- 1Research Institute for Reproductive Health and Genetic Diseases, Wuxi Maternity and Child Health Care Hospital, Wuxi, Jiangsu, China
- 2Graduate School of Nanjing Medical University, Nanjing, Jiangsu, China
- 3Institute for Fetology, First Affiliated Hospital of Soochow University, Suzhou, Jiangsu, China
- 4Department of Obstetrics, Wuxi Maternity and Child Health Care Hospital Affiliated to Nanjing Medical University, Wuxi, Jiangsu, China
Exosomes carry proteins, metabolites, nucleic acids and lipids from their parent cell of origin. They are derived from cells through exocytosis, are ingested by target cells, and can transfer biological signals between local or distant cells. Therefore, exosomes are often modified in reaction to pathological processes, including infection, cancer, cardiovascular diseases and in response to metabolic perturbations such as obesity and diabetes, all of which involve a significant inflammatory aspect. Here, we discuss how immune cell-derived exosomes origin from neutrophils, T lymphocytes, macrophages impact on the immune reprogramming of diabetes and the associated complications. Besides, exosomes derived from stem cells and their immunomodulatory properties and anti-inflammation effect in diabetes are also reviewed. Moreover, As an important addition to previous reviews, we describes promising directions involving engineered exosomes as well as current challenges of clinical applications in diabetic therapy. Further research on exosomes will explore their potential in translational medicine and provide new avenues for the development of effective clinical diagnostics and therapeutic strategies for immunoregulation of diabetes.
1 Introduction
Diabetes mellitus, a group of metabolic disorders characterized by prolonged high blood sugar levels, is a global health issue affecting over 400 million people worldwide (1). This number is expected to surge to approximately 700 million by 2045 (2). The disease occurs either due to insufficient insulin production by the pancreas or the body’s inability to effectively utilize the produced insulin (3). The most common symptoms include weight loss, polydipsia, polyuria, and constant hunger. If not properly managed, diabetes mellitus can lead to severe complications such as kidney failure, unhealed wounds, vision loss, heart attacks, nerve damage, and even increase the risk of cancer (4). There are three main types of diabetes: type 1 diabetes mellitus (T1DM), type 2 diabetes mellitus (T2DM), and gestational diabetes mellitus. T1DM and T2DM account for 7-12% and 85-90% of global diabetes cases respectively. The rapid increase in diabetes mellitus cases worldwide underscores the disease’s significance as a public health concern.
Besides traditional treatment with insulin and oral anti-diabetic drugs, clinicians are attempting to enhance patient care through the use of cell therapies involving embryonic stem cells (ESC), induced pluripotent stem cells (iPSC), and adult mesenchymal stem cells (MSC) (5). However, there are unintended safety concerns such as immune rejection, genetic or disease transfer, and ectopic cell differentiation existing in whole-cell therapy. Recently, exosomes have been reported to play a role in multiple diseases and have been shown to be key mediators of various pathogenetic mechanisms. Compared with cell-based therapy, exosomes contain large amounts of bioactive molecules including proteins and nucleic acids. They exhibit high biocompatibility and low immunogenicity (6), and are able to circulate into distant sites and freely pass across the blood-brain barrier duo to their nanoscale size (7).
Recent studies have shown that exosomes play a role in the occurrence, development, and treatment of diabetes and its complications. However, there are few summaries from the perspective of immunity and inflammation regarding the treatment and mechanisms of exosomes from different cell sources in diabetes and its complications. This review summarizes the latest advances concerning the roles of exosomes and immune regulation/inflammation in diabetes.
2 Description of exosomes
Exosomes are small membrane-bound vesicles secreted by cells, usually between 30 and 200 nanometers in diameter. They play an important role in transmitting information between cells, regulating cell function, and participating in the occurrence and development of diseases (8). The biogenesis of exosomes involves three processes: generation, release, and uptake (9). Within the cells, membrane proteins and lipid molecules responsible for membrane synthesis are synthesized and packaged into endoplasmic reticulum vesicles. Subsequently, these vesicles fuse into polyvesicles. Vesicles in polyvesicles can further fuse to form exosomes (9). The release of exosomes is mainly accomplished through the fusion of polyvesicles with cell membranes. When the polyvesicles fuse with the cell membrane, the inner vesicles are released outside the cell to form exosomes (10). Exosomes are taken up by target cells by means of membrane fusion and endocytosis, and then release their cargo into the cytoplasm to exert their effects (11).Therefore, exosomes may manipulate recipient cells and other organs over a long distance (12).
Previous studies have demonstrated that exosomes, functioning as intercellular junctions, transport proteins, lipids, and nucleic acids to target cells. They are involved in a variety of biological processes including nucleic acid regulation, antigen presentation, metabolite transportation, and inflammation management. Furthermore, they hold potential as diagnostic and therapeutic tools for various diseases (13). Significantly, small non-coding RNAs (ncRNAs), which are approximately 19 to 24 nts in length and are a subset of nucleic acids, have garnered considerable interest within the scientific community due to their regulatory function (14). In this review, we have summarized the involvement of exosomes derived from immune cells and non-immune cells (such as stem cells) in the occurrence and intervention mechanisms of diabetes and its complications, many of which involve ncRNAs (Table 1), based on recent reports. Thus, delivery of multiple ncRNAs via exosomes may have promise over a wide range of applications.
3 Immune cell-derived exosomes and diabetes
In 1996, Raposo et al. reported that B lymphocytes secrete antigen-presenting vesicles (36). Since then, more and more studies have found that exosomes secreted by immune cells interact with cells in the immune system to regulate immune responses (37). Therefore, these membranous vesicles are being explored as potential immunotherapeutic reagents. Immune cell-derived exosomes can activate the immune system through various mechanisms (38). Firstly, they can directly activate immune cells such as dendritic cells and T cells through antigen presentation on their surface. Secondly, they can indirectly activate immune cells by releasing immune-stimulating molecules such as cytokines and chemical mediators. In addition, immunogenic exosomes may also regulate the function of immune cells by transferring immune-related nucleic acid molecules such as miRNA and mRNA. Previous studies have shown that immune-derived exosomes played a role in the development and progression of diabetes mellitus, making them a key regulator in the disease (39).
3.1 The roles of neutrophils-derived exosomes in diabetes
Polymorphonuclear neutrophils (PMNs), which make up 40-70% of all white blood cells in humans, are the most prevalent type of granulocytes. Neutrophils act as the first line of defense against invasive pathogens in the host and have a natural ability to phagocytose pathogens (40). Thus, neutrophils serve as important immune and secretory cells and play a crucial role in inflammation and infection processes (41). The status of the parent cell is reflected in the neutrophils-EXOs, which exhibit strong antibacterial ability due to the presence of components like myeloperoxidase, elastase, dermcidin, and lysozyme (42). In a recent research, investigators loaded extracellular matrix (ECM) hydrogel with vascular endothelial growth factor (VEGF)-encapsulated activated neutrophil exosome mimetics (aPMNEM) to develop VEGF-aPMNEM-ECM hybrid hydrogel for treating chronic diabetic wounds (40). Compared to directly using exosomes or using exosomes derived from other cells, this aPMNEM-ECM based biomaterial has the following advantages (1): for wound infection treatment, aPMNEM can play an antibacterial role via bactericidal-associated proteins (2); as a carrier, aPMNEM can deliver cytokines, and protect them from degradation (3); as a hermosensitive material, ECM can function as an in situ gel in vivo and increase the residence of aPMNEM. The study not only provided a functional biomaterial for the regeneration of chronic diabetic wounds but also created a promising platform for cytokine therapy, which can potentially be used to treat different diseases by loading various available cytokines in aPMNEM-ECM (40).
3.2 The roles of T lymphocytes-derived exosomes in diabetes
Type 1 diabetes mellitus is an autoimmune disorder characterized by infiltration of the islets of Langerhans by immune cells and by selective elimination of the insulin-secreting β cells (43). Regazzi’s team reported that miR-142-3p, miR-142-5p and miR-155 are particularly enriched in T lymphocytes of 8 weeks NOD mice with respect to mouse pancreatic islets (44). In type 1 diabetes, T lymphocytes-EXOs carrying specific microRNAs that induce chemokine expression and apoptosis in recipient pancreatic β cells. The inactivation of miR-142-3p/-5p and miR-155 in β cells leads to increased insulin levels, decreased insulitis scores, reduced inflammation, and provides protection against diabetes development in NOD mice (44).
3.3 The roles of macrophages-derived exosomes in diabetes
Macrophage-derived exosomes have been shown to have diverse functions in immune regulation, tissue repair, and communication between cells (45). Based on the functional profiles, macrophages are divided into two sub-populations: type 1 macrophages (M1, pro-inflammation) and type 2 macrophages (M2, anti-inflammation) (46). M1 macrophages play a role in the early phase of inflammation and are linked to tissue damage and pro-inflammatory activities, whereas M2 macrophages release cytokines that suppress inflammation and have anti-inflammatory effects (47). Recent studies have shown that the macrophages-EXOs contribute to the progression of diabetes (48) (Figure 1).
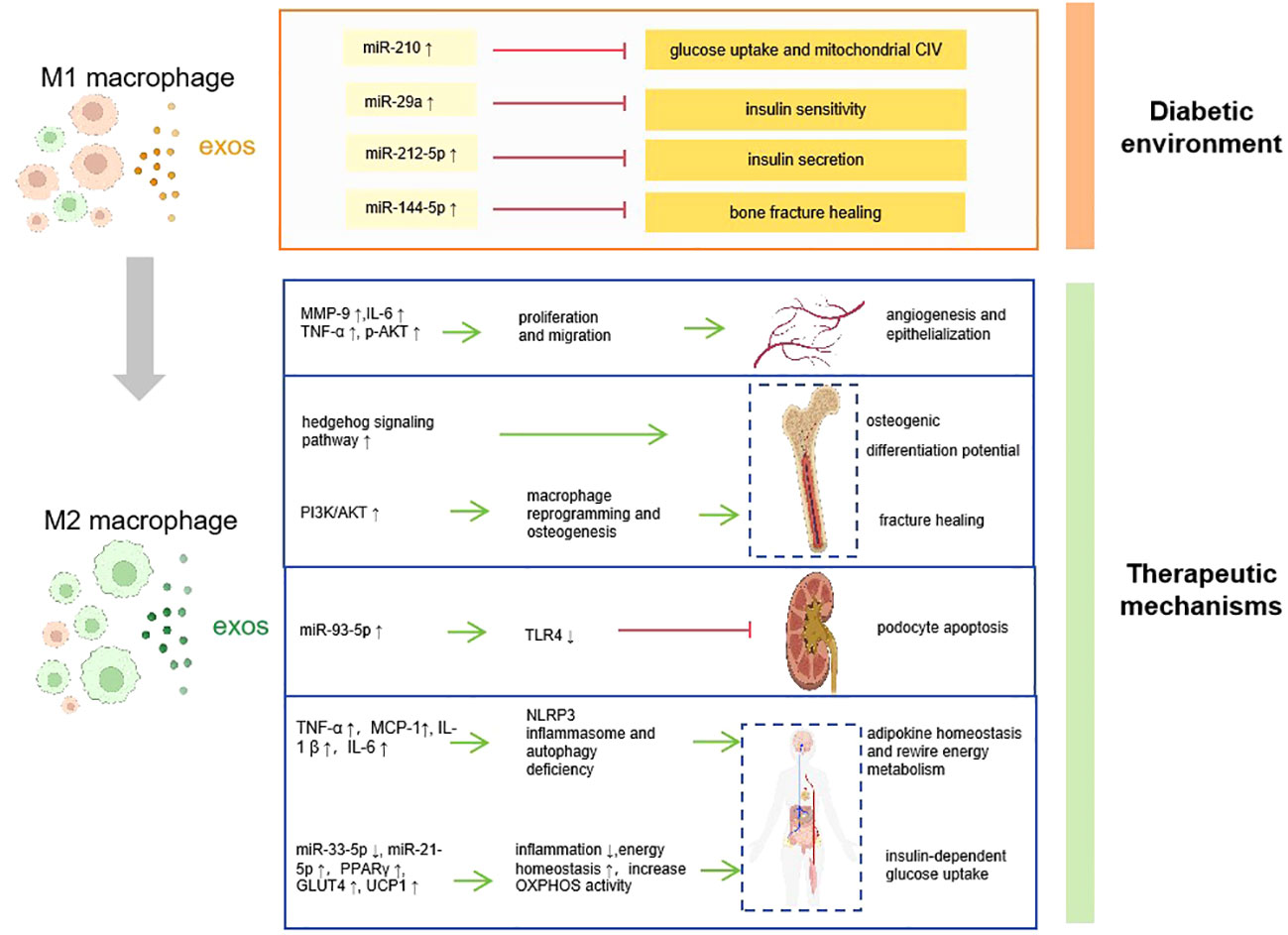
Figure 1 How macrophage derived-exosomes contribute to the pathogenesis, complications, and therapy of diabetes. Diabetic environment induce macrophage to M1 polarization, and the M1 macrophage secret exosomes which contains abnormal ncRNAs that promote diabetes and associated complications. Converting the ratio of M1/M2 macrophage polarization is supposed to be a therapeutic application, which accelerates diabetes recovery via various mechanisms. CIV, complex IV; MMP-9, matrix metalloproteinase-9; IL-6, interleukin-6; TNF-α, tumor necrosis factor-α; p-AKT, phospho-Akt; PI3K, phosphoinositide 3-kinase; TLR4, toll-like receptor 4; MCP-1, monocyte chemotactic protein-1; interleukin-1β; NLRP3, NOD-like receptor thermal protein domain associated protein 3; PPARγ, peroxisome proliferator-activated receptor γ; GLUT4, glucose transporter type 4; UCP1, uncoupling protein 1; OXPHOX, oxidative phosphorylation:inhibit
:promote.
