- 1The Second Clinical Medical College, Tongji Medical College, Huazhong University of Science and Technology, Wuhan, Hubei, China
- 2Department and Institute of Infectious Disease, Tongji Hospital, Tongji Medical College and State Key Laboratory for Diagnosis and Treatment of Severe Zoonotic Infectious Disease, Huazhong University of Science and Technology, Wuhan, Hubei, China
Since the approval for the treatment of melanoma in 2014, immune checkpoint inhibitors (ICIs) have revolutionized the therapy pattern across various malignancies. Coinciding with their frequent usage, their adverse effects, including fever, cannot be neglected. In the context of cancer diseases and cancer treatments, fever of unknown origin (FUO), which has long posed a challenge for clinicians in terms of diagnosis and management, brings forth new connotation and significance. In this paper review, we present the concept of ICIs-associated FUO, consider activated immune system and elevated cytokines as common mechanisms by which ICIs induce fever and various immune-related adverse events (irAEs), summarize and compare the primary etiologies of ICI-associated FUO, and compare it with conventional types of FUO.
1 Introduction
Cancer cells attenuate the anti-tumor immune response, promote their proliferation and metastasis through the expression of immune checkpoint proteins (1). Immune-checkpoint inhibitors (ICIs), which reverse aforementioned tumor-mediated immune evasion by blocking immune checkpoints, have revolutionized the treatment of malignant tumors since the approval in 2014 (2). Currently, FDA-approved ICIs comprise monoclonal antibodies targeting the PD-1 - PD-L1 axis or CTLA-4 - CD28 axis (3, 4) (Table 1). Concomitant with the surge of ICIs usage, immune-related adverse events (irAEs) have garnered increasing attention. The irAEs involve respiratory, digestive, nervous, hematological, and endocrine systems (5), and imbalances in their immune status may be accompanied by fever.
For over a century, fever of unknown origin (FUO) has been a diagnostic and therapeutic challenge for clinicians. FUO, characterized by the inability to clarify the cause of fever despite reasonable investigations in inpatient or outpatient settings, lacks consensus regarding specific febrile temperatures or fever duration (6). In general, FUO are classified as classic FUO, nosocomial FUO, immunodeficiency-associated FUO, and travel-associated FUO. Given that 1) ICIs are administered more frequently in cancer therapy; 2) pyrexia stands as one of the most common adverse events of ICIs (7, 8); 3) patients treated with ICIs face challenges in identifying and discriminating the etiology of FUO, we believe that it is of crucial clinical significance to list ICIs-associated FUO as a separate category of FUO, which is defined as fever during ICIs administration in cancer patients with immune activation, cytokines secretion or pathogen reactivation.
In this review, we describe the mechanisms by which ICIs leads to fever, summarize the main etiologies, pathogenesis and characteristics of ICIs-associated FUO and make comparison among them, and compare ICIs-associated FUO with conventional types.
2 Mechanisms of post-treatment pyrexia associated with ICIs
2.1 Endogenous pyrogens and other fever-related cytokines
Fever is caused by the activation of pyrogenic agents, which activate endogenous pyrogen (EP)-producing cells to generate and release EP. This results in an upward adjustment of the body temperature set point (SP), leading to regulated temperature elevation (6). EP mainly consists of cytokines such as interleukin (IL), tumor necrosis factor (TNF) and interferon (IFN), and the levels of these cytokines usually alter after immunotherapy (9, 10). IL-1 and IL-6 are considered to be the most important EP (9, 11), whose production and release lead to the release of prostaglandin E (PGE) from brain endothelial cells, which serves as a positive regulatory mediator of fever (6). Research has shown that blocking PGE production or attenuating its function can mitigate fever symptoms (12–14), which lays a theoretic foundation for aspirin and ibuprofen.
IFN was first found to induce fever in clinical trials, and subsequent studies have demonstrated that it entailed fever not due to contamination with endotoxins or its impact on the IL-1 pathway (15), but rather through its direct action on the thermoregulatory center. As a pro-inflammatory cytokine, TNF can result in fever through RANKL/RANK-COX2-PEG2-EP3R pathway and other experiments have proved that it can also stimulate IL-1 production both in vivo and in vitro (15, 16).
IL-2 and IL-8 have also been viewed as cytokines highly associated with fever, although there still remains controversy regarding whether they are EP in the strict sense. It has been suggested that IL-2 does not stimulate thermoregulatory center directly, but induces fever indirectly by eliciting other EP, such as circulating TNF (17). IL-8 is also thought to be associated with fever and can be utilized as a biomarker for pyrexia, infection, septicemia and neutropenia in cancer patients (17).
2.2 Alteration in cytokines’ level following ICIs treatment
Both PD-1/PD-L1 inhibitors and CTLA-4 inhibitors contribute to upregulation in the production and release of pro-inflammatory cytokines and even leads to the occurrence of cytokine release syndrome (CRS) in severe cases (18, 19). For instance, CTLA-4 monoclonal antibody leads to the release of TNF, IFN-γ and IL-2, which in turn further promotes T cell proliferation and activation (2). Experiments in vitro have demonstrated that CTLA-4 monoclonal antibody administered in melanoma cell lines will promote TNF-α production from NK cells (2). ICIs accelerate the production of IFN-γ, which increases tumor immunogenicity, curbs the proliferation and infiltration of cancer cells, attracts immune cells to malignant disease sites, and enhances the cytotoxic function of NK cells and CTLs (20). It has been demonstrated that PD-1/PD-L1 inhibitors can promote tumor-associated macrophages (TAMs) to produce IL-6, and IL-6 inhibitors can elevate Th1 responses and defers melanoma progression (21). As immune checkpoint molecules including PD-1 - PD-L1 lead to a decrease in IL-2 release, ICIs may increase IL-2 secretion to augment immune response (22). To the best of our knowledge, it is commonplace for patients to experience elevated levels of certain cytokines after ICIs treatment (Table 2), although the mechanisms of each cytokine’s alteration has not been thoroughly investigated and elucidated (20, 31).
To summarize, levels of IL-1, IL-2, IL-6, IL-8, TNF, and IFN in patients may undergo elevation after ICIs treatment. And these cytokines can function as EP or febrile activators, acting on the preoptic anterior hypothalamus (POAH) through blood-brain barrier (BBB) or organum vasculosum laminae terminalis (OVLT) (32). Consequently, the SP rises due to the interaction between the positive thermoregulatory center POAH and negative thermoregulatory centers including ventral septal area (VSA) and medial amygdaloid nucleus (MAN) (33–36). This eventually leads to the feverish state characterized by reduced heat dissipation and increased heat production. The possible mechanism of fever induced by ICIs therapy is illustrated in (Figure 1).
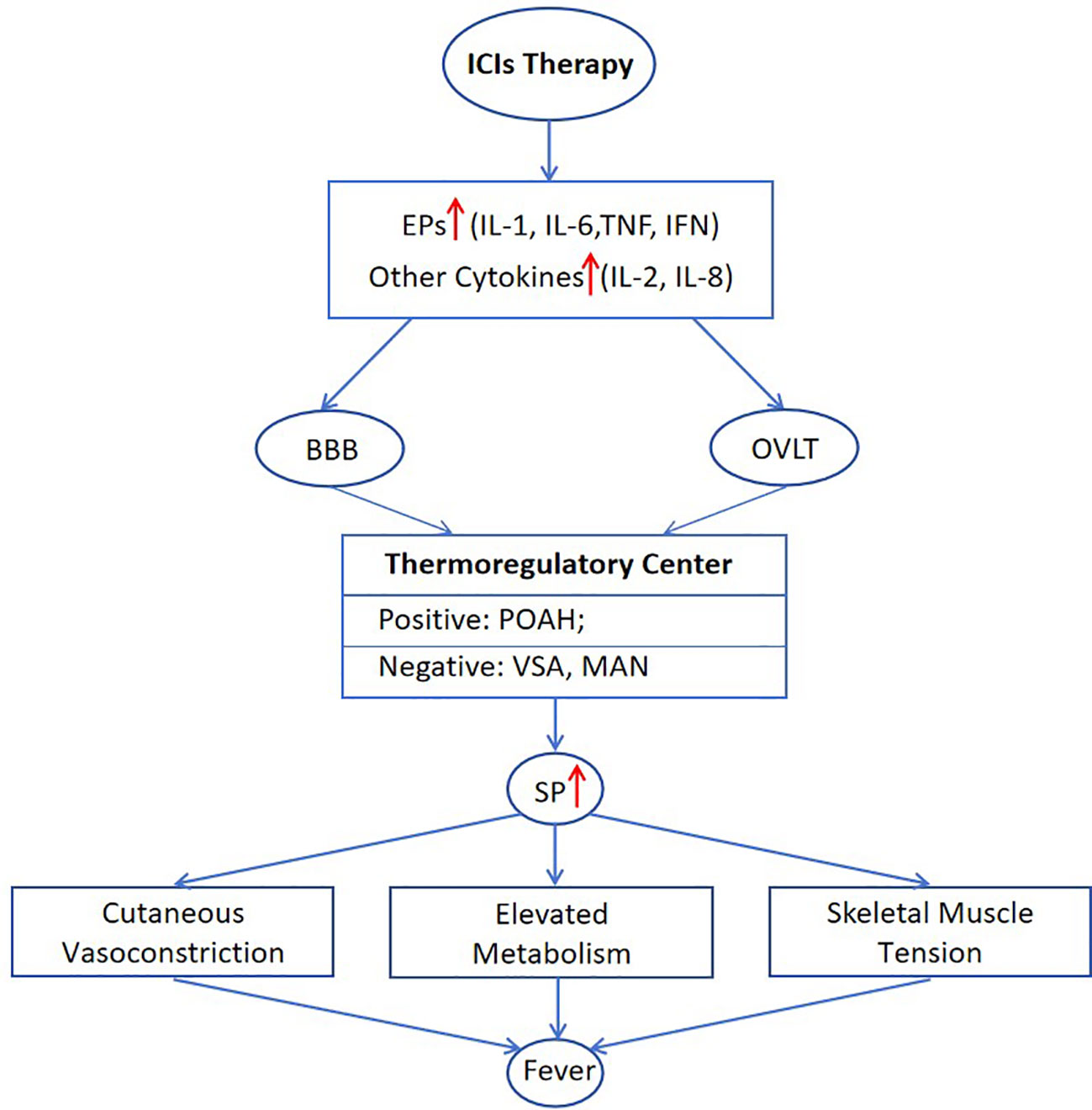
Figure 1 Mechanisms of post-treatment pyrexia associated with ICIs. BBB, blood-brain barrier; MAN, medial amygdaloid nucleus; OVLT, organum vasculosum laminae terminalis; POAH, preoptic anterior hypothalamus; SP, set point; VSA, ventral septal area.
