- 1College of Veterinary Medicine, Hunan Agricultural University, Changsha, China
- 2Hunan Province Key Laboratory of Traditional Chinese Veterinary Medicine, Hunan Agricultural University, Changsha, China
- 3DHN Business Division, Wens Foodstuff Group Co., Ltd., Zhaoqing, China
Introduction: Taraxacum mongolicum (TM) is a kind of medicinal and edible homologous plant which is included in the catalogue of feed raw materials in China. It is rich in polyphenols, flavonoids, polysaccharides and other active substances, and shows many benefits to livestock, poultry and aquatic products. The study aimed to assess the potential of TM aqueous extract (TMAE) as a substitute for poultry AGPs.
Methods: A total of 240 one-day-old Arbor Acker broilers were randomly assigned to four groups and fed a basal diet (Con) supplemented with 500, 1000, and 2000 mg/kg TMAE (Low, Medium, and High groups). The growth performance of the broilers was measured on day 21 and day 42. At the end of the trial, the researchers measured slaughter performance and collected serum, liver, spleen, ileum, and intestinal contents to investigate the effects of TMAE on serum biochemistry, antioxidant capacity, immune function, organ coefficient, intestinal morphology, flora composition, and short-chain fatty acids (SCFAs).
Results: The results showed that broilers treated with TMAE had a significantly higher average daily gain from 22 to 42 days old compared to the Con group. Various doses of TMAE resulted in different levels of improvement in serum chemistry. High doses increased serum alkaline phosphatase and decreased creatinine. TMAE also increased the antioxidant capacity of serum, liver, and ileum in broilers. Additionally, middle and high doses of TMAE enhanced the innate immune function of the liver (IL-10) and ileum (Occludin) in broilers. Compared to the control group, the TMAE treatment group exhibited an increase in the ratio of villi length to villi crypt in the duodenum. TMAE increased the abundance of beneficial bacteria, such as Alistipes and Lactobacillus, while reducing the accumulation of harmful bacteria, such as Colidextracter and Sellimonas. The cecum's SCFAs content increased with a medium dose of TMAE. Supplementing broiler diets with TMAE at varying doses enhanced growth performance and overall health. The most significant benefits were observed at a dose of 1000 mg/kg, including improved serum biochemical parameters, intestinal morphology, antioxidant capacity of the liver and ileum, immune function of the liver and ileum, and increased SCFAs content. Lactobacillus aviarius, norank_f_norank_o__Clostridia_UCG-014, and Flavonifractor are potentially dominant members of the intestinal microflora.
Conclusion: In conclusion, TMAE is a promising poultry feed additive and 1000 mg/kg is an effective reference dose.
1 Introduction
For over 60 years, antibiotic growth promoters (AGPs) have been used in livestock and poultry farming to prevent disease, promote growth, and reduce costs (1, 2). However, prolonged exposure to sub-therapeutic doses has significantly increased bacterial resistance to antibiotics, leading to pandemics in farmed animals, humans, and the environment. This poses significant obstacles and problems for livestock development and public health (3–5). In July 2020, China joined the United States, the European Union, and other regions in officially entering the era of feed prohibition (6). Commercial broilers often experience oxidative stress and intestinal flora disorder due to the imbalance between genetic improvement of carcass development and intestinal system development (7). Therefore, low-cost and effective alternatives to AGPs are crucial for the sustainable development of livestock production worldwide. Antibacterial peptides, probiotics, acidifiers, and plant active ingredients have been extensively researched and are considered promising alternatives to AGPs (8–11). However, the EU’s decades of experience in antibiotic-free farming demonstrate that AGPs cannot be replaced by a single product and require a more comprehensive strategy (12–14).
Plant-derived polyphenols and polysaccharides have been shown to provide various benefits to livestock and poultry, including immunomodulation, antioxidant, antibacterial, and anti-inflammatory effects (15–18). Certain phenolic compounds, such as flavonoids and functional polysaccharides, are not easily broken down and absorbed in the stomach and small intestine. Instead, they can reach the cecum intact and be fermented and utilized by the abundant intestinal microorganisms (19, 20). The phenolic compounds break down into smaller molecular weight phenols when absorbed by the body, providing a wide range of antioxidant effects. Additionally, during the decomposition process, they can affect the intestinal flora (21, 22). Some microbial species in the gut utilize functional polysaccharides as nutrients and ferment them to produce short-chain fatty acids, which positively impact the intestinal environment and help maintain its health (23, 24).
Taraxacum mongolicum (TM) is a perennial herb of the Asteraceae family that is distributed worldwide. It is commonly used in Chinese medicine to clear away heat and toxic materials, reduce swelling and disperse masses, and promote diuresis and treat stranguria. Recent pharmacological studies have demonstrated that it possesses antibacterial, antioxidant, antiviral, liver-protecting, and choleretic effects (25–28). It is commonly utilized for the prevention and treatment of respiratory and digestive tract ailments (29–31). TM is used as a vegetable and a substitute for tea in some countries due to its nutritional elements, such as vitamins, amino acids, minerals, and active secondary metabolites, such as polyphenols and sterols (32–34). In China, TM is also included in the list of feed ingredients. The study demonstrated that adding 500 mg/kg of dandelion powder to the broiler diet improved growth performance by enhancing the intestinal barrier and microbial composition (35). Additionally, Yang et al. (36) discovered that the water-soluble components of dandelion have a superior antioxidant effect and are more cost-effective and environmentally friendly as feed additives. However, the impact of TM aqueous extract (TMAE) on growth promotion in broilers and its possible mechanism of action have not been systematically evaluated. The International Symposium on Antibiotic Alternatives, held by the World Organization for Animal Health, emphasized the importance of considering the impact of the product on both the intestinal flora (biological barrier) and the intestinal barrier (physical and chemical barrier) (14). The hypothesis was that TMAE would enhance the antioxidant capacity, gut barrier function, gut microbiota composition, and short-chain fatty acids (SCFAs) in broilers, leading to improved growth performance. Thus, this study aimed to investigate the effects of dietary supplementation with TMAE on the growth performance, antioxidant capacity, intestinal barrier function, and intestinal microflora of broilers.
2 Materials and methods
2.1 Plant samples
The dried whole herb Taraxacum mongolicum Hand. - Mazz was purchased from Bozhou Medicinal Materials Market, Anhui Province and identified by Professor Yang Guangmin of Hunan University of Traditional Chinese Medicine. The samples are stored in Hunan Province Key Laboratory of Traditional Chinese Veterinary Medicine, Hunan Agricultural University.
2.2 Preparation of TMAE
The TMAE extract was prepared following the method described by Tan et al. (37). The extraction was carried out at 100 °C, three times for 50 minutes each, with a solid-liquid ratio of 1:8. The resulting filtrate was combined, concentrated, and dried to obtain the TMAE extract. The extract samples were analyzed for their contents of soluble sugars, flavonoids, and total phenols using chemical chromogenic methods, specifically the anthrone-sulfuric acid, alkaline nitrite-aluminum ions, and Folin-Ciocalteu methods. The commercial assay kits used in the analysis were provided by Beijing Boxbio Science & Technology Co., Ltd., China.
2.3 UHPLC-Q/TOF-MS analysis
Dissolve 4 mg of TMAE in 1 mL of 80% methanol (Merck) solution. After mixing, centrifuge the solution at 8000 r/min for 10 min and collect the supernatant. The supernatant samples were filtered using nylon membranes (13 mm × 0.22 μm, ANPEL Laboratory Technologies Inc.) and separated on an Agilent 1290 Infinity UHPLC (Agilent Technologies, Santa Clara, CA, USA) in series with an Agilent 6530 Accurate-Mass Q-TOF LC/MS (Agilent Technologies, Santa Clara, CA, USA) system equipped with Agilent Eclipse XDB-C18 (4.6 × 150 mm, 5 μm). The UHPLC-Q/TOF-MS test conditions are based on the method of Pieczykolan, A. et al. (38) with some modifications. The mobile phases used in this experiment were water containing 0.1% formic acid (solvent A) and acetonitrile containing 0.1% formic acid (solvent B). The gradient elution program followed the schedule below: 0-1.5 min, 13% B; 2-15 min, 20% B; 16-23 min, 25% B; 25-28 min, 33% B; 30-33 min, 60% B; 34-37 min, 13% B. The flow rate was 0.35 mL/min, and the column temperature was maintained at 25°C. The negative ion source voltage was set at -4.5 kV, the capillary temperature at 500°C, the spray gas at 55 psi, and the Curtain Gas at 30 psi. The mass-to-charge ratios of primary and secondary fragments of isolated compounds were screened in the MS-DIAL database and literature reports to identify compounds in extracts (39, 40).
2.4 Birds and experimental design
Prior to the experiment, the breeding ground and utensils were disinfected using formaldehyde fumigation. A total of 240 1-day-old Arbor Acres (AA) white feather broilers were randomly divided into four groups, with six replicates of ten broilers per replicate. The control group (Con) was fed a diet without TMAE, while the TMAE treatment groups were fed a basal diet supplemented with 500, 1000, and 2000 mg/kg TMAE (Low, Medium, and High). The experiment lasted for 42 days. The chickens were raised in 3-layer vertical cages, with 10 chickens in each cage. The temperature in the house was manually controlled at 37-32°C during the first week and decreased by 2°C per week from the second week. The relative humidity was maintained at 55%-70%, and the chickens had access to food and water at all times. Standardized daily management procedures for feeding and epidemic prevention were followed throughout the trial. The basic diet for white feather broilers is prepared using corn, soybean meal, soybean oil, and other raw materials in accordance with the nutritional requirements outlined in the Chicken Feeding Standard (NY/T 33-2004) (41). The feed is in powder form. Table 1 shows the composition and nutritional levels of the basic diet. The study received approval from the Animal Ethics Committee of Hunan Agricultural University (Ethic approval number: CACAHU 20220922-1).
2.5 Sample collection
Body weight (BW), average daily gain (ADG), average daily feed intake (ADFI), and feed-to-gain ratio (F/G) were measured for each cage at 21 and 42 days of age. The chickens were fasted overnight but allowed to drink water. On day 42, the chickens were euthanized, and blood was collected using inert separating gel coagulation blood collection tubes. The blood was then centrifuged at 4 °C and 1500×g for 10 min, and the serum was collected and stored in centrifuge tubes. The thymus, spleen, and bursa of Fabricius were dissected, separated, and weighed after removing surface blood and water with absorbent paper. Tissue samples from the duodenum, jejunum, ileum, and cecum were collected and preserved in 4% paraformaldehyde fixative. The liver, duodenum, jejunum, and ileum samples were wrapped in tinfoil paper, frozen in liquid nitrogen, and stored in a -80 °C refrigerator. The contents of the duodenum, jejunum, ileum, and cecum were collected under aseptic conditions and stored in 2 mL cryotubes. The cryotubes were then frozen in liquid nitrogen and stored in a refrigerator at -80 °C. The slaughter performance was determined using the method specified in Poultry Production Performance Terminology and Measurement Statistical Methods (NY/T 823-2020) (42), and the calculation formula for each index is as follows: Carcass percentage, semi-eviscerated percentage, and eviscerated percentage are calculated as a percentage of broiler live weight. Carcass percentage is determined by dividing the carcass weight by the broiler live weight and multiplying by 100. Similarly, semi-eviscerated percentage is calculated by dividing the semi-eviscerated weight by the broiler live weight and multiplying by 100, while eviscerated percentage is calculated by dividing the eviscerated weight by the broiler live weight and multiplying by 100. The abdominal fat percentage (%) can be calculated by dividing the abdominal fat weight by the sum of the eviscerated weight and abdominal fat weight, and then multiplying the result by 100. Similarly, the breast muscle percentage (%) can be calculated by dividing the bilateral breast muscle weight by the eviscerated weight and multiplying the result by 100. Finally, the leg muscle percentage (%) can be calculated by dividing the bilateral leg muscle weight by the eviscerated weight and multiplying the result by 100.
