- 1Millennium Institute on Immunology and Immunotherapy. Facultad de Ciencias Biológicas, Pontificia Universidad Católica de Chile, Santiago, Chile
- 2Instituto de Ciencias Biomédicas, Facultad de Ciencias de la Salud, Universidad Autónoma de Chile, Santiago, Chile
- 3Millennium Institute on Immunology and Immunotherapy, Departamento de Ciencias Biológicas, Facultad de Ciencias de la Vida, Universidad Andrés Bello, Santiago, Chile
- 4Departamento de Endocrinología, Facultad de Medicina, Pontificia Universidad Católica de Chile, Santiago, Chile
The COVID-19 pandemic continues to cause severe global disruption, resulting in significant excess mortality, overwhelming healthcare systems, and imposing substantial social and economic burdens on nations. While most of the attention and therapeutic efforts have concentrated on the acute phase of the disease, a notable proportion of survivors experience persistent symptoms post-infection clearance. This diverse set of symptoms, loosely categorized as long COVID, presents a potential additional public health crisis. It is estimated that 1 in 5 COVID-19 survivors exhibit clinical manifestations consistent with long COVID. Despite this prevalence, the mechanisms and pathophysiology of long COVID remain poorly understood. Alarmingly, evidence suggests that a significant proportion of cases within this clinical condition develop debilitating or disabling symptoms. Hence, urgent priority should be given to further studies on this condition to equip global public health systems for its management. This review provides an overview of available information on this emerging clinical condition, focusing on the affected individuals’ epidemiology, pathophysiological mechanisms, and immunological and inflammatory profiles.
1 Introduction
The ongoing coronavirus disease 2019 (COVID-19) pandemic, caused by the severe acute respiratory syndrome coronavirus 2 (SARS-CoV-2) (1), has resulted in over 771 million confirmed cases and more than 6.9 million deaths globally (2). It has also led to significant morbidity, adverse mental health outcomes (3–7), and substantial socioeconomic disruption (8–11). While efforts have primarily focused on the acute phase of the disease, emerging evidence of significant and concerning post-acute effects on COVID-19 survivors introduces an additional dimension to the pandemic, potentially with long-term implications. These post-acute effects are known as long COVID or by various other names such as post-COVID conditions (PCC), post-acute sequelae of COVID-19 (PASC), chronic COVID syndrome (CCS), and long-haul COVID, and they define a broad spectrum of lingering symptoms experienced by a proportion of COVID-19 survivors after clearing the acute SARS-CoV-2 infection (12). According to the United States (US) Centers for Disease Control and Prevention (CDC), post-COVID-19 conditions have various health consequences four or more weeks after SARS-CoV-2 infection. The term is loosely defined due to the diverse and heterogeneous nature of the long COVID symptoms. These may include respiratory issues like persistent breathlessness, dyspnea, tiredness, headache, fever, and multi-system complaints. Additionally, these symptoms may occur concurrently or intermittently (12, 13). Nevertheless, in certain instances, the clinical manifestations observed almost four weeks following SARS-CoV-2 infection might derive from persistent symptoms originating in the acute phase of COVID-19 (14). In light of this, the significance of defining long COVID symptoms to distinguish between patients experiencing a gradual recovery and those exhibiting symptoms that accurately align with their medical condition, has been proposed (14).
This review provides a comprehensive overview of long COVID’s clinical and biological aspects. It emphasizes the epidemiology, clinical presentation, and system-specific manifestations, focusing on the immune response and inflammation.
2 Epidemiology of long COVID
Following the 2003 SARS epidemic caused by SARS-CoV-1, some survivors experienced reduced functional capacity and could not return to work (15, 16). The prevalence of long COVID in the population remains uncertain, ranging from 10% to 60% for COVID-19 survivors and potentially exceeding 90% for severe cases requiring hospitalization (17–23). It is estimated that one-fifth to one-third of survivors may experience activity-limiting symptoms for weeks after the acute phase (20, 21, 24–28). Notably, post-COVID-19 persistent symptoms appear more common than other respiratory infections (19, 29). Studies in Italy found that most patients surviving COVID-19-related hospitalizations faced impaired physical functioning after discharge (19).
Furthermore, a case series of 143 COVID-19 survivors found that only 12.6% were symptom-free after an average of 60 days from disease onset (27). Additionally, 44.1% reported a declining quality of life due to fatigue and dyspnea (27) (Figure 1). Long-term COVID development can be influenced by different SARS-CoV-2 variants, the number of vaccine doses received, and the time elapsed since vaccination (30). Studies indicate that Omicron cases were less likely to experience long COVID (31, 32). For instance, a study in China reported that 40.4% of patients with the Delta variants had clinical sequelae 3 to 24 months after discharge, compared to only 8.89% who had Omicron (32). Similarly, a study in the United Kingdom showed a reduction in long COVID cases from 10.8% for the Delta variant to 4.5% for the Omicron variant (31). Moreover, Omicron cases were less likely to experience long COVID regardless of their vaccination regimen (31, 32). However, early studies on this matter often had limited sample sizes and focused on specific populations, so further research is needed to reach more definitive conclusions.
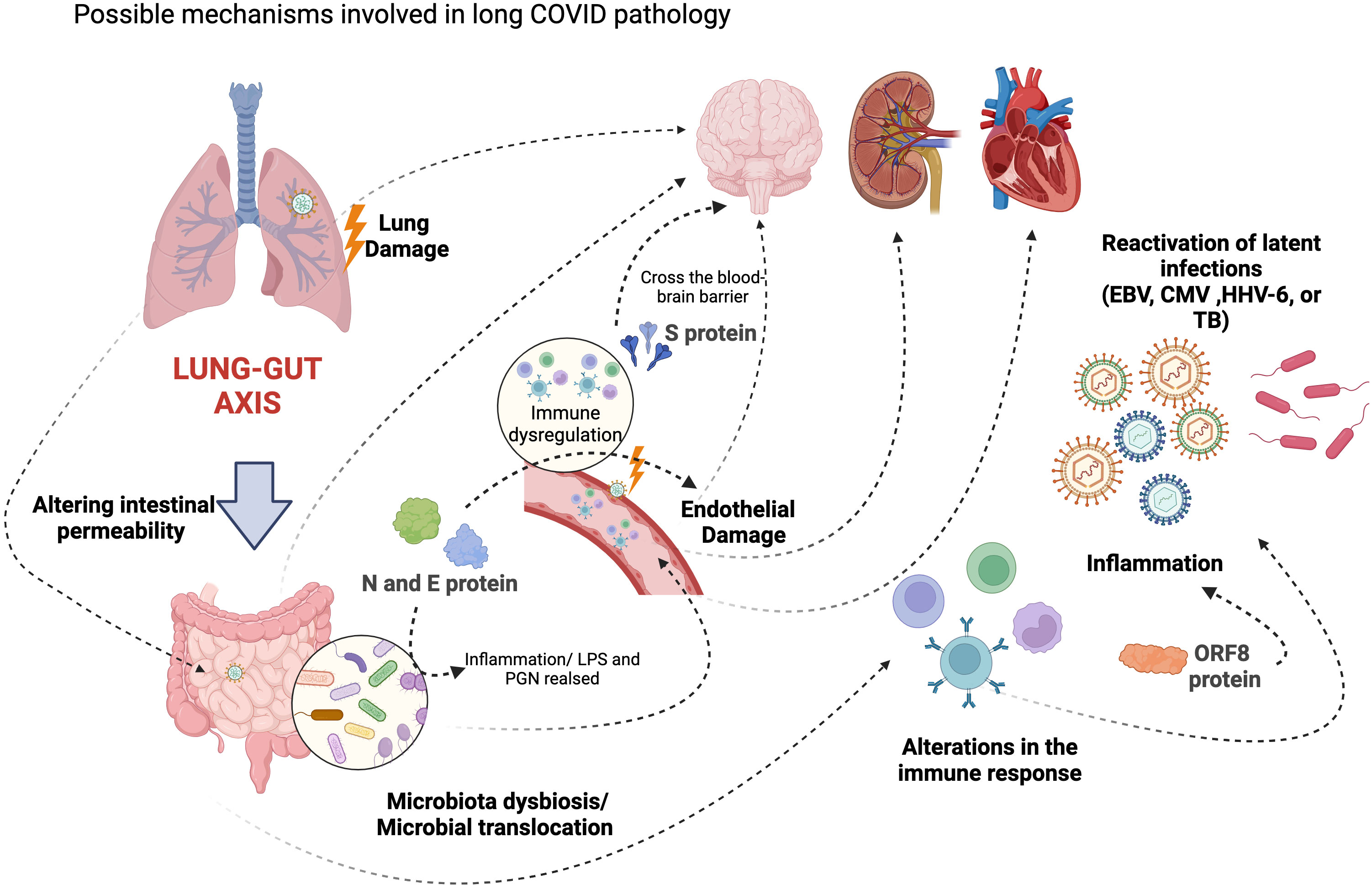
Figure 1 Possible mechanisms involved in the pathologies associated with long COVID. The figure summarizes the possible mechanisms involved in long COVID pathology. Immune dysregulation, autoimmunity, endothelial dysfunction, and hidden viral persistence in various organs are possible underlying pathophysiological mechanisms involved in long COVID. Viral persistence can lead to intestinal microbiota dysbiosis, which alters the immune response. It has been reported that changes in the intestinal microbiota can lead to neurological alterations. On the other hand, the spike protein could cross the blood-brain barrier, contributing to neurological sequelae. Additionally, nucleocapsid and envelope proteins can cause damage to endothelial cells, which can induce cytokines and activate immune responses. In addition, immune dysregulation can reactivate other viruses, such as EBV, HHV-6, and CMV, and bacterial diseases, such as TB. (Created with BioRender; License # IY26FE9X7C).
The health agencies estimate that around 1 out of 5 COVID-19 survivors may develop long COVID, marking some of the most robust evidence of its prevalence thus far (22). The CDC conducted a multi-state telephone survey of symptomatic adult COVID-19 survivors in the US to assess the prevalence of disabling or activity-limiting symptoms after COVID-19. The survey found that 35% had not returned to their normal state of health after 2–3 weeks of diagnosis. It has also been reported that one in five patients aged 18–34 with no chronic medical conditions after COVID-19 did not return to their normal state of health (29). While information on the duration of long COVID is limited, reported cases have extended from weeks to over a year (18, 33). However, recent studies indicate a decline in the incidence of long COVID cases post-pandemic, and this reduction has been associated with the advancement of vaccination (34, 35) Additionally, the Wuhan variant has been associated with diverse physical symptoms and emotional and behavioral changes, indicating a substantial impact on long-term health outcomes (36). In contrast, the Omicron variant has shown fewer post-infection effects, resembling common seasonal viral illnesses. These observations suggest that the Omicron and subsequent variants may not have the same long-term health consequences as earlier SARS-CoV-2 variants (36).
Certain risk factors, including age, gender, cardiovascular disease, and diabetes, have been associated with long COVID (37–43), which are also risk factors for severe acute COVID-19 (44, 45) Although some studies suggested that long COVID incidence may correlate with COVID-19 disease severity, age, and comorbidities (38, 46–48), others did not show such a relationship (23, 49). Notably, long COVID can emerge after mild and even asymptomatic COVID-19 cases (50). Observational evidence indicates that the severity of long COVID symptoms does not necessarily correlate with COVID-19 disease severity (50). This insight is derived from a study utilizing data from the UK Biobank, an extensive health database with measurements from thousands of volunteers before and after the pandemic’s start (51). On the other hand, an association between disease severity and the intensity of lingering respiratory symptoms has also been reported (52). Available information suggests a possible link between COVID-19 severity and long COVID incidence (53), although this association may not be statistically significant in large-scale studies and thus requires further investigation.
Female gender has been identified as a risk factor for developing post-COVID-19 symptoms (54–58). Studies indicate that women (<50 years old) were more likely to report fatigue and dyspnea after acute infection compared to men of the same age (55, 56, 59). Additionally, women may be up to three times more likely to be diagnosed with long COVID, regardless of age (60). This could be attributed to biological differences in the expression of angiotensin-converting enzyme-2 (ACE2) and transmembrane serine protease 2 (TMPRSS2) between genders (61), as well as immunological variations (62).
The role of persistent infection in long COVID remains uncertain. Various hypotheses regarding the mechanisms involved in its pathogenesis have been proposed, including circulating viral antigens or reservoirs in tissues (Figure 1) (30). Clinical and epidemiological surveillance data show that individuals may have detectable viral loads yielding positive PCR results for three months or even longer after infection. Although these individuals are usually non-infectious, their persistently detectable SARS-CoV-2 genetic material may contribute to their long-term symptoms (63–65). On the other hand, emerging research has reported the detection of persistent circulating spike protein in the bloodstream of some long COVID patients, even though they have undetectable levels of viral genetic material after the acute phase of COVID-19 (66). It has been suggested that the spike protein may go across the blood-brain barrier, triggering inflammation (67). Moreover, the persistence of nucleocapsid and envelope proteins in the liver, gallbladder, lymph nodes, and intestine has been associated with endothelial cell damage, leading to cytokine release and immune response activation (68).
Although chronically SARS-CoV-2-positive patients are generally considered non-infectious, persistent viral shedding has been reported (24). This poses a concerning risk of potential new variant emergence (69, 70). Chronic infections have led to mutations that could facilitate SARS-CoV-2 evolution and the emergence of new variants (70–73). As a result, epidemiologists and infectious disease specialists have hypothesized that SARS-CoV-2 variants like Omicron may have originated through natural selection in chronically infected hosts (69). Emerging observational data suggests that vaccination against COVID-19 may help resolve chronic infections, emphasizing the potential of vaccines in preventing the emergence of new variants (72, 74). It is important to note that while the quality of the evidence is somewhat limited, it indicates a positive impact of vaccinations in mitigating the ongoing emergence of variants.
Since the widespread availability of COVID-19 vaccines in early 2021, extensive scientific scrutiny of their impact on the risk of developing long COVID and the immune profile following a post-breakthrough SARS-CoV-2 infection (15, 54, 75–89). Studies have also investigated the severity of symptoms in vaccinated individuals who develop long COVID (82–86). Initial reports have suggested that vaccination may confer protective effects against long COVID incidences (83, 85, 90). However, recent extensive cohort studies show that vaccines provide only partial protection in case of a breakthrough infection (91, 92). One of these studies, conducted using the US Department of Veteran Affairs healthcare database with substantial sample size, provides robust evidence that vaccination’s impact in reducing the incidence of long COVID is partial (82). Available data conclusively demonstrate that vaccines diminish the risk of long COVID by decreasing the likelihood of experiencing a SARS-CoV-2 infection (84, 87, 90). Other studies have shown an almost complete reduction of long COVID symptoms after vaccination, mainly for severe manifestations like fatigue (93, 94). In this context, booster doses may be considered a preventive measure against long COVID, helping to maintain a relatively low infection risk and providing protection against concerning variants (86, 95, 96).
The FDA-approved antivirals Nirmatrelvir and Molnupiravir effectively treat acute COVID-19 (97, 98). Nirmaterlvir targets the SARS-CoV-2 type 3-chymotrypsin cysteine protease enzyme (99), while Molnupiravir heightens the frequency of viral RNA mutations (98), both leading to alterations in viral replication (41, 98). Administering these antivirals during the acute phase is linked to a reduced risk of at least eight of the thirteen post-acute sequelae, including arrhythmia, pulmonary embolism, deep vein thrombosis, fatigue and malaise, liver disease, acute kidney injury, muscle pain, and neurocognitive impairment (100, 101). Their use is associated with decreased risk of long COVID in unvaccinated individuals, vaccinated individuals, and those with both a primary SARS-CoV-2 infection and reinfection (100, 101).
Immunocompromised individuals show a notably diminished humoral immune response to vaccination, underscoring the necessity of booster shots in averting COVID-19 and mitigating the onset of long COVID within this group of patients (79). In immunocompromised children, SARS-CoV-2 infection was observed to be more asymptomatic than their immunocompetent counterparts (102). Moreover, there was a lower reported frequency of long COVID among immunocompromised children relative to immunocompetent controls (102). This is crucial, especially given the limited but strong evidence suggesting that persistent infection in these individuals may contribute to the emergence of new variants, as discussed earlier (69–72). However, it is worth noting that additional protection in the case of a breakthrough infection remains a subject of scientific debate. Positively, evidence indicates that vaccination may alleviate long COVID symptoms in previously unvaccinated patients (74, 83, 93, 94, 103, 104).
Long COVID is not exclusive to adults, as it has also been observed in the pediatric population (105). For example, a retrospective cohort study found that 51% of pediatric patients from a sample of children who recovered from COVID-19 experienced persistent symptoms 1-3 months after infection. The most common manifestations in this study were fatigue, loss of taste or smell, and headaches (106). Similarly, a small case report involving Swedish children who experienced mild to moderate COVID-19 without hospitalization revealed that all five children had persistent symptoms after 6-8 months, significantly hindering their daily activities and severely impacting their ability to attend school (107).
3 Pathophysiology of long COVID
Long COVID presents a wide range of symptoms, as mentioned before (Figure 2) (12, 13, 22, 108, 109). Despite its clinical presentation, the precise pathophysiological mechanisms remain unclear. Its involvement across multiple organs suggests potential mechanisms, including direct viral tropism, cytotoxic viral proteins, and immunopathology (Figure 2). Some studies propose that long COVID symptoms may result from persistent effects of the acute phase of COVID-19 and that different mechanisms may cause the different symptoms described (14, 27, 66, 67). Additionally, immune system activation can lead to an autoimmune response or prolonged, nonspecific inflammation, causing further harm to host cells (Figure 2) (24, 110–115).
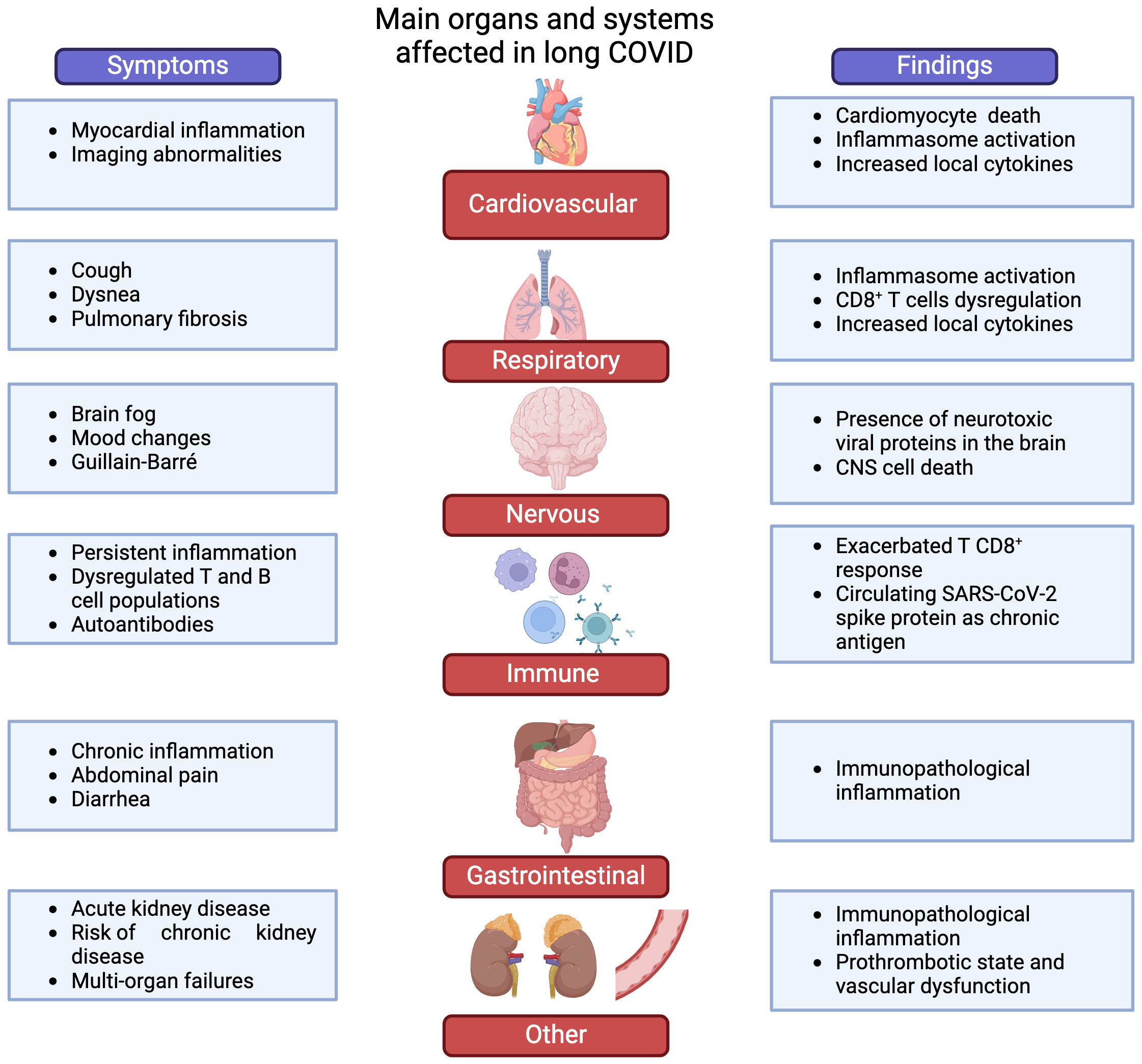
Figure 2 Main systems involved in long COVID. The figure summarizes common complications and some putative mechanisms involved in long COVID. It is estimated that 1 in 5 COVID-19 survivors develop long-lasting symptoms compatible with long COVID, which are diverse and heterogeneous. These complications can be classified into six main groups: cardiovascular, respiratory manifestations, neurological, alterations in immunity, gastrointestinal manifestations, and others. The mechanisms underlying these complications related to long COVID are still poorly understood, and relevant findings are summarized on the right side of the figure. (Created by BioRender; License # QU26HKW75S).