3 Etiology of fever of unknown origin induced by ICIs
Fever, caused by a wide variety of reasons, is frequently reported in cancer patients (37), and fever is one of the most prevalent adverse events after ICIs remedy (7, 8). When immunotherapy is combined with other therapy patterns, the incidence of fever increases remarkably. For example, the rate of febrile events lifts in the combination of PD-1/PD-L1 inhibitors and chemotherapy, compared with chemotherapy alone, as shown in a meta-analysis with rates of 18.8% (85 out of 452) and 10.7% (47 out of 438) respectively (37). In NSCLC, the incidence of fever is as high as 32.5% - 38.1% in patients receiving ICIs plus chemotherapy or radiotherapy (38).
Theoretically, adverse events following ICIs treatment involve nearly every system. Considering their incidence, severity, and their association with immune dysregulation and fever, we focus on the following complications, including pneumonitis, tuberculosis (TB), colitis, hepatitis, and hematological irAEs (Haem irAEs) (5). Therefore, we aim to review fever of unknown origin associated with ICIs according to above etiologies and compare their differences.
3.1 Pneumonitis
The prevalence of checkpoint inhibitor pneumonitis (CIP) ranges from 3.5% - 19% (39), with a mortality rate of 10% - 17% (40). The potential mechanisms by which immunotherapy leads to pneumonitis have been summarized in the following four points (41). Firstly, ICIs lead to disorder in the quantity of T cell subsets, manifested as a significant increase in CD4+ T cells, with Th1 cell infiltration playing a predominant role. There is also an increase in the infiltration of CD8+ T cells, particularly those expressing PD-1, TIM-3, and TIGIT. The reduced number and inhibited function of Tregs can also contribute to CIP. Secondly, elevated levels of autoantibodies, including rheumatoid factor (RF), antinuclear antibody, antithyroglobulin, antithyroid peroxidase and CD74 autoantibodies, also contribute to CIP (40). Thirdly, consistent with what we mentioned earlier, the increase in various inflammatory cytokines such as IL-1, IL-2, IL-13, G-CSF, GM-CSF is closely associated with high-grade irAEs. In terms of CIP, the imbalance in C-reactive protein (CRP), IL-6 and IL-17 is closely associated with disease development. Fourthly, different therapy modalities exert enormous impact. Anti-PD-1 therapy has a higher incidence rate than anti-PD-L1 and anti-CTLA-4 therapy, and combination with chemotherapy or radiotherapy raises the incidence through various mechanisms (41, 42). Additionally, intestinal flora, non-coding RNA and other immune cells (B cells, NK, DC) are also relevant to the development of CIP.
The clinical presentation of CIP mainly includes dyspnea, decreased activity tolerance, and cough, whereas fever (12%) and chest pain (7%) are less common (42, 43). Consequently, case reports of CIP reveal that fever is not the essential factor for the diagnosis of CIP. In patients who claimed pyrexia, the onset of fever occurred between week 2 and week 32 after immunotherapy, with peak temperatures ranging from 38.1°C to 40 °C (43–48). However, the fever type and daily variation of body temperature were not thoroughly documented. Organizing pneumonia (OP) is the main radiological manifestation of CIP, and bronchoscopy and bronchoalveolar lavage fluid (BALF) can be utilized for differential diagnosis (39). Biomarkers including IL-17A and IL-35 from BALF may be associated with the occurrence and severity of CIP (39).
3.2 Tuberculosis
Patients undergoing ICIs administration are eight times more likely to contract TB than the general population, according to an observational study (49). A Meta-analysis revealed that patients receiving PD-1/PD-L1 in developed Asian countries were 35 times more likely to develop TB than general population (50). Hence several reports have suggested that TB screening should be performed prior to ICIs treatment (51, 52). PD-1 and PD-L1 inhibitors are associated with TB reactivation, whereas CTLA-4 inhibitors appeared to have no impact (52, 53). Immune checkpoints are closely related to TB infection, as their expression is upregulated when TB is active and is downregulated when TB is curbed. Defects in PD-1/PD-L1 exacerbate TB infection, which is associated with increased infiltration of inflammatory cells and cytokines. Thus, the expression of immune checkpoints may be a necessary condition for maintaining lymphocyte function as protective responses against TB infection (54).
Upon infection with mycobacterium tuberculosis (MTB), body’s intrinsic immune cells, mainly macrophages, recognize TB through pattern recognition receptor (PRR). Cytokines including IL-1, IL-6, TNF-α will be secreted by macrophages to guide the accumulation of lymphocytes and monocytes at the location of MTB. Among them, CD4+ Th1 T cells release loads of cytokines, encompassing TNF-α, IFN-γ, IL-2, etc., gradually forming tuberculous granuloma. After that, the body represents a state of latent infection, during which PD-1 expression is elevated, inducing T cells’ apoptosis. Nevertheless, ICIs block PD-1/PD-L1, restoring lymphocytes’ function and releasing inflammatory cytokines such as IFN-γ and TNF-α. Excessive inflammatory cells and cytokines destroy the extracellular matrix, favoring the growth of MTB, which breaks the state of latent infection and leads to the reactivation of TB (53).
TB infection following ICIs therapy lacks specificity, and is usually characterized by cough, expectoration, breathlessness, fever and weight loss (49, 53). Imaging manifestations, from chest X-ray and contrast CT, support the diagnosis with features like new consolidation, patchy opacity/nodules, or the tree-in-bud sign, especially in the upper lobes (49). However, confirmation is achieved by culture of MTB or DNA detection by polymerase chain reaction (PCR) (52, 55). Besides, interferon-gamma release assay (IGRA) testing is recommended to assess the risk of developing active TB for relevant patients (56). In case reports, we find many patients are diagnosed with TB despite the absence of symptoms of shortness of breath, cough, fever, night sweats (55, 57). In case reports with detailed fever data, we found that the fever of TB infection following ICIs therapy occurred between week 13 and week 87, with peak temperatures between 38.6°C and 39.2°C (58–61).
3.3 Hepatitis
The incidence rate of developing immune-mediated hepatitis (IMH) in patients receiving immunotherapy ranges from 5% to 10% (62). The risk of liver toxicity is higher with CTLA-4 inhibitors, reaching up to 15%, and the figure is even higher when multiple ICIs are used in combination (63). Possible mechanisms include cytotoxicity due to complement activation, but such theory fails to elaborate why the liver would be a specific target (62). It has been proposed that ICIs block immunosuppressive signals and stimulate T cells’ function, activation and proliferation. Activated T cells, under the influence of adhesion molecules, adhere to the hepatic sinusoids. Fas receptors on activated T cells bind to FasL expressed on liver sinusoidal endothelial cells and Kupffer cells, resulting in apoptosis of activated T cells. Kupffer cells, activated by Fas/FasL binding and IFN-γ secreted by T cells, release TNF-α to render hepatocytes more sensitive to IFN-γ-mediated apoptosis, resulting in apoptosis and injury of hepatocytes ultimately (62).
Although most IMH cases are asymptomatic, a small fraction of them may exhibit fatigue (17.1%), abdominal discomfort (14.0%), fever (14.0%), rash (4.3%), and jaundice (3.7%) (64, 65). Reports indicate that fever is more prevalent in CTLA-4 inhibitors treatment than PD-1/PD-L1 inhibitors treatment (66). Elevated ALT or AST exceeding twice the upper limit of normal is regarded as an indicator of IMH (64). Imaging findings manifest hepatomegaly, peri-portal edema and lymphadenopathy, which are non-specific (62). The histological pattern of PD1/PD-L1-associated IMH tend to exhibit lobular hepatitis, whereas CTLA-4-associated IMH is more inclined to granulomatous hepatitis (66). Fever of IMH typically appears between day 3 and week 26 and ranges 38°C to 40°C (66, 67).
3.4 Colitis
The incidence of immune-mediated colitis (IMC) is approximately 3.6% (68). Statistics indicate that the incidence of all-grade colitis after PD-1/PD-L1 treatment is around 1% - 1.6% while the figure for CTLA-4 treatment can be as high as 8.8% - 9.1% (68). The inflammation in the colonic mucosa is attributed to higher level of cytokines released by CD4+ T cells and the mucosal infiltration of CD8+ T cells with enhanced cytotoxicity and proliferative state (69). IL-1, IL-10, IL-17 and transforming growth factor-β1 (TGF-β1) may serve as biomarkers of IMC (68).
