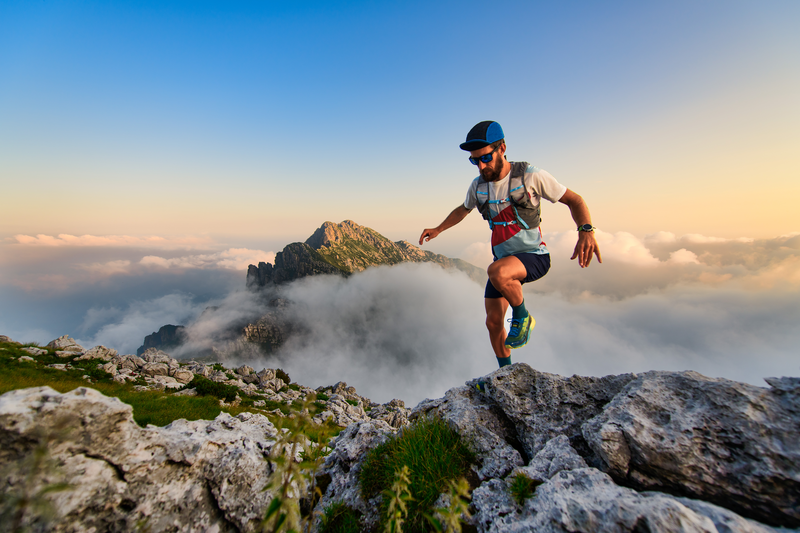
94% of researchers rate our articles as excellent or good
Learn more about the work of our research integrity team to safeguard the quality of each article we publish.
Find out more
REVIEW article
Front. Immunol. , 10 April 2024
Sec. Molecular Innate Immunity
Volume 15 - 2024 | https://doi.org/10.3389/fimmu.2024.1352946
Macrophages are crucial cells in the human body’s innate immunity and are engaged in a variety of non-inflammatory reactions. Macrophages can develop into two kinds when stimulated by distinct internal environments: pro-inflammatory M1-like macrophages and anti-inflammatory M2-type macrophages. During inflammation, the two kinds of macrophages are activated alternatively, and maintaining a reasonably steady ratio is critical for maintaining homeostasis in vivo. M1 macrophages can induce inflammation, but M2 macrophages suppress it. The imbalance between the two kinds of macrophages will have a significant impact on the illness process. As a result, there are an increasing number of research being conducted on relieving or curing illnesses by altering the amount of macrophages. This review summarizes the role of macrophage polarization in various inflammatory diseases, including autoimmune diseases (RA, EAE, MS, AIH, IBD, CD), allergic diseases (allergic rhinitis, allergic dermatitis, allergic asthma), atherosclerosis, obesity and type 2 diabetes, metabolic homeostasis, and the compounds or drugs that have been discovered or applied to the treatment of these diseases by targeting macrophage polarization.
Macrophages, as defined by Ilya Metchnikov in the nineteenth century, are phagocytic immune cells (1). It is a key component of innate immunity and plays a vital role in maintaining homeostasis and in recognizing and eliminating foreign pathogens. Research into the involvement of macrophages in the inflammatory response is burgeoning. Macrophages exhibit polarization and differentiation into distinct phenotypes in diverse inflammatory milieus, influenced by a plethora of factors. When exposed to lipopolysaccharide (LPS), interferon-α (INF-α), IL-12, IL-23, and other stimuli, resting macrophages (M0) were polarized into pro-inflammatory M1-like macrophages (M1). Conversely, IL-4 and IL-10 increased the development of anti-inflammatory M2 like macrophages (M2) (2, 3). According to various cytokines, M2 macrophages may be split into four different subtypes: M2a is generated by IL-4,IL-13 etc., M2b by immunoglobulin complexes and TLR agonists, M2c by IL-10,TGF-ß etc., and M2d is produced by M1 under the regulation of the activated adenosine 2A receptor (A2AR) (3). Among them, M2a, M2b and M2c were first proposed by Mantovani et al. according to different activation modes, while M2d was found to be a macrophage activated by toll-like receptors and specifically expressing vascular endothelial growth factor (VEGF) and IL-10 (4). M1 and M2 will coexist and be in balance under normal in vivo circumstances. When regional cytokines alter, M1 or M2 undergo reversible functional changes, allowing them to respond to inflammation in vivo (5). Both types of macrophages have their own specific surface biomarkers, among which the main markers of M1 are CD80, CD86, TLR-4, etc., and the main markers of M2 are CD163, CD206, CD209, etc (6) (Figure 1). By secreting distinct cytokines, the four subtypes of M2-like macrophages also play distinct roles in different forms of inflammation and illnesses. The main receptors on the surface of M2a include MHCII, MR, Arg1, CD206, which can secrete IL-10, TGF-β, IGF, Fibronectin, CCL22, CCL17, etc., which can promote wound healing and fibrosis (7). MHCII, MR, and CD86 are examples of M2b surface receptors that can secrete IL-1, IL-6, CCL1, CCL20, and other substances. M2b exhibits potent anti-inflammatory and immunomodulatory properties. With MR And CD163 as surface markers, M2c can secrete TGF-β, IL-10, metalloproteinases (MMPs), CCL18, etc (8, 9). At the same time, it can play a role in phagocytosis, immunosuppression, angiogenesis and tissue fibrosis development. Finally, M2d is a macrophage that is activated by toll-like receptors and specifically expresses vascular endothelial growth factor (VEGF) and IL-10, also known as TAM, which is involved in angiogenesis and tumor progression (10–12). The table below summarizes a few M1 and M2 cell surface indicators as well as cytokines that encourage M0 polarization in both directions (Table 1).
Figure 1 Diagram of macrophage polarization. There are two main forms of polarization of macrophages, M1-like and M2-like, which can be polarized into two types respectively under different cell stimulation conditions. M2-like macrophages can also be divided into four subtypes, M2a, M2b, M2c and M2d. Different macrophages have different markers on their surface, which can be used to identify the difference. The two types of macrophages secrete different cytokines during the disease process, resulting in two opposite effects.
By triggering the niacinamide adenine phosphate dinucleotide (NADPH) oxidase system and generating reactive oxygen species (ROS), M1-like macrophages aid in the removal of pathogens during infection. Consequently, ROS-induced tissue damage is mediated by M1-like macrophages, which also hinder wound healing and tissue regeneration. These macrophages have strong antibacterial and anticancer activity. On the other hand, mechanisms that restrict chronic inflammatory responses regulate the anti-inflammatory effects of M2 macrophages. M2 macrophages are involved in Th2 response, parasite removal, and inflammation inhibition. They also have a strong phagocytic capacity to remove debris and apoptotic cells, promote tissue repair and wound healing, angiogenesis, and fibrosis (16–18).
When the body is injured or exposed to an external bacterial infection, immune cells initiate the process of inflammation as a reaction. During this process, the body will mend itself by releasing numerous substances and recruiting immune cells. The duration of the inflammatory process varies from minutes to years (3, 19). An overly prolonged inflammatory response can lead to an imbalance between M1-like and M2-like macrophages, which can cause a number of problems, such as autoimmune diseases, diabetes, allergic diseases, atherosclerosis, obesity, metabolic homeostasis, and so on.
As a pivotal cell in innate immunity, macrophages play a significant role in various types of inflammation. This article primarily aims to offer an overview of inflammatory disorders closely linked to macrophage polarization and to delineate the current clinical approaches employed in treating these diseases.
Autoimmune diseases are mainly caused by an immune response that misidentifies the host as an antigen (20). Over 80 autoimmune diseases have been identified, with nearly 5% of the world’s population affected (21). Due to the unknown pathogenic mechanisms of many diseases and the limited treatment options available, numerous drugs can lead to severe side effects, making autoimmune diseases a challenging condition for both doctors and patients (22, 23). Increased M1/M2 ratio is linked to a number of autoimmune illnesses, including rheumatoid arthritis, multiple sclerosis and experimental autoimmune encephalomyelitis, autoimmune hepatitis, inflammatory bowel disease and etc (24–26). The basic explanation for how macrophage polarization can contribute to some autoimmune disorders is that M1-like macrophages can create reactive oxygen species, which are essential to the autoimmune damage and repair processes (9).
Rheumatoid arthritis (RA) is a systemic chronic autoimmune illness that is the most prevalent autoimmune rheumatic disease, is more frequent in the elderly, and affects women more than males (27, 28). The primary feature of rheumatoid arthritis (RA) is its ability to induce synovitis in multiple joints, leading to joint swelling and other symptoms. However, prolonged illness can lead to cartilage destruction, joint necrosis, and ultimately disability (29). There are medications available to treat RA, such as nonsteroidal anti-inflammatory medicines, glucocorticoids, and disease-modifying anti-rheumatic drugs, however these medications must be taken for lengthy periods of time or at high dosages to be effective (30, 31). Nevertheless, this type of medication has also resulted in serious adverse reactions, indicating the need for new RA treatment strategies. Macrophages are important in the pathophysiology of RA. TNF-α, IL-6, and other inflammatory cytokines can be produced by M1-like macrophages, aggravating or maintaining inflammation, as a result, M1-like macrophages are also regarded as an important treatment target for RA (32, 33).
High expression of M1-like macrophages is the main manifestation in rheumatoid arthritis, and macrophages with high expression can be detected in synovial tissue and peripheral blood of RA. M1-like macrophages in synovial tissue or peripheral blood primarily express major histocompatibility complex (MHC) class II, along with phenotypic markers such as CD80 and CD38, as well as inflammatory proteins like IL-6 and TNF-α. This enables them to present antigens to lymphocytes. Additionally, they can be recognized by T helper cells. Upon this recognition, they activate B lymphocytes to produce antibodies that are specific to the antigens presented by the macrophages, thereby thwarting pathogen invasion (32, 34). In RA, there is a response to invading pathogens, but persistent inflammation may lead to chronic maladaptive immune responses, leading to deterioration of the condition. The nuclear factor kappa B (NF-kB), stress-activated protein kinases/mitogen-activated protein kinases (SAPK/MAPK), and Janus kinase/signal transducer and activators of transcription (JAK/STAT) signaling pathways are connected to M1-like macrophage-induced inflammation in RA (35–37). These signaling pathways are primarily activated by pro-inflammatory cytokines, increasing macrophage survival (Figure 2).
Figure 2 The mechanism of macrophage polarization in RA. In RA, the inflammatory environment will cause macrophages to polarize toward M1 and M2. In the area affected by RA, M1 is significantly more than M2. M1 can secrete inflammatory factors such as TNF-β and IL-6 to aggravate the disease, while M2 can secrete anti-inflammatory factors such as IL-10 to alleviate the disease.
An important indicator of RA remission is an increase in M2-like macrophages with anti-inflammatory properties, which indicates that the conversion of M1-like macrophages into M2-like macrophages is of the utmost significance for RA treatment. Although there is no drug on the market that specifically targets macrophages to treat RA, in vitro research has revealed that CTLA4-Ig (abatacept) can lower some cytokines made by M1-like macrophages, additionally, after receiving CTLA4-Ig, macrophages transform into anti-inflammatory M2-like macrophages (38). In addition, folic acid modified silver nanoparticles (FA-AgNPS) have been used for the treatment of RA specifically. By entering cells and releasing Ag, FA-AgNPS promote reactive oxygen species (ROS) clearance, M1-like macrophage apoptosis, and M2-like macrophage polarization (29). In other experiments, plasmids constructed with IL-10 pDNA and betamethasone sodium phosphate (BSP) were introduced into the biomimetic vector M2 exosome (M2 Exo), M2 Exo/pDNA/BSP significantly promoted the polarization of M1 to M2 (39). Targeted macrophage polarization therapy for RA remains to be studied, which is still a hot research direction in the future.
One dangerous condition affecting the central nervous system is multiple sclerosis (MS), experimental autoimmune encephalomyelitis (EAE) is frequently utilized as an animal model of MS in pathological research because it shares many of the same clinical traits as MS (40, 41). It is an autoimmune-related neurodegenerative disease that can result in demyelination, inflammatory lesions of the CNS, and other symptoms. MS is a substantial contribution to non-traumatic disability in young individuals due to its chronic, localized, repeating, and diffuse nature (42, 43). The pathological phase of MS is associated with blood-brain barrier damage, T cell and macrophage infiltration into the CNS, activation of local microglia cells, and other variables. These acts, when combined, generate an inflammatory reaction that damages the neurological system (44, 45). Infiltrating macrophages and microglia have been identified as the primary effector of the inflammatory response in MS and EAE (46). Alterations in macrophages can influence a variety of inflammatory variables, resulting in alterations in the cellular environment, and the polarization balance of macrophages is critical in MS and other central nervous system illnesses (47).
