- 1Department of Internal Medicine IV (Gastroenterology, Hepatology, and Infectious Diseases), Jena University Hospital, Jena, Germany
- 2Department of Sports Medicine and Health Promotion, Friedrich-Schiller-University Jena, Jena, Germany
- 3Center for Sepsis Control and Care (CSCC), Jena University Hospital/Friedrich-Schiller-University Jena, Jena, Germany
- 4Center for Interdisciplinary Prevention of Diseases Related to Professional Activities, Jena, Germany
Like other infections, a SARS-CoV-2 infection can also trigger Post-Acute Infection Syndromes (PAIS), which often progress into myalgic encephalomyelitis/chronic fatigue syndrome (ME/CFS). ME/CFS, characterized by post-exercise malaise (PEM), is a severe multisystemic disease for which specific diagnostic markers or therapeutic concepts have not been established. Despite numerous indications of post-infectious neurological, immunological, endocrinal, and metabolic deviations, the exact causes and pathophysiology remain unclear. To date, there is a paucity of data, that changes in the composition and function of the gastrointestinal microbiota have emerged as a potential influencing variable associated with immunological and inflammatory pathways, shifts in ME/CFS. It is postulated that this dysbiosis may lead to intestinal barrier dysfunction, translocation of microbial components with increased oxidative stress, and the development or progression of ME/CFS. In this review, we detailed discuss the findings regarding alterations in the gastrointestinal microbiota and its microbial mediators in ME/CFS. When viewed critically, there is currently no evidence indicating causality between changes in the microbiota and the development of ME/CFS. Most studies describe associations within poorly defined patient populations, often combining various clinical presentations, such as irritable bowel syndrome and fatigue associated with ME/CFS. Nevertheless, drawing on analogies with other gastrointestinal diseases, there is potential to develop strategies aimed at modulating the gut microbiota and/or its metabolites as potential treatments for ME/CFS and other PAIS. These strategies should be further investigated in clinical trials.
Introduction
Chronic fatigue syndrome/myalgic encephalomyelitis (CFS/ME) is a complex and disabling illness with an unclear etiology and pathogenesis and is characterized by persistent and disabling fatigue, exercise intolerance, post-exertional malaise, cognitive difficulties, and musculoskeletal/joint pain (1). Various triggers for ME-CFS are known, with infections being the most common occurrence (2). Infection with the SARS-CoV-2 virus is currently understood to be the most prevalent cause of this clinical picture. Consequently, the global COVID-19 pandemic has led to a significant increase in ME/CFS patients, affecting millions of individuals, including children and young adults (3, 4). A global prevalence of ME/CFS of approximately 0.8–3.3% was described in a meta-analysis by Johnston and Brenu; these estimates are based on clinical studies and self-assessments from those affected (5). However, studies by the National Institute for Health and Care Excellence (NICE) indicating a prevalence of 0.2-0.4% of the world’s population suffering from ME/CFS appear to be more reliable (6) The question remains whether the percentage is 0.2% or 3.3%. Given the lack of proven diagnostic markers and specific therapy for ME/CFS, coupled with treatment costs for ME/CFS patients being 50% higher than those for patients with conditions like multiple sclerosis or systemic lupus erythematosus (SLE) (7), there is a significant challenge for our healthcare systems.
CFS/ME manifests a wide range of symptoms, including fatigue, cognitive dysfunction, sleep disturbances, orthostatic intolerance, myalgia, and neuro-immuno-endocrine dysfunctions, which vary from patient to patient and can fluctuate over time. However, the hallmark of the disease is post-exertional malaise (PEM). PEM is a highly disabling and sometimes progressive symptom that occurs after physical or mental exertion or overload with a patient-specific threshold. The quality of life of those affected by ME/CFS can be severely limited: long courses and limited participation in social and professional life are not uncommon (8). A Danish study shows that ME/CFS patients have a lower quality of life than patients with cancer, stroke or multiple sclerosis (7) (9). The etiology of ME/CFS is not yet fully understood despite numerous efforts. It is conceivable that various molecular damage patterns lead to different clinical presentations, resulting in heterogeneity within the disease.
Several pathophysiological signatures in ME/CFS are discussed: e.g. energy metabolism and mitochondrial dysregulation (10, 11) and neuroendocrinological processes (12) have been implicated in ME/CFS. Furthermore, the involvement of gastrointestinal process, e.g. gut microbiota, enteric dysbiosis, and bacterial translocation has been suggested (13–15). Given this complexity, the classification as a syndrome is justified, and patient subtyping becomes a central task (16). Especially the gastrointestinal tract, skin, urogenital tract, and bronchopulmonary system, serve as habitats for microorganisms. These microorganisms, collectively referred to as the microbiota, represent a diverse community primarily composed of prokaryotic bacteria, archaea, microeukaryotes, and viruses. The microbiota interacts directly with the individual, governing physiological functions from maintaining local barrier homeostasis to regulating metabolism, hematopoiesis, immunity, and other systemic control circuits. In light of this, the term ‘holobiontic concept’ was recently introduced to better emphasize the significance of interactions between microbiota and humans (17). Studies by the American Human Microbiome Project and the European MetaHIT project demonstrate that the inter-individual variability, or beta diversity, of the gastrointestinal microbiota is considerably high in healthy individuals. Thus, there is no uniform ‘standard’ or ‘normal’ intestinal microbiota that can be defined. The stability of the gastrointestinal ecosystem appears to depend on the totality of microorganisms present, encompassing all required functions, with individual microorganisms being replaceable (18). Despite the substantial interindividual diversity of the microbiota, there is a high stability and convergence at the functional level (19). The intestinal microbial ecosystem exhibits functional redundancy, implying that various combinations of metabolically active bacterial species can fulfill the same function, allowing fundamentally different bacteria to perform similar or identical functions (20). In addition to bacteria and archaea, the microbiota contains approximately 109 viruses per gram of stool. Sequencing studies in recent years have shown impressive inter-individual variability (beta diversity) with low diversity within the individual (alpha diversity). The enteric virome appears to be specific to each individual, with little or no convergence even in monozygotic twins or family members living in the same household. Most of the viral microbiota consists of previously uncharacterized viruses.
Microbiome research has evolved enormously during the past two decades and represents a new paradigm from which to approach many of the common diseases. It is well accepted, that the disturbances of the microbiota (dysbiosis) and its metabolome modulates host metabolism, inflammation, and immunity and plays a significant role in a number of gastrointestinal and extra-gastrointestinal diseases (21, 22). Although the changes underlying these diseases are becoming better understood, they remain far from sufficiently clarified. For various diseases such as chronic inflammatory bowel disease (IBD), Clostridioides difficile infections (CDI), gastrointestinal tumor diseases, liver diseases, and irritable bowel syndrome (IBS), it is evident that the gastrointestinal microbiota is characterized by reduced diversity (23, 24). The loss of this diversity is likely a potential risk factor for the development of these diseases.
There is growing evidence that the following gastrointestinal factors should be considered in ME/CFS:
● Gut dysbiosis: gut dysbiosis, which refers to an imbalance or disruption in the composition of the gut microbiota.
● Gut-brain axis: The gut-brain axis is a bidirectional communication system between the gut and the brain
● Gut permeability and bacterial translocation: Increased gut permeability, also known as “leaky gut,”.