Symptoms of IMC include abdominal pain (20%), abdominal bloating, nausea and vomiting (15%), fever (12%), along with the presence of mucus and blood in stool, and signs of peritoneal irritation (68, 70). Endoscopy is considered the gold standard for diagnosing IMC. Laboratory tests, including complete blood count, CRP and erythrocyte sedimentation rate, stool testing for infectious pathogens, and virus or parasites infections are used as adjuncts in the diagnostic process (68). Pathological biopsy lacks specificity due to its diverse histologic manifestations, but CT can evaluate the degree of inflammatory changes in IMC, which includes bowel wall thickening, mesenteric engorgement, fat stranding, and fluid-filled bowel (68). It has been observed that fever onset occurs relatively early, ranging from day 5 to week 9, with reports extending to week 43 or week 147. The peak temperature typically ranges from 37.2°C to 39°C (70–77).
3.5 Neutropenia
Patients receiving ICIs treatment exhibit reduced risk of neutropenia compared to those undergoing chemotherapy. Despite this, neutropenia remains one of the most common Haem irAEs, accounting for approximately one-fourth of hematologic complications (78, 79). Notably, neutropenia induced by ICIs, although rare, mostly presents as grade 4 (absolute neutrophil count < 500 cells/µL), posing a risk of fatal septic shock and is thus described as a life-threatening side effect (80, 81). In such cases, a substantial number of patients must permanently discontinue ICIs treatment (81, 82). A retrospective study based on FAERS database highlighted the incidence and severity of neutropenia, identifying fever and neutropenia as common fatal adverse events in PD-L1 monotherapy and the most prevalent fatal adverse events in PD-L1 combined with bevacizumab (83). The study highlights that combination therapies, the current research hot spot, can help patients delay tumor progression and reduce mortality compared with monotherapy (84). However, it also comes with a significantly increased incidence of neutropenia and fever. Therefore, a deeper understanding of ICIs-induced neutropenia could assist clinicians in more effectively managing irAEs and making informed treatment decisions. The mechanism underlying ICIs-induced neutropenia remains to be explored. According to the current research, we summarize that a portion of neutropenia may occur due to the activation of T cells, leading to widespread infiltration of cytotoxic T cells into the bone marrow tissue. This type is also referred to as the central type. Correspondingly, the peripheral type may arise due to the production of anti-neutrophil antibodies, impeding granulocytes’ maturation (85, 86). Therefore, immunological examination of anti-neutrophil antibodies and bone marrow examination can help identify potential causes.
In cases of ICIs-induced neutropenia, patients may not exhibit any symptoms, with abnormalities often detected through laboratory tests. Due to the role of neutrophils in innate immune defense, patients are susceptible to infection-related fever, and the proportion of febrile neutropenia typically usually exceeds 50% (85, 87, 88). While most fevers observed in ICIs-related trials are categorized as grade 1 or 2 adverse reactions, febrile neutropenia is consistently described as grade 3-5, which is most likely to result in the discontinuation of ICIs therapy (37). In a retrospective study involving 35 patients with Haem irAEs registered in three pharmacovigilance databases, 9 patients developed neutropenia, of which 6 patients (66.7%) progressed to febrile neutropenia due to infection. Notably, 2 deaths associated with ICIs were both attributed to febrile neutropenia (78). Despite the effectiveness of steroids in alleviating Haem irAEs, it is recommended to administer G-CSF and antibiotics to patients with febrile neutropenia instead of choosing systemic steroid treatment. The onset of febrile neutropenia commonly occurs in the third or fourth cycle of treatment, typically around 6 to 12 weeks after the initial ICIs treatment. The peak temperature of patients generally exceeds 38°C, with most cases ranging between 38°C and 39°C (89–96).
3.6 Cytokine release syndrome
Cytokine release syndrome (CRS) is relatively rare in patients treated with ICIs, as evidenced by an incidence of approximately 4.6% reported in a single-center retrospective study (19, 97, 98). However, once it occurs, it tends to be persistently severe and potentially life-threatening (99, 100). CRS, as a systemic inflammatory response mediated by inflammatory cytokines, is directly triggered by immunotherapy. It was initially used to describe the toxic side effects associated with the anti-T-cell antibody OKT3. Subsequently, it has been observed in various immune-related therapies, including anti-thymocyte globulin (ATG), rituximab, chimeric antigen receptor (CAR) T cell therapy (101–103). Thus, with the greater success of immunotherapy, there is growing interest in investigating the mechanisms, diagnosis, and treatment of CRS. T cells activated during immunotherapy can produce inflammatory cytokines such as IFN-γ, TNF, and GM-CSF. Furthermore, the lysis of tumor cells is also attributed to the elevated level of these cytokines, which can activate macrophages to secrete inflammatory mediators, including the crucial cytokines IL-6 and IL-1 (104, 105). IL-6 has been proven to actively participate in the systemic pathological response in CRS patients. It initiates the subsequent release of cytokines by signaling to non-immune tissues such as endothelial cells, triggering cascade reaction that results in severe symptoms, including vascular leakage, circulation failure, complement activation, and disseminated intravascular coagulation (106). In addition, IL-1 can also contribute to downstream cascade reaction, resulting in the release of large amounts of cytokines. Moreover, IL-1 can signal to the hypothalamus and pituitary gland to induce fever and has been found a strong association with neurotoxicity (104, 107). Given the critical role of IL-6 and IL-1 in CRS, the corresponding IL-6R inhibitors (Tocilizumab) and IL-1R inhibitors (Anakinra) have been identified for their effectiveness in treatment (19, 108, 109).
The clinical symptoms and severity of CRS exhibit wide variation. Mild cases may manifest with symptoms such as fever, headache, myalgia, and nausea, while severe cases can present with hypotension, cardiac dysfunction, cerebral edema, myocarditis, hepatic and renal failure, and other organ-related severe symptoms (19, 110). Due to the nonspecific feature of CRS symptoms, there may be overlapping symptoms with tumor lysis syndrome, infections, and neutropenia, which need to be carefully identified and considered for attribution (110). Fever, a hallmark of CRS, typically occurs around 11.0 days after the initiation of ICIs. Interestingly, patients with severe CRS may present with fever significantly later (111). CRS patients generally experience high fever, and a review of published case reports indicates that peak temperatures induced by ICIs range from 38.5°C to 40.3°C, with most cases exceeding 39°C (99, 100, 109, 112–114).
3.7 Hemophagocytic lymphohistiocytosis
Hemophagocytic lymphohistiocytosis (HLH) is a rare but critical immune complication characterized by an overactive immune system that results in multi-system damage (115–117). The observational study of the VigiBase database reveals an incidence of ICIs associated with HLH at approximately 0.08% (118). However, the incidence of life-threatening or fatal conditions in these HLH patients alarmingly reaches 44% (118). Similar to CRS, HLH is attributed to a systemic inflammatory response. The key pathophysiological process involves the over-activation of CD8+ T-cells and macrophages, leading to the secretion of substantial amounts of pro-inflammatory cytokines, including IFN-γ, TNF-α, IL-1, IL-4, IL-6, and IL-8. The excessive cytokine levels in serum lead to organ damage and systemic organ failure (119, 120). Current research highlights IFN-γ as a pivotal player in HLH. This inflammatory factor not only induces fever and activates macrophages but also hampers bone marrow and blood cell production, leading to cytopenia and lymph node disease (121, 122). Consequently, targeting IFN-γ emerges as a potential strategy to improve the prognosis of HLH patients.
According to the HLH-2004 and Hscore diagnostic criteria, common symptoms in patients with HLH include fever and splenomegaly (123, 124). Typical laboratory findings include 2 or 3 lineages of peripheral blood cytopenias, hypertriglyceridemia, hypofibrinogenemia, elevated serum ferritin, and sCD25 (125). The biopsy can reveal hemophagocytosis in the bone marrow, spleen, or lymph nodes (126). It is worth noting that many symptoms overlap between CRS and HLH. Severe CRS may present similar clinical and laboratory manifestations of HLH, including elevated serum ferritin and triglycerides, which makes it challenging to distinguish from primary HLH (127). Despite theoretical differences in cytokine levels, such as the higher levels of IL-6 in CRS patients, the challenge lies in the variability of baseline inflammatory cytokine levels in cancer patients, making it unreliable as a discriminatory factor (110). It has been suggested in the literature that CRP, an IL-6 reactant produced by the liver, can serve as a reliable surrogate for IL-6 bioactivity, aiding in the differentiation between the two complications (19, 128). ICIs-induced HLH typically occurs between 3 to 15 weeks after the initiation of ICIs, with a median of 7 weeks, often observed during the third or fourth cycle of treatment (118, 129). One of the diagnostic criteria for HLH is the presence of a fever higher than 38.5°C. According to our review, patients with ICIs-induced HLH frequently exhibit hyperthermia, with peak temperatures ranging between 38.6°C and 40.5°C (115–117, 124, 130, 131).
4 Comparison between ICIs-related FUO with other FUO types
It is generally accepted that FUO is mainly categorized into classic FUO, nosocomial FUO, and immunodeficiency-associated FUO and others (6). In this review, we aim to summarize a novel type FUO, namely ICIs-associated FUO. First and foremost, we make comparison of the etiology, incidence, clinical presentation, diagnostic methods and fever condition in various types of ICI-associated FUO (Table 3). The comparison of their time to onset of fever and peak body temperature is illustrated in (Figure 2). It can be noted that patients with CRS and HLH tend to experience higher febrile temperatures, whereas patients with TB, IMC, and neutropenia typically present febrile temperatures below 39°C. The majority of patients develop fever symptom within 25 weeks of the initiation of ICIs, with TB being the exception, possibly because reactivation of TB is associated with the patient’s latent infection status and the nutritional and immune condition, which warrants further investigation. Notably, CRS, an acute systemic inflammatory response, has the earliest onset time of fever among all irAEs. Moreover, through the in-depth exploration of the pathogenic mechanisms, we summarized immune activation, elevation of cytokines, and infection or reactivation of pathogens as crucial features of ICI-associated FUO. Consequently, laboratory tests of cytokines and pathogens can serve as two crucial clues for diagnosis in clinical practice.
