- 1INSERM, UMR_S1256, NGERE – Nutrition, Genetics, and Environmental Risk Exposure, Faculty of Medicine of Nancy, University of Lorraine, Vandoeuvre-lès-Nancy, France
- 2Department of Molecular Medicine and Personalized Therapeutics, Department of Biochemistry, Molecular Biology, Nutrition, and Metabolism, University Hospital of Nancy, Vandoeuvre-lès-Nancy, France
Background: Anaphylaxis manifests as a severe immediate-type hypersensitivity reaction initiated through the immunological activation of target B-cells by allergens, leading to the release of mediators. However, the well-known underlying pathological mechanisms do not fully explain the whole variety of clinical and immunological presentations. We performed a systemic review of proteomic and metabolomic studies and analyzed the extracted data to improve our understanding and identify potential new biomarkers of anaphylaxis.
Methods: Proteomic and metabolomic studies in both human subjects and experimental models were extracted and selected through a systematic search conducted on databases such as PubMed, Scopus, and Web of Science, up to May 2023.
Results: Of 137 retrieved publications, we considered 12 for further analysis, including seven on proteome analysis and five on metabolome analysis. A meta-analysis of the four human studies identified 118 proteins with varying expression levels in at least two studies. Beside established pathways of mast cells and basophil activation, functional analysis of proteomic data revealed a significant enrichment of biological processes related to neutrophil activation and platelet degranulation and metabolic pathways of arachidonic acid and icosatetraenoic acid. The pathway analysis highlighted also the involvement of neutrophil degranulation, and platelet activation. Metabolome analysis across different models showed 13 common metabolites, including arachidonic acid, tryptophan and lysoPC(18:0) lysophosphatidylcholines.
Conclusion: Our review highlights the underestimated role of neutrophils and platelets in the pathological mechanisms of anaphylactic reactions. These findings, derived from a limited number of publications, necessitate confirmation through human studies with larger sample sizes and could contribute to the development of new biomarkers for anaphylaxis.
Systematic review registration: https://www.crd.york.ac.uk/PROSPERO/, identifier CRD42024506246.
1 Introduction
Anaphylaxis is generally considered to be the most severe manifestation of immediate hypersensitivity reactions, induced by the introduction of an allergen into the body. The exact definition of anaphylaxis and the criteria for its diagnosis remain to be subjects of ongoing debate within the scientific community (1, 2). It is an immune response (immunoglobulin E [IgE]- or non-IgE-dependent) leading to the release of mediators in target cells, especially mast cells and basophils. The term “anaphylactoid reaction” has been used to describe clinically similar outcomes produced by a non-immune response to certain antigens (3).
The exact frequency of anaphylaxis is not well-established and is often underestimated, but it appears to be increasing. Over the last decade, the incidence of hospital admissions for anaphylaxis has surged, reaching up to sevenfold in recent years (4). Prevalence depends on geographic location, gender, atopy, and socioeconomic factors (5). Drugs constitute the primary cause of anaphylaxis, with an estimated mortality rate of approximately 0.5-0.51 per million person/year, as indicated by the most recent World Allergy Organization (WAO) anaphylaxis guidance published in 2020 (6). Despite the low rate of mortality, the percentage of fatal anaphylaxis cases due to drugs is estimated around 60% of total fatal anaphylaxis cases (7, 8). The principal drugs responsible for anaphylaxis are the antibiotics including cephalosporins and penicillin, neuromuscular-blocking agents (NMBAs), non-steroidal anti-inflammatory (NSAIDs), injectable radiocontrast agents, therapeutic antibodies, and antineoplastic agents (7–9).
The severity of an anaphylactic event can be assessed using a scoring system known as the Brown severity scale. This scale consists of three levels: mild, rarely fatal, characterized by mild symptoms such as generalized erythema and urticaria; moderate, which can be life-threatening if left untreated, with major symptoms including dyspnea, sweating, and chest or throat tightness; severe, with a high risk of mortality due to the potential development of severe complications or organ failure, major symptoms being cyanosis, hypotension, and confusion (10). The variety and systemic manifestations of anaphylaxis reflects the diversity of mediators and effector cells implicated in the reaction, although some of activated pathways may overlap. Despite the varied outcomes and mechanisms, the diagnosis of anaphylaxis usually depends on the measurement of histamine and serum tryptase during the reaction. However, these biomarkers may not be altered in all cases, prompting a reconsideration and exploration of alternative biomarkers (11). The anaphylactic reaction results predominantly from an IgE-mediated release mediators initiated by the interaction between allergens, typically proteins, peptides or haptenized drug and chemicals, and allergen-specific IgE bound to high-affinity receptors (FcϵRI) on effector cells, primarily basophils and mast cells (12). Indeed, mast cells play a crucial role in the initiation of anaphylaxis (13). Cases with “mastocytosis” (high number of mastocytes) have a high incidence of anaphylaxis (14). The interaction allergen-receptor led to the release of mediators preformed and new synthesized (15). The preformed mediators include histamine, heparin, tryptase, chymase, tumor necrosis factor-alpha (TNFα), and cathepsin G. Newly formed mediators include platelet-activating factor (PAF), prostaglandin D2 (PGD2), leukotriene C4, cytokines and GM-CSF, as well as chemokines (16). While IgE-mediated release of mediators is the predominant mechanism of anaphylaxis, other non-IgE-mediated mechanisms of target cell activation and other target cells such as platelets and eosinophils have been documented (17–19). Which specific receptor is activated in which effector cells determines the type, location and magnitude of mediator release, and consequently, the type and severity of manifestations are still issues which need to be better evaluated (20).
It is very challenging to improve our knowledge on the immunologic mechanisms of anaphylaxis in humans. Predicting the onset of an anaphylactic reaction is not straightforward, and there are only a few clinical studies due to ethical concerns on provoking anaphylaxis. In the past several years, high-throughput multi-omics approaches have been developed to identify potential biomarkers of anaphylaxis, including genes, transcripts, proteins, and metabolites. However, the already known mechanisms do not fully explain the whole variety of clinical and immunological presentation. This suggests that, in addition to IgE-mediated reactions involving mast cells and basophils, other target cells and mediators likely contribute significantly in the reaction. A recent position paper in 2022, the EAACI Task Force “Omics technologies in allergic research”, has discussed the applicability of omics and the advances of these techniques in the allergy research (21). These high-performance omics technologies can allow to understand better the mechanisms and provide molecular signatures of anaphylaxis (22). Despite this evidence, only a restricted number of omics studies have been published in recent decades. The objective of our work was to conduct a systematic review of the proteomic and metabolomic studies published thus far, aiming to identify potential new cellular targets, mediators, and mechanisms of cell activation and release. This approach seeks to enhance our understanding and identify potential new biomarkers for anaphylaxis.
2 Materials and methods
2.1 Eligibility criteria
A literature search was performed up to May 2023 using keywords “Proteome and Anaphylaxis” and “Metabolome and Anaphylaxis.” The inclusion criteria comprised: 1) original articles published in English; 2) studies on patients experiencing anaphylaxis; or experimental mechanistic studies using animal models in an anaphylaxis context; 3) analyses of proteome or metabolome performed on various types of samples. Exclusion criteria included: 1) review papers and publications in languages other than English; 2) absence of data on proteome or metabolome, and data not exclusively focused on the moment of anaphylaxis.
2.2 Data extraction
Data extraction followed an organized structure, including the following information when available: author, year, geographical region, sample type, phenotype and number of cases, phenotype and number of controls, primary aim, study method and main finding. Two investigators (AAG, RS) conducted separate assessments of the titles and abstracts for all citations found during the literature search. Any disagreements were settled through consensus.
2.3 Functional and pathways enrichment analyses
We performed an enrichment analysis on proteins showing differential expression levels related to anaphylaxis using Flame tool (v2.0) (23). We included data from all human (patients and in vitro) studies and excluded data from animal model studies. In our biological interpretation, we considered the outputs with a P-value < 0.05 in the categories of gene ontology biological process (GO-BP), gene ontology molecular function (GO-MF), and pathway terms (KEGG and REACTOME).
3 Results
3.1 Anaphylaxis and proteome
3.1.1 Literature search findings
The first search approach “anaphylaxis and proteome” yielded 98 citations. After excluding 91 studies, seven remained eligible for inclusion in this review (24–30). (Figure 1 and Supplementary Table 1). Among them, four assessed the proteome in human studies (24–27) and three evaluated the proteome using an animal model (28–30) (Table 1).