3.1 Clinical presentation of the disease
The CDC lists common long COVID symptoms, including dyspnea, fatigue, post-exertional malaise, cognitive impairment, mood changes, cough, chest pain, headache, fever, lightheadedness, anosmia, and dysgeusia (13). Like the acute phase, long COVID exhibits a multi-systemic nature (108, 109, 116). Two primary symptom patterns typically emerge in long COVID cases (1): fatigue and upper respiratory symptoms and (2) multi-system symptoms (28, 43). Due to the diverse presentation of this condition, establishing precise, standardized symptoms remains challenging. However, insights from patient follow-up studies have been invaluable. An investigation revealed enduring manifestations, primarily including breathing difficulties, fatigue, muscle weakness, anxiety, and depression, with fatigue or muscle weakness affecting a significant majority (63%) of survivors. However, respiratory and multi-organ complications described in long COVID do not appear to be linked to the need for mechanical ventilation (MV) during the acute phase (117). Some authors even report complete patient recovery one year after the acute phase, even in those patients with worse respiratory mechanics during invasive MV (117). However, other studies have shown that 60 to 70% of neuropsychiatric symptoms persisted between 3 and 6 months in patients who received MV (118, 119). The emergence of psychiatric symptoms, including psychological distress, anxiety, depression, sleep disorders, and alterations, has been documented in survivors of severe illnesses (120), including those recuperated from severe infections with other coronaviruses (121, 122). These symptoms appear to be linked with post-traumatic stress, potentially stemming from the isolation these patients experienced (123). During SARS-COV-2 infection, patients supported with MV may also experience paresthesia, neuropathies, and mobility limitations, which could be connected to the loss of gray matter during the acute phase (48). It is important to note that factors, such as MV or the socioeconomic status of patients, independent of viral infection, can contribute to symptoms like anxiety and depression (124, 125).
Despite the challenges mentioned above, specific long COVID symptoms correlate with measurable clinical indicators, including mild multi-organ impairment, mainly affecting the heart, liver, and pancreas. This discovery raises concerns about potential chronic organ damage, emphasizing the need for preventive strategies and additional research to mitigate such harm. Implementing grading scales and quantification tools for evaluating long COVID symptoms in patients could offer a potential solution to enhance follow-up and improve outcomes.
Scientists have devised strategies to assess and categorize the effects of long COVID. One such tool is the post-COVID-19 functional status (PCFS), an ordinal system that evaluates the condition of COVID-19 survivors over time (126). It employs a 4-grade scale based on functional limitations, ranging from grade 1 (indicating no limitations) to grade 4 (indicating dependence on external care for survival). This tool has demonstrated reproducibility in identifying patients needing support and rehabilitation (126). Since its introduction, it has been utilized in clinical studies on long COVID (127) and independently validated (128). A core outcome set (COS) has been proposed as a clinical instrument to establish consistent clinical outcomes (129). A multinational effort has created a COS for long COVID using a systematic literature review and a modified Delphi process. This COS is designed for adult patients in various settings (129–131). It encompasses outcomes such as fatigue or exhaustion, pain, post-exertion symptoms, work or occupational and study changes, survival from acute disease, symptoms related to physical functioning, cardiovascular and nervous system symptoms or conditions, and mental and respiratory functioning (130). Due to its global applicability and rigorous methodology (130), this COS is strongly recommended for evaluating long COVID patients in clinical and research settings.
Moreover, several studies have employed established grading scales to assess quality of life, disability, and specific levels of impairment in various tissues and physiological systems among long COVID patients (26). These tools include the EuroQol 5-Dimension 5-level (EQ5D-5L) instrument for assessing quality-of-life (132), the Washington Disability Group (WG) Short Form (133), 6-minute walking distance (127), and several clinical scales for dyspnea and fatigue (127). It is relevant to mention that quantifying the severity of long COVID is crucial for differentiating individuals experiencing minor lingering symptoms from those significantly impaired. This categorization aids in selecting participants for studies and prioritizing medical interventions.
As the pandemic endures, we anticipate gaining more insights into patient cases, providing a clearer understanding of this condition. For example, a case series focusing on female patients with long COVID symptoms revealed a notably high prevalence of orthostatic intolerance syndrome. This prompted the authors to suggest that autonomic nervous system dysfunction, potentially driven by autoimmune inflammation or viral invasion, could be an underlying factor (134). A 2020 cross-sectional study examined patients four to six weeks post-discharge from the hospital following COVID-19. It documented persistent respiratory symptoms and fatigue in many survivors (135).Elevated levels of inflammation markers, including d-dimer and C reactive protein, were also noted (135). The sustained inflammation observed in long COVID patients may have broader implications, given SARS-CoV-2’s capacity to activate inflammatory responses and oncogenic pathways. There is speculation that individuals with long COVID may face an increased risk of developing cancer (136) .
3.2 Respiratory consequences associated with long COVID
SARS-CoV-2 is linked to enduring respiratory issues, such as pulmonary fibrosis, angiogenesis, and vessel remodeling (91, 137–140). Interestingly, the seriousness of respiratory problems seems connected to the neurological effects of COVID-19 (141). In long COVID, common respiratory complications comprise breathlessness, dyspnea, fatigue, and a lingering cough, most of them at four weeks after diagnosis (26, 142). Estimates for the persistence of post-COVID-19 cough vary in studies and meta-analyses, generally falling between 7% to 15% for non-hospitalized and hospitalized survivors, regardless of whether they required MV (47, 143, 144). Breathlessness has been observed more frequently in individuals hospitalized at least one year after COVID-19, and these patients present lower forced expiratory volume in 1s than long COVID patients without breathlessness (145, 146). The secondary inflammation caused by the viral infection provokes a more significant overflow obstruction in these patients. The lung injury observed in long COVID patients can be mediated by an abnormal migration of monocytes, which, during the acute phase of the infection, had increased expression of C-X-C chemokine receptor 6 (CXCR6) and remained long-term elevated (146).
On the other hand, the prevalence of fatigue can vary between 32-42% in the first six months after COVID-19. Meanwhile, dyspnea has been reported between 12-26% of the long COVID cases (147, 148). Notably, not all long COVID patients had these symptoms during the acute phase of the disease. The risk factors associated with these symptoms are the female gender and having them during the first infection (148).
Soluble markers of inflammation are elevated in long COVID patients, which include general markers like C-reactive protein, components of the complement complex, and inflammatory cytokines such as interleukin-6 (IL-6), IL-1α, transforming growth factor beta (TGF-β), chemokines, interferon (IFN)-β, IFN-γ, granulocyte colony-stimulating factor (GM-CSF), as well as autoantibodies (40, 113, 138). These findings indicate that long COVID may primarily involve inflammation. However, long COVID patients with severe lung fibrosis have been found to have lower IFN-β production compared to those without fibrosis (138). Clinical studies consistently demonstrate increased local inflammation markers aforementioned in both severe COVID-19 patients and long COVID patients with lung fibrosis (138, 149). Another study found elevated levels of several inflammatory cytokines, including TGF-β but not IFN-β, in long COVID patients with lung complications like pulmonary fibrosis (138). Similarly, an analysis of post-mortem samples from COVID-19 cases indicated elevated type-I IFN response in lung tissue due to SARS-CoV-2 infection (149). Considering its potential link to inflammation, glucocorticoid therapy could be beneficial for managing long COVID (135, 150). Furthermore, prolonged inflammation may contribute to developing long COVID, as shown by an upregulated cytokine secretome in post-acute COVID-19 survivors (151). Another study reported similar findings regarding the decline of IFN-γ-producing CD8+ T lymphocytes in long COVID patients (24). SARS-CoV-2 might utilize mechanisms to suppress the host’s type-I IFN response, which persists long after infection clearance, and this diminished type-I IFN may play a role in the persistently exacerbated inflammation (24, 152). Additionally, inflammasome activation is emerging as a critical driver of chronic COVID-19 symptoms, affecting respiratory physiology. This prompts further investigation into inflammasome modulation as a potential intervention (153–156).
It is worth noting that mechanical ventilation (MV) in non-COVID-19 pathologies has been reported to lead to respiratory complications similar to those seen in long COVID, such as barotrauma (damage to the body caused by air or water change), volutrauma (lung injury caused by excessive stretching of the alveoli due to high tidal volumes during MV), and pneumothorax (presence of air or gas in the pleural cavity, leading to lung collapse) (157–160). Mechanical ventilation-induced lung injury can generate a systemic inflammatory response affecting other organs, also reported in long COVID (161). Moreover, MV seems to contribute to the development pulmonary fibrosis in elderly patients who have recovered from COVID-19 (162). These reports suggest that MV plays a role in the respiratory complications observed in long COVID patients.
3.3 Findings related to neurological alterations
COVID-19 can have lasting neurological effects in up to 30% of hospitalized patients at 3 months post discharge (25), often marked by “brain fog,” including disorientation, dizziness, and difficulty concentrating, sometimes leading to disability (Figure 2) (163). Termed “neuro-COVID” by some experts (164, 165) SARS-CoV-2 joins a list of viruses linked to neuropsychological issues (166), including the human respiratory syncytial virus (hRSV), human immunodeficiency virus (HIV), other human coronaviruses, Zika virus, herpes simplex virus (HSV), human cytomegalovirus (HCMV) and influenza (166–169). There is concern about potential neurological damage to offspring from infections in pregnant women, possibly leading to future congenital “neuro-COVID” manifestations (170). Neurological complications have been tied to the severity of respiratory infections (16), hospital stays (171) and exacerbated immune responses (172), potentially contributing to some “neuro-COVID” symptoms (16, 166, 167, 173, 174).
Similar to acute COVID-19, sudden loss of smell and taste (anosmia and ageusia) is prevalent in long COVID, initially experienced by around 15% of COVID-19 patients (175). While most recover these senses within weeks, these symptoms may persist in long COVID cases (176). Persistent loss of smell and taste has been linked to direct viral harm to olfactory receptor neurons and immune system-induced damage (5).
To understand cognitive impairment in long COVID, examining acute COVID-19 findings is relevant. A study of 81,337 volunteers showed a significant association between symptomatic COVID-19 and reduced cognitive function, especially in cases involving MV (141). MV alone, even without COVID-19, can cause neurological complications, which may contribute to long COVID neurological manifestations (119, 177). Even fully recovered individuals displayed poorer cognitive performance than uninfected counterparts, indicating a link between symptomatic COVID-19 and cognitive impairment (141).
An extensive study utilizing the UK Biobank repository (50) found significant correlations between COVID-19 infection and brain imaging abnormalities, even in mild cases (48). A recent cross-sectional study identified neurocognitive impairments in COVID-19 survivors months after infection, impacting functioning and quality of life and correlating with disease severity and comorbidities (178).
The exact pathophysiological mechanisms behind SARS-CoV-2-induced neurological alterations remain unclear. Nevertheless, emerging evidence suggests the virus breaches the blood-brain barrier (BBB) and directly damages the central nervous system (CNS), possibly triggering neuropsychological effects as seen in severe cases or post-mortem findings (179–182). Besides the elevated local inflammation, the direct invasion potentially accounts for the neuropsychological aftermath experienced by numerous COVID-19 survivors (Figure 2). This phenomenon could provoke a difunctional neurological signaling leading to the symptoms observed in long COVID cases (Figure 1). Notably, coronaviruses, including SARS-CoV-1, have been noted for their neurotropic tendencies, supporting the plausibility of direct neurological invasion by SARS-CoV-2 (142, 179, 181, 183). SARS-CoV-2’s presence in the CNS, extracted from cerebrospinal fluid during pandemic studies, might contribute to enduring neuropsychiatric complications (184). Animal models indicate that neurological coronavirus invasion could lead to long-lasting neurological repercussions in humans (185, 186). These models could partially elucidate the lingering cognitive impairment in many long COVID cases in humans.
An additional approach to elucidate the mechanism of SARS-CoV-2-induced neurological damage lies in identifying cytotoxic proteins expressed by the virus (5, 187). An analysis of SARS-CoV-2 proteins found some—specifically ORF6 and ORF10—possessed amyloidogenic properties and highly cytotoxic effects in neurons, potentially underpinning neurological alterations in long COVID and posing a risk for neurodegenerative disease (188).
Recently reported cases following SARS-CoV-2 infections include acute sensory and motor axonal neuropathy and acute inflammatory demyelinating polyradiculoneuropathy (Figure 2) (165, 189–191). These are subtypes of Guillain-Barré syndrome (GBS), where exposure to foreign peptides resembling those in peripheral nerves can lead to neuropathy (192, 193). Interestingly, SARS-CoV-2 contains hexapeptides, rich in lysine (KDKKKK) and glutamic acid (EIPKEE) amino acids, resembling human heat shock proteins 60 and 90 (192), potentially triggering an autoimmune response and neuropathy (5, 49, 141, 178, 194). It is important to mention that it is possible that neurological manifestations can be due to an immune pathology and not to CNS viral infection.
3.4 Cardiovascular complications found in patients with long COVID
Cardiovascular issues are recognized among the potential outcomes in long COVID cases (12, 13). Symptoms encompass chest pain, postural tachycardia syndrome, and left or right ventricular dysfunction, among other clinical signs (195). Additionally, irregularities in electrocardiograms (ECG) and Holter-ECG, temporary or lasting, have been observed in specific long COVID individuals, occurring in 1% to 27.5% of patients hospitalized due to cardiovascular problems (195). Another study revealed that the risks and associated burdens were apparent, particularly among individuals not hospitalized during the acute phase of the disease—this category comprising most COVID-19 cases. Furthermore, it was noted that the risks and associated burdens demonstrated a gradual escalation across the severity spectrum of the acute phase of COVID-19, ranging from non-hospitalized individuals to those requiring hospitalization and eventually those admitted to intensive care (196). Most cardiovascular issues noted in long COVID align with observations from the acute phase, including myocardial injury (Figure 2) (197, 198)), with cardiovascular disease emerging as a severe disease risk factor. Research reveals that 24.4% of hospitalized COVID-19 patients faced cardiac injury, which is strongly linked to higher mortality rates (199). These cardiovascular complications might persist in some patients post-recovery (198). Studies examining cardiac impairment after COVID-19 recovery found that 78% of survivors displayed cardiovascular magnetic resonance (CMR) abnormalities, with 60% showing persistent myocardial inflammation (200). These findings are noteworthy alongside evidence of COVID-19’s direct invasion of heart tissue (201) and in vitro studies replicating myocarditis (202). While mechanisms behind sustained heart inflammation remain partly unknown, overactive innate immune responses, like those in COVID-19-related lung damage, are suggested as plausible causes (156). The prevalence of these findings highlights the likelihood of COVID-19 survivors coping with cardiovascular issues, emphasizing the need for extended efforts in diagnosis and tailored management.
Histological and molecular findings from severe COVID-19 cases indicate significant local heart inflammation, suggesting a potentially similar condition in individuals with long COVID, leading to lasting myocardial injury (149, 195, 203). Heart inflammation has been linked to COVID-19 symptoms and mortality (204, 205). However, not all data confirm a significant prevalence of myocarditis in patients with sustained cardiovascular symptoms post-COVID-19 (206).
While persistent myocardial inflammation indicates long COVID, in some cases, comprehensive research is crucial to assess this population’s underlying pathophysiological mechanisms and cardiovascular risk profile. Investigations into post-COVID-19 cardiovascular damage remain crucial for a deeper understanding of adverse cardiovascular outcomes among survivors. Additionally, the vascular endothelium’s role in connecting inflammation and coagulation contributes to a prothrombotic state and vascular dysfunction in long COVID subjects (207).
3.5 Damage to endothelial barriers and viral persistence
The endothelium, crucial in hemostasis, immune reactions, and angiogenesis, plays a pivotal role in SARS-CoV-2 infection (208). The virus’s persistence in tissues post-recovery indicates potential reservoirs in extrapulmonary organs, as seen in adults and children (209, 210). This persistence has been observed in extrapulmonary organs, even in immunocompetent individuals and post-mortem subjects, several weeks or months post-infection (211). In autopsies of deceased individuals, the viral RNA was detected in various tissues, including the CNS and endothelium, weeks or months after infection despite being undetectable in plasma (212, 213). Even patients diagnosed with long COVID months or years after the initial infection exhibited lingering SARS-CoV-2 RNA in diverse tissues like the breast, appendix, and skin (213). Furthermore, in children, the virus or its remnants have been detected for weeks to months after acute infection in tissues or biological fluids, including postmortem detection of virus RNA (214). Evidence points to the presence of SARS-CoV-2 RNA in tissues of pediatric patients diagnosed with multisystem inflammatory syndrome and the detection of viral proteins and fragments in the intestine, plasma, palatine tonsils, and adenoids (215–217). It is important to note that viral RNA contaminants may be present in the analyzed samples, and their presence does not necessarily indicate persistence.
The diverse cells expressing the ACE-2 receptor can render them vulnerable to SARS-CoV-2 infection. This broad viral target might explain acute infection symptoms, yet the exact mechanism leading to prolonged symptoms remains unclear (218, 219). Although different cell targets of infection could elucidate acute symptoms, the mechanism behind the transition from acute to prolonged symptoms remains unclear (218). Extracellular vesicles (EVs) are suspected viral reservoirs, allowing SARS-CoV-2 to travel through the circulatory system, affecting endothelial cells and platelets, potentially triggering repeated immune responses and persistent symptoms (220–222).
Viral persistence, even without replication, can damage endothelial cells by inducing cytokine secretion and activating unwanted immune responses. Besides, SARS-CoV-2 can disrupt vascular homeostasis by directly infecting endothelial cells (212). Additionally, direct endothelial cell infection disrupts vascular homeostasis, impacting platelet function and promoting blood clotting and microclot attachment to the endothelium (223, 224). Furthermore, endothelial cell infection triggers increased expression of cell adhesion molecules, such as intercellular adhesion molecule 1 (ICAM-1), vascular cell adhesion protein 1 (VCAM-1), selectins (E-selectin and P-selectin), inflammatory mediators, and procoagulant factors. Endothelial dysfunction and platelet hyperactivation can lead to exposure to phosphatidylserine (PS) in the outer cell membrane. PS exposure may directly promote some procoagulant factors within the coagulation cascade and the formation of fibrinoid microclots resistant to fibrinolysis (224, 225). Meanwhile, endothelial dysfunction may increase cell permeability and leukocyte adhesion, favoring thrombus formation (212, 226).
On the other hand, it has been documented that in SARS-CoV-2 infection, mechanical damage to the microvascular glycocalyx is induced by the direct impact of fibrinogen (207). Although most recovered patients do not have detectable viral loads, the endothelial damage might stem from residual effects, possibly due to endothelial cells’ overactivation triggered by cytokine’s sustained release (68). Elevated levels of IL-1, IL-6, IFN-β, myeloid cells, and activated T cells in plasma and tissues from discharged patients are strongly associated with long COVID (227). This condition often persists with impaired oxygen exchange and tissue hypoxia, potentially contributing to a prothrombotic state and multi-organ failure in long COVID subjects (228). Moreover, hypoxia is likely to contribute to the notable and enduring B-cell abnormalities seen in cases of acute COVID-19 pneumonia (229).
3.6 Implications of intestinal barrier alterations in long COVID
Long COVID, recognized as a multi-system ailment (22, 108, 109), extends its impact beyond the respiratory system, affecting organs not traditionally associated with respiratory viruses. One of them is the gastrointestinal complications, which include diarrhea, vomiting, and abdominal pain (29, 230–234). Gastrointestinal involvement by substantial clinical cohorts has been reported to range from about 4% to nearly 13% in all acute COVID-19 cases (235). Significantly, a thorough investigation reported a 3.8% occurrence of viral RNA in fecal samples from patients seven months after their initial COVID-19 diagnosis (236). This trend is similarly observed in the persistent gastrointestinal complications within the spectrum of long COVID symptoms (12, 13, 22, 108).
