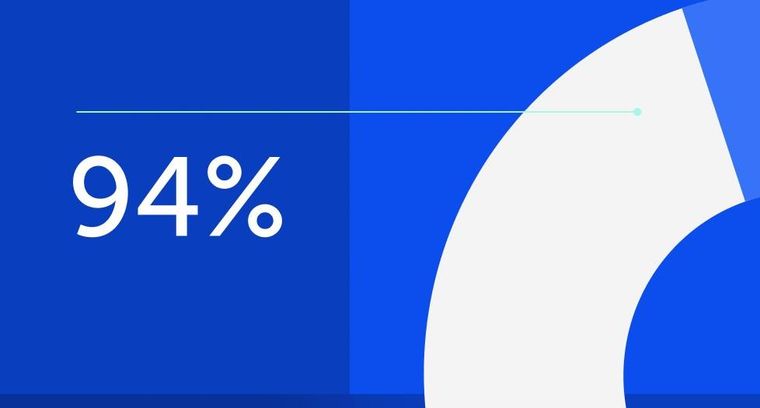
94% of researchers rate our articles as excellent or good
Learn more about the work of our research integrity team to safeguard the quality of each article we publish.
Find out more
PERSPECTIVE article
Front. Immunol., 13 February 2024
Sec. Molecular Innate Immunity
Volume 15 - 2024 | https://doi.org/10.3389/fimmu.2024.1354556
This article is part of the Research TopicThe role of the immune system in eosinophilic diseasesView all 7 articles
Heterogeneity characterises inflammatory diseases and different phenotypes and endotypes have been identified. Both innate and adaptive immunity contribute to the immunopathological mechanism of these diseases and barrier damage plays a prominent role triggering type 2 inflammation through the alarmins system, such as anti-Thymic Stromal Lymphopoietin (TSLP). Treatment with anti-TSLP monoclonal antibodies showed efficacy in severe asthma and clinical trials for other eosinophilic diseases are ongoing. The aim of this perspective review is to analyse current advances and future applications of TSLP inhibition to control barrier damage.
Human epithelium is the border between the external environment and our tissues with the role to maintain homeostasis defending from pathogens, pollutants, toxins, allergens and other external agents. This defence is provided not only by the physical barrier given by Epithelial Cells (ECs) tightness, but also through antimicrobial peptides production, mucociliary clearance, mucus production, permeability regulation (1). When damaged, pathogens, allergens and other irritant agents can penetrate the epithelium and, recognized by the Pattern Recognition Receptors, can induce innate and adaptive immune responses (1). The “barrier hypothesis”, formulated in 2017 by Pothoven and Schleimer, formulates that the epithelial damage chronologically anticipates allergic sensitization and the development of eosinophilic inflammation in many inflammatory diseases. Actually, a defective epithelial barrier has been found in many conditions such as atopic dermatitis, asthma, allergic rhinitis, chronic rhinosinusitis, eosinophilic esophagitis, coeliac disease and inflammatory bowel diseases (2). Herein, we give a general perspective on how this barrier hypothesis enriched our understanding about the pathogenesis of Type 2 (T2) diseases and how it could be exploited for their treatment.
Asthma is a chronic inflammatory airway disease clinically characterised by reversible airflow obstruction, bronchial hyperresponsiveness and respiratory symptoms (3). Although the allergic and eosinophilic represent the most frequent phenotypes, T2-low or non-T2 mediated asthma is also reported (4, 5).
Recent evidence showed that all asthma phenotypes share a loss of cohesion between the airways’ ECs with an increased permeability probably due to inflammation and to allergens-derived enzymes from House Dust Mites (HDM) or fungi capable of cleaving intercellular junctions worsening barrier damage (6).
ECs have gained increasing clinical importance as awareness about their role in initiating the inflammatory response raised (6). Nowadays, we know that ECs are not a mere physical barrier but cells capable of generating the immunological responses to external triggers. Lung ECs expresses different pattern recognition receptors as Toll-Like Receptors (TLRs), Nucleotide-binding Oligomerization Domain (NOD)-like receptors, protease activated receptors, C-type Lectin Receptors (CLRs), purinergic receptors, Retinoic acid-Inducible Gene (RIG)-I-Like Receptors (RLRs) which allow them to produce chemokines and cytokines in response to different external triggers (6). In turn, these cytokines activate many immune cells involved in asthma, such as Dendritic Cells (DCs), T helper 2 (Th2) cells, mast cells, type 2 Innate Lymphoid Cells (ILC2s), eosinophils, basophils that concur in augmenting and maintaining inflammation through the subsequent damage of the epithelial barrier (6).
Currently the best-known ECs’ cytokines are TSLP, IL-33, and IL-25. These ECs signals are rapidly released in response to various stimuli like air pollutants, bacteria, viruses and allergens such as HDM, Aspergillus fumigatus, and the cat protein Fel d1 (6). Higher concentrations of IL-33 and TSLP, but not IL-25, were shown in asthmatic patients independently of phenotype with levels inversely correlating with lung function (7). Indeed, even if their increase is much more related to allergic and eosinophilic asthma, there is evidence suggesting they can be involved even in non-allergic non-eosinophilic phenotypes. The cells source of TSLP and IL-33 have distinct cellular profiles compared to that producing IL-25, this could explain the discordant expression of alarmins, depending on the clinical context and the airway inflammatory cells sampled (8–10). In vitro findings indicate that TSLP and TLR3 ligands promote the differentiation of naive CD4+ T cells into T helper 17 (Th17)-cell via DCs activation and IL-23 production (7). Moreover, in vivo studies found out that neutrophils are a source of TSLP in bronchial biopsy tissue and, consistently with this, TSLP expression in bronchoalveolar lavages has been reported to correlate with neutrophilic inflammation (6, 7).