2.6 Determination of serum biochemistry
An automatic serum biochemistry analyzer (ZY1280, Shanghai Kehua Bioengineering Co., Ltd., China) was used to determine the levels of aspartate aminotransferase (AST), lactate dehydrogenase (LDH), albumin (ALB), urea nitrogen (BUN), uric acid (UA), triglyceride (TG), low density lipoprotein (LDL-C), alanine aminotransferase (ALT), alkaline phosphatase (ALP), total protein (TP), globulin (GLB), glucose (GLU), creatinine (CRE), total cholesterol (TC), high-density lipoprotein (HDL-C), and creatine kinase (CK). The indicators were tested using commercial kits from Shanghai Kehua Bioengineering Co., Ltd., China.
2.7 Determination of antioxidant capacity
The antioxidant capacity of TMAE, serum, liver, and ileum were tested using a commercial assay kit from Shanghai Beyotime Biotechnology Co., Ltd., China and Abbkine Scientific Co., Ltd, China. The following parameters were determined using a full-wavelength multiplate reader (Infinite® E Plex, Tecan Trading AG, Switzerland): total antioxidant capacity (T-AOC) based on ABTS and FRAP methods (43), total glutathione peroxidase (GSH-Px), superoxide dismutase (SOD), malondialdehyde (MDA), and catalase (CAT) (44).
2.8 Immune function assay
The thymus, spleen, and bursa of Fabricius were weighed, and the immune organ index was calculated using the formula: immune organ index % = 100 × immune organ weight/live body weight. Serum IgG, IgY, IgA, IgM, γ-IFN, and IL-10, as well as liver homogenate IgG and IL-10, were detected using a commercial solid-phase sandwich ELISA kit from Shanghai Enzyme-linked Biotechnology Co., Ltd., China. Serum IgG, IgY, IgA, IgM, γ-IFN, and IL-10, as well as liver homogenate IgG and IL-10, were detected using a commercial solid-phase sandwich ELISA kit from Shanghai Enzyme-linked Biotechnology Co., Ltd., China. Serum IgG, IgY, IgA, IgM, γ-IFN, and IL-10, as well as liver homogenate IgG and IL-10, were detected using a commercial solid-phase sandwich ELISA kit from Shanghai Enzyme-linked Biotechnology Co., Ltd., China. Additionally, lysozyme (LZM) and secretory IgA were measured. The ileum homogenate was analyzed for DEF β 1, Zonula Occluden-1 (ZO-1), Occludin, transferrin (TRF), Claudin-1, mucin 1 (MUC-1), mucin 2 (MUC-2), and transforming growth factor beta (TGF-β). To perform the analysis, 50 μL of either the standard or test sample was mixed with an equal volume of biotin-labeled antibody solution. The mixture was gently shaken and incubated at 37 °C for 45 minutes. Each reaction was thoroughly washed with wash solution, shaking for 30 seconds each time, and this process was repeated 4 times. To each well, add 100 μL of Horseradish Peroxidase-Streptavidin, shake well, and incubate at 37 °C for 30 minutes. After washing the reaction wells four times, add the substrate and let it react for five minutes in the dark. To terminate the reaction, add 50 μL of stop solution and measure the optical density (OD) value of each well at a wavelength of 450 nm.
2.9 Histological observation of intestinal tract
The tissues from the duodenum, jejunum, and ileum were fixed in formalin for 48 hours, rinsed with running tap water for 8 hours, dehydrated in a series of graded alcohol concentrations (70%, 80%, 90%, 95%, and 100%), transferred to xylene to make them transparent, and embedded in paraffin for pathology. The resulting paraffin blocks were cut into 6 μm sections using a microtome, stained with hematoxylin and eosin, and observed under a microscope. The study measured the length of the villi and the depth of the crypt using Olympus OlyVIA Image Viewer measurement tools (OLYMPUS Corporation, Tokyo, Japan). The length of the villi was defined as the vertical distance from the tip of the villi to the opening of the crypt, while the depth of the crypt was defined as the vertical distance from the opening of the crypt to its base.
2.10 Determination of SCFAs content
SCFAs in cecal contents were determined using the modified method of Zhang et al. (45). A 1 g sample of cecal content was weighed and mixed with 5 mL of ultrapure water for 30 min. The mixture was then centrifuged at 10000 rpm for 10 min after being left overnight at 4°C. The supernatant was transferred to a new container, and 4 mL of ultrapure water was added to the precipitate. The mixture was shaken and mixed for 30 min, then centrifuged again, and the supernatant was combined. The resulting supernatant was mixed with 25% metaphosphoric acid (v:v=9:1) to determine SCFAs. The sample was passed through a 45 μm microporous membrane and then analyzed for SCFAs, including acetic acid (aa), propionic acid (pa), isobutyric acid (iba), butyric acid (ba), isovaleric acid (iva), and valeric acid (va), using a SHIMADZU GC-2010plus gas chromatograph (SHIMADZU Corporation, Kyoto, Japan) equipped with a DB-FFAP column (0. 25 μm × 30 m × 250 μm) (Agilent Technologies Inc., Santa Clara, USA). Chromatographic conditions were as follows: The sample was heated to 70°C for 3 minutes and then programmed to reach 210°C at a rate of 5°C/min and held for 10 minutes. The vaporization chamber temperature was set to 230°C and the FID detector temperature was set to 280°C. High purity nitrogen (purity ≥ 99.999%) was used as the carrier gas with a flow rate of 1 mL/min. The injection volume was 2.0 μL with a split ratio of 50:1.
2.11 16S rRNA sequencing of intestinal microbes
The small intestine samples were prepared by mixing equal amounts of duodenum, jejunum, and ileum contents. The quality of the extracted DNA was tested using 1% agarose gel electrophoresis and the DNA concentration and purity were determined using a BioPhotometer D30 (Eppendorf, Hamburg, Germany), following the TIANamp Stool DNA Kit (TIANGEN BIOTECH (BEIJING) CO., LTD.) protocol from Beijing, China. The 16S rDNA’s V4-V16 region was amplified through PCR using 338F (5’-ACTCCTACGGGAGGCAGCAG-3’) and 806R (5’-GGACTACHVGGGTWTCTAAT-3’). The PCR products of each sample were mixed and recovered through 2% agarose gel. The products were then purified using the TIANgel Purification Kit and TIANquick Midi Purification Kit (TIANGEN BIOTECH (BEIJING) CO., LTD.), Beijing, China. The purified products were detected through 2% agarose gel electrophoresis and quantified using the Quantus™ Fluorometer (Promega, USA). The TIANSeq DirectFast Library Kit (TIANGEN BIOTECH (BEIJING) CO., LTD., Beijing, China) was used to prepare the samples. Sequencing was performed by Shanghai Majorbio Biopharm Technology Co., Ltd. (Shanghai, China) using Illumina’s MiseqPE300 platform.
Fastp (v 0.19.6) was used for quality control of raw sequencing sequences, while splicing was performed using Flash (v 1.2.11). Sequences were clustered into OTUs using Uparse (v 11) with a similarity threshold of 97%, and mosaics were rejected. The OTU representative sequences were annotated using the RDP Classifier (v 2.13) based on the silva138/16s_bacteria taxonomic database, with a confidence threshold of 0.7 for taxonomic annotation results. The results were analyzed using Majorbio Cloud Platform (https://cloud.majorbio.com). The analysis included dilution curve analysis, alpha diversity analysis (Shannon index and Simpson index), species composition analysis (community composition analysis Bar plot), beta diversity analysis (principal co-ordinates analysis, PCoA), and species difference analysis (Kruskal-Wallis test, Kruskal-Wallis H test, and LDA discriminant results table).
2.12 Data analysis
Duncan’s multiple comparison test was used to analyze the results of growth performance, slaughter performance, serum biochemistry, antioxidant capacity, immune function, intestinal morphology, and SCFAs using IBM SPSS Statistics 26.0 (IBM Corporation, Armonk, USA). A significance level of P<0.05 was considered statistically significant. The data is presented as mean ± pooled SEM. The analytical plots for gut microbiota were generated using the R package. GraphPad Prism 8.0.2 (GraphPad Software, San Diego, USA) was used to generate line graphs displaying TMAE in vitro antioxidant results.
3 Results
3.1 Active ingredient content and composition of TMAE
The chemical chromogenic method was used to determine the content of active substances in TMAE. The TMAE extract had a yield of 39.28%. The total phenols content was 0.64%, the flavonoids content was 3.22%, and the soluble sugar content was 19.03%.
Fourteen phenolic compounds were identified from TMAE by UPLC-Q-TOF-MS, including chicory acid, caffeoyl tartaric acid, caffeic acid, luteolin, and its derivatives. The main phenolic compounds of TMAE were 1,3-dihydroxyacetone dimer, L-malic acid, luteolin 7-rutinoside, and chicoric acid, as shown in Figure S1 and Table S1.
3.2 Growth performance
Table 2 shows the results of the effect of adding TMAE to the diet on the growth performance of broilers. At 21 days of age, the BW of broilers in the Con group was significantly higher than that in the TMAE groups (P<0.05). However, at 42 days of age, the BW of broilers in the TMAE groups was significantly higher than that in the Con group (P<0.05). The low-dose group showed the most significant weight gain. From 1 to 21 days of age, the average daily gain (ADG) of broilers in the Con group was significantly higher than that in the TMAE group (P<0.05). Conversely, from 22 to 42 days of age, the ADG of broilers in the TMAE group was significantly higher than that in the Con group (P<0.05).
3.3 Slaughter performance
Table 3 shows the results of the effect of TMAE on the slaughter performance of broilers. The slaughter performance of the TMAE groups was not significantly different from that of the Con group.
3.4 Serum biochemistry
The results of the effect of TMAE supplementation in the diet on serum biochemistry of broilers are shown in Table 4. The results showed that ALP in High group was significantly higher than that in Con group (P<0.05). BUN was significantly increased in Medium group (P<0.05). TG was significantly lower in Low group (P<0.05). HDL in Medium group was significantly higher than that in control group (P<0.05). The serum CRE in High group was significantly lower than that in control group (P<0.05). There was no significant effect on other indexes.