3.3.1 Exosomes derived from M1 macrophages
3.3.1.1 Impairing insulin sensitivity, secretion and glucose uptake through miRNAs
Chronic tissue inflammation caused by accumulation of M1 macrophages is an important hallmark of insulin resistance. According to prior research, the population of activated M1 macrophages residing within adipose tissue increased in obese mice, resulting in an increased ratio of M1 to M2 macrophages (49). The M1 macrophage is the predominant cell responsible for secreting exosomes containing miR-29a in obese mice (16). MiR-29a targets peroxisome proliferator-activated receptor-δ, leading to impairments of insulin sensitivity both in vitro and in vivo (16). Moreover, M1 macrophage secreted exosomal miRNA may directly give rise to beta cell impairment. Qian et al. reported that the M1 macrophage-EXOs contained miR-212-5p, which regulated the Protein Kinase B (Akt)/Glycogen synthase kinase3β (GSK-3β)/β-catenin pathway in receptor beta cells by targeting the sirtuin 2 gene to restrict insulin secretion (17). Thus, targeting miRNA or inhibiting M1 macrophage-EXOs could be manipulated to inhibit beta cell injury in T2DM.
3.3.1.2 Promoting autophagy deficiency and resistin expression
It was found that high glucose stimulation promoted the polarization of macrophages to the M1-phenotype and produced more exosomes, thereby inducing activation of NOD-like receptor thermal protein domain associated protein 3 (NLRP3) inflammasome and autophagy defects in mesangial cells, promoting development of diabetic nephropathy (50). Besides, exosomal miR-7002-5p are highly expressed in high glucose treated macrophages, which suppress autophagy activity through targeting Atg9b in mouse tubular epithelial cell and C57 mouse kidney (51). In addition to regulate functions of kidney, macrophage-derived exosomes shows impact on diabetic vascular diseases. For example, under high glucose conditions, macrophage-derived exosomal metastasis associated lung adenocarcinoma transcript 1 (MALAT1) is upregulated, inhibiting the expression of miR-150-5p and counteracting its inhibitory effect on macrophage resistance factor expression, and promoting vascular diseases. Thus, macrophage-EXOs containing MALAT1 may serve as a novel target for diabetic vascular diseases (19).
3.3.1.3 Impairing bone fracture healing
Patients with diabetes have an increased risk of nonunion and delayed union of fractures. Exosomes derived from diabetic bone marrow-derived macrophages (dBMDM-EXOs) transfer miR-144-5p to bone marrow stromal cells, inhibiting the expression of Smad1, thereby reducing bone repair and regeneration both in vivo and in vitro (18). Suppression of miR-144-5p remarkably reversed the adverse effects of dBMDM-EXOs on the osteogenic potential and the ability of fracture repair (18). However, the author didn’t test the ratio of M1/M2 or confirm the phenotype of the macrophages that transferred specific miRNAs. Given the function of M1 macrophages, they may be the predominant cell responsible for secreting exosomes containing miR-144-5p, which can lead to bone impairment.
3.3.2 Exosomes derived from M2 macrophages (M2 macrophages-EXOs)
M2 macrophages release cytokines that play a role in anti-inflammatory and tissue repair (47). Previous data validate the association between treatment of diabetic-related diseases and the exosomes secreted by M2 macrophages. For example, the M2 macrophages-EXOs reduced lipopolysaccharides-induced podocyte apoptosis by regulating the miR-93-5p/TLR4 axis, which provided a new perspective for the treatment of diabetic nephropathy patients (20). Tuan et al. Demonstrated (52) that M2 macrophage-EXOs could control chronic inflammatory diseases caused by excessive energy storage. Interleukin 4 (IL-4) stimulated THP-1 macrophage-derived extracellular vesicles can improve the homeostasis of adipose factors, retargeting the energy metabolism of macrophages and adipocytes, thereby controlling the occurrence of cardiac metabolic tissue inflammation in obesity-related diabetes.
In addition to diabetic nephropathy and cardiac diseases, M2 macrophage-EXOs are necessary for accelerating diabetic bone fracture healing. A research has shown that M2 macrophage-EXOs can activate the Hedgehog signaling pathway in BMSCs in a high glucose and high insulin microenvironment, promoting osteogenic differentiation. This suggests that they can serve as a new approach for reshaping the immune homeostasis in diabetic bone (53). Additionally, the research has demonstrated that M2 macrophage-EXOs induced the transformation of M1 macrophages into M2 macrophages by stimulating the phosphoinositide 3-kinase (PI3K)/AKT pathway, significantly reducing the proportion of M1 macrophages and regulating the bone immune microenvironment, thereby accelerating diabetic bone fracture healing (54).
4 Exosomes derived from stem cell and their effect on immune/inflammation in diabetes
In recent years, exosomes-based therapy have gained increasing attention for their comparatively high safety, biocompatibility and low immunogenicity (6). This part reviewed the exosomes from different kinds of stem cells and their main mechanisms underlying regulatory effects on inflammation/immunity in diabetes (Figure 2).
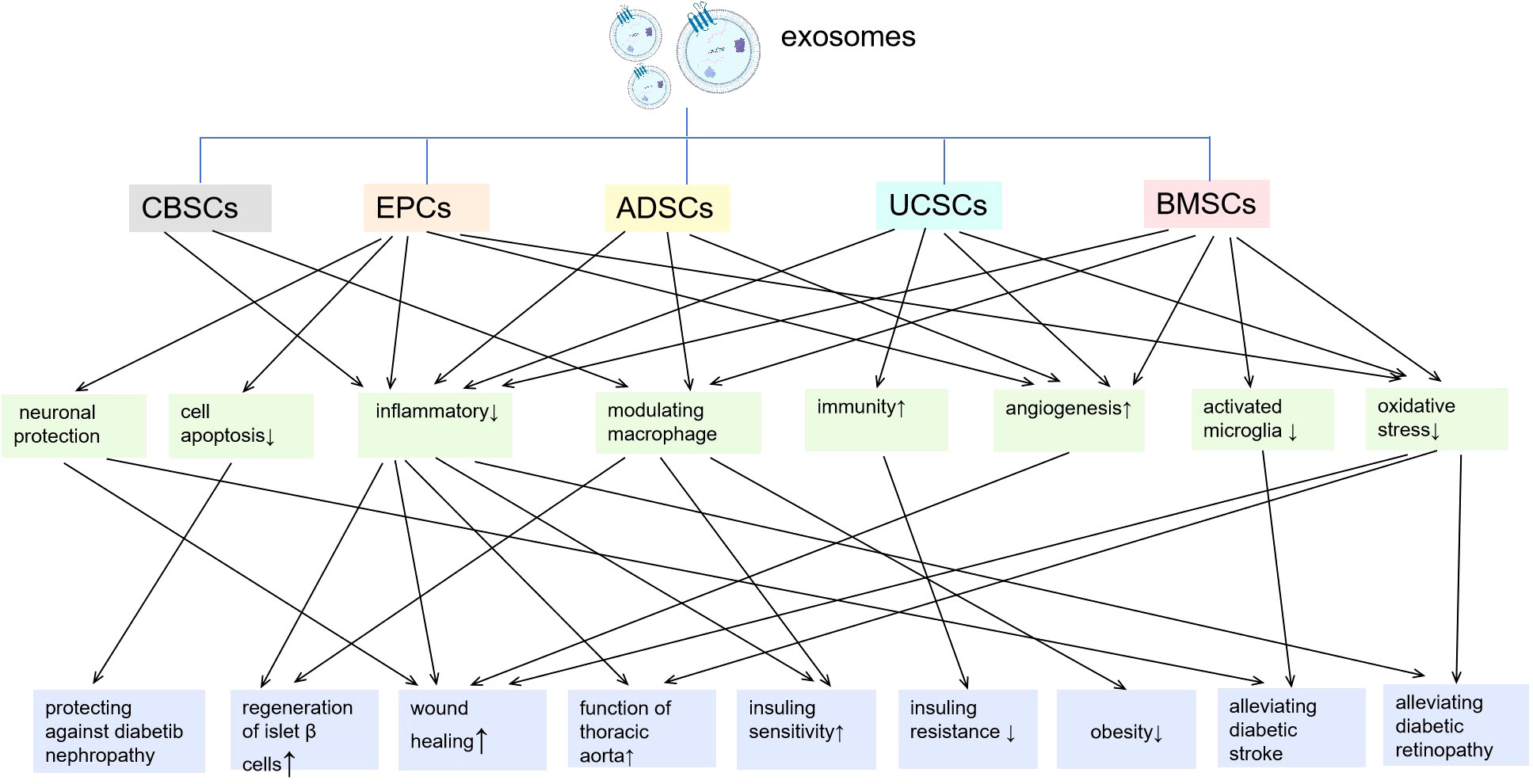
Figure 2 Exosomes from different kinds of stem cells and their main mechanisms underlying regulatory effects on inflammation/immunity in diabetes. CBSCs, cord-blood-derived stem cells; EPCs, endothelial progenitor cells; ADSCs, adipose stem cells; UCSCs, umbilical cord mesenchymal stem cells; BMSCs, bone marrow-derived mesenchymal stem cells.
4.1 Cord-blood-derived stem cells
Cord blood-derived stem cells are multipotent stem cells that exhibit a distinct phenotype characterized by both embryonic and hematopoietic markers, distinguishing them from other known stem cell types (55, 56). Phenotypic characterization reveals that CBSCs exhibit embryonic cell markers. Moreover, CBSCs exhibit minimal immunogenicity, as evidenced by their low expression of major histocompatibility complex (MHC) antigens and their inability to stimulate the proliferation of allogeneic lymphocytes (55, 57). Specifically, CBSCs adhere firmly to culture dishes, displaying a large rounded morphology, and are resistant to common detachment methods (trypsin/EDTA), facilitating the collection of suspended lymphocytes after co-culture (55, 57). Based on the unique properties of immune modulation mentioned above and their ability to adhere tightly to the surface of Petri dishes, a new technology called Stem Cell Educator (SCE) therapy was designated for use in clinical trials (58, 59). Stem Cell Educator therapy (Educator therapy) has been utilized with a closed-loop system and open-loop system. During SCE therapy, a patient’s peripheral blood mononuclear cells (PBMCs) are collected and circulated through a cell separator, where they are co-cultured with adherent human CBSCs in vitro. The resulting “educated” cells, known as CBSC-treated PBMCs, are then reintroduced into the patient’s circulation (60). These “educated” immune cells can educate other immune cells after infusion, thereby reverse the root cause(s) of the autoimmune disease and resulting in the long-lasting clinical efficacy of Educator therapy. Unlike traditional immune therapies, SCE therapy does not destroy the cells responsible for autoimmunity but modifies them (61). The clinical phase 1/2 trials indicate that SCE therapy reverses autoimmunity, promotes regeneration of islet β cells, and improves metabolic control for the treatment of Type 1 diabetes (59, 62, 63) and T2DM (59, 63).