In vivo, the development of MS and EAE is exactly complicated. M1 and M2like macrophages will arise in the body simultaneously. These cells must reduce inflammation, promote myelin repair, and phagocytosis of various debris while emitting inflammatory chemicals, causing oxidative stress, and damaging axons and myelin (48). During MS and EAE, there is a significant increase in iNOS, CD40, CD80, IL-6, and TNF-α. However, as the disease progresses, there is a shift toward M2-type macrophages taking over (47, 49) (Figure 3). It is known that taking myelin reduces inflammation and encourages the development of anti-inflammatory macrophages, demyelination is a significant inflammatory component of MS and EAE, and macrophages have a direct impact on it by removing myelin from the myelin sheath by phagocytosis (50, 51). Additionally, the blood-brain barrier’s (BBB) destruction is a significant contributor to neurological diseases, BBB can block the entry of harmful compounds into the CNS under normal physiological circumstances, whereas immune cells can pass through BBB (52). BBB is protected by microglial cells, macrophages, pericytes, etc., but when leukocyte extravasation causes inflammation, the perivascular macrophages of BBB will act as antigen-presenting cells to stimulate leukocyte recruitment to promote inflammation, this will also result in the recruitment of autoimmune T cells and accelerate the pathological process of MS through BBB (52–55). Furthermore, several additional investigations have discovered that certain proteins or non-coding RNAs can control the MS or EAE process by influencing the macrophage polarization process. For instance, studies have shown that the clinical and pathological severity of EAE is increased by the in vivo delivery of membrane protein Tim-3 antibody (56). Furthermore, circRNA_0000518 can exacerbate MS illness by promoting macrophage polarization toward M1 via the CaMKKβ/AMPK-PGC-1α pathway (45).
Figure 3 Mechanisms of macrophages in multiple sclerosis. In multiple sclerosis, microglia and macrophages play a major role in disease progression by secreting pro-inflammatory cytokines, presenting antigens, and inducing oxidative stress, leading to myelin destruction and axon damage.
There is currently no effective treatment for MS. Maintaining physiological function following deterioration and lessening the degree of deterioration are the primary goals of therapeutic therapy for MS (57). Currently, it has been shown that several substances and proteins, such as diosgenin, glatiramer acetate, neuropeptide Y, valproic acid, etc., can treat MS and EAE by decreasing M1 or boosting M2 (49, 58, 59). Furthermore, as M2 macrophage regulators or to reduce inflammatory responses, 3,4-Disubstituted Piperidine Derivatives, M2 modulator B9, Baicalein, and grape seed extract (GSE) can be utilized to treat MS and EAE (60–63). Currently, a few polarizing therapies for MS are available on the market. These therapies primarily consist of two types of substances: proteins and peptides, such as interferon beta and ethyl acetate, and small molecules, such as glucocorticoids, dimethyl fumarate, and fingolimide/sibonimide (48). Many of these have been shown to indirectly modulate the polarization of macrophages and thus can be used as drug candidates to treat MS. Despite the fact that numerous medications have been discovered to treat MS and EAE, neither of them is yet fully curable. Thus, deeper study is required to find effective clinical treatments for MS and EAE in order to eradicate this terrible illness as soon as feasible. In addition, natural compounds are also a candidate that can be used to regulate the polarization of macrophages, and more experiments are needed to verify clinical drugs for the treatment of MS.
Autoimmune hepatitis (AIH) is a chronic inflammatory disease of the liver associated with the immune system, characterized by elevated levels of transaminases and immunoglobulin G (64). It was first defined as a chronic hepatitis in young women, but in recent years, the incidence of AIH has increased, the age distribution has widened, and AIH has also surfaced in the children (65, 66). Depending on the type of antibody it expresses, AIH can be divided into two classes: AIH-1 (positive for anti-nuclear antibodies or anti-smooth muscle antibodies) and AIH-2 (positive for liver-renal microsomal antibodies type 1, anti-LkM3 or anti-liver cytoplasmic antibodies type 1) (67). Clinically, AIH has no clear particular symptoms and may even exhibit subclinical features; nonetheless, if the initial start of AIH is not treated promptly, it is very simple to progress to liver failure or hepatocellular carcinoma (65, 68, 69). Despite being an extremely damaging autoimmune illness, the pathophysiology of AIH is still unknown due to its clinical variety (70). According to study findings, macrophages can initiate and control the inflammatory response of the liver, and persistent activation of macrophages can lead to liver disease, particularly in portal vein infiltration, where macrophage accumulation in AIH is strongly associated (71–73).
The histological characteristic of AIH known as interfacial hepatitis is caused by the Infiltration of monocytes, including macrophages. This highlights the significance of macrophages in AIH. The disruption of immunological homeostasis leading to Th0 cell development into Th1 cells significantly enhances macrophage activation. In AIH, more macrophages are activated, particularly in AIH-1, and these macrophages are primarily M1-like (74–76). It was found that macrophages showed aberrant activation in liver inflammatory disorders by identifying the macrophage activation marker soluble CD163, during acute AIH, the level of sCD163 was significantly increased, suggesting that macrophages play a role in the inflammatory process of AIH, and sCD163 may also serve as a marker for subsequent AIH targeting macrophage data (77). Although there has been research on macrophage activation in AIH, there are currently few publications on the relationship between macrophage polarization and the development and treatment of AIH. In the mouse experiment, zVAD was found to alter AIH through changing macrophage death, demonstrating the importance of macrophages in AIH (78). A potential therapeutic target for AIH is Receptor-interacting protein kinase 3 (RIP3), which can contribute to macrophage activation in AIH and promote IL-6 production, in addition, enhancer of zeste homolog 2 (EZH2) -mediated H3K27me3 may also influence the progression of AIH by modulating the polarization of macrophages, EZH2 is also promising as a therapeutic target (79, 80). Furthermore, studies have shown that polyguanine (PolyG) can bind to macrophage receptors with collagen structure(MARCO), and that polyG therapy can regulate MARCO, reduce M1-like macrophage polarization, and increase M2-like macrophage polarization, thereby linking AIH, MARCO can also become a target for AIH treatment (81). Interleukin is a frequent cytokine associated with a variety of diseases. IL-34, a member of the interleukin family, was reported to stimulate M2-like polarization of macrophages to suppress AIH in Concanavalin A-induced AIH (82).
The therapy of autoimmune hepatitis has not been investigated since the pathophysiology of the disease, particularly the unique involvement of macrophages in AIH, is not fully known. Since it has been demonstrated that IKK/NF-κB signaling activates chronic liver inflammation mediated by macrophages, medications that regulate the liver NF-κB system are a desirable therapeutic option to stop the onset of certain chronic liver disorders (72, 76). However, the importance of macrophages cannot be understated. They play a role not only in autoimmune hepatitis but also in other liver diseases. When a macrophage problem arises, such as in macrophage activation syndrome, it can cause liver enlargement, persistent fever, and damage to liver cells through the release of different cytokines (76). It’s clear that macrophages are involved in liver illnesses, and treating AIH using macrophages is a promising area for further investigation.
Inflammatory bowel disease (IBD), which consists of Crohn’s disease (CD) and ulcerative colitis (UC), is a major medical challenge throughout the world, particularly in Asia, where the number of cases has increased dramatically in recent years (83, 84). UC most frequently impacts the colon and rectum, whereas CD primarily affects the small intestine and terminal colon (85). Autophagy, genetic element, as well as environmental variables such as smoking, nutrition, medicines, and intestinal microbiota, all have an influence on the development of IBD (84). IBD is most frequent in teenage adolescents and early adults, where it is typically diagnosed, and is becoming more common in youngsters (86). The earliest clinical manifestations of IBD in children and adolescents mostly include stomach discomfort, diarrhea, rectal bleeding, perianal disease, and other symptoms that have a major influence on children’s growth (86, 87). Changes in macrophage homeostasis can affect IBD, such as sphingosine 1-phosphate receptor 2 (S1PR2) and its downstream G protein RhoA/Rho kinase 1 (ROCK1) signaling pathway can aggravate IBD by regulating polarization to M1-like macrophages, in addition, Yes-related protein (YAP) can inhibit M2-like macrophages and promote the production of IL-6 in M1-like macrophages to regulate the pathological process of IBD (88, 89). Instead, under the influence of diosgenin and TNF-α, respectively, miR-125a-5p and miR-24-3p can enhance the polarization of M2-like macrophages, relieving IBD (90, 91). In this review, we summarized the mechanism of macrophage polarization in CD and UC, as well as some clinical applications.
Crohn’s disease, a chronic inflammatory illness of the gastrointestinal system in humans, may cause lesions ranging from the mouth to the anus, as well as parenteral complications (92, 93). Since the 1960s, the pathophysiology of Crohn’s disease has been thought to be due to dysfunction of macrophages. Early in the inflammatory process, macrophages take absorb antigens, produce cytokines, and when their function is compromised, they are prominent in granulomas that serve as indicators for CD (94). Furthermore, in the presence of mucosal granuloma, M1-like macrophages are much more expressed in CD patients, suggesting that studying the innate immune system is more favorable to discovering the pathogenic mechanism of CD (95). According to research, the pathophysiology of CD is more likely to be associated to innate immune system abnormalities in the intestinal mucosa. The current findings imply that Crohn’s disease-associated Escherichia coli has been shown to block NF-κB signaling and thrive in macrophages (96). Infiltrating intestinal macrophages may generate significant levels of CD14 and other components, as well as activate NFκB and release molecules that promote inflammation, which leads to CD (97). In addition, 1,25 vitamin D has been demonstrated to serve as a CD14 ligand, inhibiting the generation of pro-inflammatory molecules (97). A protective function for protein tyrosine phosphatase non-receptor type 22 (PTPN22) in CD has also been shown by other investigations. Through its own inhibitory action, PTPN22 can increase the expression in M2-like macrophages and inhibit the expression in M1-like macrophages. It may also be controlled by AKT, and further research is still needed to determine its impact on macrophage polarization in CD (98).
Ulcerative colitis is a chronic illness of the intestinal mucosa that begins in the rectal area and progresses through the colon to the proximal end (99).The main clinical symptoms of UC are hematochezia, diarrhea and abdominal pain (100). UC affects both men and women equally and tends to be highly localized, occurring more commonly in Western nations. Many factors contribute to UC, including genetic factors and environmental variables, such as health problems from the environment, which promote changes in gut flora, which in turn contribute to UC (99, 101). The polarization imbalance of macrophages is a further significant factor in UC, and more and more research is being done on modulating macrophage polarization to relieve or cure UC.
In Crohn’s disease, the innate immune response exerts a greater impact compared to adaptive immunity due to the ability of macrophages to interact with T cells and initiate adaptive immune responses. The interaction between macrophages and T cells leads to the production of different cytokines that activate M1 and M2 macrophages (102). Additionally, individuals with Crohn’s disease often experience intestinal fibrosis, which is primarily caused by CD-associated adhering invasive Escherichia coli (AIEC), a pathogenic factor that targets macrophages as its main host cells (102, 103). AIEC exacerbates Crohn’s disease by specifically targeting macrophages. However, although M1-like macrophage polarization has been reported primarily in patients with CD (95), studies have shown that simple categorization of macrophages into M1 or M2 phenotypes does not fully explain their role in Crohn’s disease; these two types of macrophages are not mutually exclusive but rather coexist within the affected tissues. Reprogramming macrophages toward an M2 phenotype may not only fail to reduce the presence of M1 macrophages but could potentially worsen the disease progression (98, 102). Therefore, further research is needed to elucidate the mechanisms underlying the involvement of macrophages in Crohn’s disease and develop effective treatment strategies.
Several drugs have been found to improve Crohn’s disease in mice. For example, Loganin, a bioactive iridoid glycoside, was orally administered to mice and was found to alleviate various pathological abnormalities. Subsequent studies revealed that Loganin could significantly reduce the number of M1 macrophages in mice by regulating the sirt1/NFkB signaling pathway (104). Another example is Tiliroside, which promotes the proteasomal degradation of HIF-1α and down-regulates HIF-1α-dependent glycolytic enzyme mRNA expression in macrophages, thereby regulating M1/M2 macrophage polarization (105).Wu-Mei-Wan, Dioscin, and Shaoyao have all been reported to relieve ulcerative colitis by suppressing M1-like macrophage polarization by different study teams (106–109).
Macrophages play a significant role in the pathogenesis of many autoimmune disorders, and an unregulated or excessive immune response is thought to be a primary pathogenic component. The development of many autoimmune disorders is associated with the change of two types of macrophages. As a result, new therapeutic strategies targeting macrophage populations can help protect or mitigate a variety of autoimmune diseases; however, because the detailed mechanisms of treatment for some of these diseases are unknown, additional research is required to determine whether subsequent treatments targeting macrophages are a first choice for clinical treatment.
