/all.15153
32. Wang X, Wang L, Wen X, Zhang L, Jiang X, He G. Interleukin-18 and il-18bp in inflammatory dermatological diseases. Front Immunol. (2023) 14:955369. doi: 10.3389/fimmu.2023.955369
33. Ware CF, Croft M, Neil GA. Realigning the light signaling network to control dysregulated inflammation. J Exp Med. (2022) 219. doi: 10.1084/jem.20220236
34. Seifeldin NS, El Sayed SB, Asaad MK, Aly AA. Role of the tumor necrosis factor family member light in the pathogenesis of atopic dermatitis. Int J Dermatol. (2015) 54:e376–82. doi: 10.1111/ijd.12851
35. Gupta RK, Figueroa DS, Fung K, Miki H, Miller J, Ay F, et al. Light signaling through ltβr and hvem in keratinocytes promotes psoriasis and atopic dermatitis-like skin inflammation. J Autoimmun. (2024) 144:103177. doi: 10.1016/j.jaut.2024.103177
36. Theill LE, Boyle WJ, Penninger JM. Rank-L and rank: T cells, bone loss, and mammalian evolution. Annu Rev Immunol. (2002) 20:795–823. doi: 10.1146/annurev.immunol.20.100301.064753
37. Lacey DL, Timms E, Tan HL, Kelley MJ, Dunstan CR, Burgess T, et al. Osteoprotegerin ligand is a cytokine that regulates osteoclast differentiation and activation. Cell. (1998) 93:165–76. doi: 10.1016/S0092-8674(00)81569-X
38. Josien R, Li HL, Ingulli E, Sarma S, Wong BR, Vologodskaia M, et al. Trance, a tumor necrosis factor family member, enhances the longevity and adjuvant properties of dendritic cells in vivo. J Exp Med. (2000) 191:495–502. doi: 10.1084/jem.191.3.495
39. He H, Suryawanshi H, Morozov P, Gay-Mimbrera J, Del Duca E, Kim HJ, et al. Single-cell transcriptome analysis of human skin identifies novel fibroblast subpopulation and enrichment of immune subsets in atopic dermatitis. J Allergy Clin Immunol. (2020) 145:1615–28. doi: 10.1016/j.jaci.2020.01.042
40. Mitamura Y, Reiger M, Kim J, Xiao Y, Zhakparov D, Tan G, et al. Spatial transcriptomics combined with single-cell rna-sequencing unravels the complex inflammatory cell network in atopic dermatitis. Allergy. (2023) 78:2215–31. doi: 10.1111/all.15781
41. Gooderham MJ, Hong HC, Eshtiaghi P, Papp KA. Dupilumab: A review of its use in the treatment of atopic dermatitis. J Am Acad Dermatol. (2018) 78:S28–s36. doi: 10.1016/j.jaad.2017.12.022
42. Jirapongsananuruk O, Donahue HL, Trumble AE, Leung DY. The modulation of cytokine and ige production by tumor necrosis factor-beta in atopic dermatitis. J Invest Dermatol. (2000) 114:200–3. doi: 10.1046/j.1523-1747.2000.00847.x
43. Saxena V, Piao W, Li L, Paluskievicz C, Xiong Y, Simon T, et al. Treg tissue stability depends on lymphotoxin beta-receptor- and adenosine-receptor-driven lymphatic endothelial cell responses. Cell Rep. (2022) 39:110727. doi: 10.1016/j.celrep.2022.110727
44. Brinkman CC, Iwami D, Hritzo MK, Xiong Y, Ahmad S, Simon T, et al. Treg engage lymphotoxin beta receptor for afferent lymphatic transendothelial migration. Nat Commun. (2016) 7:12021. doi: 10.1038/ncomms12021
45. Venuprasad K, Kong YC, Farrar MA. Control of th2-mediated inflammation by regulatory T cells. Am J Pathol. (2010) 177:525–31. doi: 10.2353/ajpath.2010.090936
46. Voisinne G, Gonzalez de Peredo A, Roncagalli R. Cd5, an undercover regulator of tcr signaling. Front Immunol. (2018) 9:2900. doi: 10.3389/fimmu.2018.02900
