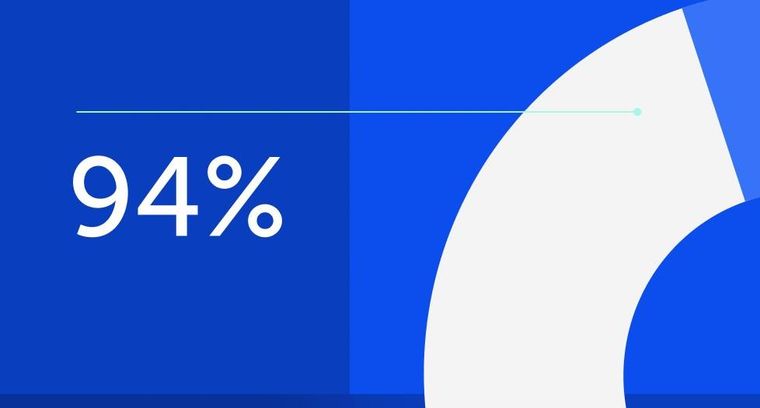
94% of researchers rate our articles as excellent or good
Learn more about the work of our research integrity team to safeguard the quality of each article we publish.
Find out more
CASE REPORT article
Front. Immunol., 25 March 2024
Sec. Viral Immunology
Volume 15 - 2024 | https://doi.org/10.3389/fimmu.2024.1357307
This article is part of the Research TopicImmunogenetics in times of COVID-19 pandemicView all 20 articles
The 2019 novel coronavirus, SARS-CoV-2, was highly prevalent in China as of December 2022, causing a range of symptoms, predominantly affecting the respiratory tract. While SARS-CoV-2 infection in children is generally mild, severe cases, especially in infants, are rare. We present a case of a previously healthy 7-month-old infant who developed cerebral infarction and coagulation dysfunction three days after COVID-19 onset. Clinically, the infant had weakness in the left limbs and pinpoint bleeding spots. A cranial magnetic resonance imaging showed ischemic strokes in the right basal ganglia and thalamus. Laboratory tests indicated thrombocytopenia and coagulation dysfunction. Inflammatory cytokines like interleukin-10 were elevated, with increased CD3+, CD4+, and CD8+ T lymphocytes but decreased CD3- CD16+ CD56+ natural killer cells. Treatment included mannitol, dexamethasone, oral aspirin, and vitamins B1 and B6 for reducing intracranial pressure, antiinflammation, anticoagulation, and nerve support, respectively. During the recovery phase, rehabilitation therapy focused on strength training, fine motor skills, and massage therapy. The infant gradually improved and successfully recovered. While rare, such cases can lead to severe complications. These combined efforts were instrumental in achieving significant functional recovery in the patient, demonstrating that even in severe instances of pediatric cerebral infarction due to COVID-19, positive outcomes are attainable with early and comprehensive medical response.
COVID-19, a globally recognized issue, arises from the widely susceptible SARS-CoV-2 virus, affecting both adults and children. Clinical symptoms are varied, ranging from fever, respiratory issues, and gastrointestinal symptoms to asymptomatic or mono-symptomatic cases like loss of smell or taste. Some patients may exhibit nonspecific neurological symptoms such as headache, dizziness, and myalgia. In most pediatric cases, the process is benign (1, 2).The risk factors for ischemic stroke increase against the backdrop of COVID-19 (3, 4).While ischemic stroke is not a common complication of COVID-19, its incidence is low in adults (5, 6) and especially rare in children (7). Ischemic stroke as a complication of COVID-19 can cause varying degrees of damage in children, ranging from hemiplegia and disability to severe cases leading to death (8, 9).
Recently, there have been reports of life-threatening COVID-19 in infants, involving cases in those as young as six months and two months old (8–10). Mierzewska-Schmidt et al. reported a rare and severe case where a previously healthy two-month-old male infant developed acute hemorrhagic necrotizing encephalitis as a result of COVID-19 (10). Fraser et al. reported a clinical case where a six- month-old infant experienced rapid progression to cerebral infarctions, pneumonia, and septic shock after COVID-19. Postmortem sputum cultures indicated Mycobacterium tuberculosis (8). Furthermore, Ghosh et al. described a case of a 6-month-old previously healthy term boy with both miliary tuberculosis and COVID-19 co-infection developed strokes, severe sepsis, and electrolyte abnormalities, cerebral infarctions, and declined rapidly within 6 days (9). The case reports suggest that infants with COVID-19 are prone to severe illness, potentially life-threatening, especially in previously healthy infants with concurrent tuberculosis. Additionally, two of the reported cases were male infants (9) (10), and the gender was not mentioned in another case (8), indicating that male infants might also be a high-risk factor for mortality due to COVID-19. Unfortunately, despite aggressive medical intervention, these infants ultimately succumbed to their conditions.
Clinically, we presented an exceptionally rare case of a previously healthy 7-month-old infant developing ischemic stroke and cerebral infarction with hemiplegia following COVID-19. The case was promptly evaluated and managed, with successful neurological rehabilitation, leading to a good recovery.