Atherosclerosis is a chronic arterial wall disease that includes both innate and adaptive immune responses. It is the major cause of many cardiovascular disorders, including coronary artery disease, carotid artery disease, and peripheral artery disease. All phases of atherosclerosis are characterized by inflammation (110, 111). Inflammation has a role in the onset and progression of atherosclerosis. Endothelial damage, lipid metabolism abnormalities, and other issues contribute to the early stages of atherosclerosis (112). When endothelial cells are stimulated, inflammatory substances such as interleukin-8, intercellular adhesion molecule-1 (ICAM-1), and vascular adhesion molecule-1 (VCAM-1) are produced, which attract inflammatory macrophages and monocytes into the artery wall, causing inflammation (113). Monocytes that enter blood vessels differentiate into macrophages, which can transform into foam cells upon absorbing oxidized low-density lipoprotein (ox-LDL), potentially resulting in apoptosis. Subsequently, as foam cells accumulate near epithelial cells, they gradually develop into atherosclerotic plaques (114).Macrophages, as important players in inflammation and immune responses, also play an important role in atherosclerosis.
The atherosclerotic necrotic core, initiated by the aggregation of foam cells, eventually forms due to subsequent inflammation. This inflammation releases more pro-inflammatory chemicals, continuously recruits additional monocytes, and directs macrophages to the lesion site (115–117). Atherosclerosis deteriorates because to the continual secretion of mechanism metalloproteinases by a significant number of macrophages in advanced atherosclerosis. MMPs destroy collagen fibers, causing plaque rupture, hemorrhage, and thrombosis (113, 118–120). Figure 4 mainly shows a progression of atherosclerosis, in which M1 plays a major role in the deterioration process, while M2-like macrophages play a role in inhibiting plaque formation. The number of M2-like macrophages decreases gradually as the disease progresses (114, 121).
Figure 4 The mechanism of macrophage polarization in atherosclerosis. The mechanism of macrophage polarization in atherosclerosis. In the early stage of atherosclerosis, endothelial cells secrete ICAM-1 and VCAM-1 after stimulation to recruit monocytes and macrophages. Monocytes will gradually differentiate into M1 and M2-like macrophages. M1 and M2 macrophages transform into foam cells after uptake of ox-LDL, which can lead to cell death. In the advanced stage of atherosclerosis, macrophages secrete MMP, which leads to the destruction of collagen fibers, plaque rupture and bleeding, when collagen fibers are degraded, resulting in thrombosis.
M1-like macrophages can be found in both early and late atherosclerosis. Many factors related to atherosclerosis, such as epigenetic modification, miRNA, autophagy and metabolic reprogramming, can also affect the polarization of macrophages (121). Plaques do not play a significant part in the condition, and the final cause of mortality is plate buildup, which leads to blood vessel obstruction. Macrophages are the most significant inflammatory cells in plaques, and the M1/M2 ratio regulates plaque growth and durability. Some investigations have shown that macrophage polarization can contribute to plaque development, providing a scientific foundation for atherosclerosis immunotherapy (122).
Because of the specialization of macrophages in the hardening of the central arteries, treating atherosclerosis by targeting macrophage polarization is a prudent strategy. Many chemicals or therapeutic treatments, including traditional Chinese medicine, have been discovered to be utilized or considered as prospective medications for the treatment of atherosclerosis by targeting macrophage polarization (123, 124). In vivo experiments in rats showed that Crocin can reduce oxidized low-density lipoprotein and inhibit atherosclerosis by inducing polarization of M2-like macrophages through vitamin D3 (125). Furthermore, ginsenosides Rb1 and Rg3 can reduce atherosclerosis by encouraging the polarization of M2-like macrophages (126). Other statins, such as sitagliptin and liraglutide for type 2 diabetes, or asperlin, have been demonstrated to reduce M1 macrophages following oral administration and are both useful in the treatment of atherosclerosis (121, 127, 128). The PI3K/Akt signaling pathway influences macrophage survival and proliferation, among other things. As a result, several research teams investigated the role of the signaling system in atherosclerosis and discovered that inhibitor therapy stimulates macrophage autophagy and greatly lowers atherosclerotic plaques in the early stage (129). Another study discovered that arsenic trioxide promotes macrophage autophagy through regulating ROS-dependent TFEB nuclear translocation and the AKT/mTOR pathway, lowering the risk of atherosclerosis (130). Other drugs that have been clinically proven to treat other diseases have also been found to regulate the phenotype of macrophages to treat atherosclerosis. For example, Kallistatin (a tissue kallikrein binding protein and serine protease inhibitor) can decrease M1-like macrophages (127, 131, 132). Many compounds that have an inhibitory effect on atherosclerosis are potential drugs for its treatment.
Based on preclinical models, plaque macrophages—the majority of plaque immune cells and the central cells of atherosclerosis—have positive effects by either decreasing the uptake of atherosclerotic plaques by macrophages, encouraging macrophage apoptosis, or polarizing macrophages more toward the M2 phenotype. It is desired to distribute therapeutic drugs selectively so they can directly modify the composition of macrophages. However, the degree and stage of the illness may determine how these therapies affect the disease’s course; if atherosclerosis is very severe, these treatments may have little or no effect.
Allergic disorders may affect people of all ages, from babies to the elderly, and they frequently have a hereditary component. Rapid allergic reaction is the most prevalent form of allergic illness; its primary categories include cutaneous allergic reaction, respiratory allergic reaction, digestive allergic reaction, and anaphylactic shock. Among these allergic diseases, the most common ones include allergic rhinitis, allergic dermatitis, allergic asthma, and anaphylactic shock.
Allergic rhinitis (AR) is a highly prevalent condition that affects both adolescents and adults, and there is a hereditary component to the sickness. Allergic rhinitis, caused by the response of immunoglobulin E (IgE) to inhaled allergens, is one of the most common chronic diseases worldwide and is a very common chronic disease in high-income countries, with incidence of as high as 50% (133). It is also frequently associated with other conditions such as asthma, sinusitis, and conjunctivitis, all of which can impose a significant burden on patients, have a significant impact on learning, sleep, and quality of life, and have a significant economic impact on education, productivity, and the use of health care resources (134, 135).
A significant level of IL-4, IL-5, TNF-α, and other expressions are observed in allergies and seasonal rhinitis. These cytokines or inflammatory factors may originate from various cells, but they all contribute to the progression of inflammation (136). Macrophages may be implicated in the etiology of AR, and two non-coding RNAs have been discovered to influence macrophage polarization in allergic rhinitis by distinct study teams. The first research discovered that lncRNA-MIR222HG was considerably down-regulated in AR, and that it triggered the TRAF6/IKK/IB/P65 signaling pathway by targeting miR146a-5p, reducing M2-like macrophage polarization in AR and promoting AR development (137). Another study discovered that the lncRNA GAS5 blocked autophagy mediated by mTORC1/ULK1/ATG13 and activated the NF-kB signaling pathway, boosting M1 macrophage polarization and exacerbating allergic rhinitis (138).
Atopic dermatitis, a chronic inflammatory dermatitis characterized by eczema lesions and extreme itching, affects people of all ages and ethnicities and is a major contributor to the global burden of skin disorders (139). Atopic dermatitis affects roughly 20% of young children, and because it is difficult to entirely treat, it places a significant social and psychological burden on patients and families (139). Atopic dermatitis is also linked to an increased risk of a variety of comorbidities, including allergic rhinitis, food allergies, mental health issues, and asthma (140).
A high level of macrophage infiltration is linked to the development of atopic dermatitis, and M2-like macrophages also play an essential role in atopic dermatitis therapy (141). As a result, some research has discovered medications that can cure or alleviate atopic dermatitis by concentrating on the unique anti-inflammatory function of M2-like macrophages. In vitro, Hsa_circ_0004287 can enhance the ubiquitination degradation of S100A8/S100A9 in an M6A-dependent manner, therefore limiting the p38/MAPK pathway and, eventually, reducing the activation of M1-like macrophages, relieving skin inflammation in atopic dermatitis animals (142). Furthermore, many investigations have indicated that the flavonoids Naringenin and Diosmetin help reduce atopic dermatitis via influencing macrophage polarization. Naringenin can suppress M1-like macrophages and increase their polarization to M2, but Diosmetin can prevent macrophages from entering atopic dermatitis areas (143, 144). Another study found that Viola yedoensis Makino formula alleviates atopic dermatitis by activating JAK2/STAT3 signaling pathway and so on promoting M2 macrophages polarization (141).
Asthma is the most prevalent chronic respiratory illness, with allergic asthma being the most frequent kind, which is often defined by environmental allergens and it is characterized by bronchospasm and inflammatory infiltration (145). Allergic asthma has a far younger average age of start than other forms of asthma, accounting for over 90% of asthma cases in children and half of asthma cases in adults (146). Allergic asthma is defined by the presence of specific IgE sensitization to one or more aeroallergens, as well as proof of an allergen as the primary cause of asthma symptoms and management (147).
Macrophages constitute a significant fraction of immune cells in the lungs and play a vital role in asthma and other respiratory disorders induced by environmental causes. ATP6V0d2, a subunit of ATP6V0D2 that is abundantly expressed in macrophages, has been demonstrated to operate as an induced feedback inhibitor of asthma disease severity by boosting Pu.1 lysosomal degradation, which may contribute to the polarization of activated macrophages (148). When exposed to an allergen, the tissue adapts to its surroundings, polarizing the macrophages. Following an allergic reaction, M1-like macrophages produce cytokines and chemokines that help pathogen clearance and the recruitment of T and B cells. According to research, M1-like macrophages have greater expression levels in non-allergic asthma and are linked to severe asthma (149). On the contrary, M2-like macrophages, which are thought to be the primary macrophage in allergic asthma, have a stronger association (149). According to research, the LncRNA AK089514/miR-125b-5p/TRAF6 axis can enhance M2 macrophage polarization, which is important in allergic asthma. This also suggests a treatment target for allergic asthma (150).
Given the unique nature of M2 macrophages in allergic asthma, many studies have made efforts to identify substances that can be used to treat or alleviate allergic asthma by altering the polarization of M2 like macrophages, including glycosidic acid binding oligomeric domain like receptor protein 3 (NLRP3), autologous motion factor receptors (AMFR), and bone marrow-specific loss of fatty acid binding protein 5 (FABP5), which have been shown to promote the polarization of M2 like macrophages, thus achieving the effect of alleviating allergic asthma and providing treatment strategies for the prevention and treatment of allergic asthma (151–153).
Other allergic diseases, such as anaphylactic shock, occur very quickly and violently. Currently, there are few studies on the polarization of macrophages in these allergic diseases, which is also a direction of future research on allergic diseases.
Since the beginning of the twenty-first century, diabetes has been a severe worldwide public health issue. While the number of patients is increasing steadily in developed countries, it is accelerating virtually uncontrollably in developing nations (154). Type 1 diabetes (T1DM), also known as insulin-dependent diabetes, and type 2 diabetes (T2DM), also known as non-insulin-dependent diabetes, are the two primary kinds of the disease (155). T2DM is the most prevalent type of diabetes, accounting for around 90% of all patients, and it is predicted that 439.9 million people will have T2DM by 2030 (156). Nowadays, people’s lives have improved tremendously as a result of the fast growth of society and the economy. Eating habits heavy in salt, sugar, and fat have significantly raised the risk of diabetes, and people are more prone to suffer from T2DM owing to circadian rhythm disruption (157–159). Obesity, traditionally described as unhealthy weight caused by excess fat, is a disorder that is closely associated to diabetes. It has been confirmed that visceral obesity is closely related to insulin resistance, and insulin resistance caused by severe obesity can lead to metabolic disorders and even increase the risk of cardiovascular disease (160). M1 macrophages are known to be mostly glycolytic, whereas M2 macrophages are associated with TCA cycling and mitochondrial oxidative phosphorylation (161). As consequently, T2DM and obesity are metabolic disorders that unavoidably have a close association with macrophage polarization.
Type 2 diabetes is highly associated to inflammation as a chronic illness, and the occurrence and development of T2DM is closely tied to patients’ daily high-sugar diet, which also leads to various T2DM comorbidities such as periodontitis, obesity, and so on. T2DM increases the risk and severity of periodontitis substantially. Previous research has shown a high number of complement components in diabetic chronic problems. In vivo experiments in mice revealed that complement 3 (C3) is overactivated in T2DM-related periodontitis. C3 can participate in the immune inflammatory response, activating the up-regulation of IL-1, IL-6, and TNF-α, thereby activating M1 macrophages and inhibiting M2 macrophages. Periodontitis induced by T2DM can be avoided by lowering the concentration of C3 (162, 163). Other research has discovered that macrophages concentrate in the adipose tissue of obese patients, and obesity is linked to insulin-resistant T2DM. Weight increase causes local aggregation of inflammation and chemokines, as well as polarization of recruited monocytes into M1-like macrophages, enhancing adipose tissue dysfunction and impairing glucose tolerance, hence encouraging the incidence and progression of T2DM (164, 165). M1-like macrophages in T2DM and obese patients can produce lactic acid by promoting glycolysis rather than converting pyruvate to acetyl-CoA, whereas M2-like macrophages produce energy-rich molecules such as ATP via fatty acid oxidation and oxidative phosphorylation, and participate in the down-regulation of tissue repair and inflammation (166, 167).