Mechanistic studies revealed that the secretion of CBSC-derived exosomes (CBSC-EXOs) enabled polarization of human blood monocytes/macrophages into M2 macrophages, thereby fundamentally correcting self-immunity and inducing immune tolerance through various molecular and cellular mechanisms (60). CBSC-EXOs preferably and quickly bind to monocytes within 2-3 h. During the coculture of CBSCs with patient’s immune cells for clinical treatment during 8-9 h, the SCE-treated monocytes may transport the CBSC-EXOs back into the body, potentially leading to additional M2 differentiation and induction of tolerance (59, 62). Therefore, Educator therapy is the leading immunotherapy to date to safely and efficiently correct autoimmunity through CBSCs mediated immune modulation and anti-inflammatory clinical effects, without the safety and ethical concerns associated with conventional immune and/or stem-cell based approaches.
4.2 Endothelial progenitor cells
Chronic diabetic foot ulceration (DFU) is among the most debilitating long-standing diabetes complications and it is also one of the main causes of physical disability. DFU is partially a result of unregulated foot wound infection caused by neuropathy, hindered angiogenesis, chronic low-grade inflammation, and peripheral vascular/arterial disease (64). Prolonged hyperglycemia intensifies the expression of inflammatory cytokines and reactive oxygen species (ROS), which severely impede angiogenesis (65–67). Thus, wound healing in diabetes always heavily relies on the formation of new blood vessels to restore reperfusion (68). EPCs are the precursors of endothelial cells, which hold great potential in treating chronic non-healing diabetic wounds because of their abilities for vascular and neuronal protection, repair and regenesis (69, 70). Nevertheless, the direct utilization of stem/progenitor cells is constrained by concerns such as potential immunological rejection, chromosomal variation, and emboli formation (71–73). Therefore, it is crucial to devise a new approach that can maximize the therapeutic benefits of stem/progenitor cells while mitigating the risks associated with their direct application.
It has been reported that the exosomes derived from EPCs (EPC-EXOs) can regulate vascular endothelial cells through miRNA. For example (21), EPC-EXOs exhibited a high expression of miRNA-221-3p. Treating skin wounds in diabetic mice with EPC-EXOs demonstrated a similar effect to that seen with miRNA-221-3p administration. MiRNA-221-3p potentially downregulated critical proteins in the AGERAGE signaling pathway, inhibiting reactive oxygen species generation and inactivating nuclear factor-kappa B (NF-kB). This process may reduce inflammatory responses, cell apoptosis, and microvascular diseases. Except for miRNA-221-3p, recent results revealed that treatment with miR-126-3 overexpressing EPC-EXOs accelerated the healing of rat skin wounds and resulted in better tissue repair with slower scar formation. In this process, the expression of caspase-1, NRLP3, interleukin-1b, inteleukin-18, PIK3R2 and SPRED1 was suppressed, promoting diabetic wound repair (22).
Exosomes derived from EPCs were reported to promote angiogenesis and the homing ability of EPCs in diabetic wound healing. Li et al. treated a diabetic rat wound model with EPC-EXOs and found that exosomes enhanced the proliferation, migration and tube formation of vascular endothelial cells in vitro. Furthermore, endothelial cells stimulated with EPC-EXOs showed increase expression of angiogenesis-related molecules such as fibroblast growth factor-1 (FGF-1), VEGFA, VEGFR-2, angiotensin I, E-selectin, Chemokine (C-X-C motif) ligand-16 (CXCR-16), endothelial nitric oxide synthase and IL-8 (74). In addition to promoting angiogenesis in wound healing, microvesicles derived from EPCs were demonstrated to be capable of changing the properties of adipose stem cells (ADSCs), thereby, improving their homing ability to migrate to the wound site. Tu TC et al. transfected exosomes derived from Alde-Low EPCs (EMVs) into human ADSCs. After receiving EMVs, the ADSCs showed a remarkable elevation in the expression of the CXCR4 chemokine receptor in vitro, and CD45+ inflammatory cells were successfully recruited to the wound sites in vivo, promoting ischemic skin repair (75).
Diabetes mellitus not only increases the risk of ischemia-reperfusion by 3-4 times compared to those without diabetes mellitus, but also exacerbates cerebral damage due to impaired endothelial function and reduced angiogenesis (23). EPCs were demonstrated to hold great potential in the treatment of stoke due to the cerebrovascular protection in the acute phase and promoting neurological recovery in chronic phases (76, 77). Previously experiment in mice indicated that enrichment of miR126 enhanced the therapeutic efficacy of EPC-EXOs on diabetic ischemic stroke by attenuating acute injury and promoting neurological function recovery (23).
Moreover, EPC-EXOs could potentially be a potential therapeutic application for treating Aherosclerosis (AS) resulting from diabetes. AS is a major macrovascular complication of diabetes mellitus characterized by inflammation and endothelial damage (78). The dysfunction of the endothelium is considered an early marker of AS. EPCs are derived from bone marrow and can differentiate into endothelium cells. In cases where ECs are damaged, EPCs may replace them to assist in the recovery from endothelial dysfunction (79). It was demonstrated that EPCs-EXOs had a significant impact on reducing D-AS plaques, lowering the levels of inflammatory factors such as intercellular cell adhesion molecule-1, IL-8, and C-reactive protein, decreasing oxidative stress factors like malondialdehyde and superoxide dismutase, and improving the function of thoracic aorta vasodilation and constriction in a mouse model of diabetic AS (80).
4.3 Mesenchymal stem cell
Mesenchymal stem cells possess various biological characteristics, such as immunomodulation, anti-inflammatory properties, and promotion of angiogenesis, making them widely used in clinical treatment and regenerative medicine (81). MSC-EXOs have been shown to be similar effective as MSCs in the treatment of diabetes and related complications (82–84), but in some contexts, they exert different biological properties (85).
4.3.1 Adipose stem cells
Adipocyte-derived stem cells have been attracting attention as an effective therapeutic tool for tissue regeneration. Exosomes derived from ADSCs (ADSC-EXOs) can ameliorate inflammation by regulating immune cells, thereby promoting the treatment of diabetes and its related complications.
4.3.1.1 ADSC-EXOs modulate macrophage polarization and immune cell activities in diabetes
Zhao et al. demonstrated that treatment with ADSC-EXOs improved metabolic homeostasis in obese mice, including enhanced insulin sensitivity (27.8% improvement), reduced obesity, and alleviated hepatic steatosis. ADSC-EXOs induced M2 macrophage polarization, reduced inflammation, and promoted Beiging in white adipose tissues (WAT) of diet-induced obese mice. Such exosomes carried active signal transducer and activator of transcription 3 (STAT3), which facilitated arginase-1 expression in macrophages, leading to the induction of anti-inflammatory M2 phenotypes. Additionally, the M2 macrophages induced by ADSC-EXOs stimulated ADSC proliferation and lactate production, thereby promoting WAT beiging and maintaining homeostasis in response to high-fat challenge (86). Luo et al. reported that overexpression of hematopoietic prostaglandin D synthase HPGDS in ADSCs accelerated chronic wound healing by improving the anti-inflammatory state and promoting M2 macrophage polarization in type 2 diabetic mice (87). As for M1 macrophages, ADSCs-EXOs play an immunosuppressive role by reducing IFN-α secretion, thus inhibiting activation of T cells, leading to enhanced aggregation capacity of M1 macrophages (88, 89). Besides, ADSC-EXOs promoted T-regulatory cell activation and facilitated wound healing by inhibiting interferon-g production and M1 macrophage accumulation in an EFGR signal-dependent manner (90).
Moreover, recent research found ADSC-EXOs to be a vital source of non-coding RNA to enhance M2 macrophage polarization and promote diabetic wound healing. For example, hypoxic treatment significantly increased circ-Snhg11 contents in ADSC-EXOs and promoted M2 polarization by inhibiting miR-144-3p expression and the STAT3 signaling pathway in skin wounds (91, 92). In another study, the in vivo experiment demonstrated that exosomes derived from miR-132-overexpressing ADSC significantly improved the survival of skin flaps and accelerated diabetic wound healing. This was achieved by reducing local inflammation, promoting angiogenesis, and stimulating M2 macrophage polarization through the NF-κB signaling pathway (25). Li et al. found that treating diabetic foot ulcer wounds with ADSC-EXOs increased miR-21-5p levels in macrophages, promoted M2 polarization, and inhibited Keuppel-like factor 6 KLF6, which has been reported to enhance the inflammatory phenotype in macrophages (26).
These findings delineate novel exosome-mediated mechanisms for ADSC-macrophage crosstalk that facilitates immune and metabolic homeostasis, thus providing potential therapy for obesity and diabetes.
4.3.1.2 ADSC-EXOs revers the inflammatory condition in wound healing
Wound healing can be delayed by chronic and excessive inflammation, therefore a well-regulated inflammation guarantees wound healing (88). ADSCs-EXOs contain immunoregulatory proteins such as tumor necrosis factor-α (TNF-α), macrophage colony-stimulating factor and retinol-binding protein 4 (93). In addition to the local effects, ADSC-EXOs can reverse the systematic inflammatory condition in diabetes models. Qiu et al. demonstrated that high glucose treatment significantly increased inflammatory factors IL-6, IL-1β, and TNF-α levels in EPCs from healthy volunteers. Such elevated levels could be partially and completely reversed by ADSC-EXOs and linc00511-overexpressing ADSCs (94). They found Exosomes from linc00511-overexpressing ADSCs promotes diabetic foot ulcers healing by accelerating angiogenesis via suppressing PAQR3-induced Twist1 ubiquitin degradation as well as suppressed inflammatory. Zhang et al. found that ADSC-EXOs significantly reduced levels of inflammatory cytokines IL-6, TNF-a, and monocyte chemotactic protein-1 (MCP-1) by decreasing ROS production and protecting mitochondrial function via sirtuin-3 (95). Wang et al. found that hypoxic ADSC-EXOs exhibited distinct miRNA expression profiles compared to ADSC-EXOs. Specifically, up-regulation of miR-21-3p, miR-126-5p, and miR-31-5p, and down-regulation of miR-99b and miR-146-a in hypoxic ADSC-EXOs promoted wound healing in diabetic mice and suppressed inflammatory factors through the PI3K/AKT signaling pathway (27). Shi reported that exosomes derived from mmu_circ_0000250-modified ADSCs promoted wound healing in diabetic mice by inducing miR-128-3p/SIRT1-mediated autophagy and improving the hyperglycemic-induced inflammatory microenvironment and recover the function of EPCs (24).
4.3.2 Umbilical cord mesenchymal stem cells
Human umbilical cord tissue (Wharton’s jelly) serves as a potent and rich source of MSCs. UCSCs-derived exosomes (UCSC-EXOs) have shown promising results in the treatment of diabetes and may become a successful strategy for treating diabetes and its complications. Injection of UCSC-EXOs significantly ameliorated hyperglycemia in rats with T2DM (96). Besides, UCSC-EXOs also contributes to the therapy of other diabetic complications, such as diabetic nephropathy, retinopathy and wound ulcer.
4.3.2.1 UCSC-EXOs increase insulin sensitivity by suppress inflammatory factors
Chronic inflammation in tissues is typically the primary cause of insulin resistance, which results in the secretion of pro-inflammatory cytokines such as tumor necrosis factor alpha (TNF-α) or IL-6 by inflammatory cells. These cytokines then inhibit the activation of the insulin signaling pathway (97, 98). It is found that injection of human UCSC-EXOs significantly ameliorated hyperglycemia in rats with T2DM. UCSC-EXOs could increase insulin sensitivity by increasing the activation of insulin/AKT signaling pathway and inhibiting the secretion of proinflammatory cytokines like TNF-α, which could reverse insulin resistance in T2DM (96).
4.3.2.2 The role of UCSC-EXOs in diabetic nephropathy
It is demonstrated that UCSC-EXOs could be a promising treatment strategy for diabetic nephropathy rats. Xiang et al. reported that UCSC-EXOs apparently reduced the levels of pro-inflammatory cytokines (IL-6, IL-1β, and TNF-α) and pro-fibrotic factor transforming growth factor β (TGF-β) in the kidney and blood of diabetic nephropathy rats. In vitro experiments showed that umbilical cord MSC conditioned medium and UCSC-EXOs decreased the production of these cytokines in high glucose injured renal tubular epithelial cells, and renal glomerular endothelial cells (99). Besides, UCSC-EXOs miR-424-5p can inhibit the activation of yes associated protein 1 in HK2 cells, reduce cell apoptosis, and epithelial-to-mesenchymal transition induced by high glucose, thereby attenuating diabetic nephropathy (28). MiR-22-3p, highly expressed in UCSC-EXOs, may play a protective role in podocytes and diabetic mice by regulating the NLRP3 inflammasome. This suggests that MSC-derived exosomes could be a promising cell-free therapeutic strategy for diabetic kidney disease (29). Another study showed that UCSC-EXOs miR-146a-5p enhanced M2 macrophage polarization by inhibiting the TRAF6/STAT1 signaling pathway, thereby protecting against diabetic nephropathy in rats (30).