A 7-month-old male infant presented with fever following his parents’ COVID-19. A throat swab PCR test confirmed the infant as SARS-CoV-2 positive. On the third day of fever, the child suddenly exhibited left-sided limb weakness, indicating possible neurological involvement. Examination revealed neurological signs: left upper limb strength at grade I, left lower limb strength at grade III, and right limb strength at grade V. The child was alert with normal mental status, muscle tone, tendon reflexes, negative bilateral Babinski signs, and scattered pinpoint bleeding spots, and no other rashes were observed. A cranial magnetic resonance imaging (MRI) indicated right basal ganglia and thalamic infarction (Figures 1A, B). Neck vascular examination showed no significant abnormalities. The complete blood count (CBC) revealed a white blood cell (WBC) count of 9.69 × 109/L, lymphocyte percentage of 90.2%, platelets at 89×109/L, and hemoglobin at 116g/L, indicating thrombocytopenia. Coagulation tests showed fibrinogen at 1.26 g/L, plasma D-dimer at 0.16mg/L, with normal prothrombin time (PT) and activated partial thromboplastin time (APTT), suggesting coagulation dysfunction. Blood gas analysis was unremarkable. Cerebrospinal fluid (CSF) analysis revealed clear transparency, negative Pandy’s test, WBC count at 1.0 × 106/L, red blood cell count at 5.0×106/L. CSF culture for five days showed no bacterial or fungal growth. Biochemical analysis of CSF indicated slightly elevated glucose at 6.63 mmol/L, chloride at 127.9 mmol/L, and total protein at 345.8 mg/L, which were higher than normal. The inflammatory marker interleukin-10 (IL-10) was elevated at 6 pg/ml, while IL-2, IL-4, IL-6, tumor necrosis factor-α (TNF-α), and interferon (IFN)-γ remained within the normal range. The proportions of immune cell markers demonstrated an increase with 71.85% of CD3+, 40.70% of CD4+, and 26.55% of CD8+ T lymphocytes, while CD3- CD16+ CD56+ natural killer cells was significantly decreased. Tests for enteroviruses, herpes simplex virus, Epstein-Barr virus, HBV virus, tuberculosis, polio, mycoplasma pneumoniae, and chlamydia yielded negative outcomes. Liver and kidney function tests, electrolytes, chest X-ray, echocardiogram, electroencephalography, and magnetic resonance angiography were all normal. Blood cultures, including those for bacteria and fungi, were negative.
Figure 1 MRI Depiction of Cerebral Infarction in a 7-Month-Old Infant Post COVID-19. (A, B) MRI reveals a patchy region in the right basal ganglia and right thalamus exhibiting long T1 and T2 signal intensities. The internal signal is heterogeneous, predominantly displaying high signal intensity on DWI and FLAIR images, indicative of infarction in these areas. No other significant abnormal MR signal abnormalities are noted. (C, D) Follow-up MRI one month post-stroke. Patchy areas exhibiting long T1 and T2 signal intensities are present in the right basal ganglia and right thalamus, characterized by short T1 signals and comparatively shorter T2 signals within the lesions. No other significant abnormal MR signal anomalies are detected. Notably, regions of encephalomalacia are evident in the right basal ganglia and right thalamus, with a discernible reduction in lesion size relative to earlier assessments.
The infant was diagnosed with cerebral infarction, hemiplegia, thrombocytopenia, and febrile infection. Upon admission, he received intravenous mannitol to reduce intracranial pressure, intravenous dexamethasone for anti-inflammation and swelling, oral aspirin for anticoagulation, and supportive therapy with vitamins B1 and B6, while closely monitoring vital signs. During hospitalization, the infant remained stable without seizures. Two days post-admission, his temperature normalized, and a repeat CBC showed WBC at 7.15×109/L, lymphocytes at 86.6%, and platelets normalized to 191×109/L. Mannitol was discontinued, and dexamethasone was stopped on the third day, continuing with aspirin and supportive therapy. The infant’s limb strength gradually improved. On the ninth day, the left upper limb strength improved to grade III, left lower limb to grade IV, and right limbs remained at grade V. Muscle tone was normal, bleeding spots receded, and coagulation function returned to normal. He was discharged for continued rehabilitation.
During rehabilitation, the infant underwent neurological tests, Bayley developmental assessment, Gross Motor Function Measure (GMFM) (Table 1), and Fine Motor Function Measure (FMFM) (Table 2). The Bayley assessment showed normal cognitive development but delayed motor skills. GMFM assessment showed delays in gross motor functions, while FMFM indicated significant differences in upper limb function between sides, with the left side markedly lagging. The infant underwent half-hour daily training in sitting, kneeling, crawling for gross motor stability and postural transition, enhancing muscle strength, stability, and coordination. Additionally, he received half-hour daily sessions of upper limb strength, grasping, coordination occupational therapy, and traditional Chinese massage to promote muscular and neural circulation and prevent muscle atrophy. After one month of rehabilitation, the infant showed marked improvement in left upper and lower limb functions, nearly reaching grade V in the left upper limb and grade V in the left lower limb, with normal muscle tone. A follow-up MRI indicated reduced size of the old lesion and resolved surrounding edema (Figures 1C, D). Tables 3, 1, and 2 summarize the clinical course and assessment results before and after rehabilitation.
Table 1 Gross Motor Function Measure (GMFM-88) assessment comparison pre- and post-rehabilitation in the infant.
Table 2 Fine Motor Function Measure Assessment comparison pre- and post-rehabilitation in the infant.
In this case, we reported a 7-month-old infant developed an ischemic stroke resulting in cerebral infarction and coagulation dysfunction following COVID-19. To the best of our knowledge, this case represents one of the youngest patients with a favorable outcome reported for ischemic stroke secondary to COVID-19.
Cerebrovascular complications associated with COVID-19 have been documented in adults, often in those with underlying cardiovascular diseases. Acute ischemic strokes following COVID-19 are exceedingly rare in children (11–13). Most children infected with COVID-19 exhibit either asymptomatic cases, mild respiratory symptoms, or fever (14). Pneumonia is one of the most common complication following COVID-19 (15). However, in this particular case, the infant displayed symptoms of fever but notably did not exhibit any signs of pneumonia. The complications of COVID-19 in children are non-specific neurological symptoms and cerebrovascular events are very rare (7, 16). In infants, however, complications can be much more severe, sometimes resulting in life-threatening ischemic strokes (8–10). We reported a rare case of an ischemic stroke and cerebral infarction leading to hemiplegia in an infant following COVID-19.
