- 1Department of Microbiology and Immunology, Indiana University School of Medicine, Indianapolis, IN, United States
- 2Department of Anesthesia, Stark Neurosciences Research Institute, Indiana University School of Medicine, Indianapolis, IN, United States
- 3Division of Hematology/Oncology, Department of Medicine, Indiana University School of Medicine, Indianapolis, IN, United States
- 4Children’s Health Services Research, Department of Pediatrics, Indiana University School of Medicine, Indianapolis, IN, United States
- 5Indiana Hemophilia and Thrombosis Center, Indianapolis, IN, United States
- 6Chronic Pain and Fatigue Research Center, Department of Anesthesiology, University of Michigan Medical School, Ann Arbor, MI, United States
- 7Susan Samueli Integrative Health Institute, and Department of Anesthesiology and Perioperative Care, School of Medicine, University of California at Irvine, Irvine, CA, United States
This study aimed to comprehensively analyze inflammatory and autoimmune characteristics of patients with sickle cell disease (SCD) at a steady-state condition (StSt) compared to healthy controls (HCs) to explore the pathogenesis of StSt and its impact on patients’ well-being. The study cohort consisted of 40 StSt participants and 23 HCs enrolled between July 2021 and April 2023. StSt participants showed elevated white blood cell (WBC) counts and altered hematological measurements when compared to HCs. A multiplex immunoassay was used to profile 80 inflammatory cytokines/chemokines/growth factors in plasma samples from these SCD participants and HCs. Significantly higher plasma levels of 35 analytes were observed in SCD participants, with HGF, IL-18, IP-10, and MCP-2 being among the most significantly affected analytes. Additionally, autoantibody profiles were also altered, with elevated levels of anti-SSA/Ro60, anti-Ribosomal P, anti-Myeloperoxidase (MPO), and anti-PM/Scl-100 observed in SCD participants. Flow cytometric analysis revealed higher rates of red blood cell (RBC)/reticulocyte-leukocyte aggregation in SCD participants, predominantly involving monocytes. Notably, correlation analysis identified associations between inflammatory mediator levels, autoantibodies, RBC/reticulocyte-leukocyte aggregation, clinical lab test results, and pain crisis/sensitivity, shedding light on the intricate interactions between these factors. The findings underscore the potential significance of specific biomarkers and therapeutic targets that may hold promise for future investigations and clinical interventions tailored to the unique challenges posed by SCD. In addition, the correlations between vaso-occlusive crisis (VOC)/pain/sensory sensitivity and inflammation/immune dysregulation offer valuable insights into the pathogenesis of SCD and may lead to more targeted and effective therapeutic strategies.
Clinical Trial Registration: ClinicalTrials.gov, Identifier: NCT05045820.
Introduction
Sickle cell disease (SCD) is a lifelong illness that affects multiple organ systems and can cause a range of complications, including acute and chronic pain, anemia, stroke, pulmonary hypertension, and organ damage (1, 2). The clinical manifestations of SCD can be broadly categorized into two phases: the steady-state (StSt) phase, characterized by mild to no symptoms associated with chronic hemolysis and persistent pain (3), and the severe pain and other clinical complications in the acute hemolytic/vaso-occlusive crisis (VOC) phase (4, 5). The acute VOC phase is marked by the sudden onset of severe pain, possibly accompanied with acute chest syndrome and stroke due to the blockage of small blood vessels by sickle-shaped red blood cells (RBCs). Individuals with SCD requiring high doses of opioids to manage VOC confront a series of substantial risks, such as an increased vulnerability to overdose and mortality, the potential development of opioid-induced hyperalgesia, and a compromised quality of life (QoL). Addressing these challenges becomes paramount, emphasizing the urgent need for evidence-based, effective, and safe pain management therapies tailored specifically for SCD. Indeed, pain is the hallmark of SCD, manifesting anywhere in the body and profoundly impacting patients’ QoL (6–8). SCD pain can manifest as acute recurrent painful crises associated with VOCs as well as chronic pain with or without nerve damage (7). The painful crisis, which evolves through four phases—prodromal, initial, established, and resolving—is a leading cause of hospitalization and emergency department treatments in SCD (7).
SCD is characterized by the presence of sickle-shaped RBCs with altered biophysical and biochemical properties (9). Unlike normal RBCs, which have a biconcave disc shape for flexibility and deformability, sickle-shaped RBCs lose their ability to deform and navigate through small blood vessels efficiently, leading to blockages and reduced blood flow. To compensate for the RBC loss, bone marrow produces more immature RBCs known as reticulocytes, releasing them into circulation and leading to elevated reticulocyte levels in the blood. In SCD participants, reticulocytes express higher levels of surface adhesion molecules such as Lutheran/basal cell adhesion molecule (Lu/BCAM) and alpha-4 beta-1 (α4β1) integrin (10–15). These adhesion molecules facilitate the binding of reticulocytes to the endothelium lining the blood vessels, causing activation of endothelial cells (ECs) and the release of inflammatory molecules (16–18). Moreover, reticulocytes in SCD interact with circulating leukocytes, including polymorphonuclear neutrophils (PMNs) and monocytes, leading to the formation of aggregates and increasing the occurrence of VOCs (19–21). These interactions are primarily mediated by α4β1 integrin and Lu/BCAM, reinforcing their adhesion to the endothelium (9, 22). In vitro studies have demonstrated that sickle-shaped RBCs can also bind to peripheral leukocytes, especially monocytes, via erythroid LW/ICAM-4 and CD44 receptors. These RBC/reticulocyte-leukocyte aggregates interact with ECs, resulting in EC activation (21). Thus, SCD is associated with increased adhesion of RBC/reticulocytes to leukocytes and ECs, which contribute to the complex pathophysiology underlying SCD pain (10–18).
Chronic inflammation persists in SCD due to continuous activation of immune cells or autoimmune responses triggered by various factors, such as hemolysis, vaso-occlusion, and sickle-shaped RBC aggregates. Hemolysis is a primary inflammatory trigger in SCD (17). Sickle-shaped RBCs are more fragile and prone to rupture, leading to the chronic release of hemoglobin and other cellular components. Hemoglobin is a potent pro-inflammatory molecule that activates immune cells such as monocytes, macrophages, and dendritic cells (DCs), prompting them to produce pro-inflammatory cytokines such as IL-1β (23), IL-6 (24), and TNF-α (23). Additionally, heme, an iron-containing component of hemoglobin, can further induce the production of pro-inflammatory cytokines, chemokines, and adhesion molecules by activating NF-κB and TLR4 signaling pathways, and promote the recruitment of leukocytes and platelets to sites of inflammation, exacerbating the inflammatory response (25). In addition, vaso-occlusion of small blood vessels by sickle-shaped RBCs leads to tissue ischemia and subsequent hypoxia, activating ECs. The activated ECs produce and release pro-inflammatory cytokines and adhesion molecules (26, 27), which recruit lymphocytes to the site of inflammation, further perpetuating the inflammatory response. Furthermore, chronic activation of the coagulation system in SCD participants results in the release of pro-inflammatory mediators such as thrombin, which can activate ECs and promote the recruitment of inflammatory cells. SCD is also associated with oxidative stress, which contributes to inflammation by activating the NF-κB pathway, leading to the production of pro-inflammatory cytokines and chemokines. Finally, the impact of autoantibodies on inflammation in SCD participants is noteworthy. Elevated inflammatory mediators, such as the B-cell activating factor (BAFF), can lead to less efficient negative selection of autoreactive B cells (28) and promote polyclonal B cell activation, leading to the production of autoantibodies (29). These autoantibodies exacerbate inflammation by forming immune complexes that activate pro-inflammatory pathways, recruit immune cells, and contribute to tissue damage. Highlighting the complex pathophysiology that contributes to inflammation in SCD, a recent study quantified serum levels of 27 inflammatory cytokines, chemokine, and growth factors in 27 individuals in StSt, 22 individuals in VOC, and 53 healthy individuals (30). The study found that both pro- and anti-inflammatory cytokines are involved in the inflammatory response during SCD, regardless of clinical phase (30). This suggests that the dysregulation of cytokine production and balance may play a role in the disease’s pathophysiology.
The current study represents the initial phase of an ongoing clinical trial (ClinicalTrials.gov, NCT05045820) focused on investigating the clinical efficacy and neurobiological mechanisms of acupuncture analgesia in SCD participants, for which recruitment is ongoing. The primary objective of the current study was to characterize the pain and the underlying immune and inflammatory abnormalities in SCD participants. To achieve this objective, we examined plasma levels of inflammatory cytokines, chemokines, growth factors, soluble receptors, and effector molecules, as well as the profiles of autoantibodies and the aggregation between RBCs/reticulocytes and leukocytes. Moreover, we investigated the association between these analytes and VOCs, patient reported pain- and QoL-related outcomes, and sensory sensitivity. Our long-term goal is to identify potential mechanisms and therapeutic targets in SCD, gaining an improved understanding of the mechanisms behind acupuncture intervention.
Materials and methods
Study participants
This work constitutes an initial part of an ongoing randomized clinical trial in SCD that commenced on June 29, 2021, and is scheduled to conclude on May 31, 2026. The primary inclusion criteria for participant enrollment included: 1) has been diagnosed with SCD, 2) experiencing chronic pain within the last 6 months or encountered at least one VOC within the past 12 months, 3) no recent changes in stimulant medication dosage or initiation, 4) willingness to continue their ongoing treatments, and 5) an agreement to limit using any new medications or treatment methods for pain management throughout the study. The major exclusion criteria included: 1) individuals with COVID-19 suspected or confirmed, 2) recent or ongoing pain management using acupuncture or acupuncture-related techniques within the last 6-months, and 3) presence of a concurrent autoimmune or inflammatory disease such as rheumatoid arthritis, systemic lupus erythematosus (SLE), and inflammatory bowel disease. In addition, participants who received a blood transfusion within the 90 days prior to recruitment were excluded for analyses. Age-, gender-, and ethnicity-matched health subjects without SCD were recruited as healthy controls (HCs). Detailed information regarding the inclusion and exclusion criteria can be found on ClinicalTrials.gov (NCT05045820).
Peripheral blood samples were collected from 40 SCD participants, comprising 17 males and 23 females, with ages ranging from 14 to 73 years. All participants were Black/African American. SCD participants and HCs were enrolled in this study through Indiana University Health hospitals in Indianapolis, the Indiana Hemophilia & Thrombosis Center, community hospitals, and other resources between July 2021 to April 2023. Peripheral blood was collected at StSt phase in heparin-coated tubes (BD Biosciences, Franklin Lakes, NJ) and subsequently separated into plasma and peripheral blood mononuclear cells (PBMCs). PBMCs were either directly used or cryopreserved in liquid nitrogen until use. Plasma samples were stored at -80°C until use. To establish a comparison, we included PBMC and plasma samples from 23 healthy volunteers, matched in terms of age, sex, and race, to serve as HCs. Detailed demographic and clinical characteristics of both SCD participants and HCs are summarized in Table 1. This study was performed with the approval of the Institutional Review Boards (IRB) at Indiana University School of Medicine, and each participant provided written informed consent during the screening visit prior to the subsequent study procedure.
