- Institute for Translational Medicine, The Affiliated Hospital of Qingdao University, College of Medicine, Qingdao University, Qingdao, China
Notch signaling pathway is a highly conserved system of cell-to-cell communication that participates in various biological processes, such as stem cell maintenance, cell fate decision, cell proliferation and death during homeostasis and development. Dysregulation of Notch signaling has been associated with many aspects of cancer biology, such as maintenance of cancer stem-like cells (CSCs), cancer cell metabolism, angiogenesis and tumor immunity. Particularly, Notch signaling can regulate antitumor or pro-tumor immune cells within the tumor microenvironment (TME). Currently, Notch signaling has drawn significant attention in the therapeutic development of cancer treatment. In this review, we focus on the role of Notch signaling pathway in remodeling tumor immune microenvironment. We describe the impact of Notch signaling on the efficacy of cancer immunotherapies. Furthermore, we summarize the results of relevant preclinical and clinical trials of Notch-targeted therapeutics and discuss the challenges in their clinical application in cancer therapy. An improved understanding of the involvement of Notch signaling in tumor immunity will open the door to new options in cancer immunotherapy treatment.
1 Introduction
Notch proteins are type I transmembrane proteins that were originally discovered in Drosophila melanogaster (1). Notch receptors are composed of an extracellular domain that combines with ligands expressed on a neighboring cell, a transmembrane region and an intracellular region that conveys the signal (2). Notch signaling pathway is initiated by binding of canonical ligands (Delta-like ligand 1 (Dll1), Dll3, Dll4, Jagged1 (Jag1), Jag2) to Notch receptors (Notch1, Notch2, Notch3 and Notch4) (3, 4). Although the core Notch signaling cascade seems to be relatively simple, it is actually a complicated and adaptable machinery, which can induce widespread cellular responses (5). Notch signaling is an evolutionarily conserved system of cell-to-cell communication between adjacent cells, affecting many biological processes such as cell fate determination, cell proliferation and differentiation (4, 6). Dysregulation of Notch signaling is involved in various human pathologies, especially cancer (7). Notch signaling pathway participates in many aspects of cancer biology, encompassing stem cell maintenance, cancer angiogenesis, invasion and metastasis (8–11). Notch signaling can play both tumor-promoting and tumor-suppressing roles in diverse types of cancer (12). A growing body of evidence manifests that Notch signaling exerts double-edged sword effects on tumor immunity by regulating the abundance and functionality of diverse immune cells including myeloid-derived suppressor cells (MDSCs), tumor-associated macrophages (TAMs), dendritic cells (DCs) and T cells (13). Accordingly, manipulation of Notch signaling may hold promise as a powerful new approach for cancer treatment (14). Considerable effort has been devoted to designing Notch-targeting therapies. The past decade has seen the emergence of various types of agents therapeutically inhibiting Notch pathway including ligand/receptor-targeted antibodies, γ-secretase inhibitor (GSI) and small molecule inhibitor (5). Nevertheless, due to the intricate roles of Notch signaling in cancer pathogenesis, the goal of developing tumor-selective Notch-directed therapies for clinical use is still challenging.
In the present review, we provide an up-to-date overview of current findings of Notch signaling in cancer and its crosstalk with tumor immune microenvironment. Moreover, the effects of Notch signaling on cancer immunotherapy responses are highlighted. In addition, we summarize recent preclinical and clinical trials of Notch-targeted therapies to offer new ideas and directions for more precise cancer immunotherapy. A better understanding of Notch signaling in cancer will accelerate the research and development of safe and efficacious Notch-targeting drugs for cancer intervention.
2 Roles of Notch signaling in cancer pathogenesis
Notch signaling pathway is an ancient and highly conserved signaling cascade that is crucial for many biological processes (15). Aberrant Notch signaling pathway is associated with both noncancerous and cancerous diseases (16). The two core players in this signaling pathway are Notch receptors and their cognate ligands (17). In mammals, there are four Notch receptors (Notch1, Notch2, Notch3 and Notch4) and five Notch ligands (Dll1, Dll3, Dll4, Jag1 and Jag2) (18, 19). Notch receptors contain extracellular epidermal growth factor (EGF)-like domains mediating their association with Notch ligands (20). Notch ligands harbor a conserved Delta-Serrate ligand (DSL) domain executing ligand-receptor interaction (21). In signal-sending cells, Notch ligands are distributed on the cell membrane and interact with Notch receptors on signal-receiving cells (22) (Figure 1). In signal-receiving cells, Notch receptors are synthesized as precursor proteins by ribosomes bound to the endoplasmic reticulum (ER) and subsequently transferred to the Golgi apparatus (23). During this process, Notch receptors are glycosylated at the EGF-like repeat domain (24). In the Golgi apparatus, Notch receptors are cleaved by furin-like proteases at Site 1 (S1), generating a non-covalently associated heterodimer made up of an extracellular subunit and a transmembrane subunit (25). The N-terminal fragment of Notch receptors is O-glycosylated with the action of glycosyltransferase enzymes (e.g., members of the Fringe family) and transferred to the cell membrane (21). Notch receptors on the signal-receiving cell are activated by binding to Notch ligands on the cell surface of an adjacent signal-sending cell (26). Upon bound with ligands, Notch receptors are cleaved at Site 2 (S2) by a disintegrin and metalloprotease 10 (ADAM10) (27). The product of S2 cleavage is composed of a transmembrane domain and an intracellular domain (Notch extracellular truncation (NEXT)) (28). NEXT then undergoes proteolytic cleavage by γ-secretase at Site 3 (S3), liberating the Notch intracellular domain (NICD) that functions to regulate gene transcription (29). S3 processing can occur both on the plasma membrane and in the endosome after NEXT is endocytosed (30). After being released, NICD migrates into the cell nucleus where it forms a Notch transcriptional activation complex (NTC) with the DNA binding factor recombinant signal-binding protein for immunoglobulin κJ region (RBPJ) and transcriptional coactivators of the Mastermind-like (MAML) family (31). Following recruitment of transcriptional coregulators, NTC associates with Notch regulatory elements (NREs), fostering transcription of Notch target genes (4). The most conserved targets of Notch signaling include members of hairy and enhancer of split (HES) family and HES related with YRPW motif (HEY) family (32). In the absence of NICD, RBPJ combines with a variety of transcription repressors (e.g., four and a half LIM domain protein 1 (FHL1) and silencing mediator of retinoid and thyroid hormone receptor (SMRT)/histone deacetylase 1 (HDAC1)-associated repressor protein (SHARP)), enabling RBPJ to restrict the transcription of Notch target genes (33). The abundance of Notch ligands and receptors presented on cell membrane, the type of ligands, and glycosylation of the EGF domain affect ligand-receptor binding and the amount of released NICD (34).
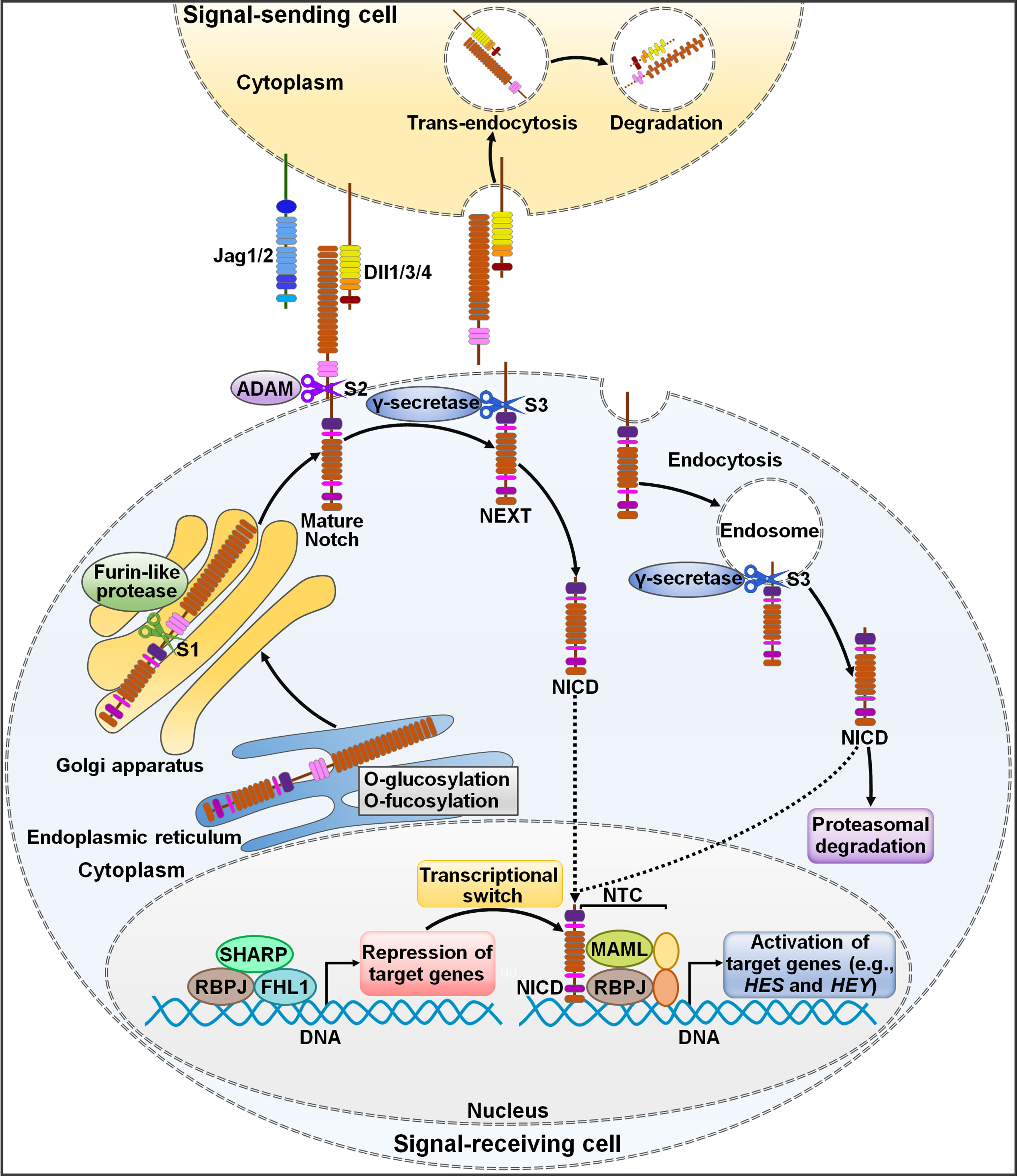
Figure 1 Schematic representation of Notch signaling pathway. The newly translated Notch receptor undergoes protein folding and glycosylation in endoplasmic reticulum. The cleavage of Notch receptor by furin-like protease at S1 occurs in the Golgi apparatus, leading to the biogenesis of mature Notch receptor. It is then transported to the cell membrane as a heterodimer. Notch ligands from signal-sending cells bind to the Notch receptor of signal-receiving cells. Endocytosis and membrane trafficking coordinate the availability of ligands and receptors on the cell surface. Ligand endocytosis may also produce mechanical force to induce structural changes in the bound Notch receptor. This conformational alteration exposes S2 in Notch for sequential cleavages by ADAM, generating a membrane-anchored Notch extracellular truncation fragment (NEXT). γ-Secretase cleaves the Notch transmembrane domain in NEXT at S3, finally releasing the Notch intracellular domain (NICD) from the cell membrane. γ-Secretase-mediated cleavage can occur at the cell surface or in the endosome. NICD translocates to the nucleus and binds to RBPJ, MAML and other proteins to form a NTC that facilitates the transcription of many genes (e.g., HES and HEY). In the absence of NICD, RBPJ may associate with corepressor proteins to suppress target gene transcription. NICD binding may induce allosteric changes in RBPJ that causes displacement of transcriptional repressors. The transcriptional activator MAML recognizes the NICD/RBPJ interface, following which other coactivators are recruited to activate transcription of downstream target genes. Jag1/2, Jagged1/2; Dll1/3/4, Delta-like ligand 1/3/4; ADAM, a disintegrin and metalloproteinase; S1, Site 1; S2, Site 2; S3, Site 3; NEXT, Notch extracellular truncation; NICD, Notch intracellular domain; NTC, Notch transcriptional activation complex; SHARP, silencing mediator of retinoid and thyroid hormone receptor/histone deacetylase 1-associated repressor protein; RBPJ, recombinant signal-binding protein for immunoglobulin κJ region; FHL1, four and a half LIM domain protein 1; MAML, mastermind-like; HES, hairy and enhancer of split; HEY, hairy and enhancer of split related with YRPW motif.