Evidence suggests that ACE2, present in gut epithelial cells, serves as the entry point for the virus (235). The SARS-CoV-2 spike protein targets this enzyme, a crucial receptor facilitating viral entry (228, 237–240). This provides a plausible pathway for viral-induced damage. Viral RNA has been detected in stool samples, and the virus has been found to show tropism for intestinal epithelial cells, as seen in SARS-CoV-1 and Middle East respiratory syndrome coronavirus (MERS-CoV) infections (241–243). The structural similarity between the spike proteins of SARS-CoV-1 and SARS-CoV-2 and the presence of ACE2 in intestinal epithelial cells support the hypothesis of direct viral infection followed by an immune-driven inflammatory response, contributing to gastrointestinal manifestations. Studies have shown the presence of the SARS-CoV-2 nucleoprotein within the epithelial cells of hospitalized COVID-19 patients (235, 244). Another study demonstrates the presence of viruses in upper and lower gastrointestinal biopsies, which implies that gastrointestinal epithelium cells may be reservoirs of the virus even after the acute phase (245). Additionally, viruses have been identified in upper and lower gastrointestinal biopsies, indicating that gastrointestinal epithelial cells may serve as reservoirs for the virus even after the acute phase, with minimal inflammatory infiltrate observed (236, 245, 246). However, the virus in the stool does not appear to be linked to long-term COVID or the virus persistence in intestinal cells (247).
Bidirectional communication exists between the intestine and the lung (248). An intact intestinal barrier modulates pulmonary immune responses and the lung microbiome (Figure 1) (249, 250). In cases of acute SARS-CoV-2 infection, as in other respiratory infections, intestinal changes may be triggered due to the virus’s impact on intestinal permeability and promoting bacterial translocation (227, 248). Microbial translocation, defined as the migration of bacteria or their byproducts from the gut to extraintestinal and systemic circulation due to changes in gut mucosal integrity, can occur with or without viral infection (251). This phenomenon may impact systemic inflammation and, indirectly, affect the intestine by altering products, metabolites, and lipids associated with the intestinal microbiota (252). Numerous components of the immune system, including antiviral peptides, inflammatory mediators, immune cell chemotaxis, and secretory immunoglobulins, could be adversely affected (253). Some members of the gut microbiome produce enzymes that degrade glycans, and when these enzymes enter the circulation, they can modify the glycosylation of plasma glycoproteins, including antibodies. Glycosylation reactions regulate immune responses, including complement activation (253). Systemic inflammation, stemming from SARS-CoV-2 infection or direct viral effects on intestinal cells, can lead to changes in intestinal structure and the rupture of the epithelial barrier (250). Severely ill COVID-19 patients often display increased plasma levels of indicators of intestinal permeability, such as zonulin, a mediator of tight junctions in the digestive tract, fatty acid binding protein 2 (FABP2), lipopolysaccharide (LPS), and peptidoglycan (PGN), indicating a compromised intestinal barrier (251, 253).
Furthermore, patients with COVID-19 have consistently shown elevated levels of gut permeability and intestinal damage compared to healthy controls throughout the disease (251). The acute exacerbated immune responses triggered by SARS-CoV-2 is linked to a Th17 immune response, which leads to vascular permeability and leakage, resembling the immune response observed in cases of bacterial translocation (254). The available evidence suggests that the cytokine storm induced by SARS-CoV-2 is responsible for intestinal damage and may be connected to the manifestations seen in long COVID.
Intestinal damage may have connections to the manifestations observed in long COVID. Emerging evidence also hints at a potential link between gut microbiota dysbiosis and the prolonged complications of COVID‐19 (see Figure 1). Maintaining intestinal microbiota balance is crucial for various functions, such as extracting substrates from the diet for energy production, obtaining vitamins and short-chain fatty acids, and regulating the balance of Th17 and T reg responses to prevent intestinal inflammation (255, 256). Dysbiosis refers to significant alterations in microbial diversity and relative abundance (256). In individuals with severe SARS-CoV-2 infection, a relationship between intestinal microflora and lung microorganisms has been established. Opportunistic fungal and bacterial pathogens, including Aspergillus, Candida, Actinomyces, Streptococcus, Veillonella, Rothia, and Clostridium, have been found to replace commensal microbes like Bifidobacterium romboutsia, Proteobacteria, Collinsella, Actinobacteria and Bacteroides (257–259). Another study showed that changes in microbial diversity induced by inflammation during viral infection can persist for months, leading to alterations in the metabolomic profile (260). These changes involve the suppression of anti-inflammatory bacteria like Faecalibacterium prausnitzii, Eubacterium rectale, and Bifidobacterium adolescentis, and the enrichment of pathogens, including Rothia, Erysipelatoclostridium, Ruminococcus gnavus, Ruminococcus torques, and Bacteroides dorei (260).
The persistence of microbiota dysbiosis in the gastrointestinal tract after the resolution of the disease could contribute to the prolonged symptoms, emphasizing the need to comprehend how gut microorganisms are involved in inflammation and COVID-19. Furthermore, exploring the involvement of dysbiosis in neurological disorders is essential, given the previously reported relationship between the human intestinal microbiome and the CNS (261). Alternatively, SARS-CoV-2 might infect and replicate within intestinal bacteria (262). This could lead to unique viral persistence and contribute to intestinal dysbiosis in long COVID. The bacteriological impact of SARS-CoV-2 might directly encourage the proliferation of specific bacteria, upsetting the balance in the intestinal microbiota (262).
3.7 Impact on the renal system
In terms of renal complications, many COVID-19 survivors, particularly those with severe pneumonia, have experienced acute kidney injury marked by a sudden decline in kidney function (263–265). About 30% of patients following acute SARS-CoV-2 infection experience renal dysfunction (266). This includes diverse conditions such as acute tubular necrosis, glomerular lesions, focal and segmental glomerulosclerosis, and renal infarction, some of which can lead to fatal outcomes (267–269). A retrospective study in China even linked kidney issues to fatal COVID-19 outcomes (244). Consequently, kidney problems have become a significant aspect of long COVID, characterized by recurrent episodes of acute kidney injury and an increased risk of chronic kidney disease (82, 91, 108, 264), as illustrated in Figure 2.
While there is evidence of ACE2 expression in kidney tissue, which hints at the possibility of direct viral infection (270–272), there is no confirmed kidney tropism by SARS-CoV-2, and this remains a hypothetical scenario. Other mechanisms, such as respiratory complications leading to hypoxemia and immune-driven inflammation, may contribute to kidney manifestations (273, 274). It is important to note that just as kidney injury raises the risk of fatal COVID-19 (38, 44, 275), the reverse relationship is observed: COVID-19 heightens the risk of developing chronic kidney disease (91). These findings underscore the importance of preparing healthcare systems for potential increases in kidney disease cases as long as COVID affects individuals in the months ahead.
4 Reactivation of latent infections
Reactivation of latent viral infections, including Epstein-Barr virus (EBV), human herpesvirus 6 (HHV-6), and human cytomegalovirus (HCMV), is a common occurrence in states of compromised immunity. Human herpesviruses like EBV and HHV-6 are prevalent globally, with over 90% of adults carrying one or more of these viruses (276, 277). Among the risk factors for viral reactivation are stress and immunosuppression, and also this reactivation has been documented in severe illness, particularly among ICU patients (278). Patients with COVID-19, whether experiencing mild or severe cases, have reported the reactivation of latent herpesviruses (279–282). However, the reactivation tends to be more severe in individuals with a critical illness and less pronounced in those with milder cases (279–282). The stress and the notable increase in cytokines and other inflammatory molecules is mainly caused by viral proteins, including ORF8, a unique accessory protein specific of SARS-CoV-2 are believed to contribute to the reactivation of these viruses (283, 284). Furthermore, this reactivation may enhance SARS-CoV-2’s entry into cells, potentially increasing viral load and symptom severity (285). While causal links have not been definitively established, it has been speculated that persistent EBV, HHV-6, and SARS-CoV-2 infections could fuel the chronic inflammation associated with long COVID (281, 286, 287). A study that compared patients with long COVID with 1 or more than 5 symptoms to those without long COVID found higher Epstein-Barr virus nuclear antigen (EBNA) IgG levels. More specifically, these patients have more neurocognitive symptoms (276). Interestingly, long COVID patients who reported preexisting autoimmune diseases like thyroiditis and have experienced fatigue showed higher levels of early antigen diffuse component (EA-D) IgG (276).
Additionally, SARS-CoV-2 has been suggested as a risk factor for various cancers (288), raising the possibility that latent herpesvirus reactivation may play a role in these oncogenic processes. This emphasizes the need to closely monitor reactivated viral infections in patients with current or past SARS-CoV-2 infections.
Moreover, the reactivation of infections has been associated with neurological complications. Long COVID shares similarities with myalgic encephalomyelitis/chronic fatigue syndrome (ME/CFS) due to comparable manifestations (289). ME/CFS is characterized by systemic exertion intolerance with neurological and immunological components, and viral infections can be a trigger (289, 290). In survivors of SARS-CoV-1, 27% reported ME/CFS symptoms four years after infection, a trend observed in long COVID patients (290). Opportunistic viruses like EBV, HHV-6, and HCMV have been linked to the development of ME/CFS (291). Consequently, reactivation of these viruses has been associated with long COVID symptoms (292, 293). A study found that long COVID patients had higher EBV nuclear antigen (NA) immunoglobulin G (IgG) levels compared to those without the condition (293). Also, EBV early antigen-diffuse (EA-D) IgG was correlated to long COVID manifestations, with fatigue and insomnia being the most frequently reported (292). Furthermore, the reactivation of EBV and HHV-6 was detected in long COVID patients who reported symptoms like sleep disorders, persistent cough, anosmia/ageusia, hair loss, shortness of breath, and chest pain (294).
Reactivation of latent infections related to COVID-19 extends beyond viral diseases. Notably, cases of tuberculosis (TB) reactivation following SARS-CoV-2 infection have been documented (295–299). The possible immunosuppressive effects of the disease, which remains controversial, and the treatments may contribute to infectious disease reactivation. Alarmingly, the WHO’s annual tuberculosis report indicates an increase in TB incidence since the beginning of the pandemic, exacerbating a condition that already imposes a significant global healthcare and economic burden (300).
5 Immune response during long COVID
Understanding the immune response to long COVID necessitates insight into the acute phase of the disease. The host immune reaction, encompassing innate and adaptive components, plays a crucial role in COVID-19. This involves the release of substantial quantities of pro-inflammatory cytokines and inflammatory cells such as neutrophils and macrophages (155, 301, 302) along with the generation of virus-specific CD4+ T cells, CD8+ T cells, and B cells (303–308). The impact of this immune response on the clinical manifestations of COVID-19 has been reported. Clinical research has indicated that in numerous severe cases, immunopathology —organ and tissue damage resulting from an imbalanced immune response—may be a primary factor in life-threatening injuries for patients (150, 301, 309–314). Acute exacerbated immune responses, mainly the self-sustaining inflammatory chain reaction are commonly observed in critical COVID-19 cases (301, 303, 309, 310). Additionally, markers of inflammation in the blood linked to immunopathology and multi-organ damage have been identified, including interferons, neutrophil markers, and other inflammatory cytokines (314). Immunopathology has also been demonstrated to play a substantial role in severe acute pediatric cases, with a relatively common manifestation being the severe, and at times fatal, systemic inflammatory condition known as multi-system inflammation in children (MIS-C). This syndrome involves exacerbated respiratory and cardiovascular tissue inflammation accompanied by fever (264, 265, 270–274).
Persistent immune dysregulation is suspected to play a role in specific chronic manifestations of long COVID (Figure 2) (24). Evidence indicates that patients exhibiting these manifestations display altered immune mediators, both cellular and humoral, with a notable focus on modified T-cell populations (21, 104, 108). It is plausible to hypothesize that these altered populations may contribute to persistent immunopathology, potentially accounting for long-term symptoms characterized by inflammation, such as chronic fever and muscle and joint pain (20, 164, 311, 312). However, it is crucial to emphasize that the connection between acute and chronic immune regulation post-SARS-CoV-2 infection is not yet fully elucidated.
Clinical studies utilizing blood samples from COVID-19-convalescent volunteers have demonstrated that SARS-CoV-2 infection strongly induces coronavirus-specific T lymphocytes and memory B cells (315–319). Besides, evidence suggests that these lymphocytes may remain persistently elevated, potentially explaining at least some clinical manifestations of long COVID (113, 150). Additionally, it has been observed that in COVID-19-convalescent patients, there are broad alterations in the transcriptome of peripheral blood mononuclear cells (PBMCs) in those with clinical presentation compatible with long COVID (320). Significant differences in innate and adaptive immune cell populations in COVID-19 convalescent individuals compared to uninfected controls were observed (320). Furthermore, a clinical study employing a high-dimensional characterization of the lungs of COVID-19 convalescent subjects found an enrichment of T and B cells in the respiratory tract compared to blood levels (110). Additionally, it was observed that the gastrointestinal manifestations of long COVID were linked to the expansion of SARS-CoV-2-specific CD8+ and CD4+ T cell populations (41).
Dysregulated tissue-resident T cells have been suggested as potential drivers of chronic lung pathology post-respiratory infections (321, 322), implying that long COVID pathology may stem from a dysregulated cellular response (315). CD4+ T cell responses during COVID-19 are correlated with disease severity, suggesting that a more severe acute SARS-CoV-2 infection could lead to a greater degree of T cell response dysregulation, potentially explaining chronic manifestations (24). Conversely, there was no correlation between immune dysregulation and persistent viral load (24), indicating that COVID-19 induces alterations in cell immunity regardless of chronic viral load, which could be a key pathophysiological trigger of long COVID (320, 323).
On the other hand, studies have shown that COVID-19 can induce significant decreases in several components of the cellular immune response, including cytokines, complements, and immune cells (303, 324, 325). Recent research showed marked reductions in counts and percentages of total lymphocytes, total T cells, CD4+ T cells, CD8+ T cells, B cells, and natural killer cells (NK) without changes in the typical TCD4:TCD8 ratio of approximately 1:1 in healthy subjects whether vaccinated or unvaccinated, who are COVID-19 survivors (324) (Figure 1). Substantial dysregulations in CD8+ T cells expressing degranulation markers have been reported after SARS-CoV-2 infection (24). Furthermore, a machine-learning-assisted clinical characterization of the immune response of healthy volunteers, COVID-19 patients, and long COVID patients revealed significantly elevated B cell and CD14+ CD16+ CCR5+ monocyte levels, along with reduced T regulatory cells (Treg) and programmed cell death protein 1(PD-1)-expressing CD4+ and CD8+ T cells in the long COVID group compared to healthy controls (325). Moreover, immunological characterization of long COVID patients found a persistent increase of CD16+ monocyte levels and sustained expression of the SARS-CoV-2 S1 protein in CD14lo, CD16+ monocytes (326). While the precise role of this expression remains uncertain, it is speculated that it could contribute to chronic vascular inflammation (326). These observations may suggest that if these alterations in cellular immunity persist, they could at least partially contribute to the symptoms present in long COVID. Consistent with this, significantly higher SARS-CoV-2-specific IFN-γ-producing CD4+ and CD8+ T cells were found in subjects with prior pulmonary disease, indicating that this condition may lead to a higher likelihood of future persistent cellular immune dysregulation after experiencing COVID-19 (24). Furthermore, a study found increased levels of activated CD4+ and CD8+ T-cells after 3 months of recovery from mild, moderate, and severe COVID-19. Higher plasma levels of T-cell-related IL-4, IL-7, IL-17, and tumor necrosis factor-alpha (TNF-α) were observed to compared mild and moderate patients (327). Despite all this evidence, a study evaluating SARS-CoV-2 cellular immunity in health workers with confirmed infection, with or without persistent symptoms, found no differences in viral neutralization or T-cell responses (328). Recently, it was observed that T cells from COVID-19 patients exhibited significantly elevated levels of IL-2 production in response to stimulation with SARS-CoV-2 peptides compared to an unexposed control group (329).
SARS-CoV-2 infection triggers a strong induction of virus-specific B cells, resulting in abundant production of IgG antibodies primarily targeting the receptor-binding domain of the spike protein (S-RBD) and the nucleocapsid protein (NP) (308, 317, 330). Studies of individuals recovering from COVID-19 consistently show elevated IgG levels persisting for up to 24 weeks post-infection (315, 320). These humoral responses correlated with disease severity and the number of post-acute symptoms (20, 320, 331). Similar patterns emerge in cellular immunity. However, the precise implications of the upregulation of both humoral and cellular responses in the pathogenesis of long COVID remain unclear. Notably, some studies find no clear correlation between the humoral response and the incidence and severity of long COVID manifestations (108, 328). It is crucial to note that elevated antibody titers do not always indicate the presence of symptoms. COVID-19 vaccinations have been associated with a sustained upregulation of both humoral and cellular immune responses, with a notable absence of chronic adverse effects in most cases (54, 78, 80, 96, 332–338). Interestingly, comorbidities, particularly diabetes, chronic heart disease, and hypertension, correlate with higher convalescent antibody titers after COVID-19 (20).
On the other hand, alterations have been observed in the development of B cells, wherein antibody-secreting cells are produced extrafollicularly (339, 340). This pathway has been previously described in individuals with lupus (341). While responses directed against the virus are initiated, they can also give rise to autoreactive humoral responses (autoantibodies), which persist for months in individuals experiencing post-COVID-19 sequelae (339, 340). Autoantibodies have emerged as potentially significant contributors to COVID-19 severity (41). Reports suggest these molecules might be responsible for up to a fifth of COVID-19-related deaths (342). Autoantibody concentrations have been positively correlated with age and the male sex, potentially explaining the higher severity and case-fatality ratio observed in these (342, 343). Additionally, 10% of individuals with acute COVID-19 infections had significant concentrations of autoantibodies that directly inhibit type-I IFN (342), but these antibodies were not found in significant concentrations in asymptomatic COVID-19 patients (343).
Like in the acute phase of COVID-19, autoantibodies in long COVID patients have been reported (344). A comprehensive multi-omics study reported the presence of autoantibodies predate SARS-CoV-2 infection and can be one of many factors predicting the incidence of long COVID (Figure 1) (40). Recent multiparameter analyses of long COVID highlight the significance of specific autoantibody production as a predictor among various risk factors for long COVID incidence (40). Furthermore, persistent circulation of the SARS-CoV-2 spike protein has been reported, potentially serving as a mechanistic link to long COVID symptoms (65, 67). Another category of autoantibodies targets G-protein coupled receptors (GPCRs), which have been implicated in cardiovascular and neurological development (345). In long COVID patients, α1 adrenergic receptor (α1 AdR), β1 AdR, and β2 AdR and the muscarinic acetylcholine receptors M2 (M2 AChR), M3 AChR, and M4 AChR were the most found and correlates with the severity of the neurological symptoms (345–347).
Despite these findings, available data on the humoral response during long COVID fail to provide conclusive evidence that can significantly contribute to our understanding of the pathophysiological mechanisms underlying this condition, underscoring the need for further research.
6 Discussion
Long COVID is a newly recognized clinical condition, and numerous uncertainties persist. Consequently, investigations into this emerging condition are only beginning to uncover potential underlying mechanisms (23, 24, 65, 67, 110, 112, 266, 302, 308, 315, 316, 323, 348). Public health authorities like the CDC actively conduct short-term and multi-year studies to enhance our understanding of this condition. Available data underscores that this clinical phenomenon is intricate and diverse, affecting multiple bodily systems and exhibiting persistence over months to years in some instances, summarized in Table 1 (23, 49, 139, 151, 164, 320, 350). As mentioned earlier, the definition of persistent symptoms for the post-COVID diagnosis is crucial in discerning whether they are indicative of long COVID or if the recovery process is simply slower than anticipated. Prolonged inflammation has been proposed as a potential driver of long-term manifestations following COVID-19. Indeed, the role of sustained inflammation in developing cardiovascular, respiratory, immunological, and neurological complications after COVID-19 has been elucidated (23, 91, 110, 112, 155, 355). National healthcare systems should be understanding the likelihood that some COVID-19 survivors may transition into patients with chronic disabilities. Simultaneously, the possibility of chronically ill COVID-19 survivors potentially contributing to the emergence of novel variants of concern due to persistent viral loads necessitates careful attention (66, 71). Current evidence on the existence of different subtypes of long COVID remains inconclusive. However, two studies have progressed in subtyping this condition (339, 358). Using semantic phenotypic grouping, the first study successfully stratified the disease into six distinct groups (358). These groups exhibit unique profiles of phenotypic abnormalities, encompassing specific pulmonary, neuropsychiatric, and cardiovascular manifestations, with one group exhibiting extensive and severe symptoms correlated with increased mortality (358). The second study, employing proteomic techniques, further subclassifies long COVID into two conditions. These conditions are characterized by the presence or absence of broad inflammatory signatures indicating increased neutrophil activity and qualitative changes in memory and B cell responses (339). Characterizing and subclassifying long COVID can potentially improve the diagnosis and treatment strategies for this ailment. Therefore, long-term studies must delve into the epidemiology, mechanisms, pathophysiology, and possible management of long COVID to address this emerging crisis with the utmost diligence from healthcare institutions. Concurrently, public health organizations should formulate comprehensive management strategies that recognize the diverse manifestations characteristic of it and address its manifold consequences.