Numerous evidences demonstrated that some chemicals induce TSLP production and may potentially augment T2 allergic responses. In murine allergic asthma model, repeated intranasal exposure to Cigarette Smoke Extract (CSE) induced TSLP production in bronchial epithelial cells and the administration of an anti-TSLP antibody to the same model attenuated CSE-enhanced leukocyte infiltration (11).
CSE induced TSLP production in vivo via oxidative stress and Tumour Necrosis Factor (TNF)-a receptor 1 activation. Oxidative stress also induced the production of oxidized lipids, which triggered TSLP production via stimulating TLR4 (12). CSE was shown to increase the expression of TSLP and TSLP-Receptor mRNA in human Airway Smooth Muscle Cells (ASMCs), and this response was not attributed to nicotine or Reactive Oxygen Species (ROS) (13).
Atopic Dermatitis (AD) is the most common chronic inflammatory skin disease affecting infants, children or that can develop in adolescents and adults as well. Pathophysiology of AD is multifactorial and association with comorbidities as food allergy, asthma and allergic rhinitis is common (14).
Although the underlying mechanisms are not fully understood, evidence suggests that AD is an inflammatory skin disease that releases systemic components able to progress to the lungs if left untreated (level shift) (15). AD is characterised by an interaction between impaired epidermal barrier function, skin inflammation, and skin microbiota dysbiosis. There is undoubtedly a relationship between AD genetic susceptibility, microbiota-related epigenetics, and how barrier restoration and microbiota manipulations affect AD (16).
A marked difference in skin microbiota biodiversity has been found in some studies between patients with AD and those with non-atopic dermatitis (17), as already described in asthma (18).
Scientific attention to the barrier mechanism is increasing and it is well described in several recent articles (19). A recent study described that skin-based impedance spectroscopy may offer an in vivo technique for assessing the integrity of all components of the epithelial barrier, also valuable for the assessment of AD severity, progression and therapy efficacy (20). Latest findings showed that TSLP induces the immune responses via activation of DCs and mast cells; therefore, TSLP is considered a key molecule in AD pathophysiology (21). Serum levels of TSLP in adults and children with AD are significantly higher than those in healthy people. In addition, TSLP gene polymorphisms were demonstrated closely related with an increased risk of developing AD and disease progression (22).
The epithelium of the esophagus protects the deepest mucous and submucous layers from triggers like environmental toxins and allergens. Maintenance of the normal esophageal barrier depends on the correct balance of epithelial, narrow differentiation proteins junctions, adherens junctions and desmosomes (23). Genetic predisposition and environmental factors contribute to the barrier damage balance (24). In Eosinophilic Esophagitis (EoE), allergens cause damage inducing defective esophageal barrier. Basal cell hyperplasia and dilated intercellular spaces have been described in EoE epithelial cells as hallmarks of barrier damage and an important feature of disease (25). Following exposure to food allergens and proteases, esophageal epithelium secretes TSLP and IL-33 inducing Th2 cells to release IL-4, IL-5, and IL-13 that affect: proteins such as the Desmosome Desmoglein-1 (DSG1), epithelial differentiation proteins filaggrin and involucrin, eosinophils, mast cells and basophils infiltration (25–28).
A dysregulation of the protease and antiprotease system is also present (29). A dysfunctional barrier predisposes to a sensitization of food allergenic proteases and other triggers.
A dysregulated neural control of the esophagus is present and directly related to dysphagia in these patients. Nociceptive sensitization within the esophageal mucosa, resulting from inflammation, leads to motor pain, dysfunction and possibly tissue remodelling as in other respiratory and skin diseases, remodelling is mainly inflammation-driven in EoE (30, 31).
Chronic Rhinosinusitis (CRS) is an inflammatory condition of nasal mucosa divided into CRS with and without Nasal Polyps (NP) (32). The nasal mucosa is the first site for inhaled antigens exposure. Nasal epithelium maintains the immunological barrier and is a metabolically active organ able to produce various protective proteins and secretory IgA with a crucial role in contrasting allergens, microbes and other noxious substances penetration (33). The integrity of the nasal epithelial barrier is essential for ensuring its protective function. The epithelial barrier damage is a feature of the disease and contributes to NP development (34). Epithelial tight junctions are altered in NP: a down-regulation of the expression of ZO-1, E-cadherin, and occludin has been observed (35). Moreover, the epithelium repairment is altered in patients with NP (36).
Examination of histological samples from nasal mucosa epithelium or nasal cytology allows the evaluation of the barrier damage directly or indirectly (37). In a cytological context, the Hyperchromatic Supranuclear Stria (SNS) has been reported as a specific marker for the anatomic and functional integrity of ciliated cells. The absence of the SNS is considered a useful prognostic sign of loss of mucosal barrier integrity in nasal disorders (38).