Notch signaling is implicated in various aspects of cancer biology, such as the maintenance of cancer stem-like cells (CSCs), angiogenesis and tumor immunity (35, 36). Notch signaling acts as an oncogenic factor, while in some cancers its role may be tumor suppressive (37, 38). For instance, Dll1-mediated Notch signaling promoted the progression of breast cancer and glioma (39, 40). This signaling pathway exerted anticarcinogenic activities in lung cancer, osteosarcoma and pancreatic carcinoma (41–43). Notch signaling is necessary for CSC maintenance in diverse cancers, which can increase cancer heterogeneity, stemness, metastasis and resistance to anticancer therapies (35, 44). Notch signaling-mediated intercellular interactions contribute to induction of epithelial-mesenchymal transition (EMT) and remodeling of the tumor stroma (45, 46). Notch signaling has been implicated in vascular development and physiology (47). Accumulating evidence indicates that Notch ligand Dll4 is highly expressed in tumor vasculature and has a role in tumor angiogenesis (48, 49). Targeting Dll4 represents a prospective antiangiogenic strategy in cancer. Dll4 deficiency disturbed the dynamic balance of tumor angiogenesis and overcame resistance to vascular endothelial growth factor (VEGF) blockade in cancer cell line-based xenograft models (50, 51). Furthermore, Notch signaling has emerged as an important regulator of tumor immunity, resulting in tumor-promoting or tumor-antagonizing outcomes, which may depend on mobilization of Notch signaling in each form of tumor-infiltrating immune cells (52–54).
3 The interplay between Notch signaling and tumor immune microenvironment
A growing body of evidence indicates that Notch signaling pathway can regulate the activity of various immune cells within tumor microenvironment (TME), such as MDSCs, TAMs, regulatory T cells (Tregs), cancer-associated fibroblasts (CAFs), DCs, neutrophils and T cells (Figure 2). Accordingly, Notch signaling is involved in cancer development through multifarious mechanisms, which include induction of cancer immunosuppression, promotion of tumor-supportive microenvironment, and regulation of adaptive antitumor immunity.
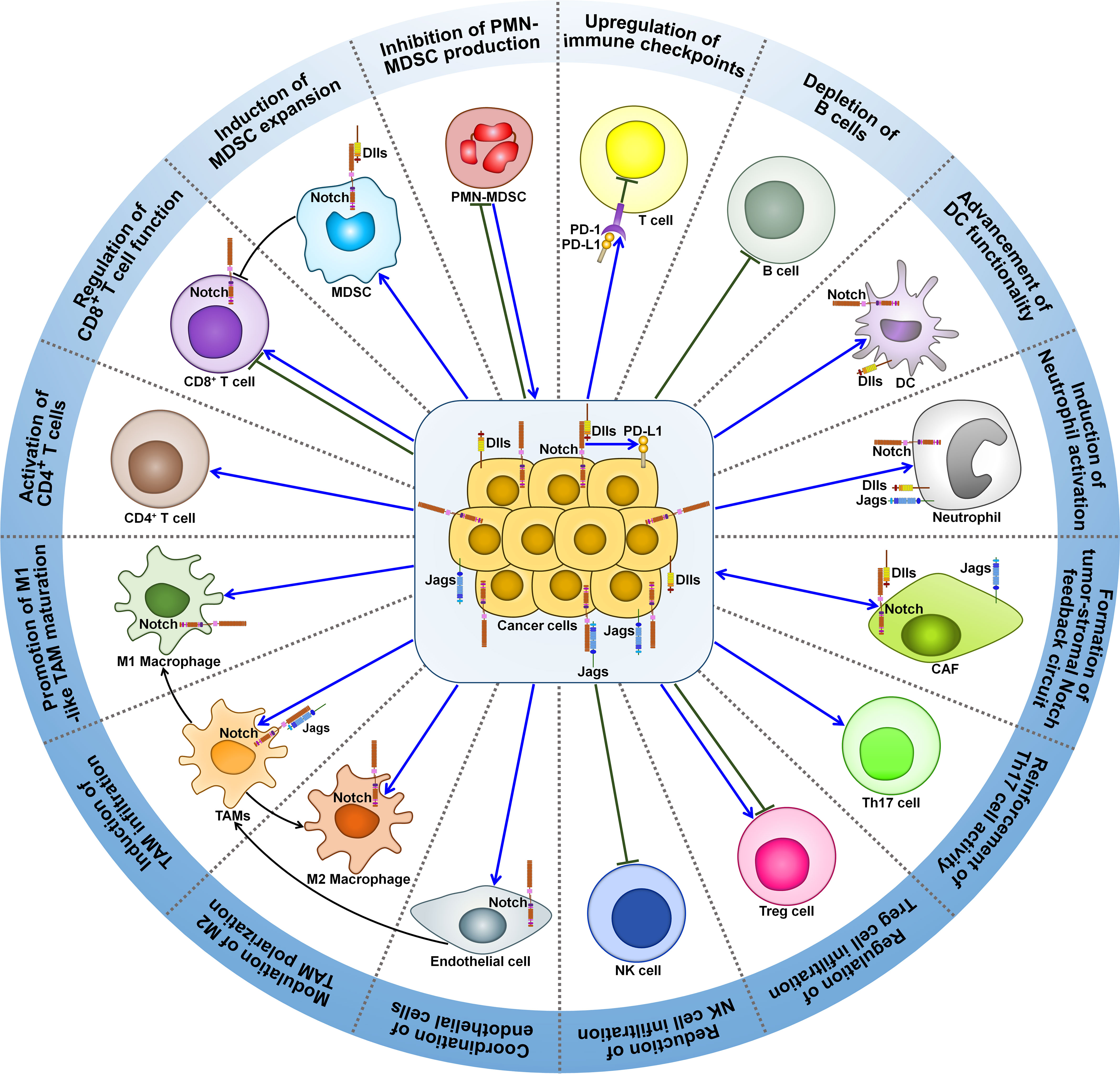
Figure 2 Roles of Notch signaling pathway in tumor immune microenvironment. Notch signaling can affect the activity of various immune cells within tumor microenvironment. Notch signaling suppresses PMN-MDSC production and induces MDSC expansion, contributing to tumor immunosuppression. Intriguingly, PMN-MDSCs can elicit Jag/Notch signal transduction pathway in cancer cell. The crosstalk between cancer cells and PMN-MDSCs finally enhances cancer metastasis. Notch signaling play dual roles in regulating T cell-mediated immune responses in cancer. Notch pathway can affect TAM recruitment and polarization. Cancer cells trigger the activation of Notch signaling in endothelial cells, which mediates the education of TAMs by cancer cells. This leads to formation of immunosuppressive microenvironment and impairment of T cell-mediated immunity. Notch activation reduces the intratumoral infiltration of NK cells, while it has opposite effects on Treg frequency and activity. Notch signaling leads to IL-17-driven inflammation via Th17 recruitment. Notch signaling mediates the communication between cancer cells and CAFs. On one hand, activated Notch pathway in CAFs causes compromised antitumor immunity and supports cancer development. On the other hand, increased activity of Notch pathway in CAFs can inhibit cancer progression. Cancer cells attract neutrophils into tumor microenvironment and motivate Notch signaling in recruited neutrophils. Notch-activated neutrophils suppress the cytotoxic activity of CD8+ T cells. Notch signaling fosters DC terminal differentiation and drives antigen cross-presentation to CD8+ T cells. Consequently, Notch-primed DCs retard cancer progression. Notch mutation is associated with a decline in B cell population in tumor microenvironment. Furthermore, Notch activation upregulates immune checkpoints (PD-1 and PD-L1) to blunt adaptive antitumor immunity. MDSC, myeloid-derived suppressor cell; Dlls, Delta-like ligands; PMN-MDSC, polymorphonuclear-myeloid-derived suppressor cell; PD-1, programmed cell death protein-1; PD-L1, programmed cell death protein-ligand 1; DC, dendritic cell; Jags, Jaggeds; CAF, cancer-associated fibroblast; Th17, T helper 17; Treg cell, regulatory T cell; NK cell, natural killer cell; TAMs, tumor-associated macrophages.
3.1 Regulation of immunosuppression
3.1.1 Myeloid-derived suppressor cells
Notch1 signaling plays a critical role in tumor-induced immunosuppression. On one hand, Notch1 contributes to tumor immunosuppression through promotion of MDSCs. Notch-activated macrophages increased the liberation of C-C motif chemokine ligand 2 (CCL2) and interleukin-10 (IL-10) to induce MDSC recruitment in glioma (55). The MDSC-rich environment educated T cells to be immunosuppressive and attenuated the efficacy of oncolytic herpes simplex virus-1 (oHSV-1)-based virotherapy. Conversely, pharmacologic blockade of Notch reversed the immunosuppressive brakes and potentiated CD8+ T cell-driven antitumor immune responses, thereby improving oncolytic virus-induced therapeutic benefit. Deregulation of Notch signaling in immature T cells induced the expansion of CD11b+Gr-1+ MDSCs in T-cell acute lymphoblastic leukemia (T-ALL) through IL-6 (56). Depletion of MDSCs inhibited T-ALL cell proliferation and expansion. Notch3 increased the expression of various cytokines such as CCL2, colony-stimulating factor-1 (CSF-1) and C-X-C motif chemokine ligand 12 (CXCL12), which promoted recruitment of macrophages and MDSCs to the TME of colorectal cancer (CRC) (57). Therefore, Notch3 accelerated CRC development by favoring immunosuppressive TME. MDSCs were able to stimulate signal transducer and activator of transcription 3 (STAT3) and Notch in breast cancer cells through IL-6 and nitric oxide (NO), respectively (58). Notch activation supported sustained STAT3 activation via IL-6. The crosstalk between Notch and STAT3 signaling pathways mediated MDSC-induced breast cancer stemness. Notch signaling was strongly inhibited in MDSCs derived from colon carcinoma- and melanoma-bearing mice (59). Notch signaling suppressed the production of polymorphonuclear (PMN)-MDSCs but increased the generation of mononuclear MDSCs. Depletion of Notch signaling reduced the inhibitory effect of MDSCs on T cell function. Manipulation of Notch signaling cascade may represent a potential therapeutic option for cancer patients. PMN-MDSC-derived reactive oxygen species (ROS) activated Notch1 in circulating tumor cells (CTCs) isolated from patients with breast cancer or melanoma, which enabled CTCs to respond to Jag1-mediated Notch activation (60). The crosstalk between PMN-MDSCs and CTCs enhanced the dissemination and metastatic potentials of CTCs. On the other hand, Notch signaling counteracts MDSC-mediated immunosuppression. Activation of Notch/RBPJ signaling pathway blocked monocarboxylate transporter 2 (MCT2)-mediated lactate import in myeloid cells through its downstream molecule HES1 (61). This resulted in the suppression of MDSC differentiation and promotion of M1-like TAM maturation, thereby restricting lung cancer progression. MDSCs decreased the expression of Notch1 and Notch2 in T cells in a NO-dependent manner (62). Notch1 increased the cytotoxic effects of CD8+ T cells and induced their conversion into a memory phenotype. Restorement of Notch1 signaling in CD8+ T cells overcame MDSC-induced T cell suppression and improved the beneficial effects of T cell-based immunotherapy in lung cancer-bearing mice.