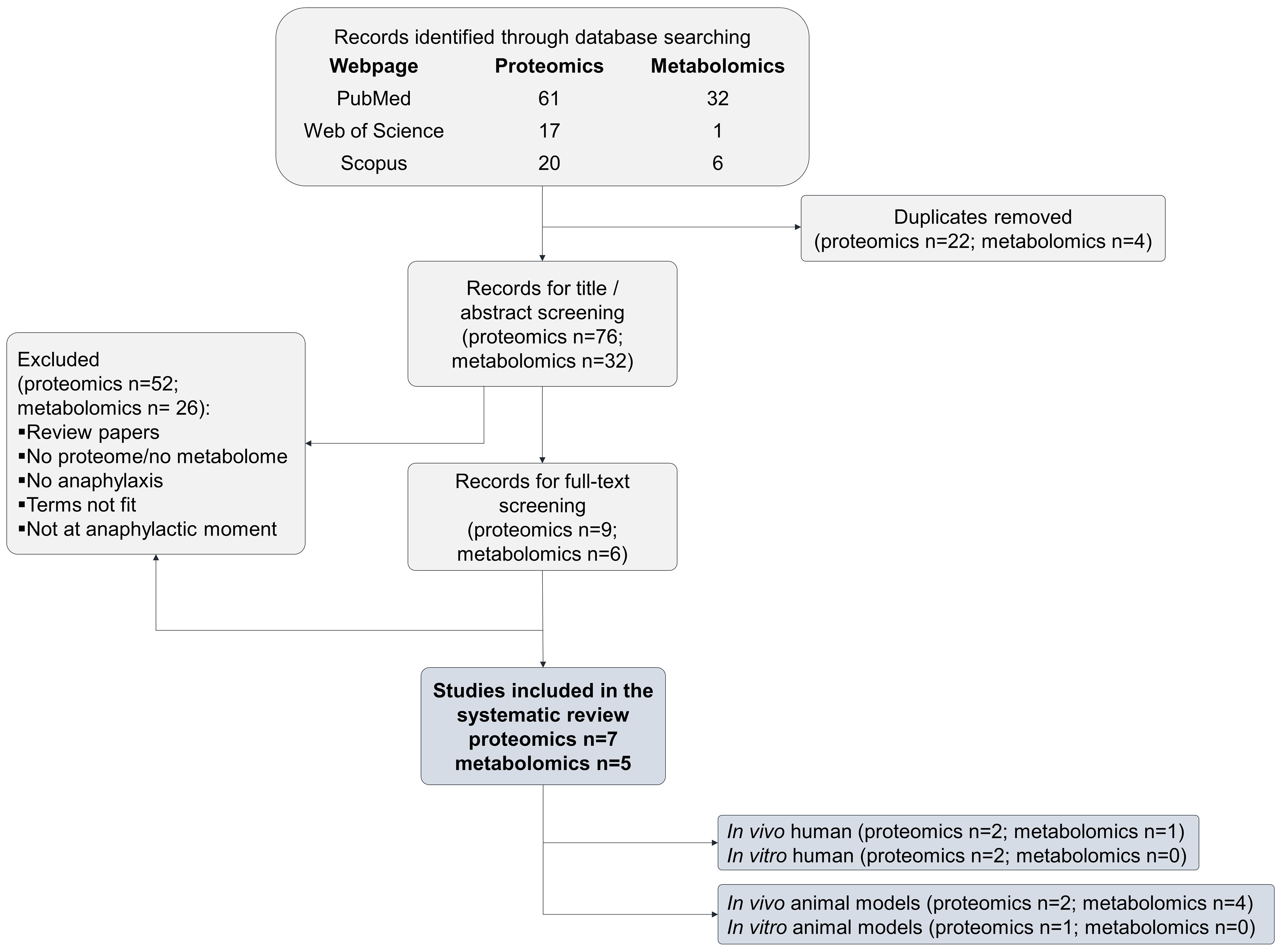
Figure 1 PRISMA flow diagram of the selection process for eligible articles in the systematic review.
1. Human studies:
Two studies assessed the proteome change in anaphylaxis in patients (24, 25). In the first study, the authors aimed to pinpoint specific biomarkers in plasma for predicting individuals with mastocytosis at a heightened risk of experiencing anaphylaxis. The study included 19 patients, with 11 allergic asthma patients as the control group and 13 volunteers without atopy. Using Proximity Extension Assay with Olink Proseek Multiplex panels, a total of 248 plasma proteins were analyzed. Among them, four proteins showed significant differences between patients with mastocytosis and anaphylaxis compared to those without anaphylaxis. Specifically, galectin-3 (Gal-3) was upregulated, while allergin-1 (MILR1), tryptase (TPSAB1), and pregnancy-associated plasma protein-A (PAPPA) were downregulated (24). In the second study conducted by Nunez-Borque et al. in 2021, the focus was on evaluating extracellular vesicles as a potential marker of anaphylaxis. Vesicles were purified from 43 patients at the initial phase of anaphylaxis and under normal conditions. In total, 83 proteins were increased significantly and 16 ones were decreased during the acute phase of anaphylaxis comparing to baseline. Three proteins, namely Cell Division Cycle 42 (CDC42), Ficolin-2 (FCN2), and S100 calcium-binding protein A9 (S100A9), were selected and replicated in other cohort with more samples (25).
Two other studies, conducted in vitro using human cells, have shown the changes in the proteome in anaphylaxis (26, 27). The first study demonstrated alterations in the protein expression pattern of human microvascular endothelial cells (ECs) using liquid chromatography coupled to mass spectrometry. In this study, ECs were exposed to sera from individuals experiencing anaphylaxis (EC-anaphylaxis) or from control (EC-control) for a duration of two hours, and the cellular responses were subsequently analyzed. The anaphylactic reactions in patients were triggered by antibiotics or NSAIDs. A total of 1,069 proteins exhibited significant differences between the EC-anaphylaxis and EC-control groups. Notably, 47 proteins showed the most substantial differences, with 38 being increased and 9 decreased. Functional enrichment analysis indicated the involvement of these proteins in blood coagulation, the complement cascade, transport, and extracellular matrix processes (26). In the second study, the aim was to elucidate the mechanism of Tween-80 in the anaphylactoid reaction using LAD2 mast cells. Cells were stimulated with Tween-80 for 30 minutes, and subsequently, label-free LCMS/MS-based proteomics was conducted. Bioinformatics analysis revealed that 313 proteins were upregulated, 111 proteins were downregulated in the Tween-80 group, and an additional 882 proteins were exclusively identified in Tween-80-treated cells. These proteins were implicated in different biological processes, including cellular processes, biological regulation, and metabolic processes, among others. Furthermore, several KEGG pathways involved in the anaphylactoid reactions were identified, such as endocytosis, cell adhesion molecules (CAMs), NF-κB signaling Pathway, and the calcium signaling pathway (27).
2. Animal model studies:
The proteome alteration under anaphylaxis condition has been assessed in two studies using an animal model (28, 29). The first study focused on anaphylactoid reaction to Xuesaitong injection (XSTI) that is a natural compound used in the Traditional Chinese Medicine, using a rat model. Samples were collected after 30 min of XSTI injection in two independent experiments. Proteome analysis allowed to identify 13 differentially expressed proteins in the XSTI group. Among these, F13b, Prdx2, Gpx1, Pklr, Ass1, and Asl were up-expressed, while F12, Cndp1, MYLPF, DCD, Kng1, C2, and C6 were down-expressed in the XSTI group. These findings suggest that XSTI triggers an anaphylactoid reaction through direct stimulation, complement activation, and involvement of the kallikrein–kinin pathway (28). In the second study, the aim was to discover protein biomarkers associated with food allergy in mice subjected to ovomucoid exposure (OVM). By Two-Dimensional Difference Gel Electrophoresis, three proteins Hp, SAA2, and PrxII were recognized as being upregulated during OVM-induced anaphylaxis. Although these proteins were detected during anaphylaxis, the authors propose that i) they could have been elevated before the challenge, possibly because of repeated gavages during sensitization and ii) it is yet uncertain if these proteins are implicated in the mechanism of anaphylaxis or if they are nonspecific proteins associated with bystander injury (29).
Finally, in a study by Hao et al. in 2022, potential anaphylactic effect of saponins were inquired using the RBL-2H3 rat cells. The study investigated differentially expressed proteins caused by several saponins, used in Chinese medicine and possessing distinct anaphylactic capabilities, following a 30-minute incubation with cells. The results indicated that the anaphylactoid reaction of saponins with strong anaphylactic abilities was associated with biological processes responding to organic cyclic compounds and cellular components of the endoplasmic reticulum and Golgi apparatus, which are closely linked to anaphylactoid reactions. Four downregulated proteins were uniquely identified as differentially expressed in the strong anaphylactic groups, suggesting their potential key roles in the anaphylactoid reactions of these cells. These proteins include A0A0G2JWQ0 (Gtf2h1), D3ZL85 (Hccs), D4A5G8 (Pdha1l1), and Q8K3F0 (UniProtKB unreviewed/TrEMBL) (30).