Experiments in mice demonstrated that high-intensity interval training (HIIT) can reduce the polarization of M1-like macrophages, which is one of the primary mechanisms of M2-like macrophage polarization, and can therefore improve liver inflammation and lipid metabolism disorders in mice with T2DM. HIIT also demonstrated that mRNA expression levels of ROR and KLF4 were highly elevated in mice (168). Additionally, activation of the prostaglandin E2 receptor EP4 can shift the polarization of adipose tissue macrophages to an anti-inflammatory M2-like phenotype, limiting the inflammatory response of islets and maintaining beta cell function (169). Incidentally, in mice, Hyperoside was also found to inhibit T2DM-induced diabetic nephropathy by promoting the formation of M2 macrophages in mice (170).
Obesity is increasingly prevalent in humans and is closely associated with the innate immune system. However, the mechanism of macrophage polarization in obesity differs significantly from that in type 2 diabetes mellitus (T2DM). Adipose tissue is susceptible to various inflammatory conditions in obese individuals as it serves as a major energy storage organ and a key site for inflammatory responses (171, 172). In obese patients, macrophages account for half of the innate immune cells in adipose tissue and are more likely to be polarized into M1-like macrophages. IL-1β, which is released by macrophages, can operate as an inflammatory cytokine, activating the NF-κB signaling pathway, phosphorylating the serine of insulin receptors, causing insulin resistance, and further promoting the progression of hyperglycemia in the body (171–174) (Figure 5). Moreover, studies have also found that miR-34a secreted by adipocytes can inhibit M2 polarization by inhibiting the expression of Kruppel-like factor 4 (Klf4), thus promoting obesity-induced inflammation (175). Recently, it has been found that autophagy can regulate immune response and macrophage polarization, while obesity can reduce the level of autophagy, make the polarization abnormal, and up-regulate the inflammatory response. It can be seen that obesity has a great impact on inflammatory diseases (175, 176).
Figure 5 The role of macrophage polarization in obesity and T2DM. In normal people, fat cells are smaller, and the main macrophages are M2-like macrophages. When the body weight increases, the size of fat cells also increases, and M1-like macrophages are mainly in this case, and inflammation will secrete IL-1, IL-6 and other substances, which further promote the production of M1-like macrophages, but inhibit M2-like macrophages. It also produces IL-1β, which activates the NFkB pathway, leads to insulin resistance, and further promotes obesity or T2DM.
Some studies have also found ways to alleviate obesity and T2DM by controlling or regulating the polarization of macrophages. For example, IL-25 can stimulate the polarization of M2-like macrophages, thereby promoting mitochondrial respiratory ability and lipolysis in adipose tissue to combat obesity (177). Sodium-glucose cotransporter (SGLT) 2 inhibitors were also found to improve obesity-related insulin resistance by regulating the balance of M1/M2 macrophages (178). In addition to this, activation of the SCAP-SREBP-1a pathway in macrophages may provide a novel therapeutic strategy to improve obesity by controlling cholesterol homeostasis in adipose tissue macrophages (179). At the same time, since obesity can also cause many complications, growth arrest-specific 6 (GAS6) can restore the phagocytic ability of macrophages and reduce the level of TUNEL and Caspase-3 positive cells, maintain cartilage thickness and prevent the progression of obesity-related arthritis. Therefore, targeting macrophage-associated ectocytosis or intraarticular injection of GAS6 is a potential therapeutic strategy for obesity-related OA (180).
Elevated glucose levels have a direct impact on macrophage polarization toward M1, and they can also stimulate the production of pro-inflammatory markers in macrophages. A high glucose concentration has a direct impact on macrophage polarization toward the M1 phenotype. With regard to the treatment and prevention of diabetes mellitus and its complications, the method of causing tissue macrophages to repolarize from a pro-inflammatory M1 phenotype to an anti-inflammatory healing M2 phenotype offers substantial potential.
Metabolic homeostasis refers to the state in which the levels of intracellular metabolic substances, enzyme activity, and energy quality remain stable over time. Various factors such as environmental conditions, diet, physiological state, and other external influences play a crucial role in maintaining intracellular metabolic balance (181). In vivo, metabolic homeostasis is largely determined by the balance of metabolic pathways such as glycogen and glucose metabolism, and it is also linked to obesity and diabetes. The alternating activation and maintenance of relative balance of M1 and M2-like cells contributes to the maintenance of tissue homeostasis and host defense (182). Obesity is linked to metabolic homeostasis because numerous foods can directly engage in the signaling pathway of inflammation, and excess nutrition can readily trigger inflammation (182).
Numerous cytokines have been identified to impact cell metabolism and macrophage polarization, thereby playing a significant role in maintaining cellular homeostasis. Signal transducer and activator of transcription 6 are transcription factors that are activated by IL-4 receptor signaling. It can improve mitochondrial oxidative metabolism and hence macrophage activation by inducing the expression of peroxisome proliferator-activated receptor-coactivator 1 (183). Furthermore, Kruppel-like factor 4 can increase M2-like macrophage polarization, hence enhancing metabolic homeostasis (184). Beige fat in the human body may use energy while also resisting cold and obesity. IL-25 has been shown in studies to stimulate the production of Beige fat in cold environments, which has benefits for maintaining metabolic homeostasis, and IL-25 can cause the creation of Beige fat by alternatively activating M1 and M2 macrophages (185). It is clear that macrophage polarization is vital in metabolic balance. Other research has discovered that glucocorticoid receptor interaction protein (GRIP) 1, a major regulator of immune metabolism, can also govern macrophage polarization via several transcriptional pathways, promoting metabolic equilibrium (186).
In the human body’s metabolic organs, parenchymal and stromal cells play a role in preserving metabolic equilibrium. Macrophages are triggered in the body when there is an imbalance in nutrition due to bacterial and viral infections or symptoms like obesity. Obesity and type 2 diabetes are tightly linked to metabolic homeostasis. On the other hand, non-obese people have more M2 macrophages than M1 macrophages, and weight reduction is associated with the conversion of M1 to M2. Therefore, metabolic homeostasis can be regulated through the regulation of macrophages, but if you can lose weight with reasonable exercise at the beginning, you can reduce the inflammation of the body. If it is already very serious, weight loss coupled with drug treatment will also have a good effect.
Macrophages are myeloid cells involved in innate immune responses, which originate from monocyte precursors in the blood and play a key role in tissue homeostasis under normal physiological conditions as well as after tissue injury (187). Macrophages are distributed in various tissues across the body and play a critical role in preserving internal balance and combating invading pathogens. Macrophages exhibit diverse morphological and functional characteristics as they undergo polarization in response to specific cytokines and growth factors present in the various tissues they inhabit (188). Macrophages are very heterogeneous and are composed of macrophages from a variety of different sources. So far, studies have found that macrophages can mainly differentiate into two phenotypes, pro-inflammatory M1-like and anti-inflammatory M2-like (5). During the inflammatory process, two types of macrophages undergo alternate transformations, and their proportions constantly change with the progression of the disease. This dynamic shift underscores the crucial role of macrophages in disease pathogenesis, making them an attractive target for therapeutic interventions. In vivo, macrophages have three main functions: phagocytosis, exogenous antigen presentation, and immune regulation. Both kinds of macrophages are stimulated alternatively during the inflammatory process. M1-like macrophages produce more and emit pro-inflammatory signals such as interleukin, restricting cell proliferation and causing tissue damage; subsequently, M2-like macrophages secrete more and play a mending function, aiding in inflammation reduction and wound healing. When the polarization of macrophages in the body is disrupted, it causes an imbalance in the M1/M2 ratio, which worsens inflammation in the body. A huge quantity of pro-inflammatory factors will set off a chain of events that will cause tissue and organ damage in the body (189, 190). As a result, if macrophage polarization can be properly regulated or changed, it will play an essential role in easing or curing a variety of disorders.
This review focuses on the role of macrophage polarization in various inflammatory diseases. The presence of abundant monocytes and macrophages in these diseases clearly underscores the pivotal role that macrophages play in inflammatory conditions. The various activation stages of macrophages are critical for the evolution of inflammation in atherosclerosis, metabolic balance, allergy illnesses, autoimmune disorders, type 2 diabetes, and other inflammatory diseases. In the early stages of many inflammatory disorders, M1-like is prominent, whereas in the later stages, M2-like is dominating. Several natural chemicals or molecules that can promote the polarization of macrophages to M2-like state have been found via ongoing research to regulate macrophage polarization. The polarization of macrophages can be harnessed to cure or alleviate numerous illnesses. Despite studies on macrophages in a variety of inflammatory diseases, research on their role in several allergy disorders remains limited. Many more studies are necessary for a complete understanding these diseases. Thus, future study on the impact of macrophage polarization on inflammatory disorders remains crucial.
Researchers are always looking for medications and chemicals that can modulate macrophage polarization in order to cure or relieve illness. Macrophages are an appropriate target for the remission or treatment of a variety of inflammatory illnesses. Some beneficial Chinese medicines, compounds, or other molecules have been discovered to have a regulatory effect on macrophage polarization. However, further research is required to develop specific drugs for clinical application. Moreover, further investigation is needed into the distinct mechanism of macrophage polarization in different inflammatory disorders. In various diseases, macrophages serve as a specific target, making it crucial and promising to explore the potential of treating other illnesses by modulating the polarization process of macrophages. In the last few decades, a wide range of disease models with inflammatory components—including cancer, joint sclerosis, kidney disease, and autoimmune—have been used to investigate therapeutic diagnostics related to macrophages. Future research will likely focus on using macrophages to cure diseases, so it is imperative that we learn more about the variations in macrophage formation, phenotype, and function. Researching the roles played by various macrophage subtypes in a range of illnesses and cancers can improve our understanding of how diseases arise and progress, influence treatment outcomes, and produce double the impact for half the work.
In recent years, significant progress has been made in the study of macrophages from the aspects of transcriptomics, transgenetics and epigenetics, among which the research results on macrophages in various inflammatory diseases are also of great significance for the clinical treatment of these diseases. However, due to the immature understanding of the mechanism of inflammatory diseases, the research on the treatment of macrophage polarization has been hindered. In addition, some people have proposed miRNA-based treatment methods, but these methods still need some basic research and other theories to verify. Despite the existing challenges in treating macrophage polarization, the extensive potential of this approach is evident from research findings, including validated methods in animal models. Subsequent studies should prioritize gaining a deeper insight into the disease pathology and the dynamics of macrophage polarization within the disease context. It is foreseeable that the clinical application of macrophage-based therapies for inflammatory diseases will undoubtedly become a reality in the future.
ML: Writing – original draft, Writing – review & editing. FZ: Data curation, Formal Analysis, Writing – original draft. HC: Investigation, Writing – original draft. MS: Resources, Writing – original draft. YW: Funding acquisition, Supervision, Visualization, Writing – original draft, Writing – review & editing.
The author(s) declare financial support was received for the research, authorship, and/or publication of this article. This research Supported by the Zunyi City Science and Technology Fund (NO.2023-39).
The authors declare that the research was conducted in the absence of any commercial or financial relationships that could be construed as a potential conflict of interest.
All claims expressed in this article are solely those of the authors and do not necessarily represent those of their affiliated organizations, or those of the publisher, the editors and the reviewers. Any product that may be evaluated in this article, or claim that may be made by its manufacturer, is not guaranteed or endorsed by the publisher.
LPS, lipopolysaccharide; INF-α, interferon-α; A2AR, adenosine 2A receptor; NADPH, niacinamide adenine phosphate dinucleotide; ROS, reactive oxygen species; RA, Rheumatoid arthritis; MHC, major histocompatibility complex; NF-kB, nuclear factor kappa B; SAPK/MAPK, stress-activated protein kinases/mitogen-activated protein kinases; JAK/STAT, Janus kinase/signal transducer and activators of transcription; FA-AgNPS, folic acid modified silver nanoparticles; CTLA4, Cytotoxic T lymphocyte-associated protein 4; BSP, betamethasone sodium phosphate; Exo, exosome; MS, multiple sclerosis; EAE, experimental autoimmune encephalomyelitis; BBB, blood-brain barrier; CNS, Central nervous system; GSE, grape seed extract; AIH, Autoimmune hepatitis; EZH2,enhancer of zeste homolog 2; MARCO, macrophage receptors; IBD, Inflammatory bowel disease; CD, Crohn’s disease; UC, ulcerative colitis; S1PR2, sphingosine 1-phosphate receptor 2; ROCK1, G protein RhoA/Rho kinase 1; YAP, Yes-related protein; PTPN22, protein tyrosine phosphatase non-receptor type 22; LDL, Low-density lipoprotein; AR, Allergic rhinitis; NLRP3, oligomeric domain like receptor protein 3; FABP5, fatty acid binding protein 5; T1DM, Type 1 diabetes; T2DM, type 2 diabetes; C3, complement 3; HIIT, high-intensity interval training.