In this mini review, we explore the proposed pathways between the gastrointestinal microbiome and ME/CFS. Overall, it is difficult to evaluate findings that were made in patients with “real” ME/CFS and that were found in patients with chronic fatigue, e.g. in the context of chronic IBD. In our review, we focus on findings of disruptions in the intestinal microbiome, with changes in the intestinal barrier leading to chronic inflammation with increased reactive oxygen species (ROS). We discuss the potential for therapeutic modulation of the microbiome in ME/CFS patients, although there is currently no convincing evidence for defined therapeutic concepts. While several questions remain unanswered, insights into microbiome modulation from studies in other diseases may have implications for modern therapeutic approaches to ME/CFS.
Intestinal dysbiosis in ME/CFS
Changes in the intestinal microbiome that are associated with reduced diversity, a loss of intestinal commensal microorganisms and an increase in “pro-inflammatory” species are referred to as dysbiosis and significantly lead to an altered host response or immune modulation of the intestine in chronic fatigue syndrome patients (15). With the start of next-generation sequencing of stool samples, it was possible to prove that intestinal dysbiosis contributes to IBD (25–27) and could act as a driving force in the development of neurodegenerative diseases (such as Parkinson’s disease (28), Alzheimer’s disease (29), multiple sclerosis (30), amyotrophic lateral sclerosis (31) and Huntington’s disease (32). It is also suspected of triggering ME/CFS and long-COVID syndrome as a potentially reversible disease (33). Interestingly, a large proportion of ME/CFS patients (35 to 90%) have gastrointestinal complaints, which are often associated with the comorbidities IBS and IBD (34). Various studies have demonstrated intestinal dysbiosis in ME/CFS (Table 1) (13, 33, 34, 36–44), although uniform microbiome signatures are non-existent. This is mainly due to the high intra- and inter-individual variability of the microbiome. During homeostasis, the six phyla “Firmicutes, Bacteroidetes, Actinobacteria, Proteobacteria, Fusobacteria and Verrucomicrobia” (45) predominate; ME/CFS is often associated with a decrease in the Firmicutes phylum and an increase in the Bacteroidetes phylum. However, no specific microorganism has been identified aetiologically. Consequently, it is unclear to what extent the intestinal microbiome is pathogenetically responsible for the development of ME/CFS. Recent research point toward an involvement of the microbiota-immune-axis in ME/CFS (15). These microbiota-immune axis should be addressed by comparative studies characterized by long-term fatigue symptoms, including IBD, post-acute infection syndromes (e.g. post–COVID-19 condition). However, it is postulated that intestinal dysbiosis leads to immunometabolic alterations (e.g. reduced production of antimicrobial peptides, short-chain fatty acids (SCFAs), altered tryptophan/kynurenine pathway), which causes a disturbed intestinal barrier, increased bacterial translocation, consecutive systemic inflammation as well as neuroinflammation and neuroimmune dysfunction (Figure 1) (14, 46, 47). Further large cohort studies are lacking in order to better understand causal or functional relationships between the microbiome, neuroinflammation and neurocognitive diseases.
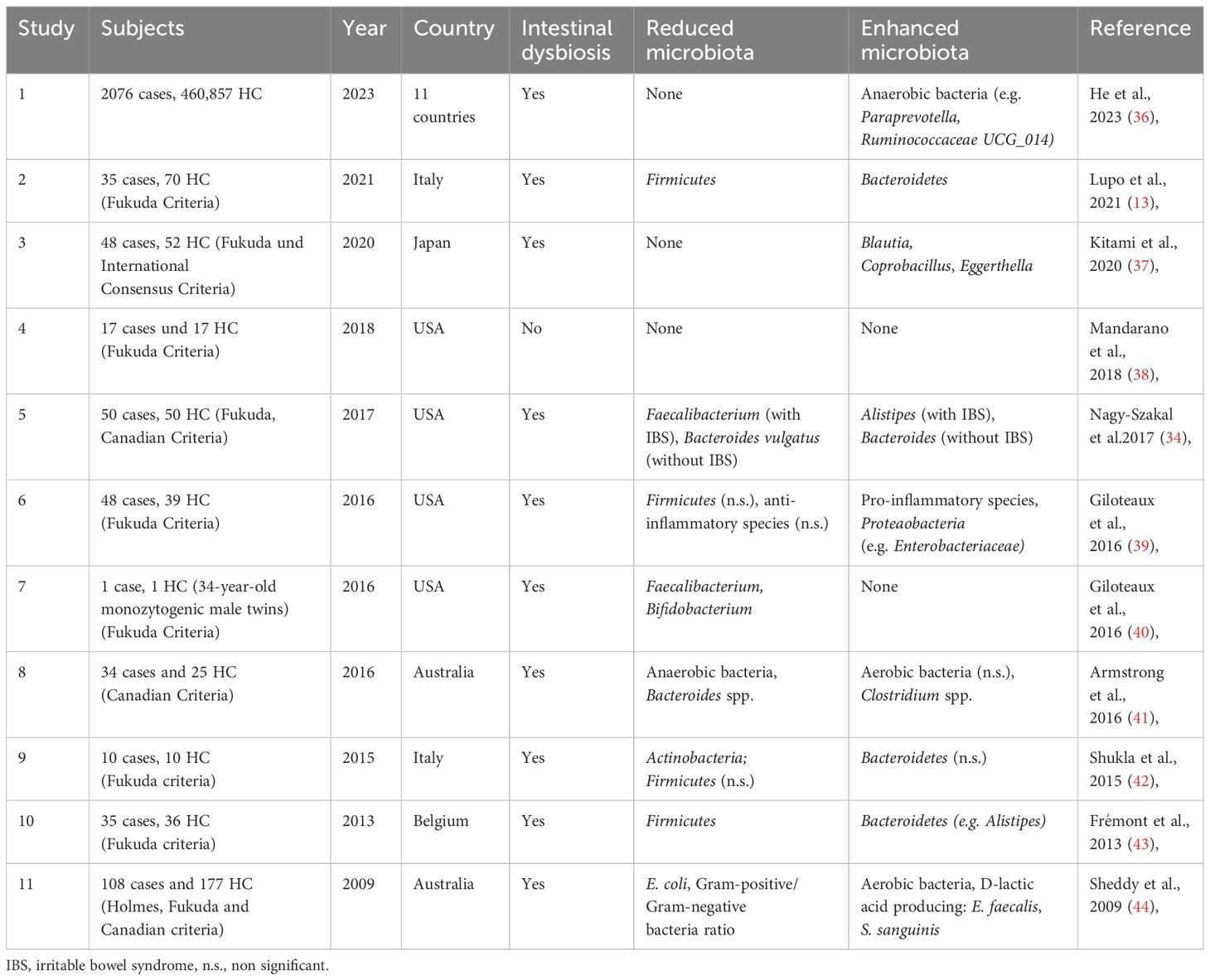
Table 1 Overview of microbial changes in patients with ME/CFS cases compared to healthy controls (HC) [modified after (33, 35)].
