- 1National-Local Joint Engineering Laboratory of Aquatic Animal Genetic Breeding and Nutrition (Zhejiang), School of Life Science, Huzhou University, Huzhou, China
- 2Zhejiang Provincial Key Laboratory of Aquatic Resources Conservation and Development, School of Life Science, Huzhou University, Huzhou, China
This study aimed to assess the impact of dietary selenoprotein extracts from Cardamine hupingshanensis (SePCH) on the growth, hematological parameters, selenium metabolism, immune responses, antioxidant capacities, inflammatory reactions and intestinal barrier functions in juvenile largemouth bass (Micropterus salmoides). The base diet was supplemented with four different concentrations of SePCH: 0.00, 0.30, 0.60 and 1.20 g/Kg (actual selenium contents: 0.37, 0.59, 0.84 and 1.30 mg/kg). These concentrations were used to formulate four isonitrogenous and isoenergetic diets for juvenile largemouth bass during a 60-day culture period. Adequate dietary SePCH (0.60 and 1.20 g/Kg) significantly increased weight gain and daily growth rate compared to the control groups (0.00 g/Kg). Furthermore, 0.60 and 1.20 g/Kg SePCH significantly enhanced amounts of white blood cells, red blood cells, platelets, lymphocytes and monocytes, and levels of hemoglobin, mean corpuscular volume and mean corpuscular hemoglobin in the hemocytes. In addition, 0.60 and 1.20 g/Kg SePCH increased the mRNA expression levels of selenocysteine lyase, selenophosphate synthase 1, 15 kDa selenoprotein, selenoprotein T2, selenoprotein H, selenoprotein P and selenoprotein K in the fish liver and intestine compared to the controls. Adequate SePCH not only significantly elevated the activities of antioxidant enzymes (Total superoxide dismutase, catalase, glutathione reductase, glutathione peroxidase), the levels of total antioxidant capacity and glutathione, while increased mRNA transcription levels of NF-E2-related factor 2, Cu/Zn-superoxide dismutase, catalase, glutathione reductase and glutathione peroxidase. However, adequate SePCH significantly decreased levels of malondialdehyde and H2O2 and the mRNA expression levels of kelch-like ECH-associated protein 1a and kelch-like ECH-associated protein 1b in the fish liver and intestine compared to the controls. Meanwhile, adequate SePCH markedly enhanced the levels of immune factors (alkaline phosphatase, acid phosphatase, lysozyme, complement component 3, complement component 4 and immunoglobulin M) and innate immune-related genes (lysozyme, hepcidin, liver-expressed antimicrobial peptide 2, complement component 3 and complement component 4) in the fish liver and intestine compared to the controls. Adequate SePCH reduced the levels of pro-inflammatory cytokines (tumour necrosis factor-α, interleukin 8, interleukin 1β and interferon γ), while increasing transforming growth factor β1 levels at both transcriptional and protein levels in the liver and intestine. The mRNA expression levels of mitogen-activated protein kinase 13 (MAPK 13), MAPK14 and nuclear factor kappa B p65 were significantly reduced in the liver and intestine of fish fed with 0.60 and 1.20 g/Kg SePCH compared to the controls. Histological sections also demonstrated that 0.60 and 1.20 g/Kg SePCH significantly increased intestinal villus height and villus width compared to the controls. Furthermore, the mRNA expression levels of tight junction proteins (zonula occludens-1, zonula occludens-3, Claudin-1, Claudin-3, Claudin-5, Claudin-11, Claudin-23 and Claudin-34) and Mucin-17 were significantly upregulated in the intestinal epithelial cells of 0.60 and 1.20 g/Kg SePCH groups compared to the controls. In conclusion, these results found that 0.60 and 1.20 g/Kg dietary SePCH can not only improve growth, hematological parameters, selenium metabolism, antioxidant capacities, enhance immune responses and intestinal functions, but also alleviate inflammatory responses. This information can serve as a useful reference for formulating feeds for largemouth bass.
1 Introduction
As an essential trace element, selenium (Se) has garnered significant attention for its diverse biological functions, encompassing the enhancement of growth, promotion of normal development and provision of antioxidant and anti-inflammatory activities in both humans and animals (1–3). Selenium sources are classified into two categories based on their composition and origin: inorganic Se (such as Sodium Selenite) and organic Se compounds like selenomethionine (SeMet), selenoyeast, selenocysteine (SeCys) and methylselenocysteine (4, 5). It is widely recognized that Se fulfills its biological roles by becoming integrated into various selenoproteins, including selenocysteine lyase (SCLY), selenophosphate synthase 1 (SPS1), selenoprotein P (SEPP), selenoprotein T (SEPT), selenoprotein W (SEPW), selenoprotein H (SEPH), selenoprotein K (SEPK), 15kDa selenoprotein (SEP15) and glutathione peroxidase (GPx), among others (2, 5). Depending on the dosage at which it is administered, Se assumes distinct physiological functions in both humans and animals (2, 6). Numerous studies have substantiated that Se deficiency or excess can lead to growth retardation, oxidative stress, compromised immunity and dysfunction of the intestinal barrier in animals (1–3, 6). Consequently, organic Se is often included in diets to satisfy the physiological requirements for normal growth and health in animals due to its superior bioavailability and reduced toxicity (1, 2, 6, 7). Nevertheless, limited information is available regarding the utilization of organic Se from plants, which are widely available and more cost-effective, in animals.
Numerous studies have identified the potential of organic selenium (Se) to exhibit antioxidant and immune regulatory effects in both humans and animals (4, 8, 9). Excessive production or content of reactive oxygen species (ROS) during metabolic imbalances and immune stimulation and/or responses can lead to oxidative stress and disruption of physiological functions, which is often indicated by the antioxidant response in animals under ROS overload (10, 11). Selenium is involved in the formation of a range of selenoproteins (SPS1, SEP15, SEPT, SEPH, SEPK and GPx), where it exists in the form of SeCys and SeMet, playing pivotal antioxidant roles in animals (5). It is widely recognized that Se is an essential and integral component of selenoproteins (2). These selenoproteins play crucial roles in antioxidant defense by catalyzing the reduction of peroxides with the assistance of glutathione, thus counteracting peroxidation processes (8, 11). Studies have consistently demonstrated that Se deficiency can disrupt the antioxidant system, diminish the capacity to eliminate ROS and induce cellular damage in organisms (1, 12). Adequate Se levels can modulate intracellular redox status by regulating the expression and activities of redox-targeted proteins and enzymes, including superoxide dismutase (SOD), thioredoxin (Trx), catalase (CAT), GPx, glutathione reductase (GR), peroxiredoxin (Prx), glutathione S-transferase (GST), SEPH and SEPK, among others (4, 7). These regulatory mechanisms are primarily governed by the well-established Nrf2/Keap1 signaling pathway in human, mammals, and other animals (1, 13).
Furthermore, there exists a close relationship between the immune status of animals and hematological parameters, including white blood cells (WBC), neutrophils (NEU), red blood cells (RBC), hemoglobin (HGB), monocytes (MON), platelets (PLT), lymphocytes (LYM), mean corpuscular hemoglobin (MCH), mean corpuscular volume (MCV), mean corpuscular hemoglobin concentration (MCHC) and others (14, 15). Previous studies have highlighted that elevated levels of RBC, WBC, HGB and LYM can serve as indicators of improved immunity and can effectively enhance fish health by promoting the hematopoietic system through the provision of adequate nutrients (3, 16). Furthermore, immune capacities are intricately linked to antibacterial peptides, immune factors, immunoglobulins (Igs) and selenoproteins found in these immune tissues or organs of animals and fish (8, 17). Among these parameters, selenoproteins can enhance the immune process by regulating the synergistic interactions among these immune cells and inhibiting the expression and contents of inflammatory factors, include tumour necrosis factor-α (TNF-α), interleukins (ILs) and interferons (IFNs), etc. (10, 11). Previous research has proved Se deficiency can impair immune functions by reducing the production or contents of antibacterial compounds and immunoglobulins, as well as mRNA expression levels of antimicrobial peptides and selenoproteins (12, 17). Despite the numerous reports on the antioxidant and immune capacities mediated by sodium selenite and Se yeast, limited information is available concerning the comprehensive relationship between hematological parameters, antioxidant capacities, immune functions and dietary organic Se derived from plant sources in animals and fish species.
Moreover, previous studies have demonstrated Se deficiency can exacerbate inflammatory responses in humans and animals by modulating the levels of pro-inflammatory cytokines and anti-inflammatory cytokines, which include ILs, IFNs, TNF-α, transforming growth factor β1 (TGF-β1) and others (11, 12). Consequently, the overproduction of these inflammatory cytokines (e.g. TNF-α, IL-8, IL-1β and IFN-γ) can lead to cell damage and a decline in immune function in various tissues (10, 11). The content or production of these cytokines is primarily regulated by the p38 MAPK/NF-kB signaling transduction pathway in both humans and animals (4, 18, 19). However, these inflammatory processes can typically be mitigated by elevating the levels or contents of anti-inflammatory cytokines, such as TGF-β1 and IL-10 (11, 20). Furthermore, Se deficiency can severely impair the structure of mucosal cells and the physical barrier of the intestine, thereby limiting digestion and absorption and increasing the risk of disease in animals (21, 22). It is well-established that the intestinal integrity and functions are closely linked to the increased expression levels of tight junction proteins (TJs), including occludin (OCLN), zonula occludens (ZOs) and claudins (CLDNs) in the epithelial cells of the intestines in both humans and animals (23, 24). These proteins play a vital role in forming a protective gel that maintains the integrity and function of the intestinal barrier, accomplished through mucin-linked carbohydrates (22). Furthermore, as the key component of intestinal mucus, mucins (MUCs) are mainly produced by goblet cells to protect the epithelium from bacteria or hazardous materials (25). Consequently, MUCs serve critical structural and functional roles within the intestinal epithelial tissue and are essential for preserving intestinal health and immune function (26). Despite the fact that adequate inorganic Se and yeast-Se have been shown to alleviate inflammatory responses, protect the integrity of the intestinal structure and enhance intestinal barrier functions in animals (9, 24), there is limited information available about the effects of organic plant-derived Se on inflammatory responses and intestinal barrier functions in fish up to this point.
Cardamine hupingshanensis, a member of the cruciferous plant genus, is a distinctive organic Se source known for its elevated Se content across all its tissues (27–29). The primary bioactive compound within C. hupingshanensis is selenocysteine, which plays a pivotal role in selenoprotein synthesis, thereby contributing to a range of physiological functions (28, 29). Consequently, selenoprotein extracts from C. hupingshanensis (SePCH) constitute an innovative organic Se source endowed with robust antioxidant and immune-enhancing properties. However, there has been limited exploration into the use of SePCH as a dietary organic Se supplement in fish feed. As a carnivorous fish species, largemouth bass (Micropterus salmoides) has gained widespread cultivation in China owing to its rapid growth, robust adaptability and superior meat quality, thus establishing itself as one of the foremost commercial freshwater species in the country, with an output of 0.8 million tons in 2022 (30). While yeast-Se requirements have been studied in largemouth bass (1), the current knowledge landscape lacks insights into the SePCH requirements for this economically significant species in freshwater aquaculture. Consequently, this experiment is designed to evaluate the impacts of SePCH on largemouth bass, focusing on gene expression and protein production. Furthermore, we aim to elucidate the underlying mechanisms through which dietary SePCH influences intestinal barrier functions and the immune system, accomplished by analyzing hematological parameters, inflammation, oxidative stress and the transcription variations of TJs and MUCs. These findings provide valuable information for optimizing the formulation of compound feeds tailored to largemouth bass.
2 Materials and methods
The care and ethical treatment of the experimental animals strictly adhered to the “Guidance of the Care and Use of Laboratory Animals.” The research protocol involving the experimental subjects received approval from the National-Local Joint Engineering Laboratory of Aquatic Animal Genetic Breeding and Nutrition (Zhejiang).
2.1 Feed composition and experimental diets
The primary protein sources in these artificial diets were casein and fish meal, while rapeseed oil served as the principal lipid source. The base diet was supplemented with 0.00, 0.30, 0.60 and 1.20 g/Kg of SePCH to form four isonitrogenous and isoenergetic diets (see Table 1 for details). The actual selenium contents are 0.37, 0.59, 0.84 and 1.30 mg/kg in these four different diets, respectively, measured with iCAPRQ ICP-MS (thermo, USA). All components underwent filtration using a 60-μm mesh and were blended according to the specifications provided in Table 1. Subsequently, these mixture underwent processing using a F-26 dual-screw extruder (Machinery Factory of South China University of Technology, Guangzhou, China), and made into pellets with 1.5 × 2.5 mm following the procedures outlined by Wu et al. (16) and Jia et al. (31). These pellets were dried at 35°C in one airflow drying oven for at least 24 hours and stored at -20°C until further use.
2.2 Fish and feeding trial
The feeding trial took place at the Aquatic animal culture center, Huzhou University. Healthy juvenile largemouth bass were procured from Zhejiang Deqing Longshengli Aquatic Hatchery (Huzhou, China) and acclimated in a recirculating aquaculture tanks for a period of two weeks prior to the experiment. During this acclimation period, they were provided with a basal diet devoid of SePCH. A total of 300 juvenile largemouth bass, initially weighing (5.04 ± 0.02)g, were selected and randomly allocated to 12 aquaculture tanks. There were 4 different experimental groups in these tanks, each with 3 replicates. Within each replicate, 25 fish were reared for a duration of 60 days. The daily feed intake during the culture period was maintained at 3% of the total fish weight and was administered at 8:00 and 17:00, respectively. Tank water was exchanged every other day, and thorough suctioning was performed to ensure water quality. Throughout the experimental period, water temperature was mainly stayed at 26.5-28.5°C, with natural lighting conditions and dissolved oxygen levels exceeding 5.8 mg/L.
2.3 Sample collection and detection of growth performances
After a 24-hour fasting period, fish from these 12 tanks were counted at the end of feeding trial. Subsequently, they were weighed to evaluate weight gain (WG) and daily growth rate (DGR) after being anesthetized with tricaine methane sulfonate (100 ppm) (MS-222, Sigma, St. Louis, MO, USA). Samples were then collected on ice for further analyses. Blood for hematological analysis was obtained from the caudal tail veins of fifteen fish randomly selected from each tank. Approximately 0.3 mL of blood was selected in 2 ml heparinized Eppendorf tubes. Liver and intestine specimens were also collected and promptly flash-frozen in liquid nitrogen and then deposited at -80 °C for the next measurements and analysis of relative enzyme activity and gene transcription variations.
