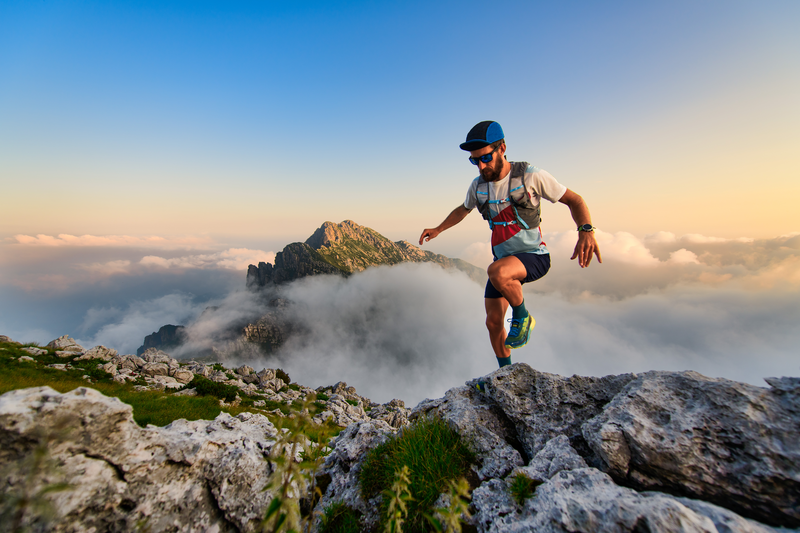
95% of researchers rate our articles as excellent or good
Learn more about the work of our research integrity team to safeguard the quality of each article we publish.
Find out more
REVIEW article
Front. Immunol. , 01 February 2024
Sec. Autoimmune and Autoinflammatory Disorders : Autoimmune Disorders
Volume 15 - 2024 | https://doi.org/10.3389/fimmu.2024.1291556
This article is part of the Research Topic The mechanisms of autoimmune diseases and chronic inflammatory diseases under exposure to environmental pollutants View all 5 articles
Vitiligo is an acquired autoimmune dermatosis characterized by patchy skin depigmentation, causing significant psychological distress to the patients. Genetic susceptibility, environmental triggers, oxidative stress, and autoimmunity contribute to melanocyte destruction in vitiligo. Due to the diversity and complexity of pathogenesis, the combination of inhibiting melanocyte destruction and stimulating melanogenesis gives the best results in treating vitiligo. The aryl hydrocarbon receptor (AhR) is a ligand-activated transcription factor that can regulate the expression of various downstream genes and play roles in cell differentiation, immune response, and physiological homeostasis maintenance. Recent studies suggested that AhR signaling pathway was downregulated in vitiligo. Activation of AhR pathway helps to activate antioxidant pathways, inhibit abnormal immunity response, and upregulate the melanogenesis gene, thereby protecting melanocytes from oxidative stress damage, controlling disease progression, and promoting lesion repigmentation. Here, we review the relevant literature and summarize the possible roles of the AhR signaling pathway in vitiligo pathogenesis and treatment, to further understand the links between the AhR and vitiligo, and provide new potential therapeutic strategies.
Vitiligo is a common depigmentation disease characterized by white macules and patches with distinct margins (1). It was reported that approximately 0.5-2% of the population worldwide is affected, and more than half of the patients develop the disease between the ages of 10 and 30 years (2). Although genetic susceptibility, environmental triggers, oxidative stress, autoimmunity, and neural hypothesis are recognized as the pathogenic factors (3), the pathogenesis of vitiligo is incompletely clarified and there is no satisfactory therapy available right now.
Aryl hydrocarbon receptor (AhR) is a nuclear transcription factor activated by exogenous and endogenous ligands. In the canonical pathway, cytoplasmic AhR binds ligands and translocates into the nucleus, subsequently combining with the aryl hydrocarbon receptor nuclear translocator (ARNT). The AhR/ARNT complex recognizes xenobiotic regulative elements (XREs) and mediates numerous toxicological or biological effects by modulating the transcription of various downstream genes (4). Besides, AhR can also regulate the gene expression through non-canonical signaling pathways, such as nuclear factor-κB (NF- κB), Krüppel-like factor 6 (KLF6), and the estrogen receptor (ESR) (5–7). Accumulating evidence has evinced that AhR is highly expressed in the skin and plays important roles in regulating epidermal barrier differentiation, cellular homeostasis, pigment synthesis, and skin immunity (8–10). In recent years, the role of AhR in the pathogenesis of various skin diseases such as non-melanoma skin cancer, melanoma, psoriasis, atopic dermatitis, acne, and hidradenitis suppurativa has been reported (11–16). The AhR agonist tapinarof has been used to treat psoriasis and atopic dermatitis (17–20).
Recent studies identified abnormal AhR expression in vitiligo (21–23). Rekik et al. found decreased AhR transcription in the lesional skin of vitiligo (21). Wang et al. showed that decreased AhR expression in peripheral blood mononuclear cells of vitiligo patients was closely associated with the disease severity (22). In addition, the relation between AhR gene functional mutations and vitiligo susceptibility has been suggested (23). Current opinions reveal that AhR signaling pathway is essential for vitiligo treatment through antioxidant, immune modulation, and melanogenesis effects (24–26). Activation of AhR signaling conduces to progression control and repigmentation, showing great potential in vitiligo treatment. Here, we review the relevant literature, summarize the possible roles of AhR pathway in vitiligo pathogenesis, and conclude the current AhR agonists and antagonists studied in vitiligo, to advance our knowledge and provide new insight into potential therapy.
Oxidative stress is recognized as the critical triggering factor in melanocyte death and the initial event of autoimmune response in vitiligo (27). The excessive reactive oxygen species (ROS) induced by exogenous or endogenous stimuli cause DNA damage, mitochondrial dysfunction, lipid peroxidation, and endoplasmic reticulum stress, ultimately leading to melanocyte death and autoantigen production which can activate adaptive immunity and enhance the attacks on melanocytes (28, 29).
Nuclear factor erythroid 2-related factor 2 (Nrf2) is a transcription factor and makes a significant contribution to redox homeostasis (30). When the Nrf2 is activated, the Keap1-Nrf2 complex breaks down, and the released Nrf2 binds to antioxidant-response elements (AREs), transcribing and upregulating the expression of several antioxidant enzymes (31). Recent opinions suggest that the Nrf2-ARE signal transduction is disordered in vitiligo melanocytes, causing antioxidant incapacity and melanocyte damage (32). Aspirin, simvastatin, and ginsenoside Rk1 can activate Nrf2 and protect melanocytes against oxidative stress damage, suggesting the Nrf2-ARE pathway could be a significant target for vitiligo therapy (33–35).
AhR has been recognized as a master switch for antioxidation. It has been reported that the inhibition of the AhR pathway enhanced H2O2-induced oxidative stress damage and apoptosis in melanocytes (36). It has also been verified that AhR signaling pathway activation can protect cells from oxidative damage via Nrf2-dependent antioxidant system (30, 37). Nrf2 can be either directly activated by ROS or other pathways such as AhR. In fact, Nrf2 has been identified as one of the target genes of AhR. AhR activation directly modulates the Nrf2 pathway and upregulates the expression of antioxidative enzymes, such as heme oxygenase-1 (HO-1) and NAD(P)H:quinone oxidoreductase 1 (NQO1), which are key molecules in maintaining the redox homeostasis of melanocytes (38). Whether AhR activation could trigger the Nrf2 pathway may depend on the ligand and cell type. The ligand, such as ketoconazole, has been proven to differentially activate the AhR/Nrf2 pathway, which inhibited BaP-induced ROS in keratinocytes (39). Besides, AhR signaling pathway is also involved in repairing mitochondrial oxidative damage, which plays a key role in mediating melanocyte apoptosis and death. AhR can upregulate Nrf1 and its downstream TFAM expression to promote mitochondrial biogenesis and avoid oxidative damage in mitochondria and melanocytes (36). In addition, AhR signaling probably crosstalk with PI3K/Akt and Wnt/β-catenin pathway as well, which are related to reducing oxidative stress-induced melanocyte destruction (35, 40, 41). AhR can upregulate the pathways of PI3K/Akt and Wnt/β-catenin, and activate their downstream signal Nrf2, thereby reducing oxidative stress (Figure 1).
Figure 1 The protective effects of AhR signaling in melanocytes under oxidative stress. AhR activation upregulates Nrf2/ARE pathway and increases the expression of antioxidative enzymes. Meanwhile, AhR upregulates Nrf1 expression and promotes mitochondrial biogenesis, ultimately reducing oxidative stress-induced mitochondria and melanocyte destruction. AhR, aryl hydrocarbon receptor; Hsp90, heat shock protein 90; XAP2, hepatitis B virus X-associated protein 2; p23: co-chaperone protein; ARNT, aryl hydrocarbon receptor nuclear translocator; XRE, xenobiotic regulative element; ROS, reactive oxygen species; Nrf2, nuclear factor erythroid 2-related factor 2; Keap1, Kelch-like ECH-associated protein 1; ARE, antioxidant response element; HO-1, heme oxygenase-1; NQO1, NAD(P)H:quinone oxidoreductase 1; Nrf1, nuclear respiratory factor 1; ↑, upregulation.