3.1.2 Tumor-associated macrophages
TAMs play dual roles in remodeling of tumor immunosuppressive microenvironment. Notch induced the expression of IL-1β and CCL2, which then recruited TAMs to the tumor, contributing to breast cancer progression (63). High expression of Jag1/Notch1 were positively linked with infiltration levels of M2-like TAMs, contributing to poor prognosis in lung adenocarcinoma (LUAD) (64). Mechanistically, Notch signaling recruited macrophages into LUAD tissues through induction the secretion of CCL2 and IL-1β and promoted their polarization into M2 type. Jag1-mediated Notch signaling increased the secretion of IL-4 and IL-6 in breast cancer cells, leading to M2 polarization of TAMs and breast cancer development (65). Epithelial ovarian cancer (EOC) cells induced the activation of Notch pathway in endothelial cells to upregulate CD44 in TAMs, propelling their education by EOC cells (66). This resulted in formation of immunosuppressive microenvironment and impairment of T cell-mediated immunity, hence accelerating EOC progression. Notch signaling was activated in TAMs in the TME of pancreatic ductal adenocarcinoma (PDAC) (67). Notch activation favored TAM polarization into an immunosuppressive M2-like phenotype characterized by high levels of immunosuppressive cytokines (IL-10, transforming growth factor-β1 (TGF-β1) and tumor necrosis factor-α (TNF-α)), arginase 1 and immune checkpoint molecules. Notch inhibition cooperated with programmed cell death protein-1 (PD-1) blockade to induce CD8+ T cell-mediated antitumor immunity in PDAC. The combined treatment achieved a stronger anticancer effect than single treatment. Activation of Notch in triple negative breast cancer (TNBC) induced the expression of IL-1β and CCL2 to attract TAMs into the tumor, culminating in cancer development and immune evasion (68). Notch signaling in PDAC cells fostered the recruitment and activation of macrophages to a M2-like phenotype through upregulation of IL-8, CCL2, IL-1α and urokinase-type plasminogen activator (uPA) (69). In turn, activated TAMs then secreted IL-6 to enhance PDAC cell metastasis. Interruption of Notch-dependent circuit holds potential as a therapeutic option for PDAC treatment. Intriguingly, Notch signaling also limits the immunosuppressive function of TAMs. Myeloid-specific Notch activation remarkably reduced the infiltration of pro-tumorigenic M2 macrophages into PDAC tissues, which led to upregulated antigen presentation and enhanced cytotoxic T effector function (70). Notch signaling in myeloid cells suppressed the expansion of Kupffer cell-like TAMs (kclTAMs), thus inhibiting hepatocellular carcinoma (HCC) progression (71).
3.1.3 Regulatory T cells
Notch1 overexpression favored melanoma growth in vivo (72). In terms of mechanism, Notch1 suppressed the intratumoral infiltration of CD8+ cytotoxic T lymphocytes (CTLs) and natural killer (NK) cells while increased the population of Tregs and MDSCs via upregulation of TGF-β1. It also inhibited T cell proliferation and activation and increased PD-1 expression on CD4+ and CD8+ T cells. High expression of Dll3 correlated with poor prognosis in patients with invasive breast cancer (73). Patients with high Dll3 expression had high proportions of T follicular helper (Tfh) cells and Tregs and low proportions of M2 macrophages and resting mast cells. The accumulation of tumor-promoting immune cells is likely to facilitate breast cancer pathogenesis. The infiltration of Tregs and T helper 17 (Th17) cells in the TME was increased with the development of gastric cancer, leading to an imbalance between Tregs and Th17 (74). CD4+CD25+CD127dim/- Tregs expressing high levels of forkhead box protein P3 (FoxP3) function to maintain peripheral tolerance in cancer by inhibiting the activation of tumor-specific effector T cells (75). Retinoic acid-related orphan receptor γt (RORγt), a key transcription factor of IL-17, is expressed in Th17 cells and correlates with IL-17-driven inflammation (76). Notch signaling pathway was activated in gastric cancer (77). Inhibition of Notch signaling reduced the expression of transcription factors FoxP3/RORγt without affecting Treg/Th17 balance. Notch inhibition blunted the suppressive function of CD4+CD25+CD127dim/- Tregs and IL-17 production by Th17 in gastric cancer. Altogether, Notch signaling played a critical role in regulating the activity of Tregs and Th17 cells during GC progression. Another study indicated that high Notch3 expression was associated with decreased infiltration of activated CD8+ T cells and emergence of immunosuppressive cells (e.g., Tregs and M2 macrophages) in the TME of gastric cancer (78). High Notch3 expression also contributed to upregulation of immune checkpoint genes (e.g., CD200, CD274, CD276, CTLA4 and HAVCR2), leading to impaired immune responses. Altogether, Notch3 was involved in immune tolerance of gastric cancer, highlighting its potential as a therapeutic target for the treatment of gastric cancer.
3.2 Modification of tumor-supportive microenvironment
3.2.1 Cancer-associated fibroblasts
Jag1/Notch2 signaling-mediated cell-to-cell contact between CAFs and lung cancer cells facilitated vascular mimicry (VM) generation and permeability (79). The loose junctions of VM networks fostered cancer intravasation and neutrophil penetration, leading to enhanced N2 neutrophil infiltration in lung cancer tissues. Blockade of Jag1/Notch2 interaction inhibited VM formation and lung cancer growth in a murine tumor model. Notch1 activation in TNBC cells promoted contact-dependent induction of CXCL8 in inflammation-induced TNBC-stromal interaction network, leading to TNBC metastasis and progression (80). CAF-derived stanniocalcin 1 (STC1) increased the stemness of HCC cells (81). Mechanistically, STC1 directly combined with Notch1 receptors to motivate the Notch signaling pathway. Moreover, Notch1 was capable of regulating STC1 at the transcriptional level, constituting a tumor-stromal amplifying STC1-Notch1 feedforward signal. Radiation induced the expansion of Dll1-overexpressing breast cancer CSCs, which impelled IL-6-dependent recruitment of CAFs within the TME (82). After that, Dll1-mediated Notch signaling in CAFs triggered Wnt signaling in Dll1-overexpressing CSCs to increase radioresistance in breast cancer. Moreover, blocking Dll1 and IL-6 sensitized Dll1-ovexpressing breast cancer cells to radiotherapy.
In contrast, Notch-activated CAFs can exert tumor-suppressive actions. Apoptotic lung cancer cells activated Notch1/Wnt-induced signaling protein-1 (WISP-1) signaling pathway in CAFs, thus inhibiting the migration and invasion of lung cancer cells (83). Intracellular Notch1 signaling in CAFs reduced the plasticity and stemness of melanoma stem/initiating cells (MICs), hence affecting cancer heterogeneity and aggressiveness (84). Increased activity of Notch pathway in melanoma-associated fibroblasts strikingly retarded cancer growth and angiogenesis (85). Activating Notch signaling in melanoma-associated fibroblasts may be a promising therapeutic strategy for melanoma treatment.
3.2.2 Neutrophils
Previously, Wood et al. (86) reported that neutrophils with activated Notch and TGF-β signaling cascades were enriched in metastatic CRC tissues, suggesting the potential implication of neutrophils in formation of a pre-metastatic niche. Radiation exposure in lung tissues induced accumulation and activation of neutrophils, contributing to a series of tissue perturbations including persistent Notch activation in epithelial cells (87). Such changes facilitated a metastatic niche within irradiated lung tissues, accelerating the growth of arriving breast cancer cells. Notch ligands expressed by cancer cells or tumor-associated neutrophils (TANs) activated Notch1 receptors on endothelial cells (88). Endothelial Notch1 hyperactivation facilitated neutrophil infiltration and metastasis through upregulation of vascular cell adhesion molecule 1 (VCAM1), forming a pre-metastatic niche to drive cancer colonization. These events promoted circulating cancer cell migration, intravasation and homing at distant sites.
3.3 Coordination of adaptive antitumor immunity
3.3.1 Dendritic cells
Jag2-induced Notch signaling could enhanced NK cell cytotoxicity mediated by DCs (89). This caused increased effector activity of cytotoxic T cells. Thus, overexpression of Jag2 retarded the proliferation of B cell lymphoma cells. Notch2 signaling was implicated in conventional DC (cDC)-mediated antitumor immunity (90). Depletion of Notch2 signaling dampened cDC terminal differentiation and inhibited antigen cross-presentation to CD8+ T cells through downregulation of chemokine receptor 7 (CCR7). Consequently, Notch-primed DCs inhibited the development of inflammation-associated colon cancer. Notch signaling in DCs prevents cancer progression by eliciting antitumor immune responses.
3.3.2 Neutrophils
Unlike DCs, neutrophils function to impair T cell-mediated antitumor immune responses. Epithelial Notch1 induced TGF-β-dependent neutrophil recruitment into the TME of CRC, contributing to decreased infiltration of CD8+ T cells and cancer metastasis (91). EOC cells recruited TANs into the TME and triggered Jag2 expression in TANs via IL-8 release (92). Jag2-activated TANs dampened cytotoxic activity of CD8+ T cells. Blockade of Notch signaling inhibited EOC growth and strengthened the killing effect of CD8+ T cells.
3.3.3 T cells
Notch signaling facilitates adaptive immune evasion in cancer. B cells, CD45+ cells, DCs, macrophages, mast cells, neutrophils and T cells were significantly reduced in Notch1-mutant relapsed T1-2N0 laryngeal cancer in comparison with wild-type cancer samples (93). Notch1-mutant recurrent tumors exhibited an immunosuppressive phenotype characterized by decreased B cells score, T cells score and tumor-infiltrating lymphocytes (TILs) score. Notch1 mutation leads to compromised immune response and immunosurveillance escape in recurrent laryngeal cancer. Tumor-derived Jag1 drove tumorigenesis through macrophage recruitment and functional repression of CD8+ T cells in breast cancer-bearing mice models (94). Jag1-induced Notch activation fostered the production of multiple cytokines including IL-6 and WISP-1, contributing to the recruitment of macrophages into the TME. Following recruitment, Notch-activated macrophages interacted with tumor-infiltrating T cells to suppress their proliferation and tumor-killing activity by enhancing CD14 and CD93 secretion. Combination of PD-1 blockade and a Notch inhibitor produced significantly greater inhibition of breast cancer growth than either individual agent. Jag1-mediated Notch activation propels adaptive immune evasion of cancer cells and offers novel therapeutic opportunities for cancer treatment. Inhibition of Notch activation reduced the production of proinflammatory cytokines (e.g., CCL2 and IL-1β) in TNBC (95). This triggered a switch to an immune-inflamed tumor phenotype characterized by a reduction in immunosuppressive Tregs and M2-polarized TAMs and increased infiltration of cytotoxic T cells into tumors. Notch signaling can program tumor immune landscape to facilitate TNBC immune evasion. Notch1 signaling decreased the expression of human leukocyte antigen (HLA) class II genes through downregulation of class II transactivator (CIITA) in chronic lymphocytic leukemia (CLL) cells (96). Notch1 also increased the expression of programmed cell death protein-ligand 1 (PD-L1) and promoted the exhaustion of CD8+ T cells. Notch1 signaling facilitated CLL escape from immune surveillance by blocking antigen presentation and inhibiting T cell activation.
Euchromatic histone methyltransferase 2 (EHMT2)-mediated activation of Notch1 signaling increased PD-L1 expression and reduced major histocompatibility complex-I (MHC-I) expression to dampen T cell immune responses, culminating in stemness maintenance of glioma stem cells (GSCs) (97). The binding of Jag1 expressed on tumor epithelial cells to Notch receptors on TAMs in PDAC microenvironment favored the activation of Notch signaling, which induced TAM polarization into an immunosuppressive phenotype (67). TAMs secreted immunosuppressive mediators (e.g., IL-10 and TGF-β) to suppress CD8+ T cell function. Furthermore, pharmacological inhibition of Notch signaling combined with PD-1 blockade led to reduced macrophage recruitment and increased CD8+ T cell activation, which led to tumor inhibition.