2.4 Hematological analyses
TEK 8500VET automatic blood analyzer provided by Jiangxi Tekang Technology (Nanchang, China) was employed for hematological analysis, which encompassed assessments of total cell counts and various cell categories. Parameters examined included counts of WBC, RBC, HGB, PLT, MCV, MCH, MCHC, MON, LYM and NEU. Fifteen duplicate blood samples from each group were subjected to analysis
2.5 Measurements of antioxidant enzyme activities and immunological parameters
To assess antioxidant enzyme activities’ variation in these fish liver and intestine, frozen tissue samples were initially pulverized using liquid nitrogen. Subsequently, the samples were homogenized with a 0.9% NaCl solution at a volume ratio of 1:9 (w/v) at 4°C. Following homogenization, the suspensions were subjected to centrifugation at 3000 rpm for 20 minutes at 4°C, and the resulting supernatants were collected. Coomassie Brilliant Blue technique was used to determine the protein concentrations of these supernatants. Activities or contents of SOD, CAT, GPx, GST, GR, glutathione (GSH), malondialdehyde (MDA), hydrogen peroxide (H2O2), total antioxidant capacity (T-AOC) and Kelch-like ECH-associated protein 1a (Keap1a) were determined with commercially available kits in accordance with these corresponding instructions provided by Jiancheng Bioengineering (Jiancheng, Nanjing, China). The activities or the levels of lysozyme, complement component 3 (C3), C4, acid phosphatase (ACP), IgM, alkaline phosphatase (ALP), TNF-α, IL-1β, IL-8, IFN-γ and TGF-β1 were also measured using diagnostic assay kits following these corresponding instructions provided by Henyuan Biotech (Shanghai, China). Each measurement was carried out in triplicate.
2.6 Measurements of genes expression
The total RNA were extracted from fish liver and intestine samples using the TRIzol reagent (Invitrogen, USA). The concentration and integrity of these RNA samples were detected through spectrophotometry and electrophoresis following the procedures outlined by Wu et al. (32). After treatment with DNase I treatment (Takara, Dalian, China), 10 µg of total RNA samples were reverse transcribed with SuperScript™ II RT (Takara, Dalian, China) in 75 µL reactions. Primers for quantitative real-time PCR were designed according to largemouth bass genes (Table 2). Primer synthesis was carried out by Biosune Co. (Shanghai, China). β-actin was served as the internal reference. Quantitative PCR (qPCR) assays were conducted following the methods described by Wu et al. (16) and Yang et al. (33). Each analysis was performed carried out in at least triplicate.
2.7 Measurements of histomorphometry
Posterior intestine samples were firstly rinsed with 0.6% saline and then fixed in 4% paraformaldehyde for 48 hours. Subsequently, the samples underwent dehydration in a series of ethanol solutions with increasing concentrations, clearing with xylene and embedding in paraffin wax. The hematoxylin and eosin (H&E) staining method was used to generate and stain histological sections. Photographic documentation of the intestine was carried out by capturing micrographs at a final magnification of 100× using a digital camera. These acquired images were then subjected to analysis using K-Viewe 1.5 software (https://kv.kintoneapp.com/en/user/, accessed on 16 June 2023) to quantify villus height, villi width, muscle thickness and crypt depth. All measurements were performed with a minimum of three replicates.
2.8 Statistical analyses
The following equations were used to calculate animal-specific parameters:
The results derived from the analysis were presented as mean ± standard deviation (SD). To compare these various groups, a one-way analysis of variance (ANOVA) was performed with SPSS 22.0 software (IBM, Chicago, IL, USA). For additional comparisons among the diverse dietary treatments, Duncan’s multiple range test was employed with a multiple comparison test. Additionally, to investigate any significant linear or quadratic effects, orthogonal polynomial comparisons were conducted. p< 0.05 was utilized to determine the presence of statistical differences.
3 Results
3.1 Growth performance and hematological indices
Following an 8-week cultivation period, the dietary inclusion of SePCH exhibited a beneficial impact on the growth parameters of largemouth bass. Evaluation of the growth parameters revealed that supplementation with 0.60 and 1.20 g/Kg SePCH markedly increased both WG and DGR compared to the control groups (0.00 g/Kg SePCH) (p< 0.05) (Figure 1). Furthermore, several hematological indicators showed significant improvements in the 0.60 and 1.20 g/Kg SePCH groups compared to the SePCH deficient groups or control groups (p< 0.05). Specifically, counts or levels of WBC, MCV, MCH and MON were markedly enhanced in 0.60 and 1.20 g/Kg SePCH groups compared to the control groups (p< 0.05). Additionally, LYM counts notably increased in 0.30, 0.60 and 1.20 g/Kg SePCH groups compared to the controls (p< 0.05). As the levels of SePCH supplementation increased, RBC, HGB and PLT levels also increased significantly, reaching their highest levels in the 0.60 g/Kg SePCH groups (p< 0.05), although no marked difference was observed between the 0.60 and 1.20 g/Kg SePCH groups. Moreover, no significant changes were obtained in terms of MCHC and NEU values across these four experimental groups (Table 3).
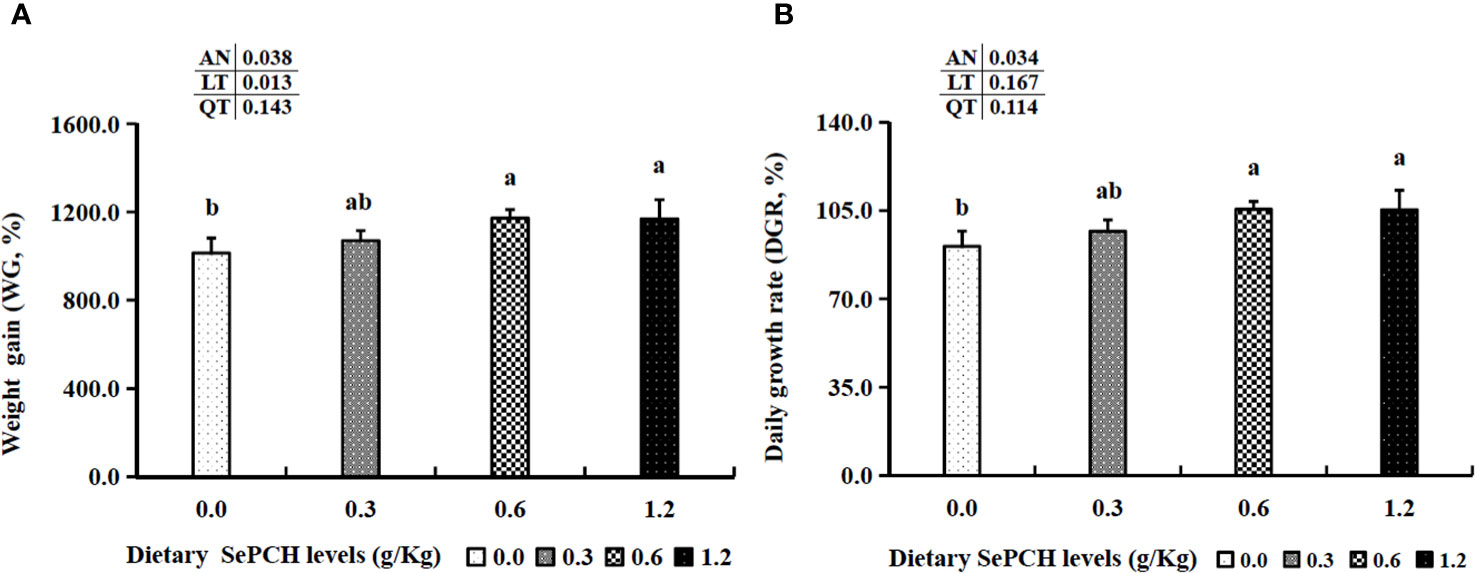
Figure 1 Effect of dietary SePCH on weight gain (%) (A) and daily growth rate (%) (B) of juvenile largemouth bass. Bars with different letters indicate significant differences (p< 0.05), while that with the same letter or no letter superscripts indicate no significant differences (p > 0.05), AN, ANOVA; LT, linear trend; QT, quadratic trend. The same as the following Figures.
3.2 Analyses of antioxidants and oxidants in fish liver and intestine
Significant variations were observed in the antioxidative indices among the different test groups of fish. In comparison to the 0.00 and 0.30 g/Kg groups, the activities of T-SOD, CAT, GPx and GR were markedly higher in the fish liver of 0.60 and 1.20 g/Kg SePCH groups (p< 0.05), with no notable difference in 0.60 and 1.20 g/Kg groups. Conversely, MDA contents exhibited a significant reduction in the 0.60 and 1.20 g/Kg SePCH groups (p< 0.05) compared to the controls (p< 0.05). The contents of H2O2 showed a significant decrease in the 0.60 and 1.20 g/Kg SePCH groups compared to the 0.00 and 0.30 g/Kg SePCH groups (p< 0.05), with no significant difference between 0.00 and 0.30 g/Kg SePCH groups. Compared to the 0.00 and 0.30 g/Kg groups, the contents of Keap1a were markedly decreased in the 0.60 and 1.20 g/Kg SePCH groups (p< 0.05), with no significant difference between the two groups. However, the contents of T-AOC and GSH were markedly increased with the increase in SePCH addition, reached the highest levels in the 0.60 g/Kg SePCH groups (p< 0.05), and no marked difference was obtained between the 0.60 g/Kg and 1.20 g/Kg SePCH groups. However, GST activities presented no notable difference among all these four fish groups (Table 4).
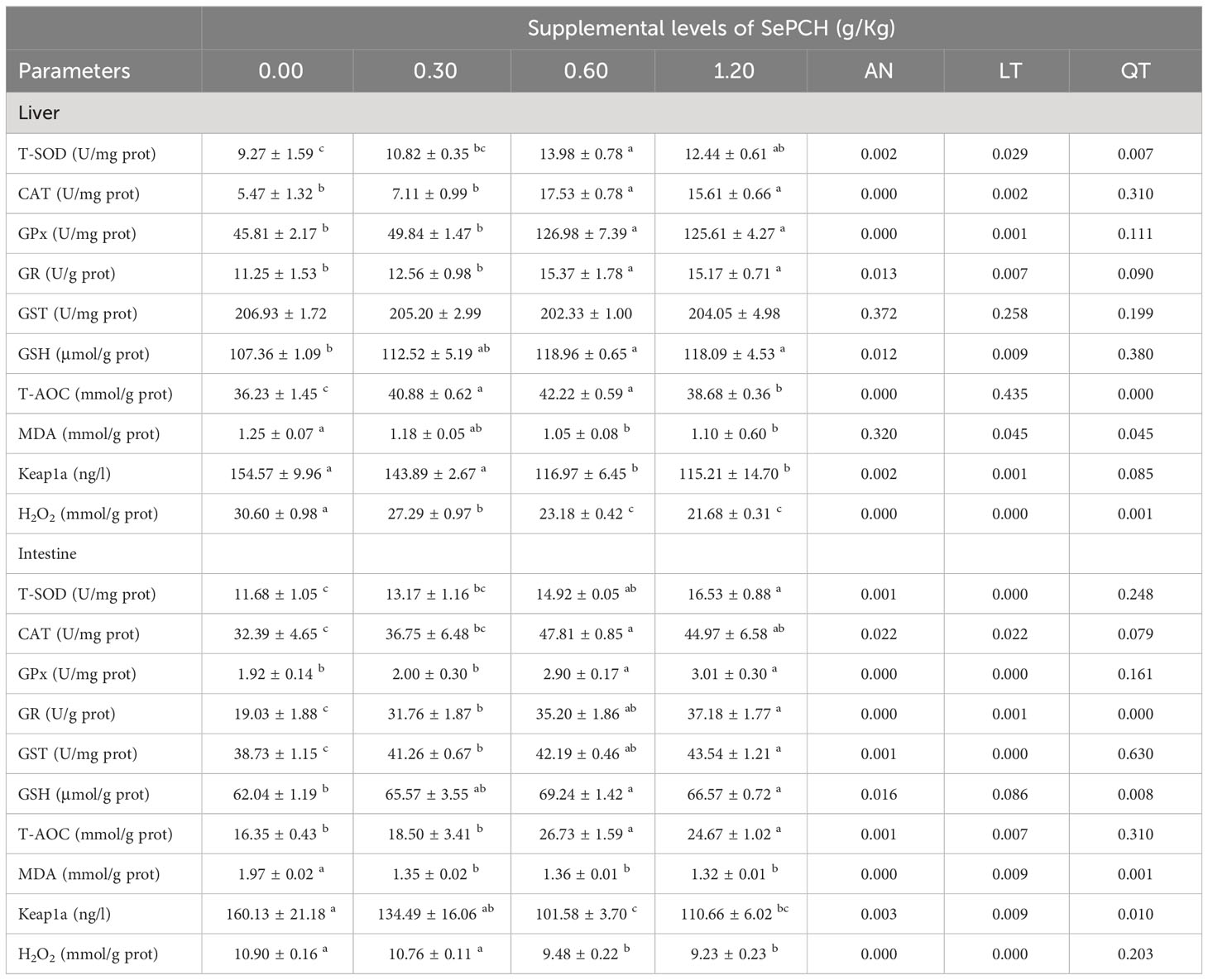
Table 4 Effects of dietary SePCH on the antioxidative and oxidative indices in the liver and intestine of largemouth bass.
Activities of T-SOD and CAT were markedly enhanced in the fish intestine of 0.60 and 1.20 g/Kg SePCH groups in comparison with the controls (p< 0.05), although there were no notable difference between these two groups. Meanwhile, GPx activities and T-AOC contents significantly increased in the 0.60 and 1.20 g/Kg SePCH groups compared to the 0.00 and 0.30 g/Kg SePCH groups (p< 0.05), although no marked difference was observed between the 0.60 and 1.20 g/Kg SePCH groups. The activities of GR and GST exhibited a progressive rise that was positively linked to the doses of SePCH (p< 0.05), reaching its maximum in the 1.20 g/Kg SePCH groups with no significant difference between the 0.60 and 1.20 g/Kg SePCH groups. Compared to the 0.00 g/Kg SePCH groups, the contents of GSH significantly increased in the 0.60 and 1.20 g/Kg SePCH groups (p< 0.05), with no marked difference between 0.60 and 1.20 g/Kg groups. However, in comparison to the 0.00 g/Kg SePCH groups, the contents of MDA were significantly decreased in the 0.30, 0.60 and 1.20 g/Kg SePCH groups (p< 0.05), and there were no marked difference between these three groups. Meanwhile, the 0.60 g/Kg SePCH groups exhibited the lowest Keap1a levels (p< 0.05) compared to the 0.00 and 0.30 g/Kg SePCH groups, although there was no notable difference between the 0.60 and 1.20 g/Kg SePCH groups. H2O2 contents in the intestine of 0.60 and 1.20 g/Kg SePCH groups were markedly lowed in comparison with those in the 0.00 and 0.30 g/Kg SePCH groups (Table 4).