3.1.2 Meta-analysis of proteome data
Only the four human studies were included in this analysis (24–27). 118 proteins with different significant levels of expression were found in at least two studies (Figure 2 and Table 2), of which, two proteins were found alerted in three studies, Fibronectin (FINC, Gene name = FN1), Integrin beta-1 (ITB1, Gene name = ITGB1). Functional Enrichment Analysis using Flame showed 96 significant enriched GO_BP (Supplementary Table 2). Figure 3 shows an integrated network containing the ten top enriched terms connected to their overlapping proteins. It is involved in “GO:0002283~ neutrophil activation involved in immune response, GO:0002446~neutrophil mediated immunity, GO:0043312~neutrophil degranulation, GO:0002576~platelet degranulation”. In the same way, this analysis shows 21 significant enriched GO_MF (Supplementary Table 2). Figure 4 visualizes the connections between the top ten enriched terms and their proteins. It is included “GO:0003723~RNA binding, GO:0045296~cadherin binding, GO:0004866~ endopeptidase inhibitor activity, GO:0003697~single-stranded DNA binding, GO:0050543~ icosatetraenoic acid binding, GO:0050544~arachidonic acid binding”. In addition, pathway analysis identified enrichment for pathways related to innate and adaptive immune system, neutrophil degranulation, and platelet activation (Figure 5). Supplementary Table 2 presents enriched pathways, including the genes mapped to each pathway and their respective significance degrees.
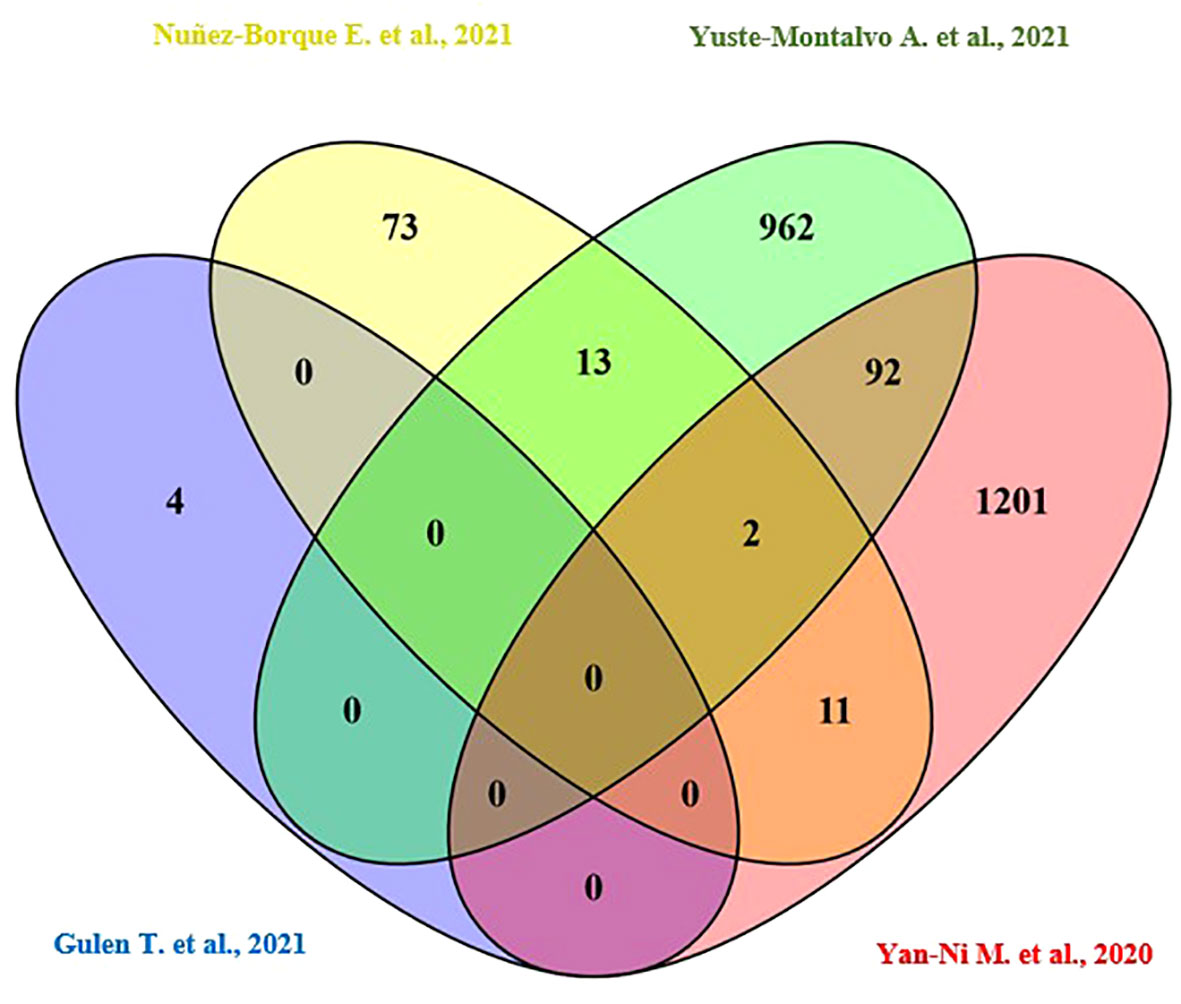
Figure 2 A Venn diagram is used to represent the number of common proteins with differential expression levels, revealing that 118 proteins were found in at least two human studies.
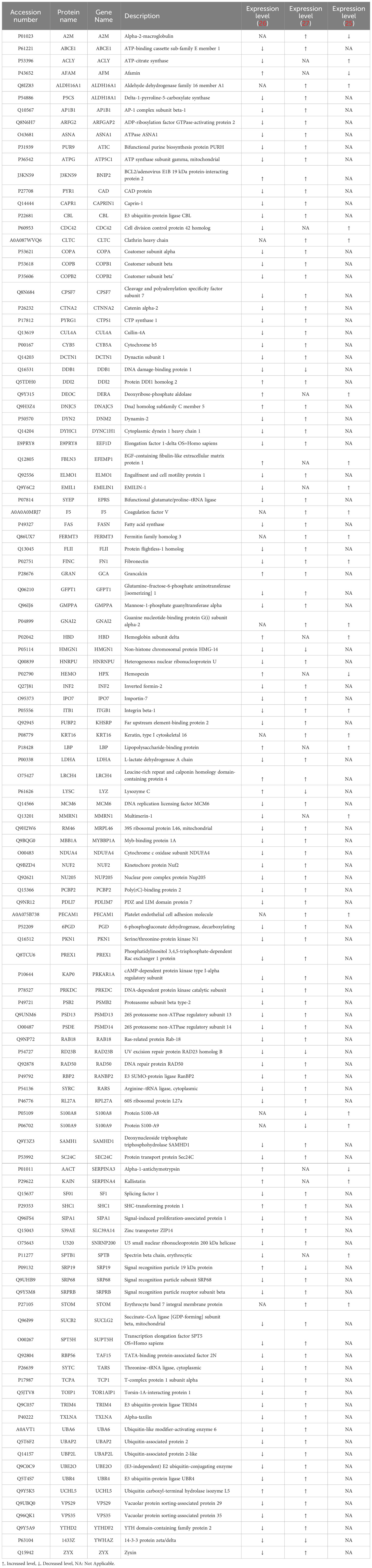
Table 2 List of proteins with altered expression levels in anaphylaxis conditions reported in at least two human studies.
