- 1Beijing Engineering Research Center of Protein and Antibody, Sinocelltech Ltd., Beijing, China
- 2Cell Culture Engineering Center, Chinese Academy of Medical Sciences & Peking Union Medical College, Beijing, China
The development of human papillomavirus (HPV) vaccines has made substantive progress, as represented by the approval of five prophylactic vaccines since 2006. Generally, the deployment of prophylactic HPV vaccines is effective in preventing newly acquired infections and incidences of HPV-related malignancies. However, there is still a long way to go regarding the prevention of all HPV infections and the eradication of established HPV infections, as well as the subsequent progression to cancer. Optimizing prophylactic HPV vaccines by incorporating L1 proteins from more HPV subtypes, exploring adjuvants that reinforce cellular immune responses to eradicate HPV-infected cells, and developing therapeutic HPV vaccines used either alone or in combination with other cancer therapeutic modalities might bring about a new era getting closer to the vision to get rid of HPV infection and related diseases. Herein, we summarize strategies for the development of HPV vaccines, both prophylactic and therapeutic, with an emphasis on the selection of antigens and adjuvants, as well as implications for vaccine efficacy based on preclinical studies and clinical trials. Additionally, we outline current cutting-edge insights on formulation strategies, dosing schedules, and age expansion among HPV vaccine recipients, which might play important roles in addressing barriers to vaccine uptake, such as vaccine hesitancy and vaccine availability.
1 Introduction
Human papillomavirus (HPV) infection can cause multiple types of clinical manifestations or diseases such as genital warts, respiratory papillomatosis, head and neck cancer (mostly oropharyngeal squamous cell carcinoma), anal cancer, penile cancer, vulvar cancer, vaginal cancer, and cervical cancer (1, 2). Among these HPV-associated cancers, cervical cancer contributes to the highest proportion and ranks as the fourth most commonly diagnosed cancer and the fourth leading cause of cancer death in women (3, 4).
Despite the high efficacy demonstrated by prophylactic HPV vaccines and the benefit of cervical screening across the female population, the number of newly diagnosed cervical cancer patients in 2018 was nearly 570,000 cases, of which 311,000 cases died from this disease worldwide (5). Globally, in 2020, the estimated new cancer cases would extend to 660,000, with about 350,000 deaths (6).
Addressing barriers to vaccine uptake, such as vaccine hesitancy and vaccine availability, might be an effective approach for improving HPV vaccine coverage (7, 8). Furthermore, efforts to improve the efficacy of HPV vaccines, both prophylactic and therapeutic, could also play important roles in tackling HPV infection and the incidence of HPV-related cancers or genital warts. In this review, we summarize the status and prospects for the development of prophylactic and therapeutic HPV vaccines. Exploration of the dosing schedules, formulation strategies, and age expansion of vaccine recipients will also be discussed.
2 Characteristics of human papillomavirus
2.1 Classification of HPV
HPV is an essential member of the Papillomaviridae family, encompassing a wide range of primitive DNA viruses distributed among various host species (9). Phylogenetically, HPVs are categorized into five genera: Alpha, Beta, Gamma, Mu, and Nu, based on the homology of the nucleotide sequence in the L1 gene (9, 10). Alpha and Beta HPVs are the most commonly studied and have distinctive biological properties. Beta viruses frequently infect cutaneous epithelium and are involved in non-melanoma skin cancers, whereas Alpha genera-HPVs infect both mucosal and genital epithelium and are the causes of many anogenital cancers in humans and primates (11, 12). The Alpha HPVs are further grouped into low-risk HPVs (LR-HPVs) and high-risk HPVs (HR-HPVs) depending on their capacity to cause cancer. The LR-HPVs include HPV-6/11/42/43/44. HPV 6 and 11 cause most cases of genital warts (13). HPV-16/18/31/33/35/39/45/51/52/56/58/59/68 are members of HR-HPVs (14, 15).
2.2 Genomic characterization of HPV
The HPV viral particles are composed of a single double-stranded DNA molecule, with approximately 8,000 base pairs (bp), and a protein capsid containing 72 pentameric capsomers (16). The genomes of all HPV types contain about eight open-reading frames (ORFs) that can be functionally divided into the early (E), the late (L), and the long control region (LCR) (Figure 1). Specifically, the E gene encodes the early proteins (E1–E7) responsible for viral replication and oncogenesis. The L gene encodes the structural proteins (L1–L2) necessary for virion assembly. The non-coding region is thought to be the most highly variable part of the viral genome (17). The structural capsid proteins of HPVs are composed of two key proteins, major basic L1 and minor basic L2, respectively encoded by L1 and L2 genes. L1 genomes display differently amongst HPV types, while the L2 genomic sequence is relatively conserved (18). The HPV capsid is made up of 360 copies of major (L1) and 72 copies of minor (L2) capsid proteins (19). The L1 protein is self-assembled into the virus-like particles (VLP), while the L2 protein, as a minor structural molecule, is unable to form VLPs. Although the L2 protein is not responsible for binding to and entering cells during the process of infection, it plays a key role in enhancing L1 assembly into VLPs and viral genome encapsidation (20). Once entering host cells, the viral E6 and E7 oncoproteins are responsible for viral oncogenesis by affecting the function of tumor suppressors p53 and pRB (21). HPV types that cause genital warts are called low-risk, while those types that cause cervical cancer are considered high-risk (22). High-risk HPV genomes are commonly integrated into the host genome in most progressive cancer cases. Conversely, low-risk HPV genomes are commonly identified extra-chromosomally in benign and low-grade lesions, which are rarely found in tumors (23).
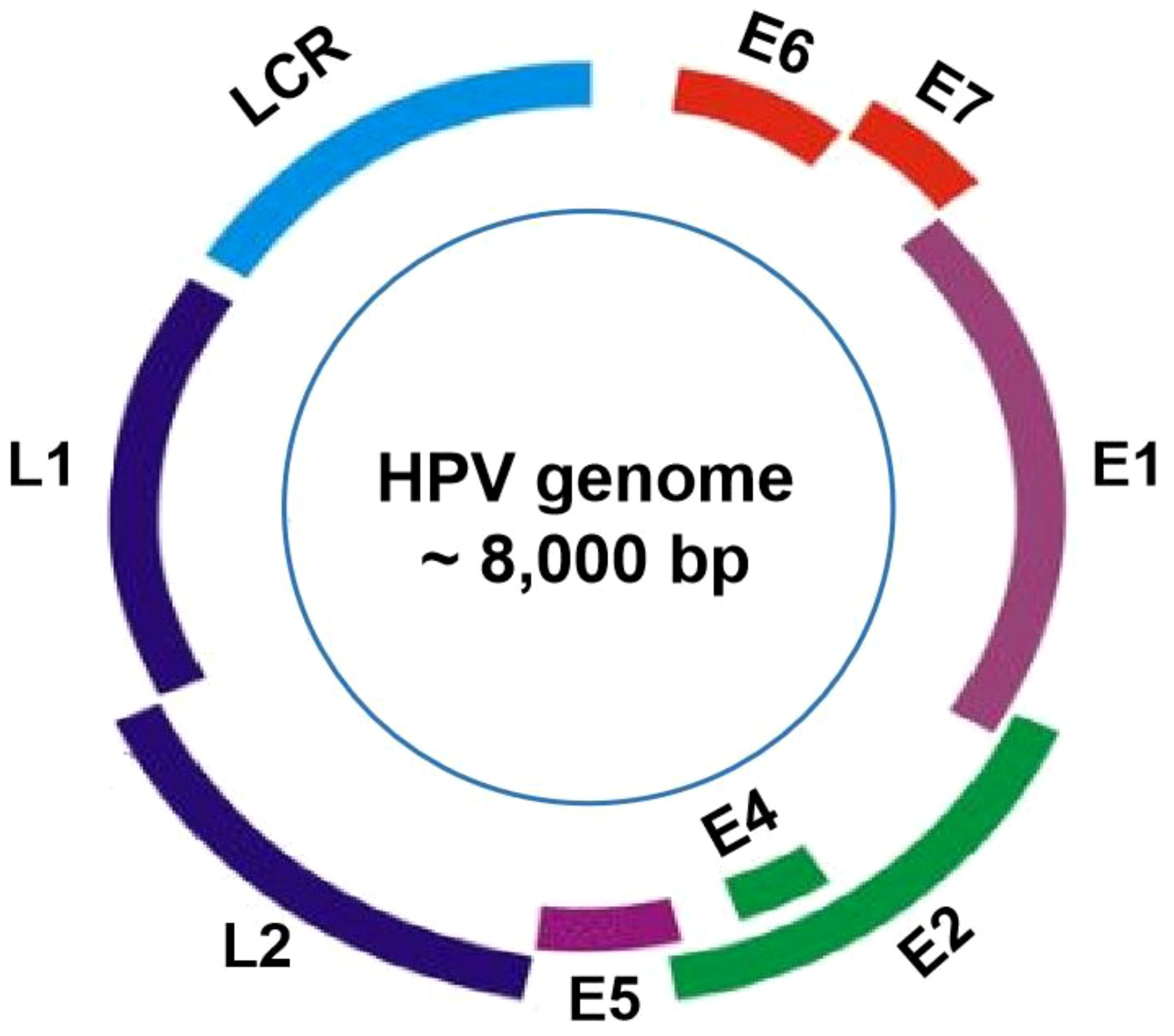
Figure 1 HPV genome organization. The genome of HPVs, about 8000 base pairs in length, is consists of approximately eight open reading frames (ORFs), which can be functionally categorized into three main regions: the E region, the L region, and the long control region (LCR).
3 Current status of HPV vaccines
3.1 Currently available prophylactic HPV vaccines
Currently, five commercial HPV vaccines targeting high-risk HPV types have been approved for prophylactic use. All licensed vaccines contain L1 VLP. One of the primary distinctions among these authorized HPV vaccines is their level of valency.
Cervarix® was licensed by the European Medicines Agency (EMA) in 2007 and by the U.S. Food and Drug Administration (FDA) in 2009. Cervarix® is effective in defending against HPV-16 and HPV-18, responsible for nearly 70% of cervical cancers (24). Cervarix® is formulated in a proprietary AS04 adjuvant containing aluminum hydroxide combined with a Toll-like receptor 4 (TLR4) ligand, 3-O-desacyl-4’-monophosphoryl lipid A (MPL) (25, 26). It is indicated for use in females aged 10 through 25 years for the prevention of persistent infection, premalignant cervical lesions, and cervical cancer caused by HPVs in the US.
Gardasil®, a quadrivalent HPV vaccine, is the first commercially available HPV vaccine approved in 2006 for people 9 through 26 years of age for the prevention of diseases caused by HPV infection (27). Aside from HPV-16 and 18, Gardasil® also protects against HPV-6 and -11 infections, which cause approximately 90% of genital warts. Gardasil® is adsorbed on an amorphous aluminum hydroxyphosphate sulfate adjuvant (28).
Gardasil 9®, licensed by the FDA in 2014, offers broader protection by covering five additional HPV types (HPV-31, 33, 45, 53, and 58) that might account for about 20% of cervical cancer cases (29). Gardasil 9® is indicated for females aged 9 through 45 years for the prevention of HPV-associated diseases. Additionally, Gardasil 9® is indicated in males 9 through 45 years of age for the prevention of anal, oropharyngeal, head neck cancers, anal precancerous, dysplastic lesions, and genital warts caused by HR-HPVs (30). Gardasil 9® is formulated with amorphous aluminum hydroxyphosphate sulfate (AAHS) as an adjuvant (31).