3.3 Analysis of immune parameters in fish liver and intestine
Lysozyme activities and IgM contents in the fish liver of 0.60 and 1.20 g/Kg SePCH groups were significantly heightened in comparison with the controls (p< 0.05), with no noticeable difference compared to the 0.30 g/Kg SePCH groups (Table 5). The activities of ACP reached the maximum in the 1.20 g/Kg SePCH groups and noticeably higher than those in the SePCH deficient groups (p< 0.05). The activities of ALP were significantly higher in the 0.60 g/Kg SePCH groups than these in other three groups (p< 0.05). Meanwhile, the levels of C3 and C4 in the 0.60 and 1.20 g/Kg SePCH groups were markedly higher than those in the 0.00 and 0.30 g/Kg SePCH groups (p< 0.05), although no marked difference was shown between the 0.60 and 1.20 g/Kg SePCH groups.
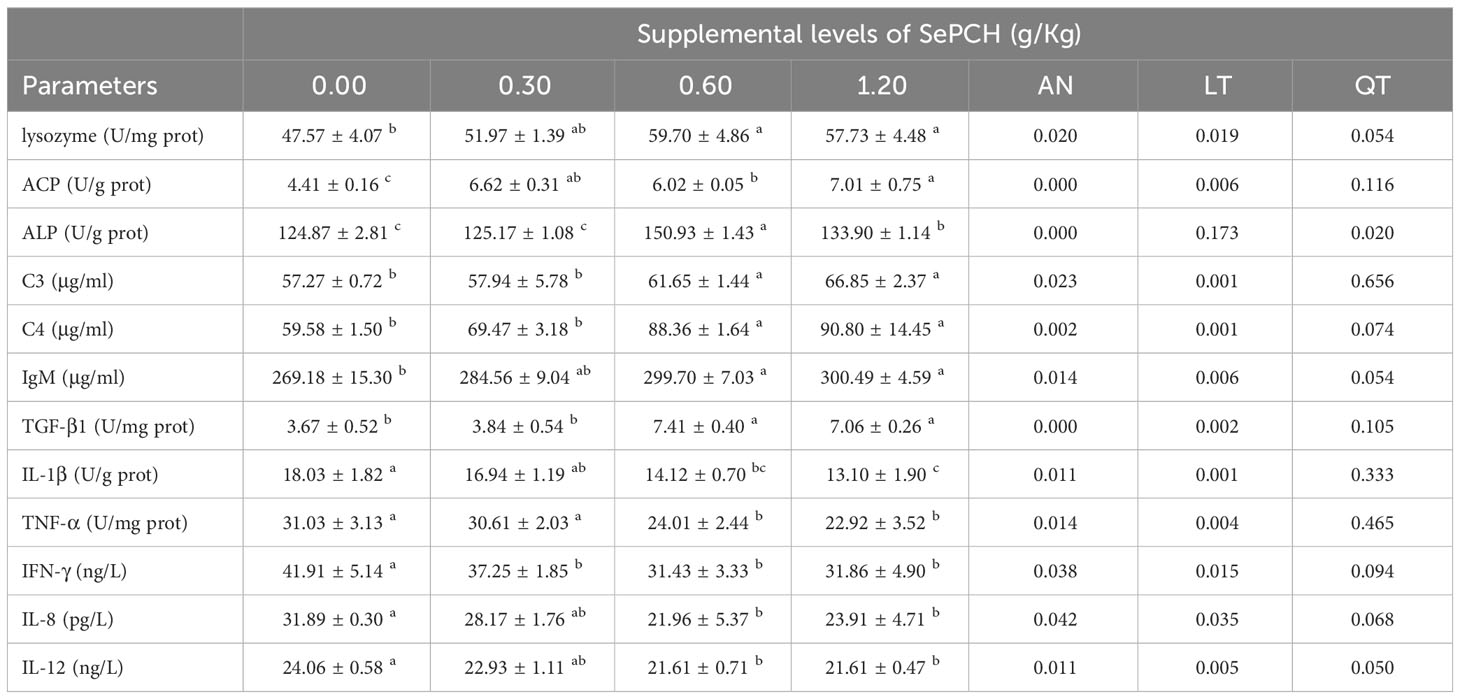
Table 5 Effect of dietary SePCH on the immune parameters and the anti-inflammatory and pro-inflammatory cytokines in the liver of juvenile largemouth bass.
Regarding anti-inflammatory factors, the levels of TGF-β1 displayed a significant increase in the fish liver of 0.60 and 1.20 g/Kg SePCH groups compared to the 0.00 and 0.30 g/Kg SePCH groups (p< 0.05), although no notable difference was presented between the 0.60 and 1.20 g/Kg SePCH groups (Table 5). However, concerning pro-inflammatory factors, the contents of IL-1β, IL-8 and IL-12 significantly decreased in the 0.60 and 1.20 g/Kg SePCH groups compared to the 0.00 g/Kg SePCH groups (p< 0.05), although there were no noticeable differences in 0.00 and 0.30 g/Kg SePCH groups. The levels of TNF-α showed a significant reduction in the 0.60 and 1.20 g/Kg SePCH groups compared to the 0.00 and 0.30 g/Kg SePCH groups (p< 0.05), although no marked difference was shown between the 0.60 and 1.20 g/Kg SePCH groups. Meanwhile, compared to the control groups, the contents of IFN-γ were noticeably reduced in the 0.30, 0.60 and 1.20 g/Kg SePCH groups (p< 0.05), and no marked differences were observed among those three groups.
Immunological parameters were also evaluated in the intestinal tissues of our experimental fish (Table 6). In comparison to the 0.00 and 0.30 g/Kg SePCH groups, the activities of lysozyme and IgM showed a noticeable increase in the 0.60 and 1.20 g/Kg SePCH groups (p< 0.05), and no marked difference was presented between the 0.60 and 1.20 g/Kg groups. Meanwhile, compared to the 0.00 g/Kg SePCH groups, the activities of ACP and ALP were markedly enhanced in the 0.60 and 1.20 g/Kg SePCH groups (p< 0.05), although no marked differences were obtained among the 0.30, 0.60 and 1.20 g/Kg SePCH groups. The contents of C3 in the intestine were markedly elevated in the 0.30 g/Kg to 1.20 g/Kg SePCH groups in comparison with the controls (p< 0.05). At the SePCH additive levels of 1.20 g/Kg, the levels of C4 were notably higher than these in other three groups (p< 0.05).
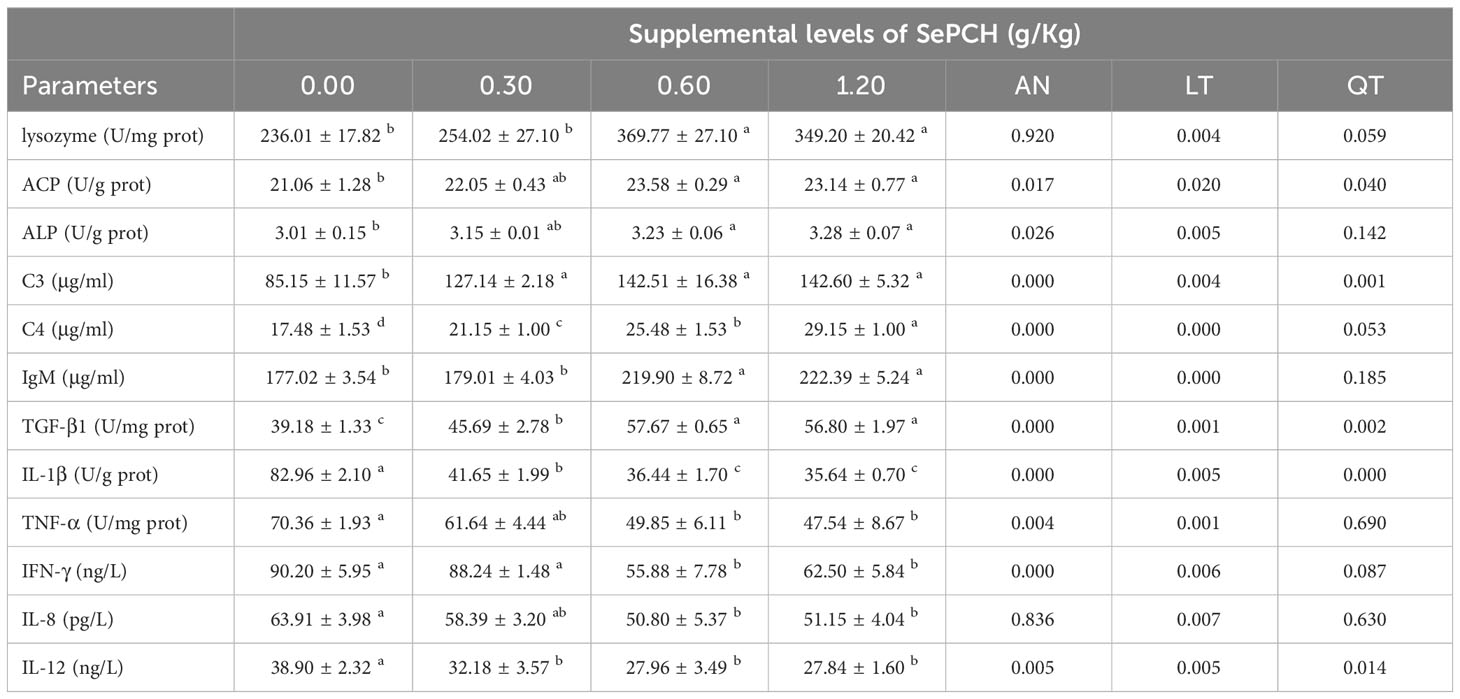
Table 6 Effect of dietary SePCH on the immune parameters and the anti-inflammatory and pro-inflammatory cytokines in the intestine of juvenile largemouth bass.
Regarding intestinal anti-inflammatory factors, the levels of TGF-β1 in the 0.60 and 1.20 g/Kg SePCH groups were noticeably higher than those in the 0.00 and 0.30 g/Kg SePCH groups (p< 0.05), and no difference was shown in the 0.60 and 1.20 g/Kg groups (Table 6). However, in comparison to the controls, the contents of IL-8 and TNF-α were markedly decreased in the 0.60 and 1.20 g/Kg SePCH groups in comparison to the controls (p< 0.05), and no marked difference was presented among the 0.30, 0.60 and 1.20 g/Kg SePCH groups. The contents of IFN-γ and IL-1β in the 0.60 and 1.20 g/Kg SePCH groups exhibited significant reductions compared to the 0.00 and 0.30 g/Kg SePCH groups (p< 0.05), with no notable difference between the 0.60 and 1.20 g/Kg groups. Similarly, compared to the controls, the contents of IL-12 significantly decreased with SePCH addition from 0.30 g/Kg to 1.20 g/Kg (p< 0.05), and no marked difference was presented among these three groups.
3.4 Analysis of genes expression changes in fish liver
The mRNA expression levels of these functional genes involved in Se metabolism, such as SCLY and SEP15, were significantly up-regulated in the 0.60 and 1.20 g/Kg SePCH groups compared to the 0.00 and 0.30 g/Kg groups (p< 0.05), with no marked difference between 0.60 and 1.20 g/Kg SePCH groups. Moreover, the transcription levels of SEPH in the liver of fish fed with 0.60 and 1.20 g/Kg SePCH were significantly increased in comparation with the controls (p< 0.05), and no marked difference was observed compared to the 0.30 g/Kg SePCH groups. With the dosage of SePCH increased, there was a gradual increase in the mRNA transcriptional expression levels of SPS1, SEPT2, SEPP and SEPK. Additionally, the 0.60 and 1.20 g/Kg SePCH markedly increased the mRNA expression levels of SPS1, SEPT2, SEPP and SEPK compared to the 0.00 and 0.30 g/Kg SePCH groups (p< 0.05), and there was no significant difference between the 0.60 and 1.20 g/Kg SePCH (Figure 2A).
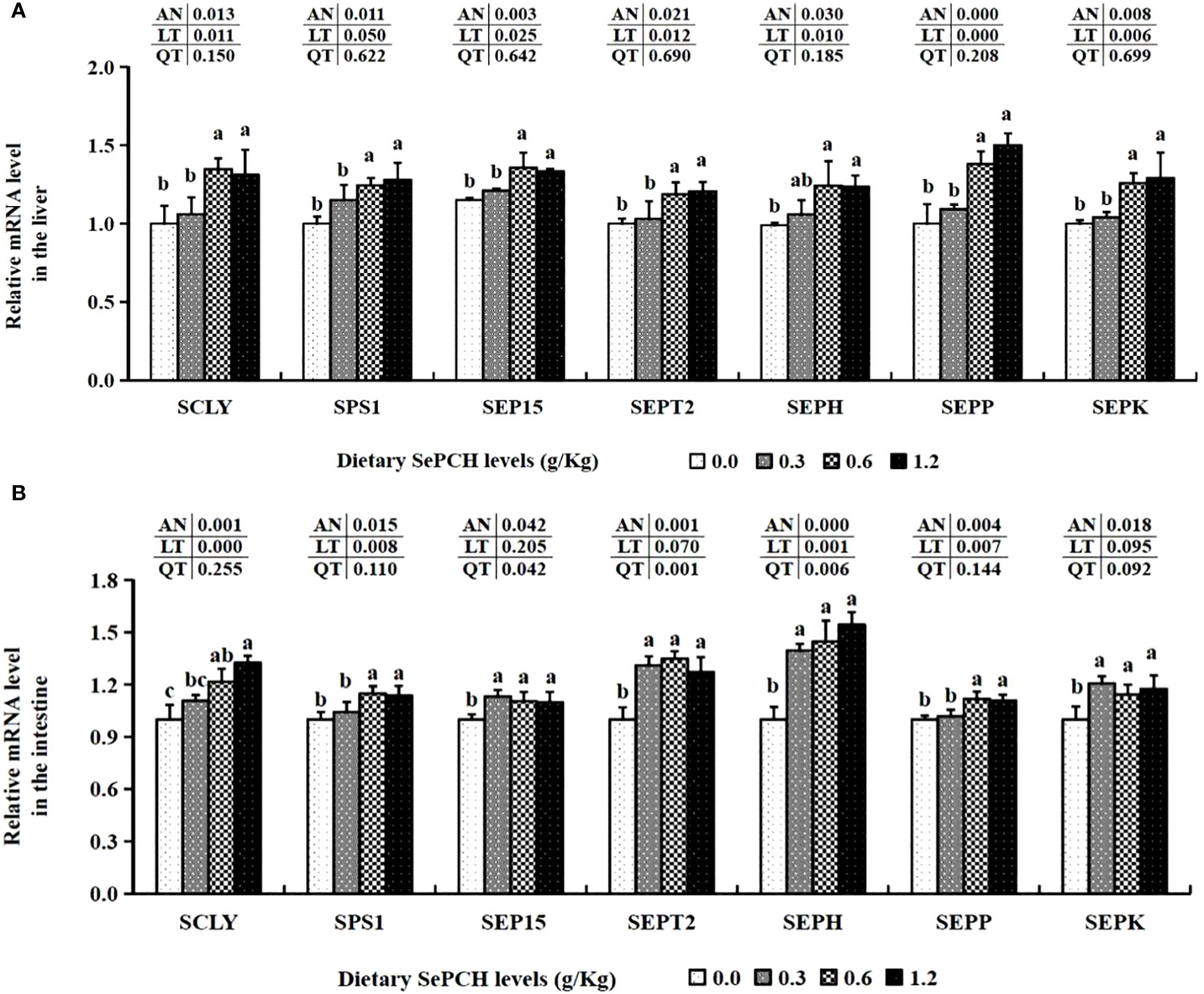
Figure 2 Effect of dietary SePCH on expression levels of selenium metabolism related genes in the liver (A) and intestine (B) of juvenile largemouth bass.