The pathogenic mechanisms leading to cerebral infarction following COVID-19 are not yet fully elucidated. Studies indicate that COVID-19 increases the risk of ischemic stroke (17). In this case, the infant developed cerebral infarction just three days after exhibiting fever from the infection, indicating rapid disease progression. Post-infection, COVID-19 may trigger cytokine cascade reactions and endothelial cell dysfunction (18, 19), leading to an inflammatory response, thrombocytopenia, and coagulation dysfunction (20). Damage to endothelial cells and coagulation dysfunction may lead to the formation of microthrombi and the development of ischemic stroke (21). Moreover, it has been reported that cytokines and the body’s immune response are correlated with the severity or risk of COVID-19 (22, 23). In severe COVID-19 cases, patients may face acute respiratory distress syndrome, multiple organ dysfunction syndrome, or even death due to a cytokine storm. This hyperinflammatory condition is characterized by the uncontrolled release of pro-inflammatory cytokines, leading to significant complications. High levels of numerous crucial pro-inflammatory cytokines, such as interleukin-1 (IL-1), IL-2, IL-6, tumor necrosis factor-α, interferon (IFN)-γ, IL-10 and so on, have been found in severe COVID-19 (24, 25). Moreover, the hyperproduction of IL-10 and IL-6 have been linked to cytokine storm-induced mortality in fatal thrombocytopenia syndrome and severe and critically ill COVID-19 patients (26). In addition, elevated IL-6 levels in serum at the time of admission have been linked to predicting a more severe progression of COVID-19 (27). Furthermore, risk factors, such as male gender, age and pre-existing comorbidities could indicate the progression of COVID-19 into a severe and critical stage (23). Indeed, infants (six-month-old, male gender) with concurrent tuberculosis and COVID-19 may face a fatal outcome (8, 9). Recently, Mierzewska-Schmidt et al. reported a case of acute hemorrhagic necrotizing encephalitis in a previously healthy, 2-month-old male infant with SARS-CoV-2 infection (10), suggested that even in the absence of underlying diseases, SARS-CoV-2 infection in younger male infants can potentially lead to a fatal outcome. We reported a case of a 7-month-old male infant presenting with thrombocytopenia, coagulation dysfunction, and elevated cytokines, such as IL-10, indicating that our case were a severe instance of pediatric COVID-19. Notably, in our case, only IL-10 level was elevated, while other cytokines, such as IL-6, remained within normal range. This unique cytokine profile indicates a distinct immune response, offering valuable insights into the complex role of cytokines in COVID-19 and its neurological effects. Furthermore, the proportions of immune cells in our patient also underwent changes. CD3+, CD4+, and CD8+ T lymphocytes was significantly increased while CD3- CD16+ CD56+ natural killer cells was significantly decreased, these results may be related to the severity of the disease (28). Fortunately, our case involved no underlying diseases, including the absence of tuberculosis co-infection, and our patient was relatively older compared to the three infants previously reported to have succumbed to COVID-19 (8–10). The protective factors including relative older age and no underlying comorbidities could be a significant factor in the favorable outcome of our case. Other studies indicate that hypoxemia could be a key factor in the occurrence of cerebral infarctions associated with COVID-19. Hypoxemia results in reduced oxygen supply to tissues, causing ischemia and hypoxia, and leads to the accumulation of red blood cells, increasing blood viscosity. This heightened blood viscosity can exacerbate the reduction in tissue oxygenation and nutrition, thereby perpetuating a detrimental cycle (29). A significant correlation between elevated whole blood viscosity and increased mortality rates was determined in patients with COVID-19 (30).Alterations in blood viscosity can profoundly impact blood flow dynamics, potentially tripling the risk of thrombus formation in both arteries and veins (31). Although the infant in this case exhibited respiratory symptoms due to COVID-19, there was no involvement of the lungs or signs of hypoxemia. Therefore, there is currently no basis to attribute the occurrence of post-COVID-19 cerebral infarction to hypoxemia. Furthermore, COVID-19 itself exhibits neuro-invasive and neurotropic characteristics, implying that the virus can directly or indirectly invade the central nervous system (32, 33). Several hypothesized pathways for the neuroinvasion of SARS-CoV-2 have been proposed, encompassing mechanisms like transsynaptic transfer through infected neurons, invasion through the olfactory nerves, infection of the vascular endothelium, and the migration of leukocytes across the blood-brain barrier (BBB) (34). The primary host cell receptor for the SARS-CoV-2 is the angiotensin-converting enzyme 2 (ACE2). ACE2 is expressed in both neurons and glial cells. The binding of SARS-CoV-2 to ACE2 can lead to vasoconstriction and cellular damage (35, 36). The spike protein of SARS-CoV-2 can also cause dysfunction in cerebral vascular endothelium by activating Ras homolog family member A, a key molecule involved in regulating the dynamics of endothelial cell cytoskeleton and tight junction complexes (37). This activation can subsequently compromise the integrity of BBB (38) and the blood-cerebrospinal fluid barrier (39). This might also explain why the primary manifestation in this infant post-infection was ischemic stroke. The aforementioned pathophysiological mechanisms are consistent with the clinical presentation observed in this case post-infection: an inflammatory response, a decline in platelets, scattered pinpoint bleeding spots throughout the body, coagulation dysfunction, leading to a right-sided cerebral infarction, and consequently, limb weakness and neurological changes in the left side of the body.