3.5 Antioxidant capacity
The in vitro total antioxidant capacity of TMAE was determined by measuring its ABTS free radical scavenging capacity and iron ion reducing antioxidant capacity. The study found that the free clearance capacity of ABTS reached its maximum at a concentration of 1.5 mg/mL of TMAE, resulting in a Trolox-Equivalent Antioxidant Capacity (TEAC) of 1.54 mM Trolox/1.5 mg/mL (Figure 1A). Additionally, the antioxidant capacity of TMAE increased with concentration in the tested range for the FRAP test, but the upward trend stabilized after reaching 2 mg/kg. The FRAP assay revealed a maximum antioxidant capacity of approximately 1.02 mM FeSO4/2 mg/mL (Figure 1B). The TMAE groups exhibited significantly higher serum total antioxidant capacity and CAT activity compared to the Con group (P<0.05). Additionally, the liver in the Low group showed significantly higher ABTS free radical scavenging ability than the control group (P<0.05). The liver’s total antioxidant capacity (FRAP) significantly increased (P<0.05) in the TMAE treatment group, while the content of MDA significantly decreased (P<0.05). The activity of CAT in the liver of broilers in the Medium group also significantly increased (P<0.05). Additionally, GSH-Px activities in the liver and ileum of broilers in the High group significantly increased (P<0.05) (Table 5).
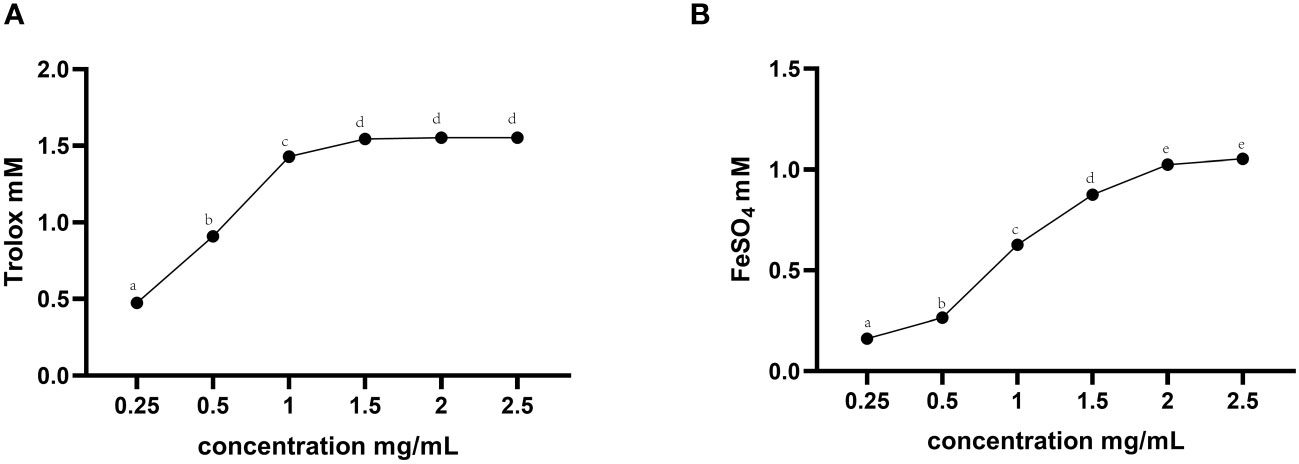
Figure 1 Total antioxidant capacity results for TMAE. (A) ABTS free radical scavenging ability; (B) Iron ion reduction antioxidant capacity. Data in the same row with no or same superscript letters indicate non-significant differences (P > 0.05), and different letters indicate significant differences (P < 0.05).
3.6 Immune function
The study results suggest that dietary supplementation with TMAE did not significantly affect immune organ indices in broilers (refer to Table 6). Furthermore, the ELISA test results of serum, liver, and ileum tissue samples (refer to Table 7) showed that TMAE treatment did not significantly affect serum immune indexes of broilers. However, the medium and high doses of TMAE significantly increased IL-10 content in the liver and Occludin content in the ileum (P<0.05). The group with higher levels also showed a significant increase in the expression of LZM, sIgA, TRF, ZO-1, and DEF β1 in the ileum (P<0.05).
3.7 Histological morphology of small intestine
Table 8 shows the effect of TMAE supplementation on small bowel morphology. Compared to the Con group, the Low, Medium, and High groups had significantly increased villus length and villus-to-crypt ratio in the duodenum (P<0.05). Additionally, the Low group had significantly increased villus length and villus-to-crypt ratio in the ileum (P<0.05).
3.8 SCFAs
Table 9 displays the SCFAs content in the cecal contents of broilers. The content of aa and ba in the cecal contents was significantly increased in the Medium group (1000 mg/kg) with the addition of TMAE compared to the Con group (P<0.05).
3.9 Gut microbiota composition
3.9.1 Microbiological analysis of small intestine
The results of the α diversity analysis (Figures 2A, B) indicate that at the OUT level, the Shannon index of microorganisms in the small intestine of the Con group was significantly higher than that of the TMAE treatment groups (P<0.05). Additionally, the Simpson index was significantly lower in the Con group compared to the TMAE treatment group (P<0.05). To analyze the effect of dietary TMAE supplementation on microbial community composition, we analyzed the microbial community of each dose group at the phylum, genus, and species levels. Firmicutes was the dominant phylum in all small intestine samples, accounting for over 99% (Figure 2C). At the genus level, the intestinal flora was relatively simple, with Lactobacillus being the main genus, accounting for over 98% (Figure 2D). At the species level, it was observed that Lactobacillus aviarius was gradually enriched in the small intestine with the addition of TMAE (Figure 2E). Cluster analysis of the population was performed using PCoA (Figure 2F), which showed that dietary TMAE supplementation did not have a significant effect on the microbial composition of the small intestine at the genus level or higher.
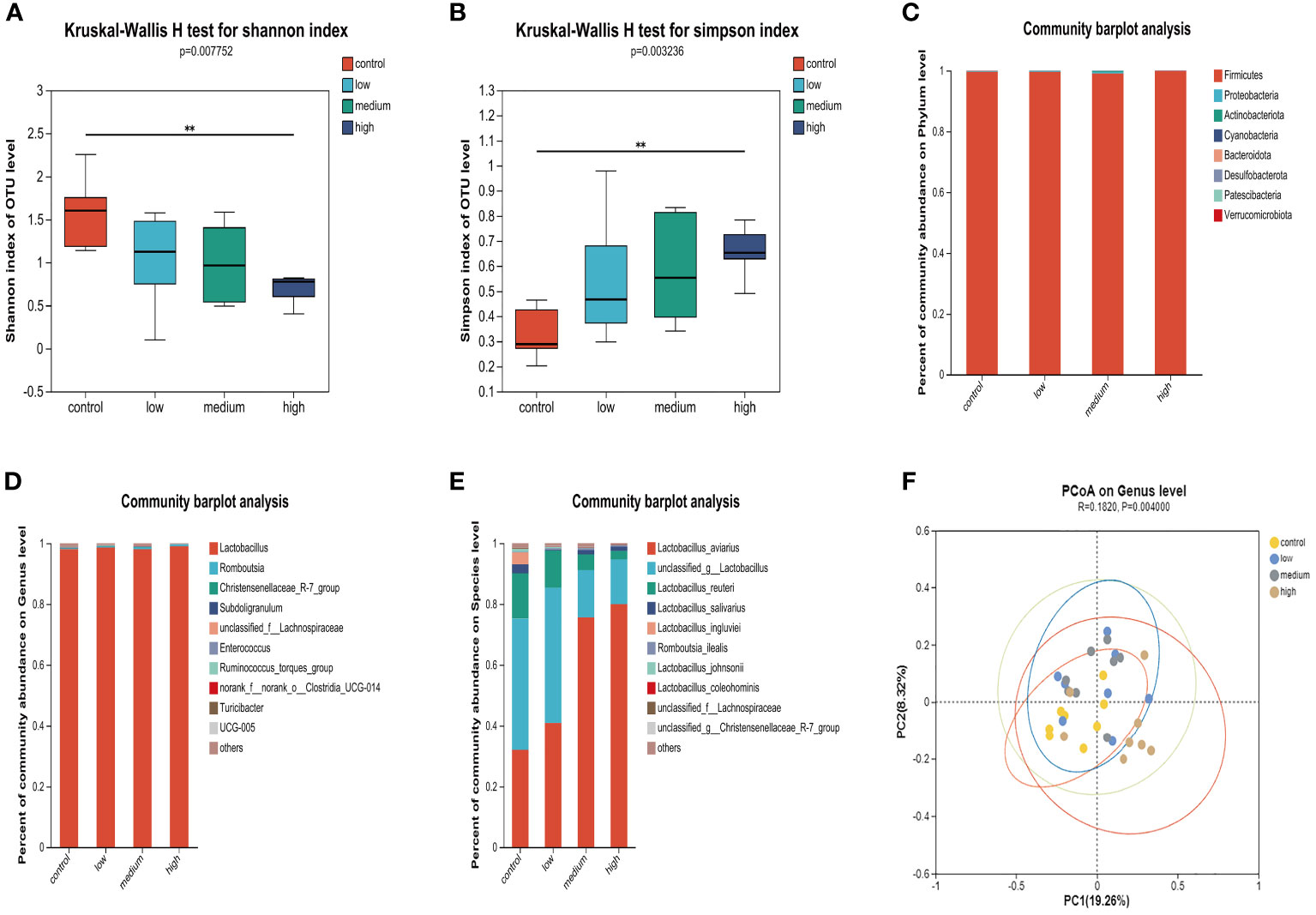
Figure 2 Effect of TMAE supplementation on small intestinal microflora of broilers. (A, B) Effect of TMAE on intestinal microbial alpha diversity (Shannon and Simpson index); (C–E) Colony composition of small intestinal microorganisms at phylum, genus and species levels after TMAE addition; (F) Intestinal microbial beta diversity analysis.
3.9.2 Microbiological analysis of cecum
The α diversity of the cecal microbiota was not significantly affected (P>0.05) by the addition of TMAE to the diet (Figures 3A, B). The dominant flora in the cecum consisted mainly of Firmicutes and Bacteroidetes, which accounted for more than 97% of the total. The proportion of Bacteroidetes increased in the low and medium dose groups of TMAE compared to the Con group (Figure 3D). The cecal flora is primarily composed of Alistipes, norank_f:norank_o:Clostridia_UCG-014, Lactobacillus, norank_o:Clostridia_vadin BB60_group, Faecalibacterium, Ruminococcus_torques_group, unclassified_f_Lachnospiraceae, UCG-005, Christensenellaceae_R-7_group, and Romboutsia (Figure 3E). The species-level composition is generally consistent with that of the genus level (Figure 3F). PCoA analysis revealed that the cecal microbiota of the Con and TMAE treated groups formed two distinct clusters (Figure 3C), indicating significant differences in microbial communities. This suggests that dietary supplementation with TMAE had varying effects on the cecal microflora. The Kruskal-Wallis H test (Figure 3G) was used to explore the flora that differed significantly between the different groups at the genus level. The relative abundance of norank_f:norank_o:Clostridia_UCG-014 and norank_f:UCG-010 was found to be significantly increased (P<0.05) using the Kruskal-Wallis H test and LEfSe Multi-level Species Difference Discriminant Analysis (LDA Linear Discriminant). The abundance of Butyricicoccus, Colidextrebacter, Sellimonas, and Fournierella was significantly decreased (P<0.05) in the TMAE-treated group compared to the control group. Parabacteroides showed significant decreases and increases in the low and medium dose groups (P<0.01), but not in the high dose group (Figure 3H). Flavonifractor increased significantly in the medium dose group (P<0.05).