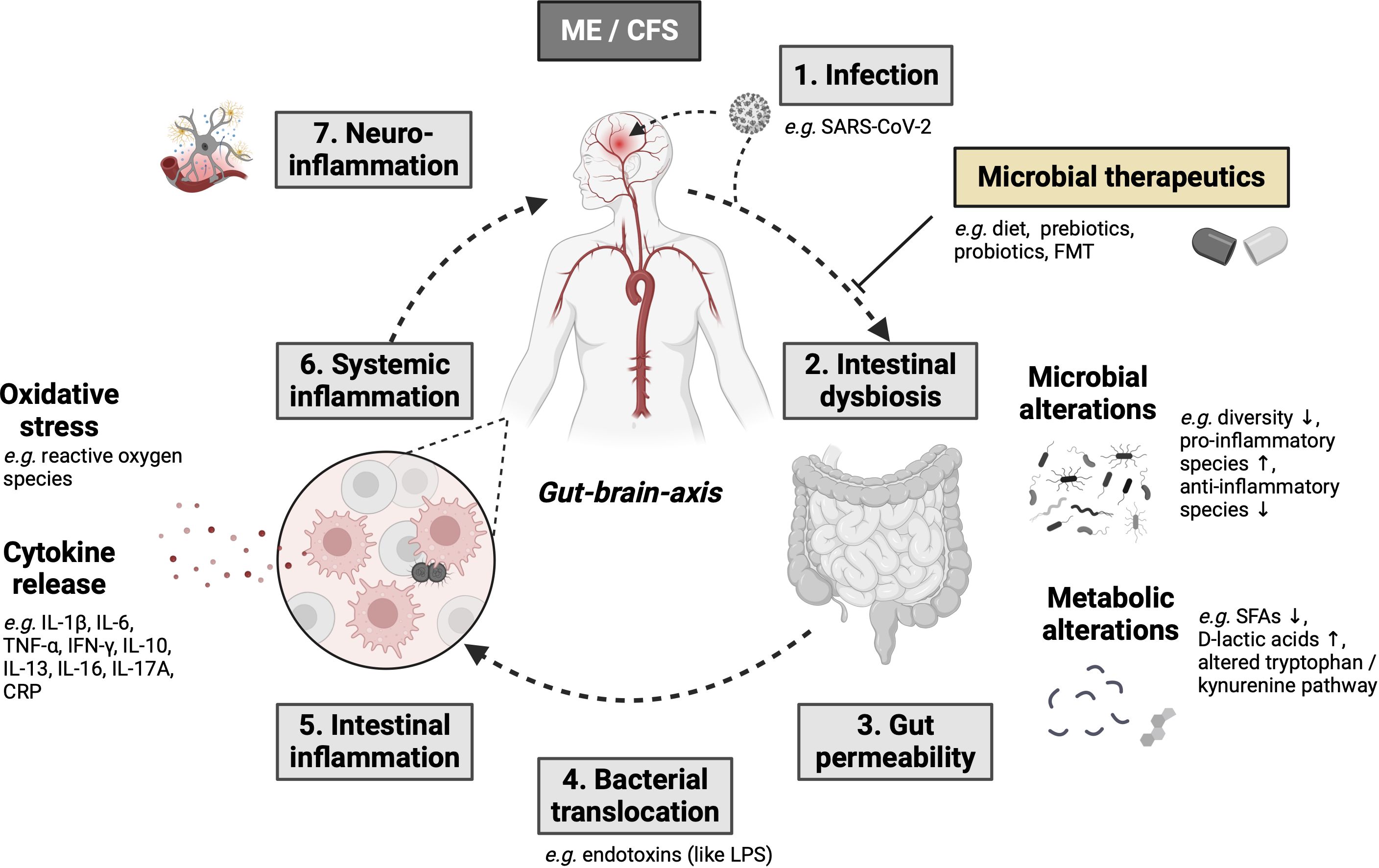
Figure 1 The gut-brain and microbiota-immune-axis as major molecular pathomechanisms resulting in ME/CFS development with potential treatment options based on the intestinal microbiome (modified after 26) (14, 33, 46, 47) Created with BioRender.com. Arrow up means "increase", arrow down means "decrease".
Intestinal barrier integrity in ME/CFS
The gastrointestinal barrier ensures the selective absorption of water, electrolytes, and nutrients while preventing the translocation of pathogenic organisms or their components from the intestinal lumen into the mucosa and its compartments. This barrier is a complex morphological-functional mechanism that involves the gut microbial barrier, mucus, gastrointestinal motility, secretion, epithelial barrier, and the immune system (both innate and adaptive). The intestinal microbiome significantly influences the integrity of this barrier in various ways. For instance, it can modulate immunological cascades or produce metabolites such as SCFAs. Disturbances in this delicate balance result in increased intestinal permeability (48). It is well known, that dysbiosis in the intestinal microbiome, triggered by antibiotic therapy, inflammatory diseases, or repeated or excessive alcohol consumption, can lead to a loss of integrity in the intestinal barrier, which is then referred to as “leaky gut”.
Undoubtedly, a leaky gut can trigger systemic chronic inflammatory reactions; inflammatory processes in the liver are a typical example (49, 50). This raises the question of whether a leaky gut is also important in ME/CFS. Various findings suggest this (42, 51–53). In a controlled study, Shukla and colleagues were able to detect a pathological bacterial translocation of 6 of 9 main bacterial genera after 72 hours after a defined stress test compared to 2 of 9 in controls (42). Moreover, there are increased specific IgA and IgA levels against LPS, a bacterial marker for gram-negative bacterial translocation, in patients with ME/CFS. This correlates with the severity of the disease (52, 53). Furthermore, there is evidence suggesting that the heightened translocation of LPS, leading to gut-derived inflammation, induces systemic inflammation and reactive nitrogen species (RNS) and reactive oxygen species (ROS) — a potential pathway in ME/CFS. Several studies report increased oxidative stress in ME/CFS (for review, see Missailidis et al.) (54).