Additionally, this case involves right basal ganglia and thalamic infarction. Previous studies have also reported rare instances of right cerebral infarction due to COVID-19 in adolescents aged 10 and 11 years (40, 41). Furthermore, in a reported case of infant fatality due to COVID-19, similar involvement of the basal ganglia and thalamus has been documented (10). Research indicates that central nervous system infections resulting from COVID-19 predominantly present as focal lesions in medium-sized vessels, often leading to basal ganglia infarctions (42, 43). This pattern is consistent with the infarction locations observed in our case, suggesting a predilection for the basal ganglia in COVID-19-related cerebral infarctions in pediatric patients. However, some cases have reported involvement of the right middle cerebral artery and right posterior cerebral artery territories, as well as the bilateral superior cerebellar arteries (8, 44). The detailed mechanisms underlying these observations warrant further exploration. We hypothesize that the susceptibility of COVID-19 to affect these regions might be related to the abundant blood flow in these areas. The brain’s blood flow is particularly abundant in areas with high metabolic demands and dense neuronal networks. One such region is the basal ganglia, which is involved in various complex brain functions including motor control, cognition, and emotion. The basal ganglia receive a rich blood supply primarily from the branches of the middle cerebral artery, making them susceptible to ischemic events in conditions that affect cerebral circulation (45). Kaneko et al. showed that ACE2 gene expression and protein levels in human brain were progressively increased by vessel size and flow rates, indicating that brain endothelial cells are susceptible to direct SARS-CoV-2 infection through flow-dependent expression of ACE2 (46). Interestingly, Lindskog et al. revealed that ACE2 expression in the normal brain is limited to the choroid plexus and ependymal cells, with minimal expression elsewhere (47). Chen et al. leveraged public brain transcriptome databases to demonstrate ACE2 expression in specific brain regions, notably the choroid plexus and thalamus’s paraventricular nuclei. Their analysis revealed ACE2’s presence across various cell types, including neurons and non-neuronal cells such as astrocytes, oligodendrocytes, and endothelial cells, particularly in the human middle temporal gyrus and posterior cingulate cortex (48). Hernández and colleagues discovered differential expression of ACE2 in the rat brain, noting its widespread presence in brain vasculature. They observed the highest concentration of ACE2-expressing capillaries in areas critical for sensory processing and autonomic regulation, including the olfactory bulb and various hypothalamic and brainstem nuclei. Additionally, ACE2 expression was identified in astrocytes, pericytes, and endothelial cells—integral components of the blood-brain barrier (49). These studies suggest the variable expression of ACE2 in the brain could provide a pathophysiological basis for COVID-19’s invasion of neural pathways. This may also be linked to the occurrence of COVID-19-related neurological symptoms, offering insights into how the virus impacts the nervous system. Crucially, COVID-19 markedly influences ACE2 expression within the brain, notably enhancing ACE2 levels in endothelial cells, predominantly in the white matter. This upregulation is most pronounced in patients with severe neurological symptoms, suggesting a direct correlation between ACE2 levels and the intensity of COVID-19’s neurological effects (47). Therefore, it is speculated that the ACE-2 receptor may be relatively highly expressed in the right basal ganglia and thalamic regions of infants, compared to other areas of the brain. This may help elucidate why reports of infant cerebral infarction cases due to COVID-19, including our own, commonly involve the basal ganglia and thalamus. Certainly, further research is necessary to validate this assertion. Interestingly, Heine et al. have recently found that specific structural imaging alterations in the thalamus and basal ganglia are associated with the prolonged fatigue experienced by patients suffering from post-COVID syndrome (50). This serves as further significant evidence of COVID-19 affecting the basal ganglia and hypothalamus.
Specific predictors for early detection of cerebral infarction as a complication of COVID-19 may enhance the prognosis of affected children. It has been reported that a significant increase in Factor VIII is associated with the hypercoagulable state related to COVID-19 (51). Additionally, significantly elevated levels of D-dimer and decreased fibrinogen can serve as early warning indicators (52). In our case, despite normal D-dimer levels, cerebral infarction still occurred, suggesting that normal D-dimer levels alone cannot completely rule out the risk of stroke. This might require a combined assessment with fibrinogen levels, where normal D-dimer levels could be misleading. Routine tests including CBC, platelet count, PT, APTT, and fibrinogen are helpful in identifying potential risk in patients (53). Therefore, for infants with persistent fever exceeding three days and any abnormalities in platelet counts, heightened vigilance for hypercoagulability and the potential for cerebral infarction is warranted. Timely coagulation function tests, and when necessary, early utilization of cerebral and neurovascular imaging to assess the possibility of stroke, can have a positive impact on the prognosis of the disease.
In treatment, anticoagulants, anti-inflammatory therapies, antiviral drugs, angiotensin-converting enzyme inhibitors, and angiotensin receptor blockers are recommended. Early use of aspirin is associated with lower mortality rates (54). In this case, our treatment encompassed acute phase management with measures to reduce intracranial pressure, anti-inflammatory and anticoagulation therapies, alongside supportive treatments (55). During recovery, we employed rehabilitation training (56, 57) combined with traditional Chinese massage therapy (58, 59). Furthermore, it should be highlighted that, as per the latest consensus statement from Chinese experts on diagnosing, treating, and preventing COVID-19 in children, there are currently no specific antiviral drugs authorized for pediatric patients with SARS-CoV-2 infection in China (60). Consequently, despite the case’s severity, antiviral medications were not utilized. One month into rehabilitation, significant improvements were observed in the infant’s left upper and lower limb functions, with left upper limb strength nearly reaching grade V and left lower limb strength at grade V, while right limb strength remained at grade V, and muscle tone was normal. Assessments using the GMFM and FMFM showed marked improvements post-rehabilitation, and follow-up cranial MRI indicated significant resolution of the lesions. This suggests that prompt treatment in the acute phase, coupled with effective rehabilitation during recovery, can markedly enhance the prognosis of infantile patients suffering from COVID-19-induced basal ganglia and thalamic infarction.