The involvement of autoimmunity is crucial in the development of vitiligo (42, 43). Endogenous and exogenous stress signals stimulate melanocytes to release heat shock protein 70 (HSP70). HSP70 stimulates the secretion of IFN-α by dendritic cells, which induces keratinocytes to produce chemokines CXCL9 and CXCL10 and recruits CD8+T cells expressing CXCR3 receptor, ultimately leading to the targeted autoimmune destruction of melanocytes (44). Recently, multiple cytokine subsets along with regulatory T (Treg) cell imbalance were found in vitiligo. Czarnowicki et al. found that the levels of T-helper (Th) 1, Th2, Th9, Th17, and Th22 cells were significantly increased in the peripheral blood of vitiligo patients, along with Treg cell counts decreased, suggesting multidimensional immune disorders in vitiligo (45). AhR signaling plays important roles in modulating T cell differentiation and function, which is likely to be an appealing target to combat abnormal autoimmune in vitiligo (46) (Figure 2).
Figure 2 Roles of AhR signaling in adaptive immune modulation. AhR induces PD-1 expression in CD8+T cells by the activation of Kyn-AhR and STAT5-5-HTP-AhR pathways and simultaneously promotes Treg cell differentiation, together inhibiting abnormal CD8+T cell activation in vitiligo. In addition, AhR is also involved in the differentiation of Th9, Th17, Th22, and TRM cells. Kyn, kynurenine; AhR, aryl hydrocarbon receptor; PD-1, programmed cell death protein-1; STAT, signal transducer and activator of transcription; 5-HTP, 5-hydroxytryptophan; IL, interleukin; FoxP3, forkhead box P3; Smad1, decapentaplegic homolog 1; c-maf, musculoaponeurotic fibrosarcoma; CD39, cluster of differentiation 39; Treg, regulatory T; RORγt, RAR-related orphan receptor γt; Th, T helper; Blimp1, B lymphocyte induced maturation protein 1; BATF, Basic Leucine Zipper ATF-Like Transcription Factor.
CD8+ T cells are vital to melanocyte destruction and disease progression. It is well known that IFN-γ-CXCL9/10-CXCR3-CD8+ T cell axis is crucial in the destruction of melanocytes (47, 48). CD8+ T cells secrete IFN-γ, activating the Janus kinase (JAK) 1/2 dimer and inducing the production of CXCL9 and CXCL10 from surrounding keratinocytes to further recruit additional T cells to the epidermis through a positive-feedback loop (48). Targeting these molecules in this pathway, including IFN-γ, the IFN-γ receptor, JAK1 and JAK2, signal transducer and activator of transcription (STAT)1, CXCL10, and CXCR3, may be appealing therapy in vitiligo (49, 50). Increasing evidence has shown that oral and topical JAK inhibitors are efficacious and safe for vitiligo and other dermatosis (51–54). The JAK1/2 inhibitor ruxolitinib cream has been approved by FDA for treating non-segmental vitiligo patients over 12 years old (55). Other emerging JAK inhibitors, including ritlecitinib and baricitinib, are under the clinical trial stage (53, 56). Besides, it has been proved that CD8+ T cells could mediate melanocyte apoptosis by secreting perforin or granzymes and Fas-FasL mechanisms (57, 58).
AhR plays an important role in the regulation of CD8+ T cell responses (59). Lawrence et al. showed that TCDD-mediated AhR activation indirectly inhibited the primary CD8+ T cell response to the influenza virus by modulating the dendritic cell function (60). Similarly, CD8+ T cells exposed to AhR agonist TCDD during development showed a reduced response to influenza virus infection in later life (61). In addition, Liu et al. demonstrated that kynurenine (Kyn) could activate AhR and upregulate the expression of PD-1 as well as SLC7A8 and PAT4. The latter two molecules are the main Kyn transporters in CD8+ T cells, thereby forming a positive feedback loop and leading to CD8+ T cell inhibition (62). Furthermore, they also found that IL-2 could activate STAT5-5-HTP-AhR axis and induce tumor-reactive CD8+T cell exhaustion (63). All the evidence indicates that AhR could probably inhibit the function of CD8+ T cells, thus regulating abnormal autoimmunity. Besides, recent literature has shown the crosstalk between AhR and JAK-STAT pathway. Furue showed that IL-13/IL-4‒JAK‒STAT6/STAT3 axis inhibited the transcription of FLG, LOR, and IVL mediated by AhR (64). Cai et al. revealed that Benvitimod could activate AhR and inhibit the JAK/STAT3 pathway in keratinocytes (65).
Treg cells facilitate immune homeostasis by inhibiting immune activation and maintaining peripheral self‐tolerance (66). It has been reported that Treg cells suppressed autoreactive CD8+ T cells by inhibiting proliferation and cytokine secretion (67). Accumulating evidence has shown that decreased numbers and suppressive function of Treg cells resulted in an unrestricted autoimmune response and melanocyte damage in vitiligo (68–70). Chen et al. found the conversion of normal Tregs to Th1-like Tregs in the peripheral blood and lesional skin of vitiligo patients indicating the impaired suppression on CD8+ T cell proliferation and activation (71). Replenishing peripheral skin Treg cells was proved to interfere with depigmentation and normalize off-balance immune responses (72, 73).
AhR activation can mediate the differentiation of Treg cells by various mechanisms including transcription regulation and epigenetic modification (74–76). To be concrete, AhR induces CD39 and IL-10 in Treg cells, as well as leading to epigenetic changes in the forkhead box P3 (FoxP3) locus and upregulating the expression of Aiolos and Smad 1, which control the expression of FoxP3 and repress IL-2 transcription, and ultimately promoting Treg cell differentiation and combating autoreactive CD8+ T cells.
Previous research suggested that elevated Th17 cells and IL-17 levels might be correlated to the duration, extent, and activity of vitiligo (77). Il-17 produces the chemokine CCL20, attracting CD8+ T cells and killing the melanocytes (77, 78). In addition, IL-17 stimulates the endothelial expression of E- and P-selectins as well as the adhesion molecules ICAM-1 and VCAM-1, then enhancing the migration of neutrophils, which results in ROS production and subsequent autophagic cell apoptosis in vitiligo (77, 79, 80). Inhibition of IL-17 expression has been proved to improve vitiligo lesions (81).
AhR is highly expressed in Th17 cells and promotes the early differentiation of Th17 cells through multiple mechanisms (82, 83). AhR cooperates with STAT3 to promote Th17 cell generation by inducing the Aiolos expression and suppressing IL-2 expression (84). AhR also inhibits the activation of STAT1 and STAT5, which are inhibitory factors during Th17 cell differentiation (85). Meanwhile, AhR can regulate the expression of IL-17 by binding to the Il17 gene locus (86). It has been certificated that IL-6, TGF-β1 promotes regulatory/non-pathogenic Th17 cell differentiation, whereas IL-23, IL-6, TGF-β3 induced pathogenic Th17 cell differentiation and IL-17 secretion (87–89). Interestingly, TGF-β1 and IL-6 induce the expression of AhR and c-Maf, transactivating IL-10 production in non-pathogenic Th17 cells. While in pathogenic Th17 cells, AhR expression was significantly downregulated (89). It seems that AhR is involved in the differentiation of pathogenic/non-pathogenic Th17 cells, though the specific mechanism is not clarified.
CD8+ TRM cells expressing the characteristic markers CD103, CD69, and CD49a have been identified in the lesion of vitiligo patients and mouse models (90–92). TRM cells develop and persist in the skin, produce IFN-γ, perforins, and granzyme B, and destroy melanocytes, causing the recurrence of depigmentation in the same area after treatment stops (93). Targeting the IL-15, such as anti-CD122 antibodies may be a potentially effective treatment approach to prevent recurrence (94). Recent research indicated that AhR is involved in modulating the differentiation and function of CD8+ TRM cells (95, 96). AhR facilitates the persistence of TRM cells in the epidermis by regulating the downstream target Blimp1, which contributes to tissue residency (95–97). Dean et al. found that AhR promotes TRM cell differentiation while suppressing TCM cells in intestinal tissue (96). Besides, AhR also regulates CD4+ TRM cell differentiation and function, which may conduce to immune protection, and tissue remodeling in vitiligo (98).