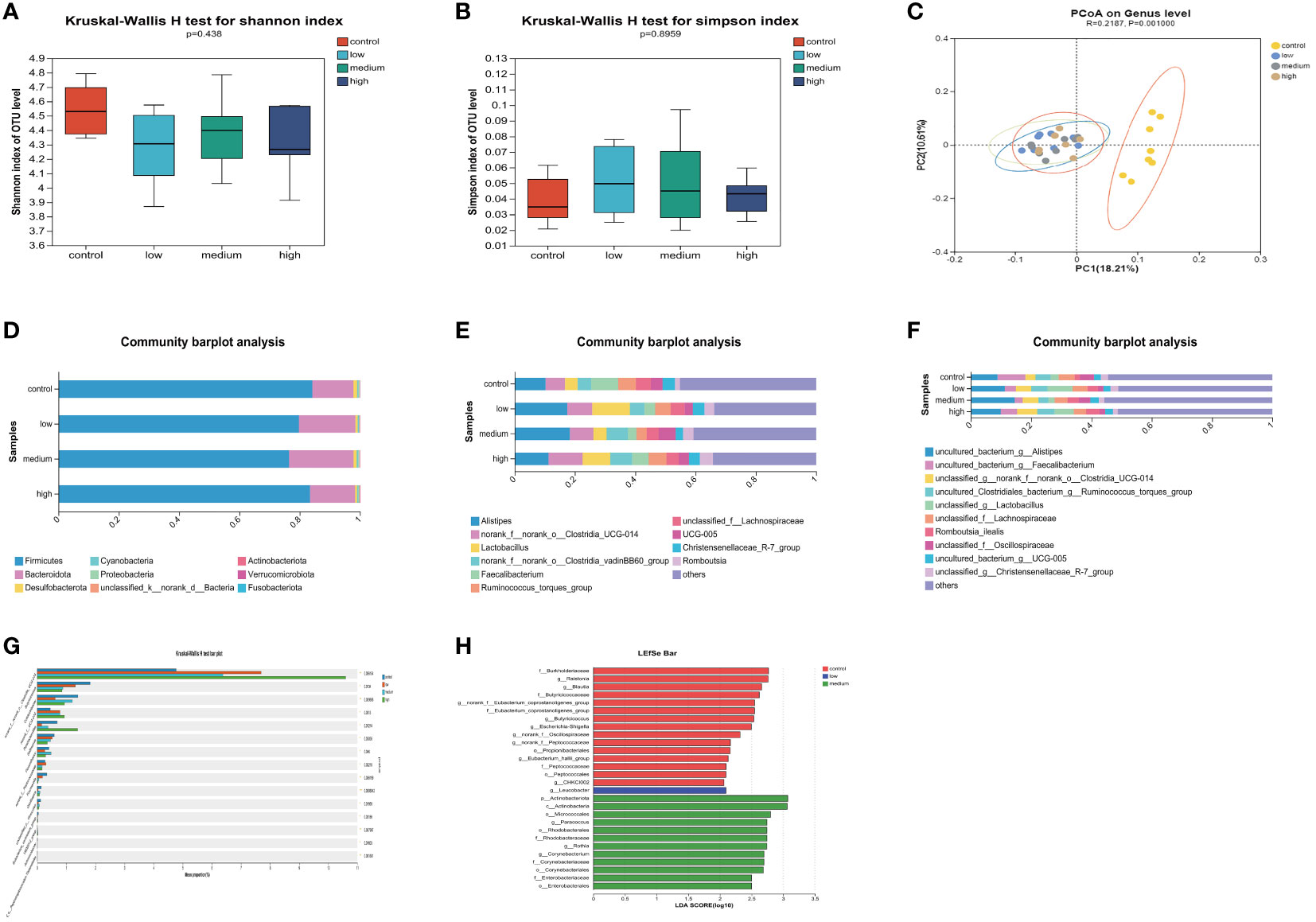
Figure 3 Effect of TMAE supplementation on cecal microorganism of broilers. (A, B) Effect of TMAE on Cecal Microbial Alpha Diversity (Shannon and Simpson index); (C) Cecal microbial beta diversity analysis; (D–F) Colony composition of cecal microorganisms at phylum, genus and species levels after TMAE addition; (G) Differentiation of enterobacteria at the genus level using the Kruskal-Wallis H test; (H) Effect of TMAE on Linear Discriminant Analysis Effect Size (LEfSe).
3.9.3 Correlation analysis between cecal microorganisms and SCFAs
Spearman correlation analysis was conducted at the genus level and a heat map was generated for the cecal microbes and SCFAs in the cecum, including aa, pa, iba, ba, iva, va (Figure 4). The abundance of norank_f_norank_o_Clostridia_UCG-014 was significantly and negatively correlated with the contents of all SCFAs (P<0.05). Additionally, the abundance of Sellimonas was significantly and positively correlated with the contents of aa and ba (P<0.05), while the abundance of Flavonifactor and Colidextribacter was significantly and positively correlated with the contents of aa, pa and ba (P<0.05). The study found significant positive correlation between the abundance of Parabacteroides and the content of pa (P <0.05), and significant negative correlation between the abundance of norank_f_norank_o_Clostridia_vadini BB60_group and the content of iva (P<0.05). Additionally, other microorganisms were found to be significantly correlated with SCFAs content at the community level.
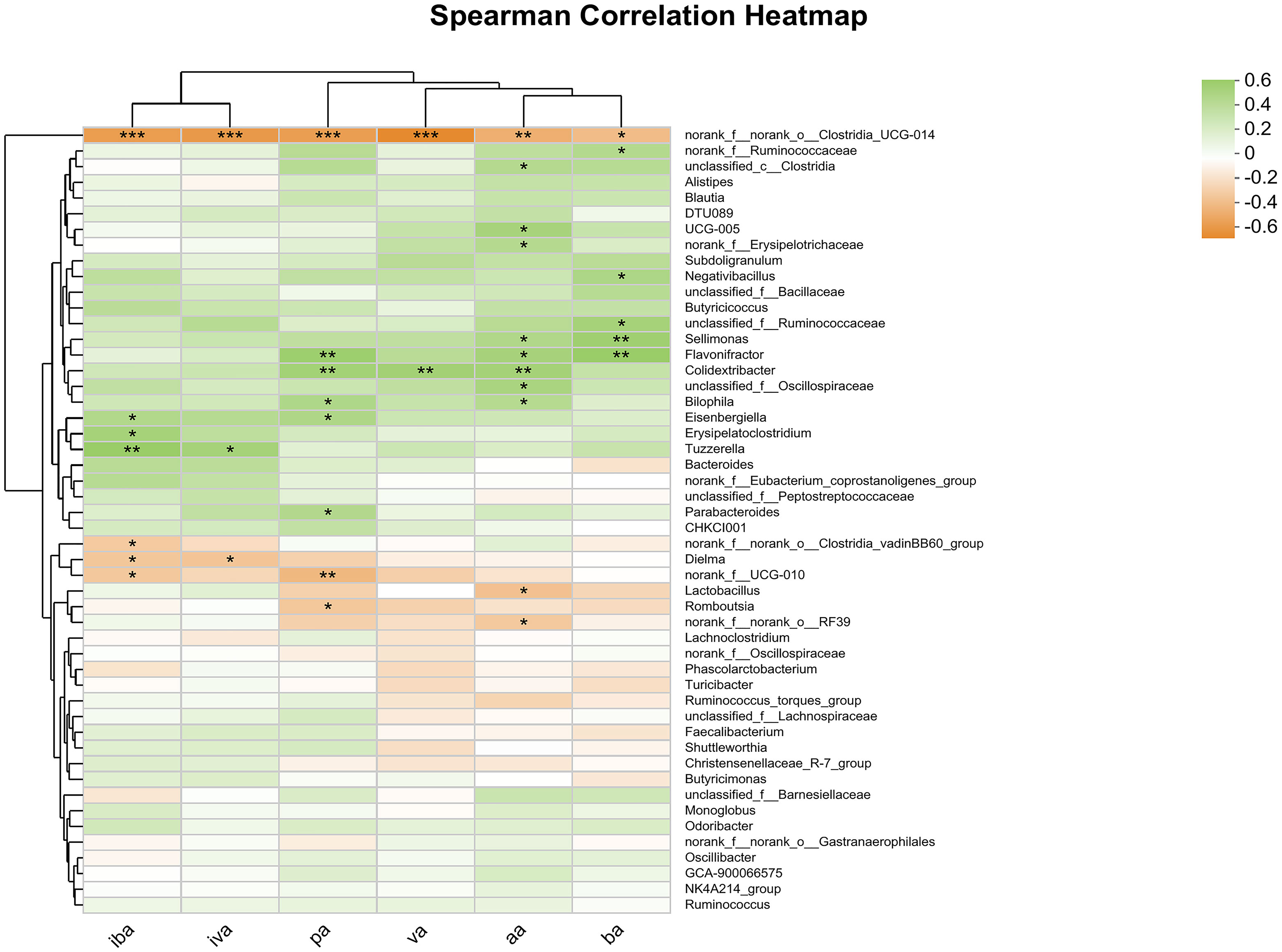
Figure 4 Heatmap of correlation analysis between cecal microorganisms and SCFAs. Statistical differences were recorded as P<0.05 (*), P<0.01 (**) and P<0.001 (***), respectively.