Notch signaling is capable of inducing T cell-mediated immune response (Figure 2). Reportedly, the transcriptional levels of Notch1/2/3 were increased in gastric cancer tissues compared with normal tissues (98). High transcription levels of Notch1/2/3/4 predicted a good prognosis in patients with gastric cancer. Upregulation of Notch family members was positively correlated with infiltration levels of CD4+ T cells, DCs, macrophages and neutrophils. Notch expression showed significant association with many immune markers in gastric cancer, including CCL2, CD163, C-X-C motif chemokine receptor 4 (CXCR4), CCR4 and IL-10. Notch1/2/3/4 might represent potential therapeutic targets for precision cancer treatment. Notch signaling pathway increased the expression of MHC-I and interferon-γ (IFN-γ)-dependent cytokines and favored the recruitment of antitumor CD8+ T cells in glioma (99). Moreover, Notch signaling promoted the conversion of TAMs into an anti-tumorigenic phenotype. Oppositely, blockade of Notch signaling facilitated tumor immune evasion and increased glioma cell aggressiveness. Collectively, Notch signaling pathway suppressed cancer development by remodeling tumor immune microenvironment. CRC patients with Notch mutation had higher infiltration levels of CD8+ T cells, M1 macrophages, neutrophils and NK cells (100). Notch signaling was positively associated with tumor immunogenicity in CRC. Notch mutant status predicted a good prognosis in CRC patients undergoing immune checkpoint inhibitor (ICI) treatment. Notch mutation is anticipated to act as a new therapeutic target and prognostic factor for CRC. Another study indicated that mutated Notch signaling correlated with an enrichment of tumor-specific CD8+ T cells and deprivation of Tregs (101). Therefore, Notch signaling pathway mutations augmented antitumor immunity in CRC. The expression levels of Notch1, Notch3 and Notch4 were remarkably decreased in prostate cancer tissues compared with normal tissues (102). The abundance of Notch family genes was connected with intratumoral infiltration of CD4+ T cells and CD8+ T cells, which influenced clinical outcomes in patients with prostate cancer. Silencing of Notch ligand Dll1 on DCs dampened antitumor immune responses in lung and pancreatic cancers by inhibiting effector CD8+ T cell function and T effector memory (Tem) cell differentiation as well as promoting the accumulation of Tregs and MDSCs (103). Consequently, pharmacological inhibition of Dll1 promoted the growth of lung and pancreatic cancers. In addition, enforced expression of Jag1 reduced Treg frequency and enhanced antitumor immunity through downregulation of PD-1 on CD8+ Tem cells. Notch ligands are crucial for triggering antitumor T cell immune responses to prevent cancer development.
3.3.4 Immune checkpoint molecules
Immune checkpoints refer to as a class of stimulatory and inhibitory molecules expressed on immune cells and cancer cells (104). They act as accessory molecules that either induce or suppress T cell activation (105). PD-1 is an inhibitory receptor expressed on T cells, while PD-L1 is a ligand of PD-1 (106). The PD-L1/PD-1 signaling cascade constitutes an adaptive immune resistance mechanism in cancer. Notch signaling was responsible for PD-L1 upregulation, contributing to immune evasion in gastric cancer (107). Notch/c-Myc/enhancer of zeste homolog 2 (EZH2) signaling induced PD-L1 expression in CLL, contributing to enhanced resistance to activated autologous T cells in a murine CLL model (108). Likewise, Notch3 was upregulated in PD-L1-overexpressing breast CSCs (109). Notch3 activation increased PD-L1 expression by regulating the activity of mammalian target of rapamycin (mTOR), which was crucial for maintaining the stemness of CSC-like cells. High expression levels of Dll3 were inversely linked with Notch1 but showed significant association with high expression of PD-L1 (110). This resulted in dysfunction of T lymphocytes. However, high Dll3 expression was associated with increased infiltration of immune cells and exerted a protective effect on both progression-free survival (PFS) and overall survival (OS) in PDAC patients.
4 Effects of Notch signaling on cancer immunotherapy response
Due to its role in tumor immunity, Notch signaling affects immunotherapy response in cancer. Reduced Notch activity in glioma impaired MHC-I expression and inhibited recruitment of IFN-γ-producing immune cells, contributing to tumor immune evasion (99). The TME of Notch-inhibited glioma was characterized by an exhaustion of T cells and homeostatic microglia-like TAMs and an enrichment of immunosuppressive TAMs. Loss of Notch activity accounted for increased resistance to CSF-1 receptor (CSF-1R) inhibition in glioma. A risk signature consisting of six Notch pathway-related genes (CNTN1, DTX3L, ENO1, GATA3, MAGEA1 and SORBS2) acted as an independent prognostic factor in bladder cancer (111). The infiltrating levels of immune cells were markedly distinct among different risk groups. The high-risk group had higher levels of macrophages and resting mast cells, while CD8+ T cells, DCs, monocytes and Tfh cells were enriched in low-risk patients. Furthermore, low-risk patients displayed a better response for immunotherapy. Thus, Notch signaling was linked with the immune status and immunotherapy response in bladder cancer.
Notch4 mutation was associated with enhanced immunogenicity and increased infiltration of TILs and CD8+ T cells in patients with diverse types of cancer (112). Patients with Notch4 mutation had a better response to ICI therapy. High-mutated Notch signaling was positively associated with increased levels of M1 macrophages, activated memory CD4+ T cells and CD8+ T cells in non-small cell lung cancer (NSCLC) (113). It also negatively correlated with M2 macrophages, quiescent mast cells and resting memory CD4+ T cells. High-mutated Notch signaling was linked with inflammatory immune microenvironment and increased immunogenicity. As expected, patients with high-mutated Notch signaling benefited more from ICI therapy than those with low quantities of mutations in Notch signaling. High-mutated Notch signaling could serve as a predictive biomarker of the response to immunotherapy in NSCLC patients. Co-occurring mutations in Notch and homologous repair (HR) pathways correlated with enhanced efficacy of ICIs and longer PFS in patients with advanced NSCLC (114). Co-occurring alteration of DNA damage response (DDR) and Notch pathways was associated with increased infiltration of CD4+ T cells in NSCLC patients (115). Mutated DDR and Notch pathways predicted superior survival outcomes in ICI-treated NSCLC patients.
Loss-of-function mutation of Notch signaling increased the expression of chemokines (e.g., CXCL1 and C-X3-C motif chemokine ligand 1 (CX3CL1)) in CRC, which then recruited both pro-tumor (e.g., MDSCs and neutrophils) and antitumor immune cells (B cells, CD4+ T cells, CD8+ T cells, DCs and NK cells) (116). Notch mutation correlated with good prognosis for CRC patients undergoing ICI therapy. Blocking Notch signaling can reinforce antitumor immunity by affecting the chemotaxis of various immune cells. Activation of TGF-β and Notch pathways was linked with response to ICI and poor therapeutic outcomes in CRC patients (117). Pharmacological blockade of TGF-β and Notch pathways overcame resistance to PD-1 inhibitor in CRC.
5 The anticancer activity of Notch-targeted treatments
As a classical signaling cascade in humans, Notch signaling is indispensable for the homeostasis and development of most tissues. Dysregulation of Notch signaling contributes to carcinogenesis and cancer pathogenesis. Many notch-directing therapies have been developed and tested in preclinical and clinical studies, including antibodies targeting Notch ligands or receptors, small molecule inhibitors of Notch receptors and GSI (Table 1).
5.1 Antibodies against Notch ligands
Jag1-targeting antibody blocked Notch signaling, suppressed TNBC CSCs and delayed tumor growth in preclinical brain metastasis xenograft models (118). Moreover, Jag1-neutralizing antibody treatment did not give rise to any detectable toxicity, suggesting its potential clinical application in cancer treatment. Jag1-based immunotherapy may represent a future treatment strategy in metastatic breast cancer. Anti-Dll1 antibody (Dl1.72) showed high affinity and specificity for Dll1 and markedly blocked Dll1/Notch signaling cascade (119). Dl1.72 inhibited the proliferation and liver metastasis of breast cancer cells in a xenograft mouse model. Dl1.72 did not cause detectable toxicity. An open-label phase 1b clinical trial was conducted in patients with platinum-resistant EOC (120). Demcizumab (Dll4-targeted IgG2 humanized monoclonal antibody) combined with paclitaxel possessed a manageable toxicity profile and resulted in clinical benefit rate of 42% in the 19 treated patients.
5.2 Antibodies against Notch receptors
Epidermal growth factor receptor (EGFR)/Notch-targeting bispecific antibodies reduced the proportion of stem-like cells, decreased the frequency of tumor-initiating cells, and overcame resistance to cetuximab and talazoparib in a NSCLC patient-derived xenograft (PDX) model (121). Moreover, combinatorial treatment of EGFR/Notch-targeting bispecific antibodies and talazoparib produced a stronger antitumor effect than the combination of cetuximab and talazoparib in epithelial tumors. Nanoparticle-mediated co-delivery of Notch1-targeting antibodies and small molecule drug ABT-737 decreased tumor burden and extended animal survival in a murine model of TNBC xenograft tumors (122). The therapeutic potential of this treatment merits thorough investigation. Tarextumab (OMP-5948), a new cross-reactive antibody that selectively targets Notch2 and Notch3, was well-tolerated in 42 enrolled patients with advanced solid tumors (123). Disease stabilization was observed in nine tarextumab-treated subjects. A randomized phase II trial demonstrated that addition of tarextumab to gemcitabine and nab-paclitaxel did not improve clinical outcomes over conventional chemotherapy in patients with untreated metastatic PDAC (124). The efficacy of tarextumab needs to be tested in further clinical studies.
5.3 Notch inhibitor
The Notch1 inhibitor ASR490 was reported to suppress the growth of breast cancer stem cells (BCSCs) and breast cancer in xenograft models (125). ASR490 may represent an attractive therapeutic agent against breast cancer, necessitating further clinical validation. Pharmacological inhibition of Notch signaling using G9 augmented antitumor immune responses and suppressed cancer growth with a favorable safety profile in a murine TNBC model (95). Notch1-selective small molecule inhibitor NADI-351 significantly restricted the growth of esophageal adenocarcinoma (EAC) with limited toxicity in PDX models through ablation of the CSC population (126). NADI-351 may be a promising therapeutic agent for cancer treatment. Both IL-17 signaling and Notch pathway accelerated PDAC progression, and IL-17 increased the activity of Notch signaling via nuclear factor-κB (NF-κB) pathway (127). Knockout of IL-17 and Notch inhibitor DAPT (N-[N-(3,5-difluorophenacetyl)-L-alanyl]-S-phenylglycine t-butyl ester) exerted synergistic effects against PDAC in vivo. The anticarcinogenic mechanism of IL-17-Notch co-inhibition needs to be adequately deciphered. Sublethal concentrations of cisplatin promoted osteosarcoma cell migration and invasion by activating the Notch signaling (128). Combination treatment of cisplatin with Notch inhibitor DAPT restrained osteosarcoma cell metastasis in xenograft tumor models.
CB-103 is an orally active small molecule that acts to impede Notch signaling (146). CB-103 combined with protein kinase B (Akt) inhibitor (MK-2206) strikingly extended OS in a xenograft model of T-ALL compared with the single agent (129). It is worthwhile to consider the further development of this combination treatment as a therapeutic option to improve clinical outcomes in T-ALL patients. In an open-label, nonrandomized, phase 1/2 dose-escalation study, CB-103 exhibited limited therapeutic benefits as monotherapy in patients with adenoid cystic carcinoma (ACC) (130). Future studies exploring the anticancer activity of CB-103 combined with current cancer treatments are required.
5.4 Antibody-drug conjugates
Notch3-targeted antibody-drug conjugates (Notch3-ADCs) outperformed conventional chemotherapy (carboplatin) and resulted in persistent cancer regressions in ovarian cancer-bearing mice (131). Continued study will be necessary to evaluate the clinical efficacy of this Notch-targeting treatment. An antibody-drug conjugate (Rova-T) comprising an anti-Dll3 antibody tethered to a chemotherapeutic drug pyrrolobenzodiazepine showed cytotoxic effects on Dll3-expressing patient-derived isocitrate dehydrogenase (IDH)-mutant glioma (132). An open-label, two-to-one randomized, phase 3 study was previously conducted to explore the anticancer benefit of Rova-T in patients with small cell lung cancer (SCLC) (133). However, patients receiving Rova-T had a shorter OS than the second-line chemotherapeutic drug topotecan.
5.5 γ-secretase inhibitor
The GSI dibenzazepine (DBZ)-mediated suppression of Notch signaling inhibited the growth of mucoepidermoid carcinoma (MEC) in vivo (134). Furthermore, co-targeting Notch and EGFR signalings exhibited an enhanced inhibitory effect on MEC growth. High Notch activity promoted bladder cancer development (135). The GSI DBZ and the mitogen-activated extracellular signal-regulated kinase (MEK) inhibitor selumetinib (AZD) were used to block Notch and MAPK signaling pathways, respectively. Combined blockade of these signaling pathways significantly repressed tumor growth in a murine xenograft model of bladder cancer. RO4929097, an oral GSI, blocked the Notch signaling pathway (136). It exerted limited inhibitory effects on the formation of brain tumor neurospheres in glioblastoma (GBM) patients in a phase 2 and pharmacodynamic trial. A phase 1b dose escalation trial of RO4929097 with exemestane indicated that this combination treatment led to a total clinical benefit rate of 20% and a PFS of 3.2 months in 14 patients with estrogen receptor α (ERα)-positive metastatic breast cancer (137). Combination of GSI and endocrine treatment warrants additional investigation in endocrine-resistant ERα-positive breast cancer. RO4929097 combined with vismodegib had good tolerability in 33 patients with advanced sarcoma (138). RO4929097 monotherapy led to a PFS of 8.9 weeks and an OS of 11.9 months. However, the administration of vismodegib did not reinforce the clinical activity of RO4929097. RO4929097 in conjunction with carboplatin and paclitaxel exhibited a good safety profile in patients with stage II-III TNBC (139). This combinatorial regimen demonstrated an overall response rate of 64% and pathologic complete response rate of 36% in TNBC patients.