Patient-reported outcome measures (PROMs)
The Patient-Reported Outcomes Measurement Information System (PROMIS)-29 Questionnaire was used to evaluate pain intensity and interference, as well as physical function (31). Neuropathic pain symptoms were evaluated using the PainDETECT Questionnaire (higher score indicated higher pain) (32, 33). The Widespread Pain Index was used to evaluate the spatial distribution of pain across the body (higher score indicated higher pain) (34, 35). In addition, depression was evaluated using the Hospital Anxiety and Depression Scale (HADS) (higher score indicated higher depression) (36). Physical function was assessed with PROMIS-29 (higher score indicated more physical dysfunction) (31). Pain-related QoL was evaluated using the Pediatric Quality of Life Inventory (PedsQL) targeting 3 different age groups (13-18, 18-25, or 25+ years old), with higher scores representing better QOL (37). The number of patient-reported VOCs in the preceding 12 months was documented (38, 39). The number of days between blood draw and the most recent or future VOCs was recorded as the time intervals after or before VOCs.
Quantitative Sensory Testing (QST)
QST is a well-established experimental protocol designed to investigate both ascending excitatory and descending inhibitory aspects of pain processing by assessing an individual’s perceptual response to various stimuli (40). QST was performed at up to three different body sites, including the primary testing site(s), which was the area(s) reported as most painful by each patient, along with the dominant-side ventral forearm and/or the dominant-side upper trapezius muscle, as described in previous studies on SCD (40, 41). HCs received primary testing at sites matched with those identified in patients, in addition to testing at the dominant forearm and/or trapezius.
Thermal (heat/cold) Detection/Pain Threshold was determined at each testing site using a TCA11 (QST-Lab, Strasbourg, France) with a thermal probe in contact with the subject’s skin surface. The thermode temperature was gradually adjusted from a baseline temperature at a controlled rate of 0.5 - 1°C/s. Subjects indicated the thermal detection threshold (when they first felt the thermal stimulus) and the hot pain threshold (when they first felt pain from the thermal stimulus). The average of three trials for each test was used for analysis.
Mechanical Detection Threshold (MDT)/Mechanical Pain Threshold (MPT) were examined using von Frey monofilaments (Stoelting, Wood Dale, IL) and calibrated pinprick stimuli (MRC Systems GmbH, Heidelberg, Germany) respectively. Each von Frey monofilament was applied three times in ascending sequence until the stimulus was detected in at least two out of three trials. The next lower von Frey monofilament was then applied, and the lowest filament to be detected at least twice was considered the detection threshold. The MPT was determined using different pinprick probes applied to the skin surface of each site. Testing started with a stimulation intensity of 8 mN and in each case, the next higher pinprick stimulator was applied until the perception of “touch” changed its quality toward an additional percept of “sharp”, “pricking,” or “stinging.” The corresponding intensity represented the first suprathreshold value. Once the first painful stimulus was perceived, the testing direction was changed step-wise toward lower stimulus intensities until the first stimulus perceived as “blunt” and no longer as being “sharp,” “pricking” or “stinging” (subthreshold value). Again, a directional change toward higher intensities occurred and the cycle was repeated until five suprathreshold and five subthreshold values were determined. An inflection point was calculated as the average value of the ten suprathreshold and subthreshold to determine the MPT.
Mechanical Temporal Summation (MTS) was assessed using a single 256 mN pinprick (MRC Systems GmbH, Heidelberg, Germany) stimulus applied in triplicate to the skin surface of the selected sites, followed by a series of 10 identical stimuli (1 Hz – metronome-guided). MTS was calculated as the average pain rating from the series of 10 stimuli minus the average pain rating from the three trials with the single stimulus.
Pressure Pain Threshold (PPT)/Pressure Pain Tolerance (PPTol) was assessed using a digital, handheld pressure algometer (Algometer II, Somedic SenseLab AB, Norra Mellby, Sweden). The pressure was manually increased at a rate of 50 kPa/s (1000 kPa max) until participants indicated that the sensation of pressure became one of faint pain (PPT) and the maximum pressure pain that the participant can tolerate (PPTol), respectively. The average of 3 trials per site was used for analysis.
Conditioned Pain Modulation (CPM): Tonic pressure pain was used as the conditioning stimulus delivered via a cuff (Hokanson, Bellevue, WA) attached to the gastrocnemius muscle of the non-dominant leg. Pressure intensities were individually calibrated for each participant to elicit moderate pain (pain rating at 40-60 on a scale of 100) (42, 43). PPT served as the test stimulus and was measured 3 times at the dominant trapezius muscle prior to and during the cuff stimulation. Pain ratings were obtained every 15 s prior to and during cuff stimulation for up to 90s. CPM magnitude was calculated as patient-reported pain ratings during conditioned cuff pressure stimuli.
Only QST results on the standard sites (forearm, trapezius) were used for correlation analysis as each SCD participant often had different primary painful site(s).
Multiplex immunoassays and enzyme-linked immunosorbent assay (ELISA)
Plasma concentrations of 80 inflammatory human cytokines, chemokines, growth factors, soluble receptors, and effector molecules and 18 human autoantibodies were simultaneously measured using the Immune Response 80-Plex Human ProcartaPlex™ Panel (Cat. #: EPX800-10080-901, Invitrogen, Carlsbad, CA) and the MILLIPLEX MAP Human Autoimmune Autoantibody Panel (HAIAB-10K, MilliporeSigma, Burlington, MA), respectively, according to the manufacturer’s instructions. The beads were read on a BioPlex 200 system (Bio-Rad, Hercules, CA). The standards at 4-fold serial dilutions were run on each plate in duplicate and used to calculate the concentrations of human cytokines, chemokines, growth factors, soluble receptors, and effector molecules using the Bio-Plex Manager Software (Bio-Rad, Hercules, CA) as previously reported (44). Plasma samples were diluted 100-fold for the autoantibody multiplex assay, and the levels of the autoantibodies were reported as mean fluorescence intensity (MFI) after background MFI subtraction. The plasma levels of C-reactive protein (CRP) were quantified using human CRP Duoset ELISA Kit (R&D Systems, Minneapolis, MN) according to the manufacturer’s instructions.
Flow cytometry
Freshly prepared PBMCs were stained with fluorochrome-conjugated antibodies against human CD45, CD71 (expressed on erythroid precursors), BCAM, and CD235ab (also known as GPA: glycophorin A, the major sialoglycoprotein on RBCs and their precursors) to measure the aggregation between RBC/erythroid precursors and CD45+ leukocytes. Cells stained with surface markers were acquired using a BD LSRFortessa flow cytometer (BD Biosciences, San Jose, CA). Flow data were analyzed using FlowJo v10 software (Tree Star, San Carlos, CA). The percentage of CD235abhi cells among the CD45+ leukocytes was defined as the RBC/reticulocyte-leukocyte aggregation rate. The association of RBCs/reticulocytes with lymphocytes or monocytes were determined based on their characteristics of FSC and SSC on flow cytometry, respectively.
Statistical analysis
Statistical analysis was performed using GraphPad Prism 10 and SPSS 29. Data were expressed as median and interquartile range (Tables 1-3). Differences between 2 groups were calculated using the Mann-Whitney test for continuous variables (Tables 1–3). χ 2 test was used for comparison between 2 groups for gender distribution (Table 1). Adjusted p values were calculated for the 80-plex and 18-plex analytes using the Holm-Šídák correction for multiple comparisons (Table 3). Inflammatory mediators that were heightened in the SCD participants were used for subsequent Spearman correlation analyses using age, gender and SCD genotype as covariables (Tables 4-6). p < 0.05 was considered statistically significant.
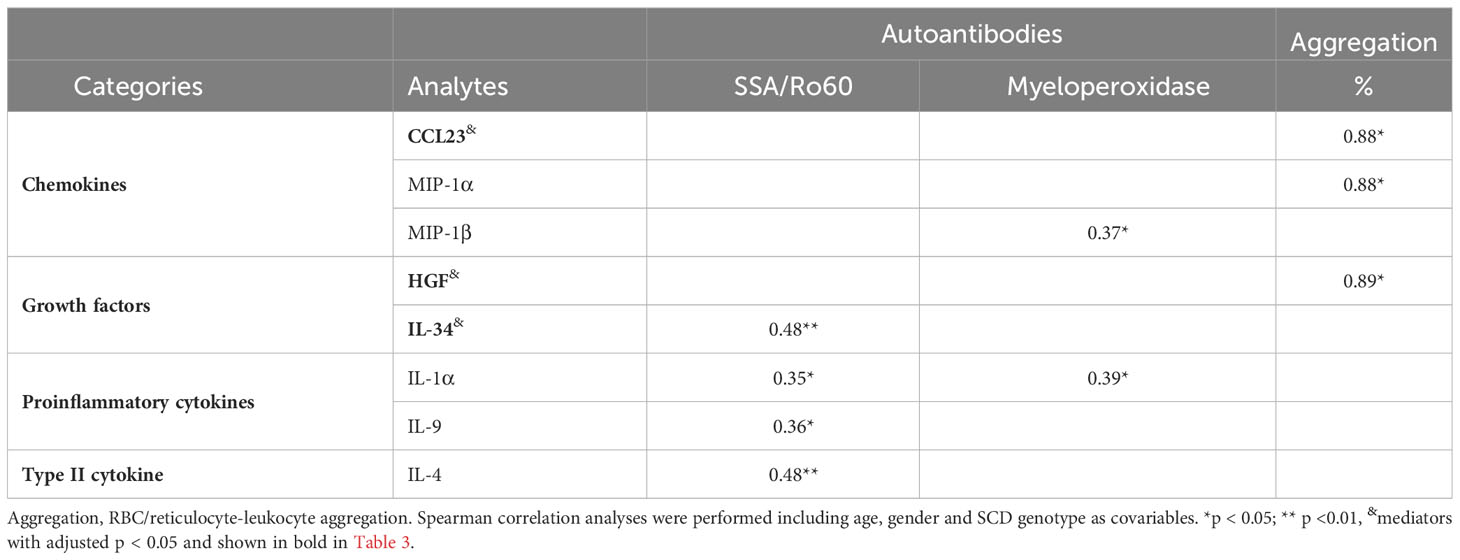
Table 4 Correlations of upregulated inflammatory mediators with altered autoantibodies and RBC/reticulocyte-leukocyte aggregation.

Table 5 Correlations of altered inflammatory mediators with time interval from after and before VOC crisis.