Notably, this report has several limitations. Firstly, it is based on a single case study. Secondly, there was a lack of focused attention on infants presenting with persistent fever exceeding three days post COVID-19. This oversight led to missed opportunities in monitoring coagulation function changes and delayed the identification of cerebral infarction risks. Prompt intervention at this stage might have mitigated the risk of subsequent cerebral infarction, despite fever being a prevalent symptom in COVID-19 cases.
This case emphasizes the need for vigilant monitoring and swift intervention in young COVID-19 patients, especially infants with prolonged fever, due to the risk of serious complications like cerebral infarction. Successful management involved prompt treatments for reducing intracranial pressure and inflammation, as well as ongoing rehabilitation, highlighting their key roles in recovery. The case demonstrates that even in severe instances of pediatric cerebral infarction following COVID-19, early and comprehensive medical care can lead to positive outcomes.
The original contributions presented in the study are included in the article/supplementary material. Further inquiries can be directed to the corresponding authors.
The studies involving humans were approved by Medical Ethics Committee of Wenling Maternal and Child Health Care Hospital. The studies were conducted in accordance with the local legislation and institutional requirements. Written informed consent for participation in this study was provided by the participants’ legal guardians/next of kin. The manuscript presents research on animals that do not require ethical approval for their study. Written informed consent was obtained from the individual(s) for the publication of any potentially identifiable images or data included in this article.
SZ: Data curation, Formal analysis, Methodology, Resources, Funding acquisition, Writing – original draft, Writing – review & editing. HC: Data curation, Investigation, Validation, Writing – review & editing. WX: Data curation, Investigation, Validation, Writing – review & editing. HL: Data curation, Investigation, Validation, Writing – review & editing. ZC: Data curation, Investigation, Validation, Writing – review & editing. JL: Conceptualization, Methodology, Supervision, Writing – review & editing, Investigation. ET: Conceptualization, Methodology, Supervision, Writing – review & editing, Formal analysis, Validation, Visualization, Writing – original draft.
The author(s) declare financial support was received for the research, authorship, and/or publication of this article. The work of the authors is supported by Social Development Science and Technology Project of Wenling City (grant no 2023S00147).
The authors declare that the research was conducted in the absence of any commercial or financial relationships that could be construed as a potential conflict of interest.
All claims expressed in this article are solely those of the authors and do not necessarily represent those of their affiliated organizations, or those of the publisher, the editors and the reviewers. Any product that may be evaluated in this article, or claim that may be made by its manufacturer, is not guaranteed or endorsed by the publisher.
1. Parri N, Lenge M, Buonsenso D. Children with covid-19 in pediatric emergency departments in Italy. N Engl J Med. (2020) 383:187–90. doi: 10.1056/NEJMc2007617
2. Götzinger F, Santiago-García B, Noguera-Julián A, Lanaspa M, Lancella L, Calò Carducci FI, et al. COVID-19 in children and adolescents in Europe: a multinational, multicentre cohort study. Lancet Child Adolesc Health. (2020) 4:653–61. doi: 10.1016/S2352-4642(20)30177-2
3. Modin D, Claggett B, Sindet-Pedersen C, Lassen MCH, Skaarup KG, Jensen JUS, et al. Acute COVID-19 and the incidence of ischemic stroke and acute myocardial infarction. Circulation. (2020) 142:2080–2. doi: 10.1161/CIRCULATIONAHA.120.050809
4. Nannoni S, de Groot R, Bell S, Markus HS. Stroke in COVID-19: A systematic review and meta-analysis. Int J Stroke. (2021) 16:137–49. doi: 10.1177/1747493020972922
5. Bekelis K, Missios S, Ahmad J, Labropoulos N, Schirmer CM, Calnan DR, et al. Ischemic stroke occurs less frequently in patients with COVID-19: A multicenter cross-sectional study. Stroke. (2020) 51:3570–6. doi: 10.1161/STROKEAHA.120.031217
6. Beyrouti R, Adams ME, Benjamin L, Cohen H, Farmer SF, Goh YY, et al. Characteristics of ischaemic stroke associated with COVID-19. J Neurol Neurosurg Psychiatry. (2020) 91:889–91. doi: 10.1136/jnnp-2020-323586
7. Ellul MA, Benjamin L, Singh B, Lant S, Michael BD, Easton A, et al. Neurological associations of COVID-19. Lancet Neurol. (2020) 19:767–83. doi: 10.1016/S1474-4422(20)30221-0
8. Fraser S, Ellsworth M, Perez N, Hamilton H, Fletcher S, Brown D, et al. Cerebral infarctions in an infant with COVID-19 delta variant infection and disseminated tuberculosis. Pediatr Neurology. (2022) 126:112–3. doi: 10.1016/j.pediatrneurol.2021.10.014
9. Ghosh A, Tchakarov A, Pérez N, Tatevian N, Bhattacharjee M. Rapidly evolving and fatal miliary tuberculosis and COVID-19 infection in an infant. Ann Clin Lab Science. (2022) 52:831–7.