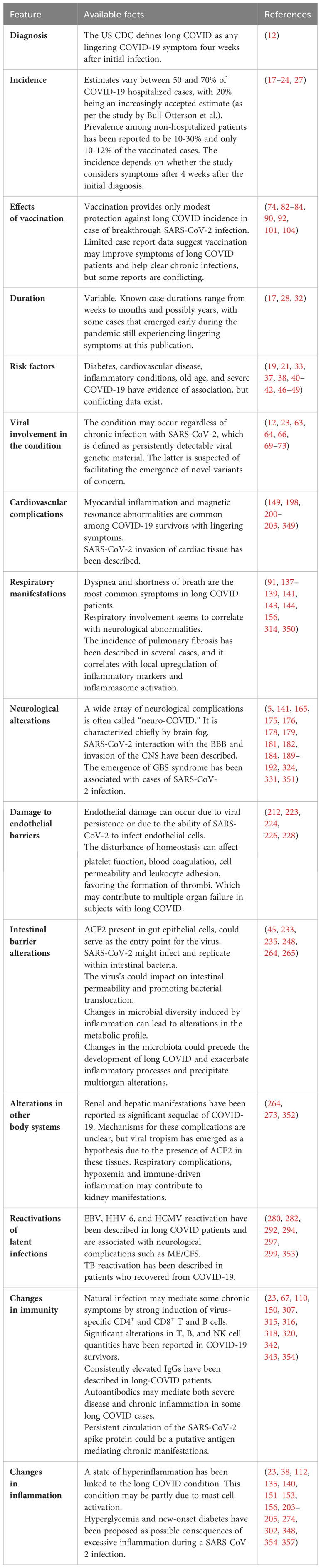
Table 1 Epidemiological, clinical, pathophysiological, and immunological characteristics of long COVID.
Author contributions
KB: Conceptualization, Writing – original draft, Writing – review & editing. BD-V: Conceptualization, Writing – original draft, Writing – review & editing. LR-G: Writing – review & editing. TR: Writing – review & editing. CR: Writing – review & editing. PG: Writing – review & editing. AK: Conceptualization, Writing – review & editing.
Funding
The author(s) declare financial support was received for the research, authorship, and/or publication of this article. This work was supported by ANID/FONDECYT grant #11221280 for KB; ANID/CONICYT National Doctoral Scholarship # 21221163 for BD-V. ANID/FONDECYT grant #1191300 for CR. ANID/FONDECYT grants #1190830, #1190864, #1170964, #1231851, and Fundación COPEC-UC for AK. Millennium Institute on Immunology and Immunotherapy (ICN09_016/ICN 2021_045; former P09/016-F).
Conflict of interest
The authors declare that the research was conducted in the absence of any commercial or financial relationships that could be construed as a potential conflict of interest.
The author(s) declared that they were an editorial board member of Frontiers, at the time of submission. This had no impact on the peer review process and the final decision.
Publisher’s note
All claims expressed in this article are solely those of the authors and do not necessarily represent those of their affiliated organizations, or those of the publisher, the editors and the reviewers. Any product that may be evaluated in this article, or claim that may be made by its manufacturer, is not guaranteed or endorsed by the publisher.
Abbreviations
COVID-19, coronavirus disease 2019; SARS-CoV-2, severe acute respiratory syndrome coronavirus 2; CDC, Centers for Disease Control and Prevention; SARS-CoV-1, severe acute respiratory syndrome coronavirus 1; ACE2, angiotensin-converting enzyme-2; TMPRSS2, transmembrane serine protease 2; MV, mechanical ventilation; COS, core outcome set; IL-6, interleukin-6; IL-1α, interleukin-1α; TGF-β, transforming growth factor beta; IFN-β, interferon (IFN)-beta; IFN-γ, interferon (IFN)-gamma; GM-CSF, granulocyte colony-stimulating factor; BBB, blood-brain barrier; CNS, central nervous system; GBS, Guillain-Barré syndrome; ECG, electrocardiograms; EVs, Extracellular vesicles; ICAM-1, intercellular adhesion molecule 1; VCAM-1, vascular cell adhesion protein 1; PS, phosphatidylserine; MERS-CoV, Middle East respiratory syndrome coronavirus; FABP2, fatty acid binding protein 2; LPS, lipopolysaccharide; PGN, eptidoglycan; EBV, Epstein-Barr virus; HHV-6, human herpesvirus 6; HCMV, human cytomegalovirus; EBNA, Epstein Barr virus nuclear antigen; EBNA, Epstein Barr virus nuclear antigen; EA-D;early antigen diffuse component; ME/CFS, myalgic encephalomyelitis/chronic fatigue syndrome; NA, myalgic encephalomyelitis/chronic fatigue syndrome; IgG, immunoglobulin G; TB, tuberculosis; Treg, T regulatory cells; TNF-α:tumor necrosis factor-alpha.
References
1. Zhou P, Yang X-L, Wang X-G, Hu B, Zhang L, Zhang W, et al. A pneumonia outbreak associated with a new coronavirus of probable bat origin. Nature. (2020) 579:270–3. doi: 10.1038/s41586-020-2012-7
2. Organization WH. WHO coronavirus disease (COVID-19) dashboard with vaccination data | WHO coronavirus (COVID-19) dashboard with vaccination data, in: World heal organ. (2022). Available online at: https://covid19.who.int/ (Accessed August 14, 2023).
3. Gold JA. Covid-19: adverse mental health outcomes for healthcare workers. BMJ. (2020) 369:m1815. doi: 10.1136/bmj.m1815
4. Jones EAK, Mitra AK, Bhuiyan AR. Impact of COVID-19 on mental health in adolescents: A systematic review. Int J Environ Res Public Health. (2021) 18:2470. doi: 10.3390/ijerph18052470
5. Lee JC, Nallani R, Cass L, Bhalla V, Chiu AG, Villwock JA. A systematic review of the neuropathologic findings of post-viral olfactory dysfunction: implications and novel insight for the COVID-19 pandemic. Am J Rhinol Allergy. (2021) 35:323–33. doi: 10.1177/1945892420957853
6. Lindert J, Jakubauskiene M, Bilsen J. The COVID-19 disaster and mental health—assessing, responding and recovering. Eur J Public Health. (2021) 31:iv31–5. doi: 10.1093/eurpub/ckab153
7. Riedel B, Horen SR, Reynolds A, Jahromi AH. Mental health disorders in nurses during the COVID-19 pandemic: implications and coping strategies. Front Public Heal. (2021) 9:707358. doi: 10.3389/fpubh.2021.707358
8. Deev O, Plíhal T. How to calm down the markets? The effects of COVID-19 economic policy responses on financial market uncertainty. Res Int Bus Financ. (2022) 60:101613. doi: 10.1016/j.ribaf.2022.101613
9. Drieskens S, Braekman E, De Ridder K, Gisle L, Charafeddine R, Hermans L, et al. Domestic violence during the COVID-19 confinement: do victims feel more socially isolated? Arch Public Heal. (2022) 80:39. doi: 10.1186/s13690-021-00765-3
10. Kondo MC, Felker-Kantor E, Wu K, Gustat J, Morrison CN, Richardson L, et al. Stress and distress during the COVID-19 pandemic: the role of neighborhood context. Int J Environ Res Public Health. (2022) 19:2779. doi: 10.3390/ijerph19052779
11. Richards F, Kodjamanova P, Chen X, Li N, Atanasov P, Bennetts L, et al. Economic burden of COVID-19: A systematic review. Clin Outcomes Res. (2022) 14:293–307. doi: 10.2147/CEOR.S338225
12. for Disease Control C, Prevention. Long COVID or post-COVID conditions (2022). Available online at: https://www.cdc.gov/coronavirus/2019-ncov/long-term-effects/index.html.
13. for Disease Control C, Prevention. Post-COVID conditions: information for healthcare providers (2021). Available online at: https://www.cdc.gov/coronavirus/2019-ncov/hcp/clinical-care/post-covid-conditions.html?CDC_AA_refVal=https%3A%2F%2Fwww.cdc.gov%2Fcoronavirus%2F2019-ncov%2Fhcp%2Fclinical-care%2Flate-sequelae.html.
14. Krishna B, Wills M, Sithole N. Long COVID: what is known and what gaps need to be addressed. Br Med Bull. (2023) 147:6–19. doi: 10.1093/BMB/LDAD016
15. Sasannejad C, Ely EW, Lahiri S. Long-term cognitive impairment after acute respiratory distress syndrome: a review of clinical impact and pathophysiological mechanisms. Crit Care. (2019) 23:352. doi: 10.1186/s13054-019-2626-z
16. Tansey CM. One-year outcomes and health care utilization in survivors of severe acute respiratory syndrome. Arch Intern Med. (2007) 167:1312. doi: 10.1001/archinte.167.12.1312
17. Ali ST, Kang AK, Patel TR, Clark JR, Perez-Giraldo GS, Orban ZS, et al. Evolution of neurologic symptoms in non-hospitalized COVID-19 “long haulers”. Ann Clin Transl Neurol. (2022) 9(7):950–61. doi: 10.1002/acn3.51570
18. Belli S, Balbi B, Prince I, Cattaneo D, Masocco F, Zaccaria S, et al. Low physical functioning and impaired performance of activities of daily life in COVID-19 patients who survived hospitalisation. Eur Respir J. (2020) 56:2002096. doi: 10.1183/13993003.02096-2020
19. Blomberg B, Mohn KG-I, Brokstad KA, Zhou F, Linchausen DW, Hansen B-A, et al. Long COVID in a prospective cohort of home-isolated patients. Nat Med. (2021) 27:1607–13. doi: 10.1038/s41591-021-01433-3
20. Bull-Otterson L, Baca S, Saydah S, Boehmer TK, Adjei S, Gray S, et al. Post–COVID conditions among adult COVID-19 survivors aged 18–64 and ≥65 years — United states, march 2020–november 2021. MMWR Morb Mortal Wkly Rep. (2022) 71:713–7. doi: 10.15585/mmwr.mm7121e1
21. Moreno-Pérez O, Merino E, Leon-Ramirez J-M, Andres M, Ramos JM, Arenas-Jiménez J, et al. Post-acute COVID-19 syndrome. Incidence and risk factors: A Mediterranean cohort study. J Infect. (2021) 82:378–83. doi: 10.1016/j.jinf.2021.01.004
22. Chen C, Haupert SR, Zimmermann L, Shi X, Fritsche LG, Mukherjee B. Global prevalence of post COVID-19 condition or long COVID: A meta-analysis and systematic review. J Infect Dis. (2022) 226(9):1593–607. doi: 10.1093/infdis/jiac136
23. Peluso MJ, Deitchman AN, Torres L, Iyer NS, Munter SE, Nixon CC, et al. Long-term SARS-CoV-2-specific immune and inflammatory responses in individuals recovering from COVID-19 with and without post-acute symptoms. Cell Rep. (2021) 36:109518. doi: 10.1016/j.celrep.2021.109518
24. Frontera JA, Yang D, Lewis A, Patel P, Medicherla C, Arena V, et al. A prospective study of long-term outcomes among hospitalized COVID-19 patients with and without neurological complications. J Neurol Sci. (2021) 426:117486. doi: 10.1016/j.jns.2021.117486
25. Sigfrid L, Drake TM, Pauley E, Jesudason EC, Olliaro P, Lim WS, et al. Long Covid in adults discharged from UK hospitals after Covid-19: A prospective, multicentre cohort study using the ISARIC WHO Clinical Characterisation Protocol. Lancet Reg Heal - Eur. (2021) 8:100186. doi: 10.1016/j.lanepe.2021.100186
26. Carfì A, Bernabei R, Landi F. Persistent symptoms in patients after acute COVID-19. JAMA. (2020) 324:603. doi: 10.1001/jama.2020.12603
27. Raveendran AV, Jayadevan R, Sashidharan S. Long COVID: an overview. Diabetes Metab Syndr Clin Res Rev. (2021) 15:869–75. doi: 10.1016/j.dsx.2021.04.007
28. Tenforde MW, Kim SS, Lindsell CJ, Rose EB, Shapiro NI, Files DC, et al. Symptom duration and risk factors for delayed return to usual health among outpatients with COVID-19 in a multistate health care systems network — United states, march–june 2020. MMWR Morb Mortal Wkly Rep. (2020) 69:993–8. doi: 10.15585/mmwr.mm6930e1
29. Rasa S, Nora-Krukle Z, Henning N, Eliassen E, Shikova E, Harrer T, et al. Chronic viral infections in myalgic encephalomyelitis/chronic fatigue syndrome (ME/CFS). J Transl Med. (2018) 16:268. doi: 10.1186/s12967-018-1644-y
30. Antonelli M, Pujol JC, Spector TD, Ourselin S, Steves CJ. Risk of long COVID associated with delta versus omicron variants of SARS-CoV-2. Lancet (London England). (2022) 399:2263–4. doi: 10.1016/S0140-6736(22)00941-2
31. Cai J, Lin K, Zhang H, Xue Q, Zhu K, Yuan G, et al. A one-year follow-up study of systematic impact of long COVID symptoms among patients post SARS-CoV-2 omicron variants infection in Shanghai, China. Emerg Microbes Infect. (2023) 12:2220578. doi: 10.1080/22221751.2023.2220578
32. Graham EL, Clark JR, Orban ZS, Lim PH, Szymanski AL, Taylor C, et al. Persistent neurologic symptoms and cognitive dysfunction in non-hospitalized Covid-19 “long haulers”. Ann Clin Transl Neurol. (2021) 8:1073–85. doi: 10.1002/acn3.51350
33. Abdelwahab N, Ingraham NE, Nguyen N, Siegel L, Silverman G, Sahoo HS, et al. Predictors of post-acute sequelae of COVID-19 development and rehabilitation: A retrospective study. Arch Phys Med Rehabil. (2022) 103:2001. doi: 10.1016/j.apmr.2022.04.009
34. Atchison CJ, Davies B, Cooper E, Lound A, Whitaker M, Hampshire A, et al. Long-term health impacts of COVID-19 among 242,712 adults in England. Nat Commun. (2023) 14:6588. doi: 10.1038/S41467-023-41879-2
35. Romero-Ibarguengoitia ME, Rodríguez-Torres JF, Garza-Silva A, Rivera-Cavazos A, Morales-Rodriguez DP, Hurtado-Cabrera M, et al. Association of vaccine status, reinfections, and risk factors with Long COVID syndrome. Sci Rep. (2024) 14:2817. doi: 10.1038/S41598-024-52925-4
36. Pazukhina E, Rumyantsev M, Baimukhambetova D, Bondarenko E, Markina N, El-Taravi Y, et al. Event rates and incidence of post-COVID-19 condition in hospitalised SARS-CoV-2 positive children and young people and controls across different pandemic waves: exposure-stratified prospective cohort study in Moscow (StopCOVID). BMC Med. (2024) 22:48. doi: 10.1186/S12916-023-03221-X
37. Bigdelou B, Sepand MR, Najafikhoshnoo S, Negrete JAT, Sharaf M, Ho JQ, et al. COVID-19 and preexisting comorbidities: risks, synergies, and clinical outcomes. Front Immunol. (2022) 13:890517. doi: 10.3389/fimmu.2022.890517
38. Hussain A, Bhowmik B, do Vale Moreira NC. COVID-19 and diabetes: Knowledge in progress. Diabetes Res Clin Pract. (2020) 162:108142. doi: 10.1016/j.diabres.2020.108142
39. Lima-Martínez MM, Boada CC, Madera-Silva MD, Marín W, Contreras M. COVID-19 y diabetes mellitus: una relación bidireccional. Clínica e Investig en Arterioscler. (2021) 33:151–7. doi: 10.1016/j.arteri.2020.10.001
40. Su Y, Yuan D, Chen DG, Ng RH, Wang K, Choi J, et al. Multiple early factors anticipate post-acute COVID-19 sequelae. Cell. (2022) 185:881–895.e20. doi: 10.1016/j.cell.2022.01.014
41. Tleyjeh IM, Kashour T, Riaz M, Amer SA, AlSwaidan N, Almutairi L, et al. Persistent COVID-19 symptoms at least one month after diagnosis: A national survey. J Infect Public Health. (2022) 15:578–85. doi: 10.1016/j.jiph.2022.04.006
42. Yong SJ. Long COVID or post-COVID-19 syndrome: putative pathophysiology, risk factors, and treatments. Infect Dis (Auckl). (2021) 53:737–54. doi: 10.1080/23744235.2021.1924397
43. Booth A, Reed AB, Ponzo S, Yassaee A, Aral M, Plans D, et al. Population risk factors for severe disease and mortality in COVID-19: A global systematic review and meta-analysis. PloS One. (2021) 16:e0247461. doi: 10.1371/journal.pone.0247461
44. Ruan Q, Yang K, Wang W, Jiang L, Song J. Clinical predictors of mortality due to COVID-19 based on an analysis of data of 150 patients from Wuhan, China. Intensive Care Med. (2020) 46:846–8. doi: 10.1007/s00134-020-05991-x
45. Kamal M, Omirah MA, Hussein A, Saeed H. Assessment and characterisation of post-COVID-19 manifestations. Int J Clin Pract. (2021) 75:e13746. doi: 10.1111/ijcp.13746
46. Stavem K, Ghanima W, Olsen MK, Gilboe HM, Einvik G. Persistent symptoms 1.5–6 months after COVID-19 in non-hospitalised subjects: a population-based cohort study. Thorax. (2021) 76:405–7. doi: 10.1136/thoraxjnl-2020-216377
47. Xie Y, Bowe B, Al-Aly Z. Burdens of post-acute sequelae of COVID-19 by severity of acute infection, demographics and health status. Nat Commun. (2021) 12:6571. doi: 10.1038/s41467-021-26513-3
48. Douaud G, Lee S, Alfaro-Almagro F, Arthofer C, Wang C, McCarthy P, et al. SARS-CoV-2 is associated with changes in brain structure in UK Biobank. Nature. (2022) 604:697–707. doi: 10.1038/s41586-022-04569-5
49. Jozuka R, Kimura H, Uematsu T, Fujigaki H, Yamamoto Y, Kobayashi M, et al. Severe and long-lasting neuropsychiatric symptoms after mild respiratory symptoms caused by COVID-19: A case report. Neuropsychopharmacol Rep. (2022) 42:114–9. doi: 10.1002/npr2.12222
51. Huang C, Wang Y, Li X, Ren L, Zhao J, Hu Y, et al. Clinical features of patients infected with 2019 novel coronavirus in Wuhan, China. Lancet. (2020) 395:497–506. doi: 10.1016/S0140-6736(20)30183-5
52. O’Mahoney LL, Routen A, Gillies C, Ekezie W, Welford A, Zhang A, et al. The prevalence and long-term health effects of Long Covid among hospitalised and non-hospitalised populations: A systematic review and meta-analysis. eClinicalMedicine. (2023) 55:101762. doi: 10.1016/j.eclinm.2022.101762
53. Abarca K, Iturriaga C, Urzúa M, Le Corre N, Pineda A, Fernández C, et al. Safety and non-inferiority evaluation of two immunization schedules with an inactivated SARS-coV-2 vaccine in adults: A randomized clinical trial. Vaccines. (2022) 10:1082. doi: 10.3390/vaccines10071082
54. Ghosn J, Piroth L, Epaulard O, Le Turnier P, Mentré F, Bachelet D, et al. Persistent COVID-19 symptoms are highly prevalent 6 months after hospitalization: results from a large prospective cohort. Clin Microbiol Infect. (2021) 27:1041.e1–1041.e4. doi: 10.1016/J.CMI.2021.03.012
55. Peghin M, Palese A, Venturini M, De Martino M, Gerussi V, Graziano E, et al. Post-COVID-19 symptoms 6 months after acute infection among hospitalized and non-hospitalized patients. Clin Microbiol Infect. (2021) 27:1507–13. doi: 10.1016/J.CMI.2021.05.033
56. Sykes DL, Holdsworth L, Jawad N, Gunasekera P, Morice AH, Crooks MG. Post-COVID-19 symptom burden: what is long-COVID and how should we manage it? Lung. (2021) 199:113–9. doi: 10.1007/s00408-021-00423-z
57. Xiong Q, Xu M, Li J, Liu Y, Zhang J, Xu Y, et al. Clinical sequelae of COVID-19 survivors in Wuhan, China: a single-centre longitudinal study. Clin Microbiol Infect. (2021) 27:89. doi: 10.1016/J.CMI.2020.09.023
58. Fernández-De-las-peñas C, Martín-Guerrero JD, Pellicer-Valero ÓJ, Navarro-Pardo E, Gómez-Mayordomo V, Cuadrado ML, et al. Female sex is a risk factor associated with long-term post-COVID related-symptoms but not with COVID-19 symptoms: the LONG-COVID-EXP-CM multicenter study. J Clin Med. (2022) 11:413. doi: 10.3390/JCM11020413
59. Bai F, Tomasoni D, Falcinella C, Barbanotti D, Castoldi R, Mulè G, et al. Female gender is associated with long COVID syndrome: a prospective cohort study. Clin Microbiol Infect. (2022) 28:611.e9–611.e16. doi: 10.1016/J.CMI.2021.11.002
60. Bwire GM. Coronavirus: why men are more vulnerable to covid-19 than women? SN Compr Clin Med. (2020) 2:874–6. doi: 10.1007/s42399-020-00341-w
61. Klein SL, Flanagan KL. Sex differences in immune responses. Nat Rev Immunol. (2016) 16:626–38. doi: 10.1038/nri.2016.90
62. Lu J, Peng J, Xiong Q, Liu Z, Lin H, Tan X, et al. Clinical, immunological and virological characterization of COVID-19 patients that test re-positive for SARS-CoV-2 by RT-PCR. eBioMedicine. (2020) 59:102960. doi: 10.1016/j.ebiom.2020.102960
63. Rhee C, Kanjilal S, Baker M, Klompas M. Duration of severe acute respiratory syndrome coronavirus 2 (SARS-coV-2) infectivity: when is it safe to discontinue isolation? Clin Infect Dis. (2021) 72:1467–74. doi: 10.1093/cid/ciaa1249
64. O’Donnell JS, Chappell KJ. Chronic SARS-coV-2, a cause of post-acute COVID-19 sequelae (Long-COVID)? Front Microbiol. (2021) 12:724654. doi: 10.3389/fmicb.2021.724654
65. Swank Z, Senussi Y, Manickas-Hill Z, Yu XG, Li JZ, Alter G, et al. Persistent circulating severe acute respiratory syndrome coronavirus 2 spike is associated with post-acute coronavirus disease 2019 sequelae. Clin Infect Dis. (2023) 76:e487–90. doi: 10.1093/CID/CIAC722
66. Callaway E. How months-long COVID infections could seed dangerous new variants. Nature. (2022) 606:452–5. doi: 10.1038/d41586-022-01613-2
67. Theoharides TC. Could SARS-coV-2 spike protein be responsible for long-COVID syndrome? Mol Neurobiol. (2022) 59:1850–61. doi: 10.1007/s12035-021-02696-0
68. Wu X, Xiang M, Jing H, Wang C, Novakovic VA, Shi J. Damage to endothelial barriers and its contribution to long COVID. Angiogenesis. (2023) 27:1–8. doi: 10.1007/S10456-023-09878-5
69. Kemp SA, Collier DA, Datir RP, Ferreira IATM, Gayed S, Jahun A, et al. SARS-CoV-2 evolution during treatment of chronic infection. Nature. (2021) 592:277–82. doi: 10.1038/s41586-021-03291-y
70. Choi B, Choudhary MC, Regan J, Sparks JA, Padera RF, Qiu X, et al. Persistence and evolution of SARS-coV-2 in an immunocompromised host. N Engl J Med. (2020) 383:2291–3. doi: 10.1056/NEJMc2031364
71. Sonnleitner ST, Prelog M, Sonnleitner S, Hinterbichler E, Halbfurter H, Kopecky DBC, et al. Cumulative SARS-CoV-2 mutations and corresponding changes in immunity in an immunocompromised patient indicate viral evolution within the host. Nat Commun. (2022) 13:2560. doi: 10.1038/s41467-022-30163-4
72. Tian F, Tong B, Sun L, Shi S, Zheng B, Wang Z, et al. N501Y mutation of spike protein in SARS-CoV-2 strengthens its binding to receptor ACE2. Elife. (2021) 10:e69091. doi: 10.7554/eLife.69091
73. Bradley RE, Ponsford MJ, Scurr MJ, Godkin A, Jolles S, Bramhall K, et al. Persistent COVID-19 infection in wiskott-aldrich syndrome cleared following therapeutic vaccination: a case report. J Clin Immunol. (2022) 42:32–5. doi: 10.1007/s10875-021-01158-5
74. Al-Aly Z, Bowe B, Xie Y. Long COVID after breakthrough SARS-CoV-2 infection. Nat Med. (2022) 28:1461–7. doi: 10.1038/s41591-022-01840-0
75. Gaete-Argel A, Saavedra-Alarcón V, Sauré D, Alonso-Palomares L, Acevedo ML, Alarcón M, et al. Impact of homologous and heterologous boosters in neutralizing antibodies titers against SARS-CoV-2 Omicron in solid-organ transplant recipients. Front Immunol. (2023) 14:1135478. doi: 10.3389/FIMMU.2023.1135478
76. Soto JA, Melo-González F, Gutierrez-Vera C, Schultz BM, Berríos-Rojas RV, Rivera-Pérez D, et al. Inactivated vaccine-induced SARS-coV-2 variant-specific immunity in children. MBio. (2022) 13:e0131122. doi: 10.1128/MBIO.01311-22
77. Mondaca S, Walbaum B, Le Corre N, Ferrés M, Valdés A, Martínez-Valdebenito C, et al. Influence of SARS-CoV-2 mRNA Vaccine Booster among Cancer Patients on Active Treatment Previously Immunized with Inactivated versus mRNA Vaccines: A Prospective Cohort Study. Vaccines. (2023) 11:1193. doi: 10.3390/VACCINES11071193
78. Melo-González F, Soto JA, González LA, Fernández J, Duarte LF, Schultz BM, et al. Recognition of variants of concern by antibodies and T cells induced by a SARS-coV-2 inactivated vaccine. Front Immunol. (2021) 12:747830. doi: 10.3389/fimmu.2021.747830
79. Balcells ME, Le Corre N, Durán J, Ceballos ME, Vizcaya C, Mondaca S, et al. Reduced immune response to inactivated severe acute respiratory syndrome coronavirus 2 vaccine in a cohort of immunocompromised patients in Chile. Clin Infect Dis. (2022) 75:e594–602. doi: 10.1093/cid/ciac167
80. Bueno SM, Abarca K, González PA, Gálvez NMSS, Soto JA, Duarte LF, et al. Safety and immunogenicity of an inactivated severe acute respiratory syndrome coronavirus 2 vaccine in a subgroup of healthy adults in Chile. Clin Infect Dis. (2021) 75:E792–804. doi: 10.1093/cid/ciab823
81. Dib M, Le Corre N, Ortiz C, García D, Ferrés M, Martinez-Valdebenito C, et al. SARS-CoV-2 vaccine booster in solid organ transplant recipients previously immunised with inactivated versus mRNA vaccines: A prospective cohort study. Lancet Reg Heal - Am. (2022) 16:100371. doi: 10.1016/j.lana.2022.100371
82. Harrison S, Walters B, Simmons Z, Cook M, Clark R. The effectiveness of vaccination against long COVID. A Rapid evidence briefing. (2022).