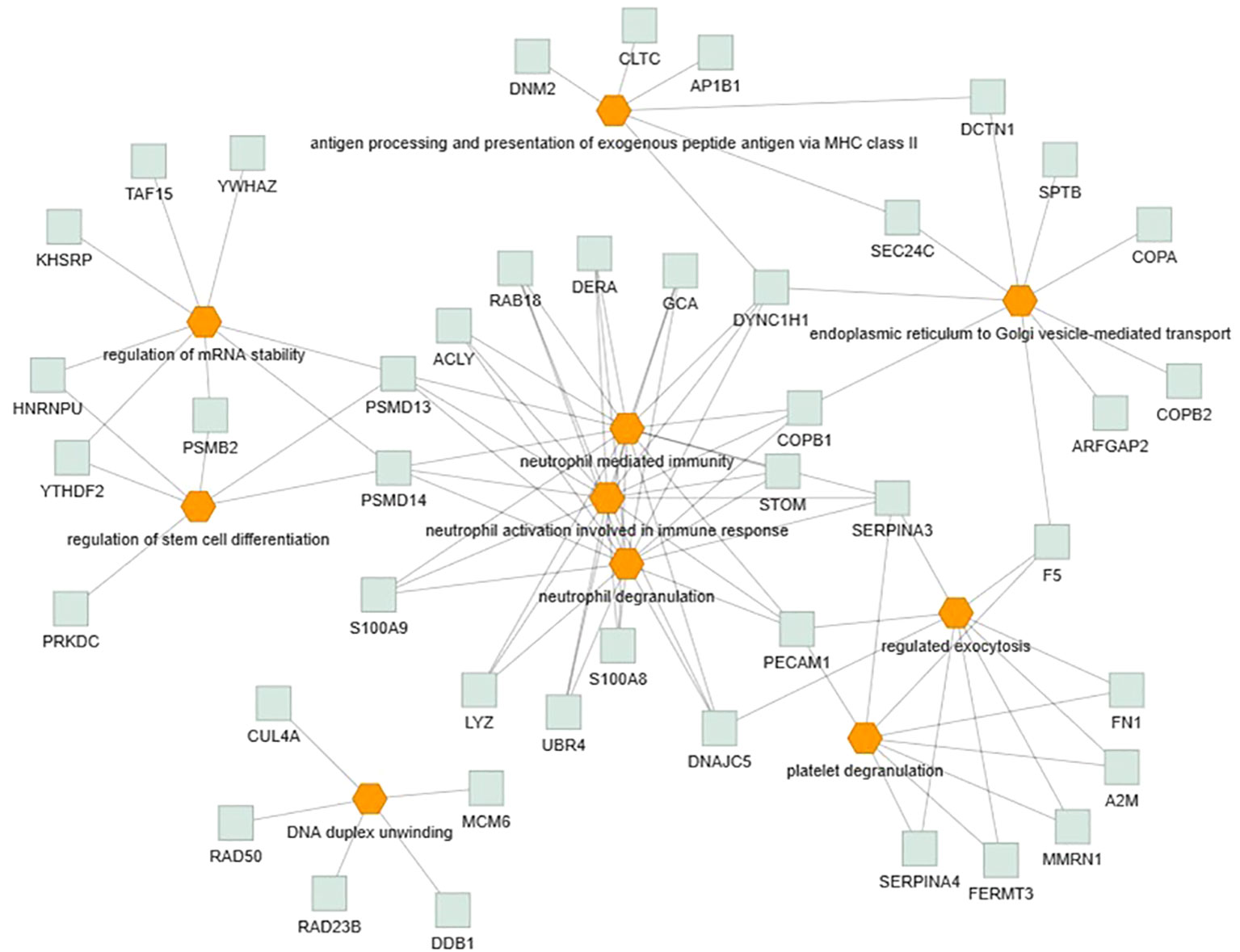
Figure 3 Functional enrichment analysis, using Flame database, of the 118 common proteins identified in this systematic review with an integrated network of the top ten enriched biological processes terms (P-value < 0.05). Orange = GO_BP, Grey = overlapping proteins within the GO term.
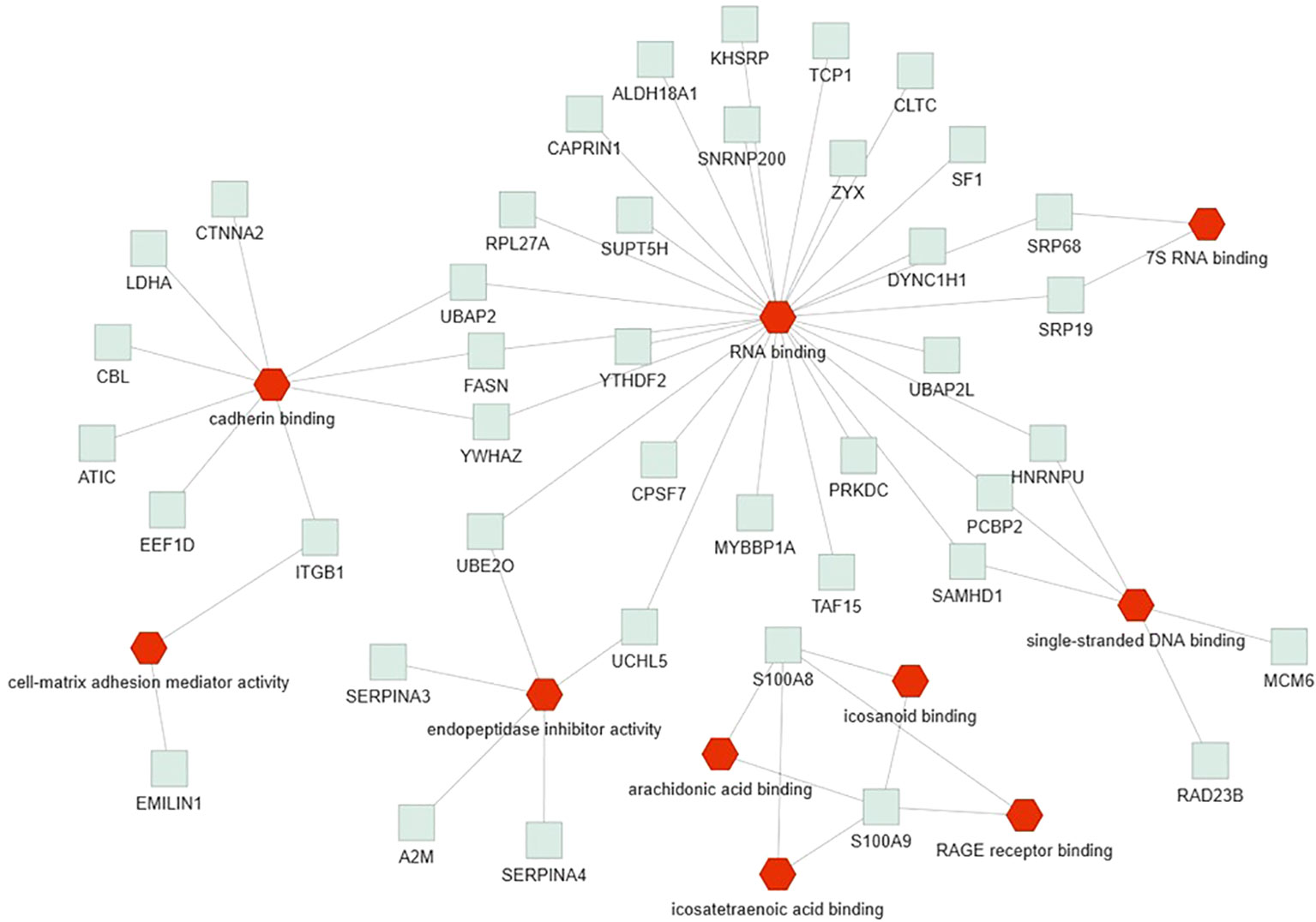
Figure 4 Functional enrichment analysis, using Flame database, of the 118 common proteins identified in this systematic review with an integrated network of the top ten enriched molecular function terms (P-value < 0.05). Red = GO_MF, Grey = overlapping proteins within the GO term.
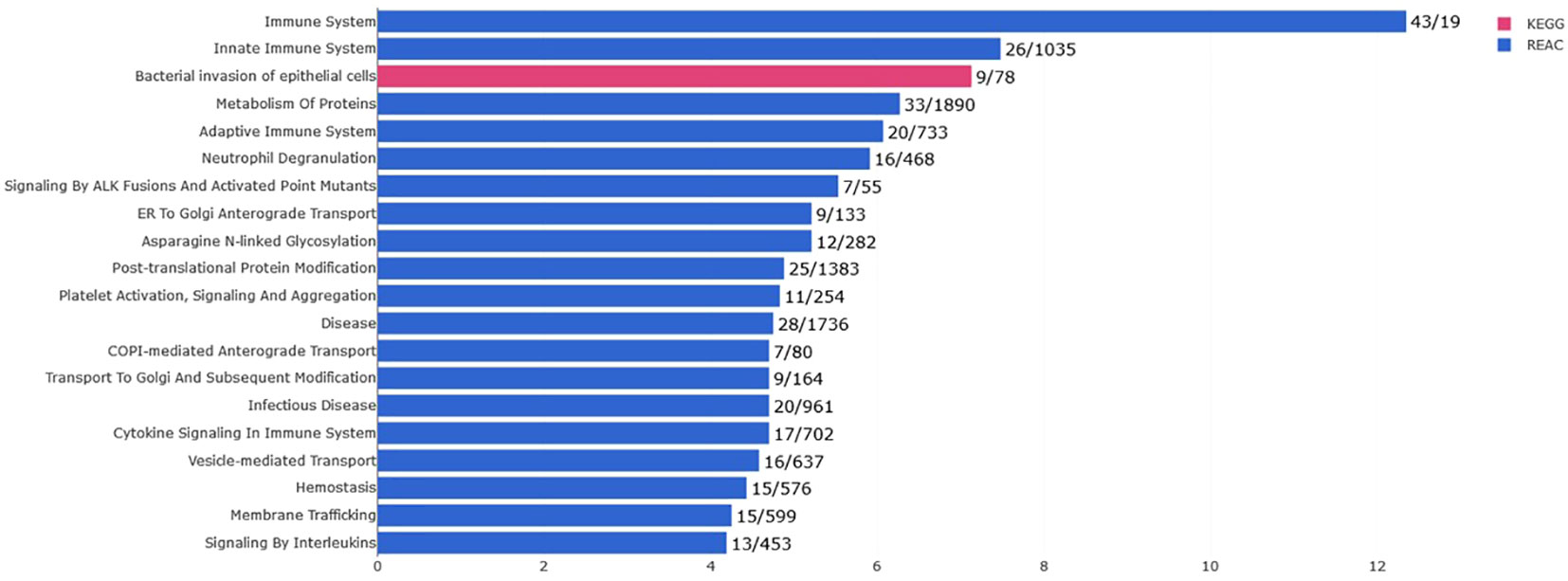
Figure 5 Pathway analysis of the 118 common proteins identified in this systematic review with the top 20 pathways (P-value < 0.05, KEGG in pink and REACTOME in blue).