The nasal microbiome also contributes to the functional sinonasal barrier. In CRS patients, the nasal microbiome appears heterogeneous, with a decrease in bacterial diversity, resulting in dysbiosis. Dysbiosis favours the relative abundance and the chronic colonisation of S. aureus. Furthermore, an increased exposure to antibiotics and steroids has been associated with a lower total bacterial biodiversity (39). The prevalence of bacterial biofilms in the paranasal sinuses in CRS has been reported in about 42–80% of patients, with a higher prevalence in Chronic Rhinosinusitis with Nasal Polyps (CRSwNP) (40). The most frequently detected organisms in CRS biofilms are S. aureus, Haemophilus influenzae, and Pseudomonas aeruginosa (41). It has been shown that S. aureus directly damages airway epithelial cells together with their repair mechanisms regardless of enterotoxins production (42). S. Aureus can be found under the epithelial surface in nasal mucosa, particularly in patients with NP (43). Damaged epithelial cells favour IL-25, IL- 33 and TSLP releasing that activate ILC2-mediated eosinophilic inflammation. In addition, the staphylococcal toxins induce a massive activation of T-naïve lymphocytes with production of T helper 1 (Th1) and Th2 cytokines (44).
The production of anti-staphylococcus IgE can directly activate the complement factors in the nasal mucosa furtherly feeding the inflammatory process (45). In asthma, S.aureus enterotoxins have been used to phenotype patients and to predict the evolution of comorbidities such as CRSwNP (46).
Given the complexity of the inflammatory cascades responsible for T2 diseases, treatment outcomes with biological drugs targeting downstream cytokines are not always satisfying. The upstream role that alarmins play makes them a promising novel therapeutic target, as they control many inflammatory patterns and cell types (Figure 1). Herein we summarise currently available or in development anti-alarmins biologics.
Figure 1 TSLP in chronic inflammation. Epithelial barrier dysfunction and epithelium immune cells’ crosstalk is remarkable in both T2 and non-T2 inflammation; TSLP plays a pleiotropic effect on innate and adaptive immune cells leading to different inflammatory pathways activation, airways remodelling and chronic tissue damage.
Tezepelumab, a fully human IgG2λ, is the first and only approved monoclonal antibody that specifically inhibits TSLP. In the phase 3 NAVIGATOR study, tezepelumab 210 mg every 4 weeks reduced asthma exacerbations independently of biomarkers level over 52 weeks. The most significant effect on exacerbation reduction was detected in patients with allergic eosinophilic asthma (71% in patients with Peripheral Blood Eosinophils (PBE) ≥300 cells/μL and allergen-sensitised; 77% in patients with PBE ≥300/μL and Fractional Exhaled Nitric Oxide (FeNO) ≥25 ppb) and unique results were shown in patients with low T2 biomarker levels (41% reduction of exacerbations in PBE <300 patients and 45% reduction in patients with triple negative signature PBE <150/μl, FeNO<25 ppb, non-allergic). Improvement on lung function, expressed by Forced Expiratory Volume in the first second (FEV1), and asthma control were also clinically significant as well as reduction of biomarkers such as eosinophils, FeNO and total IgE and cytokines IL-4, IL-5 and IL-13. Additional analysis showed that tezepelumab markedly reduced exacerbations precipitated by exposure to specific seasonal and perennial aeroallergens during periods of heightened exposure. This evidence demonstrated that the allergenic trigger could induce epithelial-driven exacerbations that tezepelumab is able to control (47). The phase 3 trial SOURCE showed a non-significant difference between treatment and placebo at 48-weeks on Oral Corticosteroids (OCS)-sparing effect (48). Further studies to reassess tezepelumab efficacy on this outcome are currently ongoing. DESTINATION, the long-term safety and tolerability phase 3 study, with a unique placebo-controlled design, showed the positive benefit-risk profile of tezepelumab over 104 weeks with less adverse event in the treatment arm than in the placebo one (49).
Tezepelumab was approved by FDA as an add-on maintenance treatment for asthma in 2021 as the only available biologic able to suppress all T2 biomarkers and with clinical efficacy not only in T2 asthma but even in non-T2 (50).
As TSLP has been found overexpressed in serum and keratinocytes of patients with AD, activates DCs to produce IL-4, IL-5, IL-13 and TNF-α and contributes to pruritus, it is reasonable to consider as a potential therapeutic target for AD. However, despite numerical results better than placebo, tezepelumab 280 mg every 2 weeks didn’t reach statistical significance in reducing Eczema Area and Severity Index (EASI)-50, EASI-75, EASI-90, SCORing Atopic Dermatitis (SCORAD) and pruritus Numeric Rating Scale (NRS) at 12 weeks in the phase IIa trial ALLEVIAD, on patients with AD (51).
Comorbidities involving both upper and lower airway diseases typically stem from a shared underlying immunologic response initiated by epithelial barrier damage, leading to “unified airway disease” definition, particularly in the context of the T2 immunologic endotype. Regarding CRSwNP, a 52-weeks clinical trial to investigate the effectiveness of tezepelumab is currently ongoing (52). Promising data in severe asthma patients with comorbid CRSwNP are already available: tezepelumab demonstrated clinically meaningful effect on sinonasal symptoms with the greatest improvements seen for the sleep, function and nasal domains of Sino-Nasal Outcome Test (SNOT)-22 questionnaire.
Itepekimab is a human IgG4P monoclonal antibody against IL-33. In a phase 2 trial on 296 patients with moderate to severe asthma randomly assigned to itepekimab, dupilumab, itepekimab plus dupilumab or placebo group, itepekimab showed loss of asthma control after discontinuation of Inhaled Corticosteroids/Long Acting Beta2-Agonists (ICS/LABA) in 22% of patients. Besides, dupilumab and itepekimab in monotherapy, but not in combination therapy, increased FEV1 as compared with placebo with mean improvement 0.16 l and 0.14 l respectively. Even if asthma control was better in dupilumab group, itepekimab treatment showed improvement in asthma control and quality of life and reduced PBE, total IgE and FeNO with statistical significance vs placebo. Hypereosinophilia was reported in dupilumab-alone group, but not in combination and itepekimab groups, suggesting that itepekimab may block downstream IL-5 signalling. No significant improvement was seen in asthma control and FEV1 in patients with PBE <300/μl, suggesting itepekimab will not be useful as non-T2 asthma treatment (53).