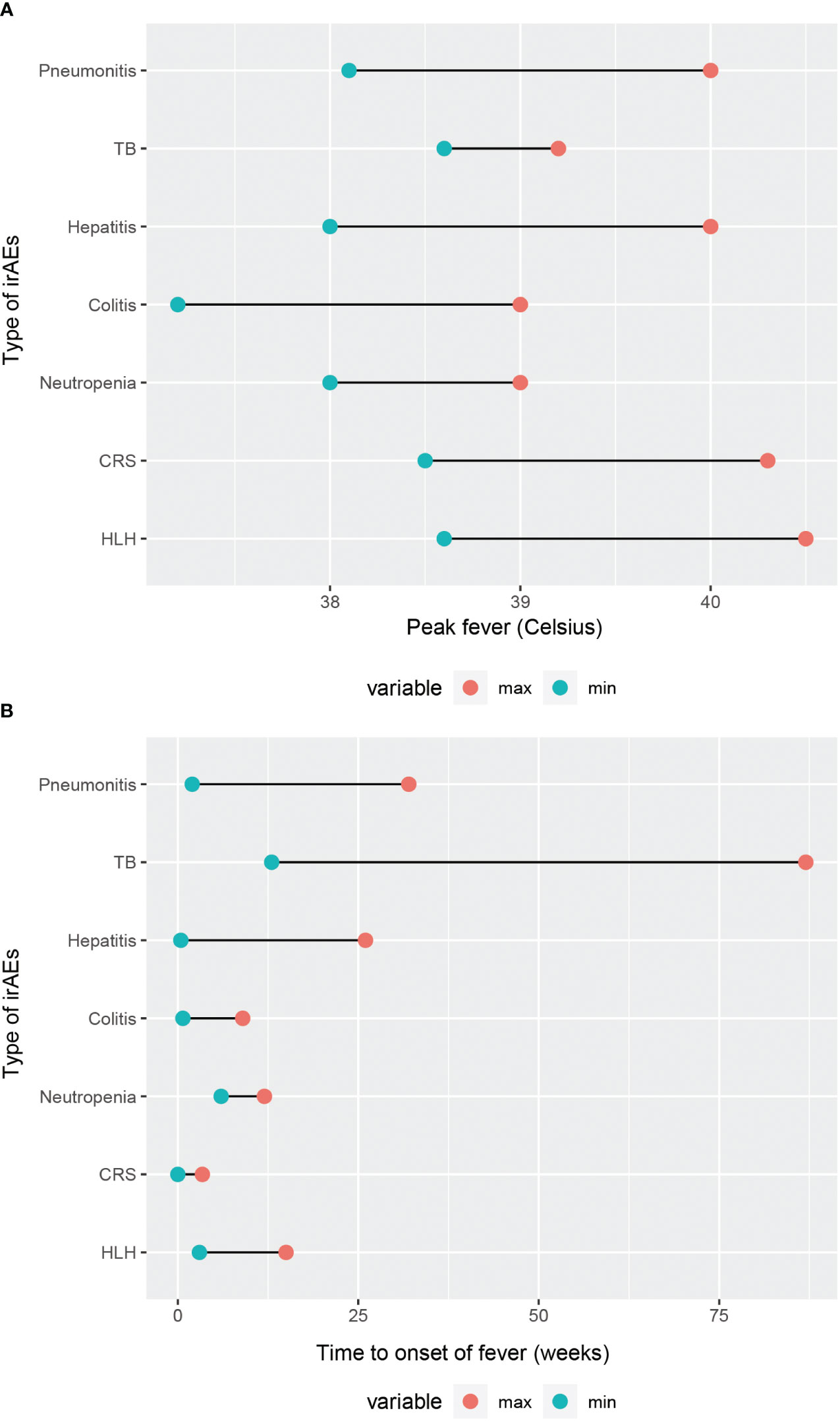
Figure 2 Comparison of fever condition in different types of irAEs. (A) Range of peak fever. (B) Time to onset of fever.
Subsequently, we conducted a comparison between ICIs-associated FUO with classic FUO, nosocomial FUO, neutropenia-associated FUO, and HIV infection-associated FUO. The main points of comparison encompass their definitions, patient distributions, primary etiologies, key elements in history inquiry and physical examination, auxiliary tests, management, and clinical course (Table 4) (6, 132–134). Instead of identifying a particular cutoff as peak temperature and duration time in the definition, we emphasize that the mechanism of ICIs-associated FUO involves autoimmune activation induced by ICIs, leading to the release of EP and other fever-associated cytokines. ICIs-associated FUO occurs in both outpatients and hospitalized patients, whose potential etiologies theoretically encompass all adverse events caused by ICIs. Therefore, during history taking, attention should be paid to the patient’s medication and treatment history, as well as their cancer conditions. Targeted physical examinations and diagnostic investigations should be carried out accordingly.
5 Discussion
It is common for cancer patients to experience fever triggered by the tumor, infection, and various treatment patterns. Drug-induced fever in cancer patients has grabbed sufficient attention, as relevant guidelines have been developed (37). It is of vital importance to clarify the causes and mechanisms of fever. The mechanisms of irAEs include augmented T cells’ response to normal and tumor tissues, elevated levels of cytokines, increased levels of autoantibodies, and enhanced complement-mediated inflammation (135). However, while this might account for the occurrence of Haem irAEs induced by ICIs, it falls short of demonstrating the specificity of adverse events occurring in individual solid organs. This review delves into the immunological mechanisms of pneumonitis, TB, hepatitis, and colitis caused by ICIs separately and finds that they all include abnormal changes in cytokines’ level. Therefore, we propose that the elevation of multiple cytokines and immune activation are a shared characteristic among them.
In addition to aforementioned compilations, there is a potential of other system disorders, such as encephalitis and meningitis, belonging to central nervous system diseases, presenting with fever (136). Because these adverse effects occur relatively less, they are inadequately documented and the potential mechanism still waits for further exploration (137, 138). Notably, they can result in poor prognosis, even fatality, which should not be overlooked in clinical practice (139).
It is noteworthy that the incidence of irAEs, the onset time of them, and the severity vary for different types of cancers and drugs. In general, the incidence and severity of adverse events at all grades are higher for anti-CTLA-4 inhibitors (135, 140). However, concerning fever solely, there seems to be a lack of extensive statistic on the incidence, onset time, and severity of fever among various irAEs caused by anti-PD-1/PD-L1 and anti-CTLA-4 inhibitors. In the section of IMH, we mention that fever is more prevalent with anti-CTLA-4 inhibitors than with anti-PD-1/PD-L1 therapy (66), but there is still a lack of statistically rigorous analyses, leaving room for future exploration. As biomarkers are a hot spot in medical research at present, exploring whether we can predict the occurrence and severity of fever in ICIs-treated patients by assessing baseline and post-treatment levels and alterations in multiple cytokines represents an avenue for future efforts. Additionally, exploring the potential for designing preventive and therapeutic measures based on these biomarkers is an area worth considering.
Consistent with the traditional view, we regard fever as an adverse symptom following ICIs treatment. In other words, we focus on the process by which ICIs induce fever, rather than the effect that fever exerts on ICIs treatment. It is reported that fever regulates the tumor immune microenvironment and enhances the immune response through heat shock protein (141). Considering that fever can promote damage to tumor DNA and induce immunogenic cell death, fever may play a positive role in suppressing tumor growth, activating the immune system and enhancing the efficacy of ICIs (138). This perspective is advantageous for a dialectical consideration of the physiological significance of ICIs-associated FUO.
Author contributions
XT: Writing – review & editing, Writing – original draft. TZ: Writing – review & editing, Writing – original draft. XD: Writing – review & editing, Supervision, Formal Analysis. DX: Writing – review & editing, Supervision, Methodology, Conceptualization.
Funding
The author(s) declare that no financial support was received for the research, authorship, and/or publication of this article.
Conflict of interest
The authors declare that the research was conducted in the absence of any commercial or financial relationships that could be construed as a potential conflict of interest.
Publisher’s note
All claims expressed in this article are solely those of the authors and do not necessarily represent those of their affiliated organizations, or those of the publisher, the editors and the reviewers. Any product that may be evaluated in this article, or claim that may be made by its manufacturer, is not guaranteed or endorsed by the publisher.