3.2 Anaphylaxis and metabolome
3.2.1 Literature search findings
The first search approach “anaphylaxis and metabolome“ yielded 39 citations, and after excluding 34 studies, five ones remained eligible for inclusion in this review (28, 31–34). (Figure 1 and Supplementary Table 3) Among them, one assessed the metabolome in human studies (31) and four in animal models (28, 32–34) (Table 3).
1. Human studies
Only one study investigated changes in the metabolome of patients with anaphylaxis, focused on reactions triggered by food or drugs (31). Serum samples were collected from adult patients at three distinct time intervals: T1 at the onset of the reaction, T2 approximately 2 to 4 hours later, and T0 after a 2 to 3-month period serving as baseline state. Metabolic alterations were measured by employing ultra-performance liquid chromatography coupled with mass spectrometry (UPLC-MS) and proton nuclear magnetic resonance spectroscopy (H-NMR). The metabolomics profiles tracked the temporal evolution of anaphylaxis by comparing metabolites at the different time points and scrutinized the severity of the response at time points. Only food-induced anaphylaxis exhibited discernible metabolic alterations at T1 vs. T2, with a remarkable stratification of patients in principal component analysis. A total of 73 metabolites were identified as significantly altered, primarily involved in phospholipid-related pathways. A notable increase in acetate, phenylalanine, lysine, creatine, and glutamine at T1 was also reported.
Regarding the association with severity, this study identified more pronounced metabolic alterations in patients with moderate reactions at T1 compared to T2. Indeed, individuals with moderate reactions displayed heightened levels of choline, lactate, acetate, arginine, glutamine, isoleucine, leucine, valine, phenylalanine, proline, and creatinine and reduced levels of phosphatidylcholines (PCs) and phosphatidylethanolamines (PEs) at T1. Conversely, an increase in PEs and PCs metabolites was observed among patients with severe reactions at T1.
In addition, the study inquired the differences in metabolite profiles between two severity levels at the basal state T0 and at T1. Comparing the severe vs moderate groups showed an alteration of seven metabolites at T1 and ten metabolites at T0. Regardless of the time point, lipids and glucose levels were elevated in the severe group; Cortisol was increased at T1 and decreased at the baseline T0 (31).
2. Animal model studies
Four articles explored changes in metabolic profiles in animal models of anaphylaxis (28, 32–34). The first study evaluated the metabolome profile in anaphylaxis induced by Qingkailing injection (QKLI-IA) in a rat model (32). QKLI-IA is a traditional Chinese medicine employed for upper respiratory tract inflammation treatment. Metabolomics analysis was performed at early (10 minutes), intermediate (30 minutes), and late (120 minutes) phase of anaphylactic reaction using ultra-performance liquid chromatography coupled to quadrupole time-of-flight mass spectrometry in both positive and negative ion modes. The results revealed 58 potentially altered metabolites during the initial stages of the reaction, with 14 of them being as top-ranked candidates by statistical analysis, including principal component analysis and receiver operator characteristic curves. These include creatine, 15-Hydroxyeicosatetraenoic acid (15-HETE), LysoPC (18:1), Sphingosine 1-phosphate (S1P), 5-Hydroxyeicosatetraenoic acid (5-HETE), 12-Hydroxyeicosatetraenoic acid (12-HETE), arachidonic acid, palmitic acid, LysoPC (20:4), PC (16:0/22:6), PC (18:2/16:0), PC (18:0/22:6), PC (18:0/20:3) and diglyceride (DG) (20:4/15:0/0:0) with sensitivity and specificity exceeding 0.85 for each. These metabolites play roles in arachidonic acid, glycerophospholipid, fatty acid, unsaturated fatty acids, and amino acid pathways. In the intermediate phase, 59 potential biomarkers were identified. Notably, 13 of them exhibited significant distinctions between allergic and control rats, including tryptophan, sphinganine, PC (18:3/19:1), PC (22:6/18:0), tyramine, LysoPC (18:4), phosphocholine, SM (d18:1/24:1), and DG (18:0/20:4), ranking as top candidates with sensitivity and specificity exceeding 0.80. These metabolites were associated with glycerophospholipid metabolism, sphingolipid, tryptophan, tyrosine, alpha-linolenic acid, and linoleic acid pathways. In the late phase of QKLI-IA, 41 potential biomarkers were identified, linked to glycerophospholipids, linoleic acid, alpha-linolenic acid, and arachidonic acid pathways. A comparative analysis across the three stages uncovered 24 metabolites associated with arachidonic acid metabolism (32). Xu et al. evaluated the responses to ovalbumin and Compound 4880 in rats after 30 minutes of injection. The study reported changes in concentrations of Arachidonic acid, leukotriene A4 (LTA4), leukotriene B4 (LTB4), 5-HPETE, and 5-OxoETE. Additionally, significant concentration changes were observed for adenosine, histamine, N-acetylhistamine, N(α)-γ-glutamylhistamine, malate, and xanthine (33).
In another study, the same authors sought to elucidate anaphylactoid reactions triggered by the injection of an herbal medicine, Xuesaitong injection (XSTI). They identified 28 metabolites with increased levels of histamine, adenosine, adenine, uric acid, glutathione, leukotriene B4 (LTB4), citric acid, hippuric acid, N-acetylhistamine, N(α)-γ-l-glutamylhistamine, valine, 3-methylhistidine, isocitrate, oxalosuccinate, fumarate, and malate, and decreased levels of alanine, arginine, tryptophan, serine, aspartic acid, arachidonic acid, α-ketoglutaric acid, octadecanoic acid, LTA4, 5-hydroperoxyeicosatetraenoic acid (5-HPETE), indoleacrylic acid, and 1-pyrroline-2-carboxylic acid in the XSTI group. These metabolites are implicated in histidine, purine, and energy metabolisms (28). Metabolites analyzed by gas chromatography-mass spectrometry were evaluated in food anaphylaxis using guinea pigs challenged for one hour. The study included three groups: control, ovalbumin-sensitized, and cattle albumin-sensitized. A total of 22 metabolites showed significant differences in the cattle albumin, while 19 differed in the ovalbumin group compared to control. Nine variables were consistently altered in both treated groups. Notably, six were provisionally identified. Glucose, fumaric acid, L-arabitol were decreased, and n-pentadecanoic acid, (R)-3-hydroxybutyric acid, and myo-inositol were increased in the treated groups. These findings suggest a potential link between anaphylaxis, energy metabolism, and signaling processes (34).
3.2.2 Meta-analysis of metabolome data
We analyzed variations in metabolites in the five identified studies while considering the heterogeneity of the model type, trigger (food or drug), and different sampling time points, resulting in the identification of 13 common metabolites (Table 4). Specifically, we observed consistent downregulation across studies by Perales-Chorda C et al. and Gao X. et al. of a metabolite, LysoPC(18:0) lysophosphatidylcholines. Furthermore, we also found upregulation in both studies by Xu Y et al. for metabolites implicated in the Krebs cycle, including isocitrate, oxalosuccinate, fumarate, and malate.