47. Tarakhovsky A, Kanner SB, Hombach J, Ledbetter JA, Müller W, Killeen N, et al. A role for cd5 in tcr-mediated signal transduction and thymocyte selection. Science. (1995) 269:535–7. doi: 10.1126/science.7542801
48. Persaud SP, Parker CR, Lo WL, Weber KS, Allen PM. Intrinsic cd4+ T cell sensitivity and response to a pathogen are set and sustained by avidity for thymic and peripheral complexes of self peptide and mhc. Nat Immunol. (2014) 15:266–74. doi: 10.1038/ni.2822
49. Azzam HS, DeJarnette JB, Huang K, Emmons R, Park CS, Sommers CL, et al. Fine tuning of tcr signaling by cd5. J Immunol. (2001) 166:5464–72. doi: 10.4049/jimmunol.166.9.5464
50. Schuster C, Kiaf B, Hatzihristidis T, Ruckdeschel A, Nieves-Bonilla J, Ishikawa Y, et al. Cd5 controls gut immunity by shaping the cytokine profile of intestinal T cells. Front Immunol. (2022) 13:906499. doi: 10.3389/fimmu.2022.906499
51. Bapat SP, Whitty C, Mowery CT, Liang Y, Yoo A, Jiang Z, et al. Obesity alters pathology and treatment response in inflammatory disease. Nature. (2022) 604:337–42. doi: 10.1038/s41586-022-04536-0
52. Noh G, Lozano F. Intravenous immune globulin effects on serum-soluble cd5 levels in atopic dermatitis. Clin Exp Allergy J Br Soc Allergy Clin Immunol. (2001) 31:1932–8. doi: 10.1046/j.1365-2222.2001.01124.x
53. Colvin RA, Campanella GS, Manice LA, Luster AD. Cxcr3 requires tyrosine sulfation for ligand binding and a second extracellular loop arginine residue for ligand-induced chemotaxis. Mol Cell Biol. (2006) 26:5838–49. doi: 10.1128/MCB.00556-06
54. Karin N. Cxcr3 ligands in cancer and autoimmunity, chemoattraction of effector T cells, and beyond. Front Immunol. (2020) 11:976. doi: 10.3389/fimmu.2020.00976
55. Nakajima S, Nomura T, Common J, Kabashima K. Insights into atopic dermatitis gained from genetically defined mouse models. J Allergy Clin Immunol. (2019) 143:13–25. doi: 10.1016/j.jaci.2018.11.014
56. Moro K, Yamada T, Tanabe M, Takeuchi T, Ikawa T, Kawamoto H, et al. Innate production of T(H)2 cytokines by adipose tissue-associated C-kit(+)Sca-1(+) lymphoid cells. Nature. (2010) 463:540–4. doi: 10.1038/nature08636
57. Laquer V, Parra V, Lacour JP, Takahashi H, Knorr J, Okragly AJ, et al. Interleukin-33 antibody failed to demonstrate benefit in a phase ii, double-blind, randomized, placebo-controlled study in adult patients with moderate-to-severe atopic dermatitis. Br J Dermatol. (2022) 187:599–602. doi: 10.1111/bjd.21631
58. Mosanya CH, Isaacs JD. Tolerising cellular therapies: what is their promise for autoimmune disease? Ann rheumatic Dis. (2019) 78:297–310. doi: 10.1136/annrheumdis-2018-214024
59. Riaz MF, Garg G, Umeano L, Iftikhar S, Alhaddad SF, Paulsingh CN, et al. Comparison of low-dose interleukin 2 therapy in conjunction with standard therapy in patients with systemic lupus erythematosus vs rheumatoid arthritis: A systematic review. Cureus. (2024) 16:e56704. doi: 10.7759/cureus.56704
60. Sanson R, Lazzara SL, Cune D, Pitasi CL, Trentesaux C, Fraudeau M, et al. Axin1 protects colon carcinogenesis by an immune-mediated effect. Cell Mol Gastroenterol Hepatol. (2023) 15:689–715. doi: 10.1016/j.jcmgh.2022.10.017
61. Xu C, Xu Z, Zhang Y, Evert M, Calvisi DF, Chen X. B-catenin signaling in hepatocellular carcinoma. J Clin Invest. (2022) 132. doi: 10.1172/JCI154515