References
1. Marin-Acevedo JA, Dholaria B, Soyano AE, Knutson KL, Chumsri S, Lou Y. Next generation of immune checkpoint therapy in cancer: new developments and challenges. J Hematol Oncol. (2018) 11:39. doi: 10.1186/s13045-018-0582-8
2. Ramos-Casals M, Brahmer JR, Callahan MK, Flores-Chávez A, Keegan N, Khamashta MA, et al. Immune-related adverse events of checkpoint inhibitors. Nat Rev Dis Primers. (2020) 6:38. doi: 10.1038/s41572-020-0160-6
3. Watanabe T, Yamaguchi Y. Cutaneous manifestations associated with immune checkpoint inhibitors. Front Immunol. (2023) 14. doi: 10.3389/fimmu.2023.1071983
4. Muhaj F, Karri PV, Moody W, Brown A, Patel AB. Mucocutaneous adverse events to immune checkpoint inhibitors. Front Allergy. (2023) 4. doi: 10.3389/falgy.2023.1147513
5. Martins F, Sofiya L, Sykiotis GP, Lamine F, Maillard M, Fraga M, et al. Adverse effects of immune-checkpoint inhibitors: epidemiology, management and surveillance. Nat Rev Clin Oncol. (2019) 16:563–80. doi: 10.1038/s41571-019-0218-0
6. Haidar G, Longo DL, Singh N. Fever of unknown origin. New Engl J Med. (2022) 386:463–77. doi: 10.1056/NEJMra2111003
7. Kong X, Chen L, Su Z, Sullivan RJ, Blum SM, Qi Z, et al. Toxicities associated with immune checkpoint inhibitors: a systematic study. Int J Surg. (2023) 109:1753–68. doi: 10.1097/JS9.0000000000000368
8. Mei T, Wang T, Deng Q, Gong Y. The safety of combining immune checkpoint inhibitors and platinum-based chemotherapy for the treatment of solid tumors: A systematic review and network meta-analysis. Front Immunol. (2023) 14. doi: 10.3389/fimmu.2023.1062679
9. Kozak W, Kluger MJ, Tesfaigzi J, Kozak A, Mayfield KP, Wachulec M, et al. Molecular mechanisms of fever and endogenous antipyresis. Ann N Y Acad Sci. (2000) 917:121–34. doi: 10.1111/j.1749-6632.2000.tb05376.x
10. Roth J, Blatteis CM. Mechanisms of fever production and lysis: lessons from experimental LPS fever. Compr Physiol. (2014) 4:1563–604. doi: 10.1002/cphy.c130033
11. Evans SS, Repasky EA, Fisher DT. Fever and the thermal regulation of immunity: the immune system feels the heat. Nat Rev Immunol. (2015) 15:335–49. doi: 10.1038/nri3843
12. Kozak W, Kluger MJ, Soszynski D, Conn CA, Rudolph K, Leon LR, et al. IL-6 and IL-1 beta in fever. Studies using cytokine-deficient (knockout) mice. Ann N Y Acad Sci. (1998) 856:33–47. doi: 10.1111/j.1749-6632.1998.tb08310.x
13. LeMay LG, Otterness IG, Vander AJ, Kluger MJ. In vivo evidence that the rise in plasma IL 6 following injection of a fever-inducing dose of LPS is mediated by IL 1 beta. Cytokine. (1990) 2:199–204. doi: 10.1016/1043-4666(90)90016-M
14. Luheshi G, Miller AJ, Brouwer S, Dascombe MJ, Rothwell NJ, Hopkins SJ. Interleukin-1 receptor antagonist inhibits endotoxin fever and systemic interleukin-6 induction in the rat. Am J Physiol. (1996) 270:E91–5. doi: 10.1152/ajpendo.1996.270.1.E91
15. Dinarello CA, Cannon JG, Wolff SM, Bernheim HA, Beutler B, Cerami A, et al. Tumor necrosis factor (cachectin) is an endogenous pyrogen and induces production of interleukin 1. J Exp Med. (1986) 163:1433–50. doi: 10.1084/jem.163.6.1433
16. Hanada R, Leibbrandt A, Hanada T, Kitaoka S, Furuyashiki T, Fujihara H, et al. Central control of fever and female body temperature by RANKL/RANK. Nature. (2009) 462:505–9. doi: 10.1038/nature08596
17. Lai J, Wu H, Qin A. Cytokines in febrile diseases. J Interferon Cytokine Res. (2021) 41:1–11. doi: 10.1089/jir.2020.0213
18. Sullivan RJ, Weber JS. Immune-related toxicities of checkpoint inhibitors: mechanisms and mitigation strategies. Nat Rev Drug Discovery. (2021) 21:495–508. doi: 10.1038/s41573-021-00259-5
19. Lee DW, Gardner R, Porter DL, Louis CU, Ahmed N, Jensen M, et al. Current concepts in the diagnosis and management of cytokine release syndrome. Blood. (2014) 124:188–95. doi: 10.1182/blood-2014-05-552729
20. Wang M, Zhai X, Li J, Guan J, Xu S, Li Y, et al. The role of cytokines in predicting the response and adverse events related to immune checkpoint inhibitors. Front Immunol. (2021) 12. doi: 10.3389/fimmu.2021.670391
21. Tsukamoto H, Fujieda K, Miyashita A, Fukushima S, Ikeda T, Kubo Y, et al. Combined blockade of IL6 and PD-1/PD-L1 signaling abrogates mutual regulation of their immunosuppressive effects in the tumor microenvironment. Cancer Res. (2018) 78:5011–22. doi: 10.1158/0008-5472.CAN-18-0118
22. Krummel MF, Allison JP. CTLA-4 engagement inhibits IL-2 accumulation and cell cycle progression upon activation of resting T cells. J Exp Med. (1996) 183:2533–40. doi: 10.1084/jem.183.6.2533
23. Sun J, Schiffman J, Raghunath A, Ng Tang D, Chen H, Sharma P. Concurrent decrease in IL-10 with development of immune-related adverse events in a patient treated with anti-CTLA-4 therapy. Cancer Immun. (2008) 8:9.
24. McNamara MJ, Hilgart-Martiszus I, Barragan Echenique DM, Linch SN, Kasiewicz MJ, Redmond WL. Interferon-γ Production by peripheral lymphocytes predicts survival of tumor-bearing mice receiving dual PD-1/CTLA-4 blockade. Cancer Immunol Res. (2016) 4:650–7. doi: 10.1158/2326-6066.CIR-16-0022
25. Boutsikou E, Domvri K, Hardavella G, Tsiouda D, Zarogoulidis K, Kontakiotis T. Tumour necrosis factor, interferon-gamma and interleukins as predictive markers of antiprogrammed cell-death protein-1 treatment in advanced non-small cell lung cancer: a pragmatic approach in clinical practice. Ther Adv Med Oncol. (2018) 10:1758835918768238. doi: 10.1177/1758835918768238
26. Ozawa Y, Amano Y, Kanata K, Hasegwa H, Matsui T, Kakutani T, et al. Impact of early inflammatory cytokine elevation after commencement of PD-1 inhibitors to predict efficacy in patients with non-small cell lung cancer. Med Oncol. (2019) 36:33. doi: 10.1007/s12032-019-1255-3
27. Agulló-Ortuño MT, Gómez-Martín Ó, Ponce S, Iglesias L, Ojeda L, Ferrer I, et al. Blood predictive biomarkers for patients with non–small-cell lung cancer associated with clinical response to nivolumab. Clin Lung Cancer. (2020) 21:75–85. doi: 10.1016/j.cllc.2019.08.006
28. Sanmamed MF, Perez-Gracia JL, Schalper KA, Fusco JP, Gonzalez A, Rodriguez-Ruiz ME, et al. Changes in serum interleukin-8 (IL-8) levels reflect and predict response to anti-PD-1 treatment in melanoma and non-small-cell lung cancer patients. Ann Oncol. (2017) 28:1988–95. doi: 10.1093/annonc/mdx190
29. Hardy-Werbin M, Rocha P, Arpi O, Taus Á, Nonell L, Durán X, et al. Serum cytokine levels as predictive biomarkers of benefit from ipilimumab in small cell lung cancer. OncoImmunology. (2019) 8:e1593810. doi: 10.1080/2162402X.2019.1593810
30. Das R, Verma R, Sznol M, Boddupalli CS, Gettinger SN, Kluger H, et al. Combination therapy with anti–CTLA-4 and anti–PD-1 leads to distinct immunologic changes in vivo. J Immunol. (2015) 194:950–9. doi: 10.4049/jimmunol.1401686
31. Mao X-C, Yang C-C, Yang Y-F, Yan L-J, Ding Z-N, Liu H, et al. Peripheral cytokine levels as novel predictors of survival in cancer patients treated with immune checkpoint inhibitors: A systematic review and meta-analysis. Front Immunol. (2022) 13. doi: 10.3389/fimmu.2022.884592
32. Elmquist JK, Scammell TE, Saper CB. Mechanisms of CNS response to systemic immune challenge: the febrile response. Trends Neurosci. (1997) 20:565–70. doi: 10.1016/S0166-2236(97)01138-7
33. Christensen JD, Hansen EW, Fjalland B. Interleukin-1 beta stimulates the release of vasopressin from rat neurohypophysis. Eur J Pharmacol. (1989) 171:233–5. doi: 10.1016/0014-2999(89)90112-X
34. Cooper KE, Naylor AM, Veale WL. Evidence supporting a role for endogenous vasopressin in fever suppression in the rat. J Physiol. (1987) 387:163–72. doi: 10.1113/jphysiol.1987.sp016568
35. Federico P, Veale WL, Pittman QJ. Vasopressin-induced antipyresis in the medial amygdaloid nucleus of conscious rats. Am J Physiol. (1992) 262:R901–8. doi: 10.1152/ajpregu.1992.262.5.R901
36. Ruwe WD. Electrical stimulation of the paraventricular nucleus attenuates pyrogen fever in the rabbit. Brain Res. (1992) 588:181–90. doi: 10.1016/0006-8993(92)91574-X
37. Liu H, Xu D, Wang W, Sun F, Zhang S, Yang X, et al. Systematic assessment of risk of fever in solid tumor patients treated with PD-1/PD-L1 inhibitors: A systematic review and meta-analysis. Front Oncol. (2020) 10. doi: 10.3389/fonc.2020.570080
38. Wu J, Ni T, Deng R, Li Y, Zhong Q, Tang F, et al. Safety and efficacy of radiotherapy/chemoradiotherapy combined with immune checkpoint inhibitors for non-small cell lung cancer: A systematic review and meta-analysis. Front Immunol. (2023) 14. doi: 10.3389/fimmu.2023.1065510
39. Gomatou G, Tzilas V, Kotteas E, Syrigos K, Bouros D. Immune checkpoint inhibitor-related pneumonitis. Respiration. (2020) 99:932–42. doi: 10.1159/000509941
40. Zhai X, Zhang J, Tian Y, Li J, Jing W, Guo H, et al. The mechanism and risk factors for immune checkpoint inhibitor pneumonitis in non-small cell lung cancer patients. Cancer Biol Med. (2020) 17:599–611. doi: 10.20892/j.issn.2095-3941.2020.0102
41. Yin J, Wu Y, Yang X, Gan L, Xue J. Checkpoint inhibitor pneumonitis induced by anti-PD-1/PD-L1 therapy in non-small-cell lung cancer: occurrence and mechanism. Front Immunol. (2022) 13. doi: 10.3389/fimmu.2022.830631
42. Wang H, Guo X, Zhou J, Li Y, Duan L, Si X, et al. Clinical diagnosis and treatment of immune checkpoint inhibitor-associated pneumonitis. Thorac Cancer. (2019) 11:191–7. doi: 10.1111/1759-7714.13240
43. Bala-Hampton JE, Bazzell AF, Dains JE. Clinical management of pneumonitis in patients receiving anti–PD-1/PD-L1 therapy. J Advanced Practitioner Oncol. (2018) 9:422–8.