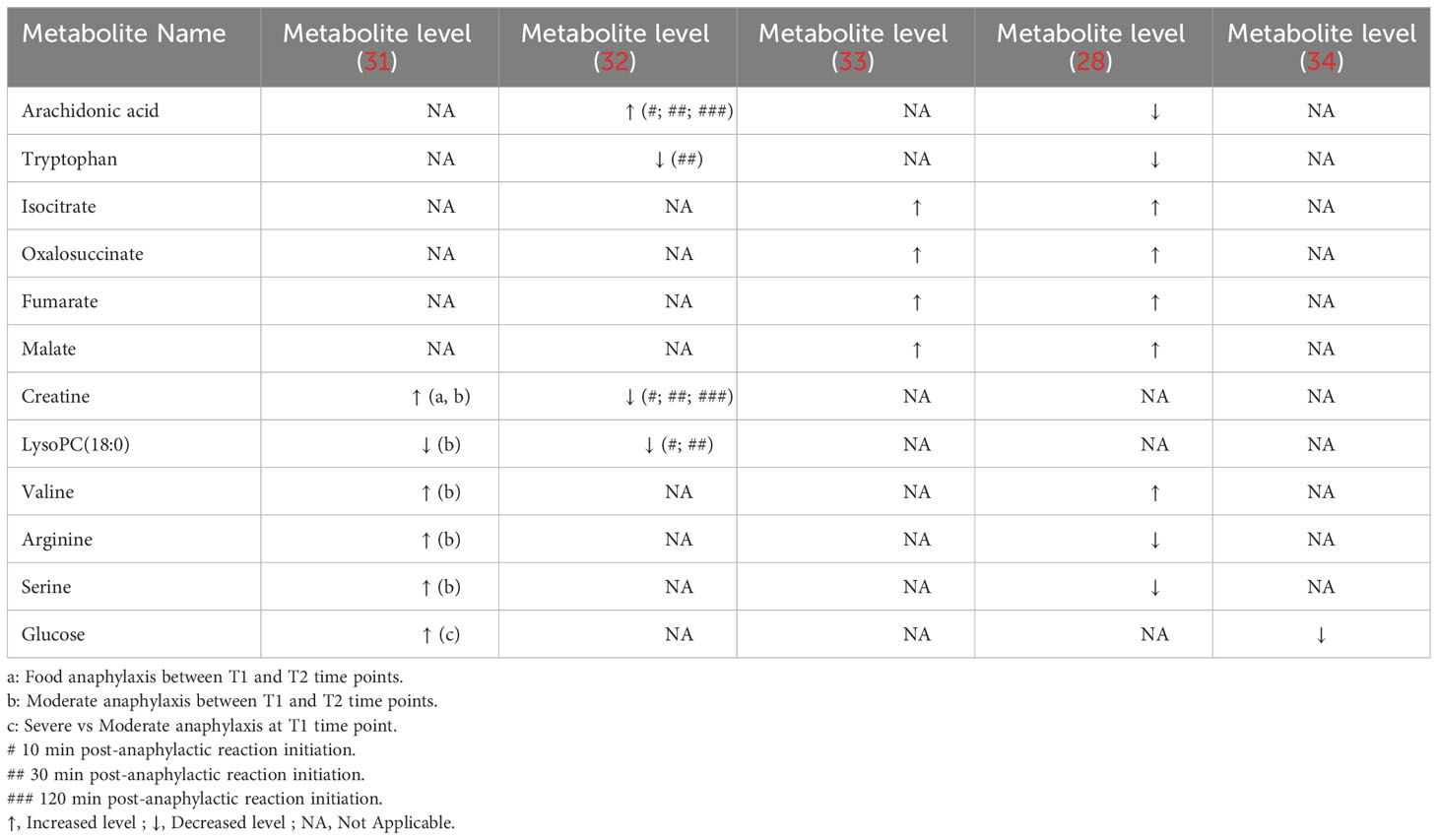
Table 4 List of metabolites with altered levels in anaphylaxis conditions reported in at least two studies.
4 Discussion
This systematic review identified solely twelve eligible publications, encompassing seven studies assessing proteome analysis in anaphylaxis context, as well as five publications that investigated the association between anaphylaxis and the metabolome. In a study conducted by Yuste-Montalvo A. et al. in 2021, protein patterns in endothelial cell model were identified after exposure to serum from patients in during the acute phase of an anaphylactic reaction (26). The study sheds light on the significance of the coagulation, fibrinolytic and complement systems in human anaphylaxis. Specifically, the activation of the coagulation and fibrinolytic systems frequently occurs simultaneously with the activation of the complement system, serving as a trigger of anaphylaxis (35). Another study suggests that the allergen, like Tween-80, could potentially trigger mediator release after endocytosis (27). This internalization process activates degranulation through the phosphoinositide phospholipase Cγ (PLCγ)/protein kinase C (PKC) pathways, inducing calcium influx and inflammatory mediators release via the NF-κB pathway (27). Indeed, endocytosis is recognized as an important mechanism for the internalization of allergic agents or stimulants by mast cells. Receptor-mediated endocytosis in mast cells involves various receptors, such as FcϵRI (36), Mas-related G protein-coupled receptors X2 (MRGPRX2) (37), and epidermal growth factor receptor (EGFR) (38). The activation of mast cells leads to: i) increases the expression of cell adhesion molecules, thereby facilitating the communication and recruitment of other cells during inflammation and hypersensitivity (39), ii) the release of both preformed and newly synthesized inflammatory mediators. Nuclear translocation, involving the Mitogen activated protein kinase (MAPK) pathway and the nuclear factor kappa light chain enhancer of activated B cells (NF-κB) pathway, plays a crucial role in the synthesis of new inflammatory mediators (40, 41).
Despite variations in research protocols, allergen types, study models, and bioinformatic analyses across the human studies included in this review, we identified 118 proteins present in at least two independent studies. The biological processes involved in this protein set are focused on immune response and neutrophil function. Data from diverse studies suggests that anaphylactic reactions cannot be exclusively attributed to mast cells and basophils as the principal cellular contributors. Neutrophils and macrophages activation has also been associated with severe allergic reactions, especially those induced by food allergens (42–44). Do et al. published a transcriptomic and epigenomic study in which they found four groups of CpGs and genes, associated with the activities of macrophage and neutrophil in immunity, in relation with the severity of reactions to peanut (43). Indeed, the activation of neutrophils can be initiated by the recognition of food antigens through the binding of IgG to Fc gamma receptors (45), resulting in the release of PAF. This mechanism implicates neutrophils in the pathogenesis of anaphylaxis (46, 47). Jönsson F. et al. provide evidence supporting the role of IgG neutrophil PAF pathway in anaphylaxis provoked by neuromuscular-blocking agents in humans. This pathway may exacerbate anaphylaxis in conjunction with the IgE pathway or potentially underlie anaphylaxis in situations where specific IgE is not present (48). When activated, neutrophils might be capable of triggering anaphylactic symptoms due to their rapid release of potent lipid and protein mediators. Additionally, they can expel their nuclear and mitochondrial DNA, in the form of Neutrophil Extracellular Traps (NETs) through a process called NETosis (49, 50). In addition, our analysis revealed that among the most significant molecular function enriched within the common proteins are icosatetraenoic acid binding and arachidonic acid (AA) binding. Furthermore, AA was significantly altered in two studies (28, 32) after 10, 30 and 120 min post-anaphylactic reaction initiation to QKLI-IA (32). AA acts as the initial substance for producing eicosanoids, powerful inflammation mediators associated with the development of diverse diseases (51, 52). Eicosanoids are primarily produced through the enzymatic actions of cyclooxygenase (also known as prostaglandin endoperoxide synthase), responsible for generating prostaglandins and thromboxane, as well as 5-lipoxygenase, leading to the synthesis of leukotrienes that can contribute to the symptoms of anaphylaxis, such as bronchoconstriction and augmented vascular permeability (52, 53). In the context of QKLI-IA drug, the dynamics of metabolic changes across different stages were illuminated, with specific biomarkers exhibiting high predictive ability. These markers also reveal insights into glycerophospholipid, sphingolipid, and tryptophan metabolism pathways (32). Altered levels of tryptophan were also found in another study in anaphylactic reactions to the XSTI drug (28). Tryptophan, an indispensable aromatic amino acid, undergoes metabolism via three primary pathways: the direct conversion by the gut microbiota, the kynurenine pathway in various immune and epithelial cells, and the transformation into serotonin (54). It is important to note that diagnosing anaphylaxis relies on clinical symptoms since there are no definitive biomarkers available. Biomarkers like serum tryptase and histamine may support the diagnosis, but they are not universally altered in all cases and have limitations in sensitivity and specificity. Despite its potential, metabolomics remains underutilized in human anaphylaxis research due to the unpredictability of anaphylactic events and ethical constraints in controlled settings.
Collectively, our systematic review enriches our understanding of anaphylactic reactions, reveals potential biomarkers, and underscores the intricate interplay between clinical manifestations and underlying metabolic processes, paving the way for improved diagnosis, prevention, and management of allergic reactions. Nevertheless, it is important to acknowledge several limitations in this review and analysis. Firstly, the number of published studies available for analysis was relatively small. Secondly, the studies exhibit distinct designs concerning sample types (e.g., patient serum, different cell cultures), allergen triggers (e.g., food, drug antigens), and bio-informatics analyses (e.g., different tools, settings, thresholds). Thirdly, a majority of these studies used small sample sizes and their results are not replicated. In future studies, systems biology network models that can integrate multiple types of omics data could guide the interpretation of proteomic and metabolomic changes associated with anaphylaxis in their metabolic context. For example, Yuste-Montalvo et al. constructed a network of protein-protein interactions from proteomics data of endothelial cells in anaphylaxis patients and controls (26). This functional enrichment analysis identified blood coagulation and the complement cascade as the main altered pathways in anaphylaxis (26).