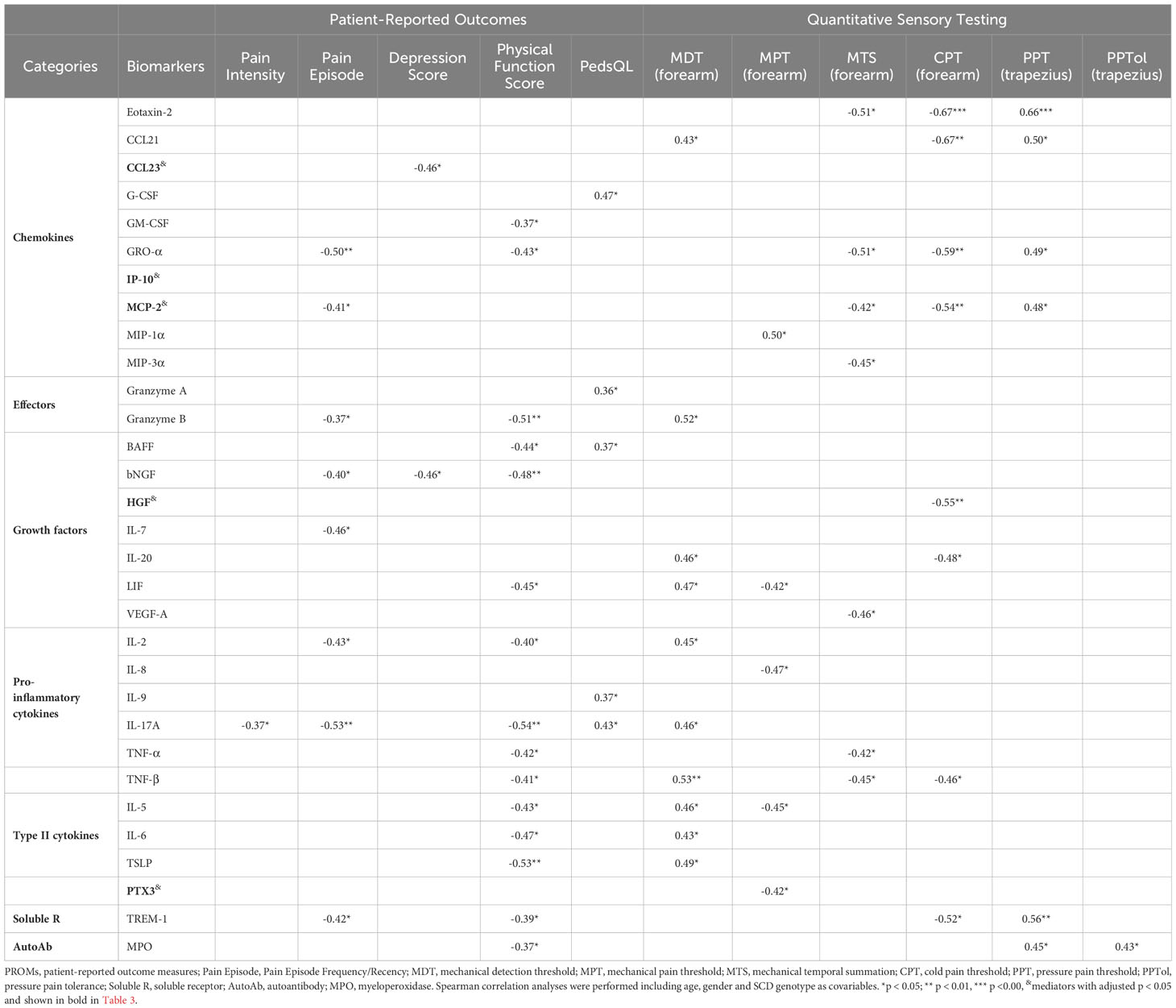
Table 6 Correlations of upregulated soluble factors/autoantibody with PROMs/Sensory sensitivity in SCD subjects.
Results
Characteristics of the study cohort: demographics, laboratory exams, patient reported outcomes and sensory sensitivity
Demographics and clinical characteristics of participants are summarized in Table 1. SCD participants and HCs showed no significant differences in terms of age and gender distribution. Compared to the HCs, SCD participants exhibited higher numbers of white blood cells (WBCs), reticulocytes, platelets, neutrophils, monocytes, and basophils but reduced RBCs, hemoglobin, and hematocrit (HCT). Additionally, SCD participants displayed elevated levels of mean corpuscular volume (MCV), mean corpuscular hemoglobin (MCH), red cell distribution width (RDW), and the systemic inflammation marker CRP than HCs (Table 1). The differences in the absolute numbers of lymphocytes and eosinophils approached a clear trend toward significance (p = 0.055 and p = 0.058, respectively) between the two groups (Table 1).
As indicated in Table 2, SCD participants reported increased pain interference and intensity, more painful body sites, reduced physical functioning, and higher levels of depression relative to HCs. Compared to HCs, SCD participants also displayed hypersensitivity to experimental mechanical (MDT, MPT, MTS), cold (CPT), and pressure stimuli (PPT and PPTol), but not hot stimuli (HDT, HPT) as measured by QST (Table 2). In addition, the CPM pain rating was similar between HCs and SCD subjects (Table 2).
SCD participants presented with elevated proinflammatory cytokines, chemokines, growth factors, effector molecules, and soluble receptors compared to HCs
SCD participants had significantly higher plasma levels of 35 analytes compared to HCs (Table 3). These elevated analytes encompassed 10 chemokines (CCL21, CCL23, Eotaxin-2, Gal-3, GRO-α, IP-10, MCP-2, MIP-1α, MIP-1β, and MIP-3α), 2 effector molecules (granzymes A and B), 9 growth factors (BAFF, bNGF, G-CSF, GM-CSF, HGF, IL-7, IL-20, IL-34, and LIF), 8 pro-inflammatory cytokines (IFN-γ, IL-1α, IL-2, IL-8, IL-9, IL-18, TNF-α, and TNF-β), 4 type II inflammatory/anti-inflammatory cytokines (IL-4, IL-5, IL-6, and TSLP), and 2 immune modulating soluble receptors (PTX3 and TREM-1) (Table 3; Supplementary Table 1). In addition, levels of the endothelial cell growth factor VEGF-A and the proinflammatory cytokine IL-17A trended higher in the SCD participants (p = 0.052 and p = 0.055, respectively) (Table 3; Supplementary Table 1). After being corrected for multiple comparisons, 9 out of the 37 altered analytes remained significantly higher in the SCD participants compared to the HCs. These included 4 chemokines (CCL23, Gal-3, IP-10, and MCP-2), 2 growth factors (HGF and IL-34), 2 proinflammatory cytokines (IFN-γ and IL-18), and the soluble receptor PTX3 (Table 3; Supplementary Table 1). Notably, among these altered analytes, HGF, IL-18, IP-10, and MCP-2 exhibited the most significant upregulation in SCD participants in comparison to HCs (Table 3; Supplementary Table 2).
Both anti-nuclear autoantibodies (ANAs) and non-ANAs were present and elevated in SCD participants
Plasma concentrations of autoantibodies against 18 human antigens were simultaneously measured using a multiplex immunoassay. We identified the presence of autoantibodies against 4 human antigens, including Sjögren’s Syndrome-related antigen A/Ro60 kDa (SSA/Ro60), ribosomal P, myeloperoxidase (MPO), and PM/Scl-100, which were significantly elevated in SCD participants when compared to HCs (Figures 1A,B). After multiple comparison corrections, SSA/Ro60 and MPO remained significantly higher in the SCD subjects (p = 0.048 and p = 0.022, respectively). It is noteworthy that while SSA/Ro60 autoantibody is typically classified as an ANA, autoantibodies against the ribosomal P, MPO, or PM/Scl-100 are not categorized as ANAs (non-ANAs). Thus, both ANAs and non-ANAs in the bloodstream were significantly elevated in SCD participants compared to HCs.
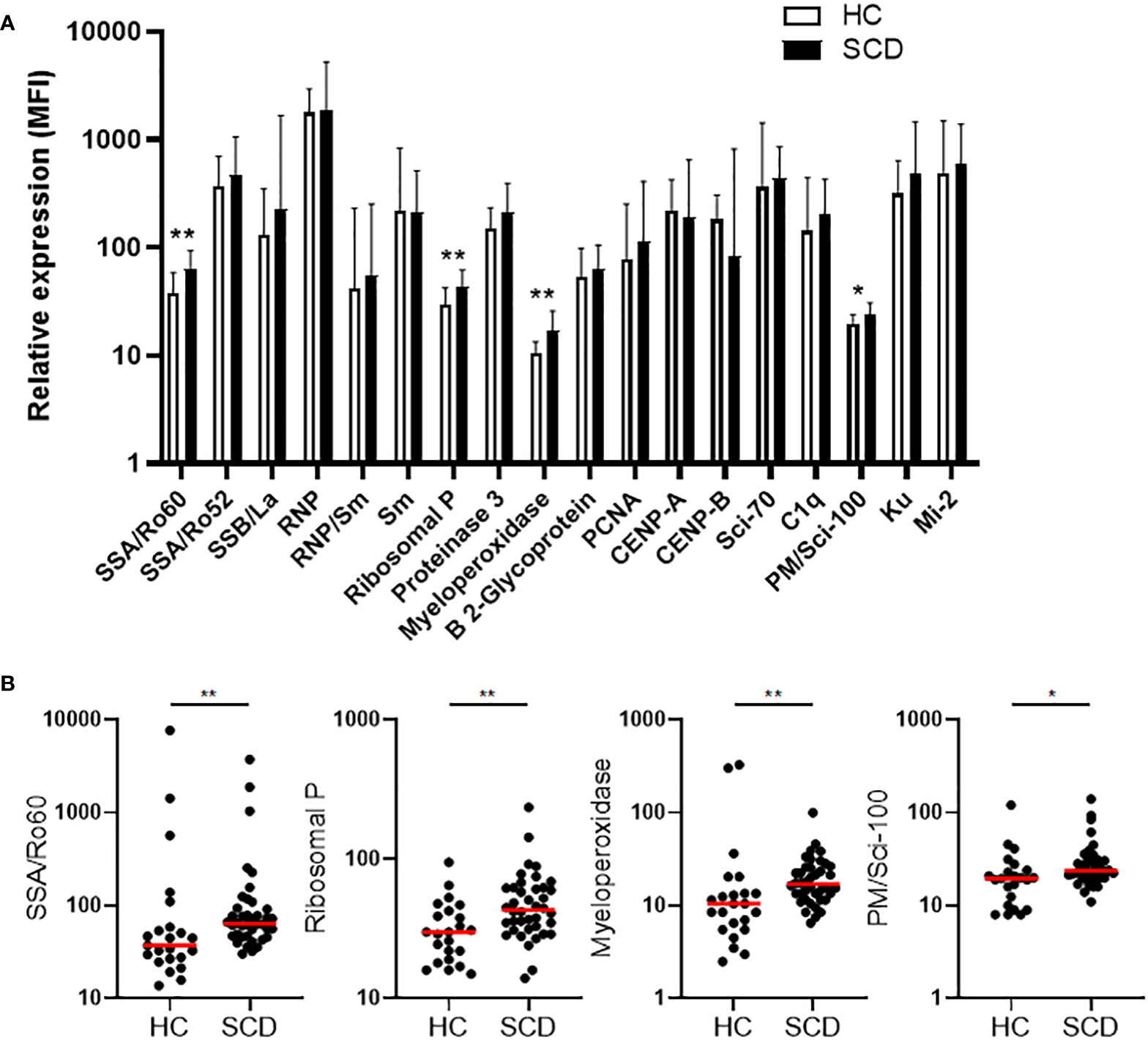
Figure 1 Comparative analysis of quantity and individual incidence of elevated autoantibodies in SCD participants versus healthy controls. (A) The levels of plasma autoantibodies against 18 autoantigens in SCD participants (n=40) versus healthy controls (HCs, n=23). The median fluorescence intensity (MFI) after background MFI subtraction was used to represent relative expression of the 18 autoantibodies in SCD and HC samples, shown as median + upper interquartile range (IQR). (B) Scatter plots demonstrating the plasma levels of 4 autoantibodies (anti-SSA/Ro60, anti-Ribosomal P, anti-Myeloperoxidase, and anti-PM/Scl-100) that were significantly elevated in SCD participants compared to healthy controls. Lines represent the median. SCD, sickle cell disease; HC, healthy control. Mann-Whitney test was used to compare the results from SCD patient versus HC samples. *p < 0.05; **p < 0.01.