10. Mierzewska-Schmidt M, Baranowski A, Szymanska K, Ciaston M, Kuchar E, Ploski R, et al. The case of fatal acute hemorrhagic necrotizing encephalitis in a two-month-old boy with Covid-19. Int J Infect Diseases: IJID: Off Publ Int Soc For Infect Diseases. (2022) 116:151–3. doi: 10.1016/j.ijid.2021.12.334
11. Niazkar HR, Zibaee B, Nasimi A, Bahri N. The neurological manifestations of COVID-19: a review article. Neurological Sciences: Off J Ital Neurological Soc Ital Soc Clin Neurophysiology. (2020) 41:1667–71. doi: 10.1007/s10072-020-04486-3
12. Yaghi S, Ishida K, Torres J, Mac Grory B, Raz E, Humbert K, et al. SARS-CoV-2 and stroke in a new york healthcare system. Stroke. (2020) 51:2002–11. doi: 10.1161/STROKEAHA.120.030335
13. Kaur S, Bansal R, Kollimuttathuillam S, Gowda AM, Singh B, Mehta D, et al. The looming storm: Blood and cytokines in COVID-19. Blood Rev. (2021) 46:100743. doi: 10.1016/j.blre.2020.100743
14. Zhu F, Ang JY. COVID-19 infection in children: diagnosis and management. Curr Infect Dis Rep. (2022) 24:51–62. doi: 10.1007/s11908-022-00779-0
15. Abbasher Hussien Mohamed Ahmed K, Hasabo EA, Haroun MS, Mah Fadelallah Eljack M, Salih EH, Altayeb YFO, et al. Clinical characteristics, complications, and predictors of outcome of hospitalized adult Sudanese patients with COVID-19 and malaria coinfection in Sudan: A multicenter retrospective cross-sectional study. J Med Virol. (2022) 94:3685–97. doi: 10.1002/jmv.27771
16. Mao L, Jin H, Wang M, Hu Y, Chen S, He Q, et al. Neurologic manifestations of hospitalized patients with coronavirus disease 2019 in wuhan, China. JAMA Neurol. (2020) 77:683–90. doi: 10.1001/jamaneurol.2020.1127
17. Luo W, Liu X, Bao K, Huang C. Ischemic stroke associated with COVID-19: a systematic review and meta-analysis. J Neurology. (2022) 269:1731–40. doi: 10.1007/s00415-021-10837-7
18. Lodigiani C, Iapichino G, Carenzo L, Cecconi M, Ferrazzi P, Sebastian T, et al. Venous and arterial thromboembolic complications in COVID-19 patients admitted to an academic hospital in Milan, Italy. Thromb Res. (2020) 191, 9–14. doi: 10.1016/j.thromres.2020.04.024
19. Rothstein A, Oldridge O, Schwennesen H, Do D, Cucchiara BL. Acute cerebrovascular events in hospitalized COVID-19 patients. Stroke. (2020) 51:e219–22. doi: 10.1161/STROKEAHA.120.030995
20. Requena M, Olivé-Gadea M, MuChada M, García-Tornel Á., Deck M, Juega J, et al. COVID-19 and stroke: incidence and etiological description in a high-volume center. J Stroke Cerebrovasc Dis. (2020) 29:105225. doi: 10.1016/j.jstrokecerebrovasdis.2020.105225
21. Iba T, Connors JM, Levy JH. The coagulopathy, endotheliopathy, and vasculitis of COVID-19. Inflammation Research: Off J Eur Histamine Res Soc … [et al.]. (2020) 69:1181–9. doi: 10.1007/s00011-020-01401-6
22. Bobcakova A, Petriskova J, Vysehradsky R, Kocan I, Kapustova L, Barnova M, et al. Immune profile in patients with COVID-19: lymphocytes exhaustion markers in relationship to clinical outcome. Front In Cell Infection Microbiol. (2021) 11:646688. doi: 10.3389/fcimb.2021.646688
23. Zhang J-J, Dong X, Liu G-H, Gao Y-D. Risk and protective factors for COVID-19 morbidity, severity, and mortality. Clin Rev In Allergy Immunol. (2023) 64, 90–107. doi: 10.1007/s12016-022-08921-5
24. Qudus MS, Tian M, Sirajuddin S, Liu S, Afaq U, Wali M, et al. The roles of critical pro-inflammatory cytokines in the drive of cytokine storm during SARS-CoV-2 infection. J Med Virol. (2023) 95:e28751. doi: 10.1002/jmv.28751
25. Mohseni Afshar Z, Barary M, Babazadeh A, Tavakoli Pirzaman A, Hosseinzadeh R, Alijanpour A, et al. The role of cytokines and their antagonists in the treatment of COVID-19 patients. Rev Med Virol. (2023) 33:e2372. doi: 10.1002/rmv.2372
26. Kang SY, Yoo JR, Park Y, Kim S-H, Heo ST, Park SH, et al. Fatal outcome of severe fever with thrombocytopenia syndrome (SFTS) and severe and critical COVID-19 is associated with the hyperproduction of IL-10 and IL-6 and the low production of TGF-β. J Med Virol. (2023) 95:e28894. doi: 10.1002/jmv.28894
27. Zobel CM, Wenzel W, Krüger JP, Baumgarten U, Wagelöhner T, Neumann N, et al. Serum interleukin-6, procalcitonin, and C-reactive protein at hospital admission can identify patients at low risk for severe COVID-19 progression. Front Microbiol. (2023) 14:1256210. doi: 10.3389/fmicb.2023.1256210
28. Li M, Guo W, Dong Y, Wang X, Dai D, Liu X, et al. Elevated exhaustion levels of NK and CD8+ T cells as indicators for progression and prognosis of COVID-19 disease. Front In Immunol. (2020) 11:580237. doi: 10.3389/fimmu.2020.580237
29. Farber PL. Can erythrocytes behavior in microcirculation help the understanding the physiopathology and improve prevention and treatment for covid-19? Clin Hemorheology Microcirculation. (2021) 78:41–7. doi: 10.3233/CH-201082