AhR signaling also regulates other T cell responses such as Th22 and Th9 cells. Th22 cells are a kind of CD4+ T cell subset that produces IL-22 in the absence of IL-17, showing a dual role in mucosal defense, tissue repair as well as inflammation and pathological change (99). Previous studies have shown that IL-22 was elevated in vitiligo and related to disease extent and activity (100, 101). AhR is the critical transcription factor for Th22 cell development (99). AhR activation enhanced IL-22 release in CD4+ T cells of vitiligo patients (102). AhR is also essential for Th9 cell development. Takami et al. indicated that exposure to AhR ligands could increase BATF expression and promote the differentiation of Th9 cells (103). Kumar et al. demonstrated the increased frequency of Th9 cells and elevated levels of IL-9 in active vitiligo patients, which contribute to reducing the IFNγ-induced oxidative stress in melanocytes (104). Thus, AhR activation may protect epidermal melanocytes from IFN-γ-induced damage by elevating the levels of IL-9.
Apart from controlling disease progression through anti-oxidative stress and immunity modulation, AhR signaling pathway activation could result in the upregulation of melanogenesis-related gene expression, and melanin content production, thus promoting melanogenesis (Figure 3).
Figure 3 The possible mechanisms of AhR signaling in melanogenesis. AhR activation directly upregulates the expression of TYR, TRPs, and MITF. AhR crosstalks with SCF/c-Kit/MAPK, Wnt/β-catenin, α-MSH/cAMP/PKA, and FAK/Src pathways, mediating melanoblast migration, maturation, and melanin synthesis. Wnt, wingless/integrated; Akt, protein kinase B; P, phosphorylation; GSK-3β, glycogen synthase kinase 3β; SCF, stem cell factor; MAPK, mitogen-activated protein kinase; α-MSH, α–melanocyte-stimulating hormone; MC1R, melanocortin 1 receptor; c-AMP, cyclic adenosine monophosphate; PKA, c-AMP dependent protein kinase A; CREB, c-AMP response element binding protein; AhR, aromatic hydrocarbon receptor; Hsp90, heat shock protein 90; XAP-2, hepatitis B virus X-associated protein 2; p23, co-chaperone protein; FAK, focal adhesion kinase; MITF, microphthalmia-associated transcription factor; TYR, tyrosinase; TRP-1, tyrosinase-related protein 1; TRP-2, tyrosinase-related protein 2.
Melanin biosynthesis occurs in melanosomes of melanocytes, and its synthesis is regulated by the expression and activity of three melanogenic enzymes, tyrosinase (TYR), tyrosinase-related protein (TRP)-1, and TRP-2 (105). It has been documented that microphthalmia-associated transcription factor (MITF) is an important transcriptional regulator of pigmentation that can activate TYR, TRP-1, and TRP-2 expression (106).
Previous studies have shown that exposure to BA or TCDD can activate AhR and significantly increase TYR activity, melanin synthesis, as well as TRP-1, TRP-2, and MITF expression (38, 107). Luecke et al. suggested that the AhR signal may play a potential role in the melanogenesis of human melanocytes. They found that there were multiple XREs in the promoter regions, introns, and 3′ noncoding regions of TYR and TRPs, implicating they were the transcriptional targets of AhR (26). Studies on human populations also demonstrated that the skin hyperpigmentation rate is related to the blood level of AhR ligand polychlorinated biphenyls (108). Thus, AhR might be involved in melanogenesis and pigmentation by upregulating the TYR activity.
Stem cell factor (SCF) and its receptor (c‐Kit) play vital roles in the survival, migration, proliferation, and differentiation of melanoblast (109). It has been confirmed that the expression of SCF, c‐Kit, and its downstream effector MITF significantly declined in vitiligo lesional and perilesional skin (110). Defects in SCF/c-Kit pathway may be related to melanocyte apoptosis in vitiligo. Furthermore, modulating SCF or c‐Kit expression, including narrow-band ultraviolet (UV) B radiation, psoralen, tacrolimus ointment, and geniposide, is the common treatment for vitiligo, which further indicates the involvement of abnormal SCF/c‐Kit signaling pathway in vitiligo (111, 112).
Jux et al. showed that UVB-induced TYR activity and melanocyte density are prominently diminished in AhR-deficient mice, which are associated with reduced SCF and c‐Kit expression in melanocytes (113). It is widely known that the promoter of c-Kit gene contains functional XREs which are addressed by AhR, suggesting that AhR signaling contributes to melanocyte homeostasis and differentiation by controlling SCF/c-Kit expression (114). Besides, AhR signaling also crosstalks with mitogen-activated protein kinase (MAPK) pathway, which is the downstream signaling of SCF/c-kit. Shi et al. revealed that PM2.5 significantly increases the phosphorylation level of MAPK proteins in an AhR-dependent manner and thereby promotes pigmentation (115). Thus, AhR signaling can upregulate the SCF/c-Kit/MAPK pathway and promote pigment synthesis.
The Wnt signaling pathway also makes significant contributions to melanocyte development and melanogenesis. Wnt1 and Wnt3a facilitate the differentiation of neural crest cells into melanocytes (116). β-catenin binds with the lymphoid enhancer-binding factor 1 (LEF1) and synergistically upregulates MITF expression and promotes melanogenesis. Accumulating evidence has shown an alteration of the Wnt/β-catenin pathway in vitiligo lesions (117). Regazzetti et al. have demonstrated the suppression of the Wnt/β-catenin pathway in melanocytes and keratinocytes under oxidative stress. It was found that the expression of Wnt pathway components such as LEF1, CDH2, and CDH3 was downregulated in the lesional skin of vitiligo (118). In addition, it was found that the expression of Wnt pathway components such as LEF1, CDH2, and CDH3 was downregulated in the lesional skin of vitiligo (118). One study showed that narrow-band UVB could activate the Wnt/β-catenin pathway and strongly promote vitiligo repigmentation (119). Moreover, the Wnt pathway also has significant effects in regulating immunity and protecting melanocytes from oxidative stress, indicating that Wnt/β-catenin signaling is likely to be a valuable target to treat vitiligo (40).
The crosstalk between AhR and Wnt/β-catenin pathway has been studied. A previous study reported that β-catenin expression was significantly increased in melanocytes treated with AhR ligands (120). The β-catenin can physically interact with AhR in DNA-binding sites (121). Our study found that the activation of AhR-dependent AKT/GSK-3β/β-catenin pathway can further upregulate MITF transcription and enhance the expression of TYR, TRP-1, and TRP-2, ultimately promoting melanogenesis (122).
α–melanocyte-stimulating hormone (α-MSH) is a key regulatory protein controlling melanocyte proliferation and melanin synthesis through cAMP/PKA/CREB/MITF, MAPK-ERK, and the Wnt signaling pathway (123–126). Melanocortin system defects have been discovered in vitiligo patients, including low α-MSH levels in serum, plasma, and lesional skin (127–130). Related synthetic analogue, such as Afamelanotide which can bind the melanocortin-1 receptor and stimulate melanogenesis, has been used as an adjuvant to increase therapeutic response to UVB phototherapy (131–133). The involvement of AhR in α-MSH-induced melanogenesis was reported. Bahraman’s study indicated that α-MSH increased melanin synthesis by upregulating the transcriptional level of AhR, CTNNB1, MITF, and TYR. Induction of α-MSH-stimulated melanogenesis may require the concomitant presence of AhR (121).
Repigmentation of vitiligo lesions hinges on melanoblast proliferation and migration from hair follicles to the epidermis. UV light therapy, including PUVA, NB-UVB, and excimer laser can stimulate melanoblast proliferation and upward migration to the epidermis, which promotes functional maturation of melanoblasts to melanin-producing melanocytes (134). AhR can regulate the early activation of focal adhesion kinase (FAK) and Src and mediate melanoblast maturation. Tomkiewicz et al. demonstrated that TCDD-mediated AhR activation resulted in the rapid activation of integrin clustering and FAK/Src, which had prominent effects on cell migration and integrin recycling (135). It suggested that AhR played a regulatory role in the migration of melanoblasts via the FAK signaling pathway. Moreover, AhR mediated the development and maturation of melanoblasts as well (136, 137). Hence, the AhR signaling pathway could promote melanin synthesis through multiple regulation mechanisms and is of great significance in repigmentation.
Vitiligo is a chronic autoimmune skin disease characterized by melanocyte destruction and skin depigmentation. Due to the diversity and complexity of pathogenesis, there is no definitive safe and efficacious treatment for vitiligo. Current therapeutic strategies mainly include topical and systemic medications, phototherapy, and surgical grafting (138, 139). However, they are not universally effective in all patients and provide only short-term benefits with a high recurrence rate after discontinuing treatment. Thus, the search for more effective, targeted therapies is necessary. Current opinions suggest that the treatment of vitiligo requires a multimodal approach targeting three different aspects at the same time. The minimization of oxidative stress, immunomodulatory and immunosuppressant action, and regeneration of melanocytes may control the excessive oxidative damage and abnormal immune response so that prevent the progress of active disease as well as promote repigmentation, giving the best results in treating vitiligo.