Microbiota-gut-brain axis in ME/CFS
The existence of a bi-directional communication and interaction between the gut and the brain is already known since the middle of the 19th century. In recent years, there is growing evidence leading to the concept of a “gut-brain axis” (55) The communication of the gut and the microbiota is of great importance for several mechanisms, physiologic and pathophysiologic. For example, Sudo et al. were able to demonstrate the importance of the intestinal microbiome for the development of the hypothalamus-pituitary-adrenal axis and on stress response using germ-free animals (56). In another study, Bercik et al. have shown, that in two different strains of germ-free mice, fecal microbiota transplantation using feces of the own strain resulted in behavior similar to the own strain, while faecal microbiota transplant (FMT) using feces of the other strain resulted in a behavior similar to the other strain (57). Although the exact physiological pathways are still matter of debate in many cases, the relevance of the gut-brain communication in ME/CFS patients is supported by several studies. Noteworthy, until today the exact pathophysiologic mechanisms how the gut-brain-axis can influence neuropsychiatric symptoms remains unclear. Evidence of this interactions resulted from different studies (58–61) Basically, it can be linked via different ways (14). These pathways include changes in the immune system, including the cellular immune system, e.g., in regulatory T-cells (62), NK-cells (63), or CD8+ T-cells (64) as well as cytokine production, e.g., increased TGF-ß production (65) and immunoglobulins (52). Noteworthy, these results are still controversial as chronic inflammation may also be caused by an underlying disease (14, 47). Neurotransmitters play an important role in gut-brain interaction (66)and play a role in the development of psychological disorders like depression (67), In ME/CFS, levels of Tryptophan as a neurotransmitter influenced by the microbiome, were linked to ME/CFS (68) Finally, the gut microbiome was shown to have direct influence on nerval stimulation of the vagal nerve, which however may also be a bi-directional influence as the vagal nerve innervates the colon (reviewed at (69))
However, even if the direct modes of action are not known until today, there are several indirect hints underlining the importance of the gut-brain-axis in neuropsychiatric symptoms in general and ME/CFS in particular. A recent study reported a reduction in ME/CFS symptoms after rectal infusion of bacteria (70). Noteworthy, the majority of the patients (52/60) suffered from concomitant IBS as underlying disease. Although, therefore, it is obvious that the underlying IBS was treated, this study gives clear evidence for the importance of gut-brain communication in ME/CFS. Additionally, the modulation of the intestinal microbiome by antibiotic or probiotic treatment showed improvement in different neuropsychiatric symptoms as another indirect hint for the relevance of the gut-brain-axis (58–61, 71)
Metagenomic profiling revealed different clusters of faecal bacterial indicative for ME/CFS, with these clusters being different in patients with or without concomitant IBS (34) In patients with IBS, increased abundance of unclassified Alistipes and decreased Faecalibacterium were reported, while in patients without IBS, increased unclassified Bacteroides abundance and decreased Bacteroides vulgatus were biomarkers of ME/CFS. Additionally, the authors also found differences in metabolomic pathways, involving unsaturated fatty acid synthesis, atrazine degradation, vitamin B6 synthesis and pyrimidine ribonucleoside degradation. As the intestinal microbiome consists of bacterial and viral species, and give the fact, that ME/CFS is, despite a high number of unexplained cases, frequently reported as post-viral sequela such as after SARS-CoV-2 (72), another study focused on viral taxa in feces, blood and saliva, however, the authors did not observe any differences between ME/CFS patients and controls (73).
Dysbiosis and the microbiota-gut-brain-axis
Dysbiosis, which refers to an imbalance or disruption in the composition of the gut microbiota, can impact the microbiota-gut-brain axis in ME/CFS (Figure 1). While the exact mechanisms are still being investigated, some potential ways how dysbiosis may influence the microbiota-gut-brain axis in ME/CFS are inflammation and immune activation, neurotransmitter signaling, metabolite generation, activation of the immune-brain axis.
● Inflammation and immune activation: Dysbiosis can lead to increased gut permeability, also known as “leaky gut,” allowing the translocation of bacteria or bacterial products from the gut into the bloodstream. This can trigger immune responses and systemic inflammation, which may affect the brain and contribute to the symptoms of ME/CFS (74).
● Neurotransmitter signaling: The gut microbiota has the ability to produce neurotransmitters and modulate their signaling. Dysbiosis can disrupt the production and balance of neurotransmitters, such as serotonin and gamma-aminobutyric acid (GABA), which play important roles in regulating mood, cognition, and other brain functions. Alterations in neurotransmitter production and signaling may contribute to the symptoms experienced by ME/CFS patients (75).
● Metabolite generation: The gut microbiota produces various metabolites such as SCFAs that can influence brain function and behavior. Dysbiosis can alter the production and availability of these metabolites, potentially affecting the gut-brain communication and contributing to the symptoms of ME/CFS.
● Activation of the immune-brain axis: Dysbiosis can activate the immune system, leading to the release of pro-inflammatory cytokines and other immune molecules. These immune molecules can communicate with the brain through various pathways, including the vagus nerve and immune cell trafficking, potentially influencing brain function and contributing to the symptoms of ME/CFS (76, 77).
Modulation of the gastrointestinal microbiome as rational therapy in ME/CFS
In ME/CFS patients, the gut microbiome has less biodiversity compared to healthy individuals and this is thought to contribute to the onset and progression of ME/CFS (39). Therefore, microbiome modulation has received attention as a new therapeutic target. There are several potential therapeutic methods including dietary exclusions or prebiotics, probiotics, FMT and other modifications. However, when evaluating microbiota-modulating studies in ME/CFS, it is crucial to consider target parameters. Endpoints such as inflammation markers, cytokines, and lymphocyte subsets are of lesser importance compared to clinically relevant endpoints, such as an improvement in the quality of life.
Probiotics, live bacteria believed to promote health, have been recognized for their beneficial effects for many decades in different indications. The use of the probiotic Escherichia coli (E. coli) Nissle 1917 is recommended in guidelines for patients with ulcerative colitis (78). A pilot study by an Italian group demonstrated that the intake of various probiotics over 8 weeks led to a modification of well-being status, as well as inflammatory and oxidative indexes in CFS/ME patients, resulting in a reduction of inflammatory parameters after probiotic intake (13)(Table 1). However, the study’s limited evidence was due to a small sample size and an uncontrolled design. In a study focused on clinical endpoints, Sullivan et al. examined the effects of Lactobacillus paracasei ssp. paracasei F19, Lactobacillus acidophilus NCFB 1748, and Bifidobacterium lactis Bb12 on fatigue and physical activity in 15 CFS patients, who met the 1994 CDC criteria for CFS (60). After 4 weeks, neurocognitive functions improved during the study period, but there were no significant changes in fatigue and physical activity scores. At the end of the study, 6 out of 15 patients reported that they had experienced improvement. A systematic review summarizes the effects of probiotic treatment on gastrointestinal symptoms and typical IBS in patients with CFS/ME. The authors found that in 25 studies (including 24 randomized control trials) the evidence available for the use of probiotic interventions in CFS/ME was poor and limited (79). Further, the high variability in probiotic formulations makes it challenging to combine the results of clinical studies.
Overall, the evidence for the use of probiotics in ME/CFS is weak. Despite numerous working groups postulating a connection between the development and progression of ME/CFS and the microbiota, it is surprising that only two studies are currently listed on ClinicalTrials.gov examining the influence of probiotics on the disease (last query: 07-Oct-2023).
In recent years, FMT has been examined as a justified alternative to classic medication concepts for a variety of gastrointestinal and neurological diseases. There is no doubt that this concept is successful in CDI, ulcerative colitis or patients with IBS and is recommended as a proven therapy for CDI in international guidelines. In recent years, FMT has emerged also as a promising therapy for ME/CFS patients. The goal of this concept is to restore a healthy gut microbiota by introducing feces from a healthy donor into the recipient’s digestive system. Since ME/CFS is a systemic disease, it is not surprising that gastrointestinal symptoms are common (80). It is known that comorbidities such as IBS or Crohn’s disease occur more frequently in ME/CFS patients, which once again points to the causal importance of the intestinal microbiome in this clinical picture (81, 82). Against this background, the effects of taking prebiotics and probiotics were compared with FMT treatment over 10 days in a non-randomized study in patients with chronic fatigue syndrome. Each patient received 10 FMT, each from a different screened donor, and the transplant was delivered via a rectal catheter, into the lower part of the sigmoid colon. The effect was measured by patient self-assessment (no improvement, “0” to maximum improvement “100”). Of the 21 patients with FMT treatment, 17 reported an improvement of 65-95%, and most importantly seven patients reported a normalization of their quality of life and performance (83). However, a recently published small randomized, double blind, placebo-controlled pilot study demonstrated that FMT was safe but did not relieve symptoms or improve the health-related quality of life of patients with CFS. The small number of study subjects limits the generalizability of these results (84). With FMT, the aim is to transfer the donor microbiome as completely and as long-term as possible. An important consideration is that this study only conducted a single FMT (84). In ulcerative colitis (85), as well as in patients with IBS, effectiveness has been shown to increase with a higher number and duration of FMT cycles. This suggests that continuous application of the donor microbiome to achieve permanent remission is a subject of discussion. To address the optimal approach for engraftment, a metagenomic analysis of stool microbiomes from donors, pre-FMT recipients, and post-FMT recipients was performed (86). Different FMT methods were compared in a systematic meta-analysis involving 24 studies (86). This groundbreaking study found that the clinical response was correlated with the extent of engraftment. Furthermore, antibiotic treatment prior to FMT significantly improved engraftment. The type of FMT administration was most strongly associated with the success of FMT, particularly a combined type of FMT administration (a combination of upper and lower gastrointestinal applications and gastrointestinal administration). Bacteroidetes and Actinobacteria spp. showed higher average engraftment rates among strains compared to Firmicutes and Proteobacteria spp., while gram-positive bacteria were less likely to have high engraftment rates than more resilient gram-negative species. These findings impressively underscore the need for characterizing and, ideally, standardizing the FMT donor stool should be sought.