Furthermore, the transcription levels of antioxidant-associated genes, such as Cu/Zn-SOD and GR, in the 0.60 g/Kg SePCH group were notably higher than these in the 0.00 and 0.30 g/Kg SePCH groups (p< 0.05), although there was no marked difference compared to the 1.20 g/Kg SePCH groups (Figure 3A). The mRNA expression levels of Mn-SOD in the 1.20 g/Kg SePCH groups were markedly higher than that in other three groups (p< 0.05), although there were no marked difference among the 0.00, 0.30 and 0.60 SePCH groups. Similarly, the mRNA levels of CAT and GPx1 were notably heightened in the 0.60 and 1.20 g/Kg dietary SePCH groups compared to the 0.00 and 0.30 g/Kg SePCH groups (p< 0.05), with no significant difference between the 0.60 and 1.20 g/Kg SePCH groups. The transcription levels of GPx3 in the 0.60 and 1.20 g/Kg SePCH groups were also notably increased compared to the SePCH deficient groups, although there were no marked difference compared to the 0.30 g/Kg SePCH groups. However, no statistical differences on GST mRNA levels were showed among these four SePCH groups (p > 0.05). Moreover, the mRNA levels of Nrf2 were notably up-regulated in the 0.60 and 1.20 g/Kg SePCH diets compared to the 0.00 and 0.30 g/Kg SePCH groups (p< 0.05). Conversely, the mRNA levels of Keap1a decreased notably in the 0.60 and 1.20 g/Kg SePCH groups compared to the 0.00 and 0.30 g/Kg SePCH groups (p< 0.05). Keap1b also showed a gradual decrease from the 0.30 g/Kg to 1.20 g/Kg SePCH groups and reached its lowest levels in the 0.60 g/Kg SePCH groups, which was notably lower than the control groups (Figure 3A).
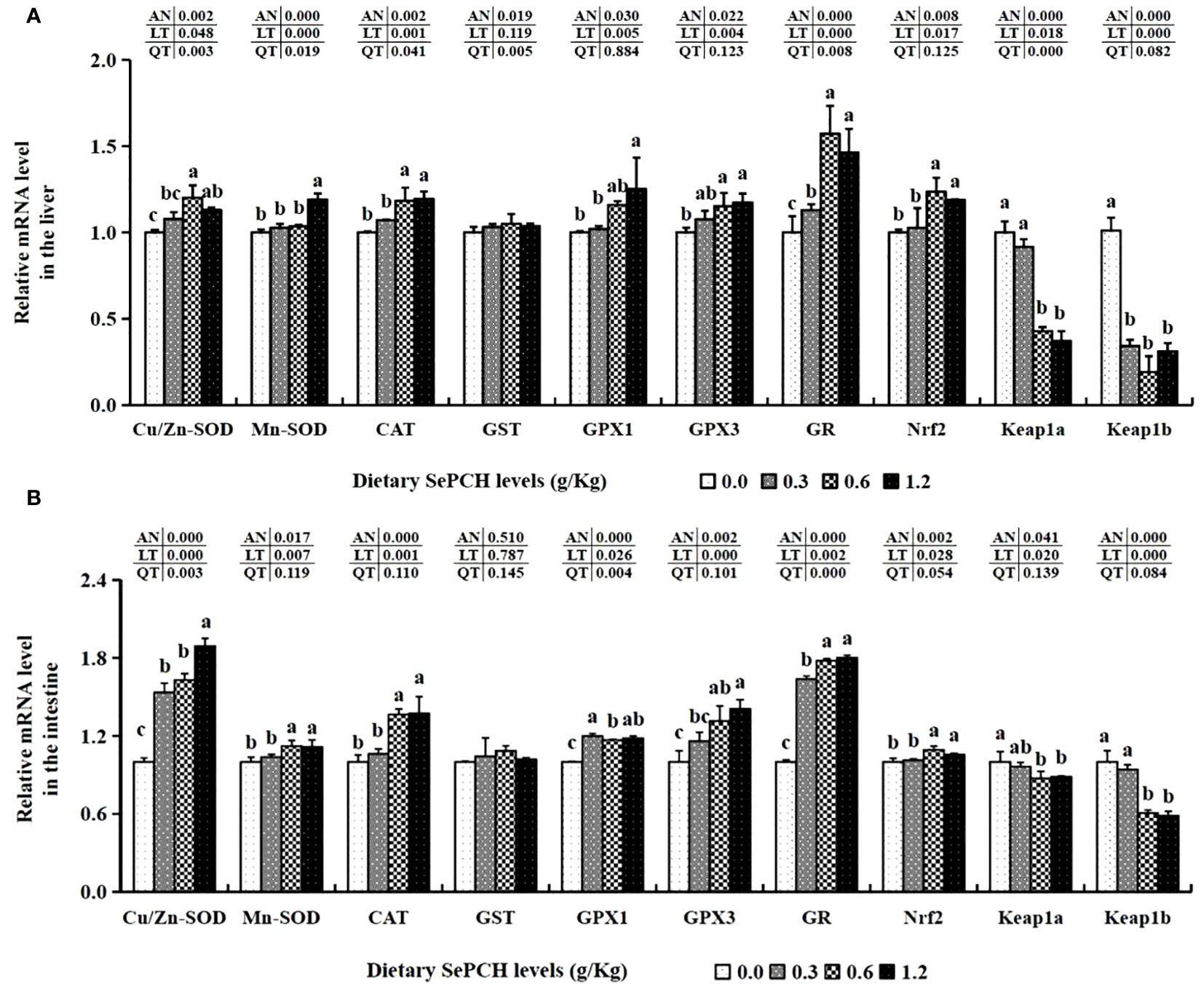
Figure 3 Effect of dietary SePCH on expression levels of antioxidant-related genes in the liver (A) and intestine (B) of juvenile largemouth bass.
The transcription levels of TGF-β1 were notably elevated in the 0.60 and 1.20 g/Kg SePCH groups compared to the 0.00 and 0.30 g/Kg SePCH groups (p< 0.05), with no marked difference between the 0.60 and 1.20 g/Kg groups (Figure 4). Additionally, the mRNA expression levels of IL-10 increased notably in the 0.30 g/Kg to 1.20 g/Kg SePCH groups in comparison with the SePCH deficient groups (p< 0.05), and there were no marked differences among these three groups. Nonetheless, 0.60 and 1.20 g/Kg SePCH exhibited a notable reduction in the transcription levels of IL-12 and IFN-γ compared to the 0.00 and 0.30 g/Kg SePCH groups (p< 0.05), and no marked difference was presented between the 0.60 and 1.20 g/Kg SePCH groups. While the transcription levels of IL-1β showed a declining trend with increasing doses of SePCH, their levels were significantly reduced only at 1.20 g/Kg SePCH compared with the other groups (p< 0.05). Compared to the controls, the transcription levels of IL-8 were significantly reduced in the 0.60 and 1.20 g/Kg SePCH groups, although no marked difference was observed between the 0.60 and 1.20 g/Kg SePCH groups (p > 0.05). At the same time, compared to the 0.00 and 0.30 g/Kg SePCH groups, the transcription levels of IFN-γ were notably reduced in the 0.60 and 1.20 g/Kg SePCH groups (p< 0.05), and there were no marked difference between the 0.60 and 1.20 g/Kg SePCH groups. Additionally, the transcription levels of NF-κB p65 and MAPK14 were notably reduced in the 0.60 an0 g/Kg SePCH groups compared to the controls (p< 0.05), and there were no statistical differences between the 0.60 and 1.20 g/Kg SePCH groups (Figure 4). With the increase of SePCH dose, MAPK13 levels showed a decreasing trend and were statistically lower than these in 0.60 g/Kg SePCH groups.
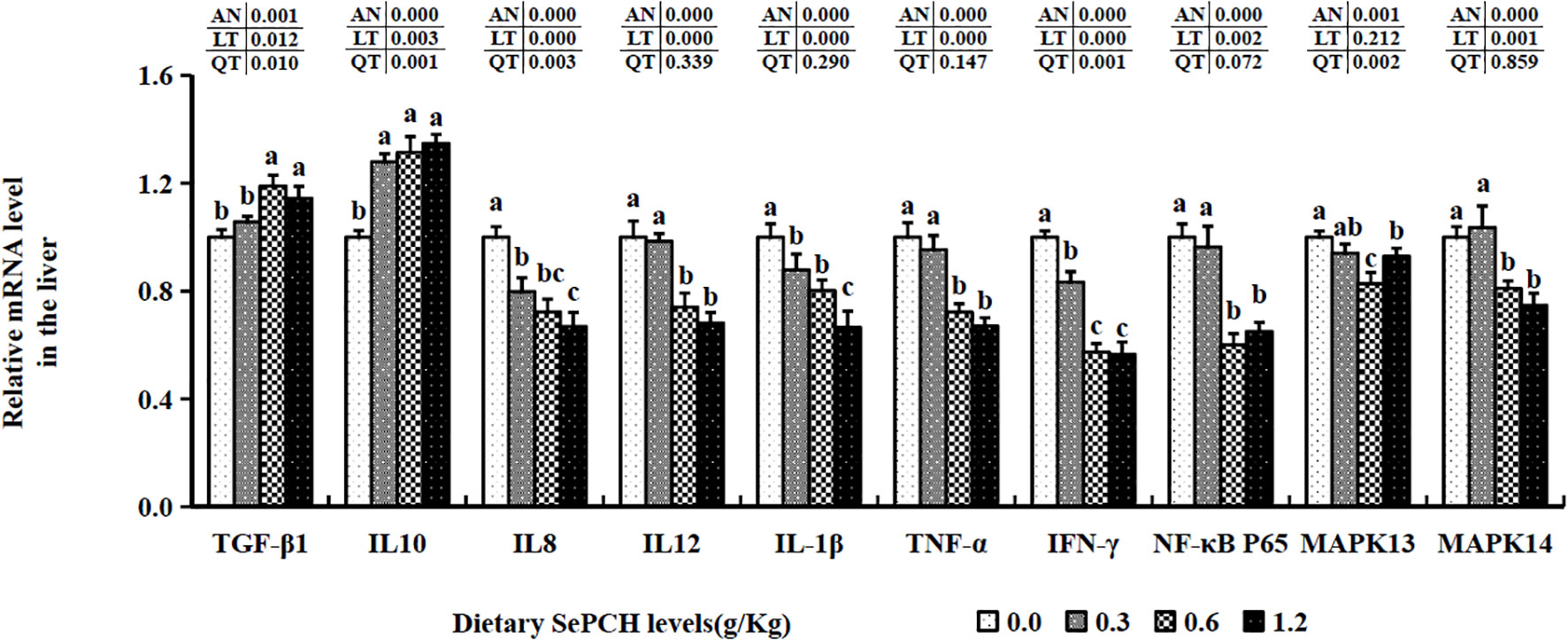
Figure 4 Effect of dietary SePCH on relative gene expression levels of anti-inflammatory, pro-inflammatory cytokines and p38 MAPK/NF-κB signaling pathway in the liver of juvenile largemouth bass.
In terms of immune-related factors, in the 0.00 to 0.60 g/Kg SePCH groups, the expression levels of lysozyme were statistically increased with the increase of SePCH dose (p< 0.05) and reached the maximum value in the 0.60 g/Kg SePCH groups, with no marked difference between the 0.60 and 1.20 g/Kg SePCH groups. When the SePCH supplementation was 0.60 and 1.20 g/Kg, the transcription levels of hepcidin (HEPC) and liver-expressed antimicrobial peptide 2 (LEAP-2) were markedly higher than those in the SePCH deficient groups (p< 0.05), with no statistical difference between them and the 0.30 g/Kg groups. Furthermore, the transcription levels of C3 and C4 were significantly up-regulated in the 0.60 and 1.20 g/Kg SePCH groups compared to those in the 0.00 and 0.30 g/Kg SePCH groups (p< 0.05), and no statistical difference was observed between 0.60 and 1.20 g/Kg SePCH groups (Figure 5A).
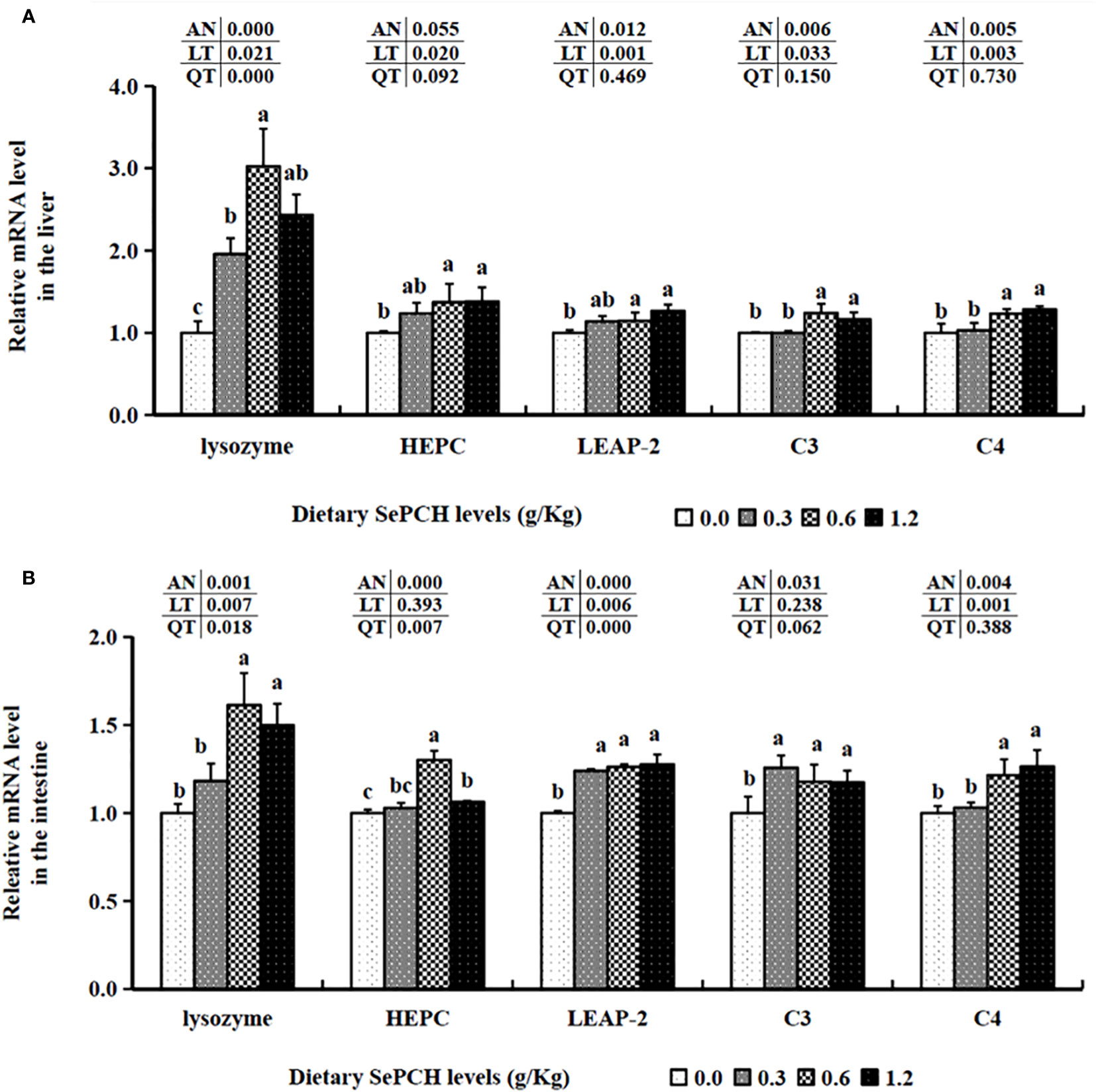
Figure 5 Effect of dietary SePCH on relative gene expression levels of immune-related factors in the liver (A) and intestine (B) of juvenile largemouth bass.