1. Blériot C, Chakarov S, Ginhoux F. Determinants of resident tissue macrophage identity and function. Immunity. (2020) 52:957–70. doi: 10.1016/j.immuni.2020.05.014
2. Abe C, Bhaswant M, Miyazawa T, Miyazawa T. The potential use of exosomes in anti-cancer effect induced by polarized macrophages. Pharmaceutics. (2023) 15(3):1024. doi: 10.3390/pharmaceutics15031024
3. Atri C, Guerfali FZ, Laouini D. Role of human macrophage polarization in inflammation during infectious diseases. Int J Mol Sci. (2018) 19(6):1801. doi: 10.3390/ijms19061801
4. Mantovani A, Sica A, Sozzani S, Allavena P, Vecchi A, Locati M. The chemokine system in diverse forms of macrophage activation and polarization. Trends Immunol Dec. (2004) 25:677–86. doi: 10.1016/j.it.2004.09.015
5. Yunna C, Mengru H, Lei W, Weidong C. Macrophage M1/M2 polarization. Eur J Pharmacol. (2020) 877:173090. doi: 10.1016/j.ejphar.2020.173090
6. Orecchioni M, Ghosheh Y, Pramod AB, Ley K. Macrophage Polarization: Different Gene Signatures in M1(LPS+) vs. Classically and M2(LPS-) vs. Alternatively Activated Macrophages. Front Immunol. (2019) 10:1084. doi: 10.3389/fimmu.2019.01084
7. Rőszer T. Understanding the mysterious M2 macrophage through activation markers and effector mechanisms. Mediators Inflamm. (2015) 2015:816460. doi: 10.1155/2015/816460
8. Wang LX, Zhang SX, Wu HJ, Rong XL, Guo J. M2b macrophage polarization and its roles in diseases. J Leukoc Biol. (2019) 106:345–58. doi: 10.1002/JLB.3RU1018-378RR
9. Funes SC, Rios M, Escobar-Vera J, Kalergis AM. Implications of macrophage polarization in autoimmunity. Immunology. (2018) 154:186–95. doi: 10.1111/imm.12910
10. Kerneur C, Cano CE, Olive D. Major pathways involved in macrophage polarization in cancer. Front Immunol. (2022) 13:1026954. doi: 10.3389/fimmu.2022.1026954
11. Gao J, Liang Y, Wang L. Shaping polarization of tumor-associated macrophages in cancer immunotherapy. Front Immunol. (2022) 13:888713. doi: 10.3389/fimmu.2022.888713
12. Xu F, Wei Y, Tang Z, Liu B, Dong J. Tumor−associated macrophages in lung cancer: Friend or foe? (Review). Mol Med Rep. (2020) 22:4107–15. doi: 10.3892/mmr.2020.11518
13. Silveira LS, Antunes Bde M, Minari AL, Dos Santos RV, Neto JC, Lira FS. Macrophage polarization: implications on metabolic diseases and the role of exercise. Crit Rev Eukaryot Gene Expr. (2016) 26:115–32. doi: 10.1615/CritRevEukaryotGeneExpr.2016015920
14. Italiani P, Boraschi D. From monocytes to M1/M2 macrophages: phenotypical vs. Functional differentiation. Front Immunol. (2014) 5:514. doi: 10.3389/fimmu.2014.00514
15. Gordon S, Martinez FO. Alternative activation of macrophages: mechanism and functions. Immunity. (2010) 32:593–604. doi: 10.1016/j.immuni.2010.05.007
16. Wynn TA, Chawla A, Pollard JW. Macrophage biology in development, homeostasis and disease. Nature. (2013) 496:445–55. doi: 10.1038/nature12034
17. Shapouri-Moghaddam A, Mohammadian S, Vazini H, Taghadosi M, Esmaeili SA, Mardani F, et al. Macrophage plasticity, polarization, and function in health and disease. J Cell Physiol. (2018) 233:6425–40. doi: 10.1002/jcp.26429
18. Horwood NJ. Macrophage polarization and bone formation: A review. Clin Rev Allergy Immunol. (2016) 51:79–86. doi: 10.1007/s12016-015-8519-2
19. Lai X, Zhong J, Zhang B, Zhu T, Liao R. Exosomal non-coding RNAs: novel regulators of macrophage-linked intercellular communication in lung cancer and inflammatory lung diseases. Biomolecules. (2023) 13:536. doi: 10.3390/biom13030536
20. Glover K, Mishra D, Singh TRR. Epidemiology of ocular manifestations in autoimmune disease. Front Immunol. (2021) 12:744396. doi: 10.3389/fimmu.2021.744396
21. Wang L, Wang FS, Gershwin ME. Human autoimmune diseases: a comprehensive update. J Internal Med. (2015) 278:369–95. doi: 10.1111/joim.12395
22. Zhang Y, Liu J, Wang C, Liu J, Lu W. Toll-like receptors gene polymorphisms in autoimmune disease. Front Immunol. (2021) 12:672346. doi: 10.3389/fimmu.2021.672346
23. Rose NR. Prediction and prevention of autoimmune disease in the 21st century: A review and preview. Am J Epidemiol. (2016) 183:403–6. doi: 10.1093/aje/kwv292
24. Triantafyllopoulou A, Franzke CW, Seshan SV, Perino G, Kalliolias GD, Ramanujam M, et al. Proliferative lesions and metalloproteinase activity in murine lupus nephritis mediated by type I interferons and macrophages. Proc Natl Acad Sci USA. (2010) 107:3012–7. doi: 10.1073/pnas.0914902107
25. Steinbach EC, Plevy SE. The role of macrophages and dendritic cells in the initiation of inflammation in IBD. Inflammatory bowel diseases. (2014) 20:166–75. doi: 10.1097/MIB.0b013e3182a69dca
26. Su Z, Zhang P, Yu Y, Lu H, Liu Y, Ni P, et al. HMGB1 facilitated macrophage reprogramming towards a proinflammatory M1-like phenotype in experimental autoimmune myocarditis development. Sci Rep. (2016) 6:21884. doi: 10.1038/srep21884
27. Firestein GS. Evolving concepts of rheumatoid arthritis. Nature. (2003) 423:356–61. doi: 10.1038/nature01661
28. Guo Q, Wang Y, Xu D, Nossent J, Pavlos NJ, Xu J. Rheumatoid arthritis: pathological mechanisms and modern pharmacologic therapies. Bone Res. (2018) 6:15. doi: 10.1038/s41413-018-0016-9
29. Yang Y, Guo L, Wang Z, Liu P, Liu X, Ding J, et al. Targeted silver nanoparticles for rheumatoid arthritis therapy via macrophage apoptosis and Re-polarization. Biomaterials. (2021) 264:120390. doi: 10.1016/j.biomaterials.2020.120390
30. Hoes JN, Jacobs JW, Buttgereit F, Bijlsma JW. Current view of glucocorticoid co-therapy with DMARDs in rheumatoid arthritis. Nat Rev Rheumatol. (2010) 6:693–702. doi: 10.1038/nrrheum.2010.179
31. Cutolo M, Campitiello R, Gotelli E, Soldano S. The role of M1/M2 macrophage polarization in rheumatoid arthritis synovitis. Front Immunol. (2022) 13:867260. doi: 10.3389/fimmu.2022.867260
32. Smolen JS, Aletaha D, Koeller M, Weisman MH, Emery P. New therapies for treatment of rheumatoid arthritis. Lancet (London England). (2007) 370:1861–74. doi: 10.1016/s0140-6736(07)60784-3
33. Udalova IA, Mantovani A, Feldmann M. Macrophage heterogeneity in the context of rheumatoid arthritis. Nat Rev Rheumatol. (2016) 12:472–85. doi: 10.1038/nrrheum.2016.91
34. Arango Duque G, Descoteaux A. Macrophage cytokines: involvement in immunity and infectious diseases. Front Immunol. (2014) 5:491. doi: 10.3389/fimmu.2014.00491
35. Takeda K, Akira S. Toll-like receptors. Curr Protoc Immunol. (2015) 109:14.12.1–14.12.10. doi: 10.1002/0471142735.im1412s109
36. Malemud CJ, Miller AH. Pro-inflammatory cytokine-induced SAPK/MAPK and JAK/STAT in rheumatoid arthritis and the new anti-depression drugs. Expert Opin Ther targets. (2008) 12:171–83. doi: 10.1517/14728222.12.2.171
37. Görtz B, Hayer S, Tuerck B, Zwerina J, Smolen JS, Schett G. Tumour necrosis factor activates the mitogen-activated protein kinases p38alpha and ERK in the synovial membrane in vivo. Arthritis Res Ther. (2005) 7:R1140–7. doi: 10.1186/ar1797
38. Cutolo M, Soldano S, Gotelli E, Montagna P, Campitiello R, Paolino S, et al. CTLA4-Ig treatment induces M1-M2 shift in cultured monocyte-derived macrophages from healthy subjects and rheumatoid arthritis patients. Arthritis Res Ther. (2021) 23:306. doi: 10.1186/s13075-021-02691-9
39. Li H, Feng Y, Zheng X, Jia M, Mei Z, Wang Y, et al. M2-type exosomes nanoparticles for rheumatoid arthritis therapy via macrophage re-polarization. J Controlled Release. (2022) 341:16–30. doi: 10.1016/j.jconrel.2021.11.019
40. Vogel DY, Vereyken EJ, Glim JE, Heijnen PD, Moeton M, van der Valk P, et al. Macrophages in inflammatory multiple sclerosis lesions have an intermediate activation status. J neuroinflammation. (2013) 10:35. doi: 10.1186/1742-2094-10-35
41. Murta V, Ferrari CC. Influence of Peripheral inflammation on the progression of multiple sclerosis: evidence from the clinic and experimental animal models. Mol Cell neurosciences. (2013) 53:6–13. doi: 10.1016/j.mcn.2012.06.004
42. Confavreux C, Vukusic S. Accumulation of irreversible disability in multiple sclerosis: from epidemiology to treatment. Clin Neurol neurosurgery. (2006) 108:327–32. doi: 10.1016/j.clineuro.2005.11.018
43. McDonald WI, Compston A, Edan G, Goodkin D, Hartung HP, Lublin FD, et al. Recommended diagnostic criteria for multiple sclerosis: guidelines from the International Panel on the diagnosis of multiple sclerosis. Ann Neurol. (2001) 50:121–7. doi: 10.1002/ana.1032
44. McGinley MP, Goldschmidt CH, Rae-Grant AD. Diagnosis and treatment of multiple sclerosis: A review. Jama. (2021) 325:765–79. doi: 10.1001/jama.2020.26858
45. Jiang F, Liu X, Cui X, Hu J, Wang L, Xue F, et al. Circ_0000518 promotes macrophage/microglia M1 polarization via the FUS/caMKKβ/AMPK pathway to aggravate multiple sclerosis. Neuroscience. (2022) 490:131–43. doi: 10.1016/j.neuroscience.2021.12.012
46. Hendriks JJ, Teunissen CE, de Vries HE, Dijkstra CD. Macrophages and neurodegeneration. Brain Res Brain Res Rev. (2005) 48:185–95. doi: 10.1016/j.brainresrev.2004.12.008
47. Leuti A, Talamonti E, Gentile A, Tiberi M, Matteocci A, Fresegna D, et al. Macrophage plasticity and polarization are altered in the experimental model of multiple sclerosis. Biomolecules. (2021) 11(6):837. doi: 10.3390/biom11060837