83. Ledford H. Do vaccines protect against long COVID? What the data say. Nature. (2021) 599:546–8. doi: 10.1038/d41586-021-03495-2
84. Strain WD, Sherwood O, Banerjee A, Van der Togt V, Hishmeh L, Rossman J. The impact of COVID vaccination on symptoms of long COVID: an international survey of people with lived experience of long COVID. Vaccines. (2022) 10:652. doi: 10.3390/vaccines10050652
85. Duarte LF, Gálvez NMS, Iturriaga C, Melo-González F, Soto JA, Schultz BM, et al. Immune profile and clinical outcome of breakthrough cases after vaccination with an inactivated SARS-coV-2 vaccine. Front Immunol. (2021) 12:742914. doi: 10.3389/fimmu.2021.742914
86. Schultz BM, Melo-González F, Duarte LF, Gálvez NMS, Pacheco GA, Soto JA, et al. A booster dose of coronaVac increases neutralizing antibodies and T cells that recognize delta and omicron variants of concern. MBio. (2022) 13:e0142322. doi: 10.1128/MBIO.01423-22
87. Reyes H, Diethelm-Varela B, Méndez C, Rebolledo-Zelada D, Lillo-Dapremont B, Muñoz SR, et al. Contribution of two-dose vaccination toward the reduction of COVID-19 cases, ICU hospitalizations and deaths in Chile assessed through explanatory generalized additive models for location, scale, and shape. Front Public Heal. (2022) 10:815036. doi: 10.3389/fpubh.2022.815036
88. Gálvez NMS, Pacheco GA, Schultz BM, Melo-González F, Soto JA, Duarte LF, et al. Differences in the immune response elicited by two immunization schedules with an inactivated SARS-CoV-2 vaccine in a randomized phase 3 clinical trial. Elife. (2022) 11:e81477. doi: 10.7554/ELIFE.81477
89. Méndez C, Peñaloza HF, Schultz BM, Piña-Iturbe A, Ríos M, Moreno-Tapia D, et al. Humoral and cellular response induced by a second booster of an inactivated SARS-CoV-2 vaccine in adults. EBioMedicine. (2023) 91:652. doi: 10.1016/J.EBIOM.2023.104563
90. Antonelli M, Penfold RS, Merino J, Sudre CH, Molteni E, Berry S, et al. Risk factors and disease profile of post-vaccination SARS-CoV-2 infection in UK users of the COVID Symptom Study app: a prospective, community-based, nested, case-control study. Lancet Infect Dis. (2022) 22:43–55. doi: 10.1016/S1473-3099(21)00460-6
91. Al-Aly Z, Xie Y, Bowe B. High-dimensional characterization of post-acute sequelae of COVID-19. Nature. (2021) 594:259–64. doi: 10.1038/s41586-021-03553-9
92. Taquet M, Dercon Q, Harrison PJ. Six-month sequelae of post-vaccination SARS-CoV-2 infection: A retrospective cohort study of 10,024 breakthrough infections. Brain Behav Immun. (2022) 103:154–62. doi: 10.1016/j.bbi.2022.04.013
93. Krishna BA, Metaxaki M, Wills MR, Sithole N. Reduced incidence of long coronavirus disease referrals to the cambridge university teaching hospital long coronavirus disease clinic. Clin Infect Dis. (2023) 76:738–40. doi: 10.1093/CID/CIAC630
94. Kuodi P, Gorelik Y, Zayyad H, Wertheim O, Wiegler KB, Abu Jabal K, et al. Association between BNT162b2 vaccination and reported incidence of post-COVID-19 symptoms: cross-sectional study 2020-21, Israel. NPJ Vaccines. (2022) 7:1–8. doi: 10.1038/s41541-022-00526-5
95. Clemens SAC, Weckx L, Clemens R, Mendes AVA, Souza AR, Silveira MBV, et al. Heterologous versus homologous COVID-19 booster vaccination in previous recipients of two doses of CoronaVac COVID-19 vaccine in Brazil (RHH-001): a phase 4, non-inferiority, single blind, randomised study. Lancet. (2022) 399:521–9. doi: 10.1016/S0140-6736(22)00094-0
96. Jara A, Undurraga EA, Zubizarreta JR, González C, Pizarro A, Acevedo J, et al. Effectiveness of homologous and heterologous booster doses for an inactivated SARS-CoV-2 vaccine: a large-scale prospective cohort study. Lancet Glob Heal. (2022) 10:e798–806. doi: 10.1016/S2214-109X(22)00112-7
97. Hammond J, Leister-Tebbe H, Gardner A, Abreu P, Bao W, Wisemandle W, et al. Oral nirmatrelvir for high-risk, nonhospitalized adults with covid-19. N Engl J Med. (2022) 386:1397–408. doi: 10.1056/NEJMoa2118542
98. Kabinger F, Stiller C, Schmitzová J, Dienemann C, Kokic G, Hillen HS, et al. Mechanism of molnupiravir-induced SARS-CoV-2 mutagenesis. Nat Struct Mol Biol 2021 289. (2021) 28:740–6. doi: 10.1038/s41594-021-00651-0
99. Owen DR, Allerton CMN, Anderson AS, Aschenbrenner L, Avery M, Berritt S, et al. An oral SARS-CoV-2 Mpro inhibitor clinical candidate for the treatment of COVID-19. Science (80-). (2021) 374:1586–93. doi: 10.1126/SCIENCE.ABL4784/SUPPL_FILE/SCIENCE.ABL4784_MDAR_REPRODUCIBILITY_CHECKLIST.PDF
100. Xie Y, Choi T, Al-Aly Z. Association of treatment with nirmatrelvir and the risk of post-COVID-19 condition. JAMA Intern Med. (2023) 183:554–64. doi: 10.1001/JAMAINTERNMED.2023.0743
101. Xie Y, Choi T, Al-Aly Z. Molnupiravir and risk of post-acute sequelae of covid-19: cohort study. BMJ. (2023) 381:e074572. doi: 10.1136/BMJ-2022-074572
102. Kuczborska K, Buda P, Książyk J. Long-COVID in immunocompromised children. Eur J Pediatr. (2022) 181:3501–9. doi: 10.1007/s00431-022-04561-1
103. Arnold DT, Milne A, Samms E, Stadon L, Maskell NA, Hamilton FW. Symptoms after COVID-19 vaccination in patients with persistent symptoms after acute infection: A case series. Ann Intern Med. (2021) 174:1334–6. doi: 10.7326/M21-1976
104. Ayoubkhani D, Bermingham C, Pouwels KB, Glickman M, Nafilyan V, Zaccardi F, et al. Trajectory of long covid symptoms after covid-19 vaccination: community based cohort study. BMJ. (2022) 377:e069676. doi: 10.1136/bmj-2021-069676
105. Izquierdo-Pujol J, Moron-Lopez S, Dalmau J, Gonzalez-Aumatell A, Carreras-Abad C, Mendez M, et al. Post COVID-19 condition in children and adolescents: an emerging problem. Front Pediatr. (2022) 10:894204. doi: 10.3389/fped.2022.894204
106. Smane L, Roge I, Pucuka Z, Pavare J. Clinical features of pediatric post-acute COVID-19: a descriptive retrospective follow-up study. Ital J Pediatr. (2021) 47:177. doi: 10.1186/s13052-021-01127-z
107. Ludvigsson JF. Case report and systematic review suggest that children may experience similar long-term effects to adults after clinical COVID-19. Acta Paediatr. (2021) 110:914–21. doi: 10.1111/apa.15673
108. Anaya J-M, Rojas M, Salinas ML, Rodríguez Y, Roa G, Lozano M, et al. Post-COVID syndrome. A case series and comprehensive review. Autoimmun Rev. (2021) 20:102947. doi: 10.1016/j.autrev.2021.102947
109. Castanares-Zapatero D, Chalon P, Kohn L, Dauvrin M, Detollenaere J, de Noordhout CM, et al. Pathophysiology and mechanism of long COVID: a comprehensive review. Ann Med. (2022) 54:1473–87. doi: 10.1080/07853890.2022.2076901
110. Cheon IS, Li C, Son YM, Goplen NP, Wu Y, Cassmann T, et al. Immune signatures underlying post-acute COVID-19 lung sequelae. Sci Immunol. (2021) 6:eabk1741. doi: 10.1126/sciimmunol.abk1741
111. Fernández-Lázaro D, Sánchez-Serrano N, Mielgo-Ayuso J, García-Hernández JL, González-Bernal JJ, Seco-Calvo J. Long COVID a new derivative in the chaos of SARS-coV-2 infection: the emergent pandemic? J Clin Med. (2021) 10:5799. doi: 10.3390/jcm10245799
112. Ong SWX, Fong S-W, Young BE, Chan Y-H, Lee B, Amrun SN, et al. Persistent symptoms and association with inflammatory cytokine signatures in recovered coronavirus disease 2019 patients. Open Forum Infect Dis. (2021) 8:ofab156. doi: 10.1093/ofid/ofab156
113. Krishna B, Lim E, Mactavous L, Jackson S, Team NBTNB, Lyons P, et al. Spontaneous, persistent T-cell dependent IFN-γ release in patients who progress to Long COVID. (2022). doi: 10.21203/RS.3.RS-2034285/V2
114. Opsteen S, Files JK, Fram T, Erdmann N. The role of immune activation and antigen persistence in acute and long COVID. J Investig Med. (2023) 71:545–62. doi: 10.1177/10815589231158041
115. Littlefield KM, Watson RO, Schneider JM, Neff CP, Yamada E, Zhang M, et al. SARS-CoV-2-specific T cells associate with inflammation and reduced lung function in pulmonary post-acute sequalae of SARS-CoV-2. PloS Pathog. (2022) 18:e1010359. doi: 10.1371/JOURNAL.PPAT.1010359
116. Chen N, Zhou M, Dong X, Qu J, Gong F, Han Y, et al. Epidemiological and clinical characteristics of 99 cases of 2019 novel coronavirus pneumonia in Wuhan, China: a descriptive study. Lancet. (2020) 395:507–13. doi: 10.1016/S0140-6736(20)30211-7
117. Daher A, Cornelissen C, Hartmann NU, Balfanz P, Müller A, Bergs I, et al. Six months follow-up of patients with invasive mechanical ventilation due to COVID-19 related ARDS. Int J Environ Res Public Health. (2021) 18. doi: 10.3390/IJERPH18115861
118. Sayde GE, Stefanescu A, Hammer R. Interdisciplinary treatment for survivors of critical illness due to COVID-19: expanding the post-intensive care recovery model and impact on psychiatric outcomes. J Acad Consult Psychiatry. (2023) 64:226–35. doi: 10.1016/J.JACLP.2023.01.009
119. Irisson-Mora I, Salgado-Cordero AM, Reyes-Varón E, Cataneo-Piña DJ, Fernández-Sánchez M, Buendía-Roldán I, et al. Comparison between the persistence of post COVID-19 symptoms on critical patients requiring invasive mechanical ventilation and non-critical patients. PloS One. (2022) 17:e0273041. doi: 10.1371/JOURNAL.PONE.0273041
120. Karnatovskaia LV, Schulte PJ, Philbrick KL, Johnson MM, Anderson BK, Gajic O, et al. Psychocognitive sequelae of critical illness and correlation with 3 months follow up. J Crit Care. (2019) 52:166–71. doi: 10.1016/J.JCRC.2019.04.028
121. Lee AM, Wong JGWS, McAlonan GM, Cheung V, Cheung C, Sham PC, et al. Stress and psychological distress among SARS survivors 1 year after the outbreak. Can J Psychiatry. (2007) 52:233–40. doi: 10.1177/070674370705200405
122. Lee SH, Shin HS, Park HY, Kim JL, Lee JJ, Lee H, et al. Depression as a mediator of chronic fatigue and post-traumatic stress symptoms in middle east respiratory syndrome survivors. Psychiatry Investig. (2019) 16:59. doi: 10.30773/pi.2018.10.22.3
123. Mateo Rodríguez E, PuChades Gimeno F, Ezzeddine Angulo A, Asensio Samper J, Saiz Ruiz C, López Alarcón MD. Postintensive care syndrome in COVID-19. Unicentric pilot study. Calm does not come after the storm. Med Clin (English ed). (2022) 159:321–6. doi: 10.1016/J.MEDCLE.2021.11.013
124. Freeman A, Tyrovolas S, Koyanagi A, Chatterji S, Leonardi M, Ayuso-Mateos JL, et al. The role of socio-economic status in depression: results from the COURAGE (aging survey in Europe). BMC Public Health. (2016) 16:1098. doi: 10.1186/S12889-016-3638-0
125. Saeidi M, Safaei A, Sadat Z, Abbasi P, Sarcheshmeh MSM, Dehghani F, et al. Prevalence of depression, anxiety and stress among patients discharged from critical care units. J Crit Care Med. (2021) 7:113. doi: 10.2478/JCCM-2021-0012
126. Klok FA, Boon GJAM, Barco S, Endres M, Geelhoed JJM, Knauss S, et al. The Post-COVID-19 Functional Status scale: a tool to measure functional status over time after COVID-19. Eur Respir J. (2020) 56:2001494. doi: 10.1183/13993003.01494-2020
127. Nopp S, Moik F, Klok FA, Gattinger D, Petrovic M, Vonbank K, et al. Outpatient pulmonary rehabilitation in patients with long COVID improves exercise capacity, functional status, dyspnea, fatigue, and quality of life. Respiration. (2022) 101:593–601. doi: 10.1159/000522118
128. MaChado FVC, Meys R, Delbressine JM, Vaes AW, Goërtz YMJ, van Herck M, et al. Construct validity of the Post-COVID-19 Functional Status Scale in adult subjects with COVID-19. Health Qual Life Outcomes. (2021) 19:40. doi: 10.1186/s12955-021-01691-2
129. Gargon E, Gorst SL, Matvienko-Sikar K, Williamson PR. Choosing important health outcomes for comparative effectiveness research: 6th annual update to a systematic review of core outcome sets for research. PloS One. (2021) 16:e0244878. doi: 10.1371/journal.pone.0244878
130. Munblit D, Nicholson T, Akrami A, Apfelbacher C, Chen J, De Groote W, et al. A core outcome set for post-COVID-19 condition in adults for use in clinical practice and research: an international Delphi consensus study. Lancet Respir Med. (2022) 10:715–24. doi: 10.1016/S2213-2600(22)00169-2
131. Williamson PR, Altman DG, Bagley H, Barnes KL, Blazeby JM, Brookes ST, et al. The COMET handbook: version 1.0. Trials. (2017) 18:280. doi: 10.1186/s13063-017-1978-4
132. Foundation ER. EQ-5D user guides (2022). Available online at: https://euroqol.org/publications/user-guides/.
133. on Disability Statistics TWG. WG short set on functioning (WG-SS) (2022). Available online at: https://www.washingtongroup-disability.com/question-sets/wg-short-set-on-functioning-wg-ss/.