3.5 Genes expression analysis in the intestine
The transcription levels of SCLY in the intestine were significantly elevated and showed a positive correlation with the dietary SePCH levels, with the highest expression observed in the 1.20 g/Kg SePCH groups, significantly surpassing that of the control groups. Furthermore, diets containing 0.60 and 1.20 g/Kg SePCH significantly increased the expression of SPS1 and SEPP in comparison with the controls (p< 0.05), with no statistical difference between the 0.60 and 1.20 g/Kg SePCH groups. Simultaneously, diets ranging from 0.30 g/Kg to 1.20 g/Kg SePCH significantly elevated the mRNA expression of SEP15, SEPT2, SEPH and SEPK compared to the SePCH deficient groups (p< 0.05), and there were no marked difference among the 0.30, 0.60 and 1.20 g/Kg SePCH groups (Figure 2B).
Gene expression assays related to antioxidants in the intestine of our experimental fish revealed a significant increase in Mn-SOD, CAT and GR at dietary SePCH levels of 0.60 and 1.20 g/Kg in comparison with the controls (p< 0.05). The transcription levels of GPx3 and Cu/Zn-SOD exhibited a constant increasing trend and reached their peak in the groups fed with 1.20 g/Kg SePCH, significantly exceeding the SePCH deficient groups (p< 0.05). Nevertheless, there were not discernible difference in GST mRNA levels in the fish intestine samples in these four experimental groups. The transcription levels of GPx1 exhibited a noteworthy increase in the 0.60 and 1.20 g/Kg SePCH groups (p< 0.05) in comparison with the controls, although there were no marked differences between the 0.60 and 1.20 g/Kg SePCH groups. The transcription levels of Nrf2 markedly increased in the groups fed with 0.60 and 1.20 g/Kg SePCH in comparison with the 0.00 and 0.30 g/Kg SePCH groups. The levels of Keap1a and Keap1b significantly decreased in 0.60 and 1.20 g/Kg dietary SePCH in comparison with the SePCH deficient groups (p< 0.05), and there were no statistical difference between the 0.60 and 1.20 g/Kg SePCH groups (Figure 3B).
The 0.60 and 1.20 g/Kg SePCH groups exhibited a marked increase in the expression levels of TGF-β1 and IL-10 in comparison with the SePCH deficient groups (p< 0.05). Importantly, the 0.60 and 1.20 g/Kg SePCH groups showed notable reductions in the transcription levels of IL-8 and IFN-γ in comparison with the SePCH deficient groups (p< 0.05). Diets containing 0.30 g/Kg to 1.20 g/Kg SePCH significantly decreased the transcription levels of TNF-α in comparison with the controls (p< 0.05), with no marked difference among the 0.30, 0.60 and 1.20 g/Kg SePCH groups. While the transcription levels of IL-12 and IL-1β declined with higher doses of SePCH, the most significant reduction occurred at 1.20 g/Kg SePCH compared to the controls, with no marked difference between the 0.60 and 1.20 g/Kg SePCH groups (Figure 6). Additionally, in the 0.60 and 1.20 g/Kg SePCH groups, the transcription levels of NF-κB p65 were considerably reduced in comparison with the SePCH deficient groups (p< 0.05). In the 0.30-1.20 g/Kg SePCH groups, the transcription levels of MAPK13 and MAPK14 were all considerably reduced in comparison with the SePCH deficient groups (Figure 6).
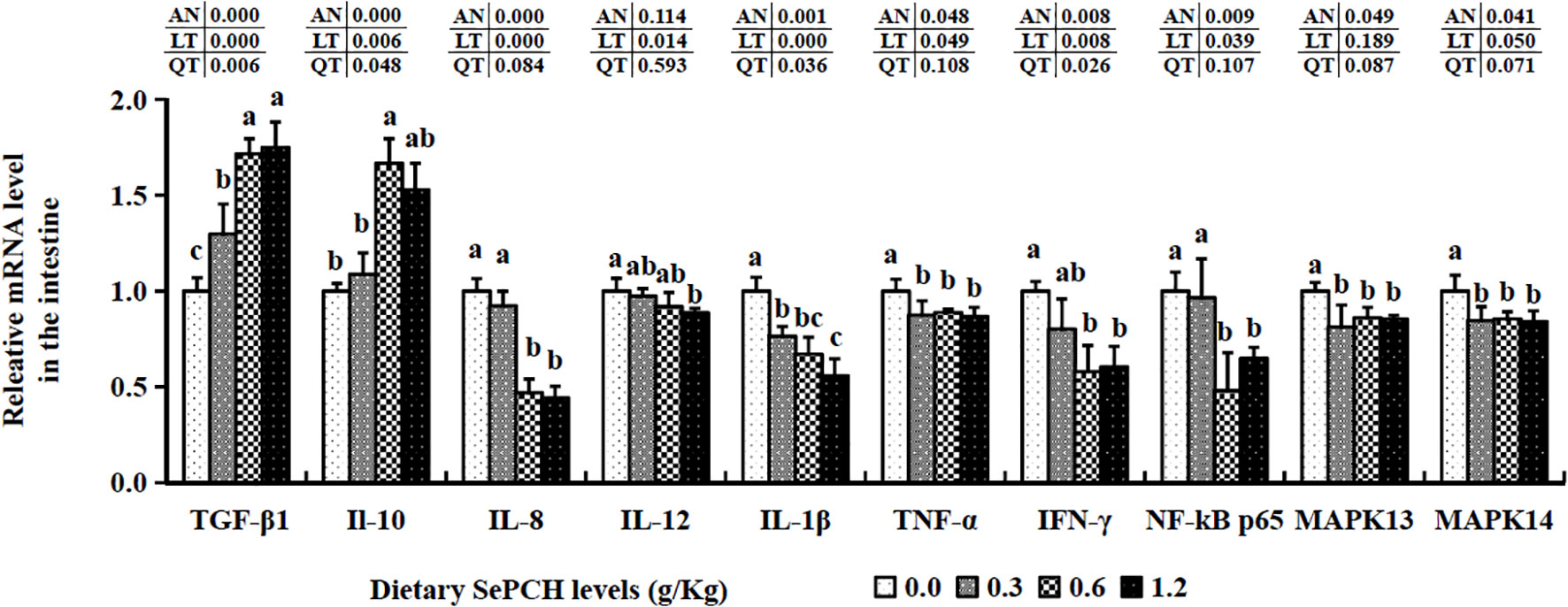
Figure 6 Effect of dietary SePCH on relative gene expression levels of anti-inflammatory, pro-inflammatory cytokines and p38 MAPK/NF-κB signaling pathway in the intestine of juvenile largemouth bass.
The transcription levels of HEPC were markedly elevated at the 0.60 g/Kg SePCH groups, significantly higher than in the 0.00, 0.30 and 1.20 g/Kg SePCH groups (Figure 5B). Additionally, in the 0.60 and 1.20 g/Kg SePCH groups, the transcription levels of lysozyme and C4 notably increased in comparison with the SePCH deficient groups (p< 0.05), with no statistical difference between the 0.60 and 1.20 g/Kg groups (p > 0.05). In the 0.30 g/Kg -1.20 g/Kg SePCH groups, the transcription levels of LEAP-2 and C3 were significantly up-regulated in comparison with the control groups (p< 0.05). All the transcription levels of CLDN-1, CLDN-11 and CLDN-34 in the intestine showed a discernible increase in the SePCH-treated groups compared to the control groups, although there were no marked differences among these three SePCH treatment groups (p > 0.05) (Figure 7). Meanwhile, the transcription levels of ZO-1, OCLN, CLDN-3 and CLDN-5 were all significantly increased at 0.60 and 1.20 g/Kg SePCH in comparison with the SePCH deficient groups. In the 0.60 g/Kg SePCH groups, the transcription levels of ZO-3 were markedly raised compared to the 0.00 and 0.30 g/Kg SePCH groups, with no discernible difference in comparison with the 1.20 g/Kg SePCH groups. In addition, mRNA expression levels of CLDN-23 exhibited a consistent increase and were notably higher in the 1.20 g/Kg SePCH groups in comparison with other three groups (p< 0.05) (Figure 7). While mRNA levels of MUC-2 and MUC-5AC consistently improved with increasing doses of SePCH, there was a significant up-regulation of MUC-2 and MUC-5AC transcription levels only in the 1.20 g/Kg SePCH groups in comparison with the SePCH deficient groups, with no statistical difference between the 0.60 and 1.20 g/Kg groups. The 0.60 and 1.20 g/Kg SePCH groups significantly up-regulated the intestinal MUC-17 genes expressions in comparison with the 0.00 and 0.30 g/Kg SePCH groups (p< 0.05) (Figure 7).
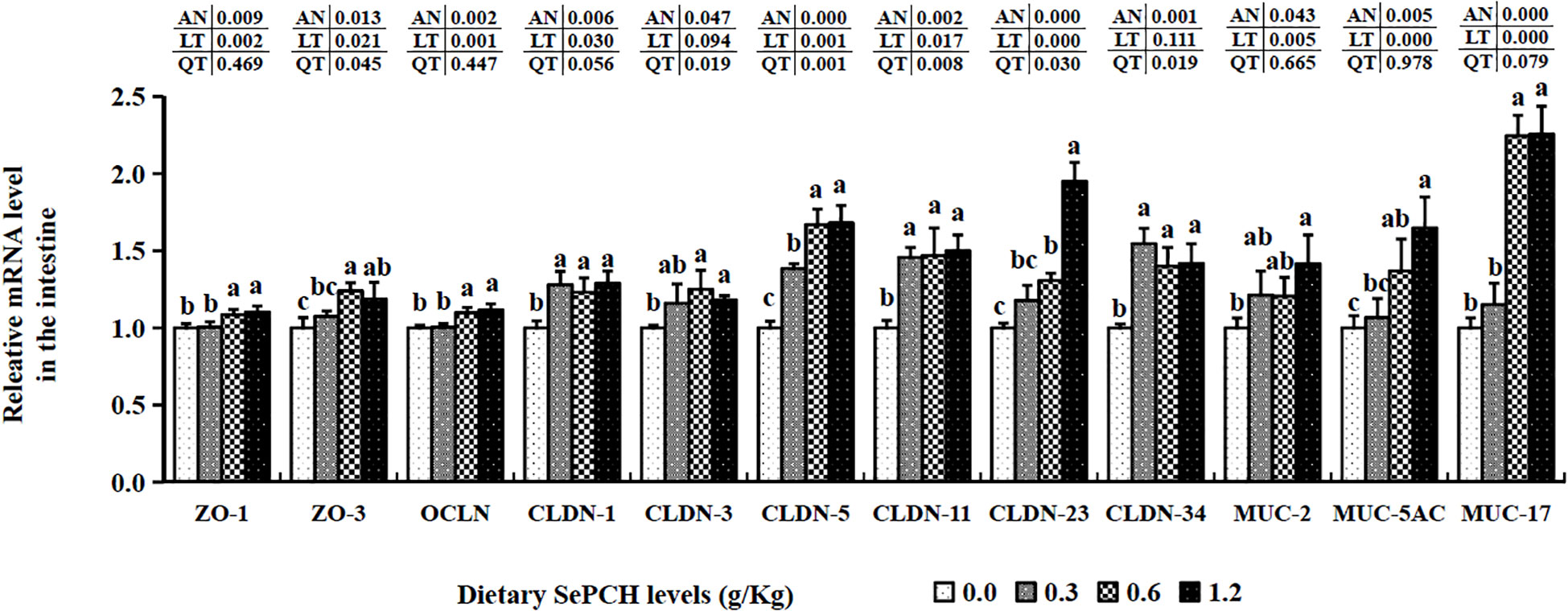
Figure 7 Effects of dietary SePCH on intestinal tight junction-related and intestinal mucins gene expression levels of juvenile largemouth bass.
3.6 Histomorphometric analysis of the intestine
Our investigation of the experimental fish involved the analysis of histological characteristics in intestinal sections (Figure 8). The villus height values in the 0.30 g/Kg to 1.20 g/Kg SePCH groups were notably greater than that in the SePCH deficient groups. Meanwhile, the intestinal morphology in the 0.30 g/Kg to 1.20 g/Kg groups showed robust development, characterized by an increased villus height, reaching its peak in the 1.20 g/Kg SePCH groups (p< 0.05). In the 0.60 and 1.20 g/Kg SePCH groups, there was a noticeable increase in villus width compared to the 0.00 g/Kg and 0.30 g/Kg groups, and there was no statistical difference between the 0.60 and 1.20 g/Kg SePCH groups (p > 0.05). However, there were no marked differences in muscle thickness and crypt depth among these four groups (Table 7 and Figure 8).