30. Choi D, Waksman O, Shaik A, Mar P, Chen Q, Cho DJ, et al. Association of blood viscosity with mortality among patients hospitalized with COVID-19. J Am Coll Cardiol. (2022) 80:316–28. doi: 10.1016/j.jacc.2022.04.060
31. Sloop GD, Pop G, Weidman JJ, St Cyr JA. COVID-19 demonstrates that inflammation is a hyperviscous state. Cureus. (2022) 14:e30603. doi: 10.7759/cureus.30603
32. Lin E, Lantos JE, Strauss SB, Phillips CD, Campion TR, Navi BB, et al. Brain Imaging of Patients with COVID-19: Findings at an Academic Institution during the Height of the Outbreak in New York City. AJNR Am J Neuroradiol. (2020) 41:2001–8. doi: 10.3174/ajnr.A6793
33. Katz JM, Libman RB, Wang JJ, Filippi CG, Sanelli P, Zlochower A, et al. COVID-19 severity and stroke: correlation of imaging and laboratory markers. AJNR Am J Neuroradiol. (2021) 42:257–61. doi: 10.3174/ajnr.A6920
34. Zubair AS, McAlpine LS, Gardin T, Farhadian S, Kuruvilla DE, Spudich S. Neuropathogenesis and neurologic manifestations of the coronaviruses in the age of coronavirus disease 2019: A review. JAMA Neurology. (2020) 77:1018–27. doi: 10.1001/jamaneurol.2020.2065
35. Zakeri A, Jadhav AP, Sullenger BA, Nimjee SM. Ischemic stroke in COVID-19-positive patients: an overview of SARS-CoV-2 and thrombotic mechanisms for the neurointerventionalist. J neurointerv Surgery. (2021) 13:202–6. doi: 10.1136/neurintsurg-2020-016794
36. Omidian N, Mohammadi P, Sadeghalvad M, Mohammadi-Motlagh H-R. Cerebral microvascular complications associated with SARS-CoV-2 infection: How did it occur and how should it be treated? BioMed Pharmacother. (2022) 154:113534. doi: 10.1016/j.biopha.2022.113534
37. Buzhdygan TP, DeOre BJ, Baldwin-Leclair A, Bullock TA, McGary HM, Khan JA, et al. The SARS-CoV-2 spike protein alters barrier function in 2D static and 3D microfluidic in-vitro models of the human blood-brain barrier. Neurobiol Dis. (2020) 146:105131. doi: 10.1016/j.nbd.2020.105131
38. DeOre BJ, Tran KA, Andrews AM, Ramirez SH, Galie PA. SARS-CoV-2 spike protein disrupts blood-brain barrier integrity via rhoA activation. J Neuroimmune Pharmacol. (2021) 16:722–8. doi: 10.1007/s11481-021-10029-0
39. Stüdle C, Nishihara H, Wischnewski S, Kulsvehagen L, Perriot S, Ishikawa H, et al. SARS-CoV-2 infects epithelial cells of the blood-cerebrospinal fluid barrier rather than endothelial cells or pericytes of the blood-brain barrier. Fluids Barriers CNS. (2023) 20:76. doi: 10.1186/s12987-023-00479-4
40. Khosravi B, Moradveisi B, Abedini M, Behzadi S, Karimi A. Stroke in a child with SARS-CoV-2 infection: A case report. ENeurologicalSci. (2021) 23:100345. doi: 10.1016/j.ensci.2021.100345
41. Scala MR, Spennato P, Cicala D, Piccolo V, Varone A, Cinalli G. Malignant cerebral infarction associated with COVID-19 in a child. Child’s Nervous System: ChNS: Off J Int Soc For Pediatr Neurosurgery. (2022) 38:441–5. doi: 10.1007/s00381-021-05273-x
42. Paterson RW, Brown RL, Benjamin L, Nortley R, Wiethoff S, Bharucha T, et al. The emerging spectrum of COVID-19 neurology: clinical, radiological and laboratory findings. Brain: J Neurology. (2020) 143:3104–20. doi: 10.1093/brain/awaa240
43. Rajapakse N, Dixit D. Human and novel coronavirus infections in children: a review. Paediatr Int Child Health. (2021) 41:36–55. doi: 10.1080/20469047.2020.1781356
44. Morgan RD, Collins RA, Nagy L. Post-COVID-19 multisystem inflammatory syndrome-related cerebral infarction in a pediatric patient managed with decompressive craniectomy. Pediatr Neurosurgery. (2023) 58:53–7. doi: 10.1159/000529682
45. Zuo L, Dong Y, Hu Y, Xiang X, Liu T, Zhou J, et al. Brain-structure changes, and cognitive impairment in basal ganglia infarcts: A pilot study. Neuropsychiatr Dis Treat. (2023) 19:1171–80. doi: 10.2147/NDT.S384726
46. Kaneko N, Satta S, Komuro Y, Muthukrishnan SD, Kakarla V, Guo L, et al. Flow-mediated susceptibility and molecular response of cerebral endothelia to SARS-CoV-2 infection. Stroke. (2021) 52:260–70. doi: 10.1161/STROKEAHA.120.032764
47. Lindskog C, Méar L, Virhammar J, Fällmar D, Kumlien E, Hesselager G, et al. Protein expression profile of ACE2 in the normal and COVID-19-affected human brain. J Proteome Res. (2022) 21:2137–45. doi: 10.1021/acs.jproteome.2c00184
48. Chen R, Wang K, Yu J, Howard D, French L, Chen Z, et al. The spatial and cell-type distribution of SARS-CoV-2 receptor ACE2 in the human and mouse brains. Front Neurol. (2020) 11:573095. doi: 10.3389/fneur.2020.573095