44. Yuan-Di W, Yuan S, Wen C, Ji Z, Xiang B, Wang B, et al. Immune checkpoint inhibitor-related chronic pneumonitis: a case report and literature review. Immunotherapy. (2023) 15:1117–23. doi: 10.2217/imt-2023-0006
45. Chen YL, Zhao J, Jia R, Wang HY, Zheng J, Bai CQ, et al. [Immune-related pneumonitis caused by programmed death-1 inhibitor Pembrolizumab: a case report and literature review]. Zhonghua Jie He He Hu Xi Za Zhi. (2017) 40:736–43. doi: 10.3760/cma.j.issn.1001-0939.2017.10.006
46. Tada K, Kurihara Y, Myojo T, Kojima F, Ishikawa Y, Yoshiyasu N, et al. Case report of nivolumab-related pneumonitis. Immunotherapy. (2017) 9:313–8. doi: 10.2217/imt-2016-0129
47. Nakashima K, Naito T, Omori S, Yoshikawa S, Endo M, Kiyohara Y, et al. Organizing pneumonia induced by nivolumab in a patient with metastatic melanoma. J Thorac Oncol. (2016) 11:432–3. doi: 10.1016/j.jtho.2015.10.004
48. Watanabe S, Kimura H, Takato H, Waseda Y, Hara J, Sone T, et al. Severe pneumonitis after nivolumab treatment in a patient with melanoma. Allergology Int. (2016) 65:487–9. doi: 10.1016/j.alit.2016.04.009
49. He Y, Peng D, Liang P, Long J, Liu A, Zeng Z. Immune checkpoint inhibitors and tuberculosis infection in lung cancer: A case series and systematic review with pooled analysis. J Clin Pharmacol. (2022) 63:397–409. doi: 10.1002/jcph.2170
50. Liu K, Wang D, Yao C, Qiao M, Li Q, Ren W, et al. Increased tuberculosis incidence due to immunotherapy based on PD-1 and PD-L1 blockade: A systematic review and meta-analysis. Front Immunol. (2022) 13. doi: 10.3389/fimmu.2022.727220
51. Langan EA, Graetz V, Allerheiligen J, Zillikens D, Rupp J, Terheyden P. Immune checkpoint inhibitors and tuberculosis: an old disease in a new context. Lancet Oncol. (2020) 21:e55–65. doi: 10.1016/S1470-2045(19)30674-6
52. Zaemes J, Kim C. Immune checkpoint inhibitor use and tuberculosis: a systematic review of the literature. Eur J Cancer. (2020) 132:168–75. doi: 10.1016/j.ejca.2020.03.015
53. Lin C, Xu G, Gao S, Feng T, Li S. Tuberculosis infection following immune checkpoint inhibitor treatment for advanced cancer: a case report and literature review. Front Immunol. (2023) 14. doi: 10.3389/fimmu.2023.1162190
54. Ahmed M, Tezera LB, Elkington PT, Leslie AJ. The paradox of immune checkpoint inhibition re-activating tuberculosis. Eur Respir J. (2022) 60:2102512. doi: 10.1183/13993003.02512-2021
55. Sirgiovanni M, Hinterleitner C, Horger M, Atique NB, Lauer UM, Zender L, et al. Long-term remission of small cell lung cancer after reactivation of tuberculosis following immune-checkpoint blockade: A case report. Thorac Cancer. (2021) 12:699–702. doi: 10.1111/1759-7714.13821
56. Fujita K, Elkington P, Redelman-Sidi G, Kanai O, Yamamoto Y, Imakita T, et al. Serial interferon-gamma release assay in lung cancer patients receiving immune checkpoint inhibitors: a prospective cohort study. Cancer Immunology Immunotherapy. (2022) 71:2757–64. doi: 10.1007/s00262-022-03198-1
57. Barber DL, Sakai S, KudChadkar RR, Fling SP, Day TA, Vergara JA, et al. Tuberculosis following PD-1 blockade for cancer immunotherapy. Sci Transl Med. (2019) 11:eaat2702. doi: 10.1126/scitranslmed.aat2702
58. Suliman AM, Bek SA, Elkhatim MS, Husain AA, Mismar AY, Eldean MZS, et al. Tuberculosis following programmed cell death receptor-1 (PD-1) inhibitor in a patient with non-small cell lung cancer. Case Rep literature review. Cancer Immunology Immunotherapy. (2020) 70:935–44. doi: 10.1007/s00262-020-02726-1
59. Lau K-S, Cheung BM-F, Lam K-O, Chan S-Y, Lam K-M, Yeung C-F, et al. Tuberculosis reactivation at ileum following immune checkpoint inhibition with pembrolizumab for metastatic nasopharyngeal carcinoma: a case report. BMC Infect Dis. (2021) 21:1148. doi: 10.1186/s12879-021-06845-7
60. Fujita K, Terashima T, Mio T. Anti-PD1 antibody treatment and the development of acute pulmonary tuberculosis. J Thorac Oncol. (2016) 11:2238–40. doi: 10.1016/j.jtho.2016.07.006
61. Tsai CC, Chen JH, Wang YC, Chang FY. Re-activation of pulmonary tuberculosis during anti-programmed death-1 (PD-1) treatment. Qjm. (2019) 112:41–2. doi: 10.1093/qjmed/hcy243
62. Hercun J, Vincent C, Bilodeau M, Lapierre P. Immune-mediated hepatitis during immune checkpoint inhibitor cancer immunotherapy: lessons from autoimmune hepatitis and liver immunology. Front Immunol. (2022) 13. doi: 10.3389/fimmu.2022.907591
63. Lleo A, Rimassa L, Colombo M. Hepatotoxicity of immune check point inhibitors: Approach and management. Digestive Liver Dis. (2019) 51:1074–8. doi: 10.1016/j.dld.2019.06.017
64. Liu Z, Zhu Y, Xie H, Zou Z. Immune-mediated hepatitis induced by immune checkpoint inhibitors: Current updates and future perspectives. Front Pharmacol. (2023) 13. doi: 10.3389/fphar.2022.1077468
65. Patrinely JR, McGuigan B, Chandra S, Fenton SE, Chowdhary A, Kennedy LB, et al. A multicenter characterization of hepatitis associated with immune checkpoint inhibitors. OncoImmunology. (2021) 10:1875639. doi: 10.1080/2162402X.2021.1875639
66. De Martin E, Michot J-M, Papouin B, Champiat S, Mateus C, Lambotte O, et al. Characterization of liver injury induced by cancer immunotherapy using immune checkpoint inhibitors. J Hepatol. (2018) 68:1181–90. doi: 10.1016/j.jhep.2018.01.033
67. Yang Y, Wu Q, Chen L, Qian K, Xu X. Severe immune-related hepatitis and myocarditis caused by PD-1 inhibitors in the treatment of triple-negative breast cancer: a case report. Ann Trans Med. (2022) 10:424–4. doi: 10.21037/atm
68. Vaziri H, Turshudzhyan A, Vecchio E. Immunotherapy-induced colitis. J Clin Gastroenterol. (2022) 56:555–64. doi: 10.1097/MCG.0000000000001705
69. Luoma AM, Suo S, Williams HL, Sharova T, Sullivan K, Manos M, et al. Molecular pathways of colon inflammation induced by cancer immunotherapy. Cell. (2020) 182:655–671.e22. doi: 10.1016/j.cell.2020.06.001
70. Marin-Acevedo JA, Harris DM, Burton MC. Immunotherapy-induced colitis: an emerging problem for the hospitalist. J Hosp Med. (2018) 13:413–8. doi: 10.12788/jhm.2925
71. Chen M, Liu M, Li C, Peng S, Li Y, Xu X, et al. Fecal microbiota transplantation effectively cures a patient with severe bleeding immune checkpoint inhibitor-associated colitis and a short review. Front Oncol. (2022) 12. doi: 10.3389/fonc.2022.913217
72. Sakellariou S, Zouki DN, Ziogas DC, Pouloudi D, Gogas H, Delladetsima I. Granulomatous colitis in a patient with metastatic melanoma under immunotherapy: a case report and literature review. BMC Gastroenterol. (2021) 21:227. doi: 10.1186/s12876-021-01812-7
73. Khalaf SA, Albarrak A, Yousef M, Tahan V. Immune checkpoint inhibitors induced colitis, stay vigilant: A case report. World J Gastrointestinal Oncol. (2020) 12:699–704. doi: 10.4251/wjgo.v12.i6.699
74. Tan S, Zhu G, Fan J, Chen J, Li X, Peng Y, et al. Immune checkpoint inhibitor-induced colitis complicated with inflammatory intestinal obstruction: a case report and literature review. Trans Cancer Res. (2022) 11:2443–8. doi: 10.21037/tcr
75. Soma K, Nishida T, Osugi N, Morimura O, Adachi S, Fujii Y, et al. Nivolumab dose escalation triggered immune checkpoint inhibitor-induced colitis after 147 weeks of prolonged stable use in a patient with lung cancer: a case report. Clin J Gastroenterol. (2021) 15:128–33. doi: 10.1007/s12328-021-01542-z
76. Nakai M, Kai Y, Suzuki K, Matsuda M, Kikukawa S, Masuda H, et al. A case of perforated immune-related colitis complicated by cytomegalovirus infection during treatment of immune-related adverse effect in lung cancer immunotherapy. Respir Med Case Rep. (2023) 41:101794. doi: 10.1016/j.rmcr.2022.101794
77. Rastogi P. Ipilimumab associated colitis: An IpiColitis case series at MedStar Georgetown University Hospital. World J Gastroenterol. (2015) 21:4373. doi: 10.3748/wjg.v21.i14.4373