Recently, two more bivalent HPV vaccines (HPV-16 and 18) have been approved in China for protection against HPV infection-induced precancerous lesions or high-grade genital lesions (32–34). Clinical data revealed that the 2-valent HPV vaccine showed favorable immunogenicity, safety, and a positive seroconvertsion rate (35–37). Both bivalent vaccines contain twice the amount of HPV-16 L1 protein as the Cervarix® vaccine and use different adjuvants and antigen expression systems. A comprehensive comparison of marketed HPV vaccines is shown in Table 1.
The immunogenicity and efficacy of five licensed HPV vaccines have been summarized elsewhere (33, 38, 39). Briefly, all marketed HPV vaccines elicit potent humoral immune responses and exhibited long-lasting protective efficacy after vaccination (34, 40–43). Serum antibody responses are the major indicators used for evaluating immunogenicity evoked by HPV vaccines in clinical trials, and comparison of vaccine-induced antibody levels or B-cell responses between HPV vaccines have been discussed (44–46). Nevertheless, definitive correlation between humoral immune responses and efficacy of HPV vaccines has not yet been reported (47). Prophylactic HPV vaccines based on L1-VLPs trigger T-cell responses, which showed no correlation with protection, probably due to the lack of L1 expression in HPV-infected cells (48). Since the protection efficacy of HPV vaccines are characterized in clinical trials by prevention of cervical cancer and intraepithelial neoplasia, immune profiles other than serum neutralizing antibody titers should be clarified, such as characteristics of Fc-effector functions, mucosal immunity and T-cell responses against HPV early proteins. For example, a comparative study between Gardasil® and Cervarix® indicated that the differences in antibody Fc-effector functions might contribute to post-infection protection (49). Immune correlate of protection by HPV vaccination needs further elucidation based on more clinical data with functional characteristic of serum or mucosal antibodies, as well as cellular immune responses (50).
Despite the high efficacy against HPV infection and HPV-related disease, low vaccination coverage of prophylactic HPV vaccines still exists. Particularly, most low- and middle-income countries incompletely implement HPV vaccination programs (51). The high cost, limited supply, and delivery requirements of the cold chain make it unavailable to people in these countries (52). Additionally, the biosafety considerations played a critical role in the successful development and deployment of HPV vaccines. Based on high quality data from very large studies, HPV vaccines exhibit favorable safety profiles (53, 54). The implementation of a robust safety monitoring system for HPV vaccines, which consistently updated data and promptly disclosed findings, would enhance accurate understanding and comprehensive awareness regarding HPV vaccine safety, mitigate vaccine hesitancy, and increase vaccine acceptance and coverage rates.
3.2 Prophylactic HPV vaccines in clinical testing
There are 12 high-risk HPV types that are involved in cancer, including types 16, 18, 31, 33, 35, 39, 45, 51, 52, 56, 58, and 59 (55). The Gardasil 9® vaccine, which contains the highest number of high-risk HPV serotypes in circulation worldwide, does not fully cover all high-risk HPV types. To achieve complete protection, the development of prophylactic HPV vaccines should further enlarge their protective extent to target more life-threatening HPV types. The following section will summarize the progress in the development of prophylactic L1-VLP-based HPV vaccines currently in clinical trials (Table 2).
All 9-valent HPV vaccines target the same highly carcinogenic HPV types (HPV-6/11/16/18/31/33/45/52/58). Shanghai Bovax Biotechnology Co., Ltd. has created a 9-valent HPV vaccine that exhibits high immunogenicity, good tolerance, and non-inferiority to Gardasil 4 in terms of both immunogenicity and safety (56). However, data regarding other HPV vaccines has not been made public. The 11-valent HPV vaccine, developed by the National Vaccine and Serum Institute (China), covered two additional HPV types (HPV-59/68) on top of the commonly used HPV types in 9-valent vaccines. The underlying development strategy might be linked to the findings that HPV-59 and 68 are potentially associated with genital cancerogenesis (57). SCT1000, developed by Sinocelltech Ltd, is a recombinant 14-valent L1-VLP-based HPV vaccine designed to prevent HPV infection and HPV-related cancers. It covers five additional HPV types (35, 39, 51, 56, and 59) compared with Gardasil 9®. SCT1000 is currently being investigated in a Phase III study for HPV infection and HPV-related carcinoma (NCT06041061). A 15-valent vaccine developed by Chengda Bio targets HPV-68, in addition to the 14 HPV types covered by SCT1000, is currently in development for the prevention of HPV-infected diseases.
3.3 Therapeutic HPV vaccines in clinical testing
Prophylactic HPV vaccines scarcely eradicate preexisting infection because L1 capsid proteins are not expressed on the surface of infected basal epithelial cells (58). Therefore, the development of prophylactic HPV vaccines is not an effective treatment approach for people already infected with HPV (59). Cellular immune responses to HPV antigens play important roles in viral clearance and anticancer immune responses. Ineffective cellular immune responses to HPV-16 E2, E6, or E7 peptides involve infection persistence or disease progression in low-grade intraepithelial lesion (LSIL) patients (60). HPV-16 E6 and E7-specific cellular immune responses facilitate the regression of HPV-16-associated lesions (61). Similarly, the percentages of IFN-γ positive enzyme-linked immunospot (ELISpot) responses to HPV-16 E6 and E7 are significantly increased among women with recently resolved HPV infection versus those with persistent HPV-16 infection in the cervix (62).
Therapeutic HPV vaccines are developed to stimulate cell-mediated immunity for the clearance of infection (63, 64). Among HPV early proteins (E1, E2, E6, and E7) and late proteins (L1, L2), HPV E6 and E7 proteins are constitutively expressed in both premalignant and invasive lesions and play key roles in the development of malignancy (65). Further, E6 and E7 can activate antigen-specific CD8+ or CD4+ T cells and finally evoke cellular immune responses (66–68). Therefore, E6 and E7 proteins are considered ideal antigens for therapeutic HPV vaccines. TLR agonists alone or mixed with other components are commonly tested adjuvants for therapeutic HPV vaccines (69–72). Recently developed new adjuvants, such as α-Galactosylceramide (α-GalCer), manganese (Mn4+)-doped silica nanoparticles (Mn4+-SNPs), and very small-size proteoliposomes (VSSP) (73–75), might play roles in reinforcing the efficacy of therapeutic HPV vaccines.
Prophylactic HPV vaccines are mainly protein-based VLPs. Therapeutic HPV vaccines are primarily based on nucleic acid, bacterial/viral vector, protein/peptide or whole cells (76). To date, no therapeutic HPV vaccine has been licensed. Safety profiles and vaccine efficacy for therapeutic HPV vaccines tested in clinical trials are summarized in Table 3. A complete regression of lesions triggered by therapeutic HPV vaccines has been reported, with a regression rate ranging from 17.4% to 89.4% in female patients (54, 78, 81, 82, 84–86, 88, 89). The clearance of HPV DNA in infected cells is considered a predictor of vaccine efficacy, as the presence of HPV DNA at the cervical site is often associated with histologic and cytological changes in cervical intraepithelial neoplasia (CIN) (77, 79–81, 83, 87, 90).
In addition, vaccine antigen-specific T-cell responses were comprehensively evaluated. In a Phase I trial, AMV002 vaccination induced E6 and/or E7 specific cellular immune response in 83.3% subjects (78). In a Phase II study of, GX-188E, 67.3% of patients showed histopathologic regression (<CIN1) and 55.8% of patients with viral clearance 36 weeks after GX-188E treatment. Importantly, E6 and E7-specific IFN-γ positive T-cell response in the patients with HPV clearance presented significant increases compared with patients without clearance (81). In a Phase I study of Candida Skin Test, a colorless extract of Candida albicans FDA-approved for use as a human adjuvant, vaccine-induced cellular immunity against HPV-16 E6 was detected in 65% of vaccine recipients. The percentages of Th1 cells and Th2 cells increased significantly, while no change was observed regarding the percentage of Tregs after two doses of vaccine administration.
Several therapeutic HPV vaccines in clinical trials indicated a correlation between vaccine efficacy and vaccine-induced cellular immune responses. The results of Phase I/II of IGMKK16E7, the first oral immunotherapeutic vaccine, showed the number of HPV-16 E7–specific interferon-γ producing cells in blood increased with response levels (stable disease, partial, and complete responses) (89). In a Phase IIb trial of VGX-3100, vaccine-induced T-cell responses to E6 were positively correlated with clinical outcomes rather than E7, while higher frequencies of HPV-specific CD8+ CD137+ T cells indicated better outcomes (82). Similarly, a recombinant MVA E2 vaccine induced antigen-specific cytotoxic T-cell responses among all vaccinated patients in a Phase III study (85). Accordingly, 90% of female and 100% of male participants exhibited complete regression of lesions 14 weeks after vaccination (85).
4 Future directions for HPV vaccine development
4.1 Alternative antigenic targets
L1 protein is a commonly used vaccine target for prophylactic HPV vaccines (91). Preliminary data indicated that L1 protein triggers both humoral and cellular immune responses (92, 93). Indeed, L1 capsomeres induced tumor regression in mice via activation of antigen-specific cytotoxic T lymphocytes (94). In HPV-positive oropharyngeal cancer (OPC) patients, E6, E7, and L1 capsid proteins were recognized by HPV-specific CD8+ and CD4+ T cells (95). However, L1-based HPV vaccines failed to be effective treatment approaches due to the lack of constitutive expression in premalignant and invasive lesions (58). Currently, many therapeutic vaccines in clinical trials primarily target E6 and E7 proteins (96). Alternative antigenic targets, such as E2 and L2, are also in clinical tests (Table 4).
HPV DNA integration and diminished E2 expression were essential for the oncogenic transition of HPV-infected cells (108). E2 controlled HPV DNA transcription and suppressed E6 and E7 expression (96). As a result, E2 was probably irrelevant to the therapeutic vaccine target. However, E2-specific T-cell responses were related to the lack of progression into high-grade intraepithelial lesions (HSILs) (60). Accordingly, more potent E2-specific T-cell responses have been reported in patients with resolved cervical dysplasia, supporting the role of E2 as a candidate for vaccine antigens in immunotherapy of pre-cancerous cervical lesions (109). E1 is continuously expressed in the basal epithelial cells after HPV infection and plays an important role in virus replication (110). E1 is crucial for carcinogenesis (111). However, E1 might not be an ideal target for vaccine antigens due to its ubiquitination and rapid degradation attributes (112, 113).
The minor capsid protein L2 is a promising candidate for an HPV vaccine since the linear neutralizing epitopes on the L2 N-terminus are well conserved across several HPV genotypes (114). In mouse models, HPV-16 L2 antigen-induced neutralizing antibodies protected against infectious HPV-16/45 challenges (115, 116). However, L2 might be less immunogenic since it is unable to form VLPs (117). Therefore, strategies to enhance the immunogenicity of L2 protein have become attractive (114, 118, 119). Notably, peptide antigens (120, 121) and HSP70-based fusion proteins are widely tested in both preclinical and clinical studies (122, 123).
4.2 Adjuvant optimization
Cervarix® and Gardasil® were formulated with AS04 and amorphous aluminum hydroxyphosphate sulfate, respectively (124, 125). Alum-based adjuvants could induce Th2-biased immune responses (126). The immunostimulant MPL, one component of the AS04 adjuvant, activates innate immune responses through Toll-like receptor 4 (TLR4) and induces a mixed Th1/Th2 immune response (127). Participants received Cervarix® displayed stronger humoral and CD4+ T cell responses against HPV-16 and 18 than patients received Gardasil® (125, 128, 129). Meanwhile, distinctive immune profiles induced by HPV vaccines formulated with an Alum-based adjuvant, or AS04, have been reported (129, 130).