5 Conclusion
Anaphylaxis is a complex and multifaceted allergic response that involves various mediators and pathways, and research in this field continues to uncover new insights. This systematic review emphasizes alterations in the proteome and metabolome under anaphylactic conditions. Several pathogenic pathways have been highlighted, including neutrophil activation, platelet degranulation, and arachidonic acid binding. However, these findings, derived from a limited number of publications, necessitate confirmation in larger human studies and could contribute to the development of new biomarkers for anaphylaxis.
Data availability statement
The original contributions presented in the study are included in the article/Supplementary Material. Further inquiries can be directed to the corresponding authors.
Author contributions
AG: Conceptualization, Formal analysis, Methodology, Writing – original draft. AH: Writing – review & editing, Supervision. R-MG-R: Writing – review & editing. JLG: Conceptualization, Funding acquisition, Supervision, Validation, Visualization, Writing – review & editing. RS: Conceptualization, Formal analysis, Methodology, Supervision, Validation, Writing – review & editing.
Funding
The author(s) declare financial support was received for the research, authorship, and/or publication of this article. This work was performed with funding from the DrNanoDALL - Funded project of EuroNanoMed EU program.
Conflict of interest
The authors declare that the research was conducted in the absence of any commercial or financial relationships that could be construed as a potential conflict of interest.
Publisher’s note
All claims expressed in this article are solely those of the authors and do not necessarily represent those of their affiliated organizations, or those of the publisher, the editors and the reviewers. Any product that may be evaluated in this article, or claim that may be made by its manufacturer, is not guaranteed or endorsed by the publisher.
Supplementary material
The Supplementary Material for this article can be found online at: https://www.frontiersin.org/articles/10.3389/fimmu.2024.1328212/full#supplementary-material
Supplementary Table 1 | Excluded proteomics studies.
Supplementary Table 2 | Functional enrichment and pathway analyses using Flame database: gene ontology biological process (GO-BP), gene ontology molecular function (GO-MF) and pathways (KEGG and REACTOME) terms with a P-value < 0.05.
Supplementary Table 3 | Excluded metabolomics studies.
References
1. Turner PJ, Worm M, Ansotegui IJ, El-Gamal Y, Rivas MF, Fineman S, et al. Time to revisit the definition and clinical criteria for anaphylaxis? World Allergy Organ J (2019) 12:100066. doi: 10.1016/j.waojou.2019.100066
2. Descotes J, Choquet-Kastylevsky G. Gell and Coombs’s classification: is it still valid? Toxicology (2001) 158:43–9. doi: 10.1016/s0300-483x(00)00400-5
3. Lieberman P, Nicklas RA, Randolph C, Oppenheimer J, Bernstein D, Bernstein J, et al. Anaphylaxis–a practice parameter update 2015. Ann Allergy Asthma Immunol (2015) 115:341–84. doi: 10.1016/j.anai.2015.07.019
4. Tejedor Alonso MA, Moro Moro M, Mugica Garcia MV. Epidemiology of anaphylaxis. Clin Exp Allergy (2015) 45:1027–39. doi: 10.1111/cea.12418
5. Lieberman P. Epidemiology of anaphylaxis. Curr Opin Allergy Clin Immunol (2008) 8:316–20. doi: 10.1097/ACI.0b013e3283036a69
6. Cardona V, Ansotegui IJ, Ebisawa M, El-Gamal Y, Fernandez Rivas M, Fineman S, et al. World allergy organization anaphylaxis guidance 2020. World Allergy Organ J (2020) 13:100472. doi: 10.1016/j.waojou.2020.100472
7. Turner PJ, Jerschow E, Umasunthar T, Lin R, Campbell DE, Boyle RJ. Fatal anaphylaxis: mortality rate and risk factors. J Allergy Clin Immunol Pract (2017) 5:1169–78. doi: 10.1016/j.jaip.2017.06.031
8. Jerschow E, Lin RY, Scaperotti MM, McGinn AP. Fatal anaphylaxis in the United States, 1999-2010: temporal patterns and demographic associations. J Allergy Clin Immunol (2014) 134:1318–28.e7. doi: 10.1016/j.jaci.2014.08.018
9. Kuruvilla M, Khan DA. Anaphylaxis to drugs. Immunol Allergy Clin North Am (2015) 35:303–19. doi: 10.1016/j.iac.2015.01.008
10. Brown SG. Clinical features and severity grading of anaphylaxis. J Allergy Clin Immunol (2004) 114:371–6. doi: 10.1016/j.jaci.2004.04.029
11. Brown SG, Blackman KE, Heddle RJ. Can serum mast cell tryptase help diagnose anaphylaxis? Emerg Med Australas (2004) 16:120–4. doi: 10.1111/j.1742-6723.2004.00562.x
12. Peavy RD, Metcalfe DD. Understanding the mechanisms of anaphylaxis. Curr Opin Allergy Clin Immunol (2008) 8:310–5. doi: 10.1097/ACI.0b013e3283036a90
13. Bischoff SC. Role of mast cells in allergic and non-allergic immune responses: comparison of human and murine data. Nat Rev Immunol (2007) 7:93–104. doi: 10.1038/nri2018
14. Schuch A, Brockow K. Mastocytosis and anaphylaxis. Immunol Allergy Clin North Am (2017) 37:153–64. doi: 10.1016/j.iac.2016.08.017
15. Ben-Shoshan M, Clarke AE. Anaphylaxis: past, present and future. Allergy (2011) 66:1–14. doi: 10.1111/j.1398-9995.2010.02422.x
16. Maruo K, Akaike T, Ono T, Okamoto T, Maeda H. Generation of anaphylatoxins through proteolytic processing of C3 and C5 by house dust mite protease. J Allergy Clin Immunol (1997) 100:253–60. doi: 10.1016/s0091-6749(97)70233-1
17. Stone SF, Brown SG. Mediators released during human anaphylaxis. Curr Allergy Asthma Rep (2012) 12:33–41. doi: 10.1007/s11882-011-0231-6
18. Finkelman FD. Anaphylaxis: lessons from mouse models. J Allergy Clin Immunol (2007) 120:506–15; quiz 516–7. doi: 10.1016/j.jaci.2007.07.033
19. Bermejo N, Gueant JL, Mata E, Gerard P, Moneret-Vautrin DA, Laxenaire MC. Platelet serotonin is a mediator potentially involved in anaphylactic reaction to neuromuscular blocking drugs. Br J Anaesth (1993) 70:322–5. doi: 10.1093/bja/70.3.322
20. Nunez-Borque E, Fernandez-Bravo S, Yuste-Montalvo A, Esteban V. Pathophysiological, cellular, and molecular events of the vascular system in anaphylaxis. Front Immunol (2022) 13:836222. doi: 10.3389/fimmu.2022.836222
21. Radzikowska U, Baerenfaller K, Cornejo-Garcia JA, Karaaslan C, Barletta E, Sarac BE, et al. Omics technologies in allergy and asthma research: An EAACI position paper. Allergy (2022) 77:2888–908. doi: 10.1111/all.15412
22. Devonshire A, Gautam Y, Johansson E, Mersha TB. Multi-omics profiling approach in food allergy. World Allergy Organ J (2023) 16:100777. doi: 10.1016/j.waojou.2023.100777
23. Karatzas E, Baltoumas FA, Aplakidou E, Kontou PI, Stathopoulos P, Stefanis L, et al. Flame (v2.0): advanced integration and interpretation of functional enrichment results from multiple sources. Bioinformatics (2023) 39. doi: 10.1093/bioinformatics/btad490
24. Gulen T, Teufelberger A, Ekoff M, Westerberg CM, Lyberg K, Dahlen SE, et al. Distinct plasma biomarkers confirm the diagnosis of mastocytosis and identify increased risk of anaphylaxis. J Allergy Clin Immunol (2021) 148:889–94. doi: 10.1016/j.jaci.2021.02.023
25. Nunez-Borque E, Fernandez-Bravo S, Pastor-Vargas C, Alvarez-Llamas G, Gutierrez-Blazquez MD, Alwashali E, et al. Proteomic profile of extracellular vesicles in anaphylaxis and their role in vascular permeability. Allergy (2021) 76:2276–9. doi: 10.1111/all.14792