78. Delanoy N, Michot J-M, Comont T, Kramkimel N, Lazarovici J, Dupont R, et al. Haematological immune-related adverse events induced by anti-PD-1 or anti-PD-L1 immunotherapy: a descriptive observational study. Lancet Haematology. (2019) 6:e48–57. doi: 10.1016/S2352-3026(18)30175-3
79. Kramer R, Zaremba A, Moreira A, Ugurel S, Johnson DB, Hassel JC, et al. Hematological immune related adverse events after treatment with immune checkpoint inhibitors. Eur J Cancer. (2021) 147:170–81. doi: 10.1016/j.ejca.2021.01.013
80. Naqash AR, Appah E, Yang LV, Muzaffar M, Marie MA, McCallen JD, et al. Isolated neutropenia as a rare but serious adverse event secondary to immune checkpoint inhibition. J ImmunoTherapy Cancer. (2019) 7:169. doi: 10.1186/s40425-019-0648-3
81. Zaremba A, Kramer R, De Temple V, Bertram S, Salzmann M, Gesierich A, et al. Grade 4 neutropenia secondary to immune checkpoint inhibition — A descriptive observational retrospective multicenter analysis. Front Oncol. (2021) 11. doi: 10.3389/fonc.2021.765608
82. Brahmer JR, Lacchetti C, Schneider BJ, Atkins MB, Brassil KJ, Caterino JM, et al. Management of immune-related adverse events in patients treated with immune checkpoint inhibitor therapy: American society of clinical oncology clinical practice guideline. J Clin Oncol. (2018) 36:1714–68. doi: 10.1200/JOP.18.00005
83. Bai S, Tian T, Pacheco JM, Tachihara M, Hu P, Zhang J. Immune-related adverse event profile of combination treatment of PD-(L)1 checkpoint inhibitors and bevacizumab in non-small cell lung cancer patients: data from the FDA adverse event reporting system. Trans Lung Cancer Res. (2021) 10:2614–24. doi: 10.21037/tlcr
84. Raghav K, Liu S, Overman MJ, Willett AF, Knafl M, Fu SC, et al. Halperin, efficacy, safety, and biomarker analysis of combined PD-L1 (Atezolizumab) and VEGF (Bevacizumab) blockade in advanced Malignant peritoneal mesothelioma. Cancer Discovery. (2021) 11:2738–47. doi: 10.1158/2159-8290.CD-21-0331
85. Finkel I, Sternschuss M, Wollner M, Shamai S, Peled N, Turgeman I, et al. Immune-related neutropenia following treatment with immune checkpoint inhibitors. J Immunother. (2020) 43:67–74. doi: 10.1097/CJI.0000000000000293
86. Michot J-M, Vargaftig J, Leduc C, Quere G, Burroni B, Lazarovici J, et al. Immune-related bone marrow failure following anti-PD1 therapy. Eur J Cancer. (2017) 80:1–4. doi: 10.1016/j.ejca.2017.04.004
87. Michot JM, Lazarovici J, Tieu A, Champiat S, Voisin AL, Ebbo M, et al. Haematological immune-related adverse events with immune checkpoint inhibitors, how to manage? Eur J Cancer. (2019) 122:72–90. doi: 10.1016/j.ejca.2019.07.014
88. Li N, Feng Y, Chen X, Li Y, Zhang C, Yin Y. Hematologic and lymphatic system toxicities associated with immune checkpoint inhibitors: a real-world study. Front Pharmacol. (2023) 14. doi: 10.3389/fphar.2023.1213608
89. Comito RR, Badu LA, Forcello N. Nivolumab-induced aplastic anemia: A case report and literature review. J Oncol Pharm Pract. (2017) 25:221–5. doi: 10.1177/1078155217726159
90. Simeone E, Grimaldi AM, Esposito A, Curvietto M, Palla M, Paone M, et al. Serious haematological toxicity during and after ipilimumab treatment: a case series. J Med Case Rep. (2014) 8:240. doi: 10.1186/1752-1947-8-240
91. Wei G, Nwakuche U, Cadavid G, Ajaz A, Seiter K, Liu D. Large granular lymphocytosis with severe neutropenia following ipilimumab therapy for metastatic melanoma. Exp Hematol Oncol. (2012) 1:3. doi: 10.1186/2162-3619-1-3
92. Okuzumi S, Kamata H, Emoto K, Shimizu T, Otake S, Irie H, et al. Successful steroid treatment of pembrolizumab-induced agranulocytosis that developed after splenectomy in a patient with non-small-cell lung cancer. Internal Med. (2023) 62:2113–21. doi: 10.2169/internalmedicine.0278-22
93. Woźniak S, Mackiewicz-Wysocka M, Krokowicz Ł, Kwinta Ł, Mackiewicz J. Febrile neutropenia in a metastatic melanoma patient treated with ipilimumab - case report. Oncol Res Treat. (2015) 38:105–8. doi: 10.1159/000377650
94. Ban-Hoefen M, Burack R, Sievert L, Sahasrabudhe D. Ipilimumab-induced neutropenia in melanoma. J Invest Med High Impact Case Rep. (2016) 4:232470961666183. doi: 10.1177/2324709616661835
95. de Naurois J, Novitzky-Basso I, Gill MJ, Marti Marti F, Cullen MH, Roila F. Management of febrile neutropenia: ESMO Clinical Practice Guidelines. Ann Oncol. (2010) 21:v252–6. doi: 10.1093/annonc/mdq196
96. Boegeholz J, Brueggen CS, Pauli C, Dimitriou F, Haralambieva E, Dummer R, et al. Challenges in diagnosis and management of neutropenia upon exposure to immune-checkpoint inhibitors: meta-analysis of a rare immune-related adverse side effect. BMC Cancer. (2020) 20:300. doi: 10.1186/s12885-020-06763-y
97. Ceschi A, Noseda R, Palin K, Verhamme K. Immune checkpoint inhibitor-related cytokine release syndrome: analysis of WHO global pharmacovigilance database. Front Pharmacol. (2020) 11. doi: 10.3389/fphar.2020.00557
98. Honjo O, Kubo T, Sugaya F, Nishizaka T, Kato K, Hirohashi Y, et al. Severe cytokine release syndrome resulting in purpura fulminans despite successful response to nivolumab therapy in a patient with pleomorphic carcinoma of the lung: a case report. J ImmunoTherapy Cancer. (2019) 7:97. doi: 10.1186/s40425-019-0582-4
99. Zhao L, Yang Y, Li W, Li T, Gao Q. Nivolumab-induced cytokine-release syndrome in relapsed/refractory Hodgkin’s lymphoma: a case report and literature review. Immunotherapy. (2018) 10:913–7. doi: 10.2217/imt-2018-0025
100. Tanaka T, Taoka M, Makimoto G, Ninomiya K, Higo H, Fujii M, et al. Severe cytokine release syndrome and immune effector cell-associated neurotoxicity syndrome in a man receiving immune checkpoint inhibitors for lung cancer: A case report. Internal Med. (2023). doi: 10.2169/internalmedicine.2429-23
101. Pihusch R, Holler E, Mühlbayer D, Göhring P, Stötzer O, Pihusch M, et al. The impact of antithymocyte globulin on short-term toxicity after allogeneic stem cell transplantation. Bone Marrow Transplant. (2002) 30:347–54. doi: 10.1038/sj.bmt.1703640
102. Winkler U, Jensen M, Manzke O, Schulz H, Diehl V, Engert A. Cytokine-release syndrome in patients with B-cell chronic lymphocytic leukemia and high lymphocyte counts after treatment with an anti-CD20 monoclonal antibody (rituximab, IDEC-C2B8). Blood. (1999) 94:2217–24. doi: 10.1182/blood.V94.7.2217.419k02_2217_2224
103. Xu X-J, Tang Y-M. Cytokine release syndrome in cancer immunotherapy with chimeric antigen receptor engineered T cells. Cancer Lett. (2014) 343:172–8. doi: 10.1016/j.canlet.2013.10.004
104. Morris EC, Neelapu SS, Giavridis T, Sadelain M. Cytokine release syndrome and associated neurotoxicity in cancer immunotherapy. Nat Rev Immunol. (2021) 22:85–96. doi: 10.1038/s41577-021-00547-6
105. Norelli M, Camisa B, Barbiera G, Falcone L, Purevdorj A, Genua M, et al. Monocyte-derived IL-1 and IL-6 are differentially required for cytokine-release syndrome and neurotoxicity due to CAR T cells. Nat Med. (2018) 24:739–48. doi: 10.1038/s41591-018-0036-4
106. Liu LL, Skribek M, Harmenberg U, Gerling M. Systemic inflammatory syndromes as life-threatening side effects of immune checkpoint inhibitors: case report and systematic review of the literature. J ImmunoTherapy Cancer. (2023) 11:e005841. doi: 10.1136/jitc-2022-005841
107. Dinarello CA. Interleukin-1 in the pathogenesis and treatment of inflammatory diseases. Blood. (2011) 117:3720–32. doi: 10.1182/blood-2010-07-273417
108. Grupp SA, Kalos M, Barrett D, Aplenc R, Porter DL, Rheingold SR, et al. Chimeric antigen receptor–modified T cells for acute lymphoid leukemia. New Engl J Med. (2013) 368:1509–18. doi: 10.1056/NEJMoa1215134
109. Amlani A, Barber C, Fifi-Mah A, Monzon J. Successful treatment of cytokine release syndrome with IL-6 blockade in a patient transitioning from immune-checkpoint to MEK/BRAF inhibition: A case report and review of literature. Oncologist. (2020) 25:e1120–3. doi: 10.1634/theoncologist.2020-0194