4.3.2.3 The role of UCSC-EXOs in wound healing and diabetic retinopathy
UCSC-EXOs serve as a novel therapeutic approach to enhance wound healing in diabetes. Studies have shown that UCSC-EXOs can induce anti-inflammatory macrophages (100), leading to a reduction in the expression of inflammatory factors such as IL-1β, IL-6, and TNF-α (101), as well as promoting angiogenesis and collagen deposition. Furthermore, UCSC-EXOs have the potential to inhibit oxidative stress injury, thereby facilitating macro-level angiogenesis and ultimately expediting the healing of diabetic wounds (101).
In addition to diabetic wounds, diabetic retinopathy is another common complication of diabetes. Previous studies have shown the therapeutic effect of UCSC-EXOs in diabetic retinopathy. For example, the administration of miR-126-expressing UCSC-EXOs significantly reduced high glucose-induced high-mobility group box 1 expression and the activity of the NLRP3 inflammasome in human retinal endothelial cells, therefore suppressing suppressed inflammation in diabetic rats (31).
At last, UCSC-EXOs treatment could be beneficial for diabetic rats to recover from the anemia-like symptoms and increase immunity by improving the erythrocytes and hemoglobin levels as well as maintaining the number of white blood cells (102). 1 mg/kg of UCSC-EXOs improved glucose tolerance in T2DM rats and ameliorate insulin resistance. Moreover, there was no significant difference in white blood cells, neutrophils, lymphocytes, monocytes, eosinophils, and basophils between the diabetic rat groups treated with both glibenclamide (one of the traditional hypoglycemic drug) and 1 mg/kg of UCSC-EXOs and the non-diabetic animal group. This finding suggests that the administration of UCSC-EXOs at 1 mg/kg could improve the immune system of diabetic rats, which is essential for reducing infections and increasing survival rates (102).
4.3.3 Bone marrow-derived mesenchymal stem cells
Bone marrow mesenchymal stem cells are multilineage progenitors with self-renewal, multidirectional differentiation, and pleiotropic paracrine functions (103). It is demonstrated that purified BMSC-derived exosomes (BMSC-EXOs) have more specific distinct benefits in damaged tissue repair than BMSCs themselves, including superior stability, tissue permeability, excellent biocompatibility, and immunomodulatory properties (104).
4.3.3.1 The role of BMSC-EXOs in diabetic wound healing
Accumulative studies have shown that BMSC-EXOs contribute to wound healing through non-coding RNAs. For example, Liu et al. found that miR-155-inhibitor-loaded BMSC-EXOs enhanced keratinocytes migration, FGF-7 recovery, and anti-inflammatory effects in vitro. Additionally, they could also be utilized to treat a diabetic wound model by promoting collagen deposition, angiogenesis, and re-epithelization. The functional coordination between miR-155-inhibitor and BMSC-EXOs played a crucial role in enhancing diabetic wound healing (32). Li reported that the injection of BMSC-EXOs overexpressing lncRNA H19 facilitated wound healing in mice with diabetic foot ulcers. Results revealed that BMSC-EXOs overexpressing lncRNA H19 led to higher level of IL-10 and lower levels of IL-1b and TNF-a, and the mechanism by which was associated with promoting fibroblast proliferation and migration, inhibiting cell apoptosis and inflammation (33). In a murine diabetic cutaneous wound model, exosomes from lncRNA KLF3-AS1-expressing BMSCs demonstrated the best effects in promoting cutaneous wound healing in diabetic mice, which were associated with minimal weight loss, increased blood vessel formation, reduced inflammation, decreased miR-383 expression, and up-regulated VEGFA (34). Except for non-coding RNAs, the anti-inflammation effect by BMSC-EXOs could induced by specific pathways that may not directly related to non-coding RNAs. Wang reported that the wounds treated with exosomes showed reduced inflammation, with decreased levels of the inflammatory cytokines TNF-α and IL-1β, and increased levels of the anti-inflammatory cytokines IL-4 and IL-10 (105). Such regenerative and anti-inflammatory effects were eliminated by Lenti-sh-Nrf2 administration, suggesting the participation of the activation of Nrf2 anti-oxidant pathway in wound healing by exosomes. In addition to miRNAs, Liu et al. reported that melatonin-pretreated BMSC-EXOs could promote diabetic wound healing by suppressing the inflammatory response, which was achieved by increasing the ratio of M2 polarization to M1 polarization through activating the phosphatase and tensin homolog/AKT signaling pathway (106).
4.3.3.2 The role of BMSC-EXOs in diabetic stroke
Diabetes increases the risk of stroke by 3-4 fold, and about 30% of stroke patients suffer from diabetes (107). Treating patients with diabetic stroke is challenging because it may cause extensive damage to the cerebral vasculature, exacerbate neurological deficits, enhance inflammatory responses, which are prone to recurrent strokes (108, 109). Therefore, it is crucial to devise therapeutic strategies specifically aimed at enhancing neurological function after stroke in individuals with diabetes. MSCs interact with and alter brain parenchymal cells via the secretion of trophic and growth factors as well as exosomes to exert therapeutic effects (110). Exosome therapy offers several advantages compared to cell therapy, as exosomes do not elicit immune rejection, do not cause vascular obstruction, and have a low risk of triggering tumors or malignant transformation (111). Besides, exosomes are more suitable for clinical use since they are relatively stable, can be obtained in large quantities from a small number of cells, and can be stored until therapeutic needed (112). Therefore, systemic administration of exosomes could be a method of delivering the active components of cell therapy to the central nervous system (113).
Studies (35, 114) have indicated that T2DM stroke was associated with increased inflammatory responses and proinflammatory microglial/macrophage phenotype. The inflammatory factor matrix metalloproteinase-9 (MMP-9) was elevated after stroke and has been implicated in aggravating blood-brain barrier disruption, neuronal death, myelin degradation and white matter injury. In addition, the inflammatory factor MCP-1 was elevated in the serum of both diabetic and stroke patients, and it aids in the accumulation of phagocytic M1 macrophages in the infarct border (115, 116). However, T2DM-BMSC-EXOs treatment has been demonstrated to significantly decrease activated microglia, M1 macrophage, and inflammatory factors MMP-9 and MCP-1 expression in the ischemic brain in T2DM stroke rats (35). Such therapeutic effects in neurological functional recovery were only induced by injection of exosomes derived from BMSCs of T2DM rats but not from BMSCs of non-diabetic animals, which may be partially mediated by decreasing miR-9 and upregulating ABCA1-IGFR1 pathway (35).
4.3.3.3 The role of BMSC-EXOs in diabetic retinopathy
BMSCs-Exos also possess other immunomodulatory properties and can suppress the activation and function of various immune cells involved in islet transplantation and diabetic retinopathy. It is reported that co-delivery of siFas and anti-miR-375 by BMSCs and derived exosomes suppressed early apoptosis of transplanted human islets, while further immune activity could be suppressed by intravenously injection of human BMSC and PBMC co-cultured exosomes. Thus, BMSC and peripheral blood mononuclear cell co-cultured exosomes performed a immunosuppressive effect for improving islet transplantation (117). Besides, BMSC-EXOs improve diabetes-induced retinal damage by inhibiting the Wnt/β-catenin signaling pathway, subsequently reducing oxidative stress, inflammation, and angiogenesis (118). BMSC-EXOs miR-146a regulates the inflammatory response of diabetic retinopathy by mediating the TLR4/MyD88/NF-κB pathway, reducing the levels of TNF-α, IL-1β, and IL-6 (119).
5 Exosomes as an innovative therapeutic tools for diabetes: current status and promising directions
5.1 Promising directions
Exosomes exhibit high biocompatibility and low immunogenicity, which makes them have great potential in delivering nucleic acid sequences and chemotherapy drugs (6). However, studies have shown that the natural half-life of most exosomes in vivo is relatively short (<6 h) (120), and the contents of natural exosomes are limited by the secreting cells, resulting in limited therapeutic effects when loaded with drug molecules. To date, increasing researches demonstrated that under certain stress or modified conditions, stem cells can produce more exosomes or exosomes with different compositions compared to basal conditions. Meanwhile, many studies demonstrated the beneficial effects of modified or pretreated stem cell-derived exosomes on preventing comorbidities or microvascular complications in diabetes. These benefits mainly stem from the following three perspectives (Table 2): a. Exosomes from genetically modified stem cells display enhanced effects on diabetic wound healing compared to wild-type exosomes; b. By adding specific drugs to the culture medium, cells may secrete exosomes that are more effective in targeting angiogenesis, anti-inflammation, promoting proliferation and migration, and inhibiting apoptosis; c. Under certain stress conditions, such as hypoxia, cells may secrete exosomes that perform better in promoting fibroblast proliferation and migration, and enhancing reepithelialization in chronic wounds. All the above demonstrated that preconditioning or pre-treatment of diabetic MSCs with various agents/stress can be used to optimize/improve cellular function prior to their use in cell therapy.
In addition to modify the donor cells that produce exosomes, direct modification to purified natural exosomes may efficiently and quickly obtain a large number of engineered exosomes, and reduce the uncertainty in the cell culture process, which is of great significance for the mass production of engineered exosomes. For example, taking advantage of natural availability and biocompatibility of exosomes as extracellular miRNA transporting particles (121), Lv et al. reported a human hASC-exos-based miRNA delivery strategy which loaded miRNA into hASC-exos by electroporation. Besides electroporation, other physical methods such as ultrasonic homogenization (128), freeze-thaw cycle (129), may also allow drugs to enter the exosomes more easily, achieving the purpose of engineering exosomes. However, such methods were usually used in treatment of cancers in vitro or in vivo in animal models, therefore, future research will focus more on the application of these methods in the treatment of diabetes and the associated complications.
Finally, in recent years, due to the high biocompatibility and modifiability, composite hydrogels loaded with exosomes and other nanoparticles have gained increasing attention in managing chronic diabetic wounds. Compared to traditional stem cell therapy, which has been shown to have short survival times, poor stability, and a high risk of immune rejection in diabetic ulcers (130), exosomes-loaded composite hydrogels have been demonstrated to possess superior functions in angiogenesis, anti-inflammatory, antibacterial, and antioxidant properties (Table 3). Since different agents have varying applicability, advantages and disadvantages for wound healing, various therapeutic agents can be incorporated inside the multifunctional hydrogel to create an outstanding drug delivery system (143). Thus, the exosomes-loaded, “all-in-one” composite hydrogels may achieve a controlled drug delivery in diabetic wound healing, prone to better drug applications.
5.2 Current challenges of clinical applications
So far, there are mainly three challenges in the clinical translations of exosomes. Firstly, minimize the therapeutic efficacy differences caused by physiological and structural variations between human and animals. Exosomes derived from various stem cell sources have been used in wound healing treatments across animal models including mice (144, 145), rats (123), rabbits (146), consistently demonstrating positive effects such as improved wound closure, reduced healing time, enhanced angiogenesis, and diminished scar formation. However, the outcomes of these preclinical studies do not necessarily translate to human skin due to significant differences in skin structure and physiology, with pig skin being the closest analogue to human skin. Porcine models have emerged as promising models to study wound healing, they possess similar anatomically and physiologically characteristics to humans, including a relatively thick epidermis, distinct rete pegs, dermal papillae, and dense elastic fibers in the dermis (147), porcine collagen (148) et al. In contrast to rodent, rabbit, and canine skin, which exhibits loos adherence to the subcutaneous fascia, porcine skin closely adheres to the underlying structures, resembling human skin (149). The turnover time of pig epidermis is similar to the human epidermis (150). Moreover, the immune cells in pig skin resemble those found in human skin (151). According to research by Sullivan and colleagues, pig models were 78% concordant with human studies. This result exceeded other small-mammal and in vitro models, which were only 53% and 57% concordant (152). Therefore, it is crucial to validate the biological effects of exosomes on wound healing using a pig model.
Secondly, the clinical translation of engineered extracellular vesicles is urgently needed. So far, clinical applications of these exosomes are limited to only a few clinical trials exploring the therapeutic effects of stem cell-derived exosomes for diabetes and its complications, such as wound healing. According to data from ClinicalTrials.gov, to date, three completed clinical trials have utilized exosomes derived from plasma (NCT02565264), adipose tissue (NCT05475418), and mesenchymal stem cells (NCT05813379) for wound healing. Another (NCT04134676) has explored the use of stem cell-conditioned medium for chronic ulcer wounds. Apart from wound treatment, very few clinical trials have investigated the use of exosomes for other diabetic conditions [only one for Type 1 diabetes (NCT02138331)].