4 Discussion
Although the use of APGs has significant beneficial effects on growth performance and disease prevention in farmed animals, their use is partially restricted and prohibited worldwide due to the increasing problem of bacterial resistance. Studies have reported the beneficial effects of plant polyphenols, flavonoids and polysaccharides on livestock and poultry breeding have been studied and reported (46–48). The results showed that TMAE supplementation could increase the daily gain, improve the serum chemical index, enhance the antioxidant capacity of serum, liver and ileum, enhance the immune function of liver and intestine, and then improve the growth performance of broilers. It indicated that the growth promotion effect of TMAE on broilers was related to a wide range of biological effects. We found that TMAE supplementation had a negative effect on broiler weight gain compared to Con at 1 - 21 days of age. We hypothesized that certain components of TMAE inhibit digestive enzyme activity, which can lead to reduced nutrient uptake in broilers. Digestive enzymes play a crucial role in mediating and limiting energy and nutrient uptake in birds (49). Research has demonstrated that phenolic compounds, including kaempferol and luteolin derivatives, found in dandelion can hinder pancreatic lipase activity (50). Furthermore, dandelion polysaccharides exhibit significant activity in inhibiting α-amylase and α-glucosidase (51). Broiler gut digestion is not optimal in the early stages of growth. Bacteroides, which are responsible for digesting complex carbohydrates in the caecum, begin to proliferate at 14 days (52). During prophase, broilers experienced weight gain inhibition due to their inability to fully digest and utilize polysaccharides in TMAE. The study found that dietary supplementation with TMAE led to an increase in daily weight gain during the 22 - 42 day period. This is a critical stage where productivity increases significantly, resulting in a terminal body weight that exceeds the Con group. The limited energy of TMAE may be the reason for its effect on broilers in the early stages of production. However, the cecal flora in the later stages is capable of fermenting polysaccharides into low molecular weight organic matter that can be utilized. As a result, broilers overcome the energy limitation and exhibit compensatory growth response (53). Broilers consume excessive amounts of energy to maintain compensatory growth. This explains their increased feed intake. In addition, TMAE contains soluble carbohydrates, L-malic acid and 1,3-Dihydroxyacetone dimer, etc., which have obvious sweet smell, improve feed palatability and stimulate appetite of broilers (30, 54–56). The results of serum biochemistry showed that the ALP content of High group was significantly increased (P<0.05). During bone growth and development, bone cells became active and produced a large number of ALP into the blood, promoting bone growth and development (57, 58). At this dose, TMAE also reduced CRE in broiler serum (P<0.05) and increased BUN at Medium dose, but the study showed that except UA, other biochemical indicators reflecting renal function lacked specificity in the assessment of renal health in birds, which was closely related to the special physiological system of birds (59). Medium treatment also significantly increased the level of HDL (P<0.05), and enhanced the conversion of cholesterol to bile acids in liver, which increased the oxidative utilization of fatty acids in muscle tissue and improved growth performance (60, 61). The level of TG in serum can reflect the ability of lipid metabolism of broilers. The study found that Low dose TMAE can significantly reduce the level of TG in serum of broilers. TM polysaccharide may contribute to improving the ability of lipid metabolism of broilers (62).
Long-term intake of high-energy-density feed during breeding will cause peroxidation in livestock and poultry (blood and tissues), and then affect the occurrence and process of inflammation (63–65). Exogenous antioxidant supplementation in the right amount can enhance the capacity of the antioxidant system in vivo, on the contrary, excessive antioxidants can also disrupt homeostasis (66, 67). TMAE has been identified in a large number of polyphenols (including flavonoids) and polysaccharides (68), compared with other extracts with stronger antioxidant capacity and extraction solvent is more economical and environmentally friendly (36). The polyphenols of TM have also been shown to have good anti-inflammatory activity (69). The antioxidant activity of TMAE in vitro was also confirmed in this study. In vivo test results showed that TMAE could significantly increase serum T-AOC and CAT content, and significantly reduce MDA content in broilers. Medium and/or High dose could significantly increase the activities of CAT and GSH-Px in liver and ileum. The antioxidant potential of TM is also partially supported by the studies of Zhao et al. and Du et al. (70, 71)., whose results show that TM polysaccharide and flavonoid extracts can improve the defense capacity of antioxidant system in vivo, including the effects on CAT, SOD, T-AOC, etc. Due to the absorption properties of polyphenols (flavonoids) and polysaccharides, we focused on the effects of TMAE on the circulatory, hepatic and intestinal innate immune systems. IL-10 has extensive inflammatory and immunosuppressive properties and plays an important role in maintaining innate immunity and inflammatory homeostasis in vivo (72, 73). The intestine is the largest immune organ in chicken, and the immune lymphocytes are the densest in the ileum. At the same time, studies have shown that bile acids play an important role in mucosal immune regulation, and bile acids are mainly absorbed in the ileum (74, 75), so this study focused on the mucosal innate immunity of the ileum. Intestinal epithelial cells and mucosa, as the first physical barrier against external factors, are important components of ileal immunity, while Occludin and ZO-1 are essential to maintain the integrity of intestinal mucosal barrier (76). Meanwhile, intestinal antimicrobial effectors TRF, LZM and DEF β1 are important components of intestinal chemical barrier, which can prevent the infection of pathogenic microorganisms (77–79). sIgA, as the major immunoglobulin in intestinal mucosa, can mediate mucosal immune defense against various endogenous and exogenous pathogens (80). In this study, we found that high dose of TMAE can significantly increase the expression of LZM, Occludin, sIgA, TRF, ZO-1, DEF β1 and other immune indicators in the ileum of broilers, significantly improve the physical and chemical barrier defense effect of ileum mucosa, and enhance the intestinal innate immune function of broilers. Mao et al. also found that TM powder could increase the gene expression level of ZO-1 (35). In addition, the histological observation of intestinal morphology showed that low dose of TMAE could effectively increase the villus height and villus/crypt ratio of duodenum and ileum of broilers, and enhance the absorption capacity of broiler intestine, which confirmed the results of growth performance test of broilers.
Intestinal symbiotic flora can ferment and decompose nutrients for absorption and utilization by the body, and also exist as a biological barrier of intestinal innate immunity, maintaining host immune homeostasis through competition (81, 82). Dietary supplementation with different doses of TMAE had different beneficial effects on broilers, and we evaluated the intervention and regulation of different doses of TMAE on intestinal microflora. The results of alpha diversity analysis based on OUT showed that TMAE supplementation significantly reduced the diversity of broiler intestinal microbiota, but beta diversity analysis and microbiota composition analysis showed that TMAE did not change the composition of intestinal microbiota. We also found that Lactobacillus aviarius was gradually enriched with the increase of TMAE addition. Lactobacillus aviarius is one of the most common lactobacilli in poultry intestine (83), which can improve intestinal immunity (84). The glucanotransferase secreted by Lactobacillus aviarius also has the ability to digest and decompose starch to produce linear α-glucan products (soluble dietary fiber), and can also improve feed utilization rate and promote growth (85, 86). The increase of relative abundance of Lactobacillus aviarius may be one of the important reasons why TMAE affects the immune and growth performance of ileum TMAE could increase the relative abundance of Bacteroidetes in caecum. Studies have shown that Bacteroidetes has the ability to rapidly degrade polysaccharides, and can encode a variety of enzymes, such as polysaccharide lyases and glycosidases, to hydrolyze polysaccharides that are difficult to digest to provide energy for the body (87, 88). The results indicated that the polysaccharides in TMAE could affect the composition of intestinal flora in cecum of broilers and increase the relative abundance of Bacteroides. At the genus level, TMAE increased the abundance of Alistipes and Lactobacillus, and the abundance of Alistipes was closely related to body weight, energy metabolism and fat deposition in broilers (89–91). Alistipes are involved in the metabolism of SCFAs, and high fat animal diets can increase the relative abundance of the genus, thereby improving lipid metabolism by modulating acetate production (92). In addition, Alistipes also had a positive effect on bone development (93), which was consistent with the results of this study that TMAE increased serum alkaline phosphatase content and promoted bone development in broilers. Alistipes are also associated with immune regulation and health homeostasis and are considered potentially beneficial bacteria (94). Lactobacillus is ubiquitous in the intestinal tract of animals. It can improve the health of animals by inhibiting bacteria, improving the ecological environment of intestinal microorganisms, strengthening the barrier layer of intestinal epithelial cells and improving the immune function of animals (95, 96). Studies have shown that the prebiotic properties of polyphenols and polysaccharides can increase the abundance of probiotics such as Lactobacillus and Bifidobacterium. Clostridia, the most significant differential microbiota produced by TMAE supplementation, not only helps digest food, strengthens the intestinal mucosal barrier, but also provides infection resistance (97, 98). The addition of TMAE could increase the proportion of beneficial bacteria in the cecum of broilers, and reduce the proportion of harmful bacteria such as Colidotribacter and Sellimonas related to intestinal inflammation (99, 100).
SCFAs are secondary products produced by fermentation of carbohydrates by cecal microorganisms, which can provide necessary energy for host metabolism, improve digestive enzyme activity, inhibit the growth of pathogenic bacteria, and stimulate immune system (101, 102), and play an extremely important role in maintaining intestinal health and function. Aa has the function of regulating intestinal pH and promoting the production of ba, which can promote the regeneration and differentiation of intestinal epithelial cells and improve intestinal morphology (103, 104). The results of this study showed that medium dose of TMAE could significantly increase the content of aa and ba in the cecum of broilers. Correlation heat maps showed that the abundance of Flavonifractor was significantly positively correlated with aa and ba content, and it was mainly enriched in the cecal samples of the middle dose group of TMAE. norank_f:norank_o:Clostridia_UCG-014 was the most abundant in the high dose group and was significantly negatively correlated with all SCFAs, and the content of all SCFAs in the high dose group decreased, which was also consistent with the results. It has been reported that when the host is in immune activation state, intestinal commensal bacteria can change intestinal metabolism, reduce SCFAs and increase the concentration of aromatic metabolites (105). Therefore, it can be speculated that Clostridia plays an important role in the activation of ileal innate immunity exhibited after high dose TMAE supplementation. In conclusion, dietary supplementation of TMAE in broilers showed varying degrees of health benefits (improvements in serum biochemistry, antioxidant capacity, immune function, and intestinal morphology) and improved growth performance. These effects may be related to the regulation of intestinal microbiota and SCFAs by TMAE, and it is considered that TMAE may be a potential poultry feed additive capable of replacing APGs. Dietary addition of TMAE at 1000 mg/kg may have a broader beneficial biological effect.
Conclusions
Supplementing broiler diets with TMAE at varying doses was found to enhance growth performance and overall health. The most significant benefits were observed at a dose of 1000 mg/kg, including improved serum biochemical parameters, intestinal morphology, antioxidant capacity of the liver and ileum, immune function of the liver and ileum, and increased SCFAs content. Lactobacillus aviarius, norank_f_norank_o:Clostridia_UCG-014, and Flavonifractor may have a dominant role in the microflora of the intestine and cecum.
Data availability statement
The raw data supporting the conclusions of this article will be made available by the authors, without undue reservation. Accession number of NIH BioProject: PRJNA1052070.
Ethics statement
The animal study was approved by Animal Ethics Committee of Hunan Agricultural University. The study was conducted in accordance with the local legislation and institutional requirements.
Author contributions
ZD: Conceptualization, Data curation, Formal analysis, Methodology, Software, Validation, Visualization, Writing – original draft, Writing – review & editing. ZL: Methodology, Writing – review & editing. YX: Methodology, Writing – review & editing. BT: Data curation, Formal analysis, Methodology, Software, Visualization, Writing – original draft. WS: Methodology, Writing – review & editing. QA: Investigation, Resources, Supervision, Writing – review & editing. ZY: Investigation, Methodology, Project administration, Supervision, Writing – review & editing. JZ: Conceptualization, Funding acquisition, Project administration, Resources, Supervision, Writing – review & editing.