Our review shows that AhR may be a potential target for vitiligo treatment. Current AhR agonists and antagonists studied in vitiligo are summarized in Table 1. In fact, narrow-band UVB, the most widely used therapy for vitiligo, probably induces repigmentation by activating AhR signaling (113). AhR can protect melanocytes from oxidate stress damage by activating the Nrf2-ARE pathway, which is already recognized as an attractive target for vitiligo treatment. More importantly, the activation of AhR signaling inhibits abnormal immune response and drives melanogenesis by upregulating the expression of melanogenic genes and transcription factors, which contribute to disease control and repigmentation. In addition, AhR also crosstalks with the JAK-STAT pathway, which is recognized as a key new therapeutic target for vitiligo.
However, there are still some limitations. Firstly, the antioxidant response mediated by the activation of AhR/Nrf2 pathway relies on the type of AhR ligand. Ketoconazole and cynaropicrin activate the AhR signaling pathway without ROS, while Dioxins, BaP, and other polycyclic aromatic hydrocarbons induce extremely high CYP1A1 expression and ROS production. Selecting appropriate ligands or inhibiting the activity of CYP1A1 may contribute to less oxidative damage. Secondly, the function of AhR is complex. Overactivation of AhR signaling has been detected in several cancer types, including melanoma and cutaneous squamous cell carcinoma. Topical treatment may be considered to reduce the risk of concurrent cancer complications. Finally, AhR promotes the differentiation of CD8+ TRM cells in the skin, which may lead to recurrence after discontinuing treatment.
Our review summarizes recent topics on AhR and vitiligo. AhR can activate antioxidant pathways, inhibit abnormal immunity response, and upregulate melanogenesis gene, thereby protecting melanocytes from oxidative stress damage, controlling disease progression, and promoting lesion repigmentation. Further in-depth research and the development of related drugs may be beneficial for vitiligo.
YL: Conceptualization, Investigation, Visualization, Writing – original draft. YZ: Funding acquisition, Investigation, Writing – review & editing. ZC: Investigation, Writing – review & editing. XT: Investigation, Writing – review & editing. XM: Investigation, Writing – review & editing. ZW: Conceptualization, Writing – review & editing.
The author(s) declare that no financial support was received for the research, authorship, and/or publication of this article.
This study was supported by Innovative Research Team of High-level Local Universities in Shanghai (SHSMU-ZDCX20211002).
The authors declare that the research was conducted in the absence of any commercial or financial relationships that could be construed as a potential conflict of interest.
All claims expressed in this article are solely those of the authors and do not necessarily represent those of their affiliated organizations, or those of the publisher, the editors and the reviewers. Any product that may be evaluated in this article, or claim that may be made by its manufacturer, is not guaranteed or endorsed by the publisher.
1. Ezzedine K, Eleftheriadou V, Whitton M, van Geel N. Vitiligo. Lancet (2015) 386(9988):74–84. doi: 10.1016/s0140-6736(14)60763-7
2. Bergqvist C, Ezzedine K. Vitiligo: A review. Dermatology (2020) 236(6):571–92. doi: 10.1159/000506103
3. Diotallevi F, Gioacchini H, De Simoni E, Marani A, Candelora M, Paolinelli M, et al. Vitiligo, from pathogenesis to therapeutic advances: state of the art. Int J Mol Sci (2023) 24(5):4910. doi: 10.3390/ijms24054910
4. Kudo I, Hosaka M, Haga A, Tsuji N, Nagata Y, Okada H, et al. The regulation mechanisms of ahr by molecular chaperone complex. J Biochem (2017) 163(3):223–32. doi: 10.1093/jb/mvx074
5. Kim DW, Gazourian L, Quadri SA, Raphaëlle, Sherr DH, Sonenshein GE. The rela nf-κb subunit and the aryl hydrocarbon receptor (Ahr) cooperate to transactivate the C-myc promoter in mammary cells. Oncogene (2000) 19(48):5498–506. doi: 10.1038/sj.onc.1203945
6. Wilson SR, Joshi AD, Elferink CJ. The tumor suppressor kruppel-like factor 6 is a novel aryl hydrocarbon receptor DNA binding partner. J Pharmacol Exp Ther (2013) 345(3):419–29. doi: 10.1124/jpet.113.203786
7. Ohtake F, Fujii-Kuriyama Y, Kawajiri K, Kato S. Cross-talk of dioxin and estrogen receptor signals through the ubiquitin system. J Steroid Biochem Mol Biol (2011) 127(1-2):102–7. doi: 10.1016/j.jsbmb.2011.03.007
8. Fernández-Gallego N, Sánchez-Madrid F, Cibrian D. Role of ahr ligands in skin homeostasis and cutaneous inflammation. Cells (2021) 10(11):3176. doi: 10.3390/cells10113176
9. Esser C, Bargen I, Weighardt H, Haarmann-Stemmann T, Krutmann J. Functions of the aryl hydrocarbon receptor in the skin. Semin Immunopathology (2013) 35(6):677–91. doi: 10.1007/s00281-013-0394-4
10. Furue M, Takahara M, Nakahara T, Uchi H. Role of ahr/arnt system in skin homeostasis. Arch Dermatol Res (2014) 306(9):769–79. doi: 10.1007/s00403-014-1481-7
11. Napolitano M, Patruno C. Aryl hydrocarbon receptor (Ahr) a possible target for the treatment of skin disease. Med Hypotheses (2018) 116:96–100. doi: 10.1016/j.mehy.2018.05.001
12. Napolitano M, Fabbrocini G, Martora F, Picone V, Morelli P, Patruno C. Role of aryl hydrocarbon receptor activation in inflammatory chronic skin diseases. Cells (2021) 10(12):3559. doi: 10.3390/cells10123559
13. Edamitsu T, Taguchi K, Okuyama R, Yamamoto M. Ahr and nrf2 in skin homeostasis and atopic dermatitis. Antioxidants (Basel) (2022) 11(2):227. doi: 10.3390/antiox11020227
14. Furue M, Hashimoto-Hachiya A, Tsuji G. Aryl hydrocarbon receptor in atopic dermatitis and psoriasis. Int J Mol Sci (2019) 20(21):5424. doi: 10.3390/ijms20215424
15. Fabbrocini G, Kaya G, Caseiro Silverio P, De Vita V, Kaya A, Fontao F, et al. Aryl hydrocarbon receptor activation in acne vulgaris skin: A case series from the region of naples, Italy. Dermatology (2015) 231(4):334–8. doi: 10.1159/000439402
16. Yidana DB. Hidradenitis suppurativa - the role of interleukin-17, the aryl hydrocarbon receptor and the link to a possible fungal aetiology. Med Hypotheses (2021) 149:110530. doi: 10.1016/j.mehy.2021.110530
17. Peppers J, Paller AS, Maeda-Chubachi T, Wu S, Robbins K, Gallagher K, et al. A phase 2, randomized dose-finding study of tapinarof (Gsk2894512 cream) for the treatment of atopic dermatitis. J Am Acad Dermatol (2019) 80(1):89–98.e3. doi: 10.1016/j.jaad.2018.06.047
18. Paller AS, Stein Gold L, Soung J, Tallman AM, Rubenstein DS, Gooderham M. Efficacy and patient-reported outcomes from a phase 2b, randomized clinical trial of tapinarof cream for the treatment of adolescents and adults with atopic dermatitis. J Am Acad Dermatol (2021) 84(3):632–8. doi: 10.1016/j.jaad.2020.05.135
19. Lebwohl MG, Stein Gold L, Strober B, Papp KA, Armstrong AW, Bagel J, et al. Phase 3 trials of tapinarof cream for plaque psoriasis. N Engl J Med (2021) 385(24):2219–29. doi: 10.1056/NEJMoa2103629
20. Aoki V, Orfali RL. Aryl-hydrocarbon receptor agonist: A novel topical therapeutic approach for inflammatory skin diseases. J Eur Acad Dermatol Venereol (2023) 37(6):1093–4. doi: 10.1111/jdv.19080
21. Rekik R, Ben Hmid A, Lajnef C, Zamali I, Zaraa I, Ben Ahmed M. Aryl hydrocarbon receptor (Ahr) transcription is decreased in skin of vitiligo patients. Int J Dermatol (2017) 56(12):1509–12. doi: 10.1111/ijd.13761