IL-25 (also known as IL-17E) binds to a heterodimeric receptor complex composed of two subunits (IL-17RA and IL-17RB) for signal transduction. Brodalumab is an anti-IL-17RA human monoclonal antibody (IgG2) which prevents IL-17A, IL-25 and IL-17F signalling. In a phase IIa study, brodalumab showed no significant efficacy on Asthma Control Questionnaire (ACQ), lung function and asthma symptoms after 12 weeks of treatment, in patients with inadequately controlled moderate-to-severe asthma (50).
In chronic inflammatory airway diseases different endotypes have been described, with T2 or non T2 immune responses involved in the inflammatory process underlying disease heterogeneity (54–56).
The chronic inflammation characterised by an active eosinophilic infiltrate and T2 cytokines production is prevalent in chronic inflammatory airway diseases and contributes to the physiological changes (57–59).
The epithelial cells produce TSLP as a crucial initiator and regulator of innate and adaptive immune responses (60). As described above, TSLP expression from the airway epithelium induces the recruitment and infiltration of DCs (61), exerts direct effects on mast cells, basophils, eosinophils activation and potently induces IL-4, IL-5 and IL-13 production (56, 62). TSLP promotes eosinophil survival and induces significant production of IL-6, an eosinophil-derived neurotoxin, chemokines, and chemokine ligands. TSLP increases the expression of Intercellular Adhesion Molecules (ICAM)-1 and CD18 and suppresses the expression of surface L-selectin promoting eosinophil transmigration and accumulation in tissues (63) involving the Extracellular signal Regulated Kinase (ERK), p38 Mitogen-Activated Protein Kinase (MAPK), and Nuclear Factor κ light chain enhancer of activated B cells (NF-κB) signalling pathways (64). TSLP can also induce the formation of eosinophil extracellular traps, which play an important role in innate immune responses to infectious agents that significantly contribute to tissue damage in asthmatic airways (63).
In patients with allergic asthma, it was reported a correlation between the level of immunopositive staining for TSLP in bronchial biopsies and airway eosinophilia 24 hours after allergen exposure (65). In contrast, TSLP concentration in asthma patients induced sputum during virus-induced exacerbations was inversely related to the number of eosinophils suggesting that different mechanisms of action of TSLP could occur in acute exacerbations compared with chronic eosinophilic inflammation (66).
The reduction of barrier function strongly contributes to immune system activation (67): a defective epithelial barrier and a dysfunctional repair system of damaged mucosa increase the vulnerability of the sub-epithelial layer lamina propria to pathogens invasion, inducing a local immune response that, in nasal mucosa for instance, contributes to NP formation process (34).
Inflammatory infiltrate contributes to epithelial barrier damage worsening, establishing a vicious circle through secretion of several cationic proteins, including Major Basic Protein (MBP), which induce a marked decrease in the number of desmosomes and an exfoliation of epithelial cells increasing the epithelium permeability. The mucosal permeability allows allergens and other noxious substances to penetrate the barrier (37, 68).
In the last decades, the employment of monoclonal antibodies to treat moderate-to severe asthma, CRSwNP and AD has deeply modified the therapeutic approach.
It is well known that TSLP initiates T2 inflammatory responses and most recent evidence supports TSLP role in non-T2 immune responses as well (7, 56). Eosinophils, basophils, mast cells, Airway Smooth Muscle Cells (ASMCs), ILC2s, lymphocytes, DCs, hematopoietic progenitor cells, and monocytes/macrophages (69) are part of the broad spectrum of cell types that express TSLP receptor where TSLP exert a direct effect between immune and epithelial cells in the airways (7).
As described above, the role of the epithelial barrier dysfunction and epithelium immune cells’ crosstalk is remarkable in both T2 and non-T2 inflammation; different types of organs and tissues share the disruption of the epithelial barrier integrity as a source of continuous triggers for chronic inflammatory damage. By targeting the effector cells, through the blockage of IL-5 or IL-4 and IL-13, T2 inflammation could be well controlled. However, there is emerging evidence about the role of molecules active at the top of the inflammatory cascade that could be exploited as therapeutic targets in a wider range of pathologies thanks to a wider range of immunological effects.
Therefore, TSLP could be considered as a promising therapeutic option in allergic eosinophilic diseases since they are primarily epithelial-driven. Moreover, it could be considered in non-T2 inflammatory diseases, considering its contribution in various inflammatory endotypes.
Given the importance of epithelial barrier damage in promoting different inflammatory diseases, targeting epithelial cytokines could have not only a therapeutic implication but could also have a preventive role, avoiding the amplification of the signals that lead to the chronic inflammatory damage.
There’s a vicious circle of the epithelial barrier damage-inflammatory pathways activation-clinical symptoms development and, its modulation with new treatment options should be considered as a future direction in managing chronic inflammatory diseases.
The original contributions presented in the study are included in the article/supplementary material. Further inquiries can be directed to the corresponding author.
IB: Data curation, Methodology, Supervision, Writing – original draft, Writing – review & editing. SC: Data curation, Methodology, Supervision, Writing – original draft, Writing – review & editing. AL: Data curation, Writing – original draft. AP: Data curation, Supervision, Writing – review & editing. AG: Supervision, Writing – review & editing. CC: Data curation, Methodology, Project administration, Supervision, Writing – original draft, Writing – review & editing.