Thirdly, The scaling-up manufacture of “Good Manufacturing Practice” (GMP)-grade exosomes is the most difficult component in the clinical use of exosomes. Challenges in the further clinical application of exosomes include quality control, such as the cell-culture system, purification, characterization/physicochemical and biological properties of exosomes, as well as the establishment of a “gold standard” for potency assay. Thus, advances in scaling-up technology for GMP-compliant exosomes manufacturing will enhance the clinical applications of these entities for diabetes and the related complications in the near future.
6 Concluding remarks and future perspectives
As a promising candidate for novel cell free therapy, exosomes may be widely used as an alternative to stem cells in management of a variety of immunity-related diseases or inflammation response for maintenance of the microenvironment for tissue homeostasis and tissue regeneration upon injury. In this review article, we describe how immune cell-derived exosomes origin from neutrophils, T lymphocytes and macrophages impact on diabetes and the associated complications. We also discuss the stem cell-derived exosomes and their role in immunomodulatory and inflammation in the progress of diabetic complications. In addition, promising directions involving engineered exosomes as well as current challenges of clinical applications are reviewed. The enhanced properties of engineered exosomes have been verified in lab, which proves that they have great clinical application prospects. However, there is still a long way to go before commercial exosome products are ready for the market, due to the lack of clinical trials and quality control for scaling-up manufacture.
In addition to the above challenges, some questions remain unanswered, which needs more attention to be paid to in the future. For example, how do exosomes transferred specific miRNAs target the genes in recipient cells? Besides, studies about gestational diabetes mellitus (GDM) are still limited. Although researchers have found that some exosomal non-coding RNAs in peripheral blood may be early diagnostic markers for GDM, it is unknown how exosomes interact with the immune system and contribute to the pathophysiology of GDM. Nevertheless, we remain confident that the hurdles facing these innovative approaches will be surmounted and that they will do influence the treatment of diabetes.
Author contributions
NL: Conceptualization, Funding acquisition, Writing – original draft, Writing – review & editing. LH: Data curation, Resources, Writing – original draft. JL: Data curation, Investigation, Project administration, Writing – original draft. YY: Data curation, Formal analysis, Methodology, Writing – original draft. ZB: Writing – review & editing. ZX: Conceptualization, Supervision, Writing – review & editing. DC: Supervision, Writing – review & editing. JT: Conceptualization, Funding acquisition, Investigation, Validation, Writing – review & editing. YG: Funding acquisition, Project administration, Resources, Writing – review & editing.
Funding
The author(s) declare financial support was received for the research, authorship, and/or publication of this article. This work was supported by Top Talent Support Program for young and middle-aged people of Wuxi Health Committee, Youth Project of Wuxi Municipal Health Commission (Q202104), Research Project of Wuxi Municipal Health Commission (M202217) and the Taihu Rencai Project.
Conflict of interest
The authors declare that the research was conducted in the absence of any commercial or financial relationships that could be construed as a potential conflict of interest.
The reviewer J-HC declared a shared parent affiliation with the authors NL, YY, ZX, DC, and YG to the handling editor at the time of review.
Publisher’s note
All claims expressed in this article are solely those of the authors and do not necessarily represent those of their affiliated organizations, or those of the publisher, the editors and the reviewers. Any product that may be evaluated in this article, or claim that may be made by its manufacturer, is not guaranteed or endorsed by the publisher.
References
1. Punthakee Z, Goldenberg R, Katz P. Definition, classification and diagnosis of diabetes, prediabetes and metabolic syndrome. Can J Diabetes. (2018) 42 Suppl 1:S10–s5. doi: 10.1016/j.jcjd.2017.10.003
2. Kamal MM, Kassem DH. Therapeutic potential of wharton’s jelly mesenchymal stem cells for diabetes: achievements and challenges. Front Cell Dev Biol. (2020) 8:16. doi: 10.3389/fcell.2020.00016
3. Jia G, Whaley-Connell A, Sowers JR. Diabetic cardiomyopathy: a hyperglycaemia- and insulin-resistance-induced heart disease. Diabetologia. (2018) 61:21–8. doi: 10.1007/s00125-017-4390-4
4. Xiao Y, Zheng L, Zou X, Wang J, Zhong J, Zhong T. Extracellular vesicles in type 2 diabetes mellitus: key roles in pathogenesis, complications, and therapy. J Extracell Vesicles. (2019) 8:1625677. doi: 10.1080/20013078.2019.1625677
5. Päth G, Perakakis N, Mantzoros CS, Seufert J. Stem cells in the treatment of diabetes mellitus - Focus on mesenchymal stem cells. Metabol: Clin Experimental. (2019) 90:1–15. doi: 10.1016/j.metabol.2018.10.005
6. Zhang Y, Yu M, Tian W. Physiological and pathological impact of exosomes of adipose tissue. Cell Proliferation. (2016) 49:3–13. doi: 10.1111/cpr.12233
7. Casado-Díaz A, Quesada-Gómez JM, Dorado G. Extracellular vesicles derived from mesenchymal stem cells (MSC) in regenerative medicine: applications in skin wound healing. Front Bioeng Biotechnol. (2020) 8:146. doi: 10.3389/fbioe.2020.00146
8. Pegtel DM, Gould SJ. Exosomes. Annu Rev Biochem. (2019) 88:487–514. doi: 10.1146/annurev-biochem-013118-111902
9. Doyle LM, Wang MZ. Overview of extracellular vesicles, their origin, composition, purpose, and methods for exosome isolation and analysis. Cells. (2019) 8. doi: 10.3390/cells8070727
10. Hessvik NP, Llorente A. Current knowledge on exosome biogenesis and release. Cell Mol Life Sci: CMLS. (2018) 75:193–208. doi: 10.1007/s00018-017-2595-9
11. Kalluri R, LeBleu VS. The biology, function, and biomedical applications of exosomes. Sci (New York NY). (2020) 367. doi: 10.1126/science.aau6977
12. Wu Y, Deng W, Klinke DJ 2nd. Exosomes: improved methods to characterize their morphology, RNA content, and surface protein biomarkers. Analyst. (2015) 140:6631–42. doi: 10.1039/C5AN00688K
13. Luo X, Li Y, Hua Z, Xue X, Wang X, Pang M, et al. Exosomes-mediated tumor metastasis through reshaping tumor microenvironment and distant niche. J Controlled Release. (2023) 353:327–36. doi: 10.1016/j.jconrel.2022.11.050
14. Zhang J, Li S, Li L, Li M, Guo C, Yao J, et al. Exosome and exosomal microRNA: trafficking, sorting, and function. Genomics Proteomics Bioinf. (2015) 13:17–24. doi: 10.1016/j.gpb.2015.02.001
15. Tian F, Tang P, Sun Z, Zhang R, Zhu D, He J, et al. miR-210 in exosomes derived from macrophages under high glucose promotes mouse diabetic obesity pathogenesis by suppressing NDUFA4 expression. J Diabetes Res. (2020) 2020:6894684. doi: 10.1155/2020/6894684
16. Liu T, Sun YC, Cheng P, Shao HG. Adipose tissue macrophage-derived exosomal miR-29a regulates obesity-associated insulin resistance. Biochem Biophys Res Commun. (2019) 515:352–8. doi: 10.1016/j.bbrc.2019.05.113
17. Qian B, Yang Y, Tang N, Wang J, Sun P, Yang N, et al. M1 macrophage-derived exosomes impair beta cell insulin secretion via miR-212-5p by targeting SIRT2 and inhibiting Akt/GSK-3β/β-catenin pathway in mice. Diabetologia. (2021) 64:2037–51. doi: 10.1007/s00125-021-05489-1
18. Zhang D, Wu Y, Li Z, Chen H, Huang S, Jian C, et al. MiR-144-5p, an exosomal miRNA from bone marrow-derived macrophage in type 2 diabetes, impairs bone fracture healing via targeting Smad1. J Nanobiotechnol. (2021) 19:226. doi: 10.1186/s12951-021-00964-8
19. Shyu KG, Wang BW, Fang WJ, Pan CM, Lin CM. Exosomal MALAT1 Derived from High Glucose-Treated Macrophages Up-Regulates Resistin Expression via miR-150-5p Downregulation. Int J Mol Sci. (2022) 23. doi: 10.3390/ijms23031095
20. Wang Z, Sun W, Li R, Liu Y. miRNA-93-5p in exosomes derived from M2 macrophages improves lipopolysaccharide-induced podocyte apoptosis by targeting Toll-like receptor 4. Bioengineered. (2022) 13:7683–96. doi: 10.1080/21655979.2021.2023794
21. Xu J, Bai S, Cao Y, Liu L, Fang Y, Du J, et al. miRNA-221-3p in endothelial progenitor cell-derived exosomes accelerates skin wound healing in diabetic mice. Diabetes Metab Syndrome Obesity: Targets Ther. (2020) 13:1259–70. doi: 10.2147/DMSO.S243549
22. Xiong W, Zhang X, Zhou J, Chen J, Liu Y, Yan Y, et al. Astragaloside IV promotes exosome secretion of endothelial progenitor cells to regulate PI3KR2/SPRED1 signaling and inhibit pyroptosis of diabetic endothelial cells. Cytotherapy. (2024) 26:36–50. doi: 10.1016/j.jcyt.2023.08.013
23. Wang J, Chen S, Zhang W, Chen Y, Bihl JC. Exosomes from miRNA-126-modified endothelial progenitor cells alleviate brain injury and promote functional recovery after stroke. CNS Neurosci Ther. (2020) 26:1255–65. doi: 10.1111/cns.13455
24. Shi R, Jin Y, Hu W, Lian W, Cao C, Han S, et al. Exosomes derived from mmu_circ_0000250-modified adipose-derived mesenchymal stem cells promote wound healing in diabetic mice by inducing miR-128-3p/SIRT1-mediated autophagy. Am J Physiol Cell Physiol. (2020) 318:C848–c56. doi: 10.1152/ajpcell.00041.2020
25. Ge L, Wang K, Lin H, Tao E, Xia W, Wang F, et al. Engineered exosomes derived from miR-132-overexpresssing adipose stem cells promoted diabetic wound healing and skin reconstruction. Front Bioeng Biotechnol. (2023) 11:1129538. doi: 10.3389/fbioe.2023.1129538
26. Li J, Wei C, Yang Y, Gao Z, Guo Z, Qi F. Apoptotic bodies extracted from adipose mesenchymal stem cells carry microRNA-21-5p to induce M2 polarization of macrophages and augment skin wound healing by targeting KLF6. Burns. (2022) 48:1893–908. doi: 10.1016/j.burns.2021.12.010
27. Wang J, Wu H, Peng Y, Zhao Y, Qin Y, Zhang Y, et al. Hypoxia adipose stem cell-derived exosomes promote high-quality healing of diabetic wound involves activation of PI3K/Akt pathways. J Nanobiotechnol. (2021) 19:202. doi: 10.1186/s12951-021-00942-0
28. Cui C, Zang N, Song J, Guo X, He Q, Hu H, et al. Exosomes derived from mesenchymal stem cells attenuate diabetic kidney disease by inhibiting cell apoptosis and epithelial-to-mesenchymal transition via miR-424-5p. FASEB J. (2022) 36:e22517. doi: 10.1096/fj.202200488R