22. Wang X, Li K, Liu L, Shi Q, Song P, Jian Z, et al. Ahr promoter variant modulates its transcription and downstream effectors by allele-specific ahr-sp1 interaction functioning as a genetic marker for vitiligo. Sci Rep (2015) 5:13542. doi: 10.1038/srep13542
23. Wang XW, Li K, Guo S, Qiang HN, Liu L, Song P, et al. The association of functional polymorphisms in the aryl hydrocarbon receptor (Ahr) gene with the risk of vitiligo in han chinese populations. Br J Dermatol (2012) 166(5):1081–7. doi: 10.1111/j.1365-2133.2011.10798.x
24. Dietrich C. Antioxidant functions of the aryl hydrocarbon receptor. Stem Cells Int (2016) 2016:7943495. doi: 10.1155/2016/7943495
25. Trikha P, Lee DA. The role of ahr in transcriptional regulation of immune cell development and function. Biochim Biophys Acta Rev Cancer (2020) 1873(1):188335. doi: 10.1016/j.bbcan.2019.188335
26. Luecke S, Backlund M, Jux B, Esser C, Krutmann J, Rannug A. The aryl hydrocarbon receptor (Ahr), a novel regulator of human melanogenesis. Pigment Cell Melanoma Res (2010) 23(6):828–33. doi: 10.1111/j.1755-148X.2010.00762.x
27. Chang WL, Ko CH. The role of oxidative stress in vitiligo: an update on its pathogenesis and therapeutic implications. Cells (2023) 12(6):936. doi: 10.3390/cells12060936
28. Chen J, Li S, Li C. Mechanisms of melanocyte death in vitiligo. Med Res Rev (2021) 41(2):1138–66. doi: 10.1002/med.21754
29. Xuan Y, Yang Y, Xiang L, Zhang C. The role of oxidative stress in the pathogenesis of vitiligo: A culprit for melanocyte death. Oxid Med Cell Longev (2022) 2022:8498472. doi: 10.1155/2022/8498472
30. Grishanova AY, Perepechaeva ML. Aryl hydrocarbon receptor in oxidative stress as a double agent and its biological and therapeutic significance. Int J Mol Sci (2022) 23(12):6719. doi: 10.3390/ijms23126719
31. Baird L, Yamamoto M. The molecular mechanisms regulating the keap1-nrf2 pathway. Mol Cell Biol (2020) 40(13):e00099–20. doi: 10.1128/MCB.00099-20
32. Jian Z, Li K, Song P, Zhu G, Zhu L, Cui T, et al. Impaired activation of the nrf2-are signaling pathway undermines H2o2-induced oxidative stress response: A possible mechanism for melanocyte degeneration in vitiligo. J Invest Dermatol (2014) 134(8):2221–30. doi: 10.1038/jid.2014.152
33. Jian Z, Tang L, Yi X, Liu B, Zhang Q, Zhu G, et al. Aspirin induces nrf2-mediated transcriptional activation of haem oxygenase-1 in protection of human melanocytes from H2 O2 -induced oxidative stress. J Cell Mol Med (2016) 20(7):1307–18. doi: 10.1111/jcmm.12812
34. Chang Y, Li S, Guo W, Yang Y, Zhang W, Zhang Q, et al. Simvastatin protects human melanocytes from H2o2-induced oxidative stress by activating nrf2. J Invest Dermatol (2017) 137(6):1286–96. doi: 10.1016/j.jid.2017.01.020
35. Xiong J, Yang J, Yan K, Guo J. Ginsenoside rk1 protects human melanocytes from H(2)O(2)−Induced oxidative injury via regulation of the pi3k/akt/nrf2/ho−1 pathway. Mol Med Rep (2021) 24(5):821. doi: 10.3892/mmr.2021.12462
36. Wang X, Li S, Liu L, Jian Z, Cui T, Yang Y, et al. Role of the aryl hydrocarbon receptor signaling pathway in promoting mitochondrial biogenesis against oxidative damage in human melanocytes. J Dermatol Sci (2019) 96(1):33–41. doi: 10.1016/j.jdermsci.2019.09.001
37. Tan YQ, Wang YN, Feng HY, Guo ZY, Li X, Nie XL, et al. Host/microbiota interactions-derived tryptophan metabolites modulate oxidative stress and inflammation via aryl hydrocarbon receptor signaling. Free Radic Biol Med (2022) 184:30–41. doi: 10.1016/j.freeradbiomed.2022.03.025
38. Furue M, Tsuji G. Chloracne and hyperpigmentation caused by exposure to hazardous aryl hydrocarbon receptor ligands. Int J Environ Res Public Health (2019) 16(23):4864. doi: 10.3390/ijerph16234864
39. Tsuji G, Takahara M, Uchi H, Matsuda T, Chiba T, Takeuchi S, et al. Identification of ketoconazole as an ahr-nrf2 activator in cultured human keratinocytes: the basis of its anti-inflammatory effect. J Invest Dermatol (2012) 132(1):59–68. doi: 10.1038/jid.2011.194
40. Lin X, Meng X, Lin J. The possible role of wnt/β-catenin signalling in vitiligo treatment. J Eur Acad Dermatol Venereol (2023) 37(11):2208–21. doi: 10.1111/jdv.19022
41. Al-Dhfyan A, Alhoshani A, Korashy HM. Aryl hydrocarbon receptor/cytochrome P450 1a1 pathway mediates breast cancer stem cells expansion through pten inhibition and β-catenin and akt activation. Mol Cancer (2017) 16(1):14. doi: 10.1186/s12943-016-0570-y
42. Roberts GHL, Santorico SA, Spritz RA. Deep genotype imputation captures virtually all heritability of autoimmune vitiligo. Hum Mol Genet (2020) 29(5):859–63. doi: 10.1093/hmg/ddaa005
43. Khaitan BK, Sindhuja T. Autoimmunity in vitiligo: therapeutic implications and opportunities. Autoimmun Rev (2022) 21(1):102932. doi: 10.1016/j.autrev.2021.102932
44. Marchioro HZ, Silva de Castro CC, Fava VM, Sakiyama PH, Dellatorre G, Miot HA. Update on the pathogenesis of vitiligo. Bras Dermatol (2022) 97(4):478–90. doi: 10.1016/j.abd.2021.09.008
45. Czarnowicki T, He H, Leonard A, Kim HJ, Kameyama N, Pavel AB, et al. Blood endotyping distinguishes the profile of vitiligo from that of other inflammatory and autoimmune skin diseases. J Allergy Clin Immunol (2019) 143(6):2095–107. doi: 10.1016/j.jaci.2018.11.031
46. Hao N, Whitelaw ML. The emerging roles of ahr in physiology and immunity. Biochem Pharmacol (2013) 86(5):561–70. doi: 10.1016/j.bcp.2013.07.004
47. Sabat R, Wolk K, Loyal L, Döcke WD, Ghoreschi K. T cell pathology in skin inflammation. Semin Immunopathol (2019) 41(3):359–77. doi: 10.1007/s00281-019-00742-7
48. Frisoli ML, Essien K, Harris JE. Vitiligo: mechanisms of pathogenesis and treatment. Annu Rev Immunol (2020) 38:621–48. doi: 10.1146/annurev-immunol-100919-023531
49. Martins C, Migayron L, Drullion C, Jacquemin C, Lucchese F, Rambert J, et al. Vitiligo skin T cells are prone to produce type 1 and type 2 cytokines to induce melanocyte dysfunction and epidermal inflammatory response through jak signaling. J Invest Dermatol (2022) 142(4):1194–205.e7. doi: 10.1016/j.jid.2021.09.015
50. Cunningham KN, Rosmarin D. Vitiligo treatments: review of current therapeutic modalities and jak inhibitors. Am J Clin Dermatol (2023) 24(2):165–86. doi: 10.1007/s40257-022-00752-6
51. Rosmarin D, Passeron T, Pandya AG, Grimes P, Harris JE, Desai SR, et al. Two phase 3, randomized, controlled trials of ruxolitinib cream for vitiligo. N Engl J Med (2022) 387(16):1445–55. doi: 10.1056/NEJMoa2118828
52. Rosmarin D, Pandya AG, Lebwohl M, Grimes P, Hamzavi I, Gottlieb AB, et al. Ruxolitinib cream for treatment of vitiligo: A randomised, controlled, phase 2 trial. Lancet (2020) 396(10244):110–20. doi: 10.1016/s0140-6736(20)30609-7
53. Ezzedine K, Peeva E, Yamaguchi Y, Cox LA, Banerjee A, Han G, et al. Efficacy and safety of oral ritlecitinib for the treatment of active nonsegmental vitiligo: A randomized phase 2b clinical trial. J Am Acad Dermatol (2023) 88(2):395–403. doi: 10.1016/j.jaad.2022.11.005
54. Vu M, Heyes C, Robertson SJ, Varigos GA, Ross G. Oral tofacitinib: A promising treatment in atopic dermatitis, alopecia areata and vitiligo. Clin Exp Dermatol (2017) 42(8):942–4. doi: 10.1111/ced.13290
55. Sheikh A, Rafique W, Owais R, Malik F, Ali E. Fda approves ruxolitinib (Opzelura) for vitiligo therapy: A breakthrough in the field of dermatology. Ann Med Surg (Lond) (2022) 81:104499. doi: 10.1016/j.amsu.2022.104499
56. Efficacy and Safety of Total Glucosides of Paeony Combined With NB-UVB on Treating Vitiligo. Available from: https://www.clinicaltrials.gov/ct2/show/NCT04822584.