Funding
The author(s) declare financial support was received for the research, authorship, and/or publication of this article. This research was funded by Hunan Provincial Key Research and Development Project, grant number 2023NK2021 and China Agriculture Research System, grant number CARS-21.
Acknowledgments
We thank the students in the team for their contributions to the sample collection process.
Conflict of interest
QA was employed by DHN Business Division, but the sponsors had no role in the design, execution, interpretation, or writing of the study.
The remaining authors declare that the research was conducted in the absence of any commercial or financial relationships that could be construed as a potential conflict of interest.
The reviewer ZS declared a shared affiliation with the authors ZD, ZL, YX, BT, WS, ZY and JZ to the handling editor at time of review.
Publisher’s note
All claims expressed in this article are solely those of the authors and do not necessarily represent those of their affiliated organizations, or those of the publisher, the editors and the reviewers. Any product that may be evaluated in this article, or claim that may be made by its manufacturer, is not guaranteed or endorsed by the publisher.
Supplementary material
The Supplementary Material for this article can be found online at: https://www.frontiersin.org/articles/10.3389/fimmu.2024.1354040/full#supplementary-material
References
1. Van TTH, Yidana Z, Smooker PM, Coloe PJ. Antibiotic use in food animals worldwide, with a focus on Africa: Pluses and minuses. J Glob Antimicrob Resist. (2020) 20:170–7. doi: 10.1016/j.jgar.2019.07.031
2. Abd El-Hack ME, El-Saadony MT, Shafi ME, Qattan SYA, Batiha GE, Khafaga AF, et al. Probiotics in poultry feed: A comprehensive review. J Anim Physiol Anim Nutr (Berl). (2020) 104:1835–50. doi: 10.1111/jpn.13454
3. Jimenez CEP, Keestra S, Tandon P, Cumming O, Pickering AJ, Moodley A, et al. Biosecurity and water, sanitation, and hygiene (WASH) interventions in animal agricultural settings for reducing infection burden, antibiotic use, and antibiotic resistance: a One Health systematic review. Lancet Planet Health. (2023) 7:e418–34. doi: 10.1016/S2542-5196(23)00049-9
4. Olaru ID, Walther B, Schaumburg F. Zoonotic sources and the spread of antimicrobial resistance from the perspective of low and middle-income countries. Infect Dis Poverty. (2023) 12:59. doi: 10.1186/s40249-023-01113-z
5. Avillan JJ, Ahmadvand P, Lu S-Y, Horton J, Liu J, Lofgren E, et al. Excreted antibiotics may be key to emergence of increasingly efficient antibiotic resistance in food animal production. Appl Environ Microbiol. (2022) 88:e0079122. doi: 10.1128/aem.00791-22
6. Gao J, Yang Z, Zhao C, Tang X, Jiang Q, Yin Y. A comprehensive review on natural phenolic compounds as alternatives to in-feed antibiotics. Sci China Life Sci. (2022) 66:1518–34. doi: 10.1007/s11427-022-2246-4
7. Lilburn MS, Loeffler S. Early intestinal growth and development in poultry. Poult Sci. (2015) 94:1569–76. doi: 10.3382/ps/pev104
8. Zhang Z-L, Meng Y-Q, Li J-J, Zhang X-X, Li J-T, Xu J-R, et al. Effects of antimicrobial peptides from dietary Hermetia illucens larvae on the growth, immunity, gene expression, intestinal microbiota and resistance to Aeromonas hydrophila of juvenile red claw crayfish (Cherax quadricarinatus). Fish Shellfish Immunol. (2024) 147:109437. doi: 10.1016/j.fsi.2024.109437
9. Zhang N, Ding J, Li J, Wang L, Wei Y. Effects of Bacillus pumilus on growth performance, serum indexes and muscle development-related gene expression of weanling Jintang black goat. Benef Microbes. (2023) 14:493–501. doi: 10.1163/18762891-20220093
10. Choi H, Chen Y, Longo F, Kim SW. Comparative effects of benzoic acid and sodium benzoate in diets for nursery pigs on growth performance and acidification of digesta and urine. J Anim Sci. (2023) 101:skad116. doi: 10.1093/jas/skad116
11. Biswas S, Ahn JM, Kim IH. Assessing the potential of phytogenic feed additives: A comprehensive review on their effectiveness as a potent dietary enhancement for nonruminant in swine and poultry. J Anim Physiol Anim Nutr (Berl). (2024) 1–13. doi: 10.1111/jpn.13922
12. Thirumeignanam D, Chellapandian M, Arulnathan N, Parthiban S, Kumar V, Vijayakumar MP, et al. Evaluation of natural antimicrobial substances blend as a replacement for antibiotic growth promoters in broiler chickens: Enhancing growth and managing intestinal bacterial diseases. Curr Microbiol. (2024) 81:55. doi: 10.1007/s00284-023-03573-w
13. Allen HK, Levine UY, Looft T, Bandrick M, Casey TA. Treatment, promotion, commotion: antibiotic alternatives in food-producing animals. Trends Microbiol. (2013) 21:114–9. doi: 10.1016/j.tim.2012.11.001
14. Lillehoj H, Liu Y, Calsamiglia S, Fernandez-Miyakawa ME, Chi F, Cravens RL, et al. Phytochemicals as antibiotic alternatives to promote growth and enhance host health. Vet Res. (2018) 49:76. doi: 10.1186/s13567-018-0562-6
15. Pan S, Yan J, Xu X, Chen Y, Chen X, Li F, et al. Current development and future application prospects of plants-derived polyphenol bioactive substance curcumin as a novel feed additive in livestock and poultry. Int J Mol Sci. (2022) 23:11905. doi: 10.3390/ijms231911905
16. El-Shall NA, Jiang S, Farag MR, Azzam M, Al-Abdullatif AA, Alhotan R, et al. Potential of Spirulina platensis as a feed supplement for poultry to enhance growth performance and immune modulation. Front Immunol. (2023) 14:1072787. doi: 10.3389/fimmu.2023.1072787
17. Liu J, Wang H, Luo J, Chen T, Xi Q, Sun J, et al. Synergism of fermented feed and ginseng polysaccharide on growth performance, intestinal development, and immunity of Xuefeng black-bone chickens. BMC Vet Res. (2024) 20:13. doi: 10.1186/s12917-023-03859-y
18. Hu Y, Wei J, Yuan Y, Wei H, Zhou Y, Xiao N, et al. Intervention effects of fructooligosaccharide and astragalus polysaccharide, as typical antibiotic alternatives, on antibiotic resistance genes in feces of layer breeding: advantages and defects. J Hazard Mater. (2024) 465:133172. doi: 10.1016/j.jhazmat.2023.133172
19. Luzardo-Ocampo I, Campos-Vega R, Gaytán-Martínez M, Preciado-Ortiz R, Mendoza S, Loarca-Piña G. Bioaccessibility and antioxidant activity of free phenolic compounds and oligosaccharides from corn (Zea mays L.) and common bean (Phaseolus vulgaris L.) chips during in vitro gastrointestinal digestion and simulated colonic fermentation. Food Res Int. (2017) 100:304–11. doi: 10.1016/j.foodres.2017.07.018
20. Zhao Q, Wang Z, Wang X, Yan X, Guo Q, Yue Y, et al. The bioaccessibility, bioavailability, bioactivity, and prebiotic effects of phenolic compounds from raw and solid-fermented mulberry leaves during in vitro digestion and colonic fermentation. Food Res Int. (2023) 165:112493. doi: 10.1016/j.foodres.2023.112493
21. Murota K, Nakamura Y, Uehara M. Flavonoid metabolism: the interaction of metabolites and gut microbiota. Biosci Biotechnol Biochem. (2018) 82:600–10. doi: 10.1080/09168451.2018.1444467
22. Ross JA, Kasum CM. Dietary flavonoids: bioavailability, metabolic effects, and safety. Annu Rev Nutr. (2002) 22:19–34. doi: 10.1146/annurev.nutr.22.111401.144957
23. Zhang D, Liu J, Cheng H, Wang H, Tan Y, Feng W, et al. Interactions between polysaccharides and gut microbiota: A metabolomic and microbial review. Food Res Int. (2022) 160:111653. doi: 10.1016/j.foodres.2022.111653
24. Yao Y, Yan L, Chen H, Wu N, Wang W, Wang D. Cyclocarya paliurus polysaccharides alleviate type 2 diabetic symptoms by modulating gut microbiota and short-chain fatty acids. Phytomedicine. (2020) 77:153268. doi: 10.1016/j.phymed.2020.153268
25. Cai X, Shao Y, Wang Z, Xu Y, Ren Z, Fu L, et al. Antiviral activity of dandelion aqueous extract against pseudorabies virus both. Vitro vivo. Front Vet Sci. (2022) 9:1090398. doi: 10.3389/fvets.2022.1090398
26. Xu X, Wang X, Xie P. Screening and unveiling antibacterial mechanism of dandelion phenolic extracts against Staphylococcus aureus by inhibiting intracellular Na+-K+ ATPase based on molecular docking and molecular dynamics simulation. J Biomol Struct Dyn. (2024) 4:1–12. doi: 10.1080/07391102.2023.2300123
27. Li Z, Li X, Shi P, Li P, Fu Y, Tan G, et al. Modulation of acute intestinal inflammation by dandelion polysaccharides: An in-depth analysis of antioxidative, anti-inflammatory effects and gut microbiota regulation. Int J Mol Sci. (2024) 25:1429. doi: 10.3390/ijms25031429
28. Ge B, Sang R, Wang W, Yan K, Yu Y, Kong L, et al. Protection of taraxasterol against acetaminophen-induced liver injury elucidated through network pharmacology and in vitro and in vivo experiments. Phytomedicine. (2023) 116:154872. doi: 10.1016/j.phymed.2023.154872
29. Li Y, Chen Y, Sun-Waterhouse D. The potential of dandelion in the fight against gastrointestinal diseases: A review. J Ethnopharmacol. (2022) 293:115272. doi: 10.1016/j.jep.2022.115272
30. Valussi M. Functional foods with digestion-enhancing properties. Int J Food Sci Nutr. (2012) 63 Suppl 1:82–9. doi: 10.3109/09637486.2011.627841
31. Popovych V, Koshel I, Malofiichuk A, Pyletska L, Semeniuk A, Filippova O, et al. A randomized, open-label, multicenter, comparative study of therapeutic efficacy, safety and tolerability of BNO 1030 extract, containing marshmallow root, chamomile flowers, horsetail herb, walnut leaves, yarrow herb, oak bark, dandelion herb in the treatment of acute non-bacterial tonsillitis in children aged 6 to 18 years. Am J Otolaryngol. (2019) 40:265–73. doi: 10.1016/j.amjoto.2018.10.012
32. Guarrera PM, Savo V. Wild food plants used in traditional vegetable mixtures in Italy. J Ethnopharmacol. (2016) 185:202–34. doi: 10.1016/j.jep.2016.02.050
33. Lai G-H, Chao J, Lin M-K, Chang W-T, Peng W-H, Sun F-C, et al. Rapid and sensitive identification of the herbal tea ingredient Taraxacum formosanum using loop-mediated isothermal amplification. Int J Mol Sci. (2015) 16:1562–75. doi: 10.3390/ijms16011562
34. Gamboa-Gómez CI, Rocha-Guzmán NE, Gallegos-Infante JA, Moreno-Jiménez MR, Vázquez-Cabral BD, González-Laredo RF. Plants with potential use on obesity and its complications. EXCLI J. (2015) 14:809–31. doi: 10.17179/excli2015-186
35. Mao J, Wang Y, Wang W, Duan T, Yin N, Guo T, et al. Effects of Taraxacum mongolicum Hand.-Mazz. (dandelion) on growth performance, expression of genes coding for tight junction protein and mucin, microbiota composition and short chain fatty acids in ileum of broiler chickens. BMC Vet Res. (2022) 18:180. doi: 10.1186/s12917-022-03278-5
36. Yang Z, Dong Z, Wu H, Tan B, Zeng J. Analysis of the material basis and mechanism of action of antioxidant function of Taraxacum mongolicum based on network pharmacology. Acta Veterinaria Zootechnica Sin. (2023) 54:2170–85. doi: 10.11843/j.issn.0366-6964.2023.05.037
37. Tan B, Dong Z, Yang Z, Liu X, Zeng J. Study of HPLC characteristic chromatogram of Taraxacum mongolicum aqueous extract and its cluster analysis and principal component analysis. Feed Industry. (2023) 44:89–98. doi: 10.13302/j.cnki.fi.2023.08.016
38. Pieczykolan A, Pietrzak W, Dos Santos Szewczyk K, Gawlik-Dziki U, Nowak R. LC-ESI-MS/MS polyphenolic profile and in vitro study of cosmetic potential of aerva lanata (L.) juss. Herb extracts. Molecules. (2022) 27:1259. doi: 10.3390/molecules27041259
39. Lis B, Jedrejek D, Rywaniak J, Soluch A, Stochmal A, Olas B. Flavonoid preparations from taraxacum officinale L. Fruits-A phytochemical, antioxidant and hemostasis studies. Molecules. (2020) 25:5402. doi: 10.3390/molecules25225402
40. Tsugawa H, Ikeda K, Takahashi M, Satoh A, Mori Y, Uchino H, et al. A lipidome atlas in MS-DIAL 4. Nat Biotechnol. (2020) 38:1159–63. doi: 10.1038/s41587-020-0531-2
41. Wen J, Cai H, Guo Y, Qi G, Chen J, Zhang G, et al. Agricultural industry standard of the People’s Republic of China—Feeding Standard of chicken (NY/T33-2004). Beijing: Ministry of Agriculture of the People’s Republic of China. (2004) 2004:34.