29. Wang Y, Liu J, Wang H, Lv S, Liu Q, Li S, et al. Mesenchymal stem cell-derived exosomes ameliorate diabetic kidney disease through the NLRP3 signaling pathway. Stem Cells (Dayton Ohio). (2023) 41:368–83. doi: 10.1093/stmcls/sxad010
30. Zhang Y, Le X, Zheng S, Zhang K, He J, Liu M, et al. MicroRNA-146a-5p-modified human umbilical cord mesenchymal stem cells enhance protection against diabetic nephropathy in rats through facilitating M2 macrophage polarization. Stem Cell Res Ther. (2022) 13:171. doi: 10.1186/s13287-022-02855-7
31. Zhang W, Wang Y, Kong Y. Exosomes derived from mesenchymal stem cells modulate miR-126 to ameliorate hyperglycemia-induced retinal inflammation via targeting HMGB1. Invest Ophthalmol Visual Sci. (2019) 60:294–303. doi: 10.1167/iovs.18-25617
32. Gondaliya P, Sayyed AA, Bhat P, Mali M, Arya N, Khairnar A, et al. Mesenchymal stem cell-derived exosomes loaded with miR-155 inhibitor ameliorate diabetic wound healing. Mol Pharmaceutics. (2022) 19:1294–308. doi: 10.1021/acs.molpharmaceut.1c00669
33. Li B, Luan S, Chen J, Zhou Y, Wang T, Li Z, et al. The MSC-Derived Exosomal lncRNA H19 Promotes Wound Healing in Diabetic Foot Ulcers by Upregulating PTEN via MicroRNA-152-3p. Mol Ther Nucleic Acids. (2020) 19:814–26. doi: 10.1016/j.omtn.2019.11.034
34. Han ZF, Cao JH, Liu ZY, Yang Z, Qi RX, Xu HL. Exosomal lncRNA KLF3-AS1 derived from bone marrow mesenchymal stem cells stimulates angiogenesis to promote diabetic cutaneous wound healing. Diabetes Res Clin Practice. (2022) 183:109126. doi: 10.1016/j.diabres.2021.109126
35. Venkat P, Zacharek A, Landschoot-Ward J, Wang F, Culmone L, Chen Z, et al. Exosomes derived from bone marrow mesenchymal stem cells harvested from type two diabetes rats promotes neurorestorative effects after stroke in type two diabetes rats. Exp Neurol. (2020) 334:113456. doi: 10.1016/j.expneurol.2020.113456
36. Raposo G, Nijman HW, Stoorvogel W, Liejendekker R, Harding CV, Melief CJ, et al. B lymphocytes secrete antigen-presenting vesicles. J Exp Med. (1996) 183:1161–72. doi: 10.1084/jem.183.3.1161
37. Zhao Y, Liu T, Zhou M. Immune-cell-derived exosomes for cancer therapy. Mol Pharmaceutics. (2022) 19:3042–56. doi: 10.1021/acs.molpharmaceut.2c00407
38. Veerman RE, Güçlüler Akpinar G, Eldh M, Gabrielsson S. Immune cell-derived extracellular vesicles - functions and therapeutic applications. Trends Mol Med. (2019) 25:382–94. doi: 10.1016/j.molmed.2019.02.003
39. Ma X, Yao M, Gao Y, Yue Y, Li Y, Zhang T, et al. Functional immune cell-derived exosomes engineered for the trilogy of radiotherapy sensitization. Adv Sci (Weinheim Baden-Wurttemberg Germany). (2022) 9:e2106031. doi: 10.1002/advs.202106031
40. Yu Y, Jin H, Li L, Zhang X, Zheng C, Gao X, et al. An injecta, activated neutrophil-derived exosome mimetics/extracellular matrix hybrid hydrogel with antibacterial activity and wound healing promotion effect for diabetic wound therapy. J Nanobiotechnol. (2023) 21:308. doi: 10.1186/s12951-023-02073-0
41. Glennon-Alty L, Hackett AP, Chapman EA, Wright HL. Neutrophils and redox stress in the pathogenesis of autoimmune disease. Free Radical Biol Med. (2018) 125:25–35. doi: 10.1016/j.freeradbiomed.2018.03.049
42. Kolonics F, Szeifert V, Timár CI, Ligeti E, Lőrincz ÁM. The functional heterogeneity of neutrophil-derived extracellular vesicles reflects the status of the parent cell. Cells. (2020) 9. doi: 10.3390/cells9122718
43. Eizirik DL, Colli ML, Ortis F. The role of inflammation in insulitis and beta-cell loss in type 1 diabetes. Nat Rev Endocrinol. (2009) 5:219–26. doi: 10.1038/nrendo.2009.21
44. Guay C, Kruit JK, Rome S, Menoud V, Mulder NL, Jurdzinski A, et al. Lymphocyte-derived exosomal microRNAs promote pancreatic β Cell death and may contribute to type 1 diabetes development. Cell Metab. (2019) 29:348–61.e6. doi: 10.1016/j.cmet.2018.09.011
45. Robbins PD, Morelli AE. Regulation of immune responses by extracellular vesicles. Nat Rev Immunol. (2014) 14:195–208. doi: 10.1038/nri3622
46. Orecchioni M, Ghosheh Y, Pramod AB, Ley K. Macrophage Polarization: Different Gene Signatures in M1(LPS+) vs. Classically and M2(LPS-) vs. Alternatively Activated Macrophages. Front Immunol. (2019) 10:1084. doi: 10.3389/fimmu.2019.01084
47. Gharavi AT, Hanjani NA, Movahed E, Doroudian M. The role of macrophage subtypes and exosomes in immunomodulation. Cell Mol Biol Lett. (2022) 27:83. doi: 10.1186/s11658-022-00384-y
48. Shan X, Zhang C, Mai C, Hu X, Cheng N, Chen W, et al. The biogenesis, biological functions, and applications of macrophage-derived exosomes. Front Mol Biosci. (2021) 8:715461. doi: 10.3389/fmolb.2021.715461
49. Stefanovic-Racic M, Yang X, Turner MS, Mantell BS, Stolz DB, Sumpter TL, et al. Dendritic cells promote macrophage infiltration and comprise a substantial proportion of obesity-associated increases in CD11c+ cells in adipose tissue and liver. Diabetes. (2012) 61:2330–9. doi: 10.2337/db11-1523
50. Liu Y, Li X, Zhao M, Wu Y, Xu Y, Li X, et al. Macrophage-derived exosomes promote activation of NLRP3 inflammasome and autophagy deficiency of mesangial cells in diabetic nephropathy. Life Sci. (2023) 330:121991. doi: 10.1016/j.lfs.2023.121991
51. Zhao J, Chen J, Zhu W, Qi XM, Wu YG. Exosomal miR-7002-5p derived from highglucose-induced macrophages suppresses autophagy in tubular epithelial cells by targeting Atg9b. FASEB J. (2022) 36:e22501. doi: 10.1096/fj.202200550RR
52. Phu TA, Ng M, Vu NK, Bouchareychas L, Raffai RL. IL-4 polarized human macrophage exosomes control cardiometabolic inflammation and diabetes in obesity. Mol Ther. (2022) 30:2274–97. doi: 10.1016/j.ymthe.2022.03.008
53. Zhang C, Bao LR, Yang YT, Wang Z, Li Y. [Role of M2 macrophage exosomes in osteogenic differentiation of mouse bone marrow mesenchymal stem cells under high-glucose and high-insulin]. Sichuan da xue xue bao Yi xue ban = J Sichuan Univ Med Sci Edition. (2022) 53:63–70. doi: 10.12182/20220160207
54. Wang Y, Lin Q, Zhang H, Wang S, Cui J, Hu Y, et al. M2 macrophage-derived exosomes promote diabetic fracture healing by acting as an immunomodulator. Bioactive Mater. (2023) 28:273–83. doi: 10.1016/j.bioactmat.2023.05.018
55. Zhao Y, Wang H, Mazzone T. Identification of stem cells from human umbilical cord blood with embryonic and hematopoietic characteristics. Exp Cell Res. (2006) 312:2454–64. doi: 10.1016/j.yexcr.2006.04.008
56. Zhao Y, Mazzone T. Human cord blood stem cells and the journey to a cure for type 1 diabetes. Autoimmun Rev. (2010) 10:103–7. doi: 10.1016/j.autrev.2010.08.011
57. Zhao Y, Huang Z, Qi M, Lazzarini P, Mazzone T. Immune regulation of T lymphocyte by a newly characterized human umbilical cord blood stem cell. Immunol Lett. (2007) 108:78–87. doi: 10.1016/j.imlet.2006.10.007
58. Zhao Y, Jiang Z, Zhao T, Ye M, Hu C, Yin Z, et al. Reversal of type 1 diabetes via islet β cell regeneration following immune modulation by cord blood-derived multipotent stem cells. BMC Med. (2012) 10:3. doi: 10.1186/1741-7015-10-3
59. Zhao Y, Jiang Z, Zhao T, Ye M, Hu C, Zhou H, et al. Targeting insulin resistance in type 2 diabetes via immune modulation of cord blood-derived multipotent stem cells (CB-SCs) in stem cell educator therapy: phase I/II clinical trial. BMC Med. (2013) 11:160. doi: 10.1186/1741-7015-11-160
60. Zhao Y, Knight CM, Jiang Z, Delgado E, Van Hoven AM, Ghanny S, et al. Stem Cell Educator therapy in type 1 diabetes: From the bench to clinical trials. Autoimmun Rev. (2022) 21:103058. doi: 10.1016/j.autrev.2022.103058
61. Hu W, Song X, Yu H, Sun J, Zhao Y. Released exosomes contribute to the immune modulation of cord blood-derived stem cells. Front Immunol. (2020) 11:165. doi: 10.3389/fimmu.2020.00165
62. Delgado E, Perez-Basterrechea M, Suarez-Alvarez B, Zhou H, Revuelta EM, Garcia-Gala JM, et al. Modulation of autoimmune T-cell memory by stem cell educator therapy: phase 1/2 clinical trial. EBioMedicine. (2015) 2:2024–36. doi: 10.1016/j.ebiom.2015.11.003
63. Zhao Y, Jiang Z, Delgado E, Li H, Zhou H, Hu W, et al. Platelet-derived mitochondria display embryonic stem cell markers and improve pancreatic islet β-cell function in humans. Stem Cells Trans Med. (2017) 6:1684–97. doi: 10.1002/sctm.17-0078
64. Acosta JB, del Barco DG, Vera DC, Savigne W, Lopez-Saura P, Guillen Nieto G, et al. The pro-inflammatory environment in recalcitrant diabetic foot wounds. Int Wound J. (2008) 5:530–9. doi: 10.1111/j.1742-481X.2008.00457.x
65. Bodnár E, Bakondi E, Kovács K, Hegedűs C, Lakatos P, Robaszkiewicz A, et al. Redox profiling reveals clear differences between molecular patterns of wound fluids from acute and chronic wounds. Oxid Med Cell Longevity. (2018) 2018:5286785. doi: 10.1155/2018/5286785
66. Rabbani PS, Abdou SA, Sultan DL, Kwong J, Duckworth A, Ceradini DJ. In vivo imaging of reactive oxygen species in a murine wound model. J Visualized Experiments: JoVE. (2018) 141). doi: 10.3791/58450
67. Rabbani PS, Soares MA, Hameedi SG, Kadle RL, Mubasher A, Kowzun M, et al. Dysregulation of nrf2/keap1 redox pathway in diabetes affects multipotency of stromal cells. Diabetes. (2019) 68:141–55. doi: 10.2337/db18-0232
68. Xing Z, Zhao C, Liu H, Fan Y. Endothelial progenitor cell-derived extracellular vesicles: A novel candidate for regenerative medicine and disease treatment. Adv Healthcare Mater. (2020) 9:e2000255. doi: 10.1002/adhm.202000255
69. Critser PJ, Yoder MC. Endothelial colony-forming cell role in neoangiogenesis and tissue repair. Curr Opin Organ Transplantation. (2010) 15:68–72. doi: 10.1097/MOT.0b013e32833454b5
70. Zhang J, Chen C, Hu B, Niu X, Liu X, Zhang G, et al. Exosomes derived from human endothelial progenitor cells accelerate cutaneous wound healing by promoting angiogenesis through erk1/2 signaling. Int J Biol Sci. (2016) 12:1472–87. doi: 10.7150/ijbs.15514
71. Duscher D, Barrera J, Wong VW, Maan ZN, Whittam AJ, Januszyk M, et al. Stem cells in wound healing: the future of regenerative medicine? A mini-review. Gerontology. (2016) 62:216–25. doi: 10.1159/000381877
72. Herberts CA, Kwa MS, Hermsen HP. Risk factors in the development of stem cell therapy. J Trans Med. (2011) 9:29. doi: 10.1186/1479-5876-9-29
73. Kansu E. Thrombosis in stem cell transplantation. Hematol (Amsterdam Netherlands). (2012) 17 Suppl 1:S159–62. doi: 10.1179/102453312X13336169156735