SCD participants exhibited abnormally high rates of RBC/reticulocyte-leukocyte aggregation
RBC/reticulocyte-leukocyte aggregates in PBMCs from the SCD participants were assessed using flow cytometry (Figure 2A). Freshly isolated PBMCs were stained with antibodies again human CD45 (a marker expressed on all leucocytes), CD235ab (a marker expressed on RBCs and their precursors), BCAM, and CD71 (transferring receptor-1, a specific marker of reticulocytes among the RBC population). As depicted in Figure 2B, the percentage of total CD235abhiCD45+ aggregates were significantly higher in SCD participants compared to HCs. The percentage of CD71+ reticulocyte aggregates was significantly higher in SCD participants compared to HCs (Figure 2C, left panel). In parallel, the percentage of CD71+ reticulocytes in free fraction were also significantly higher in SCD participants compared to HCs, indicating an increase of reticulocytes in SCD participants (Figure 2C, right panel). Similarly, BCAM expression on aggregates or unaggregated RBCs was significantly higher in SCD participants compared to HCs (Figure 2D). This observation suggested that StSt PBMC samples contained higher numbers of RBCs or RBC precursors, such as reticulocytes, and higher levels of the cell adhesion molecule BCAM, thus contributing to the increased occurrence of aggregates. Compared to HCs, the percentage of CD45+ lymphocytes in the aggregates were lower in SCD participants, whereas the frequency of monocytes was significantly higher (Figure 2E). When the immune cell types in PBMCs and the aggregates in the SCD participants were analyzed and compared, monocytes were enriched within the aggregates with a corresponding decrease of lymphocytes within aggregation relative to PBMCs (Figure 2F), indicating RBCs/RBC precursors preferentially interacted with monocytes within the aggregates.
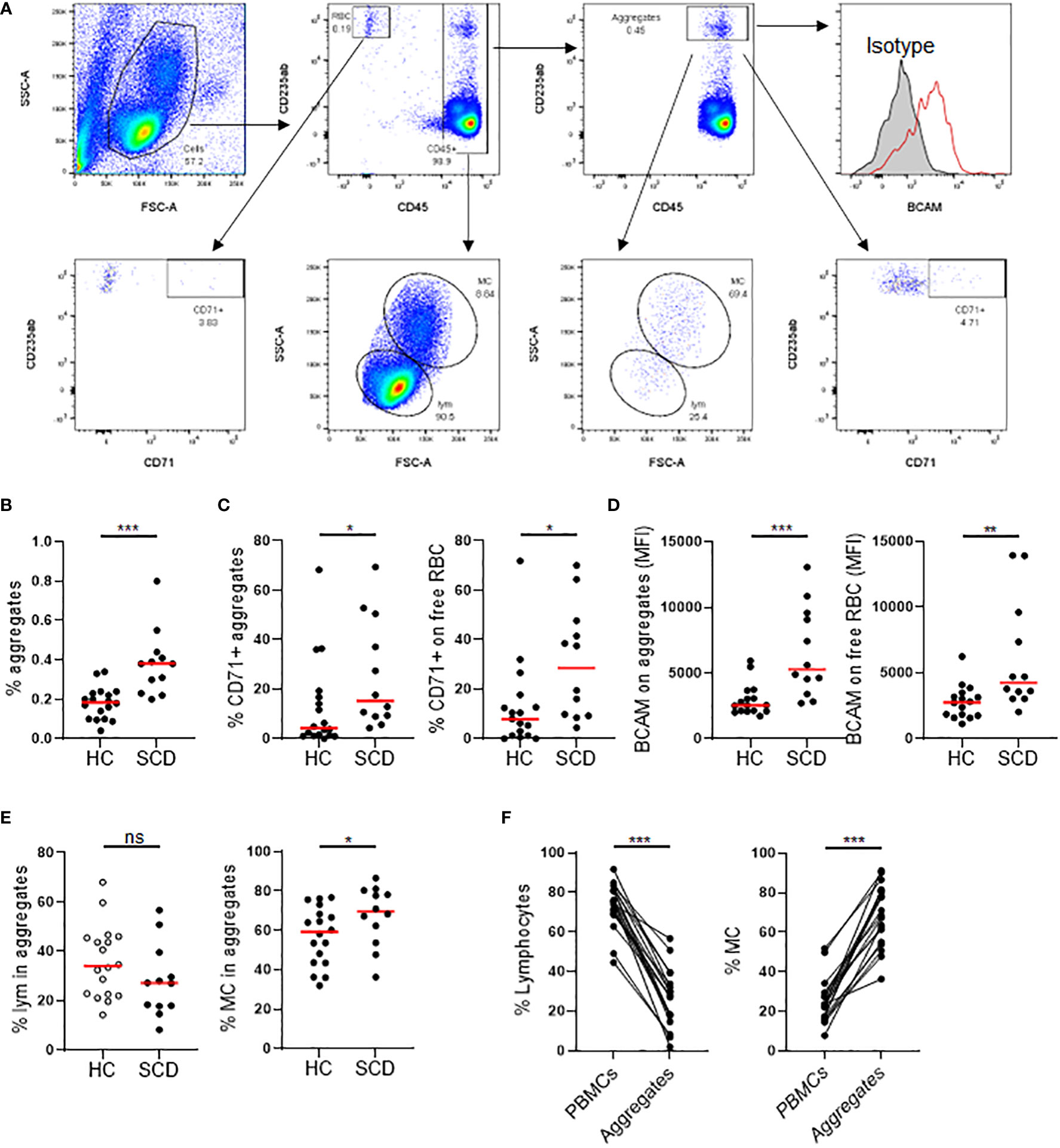
Figure 2 Comparison of RBC/reticulocyte aggregation with CD45+ PBMCs in SCD participants versus healthy controls. Freshly isolated peripheral blood mononuclear cells (PBMCs) from the SCD participants (n=12) and healthy controls (HC, n=17) were analyzed for RBC/reticulocyte aggregation with CD45+ PBMCs by flow cytometry. (A) Gating strategies for flow cytometric analysis of RBC/reticulocyte-CD45+ leukocytes aggregation. PBMCs were stained with fluorochrome-conjugated antibodies against human CD45, CD235ab, CD71, BCAM and isotype control antibodies. CD235ab+ cells that were not associated with CD45+ PBMCs were defined as RBC/reticulocyte in free fraction (RBC). Within the CD45+ cells, CD235abhi cells were defined as the RBC/reticulocyte-CD45+ leukocyte aggregates. The gate for CD71+ RBC/reticulocyte and RBC/reticulocyte-leukocyte aggregates were based on the isotype control. BCAM level was expressed as mean fluorescent intensity (MFI). Lymphocytes (LYM) and monocytes (MC) were gated based on their FCS and SSC chrematistics. (B) Scatter plots showing a significantly higher percentage of RBC/reticulocyte-CD45+ leukocyte aggregation in the SCD participants than HC. (C, D) Scatter plots displaying higher levels of CD71+ erythroid precursors (C) and the cell adhesion molecule BCAM (D) on both aggregated and unaggregated RBC/reticulocyte in the SCD participants compared to HC. (E) Scatter plots comparing the frequencies of lymphocytes and monocytes within RBC/reticulocyte-CD45+ leukocyte aggregates. (F) Before and after plots showing the percentages of lymphocytes (left) were reduced while those of monocytes (right) were heightened in the aggregates compared to PBMCs in the SCD subjects. Mann-Whitney test was used to compare the results from SCD patient versus HC samples. Wilcoxon test was used to calculate the differences between aggregates and PBMCs. *p < 0.05; **p < 0.01; ***p < 0.001. ns, not significant.
Comprehensive analysis revealed correlations of inflammatory mediators with autoantibodies and RBC/reticulocyte-leukocyte aggregation in SCD participants
Next, we evaluated correlations between the 37 elevated inflammatory mediators (9 analytes with adjusted p<0.05 bolded) that were listed in Table 3 and altered autoantibodies targeting SSA/Ro60, ribosomal P, MPO, and PM/Scl-100 and RBC/reticulocyte-leukocyte aggregation in StSt participants (Table 4). The elevated plasma level of IL-4, a type II cytokine known for facilitating the development of B cells into antibody-producing plasma cells, exhibited significant positive associations with SSA/Ro60 autoantibodies (Table 4). These autoantibodies are a type of ANAs commonly linked to autoimmune diseases such as SLE (45). IL-4 also exhibited a trend toward correlation with ribosomal P and MPO autoantibodies (r = 0.31, p = 0.076 for both). Additionally, SSA/Ro60 autoantibodies showed significant associations with multiple inflammatory mediators, including the growth factor IL-34 and 2 proinflammatory cytokines (IL-1α and IL-9). Furthermore, SSA/Ro60 autoantibodies trended to positively correlate with another type II cytokine IL-5 (r = 0.33, p = 0.062), the effector molecule granzyme A (r = 0.34, p = 0.055), and the growth factor VEGF-A (r = 0.33, p = 0.063). Both IL-1α and MIP-1β positively correlated with the non-ANA autoantibodies against MPO. Conversely, none of the 37 elevated inflammatory mediators were found to be associated with anti-PM/Scl-100 autoantibodies (data not shown).