57. Deng Q, Zou P, Du P, Shi Y, Pi Z, Xiao Y, et al. Overexpressed perforin contributes to the melanocyte destruction via epigenetic regulation in patients with vitiligo. Int Immunopharmacol (2023) 114:109574. doi: 10.1016/j.intimp.2022.109574
58. Jimbo H, Nagai H, Fujiwara S, Shimoura N, Nishigori C. Fas-fasl interaction in cytotoxic T cell-mediated vitiligo: the role of lesional expression of tumor necrosis factor-αand interferon-Γ in fas-mediated melanocyte apoptosis. Exp Dermatol (2020) 29(1):61–70. doi: 10.1111/exd.14053
59. Shinde R, McGaha TL. The aryl hydrocarbon receptor: connecting immunity to the microenvironment. Trends Immunol (2018) 39(12):1005–20. doi: 10.1016/j.it.2018.10.010
60. Lawrence BP, Roberts AD, Neumiller JJ, Cundiff JA, Woodland DL. Aryl hydrocarbon receptor activation impairs the priming but not the recall of influenza virus-specific cd8+ T cells in the lung. J Immunol (2006) 177(9):5819–28. doi: 10.4049/jimmunol.177.9.5819
61. Winans B, Nagari A, Chae M, Post CM, Ko CI, Puga A, et al. Linking the aryl hydrocarbon receptor with altered DNA methylation patterns and developmentally induced aberrant antiviral cd8+ T cell responses. J Immunol (2015) 194(9):4446–57. doi: 10.4049/jimmunol.1402044
62. Liu Y, Liang X, Dong W, Fang Y, Lv J, Zhang T, et al. Tumor-repopulating cells induce pd-1 expression in cd8(+) T cells by transferring kynurenine and ahr activation. Cancer Cell (2018) 33(3):480–94.e7. doi: 10.1016/j.ccell.2018.02.005
63. Liu Y, Zhou N, Zhou L, Wang J, Zhou Y, Zhang T, et al. Il-2 regulates tumor-reactive cd8(+) T cell exhaustion by activating the aryl hydrocarbon receptor. Nat Immunol (2021) 22(3):358–69. doi: 10.1038/s41590-020-00850-9
64. Furue M. Regulation of skin barrier function via competition between ahr axis versus il-13/il-4‒Jak‒Stat6/stat3 axis: pathogenic and therapeutic implications in atopic dermatitis. J Clin Med (2020) 9(11):3741. doi: 10.3390/jcm9113741
65. Cai Z, Zeng Y, Shi X, Zhang X, Zhu H, Wang W. Benvitimod inhibits mcm6-meditated proliferation of keratinocytes by regulating the jak/stat3 pathway. J Dermatol Sci (2023) 109(2):71–9. doi: 10.1016/j.jdermsci.2023.01.010
66. Sakaguchi S, Mikami N, Wing JB, Tanaka A, Ichiyama K, Ohkura N. Regulatory T cells and human disease. Annu Rev Immunol (2020) 38:541–66. doi: 10.1146/annurev-immunol-042718-041717
67. Maeda Y, Nishikawa H, Sugiyama D, Ha D, Hamaguchi M, Saito T, et al. Detection of self-reactive cd8⁺ T cells with an anergic phenotype in healthy individuals. Science (2014) 346(6216):1536–40. doi: 10.1126/science.aaa1292
68. Lili Y, Yi W, Ji Y, Yue S, Weimin S, Ming L. Global activation of cd8+ Cytotoxic T lymphocytes correlates with an impairment in regulatory T cells in patients with generalized vitiligo. PloS One (2012) 7(5):e37513. doi: 10.1371/journal.pone.0037513
69. Dwivedi M, Laddha NC, Arora P, Marfatia YS, Begum R. Decreased regulatory T-cells and cd4(+) /cd8(+) ratio correlate with disease onset and progression in patients with generalized vitiligo. Pigment Cell Melanoma Res (2013) 26(4):586–91. doi: 10.1111/pcmr.12105
70. Richetta A, D'Epiro S, Salvi M, Campoli M, Giancristoforo S, Mattozzi C, et al. Serum levels of functional T-regs in vitiligo: our experience and mini-review of the literature. Eur J Dermatol (2013) 23(2):154–9. doi: 10.1684/ejd.2013.1986
71. Chen J, Wang X, Cui T, Ni Q, Zhang Q, Zou D, et al. Th1-like treg in vitiligo: an incompetent regulator in immune tolerance. J Autoimmun (2022) 131:102859. doi: 10.1016/j.jaut.2022.102859
72. Mukhatayev Z, Dellacecca ER, Cosgrove C, Shivde R, Jaishankar D, Pontarolo-Maag K, et al. Antigen specificity enhances disease control by tregs in vitiligo. Front Immunol (2020) 11:581433. doi: 10.3389/fimmu.2020.581433
73. Le Poole IC, Mehrotra S. Replenishing regulatory T cells to halt depigmentation in vitiligo. J Investig Dermatol Symp Proc (2017) 18(2):S38–s45. doi: 10.1016/j.jisp.2016.10.023
74. Quintana FJ, Basso AS, Iglesias AH, Korn T, Farez MF, Bettelli E, et al. Control of T(Reg) and T(H)17 cell differentiation by the aryl hydrocarbon receptor. Nature (2008) 453(7191):65–71. doi: 10.1038/nature06880
75. Singh NP, Singh UP, Singh B, Price RL, Nagarkatti M, Nagarkatti PS. Activation of aryl hydrocarbon receptor (Ahr) leads to reciprocal epigenetic regulation of foxp3 and il-17 expression and amelioration of experimental colitis. PloS One (2011) 6(8):e23522. doi: 10.1371/journal.pone.0023522
76. Gandhi R, Kumar D, Burns EJ, Nadeau M, Dake B, Laroni A, et al. Activation of the aryl hydrocarbon receptor induces human type 1 regulatory T cell-like and foxp3(+) regulatory T cells. Nat Immunol (2010) 11(9):846–53. doi: 10.1038/ni.1915
77. Singh RK, Lee KM, Vujkovic-Cvijin I, Ucmak D, Farahnik B, Abrouk M, et al. The role of il-17 in vitiligo: A review. Autoimmun Rev (2016) 15(4):397–404. doi: 10.1016/j.autrev.2016.01.004
78. Le TVT, Ngoc Phan H, Dang TN, Pham LD. Increased circulatory interleukin-17a levels in patients with progressive and leukotrichial vitiligo. Dermatol Res Pract (2021) 2021:5524566. doi: 10.1155/2021/5524566
79. Griffin GK, Newton G, Tarrio ML, Bu DX, Maganto-Garcia E, Azcutia V, et al. Il-17 and tnf-αSustain neutrophil recruitment during inflammation through synergistic effects on endothelial activation. J Immunol (2012) 188(12):6287–99. doi: 10.4049/jimmunol.1200385
80. Zhou J, An X, Dong J, Wang Y, Zhong H, Duan L, et al. Il-17 induces cellular stress microenvironment of melanocytes to promote autophagic cell apoptosis in vitiligo. FASEB J (2018) 32(9):4899–916. doi: 10.1096/fj.201701242RR
81. Kim HJ, Del Duca E, Pavel AB, Singer GK, Abittan BJ, Chima MA, et al. Apremilast and narrowband ultraviolet B combination therapy suppresses th17 axis and promotes melanogenesis in vitiligo skin: A randomized, split-body, pilot study in skin types iv-vi. Arch Dermatol Res (2023) 315(2):215–21. doi: 10.1007/s00403-022-02343-1
82. Zhou L. Ahr function in lymphocytes: emerging concepts. Trends Immunol (2016) 37(1):17–31. doi: 10.1016/j.it.2015.11.007
83. Stockinger B, Di Meglio P, Gialitakis M, Duarte JH. The aryl hydrocarbon receptor: multitasking in the immune system. Annu Rev Immunol (2014) 32:403–32. doi: 10.1146/annurev-immunol-032713-120245
84. Quintana FJ, Jin H, Burns EJ, Nadeau M, Yeste A, Kumar D, et al. Aiolos promotes th17 differentiation by directly silencing il2 expression. Nat Immunol (2012) 13(8):770–7. doi: 10.1038/ni.2363
85. Kimura A, Naka T, Nohara K, Fujii-Kuriyama Y, Kishimoto T. Aryl hydrocarbon receptor regulates stat1 activation and participates in the development of th17 cells. Proc Natl Acad Sci U.S.A. (2008) 105(28):9721–6. doi: 10.1073/pnas.0804231105
86. Cui G, Qin X, Wu L, Zhang Y, Sheng X, Yu Q, et al. Liver X receptor (Lxr) mediates negative regulation of mouse and human th17 differentiation. J Clin Invest (2011) 121(2):658–70. doi: 10.1172/jci42974
87. Wu B, Zhang S, Guo Z, Bi Y, Zhou M, Li P, et al. The tgf-β Superfamily cytokine activin-a is induced during autoimmune neuroinflammation and drives pathogenic th17 cell differentiation. Immunity (2021) 54(2):308–23.e6. doi: 10.1016/j.immuni.2020.12.010