74. Li X, Jiang C, Zhao J. Human endothelial progenitor cells-derived exosomes accelerate cutaneous wound healing in diabetic rats by promoting endothelial function. J Diabetes its Complications. (2016) 30:986–92. doi: 10.1016/j.jdiacomp.2016.05.009
75. Tu TC, Yamashita T, Kato T, Nagano M, Trinh NT, Hamada H, et al. Microvesicles derived from Alde-Low EPCs support the wound healing capacity of AT-MSCs. Biochem Biophys Res Commun. (2016) 477:68–75. doi: 10.1016/j.bbrc.2016.06.022
76. Chen J, Chen J, Chen S, Zhang C, Zhang L, Xiao X, et al. Transfusion of CXCR4-primed endothelial progenitor cells reduces cerebral ischemic damage and promotes repair in db/db diabetic mice. PloS One. (2012) 7:e50105. doi: 10.1371/journal.pone.0050105
77. Chen J, Xiao X, Chen S, Zhang C, Chen J, Yi D, et al. Angiotensin-converting enzyme 2 priming enhances the function of endothelial progenitor cells and their therapeutic efficacy. Hypertension (Dallas Tex: 1979). (2013) 61:681–9. doi: 10.1161/HYPERTENSIONAHA.111.00202
78. Tang N, Jiang S, Yang Y, Liu S, Ponnusamy M, Xin H, et al. Noncoding RNAs as therapeutic targets in atherosclerosis with diabetes mellitus. Cardiovasc Ther. (2018) 36:e12436. doi: 10.1111/1755-5922.12436
79. Zhang Z, Dong J, Lobe CG, Gong P, Liu J, Liao L. CCR5 facilitates endothelial progenitor cell recruitment and promotes the stabilization of atherosclerotic plaques in ApoE-/- mice. Stem Cell Res Ther. (2015) 6:36. doi: 10.1186/s13287-015-0026-0
80. Bai S, Yin Q, Dong T, Dai F, Qin Y, Ye L, et al. Endothelial progenitor cell-derived exosomes ameliorate endothelial dysfunction in a mouse model of diabetes. Biomed Pharmacother = Biomed Pharmacother. (2020) 131:110756. doi: 10.1016/j.biopha.2020.110756
81. Liang X, Ding Y, Zhang Y, Tse HF, Lian Q. Paracrine mechanisms of mesenchymal stem cell-based therapy: current status and perspectives. Cell Transplantation. (2014) 23:1045–59. doi: 10.3727/096368913X667709
82. Mathew B, Ravindran S, Liu X, Torres L, Chennakesavalu M, Huang CC, et al. Mesenchymal stem cell-derived extracellular vesicles and retinal ischemia-reperfusion. Biomaterials. (2019) 197:146–60. doi: 10.1016/j.biomaterials.2019.01.016
83. Shabbir A, Cox A, Rodriguez-Menocal L, Salgado M, Van Badiavas E. Mesenchymal stem cell exosomes induce proliferation and migration of normal and chronic wound fibroblasts, and enhance angiogenesis in vitro. Stem Cells Dev. (2015) 24:1635–47. doi: 10.1089/scd.2014.0316
84. Nakano M, Kubota K, Kobayashi E, Chikenji TS, Saito Y, Konari N, et al. Bone marrow-derived mesenchymal stem cells improve cognitive impairment in an Alzheimer’s disease model by increasing the expression of microRNA-146a in hippocampus. Sci Rep. (2020) 10:10772. doi: 10.1038/s41598-020-67460-1
85. Pomatto M, Gai C, Negro F, Cedrino M, Grange C, Ceccotti E, et al. Differential therapeutic effect of extracellular vesicles derived by bone marrow and adipose mesenchymal stem cells on wound healing of diabetic ulcers and correlation to their cargoes. Int J Mol Sci. (2021) 22. doi: 10.3390/ijms22083851
86. Zhao H, Shang Q, Pan Z, Bai Y, Li Z, Zhang H, et al. Exosomes from adipose-derived stem cells attenuate adipose inflammation and obesity through polarizing M2 macrophages and beiging in white adipose tissue. Diabetes. (2018) 67:235–47. doi: 10.2337/db17-0356
87. Ouyang L, Qiu D, Fu X, Wu A, Yang P, Yang Z, et al. Overexpressing HPGDS in adipose-derived mesenchymal stem cells reduces inflammatory state and improves wound healing in type 2 diabetic mice. Stem Cell Res Ther. (2022) 13:395. doi: 10.1186/s13287-022-03082-w
88. Sugimoto MA, Sousa LP, Pinho V, Perretti M, Teixeira MM. Resolution of inflammation: what controls its onset? Front Immunol. (2016) 7:160. doi: 10.3389/fimmu.2016.00160
89. Lipsky PE. Systemic lupus erythematosus: an autoimmune disease of B cell hyperactivity. Nat Immunol. (2001) 2:764–6. doi: 10.1038/ni0901-764
90. Nosbaum A, Prevel N, Truong HA, Mehta P, Ettinger M, Scharschmidt TC, et al. Cutting edge: regulatory T cells facilitate cutaneous wound healing. J Immunol (Baltimore Md: 1950). (2016) 196:2010–4. doi: 10.4049/jimmunol.1502139
91. Lin CW, Hung CM, Chen WJ, Chen JC, Huang WY, Lu CS, et al. New horizons of macrophage immunomodulation in the healing of diabetic foot ulcers. Pharmaceutics. (2022) 14. doi: 10.3390/pharmaceutics14102065
92. Shi R, Jin Y, Zhao S, Yuan H, Shi J, Zhao H. Hypoxic ADSC-derived exosomes enhance wound healing in diabetic mice via delivery of circ-Snhg11 and induction of M2-like macrophage polarization. Biomed Pharmacother = Biomed Pharmacother. (2022) 153:113463. doi: 10.1016/j.biopha.2022.113463
93. Blazquez R, Sanchez-Margallo FM, de la Rosa O, Dalemans W, Alvarez V, Tarazona R, et al. Immunomodulatory potential of human adipose mesenchymal stem cells derived exosomes on in vitro stimulated T cells. Front Immunol. (2014) 5:556. doi: 10.3389/fimmu.2014.00556
94. Qiu J, Shu C, Li X, Ye C, Zhang WC. Exosomes from linc00511-overexpressing ADSCs accelerates angiogenesis in diabetic foot ulcers healing by suppressing PAQR3-induced Twist1 degradation. Diabetes Res Clin Practice. (2021) 180:109032. doi: 10.1016/j.diabres.2021.109032
95. Zhang Y, Bai X, Shen K, Luo L, Zhao M, Xu C, et al. Exosomes derived from adipose mesenchymal stem cells promote diabetic chronic wound healing through SIRT3/SOD2. Cells. (2022) 11. doi: 10.3390/cells11162568
96. Sun Y, Shi H, Yin S, Ji C, Zhang X, Zhang B, et al. Human mesenchymal stem cell derived exosomes alleviate type 2 diabetes mellitus by reversing peripheral insulin resistance and relieving β-cell destruction. ACS Nano. (2018) 12:7613–28. doi: 10.1021/acsnano.7b07643
97. De Taeye BM, Novitskaya T, McGuinness OP, Gleaves L, Medda M, Covington JW, et al. Macrophage TNF-alpha contributes to insulin resistance and hepatic steatosis in diet-induced obesity. Am J Physiol Endocrinol Metab. (2007) 293:E713–25. doi: 10.1152/ajpendo.00194.2007
98. Ying W, Riopel M, Bandyopadhyay G, Dong Y, Birmingham A, Seo JB, et al. Adipose tissue macrophage-derived exosomal miRNAs can modulate in Vivo and in Vitro insulin sensitivity. Cell. (2017) 171:372–84.e12. doi: 10.1016/j.cell.2017.08.035
99. Xiang E, Han B, Zhang Q, Rao W, Wang Z, Chang C, et al. Human umbilical cord-derived mesenchymal stem cells prevent the progression of early diabetic nephropathy through inhibiting inflammation and fibrosis. Stem Cell Res Ther. (2020) 11:336. doi: 10.1186/s13287-020-01852-y
100. Teng L, Maqsood M, Zhu M, Zhou Y, Kang M, Zhou J, et al. Exosomes derived from human umbilical cord mesenchymal stem cells accelerate diabetic wound healing via promoting M2 macrophage polarization, angiogenesis, and collagen deposition. Int J Mol Sci. (2022) 23. doi: 10.3390/ijms231810421
101. Yan C, Xv Y, Lin Z, Endo Y, Xue H, Hu Y, et al. Human umbilical cord mesenchymal stem cell-derived exosomes accelerate diabetic wound healing via ameliorating oxidative stress and promoting angiogenesis. Front Bioeng Biotechnol. (2022) 10:829868. doi: 10.3389/fbioe.2022.829868
102. Yap SK, Tan KL, Abd Rahaman NY, Saulol Hamid NF, Ooi J, Tor YS, et al. Human umbilical cord mesenchymal stem cell-derived small extracellular vesicles ameliorated insulin resistance in type 2 diabetes mellitus rats. Pharmaceutics. (2022) 14. doi: 10.3390/pharmaceutics14030649
103. Mohamed-Ahmed S, Fristad I, Lie SA, Suliman S, Mustafa K, Vindenes H, et al. Adipose-derived and bone marrow mesenchymal stem cells: a donor-matched comparison. Stem Cell Res Ther. (2018) 9:168. doi: 10.1186/s13287-018-0914-1
104. Zhou C, Zhang B, Yang Y, Jiang Q, Li T, Gong J, et al. Stem cell-derived exosomes: emerging therapeutic opportunities for wound healing. Stem Cell Res Ther. (2023) 14:107. doi: 10.1186/s13287-023-03345-0
105. Wang L, Cai Y, Zhang Q, Zhang Y. Pharmaceutical activation of nrf2 accelerates diabetic wound healing by exosomes from bone marrow mesenchymal stem cells. Int J Stem Cells. (2022) 15:164–72. doi: 10.15283/ijsc21067
106. Liu W, Yu M, Xie D, Wang L, Ye C, Zhu Q, et al. Melatonin-stimulated MSC-derived exosomes improve diabetic wound healing through regulating macrophage M1 and M2 polarization by targeting the PTEN/AKT pathway. Stem Cell Res Ther. (2020) 11:259. doi: 10.1186/s13287-020-01756-x
107. Ergul A, Hafez S, Fouda A, Fagan SC. Impact of comorbidities on acute injury and recovery in preclinical stroke research: focus on hypertension and diabetes. Trans Stroke Res. (2016) 7:248–60. doi: 10.1007/s12975-016-0464-8
108. Callahan A, Amarenco P, Goldstein LB, Sillesen H, Messig M, Samsa GP, et al. Risk of stroke and cardiovascular events after ischemic stroke or transient ischemic attack in patients with type 2 diabetes or metabolic syndrome: secondary analysis of the Stroke Prevention by Aggressive Reduction in Cholesterol Levels (SPARCL) trial. Arch Neurol. (2011) 68:1245–51. doi: 10.1001/archneurol.2011.146
109. Chen J, Cui X, Zacharek A, Cui Y, Roberts C, Chopp M. White matter damage and the effect of matrix metalloproteinases in type 2 diabetic mice after stroke. Stroke. (2011) 42:445–52. doi: 10.1161/STROKEAHA.110.596486
110. Otero-Ortega L, Laso-García F, Gómez-de Frutos M, Fuentes B, Diekhorst L, Díez-Tejedor E, et al. Role of exosomes as a treatment and potential biomarker for stroke. Trans Stroke Res. (2019) 10:241–9. doi: 10.1007/s12975-018-0654-7
111. Xin H, Li Y, Chopp M. Exosomes/miRNAs as mediating cell-based therapy of stroke. Front Cell Neurosci. (2014) 8:377. doi: 10.3389/fncel.2014.00377
112. Codispoti B, Marrelli M, Paduano F, Tatullo M. NANOmetric BIO-banked MSC-derived exosome (NANOBIOME) as a novel approach to regenerative medicine. J Clin Med. (2018) 7. doi: 10.3390/jcm7100357
113. Moon GJ, Sung JH, Kim DH, Kim EH, Cho YH, Son JP, et al. Application of mesenchymal stem cell-derived extracellular vesicles for stroke: biodistribution and microRNA study. Trans Stroke Res. (2019) 10:509–21. doi: 10.1007/s12975-018-0668-1
114. Ma S, Wang J, Wang Y, Dai X, Xu F, Gao X, et al. Diabetes mellitus impairs white matter repair and long-term functional deficits after cerebral ischemia. Stroke. (2018) 49:2453–63. doi: 10.1161/STROKEAHA.118.021452
115. Bose S, Cho J. Role of chemokine CCL2 and its receptor CCR2 in neurodegenerative diseases. Arch Pharmacal Res. (2013) 36:1039–50. doi: 10.1007/s12272-013-0161-z