134. Dani M, Dirksen A, Taraborrelli P, Torocastro M, Panagopoulos D, Sutton R, et al. Autonomic dysfunction in ‘long COVID’: rationale, physiology and management strategies. Clin Med (Northfield Il). (2021) 21:e63–7. doi: 10.7861/clinmed.2020-0896
135. Mandal S, Barnett J, Brill SE, Brown JS, Denneny EK, Hare SS, et al. ‘Long-COVID’: a cross-sectional study of persisting symptoms, biomarker and imaging abnormalities following hospitalisation for COVID-19. Thorax. (2021) 76:396–8. doi: 10.1136/thoraxjnl-2020-215818
136. Saini G, Aneja R. Cancer as a prospective sequela of long COVID-19. BioEssays. (2021) 43:2000331. doi: 10.1002/bies.202000331
137. Davis HE, Assaf GS, McCorkell L, Wei H, Low RJ, Re’em Y, et al. Characterizing long COVID in an international cohort: 7 months of symptoms and their impact. EClinicalMedicine. (2021) 38:101019. doi: 10.1016/j.eclinm.2021.101019
138. Colarusso C, Maglio A, Terlizzi M, Vitale C, Molino A, Pinto A, et al. Post-COVID-19 patients who develop lung fibrotic-like changes have lower circulating levels of IFN-β but higher levels of IL-1α and TGF-β. Biomedicines. (2021) 9:1931. doi: 10.3390/biomedicines9121931
139. Wang F, Kream RM, Stefano GB. Long-term respiratory and neurological sequelae of COVID-19. Med Sci Monit. (2020) 26:1–10. doi: 10.12659/MSM.928996
140. Ackermann M, Mentzer SJ, Kolb M, Jonigk D. Inflammation and intussusceptive angiogenesis in COVID-19: everything in and out of flow. Eur Respir J. (2020) 56:2003147. doi: 10.1183/13993003.03147-2020
141. Hampshire A, Trender W, Chamberlain SR, Jolly AE, Grant JE, Patrick F, et al. Cognitive deficits in people who have recovered from COVID-19. EClinicalMedicine. (2021) 39:101044. doi: 10.1016/j.eclinm.2021.101044
142. Song W-J, Hui CKM, Hull JH, Birring SS, McGarvey L, Mazzone SB, et al. Confronting COVID-19-associated cough and the post-COVID syndrome: role of viral neurotropism, neuroinflammation, and neuroimmune responses. Lancet Respir Med. (2021) 9:533–44. doi: 10.1016/S2213-2600(21)00125-9
143. Chopra V, Flanders SA, O’Malley M, Malani AN, Prescott HC. Sixty-day outcomes among patients hospitalized with COVID-19. Ann Intern Med. (2021) 174:576–8. doi: 10.7326/M20-5661
144. Petersen MS, Kristiansen MF, Hanusson KD, Danielsen ME, á Steig B, Gaini S, et al. Long COVID in the Faroe Islands: A longitudinal study among nonhospitalized patients. Clin Infect Dis. (2021) 73:e4058–63. doi: 10.1093/cid/ciaa1792
145. Evans RA, Leavy OC, Richardson M, Elneima O, McAuley HJC, Shikotra A, et al. Clinical characteristics with inflammation profiling of long COVID and association with 1-year recovery following hospitalisation in the UK: a prospective observational study. Lancet Respir Med. (2022) 10:761–75. doi: 10.1016/S2213-2600(22)00127-8
146. Scott NA, Pearmain L, Knight SB, Brand O, Morgan DJ, Jagger C, et al. Monocyte migration profiles define disease severity in acute COVID-19 and unique features of long COVID. Eur Respir J. (2023) 61:2202226. doi: 10.1183/13993003.02226-2022
147. Zheng B, Daines L, Han Q, Hurst JR, Pfeffer P, Shankar-Hari M, et al. Prevalence, risk factors and treatments for post-COVID-19 breathlessness: a systematic review and meta-analysis. Eur Respir Rev. (2022) 31. doi: 10.1183/16000617.0071-2022
148. Fernández-de-las-Peñas C, Cancela-Cilleruelo I, Rodríguez-Jiménez J, Fuensalida-Novo S, Martín-Guerrero JD, Pellicer-Valero OJ, et al. Trajectory of post-COVID self-reported fatigue and dyspnoea in individuals who had been hospitalized by COVID-19: the LONG-COVID-EXP multicenter study. Biomed. (2023) 11:1863. doi: 10.3390/BIOMEDICINES11071863
149. Delorey TM, Ziegler CGK, Heimberg G, Normand R, Yang Y, Segerstolpe Å, et al. COVID-19 tissue atlases reveal SARS-CoV-2 pathology and cellular targets. Nature. (2021) 595:107–13. doi: 10.1038/s41586-021-03570-8
150. Merad M, Blish CA, Sallusto F, Iwasaki A. The immunology and immunopathology of COVID-19. Science (80-). (2022) 375:1122–7. doi: 10.1126/science.abm8108
151. Montefusco L, Ben Nasr M, D’Addio F, Loretelli C, Rossi A, Pastore I, et al. Acute and long-term disruption of glycometabolic control after SARS-CoV-2 infection. Nat Metab. (2021) 3:774–85. doi: 10.1038/s42255-021-00407-6
152. Hadjadj J, Yatim N, Barnabei L, Corneau A, Boussier J, Smith N, et al. Impaired type I interferon activity and inflammatory responses in severe COVID-19 patients. Science (80-). (2020) 369:718–24. doi: 10.1126/science.abc6027
153. Del Valle DM, Kim-Schulze S, Huang H-H, Beckmann ND, Nirenberg S, Wang B, et al. An inflammatory cytokine signature predicts COVID-19 severity and survival. Nat Med. (2020) 26:1636–43. doi: 10.1038/s41591-020-1051-9
154. Lucas C, Wong P, Klein J, Castro TBR, Silva J, Sundaram M, et al. Longitudinal analyses reveal immunological misfiring in severe COVID-19. Nature. (2020) 584:463–9. doi: 10.1038/s41586-020-2588-y
155. Rodrigues TS, de Sá KSG, Ishimoto AY, Becerra A, Oliveira S, Almeida L, et al. Inflammasomes are activated in response to SARS-CoV-2 infection and are associated with COVID-19 severity in patients. J Exp Med. (2021) 218:e20201707. doi: 10.1084/jem.20201707
156. Sefik E, Qu R, Junqueira C, Kaffe E, Mirza H, Zhao J, et al. Inflammasome activation in infected macrophages drives COVID-19 pathology. Nature. (2022) 606:585–93. doi: 10.1038/s41586-022-04802-1
157. Khan W, Safi A, Muneeb M, Mooghal M, Aftab A, Ahmed J. Complications of invasive mechanical ventilation in critically Ill Covid-19 patients - A narrative review. Ann Med Surg. (2022) 80:104201. doi: 10.1016/J.AMSU.2022.104201
158. Steinbeis F, Thibeault C, Doellinger F, Ring RM, Mittermaier M, Ruwwe-Glösenkamp C, et al. Severity of respiratory failure and computed chest tomography in acute COVID-19 correlates with pulmonary function and respiratory symptoms after infection with SARS-CoV-2: An observational longitudinal study over 12 months. Respir Med. (2022) 191:106709. doi: 10.1016/J.RMED.2021.106709
159. Ibrahim GS, Alkandari BM, Shady IAA, Gupta VK, Abdelmohsen MA. Invasive mechanical ventilation complications in COVID-19 patients. Egypt J Radiol Nucl Med. (2021) 52:226. doi: 10.1186/S43055-021-00609-8
160. Pierson DJ. Complications associated with mechanical ventilation. Crit Care Clin. (1990) 6:711–24. doi: 10.1016/S0749-0704(18)30362-2
161. Beitler JR, Malhotra A, Thompson BT. Ventilator-induced lung injury. Clin Chest Med. (2016) 37:633–46. doi: 10.1016/J.CCM.2016.07.004
162. Farghaly S, Badedi M, Ibrahim R, Sadhan MH, Alamoudi A, Alnami A, et al. Clinical characteristics and outcomes of post-COVID-19 pulmonary fibrosis: A case-control study. Med (Baltimore). (2022) 101:E28639. doi: 10.1097/MD.0000000000028639
163. Rogers JP, Chesney E, Oliver D, Pollak TA, McGuire P, Fusar-Poli P, et al. Psychiatric and neuropsychiatric presentations associated with severe coronavirus infections: a systematic review and meta-analysis with comparison to the COVID-19 pandemic. Lancet Psychiatry. (2020) 7:611–27. doi: 10.1016/S2215-0366(20)30203-0
164. Baig AM. Deleterious outcomes in long-hauler COVID-19: the effects of SARS-coV-2 on the CNS in chronic COVID syndrome. ACS Chem Neurosci. (2020) 11:4017–20. doi: 10.1021/acschemneuro.0c00725
166. Bohmwald K, Gálvez NMS, Ríos M, Kalergis AM. Neurologic alterations due to respiratory virus infections. Front Cell Neurosci. (2018) 12:386. doi: 10.3389/fncel.2018.00386
167. Bohmwald K, Andrade CA, Kalergis AM. Contribution of pro-inflammatory molecules induced by respiratory virus infections to neurological disorders. Pharmaceuticals. (2021) 14:340. doi: 10.3390/ph14040340
168. Uribe FR, González VPI, Kalergis AM, Soto JA, Bohmwald K. Understanding the neurotrophic virus mechanisms and their potential effect on systemic lupus erythematosus development. Brain Sci. (2024) 14:59. doi: 10.3390/BRAINSCI14010059
169. Camacho-Concha N, Santana-Román ME, Sánchez NC, Velasco I, Pando-Robles V, Pedraza-Alva G, et al. Insights into zika virus pathogenesis and potential therapeutic strategies. Biomedicines. (2023) 11:3316. doi: 10.3390/BIOMEDICINES11123316
170. Riedel C, Rivera JC, Canedo-Marroquín G, Kalergis AM, Opazo MC. Respiratory viral infections during pregnancy: effects of SARS-CoV-2 and other related viruses over the offspring. J Dev Orig Health Dis. (2022) 13:3–8. doi: 10.1017/S2040174420001373
171. González-López A, Albaiceta GM, Talbot K. Newly identified precipitating factors in mechanical ventilation-induced brain damage: implications for treating ICU delirium. Expert Rev Neurother. (2014) 14:583–8. doi: 10.1586/14737175.2014.915743
172. Stephenson J, Nutma E, van der Valk P, Amor S. Inflammation in CNS neurodegenerative diseases. Immunology. (2018) 154:204–19. doi: 10.1111/imm.12922
173. Andrade CA, Kalergis AM, Bohmwald K. Potential neurocognitive symptoms due to respiratory syncytial virus infection. Pathogens. (2021) 11:47. doi: 10.3390/pathogens11010047
174. Espinoza JA, Bohmwald K, Cespedes PF, Gomez RS, Riquelme SA, Cortes CM, et al. Impaired learning resulting from Respiratory Syncytial Virus infection. Proc Natl Acad Sci. (2013) 11:9112–7. doi: 10.1073/pnas.1217508110
175. Santos REA, da Silva MG, do Monte Silva MCB, Barbosa DAM, do Vale Gomes AL, Galindo LCM, et al. Onset and duration of symptoms of loss of smell/taste in patients with COVID-19: A systematic review. Am J Otolaryngol. (2021) 42:102889. doi: 10.1016/j.amjoto.2020.102889
176. Lee Y, Min P, Lee S, Kim S-W. Prevalence and duration of acute loss of smell or taste in COVID-19 patients. J Korean Med Sci. (2020) 35:e174. doi: 10.3346/jkms.2020.35.e174
177. Albaiceta GM, Brochard L, Dos Santos CC, Fernández R, Georgopoulos D, Girard T, et al. The central nervous system during lung injury and mechanical ventilation: a narrative review. Br J Anaesth. (2021) 127:648–59. doi: 10.1016/J.BJA.2021.05.038
178. Ferrando SJ, Dornbush R, Lynch S, Shahar S, Klepacz L, Karmen CL, et al. Neuropsychological, medical, and psychiatric findings after recovery from acute COVID-19: A cross-sectional study. J Acad Consult Psychiatry. (2022) 63(5):474–84. doi: 10.1016/j.jaclp.2022.01.003
179. Yeh EA, Collins A, Cohen ME, Duffner PK, Faden H. Detection of coronavirus in the central nervous system of a child with acute disseminated encephalomyelitis. Pediatrics. (2004) 113:e73–e76. doi: 10.1542/PEDS.113.1.E73
180. Erickson MA, Rhea EM, Knopp RC, Banks WA. Interactions of SARS-coV-2 with the blood–brain barrier. Int J Mol Sci. (2021) 22:2681. doi: 10.3390/ijms22052681
181. Matschke J, Lütgehetmann M, Hagel C, Sperhake JP, Schröder AS, Edler C, et al. Neuropathology of patients with COVID-19 in Germany: a post-mortem case series. Lancet Neurol. (2020) 19:919–29. doi: 10.1016/S1474-4422(20)30308-2
182. Song E, Zhang C, Israelow B, Lu-Culligan A, Prado AV, Skriabine S, et al. Neuroinvasion of SARS-CoV-2 in human and mouse brain. J Exp Med. (2021) 218:e20202135. doi: 10.1084/jem.20202135
183. Lau K-K, Yu W-C, Chu C-M, Lau S-T, Sheng B, Yuen K-Y. Possible central nervous system infection by SARS coronavirus. Emerg Infect Dis. (2004) 10:342–4. doi: 10.3201/eid1002.030638
184. Moriguchi T, Harii N, Goto J, Harada D, Sugawara H, Takamino J, et al. A first case of meningitis/encephalitis associated with SARS-Coronavirus-2. Int J Infect Dis. (2020) 94:55–8. doi: 10.1016/j.ijid.2020.03.062
185. Wege H, Schluesener H, Meyermann R, Barac-Latas V, Suchanek G, Lassmann H. Coronavirus infection and demyelination. Development of inflammatory lesions in Lewis rats. Adv Exp Med Biol. (1998) 440:437–44.
186. Wu GF, Perlman S. Macrophage Infiltration, but Not Apoptosis, Is Correlated with Immune-Mediated Demyelination following Murine Infection with a Neurotropic Coronavirus. J Virol. (1999) 73:8771–80. doi: 10.1128/JVI.73.10.8771-8780.1999
187. da Silva MM, de Lucena ASL, de Sá Leitão Paiva Júnior S, De Carvalho VMF, de Oliveira PSS, da Rosa MM, et al. Cell death mechanisms involved in cell injury caused by SARS-CoV-2. Rev Med Virol. (2022) 32:e2292. doi: 10.1002/rmv.2292
188. Charnley M, Islam S, Bindra GK, Engwirda J, Ratcliffe J, Zhou J, et al. Neurotoxic amyloidogenic peptides in the proteome of SARS-COV2: potential implications for neurological symptoms in COVID-19. Nat Commun. (2022) 13:3387. doi: 10.1038/s41467-022-30932-1
189. Agosti E, Giorgianni A, D’Amore F, Vinacci G, Balbi S, Locatelli D, et al. Is Guillain-Barrè syndrome triggered by SARS-CoV-2? Case report and literature review. Neurol Sci. (2021) 42:607–12. doi: 10.1007/s10072-020-04553-9
190. Bigaut K, Mallaret M, Baloglu S, Nemoz B, Morand P, Baicry F, et al. Guillain-Barré syndrome related to SARS-CoV-2 infection. Neurol Neuroimmunol Neuroinflamm. (2020) 7:1351–1354. doi: 10.1212/NXI.0000000000000785
191. Ottaviani D, Boso F, Tranquillini E, Gapeni I, Pedrotti G, Cozzio S, et al. Early Guillain-Barré syndrome in coronavirus disease 2019 (COVID-19): a case report from an Italian COVID-hospital. Neurol Sci. (2020) 41:1351–4. doi: 10.1007/s10072-020-04449-8
192. Lucchese G, Flöel A. SARS-CoV-2 and Guillain-Barré syndrome: molecular mimicry with human heat shock proteins as potential pathogenic mechanism. Cell Stress Chaperones. (2020) 25:731–5. doi: 10.1007/s12192-020-01145-6
193. Yuki N, Hartung H-P. Guillain-barré syndrome. N Engl J Med. (2012) 366:2294–304. doi: 10.1056/NEJMra1114525
194. Lee J-G, Huang W, Lee H, van de Leemput J, Kane MA, Han Z. Characterization of SARS-CoV-2 proteins reveals Orf6 pathogenicity, subcellular localization, host interactions and attenuation by Selinexor. Cell Biosci. (2021) 11:58. doi: 10.1186/s13578-021-00568-7
195. Gyöngyösi M, Alcaide P, Asselbergs FW, Brundel BJJM, Camici GG, Da Costa Martins P, et al. Long COVID and the cardiovascular system-elucidating causes and cellular mechanisms in order to develop targeted diagnostic and therapeutic strategies: a joint Scientific Statement of the ESC Working Groups on Cellular Biology of the Heart and Myocardial and Pericardial Diseases. Cardiovasc Res. (2023) 119:336–56. doi: 10.1093/CVR/CVAC115
196. Xie Y, Xu E, Bowe B, Al-Aly Z. Long-term cardiovascular outcomes of COVID-19. Nat Med. (2022) 28:583–90. doi: 10.1038/s41591-022-01689-3
197. Azevedo RB, Botelho BG, de Hollanda JVG, Ferreira LVL, de Andrade LZJ, Oei SSML, et al. Covid-19 and the cardiovascular system: a comprehensive review. J Hum Hypertens. (2021) 35:4–11. doi: 10.1038/s41371-020-0387-4
198. Tajbakhsh A, Hayat SMG, Taghizadeh H, Akbari A, inabadi M, Savardashtaki A, et al. COVID-19 and cardiac injury: clinical manifestations, biomarkers, mechanisms, diagnosis, treatment, and follow up. Expert Rev Anti Infect Ther. (2021) 19:345–57. doi: 10.1080/14787210.2020.1822737
199. Zou F, Qian Z, Wang Y, Zhao Y, Bai J. Cardiac injury and COVID-19: A systematic review and meta-analysis. CJC Open. (2020) 2:386–94. doi: 10.1016/j.cjco.2020.06.010
200. Puntmann VO, Carerj ML, Wieters I, Fahim M, Arendt C, Hoffmann J, et al. Outcomes of cardiovascular magnetic resonance imaging in patients recently recovered from coronavirus disease 2019 (COVID-19). JAMA Cardiol. (2020) 5:1265. doi: 10.1001/jamacardio.2020.3557
201. Lindner D, Fitzek A, Bräuninger H, Aleshcheva G, Edler C, Meissner K, et al. Association of cardiac infection with SARS-coV-2 in confirmed COVID-19 autopsy cases. JAMA Cardiol. (2020) 5:1281. doi: 10.1001/jamacardio.2020.3551
202. Bailey AL, Dmytrenko O, Greenberg L, Bredemeyer AL, Ma P, Liu J, et al. SARS-coV-2 infects human engineered heart tissues and models COVID-19 myocarditis. JACC Basic to Transl Sci. (2021) 6:331–45. doi: 10.1016/j.jacbts.2021.01.002
203. Khismatullin RR, Ponomareva AA, Nagaswami C, Ivaeva RA, Montone KT, Weisel JW, et al. Pathology of lung-specific thrombosis and inflammation in COVID-19. J Thromb Haemost. (2021) 19:3062–72. doi: 10.1111/jth.15532
204. Inciardi RM, Lupi L, Zaccone G, Italia L, Raffo M, Tomasoni D, et al. Cardiac involvement in a patient with coronavirus disease 2019 (COVID-19). JAMA Cardiol. (2020) 5:819. doi: 10.1001/jamacardio.2020.1096
205. Shi S, Qin M, Shen B, Cai Y, Liu T, Yang F, et al. Association of cardiac injury with mortality in hospitalized patients with COVID-19 in wuhan, China. JAMA Cardiol. (2020) 5:802. doi: 10.1001/jamacardio.2020.0950
206. Breitbart P, Koch A, Schmidt M, Magedanz A, Lindhoff-Last E, Voigtländer T, et al. Clinical and cardiac magnetic resonance findings in post-COVID patients referred for suspected myocarditis. Clin Res Cardiol. (2021) 110:1832–40. doi: 10.1007/s00392-021-01929-5
207. Druzak S, Iffrig E, Roberts BR, Zhang T, Fibben KS, Sakurai Y, et al. Multiplatform analyses reveal distinct drivers of systemic pathogenesis in adult versus pediatric severe acute COVID-19. Nat Commun. (2023) 14:1638. doi: 10.1038/S41467-023-37269-3
208. Vassiliou AG, Vrettou CS, Keskinidou C, Dimopoulou I, Kotanidou A, Orfanos SE. Endotheliopathy in acute COVID-19 and long COVID. Int J Mol Sci. (2023) 24:8237. doi: 10.3390/IJMS24098237
209. Neurath MF, Uberla K, Ng SC. Gut as viral reservoir: lessons from gut viromes, HIV and COVID-19. Gut. (2021) 70:1605–8. doi: 10.1136/GUTJNL-2021-324622
210. Ccl C, Goh D, Lim X. Correction: Residual SARS-CoV-2 viral antigens detected in GI and hepatic tissues from five recovered patients with COVID-19. Gut. (2022) 71:e9–9. doi: 10.1136/gutjnl-2021-324280corr1
211. Buonsenso D, Martino L, Morello R, Mariani F, Fearnley K, Valentini P. Viral persistence in children infected with SARS-CoV-2: current evidence and future research strategies. Lancet Microbe. (2023) 4:e745–56. doi: 10.1016/S2666-5247(23)00115-5
212. Varga Z, Flammer AJ, Steiger P, Haberecker M, Andermatt R, Zinkernagel AS, et al. Endothelial cell infection and endotheliitis in COVID-19. The Lancet. (2020) 395:1417–8. doi: 10.1016/S0140-6736(20)30937-5
213. Stein SR, Ramelli SC, Grazioli A, Chung JYJE, Singh M, Yinda CK, et al. SARS-CoV-2 infection and persistence in the human body and brain at autopsy. Nature. (2022) 612:758–63. doi: 10.1038/s41586-022-05542-y.