The author(s) declare that no financial support was received for the research, authorship, and/or publication of this article.
The authors declare that the research was conducted in the absence of any commercial or financial relationships that could be construed as a potential conflict of interest.
The handling editor EH declared a past co-authorship with the authors IB, SC, AG, and CC.
All claims expressed in this article are solely those of the authors and do not necessarily represent those of their affiliated organizations, or those of the publisher, the editors and the reviewers. Any product that may be evaluated in this article, or claim that may be made by its manufacturer, is not guaranteed or endorsed by the publisher.
1. Pothoven KL, Schleimer RP. The barrier hypothesis and Oncostatin M: Restoration of epithelial barrier function as a novel therapeutic strategy for the treatment of type 2 inflammatory disease. Tissue Barriers (2017) 5:e1341367. doi: 10.1080/21688370.2017.1341367
2. Akdis CA. Does the epithelial barrier hypothesis explain the increase in allergy, autoimmunity and other chronic conditions? Nat Rev Immunol (2021) 21:739–51. doi: 10.1038/s41577-021-00538-7
3. Pelaia C, Crimi C, Vatrella A, Tinello C, Terracciano R, Pelaia G. Molecular targets for biological therapies of severe asthma. Front Immunol (2020) 11. doi: 10.3389/fimmu.2020.603312
4. Habib N, Pasha MA, Tang DD. Current understanding of asthma pathogenesis and biomarkers. Cells (2022) 11:2764. doi: 10.3390/cells11172764
5. Schleich F, Bougard N, Moermans C, Sabbe M, Louis R. Cytokine-targeted therapies for asthma and COPD. Eur Respir Rev (2023) 32:220193. doi: 10.1183/16000617.0193-2022
6. Hammad H, Lambrecht BN. The basic immunology of asthma. Cell (2021) 184:1469–85. doi: 10.1016/j.cell.2021.02.016
7. Gauvreau GM, Sehmi R, Ambrose CS, Griffiths JM. Thymic stromal lymphopoietin: its role and potential as a therapeutic target in asthma. Expert Opin Ther Targets (2020) 24(8):777–92. doi: 10.1080/14728222.2020.1783242
8. Bleck B, Kazeros A, Bakal K, Garcia-Medina L, Adams A, Liu M, et al. Coexpression of type 2 immune targets in sputum-derived epithelial and dendritic cells from asthmatic subjects. J Allergy Clin Immunol (2015) 136:619–27. doi: 10.1016/j.jaci.2014.12.1950
9. Glück J, Rymarczyk B, Kasprzak M, Rogala. B. Increased levels of interleukin-33 and thymic stromal lymphopoietin in exhaled breath condensate in chronic bronchial asthma. Int Arch Allergy Immunol (2016) 169:51–6. doi: 10.1159/000444017
10. Cheng D, Xue Z, Yi L, Shi H, Zhang K, Huo X, et al. Epithelial interleukin-25 is a key mediator in Th2-high, corticosteroid-responsive asthma. Am J Respir Crit Care Med (2014) 190:639–48. doi: 10.1164/rccm.201403-0505OC
11. Nakamura Y, Miyata M, Ohba T, Ando T, Hatsushika K, Suenaga F, et al. Cigarette smoke extract induces thymic stromal lymphopoietin expression, leading to T(H)2-type immune responses and airway inflammation. J Allergy Clin Immunol (2008) 122:1208–14. doi: 10.1016/j.jaci.2008.09.022
12. Tang H, Cao W, Kasturi SP, Ravindran R, Nakaya HI, Kundu K, et al. The Th2 response to cysteine proteases requires dendritic cell-basophil cooperation via ROS-mediated signaling. Nat Immunol (2010) 11:608–17. doi: 10.1038/ni.1883
13. Smelter DF, Sathish V, Thompson MA, Pabelick CM, Vassallo R, Prakash YS. Thymic stromal lymphopoietin in cigarette smoke-exposed human airway smooth muscle. J Immunol (2010) 185:3035–40. doi: 10.4049/jimmunol.1000252
14. Adam DN, Gooderham MJ, Beecker JR, Hong CH, Jack CS, Jain V, et al. Expert consensus on the systemic treatment of atopic dermatitis in special populations. J Eur Acad Dermatol Venereol (2023) 37(6):1135–48. doi: 10.1111/jdv.18922
15. Akdis CA, Arkwright PD, Brüggen MC, Busse W, Gadina M, Guttman-Yassky E, et al. Type 2 immunity in the skin and lungs. Allergy (2020) 75(7):1582–605. doi: 10.1111/all.14318
16. Ramírez-Marín HA, Silverberg JI. Differences between pediatric and adult atopic dermatitis. Pediatr Dermatol (2022) 39(3):345–53. doi: 10.1111/pde.14971
17. Weidinger S, Beck LA, Bieber T, Kabashima K, Irvine AD. Atopic dermatitis. Nat Rev Dis Primers (2018) 4(1):1. doi: 10.1038/s41572-018-0001-z
18. Caruso C, Colantuono S, Nicoletti A, Arasi S, Firinu D, Gasbarrini A, et al. Metabolomics, microbiota, and in vivo and in vitro biomarkers in type 2 severe asthma: A perspective review. Metabolites (2021) 11(10):647. doi: 10.3390/metabo11100647