Adjuvants may affect the strength and durability of antigen-specific immune responses. Exploratory studies of novel adjuvants used alone or in combination have highlighted their potential use in HPV vaccines (Table 4). Bacterial-derived components, such as Beclin-1 (105), AS04 (NLX+Alum) (25), CIA06B (nontoxic derivative of lipopolysaccharide (CIA05) + Alum) (100), rough LPS (R-LPS) (98), and bacterial enzymatic combinatorial chemistry (BECC)-derived TLR4 agonists/Alum (70), are widely utilized in exploratory studies.
In addition, novel molecules with distinctive contributions to immunogenicity are being evaluated in preclinical studies of HPV vaccines, such as CpG oligodeoxynucleotide (CpG) (71, 104), polyinosinic-polycytidylic acid (Poly I:C) (104), α-Galactosylceramide (α-GalCer) (73), very small size proteoliposomes (VSSP) (75), poly di (carboxylatoethylphenoxy) phosphazene (PCEP) (99), c-di-AMP (101), S-540956 (a CpG Oligonucleotide) (72), the metal ions like manganese (Mn4+)-doped silica nanoparticles (Mn4+-SNPs) (74), plant extracts like Bai Hua She She Cao (BHSSC) (102), and mRNA-encoded STINGV155M (106), Candida skin test reagent (Extract of Candida albicans) (103).
Although many novel adjuvants have demonstrated their unique efficacy in modulating immune responses in mouse models, only a few have been licensed for use in humans to date, primarily due to safety concerns. Therefore, novel HPV vaccine adjuvants require further safety and efficacy validation in clinical trials.
4.3 Combination with immune checkpoint blockade therapy
HPVs employ multiple strategies to evade the host’s immune response, including interference with antigen presentation, downregulation of antigen production, inhibition of antiviral molecules, suppression of type 1 T helper (Th1) cell immune responses, and promotion of regulatory T cell (Treg) responses (84, 131). Therapeutic HPV vaccine-evoked antigen-specific T cells are the key to tumor cell elimination and rely primarily on the loss of inhibitory signals in the tumor microenvironment (TME). Thus, the combination of therapeutic HPV vaccines and immune checkpoint inhibitors may show synergistic effects in tumor regression.
Preclinical studies have demonstrated that therapeutic HPV vaccines, such as adenovirus delivery vectors carrying modified HPV-16 E6 and E7 genes, HPV-16 E7 long peptide with DCs, and the Lm-LLO fused-E6 vaccine (Lm-LLO-E6), significantly promoted tumor regression and survival rate in tumor-bearing mouse models when used with a programmed death-ligand 1 (PD-L1) inhibitor (132–134). The clinical trials of therapeutic HPV vaccines combined with ICB therapeutic modalities are mainly in Phase I/II. Preliminary data show good efficacy and safety, and further verification in later clinical trials is needed. The current status of combination trials is listed in Table 5.
4.4 HPV L1 VLP vaccine as adjuvant therapy
L1 VLPs-based HPV vaccines have been approved primarily for the prevention of cervical cancer in populations without preexisting HPV infection. Studies have suggested an indispensable role of HPV L1 VLP vaccines as adjuvant therapy after surgical treatment for patients suffering from HPV-related clinical diseases such as recurrent respiratory papillomatosis (RRP) (140) and cervical intraepithelial neoplasia (CIN) (141). The data reported that surgical intervention and HPV vaccination combination therapy can effectively reduce the recurrence and severity of respiratory papillomatosis (142). Likewise, another study has reported that HPV vaccination significantly prolongs the intervals between surgical procedures and reduces the number of procedures in the majority of RRP patients (143). Most CINs are caused by HPV infection (144) and might progress to cervical cancer (145). CIN 1 rarely progresses to malignancy with a high potential for regression. Instead, CIN 2 and 3, as the high-grade lesions, become highly cancerous with a lower potential for regression (146, 147). Studies have reported that vaccination with the quadrivalent HPV vaccine in patients after loop electrosurgical excision procedure (LEEP) treatment significantly prevents the recurrence of CIN2–3 related to vaccine HPV types compared with non-HPV-vaccinated patients (148). These data demonstrate that HPV vaccination plays a critical role as adjuvant therapy in HPV-related disorders other than cancer.
4.5 Exploration in formulation strategy, dosing regimens, and expansion of vaccine recipients
4.5.1 Alternative strategies for HPV vaccine formulation
High cost, cold chain storage and delivery, and requirements for trained vaccinators limit vaccination coverage, which prompts the development of nano- or micro-particulate vaccines (149). Nanotechnology has been extensively used in vaccine development via the utility of advanced nano-based particles or materials designed for antigen delivery or as adjuvants to enhance immune responses (150). Archaeosomes are commonly used in cancer vaccines as delivery tools. HPV DNA vaccines containing the truncated L1, E6, and E7 genes in combination with archaeosomes induce strong cytolytic immune responses to eliminate tumor cells (151). Gold nanoparticles can also be potentially used in the HPV vaccine to strengthen cytotoxic immune responses by increasing oxidative stress (152). Chitosan is also used as a nanoparticle adjuvant due to its non-toxic, highly biocompatible, low susceptibility, and biodegradation properties (153). Chitosan-based DNA vaccines expressing HPV-16 E7 significantly promote T cell-mediated immune response and antibody production against HPV-induced tumors (154). Hence, the nanotechnology-created HPV vaccine would provide more options for the treatment of HPV-related diseases. Additionally, HPV protein antigens can be formulated into glassy microspheres using spray-dried techniques and then coated by atomic layer deposition (ALD) with nanometer-thin protective layers of alumina to improve their thermostability (155). Given the advantage of nanotechnology utilization in HPV vaccine formulation, it would provide more options for patients in prevention or treatment of HPV-related diseases. However, the current nanoparticle agents for the HPV vaccine are still far away for human use, with uncertainty concerning. Further studies regarding the safety, stability, potency, and rapid production of HPV are required to be explored.
Oral administration of the HPV vaccine is easy, with favorable safety profiles and potential long-lasting protective effects in the intestine (156). Oral vaccination could diminish vaccine hesitancy and boost vaccine coverage (157). A clinical study has reported that an oral therapeutic vaccine has demonstrated safety and potency to induce persistent immunity (90). The combinational application of a nanotechnology-based DNA vaccine and a VLP vaccine could potently stimulate both humoral and cellular immunity, which has been investigated for HIV infection (158). Oral films have been extensively used in formulations for the administration of many drugs (159). Film-based HPV vaccines would be attractive as a novel type of HPV vaccine to treat HPV-related carcinogenesis. Film materials make the administration of the HPV vaccine easy and reduce the risk of choking or suffocation in pediatric, geriatric, and psychiatric patients (160). The film dosage forms incorporated with DNA- and VLP-based vaccines offer a promising approach for future HPV development. While intramuscular injection of HPV vaccines has shown clinical effectiveness, the efficacy of orally administered HPV vaccines remains uncertain. Additional research is needed to investigate the bioavailability, immune response, and safety aspects of orally administered HPV vaccines in preclinical studies and clinical trials.
4.5.2 Dosing regimens
For novel HPV vaccines, reducing the vaccination frequency would be a wise strategy. To achieve this, participants will be administered higher concentrations of HPV antigens. A recent study demonstrated the efficacy of a single dose of the HPV vaccine in young women in Africa (161). Another report has shown one dose of quadrivalent HPV vaccination had comparable effectiveness as two or three doses in preventing cervical intraepithelial neoplasia (162). More studies to compare the efficacy among the one-dose, two-dose, and three-dose regimens are presented in Table 6. This information indicated that the potency induced by one dose vaccination was almost similar to that of two or three doses in preventing HPV infection. Additionally, self-boosting vaccination for HPV could reduce the dosing frequency and improve its lasting effectiveness by loading HPV antigens into microparticles. This could be released over time, continuously boosting its immune response (167, 168).
The current HPV dose schedule recommended by the WHO Strategic Advisory Group of Experts on Immunization (SAGE) is as follows: 1) One or two-dose schedule for the primary target of girls aged 9–14 years; 2) One or two-dose schedule for young women aged 15–20 years; 3) Two doses with a 6-month interval for women older than 21 years (169). Some experts suggest that single-dose vaccination is less expensive, supports more compliance, and is therefore logistically easier compared with multiple doses, making it more feasible to vaccinate more women in low- and middle-income countries (170). Similarly, the Joint Committee on Vaccination and Immunization (JCVI) statement also considers the potential change to a one-dose schedule for the routine HPV immunization program (171).
4.5.3 Expansion of vaccine recipients
In 2018, the FDA approved the expanded use of Gardasil 9® to include individuals 27 through 45 years old (172). According to the WHO guidelines, HPV vaccination is usually recommended for boys and girls aged 11 to 12, but can be given as early as age 9 years for the first dose before sexual contact and exposure to HPV. Children between the ages of 11 to 12 years are suggested to get 2 doses of the HPV vaccine 6 to 12 months apart. For teens and young adults at ages 15 through 26, they need three doses of the HPV vaccine. Early prevention with the HPV vaccine is a safe and effective way to reduce HPV infection (173). Studies have demonstrated that adolescents who initiated the HPV vaccine series at age 9 or 10 were 22 times more likely to complete the two-dose series by age 15 than those who started the series at age 11 or 12 (174). To date, the safety and efficacy of the HPV vaccine, for example, Gardasil®, in children below 9 years of age have not been established. Thus, whether HPV vaccination programs are adopted for children below 9 years old requires further investigation. A quality improvement initiative performed in the Nationwide Children’s Hospital system utilizing electronic medical record alerts has shown rapid uptake of the HPV vaccine before age 11, suggesting a willingness by parents and providers to initiate the vaccine earlier than previously recommended (175). On the other hand, parents have worried that early HPV vaccination in adolescents would increase sexual behavior, thereby increasing the risks of HPV infection. However, a study reported that HPV vaccination has not increased sexual activity or accelerated sexual debut in a college-aged cohort of men and women (176). Experts believe that early HPV vaccination against HPV can not only increase completion rates but also reduce cancer mortality.
HPV vaccination has been expanded to young males since 2009 in the United States (177), and gender-neutral (GN) HPV vaccination has been adopted in 33 out of 107 countries as of 2019, with 4% of males worldwide get vaccinated (178). Data from a community randomized clinical trial (NCT000534638) indicated the advantage of GN over girls-only (GO) vaccination against HPV infection (179). Vaccination in both genders might help to build resilient cervical cancer prevention (180). Despite preliminary scientific evidences and potential public health benefits, more issues should take into consideration regarding the deployment of HPV vaccination program in the future. Due to limited vaccine supply, the WHO recommended to postpone the GN vaccination policy in 2019 (181). Factors affecting HPV vaccination in males have been reviewed by several reports, including limited economic resources, as well as ethical and legal considerations (177, 182, 183).