49. Hernández VS, Zetter MA, Guerra EC, Hernández-Araiza I, Karuzin N, Hernández-Pérez OR, et al. ACE2 expression in rat brain: Implications for COVID-19 associated neurological manifestations. Exp Neurol. (2021) 345:113837. doi: 10.1016/j.expneurol.2021.113837
50. Heine J, Schwichtenberg K, Hartung TJ, Rekers S, Chien C, Boesl F, et al. Structural brain changes in patients with post-COVID fatigue: a prospective observational study. EClinicalMedicine. (2023) 58:101874. doi: 10.1016/j.eclinm.2023.101874
51. Panigada M, Bottino N, Tagliabue P, Grasselli G, Novembrino C, Chantarangkul V, et al. Hypercoagulability of COVID-19 patients in intensive care unit: A report of thromboelastography findings and other parameters of hemostasis. J Thromb Haemost. (2020) 18:1738–42. doi: 10.1111/jth.14850
52. Chavda V, Chaurasia B, Fiorindi A, Umana GE, Lu B, Montemurro N. Ischemic stroke and SARS-CoV-2 infection: the bidirectional pathology and risk morbidities. Neurol Int. (2022) 14:391–405. doi: 10.3390/neurolint14020032
53. Fatehi P, Hesam-Shariati N, Abouzaripour M, Fathi F, Hesam Shariati MB. Acute ischemic and hemorrhagic stroke and COVID-19: case series. SN Compr Clin Med. (2020) 2:2396–401. doi: 10.1007/s42399-020-00559-8
54. Chow JH, Rahnavard A, Gomberg-Maitland M, Chatterjee R, Patodi P, Yamane DP, et al. Association of early aspirin use with in-hospital mortality in patients with moderate COVID-19. JAMA Netw Open. (2022) 5:e223890. doi: 10.1001/jamanetworkopen.2022.3890
55. Mosconi MG, Paciaroni M, Ageno W. Investigational drugs for ischemic stroke: what’s in the clinical development pipeline for acute phase and prevention? Expert Opin Investig Drugs. (2022) 31:645–67. doi: 10.1080/13543784.2022.2072725
56. Novak I, Morgan C, Adde L, Blackman J, Boyd RN, Brunstrom-Hernandez J, et al. Early, accurate diagnosis and early intervention in cerebral palsy: advances in diagnosis and treatment. JAMA Pediatr. (2017) 171:897–907. doi: 10.1001/jamapediatrics.2017.1689
57. Morgan C, Fetters L, Adde L, Badawi N, Bancale A, Boyd RN, et al. Early intervention for children aged 0 to 2 years with or at high risk of cerebral palsy: international clinical practice guideline based on systematic reviews. JAMA Pediatr. (2021) 175:846–58. doi: 10.1001/jamapediatrics.2021.0878
58. Zhang C, Xiong G, Wang J, Shi X, Guo T, Jin Y, et al. A multicenter, randomized controlled trial of massage in children with pediatric cerebral palsy: Efficacy of pediatric massage for children with spastic cerebral palsy. Med (Baltimore). (2021) 100:e23469. doi: 10.1097/MD.0000000000023469
59. Wang X, Teh SH, Du X-G, Wang X-H. Acupuncture and tuina treatment for gross motor function in children with spastic cerebral palsy: A monocentric clinical study. Neuropsychiatr Dis Treat. (2023) 19:1875–86. doi: 10.2147/NDT.S424102
60. Jiang R-M, Xie Z-D, Jiang Y, Lu X-X, Jin R-M, Zheng Y-J, et al. Diagnosis, treatment and prevention of severe acute respiratory syndrome coronavirus 2 infection in children: experts’ consensus statement updated for the Omicron variant. World J Pediatr. (2023) 1–15. doi: 10.1007/s12519-023-00745-3
Keywords: COVID-19, cerebral infarction, infant, hemiplegia, rehabilitation
Citation: Zheng S, Chen H, Xu W, Li H, Chen Z, Li J and Tao E (2024) Case report: unprecedented case of infantile cerebral infarction following COVID-19 and favorable outcome. Front. Immunol. 15:1357307. doi: 10.3389/fimmu.2024.1357307
Received: 17 December 2023; Accepted: 12 March 2024;
Published: 25 March 2024.
Edited by:
Gabriela Angélica Martínez-Nava, National Institute of Rehabilitation Luis Guillermo Ibarra Ibarra, MexicoReviewed by:
Debashis Dutta, University of Nebraska Medical Center, United StatesCopyright © 2024 Zheng, Chen, Xu, Li, Chen, Li and Tao. This is an open-access article distributed under the terms of the Creative Commons Attribution License (CC BY). The use, distribution or reproduction in other forums is permitted, provided the original author(s) and the copyright owner(s) are credited and that the original publication in this journal is cited, in accordance with accepted academic practice. No use, distribution or reproduction is permitted which does not comply with these terms.
*Correspondence: Enfu Tao, dGFvZW5mdUB6anUuZWR1LmNu; Jianhua Li, empkeHN5Zmtma0AxMjYuY29t
Disclaimer: All claims expressed in this article are solely those of the authors and do not necessarily represent those of their affiliated organizations, or those of the publisher, the editors and the reviewers. Any product that may be evaluated in this article or claim that may be made by its manufacturer is not guaranteed or endorsed by the publisher.
Research integrity at Frontiers
Learn more about the work of our research integrity team to safeguard the quality of each article we publish.