Conclusion
In patients with ME/CFS, various studies have yielded inconclusive results regarding changes in the gastrointestinal processes where microbiota is involved. Whether these divergent findings are due to different molecular phenotypes of patients with ME/CFS remains speculative. Presently, dysbiosis in ME/CFS is to be understood as an association; causality is not proven from a critical perspective. Nevertheless, the growing comprehension of the interactions between the microbiome and the host presents an intriguing pathophysiological concept, forming the foundation for rational future therapeutic approaches. A randomized controlled study involving well-defined ME/CFS patients, encompassing post-exertional malaise (PEM), and employing repeated and long-term faecal microbiota transplantation (FMT), appears to be the most promising approach to establish causality. Such understanding of interactions will lead to concepts that help overcome therapeutic nihilism.
Author contributions
AS: Conceptualization, Writing – original draft, Writing – review & editing. SQ: Writing – original draft, Writing – review & editing. CP: Writing – original draft, Writing – review & editing. PR: Writing – original draft, Writing – review & editing.
Funding
The author(s) declare that no financial support was received for the research, authorship, and/or publication of this article.
Conflict of interest
The authors declare that the research was conducted in the absence of any commercial or financial relationships that could be construed as a potential conflict of interest.
Publisher’s note
All claims expressed in this article are solely those of the authors and do not necessarily represent those of their affiliated organizations, or those of the publisher, the editors and the reviewers. Any product that may be evaluated in this article, or claim that may be made by its manufacturer, is not guaranteed or endorsed by the publisher.
References
1. Bateman L, Bested AC, Bonilla HF, Chheda BV, Chu L, Curtin JM, et al. Myalgic encephalomyelitis/chronic fatigue syndrome: Essentials of diagnosis and management. Mayo Clin Proc. (2021) 96:2861–78. doi: 10.1016/j.mayocp.2021.07.004
2. Choutka J, Jansari V, Hornig M, Iwasaki A. Unexplained post-acute infection syndromes. Nat Med. (2022) 28:911–23. doi: 10.1038/s41591-022-01810-6
3. Nacul L, Authier FJ, Scheibenbogen C, Lorusso L, Helland IB, Martin JA, et al. European network on myalgic encephalomyelitis/chronic fatigue syndrome (EUROMENE): Expert consensus on the diagnosis, service provision, and care of people with ME/CFS in Europe. Medicina (Mex). (2021) 57:510. doi: 10.3390/medicina57050510
4. Royston AP, Rai M, Brigden A, Burge S, Segal TY, Crawley EM. Severe myalgic encephalomyelitis/chronic fatigue syndrome in children and young people: a British Paediatric Surveillance Unit study. Arch Dis Child. (2023) 108:230–5. doi: 10.1136/archdischild-2022-324319
5. Johnston S, Brenu EW, Staines D, Marshall-Gradisnik S. The prevalence of chronic fatigue syndrome/ myalgic encephalomyelitis: a meta-analysis. Clin Epidemiol. (2013) 5:105–10. doi: 10.2147/CLEP
6. NICE. Myalgic encephalomyelitis (or encephalopathy)/chronic fatigue syndrome: diagnosis and management. London: National Institute for Health and Care Excellence (NICE) (2021). Available at: http://www.ncbi.nlm.nih.gov/books/NBK579533/.
7. Valdez AR, Hancock EE, Adebayo S, Kiernicki DJ, Proskauer D, Attewell JR, et al. Estimating prevalence, demographics, and costs of ME/CFS using large scale medical claims data and machine learning. Front Pediatr. (2019) 6:412. doi: 10.3389/fped.2018.00412
8. Vyas J, Muirhead N, Singh R, Ephgrave R, Finlay AY. Impact of myalgic encephalomyelitis/chronic fatigue syndrome (ME/CFS) on the quality of life of people with ME/CFS and their partners and family members: an online cross-sectional survey. BMJ Open. (2022) 12:e058128. doi: 10.1136/bmjopen-2021-058128
9. Falk Hvidberg M, Brinth LS, Olesen AV, Petersen KD, Ehlers L. The health-related quality of life for patients with myalgic encephalomyelitis / chronic fatigue syndrome (ME/CFS). PLoS One. (2015) 10:e0132421. doi: 10.1371/journal.pone.0132421
10. Fluge Ø, Mella O, Bruland O, Risa K, Dyrstad SE, Alme K, et al. Metabolic profiling indicates impaired pyruvate dehydrogenase function in myalgic encephalopathy/chronic fatigue syndrome. JCI Insight. (2016) 1(21):e89376. doi: 10.1172/jci.insight.89376
11. Booth NE, Myhill S, McLaren-Howard J. Mitochondrial dysfunction and the pathophysiology of Myalgic Encephalomyelitis/Chronic Fatigue Syndrome (ME/CFS). Int J Clin Exp Med. (2012) 5:208–20.