48. Kuntzel T, Bagnard D. Manipulating macrophage/microglia polarization to treat glioblastoma or multiple sclerosis. Pharmaceutics. (2022) 14(2):34. doi: 10.3390/pharmaceutics14020344
49. Chu F, Shi M, Zheng C, Shen D, Zhu J, Zheng X, et al. The roles of macrophages and microglia in multiple sclerosis and experimental autoimmune encephalomyelitis. J Neuroimmunol. (2018) 318:1–7. doi: 10.1016/j.jneuroim.2018.02.015
50. Prineas JW, Parratt JDE. Multiple sclerosis: microglia, monocytes, and macrophage-mediated demyelination. J neuropathol Exp Neurol. (2021) 80:975–96. doi: 10.1093/jnen/nlab083
51. Haidar M, Loix M, Vanherle S, Dierckx T, Vangansewinkel T, Gervois P, et al. Targeting lipophagy in macrophages improves repair in multiple sclerosis. Autophagy. (2022) 18:2697–710. doi: 10.1080/15548627.2022.2047343
52. Ortiz GG, Pacheco-Moisés FP, Macías-Islas M, et al. Role of the blood-brain barrier in multiple sclerosis. Arch Med Res. (2014) 45:687–97. doi: 10.1016/j.arcmed.2014.11.013
53. Minagar A, Shapshak P, Fujimura R, Ownby R, Heyes M, Eisdorfer C. The role of macrophage/microglia and astrocytes in the pathogenesis of three neurologic disorders: HIV-associated dementia, Alzheimer disease, and multiple sclerosis. J neurological Sci. (2002) 202:13–23. doi: 10.1016/s0022-510x(02)00207-1
54. Bogie JF, Stinissen P, Hendriks JJ. Macrophage subsets and microglia in multiple sclerosis. Acta neuropathologica. (2014) 128:191–213. doi: 10.1007/s00401-014-1310-2
55. Kamma E, Lasisi W, Libner C, Ng HS, Plemel JR. Central nervous system macrophages in progressive multiple sclerosis: relationship to neurodegeneration and therapeutics. J neuroinflammation. (2022) 19:45. doi: 10.1186/s12974-022-02408-y
56. Ortiz GG, Pacheco-Moisés FP, Macías-Islas MÁ, Flores-Alvarado LJ, Mireles-Ramírez MA, González-Renovato ED, et al. Th1-specific cell surface protein Tim-3 regulates macrophage activation and severity of an autoimmune disease. Nature. (2002) 415:536–41. doi: 10.1038/415536a
57. Cheng Y, Sun L, Xie Z, Fan X, Cao Q, Han J, et al. Diversity of immune cell types in multiple sclerosis and its animal model: Pathological and therapeutic implications. J Neurosci Res. (2017) 95:1973–83. doi: 10.1002/jnr.24023
58. Luo C, Jian C, Liao Y, Huang Q, Wu Y, Liu X, et al. The role of microglia in multiple sclerosis. Neuropsychiatr Dis Treat. (2017) 13:1661–7. doi: 10.2147/ndt.s140634
59. Blonda M, Amoruso A, Grasso R, Di Francescantonio V, Avolio C. Multiple sclerosis treatments affect monocyte-derived microvesicle production. Front neurol. (2017) 8:422. doi: 10.3389/fneur.2017.00422
60. Che J, Li D, Hong W, Wang L, Guo Y, Wu M, et al. Phenotypic screening-based identification of 3,4-disubstituted piperidine derivatives as macrophage M2 polarization modulators: an opportunity for treating multiple sclerosis. J Medicinal Chem. (2019) 62:3268–85. doi: 10.1021/acs.jmedchem.8b01635
61. Che J, Li D, Hong W, Wang L, Guo Y, Wu M, et al. Discovery of new macrophage M2 polarization modulators as multiple sclerosis treatment agents that enable the inflammation microenvironment remodeling. Eur J Medicinal Chem. (2022) 243:114732. doi: 10.1016/j.ejmech.2022.114732
62. Ma X, Wang S, Li C, Jia X, Wang T, Leng Z, et al. Baicalein inhibits the polarization of microglia/macrophages to the M1 phenotype by targeting STAT1 in EAE mice. Int Immunopharmacol. (2022) 113:109373. doi: 10.1016/j.intimp.2022.109373
63. Wang Q, Chen YY, Yang ZC, Yuan HJ, Dong YW, Miao Q, et al. Grape seed extract attenuates demyelination in experimental autoimmune encephalomyelitis mice by inhibiting inflammatory response of immune cells. Chin J Integr Med. (2023) 29:394–404. doi: 10.1007/s11655-022-3587-7
64. EASL Clinical Practice Guidelines. Autoimmune hepatitis. J Hepatol. (2015) 63:971–1004. doi: 10.1016/j.jhep.2015.06.030
65. Lohse AW, Mieli-Vergani G. Autoimmune hepatitis. J Hepatol. (2011) 55:171–82. doi: 10.1016/j.jhep.2010.12.012
66. Strassburg CP. Autoimmune hepatitis. Best Pract Res Clin Gastroenterol. (2010) 24:667–82. doi: 10.1016/j.bpg.2010.07.011
67. Manns MP, Lohse AW, Vergani D. Autoimmune hepatitis–update 2015. J Hepatol. (2015) 62:S100–11. doi: 10.1016/j.jhep.2015.03.005
68. Muratori L, Lohse AW, Lenzi M. Diagnosis and management of autoimmune hepatitis. BMJ (Clinical Res ed). (2023) 380:e070201. doi: 10.1136/bmj-2022-070201
69. Sucher E, Sucher R, Gradistanac T, Brandacher G, Schneeberger S, Berg T. Autoimmune hepatitis-immunologically triggered liver pathogenesis-diagnostic and therapeutic strategies. J Immunol Res. (2019) 2019:9437043. doi: 10.1155/2019/9437043
70. Sahebjam F, Vierling JM. Autoimmune hepatitis. Front Med. (2015) 9:187–219. doi: 10.1007/s11684-015-0386-y
71. Szabo G, Mandrekar P, Dolganiuc A. Innate immune response and hepatic inflammation. Semin liver Dis. (2007) 27:339–50. doi: 10.1055/s-2007-991511
72. Sunami Y, Leithäuser F, Gul S, Fiedler K, Güldiken N, Espenlaub S, et al. Hepatic activation of IKK/NFκB signaling induces liver fibrosis via macrophage-mediated chronic inflammation. Hepatol (Baltimore Md). (2012) 56:1117–28. doi: 10.1002/hep.25711
73. Bartneck M, Fech V, Ehling J, Govaere O, Warzecha KT, Hittatiya K, et al. Histidine-rich glycoprotein promotes macrophage activation and inflammation in chronic liver disease. Hepatol (Baltimore Md). (2016) 63:1310–24. doi: 10.1002/hep.28418
74. Guo LP, Zhou L, Li HX, Zhang J, Wang BM. [The study of liver macrophages polarization in patients with autoimmune hepatitis]. Zhonghua Nei Ke Za Zhi. (2017) 56:763–5. doi: 10.3760/cma.j.issn.0578-1426.2017.10.011
75. Longhi MS, Ma Y, Mieli-Vergani G, Vergani D. Aetiopathogenesis of autoimmune hepatitis. J Autoimmun. (2010) 34:7–14. doi: 10.1016/j.jaut.2009.08.010
76. Tsikrikoni A, Kyriakou DS, Rigopoulou EI, Alexandrakis MG, Zachou K, Passam F, et al. Markers of cell activation and apoptosis in bone marrow mononuclear cells of patients with autoimmune hepatitis type 1 and primary biliary cirrhosis. J Hepatol. (2005) 42:393–9. doi: 10.1016/j.jhep.2004.11.023
77. Grønbaek H, Kreutzfeldt M, Kazankov K, Jessen N, Sandahl T, Hamilton-Dutoit S, et al. Single-centre experience of the macrophage activation marker soluble (s)CD163 - associations with disease activity and treatment response in patients with autoimmune hepatitis. Alimentary Pharmacol Ther. (2016) 44(10):1062–70. doi: 10.1111/apt.13801
78. Li X, Zhang Y, Wang J, Li Y, Wang Y, Shi F, et al. zVAD alleviates experimental autoimmune hepatitis in mice by increasing the sensitivity of macrophage to TNFR1-dependent necroptosis. J Autoimmun. (2022) 133:102904. doi: 10.1016/j.jaut.2022.102904
79. Zhang J, Guo L, Liu M, Jing Y, Zhou S, Li H, et al. Receptor-interacting protein kinase 3 mediates macrophage/monocyte activation in autoimmune hepatitis and regulates interleukin-6 production. United Eur Gastroenterol J. (2018) 6(5):719–28. doi: 10.1177/2050640618756124
80. Chi G, Pei JH, Li XQ. EZH2-mediated H3K27me3 promotes autoimmune hepatitis progression by regulating macrophage polarization. Int Immunopharmacol. (2022) 106:108612. doi: 10.1016/j.intimp.2022.108612
81. Cai T, Xu L, Xia D, Zhu L, Lin Y, Yu S, et al. Polyguanine alleviated autoimmune hepatitis through regulation of macrophage receptor with collagenous structure and TLR4-TRIF-NF-κB signalling. J Cell Mol Med. (2022) 26:5690–701. doi: 10.1111/jcmm.17599
82. Liu Y, Liu H, Zhu J, Bian Z. Interleukin-34 drives macrophage polarization to the M2 phenotype in autoimmune hepatitis. Pathol Res Pract. (2019) 215:152493. doi: 10.1016/j.prp.2019.152493
84. Zhang YZ, Li YY. Inflammatory bowel disease: pathogenesis. World J Gastroenterol. (2014) 20:91–9. doi: 10.3748/wjg.v20.i1.91
85. Pan Y, Wang D, Liu F. miR-146b suppresses LPS-induced M1 macrophage polarization via inhibiting the FGL2-activated NF-κB/MAPK signaling pathway in inflammatory bowel disease. Clinics (Sao Paulo Brazil). (2022) 77:100069. doi: 10.1016/j.clinsp.2022.100069
86. Rosen MJ, Dhawan A, Saeed SA. Inflammatory bowel disease in children and adolescents. JAMA Pediatr. (2015) 169:1053–60. doi: 10.1001/jamapediatrics.2015.1982
87. Oliveira SB, Monteiro IM. Diagnosis and management of inflammatory bowel disease in children. BMJ (Clinical Res ed). (2017) 357:j2083. doi: 10.1136/bmj.j2083
88. Zhou X, Li W, Wang S, Zhang P, Wang Q, Xiao J, et al. YAP aggravates inflammatory bowel disease by regulating M1/M2 macrophage polarization and gut microbial homeostasis. Cell Rep. (2019) 27(4):1176–1189.e5. doi: 10.1016/j.celrep.2019.03.028
89. Wang X, Chen S, Xiang H, Wang X, Xiao J, Zhao S, et al. S1PR2/RhoA/ROCK1 pathway promotes inflammatory bowel disease by inducing intestinal vascular endothelial barrier damage and M1 macrophage polarization. Biochem Pharmacol. (2022) 201:115077. doi: 10.1016/j.bcp.2022.115077
90. Shi L, Zhang P, Jin R, Chen X, Dong L, Chen W. Dioscin ameliorates inflammatory bowel disease by up-regulating miR-125a-5p to regulate macrophage polarization. J Clin Lab Anal. (2022) 36:e24455. doi: 10.1002/jcla.24455
91. Xu H, Fu J, Chen L, Zhou S, Fang Y, Zhang Q, et al. TNF-α Enhances the Therapeutic Effects of MenSC-Derived Small Extracellular Vesicles on Inflammatory Bowel Disease through Macrophage Polarization by miR-24-3p. Stem Cells Int. (2023) 2023:2988907. doi: 10.1155/2023/2988907