19. Schuler CF4, Billi AC, Maverakis E, Tsoi LC, Gudjonsson JE. Novel insights into atopic dermatitis. J Allergy Clin Immunol (2023) 151(5):1145–54. doi: 10.1016/j.jaci.2022.10.023
20. Rinaldi AO, Korsfeldt A, Ward S, Burla D, Dreher A, Gautschi M, et al. Electrical impedance spectroscopy for the characterization of skin barrier in atopic dermatitis. Allergy (2021) 76(10):3066–79. doi: 10.1111/all.14842
21. Luo J, Zhu Z, Zhai Y, Zeng J, Li L, Wang D, et al. The role of TSLP in atopic dermatitis: from pathogenetic molecule to therapeutical target. Mediators Inflamm (2023) 2023:7697699. doi: 10.1155/2023/7697699
22. Nygaard U, Hvid M, Johansen C, Buchner M, Fölster-Holst R, Deleuran M, et al. “TSLP, IL-31, IL-33 and sST2 are new biomarkers in endophenotypic profiling of adult and childhood atopic dermatitis,”. J Eur Acad Dermatol Venereol (2016) 30(11):1930–8. doi: 10.1111/jdv.13679
23. Orlando RC. The integrity of the esophageal mucosa. Balance between offensive and defensive mechanisms. Best Pract Res Clin Gastroenterol (2010) 24(6):873–82. doi: 10.1016/j.bpg.2010.08.008
24. Alexander ES, Martin LJ, Collins MH, Kottyan LC, Sucharew H, He H, et al. Twin and family studies reveal strong environmental and weaker genetic cues explaining heritability of eosinophilic esophagitis. J Allergy Clin Immunol (2014) 134(5):1084–1092.e1. doi: 10.1016/j.jaci.2014.07.021
25. Khokhar D, Marella S, Idelman G, Chang JW, Chehade M, Hogan SP. Eosinophilic esophagitis: Immune mechanisms and therapeutic targets. Clin Exp Allergy (2022) 52(10):1142–56. doi: 10.1111/cea.14196
26. Davis BP, Stucke EM, Khorki ME, Litosh VA, Rymer JK, Rochman M, et al. Eosinophilic esophagitis linked calpain 14 is an IL-13-induced protease that mediates esophageal epithelial barrier impairment. JCI Insight (2016) 1(4):e86355. doi: 10.1172/jci.insight.86355
27. Blanchard C, Stucke EM, Burwinkel K, Caldwell JM, Collins MH, Ahrens A, et al. Coordinate interaction between IL-13 and epithelial differentiation cluster genes in eosinophilic esophagitis. J Immunol (2010) 184:4033–41. doi: 10.4049/jimmunol.0903069
28. Sherrill JD, Kc K, Wu D, Djukic Z, Caldwell JM, Stucke EM, et al. Desmoglein-1 regulates esophageal epithelial barrier function and immune responses in eosinophilic esophagitis. Mucosal Immunol (2014) 7:718–29. doi: 10.1038/mi.2013.90
29. Azouz NP, Ynga-Durand MA, Caldwell JM, Jain A, Rochman M, Fischesser DM, et al. The antiprotease SPINK7 serves as an inhibitory checkpoint for esophageal epithelial inflammatory responses. Sci Transl Med (2018) 10(444):eaap9736. doi: 10.1126/scitranslmed.aap9736
30. Kim B, Rothenberg ME, Sun X, Bachert C, Artis D, Zaheer R, et al. Neuroimmune interplay during type 2 inflammation: symptoms, mechanisms and therapeutic targets in atopic diseases. J Allergy Clin Immunol (2023) 25:S0091-6749(23)01070-9. doi: 10.1016/j.jaci.2023.08.017
31. Reddy SB, Ketchem CJ, Dougherty MK, Eluri S, Dellon ES. Association between eosinophilic esophagitis and esophageal dysmotility: a systematic review and meta-analysis. NeurogastroenterolMotil (2023) 35:e14475. doi: 10.1111/nmo.14475
32. Akdis CA, Bachert C, Cingi C, Dykewicz MS, Hellings PW, Naclerio RM, et al. Endotypes and phenotypes of chronic rhinosinusitis: a PRACTALL document of the European Academy of Allergy and Clinical Immunology and the American Academy of Allergy, Asthma & Immunology. J Allergy Clin Immunol (2013) 131:1479–90. doi: 10.1016/j.jaci.2013.02.036
33. Schleimer RP, Kato A, Kern R, Kuperman D, Avila PC. Epithelium: at the interface of innate and adaptive immune responses. J Allergy Clin Immunol (2007) 120:1279–84. doi: 10.1016/j.jaci.2007.08.046
34. Zhang N, Van Crombruggen K, Gevaert E, Bachert C. Barrier function of the nasal mucosa in health and type-2 biased airway diseases. Allergy (2016) 71:295–307. doi: 10.1111/all.12809
35. Rogers GA, Beste KD, Parkos CA, Nusrat A, Delgaudio JM, Wise SK. Epithelial tight junction alterations in nasal polyposis. Int Forum Allergy Rhinol (2011) 1:50–4. doi: 10.1002/alr.20014
36. Yu XM, Li CW, Chao SS, Li YY, Yan Y, Zhao XN, et al. Reduced growth and proliferation dynamics of nasal epithelial stem/progenitor cells in nasal polyps in vitro. Sci Rep (2014) 4:4619. doi: 10.1038/srep04619
37. Caruso C, Giancaspro R, Guida G, Macchi A, Landi M, Heffler E, et al. Nasal cytology: A easy diagnostic tool in precision medicine for inflammation in epithelial barrier damage in the nose. A perspective mini review. Front Allergy (2022) 3:768408. doi: 10.3389/falgy.2022.768408