42. Chen K, Gao Y, Wang Z, Ding Y, Zhang X, Li H, et al. Agricultural industry standard of the People’s Republic of China—Performance ferms and measurement for poultry (NY/T 823-2004). Beijing: Ministry of Agriculture of the People’s Republic of China (2004). p. 7.
43. Zhang D-Y, Wan Y, Xu J-Y, Wu G-H, Li L, Yao X-H. Ultrasound extraction of polysaccharides from mulberry leaves and their effect on enhancing antioxidant activity. Carbohydr Polymers. (2016) 137:473–9. doi: 10.1016/j.carbpol.2015.11.016
44. Lu M-C, Zhao J, Liu Y-T, Liu T, Tao M-M, You Q-D, et al. CPUY192018, a potent inhibitor of the Keap1-Nrf2 protein-protein interaction, alleviates renal inflammation in mice by restricting oxidative stress and NF-κB activation. Redox Biol. (2019) 26:101266. doi: 10.1016/j.redox.2019.101266
45. Zhang J, Yu H, Zhang H, Zhao Q, Si W, Qin Y, et al. Dietary Epimedium extract supplementation improves intestinal functions and alters gut microbiota in broilers. J Anim Sci Biotechnol. (2023) 14:14. doi: 10.1186/s40104-022-00812-1
46. Liu A, Kim E, Cui J, Li J, Lee Y, Zhang G. Laminaria japonica polysaccharide improved the productivities and systemic health of ducks by mediating the gut microbiota and metabolome. J Agric Food Chem. (2023) 71:7382–95. doi: 10.1021/acs.jafc.2c08731
47. Zhong Y, Li L, Chen W, Xing D, Wu X. Effects of Ilicis Chinensis folium extract supplementation on growth performance, serum parameters, intestinal morphology, and antioxidant capacity of broiler chickens. BMC Vet Res. (2023) 19:94. doi: 10.1186/s12917-023-03667-4
48. Dosu G, Obanla TO, Zhang S, Sang S, Adetunji AO, Fahrenholz AC, et al. Supplementation of ginger root extract into broiler chicken diet: effects on growth performance and immunocompetence. Poultry Sci. (2023) 102:102897. doi: 10.1016/j.psj.2023.102897
49. Chen Y-H, Zhao H. Evolution of digestive enzymes and dietary diversification in birds. PeerJ. (2019) 7:e6840. doi: 10.7717/peerj.6840
50. Aabideen ZU, Mumtaz MW, Akhtar MT, Mukhtar H, Raza SA, Touqeer T, et al. Anti-obesity attributes; UHPLC-QTOF-MS/MS-based metabolite profiling and molecular docking insights of. Taraxacum officinale. Molecules. (2020) 25:4935. doi: 10.3390/molecules25214935
51. Guo H, Zhang W, Jiang Y, Wang H, Chen G, Guo M. Physicochemical, structural, and biological properties of polysaccharides from dandelion. Molecules. (2019) 24:1485. doi: 10.3390/molecules24081485
52. Feng Y, Zhang M, Liu Y, Yang X, Wei F, Jin X, et al. Quantitative microbiome profiling reveals the developmental trajectory of the chicken gut microbiota and its connection to host metabolism. iMeta. (2023) 2:e105. doi: 10.1002/imt2.105
53. Miao Z, Zhang G, Zhang J, Li J, Yang Y. Effect of early dietary energy restriction and phosphorus level on subsequent growth performance, intestinal phosphate transport, and AMPK activity in young broilers. PLoS One. (2017) 12:e0186828. doi: 10.1371/journal.pone.0186828
54. Qiu K, He W, Zhang H, Wang J, Qi G, Guo N, et al. Bio-fermented Malic acid facilitates the production of high-quality chicken via enhancing muscle antioxidant capacity of broilers. Antioxidants (Basel). (2022) 11:2309. doi: 10.3390/antiox11122309
55. Higashida M, Yoshida Y, Kawabata Y, Matsui Y, Nishimura S, Tabata S, et al. Behavioral responses to sweet compounds via T1R2-independent pathways in chickens. Poult Sci. (2022) 101:101928. doi: 10.1016/j.psj.2022.101928
56. Yoshida Y, Tanaka R, Fujishiro S, Nishimura S, Tabata S, Kawabata F. Conditioned taste aversion to L-amino acid taste stimuli and oral transcriptional changes to type 1 taste receptors T1R1 and T1R3 on chronic exposure to L-alanine solution in chickens. J Poult Sci. (2022) 59:348–56. doi: 10.2141/jpsa.0210128
57. Tang H, Ma Y, Li J, Zhang Z, Li W, Cai C, et al. Identification and genetic analysis of major gene ST3GAL4 related to serum alkaline phosphatase in chicken. Res Vet Sci. (2023) 155:115–23. doi: 10.1016/j.rvsc.2022.12.001
58. Sabir PS, Abbas KA. Effect of strontium ranelate and cerium oxide addition in the diet on bone quality and expression level of osteocalcin and alkaline phosphatase genes in broiler chicken. Vet Med Sci. (2023) 9:1726–36. doi: 10.1002/vms3.1190
59. Scope A, Schwendenwein I. Laboratory evaluation of renal function in birds. Veterinary Clinics North America: Exotic Anim Pract. (2020) 23:47–58. doi: 10.1016/j.cvex.2019.08.002
60. Yang B, Huang S, Yang N, Cao A, Zhao L, Zhang J, et al. Porcine bile acids promote the utilization of fat and vitamin A under low-fat diets. Front Nutr. (2022) 9:1005195. doi: 10.3389/fnut.2022.1005195
61. Ge XK, Wang AA, Ying ZX, Zhang LG, Su WP, Cheng K, et al. Effects of diets with different energy and bile acids levels on growth performance and lipid metabolism in broilers. Poult Sci. (2019) 98:887–95. doi: 10.3382/ps/pey434
62. Wang K, Bi C, Chen Q, Liang J, Xiao Y. Effects of Taraxacum mongolicum polysaccharides on serum biochemical and antioxidant indexes in broilers. Anim Husbandry Veterinary Med. (2015) 47:74–6.