The safety and anticancer effect of crenigacestat (LY3039478), a GSI as a selective oral Notch inhibitor, were evaluated in phase I trials (140, 141). Crenigacestat elicited limited clinical activity with a manageable safety profile in patients with advanced solid tumors. Likewise, a phase 1b study showed that crenigacestat combined with anticancer agents (abemaciclib, LY3023414, taladegib, carboplatin, cisplatin or gemcitabine) resulted in disappointing clinical efficacy in patients with advanced or metastatic solid tumors (142, 143). A multicenter, nonrandomized, open-label, dose-escalation, phase 1 trial revealed that crenigacestat showed low clinical efficacy in patients with relapsed/refractory T-ALL and T-cell lymphoblastic lymphoma (T-LBL) (144). The combination of crenigacestat and prednisone induced disease stabilization in 54.5% and 64.7% of patients with advanced or metastatic solid tumors or lymphoma that received different dosing schedules (145).
Taken together, the overall performance of Notch-directed therapies in clinical studies does not meet expectations. The clinical translation of Notch-targeted therapies has faced unneglectable challenges. A primary obstacle to their clinical use is likely to be their adverse effects. The use of Notch-targeting agents as a combination therapy or other strategies that manipulate dosing regimens would be helpful to alleviate or avoid their toxicity. The role of Notch signaling varies depending on the type of cancer and its crosstalk with other signaling cascades. Therefore, an in-depth investigation of Notch signaling in cancer biology will open the door to new options in cancer treatment.
6 Conclusions and future perspectives
Notch signaling modulates various aspects of tumor immunity including myeloid compartment functionality and T cell activation. Increasing knowledge of Notch signaling in immune cell regulation and function can shed new lights on future directions for clinical management of malignant tumors that are recalcitrant to standard therapies. Currently, the complicated roles of Notch signaling in tumor immune responses remain largely understudied. It is acknowledged that Notch signaling can regulate immune cells involved in pro-tumor or antitumor responses. Thus, Notch signaling can be tumor-promoting or tumor-suppressive, yet the inducing factors or conditions resulting in certain effects are still unclear. Relative abundance of immune cells, cytokines, chemokines, magnitude of Notch activation, and the type of Notch-activated cells may affect Notch-mediated immune regulation, which warrants future research attention. Notch signaling is generally activated among cells to mediate intercellular crosstalk, but its effects extremely rely on cancer type. The overall effect of Notch signaling on tumor immune microenvironment must be carefully detected in diverse types of cancer. In addition, Notch mutation is linked with immune cell infiltration within TME (101). It is equally important to define distinct Notch mutations and their influence on tumor immune microenvironment.
So far, some Notch-targeting drugs (e.g., Notch inhibitors, ligand/receptor-targeting antibodies or antibody-drug conjugates) have been evaluated in clinical trials. Nevertheless, there are still many obstacles, hindering the successful implementation of Notch-targeted therapeutics, such as insufficient anticancer efficacy, off-target toxicity and resistance to therapy. Pan-Notch inhibitors and limited affinity of antibody-drug conjugates might be plausible explanations for the off-target toxicity. Attenuating drug toxicities, using intermittent dosing regimens and developing multi-targeted agents are expected to be useful strategies to address this issue. Non-specific Notch inhibitors might simultaneously block Notch signaling pathway in cancer cells and immune cells, thus resulting in opposite effects on cancer control. To improve tumor targetability and agent efficacy, it is imperative to exploit new strategies for efficient delivery of Notch-targeting drugs to cancer cells. Nanocarrier systems such as dendrimers, liposomes or nanoparticles can be exploited to encapsulate, protect and target Notch-targeting drugs to the tumor site. Moreover, the perplexity of Notch signaling and bypass signaling pathways may restrict the clinical activity of Notch-targeted drugs, which highlights the need for further research on Notch regulatory networks in cancer. Notch mutation and activation status are associated with cancer pathogenesis and resistance to therapy. However, the Notch mutation and/or activation status in enrolled subjects were usually elusive in previous clinical trials. Therefore, it is necessary to monitor Notch activation status and levels in cancer patients in further clinical trials. Identifying predictive biomarkers for patient stratification is essential for the clinical development of Notch-targeting drugs. There is an urgent need to discover more predictive biomarkers for response to Notch-based therapies in cancer patients. Notch-targeted therapies can enhance response to conventional anticancer treatments (e.g., chemotherapy and immunotherapy). Notch inhibitors in conjunction with other treatment modalities can lead to synergistic anticancer effects. The combination treatment strategies hold great promise for therapeutic applications in cancer.
In conclusion, Notch signaling has emerged as a key regulator in tumor immunity. It can control the activity of various cells within TME. Therefore, targeting Notch signaling appears an effective approach to advance the clinical benefits of immunotherapy. However, much work remains to thoroughly understand Notch signaling and its effects on tumor immune microenvironment. Comprehensive insights into molecular mechanisms of Notch signaling in oncoimmunology may facilitate the development of more innovative and precise targeted therapies that will improve clinical outcomes in cancer patients.
Author contributions
MW: Conceptualization, Supervision, Visualization, Writing – original draft. FY: Investigation, Resources, Writing – review & editing. YZ: Resources, Writing – review & editing. PL: Conceptualization, Supervision, Writing – review & editing.
Funding
The author(s) declare financial support was received for the research, authorship, and/or publication of this article. This work was supported by the grant from the Natural Science Foundation of Shandong Province, China (No. ZR2021MH018).
Conflict of interest
The authors declare that the research was conducted in the absence of any commercial or financial relationships that could be construed as a potential conflict of interest.
Publisher’s note
All claims expressed in this article are solely those of the authors and do not necessarily represent those of their affiliated organizations, or those of the publisher, the editors and the reviewers. Any product that may be evaluated in this article, or claim that may be made by its manufacturer, is not guaranteed or endorsed by the publisher.
Glossary
References
1. Metz CW, Bridges CB. Incompatibility of mutant races in drosophila. Proc Natl Acad Sci United States America. (1917) 3:673–8. doi: 10.1073/pnas.3.12.673
2. Bianchi S, Dotti MT, Federico A. Physiology and pathology of notch signalling system. J Cell Physiol. (2006) 207:300–8. doi: 10.1002/jcp.20542
3. Zong D, Ouyang R, Li J, Chen Y, Chen P. Notch signaling in lung diseases: focus on notch1 and notch3. Ther Adv Respir Dis. (2016) 10:468–84. doi: 10.1177/1753465816654873
4. Bray SJ. Notch signalling in context. Nat Rev Mol Cell Biol. (2016) 17:722–35. doi: 10.1038/nrm.2016.94
5. Moore G, Annett S, McClements L, Robson T. Top notch targeting strategies in cancer: A detailed overview of recent insights and current perspectives. Cells. (2020) 9:1503. doi: 10.3390/cells9061503
6. Artavanis-Tsakonas S, Rand MD, Lake RJ. Notch signaling: cell fate control and signal integration in development. Science. (1999) 284:770–6. doi: 10.1126/science.284.5415.770
7. Guo H, Lu Y, Wang J, Liu X, Keller ET, Liu Q, et al. Targeting the notch signaling pathway in cancer therapeutics. Thorac Cancer. (2014) 5:473–86. doi: 10.1111/1759-7714.12143
8. Aster JC, Pear WS, Blacklow SC. The varied roles of notch in cancer. Annu Rev Pathol. (2017) 12:245–75. doi: 10.1146/annurev-pathol-052016-100127
9. Bolos V, Blanco M, Medina V, Aparicio G, Diaz-Prado S, Grande E. Notch signalling in cancer stem cells. Clin Trans Oncol. (2009) 11:11–9. doi: 10.1007/s12094-009-0305-2
10. Rehman AO, Wang CY. Notch signaling in the regulation of tumor angiogenesis. Trends Cell Biol. (2006) 16:293–300. doi: 10.1016/j.tcb.2006.04.003
11. Li L, Tang P, Li S, Qin X, Yang H, Wu C, et al. Notch signaling pathway networks in cancer metastasis: A new target for cancer therapy. Med Oncol. (2017) 34:180. doi: 10.1007/s12032-017-1039-6
12. Teoh SL, Das S. Notch signalling pathways and their importance in the treatment of cancers. Curr Drug Targets. (2018) 19:128–43. doi: 10.2174/1389450118666170309143419
13. Li X, Yan X, Wang Y, Kaur B, Han H, Yu J. The notch signaling pathway: A potential target for cancer immunotherapy. J Hematol Oncol. (2023) 16:45. doi: 10.1186/s13045-023-01439-z
14. Purow B. Notch inhibition as a promising new approach to cancer therapy. Adv Exp Med Biol. (2012) 727:305–19. doi: 10.1007/978-1-4614-0899-4_23
15. Aggarwal V, Tuli HS, Varol M, Tuorkey M, Sak K, Parashar NC, et al. Notch signaling: journey of an evolutionarily conserved pathway in driving tumor progression and its modulation as a therapeutic target. Crit Rev oncology/hematol. (2021) 164:103403. doi: 10.1016/j.critrevonc.2021.103403
16. Kadian LK, Gulshan G, Ahuja P, Singhal G, Sharma S, Nanda S, et al. Aberrant promoter methylation of notch1 and notch3 and its association with cervical cancer risk factors in north Indian population. Am J Trans Res. (2020) 12:2814–26.