12. Cleare AJ. The neuroendocrinology of chronic fatigue syndrome. Endocr Rev. (2003) 24:236–52. doi: 10.1210/er.2002-0014
13. Lupo GFD, Rocchetti G, Lucini L, Lorusso L, Manara E, Bertelli M, et al. Potential role of microbiome in Chronic Fatigue Syndrome/Myalgic Encephalomyelits (CFS/ME). Sci Rep. (2021) 11:7043. doi: 10.1038/s41598-021-86425-6
14. König RS, Albrich WC, Kahlert CR, Bahr LS, Löber U, Vernazza P, et al. The gut microbiome in myalgic encephalomyelitis (ME)/chronic fatigue syndrome (CFS). Front Immunol. (2022) 12:628741. doi: 10.3389/fimmu.2021.628741
15. Vogl T, Kalka IN, Klompus S, Leviatan S, Weinberger A, Segal E. Systemic antibody responses against human microbiota flagellins are overrepresented in chronic fatigue syndrome patients. Sci Adv. (2022) 8:eabq2422. doi: 10.1126/sciadv.abq2422
16. Maclachlan L, Watson S, Gallagher P, Finkelmeyer A, Jason LA, Sunnquist M, et al. Are current chronic fatigue syndrome criteria diagnosing different disease phenotypes? PLoS One. (2017) 12:e0186885. doi: 10.1371/journal.pone.0186885
17. Kundu P, Blacher E, Elinav E, Pettersson S. Our gut microbiome: The evolving inner self. Cell. (2017) 171:1481–93. doi: 10.1016/j.cell.2017.11.024
18. Lozupone CA, Stombaugh JI, Gordon JI, Jansson JK, Knight R. Diversity, stability and resilience of the human gut microbiota. Nature. (2012) 489:220–30. doi: 10.1038/nature11550
19. MetaHIT Consortium, Qin J, Li R, Raes J, Arumugam M, Burgdorf KS, et al. A human gut microbial gene catalogue established by metagenomic sequencing. Nature. (2010) 464:59–65. doi: 10.1038/nature08821
20. Moya A, Ferrer M. Functional redundancy-induced stability of gut microbiota subjected to disturbance. Trends Microbiol. (2016) 24:402–13. doi: 10.1016/j.tim.2016.02.002
21. Aden K, Rehman A, WasChina S, Pan WH, Walker A, Lucio M, et al. Metabolic functions of gut microbes associate with efficacy of tumor necrosis factor antagonists in patients with inflammatory bowel diseases. Gastroenterology. (2019) 157:1279–1292.e11. doi: 10.1053/j.gastro.2019.07.025
22. Zimmermann J, Kaleta C, WasChina S. gapseq: informed prediction of bacterial metabolic pathways and reconstruction of accurate metabolic models. Genome Biol. (2021) 22:81. doi: 10.1186/s13059-021-02295-1
23. Scher JU, Ubeda C, Artacho A, Attur M, Isaac S, Reddy SM, et al. Decreased bacterial diversity characterizes the altered gut microbiota in patients with psoriatic arthritis, resembling dysbiosis in inflammatory bowel disease. Arthritis Rheumatol. (2015) 67:128–39. doi: 10.1002/art.38892
24. Rinninella E, Raoul P, Cintoni M, Franceschi F, Miggiano GAD, Gasbarrini A, et al. What is the healthy gut microbiota composition? A changing ecosystem across age, environment, diet, and diseases. Microorganisms. (2019) 7:14. doi: 10.3390/microorganisms7010014
25. Shanahan F, Quigley EMM. Manipulation of the microbiota for treatment of IBS and IBD—Challenges and controversies. Gastroenterology. (2014) 146:1554–63. doi: 10.1053/j.gastro.2014.01.050
26. Clooney AG, Eckenberger J, Laserna-Mendieta E, Sexton KA, Bernstein MT, Vagianos K, et al. Ranking microbiome variance in inflammatory bowel disease: a large longitudinal intercontinental study. Gut. (2021) 70:499–510. doi: 10.1136/gutjnl-2020-321106
27. Kang S, Denman SE, Morrison M, Yu Z, Dore J, Leclerc M, et al. Dysbiosis of fecal microbiota in Crohnʼs disease patients as revealed by a custom phylogenetic microarray. Inflammation Bowel Dis. (2010) 16:2034–42. doi: 10.1002/ibd.21319
28. Jiang L, Li J, Tang B, Guo J. Associations between gut microbiota and Parkinson disease: A bidirectional Mendelian randomization analysis. Eur J Neurol. (2023) 30:3471–7. doi: 10.1111/ene.15848
29. Cammann D, Lu Y, Cummings MJ, Zhang ML, Cue JM, Do J, et al. Genetic correlations between Alzheimer’s disease and gut microbiome genera. Sci Rep. (2023) 13:5258. doi: 10.1038/s41598-023-31730-5
30. Chen J, Chia N, Kalari KR, Yao JZ, Novotna M, Paz Soldan MM, et al. Multiple sclerosis patients have a distinct gut microbiota compared to healthy controls. Sci Rep. (2016) 6:28484. doi: 10.1038/srep28484
31. Kim HS, Son J, Lee D, Tsai J, Wang D, Chocron ES, et al. Gut- and oral-dysbiosis differentially impact spinal- and bulbar-onset ALS, predicting ALS severity and potentially determining the location of disease onset. BMC Neurol. (2022) 22:62. doi: 10.1186/s12883-022-02586-5
32. Du G, Dong W, Yang Q, Yu X, Ma J, Gu W, et al. Altered gut microbiota related to inflammatory responses in patients with huntington’s disease. Front Immunol. (2021) 11:603594. doi: 10.3389/fimmu.2020.603594
33. Varesi A, Deumer US, Ananth S, Ricevuti G. The emerging role of gut microbiota in myalgic encephalomyelitis/chronic fatigue syndrome (ME/CFS): Current evidence and potential therapeutic applications. J Clin Med. (2021) 10:5077. doi: 10.3390/jcm10215077
34. Nagy-Szakal D, Williams BL, Mishra N, Che X, Lee B, Bateman L, et al. Fecal metagenomic profiles in subgroups of patients with myalgic encephalomyelitis/chronic fatigue syndrome. Microbiome. (2017) 5:44. doi: 10.1186/s40168-017-0261-y
35. Du Preez S, Corbitt M, Cabanas H, Eaton N, Staines D, Marshall-Gradisnik S. A systematic review of enteric dysbiosis in chronic fatigue syndrome/myalgic encephalomyelitis. Syst Rev. (2018) 7:241. doi: 10.1186/s13643-018-0909-0
36. He G, Cao Y, Ma H, Guo S, Xu W, Wang D, et al. Causal effects between gut microbiome and myalgic encephalomyelitis/chronic fatigue syndrome: A two-sample mendelian randomization study. Front Microbiol. (2023) 14:1190894. doi: 10.3389/fmicb.2023.1190894
37. Kitami T, Fukuda S, Kato T, Yamaguti K, Nakatomi Y, Yamano E, et al. Deep phenotyping of myalgic encephalomyelitis/chronic fatigue syndrome in Japanese population. Sci Rep. (2020) 10:19933. doi: 10.1038/s41598-020-77105-y
38. Mandarano AH, Giloteaux L, Keller BA, Levine SM, Hanson MR. Eukaryotes in the gut microbiota in myalgic encephalomyelitis/chronic fatigue syndrome. PeerJ. (2018) 6:e4282. doi: 10.7717/peerj.4282
39. Giloteaux L, Goodrich JK, Walters WA, Levine SM, Ley RE, Hanson MR. Reduced diversity and altered composition of the gut microbiome in individuals with myalgic encephalomyelitis/chronic fatigue syndrome. Microbiome. (2016) 4:30. doi: 10.1186/s40168-016-0171-4
40. Giloteaux L, Hanson MR, Keller BA. A pair of identical twins discordant for myalgic encephalomyelitis/chronic fatigue syndrome differ in physiological parameters and gut microbiome composition. Am J Case Rep. (2016) 17:720–9. doi: 10.12659/AJCR.900314
41. Armstrong CW, McGregor NR, Lewis DP, Butt HL, Gooley PR. The association of fecal microbiota and fecal, blood serum and urine metabolites in myalgic encephalomyelitis/chronic fatigue syndrome. Metabolomics. (2017) 13:8. doi: 10.1007/s11306-016-1145-z
42. Shukla SK, Cook D, Meyer J, Vernon SD, Le T, Clevidence D, et al. Changes in gut and plasma microbiome following exercise challenge in myalgic encephalomyelitis/chronic fatigue syndrome (ME/CFS). PLoS One. (2015) 10:e0145453. doi: 10.1371/journal.pone.0145453
43. Frémont M, Coomans D, Massart S, De Meirleir K. High-throughput 16S rRNA gene sequencing reveals alterations of intestinal microbiota in myalgic encephalomyelitis/chronic fatigue syndrome patients. Anaerobe. (2013) 22:50–6. doi: 10.1016/j.anaerobe.2013.06.002
44. Sheedy JR, Wettenhall REH, Scanlon D, Gooley PR, Lewis DP, McGregor N, et al. Increased d-lactic Acid intestinal bacteria in patients with chronic fatigue syndrome. Vivo Athens Greece. (2009) 23:621–8.