63. Çetin E, Güçlü BK. Effect of dietary L-carnitine supplementation and energy level on oxidant/antioxidant balance in laying hens subjected to high stocking density. J Anim Physiol Anim Nutr (Berl). (2020) 104:136–43. doi: 10.1111/jpn.13210
64. Hosseinian SA, Hasanzadeh F. Impact of high dietary energy on obesity and oxidative stress in domestic pigeons. Vet Med Sci. (2021) 7:1391–9. doi: 10.1002/vms3.478
65. Adebowale T, Shunshun J, Yao K. The effect of dietary high energy density and carbohydrate energy ratio on digestive enzymes activity, nutrient digestibility, amino acid utilization and intestinal morphology of weaned piglets. J Anim Physiol Anim Nutr (Berl). (2019) 103:1492–502. doi: 10.1111/jpn.13123
66. Morselli MB, Reis JH, Baldissera MD, Souza CF, Baldisserotto B, Petrolli TG, et al. Benefits of thymol supplementation on performance, the hepatic antioxidant system, and energetic metabolism in grass carp. Fish Physiol Biochem. (2020) 46:305–14. doi: 10.1007/s10695-019-00718-2
67. Berger RG, Lunkenbein S, Ströhle A, Hahn A. Antioxidants in food: mere myth or magic medicine? Crit Rev Food Sci Nutr. (2012) 52:162–71. doi: 10.1080/10408398.2010.499481
68. Liu H, Wang T. Study on water-soluble chemical constituents of Taraxacum mongolicum. J Chin Medicinal Materials. (2014) 37:989–91. doi: 10.13863/j.issn1001-4454.2014.06.029
69. Zou H, Ben T, Wu P, Waterhouse GIN, Chen Y. Effective anti-inflammatory phenolic compounds from dandelion: identification and mechanistic insights using UHPLC-ESI-MS/MS, fluorescence quenching and anisotropy, molecular docking and dynamics simulation. Food Sci Hum Wellness. (2023) 12:2184–94. doi: 10.1016/j.fshw.2023.03.031
70. Zhao L, Zhao J-L, Bai Z, Du J, Shi Y, Wang Y, et al. Polysaccharide from dandelion enriched nutritional composition, antioxidant capacity, and inhibited bioaccumulation and inflammation in Channa asiatica under hexavalent chromium exposure. Int J Biol Macromol. (2022) 201:557–68. doi: 10.1016/j.ijbiomac.2021.12.117
71. Du J-H, Xu M-Y, Wang Y, Lei Z, Yu Z, Li M-Y. Evaluation of Taraxacum mongolicum flavonoids in diets for Channa argus based on growth performance, immune responses, apoptosis and antioxidant defense system under lipopolysaccharide stress. Fish Shellfish Immunol. (2022) 131:1224–33. doi: 10.1016/j.fsi.2022.11.034
72. Saraiva M, Vieira P, O’Garra A. Biology and therapeutic potential of interleukin-10. J Exp Med. (2020) 217:e20190418. doi: 10.1084/jem.20190418
73. Ouyang W, Rutz S, Crellin NK, Valdez PA, Hymowitz SG. Regulation and functions of the IL-10 family of cytokines in inflammation and disease. Annu Rev Immunol. (2011) 29:71–109. doi: 10.1146/annurev-immunol-031210-101312
74. Chen ML, Takeda K, Sundrud MS. Emerging roles of bile acids in mucosal immunity and inflammation. Mucosal Immunol. (2019) 12:851–61. doi: 10.1038/s41385-019-0162-4
75. Mörbe UM, Jørgensen PB, Fenton TM, von Burg N, Riis LB, Spencer J, et al. Human gut-associated lymphoid tissues (GALT); diversity, structure, and function. Mucosal Immunol. (2021) 14:793–802. doi: 10.1038/s41385-021-00389-4
76. Chelakkot C, Ghim J, Ryu SH. Mechanisms regulating intestinal barrier integrity and its pathological implications. Exp Mol Med. (2018) 50:1–9. doi: 10.1038/s12276-018-0126-x
77. Yang D, Liu Z, Tewary P, Chen Q, de la Rosa G, Oppenheim JJ. Defensin participation in innate and adaptive immunity. Curr Pharm Des. (2007) 13:3131–9. doi: 10.2174/138161207782110453
78. Ragland SA, Criss AK. From bacterial killing to immune modulation: Recent insights into the functions of lysozyme. PLoS Pathog. (2017) 13:e1006512. doi: 10.1371/journal.ppat.1006512
79. Drago-Serrano ME, Campos-Rodriguez R, Carrero JC, de la Garza M. Lactoferrin and peptide-derivatives: Antimicrobial agents with potential use in nonspecific immunity modulation. Curr Pharm Des. (2018) 24:1067–78. doi: 10.2174/1381612824666180327155929
80. Muir WI, Bryden WL, Husband AJ. Immunity, vaccination and the avian intestinal tract. Dev Comp Immunol. (2000) 24:325–42. doi: 10.1016/s0145-305x(99)00081-6
81. Krajmalnik-Brown R, Ilhan Z-E, Kang D-W, DiBaise JK. Effects of gut microbes on nutrient absorption and energy regulation. Nutr Clin Pract. (2012) 27:201–14. doi: 10.1177/0884533611436116
82. Clavijo V, Flórez MJV. The gastrointestinal microbiome and its association with the control of pathogens in broiler chicken production: A review. Poult Sci. (2018) 97:1006–21. doi: 10.3382/ps/pex359
83. Feng Y, Wang Y, Zhu B, Gao GF, Guo Y, Hu Y. Metagenome-assembled genomes and gene catalog from the chicken gut microbiome aid in deciphering antibiotic resistomes. Commun Biol. (2021) 4:1305. doi: 10.1038/s42003-021-02827-2
84. Li P, Gao M, Fu J, Zhao Y, Liu Y, Yan S, et al. Construction of low intestinal bacteria model and its effect on laying performance and immune function of laying hens. Poult Sci. (2023) 102:102327. doi: 10.1016/j.psj.2022.102327
85. Hong Y, Zhou Z, Yu L, Jiang K, Xia J, Mi Y, et al. Lactobacillus salivarius and Lactobacillus agilis feeding regulates intestinal stem cells activity by modulating crypt niche in hens. Appl Microbiol Biotechnol. (2021) 105:8823–35. doi: 10.1007/s00253-021-11606-2
86. Danzeisen JL, Calvert AJ, Noll SL, McComb B, Sherwood JS, Logue CM, et al. Succession of the Turkey gastrointestinal bacterial microbiome related to weight gain. PeerJ. (2013) 1:e237. doi: 10.7717/peerj.237
87. Sun C, Fu G-Y, Zhang C-Y, Hu J, Xu L, Wang R-J, et al. Isolation and Complete Genome Sequence of Algibacter alginolytica sp. nov., a Novel Seaweed-Degrading Bacteroidetes Bacterium with Diverse Putative Polysaccharide Utilization Loci. Appl Environ Microbiol. (2016) 82:2975–87. doi: 10.1128/AEM.00204-16
88. McKee LS, La Rosa SL, Westereng B, Eijsink VG, Pope PB, Larsbrink J. Polysaccharide degradation by the Bacteroidetes: mechanisms and nomenclature. Environ Microbiol Rep. (2021) 13:559–81. doi: 10.1111/1758-2229.12980
89. Du W, Deng J, Yang Z, Zeng L, Yang X. Metagenomic analysis reveals linkages between cecal microbiota and feed efficiency in Xiayan chickens. Poultry Sci. (2020) 99:7066–75. doi: 10.1016/j.psj.2020.09.076
90. Liu X, Wang C, Wang Y, Wang C, Sun X, Zhu Y, et al. Age-associated changes in the growth development of abdominal fat and their correlations with cecal gut microbiota in broiler chickens. Poult Sci. (2023) 102:102900. doi: 10.1016/j.psj.2023.102900
91. McKenna A, Ijaz UZ, Kelly C, Linton M, Sloan WT, Green BD, et al. Impact of industrial production system parameters on chicken microbiomes: mechanisms to improve performance and reduce Campylobacter. Microbiome. (2020) 8:128. doi: 10.1186/s40168-020-00908-8
92. Jiang F, Song P, Wang H, Zhang J, Liu D, Cai Z, et al. Comparative analysis of gut microbial composition and potential functions in captive forest and alpine musk deer. Appl Microbiol Biotechnol. (2022) 106:1325–39. doi: 10.1007/s00253-022-11775-8
93. Li C, Cai H, Li S, Liu G, Deng X, Bryden WL, et al. Comparing the potential of Bacillus amyloliquefaciens CGMCC18230 with antimicrobial growth promoters for growth performance, bone development, expression of phosphorus transporters, and excreta microbiome in broiler chickens. Poult Sci. (2022) 101:102126. doi: 10.1016/j.psj.2022.102126
94. Kang Y, Kuang X, Yan H, Ren P, Yang X, Liu H, et al. A novel synbiotic alleviates autoimmune hepatitis by modulating the gut microbiota-liver axis and inhibiting the hepatic TLR4/NF-κB/NLRP3 signaling pathway. mSystems. (2023) 8:e01127-22. doi: 10.1128/msystems.01127-22
95. Azad MAK, Sarker M, Li T, Yin J. Probiotic species in the modulation of gut microbiota: An overview. BioMed Res Int. (2018) 2018:9478630. doi: 10.1155/2018/9478630
96. Heeney DD, Gareau MG, Marco ML. Intestinal Lactobacillus in health and disease, a driver or just along for the ride? Curr Opin Biotechnol. (2018) 49:140–7. doi: 10.1016/j.copbio.2017.08.004
97. Guo M, Xing D, Wang J, Zhang Y, Li Z, Jiao X. Potent intestinal mucosal barrier enhancement of nostoc commune vaucher polysaccharide supplementation ameliorates acute ulcerative colitis in mice mediated by gut microbiota. Nutrients. (2023) 15:3054. doi: 10.3390/nu15133054
98. Kim Y-G, Sakamoto K, Seo S-U, Pickard JM, Gillilland MG, Pudlo NA, et al. Neonatal acquisition of Clostridia species protects against colonization by bacterial pathogens. Science. (2017) 356:315–9. doi: 10.1126/science.aag2029
99. Yuan X, Chen B, Duan Z, Xia Z, Ding Y, Chen T, et al. Depression and anxiety in patients with active ulcerative colitis: crosstalk of gut microbiota, metabolomics and proteomics. Gut Microbes. (2021) 13:1987779. doi: 10.1080/19490976.2021.1987779
100. Leibovitzh H, Lee S-H, Xue M, Raygoza Garay JA, Hernandez-Rocha C, Madsen KL, et al. Altered gut microbiome composition and function are associated with gut barrier dysfunction in healthy relatives of patients with crohn’s disease. Gastroenterology. (2022) 163:1364–1376.e10. doi: 10.1053/j.gastro.2022.07.004
101. Morrison DJ, Preston T. Formation of short chain fatty acids by the gut microbiota and their impact on human metabolism. Gut Microbes. (2016) 7:189–200. doi: 10.1080/19490976.2015.1134082
102. Martin-Gallausiaux C, Marinelli L, Blottière HM, Larraufie P, Lapaque N. SCFA: mechanisms and functional importance in the gut. Proc Nutr Soc. (2021) 80:37–49. doi: 10.1017/S0029665120006916
103. Zhang J, Chen X, Liu P, Zhao J, Sun J, Guan W, et al. Dietary clostridium butyricum induces a phased shift in fecal microbiota structure and increases the acetic acid-producing bacteria in a weaned piglet model. J Agric Food Chem. (2018) 66:5157–66. doi: 10.1021/acs.jafc.8b01253
104. Wang RX, Lee JS, Campbell EL, Colgan SP. Microbiota-derived butyrate dynamically regulates intestinal homeostasis through regulation of actin-associated protein synaptopodin. Proc Natl Acad Sci U.S.A. (2020) 117:11648–57. doi: 10.1073/pnas.1917597117
Keywords: AGPs alternatives, antioxidant, broilers, growth performance, immune, intestinal flora, Taraxacum mongolicum
Citation: Dong Z, Liu Z, Xu Y, Tan B, Sun W, Ai Q, Yang Z and Zeng J (2024) Potential for the development of Taraxacum mongolicum aqueous extract as a phytogenic feed additive for poultry. Front. Immunol. 15:1354040. doi: 10.3389/fimmu.2024.1354040
Received: 11 December 2023; Accepted: 27 February 2024;
Published: 11 March 2024.
Edited by:
Kosuke Nishi, Ehime University, JapanReviewed by:
Zehe Song, Hunan Agricultural University, ChinaPeng Xie, Huaiyin Normal University, China
Khaled A. El-Tarabily, United Arab Emirates University, United Arab Emirates
Copyright © 2024 Dong, Liu, Xu, Tan, Sun, Ai, Yang and Zeng. This is an open-access article distributed under the terms of the Creative Commons Attribution License (CC BY). The use, distribution or reproduction in other forums is permitted, provided the original author(s) and the copyright owner(s) are credited and that the original publication in this journal is cited, in accordance with accepted academic practice. No use, distribution or reproduction is permitted which does not comply with these terms.
*Correspondence: Jianguo Zeng, emVuZ2ppYW5ndW9AaHVuYXUuZWR1LmNu
†These authors have contributed equally to this work