5 Future perspective
Prophylactic HPV vaccines have shown efficacy in preventing HPV transmission and infection, controlling the incidence of HPV-related cancers and genital warts, and improving clinical manifestations. Correlates of protection need to be better defined, such as the magnitude of humoral and cellular immune responses, specific antibody characteristics, adjuvants, and age of recipients. This information might guide the development and deployment of HPV vaccines for protection against HPV infection. Enhancing vaccine efficacy through diverse L1 antigens, potent adjuvants for cellular immunity, and therapeutic HPV vaccines targeting E6 and E7 proteins, particularly in conjunction with immune checkpoint blockade, may pave the way for eradicating HPV infections and associated cancers. As an attractive research area, therapeutic HPV vaccines in clinical trials have shown promising results in safety, HPV DNA clearance, tumor regression, and antigen-specific T-cell responses. The investigation is constantly progressing toward enlarging the protective breadth of prophylactic vaccines, with most already in the late stages of clinical trials. Promising preclinical findings support novel antigenic targets and adjuvants for vaccine design. However, confirming these findings in clinical trials is necessary. Moreover, recent progress in the exploration of vaccine formulation, immunization schedules, and age expansion may help reduce HPV transmission and infection due to increased vaccine coverage.
Author contributions
LX: Writing – review & editing, Supervision, Funding acquisition, Conceptualization. RW: Writing – review & editing, Writing – original draft. HH: Writing – original draft. CY: Writing – original draft. XL: Writing – review & editing. YW: Writing – review & editing.
Funding
The author(s) declare financial support was received for the research, authorship, and/or publication of this article. This study received funding from the Achievement Transformation Project of Administrative Commission of Zhongguancun Science Park (201905180-15). The funder was not involved in the study design, collection, analysis, interpretation of data, the writing of this article or the decision to submit it for publication.
Conflict of interest
All authors were employed by Sinocelltech Ltd.
Publisher’s note
All claims expressed in this article are solely those of the authors and do not necessarily represent those of their affiliated organizations, or those of the publisher, the editors and the reviewers. Any product that may be evaluated in this article, or claim that may be made by its manufacturer, is not guaranteed or endorsed by the publisher.
References
1. Mayeaux EJ. Reducing the economic burden of HPV-related diseases. J Am Osteopath Assoc. (2008) 108:2–7. doi: 10.7556/jaoa.2008.20005
2. Araldi RP, Sant’Ana TA, Módolo DG, de Melo TC, Spadacci-Morena DD, de Cassia Stocco R, et al. The human papillomavirus (HPV)-related cancer biology: An overview. BioMed Pharmacother. (2018) 106:1537–56. doi: 10.1016/j.biopha.2018.06.149
3. Bray F, Ferlay J, Soerjomataram I, Siegel RL, Torre LA, Jemal A. Global cancer statistics 2018: GLOBOCAN estimates of incidence and mortality worldwide for 36 cancers in 185 countries. CA Cancer J Clin. (2018) 68:394–424. doi: 10.3322/caac.21492
4. Arbyn M, Weiderpass E, Bruni L, de Sanjosé S, Saraiya M, Ferlay J, et al. Estimates of incidence and mortality of cervical cancer in 2018: a worldwide analysis. Lancet Glob Health. (2020) 8:e191–203. doi: 10.1016/S2214–109X(19)30482–6
5. De Martel C, Georges D, Bray F, Ferlay J, Clifford GM. Global burden of cancer attributable to infections in 2018: a worldwide incidence analysis. Lancet Glob Health. (2020) 8:e180–e90. doi: 10.1016/S2214–109X(19)30488–7
6. WHO. Human papillomavirus and cancer (2024). Available online at: https://www.who.int/news-room/fact-sheets/detail/human-papilloma-virus-and-cancer#:~:text=Globally%2C%20it%20is%20estimated%20that,and%20social%20and%20economic%20determinants (Accessed May 14 2024).
7. Beavis AL, Meek K, Moran MB, Fleszar L, Adler S, Rositch AF. Exploring HPV vaccine hesitant parents’ perspectives on decision-making and motivators for vaccination. Vaccine X. (2022) 12:100231. doi: 10.1016/j.jvacx.2022.100231
8. Adjei Boakye E, Nair M, Abouelella DK, Joseph CL, Gerend MA, Subramaniam DS, et al. Trends in reasons for human papillomavirus vaccine hesitancy: 2010–2020. Pediatrics. (2023) 151:e2022060410. doi: 10.1542/peds.2022–060410
9. De Villiers EM, Fauquet C, Broker TR, Bernard HU, Zur Hausen H. Classification of papillomaviruses. Virology. (2004) 324:17–27. doi: 10.1016/j.virol.2004.03.033
10. Bernard HU, Burk RD, Chen Z, Van Doorslaer K, Zur Hausen H, de Villiers E-MJV. Classification of papillomaviruses (PVs) based on 189 PV types and proposal of taxonomic amendments. Virology. (2010) 401:70–9. doi: 10.1016/j.virol.2010.02.002
11. Doorbar J, Egawa N, Griffin H, Kranjec C, Murakami I. Human papillomavirus molecular biology and disease association. Rev Med Virol. (2015) 25:2–23. doi: 10.1002/rmv.1822
12. Galloway DA, Laimins LA. Human papillomaviruses: shared and distinct pathways for pathogenesis. Curr Opin Virol. (2015) 14:87–92. doi: 10.1016/j.coviro.2015.09.001
13. Williamson AL. Recent developments in human papillomavirus (HPV) vaccinology. Viruses. (2023) 15:1440. doi: 10.3390/v15071440
14. Wheeler CM, Hunt WC, Cuzick J, Langsfeld E, Pearse A, Montoya GD, et al. A population-based study of human papillomavirus genotype prevalence in the United States: baseline measures prior to mass human papillomavirus vaccination. Int J Cancer. (2013) 132:198–207. doi: 10.1002/ijc.27608
15. Liu F, Chang L, Bai T, Liu X, Hu J. Association of human papillomavirus genotype distribution and cervical cytology: a cross-sectional study. Epidemiol Infect. (2021) 149:e95. doi: 10.1017/S0950268821000741
16. Kirnbauer R, Booy F, Cheng N, Lowy D, Schiller J. Papillomavirus L1 major capsid protein self-assembles into virus-like particles that are highly immunogenic. Proc Natl Acad Sci U.S.A. (1992) 89:12180–4. doi: 10.1073/pnas.89.24.12180
17. Graham SV. Human papillomavirus: gene expression, regulation and prospects for novel diagnostic methods and antiviral therapies. Future Microbiol. (2010) 5:1493–506. doi: 10.2217/fmb.10.107
18. Pappa KI, Kontostathi G, Lygirou V, Zoidakis J, Anagnou NP. Novel structural approaches concerning HPV proteins: Insight into targeted therapies for cervical cancer. Oncol Rep. (2018) 39:1547–54. doi: 10.3892/or.2018.6257
19. Li Z, Yan X, Yu H, Wang D, Song S, Li Y, et al. The C-terminal arm of the human papillomavirus major capsid protein is immunogenic and involved in virus-host interaction. Structure. (2016) 24:874–85. doi: 10.1016/j.str.2016.04.008
20. Wang JW, Roden RB. L2, the minor capsid protein of papillomavirus. Virology. (2013) 445:175–86. doi: 10.1016/j.virol.2013.04.017
21. Yim EK, Park JS. The role of HPV E6 and E7 oncoproteins in HPV-associated cervical carcinogenesis. Cancer Res Treat. (2005) 37:319–24. doi: 10.4143/crt.2005.37.6.319
22. Silva L, Teles AM, Santos JM, Souza de Andrade M, Medeiros R, Faustino-Rocha AI, et al. Malignancy associated with low-risk HPV6 and HPV11: a systematic review and implications for cancer prevention. Cancers (Basel). (2023) 15:4068. doi: 10.3390/cancers15164068
23. Rusan M, Li YY, Hammerman PS. Genomic landscape of human papillomavirus–associated cancers. Clin Cancer Res. (2015) 21:2009–19. doi: 10.1158/1078–0432.CCR-14–1101
24. Ahmed HG, Bensumaidea SH, Alshammari FD, Alenazi FSH, ALmutlaq BA, Alturkstani MZ, et al. Prevalence of human papillomavirus subtypes 16 and 18 among Yemeni patients with cervical cancer. Asian Pac J Cancer Prev. (2017) 18:1543–8. doi: 10.22034/APJCP.2017.18.6.1543
25. Didierlaurent AM, Morel S, Lockman L, Giannini SL, Bisteau M, Carlsen H, et al. AS04, an aluminum salt-and TLR4 agonist-based adjuvant system, induces a transient localized innate immune response leading to enhanced adaptive immunity. J Immunol. (2009) 183:6186–97. doi: 10.4049/jimmunol.0901474
26. Monie A, Hung CF, Roden R, Wu TC. Cervarix™: a vaccine for the prevention of HPV 16, 18-associated cervical cancer. Biologics. (2008) 2:107–13. doi: 10.2147/btt.s12160259
27. Siddiqui MAA, Perry CM. Human papillomavirus quadrivalent (types 6, 11, 16, 18) recombinant vaccine (Gardasil®). Drugs. (2006) 66:1263–71. doi: 10.2165/00003495–200666090–00008
28. McCormack PL. Quadrivalent human papillomavirus (types 6, 11, 16, 18) recombinant vaccine (Gardasil®): a review of its use in the prevention of premalignant anogenital lesions, cervical and anal cancers, and genital warts. Drugs. (2014) 74:1253–83. doi: 10.1007/s40265-014-0255-z
29. Signorelli C, Odone A, Ciorba V, Cella P, Audisio R, Lombardi A, et al. Human papillomavirus 9-valent vaccine for cancer prevention: a systematic review of the available evidence. Epidemiol Infect. (2017) 145:1962–82. doi: 10.1017/S0950268817000747
30. Linton D, Taylor S. The human papillomavirus vaccine expanded to middle age adults. J Nurse Pract. (2019) 15:530–1. doi: 10.1016/j.nurpra.2019.04.006
31. Soliman M, Oredein O, Dass CR. Update on safety and efficacy of HPV vaccines: focus on gardasil. Int J Mol Cell Med. (2021) 10:101–3. doi: 10.22088/IJMCM.BUMS.10.2.101
32. Tang X, Jones TE, Jiang W, Austin M, He Y, Li L, et al. Extended Human Papillomavirus genotype distribution in cervical intraepithelial neoplasia and cancer: analysis of 40,352 cases from a large academic gynecologic center in China. J Med Virol. (2023) 95:e28302. doi: 10.1002/jmv.28302
33. Li M, Zhao C, Zhao Y, Wei L. Immunogenicity, efficacy, and safety of human papillomavirus vaccine: data from China. Front Immunol. (2023) 14:1112750. doi: 10.3389/fimmu.2023.1112750
34. Li J, Shi LW, Li K, Huang LR, Li JB, Dong YL, et al. Comparison of the safety and persistence of immunogenicity of bivalent HPV16/18 vaccine in healthy 9–14-year-old and 18–26-year-old Chinese females: A randomized, double-blind, non-inferiority clinical trial. Vaccine. (2023) 41:7212–19. doi: 10.1016/j.vaccine.2023.10.041
35. Hu YM, Guo M, Li CG, Chu K, He WG, Zhang J, et al. Immunogenicity noninferiority study of 2 doses and 3 doses of an Escherichia coli-produced HPV bivalent vaccine in girls vs. 3 doses in young women. Sci China Life Sci. (2020) 63:582–91. doi: 10.1007/s11427–019-9547–7
36. Chen Q, Zhao H, Yao X, Lin Z, Li J, Lin B, et al. Comparing immunogenicity of the Escherichia coli-produced bivalent human papillomavirus vaccine in females of different ages. Vaccine. (2020) 38:6096–102. doi: 10.1016/j.vaccine.2020.07.030
37. Qiao YL, Wu T, Li RC, Hu YM, Wei LH, Li CG, et al. Efficacy, safety, and immunogenicity of an Escherichia coli-produced bivalent human papillomavirus vaccine: an interim analysis of a randomized clinical trial. J Natl Cancer Inst. (2020) 112:145–53. doi: 10.1093/jnci/djz074
38. Guo J, Guo S, Dong S. Efficacy, immunogenicity and safety of HPV vaccination in Chinese population: a meta-analysis. Front Public Health. (2023) 11:1128717. doi: 10.3389/fpubh.2023.1128717