92. Veauthier B, Hornecker JR. Crohn’s disease: diagnosis and management. Am Family physician. (2018) 98:661–9.
93. Torres J, Mehandru S, Colombel JF, Peyrin-Biroulet L. Crohn’s disease. Lancet (London England). (2017) 389:1741–55. doi: 10.1016/s0140-6736(16)31711-1
94. Caprilli R, Frieri G. The dyspeptic macrophage 30 years later: an update in the pathogenesis of Crohn’s disease. Digestive liver Dis. (2009) 41:166–8. doi: 10.1016/j.dld.2008.09.012
95. Das P, Rampal R, Udinia S, Kumar T, Pilli S, Wari N, et al. Selective M1 macrophage polarization in granuloma-positive and granuloma-negative Crohn’s disease, in comparison to intestinal tuberculosis. Intestinal Res. (2018) 16(3):426–35. doi: 10.5217/ir.2018.16.3.426
96. Rahman K, Sasaki M, Nusrat A, Klapproth JM. Crohn’s disease-associated Escherichia coli survive in macrophages by suppressing NFκB signaling. Inflammatory bowel Dis. (2014) 20:1419–25. doi: 10.1097/mib.0000000000000096
97. Dionne S, Duchatelier CF, Seidman EG. The influence of vitamin D on M1 and M2 macrophages in patients with Crohn’s disease. Innate Immun. (2017) 23:557–65. doi: 10.1177/1753425917721965
98. Tang Y, Zhao L, Lei N, Chen P, Zhang Y. Crohn’s disease patients with depression exhibit alterations in monocyte/macrophage phenotype and increased proinflammatory cytokine production. Digestive Dis (Basel Switzerland). (2020) 38:211–21. doi: 10.1159/000501122
99. Ordás I, Eckmann L, Talamini M, Baumgart DC, Sandborn WJ. Ulcerative colitis. Lancet (London England). 380(9853):1606–19. doi: 10.1016/s0140-6736(12)60150-0
100. Eisenstein M. Ulcerative colitis: towards remission. Nature. (2018) 563:S33. doi: 10.1038/d41586-018-07276-2
102. Dharmasiri S, Garrido-Martin EM, Harris RJ, Bateman AC, Collins JE, Cummings JRF, et al. Human intestinal macrophages are involved in the pathology of both ulcerative colitis and crohn disease. Inflammatory Bowel Dis. (2021) 27:1641–52. doi: 10.1093/ibd/izab029
103. Chokr D, Cornu M, Neut C, Bortolus C, Charlet R, Desreumaux P, et al. Adherent invasive Escherichia coli (AIEC) strain LF82, but not Candida albicans, plays a profibrogenic role in the intestine. Gut Pathog. (2021) 13(1):5. doi: 10.1186/s13099-021-00401-z
104. Liu S, Shen H, Li J, Gong Y, Bao H, Zhang J, et al. Loganin inhibits macrophage M1 polarization and modulates sirt1/NF-κB signaling pathway to attenuate ulcerative colitis. Bioengineered. (2020) 11(1):628–39. doi: 10.1080/21655979.2020.1774992
105. Zhuang H, Lv Q, Zhong C, Cui Y, He L, Zhang C, et al. Tiliroside ameliorates ulcerative colitis by restoring the M1/M2 macrophage balance via the HIF-1α/glycolysis pathway. Front Immunol. (2021) 12:649463. doi: 10.3389/fimmu.2021.649463
106. Yan S, Wei H, Jia R, Zhen M, Bao S, Wang W, et al. Ameliorates murine ulcerative colitis by regulating macrophage polarization. Front Pharmacol. (2022) 13:859167. doi: 10.3389/fphar.2022.859167
107. Yang Z, Lin S, Feng W, Liu Y, Song Z, Pan G, et al. A potential therapeutic target in traditional Chinese medicine for ulcerative colitis: Macrophage polarization. Front Pharmacol. (2022) 13:999179. doi: 10.3389/fphar.2022.999179
108. Wu MM, Wang QM, Huang BY, Mai CT, Wang CL, Wang TT, et al. Dioscin ameliorates murine ulcerative colitis by regulating macrophage polarization. Pharmacol Res. (2021) 172:105796. doi: 10.1016/j.phrs.2021.105796
109. Wei YY, Fan YM, Ga Y, Zhang YN, Han JC, Hao ZH. Shaoyao decoction attenuates DSS-induced ulcerative colitis, macrophage and NLRP3 inflammasome activation through the MKP1/NF-κB pathway. Phytomed: Int J phytother phytopharmacol. (2021) 92:153743. doi: 10.1016/j.phymed.2021.153743
110. Falk E. Pathogenesis of atherosclerosis. J Am Coll Cardiol. (2006) 47:C7–12. doi: 10.1016/j.jacc.2005.09.068
111. Libby P. The changing landscape of atherosclerosis. Nature. (2021) 592:524–33. doi: 10.1038/s41586-021-03392-8
112. Frostegård J. Immunity, atherosclerosis and cardiovascular disease. BMC Med. (2013) 11:117. doi: 10.1186/1741-7015-11-117
113. Zhu Y, Xian X, Wang Z, Bi Y, Chen Q, Han X, et al. Research progress on the relationship between atherosclerosis and inflammation. Biomolecules. (2018) 8(3):80. doi: 10.3390/biom8030080
114. Boyle JJ, Johns M, Kampfer T, Nguyen AT, Game L, Schaer DJ, et al. Activating transcription factor 1 directs Mhem atheroprotective macrophages through coordinated iron handling and foam cell protection. Circ Res. (2012) 110(1):20–33. doi: 10.1161/CIRCRESAHA
115. Libby P. Current concepts of the pathogenesis of the acute coronary syndromes. Circulation. (2001) 104:365–72. doi: 10.1161/01.cir.104.3.365
116. Liu X, Wu J, Tian R, Su S, Deng S, Meng X. Targeting foam cell formation and macrophage polarization in atherosclerosis: The Therapeutic potential of rhubarb. BioMed Pharmacother. (2020) 129:110433. doi: 10.1016/j.biopha.2020.110433
117. Gerasimova EV, Popkova TV, Gerasimova DA, Kirichenko TV. Macrophage dysfunction in autoimmune rheumatic diseases and atherosclerosis. Int J Mol Sci. (2022) 23(9):4513. doi: 10.3390/ijms23094513
118. Liu Y, Yu H, Zhang Y, Zhao Y. TLRs are important inflammatory factors in atherosclerosis and may be a therapeutic target. Med Hypotheses. (2008) 70:314–6. doi: 10.1016/j.mehy.2007.05.030
119. Eshghjoo S, Kim DM, Jayaraman A, Sun Y, Alaniz RC. Macrophage polarization in atherosclerosis. Genes (Basel). (2022) 13(5):756. doi: 10.3390/genes13050756
120. Bisgaard LS, Mogensen CK, Rosendahl A, Cucak H, Nielsen LB, Rasmussen SE, et al. Bone marrow-derived and peritoneal macrophages have different inflammatory response to oxLDL and M1/M2 marker expression - implications for atherosclerosis research. Sci Rep. (2016) 6:35234. doi: 10.1038/srep35234
121. Yang S, Yuan HQ, Hao YM, Ren Z, Qu SL, Liu LS, et al. Macrophage polarization in atherosclerosis. Clin Chim Acta. (2020) 501:142–6. doi: 10.1016/j.cca.2019.10.034
122. Song L, Zhang J, Ma D, Fan Y, Lai R, Tian W, et al. A bibliometric and knowledge-map analysis of macrophage polarization in atherosclerosis from 2001 to 2021. Front Immunol. (2022) 13:910444. doi: 10.3389/fimmu.2022.910444
123. Zhang Q, Liu J, Duan H, Li R, Peng W, Wu C. Activation of Nrf2/HO-1 signaling: An important molecular mechanism of herbal medicine in the treatment of atherosclerosis via the protection of vascular endothelial cells from oxidative stress. J Adv Res. (2021) 34:43–63. doi: 10.1016/j.jare.2021.06.023
124. Zhi W, Liu Y, Wang X, Zhang H. Recent advances of traditional Chinese medicine for the prevention and treatment of atherosclerosis. J Ethnopharmacol. (2023) 301:115749. doi: 10.1016/j.jep.2022.115749
125. Li J, Lei HT, Cao L, Mi YN, Li S, Cao YX. Crocin alleviates coronary atherosclerosis via inhibiting lipid synthesis and inducing M2 macrophage polarization. Int Immunopharmacol. (2018) 55:120–7. doi: 10.1016/j.intimp.2017.11.037
126. Guo M, Xiao J, Sheng X, Zhang X, Tie Y, Wang L, et al. Ginsenoside rg3 mitigates atherosclerosis progression in diabetic apoE-/- mice by skewing macrophages to the M2 phenotype. Front Pharmacol. (2018) 9:464. doi: 10.3389/fphar.2018.00464
127. Brenner C, Franz WM, Kühlenthal S, Kuschnerus K, Remm F, Gross L, et al. DPP-4 inhibition ameliorates atherosclerosis by priming monocytes into M2 macrophages. Int J Cardiol. (2015) 199:163–9. doi: 10.1016/j.ijcard.2015.07.044
128. Feng X, Chen W, Ni X, Little PJ, Xu S, Tang L, et al. Metformin, macrophage dysfunction and atherosclerosis. Front Immunol. (2021) 12:682853. doi: 10.3389/fimmu.2021.682853
129. Linton MF, Moslehi JJ, Babaev VR. Akt signaling in macrophage polarization, survival, and atherosclerosis. Int J Mol Sci. (2019) 20(11):2703. doi: 10.3390/ijms20112703
130. Fang S, Wan X, Zou X, Sun S, Hao X, Liang C, et al. Arsenic trioxide induces macrophage autophagy and atheroprotection by regulating ROS-dependent TFEB nuclear translocation and AKT/mTOR pathway. Cell Death Dis. (2021) 12(1):88. doi: 10.1038/s41419-020-03357-1
131. Bruen R, Curley S, Kajani S, Crean D, O'Reilly ME, Lucitt MB, et al. Liraglutide dictates macrophage phenotype in apolipoprotein E null mice during early atherosclerosis. Cardiovasc Diabetol. (2017) 16:143. doi: 10.1186/s12933-017-0626-3
132. Zhou Y, Chen R, Liu D, Wu C, Guo P, Lin W. Asperlin inhibits LPS-evoked foam cell formation and prevents atherosclerosis in apoE(-/-) mice. Mar Drugs. (2017) 15(11):358. doi: 10.3390/md15110358
133. Bousquet J, Anto JM, Bachert C, Baiardini I, Bosnic-Anticevich S, Walter Canonica G, et al. Allergic rhinitis. Nat Rev Dis Primers. (2020) 6(1):95. doi: 10.1038/s41572-020-00227-0
134. Schuler Iv CF, Montejo JM. Allergic rhinitis in children and adolescents. Pediatr Clin North Am. (2019) 66:981–93. doi: 10.1016/j.pcl.2019.06.004
135. Siddiqui ZA, Walker A, Pirwani MM, Tahiri M, Syed I. Allergic rhinitis: diagnosis and management. Br J Hosp Med (Lond). (2022) 83:1–9. doi: 10.12968/hmed.2021.0570
136. Howarth PH. The cellular basis for allergic rhinitis. Allergy. (1995) 50:6–10. doi: 10.1111/j.1398-9995.1995.tb02734.x
137. Wen S, Li F, Tang Y, Dong L, He Y, Deng Y, et al. MIR222HG attenuates macrophage M2 polarization and allergic inflammation in allergic rhinitis by targeting the miR146a-5p/TRAF6/NF-κB axis. Front Immunol. (2023) 14:1168920. doi: 10.3389/fimmu.2023.1168920
138. Zhu X, Sun Y, Yu Q, Wang X, Wang Y, Zhao Y. Exosomal lncRNA GAS5 promotes M1 macrophage polarization in allergic rhinitis via restraining mTORC1/ULK1/ATG13-mediated autophagy and subsequently activating NF-кB signaling. Int Immunopharmacol. (2023) 121:110450. doi: 10.1016/j.intimp.2023.110450
139. Langan SM, Irvine AD, Weidinger S. Atopic dermatitis. Lancet (London England). (2020) 396:345–60. doi: 10.1016/s0140-6736(20)31286-1
140. Weidinger S, Novak N. Atopic dermatitis. Lancet (London England). (2016) 387:1109–22. doi: 10.1016/S0140-6736(15)00149-X
141. Zeng H, Zhao B, Zhang D, Rui X, Hou X, Chen X, et al. Viola yedoensis Makino formula alleviates DNCB-induced atopic dermatitis by activating JAK2/STAT3 signaling pathway and promoting M2 macrophages polarization. Phytomed. (2022) 103:154228. doi: 10.1016/j.phymed.2022.154228
142. Yang L, Fu J, Han X, Zhang C, Xia L, Zhu R, et al. Hsa_circ_0004287 inhibits macrophage-mediated inflammation in an N(6)-methyladenosine-dependent manner in atopic dermatitis and psoriasis. J Allergy Clin Immunol. (2022) 149(6):2021–33. doi: 10.1016/j.jaci.2021.11.024
143. Karuppagounder V, Arumugam S, Thandavarayan RA, Sreedhar R, Giridharan VV, Pitchaimani V, et al. Naringenin ameliorates skin inflammation and accelerates phenotypic reprogramming from M1 to M2 macrophage polarization in atopic dermatitis NC/Nga mouse model. Exp Dermatol. (2016) 25(5):404–7. doi: 10.1111/exd.12962
144. Lee DH, Park JK, Choi J, Jang H, Seol JW. Anti-inflammatory effects of natural flavonoid diosmetin in IL-4 and LPS-induced macrophage activation and atopic dermatitis model. Int immunopharmacol. (2020) 89:107046. doi: 10.1016/j.intimp.2020.107046