45. Hou K, Wu ZX, Chen XY, Wang JQ, Zhang D, Xiao C, et al. Microbiota in health and diseases. Signal Transduct Target Ther. (2022) 7:135. doi: 10.1038/s41392-022-00974-4
46. Martín F, Blanco-Suárez M, Zambrano P, Cáceres O, Almirall M, Alegre-Martín J, et al. Increased gut permeability and bacterial translocation are associated with fibromyalgia and myalgic encephalomyelitis/chronic fatigue syndrome: implications for disease-related biomarker discovery. Front Immunol. (2023) 14:1253121. doi: 10.3389/fimmu.2023.1253121
47. VanElzakker MB, Brumfield SA, Lara Mejia PS. Neuroinflammation and cytokines in myalgic encephalomyelitis/chronic fatigue syndrome (ME/CFS): A critical review of research methods. Front Neurol. (2018) 9:1033. doi: 10.3389/fneur.2018.01033
48. Schoultz I, Keita ÅV. The intestinal barrier and current techniques for the assessment of gut permeability. Cells. (2020) 9:1909. doi: 10.3390/cells9081909
49. Keshavarzian A, Holmes EW, Patel M, Iber F, Fields JZ, Pethkar S. Leaky gut in alcoholic cirrhosis: A possible mechanism for alcohol-induced liver damage. Am J Gastroenterol. (1999) 94:200–7. doi: 10.1111/j.1572-0241.1999.00797.x
50. Trebicka J, Reiberger T, Laleman W. Gut-liver axis links portal hypertension to acute-on-chronic liver failure. Visc Med. (2018) 34:270–5. doi: 10.1159/000490262
51. Cortes Rivera M, Mastronardi C, Silva-Aldana C, Arcos-Burgos M, Lidbury B. Myalgic encephalomyelitis/chronic fatigue syndrome: A comprehensive review. Diagnostics. (2019) 9:91. doi: 10.3390/diagnostics9030091
52. Maes M, Mihaylova I, Leunis JC. Increased serum IgA and IgM against LPS of enterobacteria in chronic fatigue syndrome (CFS): Indication for the involvement of gram-negative enterobacteria in the etiology of CFS and for the presence of an increased gut–intestinal permeability. J Affect Disord. (2007) 99:237–40. doi: 10.1016/j.jad.2006.08.021
53. Maes M, Twisk FNM, Kubera M, Ringel K, Leunis JC, Geffard M. Increased IgA responses to the LPS of commensal bacteria is associated with inflammation and activation of cell-mediated immunity in chronic fatigue syndrome. J Affect Disord. (2012) 136:909–17. doi: 10.1016/j.jad.2011.09.010
54. Missailidis D, Annesley SJ, Fisher PR. Pathological mechanisms underlying myalgic encephalomyelitis/chronic fatigue syndrome. Diagnostics. (2019) 9:80. doi: 10.3390/diagnostics9030080
55. Cryan JF, Dinan TG. Mind-altering microorganisms: the impact of the gut microbiota on brain and behaviour. Nat Rev Neurosci. (2012) 13:701–12. doi: 10.1038/nrn3346
56. Sudo N, Chida Y, Aiba Y, Sonoda J, Oyama N, Yu XN, et al. Postnatal microbial colonization programs the hypothalamic-pituitary-adrenal system for stress response in mice. J Physiol. (2004) 558:263–75. doi: 10.1113/jphysiol.2004.063388
57. Bercik P, Denou E, Collins J, Jackson W, Lu J, Jury J, et al. The intestinal microbiota affect central levels of brain-derived neurotropic factor and behavior in mice. Gastroenterology. (2011) 141:599–609, 609.e1-3. doi: 10.1053/j.gastro.2011.04.052
58. Jackson ML, Butt H, Ball M, Lewis DP, Bruck D. Sleep quality and the treatment of intestinal microbiota imbalance in Chronic Fatigue Syndrome: A pilot study. Sleep Sci. (2015) 8:124–33. doi: 10.1016/j.slsci.2015.10.001
59. Wallis A, Ball M, Butt H, Lewis DP, McKechnie S, Paull P, et al. Open-label pilot for treatment targeting gut dysbiosis in myalgic encephalomyelitis/chronic fatigue syndrome: neuropsychological symptoms and sex comparisons. J Transl Med. (2018) 16:24. doi: 10.1186/s12967-018-1392-z
60. Sullivan Å, Nord CE, Evengård B. Effect of supplement with lactic-acid producing bacteria on fatigue and physical activity in patients with chronic fatigue syndrome. Nutr J. (2009) 8:4. doi: 10.1186/1475-2891-8-4
61. Rao AV, Bested AC, Beaulne TM, Katzman MA, Iorio C, Berardi JM, et al. A randomized, double-blind, placebo-controlled pilot study of a probiotic in emotional symptoms of chronic fatigue syndrome. Gut Pathog. (2009) 1:6. doi: 10.1186/1757-4749-1-6
62. Rivas JL, Palencia T, Fernández G, García M. Association of T and NK cell phenotype with the diagnosis of myalgic encephalomyelitis/chronic fatigue syndrome (ME/CFS). Front Immunol. (2018) 9:1028. doi: 10.3389/fimmu.2018.01028
63. Fletcher MA, Zeng XR, Maher K, Levis S, Hurwitz B, Antoni M, et al. Biomarkers in chronic fatigue syndrome: Evaluation of natural killer cell function and dipeptidyl peptidase IV/CD26. PLoS One. (2010) 5:e10817. doi: 10.1371/journal.pone.0010817
64. Brenu EW, Van Driel ML, Staines DR, Ashton KJ, Ramos SB, Keane J, et al. Immunological abnormalities as potential biomarkers in Chronic Fatigue Syndrome/Myalgic Encephalomyelitis. J Transl Med. (2011) 9:81. doi: 10.1186/1479-5876-9-81
65. Montoya JG, Holmes TH, Anderson JN, Maecker HT, Rosenberg-Hasson Y, Valencia IJ, et al. Cytokine signature associated with disease severity in chronic fatigue syndrome patients. Proc Natl Acad Sci. (2017) 114(34):E7150–8. doi: 10.1073/pnas.1710519114
66. Ruddick JP, Evans AK, Nutt DJ, Lightman SL, Rook GAW, Lowry CA. Tryptophan metabolism in the central nervous system: medical implications. Expert Rev Mol Med. (2006) 8:1–27. doi: 10.1017/S1462399406000068
67. Pearson-Leary J, Zhao C, Bittinger K, Eacret D, Luz S, Vigderman AS, et al. The gut microbiome regulates the increases in depressive-type behaviors and in inflammatory processes in the ventral hippocampus of stress vulnerable rats. Mol Psychiatry. (2020) 25:1068–79. doi: 10.1038/s41380-019-0380-x
68. Simonato M, Dall’Acqua S, Zilli C, Sut S, Tenconi R, Gallo N, et al. Tryptophan metabolites, cytokines, and fatty acid binding protein 2 in myalgic encephalomyelitis/chronic fatigue syndrome. Biomedicines. (2021) 9:1724. doi: 10.3390/biomedicines9111724
69. Han Y, Wang B, Gao H, He C, Hua R, Liang C, et al. Vagus nerve and underlying impact on the gut microbiota-brain axis in behavior and neurodegenerative diseases. J Inflammation Res. (2022) 15:6213–30. doi: 10.2147/JIR.S384949
70. Borody TJ, Nowak A, Finlayson S. The GI microbiome and its role in Chronic Fatigue Syndrome: A summary of bacteriotherapy. J Australas Coll Nutr Environ Med. (2012) 31:3–8.