We also analyzed the association of the inflammatory mediators with RBC/reticulocyte-leukocyte aggregation. Two chemokines (CCL23 and MIP-1α) and the growth factor HGF exhibited positive associations with the percentage of RBC/reticulocyte-leukocyte aggregates (Table 4). Together, our data demonstrate that inflammation is closely linked to autoimmunity and RBC aggregation with leukocytes in SCD participants at the steady state.
Correlation analysis uncovered significant associations between inflammatory mediators and autoantibodies with VOCs/pain/sensory sensitivity
To investigate potential underlying factors contributing to VOCs, we examined the relationships of the elevated 37 inflammatory mediators, 4 autoantibodies, or RBC/reticulocyte-leukocyte aggregates with the time intervals from blood draw for biomarker analysis to the most recent VOC episode (days after crisis) and to the future crisis (days before crisis) (Table 5). The blood samples were all taken at the steady-state with varying duration of before and after crisis time for individual SCD subjects. The median time interval for before crisis was 42 days with an interquartile of 14 and 61 days, whereas the median time interval for after crisis was 26 days with an interquartile of 15 and 123 days. After being adjusted for age, gender, and SCD genotypes, 4 inflammatory mediators (GRO-α, IL-6, IL-17A, and LIF) showed significant positive correlations with the time intervals after VOCs. In addition, the chemokine Eotaxin-2 and the cytokine IL-2 trended to positively correlate with the time intervals after VOCs (r = 0.36, p = 0.058; r = 0.37, p = 0.051 respectively). Conversely, the inflammatory cytokine IL-18 and granzyme A exhibited negative associations with the time interval before VOCs. These results suggest that specific inflammatory mediators, namely granzyme A and IL-18, might play a crucial role in the early detection or development of VOCs.
Next, we analyzed correlations of the inflammatory mediators with pain and sensory sensitivity as assessed by PROMs and QST. As presented in Table 6, several inflammatory mediators were associated with patient-reported pain intensity, physical function, and sensory sensitivity. A group of inflammatory mediators (G-CSF, granzyme A, BAFF, IL-9, and IL-17A) exhibited positive correlations with PedsQL scores. Conversely, most of the correlations between inflammatory mediators and various PROMs were negative. Specifically, 17 inflammatory mediators (CCL23, GM-CSF, GRO-α, MCP-2, Granzyme B, BAFF, bNGF, IL-7, LIF, IL-2, IL-17A, TNF-α, TNF-β, IL-5, IL-6, TSLP, and TREM-1) displayed negative associations with physical and psychological PROMs (Pain Intensity, Pain Episode, Depression Score, and Physical Function Score). Notably, 14 of the 17 factors were negatively correlated with the Physical Function Score, while 8 of them had negative correlations with Pain Episode Frequency/Recency. Moreover, 8 out of the 17 factors (Granzyme B, LIF, IL-2, IL-17A, TNF-β, IL-5, IL-6, and TSLP) showed a positive association with the mechanical threshold (MDT forearm). Two additional factors, CCL21 and IL-20, were also positively associated with the MDT forearm. Five factors (Eotaxin-2, CCL21, GRO-α, MCP-2, and TREM-1) displayed positive correlations with pressure threshold (PPT trapezius). Furthermore, a group of inflammatory factors (Eotaxin-2, CCL21, GRO-α, MCP-2, MIP-3α, HGF, IL-20, LIF, VEGF-A, IL-8, TNF-α, TNF-β, IL-5, PTX3, and TREM-1) displayed negative correlations with mechanical pain threshold (MPT forearm and MTS forearm), and sensitivity threshold to cold (CPT forearm). Of note, several inflammatory factors, including GRO-α, MCP-2, granzyme B, LIF, IL-2, IL-17A, TNF-β, IL-5, and TREM-1, showed correlations with multiple measures from both PROMs and QST.
We also assessed the relationship of PROMs and experimental sensory sensitivity with the autoantibodies and RBC/reticulocyte-leukocyte aggregations (Table 6). We found that elevated autoantibodies against MPO exhibited a negative correlation with Physical Function Score and a positive correlation with PPT (trapezius) and PPTol (trapezius). However, no correlations were detected between PROMS/QST and increased rate of RBC/reticulocyte-leukocyte aggregation, as well as BCAM and CD71 expression on the aggregates. Together, our results indicate that multiple inflammatory/autoimmune markers are inversely associated with the well-being of the SCD participants.
Discussion
This study represents an interim analysis of an ongoing clinical trial that aims at investigating the clinical efficacy and neurobiological mechanisms of acupuncture analgesia in SCD participants (NCT05045820). Results showed that SCD participants in StSt showed higher levels of sensory sensitivity, as well as increased pain intensity, pain interference, widespread pain, physical dysfunction, and depression compared to HCs (Table 2). These results highlight the significant impact of SCD on patients’ physical and emotional well-being and align with previous studies on SCD (32, 36, 40, 41, 46, 47).
We collected cross-sectional blood samples and clinical parameters to comprehensively study inflammatory mediators, autoantibody profiles, and presence of RBC/reticulocyte-leukocyte aggregates, exploring their interrelations and potential relationship with VOCs, QoL, and sensory sensitivity. Specifically, we examined the plasma levels of 80 inflammatory mediators (Supplementary Table 1), the profiles of autoantibodies against 18 human antigens (Figure 1), and the presence of RBC/reticulocyte-leukocyte aggregates in these participants (Figure 2). Moreover, we investigated the correlations between these analytes and their potential relationship with VOCs, QoL, and sensory sensitivity in SCD participants. By examining these analytes and their relationships, we sought to identify potential mechanisms and therapeutic targets for further exploration and investigation.
SCD is characterized by a persistent pro-inflammatory state that leads to elevated levels of inflammatory mediators in the bloodstream. Inflammatory mediators are vital signaling molecules that play a crucial role in the development of pain, VOCs, and the pathogenesis of SCD (8, 17, 48). A recent study measured the serum levels of 27 inflammatory mediators including 15 cytokines, 7 chemokines, and 5 growth factors in 27 StSt and 53 HCs (30). The results revealed that significantly higher levels of several cytokines, chemokines, and growth factors in SCD participants compared to HCs (30). Strikingly, SCD participants exhibited elevated serum concentrations of pro-inflammatory molecules, such as IL-1β, IL-12p70, and IL-17A, when compared to SCD participants experiencing VOC (30). Here we expanded the spectrum of analyses and observed that SCD participants exhibited higher plasma levels of 37 out of 80 analytes, including 13 pro-inflammatory/anti-inflammatory cytokines, 10 chemokines, 10 growth factors, 2 effectors, and 2 soluble receptors (Table 3; Supplementary Tables 1, 2). These findings collectively indicate a pro-inflammatory bias in SCD participants as compared to HCs.
Numerous cytokines elevated in our study, such as IL-1α, IL-4, IL-5,IL-6, IL-7, IL-8, TNF-α were previously shown to involved in VOC-related acute clinical complications such as acute chest syndrome, pulmonary hypertension, and pulmonary thrombosis (49–55). Systemic inflammation and modulation of the immune system with crosstalk of immunological molecules and immune cells were associated with the pathogenesis of persistent pain and the onset of VOCs in SCD. Plasma IFN-γ was augmented in steady-state SCD, which could modulate macrophage function and increase T helper cell expansion in SCD and most likely reflect inflammasome formation in inflammatory cells (54, 56, 57). Endothelium activation could in turn produce and release a number of potent inflammatory molecules, including IL-1α, IL-1β, IL-6, IL-8, GM-CSF, MCP-1, plasminogen activator inhibitor-1, and RANTES (54, 55, 58–60). The maturation and release of bioactive IL-1β and IL-18 (61–67), two vital immunoregulatory and proinflammatory cytokines, are governed by inflammatory caspases operating within specialized signaling platforms referred to as inflammasomes. Elevated plasma levels of IL-1β and IL-18 in SCD participants strongly suggest an aberration in the regulation of inflammasome activation within this particular group. In addition, IL-1β exerts a potent activation effect on leukocytes and ECs. Meanwhile, IL-18 plays an important role in stimulating vascular smooth muscle cell proliferation and migration, along with promoting the productions of IFN-γ, IL-2 and IL-12. These actions collectively exacerbate the inflammatory milieu in SCD participants (53, 56, 57). Notably, excessive production of IL-8 and RANTES contributes to the dehydration of sickle RBC, leading to an increase in RBC density and rigidity (68). This, in turn, enhances the adhesion of RBCs to the endothelium (69), a phenomenon closely linked to the severity of VOCs. These findings suggest that SCD participants experience persistently high levels of inflammatory mediators during StSt phases, even in the absence of severe clinical symptoms like VOCs.
Several inflammatory mediators were associated with the presence of autoantibodies and RBC/reticulocyte-leukocyte aggregation in SCD participants. Specifically, 5 inflammatory factors (MIP-1β, IL-34, IL-1α, IL-9, and IL-4) were positively associated with autoantibodies targeting 2 human antigens (SSA/Ro60 and MPO) (Table 4). Anti-SSA/Ro60 autoantibodies are typically classified as ANAs, while anti-MPO autoantibodies are categorized as non-ANAs. Thus, our results indicate that elevated inflammatory mediators are closely linked to both ANAs (anti-SSA/Ro60 autoantibodies) and non-ANAs (anti-MPO autoantibodies) in the bloodstream of SCD participants. Notably, the vast majority (4 out of 5) of inflammatory mediators displayed positive associations with ANA (SSA/Ro60) (Table 4). Given that mature RBCs in mammals lack nuclei, mitochondria, and other organelles (70), these inflammatory mediators are more likely to be implicated in autoimmune responses to cellular debris from other types of cells rather than mature RBCs. This finding suggests a potential link between ANA-associated autoimmunity and the presence of specific inflammatory mediators, which may contribute to our understanding of autoimmune processes and their association with certain cellular components in SCD.
In SCD participants, a complex and detrimental cycle is established through the interplay between inflammation and the formation of RBC/reticulocyte-leukocyte aggregates (71, 72). Inflammatory mediators disrupt immune responses, leading to an increased aggregation of RBCs/reticulocytes with leukocytes. Consequently, these aggregates exacerbate blood vessel blockages, further impeding blood flow and perpetuating the cycle of inflammation and tissue damage. This process triggers the release of additional inflammatory mediators, thus fueling a vicious circle of inflammation and VOCs. This vicious circle plays a significant role in the pathophysiology of SCD and significantly contributes to the recurrent pain crises and organ damage observed in affected individuals. We analyzed the association of inflammatory mediators with RBC/reticulocyte-leukocyte aggregation. In line with previous studies, our results also revealed 2 chemokines (CCL23 and MIP-1α) and 1 growth factor (HGF) that were associated with the aggregation (Table 4). These results provide important insights into the relationship between inflammation and RBC/reticulocyte-leukocyte aggregates that are associated with the occurrence and severity of VOCs.