39. Turner TB, Huh WK. HPV vaccines: translating immunogenicity into efficacy. Hum Vaccin Immunother. (2016) 12:1403–5. doi: 10.1080/21645515.2015.1103936
40. Hu S, Xu X, Zhu F, Hong Y, Hu Y, Zhang X, et al. Efficacy of the AS04-adjuvanted HPV-16/18 vaccine in young Chinese women with oncogenic HPV infection at baseline: post-hoc analysis of a randomized controlled trial. Hum Vaccin Immunother. (2021) 17:955–64. doi: 10.1080/21645515.2020.1829411
41. Zhao C, Zhao Y, Li J, Li M, Su Y, Mi X, et al. The eight-year long-term follow-up on the effectiveness of the quadrivalent human papillomavirus vaccine in Chinese women 20–45 years of age. Hum Vaccin Immunother. (2022) 18:2052700. doi: 10.1080/21645515.2022.2052700
42. Lv H, Wang S, Liang Z, Yu W, Yan C, Chen Y, et al. Immunogenicity and safety of the 9-valent human papillomavirus vaccine in Chinese females 9–45 years of age: A phase 3 open-label study. Vaccine. (2022) 40:3263–71. doi: 10.1016/j.vaccine.2022.02.061
43. Zhao FH, Wu T, Hu YM, Wei LH, Li MQ, Huang WJ, et al. Efficacy, safety, and immunogenicity of an Escherichia coli-produced Human Papillomavirus (16 and 18) L1 virus-like-particle vaccine: end-of-study analysis of a phase 3, double-blind, randomised, controlled trial. Lancet Infect Dis. (2022) 22:1756–68. doi: 10.1016/S1473–3099(22)00435–2
44. Prabhu PR, Carter JJ, Galloway DA. B cell responses upon human papillomavirus (HPV) infection and vaccination. Vaccines (Basel). (2022) 10:837. doi: 10.3390/vaccines10060837
45. Steele J, Mann C, Rookes S, Rollason T, Murphy D, Freeth M, et al. T-cell responses to human papillomavirus type 16 among women with different grades of cervical neoplasia. Br J Cancer. (2005) 93:248–59. doi: 10.1038/sj.bjc.6602679
46. Mariz FC, Bender N, Anantharaman D, Basu P, Bhatla N, Pillai MR, et al. Peak neutralizing and cross-neutralizing antibody levels to human papillomavirus types 6/16/18/31/33/45/52/58 induced by bivalent and quadrivalent HPV vaccines. NPJ Vaccines. (2020) 5:14. doi: 10.1038/s41541-020-0165-x
47. Joura EA, Kjaer SK, Wheeler CM, Sigurdsson K, Iversen O-E, Hernandez-Avila M, et al. HPV antibody levels and clinical efficacy following administration of a prophylactic quadrivalent HPV vaccine. Vaccine. (2008) 26:6844–51. doi: 10.1016/j.vaccine.2008.09.073
48. Meyer SI, Fuglsang K, Blaakaer J. Cell-mediated immune response: a clinical review of the therapeutic potential of human papillomavirus vaccination. Acta Obstet Gynecol Scand. (2014) 93:1209–18. doi: 10.1111/aogs.12480
49. Roy V, Jung W, Linde C, Coates E, Ledgerwood J, Costner P, et al. Differences in HPV-specific antibody Fc-effector functions following Gardasil® and Cervarix® vaccination. NPJ Vaccines. (2023) 8:39. doi: 10.1038/s41541–023-00628–8
50. Plotkin SA. Correlates of protection induced by vaccination. Clin Vaccine Immunol. (2010) 17:1055–65. doi: 10.1128/CVI.00131–10
51. Ebrahimi N, Yousefi Z, Khosravi G, Malayeri FE, Golabi M, Askarzadeh M, et al. Human papillomavirus vaccination in low-and middle-income countries: progression, barriers, and future prospective. Front Immunol. (2023) 14:1150238. doi: 10.3389/fimmu.2023.1150238
52. Madrid-Marina V, Torres-Poveda K, López-Toledo G, García-Carrancá A. Advantages and disadvantages of current prophylactic vaccines against HPV. Arch Med Res. (2009) 40:471–7. doi: 10.1016/j.arcmed.2009.08.005
53. Nicol AF, Andrade C, Russomano FB, Rodrigues LLS, Oliveira N, Provance D Jr. HPV vaccines: a controversial issue? Braz J Med Biol Res. (2016) 49:e5060. doi: 10.1590/1414–431X20155060
54. WHO. Safety of HPV vaccines (2017). Available online at: https://www.who.int/groups/global-advisory-committee-on-vaccine-safety/topics/human-papillomavirus-vaccines/safety (Accessed May 17 2024).
55. National Cancer Institute. HPV and cancer (2023). Available online at: https://www.cancer.gov/about-cancer/causes-prevention/risk/infectious-agents/hpv-and-cancer (Accessed December 5 2023).
56. Shu Y, Yu Y, Ji Y, Zhang L, Li Y, Qin H, et al. Immunogenicity and safety of two novel human papillomavirus 4-and 9-valent vaccines in Chinese women aged 20–45 years: a randomized, blinded, controlled with Gardasil (type 6/11/16/18), phase III non-inferiority clinical trial. Vaccine. (2022) 40:6947–55. doi: 10.1016/j.vaccine.2022.10.022
57. Faridi R, Zahra A, Khan K, Idrees M. Oncogenic potential of Human Papillomavirus (HPV) and its relation with cervical cancer. Virol J. (2011) 8:269. doi: 10.1186/1743–422X-8–269
58. Wang R, Pan W, Jin L, Huang W, Li Y, Wu D, et al. Human papillomavirus vaccine against cervical cancer: Opportunity and challenge. Cancer Lett. (2020) 471:88–102. doi: 10.1016/j.canlet.2019.11.039
59. Khallouf H, Grabowska AK, Riemer AB. Therapeutic vaccine strategies against human papillomavirus. Vaccines (Basel). (2014) 2:422–62. doi: 10.3390/vaccines2020422
60. Woo YL, van den Hende M, Sterling JC, Coleman N, Crawford RAF, Kwappenberg KMC, et al. A prospective study on the natural course of low-grade squamous intraepithelial lesions and the presence of HPV16 E2-, E6- and E7-specific T-cell responses. Int J Cancer. (2010) 126:133–41. doi: 10.1002/ijc.24804
61. Sarkar AK, Tortolero-Luna G, Follen M, Sastry KJ. Inverse correlation of cellular immune responses specific to synthetic peptides from the E6 and E7 oncoproteins of HPV-16 with recurrence of cervical intraepithelial neoplasia in a cross-sectional study. Gynecol Oncol. (2005) 99:S251–S61. doi: 10.1016/j.ygyno.2005.07.099
62. Farhat S, Nakagawa M, Moscicki A-B. Cell-mediated immune responses to human papillomavirus 16 E6 and E7 antigens as measured by interferon gamma enzyme-linked immunospot in women with cleared or persistent human papillomavirus infection. Int J Gynecol Cancer. (2009) 19:508–12. doi: 10.1111/IGC.0b013e3181a388c4
63. Kamolratanakul S, Pitisuttithum P. Human papillomavirus vaccine efficacy and effectiveness against cancer. Vaccines (Basel). (2021) 9:1413. doi: 10.3390/vaccines9121413
64. Vermaelen K. Vaccine strategies to improve anti-cancer cellular immune responses. Front Immunol. (2019) 10:8. doi: 10.3389/fimmu.2019.00008
65. Chabeda A, Yanez RJR, Lamprecht R, Meyers AE, Rybicki EP, Hitzeroth II. Therapeutic vaccines for high-risk HPV-associated diseases. Papillomavirus Res. (2018) 5:46–58. doi: 10.1016/j.pvr.2017.12.006
66. Yang A, Jeang J, Cheng K, Cheng T, Yang B, Wu T-C, et al. Current state in the development of candidate therapeutic HPV vaccines. Expert Rev Vaccines. (2016) 15:989–1007. doi: 10.1586/14760584.2016.1157477
67. Castro-Eguiluz D, Barquet-Muñoz SA, Arteaga-Gómez AC, Salcedo Hernández RA, Rodríguez-Trejo A, Gallardo-Rincón D, et al. Therapeutic use of human papillomavirus vaccines in cervical lesions. Rev Invest Clin. (2020) 72:239–49. doi: 10.24875/RIC.20000059
68. Kim D, Gambhira R, Karanam B, Monie A, Hung C-F, Roden R, et al. Generation and characterization of a preventive and therapeutic HPV DNA vaccine. Vaccine. (2008) 26:351–60. doi: 10.1016/j.vaccine.2007.11.019
69. Bonam SR, Partidos CD, Halmuthur SKM, Muller S. An overview of novel adjuvants designed for improving vaccine efficacy. Trends Pharmacol Sci. (2017) 38:771–93. doi: 10.1016/j.tips.2017.06.002
70. Zacharia A, Harberts E, Valencia SM, Myers B, Sanders C, Jain A, et al. Optimization of RG1-VLP vaccine performance in mice with novel TLR4 agonists. Vaccine. (2021) 39:292–302. doi: 10.1016/j.vaccine.2020.11.066
71. Maynard SK, Marshall JD, MacGill RS, Yu L, Cann JA, Cheng LI, et al. Vaccination with synthetic long peptide formulated with CpG in an oil-in-water emulsion induces robust E7-specific CD8 T cell responses and TC-1 tumor eradication. BMC Cancer. (2019) 19:1–12. doi: 10.1186/s12885-019-5725-y
72. Nakagawa T, Tanino T, Onishi M, Tofukuji S, Kanazawa T, Ishioka Y, et al. S-540956, a CpG oligonucleotide annealed to a complementary strand with an amphiphilic chain unit, acts as a potent cancer vaccine adjuvant by targeting draining lymph nodes. Front Immunol. (2021) 12:803090. doi: 10.3389/fimmu.2021.803090
73. Gableh F, Saeidi M, Hemati S, Hamdi K, Soleimanjahi H, Gorji A, et al. Combination of the toll like receptor agonist and α-Galactosylceramide as an efficient adjuvant for cancer vaccine. J BioMed Sci. (2016) 23:1–11. doi: 10.1186/s12929–016-0238–3
74. Chandra J, Teoh SM, Kuo P, Tolley L, Bashaw AA, Tuong ZK, et al. Manganese-doped silica-based nanoparticles promote the efficacy of antigen-specific immunotherapy. J Immunol. (2021) 206:987–98. doi: 10.4049/jimmunol.2000355
75. Granadillo M, Batte A, Alfonso AB, Blanco A, Urquiza D, Varas L, et al. Impact on antitumor response using a new adjuvant preparation as a component of a human papillomavirus type 16 therapeutic vaccine candidate. Vaccine. (2019) 37:3957–60. doi: 10.1016/j.vaccine.2019.05.060
76. Mo Y, Ma J, Zhang H, Shen J, Chen J, Hong J, et al. Prophylactic and therapeutic HPV vaccines: current scenario and perspectives. Front Cell Infect Microbiol. (2022) 12:909223. doi: 10.3389/fcimb.2022.909223
77. Alvarez RD, Huh WK, Bae S, Lamb LS, Conner MG, Boyer J, et al. A pilot study of pNGVL4a-CRT/E7(detox) for the treatment of patients with HPV16 + cervical intraepithelial neoplasia 2/3 (CIN2/3). Gynecol Oncol. (2016) 140:245–52. doi: 10.1016/j.ygyno.2015.11.026
78. Chandra J, Woo W, Finlayson N, Liu H, McGrath M, Ladwa R, et al. A phase 1, single centre, open label, escalating dose study to assess the safety, tolerability and immunogenicity of a therapeutic human papillomavirus (HPV) DNA vaccine (AMV002) for HPV-associated head and neck cancer (HNC). Cancer Immunol Immunother. (2021) 70:743–53. doi: 10.1007/s00262–020-02720–7
79. Hasan Y, Furtado L, Tergas A, Lee N, Brooks R, McCall A, et al. A phase 1 trial assessing the safety and tolerability of a therapeutic DNA vaccination against HPV16 and HPV18 E6/E7 oncogenes after chemoradiation for cervical cancer. Int J Radiat Oncol Biol Phys. (2020) 107:487–98. doi: 10.1016/j.ijrobp.2020.02.031
80. Hillemanns P, Denecke A, Woelber L, Böhmer G, Jentschke M, Schjetne KW, et al. A therapeutic antigen-presenting cell-targeting DNA vaccine VB10.16 in HPV16-positive high-grade cervical intraepithelial neoplasia: results from a phase I/IIa trial. Clin Cancer Res. (2022) 28:4885–92. doi: 10.1158/1078–0432.ccr-22–1927
81. Choi YJ, Hur SY, Kim T-J, Hong SR, Lee JK, Cho C-H, et al. A Phase II, Prospective, randomized, multicenter, open-label study of GX-188E, an HPV DNA vaccine, in patients with cervical intraepithelial neoplasia 3A phase II study of a therapeutic HPV DNA vaccine in CIN 3. Clin Cancer Res. (2020) 26:1616–23. doi: 10.1158/1078–0432.CCR-19–1513
82. Trimble CL, Morrow MP, Kraynyak KA, Shen X, Dallas M, Yan J, et al. Safety, efficacy, and immunogenicity of VGX-3100, a therapeutic synthetic DNA vaccine targeting human papillomavirus 16 and 18 E6 and E7 proteins for cervical intraepithelial neoplasia 2/3: a randomised, double-blind, placebo-controlled phase 2b trial. Lancet. (2015) 386:2078–88. doi: 10.1016/S0140–6736(15)00239–1
83. LARVOL. ISA201/ISA Pharma-LARVOL DELTA (2022). Available online at: https://delta.larvol.com/products/?ProductId=e78d6e97-ee75–4a91–84dd-03489e467f9e&ProductName=ISA201&Institutions=+ISA+Pharma&Index=0 (Accessed December 7 2023).