110. Shimabukuro-Vornhagen A, Gödel P, Subklewe M, Stemmler HJ, Schlößer HA, Schlaak M, et al. Cytokine release syndrome. J ImmunoTherapy Cancer. (2018) 6:56. doi: 10.1186/s40425-018-0343-9
111. Tay SH, Toh MMX, Thian YL, Vellayappan BA, Fairhurst A-M, Chan YH, et al. Cytokine release syndrome in cancer patients receiving immune checkpoint inhibitors: A case series of 25 patients and review of the literature. Front Immunol. (2022) 13. doi: 10.3389/fimmu.2022.807050
112. Dimitriou F, Matter AV, Mangana J, Urosevic-Maiwald M, Micaletto S, Braun RP, et al. Cytokine release syndrome during sequential treatment with immune checkpoint inhibitors and kinase inhibitors for metastatic melanoma. J Immunother. (2019) 42:29–32. doi: 10.1097/CJI.0000000000000236
113. Zhang X, Fu Z, Yan C. Cytokine release syndrome induced by pembrolizumab: A case report. Med. (2022) 101:e31998. doi: 10.1097/MD.0000000000031998
114. Urasaki T, Ono M, Mochizuki T, Takeda K, Nishizawa A, Fukagawa E, et al. Case report: A case of trimethoprim/sulfamethoxazole-triggered hypotensive shock: cytokine release syndrome related to immune checkpoint inhibitors and drug-induced hypersensitivity syndrome. Front Oncol. (2021) 11. doi: 10.3389/fonc.2021.681997
115. Sadaat M, Jang S. Hemophagocytic lymphohistiocytosis with immunotherapy: brief review and case report. J ImmunoTherapy Cancer. (2018) 6:49. doi: 10.1186/s40425-018-0365-3
116. Rubio-Perez J, Rodríguez-Perez ÁR, Díaz-Blázquez M, Moreno-García V, Dómine-Gómez M. Treatment-related hemophagocytic lymphohistiocytosis due to atezolizumab: a case report and review of the literature. J Med Case Rep. (2022) 16:365. doi: 10.1186/s13256-022-03585-3
117. Wei Y, He W, Sun W, Wu C, Ren D, Wang X, et al. Hemophagocytic lymphohistiocytosis in two patients following treatment with pembrolizumab: two case reports and a literature review. Trans Cancer Res. (2022) 11:2960–6. doi: 10.21037/tcr
118. Noseda R, Bertoli R, Müller L, Ceschi A. Haemophagocytic lymphohistiocytosis in patients treated with immune checkpoint inhibitors: analysis of WHO global database of individual case safety reports. J ImmunoTherapy Cancer. (2019) 7:117. doi: 10.1186/s40425-019-0598-9
119. Ramos-Casals M, Brito-Zerón P, López-Guillermo A, Khamashta MA, Bosch X. Adult haemophagocytic syndrome. Lancet. (2014) 383:1503–16. doi: 10.1016/S0140-6736(13)61048-X
120. Janka GE. Hemophagocytic syndromes. Blood Rev. (2007) 21:245–53. doi: 10.1016/j.blre.2007.05.001
121. Griffin G, Shenoi S, Hughes GC. Hemophagocytic lymphohistiocytosis: An update on pathogenesis, diagnosis, and therapy. Best Pract Res Clin Rheumatol. (2020) 34:101515. doi: 10.1016/j.berh.2020.101515
122. Xu X-J, Tang Y-M, Song H, Yang S-L, Xu W-Q, Zhao N, et al. Diagnostic accuracy of a specific cytokine pattern in hemophagocytic lymphohistiocytosis in children. J Pediatr. (2012) 160:984–990.e1. doi: 10.1016/j.jpeds.2011.11.046
123. Rajapakse P, Andanamala H. Hemophagocytic lymphohistiocytosis secondary to immune checkpoint inhibitor therapy. World J Oncol. (2022) 13:49–52. doi: 10.14740/wjon1464
124. Fardet L, Galicier L, Lambotte O, Marzac C, Aumont C, Chahwan D, et al. Development and validation of the HScore, a score for the diagnosis of reactive hemophagocytic syndrome. Arthritis Rheumatol. (2014) 66:2613–20. doi: 10.1002/art.38690
125. Diaz L, Jauzelon B, Dillies A-C, Le Souder C, Faillie J-L, Maria A, et al. Hemophagocytic lymphohistiocytosis associated with immunological checkpoint inhibitors: A pharmacovigilance study. J Clin Med. (2023) 12:1985. doi: 10.3390/jcm12051985
126. Henter JI, Horne A, Aricó M, Egeler RM, Filipovich AH, Imashuku S, et al. HLH-2004: Diagnostic and therapeutic guidelines for hemophagocytic lymphohistiocytosis. Pediatr Blood Cancer. (2006) 48:124–31. doi: 10.1002/pbc.21039
127. Kantarjian H, Stein A, Gökbuget N, Fielding AK, Schuh AC, Ribera J-M, et al. Blinatumomab versus chemotherapy for advanced acute lymphoblastic leukemia. New Engl J Med. (2017) 376:836–47. doi: 10.1056/NEJMoa1609783
128. Schultz DR, Arnold PI. Properties of four acute phase proteins: C-reactive protein, serum amyloid A protein, alpha 1-acid glycoprotein, and fibrinogen. Semin Arthritis Rheum. (1990) 20:129–47. doi: 10.1016/0049-0172(90)90055-K
129. Dupré A, Michot JM, Schoeffler A, Frumholtz L, Baroudjian B, Delyon J, et al. Haemophagocytic lymphohistiocytosis associated with immune checkpoint inhibitors: a descriptive case study and literature review. Br J Haematology. (2020) 189:985–92. doi: 10.1111/bjh.16630
130. Satzger I, Ivanyi P, Länger F, Kreipe H-H, Schaper-Gerhardt K, Beutel G, et al. Treatment-related hemophagocytic lymphohistiocytosis secondary to checkpoint inhibition with nivolumab plus ipilimumab. Eur J Cancer. (2018) 93:150–3. doi: 10.1016/j.ejca.2018.01.063
131. Nelson BE, Ejezie CL, Stephen BA, Nardo M, Campbell E, Gong J, et al. Spectrum of immune checkpoint inhibitor anemias: results from a single center, early-phase clinical trials case series experience. J Hematol. (2022) 11:113–20. doi: 10.14740/jh1006
132. Bennett JE, Blaser MJ. Mandell, Douglas, and Bennett’s principles and practice of infectious diseases. Philadelphia: Elsevier Saunders (2014) 2583–2589.
135. Postow MA, Longo DL, Sidlow R, Hellmann MD. Immune-related adverse events associated with immune checkpoint blockade. New Engl J Med. (2018) 378:158–68. doi: 10.1056/NEJMra1703481
136. Farina A, Villagrán-García M, Honnorat J. Neurological adverse events of immune checkpoint inhibitors: An update of clinical presentations, diagnosis, and management. Rev Neurol (Paris). (2023) 179:506–15. doi: 10.1016/j.neurol.2023.03.003
137. Ruggiero R, Di Napoli R, Balzano N, Ruggiero D, Riccardi C, Anatriello A, et al. Immune-related adverse events and immune checkpoint inhibitors: a focus on neurotoxicity and clinical management. Expert Rev Clin Pharmacol. (2023) 16:423–34. doi: 10.1080/17512433.2023.2211262
138. Velasco R, Villagrán M, Jové M, Simó M, Vilariño N, Alemany M, et al. Encephalitis induced by immune checkpoint inhibitors: A systematic review. JAMA Neurol. (2021) 78:864–73. doi: 10.1001/jamaneurol.2021.0249
139. Suzuki S. Encephalitis as an immune-related adverse event. J Neurol Neurosurg Psychiatry. (2020) 91:680. doi: 10.1136/jnnp-2020-323212
140. El Osta B, Hu F, Sadek R, Chintalapally R, Tang SC. A meta-analysis of immune-related adverse events (irAE) of immune checkpoint inhibitors (ICI) from cancer clinical trials. Ann Oncol. (2016) 27:vi369. doi: 10.1093/annonc/mdw378.31
Keywords: immune checkpoint inhibitors, fever, cytokines, FUO, irAEs
Citation: Tong X, Zhan T, Dong X and Xu D (2024) Fever of unknown origin associated with immune checkpoint inhibitors. Front. Immunol. 15:1364128. doi: 10.3389/fimmu.2024.1364128
Received: 01 January 2024; Accepted: 29 February 2024;
Published: 12 March 2024.
Edited by:
Wenyi Gu, The University of Queensland, AustraliaReviewed by:
Yuho Najima, Tokyo Metropolitan Komagome Hospital, JapanXiaohui Zhao, Guangzhou Medical University, China
Copyright © 2024 Tong, Zhan, Dong and Xu. This is an open-access article distributed under the terms of the Creative Commons Attribution License (CC BY). The use, distribution or reproduction in other forums is permitted, provided the original author(s) and the copyright owner(s) are credited and that the original publication in this journal is cited, in accordance with accepted academic practice. No use, distribution or reproduction is permitted which does not comply with these terms.
*Correspondence: Dong Xu, xdong@tjh.tjmu.edu.cn; Xiaoqin Dong, xiaoqind@163.com; xiaoqind@tjh.tjmu.edu.cn
†These authors have contributed equally to this work