71. Schmidtner AK, Slattery DA, Gläsner J, Hiergeist A, Gryksa K, Malik VA, et al. Minocycline alters behavior, microglia and the gut microbiome in a trait-anxiety-dependent manner. Transl Psychiatry. (2019) 9:223. doi: 10.1038/s41398-019-0556-9
72. Reuken PA, Besteher B, Finke K, Fischer A, Holl A, Katzer K, et al. Longterm course of neuropsychological symptoms and ME/CFS after SARS-CoV-2-infection: a prospective registry study. Eur Arch Psychiatry Clin Neurosci. (2023). doi: 10.1007/s00406-023-01661-3. [Epub ahead of print]
73. Briese T, Tokarz R, Bateman L, Che X, Guo C, Jain K, et al. A multicenter virome analysis of blood, feces, and saliva in myalgic encephalomyelitis/chronic fatigue syndrome. J Med Virol. (2023) 95:e28993. doi: 10.1002/jmv.28993
74. Clapp M, Aurora N, Herrera L, Bhatia M, Wilen E, Wakefield S. Gut microbiota’s effect on mental health: The gut-brain axis. Clin Pract. (2017) 7:987. doi: 10.4081/cp.2017.987
75. Loebel M, Grabowski P, Heidecke H, Bauer S, Hanitsch LG, Wittke K, et al. Antibodies to β adrenergic and muscarinic cholinergic receptors in patients with Chronic Fatigue Syndrome. Brain Behav Immun. (2016) 52:32–9. doi: 10.1016/j.bbi.2015.09.013
76. On behalf of the School of Advanced Studies of the European Headache Federation (EHF-SAS), Arzani M, Jahromi SR, Ghorbani Z, Vahabizad F, Martelletti P, et al. Gut-brain Axis and migraine headache: a comprehensive review. J Headache Pain. (2020) 21:15. doi: 10.1186/s10194-020-1078-9
77. Holzer P, Farzi A, Hassan AM, Zenz G, Jačan A, Reichmann F. Visceral inflammation and immune activation stress the brain. Front Immunol. (2017) 8:1613. doi: 10.3389/fimmu.2017.01613
78. Kucharzik T, Dignass A, Atreya R, Bokemeyer B, Esters P, Herrlinger K, et al. Aktualisierte S3-leitlinie colitis ulcerosa (Version 6.1) – februar 2023 – AWMF-registriernummer: 021-009. Z Für Gastroenterol. (2023) 61:1046–134. doi: 10.1055/a-2060-0935
79. Corbitt M, Campagnolo N, Staines D, Marshall-Gradisnik S. A systematic review of probiotic interventions for gastrointestinal symptoms and irritable bowel syndrome in chronic fatigue syndrome/myalgic encephalomyelitis (CFS/ME). Probiotics Antimicrob Proteins. (2018) 10:466–77. doi: 10.1007/s12602-018-9397-8
80. Johnston S, Staines D, Marshall-Gradisnik S. Epidemiological characteristics of chronic fatigue- syndrome/myalgic encephalomyelitis in Australian patients. Clin Epidemiol. (2016) 8:97–107. doi: 10.2147/CLEP.S96797
81. Lakhan SE, Kirchgessner A. Gut inflammation in chronic fatigue syndrome. Nutr Metab. (2010) 7:79. doi: 10.1186/1743-7075-7-79
82. Tsai SY, Chen HJ, Lio CF, Kuo CF, Kao AC, Wang WS, et al. Increased risk of chronic fatigue syndrome in patients with inflammatory bowel disease: a population-based retrospective cohort study. J Transl Med. (2019) 17:55. doi: 10.1186/s12967-019-1797-3
83. Kenyon JN, Coe S, Izadi H. A retrospective outcome study of 42 patients with Chronic Fatigue Syndrome, 30 of whom had Irritable Bowel Syndrome. Half were treated with oral approaches, and half were treated with Faecal Microbiome Transplantation. Hum Microbiome J. (2019) 13:100061. doi: 10.1016/j.humic.2019.100061
84. Salonen T, Jokinen E, Satokari R, Lahtinen P. Randomized, double-blinded, placebo-controlled pilot study: efficacy of faecal microbiota transplantation on chronic fatigue syndrome. J Transl Med. (2023) 21:513. doi: 10.1186/s12967-023-04227-y
85. Haifer C, Paramsothy S, Kaakoush NO, Saikal A, Ghaly S, Yang T, et al. Lyophilised oral faecal microbiota transplantation for ulcerative colitis (LOTUS): a randomised, double-blind, placebo-controlled trial. Lancet Gastroenterol Hepatol. (2022) 7:141–51. doi: 10.1016/S2468-1253(21)00400-3
Keywords: ME/CFS, FMT, post-COVID, gastrointestinal microbiome, fatigue
Citation: Stallmach A, Quickert S, Puta C and Reuken PA (2024) The gastrointestinal microbiota in the development of ME/CFS: a critical view and potential perspectives. Front. Immunol. 15:1352744. doi: 10.3389/fimmu.2024.1352744
Received: 08 December 2023; Accepted: 18 March 2024;
Published: 28 March 2024.
Edited by:
Scott Kenneth Durum, National Cancer Institute (NIH), United StatesReviewed by:
Camilla Tincati, University of Milan, ItalyJacqueline Margaret Cliff, Brunel University London, United Kingdom
Copyright © 2024 Stallmach, Quickert, Puta and Reuken. This is an open-access article distributed under the terms of the Creative Commons Attribution License (CC BY). The use, distribution or reproduction in other forums is permitted, provided the original author(s) and the copyright owner(s) are credited and that the original publication in this journal is cited, in accordance with accepted academic practice. No use, distribution or reproduction is permitted which does not comply with these terms.
*Correspondence: Andreas Stallmach, YW5kcmVhcy5zdGFsbG1hY2hAbWVkLnVuaS1qZW5hLmRl
†ORCID: Andreas Stallmach, orcid.org/0000-0002-6101-5244
Stefanie Quickert, orcid.org/0009-0009-9391-4111
Christian Puta, orcid.org/0000-0003-3936-4605
Philipp A. Reuken, orcid.org/0000-0002-7696-475X