84. Hatano T, Sano D, Takahashi H, Oridate N. Pathogenic role of immune evasion and integration of human papillomavirus in oropharyngeal cancer. Microorganisms. (2021) 9:891. doi: 10.3390/microorganisms9050891
85. Rosales R, López-Contreras M, Rosales C, Magallanes-Molina J-R, Gonzalez-Vergara R, Arroyo-Cazarez JM, et al. Regression of human papillomavirus intraepithelial lesions is induced by MVA E2 therapeutic vaccine. Hum Gene Ther. (2014) 25:1035–49. doi: 10.1089/hum.2014.024
86. Harper DM, Nieminen P, Donders G, Einstein MH, Garcia F, Huh WK, et al. The efficacy and safety of Tipapkinogen Sovacivec therapeutic HPV vaccine in cervical intraepithelial neoplasia grades 2 and 3: Randomized controlled phase II trial with 2.5 years of follow-up. Gynecol Oncol. (2019) 153:521–9. doi: 10.1016/j.ygyno.2019.03.250
87. Komdeur FL, Singh A, van de Wall S, Meulenberg JJM, Boerma A, Hoogeboom BN, et al. First-in-human phase I clinical trial of an SFV-based RNA replicon cancer vaccine against HPV-induced cancers. Mol Ther. (2021) 29:611–25. doi: 10.1016/j.ymthe.2020.11.002
88. Park Y-C, Ouh Y-T, Sung M-H, Park H-G, Kim T-J, Cho C-H, et al. A phase 1/2a, dose-escalation, safety and preliminary efficacy study of oral therapeutic vaccine in subjects with cervical intraepithelial neoplasia 3. J Gynecol Oncol. (2019) 30(6):e88. doi: 10.3802/jgo.2019.30.e88
89. Kawana K, Kobayashi O, Ikeda Y, Yahata H, Iwata T, Satoh T, et al. Phase I and II randomized clinical trial of an oral therapeutic vaccine targeting human papillomavirus for treatment of cervical intraepithelial neoplasia 2 and 3. JNCI Cancer Spectr. (2023) 7:pkad101. doi: 10.1093/jncics/pkad101
90. Taghinezhad-S S, Mohseni AH, Keyvani H, Razavi MR. Phase 1 safety and immunogenicity trial of recombinant lactococcus lactis expressing human papillomavirus type 16 E6 oncoprotein vaccine. Mol Ther Methods Clin Dev. (2019) 15:40–51. doi: 10.1016/j.omtm.2019.08.005
91. Schiller JT, Müller M. Next generation prophylactic human papillomavirus vaccines. Lancet Oncol. (2015) 16:e217–e25. doi: 10.1016/s1470–2045(14)71179–9
92. Shirbaghaee Z, Bolhassani A, Mirshafiey A, Motevalli F, Zohrei N. A live vector expressing HPV16 L1 generates an adjuvant-induced antibody response in-vivo. Iran J Cancer Prev. (2015) 8(6):e3991. doi: 10.17795/ijcp-3991
93. Chan PK, Liu SJ, Cheung JL, Cheung T, Yeo W, Chong P, et al. T-cell response to human papillomavirus type 52 L1, E6, and E7 peptides in women with transient infection, cervical intraepithelial neoplasia, and invasive cancer. J Med Virol. (2011) 83:1023–30. doi: 10.1002/jmv.21889
94. Öhlschläger P, Osen W, Dell K, Faath S, Garcea RL, Jochmus I, et al. Human papillomavirus type 16 L1 capsomeres induce L1-specific cytotoxic T lymphocytes and tumor regression in C57BL/6 mice. J Virol. (2003) 77:4635–45. doi: 10.1128/jvi.77.8.4635–4645.2003
95. Bhatt KH, Neller MA, Srihari S, Crooks P, Lekieffre L, Aftab BT, et al. Profiling HPV-16–specific T cell responses reveals broad antigen reactivities in oropharyngeal cancer patients. J Exp Med. (2020) 217(10):e20200389. doi: 10.1084/jem.20200389
96. Boilesen DR, Nielsen KN, Holst PJ. Novel antigenic targets of HPV therapeutic vaccines. Vaccines (Basel). (2021) 9:1262. doi: 10.3390/vaccines9111262
97. Carreño JM, Alshammary H, Tcheou J, Singh G, Raskin AJ, Kawabata H, et al. Activity of convalescent and vaccine serum against SARS-CoV-2 Omicron. Nature. (2022) 602:682–8. doi: 10.1038/s41586–022-04399–5
98. Kianmehr Z, Soleimanjahi H, Ardestani SK, Fotouhi F, Abdoli A. Influence of Brucella abortus lipopolysaccharide as an adjuvant on the immunogenicity of HPV-16 L1VLP vaccine in mice. Med Microbiol Immunol. (2015) 204:205–13. doi: 10.1007/s00430-014-0356-z
99. Valencia SM, Zacharia A, Marin A, Matthews RL, Wu C-K, Myers B, et al. Improvement of RG1-VLP vaccine performance in BALB/c mice by substitution of alhydrogel with the next generation polyphosphazene adjuvant PCEP. Hum Vaccines Immunother. (2021) 17:2748–61. doi: 10.1080/21645515.2021.1875763
100. Han JE, Wui SR, Park SA, Lee NG, Kim KS, Cho YJ, et al. Comparison of the immune responses to the CIA06-adjuvanted human papillomavirus L1 VLP vaccine with those against the licensed HPV vaccine Cervarix™ in mice. Vaccine. (2012) 30:4127–34. doi: 10.1016/j.vaccine.2012.04.079
101. Ebensen T, Delandre S, Prochnow B, Guzmán CA, Schulze K. The combination vaccine adjuvant system alum/c-di-AMP results in quantitative and qualitative enhanced immune responses post immunization. Front Cell Infect Microbiol. (2019) 9:31. doi: 10.3389/fcimb.2019.00031
102. Song Y-C, Huang H-C, Chang CY-Y, Lee H-J, Liu C-T, Lo H-Y, et al. A potential herbal adjuvant combined with a peptide-based vaccine acts against HPV-related tumors through enhancing effector and memory T-cell immune responses. Front Immunol. (2020) 11:62. doi: 10.3389/fimmu.2020.00062
103. Wang X, Che Y, Chen B, Zhang Y, Nakagawa M, Wang X. Evaluation of immune responses induced by a novel human papillomavirus type 16 E7 peptide-based vaccine with Candida skin test reagent as an adjuvant in C57BL/6 mice. Int Immunopharmacol. (2018) 56:249–60. doi: 10.1016/j.intimp.2018.01.037
104. Liu C, Chu X, Sun P, Feng X, Huang W, Liu H, et al. Synergy effects of Polyinosinic-polycytidylic acid, CpG oligodeoxynucleotide, and cationic peptides to adjuvant HPV E7 epitope vaccine through preventive and therapeutic immunization in a TC-1 grafted mouse model. Hum Vaccin Immunother. (2018) 14:931–40. doi: 10.1080/21645515.2017.1420446
105. Naziri H, Tahamtan A, Dadmanesh M, Barati M, Ghorban K. Antitumor effects of HPV DNA vaccine adjuvanted with beclin-1 as an autophagy inducer in a mice model. Iran BioMed J. (2019) 23:388. doi: 10.29252/ibj.23.6.388
106. Tse S-W, McKinney K, Walker W, Nguyen M, Iacovelli J, Small C, et al. mRNA-encoded, constitutively active STINGV155M is a potent genetic adjuvant of antigen-specific CD8+ T cell response. Mol Ther. (2021) 29:2227–38. doi: 10.1016/j.ymthe.2021.03.002
107. Tahamtan A, Ghaemi A, Gorji A, Kalhor HR, Sajadian A, Tabarraei A, et al. Antitumor effect of therapeutic HPV DNA vaccines with chitosan-based nanodelivery systems. J BioMed Sci. (2014) 21:69. doi: 10.1186/s12929–014-0069-z178
108. Bi Z, Hong W, Que H, He C, Ren W, Yang J, et al. Inactivated SARS-CoV-2 induces acute respiratory distress syndrome in human ACE2-transgenic mice. Signal Transduct Target Ther. (2021) 6:439. doi: 10.1038/s41392–021-00851–6
109. Dillon S, Sasagawa T, Crawford A, Prestidge J, Inder MK, Jerram J, et al. Resolution of cervical dysplasia is associated with T-cell proliferative responses to human papillomavirus type 16 E2. J Gen Virol. (2007) 88:803–13. doi: 10.1099/vir.0.82678–0
110. Bergvall M, Melendy T, Archambault J. The E1 proteins. Virology. (2013) 445:35–56. doi: 10.1016/j.virol.2013.07.020
111. Mirabello L, Schiffman M, Ghosh A, Rodriguez AC, Vasiljevic N, Wentzensen N, et al. Elevated methylation of HPV16 DNA is associated with the development of high grade cervical intraepithelial neoplasia. Int J Cancer. (2013) 132:1412–22. doi: 10.1002/ijc.27750
112. Malcles M-H, Cueille N, Mechali F, Coux O, Bonne-Andrea C. Regulation of bovine papillomavirus replicative helicase E1 by the ubiquitin-proteasome pathway. J Virol. (2002) 76:11350–8. doi: 10.1128/jvi.76.22.11350–11358.2002
113. Mechali F, Hsu C-Y, Castro A, Lorca T, Bonne-Andrea C. Bovine papillomavirus replicative helicase E1 is a target of the ubiquitin ligase APC. J Virol. (2004) 78:2615–9. doi: 10.1128/jvi.78.5.2615–2619.2004
114. Diamos AG, Larios D, Brown L, Kilbourne J, Kim HS, Saxena D, et al. Vaccine synergy with virus-like particle and immune complex platforms for delivery of human papillomavirus L2 antigen. Vaccine. (2019) 37:137–44. doi: 10.1016/j.vaccine.2018.11.021
115. Gambhira R, Karanam B, Jagu S, Roberts JN, Buck CB, Bossis I, et al. A protective and broadly cross-neutralizing epitope of human papillomavirus L2. J Virol. (2007) 81:13927–31. doi: 10.1128/JVI.00936–07