SCD participants experience sudden and intense episodes of VOCs, which are challenging to predict and manage (73, 74). However, understanding of the circulating markers and their pathological processes during the transitioning phase from StSt to VOC episodes is extremely limited due to the unpredictable and rapid progression of the onset of VOCs. A previous study identified PDGF-BB and IL-1Ra as potential indicators for the acute-to-chronic stage in SCD (30). Our data revealed that 2 elevated inflammatory mediators (IL-18 and granzyme A) were associated with time the intervals prior to VOC onset and 4 mediators (GRO-α, IL-6, IL-17A, and LIF) were significantly associated with time intervals after active VOCs (Table 5), suggesting that these 6 mediators hold the potential to serve as novel biomarkers for predicting VOC episodes. Of particular note, IL-18 has emerged as a critical proinflammatory regulator in both innate and adaptive immune responses (75), and blocking IL-18 has been associated with mitigating neuropathic symptoms and enhancing the analgesic activity of morphine and buprenorphine (76). Inhibition of Granzyme A has been shown to reduce the levels of IL-6 and TNF-α (77). Thus, our results underscore the significance of specific inflammatory mediators in the early detection and development of VOCs, as well as potential avenues for pain management in SCD participants experiencing VOCs.
The existing literature has extensively studied the inflammation- and immunity-based mechanisms in SCD as compared to HCs, as well as the differences between StSt and VOC phases. However, there are limited studies investigating the relationship of inflammatory markers with clinical symptoms and sensory sensitivity during StSt. In the present study, we found that multiple inflammatory molecules were negatively associated with pain intensity (higher score indicated higher pain), the frequency/recency of clinical pain episodes (higher score indicated higher frequency/recency of acute pain episodes), emotional distress (depression), and physical dysfunction (higher score indicated higher physical dysfunction) in SCD participants. Conversely, higher levels of several soluble factors correlated with higher QoL and less pain. SCD participants with less severe Pain Episodes Score (higher score indicated higher severity) were associated with higher levels of GRO-α, MCP-2, granzyme B, bNGF, IL-7, IL-2, IL-17, and TREM 1 (Table 6). Of particular interest, higher IL-17A levels were also correlated with less pain intensity, physical dysfunction and higher QoL (higher PedsQL score indicated higher QoL). In fact, elevated IL-17A is associated with absence of acute chest syndrome in SCD participants, indicating a protective role (78). Consistently, higher levels of many inflammatory markers were also associated with decreased sensory sensitivity (in other words: increased threshold/tolerance to experimental stimuli) in response to mechanical (MDT MPT, and MTS), thermal (CPT), and pressure (PPT and PPTol) stimuli. We observed that SCD participants who had higher levels of Eotaxin-2, CCL21, GRO-α, MCP-2, HGF, IL-20, TNF-β, and TREM-1 simultaneously exhibited lowered cold pain sensitivity (in other words: increased tolerance of cold-induced pain). These novel findings have not been reported in existing literature in SCD. The underlying mechanisms of these intriguing observations are unknown and warrant future investigation. We speculate that SCD participants experiencing recurrent extremely painful VOCs-related episodes developed resistance and pain inhibitory effect, thus exhibited decreased sensory sensitivity and increased tolerance to experimental pain stimuli, which therefore inhibited the antidromic release of proinflammatory mediators at periphery. This correlation profile suggested the interactive and distinct roles of inflammatory mediators in the processing of pain, and sensory sensitivity at StSt, and could be utilized for more extensive studies to explore the underlying nociceptive pathways in SCD.
In line with the correlation profile presented in Table 6, elevated anti-MPO autoantibodies were associated with decreased sensitivity and tolerance to pressure pain (PPT trapezius and PPTol trapezius). Notably, MPO plays a crucial role as a marker and modulator of inflammation and oxidative stress, primarily originating from activated leukocytes and neutrophils (79). A previous study demonstrated that increased MPO impairs EC function through vascular oxidative stress, while inhibiting MPO shows promise in improving vasodilation in mouse models of SCD (80). Furthermore, many SCD participants experience heightened cold sensitivity (81), and exposure to potential triggers, such as cold temperatures or stress, can induce vasoconstriction, potentially leading to VOC (82, 83). Therefore, elevated autoantibodies, such as anti-MPO autoantibodies (Figure 1), might contribute to vasoconstriction during the prodromal phase of VOCs while simultaneously interacting with immune- and inflammatory-targets (Table 4) to modulate pain inhibitory effect at StSt.
Inflammation, autoimmunity, and RBC/reticulocyte-leukocyte aggregations are believed to play a significant role in the pathogenesis of pain and VOCs in SCD participants (23, 84, 85). However, the direct connections of these factors with pain and VOCs in SCD participants are not fully understood. Our comprehensive analyses of these cross-sectional clinical samples provide valuable insights into the correlations between inflammatory mediators, autoantibody profiles, RBC/reticulocyte-leukocyte aggregation, clinical lab test results, and their associations with the onset of VOCs and pain sensitivity in SCD participants. This knowledge has the potential to significantly contribute to the development of suitable biomarkers/endpoints for clinical diagnosis of pain episodes in SCD participants, as well as targeted therapeutic approaches and improved management strategies for individuals affected by this condition. However, it is essential to acknowledge the limitations of our cross-sectional study, including small sample size, potential bias, confounding factors, the absence of insight into temporal trends, and the inability to establish causality. To address these limitations, we have undertaken efforts to collect longitudinal clinical samples throughout the duration of our clinical trial cohort and expand our sample size with ongoing recruitment efforts. This longitudinal approach is expected to provide a stronger foundation for establishing causal relationships between the analyzed variables. By observing changes over time, we can better infer cause-and-effect relationships of these variables, thus advancing our understanding of the mechanisms underlying the pathogenesis of SCD. Furthermore, the combination of cross-sectional and longitudinal approaches will enable us to gather complementary data, extending our investigations to address a broader range of clinical questions in both SCD participants and SCD participants experiencing acute VOC. The information gathered from these approaches will be pivotal in evaluating the clinical efficacy and neurobiological mechanisms of acupuncture analgesia in SCD participants participating in our clinical trial.
Data availability statement
The original contributions presented in the study are included in the article/Supplementary Materials. Further inquiries can be directed to the corresponding authors.
Ethics statement
The studies involving humans were approved by The Institutional Review Boards (IRB) at Indiana University School of Medicine. The studies were conducted in accordance with the local legislation and institutional requirements. Written informed consent for participation in this study was provided by the participants’ legal guardians/next of kin.
Author contributions
WL: Data curation, Investigation, Methodology, Writing – original draft, Conceptualization, Formal Analysis, Resources, Supervision, Writing – review & editing. AP: Methodology, Project administration, Investigation, Writing – review & editing. CD: Project administration, Methodology, Writing – review & editing. BR: Formal Analysis, Writing – review & editing, Data curation, Investigation, Resources. FS: Methodology, Writing – review & editing, Formal Analysis. AO: Writing – review & editing, Methodology, Project administration. RM: Writing – review & editing. NM: Resources, Writing – review & editing, Project administration. SJ: Methodology, Resources, Writing – review & editing. BH: Methodology, Resources, Writing – review & editing, Formal Analysis. AG: Resources, Writing – review & editing, Data curation, Methodology. SH: Resources, Writing – review & editing, Formal Analysis. RH: Resources, Writing – review & editing. QY: Conceptualization, Formal Analysis, Investigation, Methodology, Project administration, Resources, Supervision, Writing – original draft, Writing – review & editing. YW: Data curation, Formal Analysis, Investigation, Resources, Writing – review & editing, Conceptualization, Funding acquisition, Methodology, Project administration, Supervision, Validation, Writing – original draft.
Funding
The author(s) declare financial support was received for the research, authorship, and/or publication of this article. This work was supported by NIH K99/R00 award (Grant # 5R00AT010012 to YW) and Indiana University Health – Indiana University School of Medicine Strategic Research Initiative funding to YW.
Acknowledgments
The authors would like to thank Tyler James Barret, Nayana Dutt, Payton Mittman, Bea Paras, Ramat Gbemisola Suleiman-Oba, Amy Gao and Yongqi Yu for assisting with experimental performance, and clinical team staff at Indiana University Clinical Research Center for patient scheduling and blood draw performance.
Conflict of interest
The authors declare that the research was conducted in the absence of any commercial or financial relationships that could be construed as a potential conflict of interest.
Publisher’s note
All claims expressed in this article are solely those of the authors and do not necessarily represent those of their affiliated organizations, or those of the publisher, the editors and the reviewers. Any product that may be evaluated in this article, or claim that may be made by its manufacturer, is not guaranteed or endorsed by the publisher.
Supplementary material
The Supplementary Material for this article can be found online at: https://www.frontiersin.org/articles/10.3389/fimmu.2024.1288187/full#supplementary-material
Glossary
References
1. Ware RE, de Montalembert M, Tshilolo L, Abboud MR. Sickle cell disease. Lancet (2017) 390:311–23. doi: 10.1016/S0140-6736(17)30193-9
2. Rees DC, Williams TN, Gladwin MT. Sickle-cell disease. Lancet (2010) 376:2018–31. doi: 10.1016/S0140-6736(10)61029-X
3. Ilesanmi OO. Pathological basis of symptoms and crises in sickle cell disorder: implications for counseling and psychotherapy. Hematol Rep (2010) 2:e2. doi: 10.4081/hr.2010.e2
4. Novelli EM, Gladwin MT. Crises in sickle cell disease. Chest (2016) 149:1082–93. doi: 10.1016/j.chest.2015.12.016
5. Darbari DS, Sheehan VA, Ballas SK. The vaso-occlusive pain crisis in sickle cell disease: Definition, pathophysiology, and management. Eur J Haematol (2020) 105:237–46. doi: 10.1111/ejh.13430
6. Takaoka K, Cyril AC, Jinesh S, Radhakrishnan R. Mechanisms of pain in sickle cell disease. Br J Pain (2021) 15:213–20. doi: 10.1177/2049463720920682
7. Tran H, Gupta M, Gupta K. Targeting novel mechanisms of pain in sickle cell disease. Hematol Am Soc Hematol Educ Program (2017) 2017:546–55. doi: 10.1182/asheducation-2017.1.546
8. Ballas SK, Gupta K, Adams-Graves P. Sickle cell pain: a critical reappraisal. Blood (2012) 120:3647–56. doi: 10.1182/blood-2012-04-383430
9. Chaar V, Picot J, Renaud O, Bartolucci P, Nzouakou R, Bachir D, et al. Aggregation of mononuclear and red blood cells through an alpha4beta1-Lu/basal cell adhesion molecule interaction in sickle cell disease. Haematologica (2010) 95:1841–8. doi: 10.3324/haematol.2010.026294
10. Gee BE, Platt OS. Sickle reticulocytes adhere to VCAM-1. Blood (1995) 85:268–74. doi: 10.1182/blood.V85.1.268.bloodjournal851268
11. Joneckis CC, Ackley RL, Orringer EP, Wayner EA, Parise LV. Integrin alpha 4 beta 1 and glycoprotein IV (CD36) are expressed on circulating reticulocytes in sickle cell anemia. Blood (1993) 82:3548–55. doi: 10.1182/blood.V82.12.3548.3548
12. Brittain JE, Han J, Ataga KI, Orringer EP, Parise LV. Mechanism of CD47-induced alpha4beta1 integrin activation and adhesion in sickle reticulocytes. J Biol Chem (2004) 279:42393–402. doi: 10.1074/jbc.M407631200
13. Kumar A, Eckmam JR, Swerlick RA, Wick TM. Phorbol ester stimulation increases sickle erythrocyte adherence to endothelium: a novel pathway involving alpha 4 beta 1 integrin receptors on sickle reticulocytes and fibronectin. Blood (1996) 88:4348–58. doi: 10.1182/blood.V88.11.4348.bloodjournal88114348
14. Udani M, Zen Q, Cottman M, Leonard N, Jefferson S, Daymont C, et al. Basal cell adhesion molecule/lutheran protein. The receptor critical for sickle cell adhesion to laminin. J Clin Invest (1998) 101:2550–8. doi: 10.1172/JCI1204
15. El Nemer W, Gane P, Colin Y, Bony V, Rahuel C, Galactéros F, et al. The Lutheran blood group glycoproteins, the erythroid receptors for laminin, are adhesion molecules. J Biol Chem (1998) 273:16686–93. doi: 10.1074/jbc.273.27.16686