17. Goruganthu MUL, Shanker A, Dikov MM, Carbone DP. Specific targeting of notch ligand-receptor interactions to modulate immune responses: A review of clinical and preclinical findings. Front Immunol. (2020) 11:1958. doi: 10.3389/fimmu.2020.01958
18. Kovall RA, Gebelein B, Sprinzak D, Kopan R. The canonical notch signaling pathway: structural and biochemical insights into shape, sugar, and force. Dev Cell. (2017) 41:228–41. doi: 10.1016/j.devcel.2017.04.001
19. Kopan R, Ilagan MX. The canonical notch signaling pathway: unfolding the activation mechanism. Cell. (2009) 137:216–33. doi: 10.1016/j.cell.2009.03.045
20. Nowell CS, Radtke F. Notch as a tumour suppressor. Nat Rev Cancer. (2017) 17:145–59. doi: 10.1038/nrc.2016.145
21. Garis M, Garrett-Sinha LA. Notch signaling in B cell immune responses. Front Immunol. (2020) 11:609324. doi: 10.3389/fimmu.2020.609324
22. Zhou B, Lin W, Long Y, Yang Y, Zhang H, Wu K, et al. Notch signaling pathway: architecture, disease, and therapeutics. Signal transduction targeted Ther. (2022) 7:95. doi: 10.1038/s41392-022-00934-y
23. Bian W, Jiang H, Yao L, Hao W, Wu L, Li X. A spatially defined human notch receptor interaction network reveals notch intracellular storage and ataxin-2-mediated fast recycling. Cell Rep. (2023) 42:112819. doi: 10.1016/j.celrep.2023.112819
24. Stanley P. Regulation of notch signaling by glycosylation. Curr Opin Struct Biol. (2007) 17:530–5. doi: 10.1016/j.sbi.2007.09.007
25. Rand MD, Grimm LM, Artavanis-Tsakonas S, Patriub V, Blacklow SC, Sklar J, et al. Calcium depletion dissociates and activates heterodimeric notch receptors. Mol Cell Biol. (2000) 20:1825–35. doi: 10.1128/MCB.20.5.1825-1835.2000
26. Zamfirescu AM, Yatsenko AS, Shcherbata HR. Notch signaling sculpts the stem cell niche. Front Cell Dev Biol. (2022) 10:1027222. doi: 10.3389/fcell.2022.1027222
27. van Tetering G, Vooijs M. Proteolytic cleavage of notch: “Hit and run”. Curr Mol Med. (2011) 11:255–69. doi: 10.2174/156652411795677972
28. Groot AJ, Vooijs MA. The role of adams in notch signaling. Adv Exp Med Biol. (2012) 727:15–36. doi: 10.1007/978-1-4614-0899-4_2
29. Yamamoto S, Charng WL, Bellen HJ. Endocytosis and intracellular trafficking of notch and its ligands. Curr topics Dev Biol. (2010) 92:165–200. doi: 10.1016/S0070-2153(10)92005-X
30. Conner SD. Regulation of notch signaling through intracellular transport. Int Rev Cell Mol Biol. (2016) 323:107–27. doi: 10.1016/bs.ircmb.2015.12.002
31. Jarrett SM, Seegar TCM, Andrews M, Adelmant G, Marto JA, Aster JC, et al. Extension of the notch intracellular domain ankyrin repeat stack by nrarp promotes feedback inhibition of notch signaling. Sci Signaling. (2019) 12:eaay2369. doi: 10.1126/scisignal.aay2369
32. Gallenstein N, Tichy L, Weigand MA, Schenz J. Notch signaling in acute inflammation and sepsis. Int J Mol Sci. (2023) 24:3458. doi: 10.3390/ijms24043458
33. Yuan Z, VanderWielen BD, Giaimo BD, Pan L, Collins CE, Turkiewicz A, et al. Structural and functional studies of the rbpj-sharp complex reveal a conserved corepressor binding site. Cell Rep. (2019) 26:845–54 e6. doi: 10.1016/j.celrep.2018.12.097
34. Shen W, Huang J, Wang Y. Biological significance of notch signaling strength. Front Cell Dev Biol. (2021) 9:652273. doi: 10.3389/fcell.2021.652273
35. Akil A, Gutierrez-Garcia AK, Guenter R, Rose JB, Beck AW, Chen H, et al. Notch signaling in vascular endothelial cells, angiogenesis, and tumor progression: an update and prospective. Front Cell Dev Biol. (2021) 9:642352. doi: 10.3389/fcell.2021.642352
36. Kelliher MA, Roderick JE. Notch signaling in T-cell-mediated anti-tumor immunity and T-cell-based immunotherapies. Front Immunol. (2018) 9:1718. doi: 10.3389/fimmu.2018.01718
37. Yu XM, Jaskula-Sztul R, Georgen MR, Aburjania Z, Somnay YR, Leverson G, et al. Notch1 signaling regulates the aggressiveness of differentiated thyroid cancer and inhibits serpine1 expression. Clin Cancer Res. (2016) 22:3582–92. doi: 10.1158/1078-0432.CCR-15-1749
38. Mezquita B, Mezquita J, Barrot C, Carvajal S, Pau M, Mezquita P, et al. A truncated-flt1 isoform of breast cancer cells is upregulated by notch and downregulated by retinoic acid. J Cell Biochem. (2014) 115:52–61. doi: 10.1002/jcb.24632
39. Kumar S, Srivastav RK, Wilkes DW, Ross T, Kim S, Kowalski J, et al. Estrogen-dependent dll1-mediated notch signaling promotes luminal breast cancer. Oncogene. (2019) 38:2092–107. doi: 10.1038/s41388-018-0562-z
40. Purow BW, Haque RM, Noel MW, Su Q, Burdick MJ, Lee J, et al. Expression of notch-1 and its ligands, delta-like-1 and jagged-1, is critical for glioma cell survival and proliferation. Cancer Res. (2005) 65:2353–63. doi: 10.1158/0008-5472.CAN-04-1890
41. Biktasova AK, Dudimah DF, Uzhachenko RV, Park K, Akhter A, Arasada RR, et al. Multivalent forms of the notch ligand dll-1 enhance antitumor T-cell immunity in lung cancer and improve efficacy of egfr-targeted therapy. Cancer Res. (2015) 75:4728–41. doi: 10.1158/0008-5472.CAN-14-1154
42. Pu Y, Zhao F, Wang H, Cai S. Mir-34a-5p promotes multi-chemoresistance of osteosarcoma through down-regulation of the dll1 gene. Sci Rep. (2017) 7:44218. doi: 10.1038/srep44218
43. Rajamani D, Bhasin MK. Identification of key regulators of pancreatic cancer progression through multidimensional systems-level analysis. Genome Med. (2016) 8:38. doi: 10.1186/s13073-016-0282-3
44. You WK, Schuetz TJ, Lee SH. Targeting the dll/notch signaling pathway in cancer: challenges and advances in clinical development. Mol Cancer Ther. (2023) 22:3–11. doi: 10.1158/1535-7163.MCT-22-0243
45. Katoh M, Katoh M. Precision medicine for human cancers with notch signaling dysregulation (Review). Int J Mol Med. (2020) 45:279–97. doi: 10.3892/ijmm.2019.4418
46. Meurette O, Mehlen P. Notch signaling in the tumor microenvironment. Cancer Cell. (2018) 34:536–48. doi: 10.1016/j.ccell.2018.07.009
47. Gridley T. Notch signaling in vascular development and physiology. Dev (Cambridge England). (2007) 134:2709–18. doi: 10.1242/dev.004184
48. Ridgway J, Zhang G, Wu Y, Stawicki S, Liang WC, Chanthery Y, et al. Inhibition of dll4 signalling inhibits tumour growth by deregulating angiogenesis. Nature. (2006) 444:1083–7. doi: 10.1038/nature05313
49. Liu Z, Fan F, Wang A, Zheng S, Lu Y. Dll4-notch signaling in regulation of tumor angiogenesis. J Cancer Res Clin Oncol. (2014) 140:525–36. doi: 10.1007/s00432-013-1534-x
50. Miles KM, Seshadri M, Ciamporcero E, Adelaiye R, Gillard B, Sotomayor P, et al. Dll4 blockade potentiates the anti-tumor effects of vegf inhibition in renal cell carcinoma patient-derived xenografts. PloS One. (2014) 9:e112371. doi: 10.1371/journal.pone.0112371
51. Li JL, Sainson RC, Oon CE, Turley H, Leek R, Sheldon H, et al. Dll4-notch signaling mediates tumor resistance to anti-vegf therapy in vivo. Cancer Res. (2011) 71:6073–83. doi: 10.1158/0008-5472.can-11-1704
52. Bailis W, Yashiro-Ohtani Y, Fang TC, Hatton RD, Weaver CT, Artis D, et al. Notch simultaneously orchestrates multiple helper T cell programs independently of cytokine signals. Immunity. (2013) 39:148–59. doi: 10.1016/j.immuni.2013.07.006
53. Xu H, Zhu J, Smith S, Foldi J, Zhao B, Chung AY, et al. Notch-rbp-J signaling regulates the transcription factor irf8 to promote inflammatory macrophage polarization. Nat Immunol. (2012) 13:642–50. doi: 10.1038/ni.2304
54. Xu J, Chi F, Guo T, Punj V, Lee WN, French SW, et al. Notch reprograms mitochondrial metabolism for proinflammatory macrophage activation. J Clin Invest. (2015) 125:1579–90. doi: 10.1172/JCI76468
55. Otani Y, Yoo JY, Lewis CT, Chao S, Swanner J, Shimizu T, et al. Notch-induced mdsc recruitment after ohsv virotherapy in cns cancer models modulates antitumor immunotherapy. Clin Cancer Res. (2022) 28:1460–73. doi: 10.1158/1078-0432.CCR-21-2347
56. Grazioli P, Orlando A, Giordano N, Noce C, Peruzzi G, Abdollahzadeh B, et al. Notch-signaling deregulation induces myeloid-derived suppressor cells in T-cell acute lymphoblastic leukemia. Front Immunol. (2022) 13:809261. doi: 10.3389/fimmu.2022.809261
57. Huang K, Luo W, Fang J, Yu C, Liu G, Yuan X, et al. Notch3 signaling promotes colorectal tumor growth by enhancing immunosuppressive cells infiltration in the microenvironment. BMC Cancer. (2023) 23:55. doi: 10.1186/s12885-023-10526-w
58. Peng D, Tanikawa T, Li W, Zhao L, Vatan L, Szeliga W, et al. Myeloid-derived suppressor cells endow stem-like qualities to breast cancer cells through il6/stat3 and no/notch cross-talk signaling. Cancer Res. (2016) 76:3156–65. doi: 10.1158/0008-5472.CAN-15-2528
59. Wang SH, Lu QY, Guo YH, Song YY, Liu PJ, Wang YC. The blockage of notch signalling promoted the generation of polymorphonuclear myeloid-derived suppressor cells with lower immunosuppression. Eur J Cancer. (2016) 68:90–105. doi: 10.1016/j.ejca.2016.08.019
60. Sprouse ML, Welte T, Boral D, Liu HN, Yin W, Vishnoi M, et al. Pmn-mdscs enhance ctc metastatic properties through reciprocal interactions via ros/notch/nodal signaling. Int J Mol Sci. (2019) 20:1916. doi: 10.3390/ijms20081916
61. Zhao JL, Ye YC, Gao CC, Wang L, Ren KX, Jiang R, et al. Notch-mediated lactate metabolism regulates mdsc development through the hes1/mct2/C-jun axis. Cell Rep. (2022) 38:110451. doi: 10.1016/j.celrep.2022.110451
62. Sierra RA, Thevenot P, Raber PL, Cui Y, Parsons C, Ochoa AC, et al. Rescue of notch-1 signaling in antigen-specific cd8+ T cells overcomes tumor-induced T-cell suppression and enhances immunotherapy in cancer. Cancer Immunol Res. (2014) 2:800–11. doi: 10.1158/2326-6066.CIR-14-0021
63. Shen Q, Cohen B, Zheng W, Rahbar R, Martin B, Murakami K, et al. Notch shapes the innate immunophenotype in breast cancer. Cancer Discovery. (2017) 7:1320–35. doi: 10.1158/2159-8290.CD-17-0037
64. Yin JZ, Shi XQ, Wang MD, Du H, Zhao XW, Li B, et al. Arsenic trioxide elicits anti-tumor activity by inhibiting polarization of M2-like tumor-associated macrophages via notch signaling pathway in lung adenocarcinoma. Int Immunopharmacol. (2023) 117:109899. doi: 10.1016/j.intimp.2023.109899
65. Tao S, Chen Q, Lin C, Dong H. Linc00514 promotes breast cancer metastasis and M2 polarization of tumor-associated macrophages via jagged1-mediated notch signaling pathway. J Exp Clin Cancer Res. (2020) 39:191. doi: 10.1186/s13046-020-01676-x
66. Alsina-Sanchis E, Mulfarth R, Moll I, Bohn S, Wiedmann L, Jordana-Urriza L, et al. Endothelial rbpj is essential for the education of tumor-associated macrophages. Cancer Res. (2022) 82:4414–28. doi: 10.1158/0008-5472.CAN-22-0076