88. McGeachy MJ, Bak-Jensen KS, Chen Y, Tato CM, Blumenschein W, McClanahan T, et al. Tgf-β and il-6 drive the production of il-17 and il-10 by T cells and restrain th-17 cell–mediated pathology. Nat Immunol (2007) 8(12):1390–7. doi: 10.1038/ni1539
89. Lee Y, Awasthi A, Yosef N, Quintana FJ, Xiao S, Peters A, et al. Induction and molecular signature of pathogenic th17 cells. Nat Immunol (2012) 13(10):991–9. doi: 10.1038/ni.2416
90. Malik BT, Byrne KT, Vella JL, Zhang P, Shabaneh TB, Steinberg SM, et al. Resident memory T cells in the skin mediate durable immunity to melanoma. Sci Immunol (2017) 2(10):eaam6346. doi: 10.1126/sciimmunol.aam6346
91. Cheuk S, Schlums H, Gallais Sérézal I, Martini E, Chiang SC, Marquardt N, et al. Cd49a expression defines tissue-resident cd8(+) T cells poised for cytotoxic function in human skin. Immunity (2017) 46(2):287–300. doi: 10.1016/j.immuni.2017.01.009
92. Mueller SN, Mackay LK. Tissue-resident memory T cells: local specialists in immune defence. Nat Rev Immunol (2016) 16(2):79–89. doi: 10.1038/nri.2015.3
93. Riding RL, Harris JE. The role of memory cd8(+) T cells in vitiligo. J Immunol (2019) 203(1):11–9. doi: 10.4049/jimmunol.1900027
94. Richmond JM, Strassner JP, Zapata L Jr, Garg M, Riding RL, Refat MA, et al. Antibody blockade of il-15 signaling has the potential to durably reverse vitiligo. Sci Transl Med (2018) 10(450):eaam7710. doi: 10.1126/scitranslmed.aam7710
95. Zaid A, Mackay LK, Rahimpour A, Braun A, Veldhoen M, Carbone FR, et al. Persistence of skin-resident memory T cells within an epidermal niche. Proc Natl Acad Sci U.S.A. (2014) 111(14):5307–12. doi: 10.1073/pnas.1322292111
96. Dean JW, Helm EY, Fu Z, Xiong L, Sun N, Oliff KN, et al. The aryl hydrocarbon receptor cell intrinsically promotes resident memory cd8(+) T cell differentiation and function. Cell Rep (2023) 42(1):111963. doi: 10.1016/j.celrep.2022.111963
97. Rutishauser RL, Martins GA, Kalachikov S, Chandele A, Parish IA, Meffre E, et al. Transcriptional repressor blimp-1 promotes cd8(+) T cell terminal differentiation and represses the acquisition of central memory T cell properties. Immunity (2009) 31(2):296–308. doi: 10.1016/j.immuni.2009.05.014
98. Shah F, Patel S, Begum R, Dwivedi M. Emerging role of tissue resident memory T cells in vitiligo: from pathogenesis to therapeutics. Autoimmun Rev (2021) 20(8):102868. doi: 10.1016/j.autrev.2021.102868
99. Doulabi H, Masoumi E, Rastin M, Foolady Azarnaminy A, Esmaeili SA, Mahmoudi M. The role of th22 cells, from tissue repair to cancer progression. Cytokine (2022) 149:155749. doi: 10.1016/j.cyto.2021.155749
100. Behfarjam F, Jadali Z. Vitiligo patients show significant up-regulation of aryl hydrocarbon receptor transcription factor. Bras Dermatol (2018) 93(2):302–3. doi: 10.1590/abd1806-4841.20187183
101. Nieradko-Iwanicka B, Przybylska D, Borzęcki A. A case-control study on immunologic markers of patients with vitiligo. BioMed Pharmacother (2022) 156:113785. doi: 10.1016/j.biopha.2022.113785
102. Liu B, Xie Y, Mei X, Sun Y, Shi W, Wu Z. Reciprocal regulation of interleukin-17a and interleukin-22 secretion through aryl hydrocarbon receptor activation in cd4(+) T cells of patients with vitiligo. Exp Ther Med (2021) 21(2):158. doi: 10.3892/etm.2020.9589
103. Takami M, Fujimaki K, Nishimura MI, Iwashima M. Cutting edge: ahr is a molecular target of calcitriol in human T cells. J Immunol (2015) 195(6):2520–3. doi: 10.4049/jimmunol.1500344
104. Kumar S, Marathe S, Dhamija B, Zambare U, Bilala R, Warang S, et al. Presence and the roles of il-9/th9 axis in vitiligo. Pigment Cell Melanoma Res (2021) 34(5):966–72. doi: 10.1111/pcmr.12978
105. D’Mello SAN, Finlay GJ, Baguley BC, Askarian-Amiri ME. Signaling pathways in melanogenesis. Int J Mol Sci (2016) 17(7):1144. doi: 10.3390/ijms17071144
106. Widlund HR, Fisher DE. Microphthalamia-associated transcription factor: A critical regulator of pigment cell development and survival. Oncogene (2003) 22(20):3035–41. doi: 10.1038/sj.onc.1206443
107. Abbas S, Alam S, Singh KP, Kumar M, Gupta SK, Ansari KM. Aryl hydrocarbon receptor activation contributes to benzanthrone-induced hyperpigmentation via modulation of melanogenic signaling pathways. Chem Res Toxicol (2017) 30(2):625–34. doi: 10.1021/acs.chemrestox.6b00364
108. Leijs MM, Esser A, Amann PM, Schettgen T, Heise R, Fietkau K, et al. Expression of cyp1a1, cyp1b1 and il-1β in pbmcs and skin samples of pcb exposed individuals. Sci Total Environ (2018) 642:1429–38. doi: 10.1016/j.scitotenv.2018.06.136
109. Lan CC, Ko YC, Tu HP, Wu CS, Lee CH, Wu CS, et al. Association study between keratinocyte-derived growth factor gene polymorphisms and susceptibility to vitiligo vulgaris in a Taiwanese population: potential involvement of stem cell factor. Br J Dermatol (2009) 160(6):1180–7. doi: 10.1111/j.1365-2133.2009.09064.x
110. Kitamura R, Tsukamoto K, Harada K, Shimizu A, Shimada S, Kobayashi T, et al. Mechanisms underlying the dysfunction of melanocytes in vitiligo epidermis: role of scf/kit protein interactions and the downstream effector, mitf-M. J Pathol (2004) 202(4):463–75. doi: 10.1002/path.1538
111. Hachiya A, Kobayashi A, Ohuchi A, Takema Y, Imokawa G. The paracrine role of stem cell factor/C-kit signaling in the activation of human melanocytes in ultraviolet-B-induced pigmentation. J Invest Dermatol (2001) 116(4):578–86. doi: 10.1046/j.1523-1747.2001.01290.x
112. Lan WJ, Wang HY, Lan W, Wang KY. Geniposide enhances melanogenesis by stem cell factor/C-kit signalling in norepinephrine-exposed normal human epidermal melanocyte. Basic Clin Pharmacol Toxicol (2008) 103(1):88–93. doi: 10.1111/j.1742-7843.2008.00251.x
113. Jux B, Kadow S, Luecke S, Rannug A, Krutmann J, Esser C. The aryl hydrocarbon receptor mediates uvb radiation-induced skin tanning. J Invest Dermatol (2011) 131(1):203–10. doi: 10.1038/jid.2010.269
114. Noakes R. The aryl hydrocarbon receptor: A review of its role in the physiology and pathology of the integument and its relationship to the tryptophan metabolism. Int J Tryptophan Res (2015) 8:7–18. doi: 10.4137/ijtr.S19985
115. Shi Y, Zeng Z, Liu J, Pi Z, Zou P, Deng Q, et al. Particulate matter promotes hyperpigmentation via ahr/Mapk signaling activation and by increasing α-Msh paracrine levels in keratinocytes. Environ pollut (2021) 278:116850. doi: 10.1016/j.envpol.2021.116850
116. Dunn KJ, Brady M, Ochsenbauer-Jambor C, Snyder S, Incao A, Pavan WJ. Wnt1 and wnt3a promote expansion of melanocytes through distinct modes of action. Pigment Cell Res (2005) 18(3):167–80. doi: 10.1111/j.1600-0749.2005.00226.x
117. Zhao SJ, Jia H, Xu XL, Bu WB, Zhang Q, Chen X, et al. Identification of the role of wnt/β-catenin pathway through integrated analyses and in vivo experiments in vitiligo. Clin Cosmet Investig Dermatol (2021) 14:1089–103. doi: 10.2147/ccid.S319061
118. Regazzetti C, Joly F, Marty C, Rivier M, Mehul B, Reiniche P, et al. Transcriptional analysis of vitiligo skin reveals the alteration of wnt pathway: A promising target for repigmenting vitiligo patients. J Invest Dermatol (2015) 135(12):3105–14. doi: 10.1038/jid.2015.335
119. Nayak D, Srivastava N, Dev A, Bishnoi A, Kumaran MS, Vinay K, et al. Altered levels of lymphocyte enhancer-binding factor-1 modulates the pigmentation in acral and non-acral lesions of non-segmental vitiligo patients: A follow-up-based study in north India. Arch Dermatol Res (2023) 315(7):2003–9. doi: 10.1007/s00403-023-02585-7