145. Agache I, Palmer E, Sanver D, Kirtland M, Shamji MH. Molecular allergology approach to allergic asthma. Mol Aspects Med. (2022) 85:101027. doi: 10.1016/j.mam.2021.101027
146. Fuchs O, Bahmer T, Rabe KF, von Mutius E. Asthma transition from childhood into adulthood. Lancet Respir Med. (2017) 5:224–34. doi: 10.1016/s2213-2600(16)30187-4
147. Schatz M, Rosenwasser L. The allergic asthma phenotype. J Allergy Clin Immunol Pract. (2014) 2:645–8; quiz 649. doi: 10.1016/j.jaip.2014.09.004
148. Liu N, Feng Y, Liu H, Wu W, Liang Y, Li P, et al. ATP6V0d2 suppresses alveoli macrophage alternative polarization and allergic asthma via degradation of PU. 1 Allergy Asthma Immunol Res. (2021) 13(3):479–97. doi: 10.4168/aair.2021.13.3.479
149. Saradna A, Do DC, Kumar S, Fu QL, Gao P. Macrophage polarization and allergic asthma. Transl Res. (2018) 191:1–14. doi: 10.1016/j.trsl.2017.09.002
150. Zhu X, He L, Li X, Pei W, Yang H, Zhong M, et al. LncRNA AK089514/miR-125b-5p/TRAF6 axis mediates macrophage polarization in allergic asthma. BMC Pulm Med. (2023) 23(1):45. doi: 10.1186/s12890-023-02339-1
151. Liu Y, Gao X, Miao Y, Wang Y, Wang H, Cheng Z, et al. NLRP3 regulates macrophage M2 polarization through up-regulation of IL-4 in asthma. Biochem J. (2018) 475(12):1995–2008. doi: 10.1042/bcj20180086
152. Zhang H, Wei R, Yang X, Xu L, Jiang H, Li M, et al. AMFR drives allergic asthma development by promoting alveolar macrophage-derived GM-CSF production. J Exp Med. (2022) 219(5):e20211828. doi: 10.1084/jem.20211828
153. Yu T, Yu Y, Ma Y, Chen G. FoxO4 mediates macrophage M2 polarization by promoting LXA4R expression in an ovalbumin-induced allergic asthma model in mice. Allergol Immunopathol (Madr). (2023) 51:19–30. doi: 10.15586/aei.v51i4.847
154. Wild S, Roglic G, Green A, Sicree R, King H. Global prevalence of diabetes: estimates for the year 2000 and projections for 2030. Diabetes Care. (2004) 27:1047–53. doi: 10.2337/diacare.27.5.1047
155. Wu Y, Ding Y, Tanaka Y, Zhang W. Risk factors contributing to type 2 diabetes and recent advances in the treatment and prevention. Int J Med Sci. (2014) 11:1185–200. doi: 10.7150/ijms.10001
156. Chen L, Magliano DJ, Zimmet PZ. The worldwide epidemiology of type 2 diabetes mellitus–present and future perspectives. Nat Rev Endocrinol. (2011) 8:228–36. doi: 10.1038/nrendo.2011.183
157. Kautzky-Willer A, Harreiter J, Pacini G. Sex and gender differences in risk, pathophysiology and complications of type 2 diabetes mellitus. Endocrine Rev. (2016) 37:278–316. doi: 10.1210/er.2015-1137
158. Fletcher B, Gulanick M, Lamendola C. Risk factors for type 2 diabetes mellitus. J Cardiovasc Nurs. (2002) 16:17–23. doi: 10.1097/00005082-200201000-00003
159. Javeed N, Matveyenko AV. Circadian etiology of type 2 diabetes mellitus. Physiol (Bethesda Md). (2018) 33:138–50. doi: 10.1152/physiol.00003.2018
160. Piché ME, Tchernof A, Després JP. Obesity phenotypes, diabetes, and cardiovascular diseases. Circ Res. (2020) 126:1477–500. doi: 10.1161/circresaha.120.316101
161. Mouton AJ, Li X, Hall ME, Hall JE. Obesity, hypertension, and cardiac dysfunction: novel roles of immunometabolism in macrophage activation and inflammation. Circ Res. (2020) 126:789–806. doi: 10.1161/circresaha.119.312321
162. Ghosh P, Sahoo R, Vaidya A, Chorev M, Halperin JA. Role of complement and complement regulatory proteins in the complications of diabetes. Endocrine Rev. (2015) 36:272–88. doi: 10.1210/er.2014-1099
163. Li Y, Wang X, Wang S, Zhu C, Guo J, Li K, et al. Complement 3 mediates periodontal destruction in patients with type 2 diabetes by regulating macrophage polarization in periodontal tissues. Cell Prolif. (2020) 53(1):e12886. doi: 10.1111/cpr.12886
164. Kraakman MJ, Murphy AJ, Jandeleit-Dahm K, Kammoun HL. Macrophage polarization in obesity and type 2 diabetes: weighing down our understanding of macrophage function? Front Immunol. (2014) 5:470. doi: 10.3389/fimmu.2014.00470
165. Banu S, Sur D. Role of macrophage in type 2 diabetes mellitus: macrophage polarization a new paradigm for treatment of type 2 diabetes mellitus. Endocr Metab Immune Disord Drug Targets. (2023) 23:2–11. doi: 10.2174/1871530322666220630093359
166. Russo S, Kwiatkowski M, Govorukhina N, Bischoff R, Melgert BN. Meta-inflammation and metabolic reprogramming of macrophages in diabetes and obesity: the importance of metabolites. Front Immunol. (2021) 12:746151. doi: 10.3389/fimmu.2021.746151
167. Ren W, Xia Y, Chen S, Wu G, Bazer FW, Zhou B, et al. Glutamine metabolism in macrophages: A novel target for obesity/type 2 diabetes. Adv Nutr. (2019) 10(2):321–30. doi: 10.1093/advances/nmy084
168. Wang Y, Guo Y, Xu Y, Wang W, Zhuang S, Wang R, et al. HIIT ameliorates inflammation and lipid metabolism by regulating macrophage polarization and mitochondrial dynamics in the liver of type 2 diabetes mellitus mice. Metabolites. (2022) 13(1):14. doi: 10.3390/metabo13010014
169. Yasui-Kato M, Patlada S, Yokode M, Kamei K, Minami M. EP4 signalling is essential for controlling islet inflammation by causing a shift in macrophage polarization in obesity/type 2 diabetes. Diabetes Vasc Dis Res. (2020) 17:1479164120945675. doi: 10.1177/1479164120945675
170. Liu J, Zhang Y, Sheng H, Liang C, Liu H, Moran Guerrero JA, et al. Hyperoside suppresses renal inflammation by regulating macrophage polarization in mice with type 2 diabetes mellitus. Front Immunol. (2021) 12:733808. doi: 10.3389/fimmu.2021.733808
171. Lumeng CN, Bodzin JL, Saltiel AR. Obesity induces a phenotypic switch in adipose tissue macrophage polarization. J Clin Invest. (2007) 117:175–84. doi: 10.1172/jci29881
172. Engin AB. Adipocyte-macrophage cross-talk in obesity. Adv Exp Med Biol. (2017) 960:327–43. doi: 10.1007/978-3-319-48382-5_14
173. Xu L, Yan X, Zhao Y, Wang J, Liu B, Yu S, et al. Macrophage polarization mediated by mitochondrial dysfunction induces adipose tissue inflammation in obesity. Int J Mol Sci. (2022) 23(16):9252. doi: 10.3390/ijms23169252
174. Chylikova J, Dvorackova J, Tauber Z, Kamarad V. M1/M2 macrophage polarization in human obese adipose tissue. BioMed Pap Med Fac Univ Palacky Olomouc Czech Repub. (2018) 162:79–82. doi: 10.5507/bp.2018.015
175. Pan Y, Hui X, Hoo RLC, Ye D, Chan CYC, Feng T, et al. Adipocyte-secreted exosomal microRNA-34a inhibits M2 macrophage polarization to promote obesity-induced adipose inflammation. J Clin Invest. (2019) 129(2):834–49. doi: 10.1172/jci123069
176. Liu K, Zhao E, Ilyas G, Lalazar G, Lin Y, Haseeb M, et al. Impaired macrophage autophagy increases the immune response in obese mice by promoting proinflammatory macrophage polarization. Autophagy. (2015) 11(2):271–84. doi: 10.1080/15548627.2015.1009787
177. Feng J, Li L, Ou Z, Li Q, Gong B, Zhao Z, et al. IL-25 stimulates M2 macrophage polarization and thereby promotes mitochondrial respiratory capacity and lipolysis in adipose tissues against obesity. Cell Mol Immunol May. (2018) 15(2):493–505. doi: 10.1038/cmi.2016.71
178. Xu L, Ota T. Emerging roles of SGLT2 inhibitors in obesity and insulin resistance: Focus on fat browning and macrophage polarization. Adipocyte. (2018) 7:121–8. doi: 10.1080/21623945.2017.1413516
179. Lee JH, Lee SH, Lee EH, Cho JY, Song DK, Lee YJ, et al. SCAP deficiency facilitates obesity and insulin resistance through shifting adipose tissue macrophage polarization. J Adv Res. (2023) 45:1–13. doi: 10.1016/j.jare.2022.05.013
180. Yao Z, Qi W, Zhang H, Zhang Z, Liu L, Shao Y, et al. Down-regulated GAS6 impairs synovial macrophage efferocytosis and promotes obesity-associated osteoarthritis. Elife. (2023) 12:e83069. doi: 10.7554/eLife.83069
181. Podyma B, Parekh K, Güler AD, Deppmann CD. Metabolic homeostasis via BDNF and its receptors. Trends Endocrinol Metab. (2021) 32:488–99. doi: 10.1016/j.tem.2021.04.005
182. Meng ZX, Wang GX, Lin JD. A microRNA circuitry links macrophage polarization to metabolic homeostasis. Circulation. (2012) 125:2815–7. doi: 10.1161/circulationaha.112.111518
183. Vats D, Mukundan L, Odegaard JI, Zhang L, Smith KL, Morel CR, et al. Oxidative metabolism and PGC-1beta attenuate macrophage-mediated inflammation. Cell Metab. (2006) 4(1):13–24. doi: 10.1016/j.cmet.2006.05.011
184. Liao X, Sharma N, Kapadia F, Zhou G, Lu Y, Hong H, et al. Krüppel-like factor 4 regulates macrophage polarization. J Clin Invest. (2011) 121(7):2736–49. doi: 10.1172/jci45444
185. Li L, Ma L, Zhao Z, Luo S, Gong B, Li J, et al. IL-25-induced shifts in macrophage polarization promote development of beige fat and improve metabolic homeostasis in mice. PloS Biol. (2021) 19(8):e3001348. doi: 10.1371/journal.pbio.3001348
186. Coppo M, Chinenov Y, Sacta MA, Rogatsky I. The transcriptional coregulator GRIP1 controls macrophage polarization and metabolic homeostasis. Nat Commun. (2016) 7:12254. doi: 10.1038/ncomms12254
187. Wang N, Liang H, Zen K. Molecular mechanisms that influence the macrophage m1-m2 polarization balance. Front Immunol. (2014) 5:614. doi: 10.3389/fimmu.2014.00614
188. Boutilier AJ, Elsawa SF. Macrophage polarization states in the tumor microenvironment. Int J Mol Sci. (2021) 22(13):6995. doi: 10.3390/ijms22136995
189. Arabpour M, Saghazadeh A, Rezaei N. Anti-inflammatory and M2 macrophage polarization-promoting effect of mesenchymal stem cell-derived exosomes. Int Immunopharmacol. (2021) 97:107823. doi: 10.1016/j.intimp.2021.107823
Keywords: macrophage, M1, M2, inflammatory disease, mechanism, therapeutic application
Citation: Luo M, Zhao F, Cheng H, Su M and Wang Y (2024) Macrophage polarization: an important role in inflammatory diseases. Front. Immunol. 15:1352946. doi: 10.3389/fimmu.2024.1352946
Received: 09 December 2023; Accepted: 26 March 2024;
Published: 10 April 2024.
Edited by:
Marco Orecchioni, Augusta University, United StatesCopyright © 2024 Luo, Zhao, Cheng, Su and Wang. This is an open-access article distributed under the terms of the Creative Commons Attribution License (CC BY). The use, distribution or reproduction in other forums is permitted, provided the original author(s) and the copyright owner(s) are credited and that the original publication in this journal is cited, in accordance with accepted academic practice. No use, distribution or reproduction is permitted which does not comply with these terms.
*Correspondence: Yuanmin Wang, RHdhbmd5dWFubWluQHptdS5lZHUuY24=
Disclaimer: All claims expressed in this article are solely those of the authors and do not necessarily represent those of their affiliated organizations, or those of the publisher, the editors and the reviewers. Any product that may be evaluated in this article or claim that may be made by its manufacturer is not guaranteed or endorsed by the publisher.
Research integrity at Frontiers
Learn more about the work of our research integrity team to safeguard the quality of each article we publish.