67. Yan W, Menjivar RE, Bonilla ME, Steele NG, Kemp SB, Du W, et al. Notch signaling regulates immunosuppressive tumor-associated macrophage function in pancreatic cancer. Cancer Immunol Res. (2024) 12:91–106. doi: 10.1158/2326-6066.CIR-23-0037
68. Marini W, Wilson BE, Reedijk M. Targeting notch-driven cytokine secretion: novel therapies for triple negative breast cancer. DNA Cell Biol. (2023) 42:73–81. doi: 10.1089/dna.2022.0578
69. Geng Y, Fan J, Chen L, Zhang C, Qu C, Qian L, et al. A notch-dependent inflammatory feedback circuit between macrophages and cancer cells regulates pancreatic cancer metastasis. Cancer Res. (2021) 81:64–76. doi: 10.1158/0008-5472.CAN-20-0256
70. Cheung PF, Neff F, Neander C, Bazarna A, Savvatakis K, Liffers ST, et al. Notch-induced myeloid reprogramming in spontaneous pancreatic ductal adenocarcinoma by dual genetic targeting. Cancer Res. (2018) 78:4997–5010. doi: 10.1158/0008-5472.CAN-18-0052
71. Ye YC, Zhao JL, Lu YT, Gao CC, Yang Y, Liang SQ, et al. Notch signaling via wnt regulates the proliferation of alternative, ccr2-independent tumor-associated macrophages in hepatocellular carcinoma. Cancer Res. (2019) 79:4160–72. doi: 10.1158/0008-5472.CAN-18-1691
72. Yang Z, Qi Y, Lai N, Zhang J, Chen Z, Liu M, et al. Notch1 signaling in melanoma cells promoted tumor-induced immunosuppression via upregulation of tgf-beta1. J Exp Clin Cancer Res. (2018) 37:1. doi: 10.1186/s13046-017-0664-4
73. Yuan C, Chang K, Xu C, Li Q, Du Z. High expression of dll3 is associated with a poor prognosis and immune infiltration in invasive breast cancer patients. Trans Oncol. (2021) 14:101080. doi: 10.1016/j.tranon.2021.101080
74. Meng X, Zhu S, Dong Q, Zhang S, Ma J, Zhou C. Expression of th17/treg related molecules in gastric cancer tissues. Turkish J Gastroenterol. (2018) 29:45–51. doi: 10.5152/tjg.2018.17114
75. Nishikawa H, Sakaguchi S. Regulatory T cells in cancer immunotherapy. Curr Opin Immunol. (2014) 27:1–7. doi: 10.1016/j.coi.2013.12.005
76. Kumar R, Theiss AL, Venuprasad K. Rorgammat protein modifications and il-17-mediated inflammation. Trends Immunol. (2021) 42:1037–50. doi: 10.1016/j.it.2021.09.005
77. Yang L, Zhao KL, Qin L, Ji DX, Zhang B, Zheng PF, et al. Notch signaling pathway regulates cd4(+)Cd25(+)Cd127(Dim/-) regulatory T cells and T helper 17 cells function in gastric cancer patients. Biosci Rep. (2019) 39:BSR20182044. doi: 10.1042/BSR20182044
78. Cui Y, Li Q, Li W, Wang Y, Lv F, Shi X, et al. Notch3 is a prognostic factor and is correlated with immune tolerance in gastric cancer. Front Oncol. (2020) 10:574937. doi: 10.3389/fonc.2020.574937
79. Tsai YM, Wu KL, Liu YW, Chang WA, Huang YC, Chang CY, et al. Cooperation between cancer and fibroblasts in vascular mimicry and N2-type neutrophil recruitment via notch2-jagged1 interaction in lung cancer. Front Oncol. (2021) 11:696931. doi: 10.3389/fonc.2021.696931
80. Liubomirski Y, Lerrer S, Meshel T, Morein D, Rubinstein-Achiasaf L, Sprinzak D, et al. Notch-mediated tumor-stroma-inflammation networks promote invasive properties and cxcl8 expression in triple-negative breast cancer. Front Immunol. (2019) 10:804. doi: 10.3389/fimmu.2019.00804
81. Bai S, Zhao Y, Chen W, Peng W, Wang Y, Xiong S, et al. The stromal-tumor amplifying stc1-notch1 feedforward signal promotes the stemness of hepatocellular carcinoma. J Trans Med. (2023) 21:236. doi: 10.1186/s12967-023-04085-8
82. Nandi A, Debnath R, Nayak A, To TKJ, Thacker G, Reilly M, et al. Dll1-mediated notch signaling drives tumor cell cross-talk with cancer-associated fibroblasts to promote radioresistance in breast cancer. Cancer Res. (2022) 82:3718–33. doi: 10.1158/0008-5472.CAN-21-1225
83. Kim HJ, Yang K, Kim K, Lee YJ, Lee S, Ahn SY, et al. Reprogramming of cancer-associated fibroblasts by apoptotic cancer cells inhibits lung metastasis via notch1-wisp-1 signaling. Cell Mol Immunol. (2022) 19:1373–91. doi: 10.1038/s41423-022-00930-w
84. Du Y, Shao H, Moller M, Prokupets R, Tse YT, Liu ZJ. Intracellular notch1 signaling in cancer-associated fibroblasts dictates the plasticity and stemness of melanoma stem/initiating cells. Stem Cells. (2019) 37:865–75. doi: 10.1002/stem.3013
85. Shao H, Moller M, Cai L, Prokupets R, Yang C, Costa C, et al. Converting melanoma-associated fibroblasts into a tumor-suppressive phenotype by increasing intracellular notch1 pathway activity. PloS One. (2021) 16:e0248260. doi: 10.1371/journal.pone.0248260
86. Wood CS, Pennel KAF, Leslie H, Legrini A, Cameron AJ, Melissourgou-Syka L, et al. Spatially resolved transcriptomics deconvolutes prognostic histological subgroups in patients with colorectal cancer and synchronous liver metastases. Cancer Res. (2023) 83:1329–44. doi: 10.1158/0008-5472.CAN-22-2794
87. Nolan E, Bridgeman VL, Ombrato L, Karoutas A, Rabas N, Sewnath CAN, et al. Radiation exposure elicits a neutrophil-driven response in healthy lung tissue that enhances metastatic colonization. Nat Cancer. (2022) 3:173–87. doi: 10.1038/s43018-022-00336-7
88. Wieland E, Rodriguez-Vita J, Liebler SS, Mogler C, Moll I, Herberich SE, et al. Endothelial notch1 activity facilitates metastasis. Cancer Cell. (2017) 31:355–67. doi: 10.1016/j.ccell.2017.01.007
89. Kijima M, Yamaguchi T, Ishifune C, Maekawa Y, Koyanagi A, Yagita H, et al. Dendritic cell-mediated nk cell activation is controlled by jagged2-notch interaction. Proc Natl Acad Sci United States America. (2008) 105:7010–5. doi: 10.1073/pnas.0709919105
90. Wang L, Yu S, Chan ER, Chen KY, Liu C, Che D, et al. Notch-regulated dendritic cells restrain inflammation-associated colorectal carcinogenesis. Cancer Immunol Res. (2021) 9:348–61. doi: 10.1158/2326-6066.CIR-20-0428
91. Jackstadt R, van Hooff SR, Leach JD, Cortes-Lavaud X, Lohuis JO, Ridgway RA, et al. Epithelial notch signaling rewires the tumor microenvironment of colorectal cancer to drive poor-prognosis subtypes and metastasis. Cancer Cell. (2019) 36:319–36 e7. doi: 10.1016/j.ccell.2019.08.003
92. Yang M, Zhang G, Wang Y, He M, Xu Q, Lu J, et al. Tumour-associated neutrophils orchestrate intratumoural il-8-driven immune evasion through jagged2 activation in ovarian cancer. Br J Cancer. (2020) 123:1404–16. doi: 10.1038/s41416-020-1026-0
93. Gong XY, Chen HB, Zhang LQ, Chen DS, Li W, Chen DH, et al. Notch1 mutation associates with impaired immune response and decreased relapse-free survival in patients with resected T1-2n0 laryngeal cancer. Front Immunol. (2022) 13:920253. doi: 10.3389/fimmu.2022.920253
94. Meng J, Jiang YZ, Zhao S, Tao Y, Zhang T, Wang X, et al. Tumor-derived jagged1 promotes cancer progression through immune evasion. Cell Rep. (2022) 38:110492. doi: 10.1016/j.celrep.2022.110492
95. Jaiswal A, Murakami K, Elia A, Shibahara Y, Done SJ, Wood SA, et al. Therapeutic inhibition of usp9x-mediated notch signaling in triple-negative breast cancer. Proc Natl Acad Sci United States America. (2021) 118:e2101592118. doi: 10.1073/pnas.2101592118
96. Mangolini M, Maiques-Diaz A, Charalampopoulou S, Gerhard-Hartmann E, Bloehdorn J, Moore A, et al. Viral transduction of primary human lymphoma B cells reveals mechanisms of notch-mediated immune escape. Nat Commun. (2022) 13:6220. doi: 10.1038/s41467-022-33739-2
97. Cao Y, Liu B, Cai L, Li Y, Huang Y, Zhou Y, et al. G9a promotes immune suppression by targeting the fbxw7/notch pathway in glioma stem cells. CNS Neurosci Ther. (2023) 29:2508–21. doi: 10.1111/cns.14191
98. Hu J, Yu J, Gan J, Song N, Shi L, Liu J, et al. Notch1/2/3/4 are prognostic biomarker and correlated with immune infiltrates in gastric cancer. Aging. (2020) 12:2595–609. doi: 10.18632/aging.102764
99. Parmigiani E, Ivanek R, Rolando C, Hafen K, Turchinovich G, Lehmann FM, et al. Interferon-gamma resistance and immune evasion in glioma develop via notch-regulated co-evolution of Malignant and immune cells. Dev Cell. (2022) 57:1847–65 e9. doi: 10.1016/j.devcel.2022.06.006
100. Lin A, Yao J, Cheng Q, Liu Z, Luo P, Zhang J. Mutations status of notch signaling pathway predict prognosis of immune checkpoint inhibitors in colorectal cancer. J Inflammation Res. (2023) 16:1693–709. doi: 10.2147/JIR.S394894
101. Wang F, Long J, Li L, Zhao ZB, Wei F, Yao Y, et al. Mutations in the notch pathway are associated with enhanced anti-tumour immunity in colorectal cancer. J Cell Mol Med. (2020) 24:12176–87. doi: 10.1111/jcmm.15867
102. Qing Y, Wang Y, Hu C, Zhang H, Huang Y, Zhang Z, et al. Evaluation of notch family genes’ Expression and prognostic value in prostate cancer. Trans androl Urol. (2022) 11:627–42. doi: 10.21037/tau-22-281
103. Tchekneva EE, Goruganthu MUL, Uzhachenko RV, Thomas PL, Antonucci A, Chekneva I, et al. Determinant roles of dendritic cell-expressed notch delta-like and jagged ligands on anti-tumor T cell immunity. J immunother Cancer. (2019) 7:95. doi: 10.1186/s40425-019-0566-4
104. Huang C, Zhu HX, Yao Y, Bian ZH, Zheng YJ, Li L, et al. Immune checkpoint molecules. Possible future therapeutic implications in autoimmune diseases. J Autoimmun. (2019) 104:102333. doi: 10.1016/j.jaut.2019.102333
105. Wieder T, Eigentler T, Brenner E, Rocken M. Immune checkpoint blockade therapy. J Allergy Clin Immunol. (2018) 142:1403–14. doi: 10.1016/j.jaci.2018.02.042
106. Ghosh C, Luong G, Sun Y. A snapshot of the pd-1/pd-L1 pathway. J Cancer. (2021) 12:2735–46. doi: 10.7150/jca.57334
107. Jiang Q, Chen H, Zhou S, Zhu T, Liu W, Wu H, et al. Ubiquilin-4 induces immune escape in gastric cancer by activating the notch signaling pathway. Cell Oncol. (2023). doi: 10.1007/s13402-023-00869-8
108. Bottcher M, Bruns H, Volkl S, Lu J, Chartomatsidou E, Papakonstantinou N, et al. Control of pd-L1 expression in cll-cells by stromal triggering of the notch-C-myc-ezh2 oncogenic signaling axis. J immunother Cancer. (2021) 9:e001889. doi: 10.1136/jitc-2020-001889
109. Mansour FA, Al-Mazrou A, Al-Mohanna F, Al-Alwan M, Ghebeh H. Pd-L1 is overexpressed on breast cancer stem cells through notch3/mtor axis. Oncoimmunology. (2020) 9:1729299. doi: 10.1080/2162402X.2020.1729299
110. Lacalle-Gonzalez C, Florez-Cespedes M, Sanz-Criado L, Ochieng’ Otieno M, Ramos-Munoz E, Fernandez-Acenero MJ, et al. Dll3 is a prognostic and potentially predictive biomarker for immunotherapy linked to pd/pd-L axis and notch1 in pancreatic cancer. Biomedicines. (2023) 11:2812. doi: 10.3390/biomedicines11102812
111. Sun X, Xin S, Li W, Zhang Y, Ye L. Discovery of notch pathway-related genes for predicting prognosis and tumor microenvironment status in bladder cancer. Front Genet. (2022) 13:928778. doi: 10.3389/fgene.2022.928778