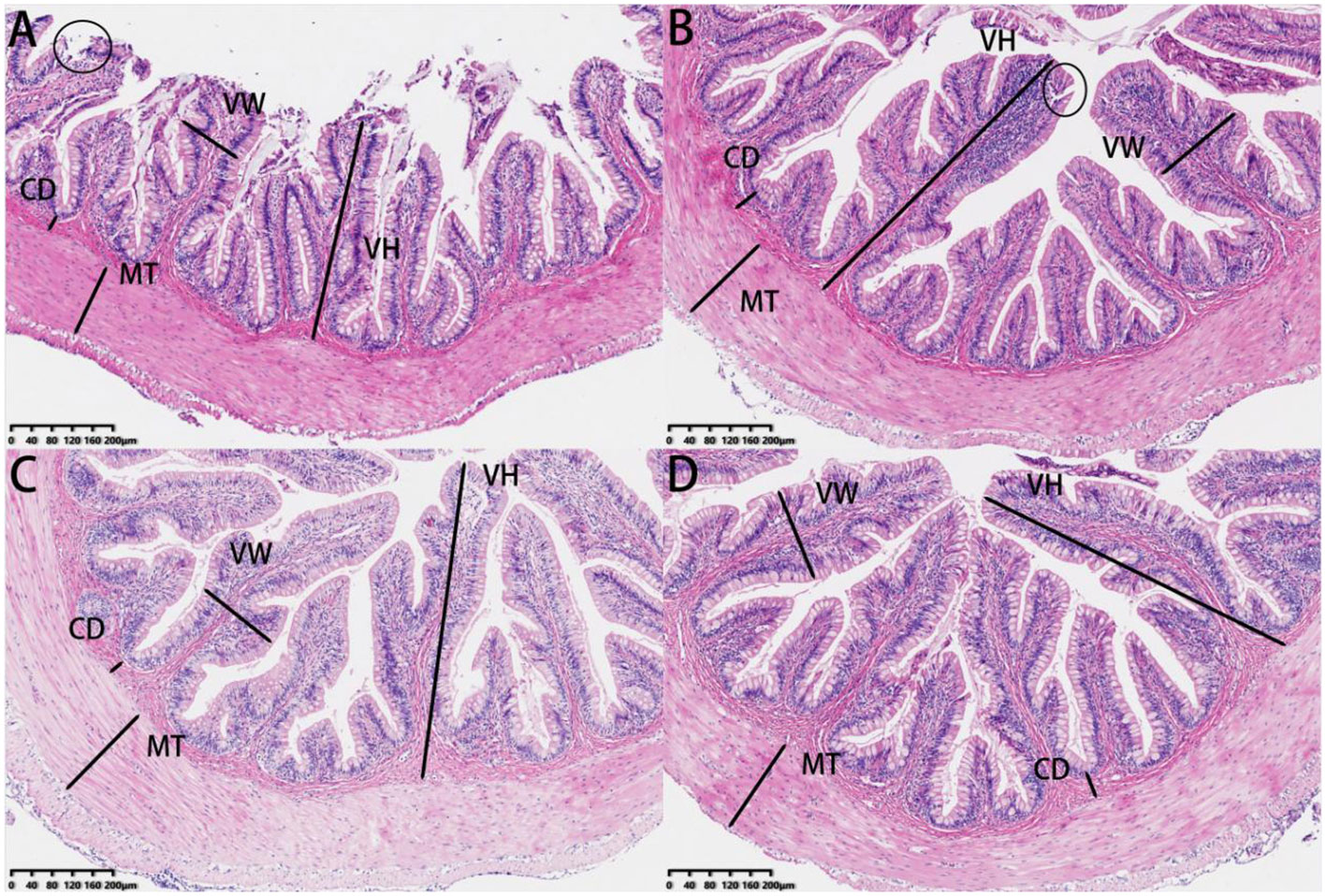
Figure 8 HE staining of the intestine sections of juvenile largemouth bass fed with diets containing 0.00 g/Kg (A), 0.30 g/Kg (B), 0.60 g/Kg (C) and 1.20 g/Kg (D) SePCH (magnification × 100). VH: villus height, VW, villus width; MT, muscular thickness; CD, crypt depth. Circles represent intestinal villi fall off.
4 Discussion
As an essential micronutrient, dietary Se plays crucial roles in promoting growth and maintaining normal physiological functions in different organisms (2, 34). Numerous studies have demonstrated that dietary selenium can influence the growth and development of various aquatic and terrestrial animal species, including largemouth bass (1), European seabass (Dicentrarchus labrax) (3), rainbow trout (Oncorhynchus mykiss) (7), common carp (Cyprinus carpio) (35), grass carp (Ctenopharyngodon idellus) (36) and chicken (37). In line with these findings, our results also presented that 0.60 and 1.20 g/Kg SePCH (equivalent to 0.84-1.30 mg/kg) notably heightened WG and DGR, highlighting the role of adequate SePCH (0.60 and 1.20 g/Kg) could enhance the growth performance of largemouth bass (1, 3). As reliable indicators, hematology parameters has been used to evaluate the healthy status and nutritional conditions in many aquatic animals (15, 16). Previous research has shown that higher levels of WBC, MON and LYM can contribute to improved immunity in animals under non-stress conditions (14, 38). In agreement with findings in common carp (39) and Nile tilapia (Oreochromis niloticus) (40), our results found that SePCH at concentrations of 0.60 and 1.20 g/Kg increased the counts of WBC, MON and LYM in the blood, suggesting enhanced cellular immune responses in largemouth bass fed with adequate SePCH. Moreover, elevated amounts of RBC, HGB and PLT can enhance oxygen-carrying capacity in animals (41). Similar with results in Nile tilapia (42), African catfish (Clarias gariepinus) (43) and European seabass (44), our study found that RBC, HGB, PLT, MCV and MCH levels were also increased by 0.60 and 1.20 g/Kg SePCH, indicating that adequate SePCH can improve oxygen-carrying capacity and cellular immune responses in largemouth bass.
As the primary form of Se found in SePCH (8), selenocysteine can undergo catalysis by SCLY to yield selenide and alanine (45). Subsequently, selenide, in combination with adenosine triphosphate (ATP), can be enzymatically converted into selenophosphate by SPS1 (8, 45). Selenophosphate plays a direct role in the synthesis of various selenoproteins, thus facilitating Se metabolism within organisms (2). Our results shown that 0.60 and 1.20 g/Kg SePCH increased the expression levels of SCLY and SPS1. This observation aligns with previous studies in black carp (Mylopharyngodon piceus) (46), Nile tilapia (47) and mice (48). It suggests that elevated SCLY and SPS1 levels induced by sufficient SePCH can enhance the synthesis of selenoproteins and then promote Se metabolism in largemouth bass. SEPP, the sole selenoprotein containing multiple selenocysteine residues, predominantly resides in the plasma, where it acts as a transporter of Se to various tissues (8, 49). Consistent with previous research in triploid rainbow trout (7) and Nile tilapia (47), our study also found higher expression levels of SEPP in fish fed with adequate SePCH, which suggested that dietary SePCH can improve Se transport via SEPP in largemouth bass. SEP15 is localized within the endoplasmic reticulum (ER) lumen, where it can assist in oxidative folding and structural maturation of N-glycosylated proteins for its oxidoreductase activity (50, 51). On the other hand, SEPH can not only play an crucial role in organ development, but also function as a regulator of redox homeostasis for suppressing DNA damage caused by oxidative stress in the nucleus (52, 53). Additionally, as a DNA-binding protein for redox response, SEPH could heighten the expression of functional genes involved in de novo GSH synthesis and phase II detoxification for maintaining cellular redox status (54, 55). SEPT is anchored to the membrane of endoplasmic reticulum (ER), where it exerts selenosulfide oxidoreductase activity via its thioredoxin-like domain, serving as a guardian of ER homeostasis (56, 57). Our results demonstrate higher levels of SEP15, SEPT2 and SEPH in fish fed with 0.60 and 1.20 g/Kg SePCH, which aligns with findings in black carp (46), rainbow trout (58) and yellow catfish (Pelteobagrus fulvidraco) (59). These findings suggest that adequate SePCH can help maintain ER redox homeostasis by increasing the levels of SEP15, SEPT2 and SEPH in largemouth bass (60). Furthermore, SEPK, an endoplasmic reticulum-membrane protein, plays a crucial role in facilitating effective Ca2+ flux during immune cell activation induced by dietary Se (61). In our study, SEPK expression levels were enhanced in the 0.60 and 1.20 g/Kg SePCH groups, consistent with findings in Nile tilapia (47), rainbow trout (58), yellow catfish (59), grass carp (62) and pig (63). These results collectively suggest that adequate SePCH can improve Se metabolism and immune activation by up-regulating the expression of these selenoproteins in largemouth bass.
As is well known, ROS are continually generated during the metabolic processes for all nutrients and can affect changes on the variations of antioxidant enzymes activities in animals and fish (64, 65). Adequate Se can mitigate excessive ROS levels and play vital antioxidant roles through numerous selenoproteins, including Se-dependent glutathione peroxidase (8, 65). In present study, the activities of T-SOD in the liver and intestine were enhanced in fish groups that received sufficient SePCH. These findings are consistent with results reported in largemouth bass (1), European seabass (3), common carp (35) and grass carp (36). Taken together, these results indicate that the higher T-SOD activities induced by adequate SePCH can catalyze the conversion of O2- to H2O2, thus mitigating oxidative stress mediated by O2- in largemouth bass. CAT and GSH-Px are the primary antioxidant enzymes responsible for decomposing oxygen radicals by catalyzing the conversion of H2O2 to H2O and O2 in animals and fish (8, 9). Similar to previous findings in largemouth bass (1), European seabass (3), Wuchang bream (Megalobrama amblycephala) (9) and common carp (35), our results shown that adequate SePCH not only increased the enzyme activities of CAT and GSH-Px but also reduced H2O2 contents compared to the control groups. This suggests that a sufficient dietary intake of SePCH can promote the breakdown of H2O2 in largemouth bass. As a dimeric disulfide oxidoreductase, GR can catalyze the reduction of GSH disulfide (GSSG) to GSH for resisting oxidative stress. This process helps maintain the redox environment in human and animal cells (66). Consistent with previous findings in various fish species (46), our study demonstrates higher GR activities and GSH levels in fish fed with adequate SePCH, indicating that sufficient SePCH can enhance cellular redox homeostasis and increase antioxidant capacities by boosting GSH synthesis in the liver and intestine of largemouth bass. Numerous studies have found that the expression of these antioxidant enzymes is primarily regulation by the classical Nrf2/Keap1 signaling transduction pathway (1, 13, 16). Nrf2 can bind Keap1 for maintaining redox homeostasis or antioxidant responses. However, when this balance is disrupted, Nrf2 translocates to the nucleus, where it activates downstream genes related to antioxidant enzymes (13, 67). In this study, we observed up-regulation of Nrf2 and its target molecules, including Cu/Zn-SOD, CAT, GPx1, GPx3 and GR, with a positive correlation to SePCH dosage. However, the expression of Keap1a and Keap1b showed the opposite trend. Similar results were also reported in largemouth bass (1), black carp (46) and chicken (13). Therefore, adequate dietary SePCH can help maintain a better redox status by promoting the transcription levels of antioxidant-related genes through the classical Nrf2/Keap1 signaling transduction pathway in largemouth bass.
ALP and ACP are two essential non-specific phosphohydrolases that can play vital roles in the immune defense through activating immune cells in animals and fish species (5, 17). Similar to findings in grass carp (17), common carp (39) and rainbow trout (68), this investigation observed elevations in ALP and ACP levels in the groups receiving adequate SePCH. Combined with the higher amounts of WBC, MON and LYM in the 0.60 and 1.20 g/Kg SePCH groups, it suggests that adequate SePCH can enhance ALP and ACP activities, thereby stimulating innate immune responses through the activation of these immune cells in largemouth bass. As an antimicrobial enzyme, lysozyme can not only catalyze the hydrolysis of glycosidic bonds in peptidoglycans in the cell walls of gram-positive bacteria, but also acts as an opsonin and play vital roles in facilitating innate immunity by activating the complement system and phagocytes (31). As key constituents in the complement system, C3 and C4 always contribute to both innate and adaptive immunity, making them crucial for host defense against pathogens (16). In this study, the levels of lysozyme, C3 and C4 were all increased in the liver and intestine of fish groups treated with adequate SePCH. These results are consistent with previous findings in black carp (46) and grass carp (17), indicating that adequate SePCH can enhance innate immunity by elevating the levels of lysozyme, C3, and C4 in largemouth bass. As a key element in the humoral immune system, IgM is the primary immunoglobulin present in fish (17). In agreement with results in Wuchang bream (9), common carp (39) and grass carp (17), the contents of IgM were significantly increased in the liver and intestine of fish fed with 0.60 and 1.20 g/Kg SePCH. This suggests that adequate SePCH can enhance humoral immunity via elevating the IgM levels in largemouth bass. HEPC and LEAP-2 are two key antimicrobial proteins expressed in the liver, and they play crucial roles in enhancing immunity by providing the first defense line against many pathogens, including bacteria, fungi, viruses and protozoans (16, 31). Similar to previous results in black carp (46) and largemouth bass (16), our study also found that the transcription levels of HEPC and LEAP-2 were both elevated in the main metabolic and immune tissues of fish fed with adequate dietary SePCH. This indicates the enhanced first-line defense properties mediated by HEPC and LEAP-2 could be enhanced by adequate dietary SePCH in largemouth bass. Taken together, these findings suggest that adequate SePCH can maintain the immunological function homeostasis and provide protective roles for the organism by up-regulating the above defense factors in largemouth bass.
The inflammatory response is a defensive reaction of the immune system to various stimuli such as injury, infection and malnutrition (11). Furthermore, there is a close relationship between the immunological status and the corresponding inflammatory responses, which are primarily controlled by proinflammatory factors (IL-1, IL-8, TNF-α and IFN-γ, etc.) and anti-inflammatory factors (TGF-β1 and IL-10, etc.) (16). In the current study, we observed that adequate SePCH reduced the levels of IL-1β, TNF-α, IL-12, IFN-γ and IL-8 and increased the levels of TGF-β1, along with changes in the transcription levels of these cytokines and IL-10 in the fish liver and intestine. These findings are consistent with previous results in grass carp (24), carp (C. carpio var. specularis) (69) and European seabass (44). Combined these above findings, it suggest that sufficient dietary SePCH can inhibit the inflammatory response by down-regulating pro-inflammatory genes in the liver and intestine of largemouth bass. It is well-known that the variations in pro-inflammatory and anti-inflammatory cytokines are primarily regulated by the classical p38 MAPK/NF-κB signaling transduction pathway in mammals and animals (4, 11). The p38 MAPK/NF-κB signaling transduction pathway has been illustrated as a crucial pathway involved in various physiological processes, including immune responses, cell survival and inflammation (70). Previous research has shown that inhibiting the p38 MAPK/NF-κB pathways can effectively decrease the transcription and expression levels of pro-inflammatory cytokines, leading to a reduction in inflammatory responses (16, 70). In this study, we observed that the transcription levels of key regulatary elements (MAPK13, MAPK14 and NF-κB p65) were all notably reduced in the liver and intestine of largemouth bass fed with 0.60 and 1.20 g/Kg SePCH in comparison with the SePCH deficient groups, which is similar to earlier studies in grass carp (24), calve (12) and common carp (C. carpio L) (71). Collectively, these results indicate that an adequate dietary SePCH can alleviate inflammatory responses by modulating the transcription levels of these pro- and anti-inflammatory genes (IL-1β, TNF-α, IFN-γ, IL-8, IL-12, TGF-β1 and IL-10) through inhibiting the p38 MAPK/NF-κB signaling transduction pathway and thus maintaining immune homeostasis in largemouth bass.