116. Hughes PM, Allegrini PR, Rudin M, Perry VH, Mir AK, Wiessner C. Monocyte chemoattractant protein-1 deficiency is protective in a murine stroke model. J Cereb Blood Flow Metab. (2002) 22:308–17. doi: 10.1097/00004647-200203000-00008
117. Wen D, Peng Y, Liu D, Weizmann Y, Mahato RI. Mesenchymal stem cell and derived exosome as small RNA carrier and Immunomodulator to improve islet transplantation. J Controlled Release. (2016) 238:166–75. doi: 10.1016/j.jconrel.2016.07.044
118. Ebrahim N, El-Halim HEA, Helal OK, El-Azab NE, Badr OAM, Hassouna A, et al. Effect of bone marrow mesenchymal stem cells-derived exosomes on diabetes-induced retinal injury: Implication of Wnt/b-catenin signaling pathway. Biomed Pharmacother = Biomed Pharmacother. (2022) 154:113554. doi: 10.1016/j.biopha.2022.113554
119. Methods In Medicine CAM. Retracted: Mesenchymal Stem Cell Exosomal miR-146a Mediates the Regulation of the TLR4/MyD88/NF-κB Signaling Pathway in Inflammation due to Diabetic Retinopathy. Comput Math Methods Med. (2023) 2023:9826235. doi: 10.1155/2023/9826235
120. Raposo G, Stoorvogel W. Extracellular vesicles: exosomes, microvesicles, and friends. J Cell Biol. (2013) 200:373–83. doi: 10.1083/jcb.201211138
121. Lv Q, Deng J, Chen Y, Wang Y, Liu B, Liu J. Engineered human adipose stem-cell-derived exosomes loaded with miR-21-5p to promote diabetic cutaneous wound healing. Mol Pharmaceutics. (2020) 17:1723–33. doi: 10.1021/acs.molpharmaceut.0c00177
122. Liang ZH, Pan NF, Lin SS, Qiu ZY, Liang P, Wang J, et al. Exosomes from mmu_circ_0001052-modified adipose-derived stem cells promote angiogenesis of DFU via miR-106a-5p and FGF4/p38MAPK pathway. Stem Cell Res Ther. (2022) 13:336. doi: 10.1186/s13287-022-03015-7
123. Li X, Xie X, Lian W, Shi R, Han S, Zhang H, et al. Exosomes from adipose-derived stem cells overexpressing Nrf2 accelerate cutaneous wound healing by promoting vascularization in a diabetic foot ulcer rat model. Exp Mol Med. (2018) 50:1–14. doi: 10.1038/s12276-018-0058-5
124. Yu M, Liu W, Li J, Lu J, Lu H, Jia W, et al. Exosomes derived from atorvastatin-pretreated MSC accelerate diabetic wound repair by enhancing angiogenesis via AKT/eNOS pathway. Stem Cell Res Ther. (2020) 11:350. doi: 10.1186/s13287-020-01824-2
125. Born LJ, Chang KH, Shoureshi P, Lay F, Bengali S, Hsu ATW, et al. HOTAIR-loaded mesenchymal stem/stromal cell extracellular vesicles enhance angiogenesis and wound healing. Adv Healthcare Mater. (2022) 11:e2002070. doi: 10.1002/adhm.202002070
126. Huang J, Yu M, Yin W, Liang B, Li A, Li J, et al. Development of a novel RNAi therapy: Engineered miR-31 exosomes promoted the healing of diabetic wounds. Bioactive Mater. (2021) 6:2841–53. doi: 10.1016/j.bioactmat.2021.02.007
127. Xiong Y, Chen L, Liu P, Yu T, Lin C, Yan C, et al. All-in-one: multifunctional hydrogel accelerates oxidative diabetic wound healing through timed-release of exosome and fibroblast growth factor. Small (Weinheim an der Bergstrasse Germany). (2022) 18:e2104229. doi: 10.1002/smll.202104229
128. Du J, Wan Z, Wang C, Lu F, Wei M, Wang D, et al. Designer exosomes for targeted and efficient ferroptosis induction in cancer via chemo-photodynamic therapy. Theranostics. (2021) 11:8185–96. doi: 10.7150/thno.59121
129. Dumontel B, Susa F, Limongi T, Vighetto V, Debellis D, Canta M, et al. Nanotechnological engineering of extracellular vesicles for the development of actively targeted hybrid nanodevices. Cell Biosci. (2022) 12:61. doi: 10.1186/s13578-022-00784-9
130. Yang J, Chen Z, Pan D, Li H, Shen J. Umbilical cord-derived mesenchymal stem cell-derived exosomes combined pluronic F127 hydrogel promote chronic diabetic wound healing and complete skin regeneration. Int J Nanomed. (2020) 15:5911–26. doi: 10.2147/IJN.S249129
131. Jiang T, Liu S, Wu Z, Li Q, Ren S, Chen J, et al. ADSC-exo@MMP-PEG smart hydrogel promotes diabetic wound healing by optimizing cellular functions and relieving oxidative stress. Mater Today Bio. (2022) 16:100365. doi: 10.1016/j.mtbio.2022.100365
132. Yuan M, Liu K, Jiang T, Li S, Chen J, Wu Z, et al. GelMA/PEGDA microneedles patch loaded with HUVECs-derived exosomes and Tazarotene promote diabetic wound healing. J Nanobiotechnol. (2022) 20:147. doi: 10.1186/s12951-022-01354-4
133. Geng X, Qi Y, Liu X, Shi Y, Li H, Zhao L. A multifunctional antibacterial and self-healing hydrogel laden with bone marrow mesenchymal stem cell-derived exosomes for accelerating diabetic wound healing. Biomater Adv. (2022) 133:112613. doi: 10.1016/j.msec.2021.112613
134. Wang Y, Cao Z, Wei Q, Ma K, Hu W, Huang Q, et al. VH298-loaded extracellular vesicles released from gelatin methacryloyl hydrogel facilitate diabetic wound healing by HIF-1α-mediated enhancement of angiogenesis. Acta Biomater. (2022) 147:342–55. doi: 10.1016/j.actbio.2022.05.018
135. Zhang Y, Li M, Wang Y, Han F, Shen K, Luo L, et al. Exosome/metformin-loaded self-healing conductive hydrogel rescues microvascular dysfunction and promotes chronic diabetic wound healing by inhibiting mitochondrial fission. Bioactive Mater. (2023) 26:323–36. doi: 10.1016/j.bioactmat.2023.01.020
136. Song Y, You Y, Xu X, Lu J, Huang X, Zhang J, et al. Adipose-derived mesenchymal stem cell-derived exosomes biopotentiated extracellular matrix hydrogels accelerate diabetic wound healing and skin regeneration. Adv Sci (Weinheim Baden-Wurttemberg Germany). (2023) 10:e2304023. doi: 10.1002/advs.202304023
137. Hu N, Cai Z, Jiang X, Wang C, Tang T, Xu T, et al. Hypoxia-pretreated ADSC-derived exosome-embedded hydrogels promote angiogenesis and accelerate diabetic wound healing. Acta Biomater. (2023) 157:175–86. doi: 10.1016/j.actbio.2022.11.057
138. Xiang K, Chen J, Guo J, Li G, Kang Y, Wang C, et al. Multifunctional ADM hydrogel containing endothelial cell-exosomes for diabetic wound healing. Mater Today Bio. (2023) 23:100863. doi: 10.1016/j.mtbio.2023.100863
139. Cheng P, Xie X, Hu L, Zhou W, Mi B, Xiong Y, et al. Hypoxia endothelial cells-derived exosomes facilitate diabetic wound healing through improving endothelial cell function and promoting M2 macrophages polarization. Bioactive Mater. (2024) 33:157–73. doi: 10.1016/j.bioactmat.2023.10.020
140. Liu K, Gong B, Li T, Lei H, Li J, Tang J, et al. Bioactive self-healing umbilical cord blood exosomes hydrogel for promoting chronic diabetic wound healing. Biochem Biophys Res Commun. (2024) 690:149241. doi: 10.1016/j.bbrc.2023.149241
141. Huang L, Shi Y, Li M, Wang T, Zhao L. Plasma exosomes loaded pH-responsive carboxymethylcellulose hydrogel promotes wound repair by activating the vascular endothelial growth factor signaling pathway in type 1 diabetic mice. J Biomed Nanotechnol. (2021) 17:2021–33. doi: 10.1166/jbn.2021.3165
142. Shang S, Zhuang K, Chen J, Zhang M, Jiang S, Li W. A bioactive composite hydrogel dressing that promotes healing of both acute and chronic diabetic skin wounds. Bioactive Mater. (2024) 34:298–310. doi: 10.1016/j.bioactmat.2023.12.026
143. Lin Y, Liu X, Liu Z, Xu Y. Visible-light-driven photocatalysis-enhanced nanozyme of tiO(2) nanotubes@MoS(2) nanoflowers for efficient wound healing infected with multidrug-resistant bacteria. Small (Weinheim an der Bergstrasse Germany). (2021) 17:e2103348. doi: 10.1002/smll.202103348
144. Qiu X, Liu J, Zheng C, Su Y, Bao L, Zhu B, et al. Exosomes released from educated mesenchymal stem cells accelerate cutaneous wound healing via promoting angiogenesis. Cell Proliferation. (2020) 53:e12830. doi: 10.1111/cpr.12830
145. Zhao G, Liu F, Liu Z, Zuo K, Wang B, Zhang Y, et al. MSC-derived exosomes attenuate cell death through suppressing AIF nucleus translocation and enhance cutaneous wound healing. Stem Cell Res Ther. (2020) 11:174. doi: 10.1186/s13287-020-01616-8
146. Zhu YZ, Hu X, Zhang J, Wang ZH, Wu S, Yi YY. Extracellular vesicles derived from human adipose-derived stem cell prevent the formation of hypertrophic scar in a rabbit model. Ann Plast Surgery. (2020) 84:602–7. doi: 10.1097/SAP.0000000000002357
147. Lindblad WJ. Considerations for selecting the correct animal model for dermal wound-healing studies. J Biomater Sci Polymer Edition. (2008) 19:1087–96. doi: 10.1163/156856208784909390
148. Heinrich W, Lange PM, Stirtz T, Iancu C, Heidemann E. Isolation and characterization of the large cyanogen bromide peptides from the alpha1- and alpha2-chains of pig skin collagen. FEBS Lett. (1971) 16:63–7. doi: 10.1016/0014-5793(71)80687-7
149. Davidson JM. Animal models for wound repair. Arch Dermatol Res. (1998) 290 Suppl:S1–11. doi: 10.1007/PL00007448
150. Hoekstra MJ, Hupkens P, Dutrieux RP, Bosch MM, Brans TA, Kreis RW. A comparative burn wound model in the New Yorkshire pig for the histopathological evaluation of local therapeutic regimens: silver sulfadiazine cream as a standard. Br J Plast Surgery. (1993) 46:585–9. doi: 10.1016/0007-1226(93)90111-N
151. Summerfield A, Meurens F, Ricklin ME. The immunology of the porcine skin and its value as a model for human skin. Mol Immunol. (2015) 66:14–21. doi: 10.1016/j.molimm.2014.10.023
Keywords: exosomes, diabetes, anti-inflammation, immune cells, clinical application
Citation: Li N, Hu L, Li J, Ye Y, Bao Z, Xu Z, Chen D, Tang J and Gu Y (2024) The Immunomodulatory effect of exosomes in diabetes: a novel and attractive therapeutic tool in diabetes therapy. Front. Immunol. 15:1357378. doi: 10.3389/fimmu.2024.1357378
Received: 18 December 2023; Accepted: 03 April 2024;
Published: 24 April 2024.
Edited by:
Zhiwen Luo, Fudan University, ChinaReviewed by:
Liang Xu, Tongji University School of Medicine, ChinaJian-Huan Chen, Jiangnan University, China
Renwen Wan, Fudan University, China
Copyright © 2024 Li, Hu, Li, Ye, Bao, Xu, Chen, Tang and Gu. This is an open-access article distributed under the terms of the Creative Commons Attribution License (CC BY). The use, distribution or reproduction in other forums is permitted, provided the original author(s) and the copyright owner(s) are credited and that the original publication in this journal is cited, in accordance with accepted academic practice. No use, distribution or reproduction is permitted which does not comply with these terms.
*Correspondence: Ying Gu, MTM4NjE4NzA0NjBAMTYzLmNvbQ==; Jiaqi Tang, dGFuZ2ppYXFpNzVAMTYzLmNvbQ==; Na Li, NTJzbXJlaGFiQDE2My5jb20=
†These authors have contributed equally to this work and share first authorship