38. Gelardi M, Cassano P, Cassano M, Fiorella M. Nasal cytology: description of a hyperchromatic supranuclear stria as a possible marker for the anatomical and functional integrity of the ciliated cell. Am J Rhinol (2003) 17:263–8. doi: 10.1177/194589240301700503
39. Feazel LM, Robertson CE, Ramakrishnan VR, Frank DN. Microbiome complexity and Staphylococcus aureus in chronic rhinosinusitis. Laryngoscope (2012) 122:467–72. doi: 10.1002/lary.22398
40. Singh P, Mehta R, Agarwal S, Mishra P. Bacterial biofilm on the sinus mucosa of healthy subjects and patients with chronic rhinosinusitis (with or without nasal polyposis). J Laryngol Otol (2015) 129:46–9. doi: 10.1017/S002221511400303X
41. Foreman A, Psaltis AJ, Tan LW, Wormald P-J. Characterization of bacterial and fungal biofilms in chronic rhinosinusitis. Am J Rhinol Allergy (2009) 23:556–61. doi: 10.2500/ajra.2009.23.3413
42. Martens K, Seys SF, Alpizar YA, Schrijvers R, Bullens DMA, Breynaert C, et al. Staphylococcus aureus enterotoxin B disrupts nasal epithelial barrier integrity. Clin Exp Immunol (2021) 51(1):87–98. doi: 10.1111/cea.13760
43. Van Zele T, Gevaert P, Watelet J-B, Claeys G, Holtappels G, Claeys C, et al. Staphylococcus aureus colonization and IgE antibody formation to enterotoxins is increased in nasal polyposis. J Allergy Clin Immunol (2004) 114:981–3. doi: 10.1016/j.jaci.2004.07.013
44. Flora M, Perrotta F, Nicolai A, Maffucci R, Pratillo A, Mollica M, et al. Staphylococcus Aureus in chronic airway diseases: An overview. Respir Med (2019) 155:66–71. doi: 10.1016/j.rmed.2019.07.008
45. Van Zele T, Coppieters F, Gevaert P, Holtappels G, Van Cauwenberge P, Bachert C. Local complement activation in nasal polyposis. Laryngoscope (2009) 119(9):1753–8. doi: 10.1002/lary.20484
46. Caruso C, Colantuono S, Ciasca G, Basile U, Di Santo R, Bagnasco D, et al. Different aspects of severe asthma in real life: Role of Staphylococcus aureus enterotoxins and correlation to comorbidities and disease severity. Allergy (2023) 78(1):131–40. doi: 10.1111/all.15466
47. Menzies-Gow A, Ambrose CS, Colice G, Hunter G, Cook B, Molfino NA, et al. Effect of tezepelumab on lung function in patients with severe, uncontrolled asthma in the phase 3 NAVIGATOR study. Adv Ther (2023) 40(11):4957–71. doi: 10.1007/s12325-023-02659-y
48. Wechsler ME, Colice G, Griffiths JM, Almqvist G, Skärby T, Piechowiak T, et al. SOURCE: a phase 3, multicentre, randomized, double-blind, placebo-controlled, parallel group trial to evaluate the efficacy and safety of tezepelumab in reducing oral corticosteroid use in adults with oral corticosteroid dependent asthma. Respir Res (2020) 21(1):264. doi: 10.1186/s12931-020-01503-z
49. Menzies-Gow A, Wechsler ME, Brightling CE, Korn S, Corren J, Israel E, et al. Long-term safety and efficacy of tezepelumab in people with severe, uncontrolled asthma (DESTINATION): a randomised, placebo-controlled extension study. Lancet Respir Med (2023) 11(5):425–38. doi: 10.1016/S2213-2600(22)00492-1
50. Chan R, Stewart K, Misirovs R, Lipworth BJ. Targeting downstream type 2 cytokines or upstream epithelial alarmins for severe asthma. J Allergy Clin Immunol Pract (2022) 10(6):1497–505. doi: 10.1016/j.jaip.2022.01.040
51. Ramírez-Jiménez F, Pavón-Romero GF, Velásquez-Rodríguez JM, López-Garza MI, Lazarini-Ruiz JF. Gutiérrez-Quiroz, K.V. et al. Biologic Therapies for Asthma and Allergic Disease: Past, Present, and Future. Pharmaceuticals (2023) 16:270. doi: 10.3390/ph16020270
52. Chiang S, Lee SE. New concepts in barrier dysfunction in CRSwNP and emerging roles of tezepelumab and dupilumab. Am J Rhinol Allergy (2023) 37(2):193–7. doi: 10.1177/19458924231154061
53. Wechsler ME, Ruddy MK, Pavord ID, Israel E, Rabe KF, Ford LB, et al. Efficacy and safety of itepekimab in patients with moderate-to-severe asthma. N Engl J Med (2021) 385(18):1656–68. doi: 10.1056/NEJMoa2024257
54. Hopp RJ. Hypersensitivity reactions: an everyday occurrence in pediatric allergy clinics. Pediatr Allergy Immunol Pulmonol (2020) 33(1):12–8. doi: 10.1089/ped.2019.1109
55. Tan BK, Klingler AI, Poposki JA, Stevens WW, Peters AT, Suh LA, et al. Heterogeneous inflammatory patterns in chronic rhinosinusitis without nasal polyps in Chicago, Illinois. J Allergy Clin Immunol (2017) 139(2):699–703. doi: 10.1016/j.jaci.2016.06.063
56. Klimek L, Hagemann J, Welkoborsky HJ, Cuevas M, Casper I, Förster-Ruhrmann U, et al. Epithelial immune regulation of inflammatory airway diseases: Chronic rhinosinusitis with nasal polyps (CRSwNP). Allergol Select (2022) 6:148–66. doi: 10.5414/ALX02296E