116. Alphs HH, Gambhira R, Karanam B, Roberts JN, Jagu S, Schiller JT, et al. Protection against heterologous human papillomavirus challenge by a synthetic lipopeptide vaccine containing a broadly cross-neutralizing epitope of L2. Proc Natl Acad Sci U.S.A. (2008) 105:5850–5. doi: 10.1073/pnas.0800868105
117. Pogoda CS, Roden RB, Garcea RL. Immunizing against anogenital cancer: HPV vaccines. PloS Pathog. (2016) 12:e1005587. doi: 10.1371/journal.ppat.1005587
118. Seitz H, Ribeiro-Müller L, Canali E, Bolchi A, Tommasino M, Ottonello S, et al. Robust in vitro and in vivo neutralization against multiple high-risk HPV types induced by a thermostable thioredoxin-L2 vaccine. Cancer Prev Res (Phila). (2015) 8:932–41. doi: 10.1158/1940–6207.CAPR-15–0164
119. Khanal S, Ferraris ED, Zahin M, Joh J, Ghim S-j, Jenson AB. Targeting synthetic Human Papillomavirus (HPV) L2 disulfide-induced N-terminus conformational epitopes for pan-HPV vaccine development. Exp Mol Pathol. (2015) 99:330–4. doi: 10.1016/j.yexmp.2015.06.021
120. Bendz H, Ruhland SC, Pandya MJ, Hainzl O, Riegelsberger S, Braüchle C, et al. Human heat shock protein 70 enhances tumor antigen presentation through complex formation and intracellular antigen delivery without innate immune signaling. J Biol Chem. (2007) 282:31688–702. doi: 10.1074/jbc.M704129200
121. Zong J, Wang C, Liu B, Liu M, Cao Y, Sun X, et al. Human hsp70 and HPV16 oE7 fusion protein vaccine induces an effective antitumor efficacy. Oncol Rep. (2013) 30:407–12. doi: 10.3892/or.2013.2445
122. Maecker HT, Ghanekar SA, Suni MA, He X-S, Picker LJ, Maino VC. Factors affecting the efficiency of CD8+ T cell cross-priming with exogenous antigens. J Immunol. (2001) 166:7268–75. doi: 10.4049/jimmunol.166.12.7268
123. Neefjes J, Ovaa H. A peptide’s perspective on antigen presentation to the immune system. Nat Chem Biol. (2013) 9:769–75. doi: 10.1038/nchembio.1391
124. Haghshenas MR, Mousavi T, Kheradmand M, Afshari M, Moosazadeh M. Efficacy of human papillomavirus l1 protein vaccines (cervarix and gardasil) in reducing the risk of cervical intraepithelial neoplasia: a meta-analysis. Int J Prev Med. (2017) 8(1):44. doi: 10.4103/ijpvm.IJPVM_413_16
125. Chen J, Ni G, Liu XS. Papillomavirus virus like particle-based therapeutic vaccine against human papillomavirus infection related diseases: immunological problems and future directions. Cell Immunol. (2011) 269:5–9. doi: 10.1016/j.cellimm.2011.03.003
126. Simon P, Buxant F, Hallez S, Burny A, Fayt I, Anaf V, et al. Cervical response to vaccination against HPV16 E7 in case of severe dysplasia. Eur J Obstet Gynecol Reprod Biol. (2003) 109:219–23. doi: 10.1016/s0301–2115(03)00093–9
127. Schwarz TF, Leo O. Immune response to human papillomavirus after prophylactic vaccination with AS04-adjuvanted HPV-16/18 vaccine: Improving upon nature. Gynecol Oncol. (2008) 110:S1–S10. doi: 10.1016/j.ygyno.2008.05.036
128. Einstein MH, Baron M, Levin MJ, Chatterjee A, Edwards RP, Zepp F, et al. Comparison of the immunogenicity and safety of Cervarix™ and Gardasil® human papillomavirus (HPV) cervical cancer vaccines in healthy women aged 18–45 years. Hum Vaccin. (2009) 5:705–19. doi: 10.4161/hv.5.10.9518
129. Herrin DM, Coates EE, Costner PJ, Kemp TJ, Nason MC, Saharia KK, et al. Comparison of adaptive and innate immune responses induced by licensed vaccines for human papillomavirus. Hum Vaccin Immunother. (2014) 10:3446–54. doi: 10.4161/hv.34408
130. Einstein MH, Levin MJ, Chatterjee A, Chakhtoura N, Takacs P, Catteau G, et al. Comparative humoral and cellular immunogenicity and safety of human papillomavirus (HPV)-16/18 AS04-adjuvanted vaccine and HPV-6/11/16/18 vaccine in healthy women aged 18–45 years: Follow-up through Month 48 in a Phase III randomized study. Hum Vaccin Immunother. (2014) 10:3455–65. doi: 10.4161/hv.36117
131. Zhou C, Tuong ZK, Frazer IH. Papillomavirus immune evasion strategies target the infected cell and the local immune system. Front Oncol. (2019) 9:682. doi: 10.3389/fonc.2019.00682
132. Liu Z, Zhou H, Wang W, Fu Y-X, Zhu M. A novel dendritic cell targeting HPV16 E7 synthetic vaccine in combination with PD-L1 blockade elicits therapeutic antitumor immunity in mice. Oncoimmunology. (2016) 5:e1147641. doi: 10.1080/2162402X.2016.1147641
133. Lin PL, Cheng YM, Wu DW, Huang YJ, Lin HC, Chen CY, et al. A combination of anti-PD-L1 mA b plus Lm-LLO-E6 vaccine efficiently suppresses tumor growth and metastasis in HPV-infected cancers. Cancer Med. (2017) 6:2052–62. doi: 10.1002/cam4.1143
134. Rice AE, Latchman Y, Balint JP, Lee JH, Gabitzsch ES, Jones FR. An HPV-E6/E7 immunotherapy plus PD-1 checkpoint inhibition results in tumor regression and reduction in PD-L1 expression. Cancer Gene Ther. (2015) 22:454–62. doi: 10.1038/cgt.2015.40
135. Youn JW, Hur S-Y, Woo JW, Kim Y-M, Lim MC, Park SY, et al. Pembrolizumab plus GX-188E therapeutic DNA vaccine in patients with HPV-16-positive or HPV-18-positive advanced cervical cancer: interim results of a single-arm, phase 2 trial. Lancet Oncol. (2020) 21:1653–60. doi: 10.1016/s1470–2045(20)30486–1
136. De Sousa LG, Rajapakshe K, Canales JR, Chin RL, Feng L, Wang Q, et al. ISA101 and nivolumab for HPV-16+ cancer: updated clinical efficacy and immune correlates of response. J Immunother Cancer. (2022) 10:e004232. doi: 10.1136/jitc-2021–004232
137. Le Tourneau C, Cassier P, Rolland F, Salas S, Limacher JM, Capitain O, et al. TG4001 (Tipapkinogene sovacivec) and avelumab for recurrent/metastatic (R/M) human papilloma virus (HPV)-16+ cancers: clinical efficacy and immunogenicity. BMJ Specialist J. (2020) 8:A841. doi: 10.1136/jitc-2020-SITC2020.0793
138. Floudas C, Strauss J, Allen C, Donahue R, Jochems C, Steinberg S, et al. Initial safety results and immune responses induced by a novel human papillomavirus (HPV)-specific gorilla adenovirus immunotherapy vaccine, PRGN-2009, in patients with advanced HPV-associated cancers. BMJ Specialist Journals. (2021) 9:A513. doi: 10.1136/jitc-2021-SITC2021.483
139. Strauss J, Floudas CS, Sater HA, Manu M, Lamping E, Francis DC, et al. Phase II evaluation of the triple combination of PDS0101, M9241, and bintrafusp alfa in patients with HPV 16 positive Malignancies. J Clin Oncol. (2021) 39:2501. doi: 10.1200/JCO.2021.39.15_suppl.2501
140. Dion GR, Teng S, Boyd LR, Northam A, Mason-Apps C, Vieira D, et al. Adjuvant human papillomavirus vaccination for secondary prevention: a systematic review. JAMA Otolaryngol Head Neck Surg. (2017) 143:614–22. doi: 10.1001/jamaoto.2016.4736
141. Di Donato V, Caruso G, Petrillo M, Kontopantelis E, Palaia I, Perniola G, et al. Adjuvant HPV vaccination to prevent recurrent cervical dysplasia after surgical treatment: a meta-analysis. Vaccines (Basel). (2021) 9:410. doi: 10.3390/vaccines9050410
142. Matsuzaki H, Makiyama K, Hasegawa H, Asai R, Morita M, Oshima T. Human papillomavirus vaccination as an adjuvant therapy for recurrent respiratory papillomatosis: additional case series. J Voice. (2021) 38:204–9. doi: 10.1016/j.jvoice.2021.07.019
143. Hočevar-Boltežar I, Matičič M, Šereg-Bahar M, Gale N, Poljak M, Kocjan B, et al. Human papilloma virus vaccination in patients with an aggressive course of recurrent respiratory papillomatosis. Eur Arch Otorhinolaryngol. (2014) 271:3255–62. doi: 10.1007/s00405-014-3143-y
144. Schiffman MH, Bauer HM, Hoover RN, Glass AG, Cadell DM, Rush BB, et al. Epidemiologic evidence showing that human papillomavirus infection causes most cervical intraepithelial neoplasia. J Natl Cancer Inst. (1993) 85:958–64. doi: 10.1093/jnci/85.12.958
145. Yang X, Cheng Y, Li C. The role of TLRs in cervical cancer with HPV infection: a review. Signal Transduct Target Ther. (2017) 2(1):e17055. doi: 10.1038/sigtrans.2017.55
146. Bansal N, Wright JD, Cohen CJ, Herzog TJ. Natural history of established low grade cervical intraepithelial (CIN 1) lesions. Anticancer Res. (2008) 28:1763–6.