16. Hebbel RP. Adhesive interactions of sickle erythrocytes with endothelium. J Clin Invest (1997) 100:S83–86.
17. Conran N, Belcher JD. Inflammation in sickle cell disease. Clin Hemorheol Microcirc (2018) 68:263–99. doi: 10.3233/CH-189012
18. Elmariah H, Garrett ME, De Castro LM, Jonassaint JC, Ataga KI, Eckman JR, et al. Factors associated with survival in a contemporary adult sickle cell disease cohort. Am J Hematol (2014) 89:530–5. doi: 10.1002/ajh.23683
19. Turhan A, Weiss LA, Mohandas N, Coller BS, Frenette PS. Primary role for adherent leukocytes in sickle cell vascular occlusion: a new paradigm. Proc Natl Acad Sci U.S.A. (2002) 99:3047–51. doi: 10.1073/pnas.052522799
20. Hofstra TC, Kalra VK, Meiselman HJ, Coates TD. Sickle erythrocytes adhere to polymorphonuclear neutrophils and activate the neutrophil respiratory burst. Blood (1996) 87:4440–7. doi: 10.1182/blood.V87.10.4440.bloodjournal87104440
21. Zennadi R, Chien A, Xu K, Batchvarova M, Telen MJ. Sickle red cells induce adhesion of lymphocytes and monocytes to endothelium. Blood (2008) 112:3474–83. doi: 10.1182/blood-2008-01-134346
22. Brittain JE, Knoll CM, Ataga KI, Orringer EP, Parise LV. Fibronectin bridges monocytes and reticulocytes via integrin alpha4beta1. Br J Haematol (2008) 141:872–81. doi: 10.1111/j.1365-2141.2008.07056.x
23. Allali S, Maciel TT, Hermine O, de Montalembert M. Innate immune cells, major protagonists of sickle cell disease pathophysiology. Haematologica (2020) 105:273–83. doi: 10.3324/haematol.2019.229989
24. Sesti-Costa R, Borges MD, Lanaro C, de Albuquerque DM, Saad STO, Costa FF. Inflammatory dendritic cells contribute to regulate the immune response in sickle cell disease. Front Immunol (2020) 11:617962. doi: 10.3389/fimmu.2020.617962
25. Adisa OA, Hu Y, Ghosh S, Aryee D, Osunkwo I, Ofori-Acquah SF. Association between plasma free haem and incidence of vaso-occlusive episodes and acute chest syndrome in children with sickle cell disease. Br J Haematol (2013) 162:702–5. doi: 10.1111/bjh.12445
26. Al-Habboubi HH, Mahdi N, Abu-Hijleh TM, Abu-Hijleh FM, Sater MS, Almawi WY. The relation of vascular endothelial growth factor (VEGF) gene polymorphisms on VEGF levels and the risk of vasoocclusive crisis in sickle cell disease. Eur J Haematol (2012) 89:403–9. doi: 10.1111/ejh.12003
27. Redha NA, Mahdi N, Al-Habboubi HH, Almawi WY. Impact of VEGFA -583C > T polymorphism on serum VEGF levels and the susceptibility to acute chest syndrome in pediatric patients with sickle cell disease. Pediatr Blood Cancer (2014) 61:2310–2. doi: 10.1002/pbc.25158
28. Lesley R, Xu Y, Kalled SL, Hess DM, Schwab SR, Shu HB, et al. Reduced competitiveness of autoantigen-engaged B cells due to increased dependence on BAFF. Immunity (2004) 20:441–53. doi: 10.1016/s1074-7613(04)00079-2
29. Mackay F, Woodcock SA, Lawton P, Ambrose C, Baetscher M, Schneider P, et al. Mice transgenic for BAFF develop lymphocytic disorders along with autoimmune manifestations. J Exp Med (1999) 190:1697–710. doi: 10.1084/jem.190.11.1697
30. Silva-Junior AL, Garcia NP, Cardoso EC, Dias S, Tarragô AM, Fraiji NA, et al. Immunological hallmarks of inflammatory status in vaso-occlusive crisis of sickle cell anemia patients. Front Immunol (2021) 12:559925. doi: 10.3389/fimmu.2021.559925
31. Curtis S, Brandow AM. Responsiveness of Patient-Reported Outcome Measurement Information System (PROMIS) pain domains and disease-specific patient-reported outcome measures in children and adults with sickle cell disease. Hematol Am Soc Hematol Educ Program (2017) 2017:542–5. doi: 10.1182/asheducation-2017.1.542
32. Brandow AM, Farley RA, Panepinto JA. Neuropathic pain in patients with sickle cell disease. Pediatr Blood Cancer (2014) 61:512–7. doi: 10.1002/pbc.24838
33. Freynhagen R, Baron R, Gockel U, Tolle TR. painDETECT: a new screening questionnaire to identify neuropathic components in patients with back pain. Curr Med Res Opin (2006) 22:1911–20. doi: 10.1185/030079906X132488
35. Dudeney J, Law EF, Meyyappan A, Palermo TM, Rabbitts JA. Evaluating the psychometric properties of the Widespread Pain Index and the Symptom Severity scale in youth with painful conditions. Can J Pain (2019) 3:137–47. doi: 10.1080/24740527.2019.1620097
36. Campbell CM, Moscou-Jackson G, Carroll CP, Kiley K, Haywood C Jr, Lanzkron S, et al. An evaluation of central sensitization in patients with sickle cell disease. J Pain (2016) 17:617–27. doi: 10.1016/j.jpain.2016.01.475
37. Panepinto JA, Torres S, Bendo CB, McCavit TL, Dinu B, Sherman-Bien S, et al. PedsQL sickle cell disease module: feasibility, reliability, and validity. Pediatr Blood Cancer (2013) 60:1338–44. doi: 10.1002/pbc.24491
38. Darbari DS, Hampson JP, Ichesco E, Kadom N, Vezina G, Evangelou I, et al. Frequency of hospitalizations for pain and association with altered brain network connectivity in sickle cell disease. J Pain (2015) 16:1077–86. doi: 10.1016/j.jpain.2015.07.005
39. Wang Y, Hardy SJ, Ichesco E, Zhang P, Harris RE, Darbari DS. Alteration of grey matter volume is associated with pain and quality of life in children with sickle cell disease. Transl Res (2022) 240:17–25. doi: 10.1016/j.trsl.2021.08.004
40. Miller RE, Brown DS, Keith SW, Hegarty SE, Setty Y, Campbell CM, et al. Quantitative sensory testing in children with sickle cell disease: additional insights and future possibilities. Br J Haematol (2019) 185:925–34. doi: 10.1111/bjh.15876
41. Darbari DS, Vaughan KJ, Roskom K, Seamon C, Diaw L, Quinn M, et al. Central sensitization associated with low fetal hemoglobin levels in adults with sickle cell anemia. Scand J Pain (2017) 17:279–86. doi: 10.1016/j.sjpain.2017.08.001
42. Nahman-Averbuch H, Yarnitsky D, Granovsky Y, Gerber E, Dagul P, Granot M. The role of stimulation parameters on the conditioned pain modulation response. Scand J Pain (2013) 4:10–4. doi: 10.1016/j.sjpain.2012.08.001
43. Schoen CJ, Ablin JN, Ichesco E, Bhavsar RJ, Kochlefl L, Harris RE, et al. A novel paradigm to evaluate conditioned pain modulation in fibromyalgia. J Pain Res (2016) 9:711–9. doi: 10.2147/JPR.S115193
44. Syed F, Li W, Relich RF, Russell PM, Zhang S, Zimmerman MK, et al. Excessive matrix metalloproteinase-1 and hyperactivation of endothelial cells occurred in COVID-19 patients and were associated with the severity of COVID-19. J Infect Dis (2021) 224:60–9. doi: 10.1093/infdis/jiab167
45. Yoshimi R, Ueda A, Ozato K, Ishigatsubo Y. Clinical and pathological roles of Ro/SSA autoantibody system. Clin Dev Immunol (2012) 2012:606195. doi: 10.1155/2012/606195
46. Reader SK, Rockman LM, Okonak KM, Ruppe NM, Keeler CN, Kazak AE. Systematic review: pain and emotional functioning in pediatric sickle cell disease. J Clin Psychol Med Settings (2020) 27:343–65. doi: 10.1007/s10880-019-09647-x
47. Sagi V, Mittal A, Tran H, Gupta K. Pain in sickle cell disease: current and potential translational therapies. Transl Res (2021) 234:141–58. doi: 10.1016/j.trsl.2021.03.007
48. Alagbe AE, Domingos IF, Adekile AD, Blotta M, Santos MNN. Anti-inflammatory cytokines in sickle cell disease. Mol Biol Rep (2022) 49:2433–42. doi: 10.1007/s11033-021-07009-1
49. Driss A, Wilson NO, Mason K, Hyacinth HI, Hibbert JM, Serjeant GR, et al. Elevated IL-1alpha and CXCL10 serum levels occur in patients with homozygous sickle cell disease and a history of acute splenic sequestration. Dis Markers (2012) 32:295–300. doi: 10.1155/2012/479275
50. Francis RB Jr., Haywood LJ. Elevated immunoreactive tumor necrosis factor and interleukin-1 in sickle cell disease. J Natl Med Assoc (1992) 84:611–5.