57. Ono S, Kabashima K. Novel insights into the role of immune cells in skin and inducible skin-associated lymphoid tissue (iSALT). Allergo J Int (2015) 24:170–9. doi: 10.1007/s40629-015-0065-1
58. Ohnmacht C. Microbiota, regulatory T cell subsets, and allergic disorders. Allergo J Int (2016) 25(5):114–23. doi: 10.1007/s40629-016-0118-0
59. Lemière C, Ernst P, Olivenstein R, Yamauchi Y, Govindaraju K, Ludwig MS, et al. Airway inflammation assessed by invasive and noninvasive means in severe asthma: eosinophilic and noneosinophilic phenotypes. J Allergy Clin Immunol (2006) 118(5):1033–9. doi: 10.1016/j.jaci.2006.08.003
60. Saenz SA, Taylor BC, Artis D. Welcome to the neighborhood: epithelial cell derived cytokines license innate and adaptive immune responses at mucosal sites. Immunol Rev (2008) 226:172–90. doi: 10.1111/j.1600-065X.2008.00713.x
61. Kamekura R, Kojima T, Koizumi J, Ogasawara N, Kurose M, Go M, et al. Thymic stromal lymphopoietin enhances tight-junction barrier function of human nasal epithelial cells. Cell Tissue Res (2009) 338(2):283–93. doi: 10.1007/s00441-009-0855-1
62. Cherry WB, Yoon J, Bartemes KR, Iijima K, Kita H. A novel IL-1 family cytokine, IL-33, potently activates human eosinophils. J Allergy Clin Immunol (2008) 121(6):1484–90. doi: 10.1016/j.jaci.2008.04.005
63. Wong CK, Hu S, Cheung PFY, Lam CW. Thymic stromal lymphopoietin induces chemotactic and prosurvival effects in eosinophils: implications in allergic inflammation. Am J Respir Cell Mol Biol (2010) 43:305–15. doi: 10.1165/rcmb.2009-0168OC
64. Cook EB, Stahl JL, Schwantes EA, Fox KE, Mathur SK. IL-3 and TNFα increase Thymic Stromal Lymphopoietin Receptor (TSLPR) expression on eosinophils and enhance TSLP-stimulated degranulation. Clin Mol Allergy (2012) 10:8. doi: 10.1186/1476-7961-10-8
65. Al-Sajee D, Sehmi R, Hawke TJ, El-Gammal A, Howie KJ, Watson RM, et al. Expression of IL-33 and TSLP and their receptors in asthmatic airways after inhaled allergen challenge. Am J Respir Crit Care Med (2018) 198:805–7. doi: 10.1164/rccm.201712-2468LE
66. Bjerregaard A, Laing IA, Poulsen N, Backer V, Sverrild A, Fally M, et al. Characteristics associated with clinical severity and inflammatory phenotype of naturally occurring virus induced exacerbations of asthma in adults. Respir Med (2017) 123:34–41. doi: 10.1016/j.rmed.2016.12.010
67. Georas SN, Rezaee F. Epithelial barrier function: at the front line of asthma immunology and allergic airway inflammation. J Allergy Clin Immunol (2014) 134:509–20. doi: 10.1016/j.jaci.2014.05.049
68. Shahana S, Kampf C, Roomans G. Effects of the cationic protein poly-L-arginine on airway epithelial cells in vitro. Mediators Inflamm (2002) 11:141–8. doi: 10.1080/09622935020138172
Keywords: type 2 inflammation, immune system, non-T2 inflammation, epithelial barrier damage, alarmins, eosinophils, tezepelumab
Citation: Baglivo I, Colantuono S, Lumaca A, Papa A, Gasbarrini A and Caruso C (2024) The last step to achieve barrier damage control. Front. Immunol. 15:1354556. doi: 10.3389/fimmu.2024.1354556
Received: 12 December 2023; Accepted: 29 January 2024;
Published: 13 February 2024.
Edited by:
Enrico Heffler, Humanitas University, ItalyReviewed by:
Giulia Scioscia, University of Foggia, ItalyCopyright © 2024 Baglivo, Colantuono, Lumaca, Papa, Gasbarrini and Caruso. This is an open-access article distributed under the terms of the Creative Commons Attribution License (CC BY). The use, distribution or reproduction in other forums is permitted, provided the original author(s) and the copyright owner(s) are credited and that the original publication in this journal is cited, in accordance with accepted academic practice. No use, distribution or reproduction is permitted which does not comply with these terms.
*Correspondence: Cristiano Caruso, Y3Jpc3RpYW5vLmNhcnVzb0Bwb2xpY2xpbmljb2dlbWVsbGkuaXQ=
†These authors have contributed equally to this work and share first authorship
Disclaimer: All claims expressed in this article are solely those of the authors and do not necessarily represent those of their affiliated organizations, or those of the publisher, the editors and the reviewers. Any product that may be evaluated in this article or claim that may be made by its manufacturer is not guaranteed or endorsed by the publisher.
Research integrity at Frontiers
Learn more about the work of our research integrity team to safeguard the quality of each article we publish.