Except as a digestive organ, the intestine also functions as a crucial immune organ, playing vital roles in barrier functions and serving as the first defense line against pathogens’ invasion in fish (31). Previous studies have demonstrated that well-developed intestinal structures are characterized by enhanced villus height and villi width (16). Our research similarly revealed that an adequate supply of SePCH could increase both villus height and villi width in largemouth bass. This suggests that a sufficient dietary intake of SePCH can promote intestinal development and growth (24). As known, the intestinal immune defense relies on the physical barrier integrity formed by the TJ complex, which includes proteins like OCLN, CLDNs and ZOs (22, 31). These proteins serve as crucial indicators for assessing the functional integrity of the intestinal barrier (16, 31). TJ proteins are crucial for maintaining the structural integrity and permeability of the intestinal lining, which is closely linked to the absorption of external nutrients (72). For instance, OCLN functions by penetrating tight junctions, lowering membrane permeability and selectively filtering certain molecules in the animal intestine (73). Meanwhile, as two peripheral membrane functional proteins, ZO-1 and ZO-3 play essential roles for establishing and maintaining tight junctions between endothelial and epithelial cells in the animal intestine (31, 74). Additionally, CLDNs, as transmembrane proteins in cellular tight junctions, are critical for maintaining cell-cell barriers, regulating intercellular communication and preserving cell polarity (22, 75). Consistent with previous findings in largemouth bass (16), grass carp (24), zebrafish (Danio rerio) (26), rainbow trout (76) and broiler (77), our results also demonstrate that SePCH at concentrations of 0.60 and 1.20 g/Kg enhances the transcription levels of OCLN, ZO-1, ZO-3, CLDN-1, CLDN-3, CLDN-5, CLDN-11, CLDN-23 and CLDN-34 in the intestine of largemouth bass. This indicates that adequate SePCH can reduce intestinal permeability and bolster the structural integrity of the intestinal barrier (16, 24, 74, 76). Furthermore, MUCs, which are mucosal surface-enriched glycoproteins, play pivotal roles in forming, maintaining and regulating the intestinal barrier and immune responses (78). In our research, we observed an increase in the transcription levels of MUCs (MUC-2, MUC-5AC and MUC-17) in largemouth bass receiving 0.60 and 1.20 g/Kg SePCH. This finding aligns with earlier results in largemouth bass (16), zebrafish (25) and turbot (23), suggesting that adequate SePCH can enhance the mucus barrier, protect the integrity and functionality of the intestinal barrier and maintain health (79). In conclusion, our results indicated that a sufficient dietary intake of SePCH (0.60 and 1.20 g/Kg) can improve intestinal structural integrity and enhance physical barrier function by up-regulating the levels of OCLN, CLDNs and ZOs in largemouth bass.
5 Conclusion
In summary, our results found that SePCH at concentrations of 0.60 and 1.20 g/Kg can present several positive effects on largemouth bass. These effects include improvements in growth, hematological indices, Se metabolism and antioxidant capacities through the Nrf2/Keap1 pathway. Additionally, SePCH enhances immunity by increasing the levels of direct defensive factors and mitigates inflammatory responses via the classical p38 MAPK/NF-κB signaling transduction pathway. Moreover, adequate dietary SePCH positively influences intestinal structural integrity and physical barrier function by modulating TJs and MUCs in largemouth bass.
Data availability statement
The original contributions presented in the study are included in the article/supplementary material. Further inquiries can be directed to the corresponding author.
Ethics statement
The animal studies were approved by the Experimental Animal Ethics Committee of Huzhou University. The studies were conducted in accordance with the local legislation and institutional requirements. Written informed consent was obtained from the owners for the participation of their animals in this study.
Author contributions
HZ: Conceptualization, Data curation, Formal analysis, Investigation, Methodology, Software, Validation, Visualization, Writing – original draft, Writing – review & editing. LZ: Data curation, Investigation, Methodology, Validation, Visualization, Writing – original draft, Writing – review & editing. PZ: Formal analysis, Methodology, Software, Validation, Visualization, Writing – original draft, Writing – review & editing, Data curation, Investigation. YX: Data curation, Formal analysis, Investigation, Methodology, Software, Validation, Writing – original draft, Writing – review & editing. XY: Data curation, Formal analysis, Investigation, Methodology, Software, Validation, Writing – original draft. XP: Data curation, Formal analysis, Investigation, Methodology, Software, Writing – original draft. YF: Data curation, Formal analysis, Investigation, Methodology, Software, Writing – original draft. JW: Formal analysis, Investigation, Methodology, Writing – original draft. HB: Resources, Writing – original draft. XS: Supervision, Writing – original draft, Writing – review & editing. JY: Supervision, Writing – review & editing. CW: Conceptualization, Funding acquisition, Methodology, Project administration, Resources, Supervision, Writing – review & editing, Data curation.
Funding
The author(s) declare financial support was received for the research, authorship, and/or publication of this article. This research was financially supported by the Key Research and Development Program of Zhejiang Province (2019C02046).
Conflict of interest
The authors declare that the research was conducted in the absence of any commercial or financial relationships that could be construed as a potential conflict of interest.
Publisher’s note
All claims expressed in this article are solely those of the authors and do not necessarily represent those of their affiliated organizations, or those of the publisher, the editors and the reviewers. Any product that may be evaluated in this article, or claim that may be made by its manufacturer, is not guaranteed or endorsed by the publisher.
References
1. Xu Z, Hu J, Zhang Y, Bai L. Evaluation of largemouth bass (Micropterus salmoide) fed selenium yeast diets: growth, histopathology, antioxidant ability, and apoptosis. Aquaculture Rep (2023) 29:101505. doi: 10.1016/j.aqrep.2023.101505
2. Roman M, Jitaru P, Barbante C. Selenium biochemistry and its role for human health. Metallomics (2014) 6:25–54. doi: 10.1039/c3mt00185g
3. Abd El-Kader MF, Fath El-Bab AF, Shoukry M, Abdel-Warith AA, Younis EM, Moustafa EM, et al. Evaluating the possible feeding strategies of selenium nanoparticles on the growth rate and wellbeing of European seabass (Dicentrarchus labrax). Aquaculture Rep (2020) 18:100539. doi: 10.1016/j.aqrep.2020.100539
4. Ojeda ML, Nogales F. Dietary selenium and its antioxidant properties related to growth, lipid and energy metabolism. Antioxidants (2022) 11:1402. doi: 10.3390/antiox11071402
5. Khalil HS, Maulu S, Verdegem M, Abdel Tawwab M. Embracing nanotechnology for selenium application in aquafeeds. Rev Aquacult (2023) 15:112–29. doi: 10.1111/raq.12705
6. Rayman MP. Selenium intake, status, and health: a complex relationship. Hormones (2020) 19:9–14. doi: 10.1007/s42000-019-00125-5
7. Teng Z, Zhang F, Wang L, Wang L, Huang T, Zhang X. Dietary selenium requirement of triploid rainbow trout (Oncorhynchus mykiss) based on selenium yeast supplementation. Aquac Res (2022) 53:3365–78. doi: 10.1111/are.15844
8. Avery J, Hoffmann P. Selenium, selenoproteins, and immunity. Nutrients (2018) 10:1203. doi: 10.3390/nu10091203
9. Guo H, Lin W, Wang L, Zhang D, Wu X, Li L, et al. The supplementation of dietary selenium yeast and green tea-derived polyphenols improves antioxidant capacity and immune response in juvenile Wuchang bream (Megalobrama amblycephala) under ammonia stress. Aquac Res (2020) 51:3790–803. doi: 10.1111/are.14724
10. Barrett CW, Short SP, Williams CS. Selenoproteins and oxidative stress-induced inflammatory tumorigenesis in the gut. Cell Mol Life Sci (2017) 74:607–16. doi: 10.1007/s00018-016-2339-2
11. Nettleford S, Prabhu K. Selenium and selenoproteins in gut inflammation—A review. Antioxidants (2018) 7:36. doi: 10.3390/antiox7030036
12. Wang S, Liu X, Lei L, Wang D, Liu Y. Selenium deficiency induces apoptosis, mitochondrial dynamic imbalance, and inflammatory responses in calf liver. Biol Trace Elem Res (2022) 200:4678–89. doi: 10.1007/s12011-021-03059-5
13. Li P, Li K, Zou C, Tong C, Sun L, Cao Z, et al. Selenium yeast alleviates ochratoxin a-induced hepatotoxicity via modulation of the PI3K/AKT and Nrf2/Keap1 signaling pathways in chickens. Toxins (2020) 12:143. doi: 10.3390/toxins12030143
14. Amira KI, Rahman MR, Sikder S, Khatoon H, Afruj J, Haque ME, et al. Data on growth, survivability, water quality and hemato-biochemical indices of Nile tilapia (Oreochromis niloticus) fry fed with selected marine microalgae. Data Brief (2021) 38:107422. doi: 10.1016/j.dib.2021.107422
15. Witeska M, Kondera E, Lugowska K, Bojarski B. Hematological methods in fish - Not only for beginners. Aquaculture (2022) 547:737498. doi: 10.1016/j.aquaculture.2021.737498
16. Wu J, Yang W, Song R, Li Z, Jia X, Zhang H, et al. Dietary soybean lecithin improves growth, immunity, antioxidant capability and intestinal barrier functions in largemouth bass (Micropterus salmoides) juveniles. Metabolites (2023) 13:512. doi: 10.3390/metabo13040512
17. Zheng L, Feng L, Jiang W, Wu P, Tang L, Kuang S, et al. Selenium deficiency impaired immune function of the immune organs in young grass carp (Ctenopharyngodon idella). Fish Shell Fish Immun (2018) 77:53–70. doi: 10.1016/j.fsi.2018.03.024
18. Lange A, Lange J, Jaskuła E. Cytokine overproduction and immune system dysregulation in alloHSCT and COVID-19 patients. Frontimmunol (2021) 12:658896. doi: 10.3389/fimmu.2021.658896
19. Lu C, Gao S, Xu G. Withdrawn: Geraniin inhibits TNF-α induced impairments of osteogenesis through NF-κB and p38 MAPK signalling pathways in bone marrow stem cells. Stroke Vasc Neurol (2017) 2:47–52. doi: 10.1136/svn-2016-000046
20. Yi P, Xu X, Qiu B, Li H. Impact of chitosan membrane culture on the expression of pro- and anti-inflammatory cytokines in mesenchymal stem cells. Exp Ther Med (2020) 20:3695–702. doi: 10.3892/etm.2020.9108
21. He X, Lin Y, Lian S, Sun D, Guo D, Wang J, et al. Selenium deficiency in chickens induces intestinal mucosal injury by affecting the mucosa morphology, SIgA secretion, and GSH-Px activity. Biol Trace Elem Res (2020) 197:660–6. doi: 10.1007/s12011-019-02017-6
22. Xie S, Liu B, Ye H, Li Q, Pan L, Zha X, et al. Dendrobium huoshanense polysaccharide regionally regulates intestinal mucosal barrier function and intestinal microbiota in mice. Carbohyd Polym (2019) 206:149–62. doi: 10.1016/j.carbpol.2018.11.002
23. Yang P, Hu H, Liu Y, Li Y, Ai Q, Xu W, et al. Dietary stachyose altered the intestinal microbiota profile and improved the intestinal mucosal barrier function of juvenile turbot (Scophthalmus maximus L.). Aquaculture (2018) 486:98–106. doi: 10.1016/j.aquaculture.2017.12.014
24. Liu S, Yu H, Li P, Wang C, Liu G, Zhang X, et al. Dietary nano-selenium alleviated intestinal damage of juvenile grass carp (Ctenopharyngodon idella) induced by high-fat diet: Insight from intestinal morphology, tight junction, inflammation, anti-oxidization and intestinal microbiota. Anim Nutr (2022) 8:235–48. doi: 10.1016/j.aninu.2021.07.001
25. Chen J, Wu S, Wu R, Ai H, Lu X, Wang J, et al. Essential oil from Artemisia argyi alleviated liver disease in zebrafish (Danio rerio) via the gut-liver axis. Fish Shellfish Immun (2023) 140:108962. doi: 10.1016/j.fsi.2023.108962
26. Zhang X, Chen J, Wang G, Chen H, Cao J, Xie L, et al. Interactive effects of fluoride and seleno-l-methionine at environmental related concentrations on zebrafish (Danio rerio) liver via the gut-liver axis. Fish Shellfish Immun (2022) 127:690–702. doi: 10.1016/j.fsi.2022.07.006
27. Zhang Z, Huang X, Li S, Zhang C, Luo K. Preparation and characterization of Zein-sulfated Cardamine hupingshanensis polysaccharide composite films. Food Sci Nurt (2021) 9:6737–45. doi: 10.1002/fsn3.2625
28. Cheng Y, Huang Y, Liu K, Pan S, Qin Z, Wu T, et al. Cardamine hupingshanensis aqueous extract improves intestinal redox status and gut microbiota in Se-deficient rats. J Sci Food Agr (2021) 101:989–96. doi: 10.1002/jsfa.10707
29. Shao S, Deng G, Mi X, Long S, Zhang J, Tang J. Accumulation and speciation of selenium in cardamine sp. in yutangba Se mining field, Enshi, China. Chin J Geochemistry (2014) 33:357–64. doi: 10.1007/s11631-014-0698-7
30. Wang D, Wu FX, Song DD, Gao HQ. China fishery statistical yearbook Vol. 25. Beijing, China: Article in China; China Agriculture Press (2022).