62. Kurogi K, Rasool MI, Alherz FA, El Daibani AA, Bairam AF, Abunnaja MS, et al. Sult genetic polymorphisms: physiological, pharmacological and clinical implications. Expert Opin Drug Metab Toxicol. (2021) 17:767–84. doi: 10.1080/17425255.2021.1940952
63. Daniels J, Kadlubar S. Sulfotransferase genetic variation: from cancer risk to treatment response. Drug Metab Rev. (2013) 45:415–22. doi: 10.3109/03602532.2013.835621
64. Masjedi A, Hajizadeh F, Beigi Dargani F, Beyzai B, Aksoun M, Hojjat-Farsangi M, et al. Oncostatin M: A mysterious cytokine in cancers. Int Immunopharmacol. (2021) 90:107158. doi: 10.1016/j.intimp.2020.107158
65. Suehiro M, Numata T, Saito R, Yanagida N, Ishikawa C, Uchida K, et al. Oncostatin M suppresses il31ra expression in dorsal root ganglia and interleukin-31-induced itching. Front Immunol. (2023) 14:1251031. doi: 10.3389/fimmu.2023.1251031
66. Tseng PY, Hoon MA. Oncostatin M can sensitize sensory neurons in inflammatory pruritus. Sci Transl Med. (2021) 13:eabe3037. doi: 10.1126/scitranslmed.abe3037
67. Kalish H, Phillips TM. Assessment of chemokine profiles in human skin biopsies by an immunoaffinity capillary electrophoresis chip. Methods. (2012) 56:198–203. doi: 10.1016/j.ymeth.2011.12.003
68. He H, Olesen CM, Pavel AB, Clausen ML, Wu J, Estrada Y, et al. Tape-strip proteomic profiling of atopic dermatitis on dupilumab identifies minimally invasive biomarkers. Front Immunol. (2020) 11:1768. doi: 10.3389/fimmu.2020.01768
69. Pavel AB, Zhou L, Diaz A, Ungar B, Dan J, He H, et al. The proteomic skin profile of moderate-to-severe atopic dermatitis patients shows an inflammatory signature. J Am Acad Dermatol. (2020) 82:690–9. doi: 10.1016/j.jaad.2019.10.039
70. Czarnowicki T, He H, Krueger JG, Guttman-Yassky E. Atopic dermatitis endotypes and implications for targeted therapeutics. J Allergy Clin Immunol. (2019) 143:1–11. doi: 10.1016/j.jaci.2018.10.032
Keywords: atopic dermatitis, cytokines, inflammation, immunotherapy, Mendelian randomization
Citation: Xuan Z, Chen X, Zhou W, Shen Y, Sun Z, Zhang H and Yao Z (2024) Exploring causal correlations between circulating cytokines and atopic dermatitis: a bidirectional two-sample Mendelian randomization study. Front. Immunol. 15:1367958. doi: 10.3389/fimmu.2024.1367958
Received: 09 January 2024; Accepted: 01 July 2024;
Published: 11 July 2024.
Edited by:
Laila Karra, University of California, San Francisco, United StatesReviewed by:
Yonghu Sun, Shandong Provincial Hospital of Dermatology, ChinaAlvarado Anabell, University of Guadalajara, Mexico
Alexandr Ceasovschih, Grigore T. Popa University of Medicine and Pharmacy, Romania
Laura Atzori, University of Cagliari, Italy
Copyright © 2024 Xuan, Chen, Zhou, Shen, Sun, Zhang and Yao. This is an open-access article distributed under the terms of the Creative Commons Attribution License (CC BY). The use, distribution or reproduction in other forums is permitted, provided the original author(s) and the copyright owner(s) are credited and that the original publication in this journal is cited, in accordance with accepted academic practice. No use, distribution or reproduction is permitted which does not comply with these terms.
*Correspondence: Hui Zhang, Y196aGFuZ2h1aUBzaW5hLmNvbQ==; Zhirong Yao, eWFvemhpcm9uZ0B4aW5odWFtZWQuY29tLmNu
†These authors have contributed equally to this work and share first authorship