26. Yuste-Montalvo A, Fernandez-Bravo S, Oliva T, Pastor-Vargas C, Betancor D, Goikoetxea MJ, et al. Proteomic and biological analysis of an in vitro human endothelial system in response to drug anaphylaxis. Front Immunol (2021) 12:692569. doi: 10.3389/fimmu.2021.692569
27. Mi YN, Yan PP, Di J, Cao L, Xiao X, Liu DZ, et al. Label-free quantitative proteomic profiling of LAD2 mast cell releasates reveals the mechanism of tween-80-induced anaphylactoid reaction. Proteomics Clin Appl (2020) 14:e1900065. doi: 10.1002/prca.201900065
28. Xu Y, Dou D, Ran X, Liu C, Chen J. Integrative analysis of proteomics and metabolomics of anaphylactoid reaction induced by Xuesaitong injection. J Chromatogr A (2015) 1416:103–11. doi: 10.1016/j.chroma.2015.09.019
29. Hobson DJ, Rupa P, Diaz GJ, Zhang H, Yang M, Mine Y, et al. Proteomic analysis of ovomucoid hypersensitivity in mice by two-dimensional difference gel electrophoresis (2D-DIGE). Food Chem Toxicol (2007) 45:2372–80. doi: 10.1016/j.fct.2007.06.039
30. Hao F, Pang X, Xu K, Wang M, Ma Z, Tan H, et al. Anaphylactic rare saponins separated from Panax notoginseng saponin and a proteomic approach to their anaphylactic mechanism. Evid Based Complement Alternat Med (2022) 2022:7565177. doi: 10.1155/2022/7565177
31. Perales-Chorda C, Obeso D, Twomey L, Rojas-Benedicto A, PuChades-Carrasco L, Roca M, et al. Characterization of anaphylaxis reveals different metabolic changes depending on severity and triggers. Clin Exp Allergy (2021) 51:1295–309. doi: 10.1111/cea.13991
32. Gao X, Qin L, Zhang Z, Zhao H, Zhou W, Xie Z, et al. Deciphering biochemical basis of Qingkailing injection-induced anaphylaxis in a rat model by time-dependent metabolomic profiling based on metabolite polarity-oriented analysis. J Ethnopharmacol (2018) 225:287–96. doi: 10.1016/j.jep.2018.07.013
33. Xu Y, Guo N, Dou D, Ran X, Liu C. Metabolomics analysis of anaphylactoid reaction reveals its mechanism in a rat model. Asian Pac J Allergy Immunol (2017) 35:224–32. doi: 10.12932/AP0845
34. Hu X, Wu GP, Zhang MH, Pan SQ, Wang RR, Ouyang JH, et al. GC-MS-based metabolic profiling reveals metabolic changes in anaphylaxis animal models. Anal Bioanal Chem (2012) 404:887–93. doi: 10.1007/s00216-012-6129-x
35. Guilarte M, Sala-Cunill A, Luengo O, Labrador-Horrillo M, Cardona V. The mast cell, contact, and coagulation system connection in anaphylaxis. Front Immunol (2017) 8:846. doi: 10.3389/fimmu.2017.00846
36. Mazucato VM, Silveira ESAM, Nicoletti LM, Jamur MC, Oliver C. GD1b-derived gangliosides modulate FcepsilonRI endocytosis in mast cells. J Histochem Cytochem (2011) 59:428–40. doi: 10.1369/0022155411400868
37. Murakami T, Suzuki K, Niyonsaba F, Tada H, Reich J, Tamura H, et al. MrgX2−mediated internalization of LL−37 and degranulation of human LAD2 mast cells. Mol Med Rep (2018) 18:4951–9. doi: 10.3892/mmr.2018.9532
38. Wee P, Wang Z. Regulation of EGFR endocytosis by CBL during mitosis. Cells (2018) 7. doi: 10.3390/cells7120257
39. Mekori YA, Metcalfe DD. Mast cell-T cell interactions. J Allergy Clin Immunol (1999) 104:517–23. doi: 10.1016/s0091-6749(99)70316-7
40. Kim HI, Hong SH, Ku JM, Kang S, Kim TY, Shin YC, et al. Tonggyu-tang, a traditional Korean medicine, suppresses pro-inflammatory cytokine production through inhibition of MAPK and NF-kappaB activation in human mast cells and keratinocytes. BMC Complement Altern Med (2017) 17:186. doi: 10.1186/s12906-017-1704-5
41. Kim SH, Shin TY. Anti-inflammatory effect of leaves of Eriobotrya japonica correlating with attenuation of p38 MAPK, ERK, and NF-kappaB activation in mast cells. Toxicol In Vitro (2009) 23:1215–9. doi: 10.1016/j.tiv.2009.07.036
42. Crespo JF, Cabanillas B. Recent advances in cellular and molecular mechanisms of IgE-mediated food allergy. Food Chem (2023) 411:135500. doi: 10.1016/j.foodchem.2023.135500
43. Do AN, Watson CT, Cohain AT, Griffin RS, Grishin A, Wood RA, et al. Dual transcriptomic and epigenomic study of reaction severity in peanut-allergic children. J Allergy Clin Immunol (2020) 145:1219–30. doi: 10.1016/j.jaci.2019.10.040
44. Tordesillas L, Rahman AH, Hartmann BM, Sampson HA, Berin MC. Mass cytometry profiling the response of basophils and the complete peripheral blood compartment to peanut. J Allergy Clin Immunol (2016) 138:1741–44.e9. doi: 10.1016/j.jaci.2016.06.048
45. Jonsson F, Mancardi DA, Zhao W, Kita Y, Iannascoli B, Khun H, et al. Human FcgammaRIIA induces anaphylactic and allergic reactions. Blood (2012) 119:2533–44. doi: 10.1182/blood-2011-07-367334
46. Jouvin-Marche E, Ninio E, Beaurain G, Tence M, Niaudet P, Benveniste J. Biosynthesis of Paf-acether (platelet-activating factor). VII. Precursors of Paf-acether and acetyl-transferase activity in human leukocytes. J Immunol (1984) 133:892–8.
47. Lotner GZ, Lynch JM, Betz SJ, Henson PM. Human neutrophil-derived platelet activating factor. J Immunol (1980) 124:676–84.
48. Jonsson F, de Chaisemartin L, Granger V, Gouel-Cheron A, Gillis CM, Zhu Q, et al. An IgG-induced neutrophil activation pathway contributes to human drug-induced anaphylaxis. Sci Transl Med (2019) 11. doi: 10.1126/scitranslmed.aat1479
49. Papayannopoulos V. Neutrophil extracellular traps in immunity and disease. Nat Rev Immunol (2018) 18:134–47. doi: 10.1038/nri.2017.105
50. Jimenez-Saiz R. Drug-induced IgG-neutrophil-mediated anaphylaxis in humans: Uncovered! Allergy (2020) 75:484–5. doi: 10.1111/all.14118
51. Yui K, Imataka G, Nakamura H, Ohara N, Naito Y. Eicosanoids derived from Arachidonic acid and their family prostaglandins and cyclooxygenase in psychiatric disorders. Curr Neuropharmacol (2015) 13:776–85. doi: 10.2174/1570159x13666151102103305
52. Serrano-Mollar A, Closa D. Arachidonic acid signaling in pathogenesis of allergy: therapeutic implications. Curr Drug Targets Inflammation Allergy (2005) 4:151–5. doi: 10.2174/1568010053586354
53. Reber LL, Hernandez JD, Galli SJ. The pathophysiology of anaphylaxis. J Allergy Clin Immunol (2017) 140:335–48. doi: 10.1016/j.jaci.2017.06.003
Keywords: anaphylaxis, omics studies, proteome, metabolome, neutrophil, platelets, arachidonic acid, icosatetraenoic acid
Citation: Gallizzi AA, Heinken A, Guéant-Rodriguez R-M, Guéant J-L and Safar R (2024) A systematic review and meta-analysis of proteomic and metabolomic alterations in anaphylaxis reactions. Front. Immunol. 15:1328212. doi: 10.3389/fimmu.2024.1328212
Received: 26 October 2023; Accepted: 11 January 2024;
Published: 07 February 2024.
Edited by:
Hong Zan, Prellis Biologics, United StatesReviewed by:
Laura Berron Ruiz, National Institute of Pediatrics, MexicoJosé Antonio Cornejo-García, University of Malaga, Spain
Copyright © 2024 Gallizzi, Heinken, Guéant-Rodriguez, Guéant and Safar. This is an open-access article distributed under the terms of the Creative Commons Attribution License (CC BY). The use, distribution or reproduction in other forums is permitted, provided the original author(s) and the copyright owner(s) are credited and that the original publication in this journal is cited, in accordance with accepted academic practice. No use, distribution or reproduction is permitted which does not comply with these terms.
*Correspondence: Jean-Louis Guéant, amVhbi1sb3Vpcy5ndWVhbnRAdW5pdi1sb3JyYWluZS5mcg==; Ramia Safar, cmFtaWEuYWxzYWllZEB1bml2LWxvcnJhaW5lLmZy
†These authors have contributed equally to this work and share last authorship