112. Long J, Wang D, Yang X, Wang A, Lin Y, Zheng M, et al. Identification of notch4 mutation as a response biomarker for immune checkpoint inhibitor therapy. BMC Med. (2021) 19:154. doi: 10.1186/s12916-021-02031-3
113. Li X, Wang Y, Li X, Feng G, Hu S, Bai Y. The impact of notch pathway alteration on tumor microenvironment and clinical survival of immune checkpoint inhibitors in nsclc. Front Immunol. (2021) 12:638763. doi: 10.3389/fimmu.2021.638763
114. Mazzotta M, Filetti M, Occhipinti M, Marinelli D, Scalera S, Terrenato I, et al. Efficacy of immunotherapy in lung cancer with co-occurring mutations in notch and homologous repair genes. J immunother Cancer. (2020) 8:e000946. doi: 10.1136/jitc-2020-000946
115. Zhang Z, Gu Y, Su X, Bai J, Guan W, Ma J, et al. Co-occurring alteration of notch and ddr pathways serves as novel predictor to efficacious immunotherapy in nsclc. Front Oncol. (2021) 11:659321. doi: 10.3389/fonc.2021.659321
116. Wang F, Huang C, Long J, Zhao ZB, Ma HQ, Yao XQ, et al. Notch signaling mutations increase intra-tumor chemokine expression and predict response to immunotherapy in colorectal cancer. BMC Cancer. (2022) 22:933. doi: 10.1186/s12885-022-10032-5
117. Bernardo M, Tolstykh T, Zhang YA, Bangari DS, Cao H, Heyl KA, et al. An experimental model of anti-pd-1 resistance exhibits activation of tgfss and notch pathways and is sensitive to local mrna immunotherapy. Oncoimmunology. (2021) 10:1881268. doi: 10.1080/2162402X.2021.1881268
118. Masiero M, Li D, Whiteman P, Bentley C, Greig J, Hassanali T, et al. Development of therapeutic anti-jagged1 antibodies for cancer therapy. Mol Cancer Ther. (2019) 18:2030–42. doi: 10.1158/1535-7163.MCT-18-1176
119. Silva G, Sales-Dias J, Casal D, Alves S, Domenici G, Barreto C, et al. Development of dl1.72, a novel anti-dll1 antibody with anti-tumor efficacy against estrogen receptor-positive breast cancer. Cancers. (2021) 13:4074. doi: 10.3390/cancers13164074
120. Coleman RL, Handley KF, Burger R, Molin GZD, Stagg R, Sood AK, et al. Demcizumab combined with paclitaxel for platinum-resistant ovarian, primary peritoneal, and fallopian tube cancer: the sierra open-label phase ib trial. Gynecologic Oncol. (2020) 157:386–91. doi: 10.1016/j.ygyno.2020.01.042
121. Fu W, Li G, Lei C, Qian K, Zhang S, Zhao J, et al. Bispecific antibodies targeting egfr/notch enhance the response to talazoparib by decreasing tumour-initiating cell frequency. Theranostics. (2023) 13:3641–54. doi: 10.7150/thno.82144
122. Valcourt DM, Dang MN, Scully MA, Day ES. Nanoparticle-mediated co-delivery of notch-1 antibodies and abt-737 as a potent treatment strategy for triple-negative breast cancer. ACS nano. (2020) 14:3378–88. doi: 10.1021/acsnano.9b09263
123. Smith DC, Chugh R, Patnaik A, Papadopoulos KP, Wang M, Kapoun AM, et al. A phase 1 dose escalation and expansion study of tarextumab (Omp-59r5) in patients with solid tumors. Investigational New Drugs. (2019) 37:722–30. doi: 10.1007/s10637-018-0714-6
124. Hu ZI, Bendell JC, Bullock A, LoConte NK, Hatoum H, Ritch P, et al. A randomized phase ii trial of nab-paclitaxel and gemcitabine with tarextumab or placebo in patients with untreated metastatic pancreatic cancer. Cancer Med. (2019) 8:5148–57. doi: 10.1002/cam4.2425
125. Saran U, Chandrasekaran B, Tyagi A, Shukla V, Singh A, Sharma AK, et al. A small molecule inhibitor of notch1 modulates stemness and suppresses breast cancer cell growth. Front Pharmacol. (2023) 14:1150774. doi: 10.3389/fphar.2023.1150774
126. Alvarez-Trotta A, Guerrant W, Astudillo L, Lahiry M, Diluvio G, Shersher E, et al. Pharmacological disruption of the notch1 transcriptional complex inhibits tumor growth by selectively targeting cancer stem cells. Cancer Res. (2021) 81:3347–57. doi: 10.1158/0008-5472.CAN-20-3611
127. Wang X, Chen H, Jiang R, Hong X, Peng J, Chen W, et al. Interleukin-17 activates and synergizes with the notch signaling pathway in the progression of pancreatic ductal adenocarcinoma. Cancer Lett. (2021) 508:1–12. doi: 10.1016/j.canlet.2021.03.003
128. Dai G, Liu G, Zheng D, Song Q. Inhibition of the notch signaling pathway attenuates progression of cell motility, metastasis, and epithelial-to-mesenchymal transition-like phenomena induced by low concentrations of cisplatin in osteosarcoma. Eur J Pharmacol. (2021) 899:174058. doi: 10.1016/j.ejphar.2021.174058
129. Cao L, Ruiz Buendia GA, Fournier N, Liu Y, Armand F, Hamelin R, et al. Resistance mechanism to notch inhibition and combination therapy in human T-cell acute lymphoblastic leukemia. Blood Adv. (2023) 7:6240–52. doi: 10.1182/bloodadvances.2023010380
130. Hanna GJ, Stathis A, Lopez-Miranda E, Racca F, Quon D, Leyvraz S, et al. A phase I study of the pan-notch inhibitor cb-103 for patients with advanced adenoid cystic carcinoma and other tumors. Cancer Res Commun. (2023) 3:1853–61. doi: 10.1158/2767-9764.CRC-23-0333
131. Geles KG, Gao Y, Giannakou A, Sridharan L, Yamin TT, Zhang J, et al. Notch3-Targeted Antibody Drug Conjugates Regress Tumors by Inducing Apoptosis in Receptor Cells and through Transendocytosis into Ligand Cells. Cell Rep Med. (2021) 2:100279. doi: 10.1016/j.xcrm.2021.100279
132. Spino M, Kurz SC, Chiriboga L, Serrano J, Zeck B, Sen N, et al. Cell surface notch ligand dll3 is a therapeutic target in isocitrate dehydrogenase-mutant glioma. Clin Cancer Res. (2019) 25:1261–71. doi: 10.1158/1078-0432.CCR-18-2312
133. Blackhall F, Jao K, Greillier L, Cho BC, Penkov K, Reguart N, et al. Efficacy and safety of rovalpituzumab tesirine compared with topotecan as second-line therapy in dll3-high sclc: results from the phase 3 tahoe study. J Thorac Oncol. (2021) 16:1547–58. doi: 10.1016/j.jtho.2021.02.009
134. Ni W, Chen Z, Zhou X, Yang R, Yu M, Lu J, et al. Targeting notch and egfr signaling in human mucoepidermoid carcinoma. Signal transduction targeted Ther. (2021) 6:27. doi: 10.1038/s41392-020-00388-0
135. Schulz GB, Elezkurtaj S, Bording T, Schmidt EM, Elmasry M, Stief CG, et al. Therapeutic and prognostic implications of notch and mapk signaling in bladder cancer. Cancer Sci. (2021) 112:1987–96. doi: 10.1111/cas.14878
136. Peereboom DM, Ye X, Mikkelsen T, Lesser GJ, Lieberman FS, Robins HI, et al. A phase ii and pharmacodynamic trial of ro4929097 for patients with recurrent/progressive glioblastoma. Neurosurgery. (2021) 88:246–51. doi: 10.1093/neuros/nyaa412
137. Means-Powell JA, Mayer IA, Ismail-Khan R, Del Valle L, Tonetti D, Abramson VG, et al. A phase ib dose escalation trial of ro4929097 (a gamma-secretase inhibitor) in combination with exemestane in patients with er + Metastatic breast cancer (Mbc). Clin Breast Cancer. (2022) 22:103–14. doi: 10.1016/j.clbc.2021.10.013
138. Gounder MM, Rosenbaum E, Wu N, Dickson MA, Sheikh TN, D’Angelo SP, et al. A phase ib/ii randomized study of ro4929097, a gamma-secretase or notch inhibitor with or without vismodegib, a hedgehog inhibitor, in advanced sarcoma. Clin Cancer Res. (2022) 28:1586–94. doi: 10.1158/1078-0432.CCR-21-3874
139. Sardesai S, Badawi M, Mrozek E, Morgan E, Phelps M, Stephens J, et al. A phase I study of an oral selective gamma secretase (Gs) inhibitor ro4929097 in combination with neoadjuvant paclitaxel and carboplatin in triple negative breast cancer. Investigational New Drugs. (2020) 38:1400–10. doi: 10.1007/s10637-020-00895-5
140. Even C, Lassen U, Merchan J, Le Tourneau C, Soria JC, Ferte C, et al. Safety and clinical activity of the notch inhibitor, crenigacestat (Ly3039478), in an open-label phase I trial expansion cohort of advanced or metastatic adenoid cystic carcinoma. Investigational New Drugs. (2020) 38:402–9. doi: 10.1007/s10637-019-00739-x
141. Doi T, Tajimi M, Mori J, Asou H, Inoue K, Benhadji KA, et al. A phase 1 study of crenigacestat (Ly3039478), the notch inhibitor, in Japanese patients with advanced solid tumors. Investigational New Drugs. (2021) 39:469–76. doi: 10.1007/s10637-020-01001-5
142. Azaro A, Massard C, Tap WD, Cassier PA, Merchan J, Italiano A, et al. A phase 1b study of the notch inhibitor crenigacestat (Ly3039478) in combination with other anticancer target agents (Taladegib, ly3023414, or abemaciclib) in patients with advanced or metastatic solid tumors. Investigational New Drugs. (2021) 39:1089–98. doi: 10.1007/s10637-021-01094-6
143. Massard C, Cassier PA, Azaro A, Anderson B, Yuen E, Yu D, et al. A phase 1b study of crenigacestat (Ly3039478) in combination with gemcitabine and cisplatin or gemcitabine and carboplatin in patients with advanced or metastatic solid tumors. Cancer chemother Pharmacol. (2022) 90:335–44. doi: 10.1007/s00280-022-04461-z
144. Borthakur G, Martinelli G, Raffoux E, Chevallier P, Chromik J, Lithio A, et al. Phase 1 study to evaluate crenigacestat (Ly3039478) in combination with dexamethasone in patients with T-cell acute lymphoblastic leukemia and lymphoma. Cancer. (2021) 127:372–80. doi: 10.1002/cncr.33188
145. Azaro A, Baldini C, Rodon J, Soria JC, Yuen E, Lithio A, et al. Phase 1 study of 2 high dose intensity schedules of the pan-notch inhibitor crenigacestat (Ly3039478) in combination with prednisone in patients with advanced or metastatic cancer. Investigational New Drugs. (2021) 39:193–201. doi: 10.1007/s10637-020-00944-z
Keywords: Notch signaling, cancer development, tumor microenvironment, cancer immunotherapy, Notch-targeted therapeutics, tumor immunity
Citation: Wang M, Yu F, Zhang Y and Li P (2024) Novel insights into Notch signaling in tumor immunity: potential targets for cancer immunotherapy. Front. Immunol. 15:1352484. doi: 10.3389/fimmu.2024.1352484
Received: 08 December 2023; Accepted: 06 February 2024;
Published: 20 February 2024.
Edited by:
Maria Pia Felli, Sapienza University of Rome, ItalyReviewed by:
Wenqing Zhang, The Ohio State University, United StatesMaria Grazia Totaro, IFOM - The FIRC Institute of Molecular Oncology, Italy
Copyright © 2024 Wang, Yu, Zhang and Li. This is an open-access article distributed under the terms of the Creative Commons Attribution License (CC BY). The use, distribution or reproduction in other forums is permitted, provided the original author(s) and the copyright owner(s) are credited and that the original publication in this journal is cited, in accordance with accepted academic practice. No use, distribution or reproduction is permitted which does not comply with these terms.
*Correspondence: Man Wang, d2FuZ21hbkBxZHUuZWR1LmNu; Peifeng Li, cGVpZmxpQHFkdS5lZHUuY24=