147. Chan J, Monk B, Brewer C, Keefe K, Osann K, McMeekin S, et al. HPV infection and number of lifetime sexual partners are strong predictors for ‘natural’regression of CIN 2 and 3. Br J Cancer. (2003) 89:1062–6. doi: 10.1038/sj.bjc.6601196
148. Kang W, Choi H, Kim S. Is vaccination with quadrivalent HPV vaccine after LEEP effective in preventing recurrence in patients with high-grade cervical intraepithelial neoplasia (CIN2–3). Gynecol Oncol. (2013) 130:264–8. doi: 10.1016/j.ygyno.2013.04.050
149. Uddin MN, Kouzi SA, Hussain MD. Strategies for developing oral vaccines for human papillomavirus (HPV) induced cancer using nanoparticle mediated delivery system. J Pharm Pharm Sci. (2015) 18:220–34. doi: 10.18433/J3RS3V
150. Kim MG, Park JY, Shon Y, Kim G, Shim G, Oh YK. Nanotechnology and vaccine development. Asian J Pharm Sci. (2014) 9:227–35. doi: 10.1016/j.ajps.2014.06.002
151. Karimi H, Soleimanjahi H, Abdoli A, Banijamali RS. Combination therapy using human papillomavirus L1/E6/E7 genes and archaeosome: A nanovaccine confer immuneadjuvanting effects to fight cervical cancer. Sci Rep. (2020) 10:5787. doi: 10.1038/s41598–020-62448–3
152. Rupar MJ, Golusinski P, Golusinski W, Masternak M. Radiotherapy. human papillomavirus and the use of nanoparticles for immunotherapy in HPV-related cancer: a review. Rep Pract Oncol Radiother. (2019) 24:544–50. doi: 10.1016/j.rpor.2019.08.006
153. Idrees H, Zaidi SZJ, Sabir A, Khan RU, Zhang X, Hassan S. A review of biodegradable natural polymer-based nanoparticles for drug delivery applications. Nanomaterials (Basel). (2020) 10:1970. doi: 10.3390/nano10101970
154. Gohar A, Ali AA, Elkhatib WF, El-Sayyad GS, Elfadil D, Noreddin AM. Combination therapy between prophylactic and therapeutic human papillomavirus (HPV) vaccines with special emphasis on implementation of nanotechnology. Microb Pathog. (2022) 171:105747. doi: 10.1016/j.micpath.2022.105747
155. Witeof AE, Meinerz NM, Walker KD, Funke HH, Garcea RL, Randolph TW. A single dose, thermostable, trivalent human papillomavirus vaccine formulated using atomic layer deposition. J Pharm Sci. (2023) 112:2223–29. doi: 10.1016/j.xphs.2023.02.007
156. Wahid R, Cannon MJ, Chow M. Virus-specific CD4+ and CD8+ cytotoxic T-cell responses and long-term T-cell memory in individuals vaccinated against polio. J Virol. (2005) 79:5988–95. doi: 10.1128/JVI.79.10.5988–5995.2005
157. Pugliese-Garcia M, Heyerdahl LW, Mwamba C, Nkwemu S, Chilengi R, Demolis R, et al. Factors influencing vaccine acceptance and hesitancy in three informal settlements in Lusaka, Zambia. Vaccine. (2018) 36:5617–24. doi: 10.1016/j.vaccine.2018.07.042
158. Uddin MN, Henry B, Carter KD, Roni MA, Kouzi SS. A novel formulation strategy to deliver combined DNA and VLP based HPV vaccine. J Pharm Pharm Sci. (2019) 22:536–47. doi: 10.18433/jpps30768
159. He M, Zhu L, Yang N, Li H, Yang Q. Recent advances of oral film as platform for drug delivery. Int J Pharm. (2021) 604:120759. doi: 10.1016/j.ijpharm.2021.120759
160. Jacob S, Nair AB, Boddu SH, Gorain B, Sreeharsha N, Shah J. An updated overview of the emerging role of patch and film-based buccal delivery systems. Pharmaceutics. (2021) 13:1206. doi: 10.3390/pharmaceutics13081206
161. Barnabas RV, Brown ER, Onono MA, Bukusi EA, Njoroge B, Winer RL, et al. Efficacy of single-dose human papillomavirus vaccination among young African women. NEJM Evid. (2022) 1:EVIDoa2100056. doi: 10.1056/EVIDoa2100056
162. Brotherton JM, Budd A, Rompotis C, Bartlett N, Malloy MJ, Andersen RL, et al. Is one dose of human papillomavirus vaccine as effective as three?: a national cohort analysis. Papillomavirus Res. (2019) 8:100177. doi: 10.1016/j.pvr.2019.100177
163. Watson-Jones D, Changalucha J, Whitworth H, Pinto L, Mutani P, Indangasi J, et al. Immunogenicity and safety of one-dose human papillomavirus vaccine compared with two or three doses in Tanzanian girls (DoRIS): an open-label, randomised, non-inferiority trial. Lancet Glob Health. (2022) 10:e1473–e84. doi: 10.1016/S2214–109X(22)00309–6
164. Rodriguez AM, Zeybek B, Vaughn M, Westra J, Kaul S, Montealegre JR, et al. Comparison of the long-term impact and clinical outcomes of fewer doses and standard doses of human papillomavirus vaccine in the United States: a database study. Cancer. (2020) 126:1656–67. doi: 10.1002/cncr.32700
165. Basu P, Malvi SG, Joshi S, Bhatla N, Muwonge R, Lucas E, et al. Vaccine efficacy against persistent human papillomavirus (HPV) 16/18 infection at 10 years after one, two, and three doses of quadrivalent HPV vaccine in girls in India: a multicentre, prospective, cohort study. Lancet Oncol. (2021) 22:1518–29. doi: 10.1016/S1470–2045(21)00453–8
166. Kreimer AR, Sampson JN, Porras C, Schiller JT, Kemp T, Herrero R, et al. Evaluation of durability of a single dose of the bivalent HPV vaccine: the CVT trial. J Natl Cancer Inst. (2020) 112:1038–46. doi: 10.1093/jnci/djaa011
167. Di J, Wang J, Wang S, Ma M, Zhang H, Liu N, et al. Self-boosting vaccination based on pulsatile antigen release from core–shell microparticles. Small. (2023) 19:2207892. doi: 10.1002/smll.202207892
168. Arinaminpathy N, Lavine JS, Grenfell BT. Self-boosting vaccines and their implications for herd immunity. Proc Natl Acad Sci U.S.A. (2012) 109:20154–9. doi: 10.1073/pnas.1209683109
169. World Health Organization. One-dose Human Papillomavirus (HPV) vaccine offers solid protection against cervical cancer (2022). Available online at: https://www.who.int/news/item/11–04-2022-one-dose-human-papillomavirus-(hpv)-vaccine-offers-solid-protection-against-cervical-cancer (Accessed December 5, 2023).
170. Prem K, Choi YH, Bénard É, Burger EA, Hadley L, Laprise J-F, et al. Global impact and cost-effectiveness of one-dose versus two-dose human papillomavirus vaccination schedules: a comparative modelling analysis. BMC Med. (2023) 21:313. doi: 10.1186/s12916–023-02988–3
171. His Majesty’s Government. Joint Committee on Vaccination and Immunisation (JCVI) statement on a one-dose schedule for the routine HPV immunisation programme (2022). Available online at: https://www.gov.uk/government/publications/single-dose-of-hpv-vaccine-jcvi-concluding-advice/jcvi-statement-on-a-one-dose-schedule-for-the-routine-hpv-immunisation-programme (Accessed December 5, 2023).
172. U.S. FOOD & DRUG. FDA approves expanded use of Gardasil 9 to include individuals 27 through 45 years old (2018). Available online at: https://www.fda.gov/news-events/press-announcements/fda-approves-expanded-use-gardasil-9-include-individuals-27-through-45-years-old (Accessed December 5, 2023).
173. Eskandari N, Yousefi Z, Aria H, Bastan R. An update on human papilloma virus (HPV) vaccines: history, types, protection, and efficacy. Front Immunol. (2021) 12:805695. doi: 10.3389/fimmu.2021.805695
174. Sauver JLS, Rutten LJF, Ebbert JO, Jacobson DJ, McGree ME, Jacobson RM. Younger age at initiation of the human papillomavirus (HPV) vaccination series is associated with higher rates of on-time completion. Prev Med. (2016) 89:327–33. doi: 10.1016/j.ypmed.2016.02.039
175. Goleman MJ, Dolce M, Morack J. Quality improvement initiative to improve human papillomavirus vaccine initiation at 9 years of age. Acad Pediatr. (2018) 18:769–75. doi: 10.1016/j.acap.2018.05.005
176. Brouwer AF, Delinger RL, Eisenberg MC, Campredon LP, Walline HM, Carey TE, et al. HPV vaccination has not increased sexual activity or accelerated sexual debut in a college-aged cohort of men and women. BMC Public Health. (2019) 19:821. doi: 10.1186/s12889–019-7134–1
177. Logel M, Laurie C, El-Zein M, Guichon J, Franco EL. A review of ethical and legal aspects of gender-neutral human papillomavirus vaccination. Cancer Epidemiol Biomarkers Prev. (2022) 31:919–31. doi: 10.1158/1055–9965.EPI-21–1256
178. Bruni L, Saura-Lázaro A, Montoliu A, Brotons M, Alemany L, Diallo MS, et al. HPV vaccination introduction worldwide and WHO and UNICEF estimates of national HPV immunization coverage 2010–2019. Prev Med. (2021) 144:106399. doi: 10.1016/j.ypmed.2020.106399
179. Lehtinen M, Söderlund-Strand A, Vänskä S, Luostarinen T, Eriksson T, Natunen K, et al. Impact of gender-neutral or girls-only vaccination against human papillomavirus—Results of a community-randomized clinical trial (I). Int J Cancer. (2018) 142:949–58. doi: 10.1002/ijc.31119
180. Man I, Georges D, Sankaranarayanan R, Basu P, Baussano I. Building resilient cervical cancer prevention through gender-neutral HPV vaccination. Elife. (2023) 12:e85735. doi: 10.7554/eLife.85735
181. WHO. Weekly epidemiological record: Meeting of the Strategic Advisory Group of Experts on Immunization, October 2019: conclusions and recommendations (2019). Available online at: https://reliefweb.int/report/world/weekly-epidemiological-record-wer-22-november-2019-vol-94-no-47–541-560-enfr (Accessed March 13, 2024).
182. Shin H, Jeon S, Cho I, Park H. Factors affecting human papillomavirus vaccination in men: systematic review. JMIR Public Health Surveill. (2022) 8:e34070. doi: 10.2196/34070
Keywords: human papillomavirus vaccine, prophylactic, therapeutic, antigens, adjuvants, formulation strategy
Citation: Wang R, Huang H, Yu C, Li X, Wang Y and Xie L (2024) Current status and future directions for the development of human papillomavirus vaccines. Front. Immunol. 15:1362770. doi: 10.3389/fimmu.2024.1362770
Received: 29 December 2023; Accepted: 11 June 2024;
Published: 25 June 2024.
Edited by:
Fabio Bagnoli, GlaxoSmithKline, ItalyReviewed by:
Srinivasa Reddy Bonam, University of Texas Medical Branch at Galveston, United StatesArash Arashkia, Pasteur Institute of Iran (PII), Iran
Copyright © 2024 Wang, Huang, Yu, Li, Wang and Xie. This is an open-access article distributed under the terms of the Creative Commons Attribution License (CC BY). The use, distribution or reproduction in other forums is permitted, provided the original author(s) and the copyright owner(s) are credited and that the original publication in this journal is cited, in accordance with accepted academic practice. No use, distribution or reproduction is permitted which does not comply with these terms.
*Correspondence: Liangzhi Xie, LX@sinocelltech.com
†These authors have contributed equally to this work