214. Ninan S, Thompson P, Gershon T, Ford N, Mills W, Jewells V, et al. Fatal pediatric COVID-19 case with seizures and fulminant cerebral edema. Child Neurol Open. (2021) 8:2329048X2110225. doi: 10.1177/2329048X211022532
215. Arostegui D, Castro K, Schwarz S, Vaidy K, Rabinowitz S, Wallach T. Persistent SARS-coV-2 nucleocapsid protein presence in the intestinal epithelium of a pediatric patient 3 months after acute infection. JPGN Rep. (2021) 3:e152. doi: 10.1097/PG9.0000000000000152
216. Sigal GB, Novak T, Mathew A, Chou J, Zhang Y, Manjula N, et al. Measurement of severe acute respiratory syndrome coronavirus 2 antigens in plasma of pediatric patients with acute coronavirus disease 2019 or multisystem inflammatory syndrome in children using an ultrasensitive and quantitative immunoassay. Clin Infect Dis. (2022) 75:1351–8. doi: 10.1093/CID/CIAC160
217. Xu Q, Milanez-Almeida P, Martins AJ, Radtke AJ, Hoehn KB, Oguz C, et al. Adaptive immune responses to SARS-CoV-2 persist in the pharyngeal lymphoid tissue of children. Nat Immunol. (2023) 24:186–99. doi: 10.1038/s41590-022-01367-z
218. Chen B, Julg B, Mohandas S, Bradfute SB. Viral persistence, reactivation, and mechanisms of long COVID. Elife. (2023) 12:e86015. doi: 10.7554/ELIFE.86015
219. Taweevisit M, Chindamporn A, Sujjavorakul K, Samransamruajkit R, Thorner PS. Multisystem inflammatory syndrome in children (MIS-C) showing disseminated aspergillosis, cytomegalovirus reactivation and persistent SARS-COV-2: Case report with autopsy review. Pathol Res Pract. (2022) 238:154106. doi: 10.1016/J.PRP.2022.154106
220. Turner S, Khan MA, Putrino D, Woodcock A, Kell DB, Pretorius E. Long COVID: pathophysiological factors and abnormalities of coagulation. Trends Endocrinol Metab. (2023) 34:321–44. doi: 10.1016/J.TEM.2023.03.002
221. Borowiec BM, Volponi AA, Mozdziak P, Kempisty B, Dyszkiewicz-Konwińska M. Small extracellular vesicles and COVID19-using the “Trojan horse” to tackle the giant. Cells. (2021) 10:3383. doi: 10.3390/CELLS10123383
222. Barberis E, Vanella VV, Falasca M, Caneapero V, Cappellano G, Raineri D, et al. Circulating exosomes are strongly involved in SARS-coV-2 infection. Front Mol Biosci. (2021) 8:632290. doi: 10.3389/FMOLB.2021.632290
223. Ali RA, Wuescher LM, Worth RG. Platelets: essential components of the immune system. Curr Trends Immunol. (2015) 16:65.
224. Pretorius E, Vlok M, Venter C, Bezuidenhout JA, Laubscher GJ, Steenkamp J, et al. Persistent clotting protein pathology in Long COVID/Post-Acute Sequelae of COVID-19 (PASC) is accompanied by increased levels of antiplasmin. Cardiovasc Diabetol. (2021) 20:172. doi: 10.1186/S12933-021-01359-7
225. Wang C, Yu C, Jing H, Wu X, Novakovic VA, Xie R, et al. Long COVID: the nature of thrombotic sequelae determines the necessity of early anticoagulation. Front Cell Infect Microbiol. (2022) 12:861703. doi: 10.3389/FCIMB.2022.861703
226. Ackermann M, Verleden SE, Kuehnel M, Haverich A, Welte T, Laenger F, et al. Pulmonary vascular endothelialitis, thrombosis, and angiogenesis in covid-19. N Engl J Med. (2020) 383:120–8. doi: 10.1056/NEJMoa2015432
227. Phetsouphanh C, Darley DR, Wilson DB, Howe A, Munier CML, Patel SK, et al. Immunological dysfunction persists for 8 months following initial mild-to-moderate SARS-CoV-2 infection. Nat Immunol. (2022) 23:210–6. doi: 10.1038/s41590-021-01113-x
228. Clausen TM, Sandoval DR, Spliid CB, Pihl J, Perrett HR, Painter CD, et al. SARS-coV-2 infection depends on cellular heparan sulfate and ACE2. Cell. (2020) 183:1043–1057.e15. doi: 10.1016/j.cell.2020.09.033
229. Kotagiri P, Mescia F, Hanson AL, Turner L, Bergamaschi L, Peñalver A, et al. The impact of hypoxia on B cells in COVID-19. EBioMedicine. (2022) 77:103878. doi: 10.1016/J.EBIOM.2022.103878
230. Carvalho-Schneider C, Laurent E, Lemaignen A, Beaufils E, Bourbao-Tournois C, Laribi S, et al. Follow-up of adults with noncritical COVID-19 two months after symptom onset. Clin Microbiol Infect. (2021) 27:258–63. doi: 10.1016/j.cmi.2020.09.052
231. D’Cruz RF, Waller MD, Perrin F, Periselneris J, Norton S, Smith L-J, et al. Chest radiography is a poor predictor of respiratory symptoms and functional impairment in survivors of severe COVID-19 pneumonia. ERJ Open Res. (2021) 7:655–2020. doi: 10.1183/23120541.00655-2020
232. Tomasoni D, Bai F, Castoldi R, Barbanotti D, Falcinella C, Mulè G, et al. Anxiety and depression symptoms after virological clearance of COVID-19: A cross-sectional study in Milan, Italy. J Med Virol. (2021) 93:1175–9. doi: 10.1002/jmv.26459
233. Wong SH, Lui RNS, Sung JJY. Covid-19 and the digestive system. J Gastroenterol Hepatol. (2020) 35:744–8. doi: 10.1111/jgh.15047
234. Zhao Y, Shang Y, Song W, Li Q, Xie H, Xu Q, et al. Follow-up study of the pulmonary function and related physiological characteristics of COVID-19 survivors three months after recovery. EClinicalMedicine. (2020) 25:100463. doi: 10.1016/j.eclinm.2020.100463
235. Xiao F, Tang M, Zheng X, Liu Y, Li X, Shan H. Evidence for gastrointestinal infection of SARS-coV-2. Gastroenterology. (2020) 158:1831–1833.e3. doi: 10.1053/j.gastro.2020.02.055
236. Natarajan A, Zlitni S, Brooks EF, Vance SE, Dahlen A, Hedlin H, et al. Gastrointestinal symptoms and fecal shedding of SARS-CoV-2 RNA suggest prolonged gastrointestinal infection. Med (New York NY). (2022) 3:371–387.e9. doi: 10.1016/J.MEDJ.2022.04.001
237. Hoffmann M, Kleine-Weber H, Schroeder S, Krüger N, Herrler T, Erichsen S, et al. SARS-coV-2 cell entry depends on ACE2 and TMPRSS2 and is blocked by a clinically proven protease inhibitor. Cell. (2020) 181:271–280.e8. doi: 10.1016/j.cell.2020.02.052
238. Jackson CB, Farzan M, Chen B, Choe H. Mechanisms of SARS-CoV-2 entry into cells. Nat Rev Mol Cell Biol. (2022) 23:3–20. doi: 10.1038/s41580-021-00418-x
239. Lupala CS, Ye Y, Chen H, Su X-D, Liu H. Mutations on RBD of SARS-CoV-2 Omicron variant result in stronger binding to human ACE2 receptor. Biochem Biophys Res Commun. (2022) 590:34–41. doi: 10.1016/j.bbrc.2021.12.079
240. Winkler ES, Chen RE, Alam F, Yildiz S, Case JB, Uccellini MB, et al. SARS-coV-2 causes lung infection without severe disease in human ACE2 knock-in mice. J Virol. (2022) 96:e0151121. doi: 10.1128/JVI.01511-21
241. Hung IFN, Cheng VCC, Wu AKL, Tang BSF, Chan KH, Chu CM, et al. Viral loads in clinical specimens and SARS manifestations. Emerg Infect Dis. (2004) 10:1550–7. doi: 10.3201/eid1009.040058
242. Leung WK, To K, Chan PKS, Chan HLY, Wu AKL, Lee N, et al. Enteric involvement of severe acute respiratory syndrome-associated coronavirus infection. Gastroenterology. (2003) 125:as0016508503012150. doi: 10.1016/S0016-5085(03)01215-0
243. Zhou J, Li C, Zhao G, Chu H, Wang D, Yan HH-N, et al. Human intestinal tract serves as an alternative infection route for Middle East respiratory syndrome coronavirus. Sci Adv. (2017) 3:eaao4966. doi: 10.1126/sciadv.aao4966
244. Lu R, Zhao X, Li J, Niu P, Yang B, Wu H, et al. Genomic characterisation and epidemiology of 2019 novel coronavirus: implications for virus origins and receptor binding. Lancet. (2020) 395:565–74. doi: 10.1016/S0140-6736(20)30251-8
245. Gaebler C, Wang Z, Lorenzi JCC, Muecksch F, Finkin S, Tokuyama M, et al. Evolution of antibody immunity to SARS-CoV-2. Nat. (2021) 591:639–44. doi: 10.1038/s41586-021-03207-w
246. Morone G, Palomba A, Iosa M, Caporaso T, De Angelis D, Venturiero V, et al. Incidence and persistence of viral shedding in COVID-19 post-acute patients with negativized pharyngeal swab: A systematic review. Front Med. (2020) 7:562. doi: 10.3389/FMED.2020.00562
247. Wu Y, Guo C, Tang L, Hong Z, Zhou J, Dong X, et al. Prolonged presence of SARS-CoV-2 viral RNA in faecal samples. Lancet Gastroenterol Hepatol. (2020) 5:434–5. doi: 10.1016/S2468-1253(20)30083-2
248. Sencio V, MaChado MG, Trottein F. The lung-gut axis during viral respiratory infections: the impact of gut dysbiosis on secondary disease outcomes. Mucosal Immunol. (2021) 14:296–304. doi: 10.1038/S41385-020-00361-8
249. Neag MA, Vulturar DM, Gherman D, Burlacu CC, Todea DA, Buzoianu AD. Gastrointestinal microbiota: A predictor of COVID-19 severity? World J Gastroenterol. (2022) 28:6328. doi: 10.3748/WJG.V28.I45.6328
250. Zhang H, Shao B, Dang Q, Chen Z, Zhou Q, Luo H, et al. Pathogenesis and mechanism of gastrointestinal infection with COVID-19. Front Immunol. (2021) 12:674074. doi: 10.3389/FIMMU.2021.674074
251. Oliva A, Miele MC, Di Timoteo F, De Angelis M, Mauro V, Aronica R, et al. Persistent systemic microbial translocation and intestinal damage during coronavirus disease-19. Front Immunol. (2021) 12:708149. doi: 10.3389/fimmu.2021.708149.
252. George V, Harrison L, Roach M, Li XD, Tierney C, Fischl MA, et al. Associations of plasma cytokine and microbial translocation biomarkers with immune reconstitution inflammatory syndrome. J Infect Dis. (2017) 216:1159–63. doi: 10.1093/INFDIS/JIX460
253. Giron LB, Dweep H, Yin X, Wang H, Damra M, Goldman AR, et al. Plasma markers of disrupted gut permeability in severe COVID-19 patients. Front Immunol. (2021) 12:686240. doi: 10.3389/fimmu.2021.779064
254. Wu D, Yang XO. TH17 responses in cytokine storm of COVID-19: An emerging target of JAK2 inhibitor Fedratinib. J Microbiol Immunol Infect. (2020) 53:368–70. doi: 10.1016/J.JMII.2020.03.005
255. Belizário JE, Faintuch J, Garay-Malpartida M. Gut microbiome dysbiosis and immunometabolism: new frontiers for treatment of metabolic diseases. Mediators Inflammation. (2018) 2018:2037838. doi: 10.1155/2018/2037838
256. Belizário JE, Faintuch J. Microbiome and gut dysbiosis. Exp Suppl. (2018) 109:459–76. doi: 10.1007/978-3-319-74932-7_13
257. Dickson RP, Schultz MJ, van der Poll T, Schouten LR, Falkowski NR, Luth JE, et al. Lung microbiota predict clinical outcomes in critically ill patients. Am J Respir Crit Care Med. (2020) 201:555–63. doi: 10.1164/rccm.201907-1487OC
258. Gu S, Chen Y, Wu Z, Chen Y, Gao H, Lv L, et al. Alterations of the gut microbiota in patients with coronavirus disease 2019 or H1N1 influenza. Clin Infect Dis. (2020) 71:2669–78. doi: 10.1093/CID/CIAA709
259. Xu R, Lu R, Zhang T, Wu Q, Cai W, Han X, et al. Temporal association between human upper respiratory and gut bacterial microbiomes during the course of COVID-19 in adults. Commun Biol. (2021) 4:1–11. doi: 10.1038/s42003-021-01796-w
260. Chen Y, Gu S, Chen Y, Lu H, Shi D, Guo J, et al. Six-month follow-up of gut microbiota richness in patients with COVID-19. Gut. (2022) 71:222–5. doi: 10.1136/GUTJNL-2021-324090
261. Irum N, Afzal T, Faraz MH, Aslam Z, Rasheed F. The role of gut microbiota in depression: an analysis of the gut-brain axis. Front Behav Neurosci. (2023) 17:1185522/BIBTEX. doi: 10.3389/fnbeh.2023.1185522
262. Brogna C, Brogna B, Bisaccia DR, Lauritano F, Marino G, Montano L, et al. Could SARS-coV-2 have bacteriophage behavior or induce the activity of other bacteriophages? Vaccines. (2022) 10:708. doi: 10.3390/VACCINES10050708
263. Palevsky PM. Endpoints for clinical trials of acute kidney injury. Nephron. (2018) 140:111–5. doi: 10.1159/000493203
264. Yende S, Parikh CR. Long COVID and kidney disease. Nat Rev Nephrol. (2021) 17:792–3. doi: 10.1038/s41581-021-00487-3
265. Pei G, Zhang Z, Peng J, Liu L, Zhang C, Yu C, et al. Renal involvement and early prognosis in patients with COVID-19 pneumonia. J Am Soc Nephrol. (2020) 31:1157–65. doi: 10.1681/ASN.2020030276
266. Davis HE, McCorkell L, Vogel JM, Topol EJ. Long COVID: major findings, mechanisms and recommendations. Nat Rev Microbiol. (2023) 21:133–46. doi: 10.1038/s41579-022-00846-2
267. Kellum JA, Olivier van Till JW, Mulligan G. Targeting acute kidney injury in COVID-19. Nephrol Dial Transplant. (2020) 35:1652–62. doi: 10.1093/NDT/GFAA231
268. Farkash EA, Wilson AM, Jentzen JM. Ultrastructural evidence for direct renal infection with SARS-coV-2. J Am Soc Nephrol. (2020) 31:1683–7. doi: 10.1681/ASN.2020040432
269. Chueh TI, Zheng CM, Hou YC, Lu KC. Novel evidence of acute kidney injury in COVID-19. J Clin Med. (2020) 9:1–14. doi: 10.3390/JCM9113547
270. Ahmadian E, Khatibi SMH, Soofiyani SR, Abediazar S, Shoja MM, Ardalan M, et al. Covid-19 and kidney injury: Pathophysiology and molecular mechanisms. Rev Med Virol. (2021) 31:e2176. doi: 10.1002/rmv.2176
271. Donoghue M, Hsieh F, Baronas E, Godbout K, Gosselin M, Stagliano N, et al. A novel angiotensin-converting enzyme–related carboxypeptidase (ACE2) converts angiotensin I to angiotensin 1-9. Circ Res. (2000) 87:e1–e9. doi: 10.1161/01.RES.87.5.e1
272. Lores E, Wysocki J, Batlle D. ACE2, the kidney and the emergence of COVID-19 two decades after ACE2 discovery. Clin Sci. (2020) 134:2791–805. doi: 10.1042/CS20200484
273. Crook H, Raza S, Nowell J, Young M, Edison P. Long covid—mechanisms, risk factors, and management. BMJ. (2021) 374:n1648. doi: 10.1136/bmj.n1648
274. Danladi J, Sabir H. Innate immunity, inflammation activation and heat-shock protein in COVID-19 pathogenesis. J Neuroimmunol. (2021) 358:577632. doi: 10.1016/j.jneuroim.2021.577632
275. Gao Y, Ding M, Dong X, Zhang J, Azkur AK, Azkur D, et al. Risk factors for severe and critically ill COVID-19 patients: A review. Allergy. (2021) 76:428–55. doi: 10.1111/all.14657
276. Peluso MJ, Deveau TM, Munter SE, Ryder D, Buck A, Beck-Engeser G, et al. Chronic viral coinfections differentially affect the likelihood of developing long COVID. J Clin Invest. (2023) 133:e163669. doi: 10.1172/JCI163669
277. Lan K, Luo MH. Herpesviruses: epidemiology, pathogenesis, and interventions. Virol Sin. (2017) 32:347. doi: 10.1007/S12250-017-4108-2
278. Mitchell BM, Bloom DC, Cohrs RJ, Gilden DH, Kennedy PGE. Herpes simplex virus-1 and varicella-zoster virus latency in ganglia. J Neurovirol. (2003) 9:194–204. doi: 10.1080/13550280390194000
279. Katz J, Yue S, Xue W. Herpes simplex and herpes zoster viruses in COVID-19 patients. Ir J Med Sci. (2022) 191:1093. doi: 10.1007/s11845-021-02714-z
280. Xu R, Zhou Y, Cai L, Wang L, Han J, Yang X, et al. Co-reactivation of the human herpesvirus alpha subfamily (herpes simplex virus-1 and varicella zoster virus) in a critically ill patient with COVID-19. Br J Dermatol. (2020) 183:1145–7. doi: 10.1111/BJD.19484
281. Kim JYH, Ragusa M, Tortosa F, Torres A, Gresh L, Méndez-Rico JA, et al. Viral reactivations and co-infections in COVID-19 patients: a systematic review. BMC Infect Dis. (2023) 23:1–25. doi: 10.1186/s12879-023-08117-y
282. Simonnet A, Engelmann I, Moreau AS, Garcia B, Six S, El Kalioubie A, et al. High incidence of Epstein-Barr virus, cytomegalovirus, and human-herpes virus-6 reactivations in critically ill patients with COVID-19. Infect Dis now. (2021) 51:296–9. doi: 10.1016/J.IDNOW.2021.01.005
283. Pona A, Jiwani RA, Afriyie F, Labbe J, Cook PP, Mao Y. Herpes zoster as a potential complication of coronavirus disease 2019. Dermatol Ther. (2020) 33:e13930. doi: 10.1111/DTH.13930
284. Busani S, Bedini A, Biagioni E, Serio L, Tonelli R, Meschiari M, et al. Two fatal cases of acute liver failure due to HSV-1 infection in COVID-19 patients following immunomodulatory therapies. Clin Infect Dis. (2021) 73:E252–5. doi: 10.1093/CID/CIAA1246
285. Perera MR, Greenwood EJD, Crozier TWM, Elder EG, Schmitt J, Crump CM, et al. Human cytomegalovirus infection of epithelial cells increases SARS-coV-2 superinfection by upregulating the ACE2 receptor. J Infect Dis. (2023) 227:543–53. doi: 10.1093/INFDIS/JIAC452
286. Vojdani A, Vojdani E, Saidara E, Maes M. Persistent SARS-coV-2 infection, EBV, HHV-6 and other factors may contribute to inflammation and autoimmunity in long COVID. Viruses. (2023) 15:400. doi: 10.3390/V15020400
287. Ahamed J, Laurence J. Long COVID endotheliopathy: hypothesized mechanisms and potential therapeutic approaches. J Clin Invest. (2022) 132. doi: 10.1172/JCI161167
288. Jafarzadeh A, Gosain R, Mortazavi SMJ, Nemati M, Jafarzadeh S, Ghaderi A. SARS-coV-2 infection: A possible risk factor for incidence and recurrence of cancers. Int J Hematol Stem Cell Res. (2022) 16:117–27. doi: 10.18502/IJHOSCR.V16I2.9205
289. Komaroff AL, Lipkin WI. ME/CFS and Long COVID share similar symptoms and biological abnormalities: road map to the literature. Front Med. (2023) 10:1187163. doi: 10.3389/FMED.2023.1187163
290. Lam MHB, Wing YK, Yu MWM, Leung CM, Ma RCW, Kong APS, et al. Mental morbidities and chronic fatigue in severe acute respiratory syndrome survivors: long-term follow-up. Arch Intern Med. (2009) 169:2142–7. doi: 10.1001/ARCHINTERNMED.2009.384
291. Shikova E, Reshkova V, Kumanova А, Raleva S, Alexandrova D, Capo N, et al. Cytomegalovirus, Epstein-Barr virus, and human herpesvirus-6 infections in patients with myalgic еncephalomyelitis/chronic fatigue syndrome. J Med Virol. (2020) 92:3682–8. doi: 10.1002/JMV.25744
292. Peluso MJ, Deveau T-M, Munter SE, Ryder D, Buck A, Beck-Engeser G, et al. Impact of pre-existing chronic viral infection and reactivation on the development of long COVID. medRxiv. (2022). doi: 10.1101/2022.06.21.22276660
293. Gold JE, Okyay RA, Licht WE, Hurley DJ. Investigation of long COVID prevalence and its relationship to epstein-barr virus reactivation. Pathog (Basel Switzerland). (2021) 10:E1–9. doi: 10.3390/PATHOGENS10060763
294. Zubchenko S, Kril I, Nadizhko O, Matsyura O, Chopyak V. Herpesvirus infections and post-COVID-19 manifestations: a pilot observational study. Rheumatol Int. (2022) 42:1523–30. doi: 10.1007/S00296-022-05146-9
295. Leonso AA, Brown K, Prol R, Rawat S, Khunger A, Bromberg R. A rare case of latent tuberculosis reactivation secondary to a COVID-19 infection. Infect Dis Rep. (2022) 14:446. doi: 10.3390/IDR14030048
296. Tang W, Leonhardt L, Pervez A, Sarvepalli S. A case of pleural tuberculosis vs latent tuberculosis reactivation as a result of COVID-19 infection and treatment. J Community Hosp Intern Med Perspect. (2022) 12:90–4. doi: 10.55729/2000-9666.1078
297. Friedman A, Degeorge KC. Reactivation of latent tuberculosis in a COVID-19 patient on corticosteroid treatment. BMJ Case Rep. (2022) 15:e247562. doi: 10.1136/BCR-2021-247562
298. Bardhan M, Hasan MM, Ray I, Sarkar A, Chahal P, Rackimuthu S, et al. Tuberculosis amidst COVID-19 pandemic in India: unspoken challenges and the way forward. Trop Med Health. (2021) 49:84. doi: 10.1186/S41182-021-00377-1
299. Alemu A, Bitew ZW, Seid G, Diriba G, Gashu E, Berhe N, et al. Tuberculosis in individuals who recovered from COVID-19: A systematic review of case reports. PloS One. (2022) 17:e0277807. doi: 10.1371/JOURNAL.PONE.0277807
300. WHO Global Tuberculosis Report 2022. 1. COVID-19 and TB. WHO rep (2022). Available online at: https://www.who.int/teams/global-tuberculosis-programme/tb-reports/global-tuberculosis-report-2022/covid-19-and-tb (Accessed October 24, 2023).