31. Jia XW, Qian PC, Wu CL, Xie YY, Yang WX, Song R, et al. Effects of dietary pantothenic acid on growth, antioxidant ability and innate immune response in juvenile black carp. Aquac. Rep (2022) 24:101131. doi: 10.1016/j.aqrep.2022.101131
32. Wu C, Ye J, Gao J, Chen L, Lu Z. The effects of dietary carbohydrate on the growth, antioxidant capacities, innate immune responses and pathogen resistance of juvenile Black carp Mylopharyngodon piceus. FISH SHELLFISH Immun (2016) 49:132–42. doi: 10.1016/j.fsi.2015.12.030
33. Yang W, Wu J, Song R, Li Z, Jia X, Qian P, et al. Effects of dietary soybean lecithin on growth performances, body composition, serum biochemical parameters, digestive and metabolic abilities in largemouth bass Micropterus salmoides. Aquaculture Rep (2023) 29:101528. doi: 10.1016/j.aqrep.2023.101528
34. Genchi G, Lauria G, Catalano A, Sinicropi MS, Carocci A. Biological activity of selenium and its impact on human health. Int J Mol Sci (2023) 24:2633. doi: 10.3390/ijms24032633
35. Saffari S, Keyvanshokooh S, Zakeri M, Johari SA, Pasha-Zanoosi H. Effects of different dietary selenium sources (sodium selenite, selenomethionine and nanoselenium) on growth performance, muscle composition, blood enzymes and antioxidant status of common carp (Cyprinus carpio). Aquac Nutr (2017) 23:611–7. doi: 10.1111/anu.12428
36. Liu LW, Liang XF, Li J, Fang JG, Yuan XC, Li J, et al. Effects of dietary selenium on growth performance and oxidative stress in juvenile grass carp (Ctenopharyngodon idellus). Aquacult Nutr (2018) 24:1296–303. doi: 10.1111/anu.12428
37. Mohammadi E, Janmohammadi H, Olyayee M, Helan JA, Kalanaky S. Nano selenium improves humoral immunity, growth performance and breast-muscle selenium concentration of broiler chickens. Anim Prod Sci (2020) 60:1902. doi: 10.1071/AN19581
38. Petrera F, Abeni F. Hematological and iron-related parameters in a dual-purpose local cattle breed compared to the specialized Italian Friesian breed during transition and lactation periods. Comp Clin Pathol (2018) 27:869–78. doi: 10.1007/s00580-018-2675-8
39. Saffari S, Keyvanshokooh S, Zakeri M, Johari SA, Pasha-Zanoosi H, Mozanzadeh MT. Effects of dietary organic, inorganic, and nanoparticulate selenium sources on growth, hemato-immunological, and serum biochemical parameters of common carp (Cyprinus carpio). Fish Physiol BiochemI (2018) 44:1087–97. doi: 10.1007/s10695-018-0496-y
40. Neamat-Allah ANF, Mahmoud EA, Abd El Hakim Y. Efficacy of dietary Nano-selenium on growth, immune response, antioxidant, transcriptomic profile and resistance of Nile tilapia (Oreochromis niloticus) against Streptococcus iniae infection. Fish Shellfish Immun (2019) 94:280–7. doi: 10.1016/j.fsi.2019.09.019
41. Morel N, Moisan M. Blood components are essential to regulate microcirculatory blood flow. Crit Care (2017) 21:49. doi: 10.1186/s13054-017-1621-5
42. Rathore SS, Murthy HS, Mamun MA, Nasren S, Rakesh K, Kumar BTN, et al. Nano-selenium supplementation to ameliorate nutrition physiology, immune response, antioxidant system and disease resistance against aeromonas hydrophila in monosex Nile Tilapia (Oreochromis niloticus). Biol Trace Elem Res (2021) 199:3073–88. doi: 10.1007/s12011-020-02416-0
43. Owolabi OD, Babarinsa MK. Assessment of growth performance, nutrient utilization and haematological profile of African catfish (Clarias gariepinus) fed with nanoselenium formulated diets. IOP Conf series. Materials Sci Eng (2020) 805:12014. doi: 10.1088/1757-899X/805/1/012014
44. Abd El-Kader MF, Fath El-Bab AF, Abd-Elghany MF, Abdel-Warith AA, Younis EM, Dawood MAO. Selenium nanoparticles act potentially on the growth performance, hemato-biochemical indices, antioxidative, and immune-related genes of European seabass (Dicentrarchus labrax). Biol Trace Elem Res (2021) 199:3126–34. doi: 10.1007/s12011-020-02431-1
45. Scortecci JF, Serro VHB, Fernandes AF, Basso LGM, Gutierrez RF, Araujo APU, et al. Initial steps in selenocysteine biosynthesis: The interaction between selenocysteine lyase and selenophosphate synthetase. Int J Biol Macromol (2020) 156:18–26. doi: 10.1016/j.ijbiomac.2020.03.241
46. Zhang C, Jia XW, Qian PC, Chen YT, Wu CL, Ye JY. Selenium-rich cardamine hupingshanensis on growth, biochemical indices, selenium metabolism, antioxidant capscities and innate immunities in juvenile black carp (mylopharyngodon piceus). Acta Hydrobiologica Sin (2022) 47:523–34. doi: 10.7541/2023.2022.0063
47. Rathore SS, Murthy HS, Girisha SK, Nithin MS, Nasren S, Mamun MAA, et al. Supplementation of nano-elenium in fish diet: Impact on selenium assimilation and immune-regulated selenoproteome expression in monosex Nile tilapia (Oreochromis niloticus). Comp Biochem Physiol Part C: Toxicol Pharmacol (2021) 240:108907. doi: 10.1016/j.cbpc.2020.108907
48. Kremer PM, Torres DJ, Hashimoto AC, Berry MJ. Disruption of selenium handling during puberty causes sex-specific neurological impairments in mice. Antioxidants (2019) 8:110. doi: 10.3390/antiox8040110
49. Schomburg L. Selenoprotein P - Selenium transport protein, enzyme and biomarker of selenium status. Free Radical Bio Med (2022) 191:150–63. doi: 10.1016/j.freeradbiomed.2022.08.022
50. Addinsall AB, Wright CR, Andrikopoulos S, van der Poel C, Stupka N. Emerging roles of endoplasmic reticulum-resident selenoproteins in the regulation of cellular stress responses and the implications for metabolic disease. Biochemj (2018) 475:1037–57. doi: 10.1042/bcj20170920
51. Labunskyy VM, Yoo M, Hatfield DL, Gladyshev VN. Sep15, a thioredoxin-like selenoprotein, is involved in the unfolded protein response and differentially regulated by adaptive and acute ER stresses. Biochemistry-us (2009) 48:8458–65. doi: 10.1021/bi900717p
52. Wu RT, Cao L, Chen BP, Cheng WH. Selenoprotein H suppresses cellular senescence through genome maintenance and redox regulation. J Biol Chem (2014) 289:34378–88. doi: 10.1074/jbc.m114.611970
53. Cui J, Zhou J, He W, Ye J, Westlake T, Medina R, et al. Targeting selenoprotein H in the nucleolus suppresses tumors and metastases by Isovalerylspiramycin I. J Exp Clin Canc Res (2022) 41:126. doi: 10.1186/s13046-022-02350-0
54. Panee J, Stoytcheva ZR, Liu W, Berry MJ. Selenoprotein H is a redox-sensing high mobility group family DNA-binding protein that up-regulates genes involved in glutathione synthesis and Phase II Detoxification*. J Biol Chem (2007) 282:23759–65. doi: 10.1074/jbc.m702267200
55. Cao L, Pechan T, Lee S, Cheng W. Identification of selenoprotein H isoforms and impact of selenoprotein H overexpression on protein but not mRNA levels of 2 other selenoproteins in 293T Cells. J Nutr (2021) 151:3329–38. doi: 10.1093/jn/nxab290
56. Anouar Y, Lihrmann I, Falluel-Morel A, Boukhzar L. Selenoprotein T is a key player in ER proteostasis, endocrine homeostasis and neuroprotection. Free Radical Bio Med (2018) 127:145–52. doi: 10.1016/j.freeradbiomed.2018.05.076
57. Pothion H, Jehan C, Tostivint H, Cartier D, Bucharles C, Falluel-Morel A, et al. Selenoprotein T: an essential oxidoreductase serving as a guardian of endoplasmic reticulum homeostasis. Antioxid Redox Sign (2020) 33:1257–75. doi: 10.1089/ars.2019.7931
58. Wang L, Zhang X, Wu L, Liu Q, Zhang D, Yin J. Expression of selenoprotein genes in muscle is crucial for the growth of rainbow trout (Oncorhynchus mykiss) fed diets supplemented with selenium yeast. Aquaculture (2018) 492:82–90. doi: 10.1016/j.aquaculture.2018.03.054
59. Ke J, Zhang D, Lei X, Liu G, Luo Z. Characterization and tissue expression of twelve selenoproteins in yellow catfish (Pelteobagrus fulvidraco) fed diets varying in oxidized fish oil and selenium levels. J Trace Elem Med Bio (2023) 79:127204. doi: 10.1016/j.jtemb.2023.127204
60. Zhang Y, Roh YJ, Han S, Park I, Lee HM, Ok YS, et al. Role of selenoproteins in redox regulation of signaling and the antioxidant system: A review. Antioxidants (2020) 9:383. doi: 10.3390/antiox9050383
61. Lv L, Chai L, Wang J, Wang M, Qin D, Song H, et al. Selenoprotein K enhances sting oligomerization to facilitate antiviral response. PloS Pathog (2023) 19:1011314. doi: 10.1371/journal.ppat.1011314
62. Chen F, Zhang Z, Wang L, Yu H, Zhang X, Rong K. Dietary selenium requirement for on-growing grass carp (Ctenopharyngodon idellus). Aquaculture (2023) 573:739572. doi: 10.1016/j.aquaculture.2023.739572
63. Lu Z, Wang P, Teng T, Shi B, Shan A, Lei XG. Effects of dietary selenium deficiency or excess on selenoprotein gene expression in the spleen tissue of pigs. Animals (2019) 9:1122. doi: 10.3390/ani9121122
64. Tolmacheva AS, Nevinsky GA. Essential protective role of catalytically active antibodies (Abzymes) with redox antioxidant functions in animals and humans. Int J Mol Sci (2022) 23:3898. doi: 10.3390/ijms23073898
65. Lennicke C, Cocheme HM. Redox metabolism: ROS as specific molecular regulators of cell signaling and function. Mol Cell (2021) 81:3691–707. doi: 10.1016/j.molcel.2021.08.018
66. Chai Y, Mieyal JJ. Glutathione and glutaredoxin—key players in cellular redox homeostasis and signaling. Antioxidants (2023) 12:1553. doi: 10.3390/antiox12081553
67. Liang H, Wu L, Hamunjo Chama MK, Ge X, Ren M, Chen X, et al. Culture salinity modulates Nrf2 antioxidant signaling pathway and immune response of juvenile genetically improved farmed tilapia (Oreochromis niloticus) under different dietary protein levels. Fish Shellfish Immun (2021) 117:220–7. doi: 10.1016/j.fsi.2021.08.014
68. Harsij M, Gholipour Kanani H, Adineh H. Effects of antioxidant supplementation (nano-selenium, vitamin C and E) on growth performance, blood biochemistry, immune status and body composition of rainbow trout (Oncorhynchus mykiss) under sub-lethal ammonia exposure. Aquaculture (2020) 521:734942. doi: 10.1016/j.aquaculture.2020.734942
69. Shang X, Wang B, Sun Q, Zhang Y, Lu Y, Liu S, et al. Selenium-enriched bacillus subtilis reduces the effects of mercury-induced on inflammation and intestinal microbes in carp (Cyprinus carpio var. specularis). Fish Physiol Biochem (2022) 48:215–26. doi: 10.1007/s10695-022-01046-8
70. Wang H, Bi C, Wang Y, Sun J, Meng X, Li J. Selenium ameliorates staphylococcus aureus-induced inflammation in bovine mammary epithelial cells by inhibiting activation of TLR2, NF-κB and MAPK signaling pathways. BMC Vet Res (2018) 14:197. doi: 10.1186/s12917-018-1508-y
71. Xu R, Cao J, Xu T, Liu T, Zhu M, Guo M. Selenium deficiency induced inflammation and apoptosis via NF-κB and MAPKs pathways in muscle of common carp (Cyprinus carpio L.). Fish Shellfish Immun (2023) 138:108847. doi: 10.1016/j.fsi.2023.108847
72. Heinemann U, Schuetz A. Structural features of tight-junction proteins. Int J Mol Sci (2019) 20:6020. doi: 10.3390/ijms20236020
73. Suzuki T. Regulation of intestinal epithelial permeability by tight junctions. Cell Mol Life Sci (2013) 70:631–59. doi: 10.1007/s00018-012-1070-x
74. Islas S, Vega J, Ponce L, Gonzalez-Mariscal L. Nuclear localization of the tight junction protein ZO-2 in epithelial cells. Exp Cell Res (2002) 274:138–48. doi: 10.1006/excr.2001.5457
75. Tamura A, Kitano Y, Hata M, Katsuno T, Moriwaki K, Sasaki H, et al. Megaintestine in claudin-15-eficient mice. Gastroenteroligy (2008) 134:523–34. doi: 10.1053/j.gastro.2007.11.040
76. Li L, Liu Z, Quan J, Sun J, Lu J, Zhao G. Dietary nano-selenium alleviates heat stress-induced intestinal damage through affecting intestinal antioxidant capacity and microbiota in rainbow trout (Oncorhynchus mykiss). Fish Shellfish Immun (2023) 133:108537. doi: 10.1016/j.fsi.2023.108537
77. Chen S, Xue Y, Shen Y, Ju H, Zhang X, Liu J, et al. Effects of different selenium sources on duodenum and jejunum tight junction network and growth performance of broilers in a model of fluorine-induced chronic oxidative stress. Poultry Sci (2022) 101:101664. doi: 10.1016/j.psj.2021.101664
78. Yang S, Yu M. Role of goblet cells in intestinal barrier and mucosal immunity. J Inflammation Res (2021) 14:3171–83. doi: 10.2147/jir.s318327
Keywords: Micropterus salmoides, selenoprotein extracts from Cardamine hupingshanensis, hematology, antioxidant capacities, immune and inflammatory responses
Citation: Zhang H, Zhao L, Zhang P, Xie Y, Yao X, Pan X, Fu Y, Wei J, Bai H, Shao X, Ye J and Wu C (2024) Effects of selenoprotein extracts from Cardamine hupingshanensis on growth, selenium metabolism, antioxidant capacity, immunity and intestinal health in largemouth bass Micropterus salmoides. Front. Immunol. 15:1342210. doi: 10.3389/fimmu.2024.1342210
Received: 21 November 2023; Accepted: 08 January 2024;
Published: 22 January 2024.
Edited by:
Hongyu Liu, Guangdong Ocean University, ChinaReviewed by:
Peng Tan, Marine Fishery Institute of Zhejiang Province, ChinaKaterina Kousoulaki, Fisheries and Aquaculture Research (Nofima), Norway
Yanjiao Zhang, Ocean University of China, China
Copyright © 2024 Zhang, Zhao, Zhang, Xie, Yao, Pan, Fu, Wei, Bai, Shao, Ye and Wu. This is an open-access article distributed under the terms of the Creative Commons Attribution License (CC BY). The use, distribution or reproduction in other forums is permitted, provided the original author(s) and the copyright owner(s) are credited and that the original publication in this journal is cited, in accordance with accepted academic practice. No use, distribution or reproduction is permitted which does not comply with these terms.
*Correspondence: Chenglong Wu, d3VjaGVuZ2xvbmcwMDdAMTYzLmNvbQ==