51. Gbotosho OT, Gollamudi J, Hyacinth HI. The role of inflammation in the cellular and molecular mechanisms of cardiopulmonary complications of sickle cell disease. Biomolecules (2023) 13(2):381. doi: 10.3390/biom13020381
52. Keikhaei B, Mohseni AR, Norouzirad R, Alinejadi M, Ghanbari S, Shiravi F, et al. Altered levels of pro-inflammatory cytokines in sickle cell disease patients during vaso-occlusive crises and the steady state condition. Eur Cytokine Netw (2013) 24:45–52. doi: 10.1684/ecn.2013.0328
53. Qari MH, Dier U, Mousa SA. Biomarkers of inflammation, growth factor, and coagulation activation in patients with sickle cell disease. Clin Appl Thromb Hemost (2012) 18:195–200. doi: 10.1177/1076029611420992
54. Pathare A, Al Kindi S, Alnaqdy AA, Daar S, Knox-Macaulay H, Dennison D. Cytokine profile of sickle cell disease in Oman. Am J Hematol (2004) 77:323–8. doi: 10.1002/ajh.20196
55. Sakamoto TM, Lanaro C, Ozelo MC, Garrido VT, Olalla-Saad ST, Conran N, et al. Increased adhesive and inflammatory properties in blood outgrowth endothelial cells from sickle cell anemia patients. Microvasc Res (2013) 90:173–9. doi: 10.1016/j.mvr.2013.10.002
56. Asare K, Gee BE, Stiles JK, Wilson NO, Driss A, Quarshie A, et al. Plasma interleukin-1beta concentration is associated with stroke in sickle cell disease. Cytokine (2010) 49:39–44. doi: 10.1016/j.cyto.2009.10.002
57. Cerqueira BA, Boas WV, Zanette AD, Reis MG, Goncalves MS. Increased concentrations of IL-18 and uric acid in sickle cell anemia: contribution of hemolysis, endothelial activation and the inflammasome. Cytokine (2011) 56:471–6. doi: 10.1016/j.cyto.2011.08.013
58. Conran N, Almeida CB, Lanaro C, Ferreira RP, Traina F, Saad ST, et al. Inhibition of caspase-dependent spontaneous apoptosis via a cAMP-protein kinase A dependent pathway in neutrophils from sickle cell disease patients. Br J Haematol (2007) 139:148–58. doi: 10.1111/j.1365-2141.2007.06748.x
59. Zachlederova M, Jarolim P. The dynamics of gene expression in human lung microvascular endothelial cells after stimulation with inflammatory cytokines. Physiol Res (2006) 55:39–47. doi: 10.33549/physiolres.930703
60. Patel N, Sundaram N, Yang M, Madigan C, Kalra VK, Malik P. Placenta growth factor (PlGF), a novel inducer of plasminogen activator inhibitor-1 (PAI-1) in sickle cell disease (SCD). J Biol Chem (2010) 285:16713–22. doi: 10.1074/jbc.M110.101691
61. Lamkanfi M. Emerging inflammasome effector mechanisms. Nat Rev Immunol (2011) 11:213–20. doi: 10.1038/nri2936
62. Rathinam VA, Fitzgerald KA. Inflammasome complexes: emerging mechanisms and effector functions. Cell (2016) 165:792–800. doi: 10.1016/j.cell.2016.03.046
63. Bauernfeind F, Hornung V. Of inflammasomes and pathogens–sensing of microbes by the inflammasome. EMBO Mol Med (2013) 5:814–26. doi: 10.1002/emmm.201201771
64. Midtbo K, Eklund D, Sarndahl E, Persson A. Molecularly distinct NLRP3 inducers mediate diverse ratios of interleukin-1beta and interleukin-18 from human monocytes. Mediators Inflammation (2020) 2020:4651090. doi: 10.1155/2020/4651090
65. de Vasconcelos NM, Lamkanfi M. Recent insights on inflammasomes, gasdermin pores, and pyroptosis. Cold Spring Harb Perspect Biol (2020) 12(5):a036392. doi: 10.1101/cshperspect.a036392
66. Chan AH, Schroder K. Inflammasome signaling and regulation of interleukin-1 family cytokines. J Exp Med (2020) 217(1):e20190314. doi: 10.1084/jem.20190314
67. Satoh T, Otsuka A, Contassot E, French LE. The inflammasome and IL-1beta: implications for the treatment of inflammatory diseases. Immunotherapy (2015) 7:243–54. doi: 10.2217/imt.14.106
68. Durpes MC, Nebor D, du Mesnil PC, Mougenel D, Decastel M, Elion J, et al. Effect of interleukin-8 and RANTES on the Gardos channel activity in sickle human red blood cells: role of the Duffy antigen receptor for chemokines. Blood Cells Mol Dis (2010) 44:219–23. doi: 10.1016/j.bcmd.2010.02.001
69. Durpes MC, Hardy-Dessources MD, El Nemer W, Picot J, Lemonne N, Elion J, et al. Activation state of alpha4beta1 integrin on sickle red blood cells is linked to the duffy antigen receptor for chemokines (DARC) expression. J Biol Chem (2011) 286:3057–64. doi: 10.1074/jbc.M110.173229
70. Bratosin D, Estaquier J, Petit F, Arnoult D, Quatannens B, Tissier JP, et al. Programmed cell death in mature erythrocytes: a model for investigating death effector pathways operating in the absence of mitochondria. Cell Death Differ (2001) 8:1143–56. doi: 10.1038/sj.cdd.4400946
71. Nader E, Romana M, Connes P. The red blood cell-inflammation vicious circle in sickle cell disease. Front Immunol (2020) 11:454. doi: 10.3389/fimmu.2020.00454
72. Jang T, Poplawska M, Cimpeanu E, Mo G, Dutta D, Lim SH. Vaso-occlusive crisis in sickle cell disease: a vicious cycle of secondary events. J Transl Med (2021) 19:397. doi: 10.1186/s12967-021-03074-z
73. Zaidi AU, Glaros AK, Lee S, Wang T, Bhojwani R, Morris E, et al. A systematic literature review of frequency of vaso-occlusive crises in sickle cell disease. Orphanet J Rare Dis (2021) 16:460. doi: 10.1186/s13023-021-02096-6
74. Veluswamy S, Shah P, Denton CC, Chalacheva P, Khoo MCK, Coates TD. Vaso-occlusion in sickle cell disease: is autonomic dysregulation of the microvasculature the trigger? J Clin Med (2019) 8(10):1690. doi: 10.3390/jcm8101690
75. Shimizu M, Takei S, Mori M, Yachie A. Pathogenic roles and diagnostic utility of interleukin-18 in autoinflammatory diseases. Front Immunol (2022) 13:951535. doi: 10.3389/fimmu.2022.951535
76. Pilat D, Piotrowska A, Rojewska E, Jurga A, Ślusarczyk J, Makuch W, et al. Blockade of IL-18 signaling diminished neuropathic pain and enhanced the efficacy of morphine and buprenorphine. Mol Cell Neurosci (2016) 71:114–24. doi: 10.1016/j.mcn.2015.12.013
77. Garzon-Tituana M, Sierra-Monzón JL, Comas L, Santiago L, Khaliulina-Ushakova T, Uranga-Murillo I, et al. Granzyme A inhibition reduces inflammation and increases survival during abdominal sepsis. Theranostics (2021) 11:3781–95. doi: 10.7150/thno.49288
78. da Silva RR, Pereira MC, Melo Rêgo MJ, Domingues Hatzlhofer BL, da Silva Araújo A, Cavalcanti Bezerra MA, et al. Evaluation of Th17 related cytokines associated with clinical and laboratorial parameters in sickle cell anemia patients with leg ulcers. Cytokine (2014) 65:143–7. doi: 10.1016/j.cyto.2013.11.012
79. Ndrepepa G. Myeloperoxidase - A bridge linking inflammation and oxidative stress with cardiovascular disease. Clin Chim Acta (2019) 493:36–51. doi: 10.1016/j.cca.2019.02.022
80. Zhang H, Xu H, Weihrauch D, Jones DW, Jing X, Shi Y, et al. Inhibition of myeloperoxidase decreases vascular oxidative stress and increases vasodilatation in sickle cell disease mice. J Lipid Res (2013) 54:3009–15. doi: 10.1194/jlr.M038281
81. Brandow AM, Hansen K, Nugent M, Pan A, Panepinto JA, Stucky CL. Children and adolescents with sickle cell disease have worse cold and mechanical hypersensitivity during acute painful events. Pain (2019) 160:407–16. doi: 10.1097/j.pain.0000000000001407
82. Chalacheva P, Kato RM, Sangkatumvong S, Detterich J, Bush A, Wood JC, et al. Autonomic responses to cold face stimulation in sickle cell disease: a time-varying model analysis. Physiol Rep (2015) 3(7):e12463. doi: 10.14814/phy2.12463
83. Shah P, Khaleel M, Thuptimdang W, Sunwoo J, Veluswamy S, Chalacheva P, et al. Mental stress causes vasoconstriction in subjects with sickle cell disease and in normal controls. Haematologica (2020) 105:83–90. doi: 10.3324/haematol.2018.211391
84. Telen MJ, Afenyi-Annan A, Garrett ME, Combs MR, Orringer EP, Ashley-Koch AE. Alloimmunization in sickle cell disease: changing antibody specificities and association with chronic pain and decreased survival. Transfusion (2015) 55:1378–87. doi: 10.1111/trf.12940
Keywords: sickle cell disease, inflammation, autoantibody, aggregate, steady-state condition, vaso-occlusive crisis, pain sensitivity
Citation: Li W, Pucka AQ, Debats C, Reyes BA, Syed F, O’Brien ARW, Mehta R, Manchanda N, Jacob SA, Hardesty BM, Greist A, Harte SE, Harris RE, Yu Q and Wang Y (2024) Inflammation and autoimmunity are interrelated in patients with sickle cell disease at a steady-state condition: implications for vaso-occlusive crisis, pain, and sensory sensitivity. Front. Immunol. 15:1288187. doi: 10.3389/fimmu.2024.1288187
Received: 03 September 2023; Accepted: 15 January 2024;
Published: 01 February 2024.
Edited by:
Kun Xiong, Central South University, ChinaReviewed by:
Erica Sparkenbaugh, University of North Carolina at Chapel Hill, United StatesNirupama Ramadas, University of North Carolina at Chapel Hill, United States
Copyright © 2024 Li, Pucka, Debats, Reyes, Syed, O’Brien, Mehta, Manchanda, Jacob, Hardesty, Greist, Harte, Harris, Yu and Wang. This is an open-access article distributed under the terms of the Creative Commons Attribution License (CC BY). The use, distribution or reproduction in other forums is permitted, provided the original author(s) and the copyright owner(s) are credited and that the original publication in this journal is cited, in accordance with accepted academic practice. No use, distribution or reproduction is permitted which does not comply with these terms.
*Correspondence: Ying Wang, eXdhMTJAaXUuZWR1; Wei Li, d2wxQGl1cHVpLmVkdQ==; Qigui Yu, YW5keXVAaXVwdWkuZWR1