120. Nakamura M, Ueda Y, Hayashi M, Kato H, Furuhashi T, Morita A. Tobacco smoke-induced skin pigmentation is mediated by the aryl hydrocarbon receptor. Exp Dermatol (2013) 22(8):556–8. doi: 10.1111/exd.12170
121. Bahraman AG, Jamshidzadeh A, Keshavarzi M, Arabnezhad MR, Mohammadi H, Mohammadi-Bardbori A. α-melanocyte-stimulating hormone triggers melanogenesis via activation of the aryl hydrocarbon receptor pathway in B16f10 mouse melanoma cells. Int J Toxicol (2021) 40(2):153–60. doi: 10.1177/1091581820987548
122. Liu B, Xie Y, Wu Z. Astragaloside iv enhances melanogenesis via the ahr-dependent akt/gsk-3β/β-catenin pathway in normal human epidermal melanocytes. Evid Based Complement Alternat Med (2020) 2020:8838656. doi: 10.1155/2020/8838656
123. Lee AY, Noh M. The regulation of epidermal melanogenesis via camp and/or pkc signaling pathways: insights for the development of hypopigmenting agents. Arch Pharm Res (2013) 36(7):792–801. doi: 10.1007/s12272-013-0130-6
124. Yuan XH, Jin ZH. Paracrine regulation of melanogenesis. Br J Dermatol (2018) 178(3):632–9. doi: 10.1111/bjd.15651
125. Bellei B, Pitisci A, Catricalà C, Larue L, Picardo M. Wnt/β-catenin signaling is stimulated by α-melanocyte-stimulating hormone in melanoma and melanocyte cells: implication in cell differentiation. Pigment Cell Melanoma Res (2011) 24(2):309–25. doi: 10.1111/j.1755-148X.2010.00800.x
126. Herraiz C, Martínez-Vicente I, Maresca V. The α-melanocyte-stimulating hormone/melanocortin-1 receptor interaction: A driver of pleiotropic effects beyond pigmentation. Pigment Cell Melanoma Res (2021) 34(4):748–61. doi: 10.1111/pcmr.12980
128. Pichler R, Sfetsos K, Badics B, Gutenbrunner S, Auböck J. Vitiligo patients present lower plasma levels of alpha-melanotropin immunoreactivities. Neuropeptides (2006) 40(3):177–83. doi: 10.1016/j.npep.2006.03.001
129. Graham A, Westerhof W, Thody AJ. The expression of alpha-msh by melanocytes is reduced in vitiligo. Ann N Y Acad Sci (1999) 885:470–3. doi: 10.1111/j.1749-6632.1999.tb08715.x
130. Abdalla MA, Nayaf MS, Hussein SZ. Correlation between serum α-msh and vitamin D levels in vitiligo patients. Iranian J Dermatol (2020) 23(4):163–7. doi: 10.22034/ijd.2020.120836
131. Toh JJH, Chuah SY, Jhingan A, Chong WS, Thng STG. Afamelanotide implants and narrow-band ultraviolet B phototherapy for the treatment of nonsegmental vitiligo in asians. J Am Acad Dermatol (2020) 82(6):1517–9. doi: 10.1016/j.jaad.2020.01.035
132. Lim HW, Grimes PE, Agbai O, Hamzavi I, Henderson M, Haddican M, et al. Afamelanotide and narrowband uv-B phototherapy for the treatment of vitiligo: A randomized multicenter trial. JAMA Dermatol (2015) 151(1):42–50. doi: 10.1001/jamadermatol.2014.1875
133. Grimes PE, Hamzavi I, Lebwohl M, Ortonne JP, Lim HW. The efficacy of afamelanotide and narrowband uv-B phototherapy for repigmentation of vitiligo. JAMA Dermatol (2013) 149(1):68–73. doi: 10.1001/2013.jamadermatol.386
134. Liao YH, Huang YT, Deng JY, Chen WS, Jee SH. Pulsed ultrasound promotes melanoblast migration through upregulation of macrophage colony-stimulating factor/focal adhesion kinase autocrine signaling and paracrine mechanisms. Pigment Cell Melanoma Res (2013) 26(5):654–65. doi: 10.1111/pcmr.12125
135. Tomkiewicz C, Herry L, Bui LC, Métayer C, Bourdeloux M, Barouki R, et al. The aryl hydrocarbon receptor regulates focal adhesion sites through a non-genomic fak/src pathway. Oncogene (2013) 32(14):1811–20. doi: 10.1038/onc.2012.197
136. Nakamura M, Nishida E, Morita A. Action spectrum of melanoblast maturation and involvement of the aryl hydrocarbon receptor. Exp Dermatol (2016) 25 Suppl 3:41–4. doi: 10.1111/exd.13088
137. Lan CC, Yu HS, Lu JH, Wu CS, Lai HC. Irradiance, but not fluence, plays a crucial role in uvb-induced immature pigment cell development: new insights for efficient uvb phototherapy. Pigment Cell Melanoma Res (2013) 26(3):367–76. doi: 10.1111/pcmr.12077
138. Searle T, Al-Niaimi F, Ali FR. Vitiligo: an update on systemic treatments. Clin Exp Dermatol (2021) 46(2):248–58. doi: 10.1111/ced.14435
139. LeWitt TM, Kundu RV. Vitiligo. JAMA Dermatol (2021) 157(9):1136. doi: 10.1001/jamadermatol.2021.1688
140. Fritsche E, Schäfer C, Calles C, Bernsmann T, Bernshausen T, Wurm M, et al. Lightening up the uv response by identification of the arylhydrocarbon receptor as a cytoplasmatic target for ultraviolet B radiation. Proc Natl Acad Sci U.S.A. (2007) 104(21):8851–6. doi: 10.1073/pnas.0701764104
141. Liu L, Yang Q, Su MW, Zhou Y. Vitiligo responds to topical aryl hydrocarbon receptor agonist tapinarof (Wbi-1001). JAAD Case Rep (2023) 39:150–1. doi: 10.1016/j.jdcr.2023.06.051
142. Schallreuter KU, Salem MA, Gibbons NC, Maitland DJ, Marsch E, Elwary SM, et al. Blunted epidermal L-tryptophan metabolism in vitiligo affects immune response and ros scavenging by fenton chemistry, part 2: epidermal H2o2/onoo(-)-mediated stress in vitiligo hampers indoleamine 2,3-dioxygenase and aryl hydrocarbon receptor-mediated immune response signaling. FASEB J (2012) 26(6):2471–85. doi: 10.1096/fj.11-201897
143. Ferreira Branquinho MS, Silva MBB, Castilho GA, Cavalcante J, Barros SBM, Clara RO, et al. Kynurenine inhibits melanogenesis in human melanocyte-keratinocyte co-cultures and in a reconstructed 3d skin model. Exp Dermatol (2022) 31(3):427–32. doi: 10.1111/exd.14486
144. Shahin Z, Leheta TM, Abdel Hay RM, Abdel Aal HM, Rashed LA. Detection of plasma and urinary monoamines and their metabolites in nonsegmental vitiligo. Acta Dermatovenerol Croat (2012) 20(1):14–20.
Keywords: aryl hydrocarbon receptor, vitiligo, oxidative stress, immunity modulation, melanogenesis
Citation: Li Y, Zeng Y, Chen Z, Tan X, Mei X and Wu Z (2024) The role of aryl hydrocarbon receptor in vitiligo: a review. Front. Immunol. 15:1291556. doi: 10.3389/fimmu.2024.1291556
Received: 09 September 2023; Accepted: 18 January 2024;
Published: 01 February 2024.
Edited by:
Nayana Prabhu, Institute of Molecular and Cell Biology (A*STAR), SingaporeReviewed by:
Maddalena Napolitano, University of Molise, ItalyCopyright © 2024 Li, Zeng, Chen, Tan, Mei and Wu. This is an open-access article distributed under the terms of the Creative Commons Attribution License (CC BY). The use, distribution or reproduction in other forums is permitted, provided the original author(s) and the copyright owner(s) are credited and that the original publication in this journal is cited, in accordance with accepted academic practice. No use, distribution or reproduction is permitted which does not comply with these terms.
*Correspondence: Zhouwei Wu, emhvdXdlaS53dUBzaGdoLmNu
†These authors have contributed equally to this work
Disclaimer: All claims expressed in this article are solely those of the authors and do not necessarily represent those of their affiliated organizations, or those of the publisher, the editors and the reviewers. Any product that may be evaluated in this article or claim that may be made by its manufacturer is not guaranteed or endorsed by the publisher.
Research integrity at Frontiers
Learn more about the work of our research integrity team to safeguard the quality of each article we publish.