301. Ramasamy S, Subbian S. Critical determinants of cytokine storm and type I interferon response in COVID-19 pathogenesis. Clin Microbiol Rev. (2021) 34:e00299–20. doi: 10.1128/CMR.00299-20
302. Patterson BK, Guevara-Coto J, Yogendra R, Francisco EB, Long E, Pise A, et al. Immune-based prediction of COVID-19 severity and chronicity decoded using machine learning. Front Immunol. (2021) 12:700782. doi: 10.3389/fimmu.2021.700782
303. Canedo-Marroquín G, Saavedra F, Andrade CA, Berrios RV, Rodríguez-Guilarte L, Opazo MC, et al. SARS-coV-2: immune response elicited by infection and development of vaccines and treatments. Front Immunol. (2020) 11:569760. doi: 10.3389/fimmu.2020.569760
304. Carvalho T, Krammer F, Iwasaki A. The first 12 months of COVID-19: a timeline of immunological insights. Nat Rev Immunol. (2021) 21:245–56. doi: 10.1038/s41577-021-00522-1
305. Dotan A, Muller S, Kanduc D, David P, Halpert G, Shoenfeld Y. The SARS-CoV-2 as an instrumental trigger of autoimmunity. Autoimmun Rev. (2021) 20:102792. doi: 10.1016/j.autrev.2021.102792
306. Jordan SC. Innate and adaptive immune responses to SARS-CoV-2 in humans: relevance to acquired immunity and vaccine responses. Clin Exp Immunol. (2021) 204:310–20. doi: 10.1111/cei.13582
307. Sánchez-Zuno GA, Matuz-Flores MG, González-Estevez G, Nicoletti F, Turrubiates-Hernández FJ, Mangano K, et al. A review: Antibody-dependent enhancement in COVID-19: The not so friendly side of antibodies. Int J Immunopathol Pharmacol. (2021) 35:205873842110501. doi: 10.1177/20587384211050199
308. Sette A, Crotty S. Adaptive immunity to SARS-coV-2 and COVID-19. Cell. (2021) 184:861–80. doi: 10.1016/j.cell.2021.01.007
309. Hu B, Huang S, Yin L. The cytokine storm and COVID-19. J Med Virol. (2021) 93:250–6. doi: 10.1002/jmv.26232
310. Ye Q, Wang B, Mao J. The pathogenesis and treatment of the `Cytokine Storm’ in COVID-19. J Infect. (2020) 80:607–13. doi: 10.1016/j.jinf.2020.03.037
311. Arish M, Qian W, Narasimhan H, Sun J. COVID-19 immunopathology: From acute diseases to chronic sequelae. J Med Virol. (2023) 95:e28122. doi: 10.1002/JMV.28122
312. Tana C, Cinetto F, Mantini C, Bernardinello N, Tana M, Ricci F, et al. Sarcoidosis and COVID-19: at the cross-road between immunopathology and clinical manifestation. Biomedicines. (2022) 10:2525. doi: 10.3390/BIOMEDICINES10102525
313. Dorward DA, Russell CD, Um IH, Elshani M, Armstrong SD, Penrice-Randal R, et al. Tissue-specific immunopathology in fatal COVID-19. Am J Respir Crit Care Med. (2021) 203:192–201. doi: 10.1164/rccm.202008-3265OC
314. Chen Y-M, Zheng Y, Yu Y, Wang Y, Huang Q, Qian F, et al. Blood molecular markers associated with COVID-19 immunopathology and multi-organ damage. EMBO J. (2020) 39:e105896. doi: 10.15252/EMBJ.2020105896
315. Gong F, Dai Y, Zheng T, Cheng L, Zhao D, Wang H, et al. Peripheral CD4+ T cell subsets and antibody response in COVID-19 convalescent individuals. J Clin Invest. (2020) 130:6588–99. doi: 10.1172/JCI141054
316. Kared H, Redd AD, Bloch EM, Bonny TS, Sumatoh H, Kairi F, et al. SARS-CoV-2–specific CD8+ T cell responses in convalescent COVID-19 individuals. J Clin Invest. (2021) 131:e145476. doi: 10.1172/JCI145476
317. Nguyen-Contant P, Embong AK, Kanagaiah P, Chaves FA, Yang H, Branche AR, et al. S protein-reactive igG and memory B cell production after human SARS-coV-2 infection includes broad reactivity to the S2 subunit. MBio. (2020) 11:e01991–20. doi: 10.1128/mBio.01991-20
318. Ni L, Ye F, Cheng M-L, Feng Y, Deng Y-Q, Zhao H, et al. Detection of SARS-coV-2-specific humoral and cellular immunity in COVID-19 convalescent individuals. Immunity. (2020) 52:971–977.e3. doi: 10.1016/j.immuni.2020.04.023
319. Toor SM, Saleh R, Nair VS, Taha RZ, Elkord E. T-cell responses and therapies against SARS-CoV-2 infection. Immunology. (2021) 162:30–43. doi: 10.1111/imm.13262
320. Ryan FJ, Hope CM, Masavuli MG, Lynn MA, Mekonnen ZA, Yeow AEL, et al. Long-term perturbation of the peripheral immune system months after SARS-CoV-2 infection. BMC Med. (2022) 20:26. doi: 10.1186/s12916-021-02228-6
321. Wang Z, Wang S, Goplen NP, Li C, Cheon IS, Dai Q, et al. PD-1 hi CD8+ resident memory T cells balance immunity and fibrotic sequelae. Sci Immunol. (2019) 4:eaaw1217. doi: 10.1126/sciimmunol.aaw1217
322. Goplen NP, Wu Y, Son YM, Li C, Wang Z, Cheon IS, et al. Tissue-resident CD8+ T cells drive age-associated chronic lung sequelae after viral pneumonia. Sci Immunol. (2020) 5:eabc4557. doi: 10.1126/sciimmunol.abc4557
323. Ganji A, Farahani I, Khansarinejad B, Ghazavi A, Mosayebi G. Increased expression of CD8 marker on T-cells in COVID-19 patients. Blood Cells Mol Dis. (2020) 83:102437. doi: 10.1016/j.bcmd.2020.102437
324. Al-Attiyah R, Safar HA, Botras L, Botras M, Al-Kandari F, Chehadeh W, et al. Immune cells profiles in the peripheral blood of patients with moderate to severe COVID-19 and healthy subjects with and without vaccination with the pfizer-bioNTech mRNA vaccine. Front Immunol. (2022) 13:851765. doi: 10.3389/fimmu.2022.851765
325. Patterson BK, Seethamraju H, Dhody K, Corley MJ, Kazempour K, Lalezari J, et al. CCR5 inhibition in critical COVID-19 patients decreases inflammatory cytokines, increases CD8 T-cells, and decreases SARS-CoV2 RNA in plasma by day 14. Int J Infect Dis. (2021) 103:25–32. doi: 10.1016/j.ijid.2020.10.101
326. Patterson BK, Francisco EB, Yogendra R, Long E, Pise A, Rodrigues H, et al. Persistence of SARS coV-2 S1 protein in CD16+ Monocytes in post-acute sequelae of COVID-19 (PASC) up to 15 months post-infection. Front Immunol. (2022) 12:746021. doi: 10.3389/fimmu.2021.746021
327. Santopaolo M, Gregorova M, Hamilton F, Arnold D, Long A, Lacey A, et al. Prolonged T-cell activation and long COVID symptoms independently associate with severe COVID-19 at 3 months. Elife. (2023) 12:e85009. doi: 10.7554/ELIFE.85009
328. Altmann DM, Reynolds CJ, Joy G, Otter AD, Gibbons JM, Pade C, et al. Persistent symptoms after COVID-19 are not associated with differential SARS-CoV-2 antibody or T cell immunity. Nat Commun. (2023) 14:1–9. doi: 10.1038/s41467-023-40460-1
329. Krishna BA, Lim EY, Mactavous L, Lyons PA, Doffinger R, Bradley JR, et al. Evidence of previous SARS-CoV-2 infection in seronegative patients with long COVID. EBioMedicine. (2022) 81:104129. doi: 10.1016/J.EBIOM.2022.104129
330. Seydoux E, Homad LJ, MacCamy AJ, Parks KR, Hurlburt NK, Jennewein MF, et al. Analysis of a SARS-coV-2-infected individual reveals development of potent neutralizing antibodies with limited somatic mutation. Immunity. (2020) 53:98–105.e5. doi: 10.1016/j.immuni.2020.06.001
331. Sedaghat Z, Karimi N. Guillain Barre syndrome associated with COVID-19 infection: A case report. J Clin Neurosci. (2020) 76:233–5. doi: 10.1016/j.jocn.2020.04.062
332. Wu Z, Hu Y, Xu M, Chen Z, Yang W, Jiang Z, et al. Safety, tolerability, and immunogenicity of an inactivated SARS-CoV-2 vaccine (CoronaVac) in healthy adults aged 60 years and older: a randomised, double-blind, placebo-controlled, phase 1/2 clinical trial. Lancet Infect Dis. (2021) 21:803–12. doi: 10.1016/S1473-3099(20)30987-7
333. Alencar CH, de Góes Cavalcanti LP, de Almeida MM, Barbosa PPL, de Sousa Cavalcante KK, de Melo DN, et al. High effectiveness of SARS-coV-2 vaccines in reducing COVID-19-related deaths in over 75-year-olds, ceará State, Brazil. Trop Med Infect Dis. (2021) 6:129. doi: 10.3390/tropicalmed6030129
334. Collier DA, Ferreira IATM, Kotagiri P, Datir RP, Lim EY, Touizer E, et al. Age-related immune response heterogeneity to SARS-CoV-2 vaccine BNT162b2. Nature. (2021) 596:417–22. doi: 10.1038/s41586-021-03739-1
335. Farias JP, de Souza da Silva P, Fogaça MMC, Santana IVR, Luiz WB, Birbrair A, et al. The COVID-19 humoral immunological status induced by coronaVac and astraZeneca vaccines significantly benefits from a booster shot with the pfizer vaccine. J Virol. (2022) 96(8):e0017722. doi: 10.1128/jvi.00177-22
336. Kang M, Yi Y, Li Y, Sun L, Deng A, Hu T, et al. Effectiveness of inactivated COVID-19 vaccines against COVID-19 pneumonia and severe illness caused by the B.1.617.2 (Delta) variant: evidence from an outbreak in guangdong, China. SSRN Electron J. (2021) 175(11):533–40. doi: 10.2139/ssrn.3895639
337. Bernal JL, Andrews N, Gower C, Gallagher E, Simmons R, Thelwall S, et al. Effectiveness of covid-19 vaccines against the B.1.617.2 (Delta) variant. N Engl J Med. (2021) 385:585–94. doi: 10.1056/NEJMoa2108891
338. Sauré D, O’Ryan M, Torres JP, Zuniga M, Santelices E, Basso LJ. Dynamic IgG seropositivity after rollout of CoronaVac and BNT162b2 COVID-19 vaccines in Chile: a sentinel surveillance study. Lancet Infect Dis. (2022) 22:56–63. doi: 10.1016/S1473-3099(21)00479-5
339. Woodruff MC, Bonham KS, Anam FA, Walker TA, Faliti CE, Ishii Y, et al. Chronic inflammation, neutrophil activity, and autoreactivity splits long COVID. Nat Commun. (2023) 14:4201. doi: 10.1038/S41467-023-40012-7
340. Woodruff MC, Ramonell RP, Nguyen DC, Cashman KS, Saini AS, Haddad NS, et al. Extrafollicular B cell responses correlate with neutralizing antibodies and morbidity in COVID-19. Nat Immunol. (2020) 21:1506–16. doi: 10.1038/s41590-020-00814-z
341. Voss LF, Howarth AJ, Wittenborn TR, Hummelgaard S, Juul-Madsen K, Kastberg KS, et al. The extrafollicular response is sufficient to drive initiation of autoimmunity and early disease hallmarks of lupus. Front Immunol. (2022) 13:1021370. doi: 10.3389/FIMMU.2022.1021370
342. Bastard P, Rosen LB, Zhang Q, Michailidis E, Hoffmann H-H, Zhang Y, et al. Autoantibodies against type I IFNs in patients with life-threatening COVID-19. Science (80-). (2020) 370:eabd4585. doi: 10.1126/science.abd4585
343. Troya J, Bastard P, Planas-Serra L, Ryan P, Ruiz M, de Carranza M, et al. Neutralizing autoantibodies to type I IFNs in > 10% of patients with severe COVID-19 pneumonia hospitalized in madrid, Spain. J Clin Immunol. (2021) 41:914–22. doi: 10.1007/s10875-021-01036-0
344. Thurner L, Fadle N, Regitz E, Preuss K-D, Neumann F, Cetin O, et al. Autoantibodies against SUMO1-DHX35 in long-COVID. J Transl Autoimmun. (2022) 5:100171. doi: 10.1016/j.jtauto.2022.100171
345. Wallukat G, Hohberger B, Wenzel K, Fürst J, Schulze-Rothe S, Wallukat A, et al. Functional autoantibodies against G-protein coupled receptors in patients with persistent Long-COVID-19 symptoms. J Transl Autoimmun. (2021) 4:100100. doi: 10.1016/J.JTAUTO.2021.100100
346. Achleitner M, Steenblock C, Dänhardt J, Jarzebska N, Kardashi R, Kanczkowski W, et al. Clinical improvement of Long-COVID is associated with reduction in autoantibodies, lipids, and inflammation following therapeutic apheresis. Mol Psychiatry. (2023) 28(7):1–6. doi: 10.1038/s41380-023-02084-1
347. Seibert FS, Stervbo U, Wiemers L, Skrzypczyk S, Hogeweg M, Bertram S, et al. Severity of neurological Long-COVID symptoms correlates with increased level of autoantibodies targeting vasoregulatory and autonomic nervous system receptors. Autoimmun Rev. (2023) 22:103445. doi: 10.1016/J.AUTREV.2023.103445
348. Zuo J, Dowell AC, Pearce H, Verma K, Long HM, Begum J, et al. Robust SARS-CoV-2-specific T cell immunity is maintained at 6 months following primary infection. Nat Immunol. (2021) 22:620–6. doi: 10.1038/s41590-021-00902-8
349. Magadum A, Kishore R. Cardiovascular manifestations of COVID-19 infection. Cells. (2020) 9:2508. doi: 10.3390/cells9112508
350. Blanco J-R, Cobos-Ceballos M-J, Navarro F, Sanjoaquin I, de las Revillas FA, Bernal E, et al. Pulmonary long-term consequences of COVID-19 infections after hospital discharge. Clin Microbiol Infect. (2021) 27:892–6. doi: 10.1016/j.cmi.2021.02.019
351. Ellul M, Varatharaj A, Nicholson TR, Pollak TA, Thomas N, Easton A, et al. Defining causality in COVID-19 and neurological disorders. J Neurol Neurosurg Psychiatry. (2020) 91:811–2. doi: 10.1136/jnnp-2020-323667
352. Yang P-CC, Jafri MS. Ca2+ signaling in T lymphocytes: the interplay of the endoplasmic reticulum, mitochondria, membrane potential, and CRAC channels on transcription factor activation. Heliyon. (2020) 6:e03526. doi: 10.1016/j.heliyon.2020.e03526
353. Al-Kayali RS, Kashkash MF, Alhussein Alhajji AH, Khouri A. Activation of tuberculosis in recovered COVID-19 patients: a case report. Ann Med Surg. (2023) 85:280–3. doi: 10.1097/MS9.0000000000000188
354. Afrin LB, Weinstock LB, Molderings GJ. Covid-19 hyperinflammation and post-Covid-19 illness may be rooted in mast cell activation syndrome. Int J Infect Dis. (2020) 100:327–32. doi: 10.1016/j.ijid.2020.09.016
355. Doykov I, Hällqvist J, Gilmour KC, Grandjean L, Mills K, Heywood WE. ‘The long tail of Covid-19’ - The detection of a prolonged inflammatory response after a SARS-CoV-2 infection in asymptomatic and mildly affected patients. F1000Research. (2021) 9:1349. doi: 10.12688/f1000research.27287.2
356. Fadini GP, Morieri ML, Longato E, Avogaro A. Prevalence and impact of diabetes among people infected with SARS-CoV-2. J Endocrinol Invest. (2020) 43:867–9. doi: 10.1007/s40618-020-01236-2
357. Sardu C, D’Onofrio N, Balestrieri ML, Barbieri M, Rizzo MR, Messina V, et al. Outcomes in patients with hyperglycemia affected by COVID-19: can we do more on glycemic control? Diabetes Care. (2020) 43:1408–15. doi: 10.2337/dc20-0723
Keywords: SARS-CoV-2, long Covid, epidemiology, pathophysiology, neurological alterations, immune response.
Citation: Bohmwald K, Diethelm-Varela B, Rodríguez-Guilarte L, Rivera T, Riedel CA, González PA and Kalergis AM (2024) Pathophysiological, immunological, and inflammatory features of long COVID. Front. Immunol. 15:1341600. doi: 10.3389/fimmu.2024.1341600
Received: 20 November 2023; Accepted: 09 February 2024;
Published: 28 February 2024.
Edited by:
Prasun K. Datta, Tulane University, United StatesReviewed by:
Zhenlong Liu, McGill University, CanadaBenjamin Anthony Krishna, University of Cambridge, United Kingdom
Matthew Woodruff, Emory University, United States
Copyright © 2024 Bohmwald, Diethelm-Varela, Rodríguez-Guilarte, Rivera, Riedel, González and Kalergis. This is an open-access article distributed under the terms of the Creative Commons Attribution License (CC BY). The use, distribution or reproduction in other forums is permitted, provided the original author(s) and the copyright owner(s) are credited and that the original publication in this journal is cited, in accordance with accepted academic practice. No use, distribution or reproduction is permitted which does not comply with these terms.
*Correspondence: Alexis M. Kalergis, YWthbGVyZ2lzQGJpby5wdWMuY2w=; YWthbGVyZ2lzQGljbG91ZC5jb20=
†These authors have contributed equally to this work